CHAPTER5 INTRODUCTION TO DEEP FOUNDATIONS 1 INTRODUCTION Deep
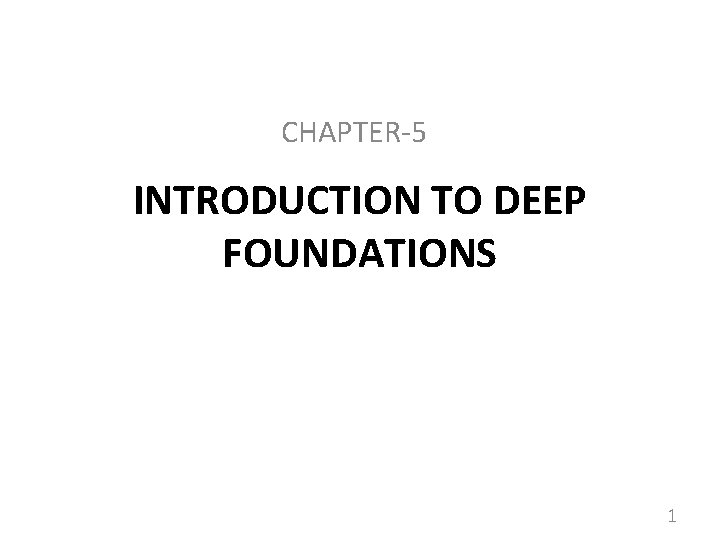
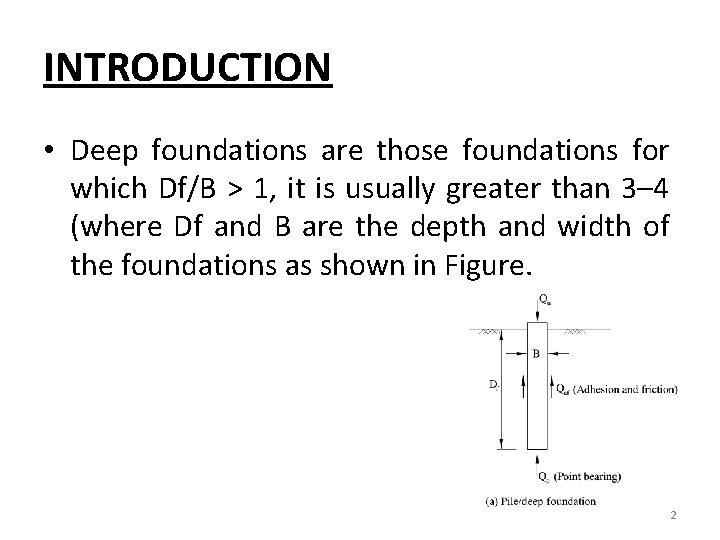
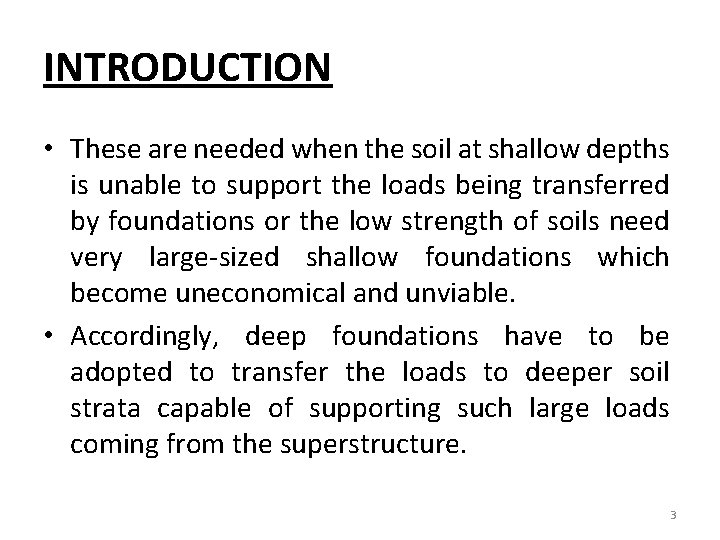
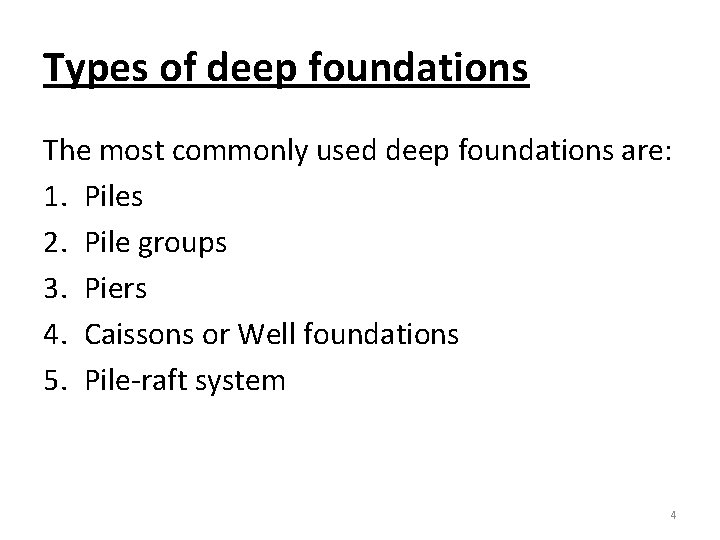
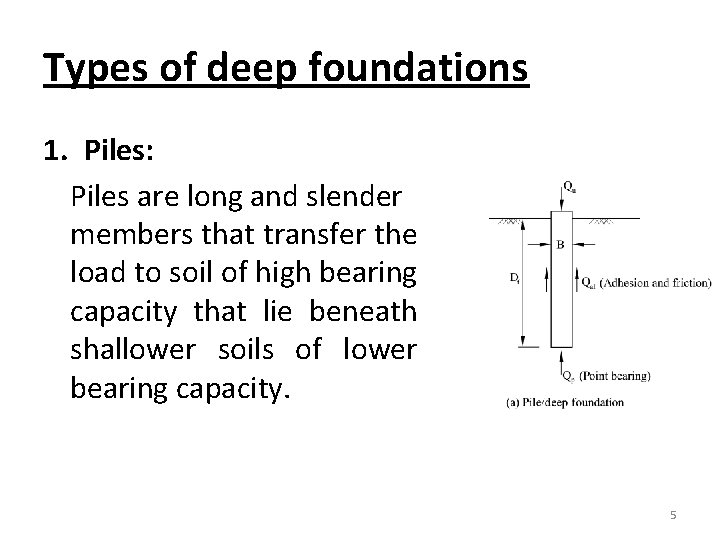
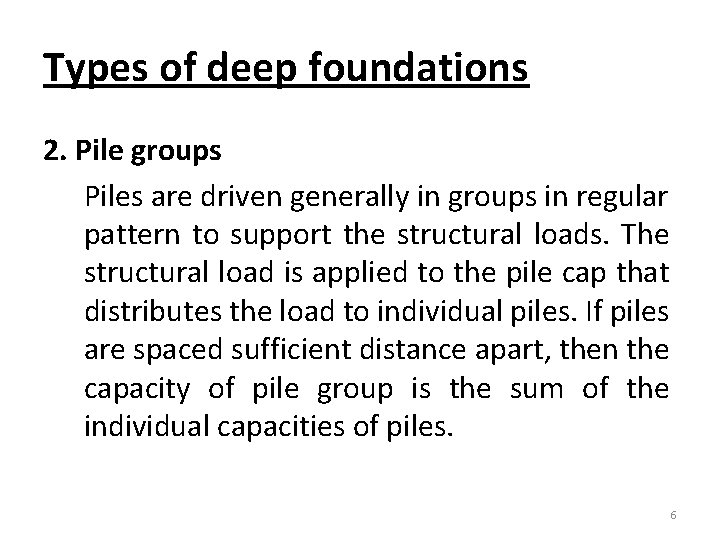
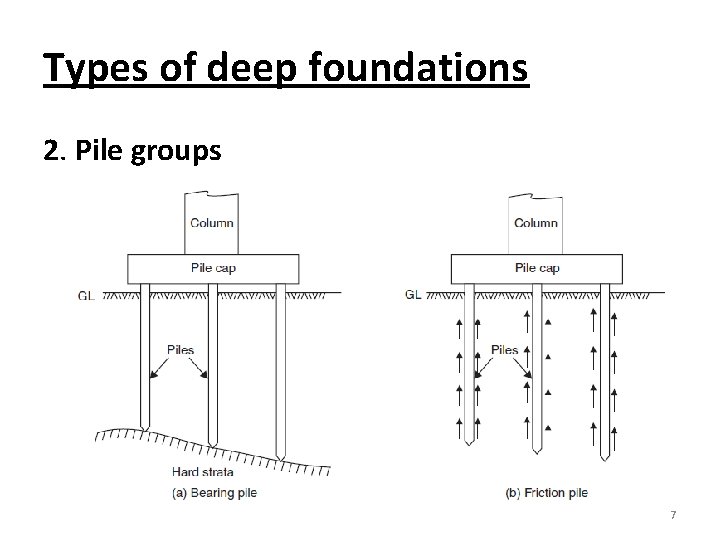
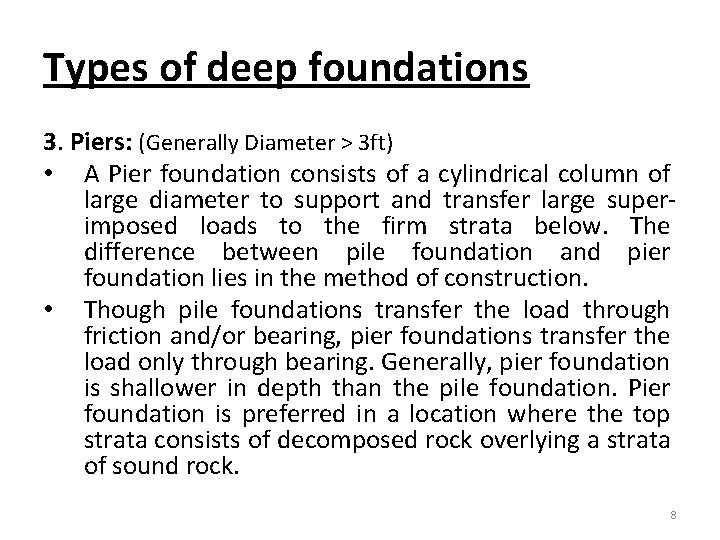
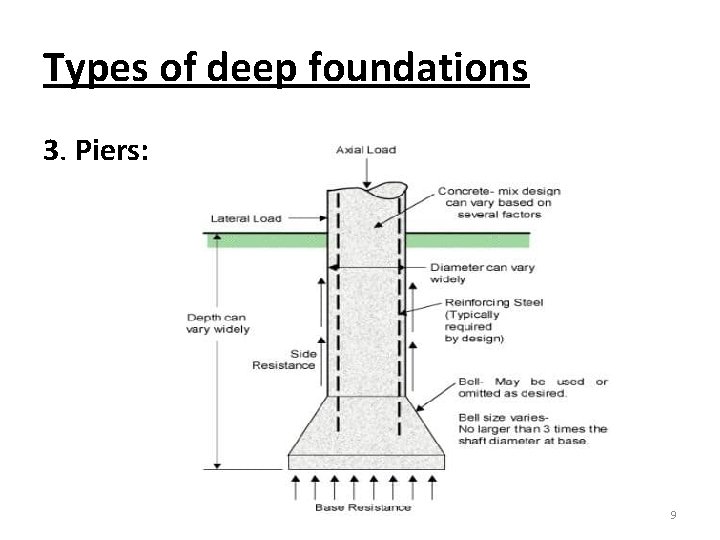
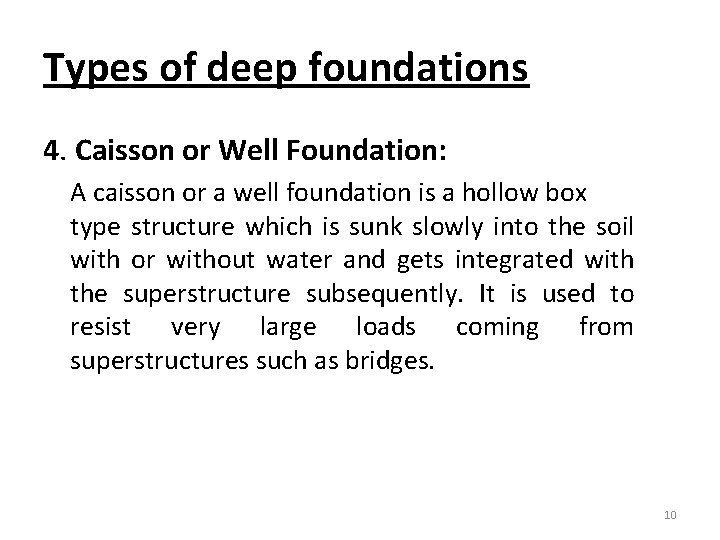
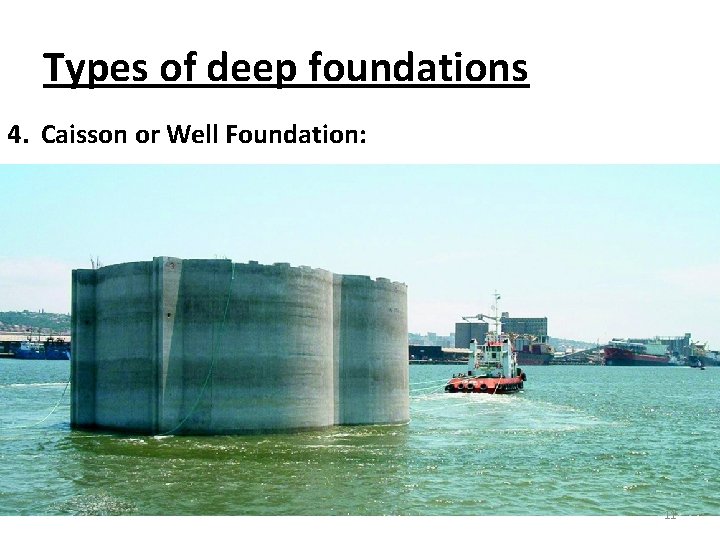
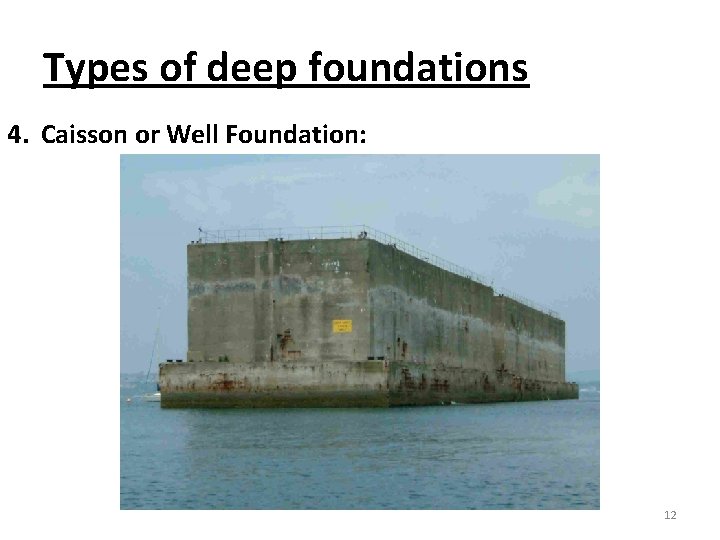
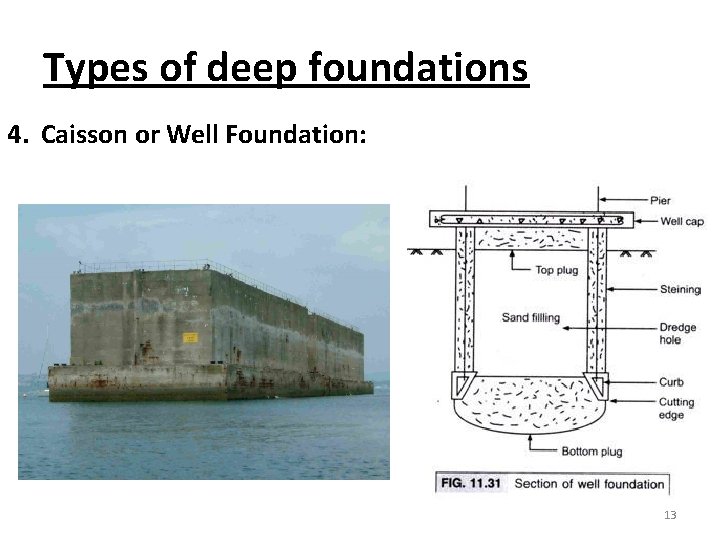
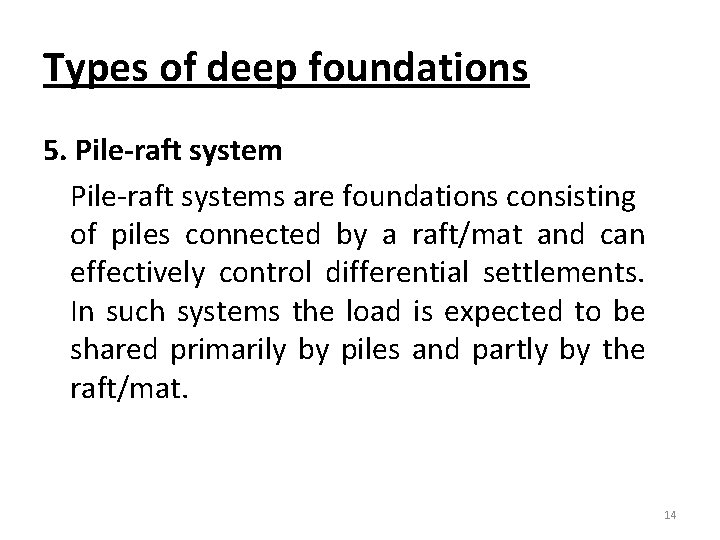
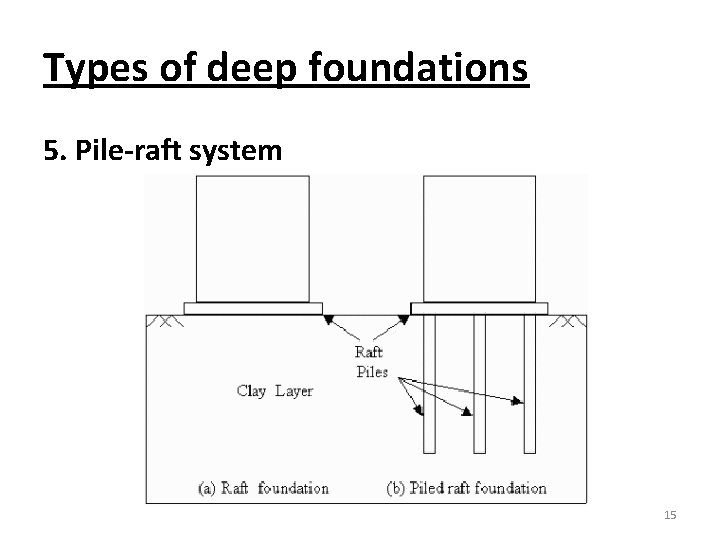
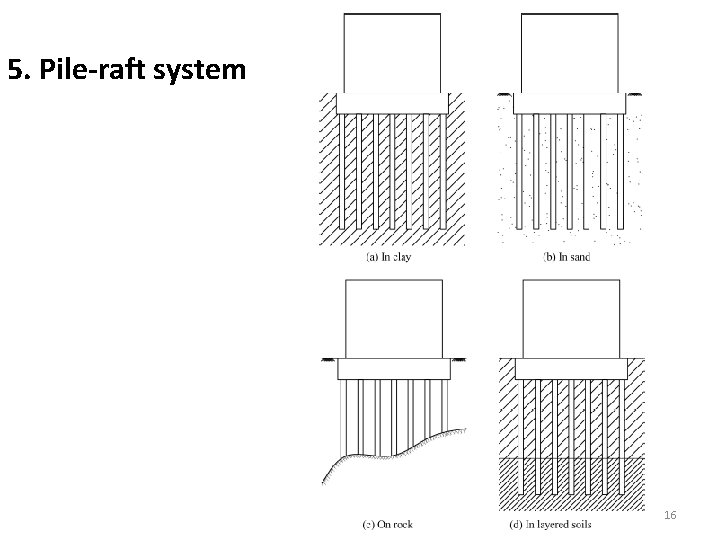
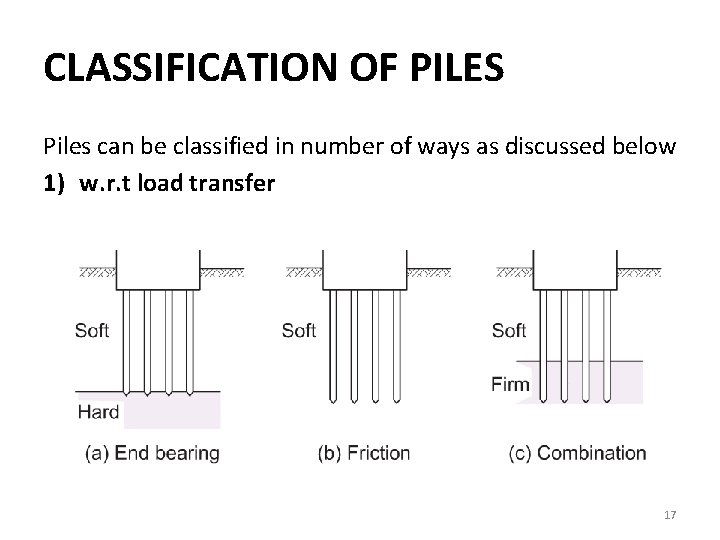
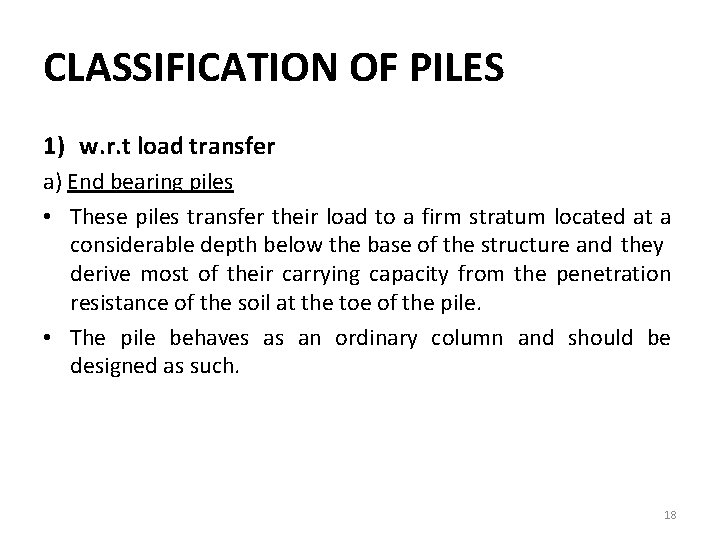
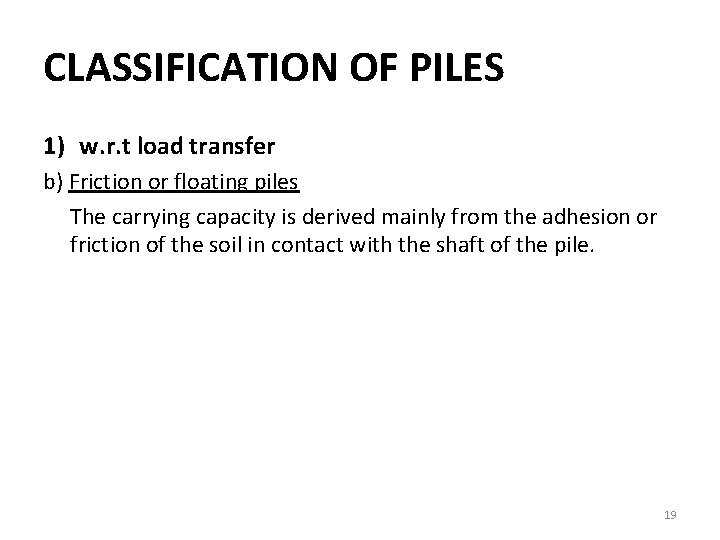
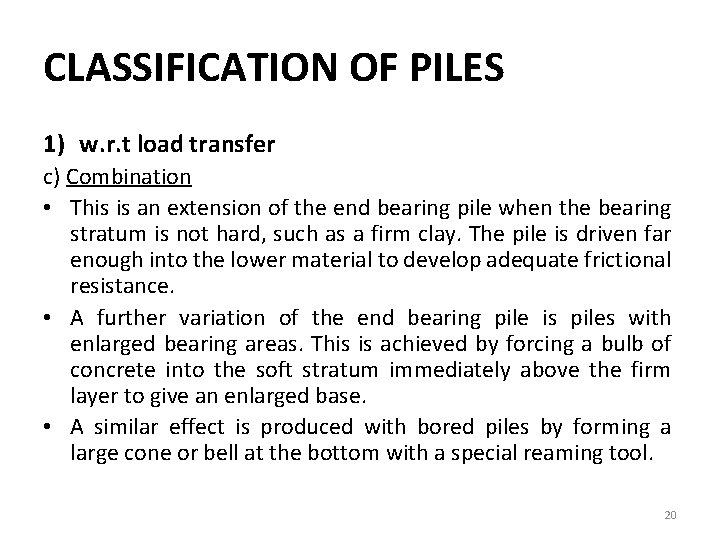
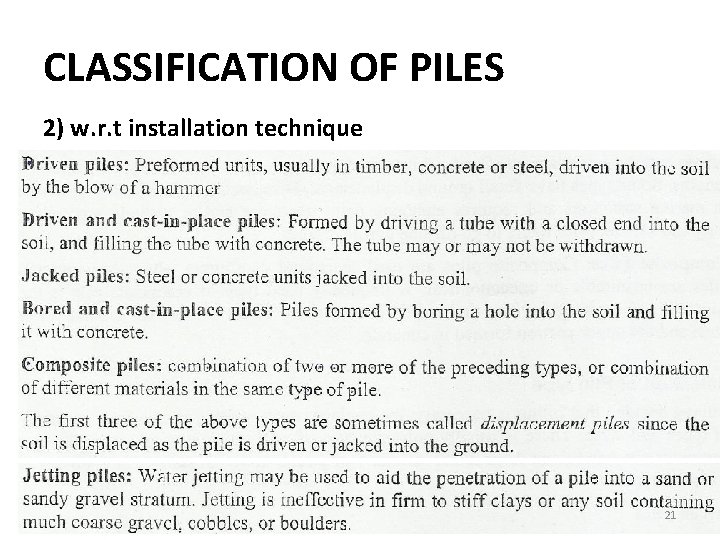
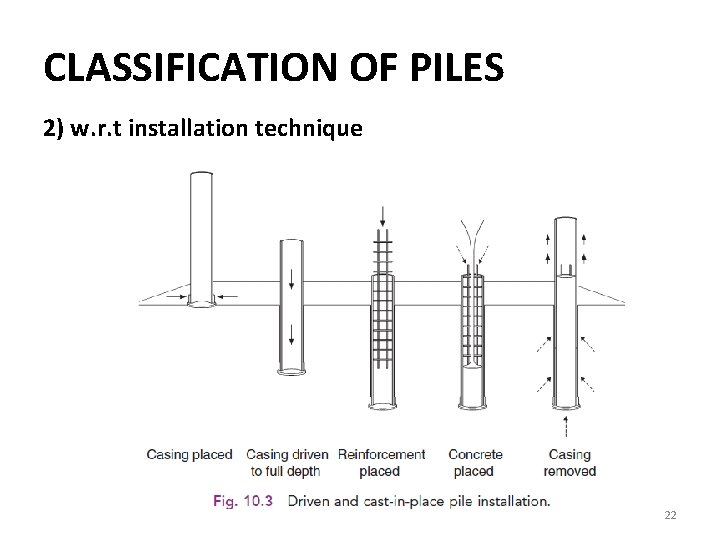
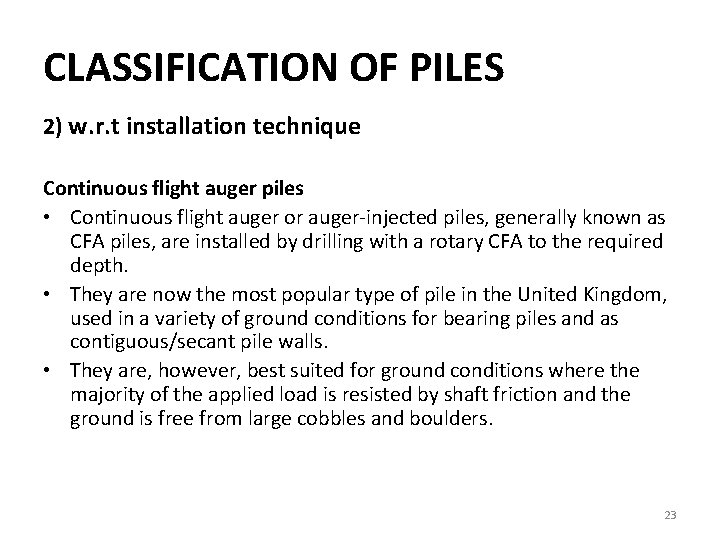
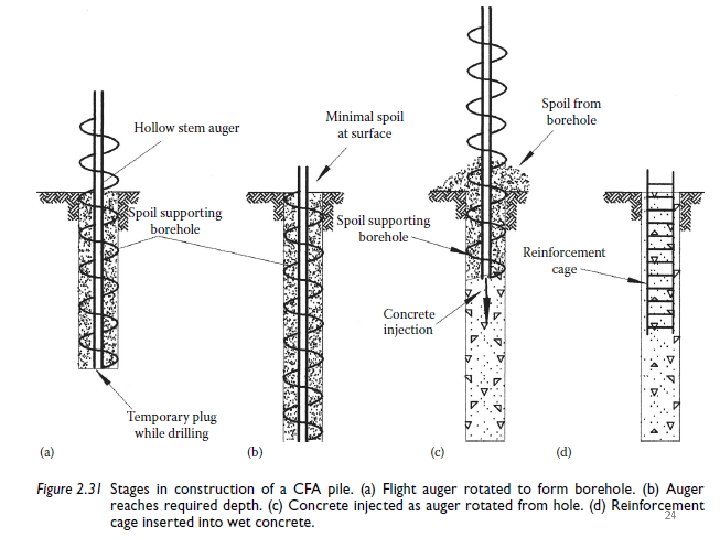
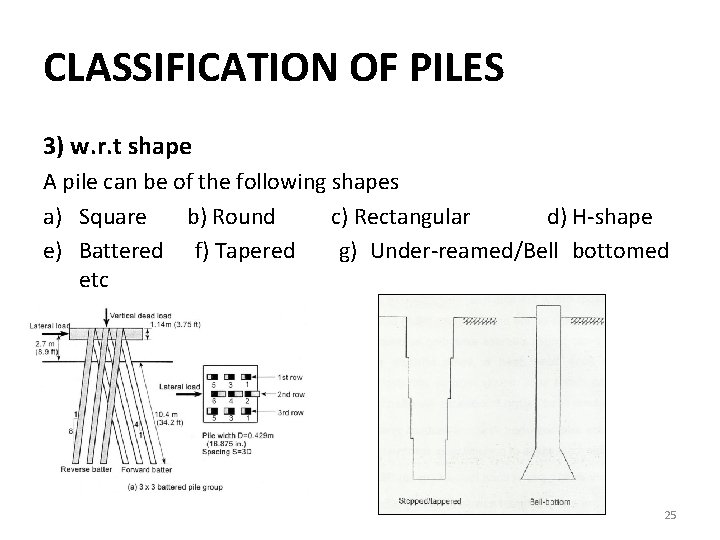
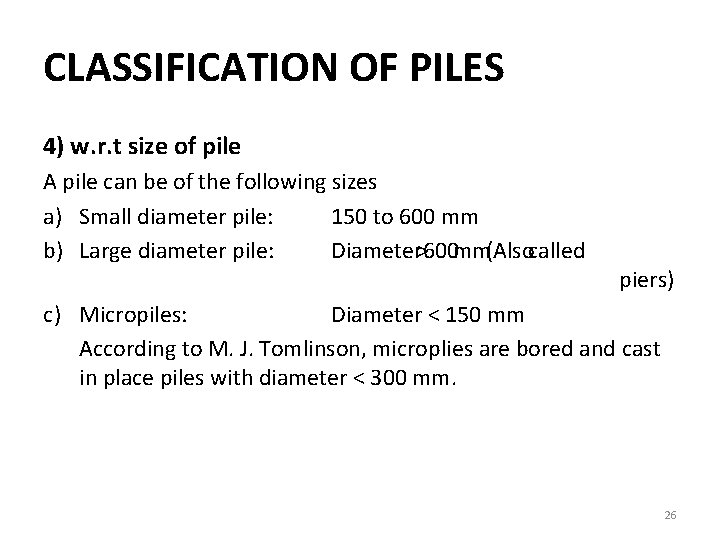
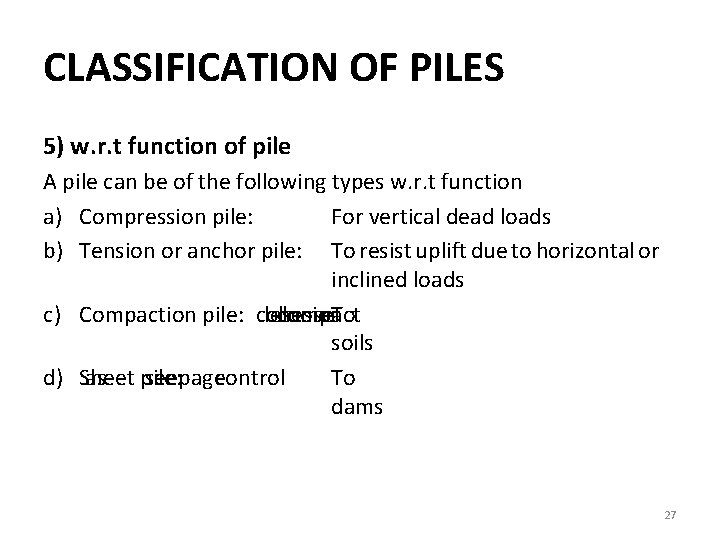
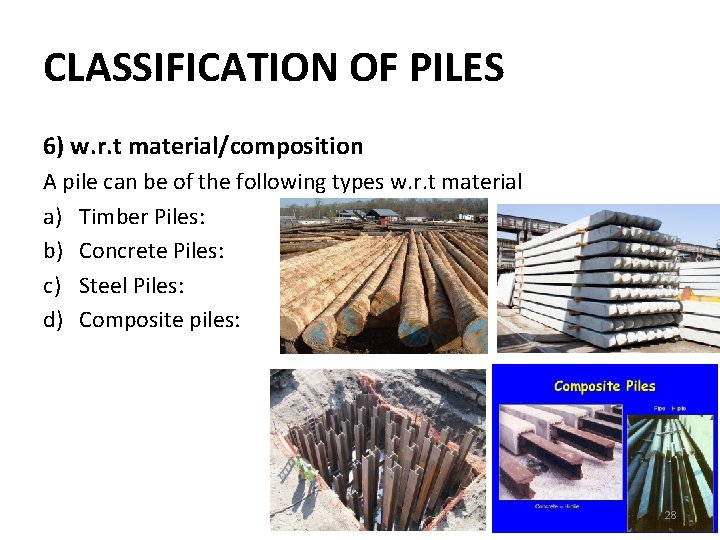
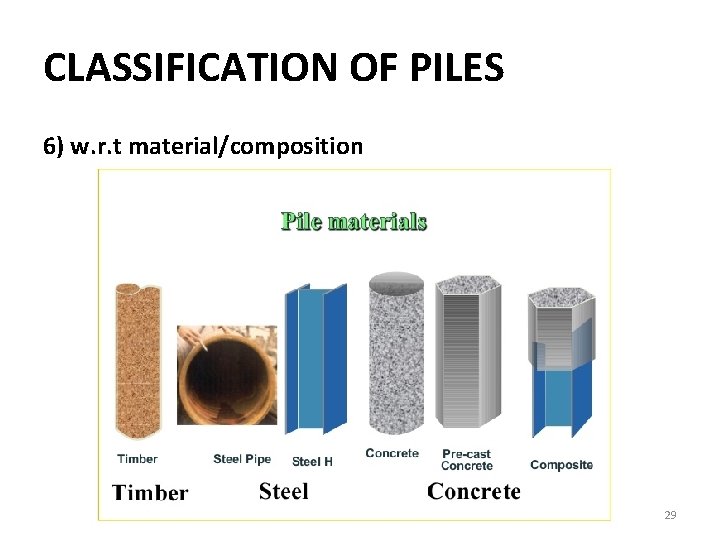
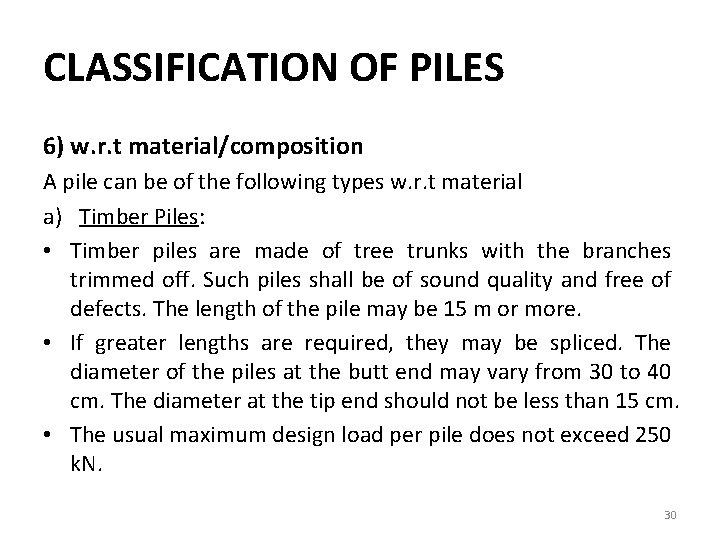
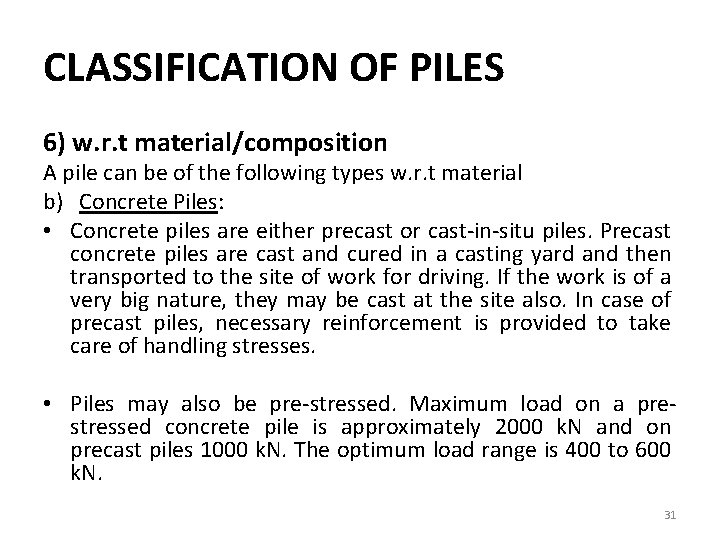
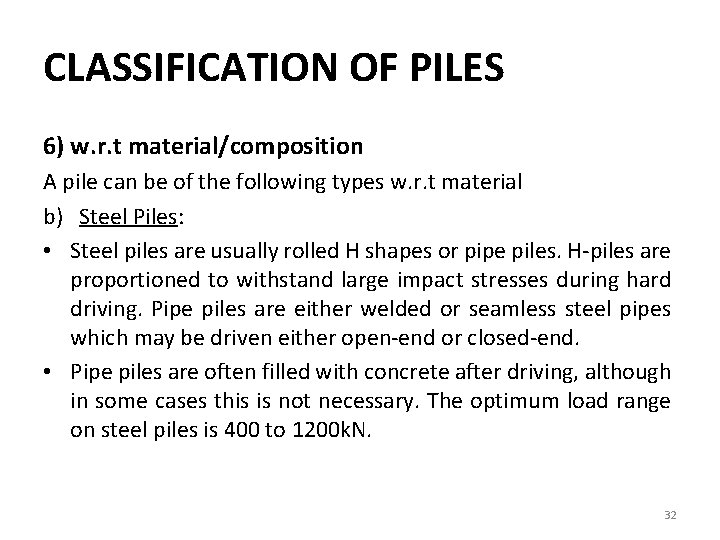
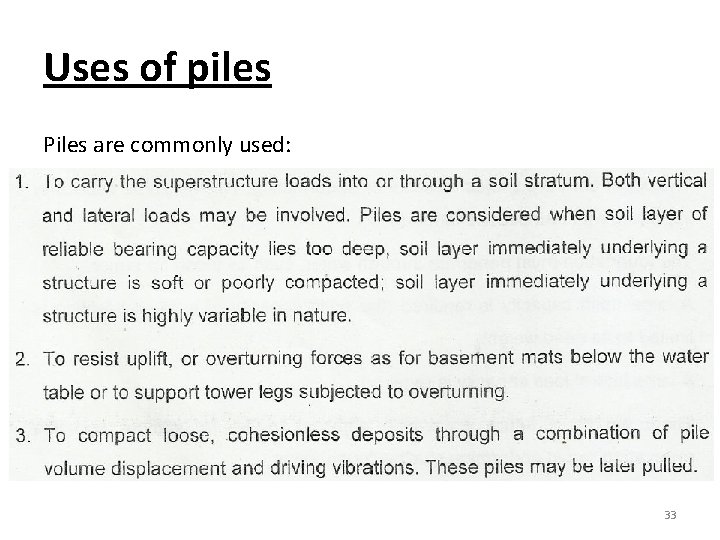
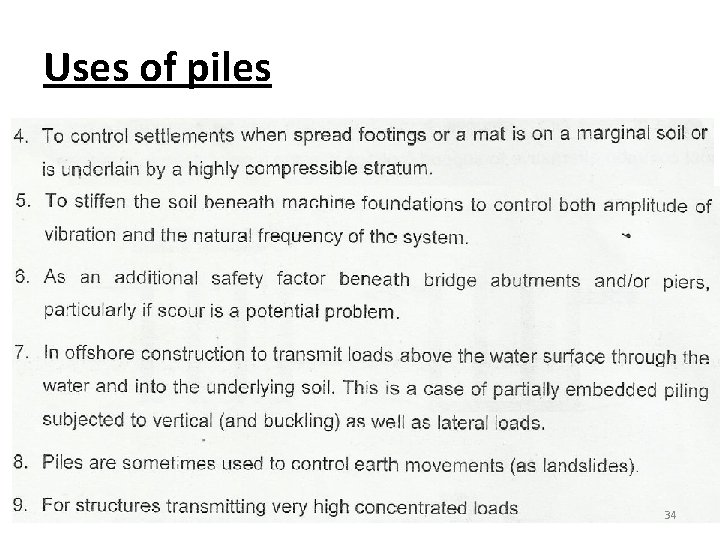
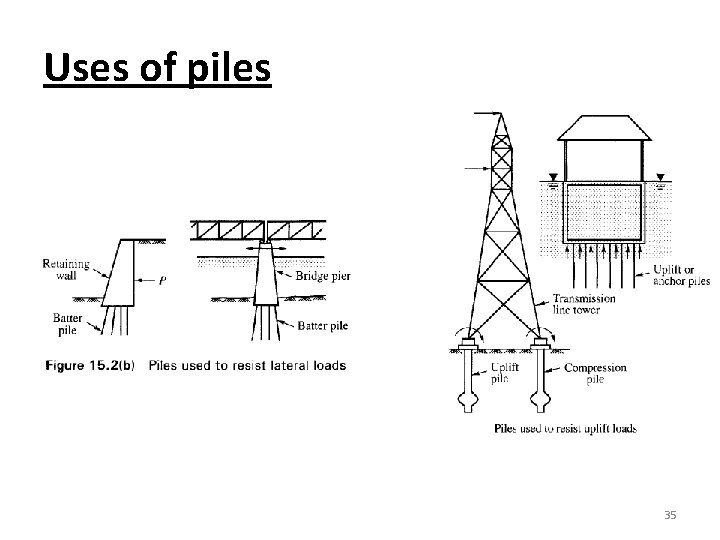
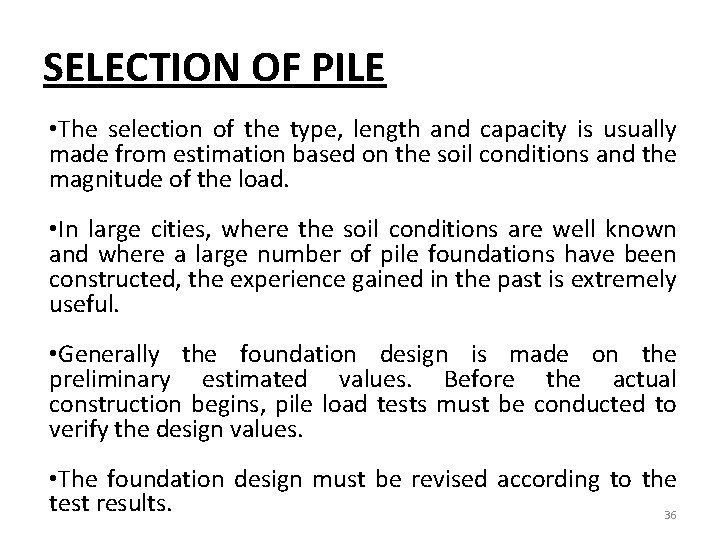
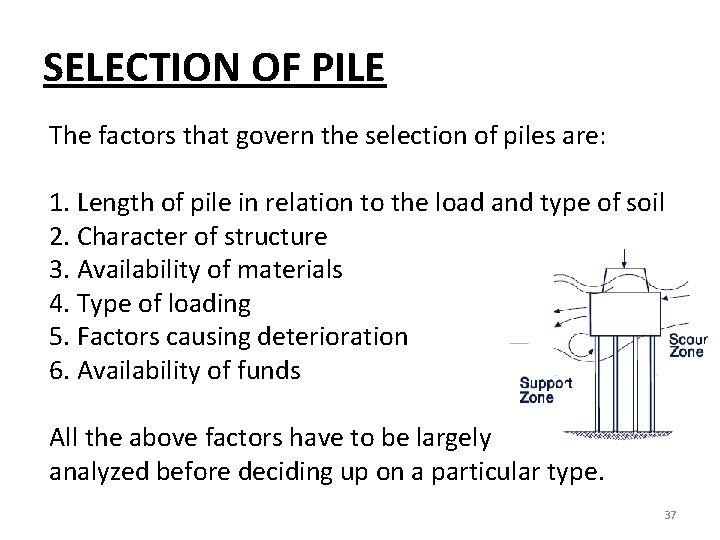
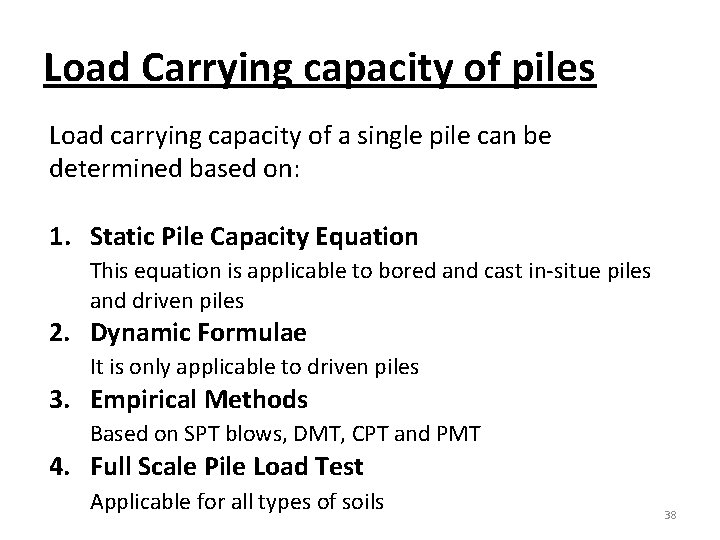
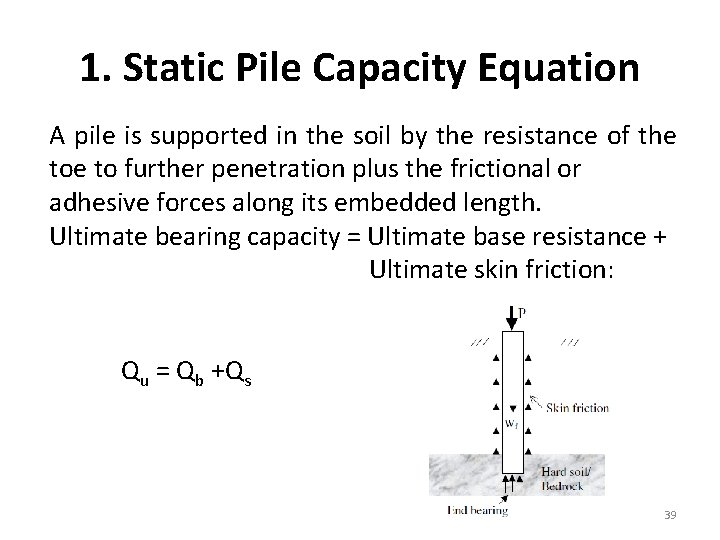
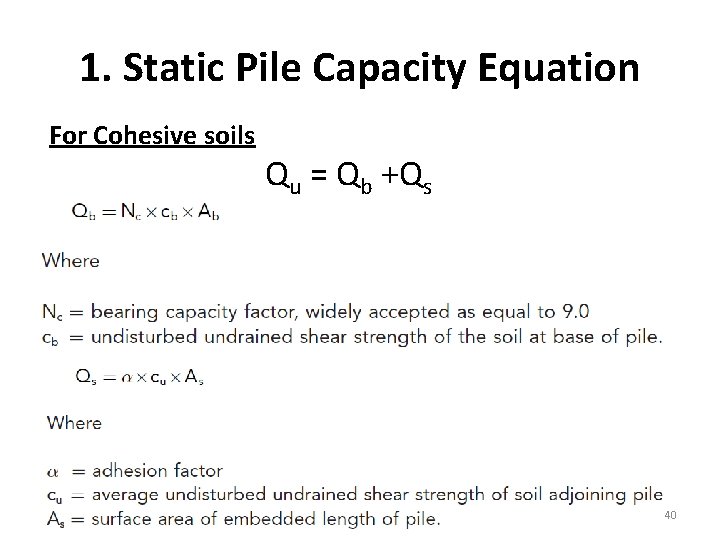
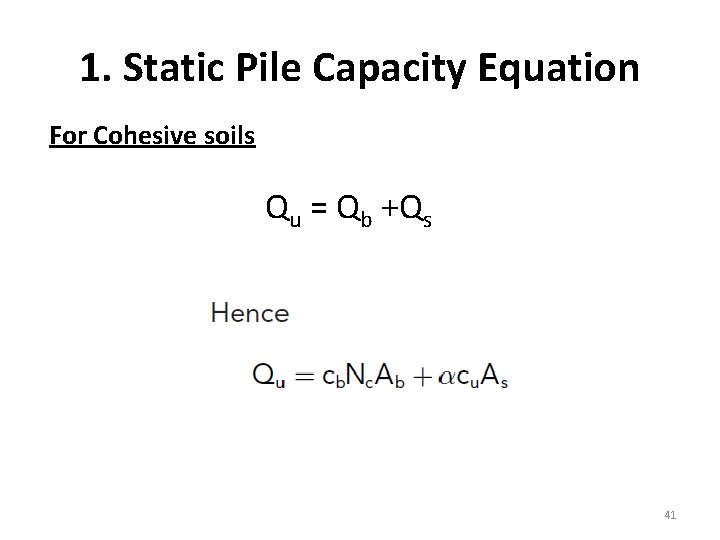
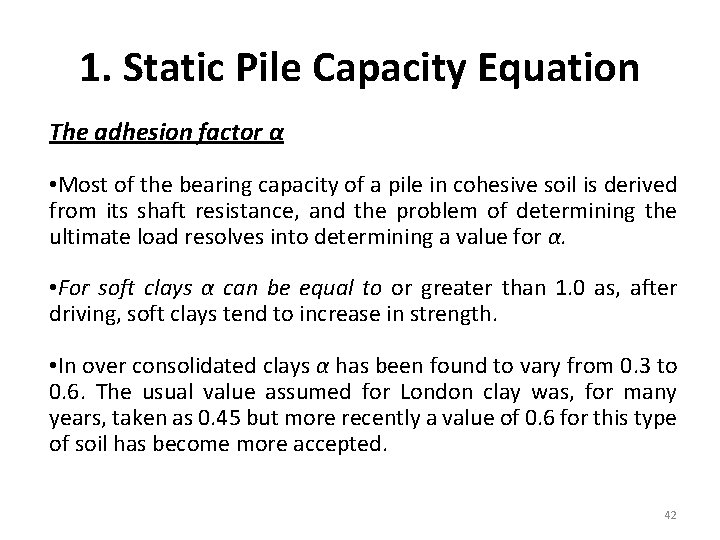
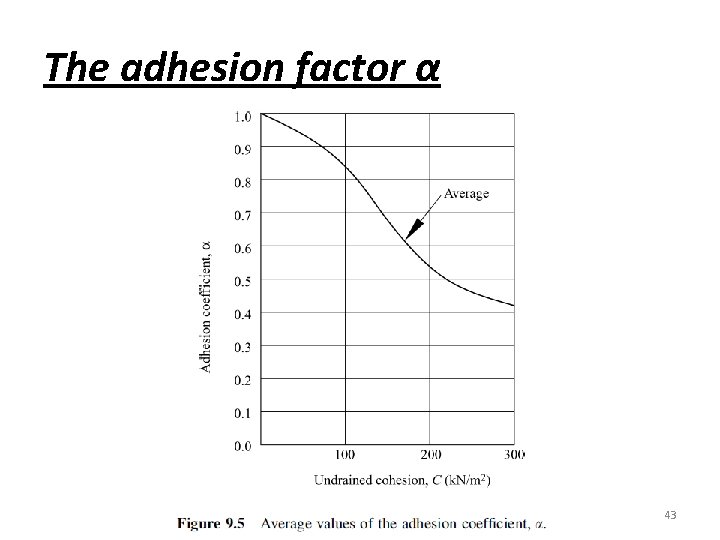
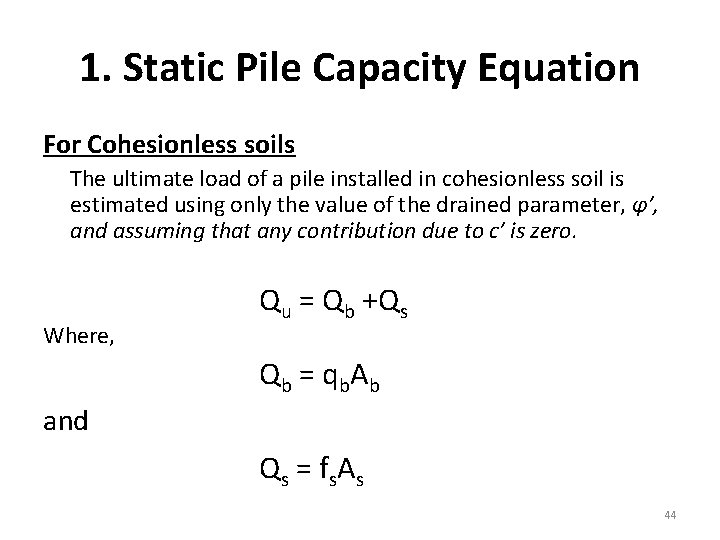
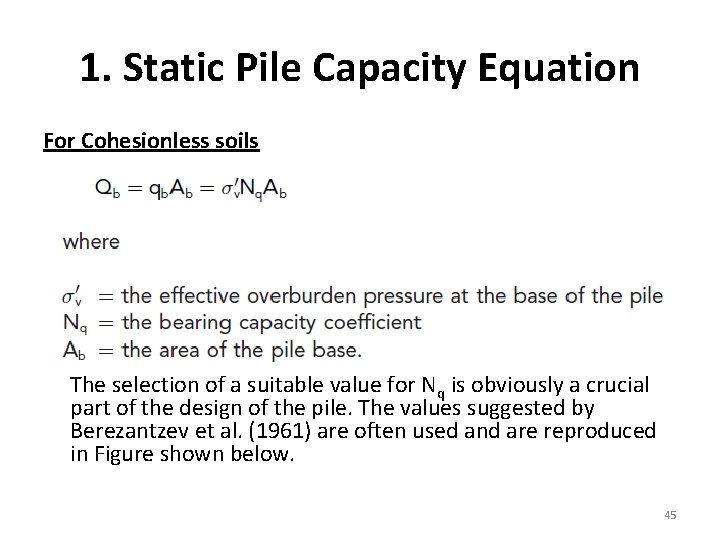
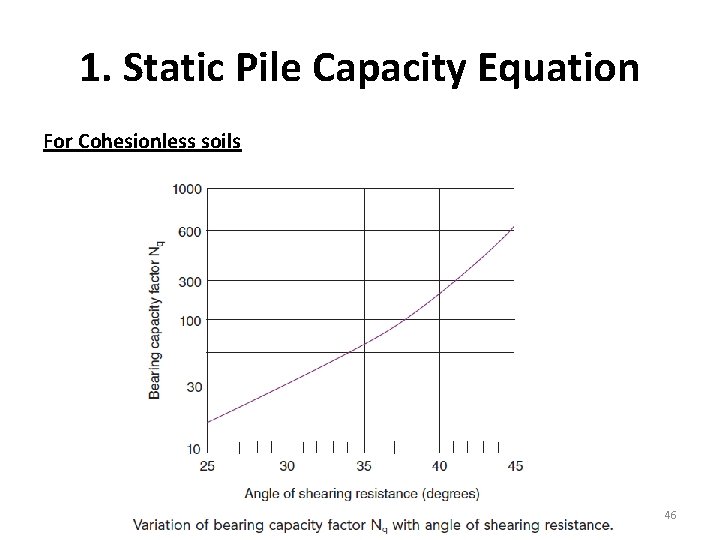
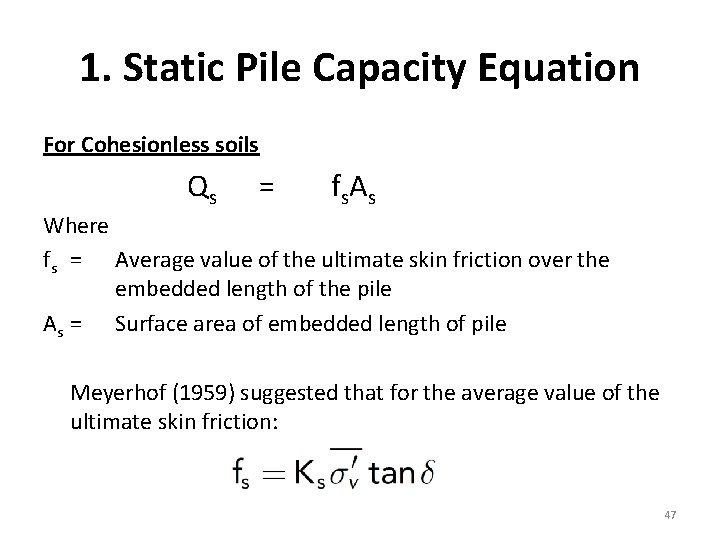
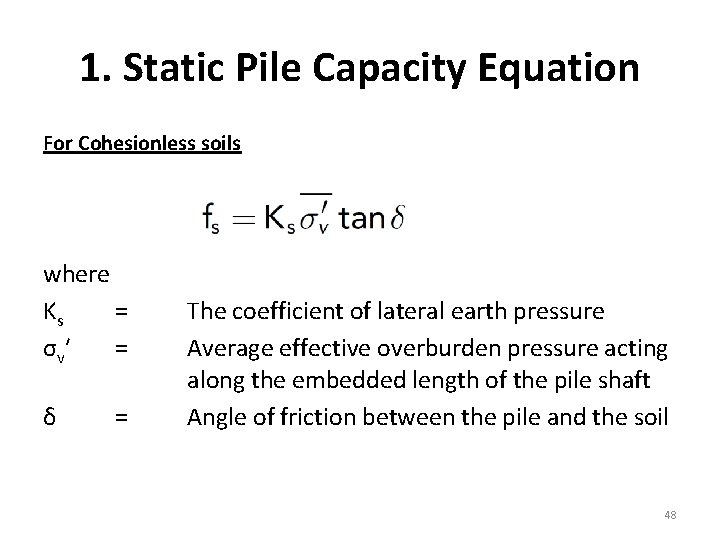
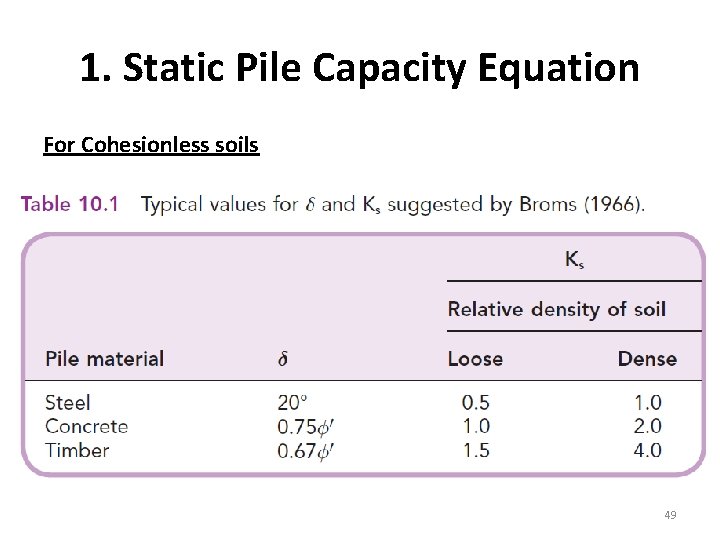
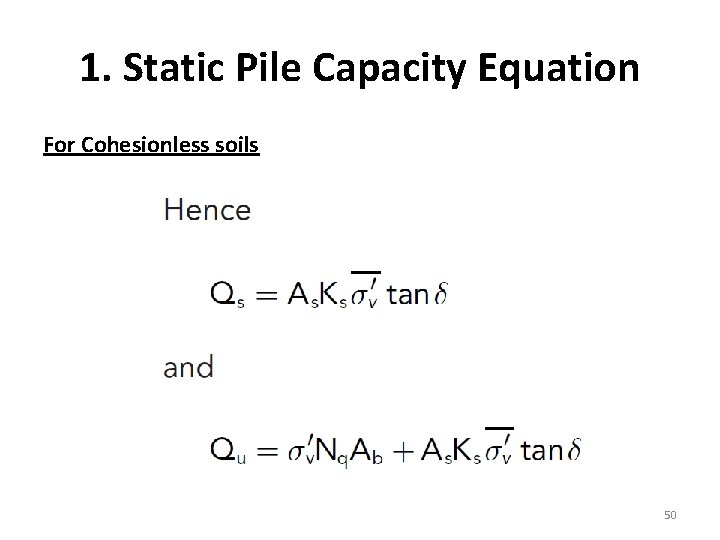
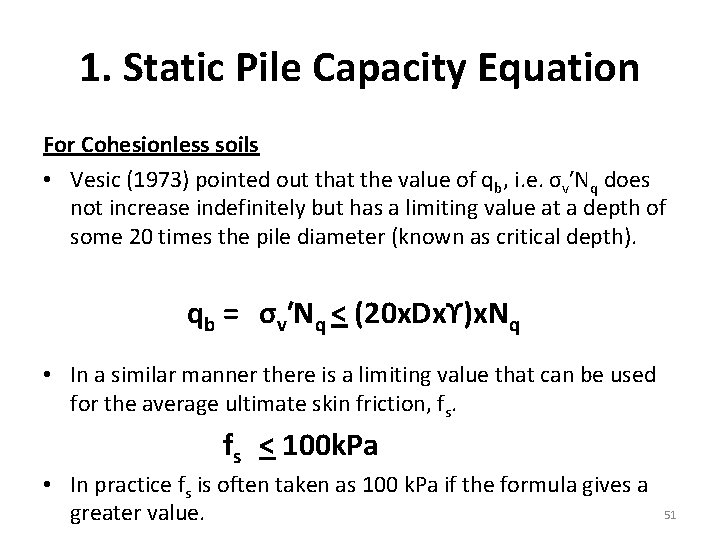
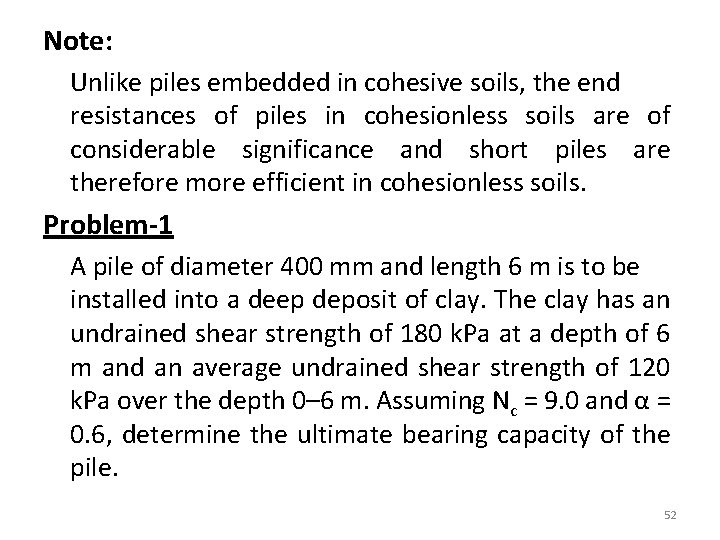
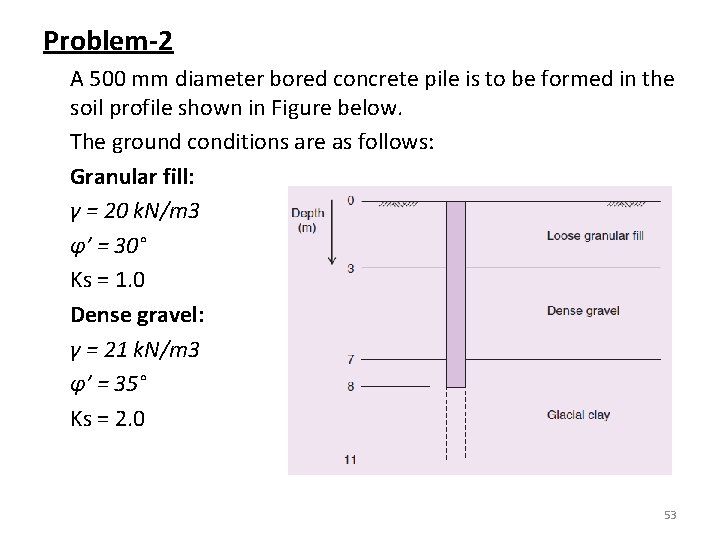
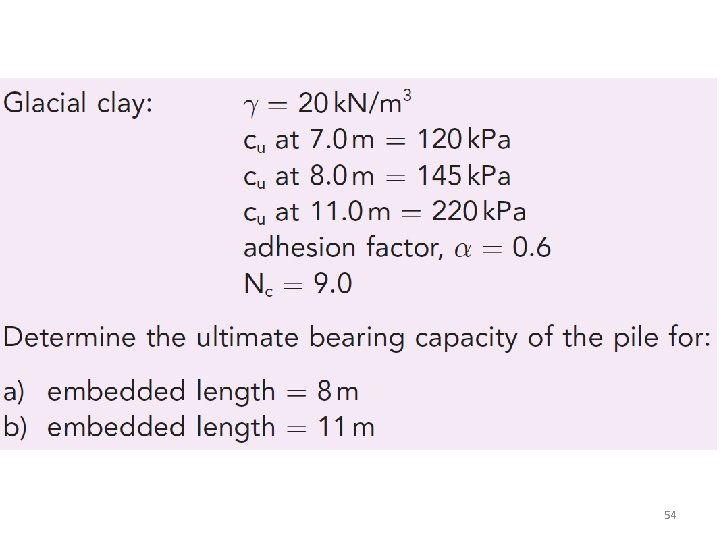
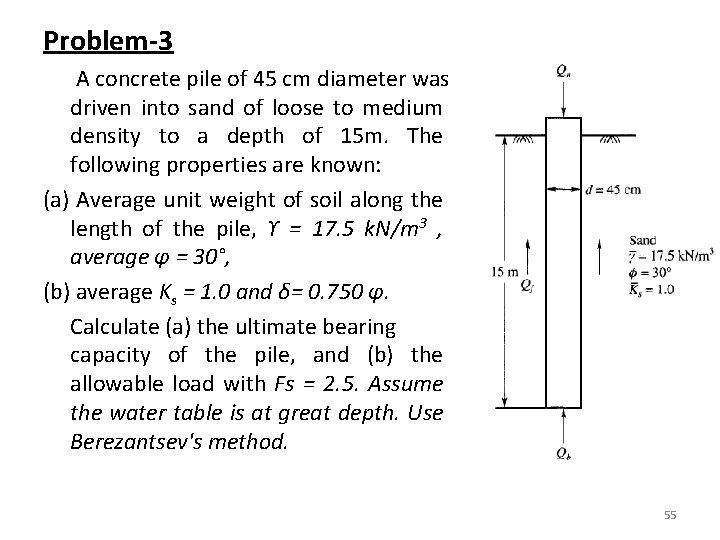
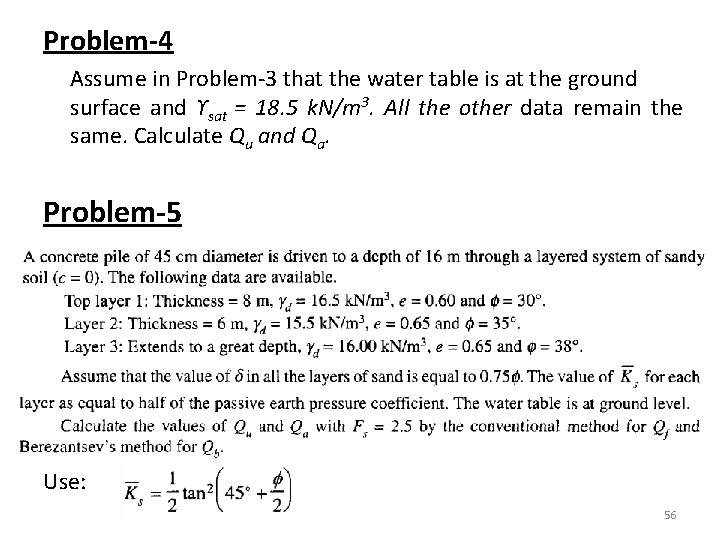
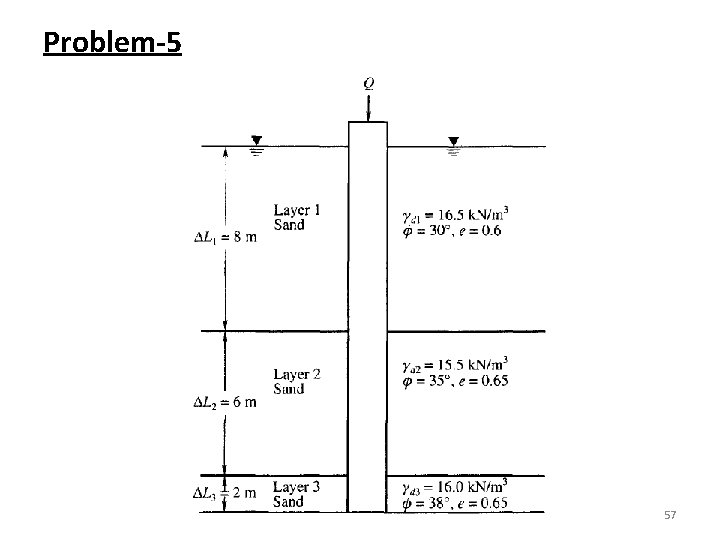
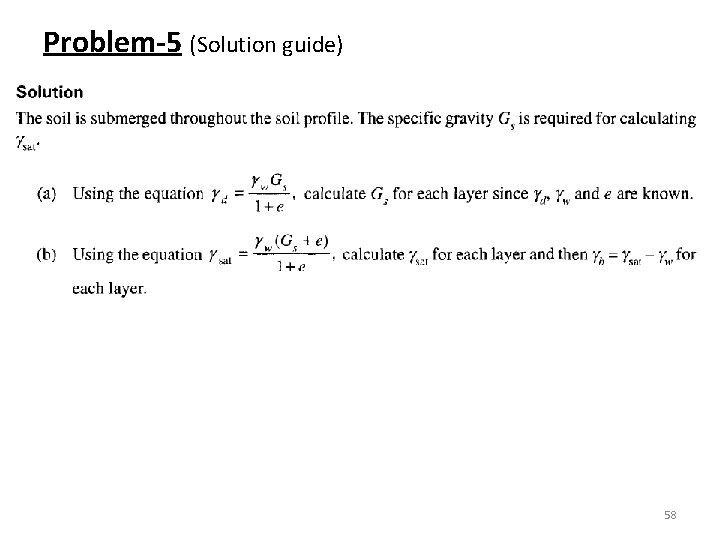
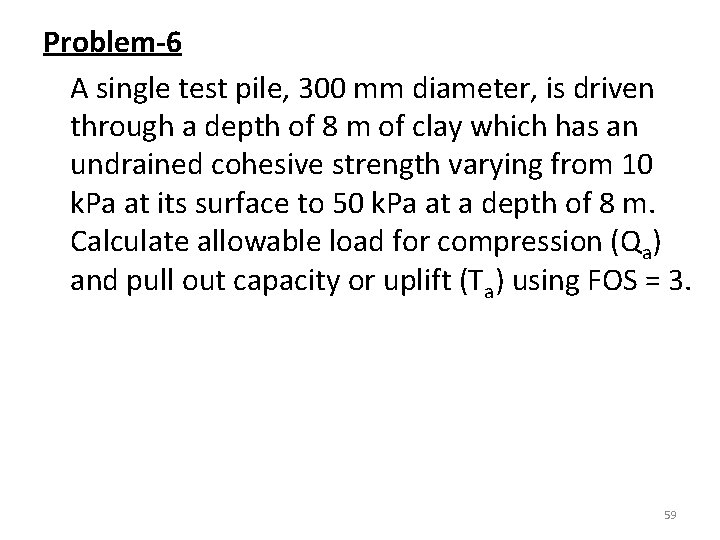
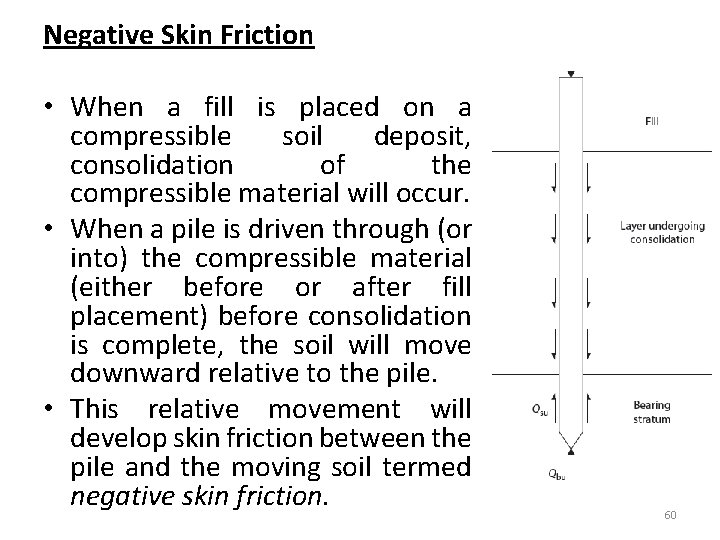
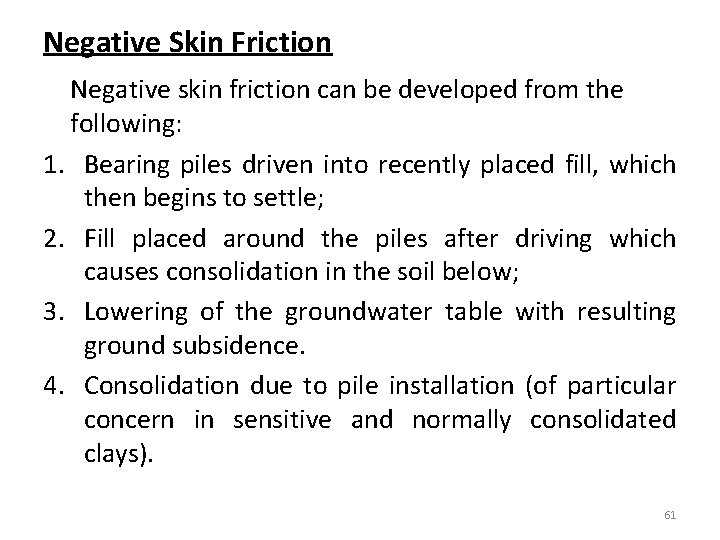
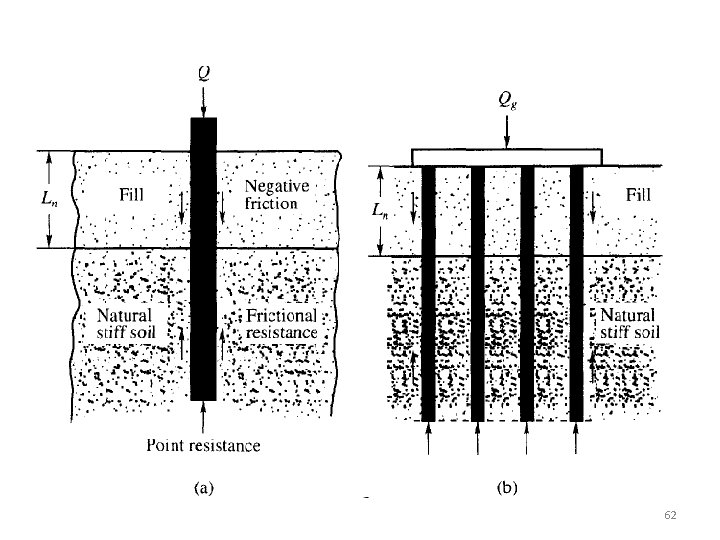
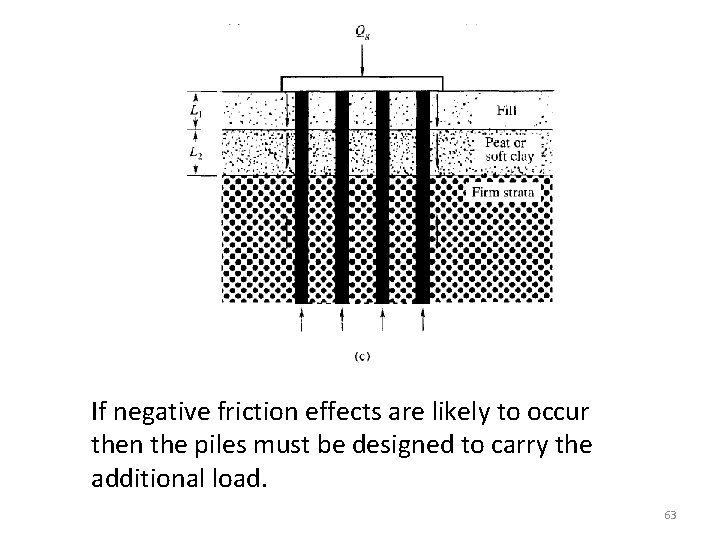
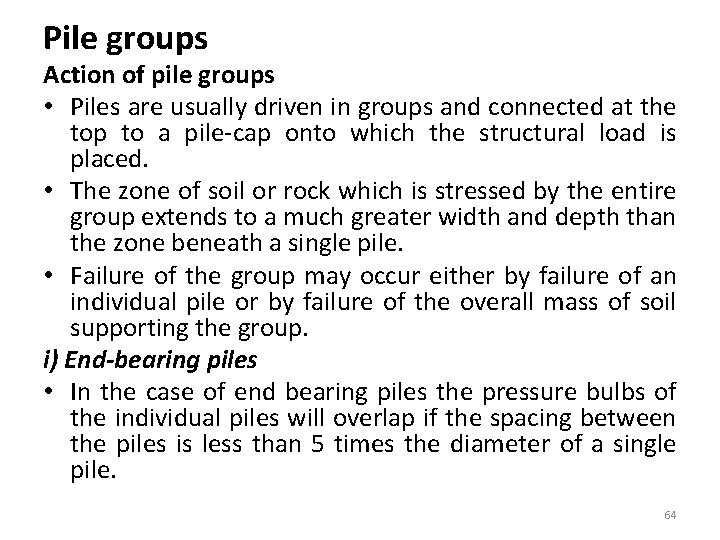
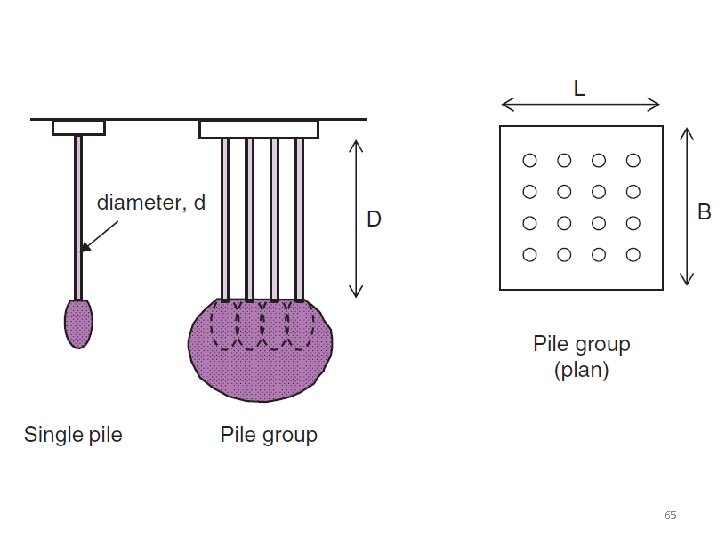
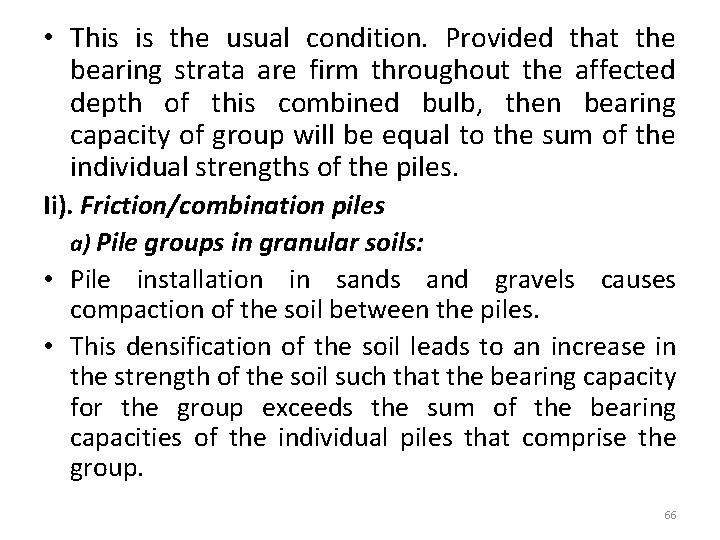
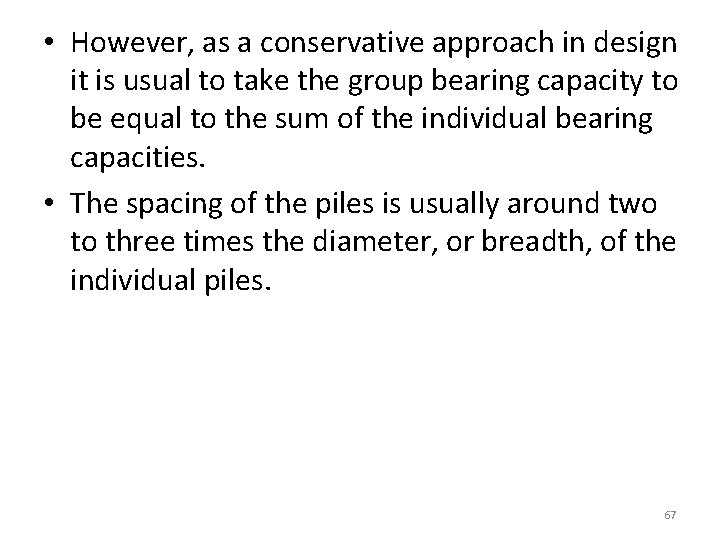
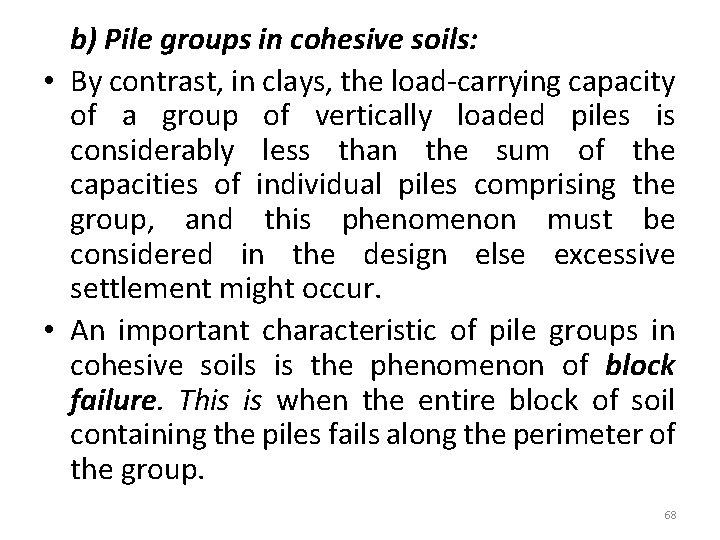
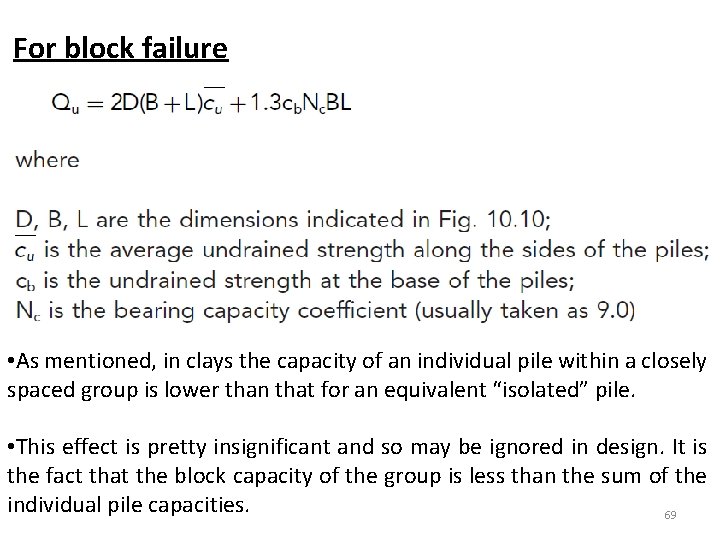
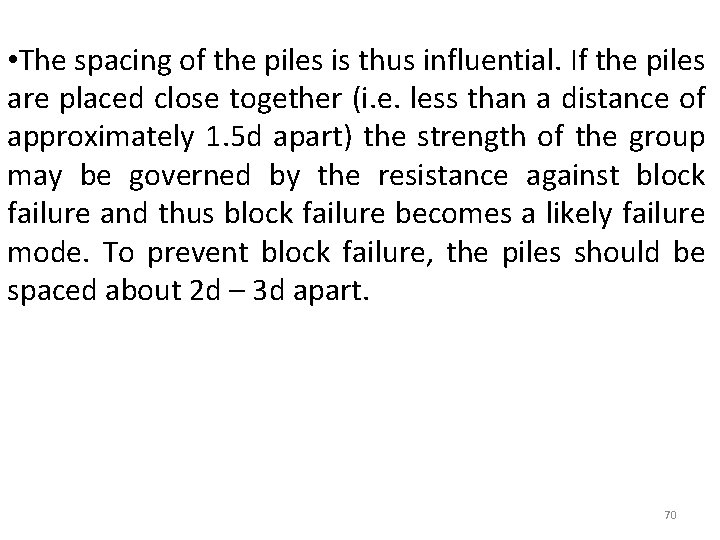
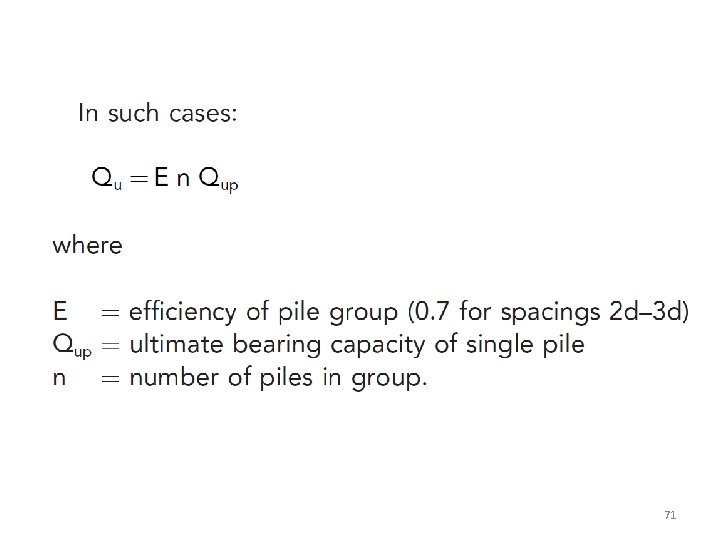
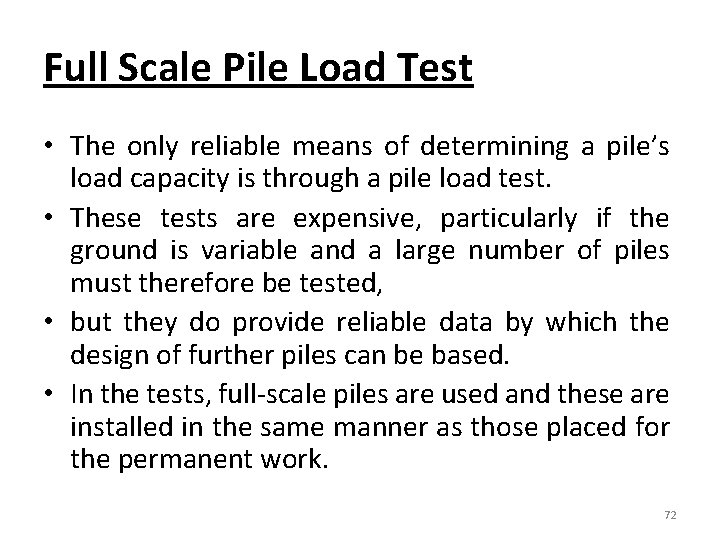
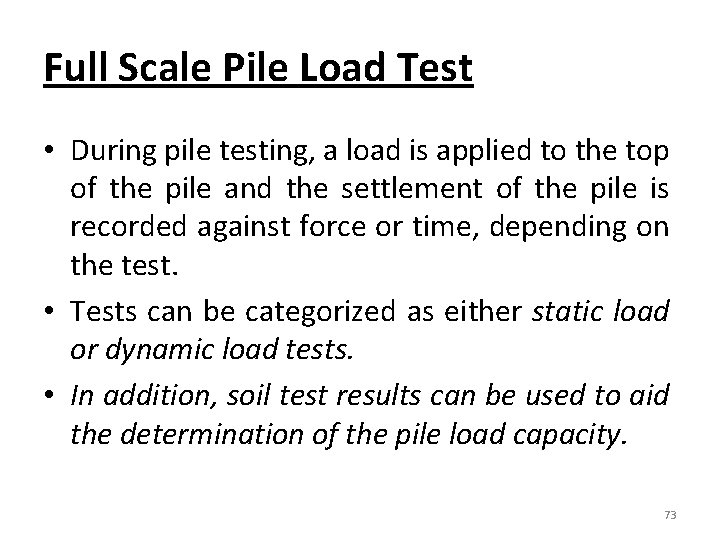
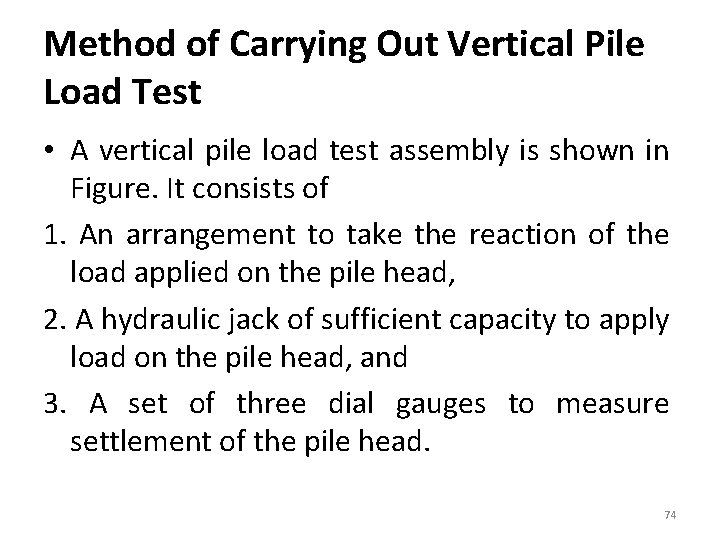
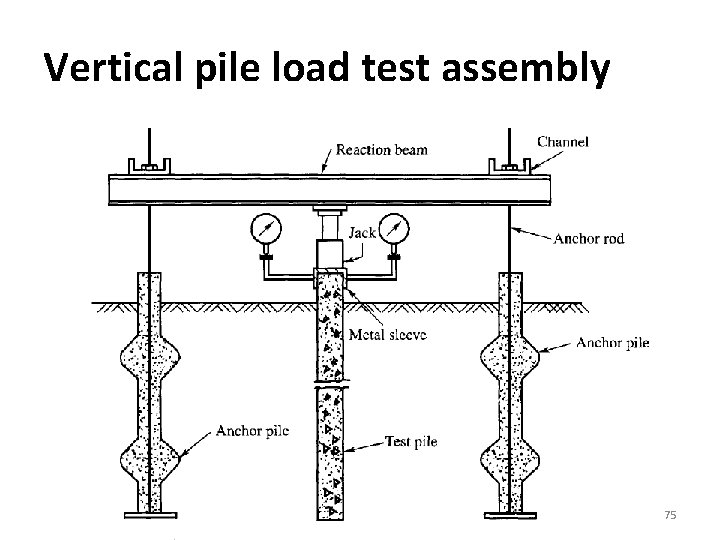
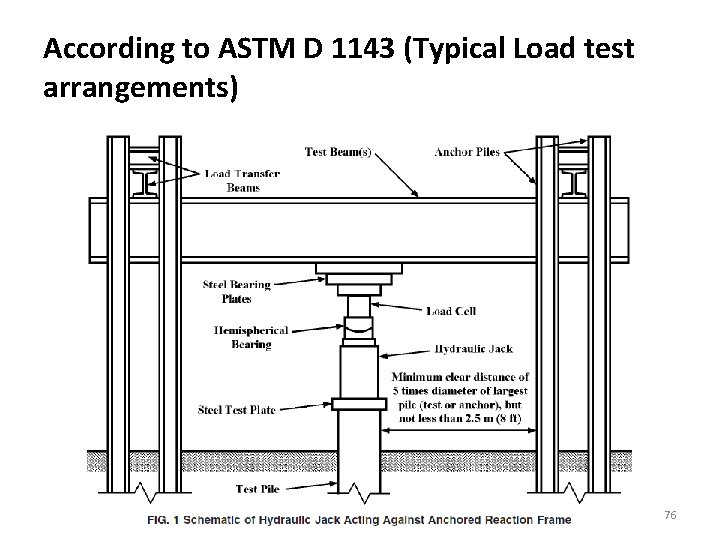
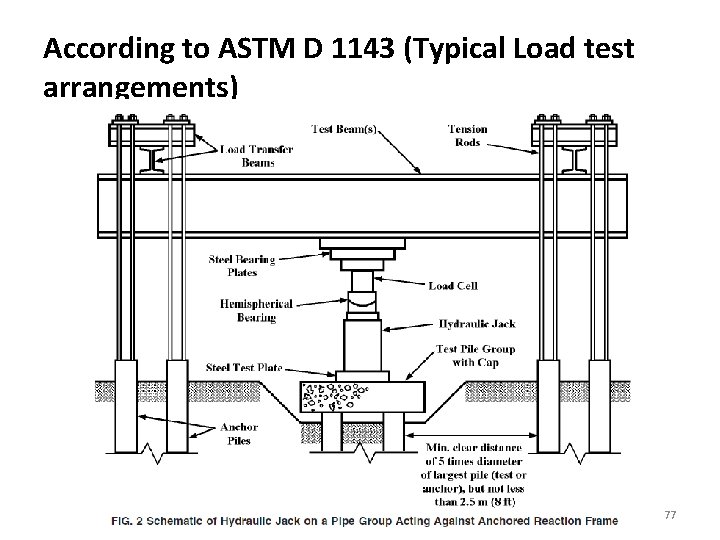
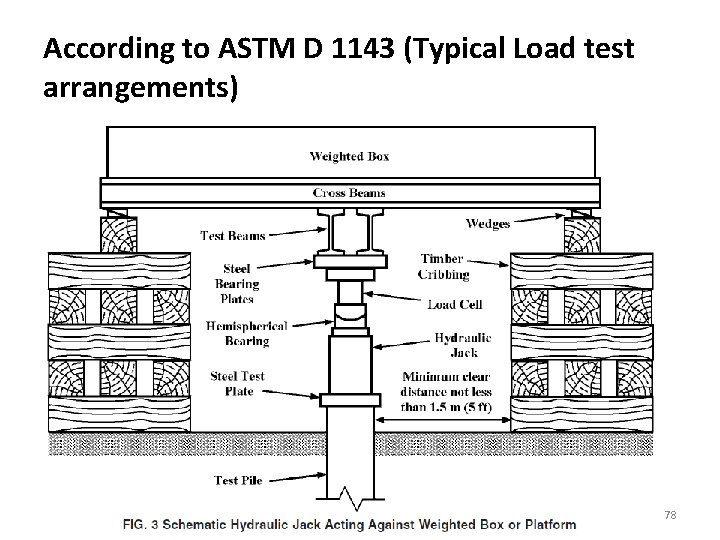
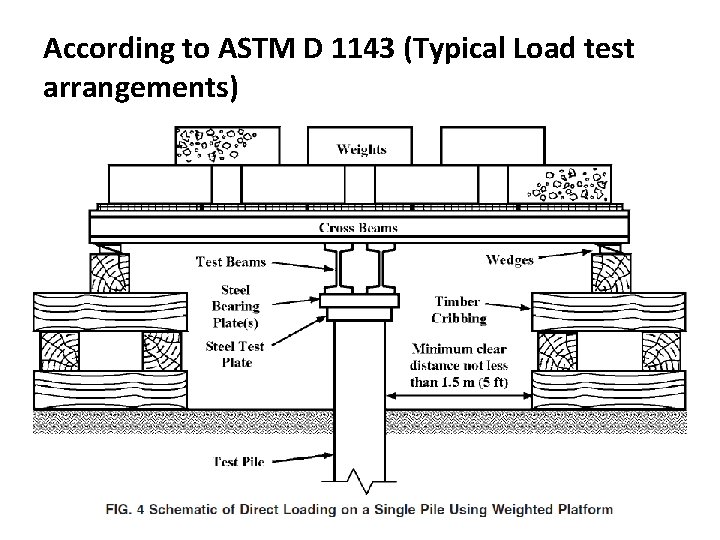
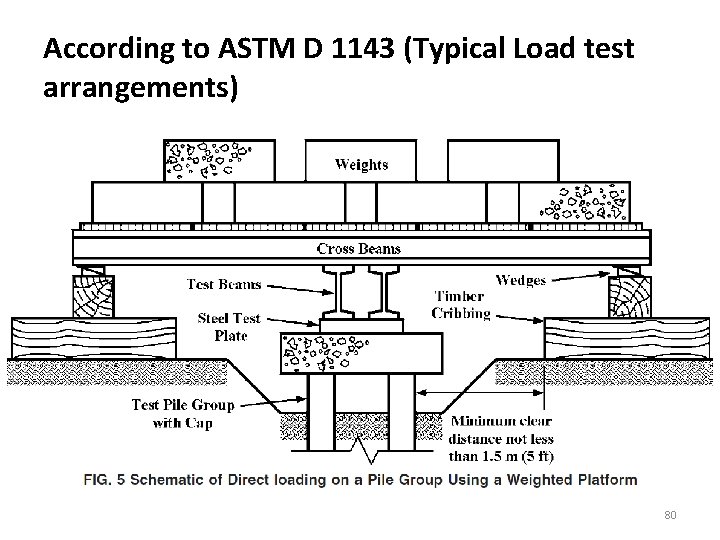
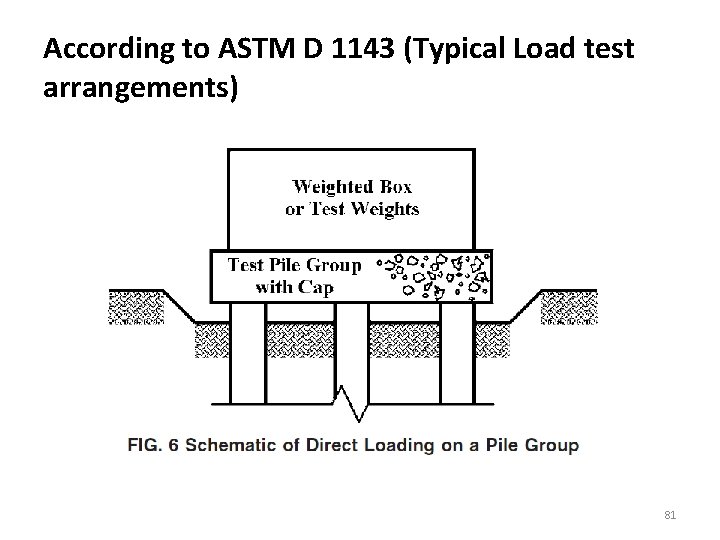
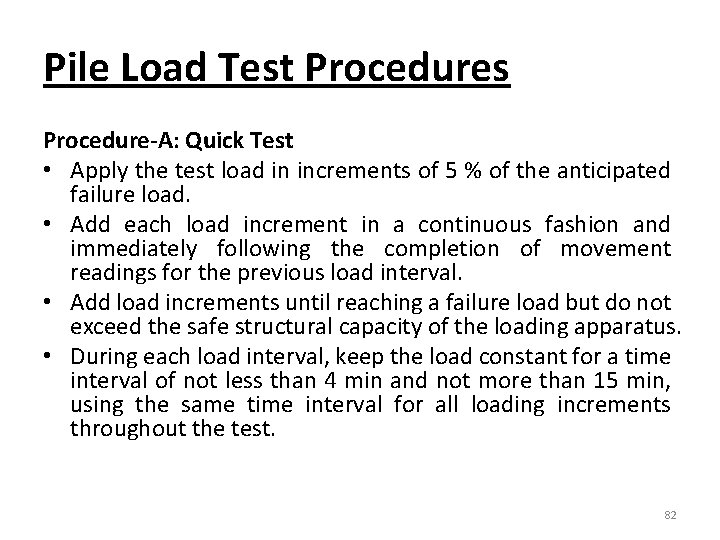
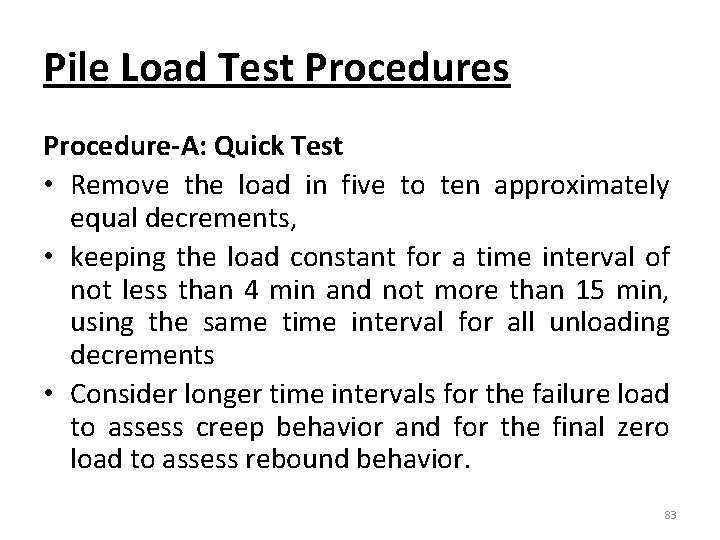
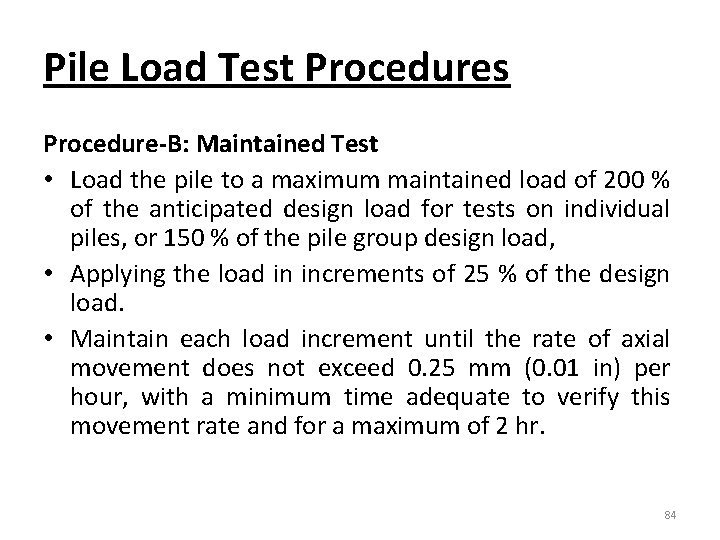
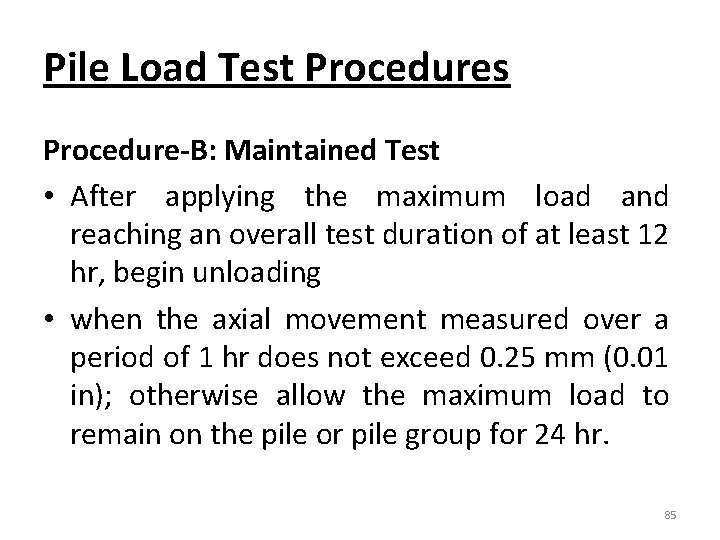
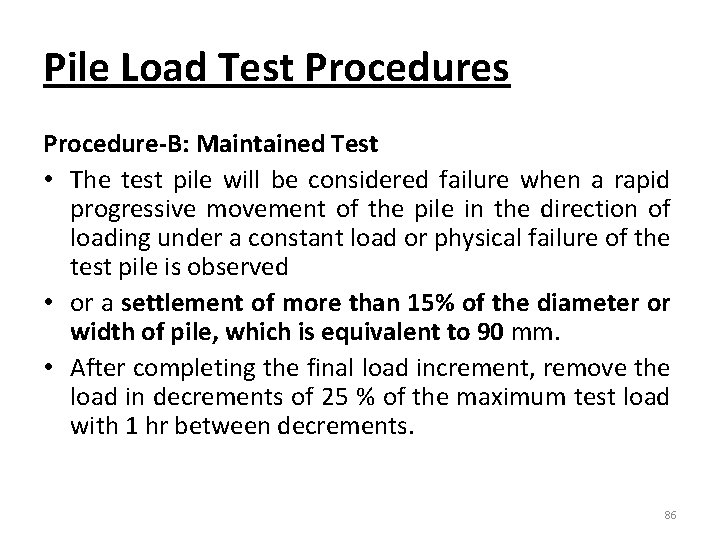
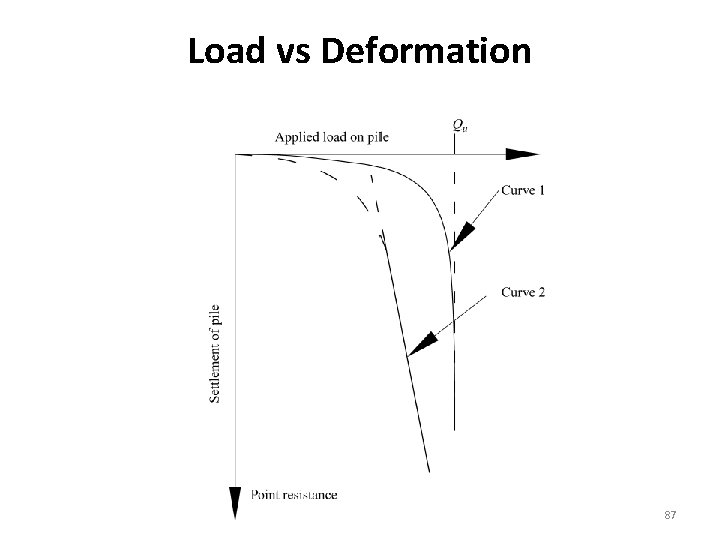
- Slides: 87
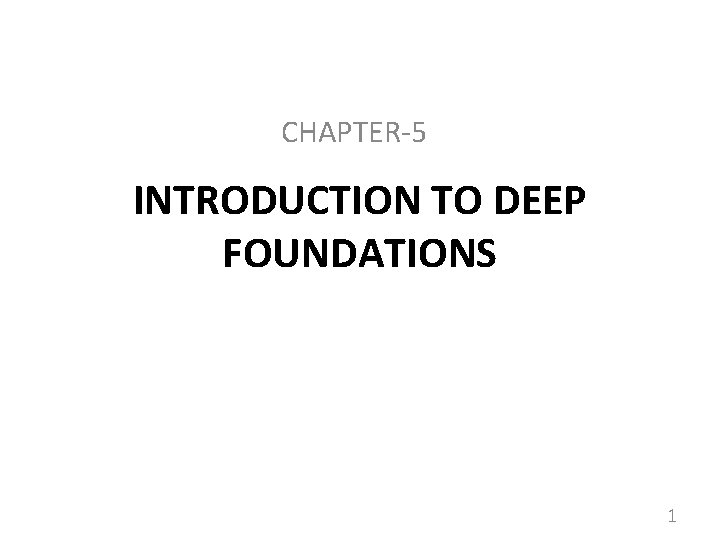
CHAPTER-5 INTRODUCTION TO DEEP FOUNDATIONS 1
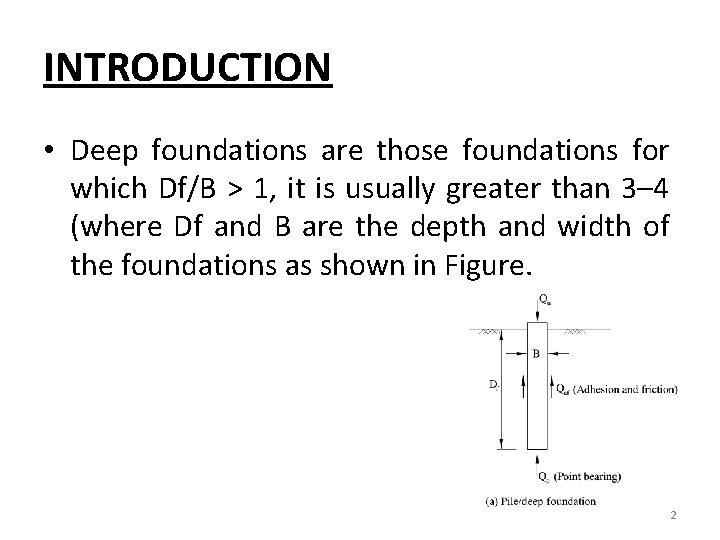
INTRODUCTION • Deep foundations are those foundations for which Df/B > 1, it is usually greater than 3– 4 (where Df and B are the depth and width of the foundations as shown in Figure. 2
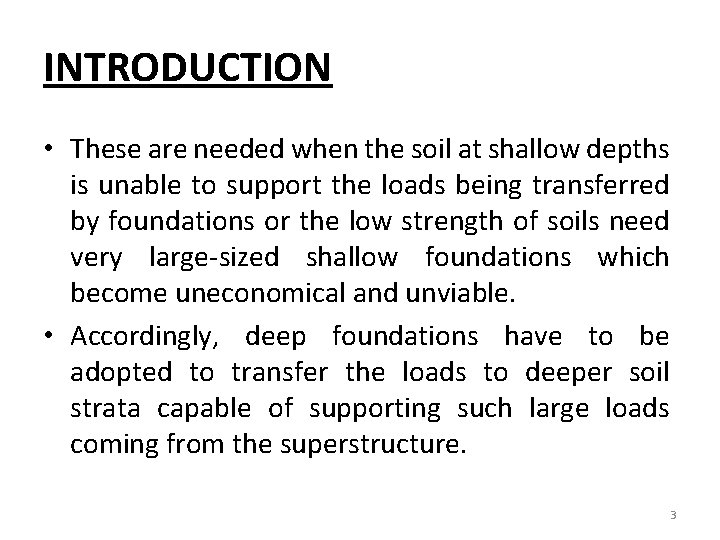
