100 340 GHz Systems Transistors and Applications M
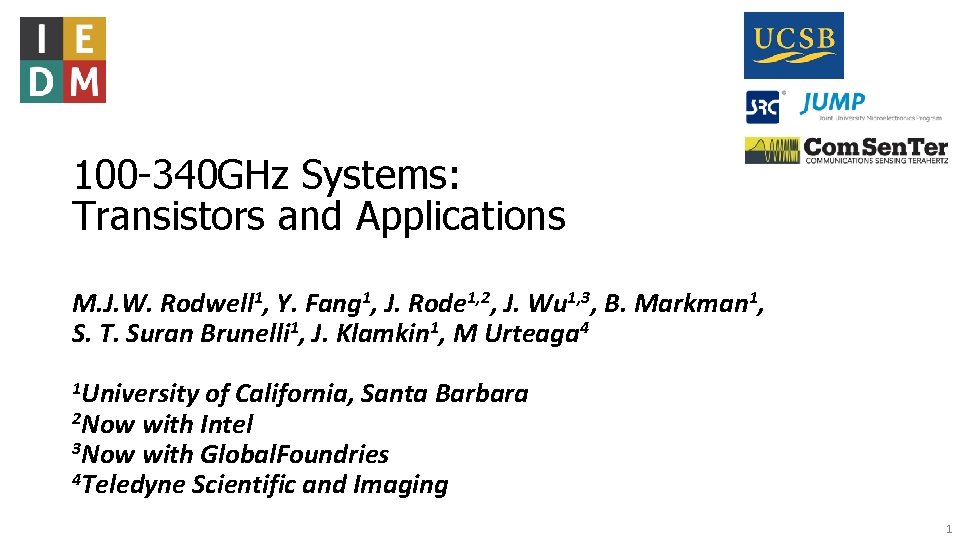
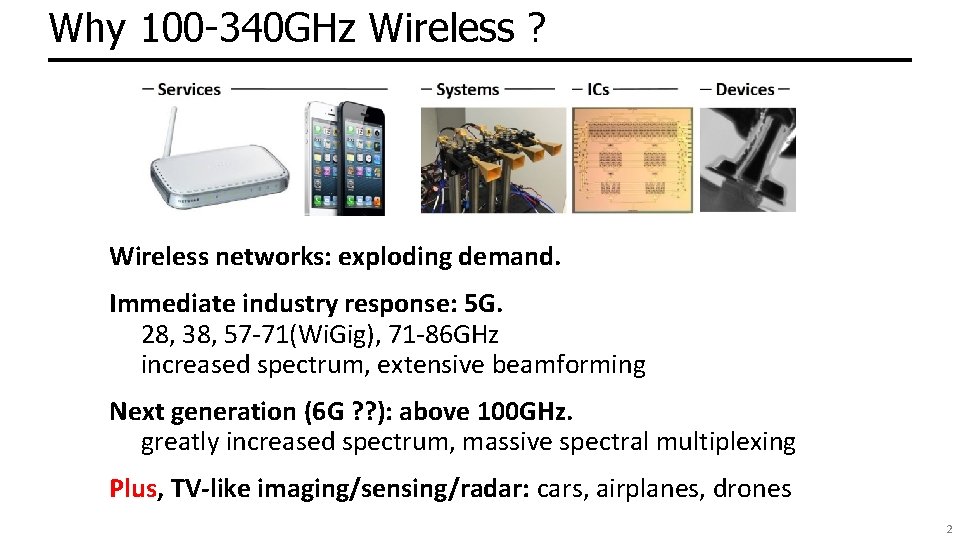
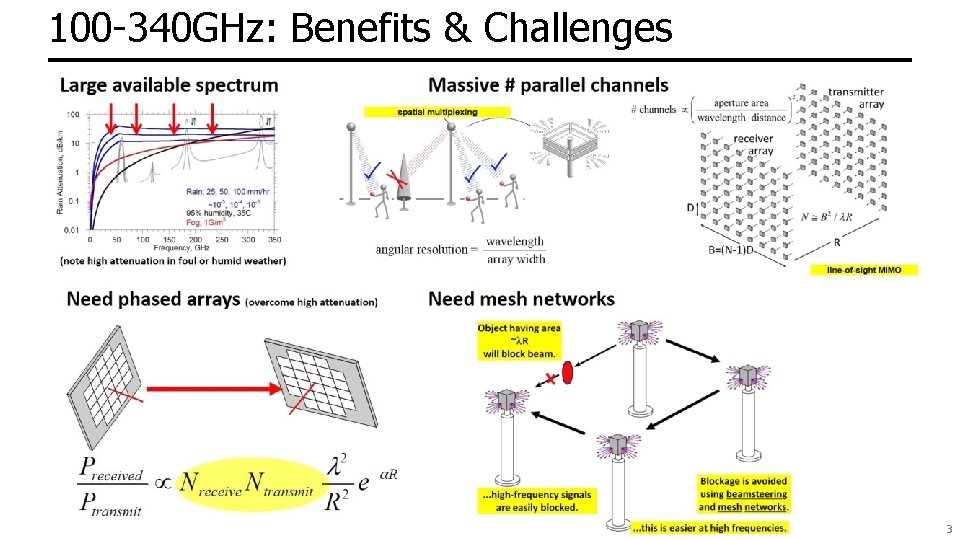
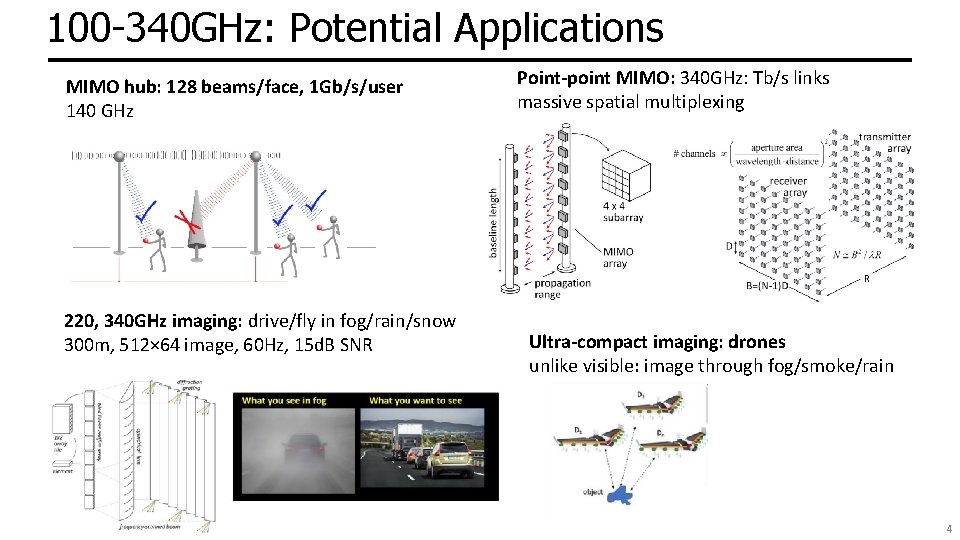
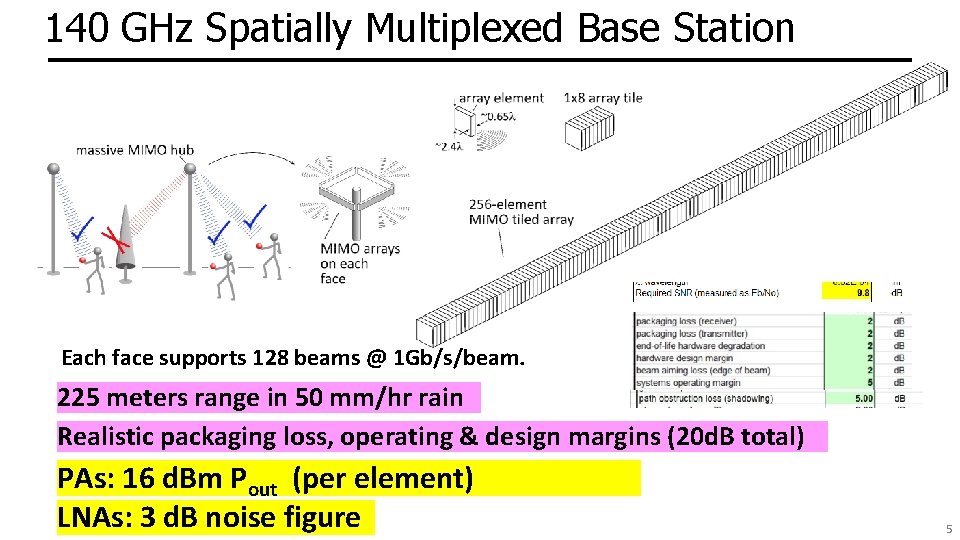
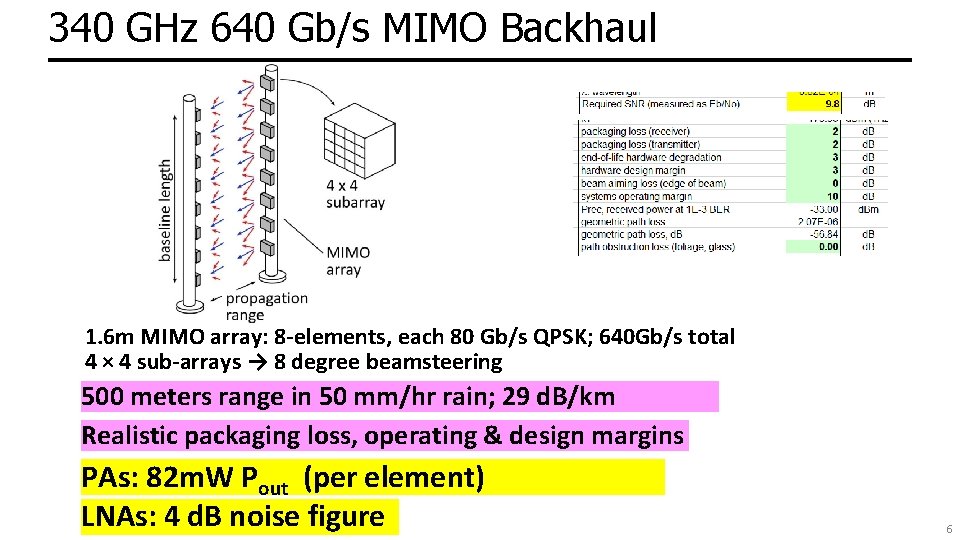
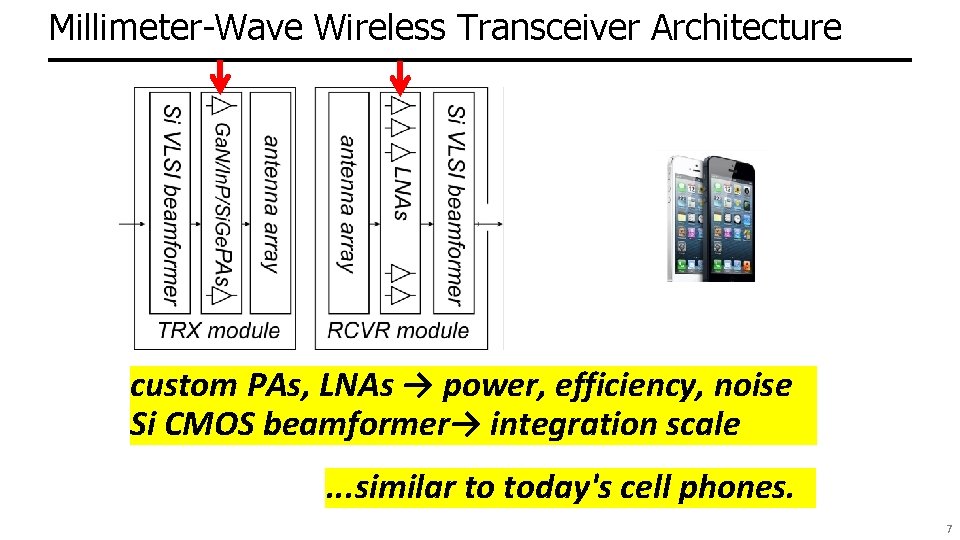
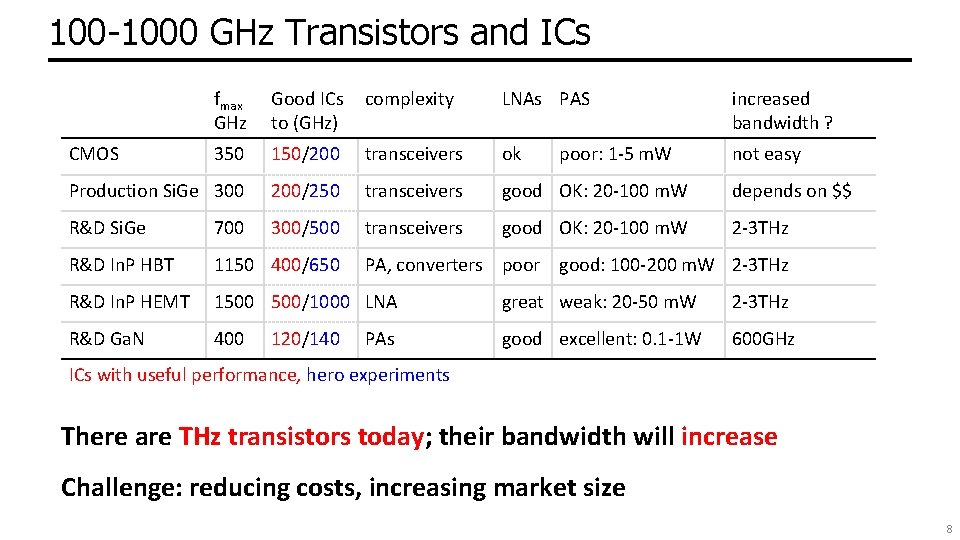
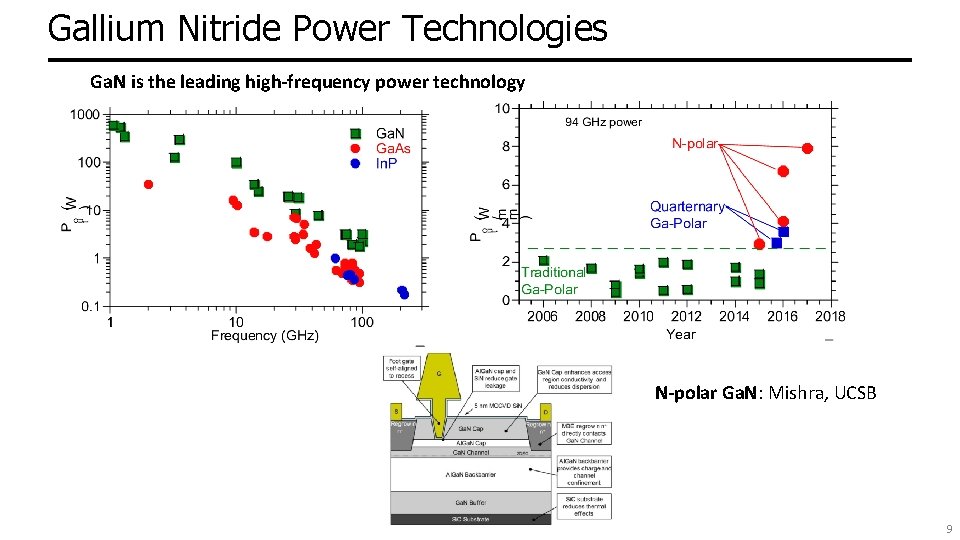
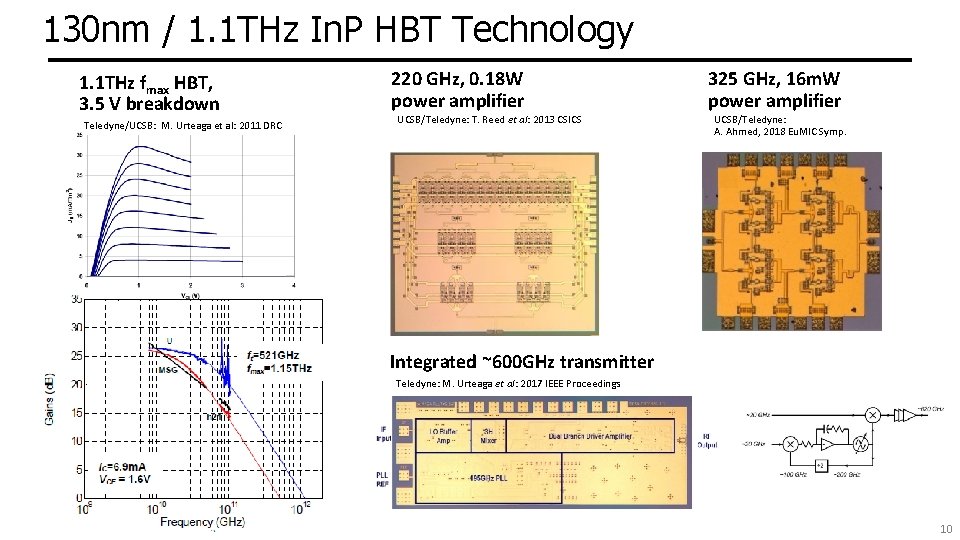
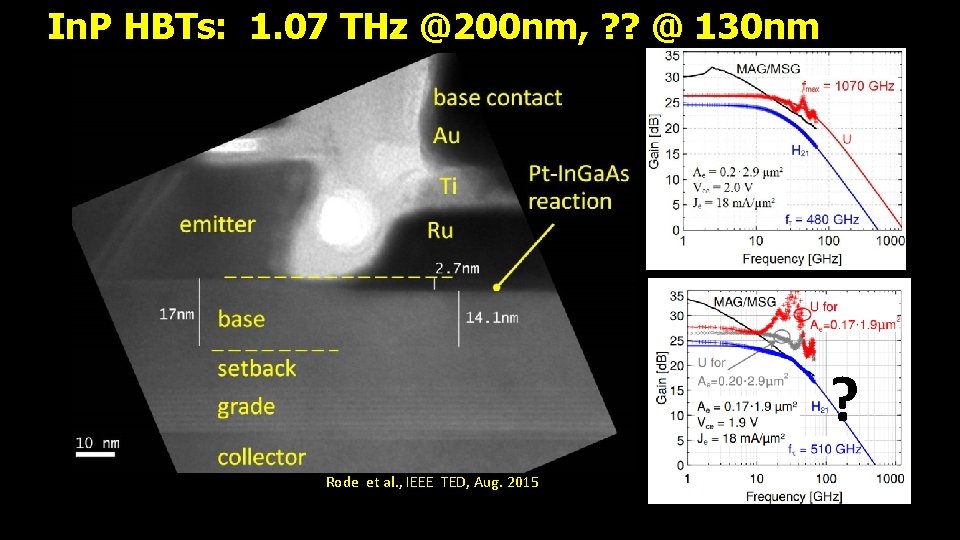
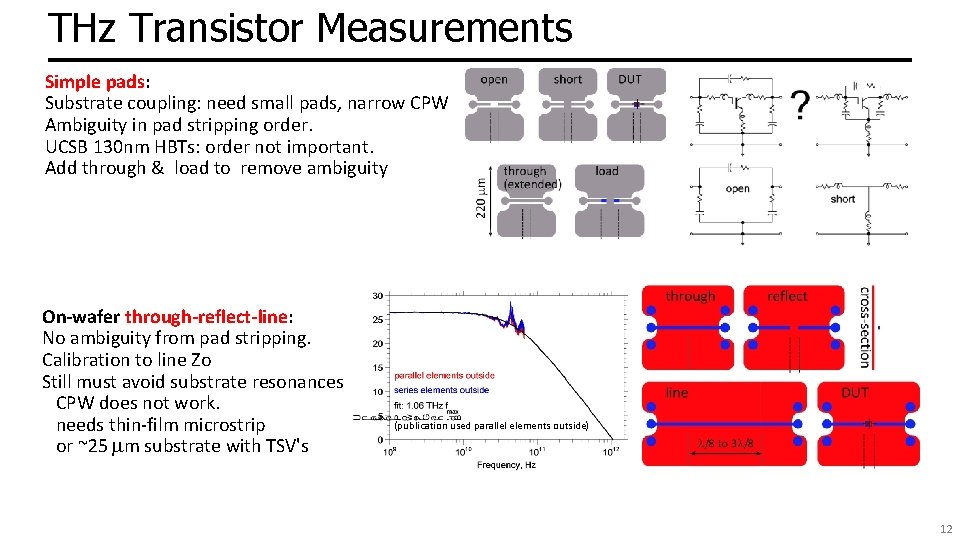
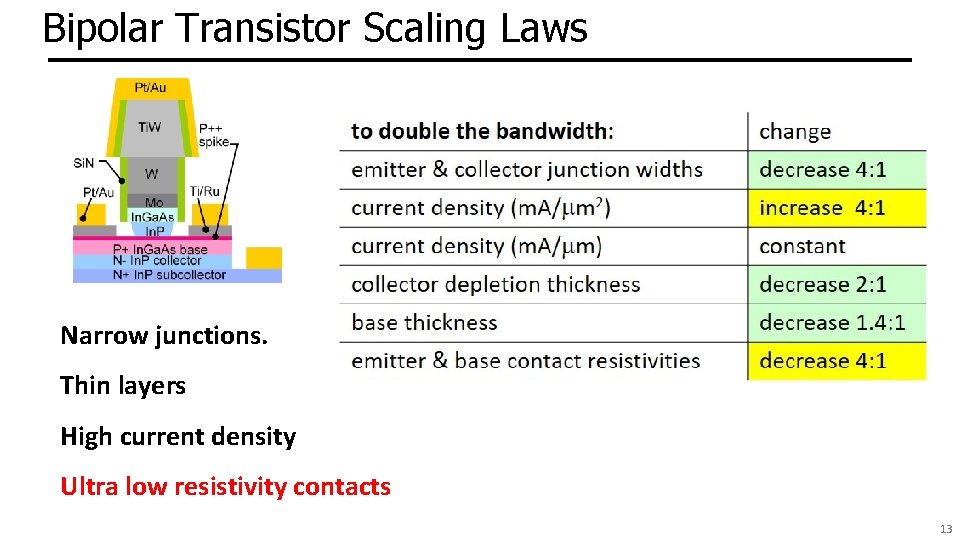
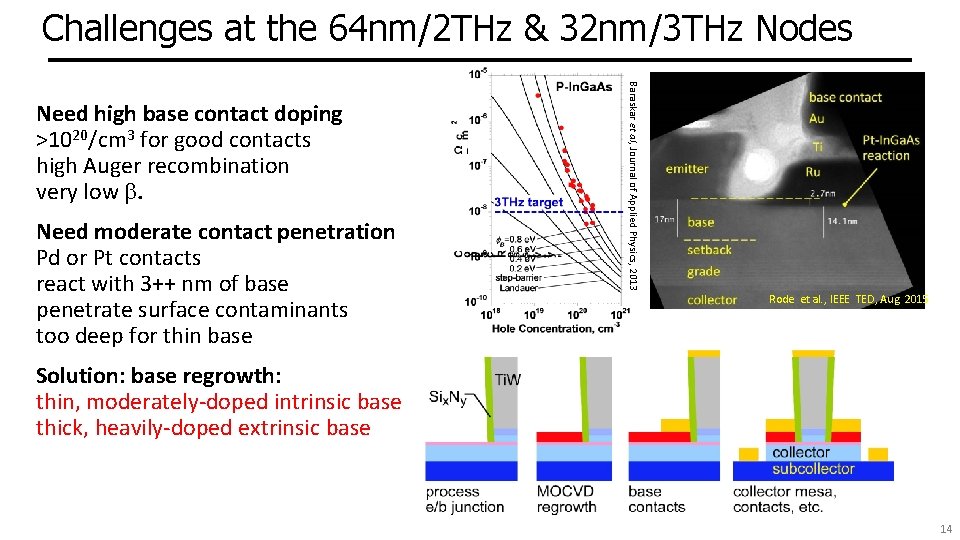
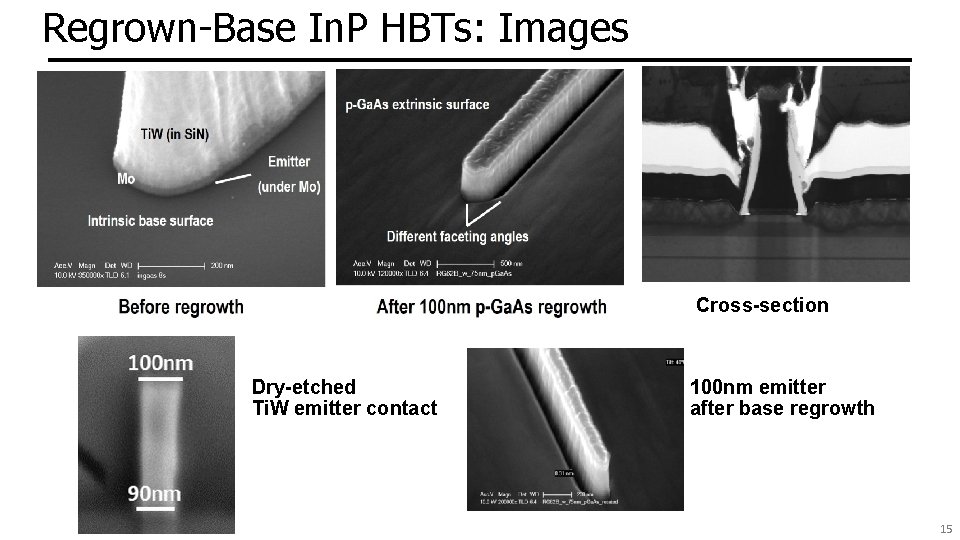
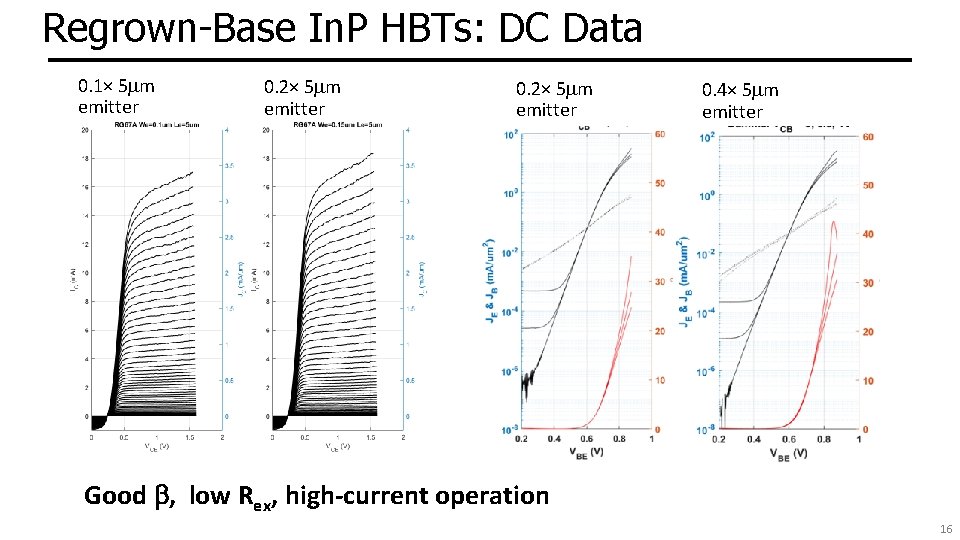
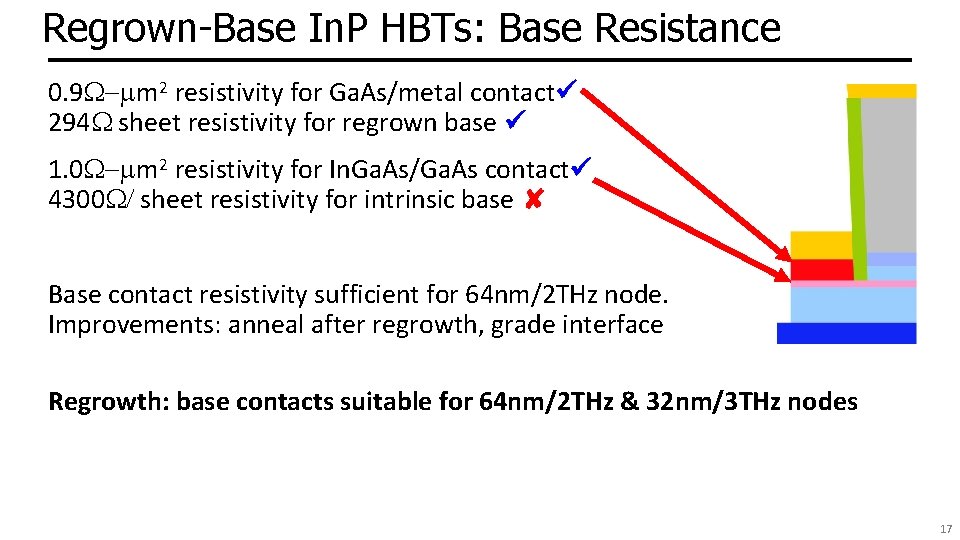
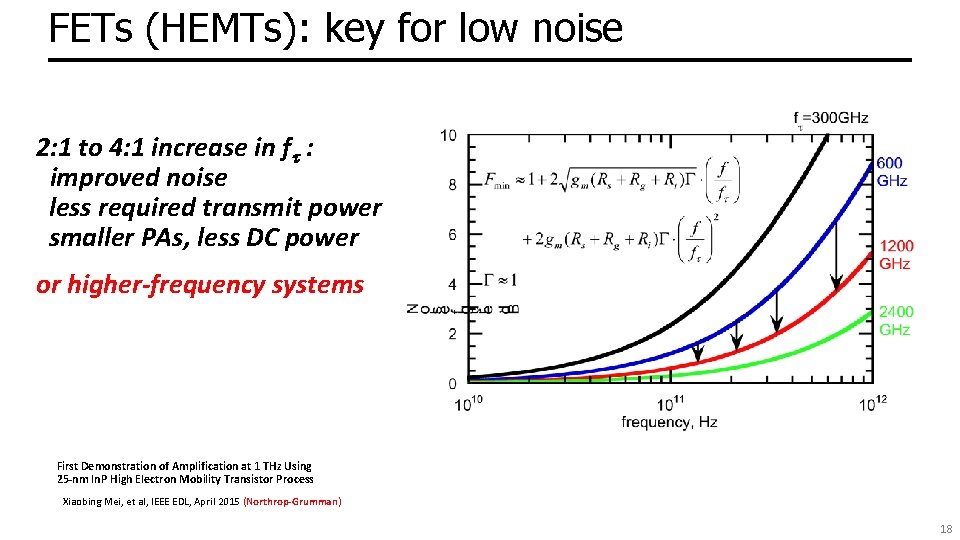
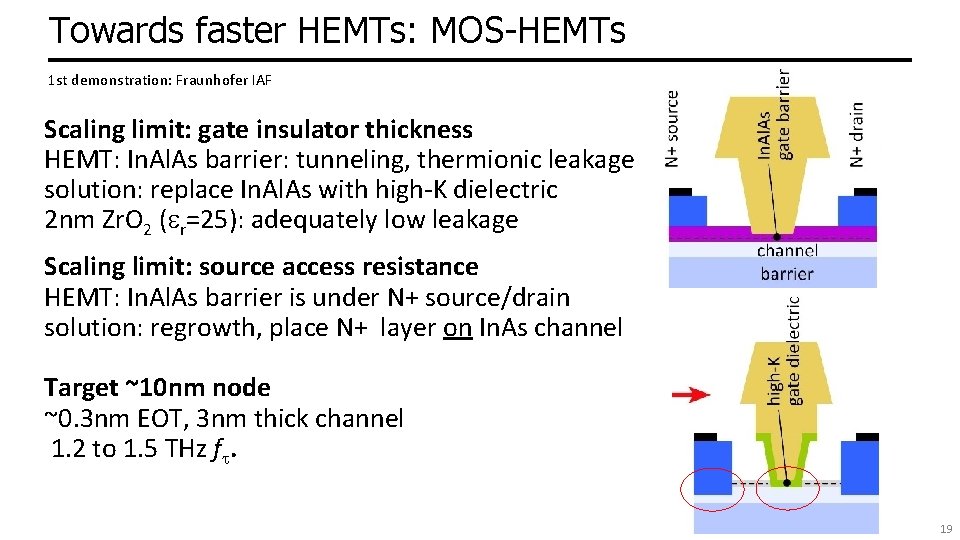
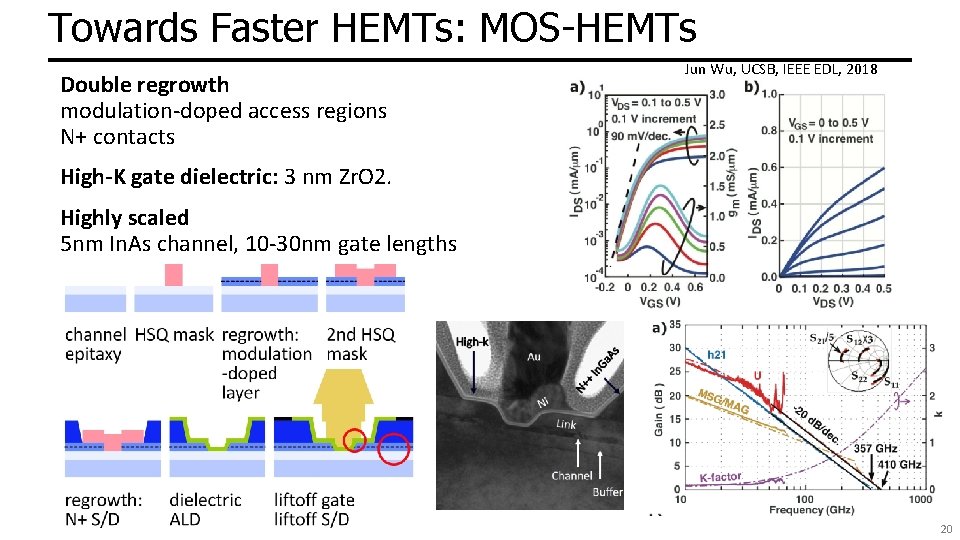
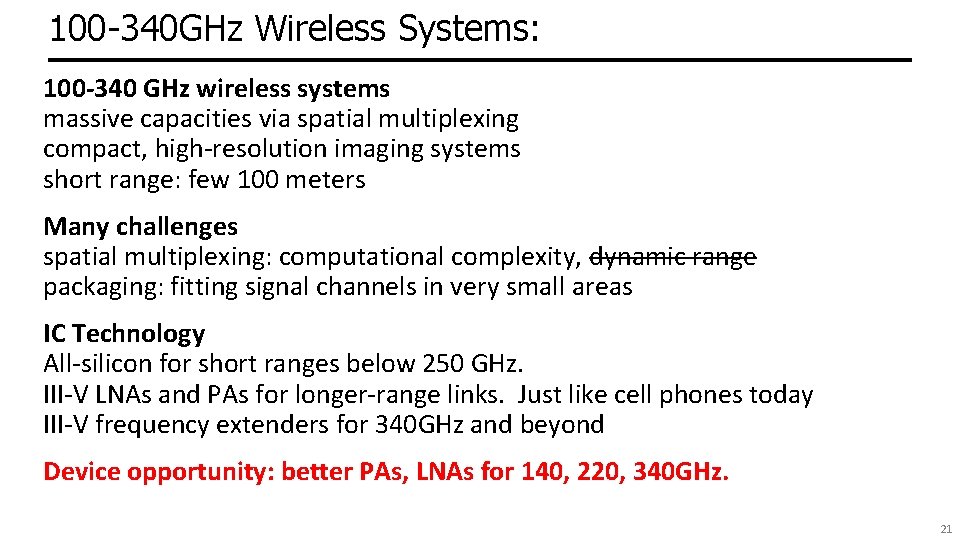
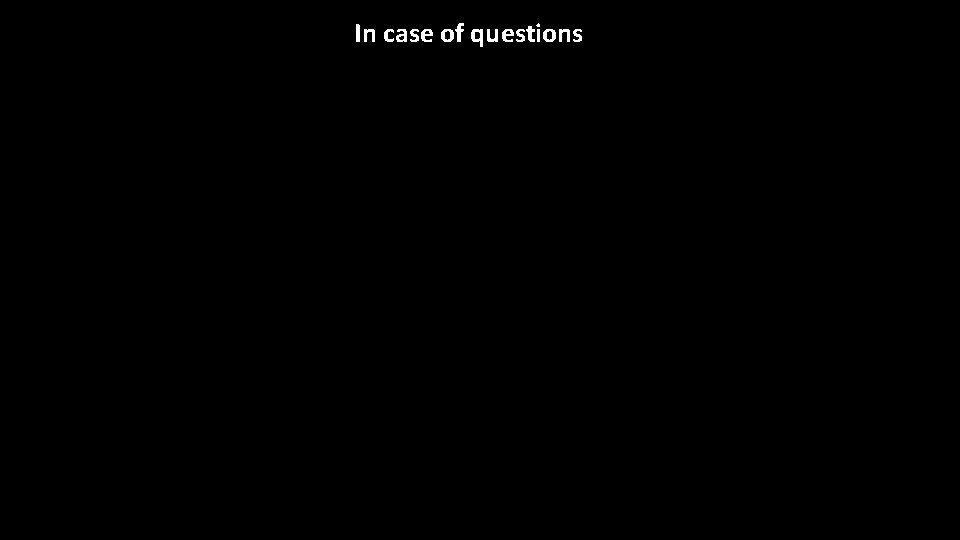
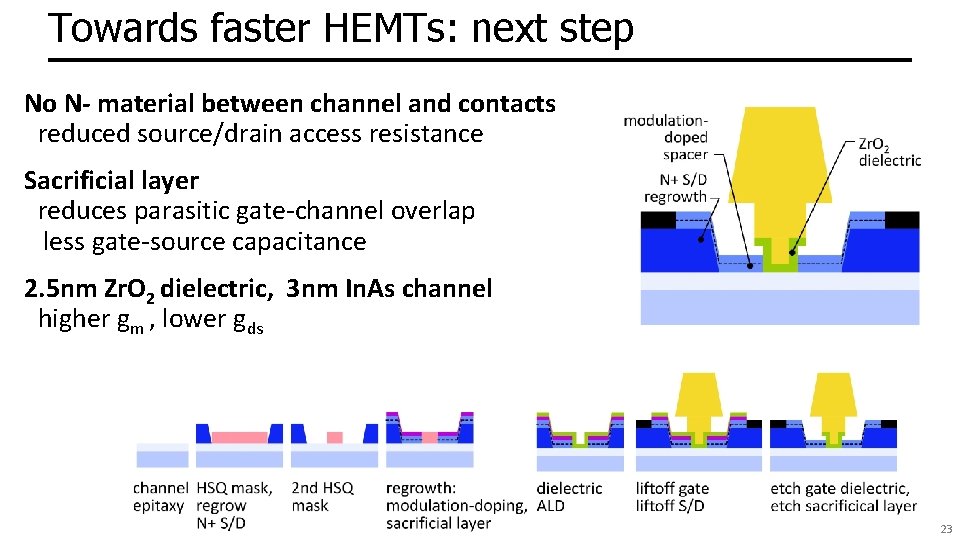
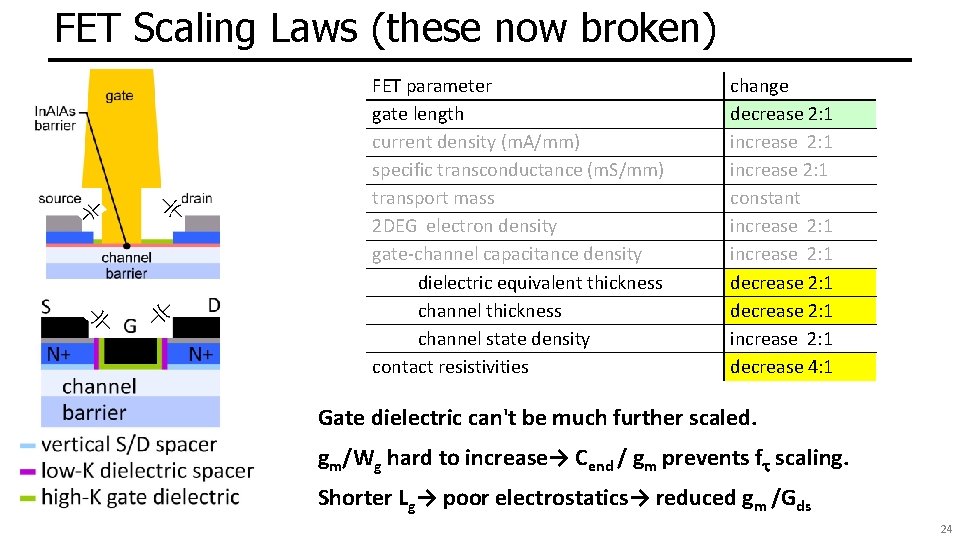
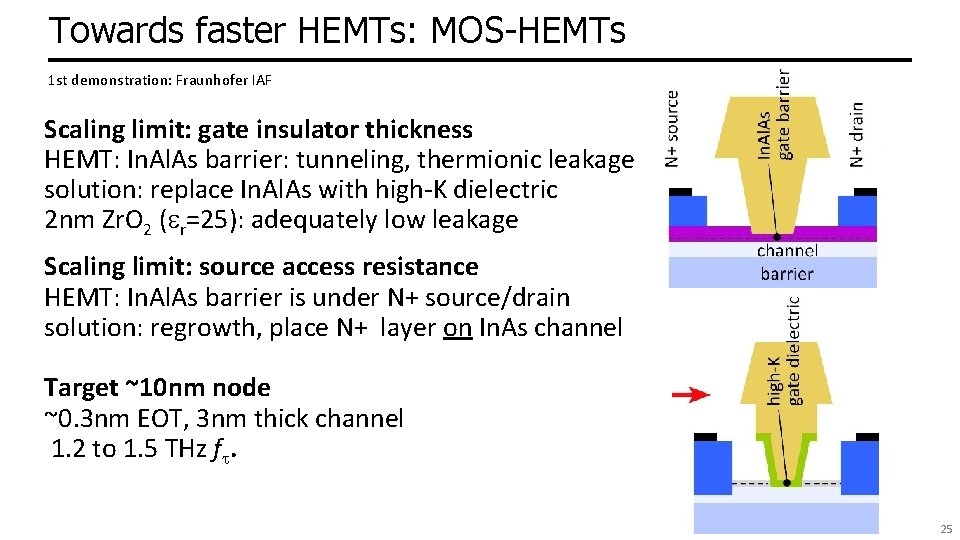
- Slides: 25
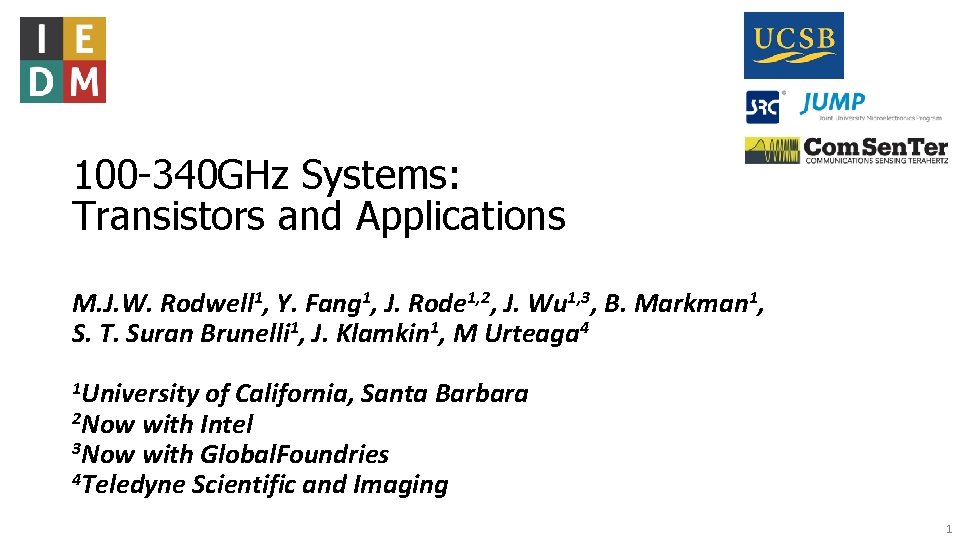
100 -340 GHz Systems: Transistors and Applications M. J. W. Rodwell 1, Y. Fang 1, J. Rode 1, 2, J. Wu 1, 3, B. Markman 1, S. T. Suran Brunelli 1, J. Klamkin 1, M Urteaga 4 1 University of California, Santa Barbara 2 Now with Intel 3 Now with Global. Foundries 4 Teledyne Scientific and Imaging 1
