Solutions of Electrolytes Lector Varikova T O Degree
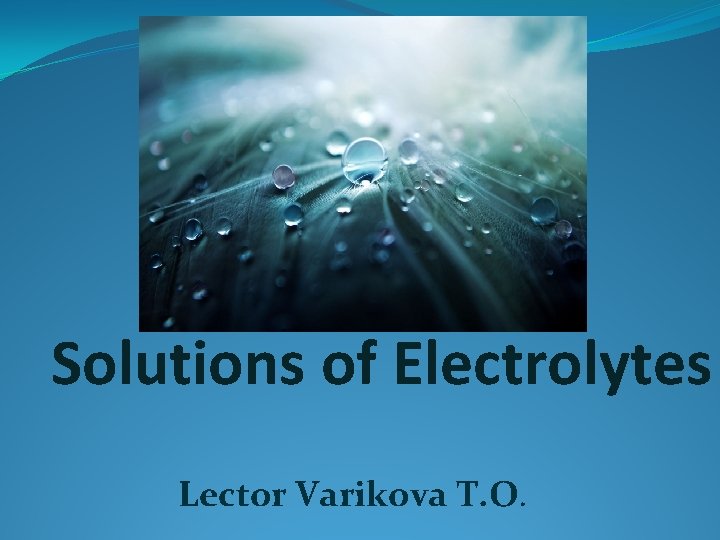
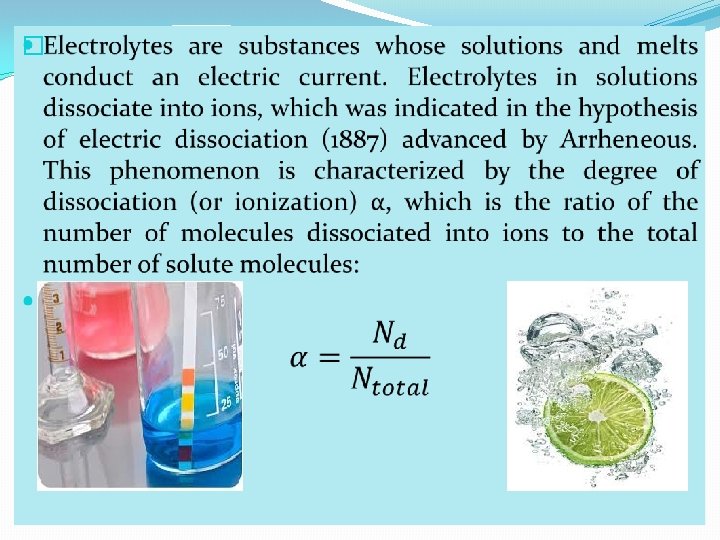
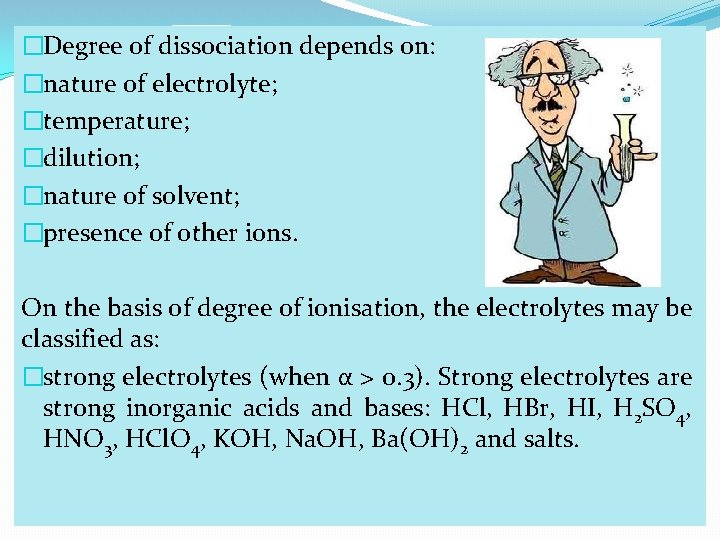
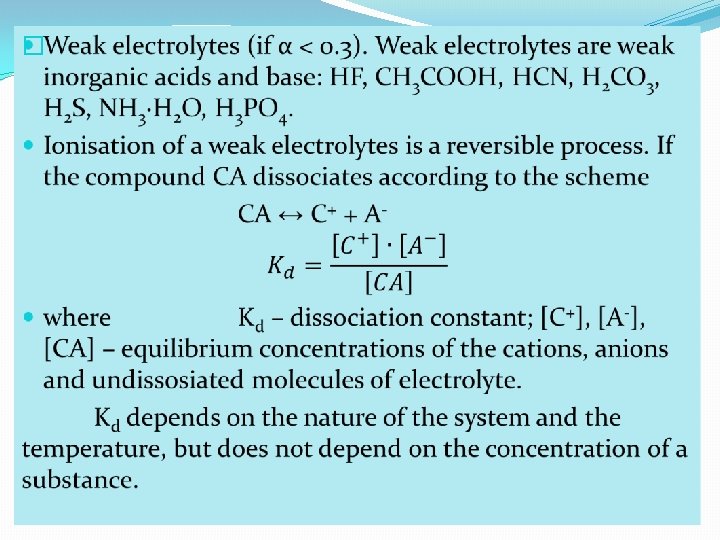
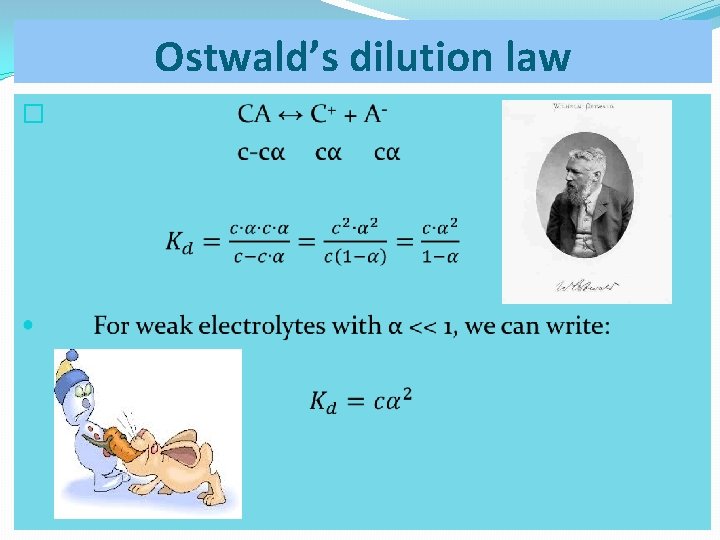
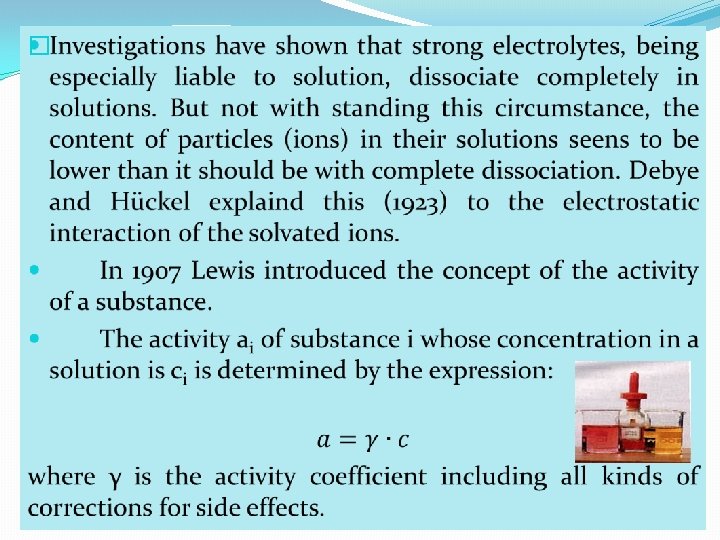
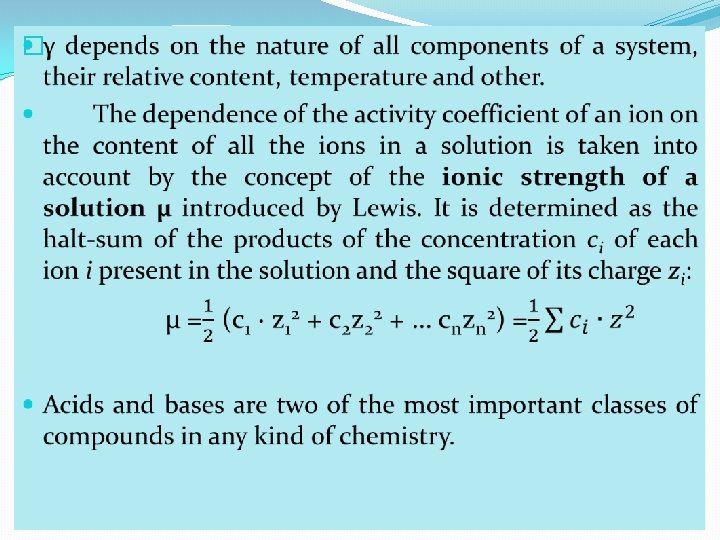
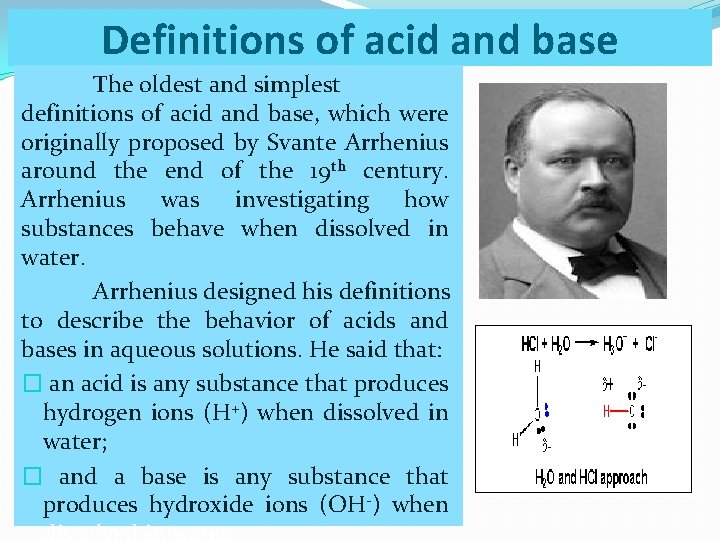
