Evaluation of 1 GHz vs 2 GHz RF
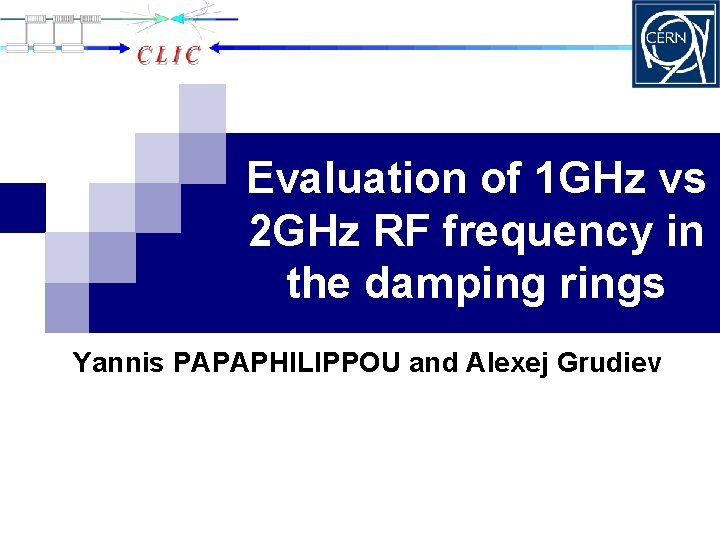
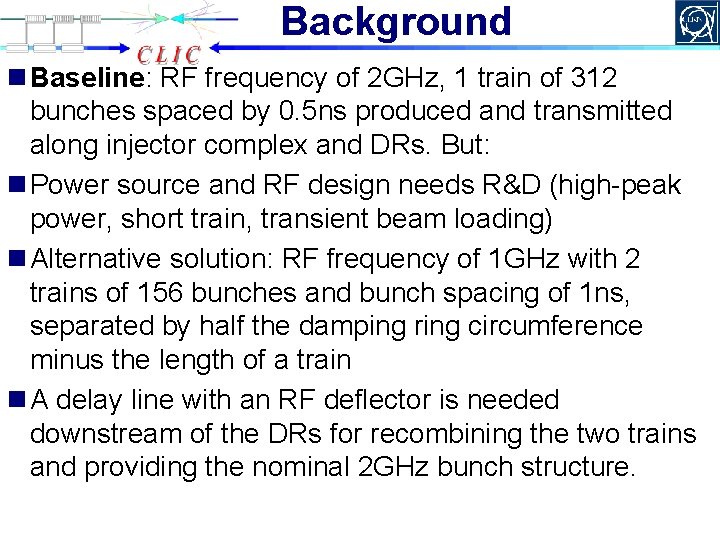
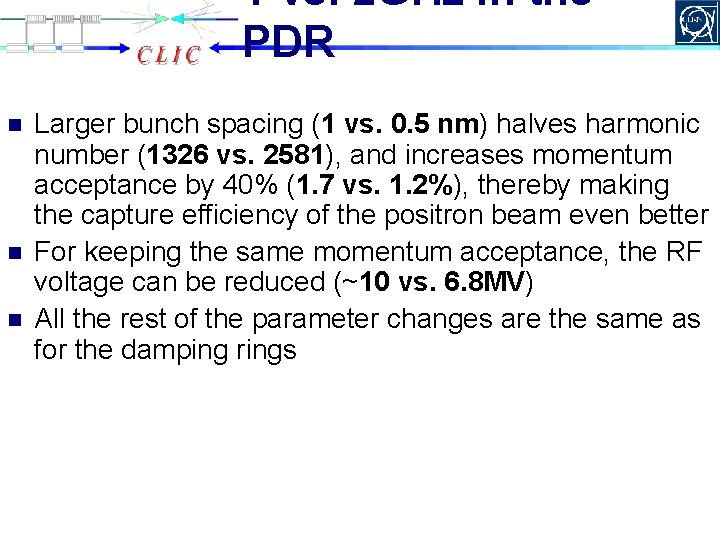
![New DR parameters Parameter DR @ 1 GHz Circumference [m] Harmonic number DR @ New DR parameters Parameter DR @ 1 GHz Circumference [m] Harmonic number DR @](https://slidetodoc.com/presentation_image/08b89c37a9faa5b08dee4568a4cafe2f/image-4.jpg)
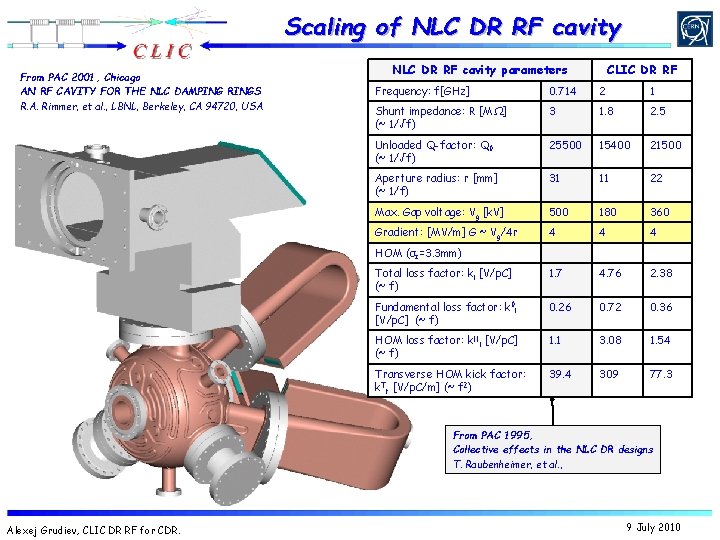
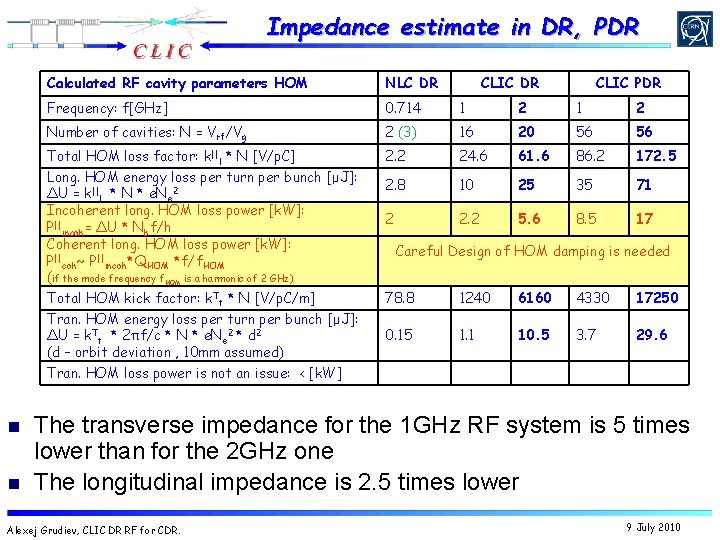
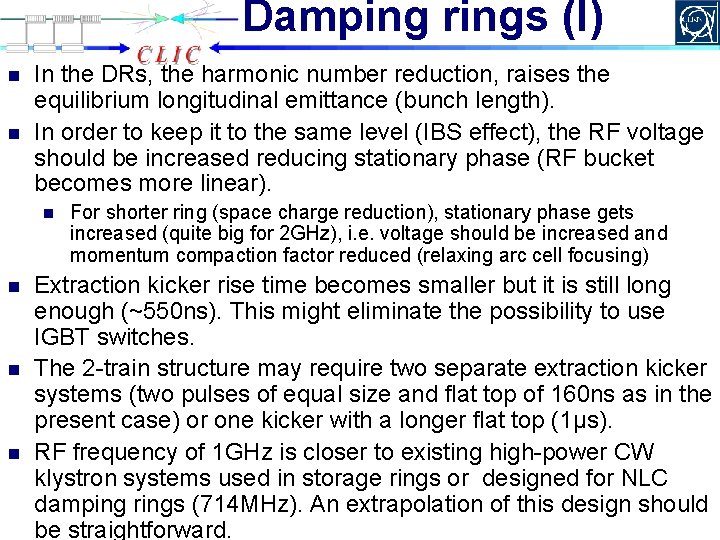
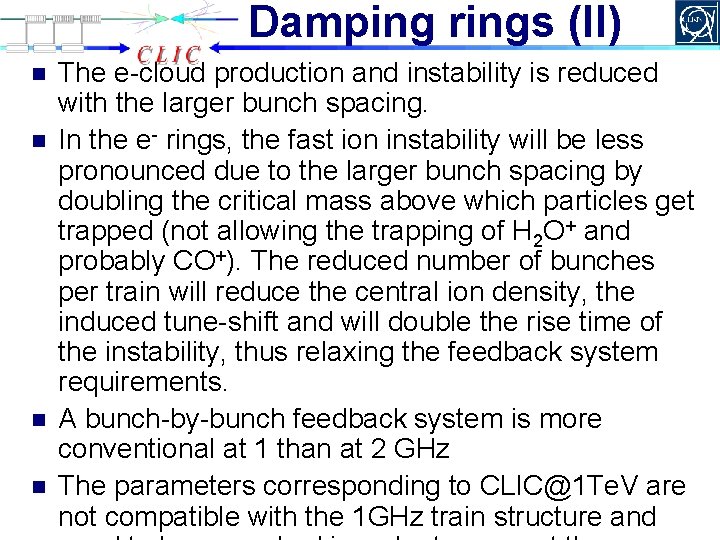
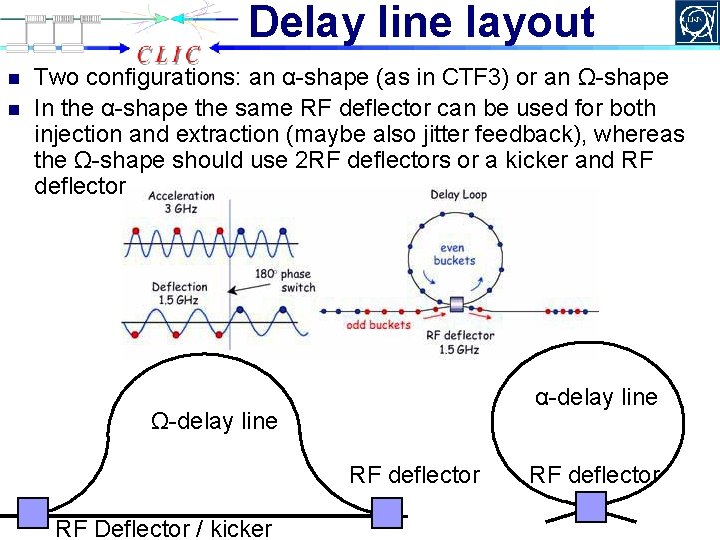
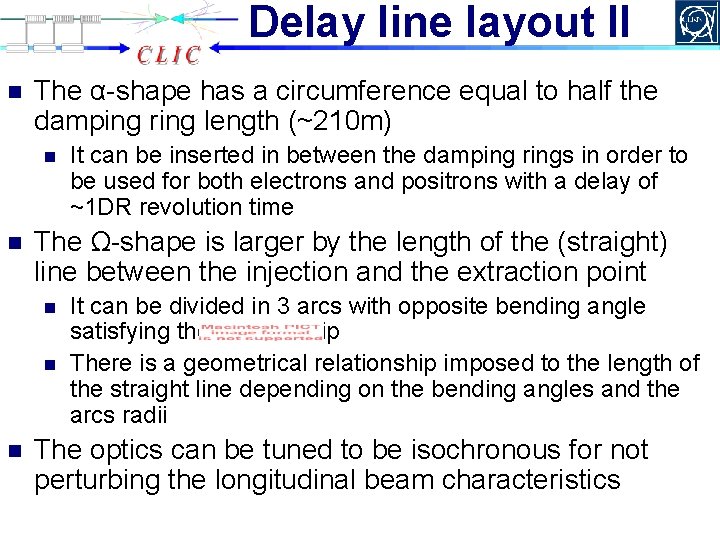
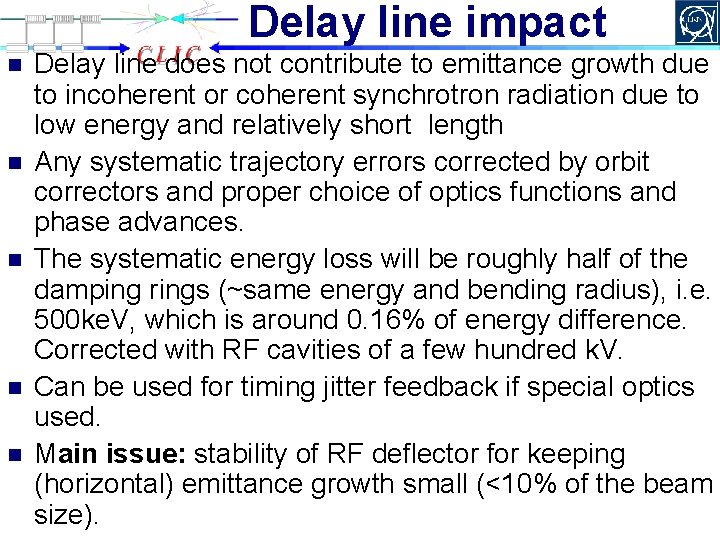
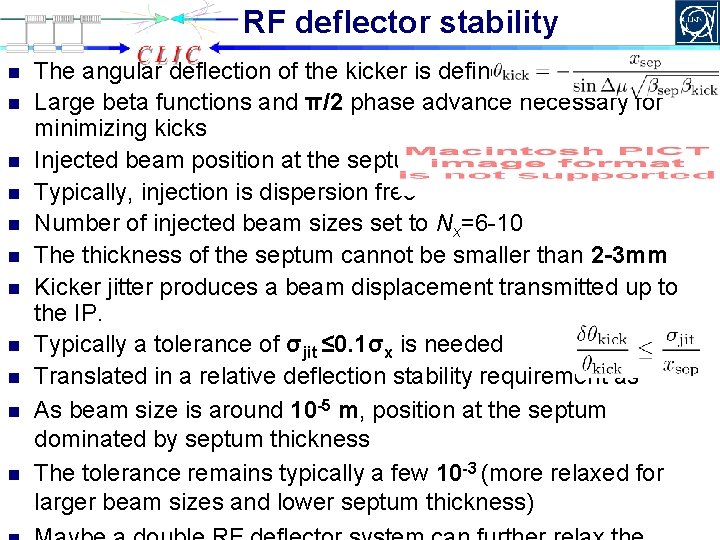
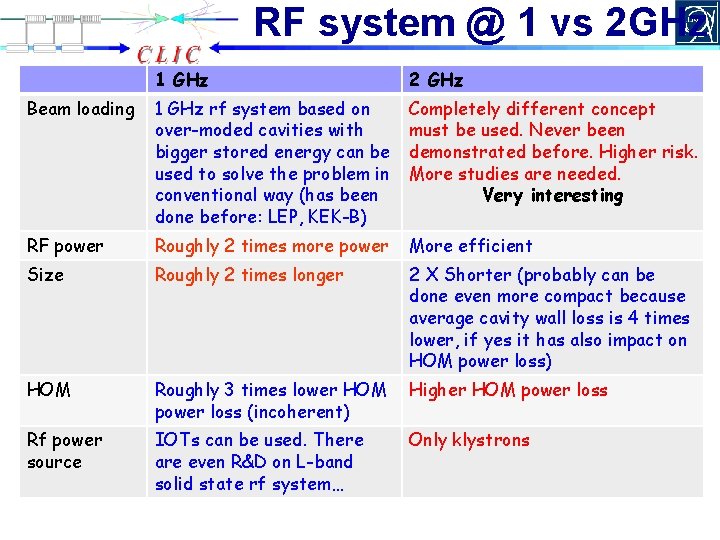
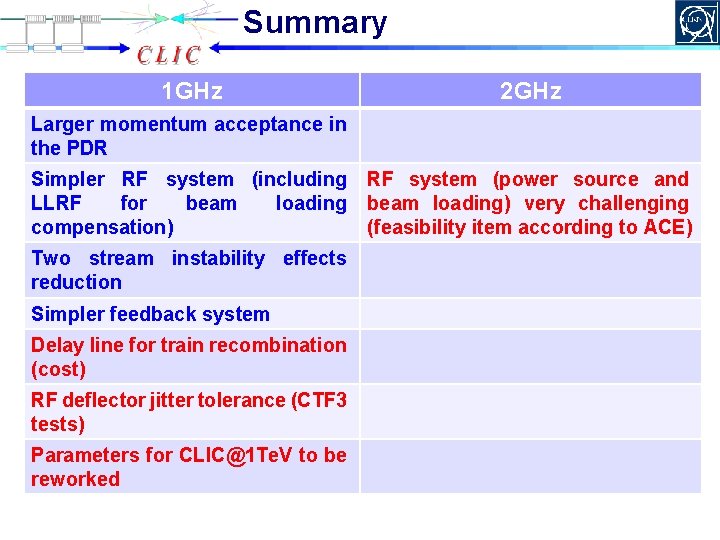
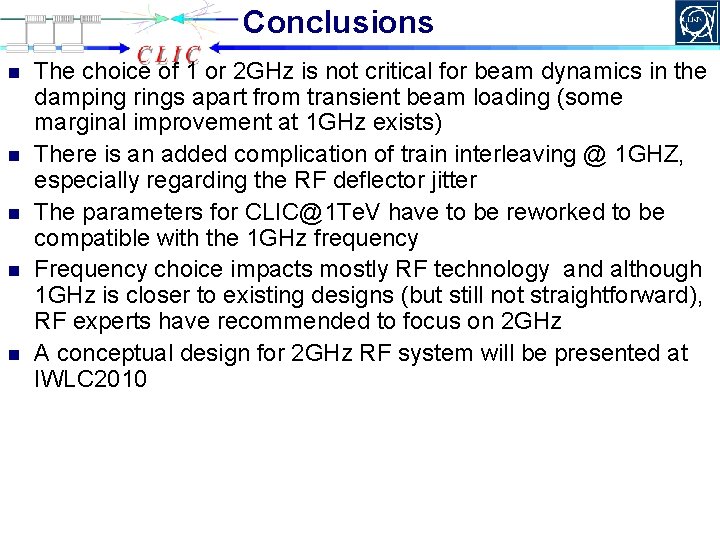
- Slides: 15
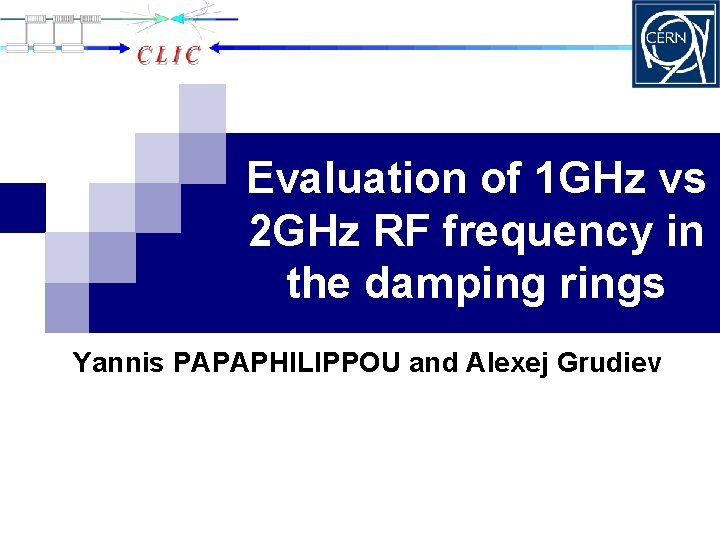
Evaluation of 1 GHz vs 2 GHz RF frequency in the damping rings Yannis PAPAPHILIPPOU and Alexej Grudiev
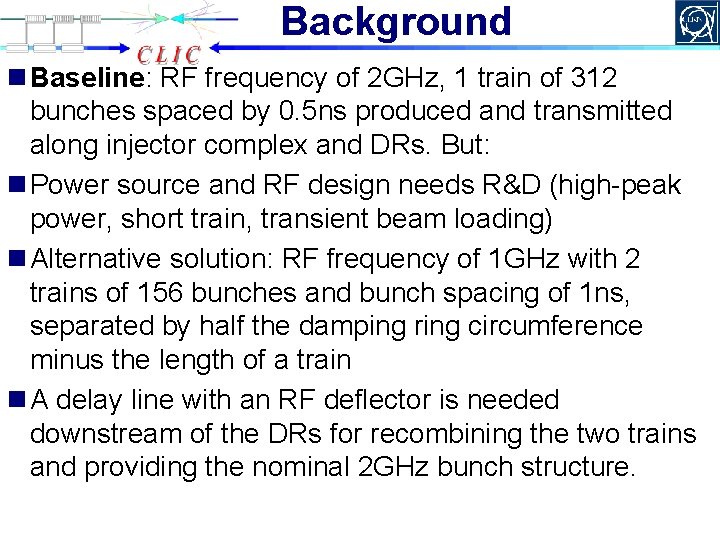
Background n Baseline: RF frequency of 2 GHz, 1 train of 312 bunches spaced by 0. 5 ns produced and transmitted along injector complex and DRs. But: n Power source and RF design needs R&D (high-peak power, short train, transient beam loading) n Alternative solution: RF frequency of 1 GHz with 2 trains of 156 bunches and bunch spacing of 1 ns, separated by half the damping ring circumference minus the length of a train n A delay line with an RF deflector is needed downstream of the DRs for recombining the two trains and providing the nominal 2 GHz bunch structure.
