Photovoltaics Fundamental concepts and novel systems First practical
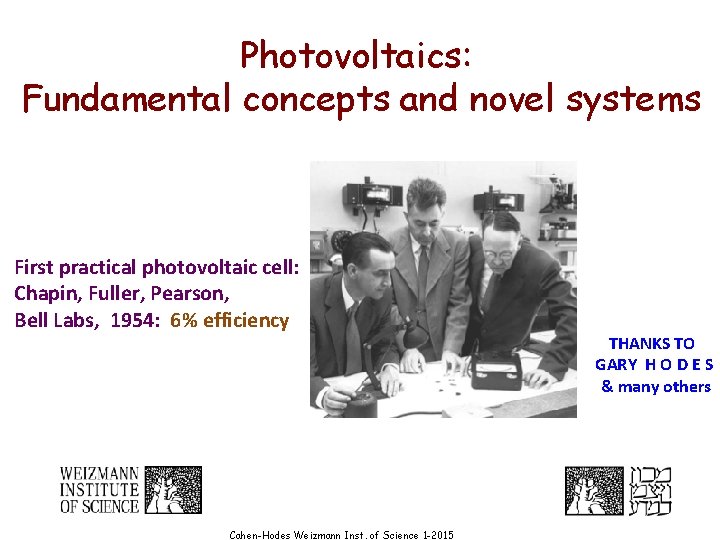
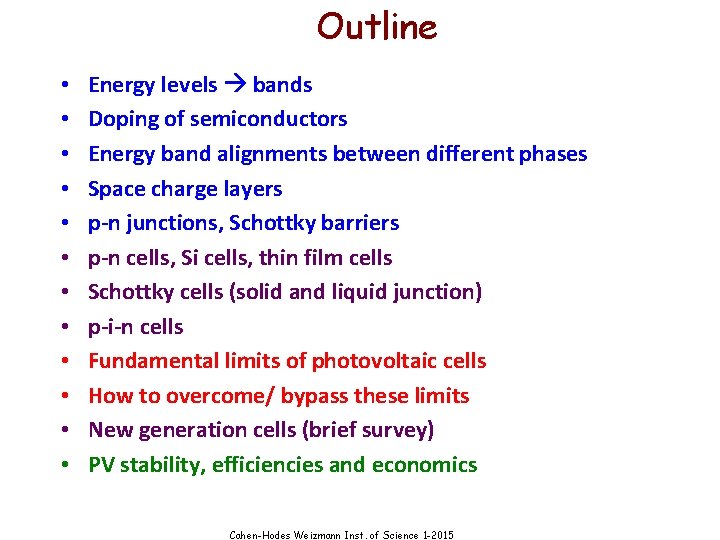
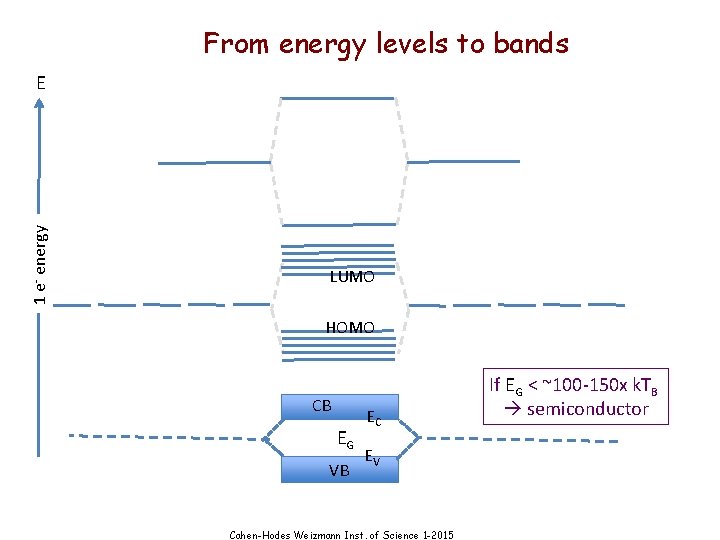
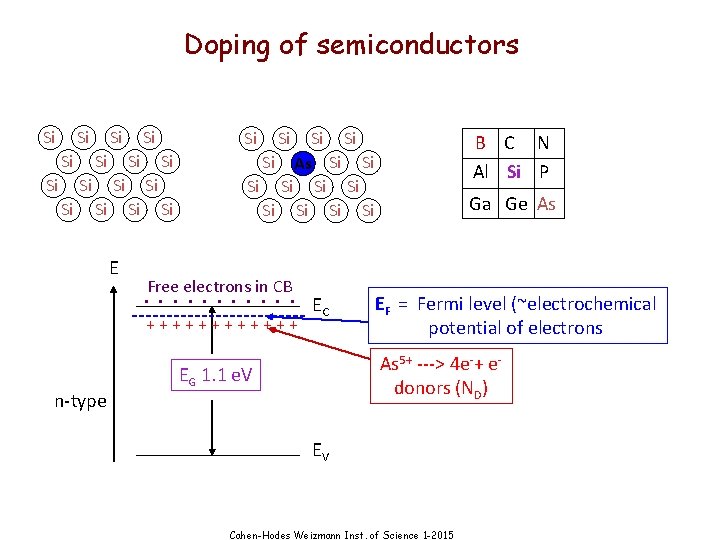
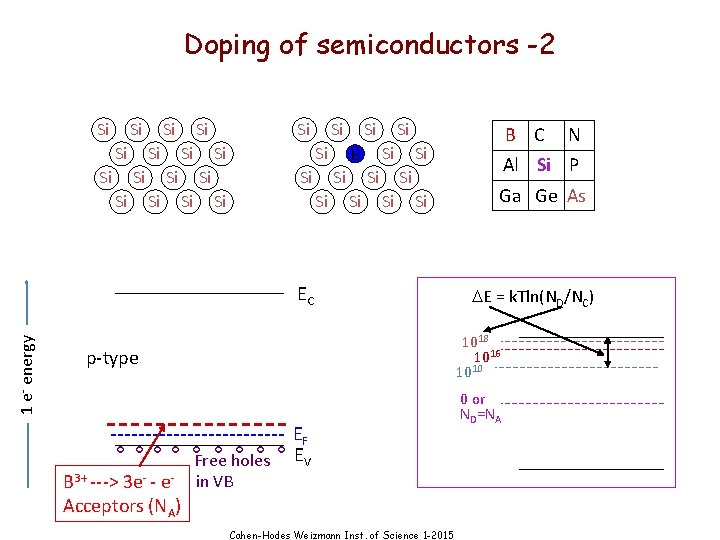
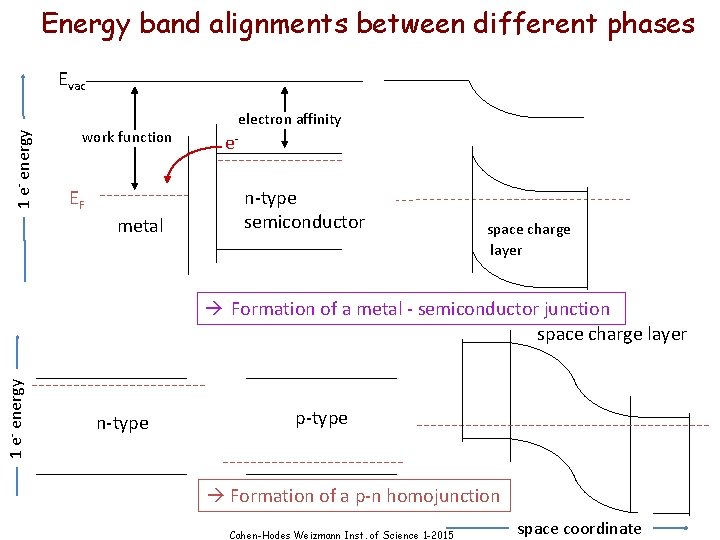
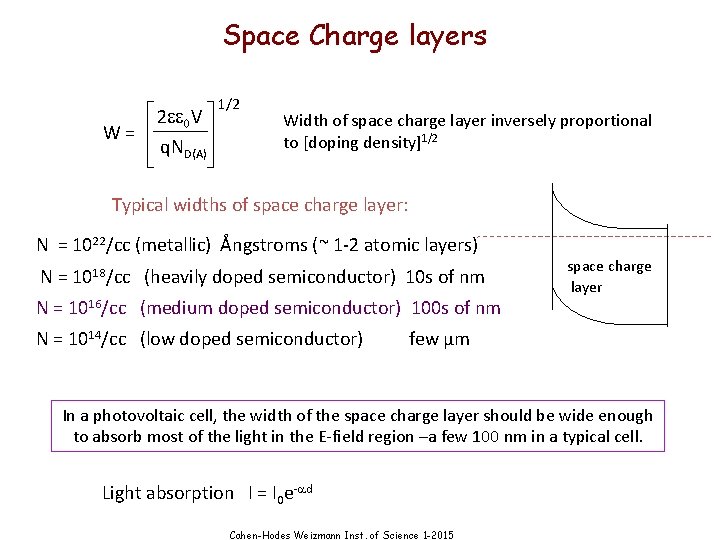
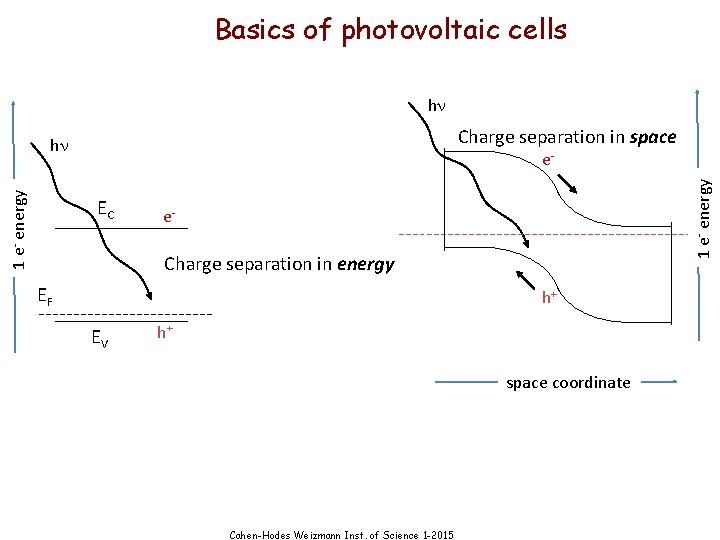
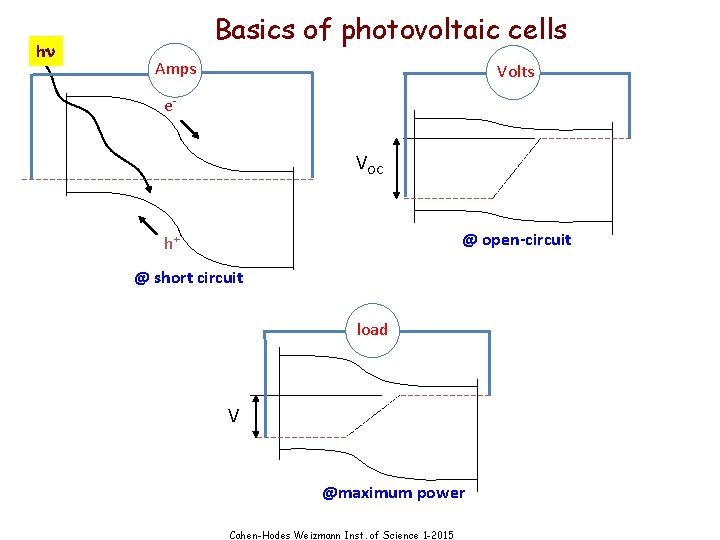
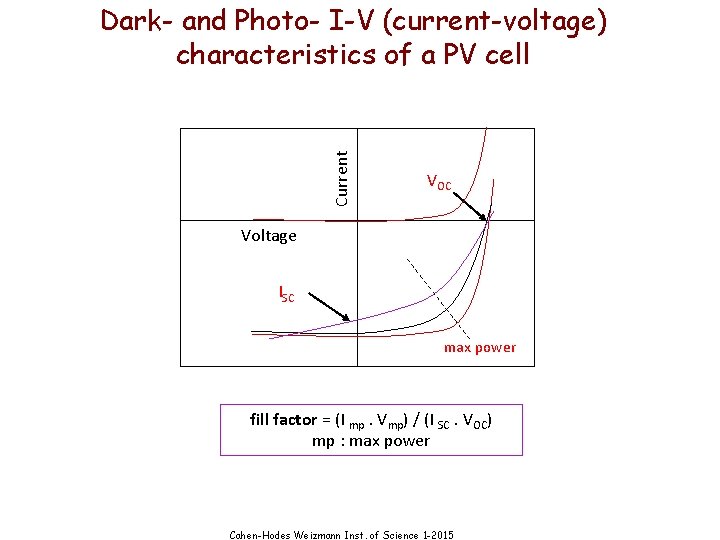
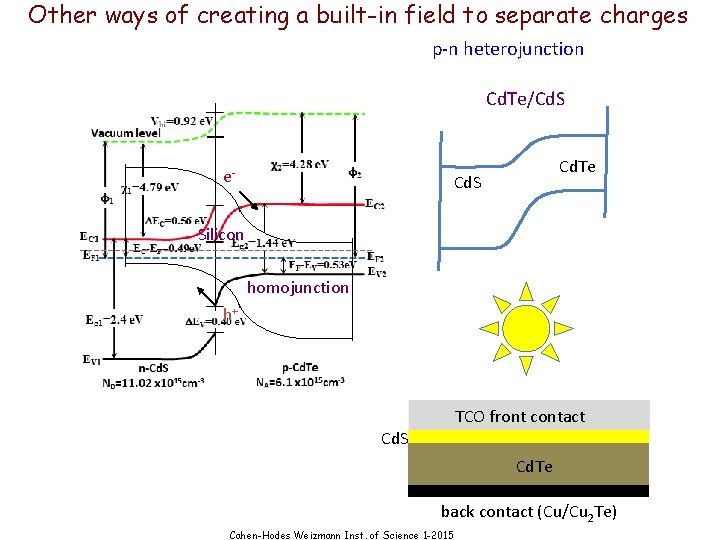
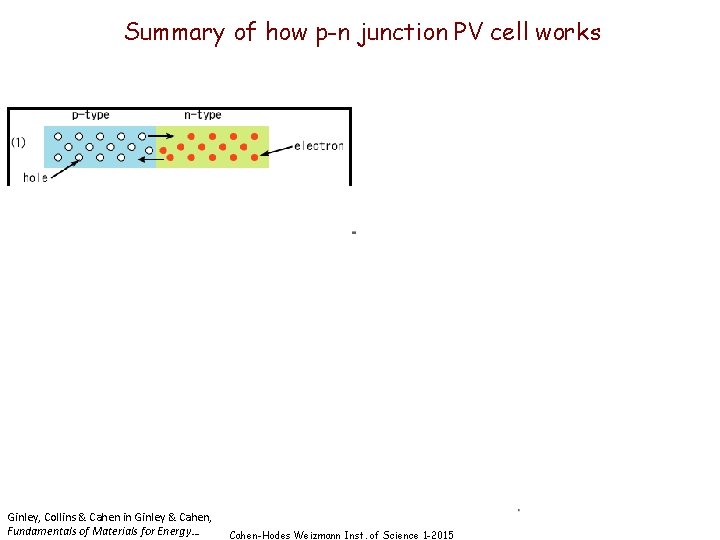
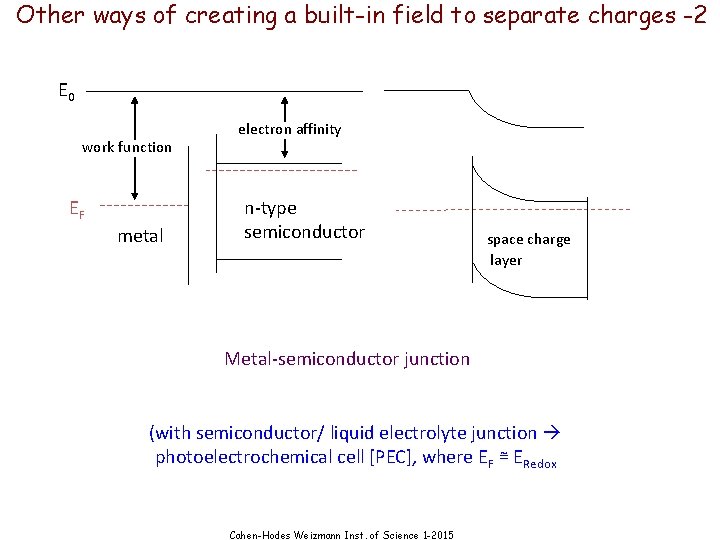
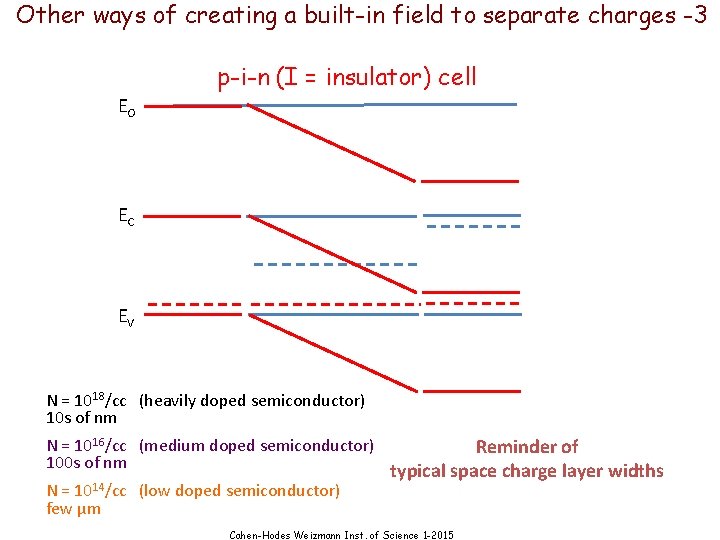
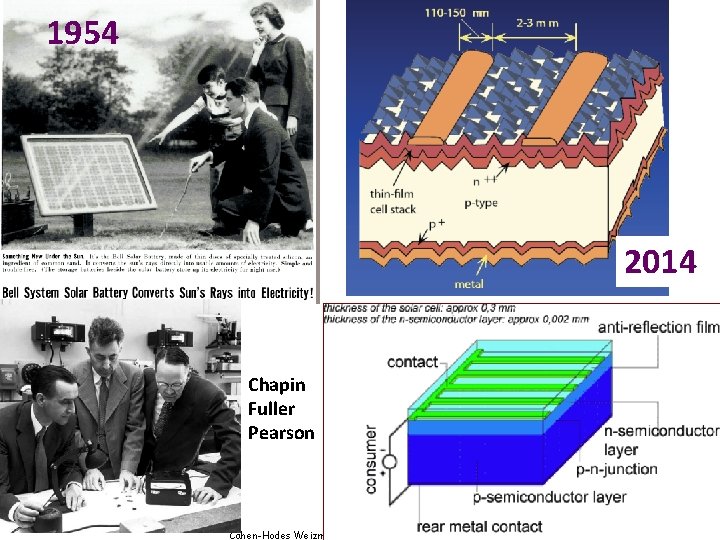
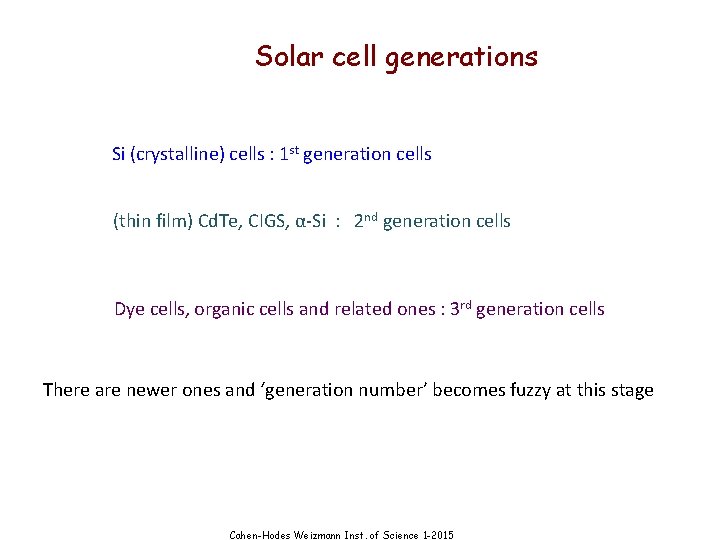
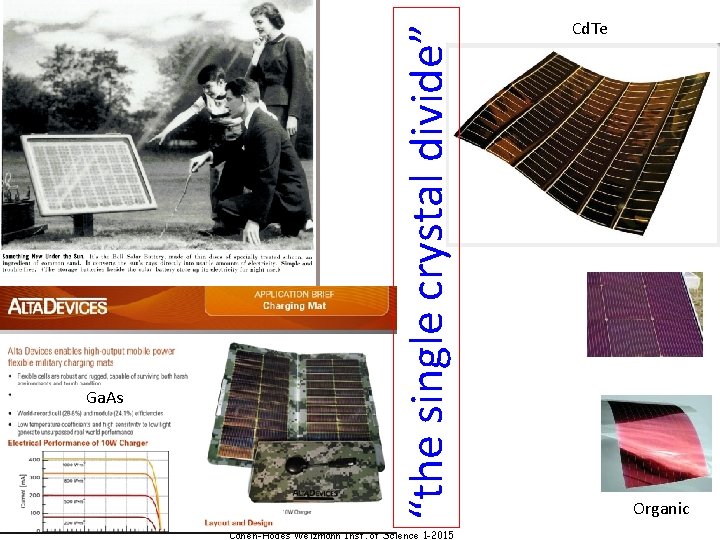
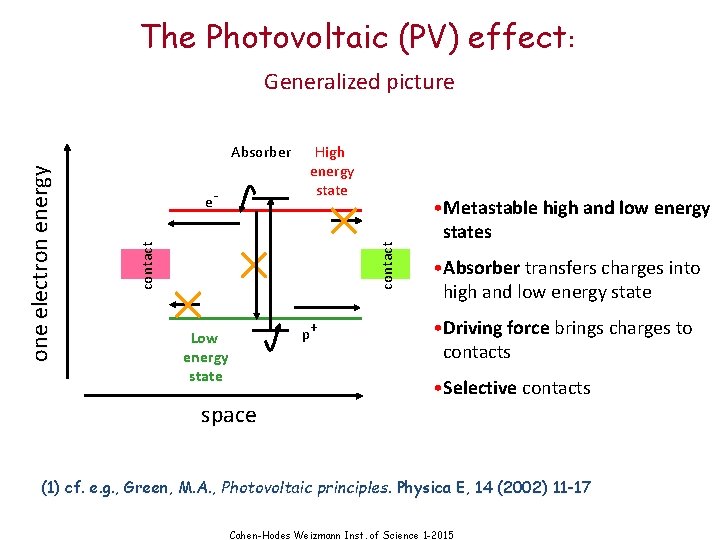
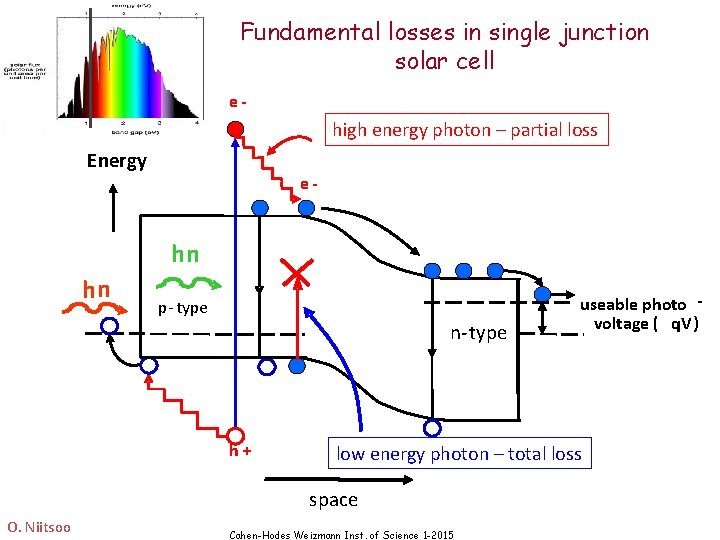
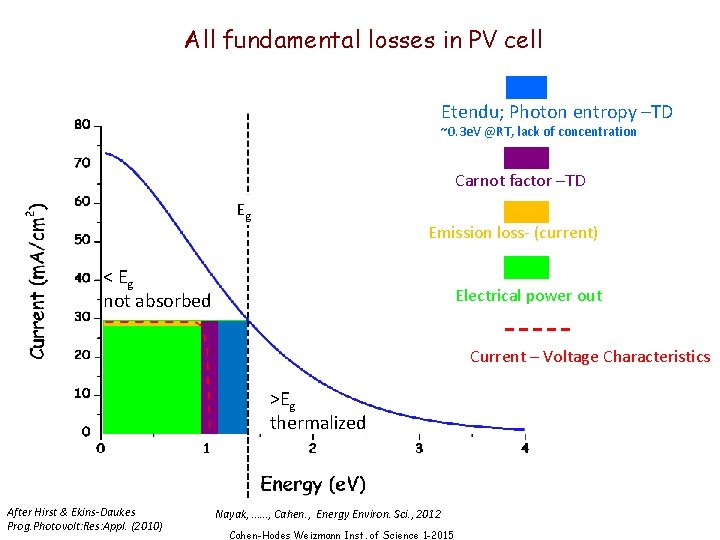
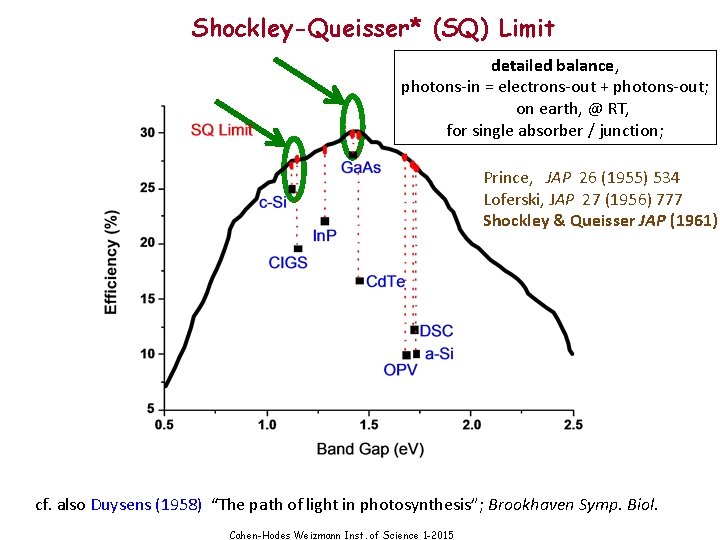
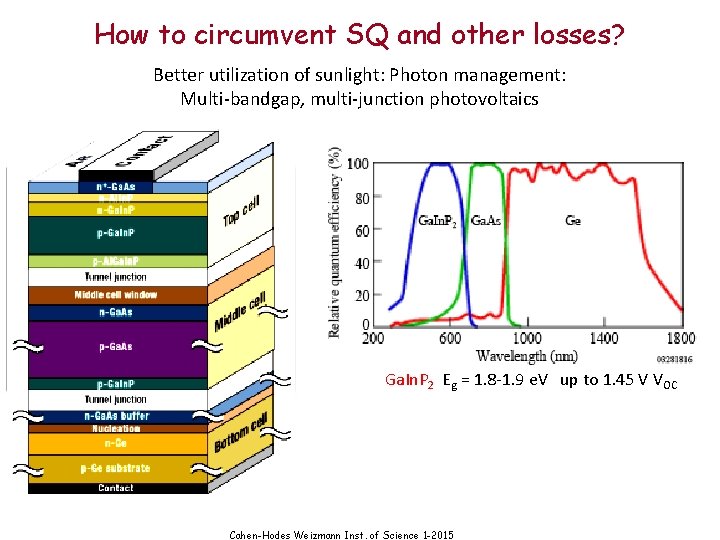
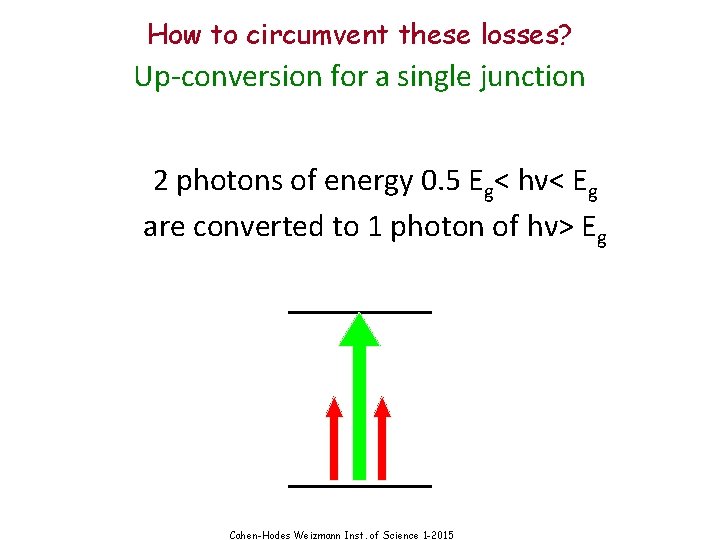
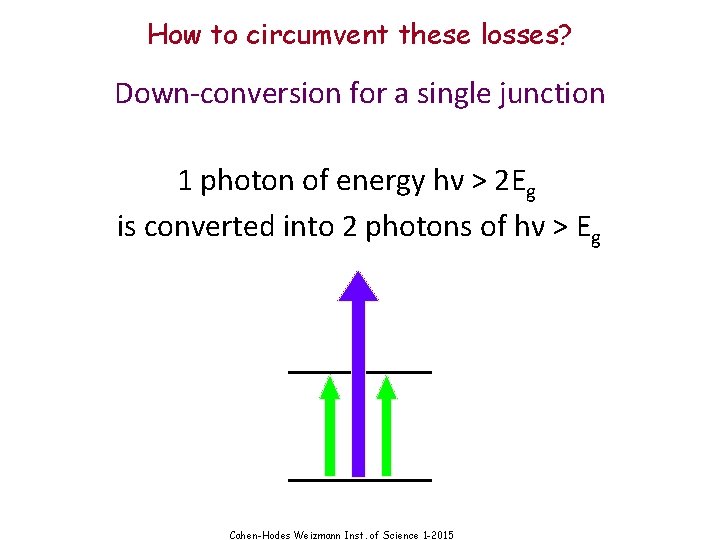
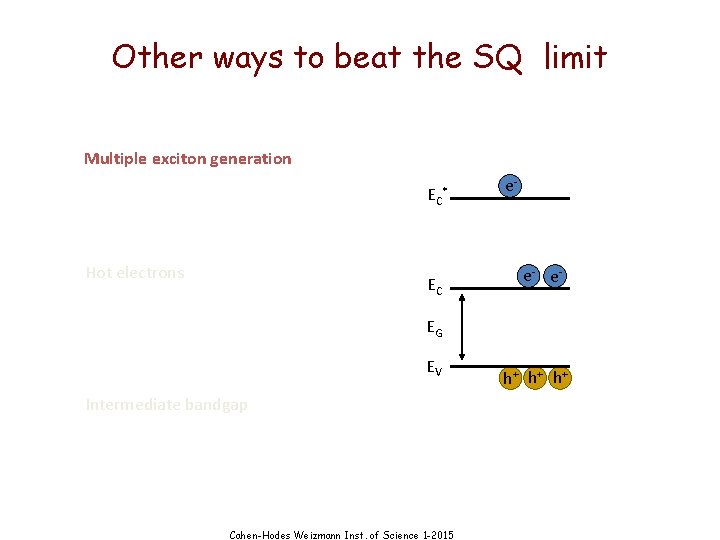
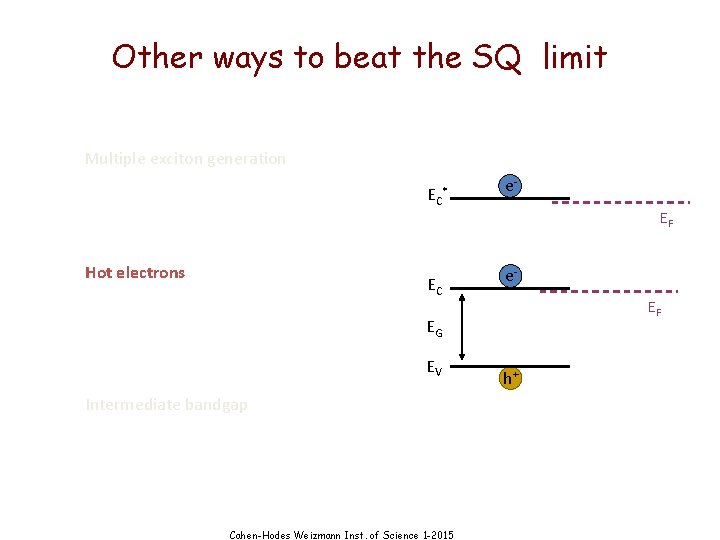
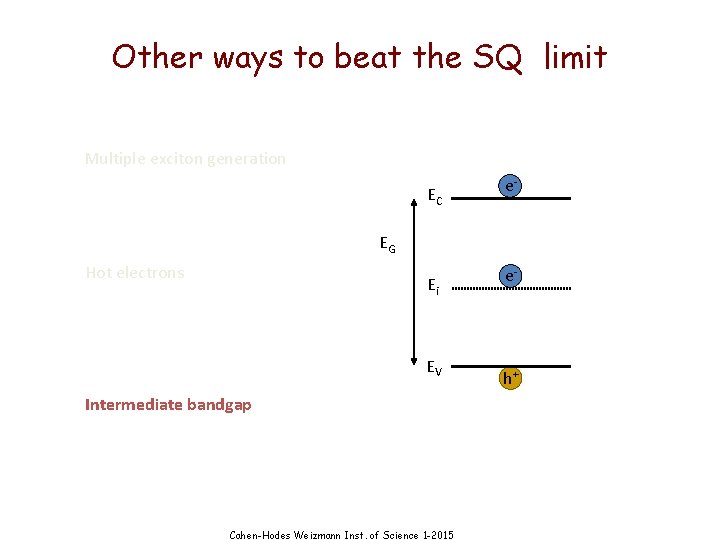
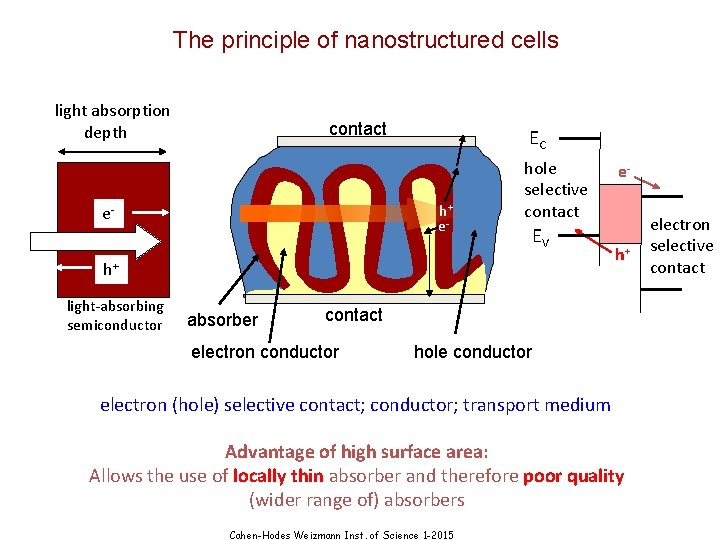
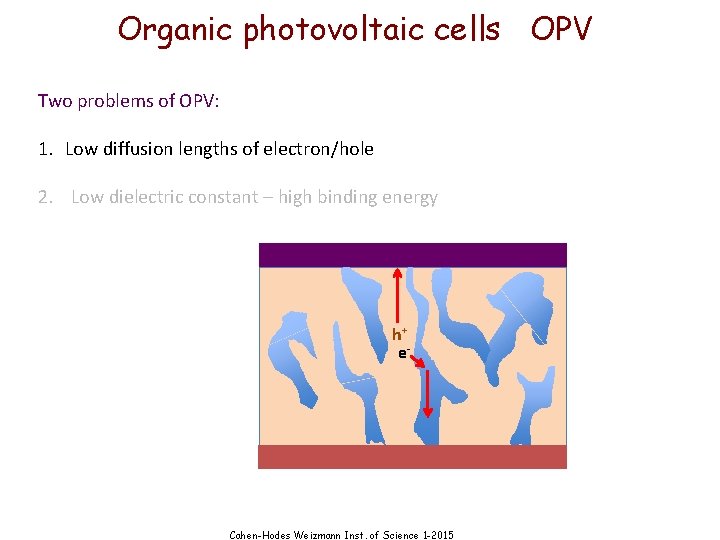
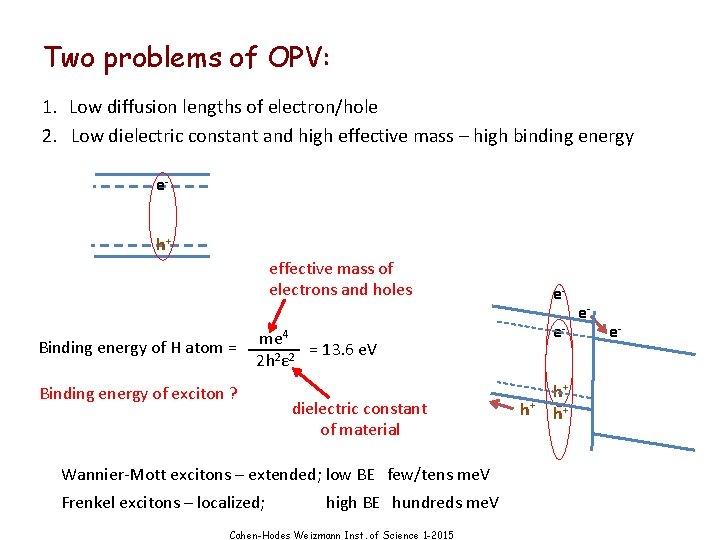
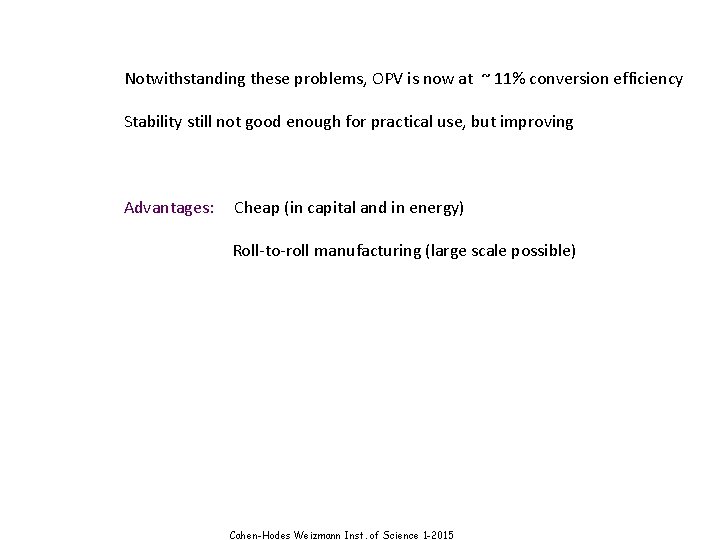
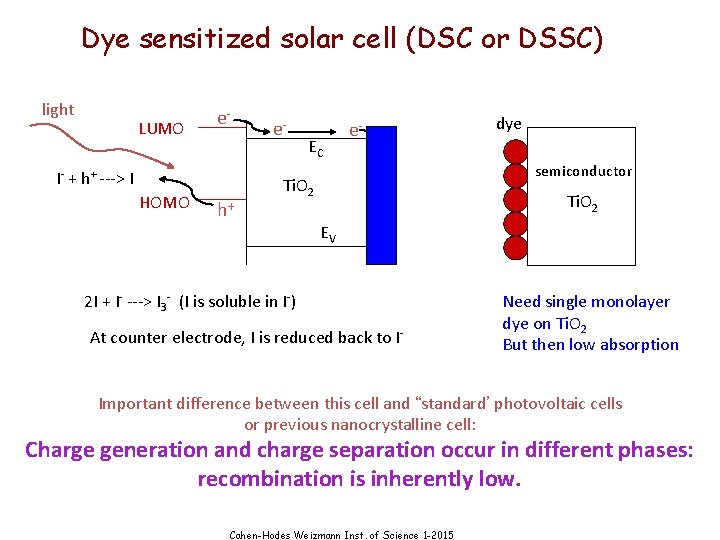
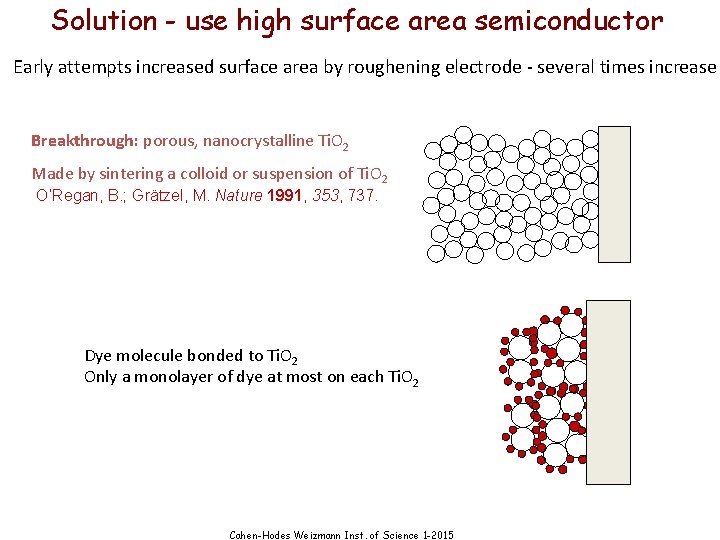
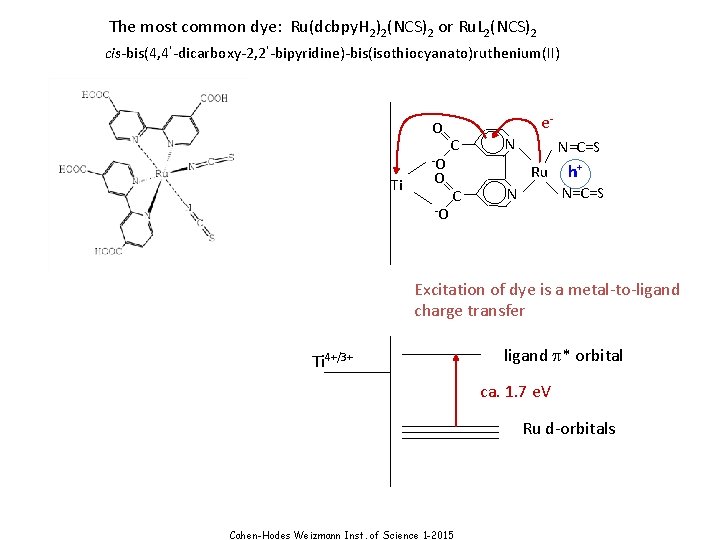
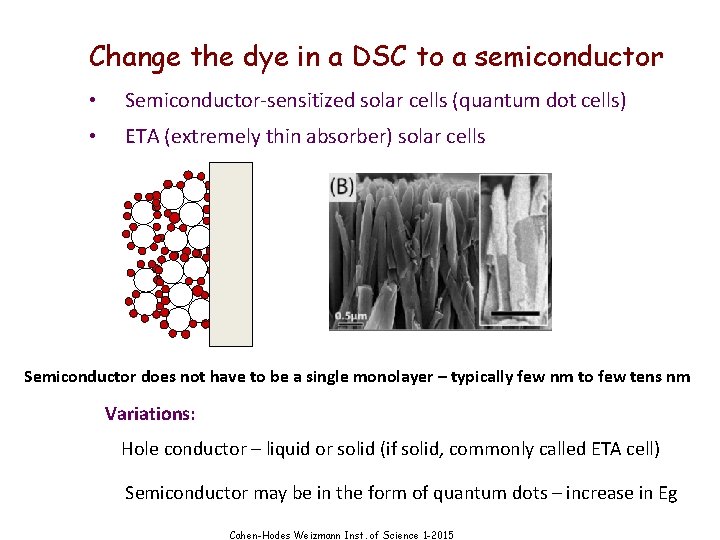
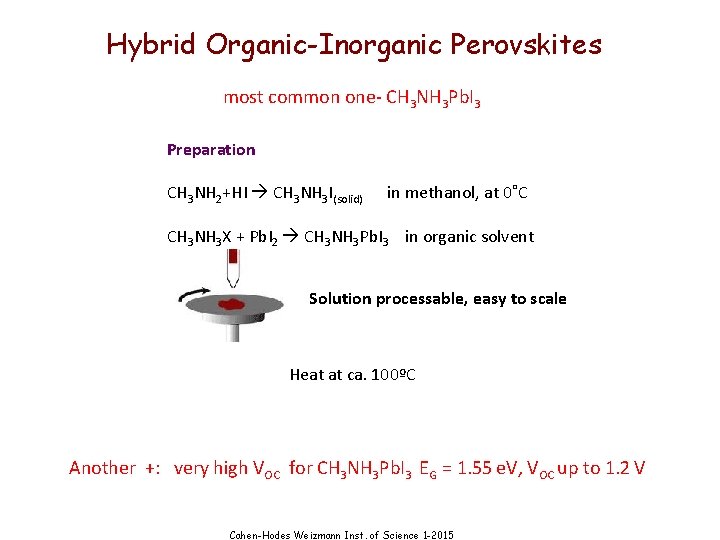
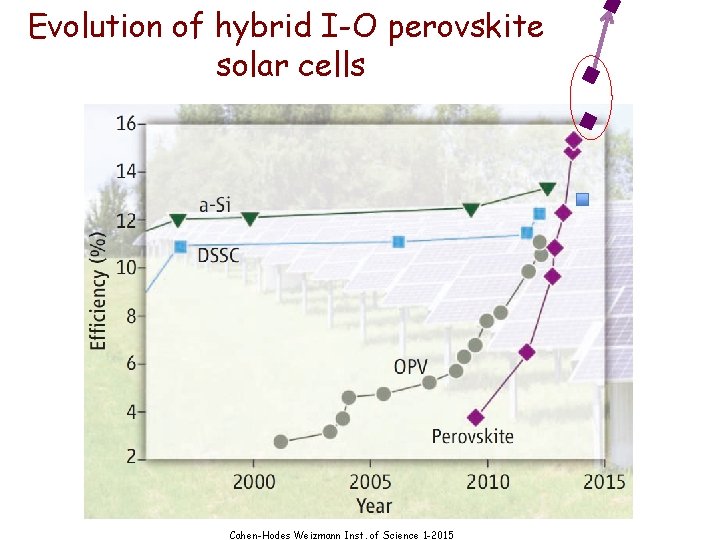
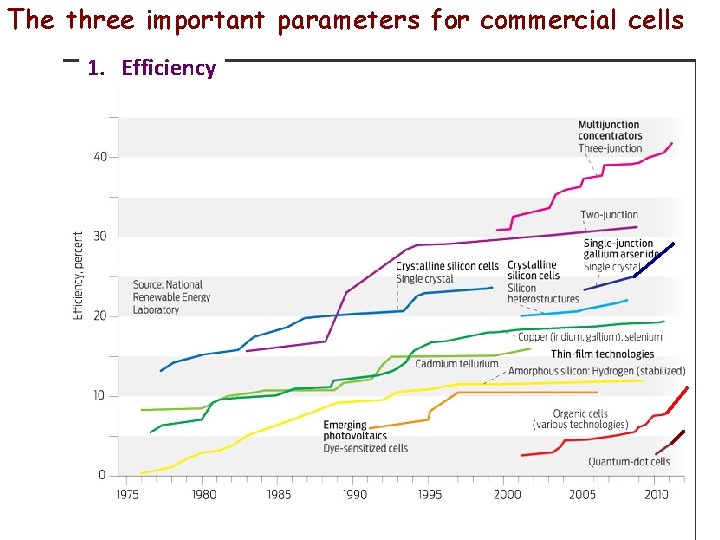
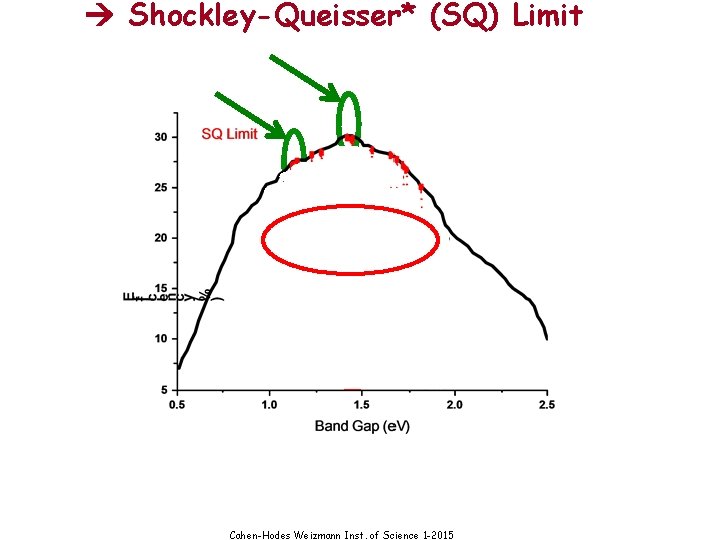
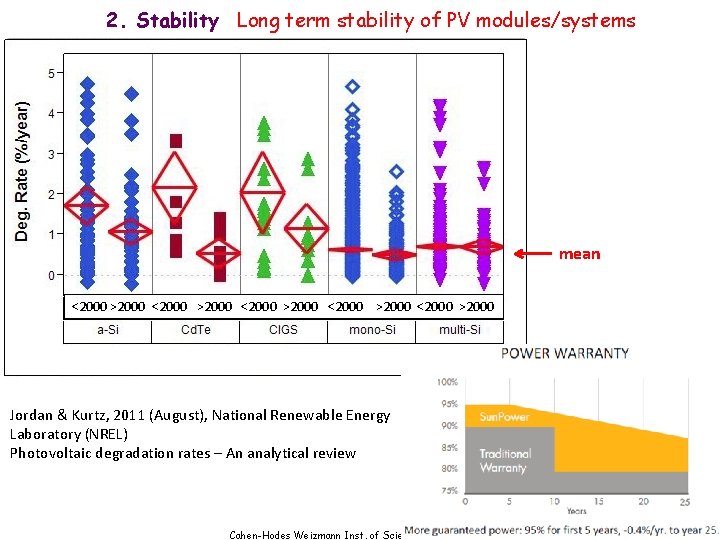
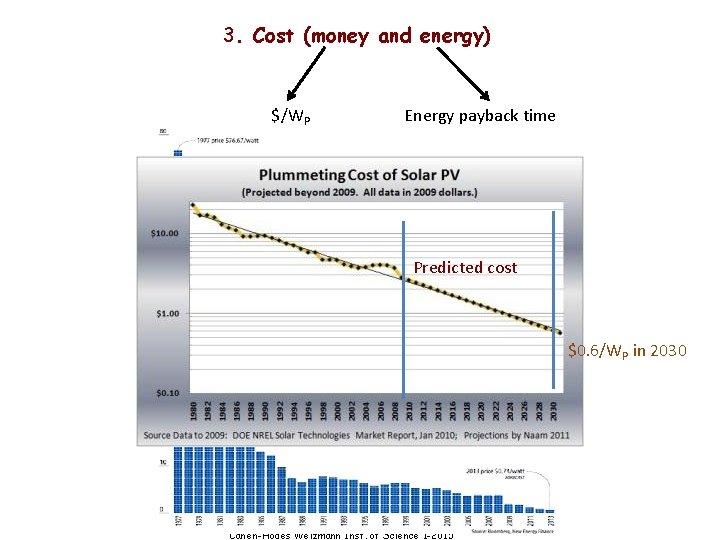
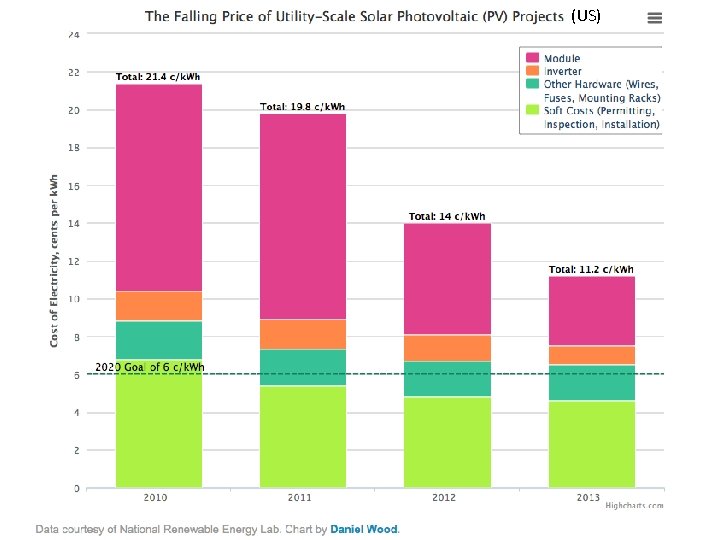
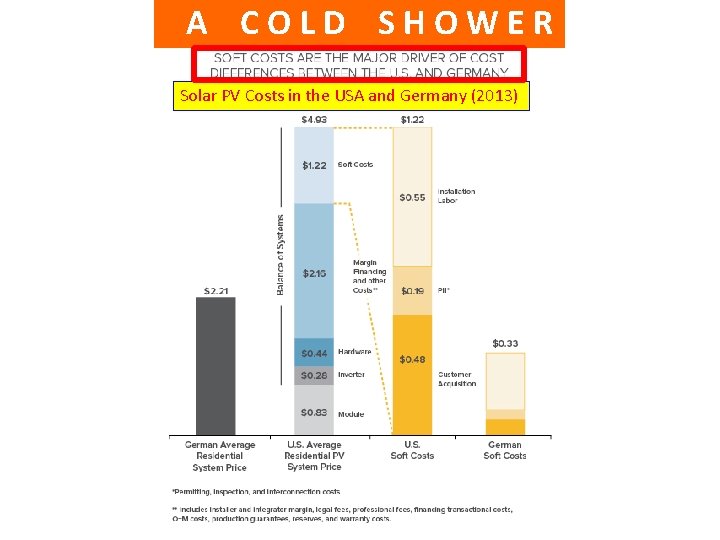
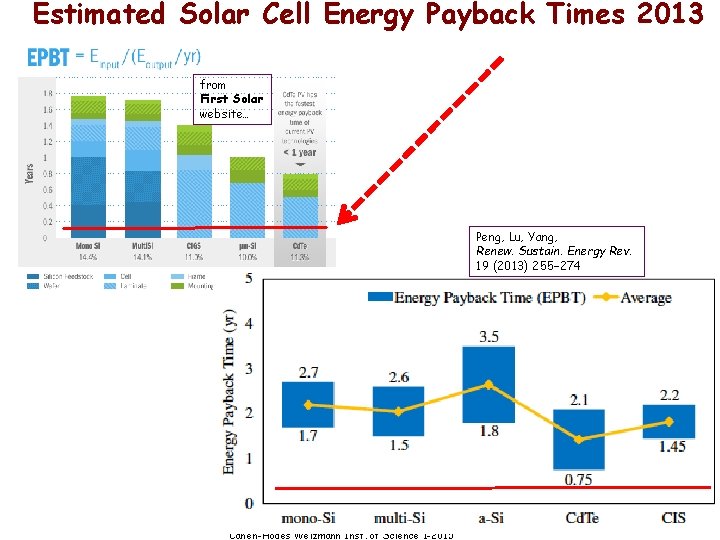
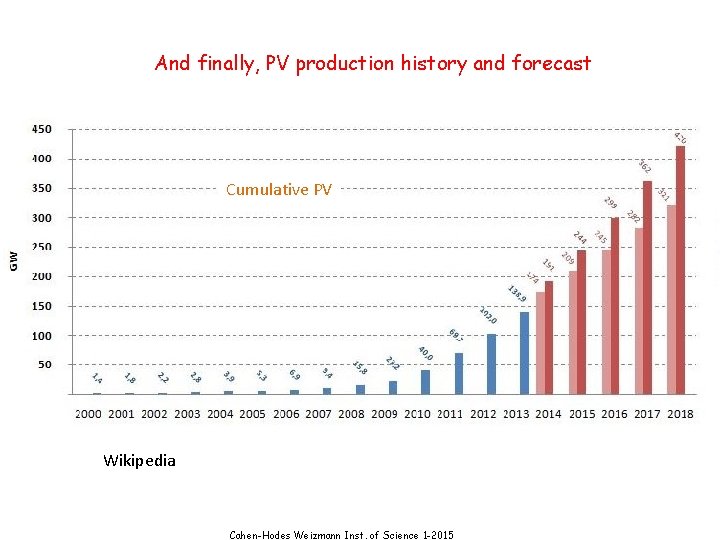
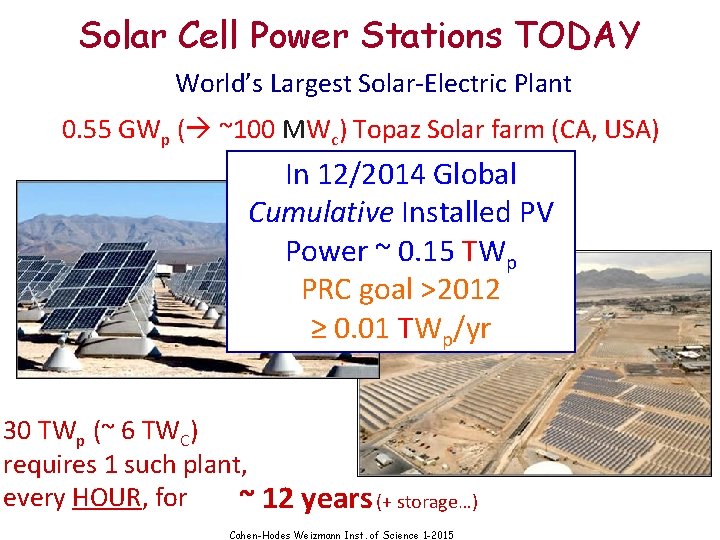
- Slides: 46
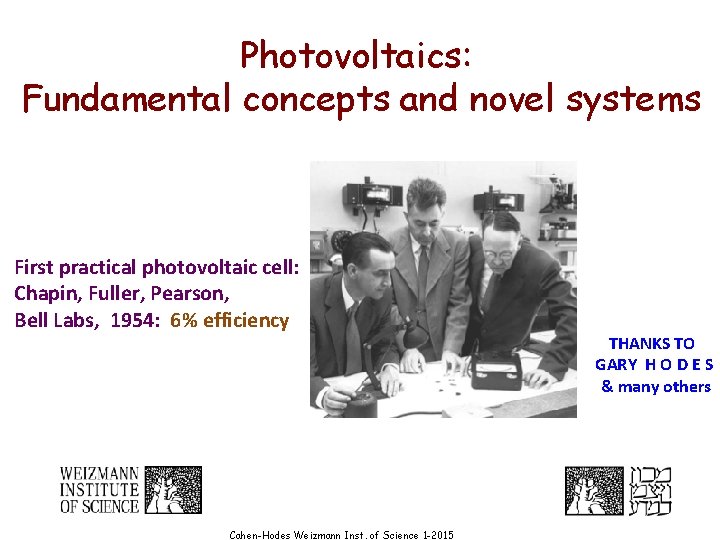
Photovoltaics: Fundamental concepts and novel systems First practical photovoltaic cell: Chapin, Fuller, Pearson, Bell Labs, 1954: 6% efficiency Cahen-Hodes Weizmann Inst. of Science 1 -2015 THANKS TO GARY H O D E S & many others
