Noctilucent Clouds Polar Mesospheric Summer Echoes and Dusty
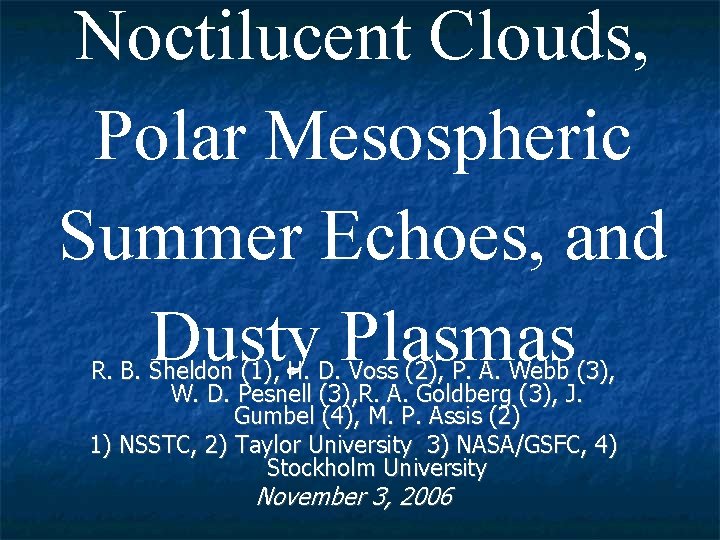
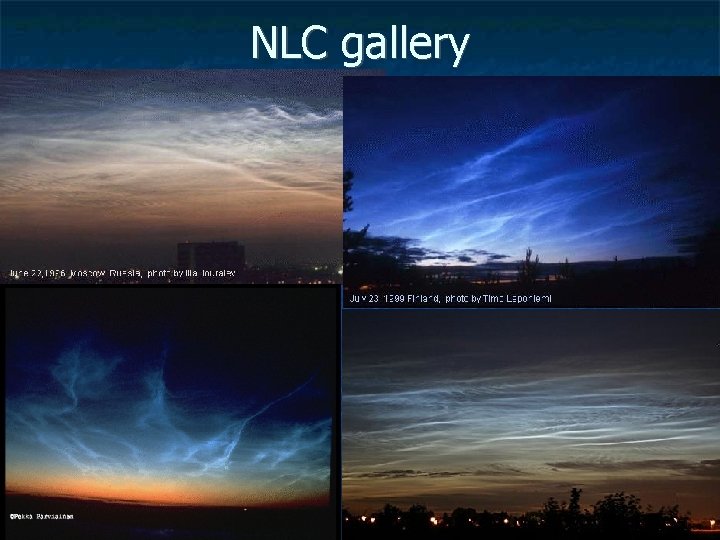
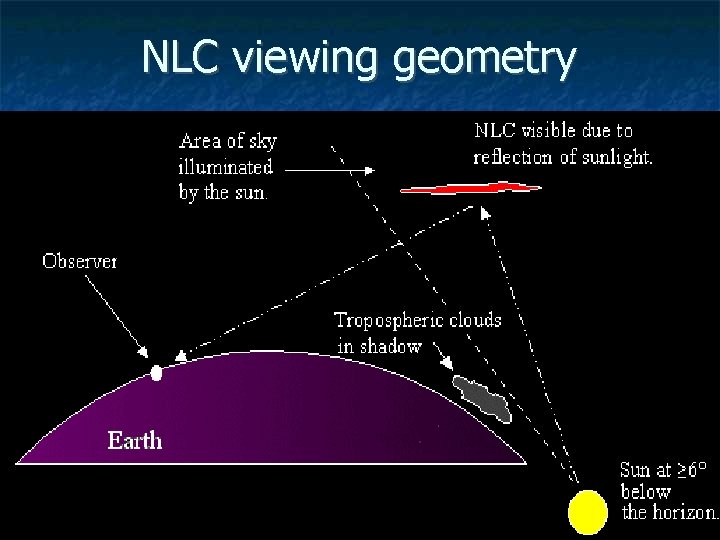
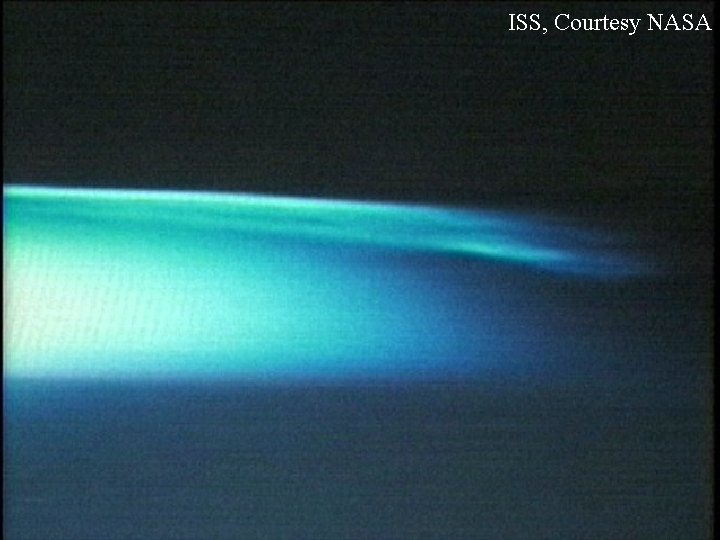
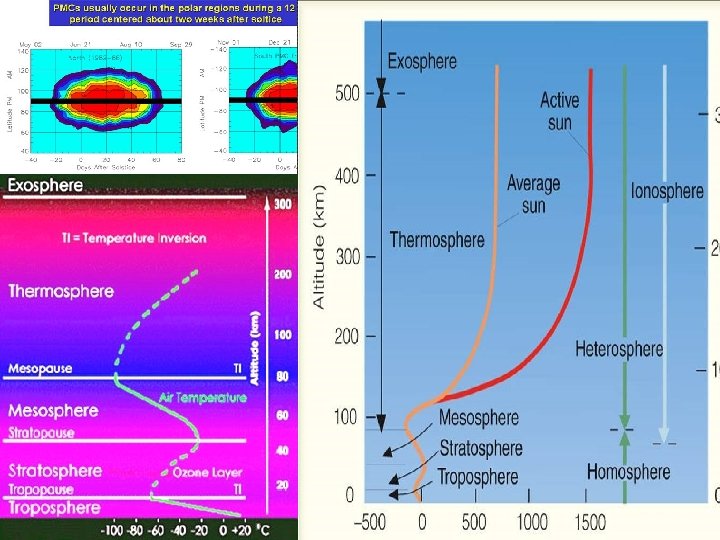
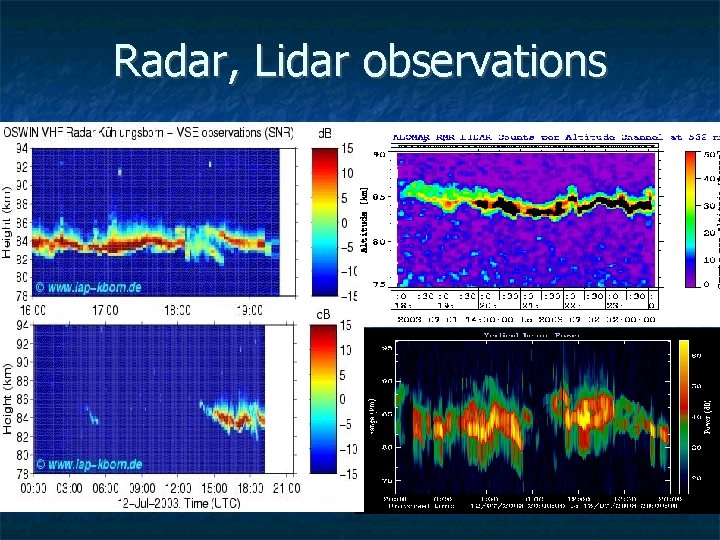
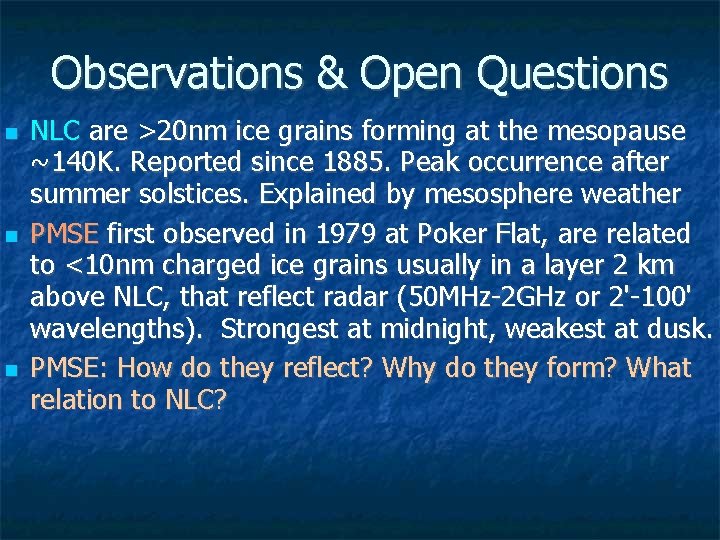
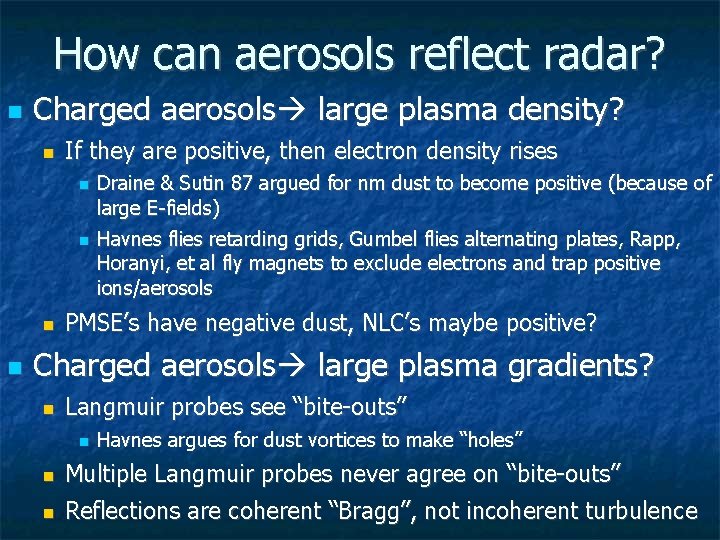
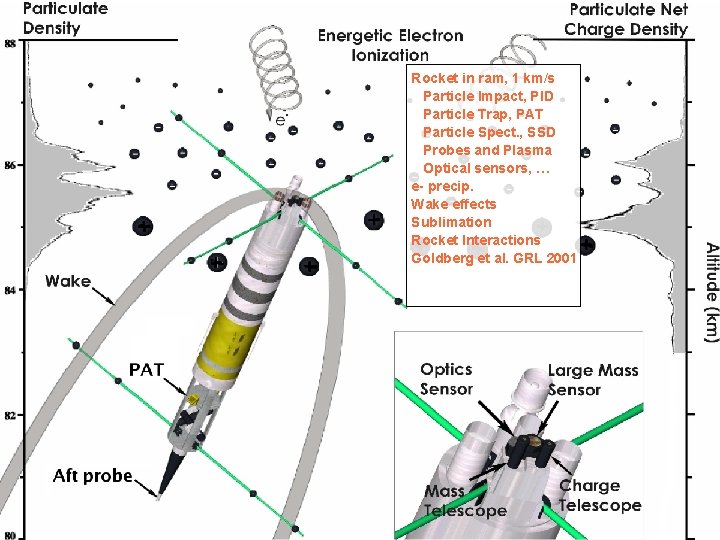
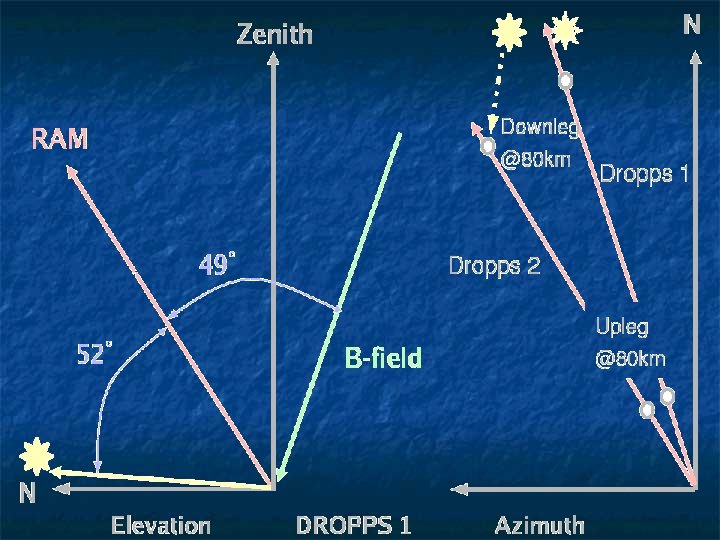
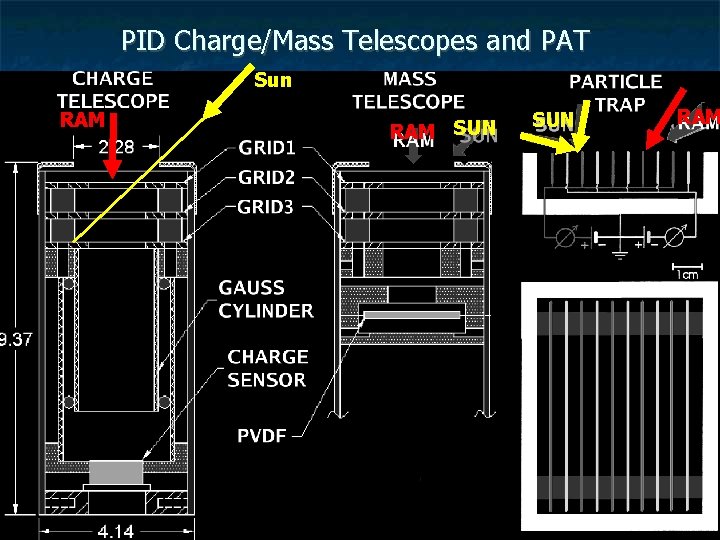
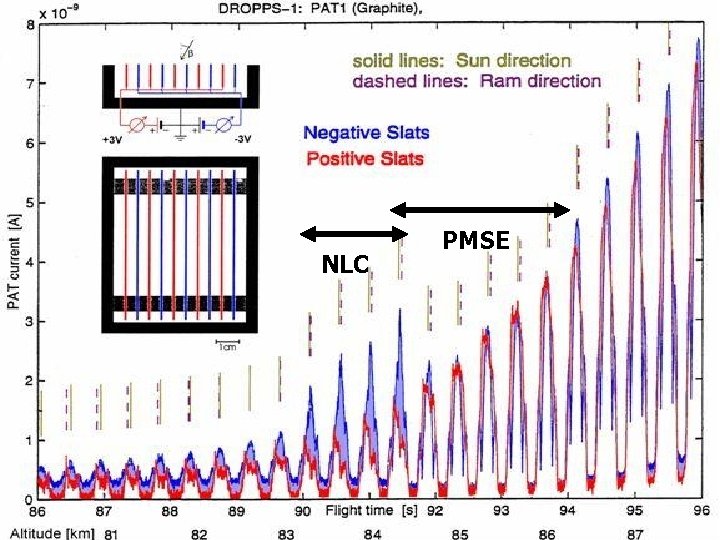
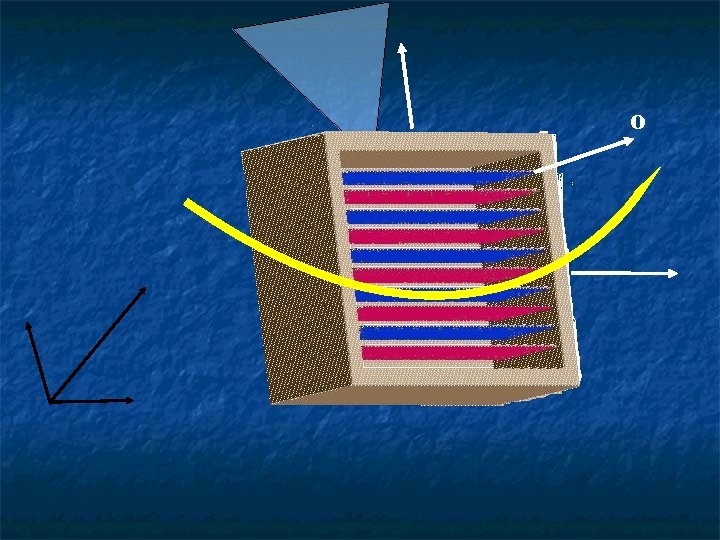
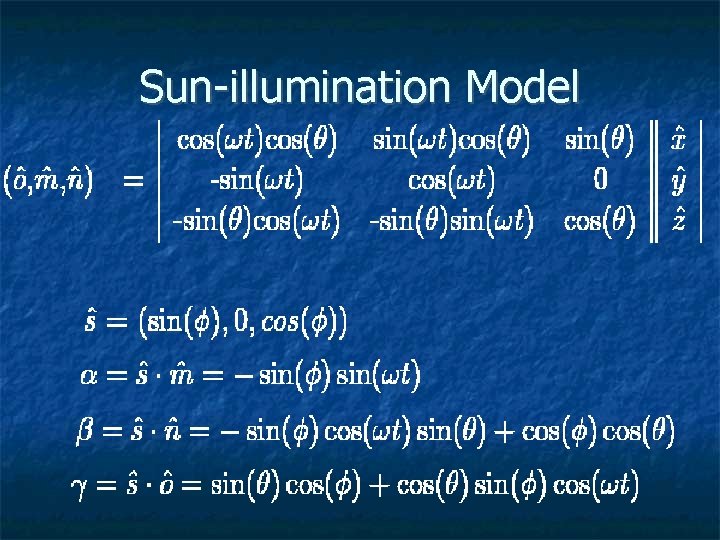
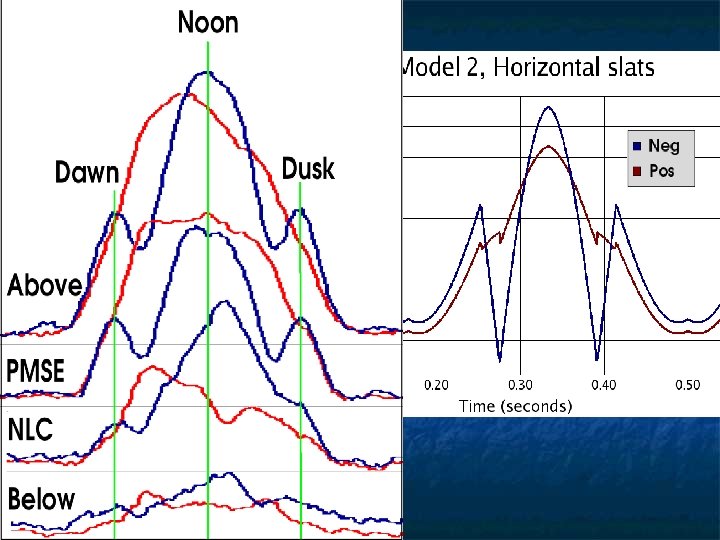
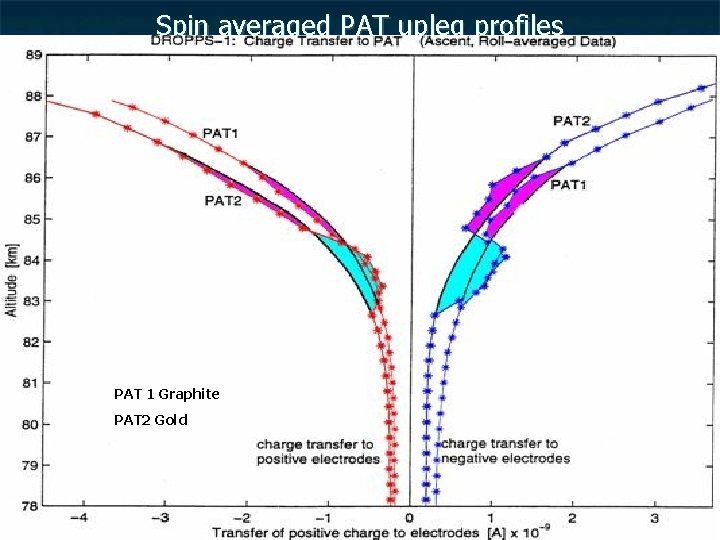
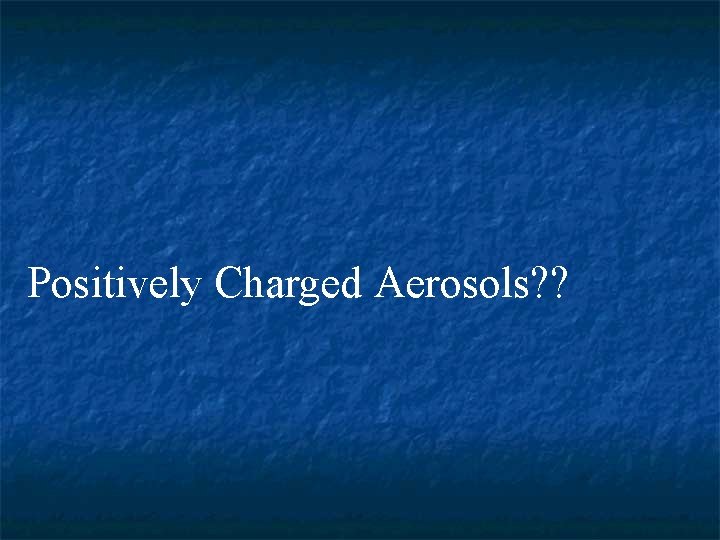
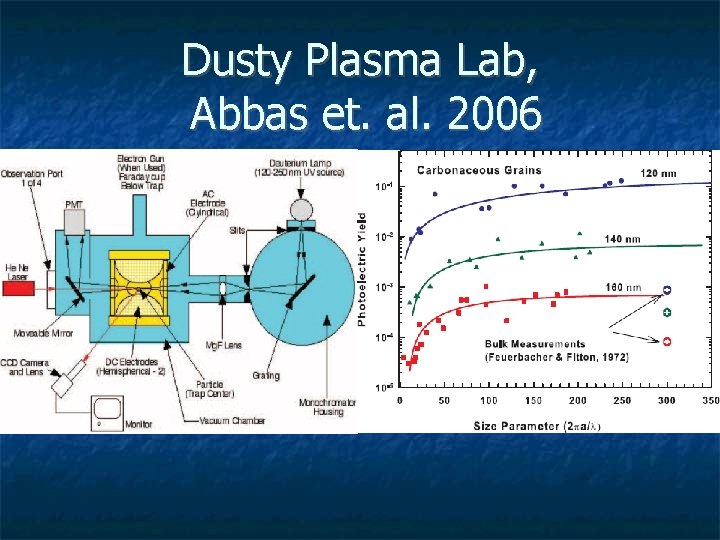
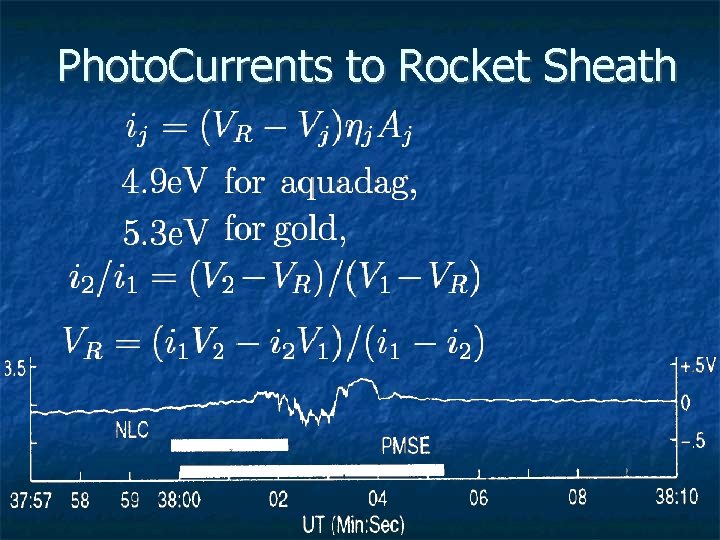
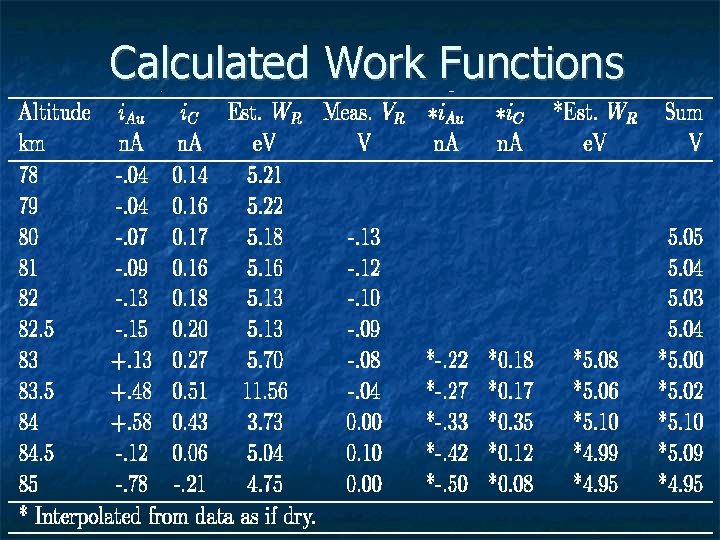
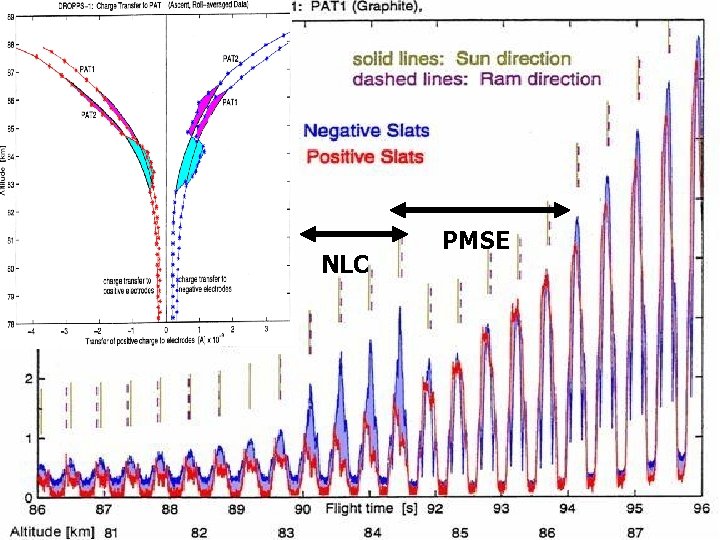
