Conditions Leading to Anomalously Early LoranC Signal Skywave
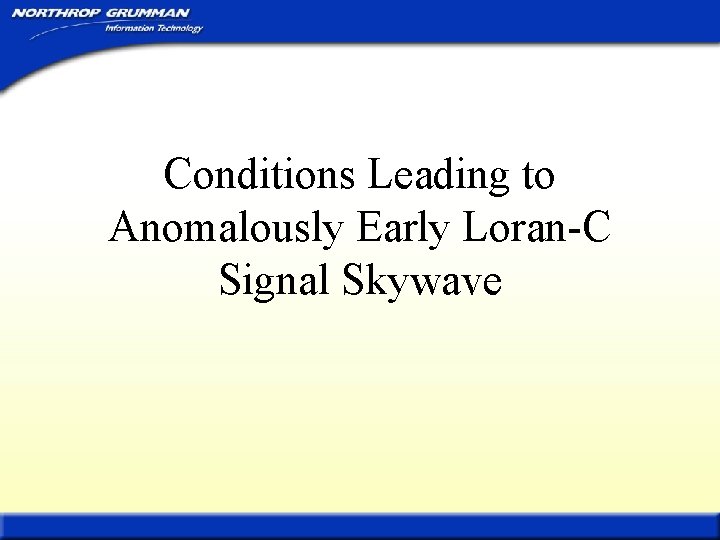
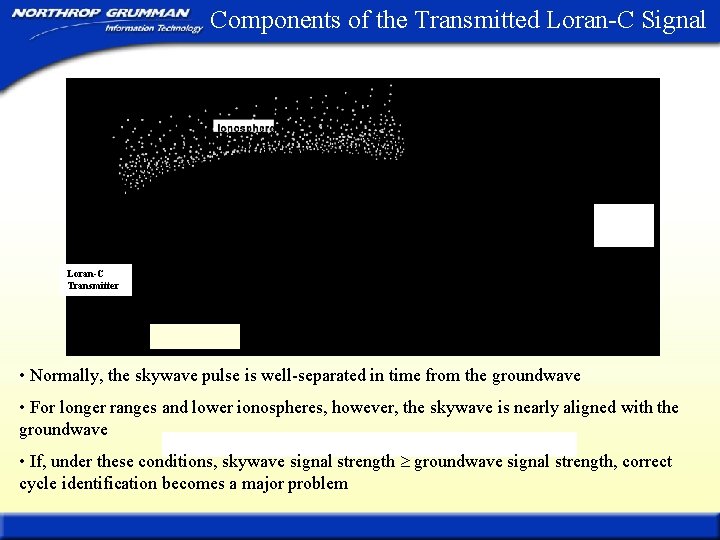
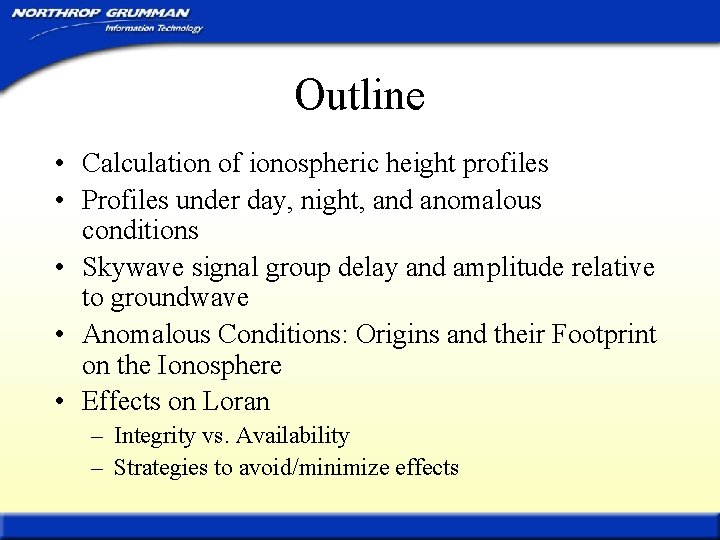
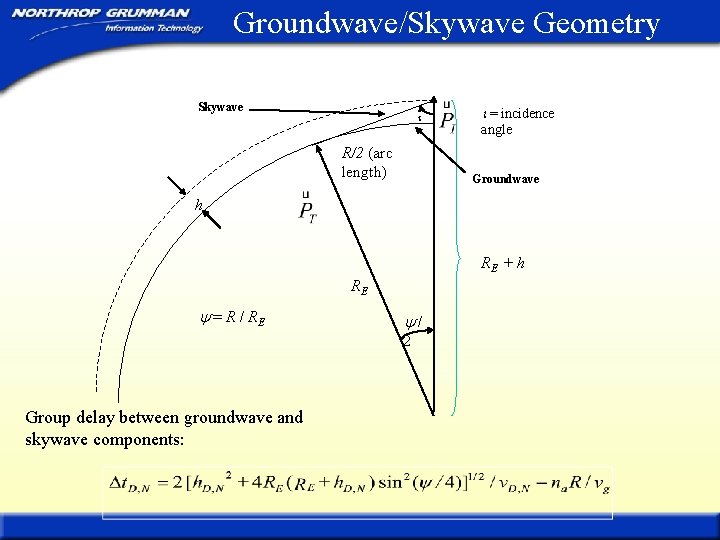
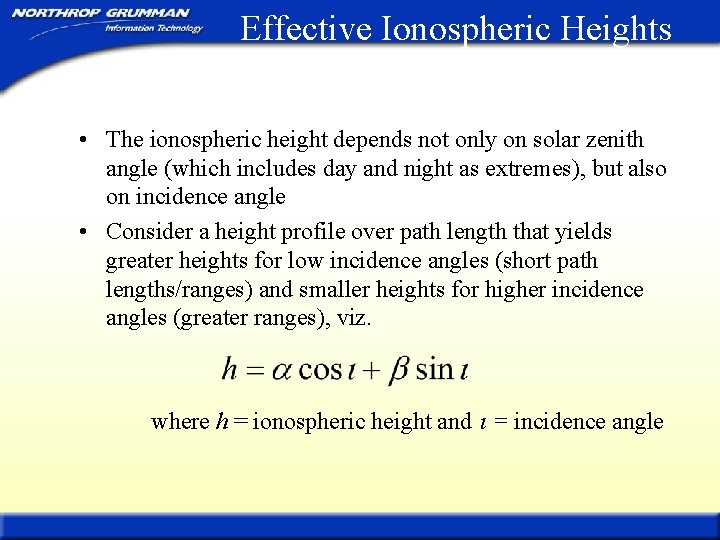
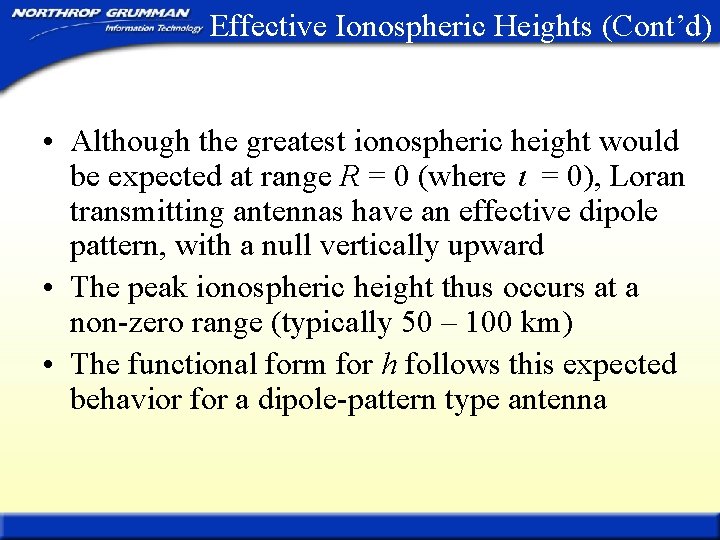
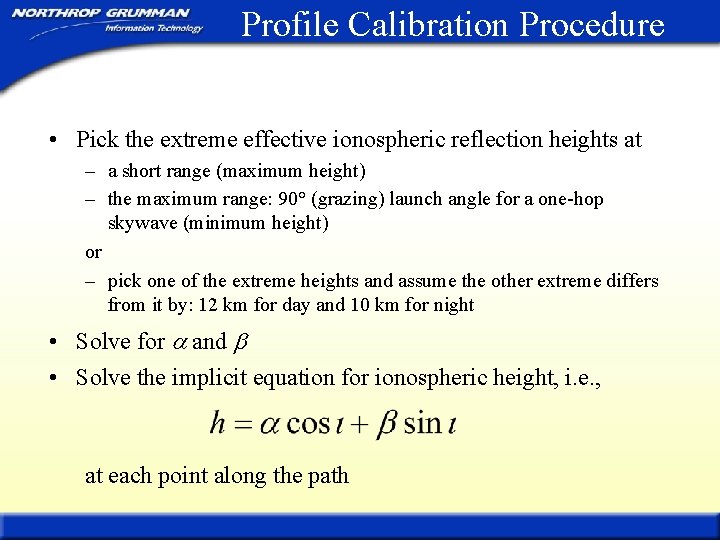
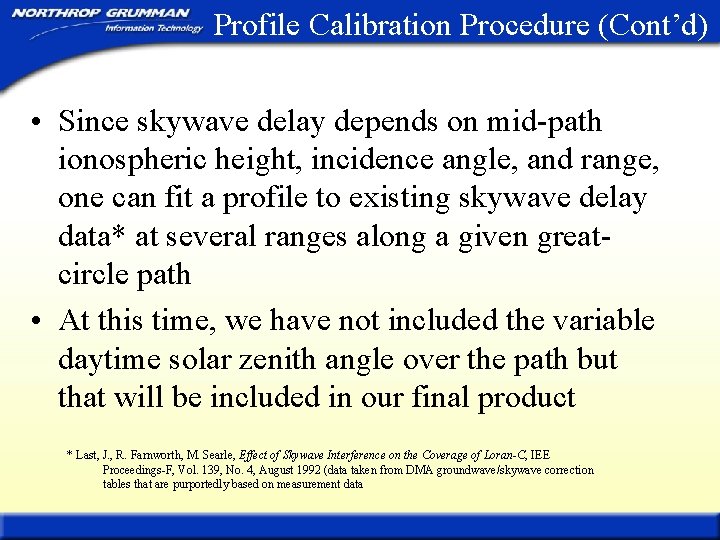
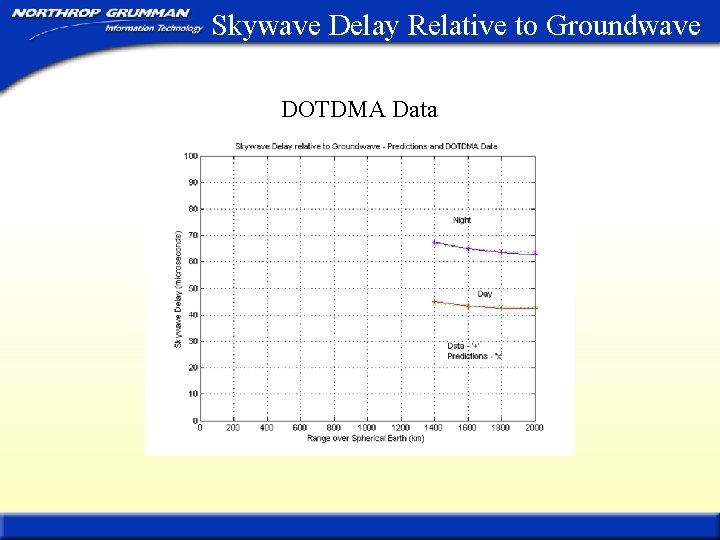
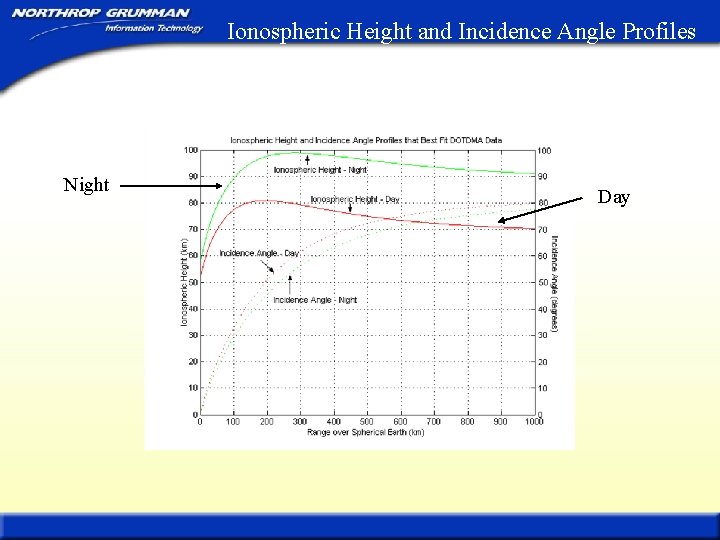
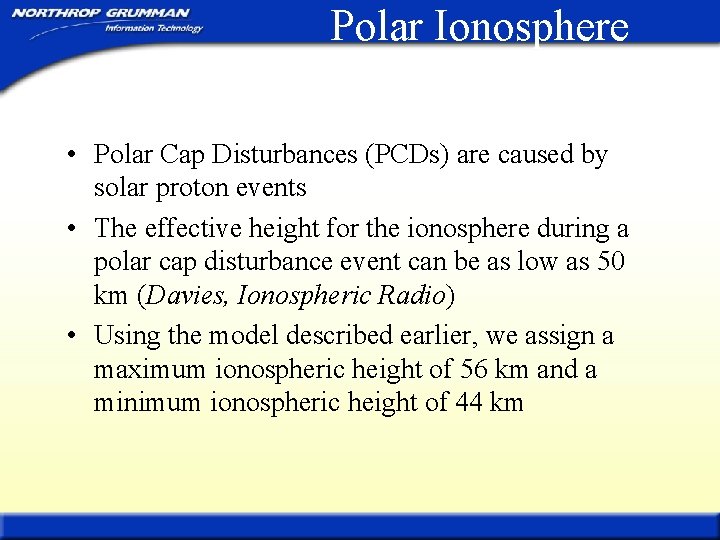
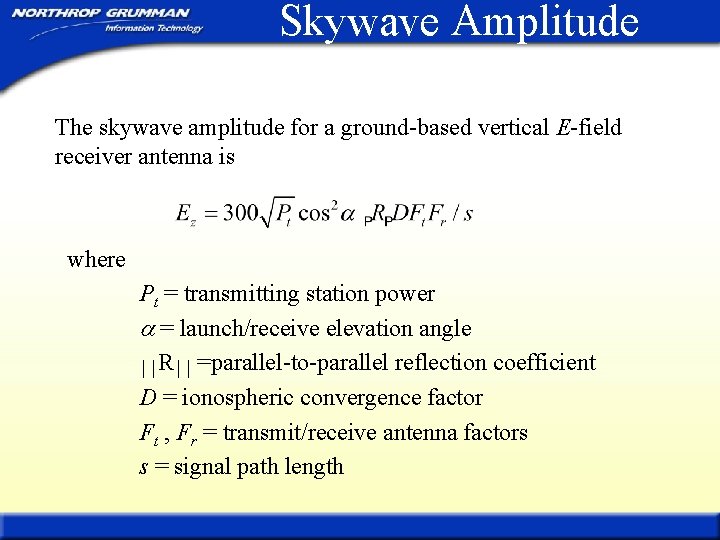
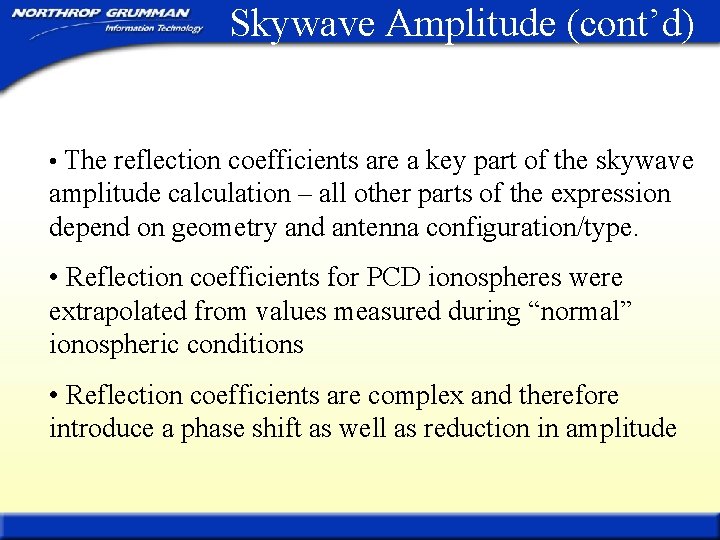
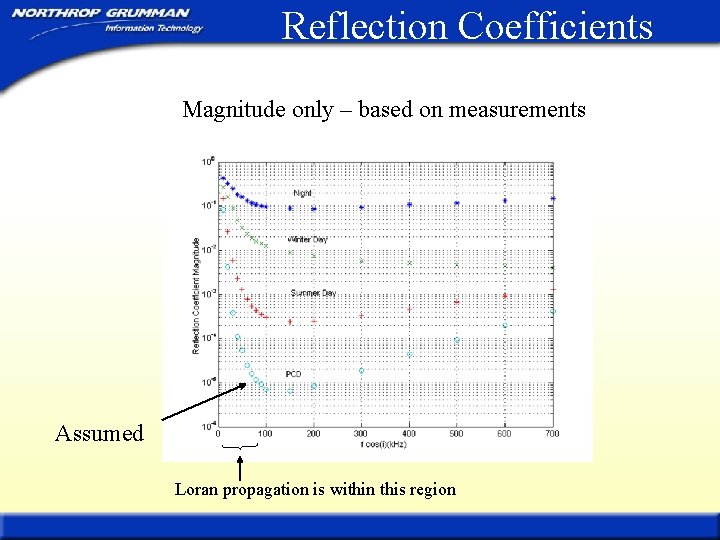
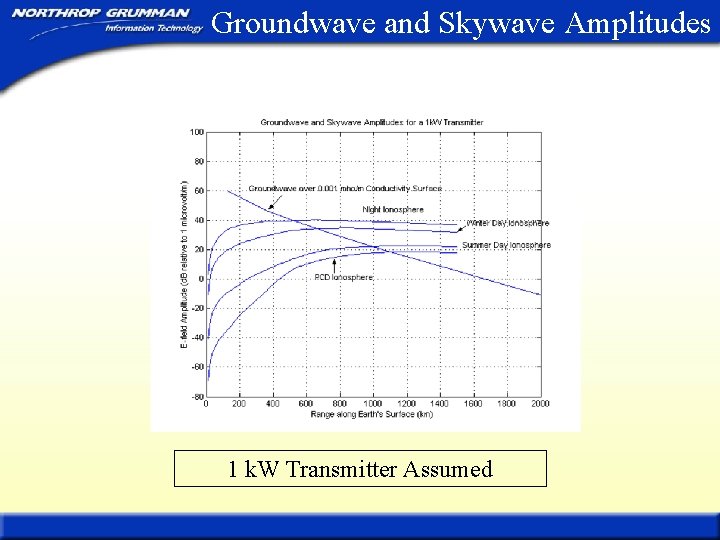
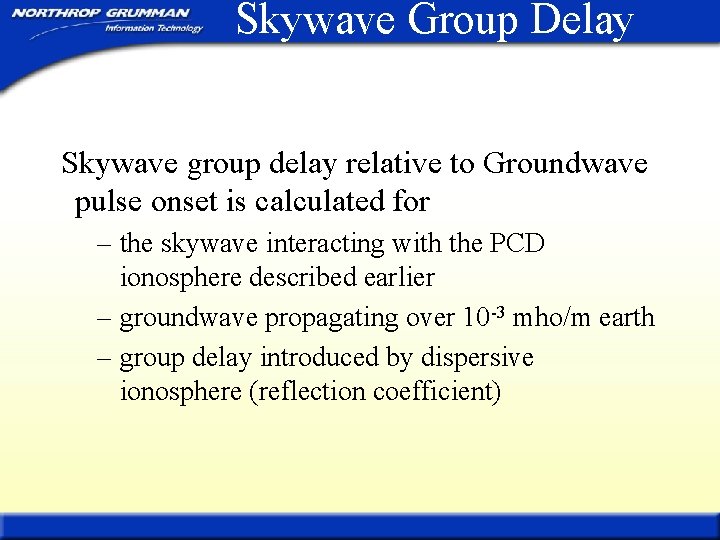
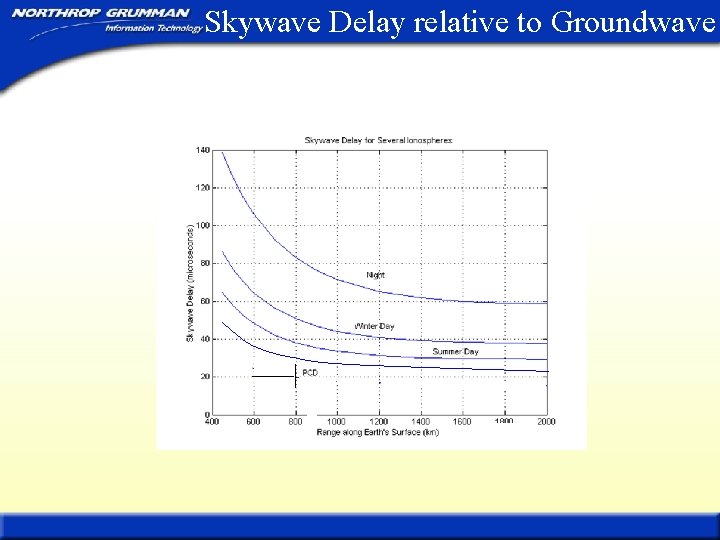
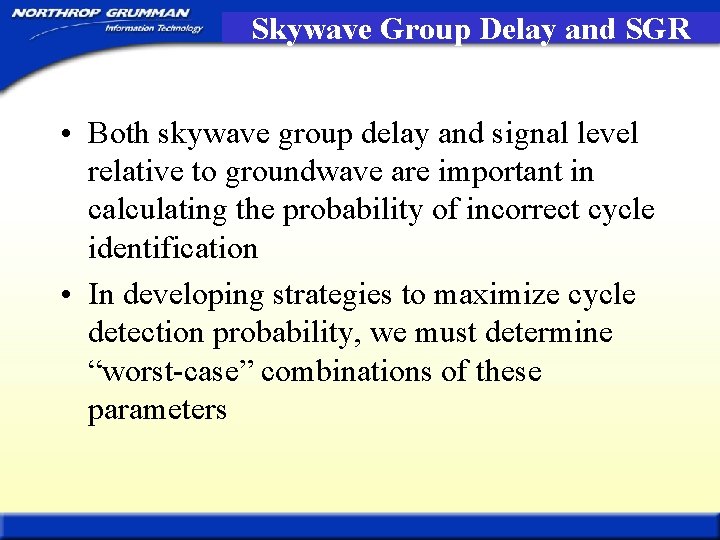
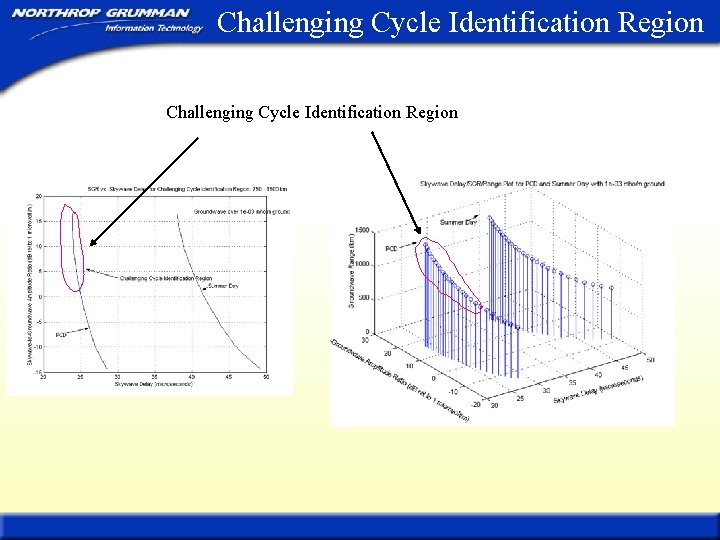
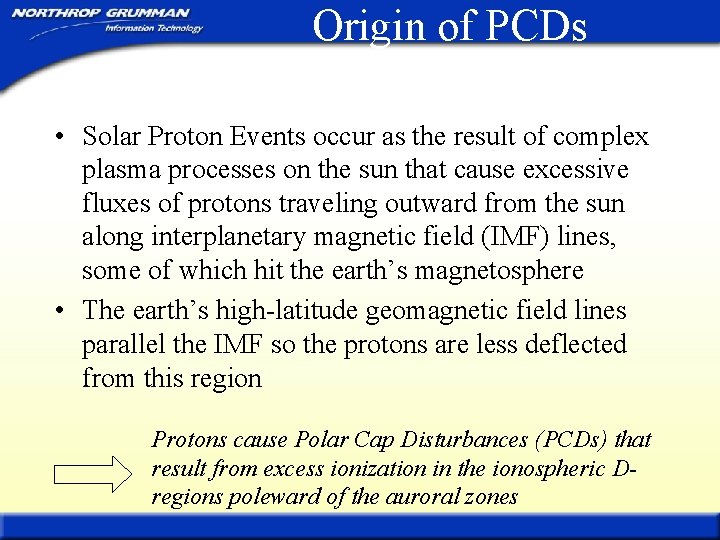
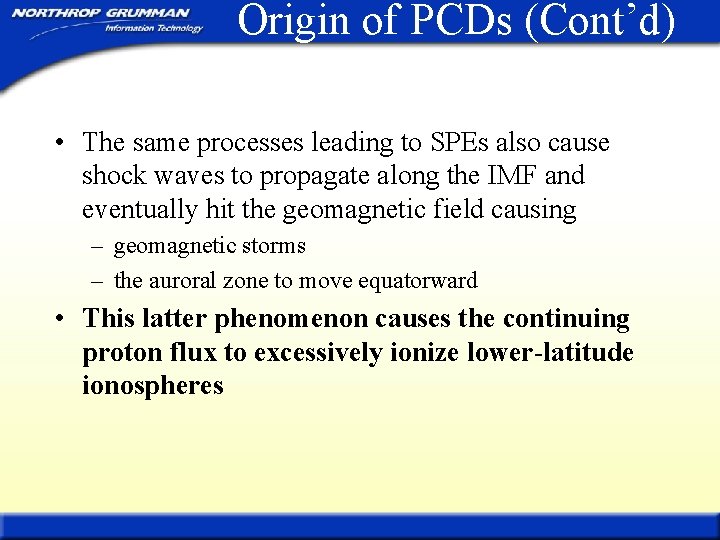
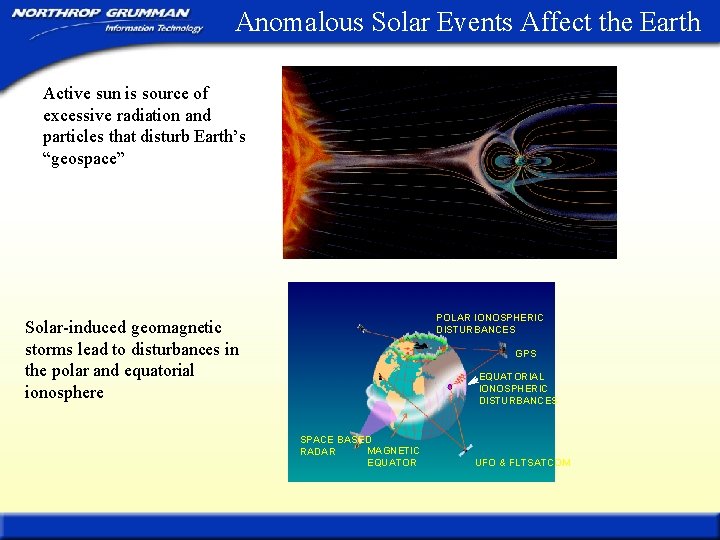
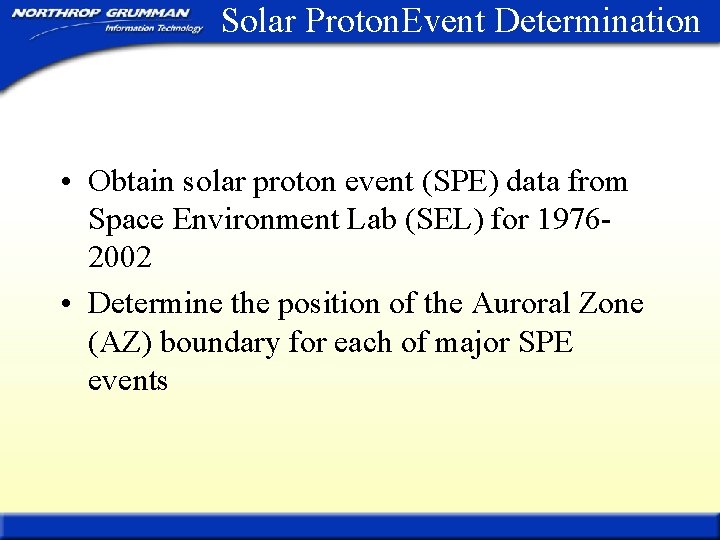
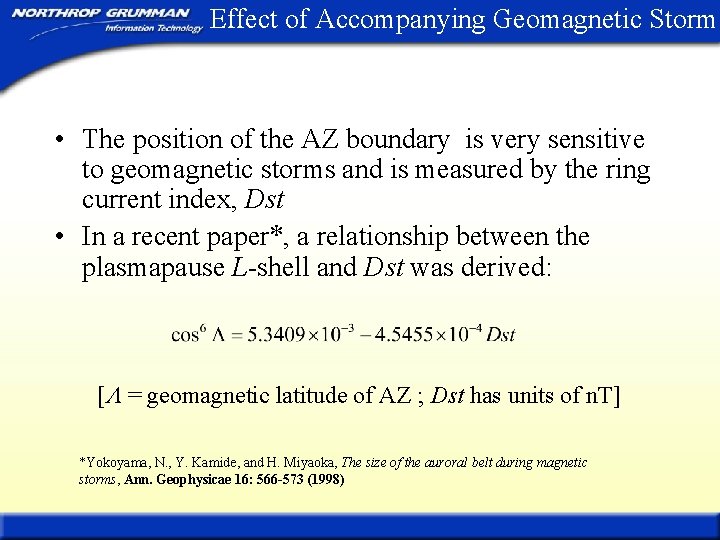
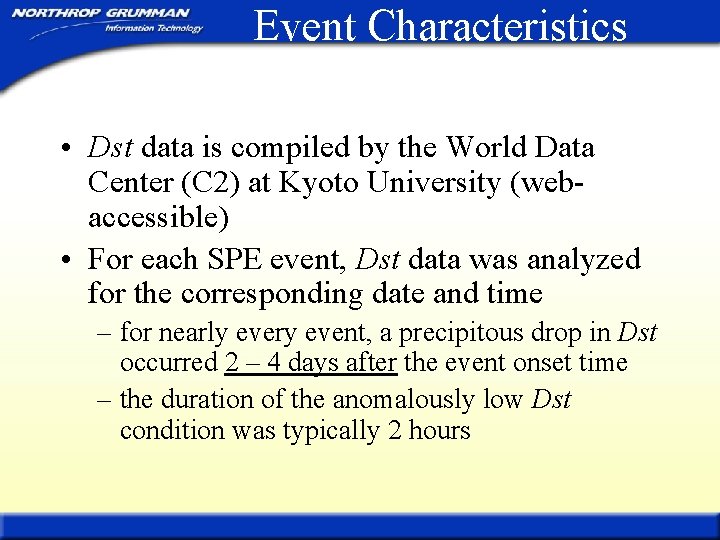
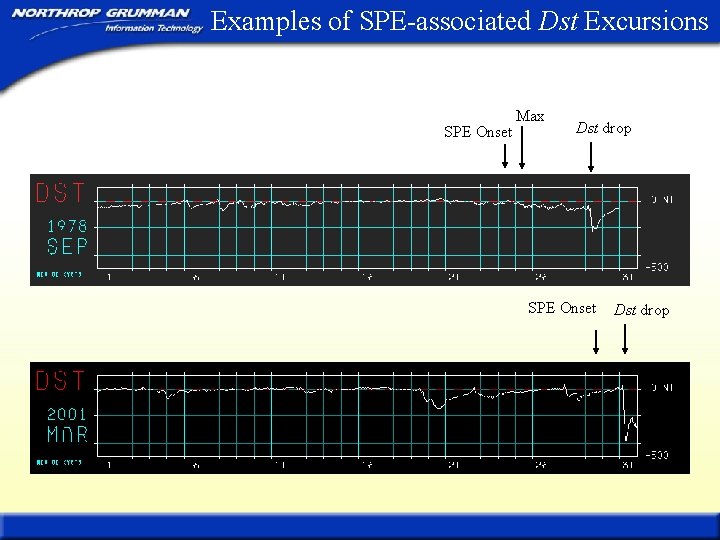
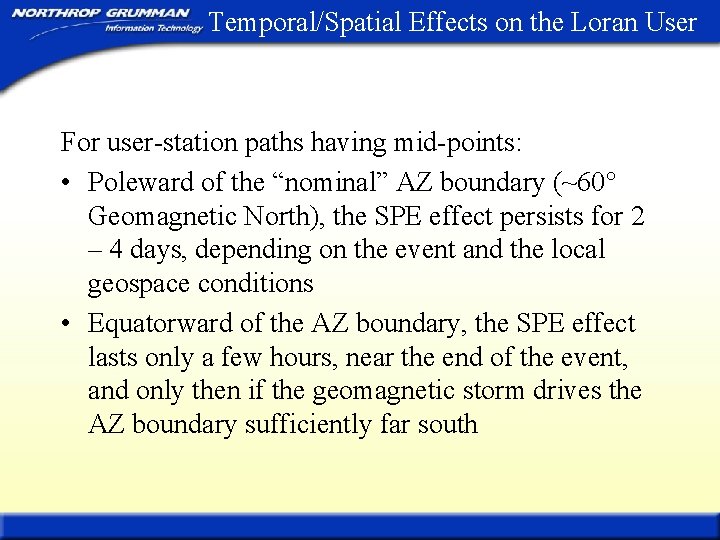
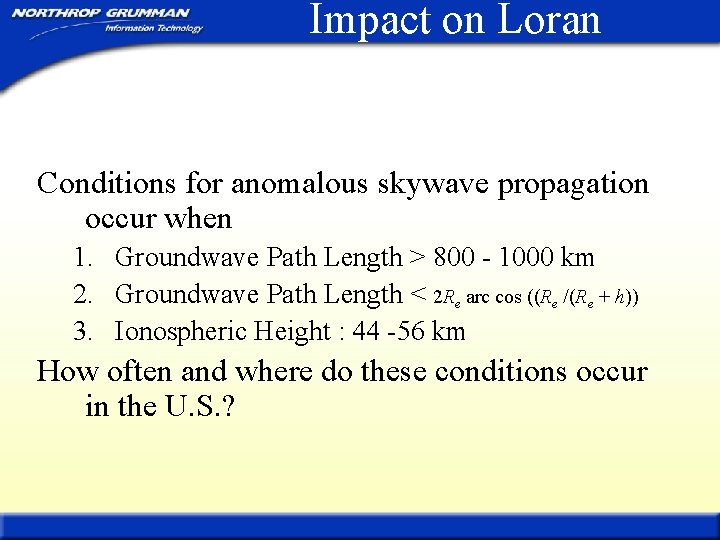
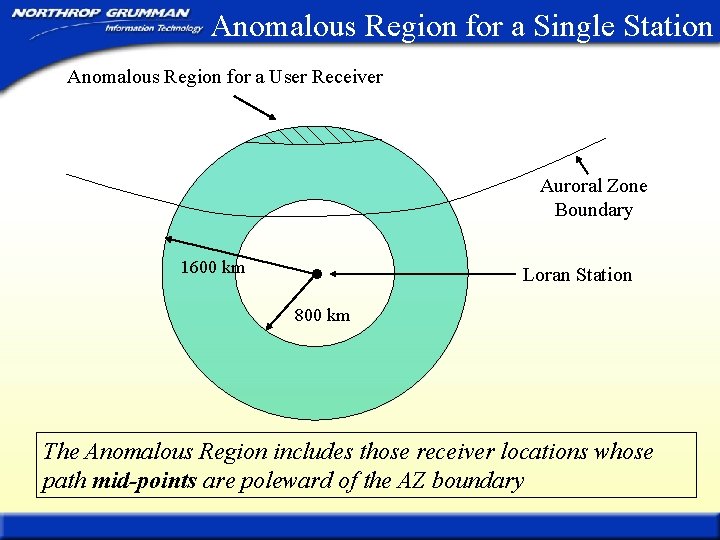
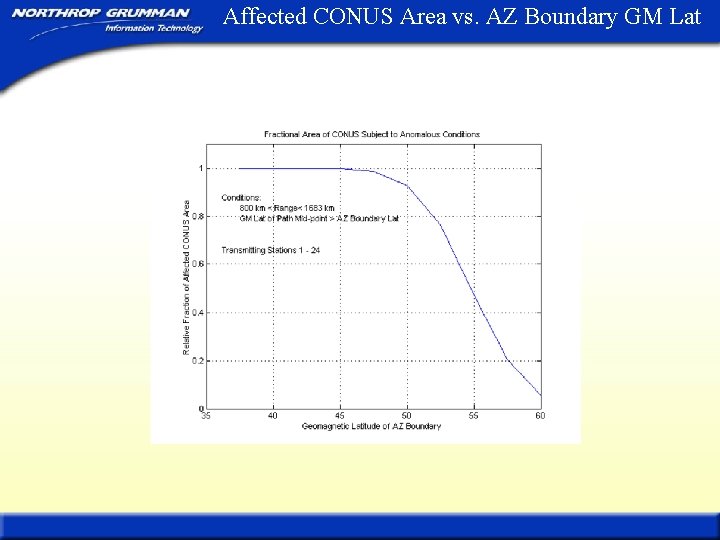
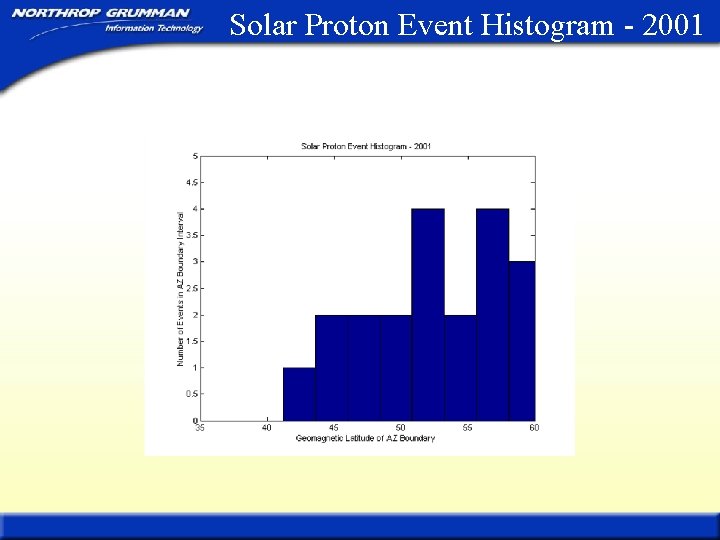
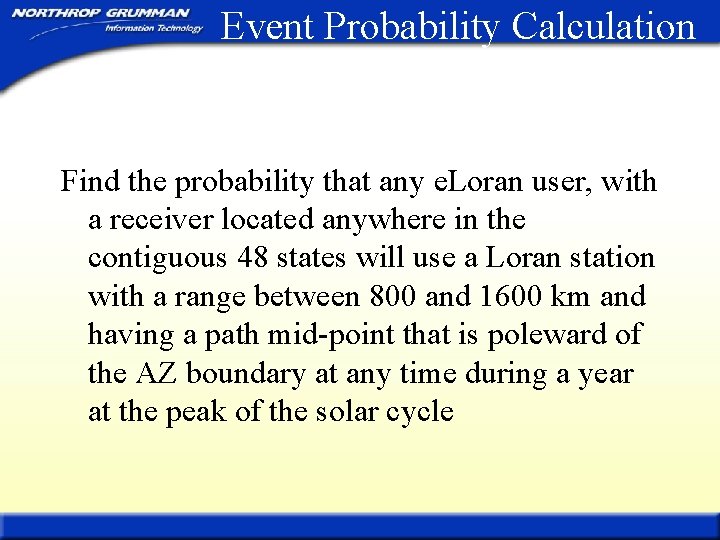
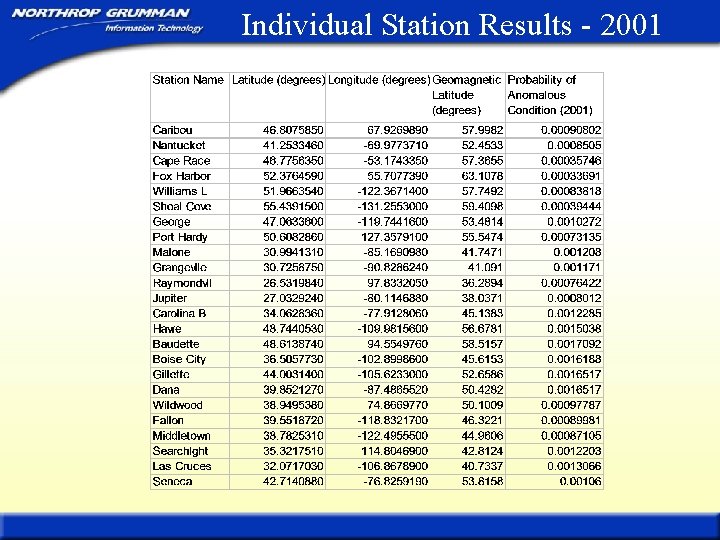
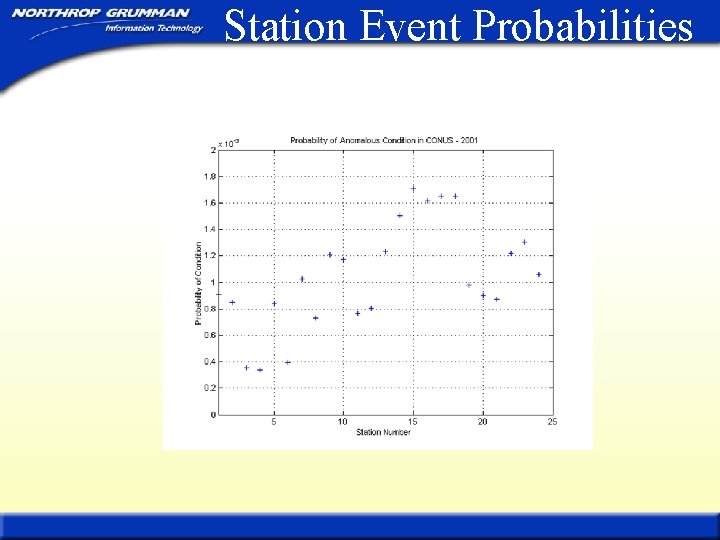
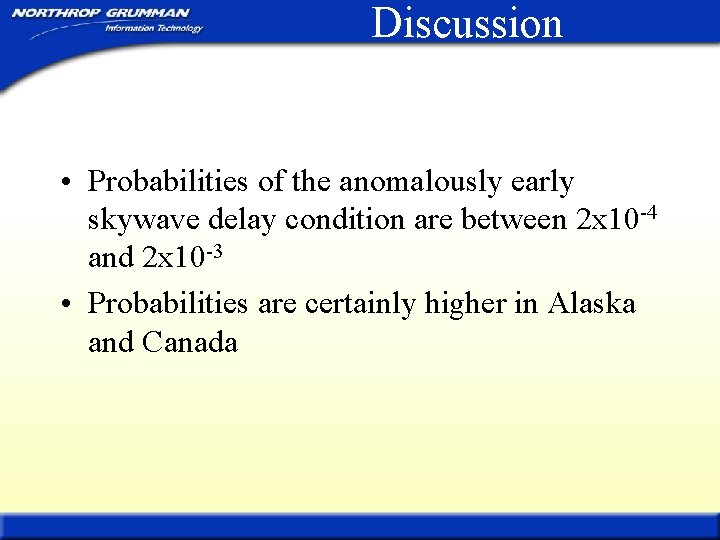
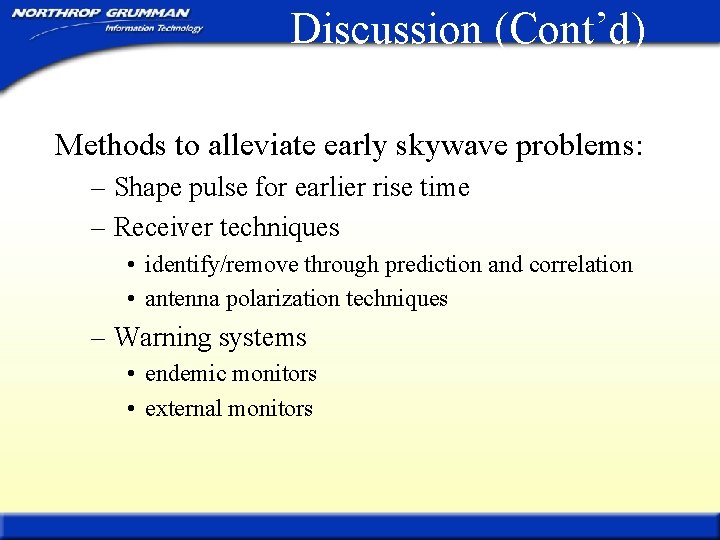