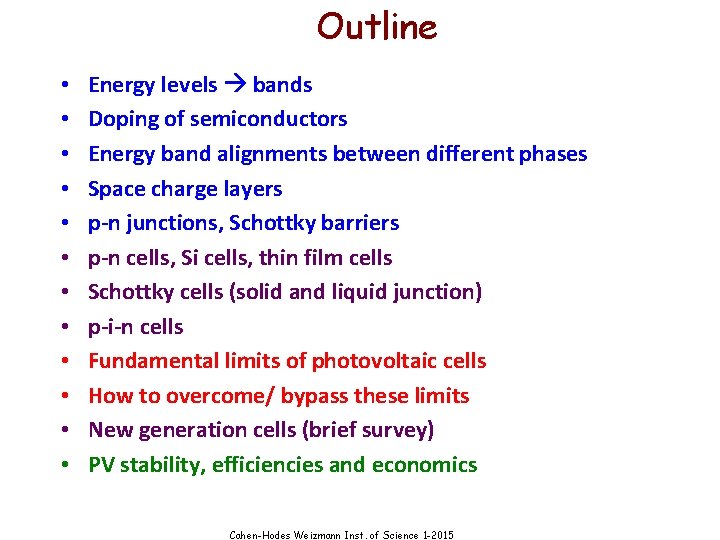
Outline • • • Energy levels bands Doping of semiconductors Energy band alignments between different phases Space charge layers p-n junctions, Schottky barriers p-n cells, Si cells, thin film cells Schottky cells (solid and liquid junction) p-i-n cells Fundamental limits of photovoltaic cells How to overcome/ bypass these limits New generation cells (brief survey) PV stability, efficiencies and economics Cahen-Hodes Weizmann Inst. of Science 1 -2015
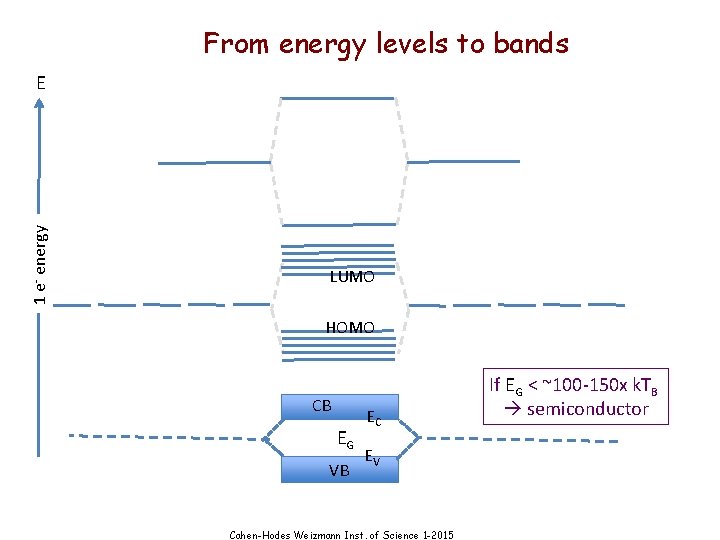
From energy levels to bands 1 e- energy E LUMO HOMO CB EG VB EC EV Cahen-Hodes Weizmann Inst. of Science 1 -2015 If EG < ~100 -150 x k. TB semiconductor
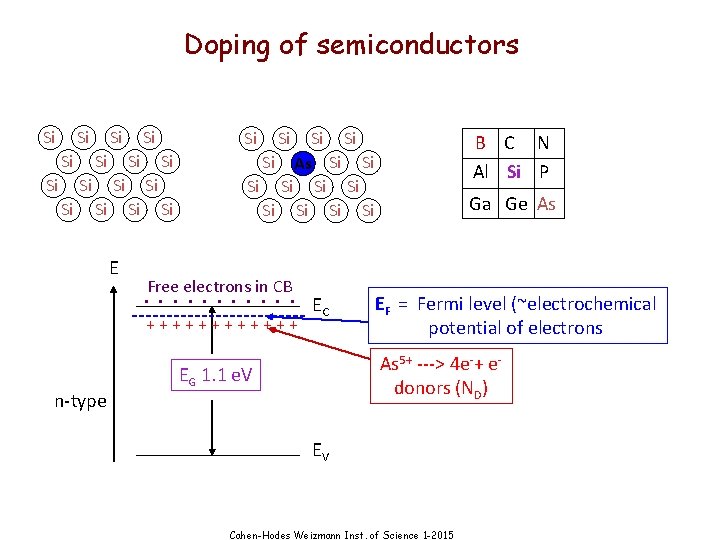
Doping of semiconductors Si Si Si Si As Si Si Si Si E Si Si Si Free electrons in CB ++++++ n-type EC B C N Al Si P Ga Ge As EF = Fermi level (~electrochemical potential of electrons As 5+ ---> 4 e-+ edonors (ND) EG 1. 1 e. V EV Cahen-Hodes Weizmann Inst. of Science 1 -2015
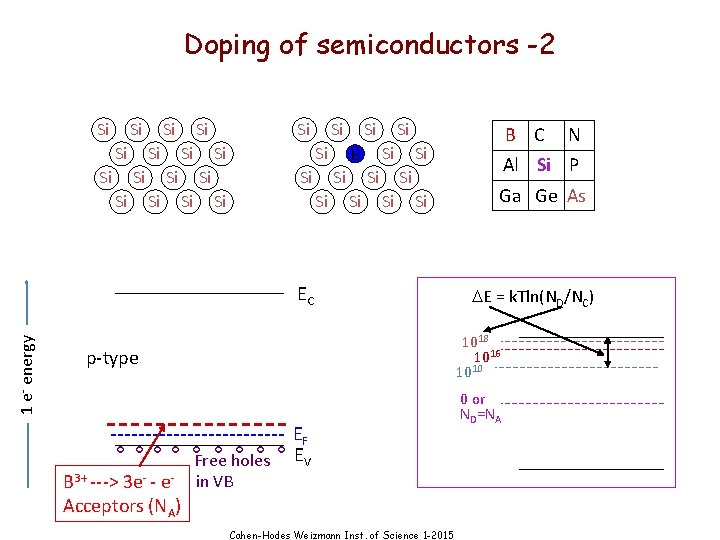
Doping of semiconductors -2 Si Si Si Si Si Si B Si Si B C N Al Si P Si Ga Ge As Si Si 1 e- energy EC 1018 1016 1010 p-type B 3+ ---> 3 e- - e. Acceptors (NA) DE = k. Tln(ND/NC) Free holes in VB EF EV Cahen-Hodes Weizmann Inst. of Science 1 -2015 0 or ND=NA
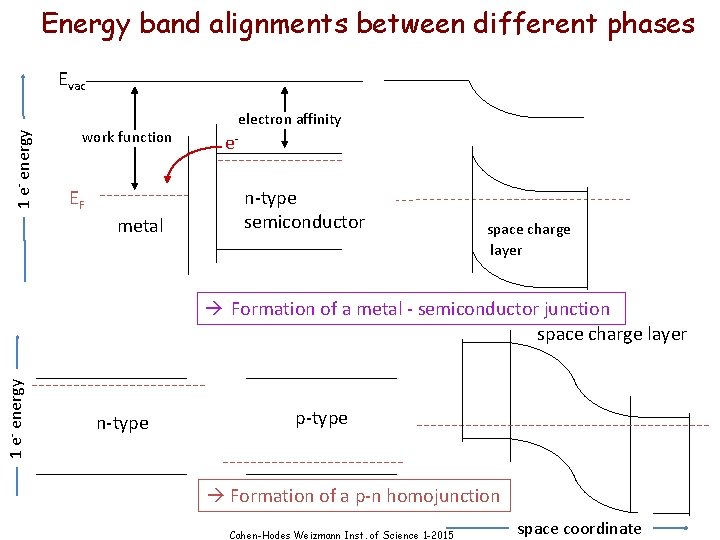
Energy band alignments between different phases 1 e- energy Evac work function EF metal electron affinity en-type semiconductor space charge layer 1 e- energy Formation of a metal - semiconductor junction space charge layer n-type p-type Formation of a p-n homojunction Cahen-Hodes Weizmann Inst. of Science 1 -2015 space coordinate
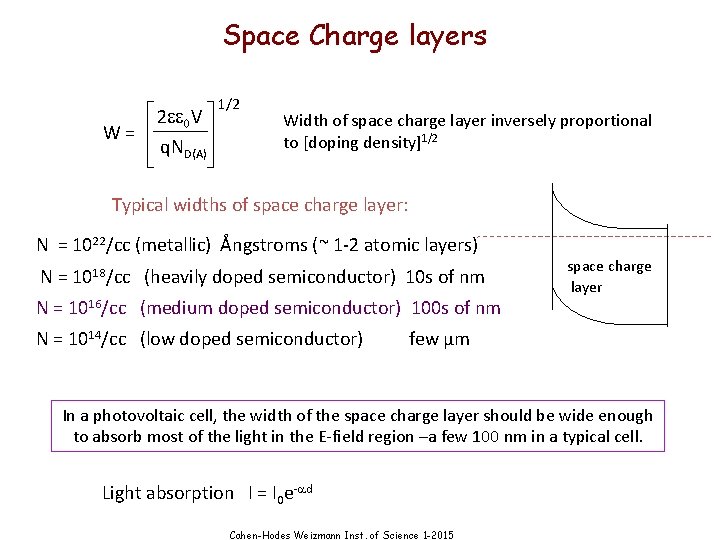
Space Charge layers W= 2 ee 0 V q. ND(A) 1/2 Width of space charge layer inversely proportional to [doping density]1/2 Typical widths of space charge layer: N = 1022/cc (metallic) Ångstroms (~ 1 -2 atomic layers) N = 1018/cc (heavily doped semiconductor) 10 s of nm N = 1016/cc (medium doped semiconductor) 100 s of nm N = 1014/cc (low doped semiconductor) space charge layer few µm In a photovoltaic cell, the width of the space charge layer should be wide enough to absorb most of the light in the E-field region –a few 100 nm in a typical cell. Light absorption I = I 0 e-ad Cahen-Hodes Weizmann Inst. of Science 1 -2015
