The i MPACT project tracker and calorimeter innovative
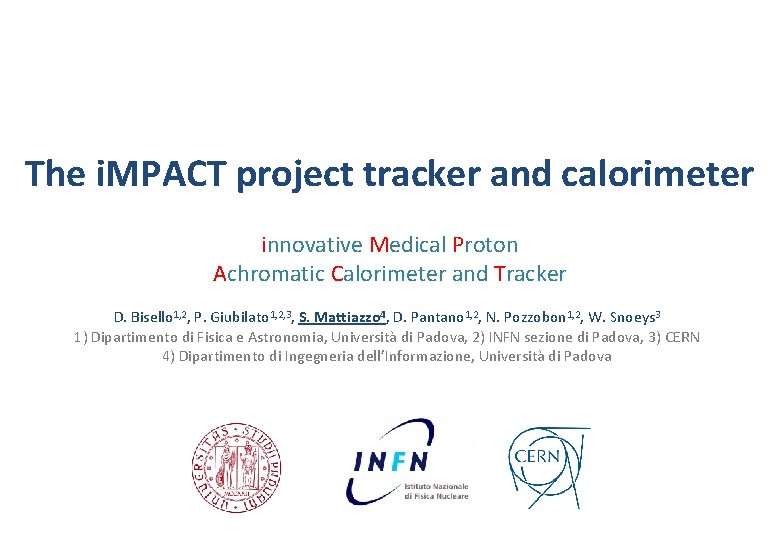
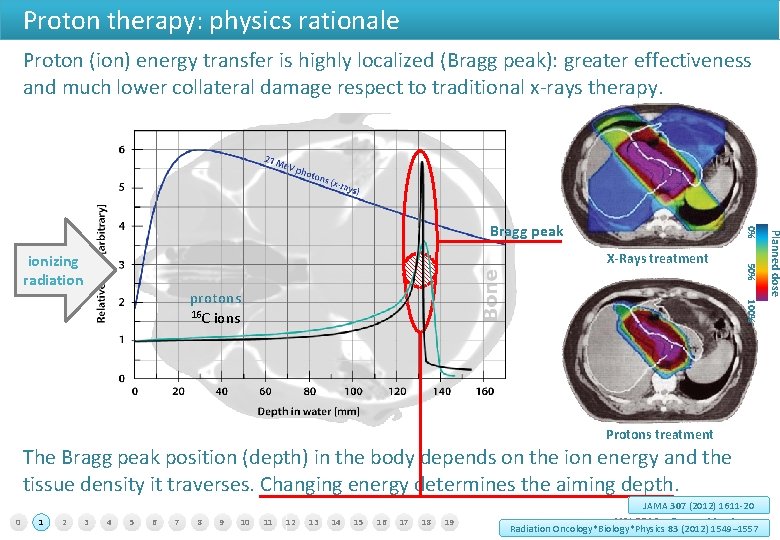
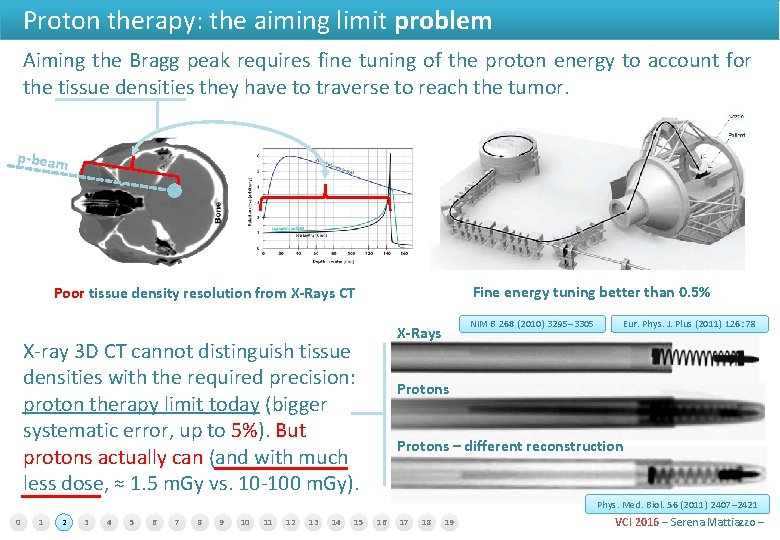
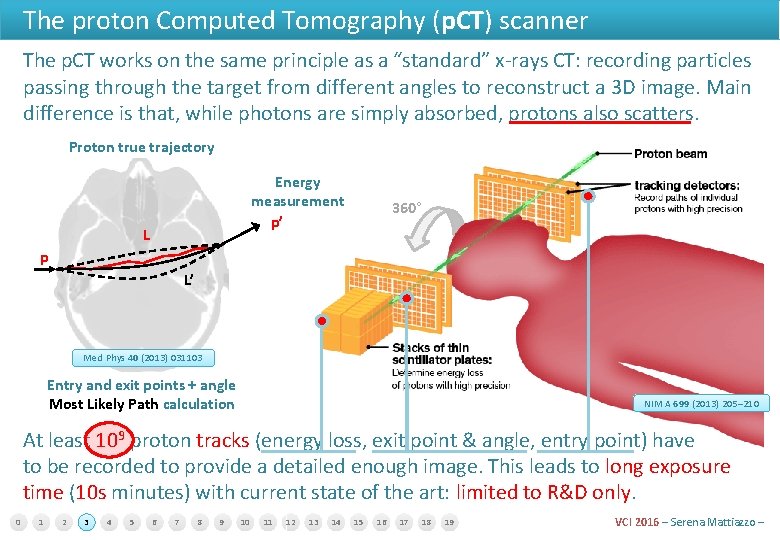
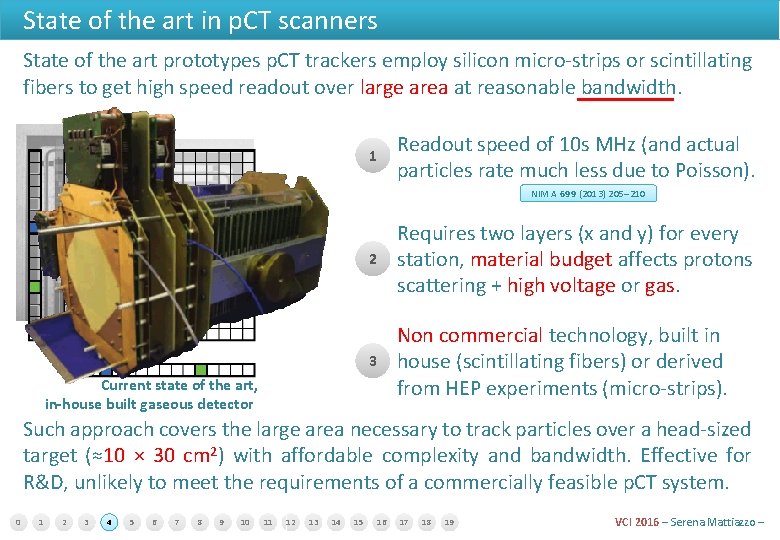
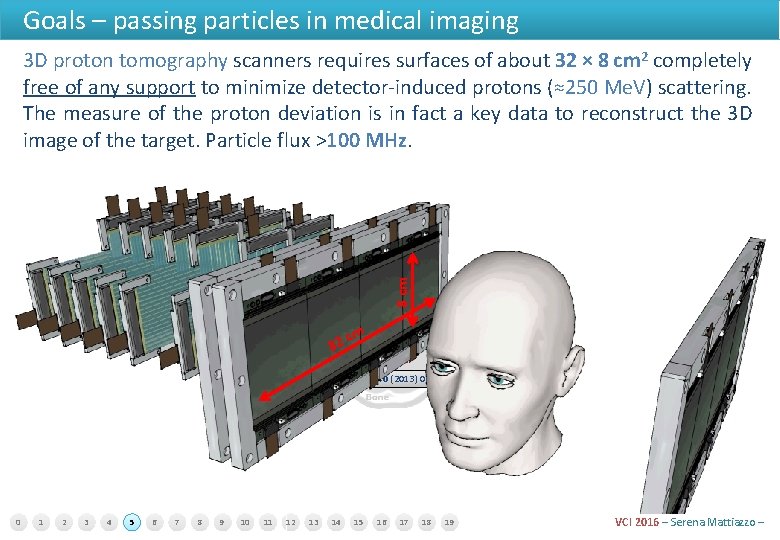
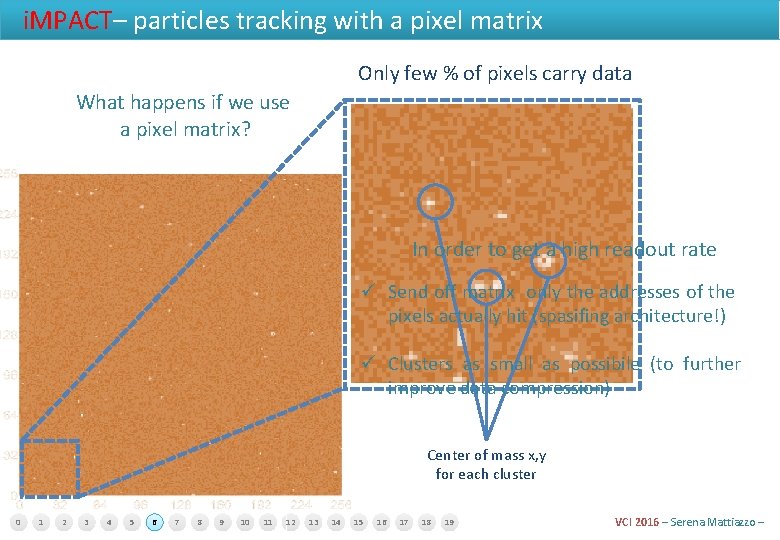
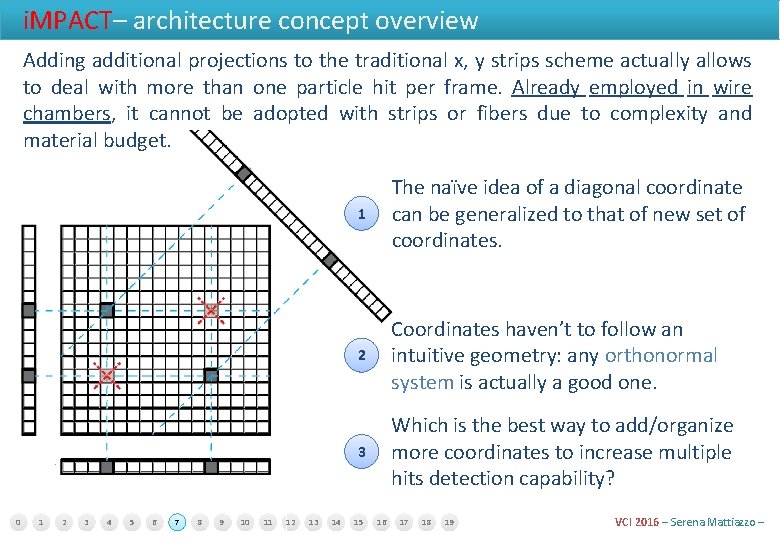
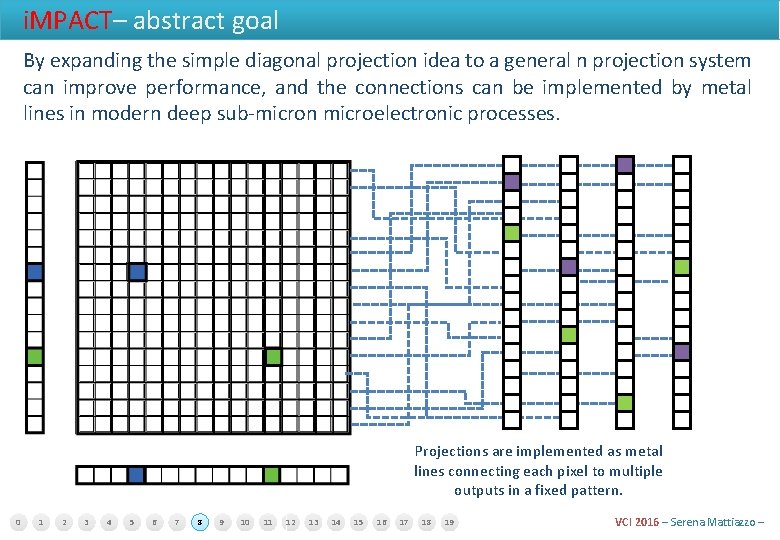
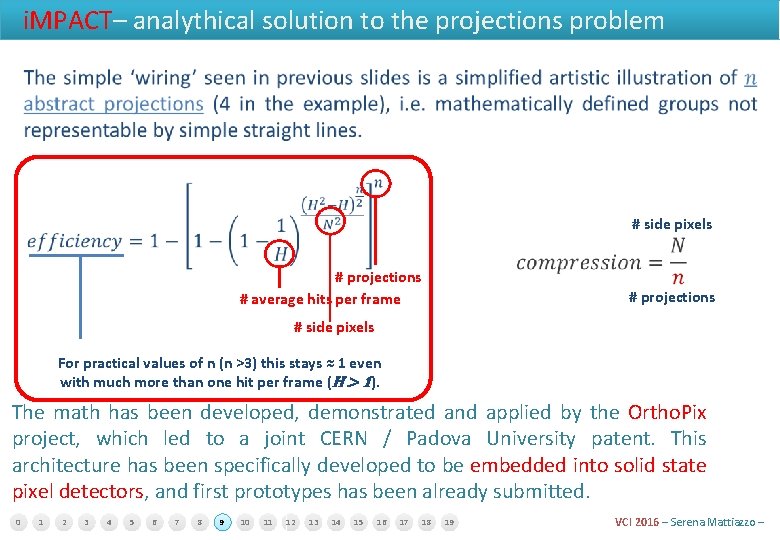
![Efficiency [%] i. MPACT– monte-carlo results from real clusters data DB 100% Matrix side Efficiency [%] i. MPACT– monte-carlo results from real clusters data DB 100% Matrix side](https://slidetodoc.com/presentation_image_h/19fbeafe0228552cc5b46a4363568437/image-11.jpg)
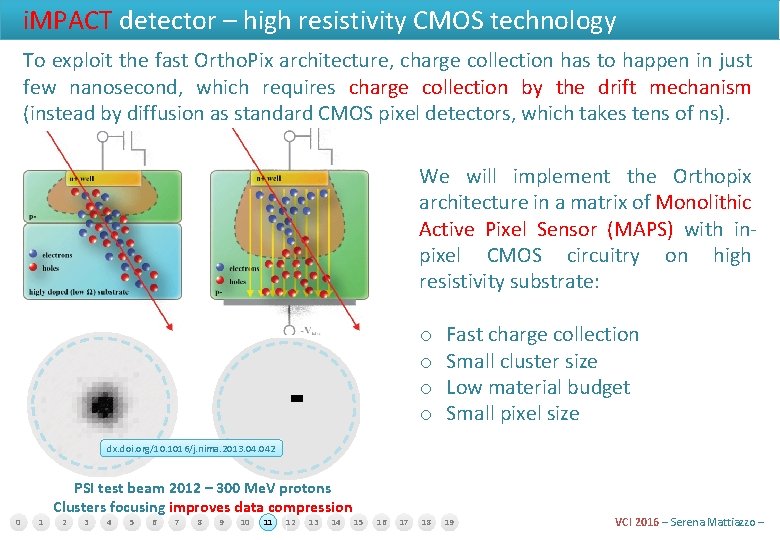
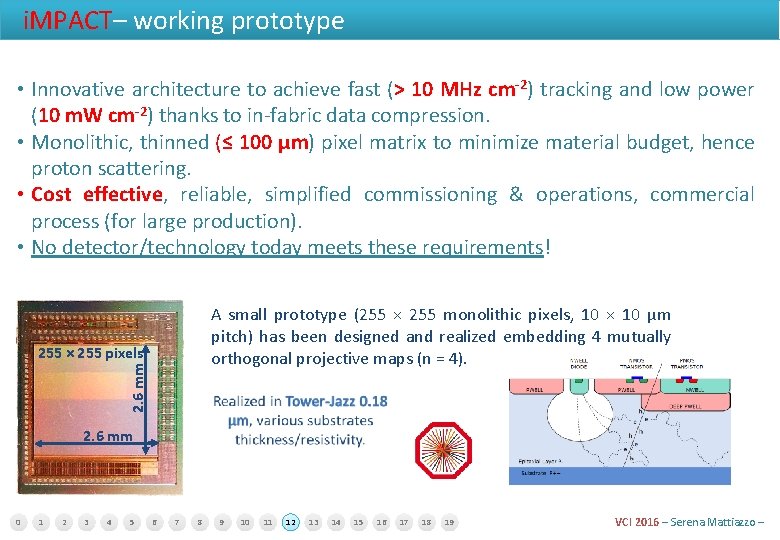
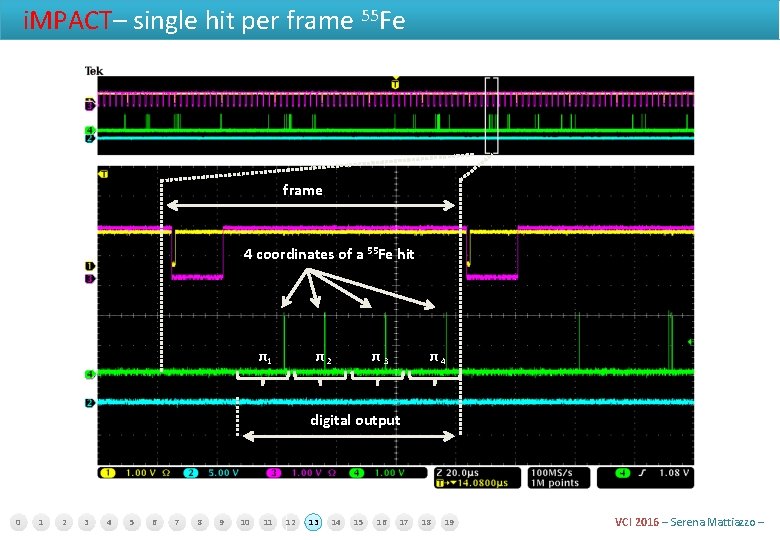
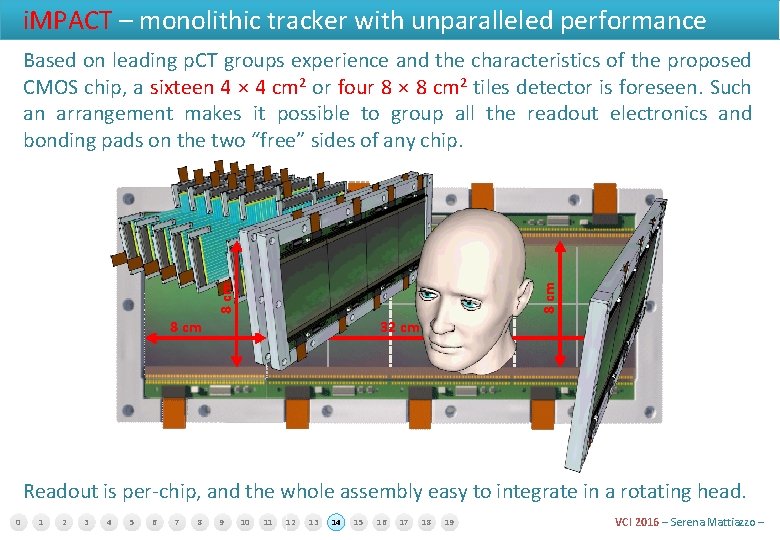
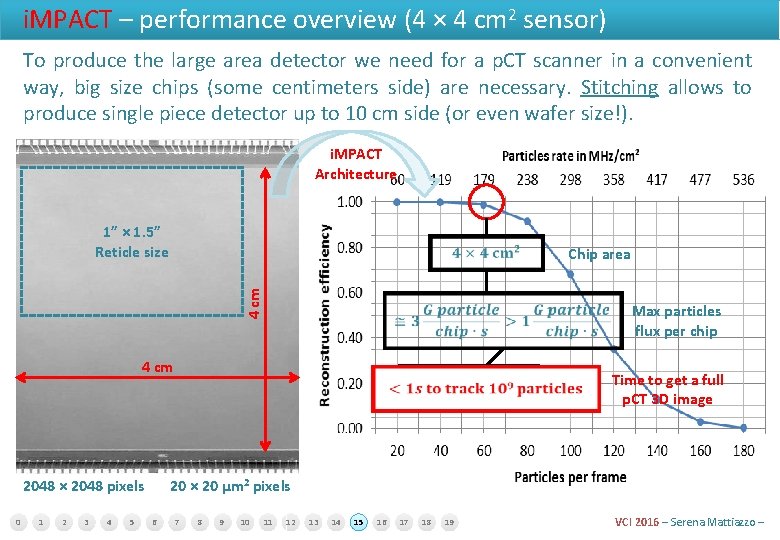
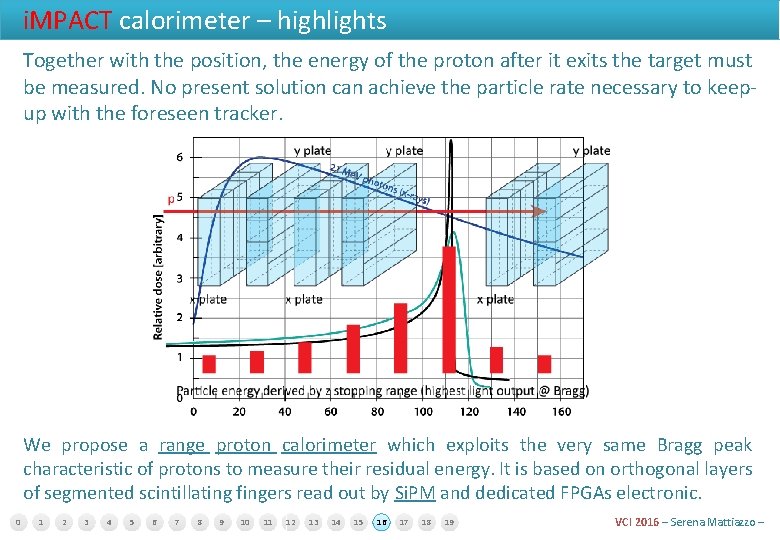
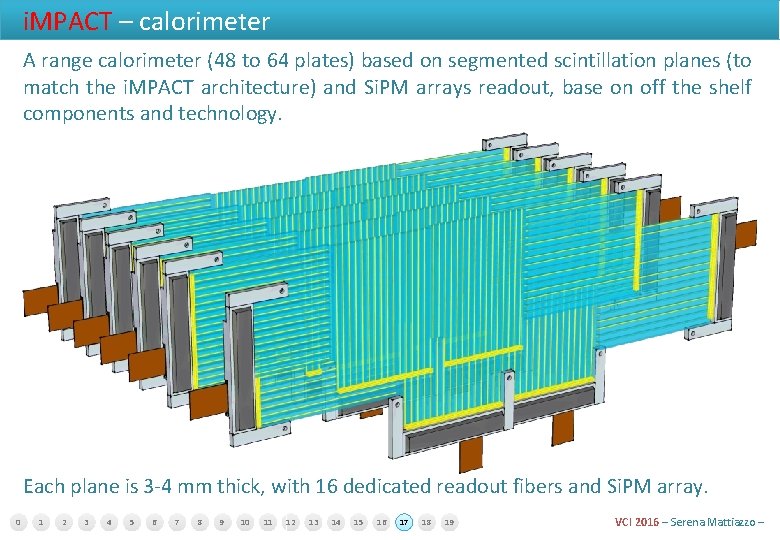
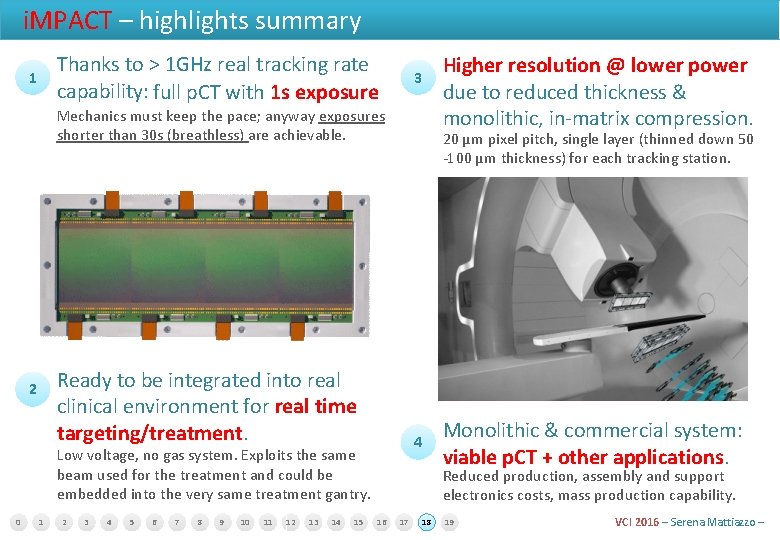
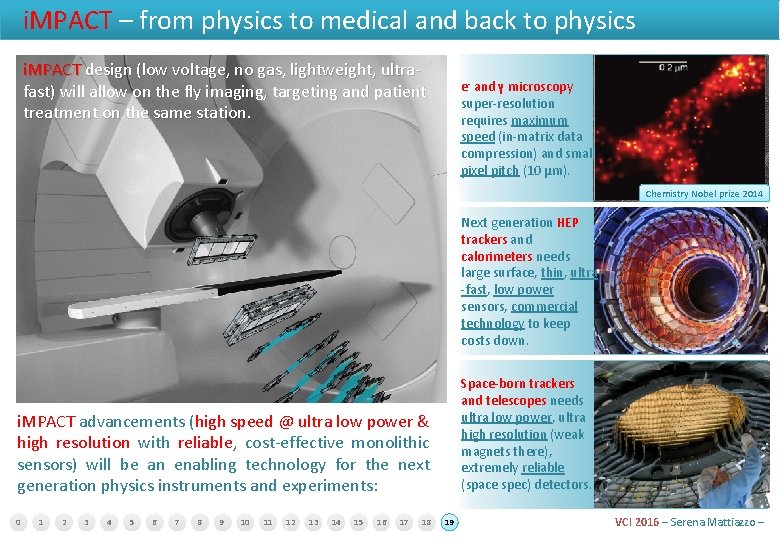
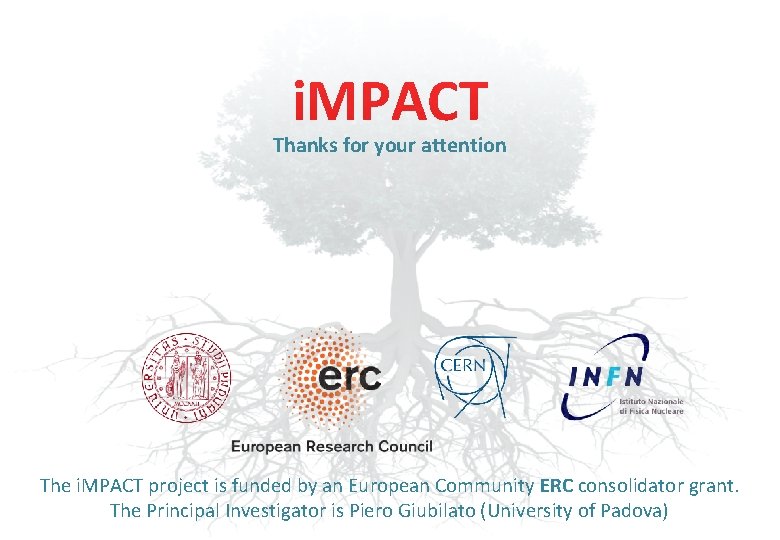
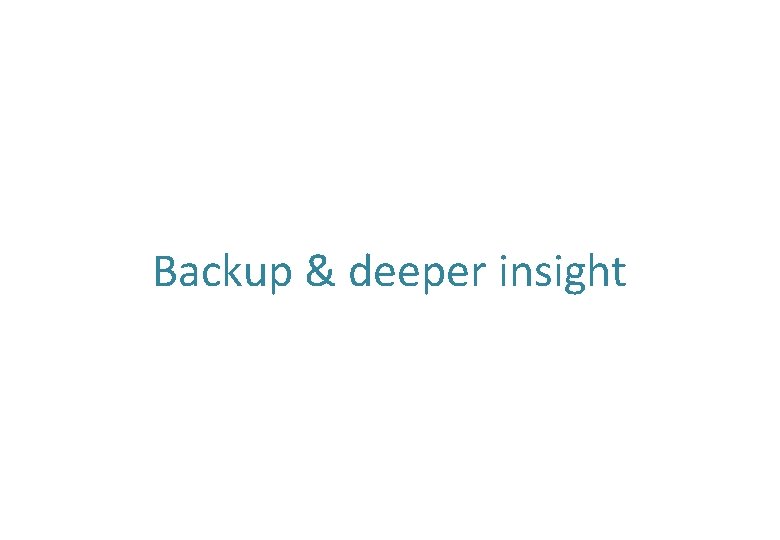
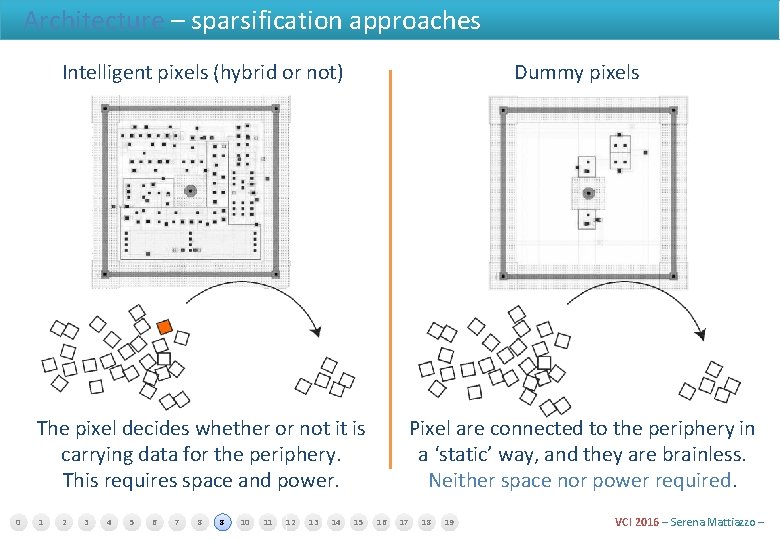
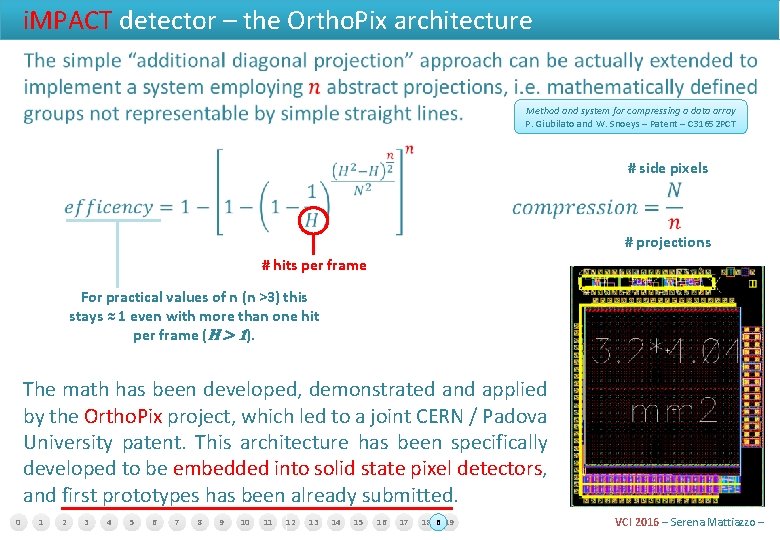
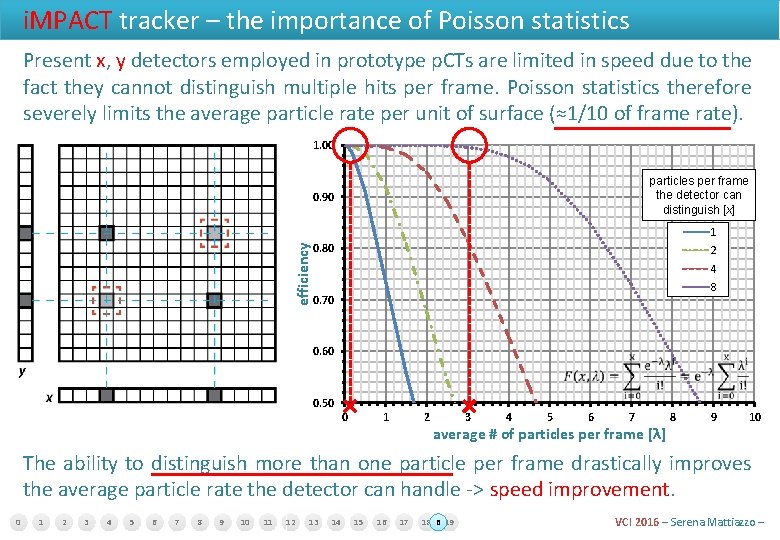
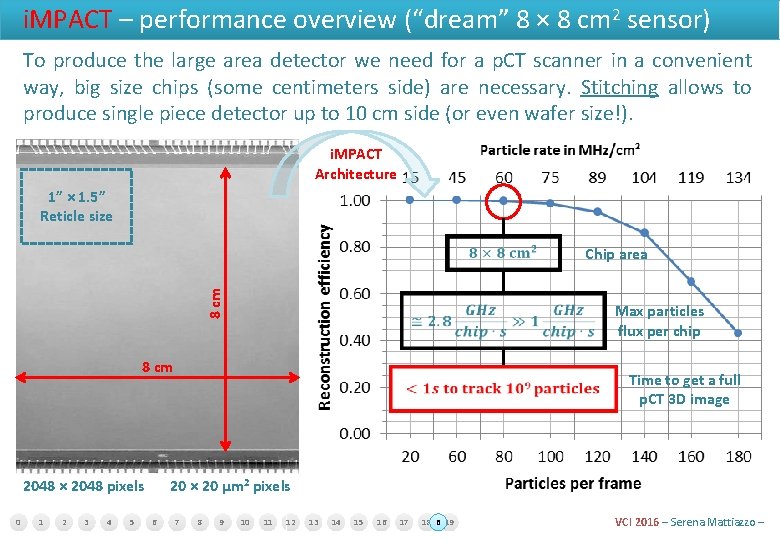
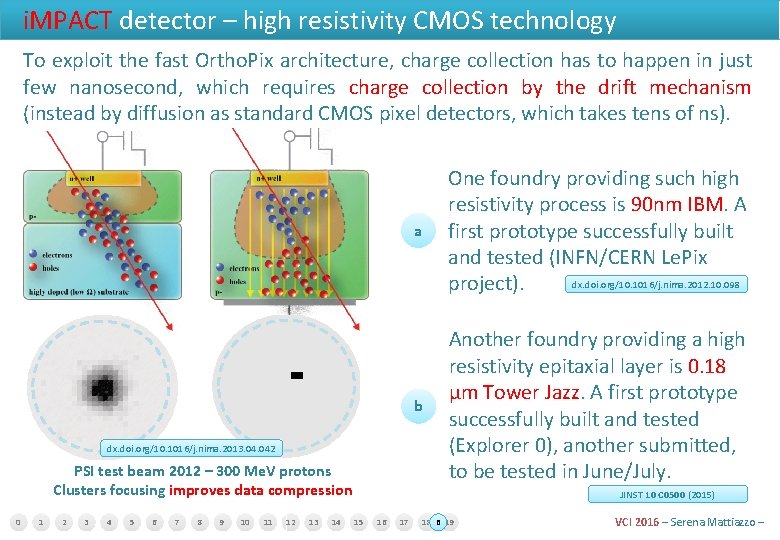
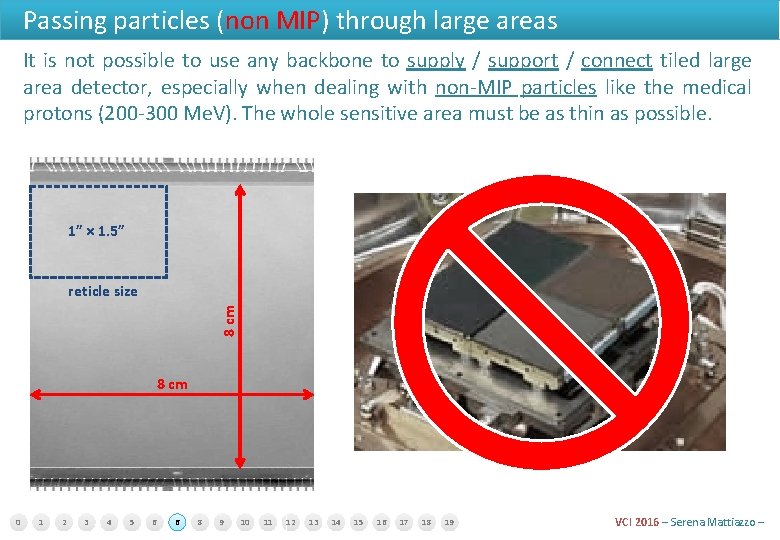
- Slides: 28
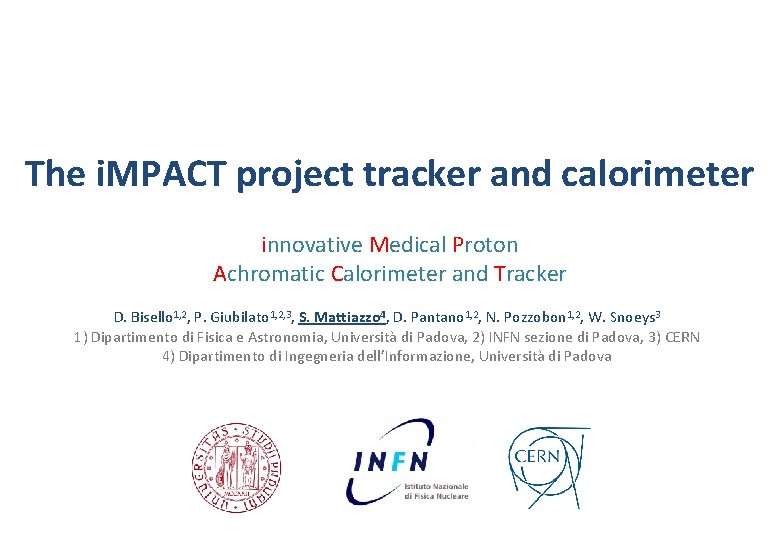
The i. MPACT project tracker and calorimeter innovative Medical Proton Achromatic Calorimeter and Tracker D. Bisello 1, 2, P. Giubilato 1, 2, 3, S. Mattiazzo 4, D. Pantano 1, 2, N. Pozzobon 1, 2, W. Snoeys 3 1) Dipartimento di Fisica e Astronomia, Università di Padova, 2) INFN sezione di Padova, 3) CERN 4) Dipartimento di Ingegneria dell’Informazione, Università di Padova
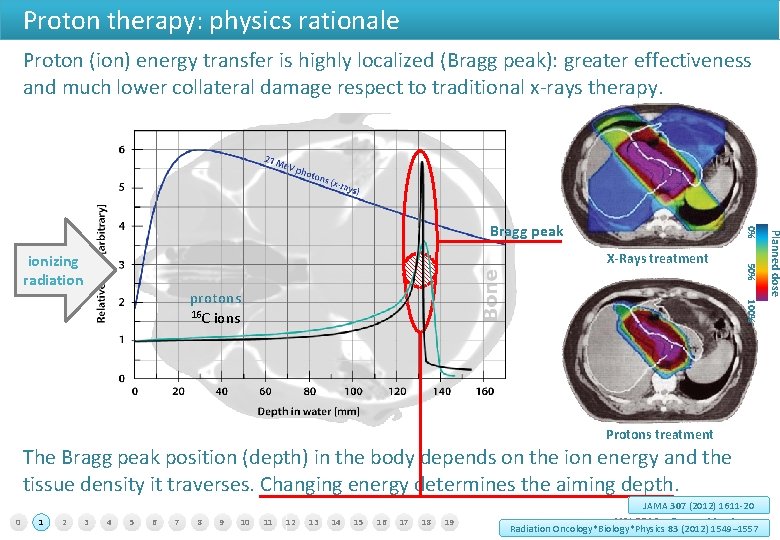
Proton therapy: physics rationale Proton (ion) energy transfer is highly localized (Bragg peak): greater effectiveness and much lower collateral damage respect to traditional x-rays therapy. 100% protons 16 C ions 50% X-Rays treatment ionizing radiation Protons treatment The Bragg peak position (depth) in the body depends on the ion energy and the tissue density it traverses. Changing energy determines the aiming depth. JAMA 307 (2012) 1611 -20 0 1 2 3 4 5 6 7 8 9 10 11 12 13 14 15 16 17 18 19 VCI 2016 – Serena Mattiazzo – Radiation Oncology*Biology*Physics 83 (2012) 1549– 1557 Planned dose 0% Bragg peak
