Exams and course evaluation 4 exams 1 st
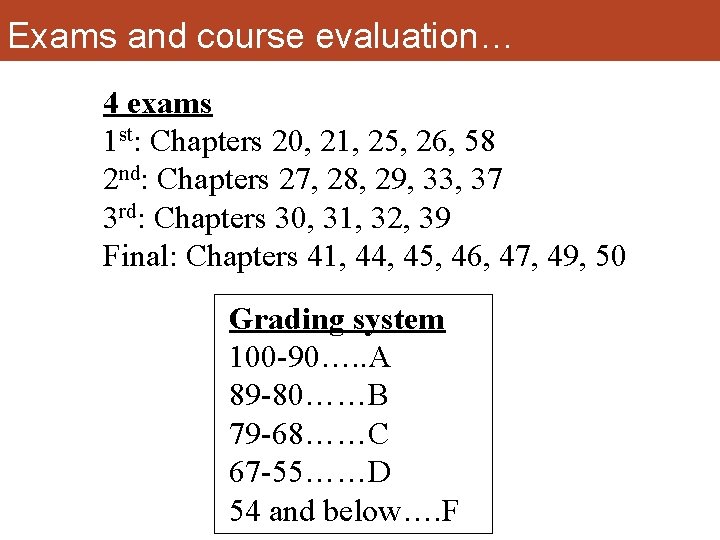
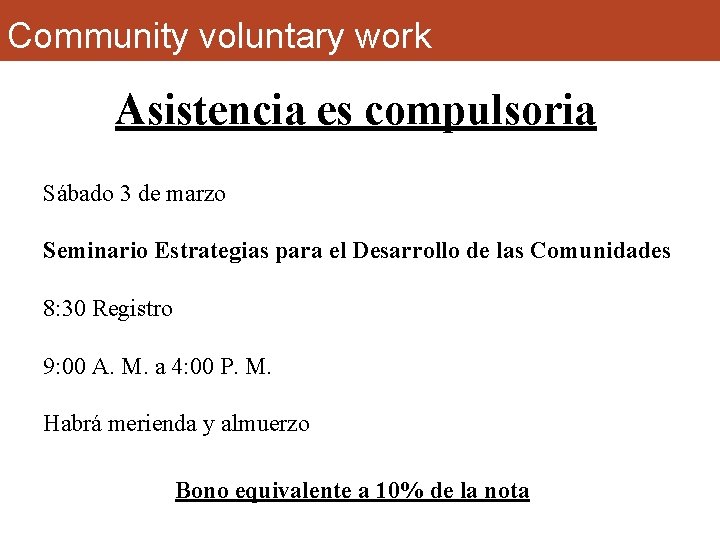
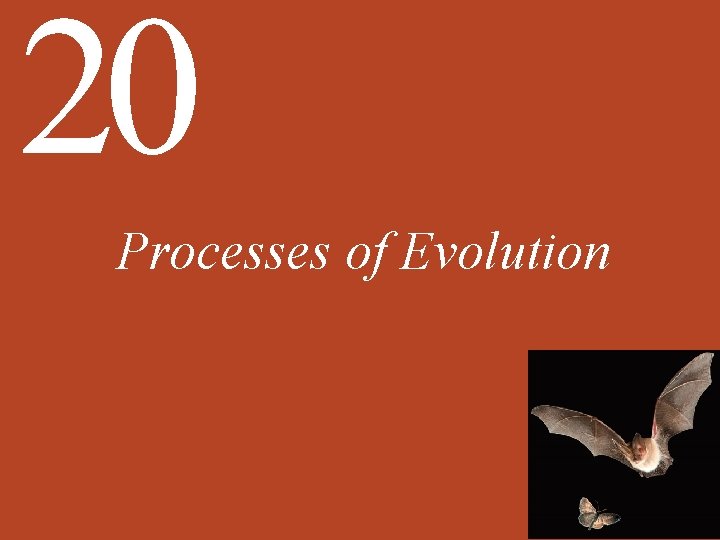
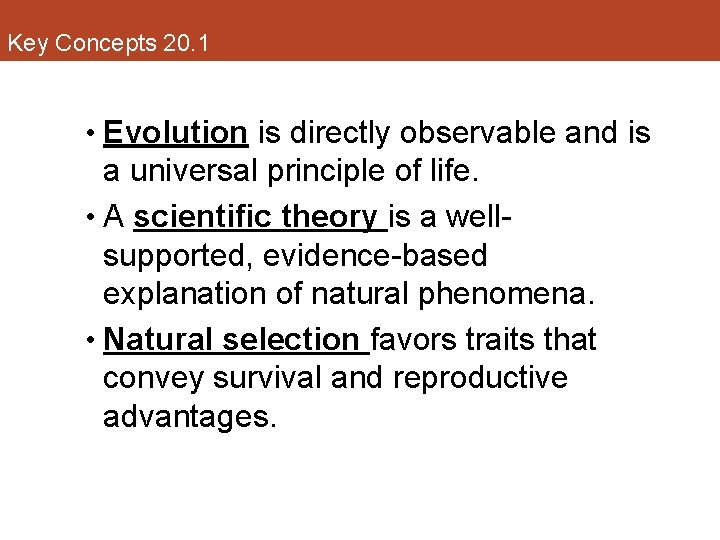
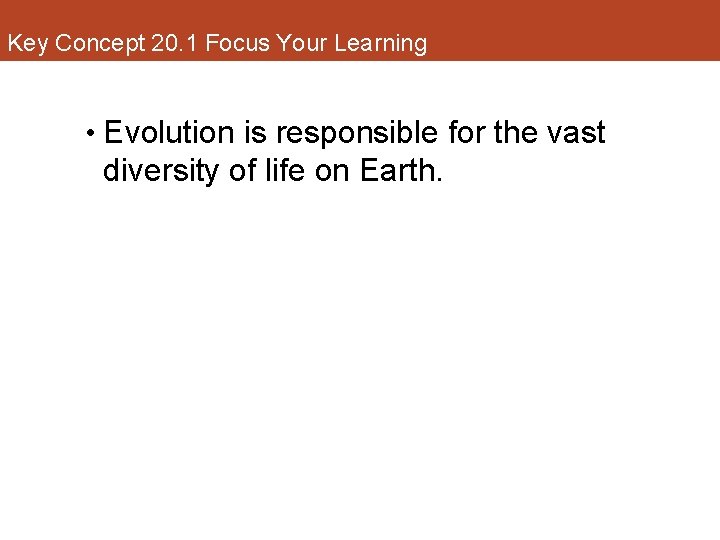
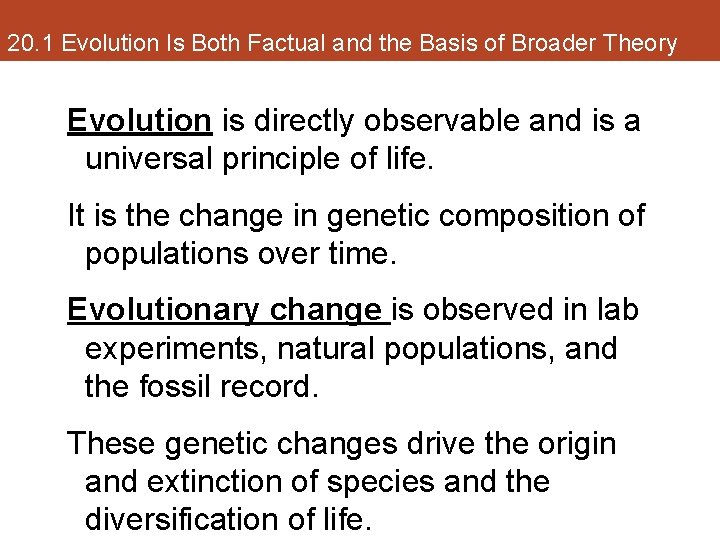
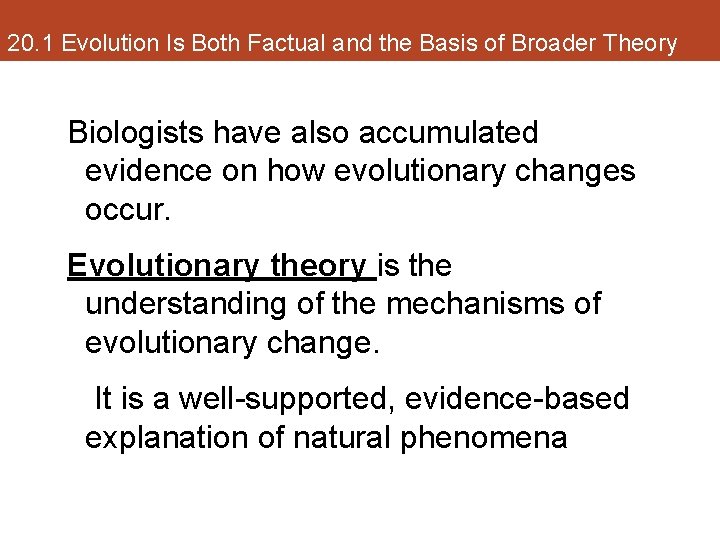
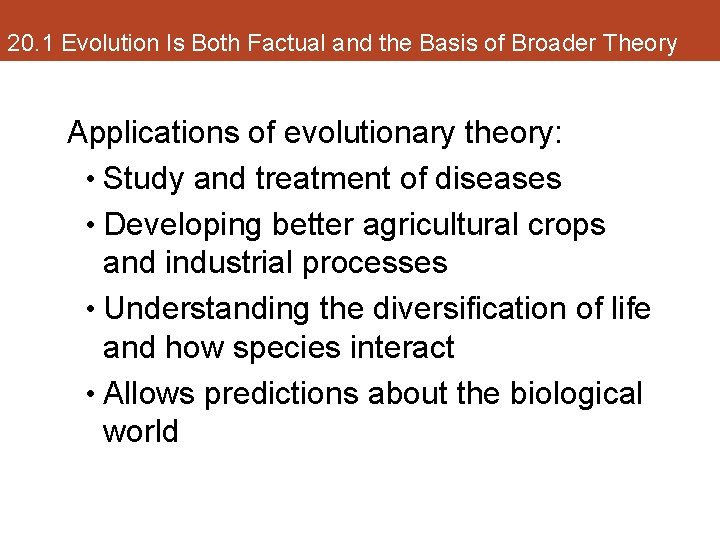
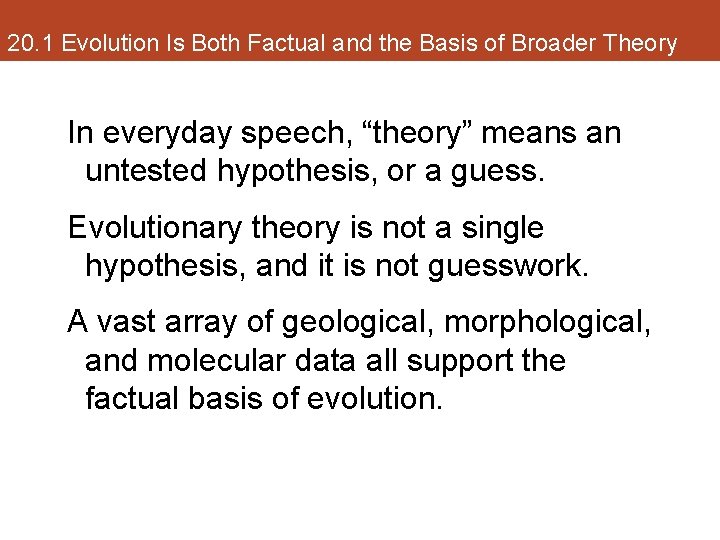
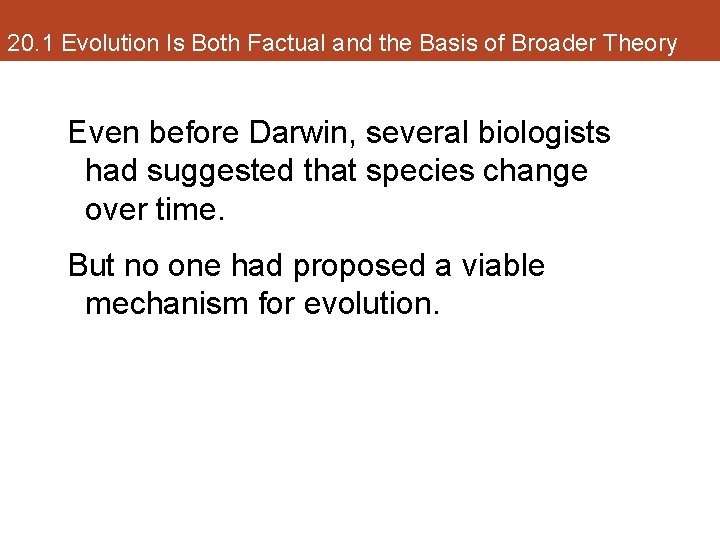
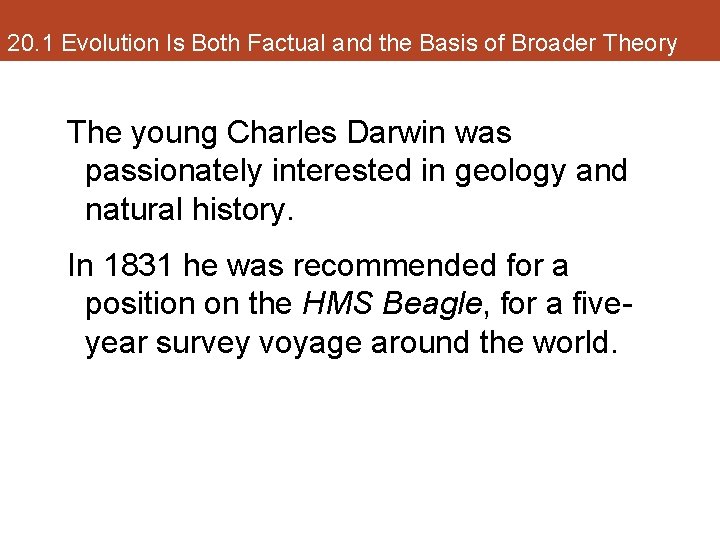
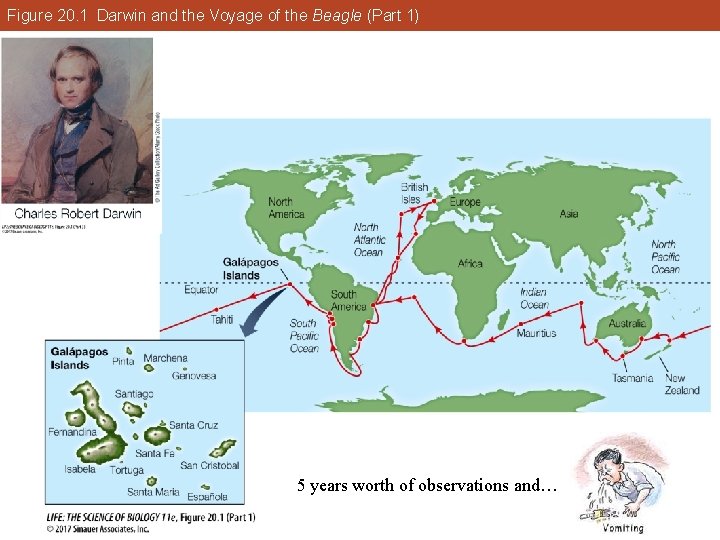
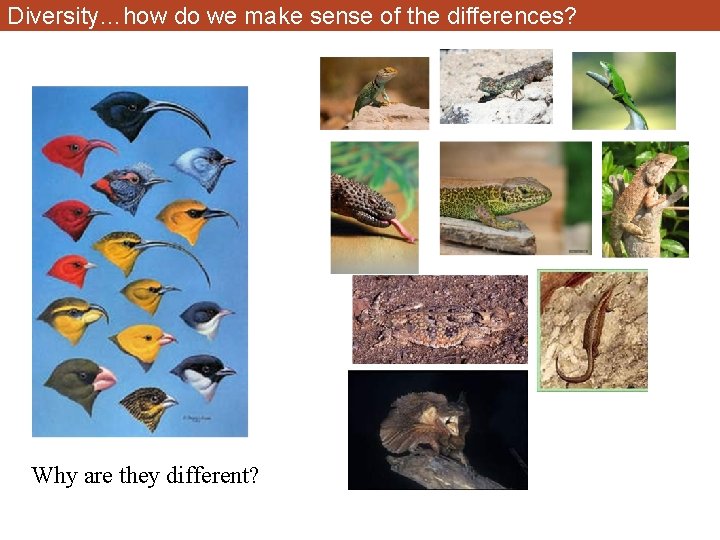
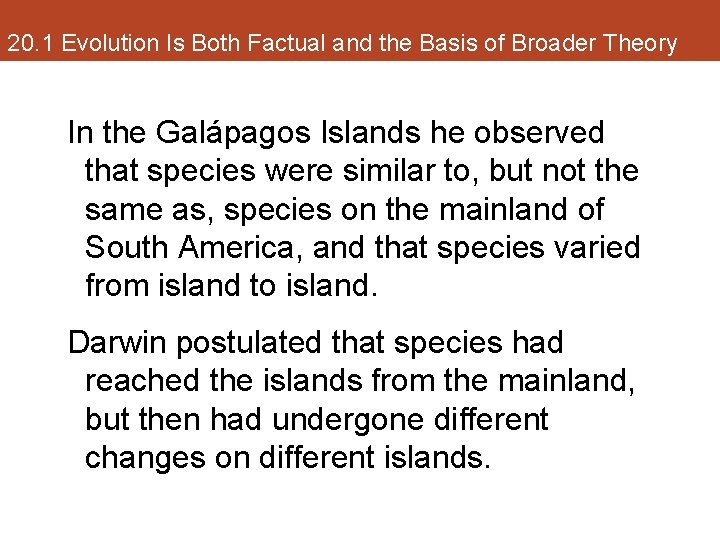
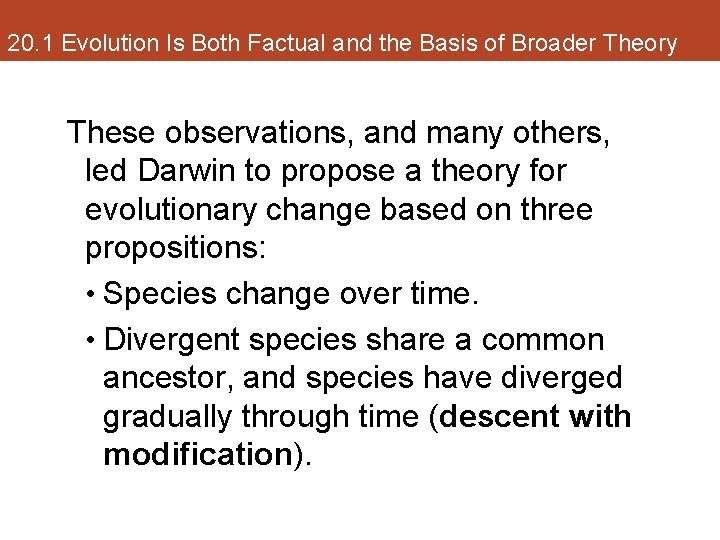
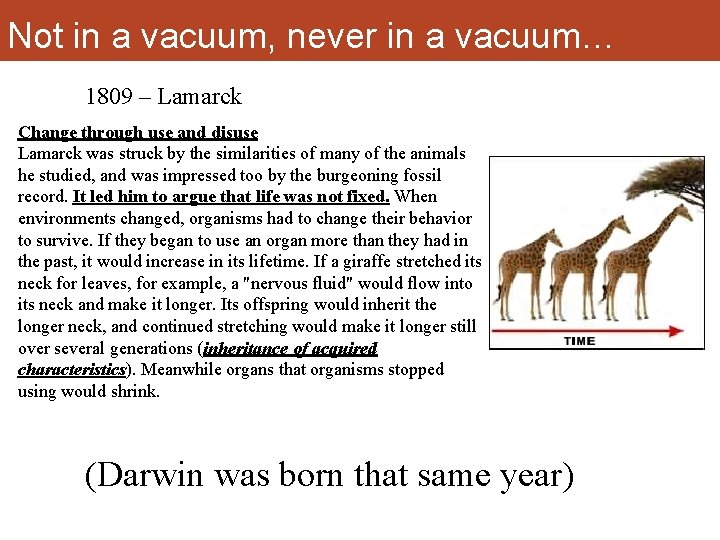
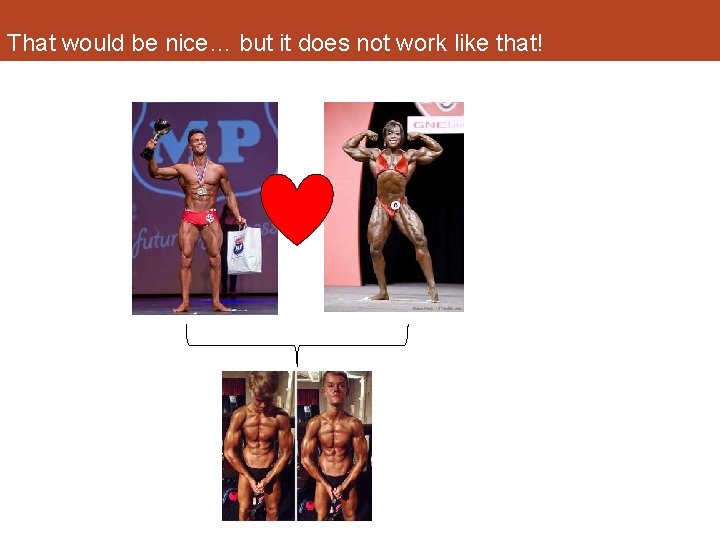
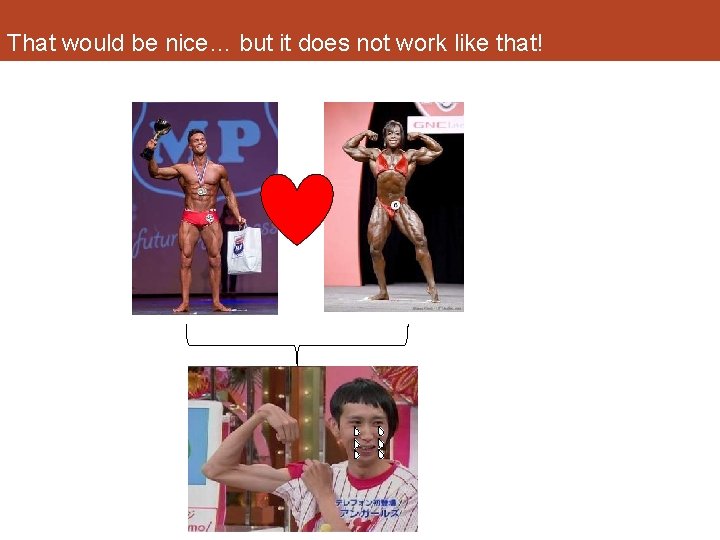
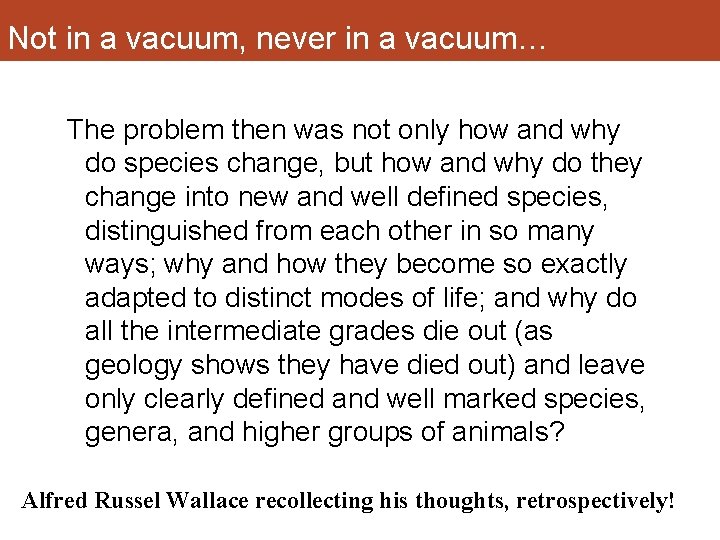
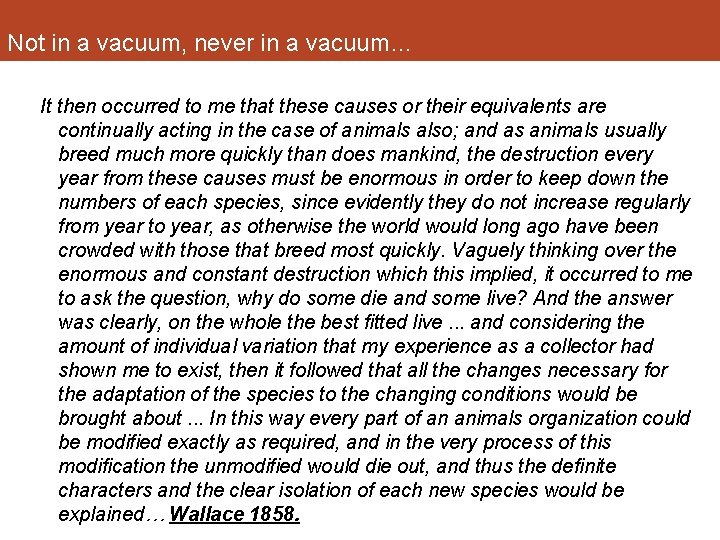
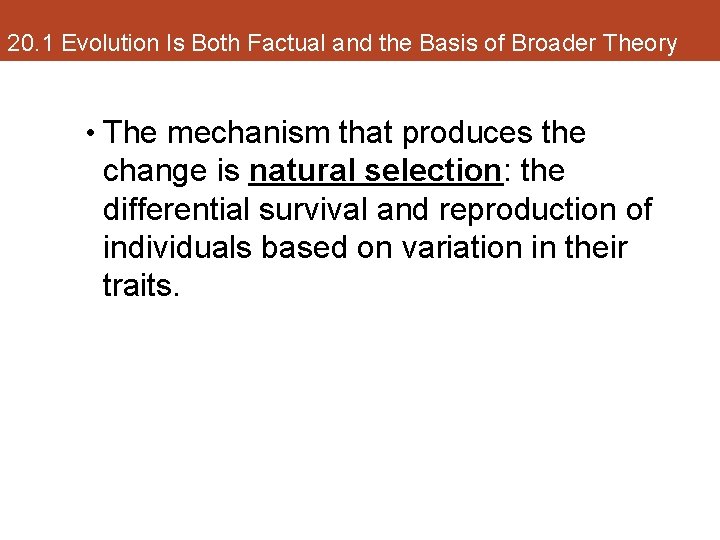
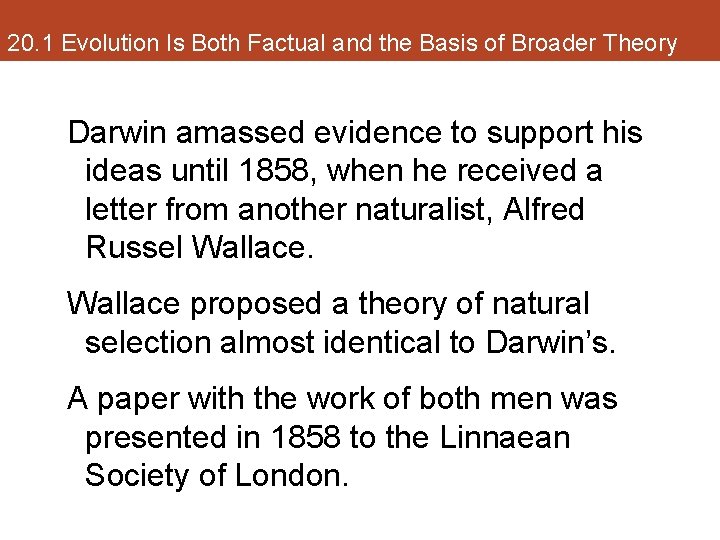
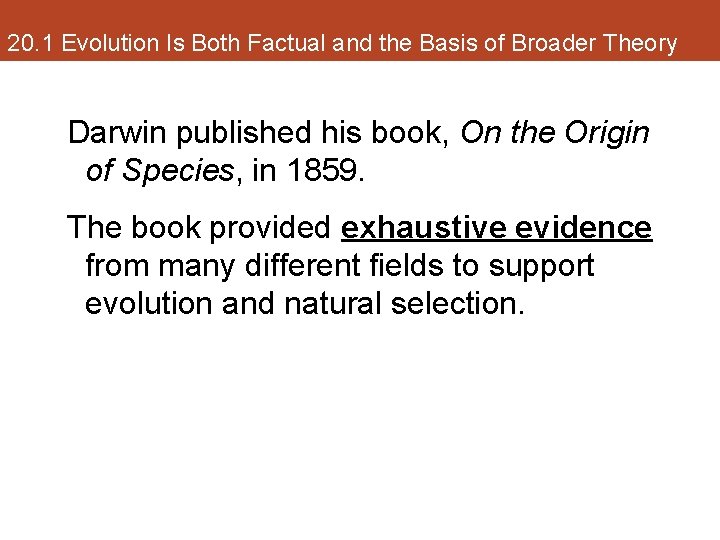
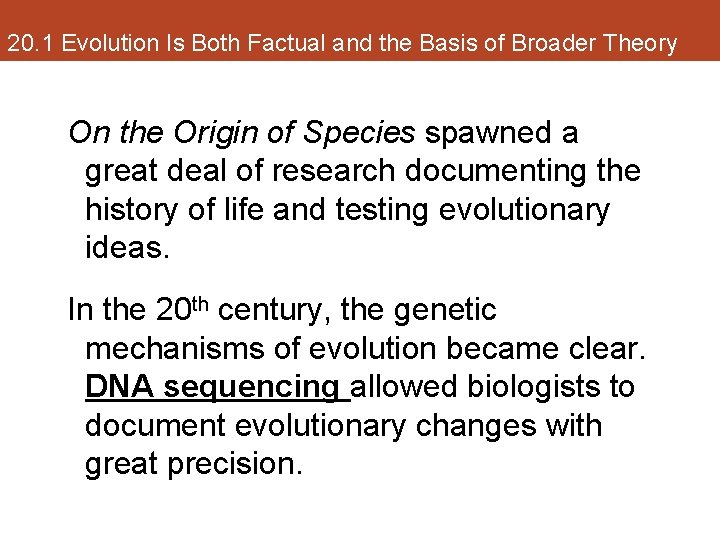
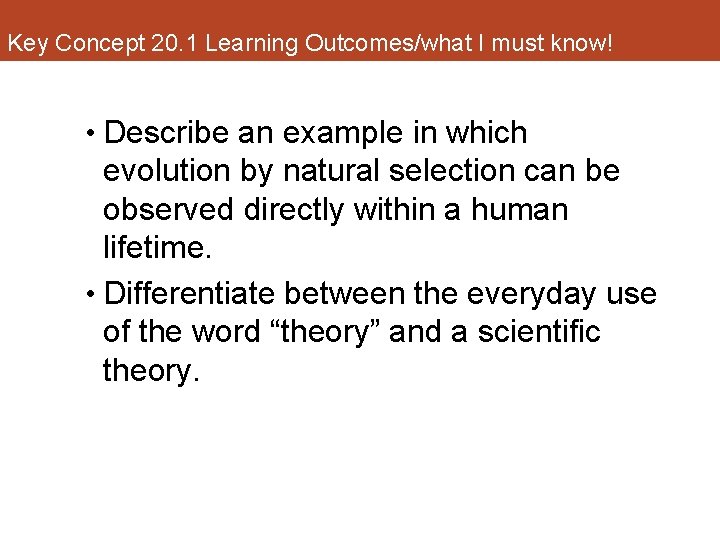
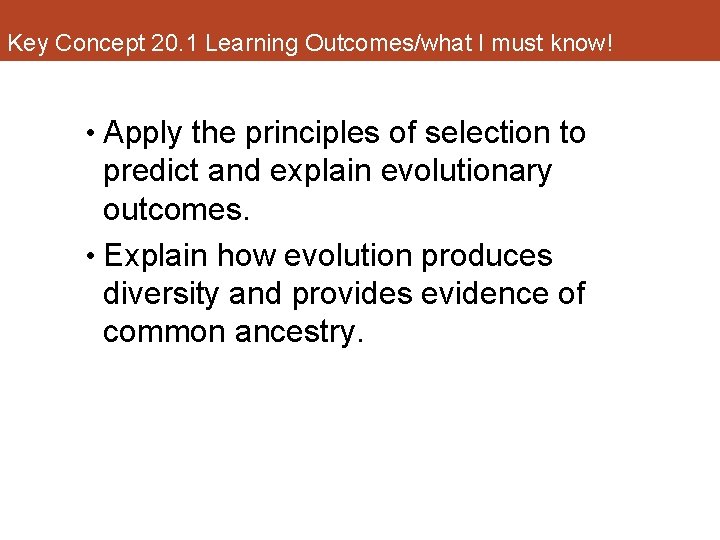
