Considerations for the implementation of experiments Manfred Grieser
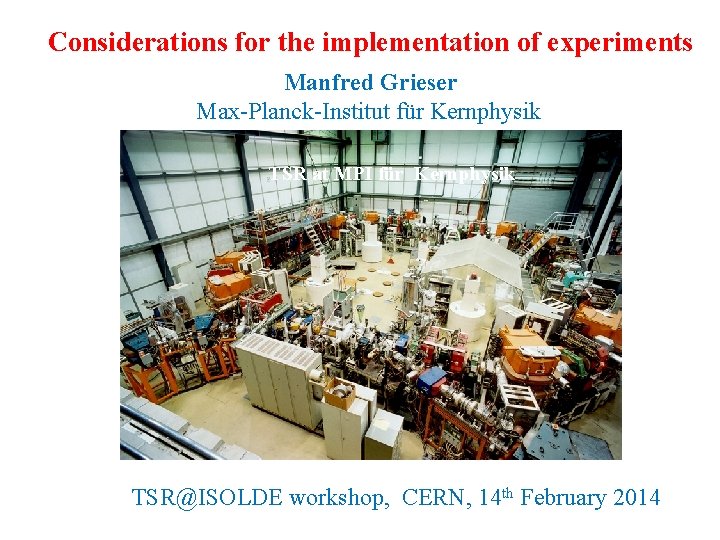
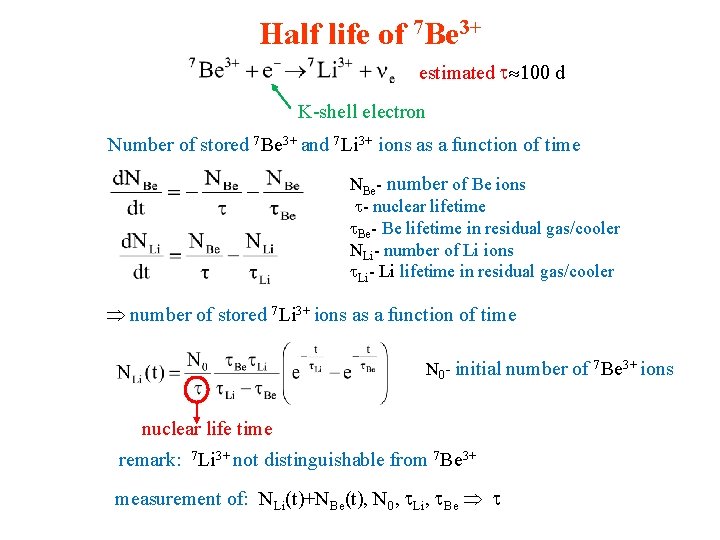
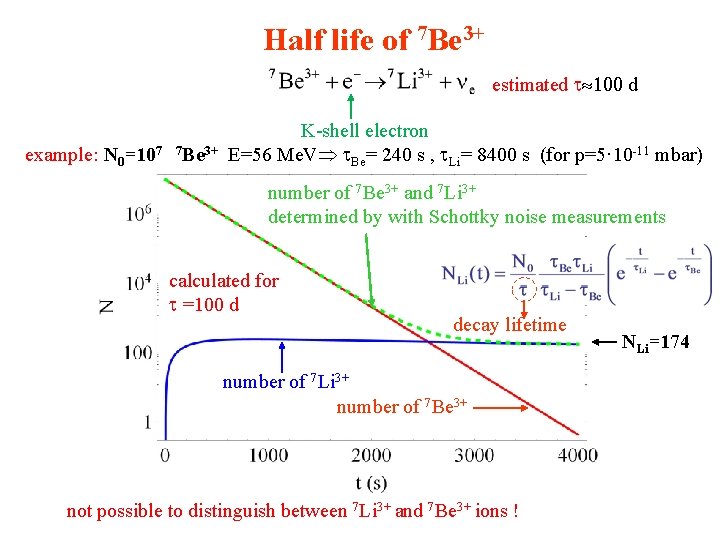
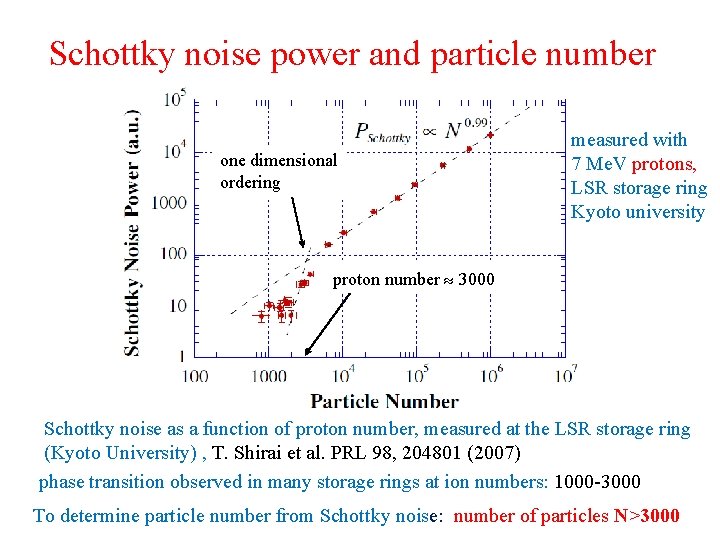
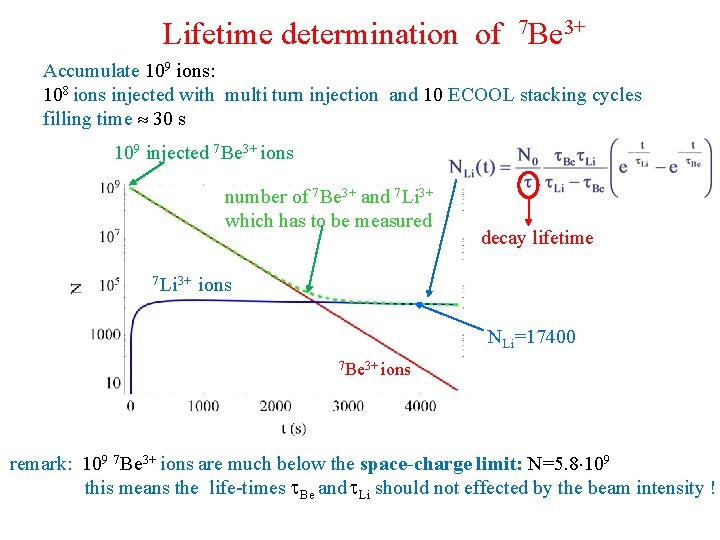
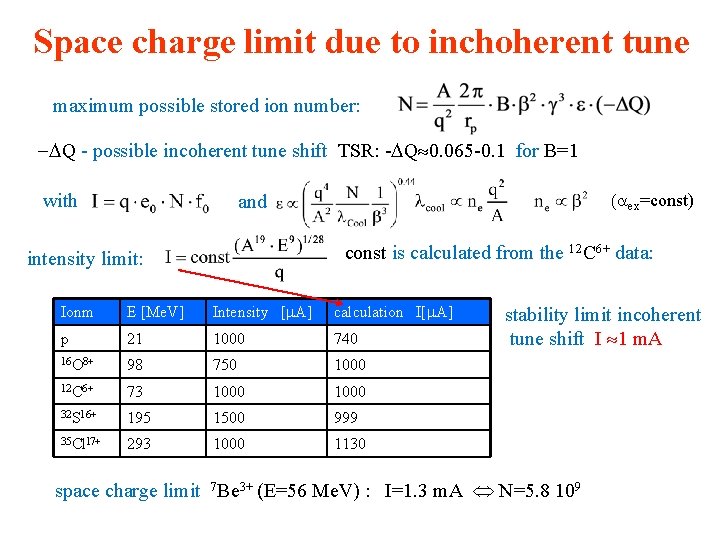
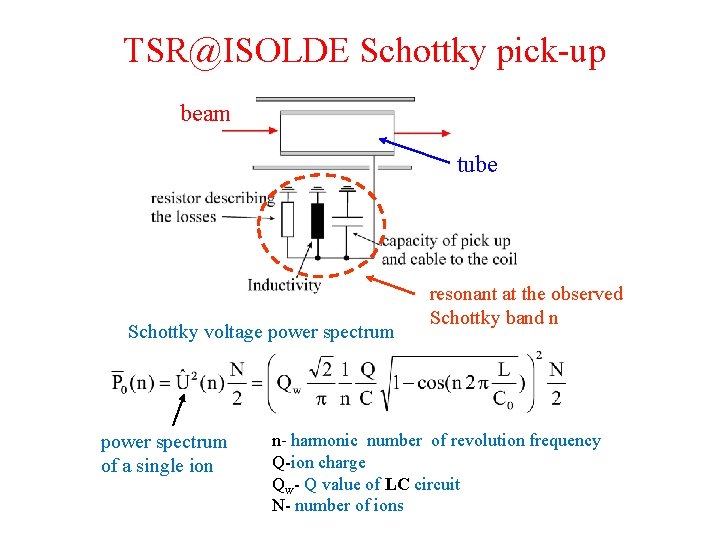
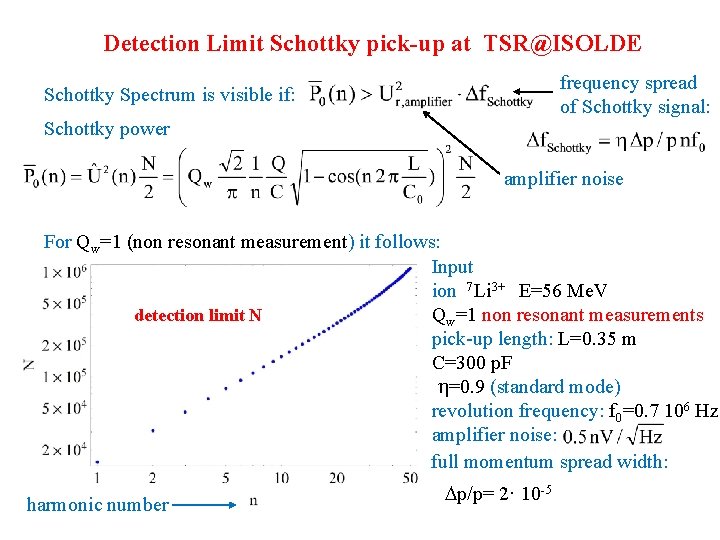
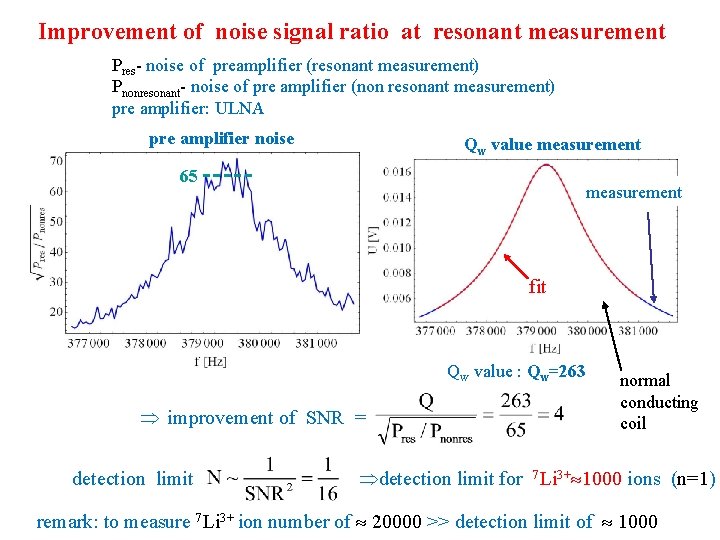
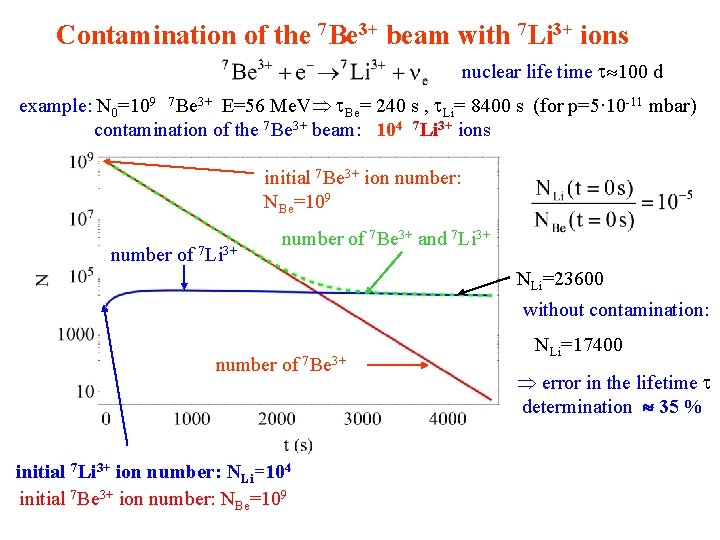
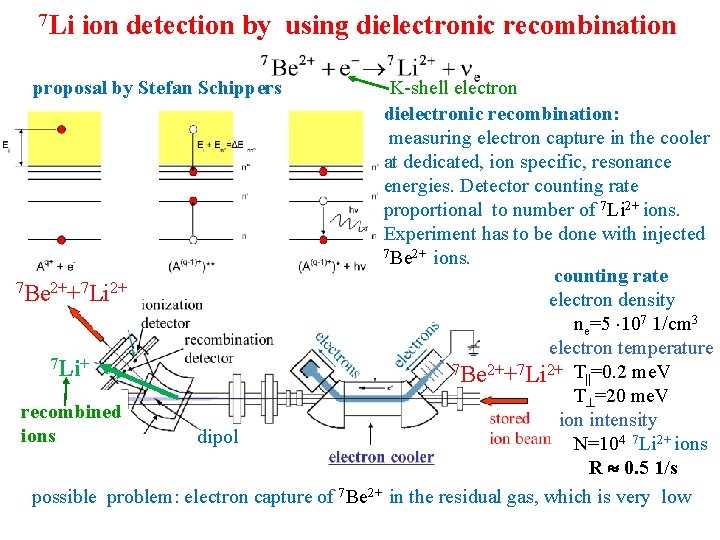
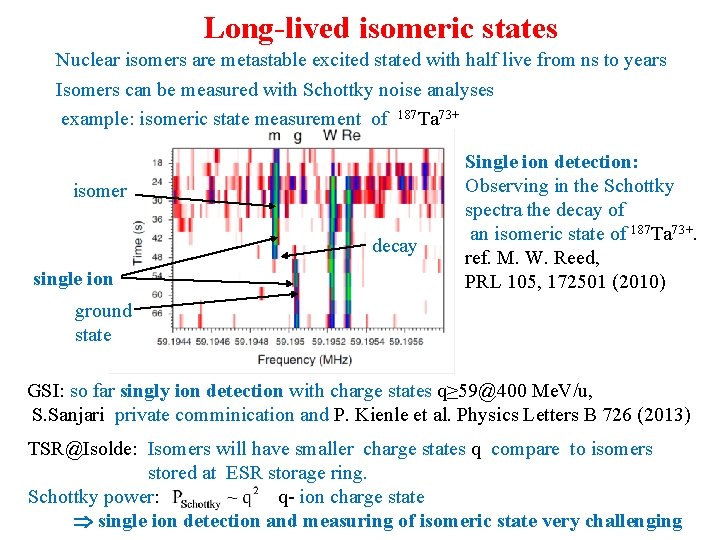
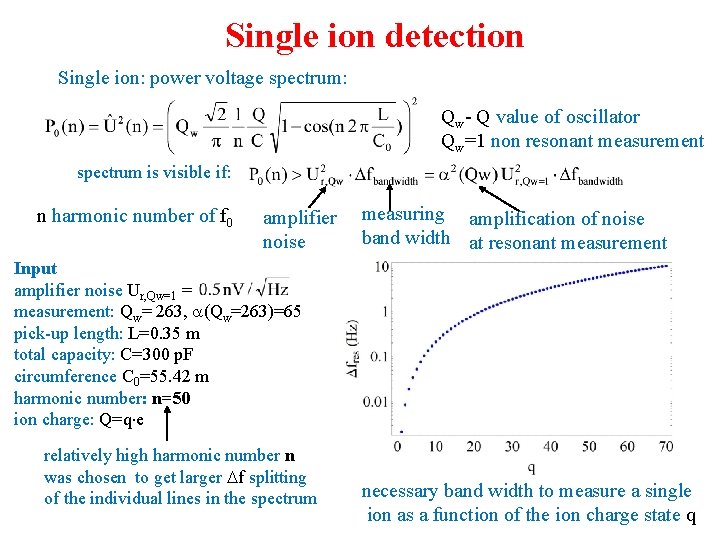
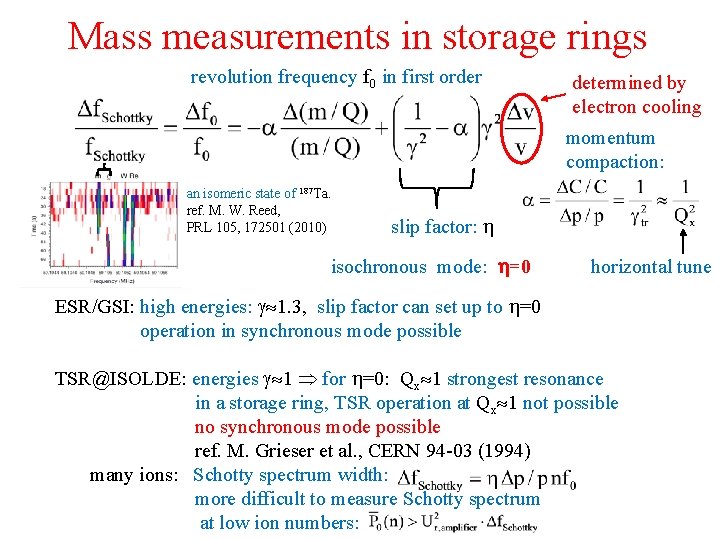
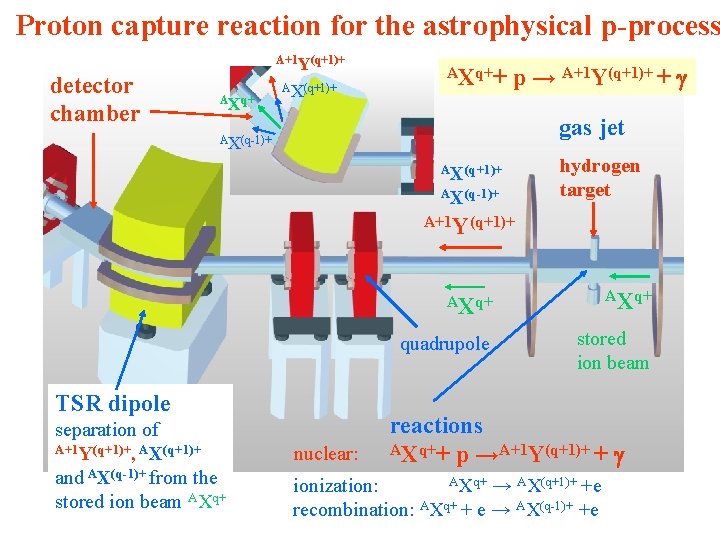
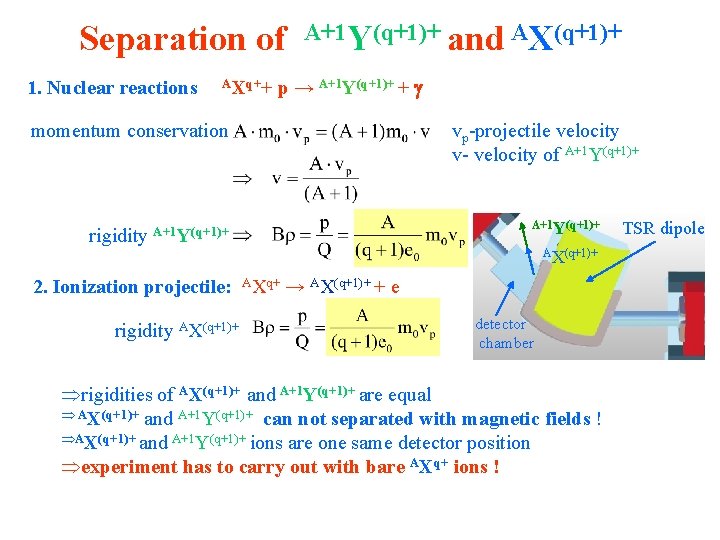
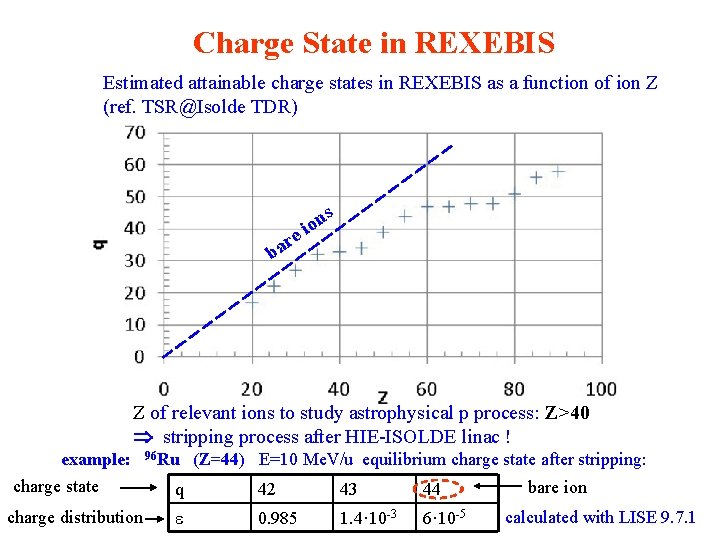
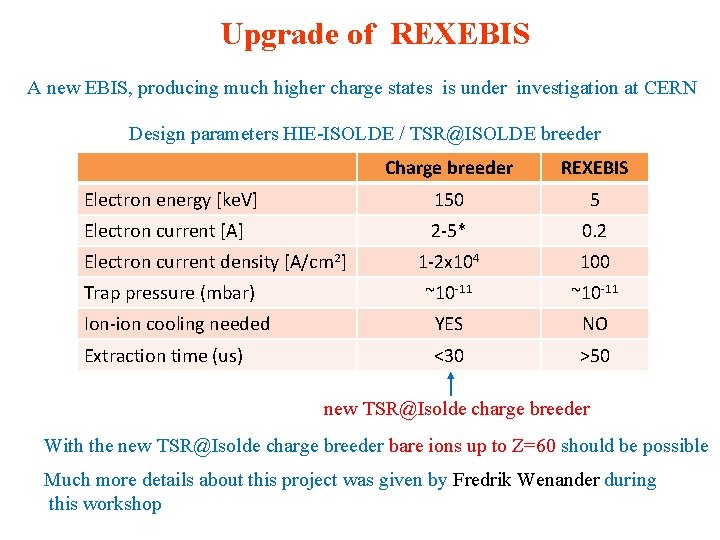
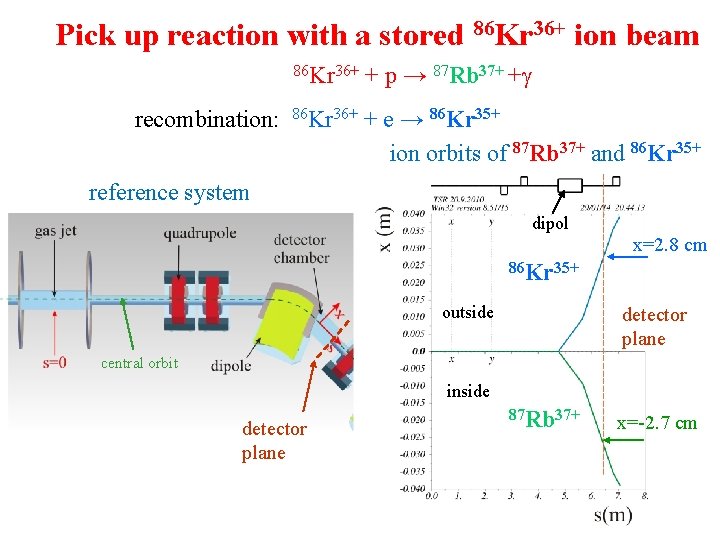
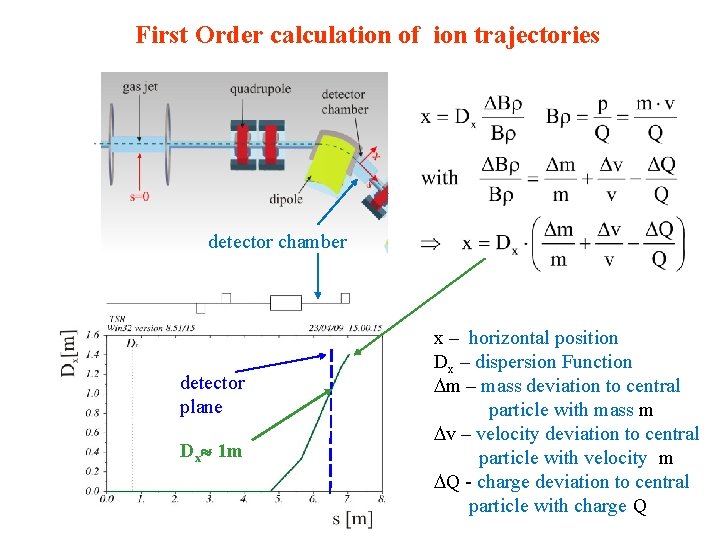
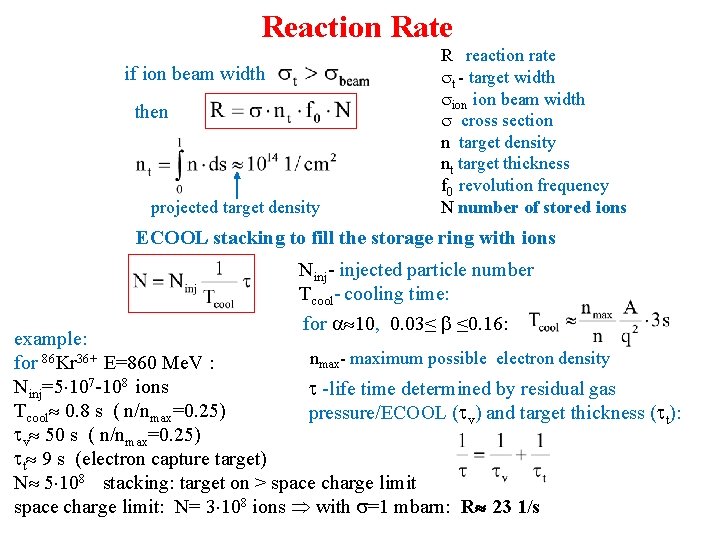
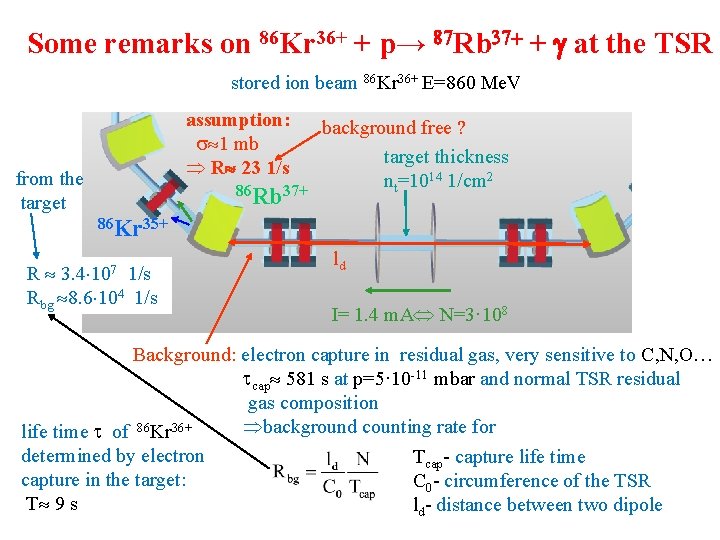
