OCCUPATIONAL ERGONOMICS Chapter 5 WORK PHYSIOLOGY WORK PHYSIOLOGY
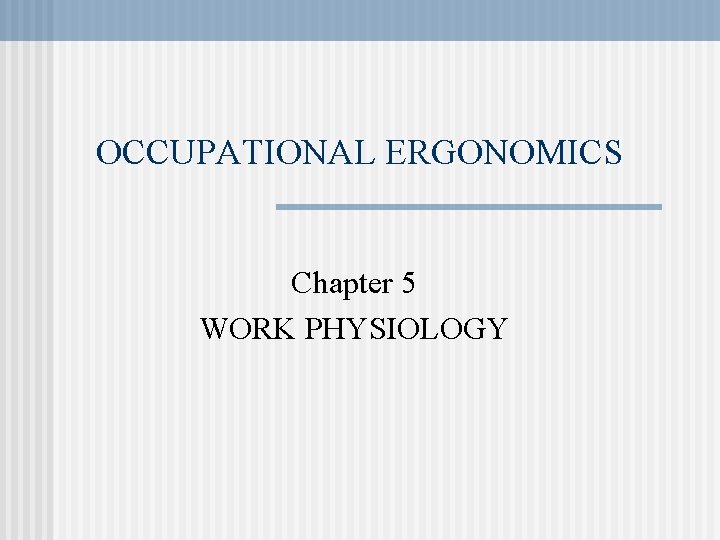
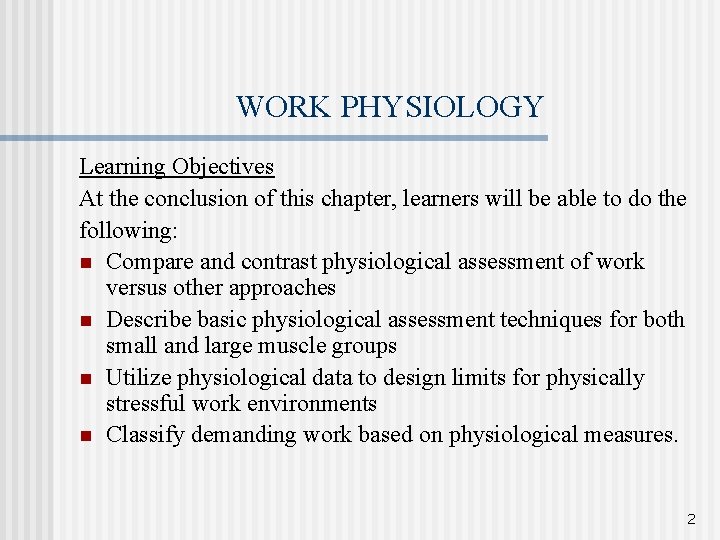
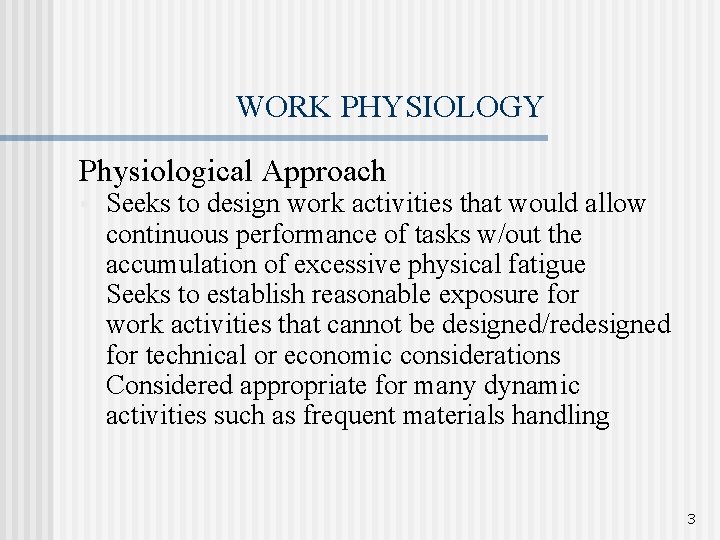
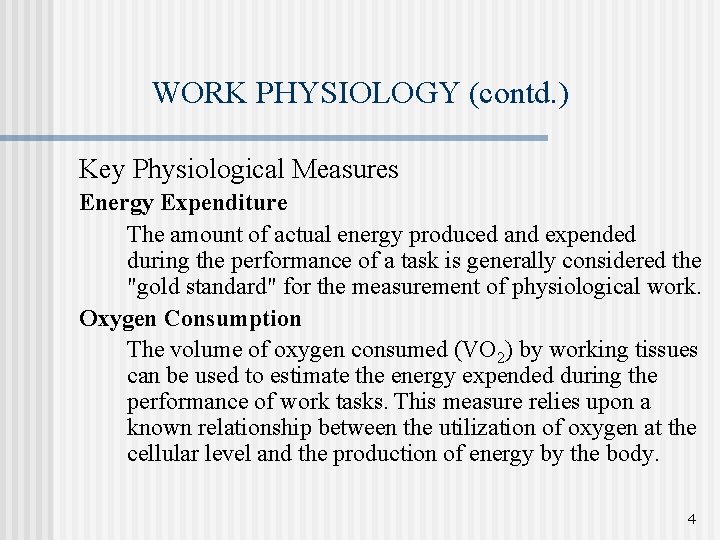
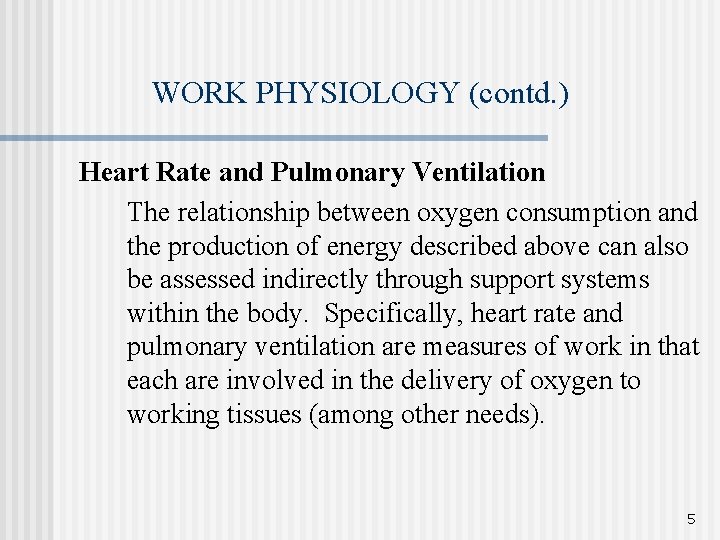
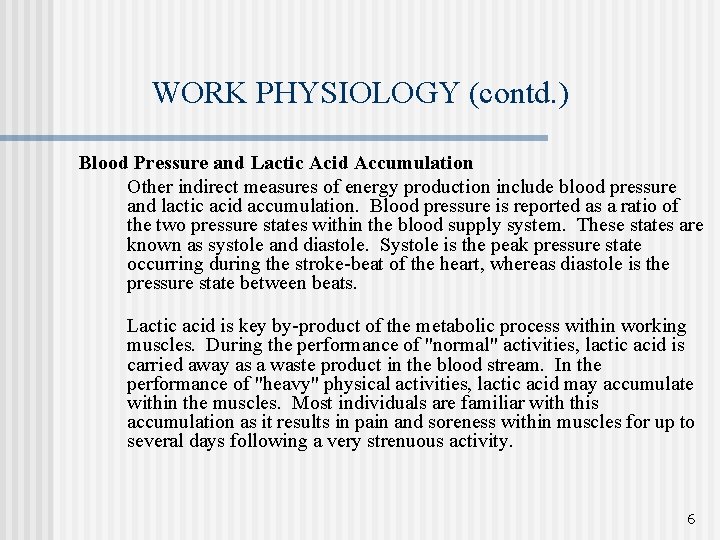
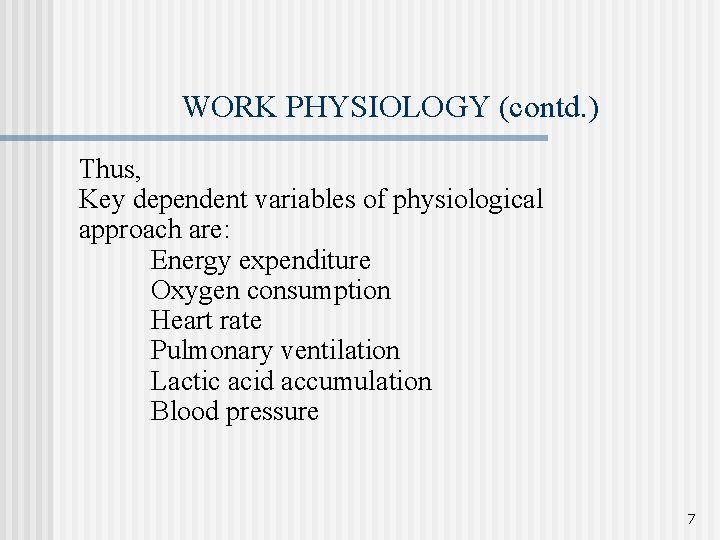
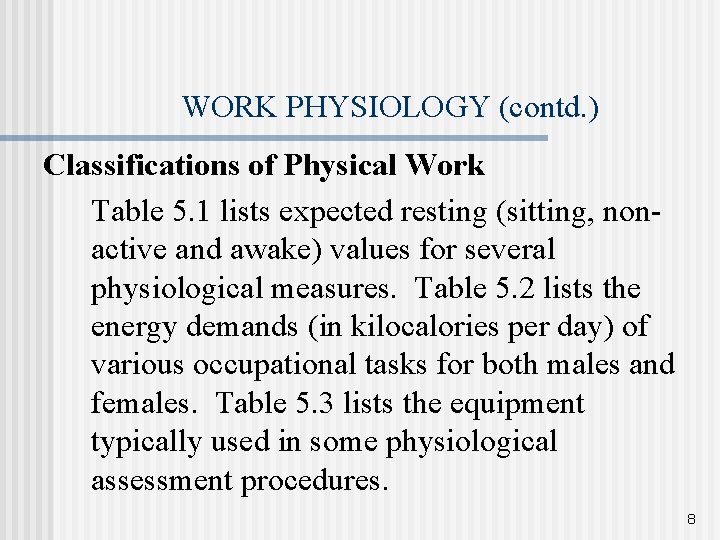
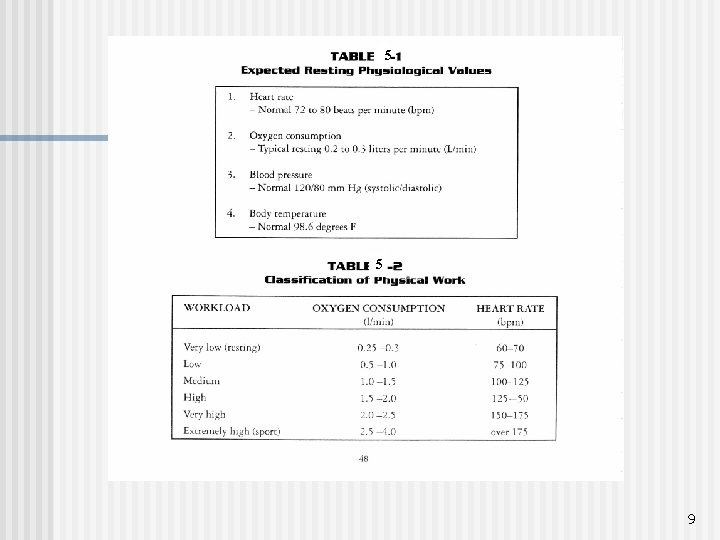
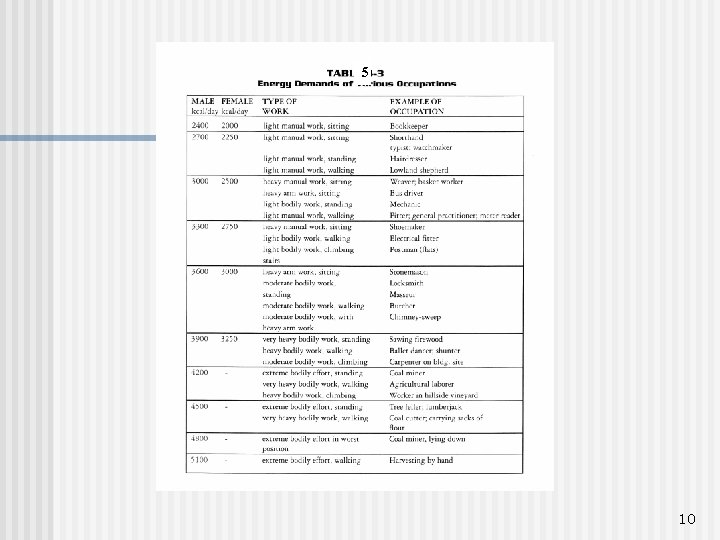
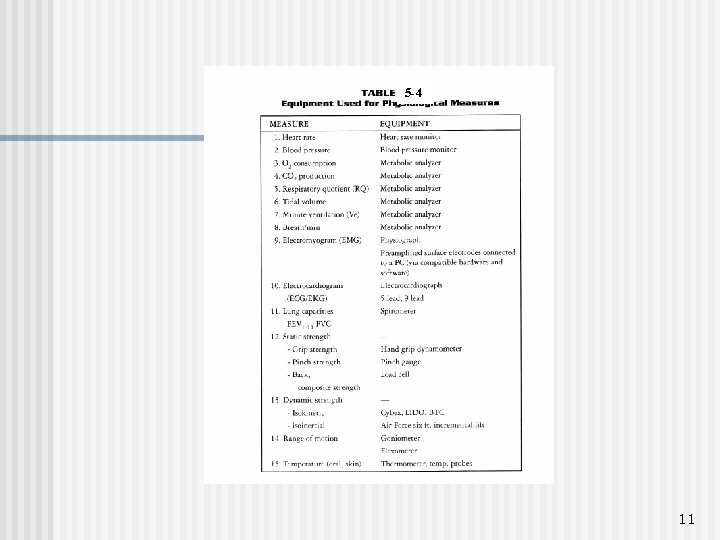
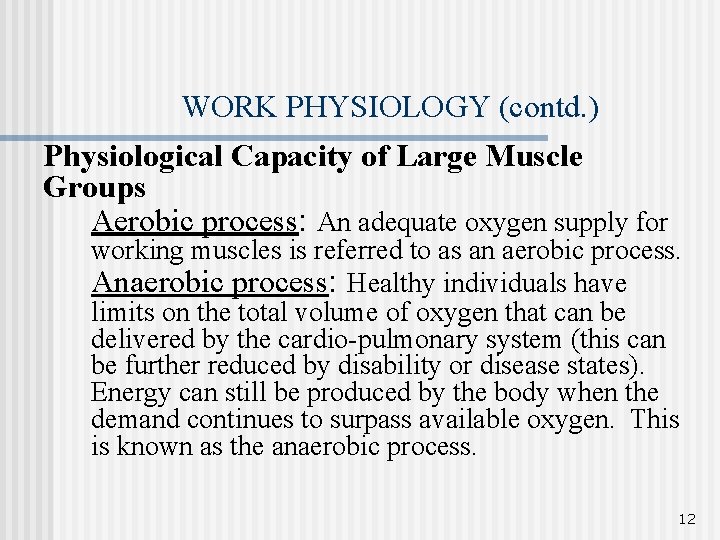
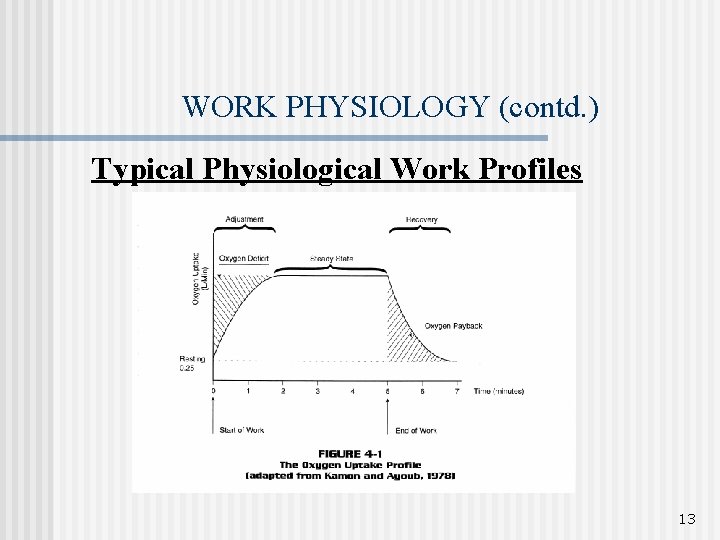
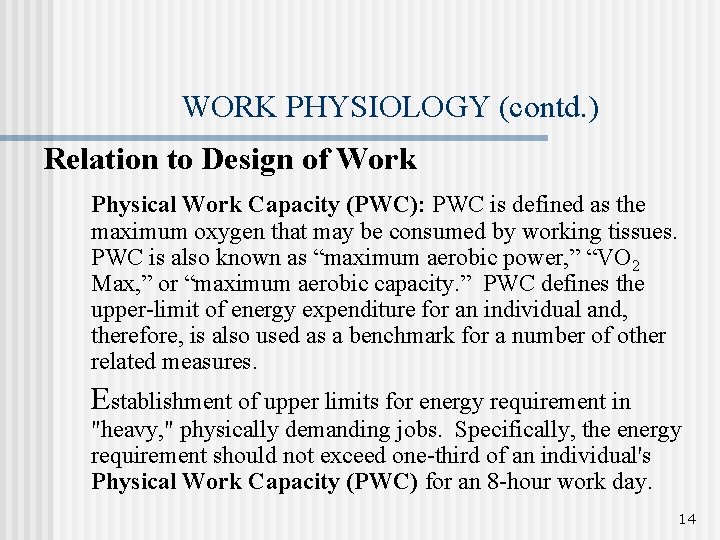
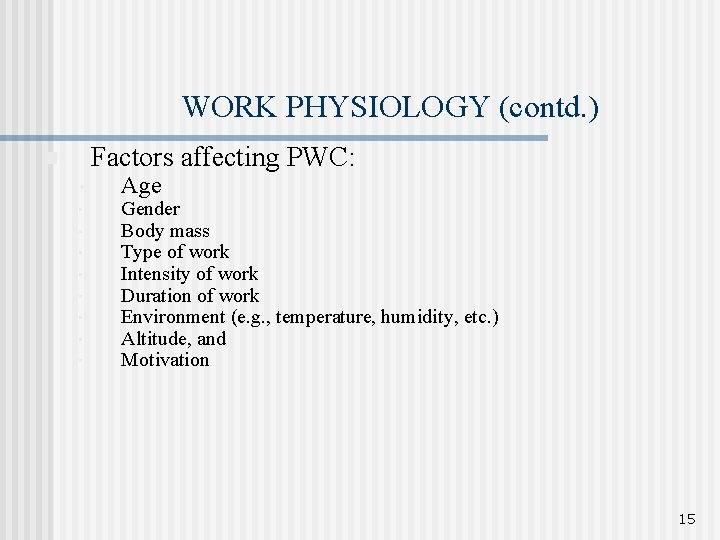
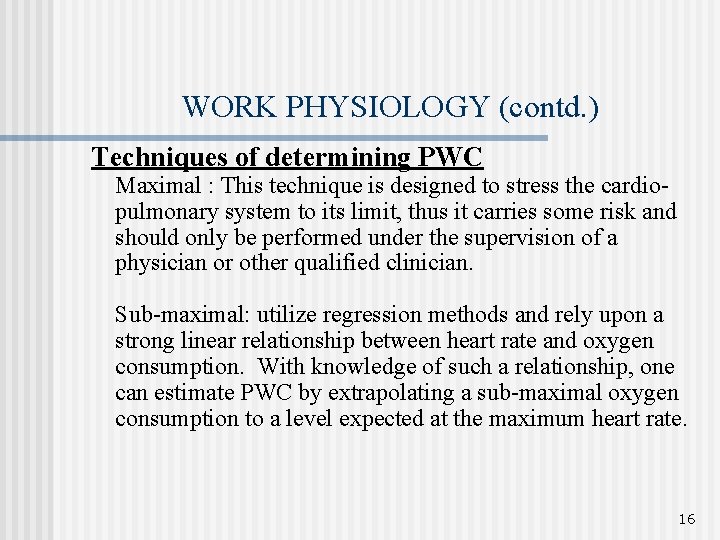
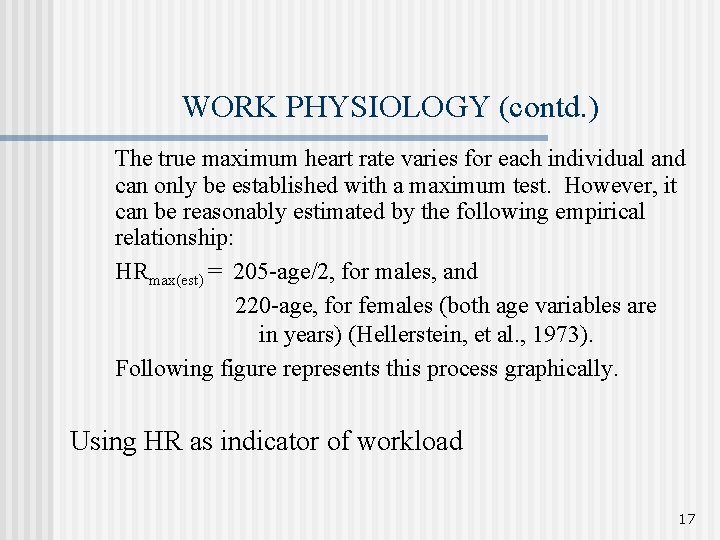
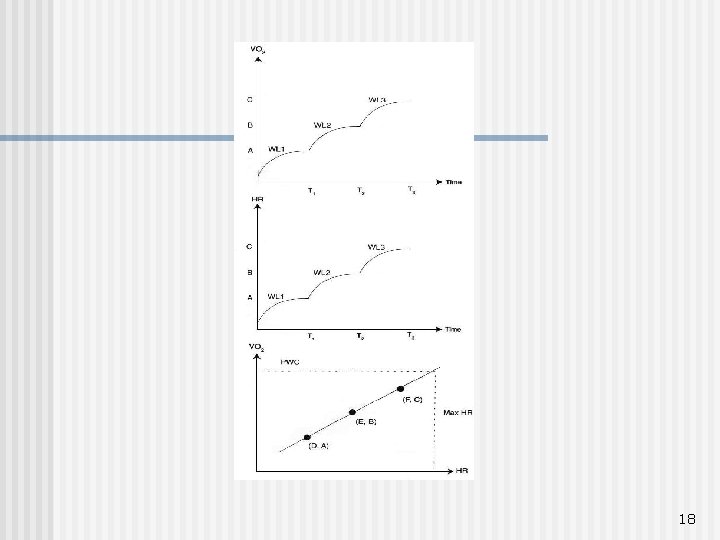
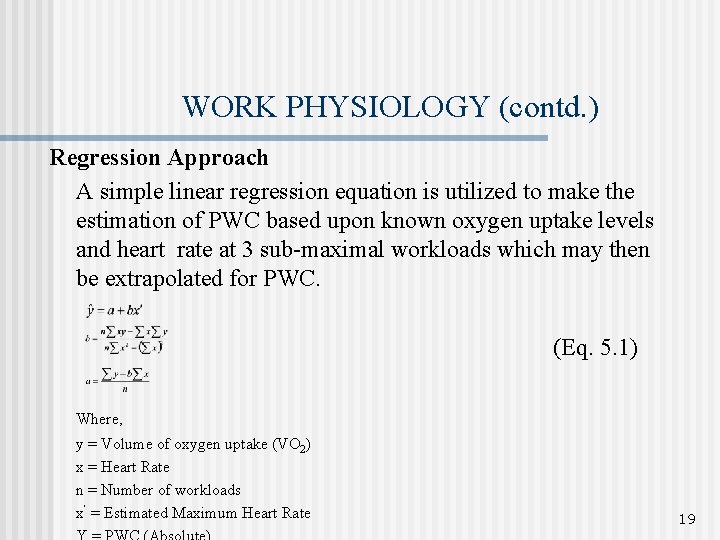
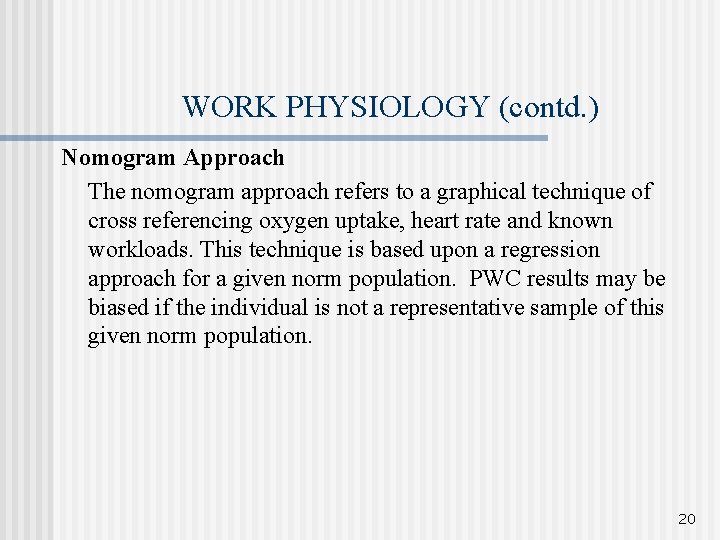
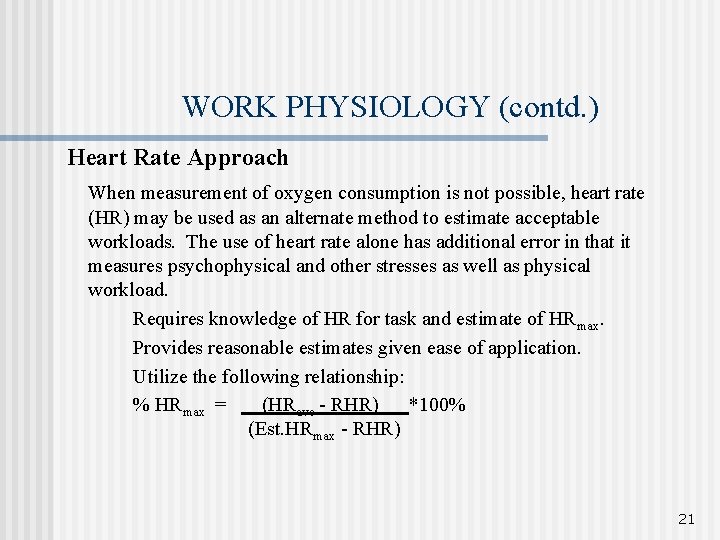
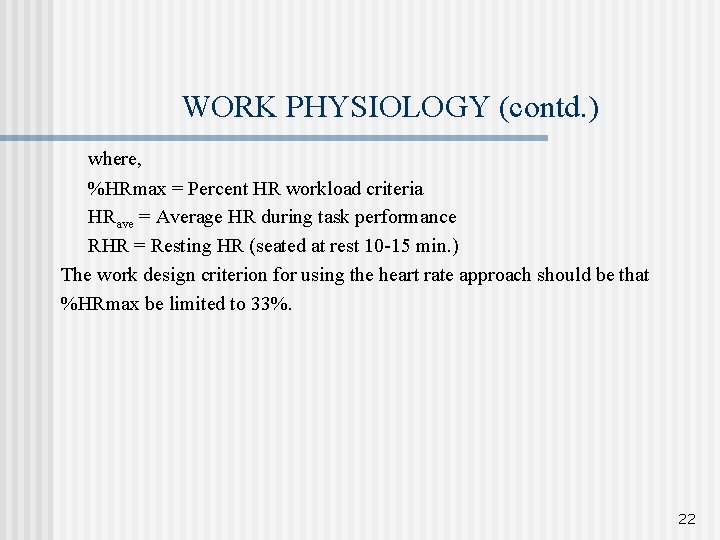
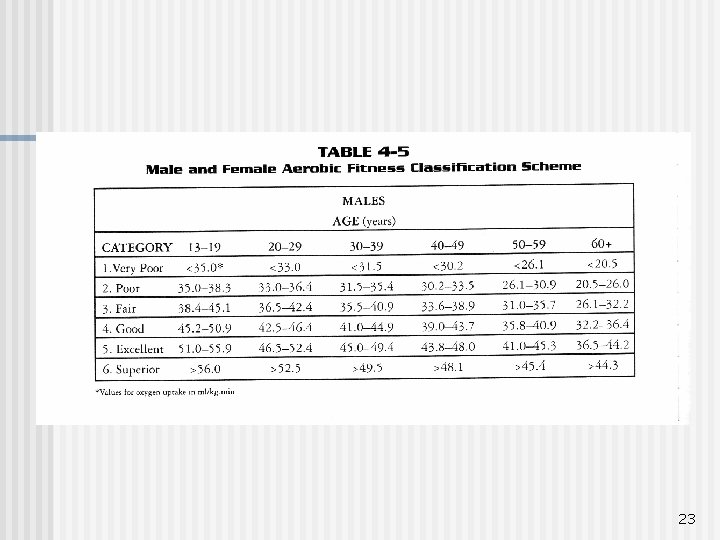
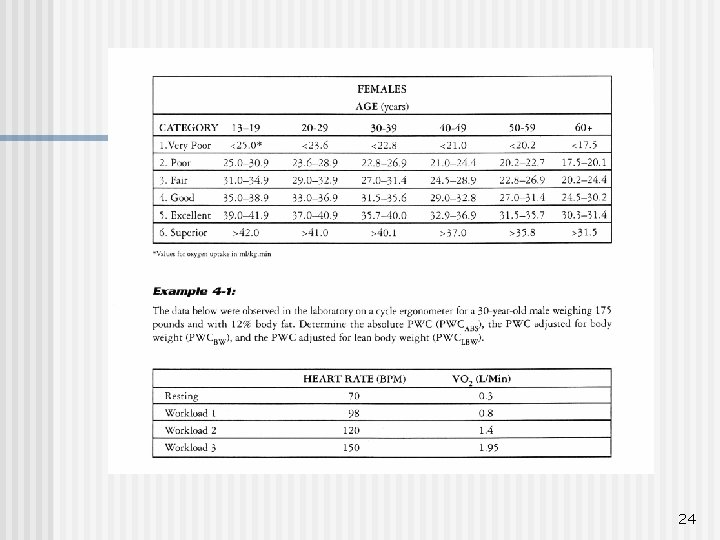
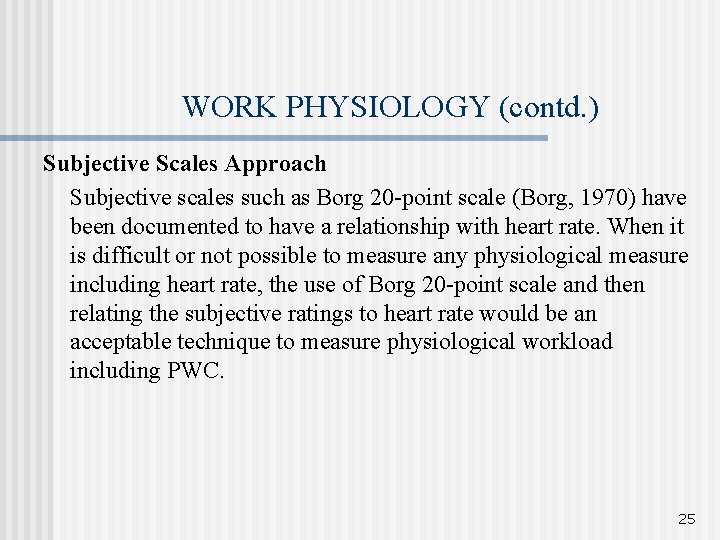
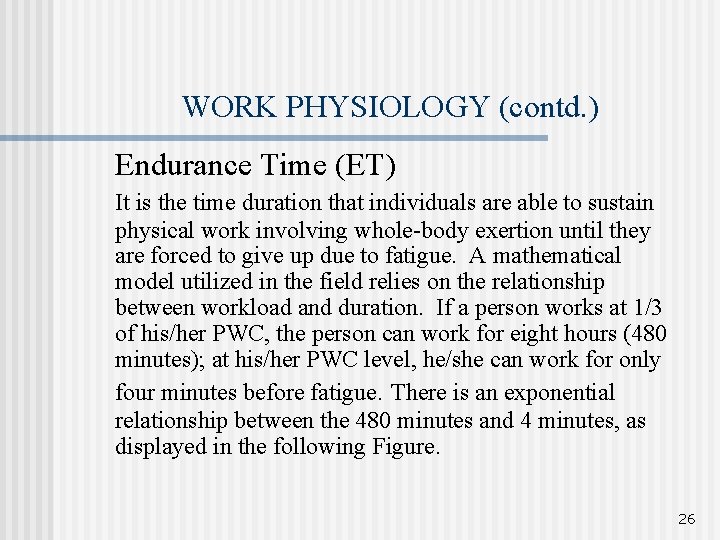
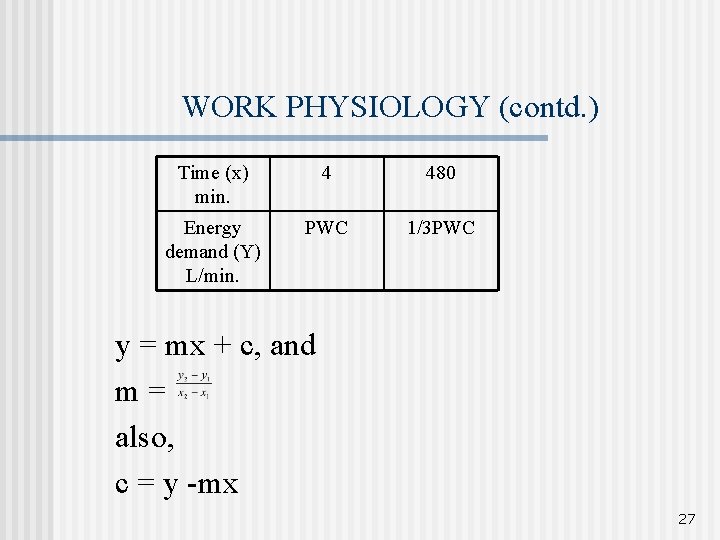
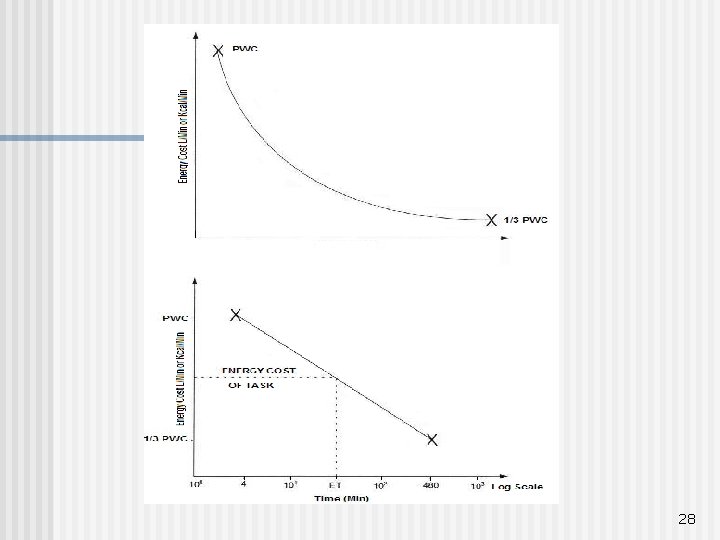
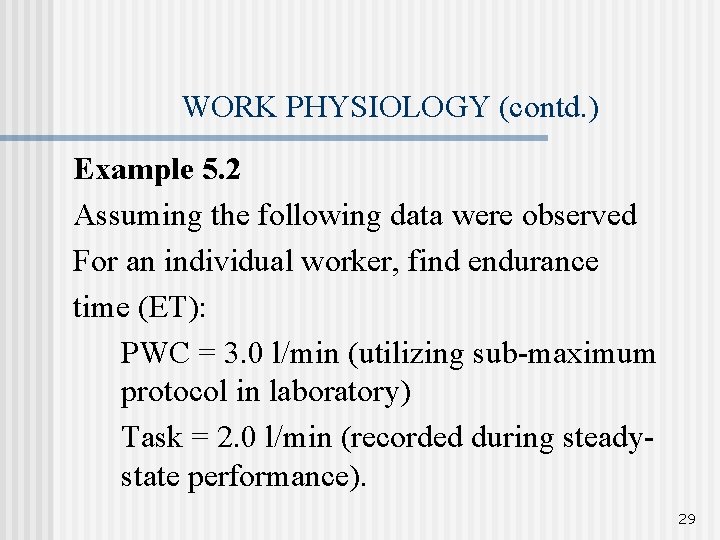
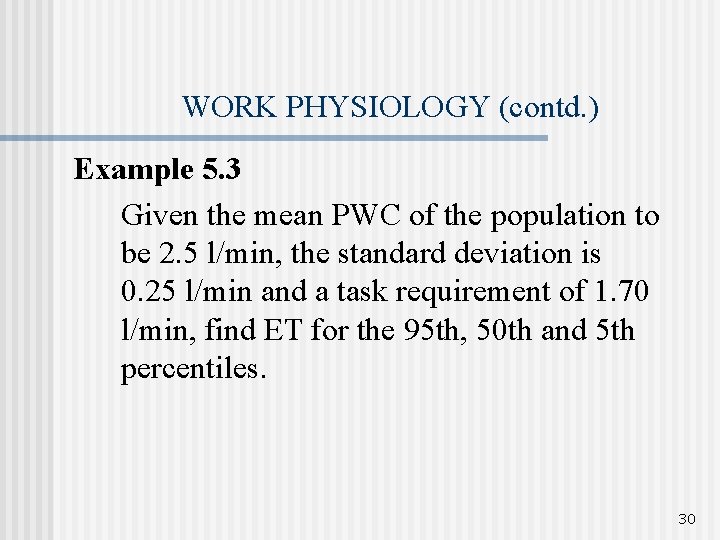
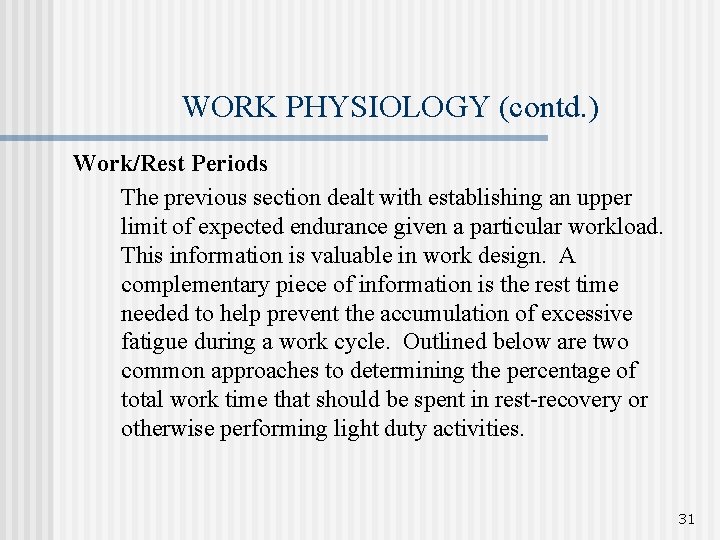
![WORK PHYSIOLOGY (contd. ) 1. Spitzer's Model (Spitzer, 1951): R% = [(M/4)] ‑ 1] WORK PHYSIOLOGY (contd. ) 1. Spitzer's Model (Spitzer, 1951): R% = [(M/4)] ‑ 1]](https://slidetodoc.com/presentation_image_h2/3a953aa76ab0867ff9ed4606dd7d13c8/image-32.jpg)
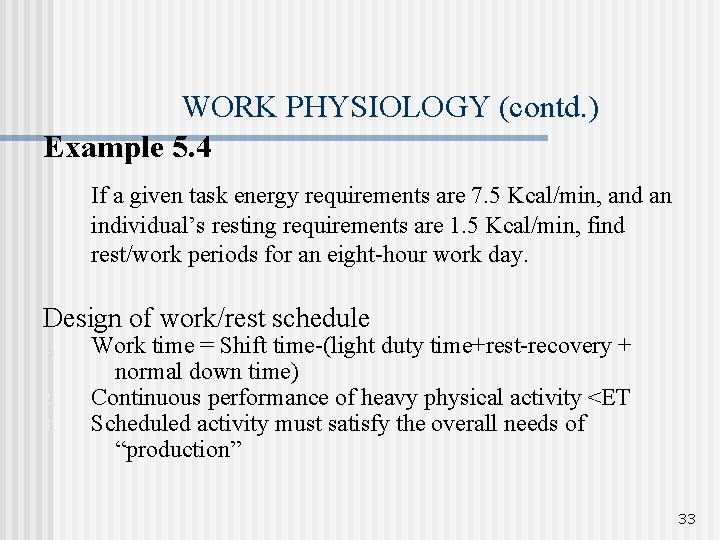
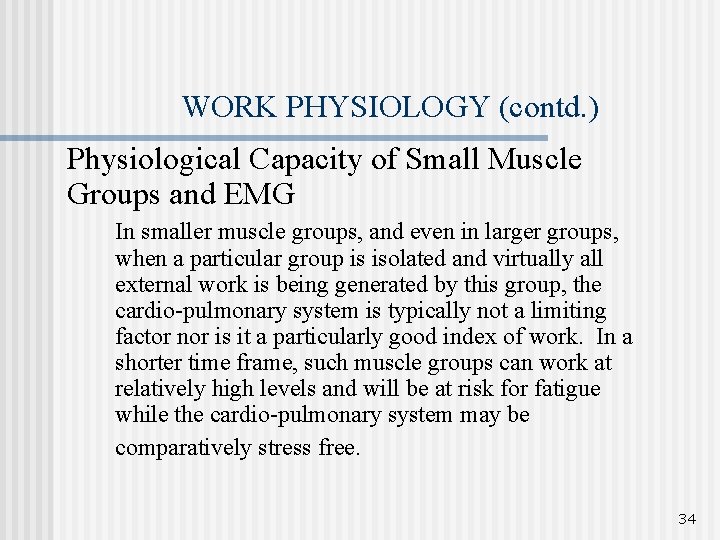
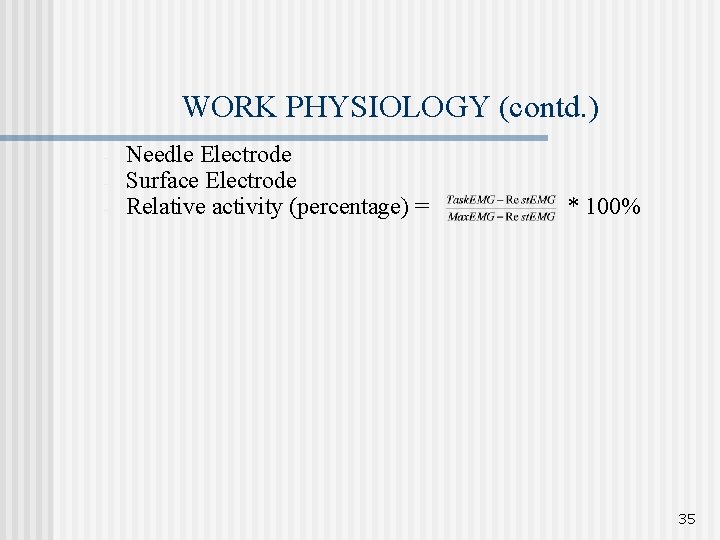
- Slides: 35
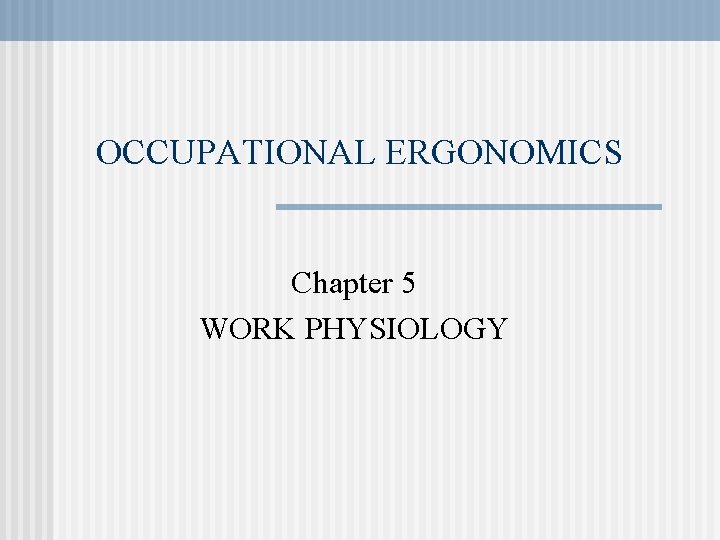
OCCUPATIONAL ERGONOMICS Chapter 5 WORK PHYSIOLOGY
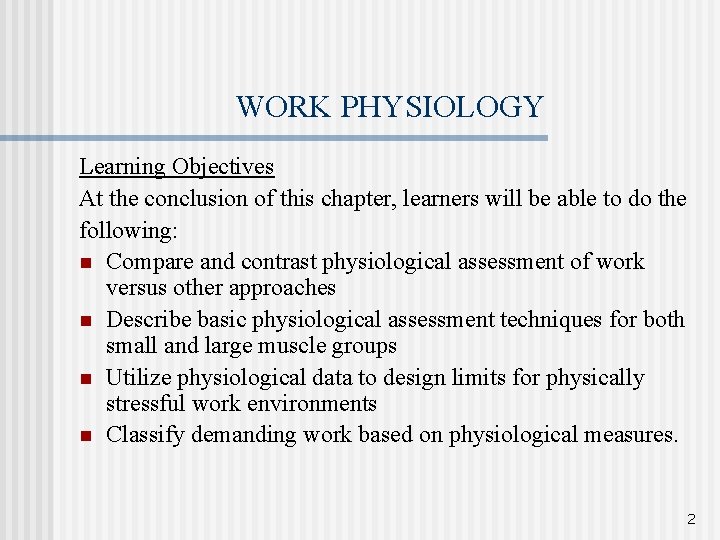