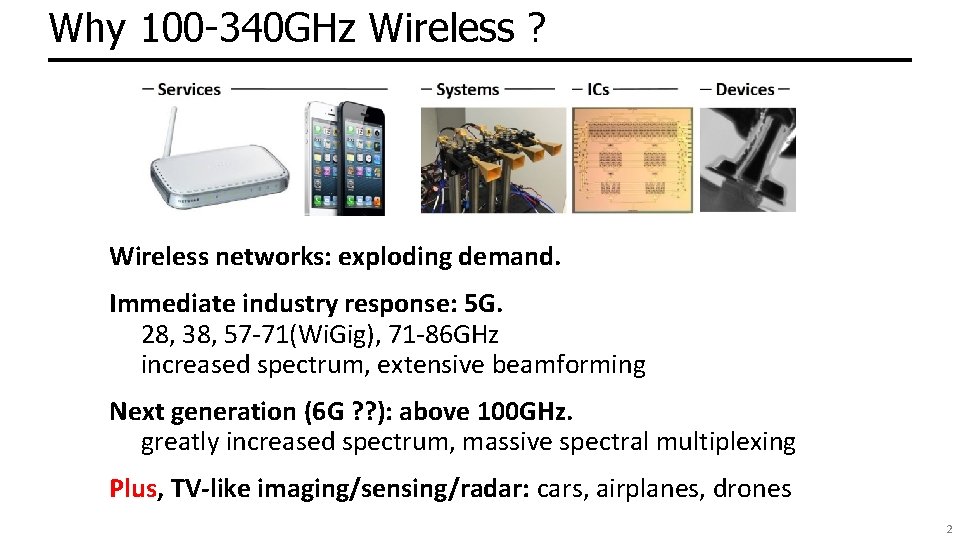
Why 100 -340 GHz Wireless ? Wireless networks: exploding demand. Immediate industry response: 5 G. 28, 38, 57 -71(Wi. Gig), 71 -86 GHz increased spectrum, extensive beamforming Next generation (6 G ? ? ): above 100 GHz. greatly increased spectrum, massive spectral multiplexing Plus, TV-like imaging/sensing/radar: cars, airplanes, drones 2
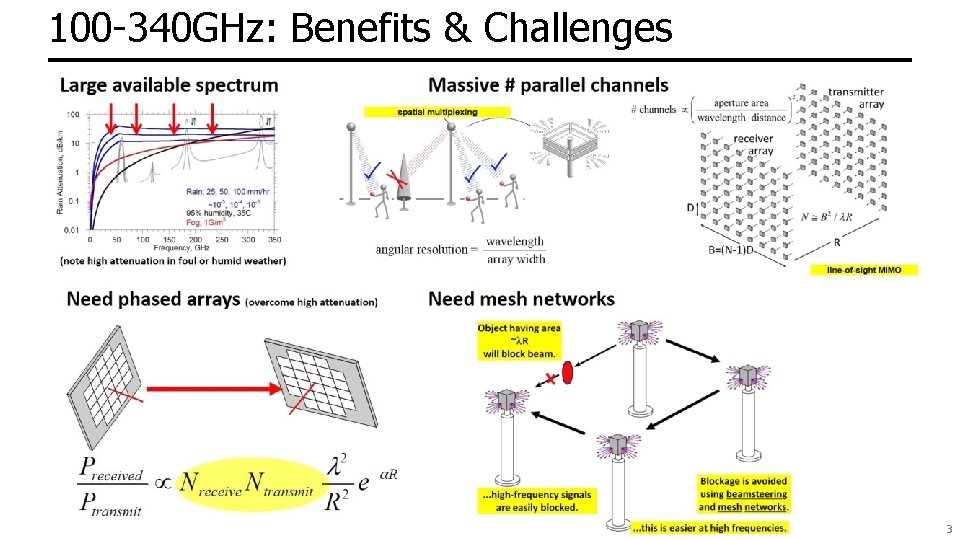
100 -340 GHz: Benefits & Challenges 3
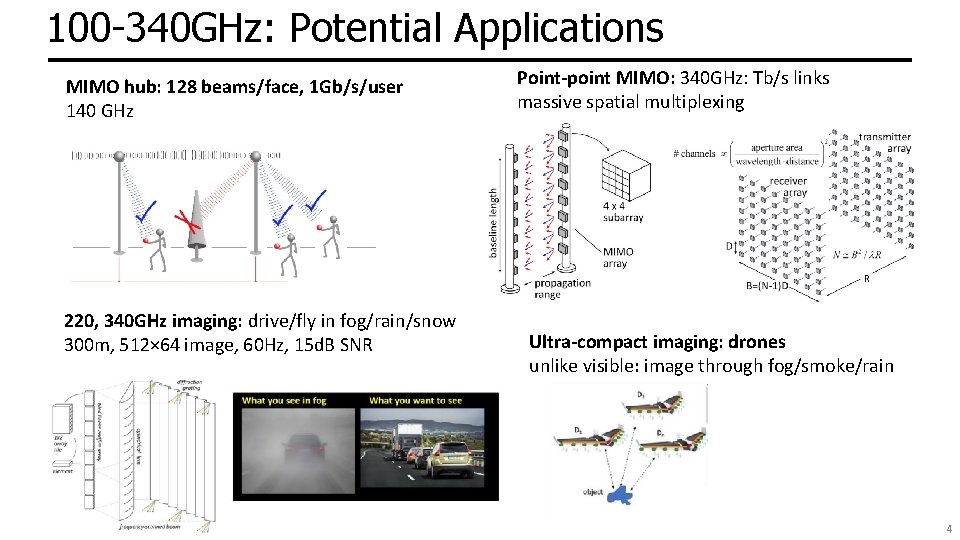
100 -340 GHz: Potential Applications MIMO hub: 128 beams/face, 1 Gb/s/user 140 GHz 220, 340 GHz imaging: drive/fly in fog/rain/snow 300 m, 512× 64 image, 60 Hz, 15 d. B SNR Point-point MIMO: 340 GHz: Tb/s links massive spatial multiplexing Ultra-compact imaging: drones unlike visible: image through fog/smoke/rain 4
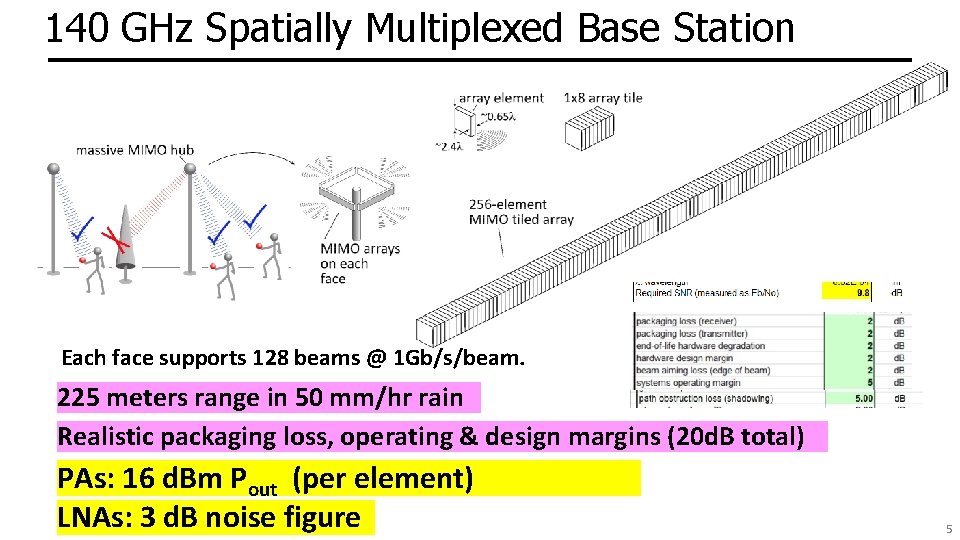
140 GHz Spatially Multiplexed Base Station Each face supports 128 beams @ 1 Gb/s/beam. 225 meters range in 50 mm/hr rain Realistic packaging loss, operating & design margins (20 d. B total) PAs: 16 d. Bm Pout (per element) LNAs: 3 d. B noise figure 5
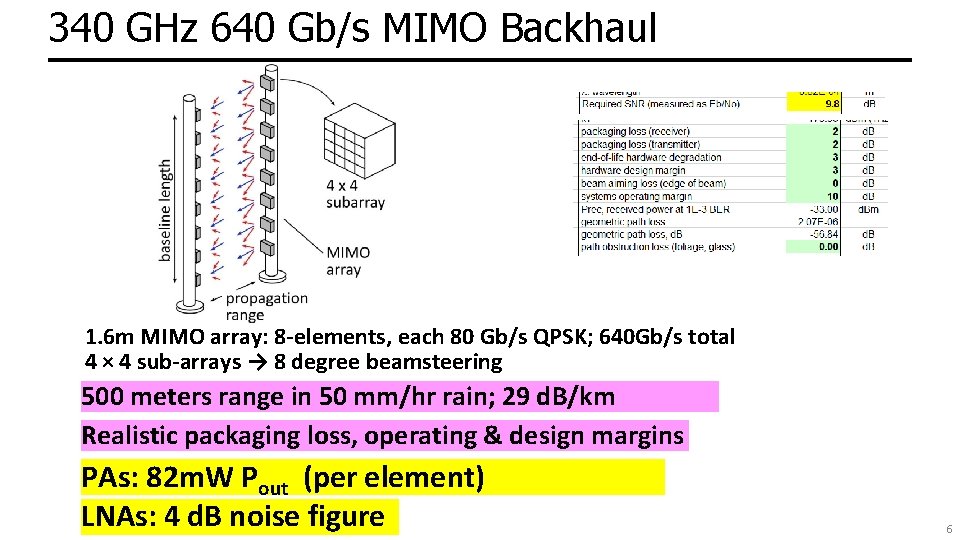