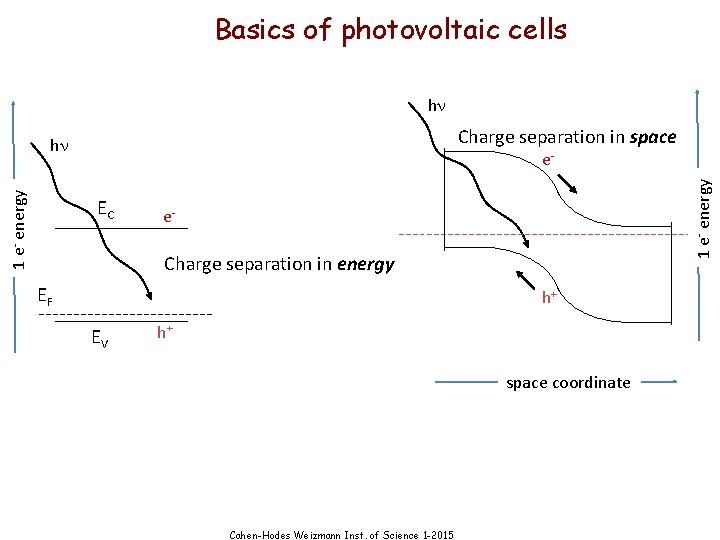
Basics of photovoltaic cells hn Charge separation in space e- EC 1 e- energy hn e- Charge separation in energy EF h+ EV h+ space coordinate Cahen-Hodes Weizmann Inst. of Science 1 -2015
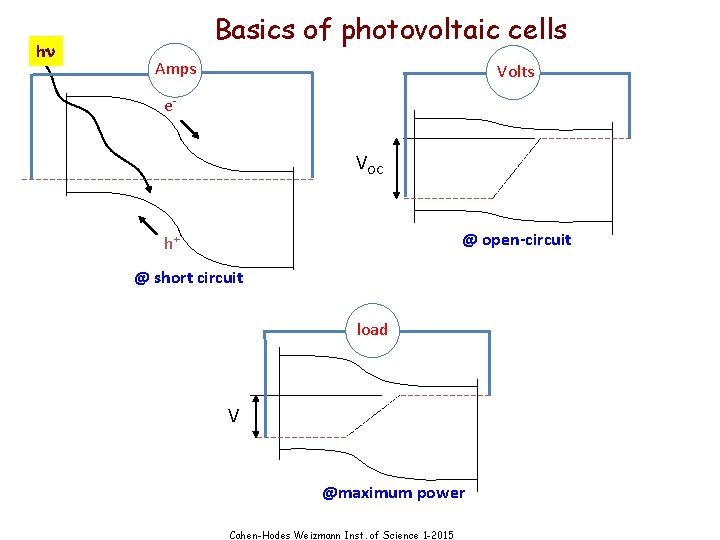
hn Basics of photovoltaic cells Amps Volts e- VOC @ open-circuit h+ @ short circuit load V @maximum power Cahen-Hodes Weizmann Inst. of Science 1 -2015
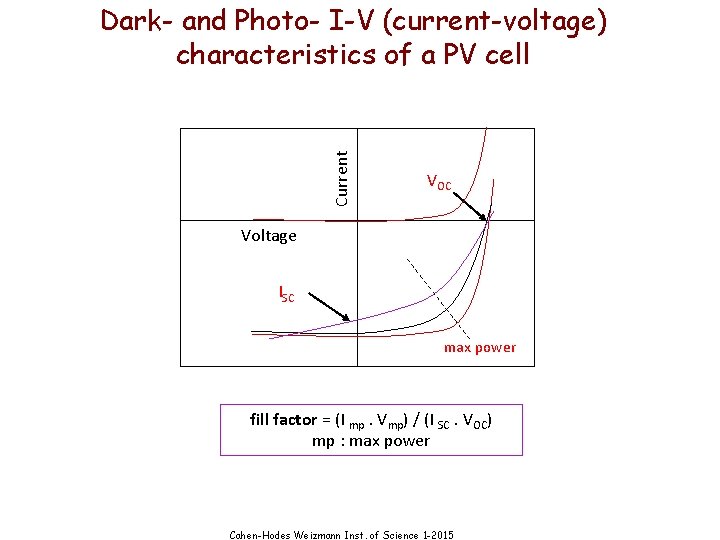
Current Dark- and Photo- I-V (current-voltage) characteristics of a PV cell VOC Voltage ISC max power fill factor = (I mp. Vmp) / (I SC. VOC) mp : max power Cahen-Hodes Weizmann Inst. of Science 1 -2015
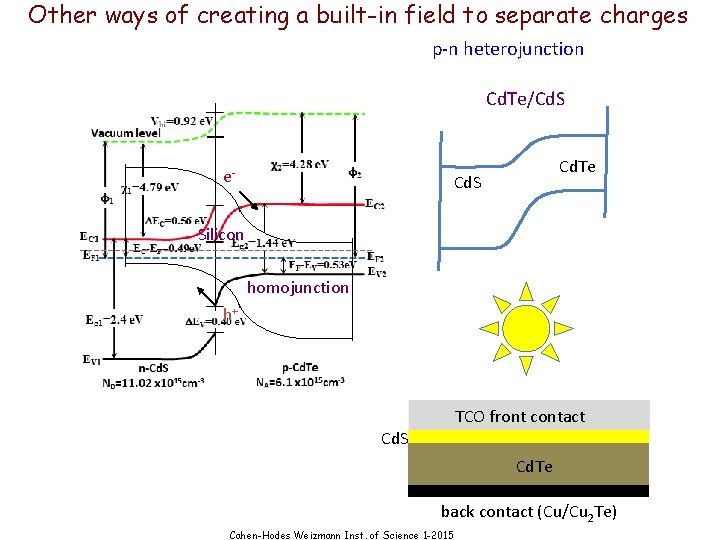
Other ways of creating a built-in field to separate charges p-n heterojunction Cd. Te/Cd. S e- Cd. Te Cd. S Silicon homojunction h+ TCO front contact Cd. S Cd. Te back contact (Cu/Cu 2 Te) Cahen-Hodes Weizmann Inst. of Science 1 -2015
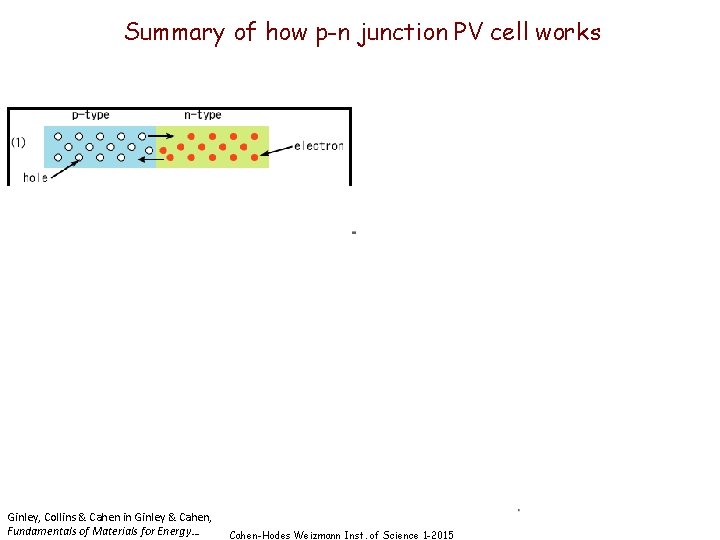
Summary of how p-n junction PV cell works 1 e- energy • Absorb light • Absorbed light creates carriers • Carrier collection, by diffusion, drift space Ginley, Collins & Cahen in Ginley & Cahen, Fundamentals of Materials for Energy… Cahen-Hodes Weizmann Inst. of Science 1 -2015
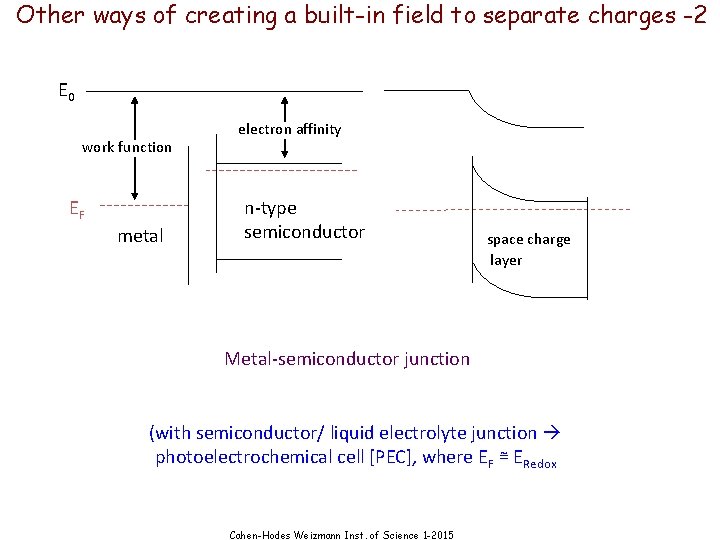
Other ways of creating a built-in field to separate charges -2 E 0 work function EF metal electron affinity n-type semiconductor space charge layer Metal-semiconductor junction (with semiconductor/ liquid electrolyte junction photoelectrochemical cell [PEC], where EF ≅ ERedox Cahen-Hodes Weizmann Inst. of Science 1 -2015
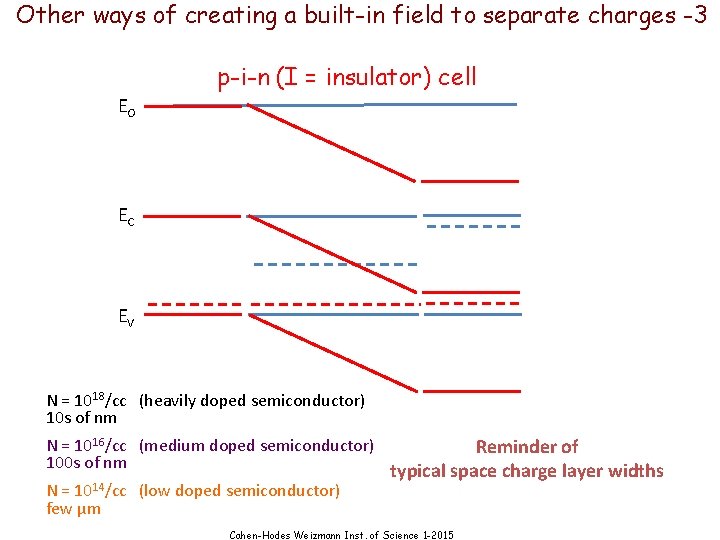
Other ways of creating a built-in field to separate charges -3 p-i-n (I = insulator) cell EO EC EV N = 1018/cc (heavily doped semiconductor) 10 s of nm N = 1016/cc (medium doped semiconductor) Reminder of 100 s of nm typical space charge layer widths 14 N = 10 /cc (low doped semiconductor) few µm Cahen-Hodes Weizmann Inst. of Science 1 -2015