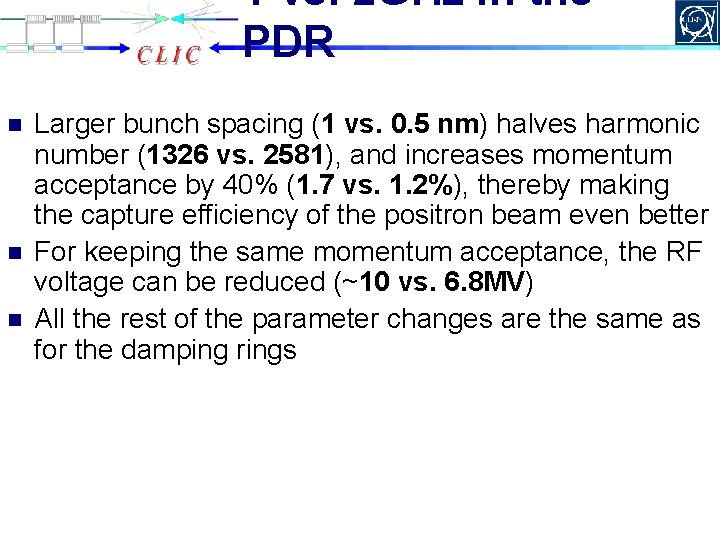
1 vs. 2 GHz in the PDR n n n Larger bunch spacing (1 vs. 0. 5 nm) halves harmonic number (1326 vs. 2581), and increases momentum acceptance by 40% (1. 7 vs. 1. 2%), thereby making the capture efficiency of the positron beam even better For keeping the same momentum acceptance, the RF voltage can be reduced (~10 vs. 6. 8 MV) All the rest of the parameter changes are the same as for the damping rings
![New DR parameters Parameter DR 1 GHz Circumference m Harmonic number DR New DR parameters Parameter DR @ 1 GHz Circumference [m] Harmonic number DR @](https://slidetodoc.com/presentation_image/08b89c37a9faa5b08dee4568a4cafe2f/image-4.jpg)
New DR parameters Parameter DR @ 1 GHz Circumference [m] Harmonic number DR @ 2 GHz 420. 56* 1402 2805 Energy Loss/turn [Me. V] 4. 20 Damping times [ms] (1. 88, 1. 91, 0. 96) Number of wigglers 52 0 -current emittances [nm, e. Vm] (280, 3. 7, 4400) 0 -current mom. spread/bunch length [%/mm] 0. 11/1. 4 RF Voltage/Stat. phase [MV/deg] 4. 9/59 4. 4/73 Momentum compaction factor 7. 6 x 10 -5 Steady state emittances [nm, e. Vm] (480, 4. 5, 5960)** St. state mom. spread/bunch length [%/mm] 0. 13/1. 6 Space charge tune-shift -(0. 006, 0. 12) Peak/Average current [A] 0. 66/0. 145 1. 3/0. 145 * The ring circumferenc e was shortened after relaxing longitudinal parameters in order to reduce space -charge ** Using Bane approximatio n. Piwinski theory gives (400, 4. 5, 540 0)