340 GHz 640 Gb/s MIMO Backhaul 1. 6 m MIMO array: 8 -elements, each 80 Gb/s QPSK; 640 Gb/s total 4 × 4 sub-arrays → 8 degree beamsteering 500 meters range in 50 mm/hr rain; 29 d. B/km Realistic packaging loss, operating & design margins PAs: 82 m. W Pout (per element) LNAs: 4 d. B noise figure 6
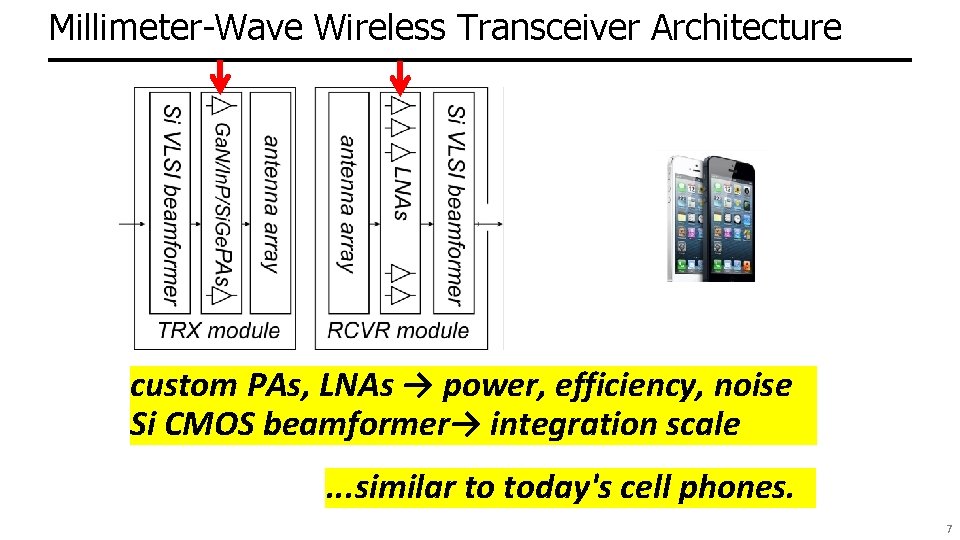
Millimeter-Wave Wireless Transceiver Architecture custom PAs, LNAs → power, efficiency, noise Si CMOS beamformer→ integration scale. . . similar to today's cell phones. 7
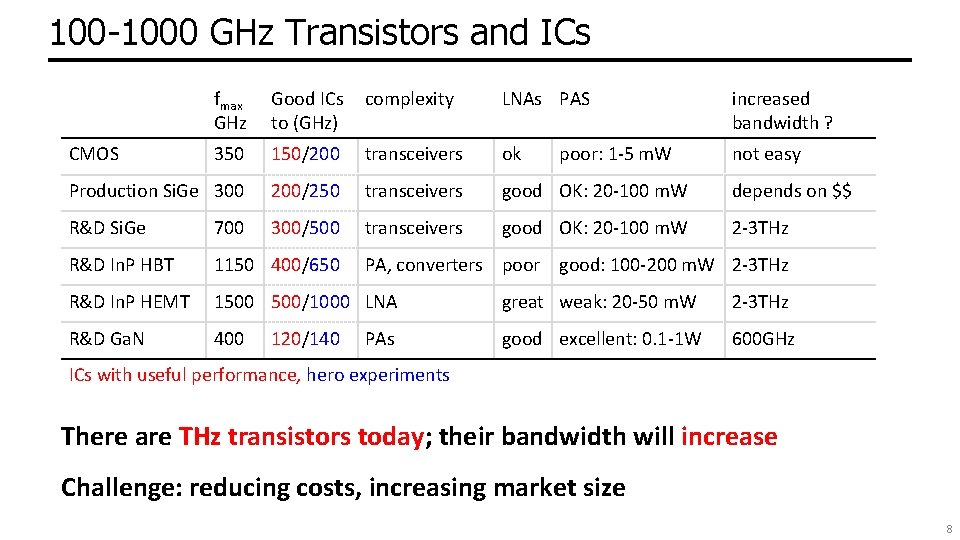
100 -1000 GHz Transistors and ICs fmax GHz Good ICs to (GHz) complexity LNAs PAS increased bandwidth ? 350 150/200 transceivers ok not easy Production Si. Ge 300 200/250 transceivers good OK: 20 -100 m. W depends on $$ R&D Si. Ge 700 300/500 transceivers good OK: 20 -100 m. W 2 -3 THz R&D In. P HBT 1150 400/650 R&D In. P HEMT 1500 500/1000 LNA great weak: 20 -50 m. W 2 -3 THz R&D Ga. N 400 good excellent: 0. 1 -1 W 600 GHz CMOS 120/140 poor: 1 -5 m. W PA, converters poor good: 100 -200 m. W 2 -3 THz PAs ICs with useful performance, hero experiments There are THz transistors today; their bandwidth will increase Challenge: reducing costs, increasing market size 8
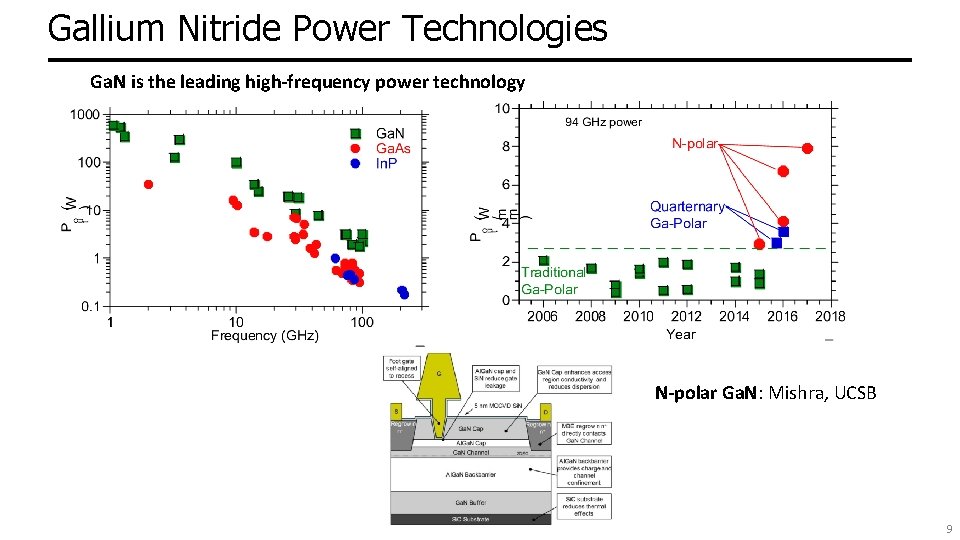
Gallium Nitride Power Technologies Ga. N is the leading high-frequency power technology N-polar Ga. N: Mishra, UCSB 9