WORK PHYSIOLOGY Learning Objectives At the conclusion of this chapter, learners will be able to do the following: n Compare and contrast physiological assessment of work versus other approaches n Describe basic physiological assessment techniques for both small and large muscle groups n Utilize physiological data to design limits for physically stressful work environments n Classify demanding work based on physiological measures. 2
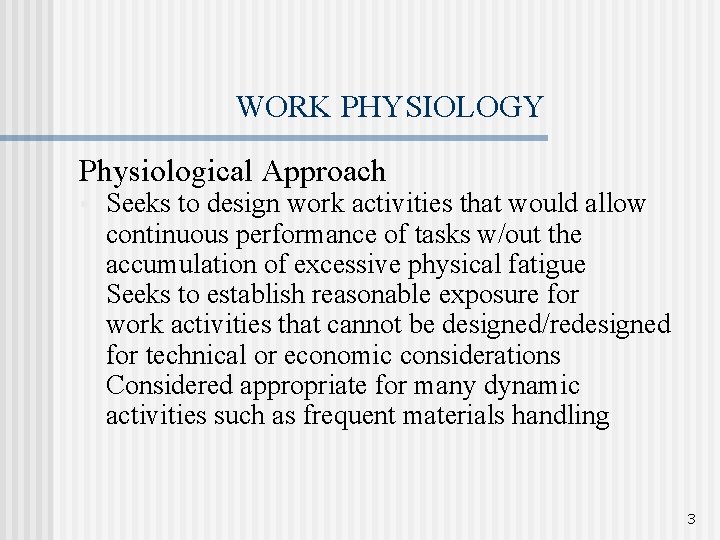
WORK PHYSIOLOGY Physiological Approach § Seeks to design work activities that would allow continuous performance of tasks w/out the accumulation of excessive physical fatigue Seeks to establish reasonable exposure for work activities that cannot be designed/redesigned for technical or economic considerations Considered appropriate for many dynamic activities such as frequent materials handling 3
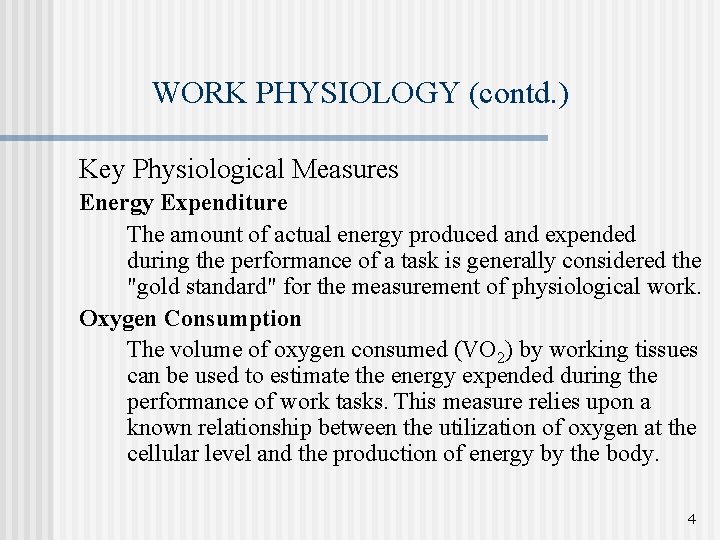
WORK PHYSIOLOGY (contd. ) Key Physiological Measures Energy Expenditure The amount of actual energy produced and expended during the performance of a task is generally considered the "gold standard" for the measurement of physiological work. Oxygen Consumption The volume of oxygen consumed (VO 2) by working tissues can be used to estimate the energy expended during the performance of work tasks. This measure relies upon a known relationship between the utilization of oxygen at the cellular level and the production of energy by the body. 4
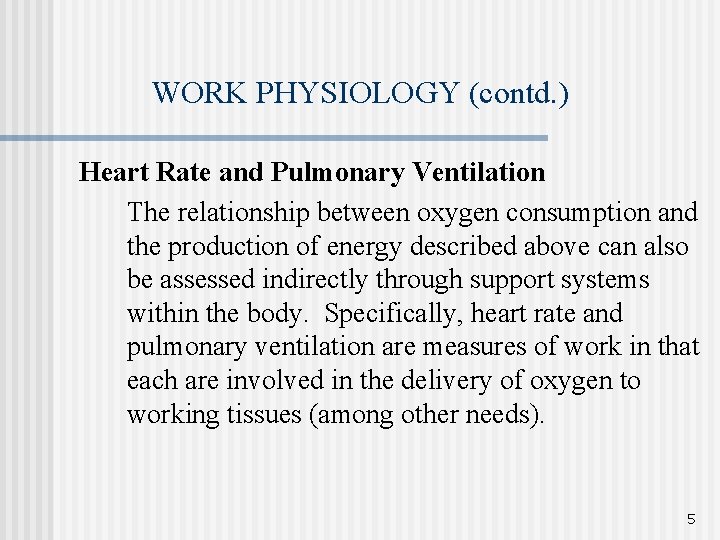
WORK PHYSIOLOGY (contd. ) Heart Rate and Pulmonary Ventilation The relationship between oxygen consumption and the production of energy described above can also be assessed indirectly through support systems within the body. Specifically, heart rate and pulmonary ventilation are measures of work in that each are involved in the delivery of oxygen to working tissues (among other needs). 5
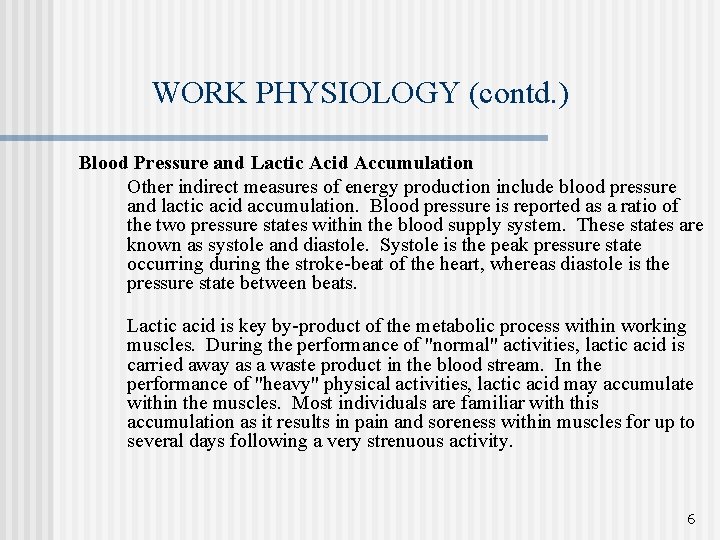