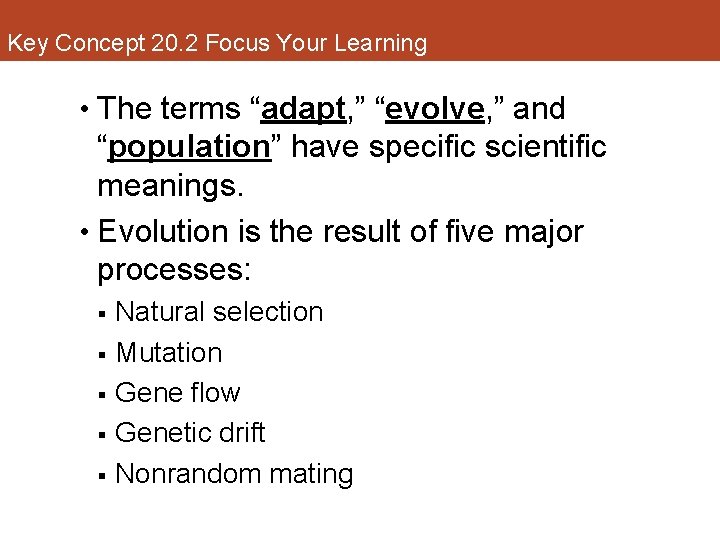
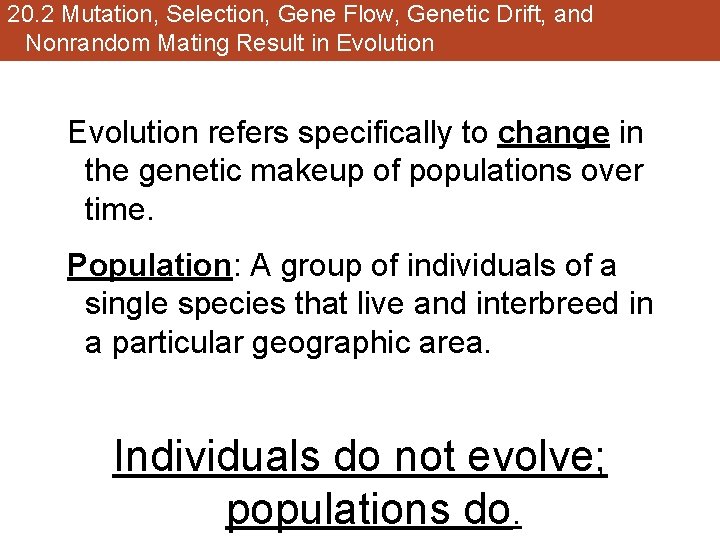
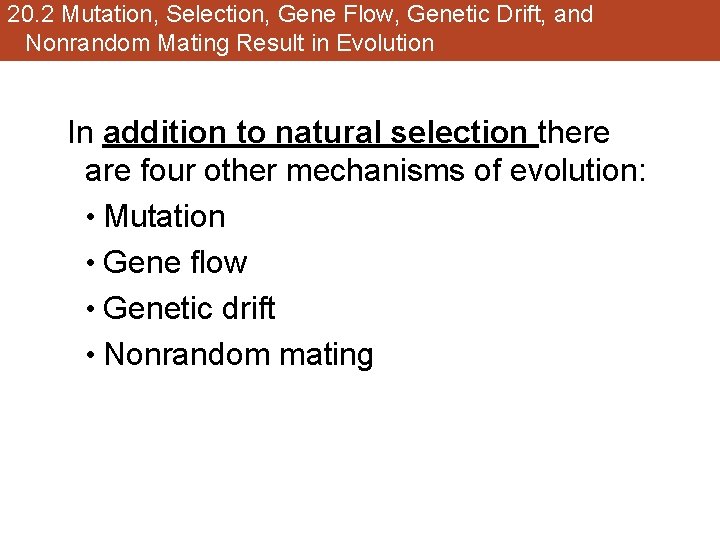
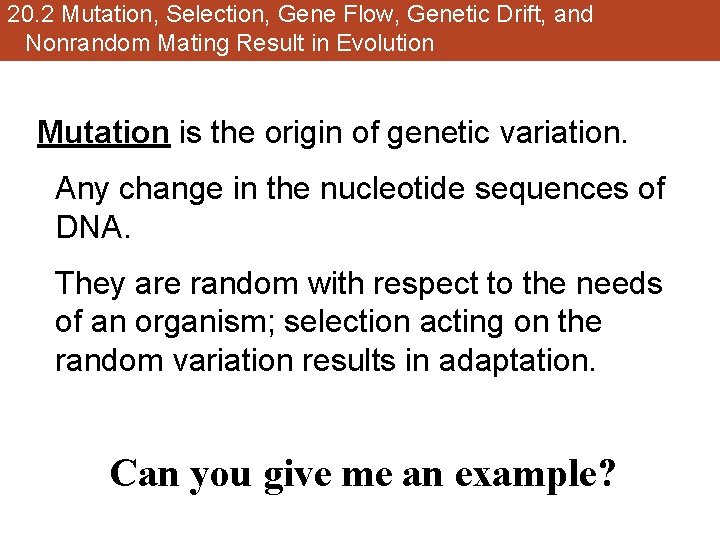
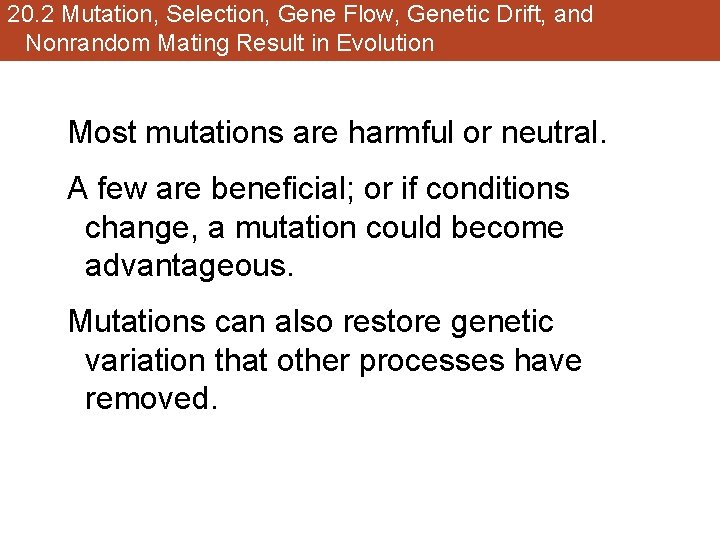
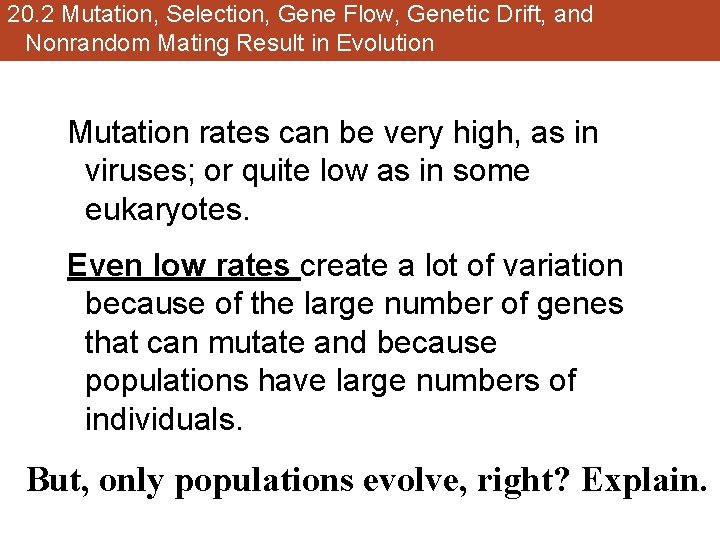
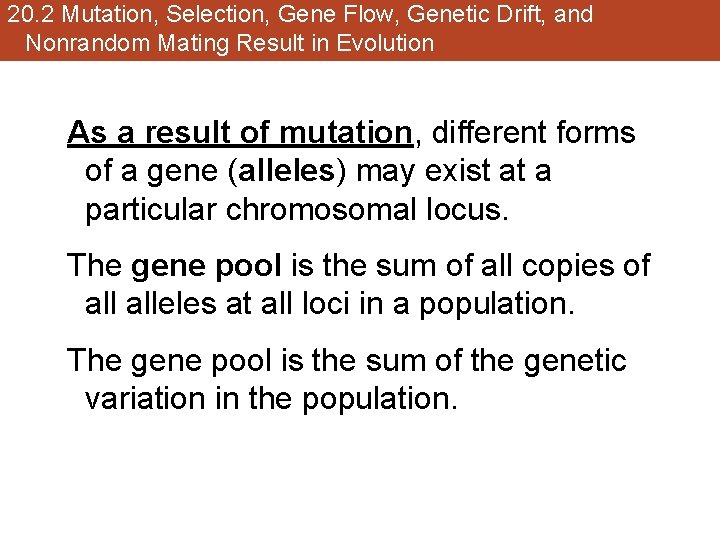
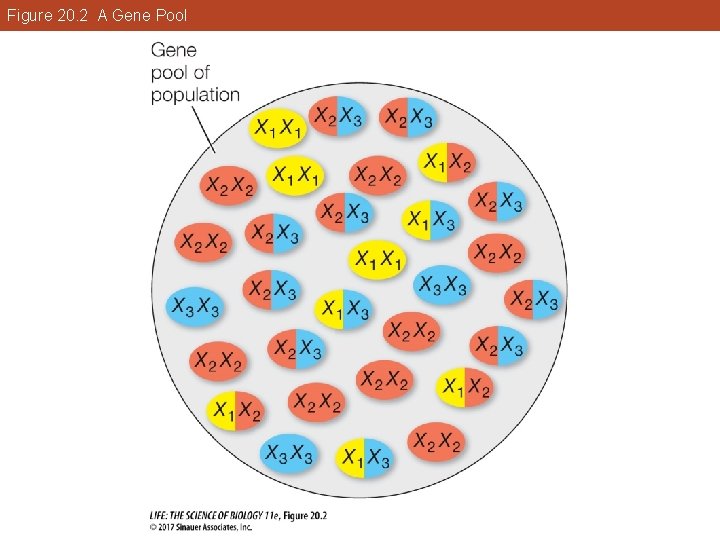
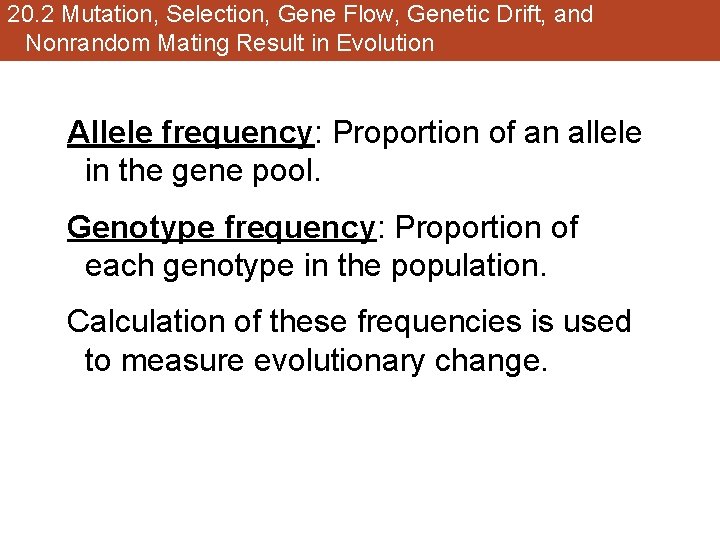
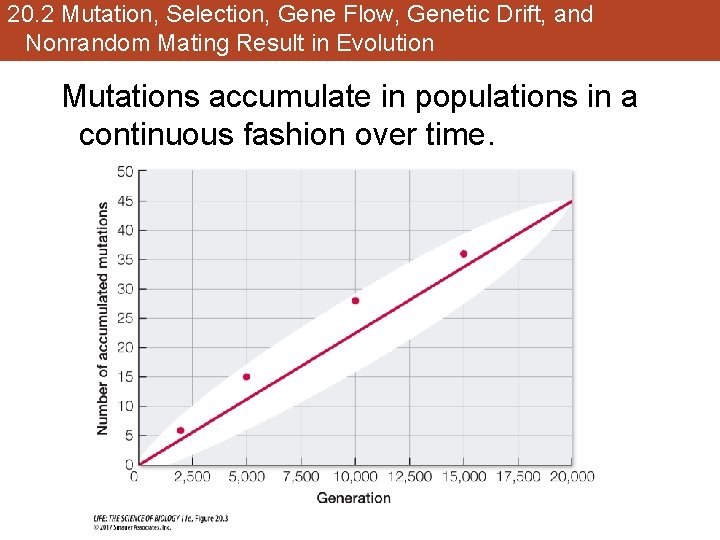
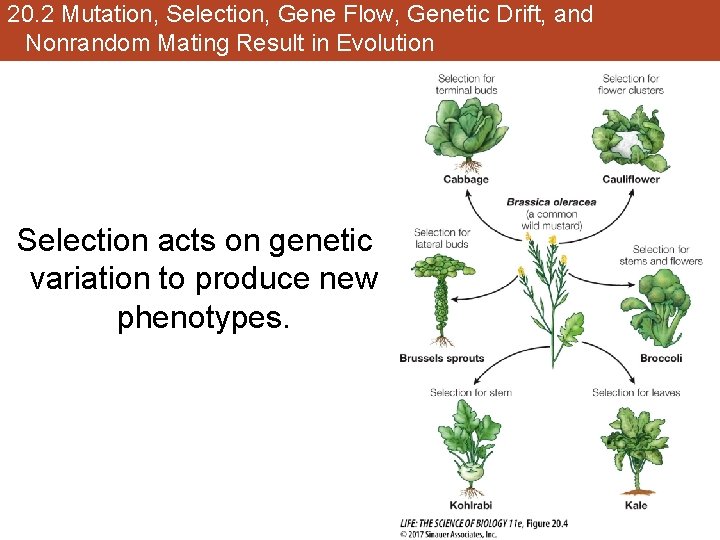
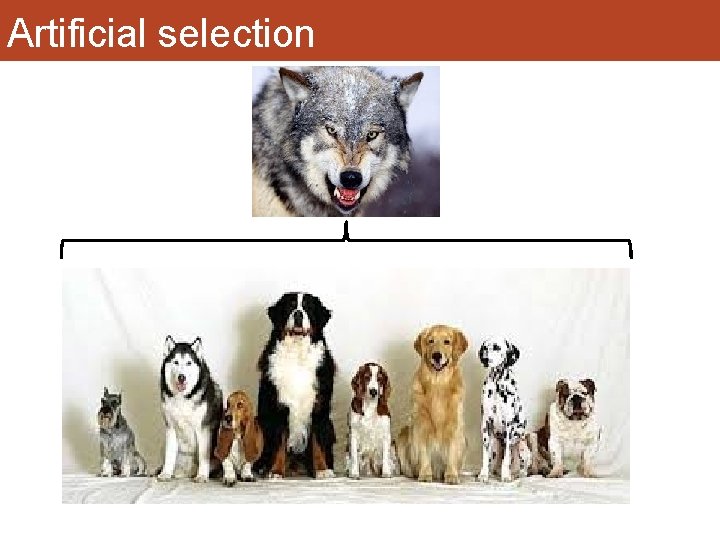
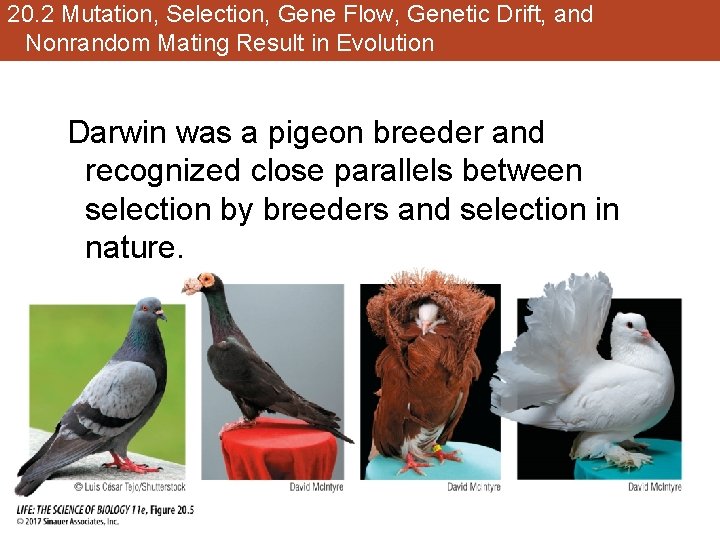
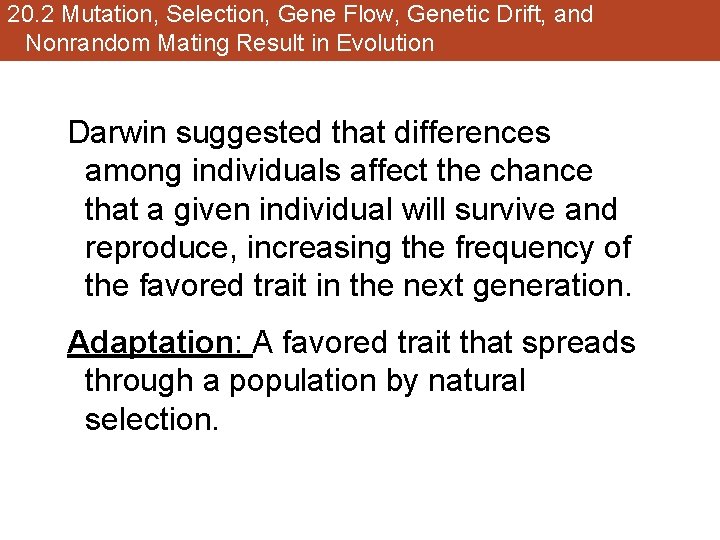
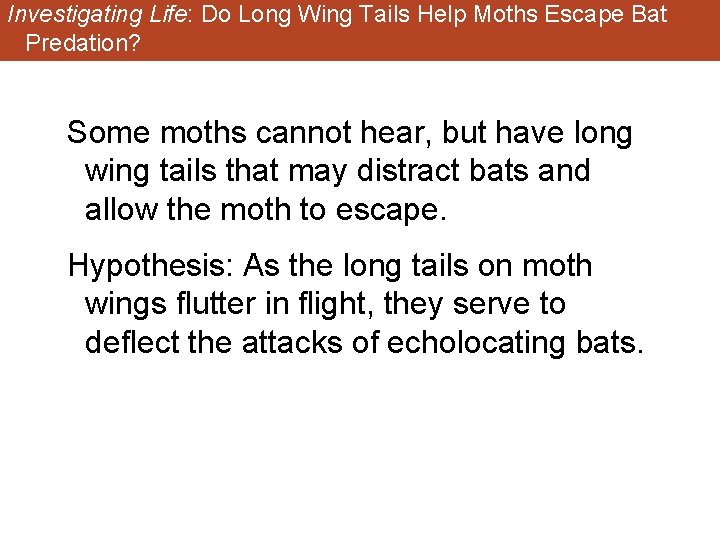
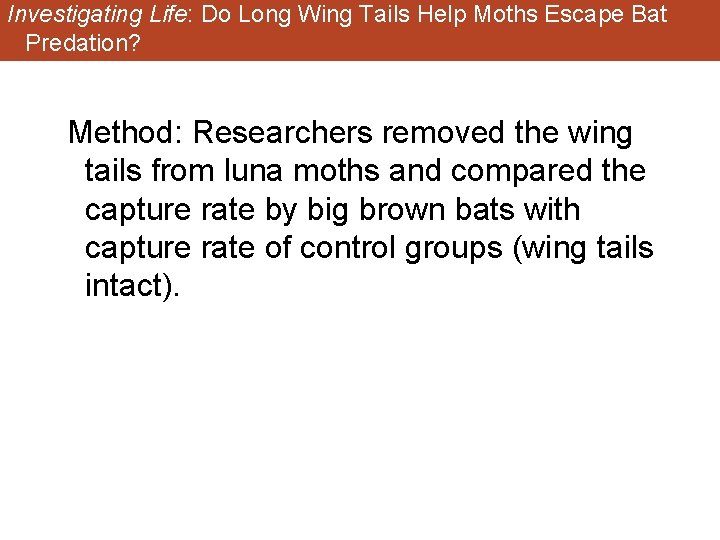
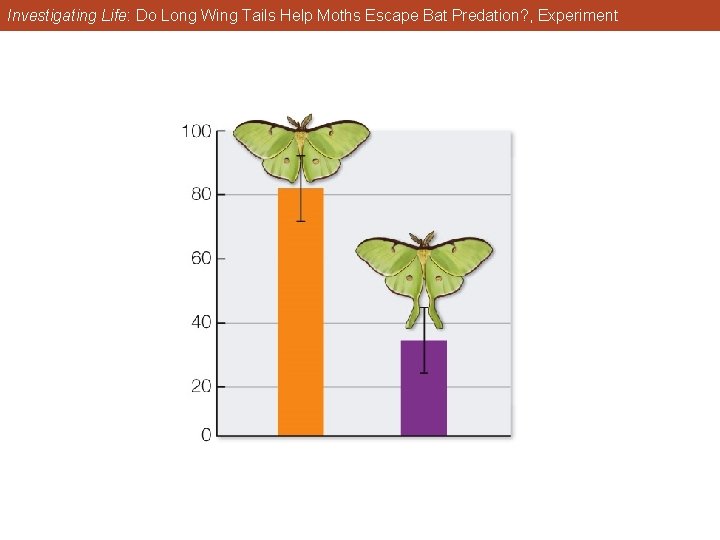
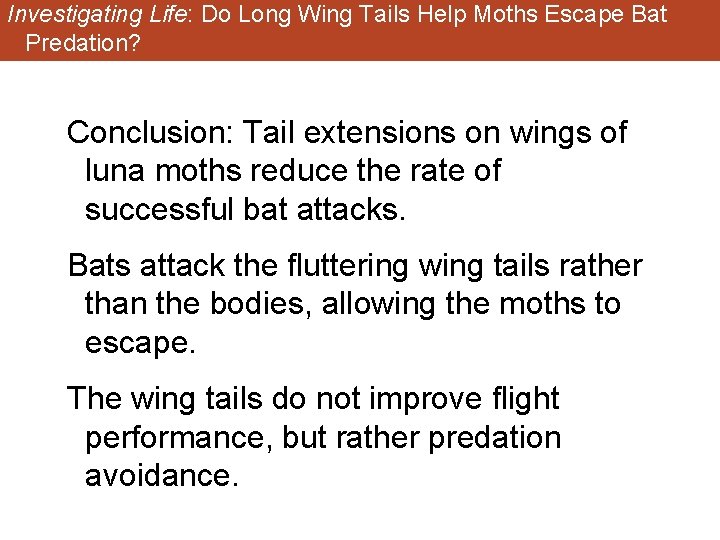
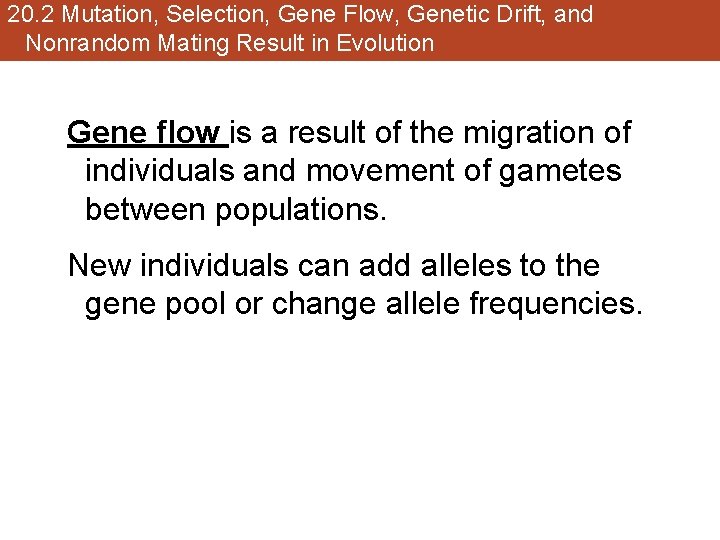
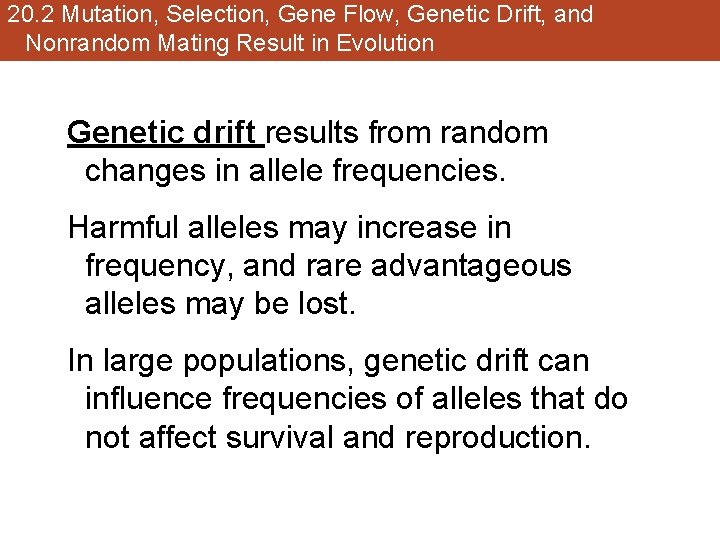
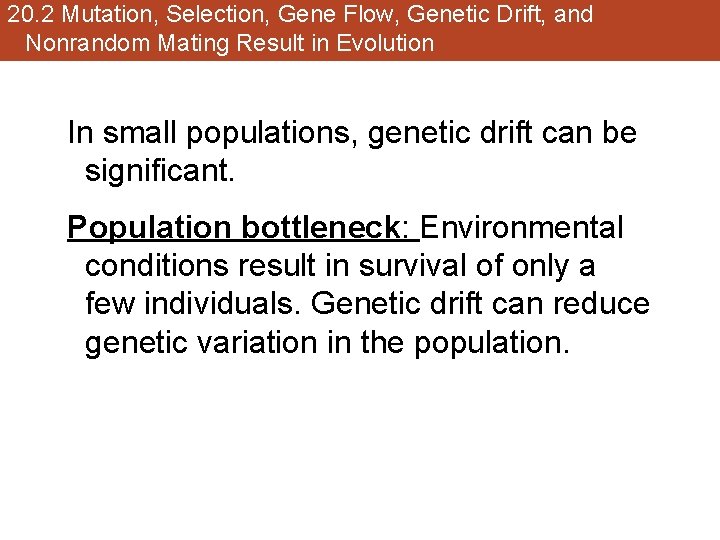
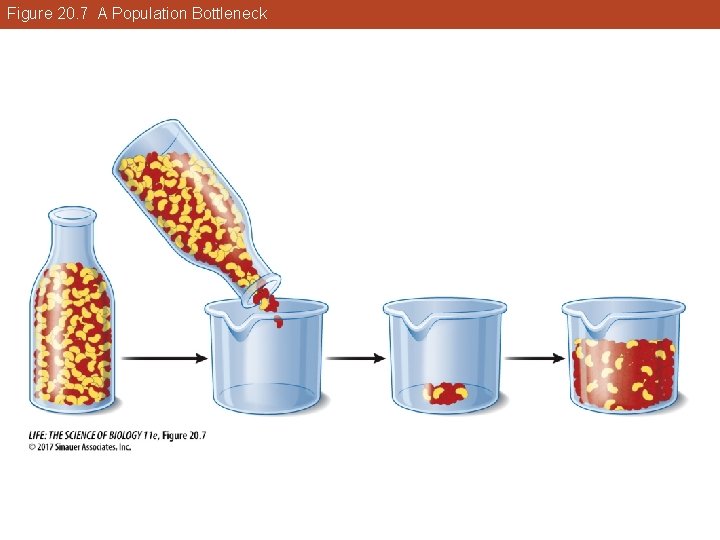
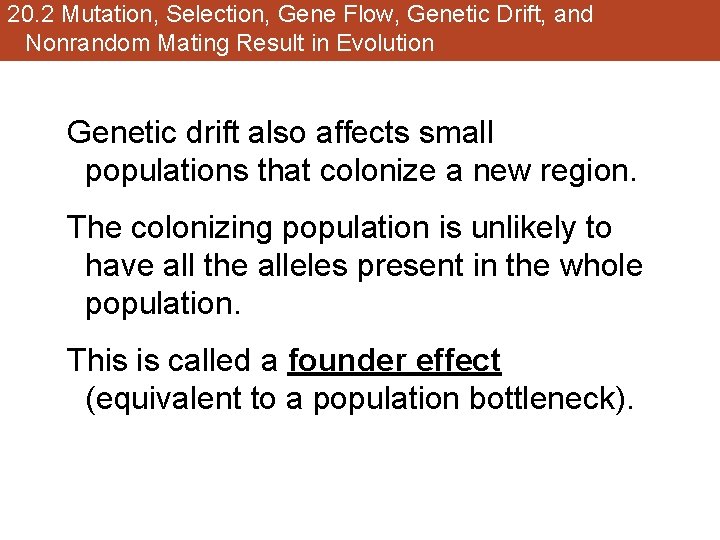
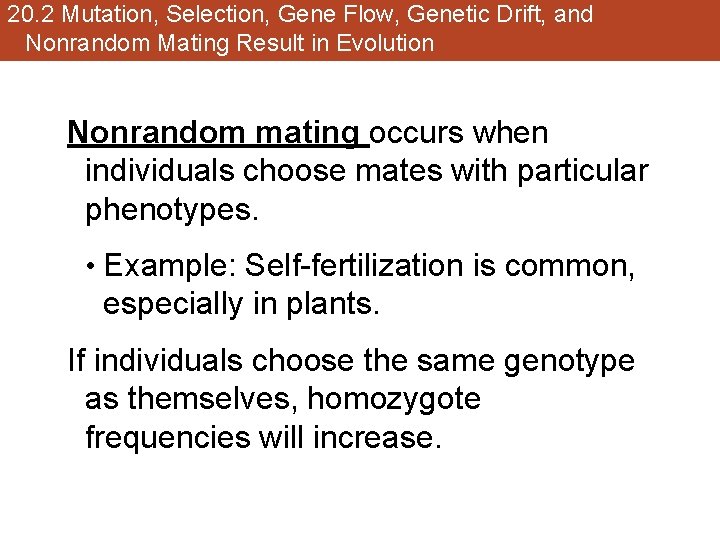
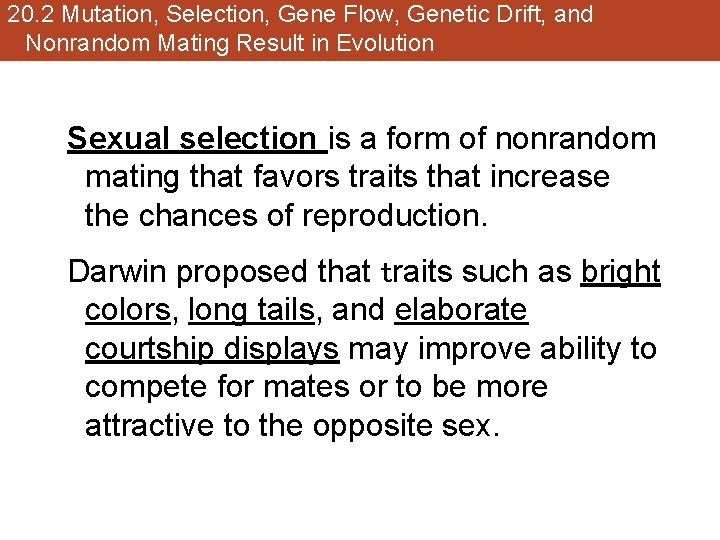
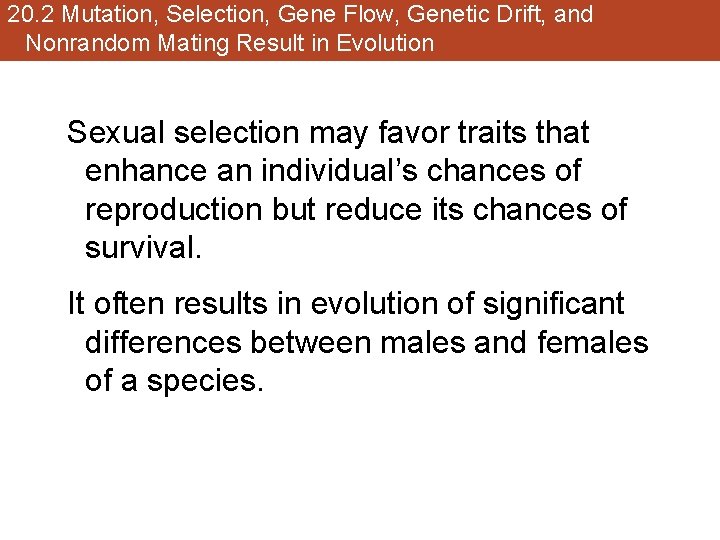
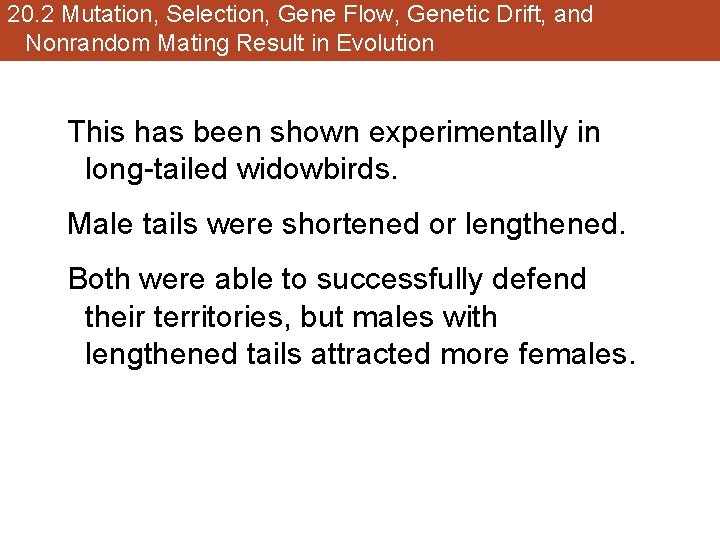
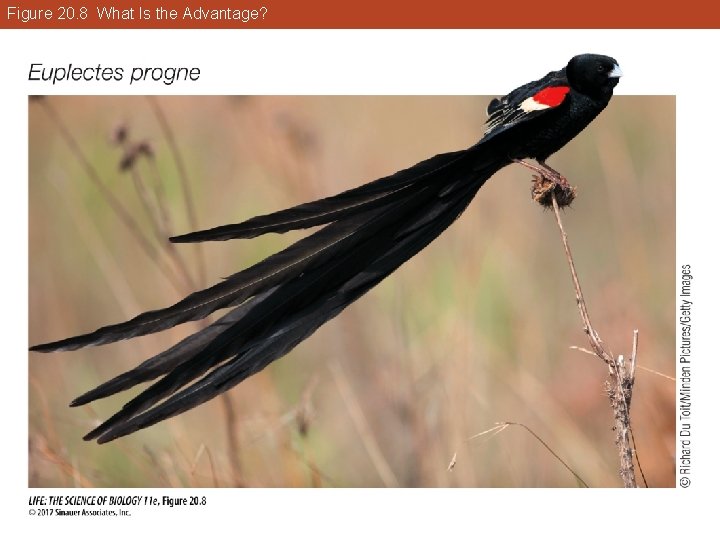