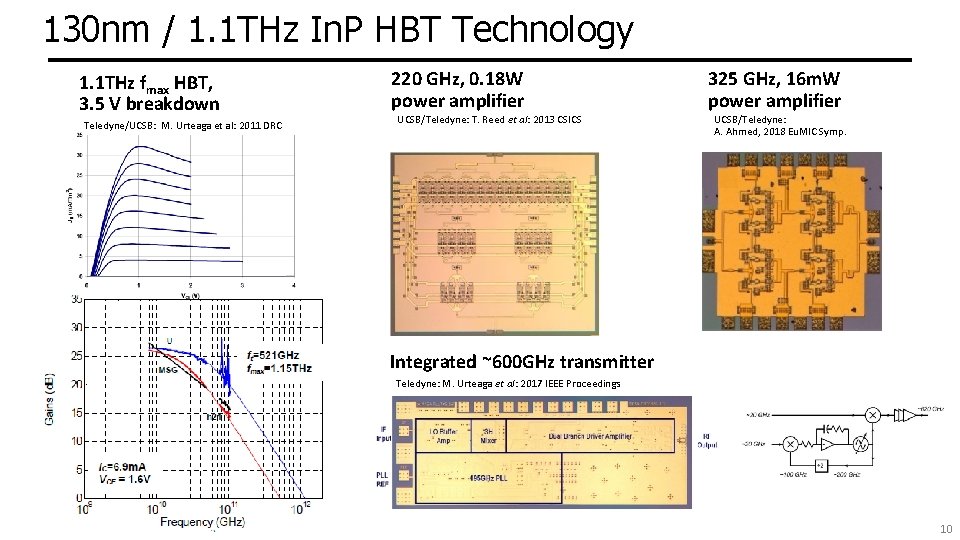
130 nm / 1. 1 THz In. P HBT Technology 1. 1 THz fmax HBT, 3. 5 V breakdown Teledyne/UCSB: M. Urteaga et al: 2011 DRC 220 GHz, 0. 18 W power amplifier UCSB/Teledyne: T. Reed et al: 2013 CSICS 325 GHz, 16 m. W power amplifier UCSB/Teledyne: A. Ahmed, 2018 Eu. MIC Symp. Integrated ~600 GHz transmitter Teledyne: M. Urteaga et al: 2017 IEEE Proceedings 10
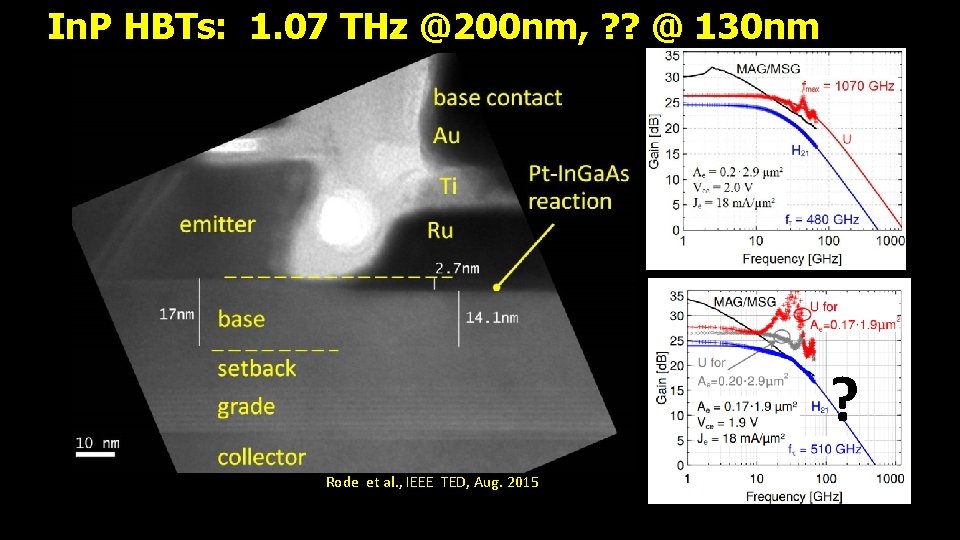
In. P HBTs: 1. 07 THz @200 nm, ? ? @ 130 nm ? Rode et al. , IEEE TED, Aug. 2015 11
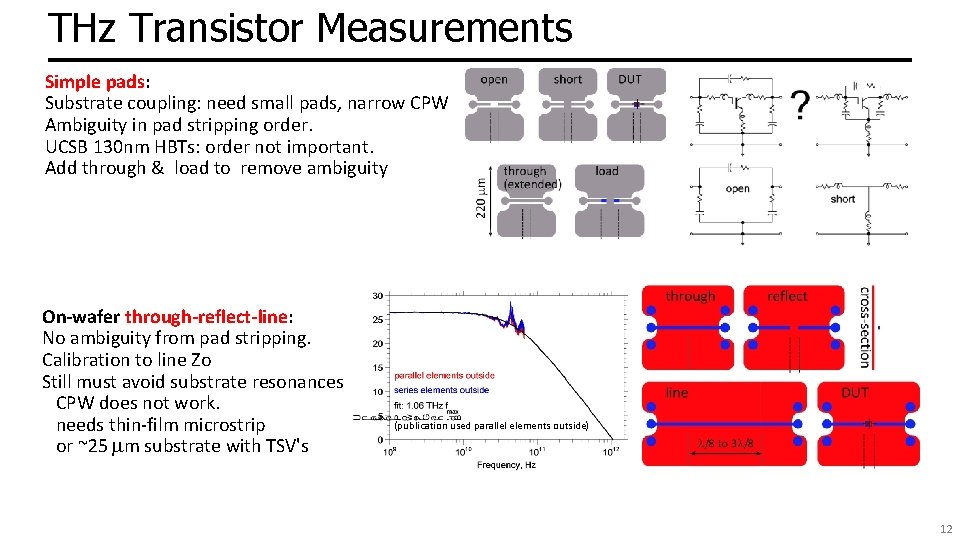
THz Transistor Measurements Simple pads: Substrate coupling: need small pads, narrow CPW Ambiguity in pad stripping order. UCSB 130 nm HBTs: order not important. Add through & load to remove ambiguity On-wafer through-reflect-line: No ambiguity from pad stripping. Calibration to line Zo Still must avoid substrate resonances CPW does not work. needs thin-film microstrip or ~25 mm substrate with TSV's (publication used parallel elements outside) 12
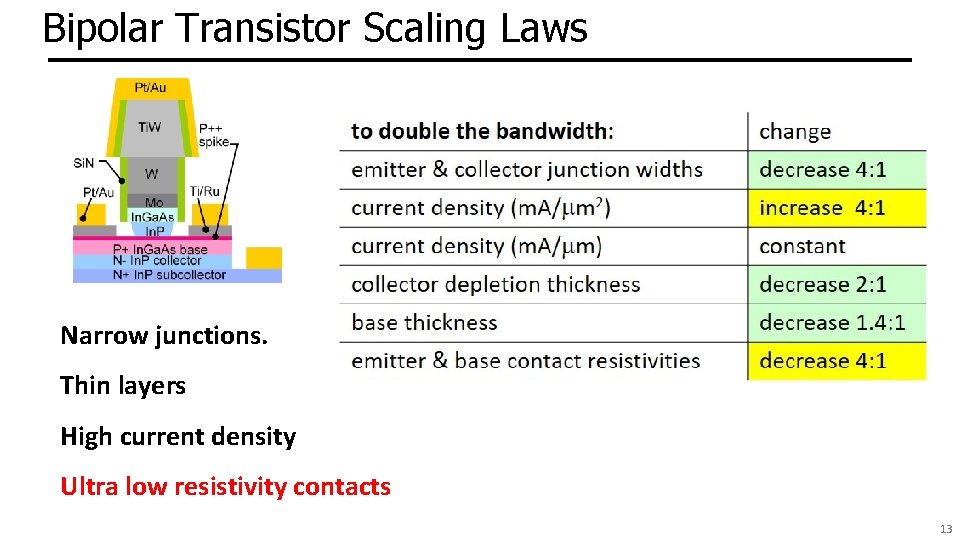
Bipolar Transistor Scaling Laws Narrow junctions. Thin layers High current density Ultra low resistivity contacts 13
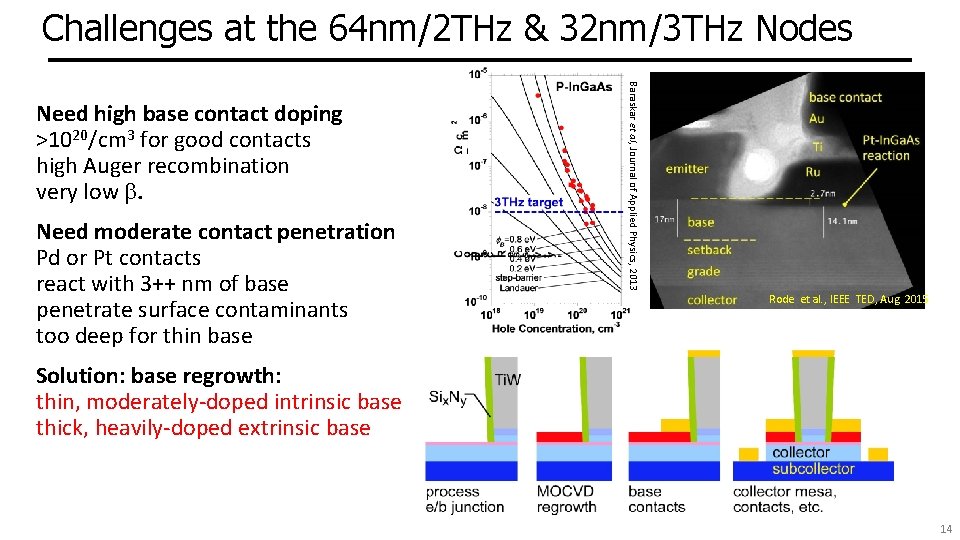