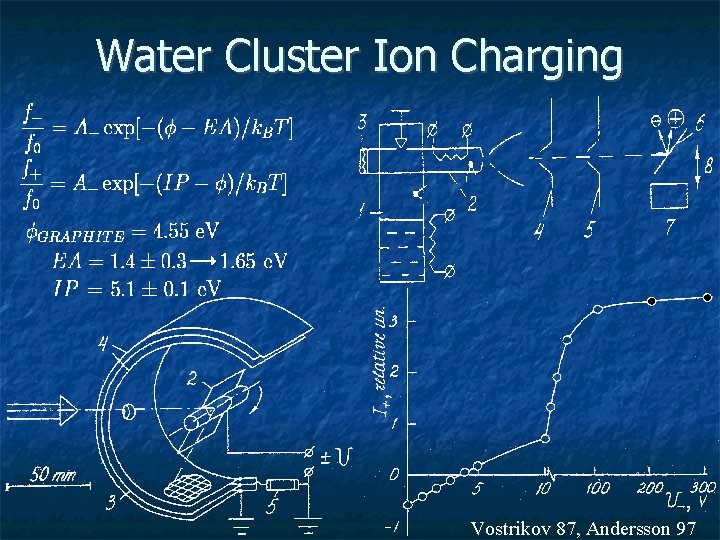
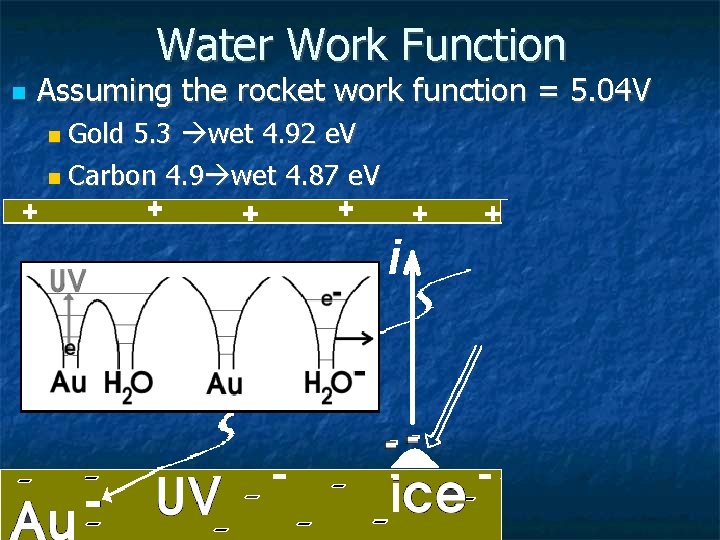
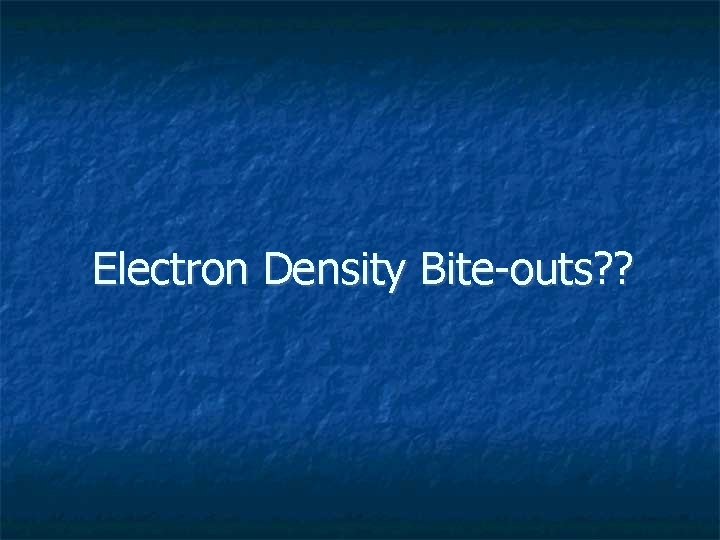
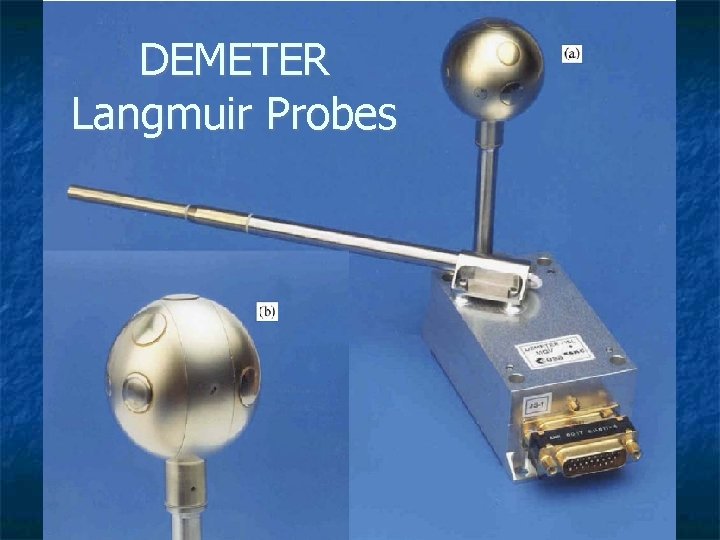
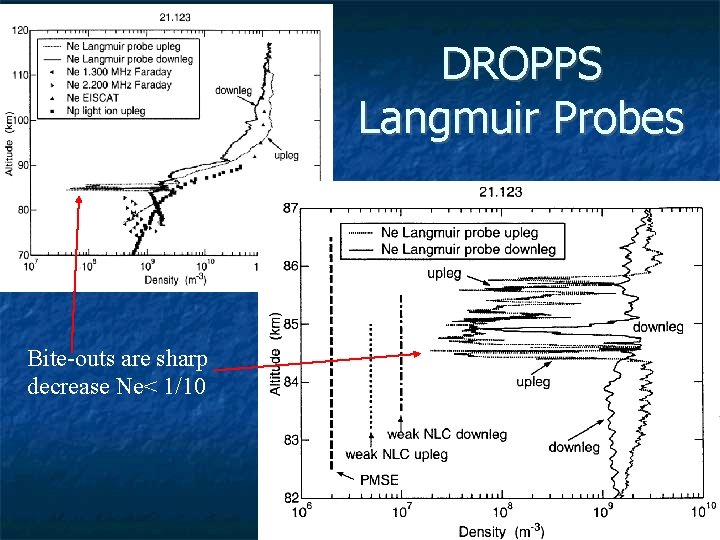
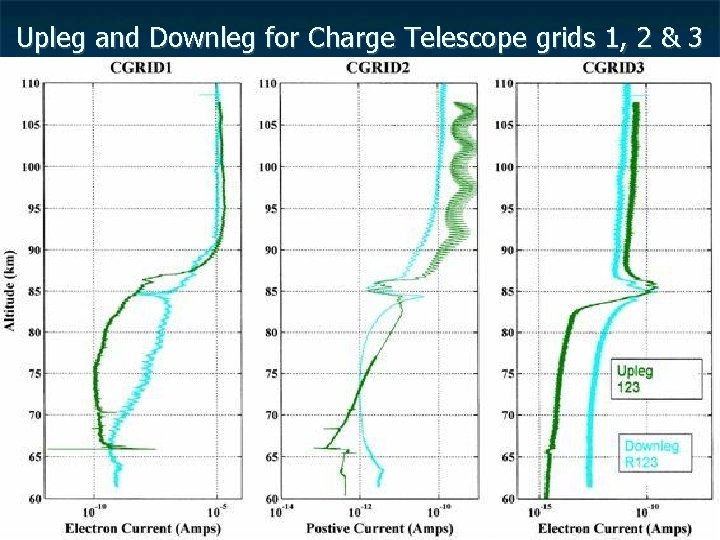
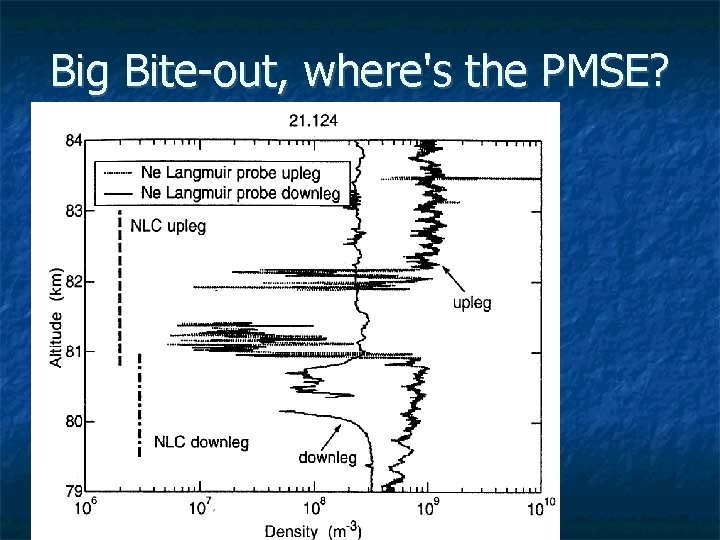
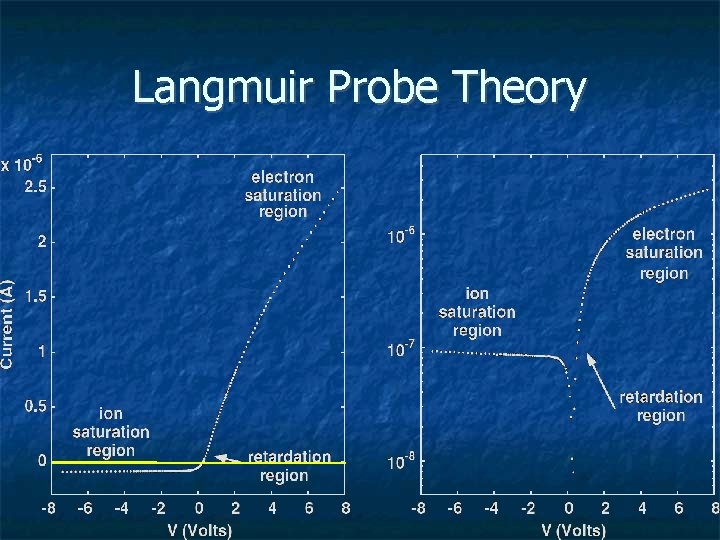
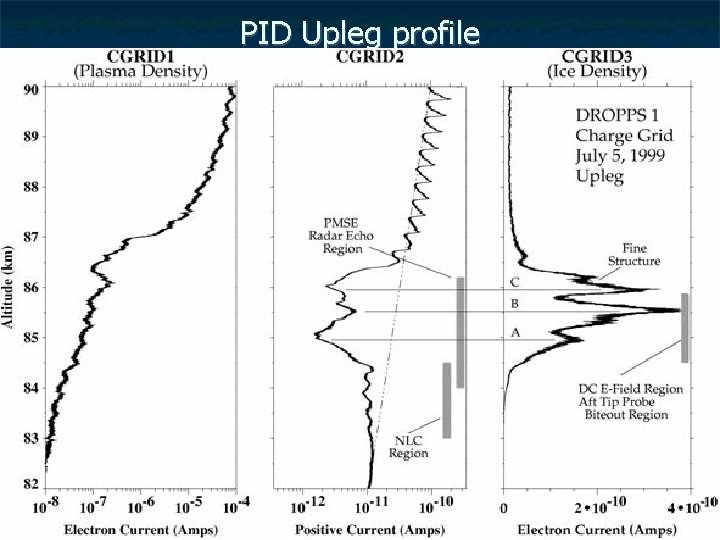
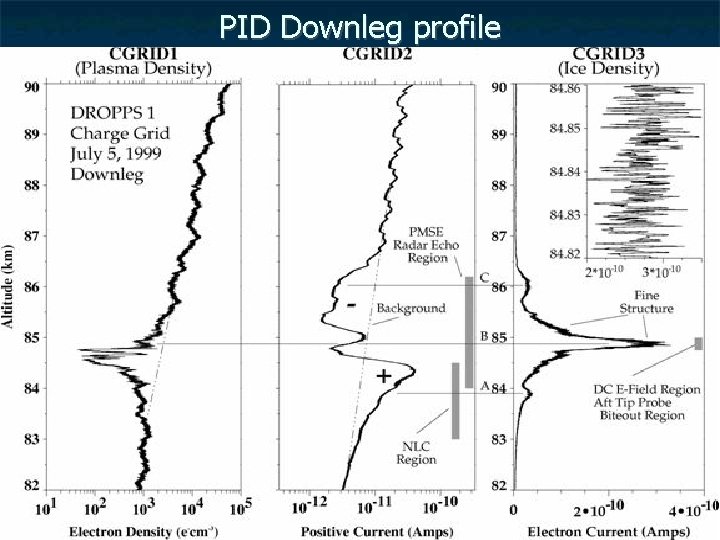
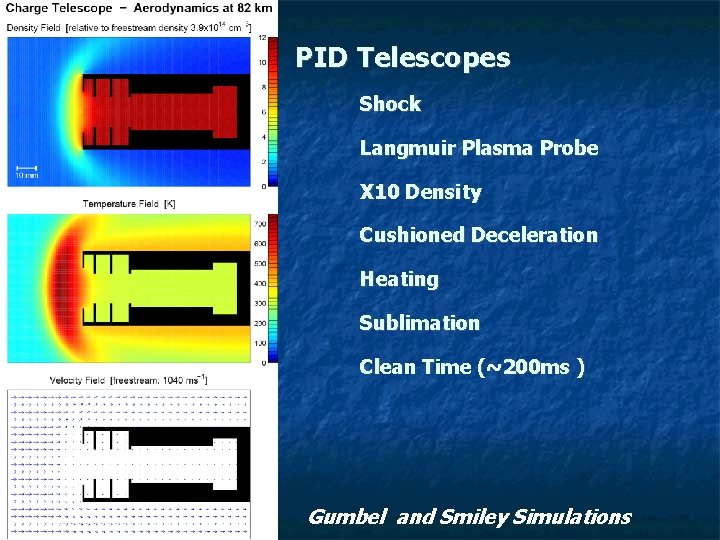
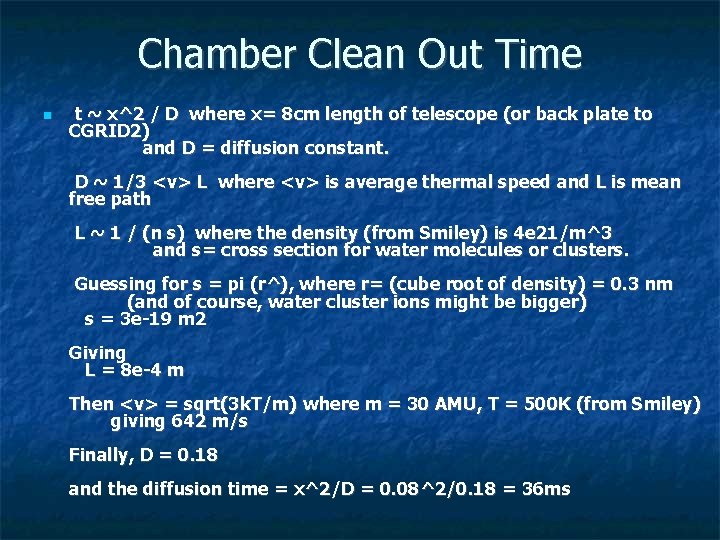
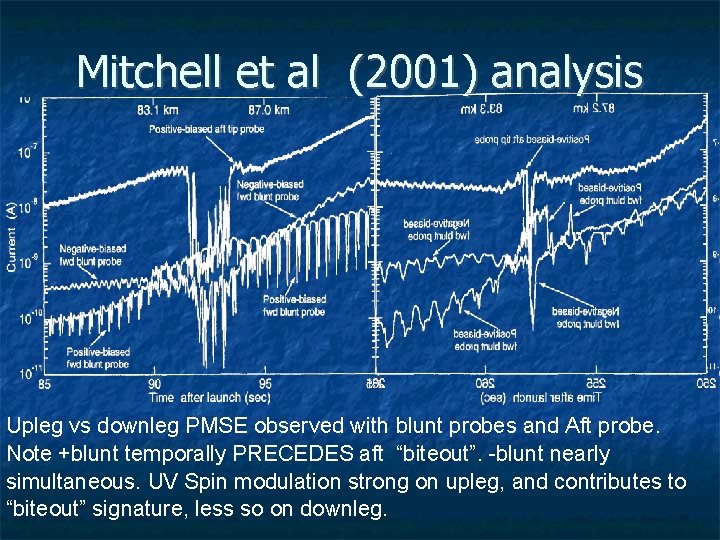
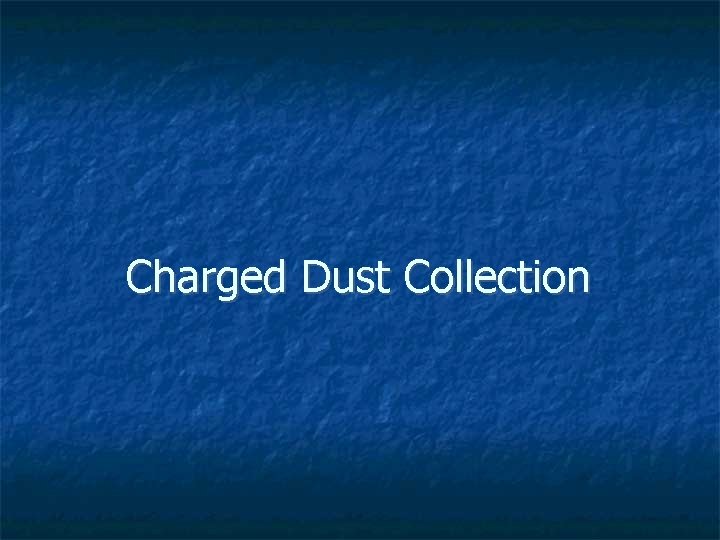
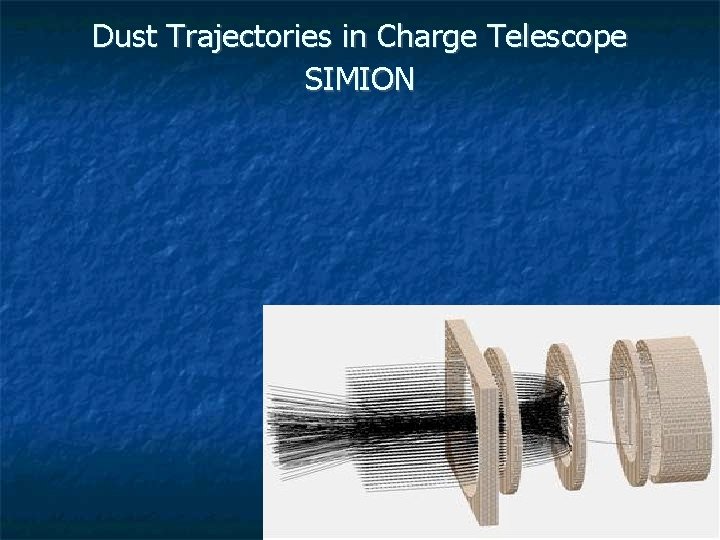
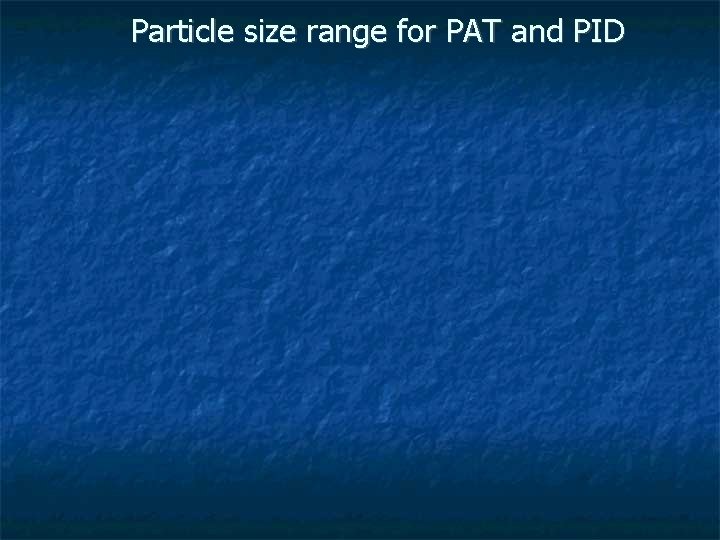
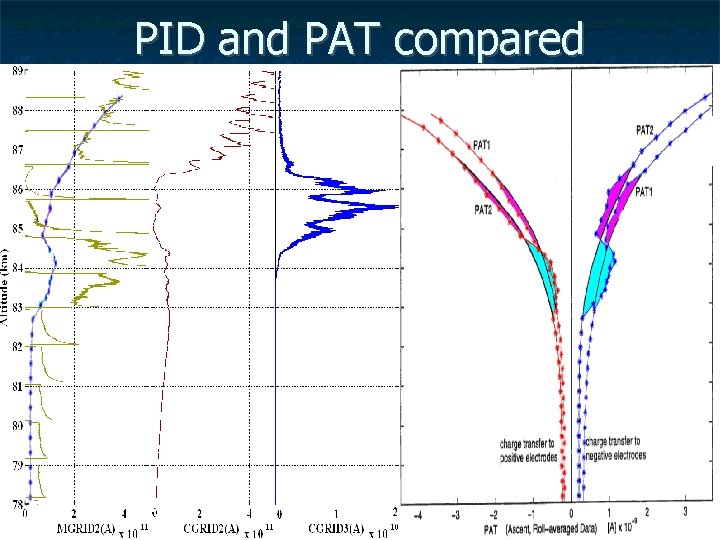
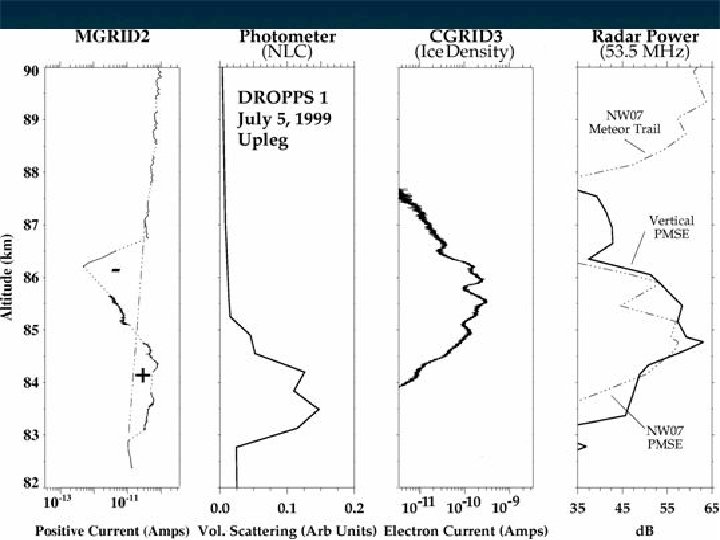
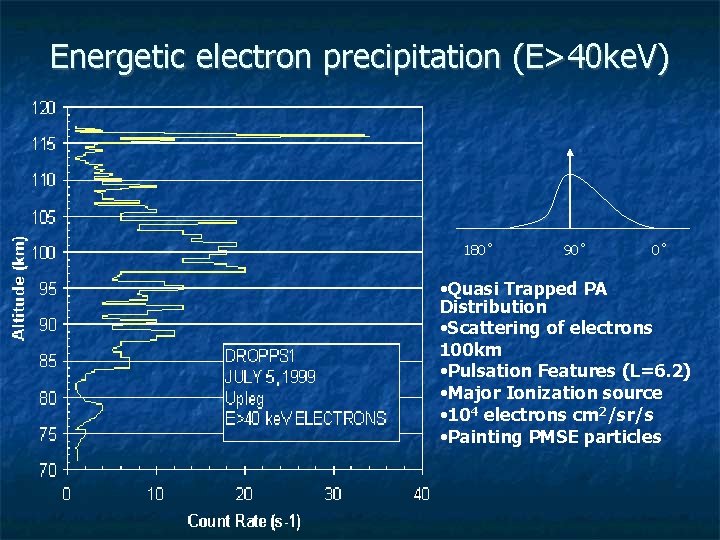
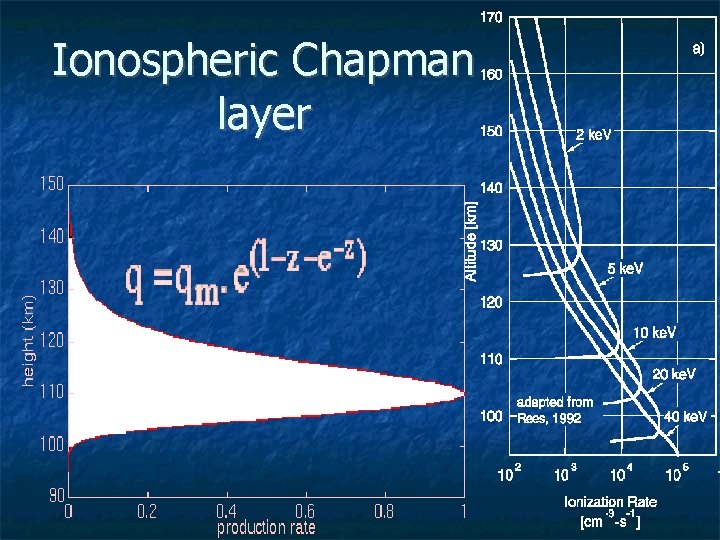
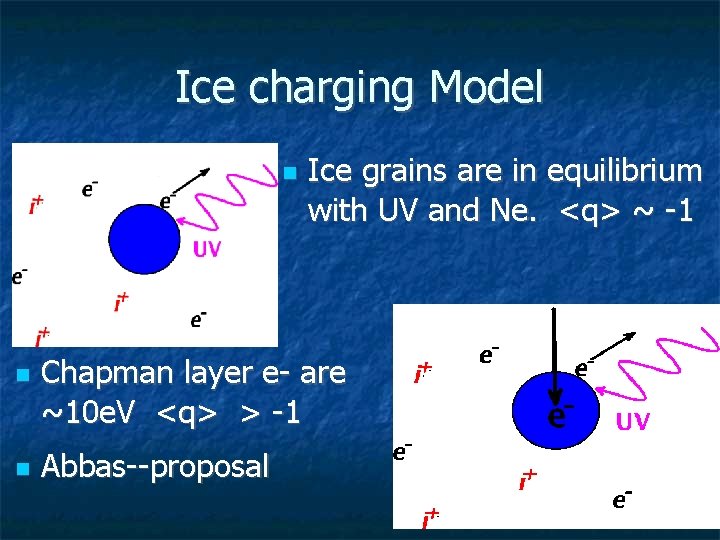
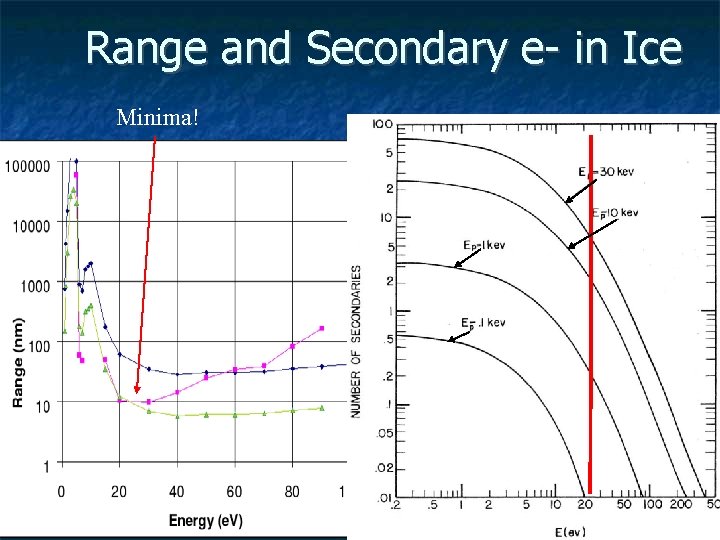
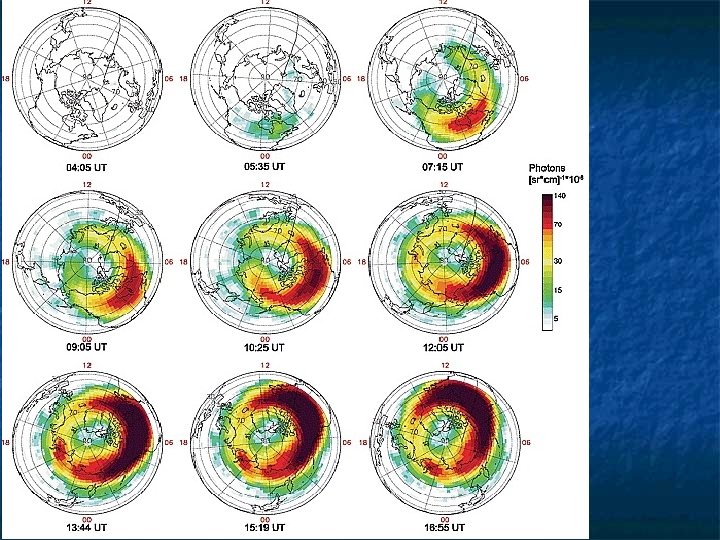
