STRUCTURE FORMATION MATTEO VIEL INAF and INFN Trieste
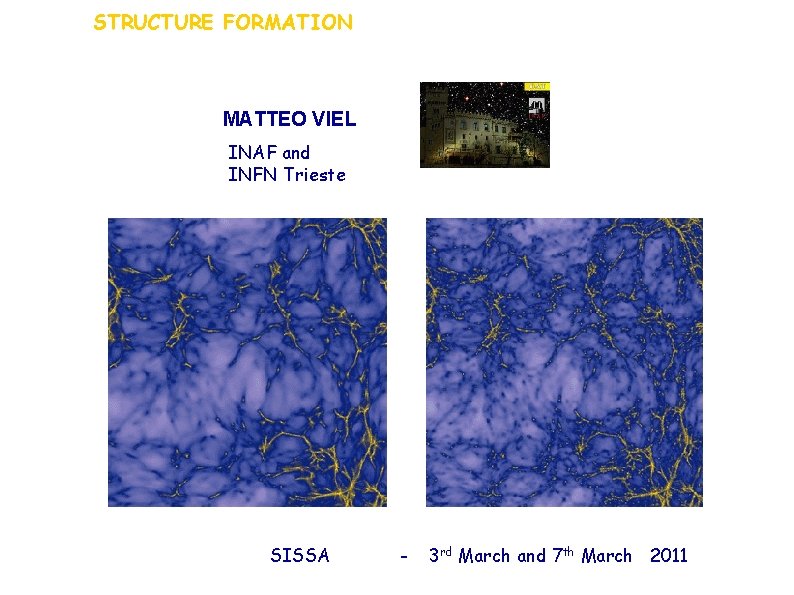
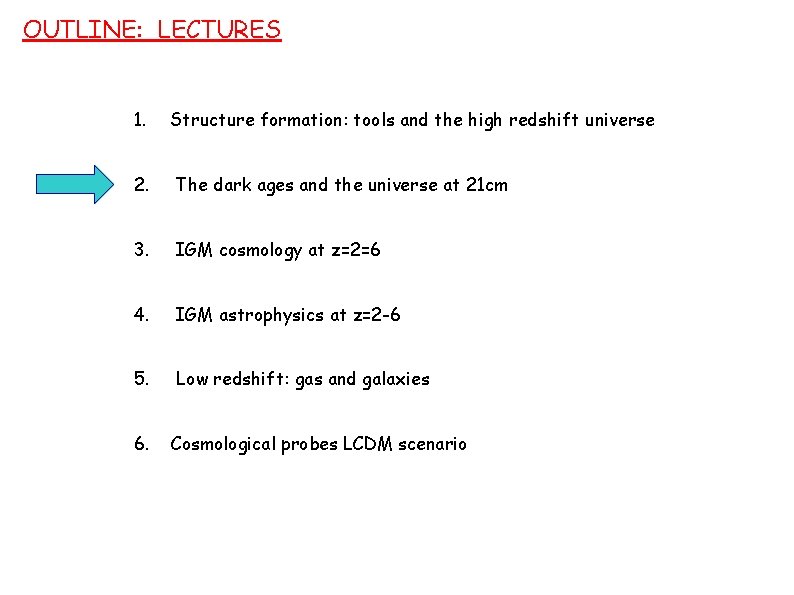
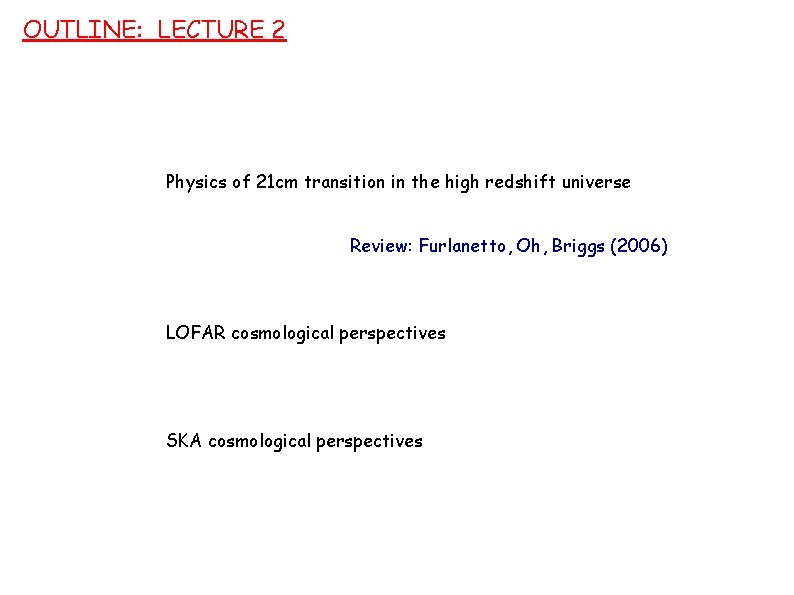
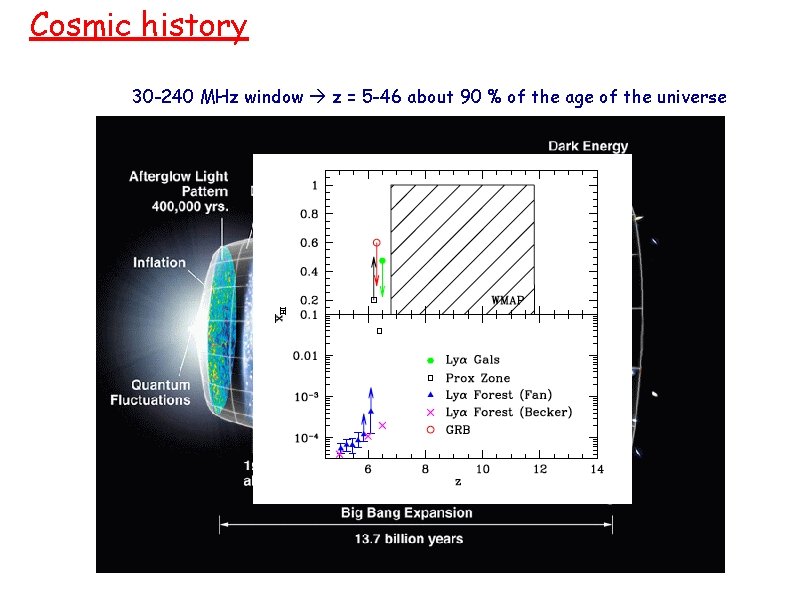
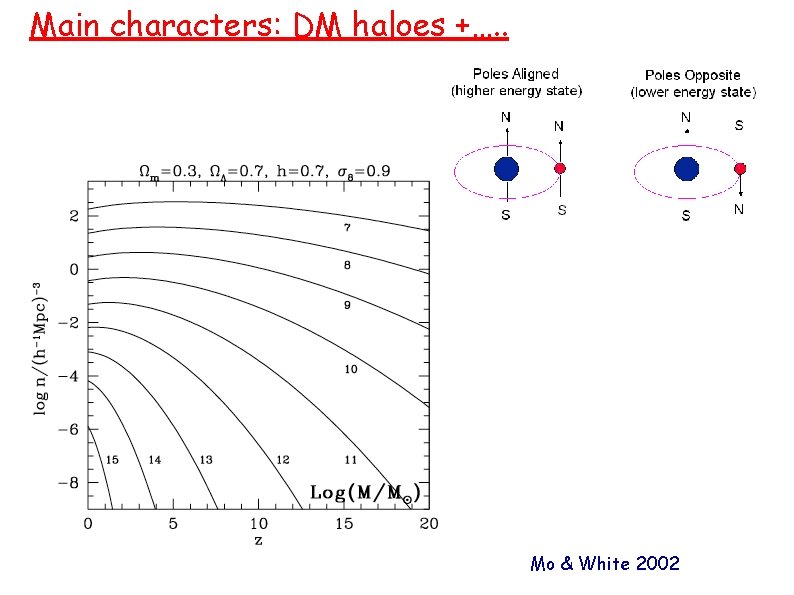
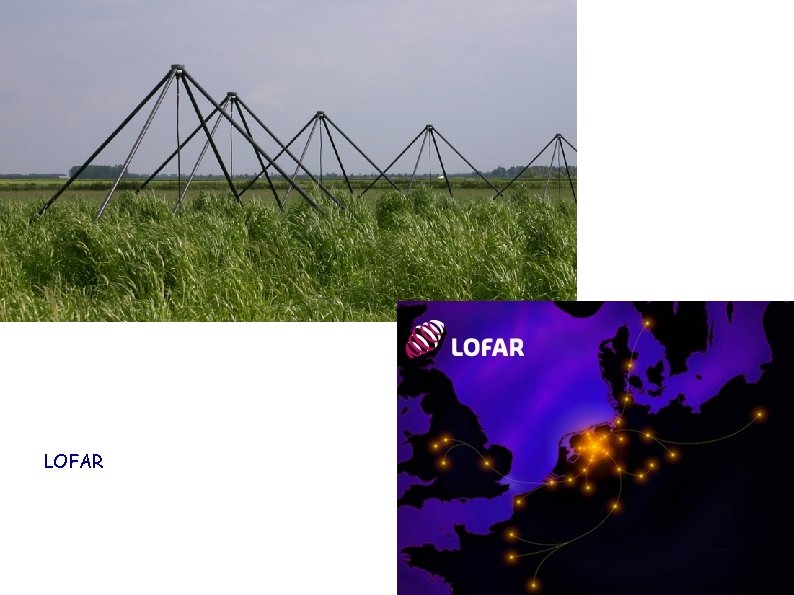
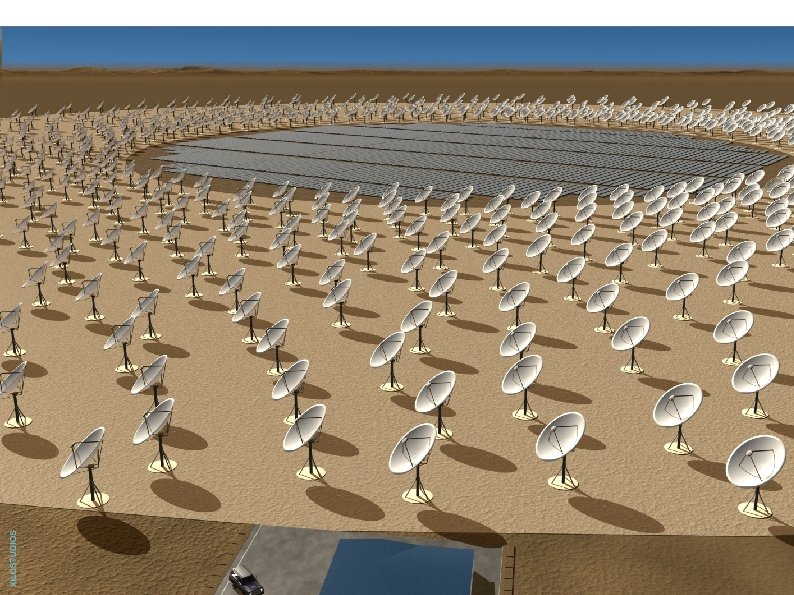
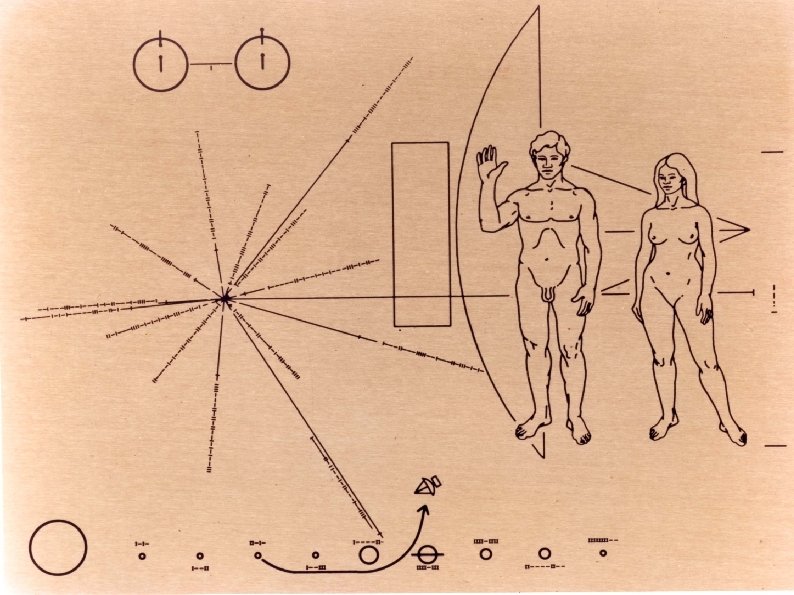
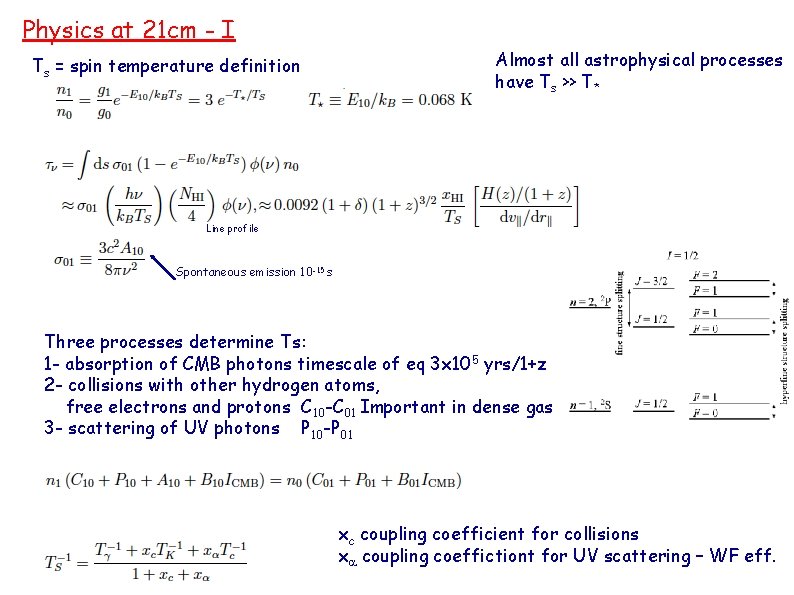
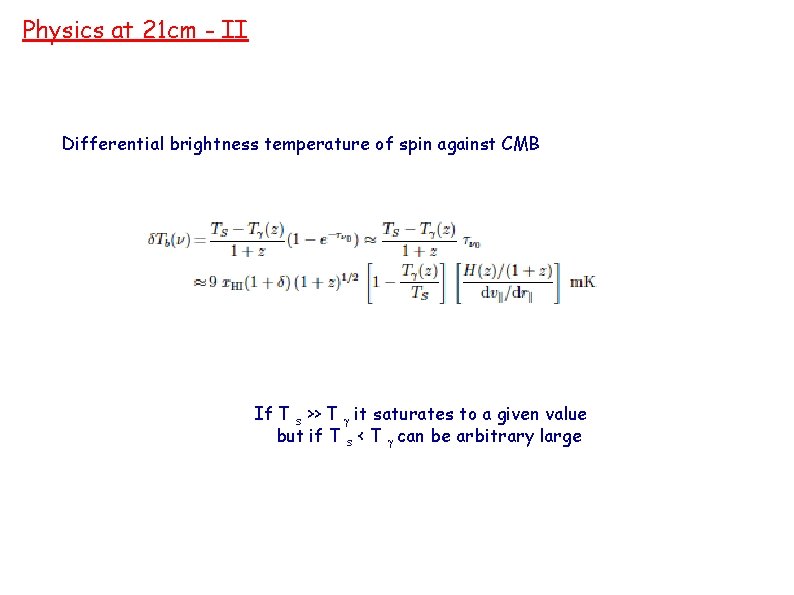
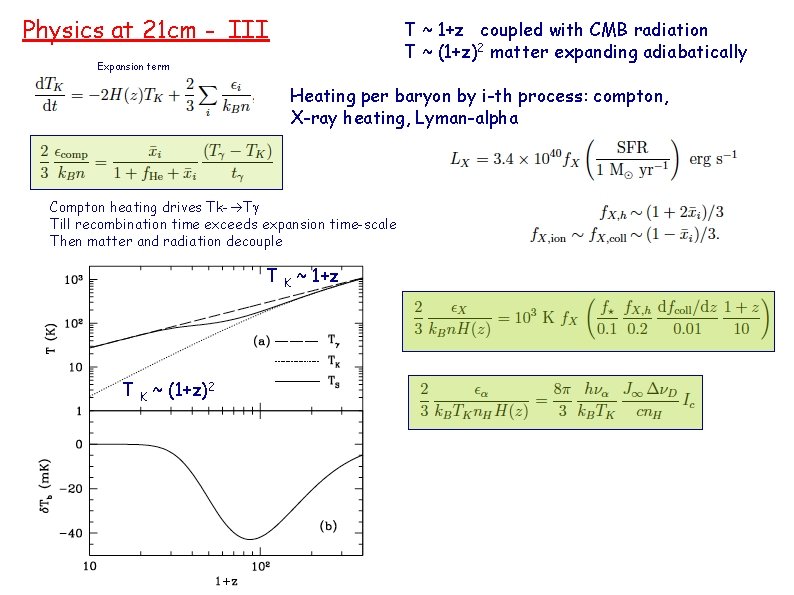
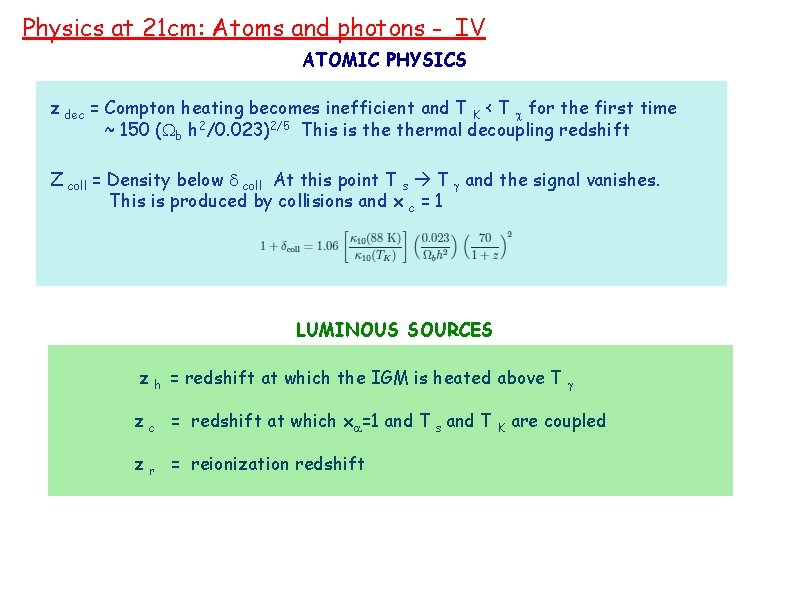
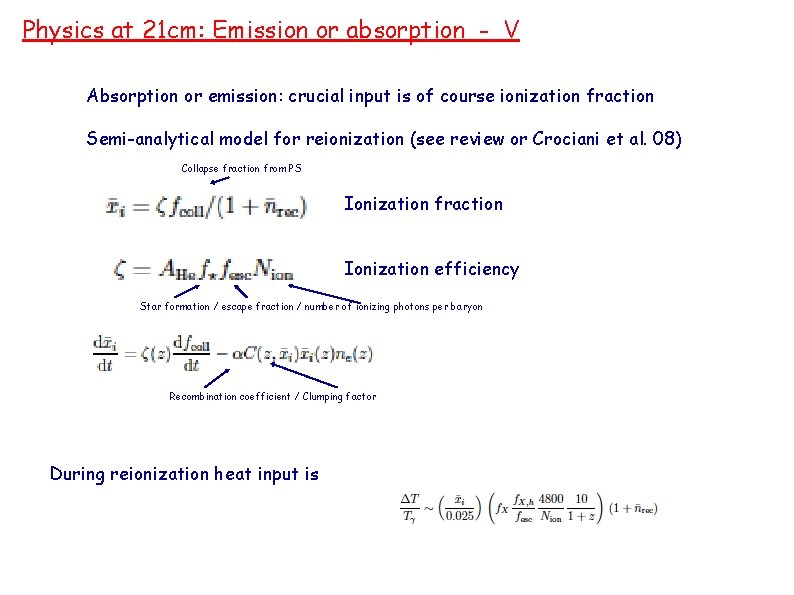
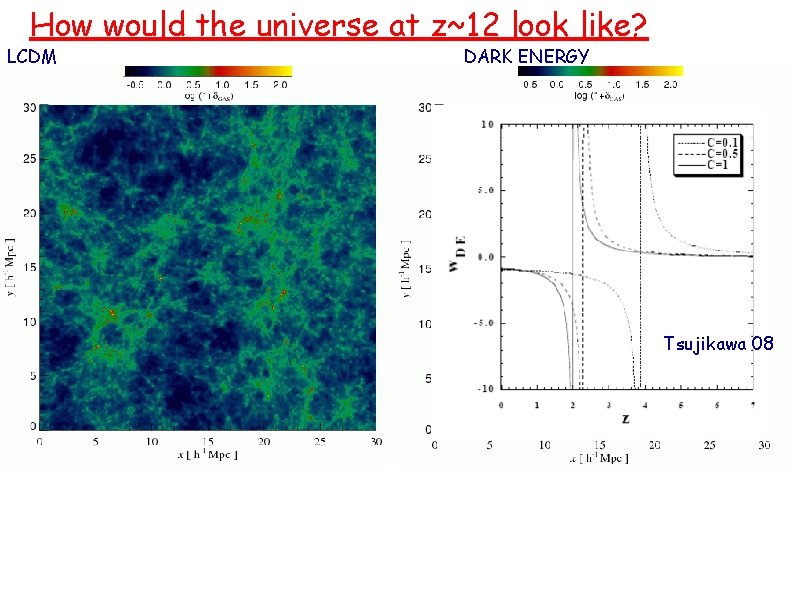
