Magnetic Force on Free Moving Charges As mentioned
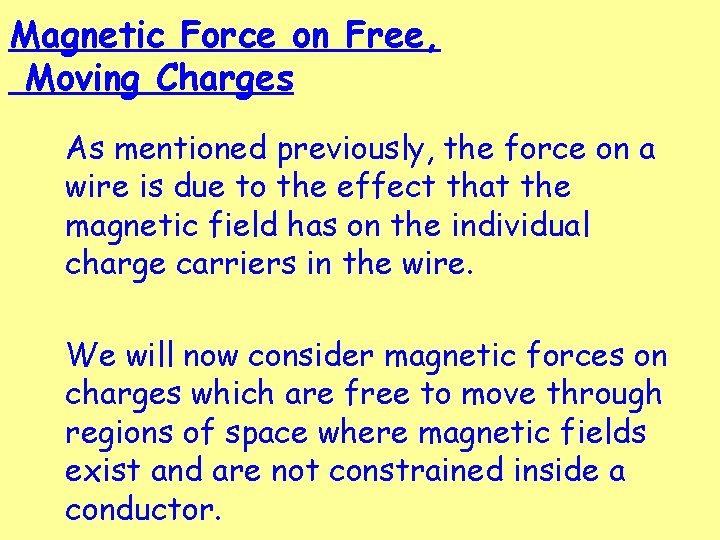
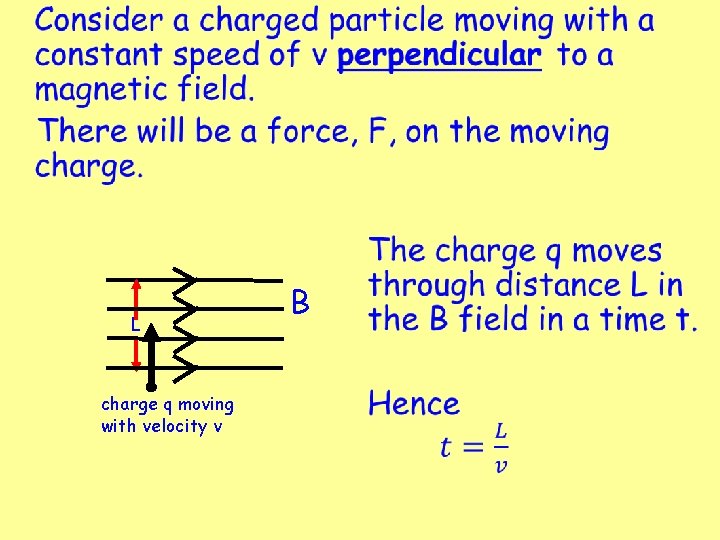
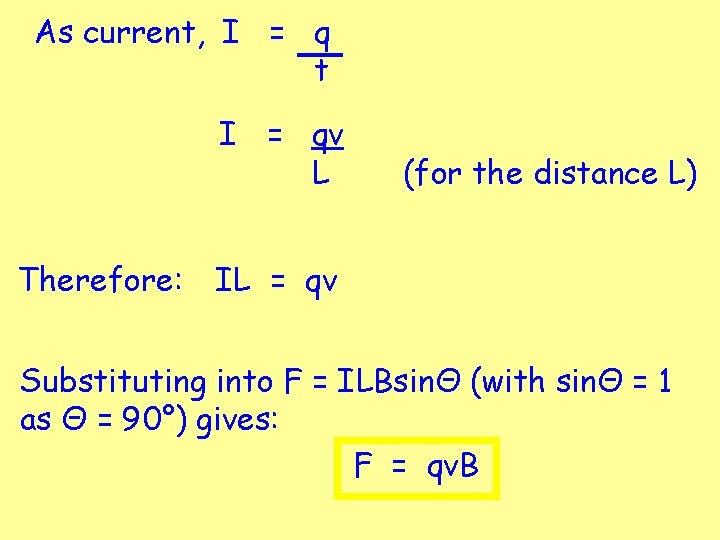
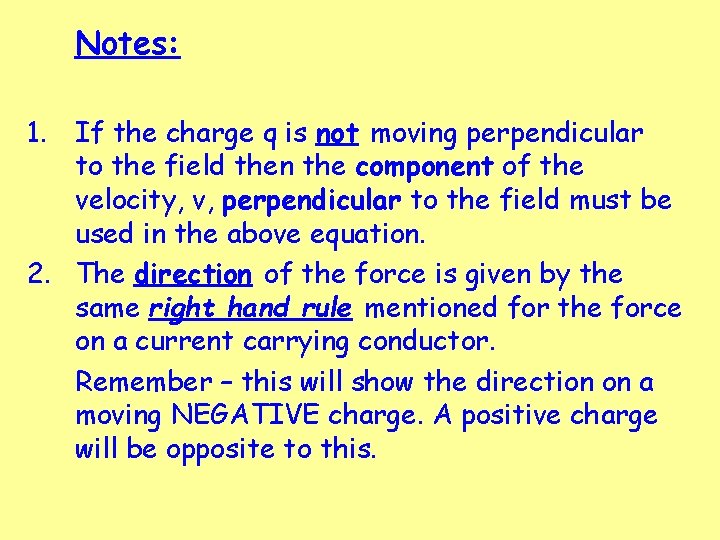
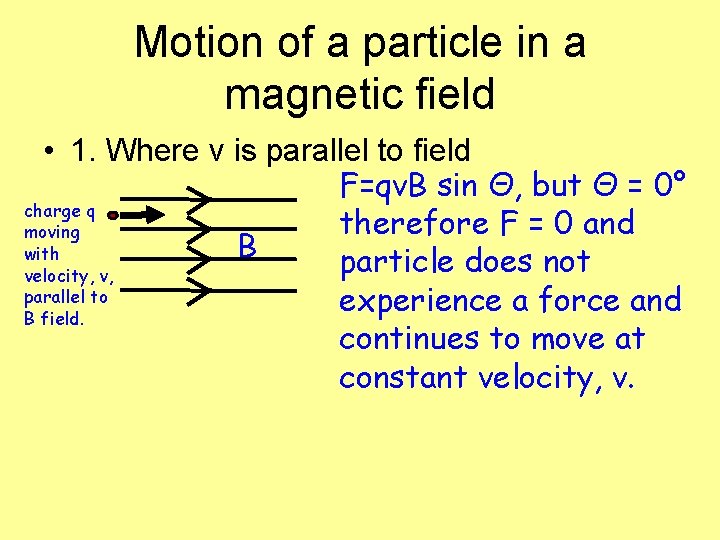
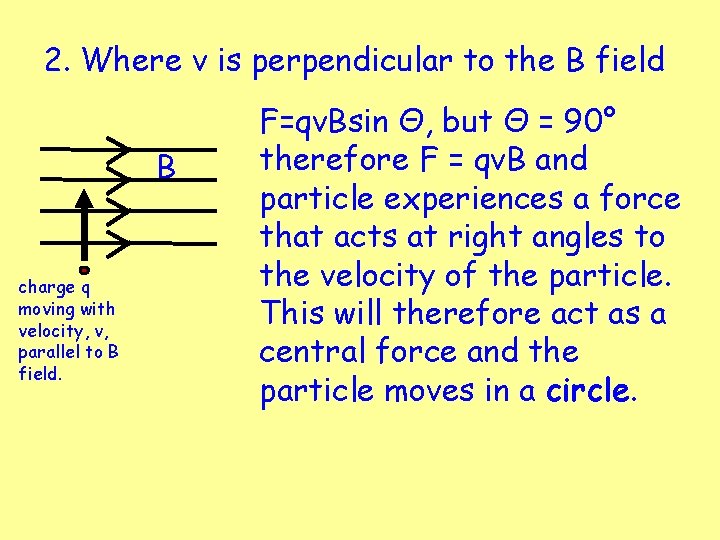