WORK PHYSIOLOGY (contd. ) Blood Pressure and Lactic Acid Accumulation Other indirect measures of energy production include blood pressure and lactic acid accumulation. Blood pressure is reported as a ratio of the two pressure states within the blood supply system. These states are known as systole and diastole. Systole is the peak pressure state occurring during the stroke-beat of the heart, whereas diastole is the pressure state between beats. Lactic acid is key by-product of the metabolic process within working muscles. During the performance of "normal" activities, lactic acid is carried away as a waste product in the blood stream. In the performance of "heavy" physical activities, lactic acid may accumulate within the muscles. Most individuals are familiar with this accumulation as it results in pain and soreness within muscles for up to several days following a very strenuous activity. 6
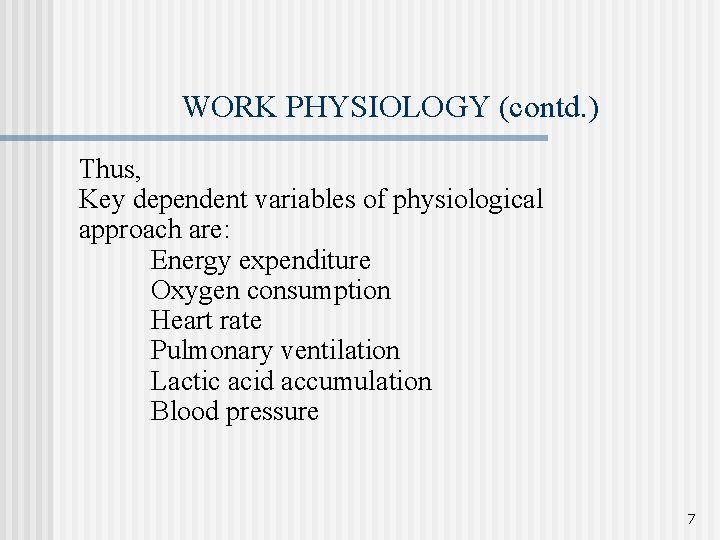
WORK PHYSIOLOGY (contd. ) Thus, Key dependent variables of physiological approach are: Energy expenditure Oxygen consumption Heart rate Pulmonary ventilation Lactic acid accumulation Blood pressure 7
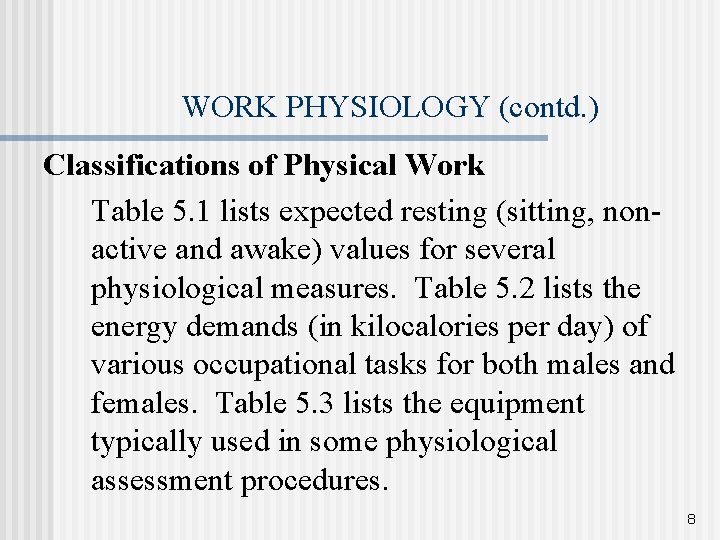
WORK PHYSIOLOGY (contd. ) Classifications of Physical Work Table 5. 1 lists expected resting (sitting, nonactive and awake) values for several physiological measures. Table 5. 2 lists the energy demands (in kilocalories per day) of various occupational tasks for both males and females. Table 5. 3 lists the equipment typically used in some physiological assessment procedures. 8
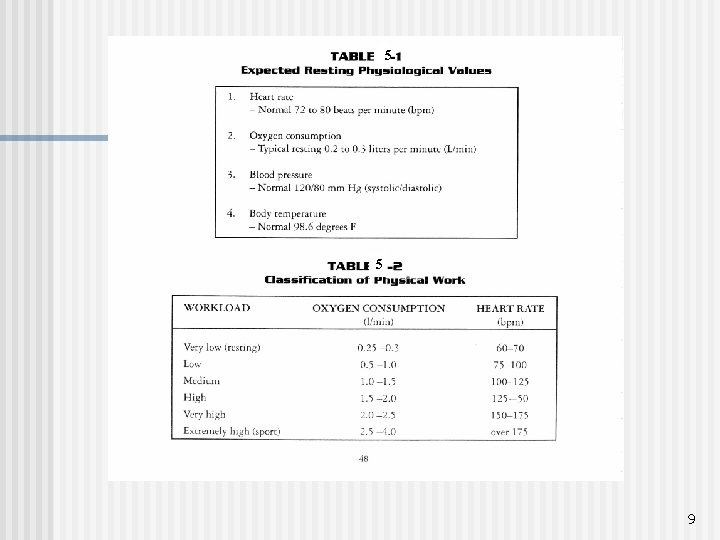
5 5 9
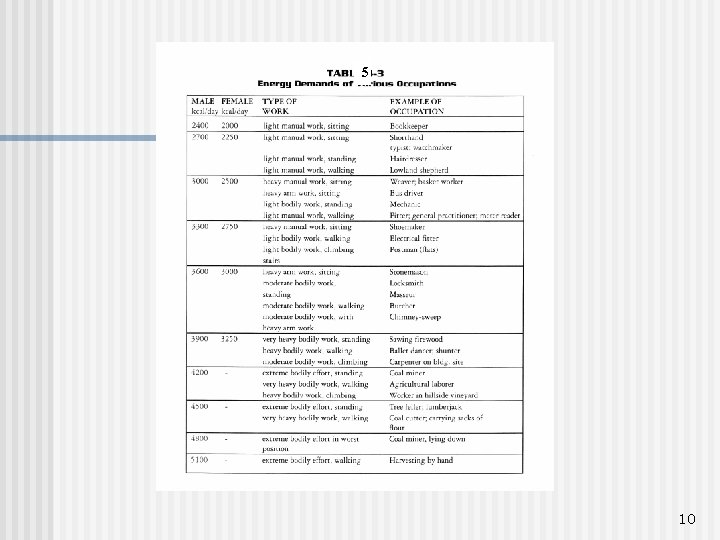
5 10
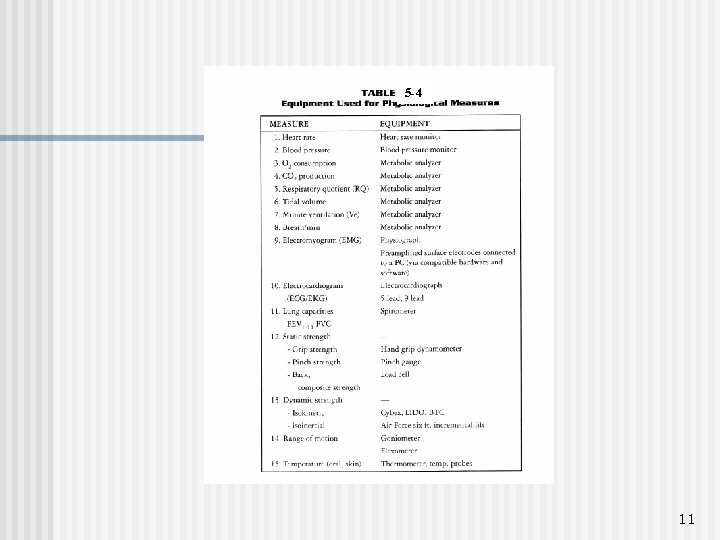
5 -4 11
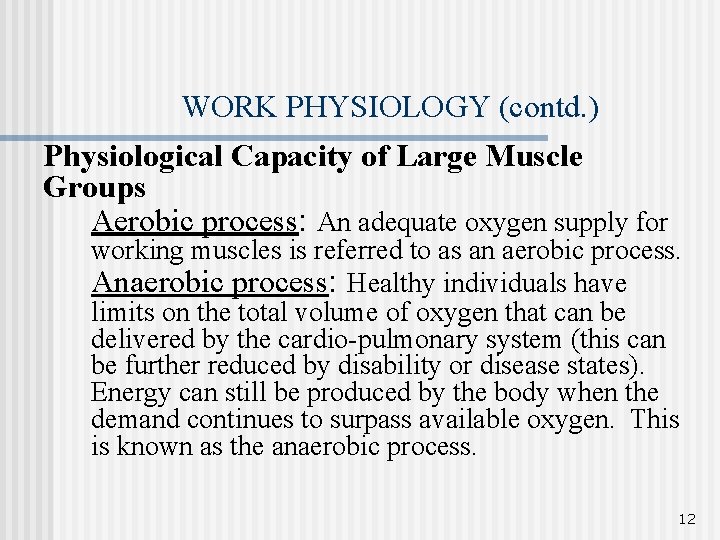