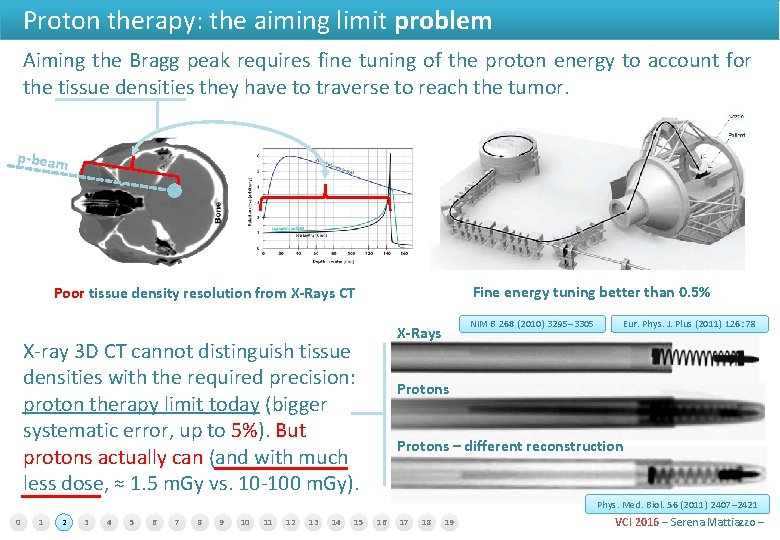
Proton therapy: the aiming limit problem Aiming the Bragg peak requires fine tuning of the proton energy to account for the tissue densities they have to traverse to reach the tumor. p-beam Fine energy tuning better than 0. 5% Poor tissue density resolution from X-Rays CT NIM B 268 (2010) 3295– 3305 X-Rays X-ray 3 D CT cannot distinguish tissue densities with the required precision: proton therapy limit today (bigger systematic error, up to 5%). But protons actually can (and with much less dose, ≈ 1. 5 m. Gy vs. 10 -100 m. Gy). Eur. Phys. J. Plus (2011) 126: 78 Protons – different reconstruction Phys. Med. Biol. 56 (2011) 2407– 2421 0 1 2 3 4 5 6 7 8 9 10 11 12 13 14 15 16 17 18 19 VCI 2016 – Serena Mattiazzo –
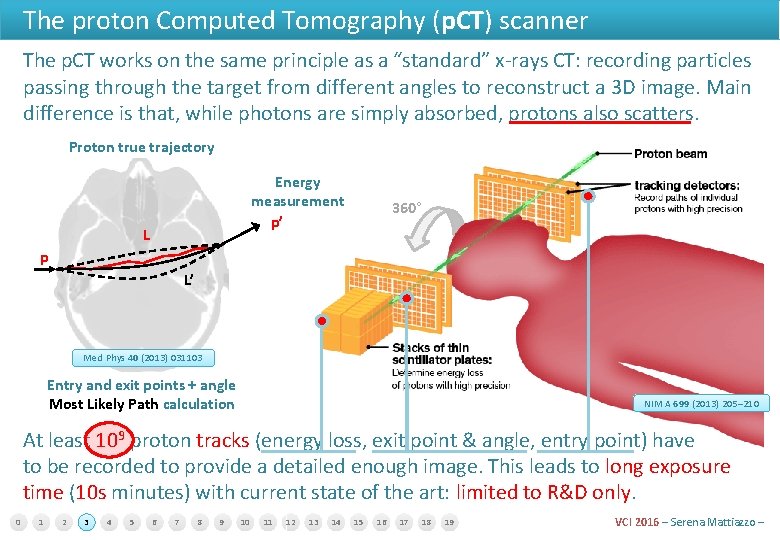
The proton Computed Tomography (p. CT) scanner The p. CT works on the same principle as a “standard” x-rays CT: recording particles passing through the target from different angles to reconstruct a 3 D image. Main difference is that, while photons are simply absorbed, protons also scatters. Proton true trajectory Energy measurement p’ L 360° p L’ Med Phys 40 (2013) 031103 Entry and exit points + angle Most Likely Path calculation NIM A 699 (2013) 205– 210 At least 109 proton tracks (energy loss, exit point & angle, entry point) have to be recorded to provide a detailed enough image. This leads to long exposure time (10 s minutes) with current state of the art: limited to R&D only. 0 1 2 3 4 5 6 7 8 9 10 11 12 13 14 15 16 17 18 19 VCI 2016 – Serena Mattiazzo –
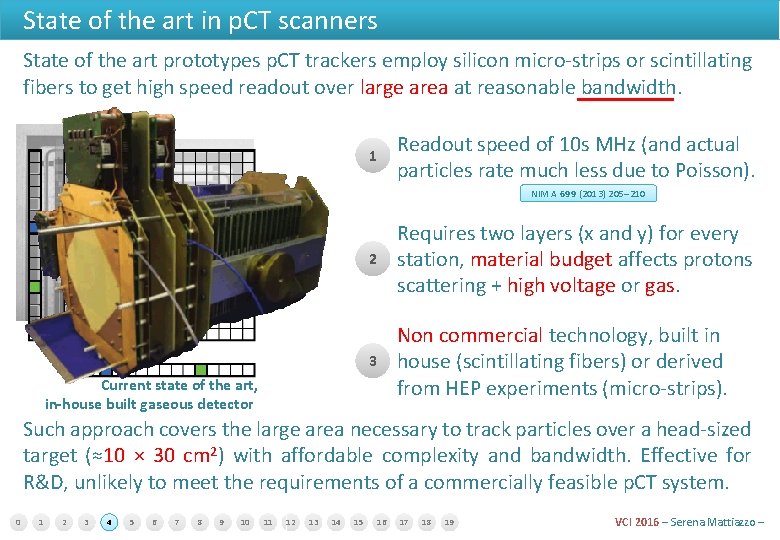
State of the art in p. CT scanners State of the art prototypes p. CT trackers employ silicon micro-strips or scintillating fibers to get high speed readout over large area at reasonable bandwidth. 1 Readout speed of 10 s MHz (and actual particles rate much less due to Poisson). NIM A 699 (2013) 205– 210 2 Requires two layers (x and y) for every station, material budget affects protons scattering + high voltage or gas. 3 Non commercial technology, built in house (scintillating fibers) or derived from HEP experiments (micro-strips). Current state of the art, in-house built gaseous detector Such approach covers the large area necessary to track particles over a head-sized target (≈10 × 30 cm 2) with affordable complexity and bandwidth. Effective for R&D, unlikely to meet the requirements of a commercially feasible p. CT system. 0 1 2 3 4 5 6 7 8 9 10 11 12 13 14 15 16 17 18 19 VCI 2016 – Serena Mattiazzo –