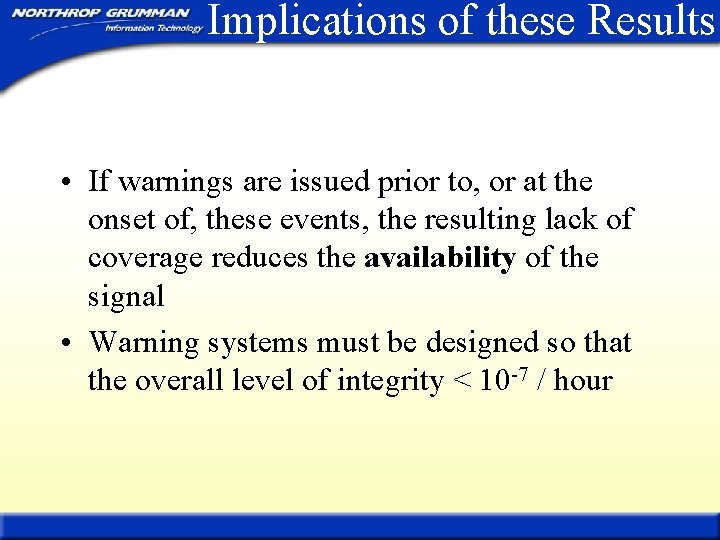
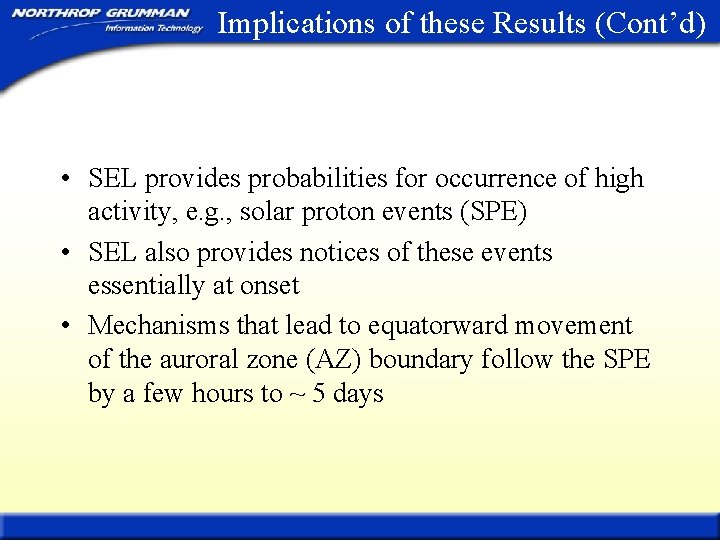
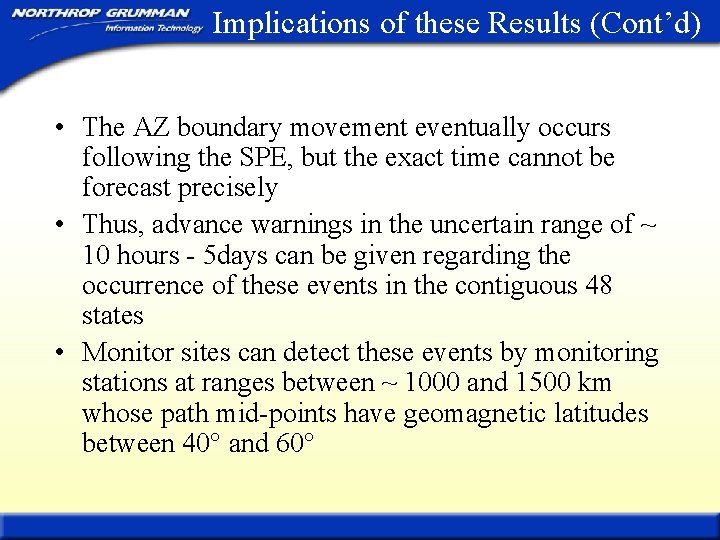
- Slides: 39
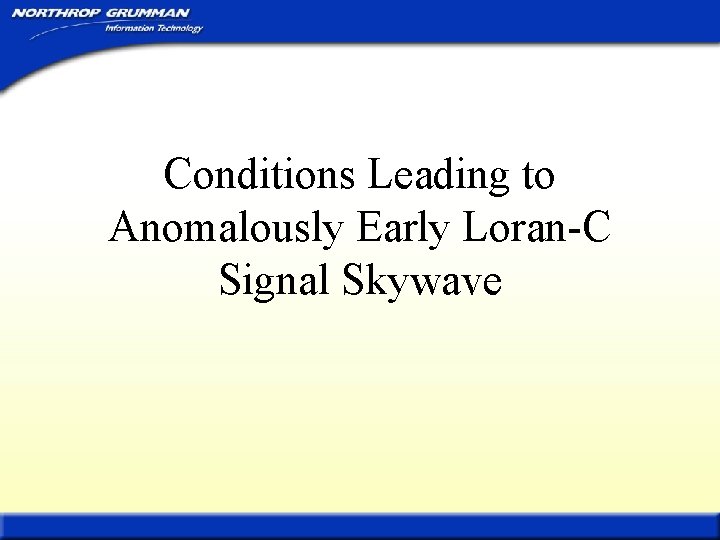
Conditions Leading to Anomalously Early Loran-C Signal Skywave
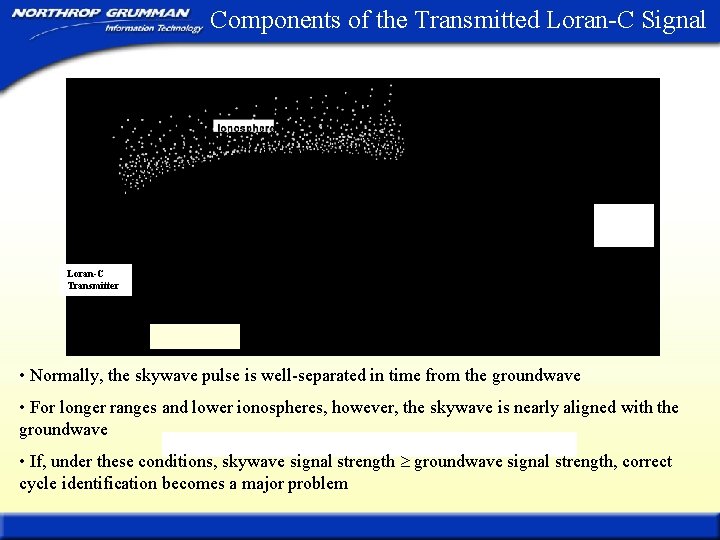
Components of the Transmitted Loran-C Signal Loran-C Transmitter • Normally, the skywave pulse is well-separated in time from the groundwave • For longer ranges and lower ionospheres, however, the skywave is nearly aligned with the groundwave • If, under these conditions, skywave signal strength groundwave signal strength, correct cycle identification becomes a major problem
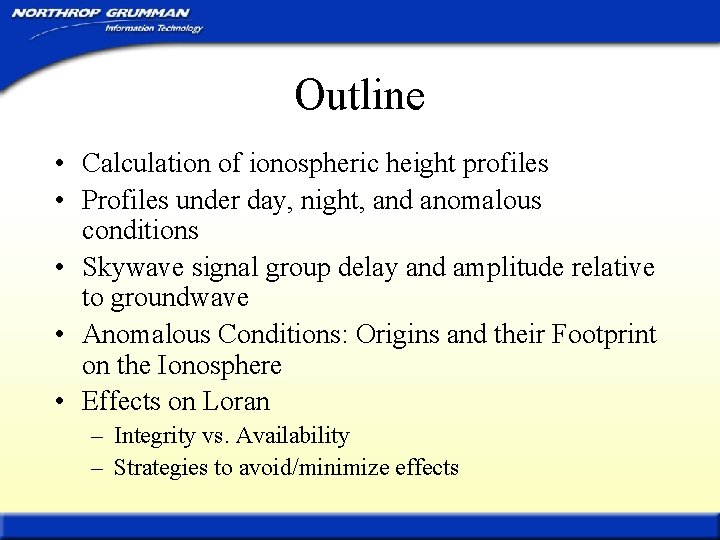
Outline • Calculation of ionospheric height profiles • Profiles under day, night, and anomalous conditions • Skywave signal group delay and amplitude relative to groundwave • Anomalous Conditions: Origins and their Footprint on the Ionosphere • Effects on Loran – Integrity vs. Availability – Strategies to avoid/minimize effects
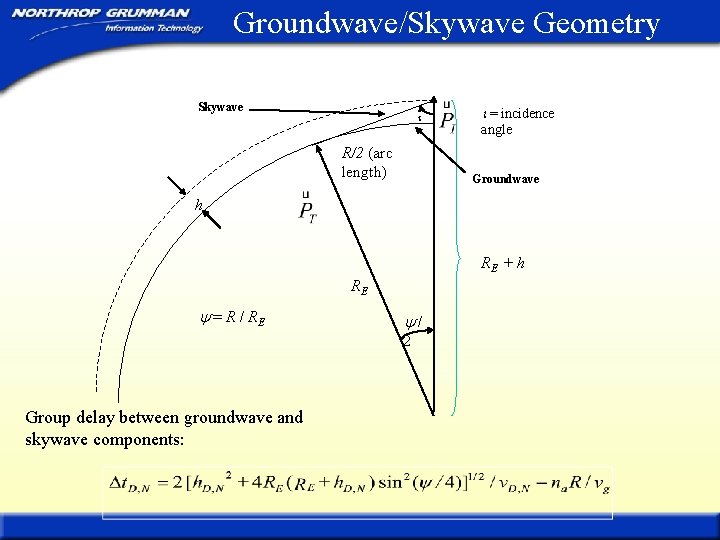
Groundwave/Skywave Geometry Skywave R/2 (arc length) = incidence angle Groundwave h RE + h RE = R / RE / 2 Group delay between groundwave and skywave components:
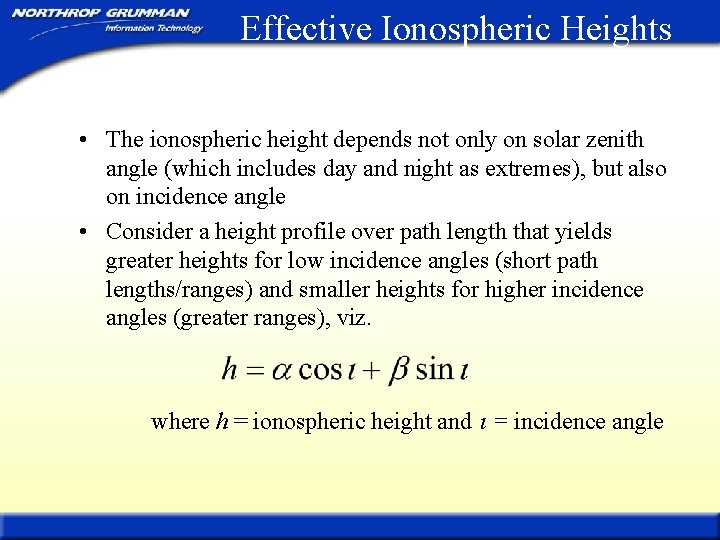
Effective Ionospheric Heights • The ionospheric height depends not only on solar zenith angle (which includes day and night as extremes), but also on incidence angle • Consider a height profile over path length that yields greater heights for low incidence angles (short path lengths/ranges) and smaller heights for higher incidence angles (greater ranges), viz. where h = ionospheric height and = incidence angle
