MODULE 4 Supply Outlook August 2016 This module
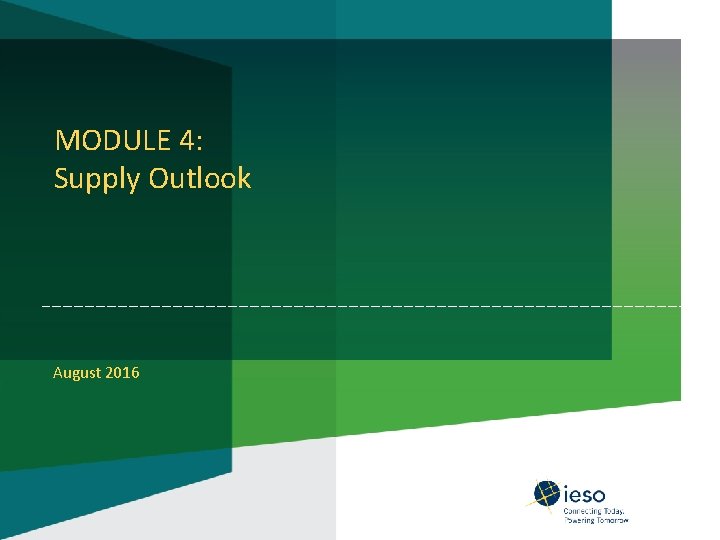
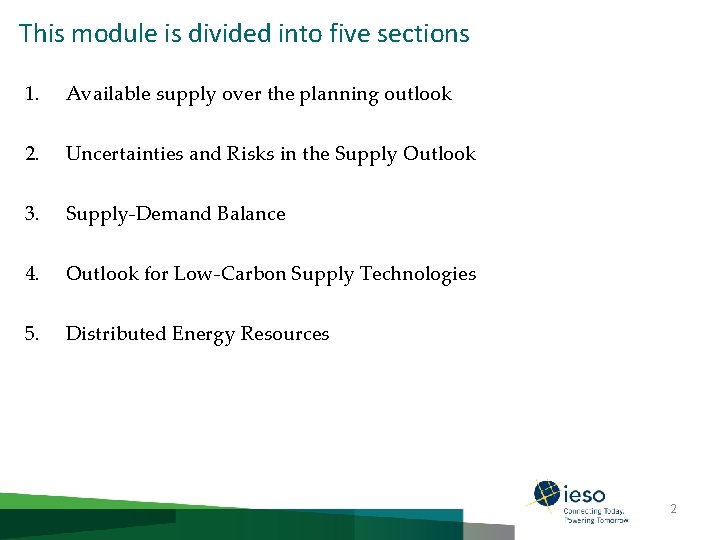
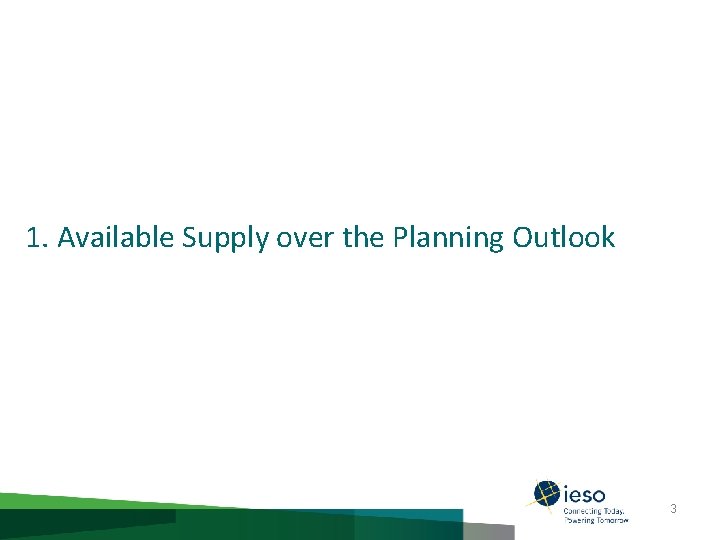
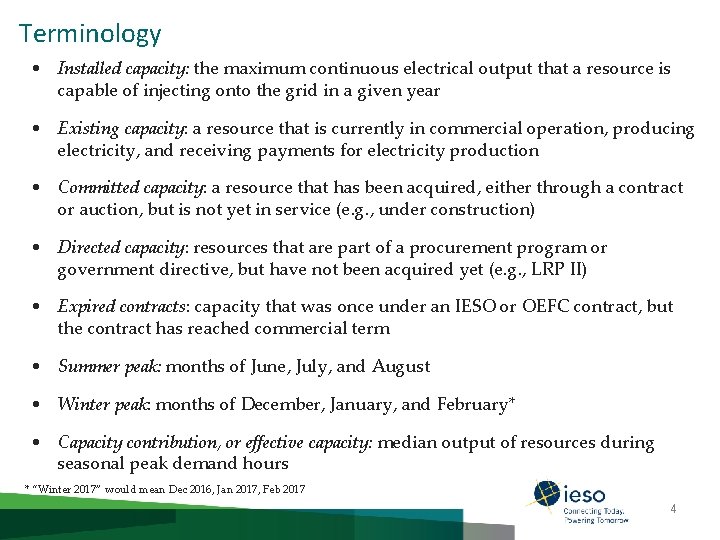
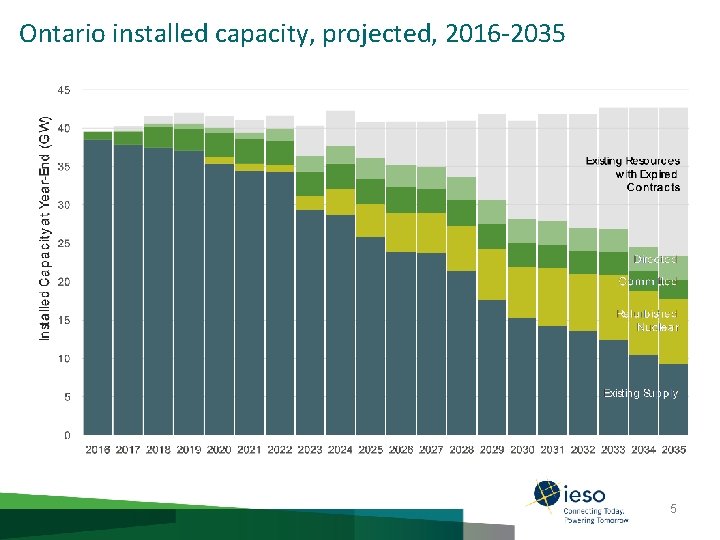
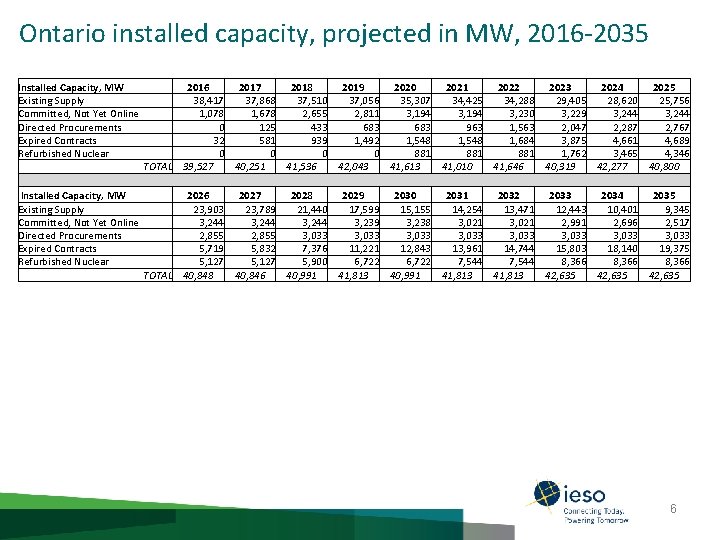
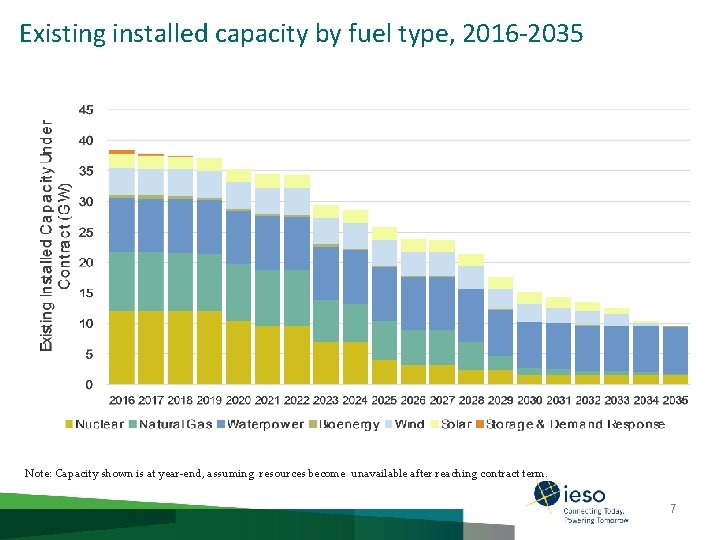
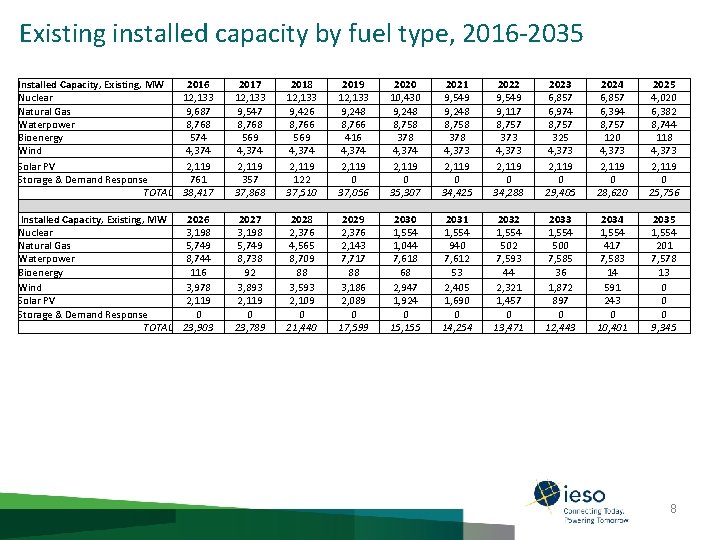
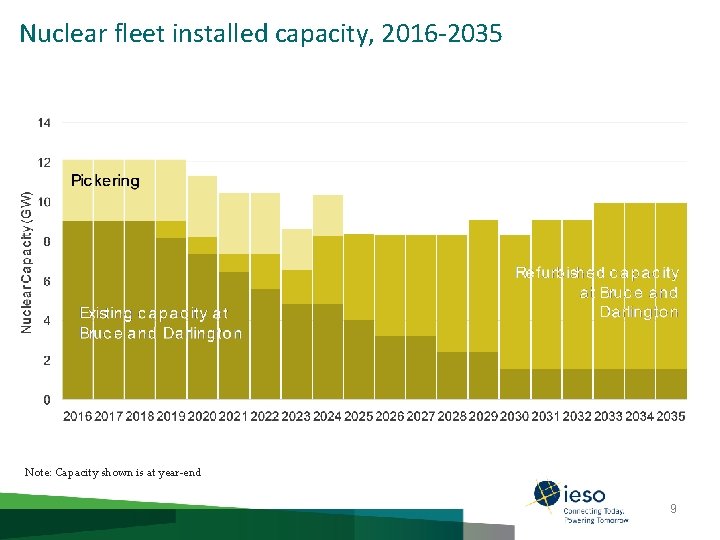
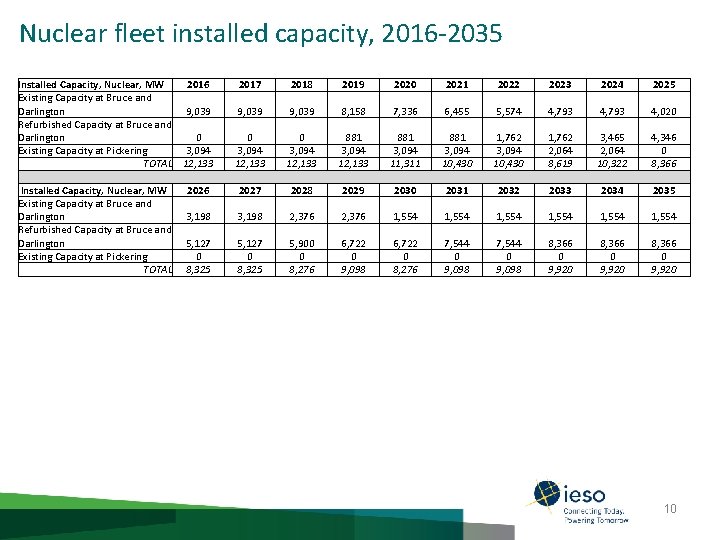
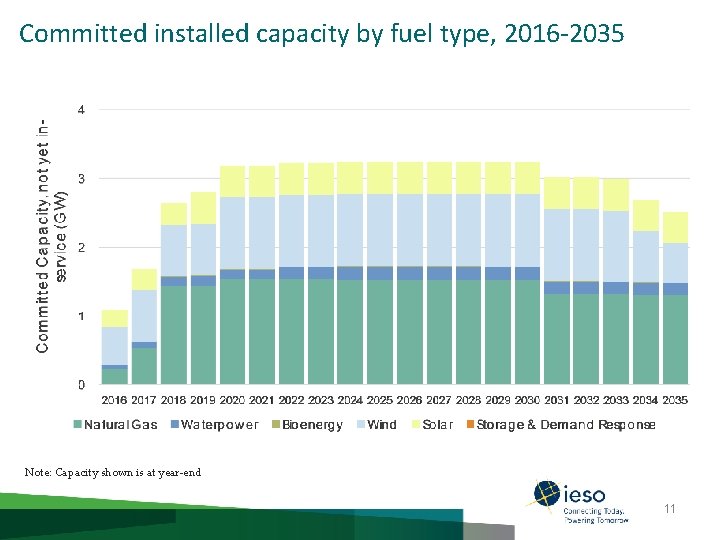
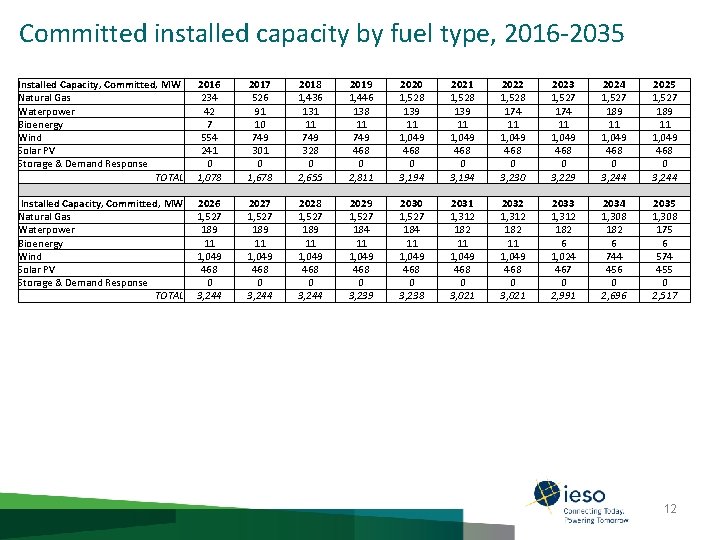
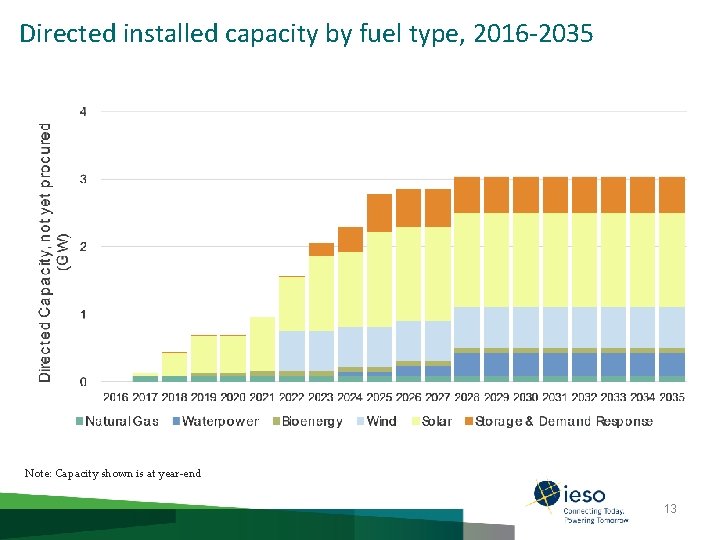
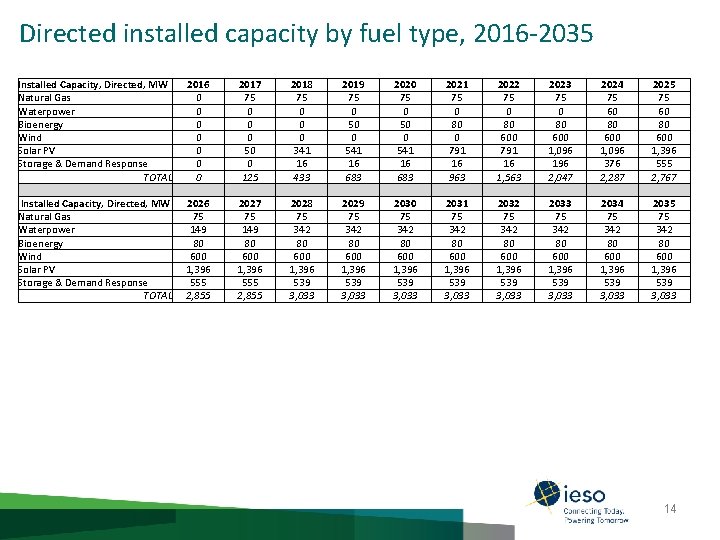
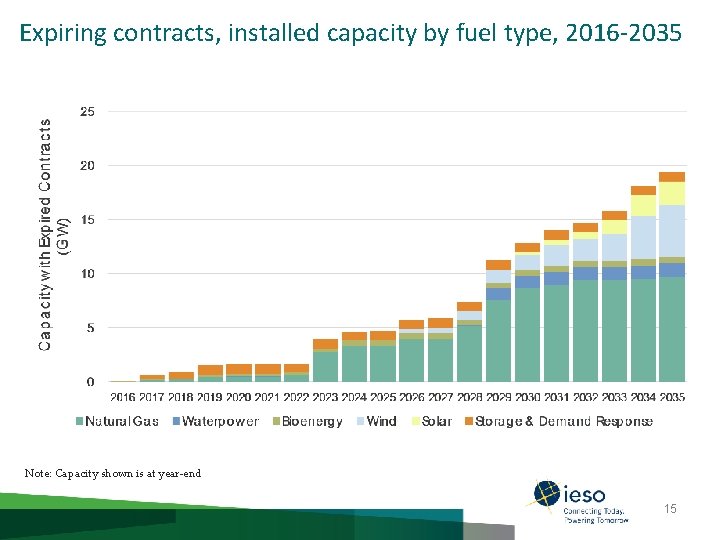
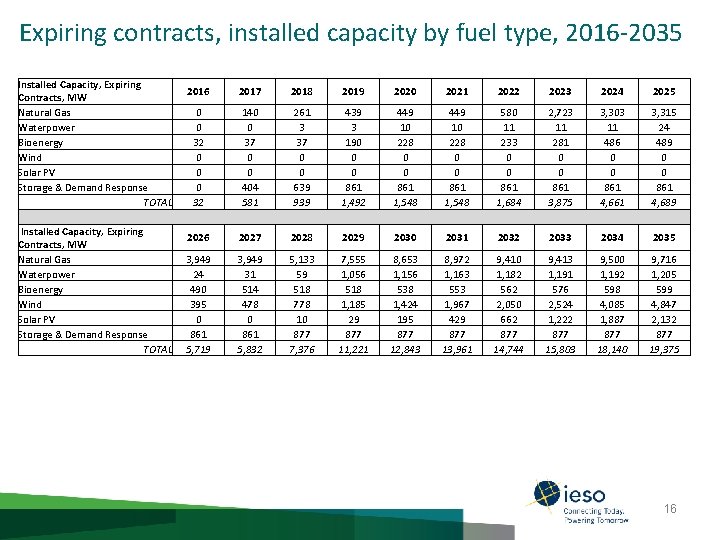
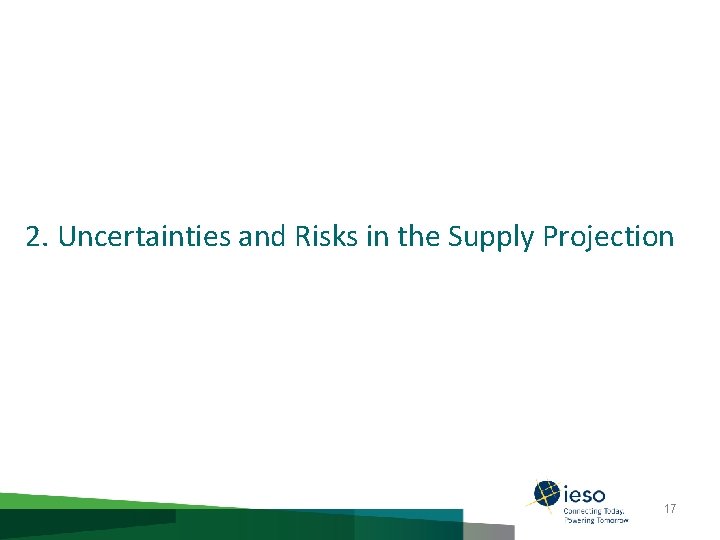
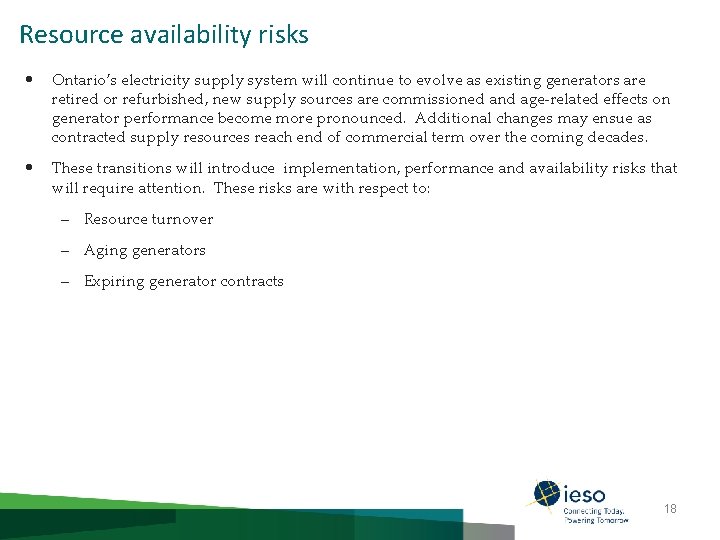
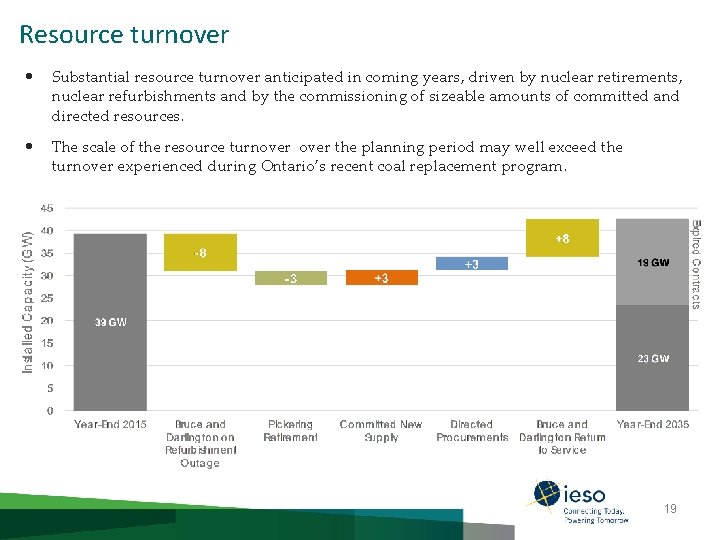
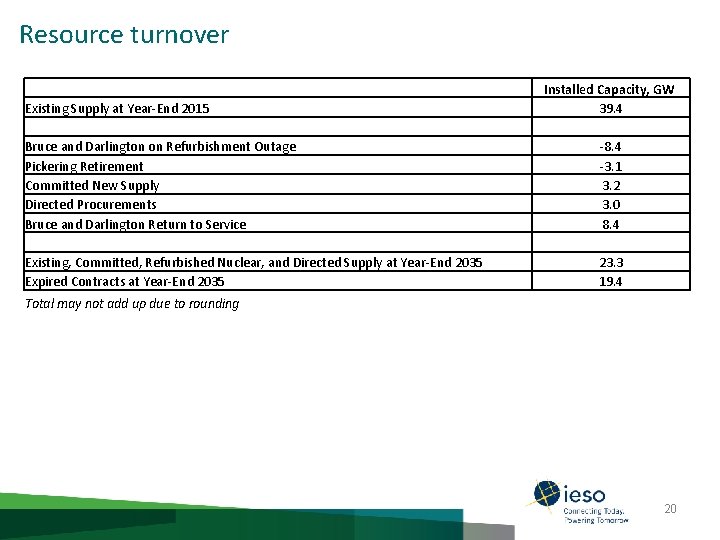
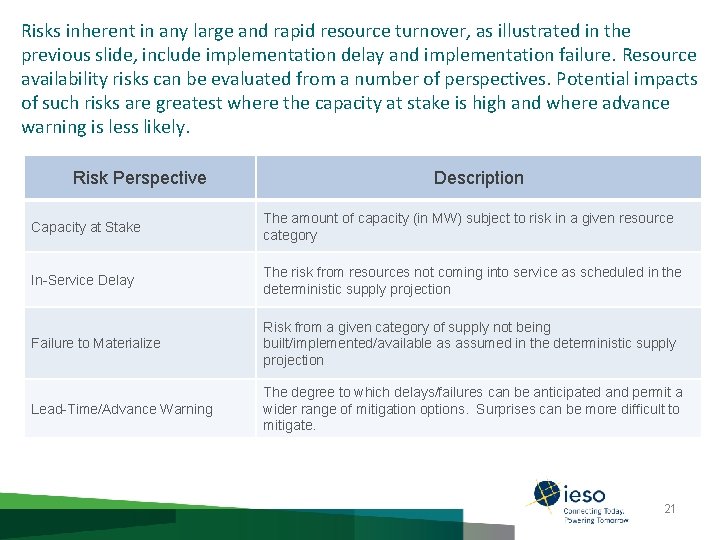
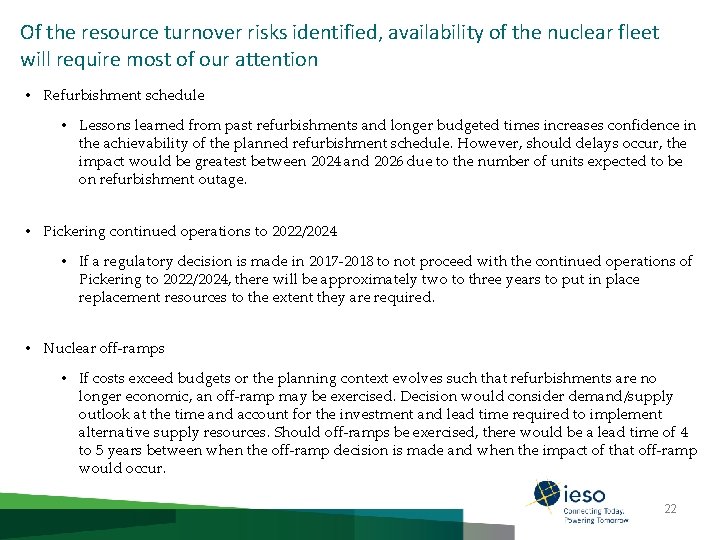
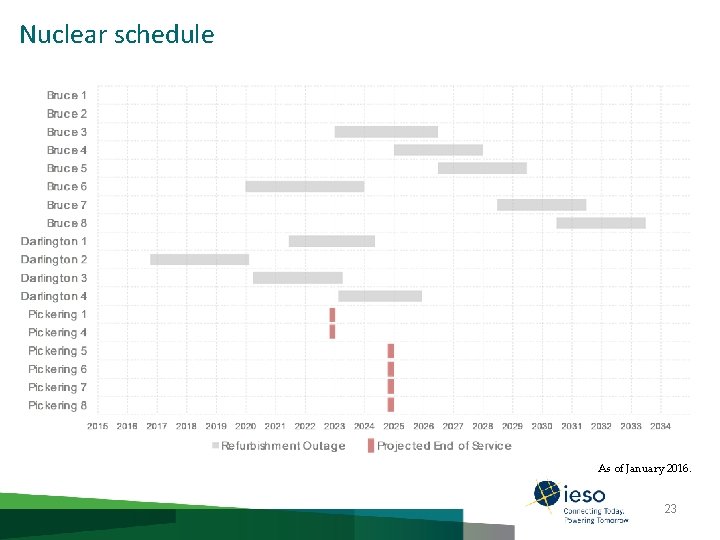
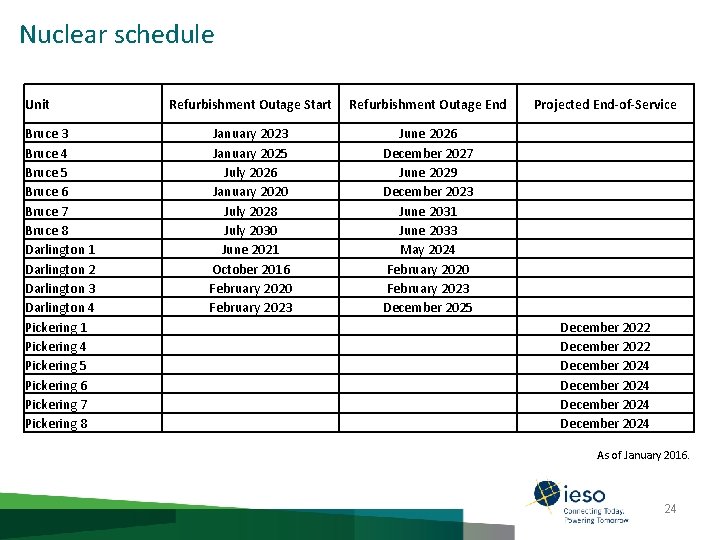
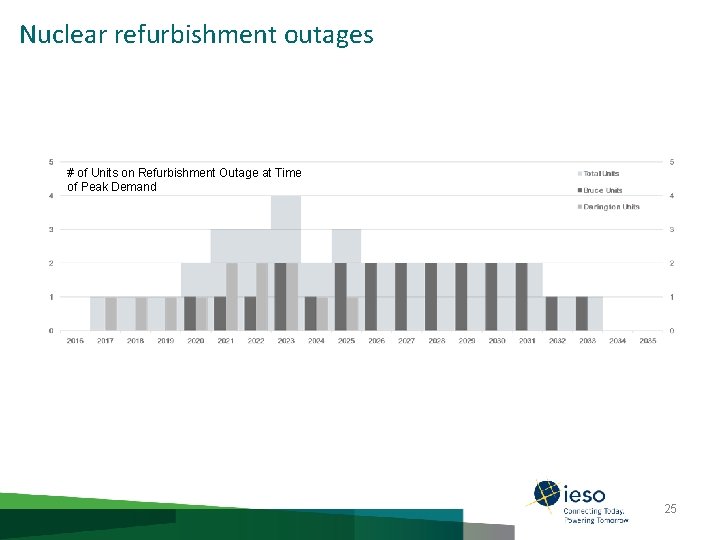
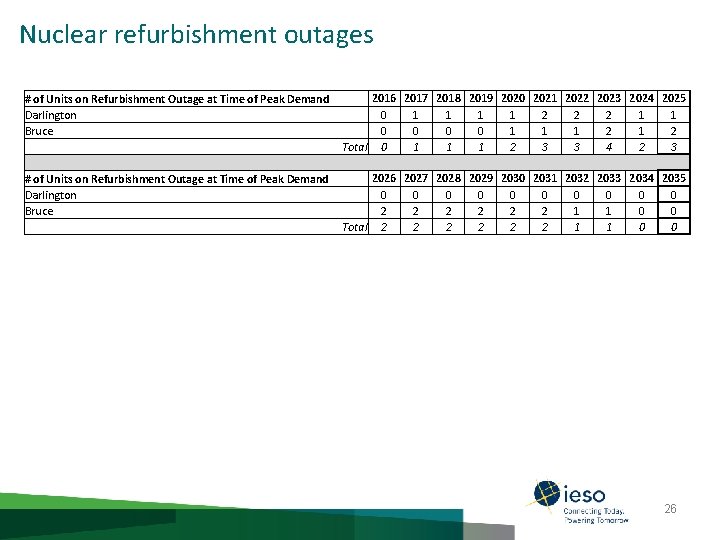
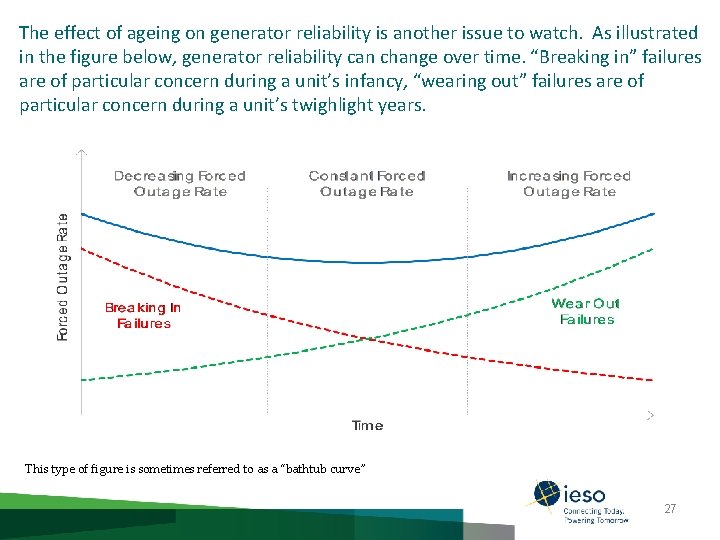
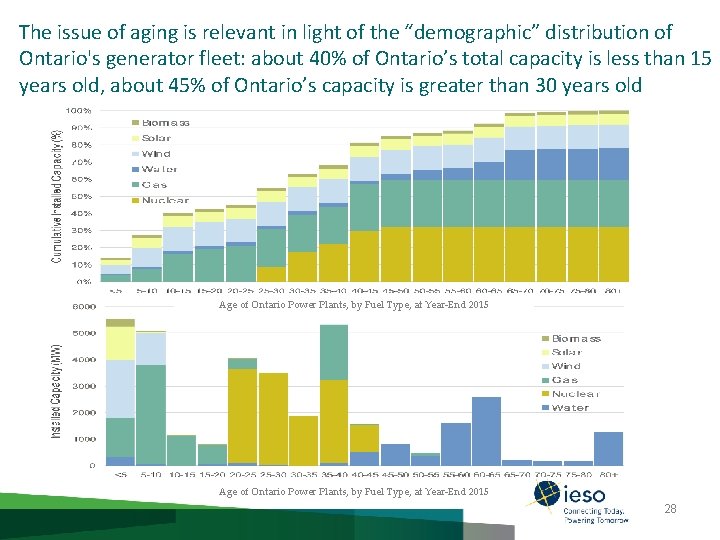
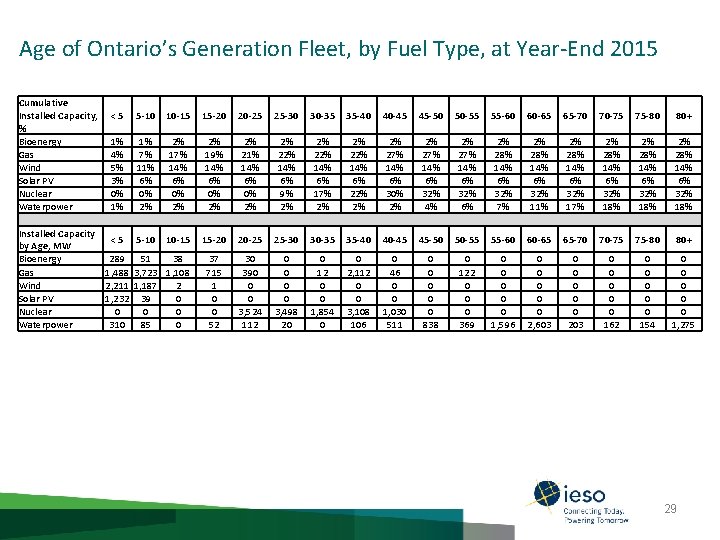
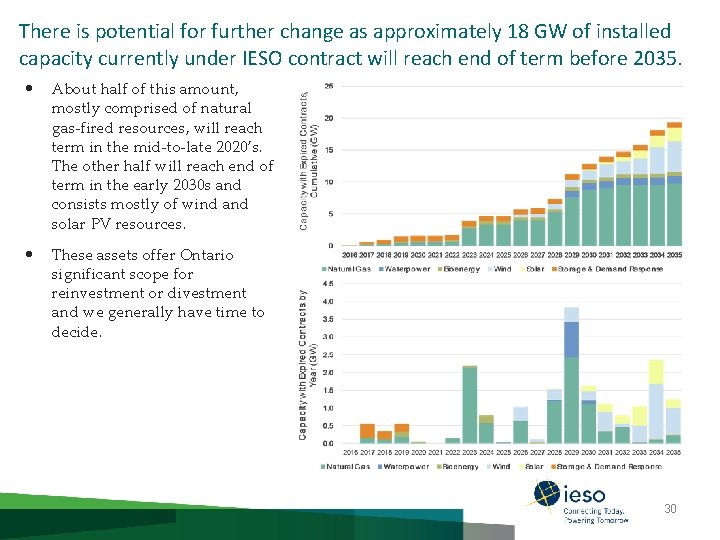
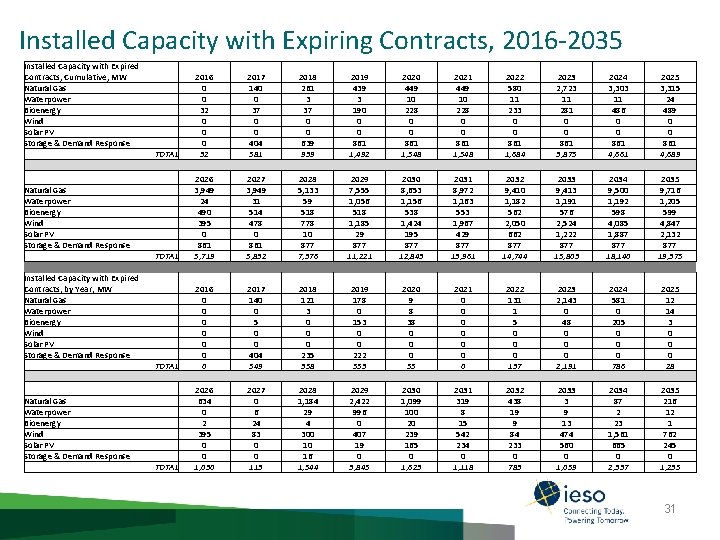
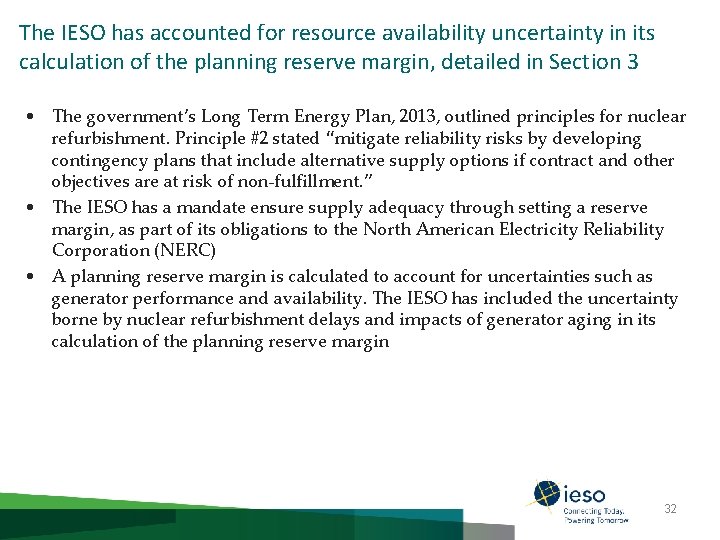
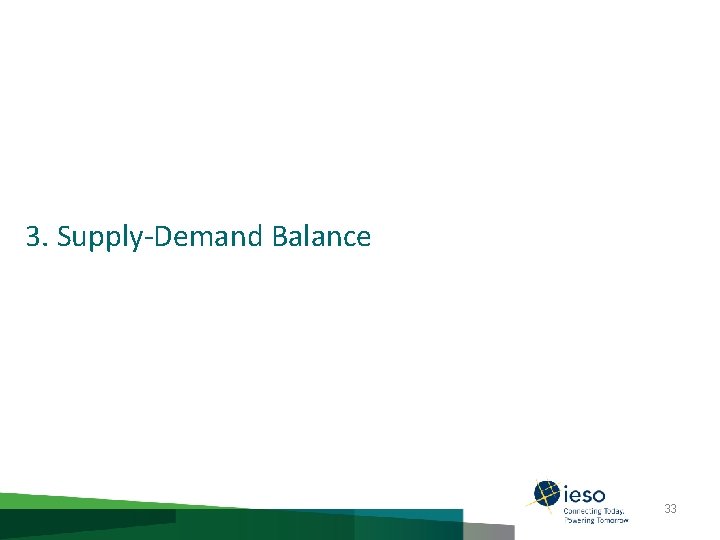
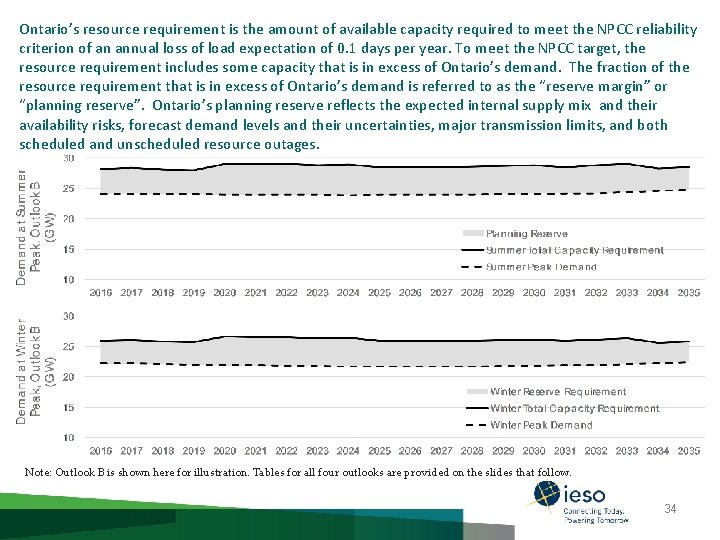
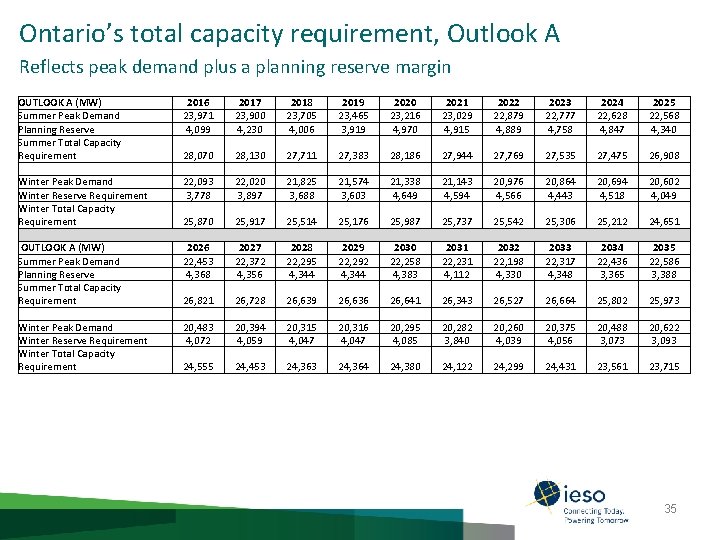
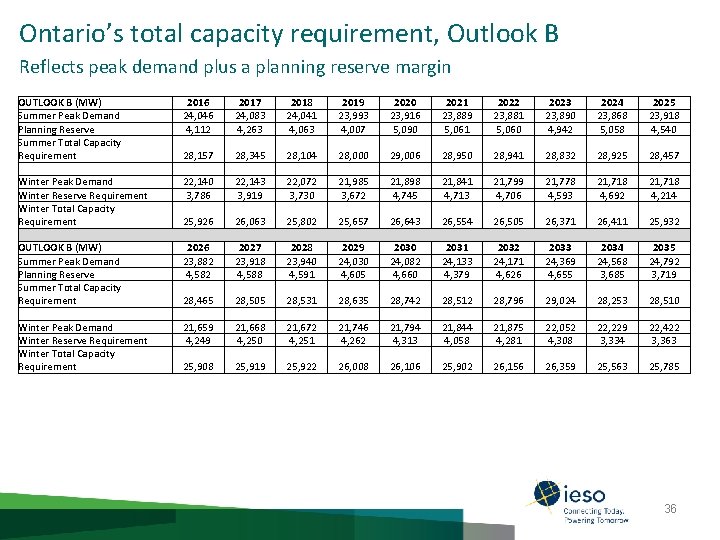
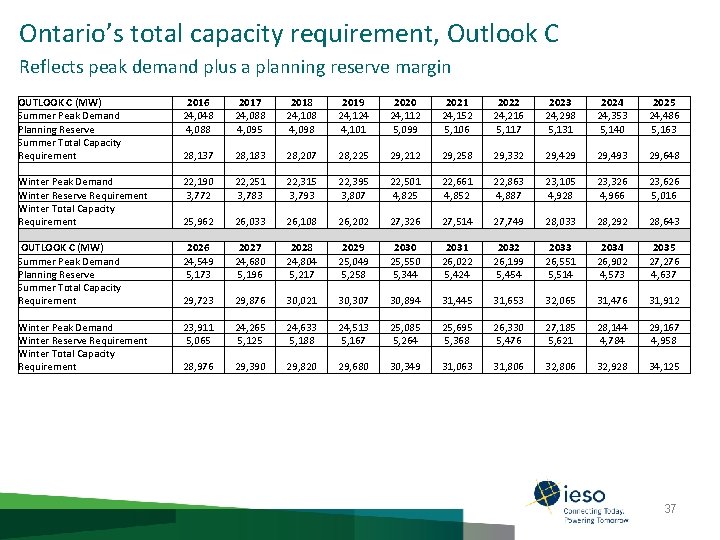
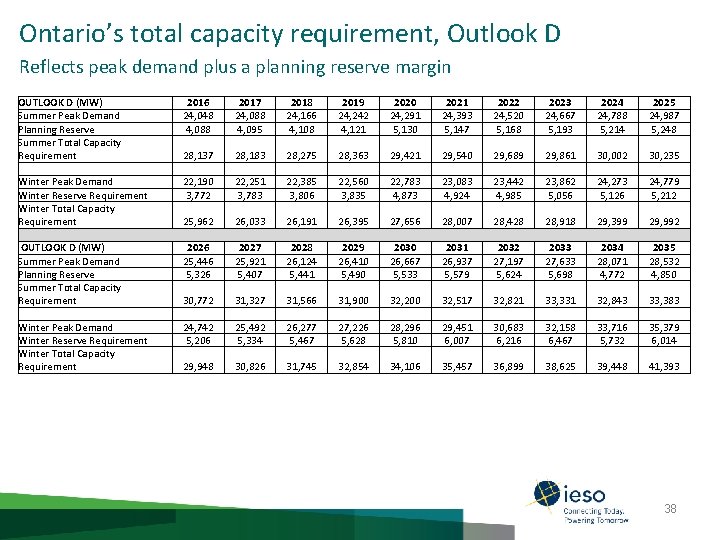
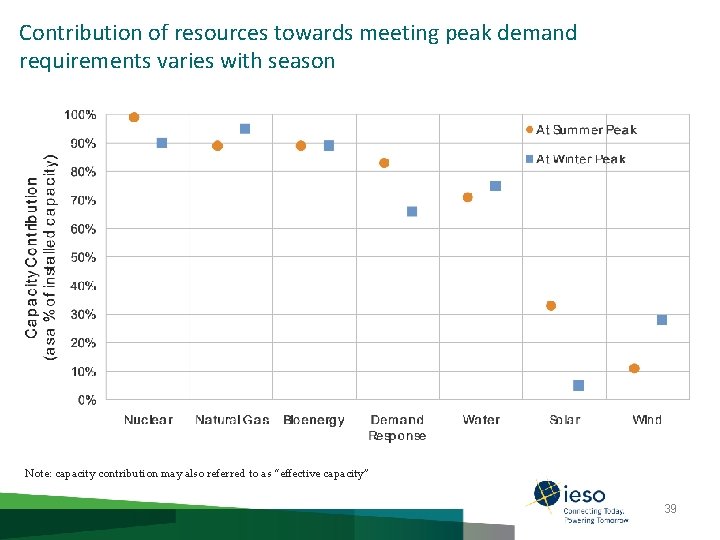
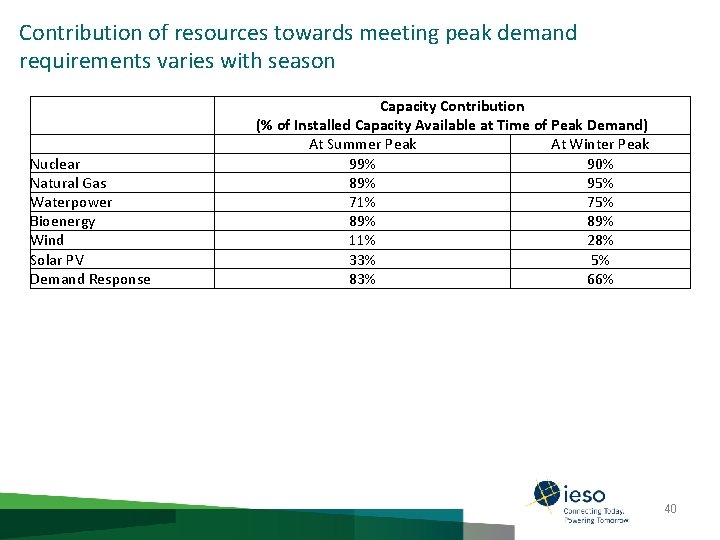
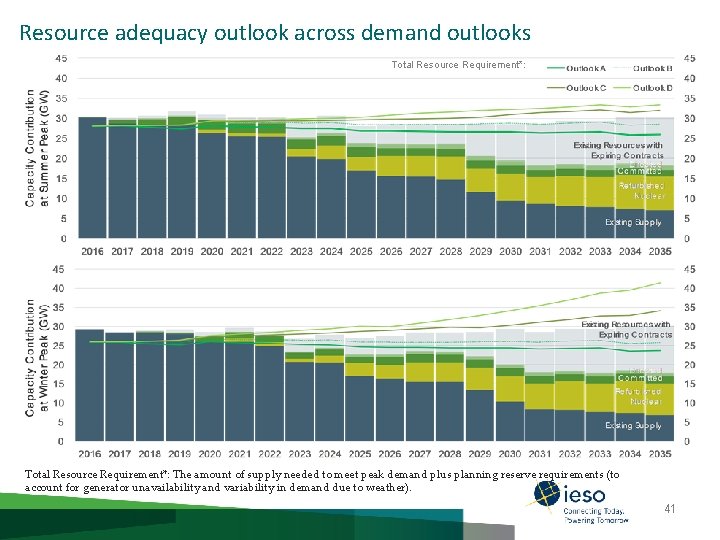
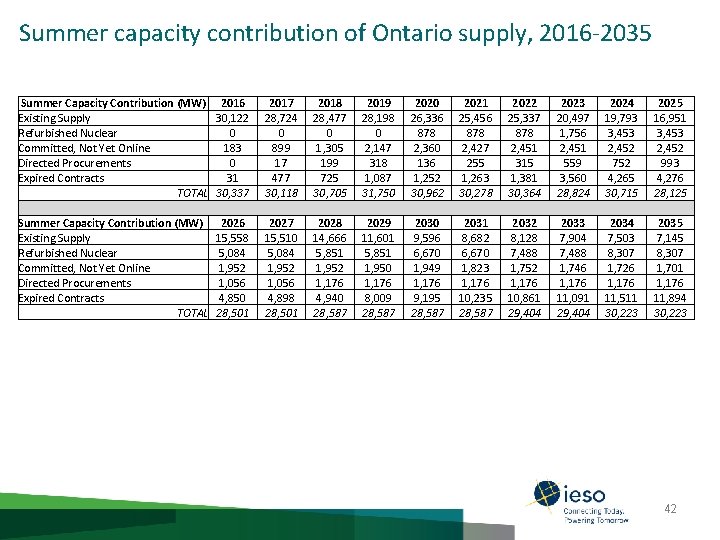
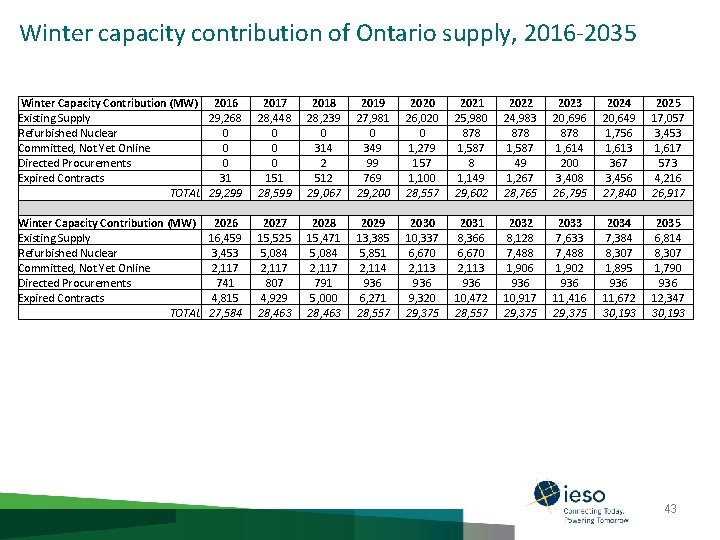
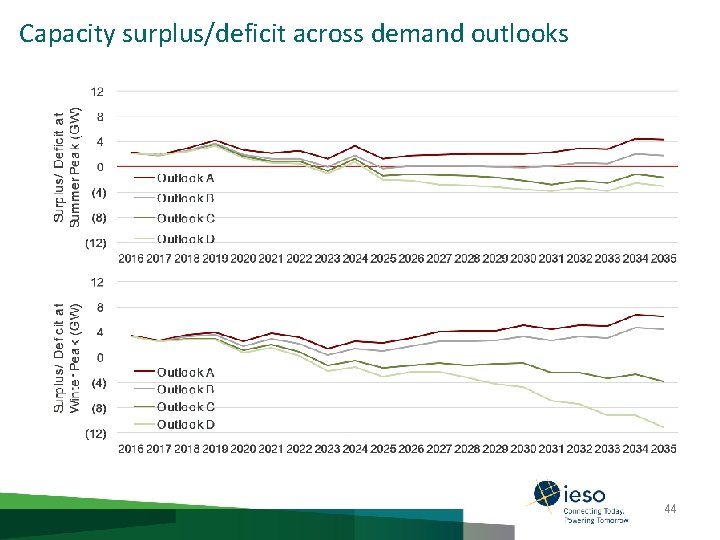
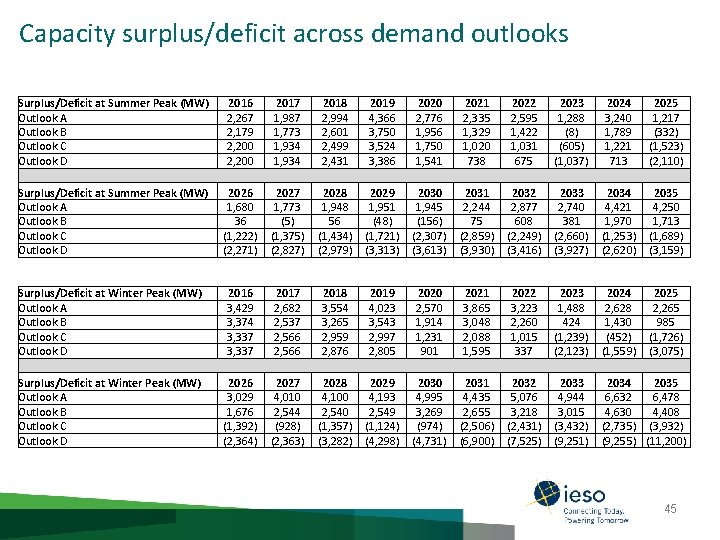
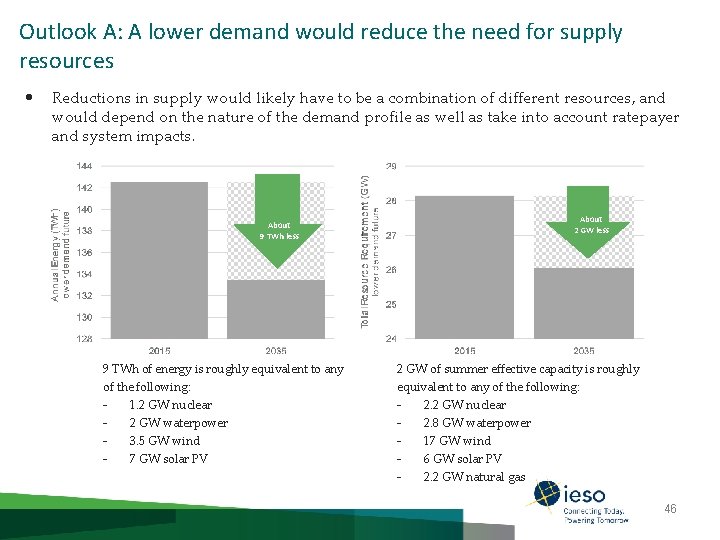
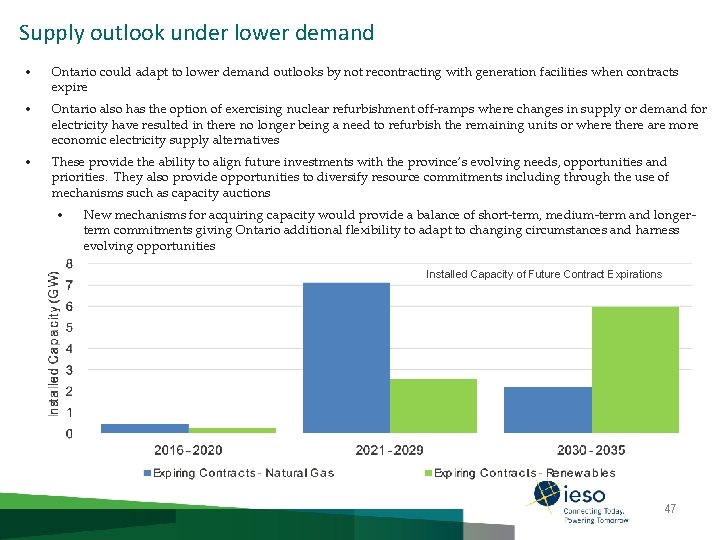
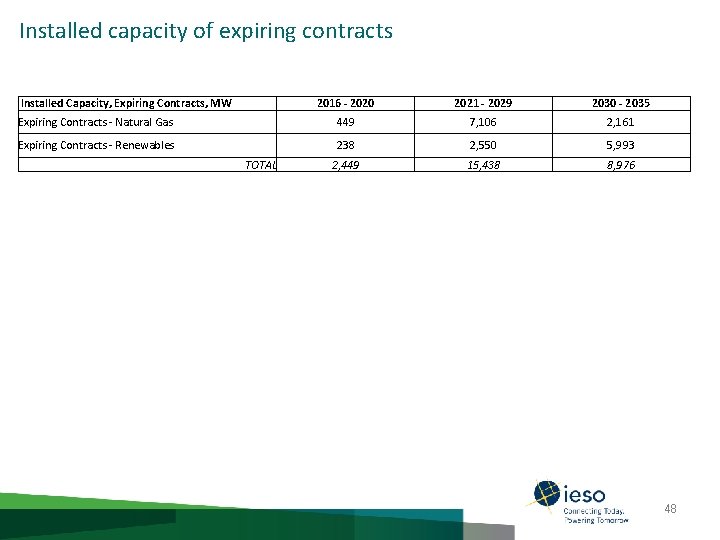
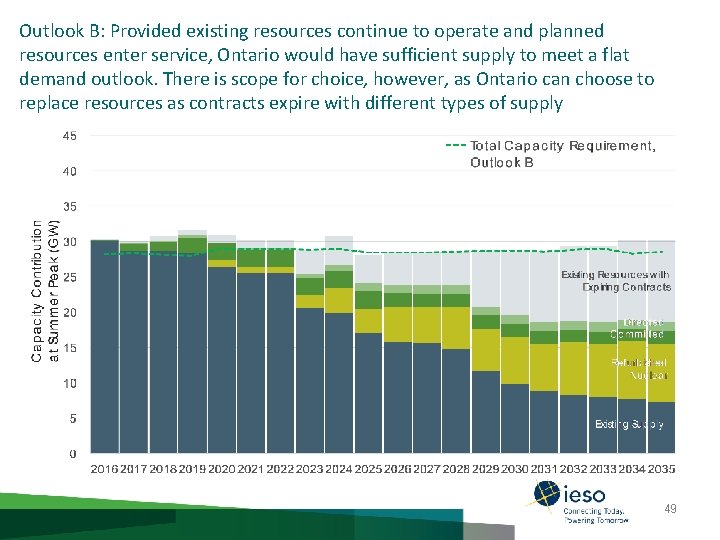
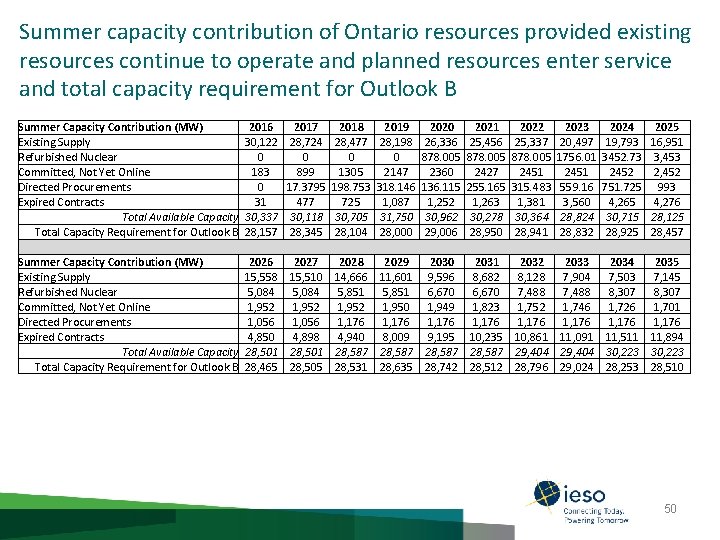
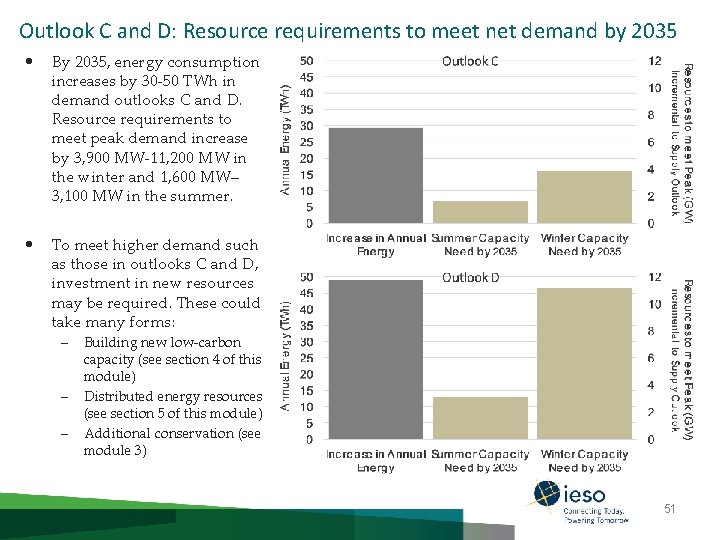
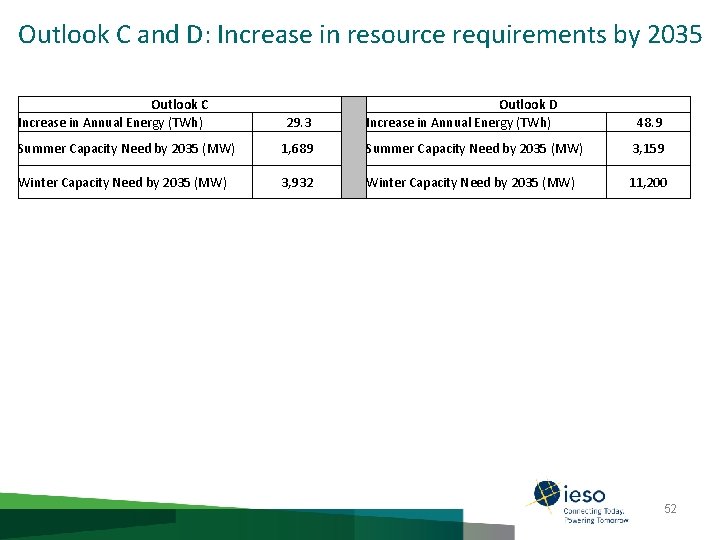
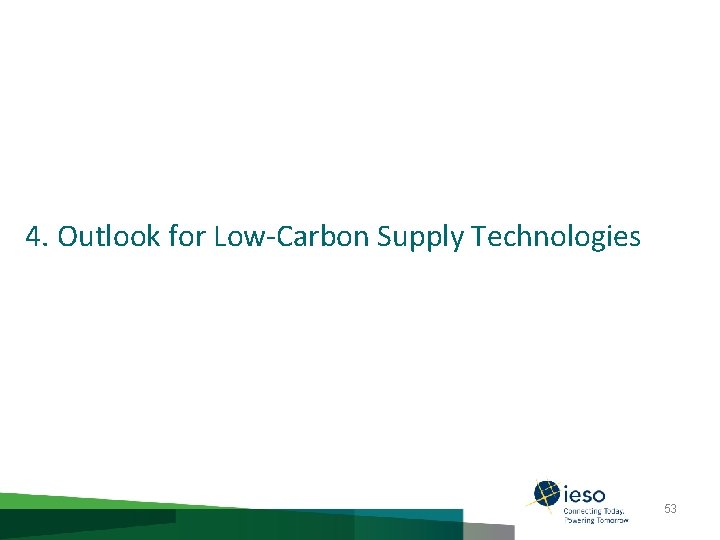
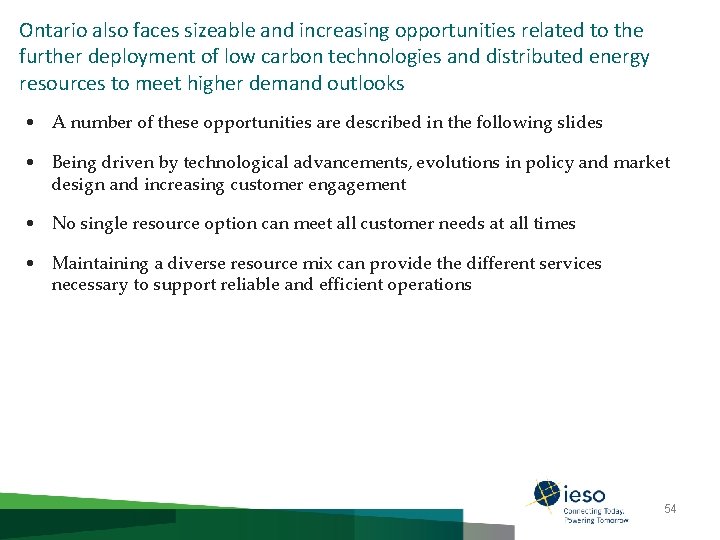
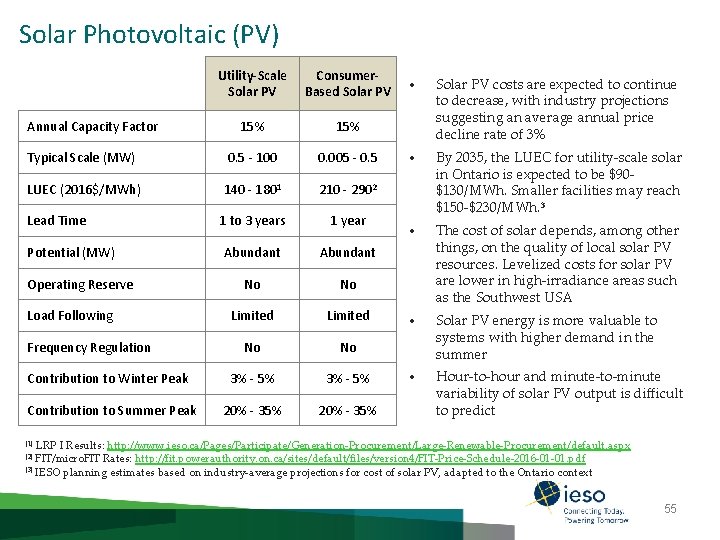
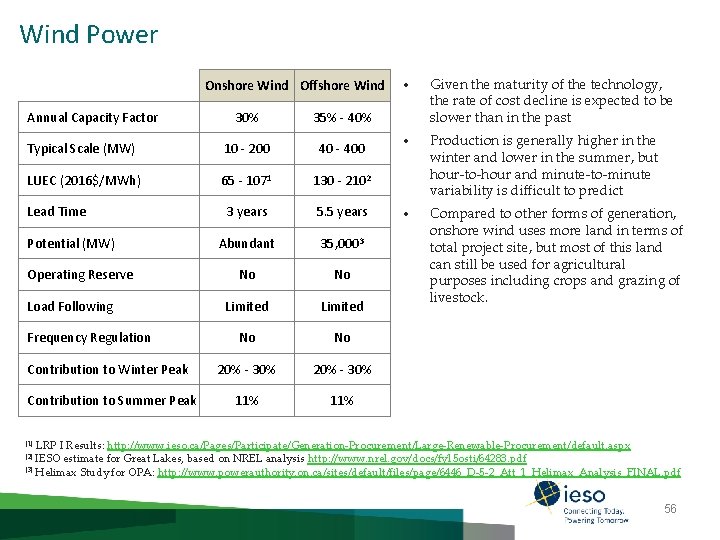
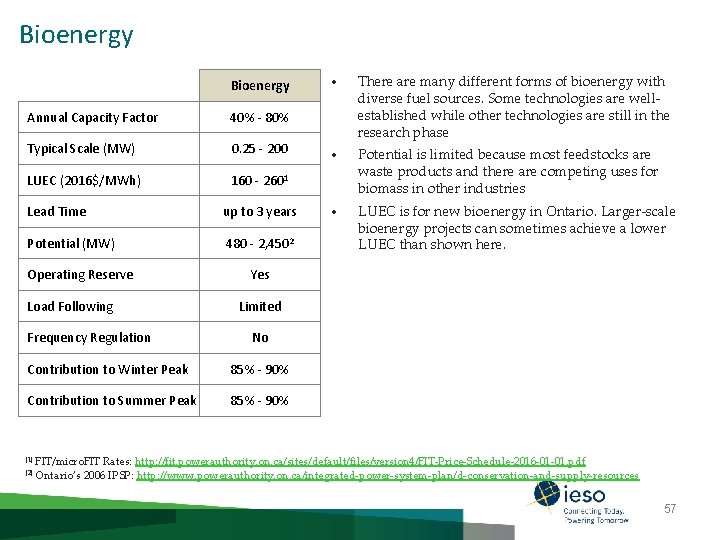
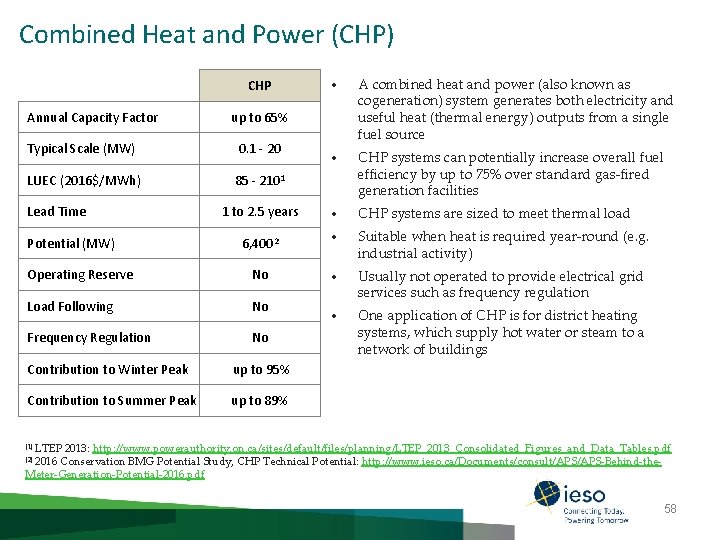
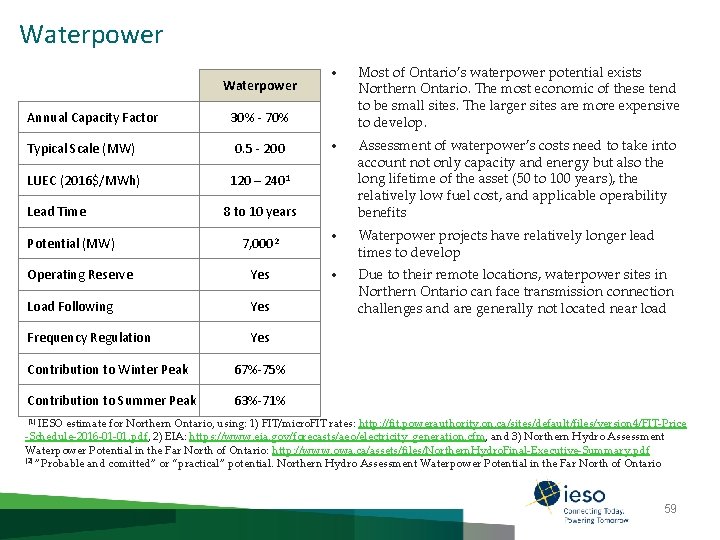
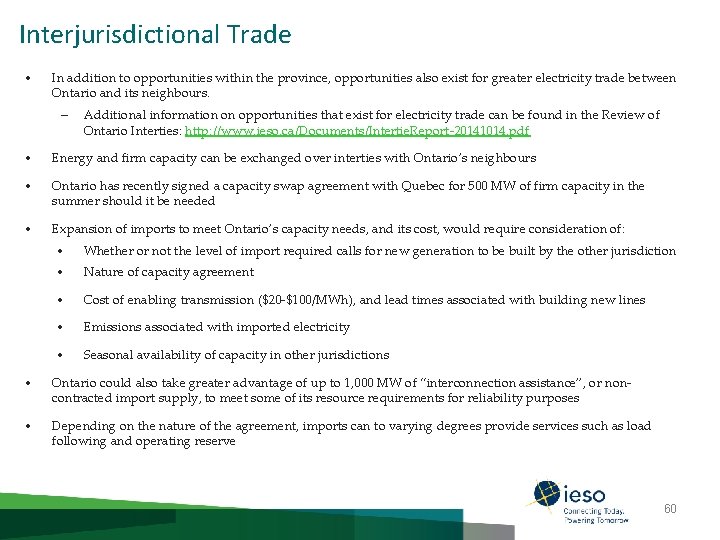
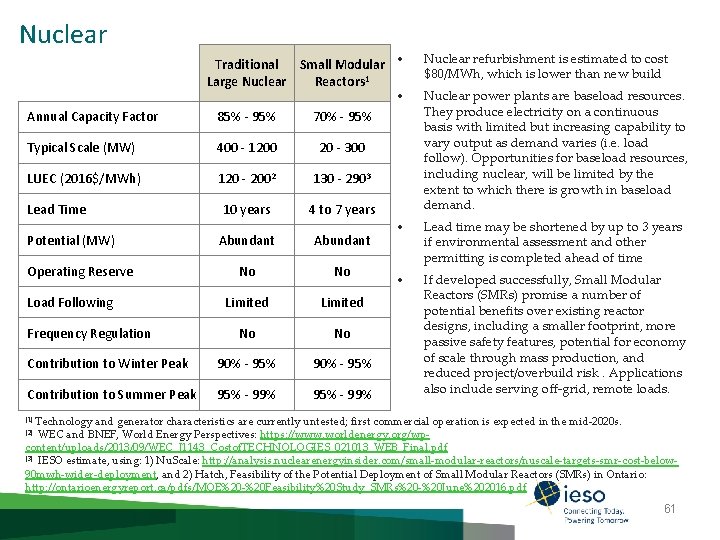
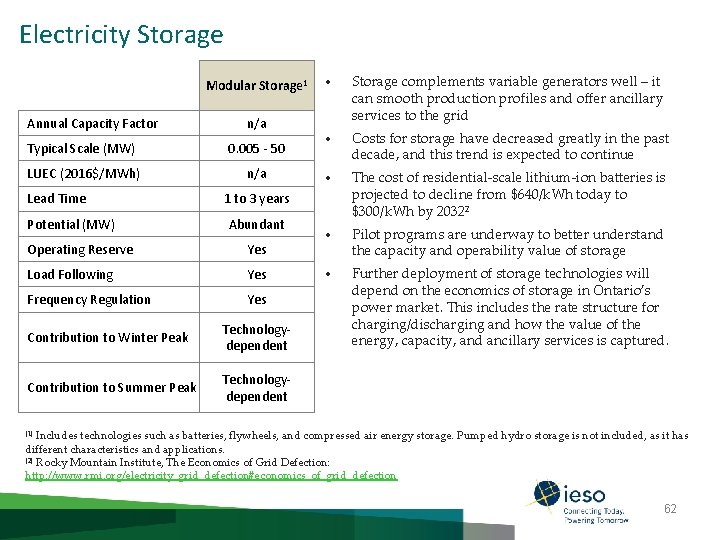
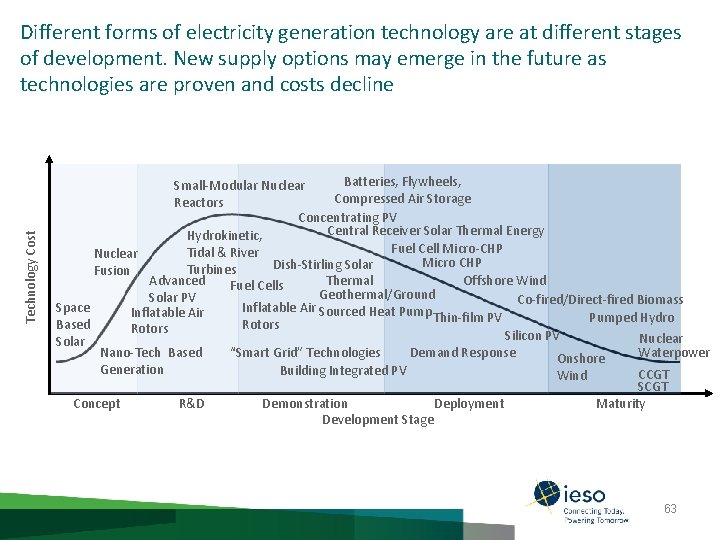
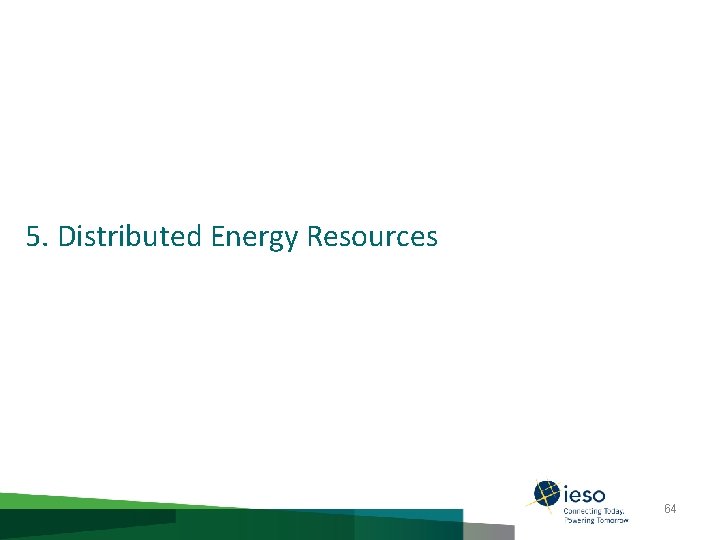
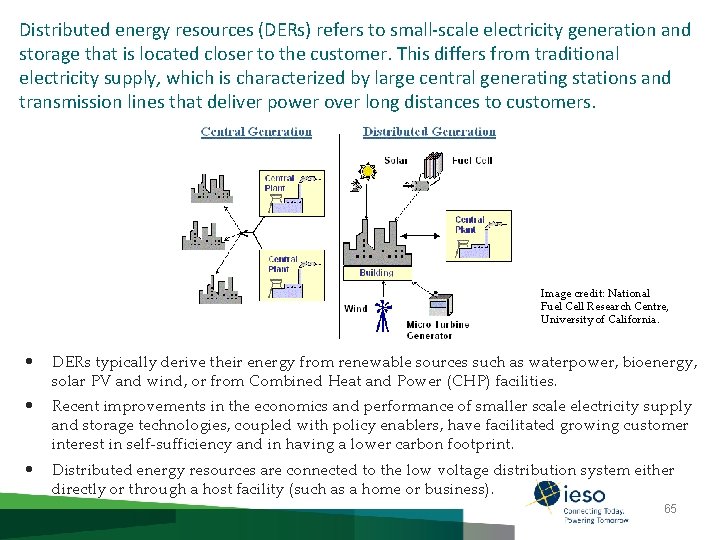
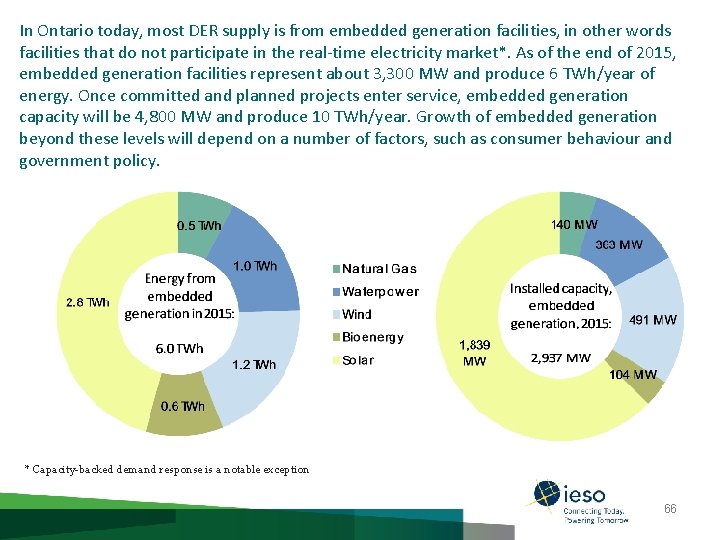
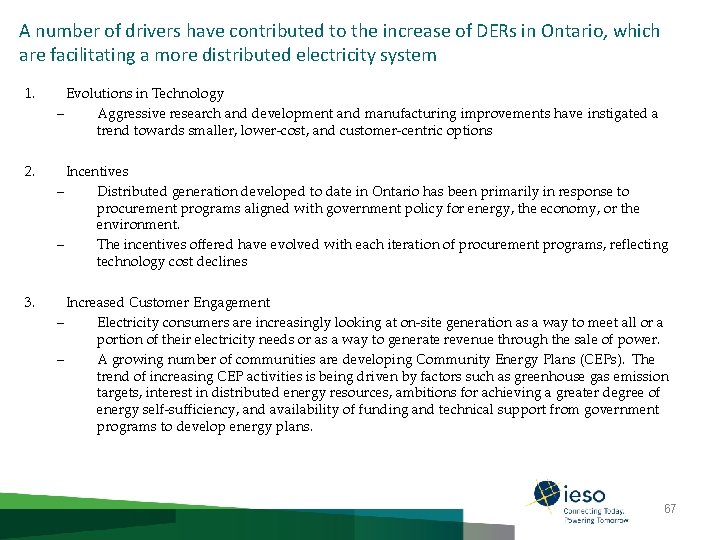
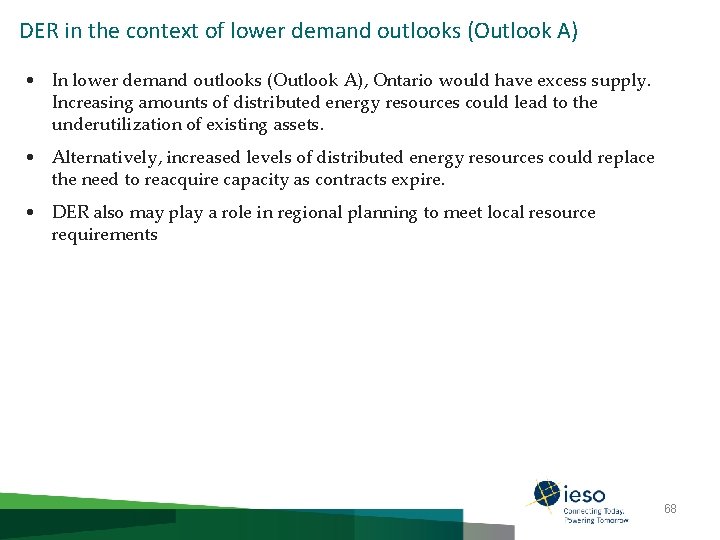
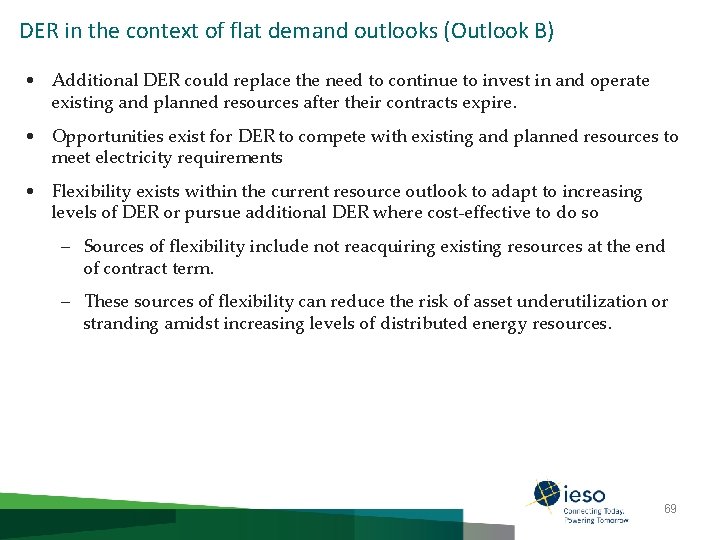
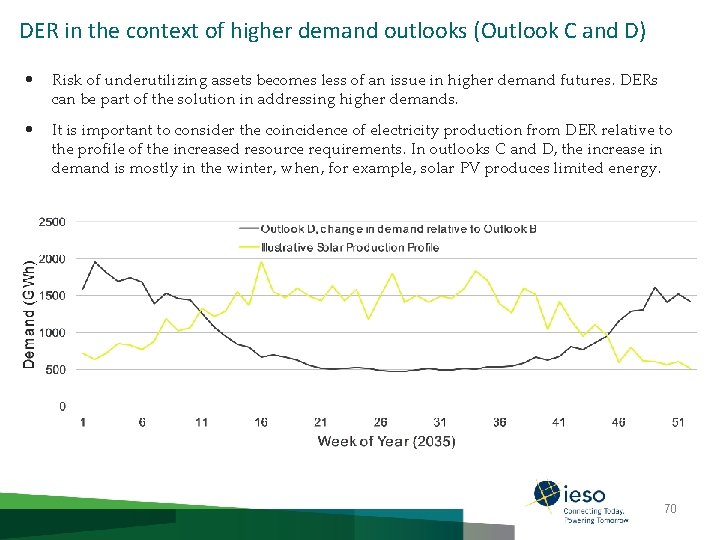
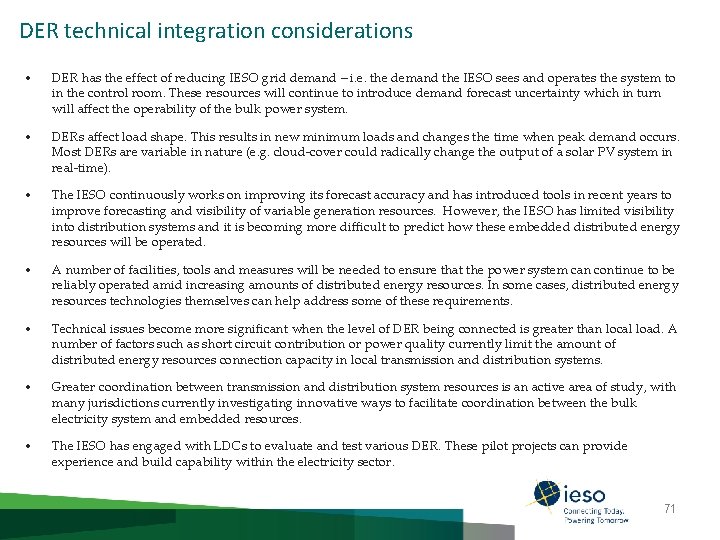
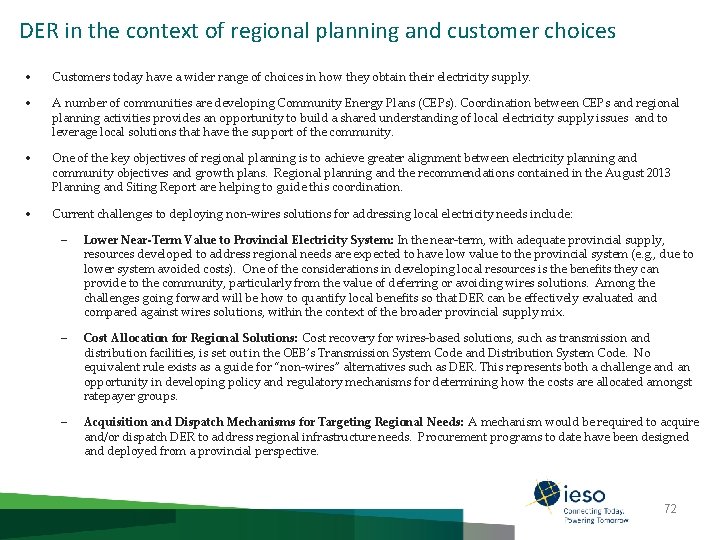
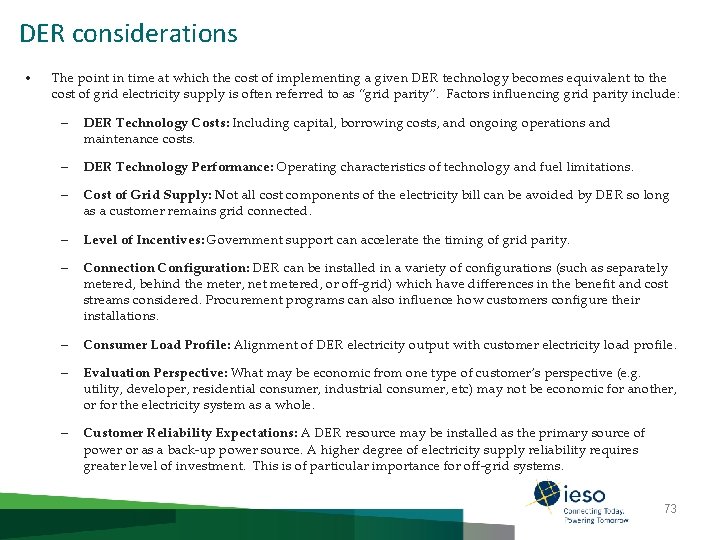
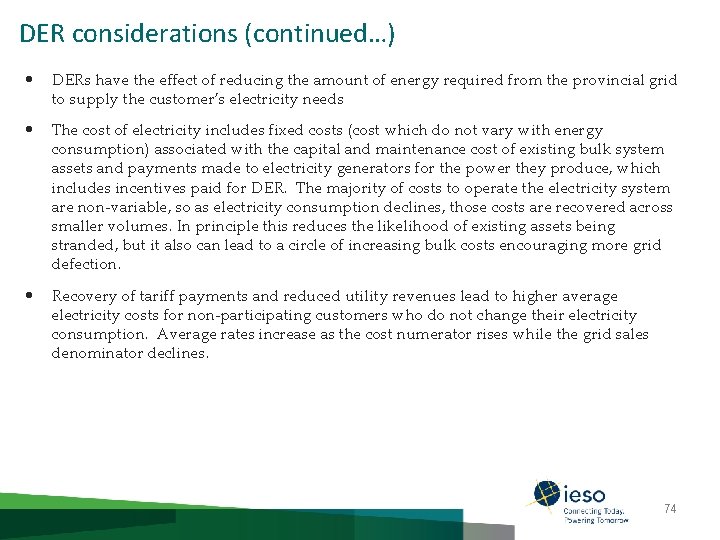
- Slides: 74
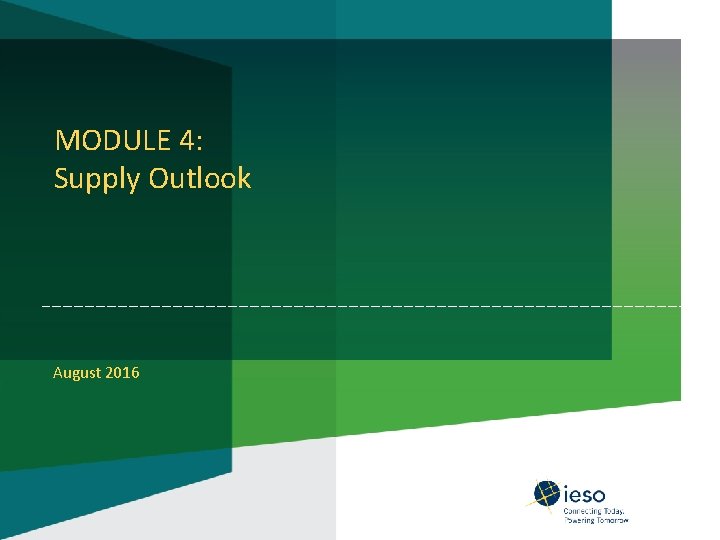
MODULE 4: Supply Outlook August 2016
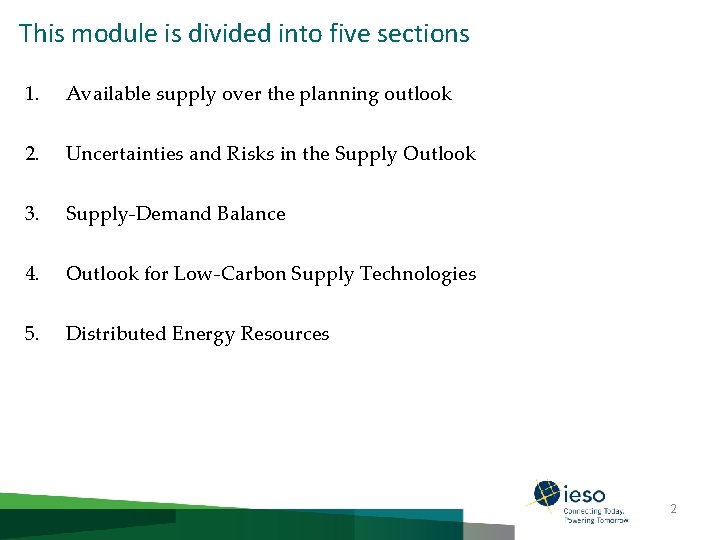
This module is divided into five sections 1. Available supply over the planning outlook 2. Uncertainties and Risks in the Supply Outlook 3. Supply-Demand Balance 4. Outlook for Low-Carbon Supply Technologies 5. Distributed Energy Resources 2
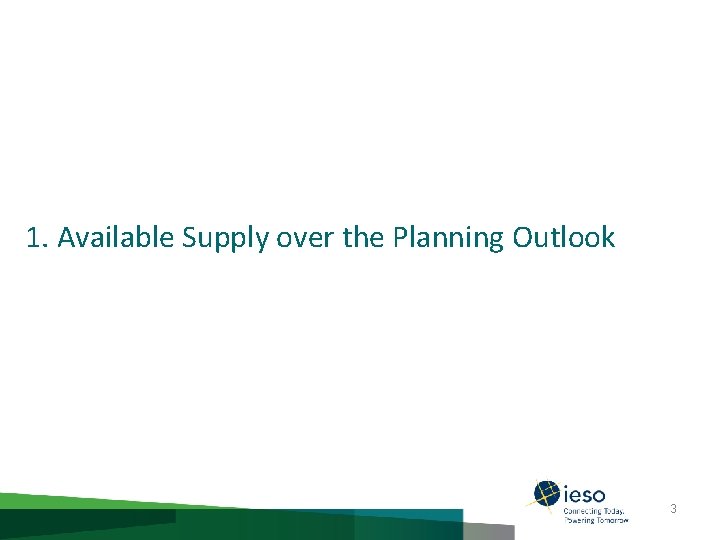
1. Available Supply over the Planning Outlook 3
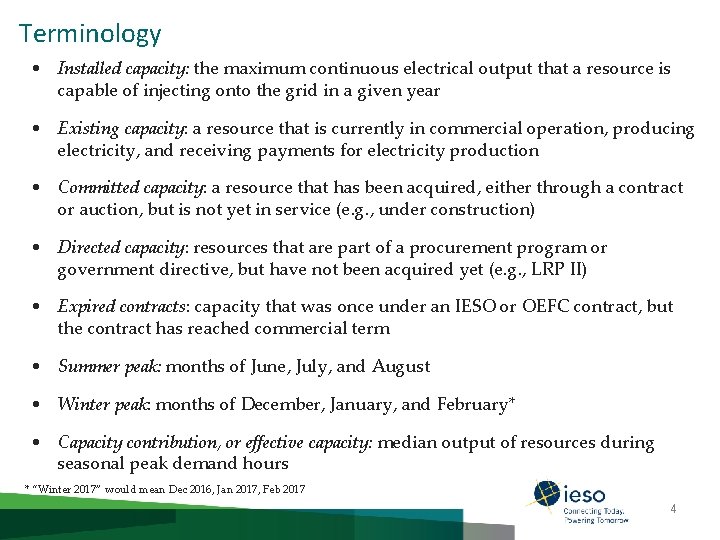
Terminology • Installed capacity: the maximum continuous electrical output that a resource is capable of injecting onto the grid in a given year • Existing capacity: a resource that is currently in commercial operation, producing electricity, and receiving payments for electricity production • Committed capacity: a resource that has been acquired, either through a contract or auction, but is not yet in service (e. g. , under construction) • Directed capacity: resources that are part of a procurement program or government directive, but have not been acquired yet (e. g. , LRP II) • Expired contracts: capacity that was once under an IESO or OEFC contract, but the contract has reached commercial term • Summer peak: months of June, July, and August • Winter peak: months of December, January, and February* • Capacity contribution, or effective capacity: median output of resources during seasonal peak demand hours * “Winter 2017” would mean Dec 2016, Jan 2017, Feb 2017 4
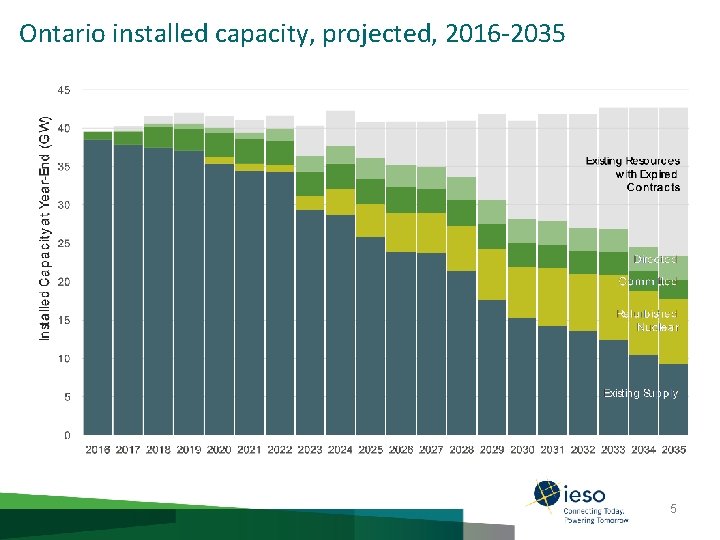
Ontario installed capacity, projected, 2016 -2035 5
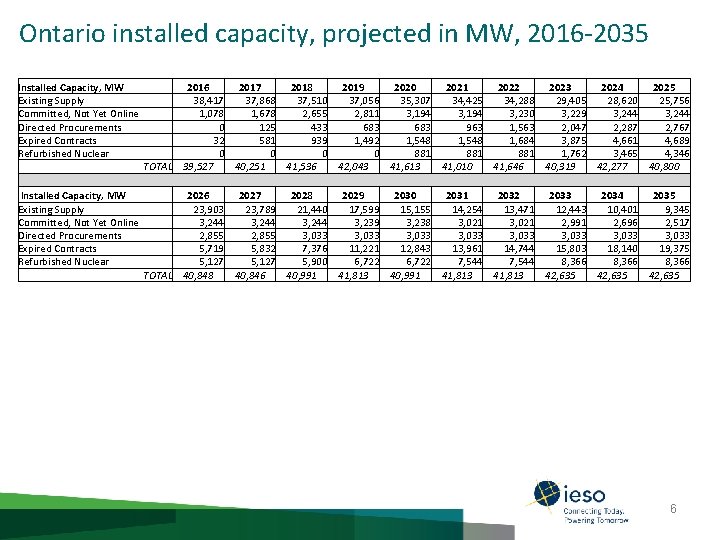
Ontario installed capacity, projected in MW, 2016 -2035 Installed Capacity, MW Existing Supply Committed, Not Yet Online Directed Procurements Expired Contracts Refurbished Nuclear 2016 2017 2018 2019 2020 2021 2022 2023 2024 2025 38, 417 37, 868 37, 510 37, 056 35, 307 34, 425 34, 288 29, 405 28, 620 25, 756 1, 078 1, 678 2, 655 2, 811 3, 194 3, 230 3, 229 3, 244 0 125 433 683 963 1, 563 2, 047 2, 287 2, 767 32 581 939 1, 492 1, 548 1, 684 3, 875 4, 661 4, 689 0 0 881 881 1, 762 3, 465 4, 346 TOTAL 39, 527 40, 251 41, 536 42, 043 41, 613 41, 010 41, 646 40, 319 42, 277 40, 800 Installed Capacity, MW Existing Supply Committed, Not Yet Online Directed Procurements Expired Contracts Refurbished Nuclear 2026 2027 2028 2029 2030 2031 2032 2033 2034 2035 23, 903 23, 789 21, 440 17, 599 15, 155 14, 254 13, 471 12, 443 10, 401 9, 345 3, 244 3, 239 3, 238 3, 021 2, 991 2, 696 2, 517 2, 855 3, 033 3, 033 5, 719 5, 832 7, 376 11, 221 12, 843 13, 961 14, 744 15, 803 18, 140 19, 375 5, 127 5, 900 6, 722 7, 544 8, 366 TOTAL 40, 848 40, 846 40, 991 41, 813 42, 635 6
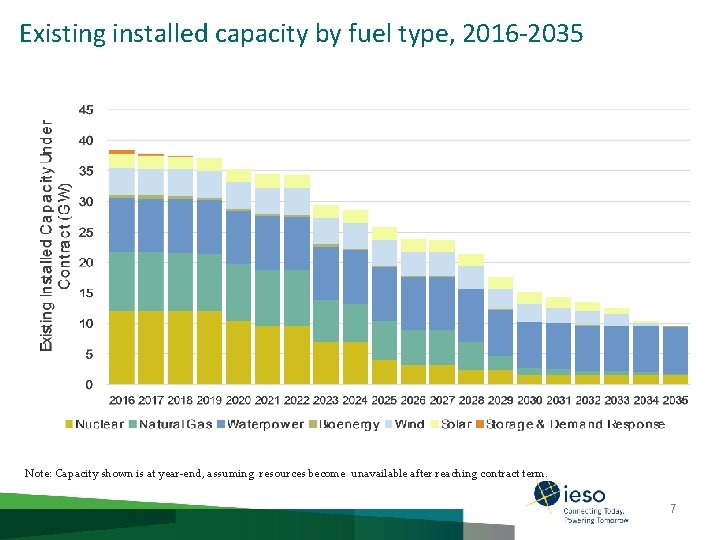
Existing installed capacity by fuel type, 2016 -2035 Note: Capacity shown is at year-end, assuming resources become unavailable after reaching contract term. 7
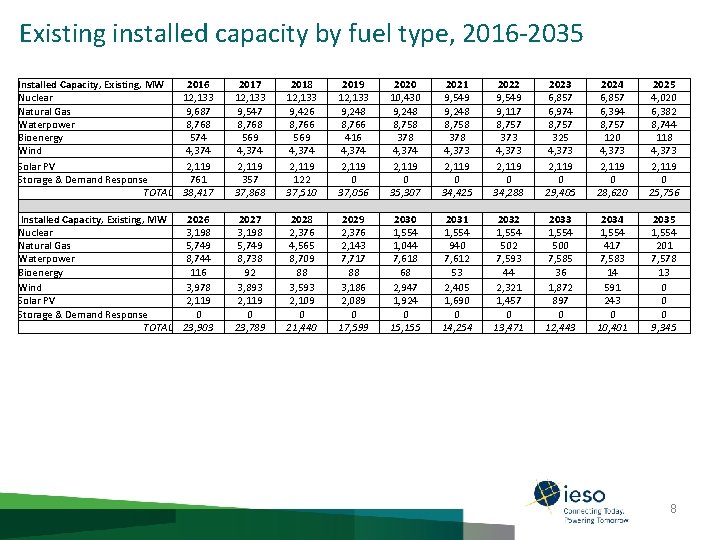
Existing installed capacity by fuel type, 2016 -2035 Installed Capacity, Existing, MW 2016 Nuclear 12, 133 Natural Gas 9, 687 Waterpower 8, 768 Bioenergy 574 Wind 4, 374 Solar PV 2, 119 Storage & Demand Response 761 TOTAL 38, 417 2017 12, 133 9, 547 8, 768 569 4, 374 2, 119 357 37, 868 2018 12, 133 9, 426 8, 766 569 4, 374 2, 119 122 37, 510 2019 12, 133 9, 248 8, 766 416 4, 374 2, 119 0 37, 056 2020 10, 430 9, 248 8, 758 378 4, 374 2, 119 0 35, 307 2021 9, 549 9, 248 8, 758 378 4, 373 2, 119 0 34, 425 2022 9, 549 9, 117 8, 757 373 4, 373 2, 119 0 34, 288 2023 6, 857 6, 974 8, 757 325 4, 373 2, 119 0 29, 405 2024 6, 857 6, 394 8, 757 120 4, 373 2, 119 0 28, 620 2025 4, 020 6, 382 8, 744 118 4, 373 2, 119 0 25, 756 Installed Capacity, Existing, MW 2026 Nuclear 3, 198 Natural Gas 5, 749 Waterpower 8, 744 Bioenergy 116 Wind 3, 978 Solar PV 2, 119 Storage & Demand Response 0 TOTAL 23, 903 2027 3, 198 5, 749 8, 738 92 3, 893 2, 119 0 23, 789 2028 2, 376 4, 565 8, 709 88 3, 593 2, 109 0 21, 440 2029 2, 376 2, 143 7, 717 88 3, 186 2, 089 0 17, 599 2030 1, 554 1, 044 7, 618 68 2, 947 1, 924 0 15, 155 2031 1, 554 940 7, 612 53 2, 405 1, 690 0 14, 254 2032 1, 554 502 7, 593 44 2, 321 1, 457 0 13, 471 2033 1, 554 500 7, 585 36 1, 872 897 0 12, 443 2034 1, 554 417 7, 583 14 591 243 0 10, 401 2035 1, 554 201 7, 578 13 0 0 0 9, 345 8
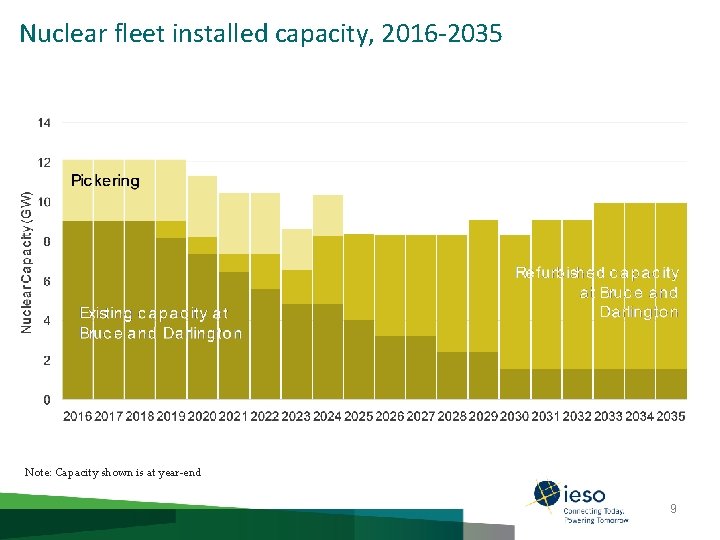
Nuclear fleet installed capacity, 2016 -2035 Note: Capacity shown is at year-end 9
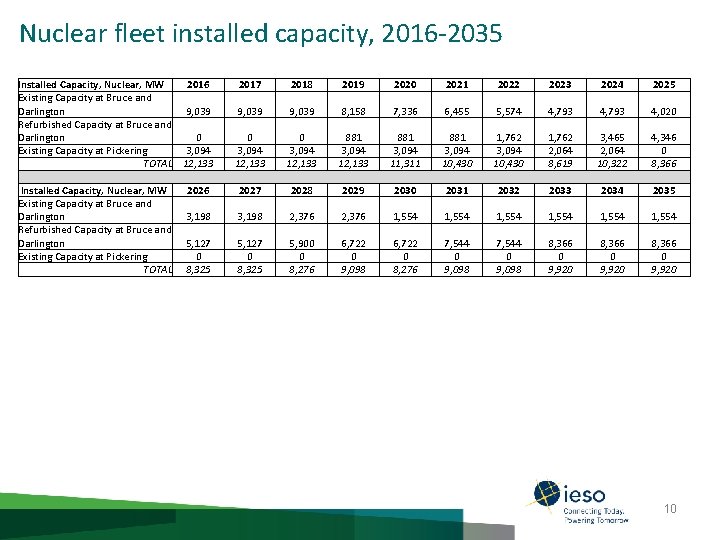
Nuclear fleet installed capacity, 2016 -2035 Installed Capacity, Nuclear, MW 2016 Existing Capacity at Bruce and Darlington 9, 039 Refurbished Capacity at Bruce and Darlington 0 Existing Capacity at Pickering 3, 094 TOTAL 12, 133 Installed Capacity, Nuclear, MW Existing Capacity at Bruce and Darlington Refurbished Capacity at Bruce and Darlington Existing Capacity at Pickering TOTAL 2017 2018 2019 2020 2021 2022 2023 2024 2025 9, 039 8, 158 7, 336 6, 455 5, 574 4, 793 4, 020 0 3, 094 12, 133 881 3, 094 11, 311 881 3, 094 10, 430 1, 762 2, 064 8, 619 3, 465 2, 064 10, 322 4, 346 0 8, 366 2027 2028 2029 2030 2031 2032 2033 2034 2035 3, 198 2, 376 1, 554 1, 554 5, 127 0 8, 325 5, 900 0 8, 276 6, 722 0 9, 098 6, 722 0 8, 276 7, 544 0 9, 098 8, 366 0 9, 920 10