INTRODUCTION • These are needed when the soil at shallow depths is unable to support the loads being transferred by foundations or the low strength of soils need very large-sized shallow foundations which become uneconomical and unviable. • Accordingly, deep foundations have to be adopted to transfer the loads to deeper soil strata capable of supporting such large loads coming from the superstructure. 3
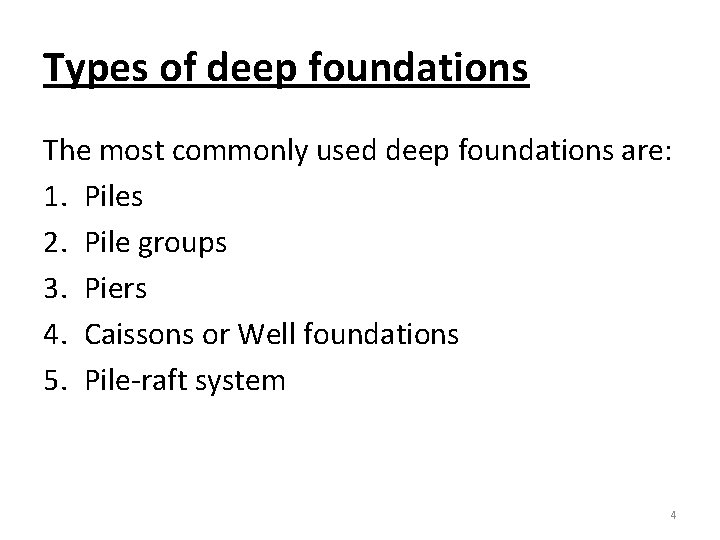
Types of deep foundations The most commonly used deep foundations are: 1. Piles 2. Pile groups 3. Piers 4. Caissons or Well foundations 5. Pile-raft system 4
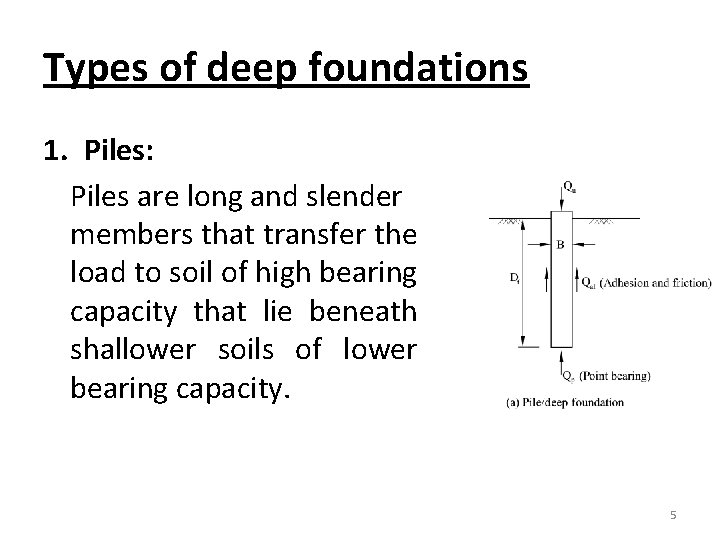
Types of deep foundations 1. Piles: Piles are long and slender members that transfer the load to soil of high bearing capacity that lie beneath shallower soils of lower bearing capacity. 5
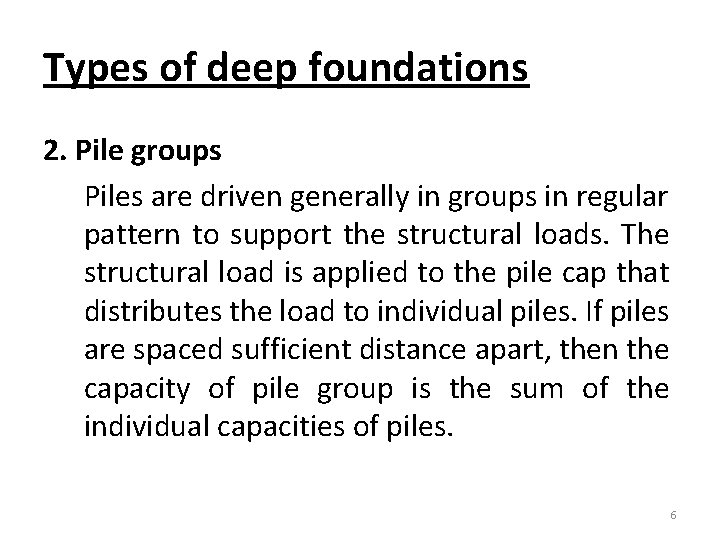
Types of deep foundations 2. Pile groups Piles are driven generally in groups in regular pattern to support the structural loads. The structural load is applied to the pile cap that distributes the load to individual piles. If piles are spaced sufficient distance apart, then the capacity of pile group is the sum of the individual capacities of piles. 6
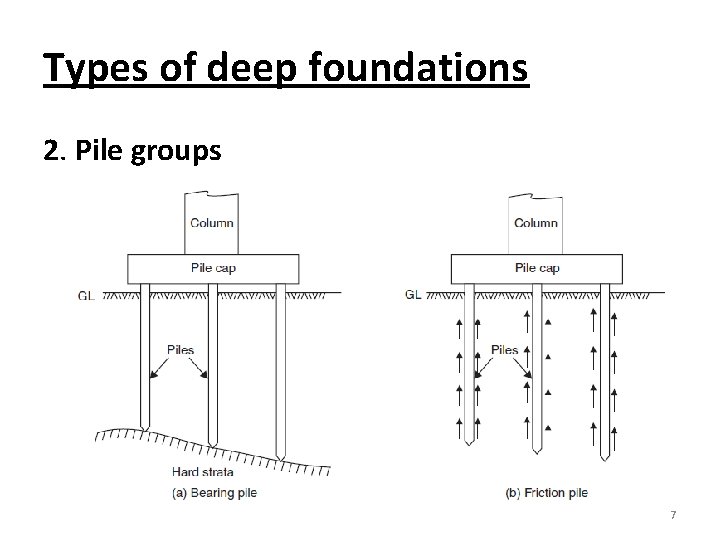
Types of deep foundations 2. Pile groups 7
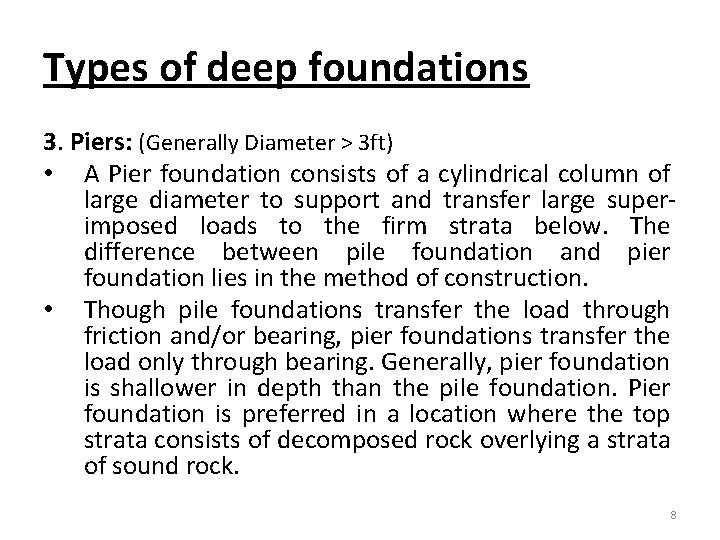
Types of deep foundations 3. Piers: (Generally Diameter > 3 ft) • A Pier foundation consists of a cylindrical column of large diameter to support and transfer large superimposed loads to the firm strata below. The difference between pile foundation and pier foundation lies in the method of construction. • Though pile foundations transfer the load through friction and/or bearing, pier foundations transfer the load only through bearing. Generally, pier foundation is shallower in depth than the pile foundation. Pier foundation is preferred in a location where the top strata consists of decomposed rock overlying a strata of sound rock. 8
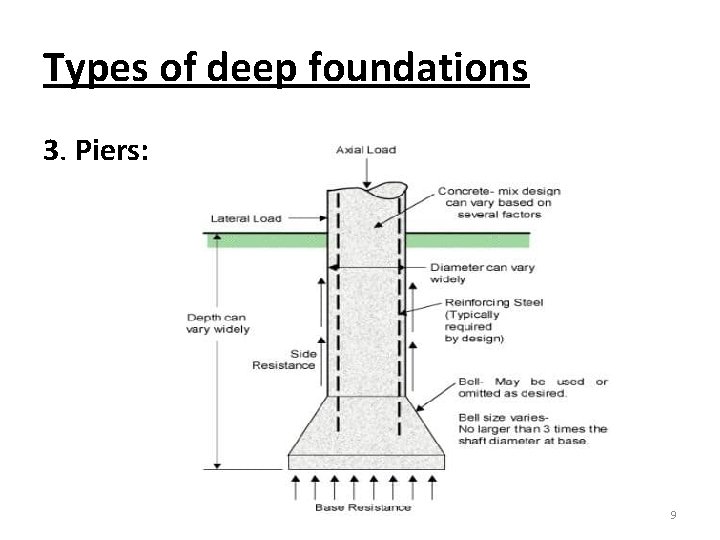
Types of deep foundations 3. Piers: 9
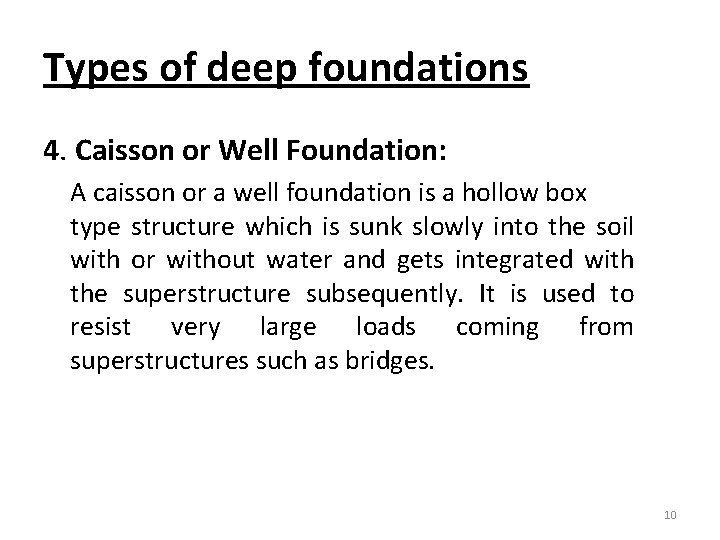
Types of deep foundations 4. Caisson or Well Foundation: A caisson or a well foundation is a hollow box type structure which is sunk slowly into the soil with or without water and gets integrated with the superstructure subsequently. It is used to resist very large loads coming from superstructures such as bridges. 10
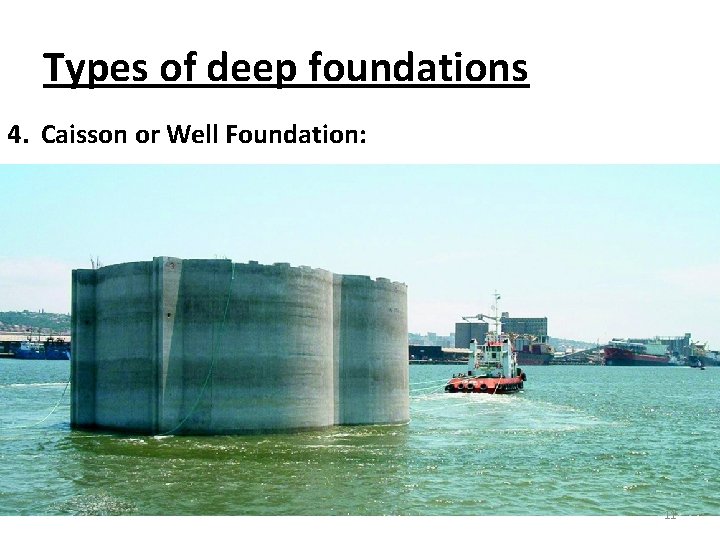
Types of deep foundations 4. Caisson or Well Foundation: 11
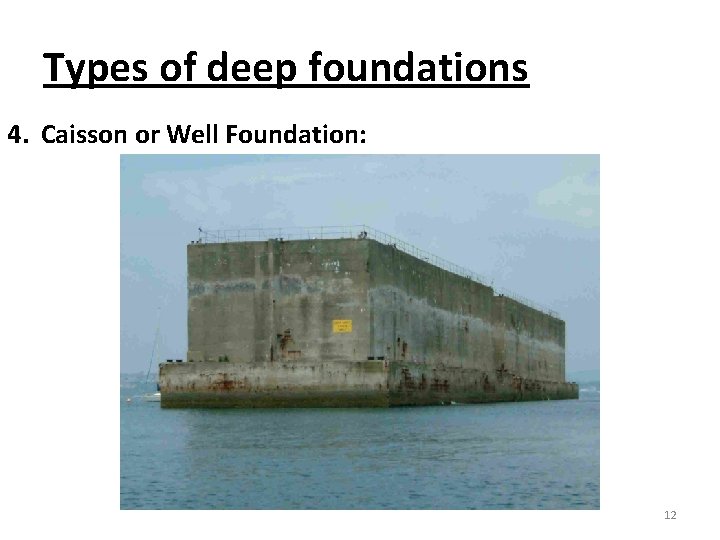
Types of deep foundations 4. Caisson or Well Foundation: 12
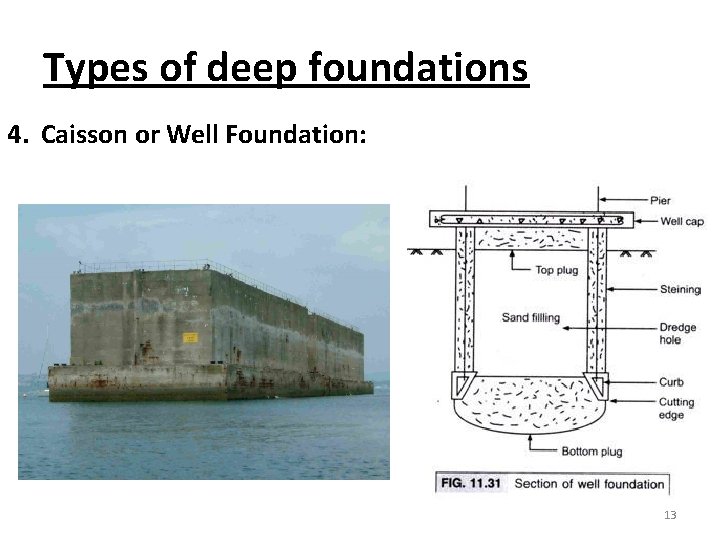
Types of deep foundations 4. Caisson or Well Foundation: 13
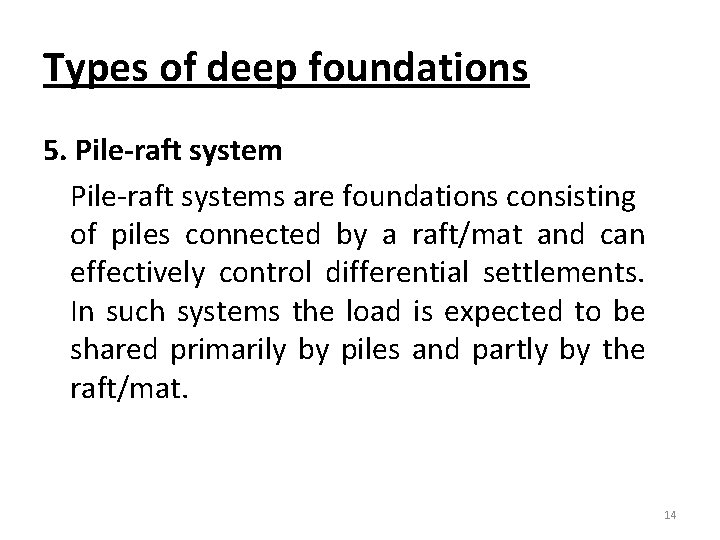
Types of deep foundations 5. Pile-raft systems are foundations consisting of piles connected by a raft/mat and can effectively control differential settlements. In such systems the load is expected to be shared primarily by piles and partly by the raft/mat. 14
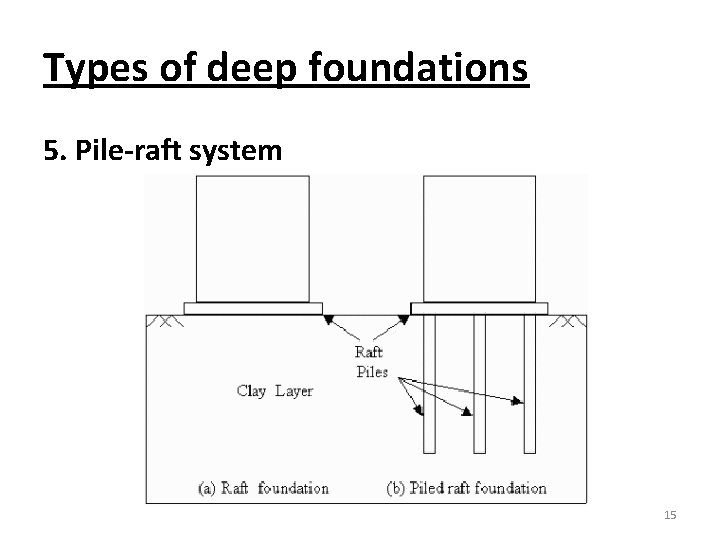
Types of deep foundations 5. Pile-raft system 15
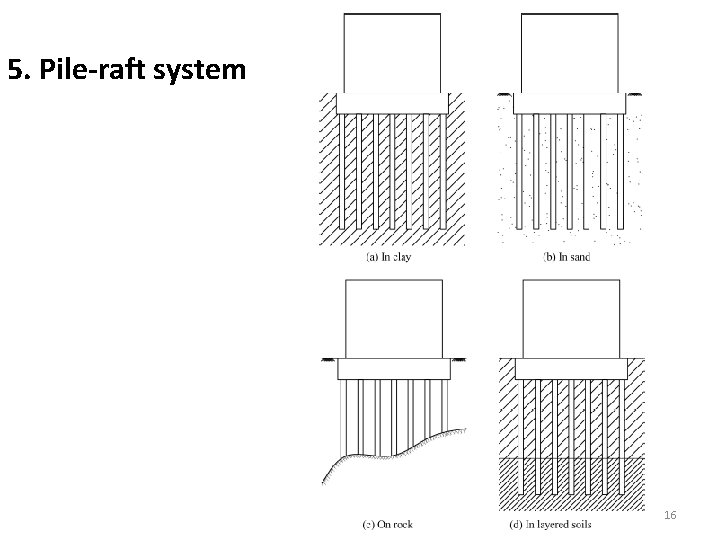
5. Pile-raft system 16
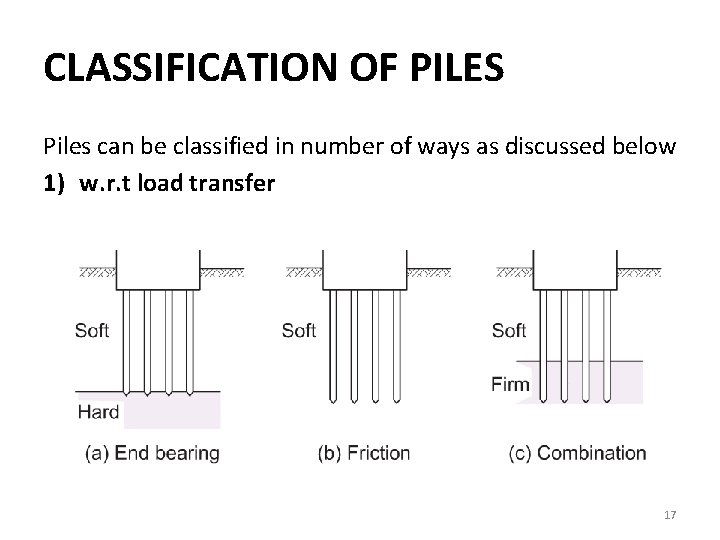
CLASSIFICATION OF PILES Piles can be classified in number of ways as discussed below 1) w. r. t load transfer 17
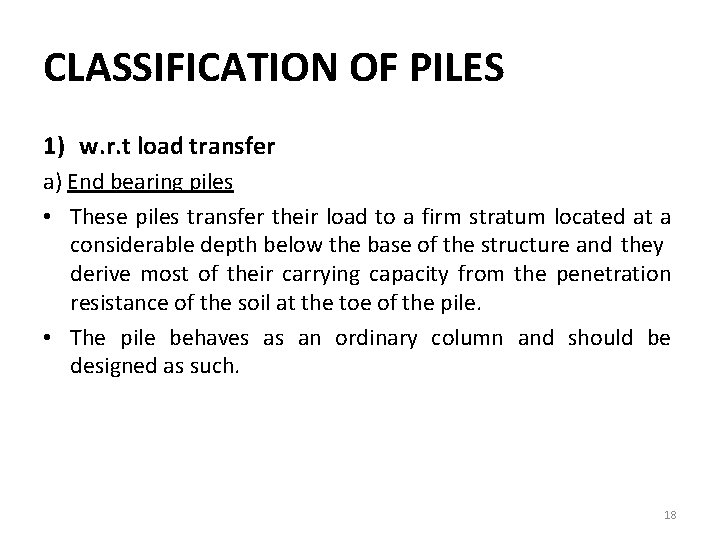
CLASSIFICATION OF PILES 1) w. r. t load transfer a) End bearing piles • These piles transfer their load to a firm stratum located at a considerable depth below the base of the structure and they derive most of their carrying capacity from the penetration resistance of the soil at the toe of the pile. • The pile behaves as an ordinary column and should be designed as such. 18
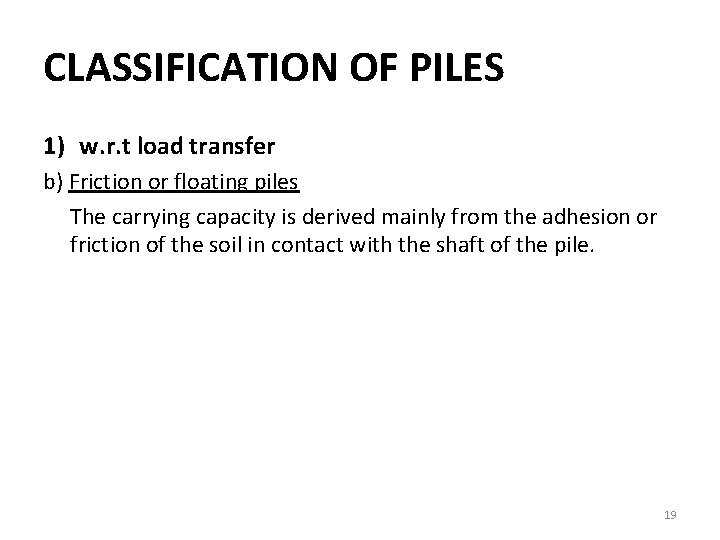
CLASSIFICATION OF PILES 1) w. r. t load transfer b) Friction or floating piles The carrying capacity is derived mainly from the adhesion or friction of the soil in contact with the shaft of the pile. 19