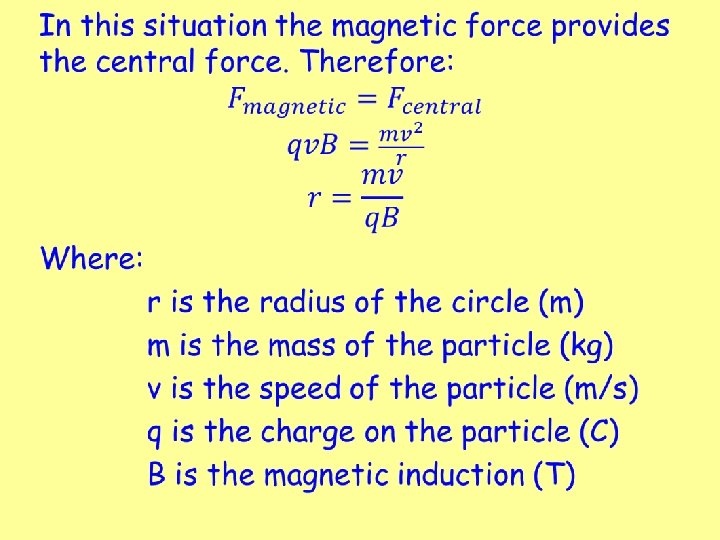
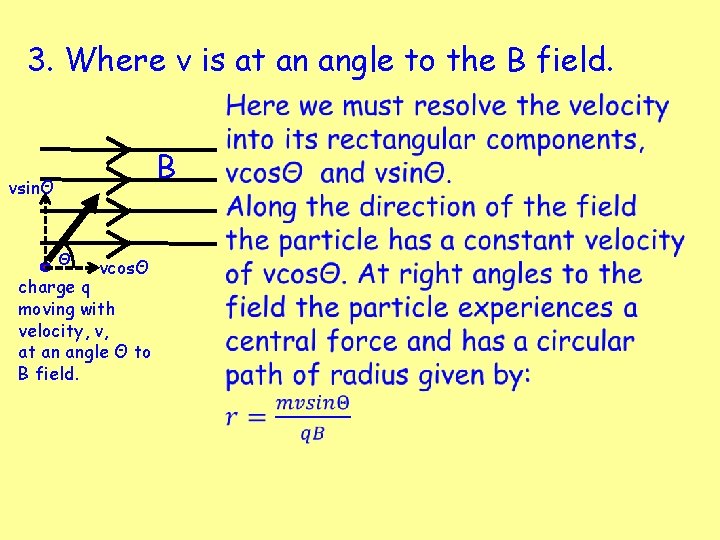
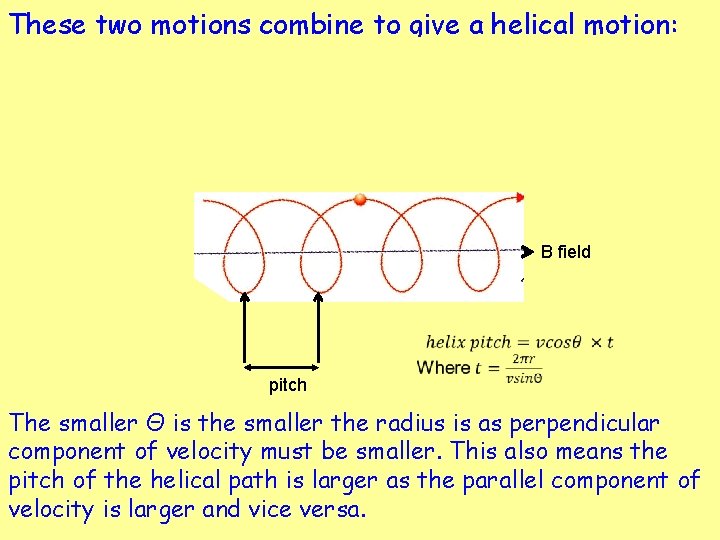
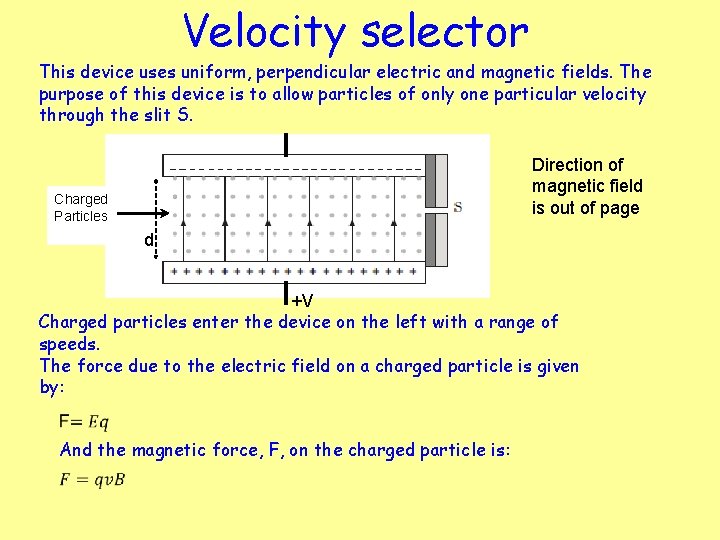
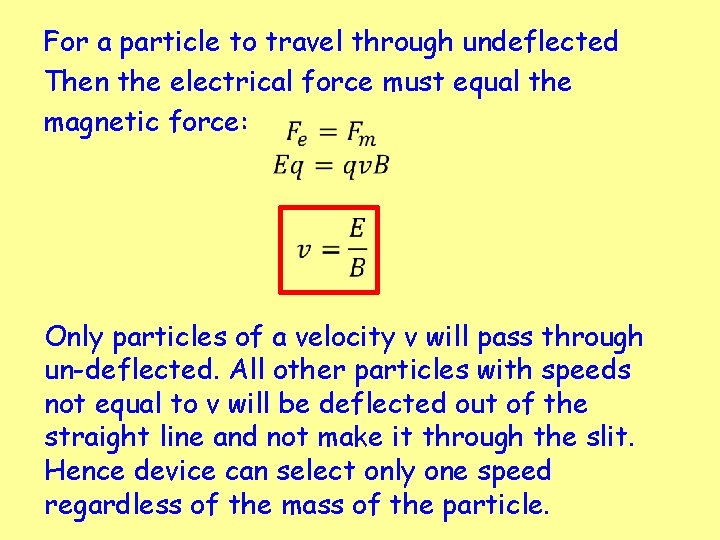
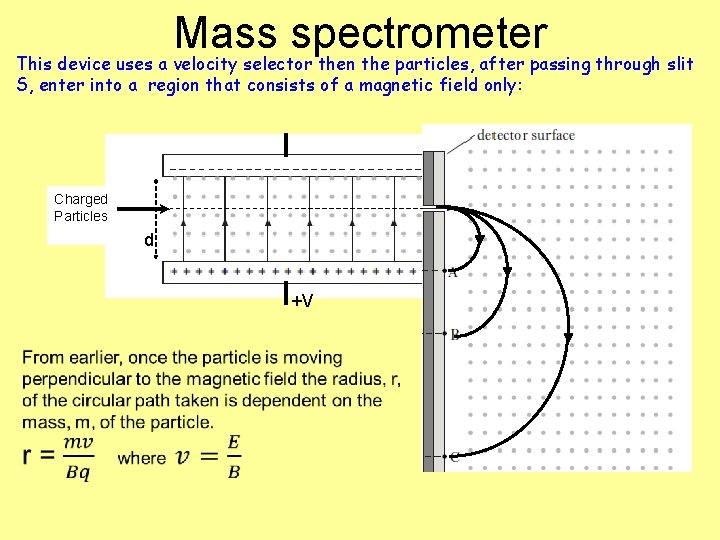
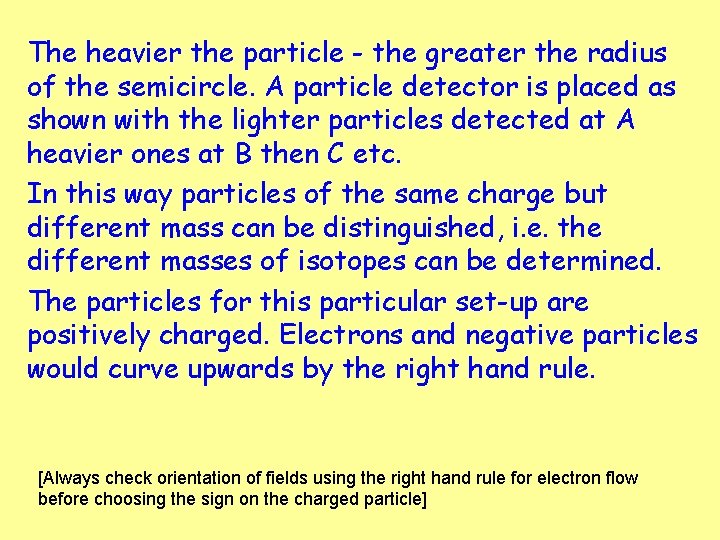
