Uncertainties Cf E Higher Physics Any measurement that
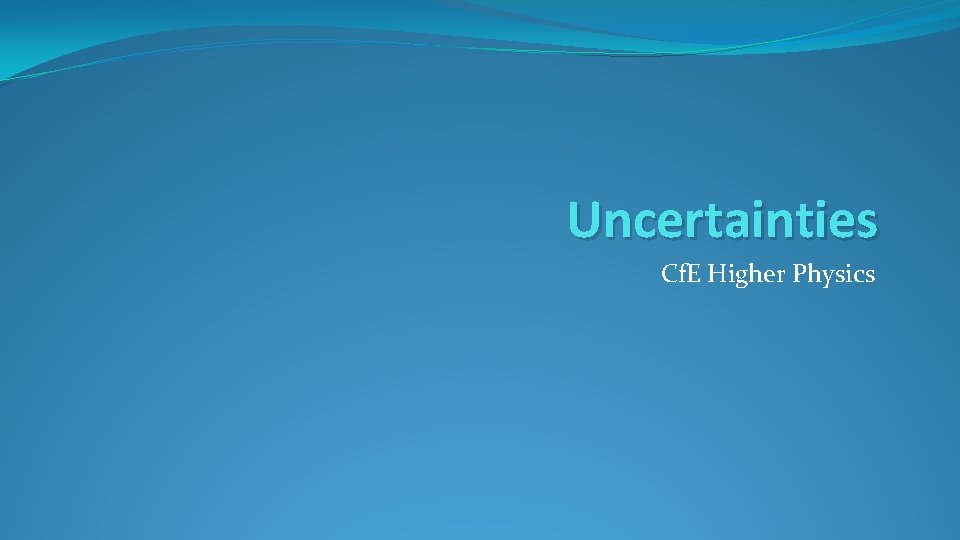
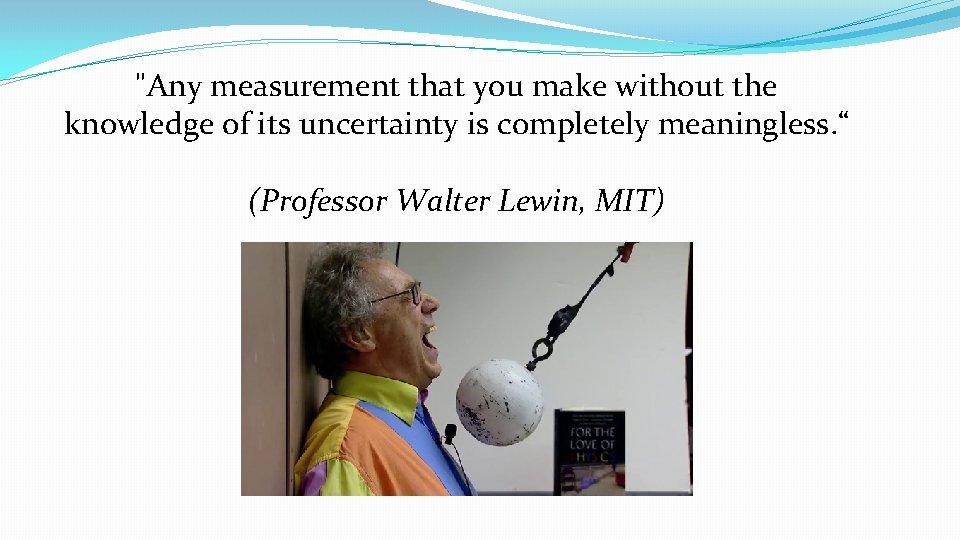
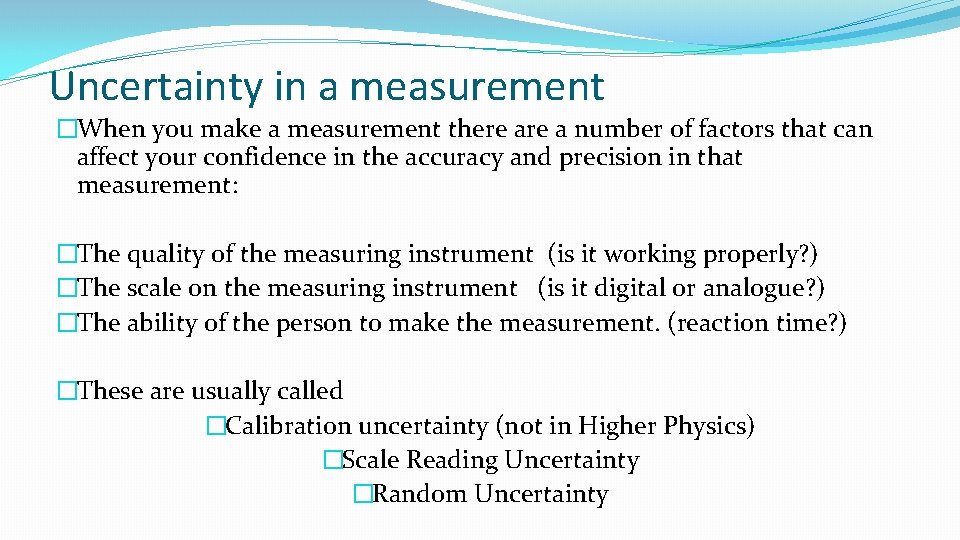
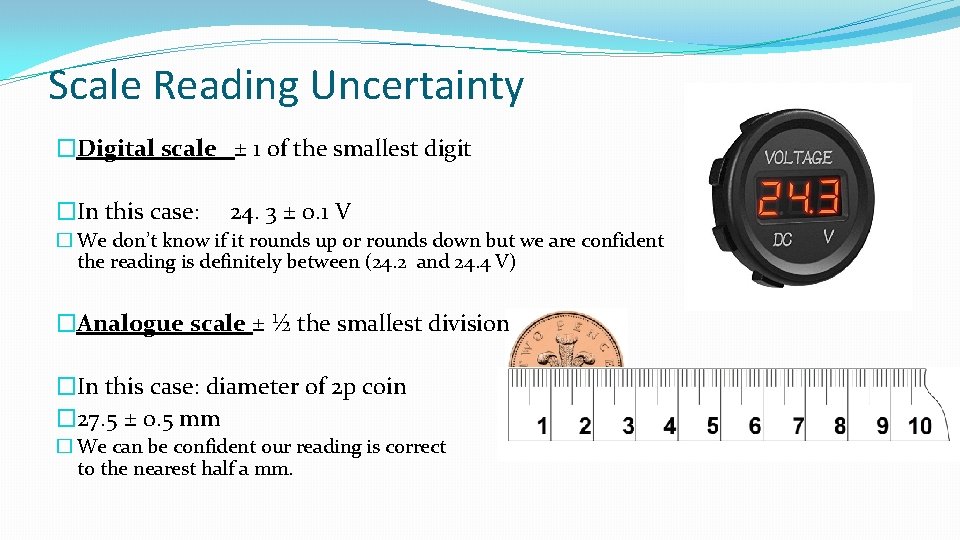
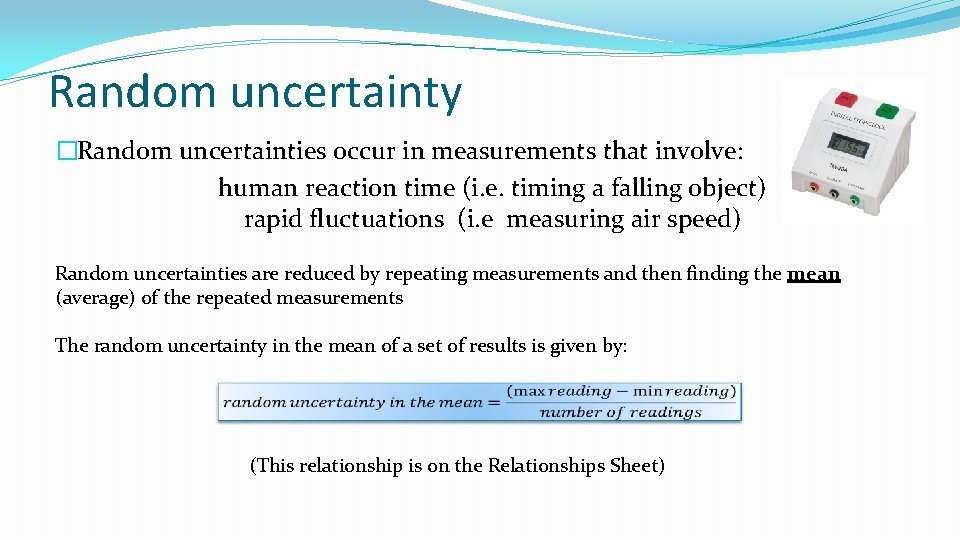
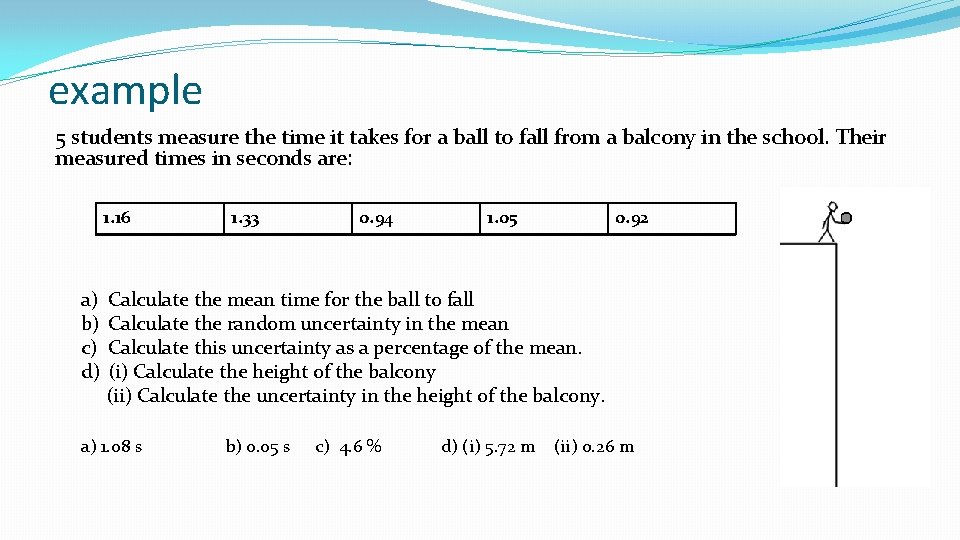
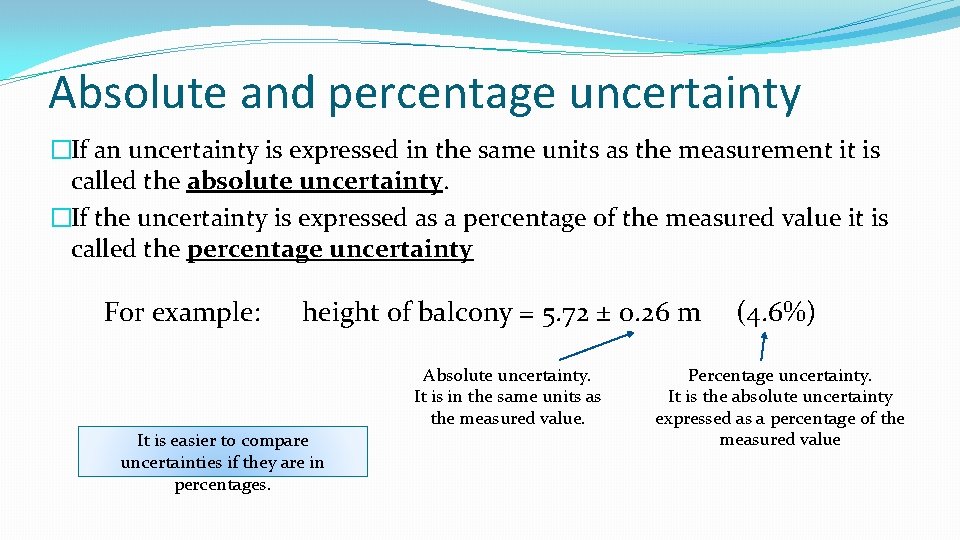
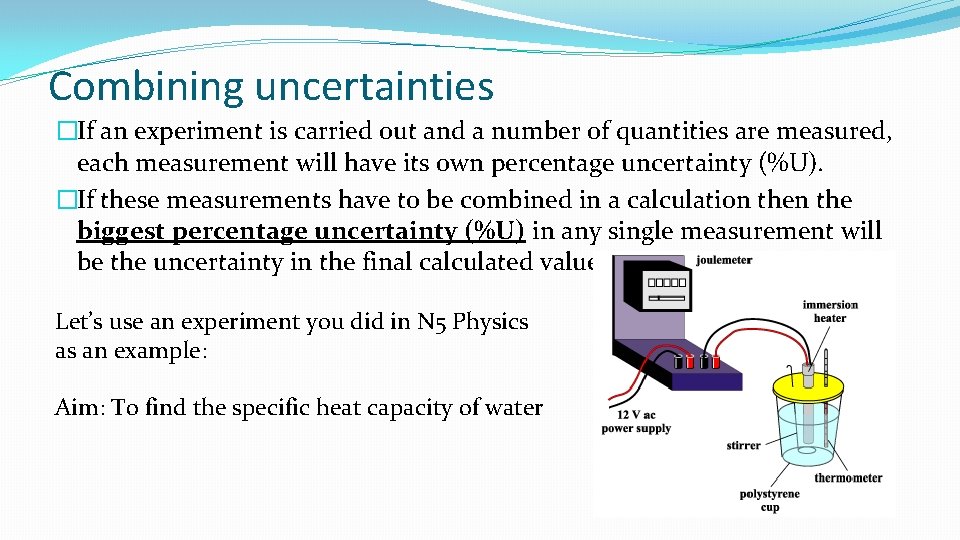
