Linear Mechanics NCEA AS 3 4 Text Chapters
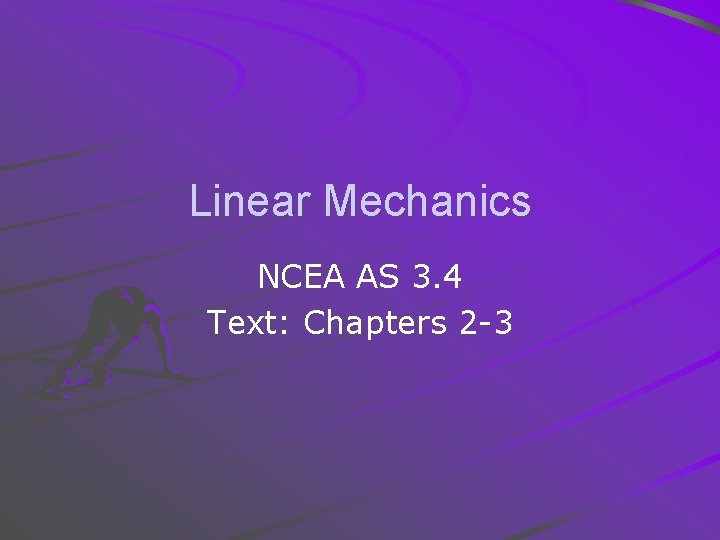
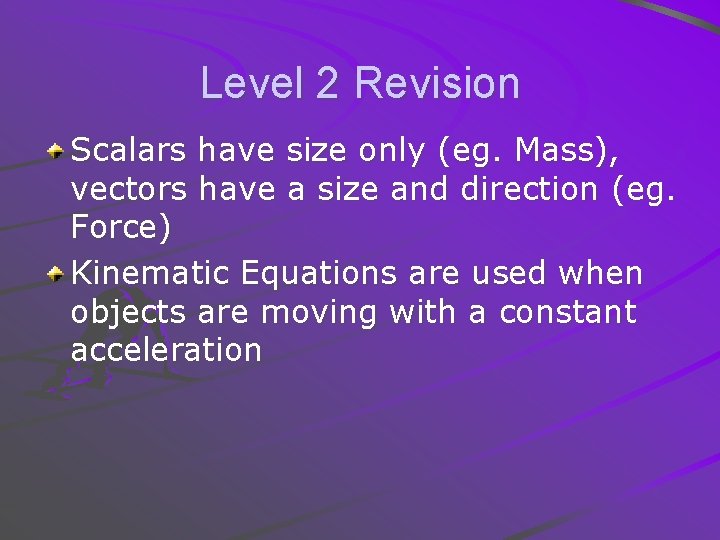
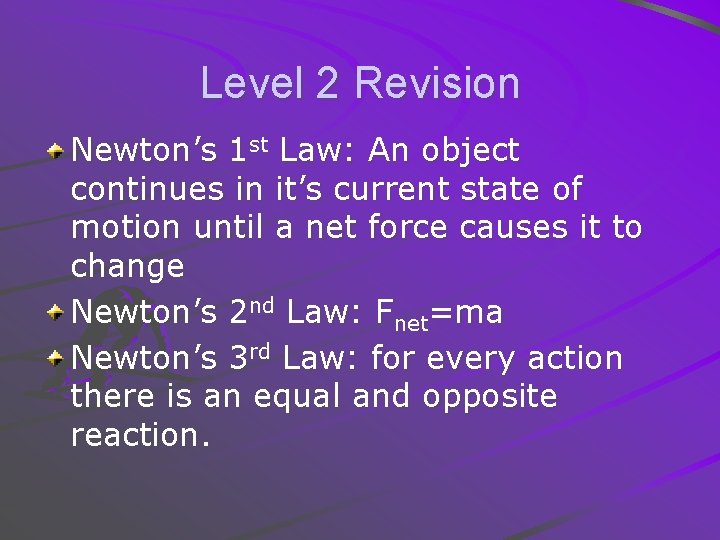
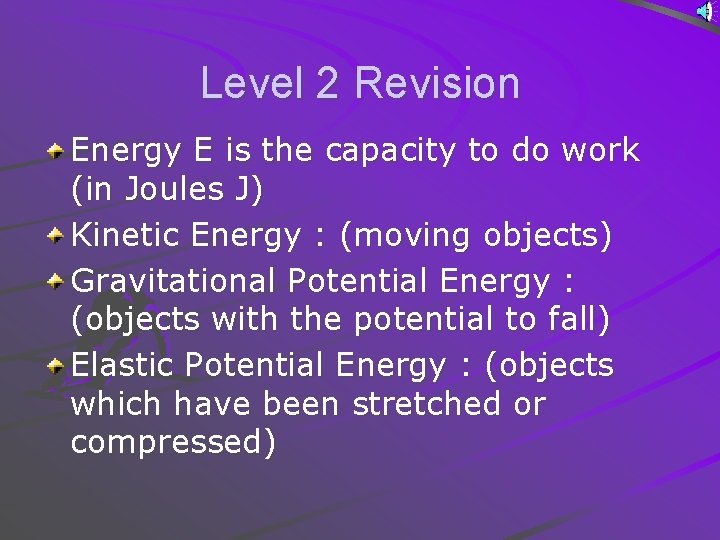
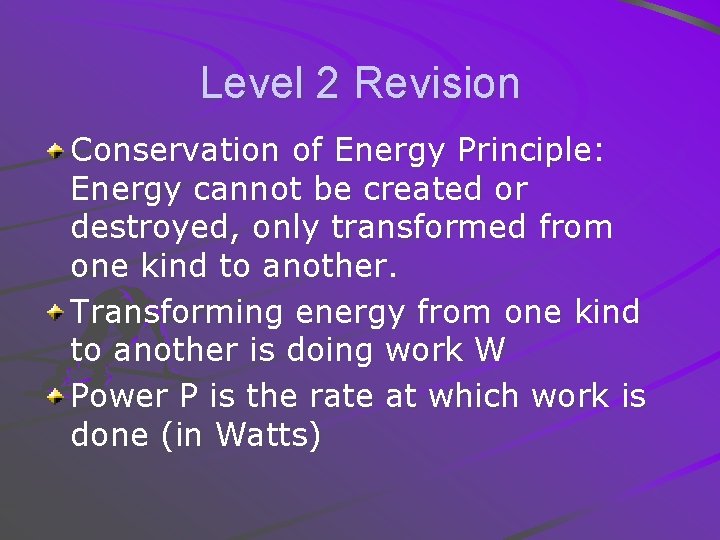
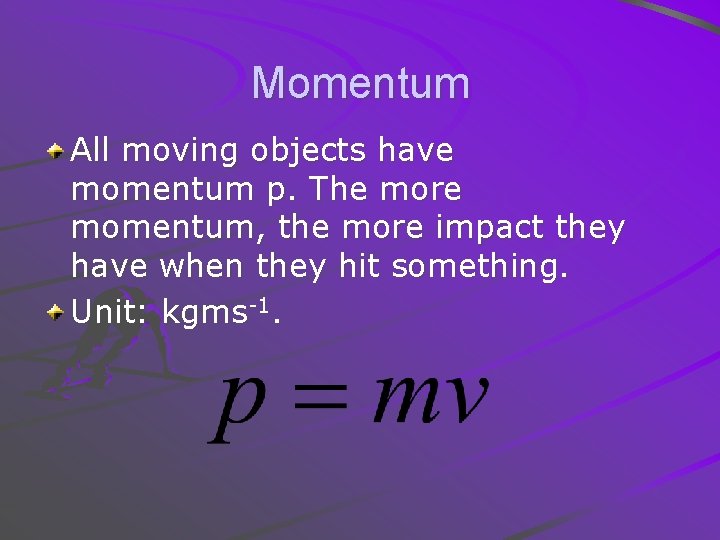
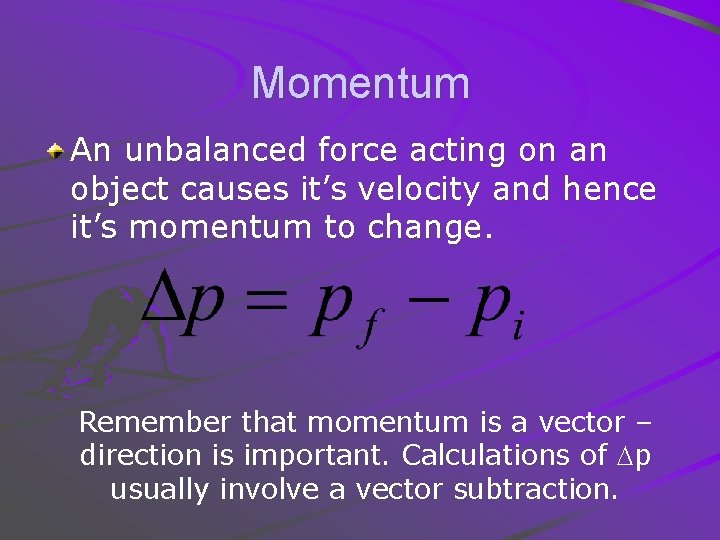
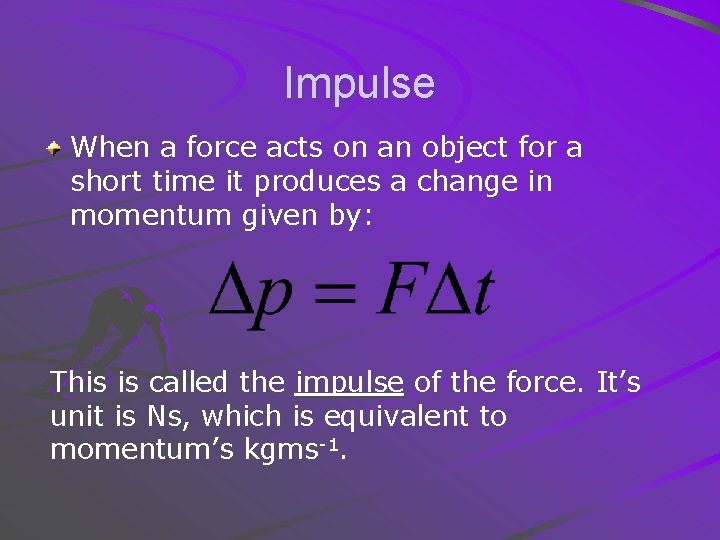
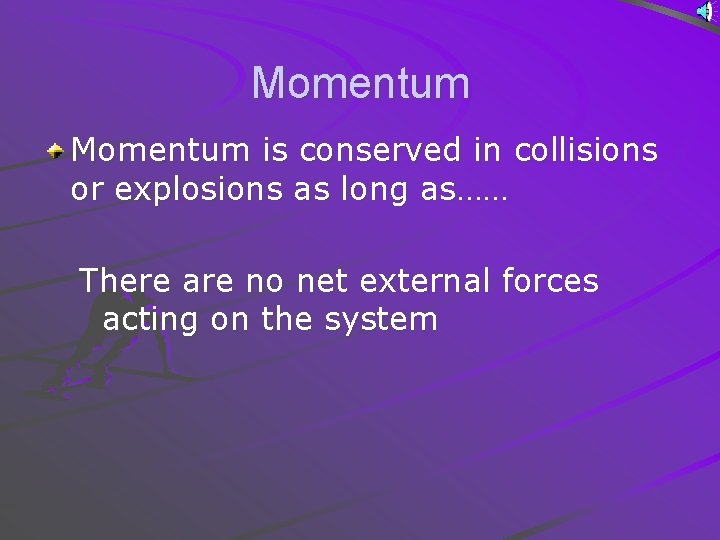
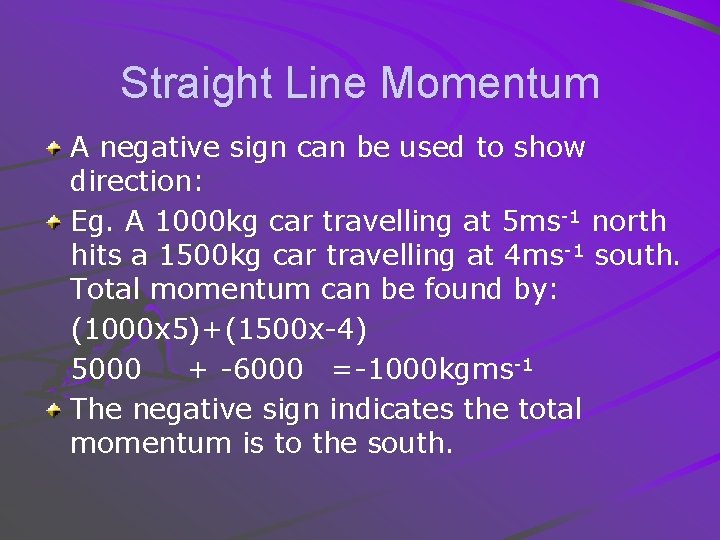
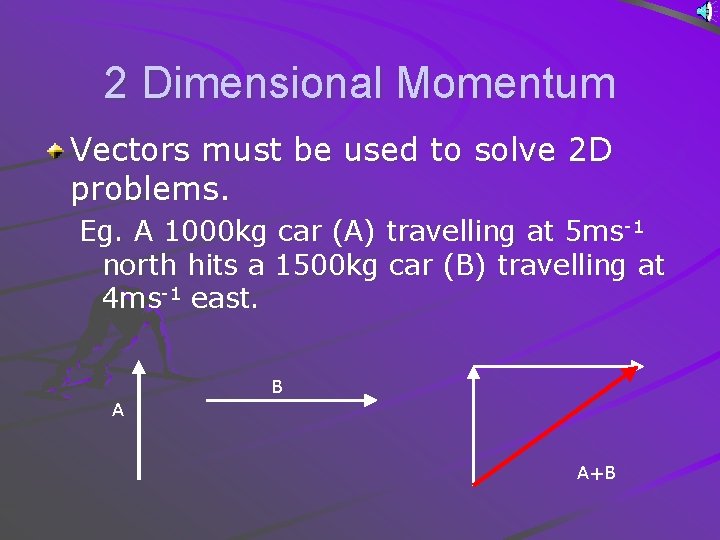