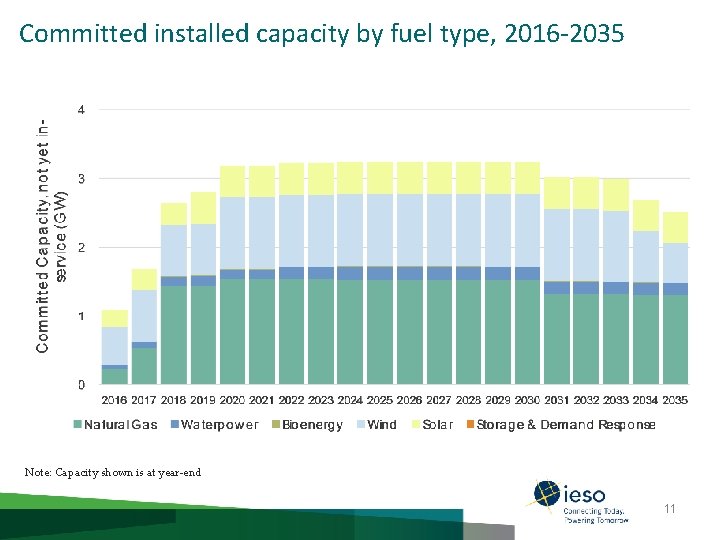
Committed installed capacity by fuel type, 2016 -2035 Note: Capacity shown is at year-end 11
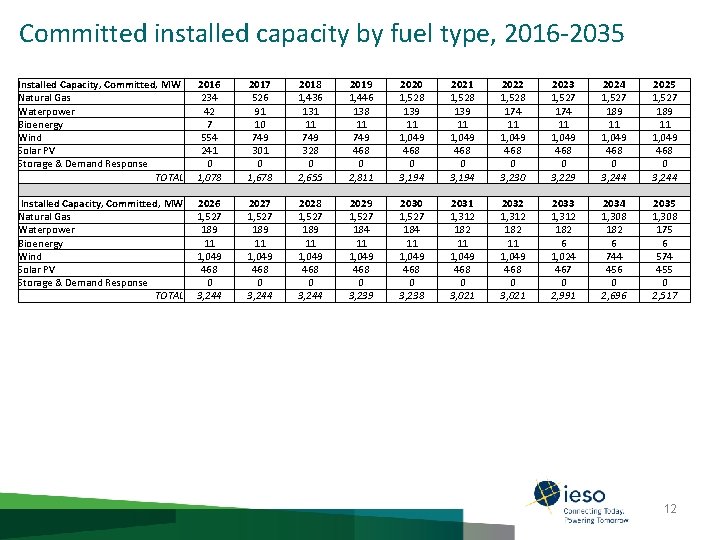
Committed installed capacity by fuel type, 2016 -2035 Installed Capacity, Committed, MW Natural Gas Waterpower Bioenergy Wind Solar PV Storage & Demand Response TOTAL 2016 234 42 7 554 241 0 1, 078 2017 526 91 10 749 301 0 1, 678 2018 1, 436 131 11 749 328 0 2, 655 2019 1, 446 138 11 749 468 0 2, 811 2020 1, 528 139 11 1, 049 468 0 3, 194 2021 1, 528 139 11 1, 049 468 0 3, 194 2022 1, 528 174 11 1, 049 468 0 3, 230 2023 1, 527 174 11 1, 049 468 0 3, 229 2024 1, 527 189 11 1, 049 468 0 3, 244 2025 1, 527 189 11 1, 049 468 0 3, 244 Installed Capacity, Committed, MW Natural Gas Waterpower Bioenergy Wind Solar PV Storage & Demand Response TOTAL 2026 1, 527 189 11 1, 049 468 0 3, 244 2027 1, 527 189 11 1, 049 468 0 3, 244 2028 1, 527 189 11 1, 049 468 0 3, 244 2029 1, 527 184 11 1, 049 468 0 3, 239 2030 1, 527 184 11 1, 049 468 0 3, 238 2031 1, 312 182 11 1, 049 468 0 3, 021 2032 1, 312 182 11 1, 049 468 0 3, 021 2033 1, 312 182 6 1, 024 467 0 2, 991 2034 1, 308 182 6 744 456 0 2, 696 2035 1, 308 175 6 574 455 0 2, 517 12
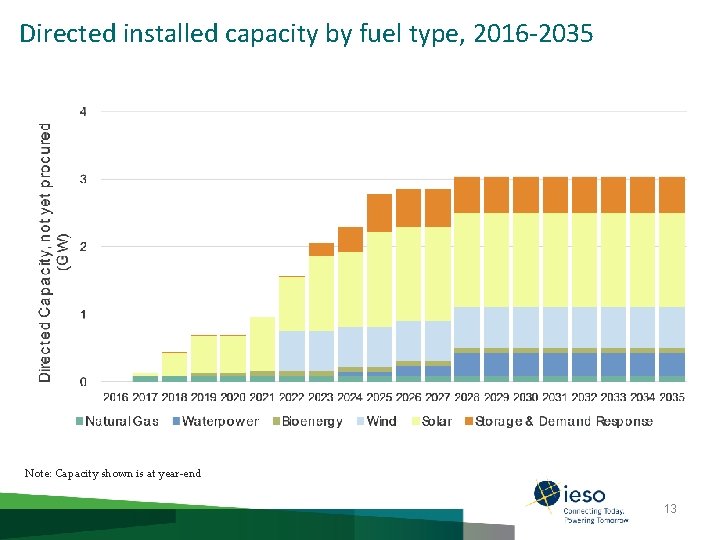
Directed installed capacity by fuel type, 2016 -2035 Note: Capacity shown is at year-end 13
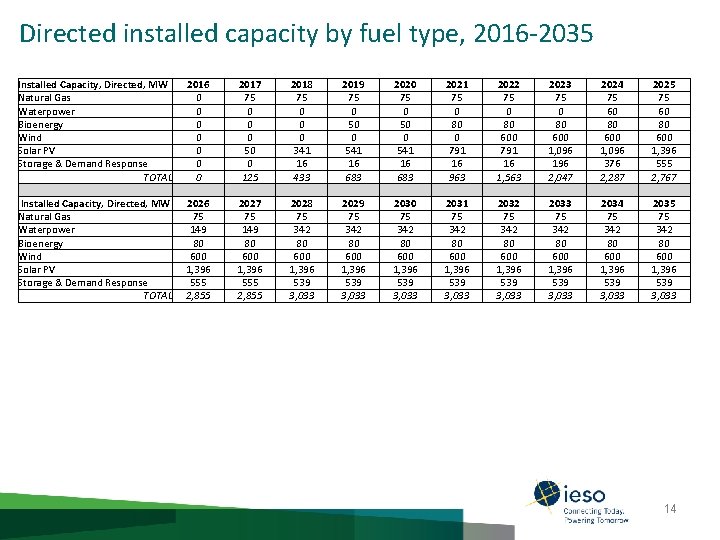
Directed installed capacity by fuel type, 2016 -2035 Installed Capacity, Directed, MW 2016 Natural Gas 0 Waterpower 0 Bioenergy 0 Wind 0 Solar PV 0 Storage & Demand Response 0 TOTAL 0 Installed Capacity, Directed, MW 2026 Natural Gas 75 Waterpower 149 Bioenergy 80 Wind 600 Solar PV 1, 396 Storage & Demand Response 555 TOTAL 2, 855 2017 75 0 0 0 50 0 125 2027 75 149 80 600 1, 396 555 2, 855 2018 75 0 0 0 341 16 433 2028 75 342 80 600 1, 396 539 3, 033 2019 75 0 50 0 541 16 683 2029 75 342 80 600 1, 396 539 3, 033 2020 75 0 50 0 541 16 683 2030 75 342 80 600 1, 396 539 3, 033 2021 75 0 80 0 791 16 963 2031 75 342 80 600 1, 396 539 3, 033 2022 75 0 80 600 791 16 1, 563 2032 75 342 80 600 1, 396 539 3, 033 2023 75 0 80 600 1, 096 196 2, 047 2033 75 342 80 600 1, 396 539 3, 033 2024 75 60 80 600 1, 096 376 2, 287 2034 75 342 80 600 1, 396 539 3, 033 2025 75 60 80 600 1, 396 555 2, 767 2035 75 342 80 600 1, 396 539 3, 033 14
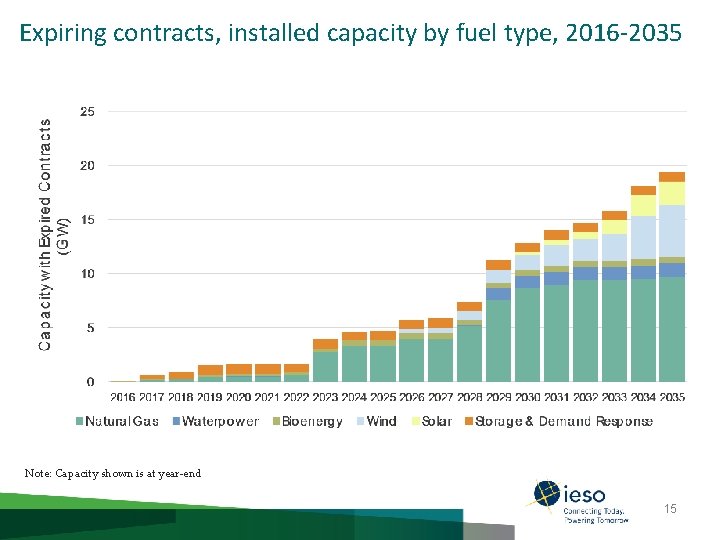
Expiring contracts, installed capacity by fuel type, 2016 -2035 Note: Capacity shown is at year-end 15
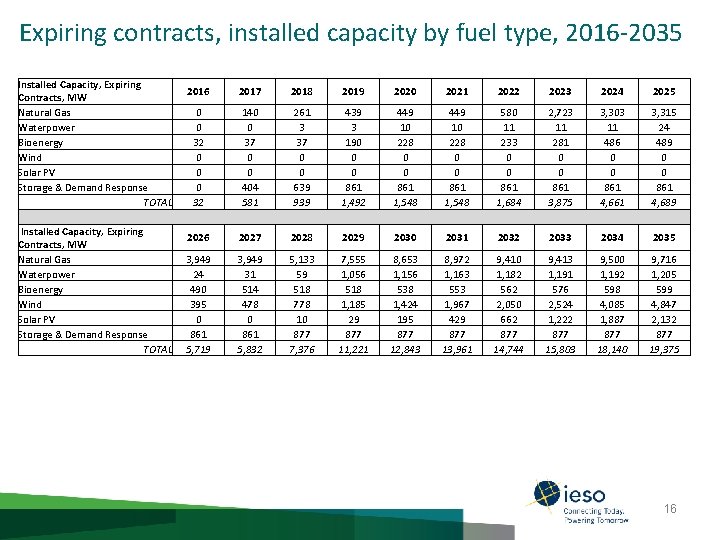
Expiring contracts, installed capacity by fuel type, 2016 -2035 Installed Capacity, Expiring Contracts, MW Natural Gas Waterpower Bioenergy Wind Solar PV Storage & Demand Response TOTAL 2016 2017 2018 2019 2020 2021 2022 2023 2024 2025 0 0 32 0 0 0 32 140 0 37 0 0 404 581 261 3 37 0 0 639 939 439 3 190 0 0 861 1, 492 449 10 228 0 0 861 1, 548 580 11 233 0 0 861 1, 684 2, 723 11 281 0 0 861 3, 875 3, 303 11 486 0 0 861 4, 661 3, 315 24 489 0 0 861 4, 689 2026 2027 2028 2029 2030 2031 2032 2033 2034 2035 3, 949 24 490 395 0 861 5, 719 3, 949 31 514 478 0 861 5, 832 5, 133 59 518 778 10 877 7, 376 7, 555 1, 056 518 1, 185 29 877 11, 221 8, 653 1, 156 538 1, 424 195 877 12, 843 8, 972 1, 163 553 1, 967 429 877 13, 961 9, 410 1, 182 562 2, 050 662 877 14, 744 9, 413 1, 191 576 2, 524 1, 222 877 15, 803 9, 500 1, 192 598 4, 085 1, 887 877 18, 140 9, 716 1, 205 599 4, 847 2, 132 877 19, 375 16
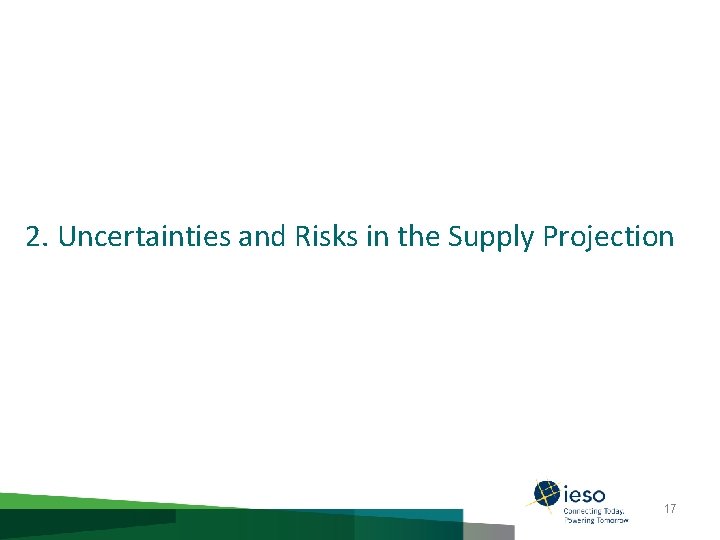
2. Uncertainties and Risks in the Supply Projection 17
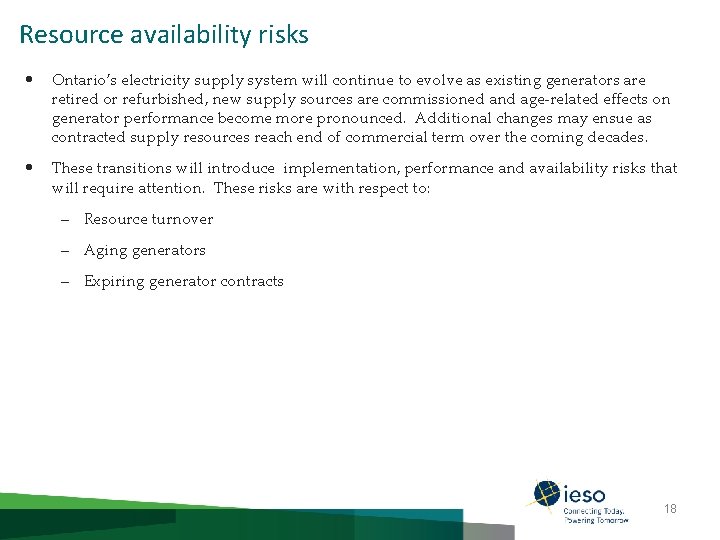
Resource availability risks • Ontario’s electricity supply system will continue to evolve as existing generators are retired or refurbished, new supply sources are commissioned and age-related effects on generator performance become more pronounced. Additional changes may ensue as contracted supply resources reach end of commercial term over the coming decades. • These transitions will introduce implementation, performance and availability risks that will require attention. These risks are with respect to: – Resource turnover – Aging generators – Expiring generator contracts 18
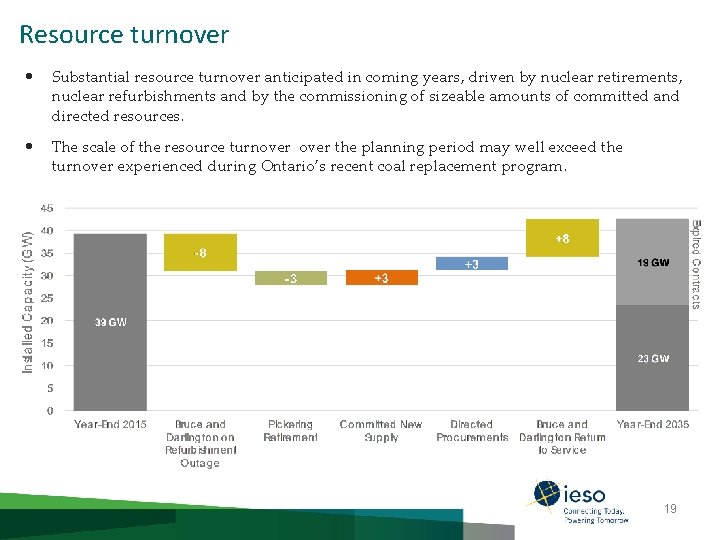
Resource turnover • Substantial resource turnover anticipated in coming years, driven by nuclear retirements, nuclear refurbishments and by the commissioning of sizeable amounts of committed and directed resources. • The scale of the resource turnover the planning period may well exceed the turnover experienced during Ontario’s recent coal replacement program. 39 GW 19
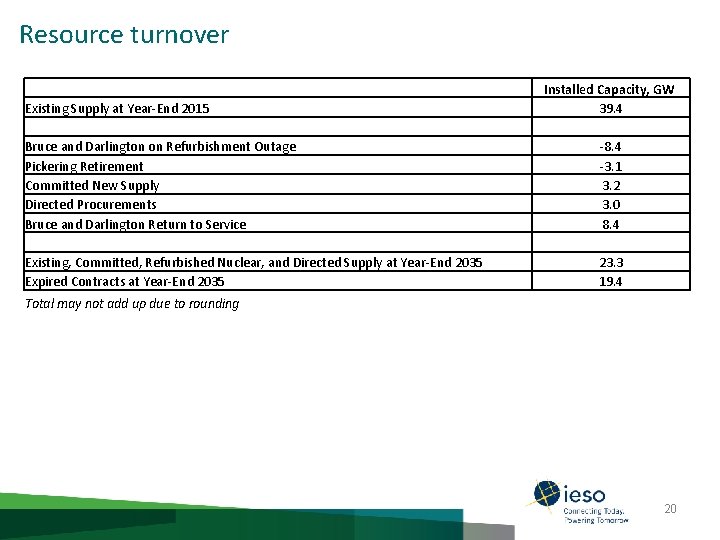
Resource turnover Existing Supply at Year-End 2015 Installed Capacity, GW 39. 4 Bruce and Darlington on Refurbishment Outage Pickering Retirement Committed New Supply Directed Procurements Bruce and Darlington Return to Service -8. 4 -3. 1 3. 2 3. 0 8. 4 Existing, Committed, Refurbished Nuclear, and Directed Supply at Year-End 2035 Expired Contracts at Year-End 2035 23. 3 19. 4 Total may not add up due to rounding 39 GW 20
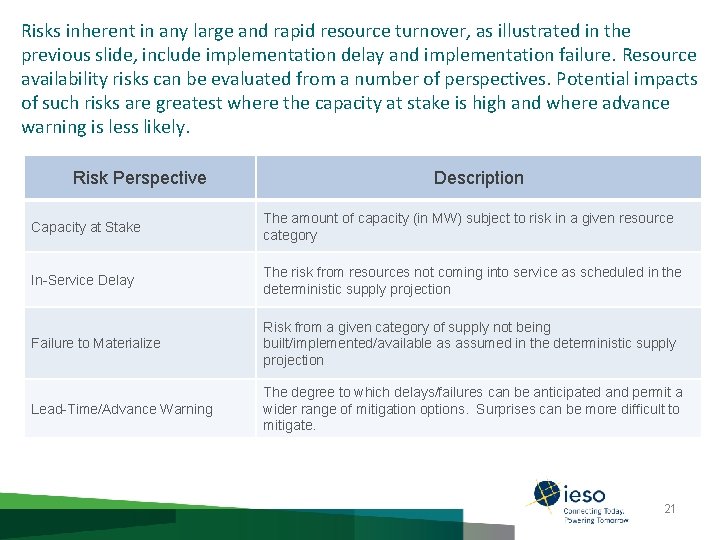
Risks inherent in any large and rapid resource turnover, as illustrated in the previous slide, include implementation delay and implementation failure. Resource availability risks can be evaluated from a number of perspectives. Potential impacts of such risks are greatest where the capacity at stake is high and where advance warning is less likely. Risk Perspective Description Capacity at Stake The amount of capacity (in MW) subject to risk in a given resource category In-Service Delay The risk from resources not coming into service as scheduled in the deterministic supply projection Failure to Materialize Risk from a given category of supply not being built/implemented/available as assumed in the deterministic supply projection Lead-Time/Advance Warning The degree to which delays/failures can be anticipated and permit a wider range of mitigation options. Surprises can be more difficult to mitigate. 21
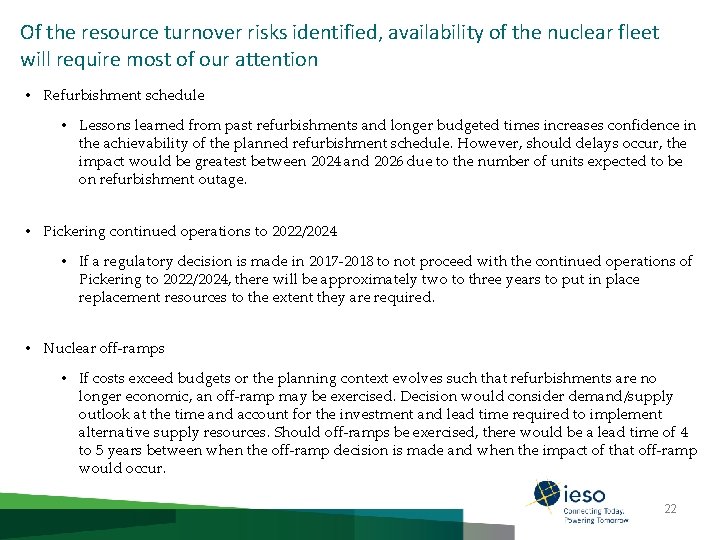
Of the resource turnover risks identified, availability of the nuclear fleet will require most of our attention • Refurbishment schedule • Lessons learned from past refurbishments and longer budgeted times increases confidence in the achievability of the planned refurbishment schedule. However, should delays occur, the impact would be greatest between 2024 and 2026 due to the number of units expected to be on refurbishment outage. • Pickering continued operations to 2022/2024 • If a regulatory decision is made in 2017 -2018 to not proceed with the continued operations of Pickering to 2022/2024, there will be approximately two to three years to put in place replacement resources to the extent they are required. • Nuclear off-ramps • If costs exceed budgets or the planning context evolves such that refurbishments are no longer economic, an off-ramp may be exercised. Decision would consider demand/supply outlook at the time and account for the investment and lead time required to implement alternative supply resources. Should off-ramps be exercised, there would be a lead time of 4 to 5 years between when the off-ramp decision is made and when the impact of that off-ramp would occur. 22
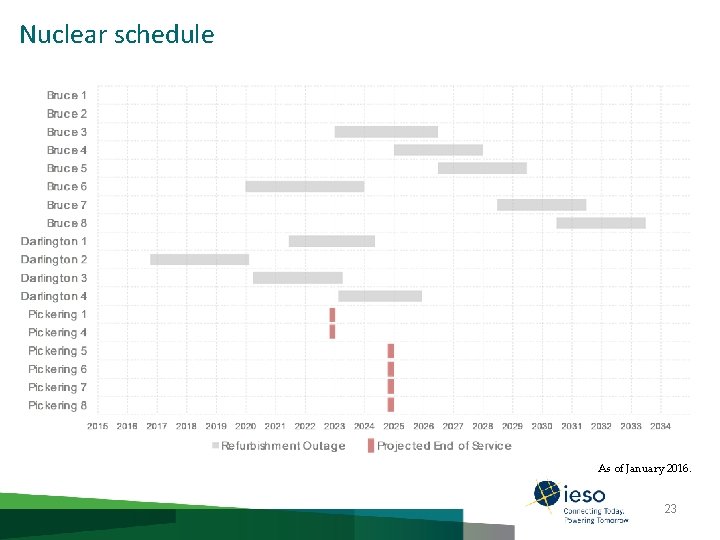
Nuclear schedule As of January 2016. 23
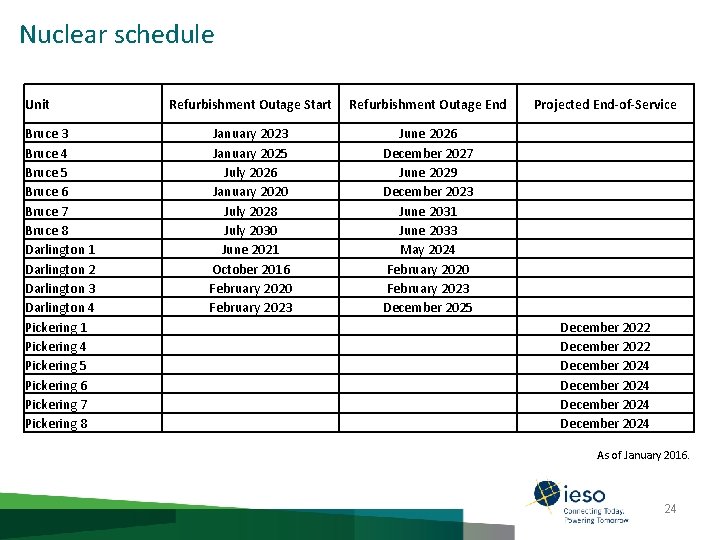
Nuclear schedule Unit Bruce 3 Bruce 4 Bruce 5 Bruce 6 Bruce 7 Bruce 8 Darlington 1 Darlington 2 Darlington 3 Darlington 4 Pickering 1 Pickering 4 Pickering 5 Pickering 6 Pickering 7 Pickering 8 Refurbishment Outage Start Refurbishment Outage End January 2023 January 2025 July 2026 January 2020 July 2028 July 2030 June 2021 October 2016 February 2020 February 2023 June 2026 December 2027 June 2029 December 2023 June 2031 June 2033 May 2024 February 2020 February 2023 December 2025 Projected End-of-Service December 2022 December 2024 As of January 2016. 24
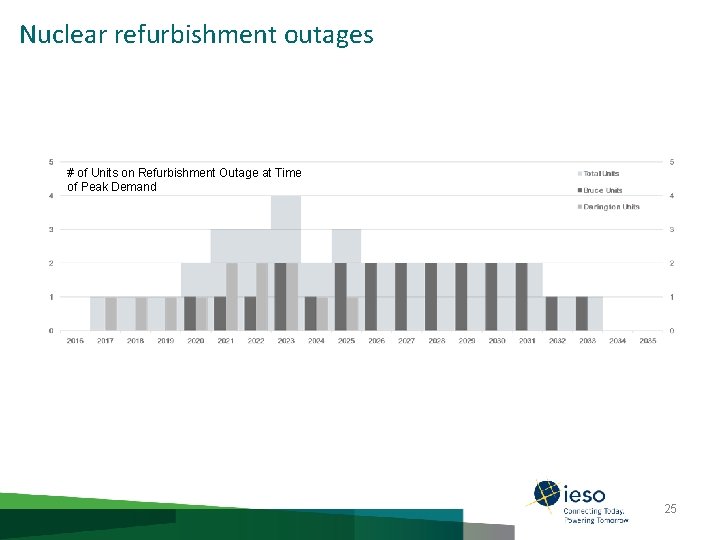
