GGR 3481408 CFree Energy Supply Chapter 1 Introduction
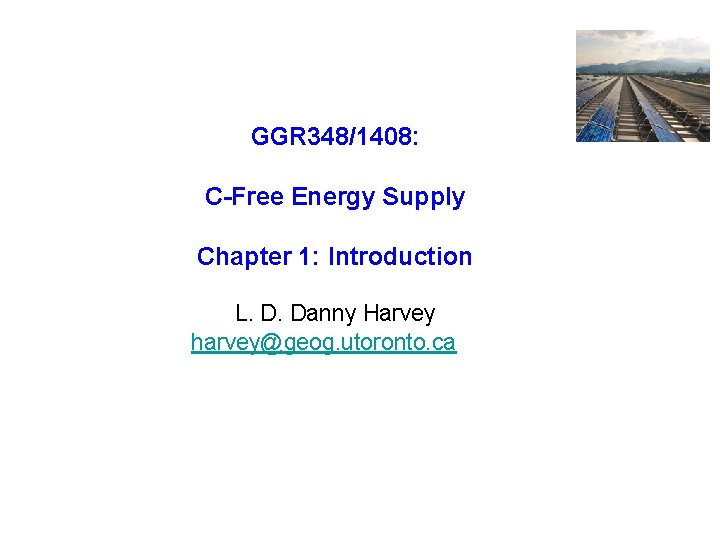
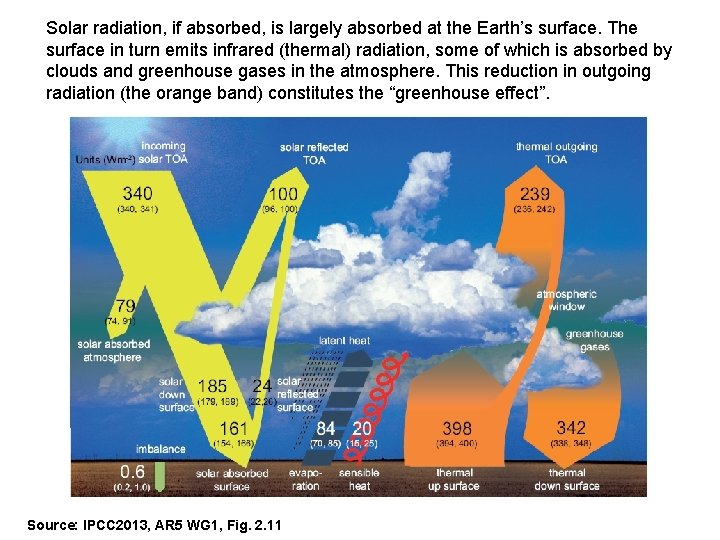
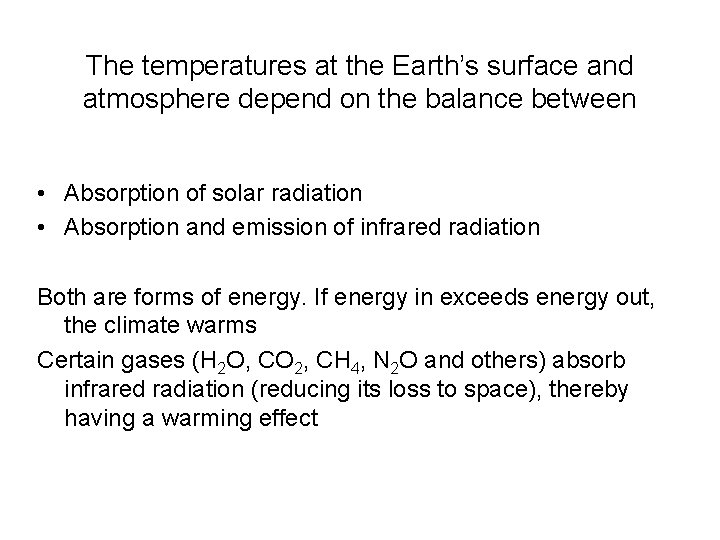
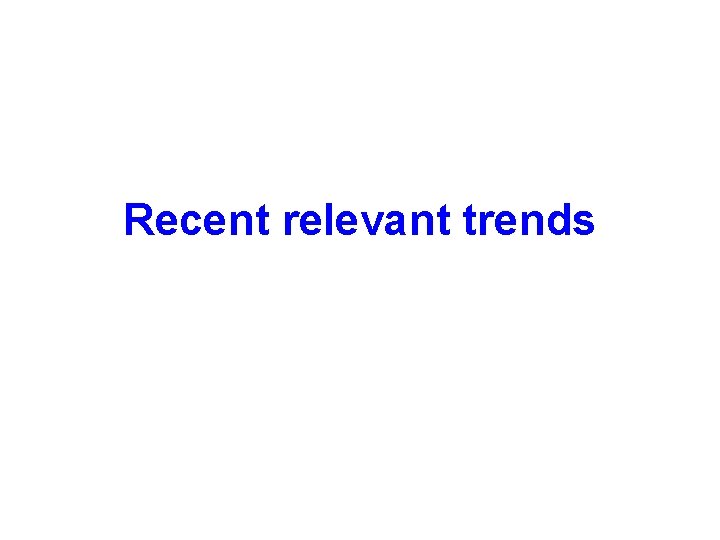
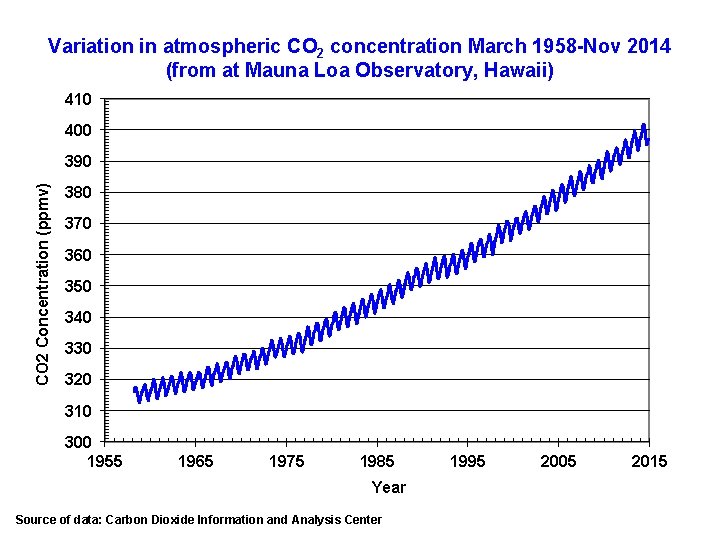
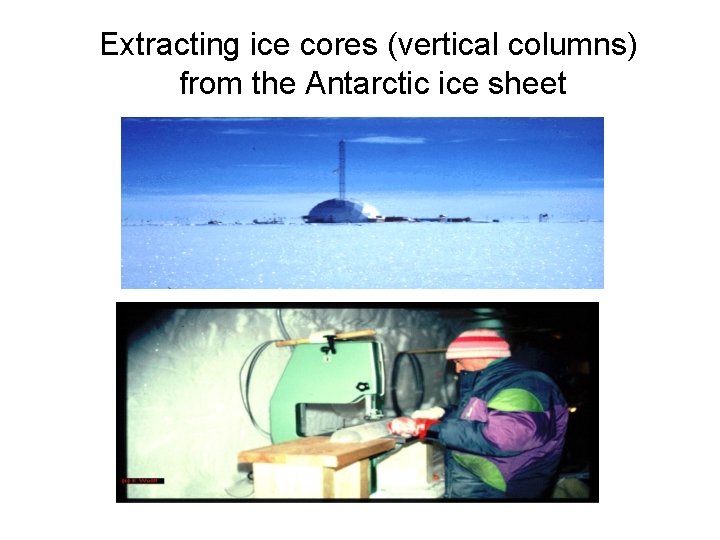
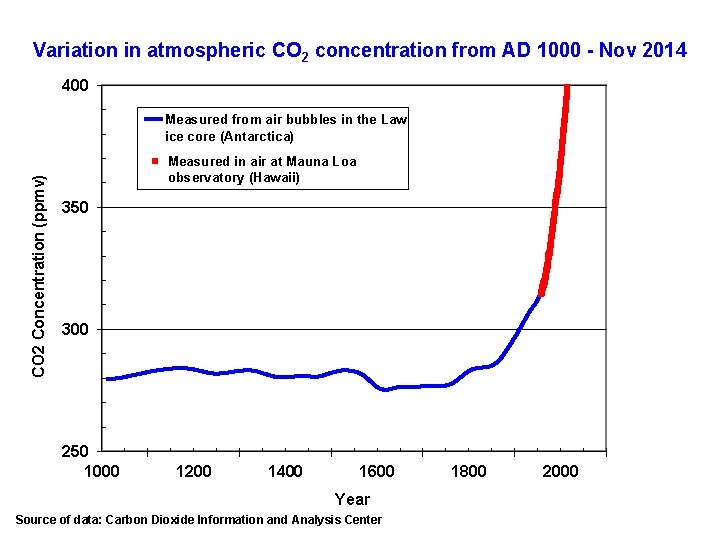
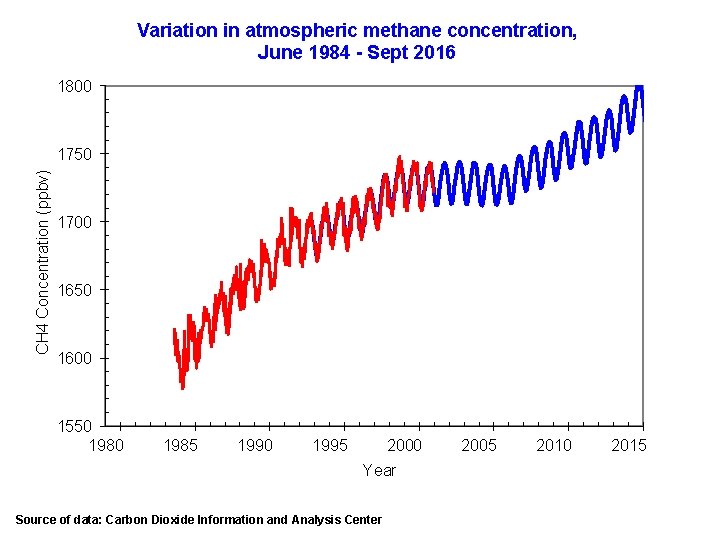
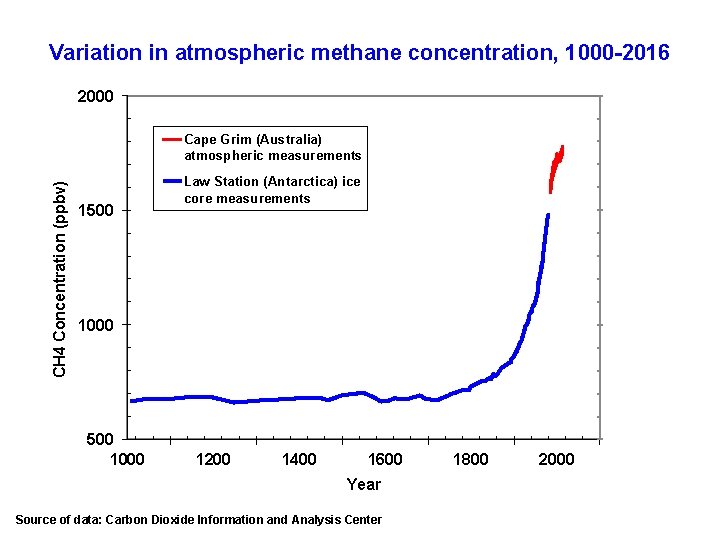
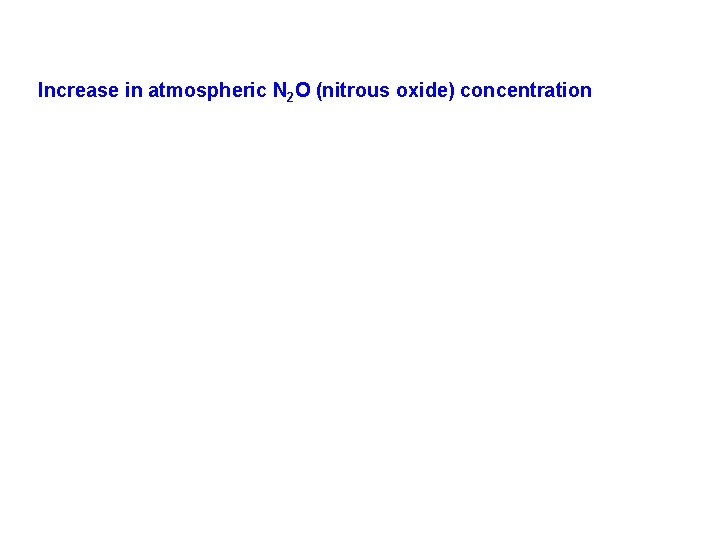
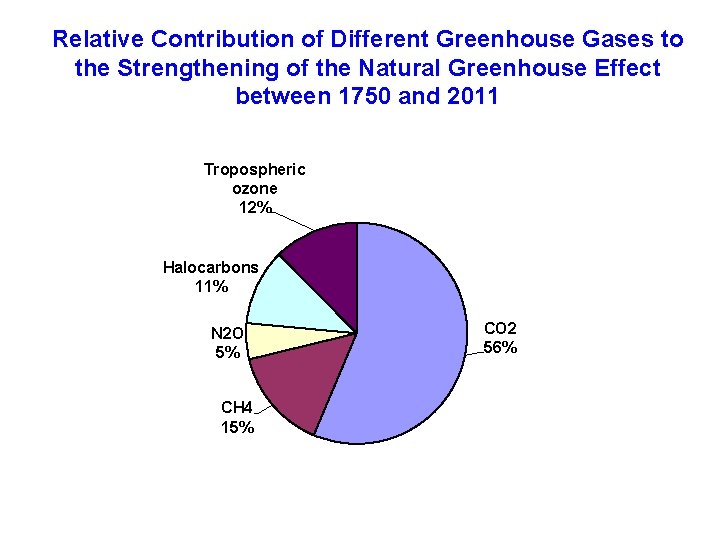
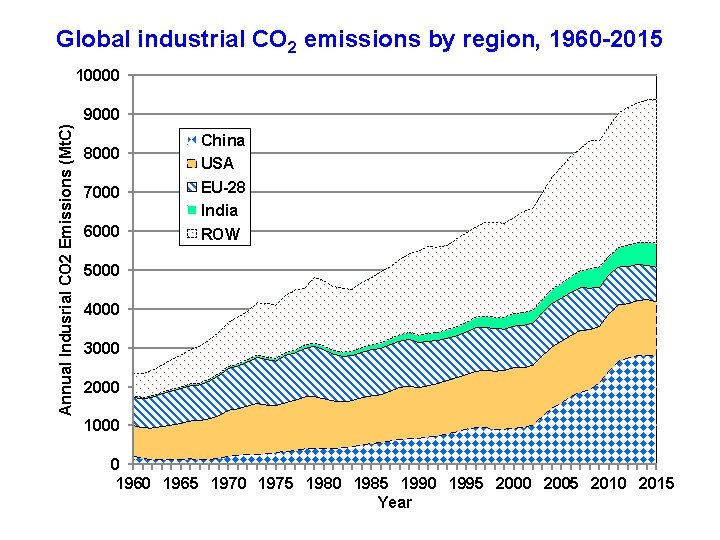
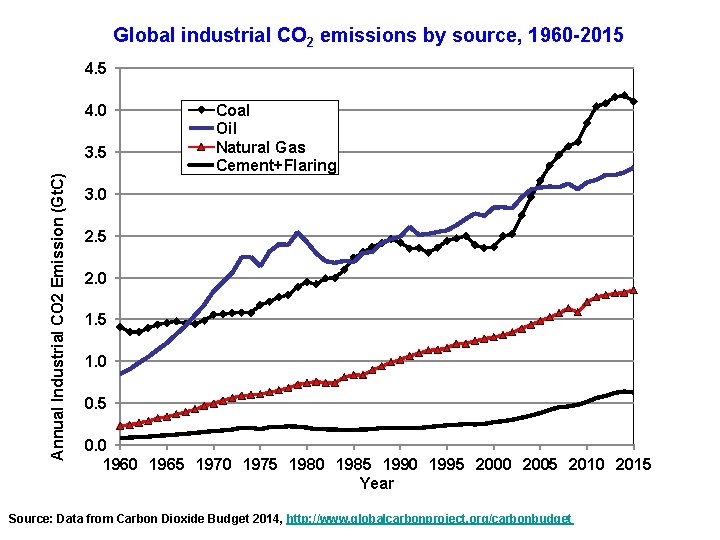
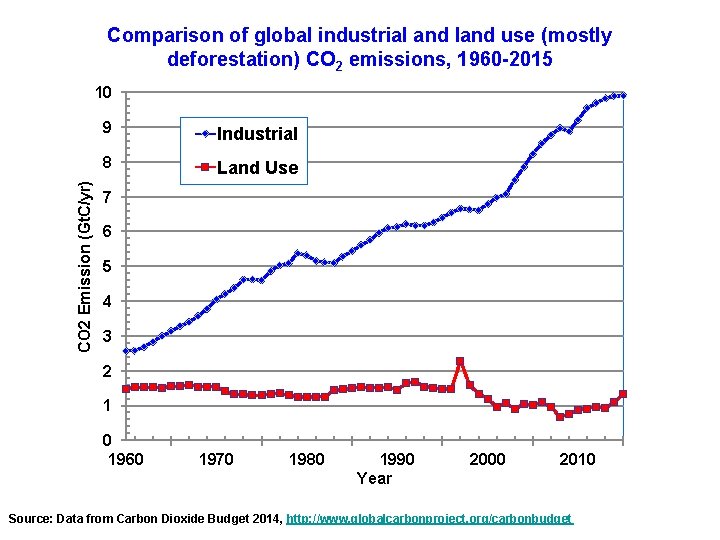
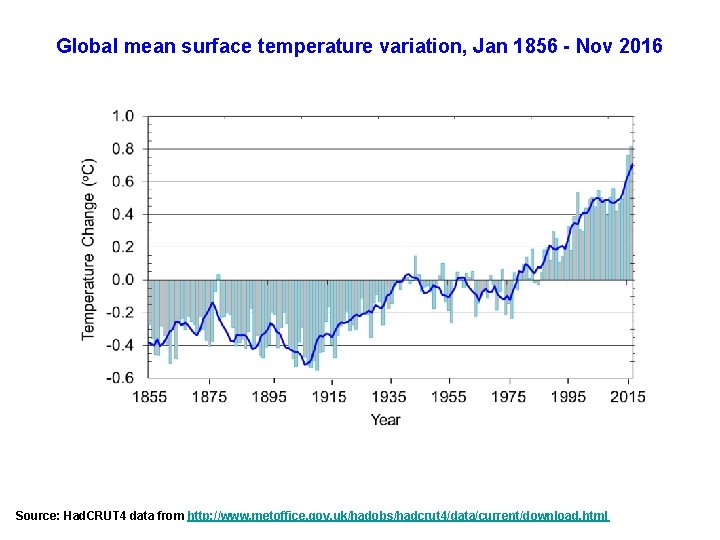
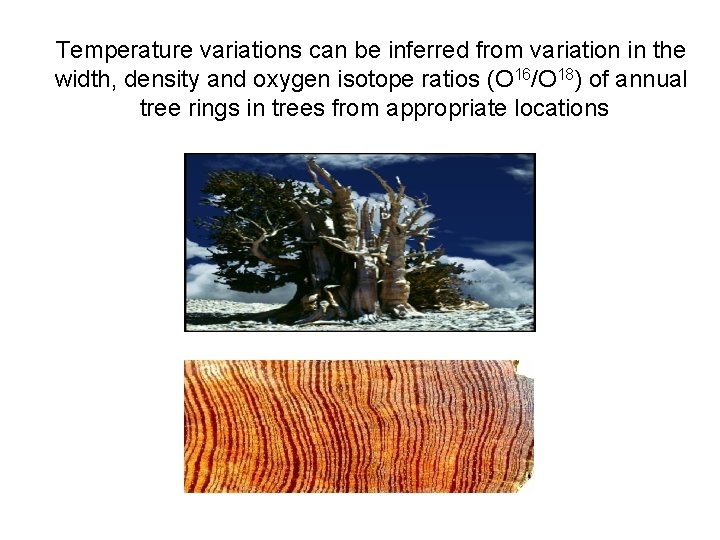
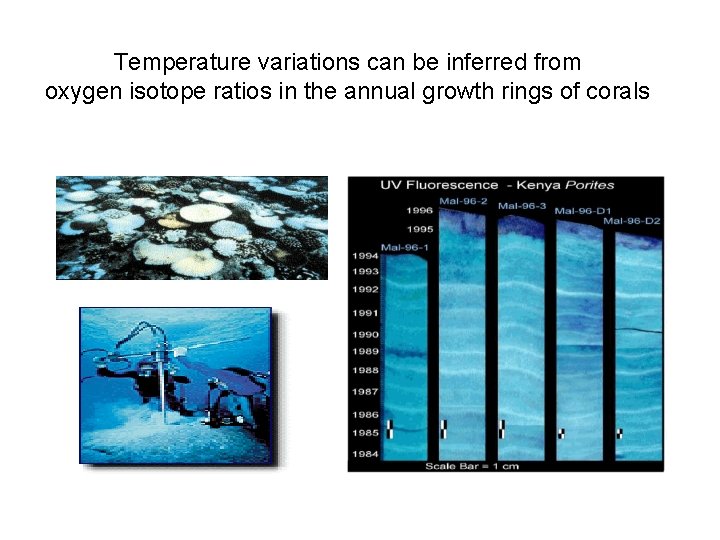
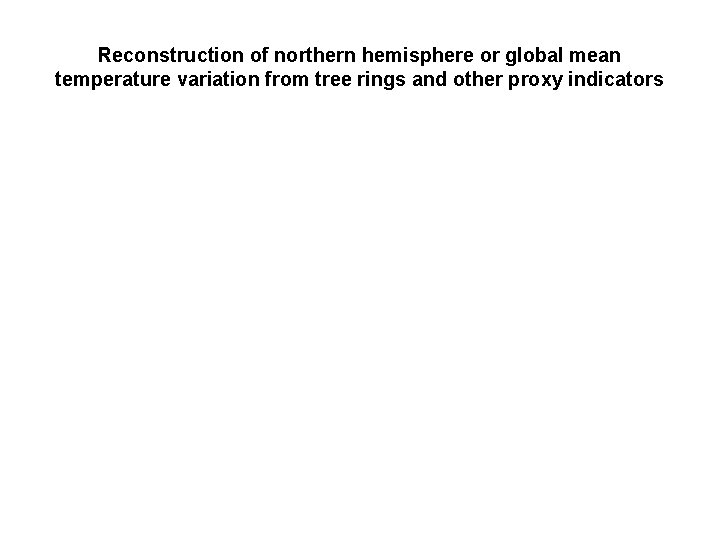
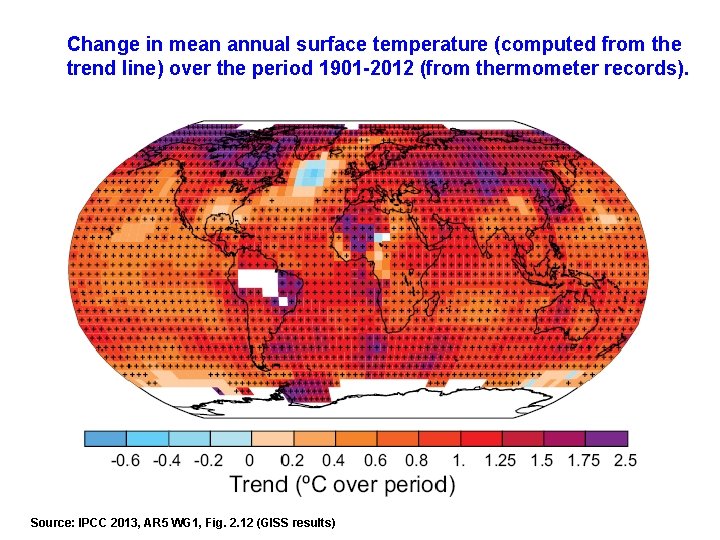
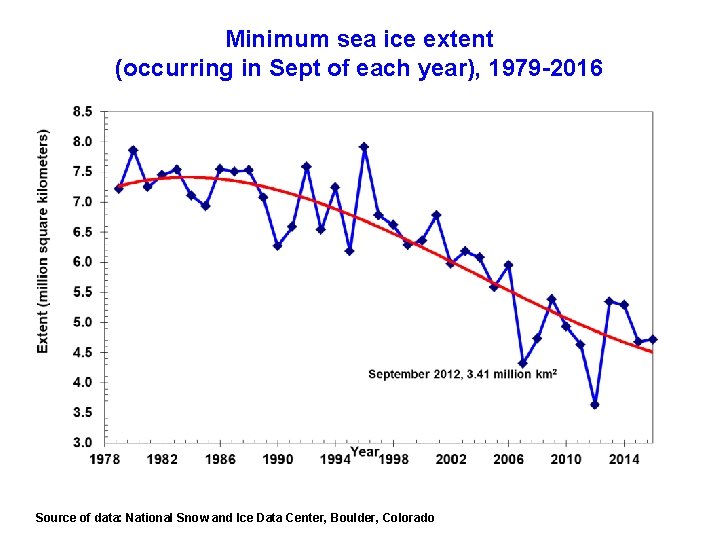
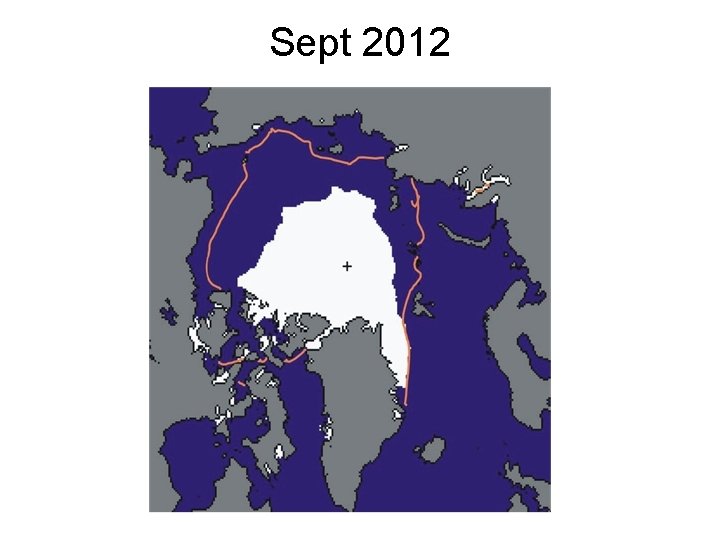
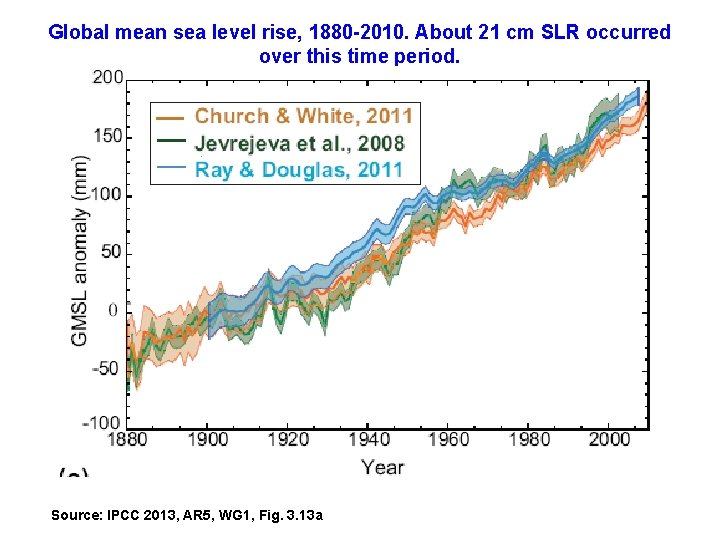
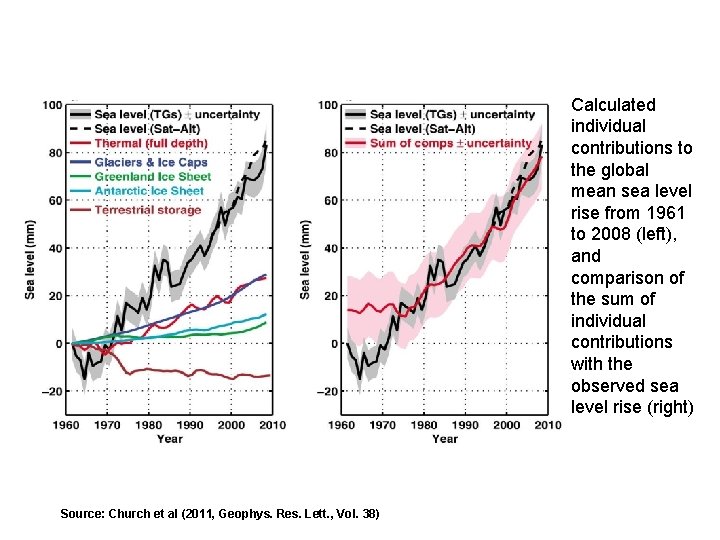
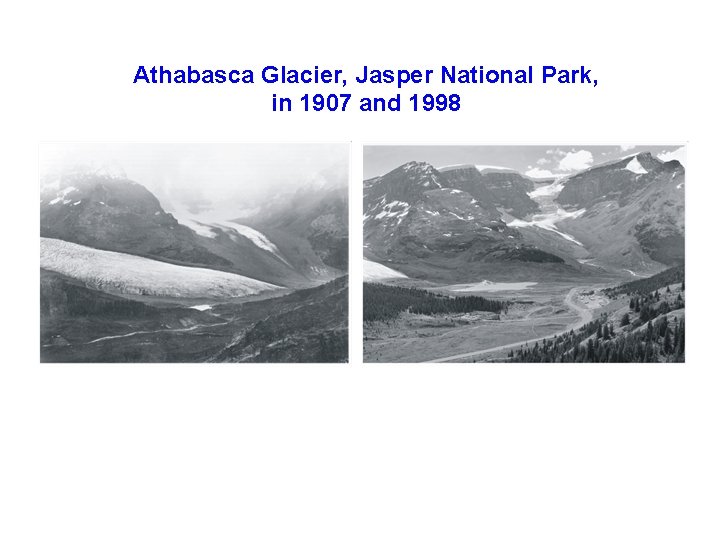
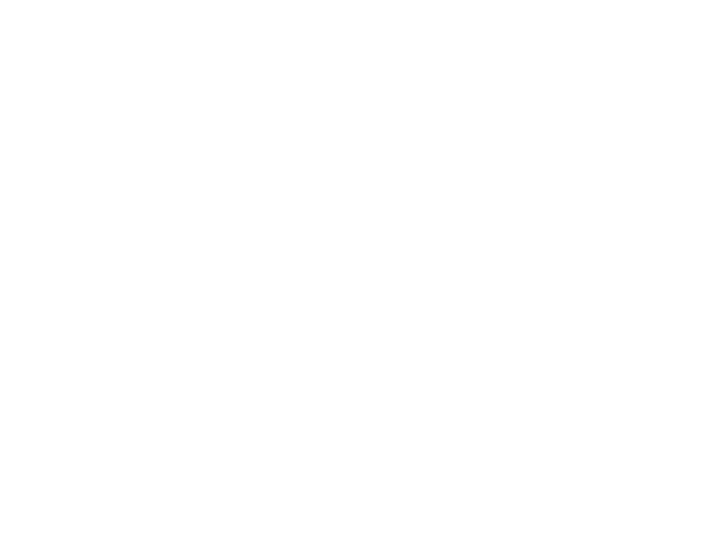
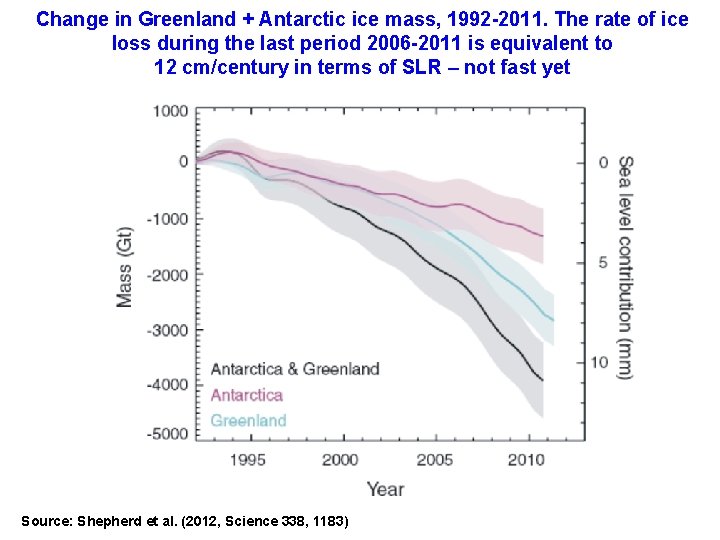
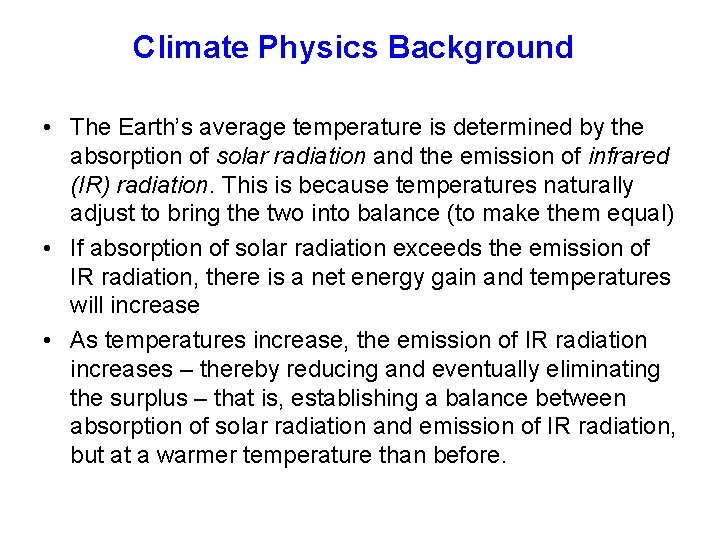
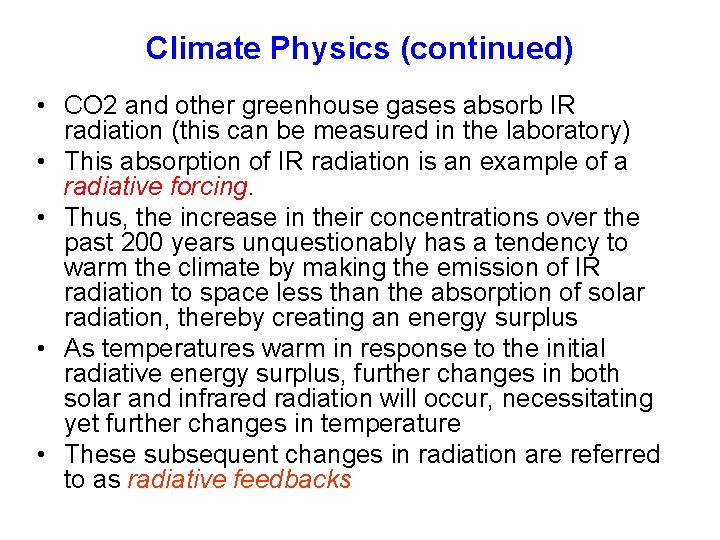
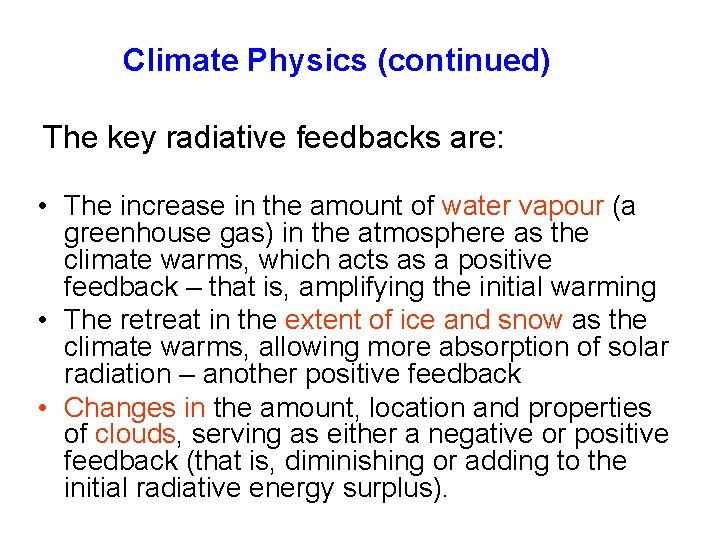
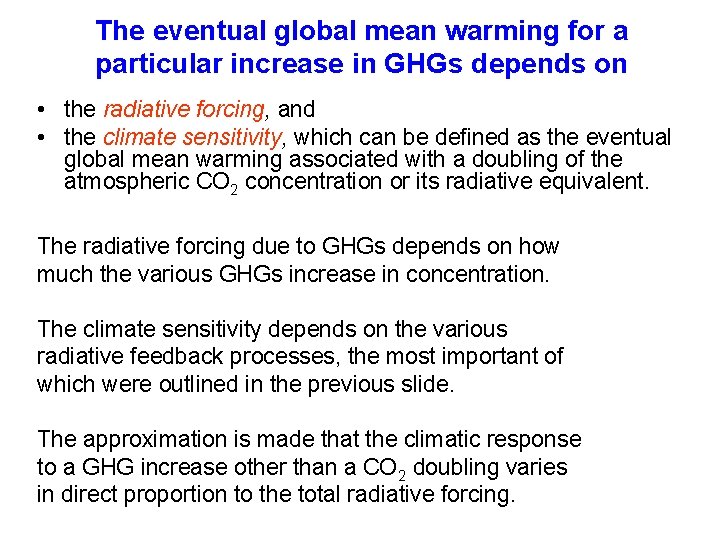
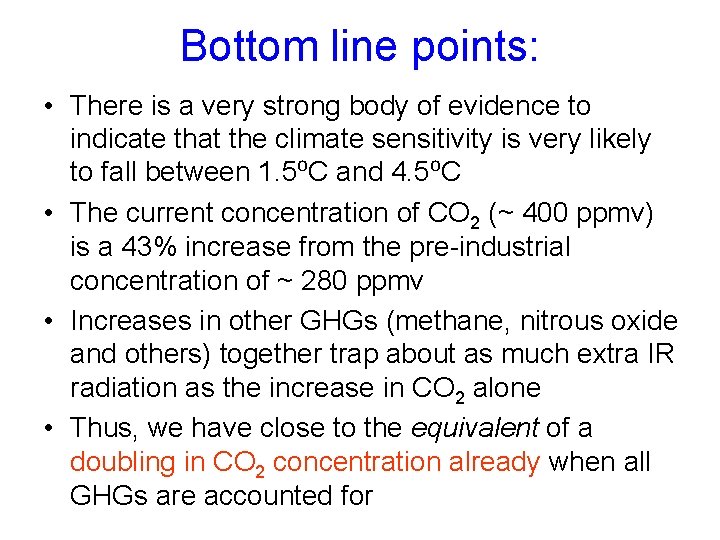
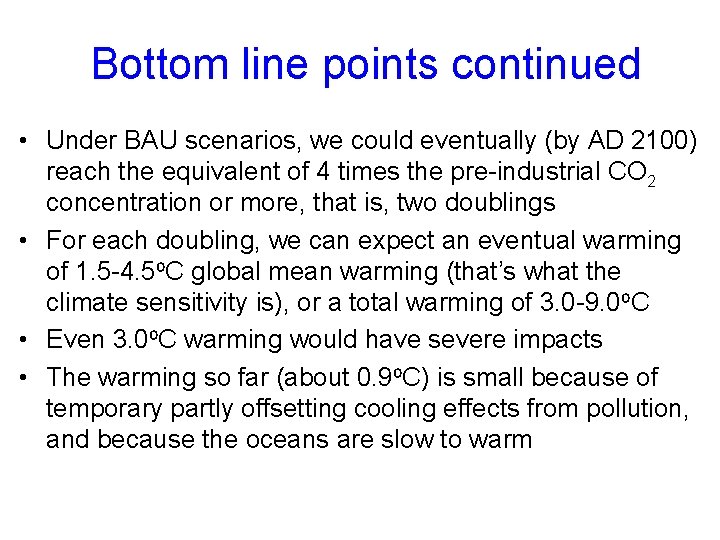
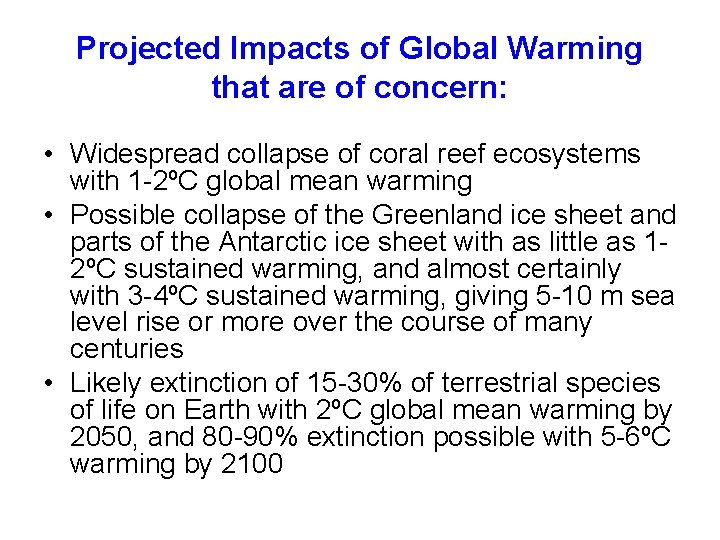
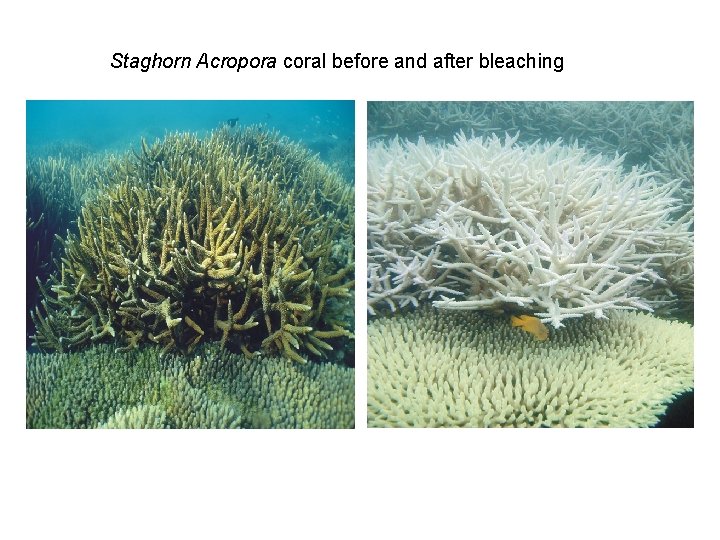
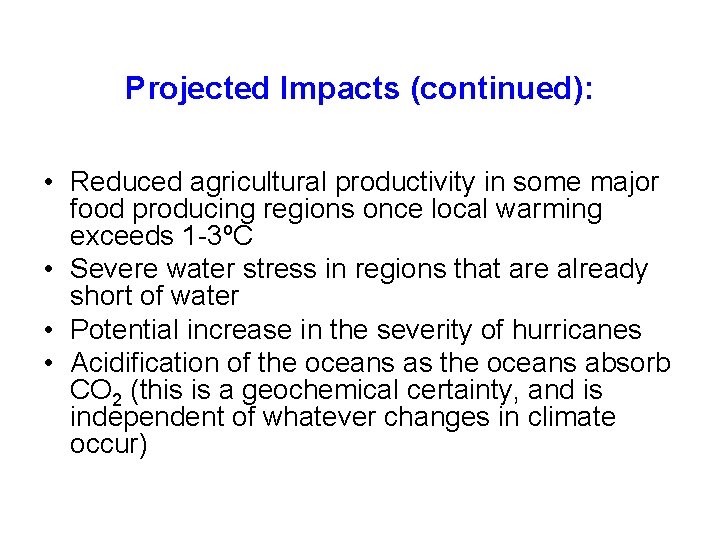
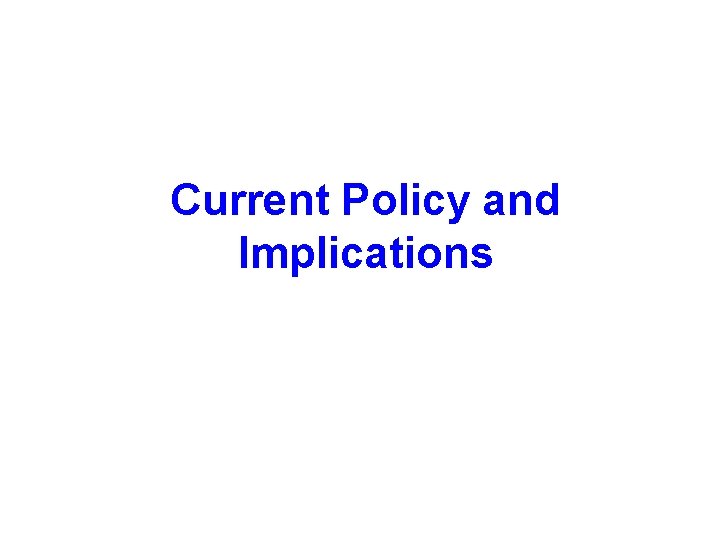
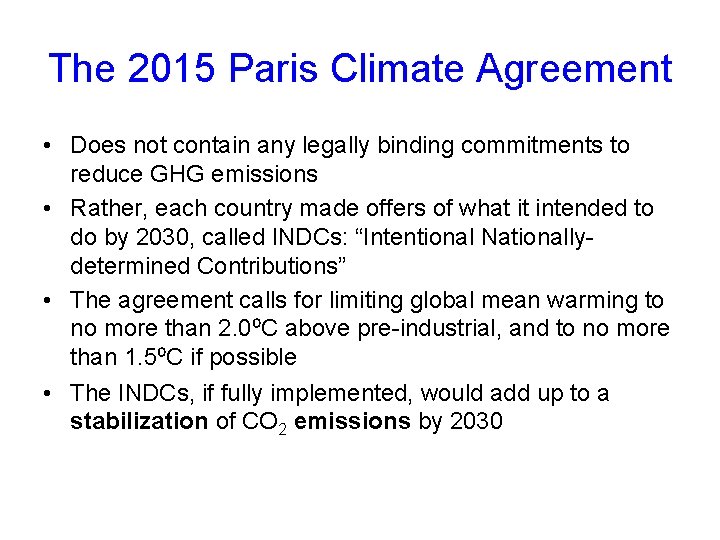
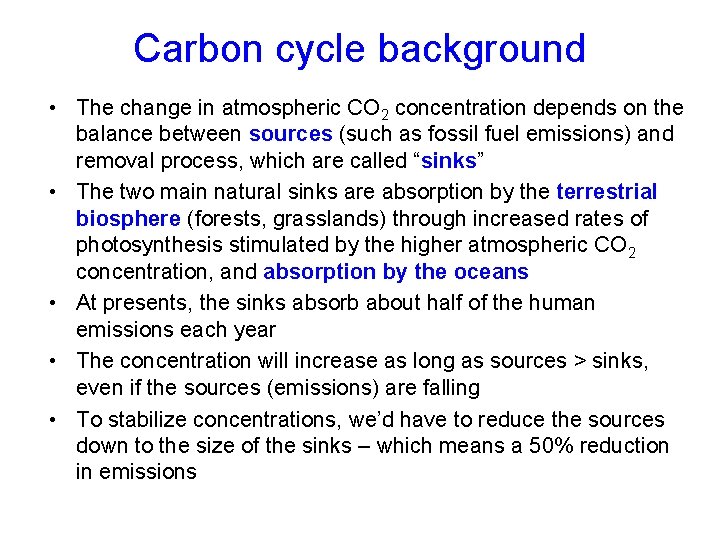
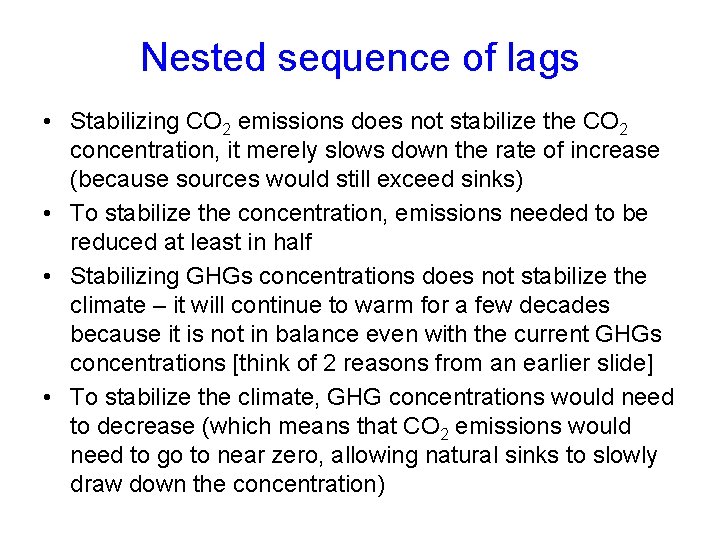
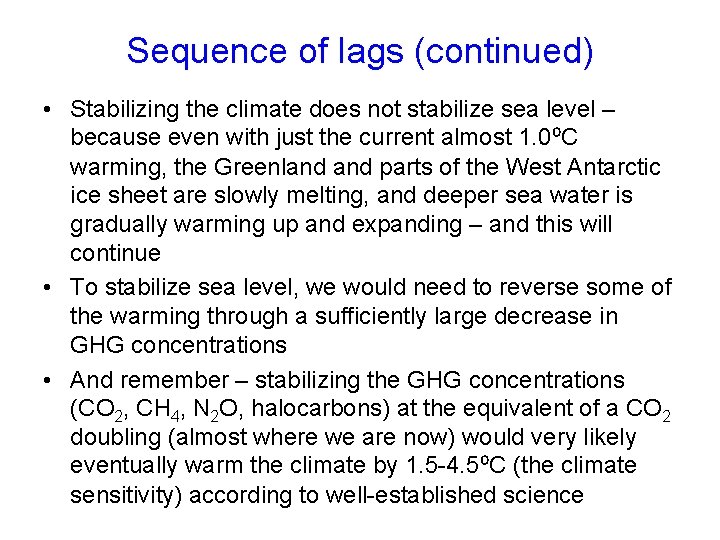
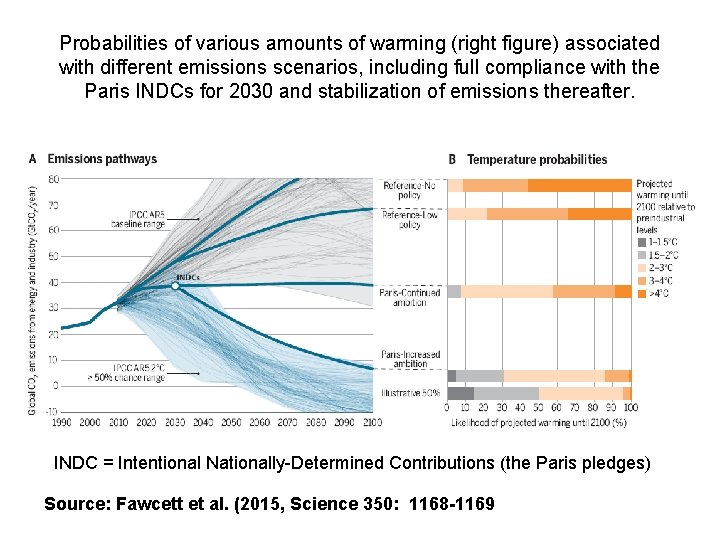
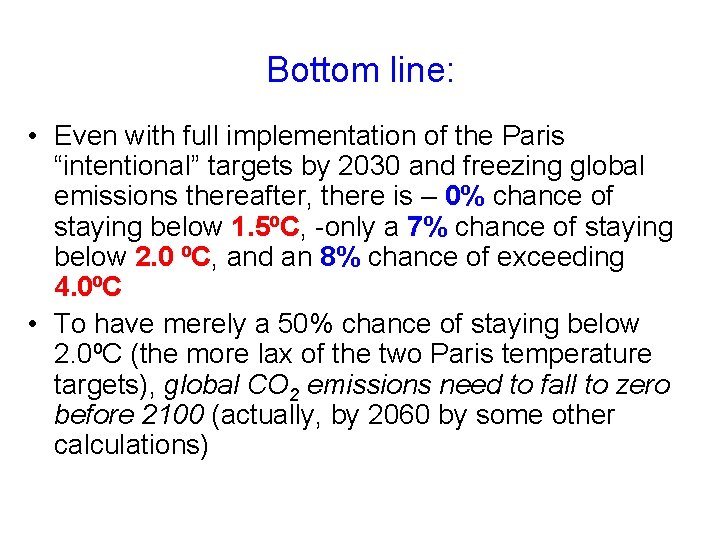
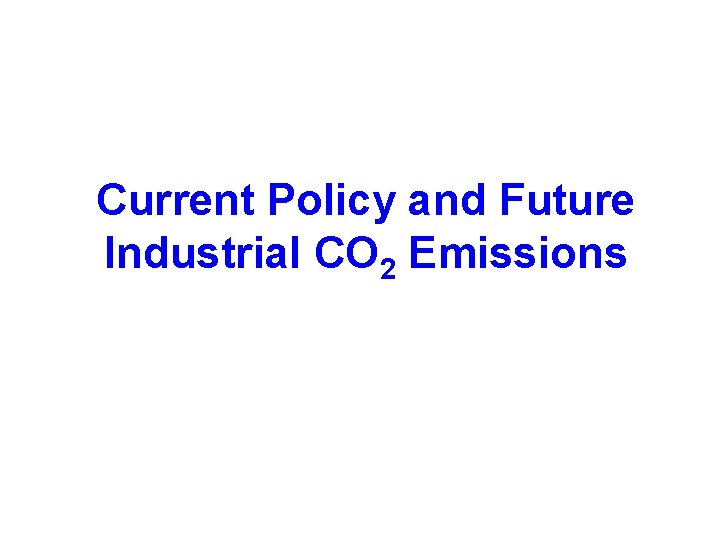
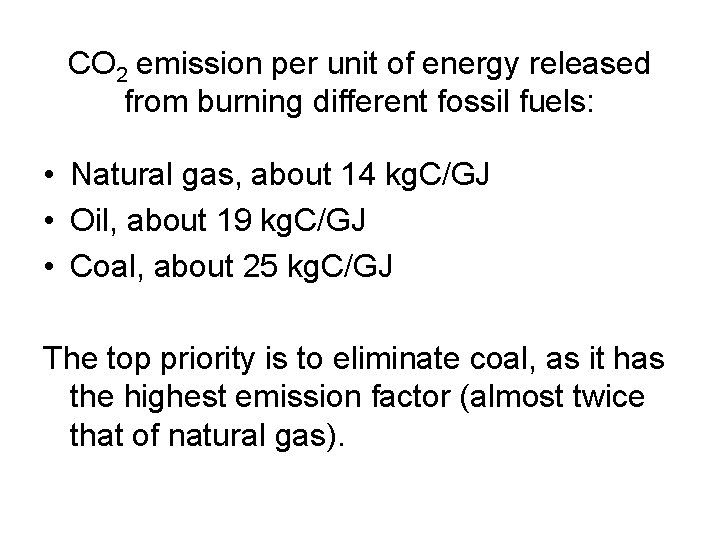
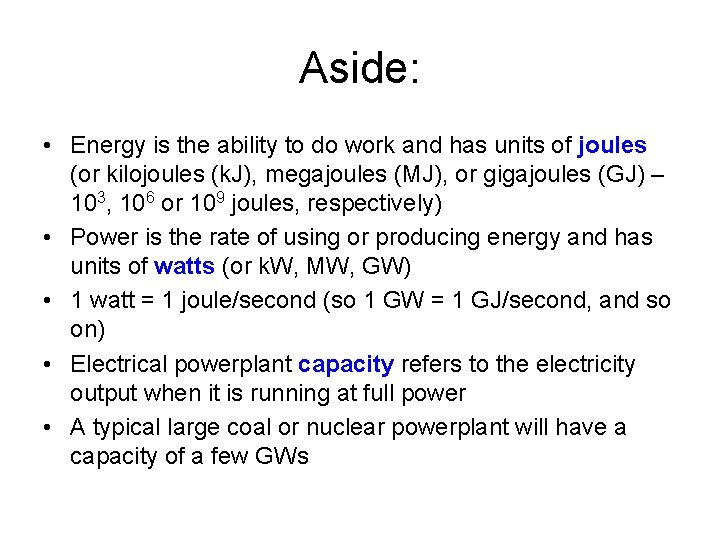
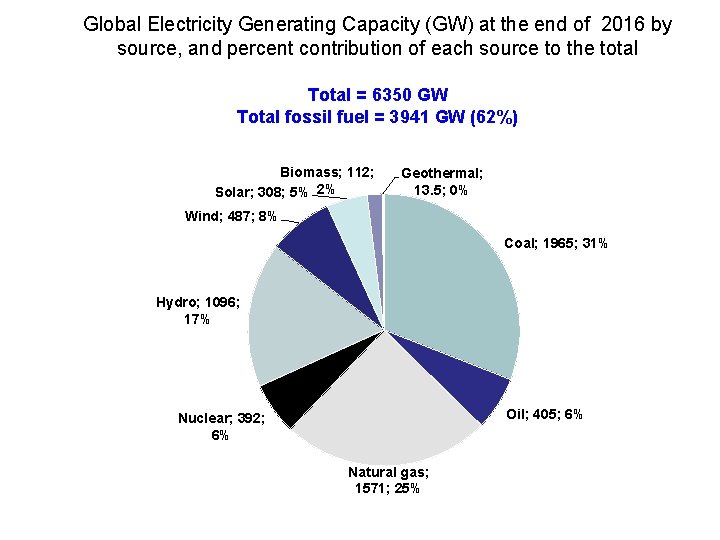
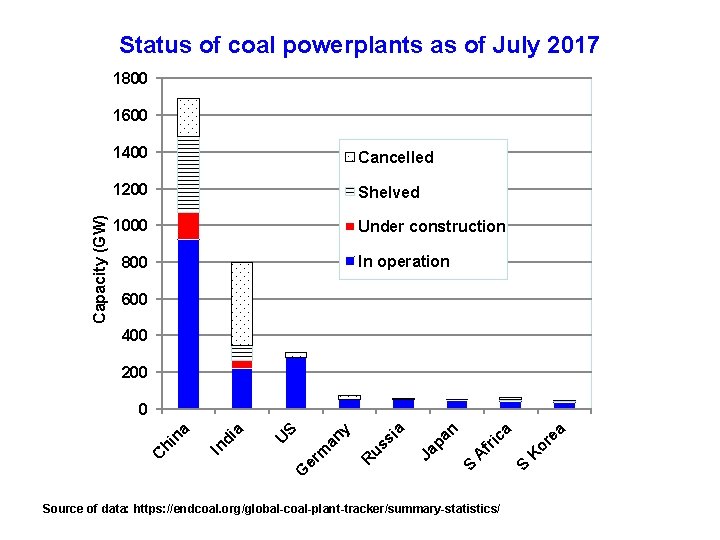
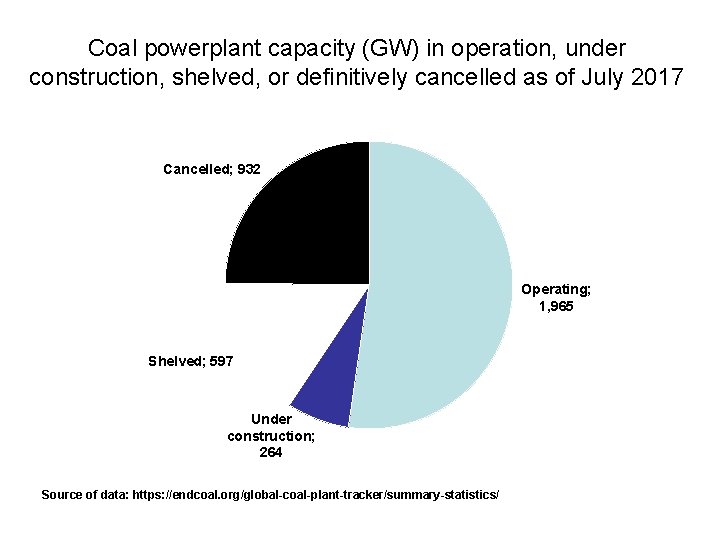
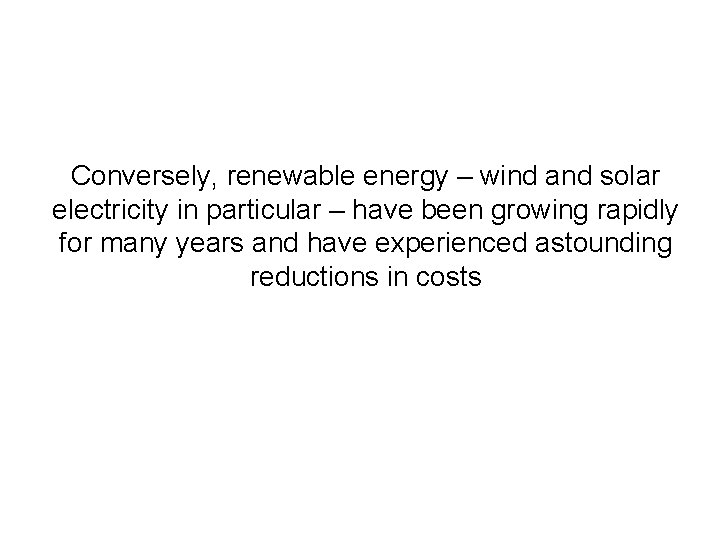
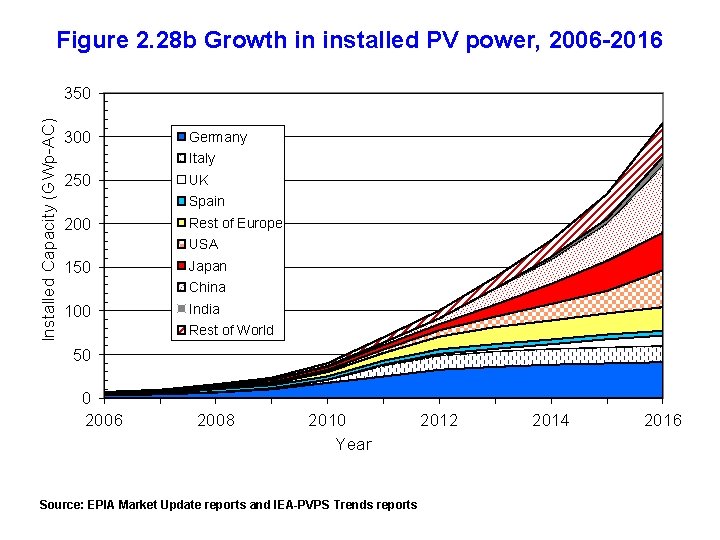
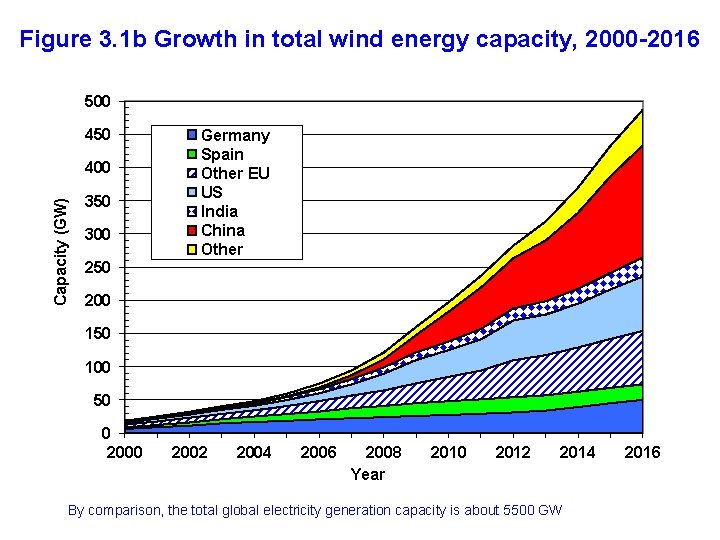
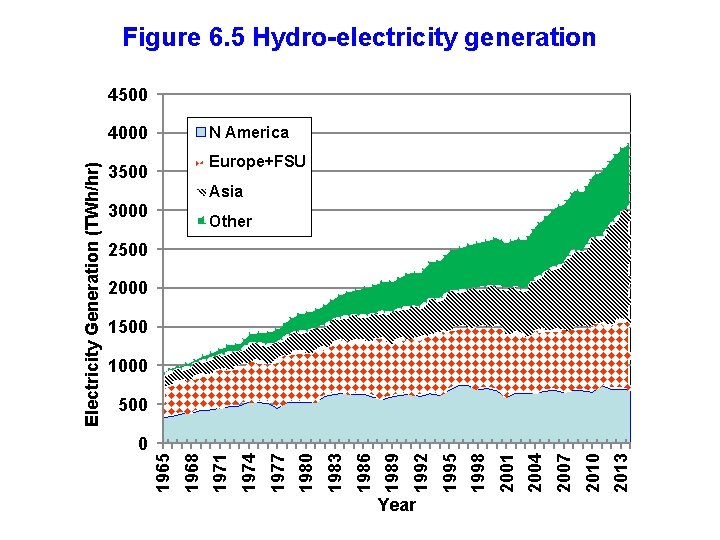
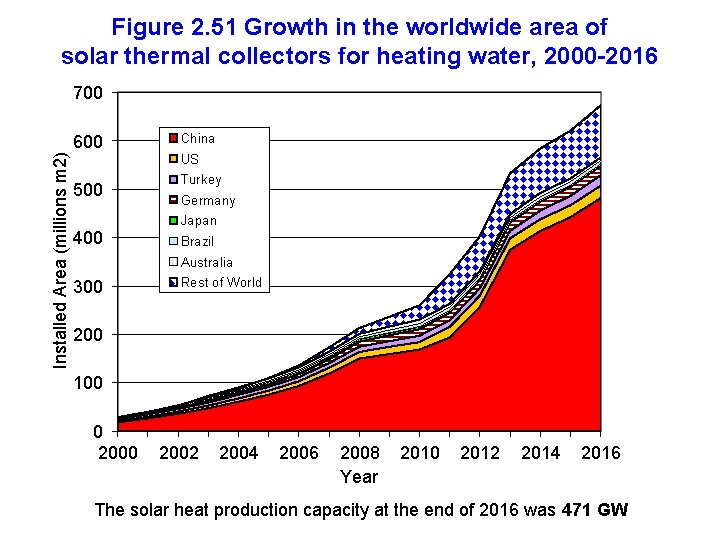
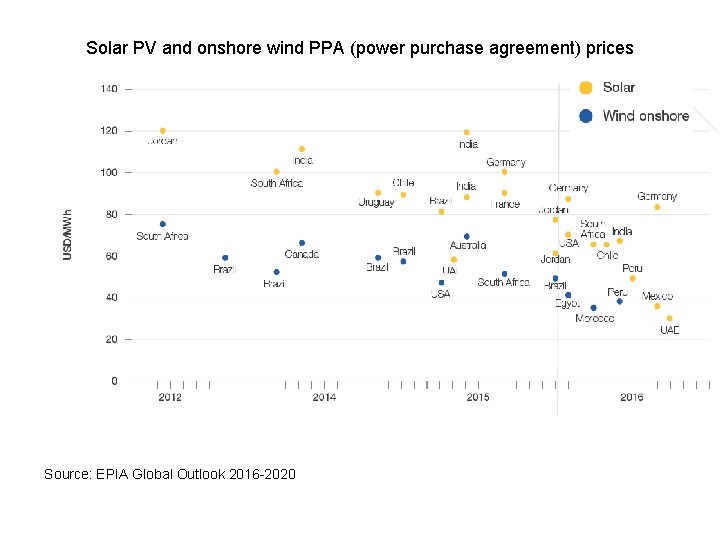
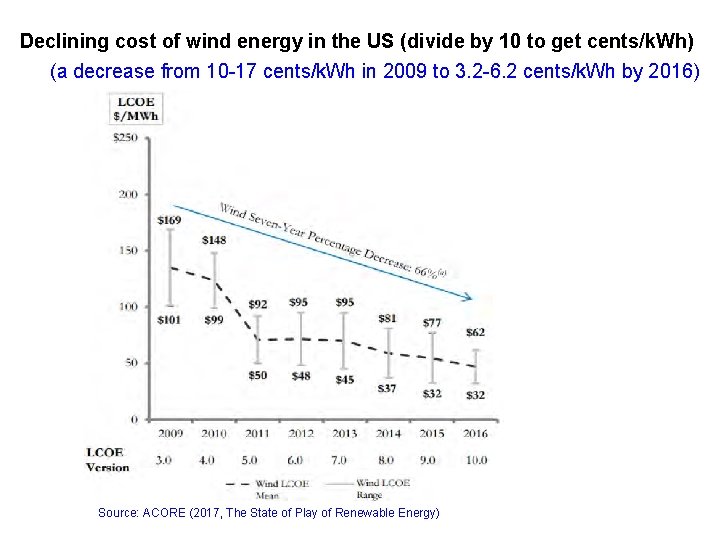
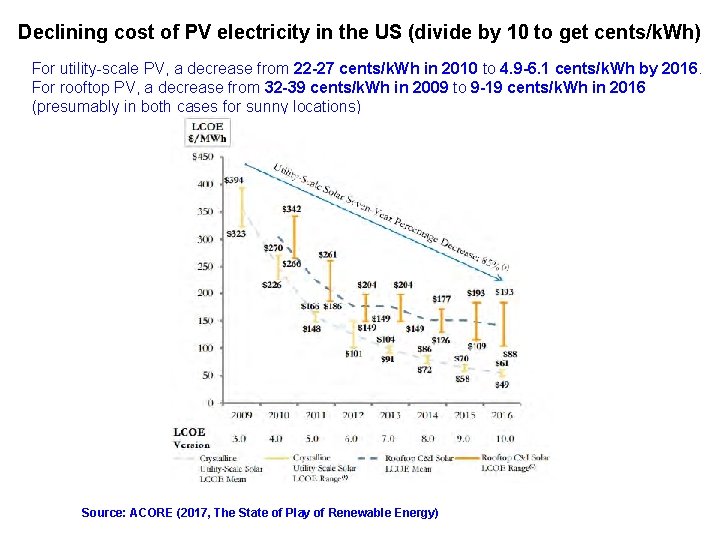
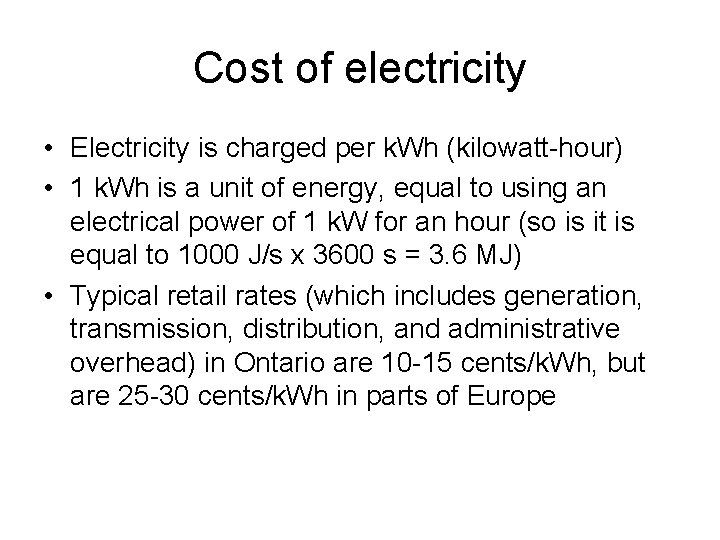
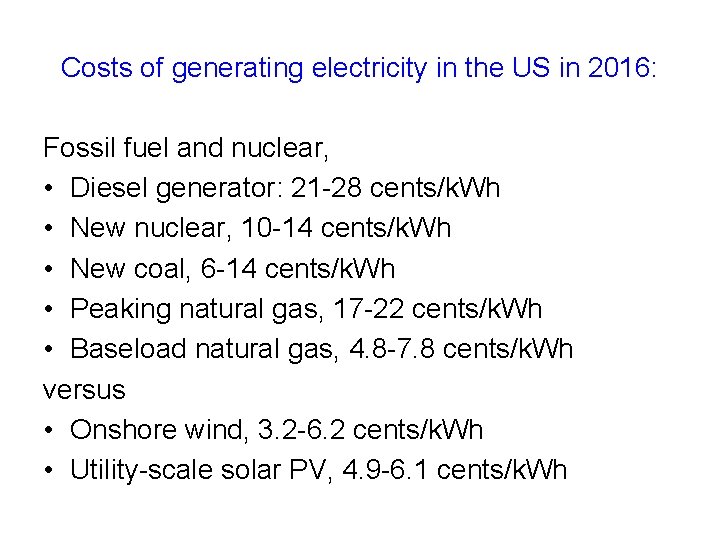
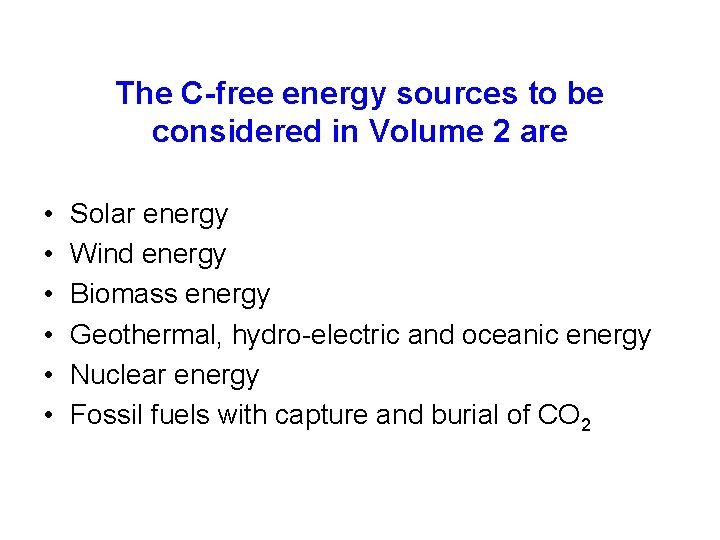
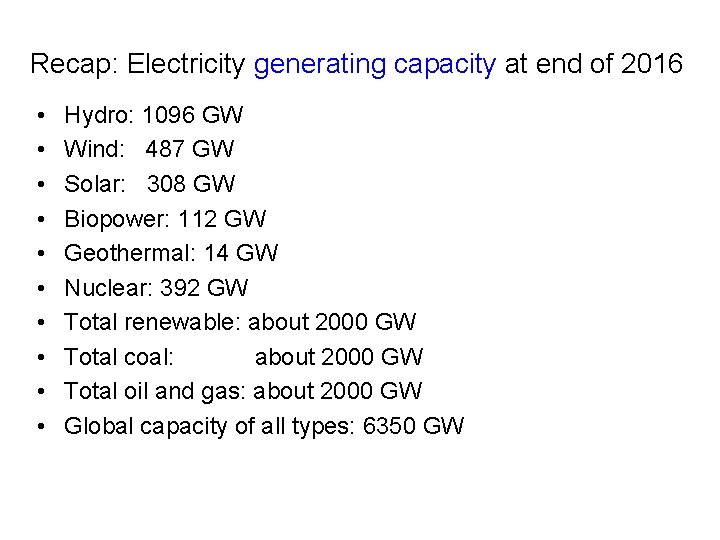
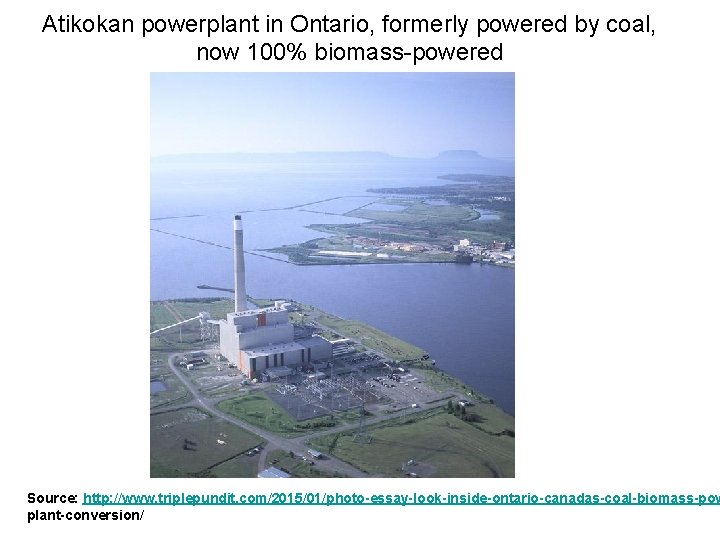
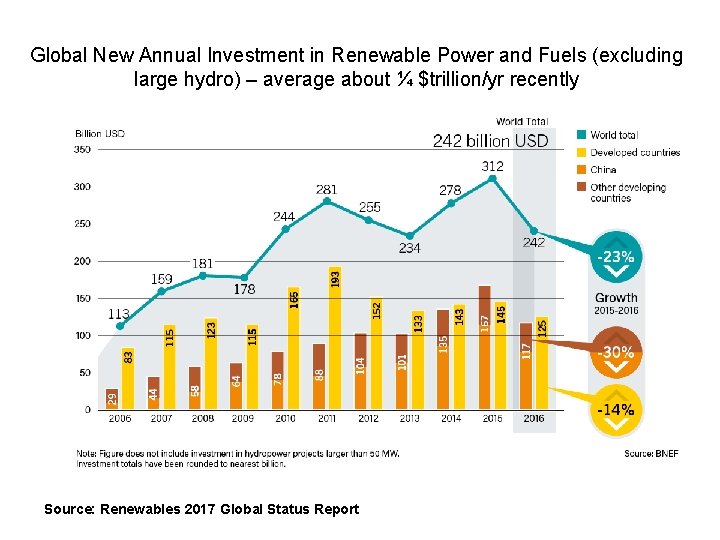
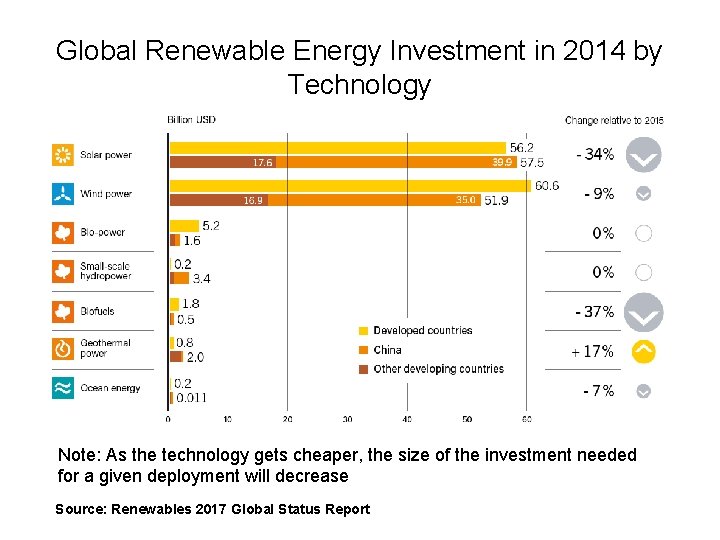
- Slides: 63
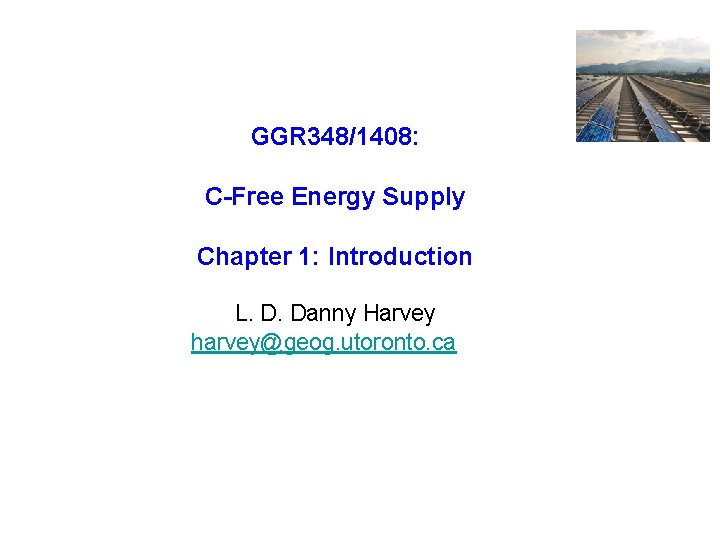
GGR 348/1408: C-Free Energy Supply Chapter 1: Introduction L. D. Danny Harvey harvey@geog. utoronto. ca
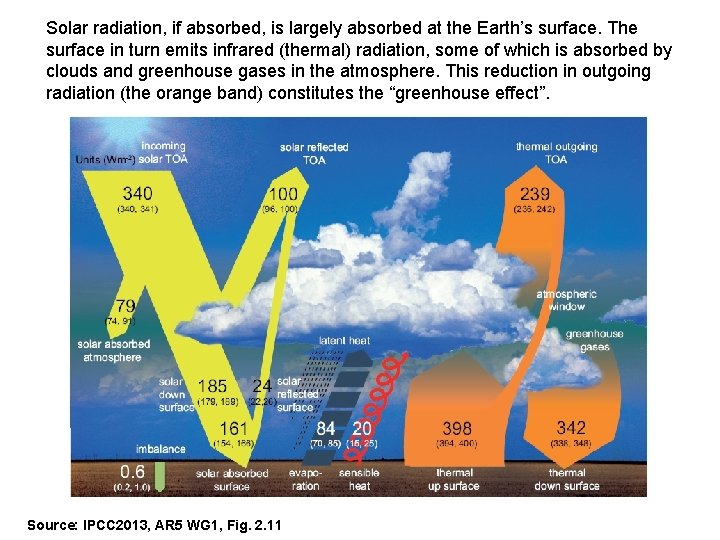
Solar radiation, if absorbed, is largely absorbed at the Earth’s surface. The surface in turn emits infrared (thermal) radiation, some of which is absorbed by clouds and greenhouse gases in the atmosphere. This reduction in outgoing radiation (the orange band) constitutes the “greenhouse effect”. Source: IPCC 2013, AR 5 WG 1, Fig. 2. 11
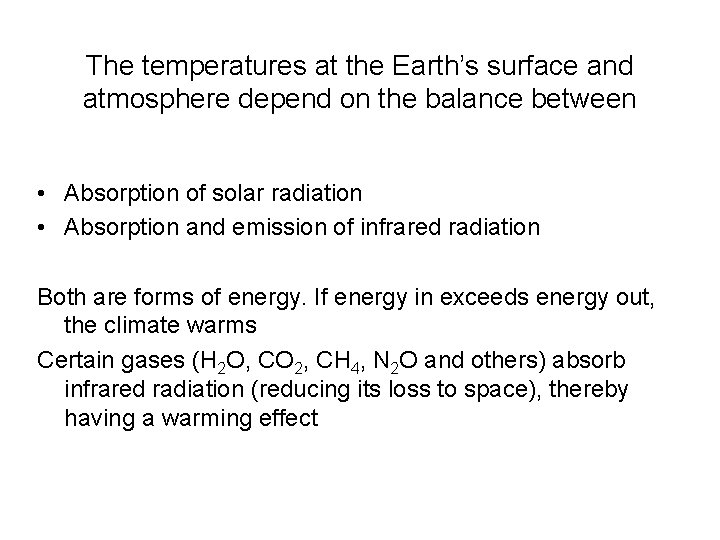