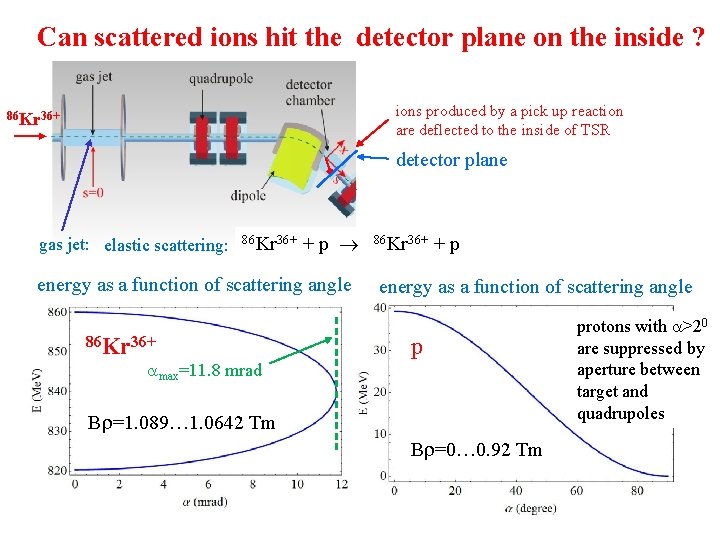
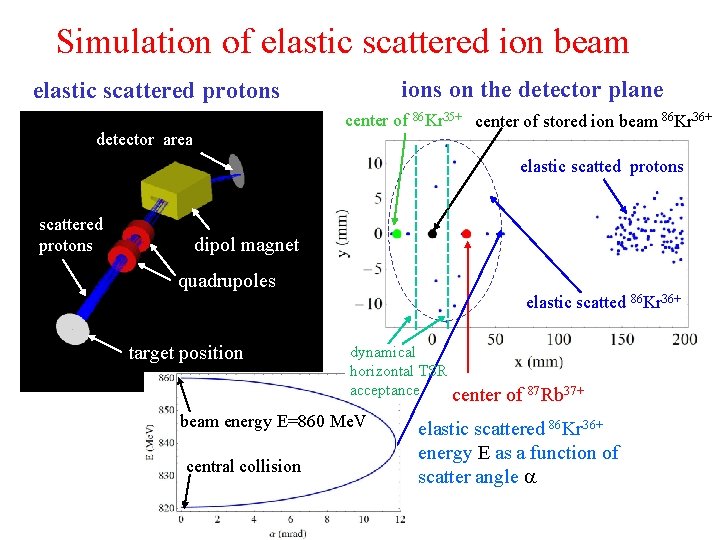
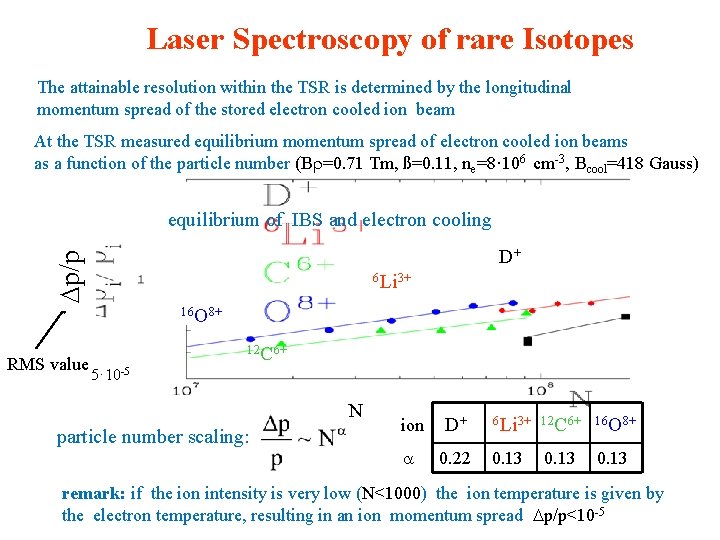
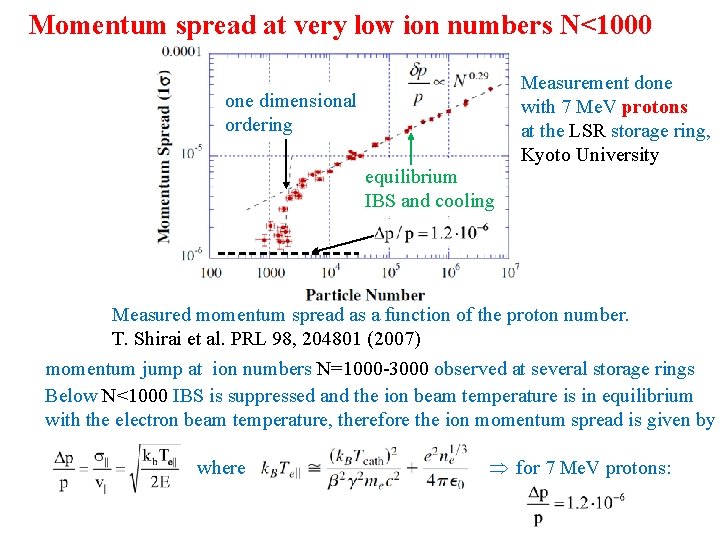
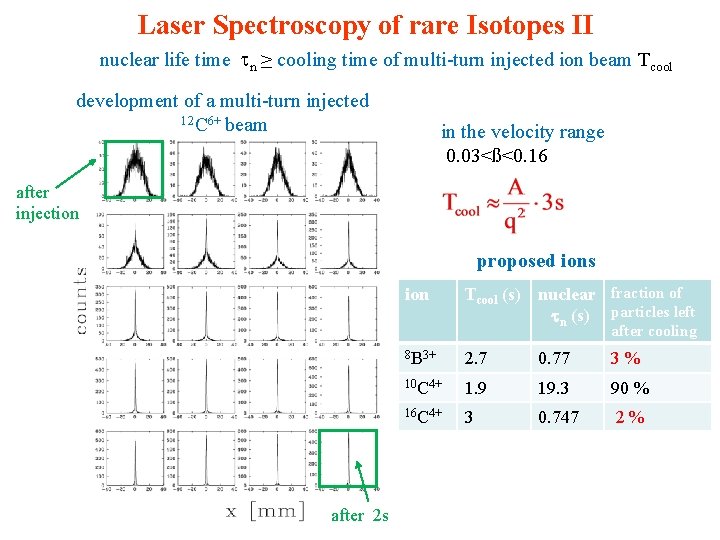
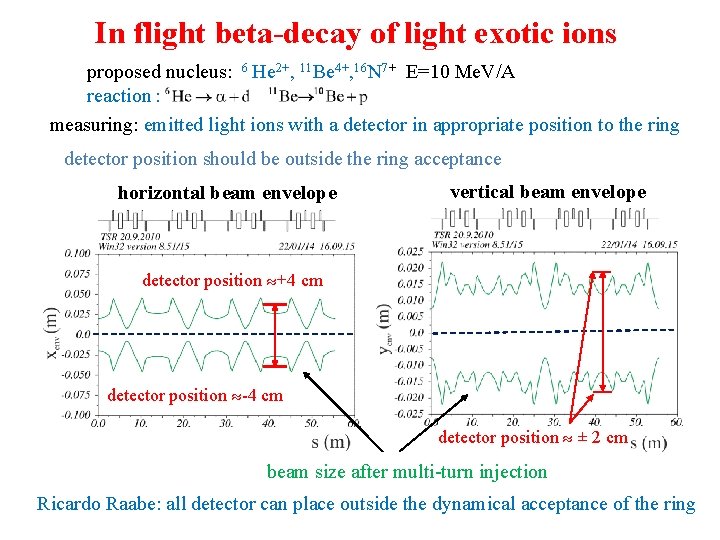
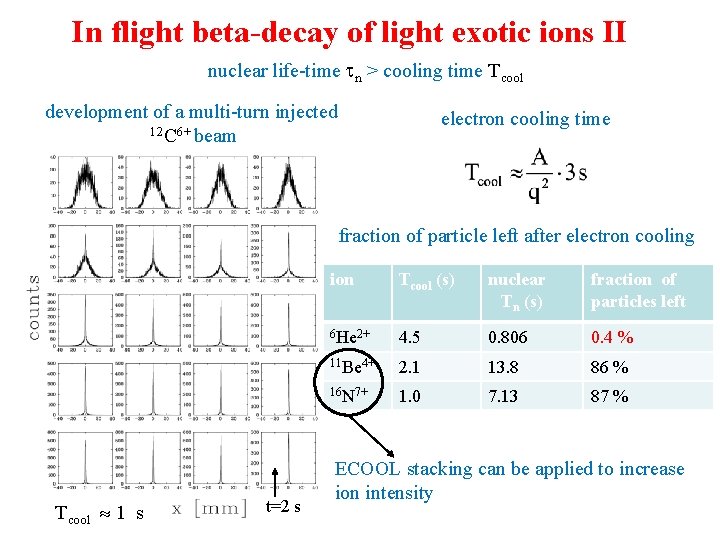
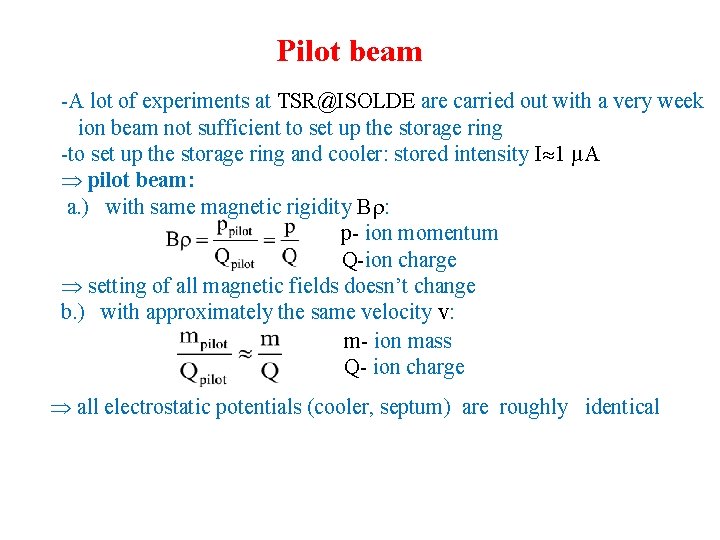
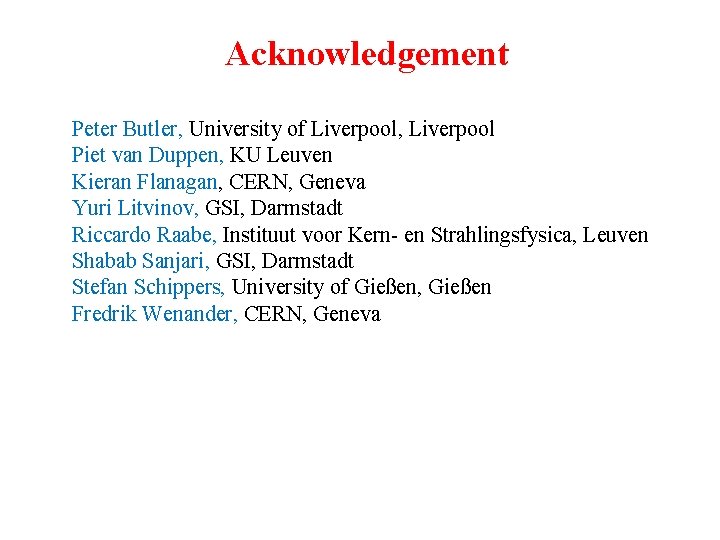
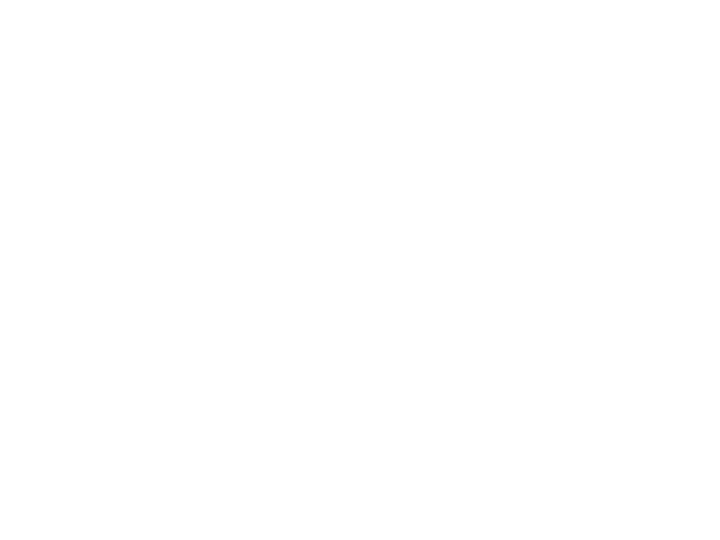
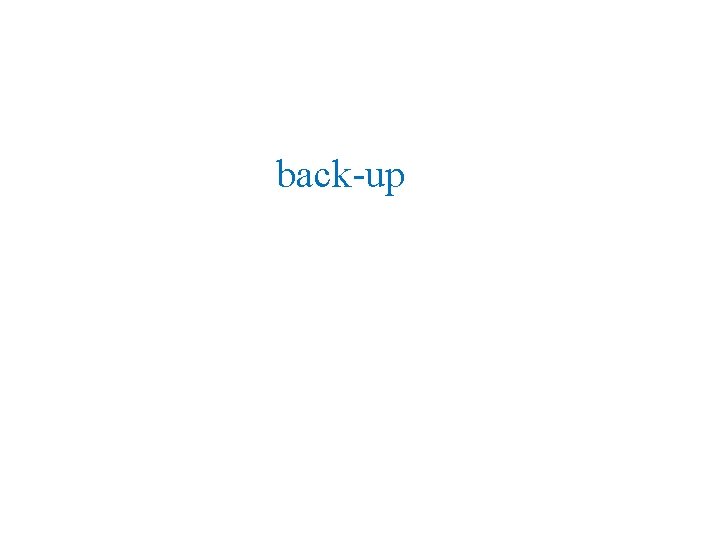
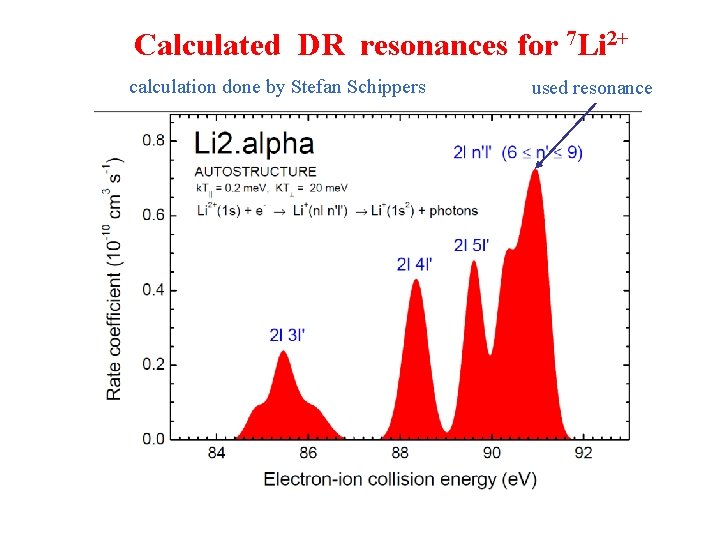
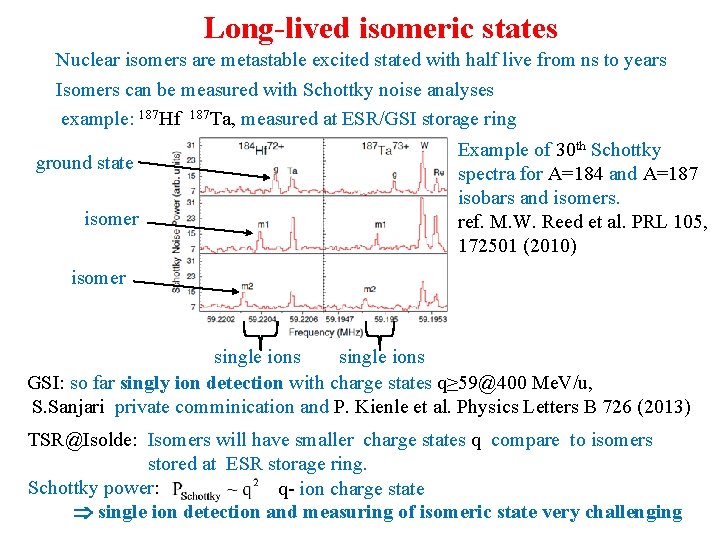
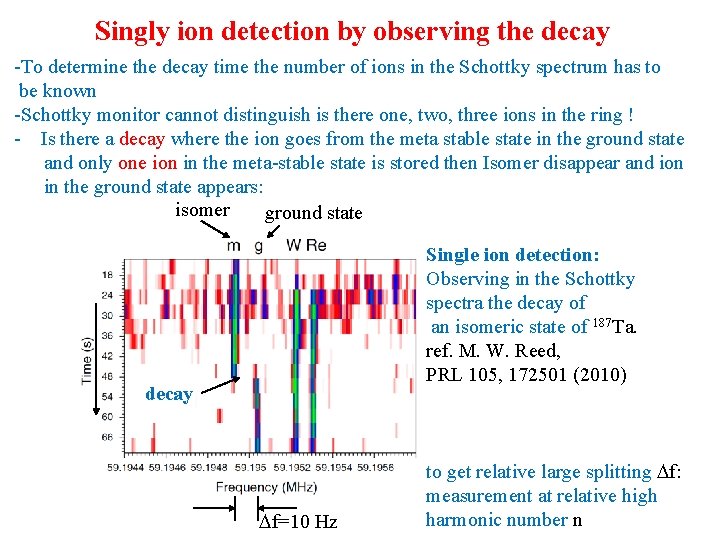
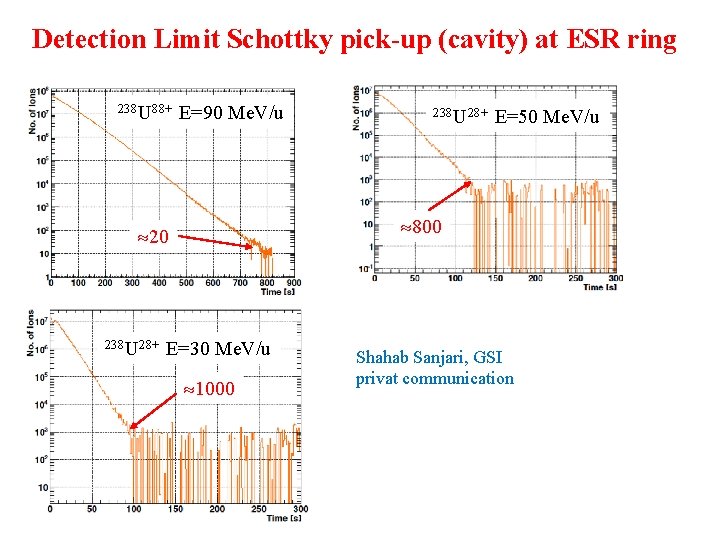
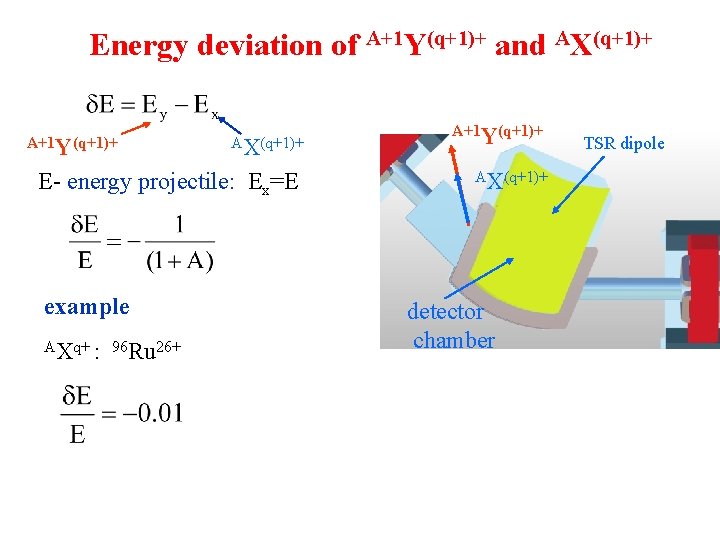
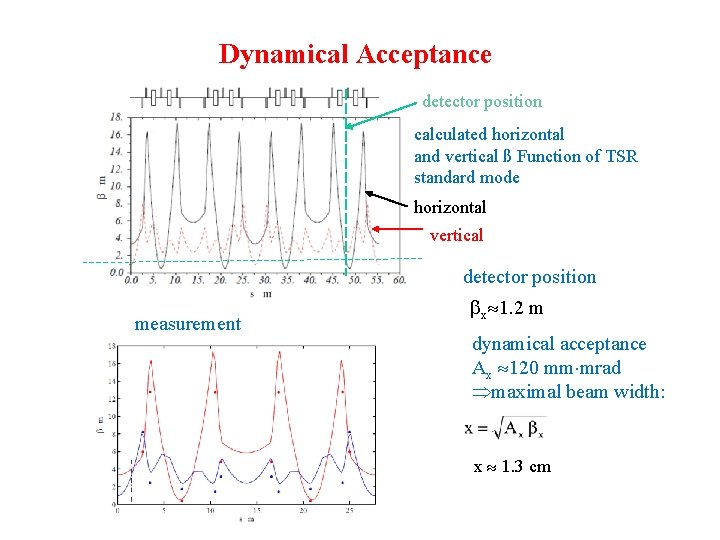
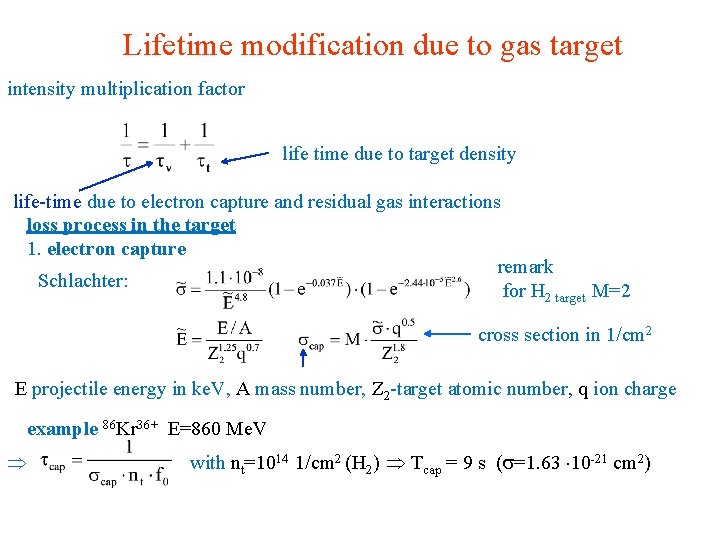
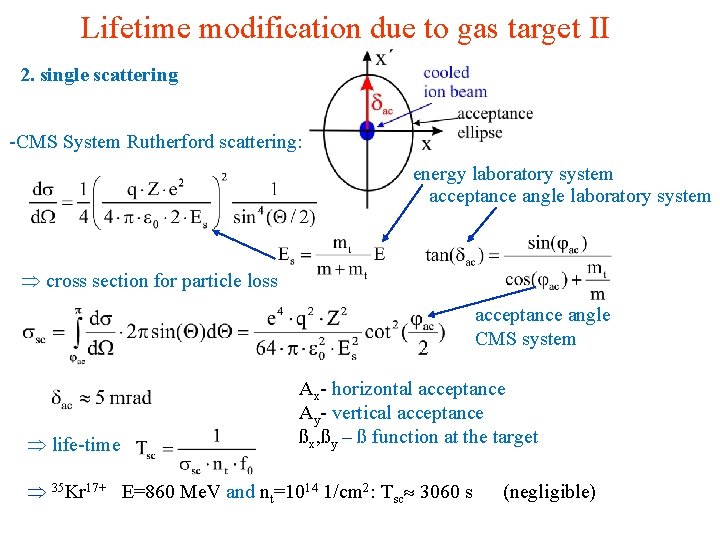
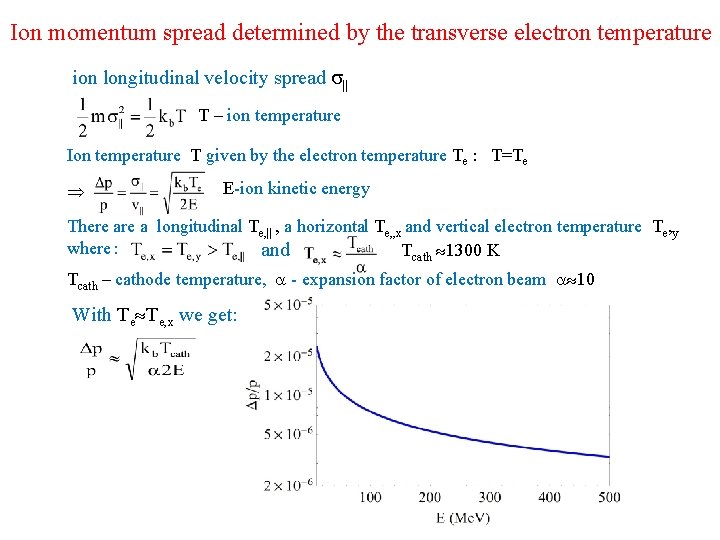
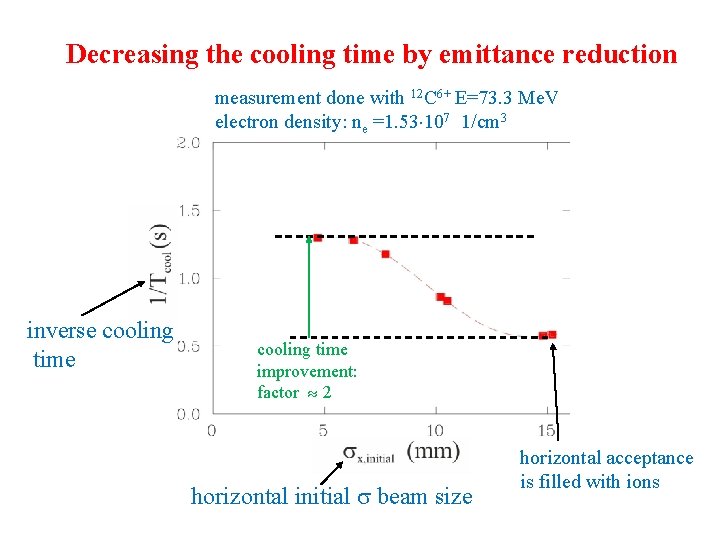
- Slides: 43
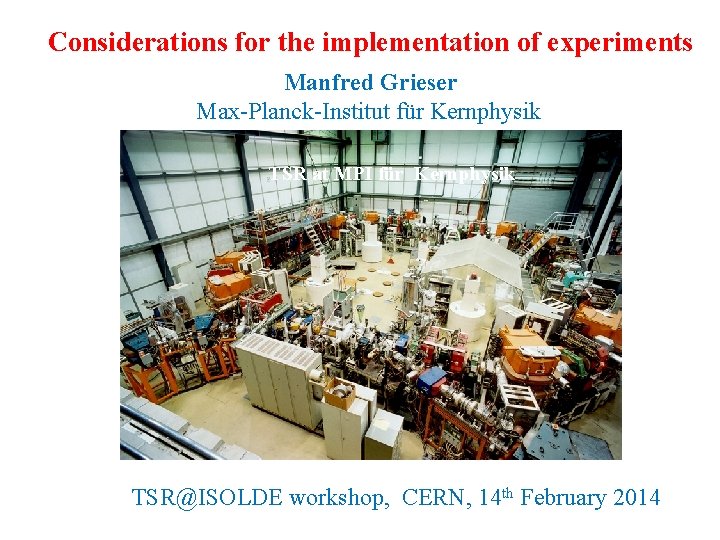
Considerations for the implementation of experiments Manfred Grieser Max-Planck-Institut für Kernphysik TSR at MPI für Kernphysik TSR@ISOLDE workshop, CERN, 14 th February 2014