Challenges at the 64 nm/2 THz & 32 nm/3 THz Nodes Need moderate contact penetration Pd or Pt contacts react with 3++ nm of base penetrate surface contaminants too deep for thin base Baraskar et al, Journal of Applied Physics, 2013 Need high base contact doping >1020/cm 3 for good contacts high Auger recombination very low b. Rode et al. , IEEE TED, Aug. 2015 Solution: base regrowth: thin, moderately-doped intrinsic base thick, heavily-doped extrinsic base 14
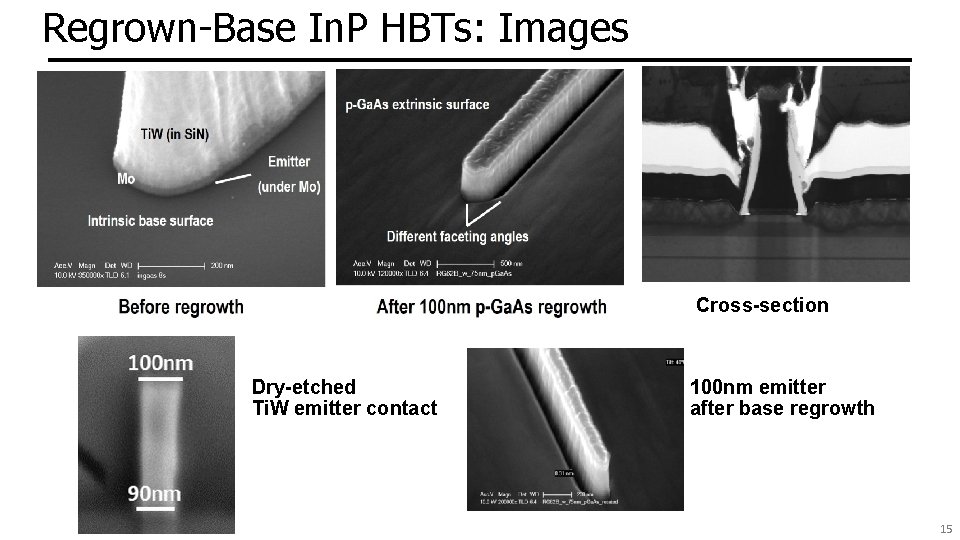
Regrown-Base In. P HBTs: Images Cross-section Dry-etched Ti. W emitter contact 100 nm emitter after base regrowth 15
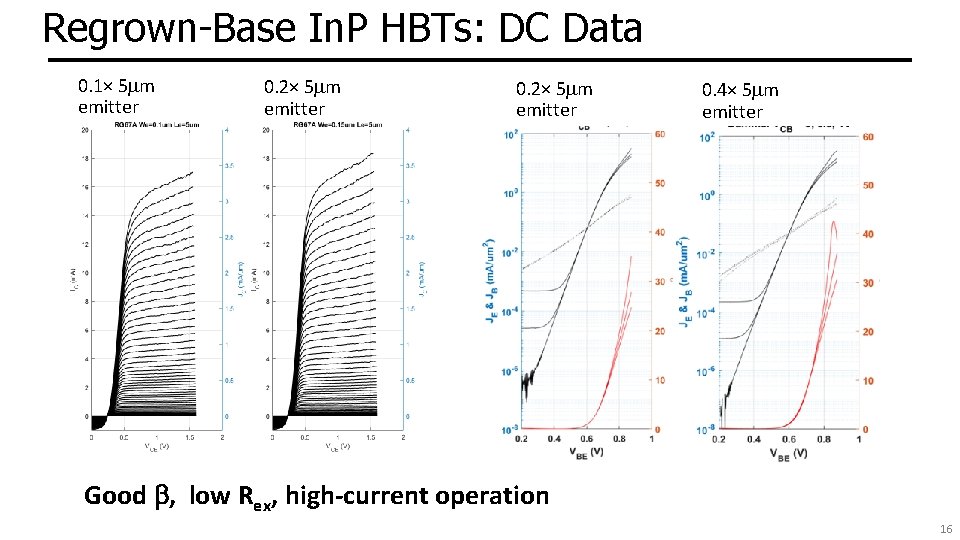
Regrown-Base In. P HBTs: DC Data 0. 1× 5 mm emitter 0. 2× 5 mm emitter 0. 4× 5 mm emitter Good b, low Rex, high-current operation 16
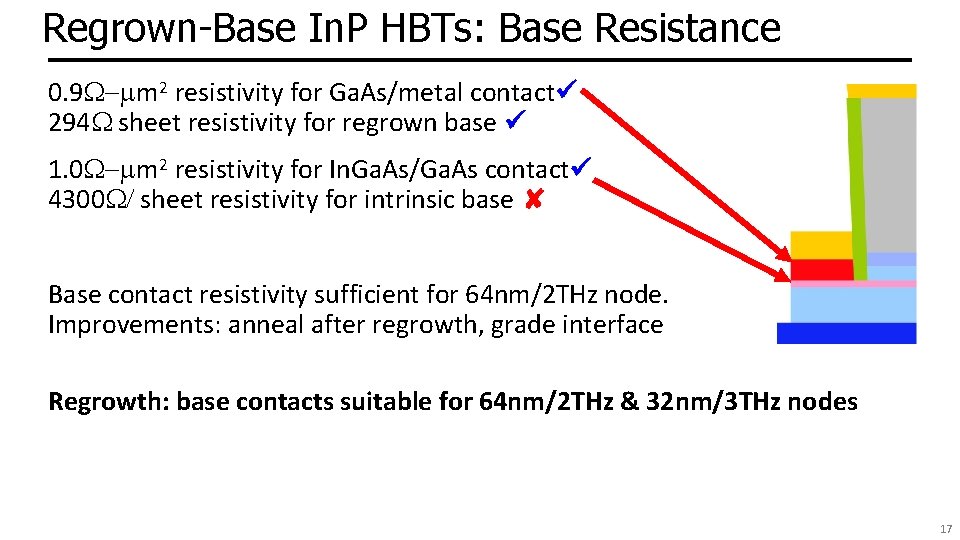
Regrown-Base In. P HBTs: Base Resistance 0. 9 W-mm 2 resistivity for Ga. As/metal contact 294 W sheet resistivity for regrown base 1. 0 W-mm 2 resistivity for In. Ga. As/Ga. As contact 4300 W/ sheet resistivity for intrinsic base ✘ Base contact resistivity sufficient for 64 nm/2 THz node. Improvements: anneal after regrowth, grade interface Regrowth: base contacts suitable for 64 nm/2 THz & 32 nm/3 THz nodes 17
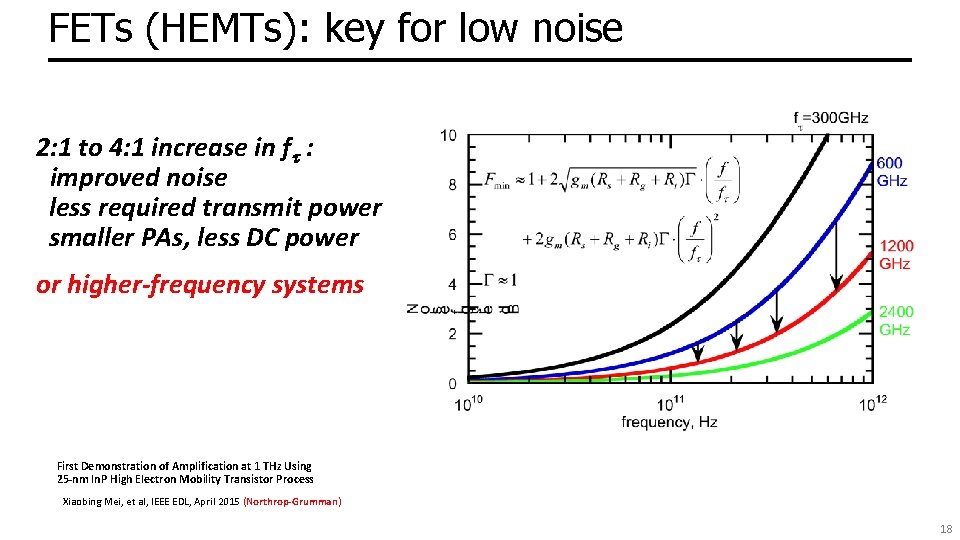