Nuclear refurbishment outages # of Units on Refurbishment Outage at Time of Peak Demand 25
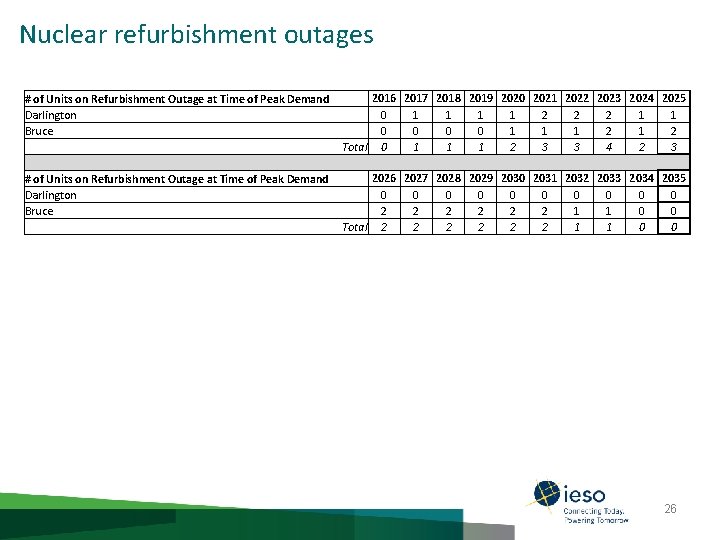
Nuclear refurbishment outages # of Units on Refurbishment Outage at Time of Peak Demand Darlington Bruce 2016 2017 2018 2019 2020 2021 2022 2023 2024 2025 0 1 1 2 2 2 1 1 0 0 1 1 1 2 Total 0 1 1 1 2 3 3 4 2 3 # of Units on Refurbishment Outage at Time of Peak Demand Darlington Bruce 2026 2027 2028 2029 2030 2031 2032 2033 2034 2035 0 0 0 0 0 2 2 2 1 1 0 0 Total 2 2 2 1 1 0 0 26
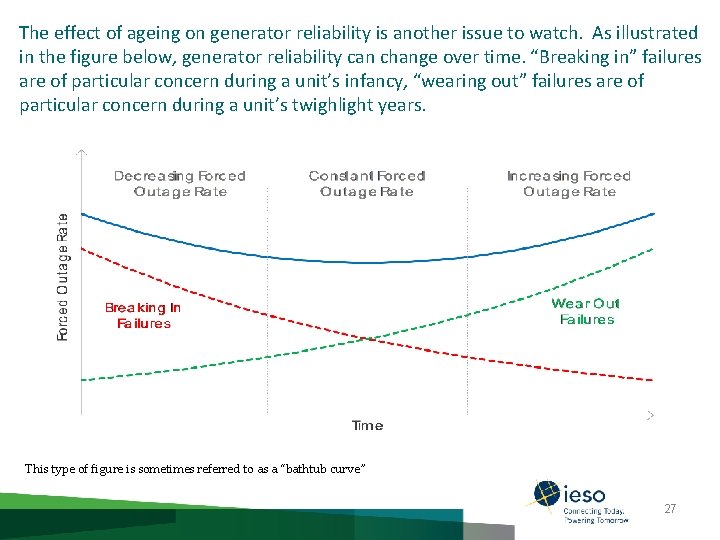
The effect of ageing on generator reliability is another issue to watch. As illustrated in the figure below, generator reliability can change over time. “Breaking in” failures are of particular concern during a unit’s infancy, “wearing out” failures are of particular concern during a unit’s twighlight years. This type of figure is sometimes referred to as a “bathtub curve” 27
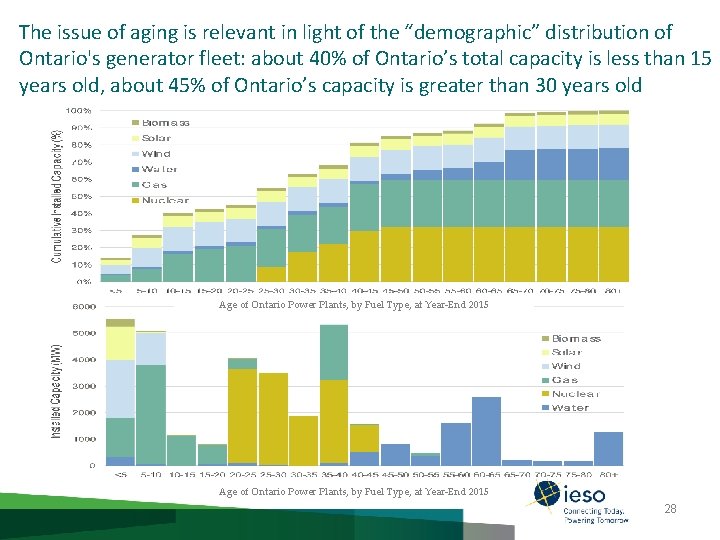
The issue of aging is relevant in light of the “demographic” distribution of Ontario's generator fleet: about 40% of Ontario’s total capacity is less than 15 years old, about 45% of Ontario’s capacity is greater than 30 years old Age of Ontario Power Plants, by Fuel Type, at Year-End 2015 28
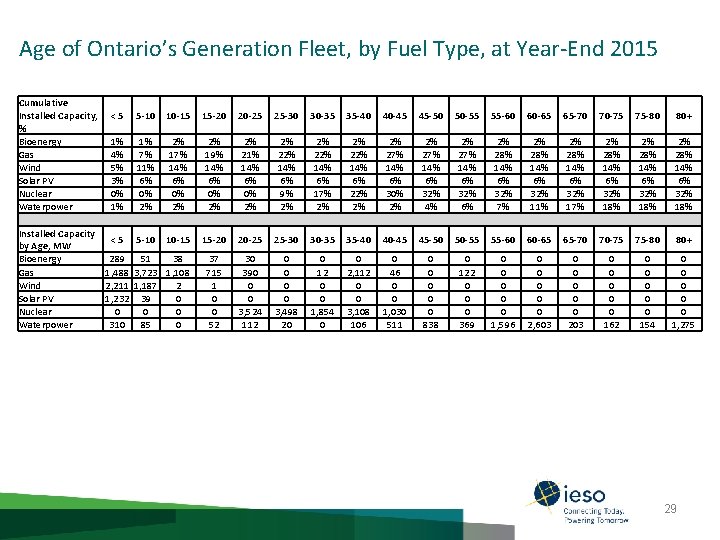
Age of Ontario’s Generation Fleet, by Fuel Type, at Year-End 2015 Cumulative Installed Capacity, % Bioenergy Gas Wind Solar PV Nuclear Waterpower < 5 5 -10 10 -15 15 -20 20 -25 25 -30 30 -35 35 -40 40 -45 45 -50 50 -55 55 -60 60 -65 65 -70 70 -75 75 -80 80+ 1% 4% 5% 3% 0% 1% 1% 7% 11% 6% 0% 2% 2% 17% 14% 6% 0% 2% 2% 19% 14% 6% 0% 2% 2% 21% 14% 6% 0% 2% 2% 22% 14% 6% 9% 2% 2% 22% 14% 6% 17% 2% 2% 22% 14% 6% 22% 2% 2% 27% 14% 6% 30% 2% 2% 27% 14% 6% 32% 4% 2% 27% 14% 6% 32% 6% 2% 28% 14% 6% 32% 7% 2% 28% 14% 6% 32% 11% 2% 28% 14% 6% 32% 17% 2% 28% 14% 6% 32% 18% 15 -20 20 -25 25 -30 30 -35 35 -40 40 -45 45 -50 50 -55 55 -60 60 -65 65 -70 70 -75 75 -80 80+ 37 715 1 0 0 52 30 390 0 0 3, 524 112 0 0 3, 498 20 0 12 0 0 1, 854 0 0 2, 112 0 0 3, 108 106 0 46 0 0 1, 030 511 0 0 0 838 0 122 0 0 0 369 0 0 0 1, 596 0 0 0 2, 603 0 0 0 203 0 0 0 162 0 0 0 154 0 0 0 1, 275 Installed Capacity < 5 5 -10 10 -15 by Age, MW Bioenergy 289 51 38 Gas 1, 488 3, 723 1, 108 Wind 2, 211 1, 187 2 Solar PV 1, 232 39 0 Nuclear 0 0 0 Waterpower 310 85 0 29
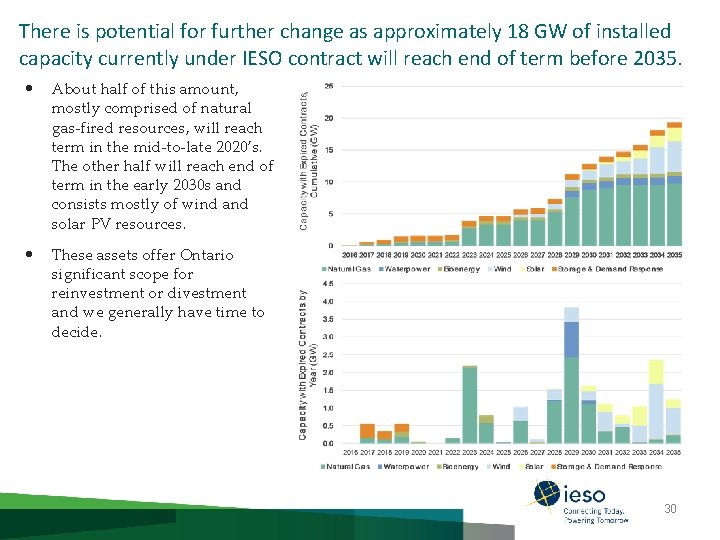
There is potential for further change as approximately 18 GW of installed capacity currently under IESO contract will reach end of term before 2035. • About half of this amount, mostly comprised of natural gas-fired resources, will reach term in the mid-to-late 2020’s. The other half will reach end of term in the early 2030 s and consists mostly of wind and solar PV resources. • These assets offer Ontario significant scope for reinvestment or divestment and we generally have time to decide. 30
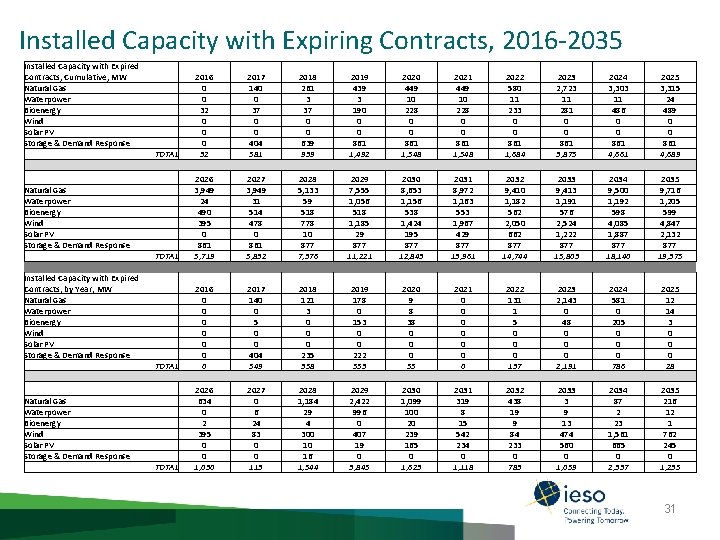
Installed Capacity with Expiring Contracts, 2016 -2035 Installed Capacity with Expired Contracts, Cumulative, MW Natural Gas Waterpower Bioenergy Wind Solar PV Storage & Demand Response TOTAL 2016 0 0 32 0 0 0 32 2017 140 0 37 0 0 404 581 2018 261 3 37 0 0 639 939 2019 439 3 190 0 0 861 1, 492 2020 449 10 228 0 0 861 1, 548 2021 449 10 228 0 0 861 1, 548 2022 580 11 233 0 0 861 1, 684 2023 2, 723 11 281 0 0 861 3, 875 2024 3, 303 11 486 0 0 861 4, 661 2025 3, 315 24 489 0 0 861 4, 689 TOTAL 2026 3, 949 24 490 395 0 861 5, 719 2027 3, 949 31 514 478 0 861 5, 832 2028 5, 133 59 518 778 10 877 7, 376 2029 7, 555 1, 056 518 1, 185 29 877 11, 221 2030 8, 653 1, 156 538 1, 424 195 877 12, 843 2031 8, 972 1, 163 553 1, 967 429 877 13, 961 2032 9, 410 1, 182 562 2, 050 662 877 14, 744 2033 9, 413 1, 191 576 2, 524 1, 222 877 15, 803 2034 9, 500 1, 192 598 4, 085 1, 887 877 18, 140 2035 9, 716 1, 205 599 4, 847 2, 132 877 19, 375 TOTAL 2016 0 0 0 0 2017 140 0 5 0 0 404 549 2018 121 3 0 0 0 235 358 2019 178 0 153 0 0 222 553 2020 9 8 38 0 0 0 55 2021 0 0 0 0 2022 131 1 5 0 0 0 137 2023 2, 143 0 48 0 0 0 2, 191 2024 581 0 205 0 0 0 786 2025 12 14 3 0 0 0 28 TOTAL 2026 634 0 2 395 0 0 1, 030 2027 0 6 24 83 0 0 113 2028 1, 184 29 4 300 10 16 1, 544 2029 2, 422 996 0 407 19 0 3, 845 2030 1, 099 100 20 239 165 0 1, 623 2031 319 8 15 542 234 0 1, 118 2032 438 19 9 84 233 0 783 2033 3 9 13 474 560 0 1, 059 2034 87 2 23 1, 561 665 0 2, 337 2035 216 12 1 762 245 0 1, 235 Natural Gas Waterpower Bioenergy Wind Solar PV Storage & Demand Response Installed Capacity with Expired Contracts, by Year, MW Natural Gas Waterpower Bioenergy Wind Solar PV Storage & Demand Response 31
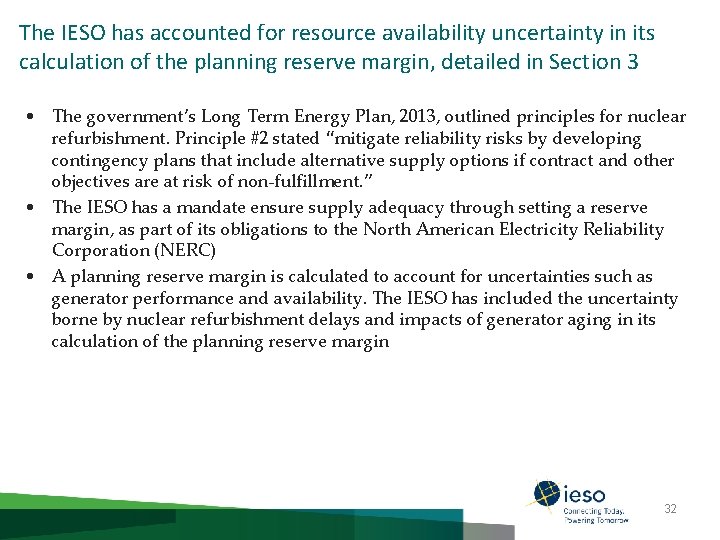
The IESO has accounted for resource availability uncertainty in its calculation of the planning reserve margin, detailed in Section 3 • The government’s Long Term Energy Plan, 2013, outlined principles for nuclear refurbishment. Principle #2 stated “mitigate reliability risks by developing contingency plans that include alternative supply options if contract and other objectives are at risk of non-fulfillment. ” • The IESO has a mandate ensure supply adequacy through setting a reserve margin, as part of its obligations to the North American Electricity Reliability Corporation (NERC) • A planning reserve margin is calculated to account for uncertainties such as generator performance and availability. The IESO has included the uncertainty borne by nuclear refurbishment delays and impacts of generator aging in its calculation of the planning reserve margin 32
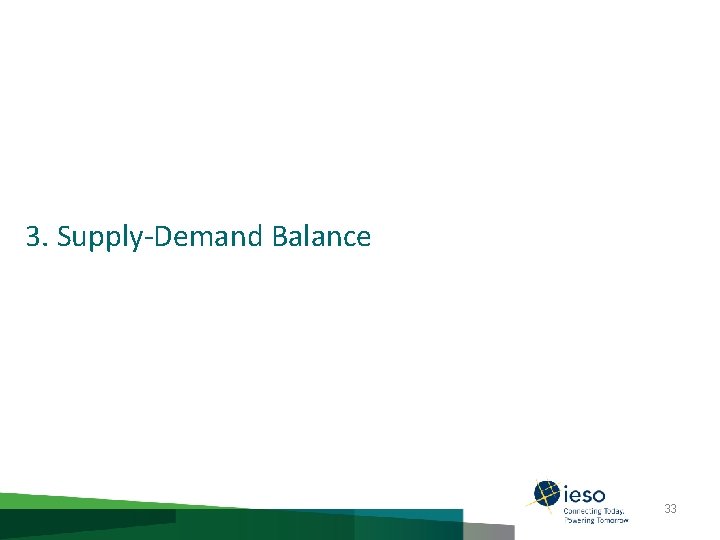
3. Supply-Demand Balance 33
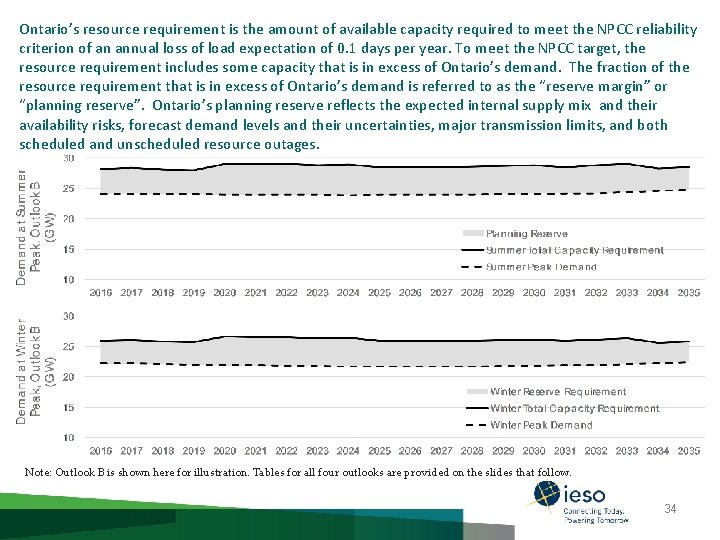
Ontario’s resource requirement is the amount of available capacity required to meet the NPCC reliability criterion of an annual loss of load expectation of 0. 1 days per year. To meet the NPCC target, the resource requirement includes some capacity that is in excess of Ontario’s demand. The fraction of the resource requirement that is in excess of Ontario’s demand is referred to as the “reserve margin” or “planning reserve”. Ontario’s planning reserve reflects the expected internal supply mix and their availability risks, forecast demand levels and their uncertainties, major transmission limits, and both scheduled and unscheduled resource outages. Note: Outlook B is shown here for illustration. Tables for all four outlooks are provided on the slides that follow. 34
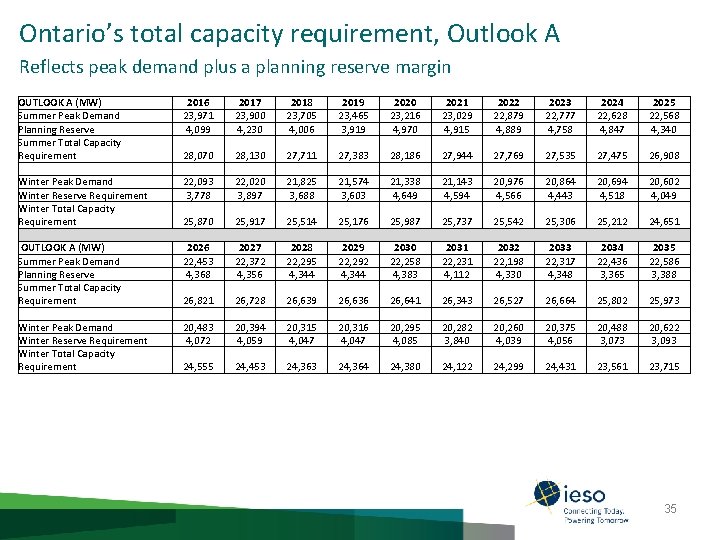
Ontario’s total capacity requirement, Outlook A Reflects peak demand plus a planning reserve margin OUTLOOK A (MW) Summer Peak Demand Planning Reserve Summer Total Capacity Requirement Winter Peak Demand Winter Reserve Requirement Winter Total Capacity Requirement 2016 23, 971 4, 099 2017 23, 900 4, 230 2018 23, 705 4, 006 2019 23, 465 3, 919 2020 23, 216 4, 970 2021 23, 029 4, 915 2022 22, 879 4, 889 2023 22, 777 4, 758 2024 22, 628 4, 847 2025 22, 568 4, 340 28, 070 22, 093 3, 778 28, 130 22, 020 3, 897 27, 711 21, 825 3, 688 27, 383 21, 574 3, 603 28, 186 21, 338 4, 649 27, 944 21, 143 4, 594 27, 769 20, 976 4, 566 27, 535 20, 864 4, 443 27, 475 20, 694 4, 518 26, 908 20, 602 4, 049 25, 870 25, 917 25, 514 25, 176 25, 987 25, 737 25, 542 25, 306 25, 212 24, 651 OUTLOOK A (MW) Summer Peak Demand Planning Reserve Summer Total Capacity Requirement Winter Peak Demand Winter Reserve Requirement Winter Total Capacity Requirement 2026 22, 453 4, 368 2027 22, 372 4, 356 2028 22, 295 4, 344 2029 22, 292 4, 344 2030 22, 258 4, 383 2031 22, 231 4, 112 2032 22, 198 4, 330 2033 22, 317 4, 348 2034 22, 436 3, 365 2035 22, 586 3, 388 26, 821 20, 483 4, 072 26, 728 20, 394 4, 059 26, 639 20, 315 4, 047 26, 636 20, 316 4, 047 26, 641 20, 295 4, 085 26, 343 20, 282 3, 840 26, 527 20, 260 4, 039 26, 664 20, 375 4, 056 25, 802 20, 488 3, 073 25, 973 20, 622 3, 093 24, 555 24, 453 24, 364 24, 380 24, 122 24, 299 24, 431 23, 561 23, 715 35
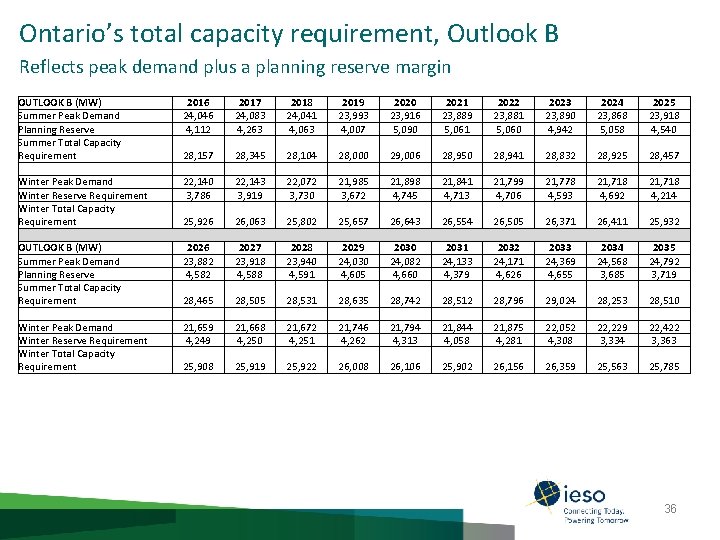
