chapter 3 Fuel cell types chapter 3 Fuel
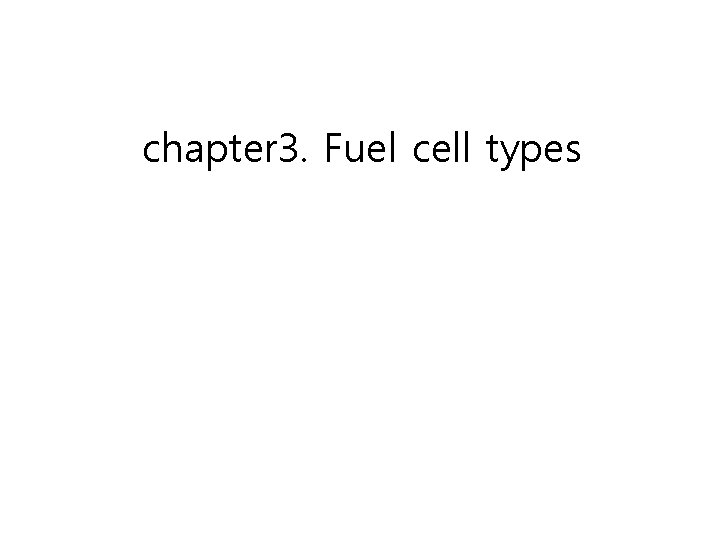
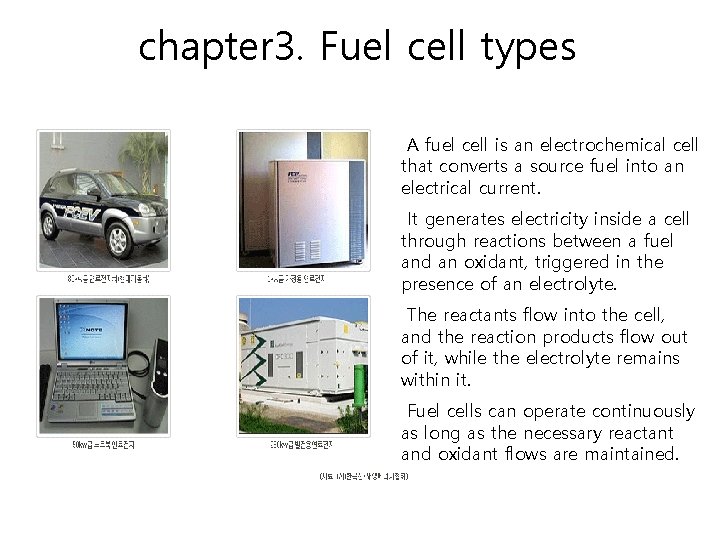
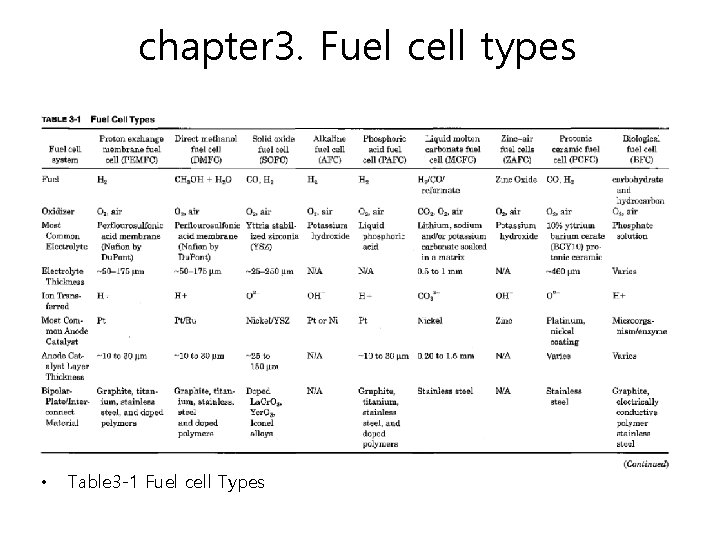
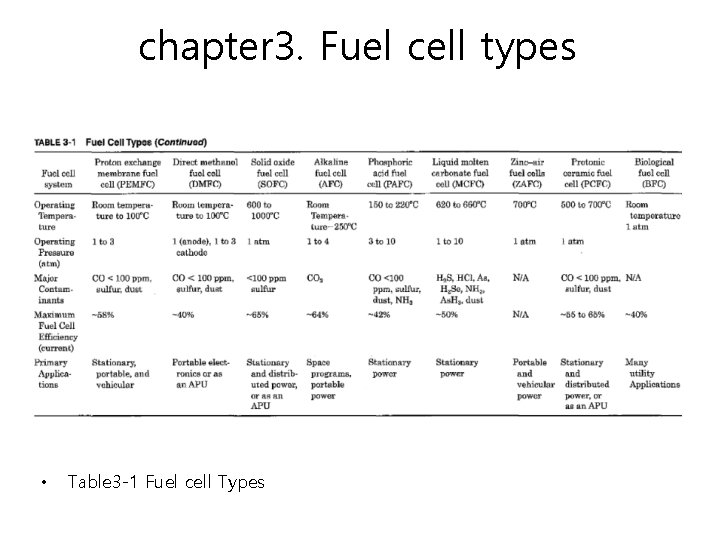
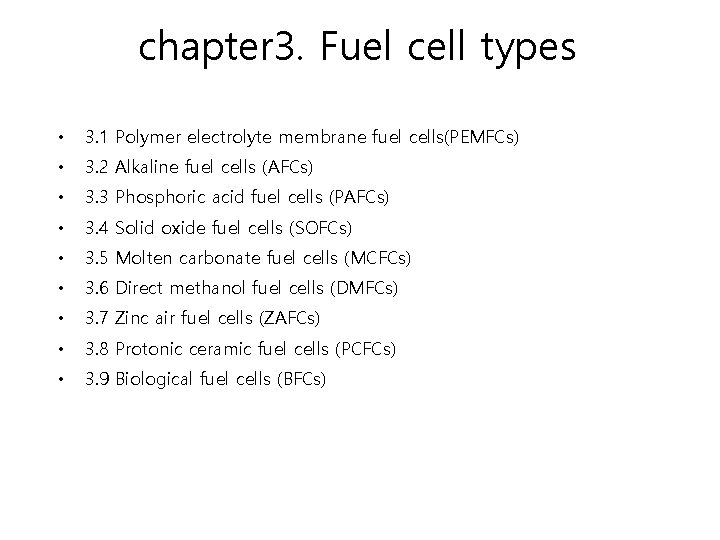
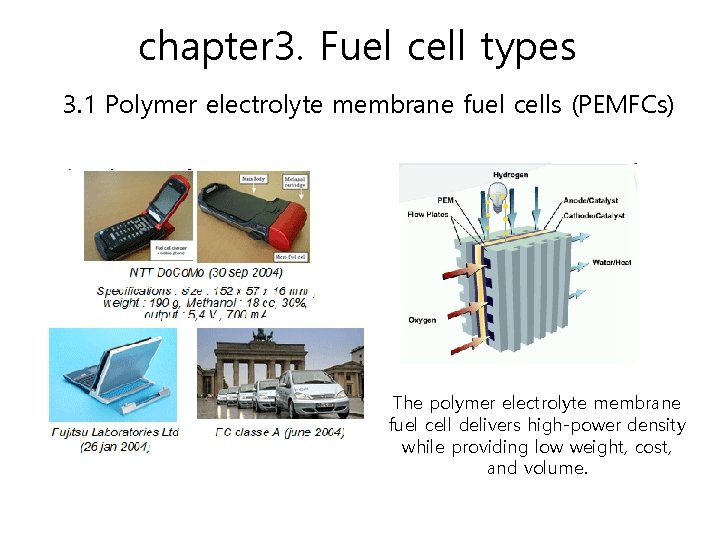
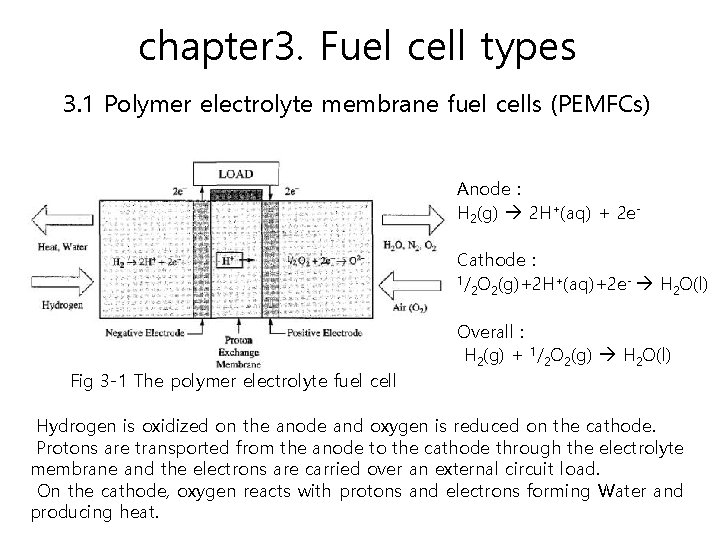
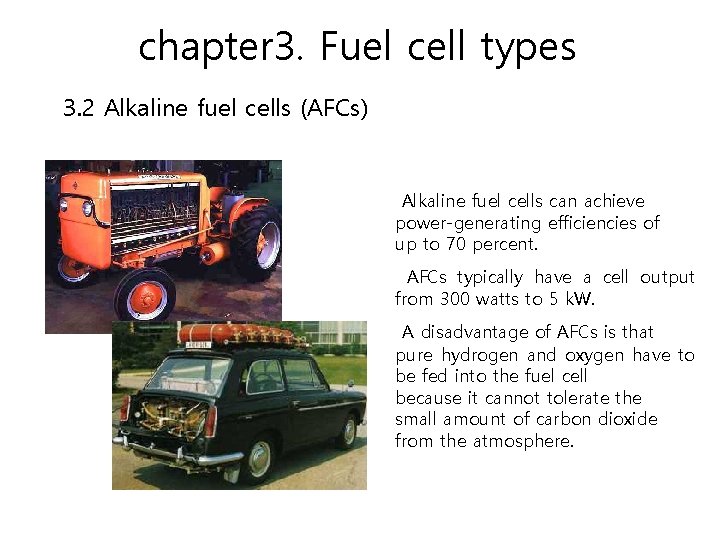
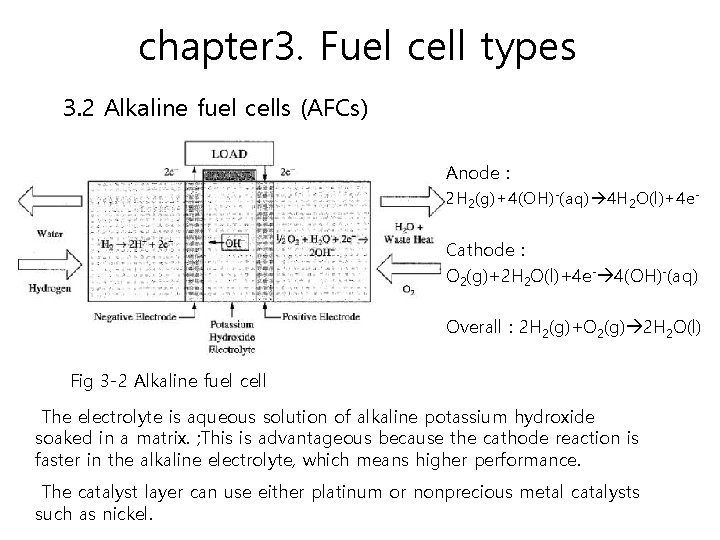
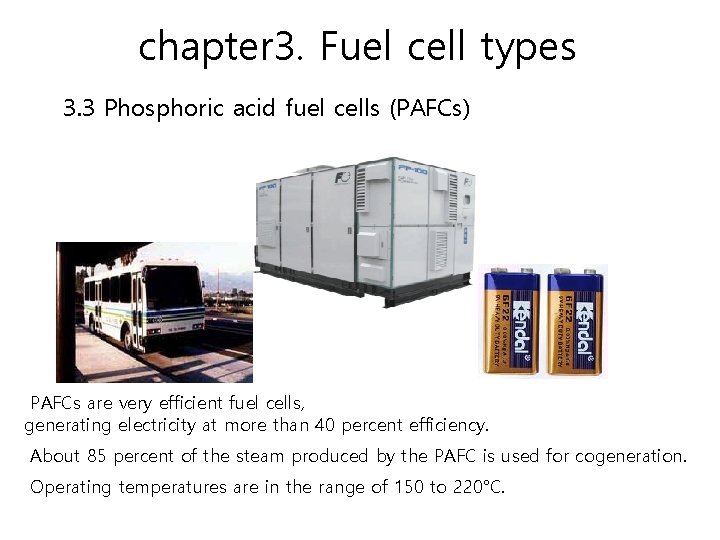
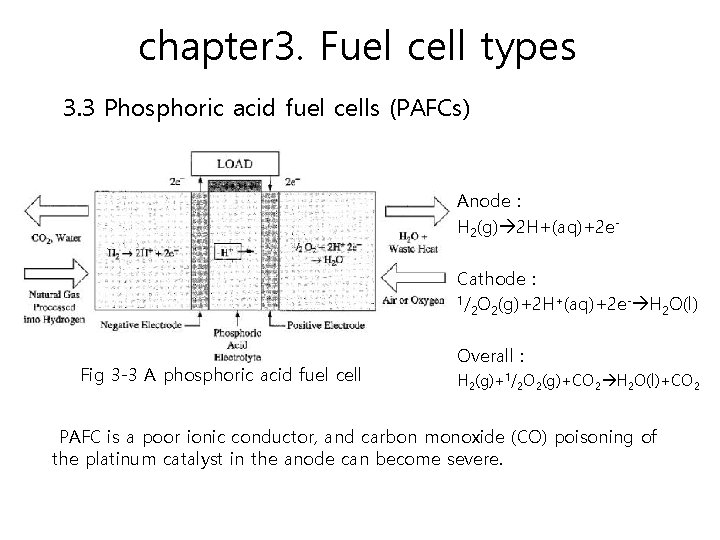
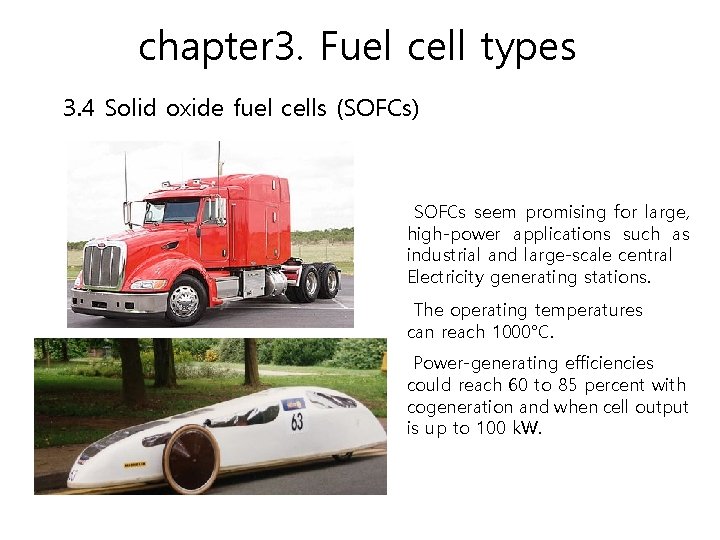
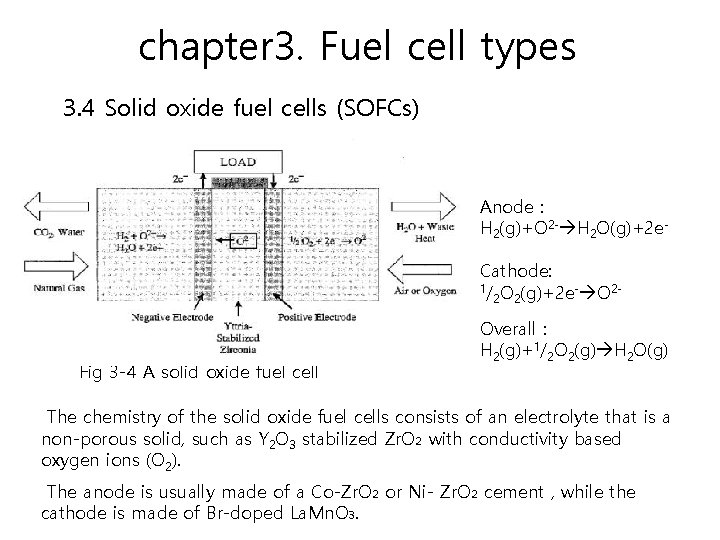
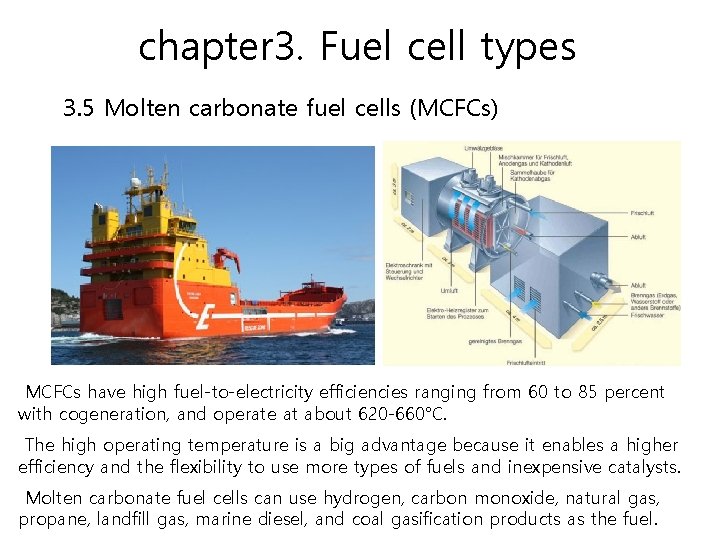
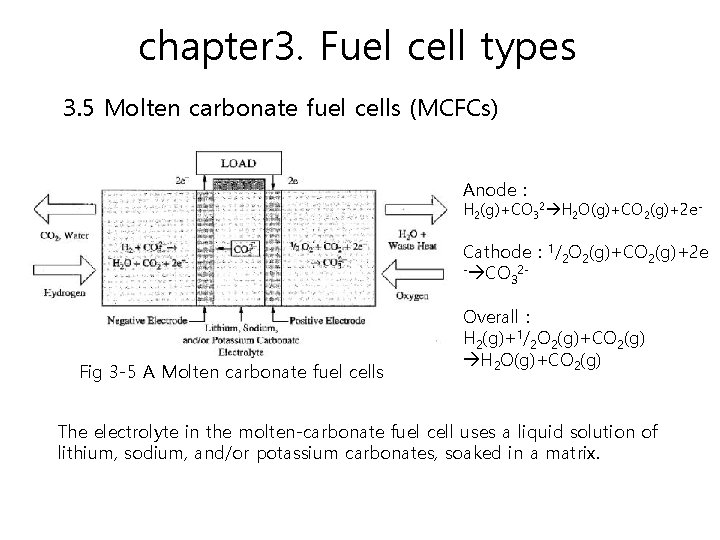
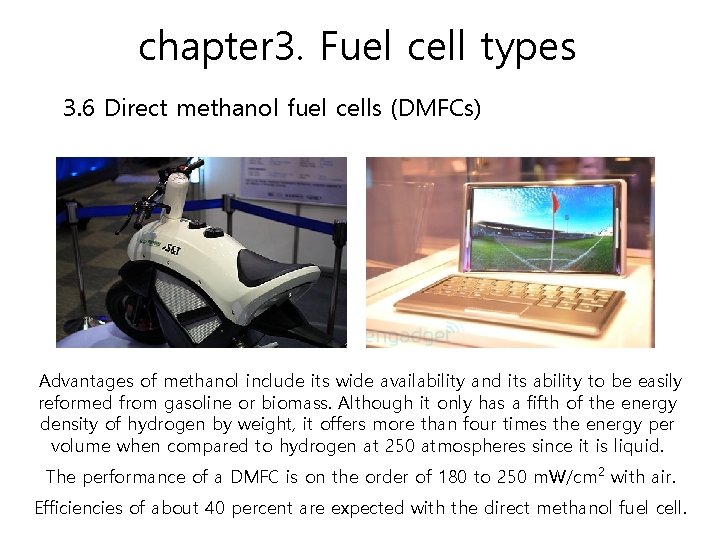
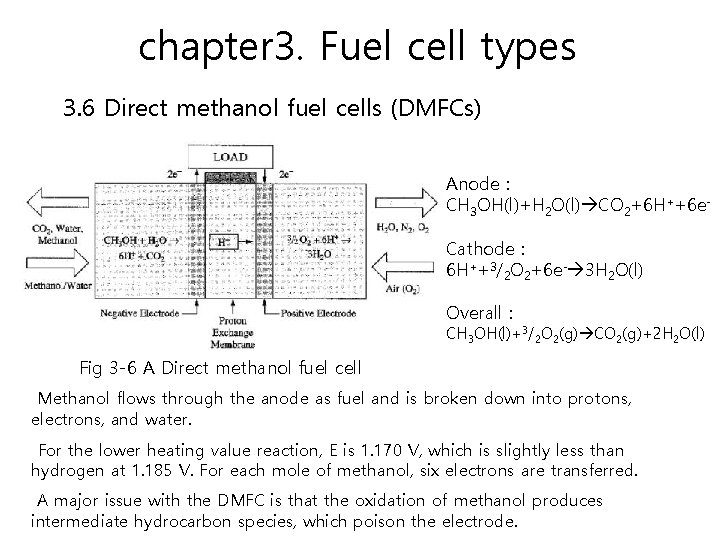
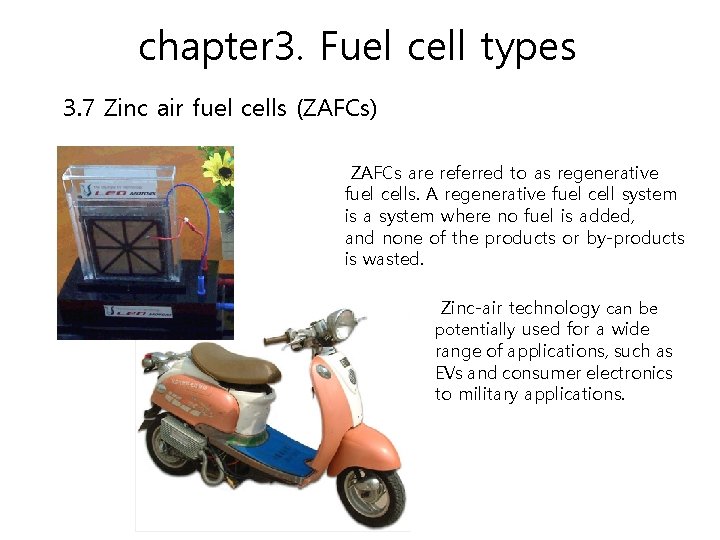
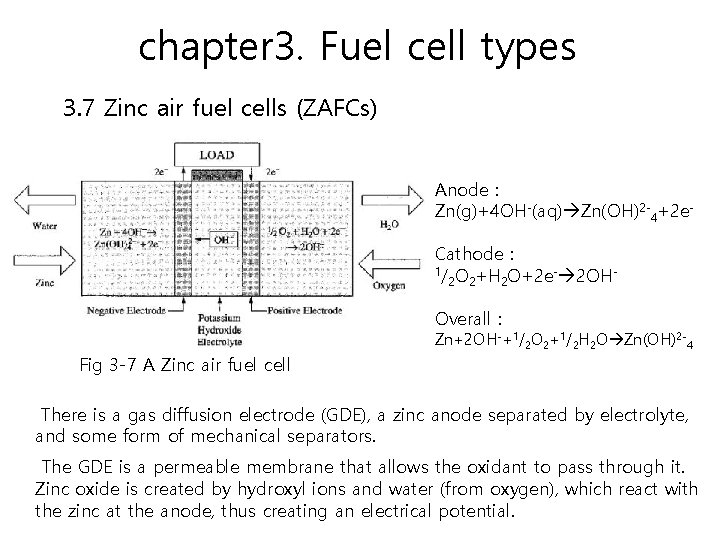