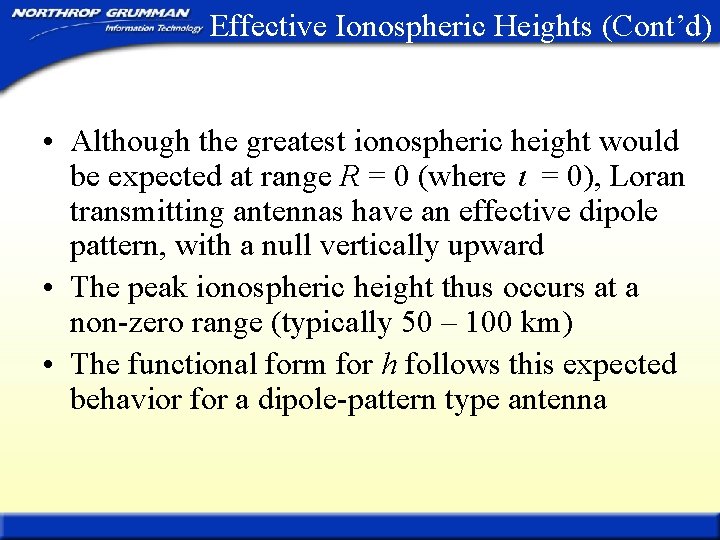
Effective Ionospheric Heights (Cont’d) • Although the greatest ionospheric height would be expected at range R = 0 (where = 0), Loran transmitting antennas have an effective dipole pattern, with a null vertically upward • The peak ionospheric height thus occurs at a non-zero range (typically 50 – 100 km) • The functional form for h follows this expected behavior for a dipole-pattern type antenna
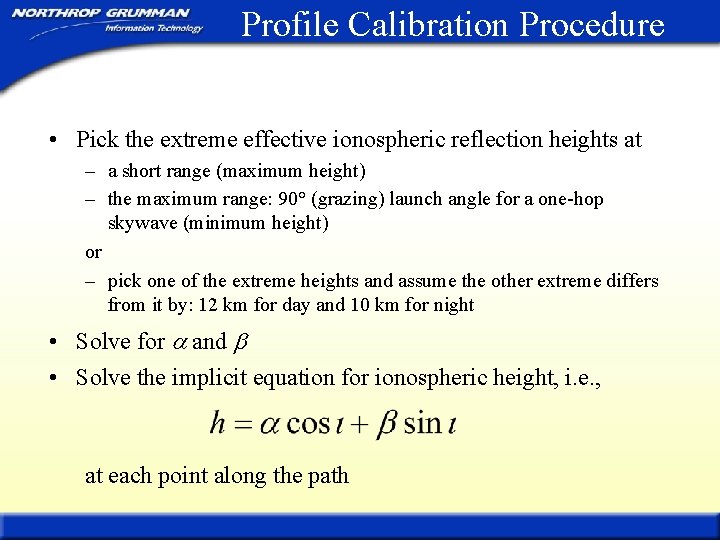
Profile Calibration Procedure • Pick the extreme effective ionospheric reflection heights at – a short range (maximum height) – the maximum range: 90° (grazing) launch angle for a one-hop skywave (minimum height) or – pick one of the extreme heights and assume the other extreme differs from it by: 12 km for day and 10 km for night • Solve for and • Solve the implicit equation for ionospheric height, i. e. , at each point along the path
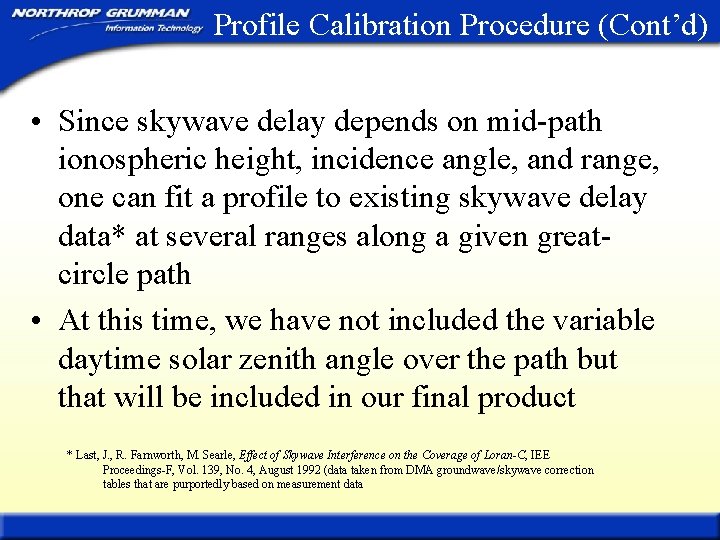
Profile Calibration Procedure (Cont’d) • Since skywave delay depends on mid-path ionospheric height, incidence angle, and range, one can fit a profile to existing skywave delay data* at several ranges along a given greatcircle path • At this time, we have not included the variable daytime solar zenith angle over the path but that will be included in our final product * Last, J. , R. Farnworth, M. Searle, Effect of Skywave Interference on the Coverage of Loran-C, IEE Proceedings-F, Vol. 139, No. 4, August 1992 (data taken from DMA groundwave/skywave correction tables that are purportedly based on measurement data
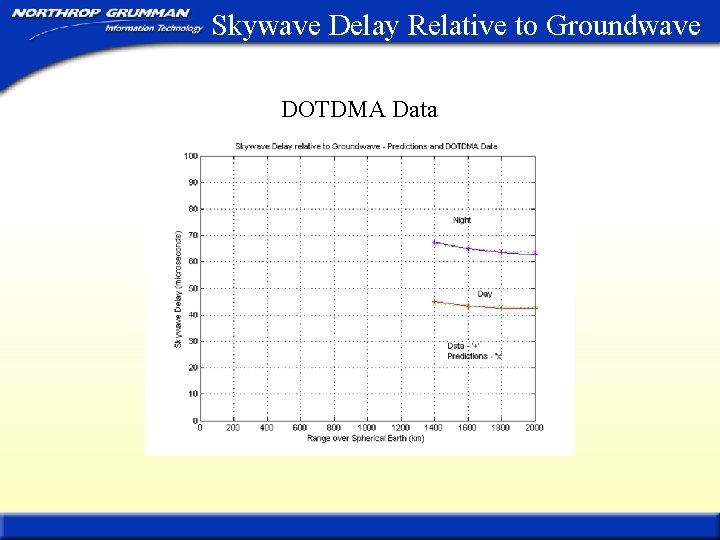
Skywave Delay Relative to Groundwave DOTDMA Data
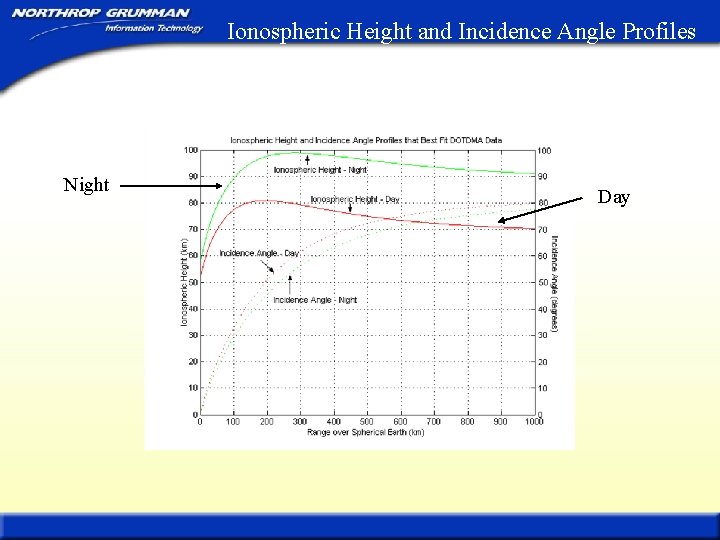
Ionospheric Height and Incidence Angle Profiles Night Day
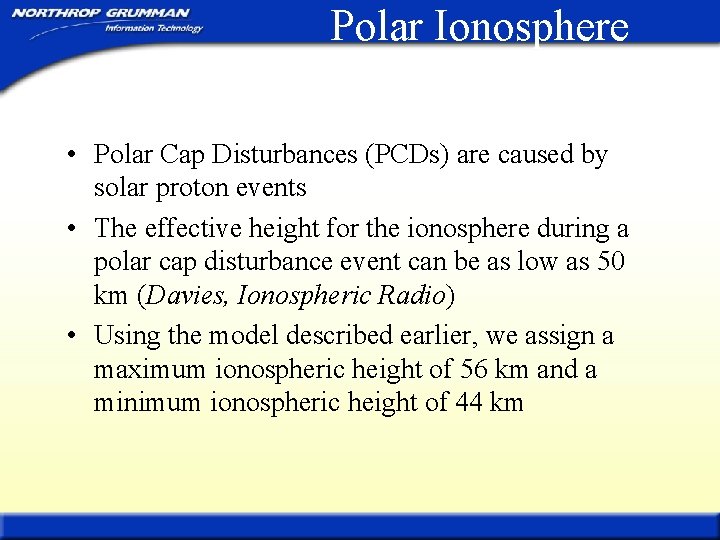
Polar Ionosphere • Polar Cap Disturbances (PCDs) are caused by solar proton events • The effective height for the ionosphere during a polar cap disturbance event can be as low as 50 km (Davies, Ionospheric Radio) • Using the model described earlier, we assign a maximum ionospheric height of 56 km and a minimum ionospheric height of 44 km
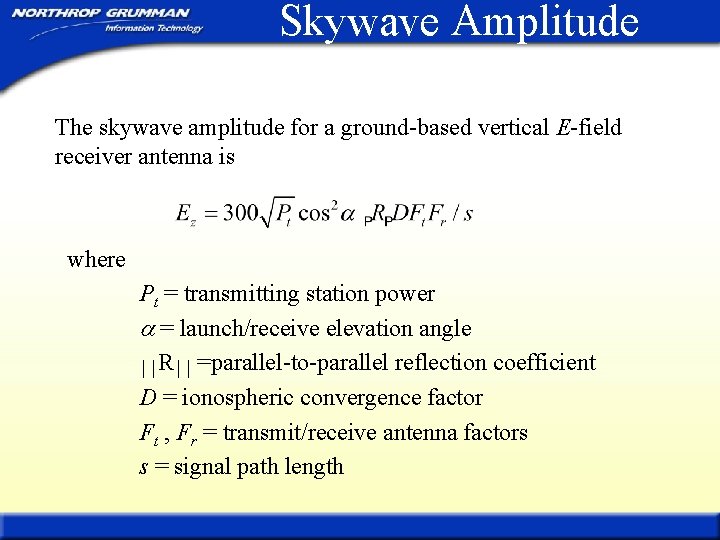
Skywave Amplitude The skywave amplitude for a ground-based vertical E-field receiver antenna is where Pt = transmitting station power = launch/receive elevation angle R =parallel-to-parallel reflection coefficient D = ionospheric convergence factor Ft , Fr = transmit/receive antenna factors s = signal path length
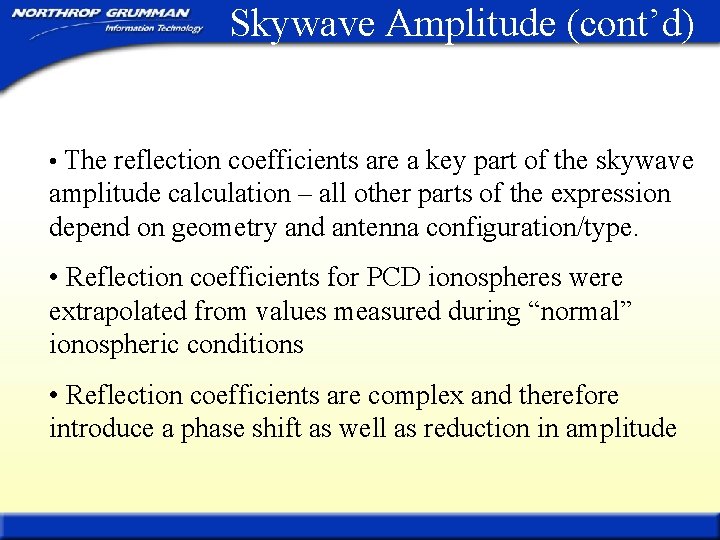
Skywave Amplitude (cont’d) • The reflection coefficients are a key part of the skywave amplitude calculation – all other parts of the expression depend on geometry and antenna configuration/type. • Reflection coefficients for PCD ionospheres were extrapolated from values measured during “normal” ionospheric conditions • Reflection coefficients are complex and therefore introduce a phase shift as well as reduction in amplitude