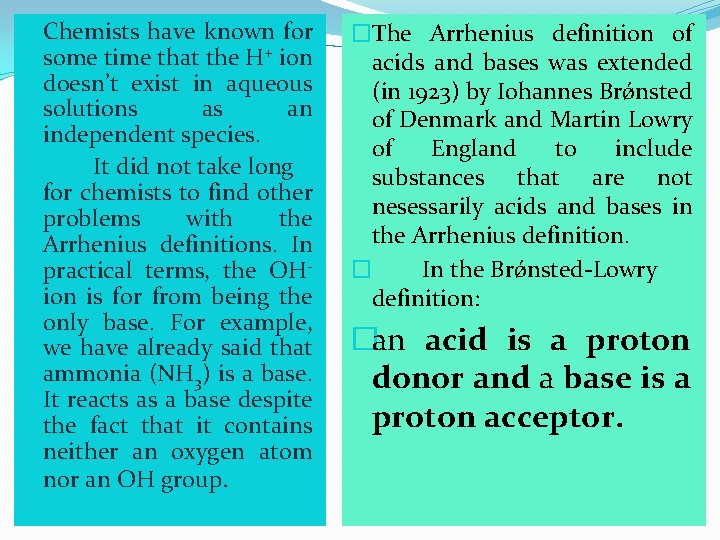
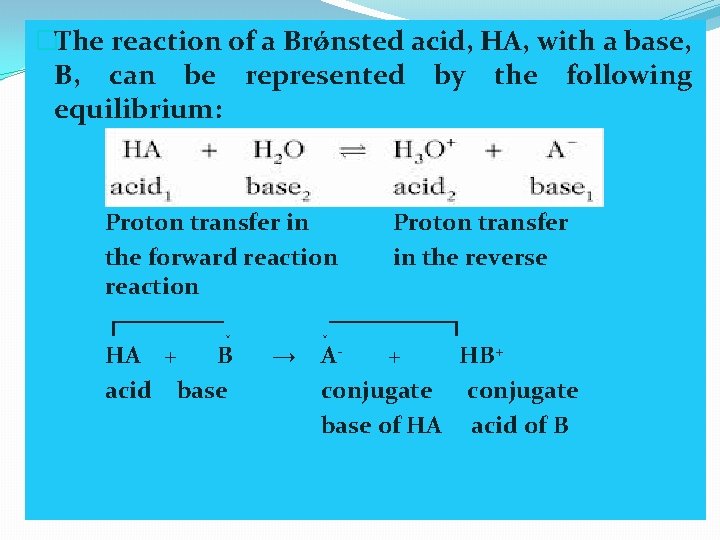
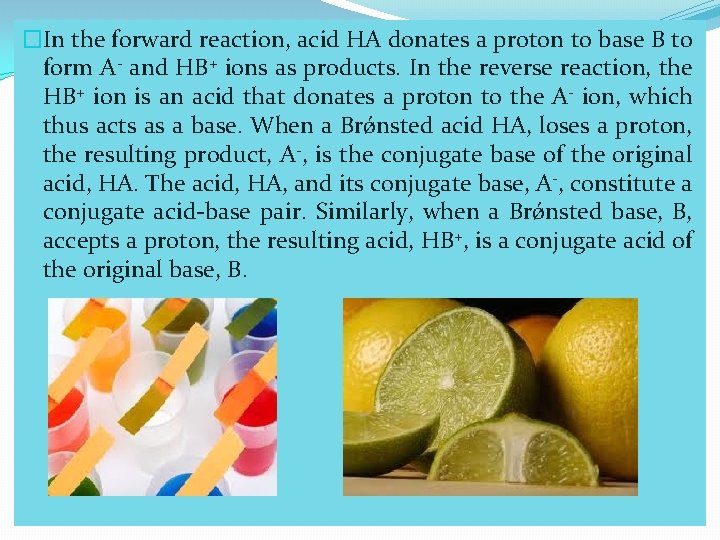
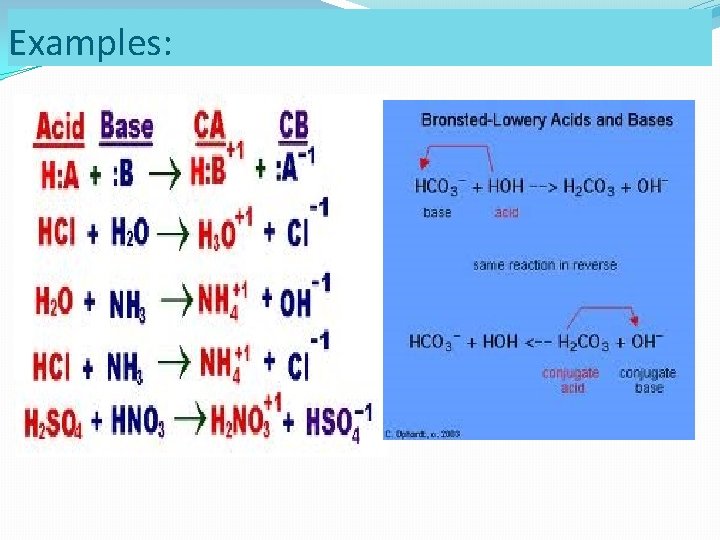
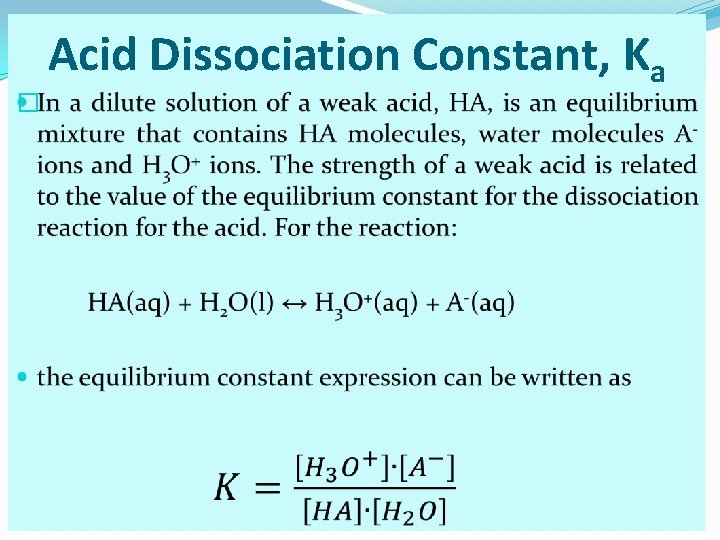
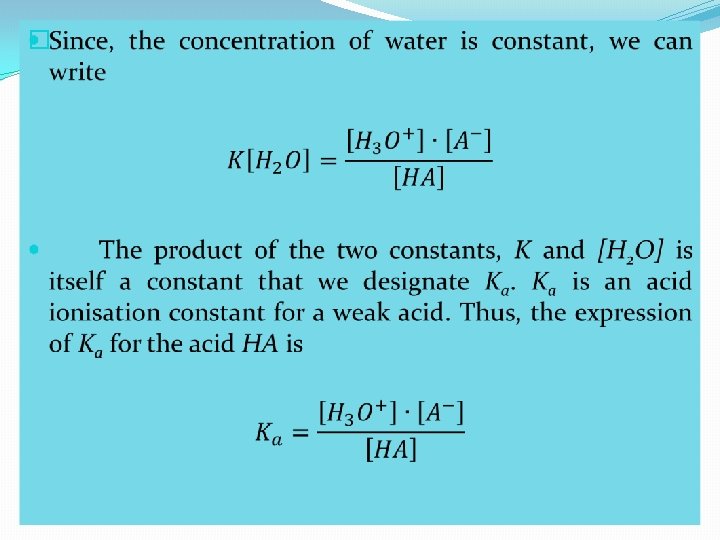
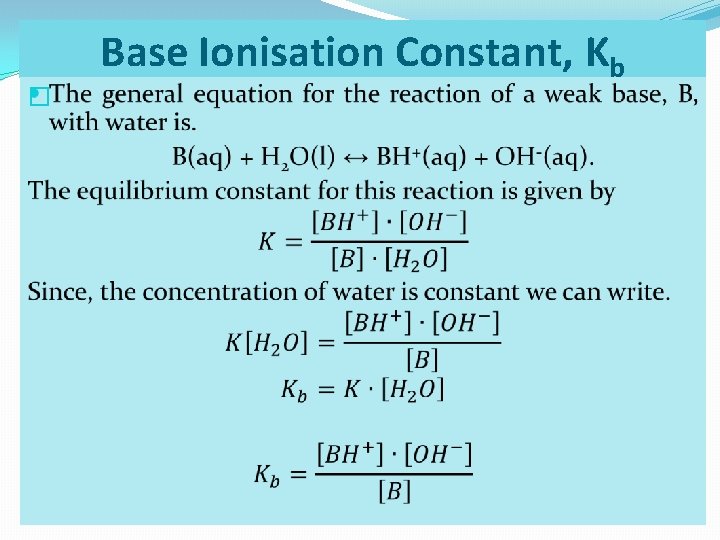
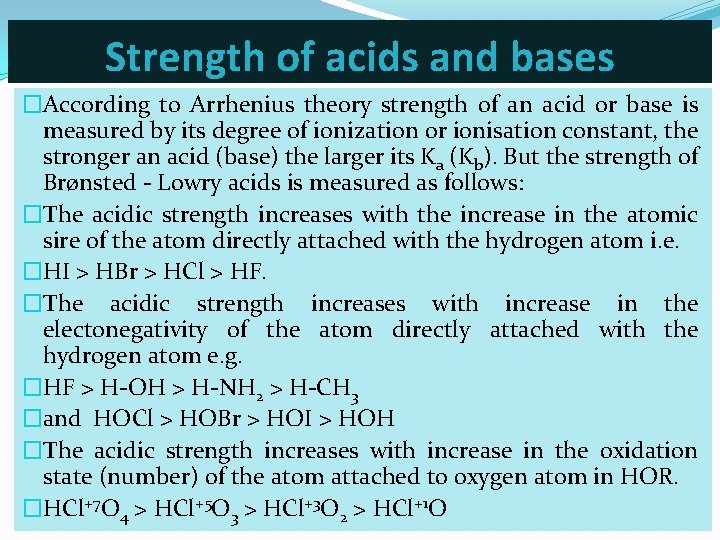
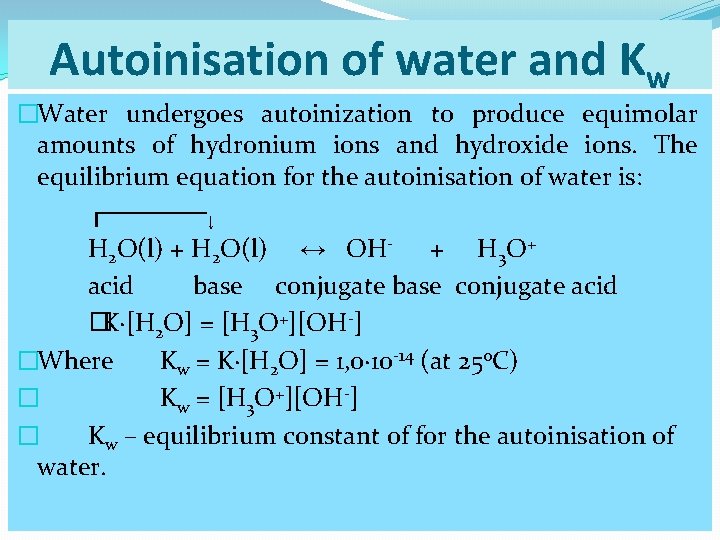