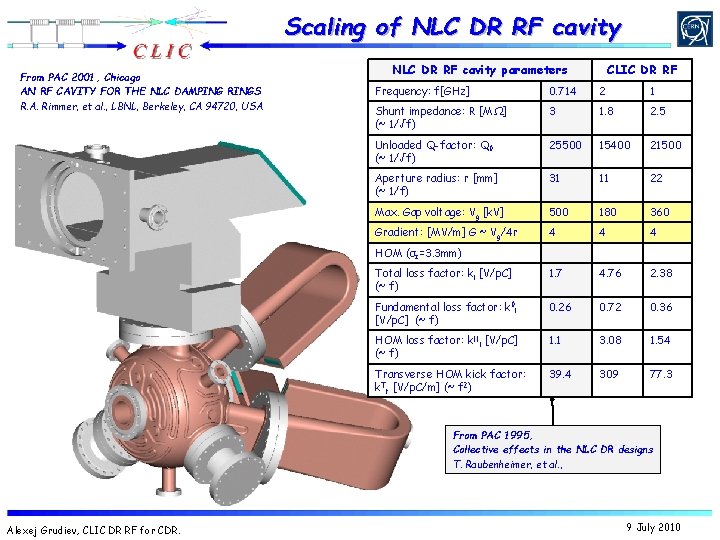
Scaling of NLC DR RF cavity From PAC 2001, Chicago AN RF CAVITY FOR THE NLC DAMPING RINGS R. A. Rimmer, et al. , LBNL, Berkeley, CA 94720, USA NLC DR RF cavity parameters CLIC DR RF Frequency: f[GHz] 0. 714 2 1 Shunt impedance: R [MΩ] (~ 1/√f) 3 1. 8 2. 5 Unloaded Q-factor: Q 0 (~ 1/√f) 25500 15400 21500 Aperture radius: r [mm] (~ 1/f) 31 11 22 Max. Gap voltage: Vg [k. V] 500 180 360 Gradient: [MV/m] G ~ Vg/4 r 4 4 4 Total loss factor: kl [V/p. C] (~ f) 1. 7 4. 76 2. 38 Fundamental loss factor: k 0 l [V/p. C] (~ f) 0. 26 0. 72 0. 36 HOM loss factor: k||l [V/p. C] (~ f) 1. 1 3. 08 1. 54 Transverse HOM kick factor: k. Tt [V/p. C/m] (~ f 2) 39. 4 309 77. 3 HOM (σz=3. 3 mm) From PAC 1995, Collective effects in the NLC DR designs T. Raubenheimer, et al. , Alexej Grudiev, CLIC DR RF for CDR. 9 July 2010
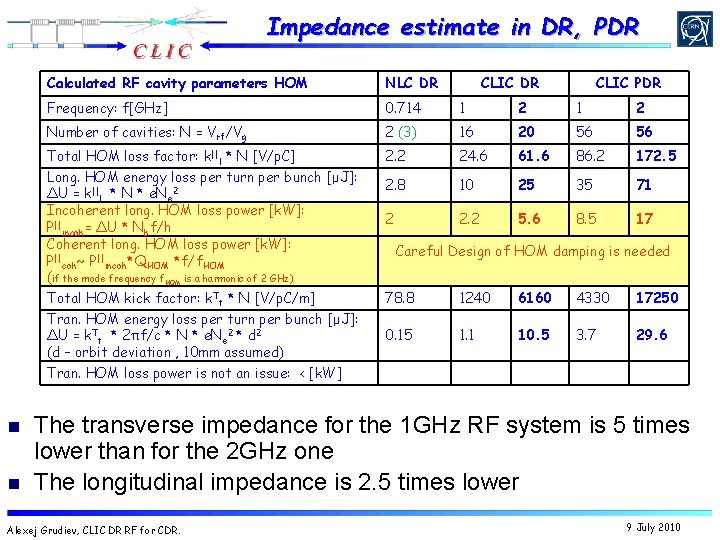
Impedance estimate in DR, PDR n n Calculated RF cavity parameters HOM NLC DR CLIC PDR Frequency: f[GHz] 0. 714 1 2 Number of cavities: N = Vrf/Vg 2 (3) 16 20 56 56 Total HOM loss factor: k||l * N [V/p. C] Long. HOM energy loss per turn per bunch [μJ]: ΔU = k||l * N * e. Ne 2 Incoherent long. HOM loss power [k. W]: P||incoh= ΔU * Nbf/h Coherent long. HOM loss power [k. W]: P||coh~ P||incoh*QHOM *f/f. HOM (if the