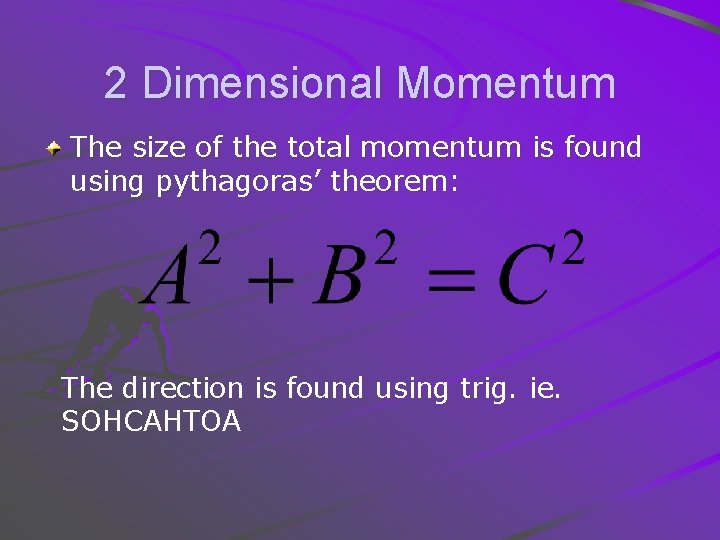
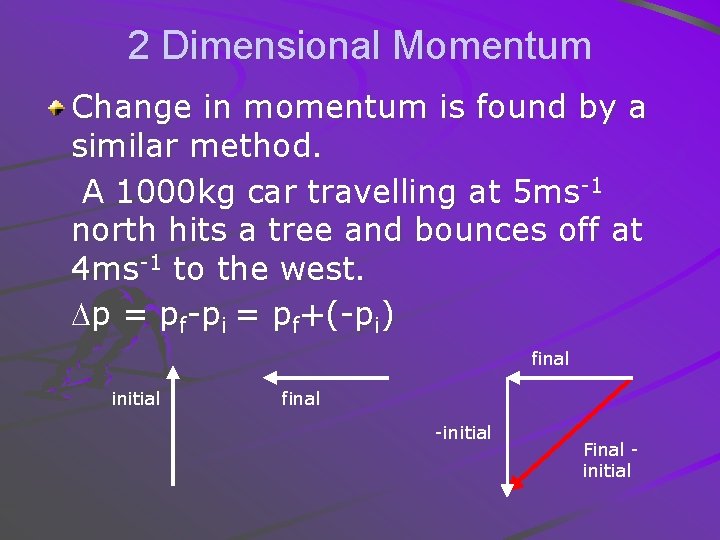
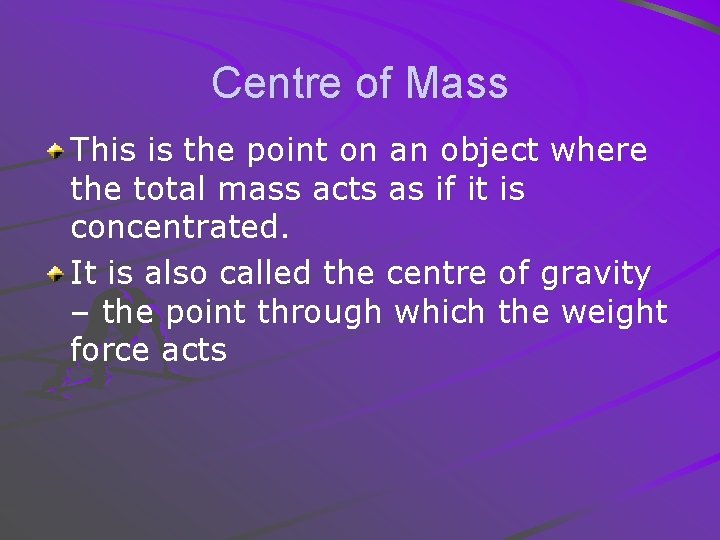
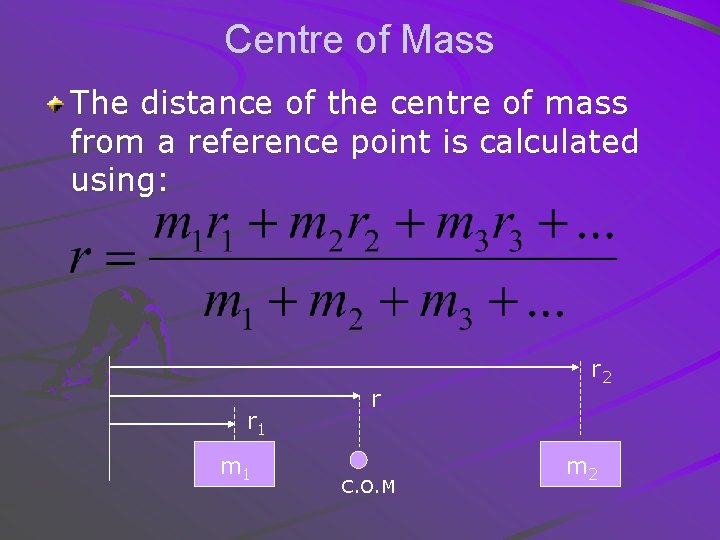
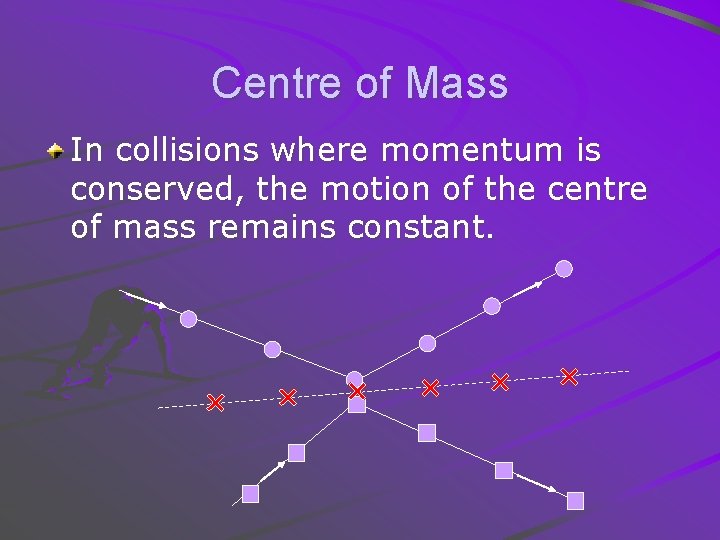
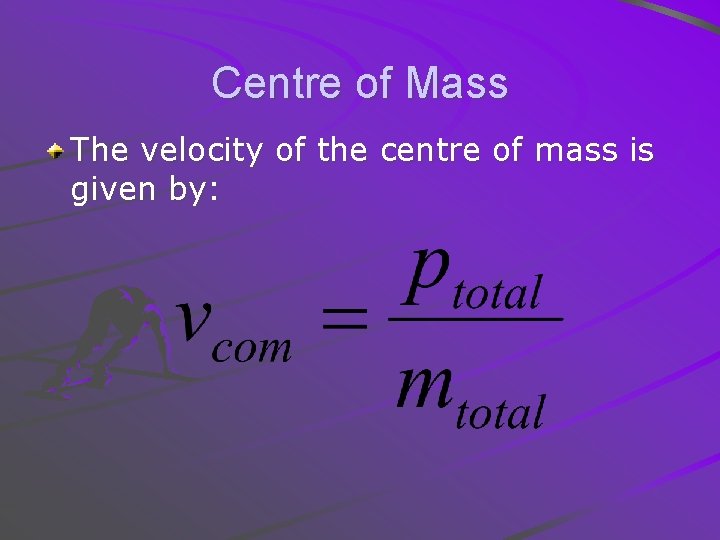
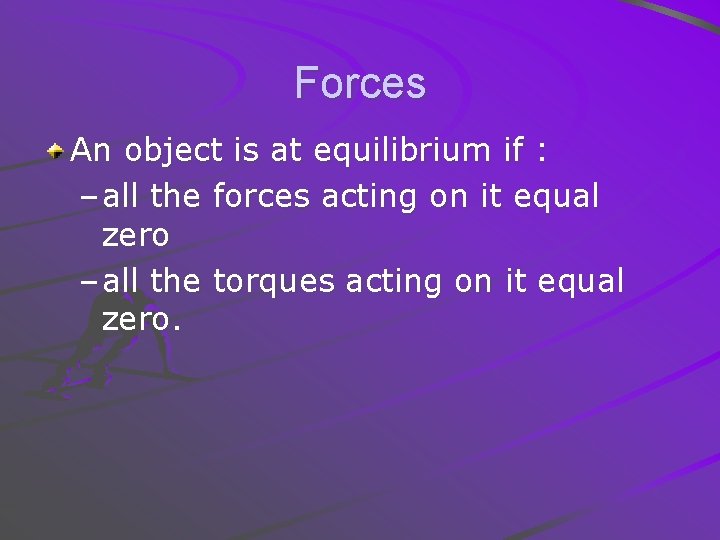
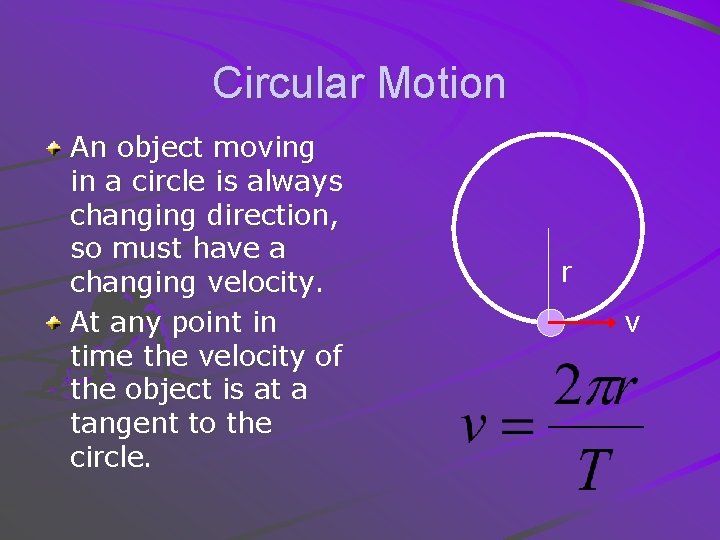
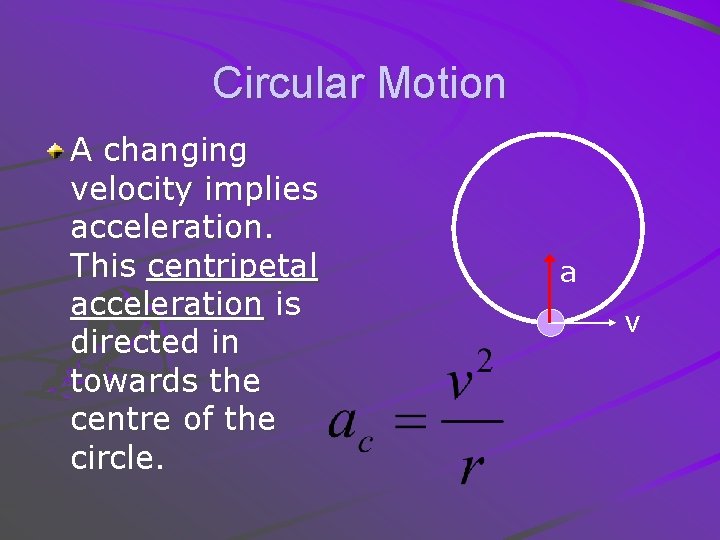
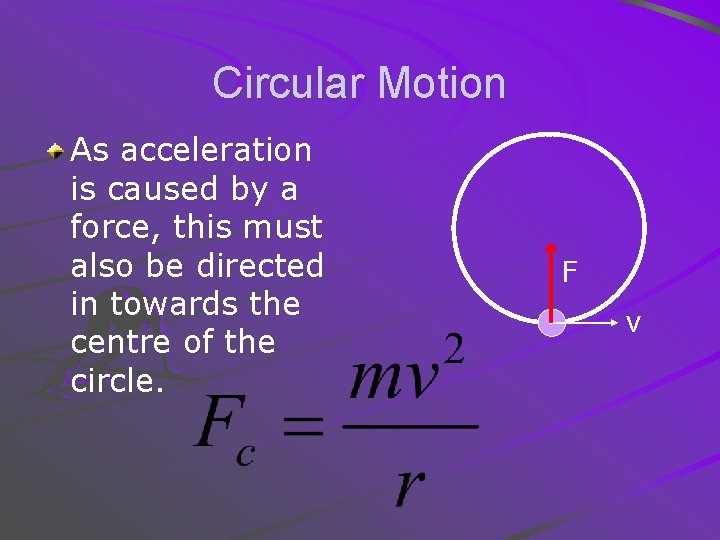
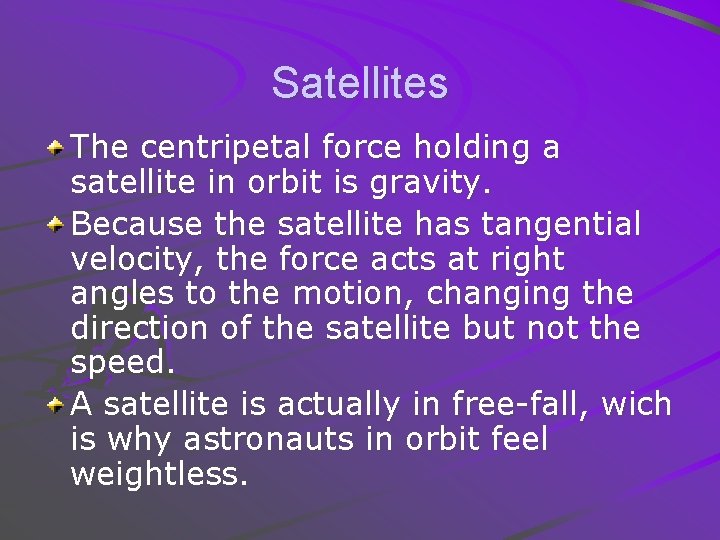