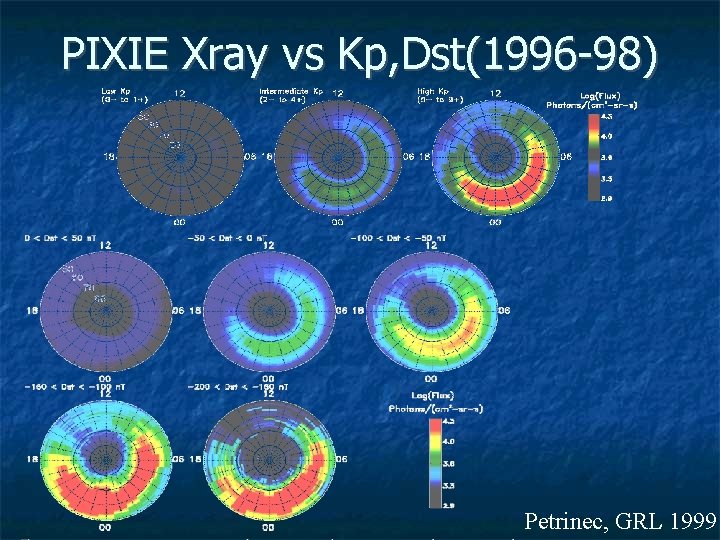
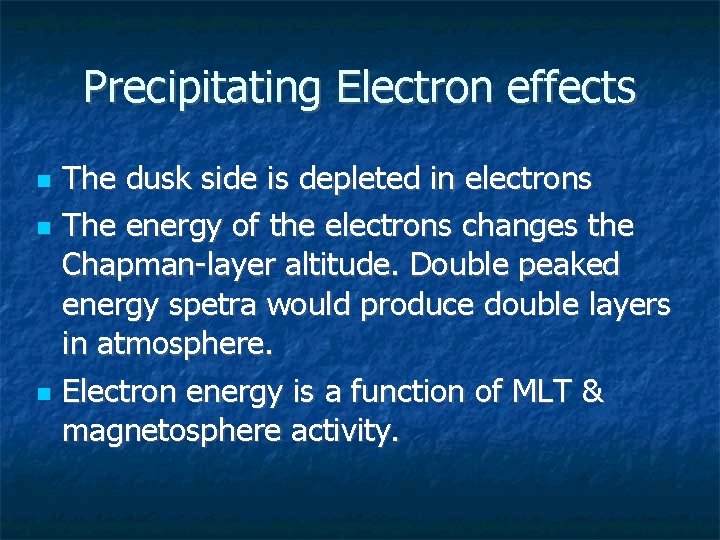
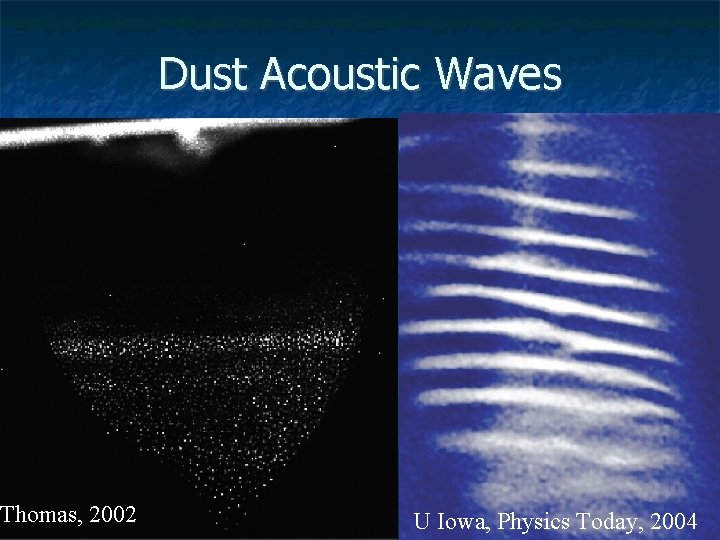
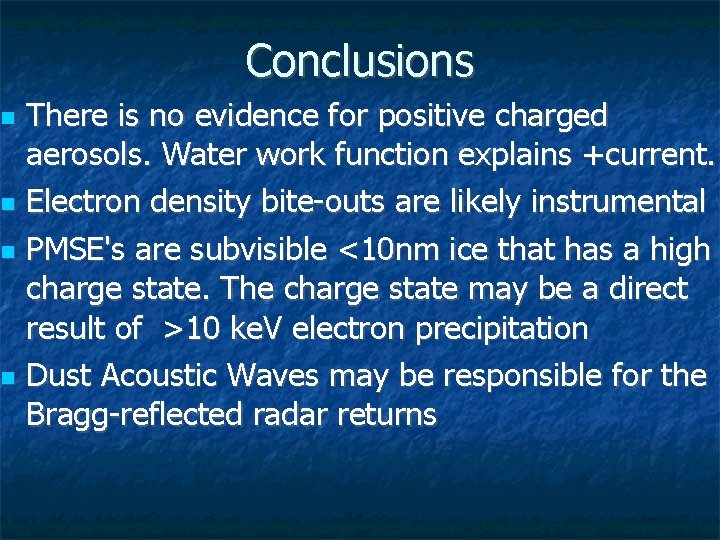
- Slides: 48
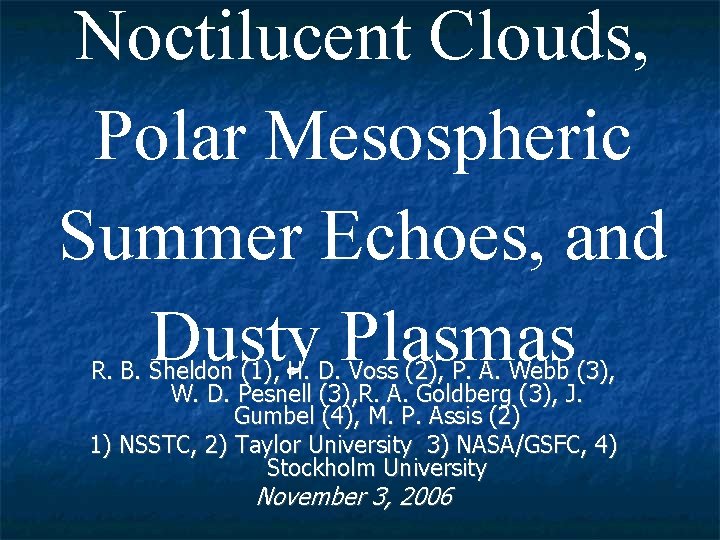
Noctilucent Clouds, Polar Mesospheric Summer Echoes, and Dusty Plasmas R. B. Sheldon (1), H. D. Voss (2), P. A. Webb (3), W. D. Pesnell (3), R. A. Goldberg (3), J. Gumbel (4), M. P. Assis (2) 1) NSSTC, 2) Taylor University 3) NASA/GSFC, 4) Stockholm University November 3, 2006
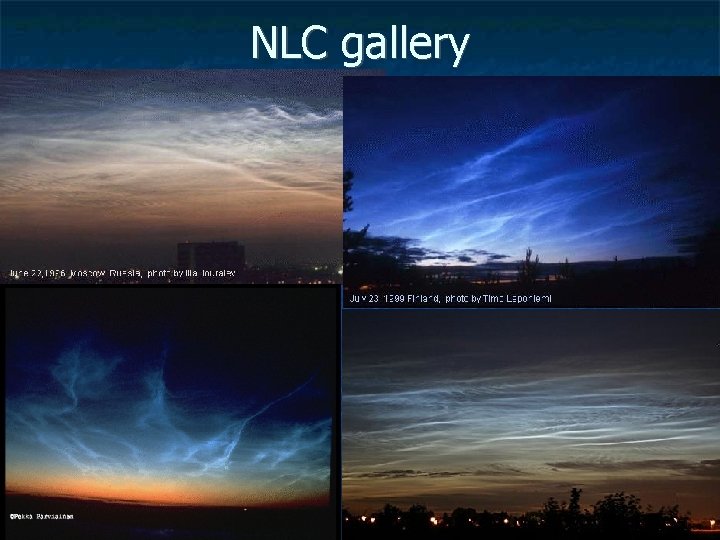
NLC gallery
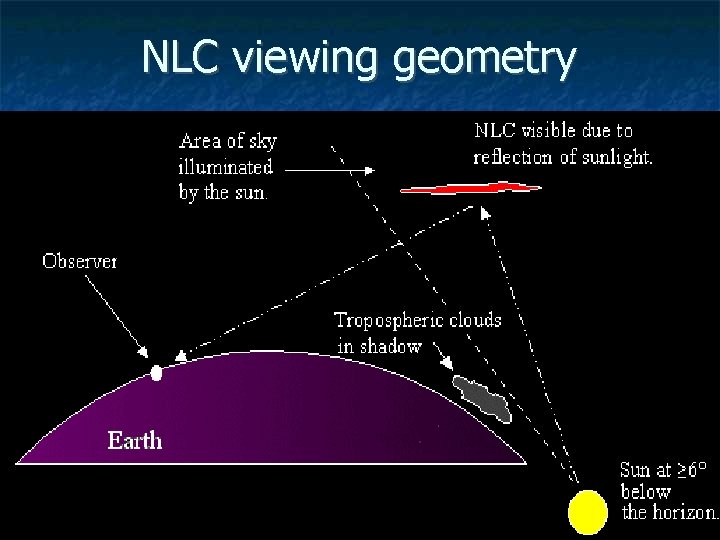
NLC viewing geometry
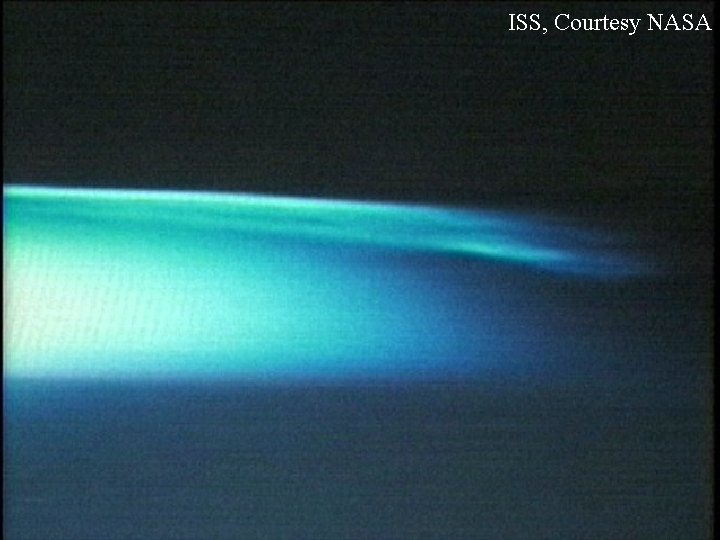
ISS, Courtesy NASA
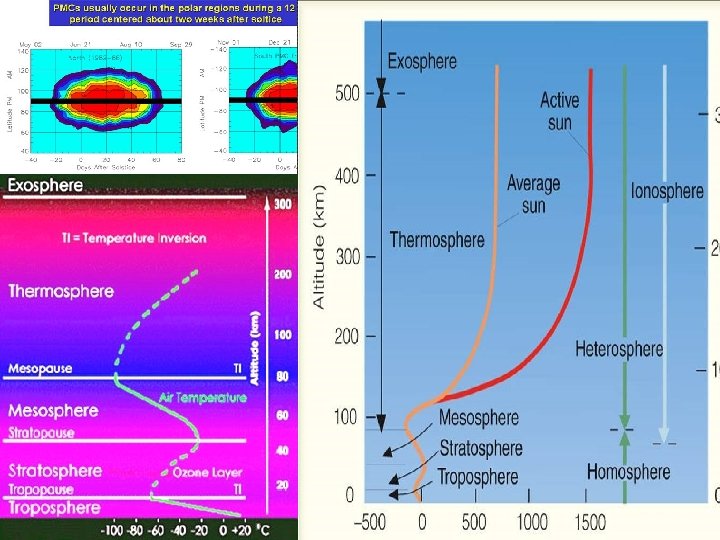
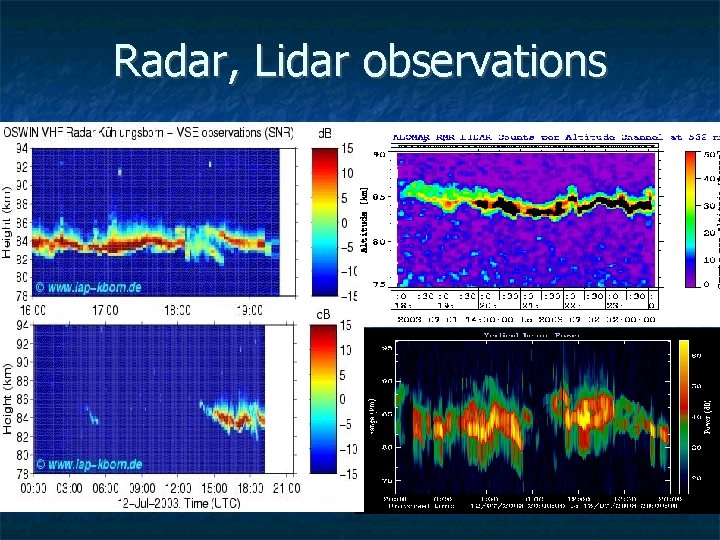
Radar, Lidar observations
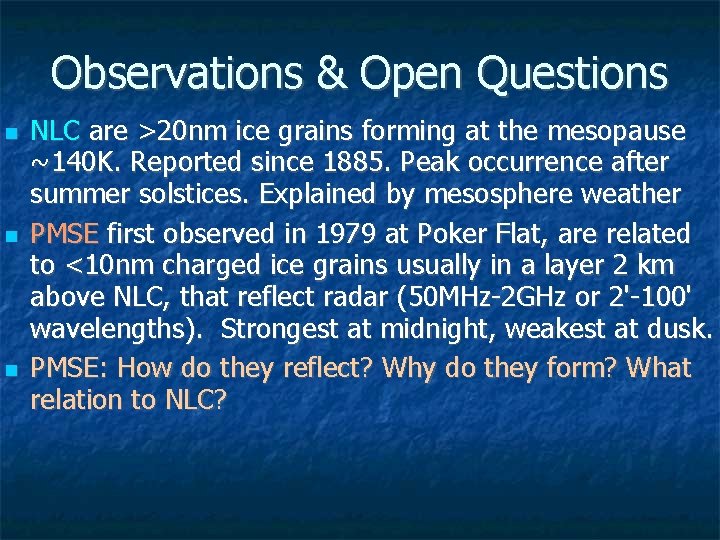