Ontario’s total capacity requirement, Outlook B Reflects peak demand plus a planning reserve margin OUTLOOK B (MW) Summer Peak Demand Planning Reserve Summer Total Capacity Requirement Winter Peak Demand Winter Reserve Requirement Winter Total Capacity Requirement 2016 24, 046 4, 112 2017 24, 083 4, 263 2018 24, 041 4, 063 2019 23, 993 4, 007 2020 23, 916 5, 090 2021 23, 889 5, 061 2022 23, 881 5, 060 2023 23, 890 4, 942 2024 23, 868 5, 058 2025 23, 918 4, 540 28, 157 22, 140 3, 786 28, 345 22, 143 3, 919 28, 104 22, 072 3, 730 28, 000 21, 985 3, 672 29, 006 21, 898 4, 745 28, 950 21, 841 4, 713 28, 941 21, 799 4, 706 28, 832 21, 778 4, 593 28, 925 21, 718 4, 692 28, 457 21, 718 4, 214 25, 926 26, 063 25, 802 25, 657 26, 643 26, 554 26, 505 26, 371 26, 411 25, 932 OUTLOOK B (MW) Summer Peak Demand Planning Reserve Summer Total Capacity Requirement Winter Peak Demand Winter Reserve Requirement Winter Total Capacity Requirement 2026 23, 882 4, 582 2027 23, 918 4, 588 2028 23, 940 4, 591 2029 24, 030 4, 605 2030 24, 082 4, 660 2031 24, 133 4, 379 2032 24, 171 4, 626 2033 24, 369 4, 655 2034 24, 568 3, 685 2035 24, 792 3, 719 28, 465 21, 659 4, 249 28, 505 21, 668 4, 250 28, 531 21, 672 4, 251 28, 635 21, 746 4, 262 28, 742 21, 794 4, 313 28, 512 21, 844 4, 058 28, 796 21, 875 4, 281 29, 024 22, 052 4, 308 28, 253 22, 229 3, 334 28, 510 22, 422 3, 363 25, 908 25, 919 25, 922 26, 008 26, 106 25, 902 26, 156 26, 359 25, 563 25, 785 36
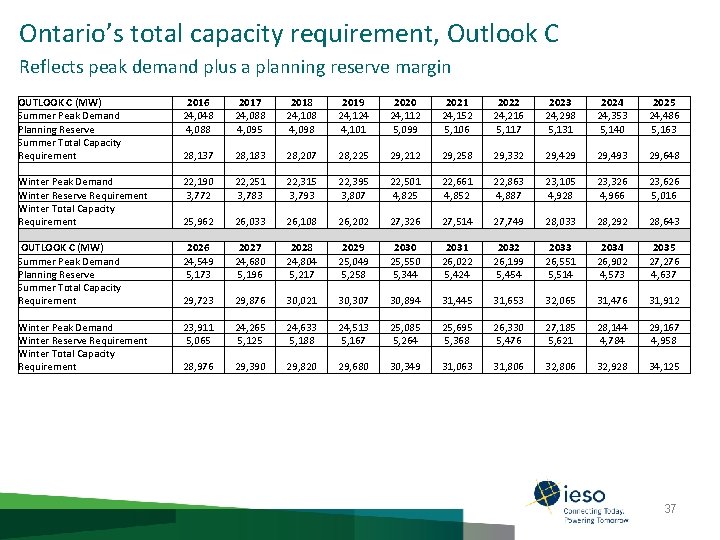
Ontario’s total capacity requirement, Outlook C Reflects peak demand plus a planning reserve margin OUTLOOK C (MW) Summer Peak Demand Planning Reserve Summer Total Capacity Requirement Winter Peak Demand Winter Reserve Requirement Winter Total Capacity Requirement 2016 24, 048 4, 088 2017 24, 088 4, 095 2018 24, 108 4, 098 2019 24, 124 4, 101 2020 24, 112 5, 099 2021 24, 152 5, 106 2022 24, 216 5, 117 2023 24, 298 5, 131 2024 24, 353 5, 140 2025 24, 486 5, 163 28, 137 22, 190 3, 772 28, 183 22, 251 3, 783 28, 207 22, 315 3, 793 28, 225 22, 395 3, 807 29, 212 22, 501 4, 825 29, 258 22, 661 4, 852 29, 332 22, 863 4, 887 29, 429 23, 105 4, 928 29, 493 23, 326 4, 966 29, 648 23, 626 5, 016 25, 962 26, 033 26, 108 26, 202 27, 326 27, 514 27, 749 28, 033 28, 292 28, 643 OUTLOOK C (MW) Summer Peak Demand Planning Reserve Summer Total Capacity Requirement Winter Peak Demand Winter Reserve Requirement Winter Total Capacity Requirement 2026 24, 549 5, 173 2027 24, 680 5, 196 2028 24, 804 5, 217 2029 25, 049 5, 258 2030 25, 550 5, 344 2031 26, 022 5, 424 2032 26, 199 5, 454 2033 26, 551 5, 514 2034 26, 902 4, 573 2035 27, 276 4, 637 29, 723 23, 911 5, 065 29, 876 24, 265 5, 125 30, 021 24, 633 5, 188 30, 307 24, 513 5, 167 30, 894 25, 085 5, 264 31, 445 25, 695 5, 368 31, 653 26, 330 5, 476 32, 065 27, 185 5, 621 31, 476 28, 144 4, 784 31, 912 29, 167 4, 958 28, 976 29, 390 29, 820 29, 680 30, 349 31, 063 31, 806 32, 928 34, 125 37
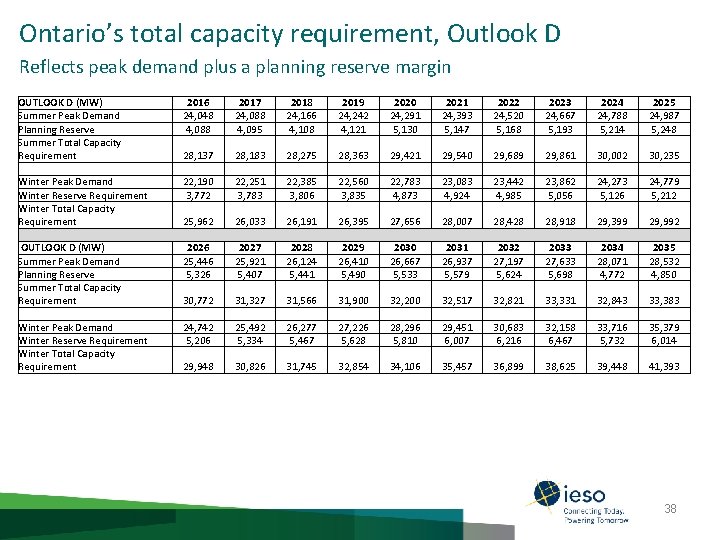
Ontario’s total capacity requirement, Outlook D Reflects peak demand plus a planning reserve margin OUTLOOK D (MW) Summer Peak Demand Planning Reserve Summer Total Capacity Requirement Winter Peak Demand Winter Reserve Requirement Winter Total Capacity Requirement 2016 24, 048 4, 088 2017 24, 088 4, 095 2018 24, 166 4, 108 2019 24, 242 4, 121 2020 24, 291 5, 130 2021 24, 393 5, 147 2022 24, 520 5, 168 2023 24, 667 5, 193 2024 24, 788 5, 214 2025 24, 987 5, 248 28, 137 22, 190 3, 772 28, 183 22, 251 3, 783 28, 275 22, 385 3, 806 28, 363 22, 560 3, 835 29, 421 22, 783 4, 873 29, 540 23, 083 4, 924 29, 689 23, 442 4, 985 29, 861 23, 862 5, 056 30, 002 24, 273 5, 126 30, 235 24, 779 5, 212 25, 962 26, 033 26, 191 26, 395 27, 656 28, 007 28, 428 28, 918 29, 399 29, 992 OUTLOOK D (MW) Summer Peak Demand Planning Reserve Summer Total Capacity Requirement Winter Peak Demand Winter Reserve Requirement Winter Total Capacity Requirement 2026 25, 446 5, 326 2027 25, 921 5, 407 2028 26, 124 5, 441 2029 26, 410 5, 490 2030 26, 667 5, 533 2031 26, 937 5, 579 2032 27, 197 5, 624 2033 27, 633 5, 698 2034 28, 071 4, 772 2035 28, 532 4, 850 30, 772 24, 742 5, 206 31, 327 25, 492 5, 334 31, 566 26, 277 5, 467 31, 900 27, 226 5, 628 32, 200 28, 296 5, 810 32, 517 29, 451 6, 007 32, 821 30, 683 6, 216 33, 331 32, 158 6, 467 32, 843 33, 716 5, 732 33, 383 35, 379 6, 014 29, 948 30, 826 31, 745 32, 854 34, 106 35, 457 36, 899 38, 625 39, 448 41, 393 38
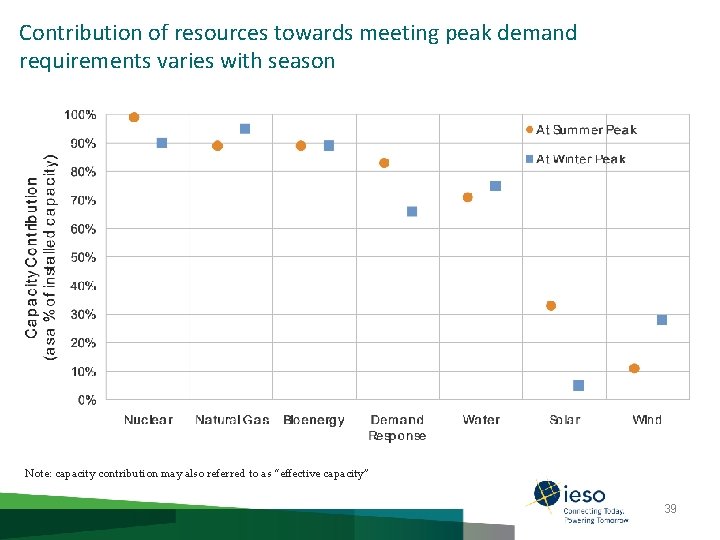
Contribution of resources towards meeting peak demand requirements varies with season Note: capacity contribution may also referred to as “effective capacity” 39
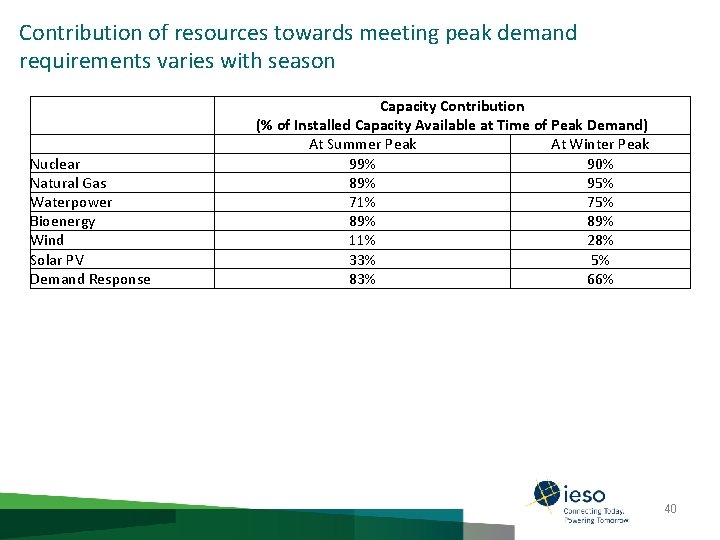
Contribution of resources towards meeting peak demand requirements varies with season Nuclear Natural Gas Waterpower Bioenergy Wind Solar PV Demand Response Capacity Contribution (% of Installed Capacity Available at Time of Peak Demand) At Summer Peak At Winter Peak 99% 90% 89% 95% 71% 75% 89% 11% 28% 33% 5% 83% 66% 40
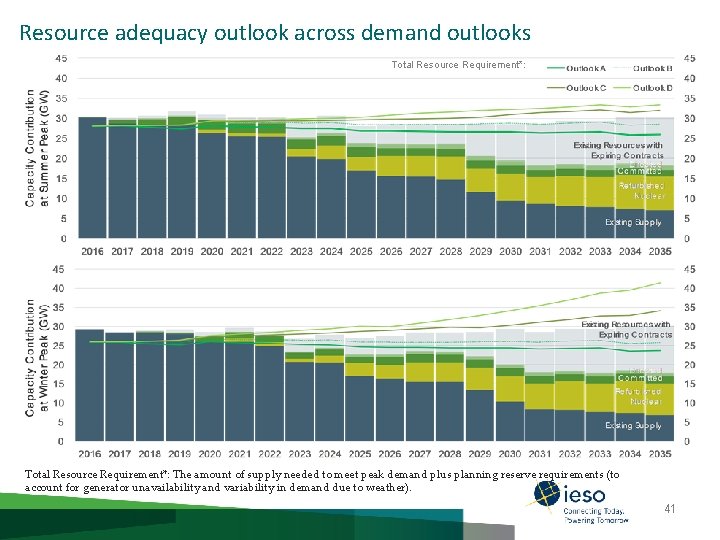
Resource adequacy outlook across demand outlooks Total Resource Requirement*: The amount of supply needed to meet peak demand plus planning reserve requirements (to account for generator unavailability and variability in demand due to weather). 41
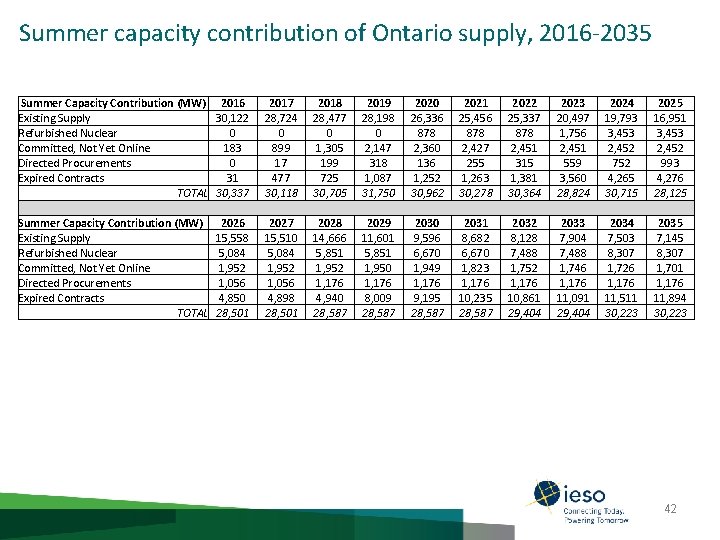
Summer capacity contribution of Ontario supply, 2016 -2035 Summer Capacity Contribution (MW) 2016 Existing Supply 30, 122 Refurbished Nuclear 0 Committed, Not Yet Online 183 Directed Procurements 0 Expired Contracts 31 TOTAL 30, 337 2017 28, 724 0 899 17 477 30, 118 2018 28, 477 0 1, 305 199 725 30, 705 2019 28, 198 0 2, 147 318 1, 087 31, 750 2020 26, 336 878 2, 360 136 1, 252 30, 962 2021 25, 456 878 2, 427 255 1, 263 30, 278 2022 25, 337 878 2, 451 315 1, 381 30, 364 2023 20, 497 1, 756 2, 451 559 3, 560 28, 824 2024 19, 793 3, 453 2, 452 752 4, 265 30, 715 2025 16, 951 3, 453 2, 452 993 4, 276 28, 125 Summer Capacity Contribution (MW) Existing Supply Refurbished Nuclear Committed, Not Yet Online Directed Procurements Expired Contracts TOTAL 2027 15, 510 5, 084 1, 952 1, 056 4, 898 28, 501 2028 14, 666 5, 851 1, 952 1, 176 4, 940 28, 587 2029 11, 601 5, 851 1, 950 1, 176 8, 009 28, 587 2030 9, 596 6, 670 1, 949 1, 176 9, 195 28, 587 2031 8, 682 6, 670 1, 823 1, 176 10, 235 28, 587 2032 8, 128 7, 488 1, 752 1, 176 10, 861 29, 404 2033 7, 904 7, 488 1, 746 1, 176 11, 091 29, 404 2034 7, 503 8, 307 1, 726 1, 176 11, 511 30, 223 2035 7, 145 8, 307 1, 701 1, 176 11, 894 30, 223 2026 15, 558 5, 084 1, 952 1, 056 4, 850 28, 501 42
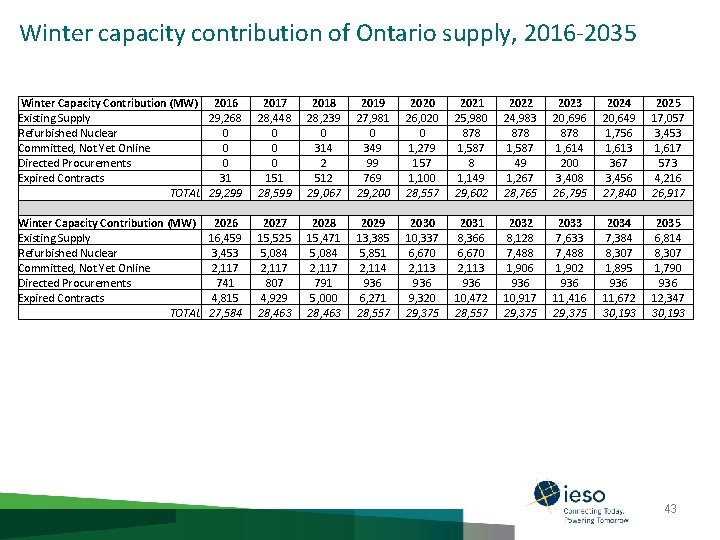
Winter capacity contribution of Ontario supply, 2016 -2035 Winter Capacity Contribution (MW) 2016 Existing Supply 29, 268 Refurbished Nuclear 0 Committed, Not Yet Online 0 Directed Procurements 0 Expired Contracts 31 TOTAL 29, 299 2017 28, 448 0 0 0 151 28, 599 2018 28, 239 0 314 2 512 29, 067 2019 27, 981 0 349 99 769 29, 200 2020 26, 020 0 1, 279 157 1, 100 28, 557 2021 25, 980 878 1, 587 8 1, 149 29, 602 2022 24, 983 878 1, 587 49 1, 267 28, 765 2023 20, 696 878 1, 614 200 3, 408 26, 795 2024 20, 649 1, 756 1, 613 367 3, 456 27, 840 2025 17, 057 3, 453 1, 617 573 4, 216 26, 917 Winter Capacity Contribution (MW) Existing Supply Refurbished Nuclear Committed, Not Yet Online Directed Procurements Expired Contracts TOTAL 2027 15, 525 5, 084 2, 117 807 4, 929 28, 463 2028 15, 471 5, 084 2, 117 791 5, 000 28, 463 2029 13, 385 5, 851 2, 114 936 6, 271 28, 557 2030 10, 337 6, 670 2, 113 936 9, 320 29, 375 2031 8, 366 6, 670 2, 113 936 10, 472 28, 557 2032 8, 128 7, 488 1, 906 936 10, 917 29, 375 2033 7, 633 7, 488 1, 902 936 11, 416 29, 375 2034 7, 384 8, 307 1, 895 936 11, 672 30, 193 2035 6, 814 8, 307 1, 790 936 12, 347 30, 193 2026 16, 459 3, 453 2, 117 741 4, 815 27, 584 43
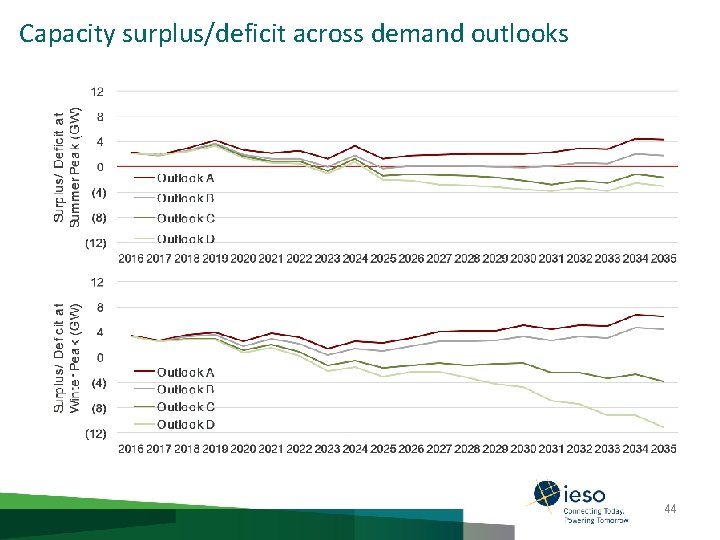
Capacity surplus/deficit across demand outlooks 44
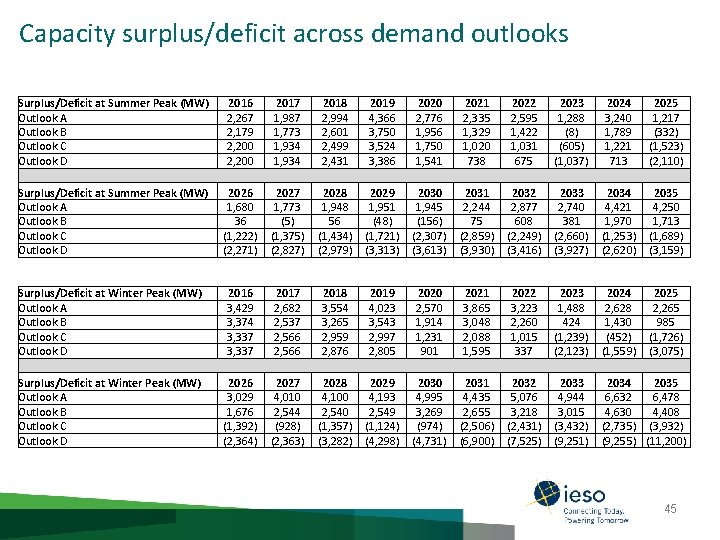