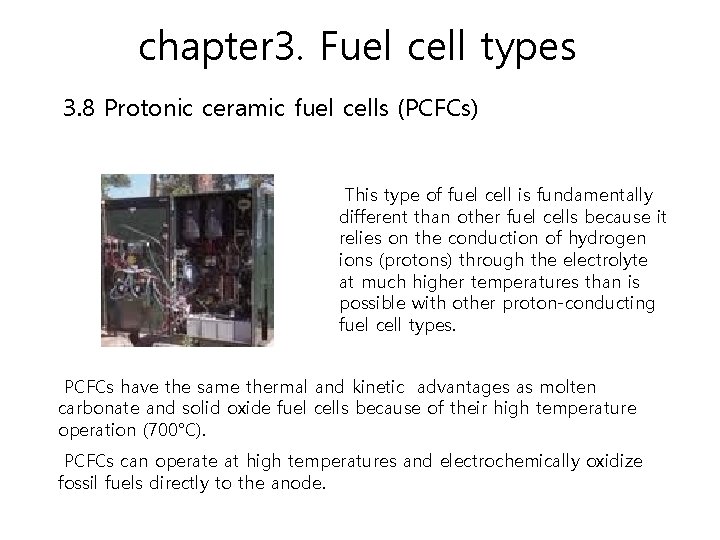
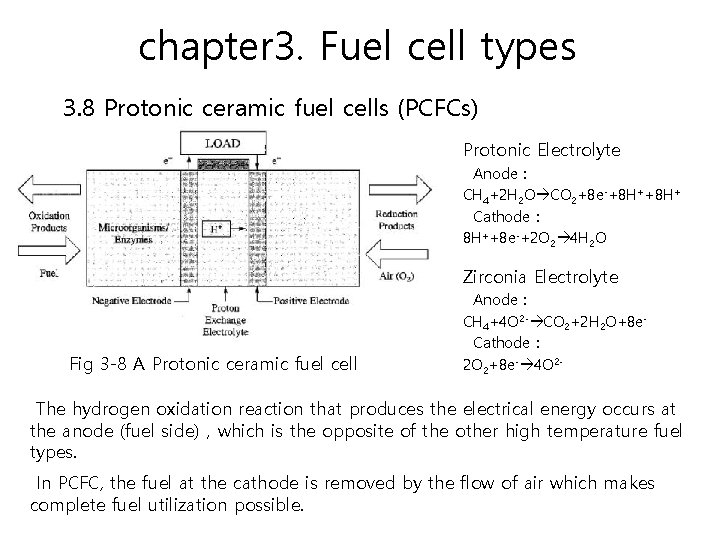
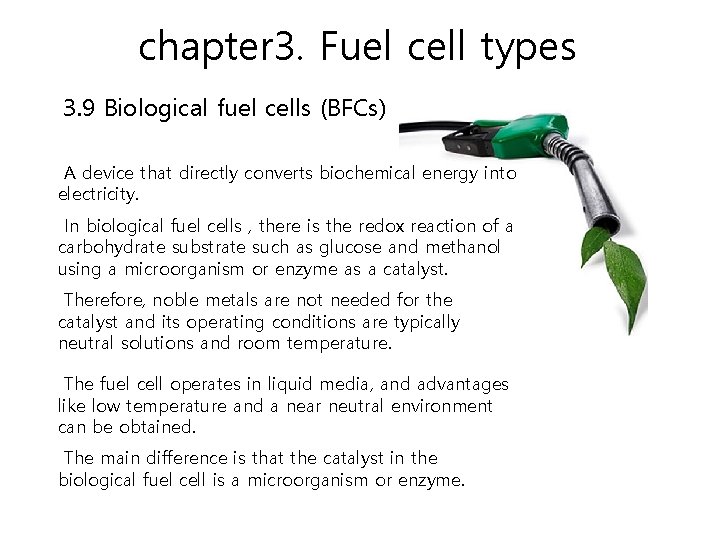
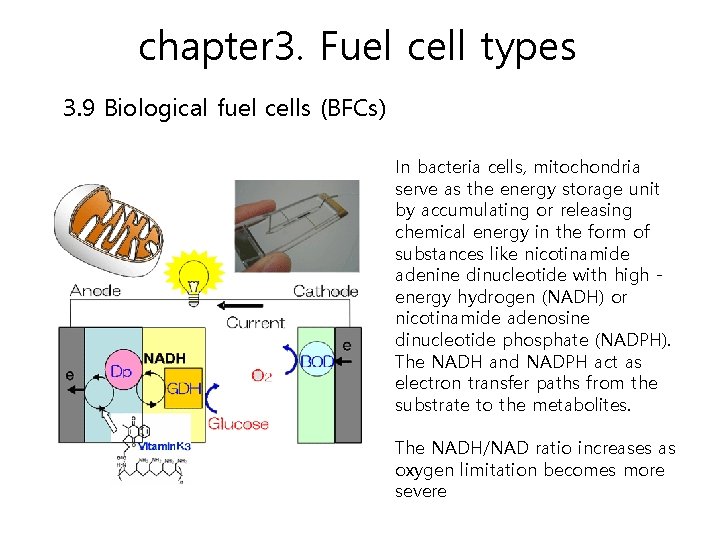
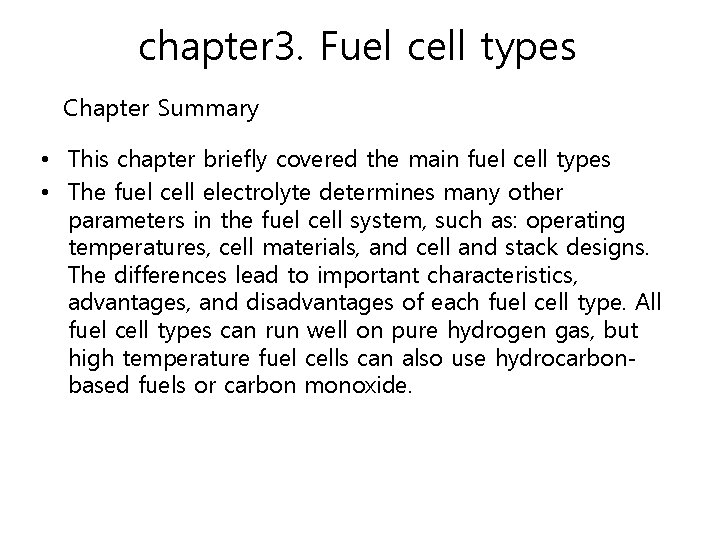
- Slides: 24
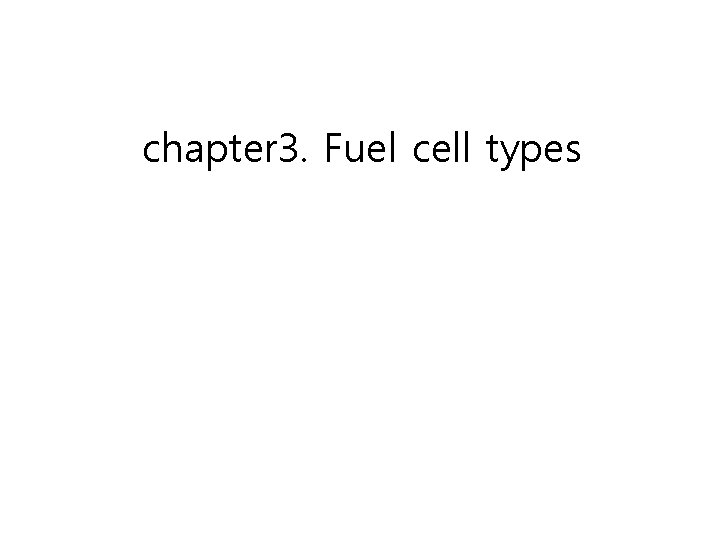
chapter 3. Fuel cell types
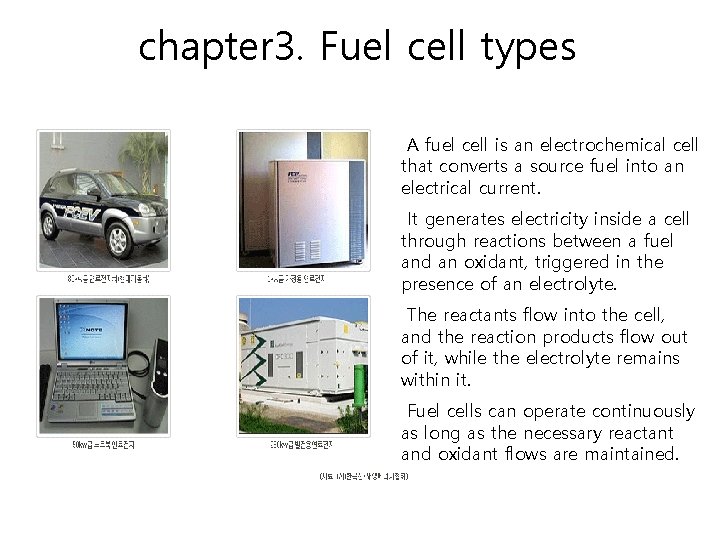
chapter 3. Fuel cell types A fuel cell is an electrochemical cell that converts a source fuel into an electrical current. It generates electricity inside a cell through reactions between a fuel and an oxidant, triggered in the presence of an electrolyte. The reactants flow into the cell, and the reaction products flow out of it, while the electrolyte remains within it. Fuel cells can operate continuously as long as the necessary reactant and oxidant flows are maintained.
