Regulation of Gene Expression Chapter 13 Regulation of
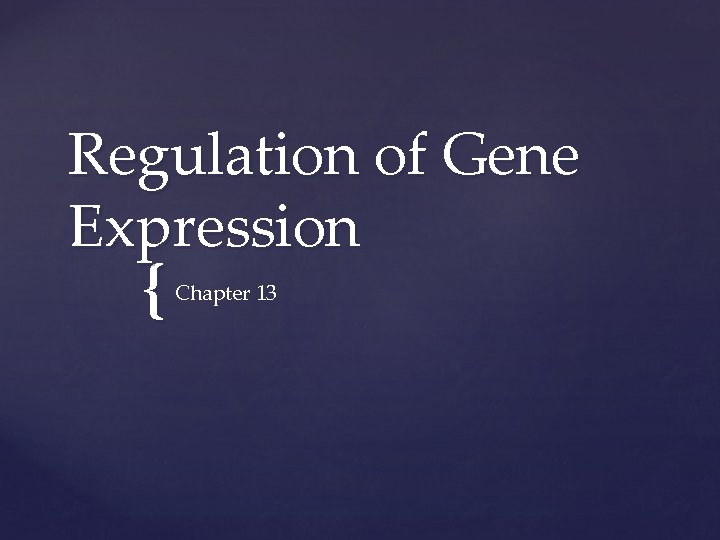
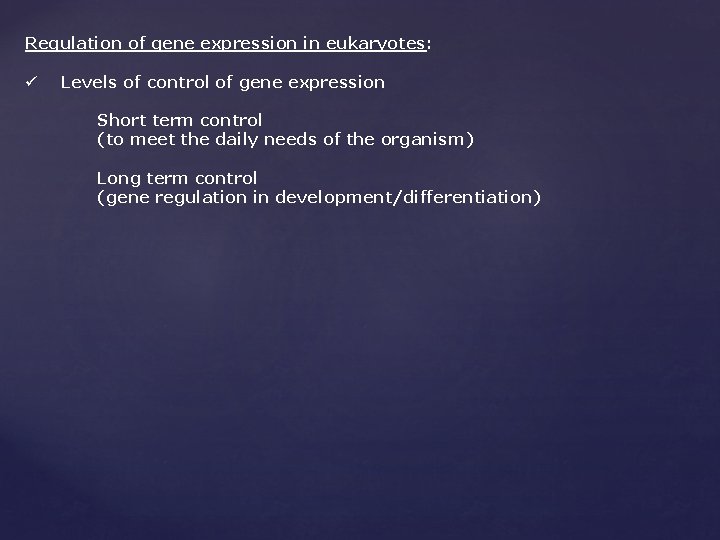
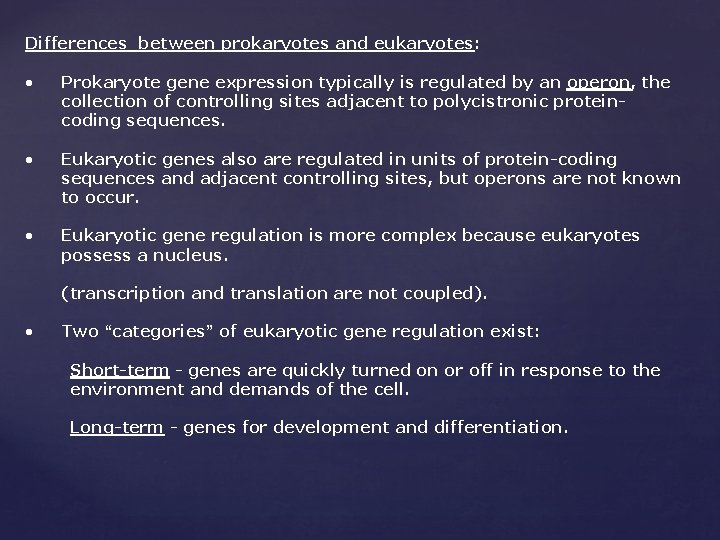
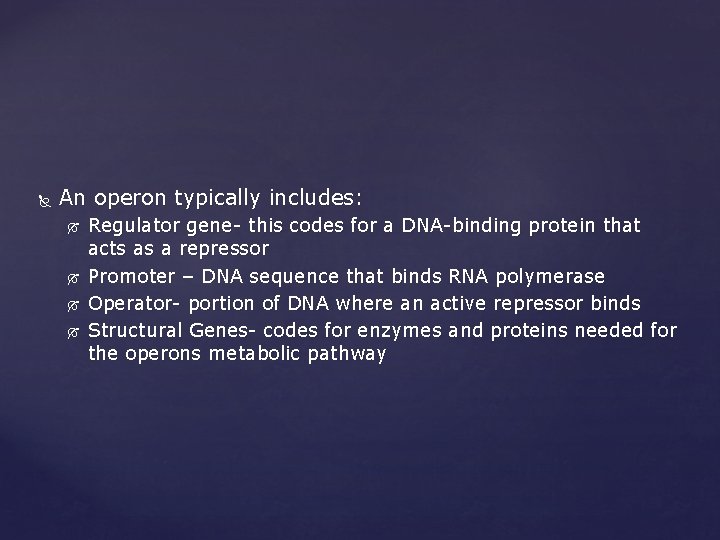
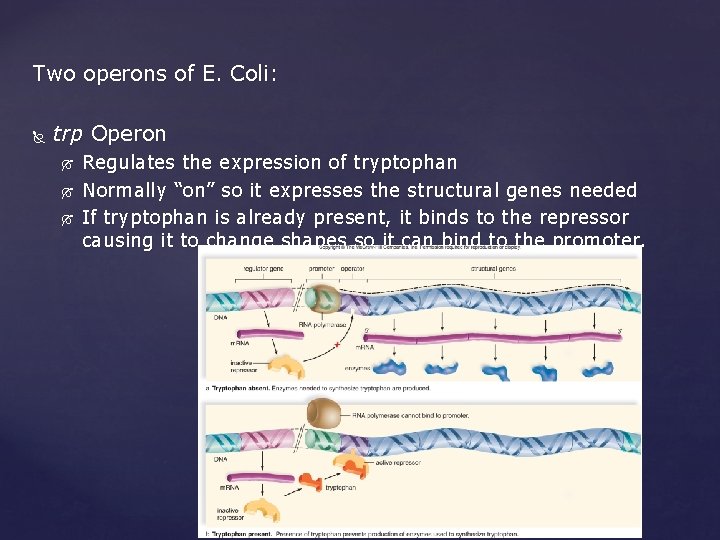
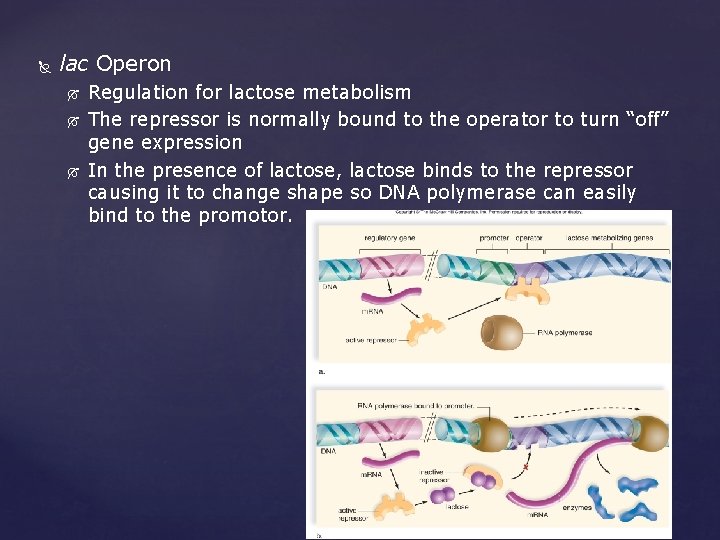
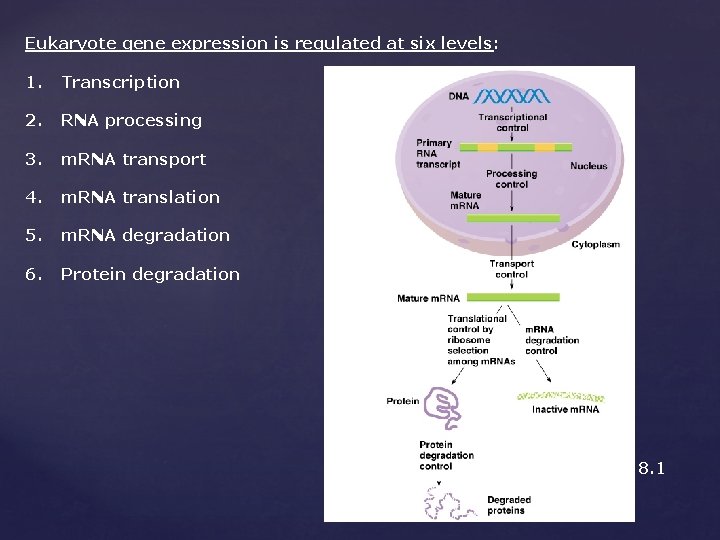
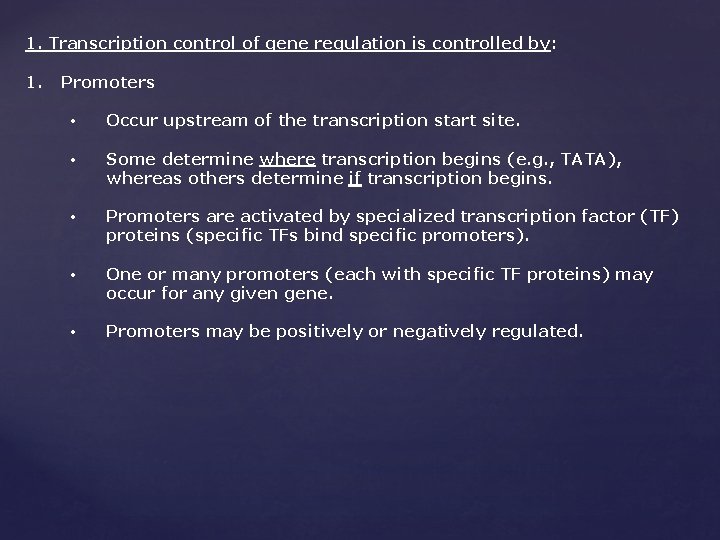
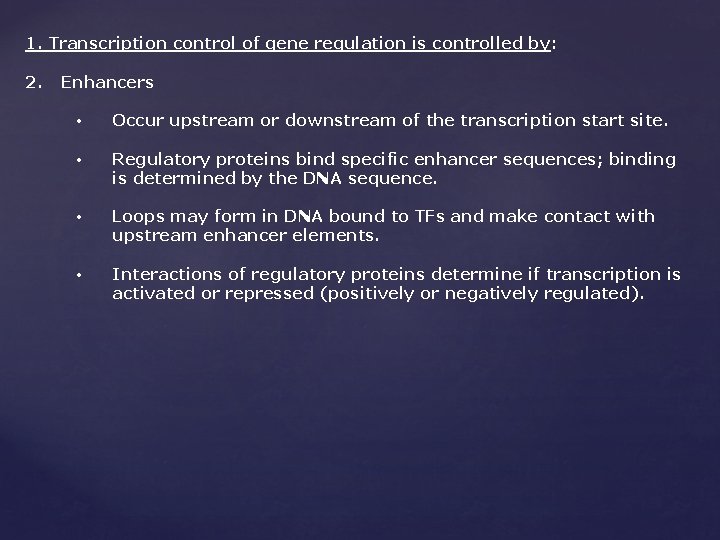
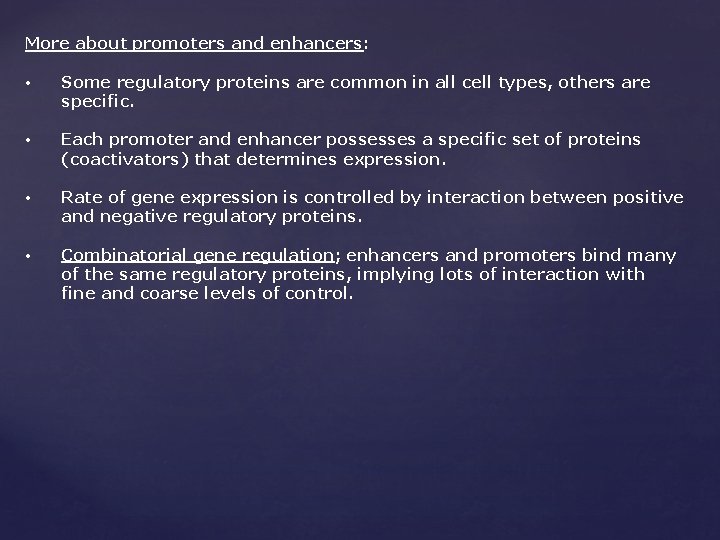
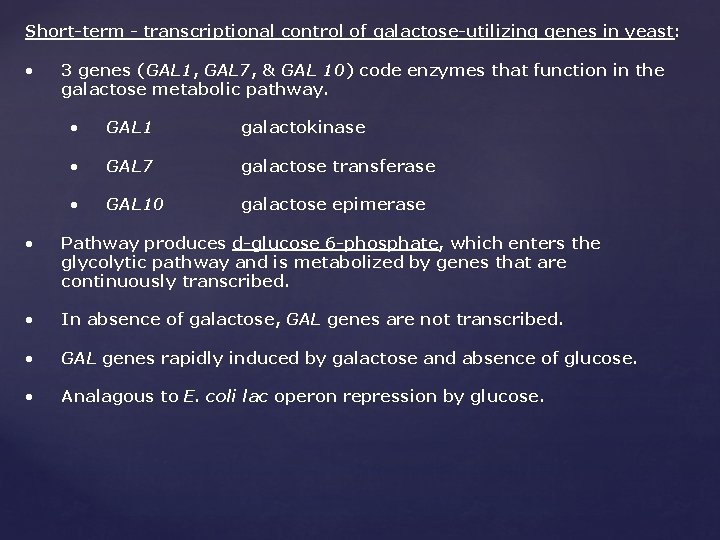
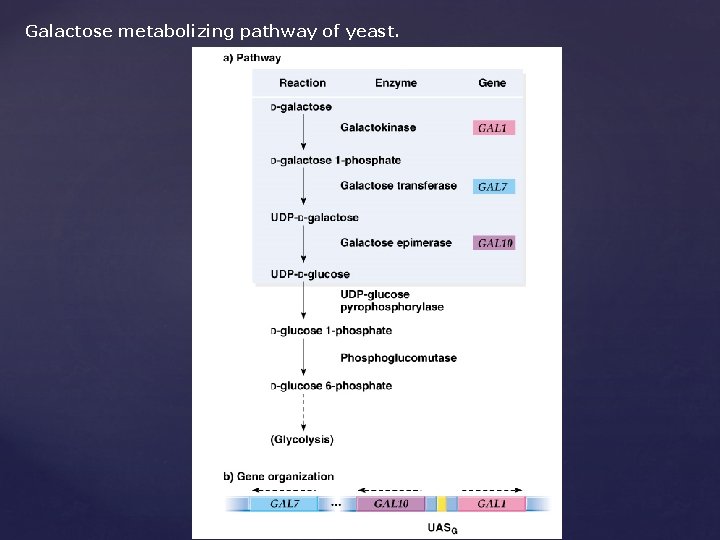