WORK PHYSIOLOGY (contd. ) Physiological Capacity of Large Muscle Groups Aerobic process: An adequate oxygen supply for working muscles is referred to as an aerobic process. Anaerobic process: Healthy individuals have limits on the total volume of oxygen that can be delivered by the cardio-pulmonary system (this can be further reduced by disability or disease states). Energy can still be produced by the body when the demand continues to surpass available oxygen. This is known as the anaerobic process. 12
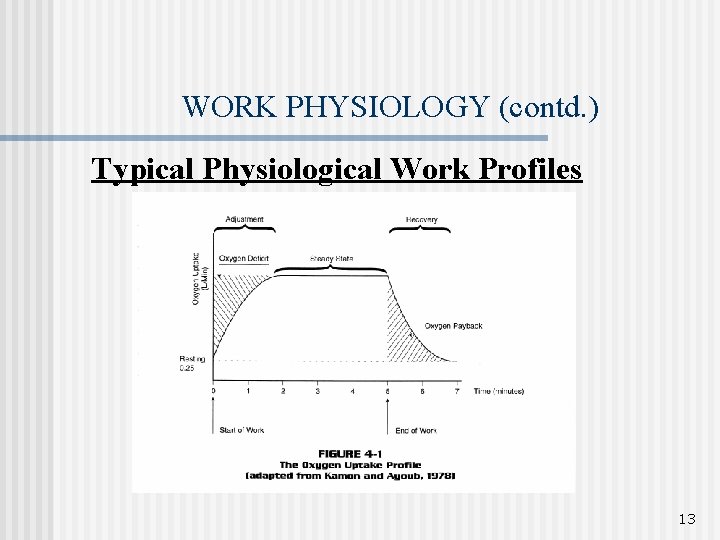
WORK PHYSIOLOGY (contd. ) Typical Physiological Work Profiles 13
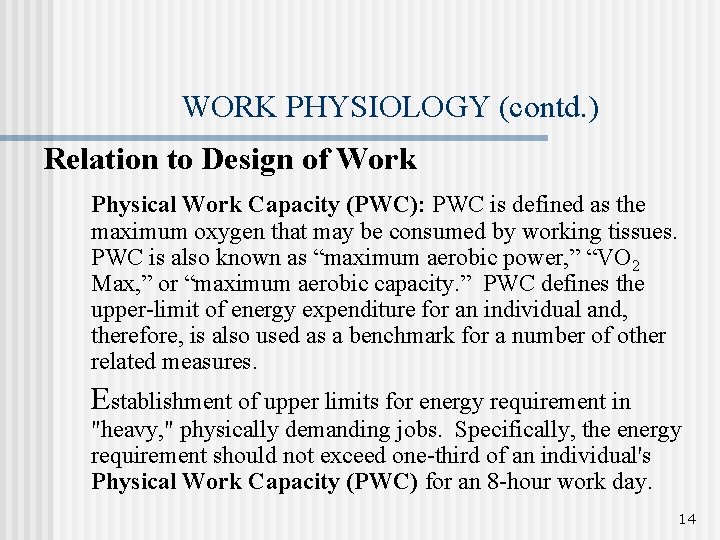
WORK PHYSIOLOGY (contd. ) Relation to Design of Work Physical Work Capacity (PWC): PWC is defined as the maximum oxygen that may be consumed by working tissues. PWC is also known as “maximum aerobic power, ” “VO 2 Max, ” or “maximum aerobic capacity. ” PWC defines the upper-limit of energy expenditure for an individual and, therefore, is also used as a benchmark for a number of other related measures. Establishment of upper limits for energy requirement in "heavy, " physically demanding jobs. Specifically, the energy requirement should not exceed one-third of an individual's Physical Work Capacity (PWC) for an 8 -hour work day. 14
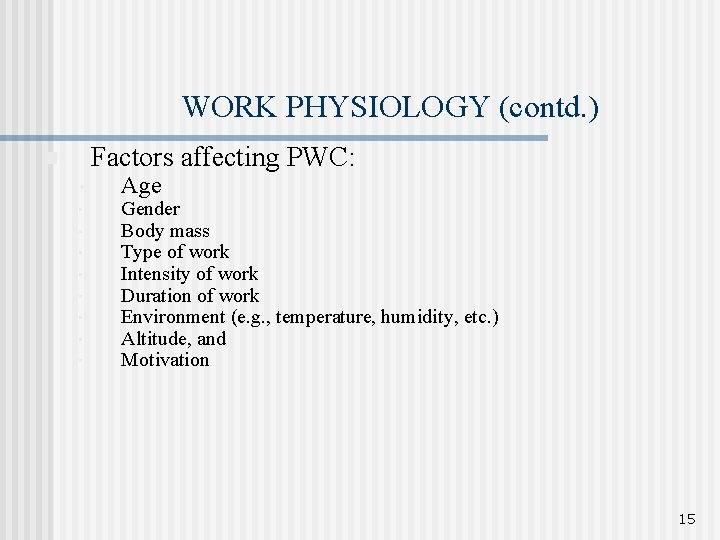
WORK PHYSIOLOGY (contd. ) Factors affecting PWC: n • • • Age Gender Body mass Type of work Intensity of work Duration of work Environment (e. g. , temperature, humidity, etc. ) Altitude, and Motivation 15
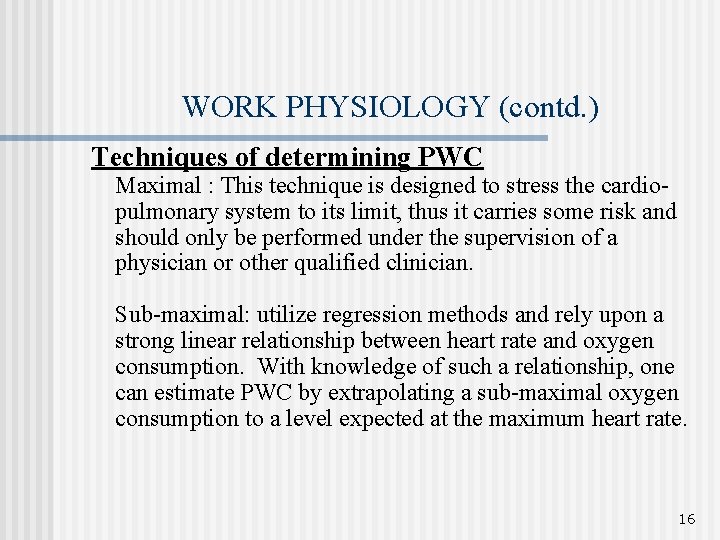
WORK PHYSIOLOGY (contd. ) Techniques of determining PWC Maximal : This technique is designed to stress the cardiopulmonary system to its limit, thus it carries some risk and should only be performed under the supervision of a physician or other qualified clinician. Sub-maximal: utilize regression methods and rely upon a strong linear relationship between heart rate and oxygen consumption. With knowledge of such a relationship, one can estimate PWC by extrapolating a sub-maximal oxygen consumption to a level expected at the maximum heart rate. 16