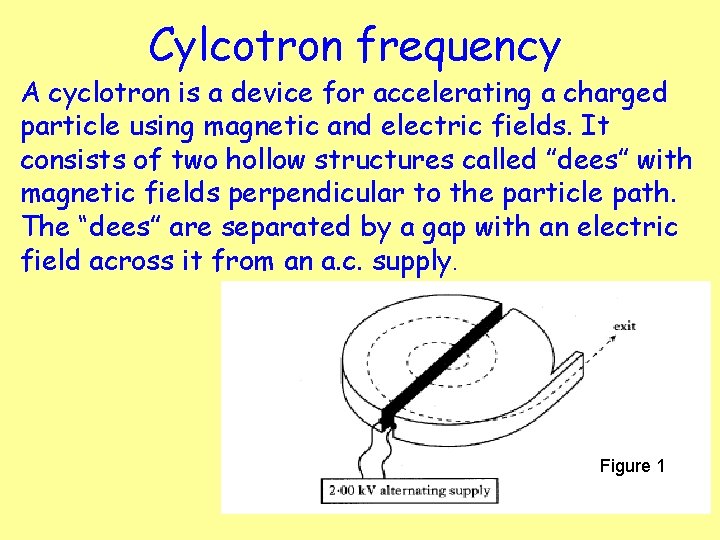
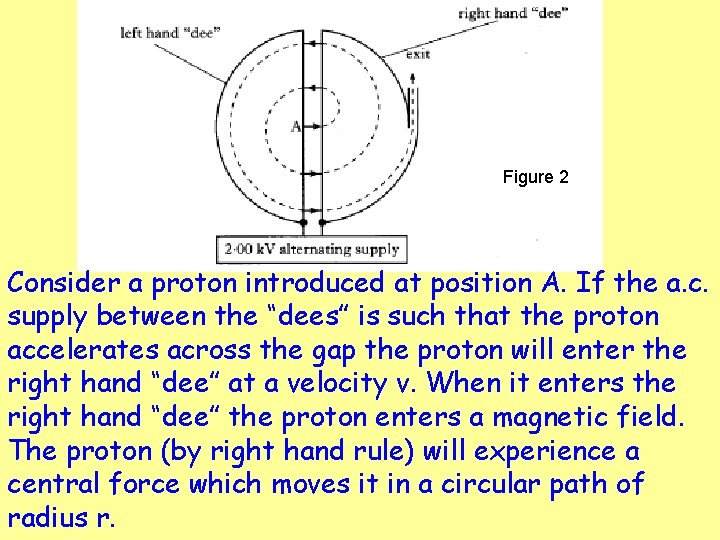
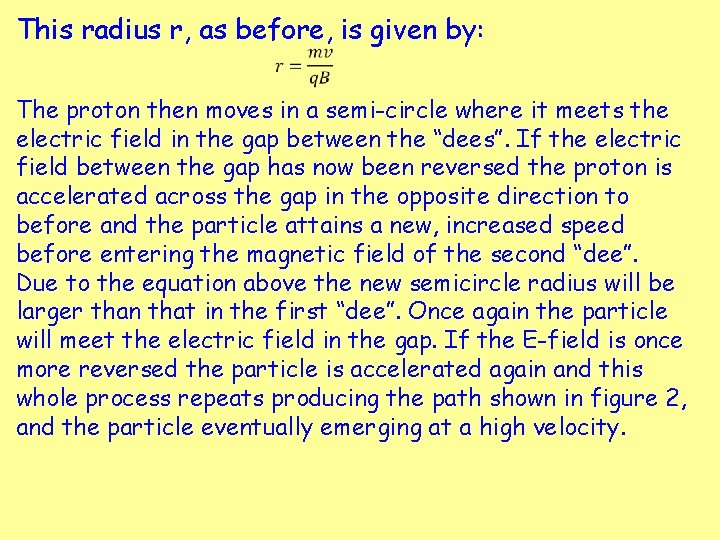
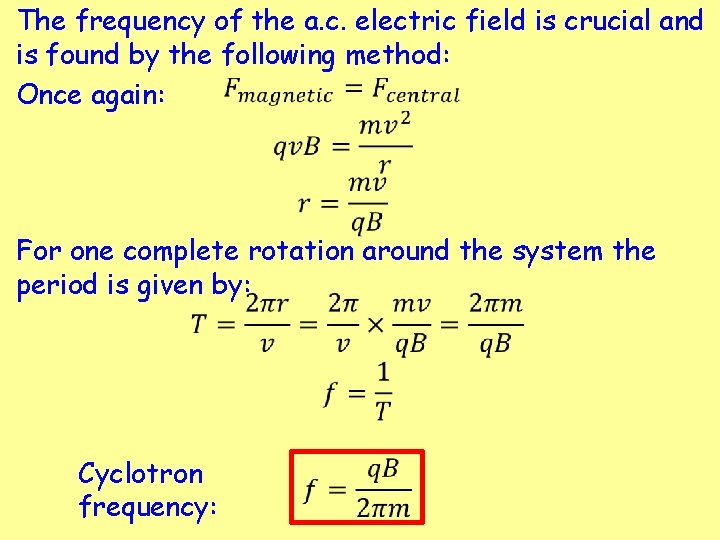
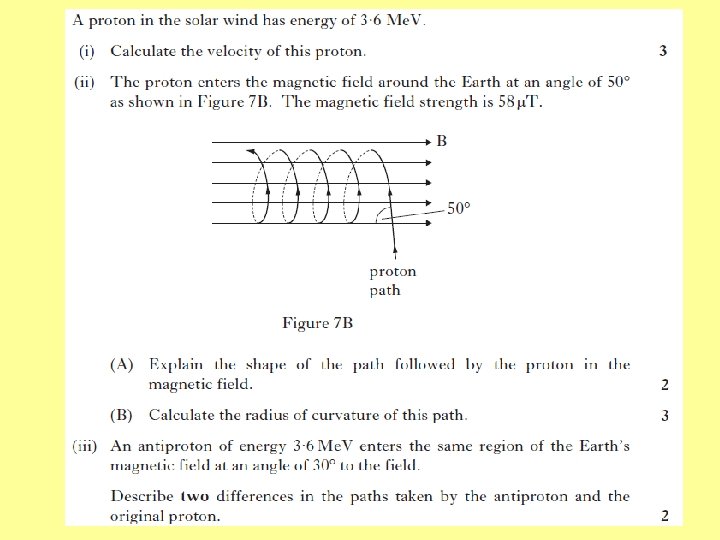
- Slides: 18
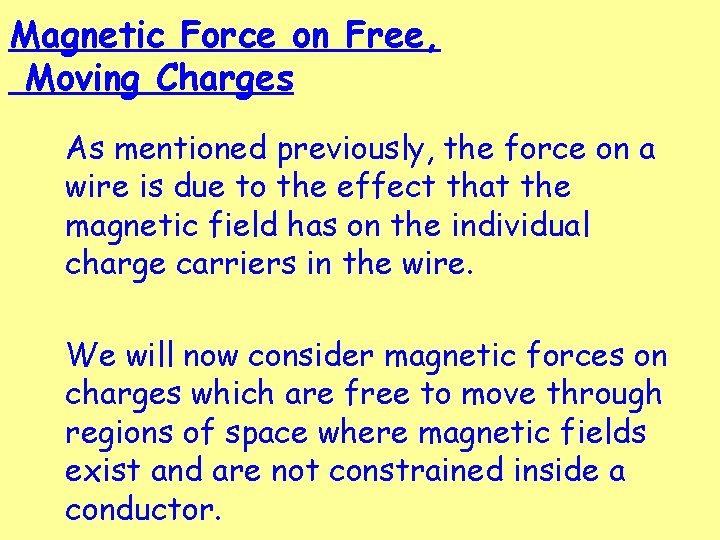
Magnetic Force on Free, Moving Charges As mentioned previously, the force on a wire is due to the effect that the magnetic field has on the individual charge carriers in the wire. We will now consider magnetic forces on charges which are free to move through regions of space where magnetic fields exist and are not constrained inside a conductor.