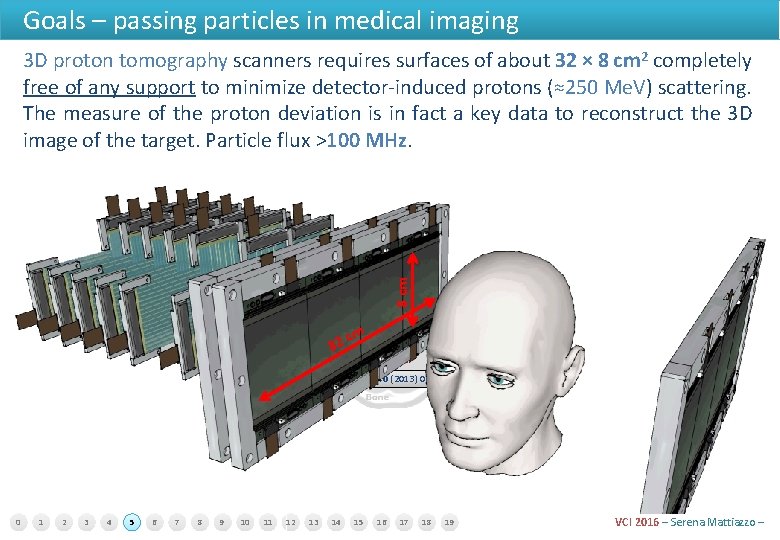
Goals – passing particles in medical imaging 8 cm 3 D proton tomography scanners requires surfaces of about 32 × 8 cm 2 completely free of any support to minimize detector-induced protons (≈250 Me. V) scattering. The measure of the proton deviation is in fact a key data to reconstruct the 3 D image of the target. Particle flux >100 MHz. p 32 cm L p’ L’ Med Phys 40 (2013) 031103 0 1 2 3 4 5 6 7 8 9 10 11 12 13 14 15 16 17 18 19 VCI 2016 – Serena Mattiazzo –
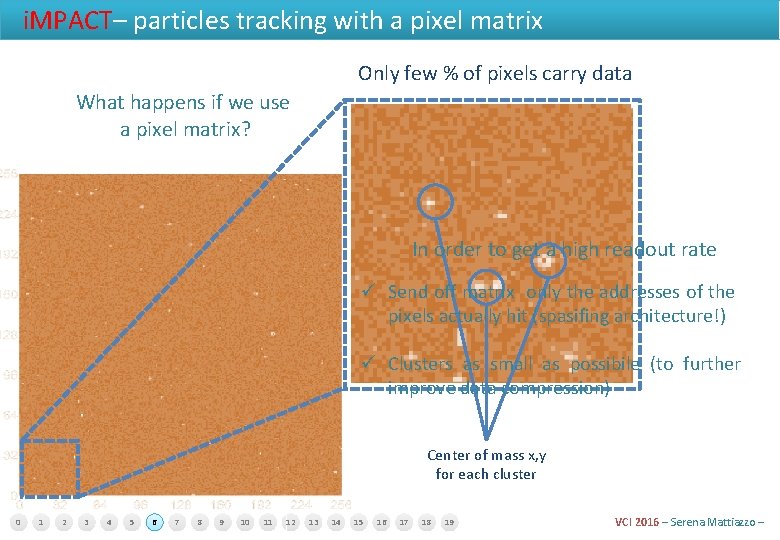
i. MPACT– particles tracking with a pixel matrix Only few % of pixels carry data What happens if we use a pixel matrix? In order to get a high readout rate ü Send off matrix only the addresses of the pixels actually hit (spasifing architecture!) ü Clusters as small as possibile (to further improve data compression) Center of mass x, y for each cluster 0 1 2 3 4 5 6 7 8 9 10 11 12 13 14 15 16 17 18 19 VCI 2016 – Serena Mattiazzo –
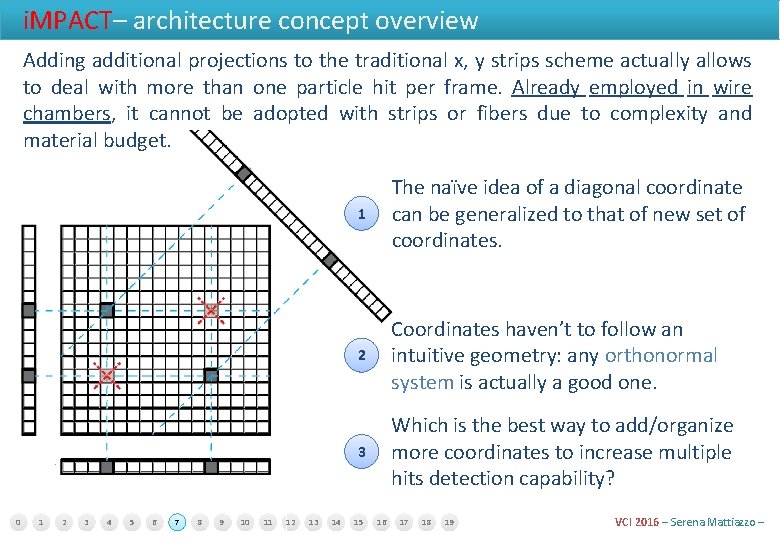
i. MPACT– architecture concept overview Adding additional projections to the traditional x, y strips scheme actually allows to deal with more than one particle hit per frame. Already employed in wire chambers, it cannot be adopted with strips or fibers due to complexity and material budget. 0 1 2 3 4 5 6 7 8 9 10 11 12 13 14 1 The naïve idea of a diagonal coordinate can be generalized to that of new set of coordinates. 2 Coordinates haven’t to follow an intuitive geometry: any orthonormal system is actually a good one. 3 Which is the best way to add/organize more coordinates to increase multiple hits detection capability? 15 16 17 18 19 VCI 2016 – Serena Mattiazzo –
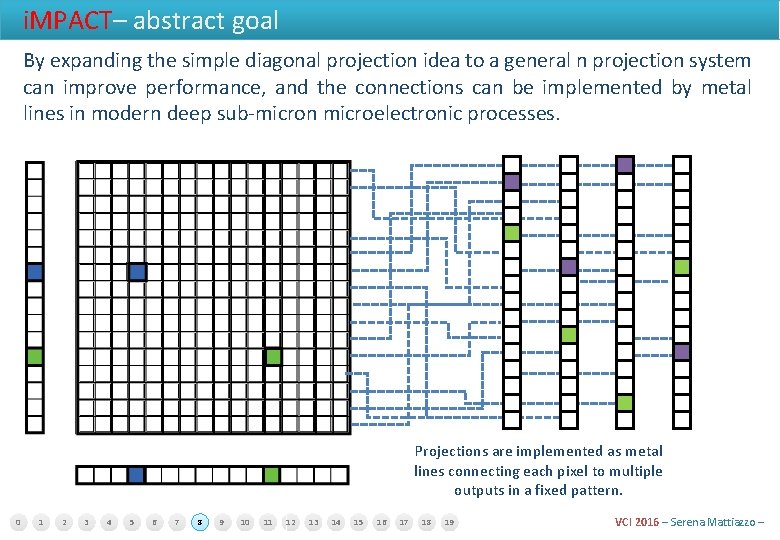
i. MPACT– abstract goal By expanding the simple diagonal projection idea to a general n projection system can improve performance, and the connections can be implemented by metal lines in modern deep sub-micron microelectronic processes. Projections are implemented as metal lines connecting each pixel to multiple outputs in a fixed pattern. 0 1 2 3 4 5 6 7 8 9 10 11 12 13 14 15 16 17 18 19 VCI 2016 – Serena Mattiazzo –