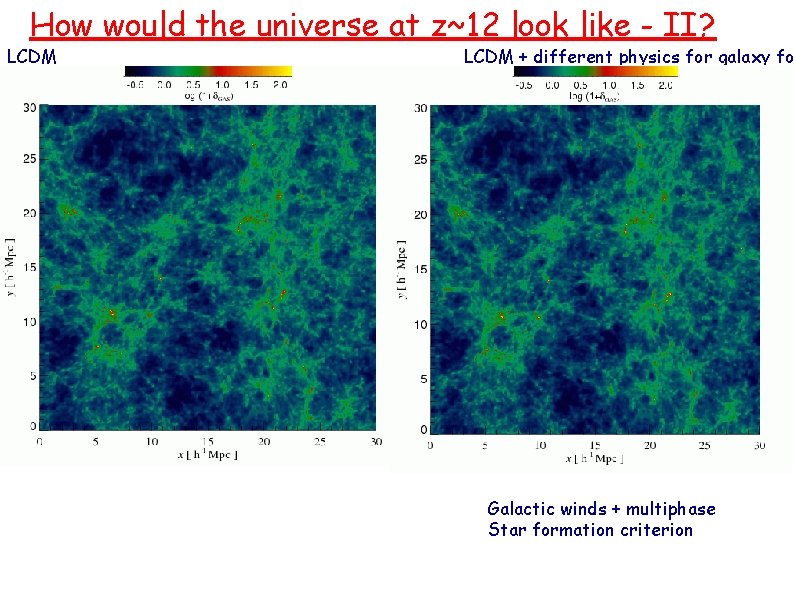
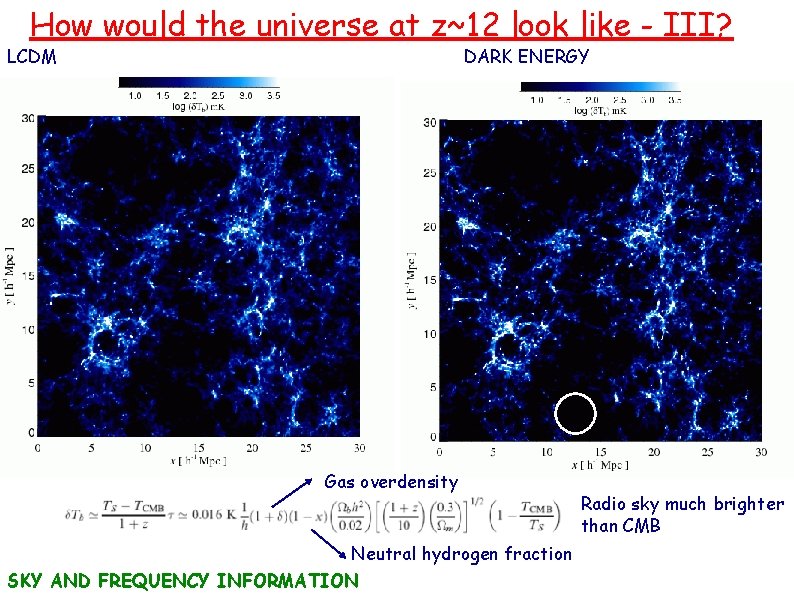
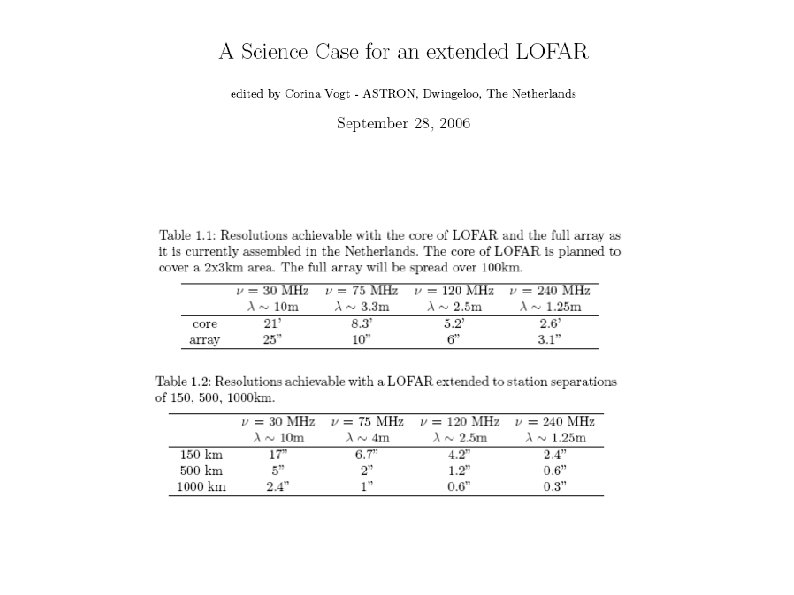
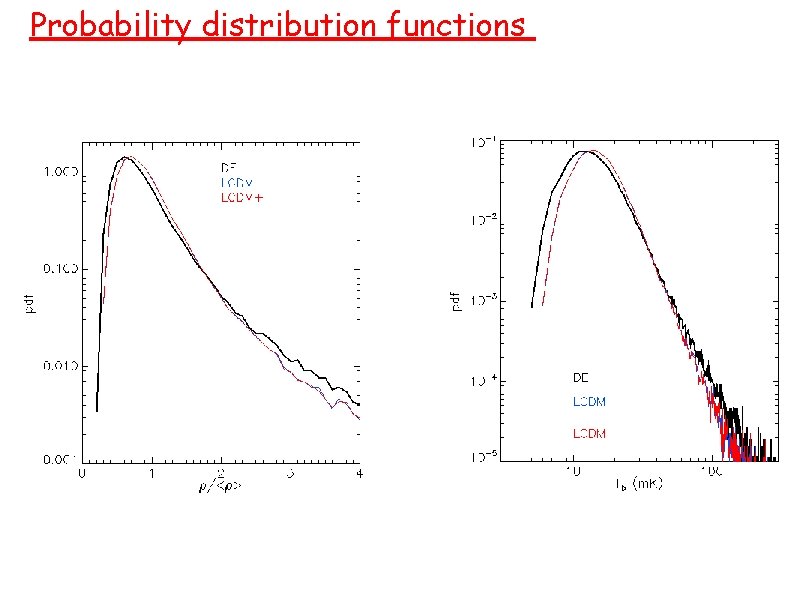
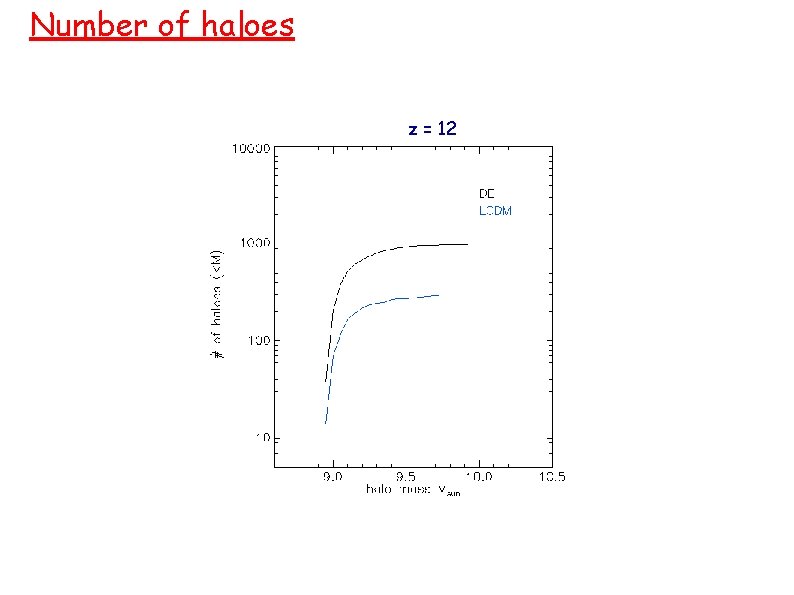
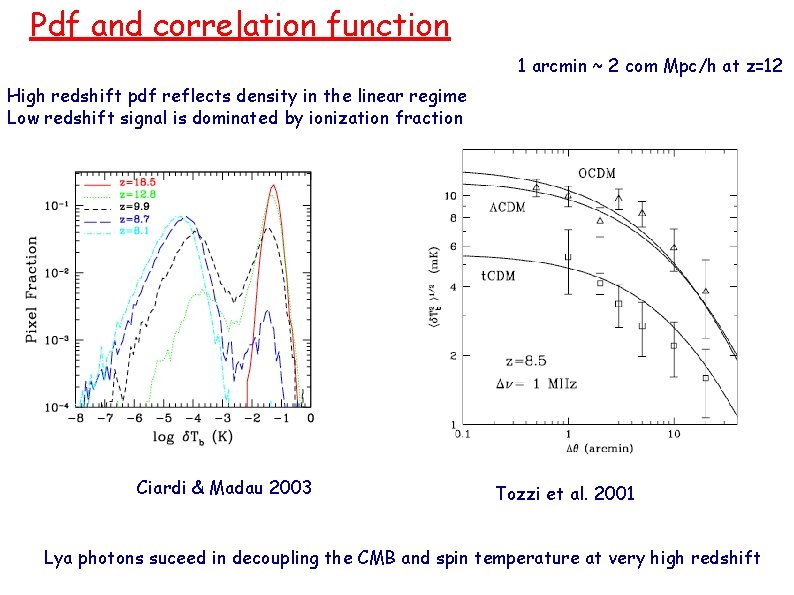
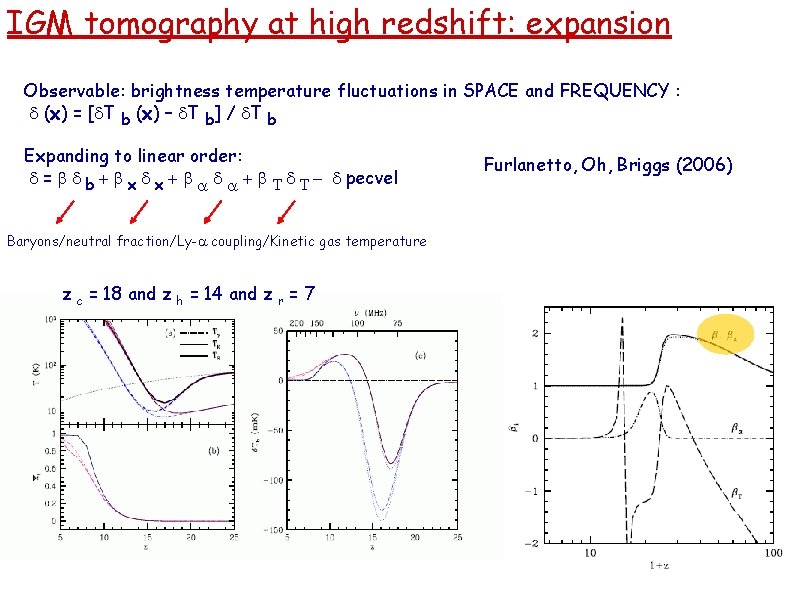
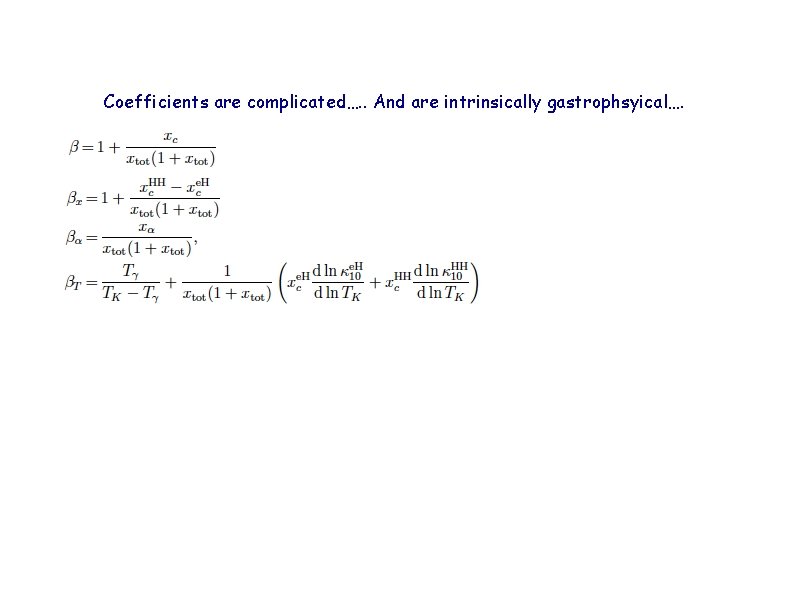
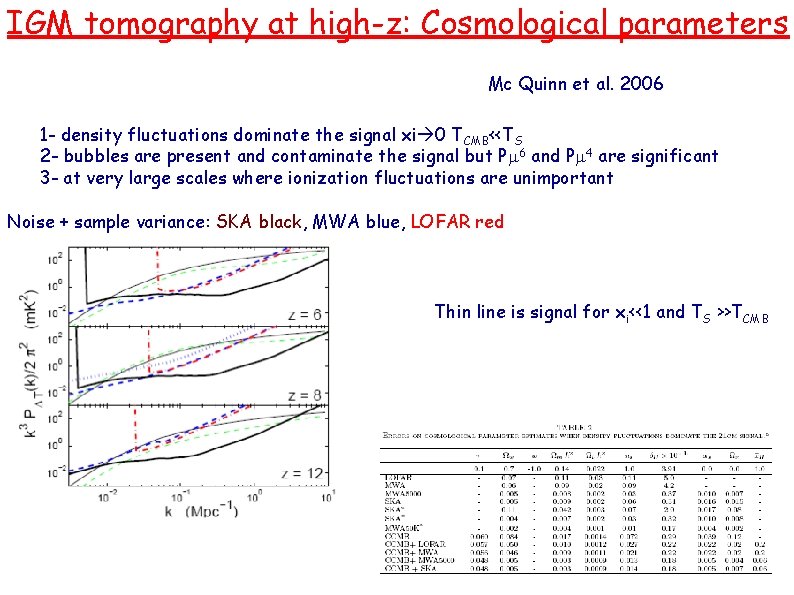
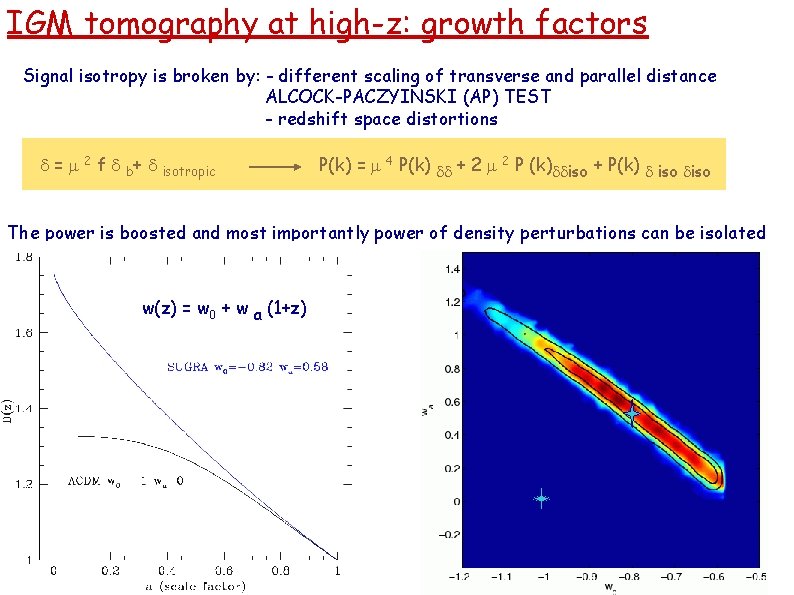
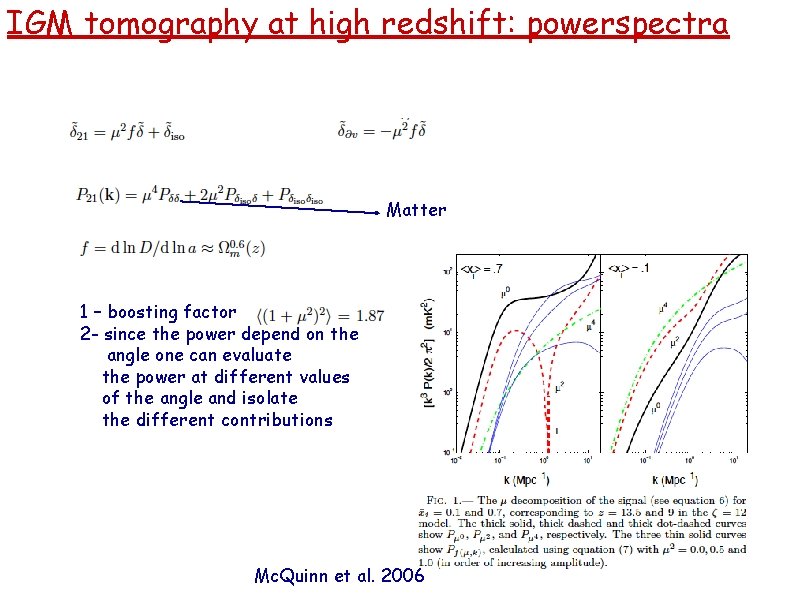
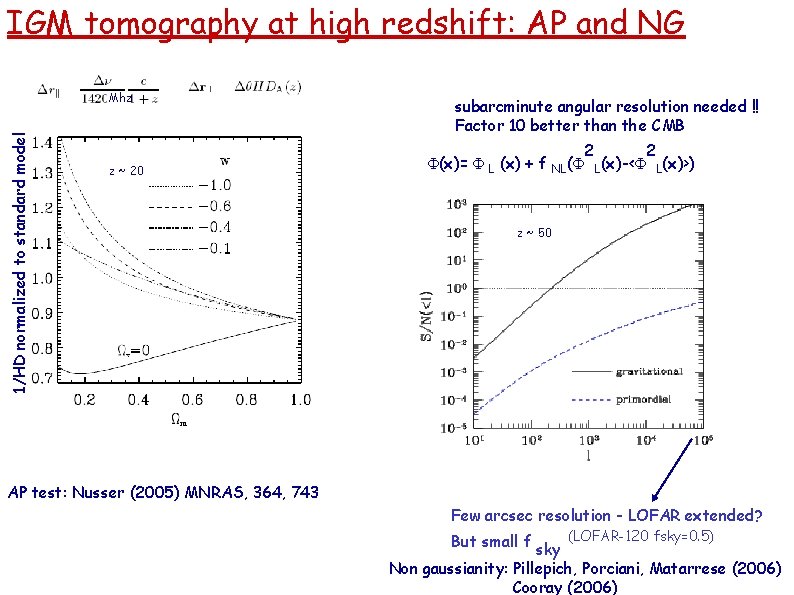
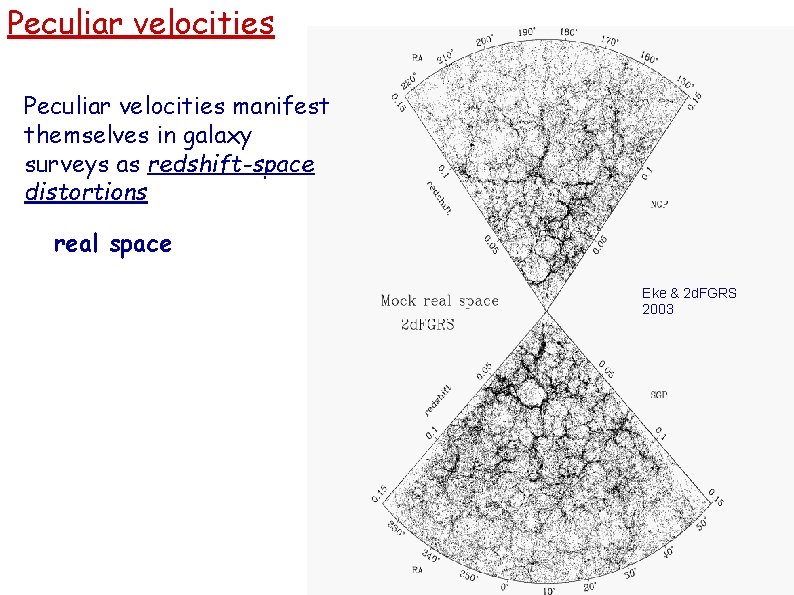
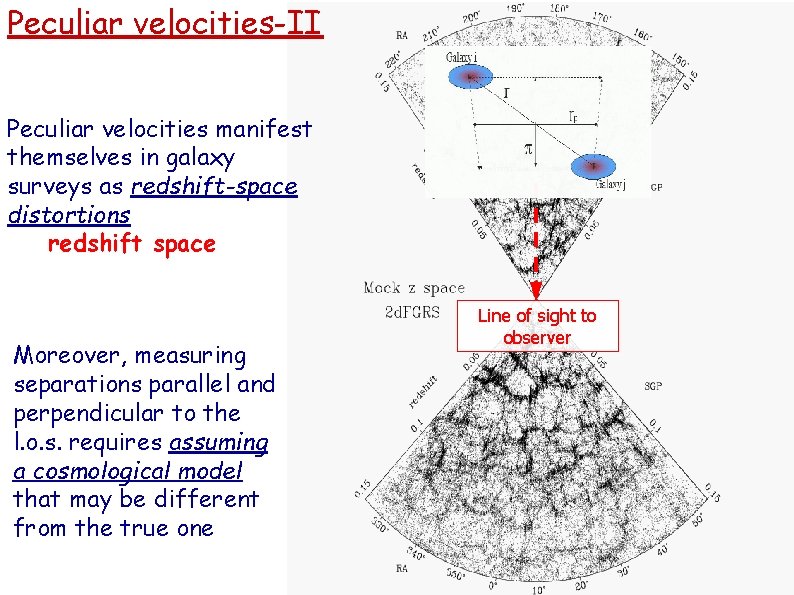
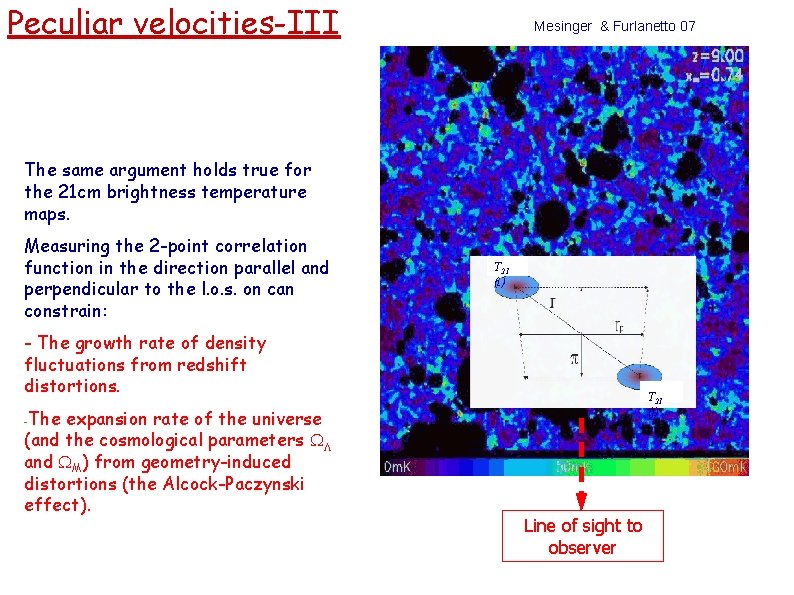