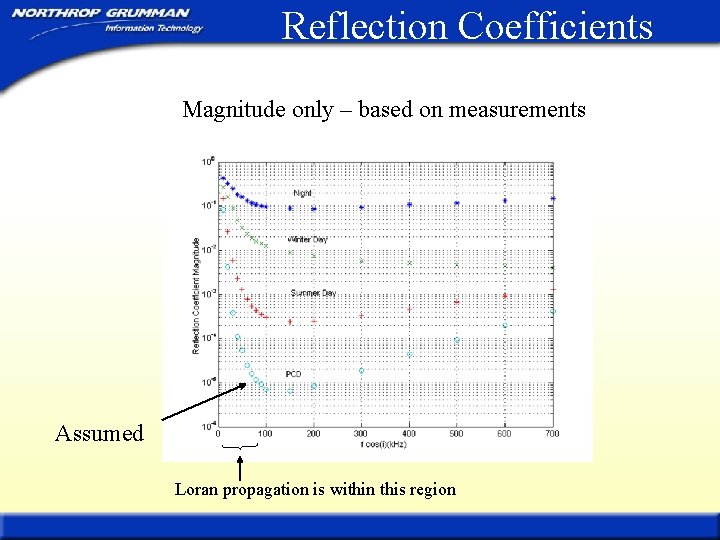
Reflection Coefficients Magnitude only – based on measurements Assumed Loran propagation is within this region
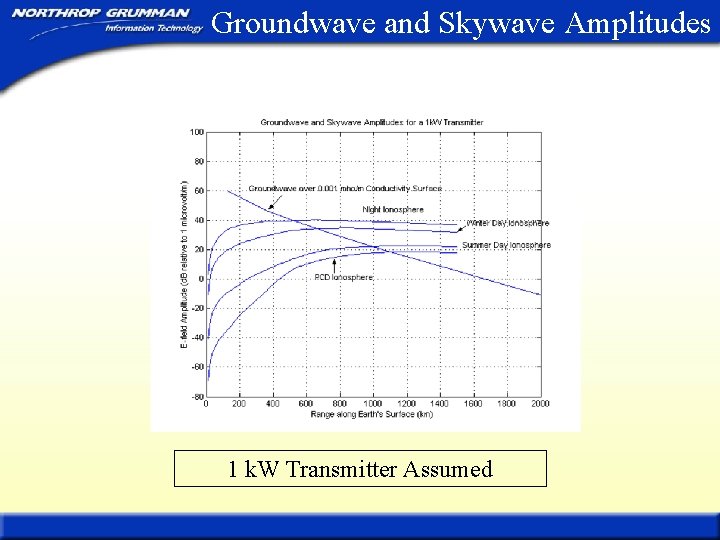
Groundwave and Skywave Amplitudes 1 k. W Transmitter Assumed
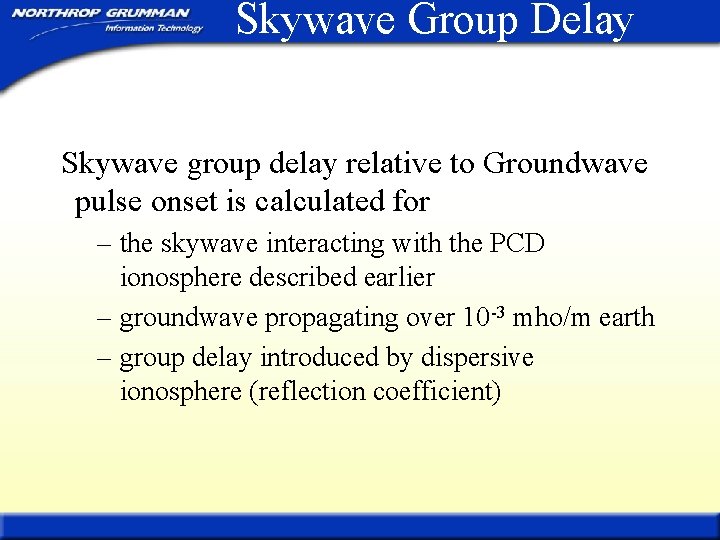
Skywave Group Delay Skywave group delay relative to Groundwave pulse onset is calculated for – the skywave interacting with the PCD ionosphere described earlier – groundwave propagating over 10 -3 mho/m earth – group delay introduced by dispersive ionosphere (reflection coefficient)
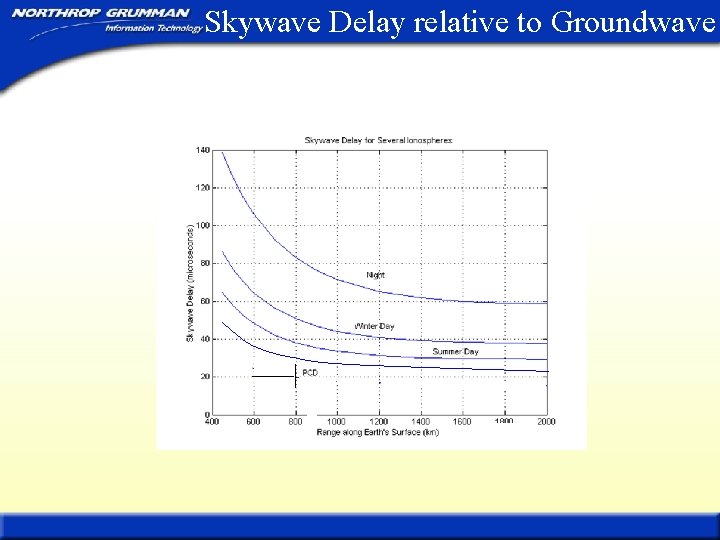
Skywave Delay relative to Groundwave
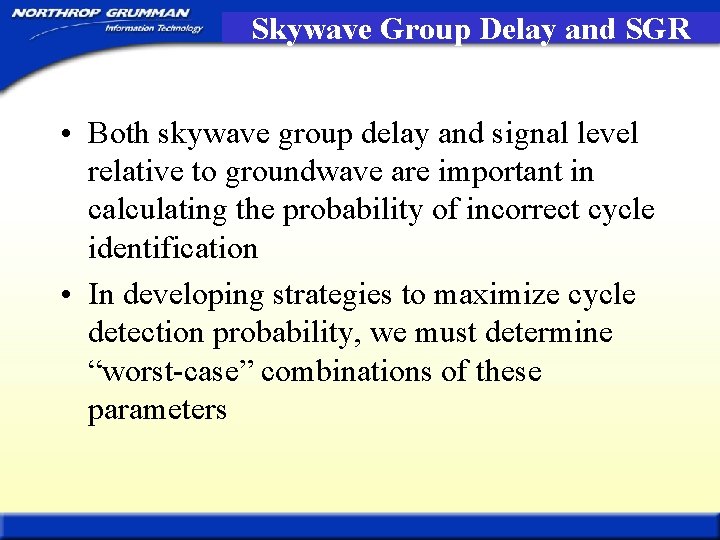
Skywave Group Delay and SGR • Both skywave group delay and signal level relative to groundwave are important in calculating the probability of incorrect cycle identification • In developing strategies to maximize cycle detection probability, we must determine “worst-case” combinations of these parameters
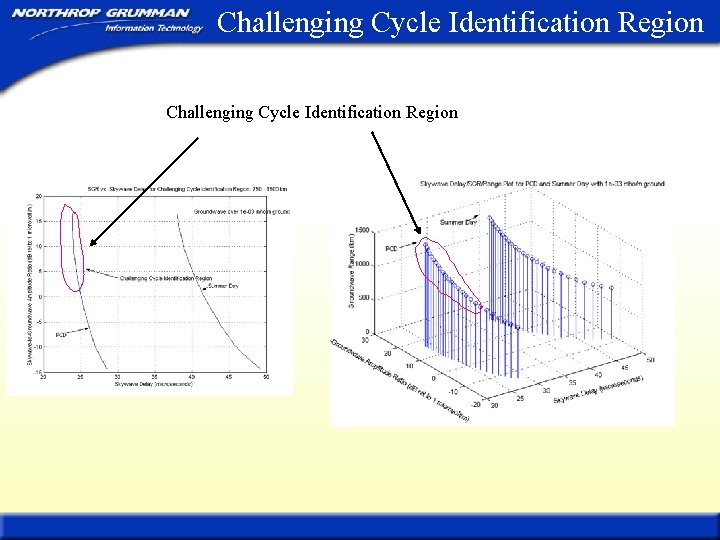
Challenging Cycle Identification Region
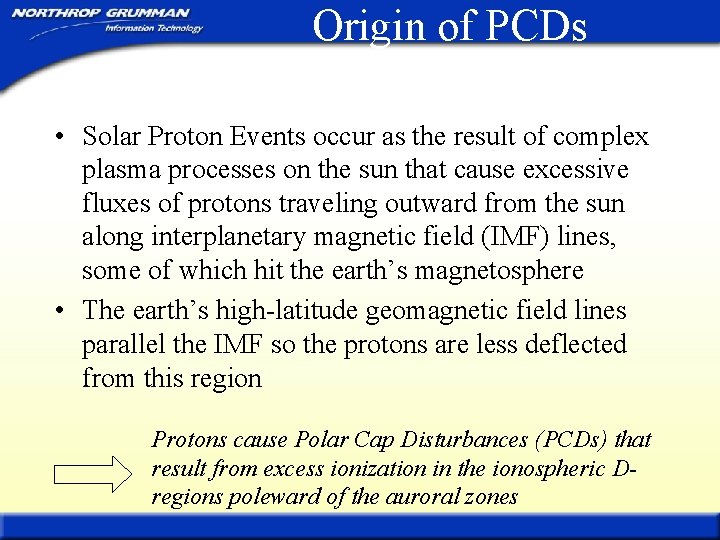
Origin of PCDs • Solar Proton Events occur as the result of complex plasma processes on the sun that cause excessive fluxes of protons traveling outward from the sun along interplanetary magnetic field (IMF) lines, some of which hit the earth’s magnetosphere • The earth’s