FETs (HEMTs): key for low noise 2: 1 to 4: 1 increase in ft : improved noise less required transmit power smaller PAs, less DC power or higher-frequency systems First Demonstration of Amplification at 1 THz Using 25 -nm In. P High Electron Mobility Transistor Process Xiaobing Mei, et al, IEEE EDL, April 2015 (Northrop-Grumman) 18
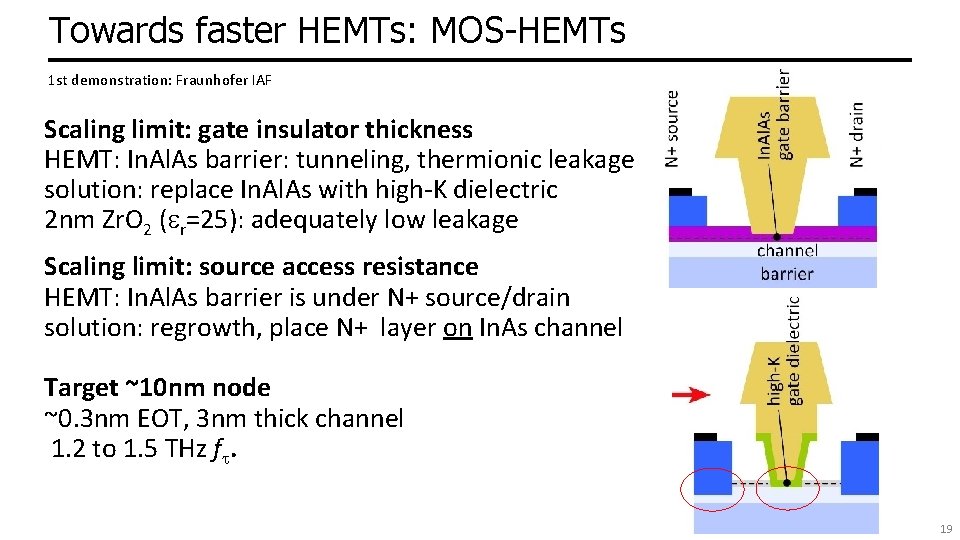
Towards faster HEMTs: MOS-HEMTs 1 st demonstration: Fraunhofer IAF Scaling limit: gate insulator thickness HEMT: In. Al. As barrier: tunneling, thermionic leakage solution: replace In. Al. As with high-K dielectric 2 nm Zr. O 2 (er=25): adequately low leakage Scaling limit: source access resistance HEMT: In. Al. As barrier is under N+ source/drain solution: regrowth, place N+ layer on In. As channel Target ~10 nm node ~0. 3 nm EOT, 3 nm thick channel 1. 2 to 1. 5 THz ft. 19
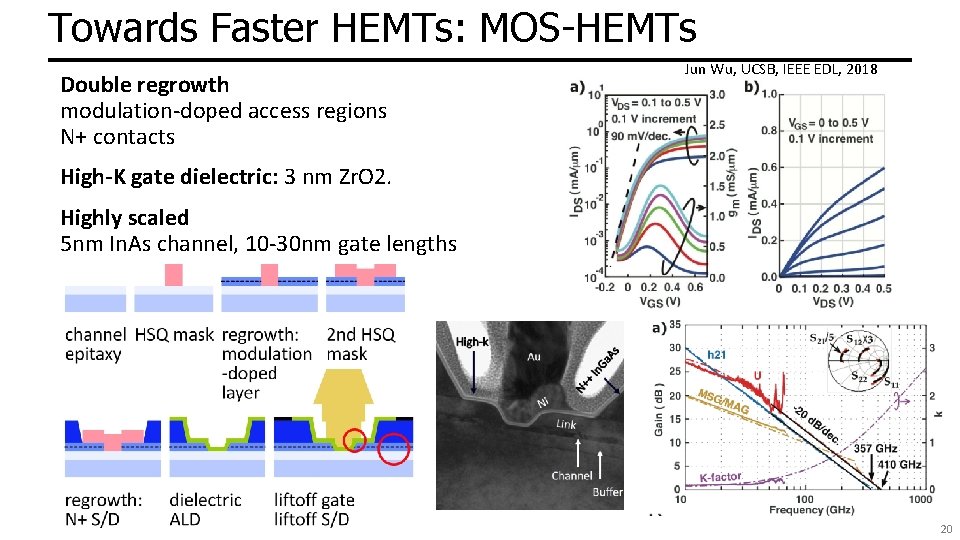
Towards Faster HEMTs: MOS-HEMTs Double regrowth modulation-doped access regions N+ contacts Jun Wu, UCSB, IEEE EDL, 2018 High-K gate dielectric: 3 nm Zr. O 2. Highly scaled 5 nm In. As channel, 10 -30 nm gate lengths 20
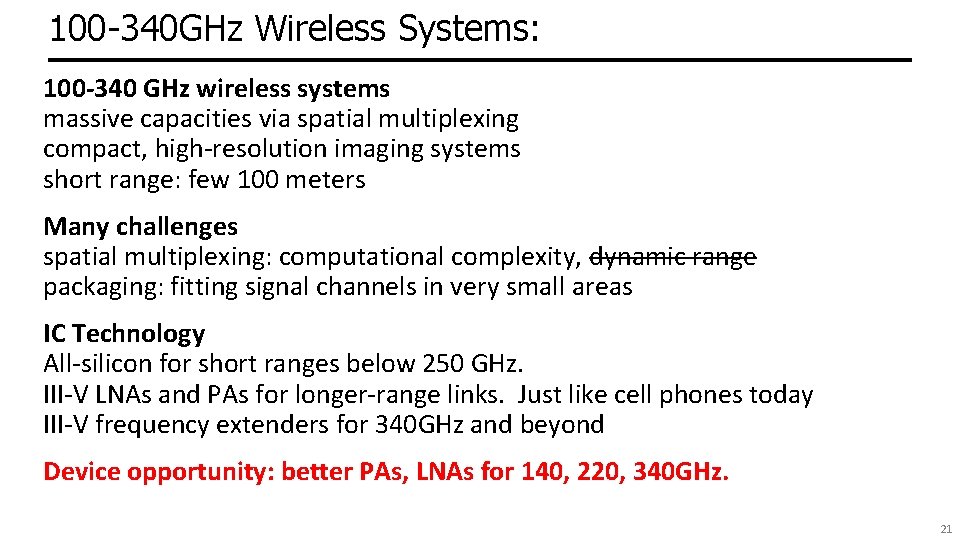
100 -340 GHz Wireless Systems: 100 -340 GHz wireless systems massive capacities via spatial multiplexing compact, high-resolution imaging systems short range: few 100 meters Many challenges spatial multiplexing: computational