Free Energy All living systems require constant input
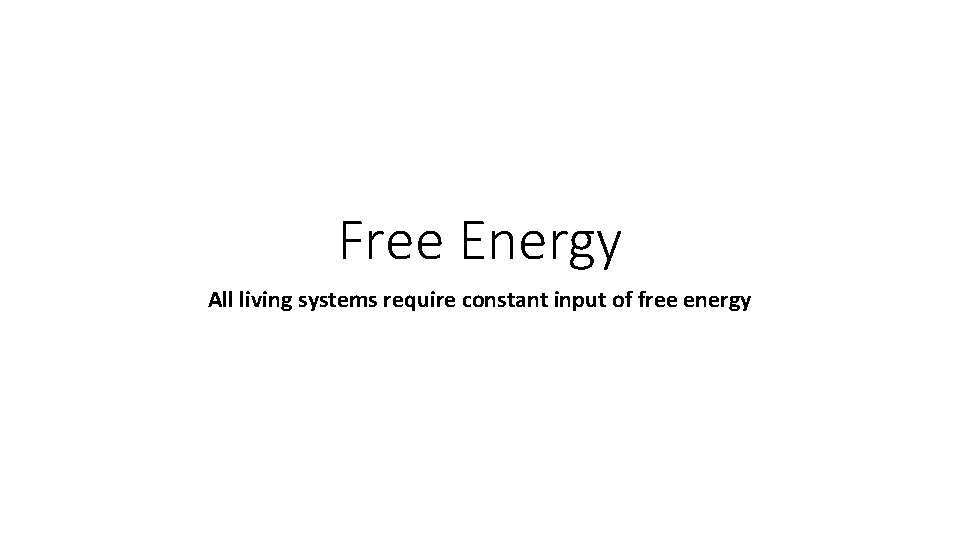
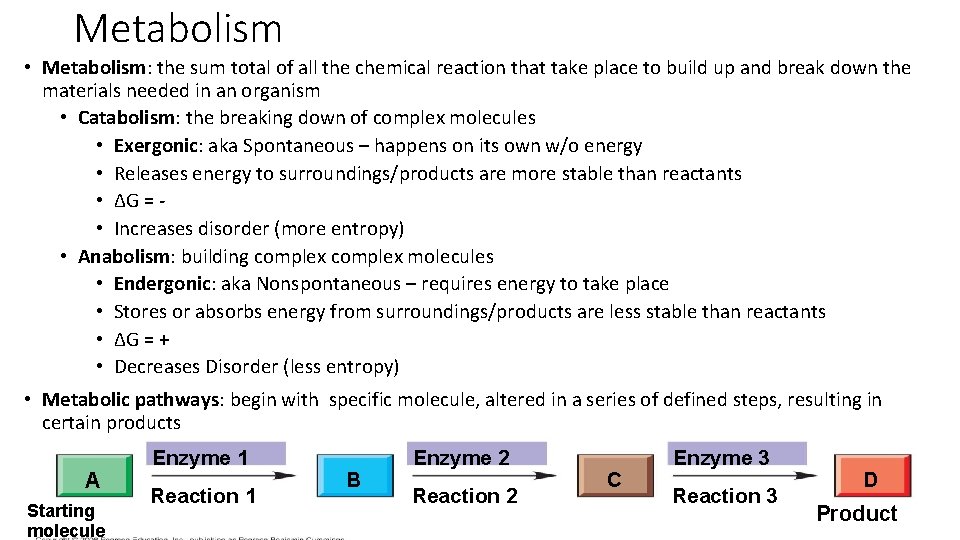
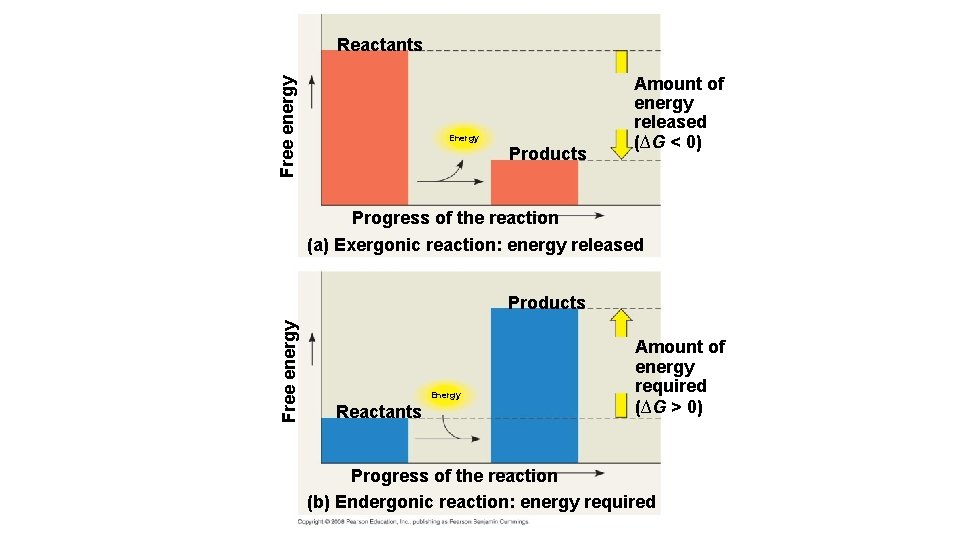
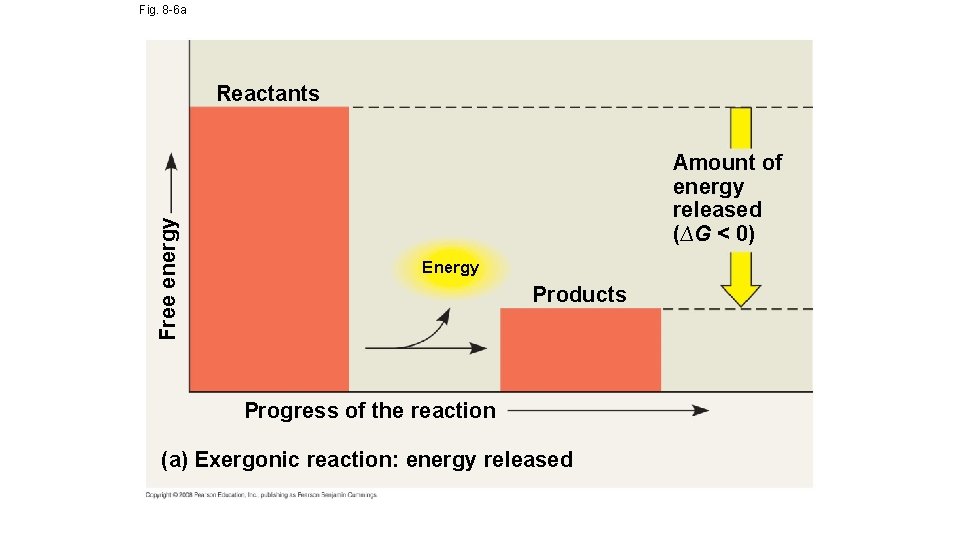
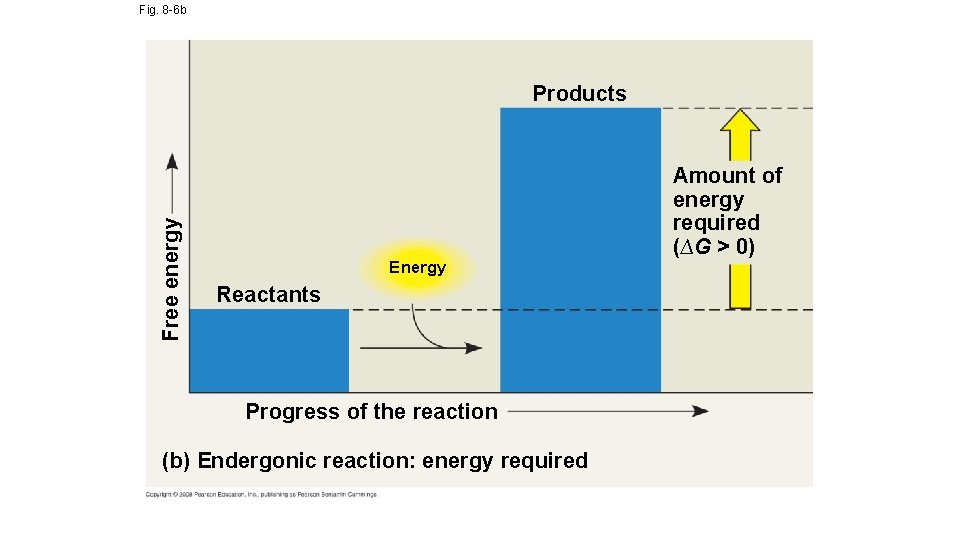
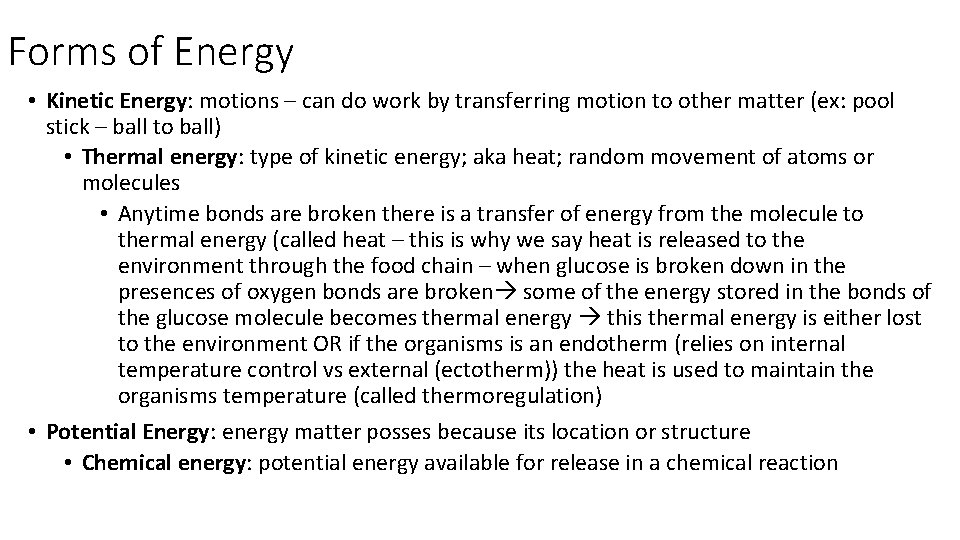
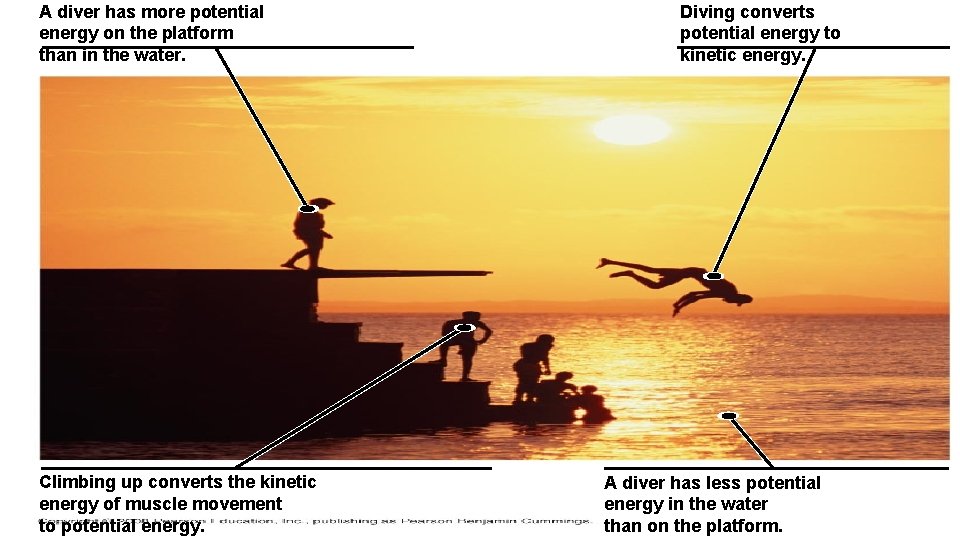
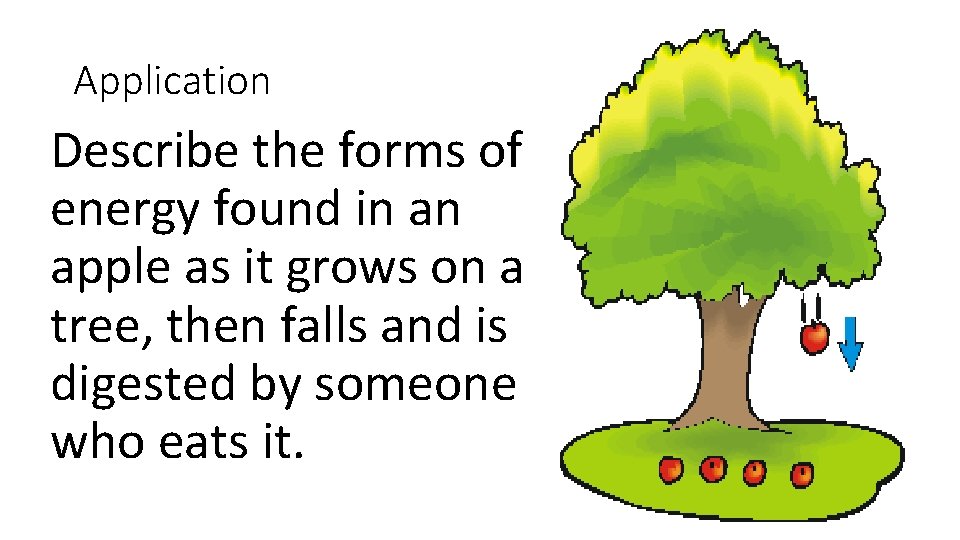
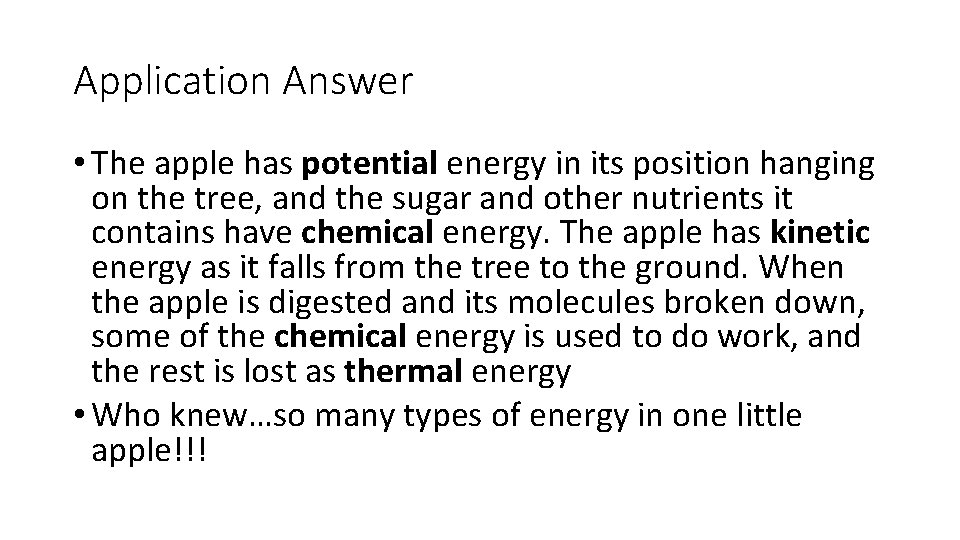
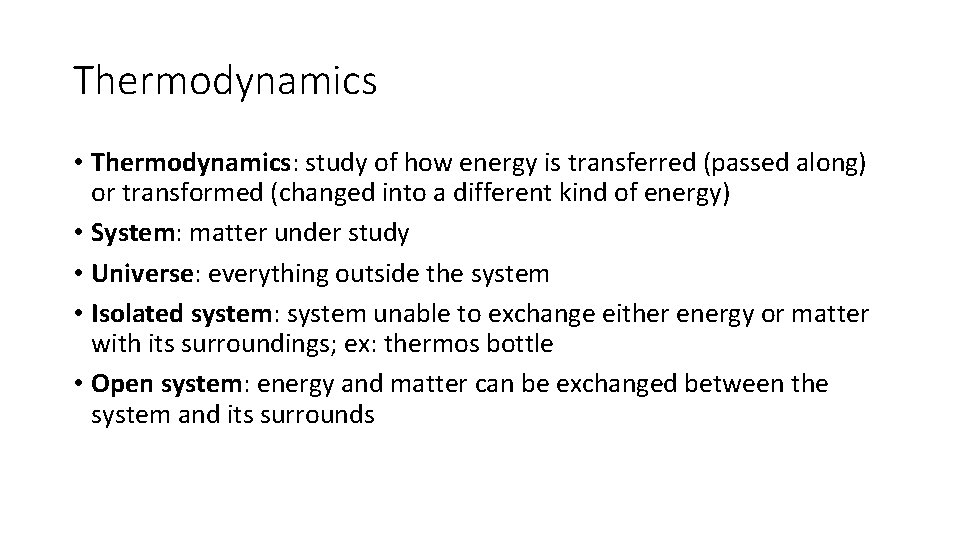
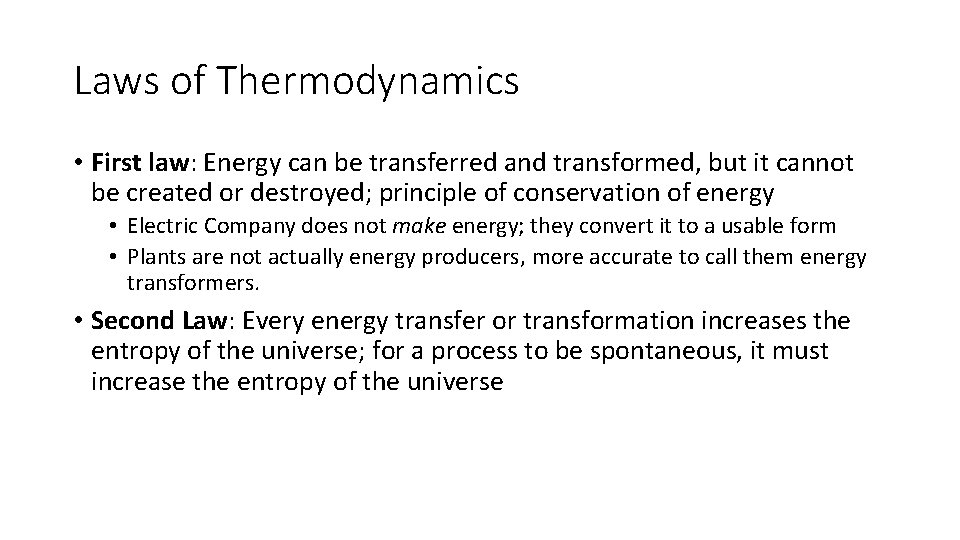
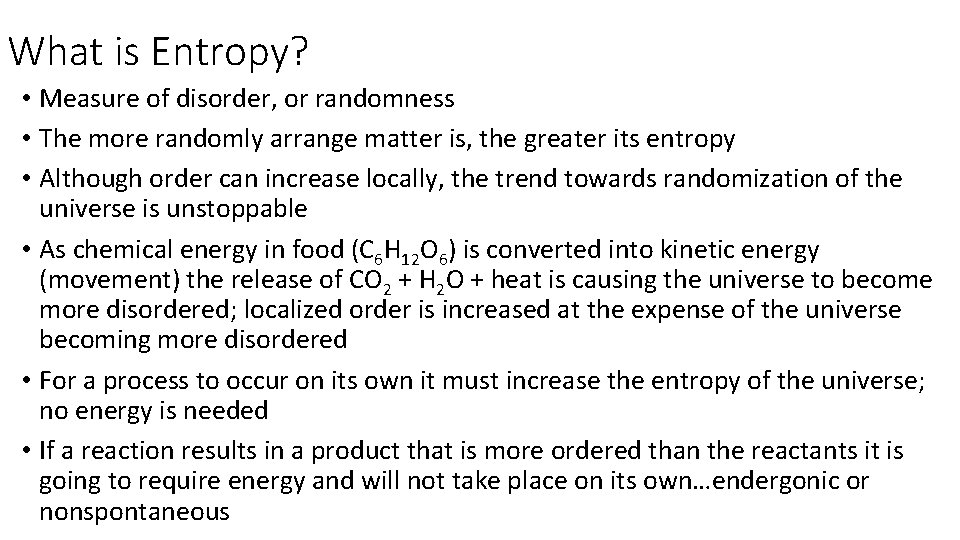
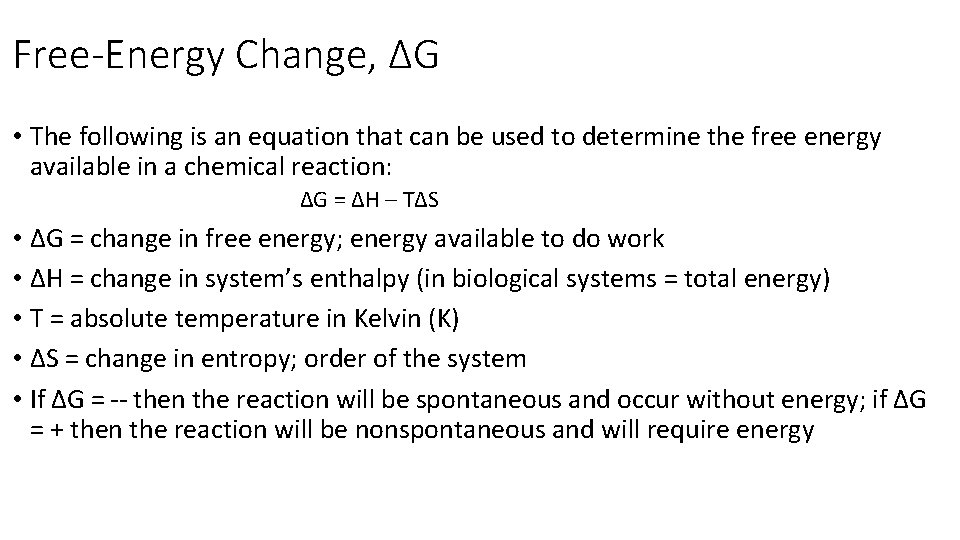
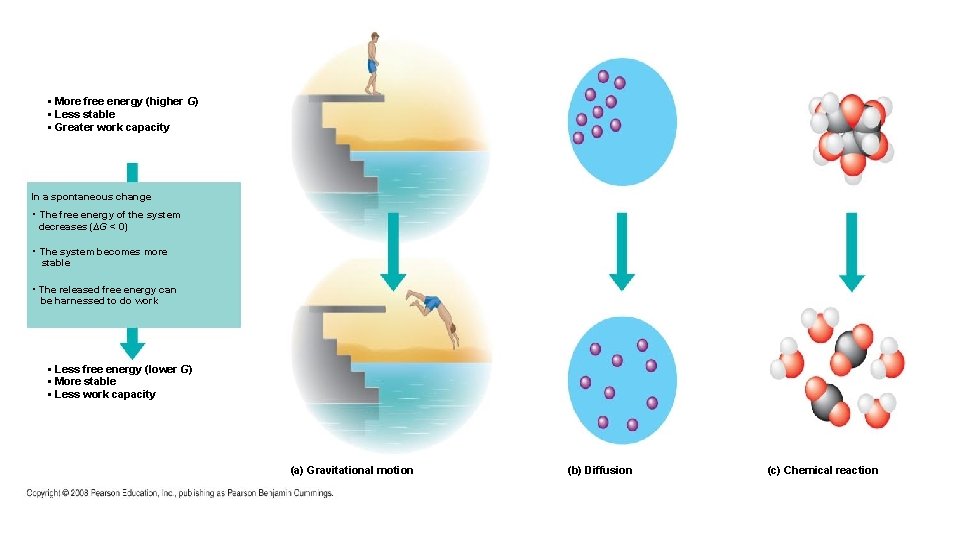
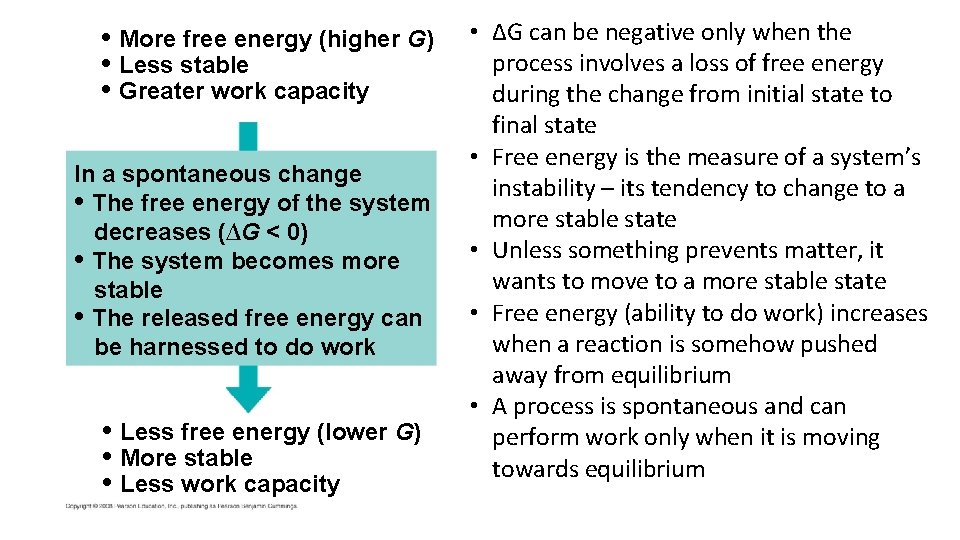
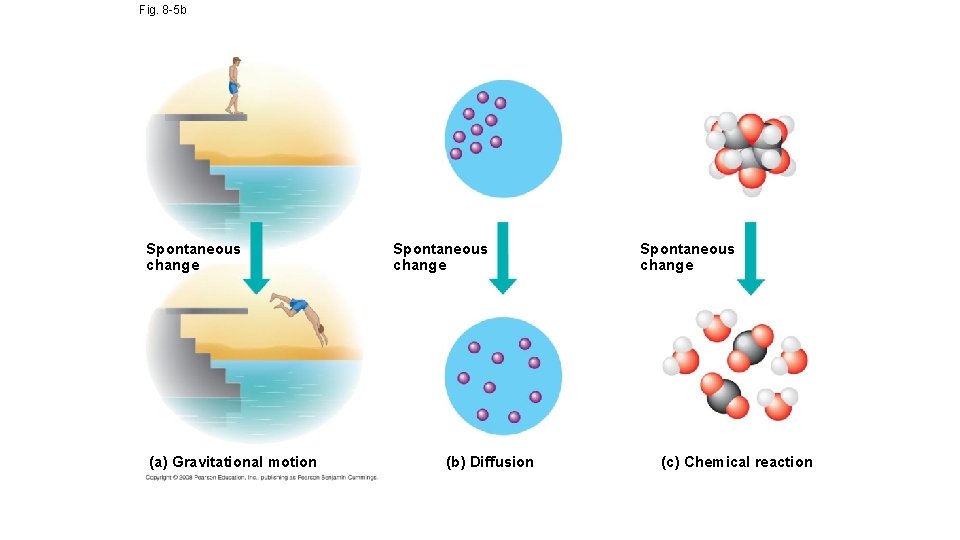
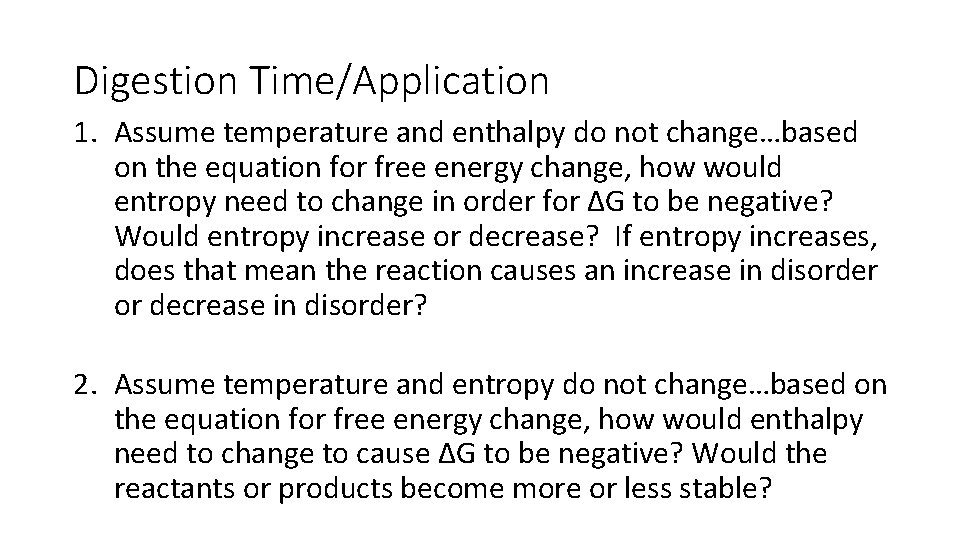
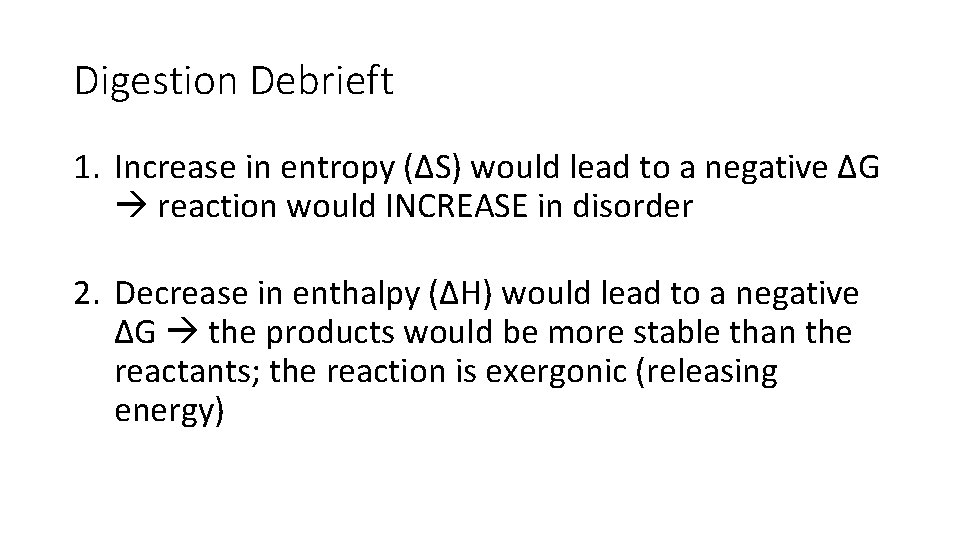
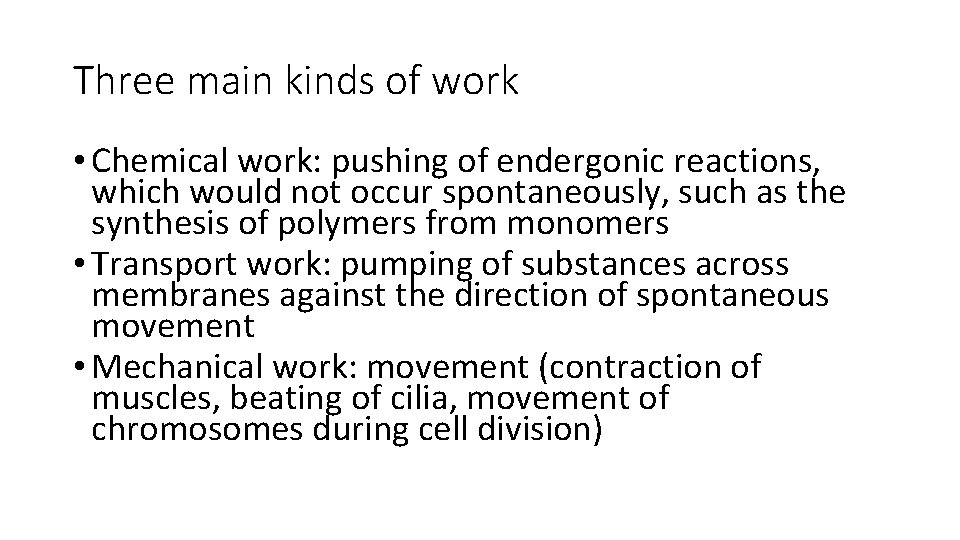
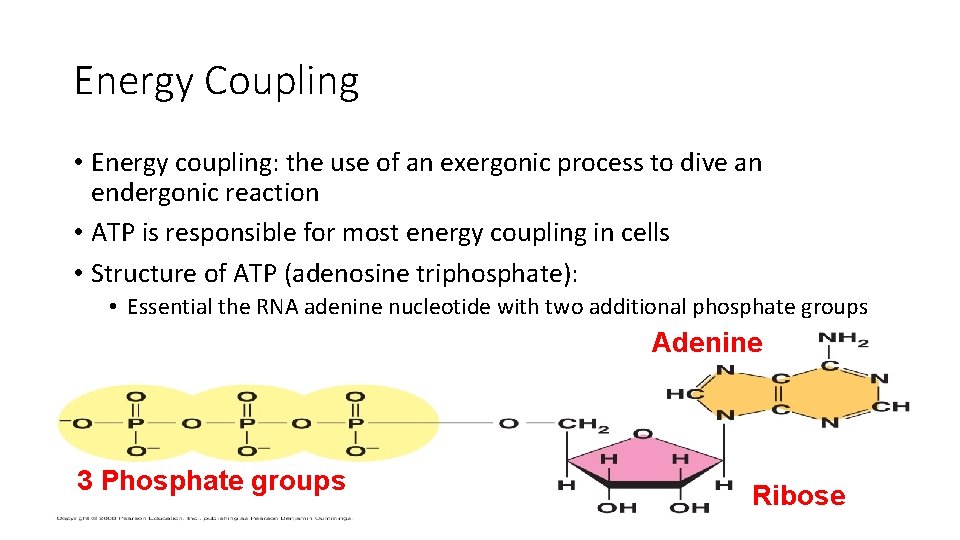
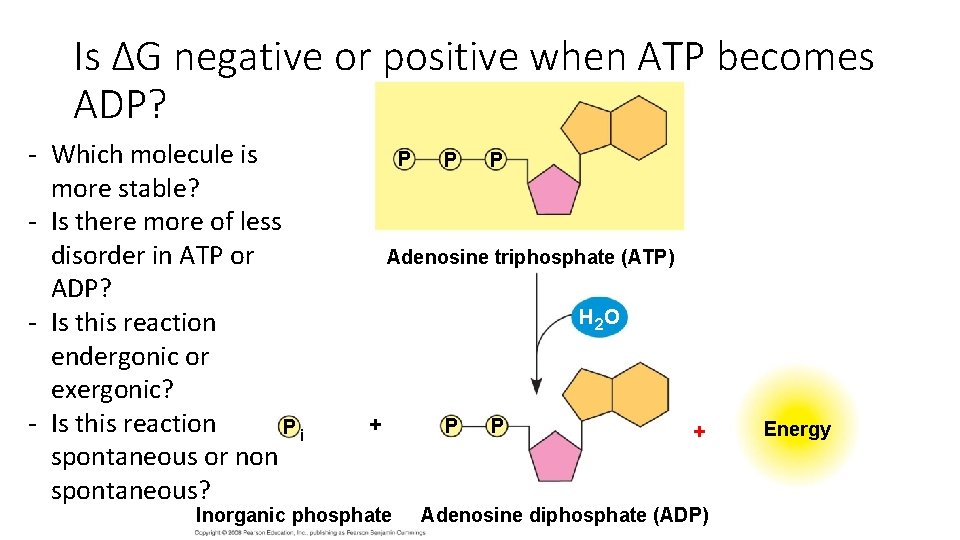
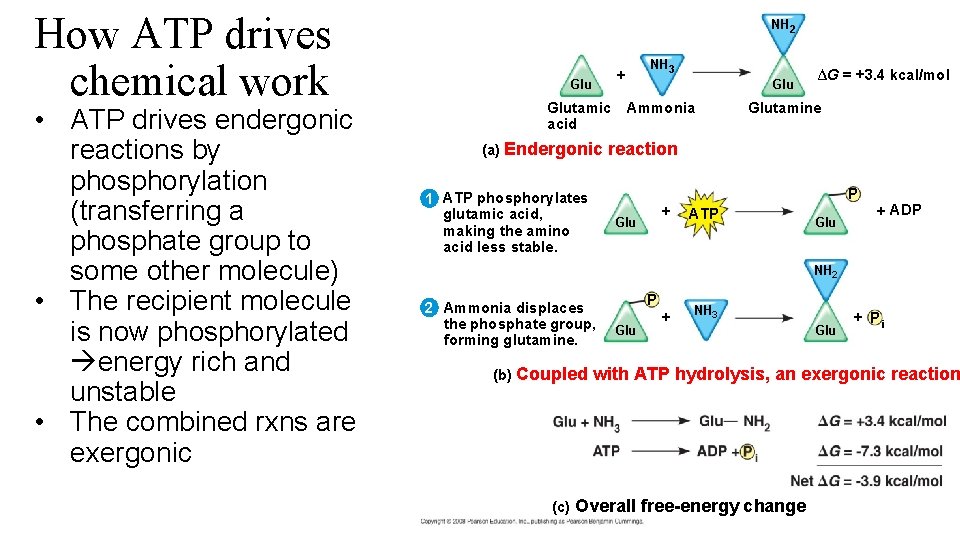