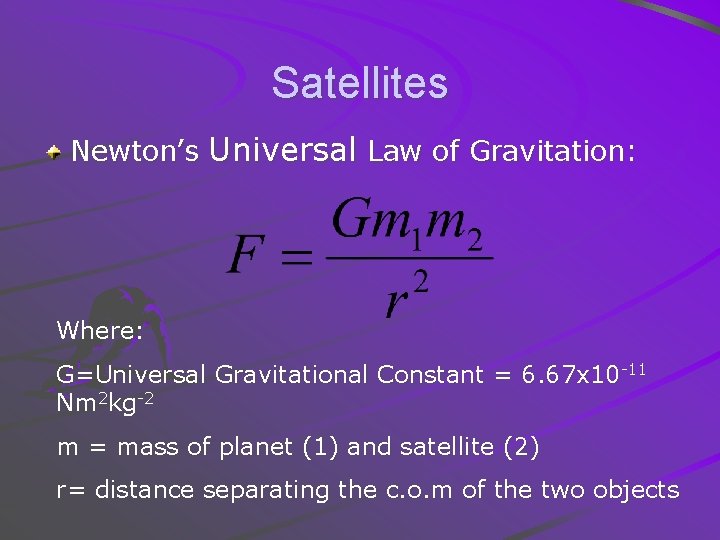
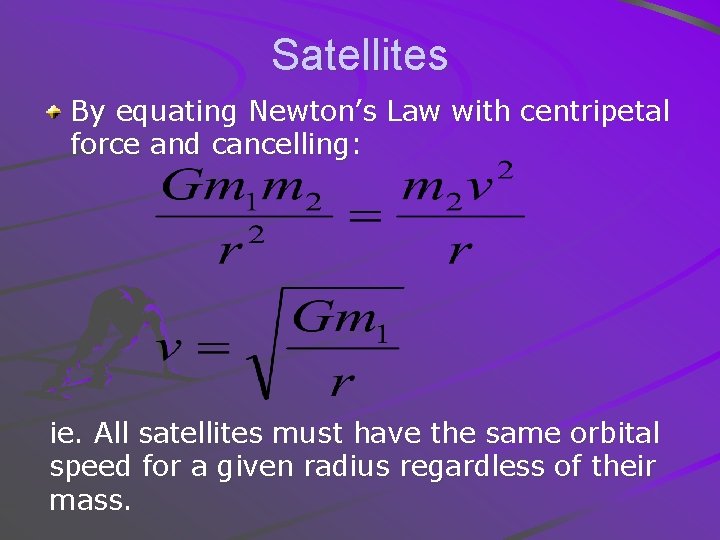
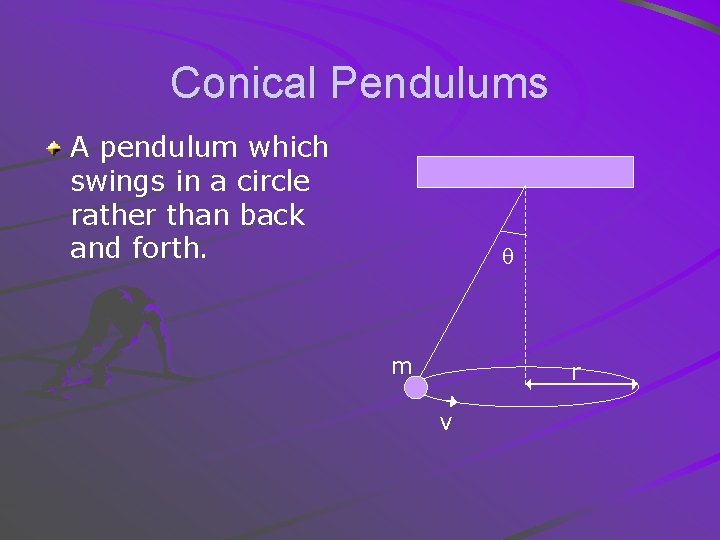
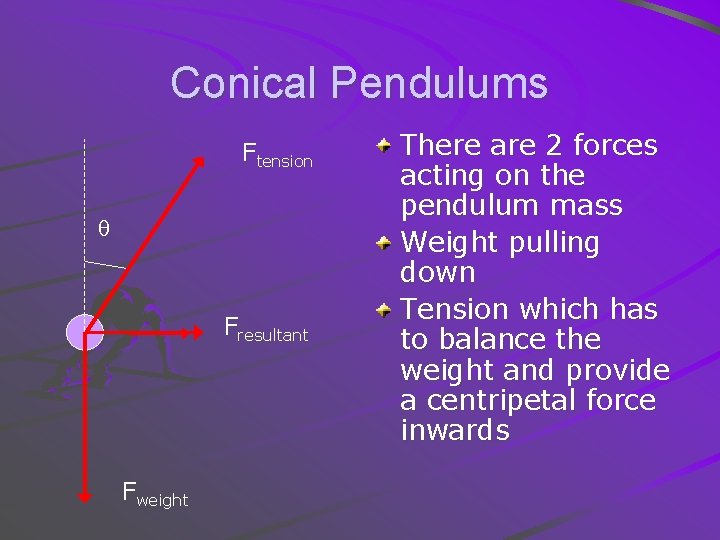
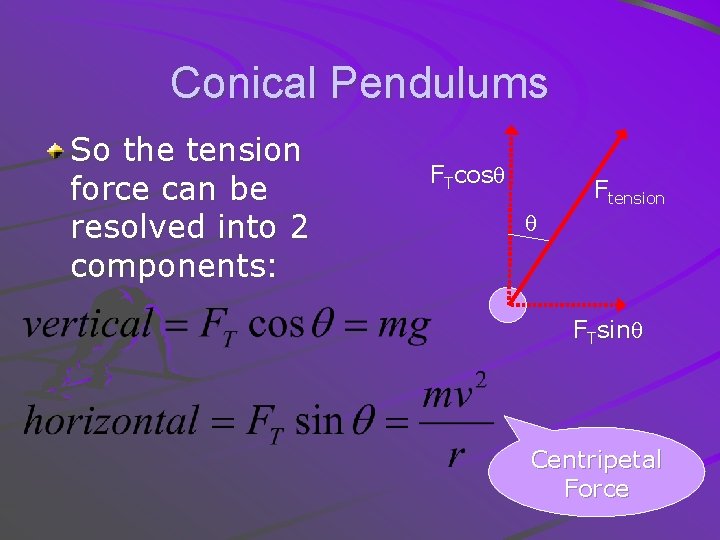
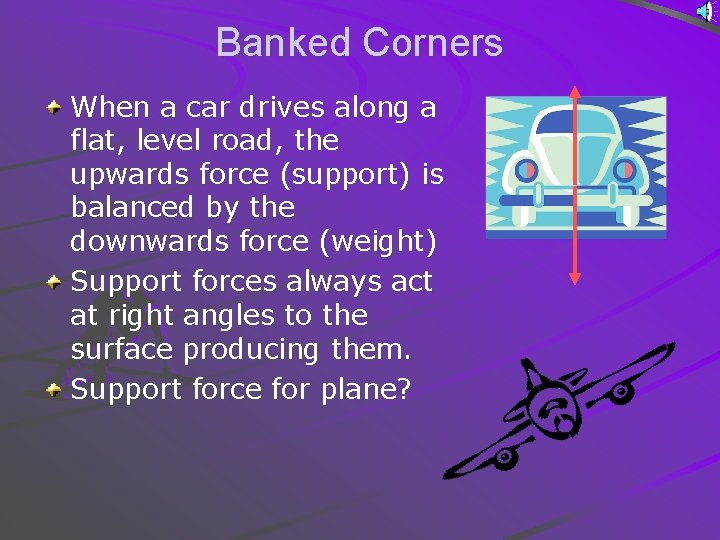
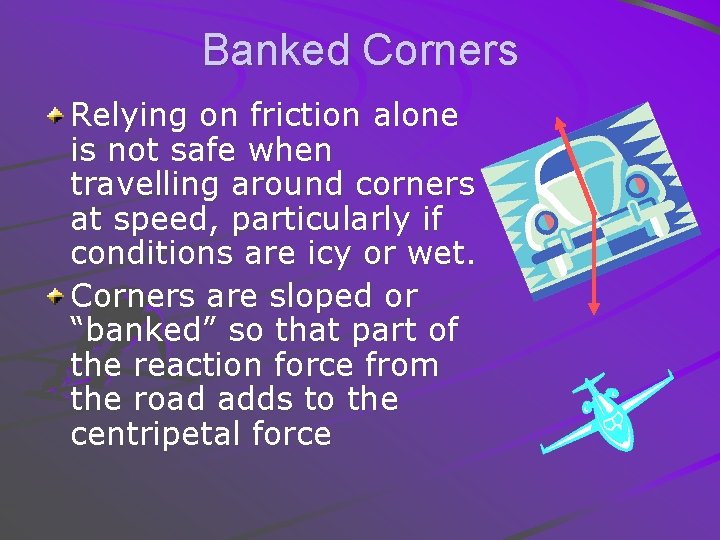
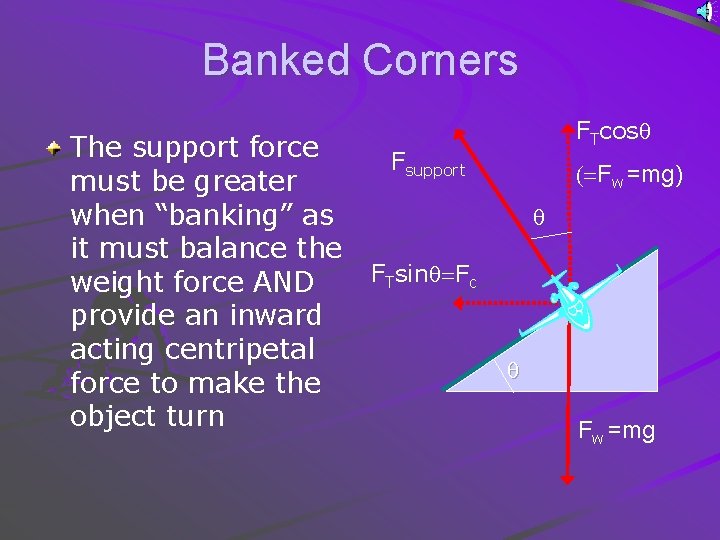
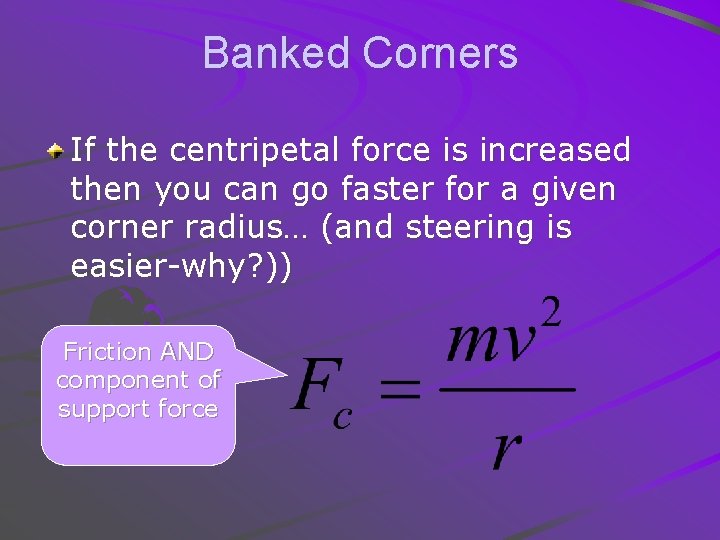
- Slides: 31
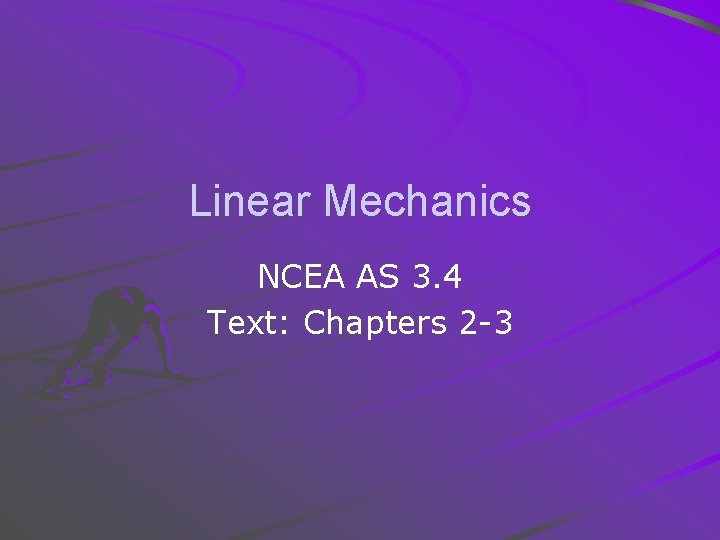
Linear Mechanics NCEA AS 3. 4 Text: Chapters 2 -3