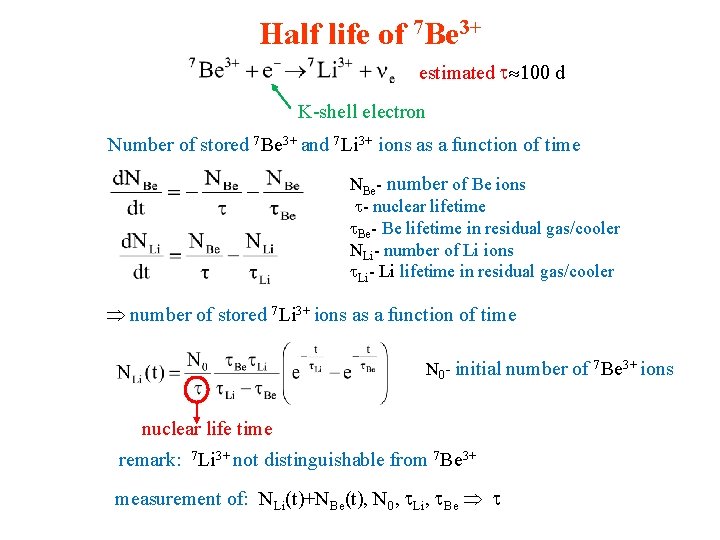
Half life of 7 Be 3+ estimated t 100 d K-shell electron Number of stored 7 Be 3+ and 7 Li 3+ ions as a function of time NBe- number of Be ions t- nuclear lifetime t. Be- Be lifetime in residual gas/cooler NLi- number of Li ions t. Li- Li lifetime in residual gas/cooler number of stored 7 Li 3+ ions as a function of time N 0 - initial number of 7 Be 3+ ions nuclear life time remark: 7 Li 3+ not distinguishable from 7 Be 3+ measurement of: NLi(t)+NBe(t), N 0, t. Li, t. Be t
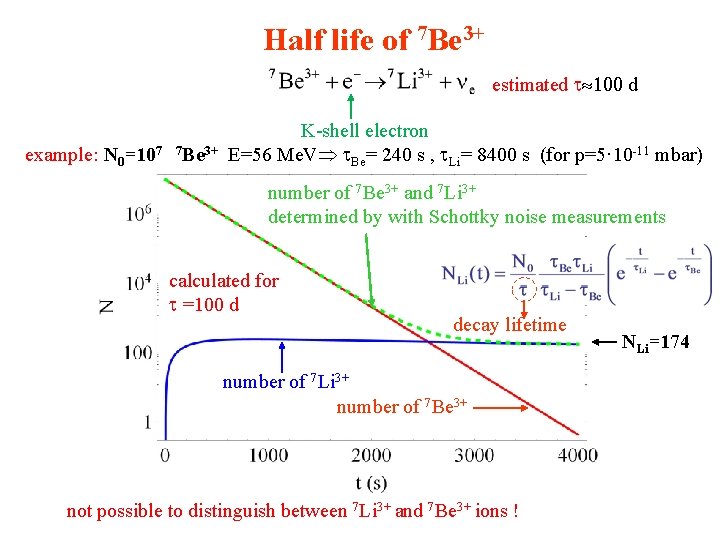
Half life of 7 Be 3+ estimated t 100 d example: N 0=107 7 Be 3+ K-shell electron E=56 Me. V t. Be= 240 s , t. Li= 8400 s (for p=5· 10 -11 mbar) number of 7 Be 3+ and 7 Li 3+ determined by with Schottky noise measurements calculated for t =100 d decay lifetime number of 7 Li 3+ number of 7 Be 3+ not possible to distinguish between 7 Li 3+ and 7 Be 3+ ions ! NLi=174