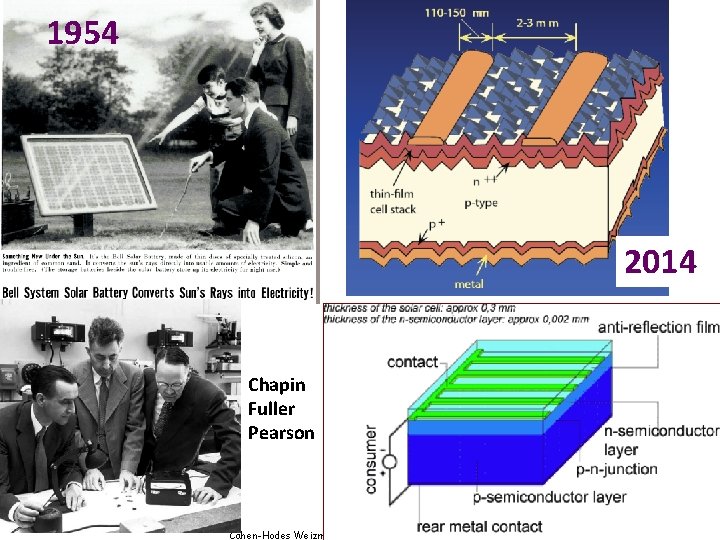
1954 2014 Chapin Fuller Pearson Cahen-Hodes Weizmann Inst. of Science 1 -2015
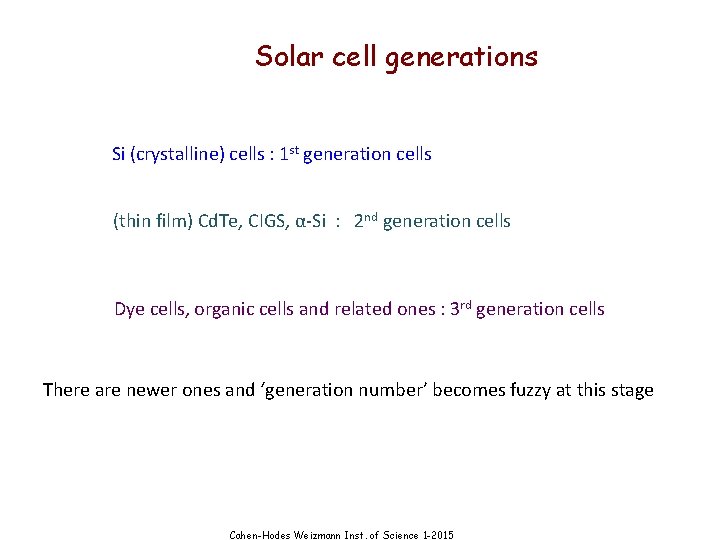
Solar cell generations Si (crystalline) cells : 1 st generation cells (thin film) Cd. Te, CIGS, α-Si : 2 nd generation cells Dye cells, organic cells and related ones : 3 rd generation cells There are newer ones and ‘generation number’ becomes fuzzy at this stage Cahen-Hodes Weizmann Inst. of Science 1 -2015
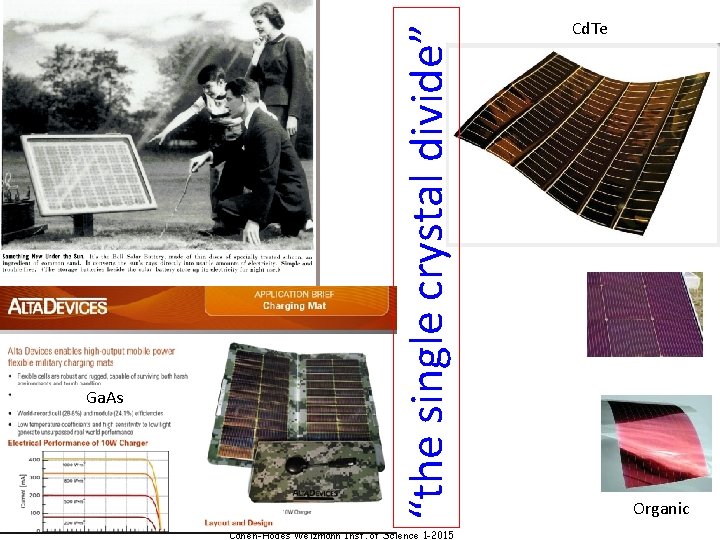
“the single crystal divide” Ga. As Cahen-Hodes Weizmann Inst. of Science 1 -2015 Cd. Te Organic
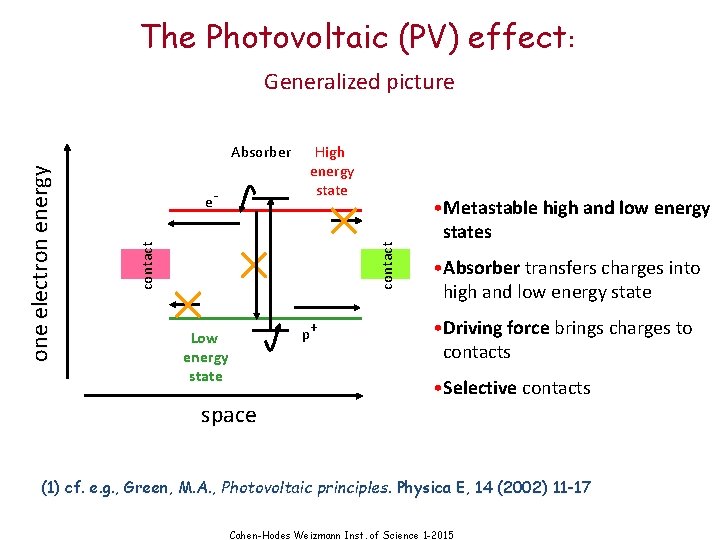
The Photovoltaic (PV) effect: Generalized picture contact e- High energy state contact one electron energy Absorber p+ Low energy state space • Metastable high and low energy states • Absorber transfers charges into high and low energy state • Driving force brings charges to contacts • Selective contacts (1) cf. e. g. , Green, M. A. , Photovoltaic principles. Physica E, 14 (2002) 11 -17 Cahen-Hodes Weizmann Inst. of Science 1 -2015
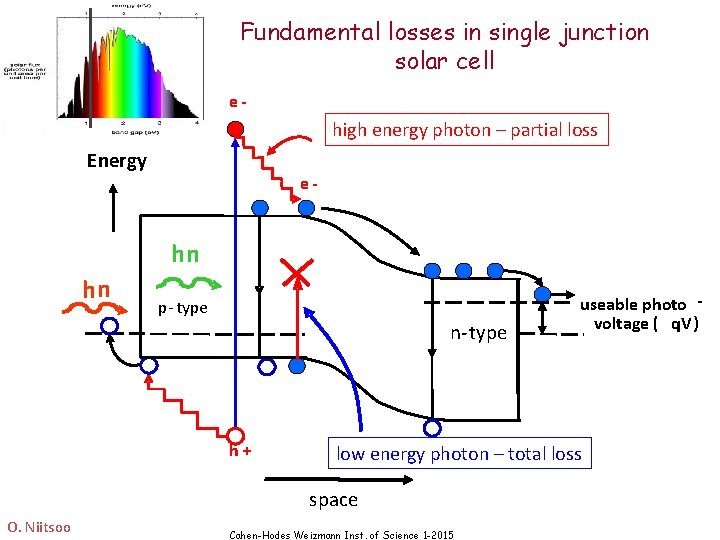
Fundamental losses in single junction solar cell e- high energy photon – partial loss Energy e- hn hn p- type n- type h+ low energy photon – total loss space O. Niitsoo useable photo voltage ( q. V) Cahen-Hodes Weizmann Inst. of Science 1 -2015
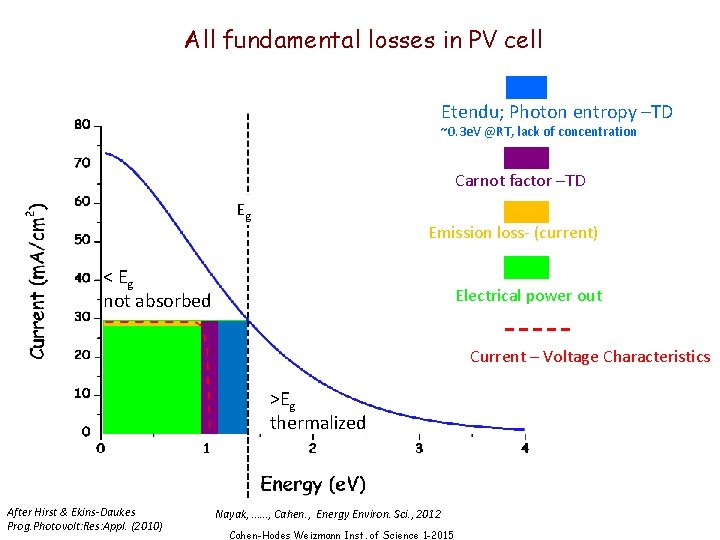
All fundamental losses in PV cell Etendu; Photon entropy –TD ~0. 3 e. V @RT, lack of concentration Carnot factor –TD Eg Emission loss- (current) < Eg not absorbed Electrical power out Current – Voltage Characteristics >Eg thermalized After Hirst & Ekins-Daukes Prog. Photovolt: Res: Appl. (2010) Nayak, ……, Cahen. , Energy Environ. Sci. , 2012 Cahen-Hodes Weizmann Inst. of Science 1 -2015
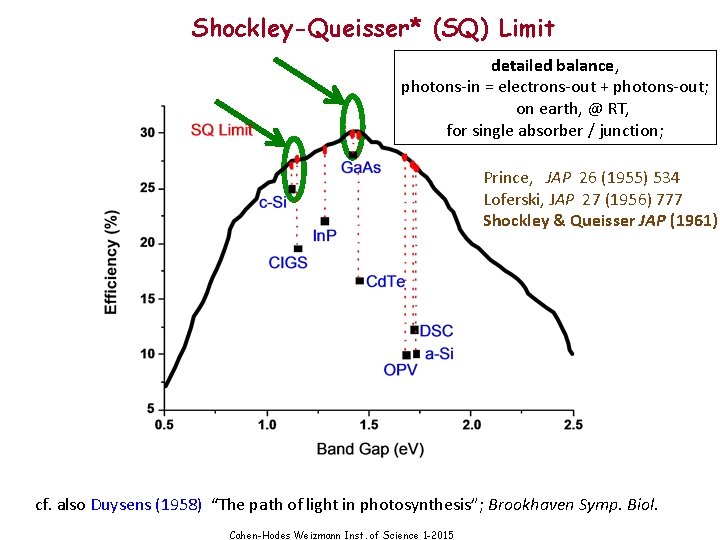
Shockley-Queisser* (SQ) Limit detailed balance, photons-in = electrons-out + photons-out; on earth, @ RT, for single absorber / junction; Prince, JAP 26 (1955) 534 Loferski, JAP 27 (1956) 777 Shockley & Queisser JAP (1961) cf. also Duysens (1958) “The path of light in photosynthesis”; Brookhaven Symp. Biol. Cahen-Hodes Weizmann Inst. of Science 1 -2015