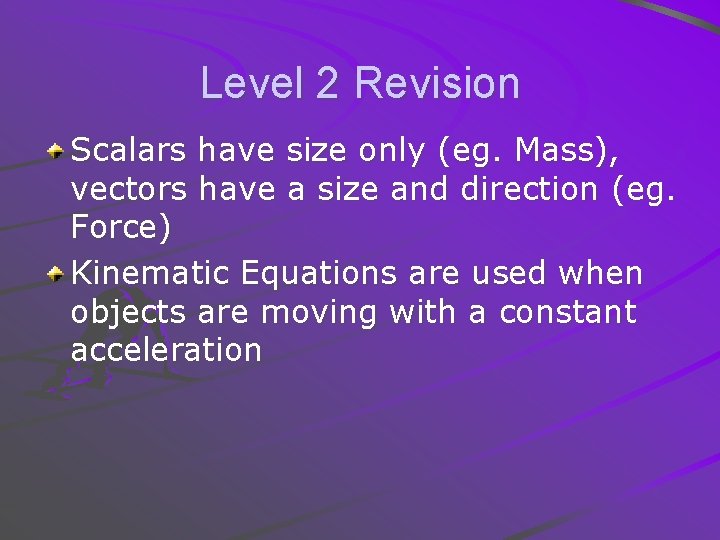
Level 2 Revision Scalars have size only (eg. Mass), vectors have a size and direction (eg. Force) Kinematic Equations are used when objects are moving with a constant acceleration
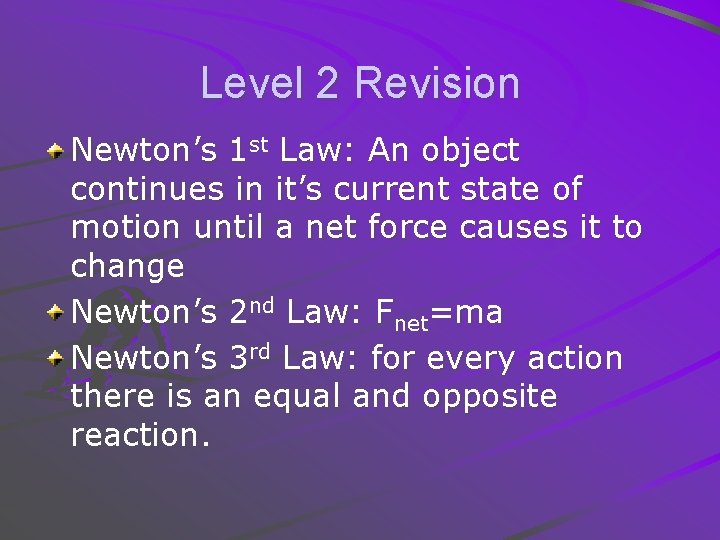
Level 2 Revision Newton’s 1 st Law: An object continues in it’s current state of motion until a net force causes it to change Newton’s 2 nd Law: Fnet=ma Newton’s 3 rd Law: for every action there is an equal and opposite reaction.