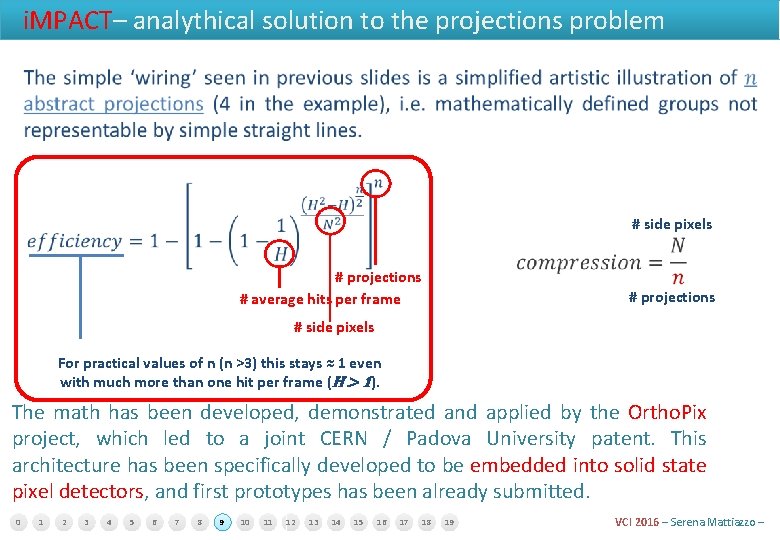
i. MPACT– analythical solution to the projections problem # projections # average hits per frame # side pixels # projections # side pixels For practical values of n (n >3) this stays ≈ 1 even with much more than one hit per frame (H > 1). The math has been developed, demonstrated and applied by the Ortho. Pix project, which led to a joint CERN / Padova University patent. This architecture has been specifically developed to be embedded into solid state pixel detectors, and first prototypes has been already submitted. 0 1 2 3 4 5 6 7 8 9 10 11 12 13 14 15 16 17 18 19 VCI 2016 – Serena Mattiazzo –
![Efficiency i MPACT montecarlo results from real clusters data DB 100 Matrix side Efficiency [%] i. MPACT– monte-carlo results from real clusters data DB 100% Matrix side](https://slidetodoc.com/presentation_image_h/19fbeafe0228552cc5b46a4363568437/image-11.jpg)
Efficiency [%] i. MPACT– monte-carlo results from real clusters data DB 100% Matrix side 90% 257 80% 529 70% 1089 60% 2209 50% 4225 40% 8281 30% 20% 10% 0% 0 50 100 150 200 250 300 1 2 3 4 5 6 7 8 400 450 500 550 λ - [average # of particles per frame] Lines are the formula, dots the monte-carlo results for a 4 projection system 0 350 9 10 11 12 13 14 15 16 17 18 19 VCI 2016 – Serena Mattiazzo –
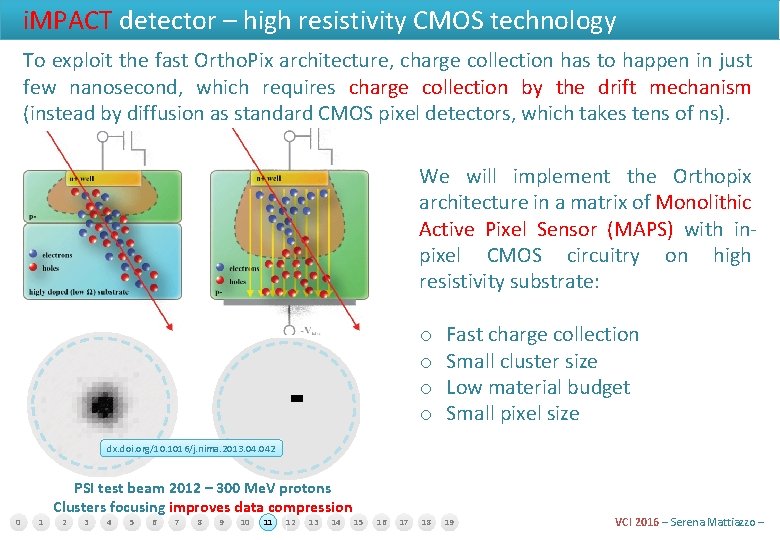
i. MPACT detector – high resistivity CMOS technology To exploit the fast Ortho. Pix architecture, charge collection has to happen in just few nanosecond, which requires charge collection by the drift mechanism (instead by diffusion as standard CMOS pixel detectors, which takes tens of ns). We will implement the Orthopix architecture in a matrix of Monolithic Active Pixel Sensor (MAPS) with inpixel CMOS circuitry on high resistivity substrate: o o Fast charge collection Small cluster size Low material budget Small pixel size dx. doi. org/10. 1016/j. nima. 2013. 042 PSI test beam 2012 – 300 Me. V protons Clusters focusing improves data compression 0 1 2 3 4 5 6 7 8 9 10 11 12 13 14 15 16 17 18 19 VCI 2016 – Serena Mattiazzo –
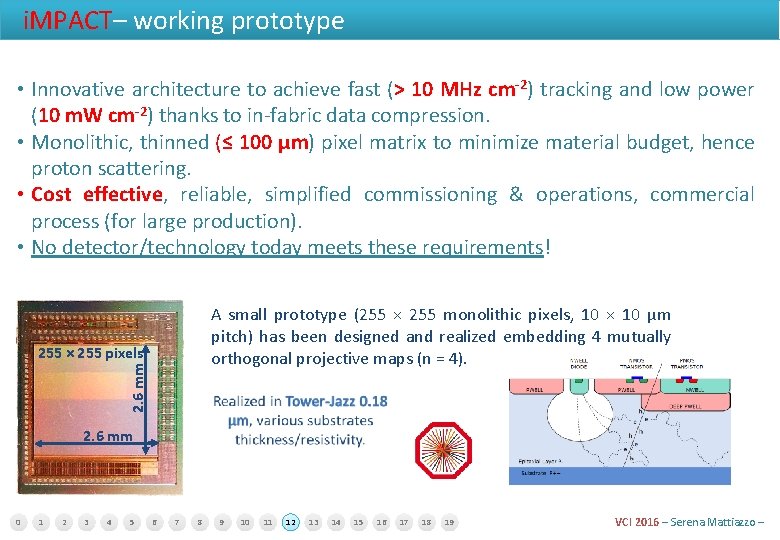