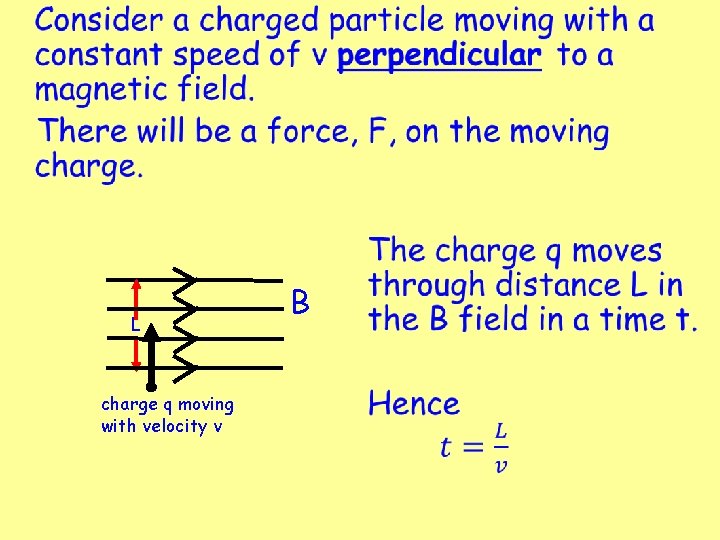
• L charge q moving with velocity v B
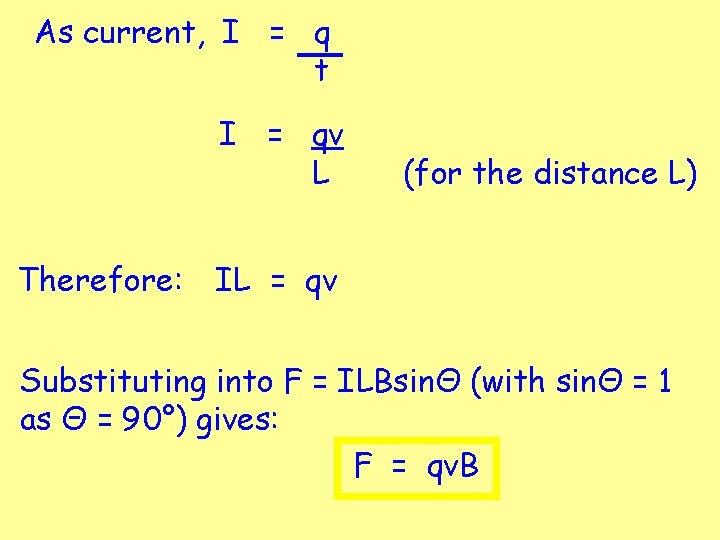
As current, I = q t I = qv L Therefore: (for the distance L) IL = qv Substituting into F = ILBsinΘ (with sinΘ = 1 as Θ = 90°) gives: F = qv. B
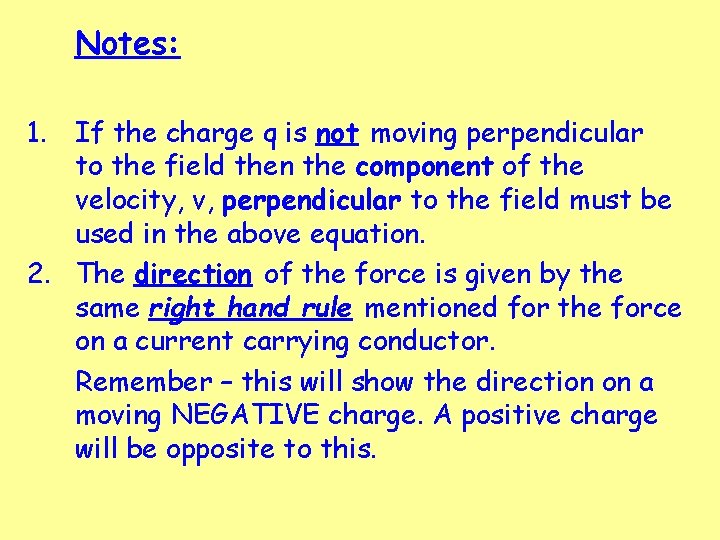
Notes: 1. If the charge q is not moving perpendicular to the field then the component of the velocity, v, perpendicular to the field must be used in the above equation. 2. The direction of the force is given by the same right hand rule mentioned for the force on a current carrying conductor. Remember – this will show the direction on a moving NEGATIVE charge. A positive charge will be opposite to this.
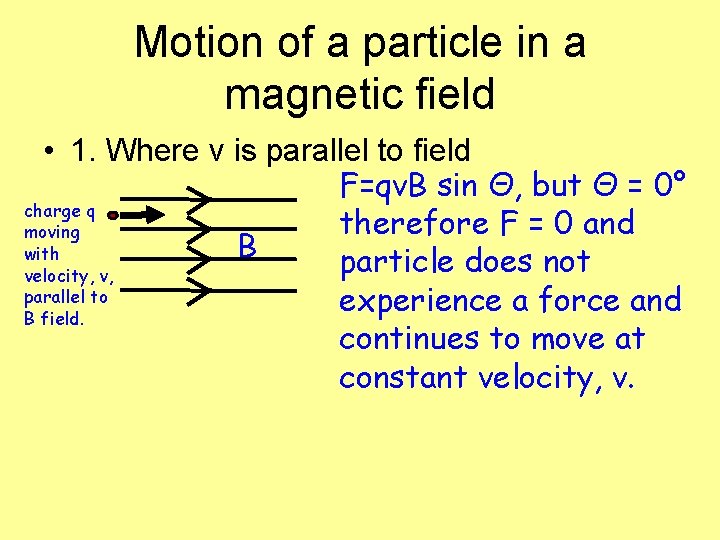
Motion of a particle in a magnetic field • 1. Where v is parallel to field F=qv. B sin Θ, but Θ = 0° charge q therefore F = 0 and moving B with particle does not velocity, v, parallel to experience a force and B field. continues to move at constant velocity, v.
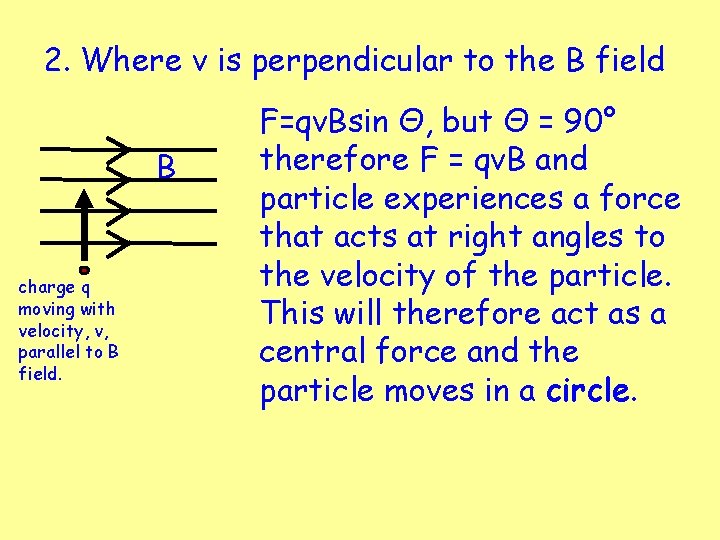
2. Where v is perpendicular to the B field B charge q moving with velocity, v, parallel to B field. F=qv. Bsin Θ, but Θ = 90° therefore F = qv. B and particle experiences a force that acts at right angles to the velocity of the particle. This will therefore act as a central force and the particle moves in a circle.