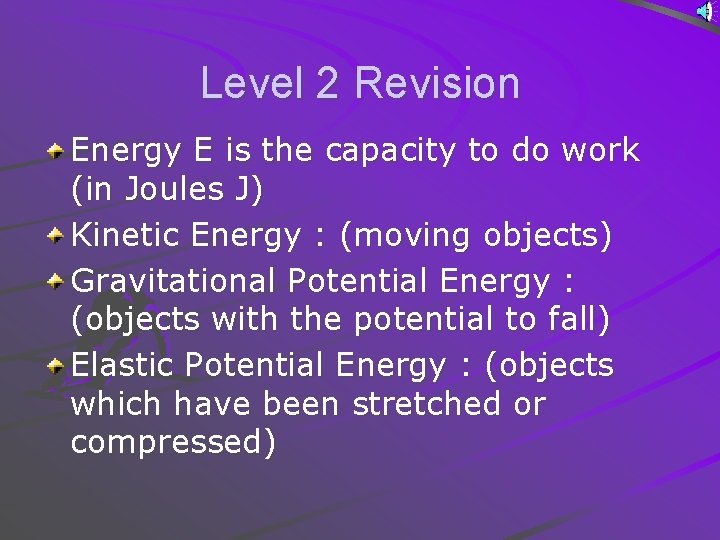
Level 2 Revision Energy E is the capacity to do work (in Joules J) Kinetic Energy : (moving objects) Gravitational Potential Energy : (objects with the potential to fall) Elastic Potential Energy : (objects which have been stretched or compressed)
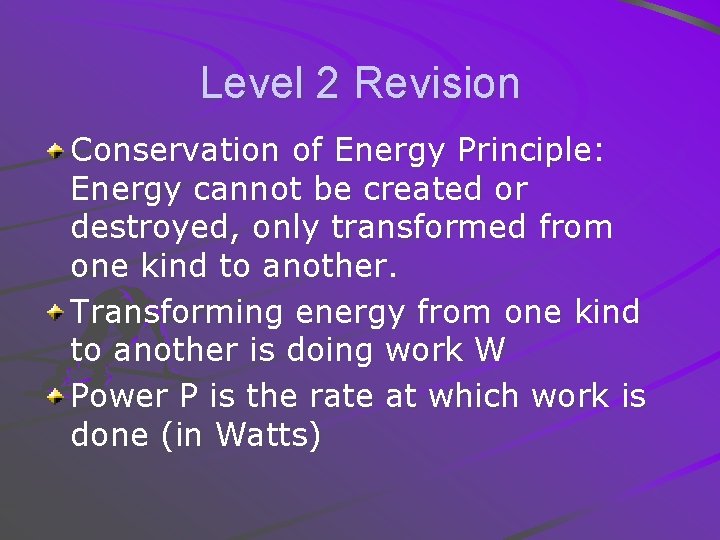
Level 2 Revision Conservation of Energy Principle: Energy cannot be created or destroyed, only transformed from one kind to another. Transforming energy from one kind to another is doing work W Power P is the rate at which work is done (in Watts)
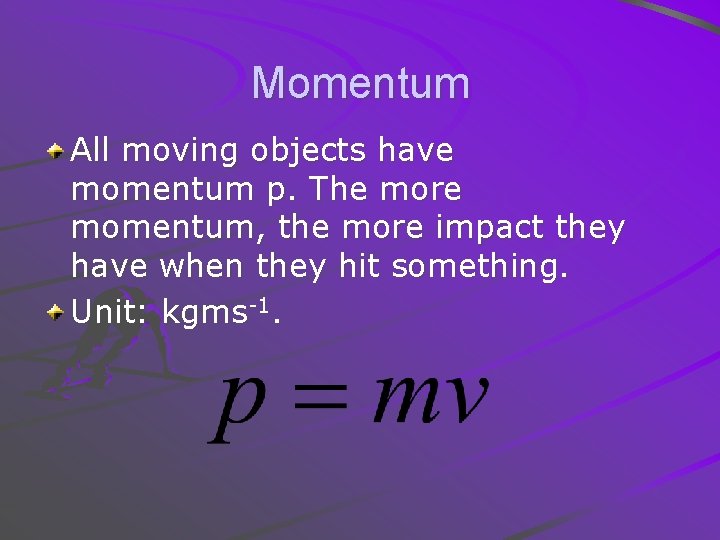
Momentum All moving objects have momentum p. The more momentum, the more impact they have when they hit something. Unit: kgms-1.
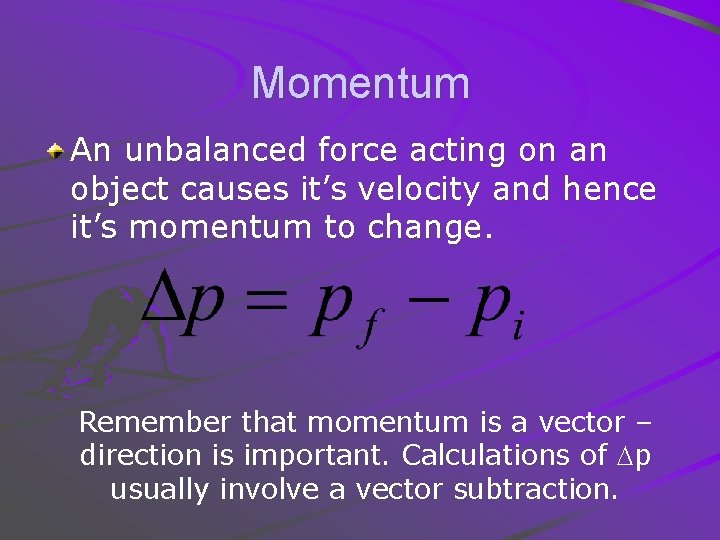
Momentum An unbalanced force acting on an object causes it’s velocity and hence it’s momentum to change. Remember that momentum is a vector – direction is important. Calculations of Dp usually involve a vector subtraction.
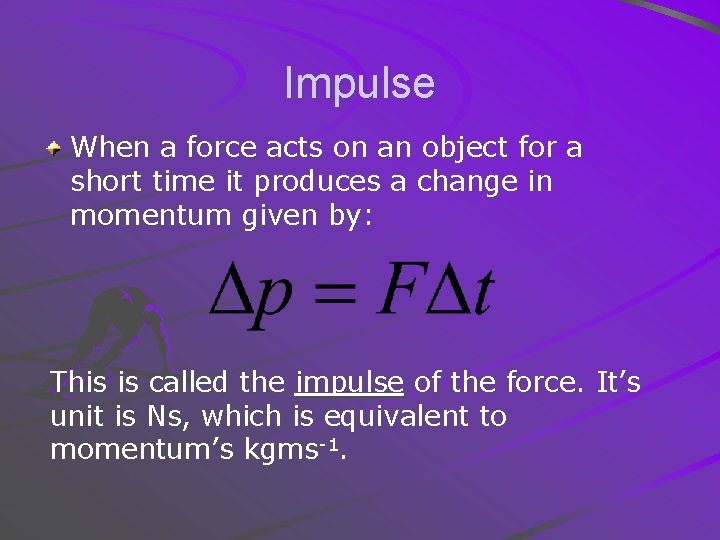
Impulse When a force acts on an object for a short time it produces a change in momentum given by: This is called the impulse of the force. It’s unit is Ns, which is equivalent to momentum’s kgms-1.
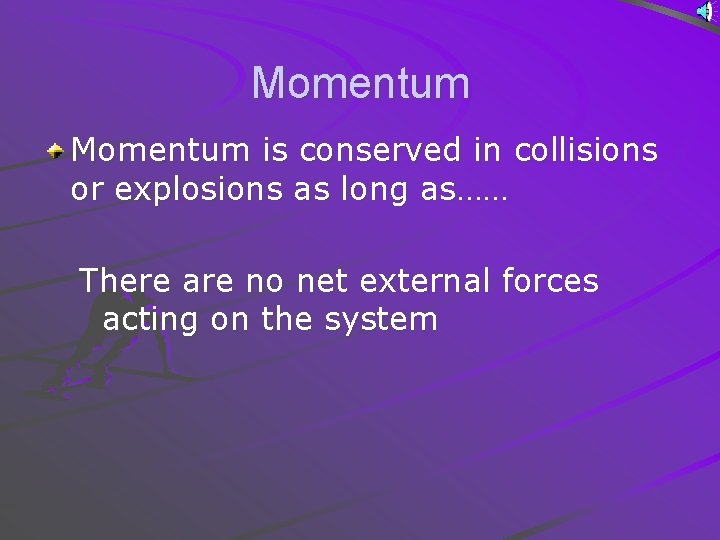
Momentum is conserved in collisions or explosions as long as…… There are no net external forces acting on the system
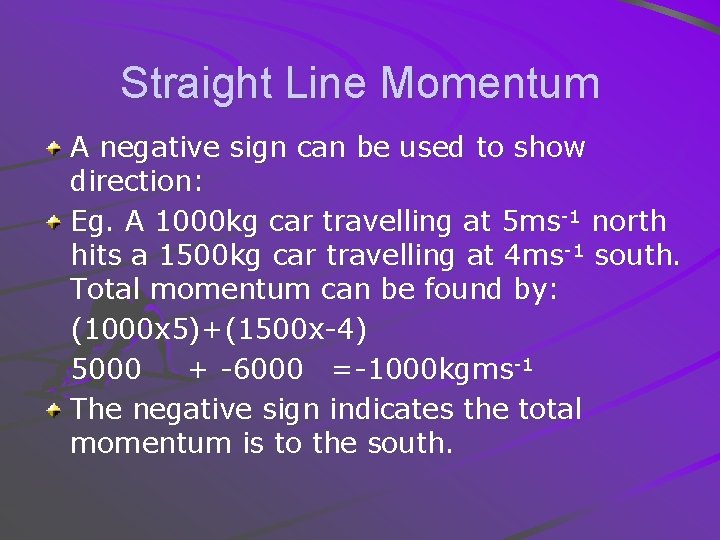
Straight Line Momentum A negative sign can be used to show direction: Eg. A 1000 kg car travelling at 5 ms-1 north hits a 1500 kg car travelling at 4 ms-1 south. Total momentum can be found by: (1000 x 5)+(1500 x-4) 5000 + -6000 =-1000 kgms-1 The negative sign indicates the total momentum is to the south.