i. MPACT– working prototype • Innovative architecture to achieve fast (> 10 MHz cm-2) tracking and low power (10 m. W cm-2) thanks to in-fabric data compression. • Monolithic, thinned (≤ 100 µm) pixel matrix to minimize material budget, hence proton scattering. • Cost effective, reliable, simplified commissioning & operations, commercial process (for large production). • No detector/technology today meets these requirements! A small prototype (255 × 255 monolithic pixels, 10 × 10 µm pitch) has been designed and realized embedding 4 mutually orthogonal projective maps (n = 4). 2. 6 mm 255 × 255 pixels 2. 6 mm 0 1 2 3 4 5 6 7 8 9 10 11 12 13 14 15 16 17 18 19 VCI 2016 – Serena Mattiazzo –
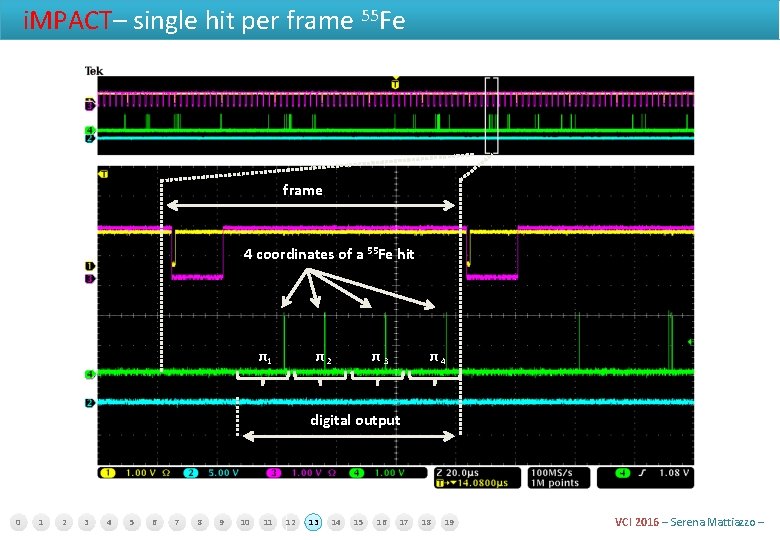
i. MPACT– single hit per frame 55 Fe frame 4 coordinates of a 55 Fe hit π1 π2 π3 π4 digital output 0 1 2 3 4 5 6 7 8 9 10 11 12 13 14 15 16 17 18 19 VCI 2016 – Serena Mattiazzo –
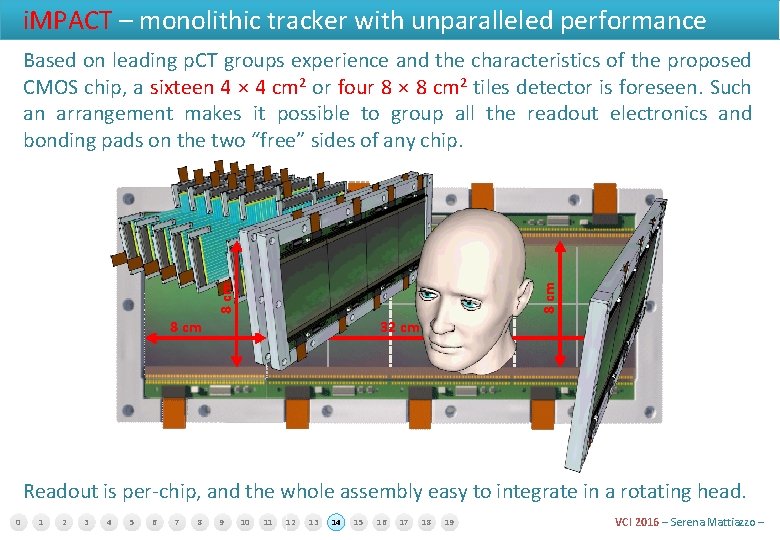
i. MPACT – monolithic tracker with unparalleled performance 8 cm Based on leading p. CT groups experience and the characteristics of the proposed CMOS chip, a sixteen 4 × 4 cm 2 or four 8 × 8 cm 2 tiles detector is foreseen. Such an arrangement makes it possible to group all the readout electronics and bonding pads on the two “free” sides of any chip. 8 cm 32 cm Readout is per-chip, and the whole assembly easy to integrate in a rotating head. 0 1 2 3 4 5 6 7 8 9 10 11 12 13 14 15 16 17 18 19 VCI 2016 – Serena Mattiazzo –
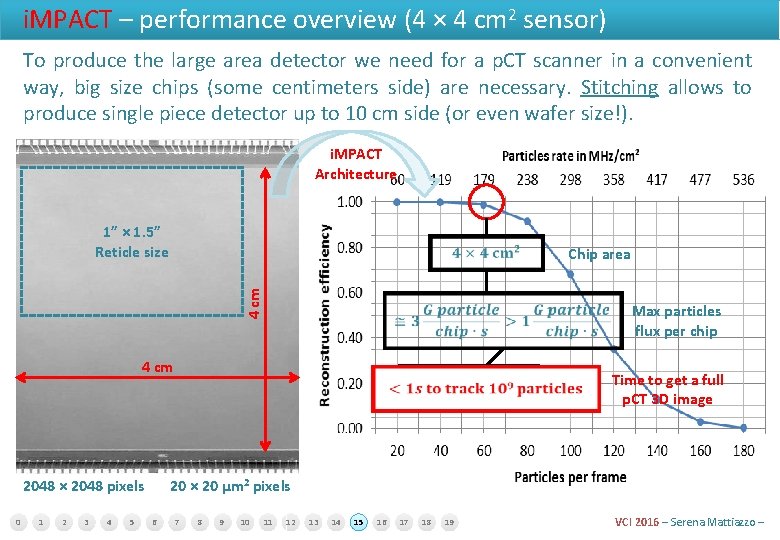
i. MPACT – performance overview (4 × 4 cm 2 sensor) To produce the large area detector we need for a p. CT scanner in a convenient way, big size chips (some centimeters side) are necessary. Stitching allows to produce single piece detector up to 10 cm side (or even wafer size!). i. MPACT Architecture 1” × 1. 5” Reticle size 4 cm 1 2 3 4 5 Max particles flux per chip Time to get a full p. CT 3 D image 20 × 20 µm 2 pixels 2048 × 2048 pixels 0 Chip area 6 7 8 9 10 11 12 13 14 15 16 17 18 19 VCI 2016 – Serena Mattiazzo –
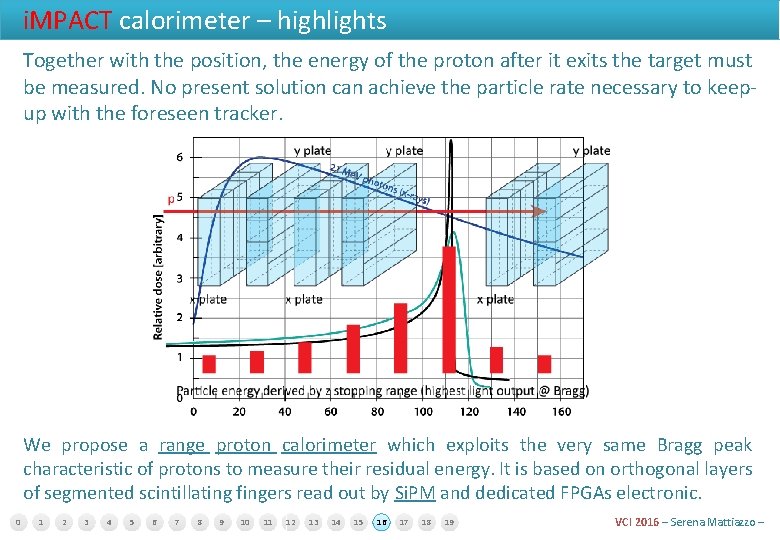
i. MPACT calorimeter – highlights Together with the position, the energy of the proton after it exits the target must be measured. No present solution can achieve the particle rate necessary to keepup with the foreseen tracker. We propose a range proton calorimeter which exploits the very same Bragg peak characteristic of protons to measure their residual energy. It is based on orthogonal layers of segmented scintillating fingers read out by Si. PM and dedicated FPGAs electronic. 0 1 2 3 4 5 6 7 8 9 10 11 12 13 14 15 16 17 18 19 VCI 2016 – Serena Mattiazzo –