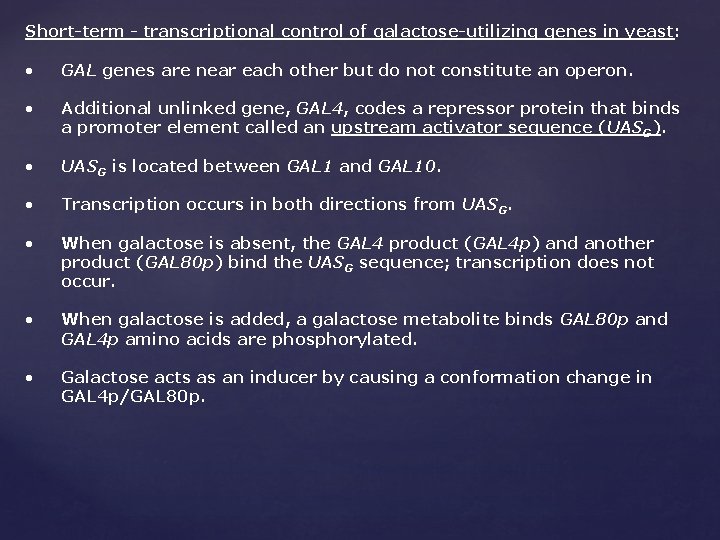
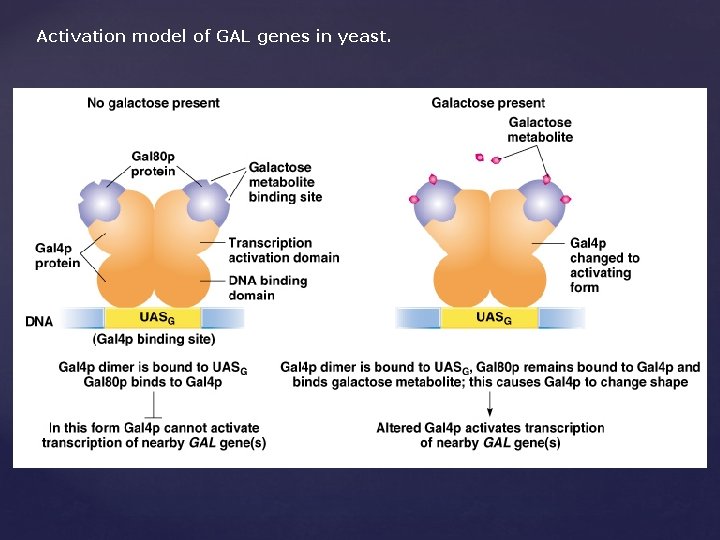
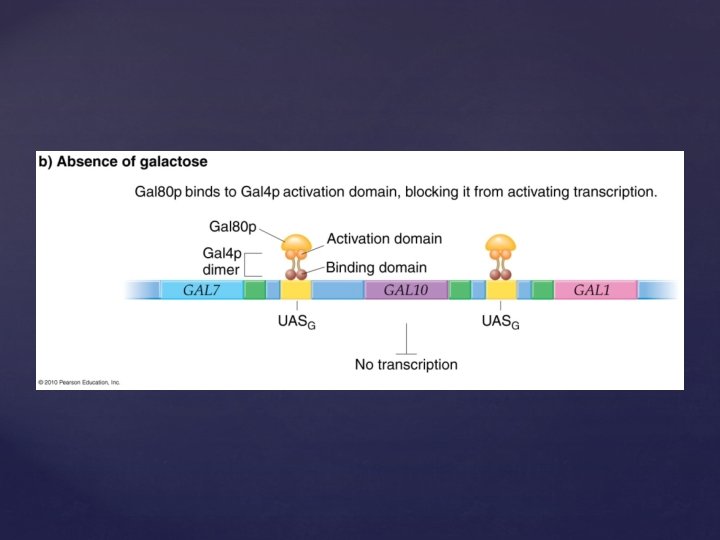
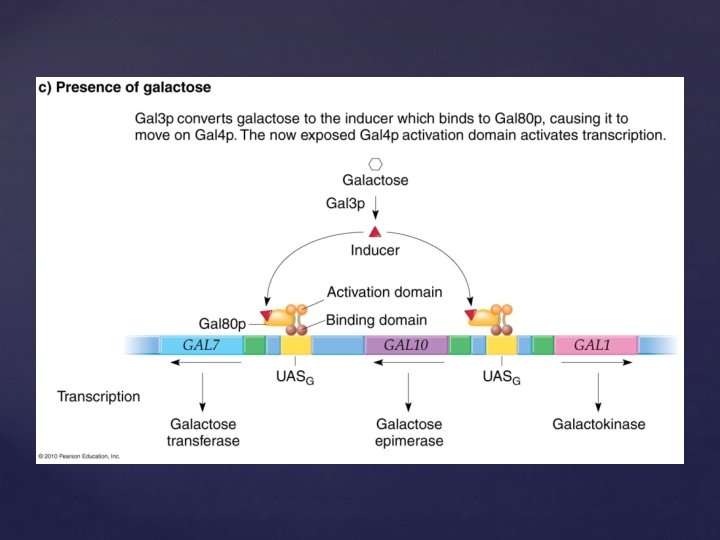
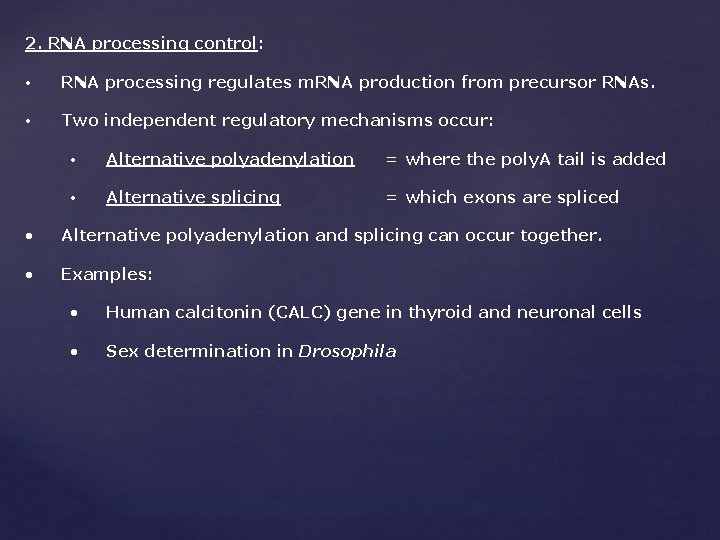
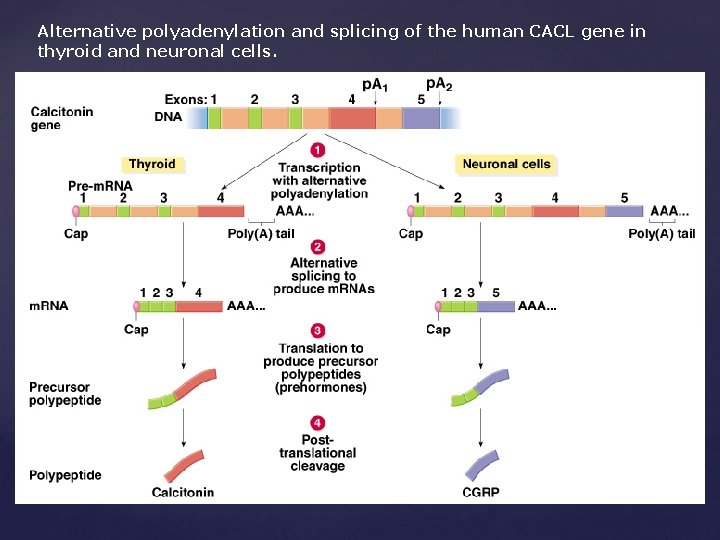
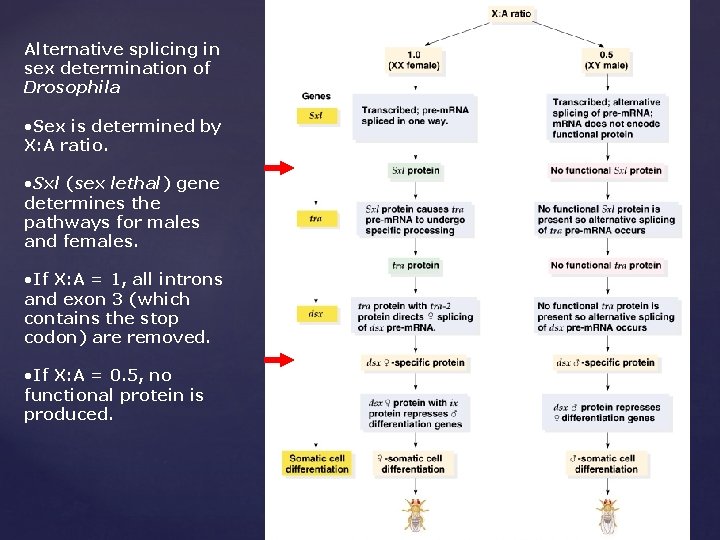
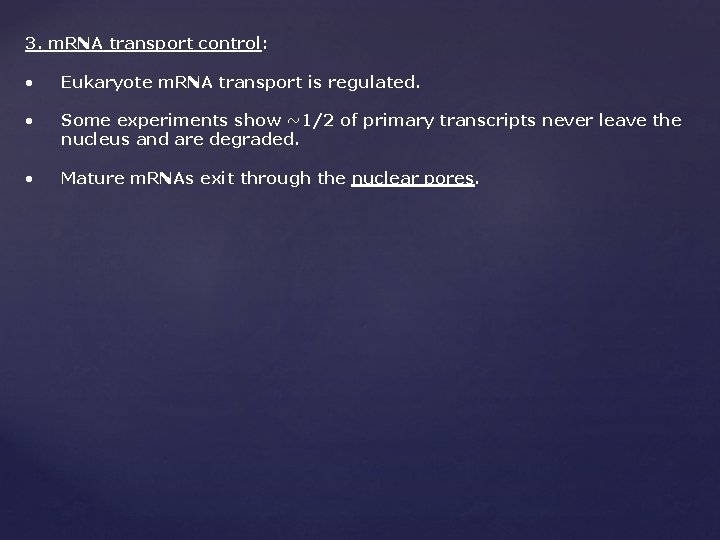
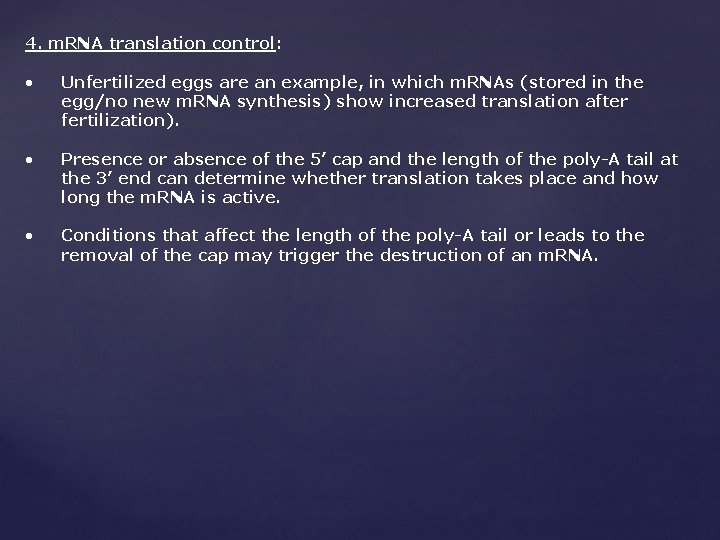
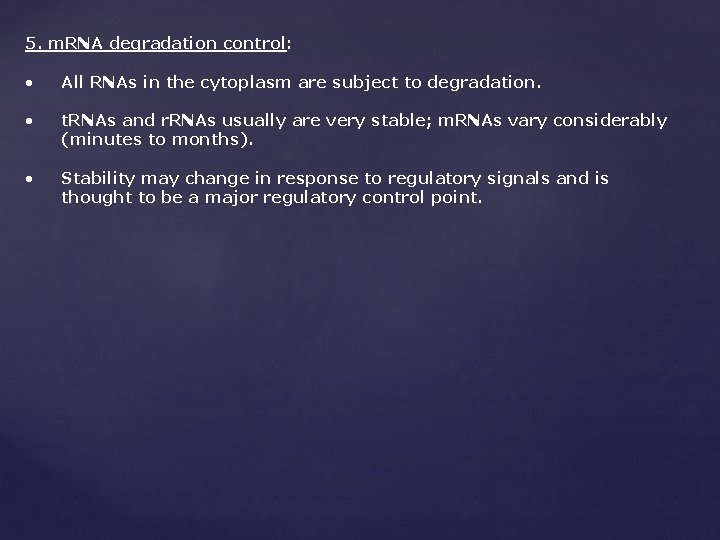
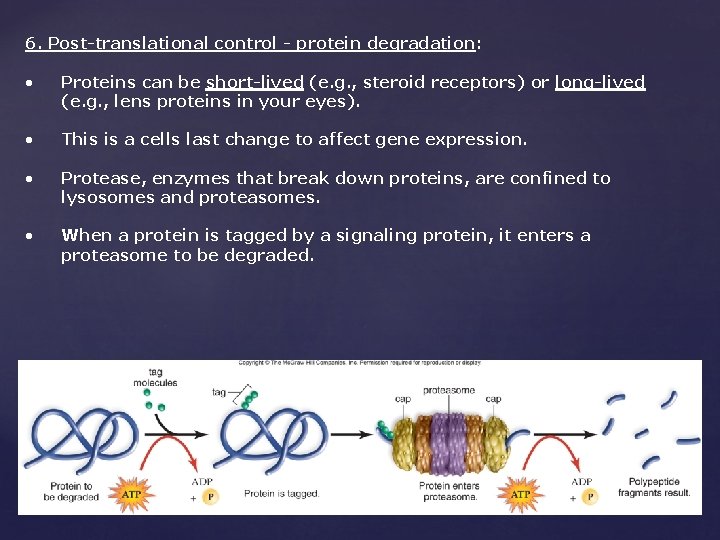
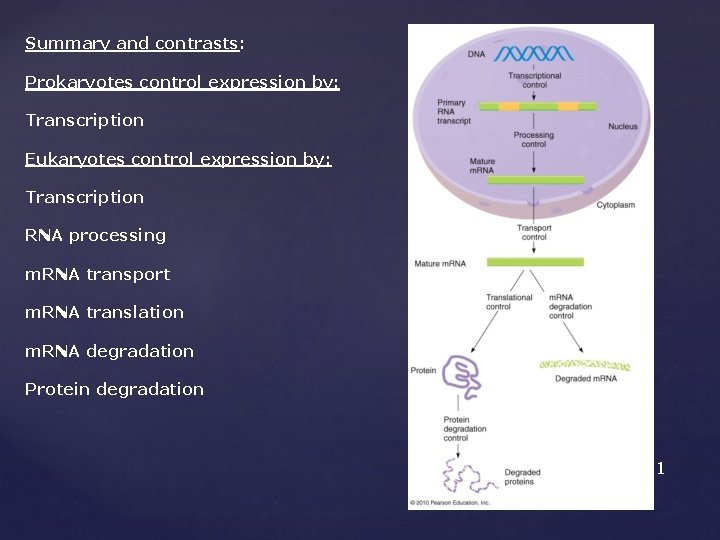
- Slides: 24