mode frequency f. HOM is a harmonic of 2 GHz) Total HOM kick factor: k. Tt * N [V/p. C/m] Tran. HOM energy loss per turn per bunch [μJ]: ΔU = k. Tt * 2πf/c * N * e. Ne 2 * d 2 (d – orbit deviation , 10 mm assumed) Tran. HOM loss power is not an issue: < [k. W] 2. 2 24. 6 61. 6 86. 2 172. 5 2. 8 10 25 35 71 2 2. 2 5. 6 8. 5 17 Careful Design of HOM damping is needed 78. 8 1240 6160 4330 17250 0. 15 1. 1 10. 5 3. 7 29. 6 The transverse impedance for the 1 GHz RF system is 5 times lower than for the 2 GHz one The longitudinal impedance is 2. 5 times lower Alexej Grudiev, CLIC DR RF for CDR. 9 July 2010
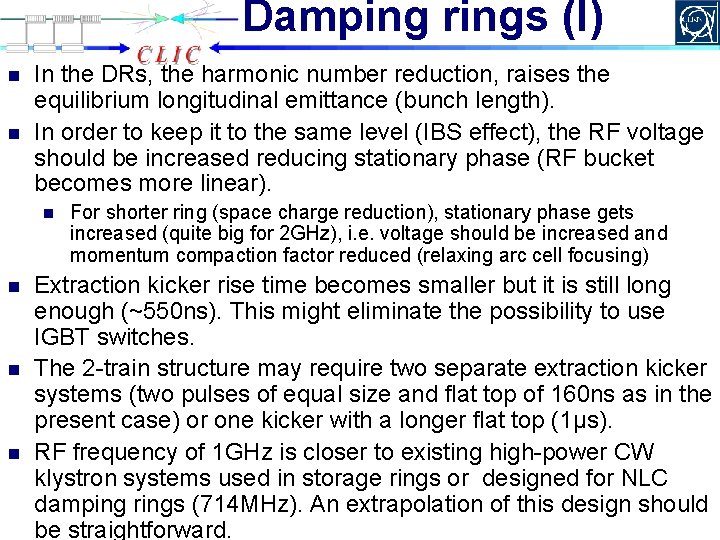
Damping rings (I) n n In the DRs, the harmonic number reduction, raises the equilibrium longitudinal emittance (bunch length). In order to keep it to the same level (IBS effect), the RF voltage should be increased reducing stationary phase (RF bucket becomes more linear). n n For shorter ring (space charge reduction), stationary phase gets increased (quite big for 2 GHz), i. e. voltage should be increased and momentum compaction factor reduced (relaxing arc cell focusing) Extraction kicker rise time becomes smaller but it is still long enough (~550 ns). This might eliminate the possibility to use IGBT switches. The 2 -train structure may require two separate extraction kicker systems (two pulses of equal size and flat top of 160 ns as in the present case) or one kicker with a longer flat top (1μs). RF frequency of 1 GHz is closer to existing high-power CW klystron systems used in storage rings or designed for NLC damping rings (714 MHz). An extrapolation of this design should be straightforward.