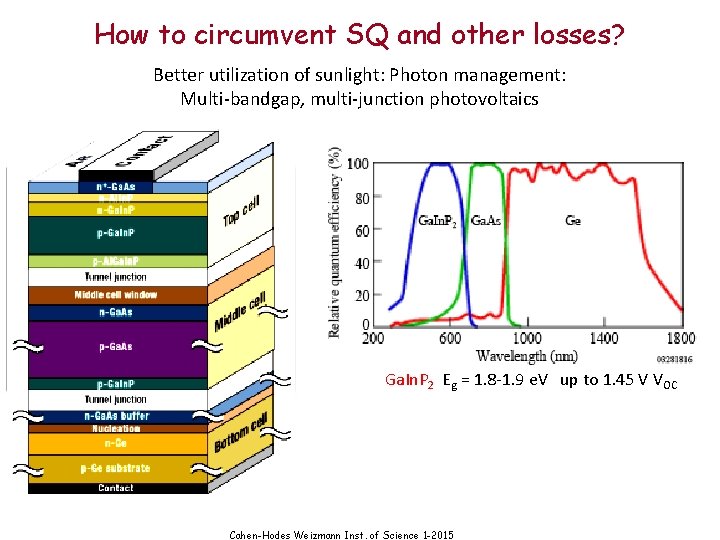
How to circumvent SQ and other losses? Better utilization of sunlight: Photon management: Multi-bandgap, multi-junction photovoltaics Ga. In. P 2 Eg = 1. 8 -1. 9 e. V up to 1. 45 V VOC Cahen-Hodes Weizmann Inst. of Science 1 -2015
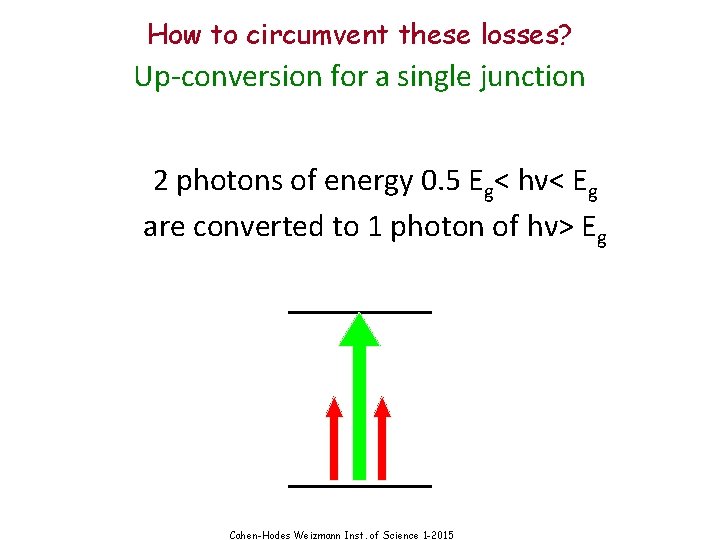
How to circumvent these losses? Up-conversion for a single junction 2 photons of energy 0. 5 Eg< hν< Eg are converted to 1 photon of hν> Eg Cahen-Hodes Weizmann Inst. of Science 1 -2015
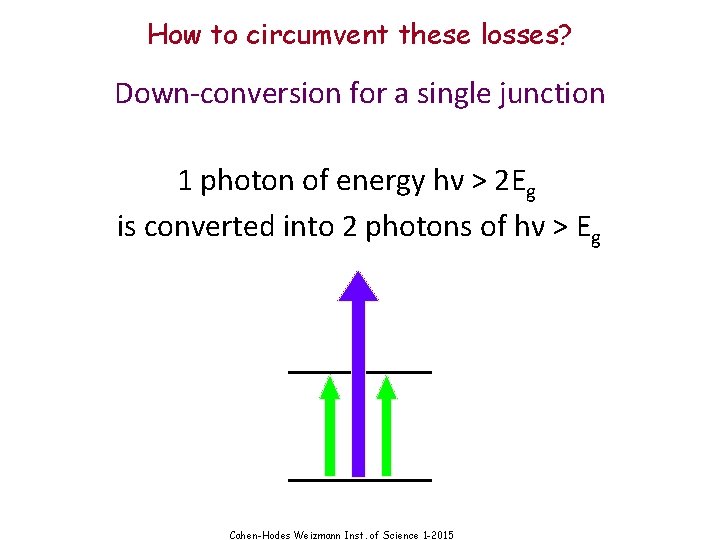
How to circumvent these losses? Down-conversion for a single junction 1 photon of energy hν > 2 Eg is converted into 2 photons of hν > Eg Cahen-Hodes Weizmann Inst. of Science 1 -2015
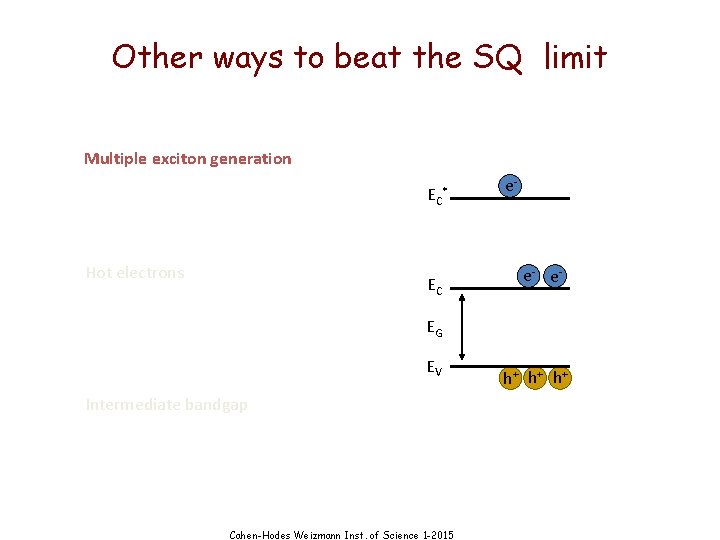
Other ways to beat the SQ limit Multiple exciton generation EC Hot electrons * EC e- e- e- EG EV Intermediate bandgap Cahen-Hodes Weizmann Inst. of Science 1 -2015 h+ h+ h+
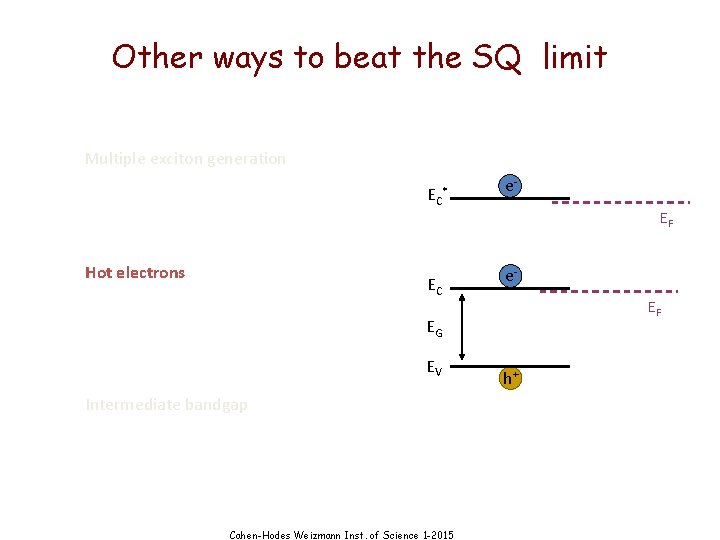
Other ways to beat the SQ limit Multiple exciton generation EC Hot electrons * EC e. EF EG EV Intermediate bandgap Cahen-Hodes Weizmann Inst. of Science 1 -2015 h+
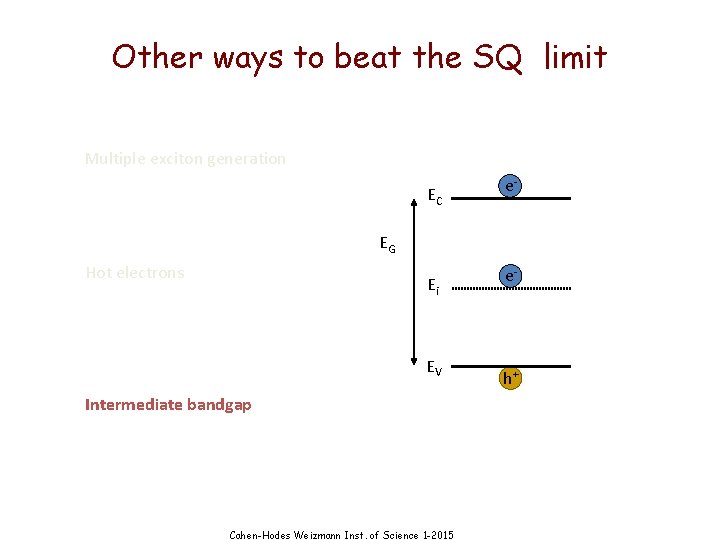
Other ways to beat the SQ limit Multiple exciton generation EC e- EG Hot electrons Ei EV Intermediate bandgap Cahen-Hodes Weizmann Inst. of Science 1 -2015 e- h+
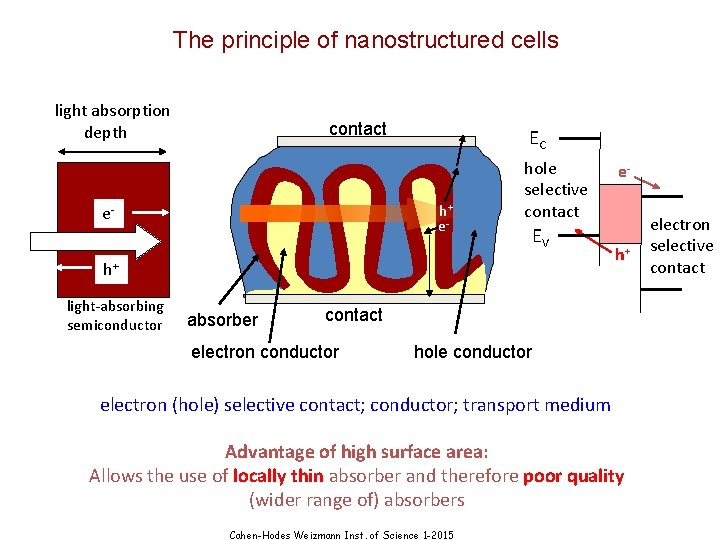
The principle of nanostructured cells light absorption depth contact EC h+ e- e- hole selective contact EV h+ light-absorbing semiconductor absorber e- h+ contact electron conductor hole conductor electron (hole) selective contact; conductor; transport medium Advantage of high surface area: Allows the use of locally thin absorber and therefore poor quality (wider range of) absorbers Cahen-Hodes Weizmann Inst. of Science 1 -2015 electron selective contact
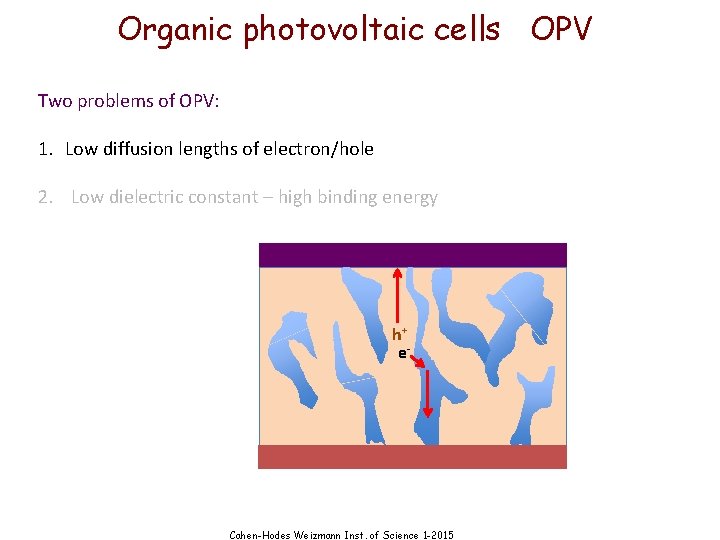
Organic photovoltaic cells OPV Two problems of OPV: 1. Low diffusion lengths of electron/hole 2. Low dielectric constant – high binding energy h+ e- Cahen-Hodes Weizmann Inst. of Science 1 -2015
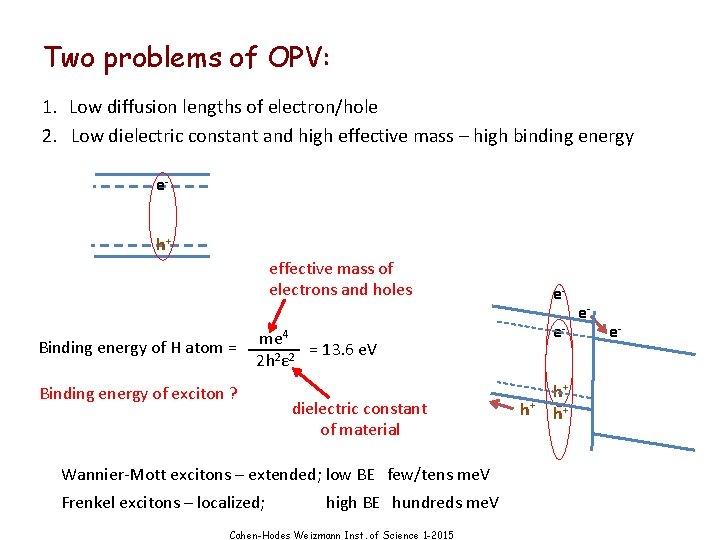