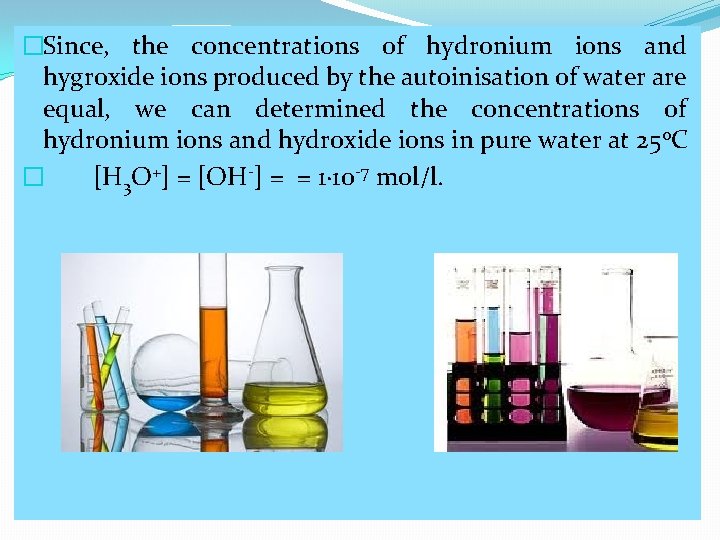
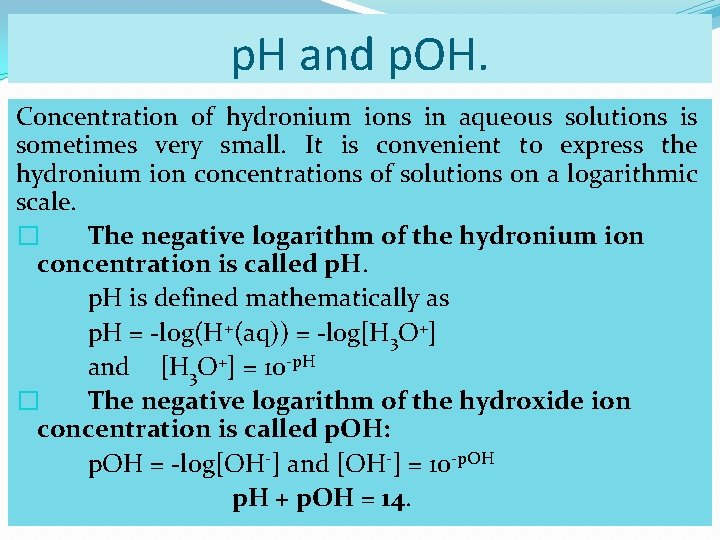
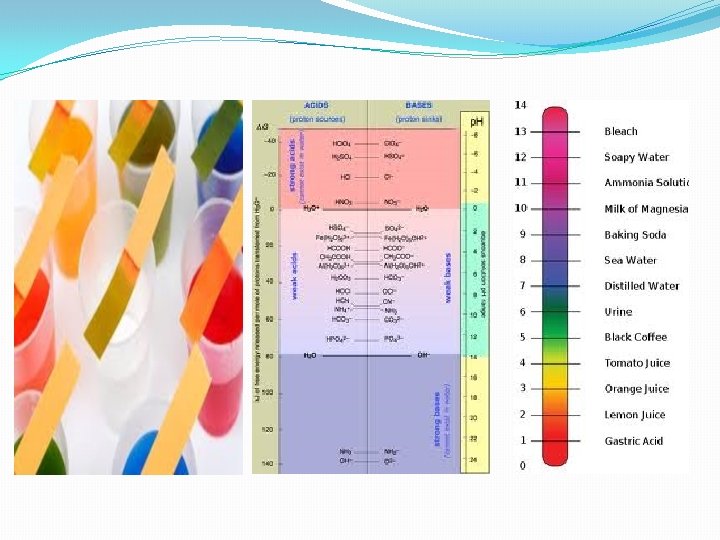
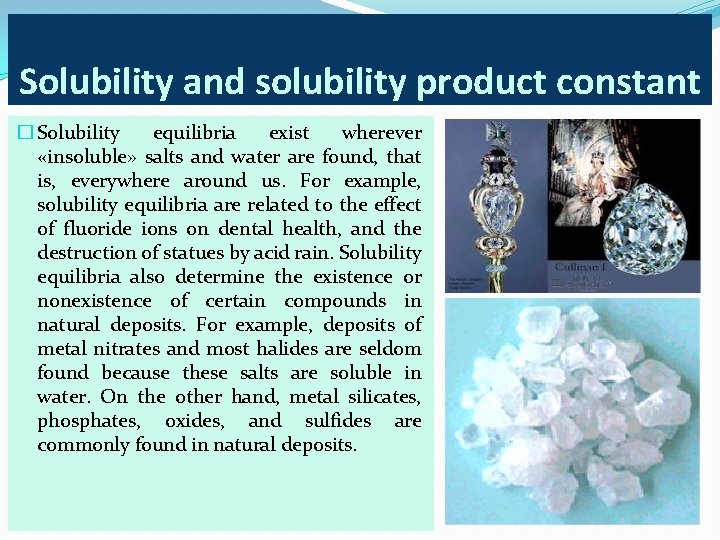
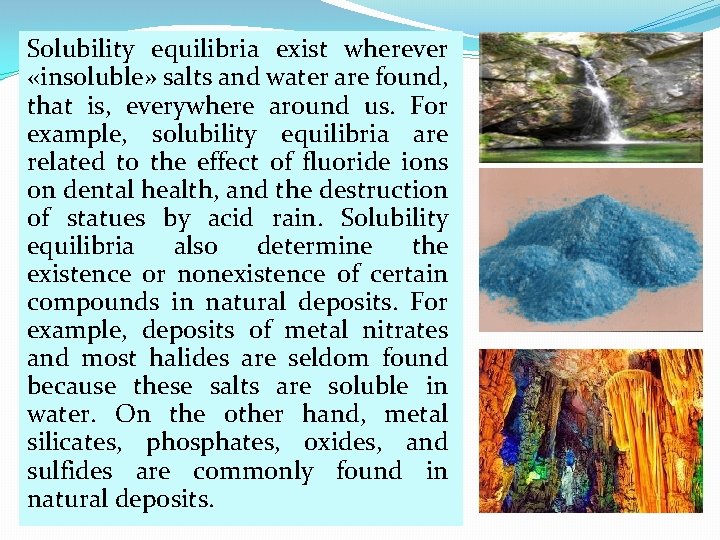
- Slides: 22
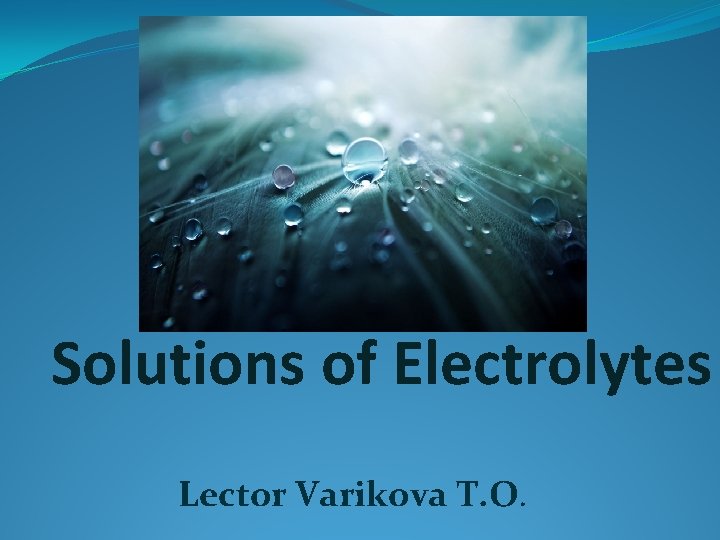
Solutions of Electrolytes Lector Varikova T. O.
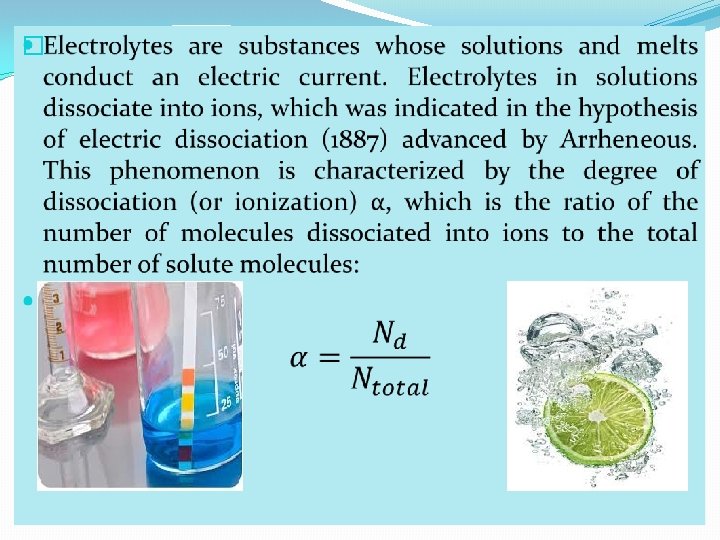
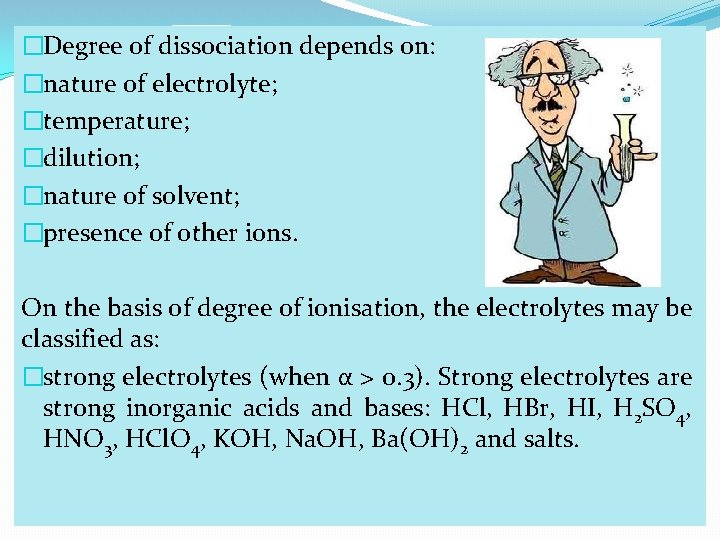
�Degree of dissociation depends on: �nature of electrolyte; �temperature; �dilution; �nature of solvent; �presence of other ions. On the basis of degree of ionisation, the electrolytes may be classified as: �strong electrolytes (when α > 0. 3). Strong electrolytes are strong inorganic acids and bases: HCl, HBr, HI, H 2 SO 4, HNO 3, HCl. O 4, KOH, Na. OH, Ba(OH)2 and salts.