high-latitude geomagnetic field lines parallel the IMF so the protons are less deflected from this region Protons cause Polar Cap Disturbances (PCDs) that result from excess ionization in the ionospheric Dregions poleward of the auroral zones
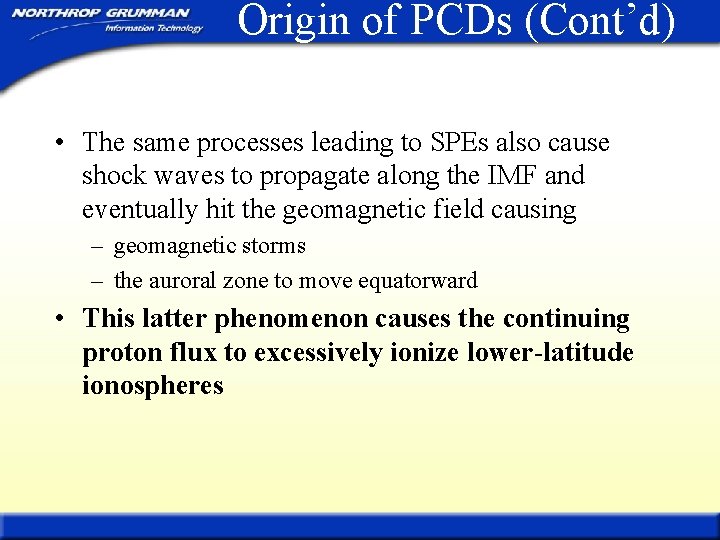
Origin of PCDs (Cont’d) • The same processes leading to SPEs also cause shock waves to propagate along the IMF and eventually hit the geomagnetic field causing – geomagnetic storms – the auroral zone to move equatorward • This latter phenomenon causes the continuing proton flux to excessively ionize lower-latitude ionospheres
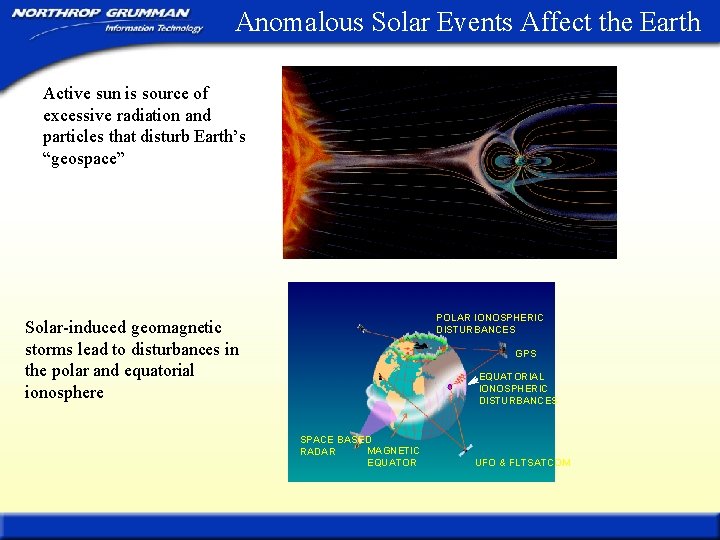
Anomalous Solar Events Affect the Earth Active sun is source of excessive radiation and particles that disturb Earth’s “geospace” POLAR IONOSPHERIC DISTURBANCES Solar-induced geomagnetic storms lead to disturbances in the polar and equatorial ionosphere GPS EQUATORIAL IONOSPHERIC DISTURBANCES SPACE BASED MAGNETIC RADAR EQUATOR UFO & FLTSATCOM
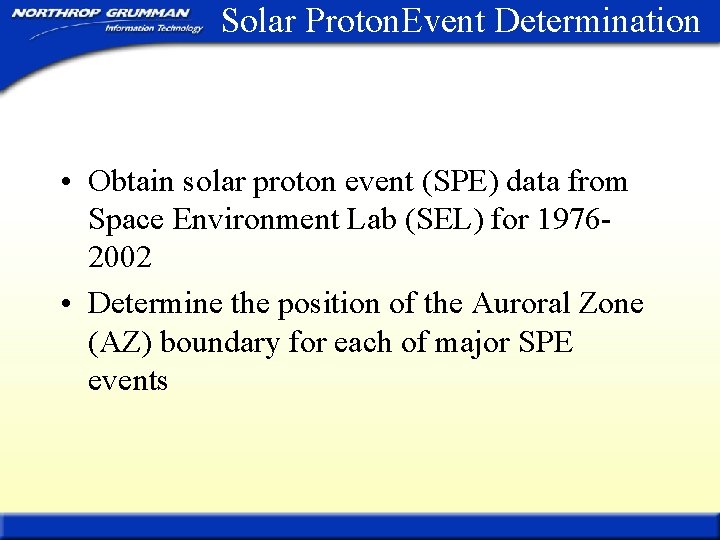
Solar Proton. Event Determination • Obtain solar proton event (SPE) data from Space Environment Lab (SEL) for 19762002 • Determine the position of the Auroral Zone (AZ) boundary for each of major SPE events
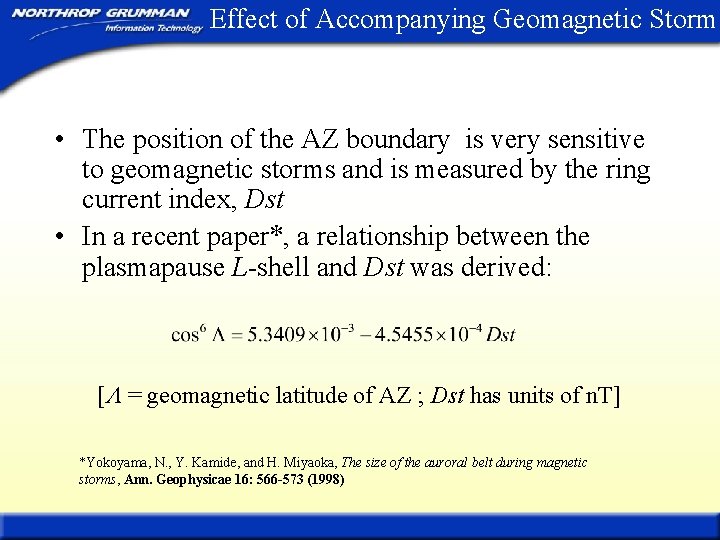
Effect of Accompanying Geomagnetic Storm • The position of the AZ boundary is very sensitive to geomagnetic storms and is measured by the ring current index, Dst • In a recent paper*, a relationship between the plasmapause L-shell and Dst was derived: [ = geomagnetic latitude of AZ ; Dst has units of n. T] *Yokoyama, N. , Y. Kamide, and H. Miyaoka, The size of the auroral belt during magnetic storms, Ann. Geophysicae 16: 566 -573 (1998)