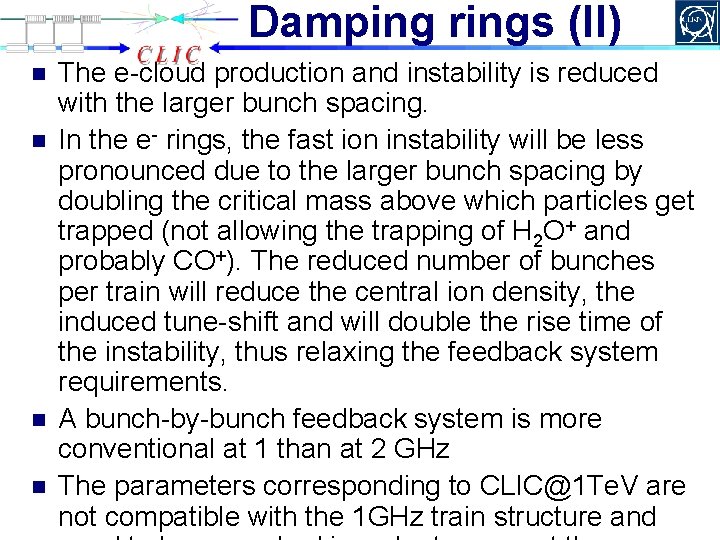
Damping rings (II) n n The e-cloud production and instability is reduced with the larger bunch spacing. In the e- rings, the fast ion instability will be less pronounced due to the larger bunch spacing by doubling the critical mass above which particles get trapped (not allowing the trapping of H 2 O+ and probably CO+). The reduced number of bunches per train will reduce the central ion density, the induced tune-shift and will double the rise time of the instability, thus relaxing the feedback system requirements. A bunch-by-bunch feedback system is more conventional at 1 than at 2 GHz The parameters corresponding to CLIC@1 Te. V are not compatible with the 1 GHz train structure and
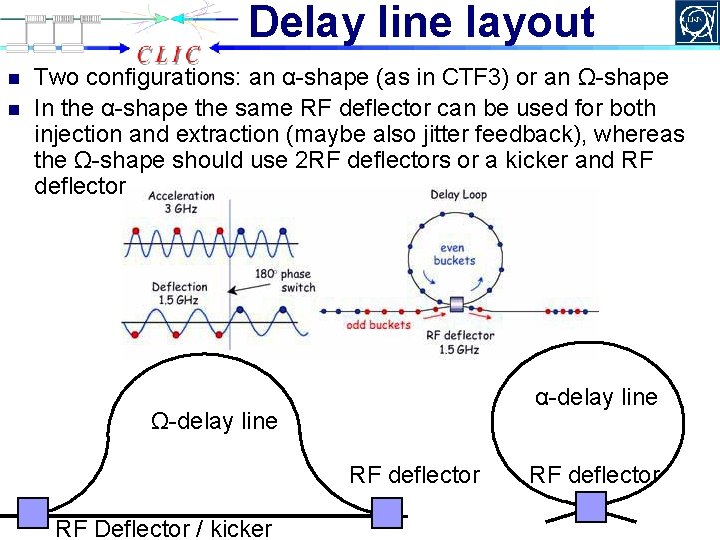
Delay line layout n n Two configurations: an α-shape (as in CTF 3) or an Ω-shape In the α-shape the same RF deflector can be used for both injection and extraction (maybe also jitter feedback), whereas the Ω-shape should use 2 RF deflectors or a kicker and RF deflector α-delay line Ω-delay line RF deflector RF Deflector / kicker RF deflector
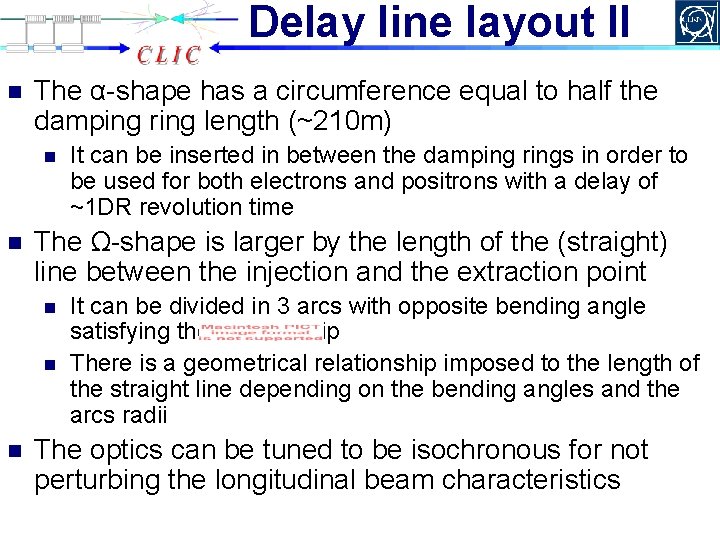