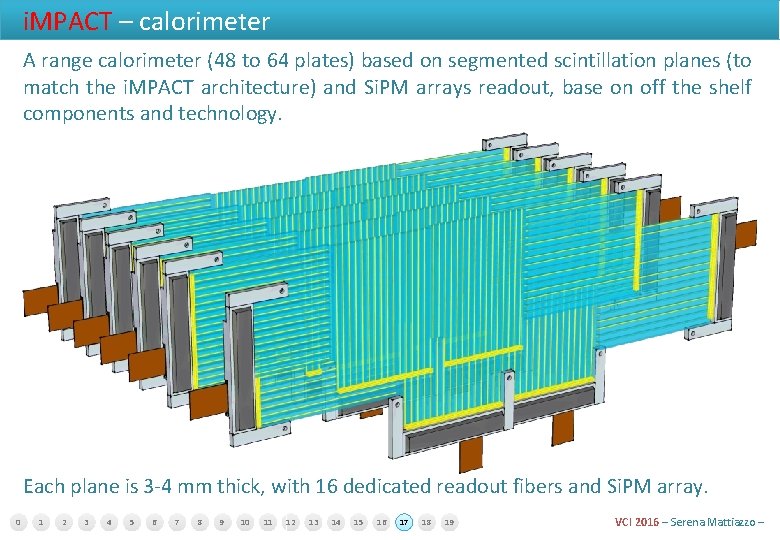
i. MPACT – calorimeter A range calorimeter (48 to 64 plates) based on segmented scintillation planes (to match the i. MPACT architecture) and Si. PM arrays readout, base on off the shelf components and technology. Each plane is 3 -4 mm thick, with 16 dedicated readout fibers and Si. PM array. 0 1 2 3 4 5 6 7 8 9 10 11 12 13 14 15 16 17 18 19 VCI 2016 – Serena Mattiazzo –
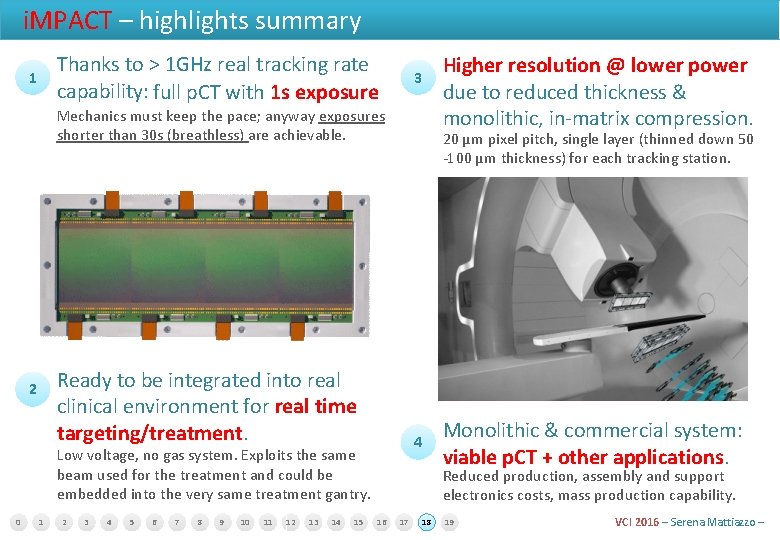
i. MPACT – highlights summary Thanks to > 1 GHz real tracking rate capability: full p. CT with 1 s exposure 1 3 Mechanics must keep the pace; anyway exposures shorter than 30 s (breathless) are achievable. 20 µm pixel pitch, single layer (thinned down 50 -100 µm thickness) for each tracking station. Ready to be integrated into real clinical environment for real time targeting/treatment. 2 4 Low voltage, no gas system. Exploits the same beam used for the treatment and could be embedded into the very same treatment gantry. 0 1 2 3 4 5 6 7 8 9 10 11 12 13 14 15 Higher resolution @ lower power due to reduced thickness & monolithic, in-matrix compression. Monolithic & commercial system: viable p. CT + other applications. Reduced production, assembly and support electronics costs, mass production capability. 16 17 18 19 VCI 2016 – Serena Mattiazzo –
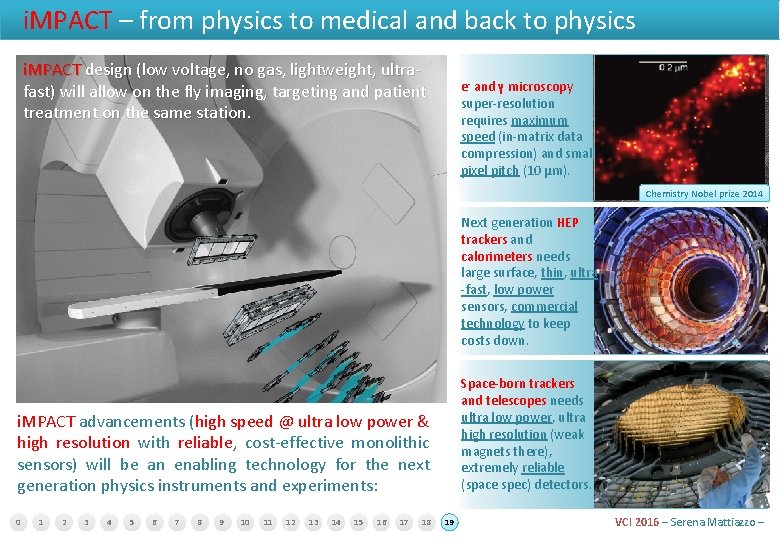
i. MPACT – from physics to medical and back to physics i. MPACT design (low voltage, no gas, lightweight, ultrafast) will allow on the fly imaging, targeting and patient treatment on the same station. e- and γ microscopy super-resolution requires maximum speed (in-matrix data compression) and small pixel pitch (10 µm). Chemistry Nobel prize 2014 Next generation HEP trackers and calorimeters needs large surface, thin, ultra -fast, low power sensors, commercial technology to keep costs down. Space-born trackers and telescopes needs ultra low power, ultra high resolution (weak magnets there), extremely reliable (space spec) detectors. i. MPACT advancements (high speed @ ultra low power & high resolution with reliable, cost-effective monolithic sensors) will be an enabling technology for the next generation physics instruments and experiments: 0 1 2 3 4 5 6 7 8 9 10 11 12 13 14 15 16 17 18 19 VCI 2016 – Serena Mattiazzo –
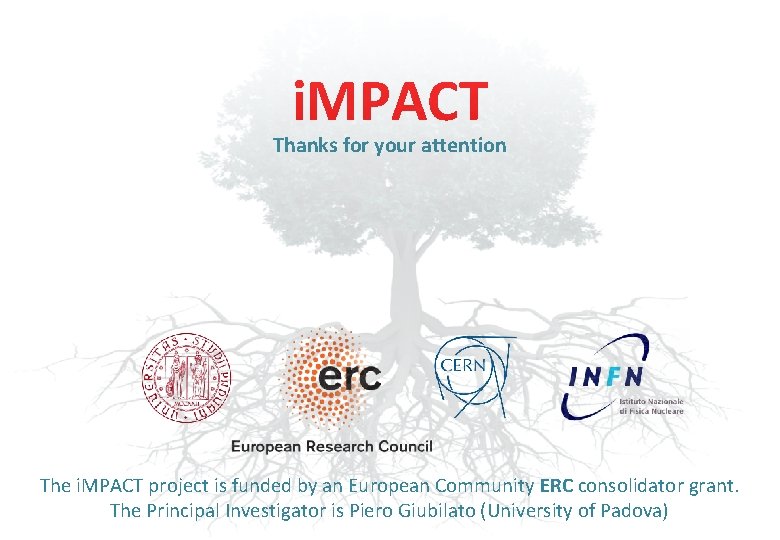