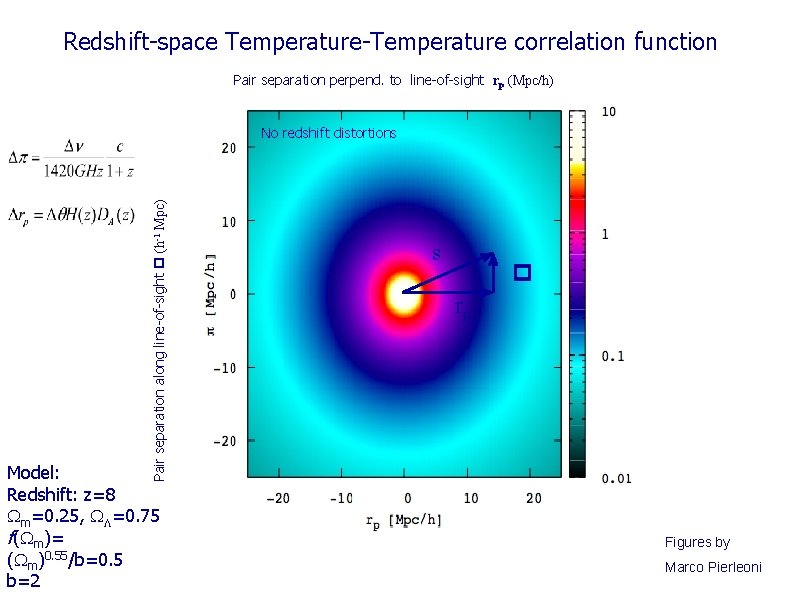
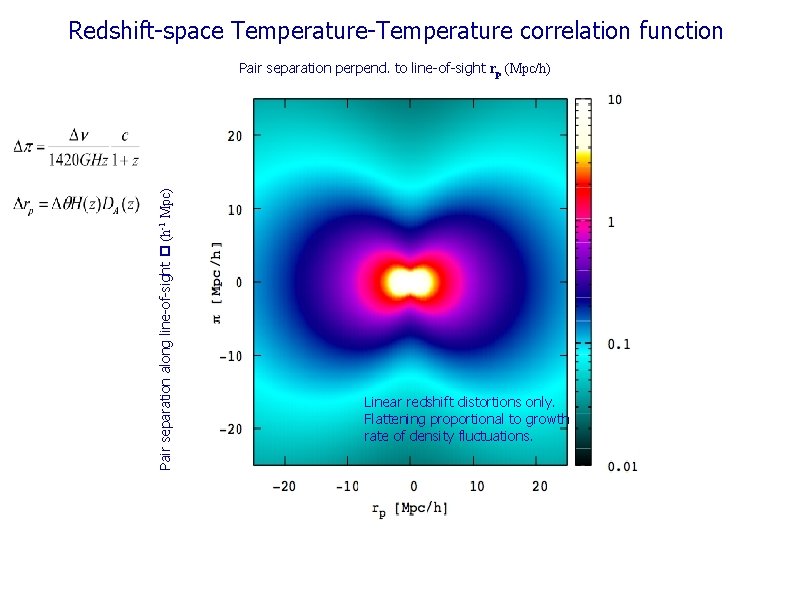
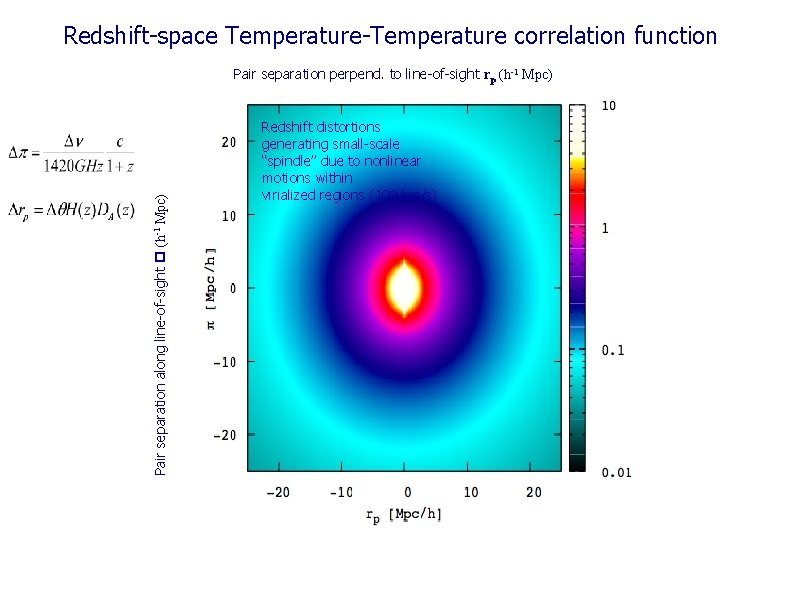
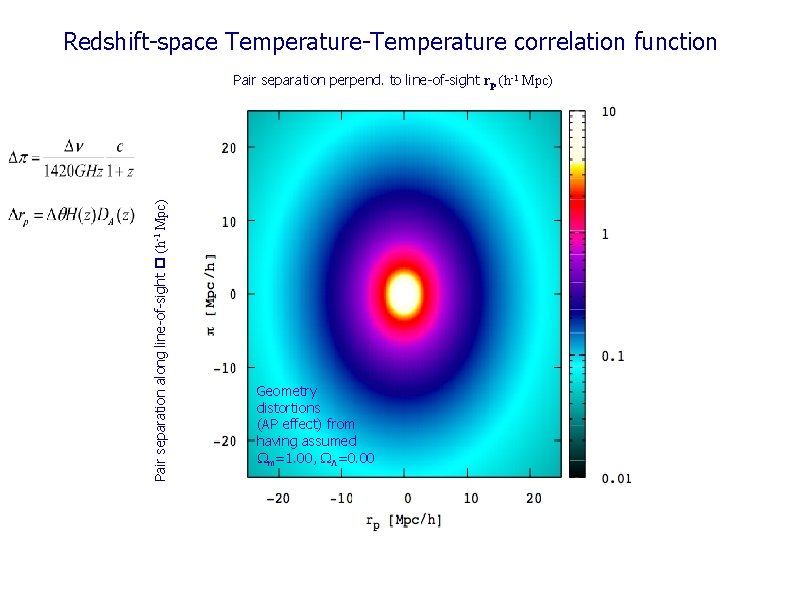
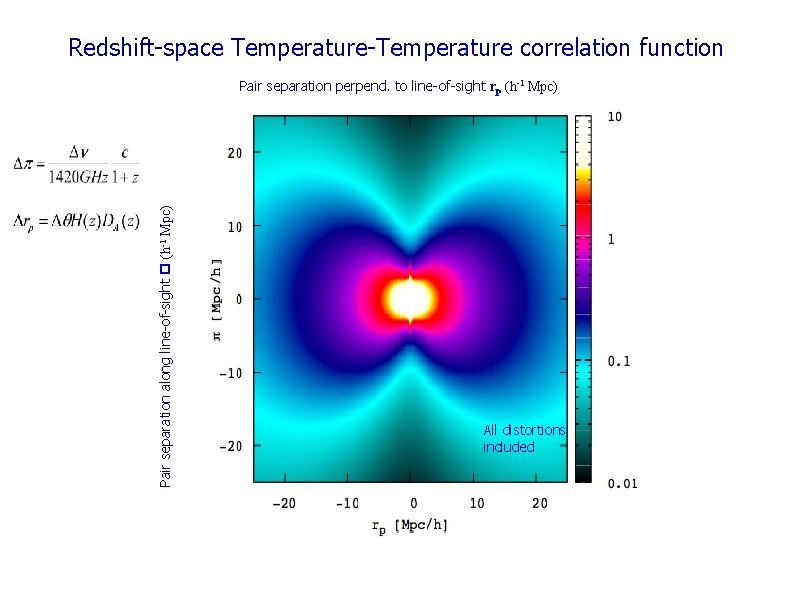
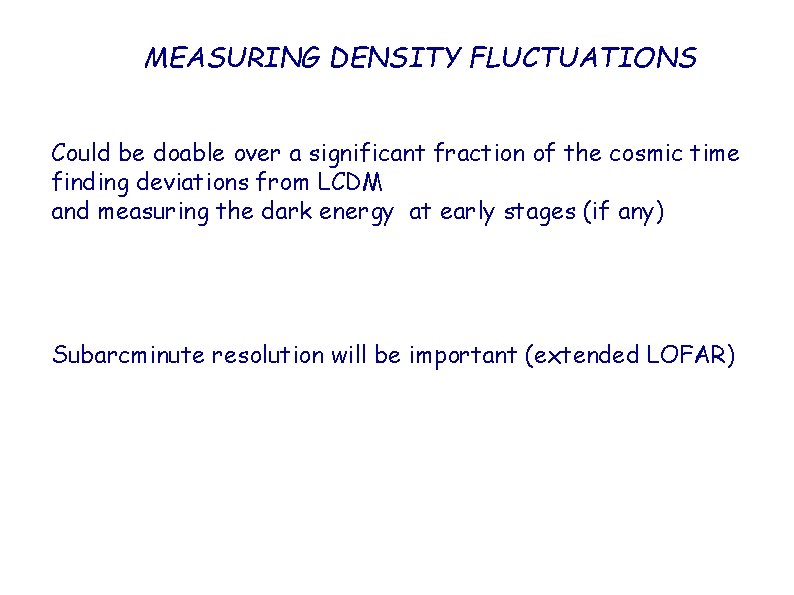
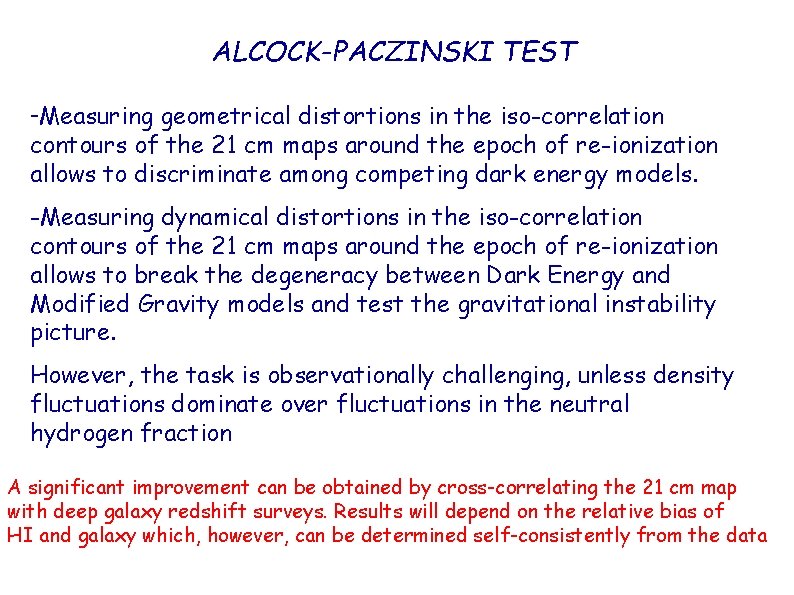
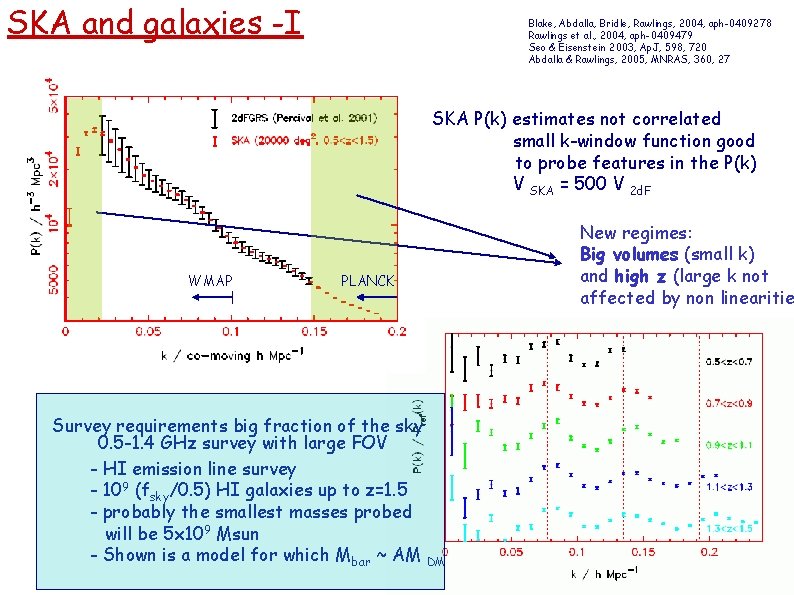
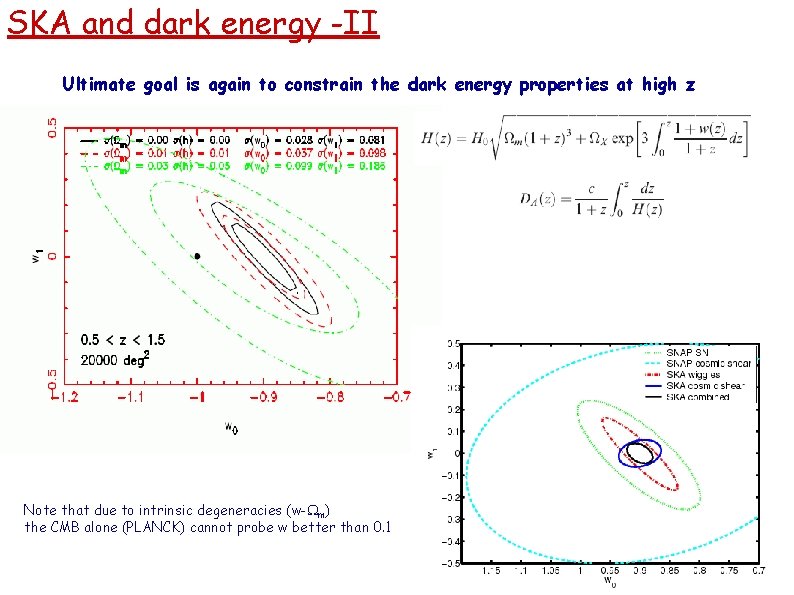
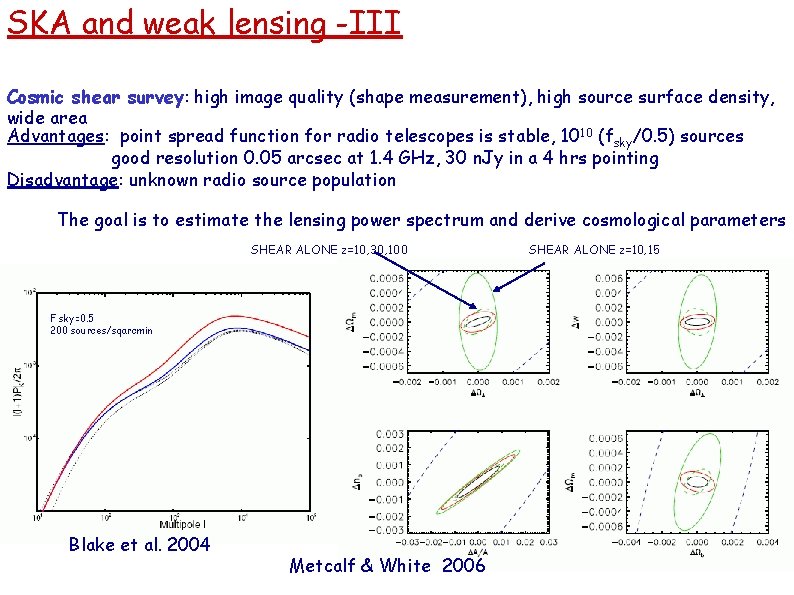
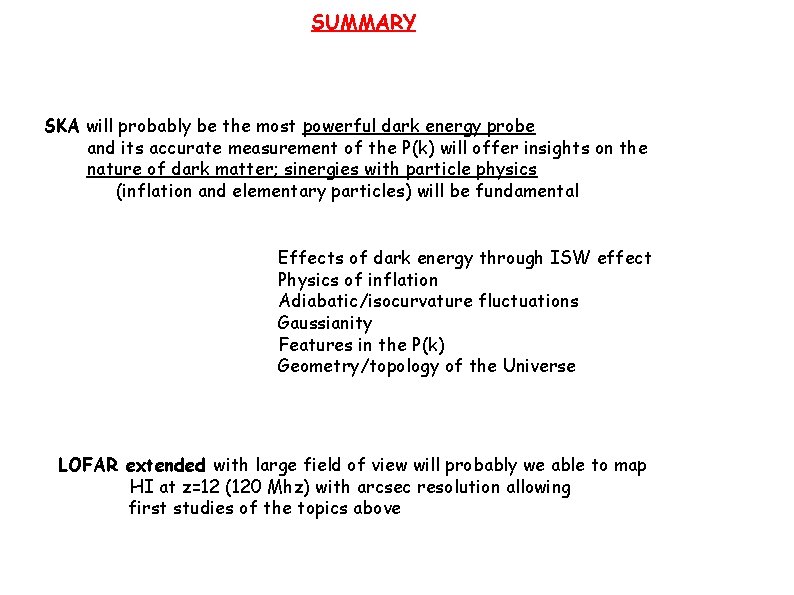
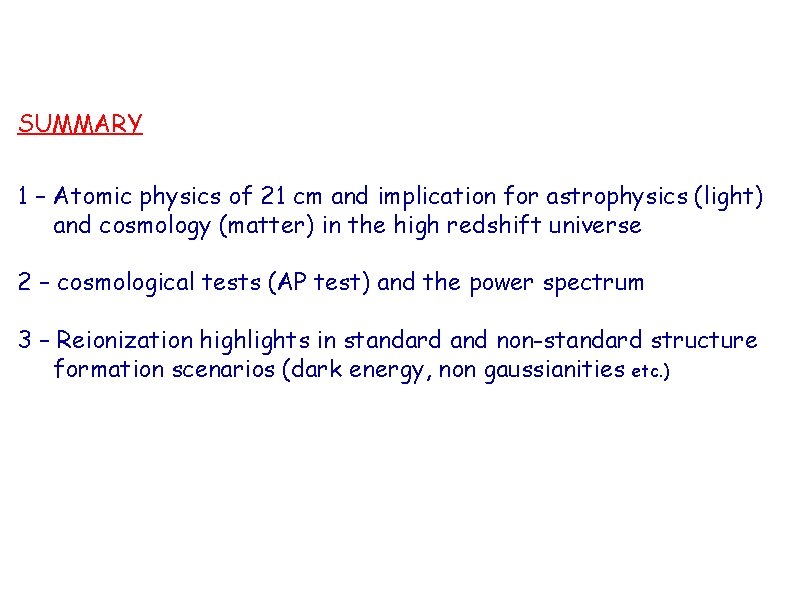
- Slides: 41
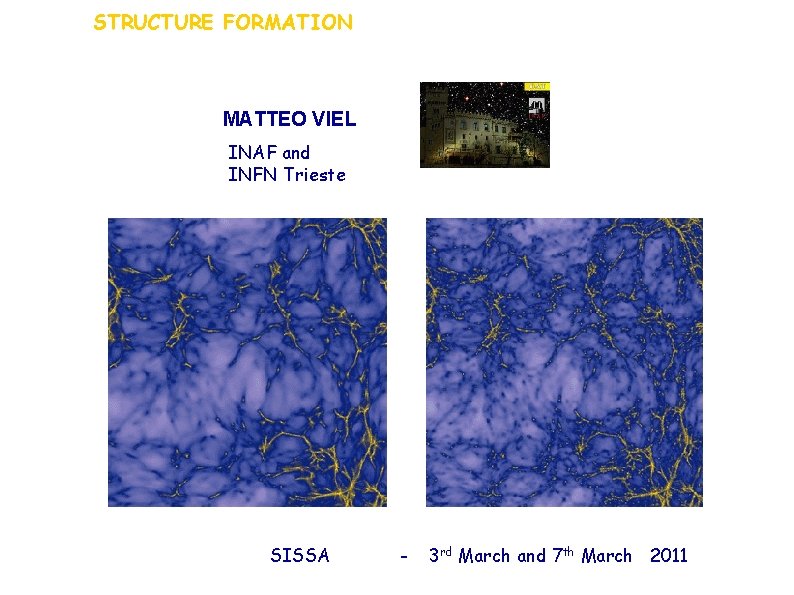
STRUCTURE FORMATION MATTEO VIEL INAF and INFN Trieste SISSA - 3 rd March and 7 th March 2011