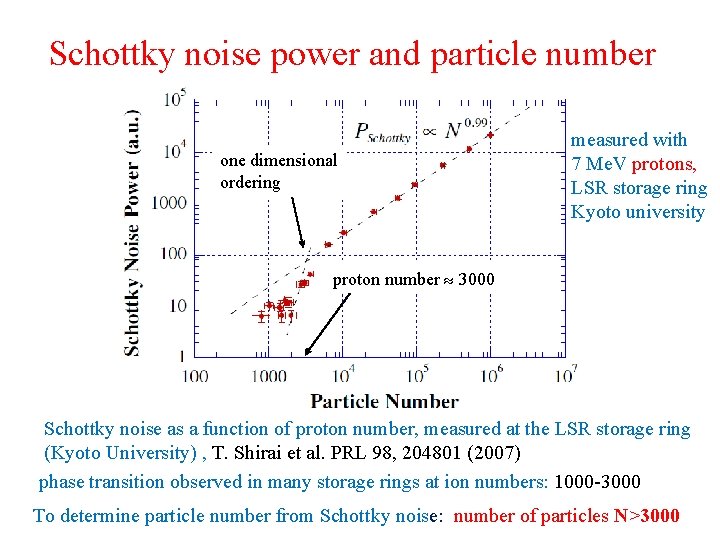
Schottky noise power and particle number one dimensional ordering measured with 7 Me. V protons, LSR storage ring Kyoto university proton number 3000 Schottky noise as a function of proton number, measured at the LSR storage ring (Kyoto University) , T. Shirai et al. PRL 98, 204801 (2007) phase transition observed in many storage rings at ion numbers: 1000 -3000 To determine particle number from Schottky noise: number of particles N>3000
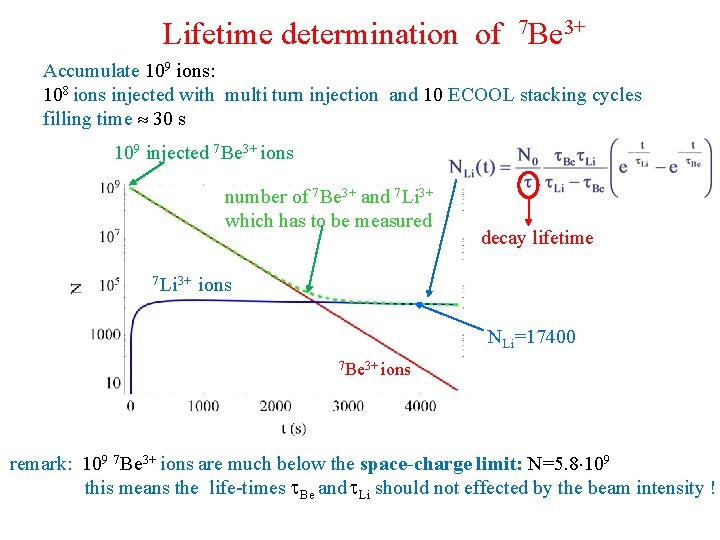
Lifetime determination of 7 Be 3+ Accumulate 109 ions: 108 ions injected with multi turn injection and 10 ECOOL stacking cycles filling time 30 s 109 injected 7 Be 3+ ions number of 7 Be 3+ and 7 Li 3+ which has to be measured 7 Li 3+ decay lifetime ions NLi=17400 7 Be 3+ ions remark: 109 7 Be 3+ ions are much below the space-charge limit: N=5. 8 109 this means the life-times t. Be and t. Li should not effected by the beam intensity !