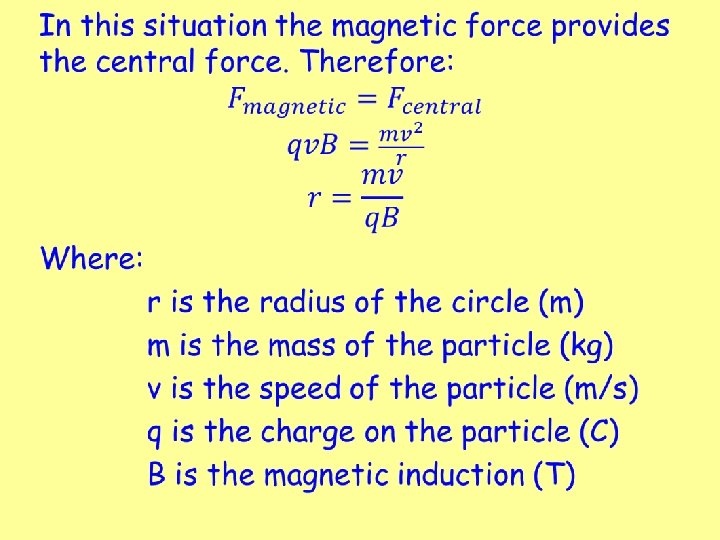
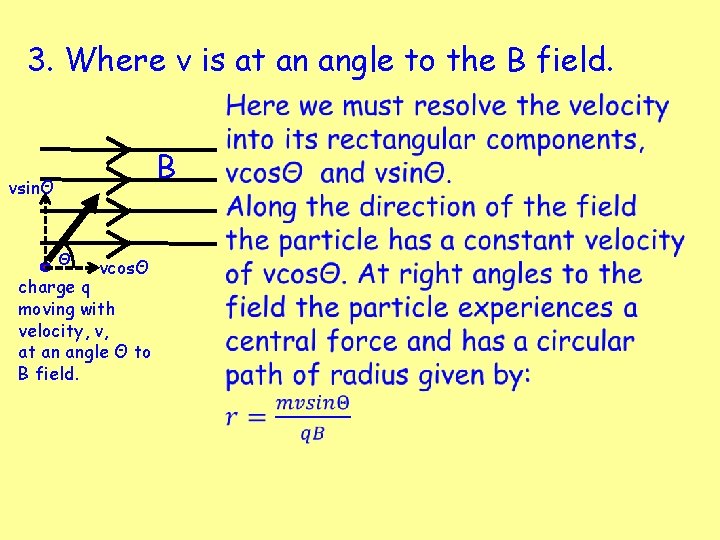
3. Where v is at an angle to the B field. B vsinΘ Θ vcosΘ charge q moving with velocity, v, at an angle Θ to B field.
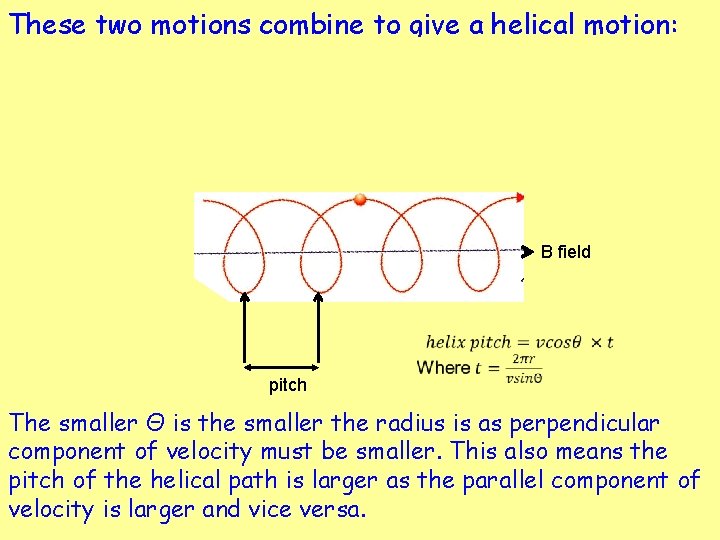
These two motions combine to give a helical motion: B field pitch The smaller Θ is the smaller the radius is as perpendicular component of velocity must be smaller. This also means the pitch of the helical path is larger as the parallel component of velocity is larger and vice versa.
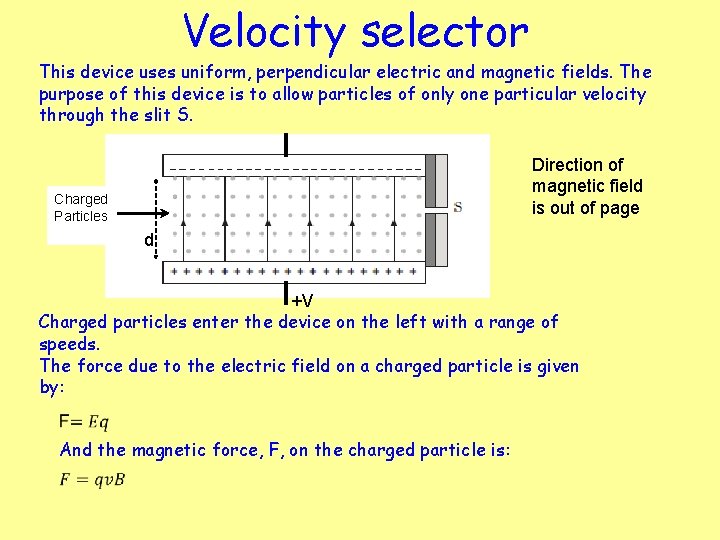
Velocity selector This device uses uniform, perpendicular electric and magnetic fields. The purpose of this device is to allow particles of only one particular velocity through the slit S. Direction of magnetic field is out of page Charged Particles d +V Charged particles enter the device on the left with a range of speeds. The force due to the electric field on a charged particle is given by: And the magnetic force, F, on the charged particle is:
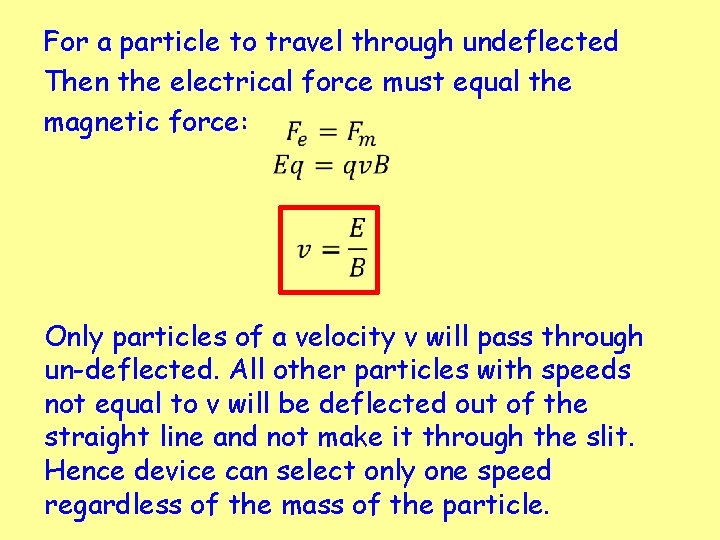
For a particle to travel through undeflected Then the electrical force must equal the magnetic force: Only particles of a velocity v will pass through un-deflected. All other particles with speeds not equal to v will be deflected out of the straight line and not make it through the slit. Hence device can select only one speed regardless of the mass of the particle.