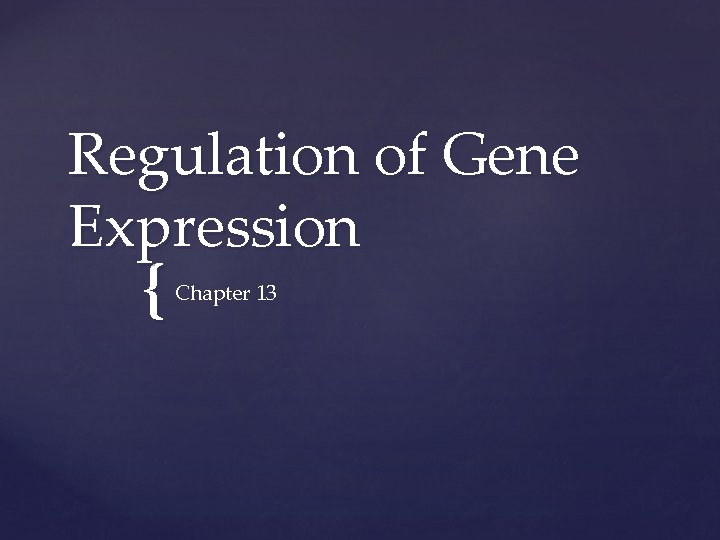
Regulation of Gene Expression { Chapter 13
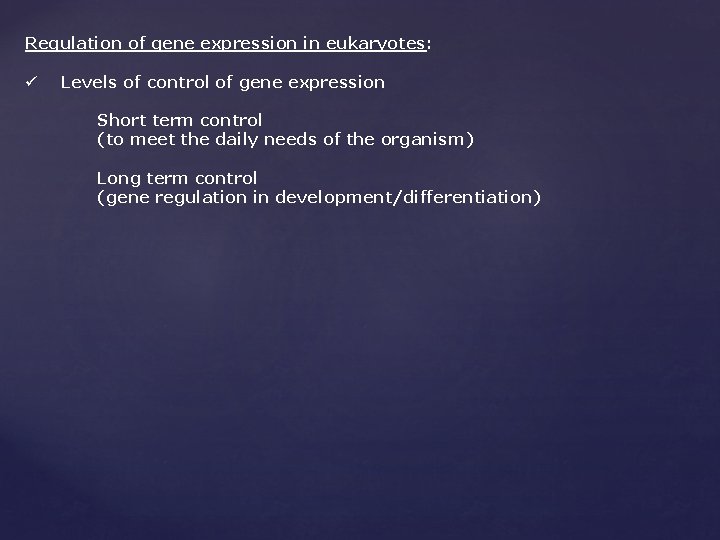
Regulation of gene expression in eukaryotes: ü Levels of control of gene expression Short term control (to meet the daily needs of the organism) Long term control (gene regulation in development/differentiation)
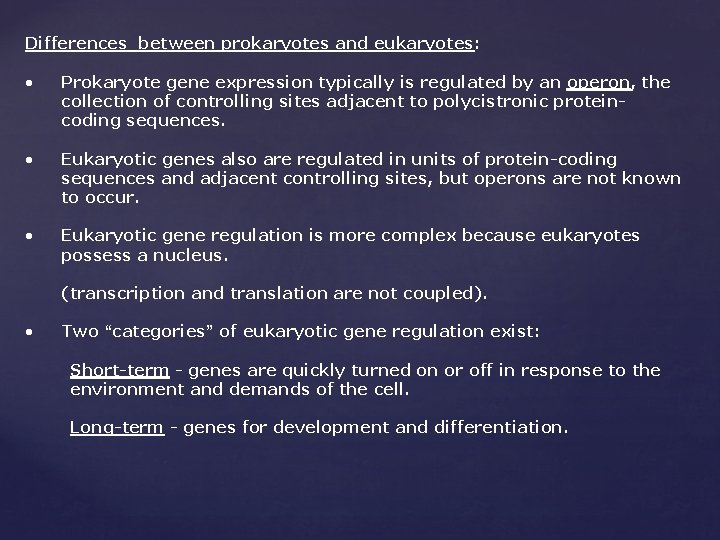
Differences between prokaryotes and eukaryotes: • Prokaryote gene expression typically is regulated by an operon, the collection of controlling sites adjacent to polycistronic proteincoding sequences. • Eukaryotic genes also are regulated in units of protein-coding sequences and adjacent controlling sites, but operons are not known to occur. • Eukaryotic gene regulation is more complex because eukaryotes possess a nucleus. (transcription and translation are not coupled). • Two “categories” of eukaryotic gene regulation exist: Short-term - genes are quickly turned on or off in response to the environment and demands of the cell. Long-term - genes for development and differentiation.