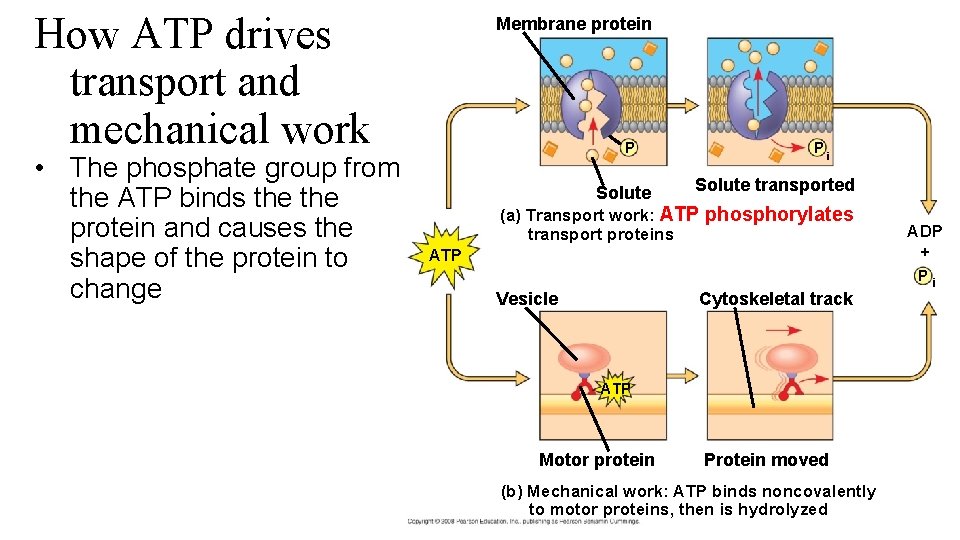
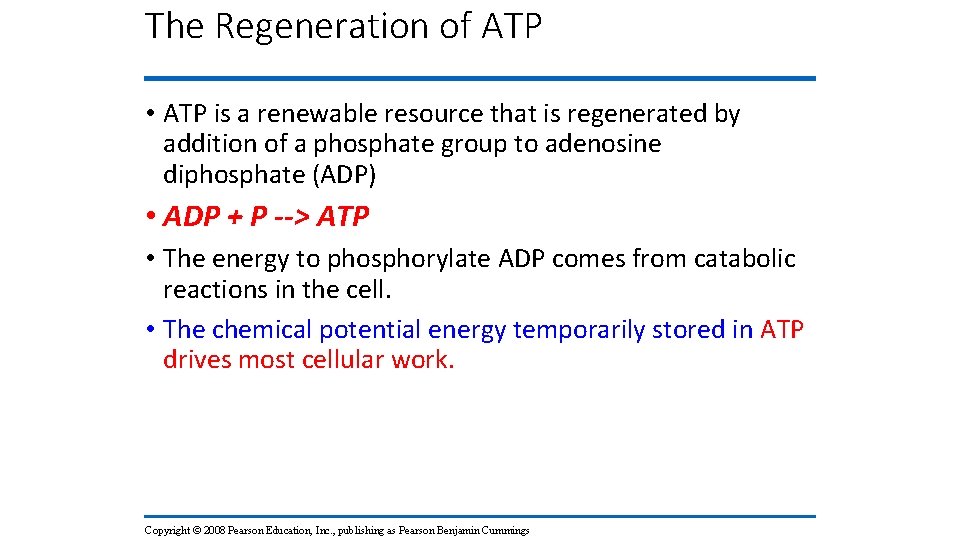
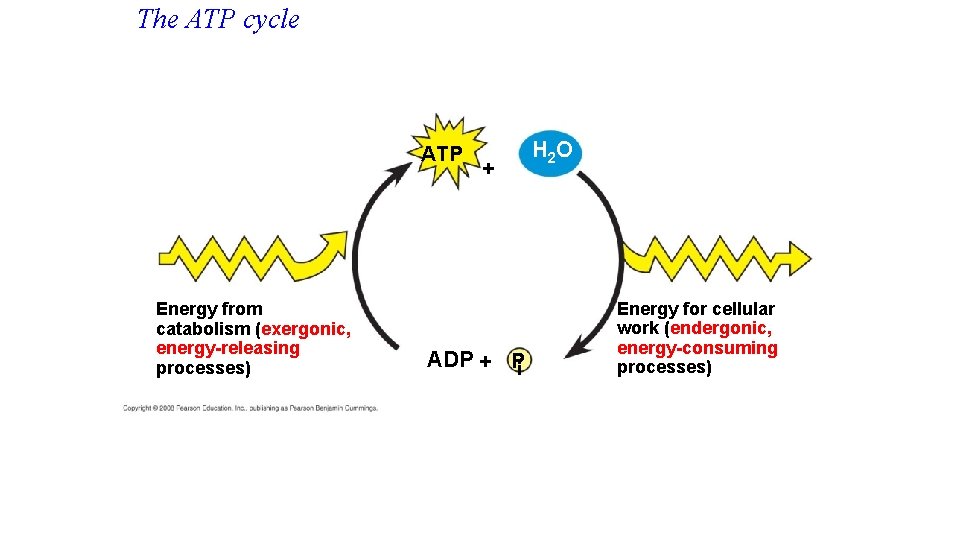
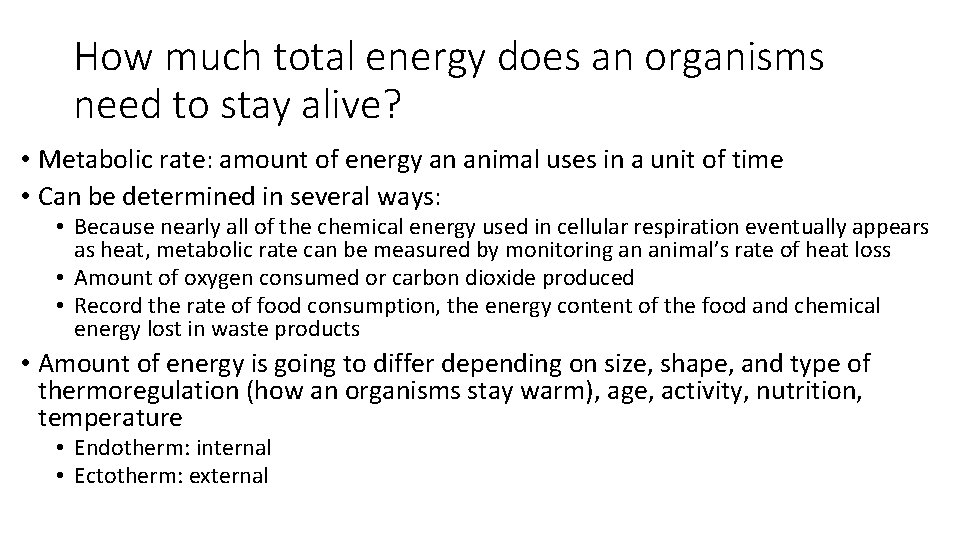
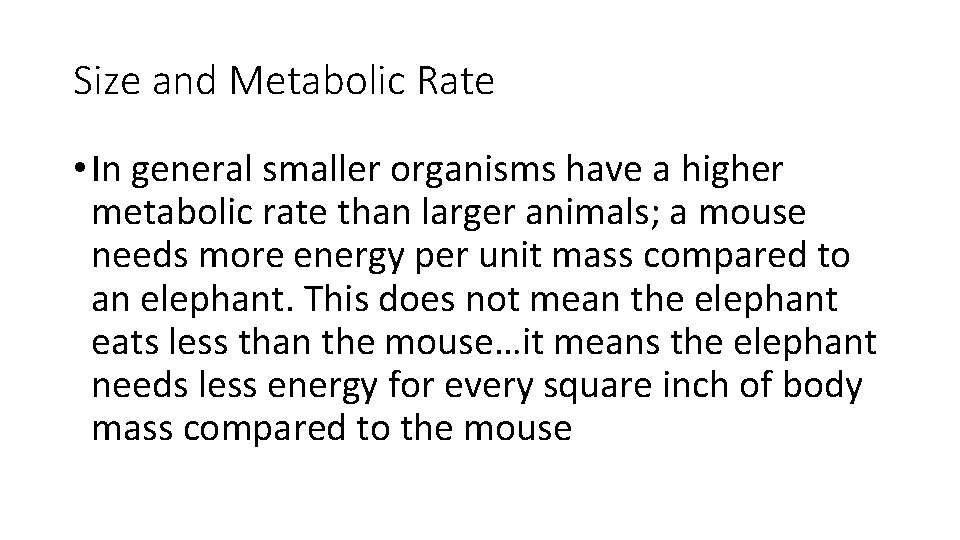
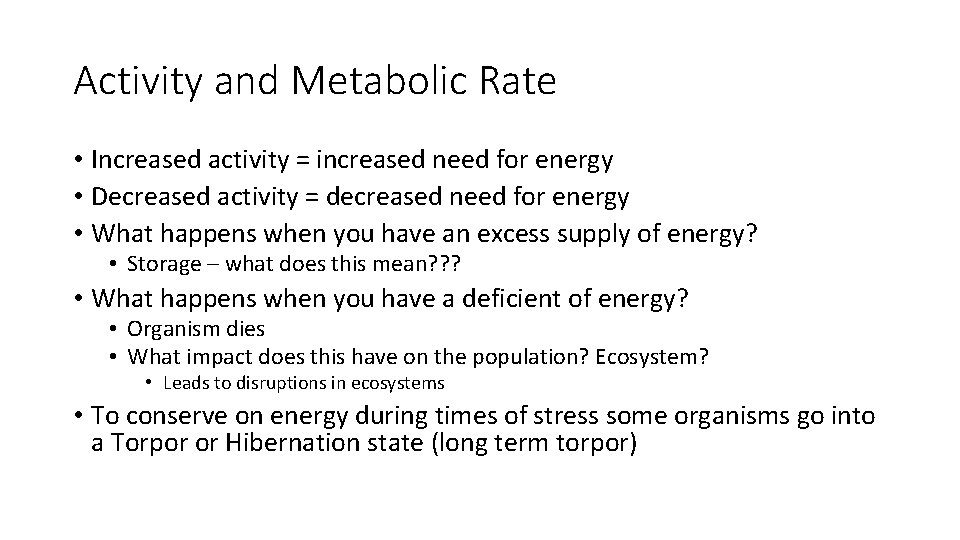
- Slides: 28
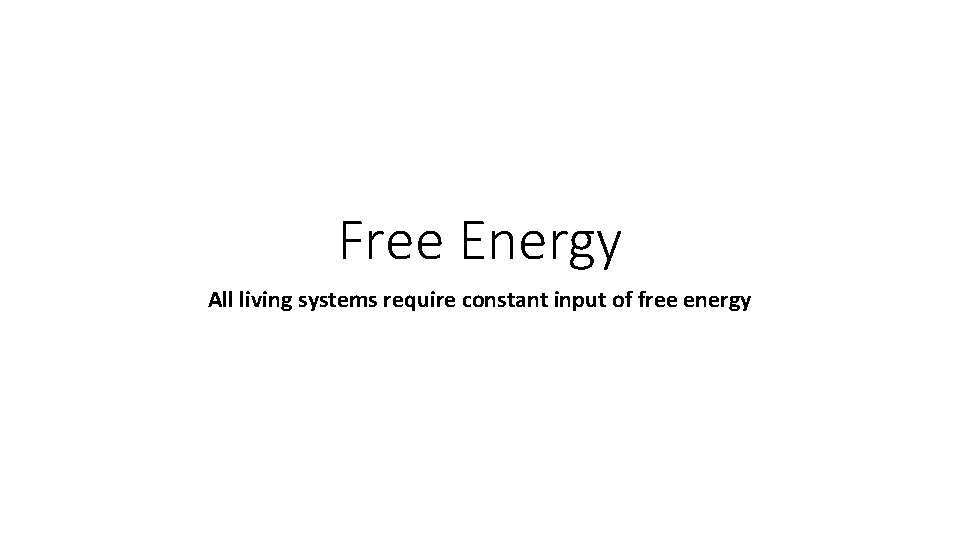
Free Energy All living systems require constant input of free energy
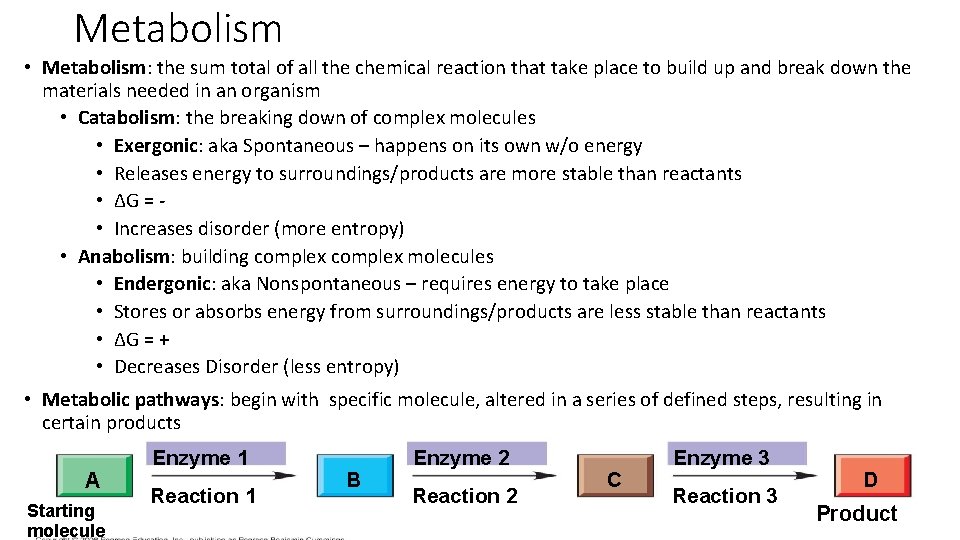
Metabolism • Metabolism: the sum total of all the chemical reaction that take place to build up and break down the materials needed in an organism • Catabolism: the breaking down of complex molecules • Exergonic: aka Spontaneous – happens on its own w/o energy • Releases energy to surroundings/products are more stable than reactants • ∆G = • Increases disorder (more entropy) • Anabolism: building complex molecules • Endergonic: aka Nonspontaneous – requires energy to take place • Stores or absorbs energy from surroundings/products are less stable than reactants • ∆G = + • Decreases Disorder (less entropy) • Metabolic pathways: begin with specific molecule, altered in a series of defined steps, resulting in certain products A Starting molecule Enzyme 1 Reaction 1 B Enzyme 2 Reaction 2 C Enzyme 3 Reaction 3 D Product
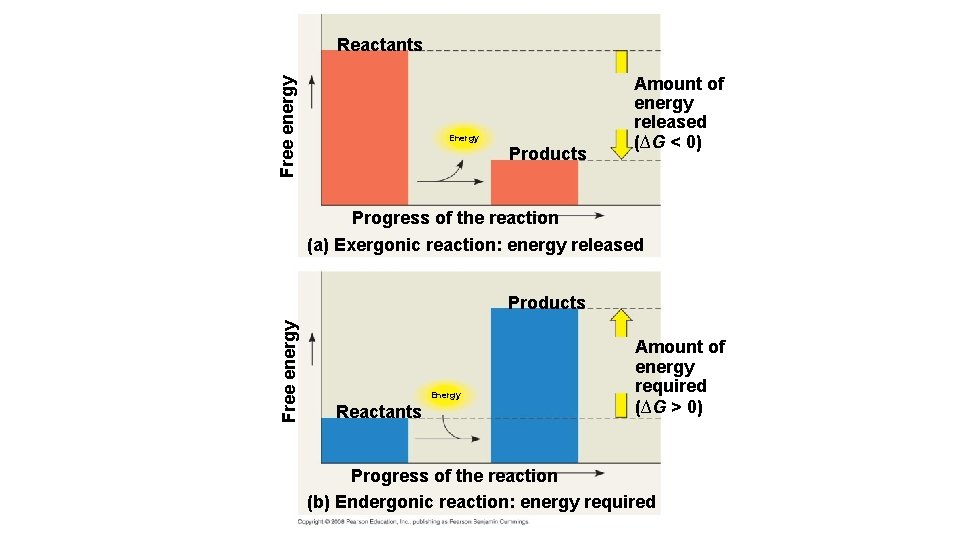
Free energy Reactants Energy Products Amount of energy released (∆G < 0) Progress of the reaction (a) Exergonic reaction: energy released Free energy Products Energy Reactants Amount of energy required (∆G > 0) Progress of the reaction (b) Endergonic reaction: energy required
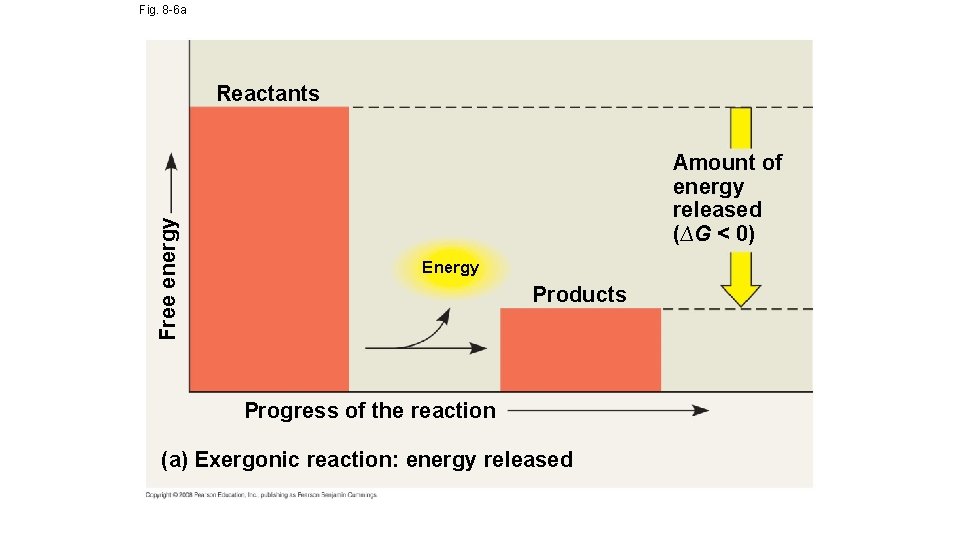
Fig. 8 -6 a Free energy Reactants Amount of energy released (∆G < 0) Energy Products Progress of the reaction (a) Exergonic reaction: energy released
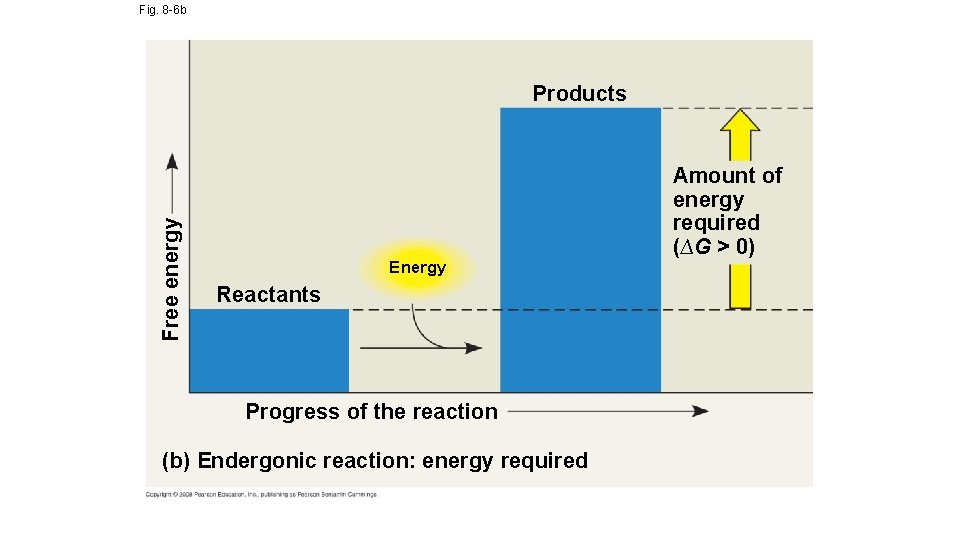
Fig. 8 -6 b Free energy Products Energy Reactants Progress of the reaction (b) Endergonic reaction: energy required Amount of energy required (∆G > 0)