The temperatures at the Earth’s surface and atmosphere depend on the balance between • Absorption of solar radiation • Absorption and emission of infrared radiation Both are forms of energy. If energy in exceeds energy out, the climate warms Certain gases (H 2 O, CO 2, CH 4, N 2 O and others) absorb infrared radiation (reducing its loss to space), thereby having a warming effect
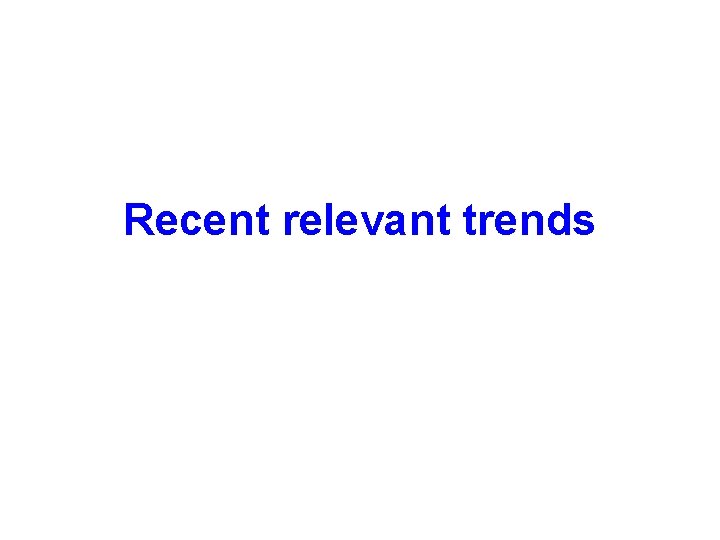
Recent relevant trends
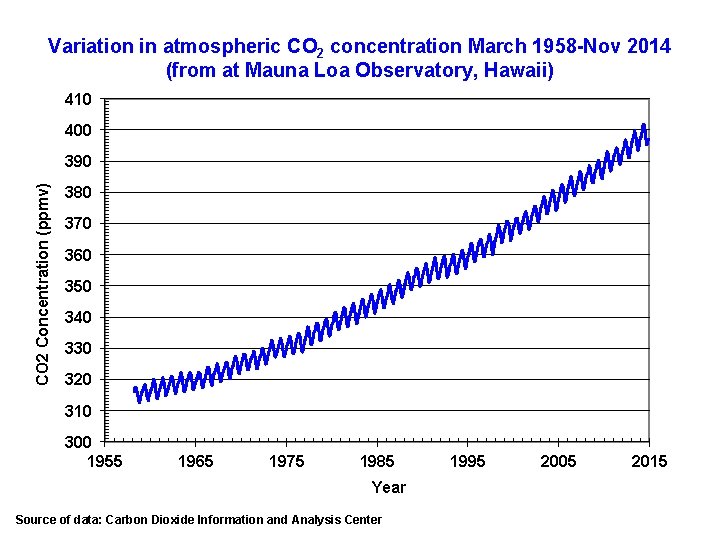
Variation in atmospheric CO 2 concentration March 1958 -Nov 2014 (from at Mauna Loa Observatory, Hawaii) 410 400 CO 2 Concentration (ppmv) 390 380 370 360 350 340 330 320 310 300 1955 1965 1975 1985 Year Source of data: Carbon Dioxide Information and Analysis Center 1995 2005 2015
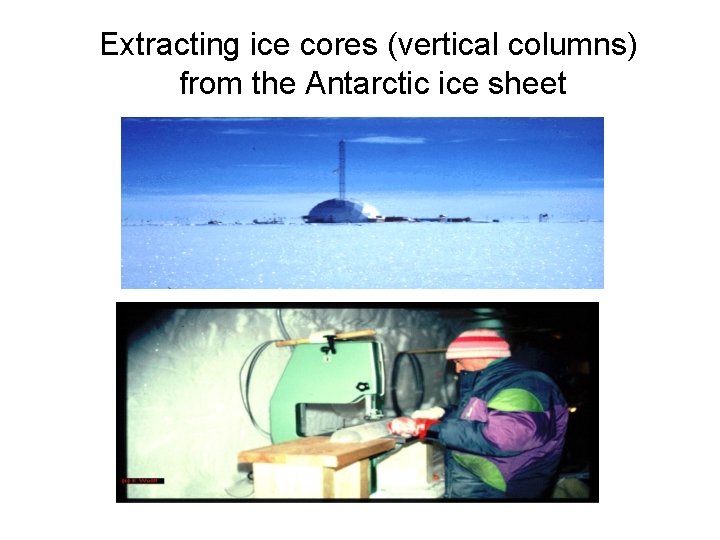
Extracting ice cores (vertical columns) from the Antarctic ice sheet
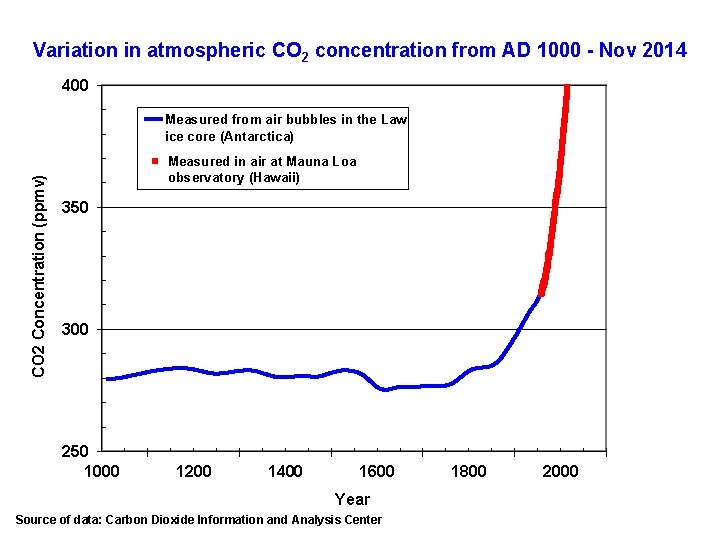
Variation in atmospheric CO 2 concentration from AD 1000 - Nov 2014 400 CO 2 Concentration (ppmv) Measured from air bubbles in the Law ice core (Antarctica) Measured in air at Mauna Loa observatory (Hawaii) 350 300 250 1000 1200 1400 1600 Year Source of data: Carbon Dioxide Information and Analysis Center 1800 2000
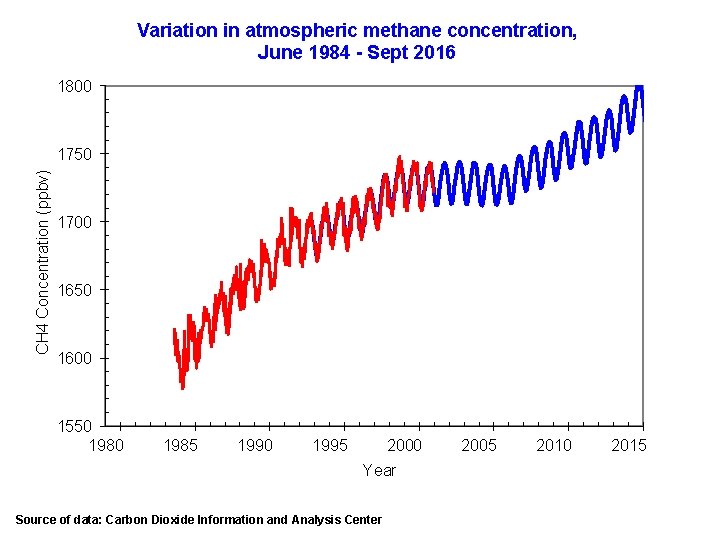
Variation in atmospheric methane concentration, June 1984 - Sept 2016 1800 CH 4 Concentration (ppbv) 1750 1700 1650 1600 1550 1985 1990 1995 2000 Year Source of data: Carbon Dioxide Information and Analysis Center 2005 2010 2015
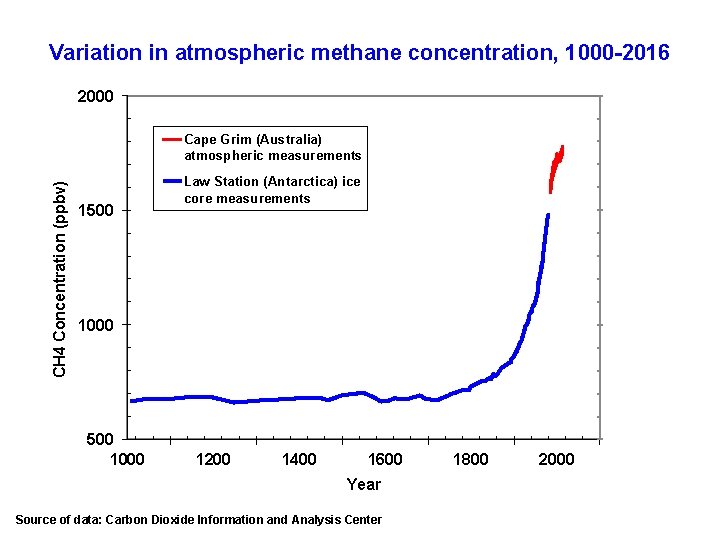
Variation in atmospheric methane concentration, 1000 -2016 2000 CH 4 Concentration (ppbv) Cape Grim (Australia) atmospheric measurements 1500 Law Station (Antarctica) ice core measurements 1000 500 1000 1200 1400 1600 Year Source of data: Carbon Dioxide Information and Analysis Center 1800 2000
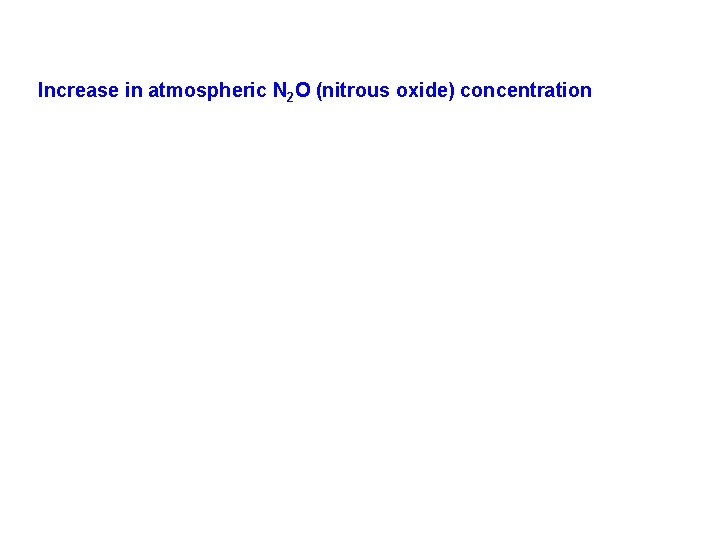
Increase in atmospheric N 2 O (nitrous oxide) concentration
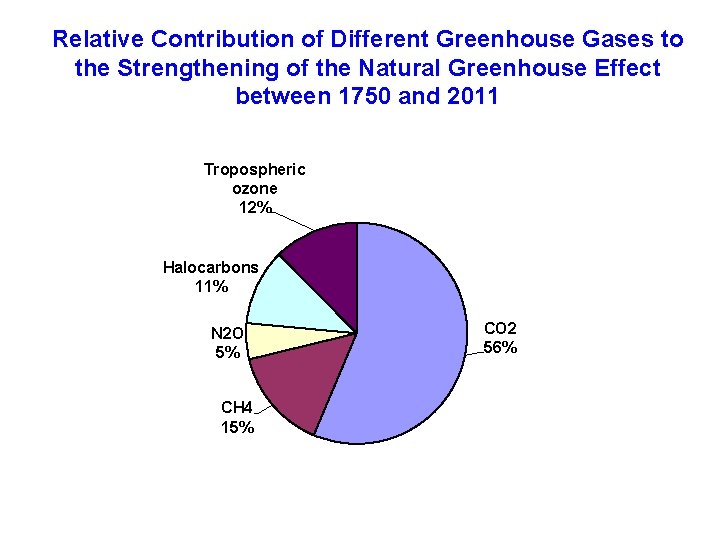
Relative Contribution of Different Greenhouse Gases to the Strengthening of the Natural Greenhouse Effect between 1750 and 2011 Tropospheric ozone 12% Halocarbons 11% N 2 O 5% CH 4 15% CO 2 56%
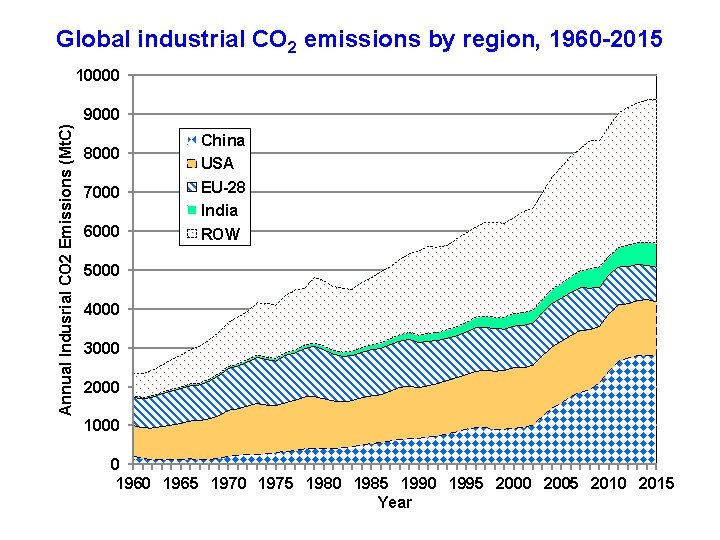
Global industrial CO 2 emissions by region, 1960 -2015 10000 Annual Indusrial CO 2 Emissions (Mt. C) 9000 8000 7000 6000 China USA EU-28 India ROW 5000 4000 3000 2000 1000 0 1965 1970 1975 1980 1985 1990 1995 2000 2005 2010 2015 Year
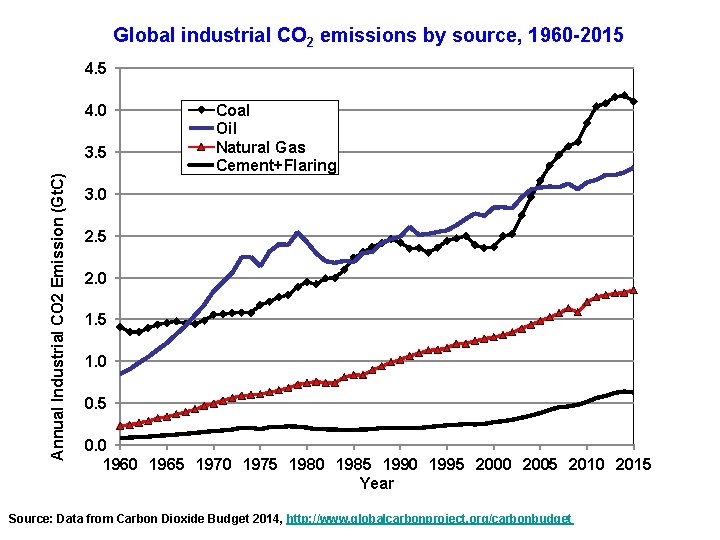
Global industrial CO 2 emissions by source, 1960 -2015 4. 0 Annual Industrial CO 2 Emission (Gt. C) 3. 5 Coal Oil Natural Gas Cement+Flaring 3. 0 2. 5 2. 0 1. 5 1. 0 0. 5 0. 0 1965 1970 1975 1980 1985 1990 1995 2000 2005 2010 2015 Year Source: Data from Carbon Dioxide Budget 2014, http: //www. globalcarbonproject. org/carbonbudget
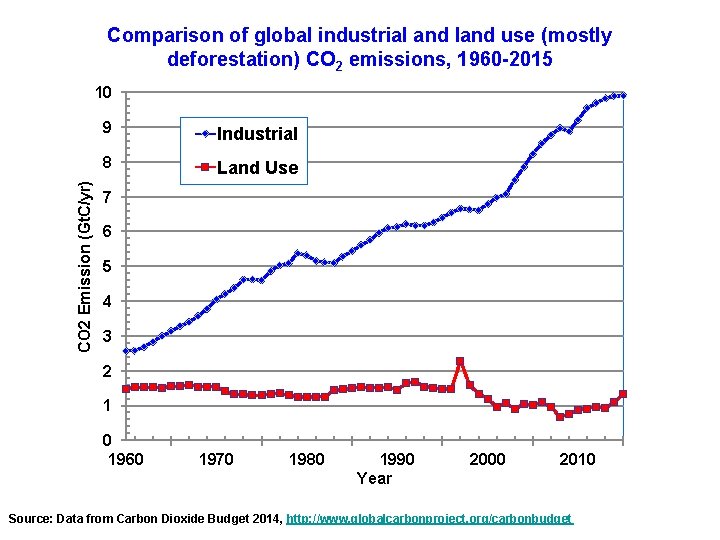
Comparison of global industrial and land use (mostly deforestation) CO 2 emissions, 1960 -2015 CO 2 Emission (Gt. C/yr) 10 9 Industrial 8 Land Use 7 6 5 4 3 2 1 0 1960 1970 1980 1990 Year 2000 2010 Source: Data from Carbon Dioxide Budget 2014, http: //www. globalcarbonproject. org/carbonbudget
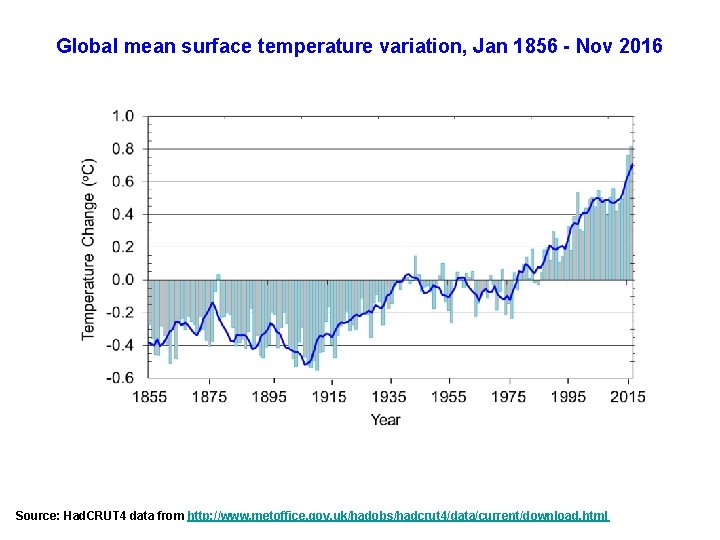
Global mean surface temperature variation, Jan 1856 - Nov 2016 Source: Had. CRUT 4 data from http: //www. metoffice. gov. uk/hadobs/hadcrut 4/data/current/download. html