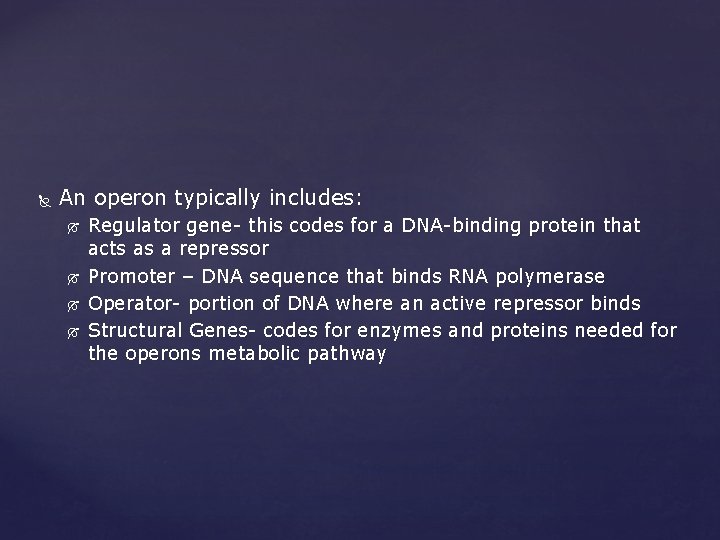
An operon typically includes: Regulator gene- this codes for a DNA-binding protein that acts as a repressor Promoter – DNA sequence that binds RNA polymerase Operator- portion of DNA where an active repressor binds Structural Genes- codes for enzymes and proteins needed for the operons metabolic pathway
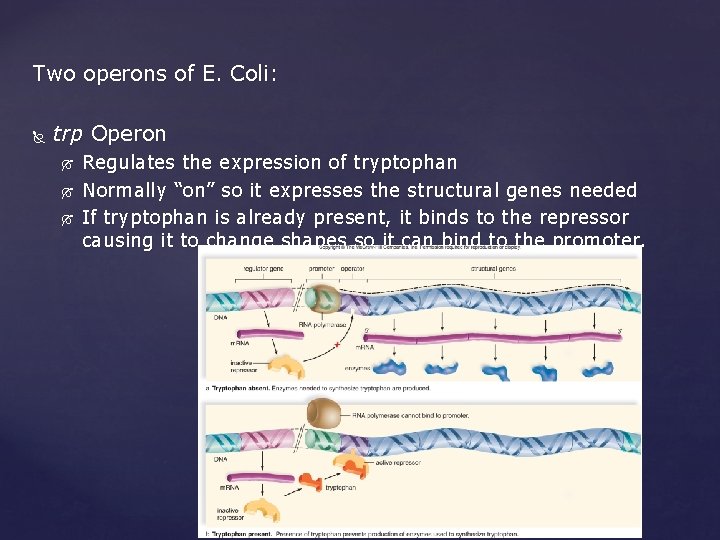
Two operons of E. Coli: trp Operon Regulates the expression of tryptophan Normally “on” so it expresses the structural genes needed If tryptophan is already present, it binds to the repressor causing it to change shapes so it can bind to the promoter.
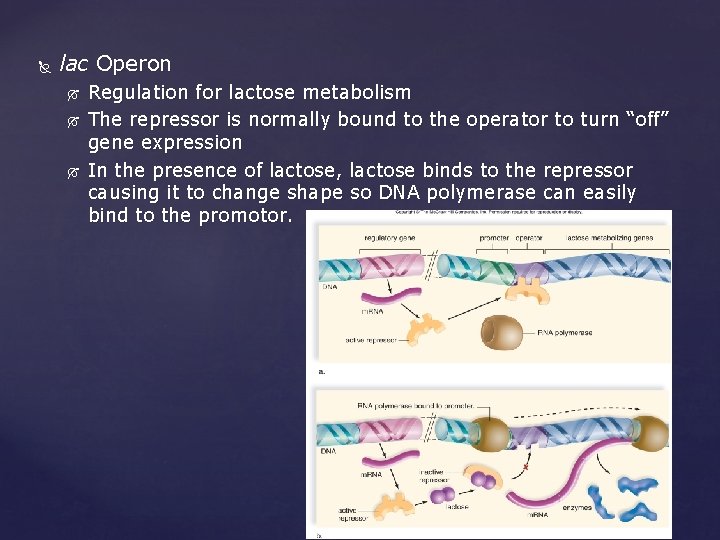
lac Operon Regulation for lactose metabolism The repressor is normally bound to the operator to turn “off” gene expression In the presence of lactose, lactose binds to the repressor causing it to change shape so DNA polymerase can easily bind to the promotor.
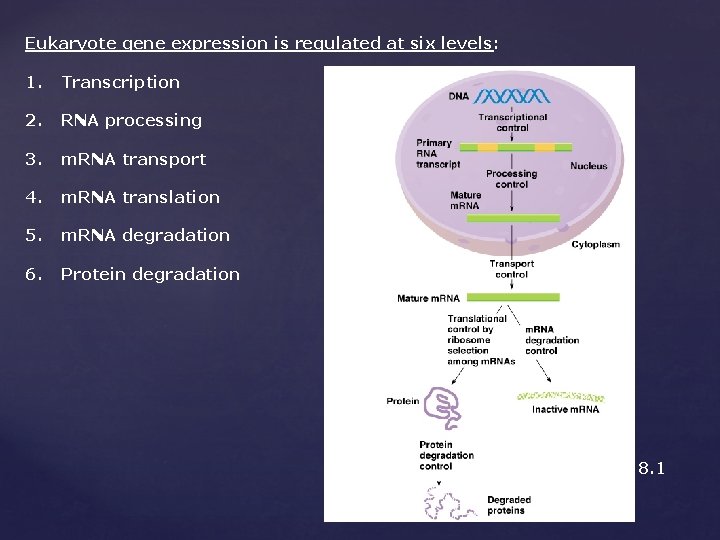
Eukaryote gene expression is regulated at six levels: 1. Transcription 2. RNA processing 3. m. RNA transport 4. m. RNA translation 5. m. RNA degradation 6. Protein degradation Fig. 18. 1
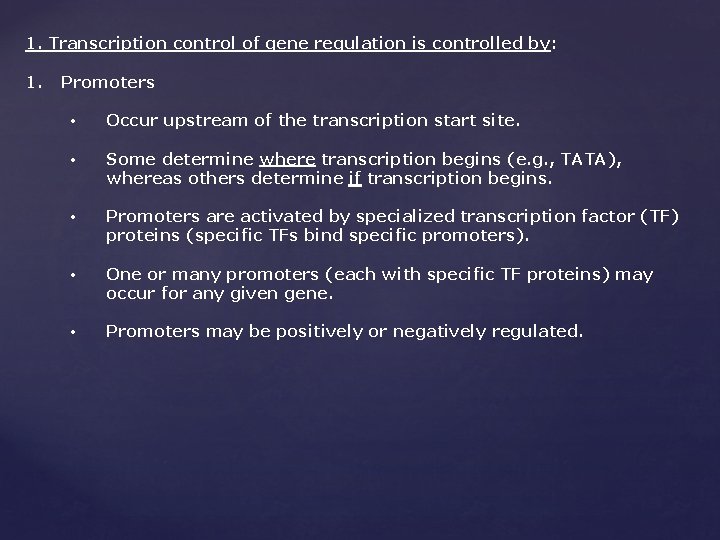
1. Transcription control of gene regulation is controlled by: 1. Promoters • Occur upstream of the transcription start site. • Some determine where transcription begins (e. g. , TATA), whereas others determine if transcription begins. • Promoters are activated by specialized transcription factor (TF) proteins (specific TFs bind specific promoters). • One or many promoters (each with specific TF proteins) may occur for any given gene. • Promoters may be positively or negatively regulated.