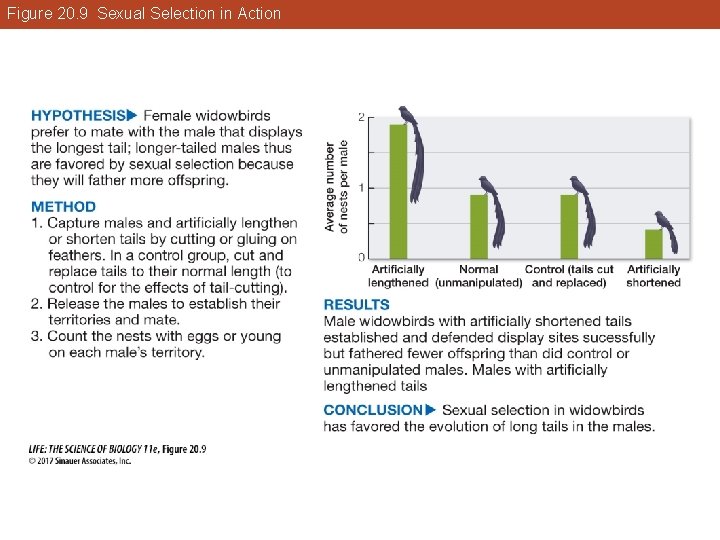
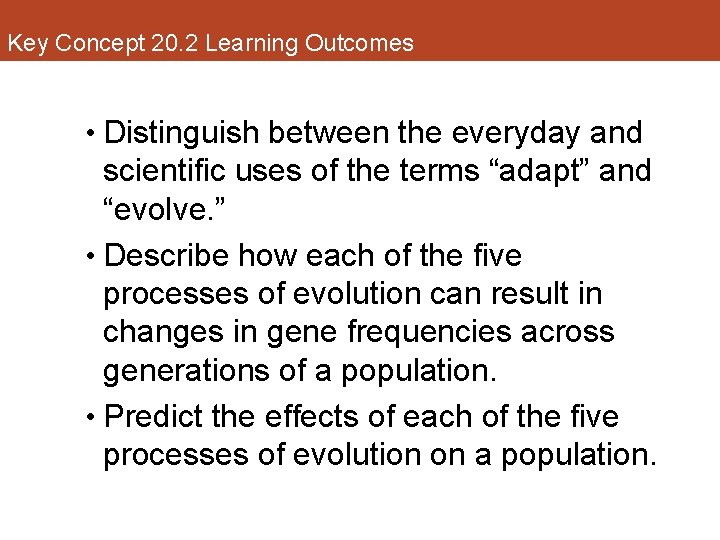
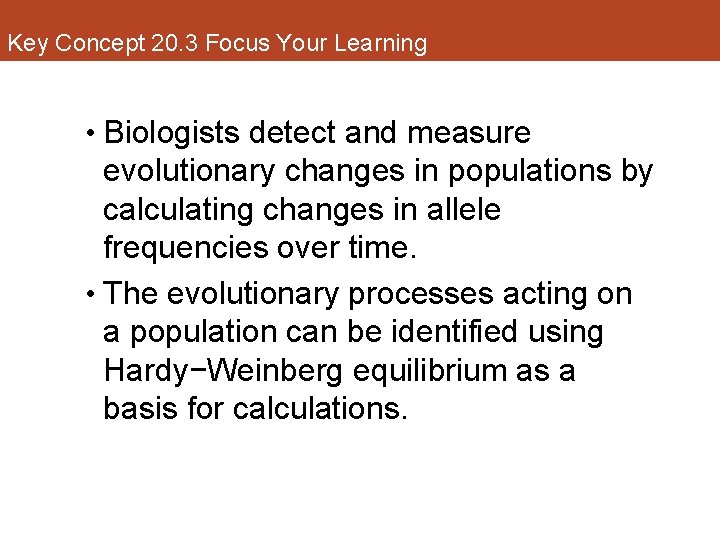
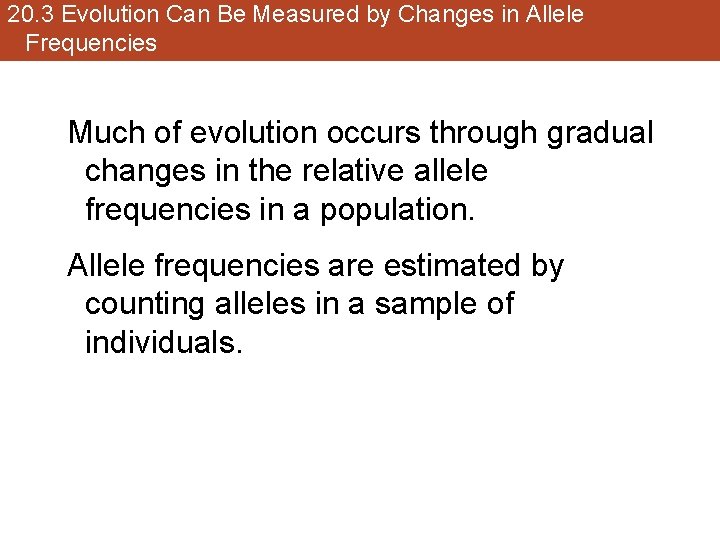
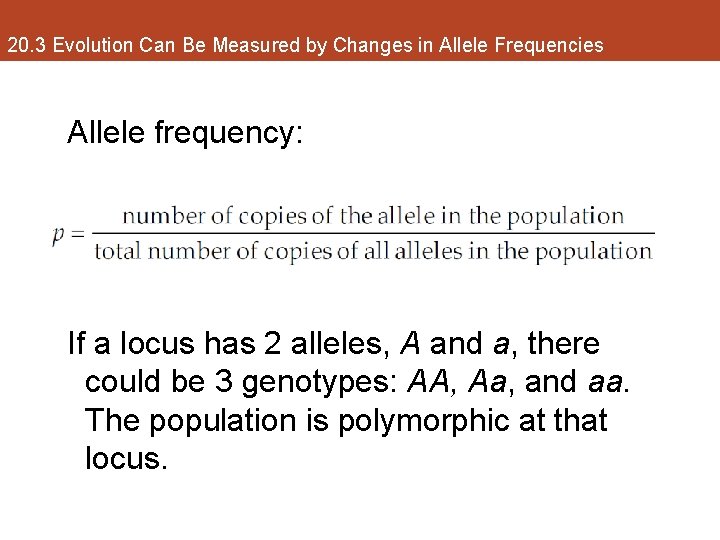
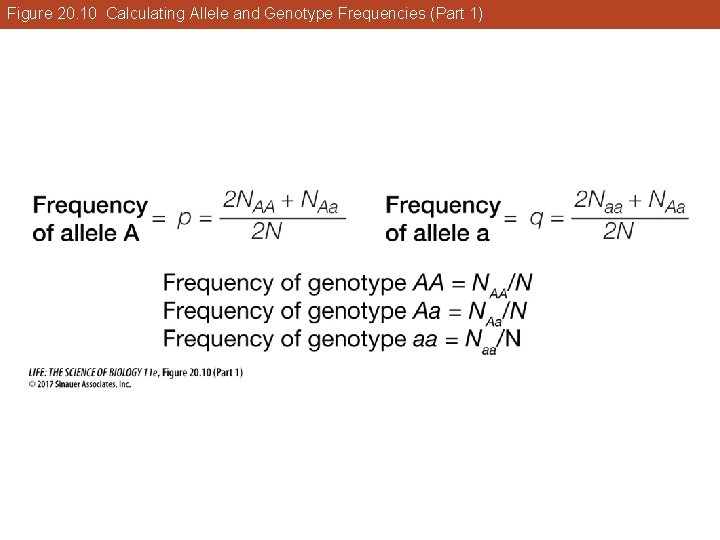
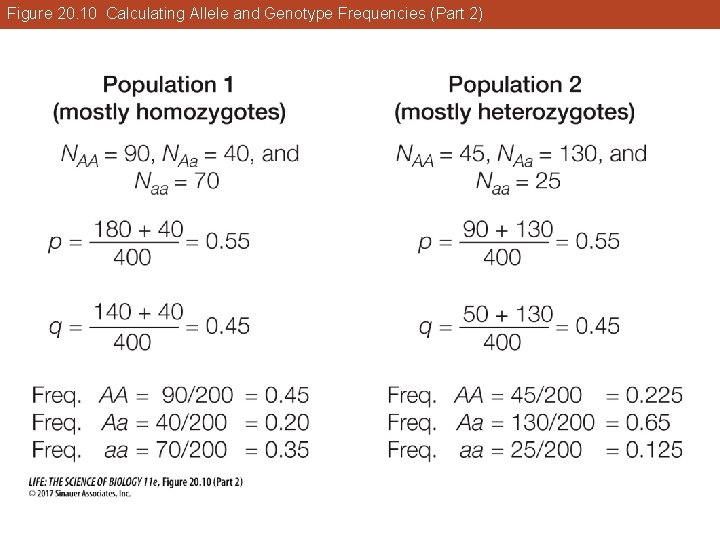
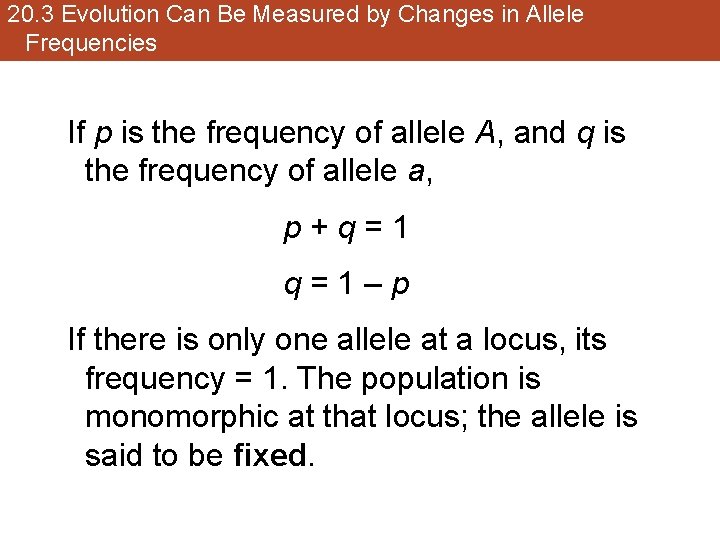
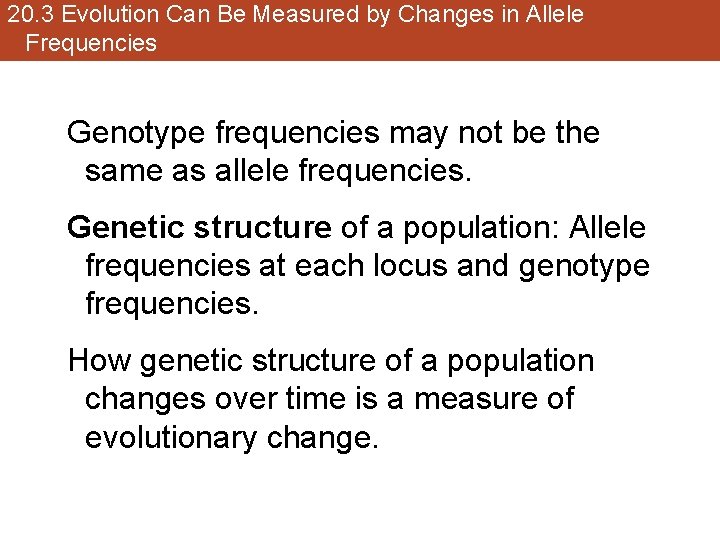
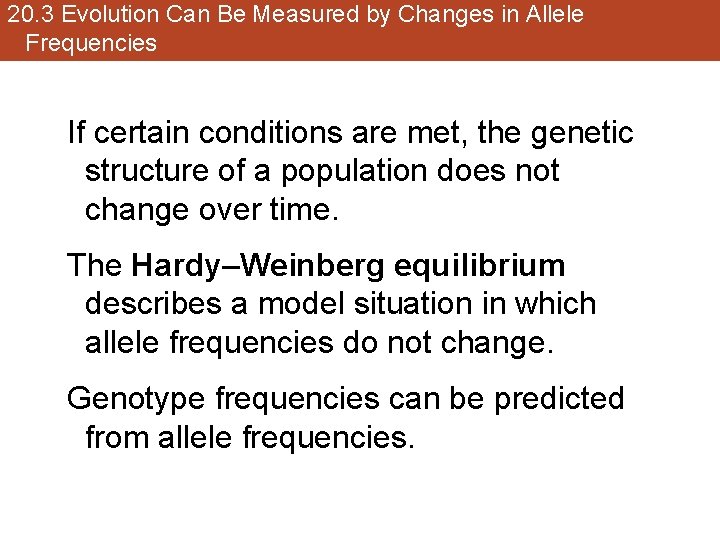
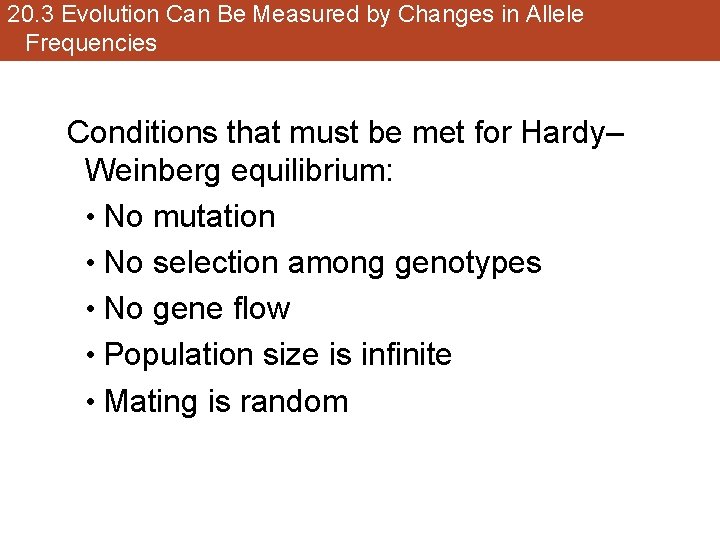
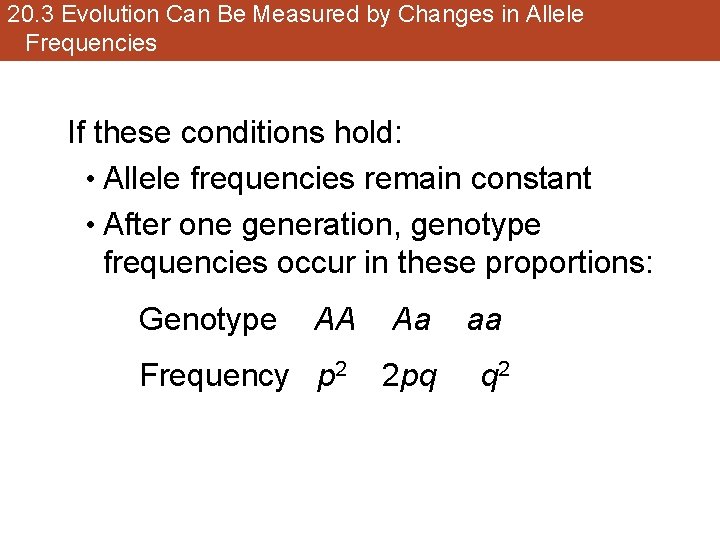
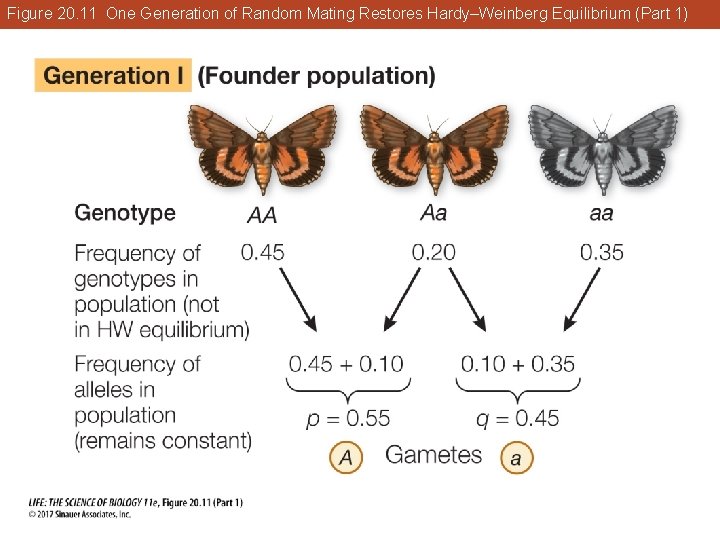
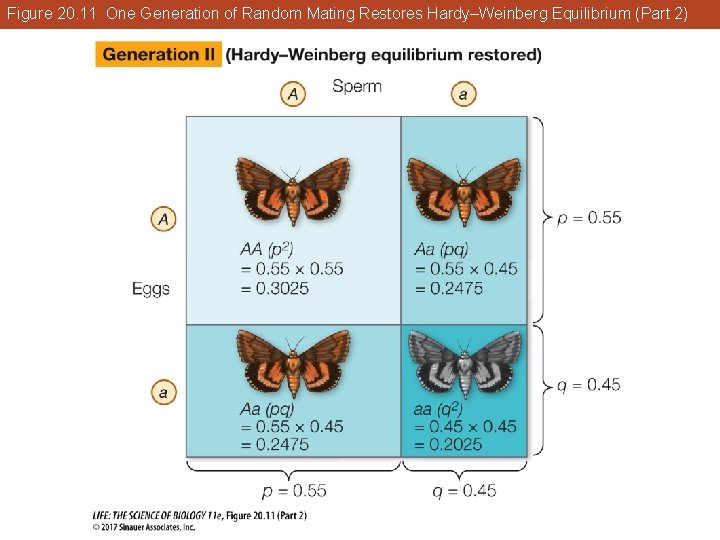
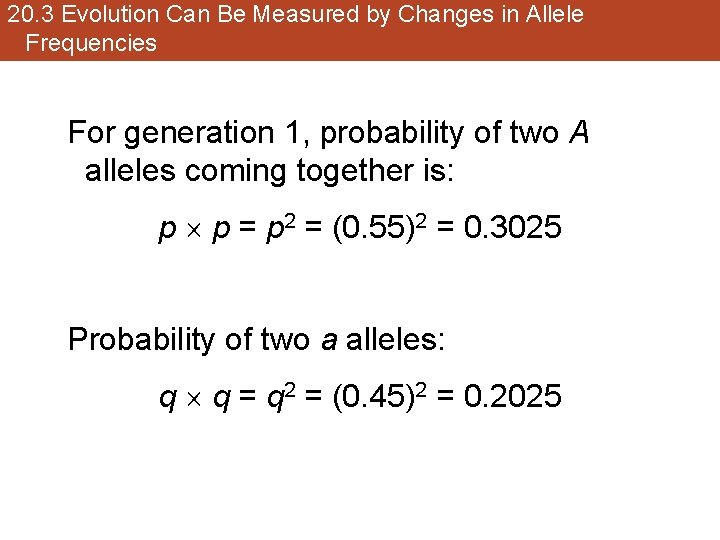
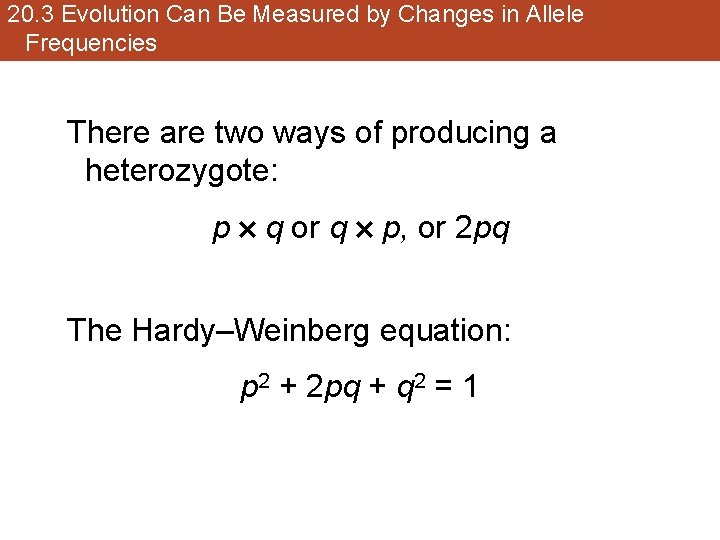
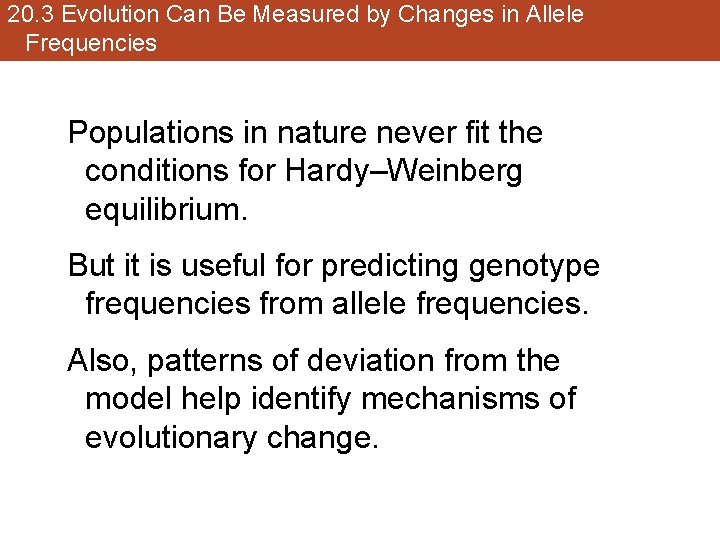
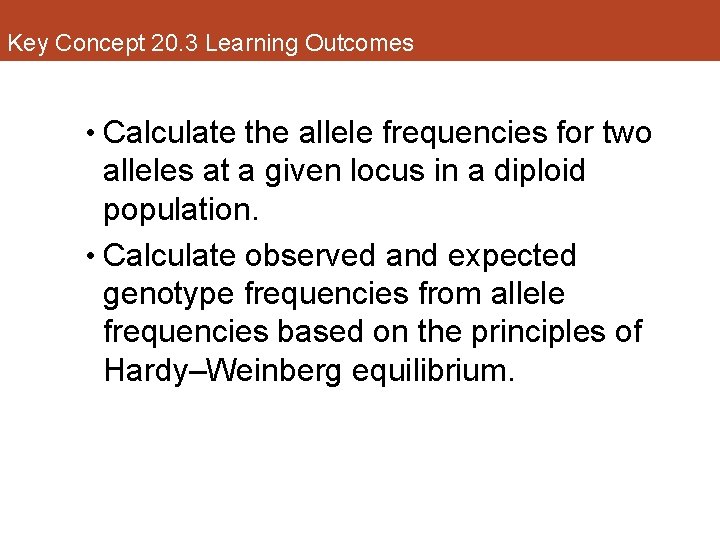
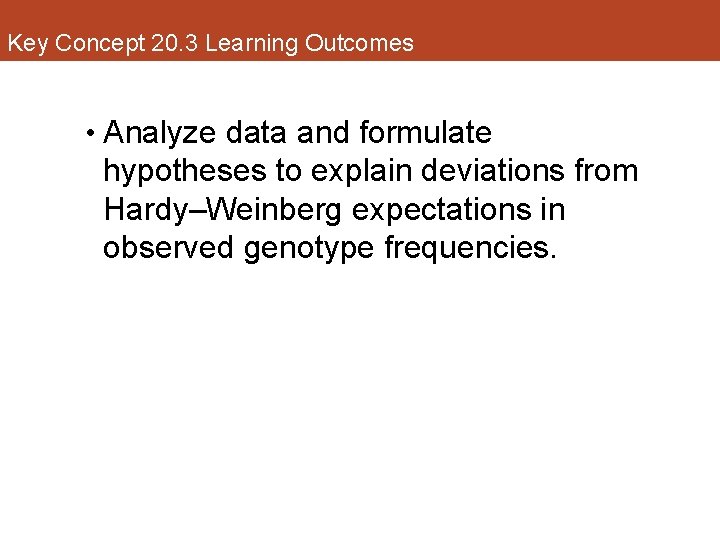
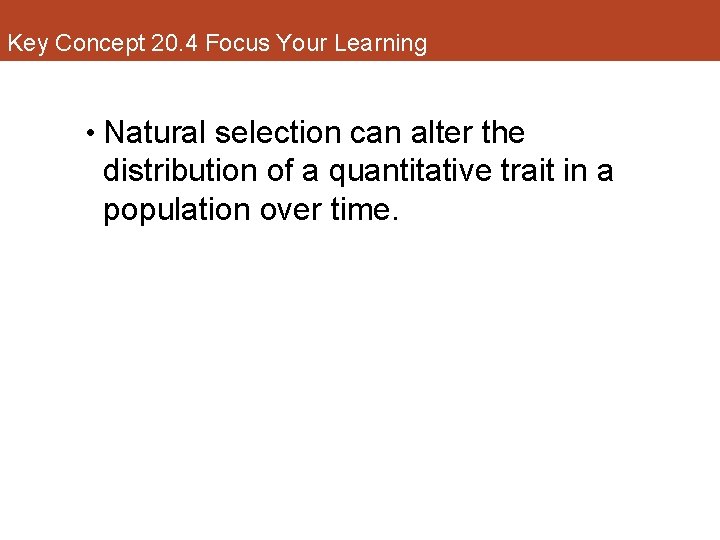
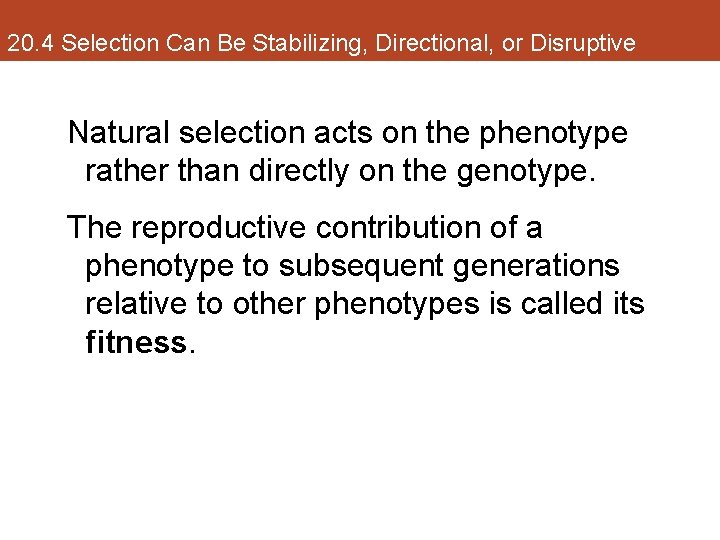
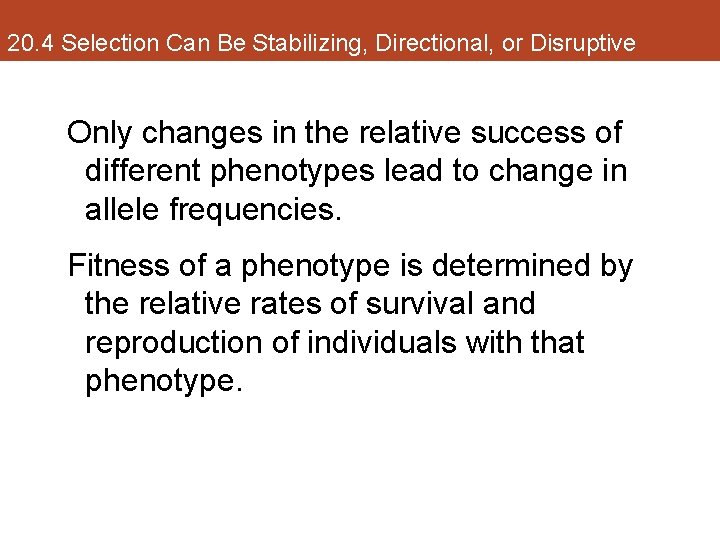
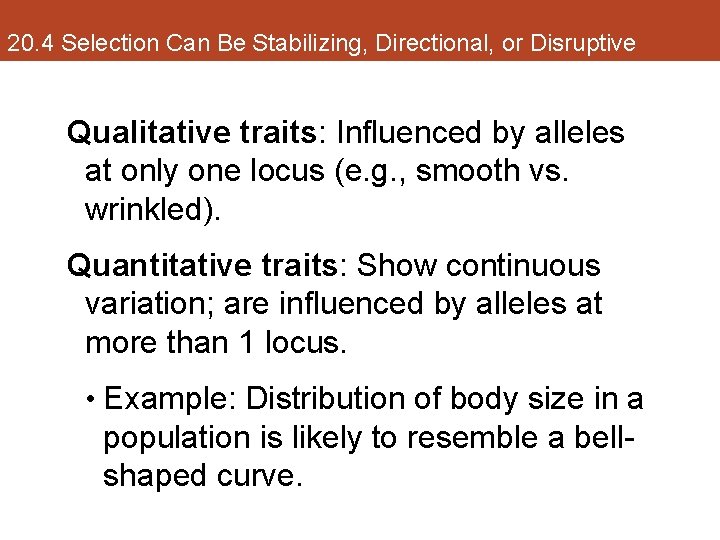
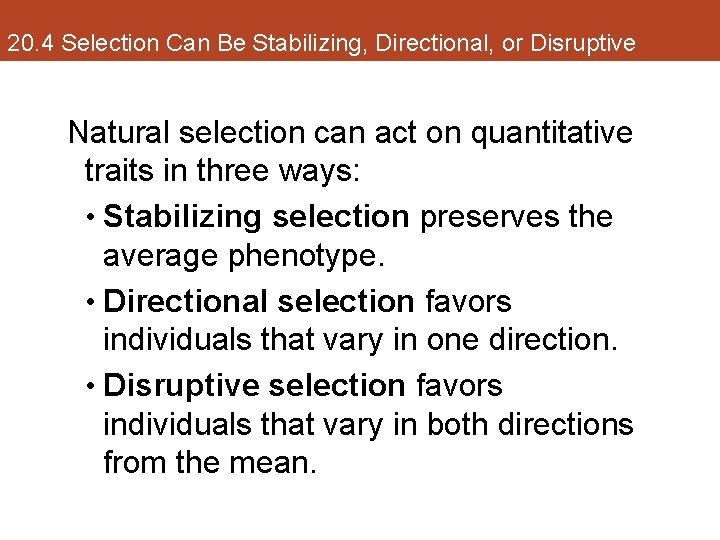
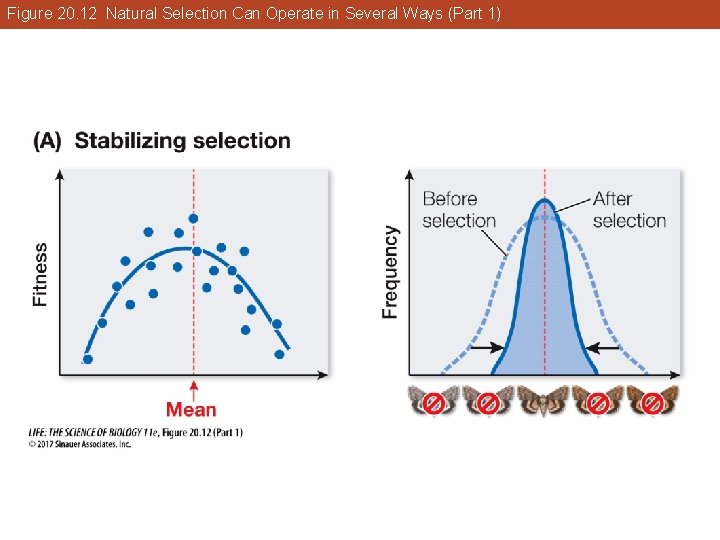
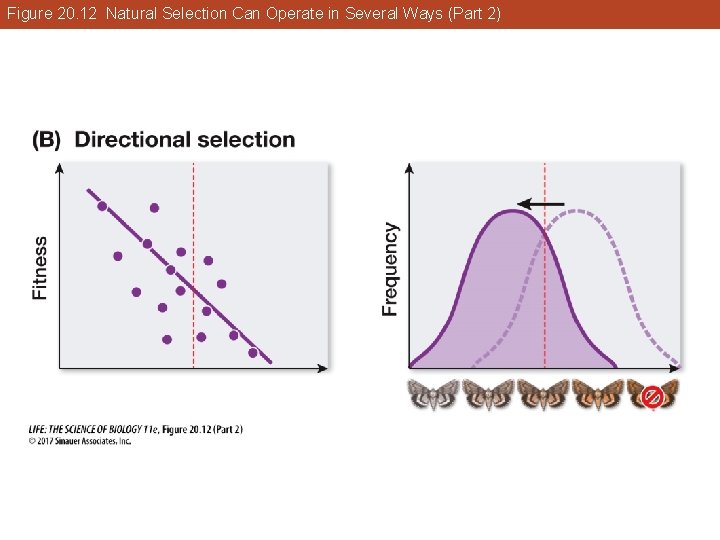
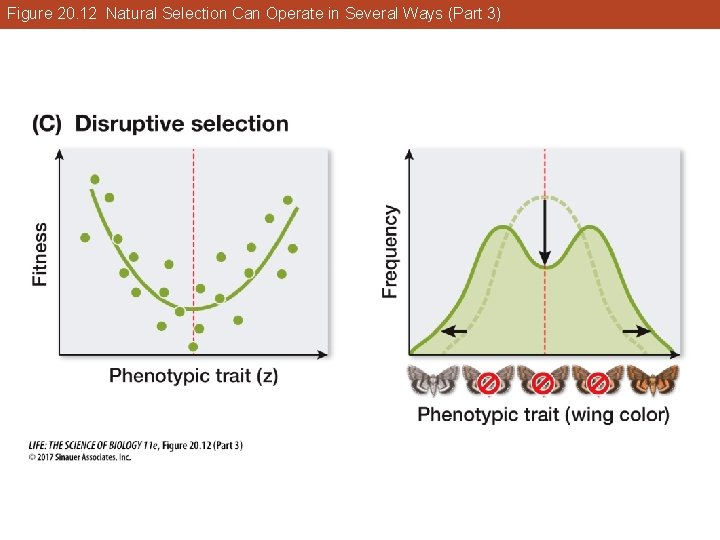