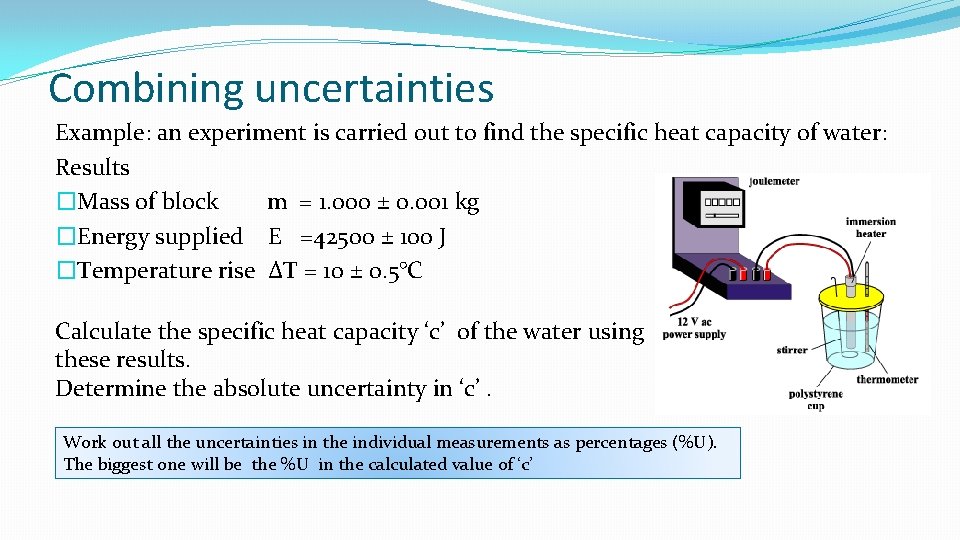
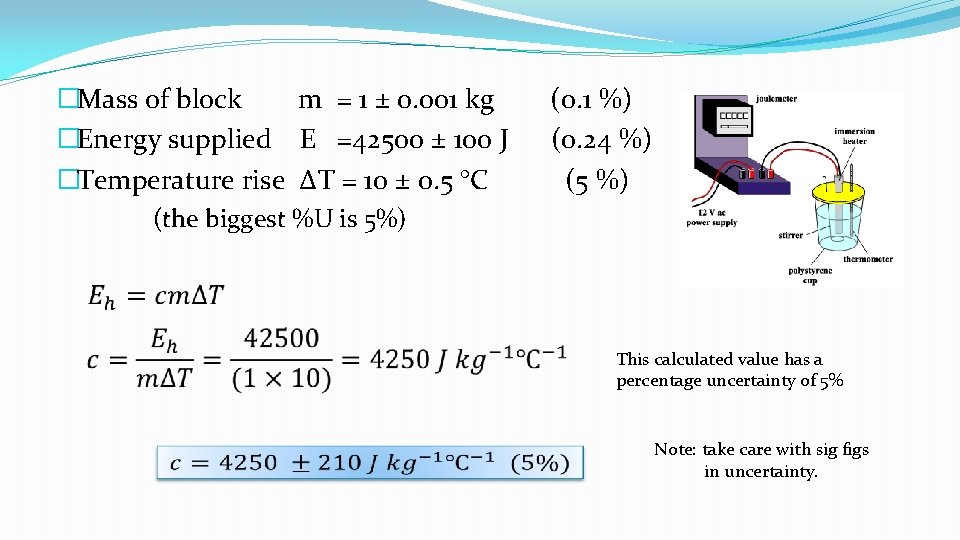
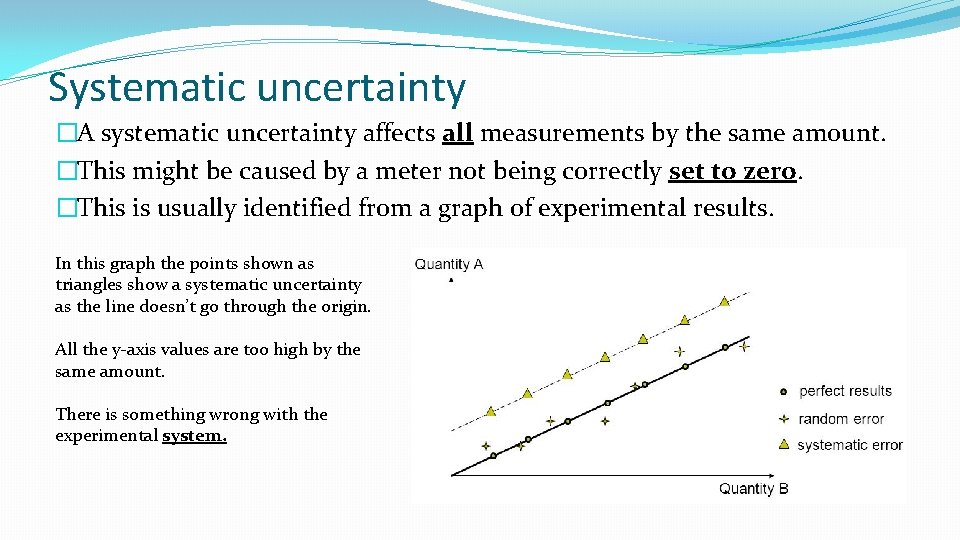
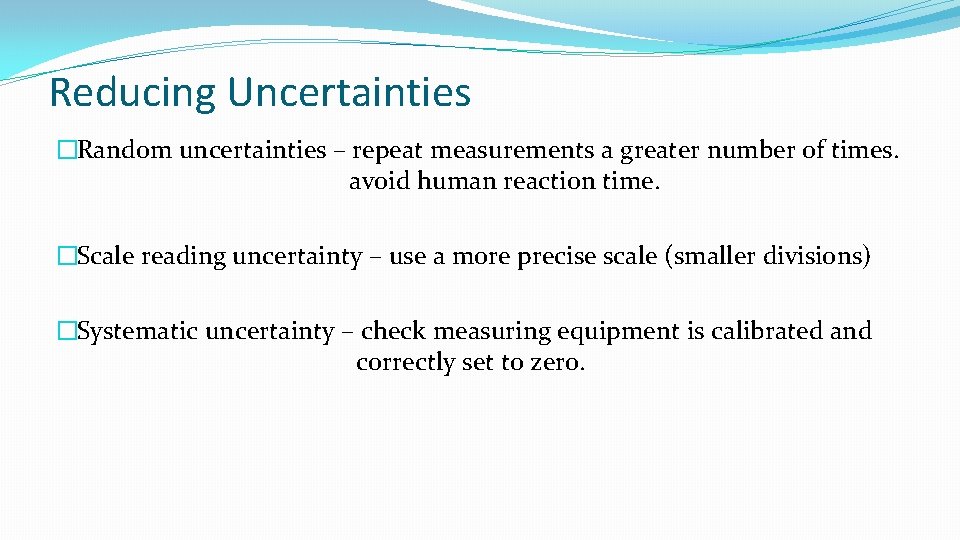
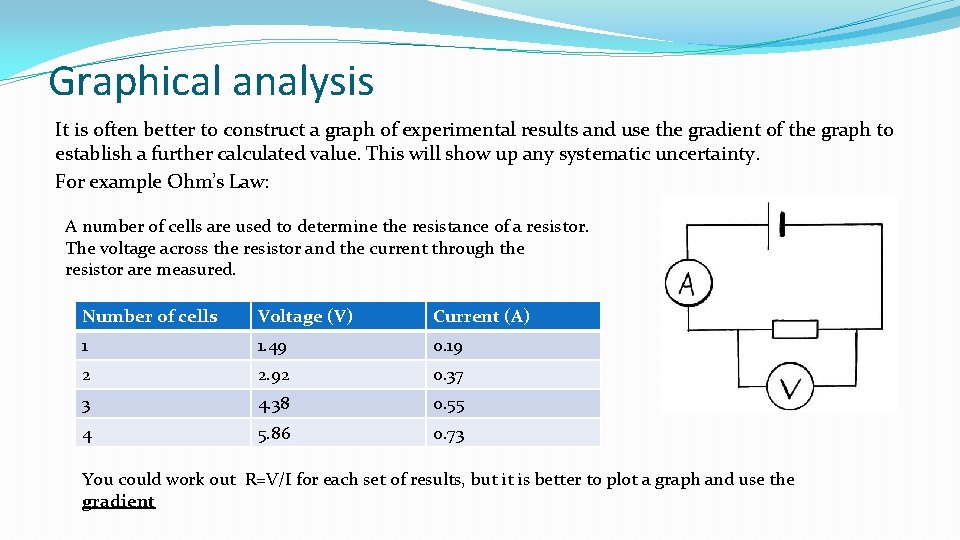
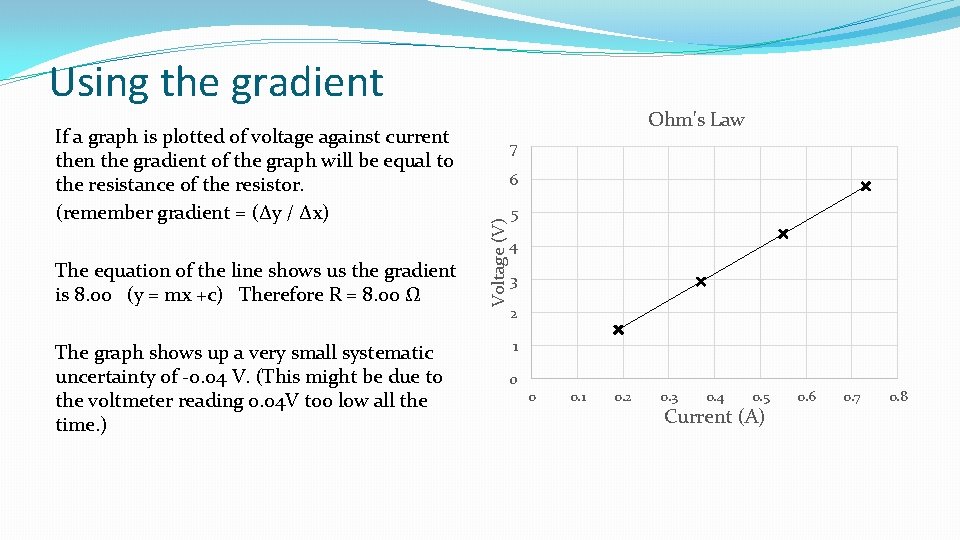
- Slides: 14
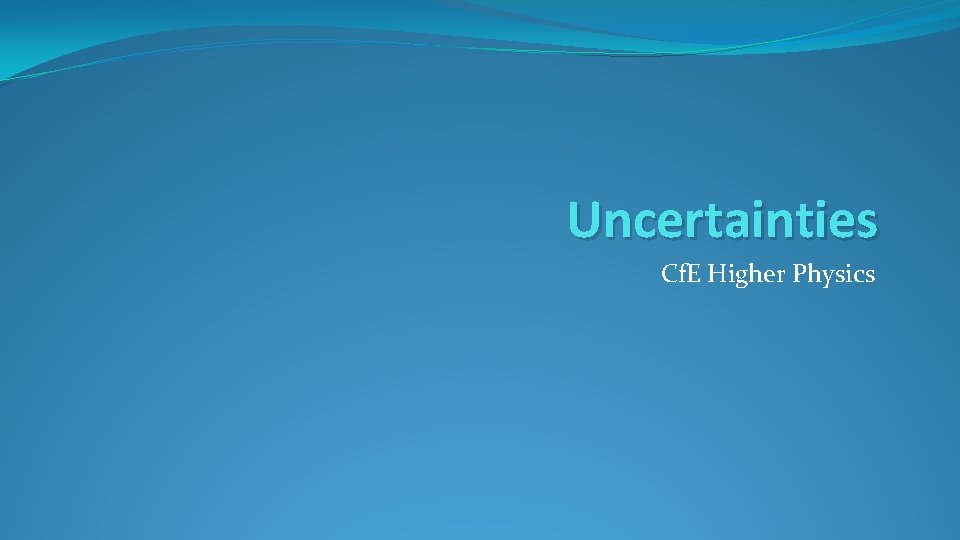
Uncertainties Cf. E Higher Physics
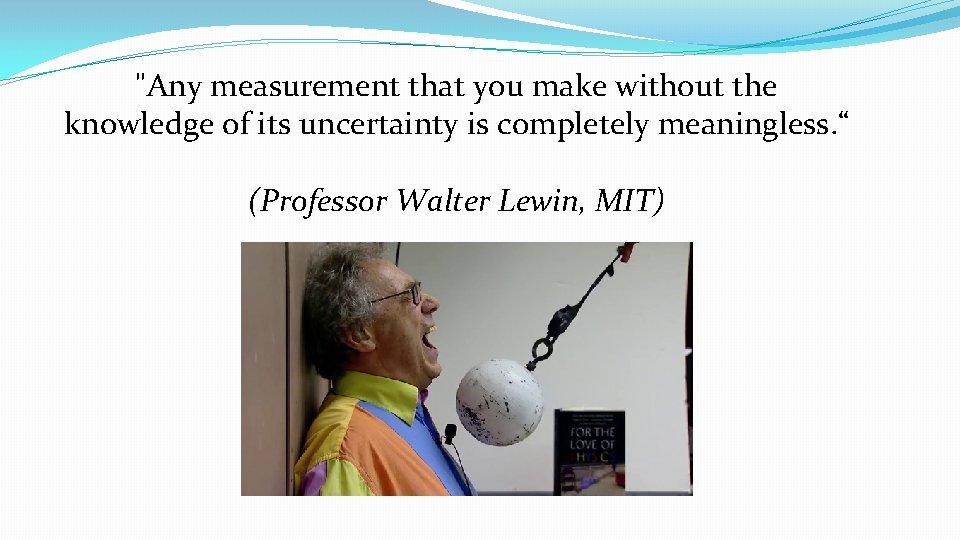