Observations & Open Questions NLC are >20 nm ice grains forming at the mesopause ~140 K. Reported since 1885. Peak occurrence after summer solstices. Explained by mesosphere weather PMSE first observed in 1979 at Poker Flat, are related to <10 nm charged ice grains usually in a layer 2 km above NLC, that reflect radar (50 MHz-2 GHz or 2'-100' wavelengths). Strongest at midnight, weakest at dusk. PMSE: How do they reflect? Why do they form? What relation to NLC?
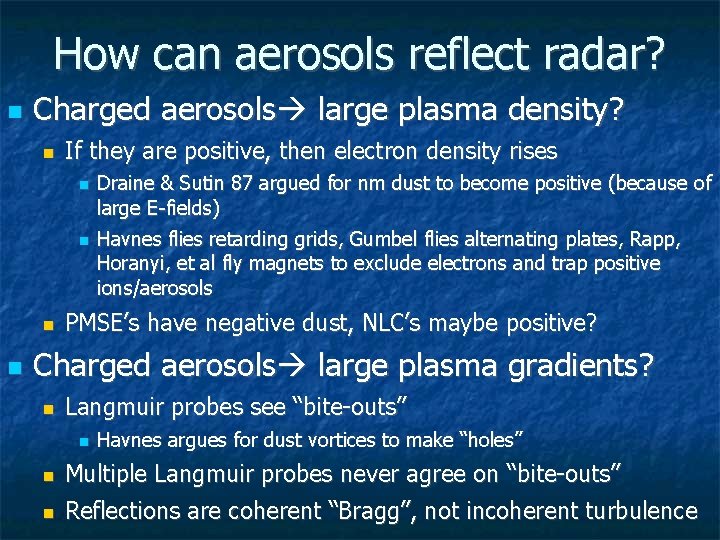
How can aerosols reflect radar? Charged aerosols large plasma density? If they are positive, then electron density rises Draine & Sutin 87 argued for nm dust to become positive (because of large E-fields) Havnes flies retarding grids, Gumbel flies alternating plates, Rapp, Horanyi, et al fly magnets to exclude electrons and trap positive ions/aerosols PMSE’s have negative dust, NLC’s maybe positive? Charged aerosols large plasma gradients? Langmuir probes see “bite-outs” Havnes argues for dust vortices to make “holes” Multiple Langmuir probes never agree on “bite-outs” Reflections are coherent “Bragg”, not incoherent turbulence