complexity, dynamic range packaging: fitting signal channels in very small areas IC Technology All-silicon for short ranges below 250 GHz. III-V LNAs and PAs for longer-range links. Just like cell phones today III-V frequency extenders for 340 GHz and beyond Device opportunity: better PAs, LNAs for 140, 220, 340 GHz. 21
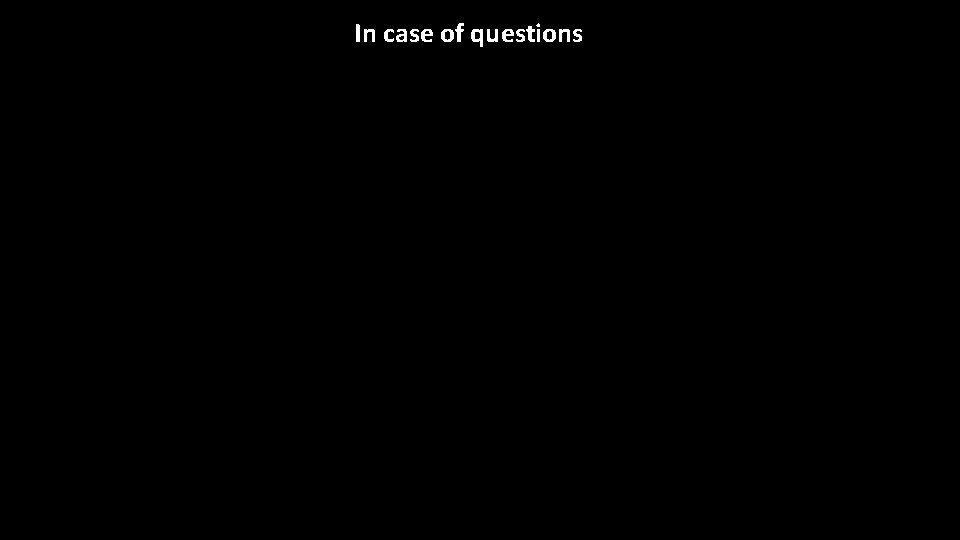
In case of questions 22
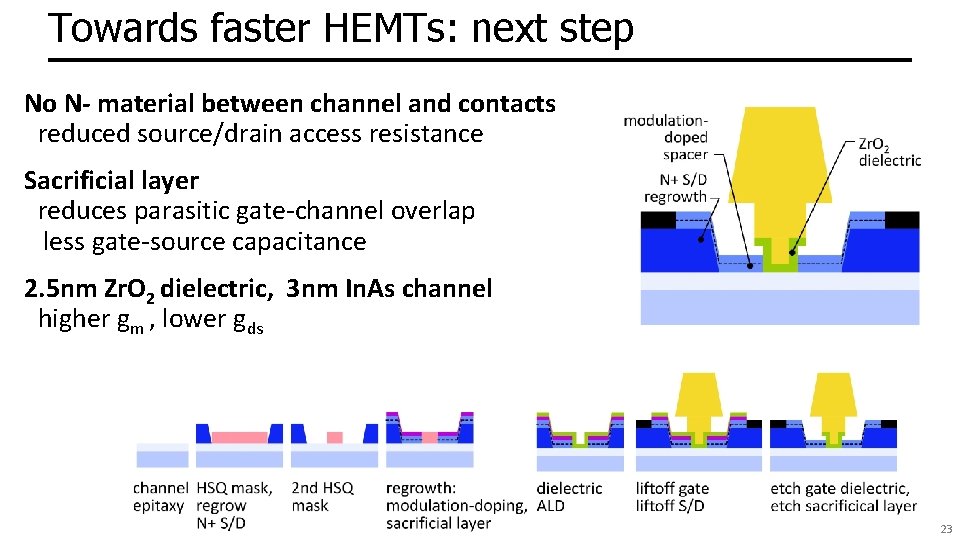
Towards faster HEMTs: next step No N- material between channel and contacts reduced source/drain access resistance Sacrificial layer reduces parasitic gate-channel overlap less gate-source capacitance 2. 5 nm Zr. O 2 dielectric, 3 nm In. As channel higher gm , lower gds 23
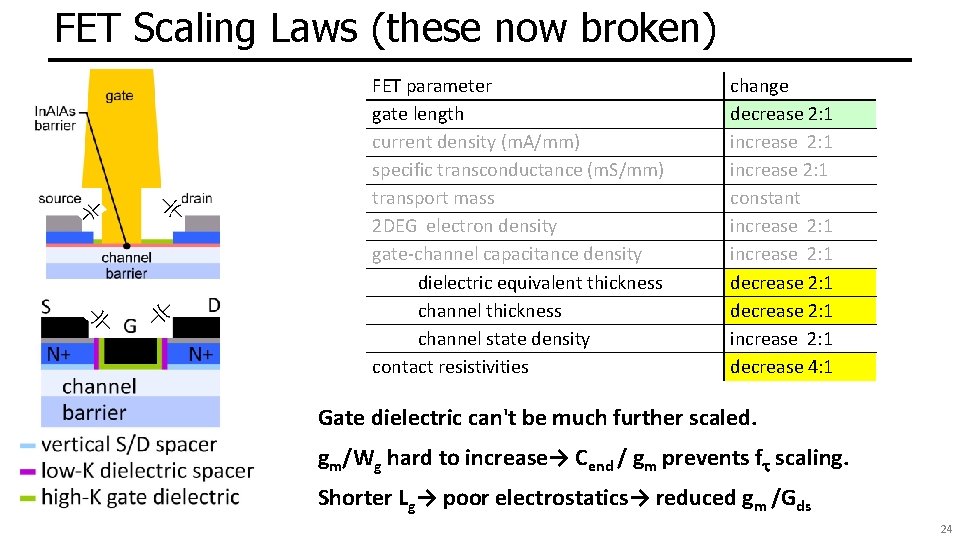
FET Scaling Laws (these now broken) FET parameter gate length current density (m. A/mm) specific transconductance (m. S/mm) transport mass 2 DEG electron density gate-channel capacitance density dielectric equivalent thickness channel state density contact resistivities change decrease 2: 1 increase 2: 1 constant increase 2: 1 decrease 2: 1 increase 2: 1 decrease 4: 1 Gate dielectric can't be much further scaled. gm/Wg hard to increase→ Cend / gm prevents ft scaling. Shorter Lg→ poor electrostatics→ reduced gm /Gds 24
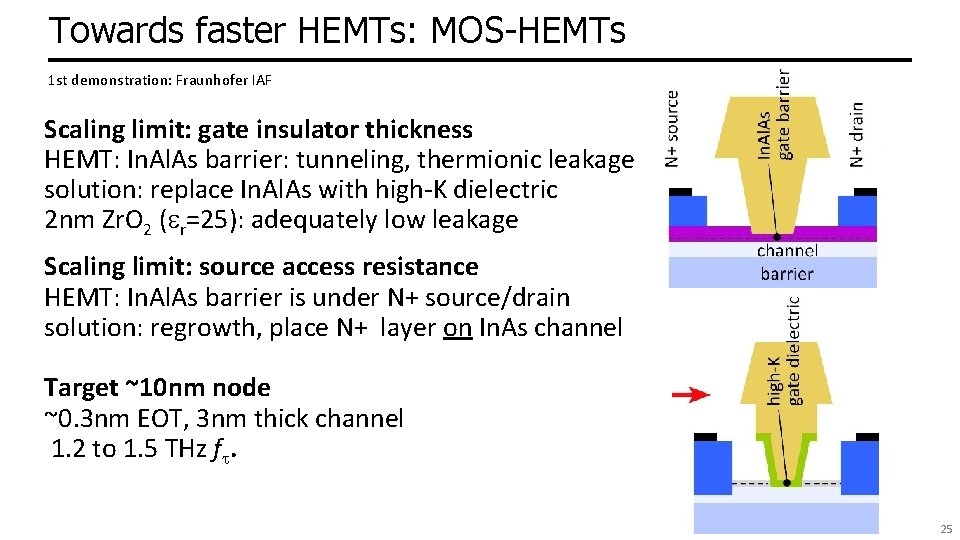