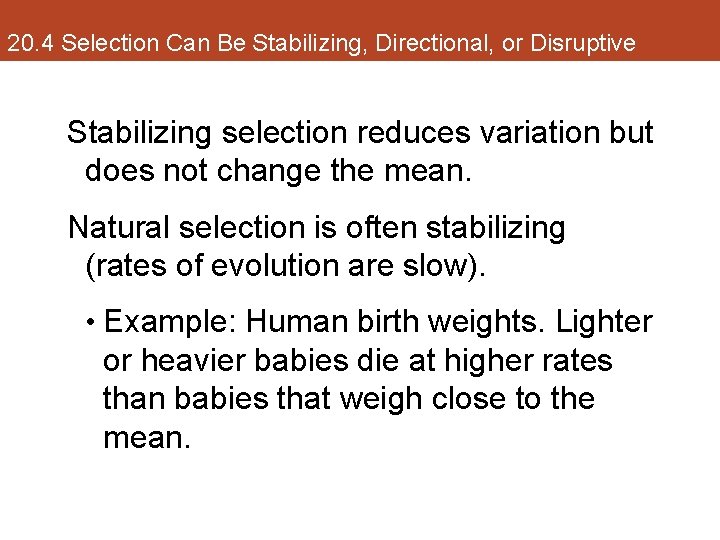
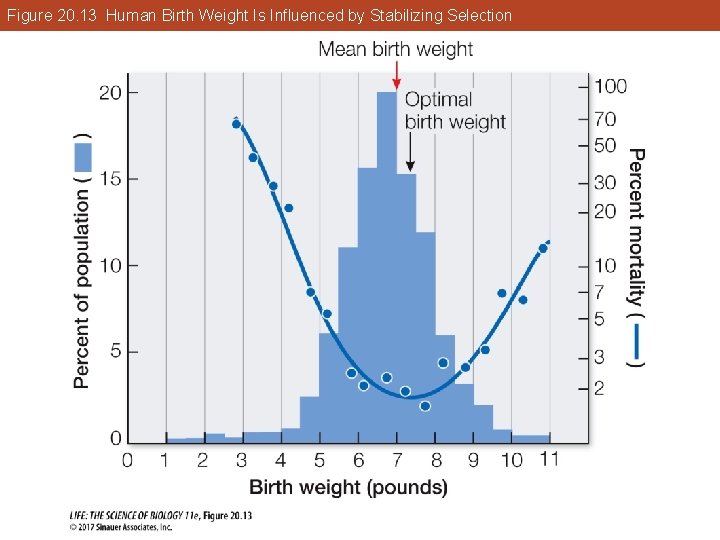
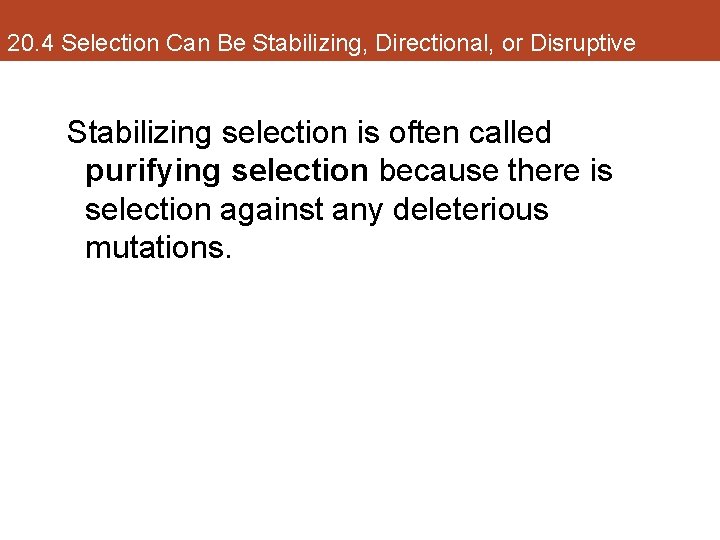
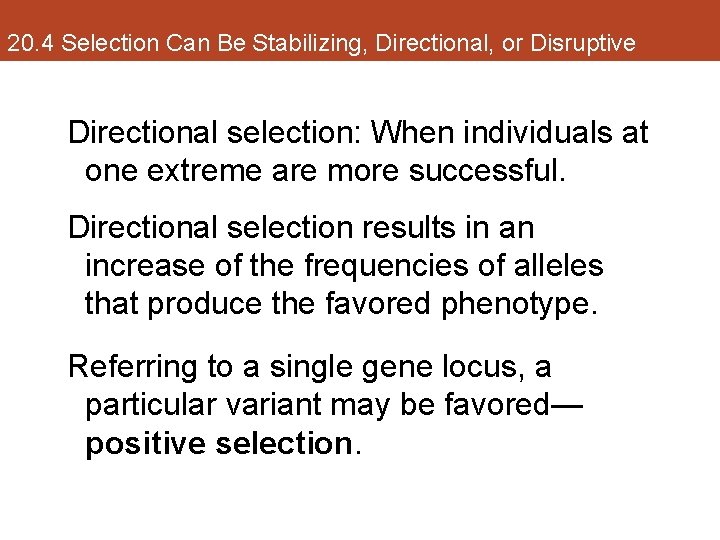
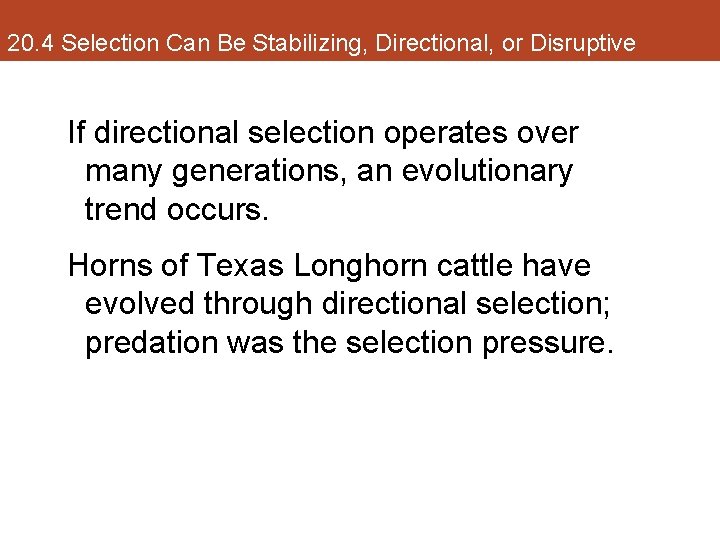
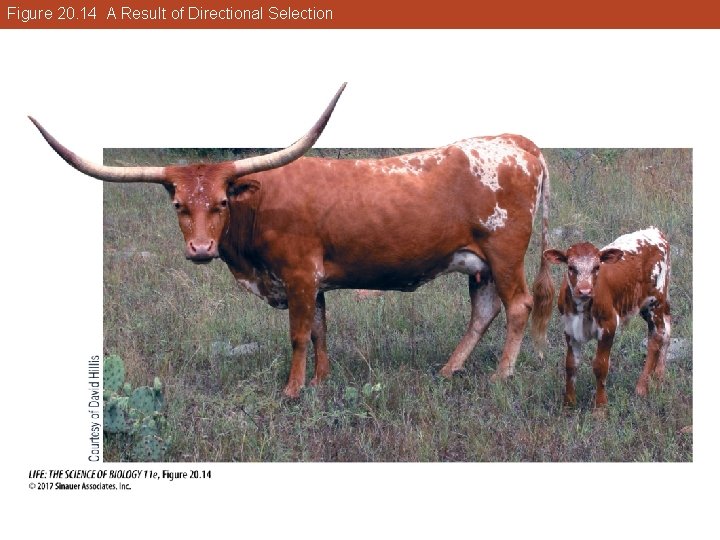
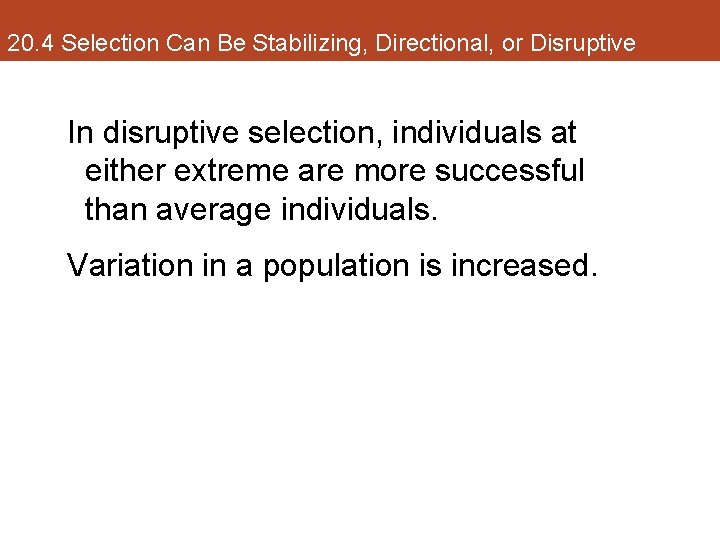
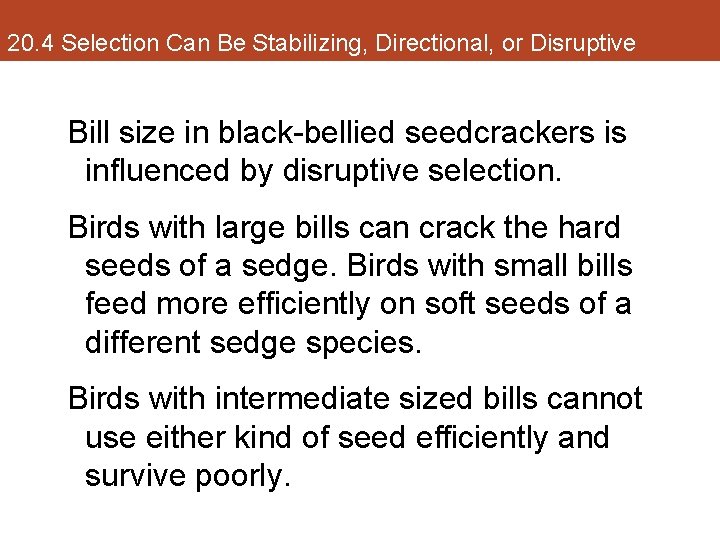
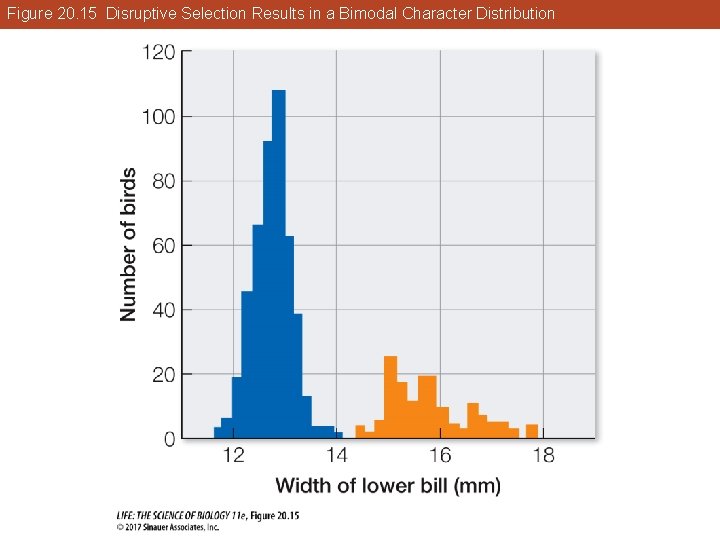
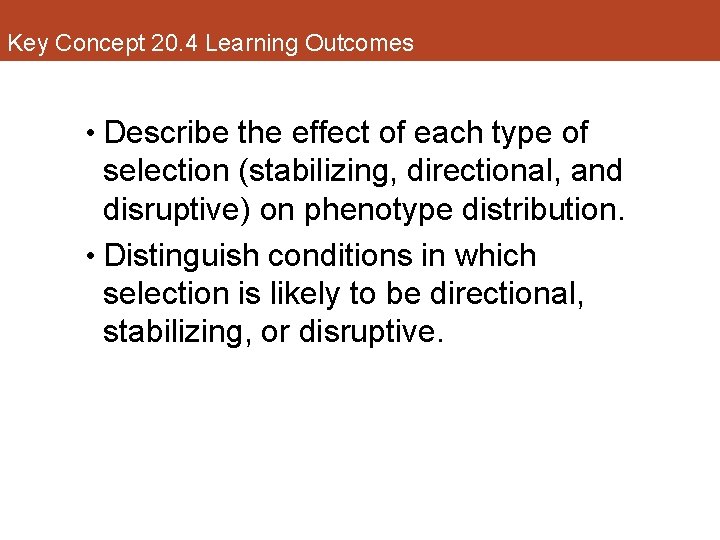
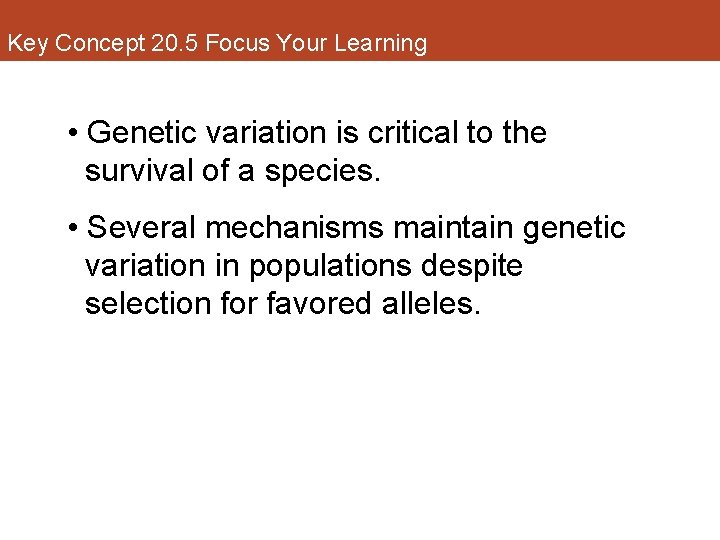
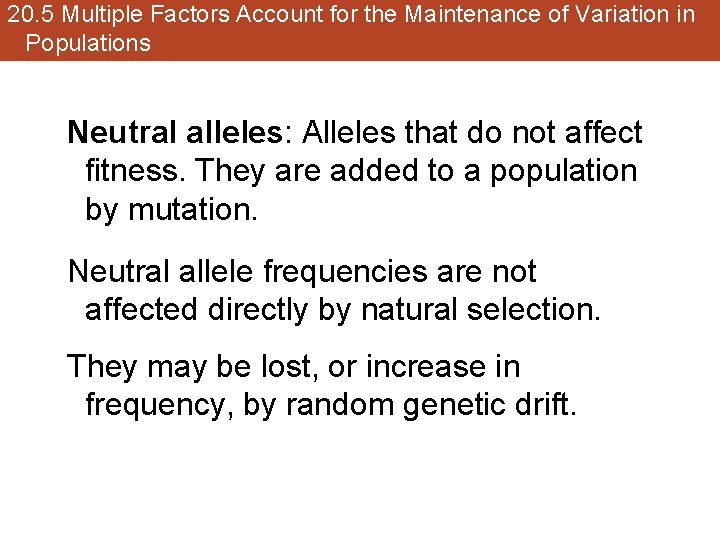
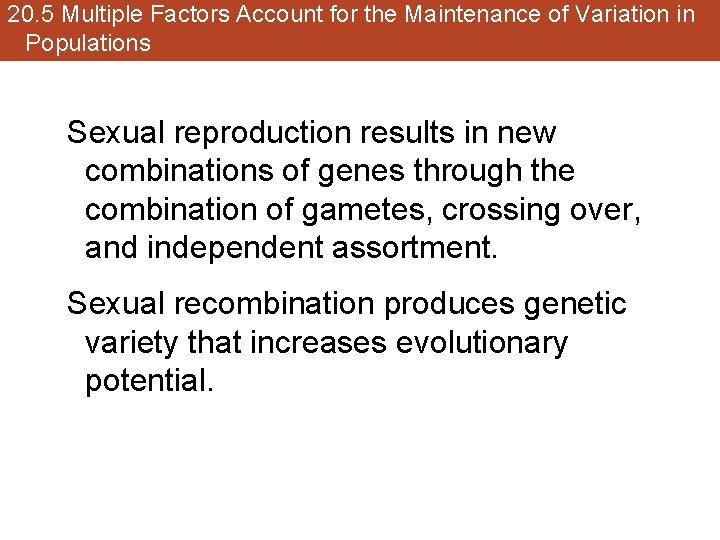
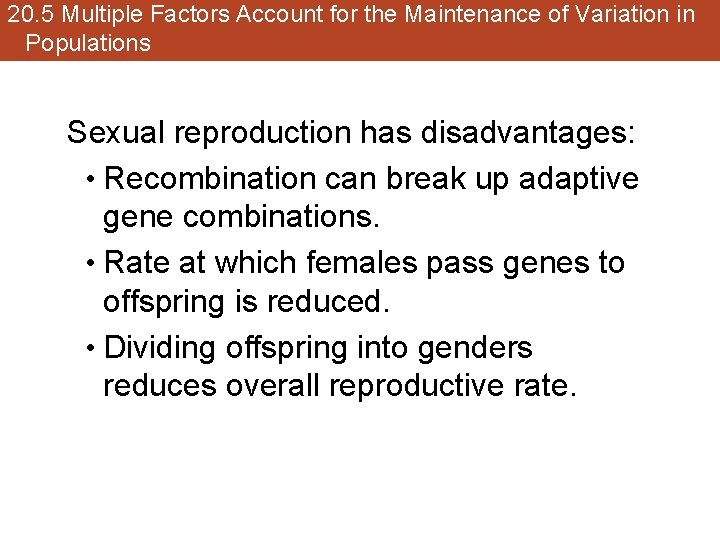
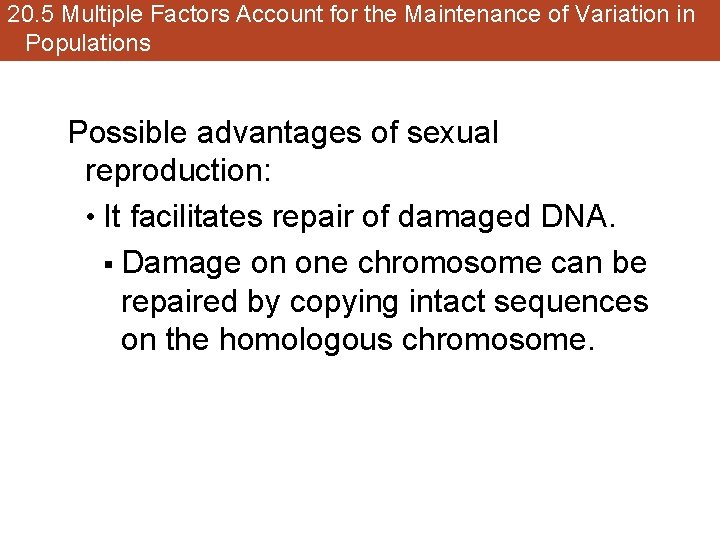
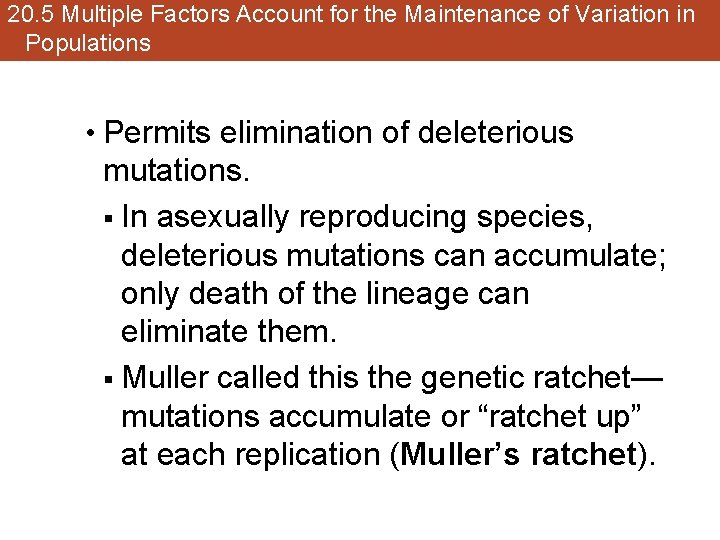
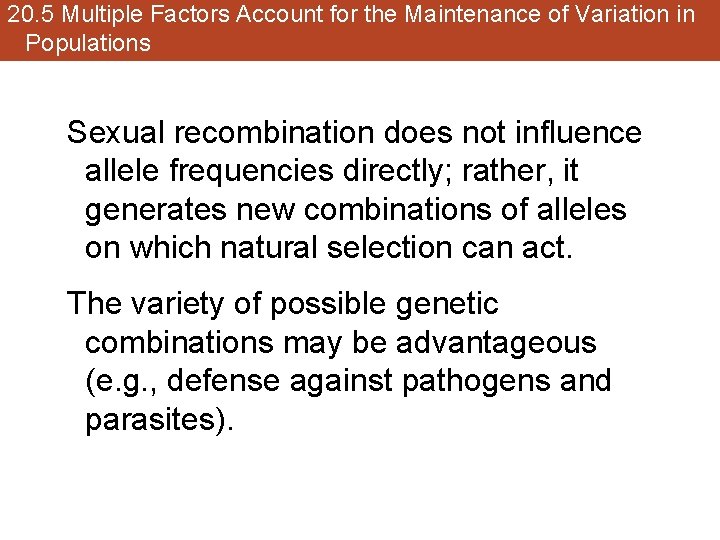
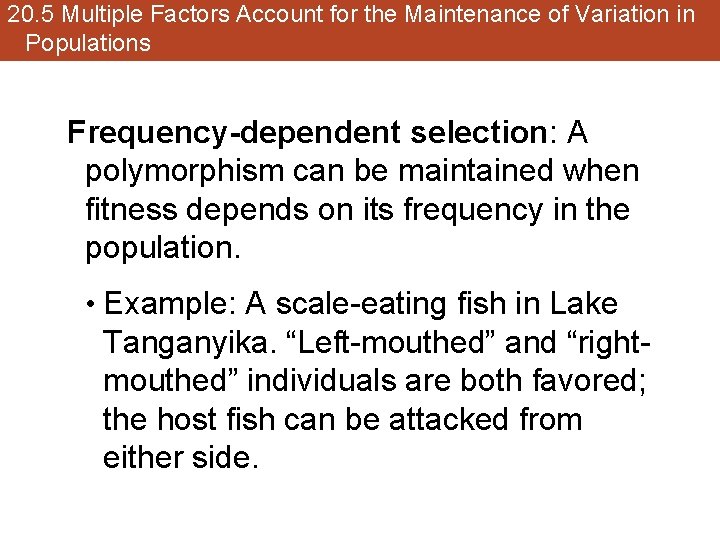
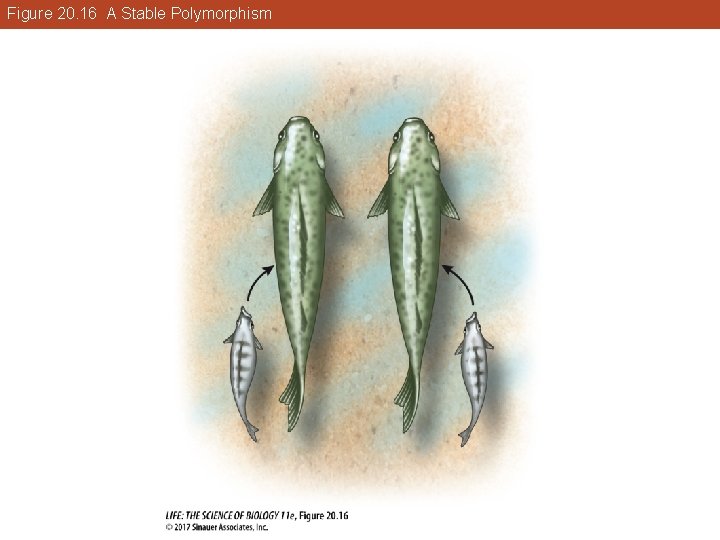
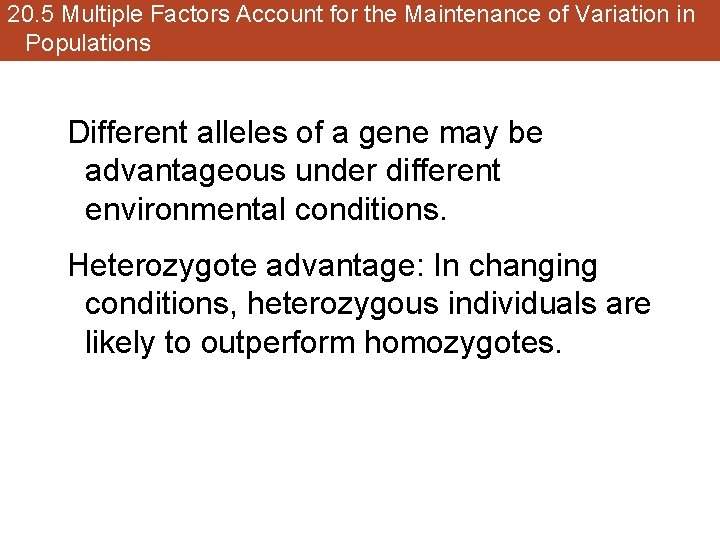
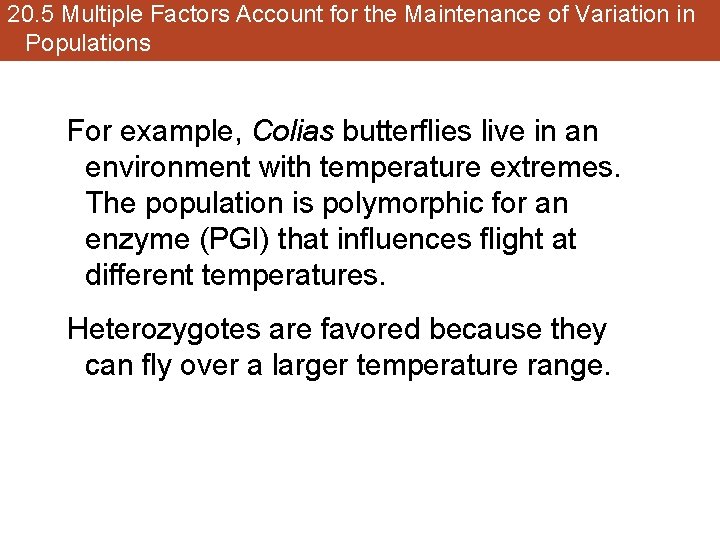
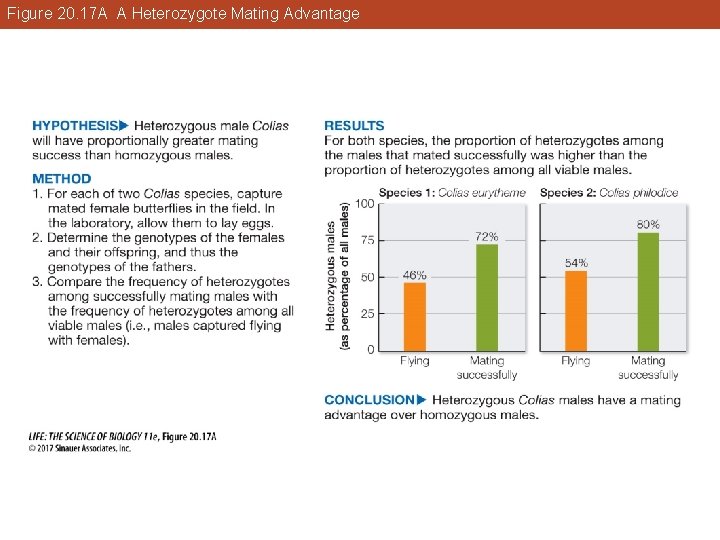
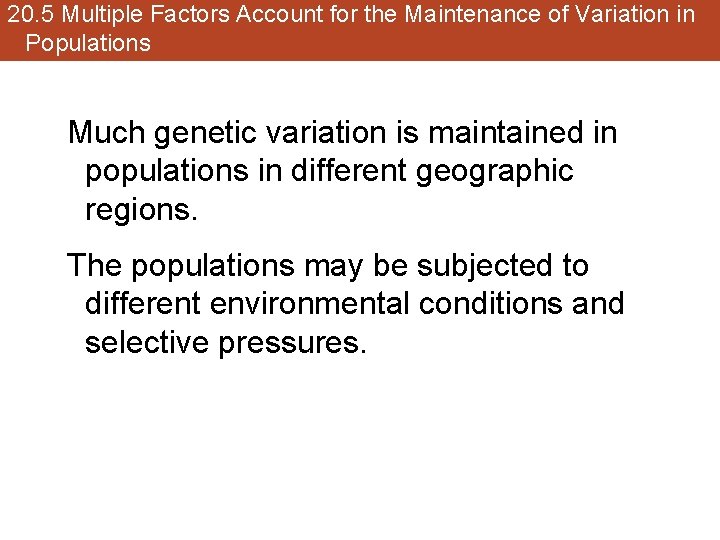
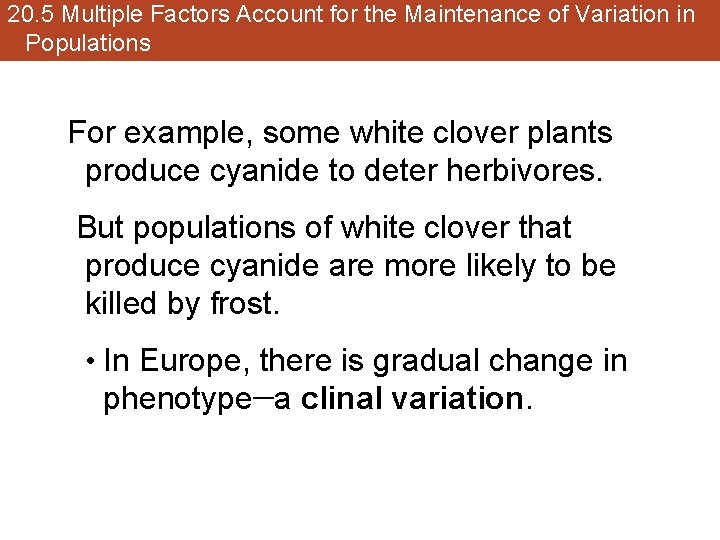
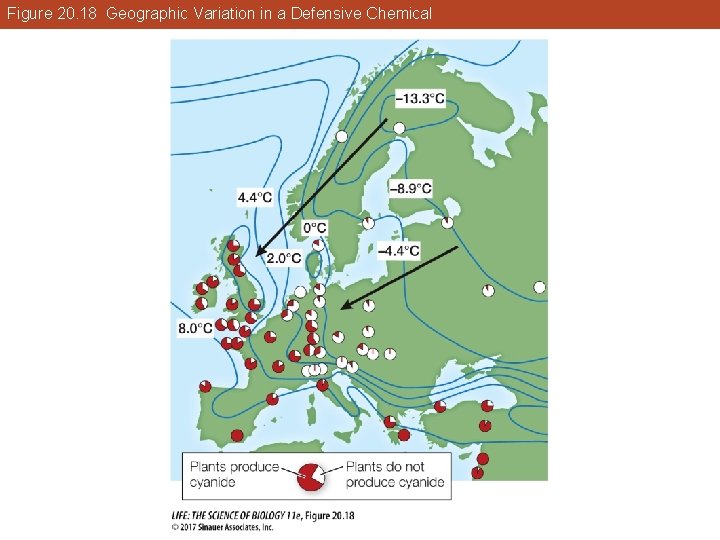
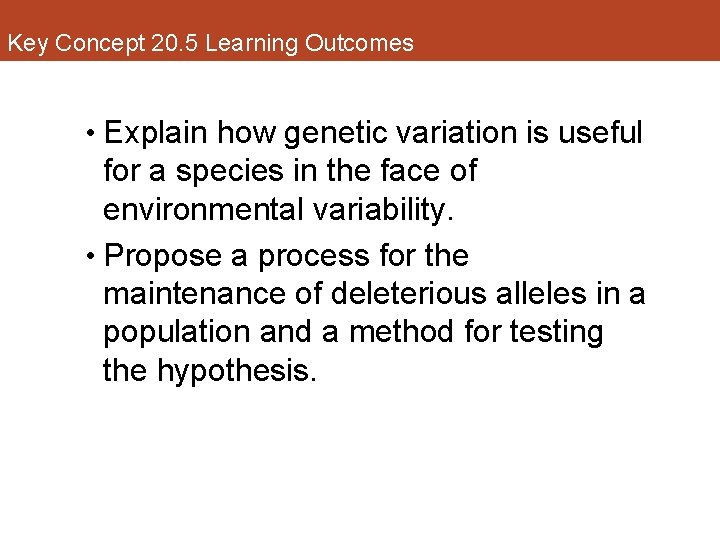
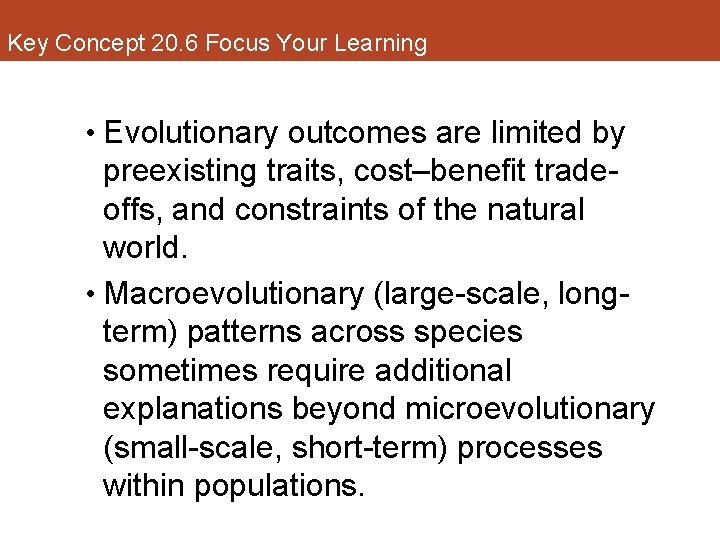