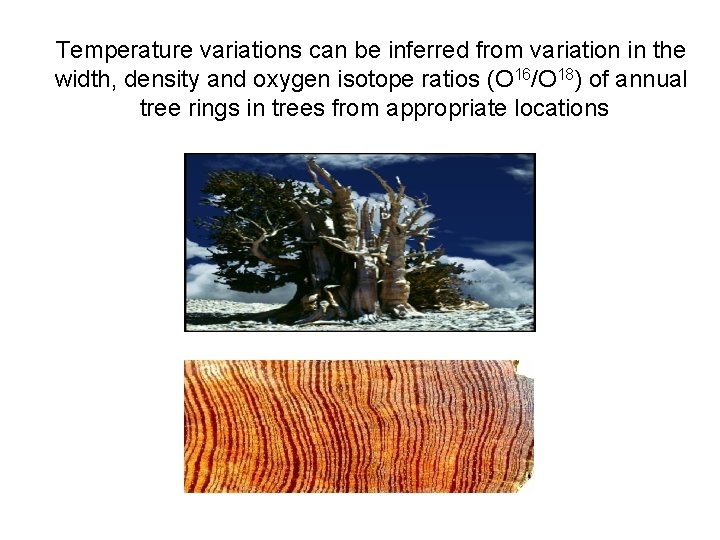
Temperature variations can be inferred from variation in the width, density and oxygen isotope ratios (O 16/O 18) of annual tree rings in trees from appropriate locations
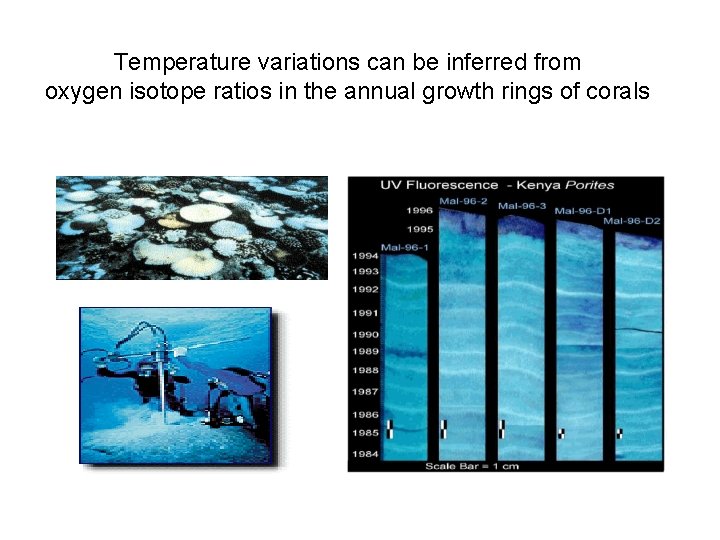
Temperature variations can be inferred from oxygen isotope ratios in the annual growth rings of corals
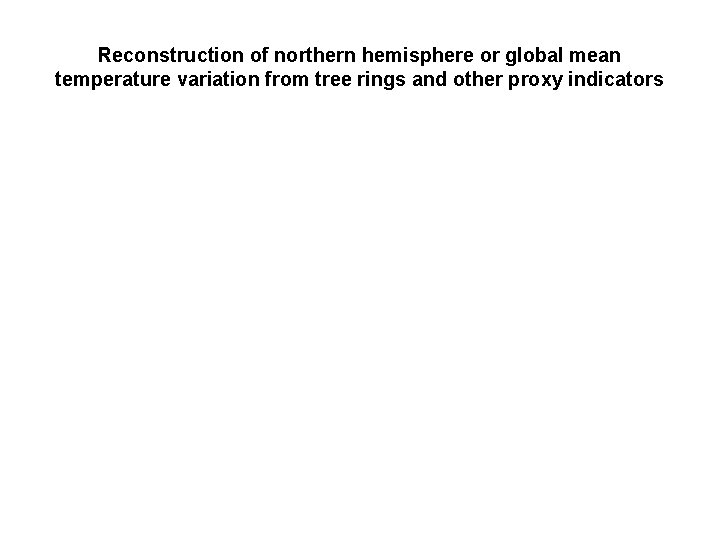
Reconstruction of northern hemisphere or global mean temperature variation from tree rings and other proxy indicators
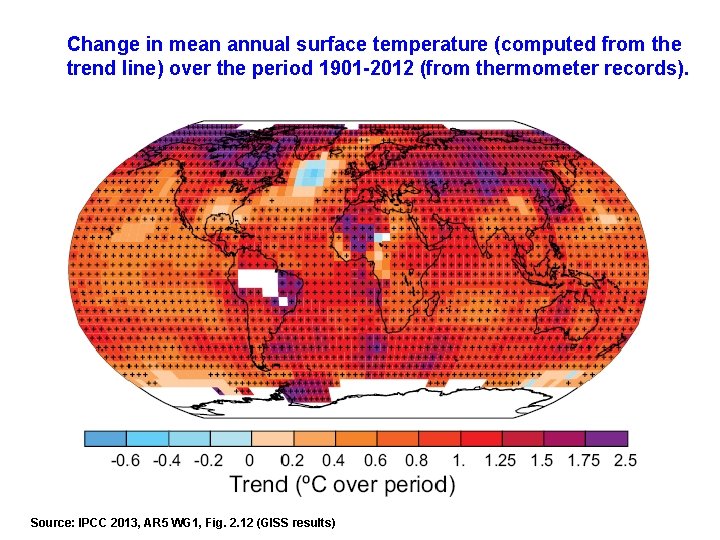
Change in mean annual surface temperature (computed from the trend line) over the period 1901 -2012 (from thermometer records). Source: IPCC 2013, AR 5 WG 1, Fig. 2. 12 (GISS results)
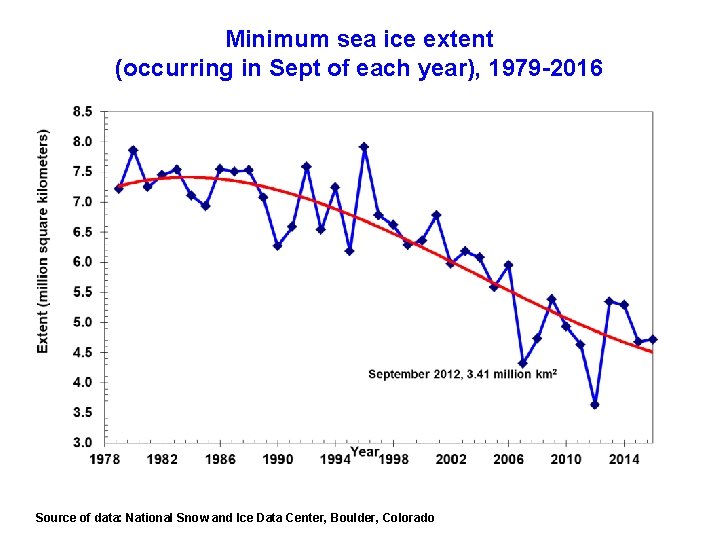
Minimum sea ice extent (occurring in Sept of each year), 1979 -2016 Source of data: National Snow and Ice Data Center, Boulder, Colorado
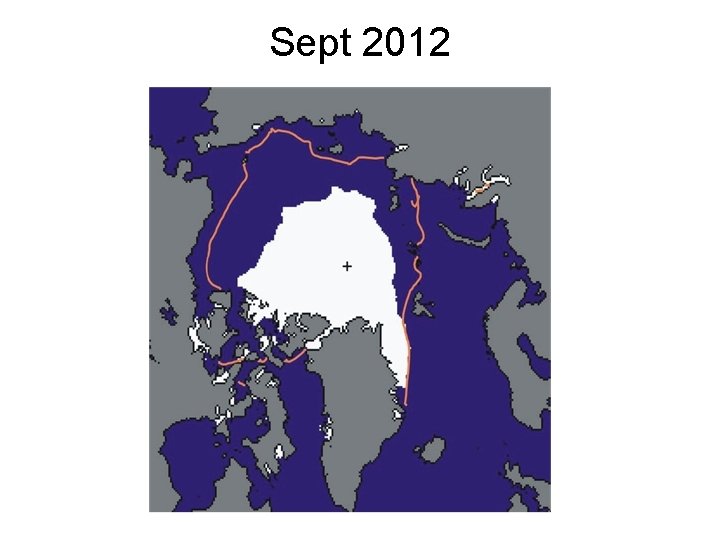
Sept 2012
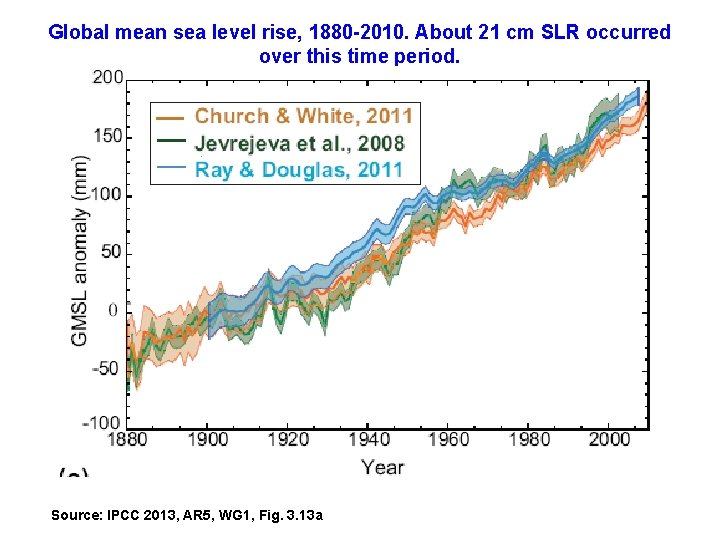
Global mean sea level rise, 1880 -2010. About 21 cm SLR occurred over this time period. Source: IPCC 2013, AR 5, WG 1, Fig. 3. 13 a
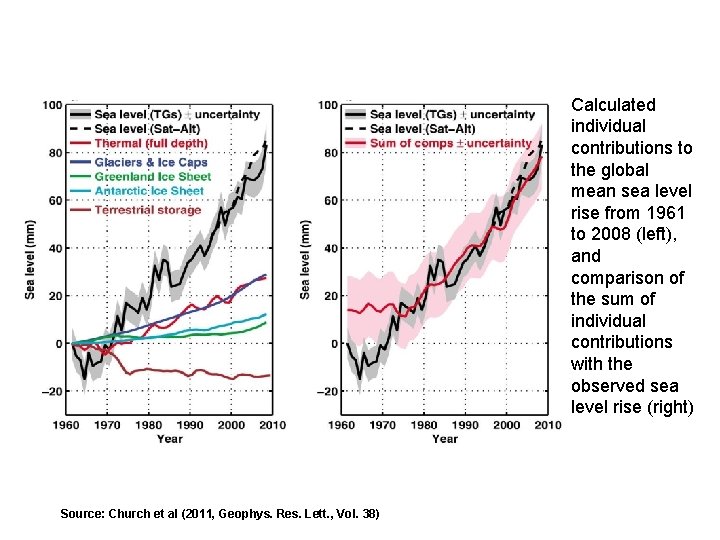
Calculated individual contributions to the global mean sea level rise from 1961 to 2008 (left), and comparison of the sum of individual contributions with the observed sea level rise (right) Source: Church et al (2011, Geophys. Res. Lett. , Vol. 38)
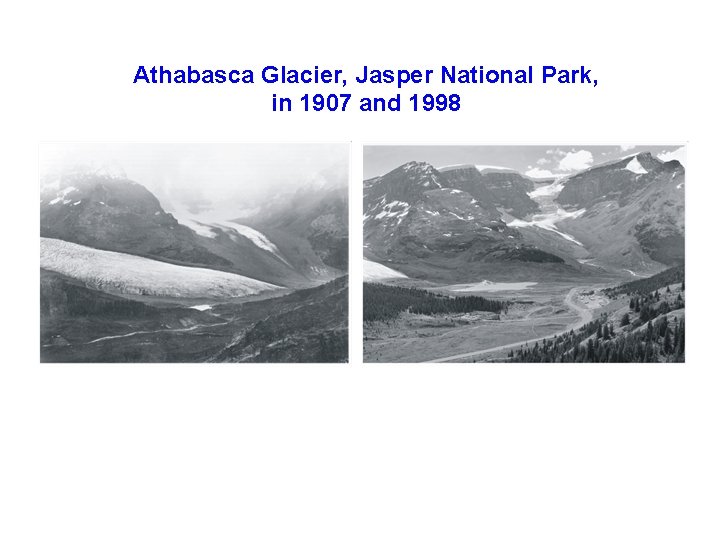
Athabasca Glacier, Jasper National Park, in 1907 and 1998
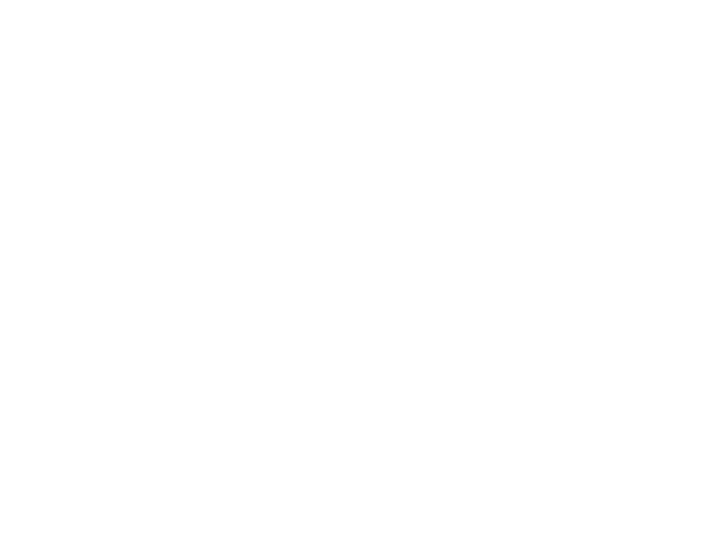
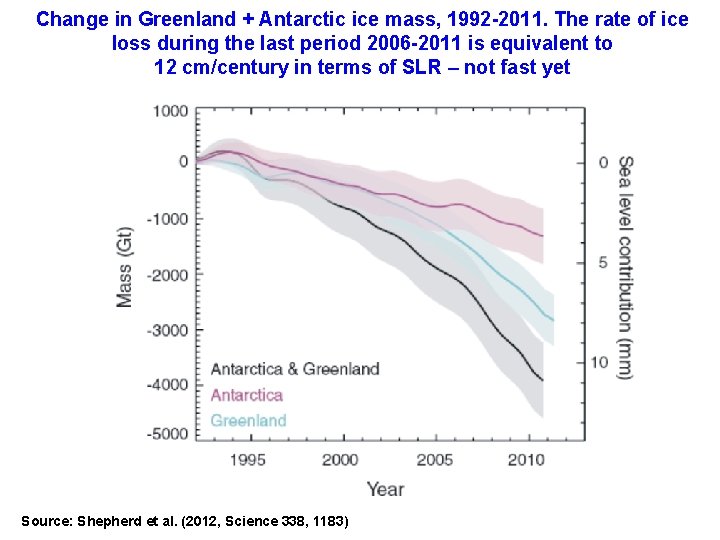
Change in Greenland + Antarctic ice mass, 1992 -2011. The rate of ice loss during the last period 2006 -2011 is equivalent to 12 cm/century in terms of SLR – not fast yet Source: Shepherd et al. (2012, Science 338, 1183)
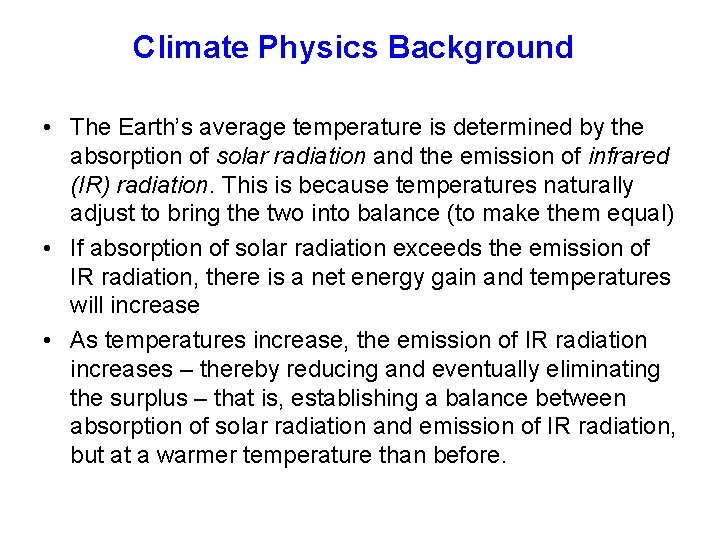
Climate Physics Background • The Earth’s average temperature is determined by the absorption of solar radiation and the emission of infrared (IR) radiation. This is because temperatures naturally adjust to bring the two into balance (to make them equal) • If absorption of solar radiation exceeds the emission of IR radiation, there is a net energy gain and temperatures will increase • As temperatures increase, the emission of IR radiation increases – thereby reducing and eventually eliminating the surplus – that is, establishing a balance between absorption of solar radiation and emission of IR radiation, but at a warmer temperature than before.
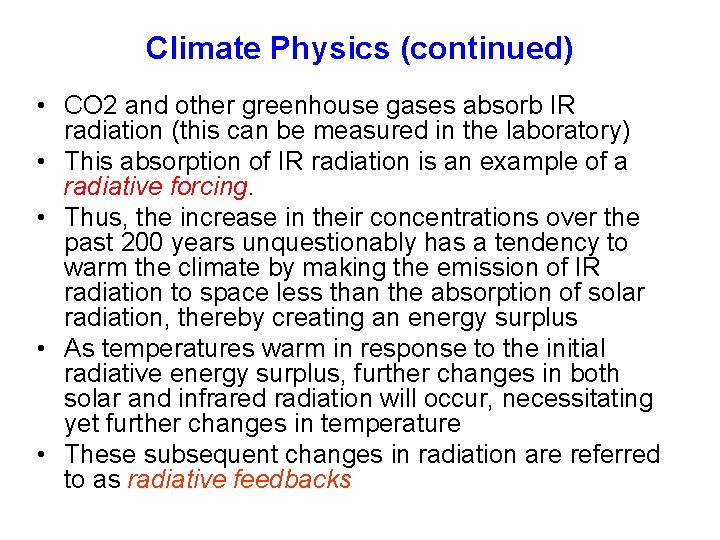
Climate Physics (continued) • CO 2 and other greenhouse gases absorb IR radiation (this can be measured in the laboratory) • This absorption of IR radiation is an example of a radiative forcing. • Thus, the increase in their concentrations over the past 200 years unquestionably has a tendency to warm the climate by making the emission of IR radiation to space less than the absorption of solar radiation, thereby creating an energy surplus • As temperatures warm in response to the initial radiative energy surplus, further changes in both solar and infrared radiation will occur, necessitating yet further changes in temperature • These subsequent changes in radiation are referred to as radiative feedbacks