Towards faster HEMTs: MOS-HEMTs 1 st demonstration: Fraunhofer IAF Scaling limit: gate insulator thickness HEMT: In. Al. As barrier: tunneling, thermionic leakage solution: replace In. Al. As with high-K dielectric 2 nm Zr. O 2 (er=25): adequately low leakage Scaling limit: source access resistance HEMT: In. Al. As barrier is under N+ source/drain solution: regrowth, place N+ layer on In. As channel Target ~10 nm node ~0. 3 nm EOT, 3 nm thick channel 1. 2 to 1. 5 THz ft. 25
100 100 100 100 100
Compaq presario pentium 2
24 ghz transverter
From 12 to 140 ghz
Logic gates truth table
How pnp transistor works as a switch
Transistor exercises
Application des transistors bipolaires
Cascaded bjt amplifier
8086 transistor count
Graphical analysis
Ujt structure
Mega giga tera peta
Transistors were used in which generation of computers
Today nanotechnology produced computer transistors
Box plot gcse
100 iops/gb and 100,000 iops per volume oci
Sap systems applications and products
Systems, applications & products in data processing
Systems applications and products
Acquiring information systems and applications
Sap now
Developing and acquiring information systems
Acquiring information systems and applications
"patch operating systems and applications using"
"patch operating systems and applications using"