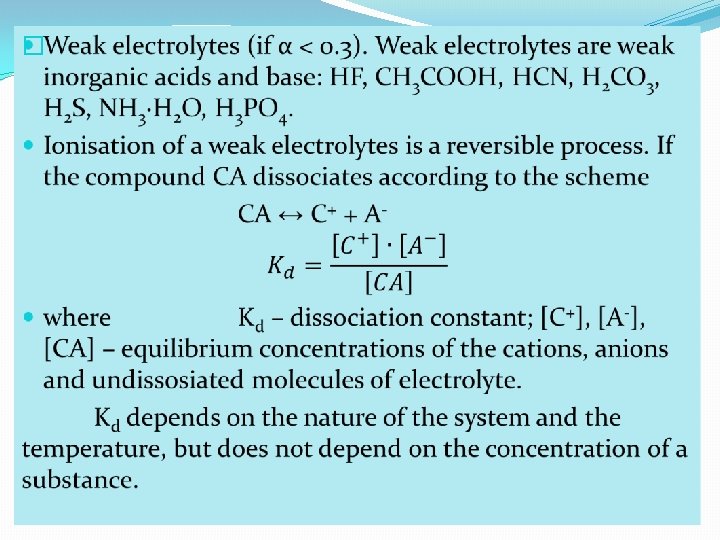
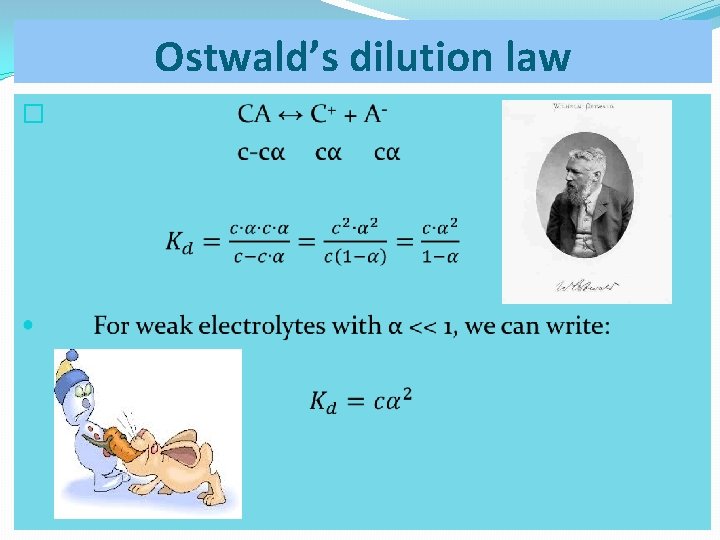
Ostwald’s dilution law �
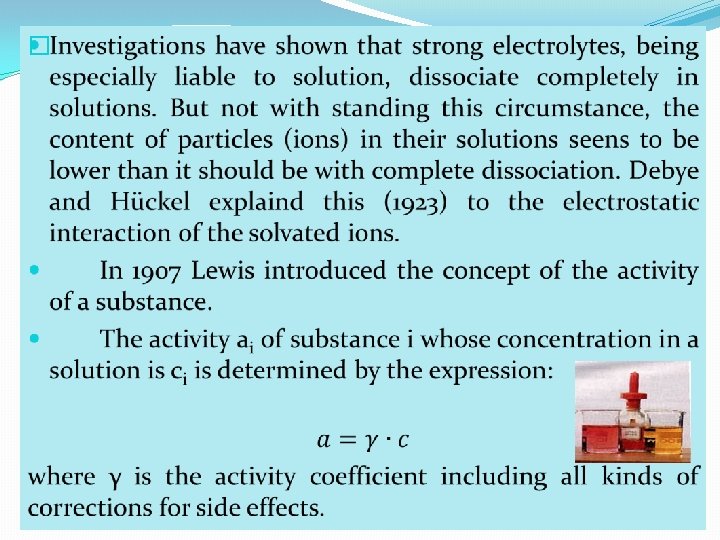
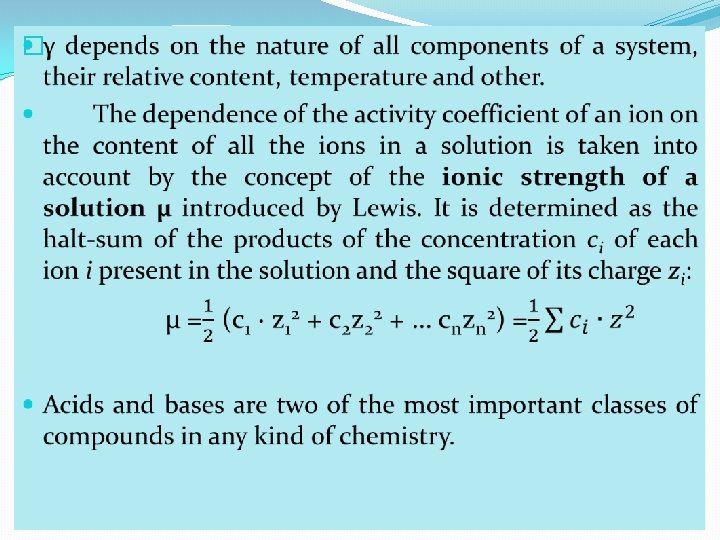
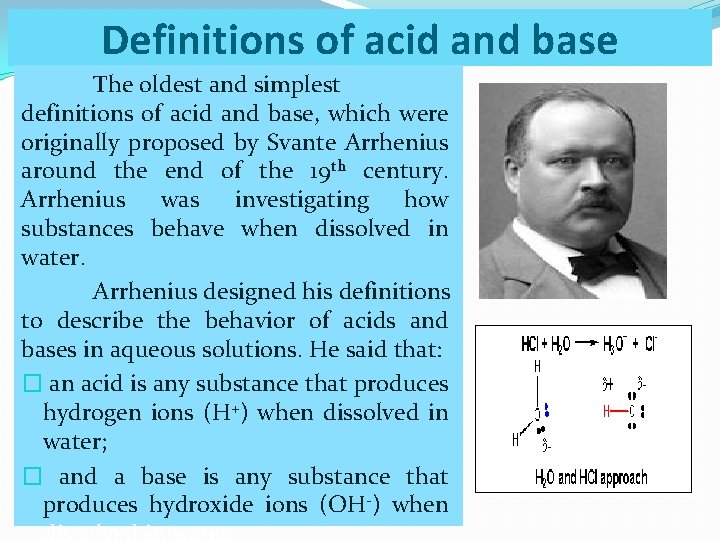
Definitions of acid and base The oldest and simplest definitions of acid and base, which were originally proposed by Svante Arrhenius around the end of the 19 th century. Arrhenius was investigating how substances behave when dissolved in water. Arrhenius designed his definitions to describe the behavior of acids and bases in aqueous solutions. He said that: � an acid is any substance that produces hydrogen ions (H+) when dissolved in water; � and a base is any substance that produces hydroxide ions (OH-) when
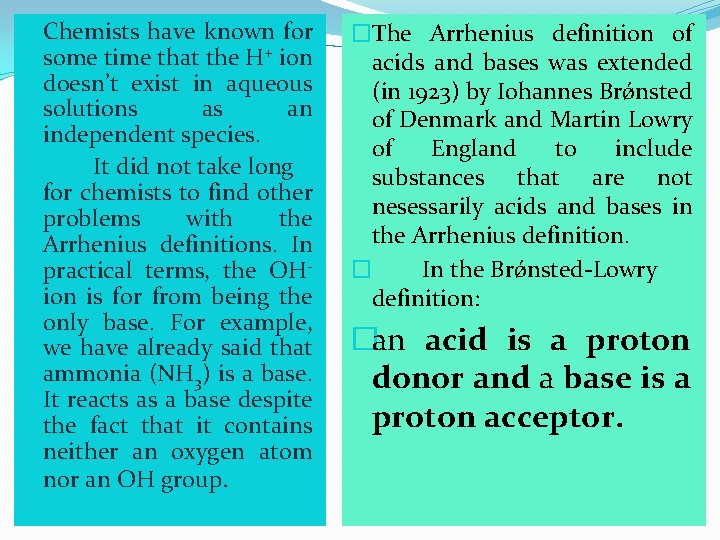
�Chemists have known for some time that the H+ ion doesn’t exist in aqueous solutions as an independent species. � It did not take long for chemists to find other problems with the Arrhenius definitions. In practical terms, the OHion is for from being the only base. For example, we have already said that ammonia (NH 3) is a base. It reacts as a base despite the fact that it contains neither an oxygen atom nor an OH group. �The Arrhenius definition of acids and bases was extended (in 1923) by Iohannes Brǿnsted of Denmark and Martin Lowry of England to include substances that are not nesessarily acids and bases in the Arrhenius definition. � In the Brǿnsted-Lowry definition: �an acid is a proton donor and a base is a proton acceptor.