"Any measurement that you make without the knowledge of its uncertainty is completely meaningless. “ (Professor Walter Lewin, MIT)
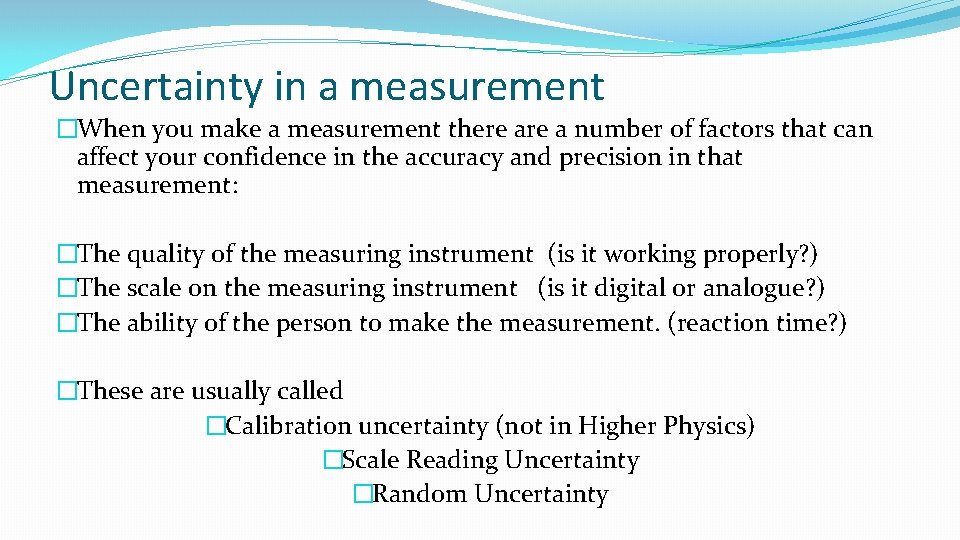
Uncertainty in a measurement �When you make a measurement there a number of factors that can affect your confidence in the accuracy and precision in that measurement: �The quality