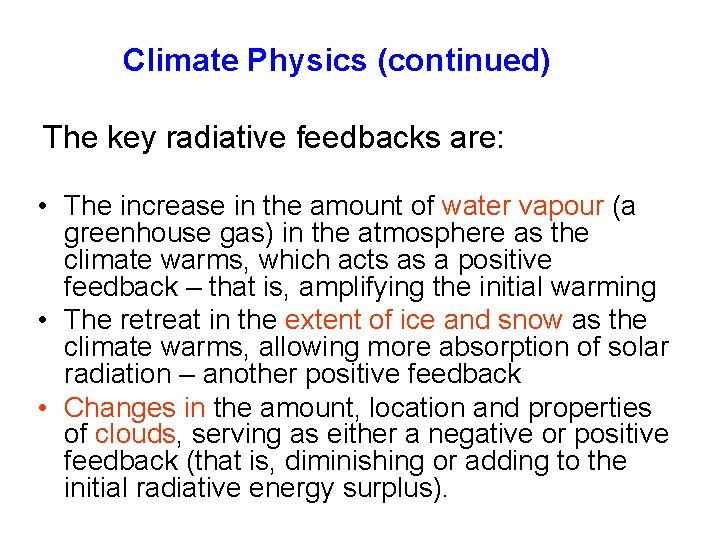
Climate Physics (continued) The key radiative feedbacks are: • The increase in the amount of water vapour (a greenhouse gas) in the atmosphere as the climate warms, which acts as a positive feedback – that is, amplifying the initial warming • The retreat in the extent of ice and snow as the climate warms, allowing more absorption of solar radiation – another positive feedback • Changes in the amount, location and properties of clouds, serving as either a negative or positive feedback (that is, diminishing or adding to the initial radiative energy surplus).
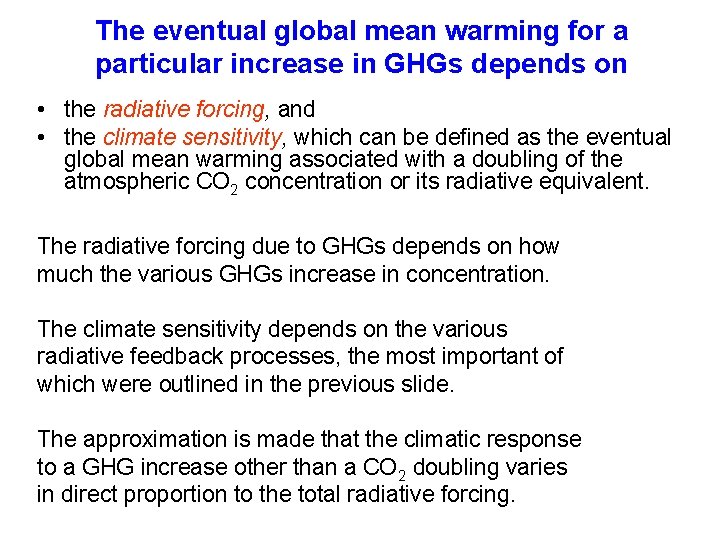
The eventual global mean warming for a particular increase in GHGs depends on • the radiative forcing, and • the climate sensitivity, which can be defined as the eventual global mean warming associated with a doubling of the atmospheric CO 2 concentration or its radiative equivalent. The radiative forcing due to GHGs depends on how much the various GHGs increase in concentration. The climate sensitivity depends on the various radiative feedback processes, the most important of which were outlined in the previous slide. The approximation is made that the climatic response to a GHG increase other than a CO 2 doubling varies in direct proportion to the total radiative forcing.
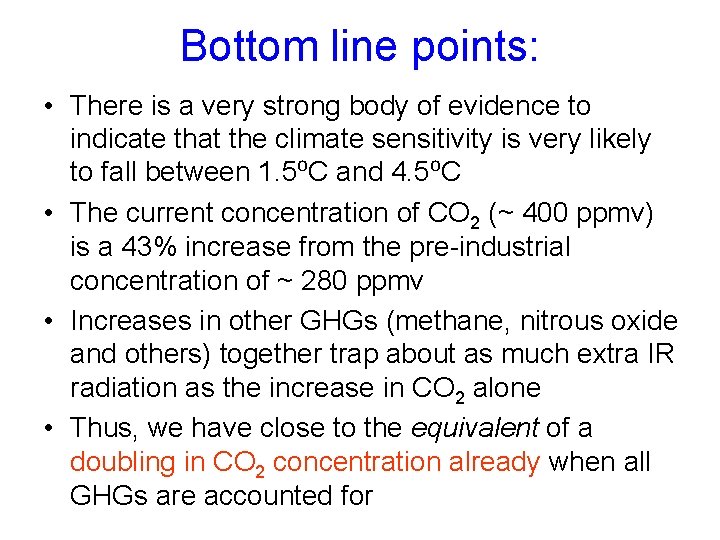
Bottom line points: • There is a very strong body of evidence to indicate that the climate sensitivity is very likely to fall between 1. 5⁰C and 4. 5⁰C • The current concentration of CO 2 (~ 400 ppmv) is a 43% increase from the pre-industrial concentration of ~ 280 ppmv • Increases in other GHGs (methane, nitrous oxide and others) together trap about as much extra IR radiation as the increase in CO 2 alone • Thus, we have close to the equivalent of a doubling in CO 2 concentration already when all GHGs are accounted for
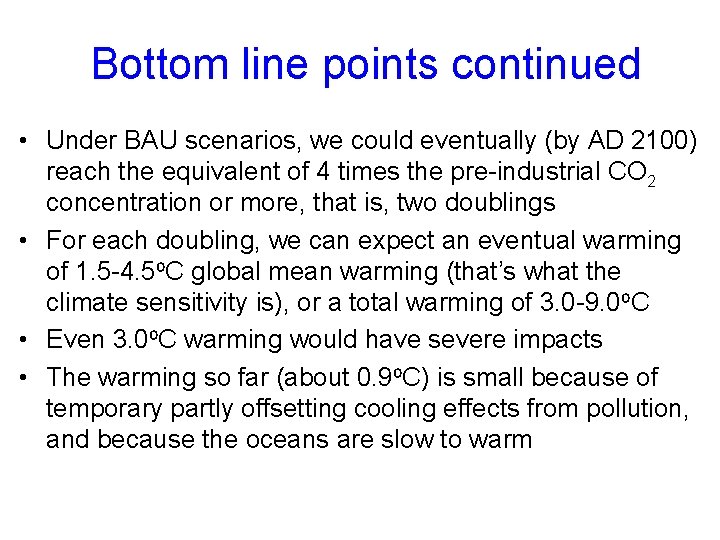
Bottom line points continued • Under BAU scenarios, we could eventually (by AD 2100) reach the equivalent of 4 times the pre-industrial CO 2 concentration or more, that is, two doublings • For each doubling, we can expect an eventual warming of 1. 5 -4. 5⁰C global mean warming (that’s what the climate sensitivity is), or a total warming of 3. 0 -9. 0⁰C • Even 3. 0⁰C warming would have severe impacts • The warming so far (about 0. 9⁰C) is small because of temporary partly offsetting cooling effects from pollution, and because the oceans are slow to warm
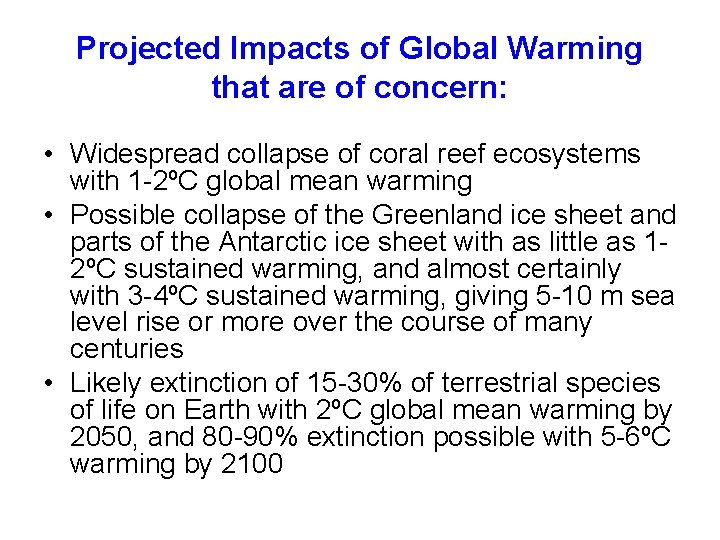
Projected Impacts of Global Warming that are of concern: • Widespread collapse of coral reef ecosystems with 1 -2ºC global mean warming • Possible collapse of the Greenland ice sheet and parts of the Antarctic ice sheet with as little as 12ºC sustained warming, and almost certainly with 3 -4ºC sustained warming, giving 5 -10 m sea level rise or more over the course of many centuries • Likely extinction of 15 -30% of terrestrial species of life on Earth with 2ºC global mean warming by 2050, and 80 -90% extinction possible with 5 -6ºC warming by 2100
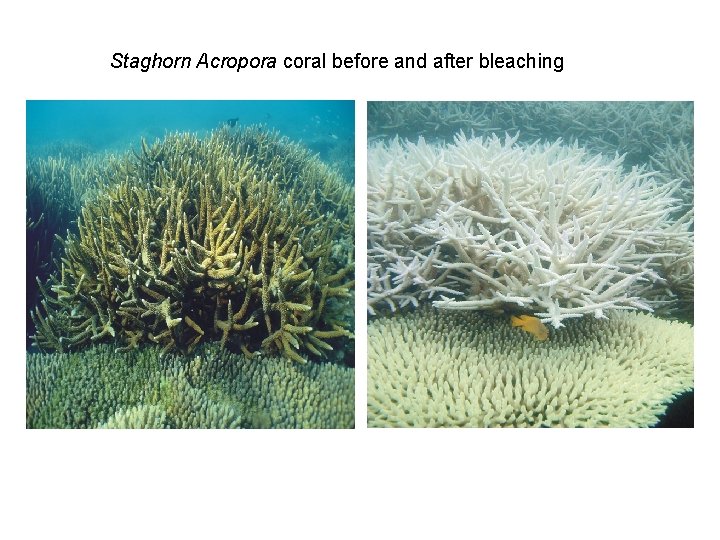
Staghorn Acropora coral before and after bleaching