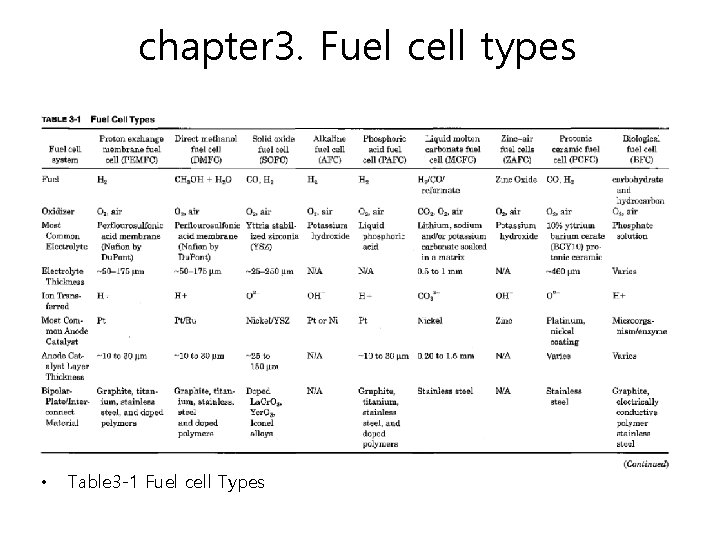
chapter 3. Fuel cell types • Table 3 -1 Fuel cell Types
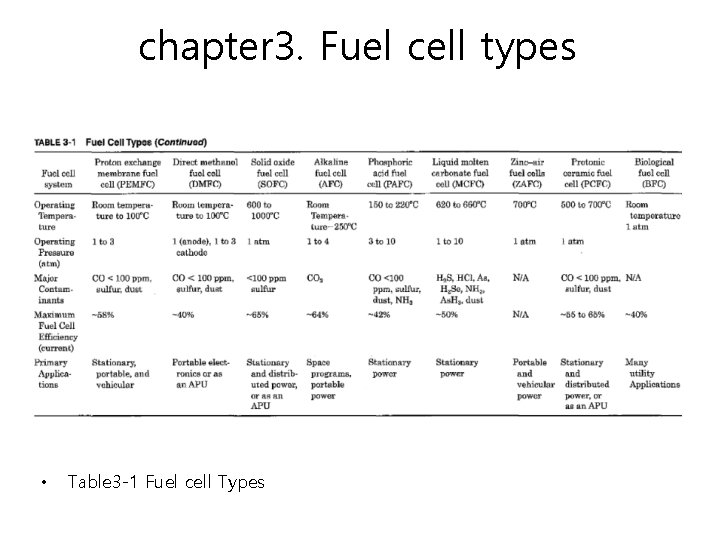
chapter 3. Fuel cell types • Table 3 -1 Fuel cell Types
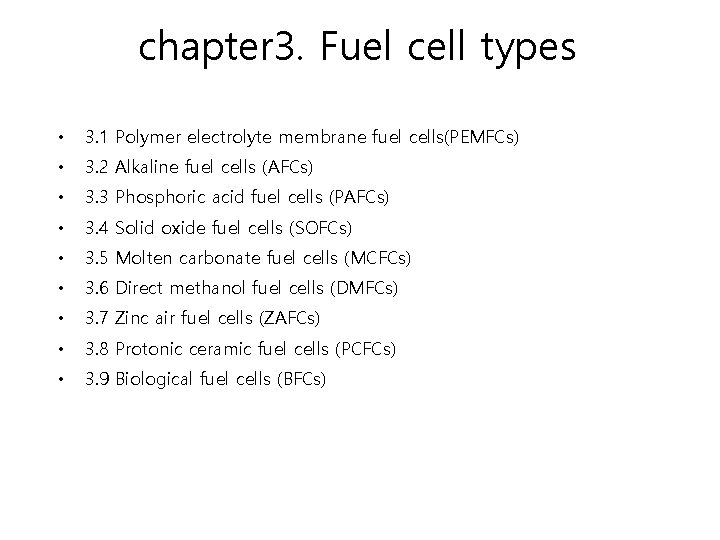
chapter 3. Fuel cell types • 3. 1 Polymer electrolyte membrane fuel cells(PEMFCs) • 3. 2 Alkaline fuel cells (AFCs) • 3. 3 Phosphoric acid fuel cells (PAFCs) • 3. 4 Solid oxide fuel cells (SOFCs) • 3. 5 Molten carbonate fuel cells (MCFCs) • 3. 6 Direct methanol fuel cells (DMFCs) • 3. 7 Zinc air fuel cells (ZAFCs) • 3. 8 Protonic ceramic fuel cells (PCFCs) • 3. 9 Biological fuel cells (BFCs)
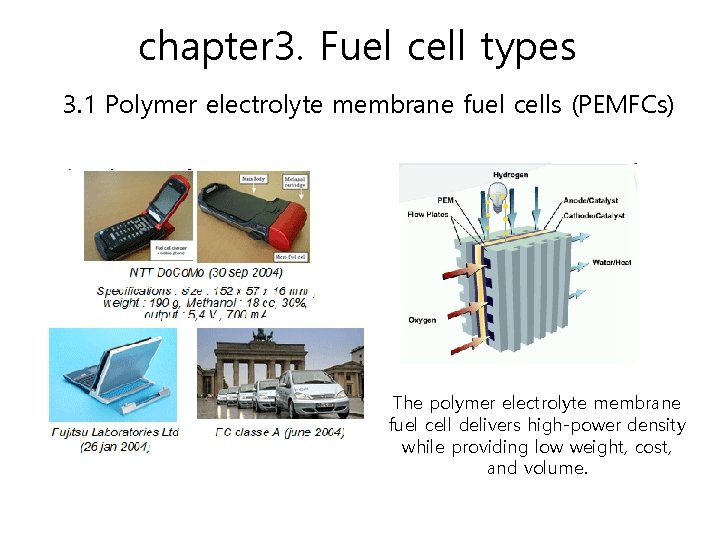
chapter 3. Fuel cell types 3. 1 Polymer electrolyte membrane fuel cells (PEMFCs) The polymer electrolyte membrane fuel cell delivers high-power density while providing low weight, cost, and volume.