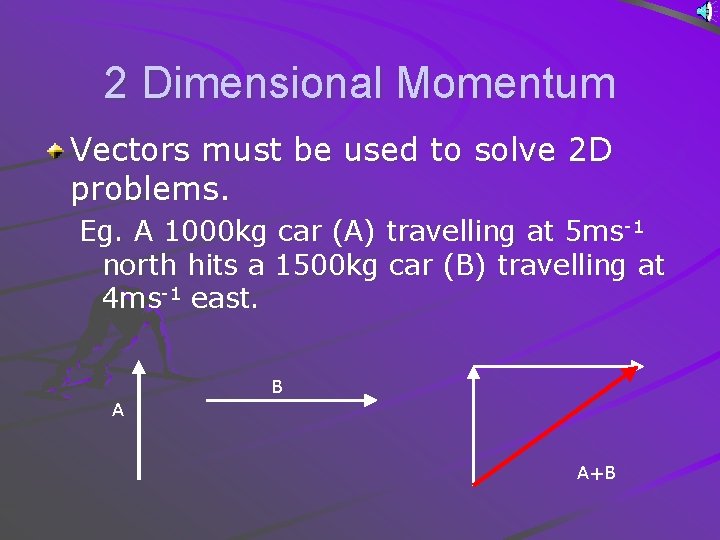
2 Dimensional Momentum Vectors must be used to solve 2 D problems. Eg. A 1000 kg car (A) travelling at 5 ms-1 north hits a 1500 kg car (B) travelling at 4 ms-1 east. B A A+B
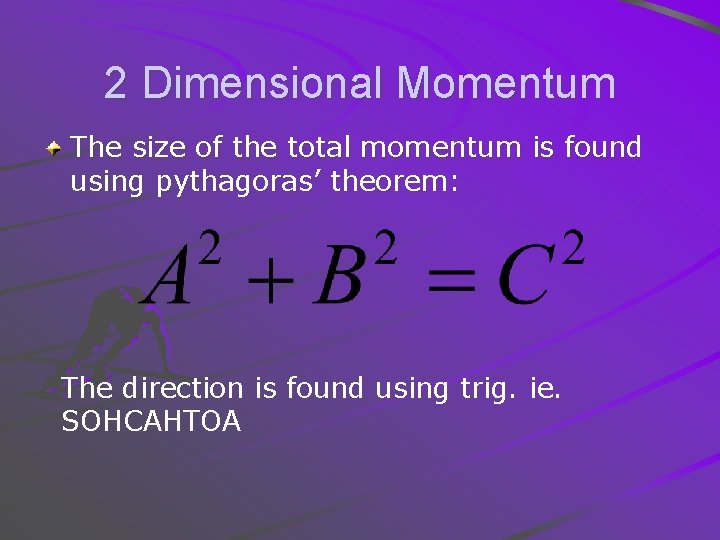
2 Dimensional Momentum The size of the total momentum is found using pythagoras’ theorem: The direction is found using trig. ie. SOHCAHTOA
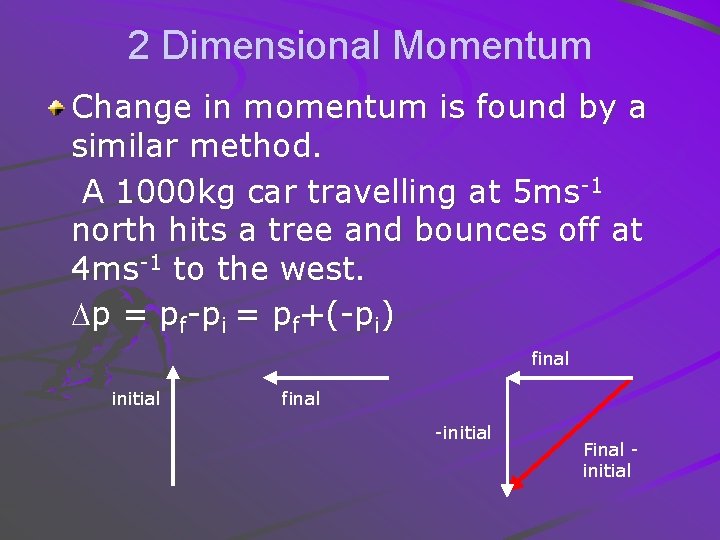
2 Dimensional Momentum Change in momentum is found by a similar method. A 1000 kg car travelling at 5 ms-1 north hits a tree and bounces off at 4 ms-1 to the west. Dp = pf-pi = pf+(-pi) final initial final -initial Final initial
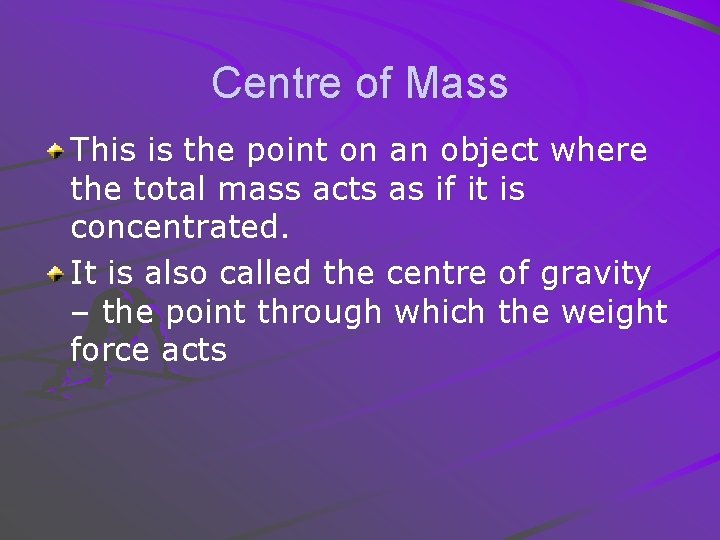
Centre of Mass This is the point on an object where the total mass acts as if it is concentrated. It is also called the centre of gravity – the point through which the weight force acts
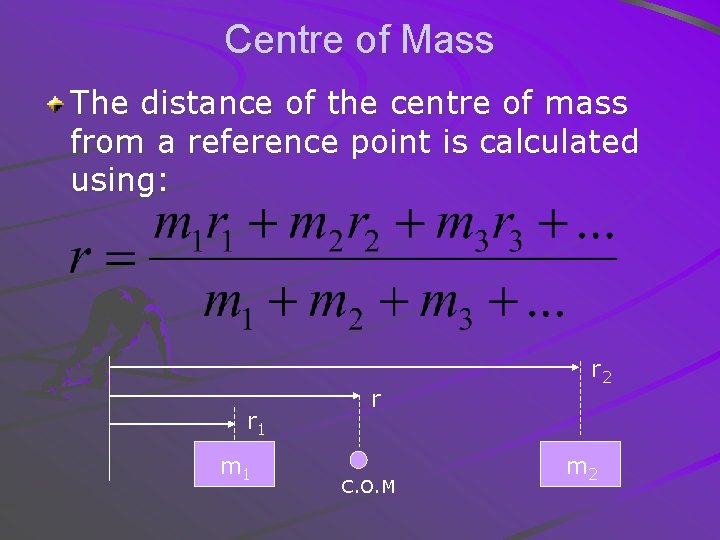
Centre of Mass The distance of the centre of mass from a reference point is calculated using: r 1 m 1 r C. O. M r 2 m 2
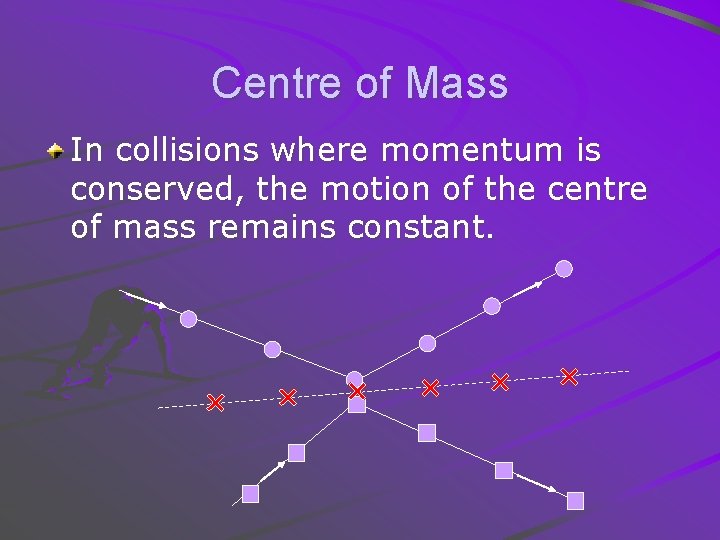
Centre of Mass In collisions where momentum is conserved, the motion of the centre of mass remains constant.