Two problems of OPV: 1. Low diffusion lengths of electron/hole 2. Low dielectric constant and high effective mass – high binding energy eh+ effective mass of electrons and holes Binding energy of H atom = ee- me 4 = 13. 6 e. V 2 h 2ε 2 Binding energy of exciton ? dielectric constant of material Wannier-Mott excitons – extended; low BE few/tens me. V Frenkel excitons – localized; high BE hundreds me. V Cahen-Hodes Weizmann Inst. of Science 1 -2015 h+ h+ h+ e- e-
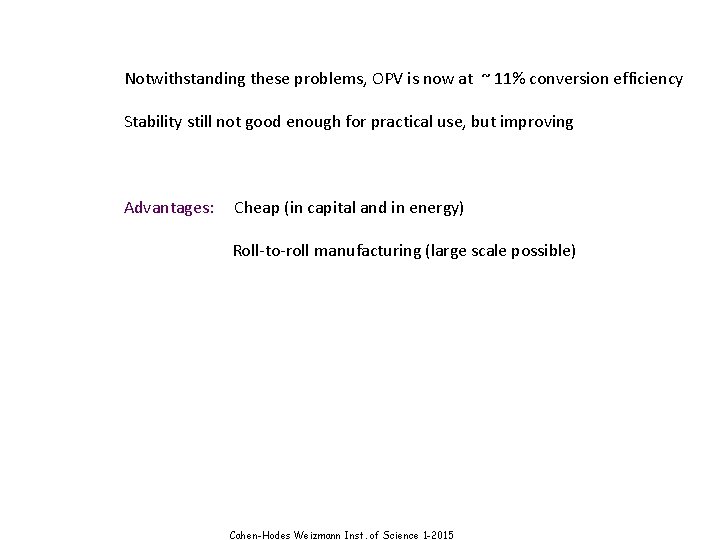
Notwithstanding these problems, OPV is now at ~ 11% conversion efficiency Stability still not good enough for practical use, but improving Advantages: Cheap (in capital and in energy) Roll-to-roll manufacturing (large scale possible) Cahen-Hodes Weizmann Inst. of Science 1 -2015
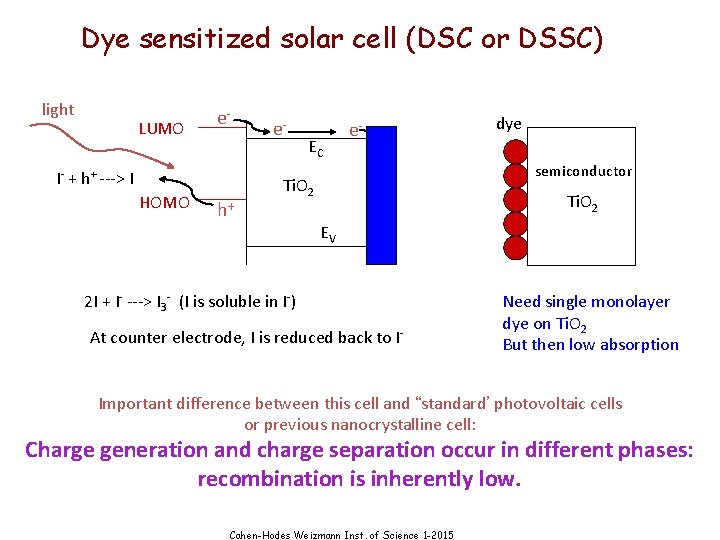
Dye sensitized solar cell (DSC or DSSC) light LUMO e- I- + h+ ---> I HOMO h+ e- EC e- dye semiconductor Ti. O 2 EV 2 I + I- ---> I 3 - (I is soluble in I-) At counter electrode, I is reduced back to I- Need single monolayer dye on Ti. O 2 But then low absorption Important difference between this cell and “standard’ photovoltaic cells or previous nanocrystalline cell: Charge generation and charge separation occur in different phases: recombination is inherently low. Cahen-Hodes Weizmann Inst. of Science 1 -2015
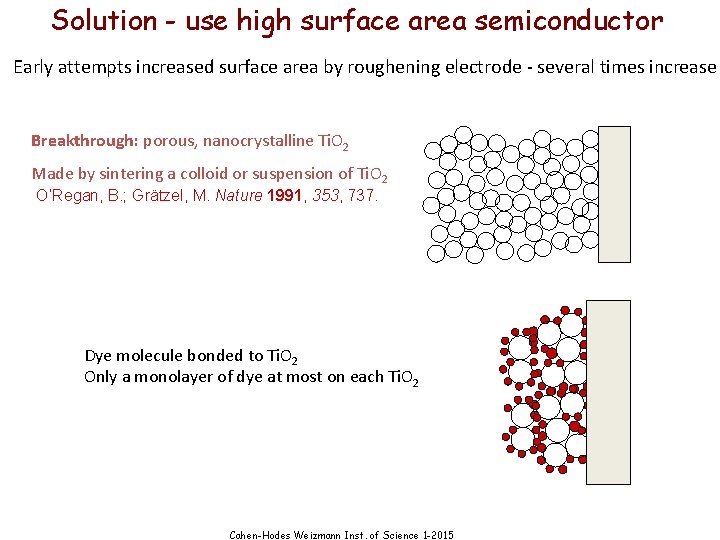
Solution - use high surface area semiconductor Early attempts increased surface area by roughening electrode - several times increase Breakthrough: porous, nanocrystalline Ti. O 2 Made by sintering a colloid or suspension of Ti. O 2 O’Regan, B. ; Grätzel, M. Nature 1991, 353, 737. Dye molecule bonded to Ti. O 2 Only a monolayer of dye at most on each Ti. O 2 Cahen-Hodes Weizmann Inst. of Science 1 -2015
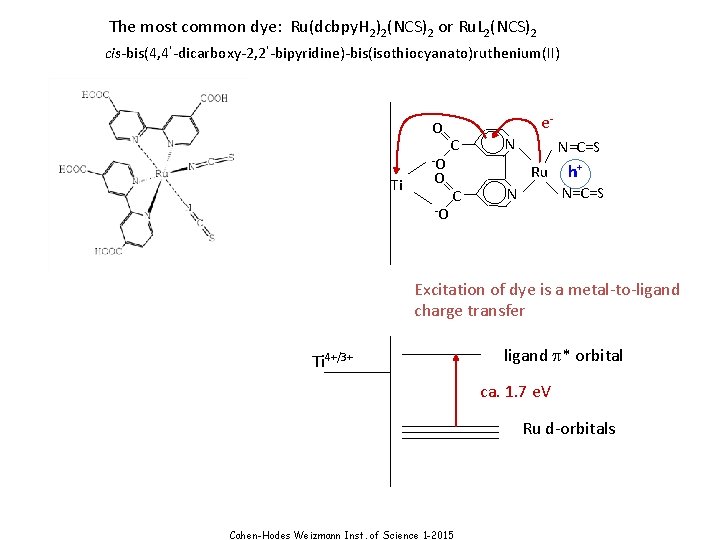
The most common dye: Ru(dcbpy. H 2)2(NCS)2 or Ru. L 2(NCS)2 cis-bis(4, 4’-dicarboxy-2, 2’-bipyridine)-bis(isothiocyanato)ruthenium(II) O -O Ti O -O e. C N N=C=S Ru C h+ N=C=S N Excitation of dye is a metal-to-ligand charge transfer Ti 4+/3+ ligand p* orbital ca. 1. 7 e. V Ru d-orbitals Cahen-Hodes Weizmann Inst. of Science 1 -2015
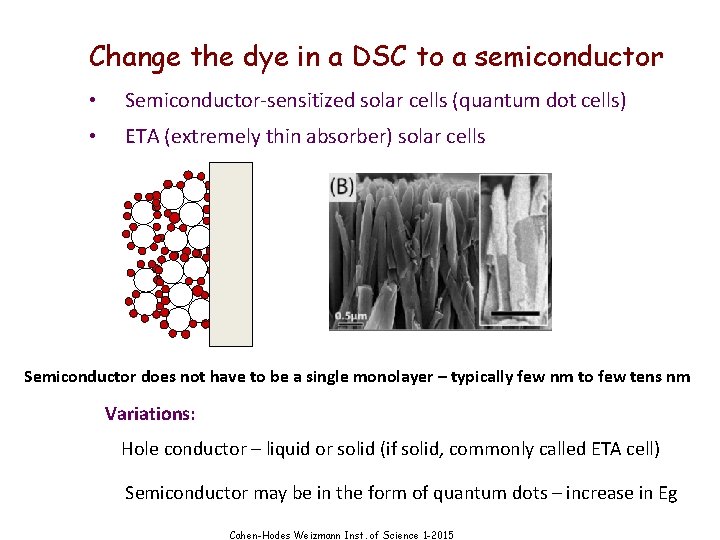