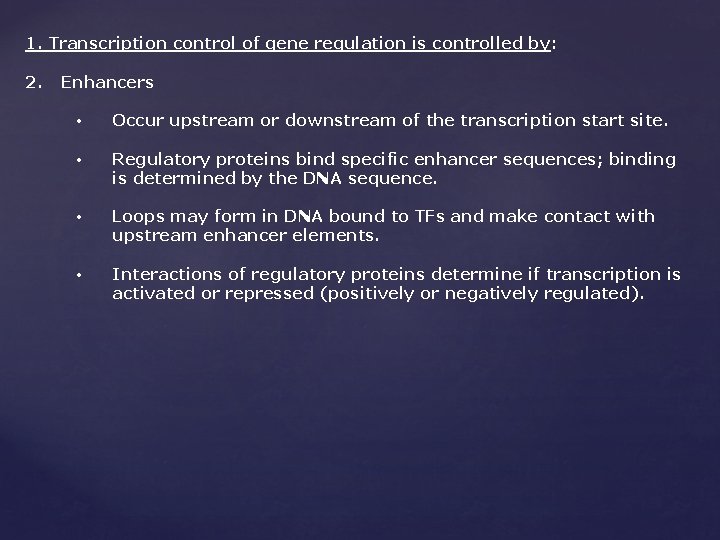
1. Transcription control of gene regulation is controlled by: 2. Enhancers • Occur upstream or downstream of the transcription start site. • Regulatory proteins bind specific enhancer sequences; binding is determined by the DNA sequence. • Loops may form in DNA bound to TFs and make contact with upstream enhancer elements. • Interactions of regulatory proteins determine if transcription is activated or repressed (positively or negatively regulated).
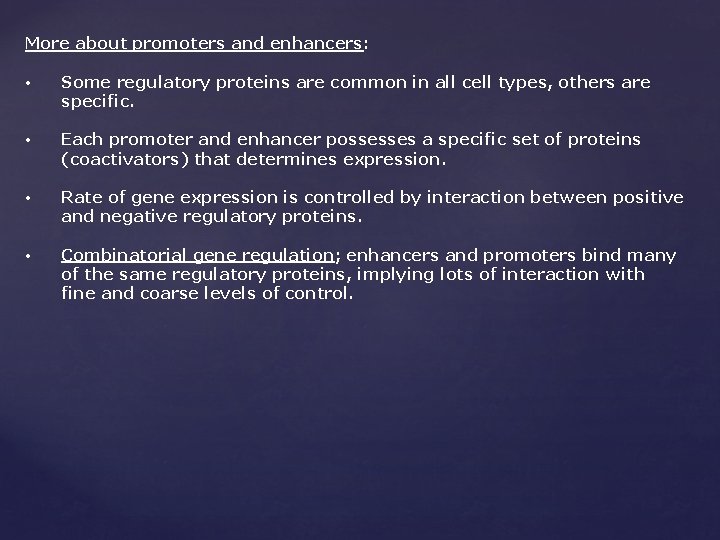
More about promoters and enhancers: • Some regulatory proteins are common in all cell types, others are specific. • Each promoter and enhancer possesses a specific set of proteins (coactivators) that determines expression. • Rate of gene expression is controlled by interaction between positive and negative regulatory proteins. • Combinatorial gene regulation; enhancers and promoters bind many of the same regulatory proteins, implying lots of interaction with fine and coarse levels of control.
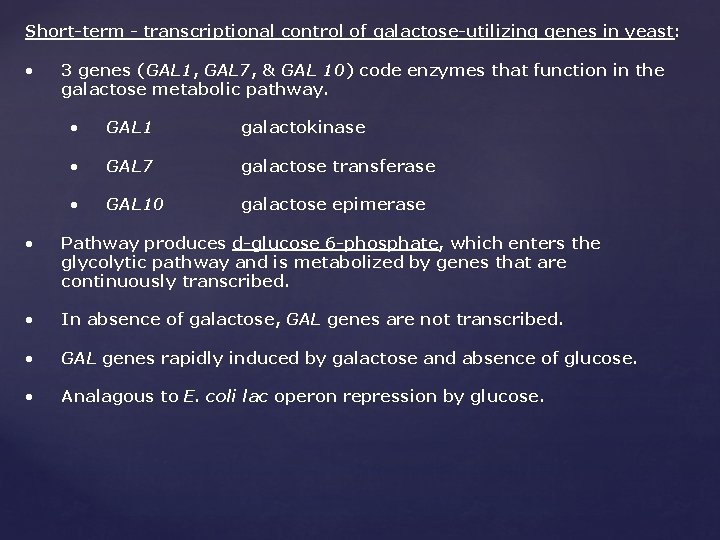
Short-term - transcriptional control of galactose-utilizing genes in yeast: • 3 genes (GAL 1, GAL 7, & GAL 10) code enzymes that function in the galactose metabolic pathway. • GAL 1 galactokinase • GAL 7 galactose transferase • GAL 10 galactose epimerase • Pathway produces d-glucose 6 -phosphate, which enters the glycolytic pathway and is metabolized by genes that are continuously transcribed. • In absence of galactose, GAL genes are not transcribed. • GAL genes rapidly induced by galactose and absence of glucose. • Analagous to E. coli lac operon repression by glucose.
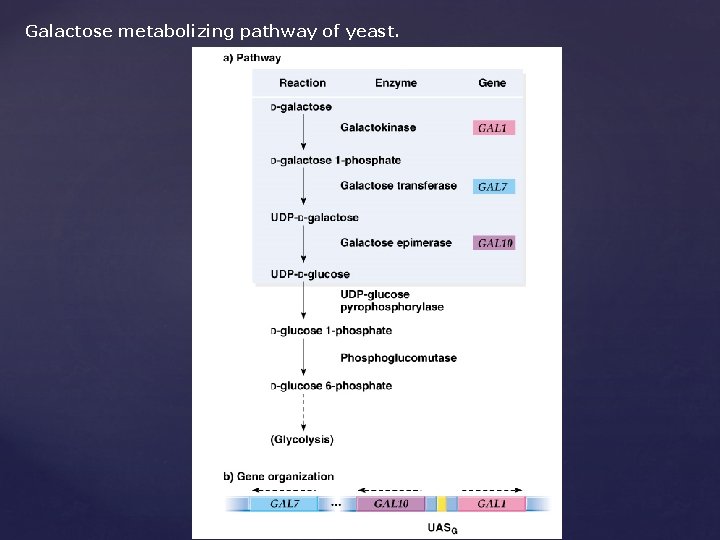
Galactose metabolizing pathway of yeast.
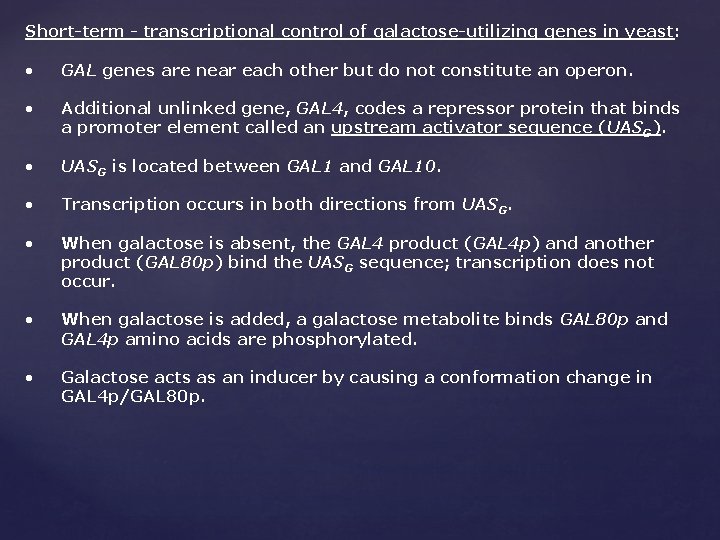
Short-term - transcriptional control of galactose-utilizing genes in yeast: • GAL genes are near each other but do not constitute an operon. • Additional unlinked gene, GAL 4, codes a repressor protein that binds a promoter element called an upstream activator sequence (UASG). • UASG is located between GAL 1 and GAL 10. • Transcription occurs in both directions from UASG. • When galactose is absent, the GAL 4 product (GAL 4 p) and another product (GAL 80 p) bind the UASG sequence; transcription does not occur. • When galactose is added, a galactose metabolite binds GAL 80 p and GAL 4 p amino acids are phosphorylated. • Galactose acts as an inducer by causing a conformation change in GAL 4 p/GAL 80 p.