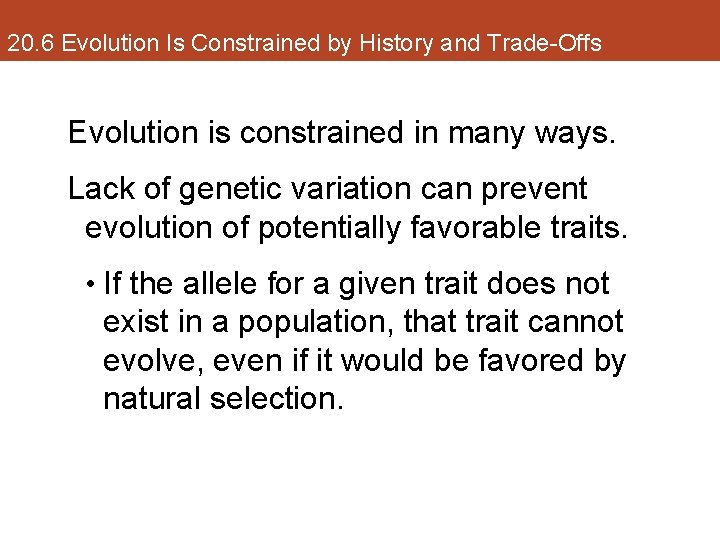
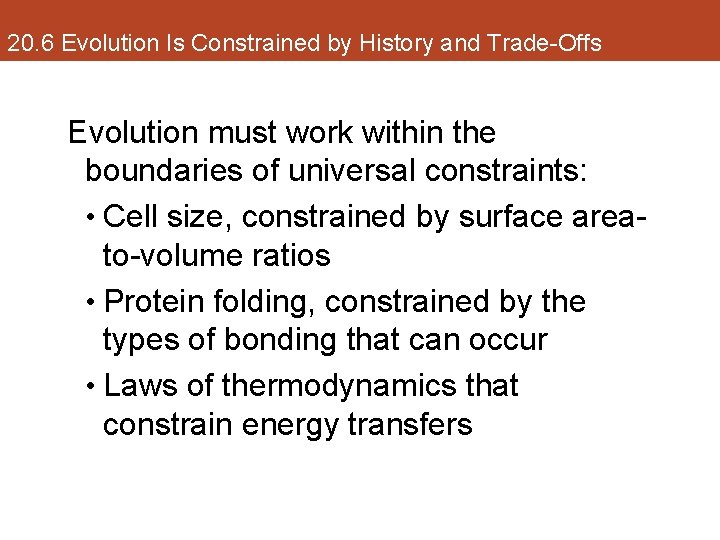
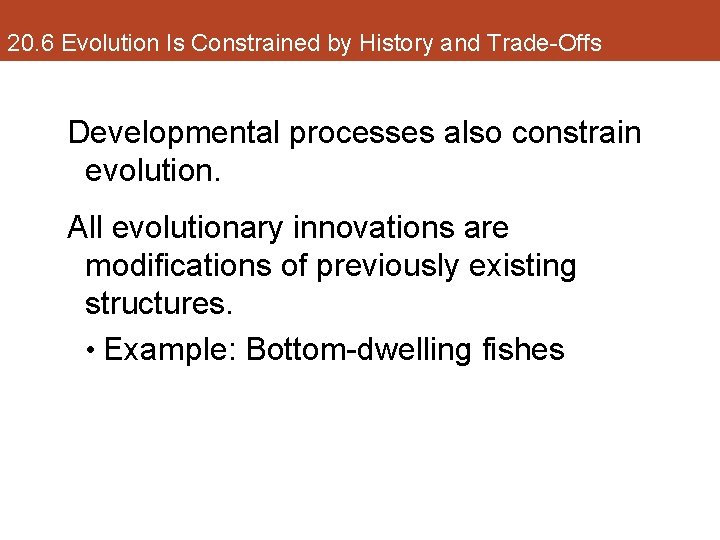
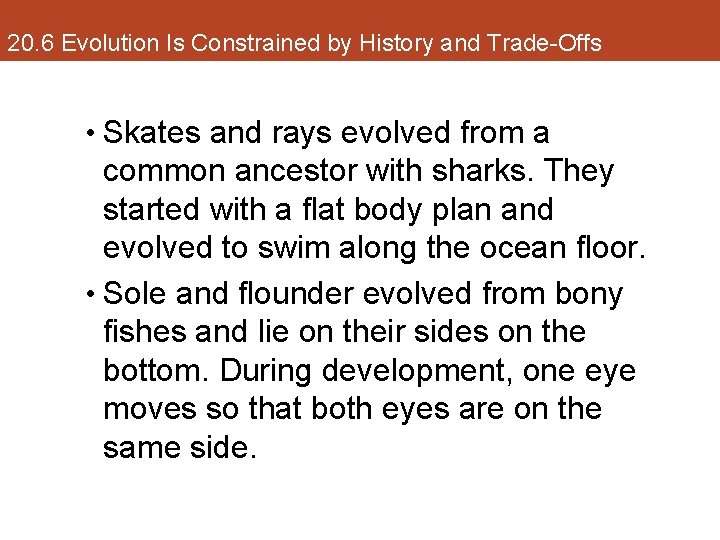
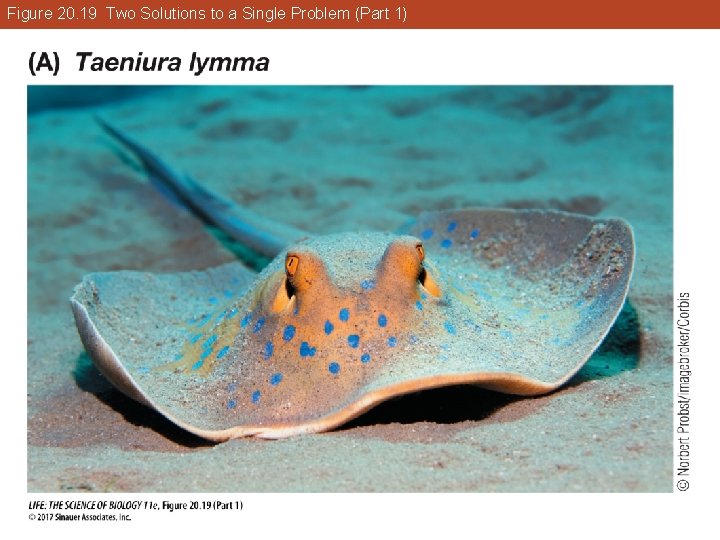
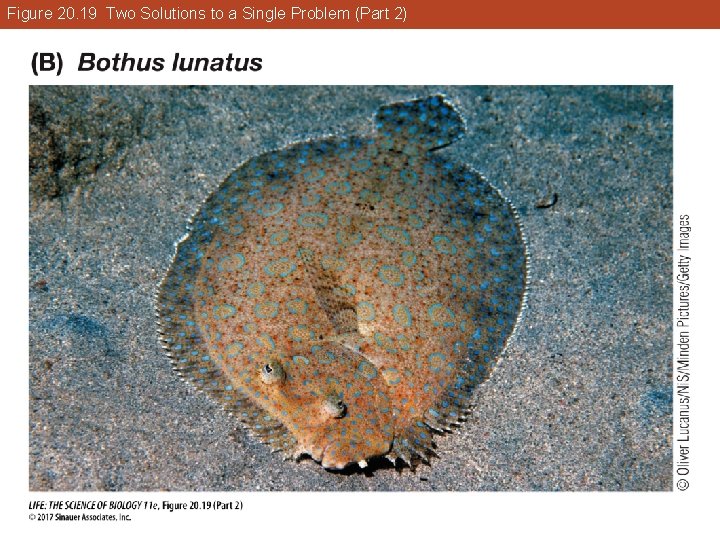
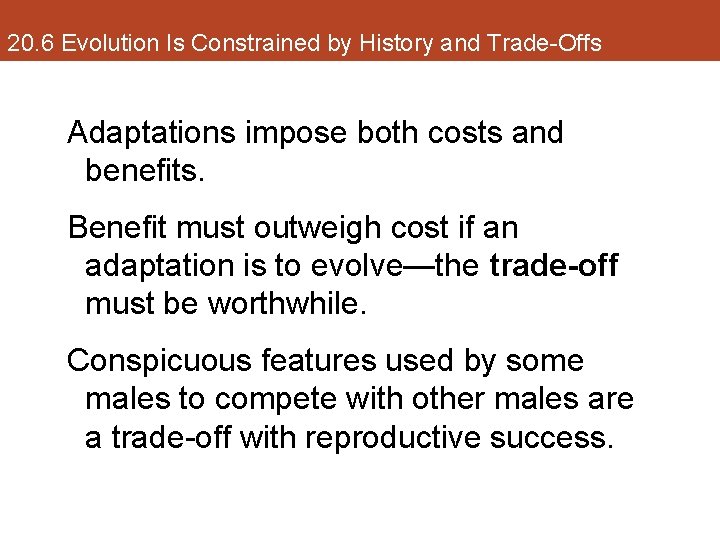
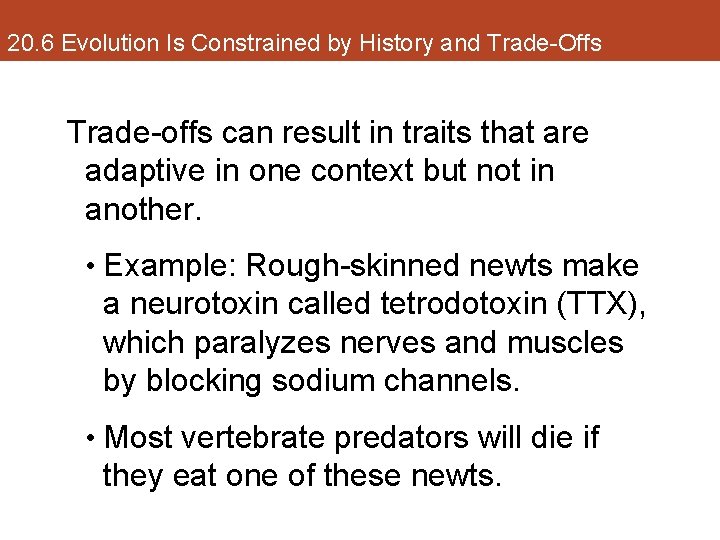
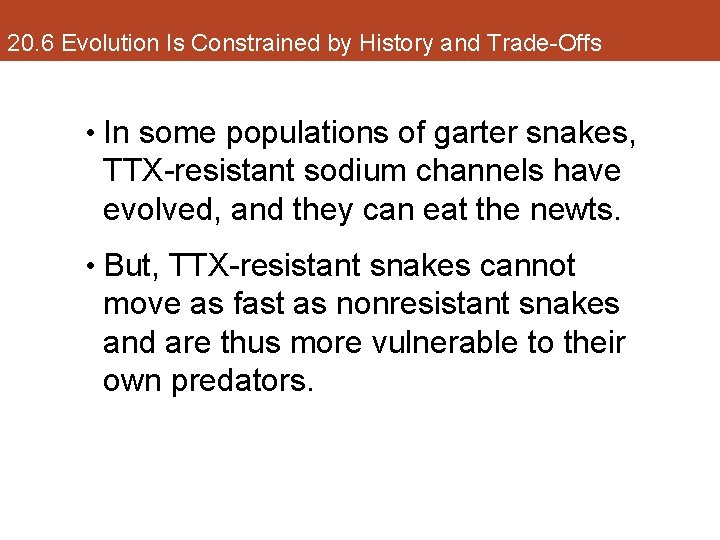
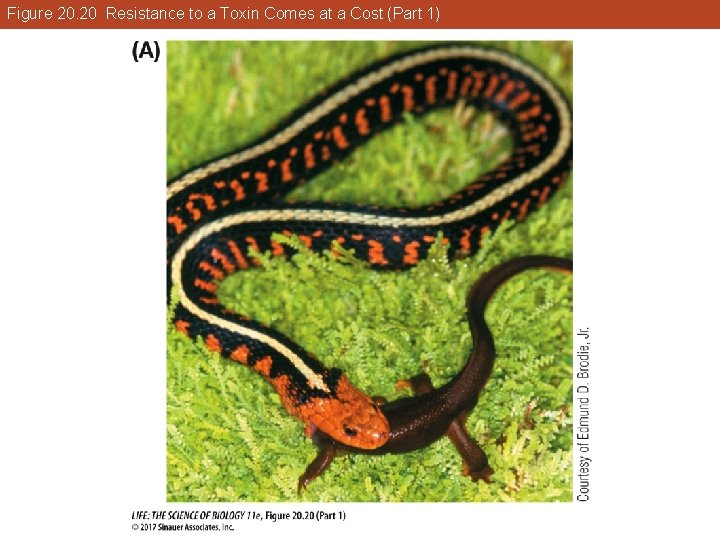
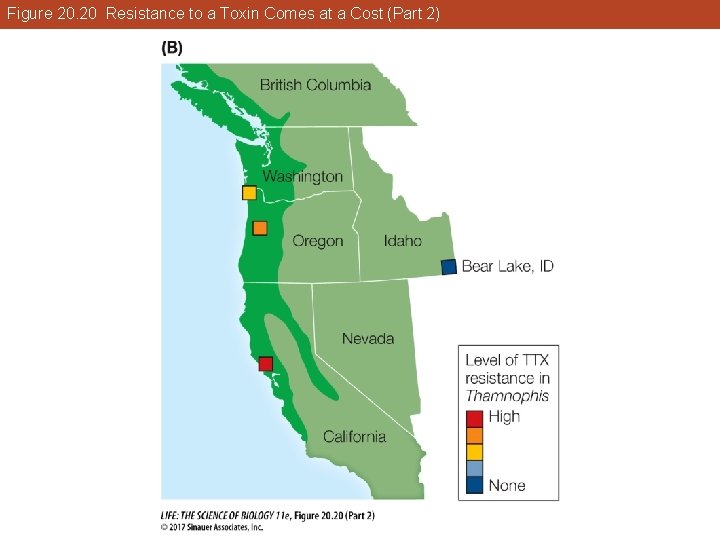
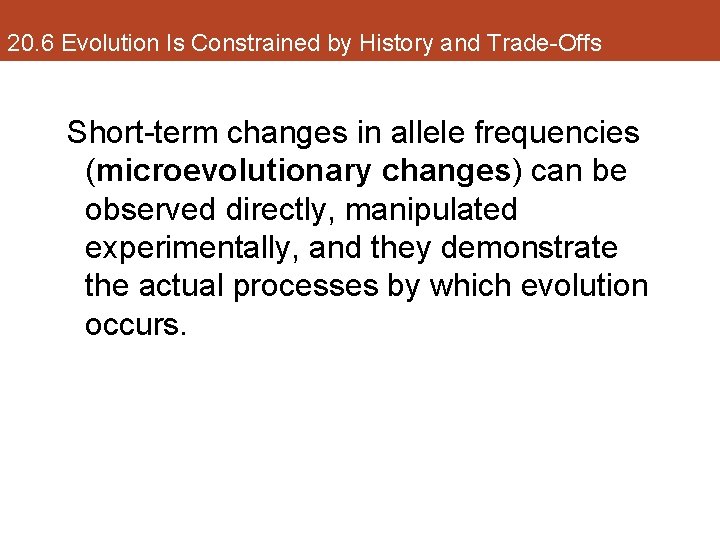
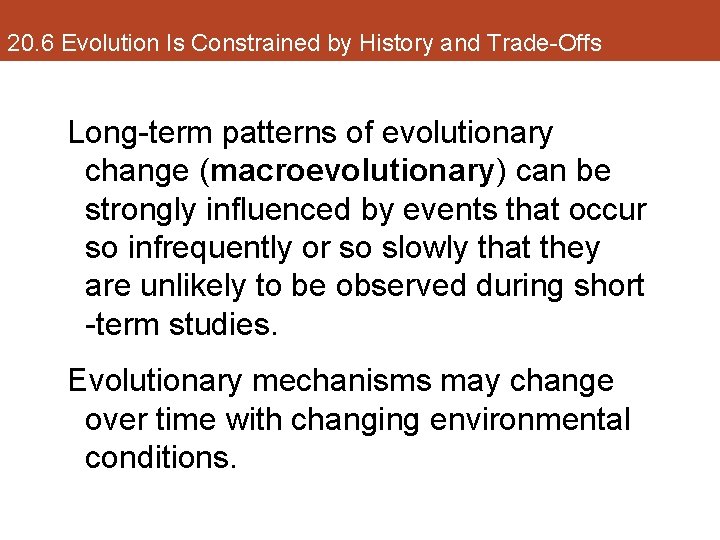
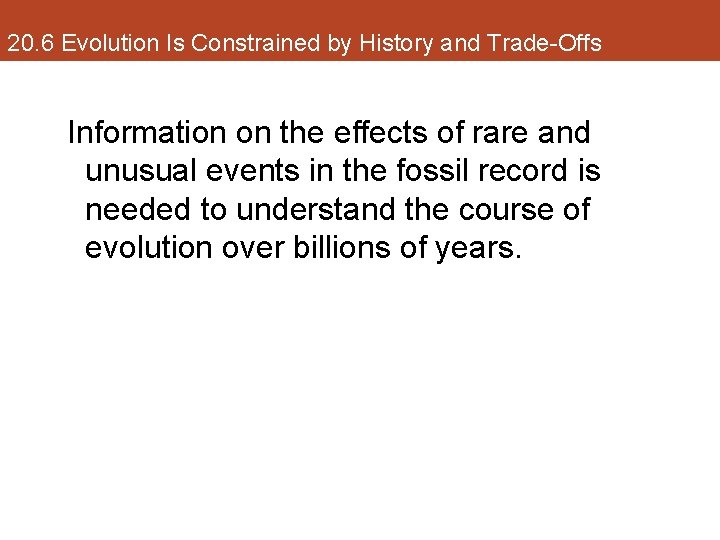
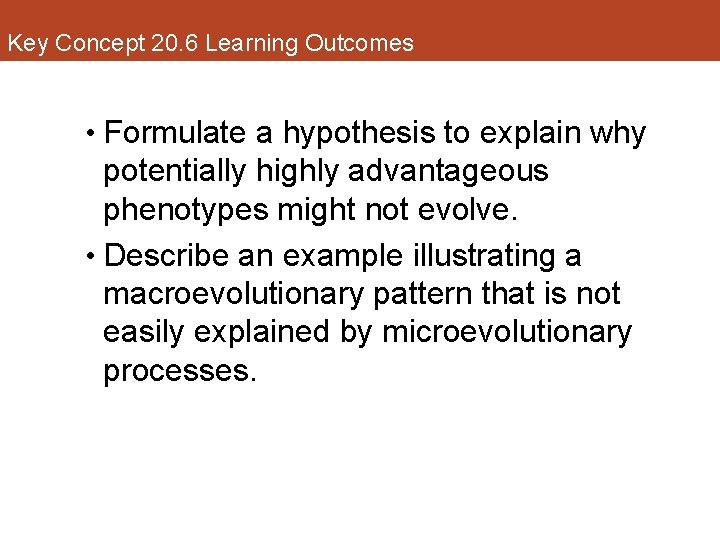
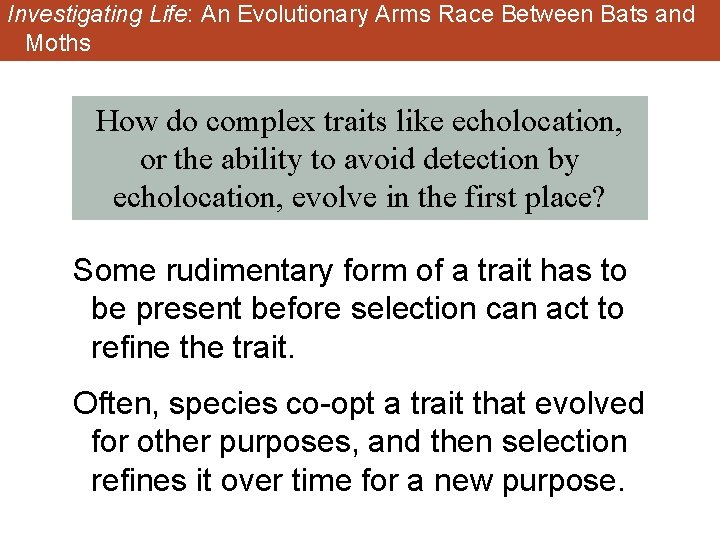
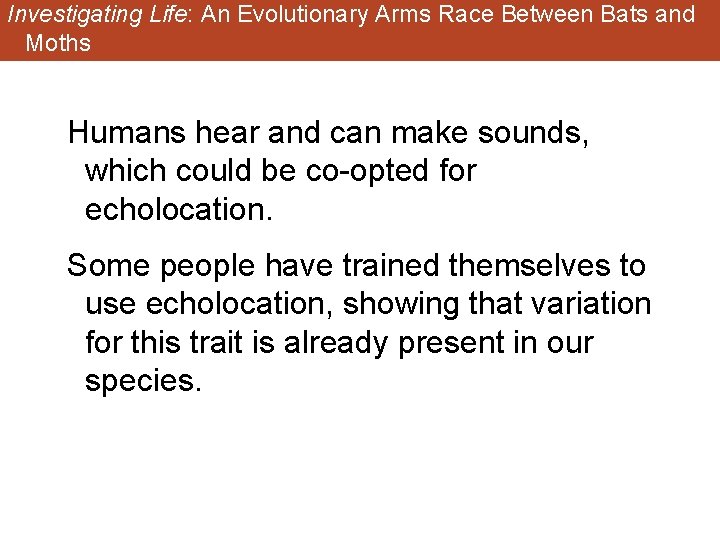
- Slides: 125
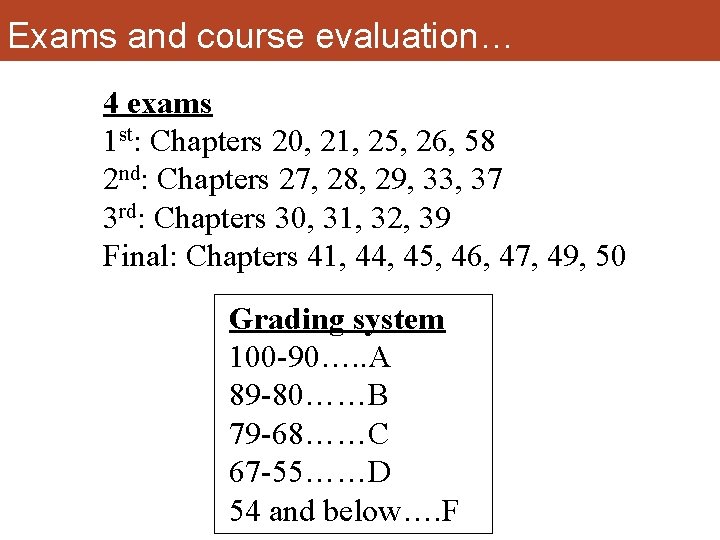
Exams and course evaluation… 4 exams 1 st: Chapters 20, 21, 25, 26, 58 2 nd: Chapters 27, 28, 29, 33, 37 3 rd: Chapters 30, 31, 32, 39 Final: Chapters 41, 44, 45, 46, 47, 49, 50 Grading system 100 -90…. . A 89 -80……B 79 -68……C 67 -55……D 54 and below…. F
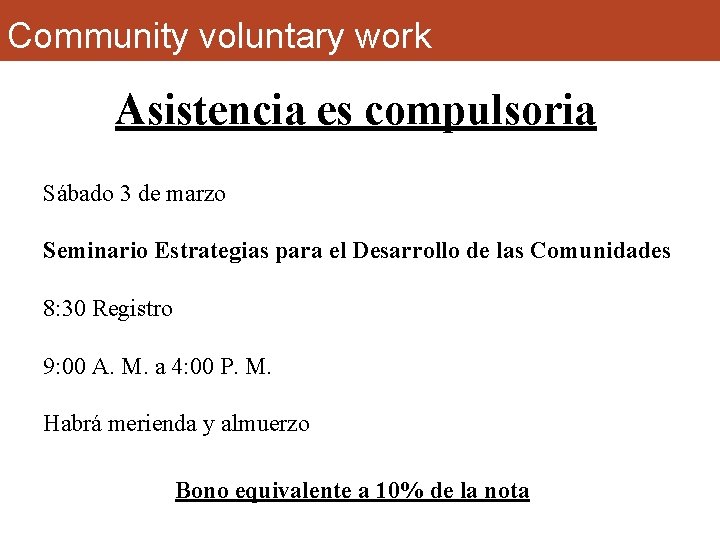