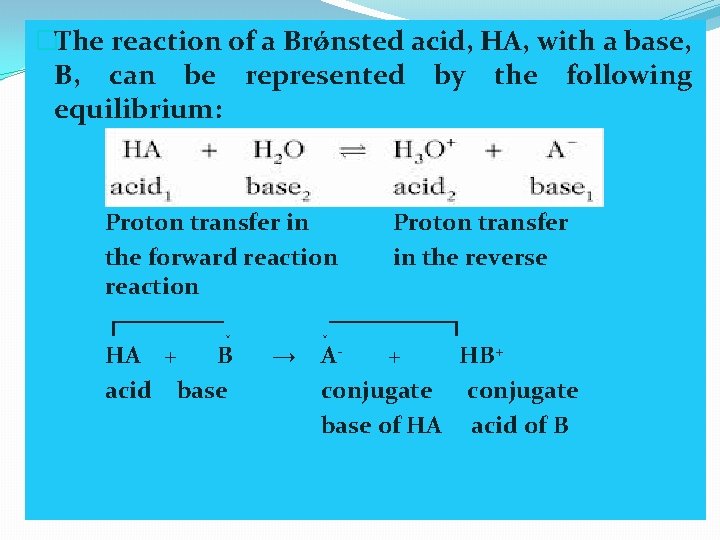
�The reaction of a Brǿnsted acid, HA, with a base, B, can be represented by the following equilibrium: Proton transfer in Proton transfer the forward reaction in the reverse reaction ┌──────↓ ↓───────┐ HA + B → A+ HB+ acid base conjugate base of HA acid of B
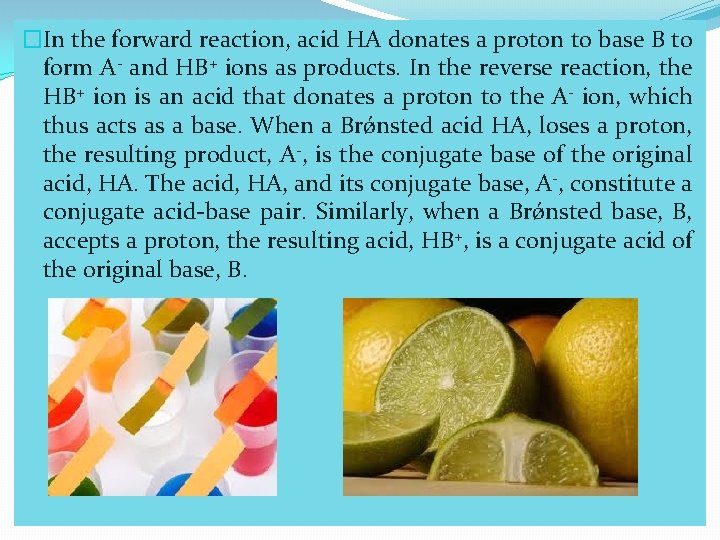
�In the forward reaction, acid HA donates a proton to base B to form A- and HB+ ions as products. In the reverse reaction, the HB+ ion is an acid that donates a proton to the A- ion, which thus acts as a base. When a Brǿnsted acid HA, loses a proton, the resulting product, A-, is the conjugate base of the original acid, HA. The acid, HA, and its conjugate base, A-, constitute a conjugate acid-base pair. Similarly, when a Brǿnsted base, B, accepts a proton, the resulting acid, HB+, is a conjugate acid of the original base, B.