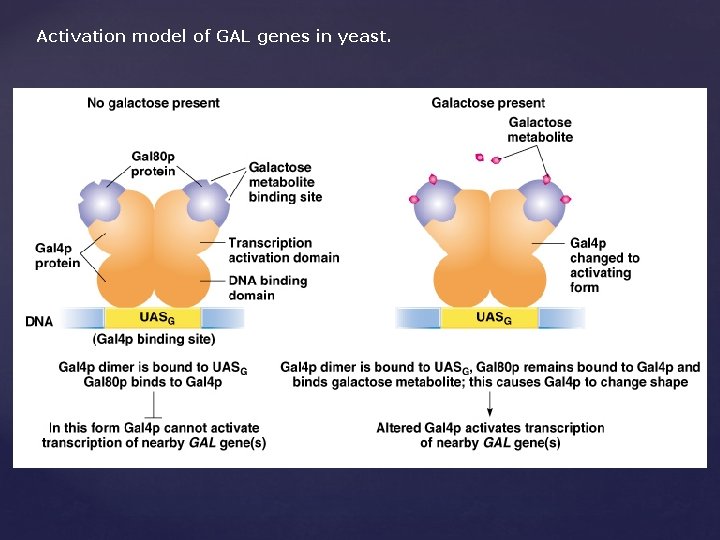
Activation model of GAL genes in yeast.
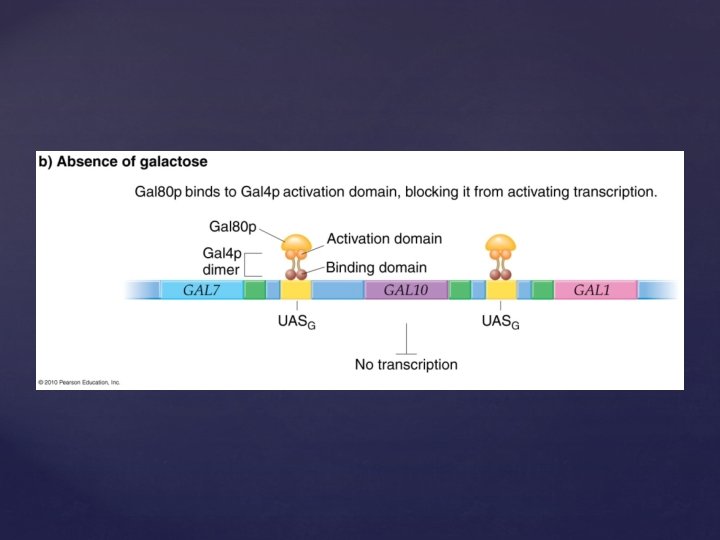
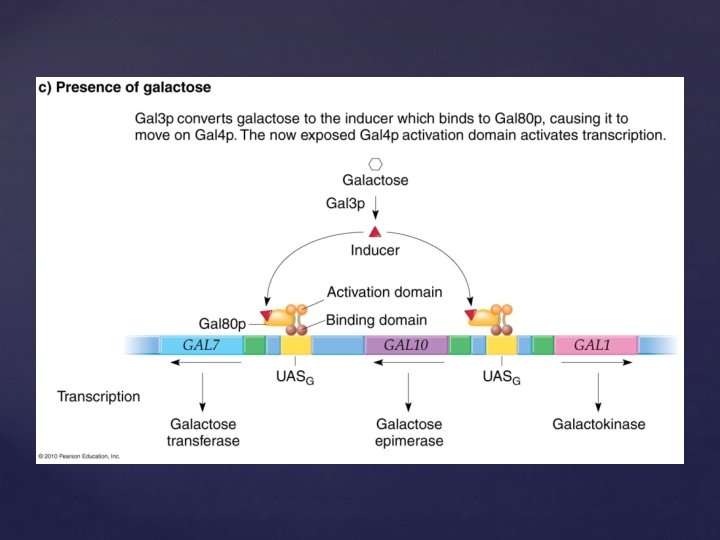
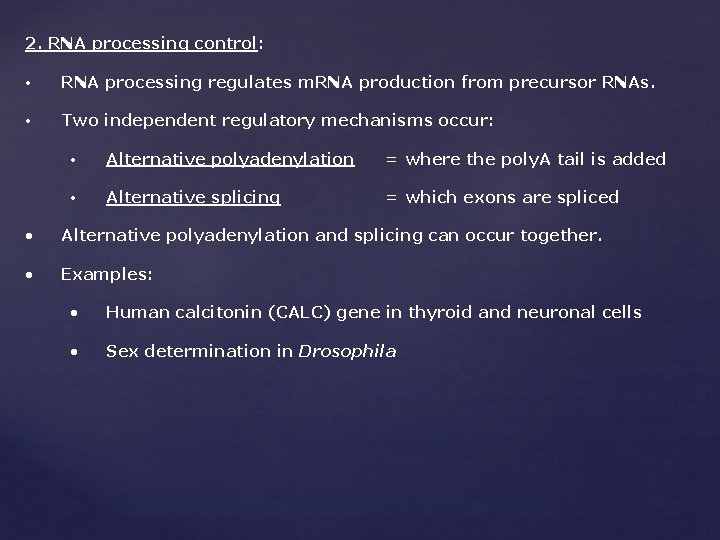
2. RNA processing control: • RNA processing regulates m. RNA production from precursor RNAs. • Two independent regulatory mechanisms occur: • Alternative polyadenylation = where the poly. A tail is added • Alternative splicing = which exons are spliced • Alternative polyadenylation and splicing can occur together. • Examples: • Human calcitonin (CALC) gene in thyroid and neuronal cells • Sex determination in Drosophila
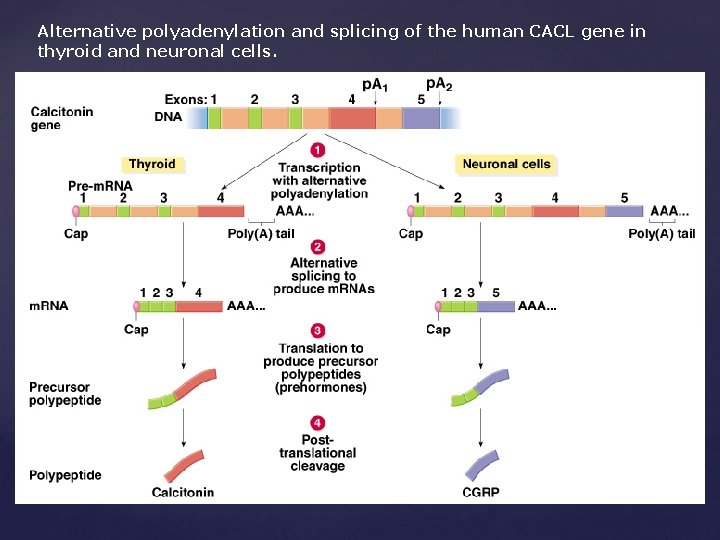
Alternative polyadenylation and splicing of the human CACL gene in thyroid and neuronal cells. Calcitonin gene -related peptide
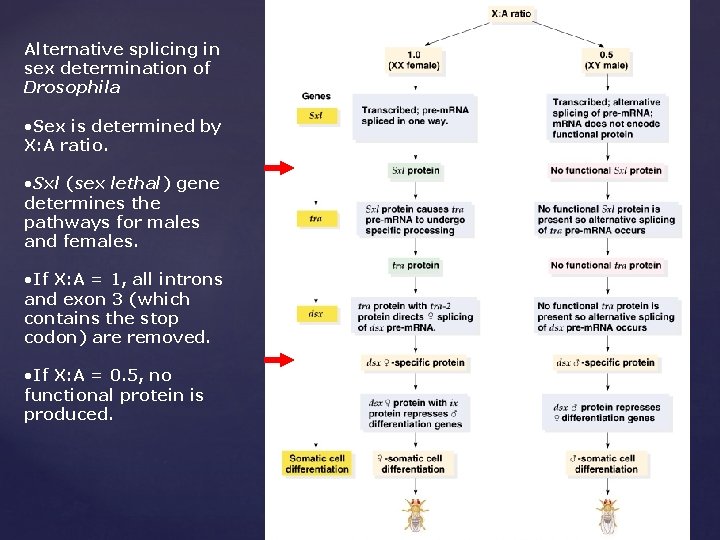
Alternative splicing in sex determination of Drosophila • Sex is determined by X: A ratio. • Sxl (sex lethal) gene determines the pathways for males and females. • If X: A = 1, all introns and exon 3 (which contains the stop codon) are removed. • If X: A = 0. 5, no functional protein is produced.