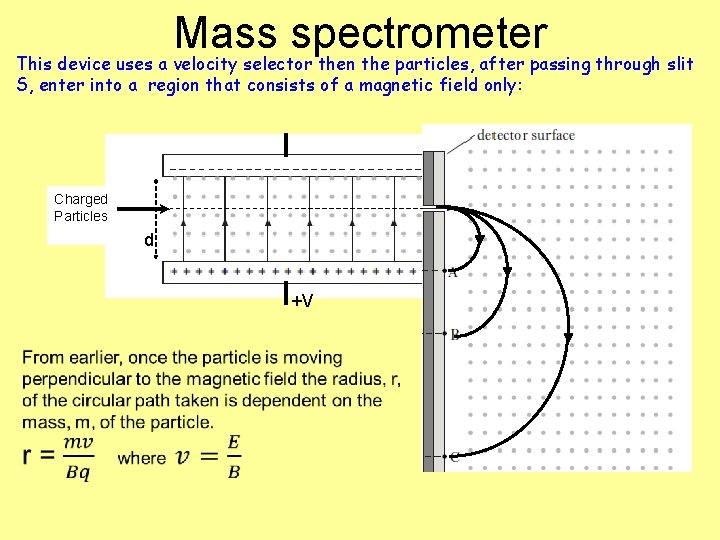
Mass spectrometer This device uses a velocity selector then the particles, after passing through slit S, enter into a region that consists of a magnetic field only: Charged Particles d +V
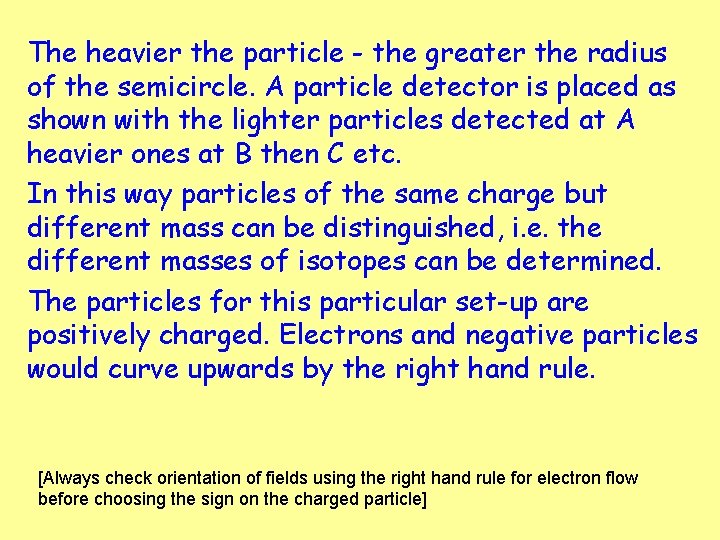
The heavier the particle - the greater the radius of the semicircle. A particle detector is placed as shown with the lighter particles detected at A heavier ones at B then C etc. In this way particles of the same charge but different mass can be distinguished, i. e. the different masses of isotopes can be determined. The particles for this particular set-up are positively charged. Electrons and negative particles would curve upwards by the right hand rule. [Always check orientation of fields using the right hand rule for electron flow before choosing the sign on the charged particle]
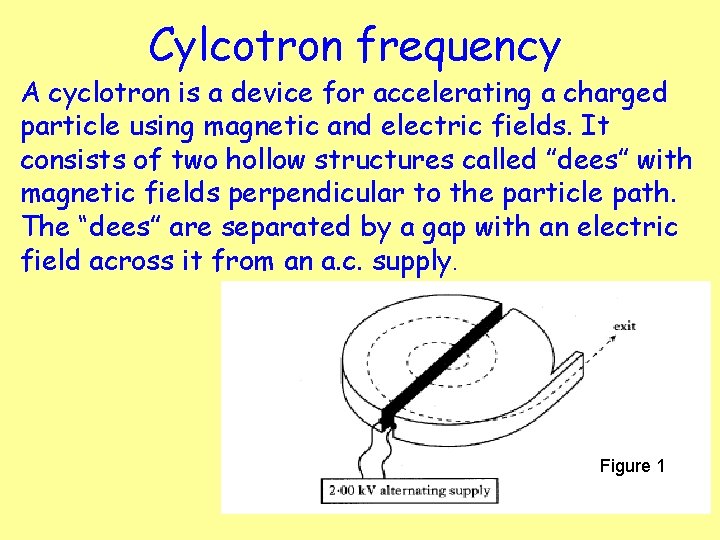
Cylcotron frequency A cyclotron is a device for accelerating a charged particle using magnetic and electric fields. It consists of two hollow structures called ”dees” with magnetic fields perpendicular to the particle path. The “dees” are separated by a gap with an electric field across it from an a. c. supply. Figure 1
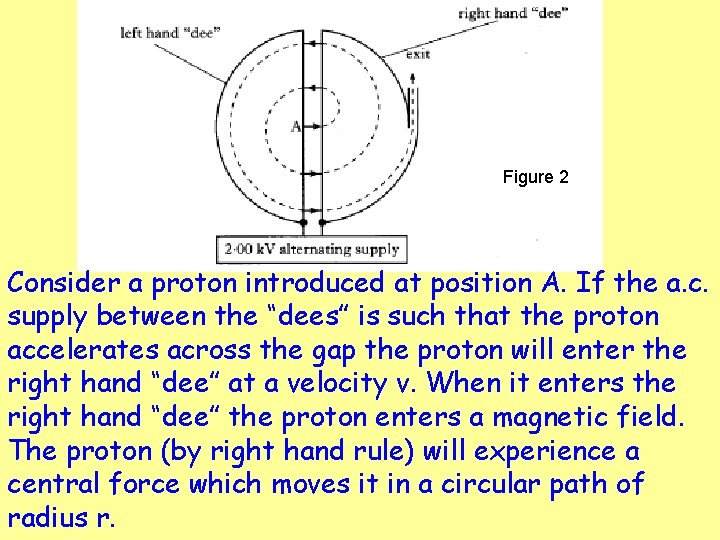
Figure 2 Consider a proton introduced at position A. If the a. c. supply between the “dees” is such that the proton accelerates across the gap the proton will enter the right hand “dee” at a velocity v. When it enters the right hand “dee” the proton enters a magnetic field. The proton (by right hand rule) will experience a central force which moves it in a circular path of radius r.