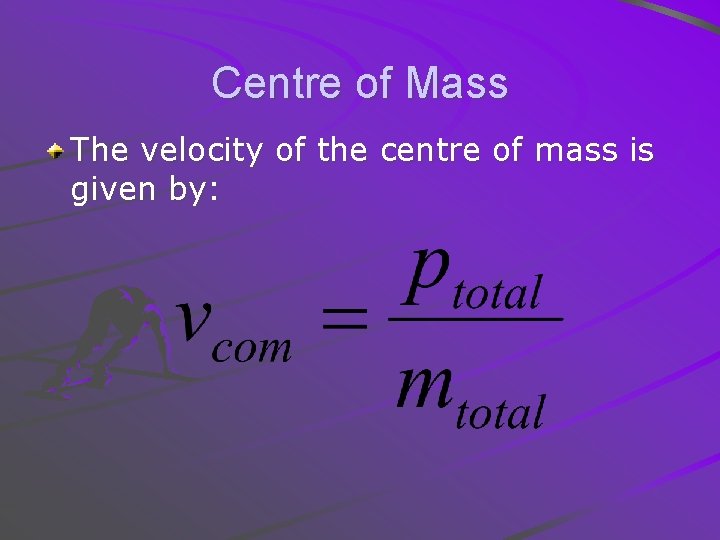
Centre of Mass The velocity of the centre of mass is given by:
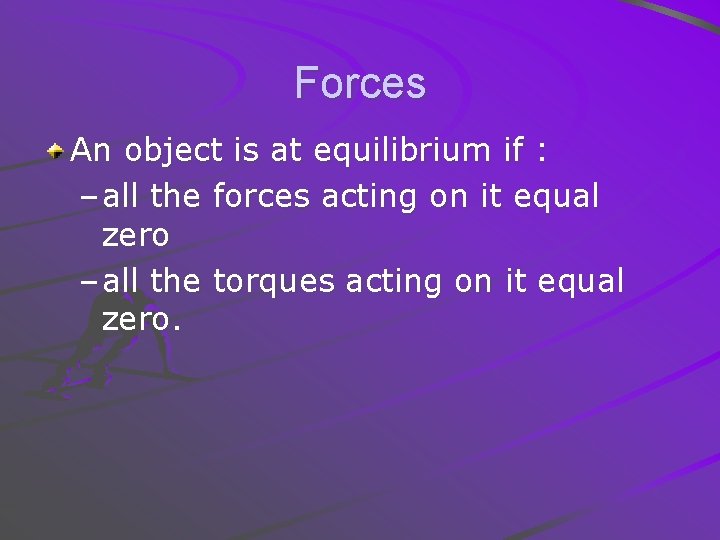
Forces An object is at equilibrium if : – all the forces acting on it equal zero – all the torques acting on it equal zero.
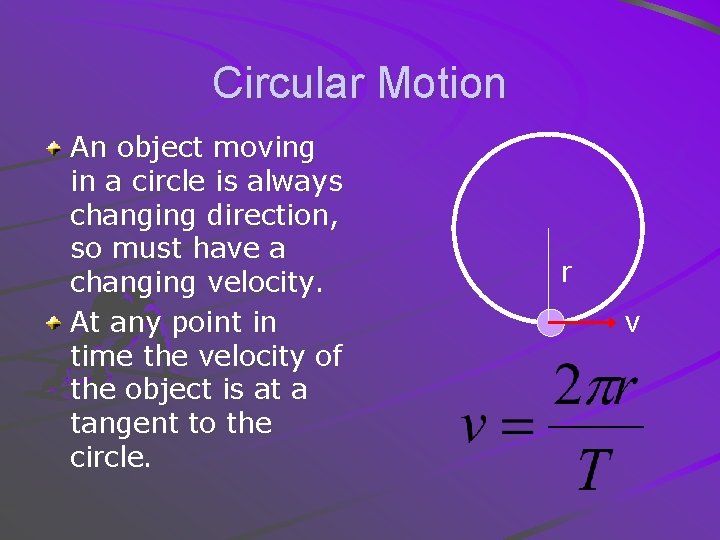
Circular Motion An object moving in a circle is always changing direction, so must have a changing velocity. At any point in time the velocity of the object is at a tangent to the circle. r v
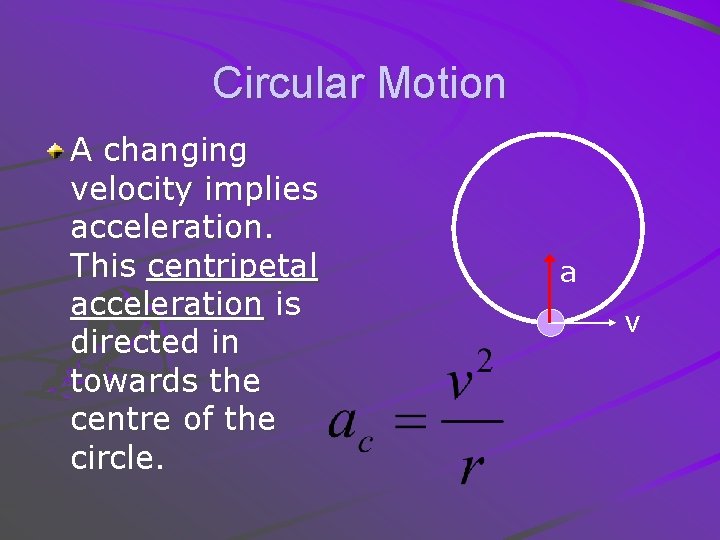
Circular Motion A changing velocity implies acceleration. This centripetal acceleration is directed in towards the centre of the circle. a v
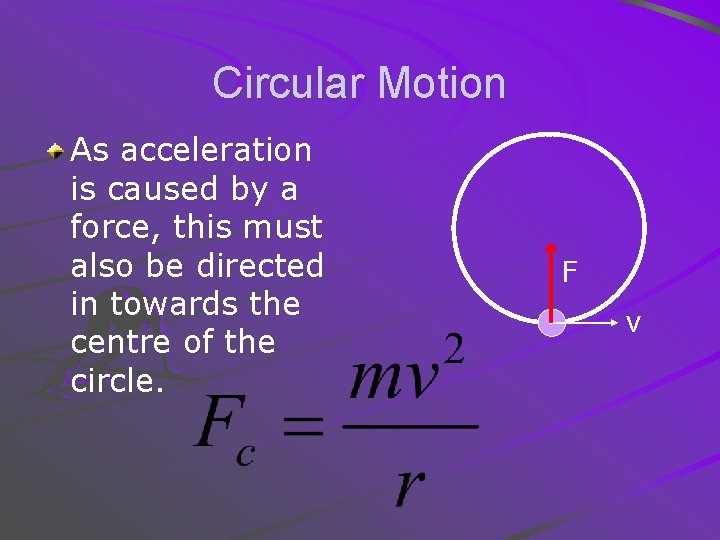
Circular Motion As acceleration is caused by a force, this must also be directed in towards the centre of the circle. F v
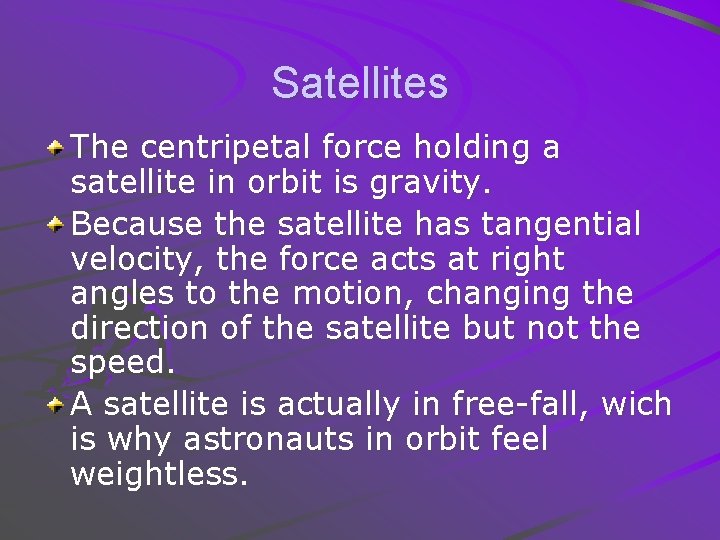
Satellites The centripetal force holding a satellite in orbit is gravity. Because the satellite has tangential velocity, the force acts at right angles to the motion, changing the direction of the satellite but not the speed. A satellite is actually in free-fall, wich is why astronauts in orbit feel weightless.
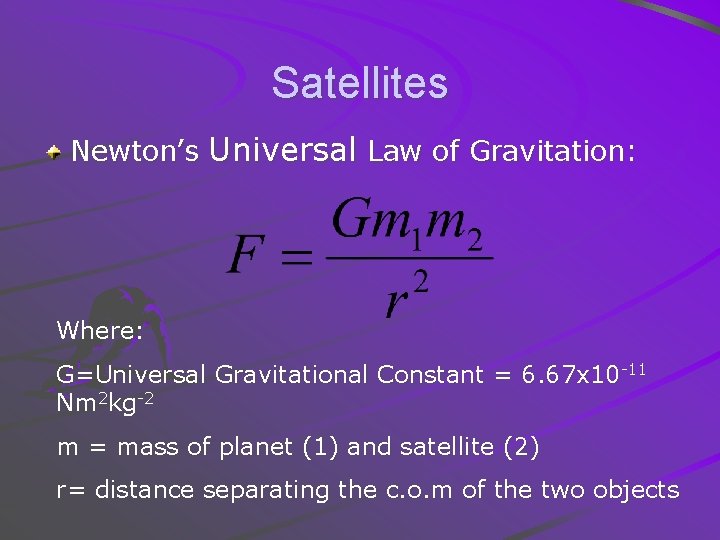
Satellites Newton’s Universal Law of Gravitation: Where: G=Universal Gravitational Constant = 6. 67 x 10 -11 Nm 2 kg-2 m = mass of planet (1) and satellite (2) r= distance separating the c. o. m of the two objects
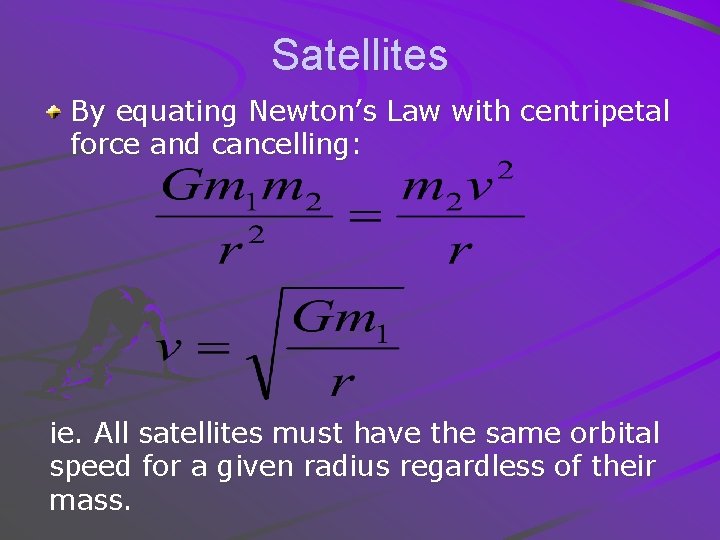
Satellites By equating Newton’s Law with centripetal force and cancelling: ie. All satellites must have the same orbital speed for a given radius regardless of their mass.