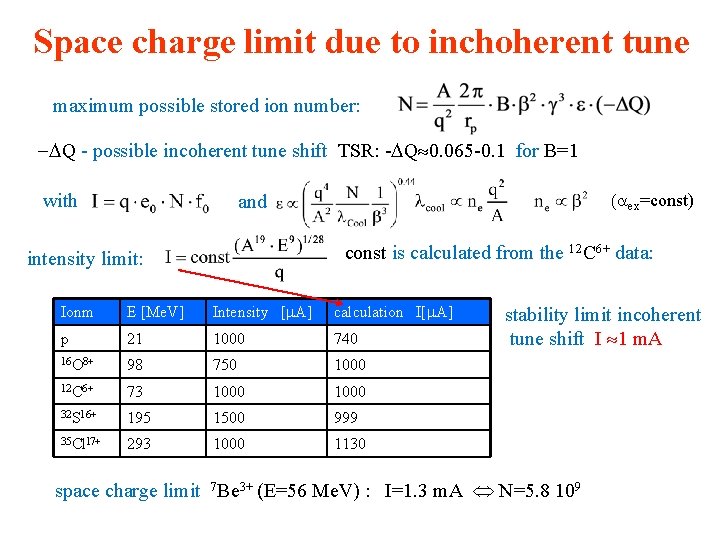
Space charge limit due to inchoherent tune maximum possible stored ion number: -DQ - possible incoherent tune shift TSR: -DQ 0. 065 -0. 1 for B=1 with (aex=const) and const is calculated from the 12 C 6+ data: intensity limit: Ionm E [Me. V] Intensity [m. A] calculation I[m. A] p 21 1000 740 16 O 8+ 98 750 1000 12 C 6+ 73 1000 32 S 16+ 195 1500 999 35 Cll 7+ 293 1000 1130 stability limit incoherent tune shift I 1 m. A space charge limit 7 Be 3+ (E=56 Me. V) : I=1. 3 m. A N=5. 8 109
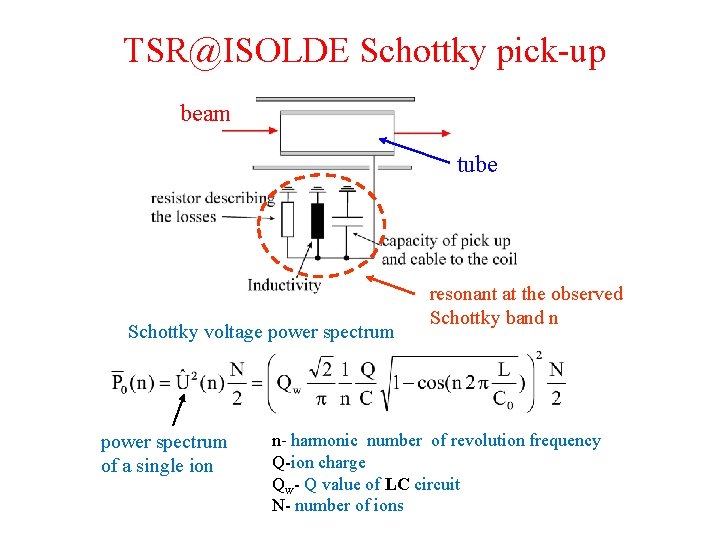
TSR@ISOLDE Schottky pick-up beam tube Schottky voltage power spectrum of a single ion resonant at the observed Schottky band n n- harmonic number of revolution frequency Q-ion charge Qw- Q value of LC circuit N- number of ions
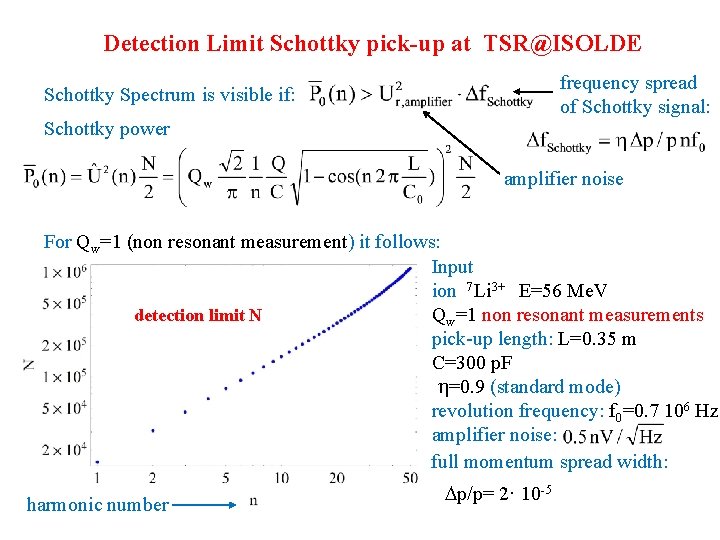
Detection Limit Schottky pick-up at TSR@ISOLDE frequency spread of Schottky signal: Schottky Spectrum is visible if: Schottky power amplifier noise For Qw=1 (non resonant measurement) it follows: Input ion 7 Li 3+ E=56 Me. V Qw=1 non resonant measurements detection limit N pick-up length: L=0. 35 m C=300 p. F h=0. 9 (standard mode) revolution frequency: f 0=0. 7 106 Hz amplifier noise: full momentum spread width: harmonic number Dp/p= 2· 10 -5
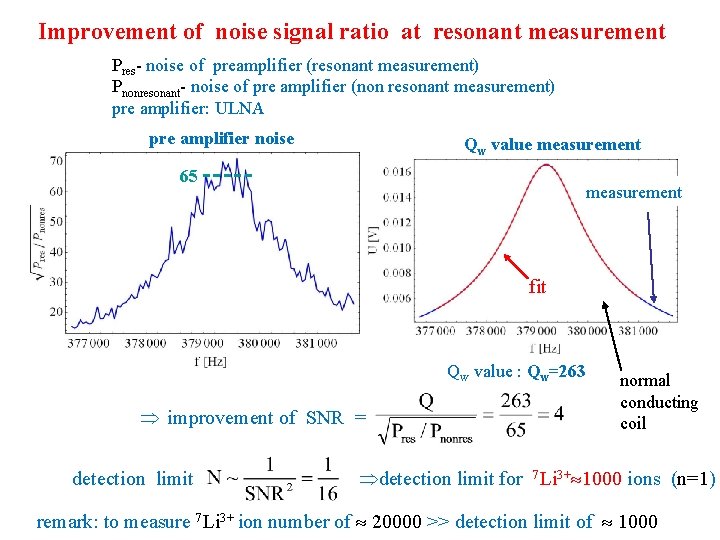
Improvement of noise signal ratio at resonant measurement Pres- noise of preamplifier (resonant measurement) Pnonresonant- noise of pre amplifier (non resonant measurement) pre amplifier: ULNA pre amplifier noise Qw value measurement 65 measurement fit Qw value : Qw=263 improvement of SNR = detection limit normal conducting coil detection limit for 7 Li 3+ 1000 ions (n=1) remark: to measure 7 Li 3+ ion number of 20000 >> detection limit of 1000
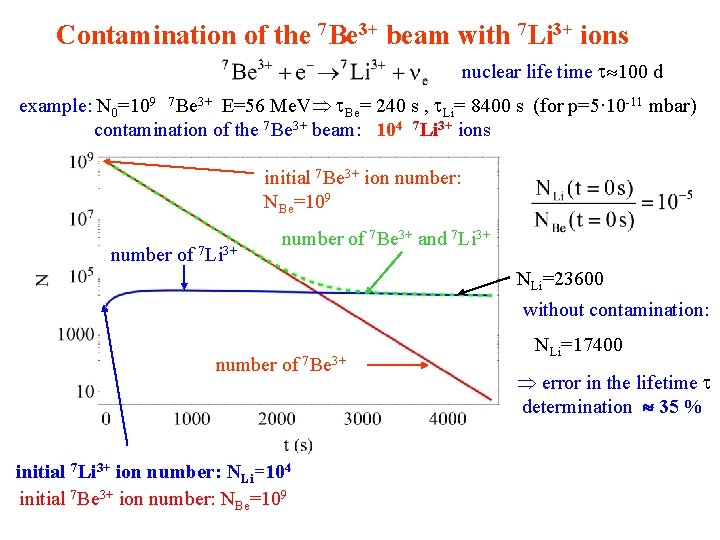
Contamination of the 7 Be 3+ beam with 7 Li 3+ ions nuclear life time t 100 d example: N 0=109 7 Be 3+ E=56 Me. V t. Be= 240 s , t. Li= 8400 s (for p=5· 10 -11 mbar) contamination of the 7 Be 3+ beam: 104 7 Li 3+ ions initial 7 Be 3+ ion number: NBe=109 number of 7 Li 3+ number of 7 Be 3+ and 7 Li 3+ NLi=23600 without contamination: number of 7 Be 3+ initial 7 Li 3+ ion number: NLi=104 initial 7 Be 3+ ion number: NBe=109 NLi=17400 error in the lifetime t determination 35 %
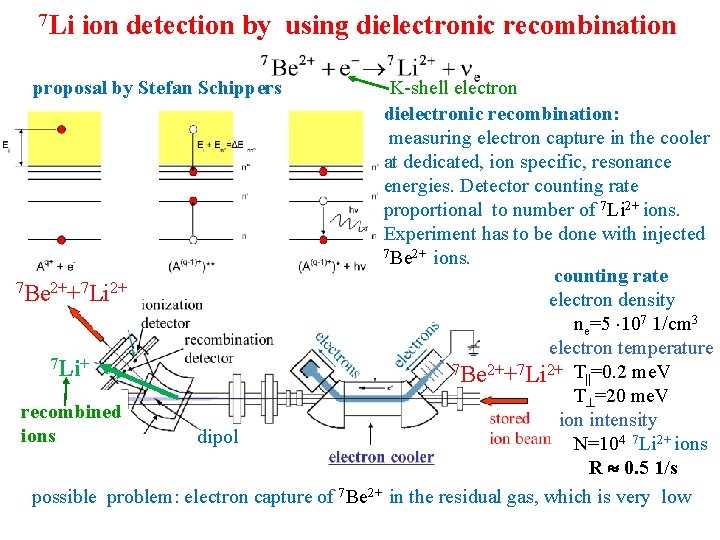
7 Li ion detection by using dielectronic recombination proposal by Stefan Schippers K-shell electron dielectronic recombination: measuring electron capture in the cooler at dedicated, ion specific, resonance energies. Detector counting rate proportional to number of 7 Li 2+ ions. Experiment has to be done with injected 7 Be 2+ ions. counting rate 7 Be 2++7 Li 2+ electron density ne=5 107 1/cm 3 electron temperature 7 Li+ 7 Be 2++7 Li 2+ T =0. 2 me. V || T =20 me. V recombined ion intensity ions dipol N=104 7 Li 2+ ions ddiipol R 0. 5 1/s possible problem: electron capture of 7 Be 2+ in the residual gas, which is very low
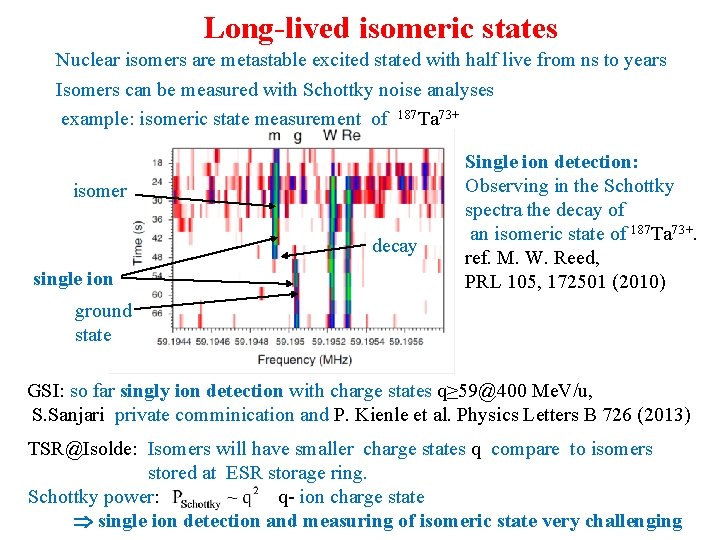
Long-lived isomeric states Nuclear isomers are metastable excited stated with half live from ns to years Isomers can be measured with Schottky noise analyses example: isomeric state measurement of 187 Ta 73+ isomer decay single ion Single ion detection: Observing in the Schottky spectra the decay of an isomeric state of 187 Ta 73+. ref. M. W. Reed, PRL 105, 172501 (2010) ground state GSI: so far singly ion detection with charge states q≥ 59@400 Me. V/u, S. Sanjari private comminication and P. Kienle et al. Physics Letters B 726 (2013) TSR@Isolde: Isomers will have smaller charge states q compare to isomers stored at ESR storage ring. q- ion charge state Schottky power: single ion detection and measuring of isomeric state very challenging
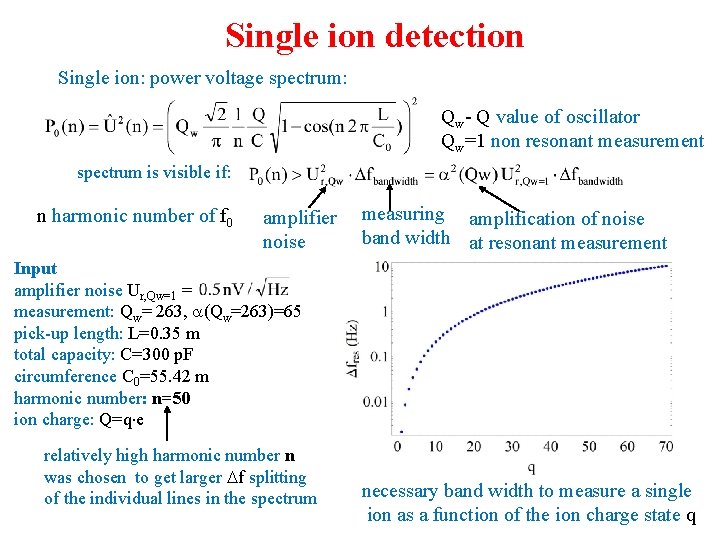
Single ion detection Single ion: power voltage spectrum: Qw- Q value of oscillator Qw=1 non resonant measurement spectrum is visible if: n harmonic number of f 0 amplifier noise measuring amplification of noise band width at resonant measurement Input amplifier noise Ur, Qw=1 = measurement: Qw= 263, a(Qw=263)=65 pick-up length: L=0. 35 m total capacity: C=300 p. F circumference C 0=55. 42 m harmonic number: n=50 ion charge: Q=q e relatively high harmonic number n was chosen to get larger Df splitting of the individual lines in the spectrum necessary band width to measure a single ion as a function of the ion charge state q
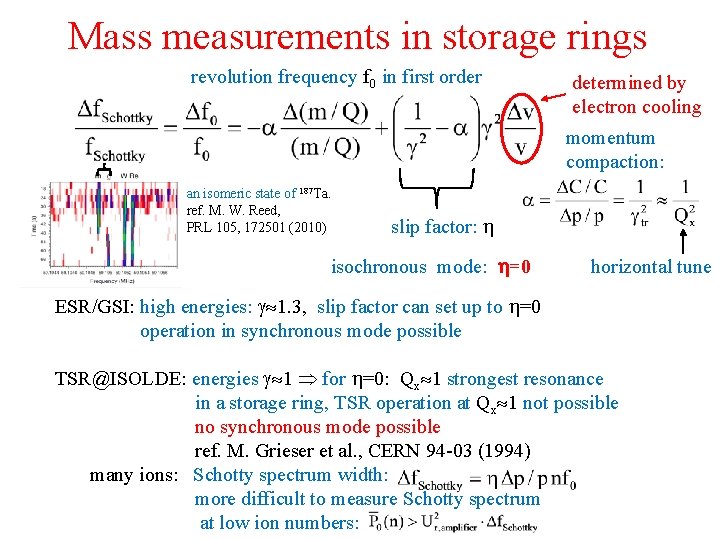