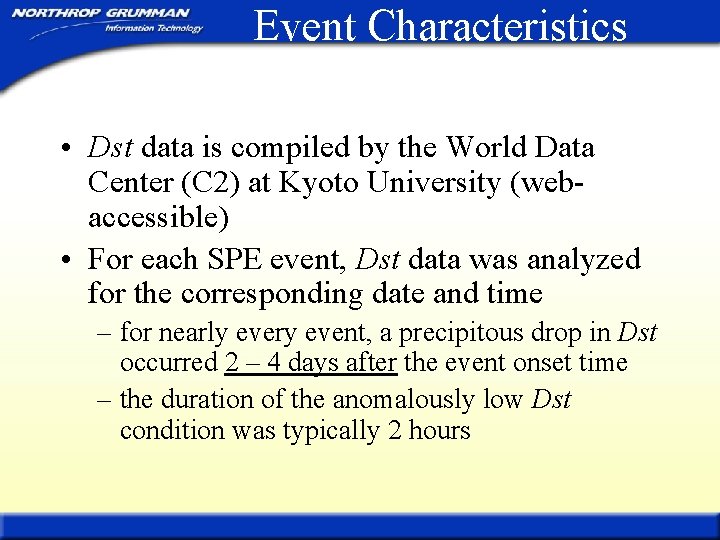
Event Characteristics • Dst data is compiled by the World Data Center (C 2) at Kyoto University (webaccessible) • For each SPE event, Dst data was analyzed for the corresponding date and time – for nearly every event, a precipitous drop in Dst occurred 2 – 4 days after the event onset time – the duration of the anomalously low Dst condition was typically 2 hours
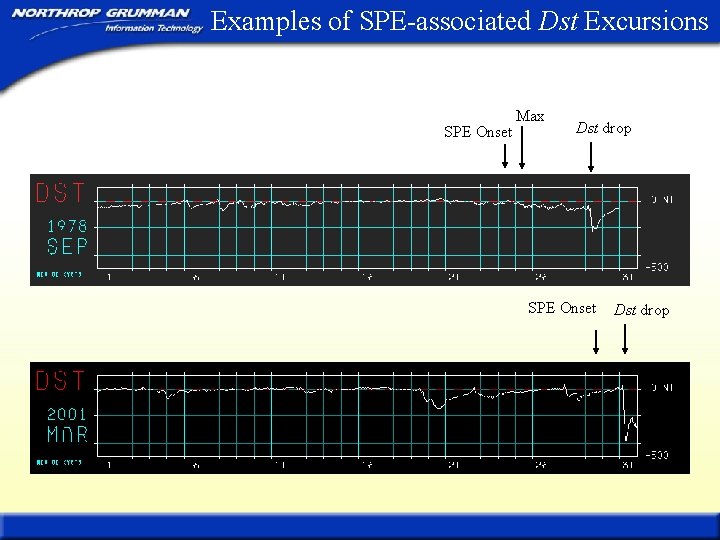
Examples of SPE-associated Dst Excursions SPE Onset Max Dst drop SPE Onset Dst drop
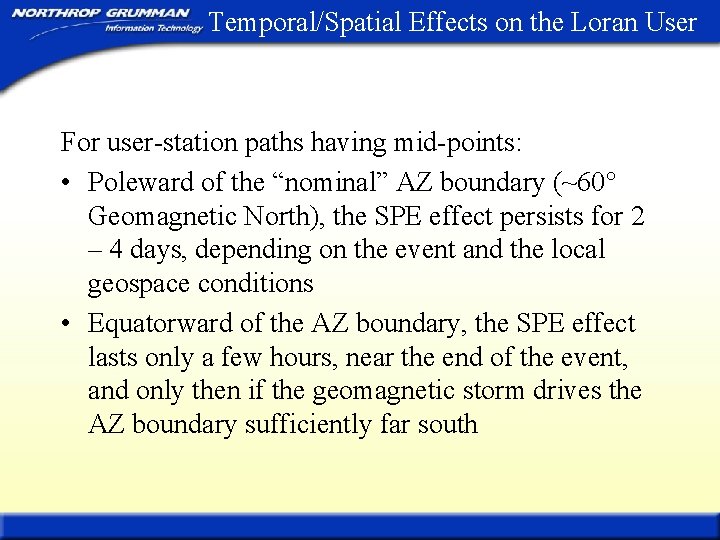
Temporal/Spatial Effects on the Loran User For user-station paths having mid-points: • Poleward of the “nominal” AZ boundary (~60° Geomagnetic North), the SPE effect persists for 2 – 4 days, depending on the event and the local geospace conditions • Equatorward of the AZ boundary, the SPE effect lasts only a few hours, near the end of the event, and only then if the geomagnetic storm drives the AZ boundary sufficiently far south
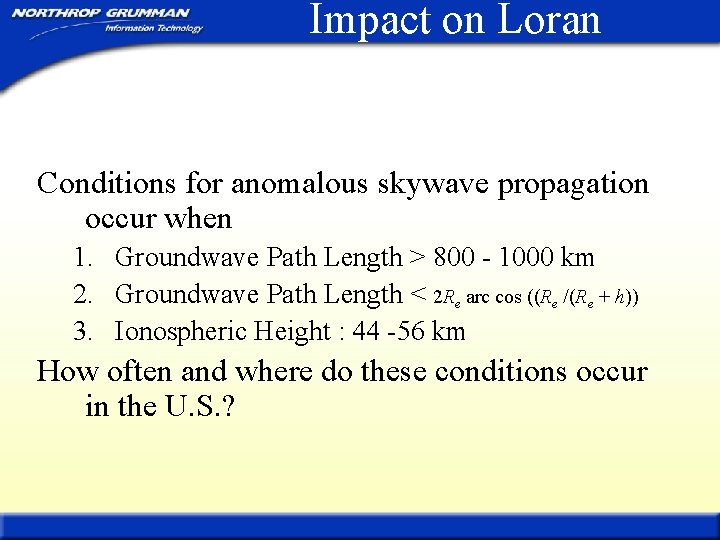
Impact on Loran Conditions for anomalous skywave propagation occur when 1. Groundwave Path Length > 800 - 1000 km 2. Groundwave Path Length < 2 Re arc cos ((Re /(Re + h)) 3. Ionospheric Height : 44 -56 km How often and where do these conditions occur in the U. S. ?