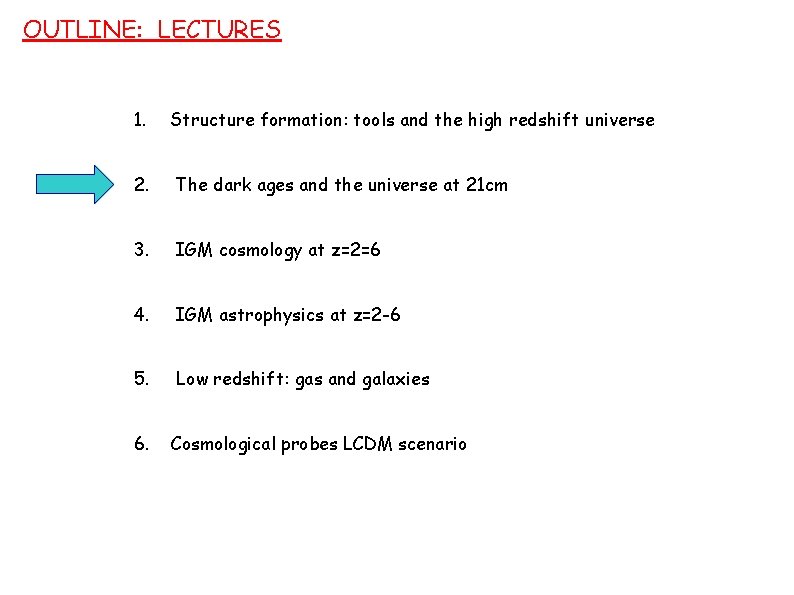
OUTLINE: LECTURES 1. Structure formation: tools and the high redshift universe 2. The dark ages and the universe at 21 cm 3. IGM cosmology at z=2=6 4. IGM astrophysics at z=2 -6 5. Low redshift: gas and galaxies 6. Cosmological probes LCDM scenario
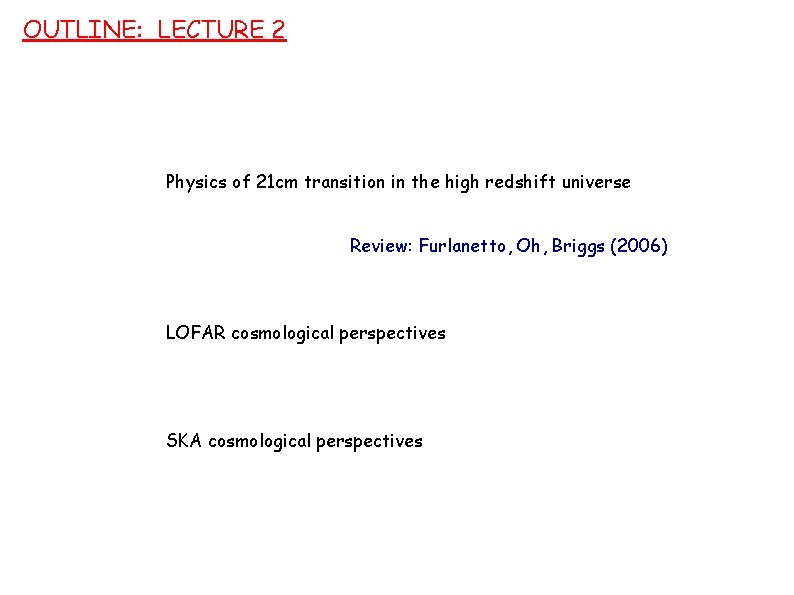
OUTLINE: LECTURE 2 Physics of 21 cm transition in the high redshift universe Review: Furlanetto, Oh, Briggs (2006) LOFAR cosmological perspectives SKA cosmological perspectives
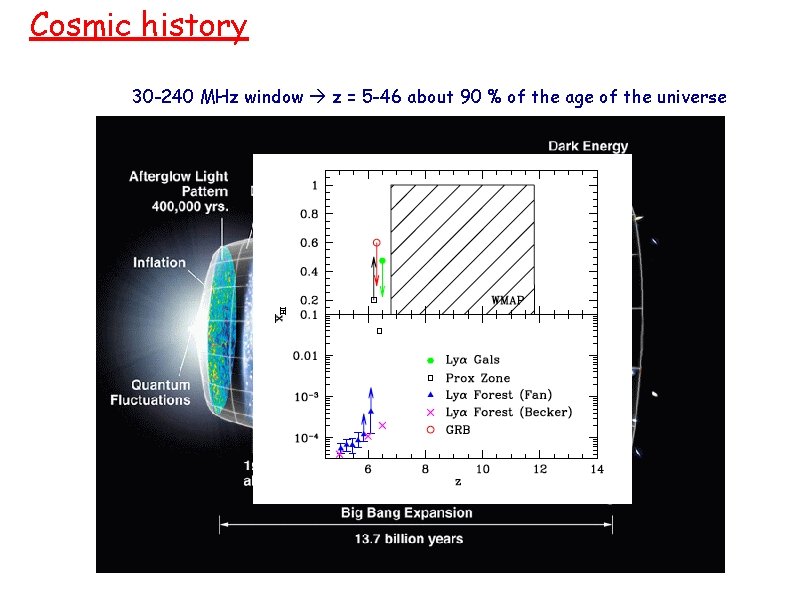
Cosmic history 30 -240 MHz window z = 5 -46 about 90 % of the age of the universe ?