Delay line layout II n The α-shape has a circumference equal to half the damping ring length (~210 m) n n The Ω-shape is larger by the length of the (straight) line between the injection and the extraction point n n n It can be inserted in between the damping rings in order to be used for both electrons and positrons with a delay of ~1 DR revolution time It can be divided in 3 arcs with opposite bending angle satisfying the relationship There is a geometrical relationship imposed to the length of the straight line depending on the bending angles and the arcs radii The optics can be tuned to be isochronous for not perturbing the longitudinal beam characteristics
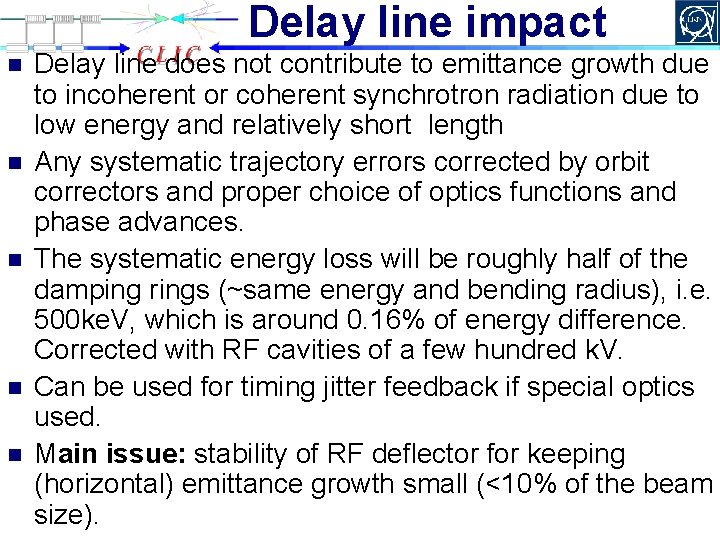
Delay line impact n n n Delay line does not contribute to emittance growth due to incoherent or coherent synchrotron radiation due to low energy and relatively short length Any systematic trajectory errors corrected by orbit correctors and proper choice of optics functions and phase advances. The systematic energy loss will be roughly half of the damping rings (~same energy and bending radius), i. e. 500 ke. V, which is around 0. 16% of energy difference. Corrected with RF cavities of a few hundred k. V. Can be used for timing jitter feedback if special optics used. Main issue: stability of RF deflector for keeping (horizontal) emittance growth small (<10% of the beam size).
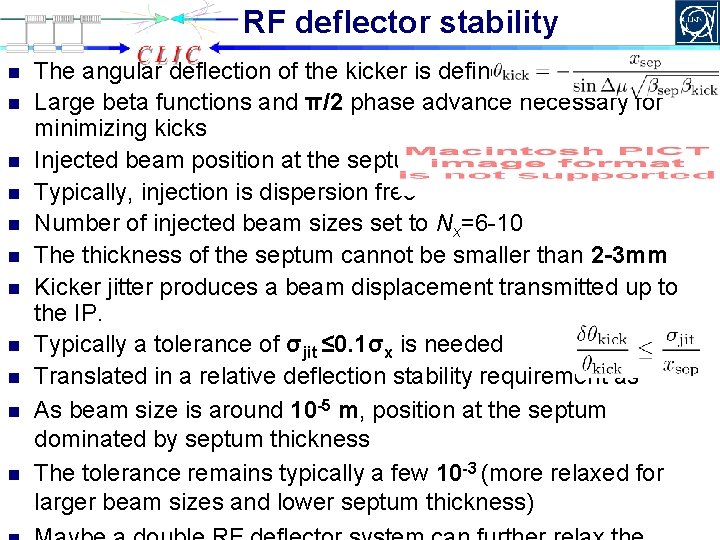