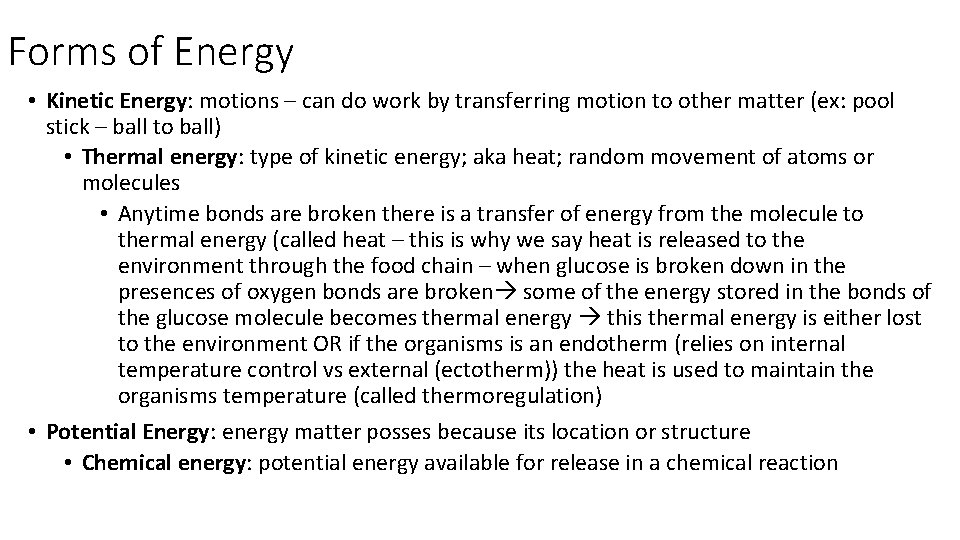
Forms of Energy • Kinetic Energy: motions – can do work by transferring motion to other matter (ex: pool stick – ball to ball) • Thermal energy: type of kinetic energy; aka heat; random movement of atoms or molecules • Anytime bonds are broken there is a transfer of energy from the molecule to thermal energy (called heat – this is why we say heat is released to the environment through the food chain – when glucose is broken down in the presences of oxygen bonds are broken some of the energy stored in the bonds of the glucose molecule becomes thermal energy this thermal energy is either lost to the environment OR if the organisms is an endotherm (relies on internal temperature control vs external (ectotherm)) the heat is used to maintain the organisms temperature (called thermoregulation) • Potential Energy: energy matter posses because its location or structure • Chemical energy: potential energy available for release in a chemical reaction