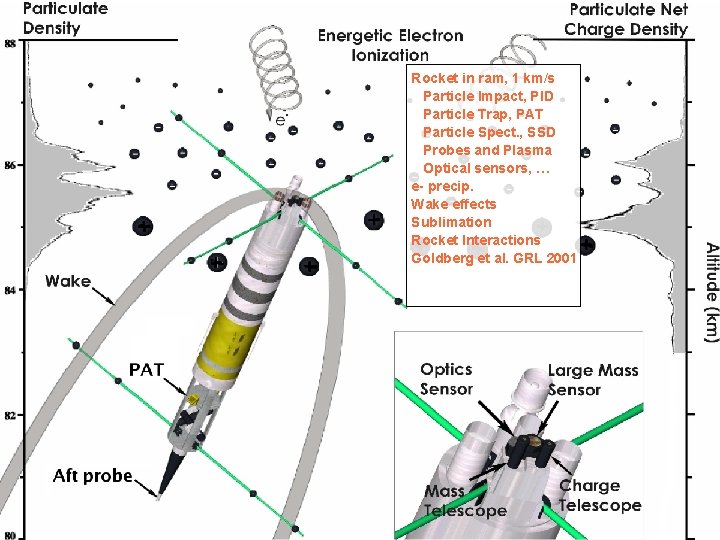
DROPPS Rocket Concept Rocket in ram, 1 km/s Particle Impact, PID Particle Trap, PAT Particle Spect. , SSD Probes and Plasma Optical sensors, … e- precip. Wake effects Sublimation Rocket Interactions Goldberg et al. GRL 2001
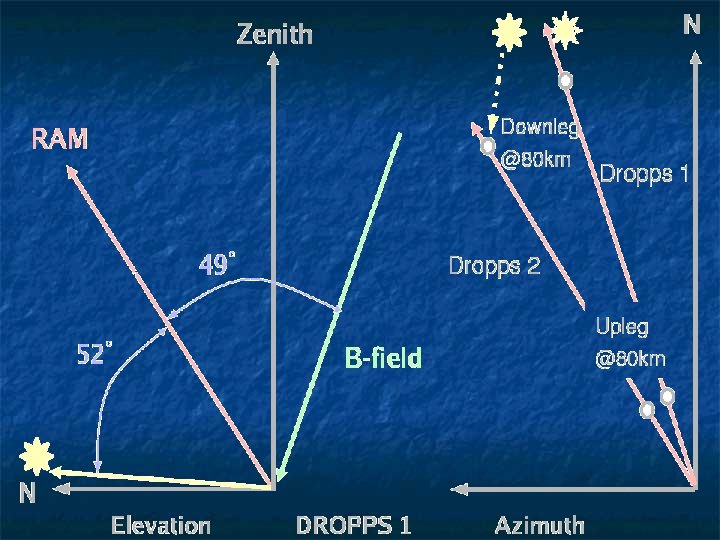
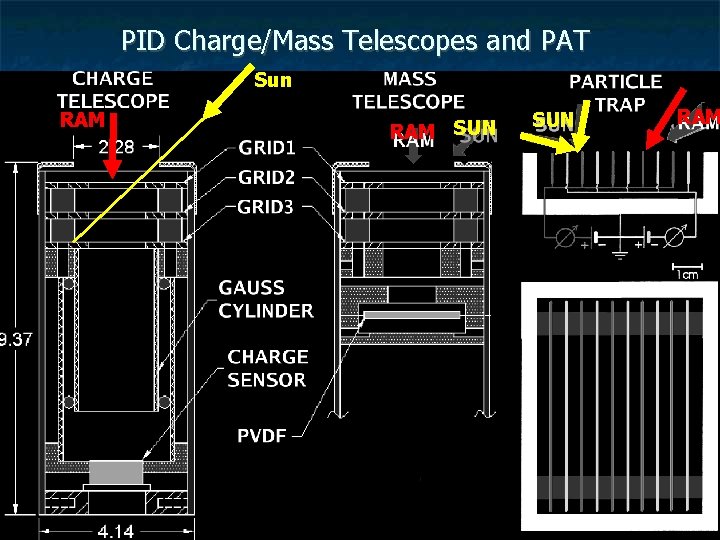
PID Charge/Mass Telescopes and PAT Sun RAM SUN RAM
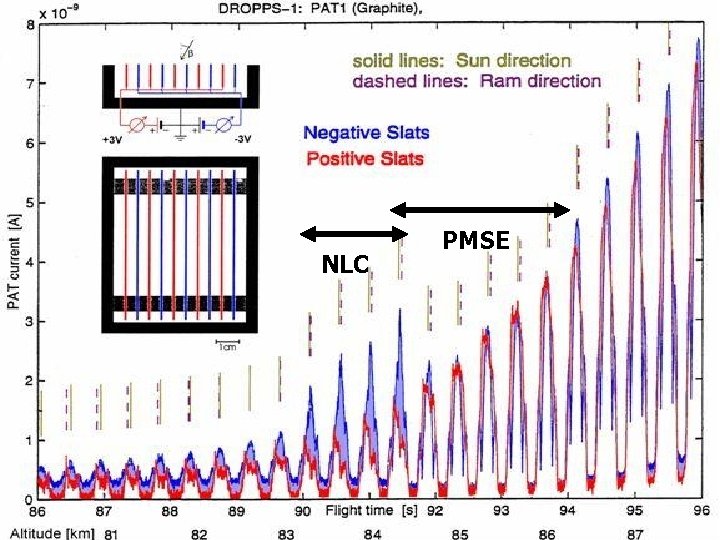
Particle Trap (PAT) instrument NLC PMSE
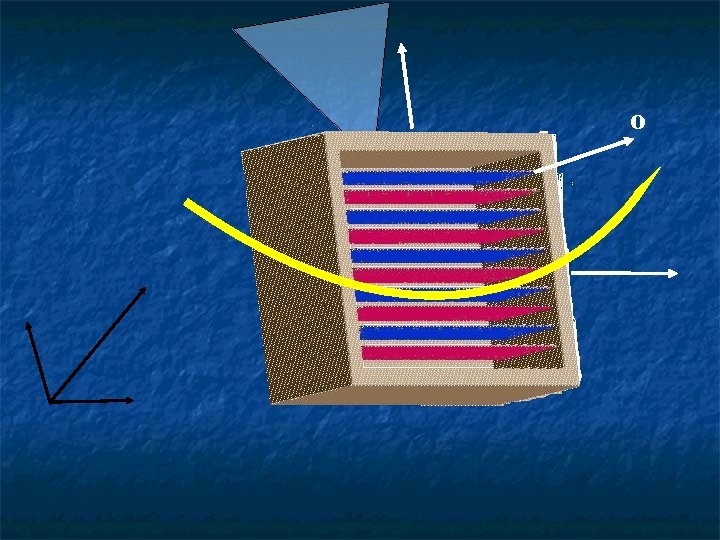
o
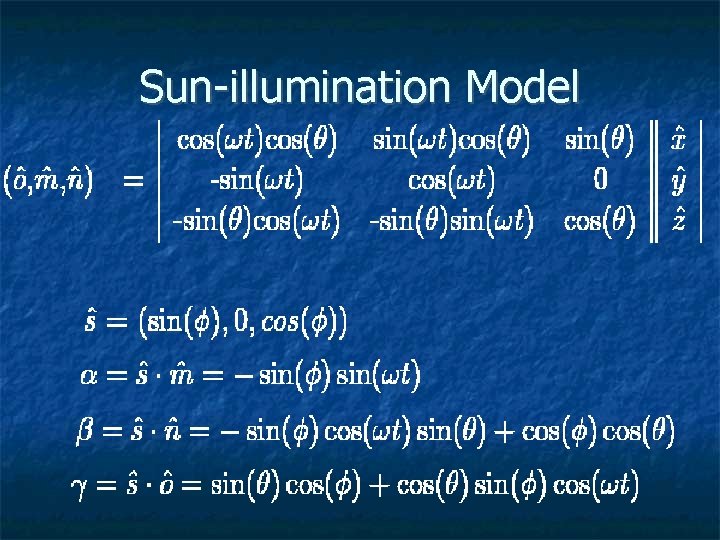
Sun-illumination Model
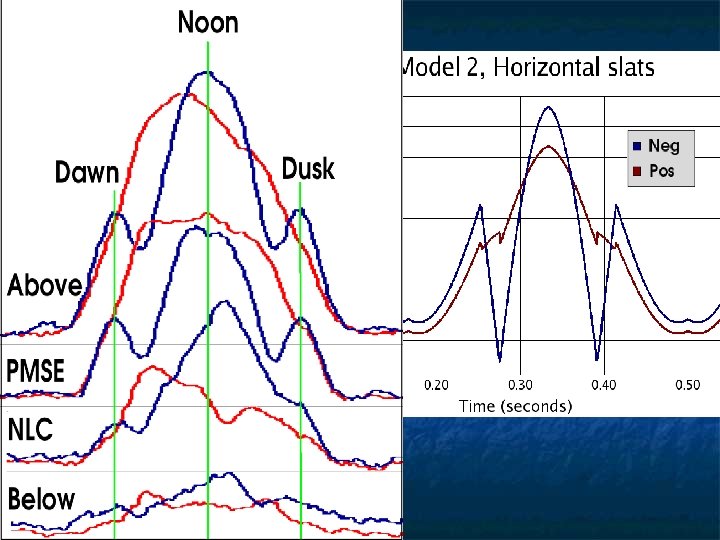
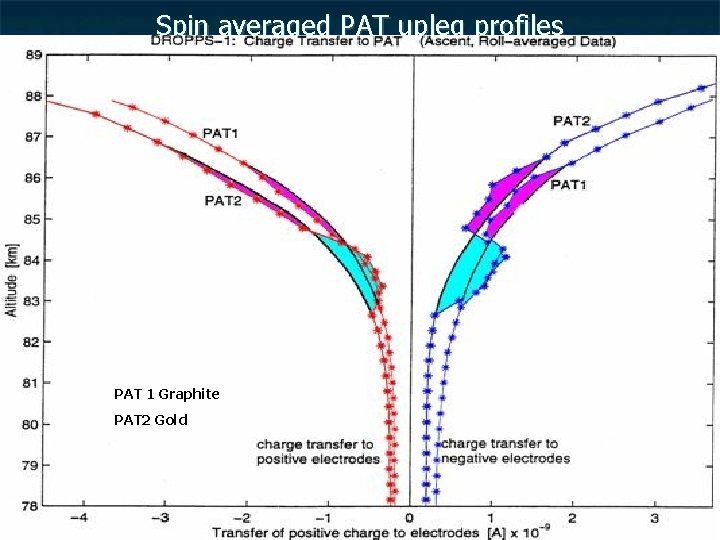
Spin averaged PAT upleg profiles PAT 1 Graphite PAT 2 Gold
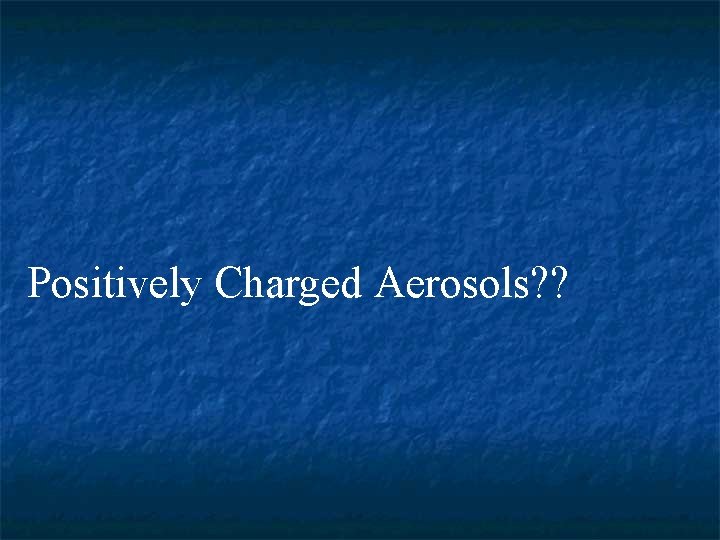
Positively Charged Aerosols? ?