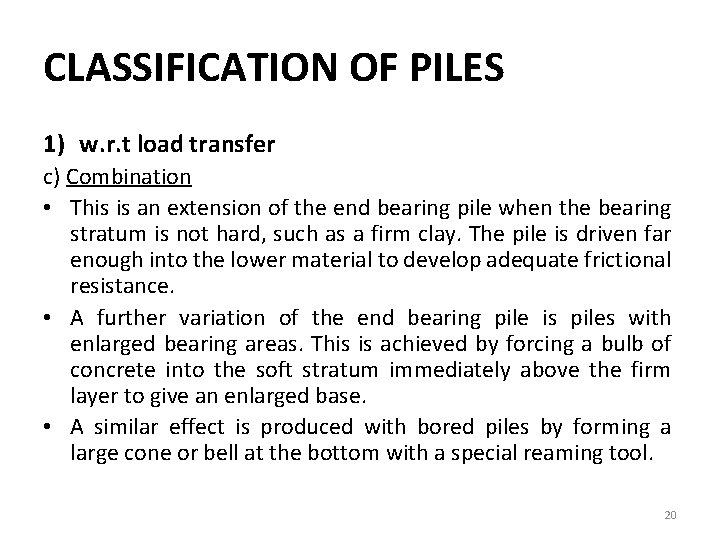
CLASSIFICATION OF PILES 1) w. r. t load transfer c) Combination • This is an extension of the end bearing pile when the bearing stratum is not hard, such as a firm clay. The pile is driven far enough into the lower material to develop adequate frictional resistance. • A further variation of the end bearing pile is piles with enlarged bearing areas. This is achieved by forcing a bulb of concrete into the soft stratum immediately above the firm layer to give an enlarged base. • A similar effect is produced with bored piles by forming a large cone or bell at the bottom with a special reaming tool. 20
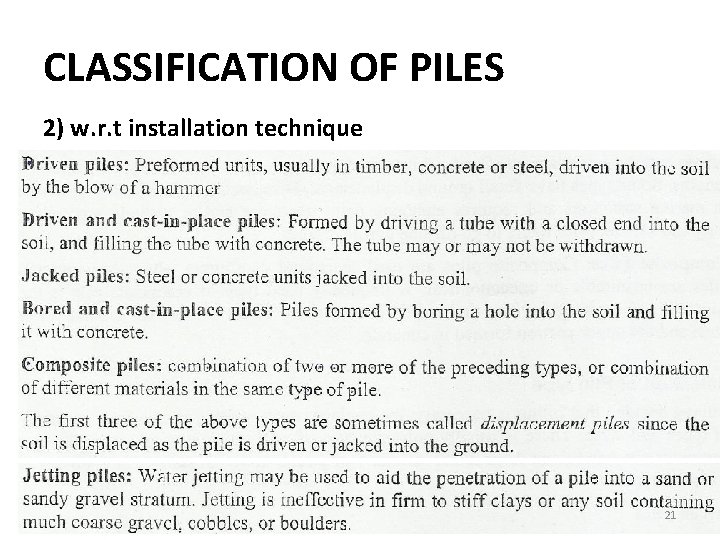
CLASSIFICATION OF PILES 2) w. r. t installation technique 21
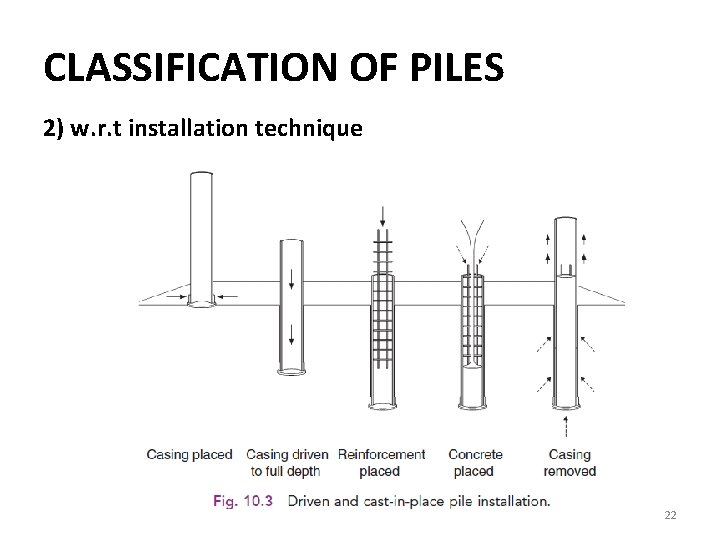
CLASSIFICATION OF PILES 2) w. r. t installation technique 22
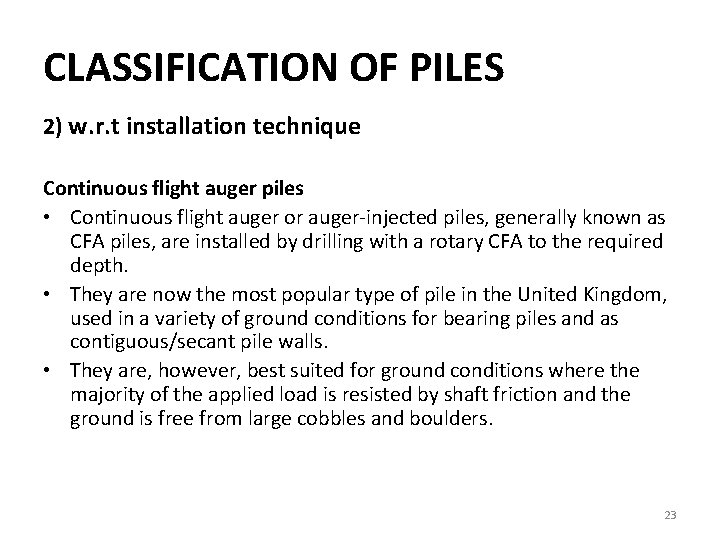
CLASSIFICATION OF PILES 2) w. r. t installation technique Continuous flight auger piles • Continuous flight auger or auger-injected piles, generally known as CFA piles, are installed by drilling with a rotary CFA to the required depth. • They are now the most popular type of pile in the United Kingdom, used in a variety of ground conditions for bearing piles and as contiguous/secant pile walls. • They are, however, best suited for ground conditions where the majority of the applied load is resisted by shaft friction and the ground is free from large cobbles and boulders. 23
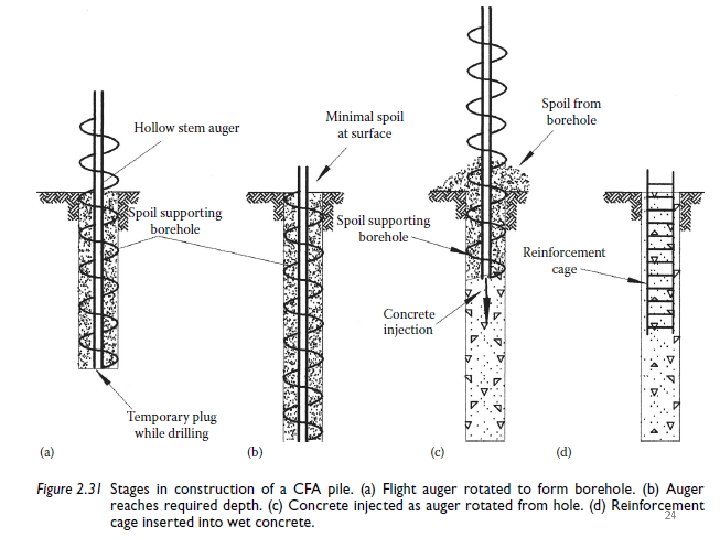
24
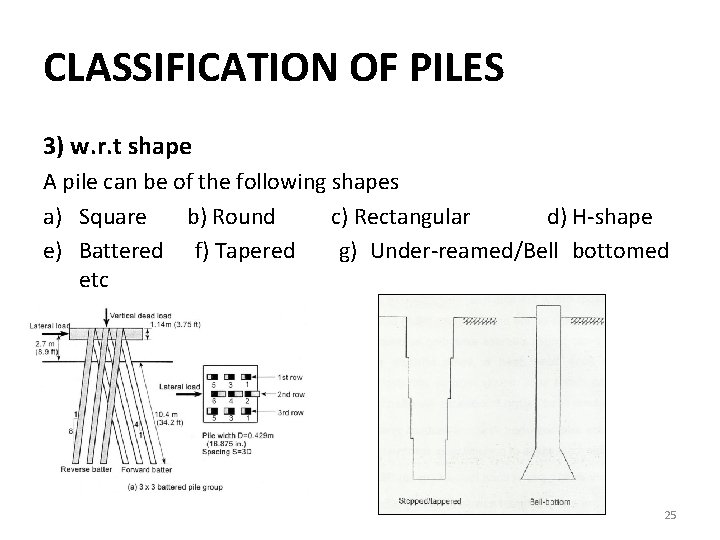
CLASSIFICATION OF PILES 3) w. r. t shape A pile can be of the following shapes a) Square b) Round c) Rectangular d) H-shape e) Battered f) Tapered g) Under-reamed/Bell bottomed etc 25
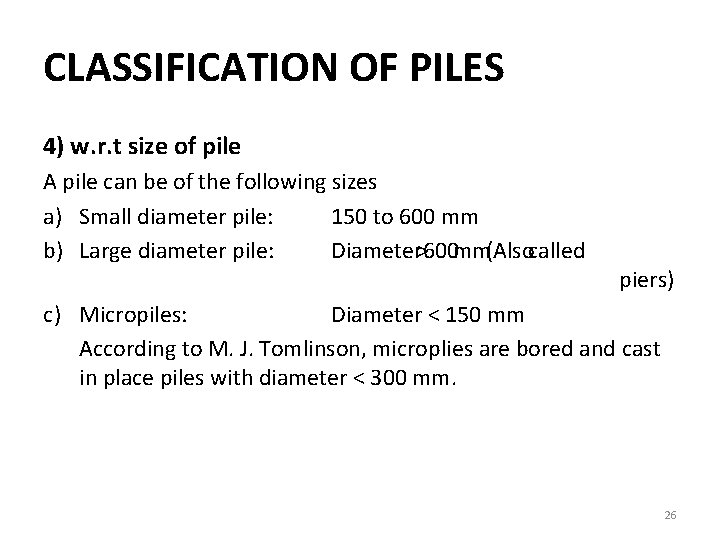
CLASSIFICATION OF PILES 4) w. r. t size of pile A pile can be of the following sizes a) Small diameter pile: 150 to 600 mm b) Large diameter pile: Diameter>600 mm(Alsocalled piers) c) Micropiles: Diameter < 150 mm According to M. J. Tomlinson, microplies are bored and cast in place piles with diameter < 300 mm. 26
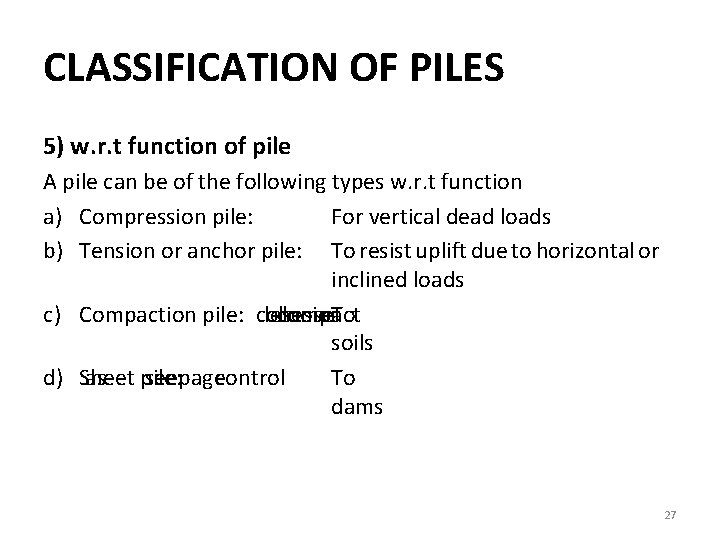
CLASSIFICATION OF PILES 5) w. r. t function of pile A pile can be of the following types w. r. t function a) Compression pile: For vertical dead loads b) Tension or anchor pile: To resist uplift due to horizontal or inclined loads c) Compaction pile: cohesion less compact loose. To soils d) Sheet as pile: seepagecontrol To dams 27
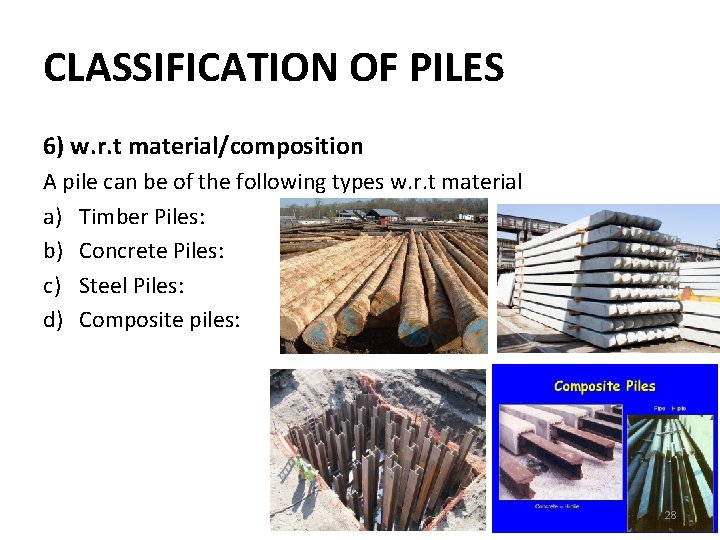
CLASSIFICATION OF PILES 6) w. r. t material/composition A pile can be of the following types w. r. t material a) Timber Piles: b) Concrete Piles: c) Steel Piles: d) Composite piles: 28
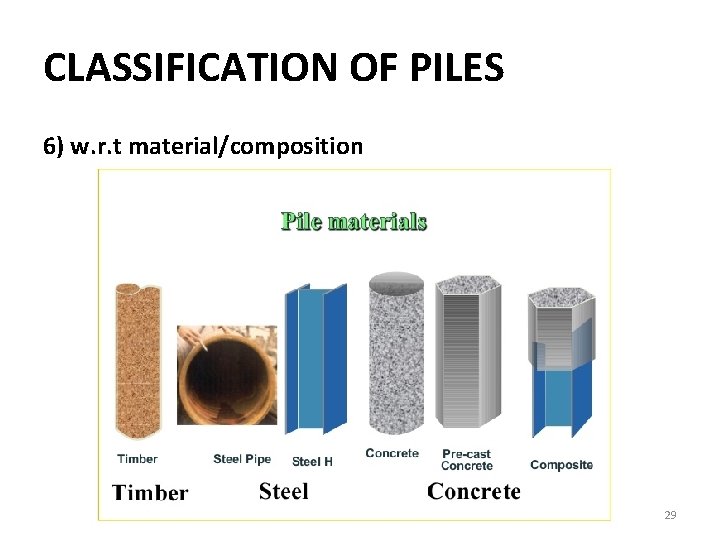
CLASSIFICATION OF PILES 6) w. r. t material/composition 29
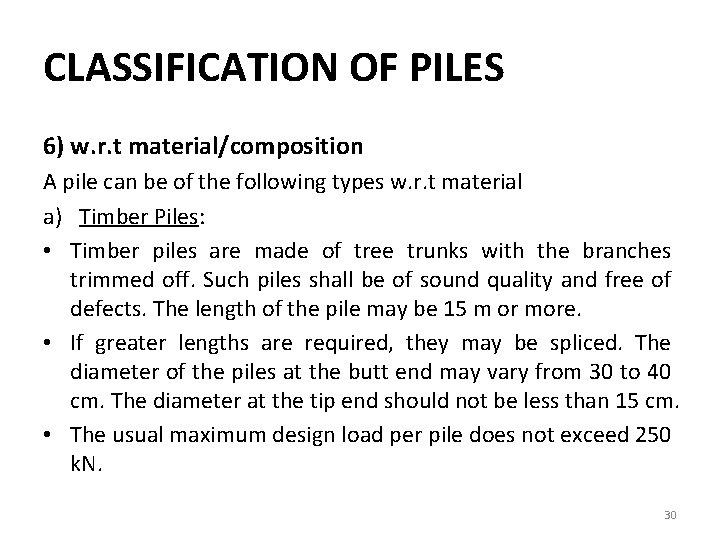
CLASSIFICATION OF PILES 6) w. r. t material/composition A pile can be of the following types w. r. t material a) Timber Piles: • Timber piles are made of tree trunks with the branches trimmed off. Such piles shall be of sound quality and free of defects. The length of the pile may be 15 m or more. • If greater lengths are required, they may be spliced. The diameter of the piles at the butt end may vary from 30 to 40 cm. The diameter at the tip end should not be less than 15 cm. • The usual maximum design load per pile does not exceed 250 k. N. 30
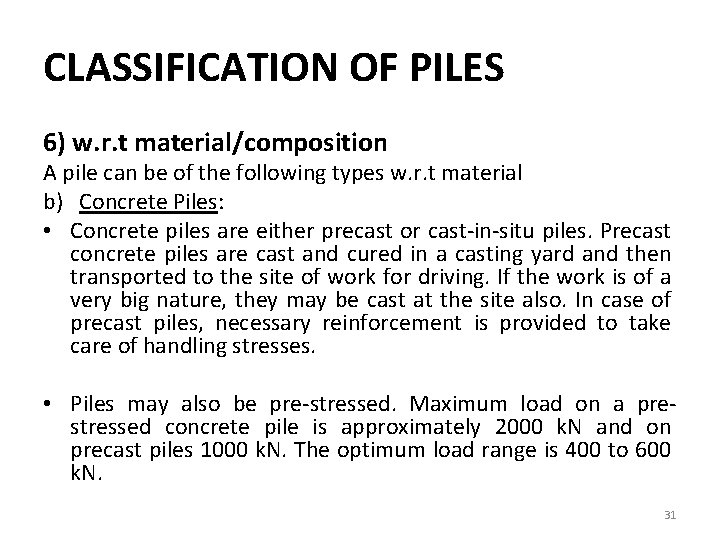
CLASSIFICATION OF PILES 6) w. r. t material/composition A pile can be of the following types w. r. t material b) Concrete Piles: • Concrete piles are either precast or cast-in-situ piles. Precast concrete piles are cast and cured in a casting yard and then transported to the site of work for driving. If the work is of a very big nature, they may be cast at the site also. In case of precast piles, necessary reinforcement is provided to take care of handling stresses. • Piles may also be pre-stressed. Maximum load on a prestressed concrete pile is approximately 2000 k. N and on precast piles 1000 k. N. The optimum load range is 400 to 600 k. N. 31