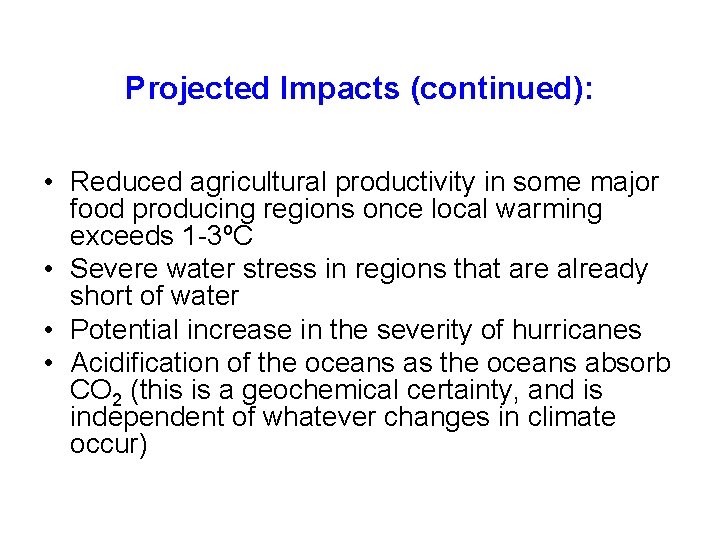
Projected Impacts (continued): • Reduced agricultural productivity in some major food producing regions once local warming exceeds 1 -3ºC • Severe water stress in regions that are already short of water • Potential increase in the severity of hurricanes • Acidification of the oceans as the oceans absorb CO 2 (this is a geochemical certainty, and is independent of whatever changes in climate occur)
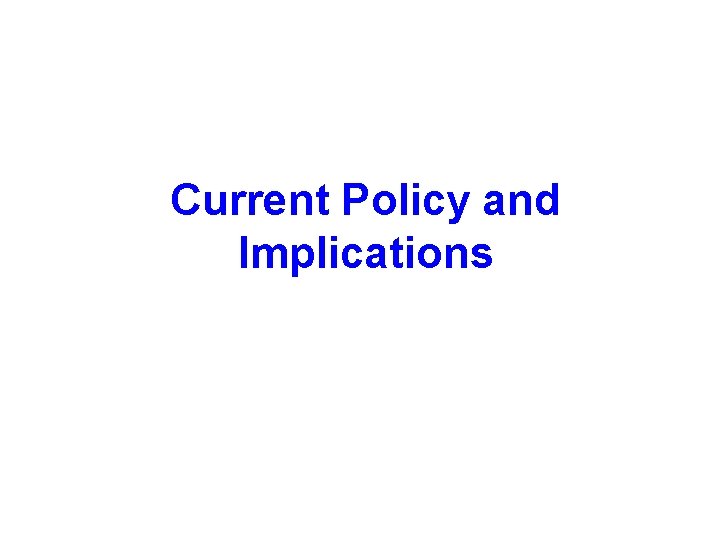
Current Policy and Implications
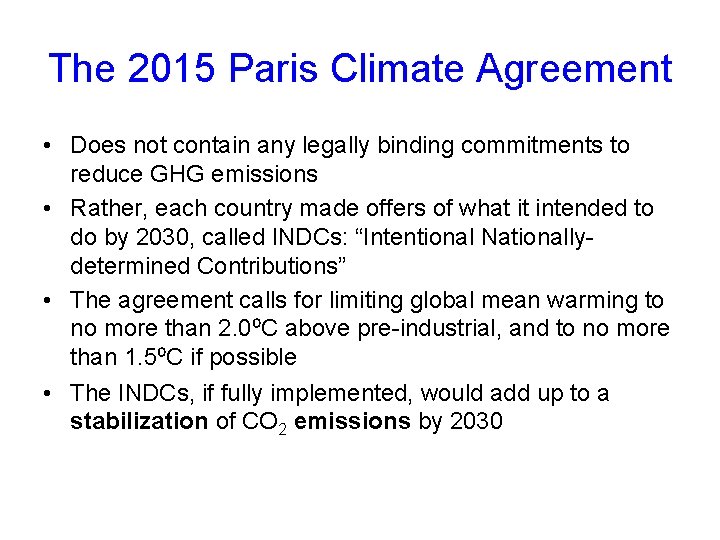
The 2015 Paris Climate Agreement • Does not contain any legally binding commitments to reduce GHG emissions • Rather, each country made offers of what it intended to do by 2030, called INDCs: “Intentional Nationallydetermined Contributions” • The agreement calls for limiting global mean warming to no more than 2. 0⁰C above pre-industrial, and to no more than 1. 5⁰C if possible • The INDCs, if fully implemented, would add up to a stabilization of CO 2 emissions by 2030
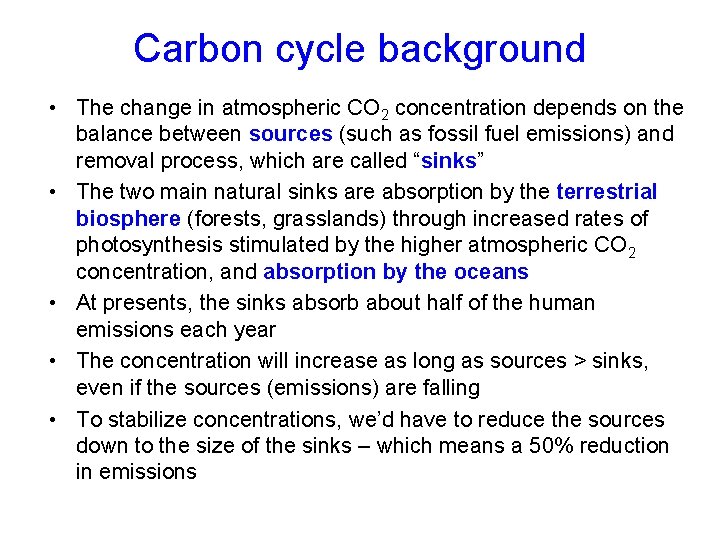
Carbon cycle background • The change in atmospheric CO 2 concentration depends on the balance between sources (such as fossil fuel emissions) and removal process, which are called “sinks” • The two main natural sinks are absorption by the terrestrial biosphere (forests, grasslands) through increased rates of photosynthesis stimulated by the higher atmospheric CO 2 concentration, and absorption by the oceans • At presents, the sinks absorb about half of the human emissions each year • The concentration will increase as long as sources > sinks, even if the sources (emissions) are falling • To stabilize concentrations, we’d have to reduce the sources down to the size of the sinks – which means a 50% reduction in emissions
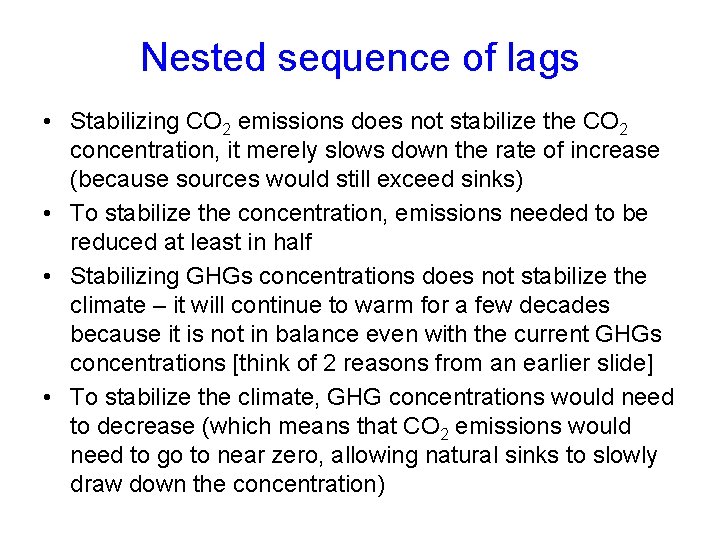
Nested sequence of lags • Stabilizing CO 2 emissions does not stabilize the CO 2 concentration, it merely slows down the rate of increase (because sources would still exceed sinks) • To stabilize the concentration, emissions needed to be reduced at least in half • Stabilizing GHGs concentrations does not stabilize the climate – it will continue to warm for a few decades because it is not in balance even with the current GHGs concentrations [think of 2 reasons from an earlier slide] • To stabilize the climate, GHG concentrations would need to decrease (which means that CO 2 emissions would need to go to near zero, allowing natural sinks to slowly draw down the concentration)
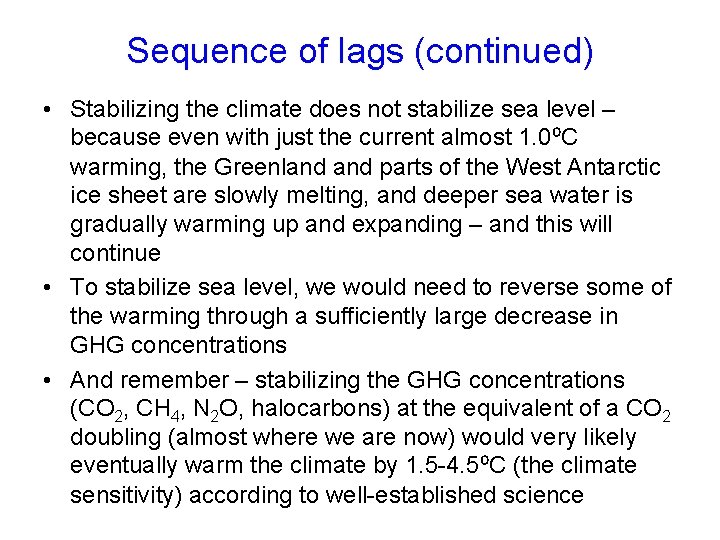
Sequence of lags (continued) • Stabilizing the climate does not stabilize sea level – because even with just the current almost 1. 0⁰C warming, the Greenland parts of the West Antarctic ice sheet are slowly melting, and deeper sea water is gradually warming up and expanding – and this will continue • To stabilize sea level, we would need to reverse some of the warming through a sufficiently large decrease in GHG concentrations • And remember – stabilizing the GHG concentrations (CO 2, CH 4, N 2 O, halocarbons) at the equivalent of a CO 2 doubling (almost where we are now) would very likely eventually warm the climate by 1. 5 -4. 5⁰C (the climate sensitivity) according to well-established science