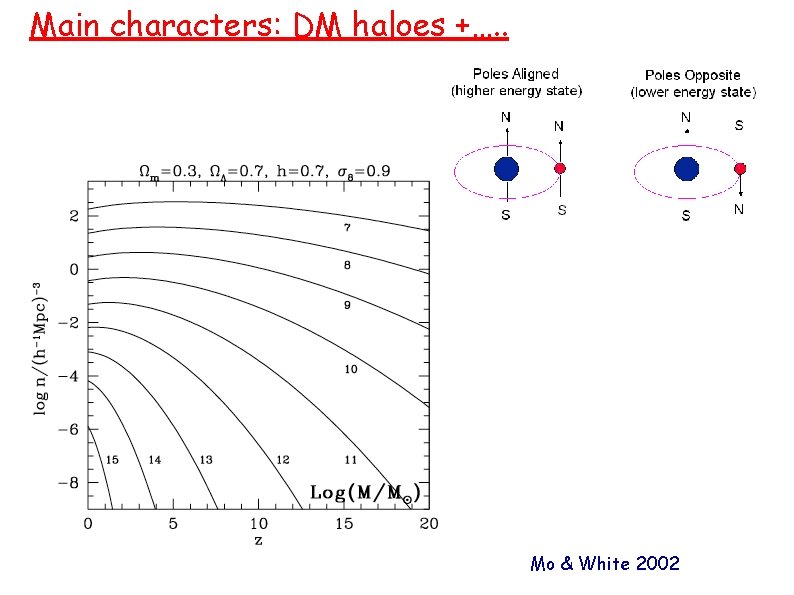
Main characters: DM haloes +…. . Mo & White 2002
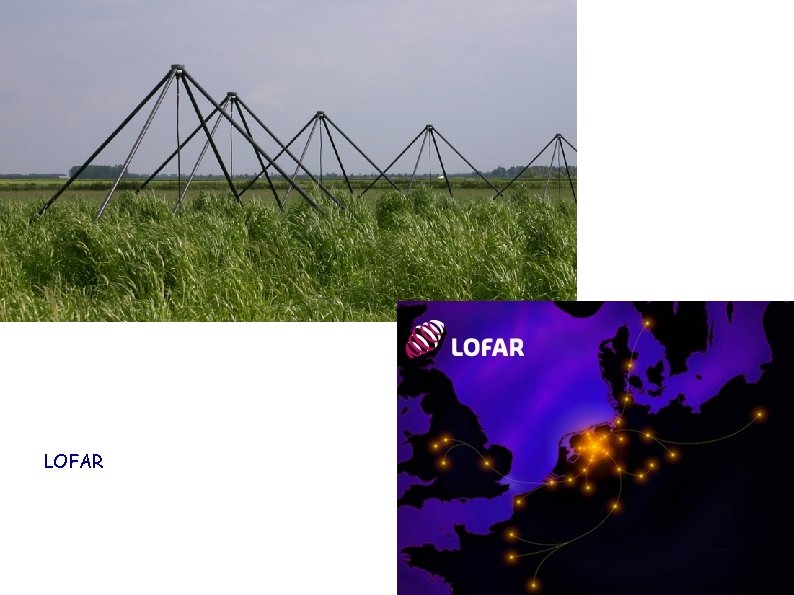
LOFAR
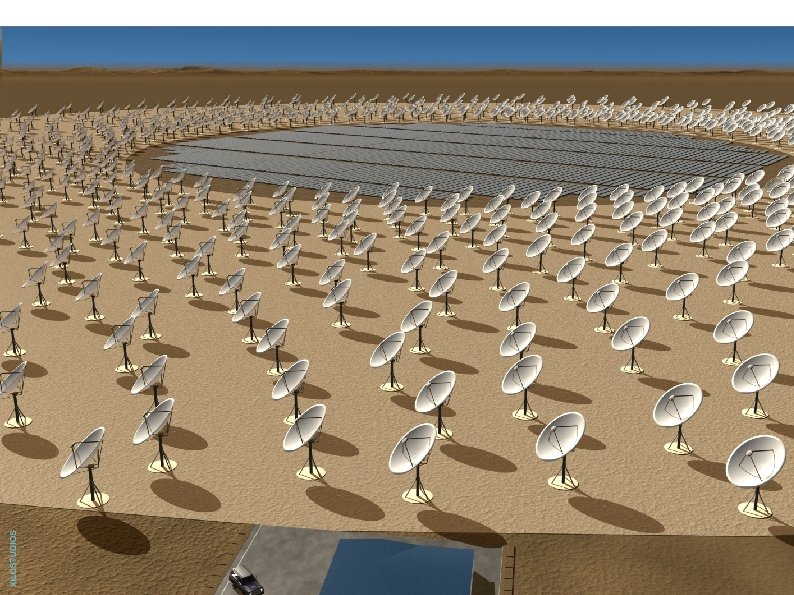
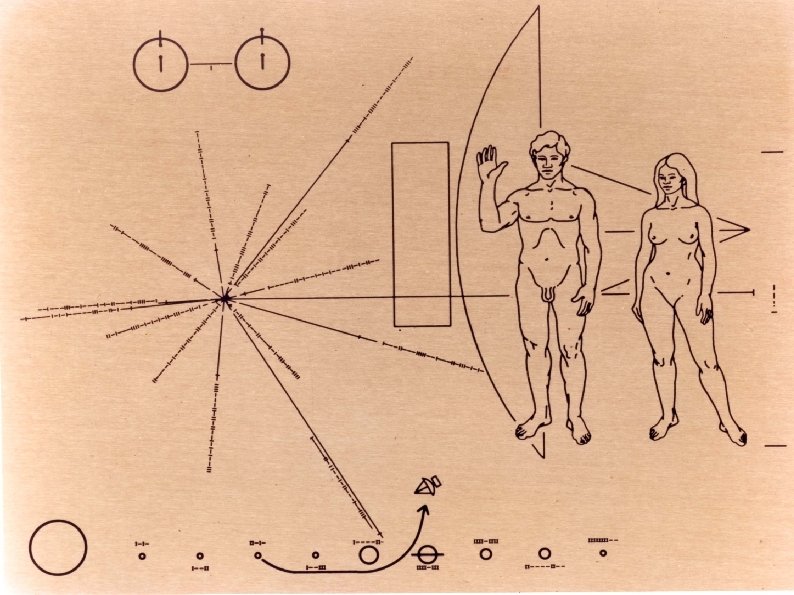
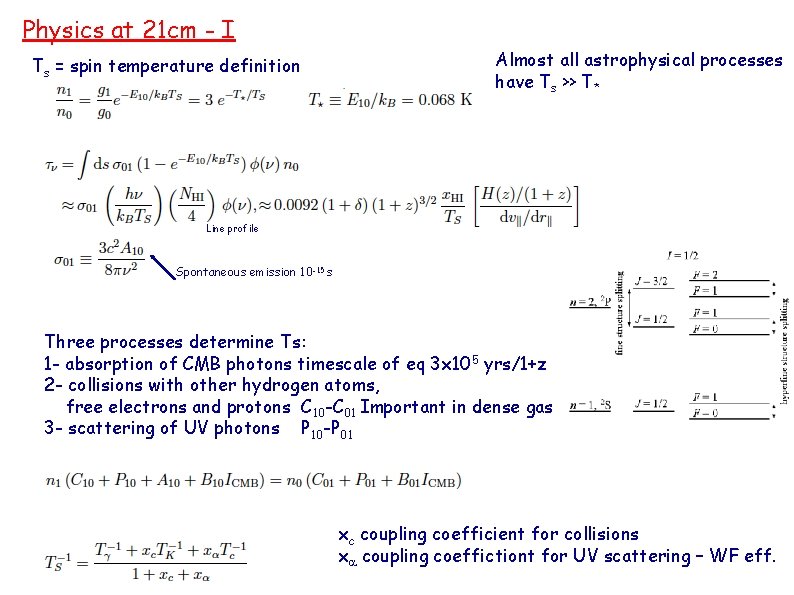
Physics at 21 cm - I Ts = spin temperature definition Almost all astrophysical processes have Ts >> T* Line profile Spontaneous emission 10 -15 s Three processes determine Ts: 1 - absorption of CMB photons timescale of eq 3 x 105 yrs/1+z 2 - collisions with other hydrogen atoms, free electrons and protons C 10 -C 01 Important in dense gas 3 - scattering of UV photons P 10 -P 01 xc coupling coefficient for collisions xa coupling coeffictiont for UV scattering – WF eff.
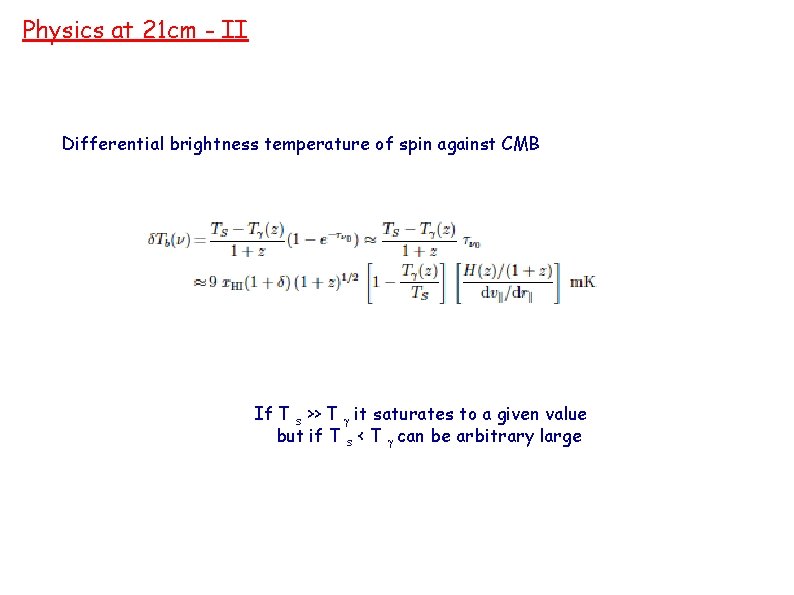
Physics at 21 cm - II Differential brightness temperature of spin against CMB If T s >> T g it saturates to a given value but if T s < T g can be arbitrary large
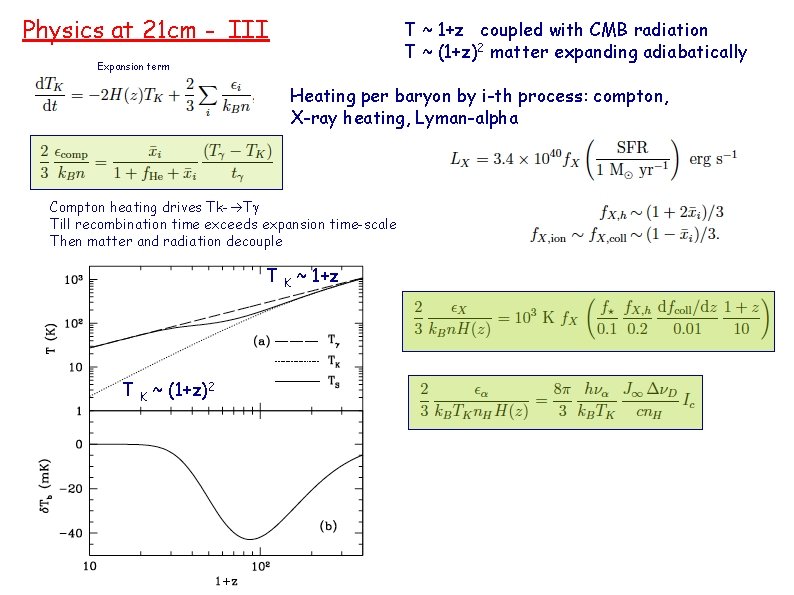
Physics at 21 cm - III T ~ 1+z coupled with CMB radiation T ~ (1+z)2 matter expanding adiabatically Expansion term Heating per baryon by i-th process: compton, X-ray heating, Lyman-alpha Compton heating drives Tk- Tg Till recombination time exceeds expansion time-scale Then matter and radiation decouple T K ~ 1+z T K ~ (1+z)2
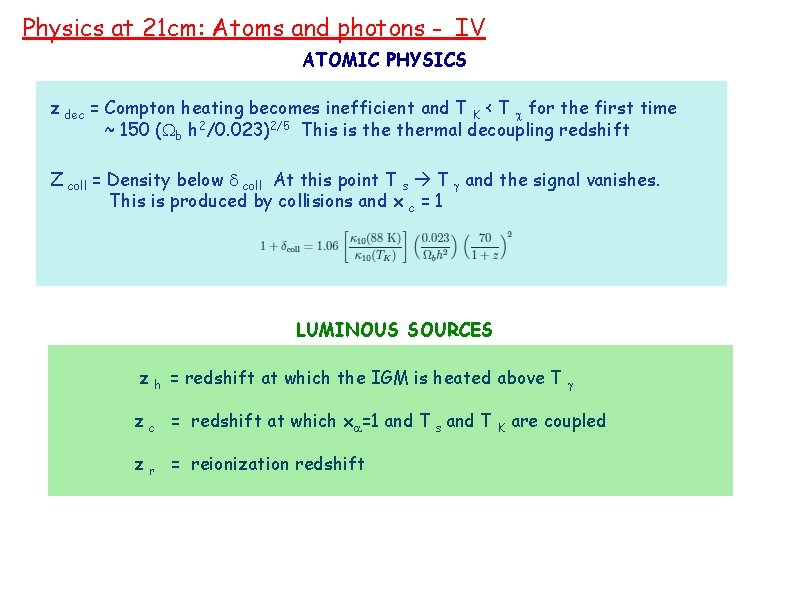
Physics at 21 cm: Atoms and photons - IV ATOMIC PHYSICS z dec = Compton heating becomes inefficient and T K < T c for the first time ~ 150 ( b h 2/0. 023)2/5 This is thermal decoupling redshift Z coll = Density below d coll At this point T s T g and the signal vanishes. This is produced by collisions and x c = 1 LUMINOUS SOURCES z h = redshift at which the IGM is heated above T z c = redshift at which xa=1 and T s and T z r = reionization redshift K g are coupled
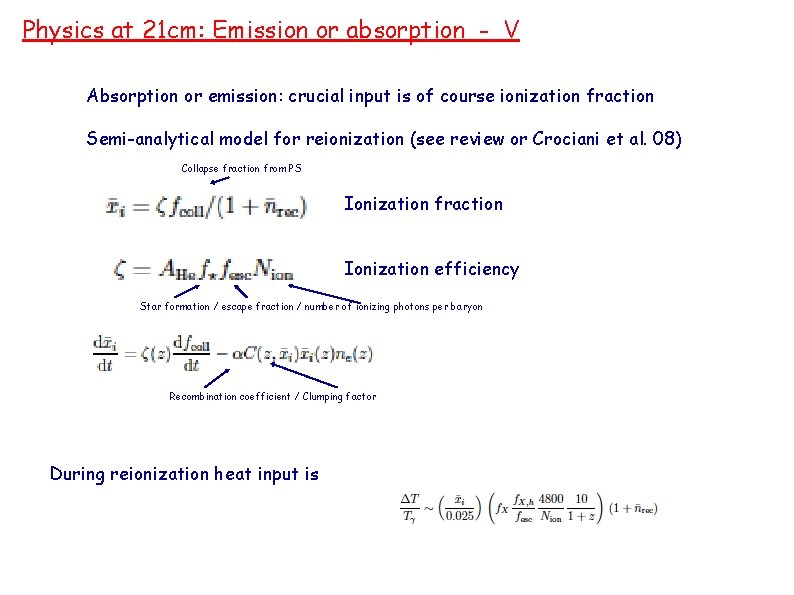