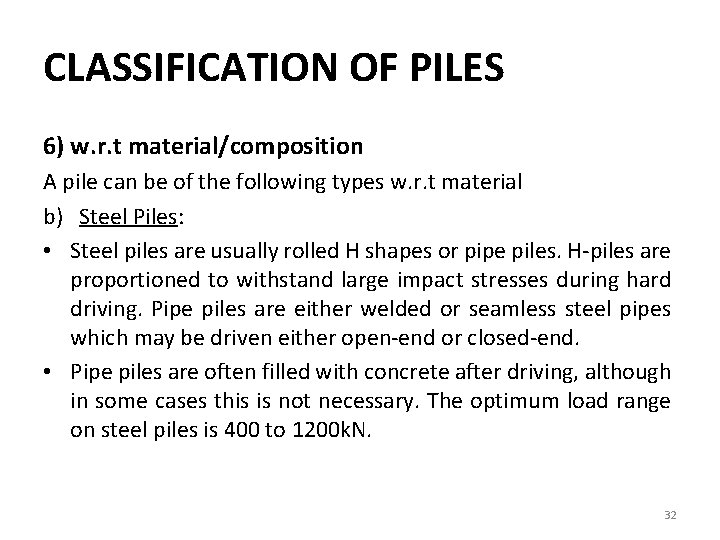
CLASSIFICATION OF PILES 6) w. r. t material/composition A pile can be of the following types w. r. t material b) Steel Piles: • Steel piles are usually rolled H shapes or pipe piles. H-piles are proportioned to withstand large impact stresses during hard driving. Pipe piles are either welded or seamless steel pipes which may be driven either open-end or closed-end. • Pipe piles are often filled with concrete after driving, although in some cases this is not necessary. The optimum load range on steel piles is 400 to 1200 k. N. 32
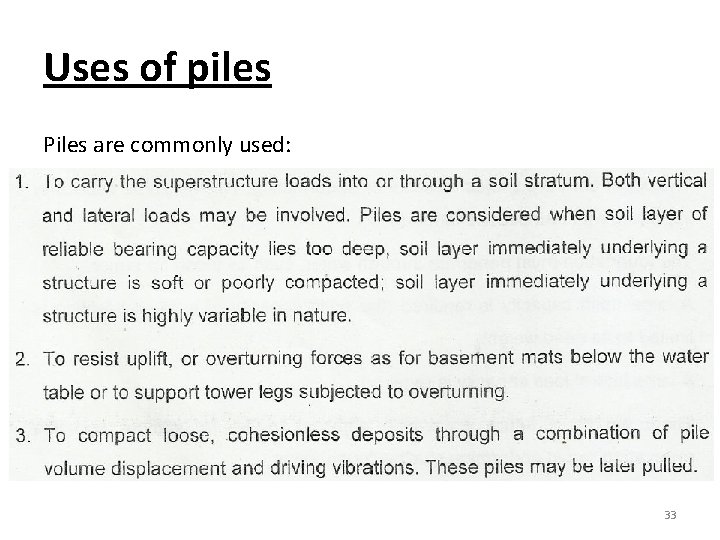
Uses of piles Piles are commonly used: 33
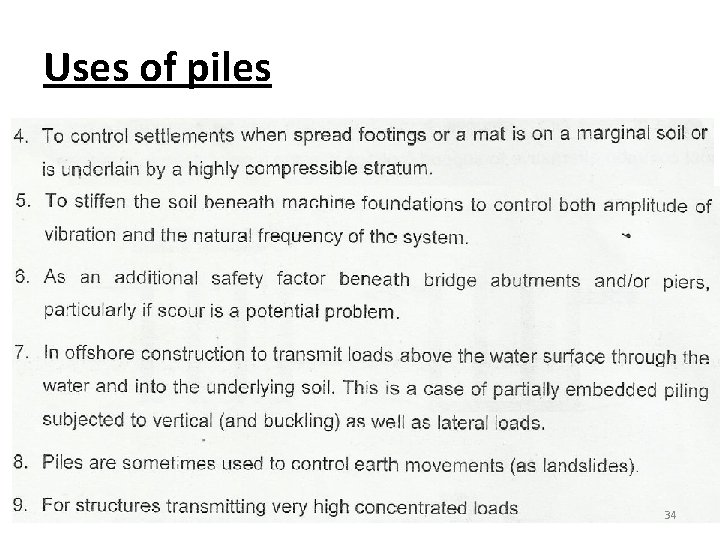
Uses of piles 34
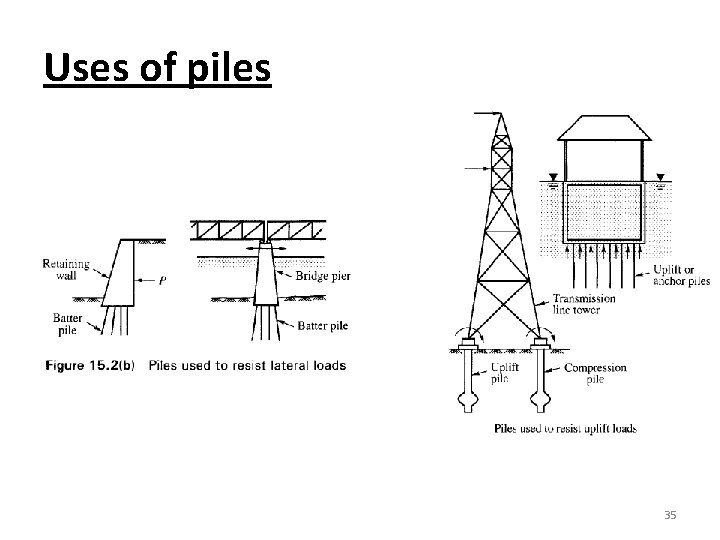
Uses of piles 35
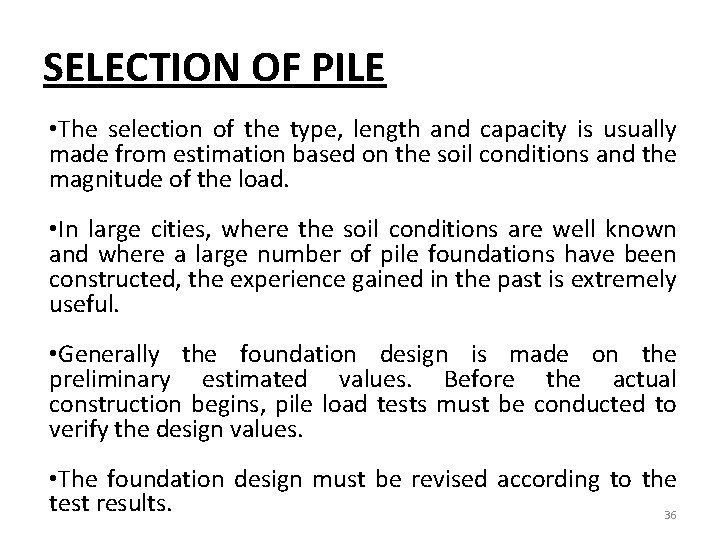
SELECTION OF PILE • The selection of the type, length and capacity is usually made from estimation based on the soil conditions and the magnitude of the load. • In large cities, where the soil conditions are well known and where a large number of pile foundations have been constructed, the experience gained in the past is extremely useful. • Generally the foundation design is made on the preliminary estimated values. Before the actual construction begins, pile load tests must be conducted to verify the design values. • The foundation design must be revised according to the test results. 36
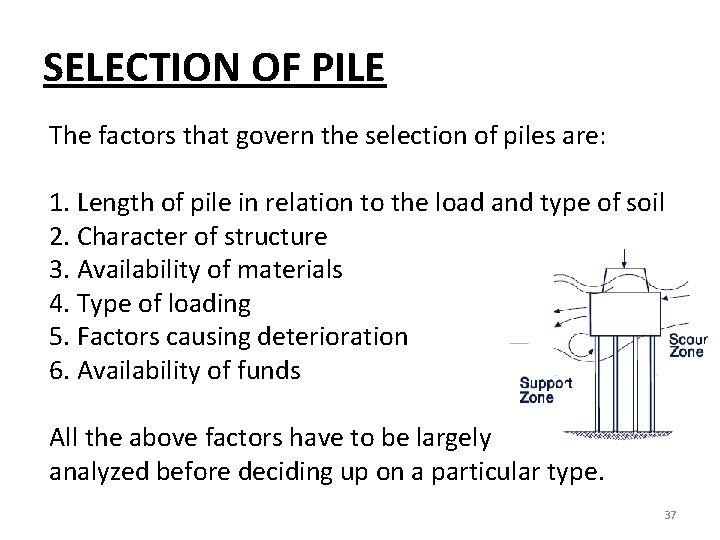
SELECTION OF PILE The factors that govern the selection of piles are: 1. Length of pile in relation to the load and type of soil 2. Character of structure 3. Availability of materials 4. Type of loading 5. Factors causing deterioration 6. Availability of funds All the above factors have to be largely analyzed before deciding up on a particular type. 37
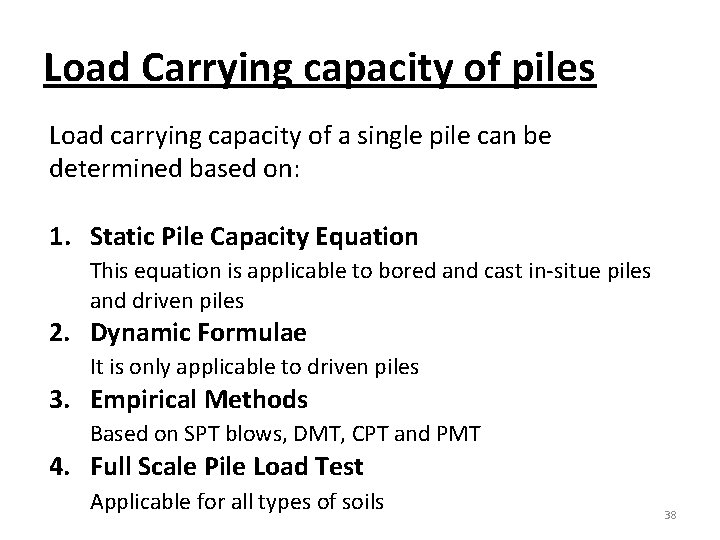
Load Carrying capacity of piles Load carrying capacity of a single pile can be determined based on: 1. Static Pile Capacity Equation This equation is applicable to bored and cast in-situe piles and driven piles 2. Dynamic Formulae It is only applicable to driven piles 3. Empirical Methods Based on SPT blows, DMT, CPT and PMT 4. Full Scale Pile Load Test Applicable for all types of soils 38
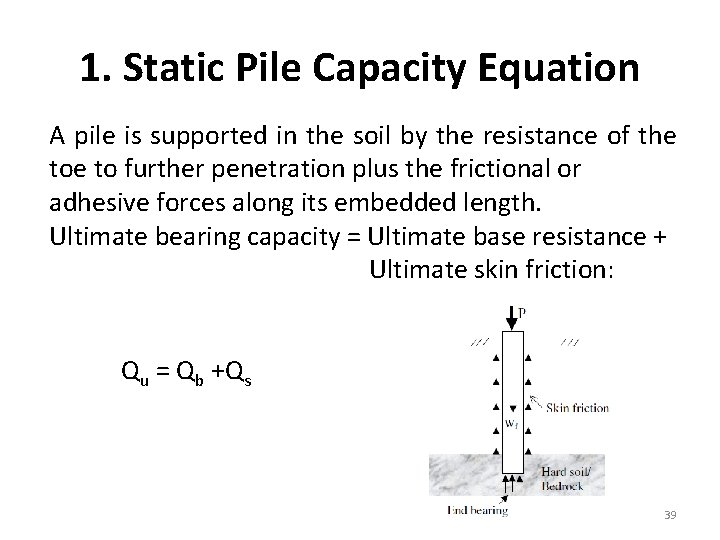
1. Static Pile Capacity Equation A pile is supported in the soil by the resistance of the to further penetration plus the frictional or adhesive forces along its embedded length. Ultimate bearing capacity = Ultimate base resistance + Ultimate skin friction: Qu = Qb +Qs 39
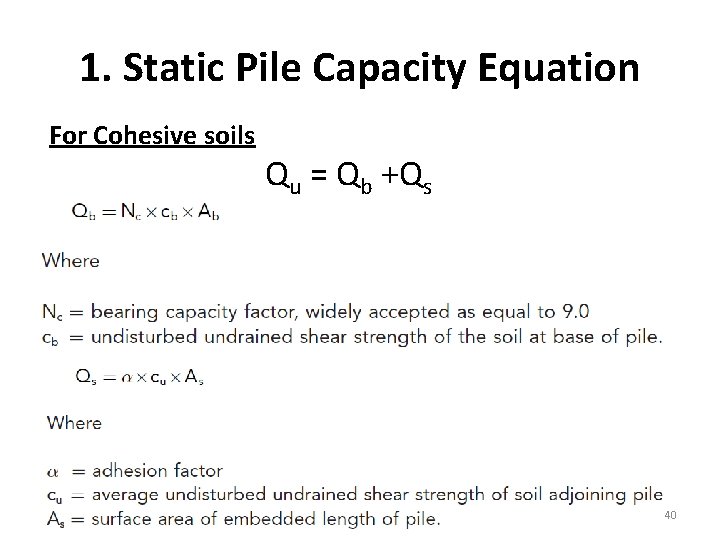
1. Static Pile Capacity Equation For Cohesive soils Qu = Qb +Qs 40
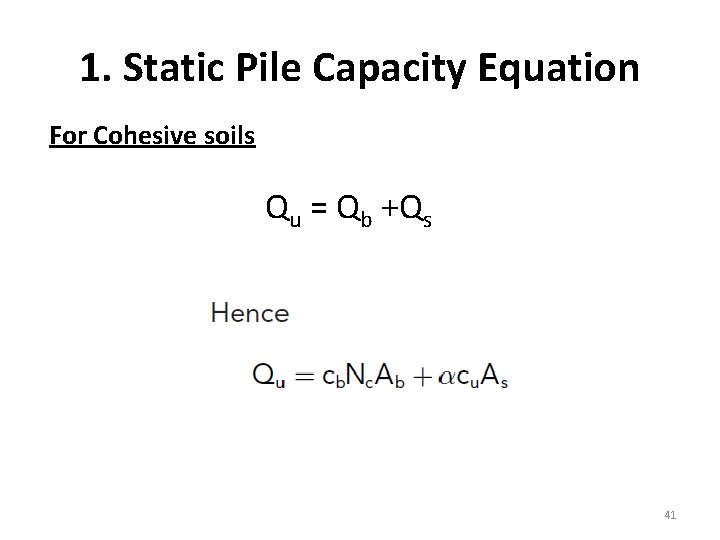
1. Static Pile Capacity Equation For Cohesive soils Qu = Qb +Qs 41
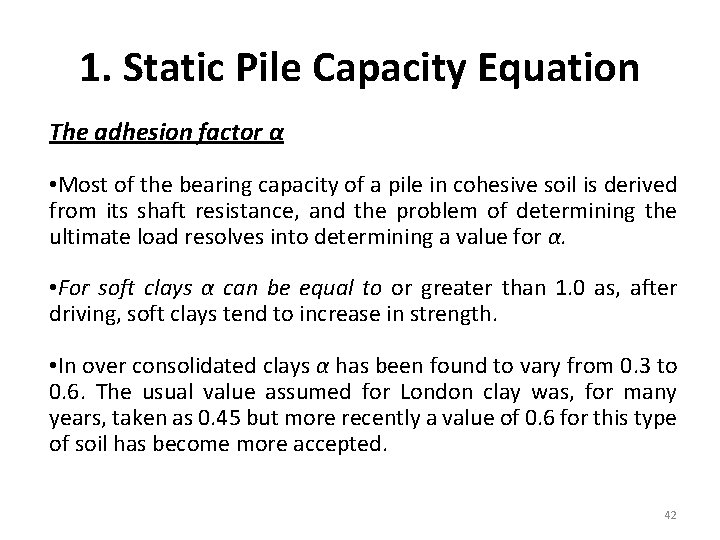
1. Static Pile Capacity Equation The adhesion factor α • Most of the bearing capacity of a pile in cohesive soil is derived from its shaft resistance, and the problem of determining the ultimate load resolves into determining a value for α. • For soft clays α can be equal to or greater than 1. 0 as, after driving, soft clays tend to increase in strength. • In over consolidated clays α has been found to vary from 0. 3 to 0. 6. The usual value assumed for London clay was, for many years, taken as 0. 45 but more recently a value of 0. 6 for this type of soil has become more accepted. 42
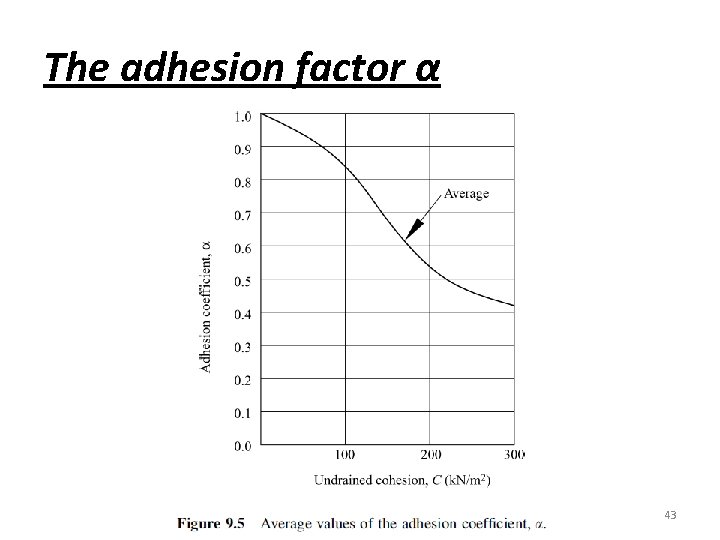
The adhesion factor α 43
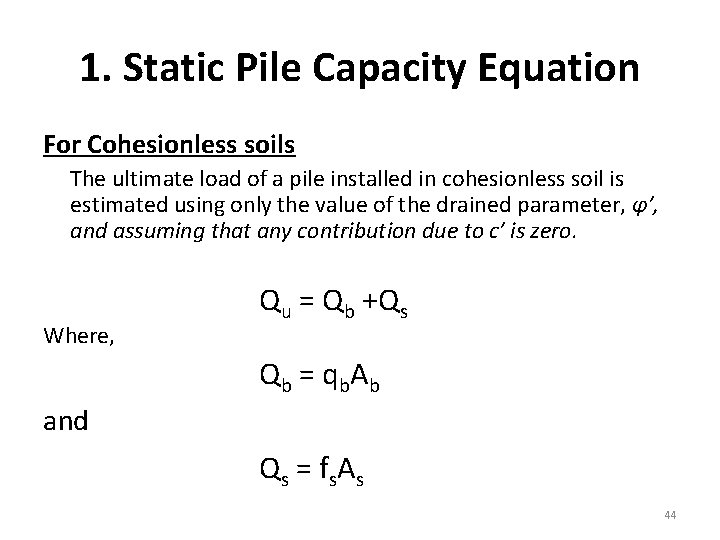
1. Static Pile Capacity Equation For Cohesionless soils The ultimate load of a pile installed in cohesionless soil is estimated using only the value of the drained parameter, φ′, and assuming that any contribution due to c′ is zero. Where, Qu = Qb +Qs Q b = q b. A b and Q s = f s. A s 44
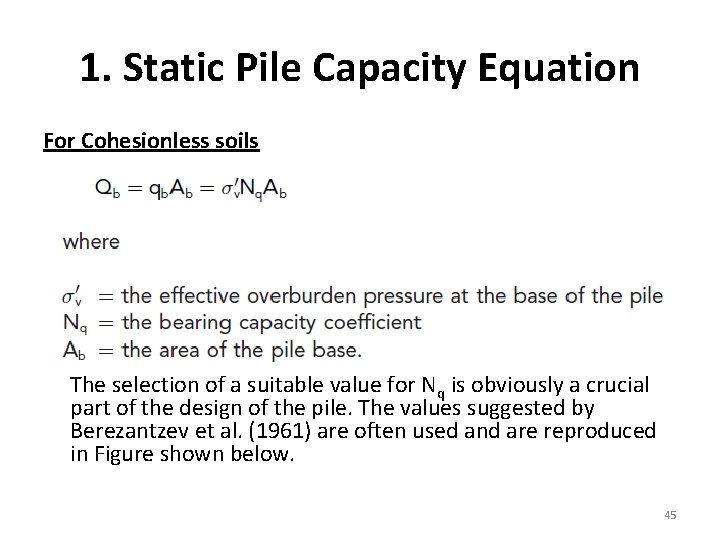