RF deflector stability n n n The angular deflection of the kicker is defined as Large beta functions and π/2 phase advance necessary for minimizing kicks Injected beam position at the septum Typically, injection is dispersion free Number of injected beam sizes set to Nx=6 -10 The thickness of the septum cannot be smaller than 2 -3 mm Kicker jitter produces a beam displacement transmitted up to the IP. Typically a tolerance of σjit ≤ 0. 1σx is needed Translated in a relative deflection stability requirement as As beam size is around 10 -5 m, position at the septum dominated by septum thickness The tolerance remains typically a few 10 -3 (more relaxed for larger beam sizes and lower septum thickness)
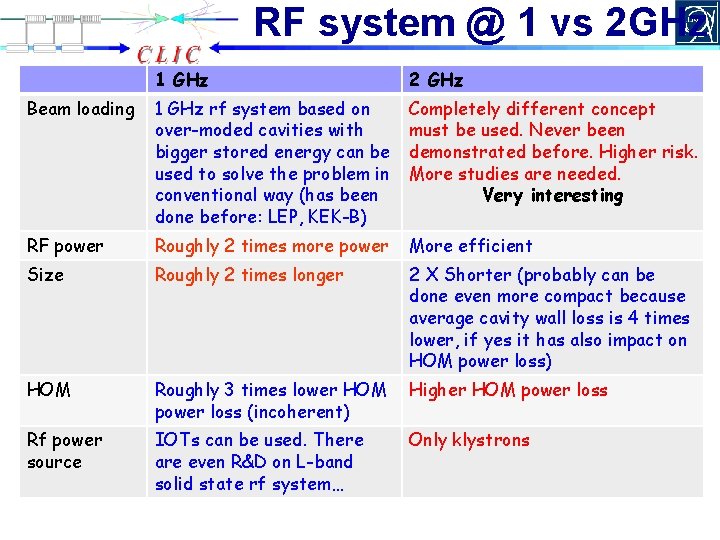
RF system @ 1 vs 2 GHz 1 GHz 2 GHz over-moded cavities with bigger stored energy can be used to solve the problem in conventional way (has been done before: LEP, KEK-B) must be used. Never been demonstrated before. Higher risk. More studies are needed. Very interesting RF power Roughly 2 times more power More efficient Size Roughly 2 times longer 2 X Shorter (probably can be done even more compact because average cavity wall loss is 4 times lower, if yes it has also impact on HOM power loss) HOM Roughly 3 times lower HOM power loss (incoherent) Higher HOM power loss Rf power source IOTs can be used. There are even R&D on L-band solid state rf system… Only klystrons 1 or 21 GHz, cons rf systempros based on and Completely different concept Beam loading
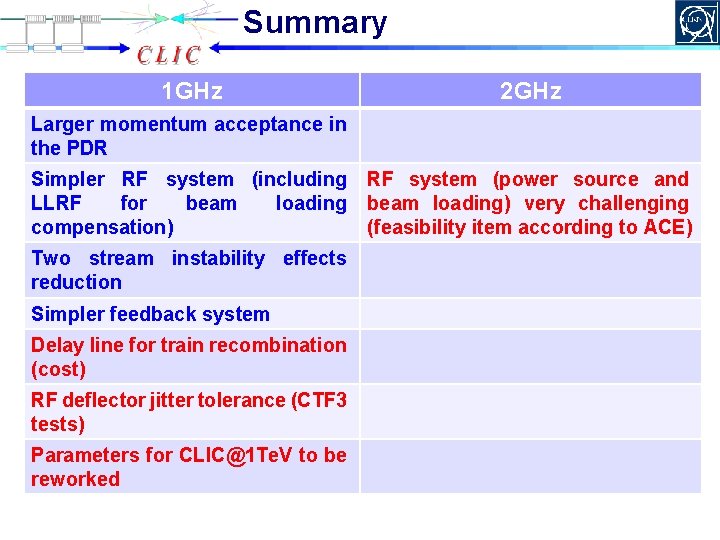