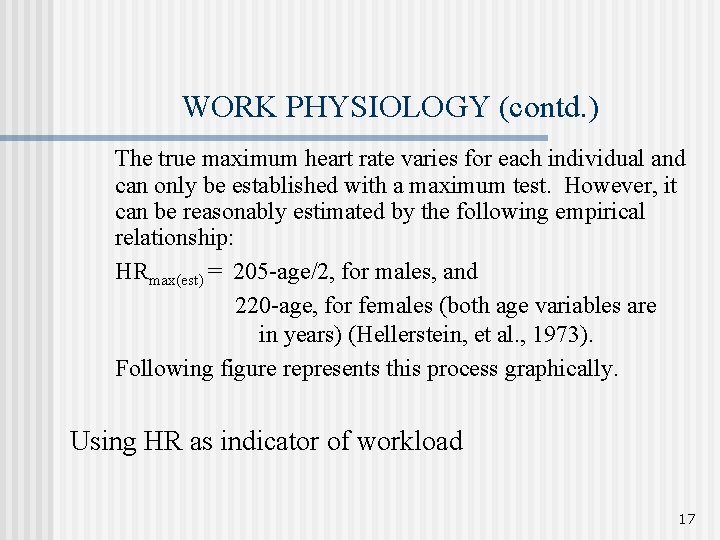
WORK PHYSIOLOGY (contd. ) The true maximum heart rate varies for each individual and can only be established with a maximum test. However, it can be reasonably estimated by the following empirical relationship: HRmax(est) = 205 -age/2, for males, and 220 -age, for females (both age variables are in years) (Hellerstein, et al. , 1973). Following figure represents this process graphically. Using HR as indicator of workload 17
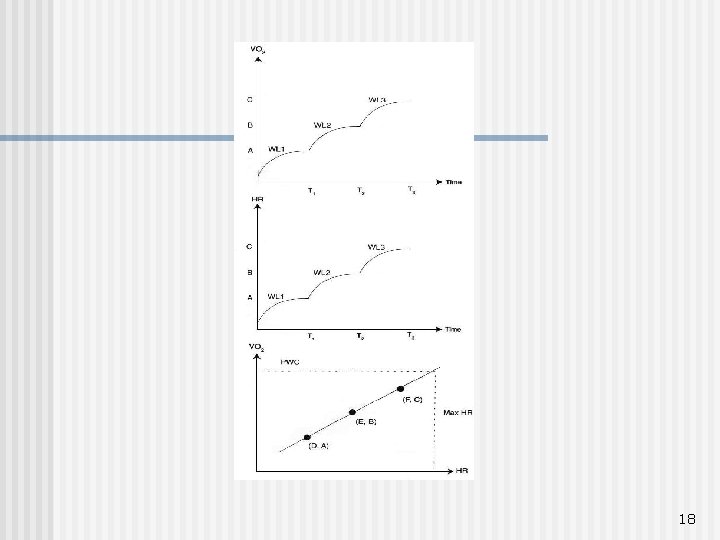
18
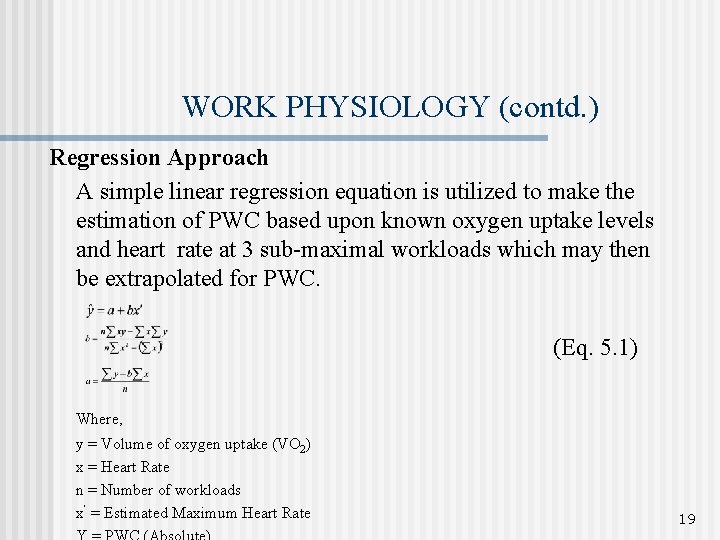
WORK PHYSIOLOGY (contd. ) Regression Approach A simple linear regression equation is utilized to make the estimation of PWC based upon known oxygen uptake levels and heart rate at 3 sub-maximal workloads which may then be extrapolated for PWC. (Eq. 5. 1) Where, y = Volume of oxygen uptake (VO 2) x = Heart Rate n = Number of workloads x = Estimated Maximum Heart Rate 19
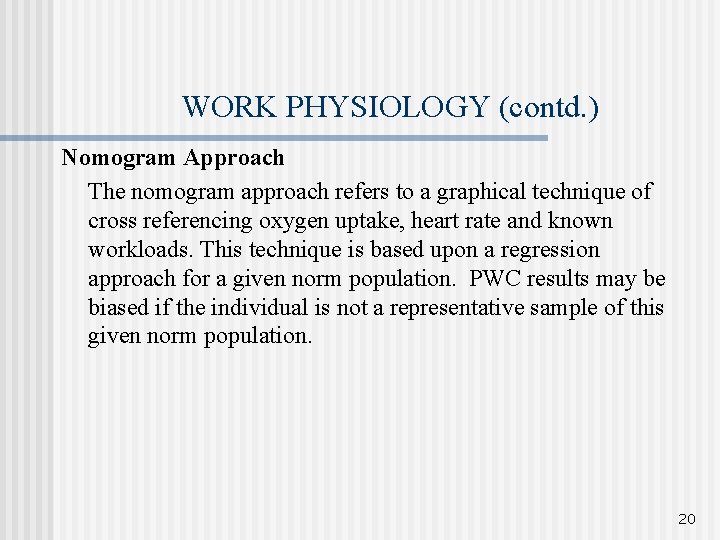
WORK PHYSIOLOGY (contd. ) Nomogram Approach The nomogram approach refers to a graphical technique of cross referencing oxygen uptake, heart rate and known workloads. This technique is based upon a regression approach for a given norm population. PWC results may be biased if the individual is not a representative sample of this given norm population. 20
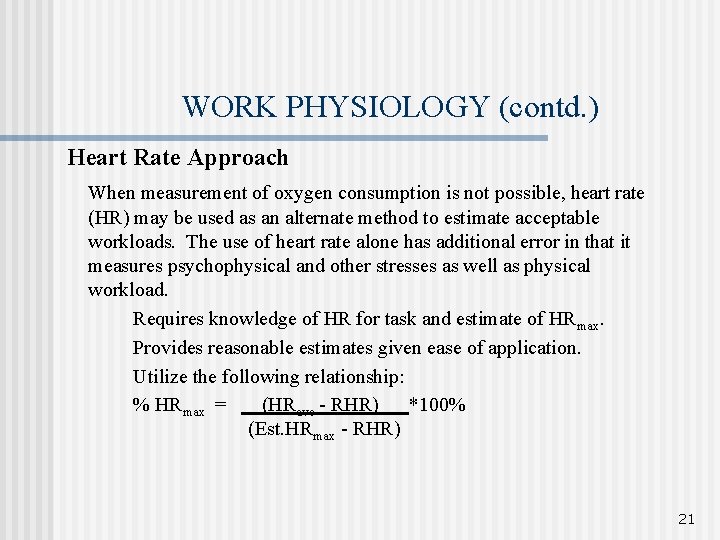
WORK PHYSIOLOGY (contd. ) Heart Rate Approach When measurement of oxygen consumption is not possible, heart rate (HR) may be used as an alternate method to estimate acceptable workloads. The use of heart rate alone has additional error in that it measures psychophysical and other stresses as well as physical workload. Requires knowledge of HR for task and estimate of HRmax. Provides reasonable estimates given ease of application. Utilize the following relationship: % HRmax = (HRave - RHR) *100% (Est. HRmax - RHR) 21