1. Static Pile Capacity Equation For Cohesionless soils The selection of a suitable value for Nq is obviously a crucial part of the design of the pile. The values suggested by Berezantzev et al. (1961) are often used and are reproduced in Figure shown below. 45
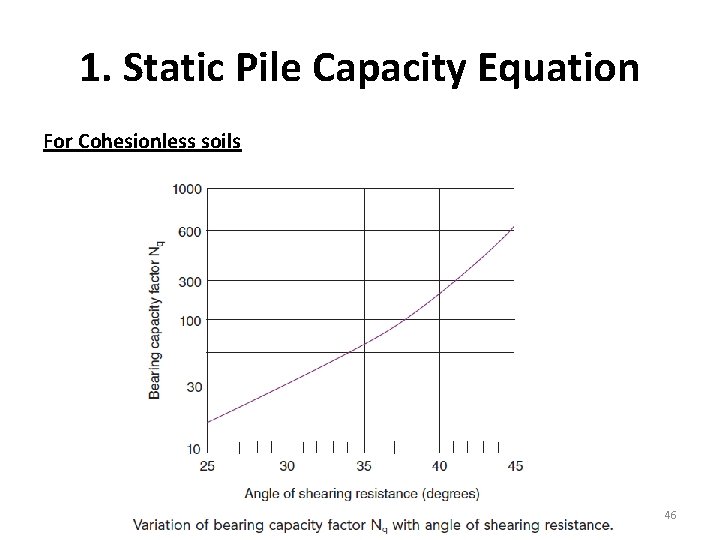
1. Static Pile Capacity Equation For Cohesionless soils 46
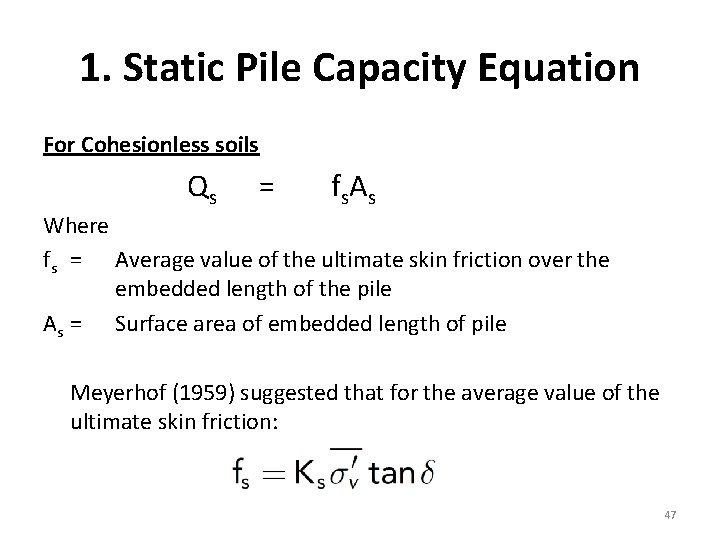
1. Static Pile Capacity Equation For Cohesionless soils Qs = f s. A s Where fs = Average value of the ultimate skin friction over the embedded length of the pile As = Surface area of embedded length of pile Meyerhof (1959) suggested that for the average value of the ultimate skin friction: 47
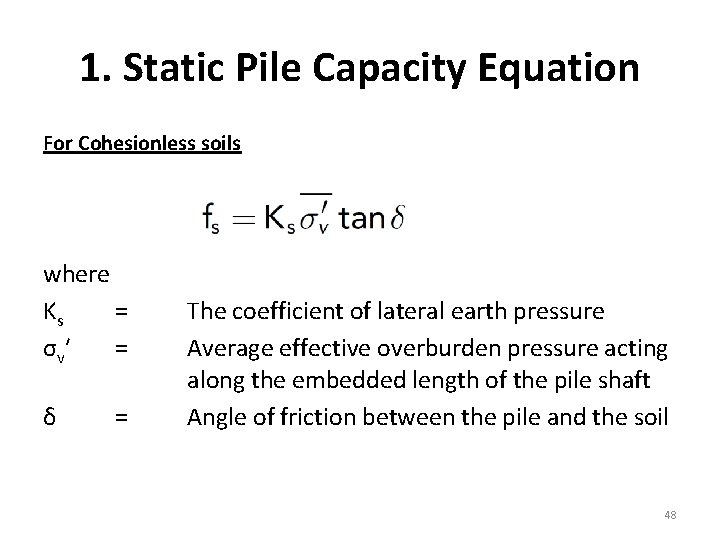
1. Static Pile Capacity Equation For Cohesionless soils where Ks = σ v′ = δ = The coefficient of lateral earth pressure Average effective overburden pressure acting along the embedded length of the pile shaft Angle of friction between the pile and the soil 48
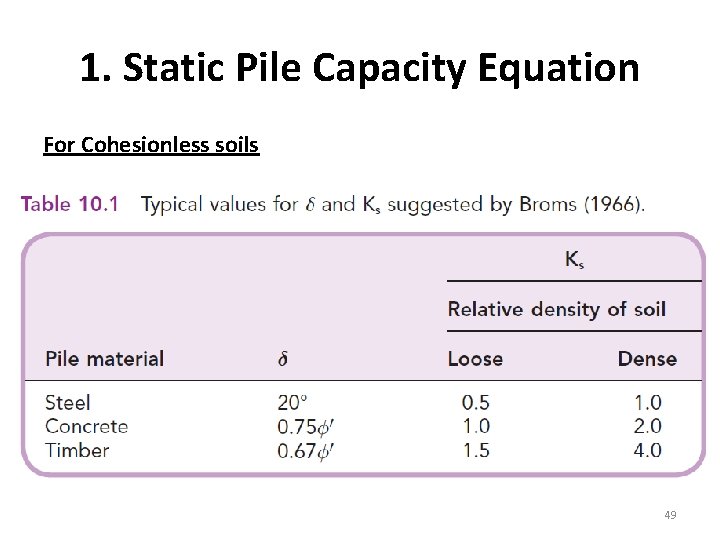
1. Static Pile Capacity Equation For Cohesionless soils 49
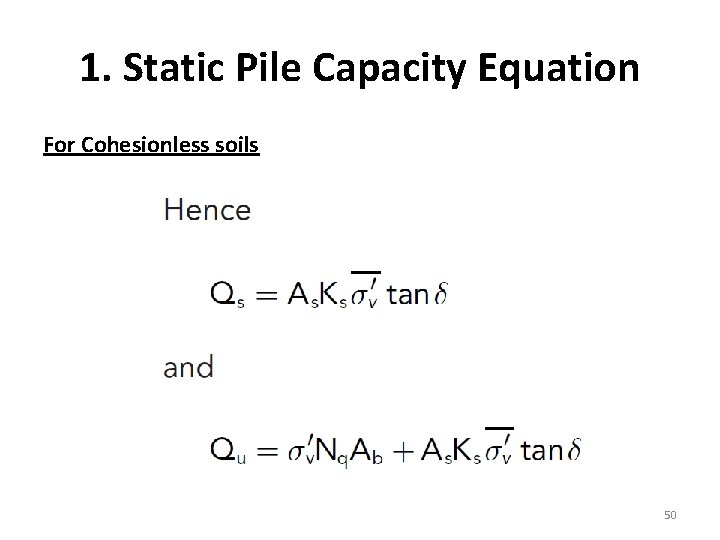
1. Static Pile Capacity Equation For Cohesionless soils 50
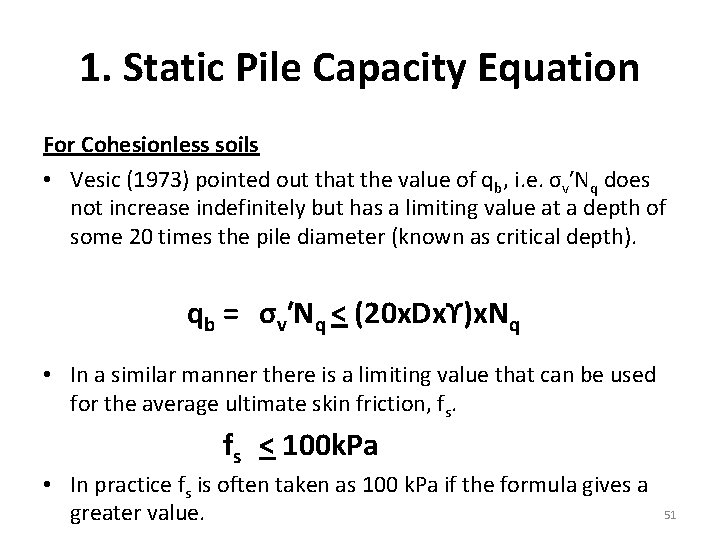
1. Static Pile Capacity Equation For Cohesionless soils • Vesic (1973) pointed out that the value of qb, i. e. σv′Nq does not increase indefinitely but has a limiting value at a depth of some 20 times the pile diameter (known as critical depth). qb = σv′Nq < (20 x. Dxϒ)x. Nq • In a similar manner there is a limiting value that can be used for the average ultimate skin friction, fs. fs < 100 k. Pa • In practice fs is often taken as 100 k. Pa if the formula gives a greater value. 51
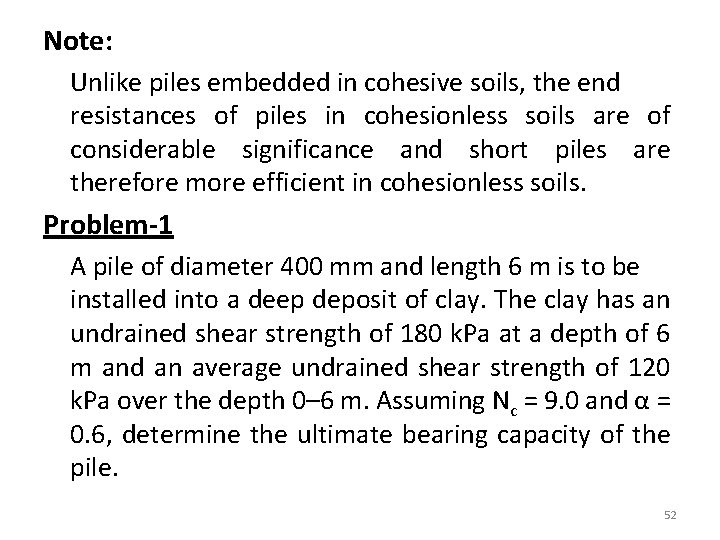
Note: Unlike piles embedded in cohesive soils, the end resistances of piles in cohesionless soils are of considerable significance and short piles are therefore more efficient in cohesionless soils. Problem-1 A pile of diameter 400 mm and length 6 m is to be installed into a deep deposit of clay. The clay has an undrained shear strength of 180 k. Pa at a depth of 6 m and an average undrained shear strength of 120 k. Pa over the depth 0– 6 m. Assuming Nc = 9. 0 and α = 0. 6, determine the ultimate bearing capacity of the pile. 52
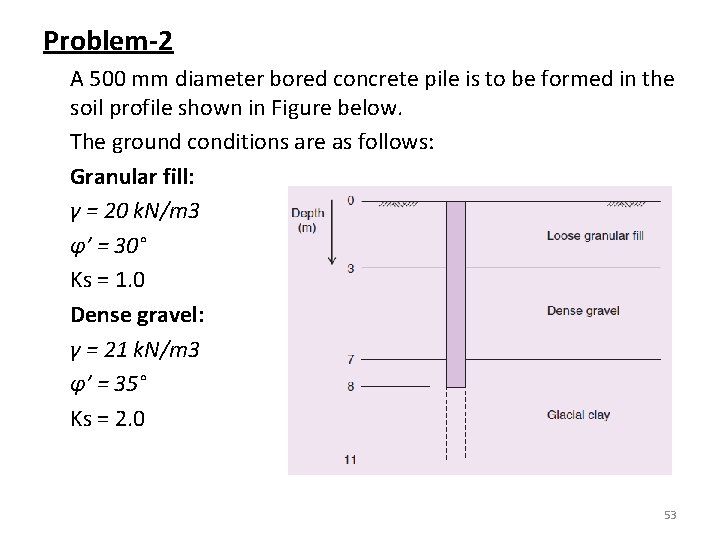
Problem-2 A 500 mm diameter bored concrete pile is to be formed in the soil profile shown in Figure below. The ground conditions are as follows: Granular fill: γ = 20 k. N/m 3 φ′ = 30° Ks = 1. 0 Dense gravel: γ = 21 k. N/m 3 φ′ = 35° Ks = 2. 0 53
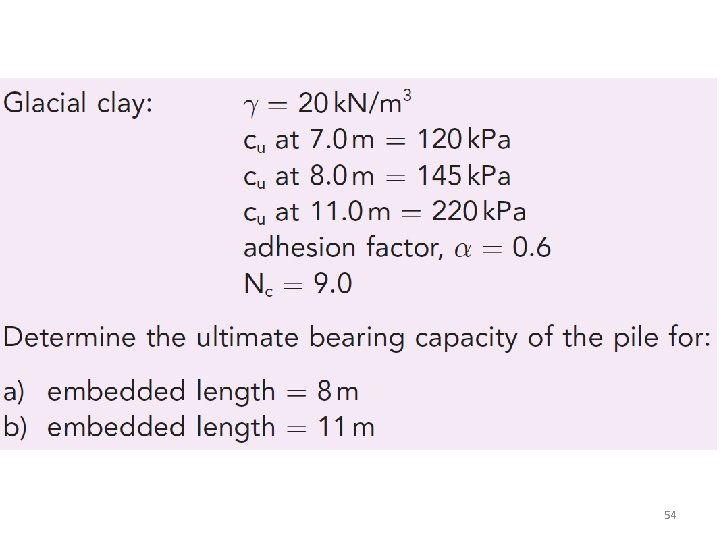
54
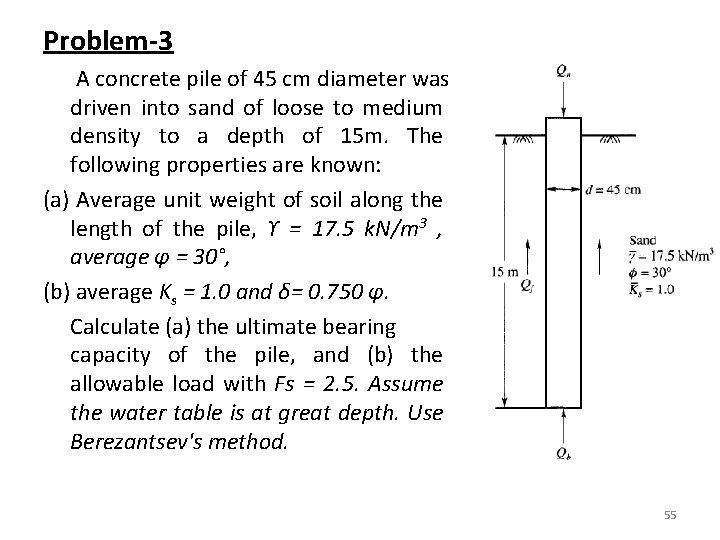
Problem-3 A concrete pile of 45 cm diameter was driven into sand of loose to medium density to a depth of 15 m. The following properties are known: (a) Average unit weight of soil along the length of the pile, ϒ = 17. 5 k. N/m 3 , average φ = 30°, (b) average Ks = 1. 0 and δ= 0. 750 φ. Calculate (a) the ultimate bearing capacity of the pile, and (b) the allowable load with Fs = 2. 5. Assume the water table is at great depth. Use Berezantsev's method. 55
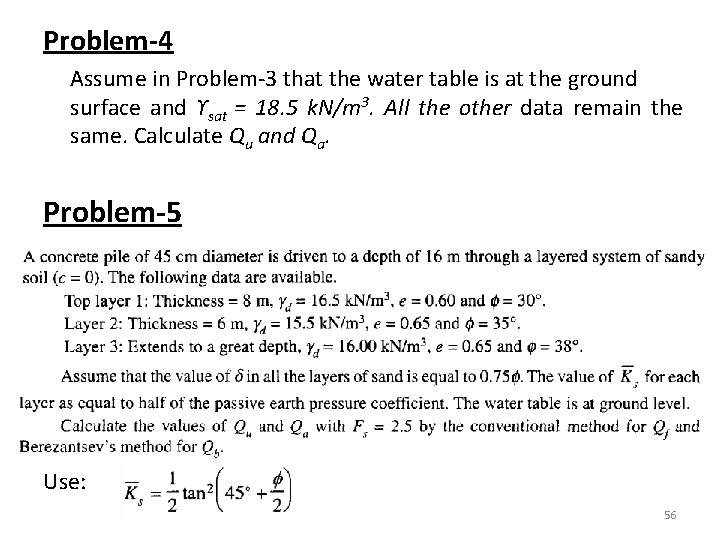
Problem-4 Assume in Problem-3 that the water table is at the ground surface and ϒsat = 18. 5 k. N/m 3. All the other data remain the same. Calculate Qu and Qa. Problem-5 Use: 56
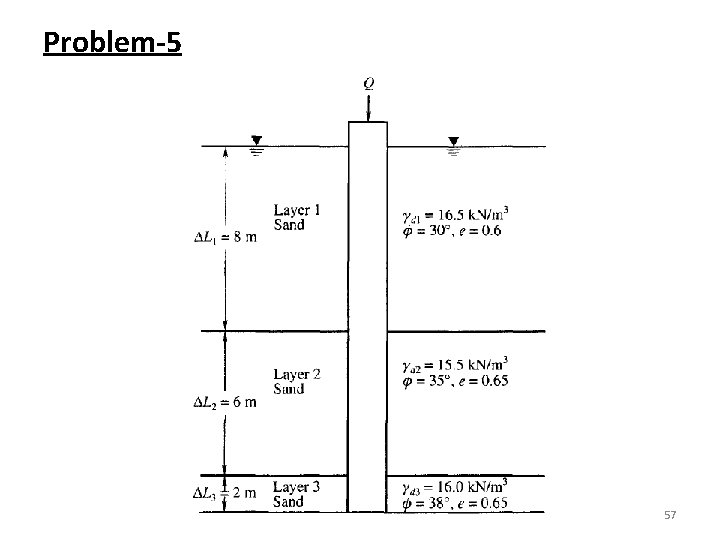
Problem-5 57
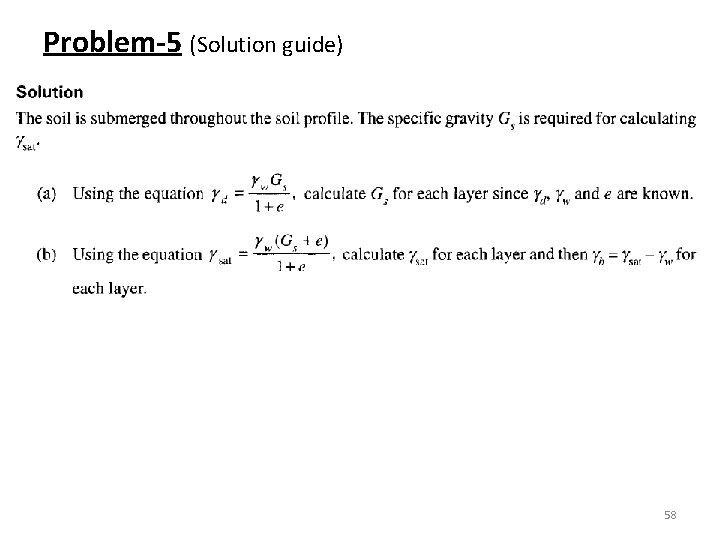
Problem-5 (Solution guide) 58
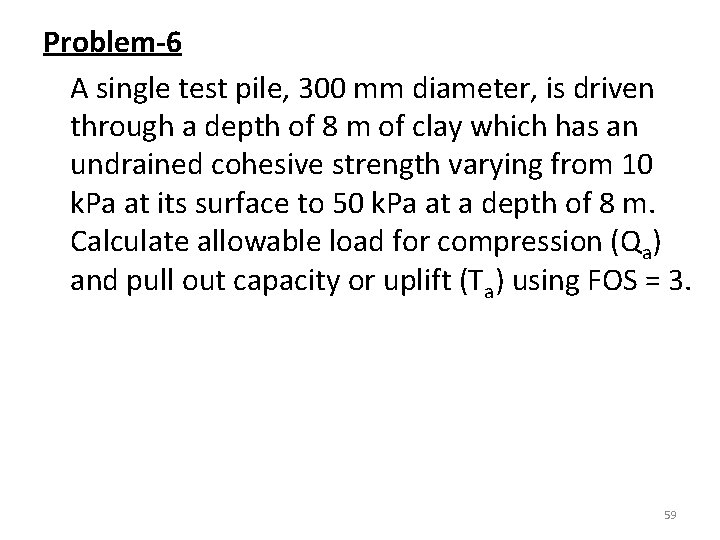
Problem-6 A single test pile, 300 mm diameter, is driven through a depth of 8 m of clay which has an undrained cohesive strength varying from 10 k. Pa at its surface to 50 k. Pa at a depth of 8 m. Calculate allowable load for compression (Qa) and pull out capacity or uplift (Ta) using FOS = 3. 59
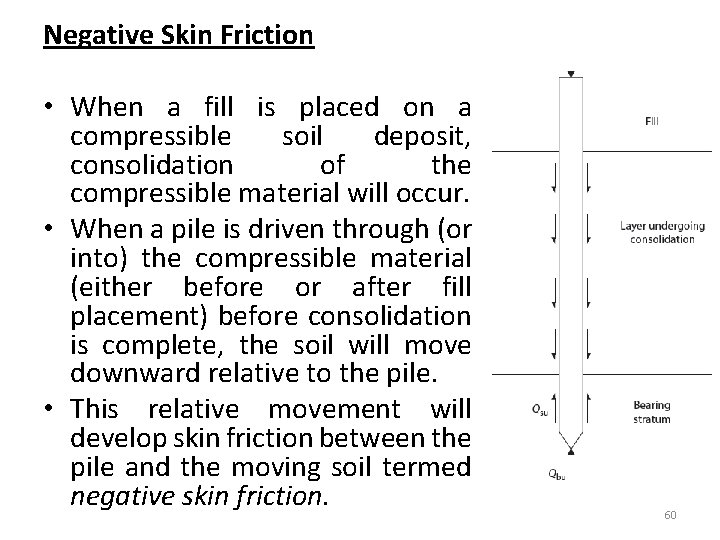