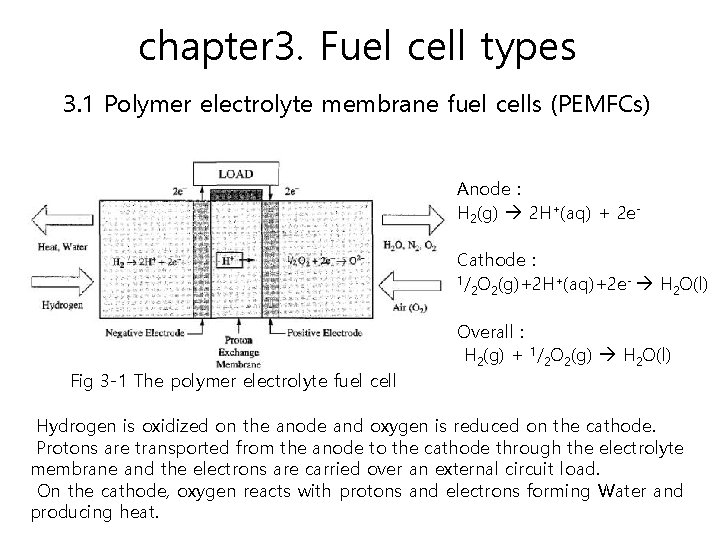
chapter 3. Fuel cell types 3. 1 Polymer electrolyte membrane fuel cells (PEMFCs) Anode : H 2(g) 2 H+(aq) + 2 e. Cathode : 1/ O (g)+2 H+(aq)+2 e- H O(l) 2 2 2 Fig 3 -1 The polymer electrolyte fuel cell Overall : H 2(g) + 1/2 O 2(g) H 2 O(l) Hydrogen is oxidized on the anode and oxygen is reduced on the cathode. Protons are transported from the anode to the cathode through the electrolyte membrane and the electrons are carried over an external circuit load. On the cathode, oxygen reacts with protons and electrons forming Water and producing heat.
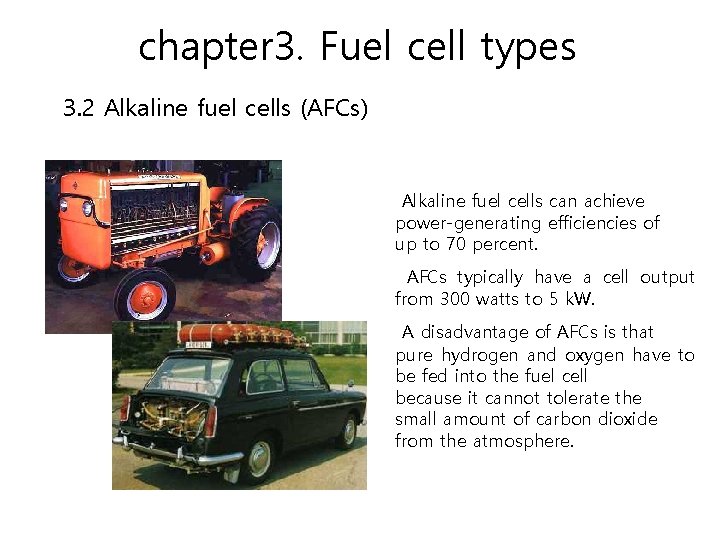