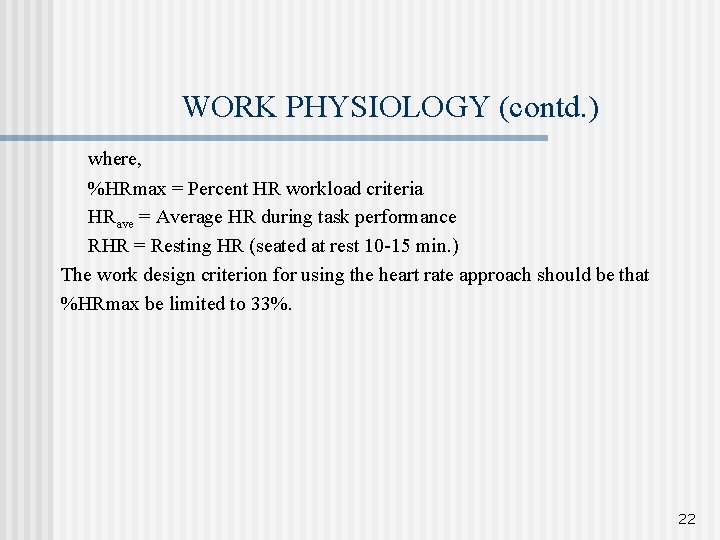
WORK PHYSIOLOGY (contd. ) where, %HRmax = Percent HR workload criteria HRave = Average HR during task performance RHR = Resting HR (seated at rest 10 -15 min. ) The work design criterion for using the heart rate approach should be that %HRmax be limited to 33%. 22
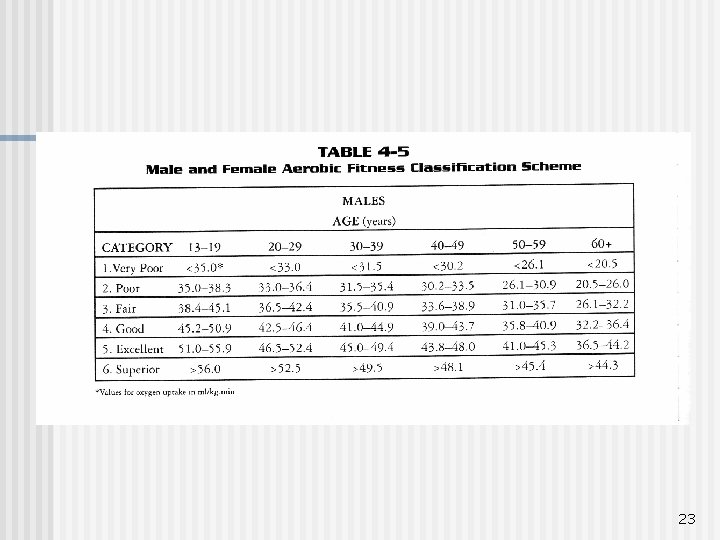
23
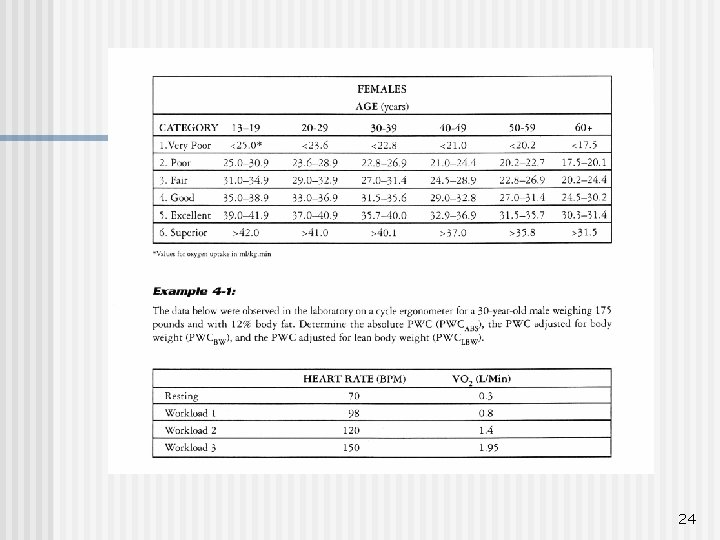
24
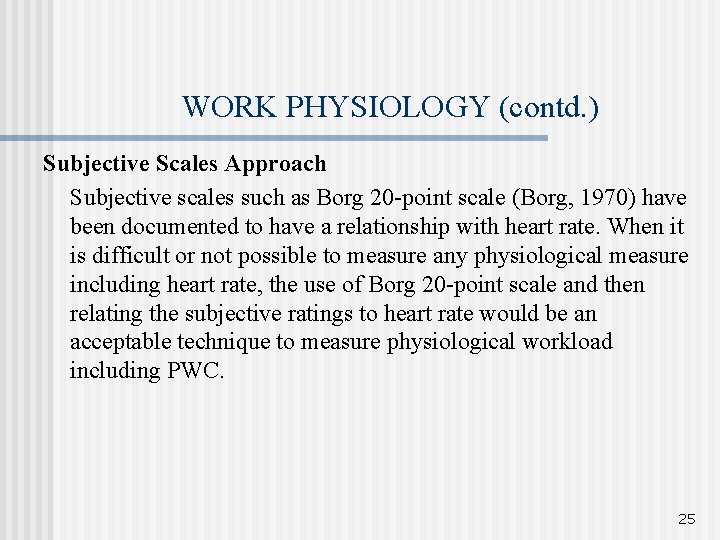
WORK PHYSIOLOGY (contd. ) Subjective Scales Approach Subjective scales such as Borg 20 -point scale (Borg, 1970) have been documented to have a relationship with heart rate. When it is difficult or not possible to measure any physiological measure including heart rate, the use of Borg 20 -point scale and then relating the subjective ratings to heart rate would be an acceptable technique to measure physiological workload including PWC. 25
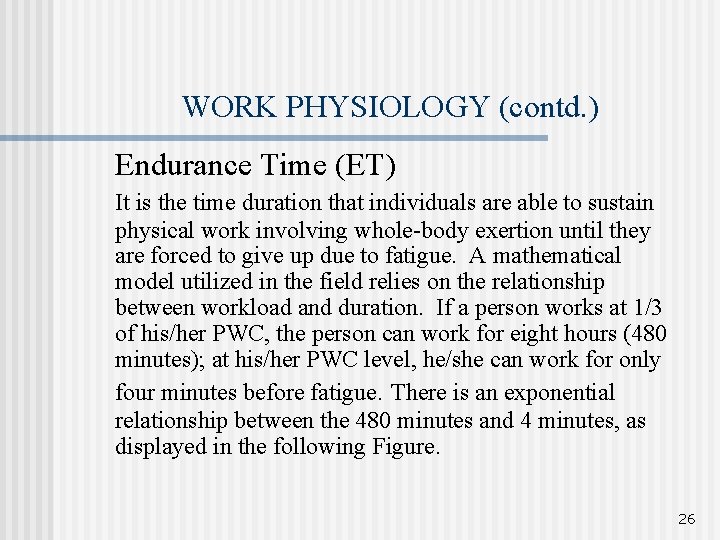
WORK PHYSIOLOGY (contd. ) Endurance Time (ET) It is the time duration that individuals are able to sustain physical work involving whole-body exertion until they are forced to give up due to fatigue. A mathematical model utilized in the field relies on the relationship between workload and duration. If a person works at 1/3 of his/her PWC, the person can work for eight hours (480 minutes); at his/her PWC level, he/she can work for only four minutes before fatigue. There is an exponential relationship between the 480 minutes and 4 minutes, as displayed in the following Figure. 26
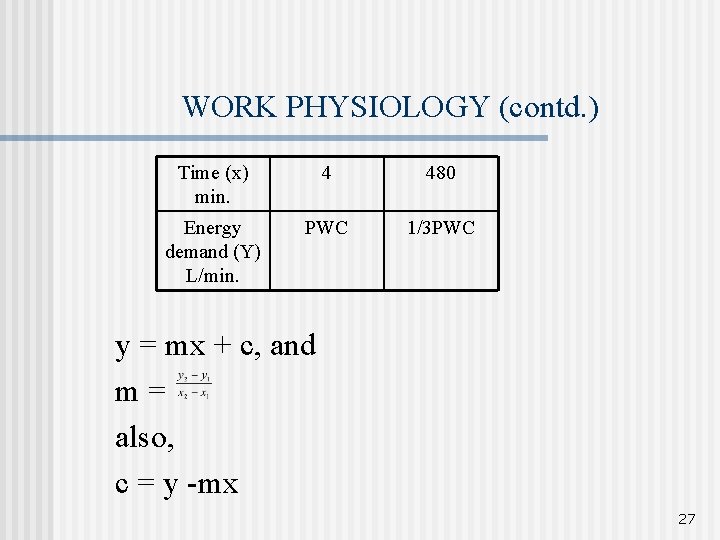
WORK PHYSIOLOGY (contd. ) Time (x) min. 4 480 Energy demand (Y) L/min. PWC 1/3 PWC y = mx + c, and m= also, c = y -mx 27
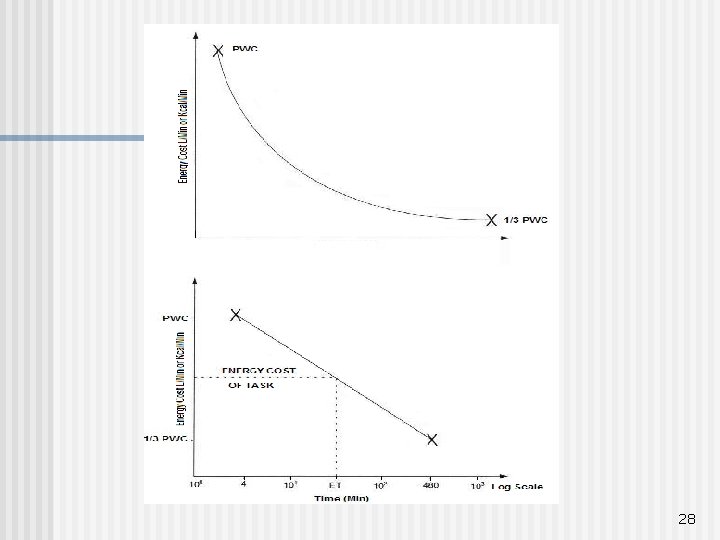
28
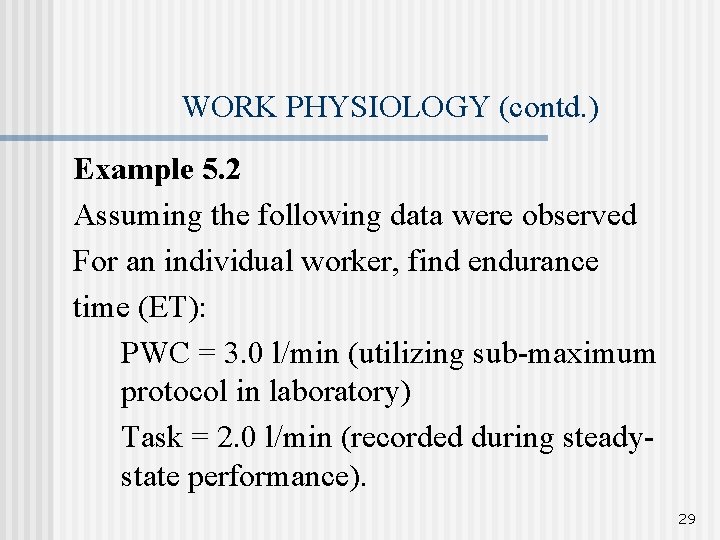