Negative Skin Friction • When a fill is placed on a compressible soil deposit, consolidation of the compressible material will occur. • When a pile is driven through (or into) the compressible material (either before or after fill placement) before consolidation is complete, the soil will move downward relative to the pile. • This relative movement will develop skin friction between the pile and the moving soil termed negative skin friction. 60
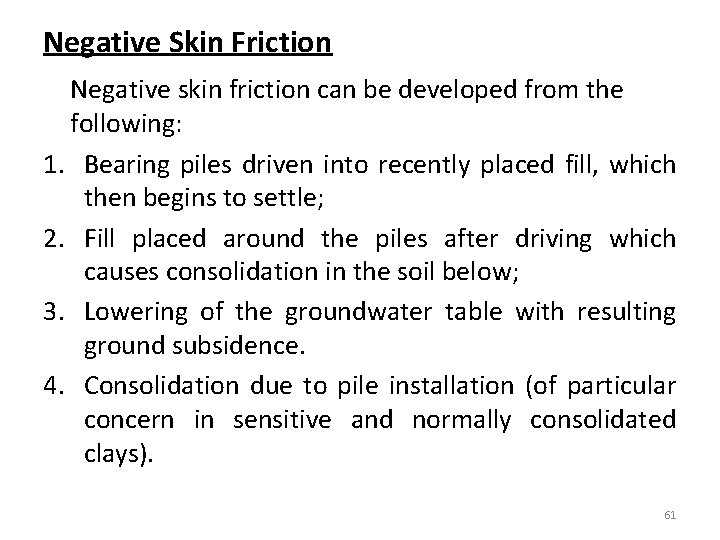
Negative Skin Friction Negative skin friction can be developed from the following: 1. Bearing piles driven into recently placed fill, which then begins to settle; 2. Fill placed around the piles after driving which causes consolidation in the soil below; 3. Lowering of the groundwater table with resulting ground subsidence. 4. Consolidation due to pile installation (of particular concern in sensitive and normally consolidated clays). 61
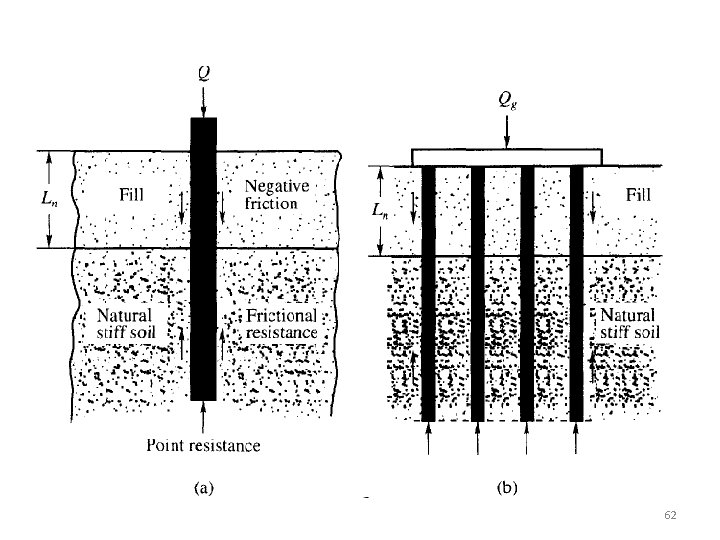
62
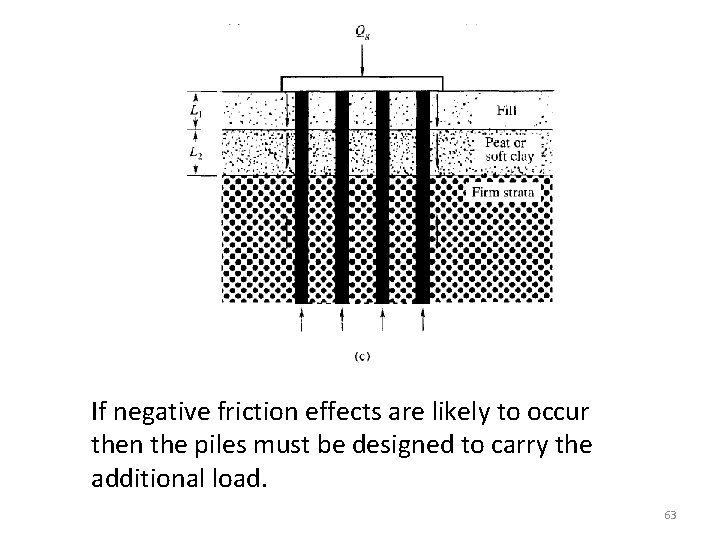
If negative friction effects are likely to occur then the piles must be designed to carry the additional load. 63
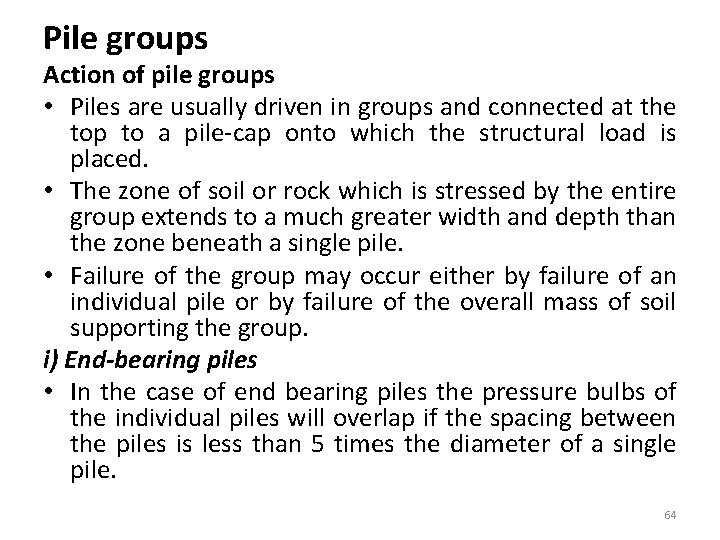
Pile groups Action of pile groups • Piles are usually driven in groups and connected at the top to a pile-cap onto which the structural load is placed. • The zone of soil or rock which is stressed by the entire group extends to a much greater width and depth than the zone beneath a single pile. • Failure of the group may occur either by failure of an individual pile or by failure of the overall mass of soil supporting the group. i) End-bearing piles • In the case of end bearing piles the pressure bulbs of the individual piles will overlap if the spacing between the piles is less than 5 times the diameter of a single pile. 64
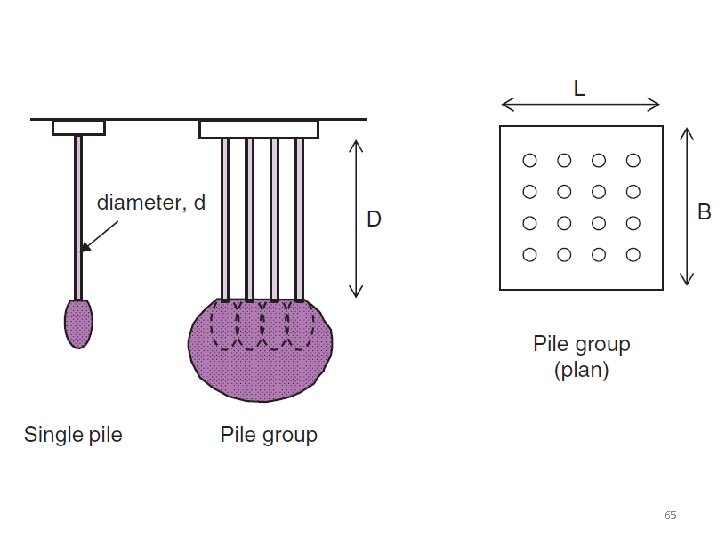
65
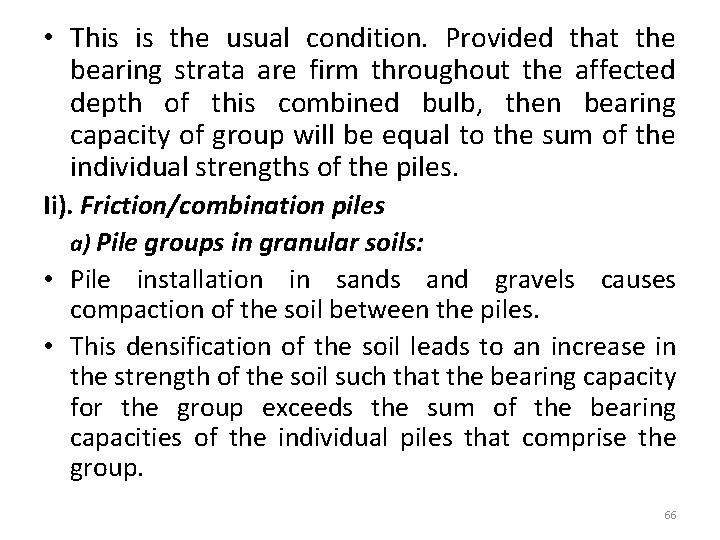
• This is the usual condition. Provided that the bearing strata are firm throughout the affected depth of this combined bulb, then bearing capacity of group will be equal to the sum of the individual strengths of the piles. Ii). Friction/combination piles a) Pile groups in granular soils: • Pile installation in sands and gravels causes compaction of the soil between the piles. • This densification of the soil leads to an increase in the strength of the soil such that the bearing capacity for the group exceeds the sum of the bearing capacities of the individual piles that comprise the group. 66
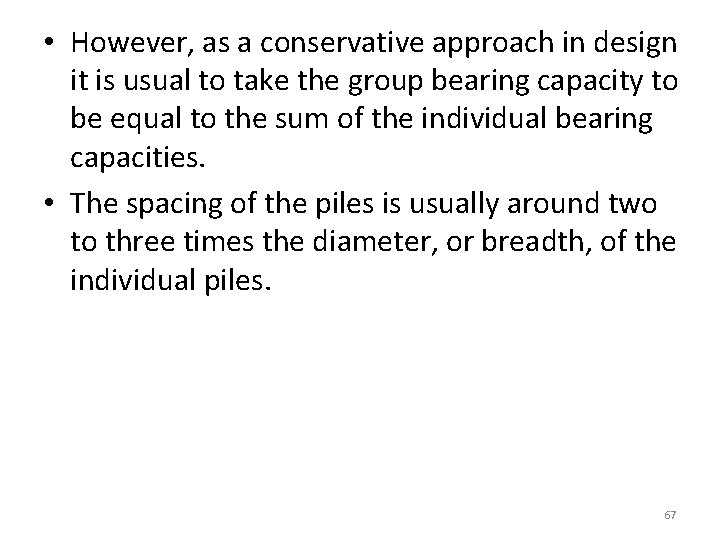
• However, as a conservative approach in design it is usual to take the group bearing capacity to be equal to the sum of the individual bearing capacities. • The spacing of the piles is usually around two to three times the diameter, or breadth, of the individual piles. 67
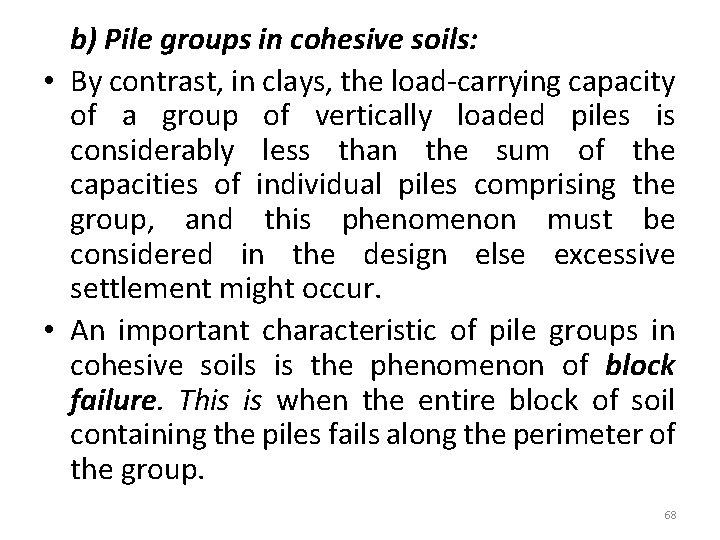
b) Pile groups in cohesive soils: • By contrast, in clays, the load-carrying capacity of a group of vertically loaded piles is considerably less than the sum of the capacities of individual piles comprising the group, and this phenomenon must be considered in the design else excessive settlement might occur. • An important characteristic of pile groups in cohesive soils is the phenomenon of block failure. This is when the entire block of soil containing the piles fails along the perimeter of the group. 68
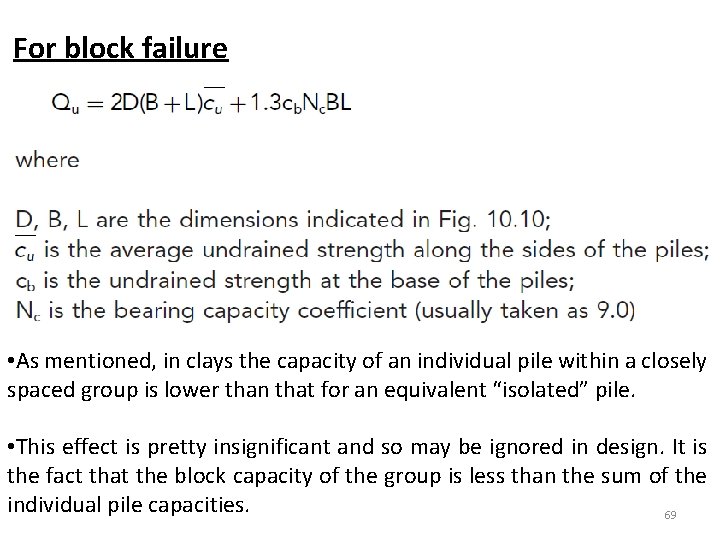
For block failure • As mentioned, in clays the capacity of an individual pile within a closely spaced group is lower than that for an equivalent “isolated” pile. • This effect is pretty insignificant and so may be ignored in design. It is the fact that the block capacity of the group is less than the sum of the individual pile capacities. 69
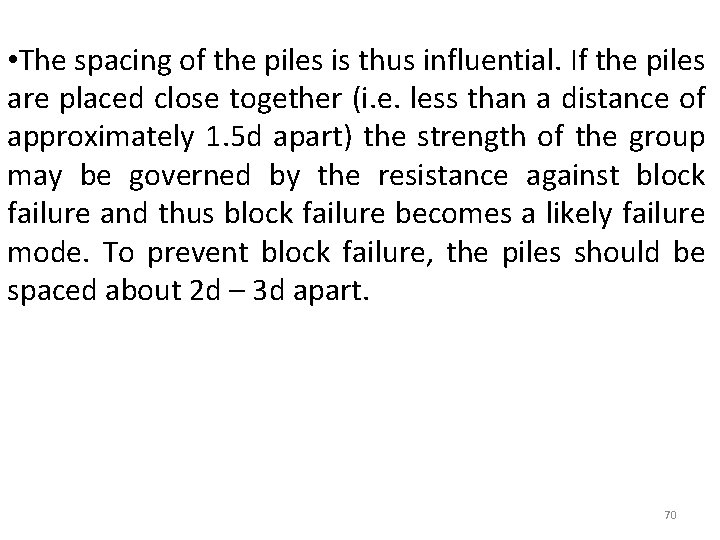
• The spacing of the piles is thus influential. If the piles are placed close together (i. e. less than a distance of approximately 1. 5 d apart) the strength of the group may be governed by the resistance against block failure and thus block failure becomes a likely failure mode. To prevent block failure, the piles should be spaced about 2 d – 3 d apart. 70