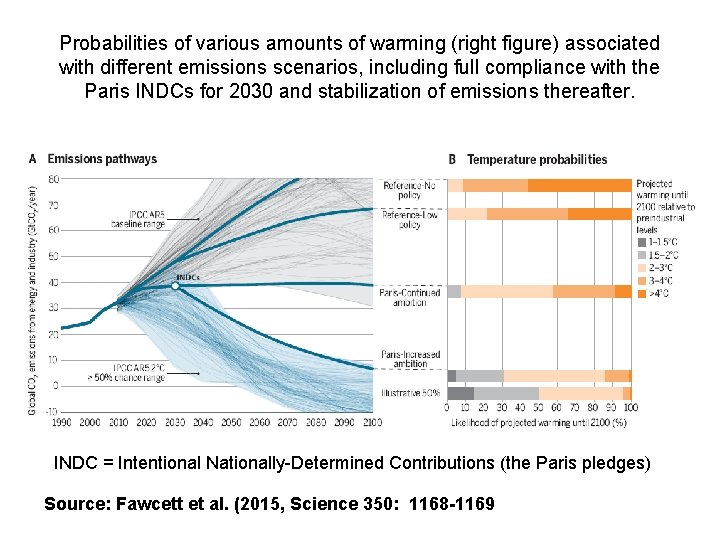
Probabilities of various amounts of warming (right figure) associated with different emissions scenarios, including full compliance with the Paris INDCs for 2030 and stabilization of emissions thereafter. INDC = Intentional Nationally-Determined Contributions (the Paris pledges) Source: Fawcett et al. (2015, Science 350: 1168 -1169
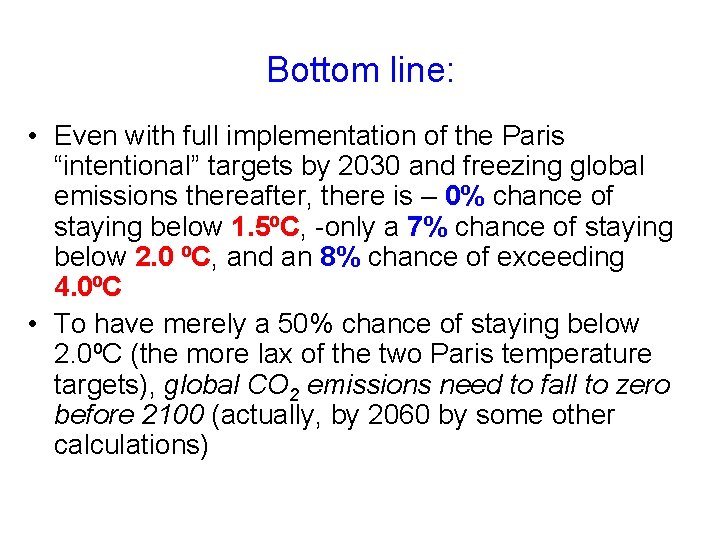
Bottom line: • Even with full implementation of the Paris “intentional” targets by 2030 and freezing global emissions thereafter, there is – 0% chance of staying below 1. 5⁰C, -only a 7% chance of staying below 2. 0 ⁰C, and an 8% chance of exceeding 4. 0⁰C • To have merely a 50% chance of staying below 2. 0⁰C (the more lax of the two Paris temperature targets), global CO 2 emissions need to fall to zero before 2100 (actually, by 2060 by some other calculations)
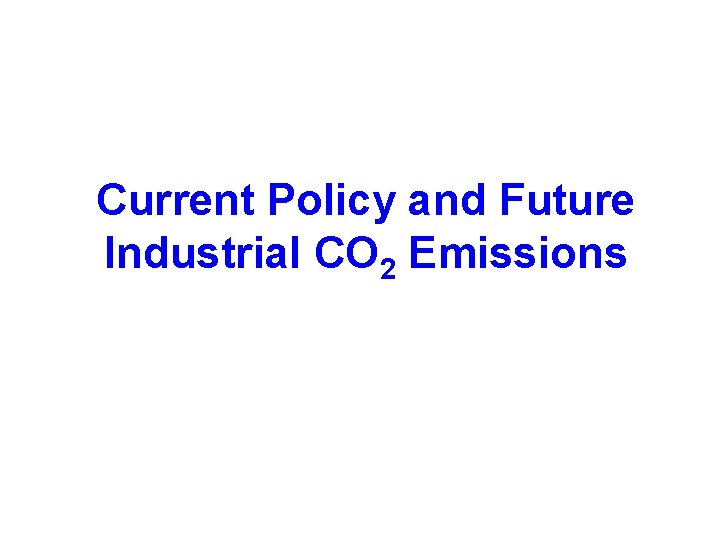
Current Policy and Future Industrial CO 2 Emissions
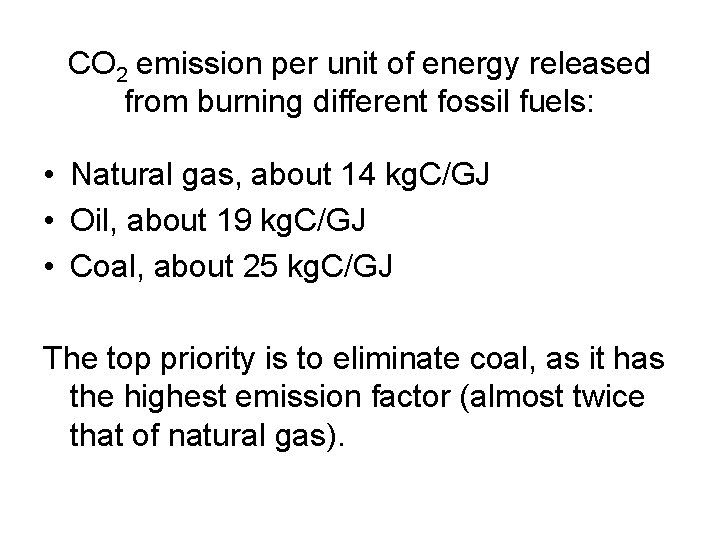
CO 2 emission per unit of energy released from burning different fossil fuels: • Natural gas, about 14 kg. C/GJ • Oil, about 19 kg. C/GJ • Coal, about 25 kg. C/GJ The top priority is to eliminate coal, as it has the highest emission factor (almost twice that of natural gas).
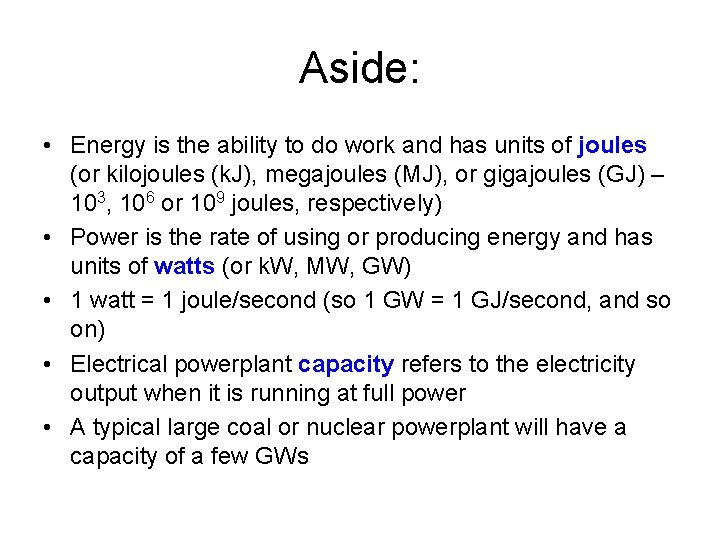
Aside: • Energy is the ability to do work and has units of joules (or kilojoules (k. J), megajoules (MJ), or gigajoules (GJ) – 103, 106 or 109 joules, respectively) • Power is the rate of using or producing energy and has units of watts (or k. W, MW, GW) • 1 watt = 1 joule/second (so 1 GW = 1 GJ/second, and so on) • Electrical powerplant capacity refers to the electricity output when it is running at full power • A typical large coal or nuclear powerplant will have a capacity of a few GWs
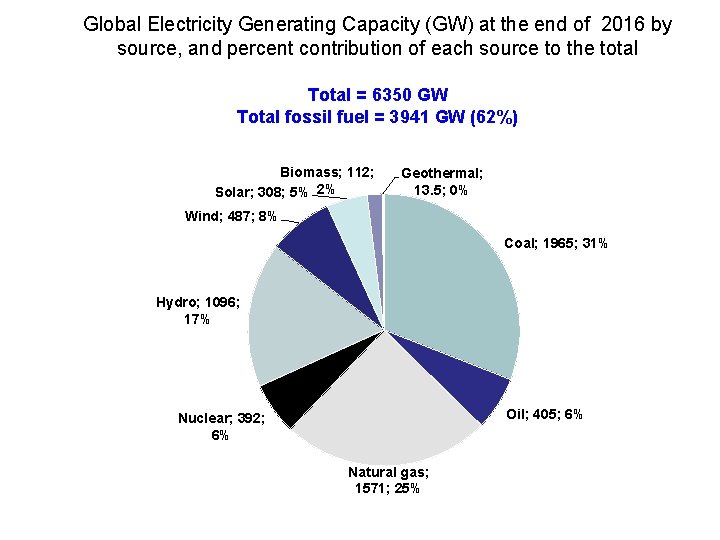
Global Electricity Generating Capacity (GW) at the end of 2016 by source, and percent contribution of each source to the total Total = 6350 GW Total fossil fuel = 3941 GW (62%) Biomass; 112; Solar; 308; 5% 2% Geothermal; 13. 5; 0% Wind; 487; 8% Coal; 1965; 31% Hydro; 1096; 17% Oil; 405; 6% Nuclear; 392; 6% Natural gas; 1571; 25%
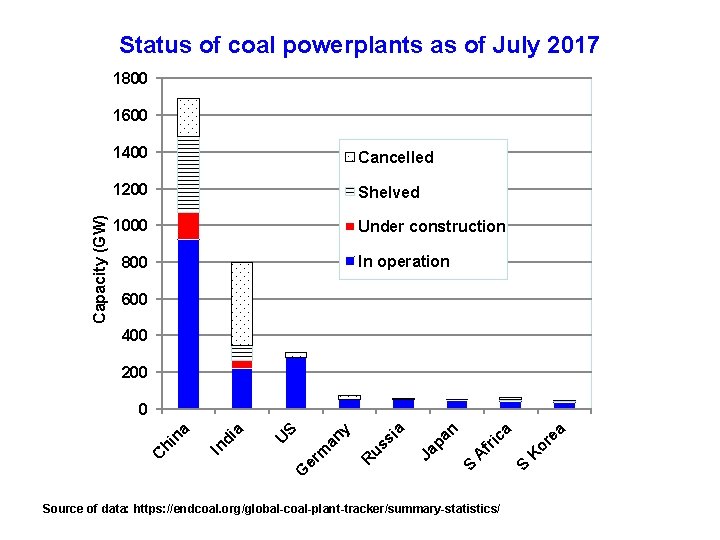
Status of coal powerplants as of July 2017 1800 Capacity (GW) 1600 1400 Cancelled 1200 Shelved 1000 Under construction In operation 800 600 400 200 Source of data: https: //endcoal. org/global-coal-plant-tracker/summary-statistics/ K S S A fr or ic ea a n Ja pa si a R us y er m an S G U a di In C hi na 0
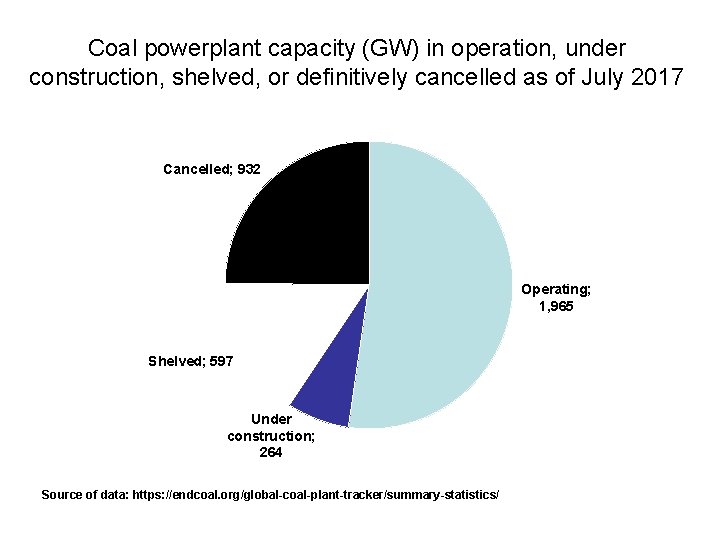
Coal powerplant capacity (GW) in operation, under construction, shelved, or definitively cancelled as of July 2017 Cancelled; 932 Operating; 1, 965 Shelved; 597 Under construction; 264 Source of data: https: //endcoal. org/global-coal-plant-tracker/summary-statistics/
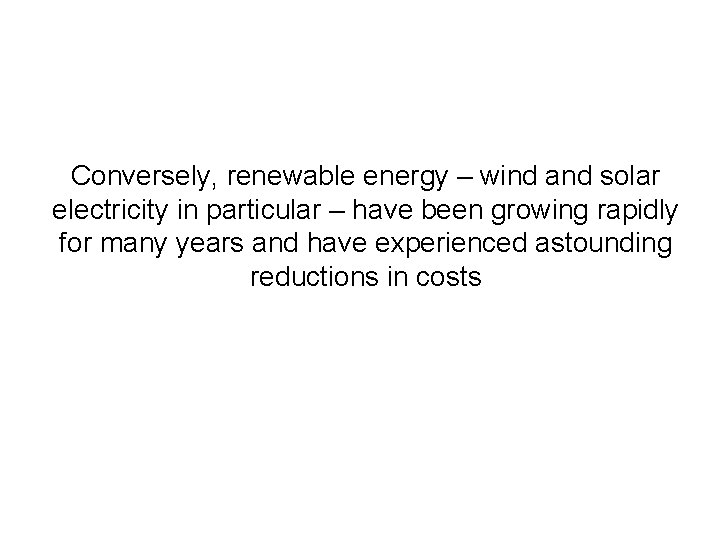
Conversely, renewable energy – wind and solar electricity in particular – have been growing rapidly for many years and have experienced astounding reductions in costs
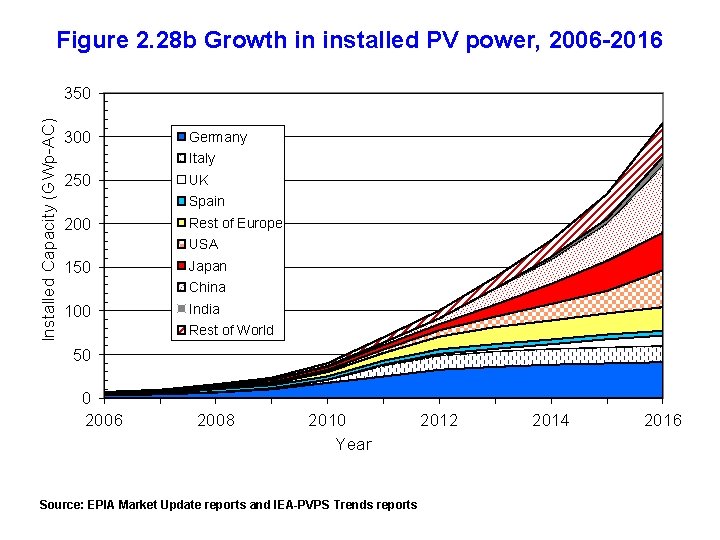