Mass measurements in storage rings revolution frequency f 0 in first order determined by electron cooling momentum compaction: an isomeric state of 187 Ta. ref. M. W. Reed, PRL 105, 172501 (2010) slip factor: h isochronous mode: h=0 horizontal tune ESR/GSI: high energies: g 1. 3, slip factor can set up to h=0 operation in synchronous mode possible TSR@ISOLDE: energies g 1 for h=0: Qx 1 strongest resonance in a storage ring, TSR operation at Qx 1 not possible no synchronous mode possible ref. M. Grieser et al. , CERN 94 -03 (1994) many ions: Schotty spectrum width: more difficult to measure Schotty spectrum at low ion numbers:
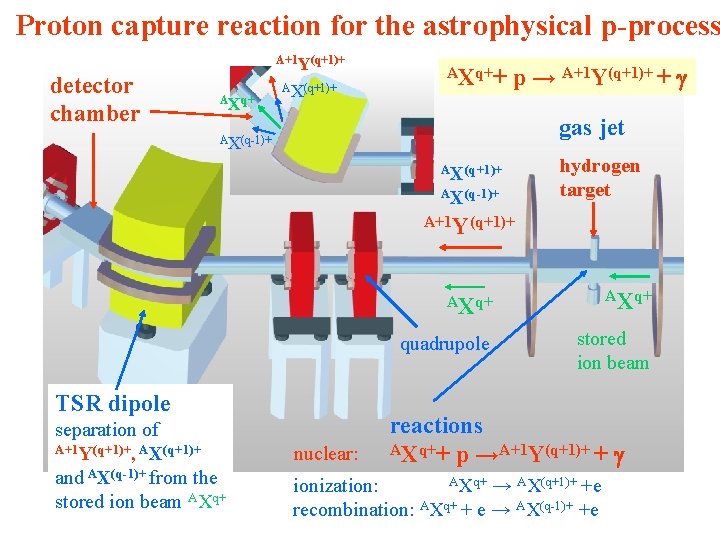
Proton capture reaction for the astrophysical p-process detector chamber A+1 Y(q+1)+ AXq+ AX(q+1)+ AXq++ p → A+1 Y(q+1)+ + g gas jet AX(q-1)+ AX(q+1)+ AX(q-1)+ hydrogen target A+1 Y(q+1)+ TSR dipole separation of A+1 Y(q+1)+, AX(q+1)+ and AX(q-1)+ from the stored ion beam AXq+ nuclear: AXq+ quadrupole stored ion beam reactions AXq++ p →A+1 Y(q+1)+ + g AXq+ → AX(q+1)+ +e ionization: recombination: AXq+ + e → AX(q-1)+ +e
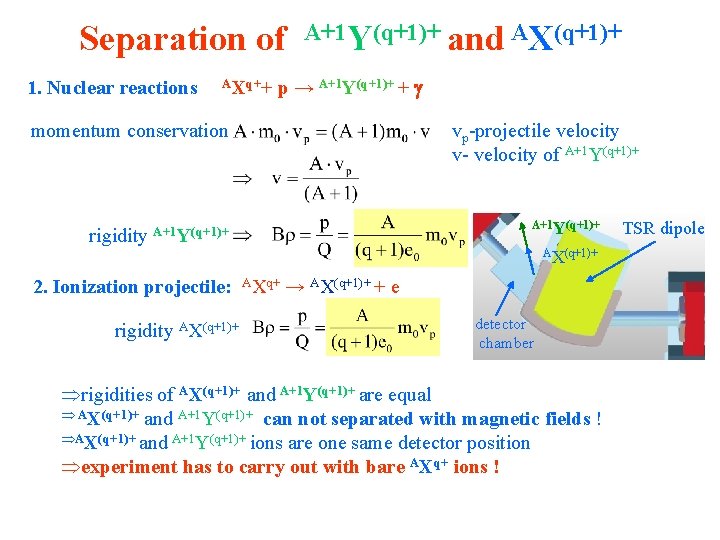
Separation of 1. Nuclear reactions AXq++ A+1 Y(q+1)+ and AX(q+1)+ p → A+1 Y(q+1)+ + g momentum conservation rigidity A+1 Y(q+1)+ vp-projectile velocity v- velocity of A+1 Y(q+1)+ AX(q+1)+ 2. Ionization projectile: AXq+ → AX(q+1)+ + e rigidity AX(q+1)+ detector chamber rigidities of AX(q+1)+ and A+1 Y(q+1)+ are equal AX(q+1)+ and A+1 Y(q+1)+ can not separated with magnetic fields ! AX(q+1)+ and A+1 Y(q+1)+ ions are one same detector position experiment has to carry out with bare AXq+ ions ! TSR dipole
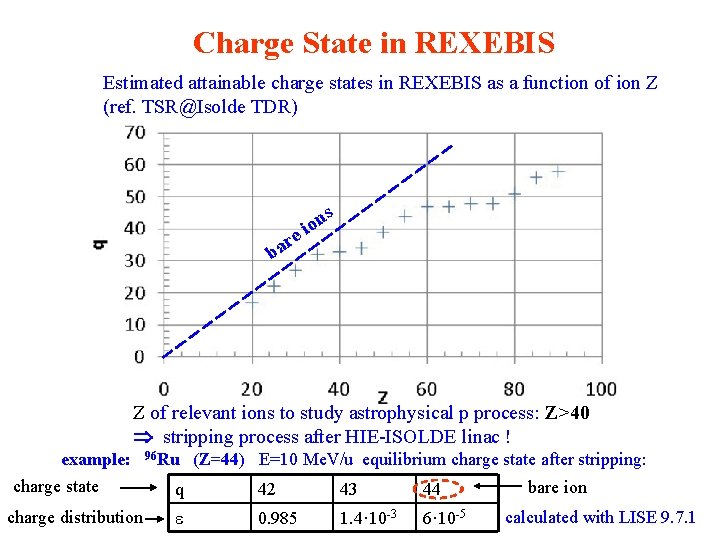
Charge State in REXEBIS Estimated attainable charge states in REXEBIS as a function of ion Z (ref. TSR@Isolde TDR) s on i e r ba Z of relevant ions to study astrophysical p process: Z>40 stripping process after HIE-ISOLDE linac ! example: charge state charge distribution 96 Ru (Z=44) E=10 Me. V/u equilibrium charge state after stripping: q 42 43 44 e 0. 985 1. 4· 10 -3 6· 10 -5 bare ion calculated with LISE 9. 7. 1
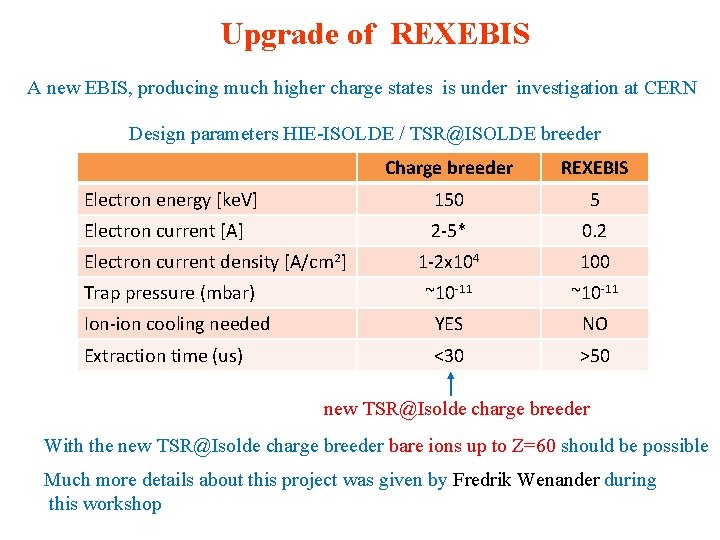
Upgrade of REXEBIS A new EBIS, producing much higher charge states is under investigation at CERN Design parameters HIE-ISOLDE / TSR@ISOLDE breeder Charge breeder REXEBIS Electron energy [ke. V] 150 5 Electron current [A] 2 -5* 0. 2 1 -2 x 104 100 ~10 -11 Ion-ion cooling needed YES NO Extraction time (us) <30 >50 Electron current density [A/cm 2] Trap pressure (mbar) new TSR@Isolde charge breeder With the new TSR@Isolde charge breeder bare ions up to Z=60 should be possible Much more details about this project was given by Fredrik Wenander during this workshop
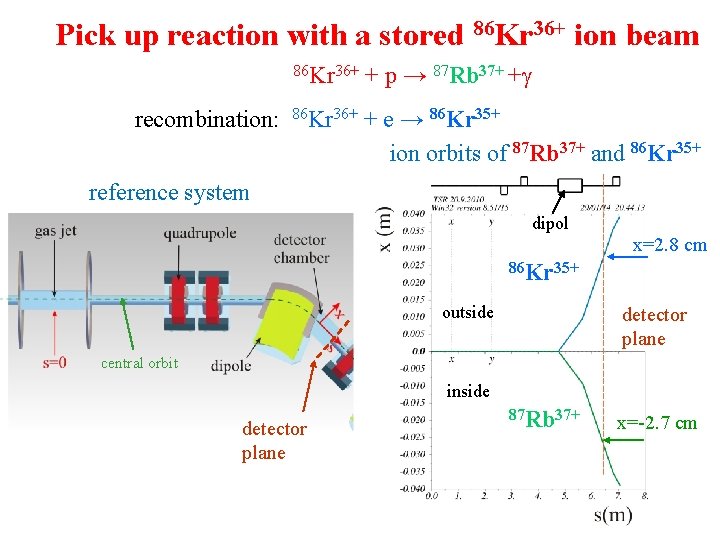
Pick up reaction with a stored 86 Kr 36+ ion beam recombination: 86 Kr 36+ + p → 87 Rb 37+ +g 86 Kr 36+ + e → 86 Kr 35+ ion orbits of 87 Rb 37+ and 86 Kr 35+ reference system dipol x=2. 8 cm 86 Kr 35+ outside detector plane central orbit inside detector plane 87 Rb 37+ x=-2. 7 cm
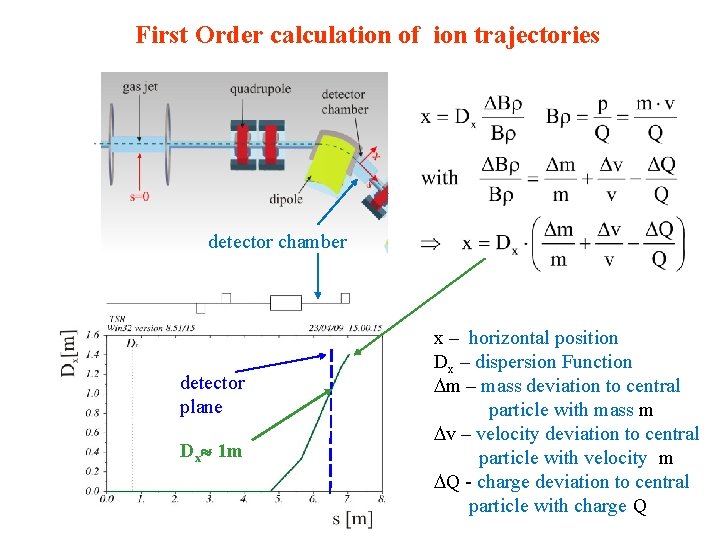
First Order calculation of ion trajectories detector chamber detector plane Dx 1 m x – horizontal position Dx – dispersion Function Dm – mass deviation to central particle with mass m Dv – velocity deviation to central particle with velocity m DQ - charge deviation to central particle with charge Q
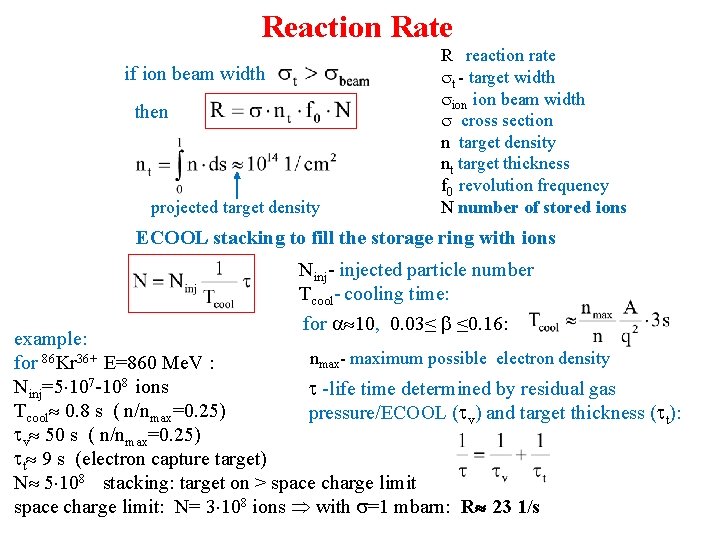
Reaction Rate if ion beam width then projected target density R reaction rate st - target width sion beam width s cross section n target density nt target thickness f 0 revolution frequency N number of stored ions ECOOL stacking to fill the storage ring with ions Ninj- injected particle number Tcool- cooling time: for a 10, 0. 03≤ b ≤ 0. 16: example: nmax- maximum possible electron density for 86 Kr 36+ E=860 Me. V : Ninj=5 107 -108 ions t -life time determined by residual gas Tcool 0. 8 s ( n/nmax=0. 25) pressure/ECOOL (tv) and target thickness (tt): tv 50 s ( n/nmax=0. 25) tt 9 s (electron capture target) N 5 108 stacking: target on > space charge limit: N= 3 108 ions with s=1 mbarn: R 23 1/s
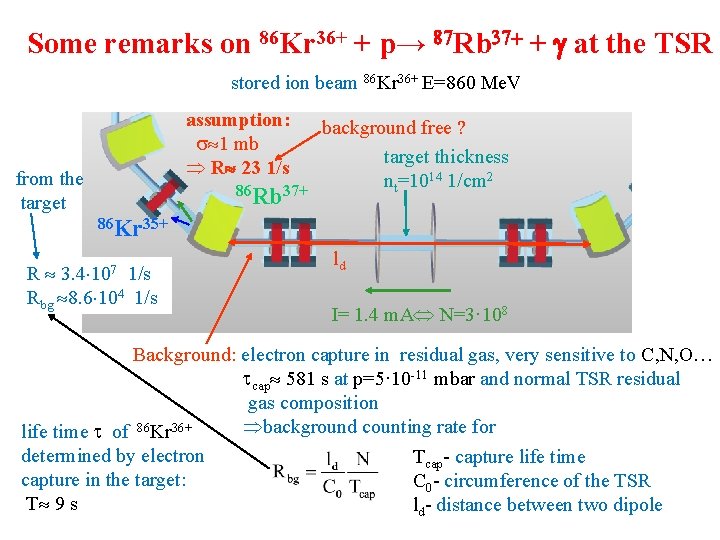
Some remarks on 86 Kr 36+ + p→ 87 Rb 37+ + g at the TSR stored ion beam 86 Kr 36+ E=860 Me. V assumption: s 1 mb R 23 1/s from the target 86 Rb 37+ background free ? target thickness nt=1014 1/cm 2 86 Kr 35+ R 1/s Rbg 8. 6 104 1/s 3. 4 107 ld I= 1. 4 m. A N=3· 108 Background: electron capture in residual gas, very sensitive to C, N, O… tcap 581 s at p=5· 10 -11 mbar and normal TSR residual gas composition background counting rate for life time t of 86 Kr 36+ determined by electron Tcap- capture life time capture in the target: C 0 - circumference of the TSR T 9 s ld- distance between two dipole
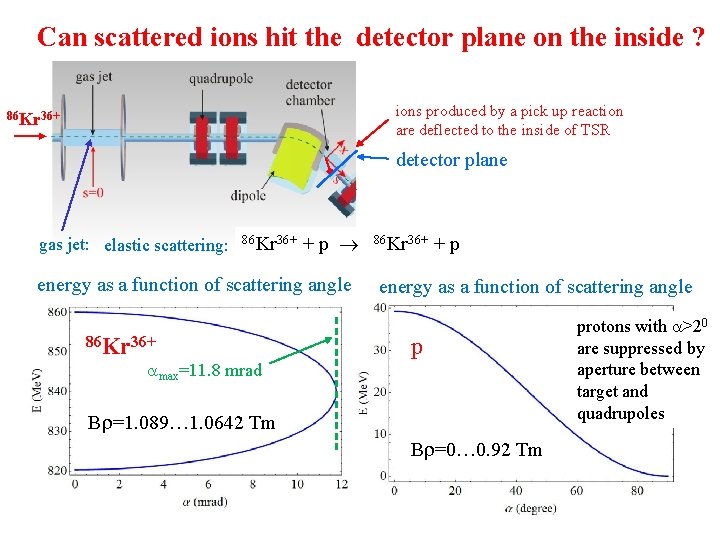
Can scattered ions hit the detector plane on the inside ? ions produced by a pick up reaction are deflected to the inside of TSR 86 Kr 36+ detector plane gas jet: elastic scattering: 86 Kr 36+ +p energy as a function of scattering angle 86 Kr 36+ amax=11. 8 mrad 86 Kr 36+ +p energy as a function of scattering angle p Br=1. 089… 1. 0642 Tm Br=0… 0. 92 Tm protons with a>20 are suppressed by aperture between target and quadrupoles