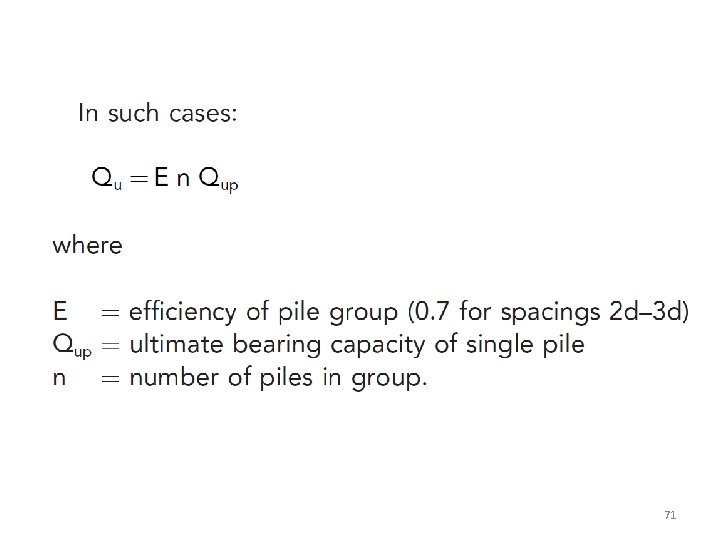
71
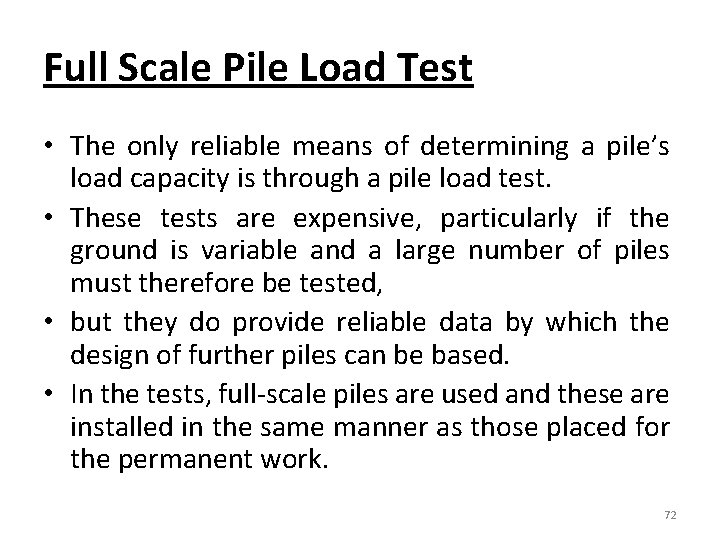
Full Scale Pile Load Test • The only reliable means of determining a pile’s load capacity is through a pile load test. • These tests are expensive, particularly if the ground is variable and a large number of piles must therefore be tested, • but they do provide reliable data by which the design of further piles can be based. • In the tests, full-scale piles are used and these are installed in the same manner as those placed for the permanent work. 72
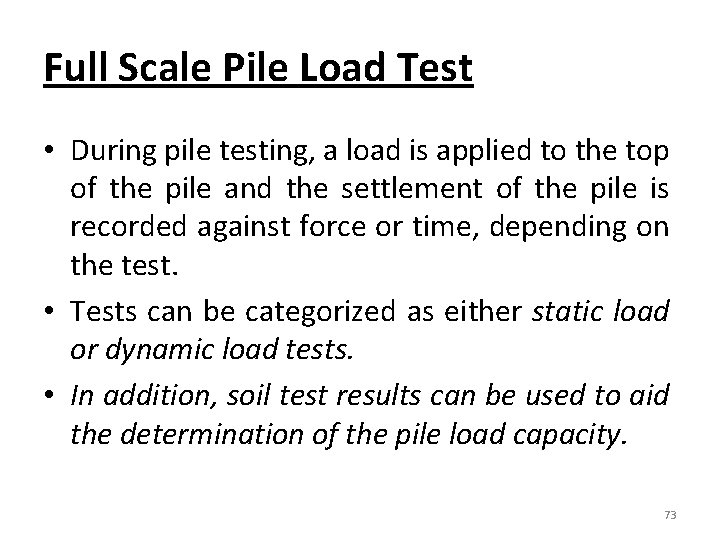
Full Scale Pile Load Test • During pile testing, a load is applied to the top of the pile and the settlement of the pile is recorded against force or time, depending on the test. • Tests can be categorized as either static load or dynamic load tests. • In addition, soil test results can be used to aid the determination of the pile load capacity. 73
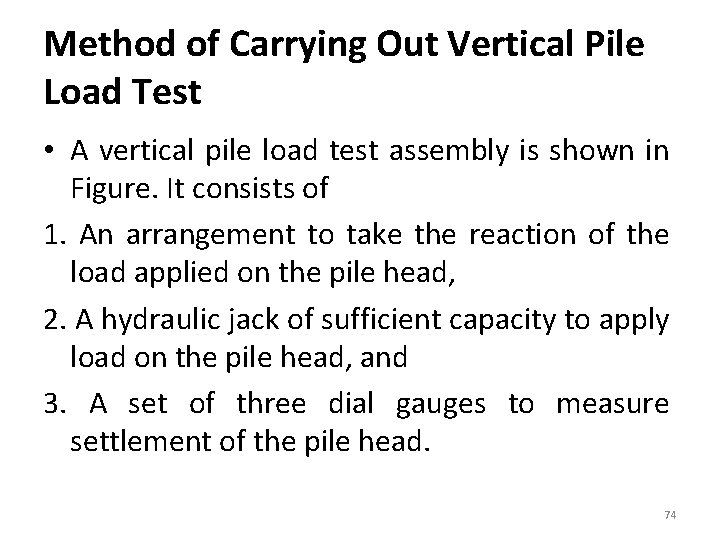
Method of Carrying Out Vertical Pile Load Test • A vertical pile load test assembly is shown in Figure. It consists of 1. An arrangement to take the reaction of the load applied on the pile head, 2. A hydraulic jack of sufficient capacity to apply load on the pile head, and 3. A set of three dial gauges to measure settlement of the pile head. 74
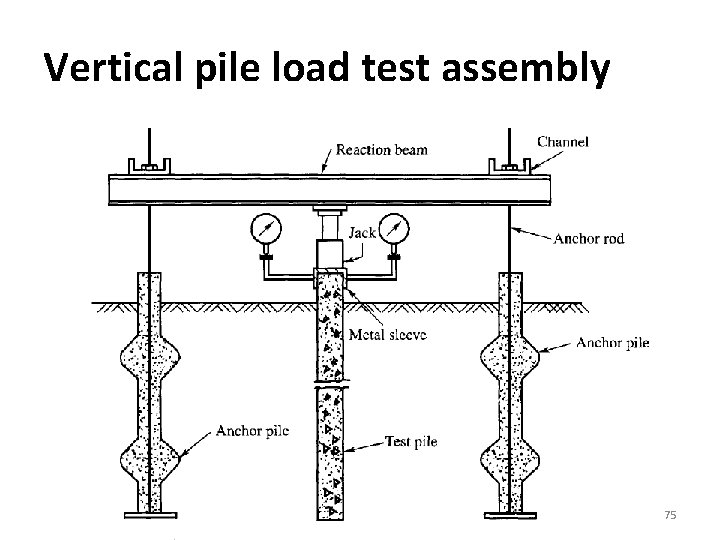
Vertical pile load test assembly 75
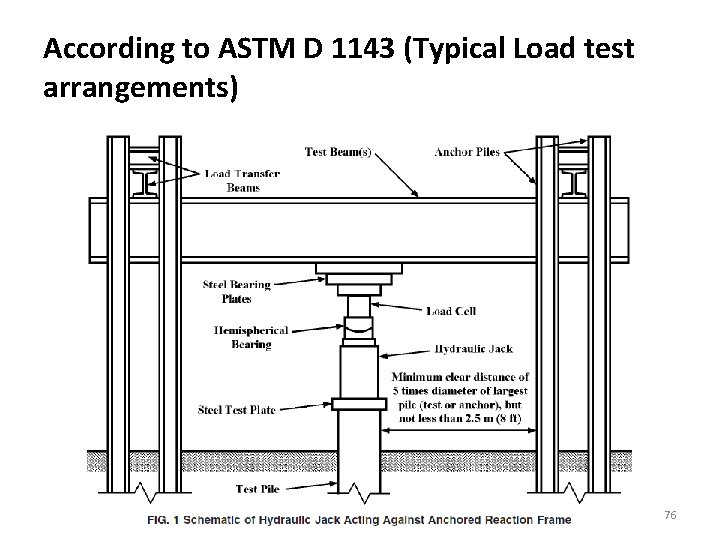
According to ASTM D 1143 (Typical Load test arrangements) 76
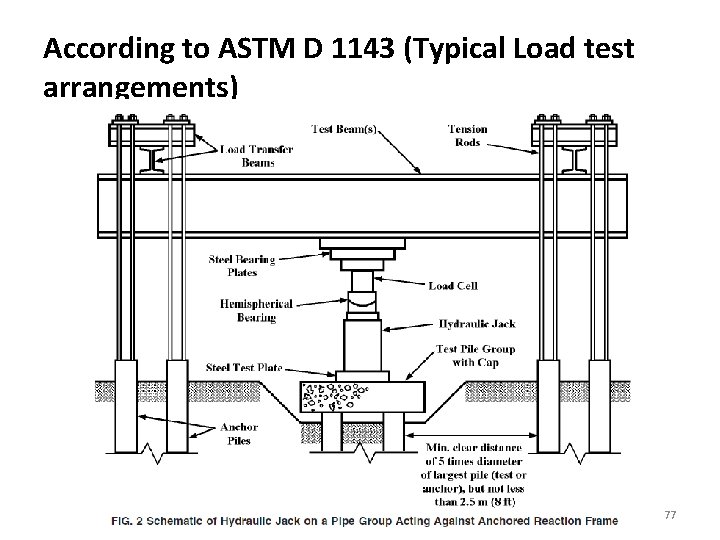
According to ASTM D 1143 (Typical Load test arrangements) 77
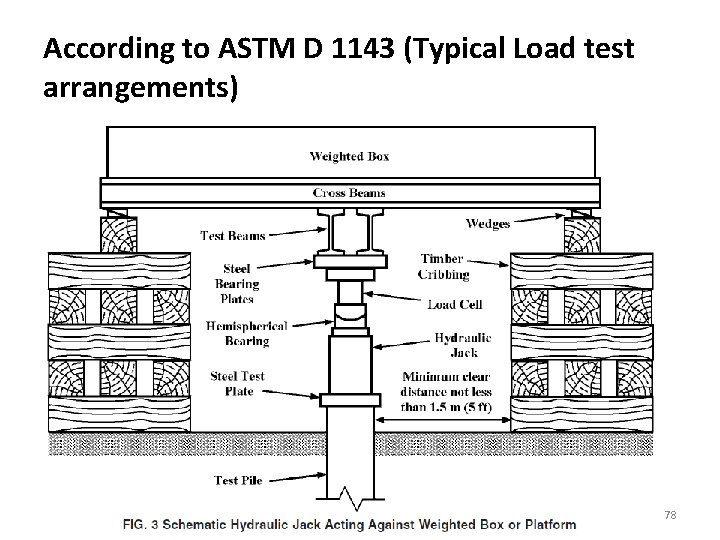
According to ASTM D 1143 (Typical Load test arrangements) 78
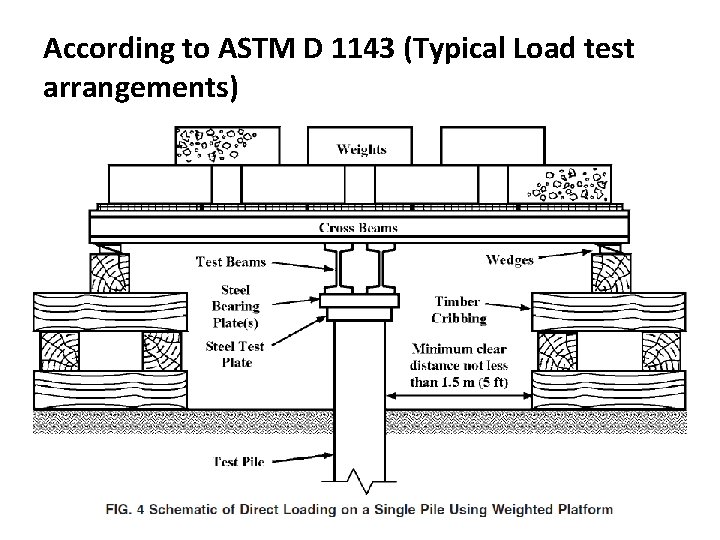
According to ASTM D 1143 (Typical Load test arrangements) 79
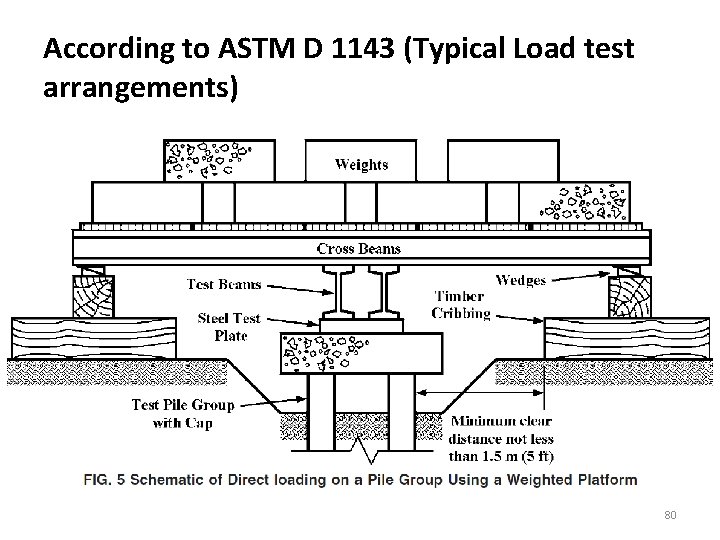
According to ASTM D 1143 (Typical Load test arrangements) 80
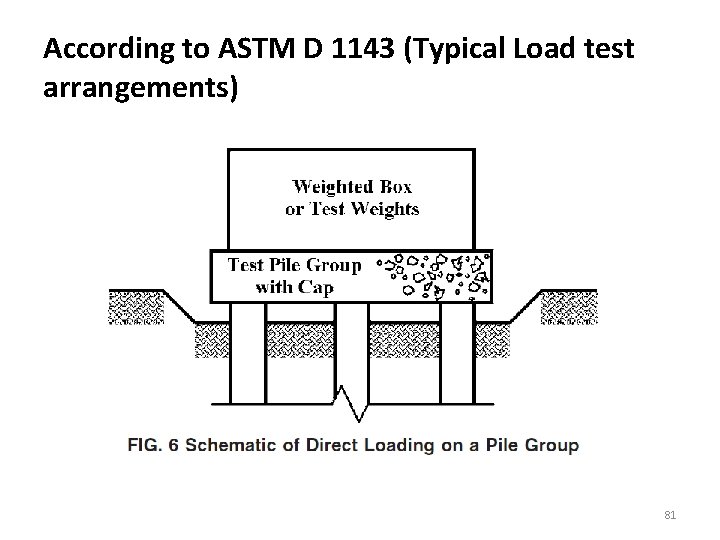
According to ASTM D 1143 (Typical Load test arrangements) 81
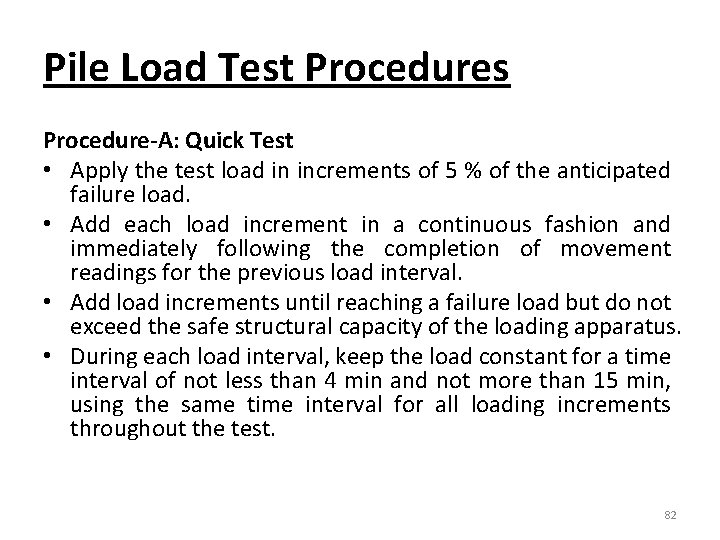
Pile Load Test Procedures Procedure-A: Quick Test • Apply the test load in increments of 5 % of the anticipated failure load. • Add each load increment in a continuous fashion and immediately following the completion of movement readings for the previous load interval. • Add load increments until reaching a failure load but do not exceed the safe structural capacity of the loading apparatus. • During each load interval, keep the load constant for a time interval of not less than 4 min and not more than 15 min, using the same time interval for all loading increments throughout the test. 82
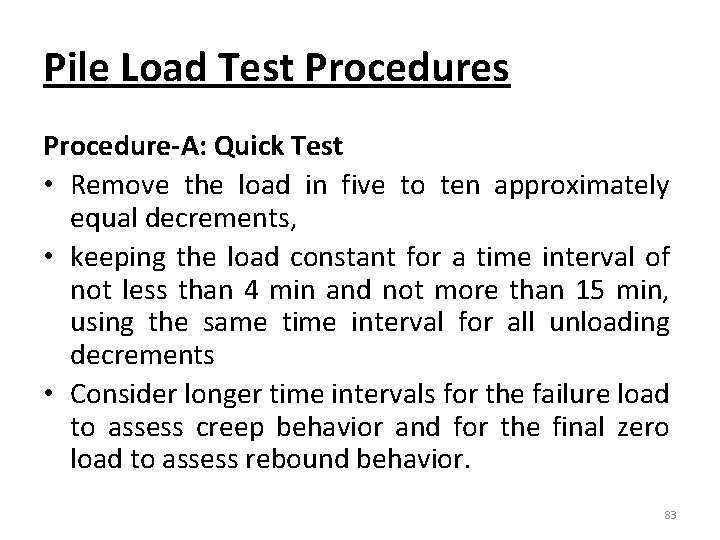
Pile Load Test Procedures Procedure-A: Quick Test • Remove the load in five to ten approximately equal decrements, • keeping the load constant for a time interval of not less than 4 min and not more than 15 min, using the same time interval for all unloading decrements • Consider longer time intervals for the failure load to assess creep behavior and for the final zero load to assess rebound behavior. 83
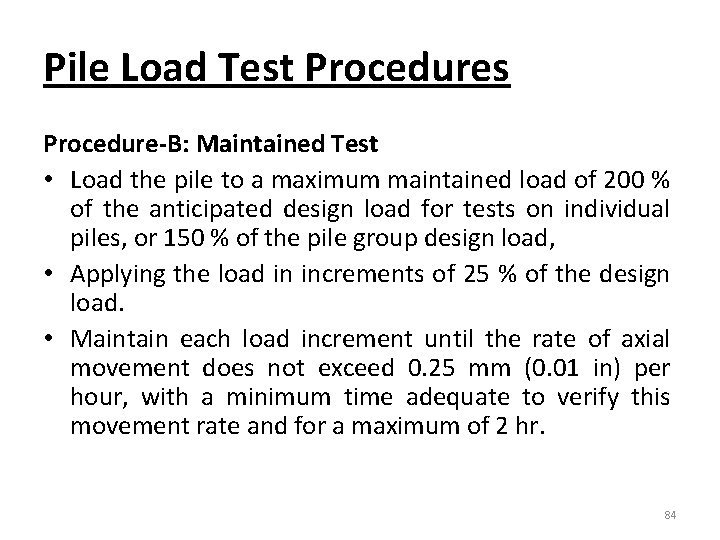
Pile Load Test Procedures Procedure-B: Maintained Test • Load the pile to a maximum maintained load of 200 % of the anticipated design load for tests on individual piles, or 150 % of the pile group design load, • Applying the load in increments of 25 % of the design load. • Maintain each load increment until the rate of axial movement does not exceed 0. 25 mm (0. 01 in) per hour, with a minimum time adequate to verify this movement rate and for a maximum of 2 hr. 84
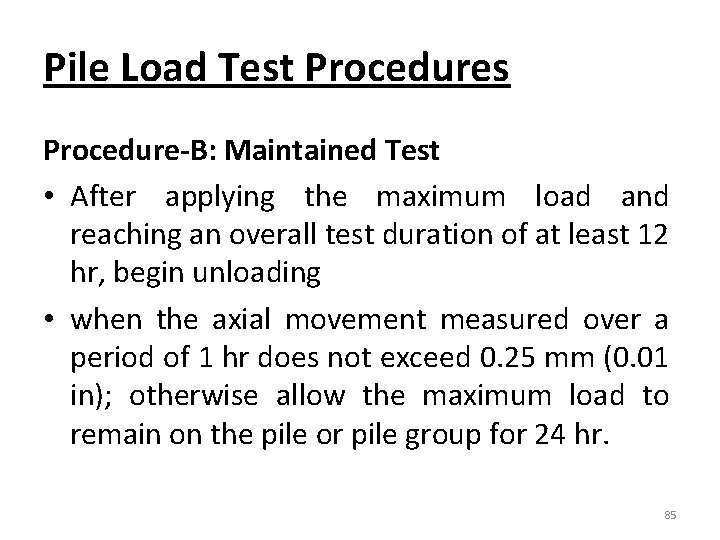
Pile Load Test Procedures Procedure-B: Maintained Test • After applying the maximum load and reaching an overall test duration of at least 12 hr, begin unloading • when the axial movement measured over a period of 1 hr does not exceed 0. 25 mm (0. 01 in); otherwise allow the maximum load to remain on the pile or pile group for 24 hr. 85