Figure 2. 28 b Growth in installed PV power, 2006 -2016 Installed Capacity (GWp-AC) 350 300 Germany Italy 250 UK Spain 200 Rest of Europe USA 150 Japan China 100 India Rest of World 50 0 2006 2008 2010 Year Source: EPIA Market Update reports and IEA-PVPS Trends reports 2012 2014 2016
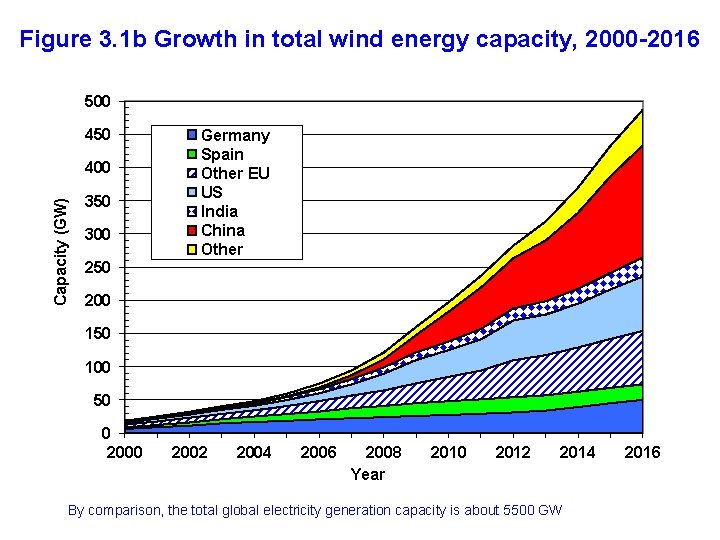
Figure 3. 1 b Growth in total wind energy capacity, 2000 -2016 500 450 Capacity (GW) 400 350 300 250 Germany Spain Other EU US India China Other 200 150 100 50 0 2002 2004 2006 2008 Year 2010 2012 2014 By comparison, the total global electricity generation capacity is about 5500 GW 2016
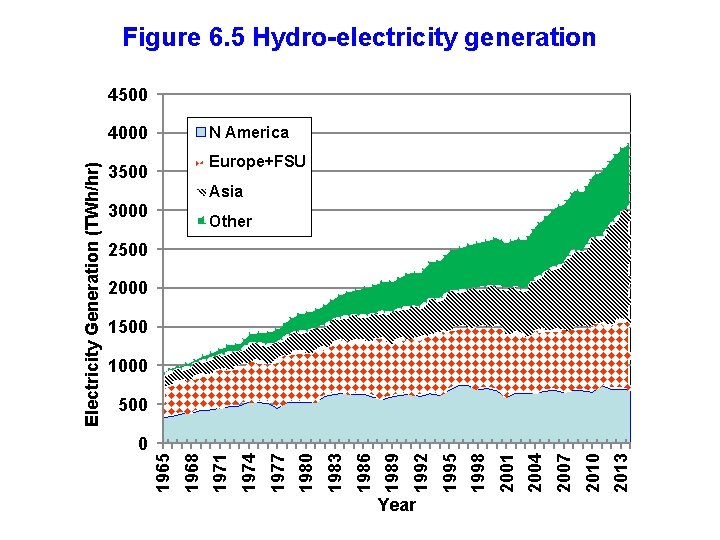
Figure 6. 5 Hydro-electricity generation 4500 N America Europe+FSU 3500 Asia 3000 Other 2500 2000 1500 1000 500 Year 2013 2010 2007 2004 2001 1998 1995 1992 1989 1986 1983 1980 1977 1974 1971 1968 0 1965 Electricity Generation (TWh/hr) 4000
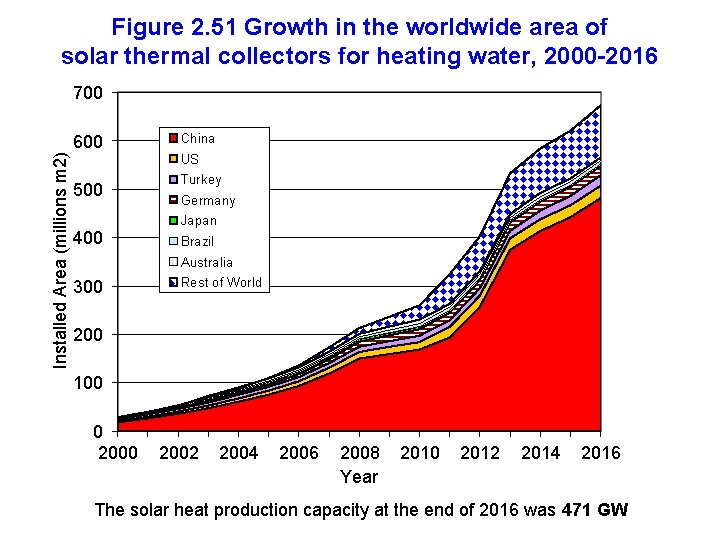
Figure 2. 51 Growth in the worldwide area of solar thermal collectors for heating water, 2000 -2016 700 Installed Area (millions m 2) 600 China US 500 400 Turkey Germany Japan Brazil Australia 300 Rest of World 200 100 0 2002 2004 2006 2008 Year 2010 2012 2014 2016 The solar heat production capacity at the end of 2016 was 471 GW
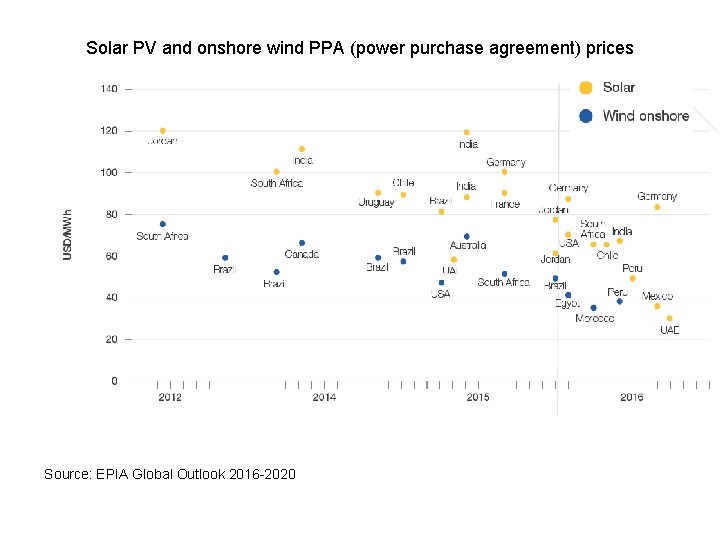
Solar PV and onshore wind PPA (power purchase agreement) prices Source: EPIA Global Outlook 2016 -2020
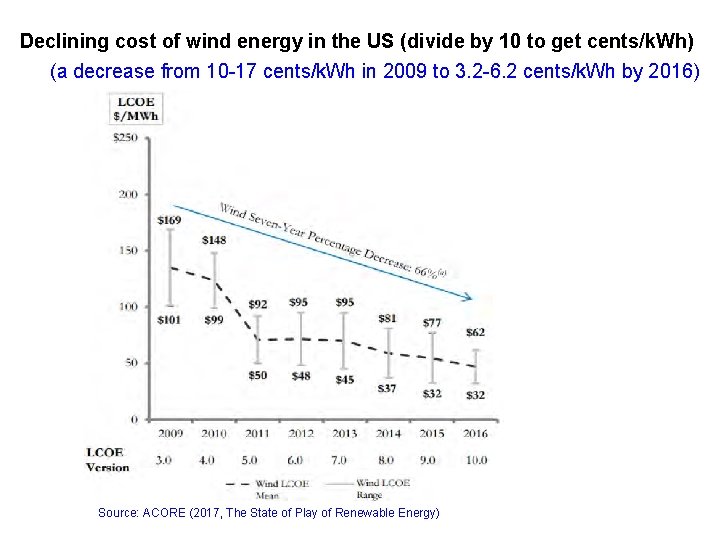
Declining cost of wind energy in the US (divide by 10 to get cents/k. Wh) (a decrease from 10 -17 cents/k. Wh in 2009 to 3. 2 -6. 2 cents/k. Wh by 2016) Source: ACORE (2017, The State of Play of Renewable Energy)
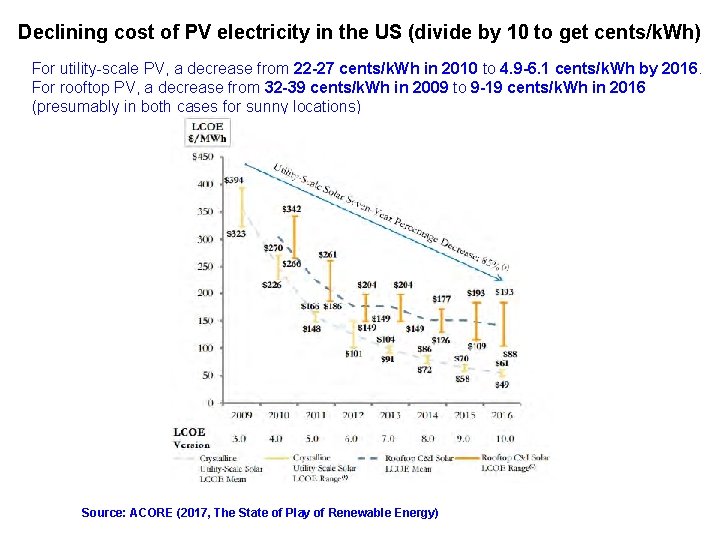
Declining cost of PV electricity in the US (divide by 10 to get cents/k. Wh) For utility-scale PV, a decrease from 22 -27 cents/k. Wh in 2010 to 4. 9 -6. 1 cents/k. Wh by 2016. For rooftop PV, a decrease from 32 -39 cents/k. Wh in 2009 to 9 -19 cents/k. Wh in 2016 (presumably in both cases for sunny locations) Source: ACORE (2017, The State of Play of Renewable Energy)
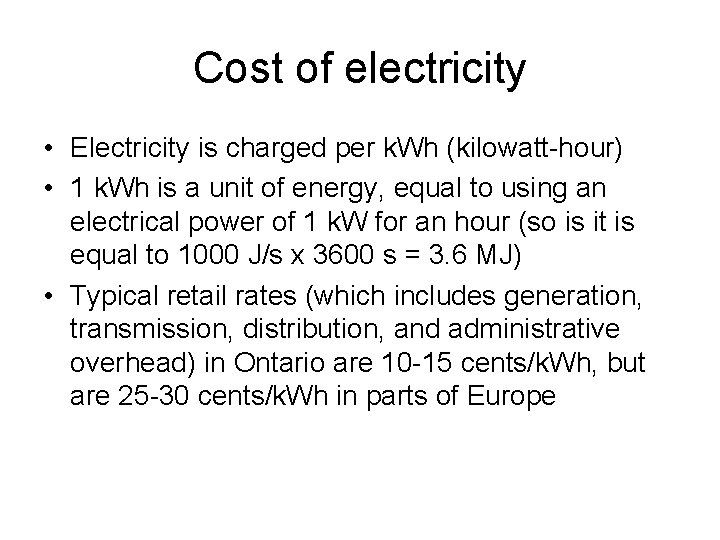
Cost of electricity • Electricity is charged per k. Wh (kilowatt-hour) • 1 k. Wh is a unit of energy, equal to using an electrical power of 1 k. W for an hour (so is it is equal to 1000 J/s x 3600 s = 3. 6 MJ) • Typical retail rates (which includes generation, transmission, distribution, and administrative overhead) in Ontario are 10 -15 cents/k. Wh, but are 25 -30 cents/k. Wh in parts of Europe
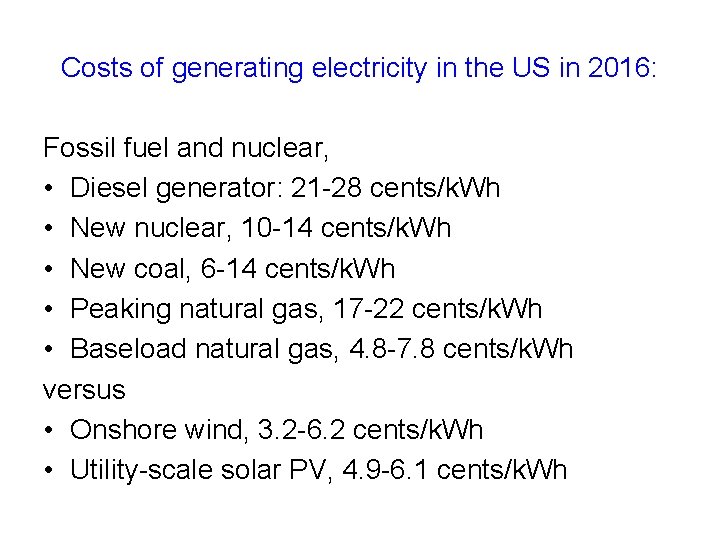
Costs of generating electricity in the US in 2016: Fossil fuel and nuclear, • Diesel generator: 21 -28 cents/k. Wh • New nuclear, 10 -14 cents/k. Wh • New coal, 6 -14 cents/k. Wh • Peaking natural gas, 17 -22 cents/k. Wh • Baseload natural gas, 4. 8 -7. 8 cents/k. Wh versus • Onshore wind, 3. 2 -6. 2 cents/k. Wh • Utility-scale solar PV, 4. 9 -6. 1 cents/k. Wh
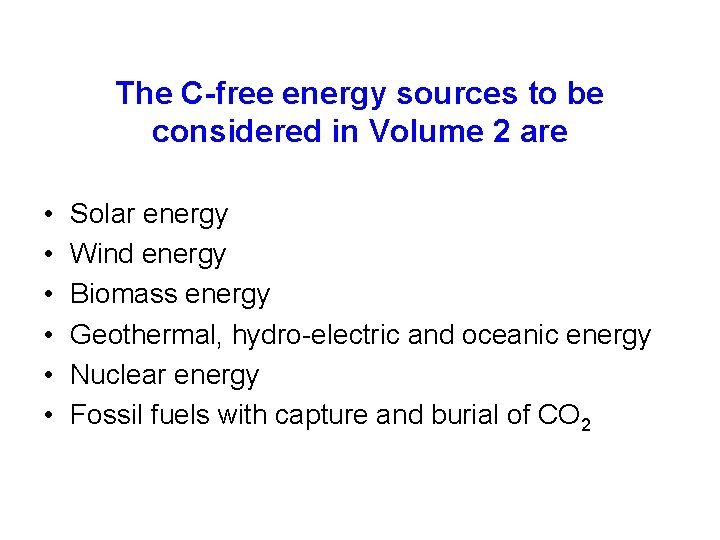
The C-free energy sources to be considered in Volume 2 are • • • Solar energy Wind energy Biomass energy Geothermal, hydro-electric and oceanic energy Nuclear energy Fossil fuels with capture and burial of CO 2
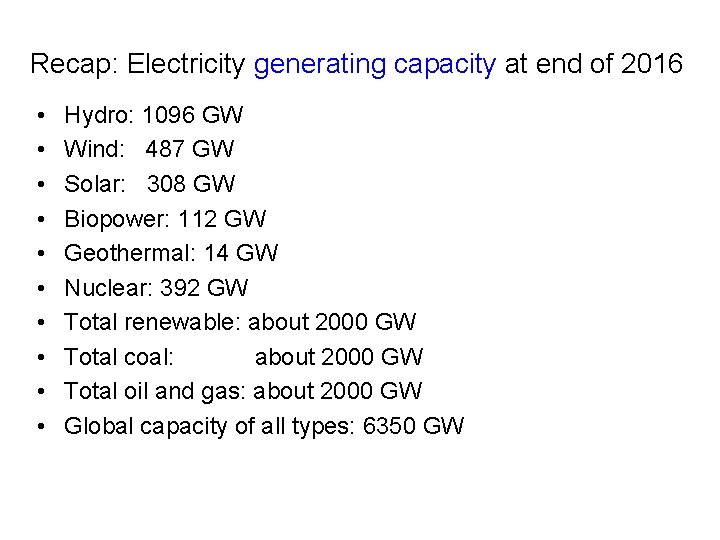
Recap: Electricity generating capacity at end of 2016 • • • Hydro: 1096 GW Wind: 487 GW Solar: 308 GW Biopower: 112 GW Geothermal: 14 GW Nuclear: 392 GW Total renewable: about 2000 GW Total coal: about 2000 GW Total oil and gas: about 2000 GW Global capacity of all types: 6350 GW