of the measuring instrument (is it working properly? ) �The scale on the measuring instrument (is it digital or analogue? ) �The ability of the person to make the measurement. (reaction time? ) �These are usually called �Calibration uncertainty (not in Higher Physics) �Scale Reading Uncertainty �Random Uncertainty
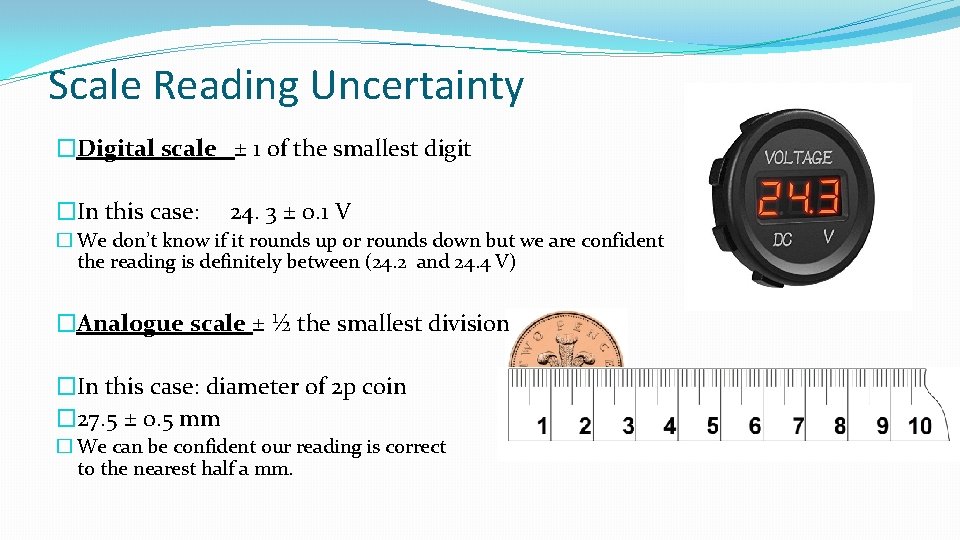
Scale Reading Uncertainty �Digital scale ± 1 of the smallest digit �In this case: 24. 3 ± 0. 1 V � We don’t know if it rounds up or rounds down but we are confident the reading is definitely between (24. 2 and 24. 4 V) �Analogue scale ± ½ the smallest division �In this case: diameter of 2 p coin � 27. 5 ± 0. 5 mm � We can be confident our reading is correct to the nearest half a mm.