WORK PHYSIOLOGY (contd. ) Example 5. 2 Assuming the following data were observed For an individual worker, find endurance time (ET): PWC = 3. 0 l/min (utilizing sub-maximum protocol in laboratory) Task = 2. 0 l/min (recorded during steadystate performance). 29
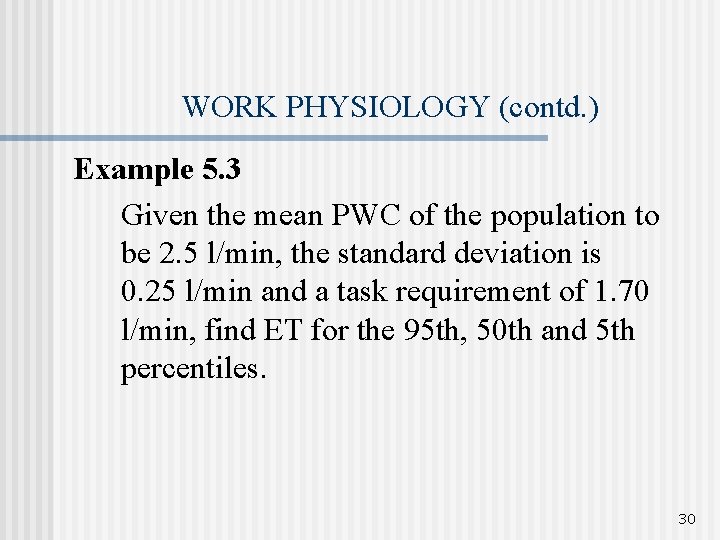
WORK PHYSIOLOGY (contd. ) Example 5. 3 Given the mean PWC of the population to be 2. 5 l/min, the standard deviation is 0. 25 l/min and a task requirement of 1. 70 l/min, find ET for the 95 th, 50 th and 5 th percentiles. 30
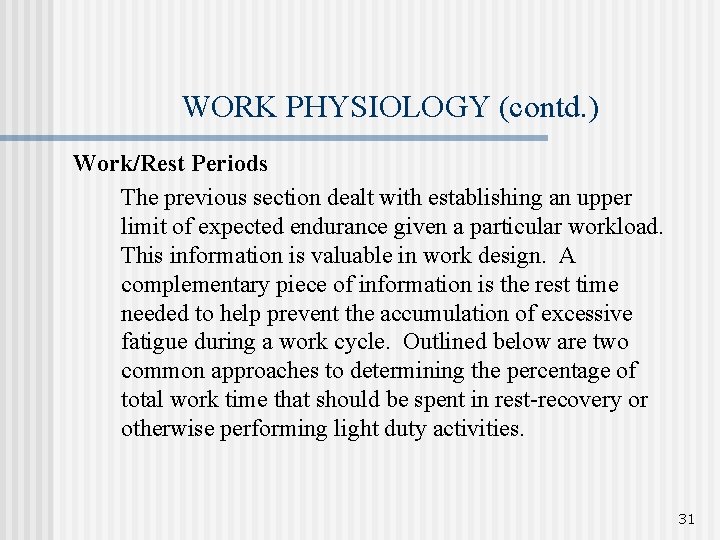
WORK PHYSIOLOGY (contd. ) Work/Rest Periods The previous section dealt with establishing an upper limit of expected endurance given a particular workload. This information is valuable in work design. A complementary piece of information is the rest time needed to help prevent the accumulation of excessive fatigue during a work cycle. Outlined below are two common approaches to determining the percentage of total work time that should be spent in rest-recovery or otherwise performing light duty activities. 31
![WORK PHYSIOLOGY contd 1 Spitzers Model Spitzer 1951 R M4 1 WORK PHYSIOLOGY (contd. ) 1. Spitzer's Model (Spitzer, 1951): R% = [(M/4)] ‑ 1]](https://slidetodoc.com/presentation_image_h2/3a953aa76ab0867ff9ed4606dd7d13c8/image-32.jpg)
WORK PHYSIOLOGY (contd. ) 1. Spitzer's Model (Spitzer, 1951): R% = [(M/4)] ‑ 1] * 100% for 4<M<8 2. Murrel's Model (Murrel, 1965): RT = [T * (M ‑ 4)] / [M ‑ 1. 5] for M≥ 8 Where (both models), R% = % of work time allowed for rest-recovery (added to work time for total shift) RT = rest-recovery time for total shift time M = net metabolic cost (kcal/min) of activity, (if not given) resting metabolism is approximately 1. 7 kcal/min for males and 1. 4 kcal/min for females T = total shift time 32
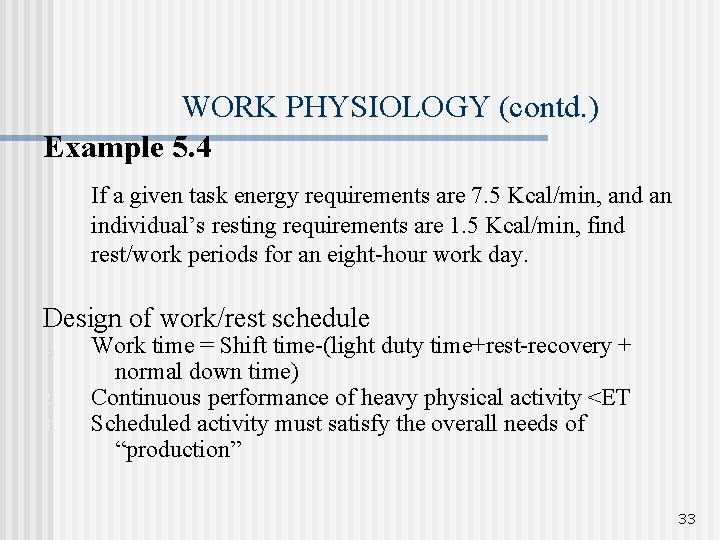