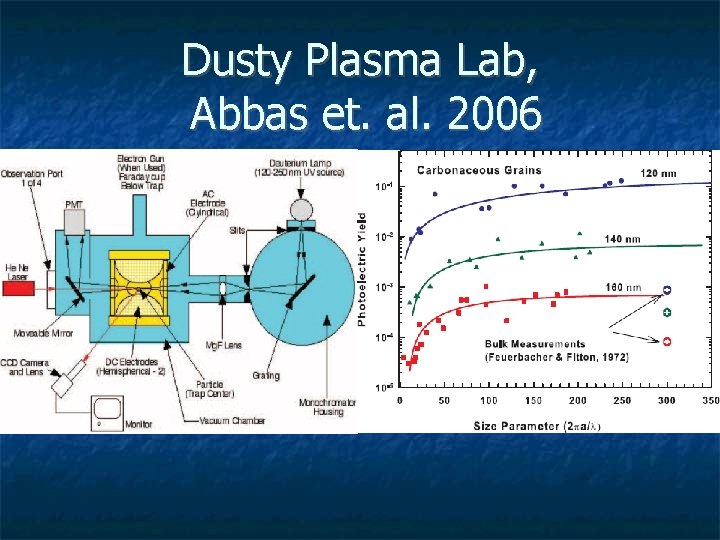
Dusty Plasma Lab, Abbas et. al. 2006
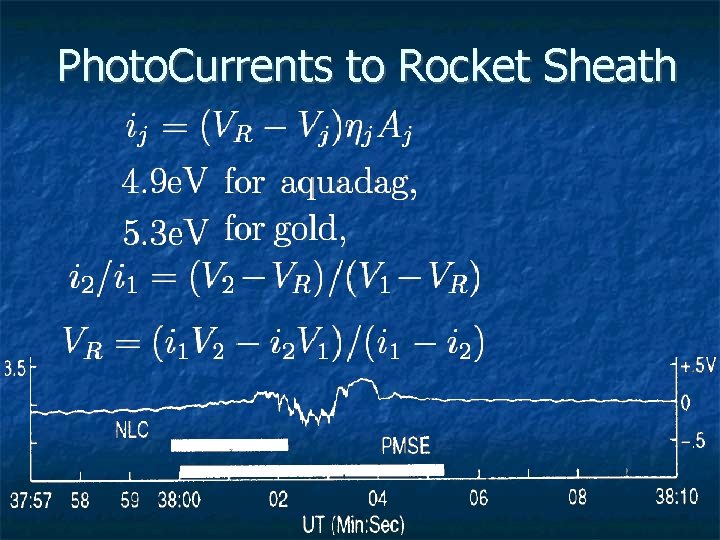
Photo. Currents to Rocket Sheath
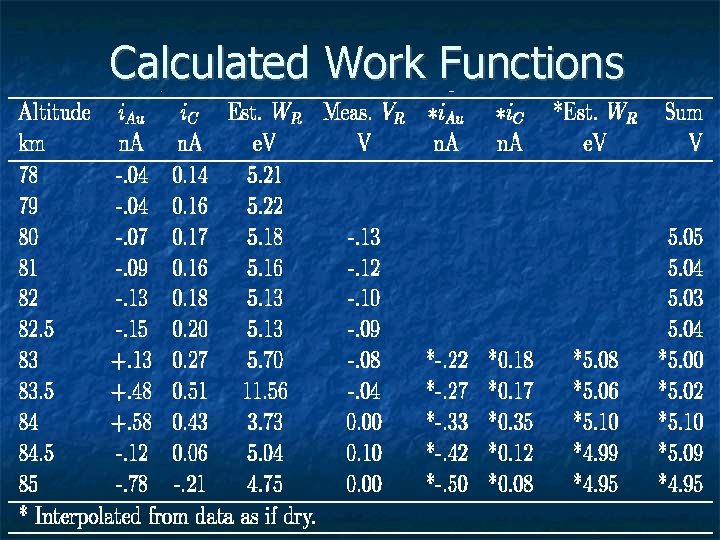
Calculated Work Functions
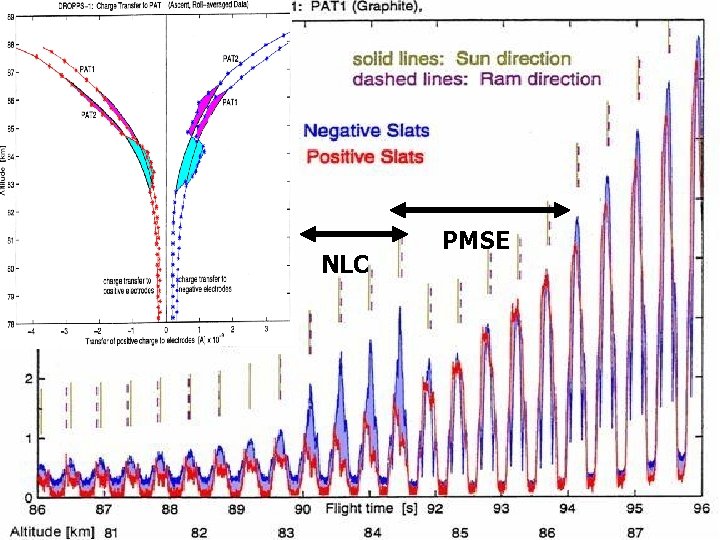
Particle Trap (PAT) instrument NLC PMSE
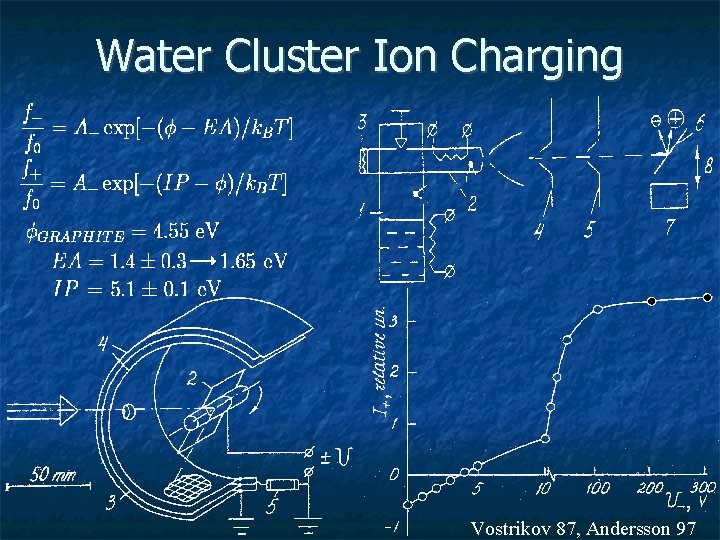
Water Cluster Ion Charging Vostrikov 87, Andersson 97
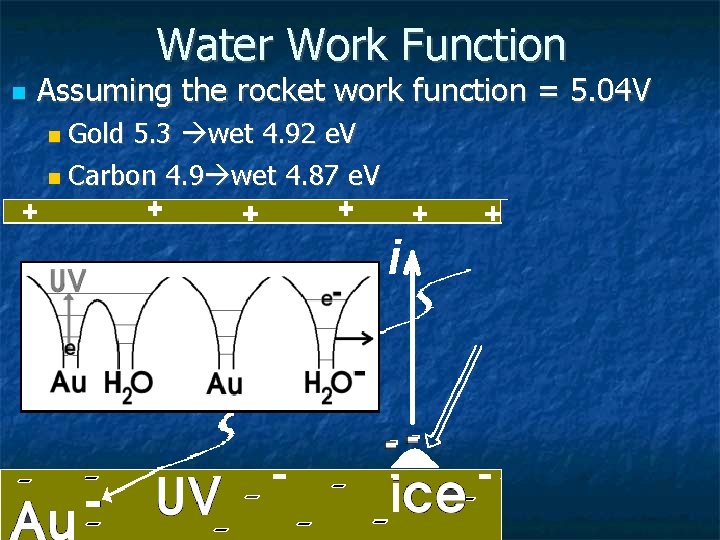
Water Work Function Assuming the rocket work function = 5. 04 V Gold 5. 3 wet 4. 92 e. V Carbon 4. 9 wet 4. 87 e. V
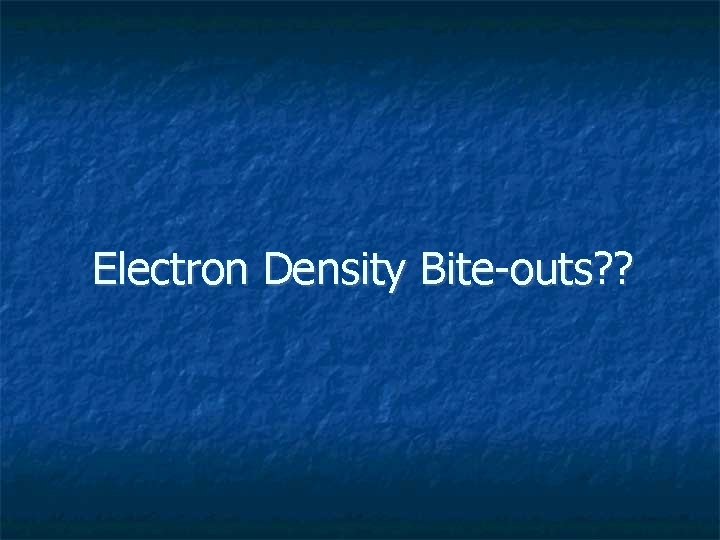
Electron Density Bite-outs? ?