Change the dye in a DSC to a semiconductor • Semiconductor-sensitized solar cells (quantum dot cells) • ETA (extremely thin absorber) solar cells Semiconductor does not have to be a single monolayer – typically few nm to few tens nm Variations: Hole conductor – liquid or solid (if solid, commonly called ETA cell) Semiconductor may be in the form of quantum dots – increase in Eg Cahen-Hodes Weizmann Inst. of Science 1 -2015
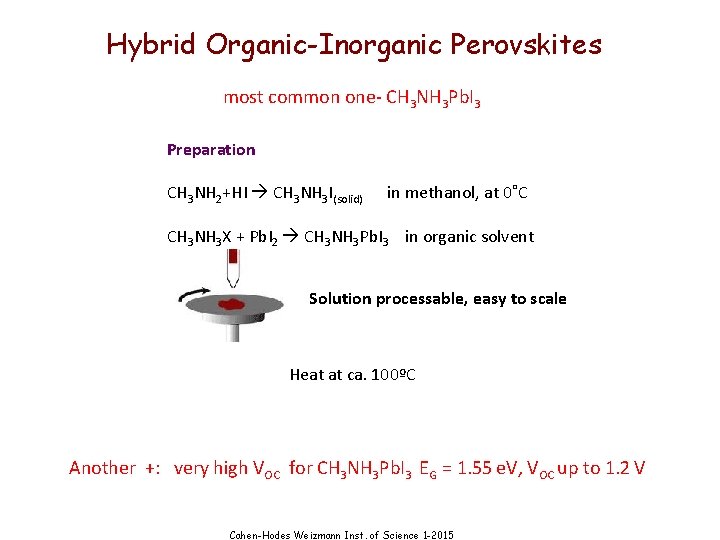
Hybrid Organic-Inorganic Perovskites most common one- CH 3 NH 3 Pb. I 3 Preparation CH 3 NH 2+HI CH 3 NH 3 I(solid) in methanol, at 0˚C CH 3 NH 3 X + Pb. I 2 CH 3 NH 3 Pb. I 3 in organic solvent Solution processable, easy to scale Heat at ca. 100ºC Another +: very high VOC for CH 3 NH 3 Pb. I 3 EG = 1. 55 e. V, VOC up to 1. 2 V Cahen-Hodes Weizmann Inst. of Science 1 -2015
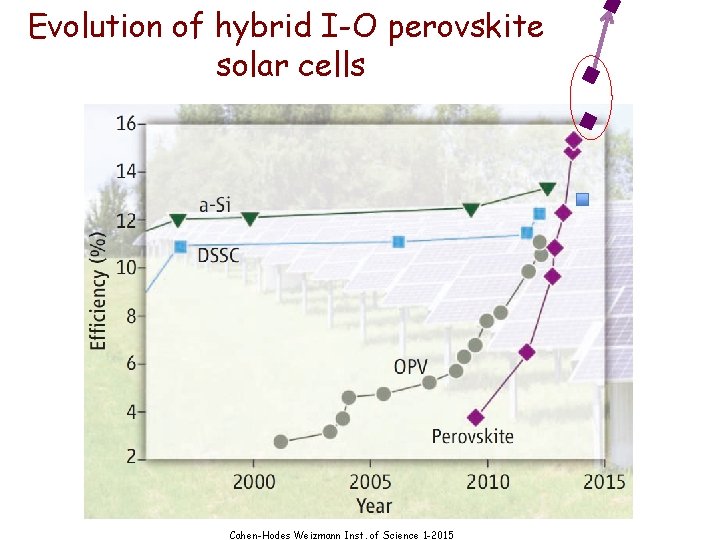
Evolution of hybrid I-O perovskite solar cells Cahen-Hodes Weizmann Inst. of Science 1 -2015
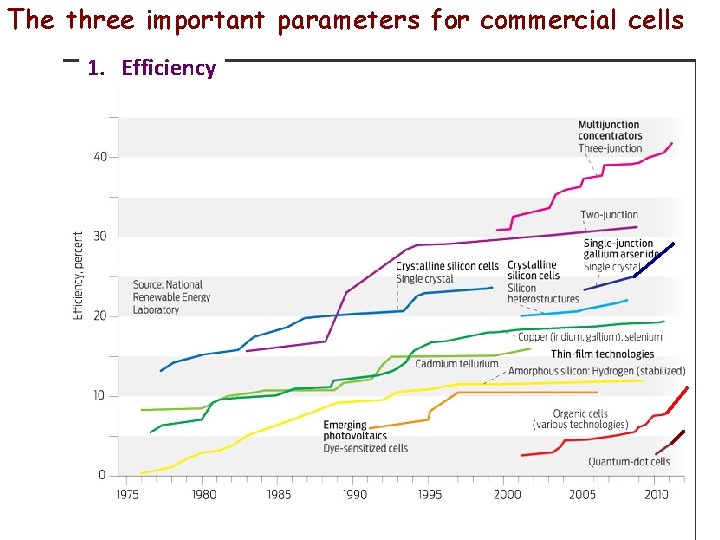
The three important parameters for commercial cells 1. Efficiency Cahen-Hodes Weizmann Inst. of Science 1 -2015
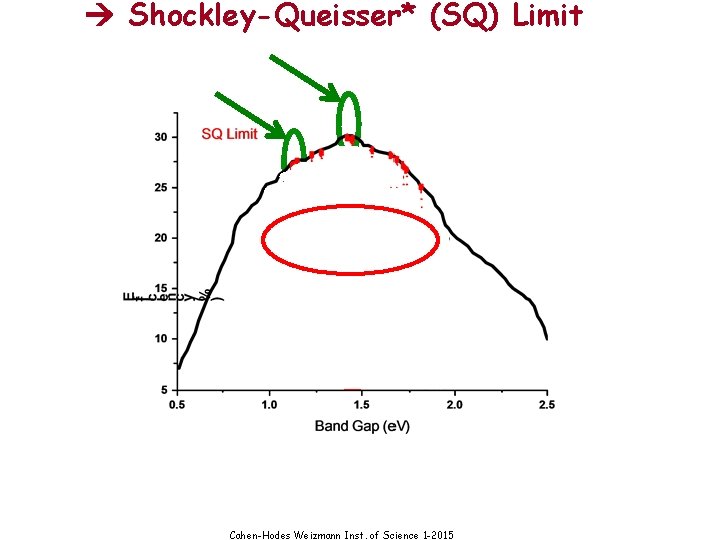
Shockley-Queisser* (SQ) Limit Cahen-Hodes Weizmann Inst. of Science 1 -2015
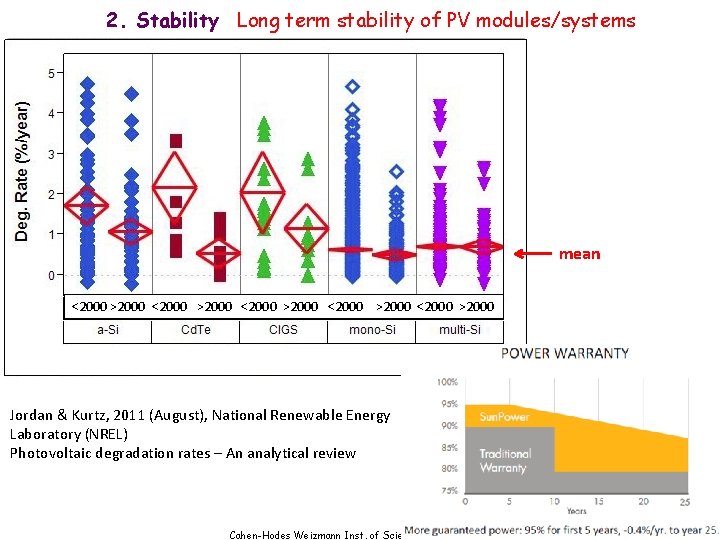
2. Stability Long term stability of PV modules/systems mean <2000 >2000 <2000 >2000 Jordan & Kurtz, 2011 (August), National Renewable Energy Laboratory (NREL) Photovoltaic degradation rates – An analytical review Cahen-Hodes Weizmann Inst. of Science 1 -2015
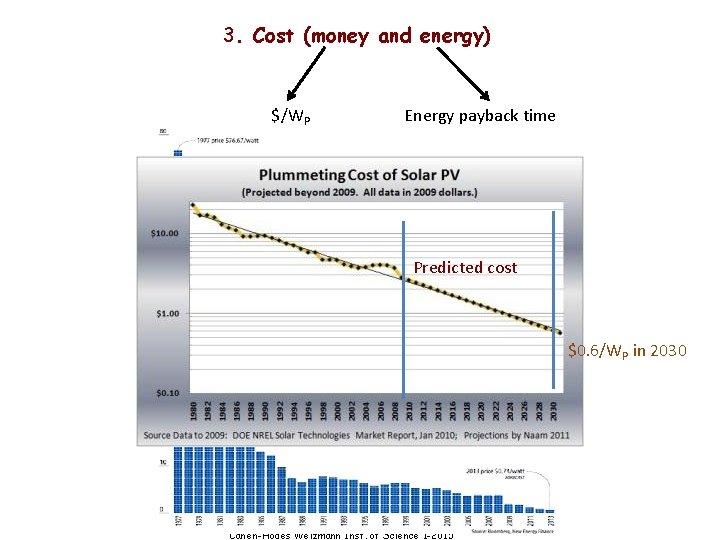
3. Cost (money and energy) $/WP Energy payback time Predicted cost $0. 6/WP in 2030 Cahen-Hodes Weizmann Inst. of Science 1 -2015
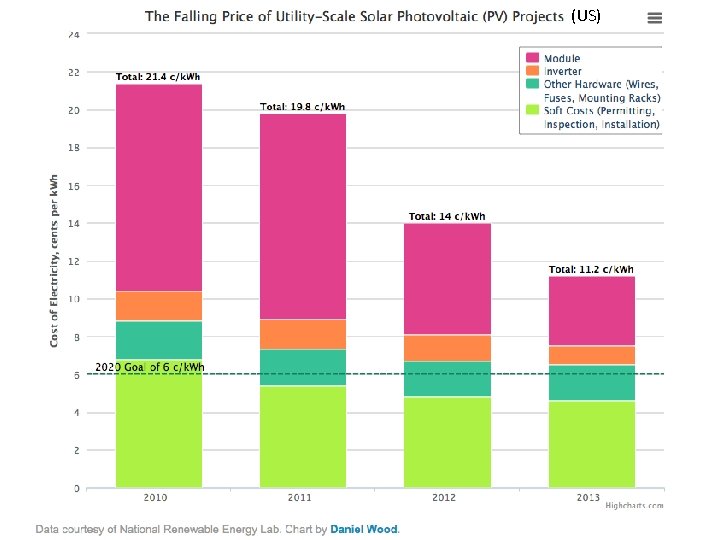
(US) Cahen-Hodes Weizmann Inst. of Science 1 -2015
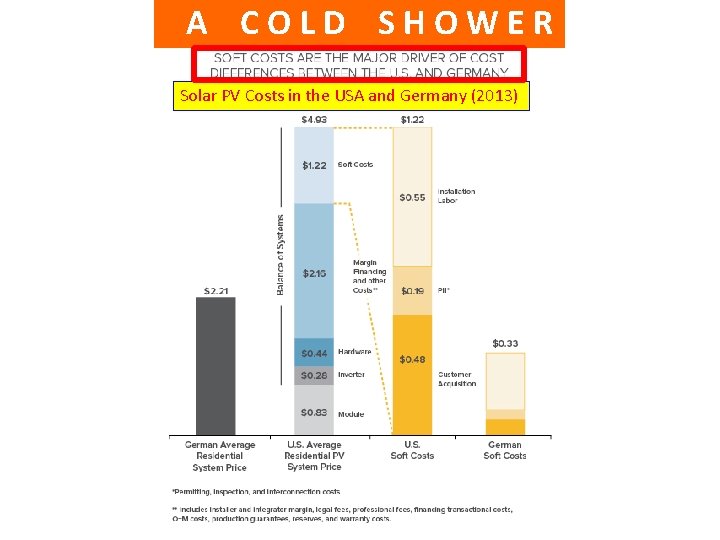
A COLD SHOWER Solar PV Costs in the USA and Germany (2013) Cahen-Hodes Weizmann Inst. of Science 1 -2015
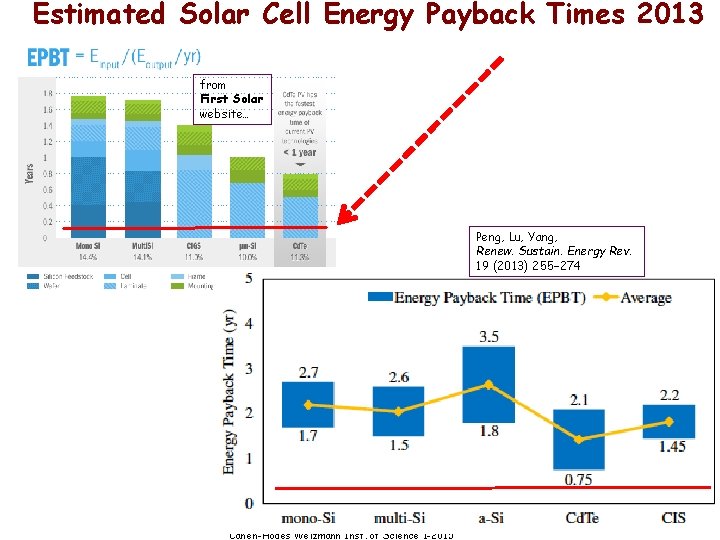
Estimated Solar Cell Energy Payback Times 2013 from First Solar website… Peng, Lu, Yang, Renew. Sustain. Energy Rev. 19 (2013) 255– 274 Cahen-Hodes Weizmann Inst. of Science 1 -2015