Capacity surplus/deficit across demand outlooks Surplus/Deficit at Summer Peak (MW) Outlook A Outlook B Outlook C Outlook D 2016 2, 267 2, 179 2, 200 2017 1, 987 1, 773 1, 934 2018 2, 994 2, 601 2, 499 2, 431 2019 4, 366 3, 750 3, 524 3, 386 2020 2, 776 1, 956 1, 750 1, 541 2021 2, 335 1, 329 1, 020 738 2022 2, 595 1, 422 1, 031 675 2023 1, 288 (8) (605) (1, 037) 2024 3, 240 1, 789 1, 221 713 2025 1, 217 (332) (1, 523) (2, 110) Surplus/Deficit at Summer Peak (MW) Outlook A Outlook B Outlook C Outlook D 2026 1, 680 36 (1, 222) (2, 271) 2027 1, 773 (5) (1, 375) (2, 827) 2028 1, 948 56 (1, 434) (2, 979) 2029 1, 951 (48) (1, 721) (3, 313) 2030 1, 945 (156) (2, 307) (3, 613) 2031 2, 244 75 (2, 859) (3, 930) 2032 2, 877 608 (2, 249) (3, 416) 2033 2, 740 381 (2, 660) (3, 927) 2034 4, 421 1, 970 (1, 253) (2, 620) 2035 4, 250 1, 713 (1, 689) (3, 159) Surplus/Deficit at Winter Peak (MW) Outlook A Outlook B Outlook C Outlook D 2016 3, 429 3, 374 3, 337 2017 2, 682 2, 537 2, 566 2018 3, 554 3, 265 2, 959 2, 876 2019 4, 023 3, 543 2, 997 2, 805 2020 2, 570 1, 914 1, 231 901 2021 3, 865 3, 048 2, 088 1, 595 2022 3, 223 2, 260 1, 015 337 2023 1, 488 424 (1, 239) (2, 123) 2024 2, 628 1, 430 (452) (1, 559) 2025 2, 265 985 (1, 726) (3, 075) Surplus/Deficit at Winter Peak (MW) Outlook A Outlook B Outlook C Outlook D 2026 3, 029 1, 676 (1, 392) (2, 364) 2027 4, 010 2, 544 (928) (2, 363) 2028 4, 100 2, 540 (1, 357) (3, 282) 2029 4, 193 2, 549 (1, 124) (4, 298) 2030 4, 995 3, 269 (974) (4, 731) 2031 4, 435 2, 655 (2, 506) (6, 900) 2032 5, 076 3, 218 (2, 431) (7, 525) 2033 4, 944 3, 015 (3, 432) (9, 251) 2034 2035 6, 632 6, 478 4, 630 4, 408 (2, 735) (3, 932) (9, 255) (11, 200) 45
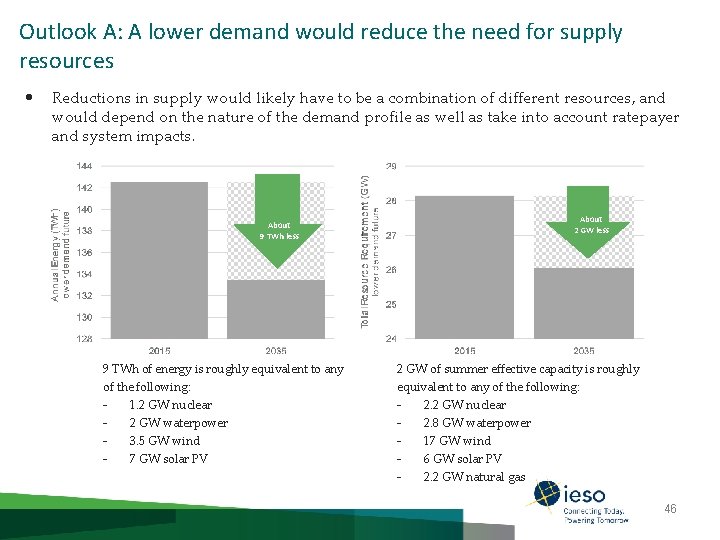
Outlook A: A lower demand would reduce the need for supply resources • Reductions in supply would likely have to be a combination of different resources, and would depend on the nature of the demand profile as well as take into account ratepayer and system impacts. About 9 TWh less About 2 GW less 9 TWh of energy is roughly equivalent to any 2 GW of summer effective capacity is roughly of the following: 1. 2 GW nuclear 2 GW waterpower 3. 5 GW wind 7 GW solar PV equivalent to any of the following: 2. 2 GW nuclear 2. 8 GW waterpower 17 GW wind 6 GW solar PV 2. 2 GW natural gas 46
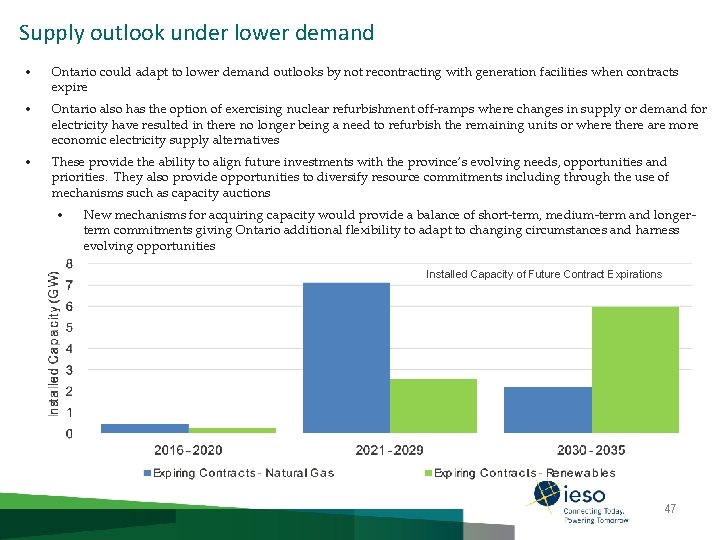
Supply outlook under lower demand • Ontario could adapt to lower demand outlooks by not recontracting with generation facilities when contracts expire • Ontario also has the option of exercising nuclear refurbishment off-ramps where changes in supply or demand for electricity have resulted in there no longer being a need to refurbish the remaining units or where there are more economic electricity supply alternatives • These provide the ability to align future investments with the province’s evolving needs, opportunities and priorities. They also provide opportunities to diversify resource commitments including through the use of mechanisms such as capacity auctions • New mechanisms for acquiring capacity would provide a balance of short-term, medium-term and longerterm commitments giving Ontario additional flexibility to adapt to changing circumstances and harness evolving opportunities Installed Capacity of Future Contract Expirations 47
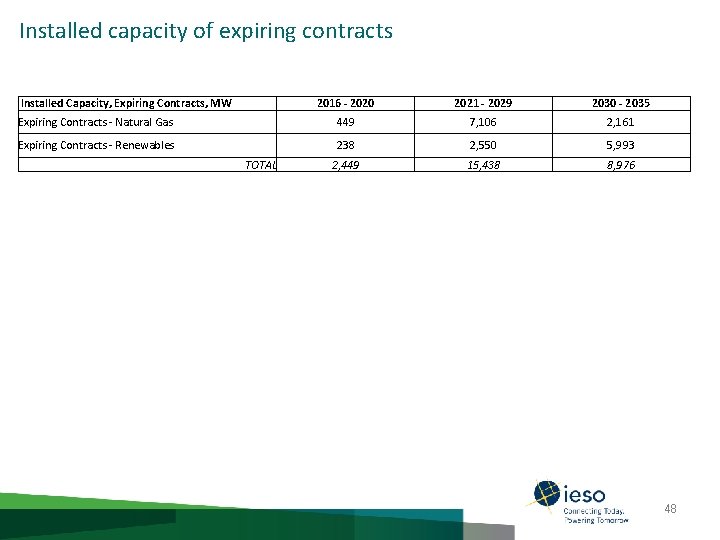
Installed capacity of expiring contracts Installed Capacity, Expiring Contracts, MW 2016 - 2020 2021 - 2029 2030 - 2035 Expiring Contracts - Natural Gas 449 7, 106 2, 161 Expiring Contracts - Renewables 238 2, 550 5, 993 2, 449 15, 438 8, 976 TOTAL 48
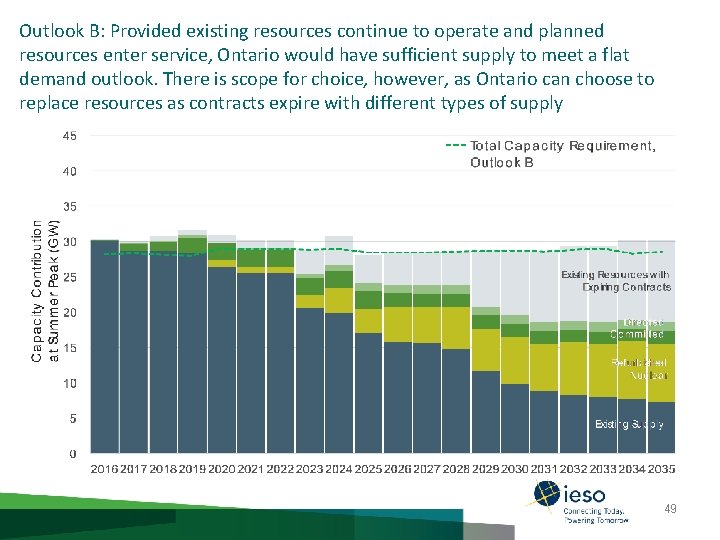
Outlook B: Provided existing resources continue to operate and planned resources enter service, Ontario would have sufficient supply to meet a flat demand outlook. There is scope for choice, however, as Ontario can choose to replace resources as contracts expire with different types of supply 49
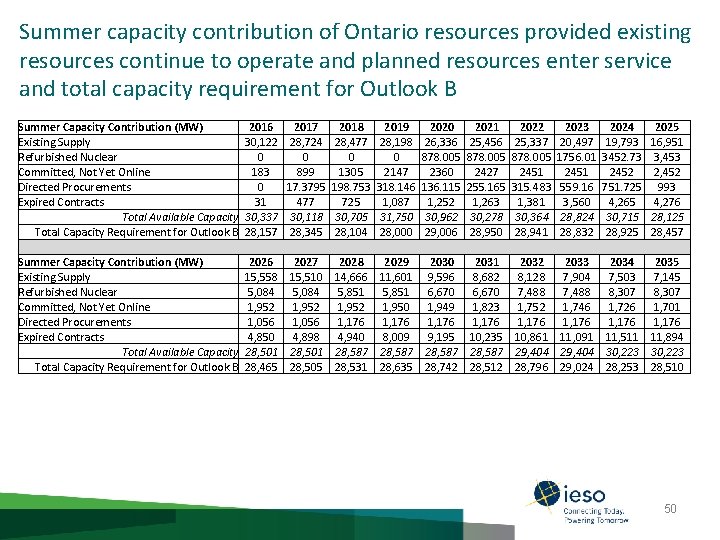
Summer capacity contribution of Ontario resources provided existing resources continue to operate and planned resources enter service and total capacity requirement for Outlook B Summer Capacity Contribution (MW) Existing Supply Refurbished Nuclear Committed, Not Yet Online Directed Procurements Expired Contracts Total Available Capacity Total Capacity Requirement for Outlook B 2016 30, 122 0 183 0 31 30, 337 28, 157 2017 28, 724 0 899 17. 3795 477 30, 118 28, 345 2018 28, 477 0 1305 198. 753 725 30, 705 28, 104 2019 28, 198 0 2147 318. 146 1, 087 31, 750 28, 000 2020 26, 336 878. 005 2360 136. 115 1, 252 30, 962 29, 006 2021 25, 456 878. 005 2427 255. 165 1, 263 30, 278 28, 950 2022 25, 337 878. 005 2451 315. 483 1, 381 30, 364 28, 941 2023 20, 497 1756. 01 2451 559. 16 3, 560 28, 824 28, 832 2024 19, 793 3452. 73 2452 751. 725 4, 265 30, 715 28, 925 2025 16, 951 3, 453 2, 452 993 4, 276 28, 125 28, 457 Summer Capacity Contribution (MW) Existing Supply Refurbished Nuclear Committed, Not Yet Online Directed Procurements Expired Contracts Total Available Capacity Total Capacity Requirement for Outlook B 2026 2027 2028 2029 2030 2031 2032 2033 2034 2035 15, 558 15, 510 14, 666 11, 601 9, 596 8, 682 8, 128 7, 904 7, 503 7, 145 5, 084 5, 851 6, 670 7, 488 8, 307 1, 952 1, 950 1, 949 1, 823 1, 752 1, 746 1, 726 1, 701 1, 056 1, 176 1, 176 4, 850 4, 898 4, 940 8, 009 9, 195 10, 235 10, 861 11, 091 11, 511 11, 894 28, 501 28, 587 29, 404 30, 223 28, 465 28, 505 28, 531 28, 635 28, 742 28, 512 28, 796 29, 024 28, 253 28, 510 50
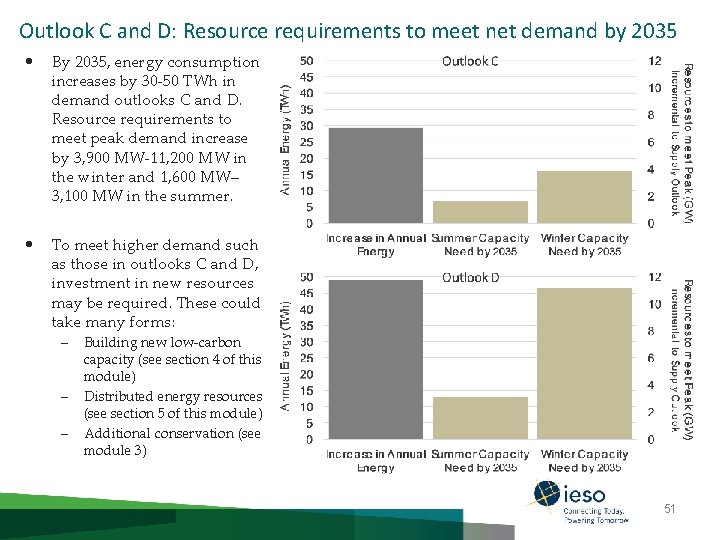
Outlook C and D: Resource requirements to meet net demand by 2035 • By 2035, energy consumption increases by 30 -50 TWh in demand outlooks C and D. Resource requirements to meet peak demand increase by 3, 900 MW-11, 200 MW in the winter and 1, 600 MW– 3, 100 MW in the summer. • To meet higher demand such as those in outlooks C and D, investment in new resources may be required. These could take many forms: – – – Building new low-carbon capacity (see section 4 of this module) Distributed energy resources (see section 5 of this module) Additional conservation (see module 3) 51
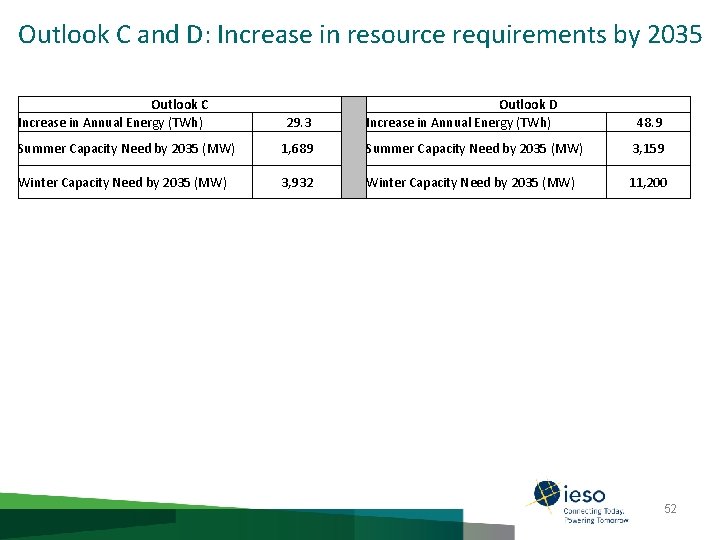
Outlook C and D: Increase in resource requirements by 2035 Outlook C Increase in Annual Energy (TWh) 29. 3 Outlook D Increase in Annual Energy (TWh) 48. 9 Summer Capacity Need by 2035 (MW) 1, 689 Summer Capacity Need by 2035 (MW) 3, 159 Winter Capacity Need by 2035 (MW) 3, 932 Winter Capacity Need by 2035 (MW) 11, 200 52
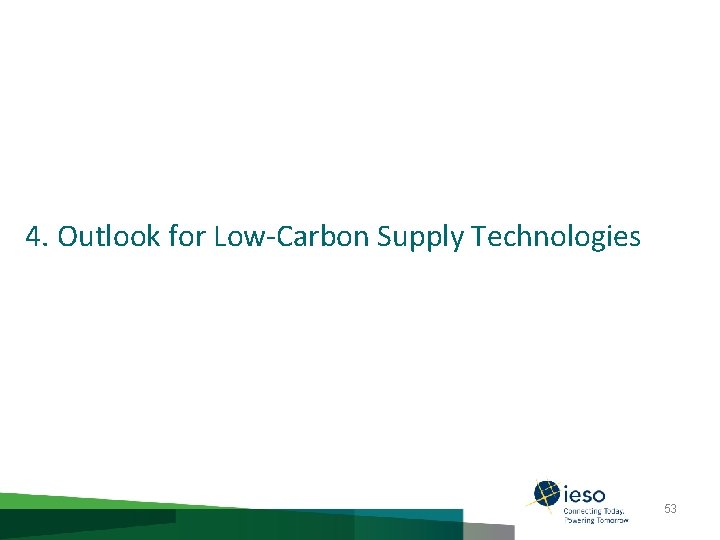
4. Outlook for Low-Carbon Supply Technologies 53
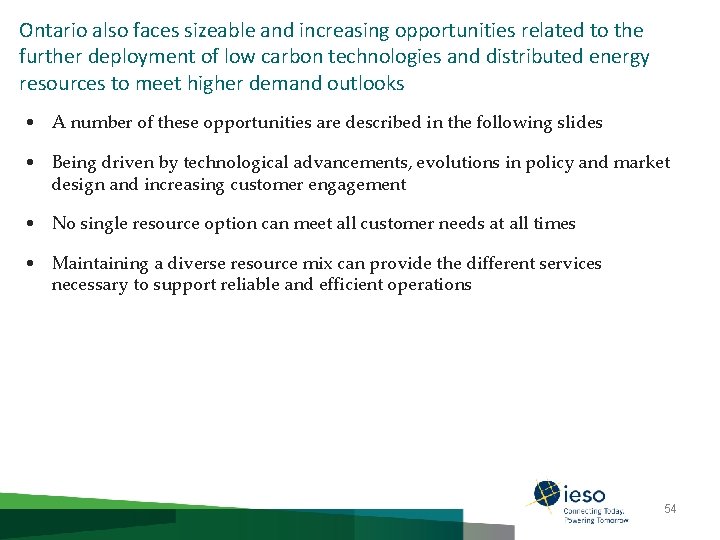
Ontario also faces sizeable and increasing opportunities related to the further deployment of low carbon technologies and distributed energy resources to meet higher demand outlooks • A number of these opportunities are described in the following slides • Being driven by technological advancements, evolutions in policy and market design and increasing customer engagement • No single resource option can meet all customer needs at all times • Maintaining a diverse resource mix can provide the different services necessary to support reliable and efficient operations 54
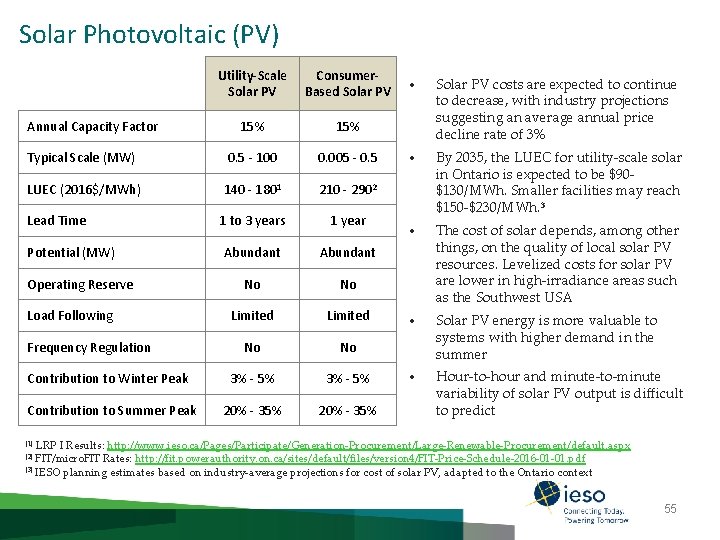
Solar Photovoltaic (PV) Utility-Scale Solar PV Consumer. Based Solar PV 15% Typical Scale (MW) 0. 5 - 100 0. 005 - 0. 5 LUEC (2016$/MWh) 140 - 1801 210 - 2902 Lead Time 1 to 3 years 1 year Abundant No No Limited No No 3% - 5% 20% - 35% Annual Capacity Factor Potential (MW) Operating Reserve Load Following Frequency Regulation Contribution to Winter Peak Contribution to Summer Peak • Solar PV costs are expected to continue to decrease, with industry projections suggesting an average annual price decline rate of 3% • By 2035, the LUEC for utility-scale solar in Ontario is expected to be $90$130/MWh. Smaller facilities may reach $150 -$230/MWh. 3 • The cost of solar depends, among other things, on the quality of local solar PV resources. Levelized costs for solar PV are lower in high-irradiance areas such as the Southwest USA • Solar PV energy is more valuable to systems with higher demand in the summer • Hour-to-hour and minute-to-minute variability of solar PV output is difficult to predict LRP I Results: http: //www. ieso. ca/Pages/Participate/Generation-Procurement/Large-Renewable-Procurement/default. aspx Rates: http: //fit. powerauthority. on. ca/sites/default/files/version 4/FIT-Price-Schedule-2016 -01 -01. pdf [3] IESO planning estimates based on industry-average projections for cost of solar PV, adapted to the Ontario context [1] [2] FIT/micro. FIT 55