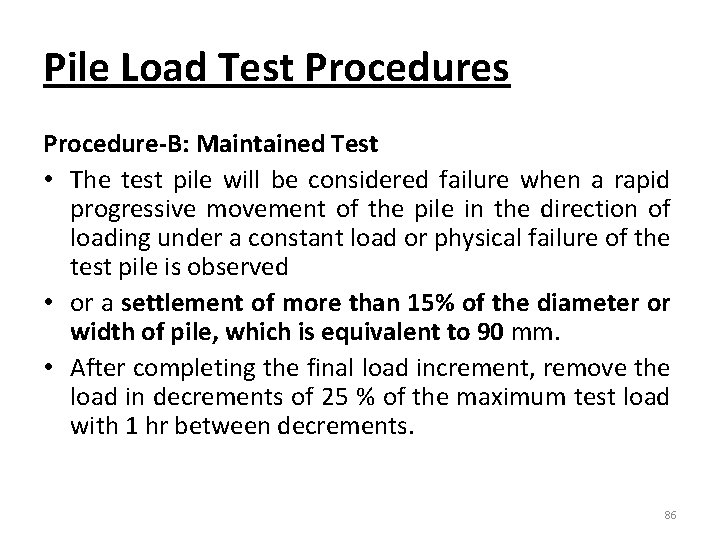
Pile Load Test Procedures Procedure-B: Maintained Test • The test pile will be considered failure when a rapid progressive movement of the pile in the direction of loading under a constant load or physical failure of the test pile is observed • or a settlement of more than 15% of the diameter or width of pile, which is equivalent to 90 mm. • After completing the final load increment, remove the load in decrements of 25 % of the maximum test load with 1 hr between decrements. 86
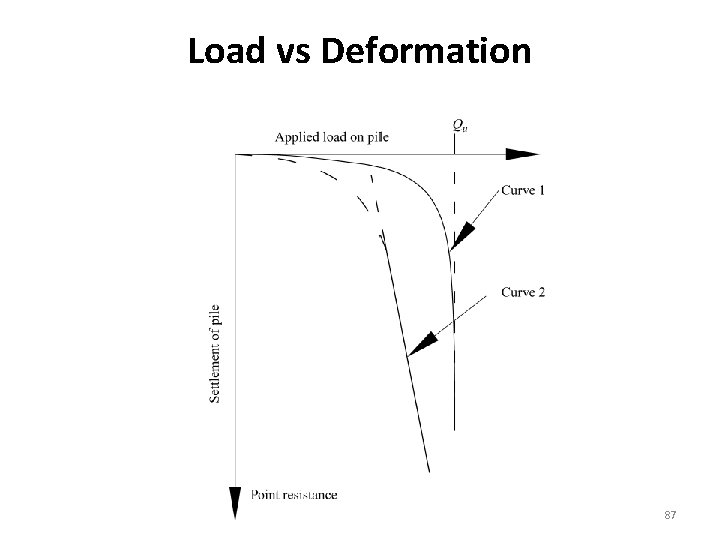
Load vs Deformation 87
Hotel math fundamentals
Deep asleep deep asleep it lies
Deep forest towards an alternative to deep neural networks
深哉深哉耶穌的愛
Idempotent law example
The foundations logic and proofs
Pre k curriculum california
What are the five psychological foundations of curriculum
New foundations anderson sc
Chapter 6 lesson 1 foundations of a healthy relationship
Indiana early learning foundations
History and geography: the foundations of culture
Biographical characteristic of an employee
Foundations of business 5th edition
Cognitive foundations of entrepreneurship
Cognitive foundations of entrepreneurship
Cy 2550 northeastern
Chapter 6 skills for healthy relationships lesson 1
Describe the care team and the chain of command
Foundations of individual behavior
California preschool learning foundations
California preschool learning foundations volume 1
Aohs foundations of anatomy and physiology 1
Aohs foundations of anatomy and physiology 2
Foundations and earth retaining structures
Preschool learning foundations volume 3
Sigma simplicity
Foundations of planning
Building the right foundations/fundamentals
Preschool learning foundations social emotional
Preschool learning foundations
Encounters and foundations to 1800
Secure foundations
Philosophical foundations of curriculum
Mathematical foundations of computer graphics and vision
Foundations of statistical natural language processing
Different foundations of curriculum
Preschool learning foundations math
4 foundations of marketing
Pencounters
Foundations and encounters early american literature
What is cyber foundations class
Cit 592
Chapter 7 organizational behavior
Historical foundations of curriculum
Foundations of software testing aditya p mathur pdf
Foundations of information systems in business
Foundations of business solutions
Foundations of government section 3
Chapter one foundations of government
Types of building foundations
Aohs foundations of anatomy and physiology 1
Aohs foundations of anatomy and physiology 1
Philosophical foundations of the american revolution
Collocation nlp
Foundations of addictions counseling 3rd edition
Foundations of faith elder cook
Design of mat
Chapter 4 foundations background to american history
Section 2 three basic reasons to save money
Foundation of organizational structure
Theoretical foundations of transcultural nursing
The speed at which the body consumes energy
Aohs foundations of anatomy and physiology 1
Chapter 1 review personal finance
Wharton business foundations capstone
Types of caisson
Foundations of privacy
Chapter 1 foundations of government vocabulary
Unit 1 foundations of american government
The generic term for the guest room sheets and pillowcases
Foundations of corporate finance
Aws academy cloud foundations (acf)
Software architecture foundations theory and practice
Sales foundations for success
Preschool learning foundations
Lotus bunkering
Software architecture foundations theory and practice
The purpose of menu
Multinational financial management shapiro
Foundations of group behavior
Chapter 1: foundations of government pdf
Foundations of american government unit 1 test review
Draw a strip foundation
Clinical supervision foundations ii
Foundations of government (chapter 1 test form a)
Batch sequential architecture
Social and emotional foundations for early learning