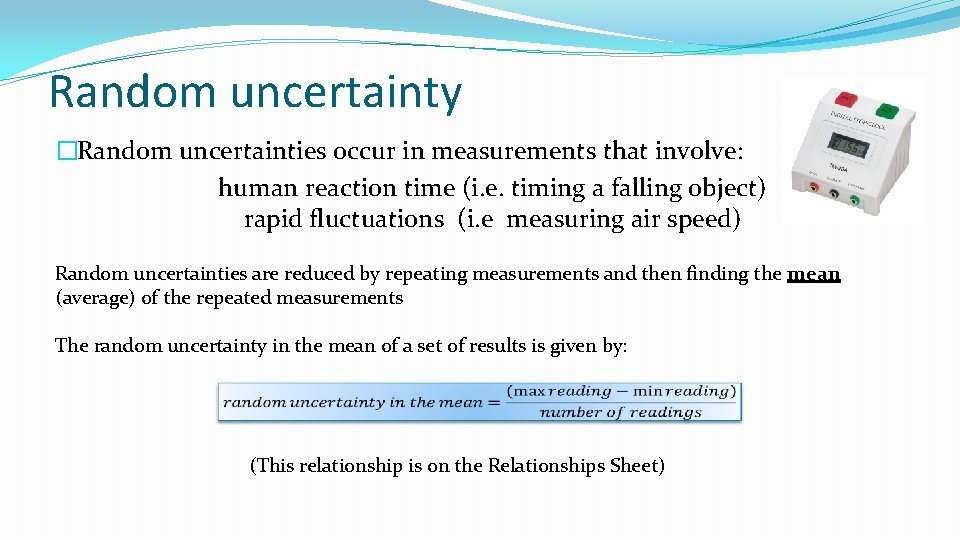
Random uncertainty �Random uncertainties occur in measurements that involve: human reaction time (i. e. timing a falling object) rapid fluctuations (i. e measuring air speed) Random uncertainties are reduced by repeating measurements and then finding the mean (average) of the repeated measurements The random uncertainty in the mean of a set of results is given by: (This relationship is on the Relationships Sheet)
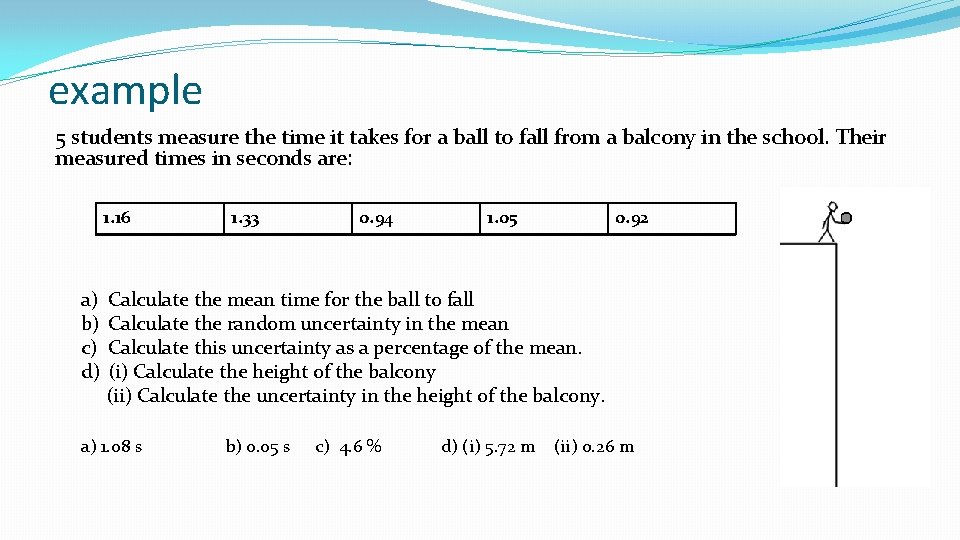
example 5 students measure the time it takes for a ball to fall from a balcony in the school. Their measured times in seconds are: 1. 16 1. 33 0. 94 1. 05 0. 92 a) Calculate the mean time for the ball to fall b) Calculate the random uncertainty in the mean c) Calculate this uncertainty as a percentage of the mean. d) (i) Calculate the height of the balcony (ii) Calculate the uncertainty in the height of the balcony. a) 1. 08 s b) 0. 05 s c) 4. 6 % d) (i) 5. 72 m (ii) o. 26 m
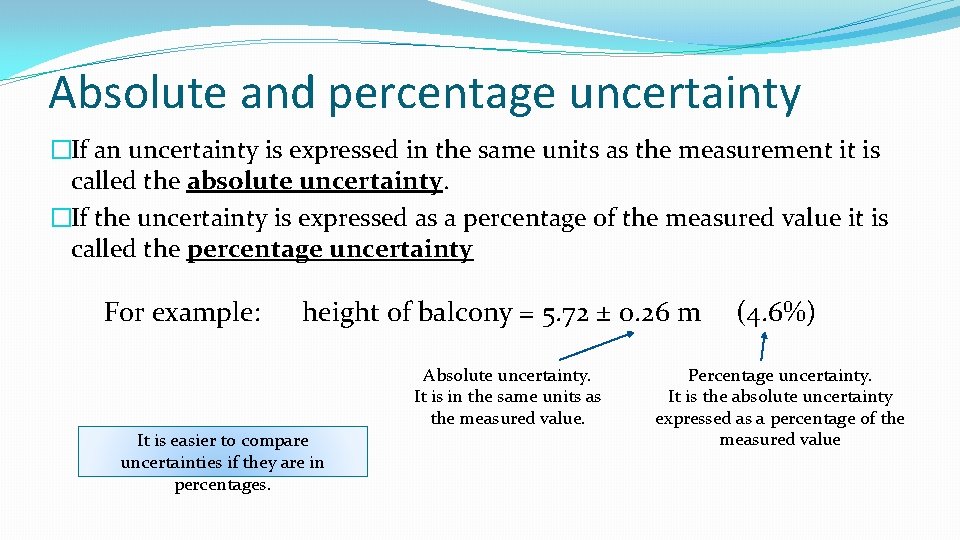
Absolute and percentage uncertainty �If an uncertainty is expressed in the same units as the measurement it is called the absolute uncertainty. �If the uncertainty is expressed as a percentage of the measured value it is called the percentage uncertainty For example: height of balcony = 5. 72 ± 0. 26 m (4. 6%) Absolute uncertainty. It is in the same units as the measured value. It is easier to compare uncertainties if they are in percentages. Percentage uncertainty. It is the absolute uncertainty expressed as a percentage of the measured value
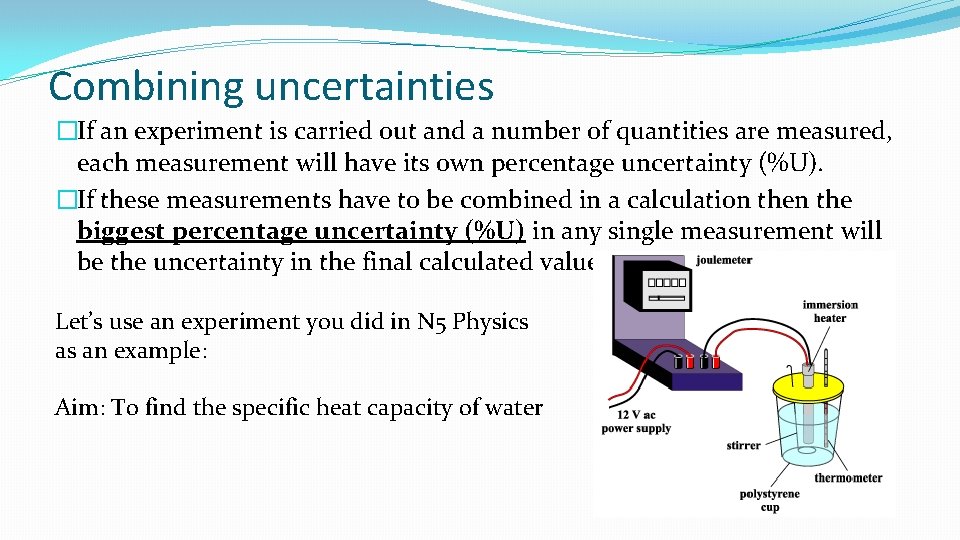