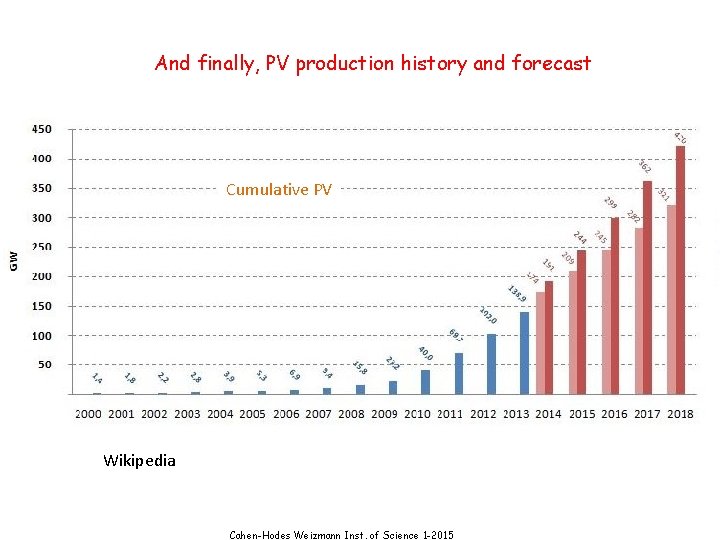
And finally, PV production history and forecast Cumulative PV Wikipedia Cahen-Hodes Weizmann Inst. of Science 1 -2015
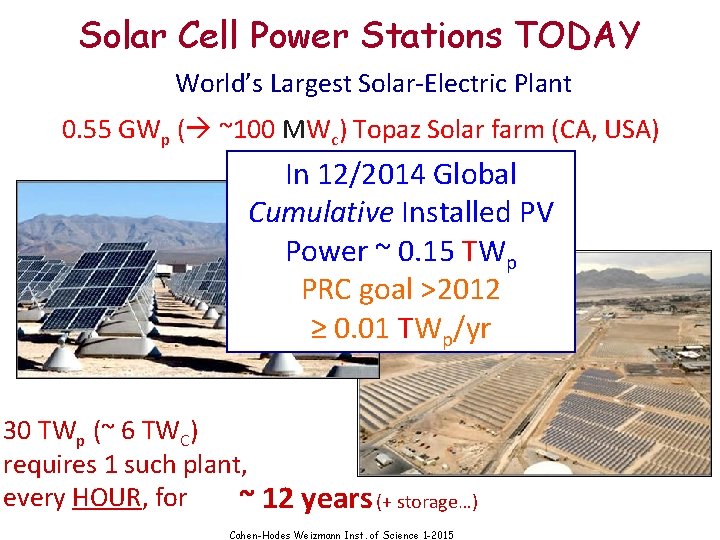
Solar Cell Power Stations TODAY World’s Largest Solar-Electric Plant 0. 55 GWp ( ~100 MWc) Topaz Solar farm (CA, USA) In 12/2014 Global Cumulative Installed PV Power ~ 0. 15 TWp PRC goal >2012 ≥ 0. 01 TWp/yr 30 TWp (~ 6 TWC) requires 1 such plant, every HOUR, for ~ 12 years (+ storage…) Cahen-Hodes Weizmann Inst. of Science 1 -2015
Graphic novel terms
Fundamental and powerful concepts
Fundamental concepts in video
Basic concepts of simulation
Time perspective in managerial economics
Fundamental concepts in sociolinguistics
Four fundamental oop concepts
Chapter p prerequisites: fundamental concepts of algebra
Chapter p prerequisites fundamental concepts of algebra
Chapter p prerequisites fundamental concepts of algebra
Occbio
Unit 1 review fundamental economic concepts answers
Fundamental concepts of managerial economics
Fundamental concepts in video
Champion of filipino students ilipino students
Fundamental model of distributed system
What are the three fundamental principles of fingerprints?
Weingarten equation in differential geometry
What is fundamental theorem
Fundamental theorem.of calculus
Database management system by korth
Operating systems concepts
Core concepts of accounting information systems
Decision support systems and intelligent systems
Difference between code first and database first approach
Breadth first vs depth first
Dicapine
Embedded systems vs cyber physical systems
Elegant systems
Summary the reading public
Literary genres venn diagram
Gothic novel characteristics
The ability to produce novel and valuable ideas is called
Extrinsic elements of prose
Who is the author of tsotsi
Reacting system
The first global economic systems answer key
Maturity continuum examples
Sdl first vs code first
Put first things first activities
Habit 3 first things first
First to file vs first to invent
First in first out
Diketahui suatu stack dengan max_stack = 6
First in first out
First come first serve
Put first things first definition