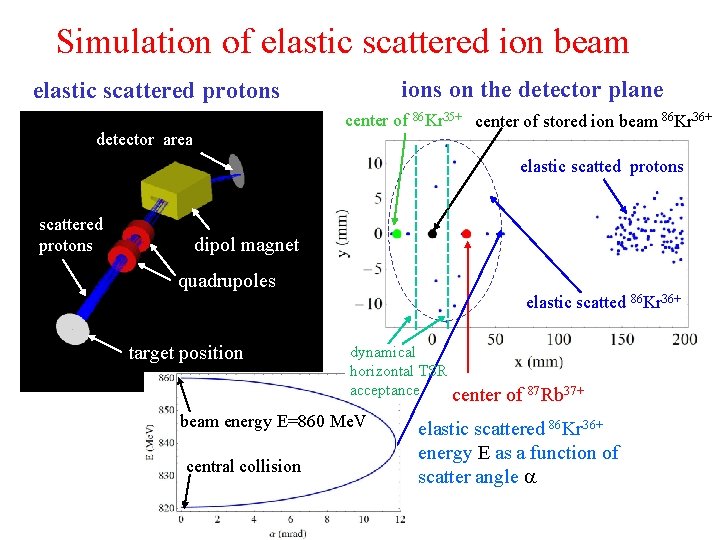
Simulation of elastic scattered ion beam ions on the detector plane elastic scattered protons center of 86 Kr 35+ center of stored ion beam 86 Kr 36+ detector area elastic scatted protons scattered protons dipol magnet quadrupoles elastic scatted 86 Kr 36+ target position dynamical horizontal TSR acceptance center beam energy E=860 Me. V central collision of 87 Rb 37+ elastic scattered 86 Kr 36+ energy E as a function of scatter angle a
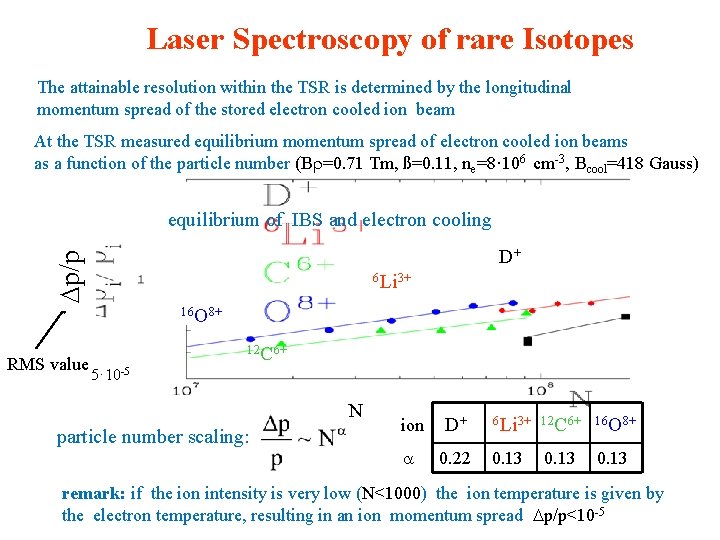
Laser Spectroscopy of rare Isotopes The attainable resolution within the TSR is determined by the longitudinal momentum spread of the stored electron cooled ion beam At the TSR measured equilibrium momentum spread of electron cooled ion beams as a function of the particle number (Br=0. 71 Tm, ß=0. 11, ne=8· 106 cm-3, Bcool=418 Gauss) equilibrium of IBS and electron cooling Dp/p D+ 6 Li 3+ 16 O 8+ RMS value 12 C 6+ 5· 10 -5 N particle number scaling: ion D+ a 0. 22 6 Li 3+ 12 C 6+ 16 O 8+ 0. 13 remark: if the ion intensity is very low (N<1000) the ion temperature is given by the electron temperature, resulting in an ion momentum spread Dp/p<10 -5
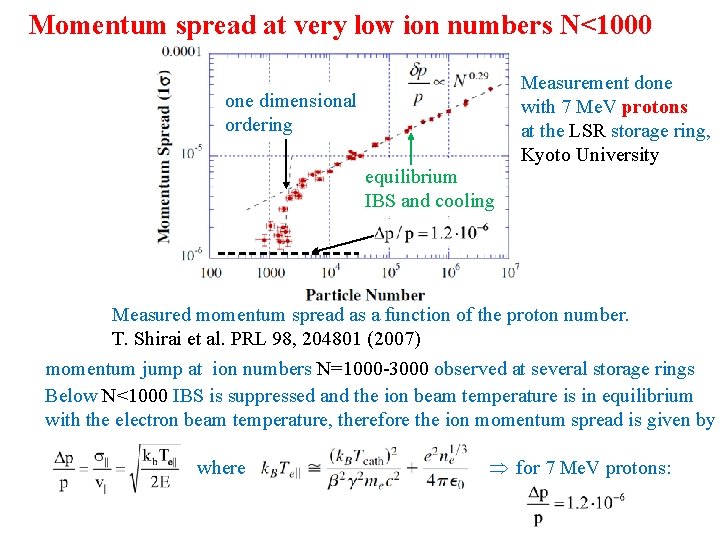
Momentum spread at very low ion numbers N<1000 one dimensional ordering equilibrium IBS and cooling Measurement done with 7 Me. V protons at the LSR storage ring, Kyoto University Measured momentum spread as a function of the proton number. T. Shirai et al. PRL 98, 204801 (2007) momentum jump at ion numbers N=1000 -3000 observed at several storage rings Below N<1000 IBS is suppressed and the ion beam temperature is in equilibrium with the electron beam temperature, therefore the ion momentum spread is given by where for 7 Me. V protons:
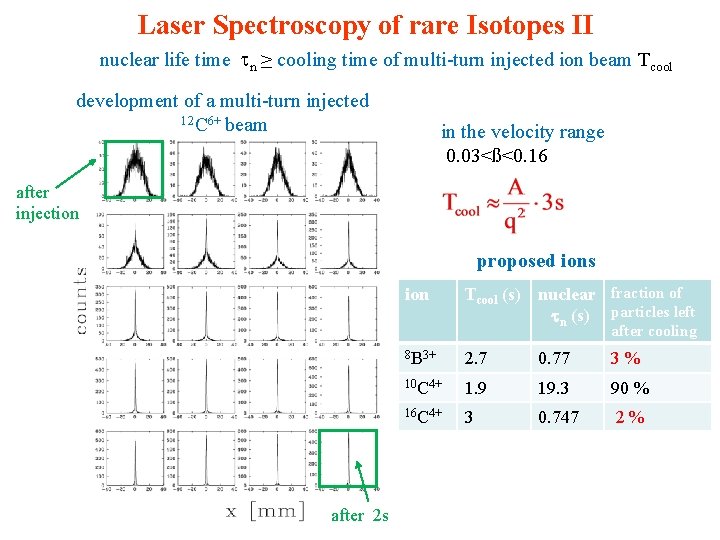
Laser Spectroscopy of rare Isotopes II nuclear life time tn ≥ cooling time of multi-turn injected ion beam Tcool development of a multi-turn injected 12 C 6+ beam in the velocity range 0. 03<ß<0. 16 after injection proposed ions ion Tcool (s) nuclear fraction of tn (s) particles left after cooling after 2 s 8 B 3+ 2. 7 0. 77 3% 10 C 4+ 1. 9 19. 3 90 % 16 C 4+ 3 0. 747 2%
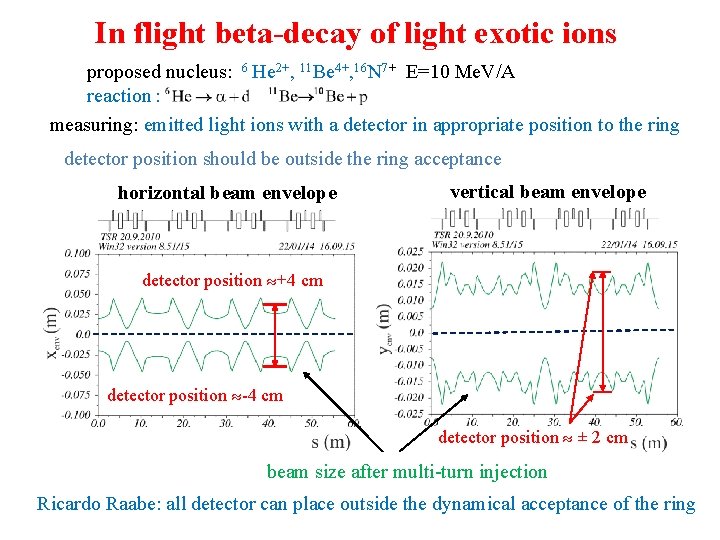
In flight beta-decay of light exotic ions proposed nucleus: 6 He 2+, 11 Be 4+, 16 N 7+ E=10 Me. V/A reaction : measuring: emitted light ions with a detector in appropriate position to the ring detector position should be outside the ring acceptance horizontal beam envelope vertical beam envelope detector position +4 cm detector position -4 cm detector position ± 2 cm beam size after multi-turn injection Ricardo Raabe: all detector can place outside the dynamical acceptance of the ring
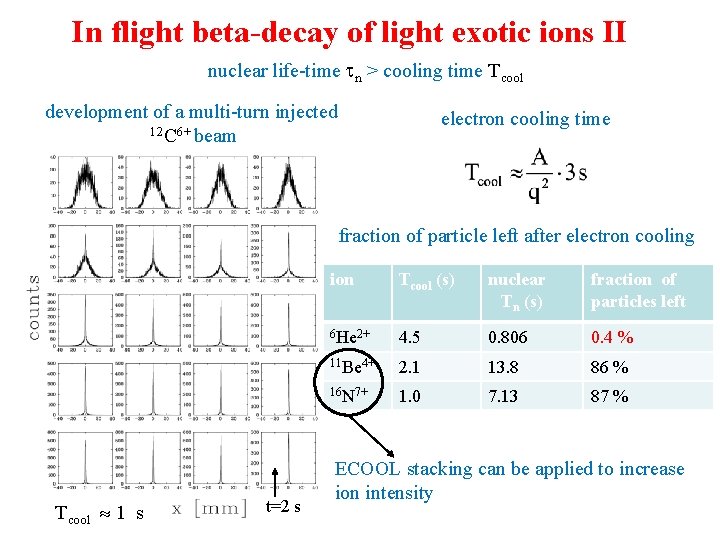
In flight beta-decay of light exotic ions II nuclear life-time tn > cooling time Tcool development of a multi-turn injected 12 C 6+ beam electron cooling time fraction of particle left after electron cooling Tcool 1 s t=2 s ion Tcool (s) nuclear Tn (s) fraction of particles left 6 He 2+ 4. 5 0. 806 0. 4 % 11 Be 4+ 2. 1 13. 8 86 % 16 N 7+ 1. 0 7. 13 87 % ECOOL stacking can be applied to increase ion intensity
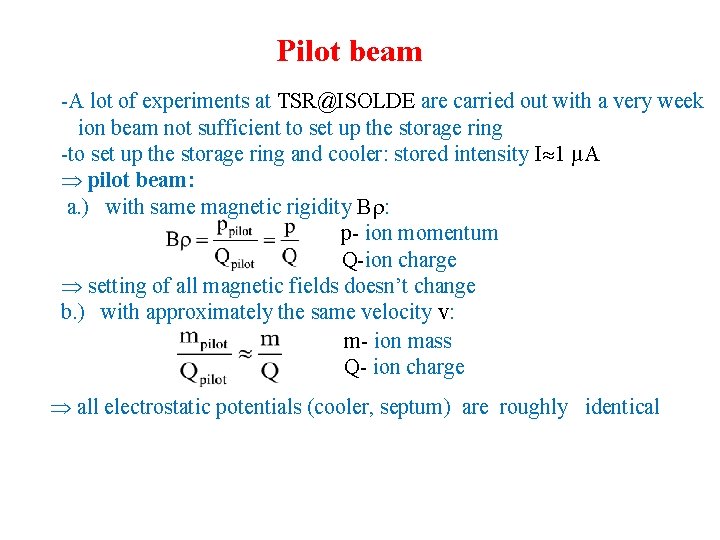
Pilot beam -A lot of experiments at TSR@ISOLDE are carried out with a very week ion beam not sufficient to set up the storage ring -to set up the storage ring and cooler: stored intensity I 1 µA pilot beam: a. ) with same magnetic rigidity Br: p- ion momentum Q-ion charge setting of all magnetic fields doesn’t change b. ) with approximately the same velocity v: m- ion mass Q- ion charge all electrostatic potentials (cooler, septum) are roughly identical
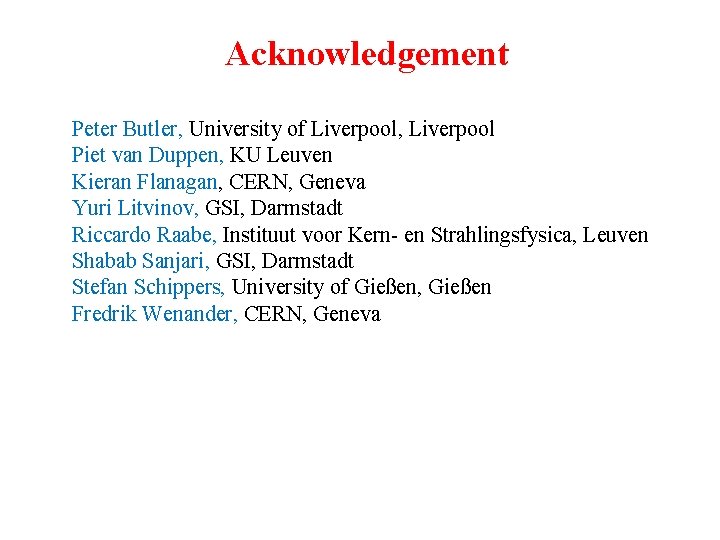
Acknowledgement Peter Butler, University of Liverpool, Liverpool Piet van Duppen, KU Leuven Kieran Flanagan, CERN, Geneva Yuri Litvinov, GSI, Darmstadt Riccardo Raabe, Instituut voor Kern- en Strahlingsfysica, Leuven Shabab Sanjari, GSI, Darmstadt Stefan Schippers, University of Gießen, Gießen Fredrik Wenander, CERN, Geneva
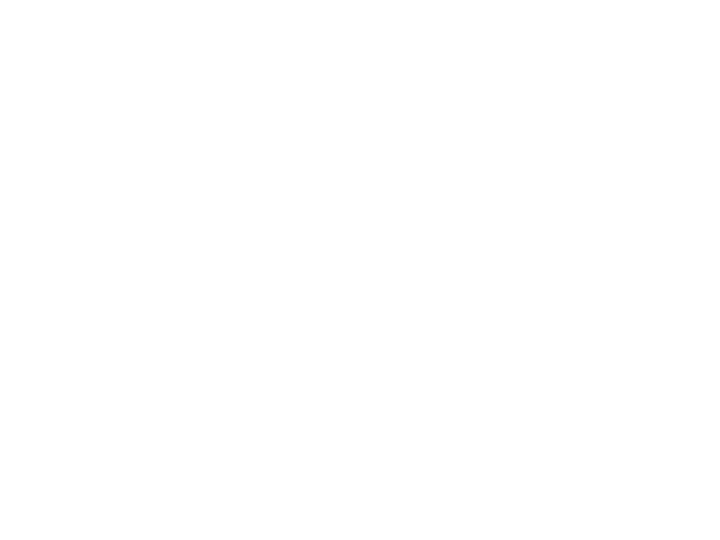
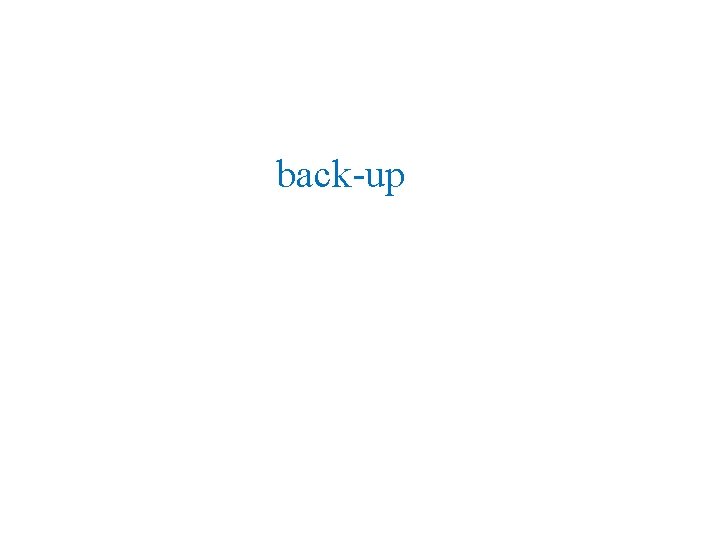
back-up
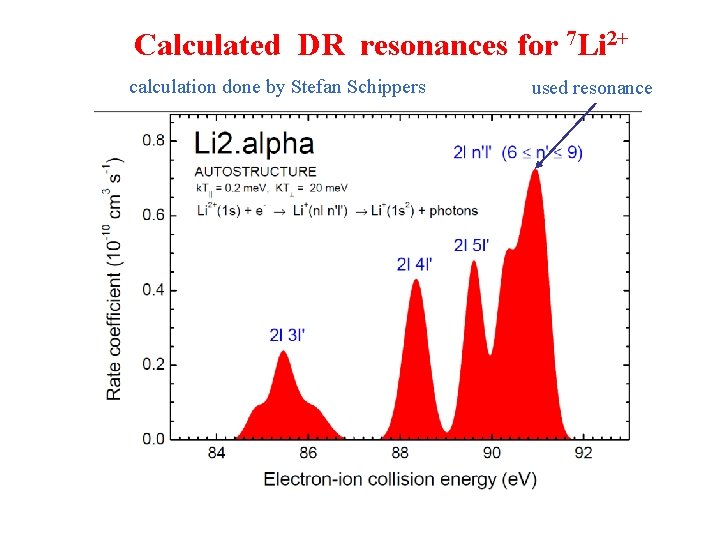
Calculated DR resonances for 7 Li 2+ calculation done by Stefan Schippers used resonance
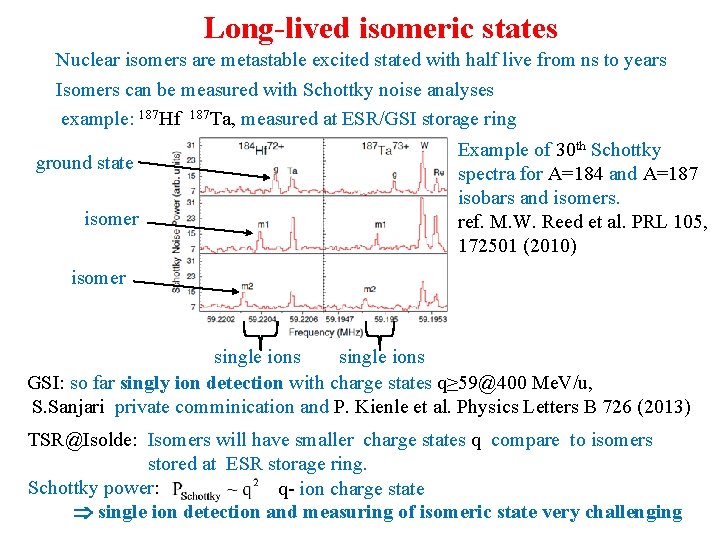