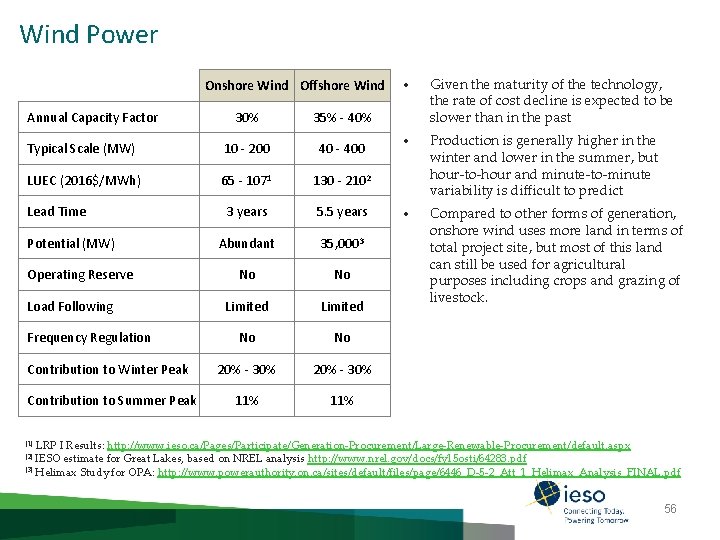
Wind Power Onshore Wind Offshore Wind Annual Capacity Factor 30% 35% - 40% Typical Scale (MW) 10 - 200 40 - 400 LUEC (2016$/MWh) 65 - 1071 130 - 2102 3 years 5. 5 years Abundant 35, 0003 No No Limited No No 20% - 30% 11% Lead Time Potential (MW) Operating Reserve Load Following Frequency Regulation Contribution to Winter Peak Contribution to Summer Peak • Given the maturity of the technology, the rate of cost decline is expected to be slower than in the past • Production is generally higher in the winter and lower in the summer, but hour-to-hour and minute-to-minute variability is difficult to predict • Compared to other forms of generation, onshore wind uses more land in terms of total project site, but most of this land can still be used for agricultural purposes including crops and grazing of livestock. LRP I Results: http: //www. ieso. ca/Pages/Participate/Generation-Procurement/Large-Renewable-Procurement/default. aspx estimate for Great Lakes, based on NREL analysis http: //www. nrel. gov/docs/fy 15 osti/64283. pdf [3] Helimax Study for OPA: http: //www. powerauthority. on. ca/sites/default/files/page/6446_D-5 -2_Att_1_Helimax_Analysis_FINAL. pdf [1] [2] IESO 56
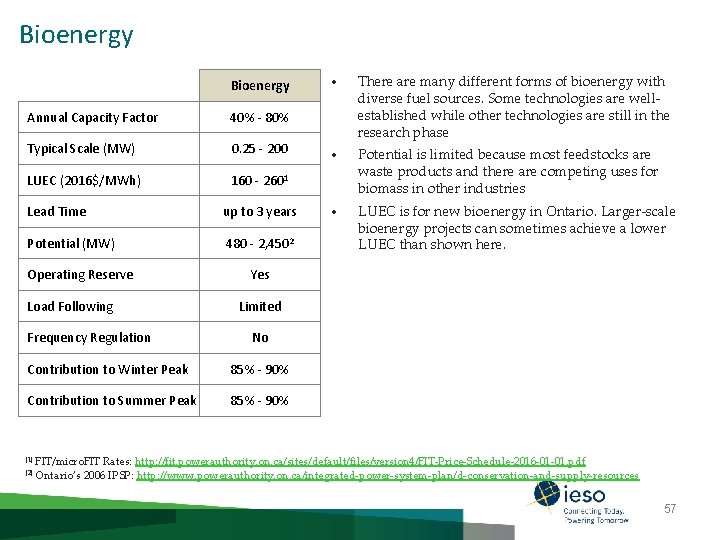
Bioenergy Annual Capacity Factor 40% - 80% Typical Scale (MW) 0. 25 - 200 LUEC (2016$/MWh) 160 - 2601 Lead Time up to 3 years Potential (MW) 480 - 2, 4502 Operating Reserve Load Following Frequency Regulation • There are many different forms of bioenergy with diverse fuel sources. Some technologies are wellestablished while other technologies are still in the research phase • Potential is limited because most feedstocks are waste products and there are competing uses for biomass in other industries • LUEC is for new bioenergy in Ontario. Larger-scale bioenergy projects can sometimes achieve a lower LUEC than shown here. Yes Limited No Contribution to Winter Peak 85% - 90% Contribution to Summer Peak 85% - 90% [1] FIT/micro. FIT [2] Ontario’s Rates: http: //fit. powerauthority. on. ca/sites/default/files/version 4/FIT-Price-Schedule-2016 -01 -01. pdf 2006 IPSP: http: //www. powerauthority. on. ca/integrated-power-system-plan/d-conservation-and-supply-resources 57
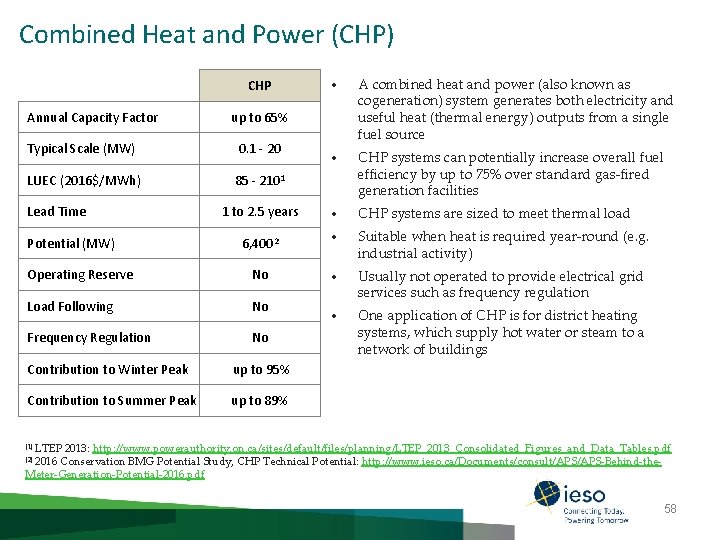
Combined Heat and Power (CHP) • A combined heat and power (also known as cogeneration) system generates both electricity and useful heat (thermal energy) outputs from a single fuel source • CHP systems can potentially increase overall fuel efficiency by up to 75% over standard gas-fired generation facilities 1 to 2. 5 years • CHP systems are sized to meet thermal load 6, 4002 • Suitable when heat is required year-round (e. g. industrial activity) Operating Reserve No • Load Following No Usually not operated to provide electrical grid services such as frequency regulation • Frequency Regulation No One application of CHP is for district heating systems, which supply hot water or steam to a network of buildings CHP Annual Capacity Factor up to 65% Typical Scale (MW) 0. 1 - 20 LUEC (2016$/MWh) 85 - 2101 Lead Time Potential (MW) Contribution to Winter Peak up to 95% Contribution to Summer Peak up to 89% [1] LTEP 2013: http: //www. powerauthority. on. ca/sites/default/files/planning/LTEP_2013_Consolidated_Figures_and_Data_Tables. pdf Conservation BMG Potential Study, CHP Technical Potential: http: //www. ieso. ca/Documents/consult/APS-Behind-the. Meter-Generation-Potential-2016. pdf [2] 2016 58
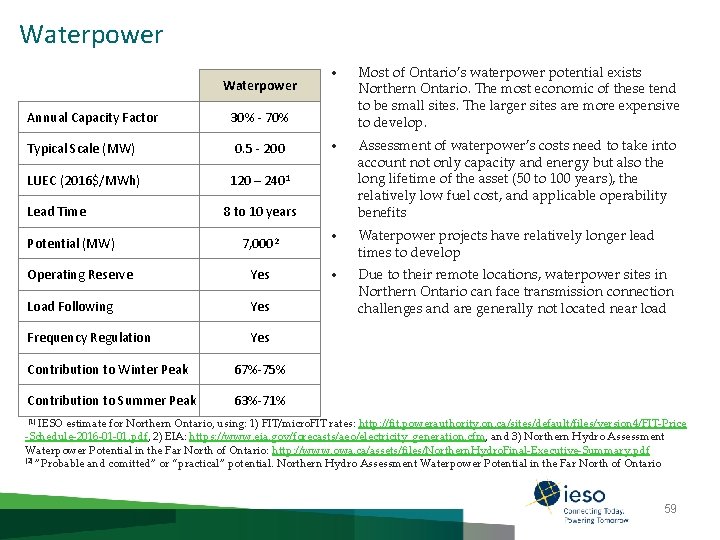
Waterpower Annual Capacity Factor 0. 5 - 200 LUEC (2016$/MWh) 120 – 2401 Potential (MW) Most of Ontario’s waterpower potential exists Northern Ontario. The most economic of these tend to be small sites. The larger sites are more expensive to develop. • Assessment of waterpower’s costs need to take into account not only capacity and energy but also the long lifetime of the asset (50 to 100 years), the relatively low fuel cost, and applicable operability benefits • Waterpower projects have relatively longer lead times to develop • Due to their remote locations, waterpower sites in Northern Ontario can face transmission connection challenges and are generally not located near load 30% - 70% Typical Scale (MW) Lead Time • 8 to 10 years 7, 0002 Operating Reserve Yes Load Following Yes Frequency Regulation Yes Contribution to Winter Peak 67%-75% Contribution to Summer Peak 63%-71% [1] IESO estimate for Northern Ontario, using: 1) FIT/micro. FIT rates: http: //fit. powerauthority. on. ca/sites/default/files/version 4/FIT-Price -Schedule-2016 -01 -01. pdf, 2) EIA: https: //www. eia. gov/forecasts/aeo/electricity_generation. cfm, and 3) Northern Hydro Assessment Waterpower Potential in the Far North of Ontario: http: //www. owa. ca/assets/files/Northern. Hydro. Final-Executive-Summary. pdf [2] ”Probable and comitted” or “practical” potential. Northern Hydro Assessment Waterpower Potential in the Far North of Ontario 59
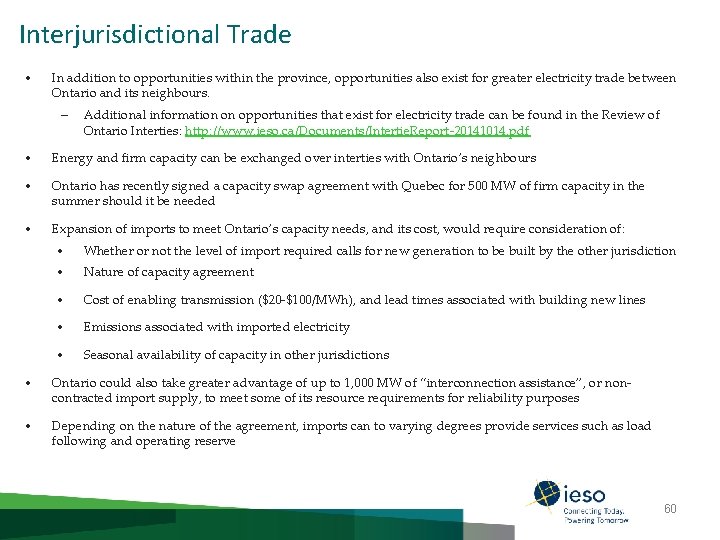
Interjurisdictional Trade • In addition to opportunities within the province, opportunities also exist for greater electricity trade between Ontario and its neighbours. – Additional information on opportunities that exist for electricity trade can be found in the Review of Ontario Interties: http: //www. ieso. ca/Documents/Intertie. Report-20141014. pdf • Energy and firm capacity can be exchanged over interties with Ontario’s neighbours • Ontario has recently signed a capacity swap agreement with Quebec for 500 MW of firm capacity in the summer should it be needed • Expansion of imports to meet Ontario’s capacity needs, and its cost, would require consideration of: • Whether or not the level of import required calls for new generation to be built by the other jurisdiction • Nature of capacity agreement • Cost of enabling transmission ($20 -$100/MWh), and lead times associated with building new lines • Emissions associated with imported electricity • Seasonal availability of capacity in other jurisdictions • Ontario could also take greater advantage of up to 1, 000 MW of “interconnection assistance”, or noncontracted import supply, to meet some of its resource requirements for reliability purposes • Depending on the nature of the agreement, imports can to varying degrees provide services such as load following and operating reserve 60
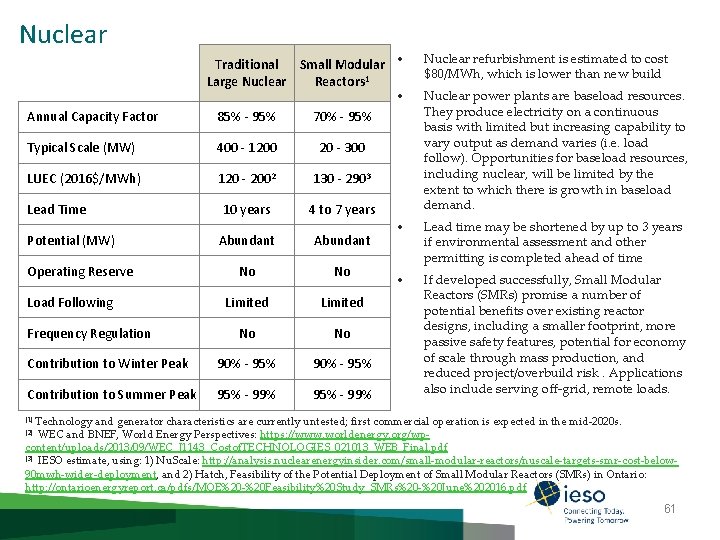
Nuclear Traditional Small Modular • Large Nuclear Reactors 1 • Annual Capacity Factor 85% - 95% 70% - 95% Typical Scale (MW) 400 - 1200 20 - 300 LUEC (2016$/MWh) 120 - 2002 130 - 2903 10 years 4 to 7 years Abundant No No Limited No No Contribution to Winter Peak 90% - 95% Contribution to Summer Peak 95% - 99% Lead Time Potential (MW) Operating Reserve Load Following Frequency Regulation Nuclear refurbishment is estimated to cost $80/MWh, which is lower than new build Nuclear power plants are baseload resources. They produce electricity on a continuous basis with limited but increasing capability to vary output as demand varies (i. e. load follow). Opportunities for baseload resources, including nuclear, will be limited by the extent to which there is growth in baseload demand. • Lead time may be shortened by up to 3 years if environmental assessment and other permitting is completed ahead of time • If developed successfully, Small Modular Reactors (SMRs) promise a number of potential benefits over existing reactor designs, including a smaller footprint, more passive safety features, potential for economy of scale through mass production, and reduced project/overbuild risk. Applications also include serving off-grid, remote loads. [1] Technology and generator characteristics are currently untested; first commercial operation is expected in the mid-2020 s. WEC and BNEF, World Energy Perspectives: https: //www. worldenergy. org/wpcontent/uploads/2013/09/WEC_J 1143_Costof. TECHNOLOGIES_021013_WEB_Final. pdf [3] IESO estimate, using: 1) Nu. Scale: http: //analysis. nuclearenergyinsider. com/small-modular-reactors/nuscale-targets-smr-cost-below 90 mwh-wider-deployment, and 2) Hatch, Feasibility of the Potential Deployment of Small Modular Reactors (SMRs) in Ontario: http: //ontarioenergyreport. ca/pdfs/MOE%20 -%20 Feasibility%20 Study_SMRs%20 -%20 June%202016. pdf [2] 61