chapter 3. Fuel cell types 3. 2 Alkaline fuel cells (AFCs) Alkaline fuel cells can achieve power-generating efficiencies of up to 70 percent. AFCs typically have a cell output from 300 watts to 5 k. W. A disadvantage of AFCs is that pure hydrogen and oxygen have to be fed into the fuel cell because it cannot tolerate the small amount of carbon dioxide from the atmosphere.
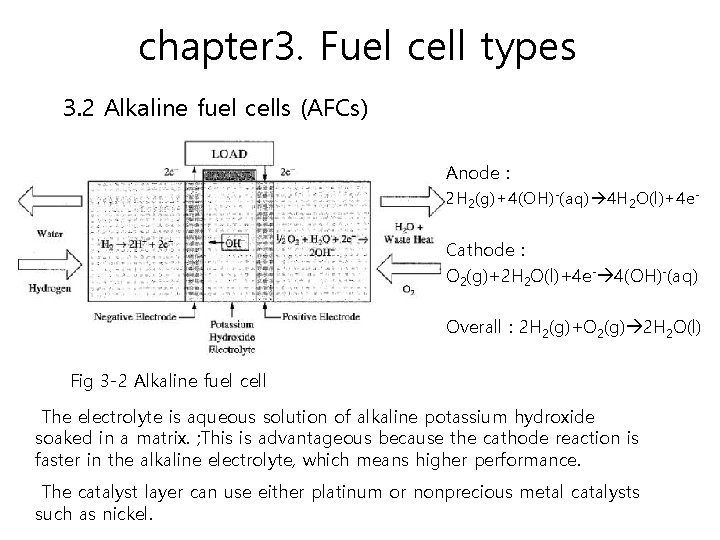
chapter 3. Fuel cell types 3. 2 Alkaline fuel cells (AFCs) Anode : 2 H 2(g)+4(OH)-(aq) 4 H 2 O(l)+4 e. Cathode : O 2(g)+2 H 2 O(l)+4 e- 4(OH)-(aq) Overall : 2 H 2(g)+O 2(g) 2 H 2 O(l) Fig 3 -2 Alkaline fuel cell The electrolyte is aqueous solution of alkaline potassium hydroxide soaked in a matrix. ; This is advantageous because the cathode reaction is faster in the alkaline electrolyte, which means higher performance. The catalyst layer can use either platinum or nonprecious metal catalysts such as nickel.