Long-lived isomeric states Nuclear isomers are metastable excited stated with half live from ns to years Isomers can be measured with Schottky noise analyses example: 187 Hf 187 Ta, measured at ESR/GSI storage ring ground state isomer Example of 30 th Schottky spectra for A=184 and A=187 isobars and isomers. ref. M. W. Reed et al. PRL 105, 172501 (2010) isomer single ions GSI: so far singly ion detection with charge states q≥ 59@400 Me. V/u, S. Sanjari private comminication and P. Kienle et al. Physics Letters B 726 (2013) TSR@Isolde: Isomers will have smaller charge states q compare to isomers stored at ESR storage ring. Schottky power: q- ion charge state single ion detection and measuring of isomeric state very challenging
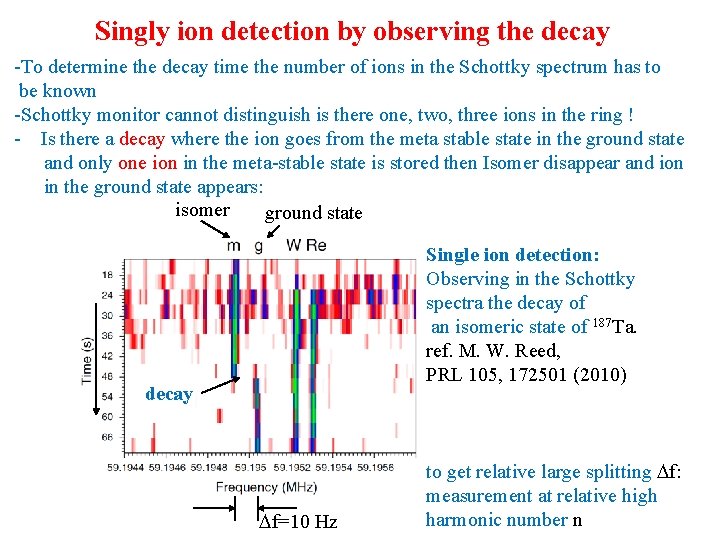
Singly ion detection by observing the decay -To determine the decay time the number of ions in the Schottky spectrum has to be known -Schottky monitor cannot distinguish is there one, two, three ions in the ring ! - Is there a decay where the ion goes from the meta stable state in the ground state and only one ion in the meta-stable state is stored then Isomer disappear and ion in the ground state appears: isomer ground state Single ion detection: Observing in the Schottky spectra the decay of an isomeric state of 187 Ta. ref. M. W. Reed, PRL 105, 172501 (2010) decay Df=10 Hz to get relative large splitting Df: measurement at relative high harmonic number n
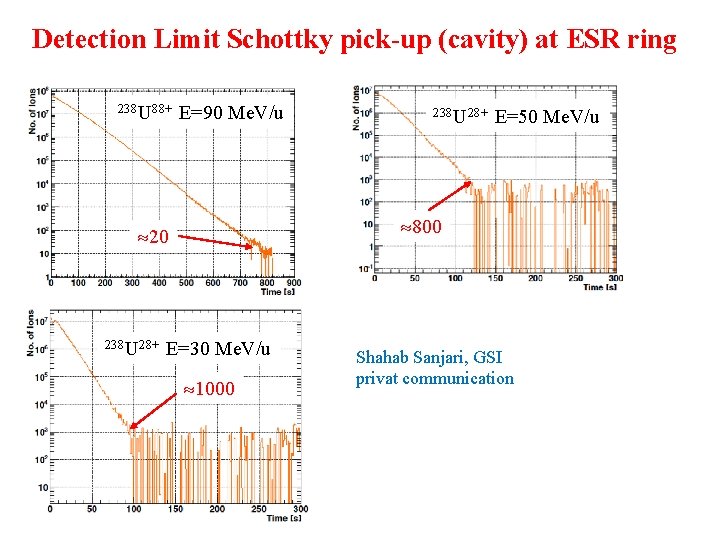
Detection Limit Schottky pick-up (cavity) at ESR ring 238 U 88+ E=90 Me. V/u E=50 Me. V/u 800 20 238 U 28+ E=30 Me. V/u 1000 Shahab Sanjari, GSI privat communication
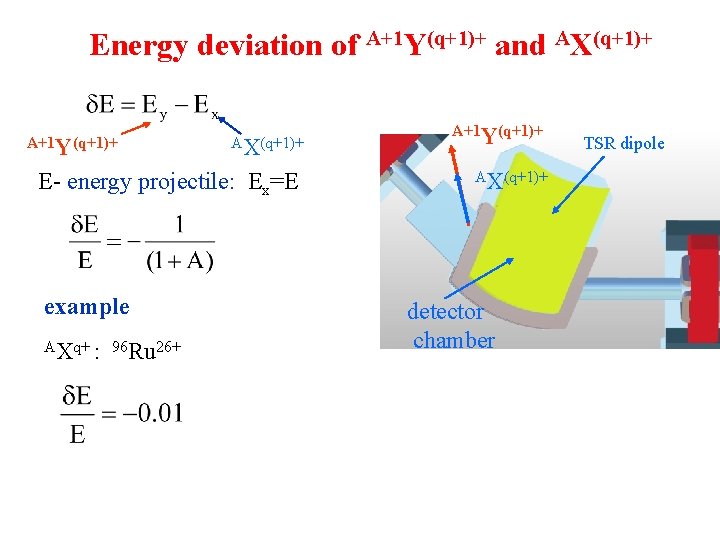
Energy deviation of A+1 Y(q+1)+ and AX(q+1)+ A+1 Y(q+1)+ AX(q+1)+ E- energy projectile: Ex=E example AXq+ : 96 Ru 26+ A+1 Y(q+1)+ AX(q+1)+ detector chamber TSR dipole
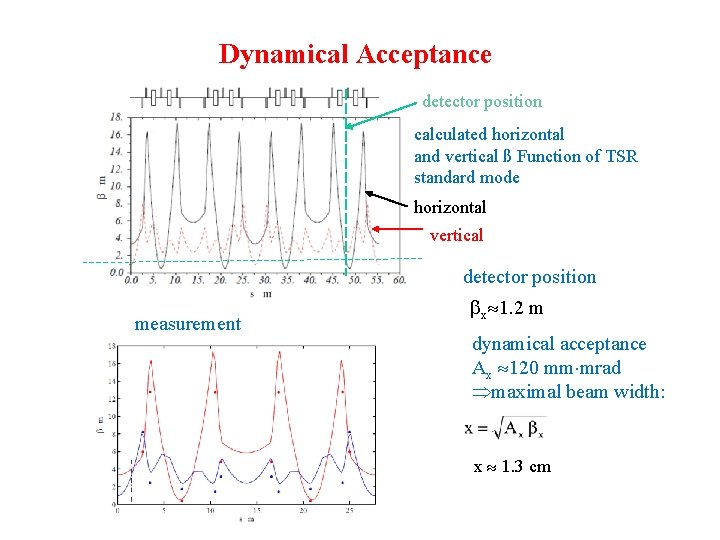
Dynamical Acceptance detector position calculated horizontal and vertical ß Function of TSR standard mode horizontal vertical detector position measurement bx 1. 2 m dynamical acceptance Ax 120 mm mrad maximal beam width: x 1. 3 cm
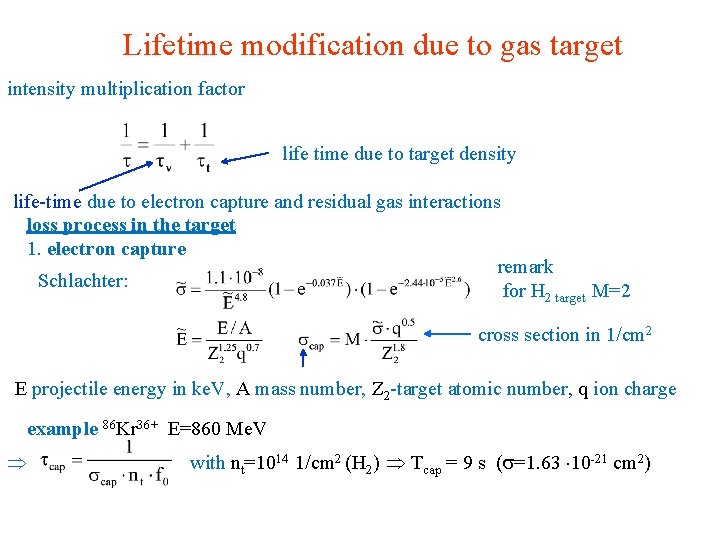
Lifetime modification due to gas target intensity multiplication factor life time due to target density life-time due to electron capture and residual gas interactions loss process in the target 1. electron capture remark Schlachter: for H 2 target M=2 cross section in 1/cm 2 E projectile energy in ke. V, A mass number, Z 2 -target atomic number, q ion charge example 86 Kr 36+ E=860 Me. V with nt=1014 1/cm 2 (H 2) Tcap = 9 s (s=1. 63 10 -21 cm 2)
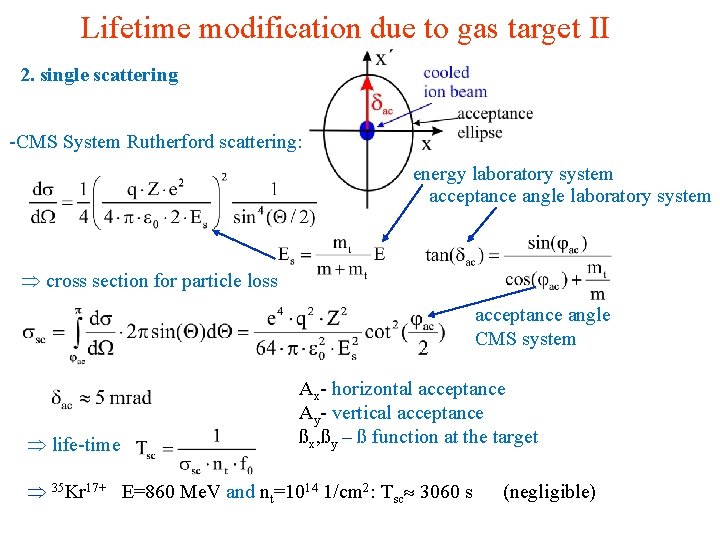
Lifetime modification due to gas target II 2. single scattering -CMS System Rutherford scattering: energy laboratory system acceptance angle laboratory system cross section for particle loss acceptance angle CMS system life-time Ax- horizontal acceptance Ay- vertical acceptance ßx, ßy – ß function at the target 35 Kr 17+ E=860 Me. V and nt=1014 1/cm 2: Tsc 3060 s (negligible)
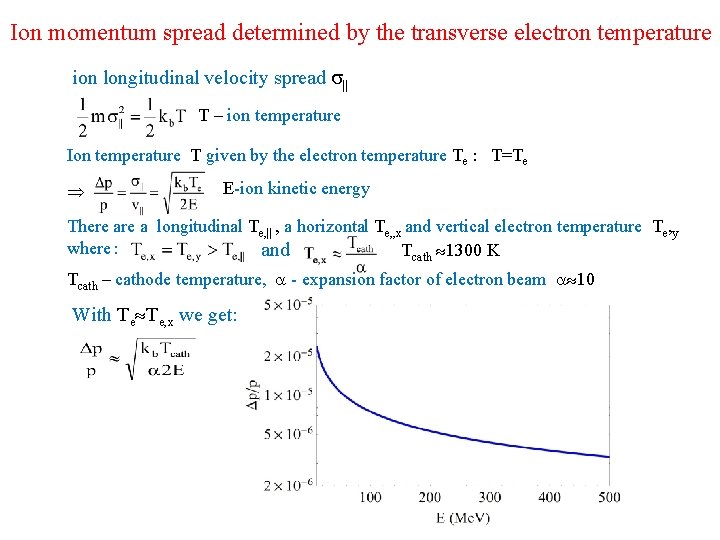
Ion momentum spread determined by the transverse electron temperature ion longitudinal velocity spread s|| T – ion temperature Ion temperature T given by the electron temperature Te : T=Te E-ion kinetic energy There a longitudinal Te, || , a horizontal Te, , x and vertical electron temperature Te, y where : and Tcath 1300 K Tcath – cathode temperature, a - expansion factor of electron beam a 10 With Te Te, x we get:
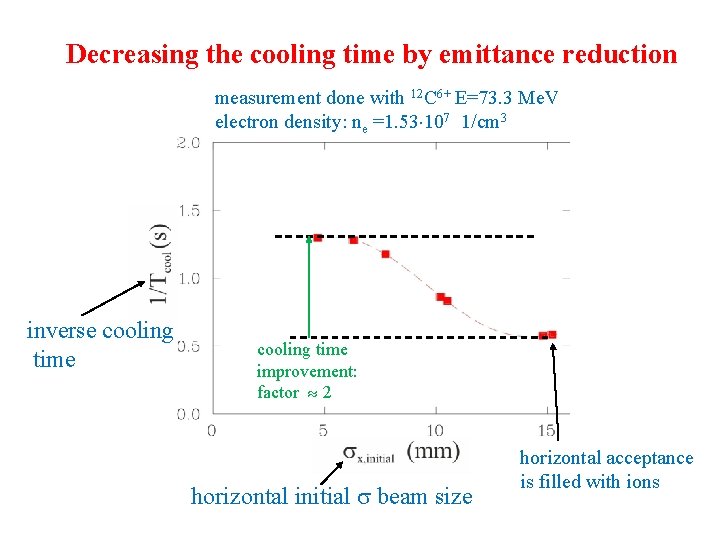
Decreasing the cooling time by emittance reduction measurement done with 12 C 6+ E=73. 3 Me. V electron density: ne =1. 53 107 1/cm 3 inverse cooling time improvement: factor 2 horizontal initial s beam size horizontal acceptance is filled with ions
Web security considerations
Writing strategies and ethical considerations
Hach wims
Quasi experimental design ethical issues
Tax considerations for setting up a new business
Azure landing zone hub and spoke
Functional cusp bevel
Advantages of venturi mask
Database design considerations
Final design icon
Types of costume design
Basal state specimen
Ethical consideration in research
Emv kiosk considerations
Emv kiosk considerations
Anatomical considerations
Eswl anesthesia considerations
Psychomotor considerations language acquisition
Ethical considerations examples
Temperature regulation pdhpe
Pricing considerations and approaches
Bioreactor considerations for animal cell culture
Azure landing zone considerations
Design considerations for mobile computing
Exchange transaction and relationship in marketing
Retromylohyoid curtain is formed by
Biopharmaceutic considerations in drug product design
Formulating objectives examples
Cloud delivery models
Consideration examples
What are the general considerations in machine design
Natalya hasan
Collaboration design considerations
Data warehouse considerations
Appendices in research
Mechanical design of transmission line
Dogso i spa
Manfred jeitler
Manfred huber uta
Manfred harth
Manfred huber
Manfred huber rate my professor
Manfred doepp
Manfred peter eisenhut