i. MPACT Thanks for your attention The i. MPACT project is funded by an European Community ERC consolidator grant. The Principal Investigator is Piero Giubilato (University of Padova)
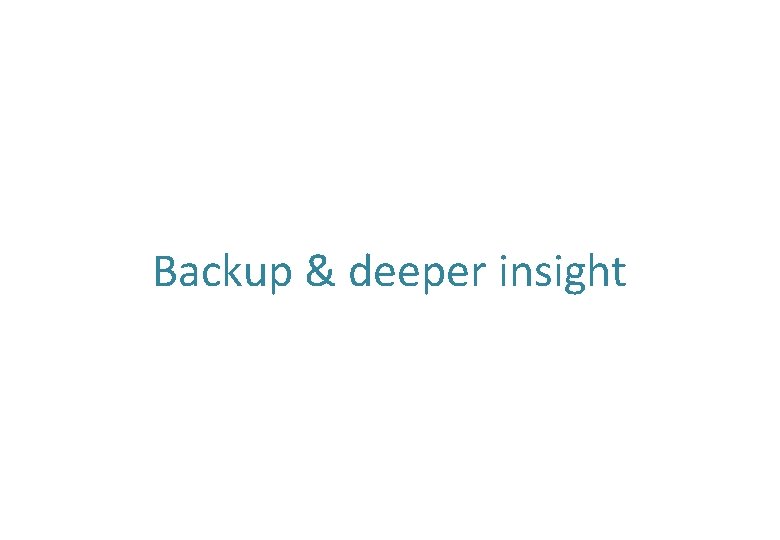
Backup & deeper insight
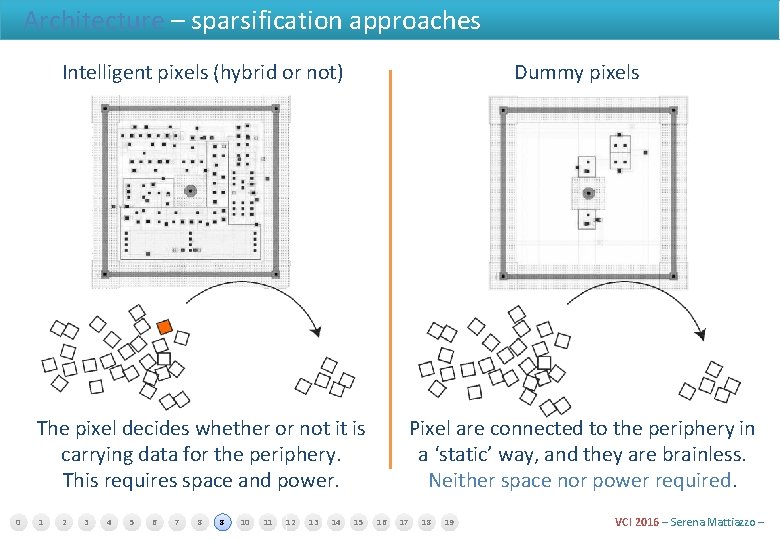
Architecture – sparsification approaches Intelligent pixels (hybrid or not) Dummy pixels The pixel decides whether or not it is carrying data for the periphery. This requires space and power. 0 1 2 3 4 5 6 7 8 9 8 10 11 12 13 14 15 Pixel are connected to the periphery in a ‘static’ way, and they are brainless. Neither space nor power required. 16 17 18 19 VCI 2016 – Serena Mattiazzo –
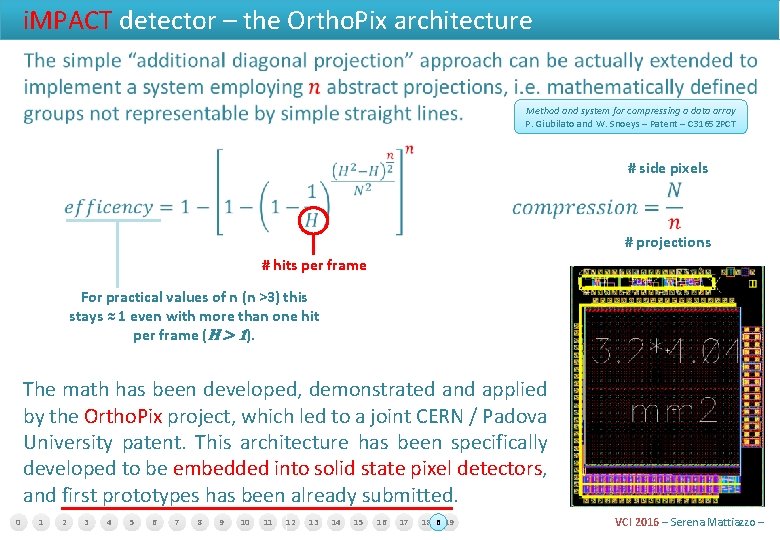
i. MPACT detector – the Ortho. Pix architecture Method and system for compressing a data array P. Giubilato and W. Snoeys – Patent – C 31652 PCT # side pixels # projections # hits per frame For practical values of n (n >3) this stays ≈ 1 even with more than one hit per frame (H > 1). The math has been developed, demonstrated and applied by the Ortho. Pix project, which led to a joint CERN / Padova University patent. This architecture has been specifically developed to be embedded into solid state pixel detectors, and first prototypes has been already submitted. 0 1 2 3 4 5 6 7 8 9 10 11 12 13 14 15 16 17 18 B 19 VCI 2016 – Serena Mattiazzo –
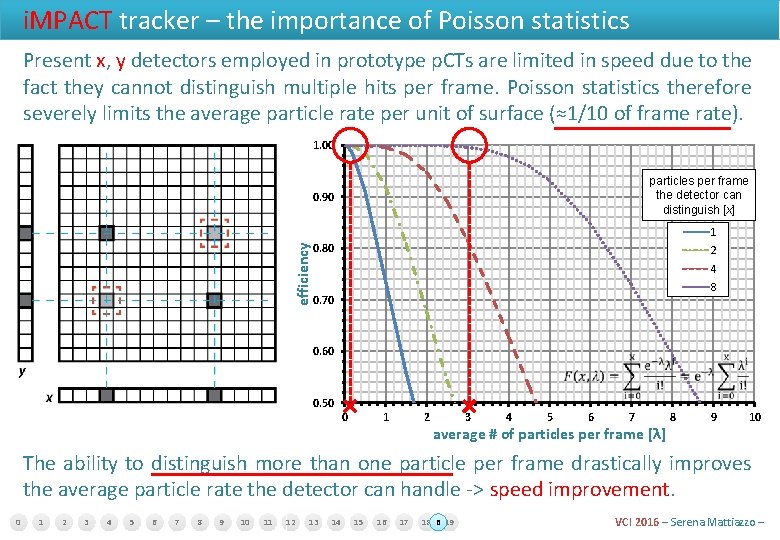
i. MPACT tracker – the importance of Poisson statistics Present x, y detectors employed in prototype p. CTs are limited in speed due to the fact they cannot distinguish multiple hits per frame. Poisson statistics therefore severely limits the average particle rate per unit of surface (≈1/10 of frame rate). 1. 00 particles per frame the detector can distinguish [x] 0. 90 efficiency 1 0. 80 2 4 8 0. 70 0. 60 0. 50 0 1 2 3 4 5 6 7 average # of particles per frame [λ] 8 9 10 The ability to distinguish more than one particle per frame drastically improves the average particle rate the detector can handle -> speed improvement. 0 1 2 3 4 5 6 7 8 9 10 11 12 13 14 15 16 17 18 B 19 VCI 2016 – Serena Mattiazzo –
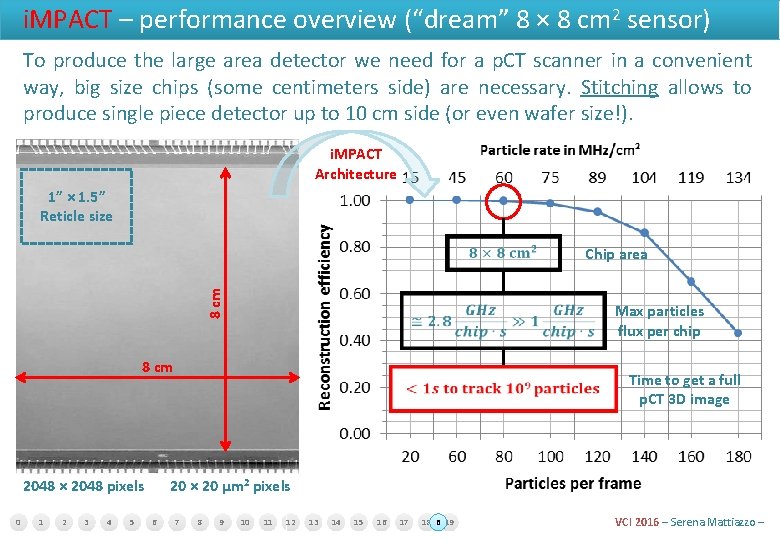