Community voluntary work Asistencia es compulsoria Sábado 3 de marzo Seminario Estrategias para el Desarrollo de las Comunidades 8: 30 Registro 9: 00 A. M. a 4: 00 P. M. Habrá merienda y almuerzo Bono equivalente a 10% de la nota
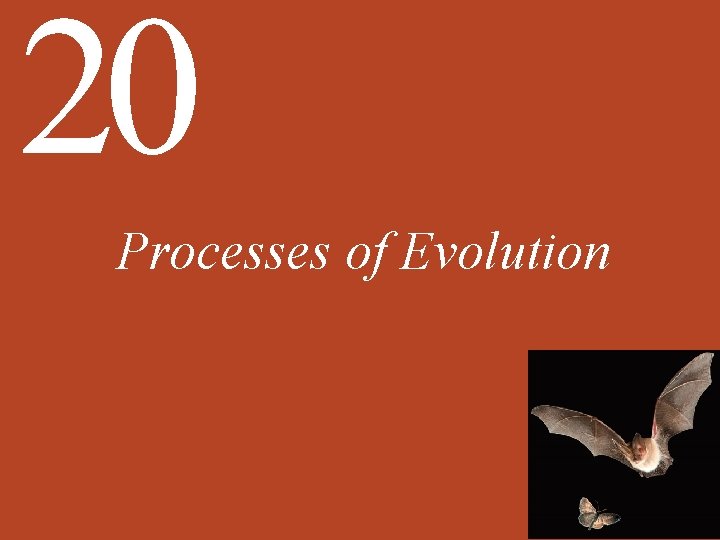
20 Processes of Evolution
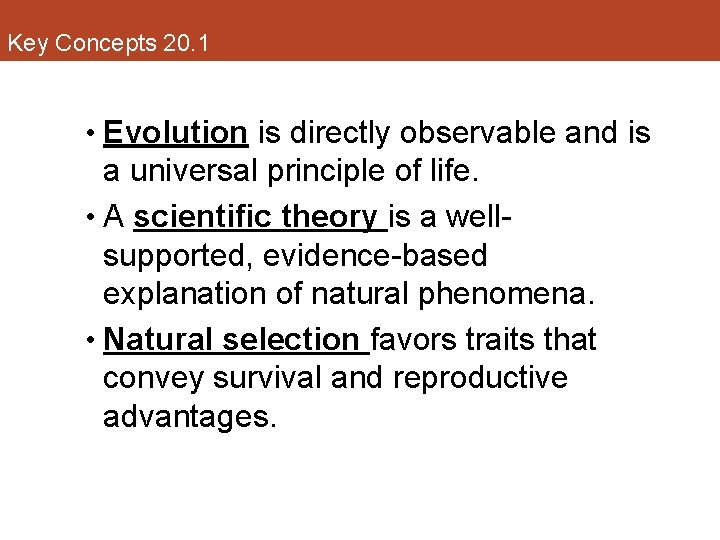
Key Concepts 20. 1 • Evolution is directly observable and is a universal principle of life. • A scientific theory is a wellsupported, evidence-based explanation of natural phenomena. • Natural selection favors traits that convey survival and reproductive advantages.
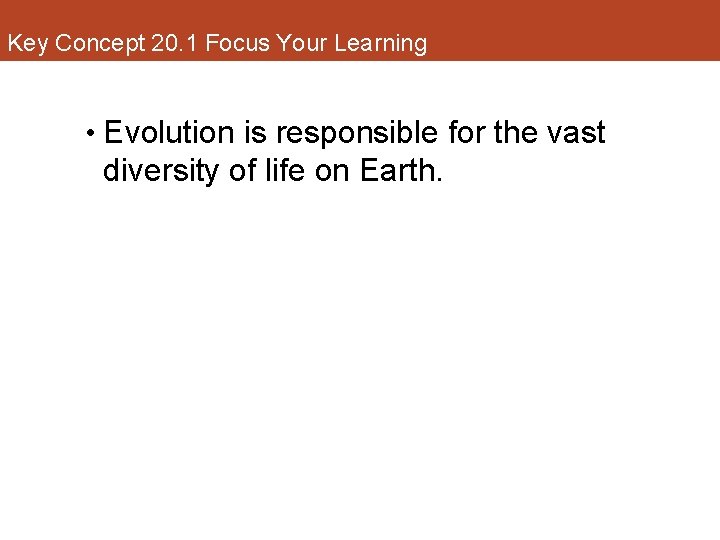
Key Concept 20. 1 Focus Your Learning • Evolution is responsible for the vast diversity of life on Earth.
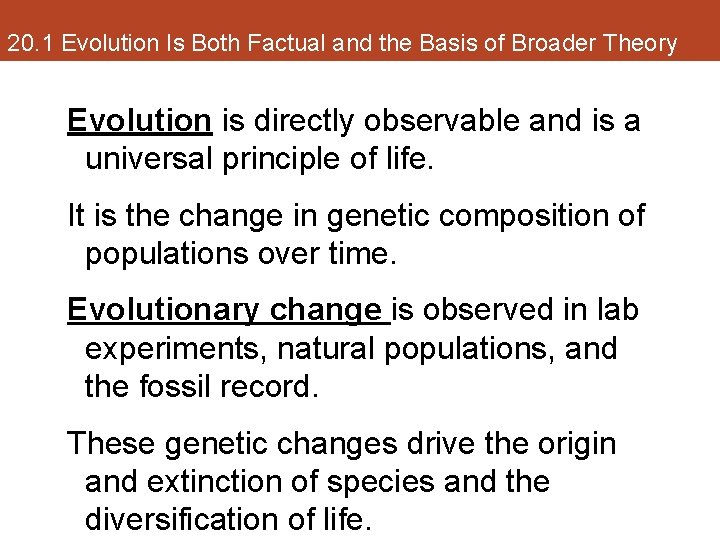
20. 1 Evolution Is Both Factual and the Basis of Broader Theory Evolution is directly observable and is a universal principle of life. It is the change in genetic composition of populations over time. Evolutionary change is observed in lab experiments, natural populations, and the fossil record. These genetic changes drive the origin and extinction of species and the diversification of life.
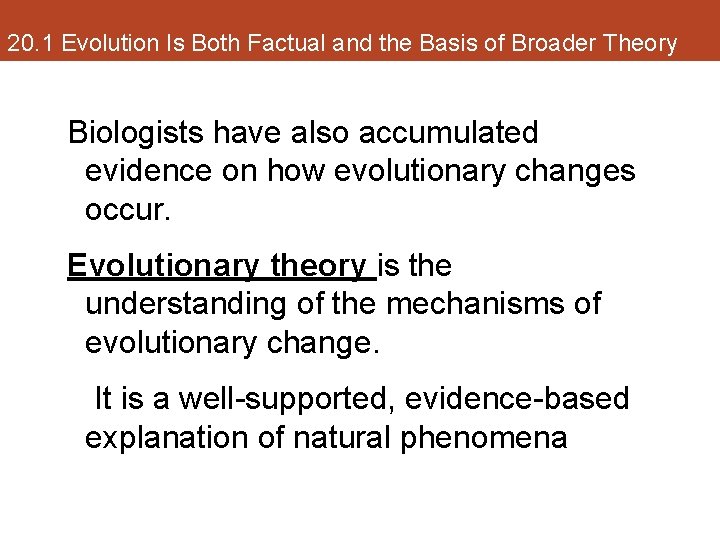