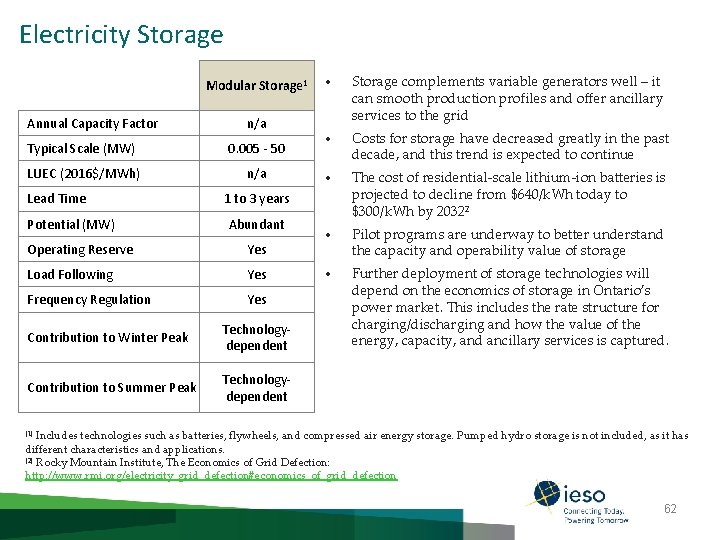
Electricity Storage Modular Storage 1 Annual Capacity Factor Typical Scale (MW) LUEC (2016$/MWh) Lead Time Potential (MW) n/a 0. 005 - 50 n/a • Storage complements variable generators well – it can smooth production profiles and offer ancillary services to the grid • Costs for storage have decreased greatly in the past decade, and this trend is expected to continue • The cost of residential-scale lithium-ion batteries is projected to decline from $640/k. Wh today to $300/k. Wh by 20322 • Pilot programs are underway to better understand the capacity and operability value of storage • Further deployment of storage technologies will depend on the economics of storage in Ontario’s power market. This includes the rate structure for charging/discharging and how the value of the energy, capacity, and ancillary services is captured. 1 to 3 years Abundant Operating Reserve Yes Load Following Yes Frequency Regulation Yes Contribution to Winter Peak Technologydependent Contribution to Summer Peak Technologydependent Includes technologies such as batteries, flywheels, and compressed air energy storage. Pumped hydro storage is not included, as it has different characteristics and applications. [2] Rocky Mountain Institute, The Economics of Grid Defection: http: //www. rmi. org/electricity_grid_defection#economics_of_grid_defection [1] 62
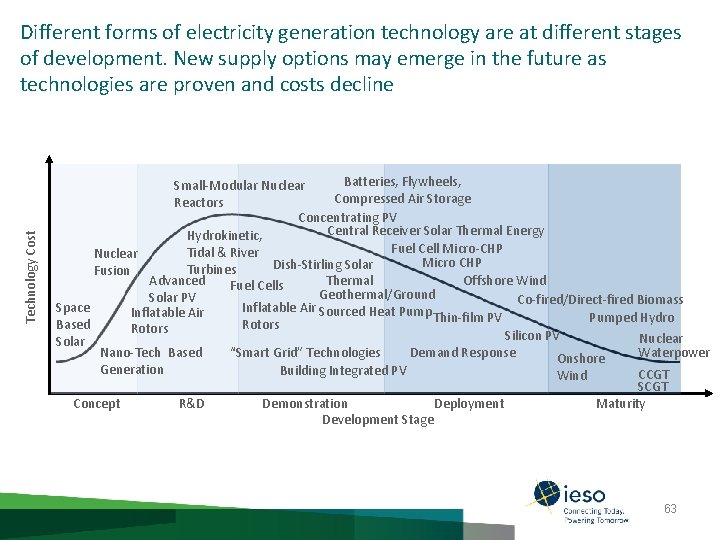
Technology Cost Different forms of electricity generation technology are at different stages of development. New supply options may emerge in the future as technologies are proven and costs decline Batteries, Flywheels, Small-Modular Nuclear Compressed Air Storage Reactors Concentrating PV Central Receiver Solar Thermal Energy Hydrokinetic, Fuel Cell Micro-CHP Tidal & River Nuclear Micro CHP Dish-Stirling Solar Turbines Fusion Advanced Offshore Wind Thermal Fuel Cells Geothermal/Ground Solar PV Co-fired/Direct-fired Biomass Space Inflatable Air Sourced Heat Pump Inflatable Air Thin-film PV Pumped Hydro Based Rotors Silicon PV Nuclear Solar “Smart Grid” Technologies Nano-Tech Based Demand Response Waterpower Onshore Generation Building Integrated PV CCGT Wind SCGT Concept R&D Demonstration Deployment Maturity Development Stage 63
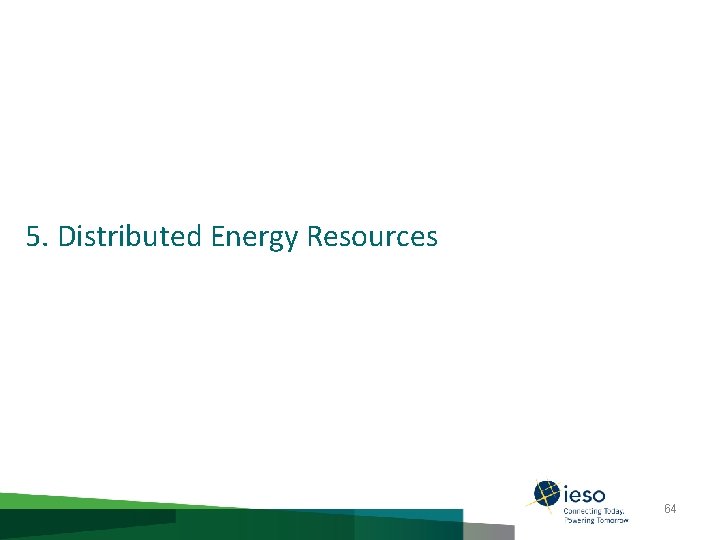
5. Distributed Energy Resources 64
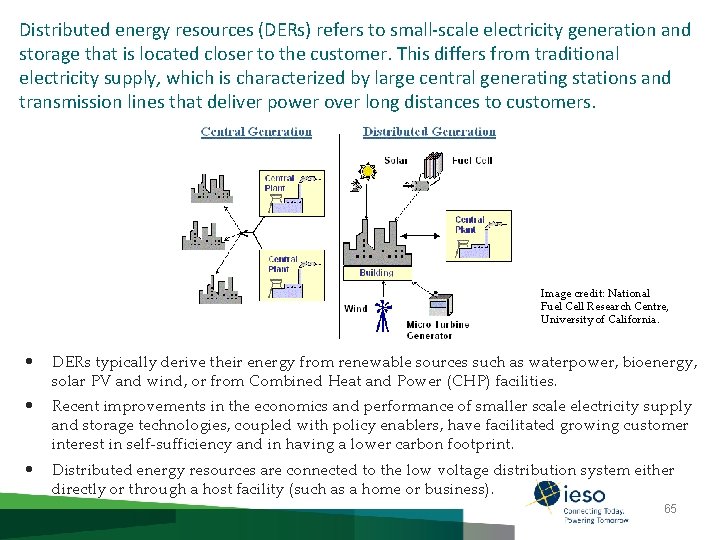
Distributed energy resources (DERs) refers to small-scale electricity generation and storage that is located closer to the customer. This differs from traditional electricity supply, which is characterized by large central generating stations and transmission lines that deliver power over long distances to customers. Image credit: National Fuel Cell Research Centre, University of California. • DERs typically derive their energy from renewable sources such as waterpower, bioenergy, solar PV and wind, or from Combined Heat and Power (CHP) facilities. • Recent improvements in the economics and performance of smaller scale electricity supply and storage technologies, coupled with policy enablers, have facilitated growing customer interest in self-sufficiency and in having a lower carbon footprint. • Distributed energy resources are connected to the low voltage distribution system either directly or through a host facility (such as a home or business). 65
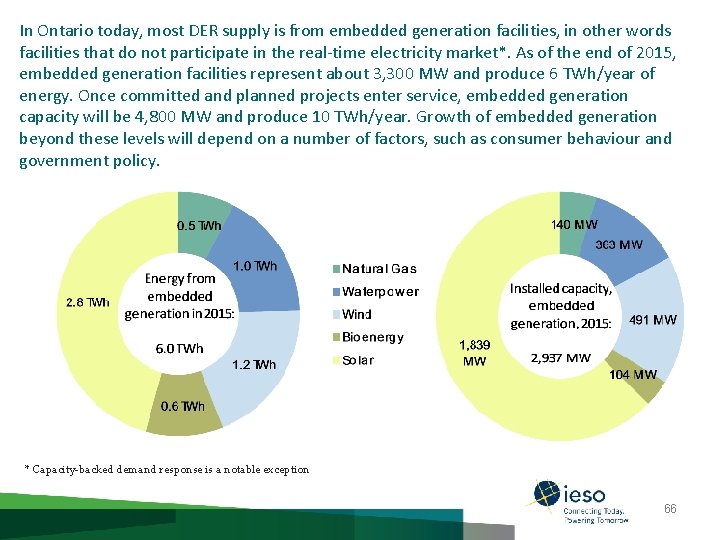
In Ontario today, most DER supply is from embedded generation facilities, in other words facilities that do not participate in the real-time electricity market*. As of the end of 2015, embedded generation facilities represent about 3, 300 MW and produce 6 TWh/year of energy. Once committed and planned projects enter service, embedded generation capacity will be 4, 800 MW and produce 10 TWh/year. Growth of embedded generation beyond these levels will depend on a number of factors, such as consumer behaviour and government policy. * Capacity-backed demand response is a notable exception 66
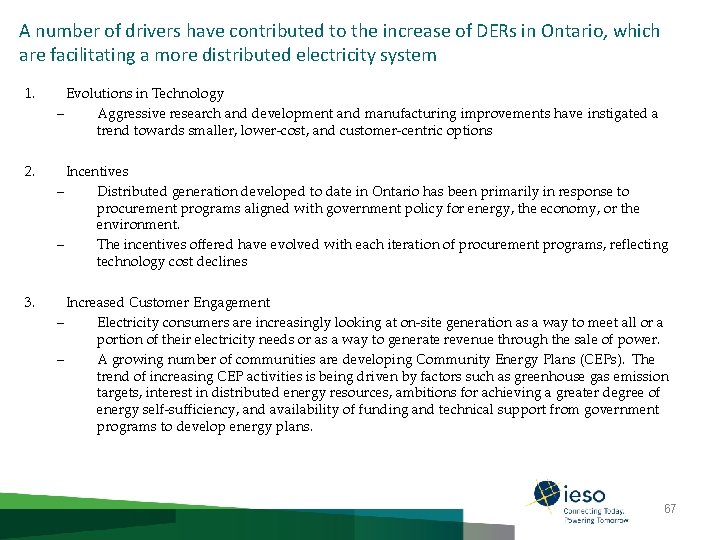
A number of drivers have contributed to the increase of DERs in Ontario, which are facilitating a more distributed electricity system 1. Evolutions in Technology – Aggressive research and development and manufacturing improvements have instigated a trend towards smaller, lower-cost, and customer-centric options 2. Incentives – Distributed generation developed to date in Ontario has been primarily in response to procurement programs aligned with government policy for energy, the economy, or the environment. – The incentives offered have evolved with each iteration of procurement programs, reflecting technology cost declines 3. Increased Customer Engagement – Electricity consumers are increasingly looking at on-site generation as a way to meet all or a portion of their electricity needs or as a way to generate revenue through the sale of power. – A growing number of communities are developing Community Energy Plans (CEPs). The trend of increasing CEP activities is being driven by factors such as greenhouse gas emission targets, interest in distributed energy resources, ambitions for achieving a greater degree of energy self-sufficiency, and availability of funding and technical support from government programs to develop energy plans. 67
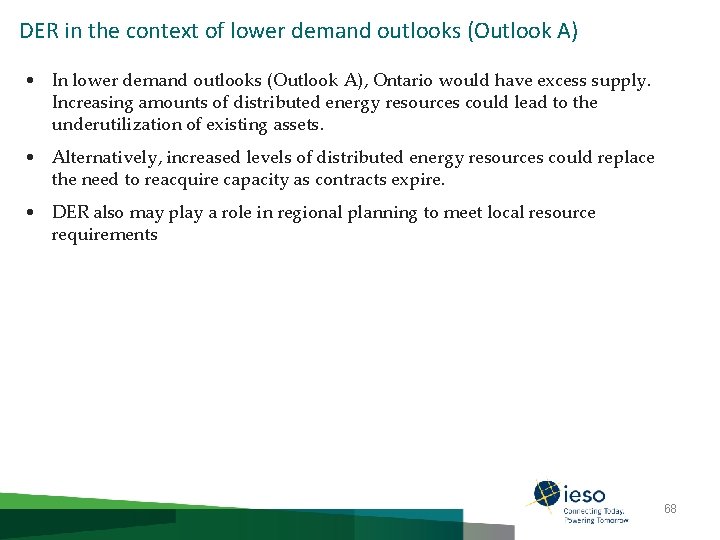
DER in the context of lower demand outlooks (Outlook A) • In lower demand outlooks (Outlook A), Ontario would have excess supply. Increasing amounts of distributed energy resources could lead to the underutilization of existing assets. • Alternatively, increased levels of distributed energy resources could replace the need to reacquire capacity as contracts expire. • DER also may play a role in regional planning to meet local resource requirements 68
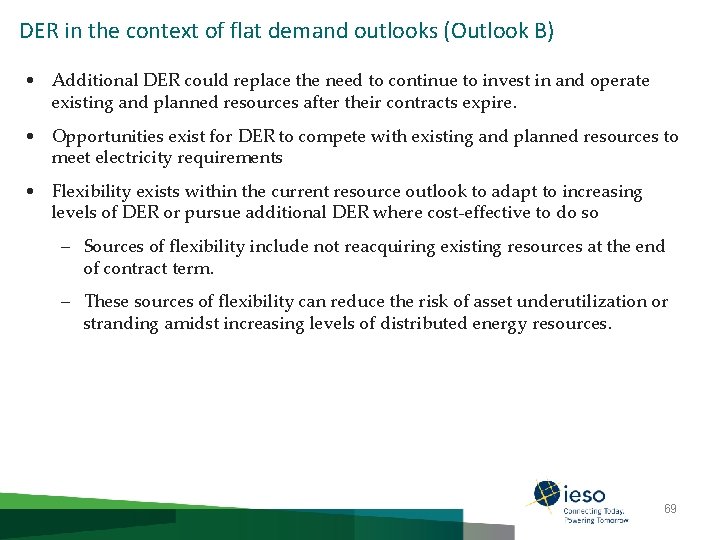
DER in the context of flat demand outlooks (Outlook B) • Additional DER could replace the need to continue to invest in and operate existing and planned resources after their contracts expire. • Opportunities exist for DER to compete with existing and planned resources to meet electricity requirements • Flexibility exists within the current resource outlook to adapt to increasing levels of DER or pursue additional DER where cost-effective to do so – Sources of flexibility include not reacquiring existing resources at the end of contract term. – These sources of flexibility can reduce the risk of asset underutilization or stranding amidst increasing levels of distributed energy resources. 69
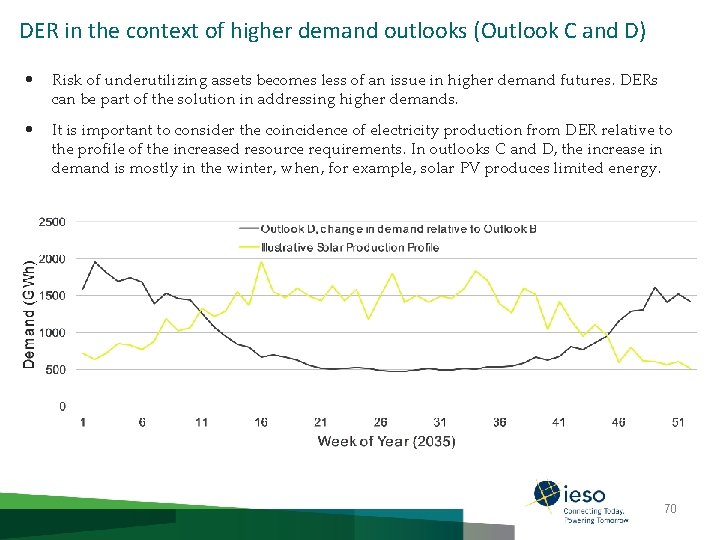
DER in the context of higher demand outlooks (Outlook C and D) • Risk of underutilizing assets becomes less of an issue in higher demand futures. DERs can be part of the solution in addressing higher demands. • It is important to consider the coincidence of electricity production from DER relative to the profile of the increased resource requirements. In outlooks C and D, the increase in demand is mostly in the winter, when, for example, solar PV produces limited energy. 70
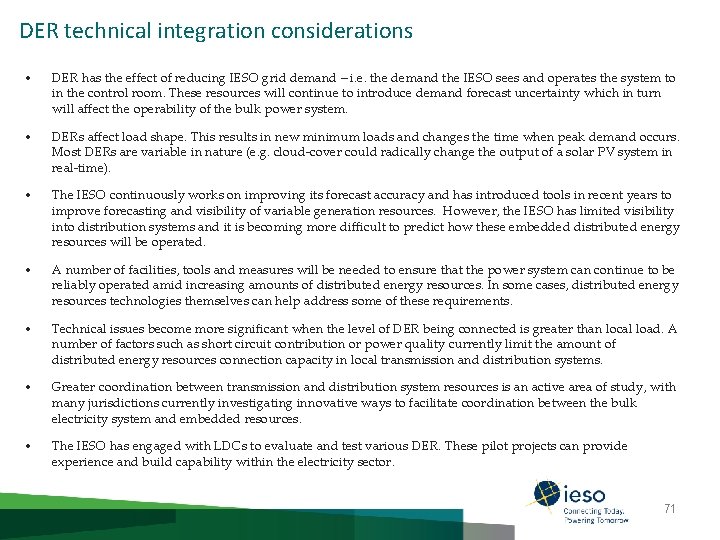