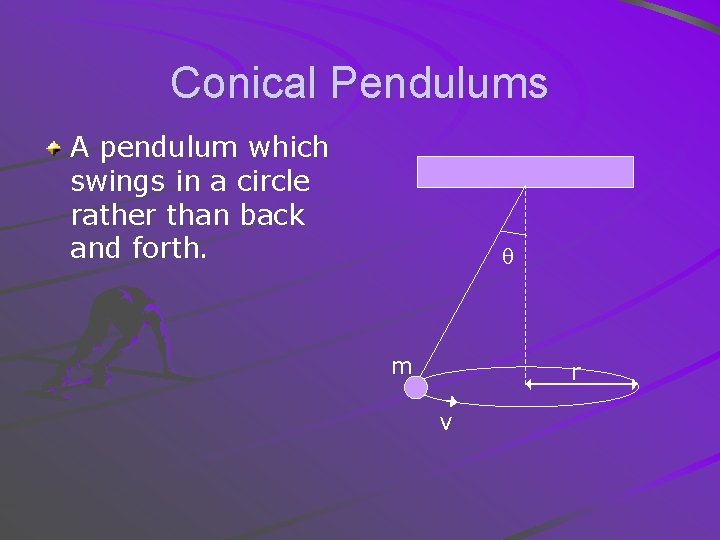
Conical Pendulums A pendulum which swings in a circle rather than back and forth. q m r v
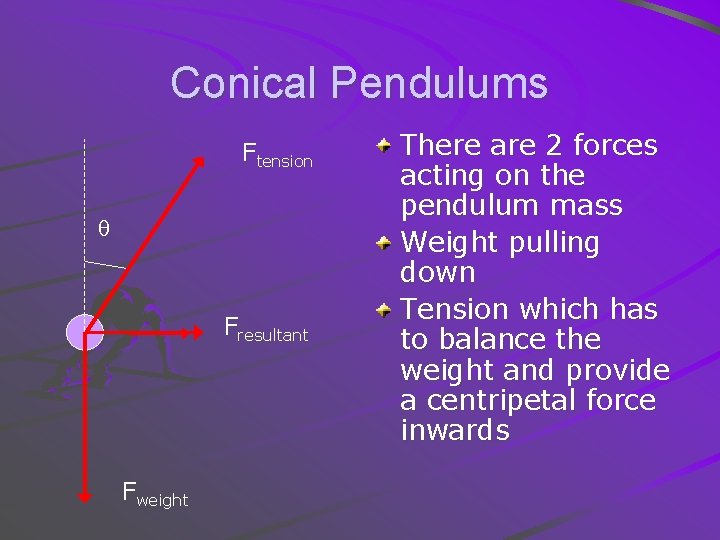
Conical Pendulums Ftension q Fresultant Fweight There are 2 forces acting on the pendulum mass Weight pulling down Tension which has to balance the weight and provide a centripetal force inwards
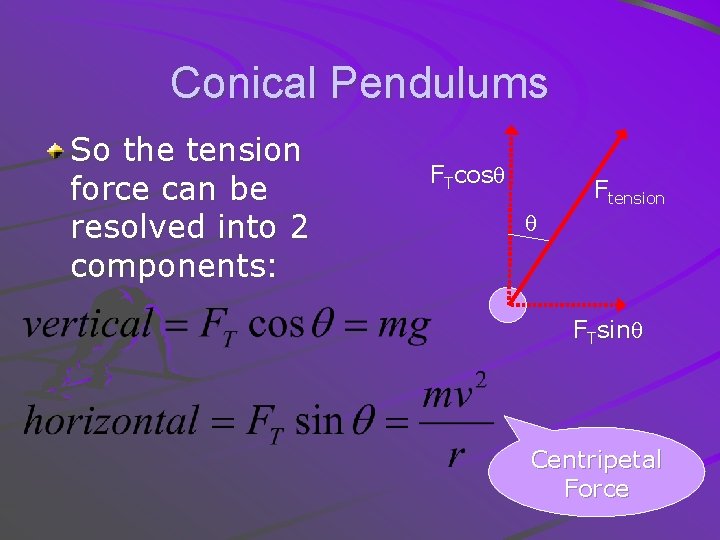
Conical Pendulums So the tension force can be resolved into 2 components: FTcosq q Ftension FTsinq Centripetal Force
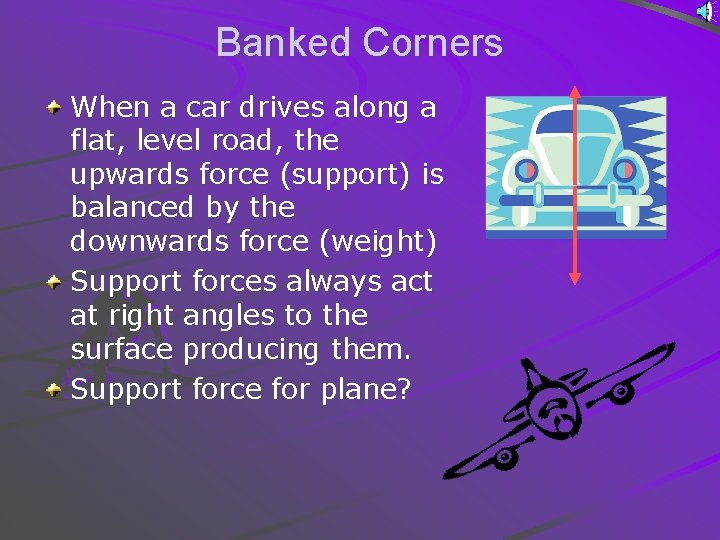
Banked Corners When a car drives along a flat, level road, the upwards force (support) is balanced by the downwards force (weight) Support forces always act at right angles to the surface producing them. Support force for plane?
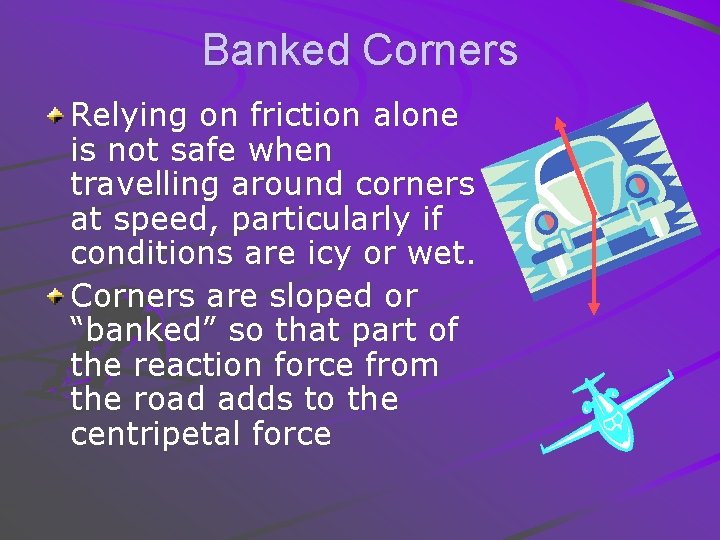
Banked Corners Relying on friction alone is not safe when travelling around corners at speed, particularly if conditions are icy or wet. Corners are sloped or “banked” so that part of the reaction force from the road adds to the centripetal force
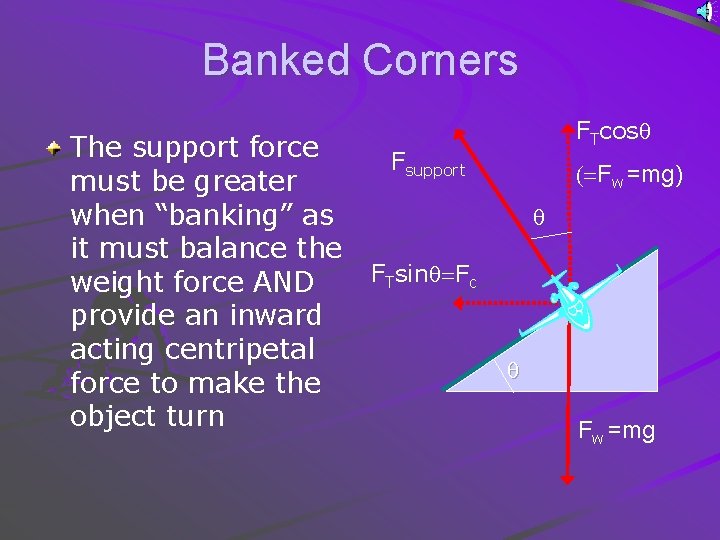
Banked Corners The support force must be greater when “banking” as it must balance the weight force AND provide an inward acting centripetal force to make the object turn FTcosq Fsupport (=Fw =mg) q FTsinq=Fc q Fw =mg
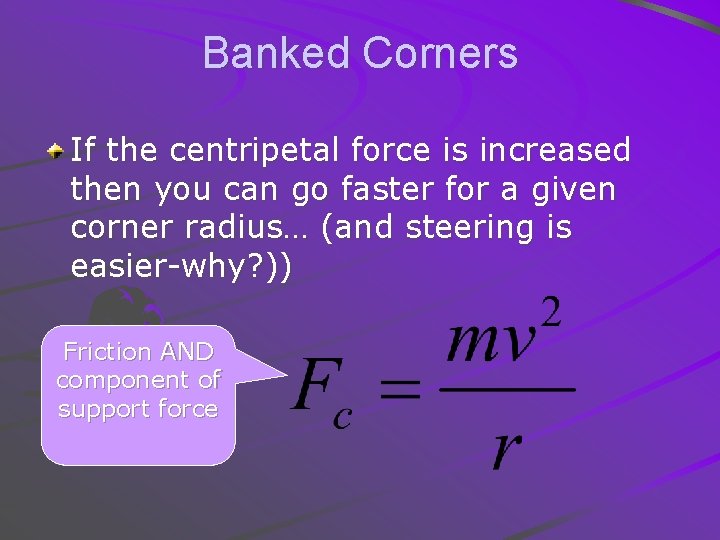
Banked Corners If the centripetal force is increased then you can go faster for a given corner radius… (and steering is easier-why? )) Friction AND component of support force
Nzqa mechanics
Example of linear text
Making connections
Linear text to nonlinear text
Ncea
As90947
Redox reactions ncea level 2
As90947
2020 waves level 2
Solubility rules ncea
90940 ncea
As91165
Ncea
Ncea
As90947
Simple multiple linear regression
Contoh soal metode biseksi dan penyelesaiannya
Definition of linear plot in literature
Contoh soal metode iterasi sederhana
Reservation table in pipeline
Define multimedia
A left linear grammar is always
Fungsi linear fungsi kuadrat dan fungsi rasional
Pengertian fungsi linear dan non linear
Linearly independent
Linear algebra linear transformation
Cara dwi koordinat
Linear impulse equation
Persamaan simultan
Linear vs nonlinear functions
Linear and nonlinear table
Difference between linear and nonlinear equation