Physics at 21 cm: Emission or absorption - V Absorption or emission: crucial input is of course ionization fraction Semi-analytical model for reionization (see review or Crociani et al. 08) Collapse fraction from PS Ionization fraction Ionization efficiency Star formation / escape fraction / number of ionizing photons per baryon Recombination coefficient / Clumping factor During reionization heat input is
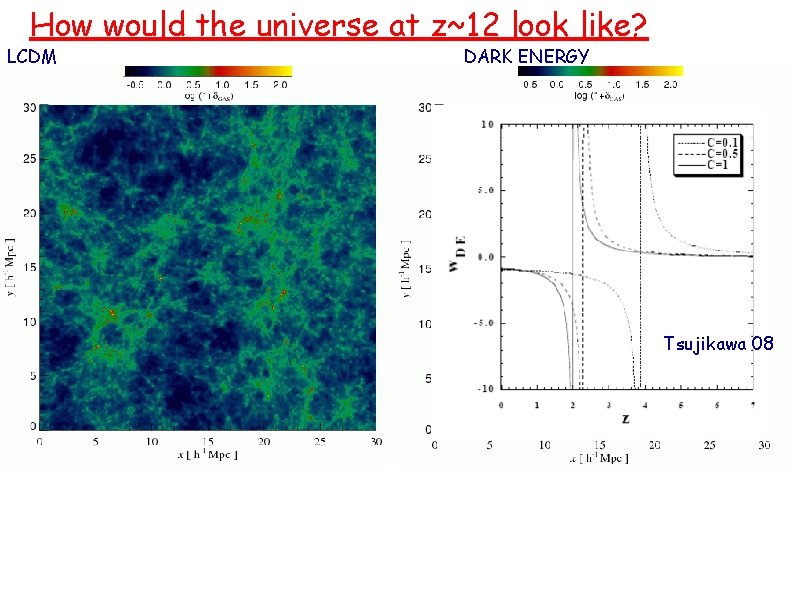
How would the universe at z~12 look like? LCDM DARK ENERGY Tsujikawa 08
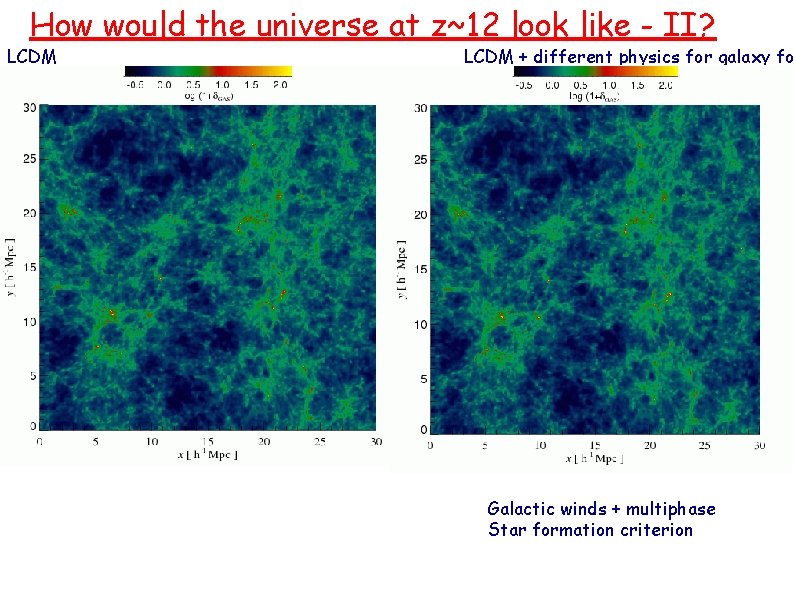
How would the universe at z~12 look like - II? LCDM + different physics for galaxy fo Galactic winds + multiphase Star formation criterion
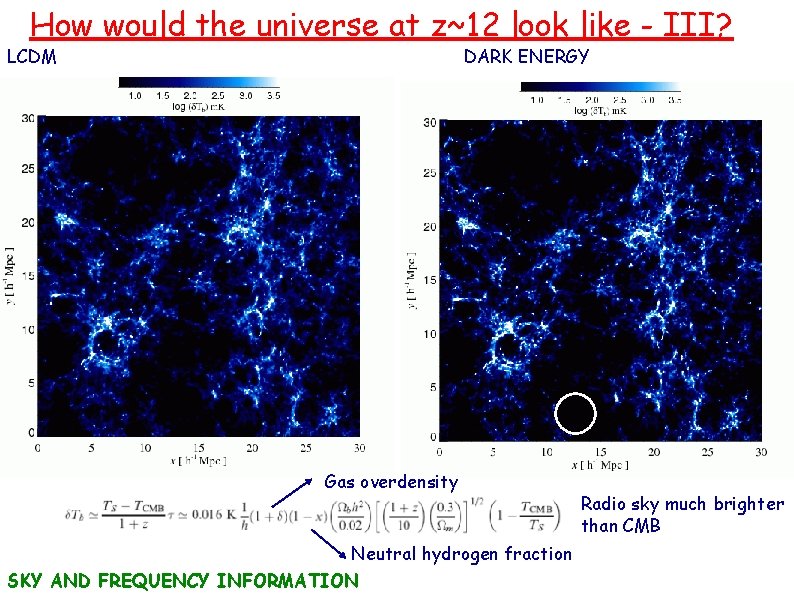
How would the universe at z~12 look like - III? LCDM DARK ENERGY Gas overdensity Neutral hydrogen fraction SKY AND FREQUENCY INFORMATION Radio sky much brighter than CMB
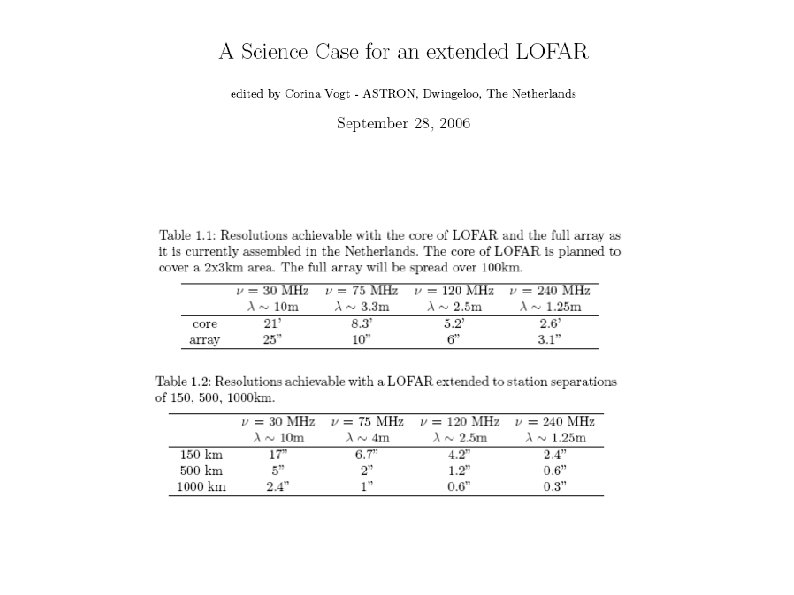
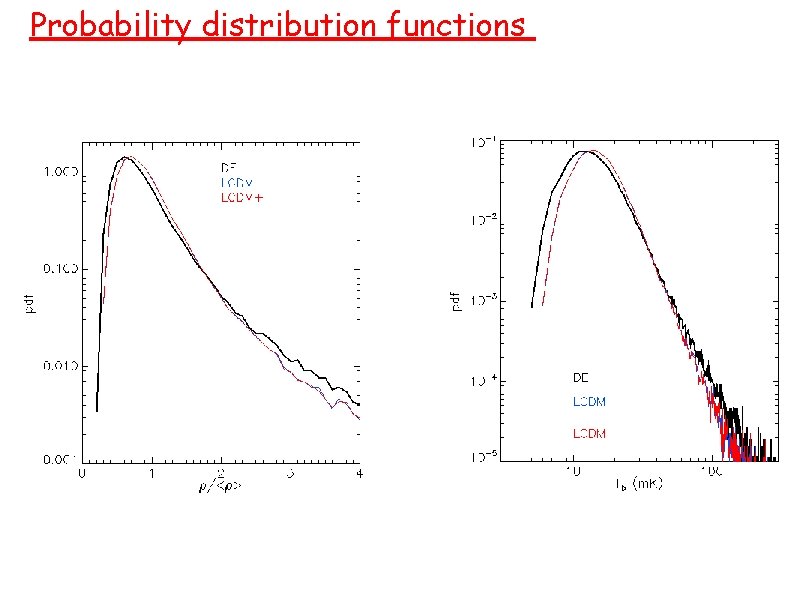
Probability distribution functions
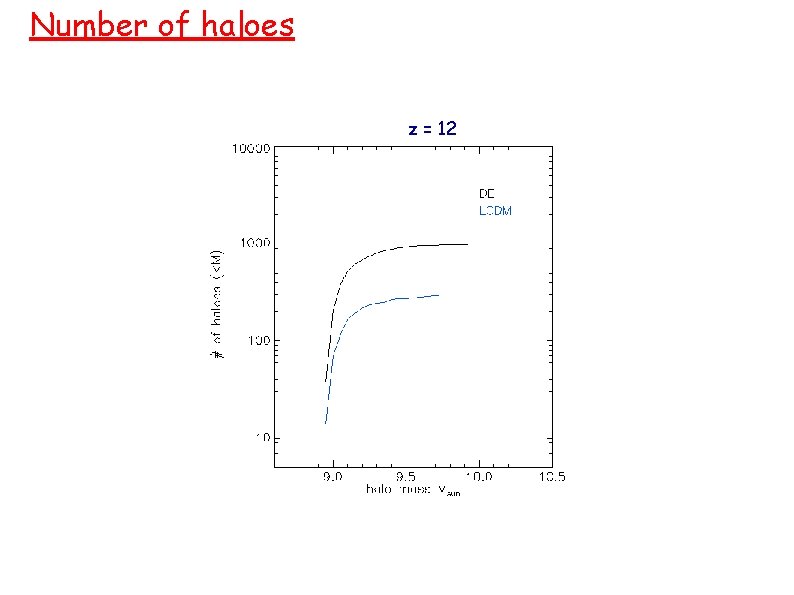
Number of haloes z = 12
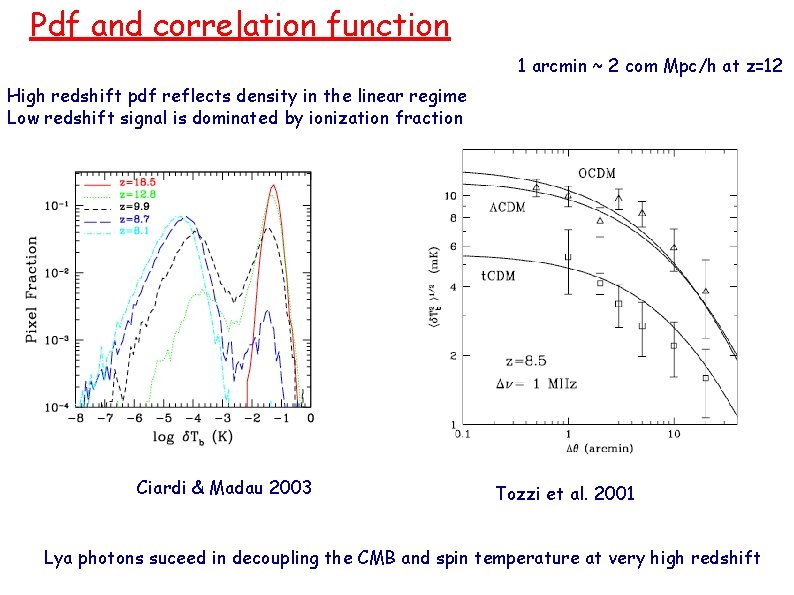
Pdf and correlation function 1 arcmin ~ 2 com Mpc/h at z=12 High redshift pdf reflects density in the linear regime Low redshift signal is dominated by ionization fraction Ciardi & Madau 2003 Tozzi et al. 2001 Lya photons suceed in decoupling the CMB and spin temperature at very high redshift
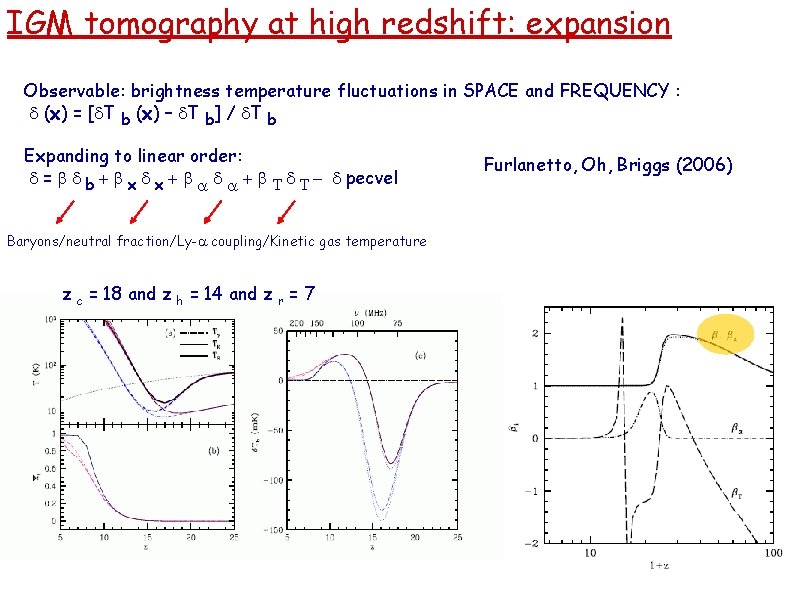
IGM tomography at high redshift: expansion Observable: brightness temperature fluctuations in SPACE and FREQUENCY : d (x) = [d. T b (x) – d. T b] / d. T b Expanding to linear order: d = b d b + b x d x + b a d a + b T d T - d pecvel Baryons/neutral fraction/Ly-a coupling/Kinetic gas temperature z c = 18 and z h = 14 and z r = 7 Furlanetto, Oh, Briggs (2006)
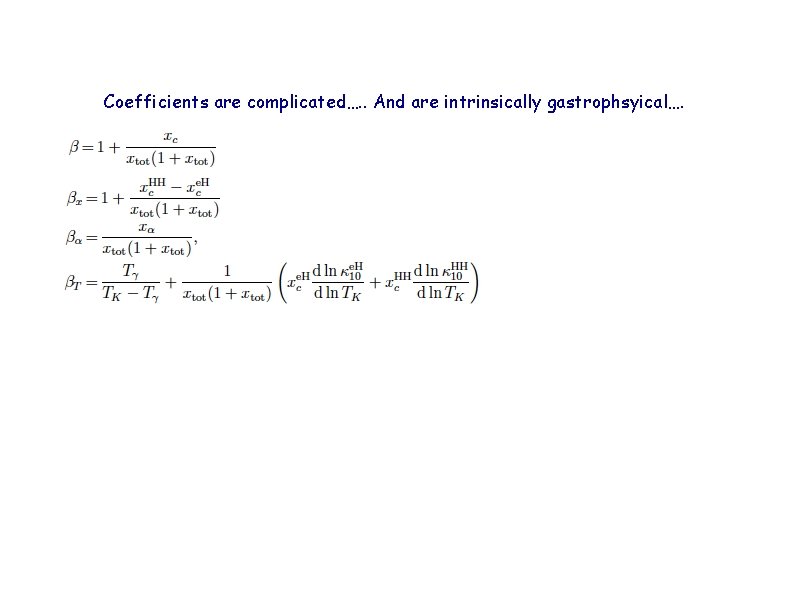
Coefficients are complicated…. . And are intrinsically gastrophsyical….