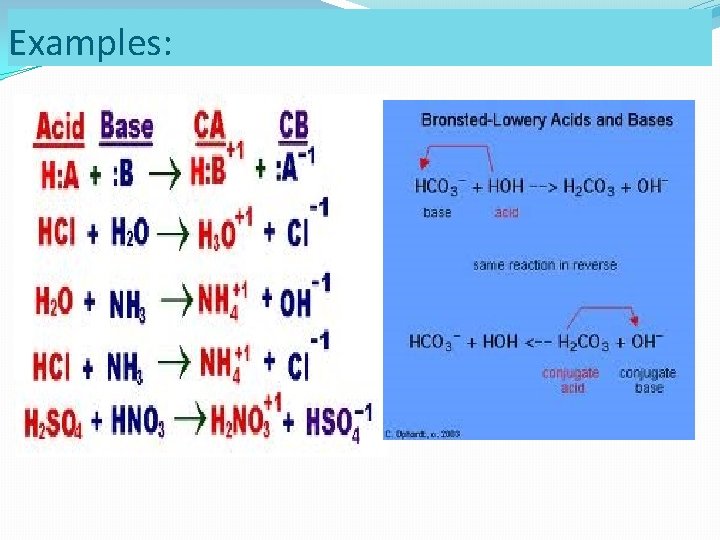
Examples:
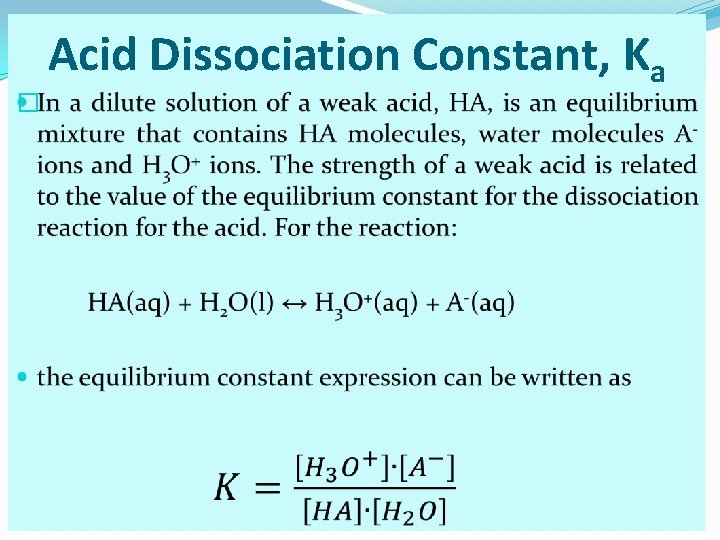
� Acid Dissociation Constant, Ka
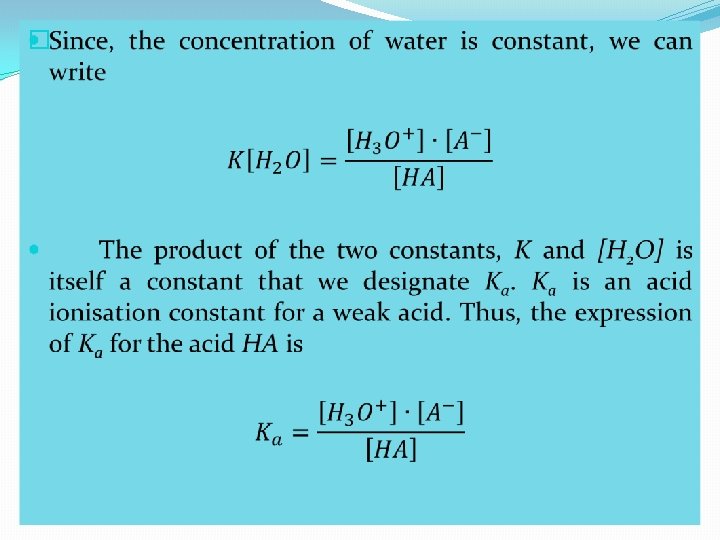
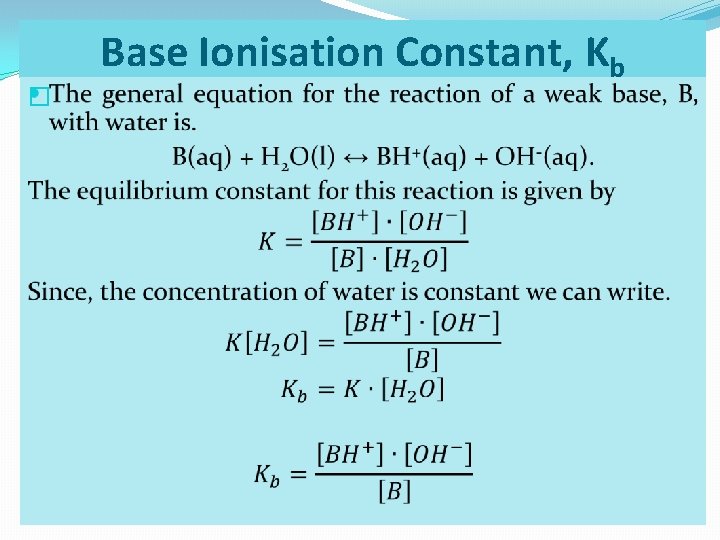
� Base Ionisation Constant, Kb
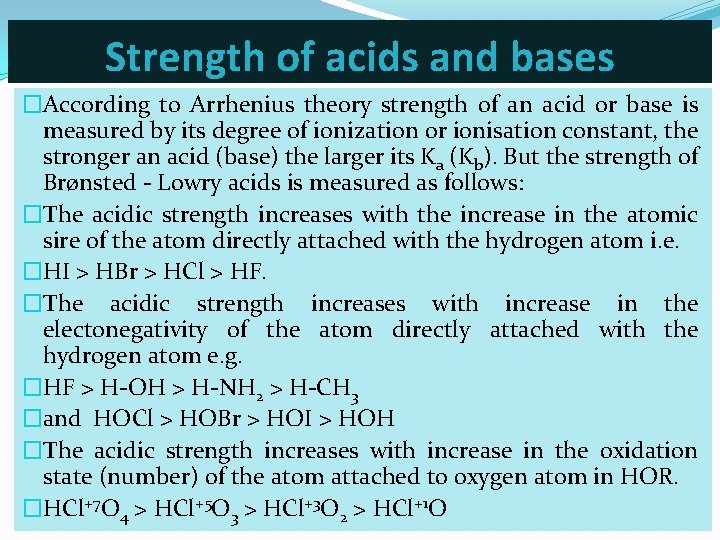
Strength of acids and bases �According to Arrhenius theory strength of an acid or base is measured by its degree of ionization or ionisation constant, the stronger an acid (base) the larger its Ka (Kb). But the strength of Brønsted - Lowry acids is measured as follows: �The acidic strength increases with the increase in the atomic sire of the atom directly attached with the hydrogen atom i. e. �HI > HBr > HCl > HF. �The acidic strength increases with increase in the electonegativity of the atom directly attached with the hydrogen atom e. g. �HF > H-OH > H-NH 2 > H-CH 3 �and HOCl > HOBr > HOI > HOH �The acidic strength increases with increase in the oxidation state (number) of the atom attached to oxygen atom in HOR. �HCl+7 O 4 > HCl+5 O 3 > HCl+3 O 2 > HCl+1 O
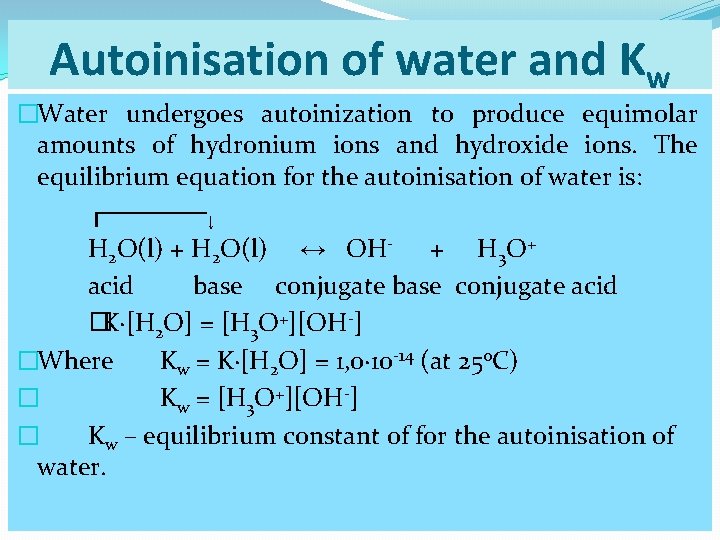
Autoinisation of water and Kw �Water undergoes autoinization to produce equimolar amounts of hydronium ions and hydroxide ions. The equilibrium equation for the autoinisation of water is: ┌──────↓ H 2 O(l) + H 2 O(l) ↔ OH- + H 3 O+ acid base conjugate acid �K∙[H 2 O] = [H 3 O+][OH-] �Where Kw = K∙[H 2 O] = 1, 0∙ 10 -14 (at 25 o. C) � Kw = [H 3 O+][OH-] � Kw – equilibrium constant of for the autoinisation of water.
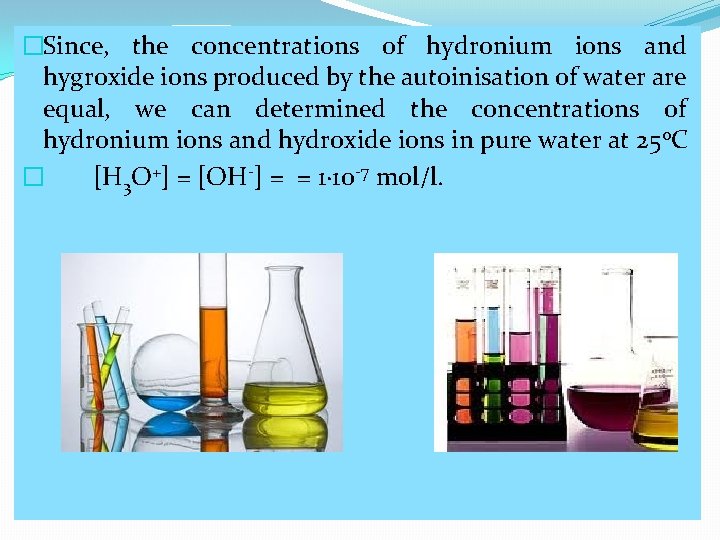
�Since, the concentrations of hydronium ions and hygroxide ions produced by the autoinisation of water are equal, we can determined the concentrations of hydronium ions and hydroxide ions in pure water at 25 o. C � [H 3 O+] = [OH-] = = 1∙ 10 -7 mol/l.