Combining uncertainties �If an experiment is carried out and a number of quantities are measured, each measurement will have its own percentage uncertainty (%U). �If these measurements have to be combined in a calculation the biggest percentage uncertainty (%U) in any single measurement will be the uncertainty in the final calculated value. Let’s use an experiment you did in N 5 Physics as an example: Aim: To find the specific heat capacity of water
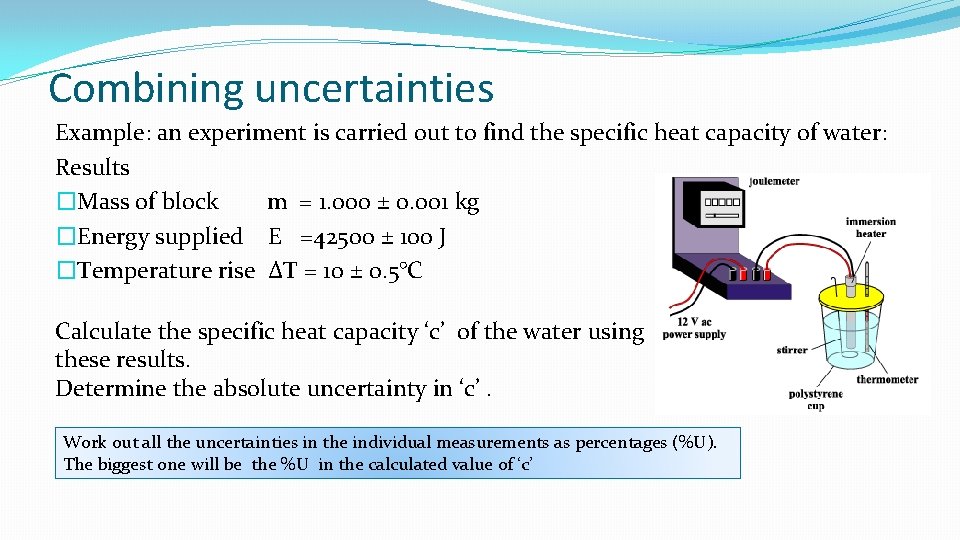
Combining uncertainties Example: an experiment is carried out to find the specific heat capacity of water: Results �Mass of block m = 1. 000 ± 0. 001 kg �Energy supplied E =42500 ± 100 J �Temperature rise ΔT = 10 ± 0. 5°C Calculate the specific heat capacity ‘c’ of the water using these results. Determine the absolute uncertainty in ‘c’. Work out all the uncertainties in the individual measurements as percentages (%U). The biggest one will be the %U in the calculated value of ‘c’
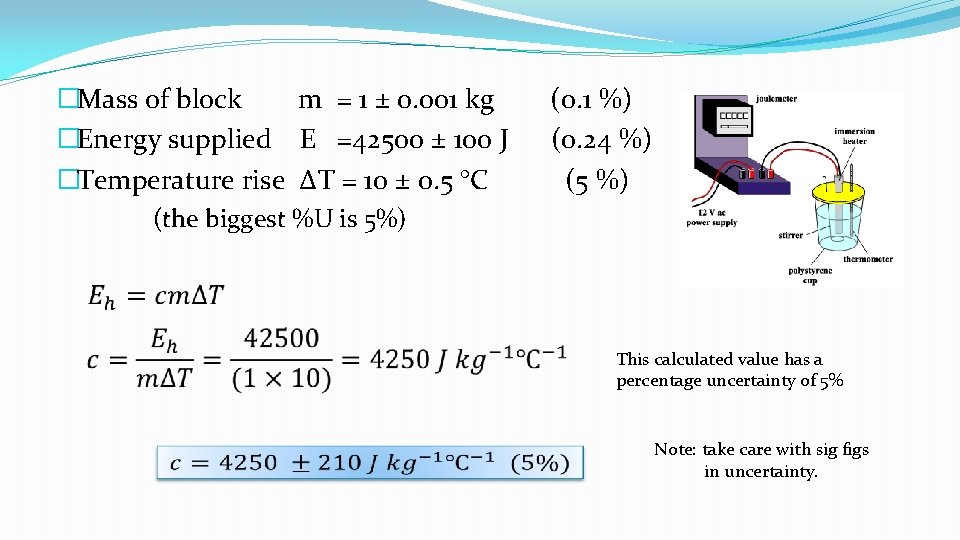
�Mass of block m = 1 ± 0. 001 kg (0. 1 %) �Energy supplied E =42500 ± 100 J (0. 24 %) �Temperature rise ΔT = 10 ± 0. 5 °C (5 %) (the biggest %U is 5%) This calculated value has a percentage uncertainty of 5% Note: take care with sig figs in uncertainty.
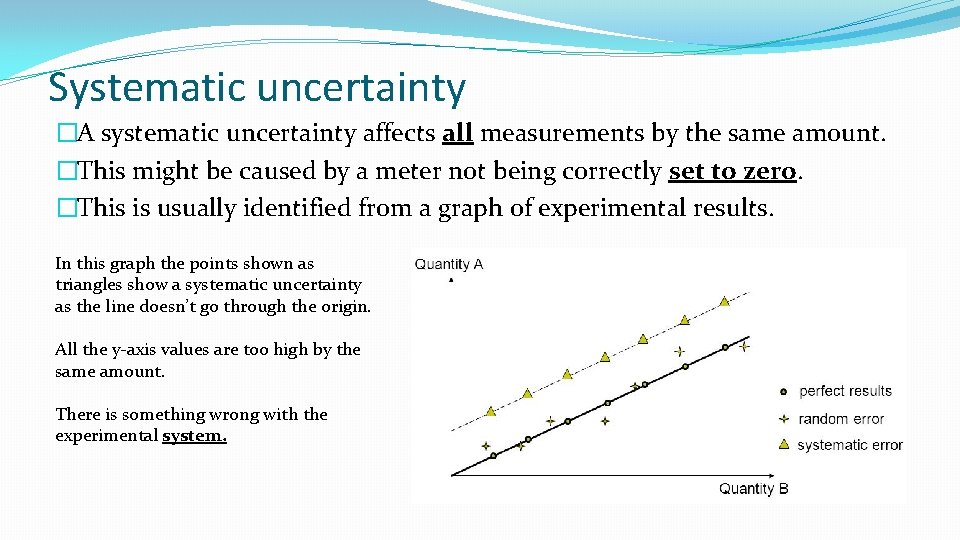
Systematic uncertainty �A systematic uncertainty affects all measurements by the same amount. �This might be caused by a meter not being correctly set to zero. �This is usually identified from a graph of experimental results. In this graph the points shown as triangles show a systematic uncertainty as the line doesn’t go through the origin. All the y-axis values are too high by the same amount. There is something wrong with the experimental system.