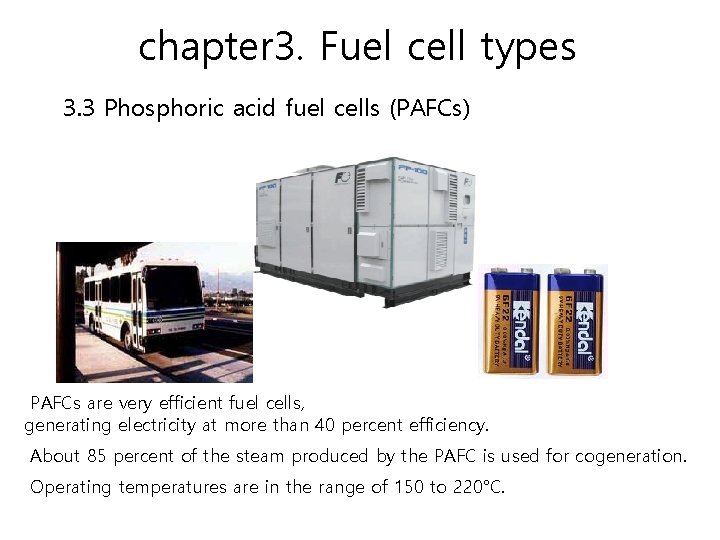
chapter 3. Fuel cell types 3. 3 Phosphoric acid fuel cells (PAFCs) PAFCs are very efficient fuel cells, generating electricity at more than 40 percent efficiency. About 85 percent of the steam produced by the PAFC is used for cogeneration. Operating temperatures are in the range of 150 to 220°C.
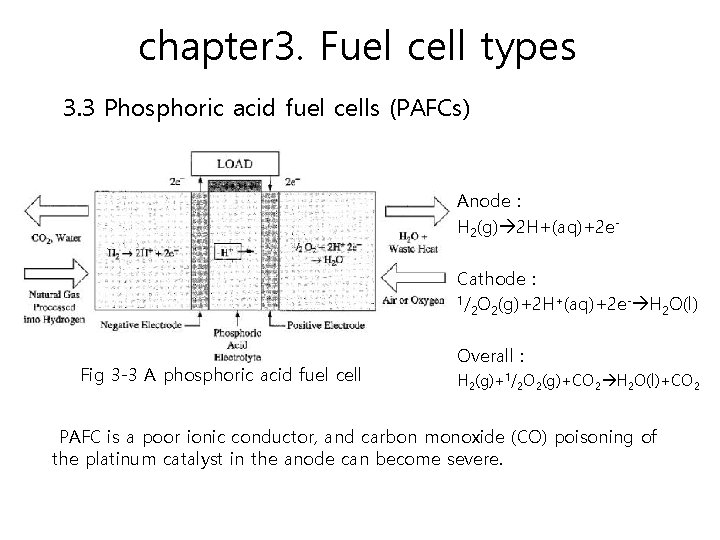
chapter 3. Fuel cell types 3. 3 Phosphoric acid fuel cells (PAFCs) Anode : H 2(g) 2 H+(aq)+2 e. Cathode : 1/ O (g)+2 H+(aq)+2 e- H O(l) 2 2 2 Fig 3 -3 A phosphoric acid fuel cell Overall : H 2(g)+1/2 O 2(g)+CO 2 H 2 O(l)+CO 2 PAFC is a poor ionic conductor, and carbon monoxide (CO) poisoning of the platinum catalyst in the anode can become severe.
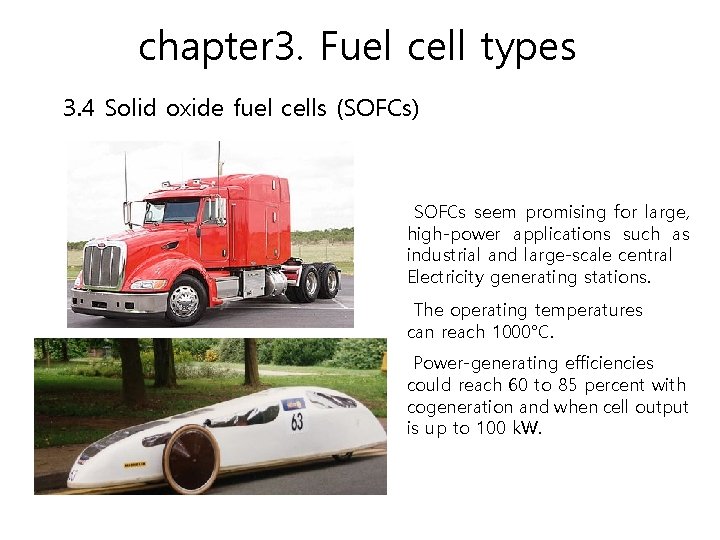
chapter 3. Fuel cell types 3. 4 Solid oxide fuel cells (SOFCs) SOFCs seem promising for large, high-power applications such as industrial and large-scale central Electricity generating stations. The operating temperatures can reach 1000°C. Power-generating efficiencies could reach 60 to 85 percent with cogeneration and when cell output is up to 100 k. W.
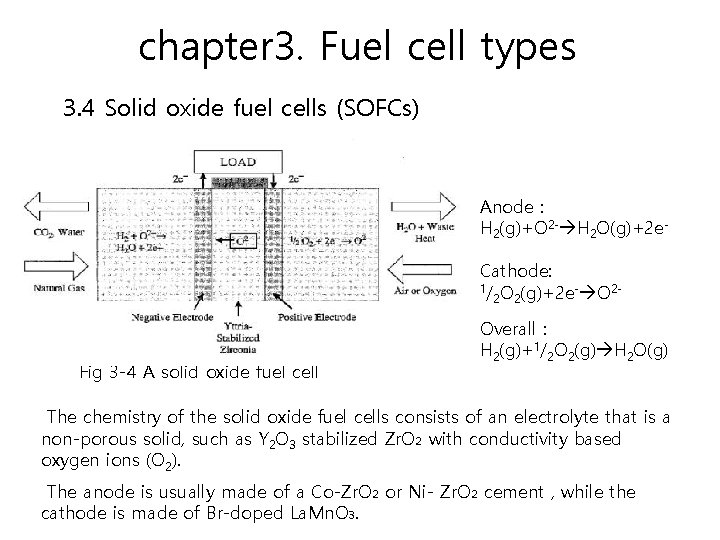
chapter 3. Fuel cell types 3. 4 Solid oxide fuel cells (SOFCs) Anode : H 2(g)+O 2 - H 2 O(g)+2 e. Cathode: 1/ O (g)+2 e- O 22 2 Fig 3 -4 A solid oxide fuel cell Overall : H 2(g)+1/2 O 2(g) H 2 O(g) The chemistry of the solid oxide fuel cells consists of an electrolyte that is a non-porous solid, such as Y 2 O 3 stabilized Zr. O₂ with conductivity based oxygen ions (O 2). The anode is usually made of a Co-Zr. O₂ or Ni- Zr. O₂ cement , while the cathode is made of Br-doped La. Mn. O₃.
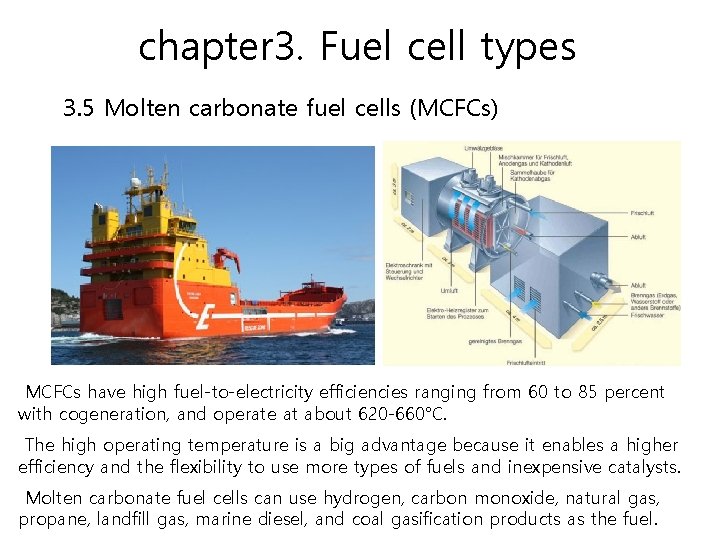
chapter 3. Fuel cell types 3. 5 Molten carbonate fuel cells (MCFCs) MCFCs have high fuel-to-electricity efficiencies ranging from 60 to 85 percent with cogeneration, and operate at about 620 -660°C. The high operating temperature is a big advantage because it enables a higher efficiency and the flexibility to use more types of fuels and inexpensive catalysts. Molten carbonate fuel cells can use hydrogen, carbon monoxide, natural gas, propane, landfill gas, marine diesel, and coal gasification products as the fuel.