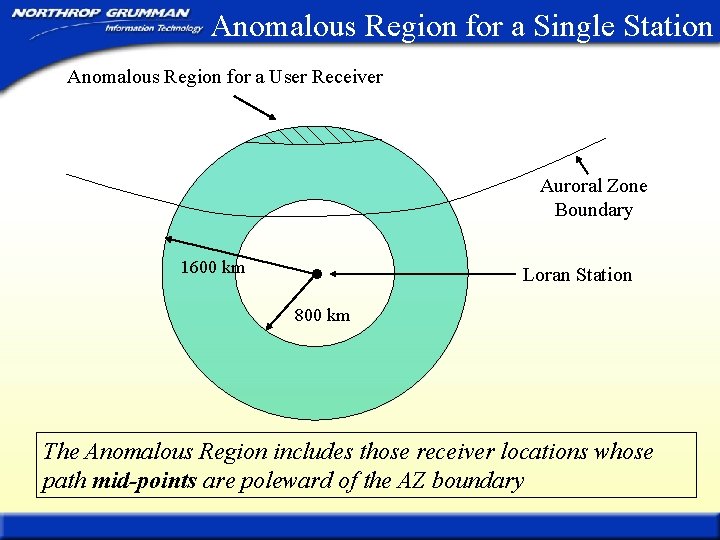
Anomalous Region for a Single Station Anomalous Region for a User Receiver Auroral Zone Boundary 1600 km Loran Station 800 km The Anomalous Region includes those receiver locations whose path mid-points are poleward of the AZ boundary
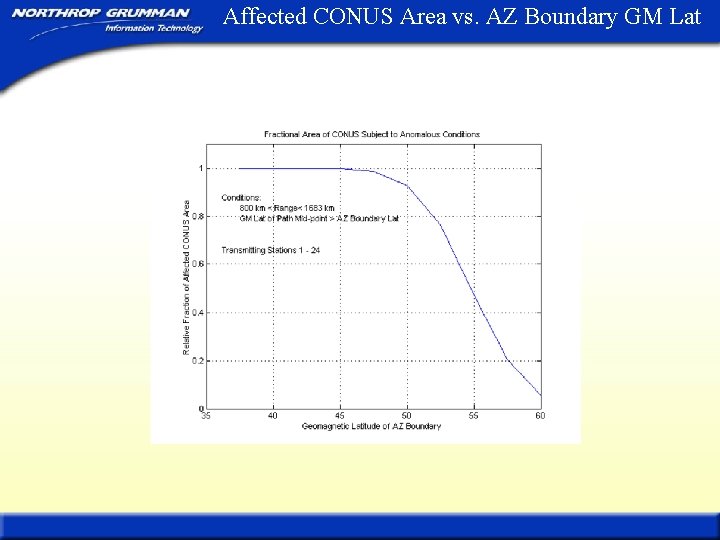
Affected CONUS Area vs. AZ Boundary GM Lat
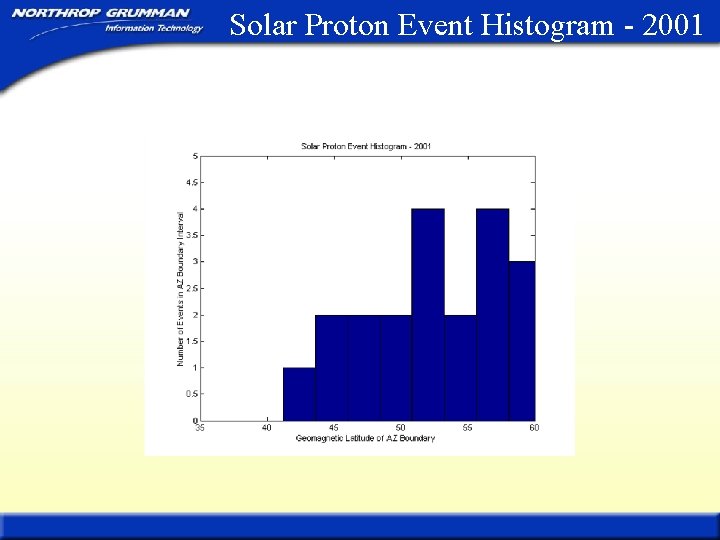
Solar Proton Event Histogram - 2001
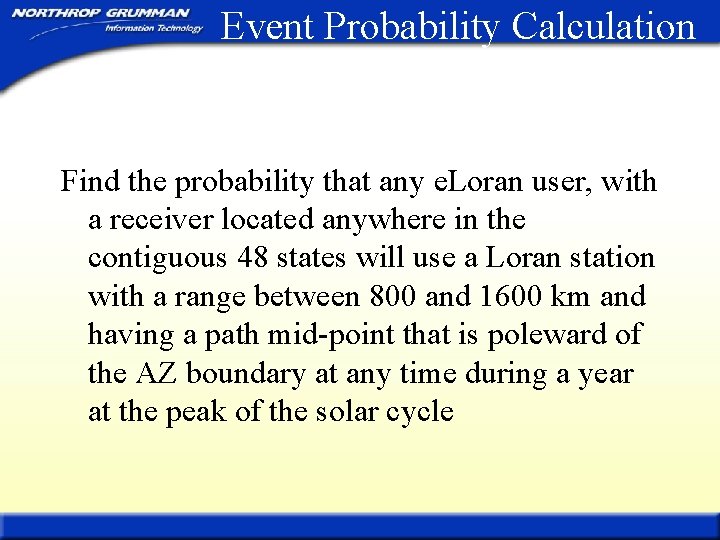
Event Probability Calculation Find the probability that any e. Loran user, with a receiver located anywhere in the contiguous 48 states will use a Loran station with a range between 800 and 1600 km and having a path mid-point that is poleward of the AZ boundary at any time during a year at the peak of the solar cycle
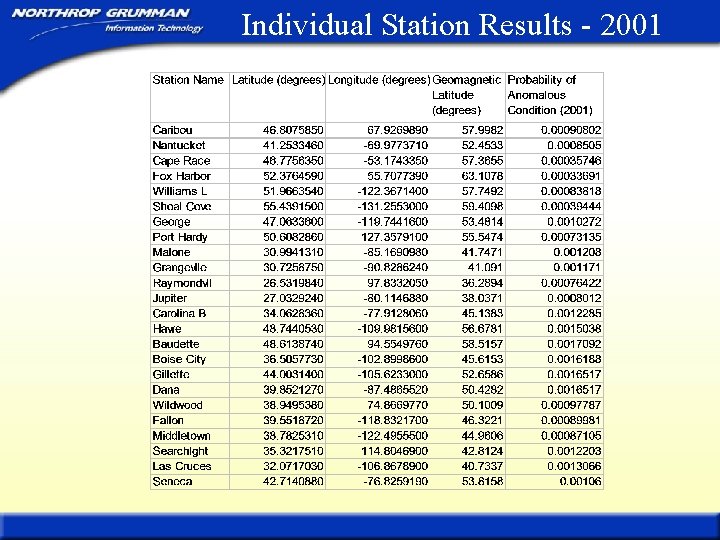
Individual Station Results - 2001
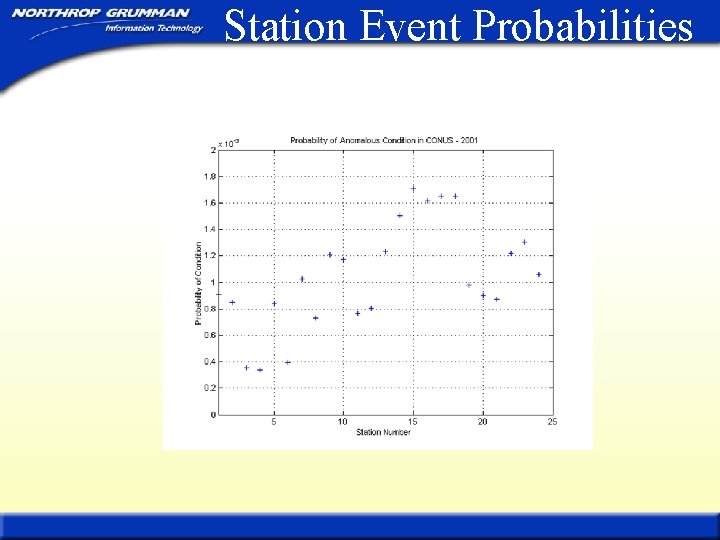
Station Event Probabilities
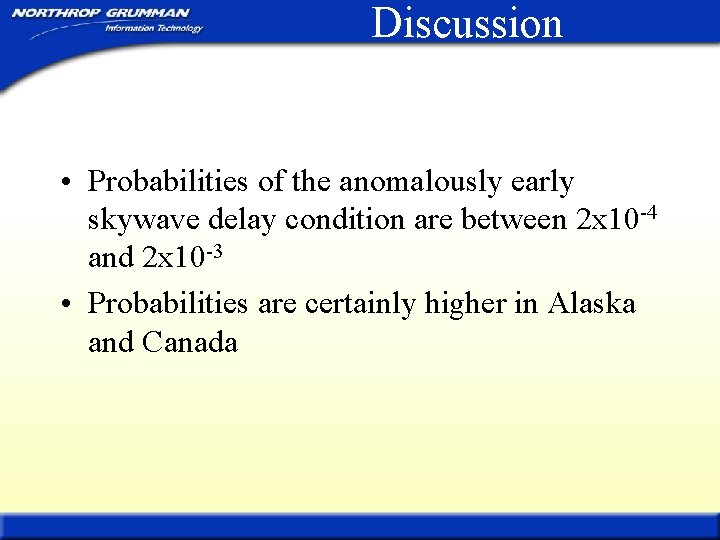
Discussion • Probabilities of the anomalously early skywave delay condition are between 2 x 10 -4 and 2 x 10 -3 • Probabilities are certainly higher in Alaska and Canada
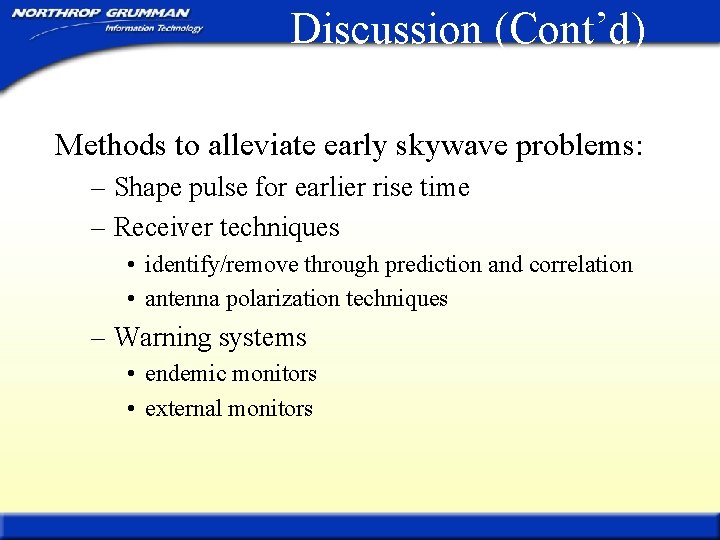
Discussion (Cont’d) Methods to alleviate early skywave problems: – Shape pulse for earlier rise time – Receiver techniques • identify/remove through prediction and correlation • antenna polarization techniques – Warning systems • endemic monitors • external monitors
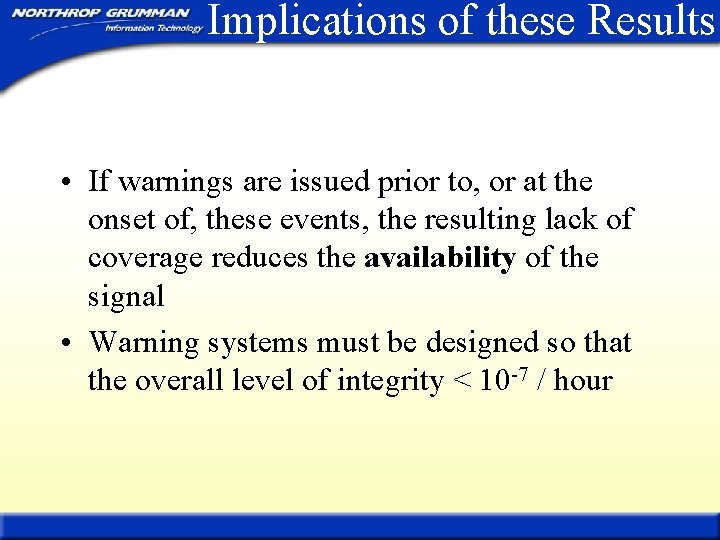
Implications of these Results • If warnings are issued prior to, or at the onset of, these events, the resulting lack of coverage reduces the availability of the signal • Warning systems must be designed so that the overall level of integrity < 10 -7 / hour
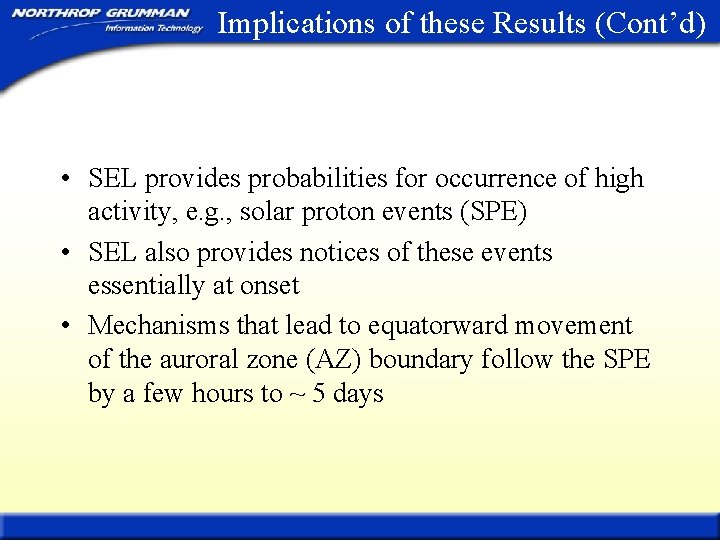
Implications of these Results (Cont’d) • SEL provides probabilities for occurrence of high activity, e. g. , solar proton events (SPE) • SEL also provides notices of these events essentially at onset • Mechanisms that lead to equatorward movement of the auroral zone (AZ) boundary follow the SPE by a few hours to ~ 5 days
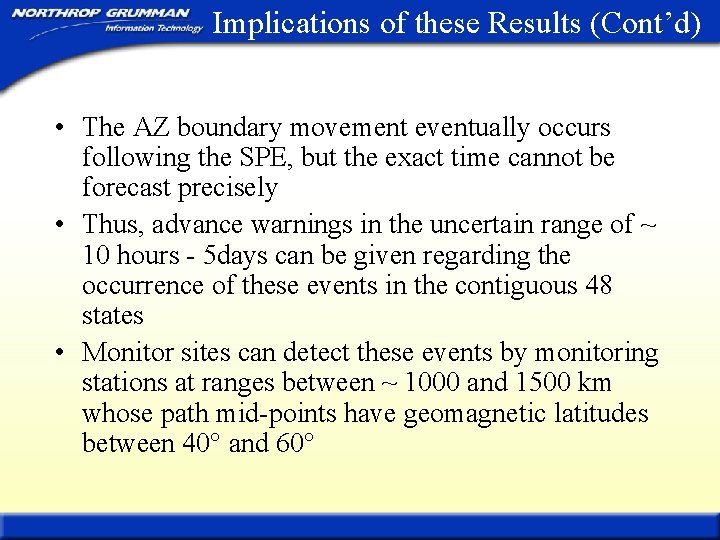
Implications of these Results (Cont’d) • The AZ boundary movement eventually occurs following the SPE, but the exact time cannot be forecast precisely • Thus, advance warnings in the uncertain range of ~ 10 hours - 5 days can be given regarding the occurrence of these events in the contiguous 48 states • Monitor sites can detect these events by monitoring stations at ranges between ~ 1000 and 1500 km whose path mid-points have geomagnetic latitudes between 40° and 60°
Skywave.zenith
Objectives of roving frame
Signs signals and roadway markings
What is the product of an even signal and odd signal?
Baseband signal and bandpass signal
Baseband signal and bandpass signal
Digital signal as a composite analog signal
Early cpr and early defibrillation can: *
Hamlet act 3 events leading to climax
End behavior how to find
Define delacroix
Sagar recruitment
Leading intercessions
Factors leading to confederation
The perils and pitfalls of leading change case solution
Leading edge detection
Technique meaning art
Leading coefficient test
Troop leading procedures
Liberty leading the people, 1830
Leading cause of preventable blindness
Dispersive model
Leading greatly
Leading nation
Leading up to the war of 1812
Paduan beberapa nada yang terdengar merdu disebut
A leading light
Leading through change presentation
Answer key
Planning organizing leading and controlling
Leading better value care
Leading coefficient quadratic equation
Provider gaps
Liberty leading the people, 1830
Leading and working in teams
Planks of leadership
Leading change why transformation efforts fail
Starbucks planning organizing leading and controlling
I can recognize polynomial function yes or no
Leading a team