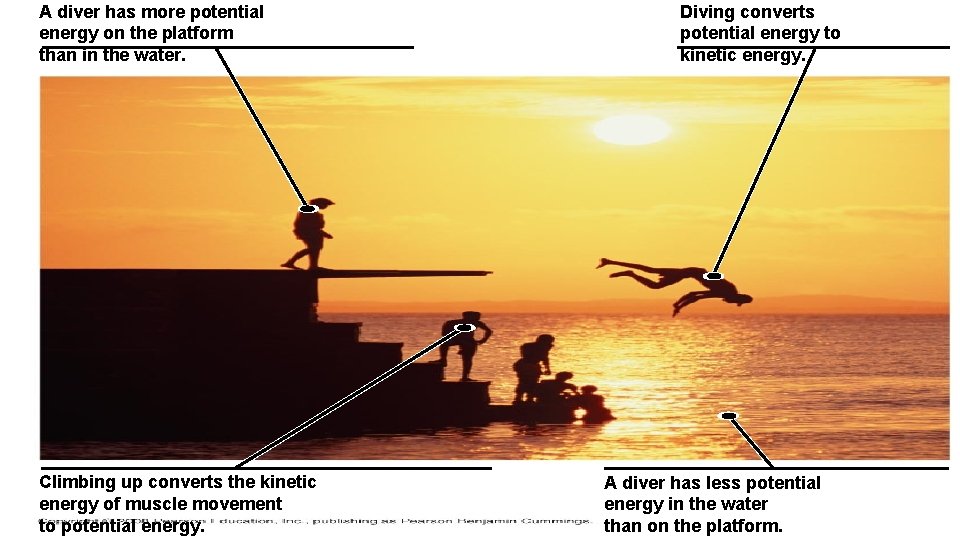
A diver has more potential energy on the platform than in the water. Climbing up converts the kinetic energy of muscle movement to potential energy. Diving converts potential energy to kinetic energy. A diver has less potential energy in the water than on the platform.
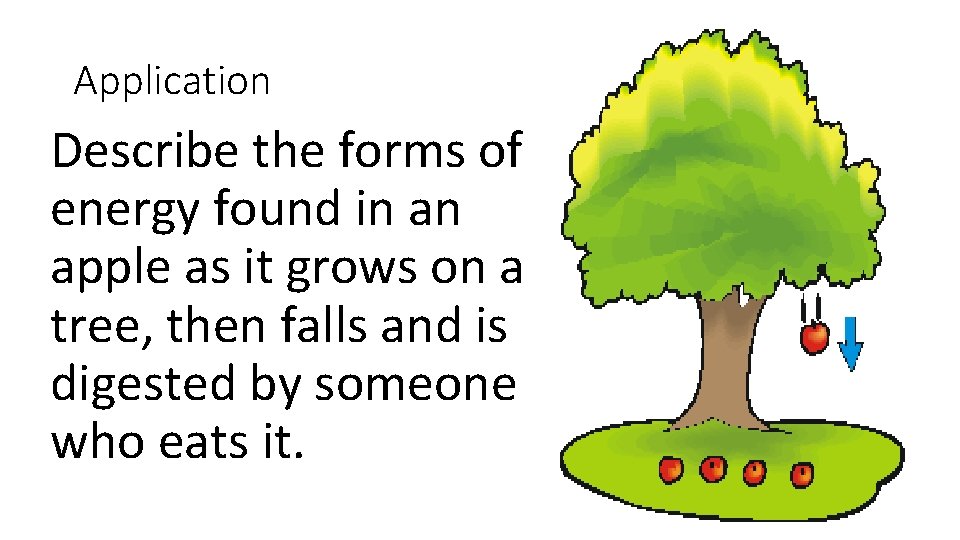
Application Describe the forms of energy found in an apple as it grows on a tree, then falls and is digested by someone who eats it.
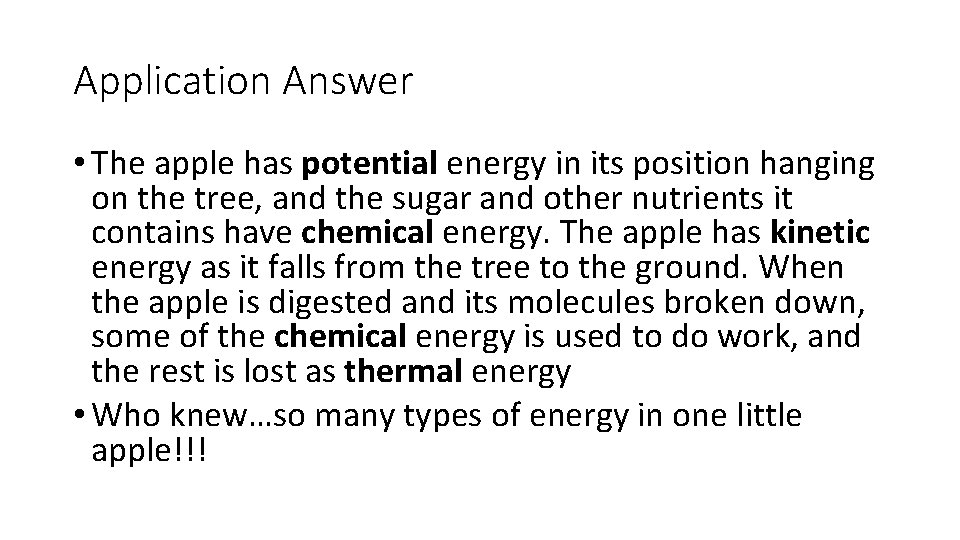
Application Answer • The apple has potential energy in its position hanging on the tree, and the sugar and other nutrients it contains have chemical energy. The apple has kinetic energy as it falls from the tree to the ground. When the apple is digested and its molecules broken down, some of the chemical energy is used to do work, and the rest is lost as thermal energy • Who knew…so many types of energy in one little apple!!!