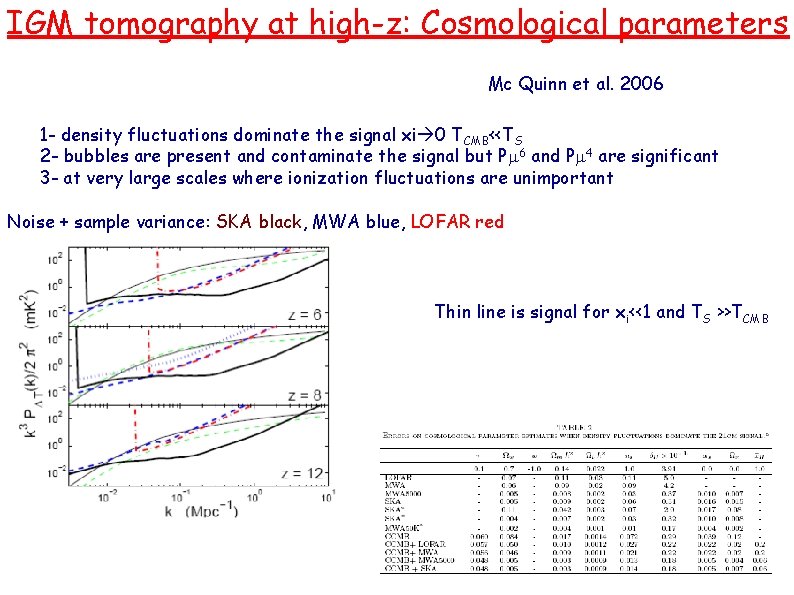
IGM tomography at high-z: Cosmological parameters Mc Quinn et al. 2006 1 - density fluctuations dominate the signal xi 0 TCMB<<TS 2 - bubbles are present and contaminate the signal but Pm 6 and Pm 4 are significant 3 - at very large scales where ionization fluctuations are unimportant Noise + sample variance: SKA black, MWA blue, LOFAR red Thin line is signal for xi<<1 and TS >>TCMB
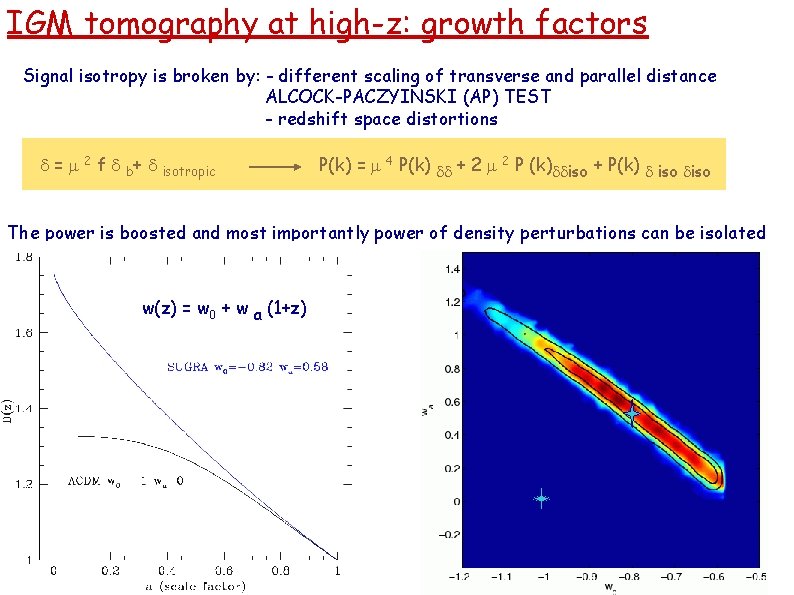
IGM tomography at high-z: growth factors Signal isotropy is broken by: - different scaling of transverse and parallel distance ALCOCK-PACZYINSKI (AP) TEST - redshift space distortions d = m 2 f d b+ d isotropic P(k) = m 4 P(k) dd + 2 m 2 P (k)ddiso + P(k) d iso diso The power is boosted and most importantly power of density perturbations can be isolated w(z) = w 0 + w a (1+z)
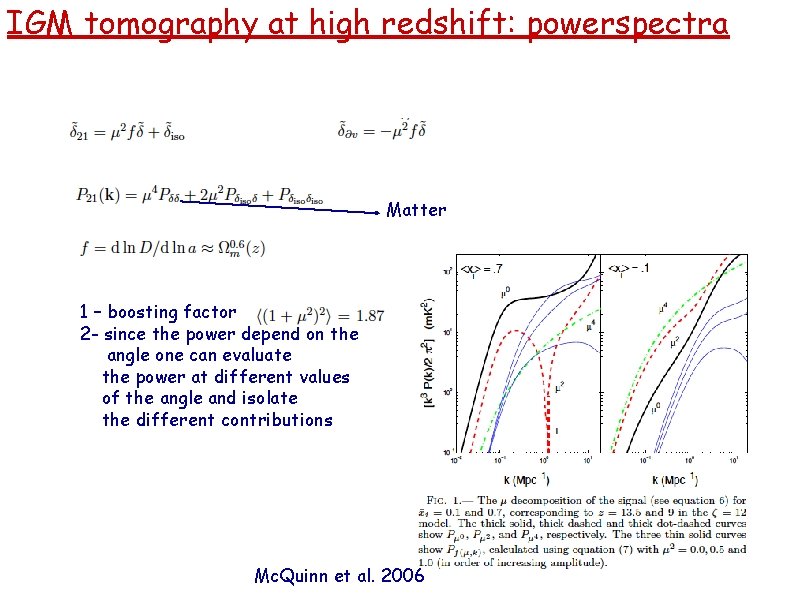
IGM tomography at high redshift: powerspectra Matter 1 – boosting factor 2 - since the power depend on the angle one can evaluate the power at different values of the angle and isolate the different contributions Mc. Quinn et al. 2006
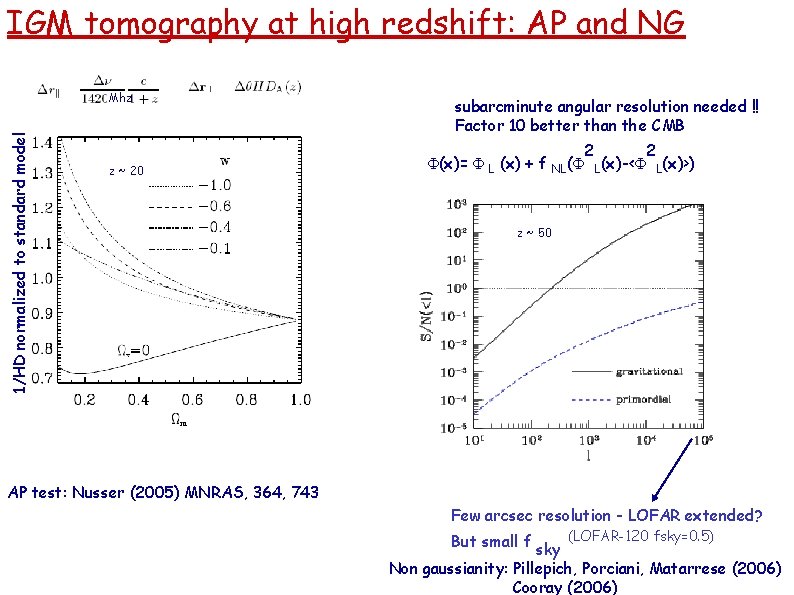
IGM tomography at high redshift: AP and NG 1/HD normalized to standard model Mhz z ~ 20 subarcminute angular resolution needed !! Factor 10 better than the CMB F(x)= F L (x) + f NL(F 2 L(x)-<F 2 L(x)>) z ~ 50 AP test: Nusser (2005) MNRAS, 364, 743 Few arcsec resolution - LOFAR extended? But small f sky (LOFAR-120 fsky=0. 5) Non gaussianity: Pillepich, Porciani, Matarrese (2006) Cooray (2006)
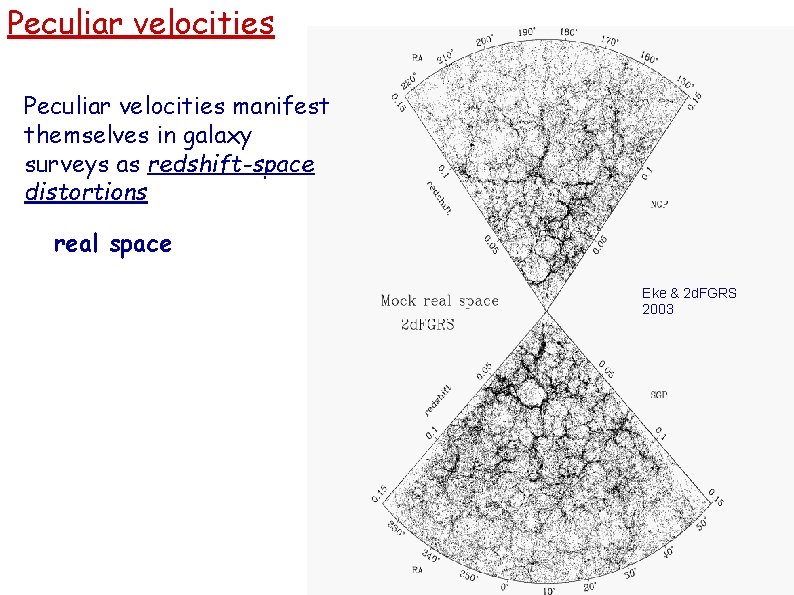
Peculiar velocities manifest themselves in galaxy surveys as redshift-space distortions real space Eke & 2 d. FGRS 2003
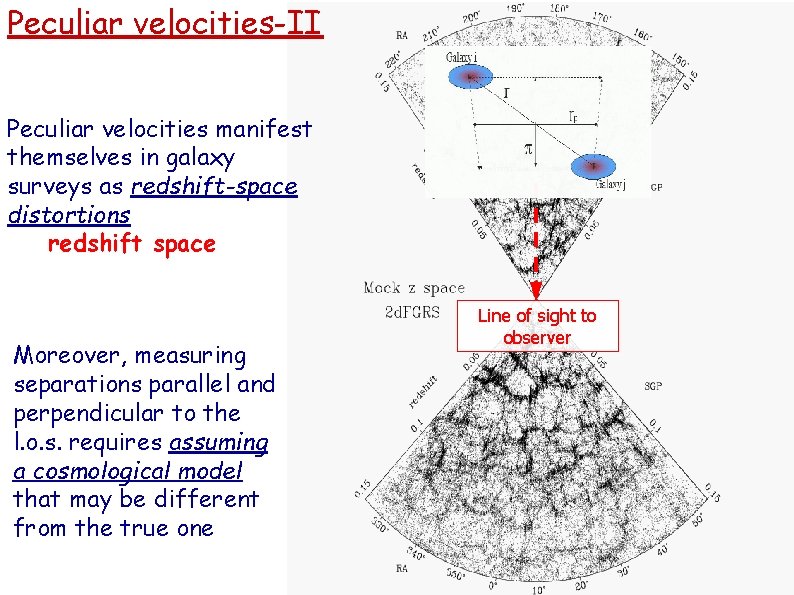
Peculiar velocities-II Peculiar velocities manifest themselves in galaxy surveys as redshift-space distortions redshift space Moreover, measuring separations parallel and perpendicular to the l. o. s. requires assuming a cosmological model that may be different from the true one Line of sight to observer
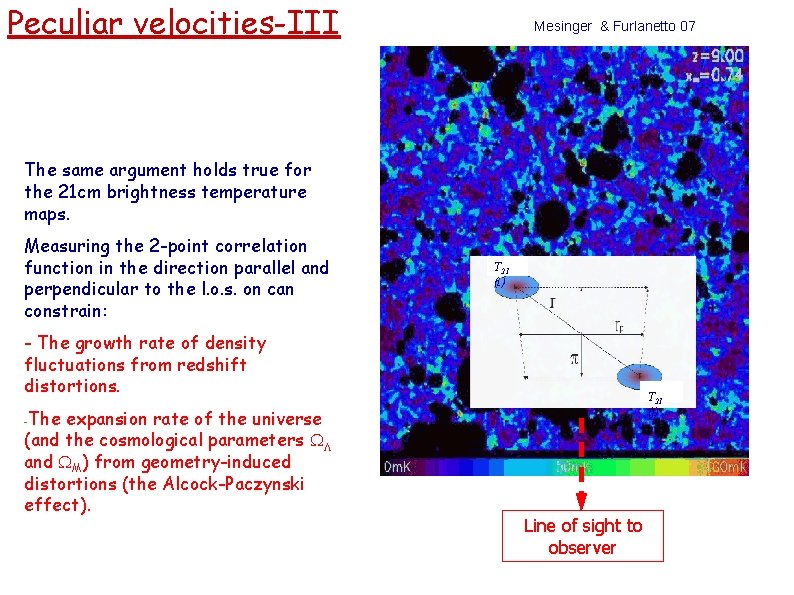
Peculiar velocities-III Mesinger & Furlanetto 07 The same argument holds true for the 21 cm brightness temperature maps. Measuring the 2 -point correlation function in the direction parallel and perpendicular to the l. o. s. on can constrain: T 21 (i) - The growth rate of density fluctuations from redshift distortions. The expansion rate of the universe (and the cosmological parameters and M) from geometry-induced distortions (the Alcock-Paczynski effect). T 21 (j) - Line of sight to observer
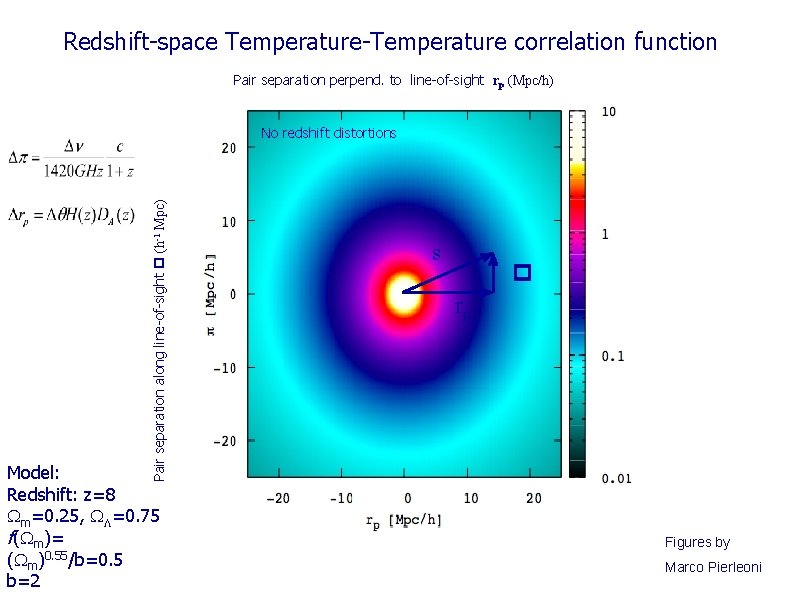
Redshift-space Temperature-Temperature correlation function Pair separation perpend. to line-of-sight rp (Mpc/h) Pair separation along line-of-sight (h-1 Mpc) No redshift distortions Model: Redshift: z=8 m=0. 25, =0. 75 f( m)= ( m)0. 55/b=0. 5 b=2 s rp Figures by Marco Pierleoni
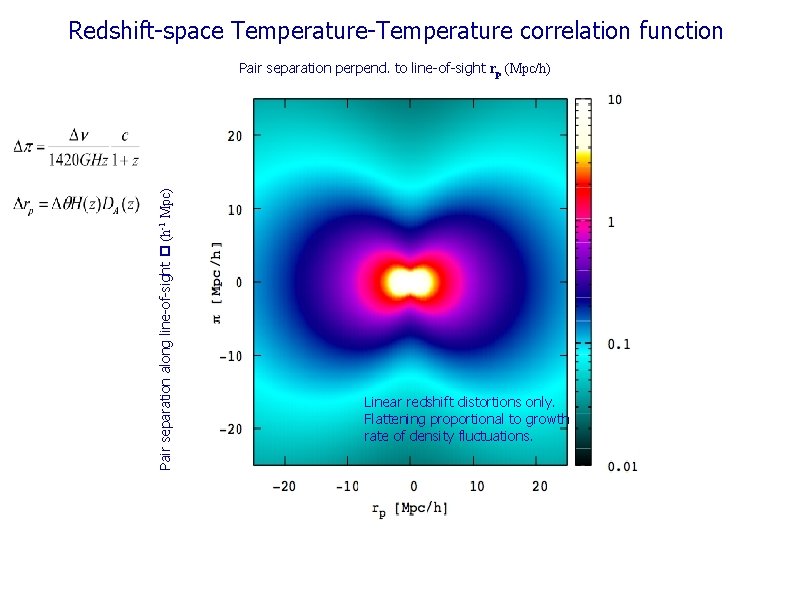
Redshift-space Temperature-Temperature correlation function Pair separation along line-of-sight (h-1 Mpc) Pair separation perpend. to line-of-sight rp (Mpc/h) Linear redshift distortions only. Flattening proportional to growth rate of density fluctuations.