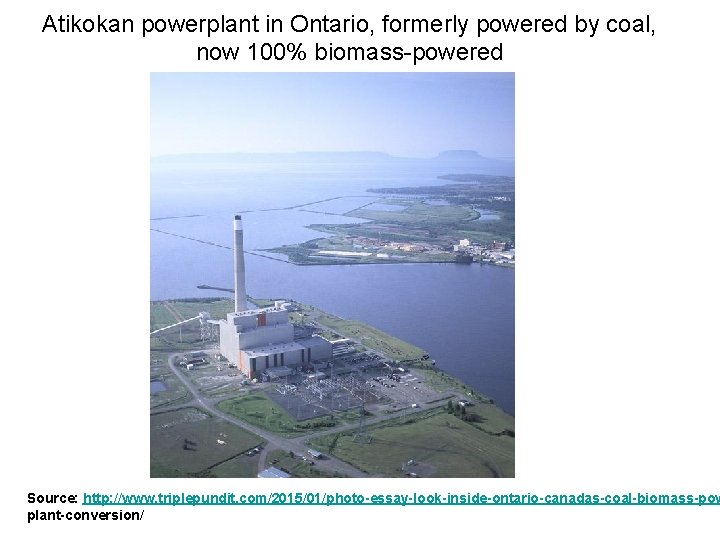
Atikokan powerplant in Ontario, formerly powered by coal, now 100% biomass-powered Source: http: //www. triplepundit. com/2015/01/photo-essay-look-inside-ontario-canadas-coal-biomass-pow plant-conversion/
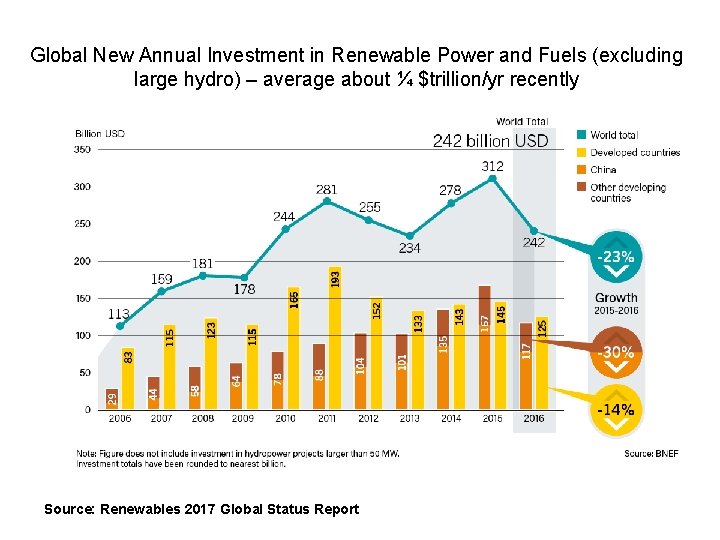
Global New Annual Investment in Renewable Power and Fuels (excluding large hydro) – average about ¼ $trillion/yr recently Source: Renewables 2017 Global Status Report
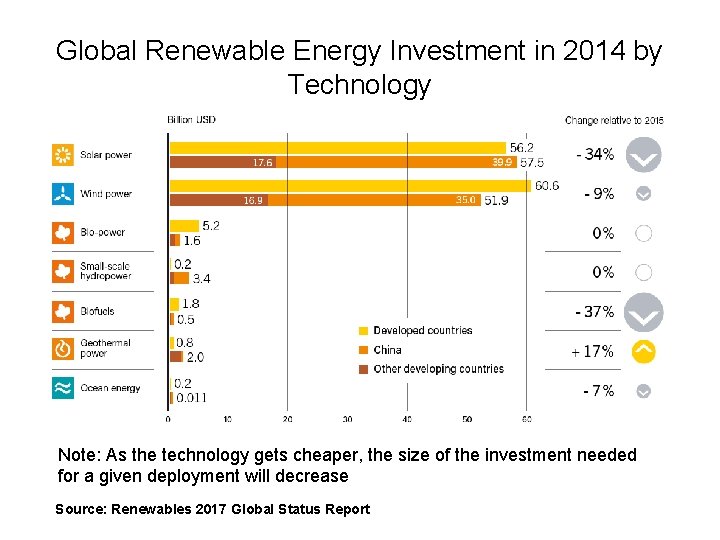
Global Renewable Energy Investment in 2014 by Technology Note: As the technology gets cheaper, the size of the investment needed for a given deployment will decrease Source: Renewables 2017 Global Status Report
Chapter 5 section 1 supply and the law of supply
Energy energy transfer and general energy analysis
Energy energy transfer and general energy analysis
Uri ng elastisidad ng supply na may parehong bahagdan
Matching supply and demand in supply chain
Chapter 7 energy conservation of energy
Where do we get a constant supply of free energy
Logistics management introduction
Introduction to operations and supply chain management
Module 5 supply and demand introduction and demand
Acdc copyright
Chapter 5 section 2 the supply curve shifts answers
Drivers of supply chain management
Chapter 6 supply chain management
Chapter 6 section 1 price supply and demand together
Understanding supply section 1
Chapter 33 aggregate demand and aggregate supply
Chapter 16 determinants of the money supply
Chapter 16 determinants of the money supply
The number of tankers needed for a fire will depend on the
Chapter 1 supply chain management
Chapter 6 supply chain management
Chapter 5 section 3 changes in supply
Drivers for lean supply chain
Chapter 16 determinants of the money supply
The formula for the simple deposit multiplier is
Chapter 6 demand supply and prices
Chapter 6 supply chain management
Chapter 5 section 3 changes in supply
Chapter 1 supply chain management
Tailored network in supply chain
Chapter 6 supply demand and government policies
Chemical potential energy images
Kinetic energy of a spring
Primary energy and secondary energy
Disadvantages of conventional energy
How to calculate gibbs free energy
Gibbs energy and equilibrium
How to increase potential energy
How are thermal energy and temperature different
A hairdryer converts ____ energy into ____ energy.
Electrical potential energy formula
How to convert mechanical energy to electrical energy
Section 3 using thermal energy answers
What is delta g
Helmholtz free energy
As nutritional energy passes through the food chain, energy
As a roller coaster goes downhill
Indirect solar energy
________ converts light energy into chemical energy. *
Gravitational potential energy formula
Potential energy examples
Electric potential formula physics
Physic energy
Potential energy to mechanical energy examples
Gravitational potential energy vs kinetic energy
Useful output power
Mass and thermal energy
Renewable energy and energy efficiency partnership
Kinetic potential
Kinetic energy example
Potential energy of spring
Kinetic energy and potential energy formula
The change in mechanical energy