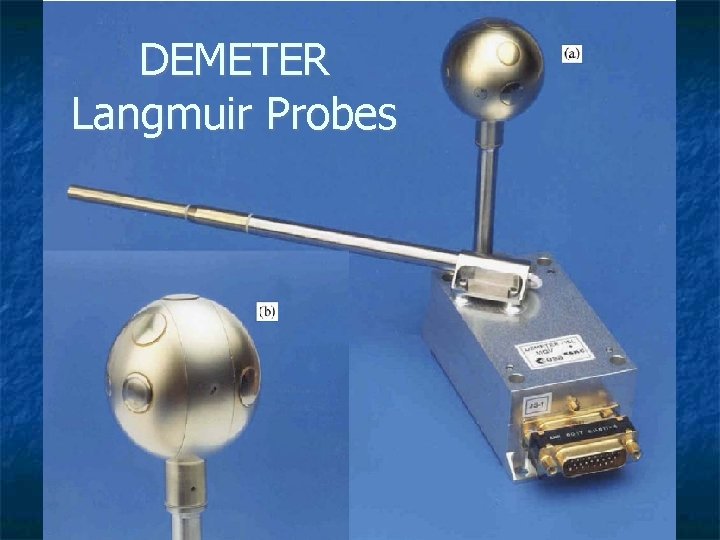
DEMETER Langmuir Probes
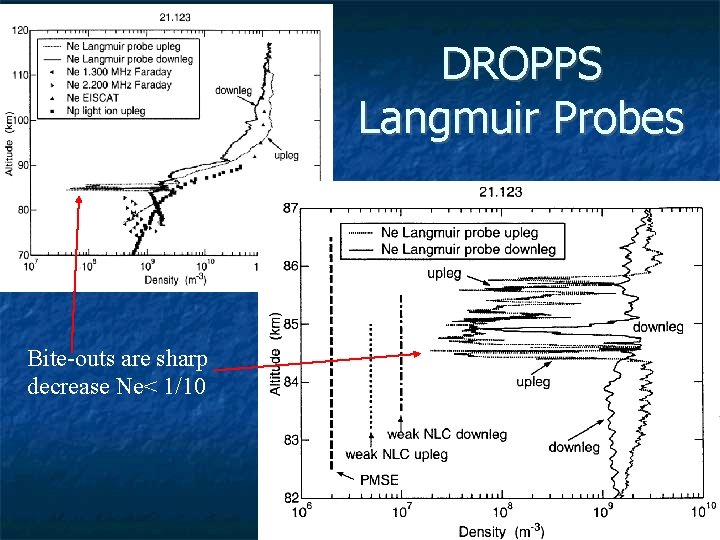
DROPPS Langmuir Probes Bite-outs are sharp decrease Ne< 1/10
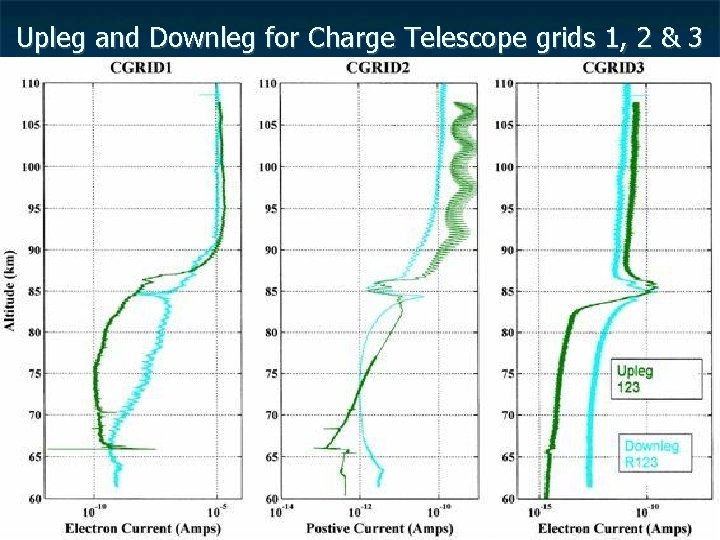
Upleg and Downleg for Charge Telescope grids 1, 2 & 3
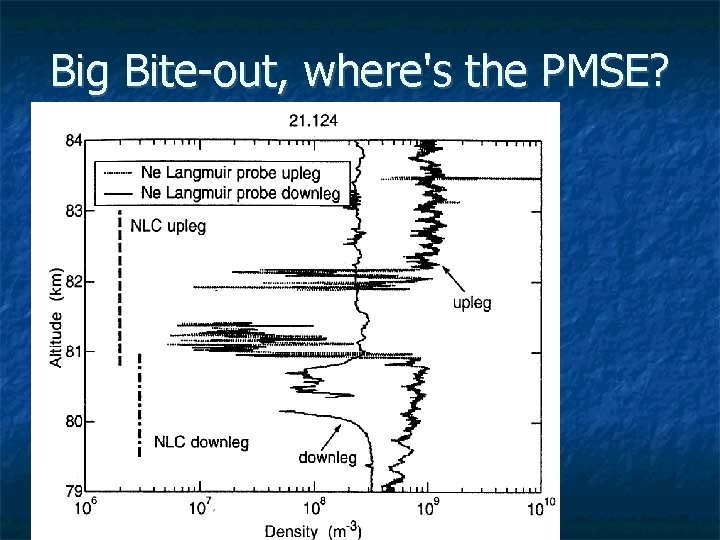
Big Bite-out, where's the PMSE?
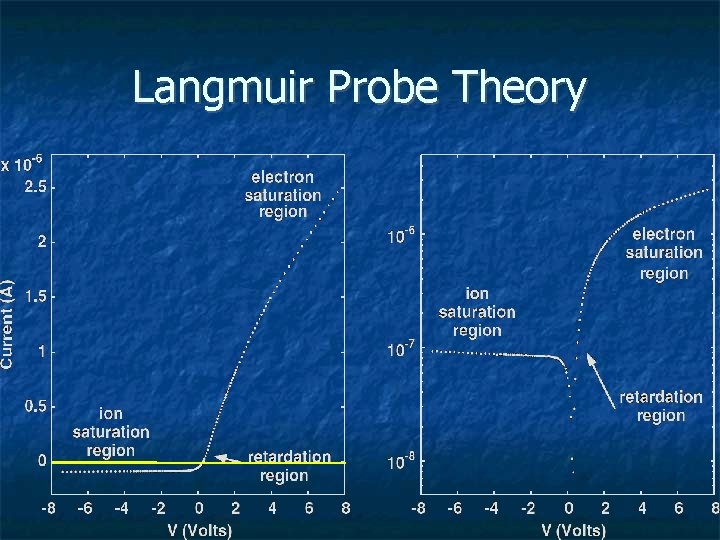
Langmuir Probe Theory
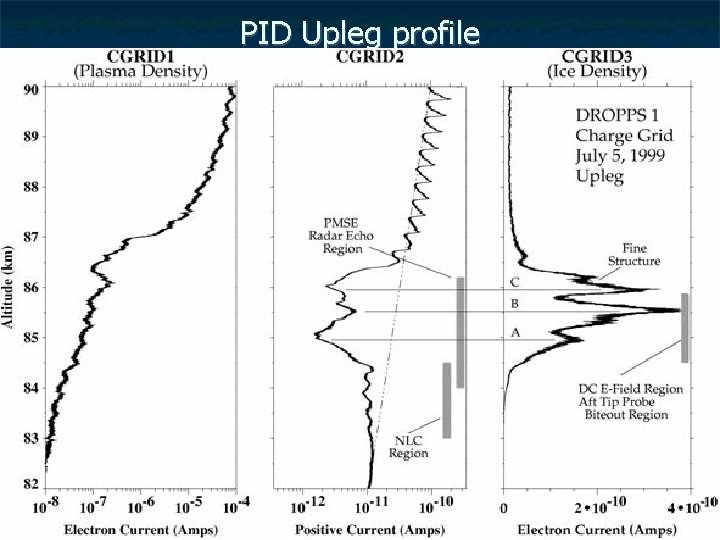
PID Upleg profile
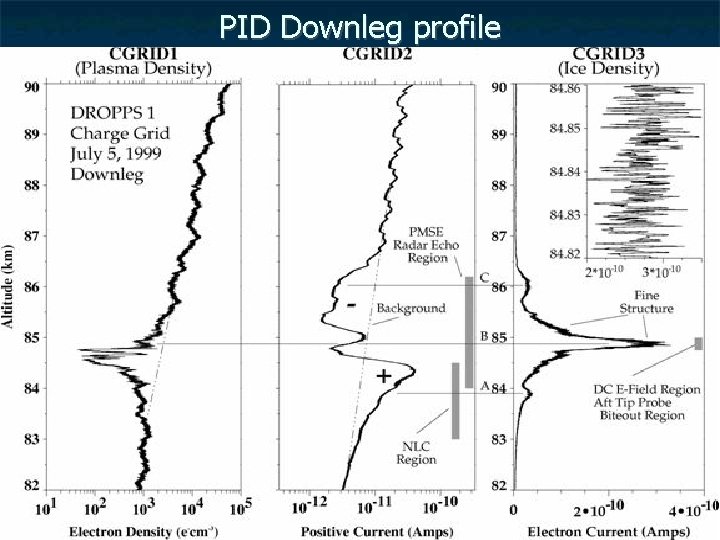
PID Downleg profile
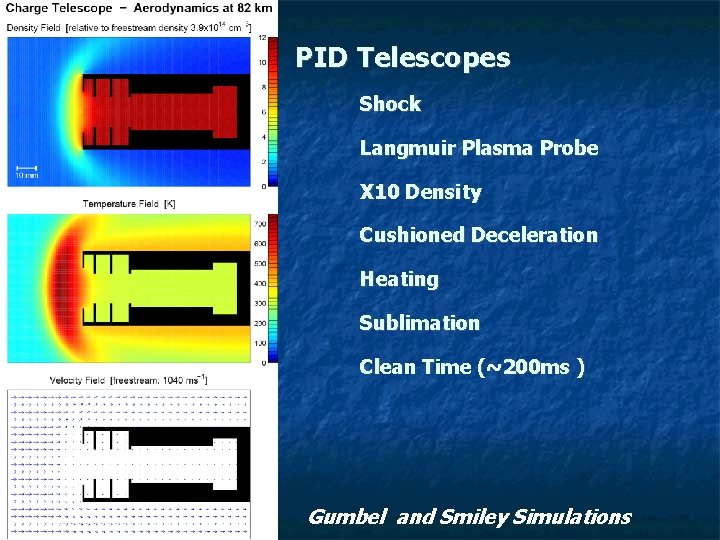
PID Telescopes Shock Langmuir Plasma Probe X 10 Density Cushioned Deceleration Heating Sublimation Clean Time (~200 ms ) Gumbel and Smiley Simulations
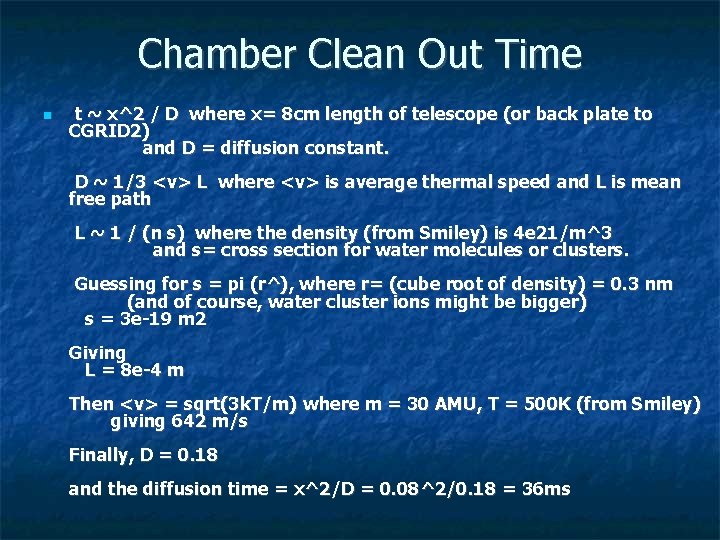
Chamber Clean Out Time t ~ x^2 / D where x= 8 cm length of telescope (or back plate to CGRID 2) and D = diffusion constant. D ~ 1/3 <v> L where <v> is average thermal speed and L is mean free path L ~ 1 / (n s) where the density (from Smiley) is 4 e 21/m^3 and s= cross section for water molecules or clusters. Guessing for s = pi (r^), where r= (cube root of density) = 0. 3 nm (and of course, water cluster ions might be bigger) s = 3 e-19 m 2 Giving L = 8 e-4 m Then <v> = sqrt(3 k. T/m) where m = 30 AMU, T = 500 K (from Smiley) giving 642 m/s Finally, D = 0. 18 and the diffusion time = x^2/D = 0. 08^2/0. 18 = 36 ms
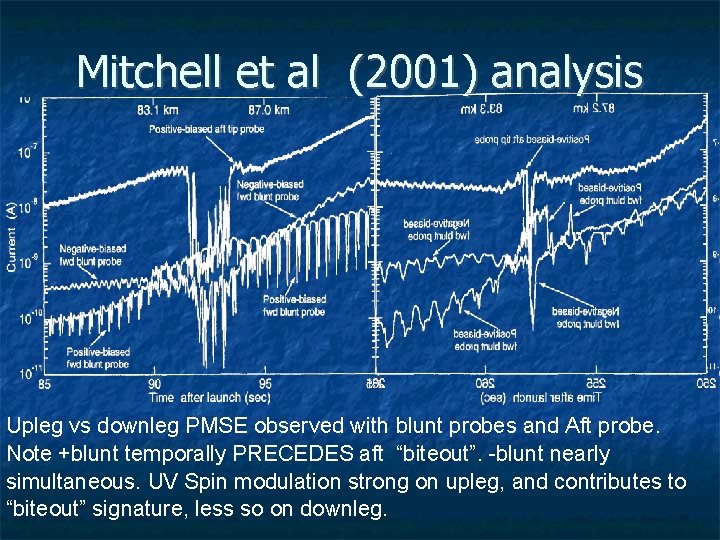
Mitchell et al (2001) analysis Upleg vs downleg PMSE observed with blunt probes and Aft probe. Note +blunt temporally PRECEDES aft “biteout”. -blunt nearly simultaneous. UV Spin modulation strong on upleg, and contributes to “biteout” signature, less so on downleg.