Summary 1 GHz 2 GHz Larger momentum acceptance in the PDR Simpler RF system (including LLRF for beam loading compensation) Two stream instability effects reduction Simpler feedback system Delay line for train recombination (cost) RF deflector jitter tolerance (CTF 3 tests) Parameters for CLIC@1 Te. V to be reworked RF system (power source and beam loading) very challenging (feasibility item according to ACE)
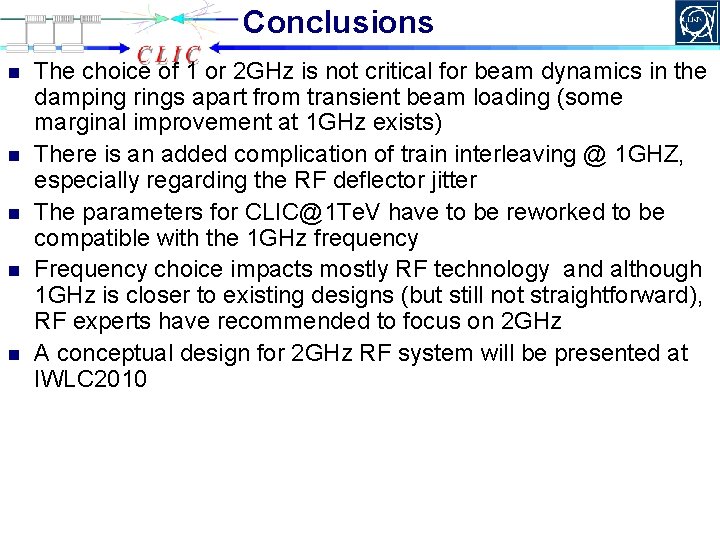
Conclusions n n n The choice of 1 or 2 GHz is not critical for beam dynamics in the damping rings apart from transient beam loading (some marginal improvement at 1 GHz exists) There is an added complication of train interleaving @ 1 GHZ, especially regarding the RF deflector jitter The parameters for CLIC@1 Te. V have to be reworked to be compatible with the 1 GHz frequency Frequency choice impacts mostly RF technology and although 1 GHz is closer to existing designs (but still not straightforward), RF experts have recommended to focus on 2 GHz A conceptual design for 2 GHz RF system will be presented at IWLC 2010
Compaq presario pentium 2
24 ghz transverter
From 12 to 140 ghz
Three phases in the channel member performance audit
Danielson framework
Evaluate schema theory with reference to research studies
Evaluation of aversion therapy
Objectives of evaluation
Evaluation of sources history
It customer service checklist
Erate 471
Evaluation standards
Randa teacher evaluation
Qps evaluation services
Oral reading contest
Evaluation plan