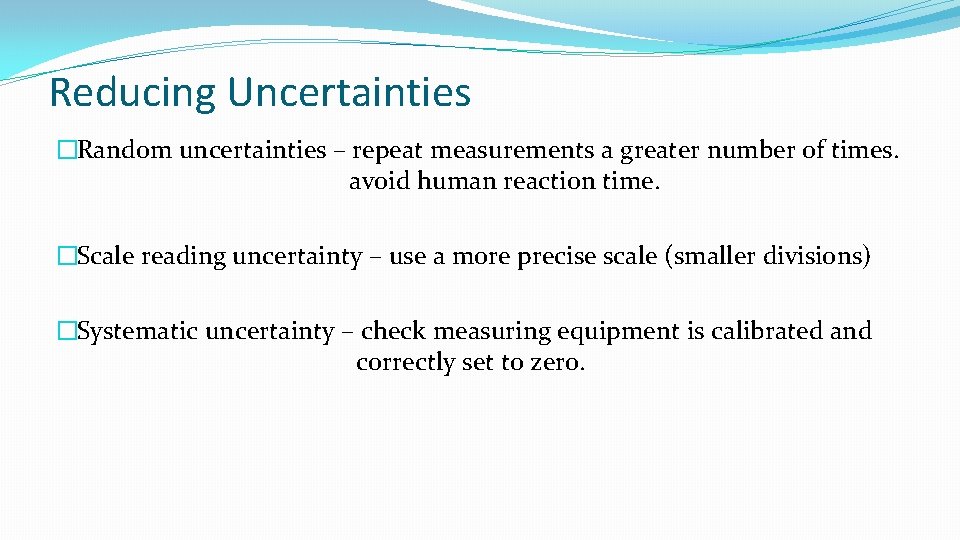
Reducing Uncertainties �Random uncertainties – repeat measurements a greater number of times. avoid human reaction time. �Scale reading uncertainty – use a more precise scale (smaller divisions) �Systematic uncertainty – check measuring equipment is calibrated and correctly set to zero.
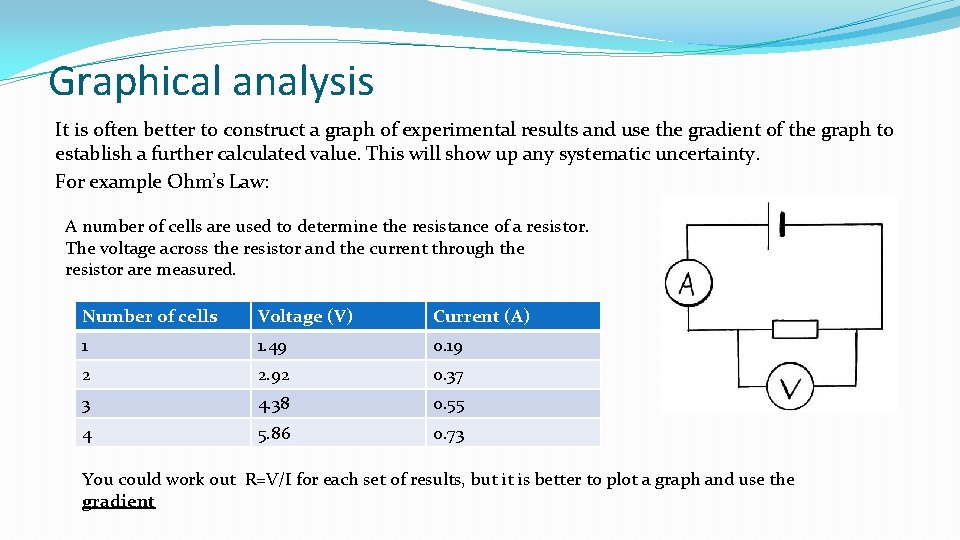
Graphical analysis It is often better to construct a graph of experimental results and use the gradient of the graph to establish a further calculated value. This will show up any systematic uncertainty. For example Ohm’s Law: A number of cells are used to determine the resistance of a resistor. The voltage across the resistor and the current through the resistor are measured. Number of cells Voltage (V) Current (A) 1 1. 49 0. 19 2 2. 92 0. 37 3 4. 38 0. 55 4 5. 86 0. 73 You could work out R=V/I for each set of results, but it is better to plot a graph and use the gradient
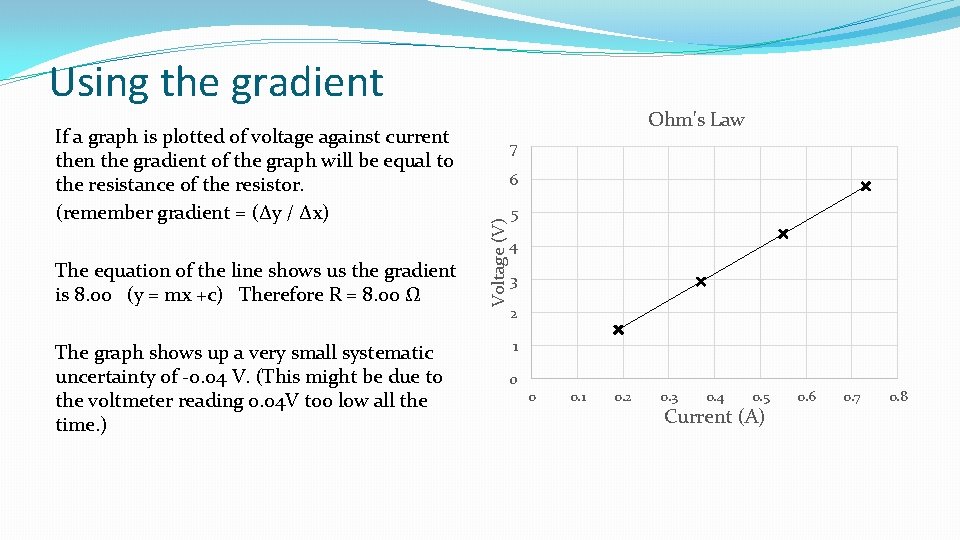
Using the gradient The equation of the line shows us the gradient is 8. 00 (y = mx +c) Therefore R = 8. 00 Ω The graph shows up a very small systematic uncertainty of -0. 04 V. (This might be due to the voltmeter reading 0. 04 V too low all the time. ) 7 6 Voltage (V) If a graph is plotted of voltage against current then the gradient of the graph will be equal to the resistance of the resistor. (remember gradient = (Δy / Δx) Ohm's Law 5 4 3 2 1 0 0 0. 1 0. 2 0. 3 0. 4 0. 5 Current (A) 0. 6 0. 7 0. 8
Random uncertainty
Advanced higher physics uncertainties
Measurements and uncertainties ib physics
Uncertainty of multiplication
Ngoại tâm thu thất chùm đôi
Premature atrial contraction
Thơ thất ngôn tứ tuyệt đường luật
Thơ thất ngôn tứ tuyệt đường luật
Walmart thất bại ở nhật
Tìm độ lớn thật của tam giác abc
Con hãy đưa tay khi thấy người vấp ngã
Tôn thất thuyết là ai
Gây tê cơ vuông thắt lưng
Sau thất bại ở hồ điển triệt
There is some cake