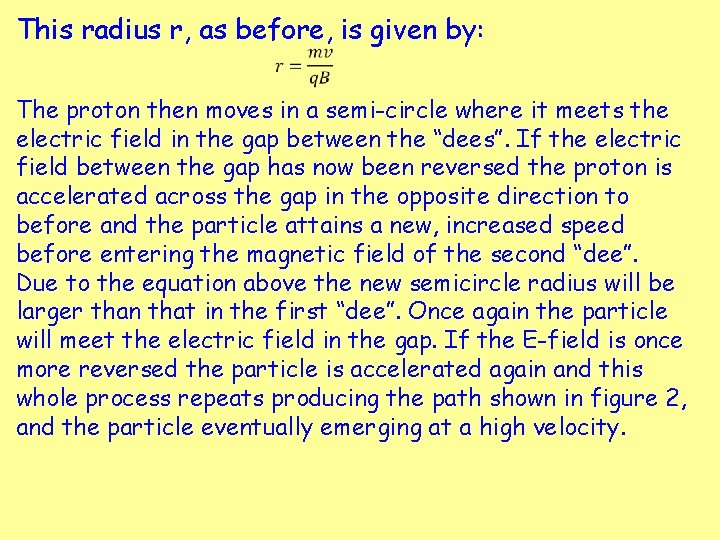
This radius r, as before, is given by: The proton then moves in a semi-circle where it meets the electric field in the gap between the “dees”. If the electric field between the gap has now been reversed the proton is accelerated across the gap in the opposite direction to before and the particle attains a new, increased speed before entering the magnetic field of the second “dee”. Due to the equation above the new semicircle radius will be larger than that in the first “dee”. Once again the particle will meet the electric field in the gap. If the E-field is once more reversed the particle is accelerated again and this whole process repeats producing the path shown in figure 2, and the particle eventually emerging at a high velocity.
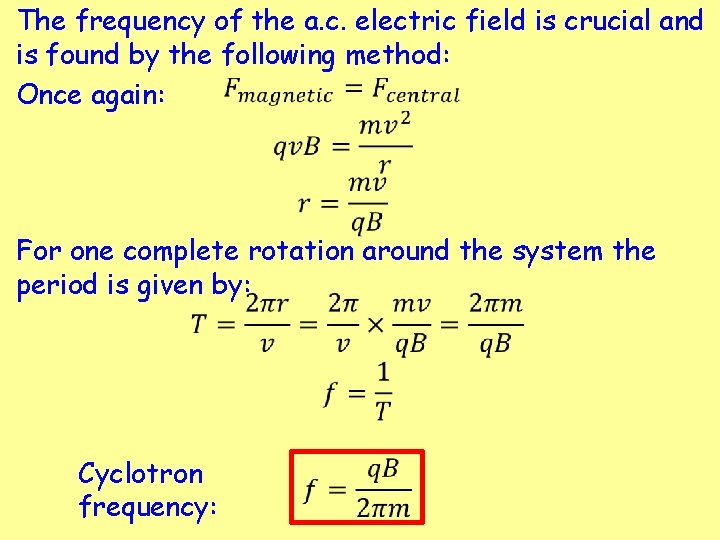
The frequency of the a. c. electric field is crucial and is found by the following method: Once again: For one complete rotation around the system the period is given by: Cyclotron frequency:
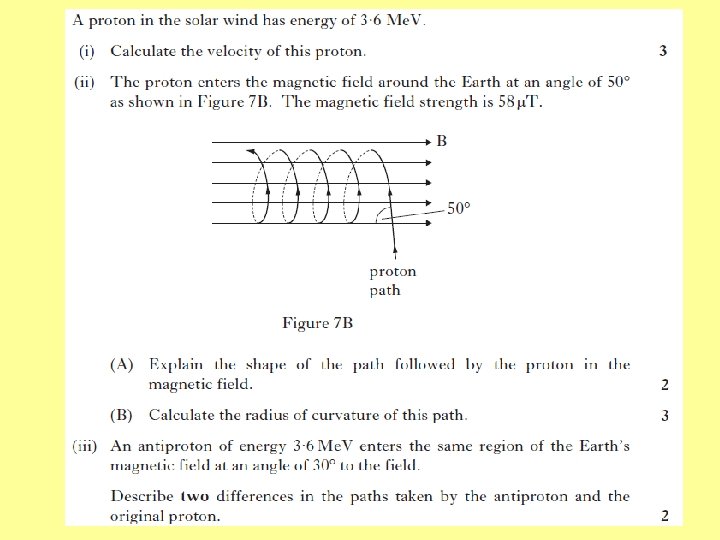
Force on a charged particle
Like charges blank and opposite charges blank
Electric field induction
Electric force meaning
What is coulomb law
Remanent magnetization
Weber flux
Magnetic moment and magnetic field relation
Four greek words for love
2 kings jonah
You never mentioned
Carp pandyas
Christmas science quiz
As we mentioned above
Which idea is presented in both passages?
Please find the below link
"as mentioned earlier"
Free moving ions
It is created by the force of moving water