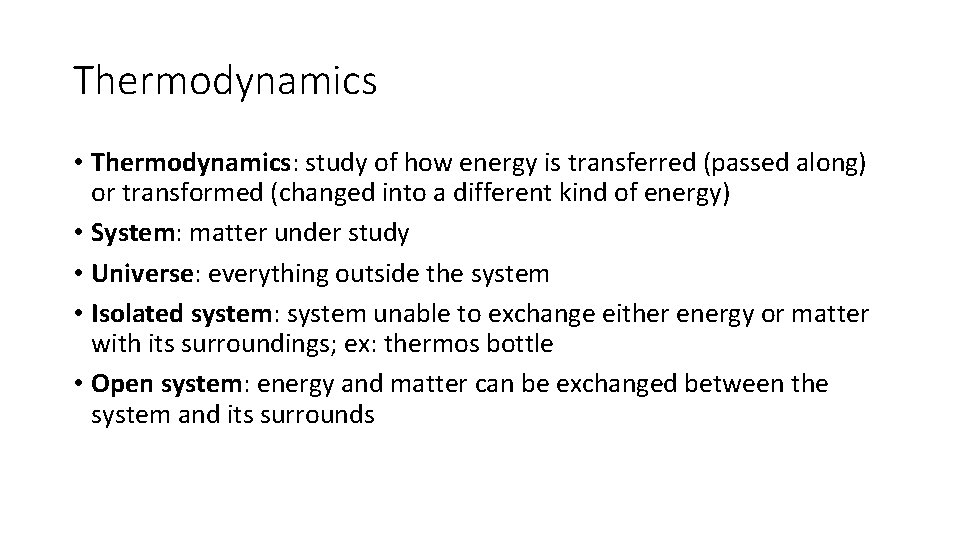
Thermodynamics • Thermodynamics: study of how energy is transferred (passed along) or transformed (changed into a different kind of energy) • System: matter under study • Universe: everything outside the system • Isolated system: system unable to exchange either energy or matter with its surroundings; ex: thermos bottle • Open system: energy and matter can be exchanged between the system and its surrounds
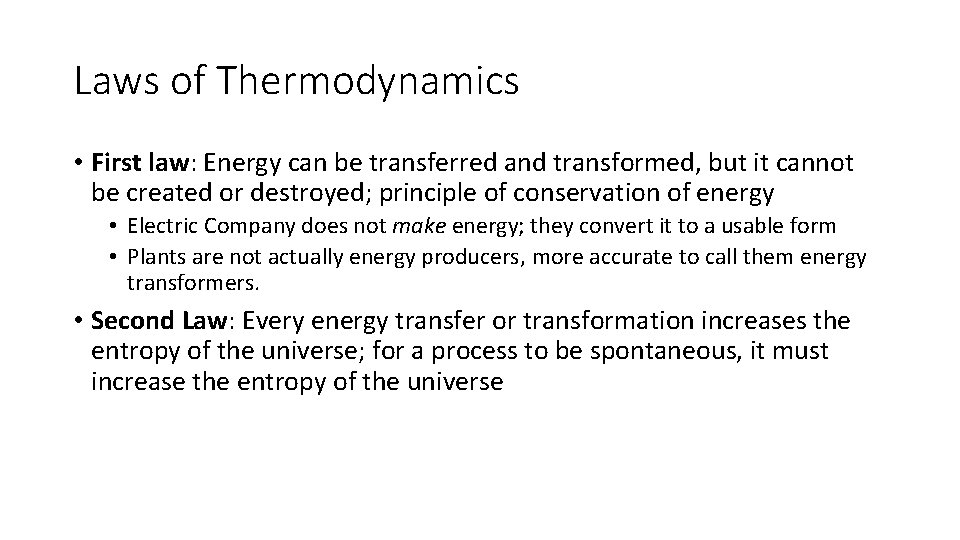
Laws of Thermodynamics • First law: Energy can be transferred and transformed, but it cannot be created or destroyed; principle of conservation of energy • Electric Company does not make energy; they convert it to a usable form • Plants are not actually energy producers, more accurate to call them energy transformers. • Second Law: Every energy transfer or transformation increases the entropy of the universe; for a process to be spontaneous, it must increase the entropy of the universe
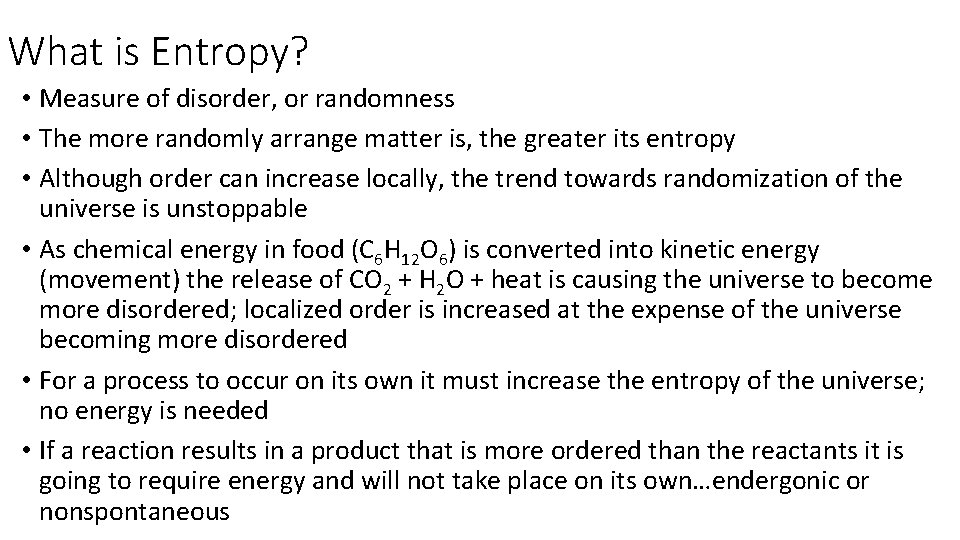
What is Entropy? • Measure of disorder, or randomness • The more randomly arrange matter is, the greater its entropy • Although order can increase locally, the trend towards randomization of the universe is unstoppable • As chemical energy in food (C 6 H 12 O 6) is converted into kinetic energy (movement) the release of CO 2 + H 2 O + heat is causing the universe to become more disordered; localized order is increased at the expense of the universe becoming more disordered • For a process to occur on its own it must increase the entropy of the universe; no energy is needed • If a reaction results in a product that is more ordered than the reactants it is going to require energy and will not take place on its own…endergonic or nonspontaneous
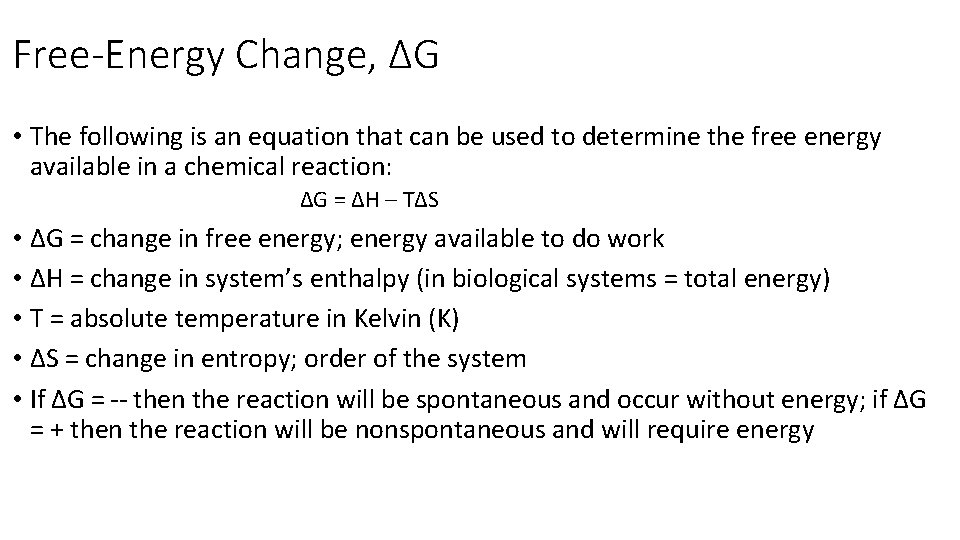
Free-Energy Change, ∆G • The following is an equation that can be used to determine the free energy available in a chemical reaction: ∆G = ∆H – T∆S • ∆G = change in free energy; energy available to do work • ∆H = change in system’s enthalpy (in biological systems = total energy) • T = absolute temperature in Kelvin (K) • ∆S = change in entropy; order of the system • If ∆G = -- then the reaction will be spontaneous and occur without energy; if ∆G = + then the reaction will be nonspontaneous and will require energy
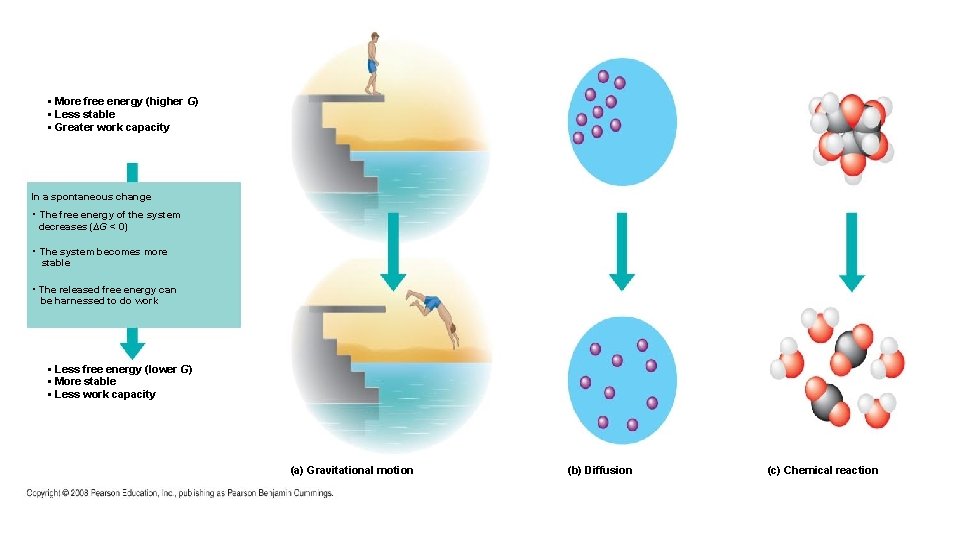
• More free energy (higher G) • Less stable • Greater work capacity In a spontaneous change • The free energy of the system decreases (∆G < 0) • The system becomes more stable • The released free energy can be harnessed to do work • Less free energy (lower G) • More stable • Less work capacity (a) Gravitational motion (b) Diffusion (c) Chemical reaction
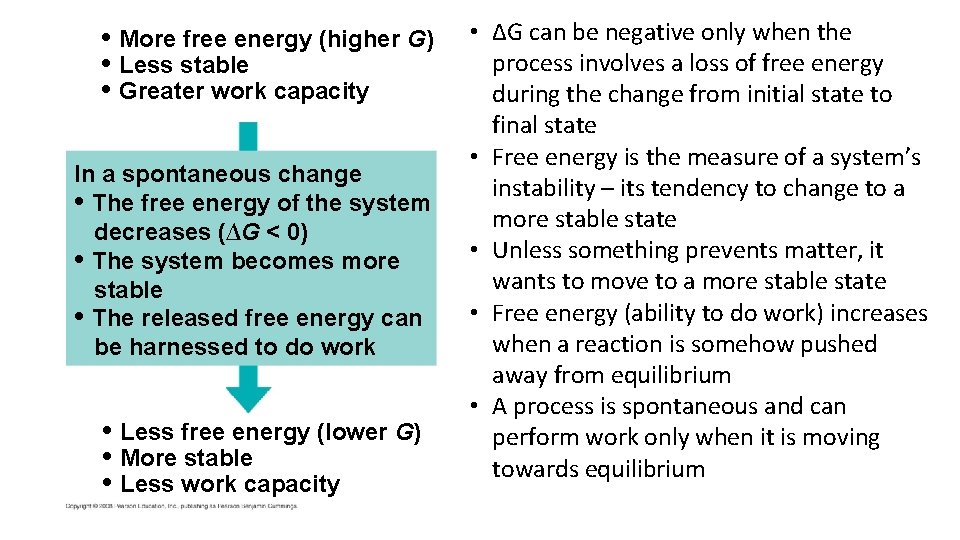
• More free energy (higher G) • Less stable • Greater work capacity In a spontaneous change • The free energy of the system decreases (∆G < 0) • The system becomes more stable • The released free energy can be harnessed to do work • Less free energy (lower G) • More stable • Less work capacity • ∆G can be negative only when the process involves a loss of free energy during the change from initial state to final state • Free energy is the measure of a system’s instability – its tendency to change to a more stable state • Unless something prevents matter, it wants to move to a more stable state • Free energy (ability to do work) increases when a reaction is somehow pushed away from equilibrium • A process is spontaneous and can perform work only when it is moving towards equilibrium
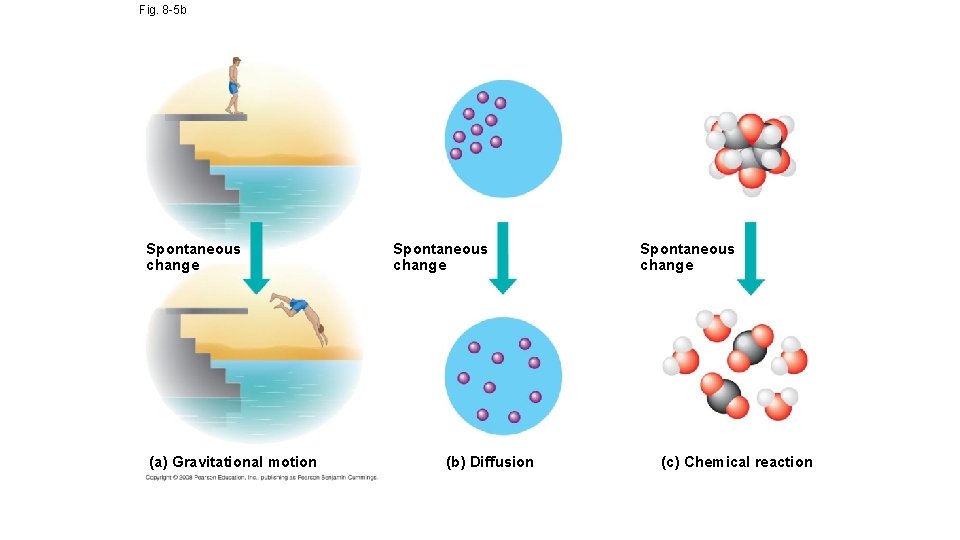
Fig. 8 -5 b Spontaneous change (a) Gravitational motion Spontaneous change (b) Diffusion Spontaneous change (c) Chemical reaction
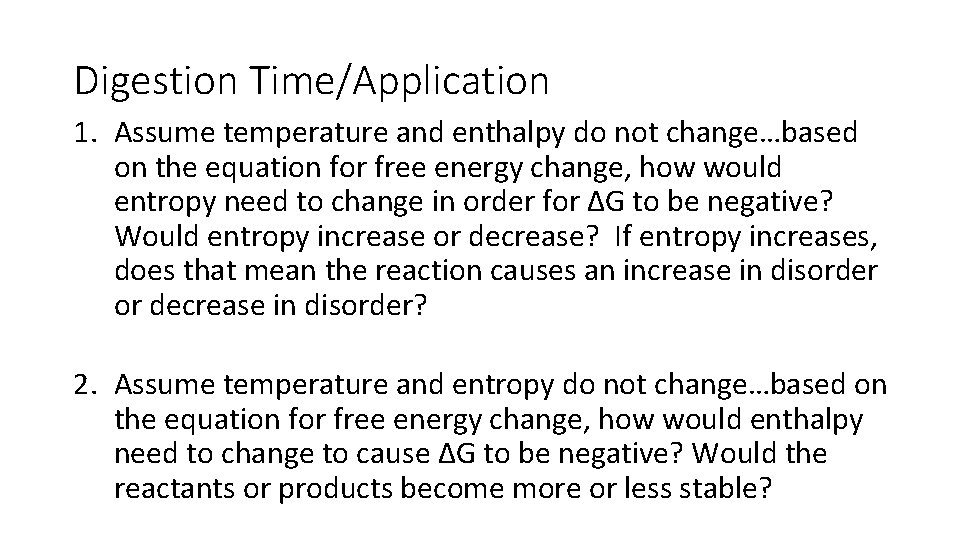
Digestion Time/Application 1. Assume temperature and enthalpy do not change…based on the equation for free energy change, how would entropy need to change in order for ∆G to be negative? Would entropy increase or decrease? If entropy increases, does that mean the reaction causes an increase in disorder or decrease in disorder? 2. Assume temperature and entropy do not change…based on the equation for free energy change, how would enthalpy need to change to cause ∆G to be negative? Would the reactants or products become more or less stable?