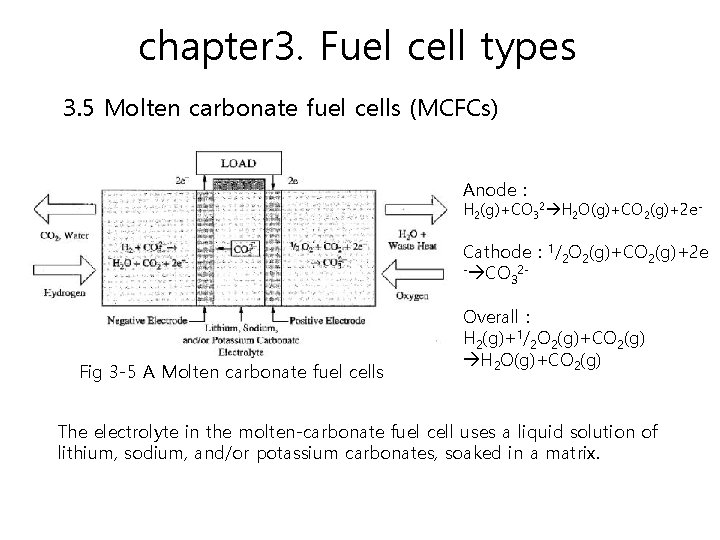
chapter 3. Fuel cell types 3. 5 Molten carbonate fuel cells (MCFCs) Anode : H 2(g)+CO 32 H 2 O(g)+CO 2(g)+2 e- Cathode : 1/2 O 2(g)+CO 2(g)+2 e - CO 23 Fig 3 -5 A Molten carbonate fuel cells Overall : H 2(g)+1/2 O 2(g)+CO 2(g) H 2 O(g)+CO 2(g) The electrolyte in the molten-carbonate fuel cell uses a liquid solution of lithium, sodium, and/or potassium carbonates, soaked in a matrix.
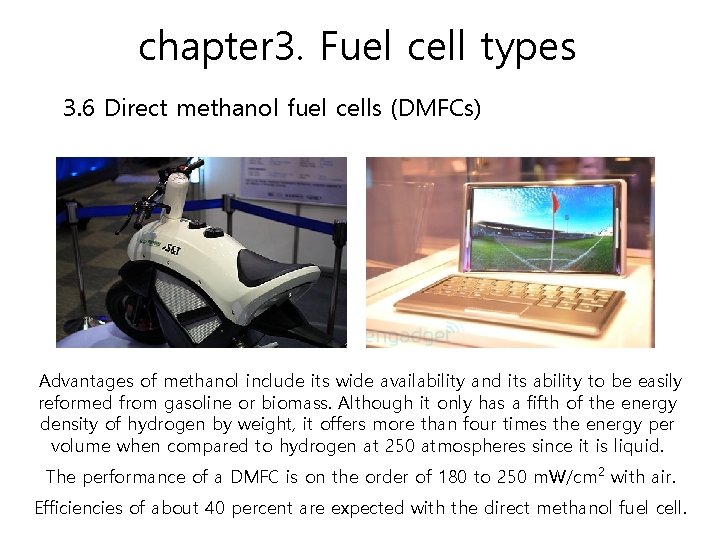
chapter 3. Fuel cell types 3. 6 Direct methanol fuel cells (DMFCs) Advantages of methanol include its wide availability and its ability to be easily reformed from gasoline or biomass. Although it only has a fifth of the energy density of hydrogen by weight, it offers more than four times the energy per volume when compared to hydrogen at 250 atmospheres since it is liquid. The performance of a DMFC is on the order of 180 to 250 m. W/cm 2 with air. Efficiencies of about 40 percent are expected with the direct methanol fuel cell.
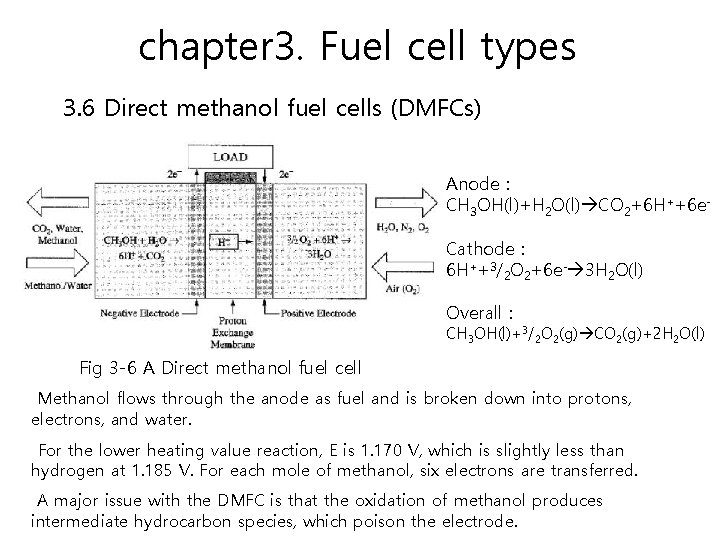
chapter 3. Fuel cell types 3. 6 Direct methanol fuel cells (DMFCs) Anode : CH 3 OH(l)+H 2 O(l) CO 2+6 H++6 e. Cathode : 6 H++3/2 O 2+6 e- 3 H 2 O(l) Overall : CH 3 OH(l)+3/2 O 2(g) CO 2(g)+2 H 2 O(l) Fig 3 -6 A Direct methanol fuel cell Methanol flows through the anode as fuel and is broken down into protons, electrons, and water. For the lower heating value reaction, E is 1. 170 V, which is slightly less than hydrogen at 1. 185 V. For each mole of methanol, six electrons are transferred. A major issue with the DMFC is that the oxidation of methanol produces intermediate hydrocarbon species, which poison the electrode.
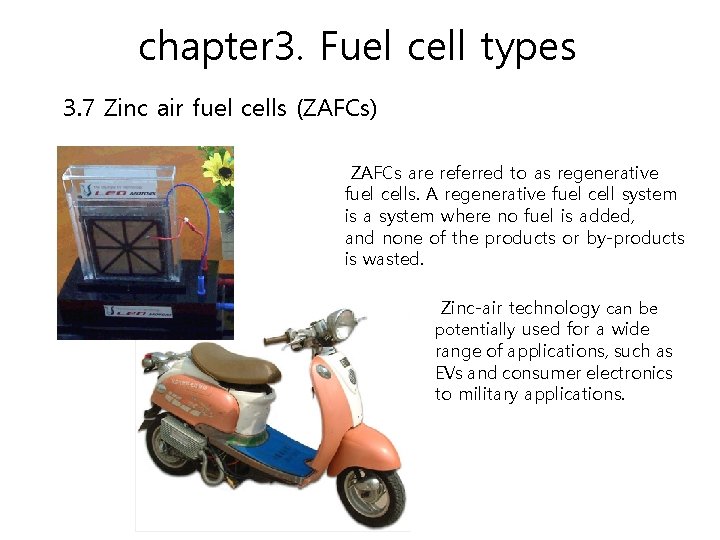
chapter 3. Fuel cell types 3. 7 Zinc air fuel cells (ZAFCs) ZAFCs are referred to as regenerative fuel cells. A regenerative fuel cell system is a system where no fuel is added, and none of the products or by-products is wasted. Zinc-air technology can be potentially used for a wide range of applications, such as EVs and consumer electronics to military applications.
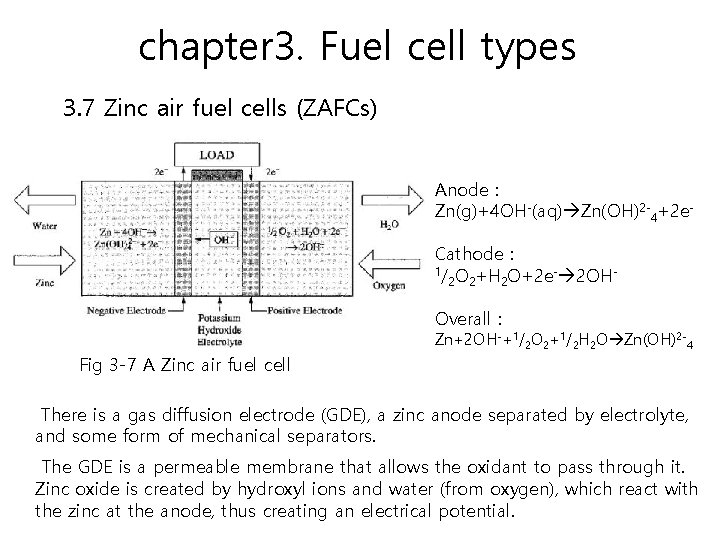
chapter 3. Fuel cell types 3. 7 Zinc air fuel cells (ZAFCs) Anode : Zn(g)+4 OH-(aq) Zn(OH)2 -4+2 e. Cathode : 1/ O +H O+2 e- 2 OH 2 2 2 Overall : Zn+2 OH-+1/2 O 2+1/2 H 2 O Zn(OH)2 -4 Fig 3 -7 A Zinc air fuel cell There is a gas diffusion electrode (GDE), a zinc anode separated by electrolyte, and some form of mechanical separators. The GDE is a permeable membrane that allows the oxidant to pass through it. Zinc oxide is created by hydroxyl ions and water (from oxygen), which react with the zinc at the anode, thus creating an electrical potential.