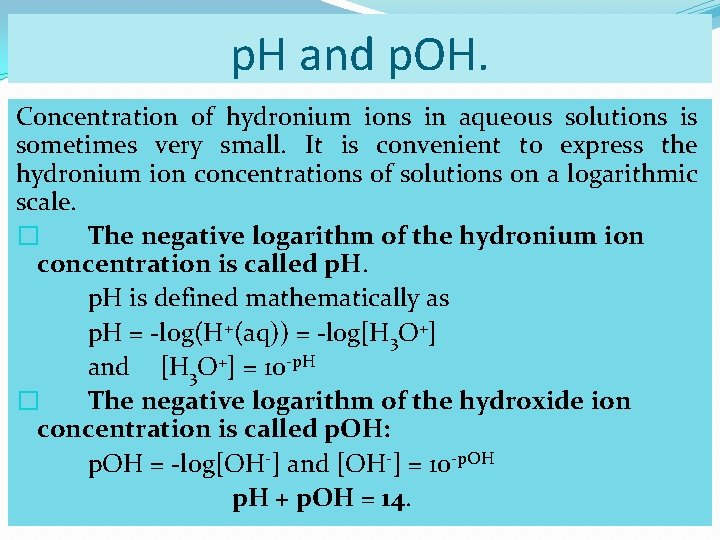
p. H and p. OH. Concentration of hydronium ions in aqueous solutions is sometimes very small. It is convenient to express the hydronium ion concentrations of solutions on a logarithmic scale. � The negative logarithm of the hydronium ion concentration is called p. H is defined mathematically as p. H = -log(H+(aq)) = -log[H 3 O+] and [H 3 O+] = 10 -p. H � The negative logarithm of the hydroxide ion concentration is called p. OH: p. OH = -log[OH-] and [OH-] = 10 -p. OH p. H + p. OH = 14.
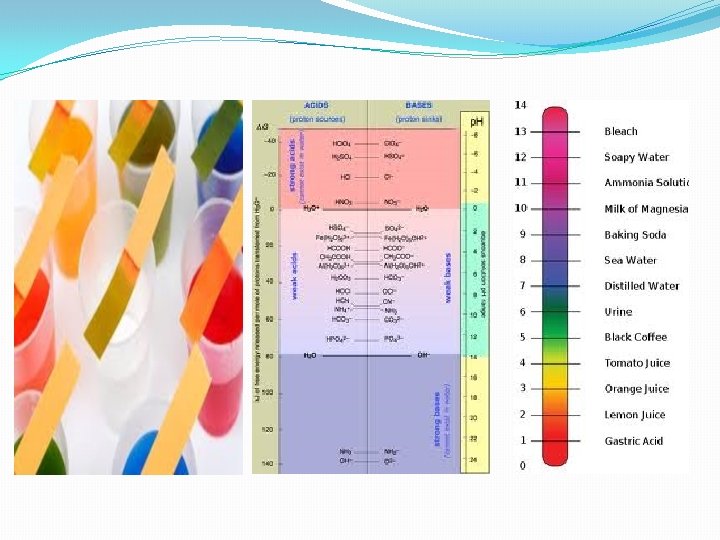
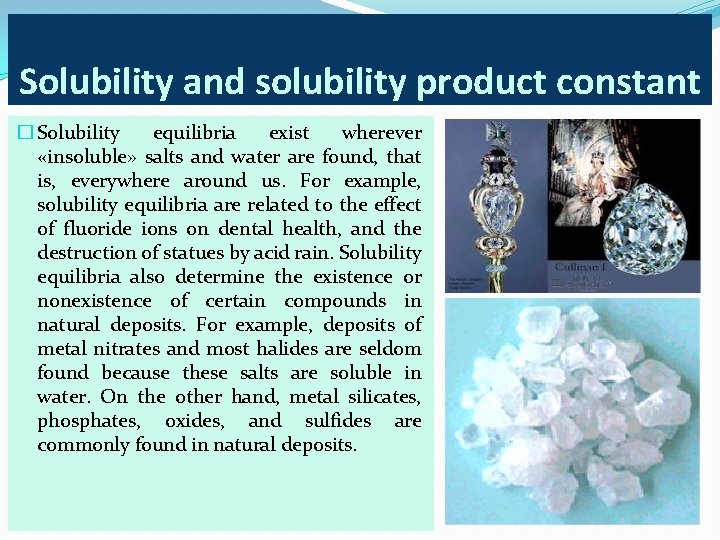
Solubility and solubility product constant � Solubility equilibria exist wherever «insoluble» salts and water are found, that is, everywhere around us. For example, solubility equilibria are related to the effect of fluoride ions on dental health, and the destruction of statues by acid rain. Solubility equilibria also determine the existence or nonexistence of certain compounds in natural deposits. For example, deposits of metal nitrates and most halides are seldom found because these salts are soluble in water. On the other hand, metal silicates, phosphates, oxides, and sulfides are commonly found in natural deposits.
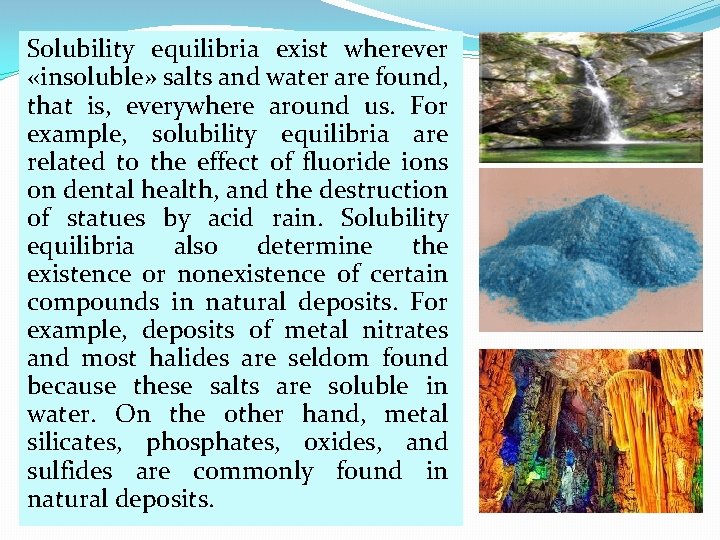
Solubility equilibria exist wherever «insoluble» salts and water are found, that is, everywhere around us. For example, solubility equilibria are related to the effect of fluoride ions on dental health, and the destruction of statues by acid rain. Solubility equilibria also determine the existence or nonexistence of certain compounds in natural deposits. For example, deposits of metal nitrates and most halides are seldom found because these salts are soluble in water. On the other hand, metal silicates, phosphates, oxides, and sulfides are commonly found in natural deposits.
Is sucrose soluble in water
Herbalife 24 line
Deficiency of carbohydrate
Weak electrolytes
Normal electrolytes values
Negative deviation from raoult's law
Diabetic ketoacidosis
Refeeding syndrome electrolytes
Tropolar
Normal values of potassium
Major intra and extracellular electrolytes
How does electrolytes affect the chemical equilibria?
Strong vs weak electrolytes
Colloid meaning
Effect of electrolytes on colloidal dispersion
Strong vs weak electrolytes
Electrolytes and nonelectrolytes
Solubility
Vacid definition
Non classical adrenal hyperplasia
Chapter 18 fluids and electrolytes
Lector lectrix l'homme à l'oreille coupée
Lector de tarjetas