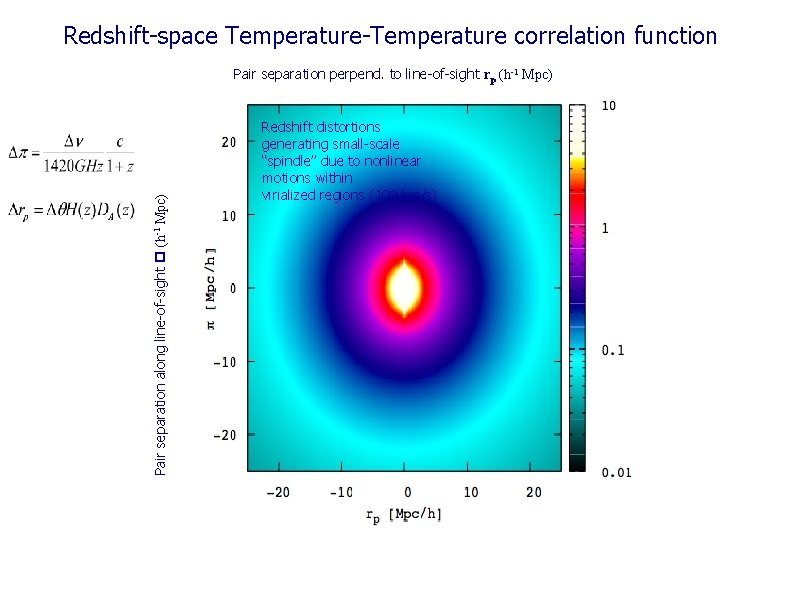
Redshift-space Temperature-Temperature correlation function Pair separation along line-of-sight (h-1 Mpc) Pair separation perpend. to line-of-sight rp (h-1 Mpc) Redshift distortions generating small-scale “spindle” due to nonlinear motions within virialized regions (100 km/s)
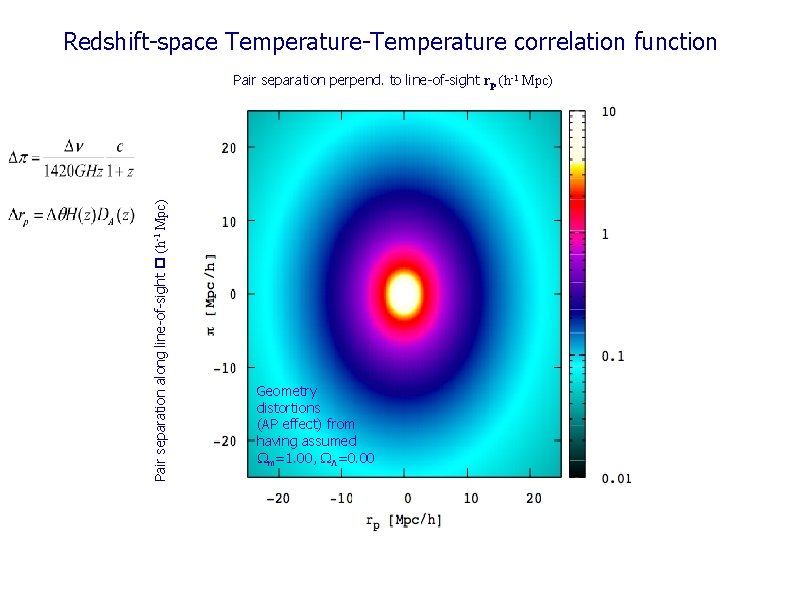
Redshift-space Temperature-Temperature correlation function Pair separation along line-of-sight (h-1 Mpc) Pair separation perpend. to line-of-sight rp (h-1 Mpc) Geometry distortions (AP effect) from having assumed m=1. 00, =0. 00
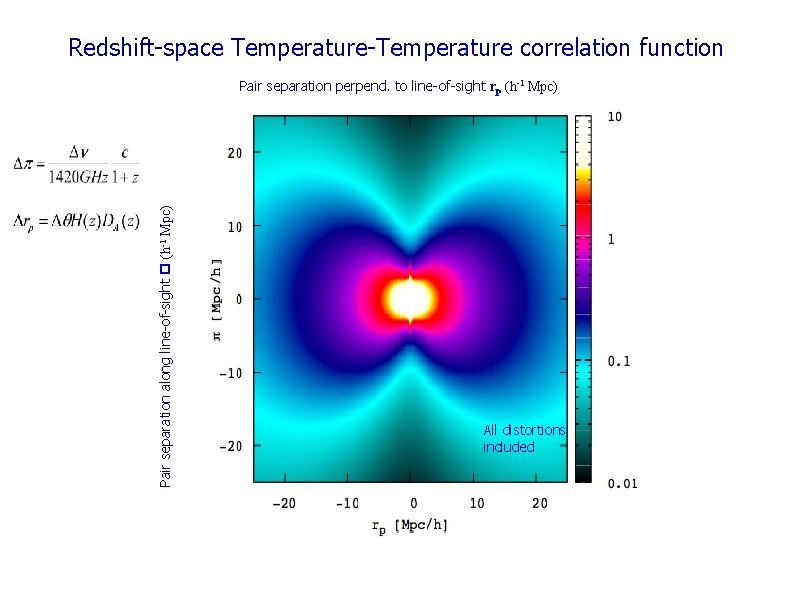
Redshift-space Temperature-Temperature correlation function Pair separation along line-of-sight (h-1 Mpc) Pair separation perpend. to line-of-sight rp (h-1 Mpc) All distortions included
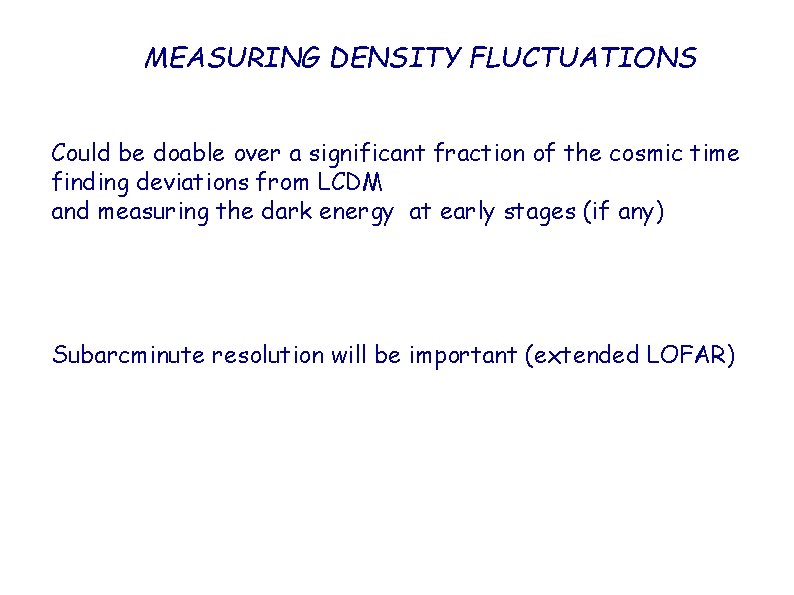
MEASURING DENSITY FLUCTUATIONS Could be doable over a significant fraction of the cosmic time finding deviations from LCDM and measuring the dark energy at early stages (if any) Subarcminute resolution will be important (extended LOFAR)
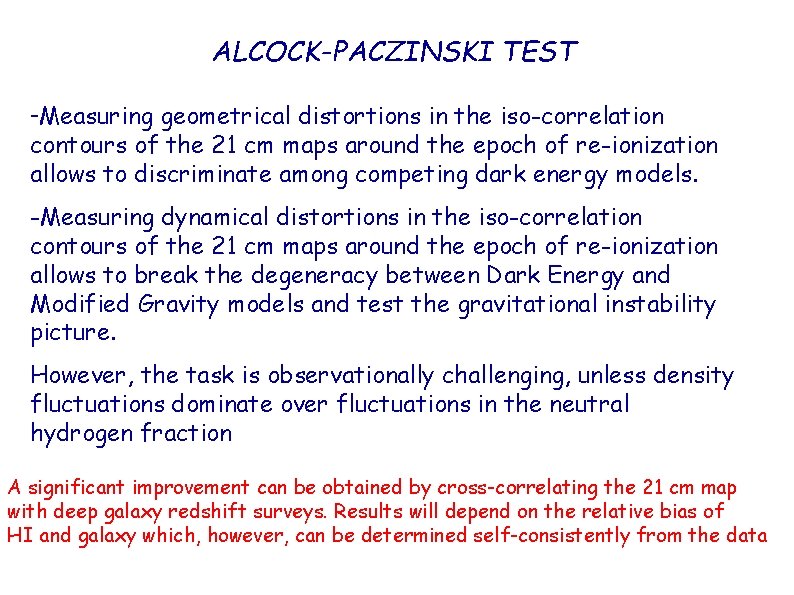
ALCOCK-PACZINSKI TEST -Measuring geometrical distortions in the iso-correlation contours of the 21 cm maps around the epoch of re-ionization allows to discriminate among competing dark energy models. -Measuring dynamical distortions in the iso-correlation contours of the 21 cm maps around the epoch of re-ionization allows to break the degeneracy between Dark Energy and Modified Gravity models and test the gravitational instability picture. However, the task is observationally challenging, unless density fluctuations dominate over fluctuations in the neutral hydrogen fraction A significant improvement can be obtained by cross-correlating the 21 cm map with deep galaxy redshift surveys. Results will depend on the relative bias of HI and galaxy which, however, can be determined self-consistently from the data
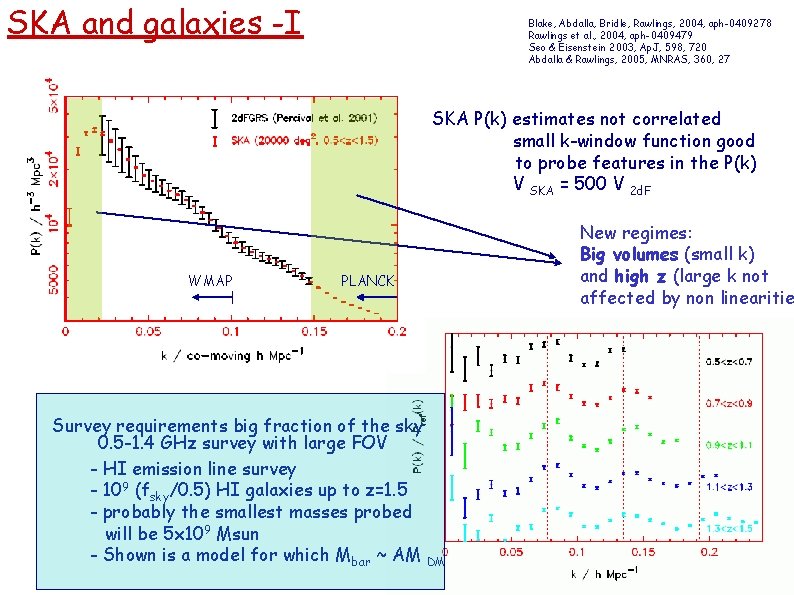
SKA and galaxies -I Blake, Abdalla, Bridle, Rawlings, 2004, aph-0409278 Rawlings et al. , 2004, aph-0409479 Seo & Eisenstein 2003, Ap. J, 598, 720 Abdalla & Rawlings, 2005, MNRAS, 360, 27 SKA P(k) estimates not correlated small k-window function good to probe features in the P(k) V SKA = 500 V 2 d. F WMAP PLANCK Survey requirements big fraction of the sky 0. 5 -1. 4 GHz survey with large FOV - HI emission line survey - 109 (fsky/0. 5) HI galaxies up to z=1. 5 - probably the smallest masses probed will be 5 x 109 Msun - Shown is a model for which Mbar ~ AM DM New regimes: Big volumes (small k) and high z (large k not affected by non linearitie
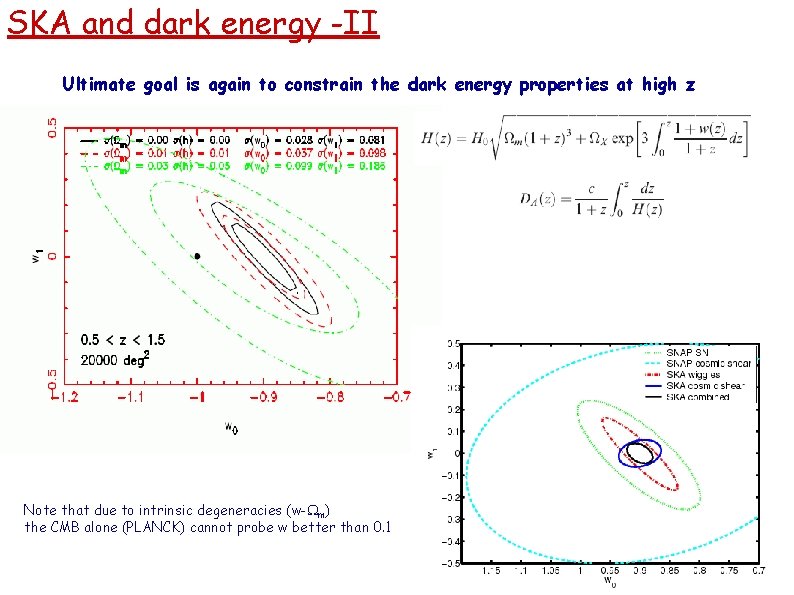
SKA and dark energy -II Ultimate goal is again to constrain the dark energy properties at high z Note that due to intrinsic degeneracies (w- m) the CMB alone (PLANCK) cannot probe w better than 0. 1
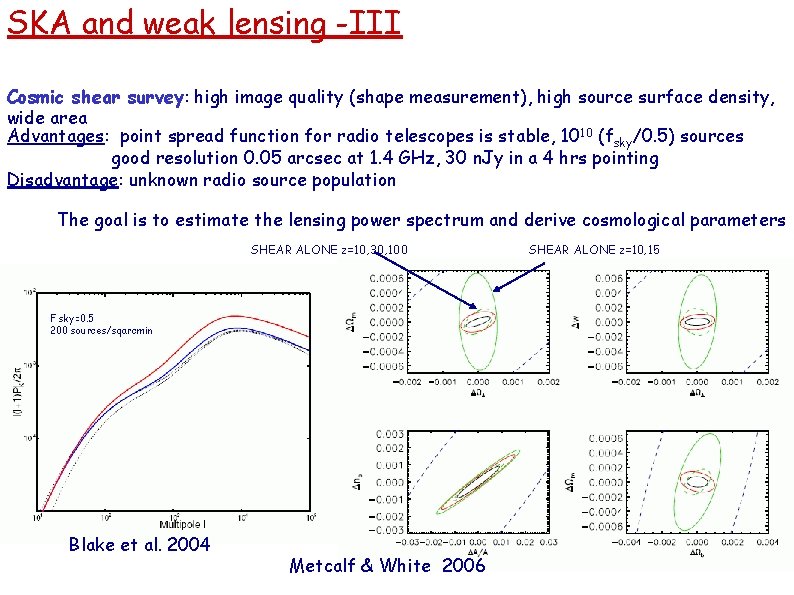
SKA and weak lensing -III Cosmic shear survey: high image quality (shape measurement), high source surface density, wide area Advantages: point spread function for radio telescopes is stable, 10 10 (fsky/0. 5) sources good resolution 0. 05 arcsec at 1. 4 GHz, 30 n. Jy in a 4 hrs pointing Disadvantage: unknown radio source population The goal is to estimate the lensing power spectrum and derive cosmological parameters SHEAR ALONE z=10, 30, 100 F sky=0. 5 200 sources/sqarcmin Blake et al. 2004 Metcalf & White 2006 SHEAR ALONE z=10, 15
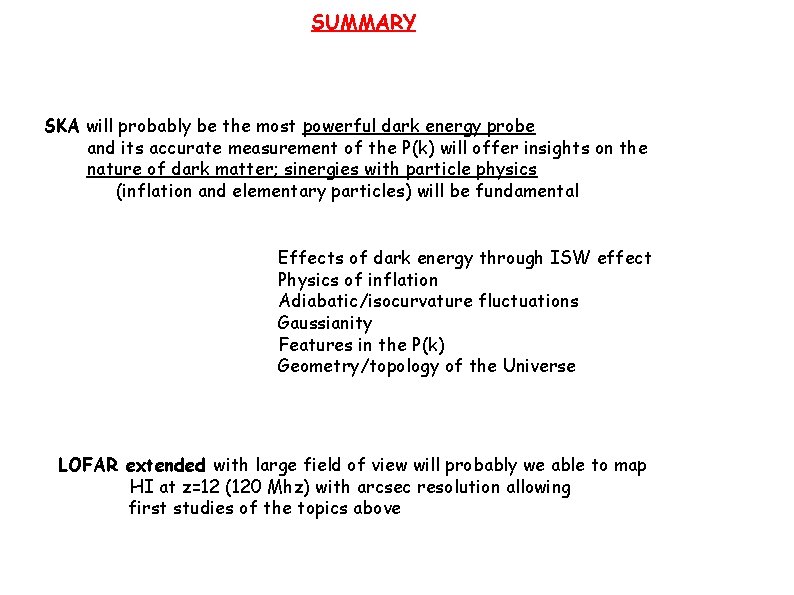
SUMMARY SKA will probably be the most powerful dark energy probe and its accurate measurement of the P(k) will offer insights on the nature of dark matter; sinergies with particle physics (inflation and elementary particles) will be fundamental Effects of dark energy through ISW effect Physics of inflation Adiabatic/isocurvature fluctuations Gaussianity Features in the P(k) Geometry/topology of the Universe LOFAR extended with large field of view will probably we able to map HI at z=12 (120 Mhz) with arcsec resolution allowing first studies of the topics above
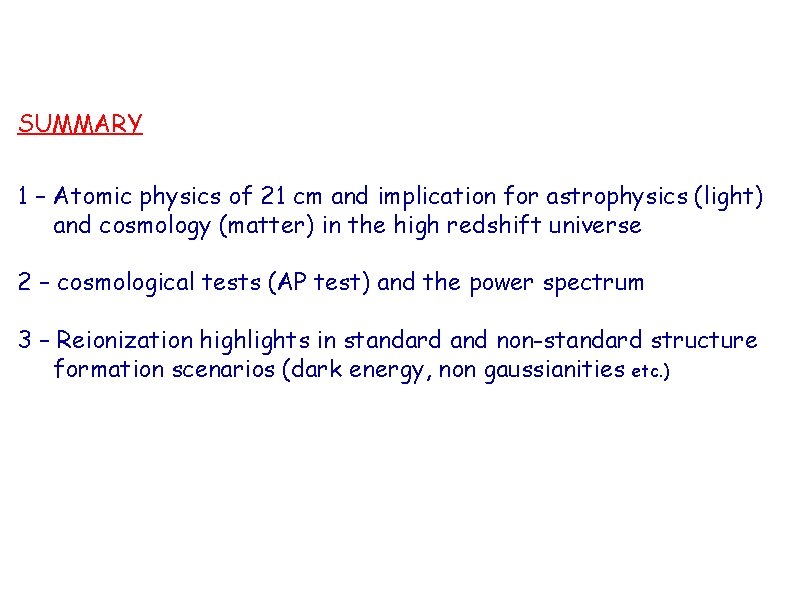
SUMMARY 1 – Atomic physics of 21 cm and implication for astrophysics (light) and cosmology (matter) in the high redshift universe 2 – cosmological tests (AP test) and the power spectrum 3 – Reionization highlights in standard and non-standard structure formation scenarios (dark energy, non gaussianities etc. )
Wem viel vergeben ist der liebt viel
Matteo viel
Matteo viel
Inaf sede centrale
Inaf padova
Viel stupnovani
Ein lächeln kostet nichts und bringt doch so viel
Wie viel uhr ist es
Wie viel verdienen hundetrainer
Wie viel darf man die parkscheibe vorstellen
Www.luftlinie.org
Kaninchen trinken viel
Wir haben viel spass gehabt
Wie viel uhr ist 17 uhr
Wie viel serben leben in kosovo
Woher kommt salz
Alg 1 wie viel prozent
Externe speichermedien referat
Wie viel platz braucht ein brief ohne bilder
Ich bin ein huhn und hab nicht viel zu tun
Saba goal zanichelli
Trieste saba testo
Intracomponente
Usmaf trieste
Synchronic
Ssd trieste
Villaggio del fanciullo trieste
Caritas trieste
Discipline storiche e filosofiche trieste
Dico al mio cuore intanto che t'aspetto
Stelle sulla terra trieste
Hotel savoia trieste
Renzo nicolini
Batiskaf trieste
Formation initiale vs formation continue
Matteo tropeano
Johannes pittore svizzero
Ilaria di matteo
Matteo ricciardi physik
Matteo 13, 1-9
Matteo belotti
Matteo magnaricotte