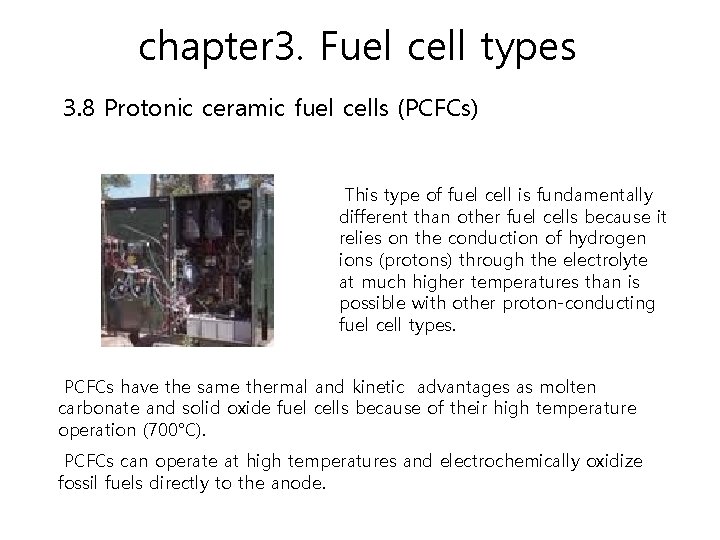
chapter 3. Fuel cell types 3. 8 Protonic ceramic fuel cells (PCFCs) This type of fuel cell is fundamentally different than other fuel cells because it relies on the conduction of hydrogen ions (protons) through the electrolyte at much higher temperatures than is possible with other proton-conducting fuel cell types. PCFCs have the same thermal and kinetic advantages as molten carbonate and solid oxide fuel cells because of their high temperature operation (700°C). PCFCs can operate at high temperatures and electrochemically oxidize fossil fuels directly to the anode.
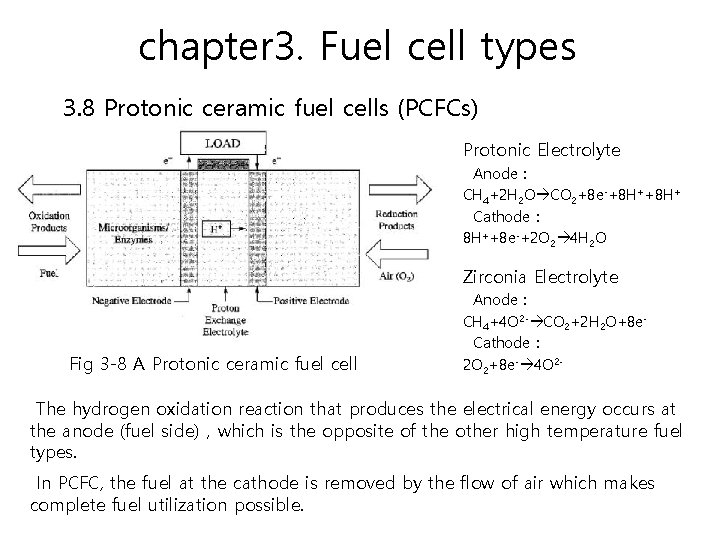
chapter 3. Fuel cell types 3. 8 Protonic ceramic fuel cells (PCFCs) Protonic Electrolyte Anode : CH 4+2 H 2 O CO 2+8 e-+8 H+ Cathode : 8 H++8 e-+2 O 2 4 H 2 O Zirconia Electrolyte Fig 3 -8 A Protonic ceramic fuel cell Anode : CH 4+4 O 2 - CO 2+2 H 2 O+8 e. Cathode : 2 O 2+8 e- 4 O 2 - The hydrogen oxidation reaction that produces the electrical energy occurs at the anode (fuel side),which is the opposite of the other high temperature fuel types. In PCFC, the fuel at the cathode is removed by the flow of air which makes complete fuel utilization possible.
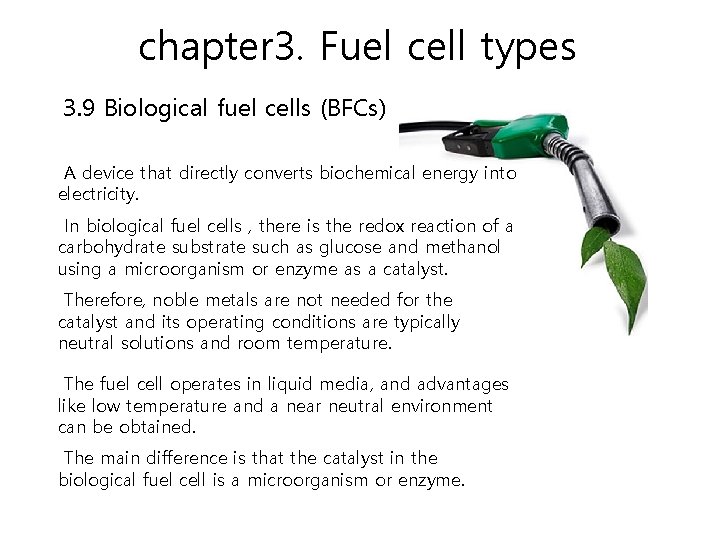
chapter 3. Fuel cell types 3. 9 Biological fuel cells (BFCs) A device that directly converts biochemical energy into electricity. In biological fuel cells , there is the redox reaction of a carbohydrate substrate such as glucose and methanol using a microorganism or enzyme as a catalyst. Therefore, noble metals are not needed for the catalyst and its operating conditions are typically neutral solutions and room temperature. The fuel cell operates in liquid media, and advantages like low temperature and a near neutral environment can be obtained. The main difference is that the catalyst in the biological fuel cell is a microorganism or enzyme.
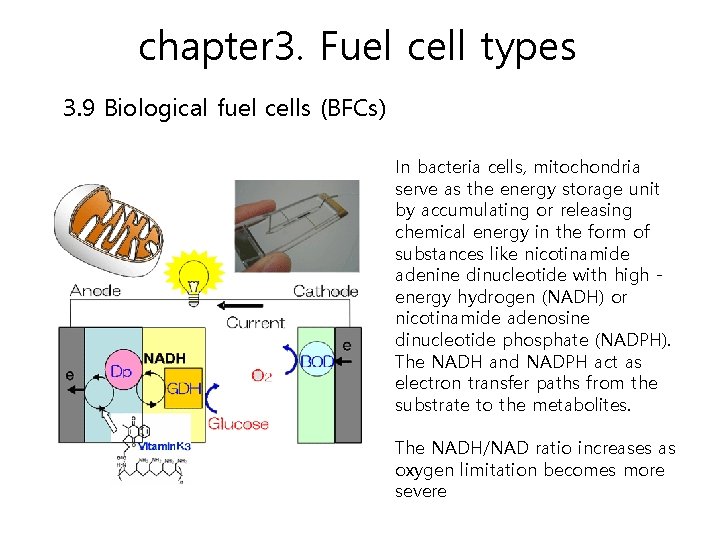
chapter 3. Fuel cell types 3. 9 Biological fuel cells (BFCs) In bacteria cells, mitochondria serve as the energy storage unit by accumulating or releasing chemical energy in the form of substances like nicotinamide adenine dinucleotide with high energy hydrogen (NADH) or nicotinamide adenosine dinucleotide phosphate (NADPH). The NADH and NADPH act as electron transfer paths from the substrate to the metabolites. The NADH/NAD ratio increases as oxygen limitation becomes more severe
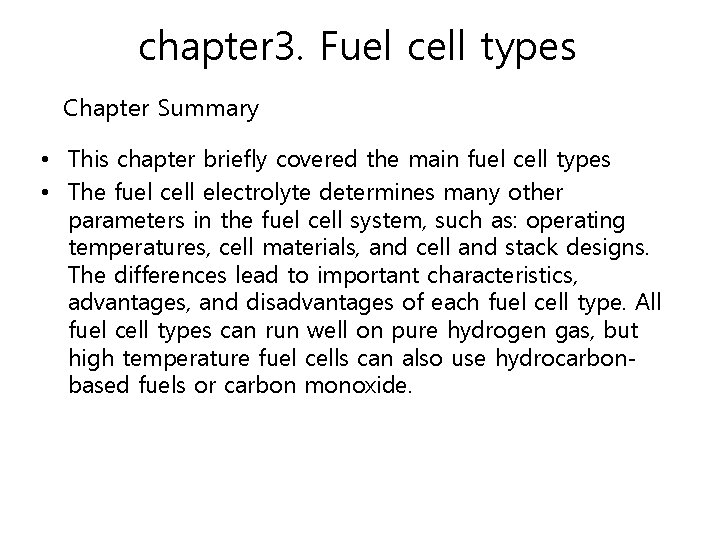
chapter 3. Fuel cell types Chapter Summary • This chapter briefly covered the main fuel cell types • The fuel cell electrolyte determines many other parameters in the fuel cell system, such as: operating temperatures, cell materials, and cell and stack designs. The differences lead to important characteristics, advantages, and disadvantages of each fuel cell type. All fuel cell types can run well on pure hydrogen gas, but high temperature fuel cells can also use hydrocarbonbased fuels or carbon monoxide.
What is fuel cell in chemistry
Fuel cell apollo
Fuel cell apollo
Roller cell fuel pump
Hydrogen cycle
Fuel cell uses
Fuel cell system
Molten carbonate fuel cell
Solid oxide fuel cell
Valuetheenergy
Fuel cell heat exchanger
Hydrogen energy advantages and disadvantages
Fuel cell cartridge
The scientist mathias schleiden studied _______ in ______.
Cell city worksheet
Difference between mercury cell and diaphragm cell
Prokaryotic vs eukaryotic cell
Prokaryotic reproduction
Venn diagram plant vs animal cells
Balancing redox reactions in acidic solution
Dry cell vs wet cell
What is an organelle
Cell wall function
Vacuole function
What is the function of vacuole in the cell