20. 1 Evolution Is Both Factual and the Basis of Broader Theory Biologists have also accumulated evidence on how evolutionary changes occur. Evolutionary theory is the understanding of the mechanisms of evolutionary change. It is a well-supported, evidence-based explanation of natural phenomena
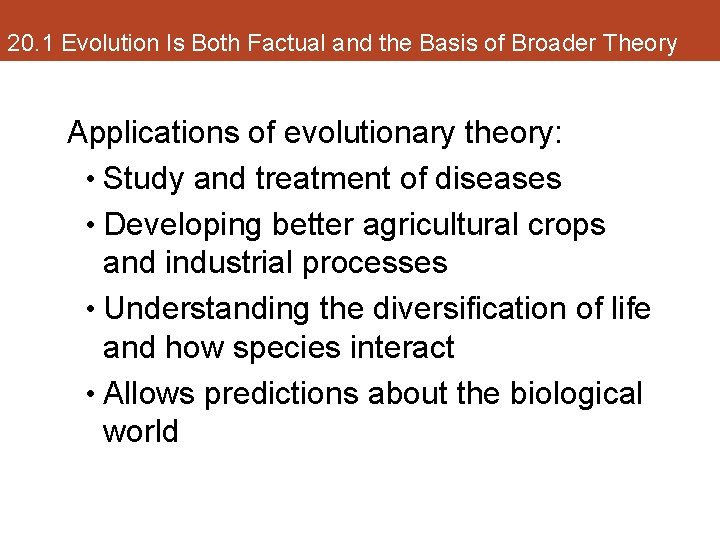
20. 1 Evolution Is Both Factual and the Basis of Broader Theory Applications of evolutionary theory: • Study and treatment of diseases • Developing better agricultural crops and industrial processes • Understanding the diversification of life and how species interact • Allows predictions about the biological world
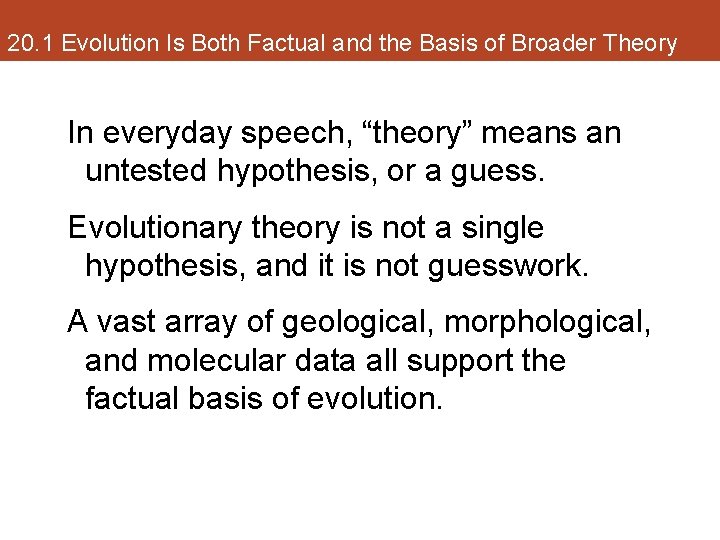
20. 1 Evolution Is Both Factual and the Basis of Broader Theory In everyday speech, “theory” means an untested hypothesis, or a guess. Evolutionary theory is not a single hypothesis, and it is not guesswork. A vast array of geological, morphological, and molecular data all support the factual basis of evolution.
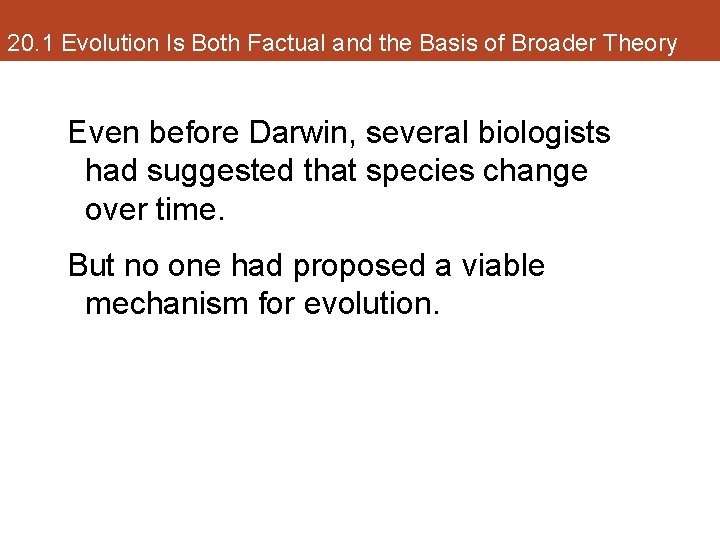