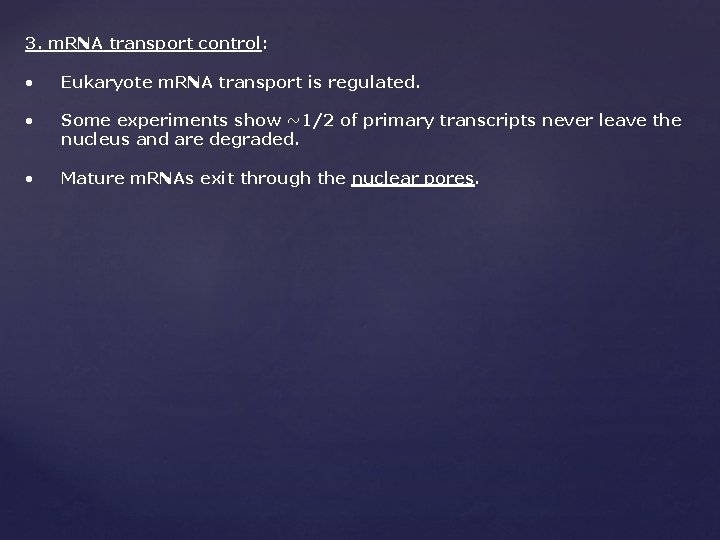
3. m. RNA transport control: • Eukaryote m. RNA transport is regulated. • Some experiments show ~1/2 of primary transcripts never leave the nucleus and are degraded. • Mature m. RNAs exit through the nuclear pores.
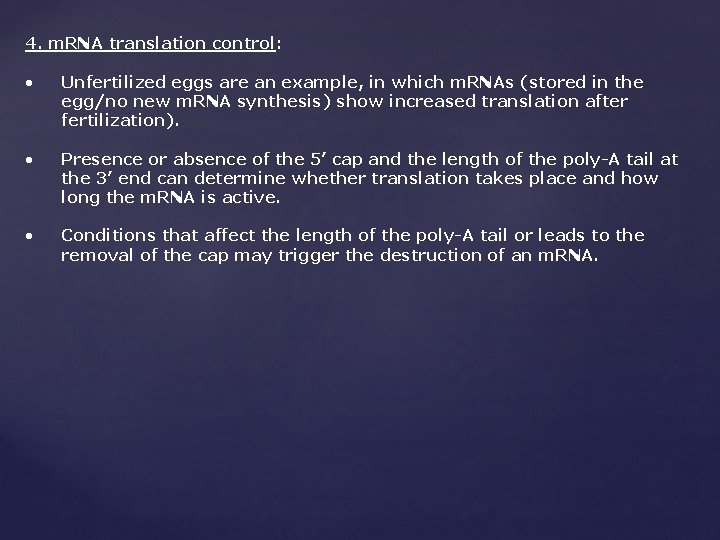
4. m. RNA translation control: • Unfertilized eggs are an example, in which m. RNAs (stored in the egg/no new m. RNA synthesis) show increased translation after fertilization). • Presence or absence of the 5’ cap and the length of the poly-A tail at the 3’ end can determine whether translation takes place and how long the m. RNA is active. • Conditions that affect the length of the poly-A tail or leads to the removal of the cap may trigger the destruction of an m. RNA.
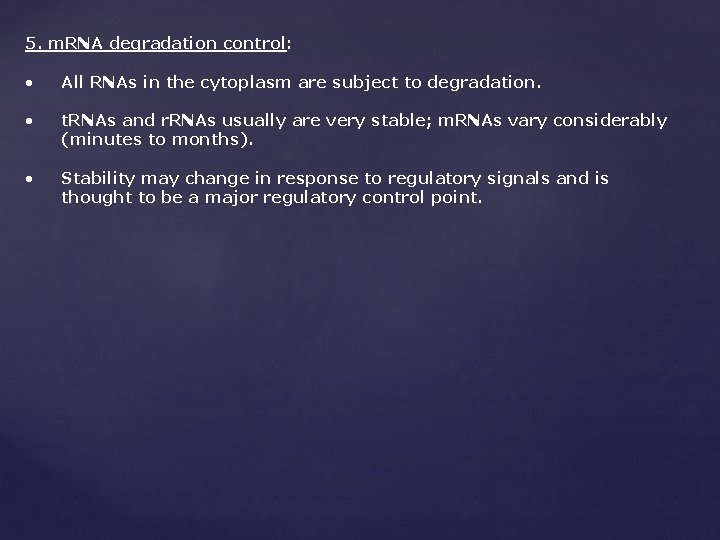
5. m. RNA degradation control: • All RNAs in the cytoplasm are subject to degradation. • t. RNAs and r. RNAs usually are very stable; m. RNAs vary considerably (minutes to months). • Stability may change in response to regulatory signals and is thought to be a major regulatory control point.
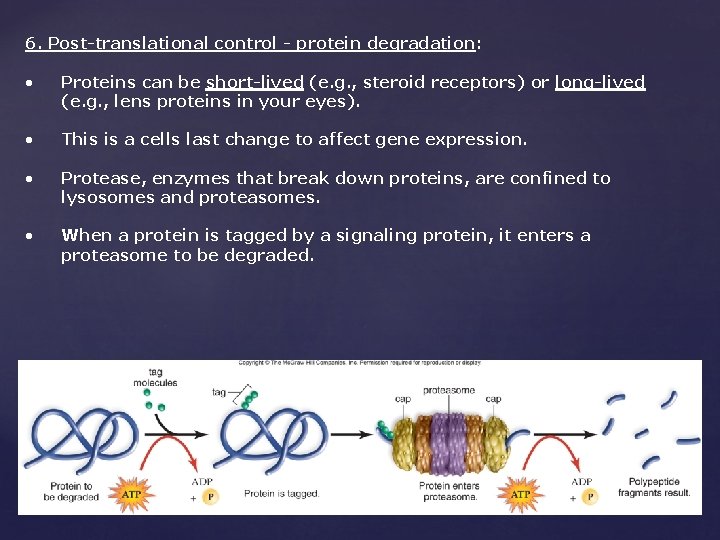
6. Post-translational control - protein degradation: • Proteins can be short-lived (e. g. , steroid receptors) or long-lived (e. g. , lens proteins in your eyes). • This is a cells last change to affect gene expression. • Protease, enzymes that break down proteins, are confined to lysosomes and proteasomes. • When a protein is tagged by a signaling protein, it enters a proteasome to be degraded.
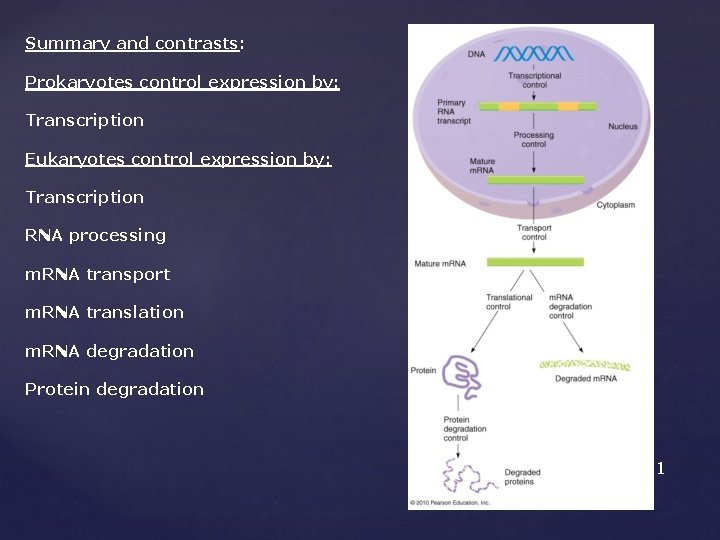
Summary and contrasts: Prokaryotes control expression by: Transcription Eukaryotes control expression by: Transcription RNA processing m. RNA transport m. RNA translation m. RNA degradation Protein degradation Fig. 18. 1
Chapter 17 from gene to protein
Chapter 18 regulation of gene expression
Chapter 18 regulation of gene expression
Chapter 18 regulation of gene expression
Chapter 18
Regulation of gene expression in bacteria
Regulation of gene expression
Chapter 12 section 4 gene regulation and mutations
Gene by gene test results
Section 4 gene regulation and mutations
Gene expression in prokaryotes and eukaryotes
Section 12-5 gene regulation answer key
Prokaryotes vs eukaryotes gene regulation
Section 12-1 dna
What is gene regulation
Gene regulation
Differential gene regulation
Negative control
Prokaryotic gene expression
Dr kevin ahern
טרנסלציה
Genetic effects on gene expression across human tissues
Fret rt pcr
Gene expression omnibus tutorial
Gene expression