i. MPACT – performance overview (“dream” 8 × 8 cm 2 sensor) To produce the large area detector we need for a p. CT scanner in a convenient way, big size chips (some centimeters side) are necessary. Stitching allows to produce single piece detector up to 10 cm side (or even wafer size!). i. MPACT Architecture 1” × 1. 5” Reticle size 8 cm 1 2 3 4 5 Max particles flux per chip Time to get a full p. CT 3 D image 20 × 20 µm 2 pixels 2048 × 2048 pixels 0 Chip area 6 7 8 9 10 11 12 13 14 15 16 17 18 B 19 VCI 2016 – Serena Mattiazzo –
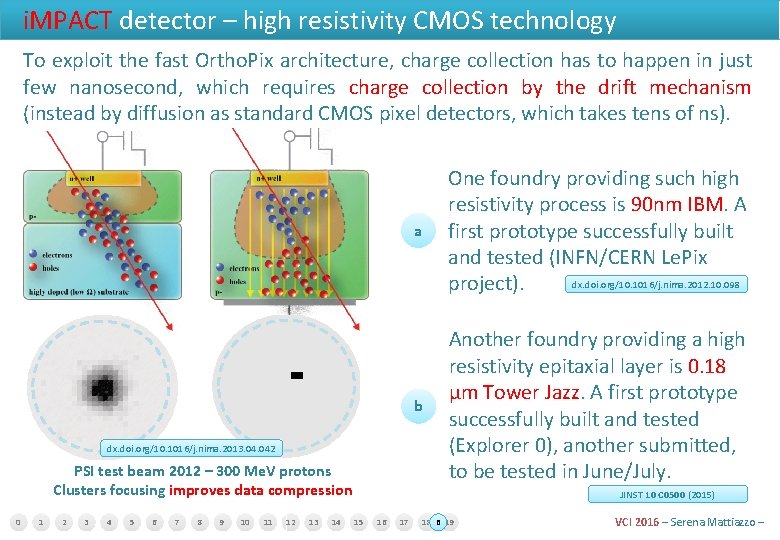
i. MPACT detector – high resistivity CMOS technology To exploit the fast Ortho. Pix architecture, charge collection has to happen in just few nanosecond, which requires charge collection by the drift mechanism (instead by diffusion as standard CMOS pixel detectors, which takes tens of ns). dx. doi. org/10. 1016/j. nima. 2013. 042 PSI test beam 2012 – 300 Me. V protons Clusters focusing improves data compression 0 1 2 3 4 5 6 7 8 9 10 11 12 13 14 a One foundry providing such high resistivity process is 90 nm IBM. A first prototype successfully built and tested (INFN/CERN Le. Pix dx. doi. org/10. 1016/j. nima. 2012. 10. 098 project). b Another foundry providing a high resistivity epitaxial layer is 0. 18 µm Tower Jazz. A first prototype successfully built and tested (Explorer 0), another submitted, to be tested in June/July. JINST 10 C 0500 (2015) 15 16 17 18 B 19 VCI 2016 – Serena Mattiazzo –
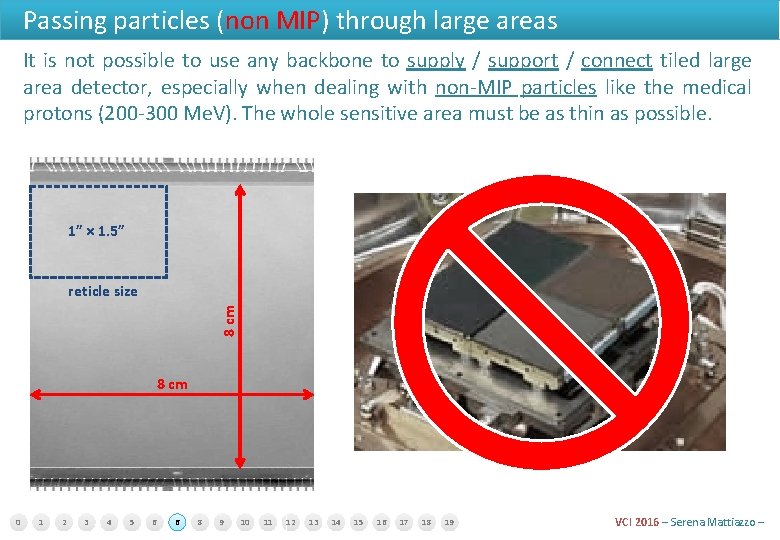
Passing particles (non MIP) through large areas It is not possible to use any backbone to supply / support / connect tiled large area detector, especially when dealing with non-MIP particles like the medical protons (200 -300 Me. V). The whole sensitive area must be as thin as possible. 1” × 1. 5” 8 cm reticle size 8 cm 0 1 2 3 4 5 6 7 6 8 9 10 11 12 13 14 15 16 17 18 19 VCI 2016 – Serena Mattiazzo –
Coffee cup calorimeter vs bomb calorimeter
Coffee cup calorimeter formula
Constant volume calorimeter
Alma project tracker
Specific heat equation units
Constant volume calorimeter
Benedict's oxy calorimeter
Accelerating rate calorimeter
Thermochemistry notes
Constant volume calorimeter
Coffee cup calorimeter equation
Combustion reaction
Identify what a bomb calorimeter measures
Hadron calorimeter
Slug calorimeter
Keith baessler
Elena rocco
Burning a match is what type of energy
Bomb calorimeter uses
Calorimeter unit
Parr calorimeter
Calorimetry equation
Calorimetry questions
Bomb calorimeter
How does a calorimeter work
Bomb calorimeter
Calorimeter constant
Inventive and innovative
List 5 innovative and 5 functional products