WORK PHYSIOLOGY (contd. ) Example 5. 4 If a given task energy requirements are 7. 5 Kcal/min, and an individual’s resting requirements are 1. 5 Kcal/min, find rest/work periods for an eight-hour work day. Design of work/rest schedule 1. 2. 3. Work time = Shift time-(light duty time+rest-recovery + normal down time) Continuous performance of heavy physical activity <ET Scheduled activity must satisfy the overall needs of “production” 33
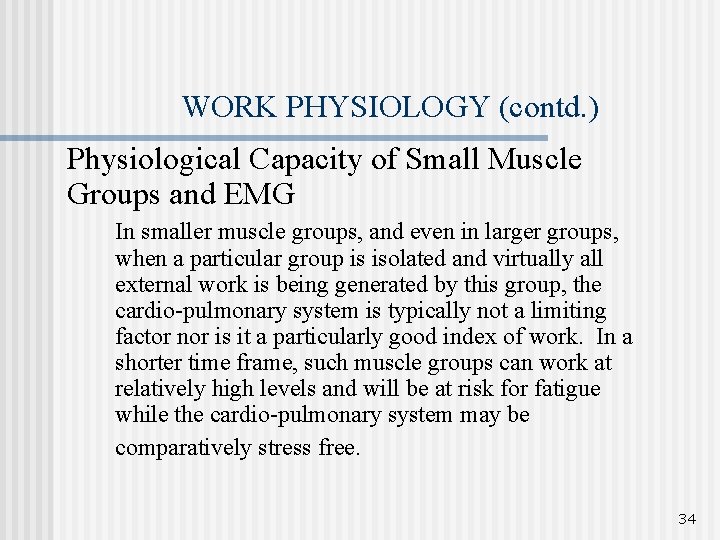
WORK PHYSIOLOGY (contd. ) Physiological Capacity of Small Muscle Groups and EMG In smaller muscle groups, and even in larger groups, when a particular group is isolated and virtually all external work is being generated by this group, the cardio-pulmonary system is typically not a limiting factor nor is it a particularly good index of work. In a shorter time frame, such muscle groups can work at relatively high levels and will be at risk for fatigue while the cardio-pulmonary system may be comparatively stress free. 34
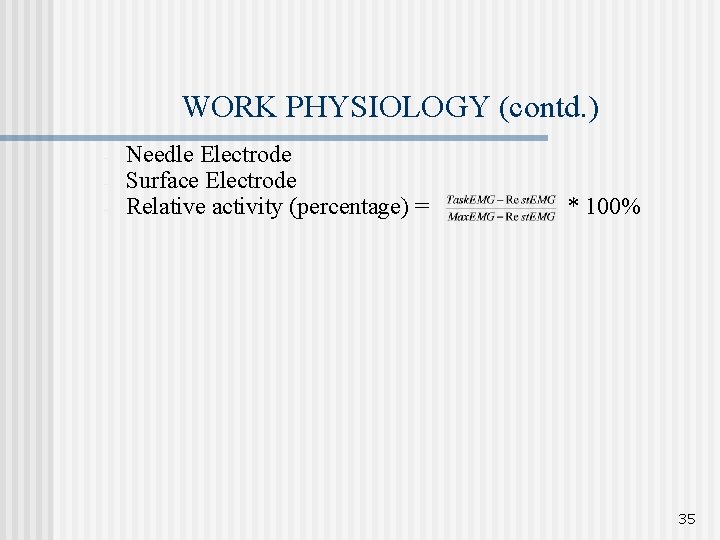
WORK PHYSIOLOGY (contd. ) - Needle Electrode Surface Electrode Relative activity (percentage) = * 100% 35
Psyc info
Jst umn
Workplace environment and ergonomics
Good chair
Ergonomics lifting techniques
Ergonomics and anthropometrics
Ergonomics session
Types of ergonomics
Objectives of ergonomics
Define ergonomics in industrial engineering
Objectives of ergonomics
Ergonomics in textile industry
Bathroom ergonomics
Differentiate the 10 principles of ergonomics
Six pillars of ergonomics
What is ergonomics
Types of ergonomics
Patient care ergonomics
Types of ergonomics
Ergo nomic
Visual display in ergonomics
Cms ergonomics
Types of ergonomics
Ergonomics awareness training for supervisors
Ergonomics awareness training for supervisors
Ergonomic tips for computer users
Controller ergonomics
Driving ergonomics checklist
Terry howerton
What do you see in this picture
Six pillars of ergonomics
Objectives of ergonomics
Ergonomics definition medical
Ergonomics in welding
Ergonomics for healthcare workers
Milady chapter 3