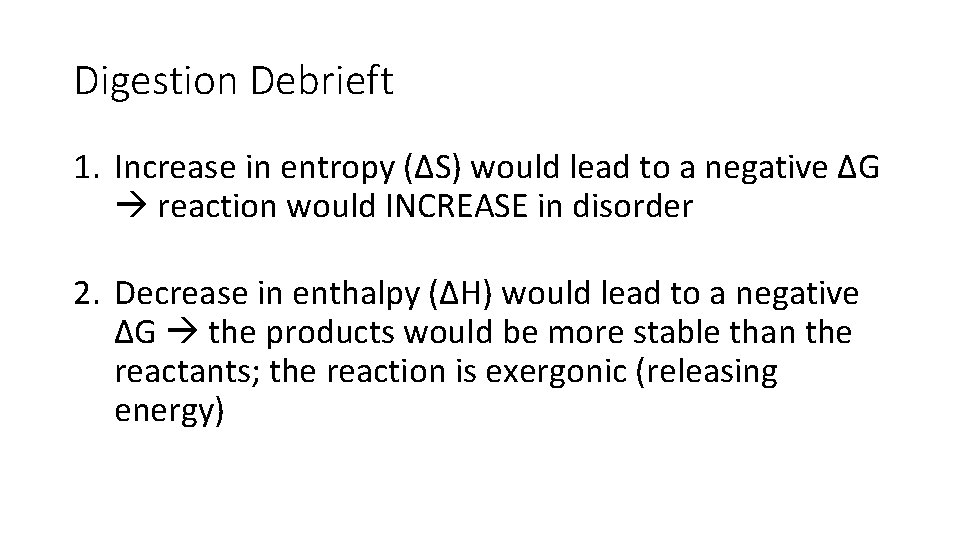
Digestion Debrieft 1. Increase in entropy (∆S) would lead to a negative ∆G reaction would INCREASE in disorder 2. Decrease in enthalpy (∆H) would lead to a negative ∆G the products would be more stable than the reactants; the reaction is exergonic (releasing energy)
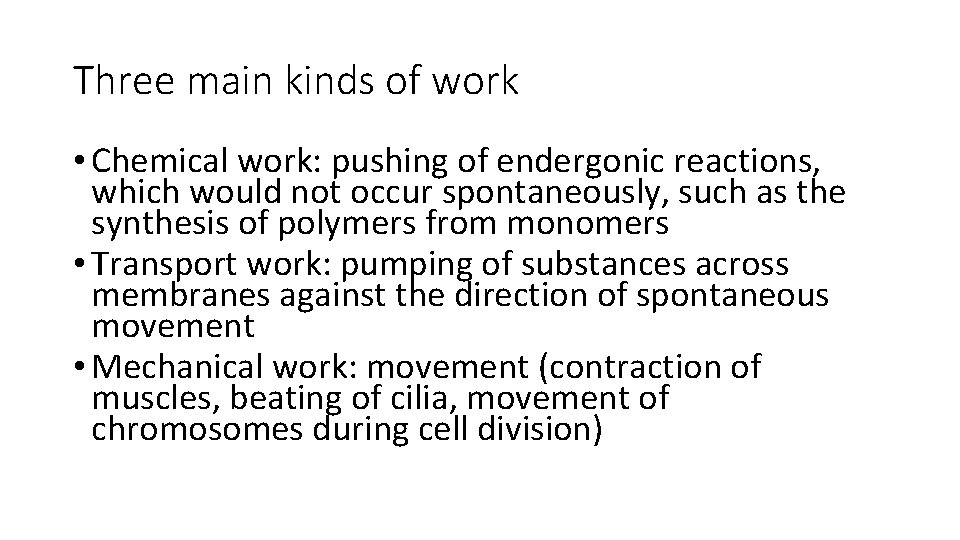
Three main kinds of work • Chemical work: pushing of endergonic reactions, which would not occur spontaneously, such as the synthesis of polymers from monomers • Transport work: pumping of substances across membranes against the direction of spontaneous movement • Mechanical work: movement (contraction of muscles, beating of cilia, movement of chromosomes during cell division)
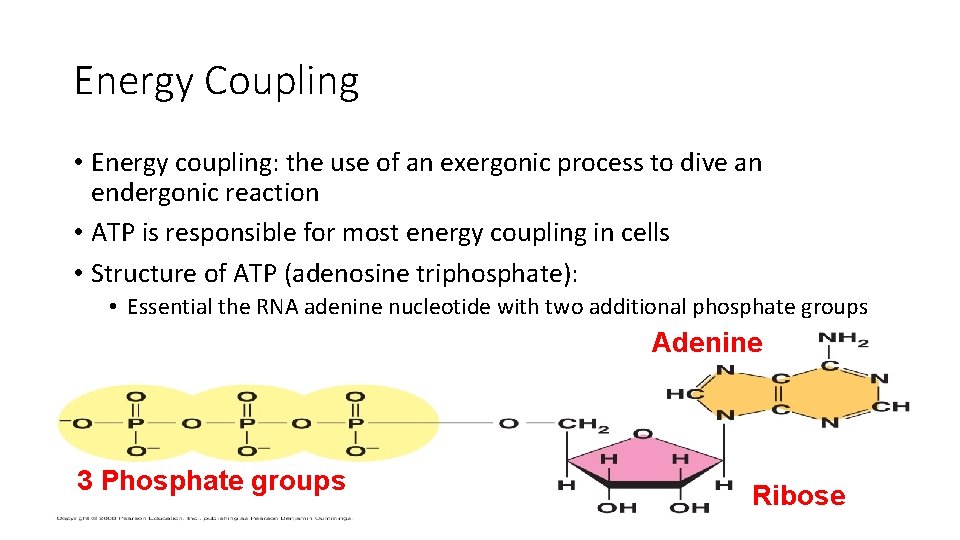
Energy Coupling • Energy coupling: the use of an exergonic process to dive an endergonic reaction • ATP is responsible for most energy coupling in cells • Structure of ATP (adenosine triphosphate): • Essential the RNA adenine nucleotide with two additional phosphate groups Adenine 3 Phosphate groups Ribose
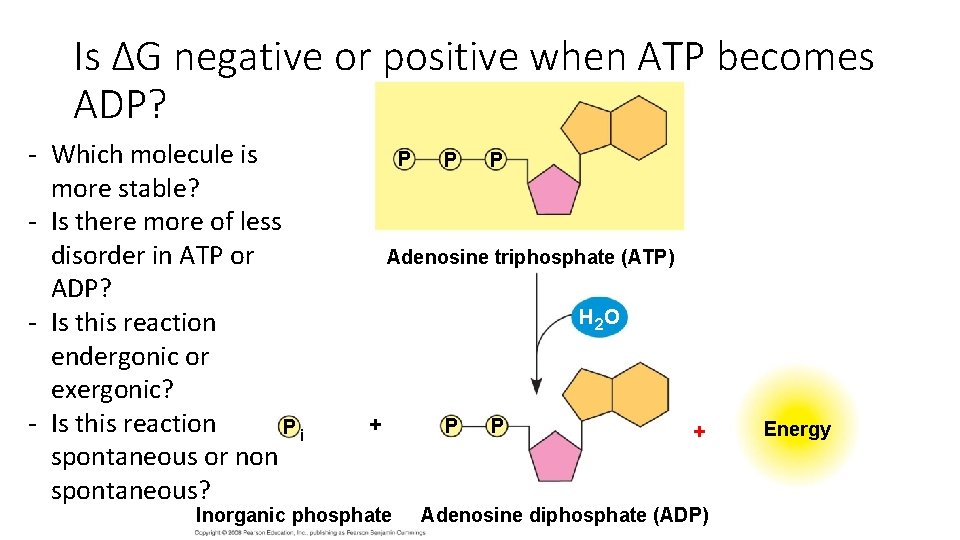
Is ∆G negative or positive when ATP becomes ADP? - Which molecule is more stable? - Is there more of less disorder in ATP or ADP? - Is this reaction endergonic or exergonic? - Is this reaction Pi spontaneous or non spontaneous? P P P Adenosine triphosphate (ATP) H 2 O + Inorganic phosphate P P + Adenosine diphosphate (ADP) Energy
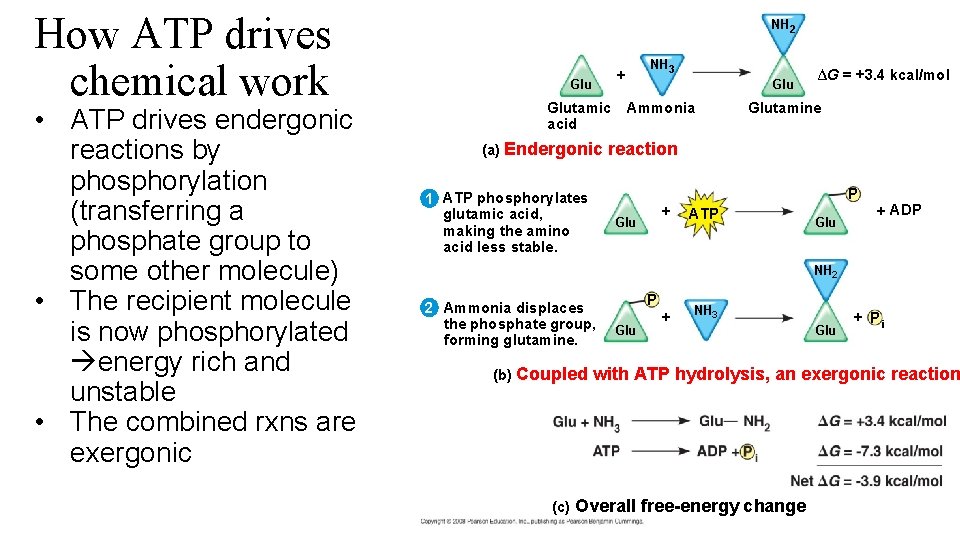
How ATP drives chemical work • ATP drives endergonic reactions by phosphorylation (transferring a phosphate group to some other molecule) • The recipient molecule is now phosphorylated energy rich and unstable • The combined rxns are exergonic NH 2 NH 3 + Glutamic acid (a) Endergonic Glu Ammonia ∆G = +3. 4 kcal/mol Glutamine reaction P 1 ATP phosphorylates glutamic acid, making the amino acid less stable. + Glu ATP Glu + ADP NH 2 2 Ammonia displaces the phosphate group, forming glutamine. (b) Coupled P Glu + NH 3 Glu + Pi with ATP hydrolysis, an exergonic reaction (c) Overall free-energy change
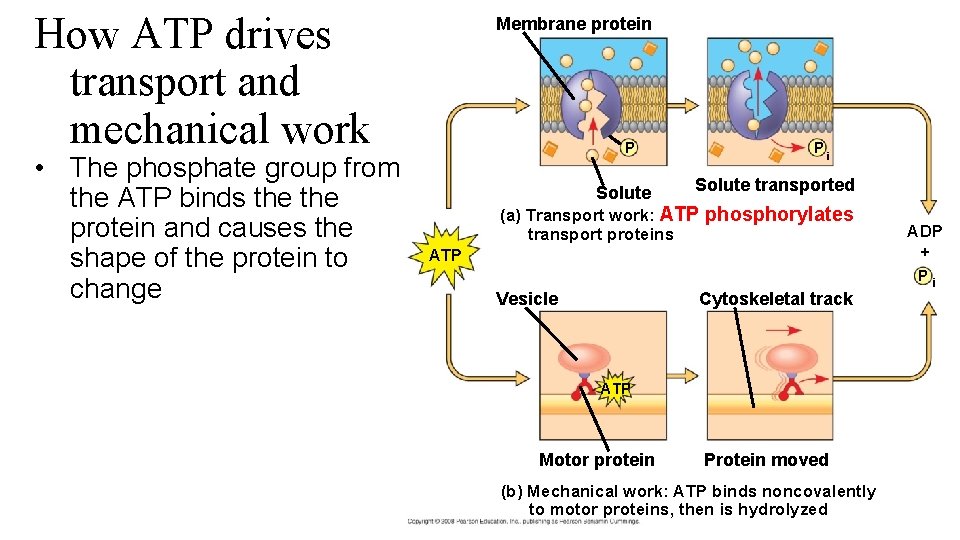
How ATP drives transport and mechanical work • The phosphate group from the ATP binds the protein and causes the shape of the protein to change Membrane protein P Solute Pi Solute transported (a) Transport work: ATP phosphorylates transport proteins ADP + ATP Vesicle Cytoskeletal track ATP Motor protein Protein moved (b) Mechanical work: ATP binds noncovalently to motor proteins, then is hydrolyzed Pi
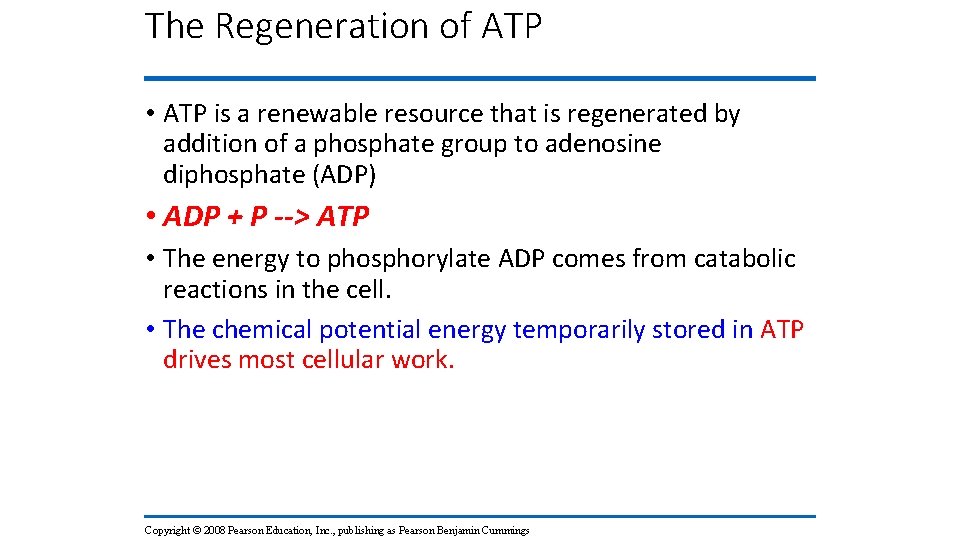
The Regeneration of ATP • ATP is a renewable resource that is regenerated by addition of a phosphate group to adenosine diphosphate (ADP) • ADP + P --> ATP • The energy to phosphorylate ADP comes from catabolic reactions in the cell. • The chemical potential energy temporarily stored in ATP drives most cellular work. Copyright © 2008 Pearson Education, Inc. , publishing as Pearson Benjamin Cummings