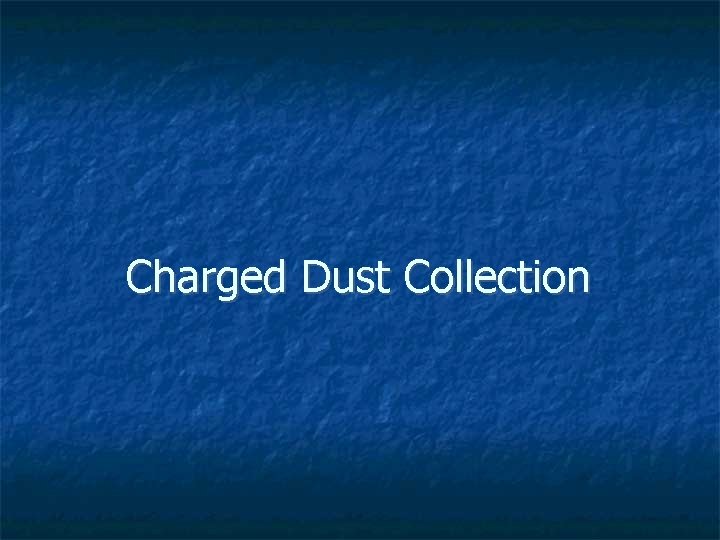
Charged Dust Collection
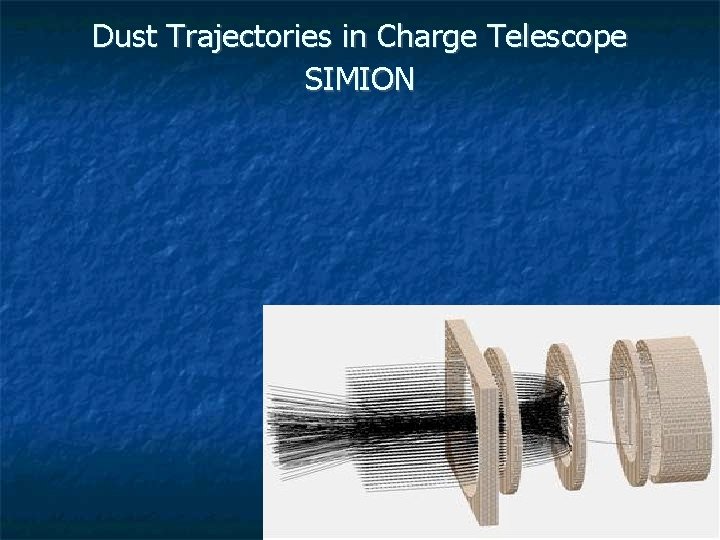
Dust Trajectories in Charge Telescope SIMION
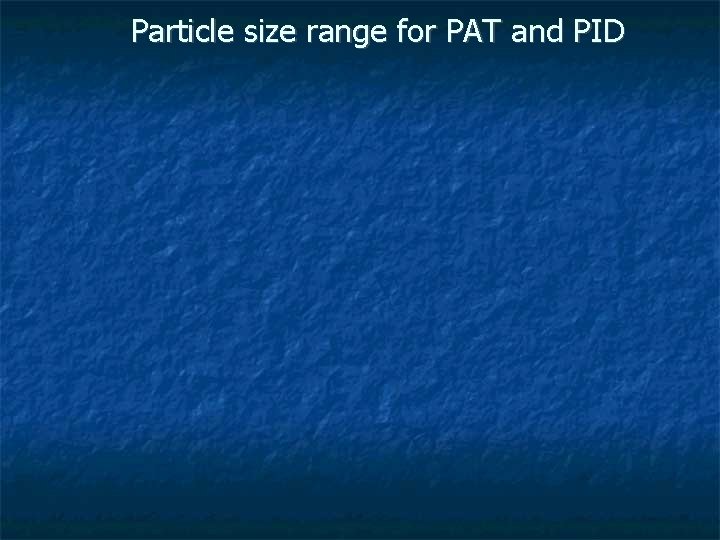
Particle size range for PAT and PID
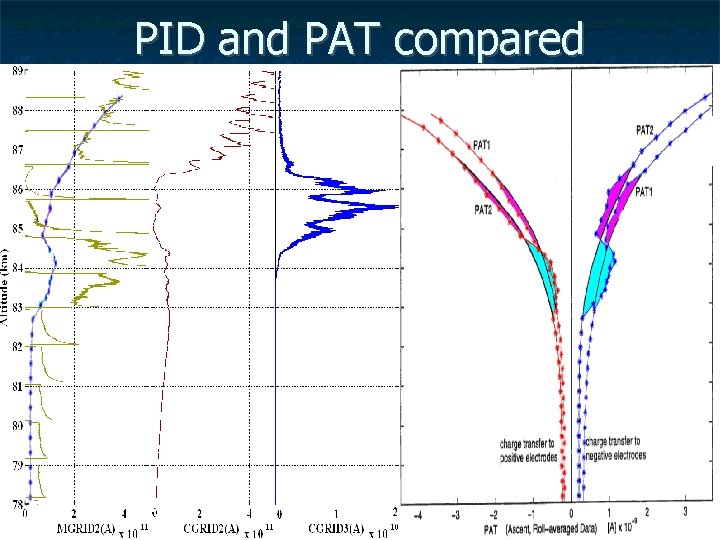
PID and PAT compared
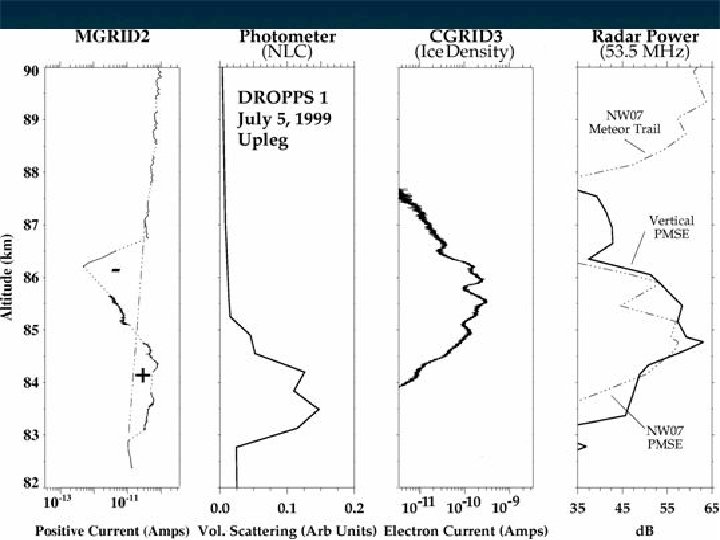
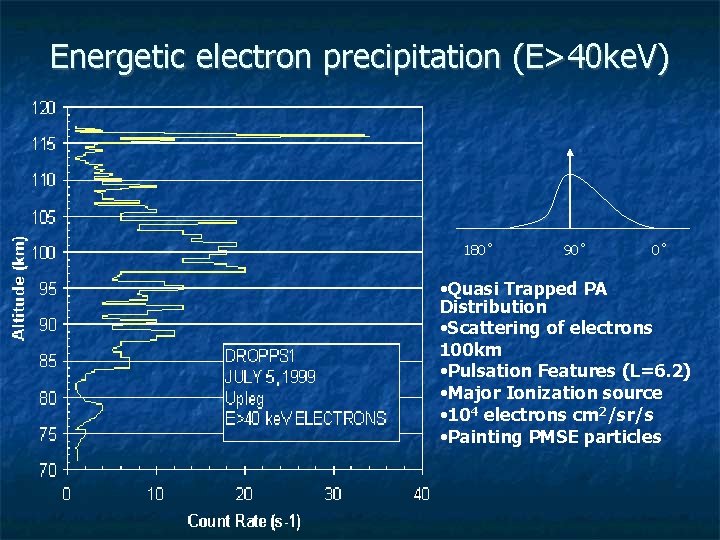
Energetic electron precipitation (E>40 ke. V) 180˚ 90˚ 0˚ • Quasi Trapped PA Distribution • Scattering of electrons 100 km • Pulsation Features (L=6. 2) • Major Ionization source • 104 electrons cm 2/sr/s • Painting PMSE particles
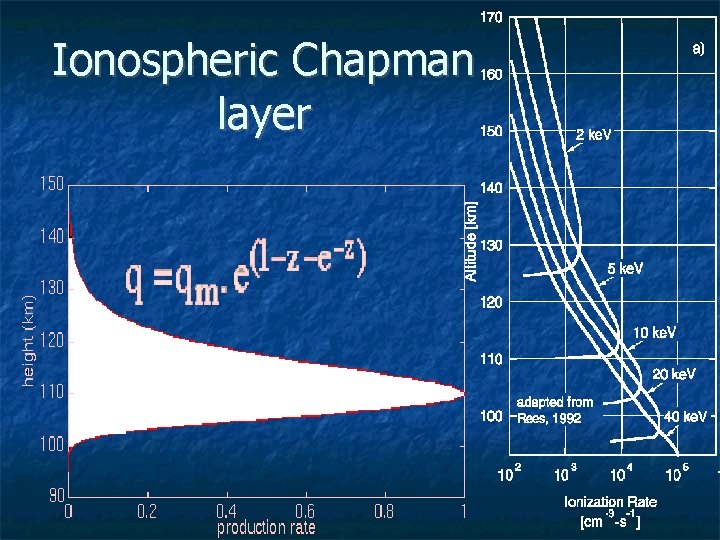
Ionospheric Chapman layer
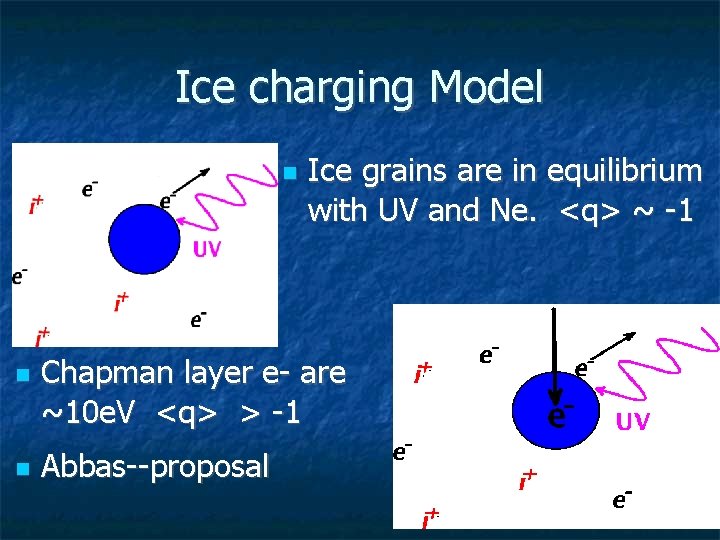
Ice charging Model Ice grains are in equilibrium with UV and Ne. <q> ~ -1 Chapman layer e- are ~10 e. V <q> > -1 Abbas--proposal
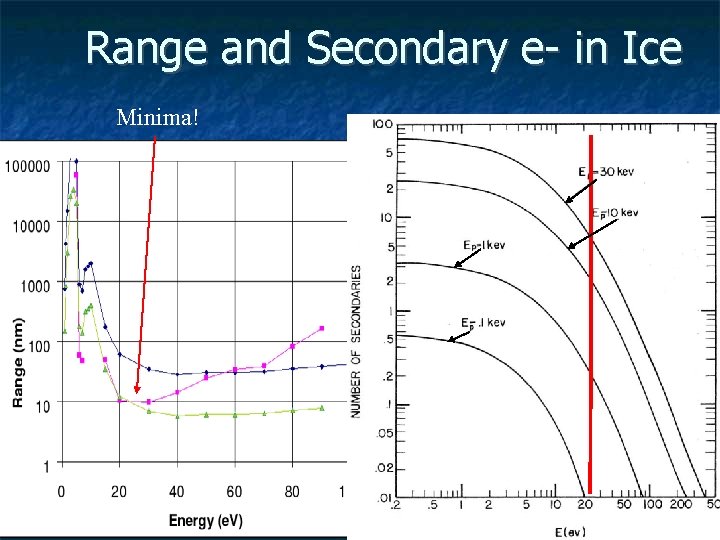
Range and Secondary e- in Ice Minima!
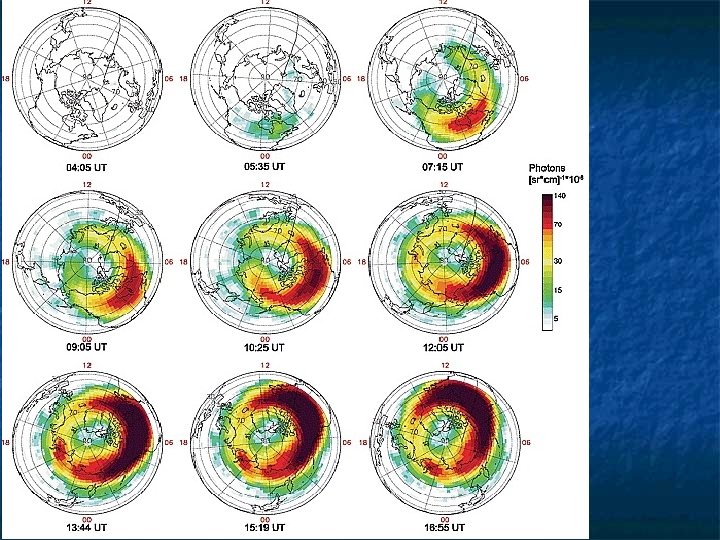
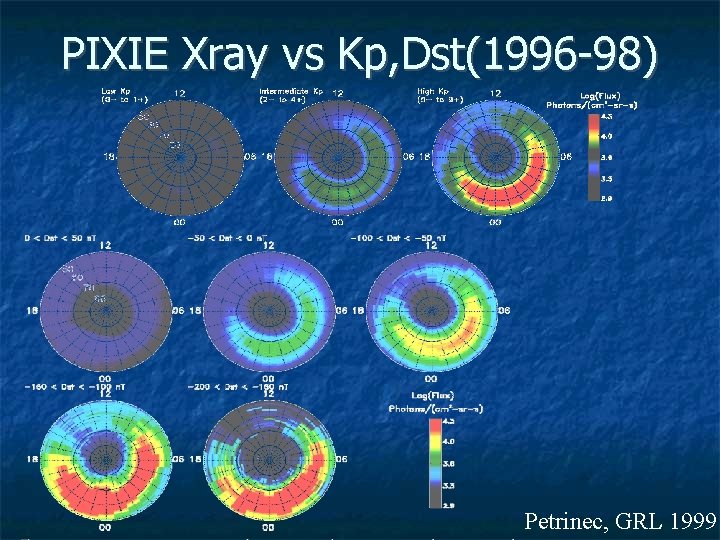
PIXIE Xray vs Kp, Dst(1996 -98) Petrinec, GRL 1999
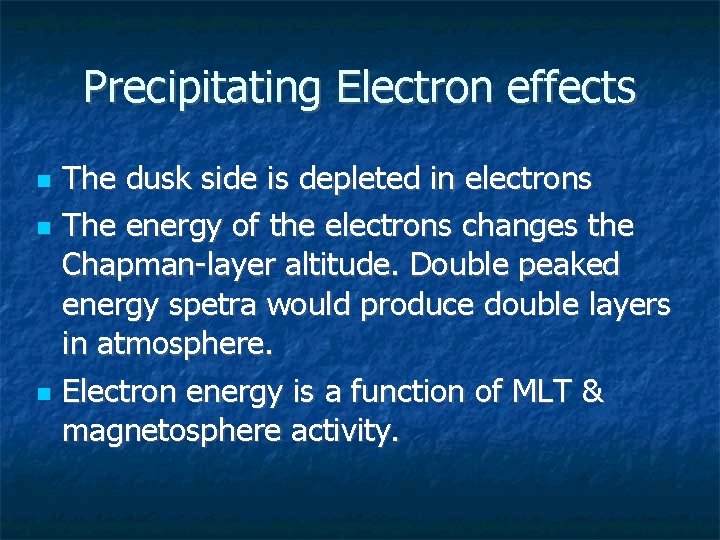
Precipitating Electron effects The dusk side is depleted in electrons The energy of the electrons changes the Chapman-layer altitude. Double peaked energy spetra would produce double layers in atmosphere. Electron energy is a function of MLT & magnetosphere activity.
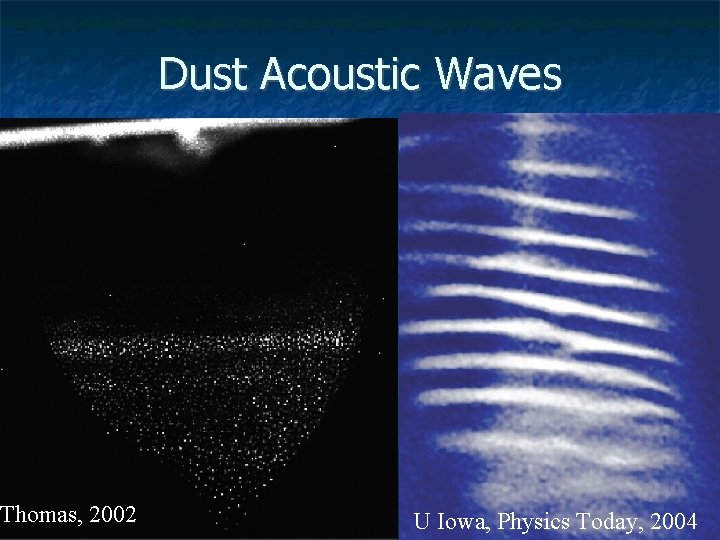
Thomas, 2002 Dust Acoustic Waves U Iowa, Physics Today, 2004
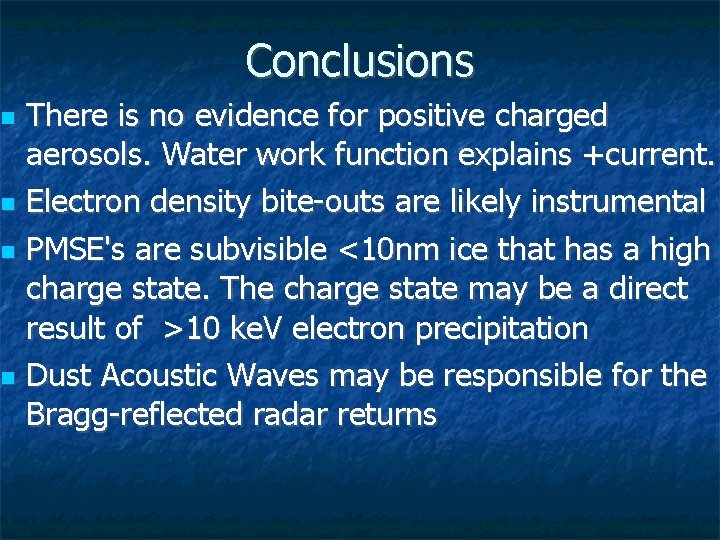
Conclusions There is no evidence for positive charged aerosols. Water work function explains +current. Electron density bite-outs are likely instrumental PMSE's are subvisible <10 nm ice that has a high charge state. The charge state may be a direct result of >10 ke. V electron precipitation Dust Acoustic Waves may be responsible for the Bragg-reflected radar returns
Polar stratospheric clouds
Polar stratospheric clouds
Polar stratospheric clouds
Atlantic revolutions and their echoes
Hydrogen or covalent bond stronger
Polar and non polar amino acids
What are polar and nonpolar dielectrics
Dusty overalls and muddy boots
Atlantic revolutions in a global context
Plural tomato
Echoes a collection of isc short stories
Como saber si un elemento es polar o apolar
Polar attraction
Enlace dativo
Dusty torus
Fission fragment rocket
Bald light bulb
Dusty torus
Compare and contrast clouds and fogs.
Every complete sentence contains two parts, a subject and a
Air that resists vertical movement is said to be
Three main clouds
Stratus cumulus cirrus
Localized convective lifting examples
Section 3 clouds and precipitation
Poem i wonder by jeannie kirby
Characteristics of clouds
Cirrus stratus cumulus cumulonimbus clouds
Moisture clouds and precipitation
Svensmark
Low-lying clouds that produce rain and snow
Pt weather chart
Why is a tornado dangerous
Clouds that look like fluffy rounded piles of cotton
Lesson 2 clouds
Lumpy
What is the mood of the treasure of lemon brown
Earth without clouds
Stratus clouds
Morning glory cloud formation
The man to send rain clouds
Green stucco house one crazy summer
Types of clouds in geography
What is it called
Red clouds war
How do clouds form
Cloud formation
What are clouds formed by
Clouds preschool etiwanda