DER technical integration considerations • DER has the effect of reducing IESO grid demand – i. e. the demand the IESO sees and operates the system to in the control room. These resources will continue to introduce demand forecast uncertainty which in turn will affect the operability of the bulk power system. • DERs affect load shape. This results in new minimum loads and changes the time when peak demand occurs. Most DERs are variable in nature (e. g. cloud-cover could radically change the output of a solar PV system in real-time). • The IESO continuously works on improving its forecast accuracy and has introduced tools in recent years to improve forecasting and visibility of variable generation resources. However, the IESO has limited visibility into distribution systems and it is becoming more difficult to predict how these embedded distributed energy resources will be operated. • A number of facilities, tools and measures will be needed to ensure that the power system can continue to be reliably operated amid increasing amounts of distributed energy resources. In some cases, distributed energy resources technologies themselves can help address some of these requirements. • Technical issues become more significant when the level of DER being connected is greater than local load. A number of factors such as short circuit contribution or power quality currently limit the amount of distributed energy resources connection capacity in local transmission and distribution systems. • Greater coordination between transmission and distribution system resources is an active area of study, with many jurisdictions currently investigating innovative ways to facilitate coordination between the bulk electricity system and embedded resources. • The IESO has engaged with LDCs to evaluate and test various DER. These pilot projects can provide experience and build capability within the electricity sector. 71
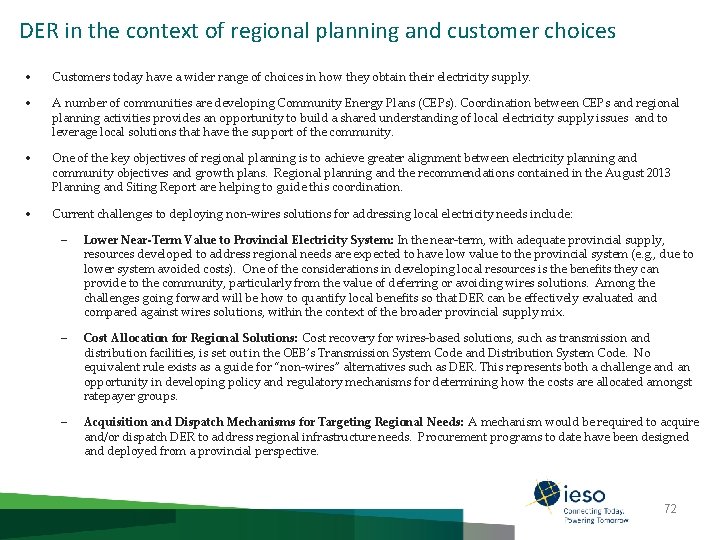
DER in the context of regional planning and customer choices • Customers today have a wider range of choices in how they obtain their electricity supply. • A number of communities are developing Community Energy Plans (CEPs). Coordination between CEPs and regional planning activities provides an opportunity to build a shared understanding of local electricity supply issues and to leverage local solutions that have the support of the community. • One of the key objectives of regional planning is to achieve greater alignment between electricity planning and community objectives and growth plans. Regional planning and the recommendations contained in the August 2013 Planning and Siting Report are helping to guide this coordination. • Current challenges to deploying non-wires solutions for addressing local electricity needs include: – Lower Near-Term Value to Provincial Electricity System: In the near-term, with adequate provincial supply, resources developed to address regional needs are expected to have low value to the provincial system (e. g. , due to lower system avoided costs). One of the considerations in developing local resources is the benefits they can provide to the community, particularly from the value of deferring or avoiding wires solutions. Among the challenges going forward will be how to quantify local benefits so that DER can be effectively evaluated and compared against wires solutions, within the context of the broader provincial supply mix. – Cost Allocation for Regional Solutions: Cost recovery for wires-based solutions, such as transmission and distribution facilities, is set out in the OEB’s Transmission System Code and Distribution System Code. No equivalent rule exists as a guide for “non-wires” alternatives such as DER. This represents both a challenge and an opportunity in developing policy and regulatory mechanisms for determining how the costs are allocated amongst ratepayer groups. – Acquisition and Dispatch Mechanisms for Targeting Regional Needs: A mechanism would be required to acquire and/or dispatch DER to address regional infrastructure needs. Procurement programs to date have been designed and deployed from a provincial perspective. 72
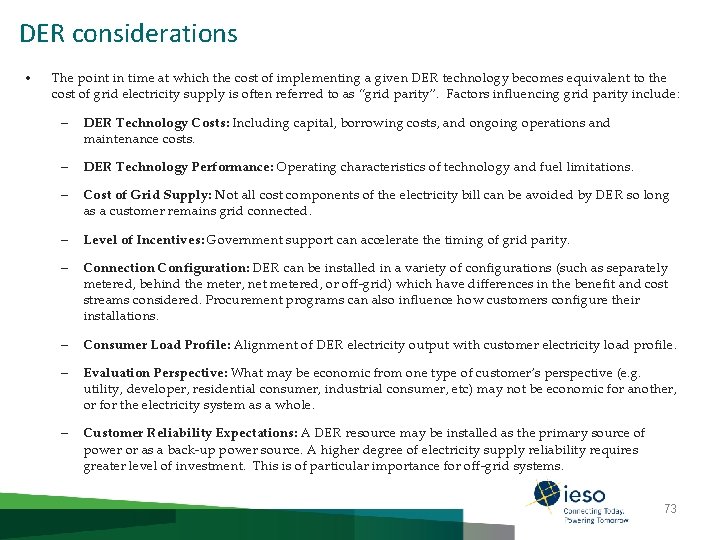
DER considerations • The point in time at which the cost of implementing a given DER technology becomes equivalent to the cost of grid electricity supply is often referred to as “grid parity”. Factors influencing grid parity include: – DER Technology Costs: Including capital, borrowing costs, and ongoing operations and maintenance costs. – DER Technology Performance: Operating characteristics of technology and fuel limitations. – Cost of Grid Supply: Not all cost components of the electricity bill can be avoided by DER so long as a customer remains grid connected. – Level of Incentives: Government support can accelerate the timing of grid parity. – Connection Configuration: DER can be installed in a variety of configurations (such as separately metered, behind the meter, net metered, or off-grid) which have differences in the benefit and cost streams considered. Procurement programs can also influence how customers configure their installations. – Consumer Load Profile: Alignment of DER electricity output with customer electricity load profile. – Evaluation Perspective: What may be economic from one type of customer’s perspective (e. g. utility, developer, residential consumer, industrial consumer, etc) may not be economic for another, or for the electricity system as a whole. – Customer Reliability Expectations: A DER resource may be installed as the primary source of power or as a back-up power source. A higher degree of electricity supply reliability requires greater level of investment. This is of particular importance for off-grid systems. 73
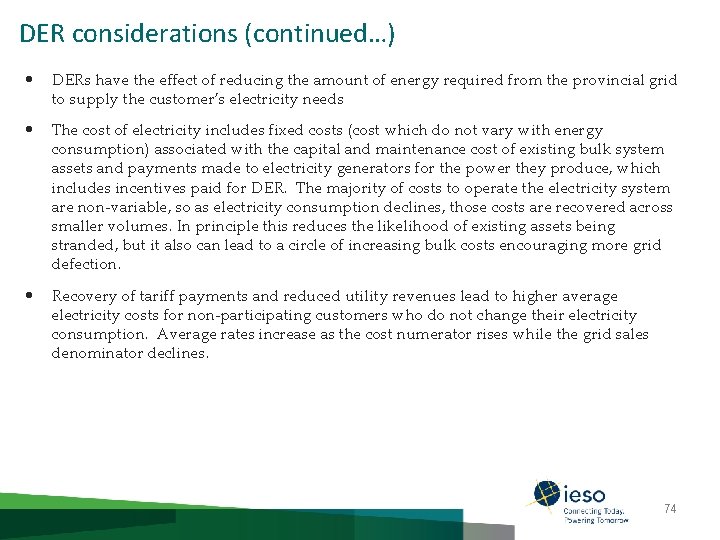
DER considerations (continued…) • DERs have the effect of reducing the amount of energy required from the provincial grid to supply the customer’s electricity needs • The cost of electricity includes fixed costs (cost which do not vary with energy consumption) associated with the capital and maintenance cost of existing bulk system assets and payments made to electricity generators for the power they produce, which includes incentives paid for DER. The majority of costs to operate the electricity system are non-variable, so as electricity consumption declines, those costs are recovered across smaller volumes. In principle this reduces the likelihood of existing assets being stranded, but it also can lead to a circle of increasing bulk costs encouraging more grid defection. • Recovery of tariff payments and reduced utility revenues lead to higher average electricity costs for non-participating customers who do not change their electricity consumption. Average rates increase as the cost numerator rises while the grid sales denominator declines. 74
Outlook for corporate incentive travel programs
Présence inconnue outlook
Outlook ordnerbereich anpassen
Imap terra outlook 2016
Chapter 5 section 1 supply and the law of supply
Elastisidad ng suplay
Matching supply with demand
Chapter8 enterprice recource planning
Module 5 supply and demand introduction and demand
Order promising module of supply chain management
C device module module 1
August šenoa budi svoj analiza
Light in august quotes
Berlin weather august
Do que miranda amiga de via chamava august
August kekulé
Diexi slides, sichuan, china, august 1933
August leyweg 4
Shawn johnstone
Dramaanalyse
Napoleon bonaparte was born on 15 august
August lösch
Kugina kuća august šenoa
August safety topics
6 months before august 31
Longest rivers in china
Mirjam nilsson
August stern
Fences character map
August prima porta
Julie august
Teori big bang
Diane august
Pinus radiata dan pinus muricata isolasi
What happened on august 23 1939
Agosto 23, 1896
August lec 250
August strindberg giftas
August strindberg fruar
Chinese names pronunciation
Monday august 26
August šenoa povjestice
2003 august 25
August safety
August robert ludwig macke
Nilsson kommunikation download
August 26 2010
Micro computer services began operations on august 1
August hlond przepowiednie
It was late summer 26 august 1910
Gottfried august bürger lenore
What is testimonial propaganda
Which country is michael jackson from
August alsina testimony free album download zip file
Dr ralph ferraro
August gailiti romaanid
August 27 2002
August kiss
August 21, 2017 plus 1260 days
šablóny powerpoint
Madonna last name
August wilson
Full moon august 2011
Timeline ng kaligirang pangkasaysayan ng el filibusterismo
August
August strindberg kända verk
Cnn 10 sep 7
Analysis of fences by august wilson
Themes of fences by august wilson
S3xt trade
August shi
February march april may june july august september
August alsina testimony album download
30th august 2005
Starr company reports the following information for august.