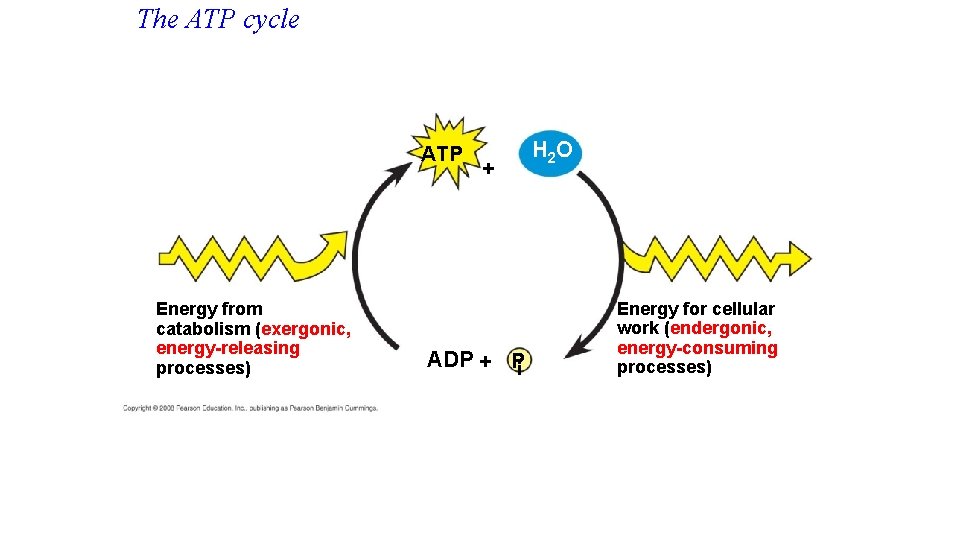
The ATP cycle ATP Energy from catabolism (exergonic, energy-releasing processes) + ADP + Pi H 2 O Energy for cellular work (endergonic, energy-consuming processes)
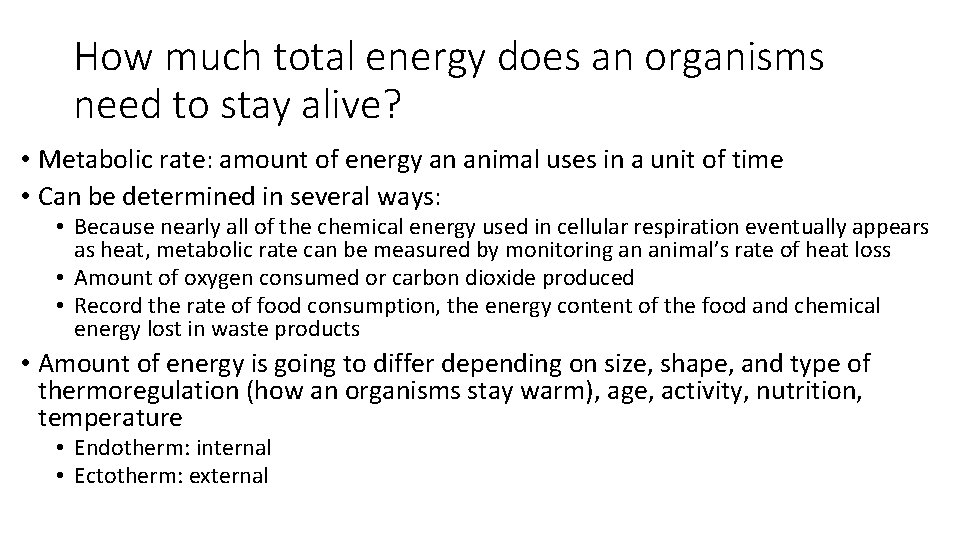
How much total energy does an organisms need to stay alive? • Metabolic rate: amount of energy an animal uses in a unit of time • Can be determined in several ways: • Because nearly all of the chemical energy used in cellular respiration eventually appears as heat, metabolic rate can be measured by monitoring an animal’s rate of heat loss • Amount of oxygen consumed or carbon dioxide produced • Record the rate of food consumption, the energy content of the food and chemical energy lost in waste products • Amount of energy is going to differ depending on size, shape, and type of thermoregulation (how an organisms stay warm), age, activity, nutrition, temperature • Endotherm: internal • Ectotherm: external
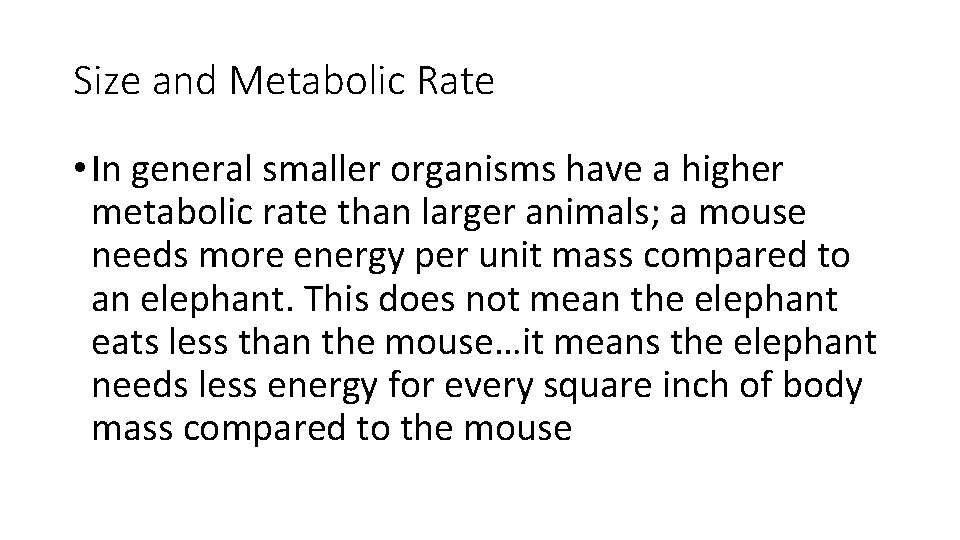
Size and Metabolic Rate • In general smaller organisms have a higher metabolic rate than larger animals; a mouse needs more energy per unit mass compared to an elephant. This does not mean the elephant eats less than the mouse…it means the elephant needs less energy for every square inch of body mass compared to the mouse
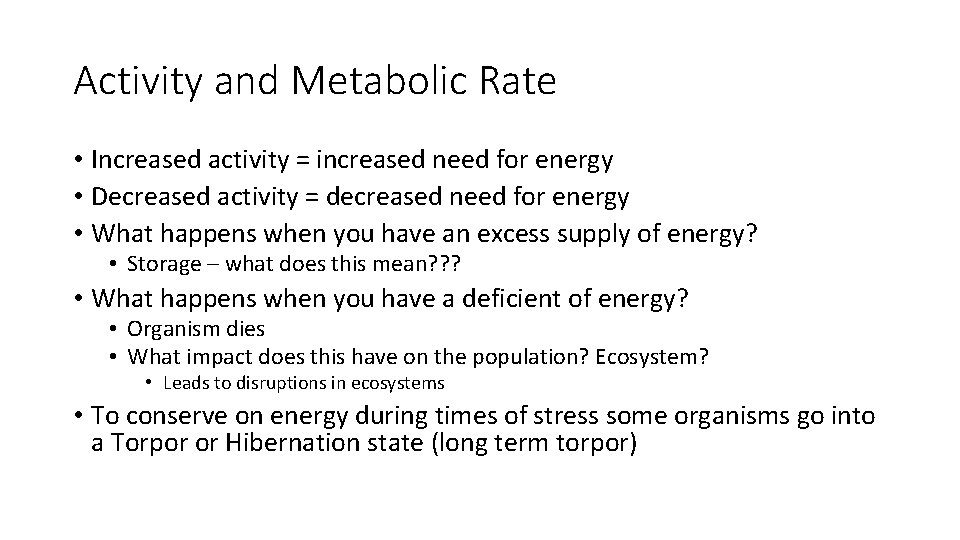
Activity and Metabolic Rate • Increased activity = increased need for energy • Decreased activity = decreased need for energy • What happens when you have an excess supply of energy? • Storage – what does this mean? ? ? • What happens when you have a deficient of energy? • Organism dies • What impact does this have on the population? Ecosystem? • Leads to disruptions in ecosystems • To conserve on energy during times of stress some organisms go into a Torpor or Hibernation state (long term torpor)
Gibbs free energy unit
Gibbs free energy equation
Calculate delta g
Helmholtz free energy and gibbs free energy
Mitochondria information
Where do we get a constant supply of free energy
3 types of active transport
Does simple diffusion require energy
Integral and peripheral proteins
Does osmosis require energy
Diffusion vs facilitated diffusion
What is useful energy
Constant pointer and pointer to constant
üyou
Constant pointer and pointer to constant
Metals tend to be
Equilibrium of chemical reactions
Big k vs little k chemistry
Complex ion formation and solubility
Constant pointer and pointer to constant
Display the address of intval using cout and intptr.
Potassium lithium
Specific cake resistance formula
Units of gas constant
Name a line containing point a
Venn diagram living and nonliving things
Is mold living or nonliving
Living non living dead
Energy energy transfer and general energy analysis