20. 1 Evolution Is Both Factual and the Basis of Broader Theory Even before Darwin, several biologists had suggested that species change over time. But no one had proposed a viable mechanism for evolution.
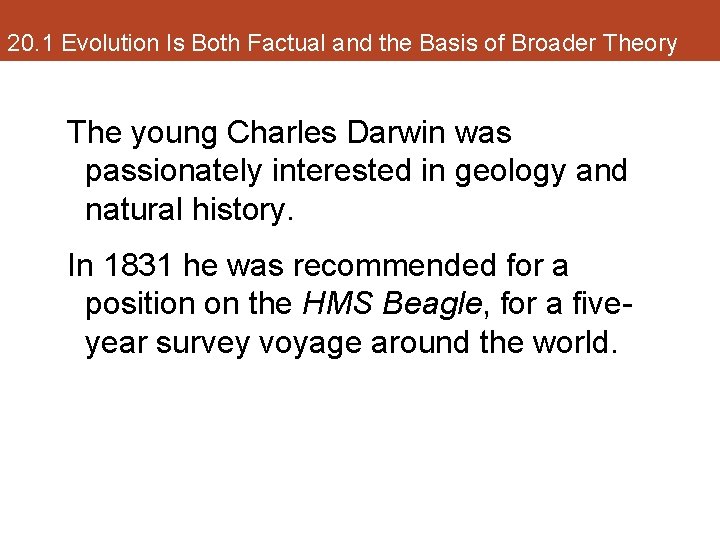
20. 1 Evolution Is Both Factual and the Basis of Broader Theory The young Charles Darwin was passionately interested in geology and natural history. In 1831 he was recommended for a position on the HMS Beagle, for a fiveyear survey voyage around the world.
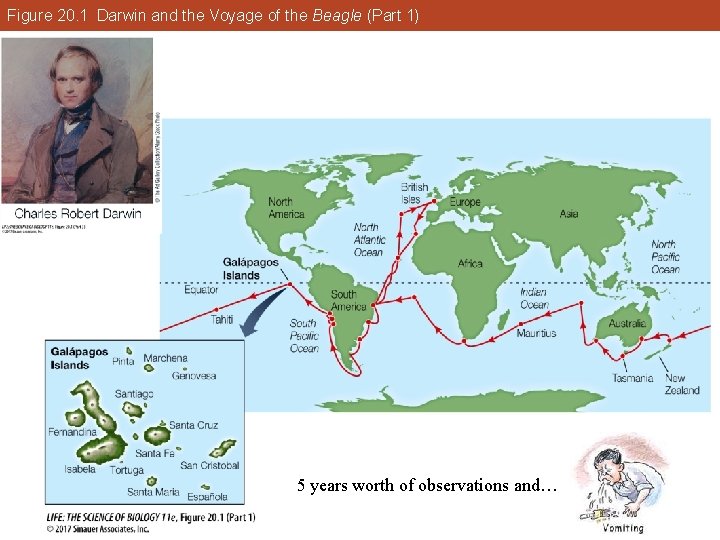
Figure 20. 1 Darwin and the Voyage of the Beagle (Part 1) 5 years worth of observations and…
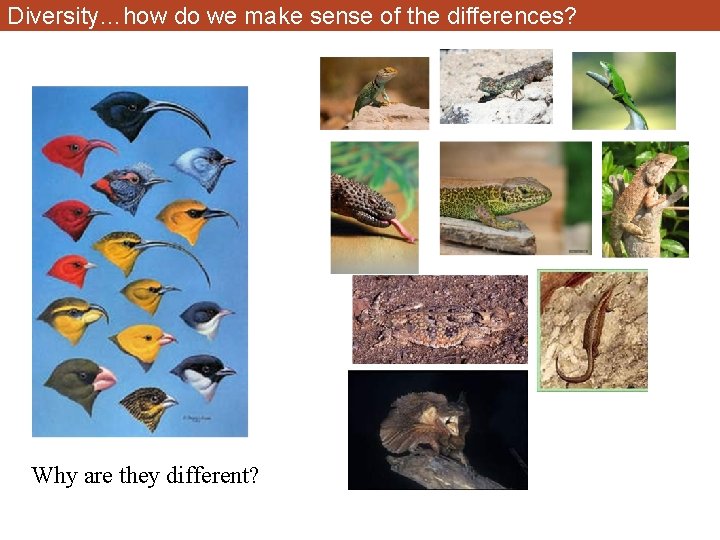
Diversity…how do we make sense of the differences? Why are they different?
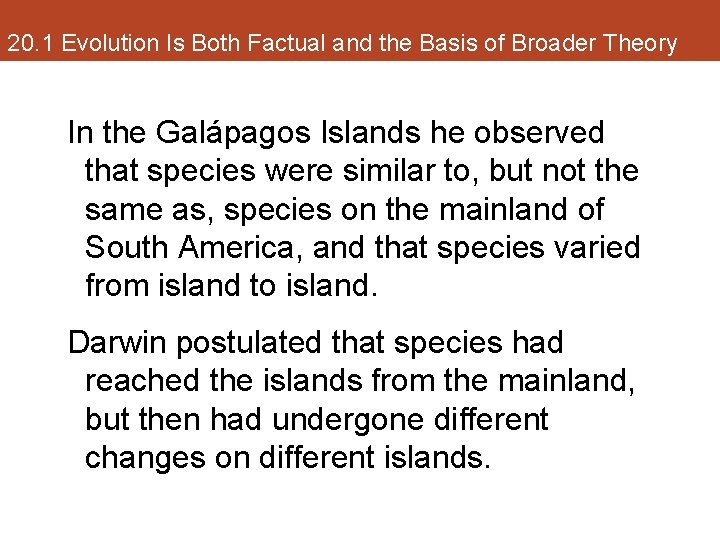
20. 1 Evolution Is Both Factual and the Basis of Broader Theory In the Galápagos Islands he observed that species were similar to, but not the same as, species on the mainland of South America, and that species varied from island to island. Darwin postulated that species had reached the islands from the mainland, but then had undergone different changes on different islands.
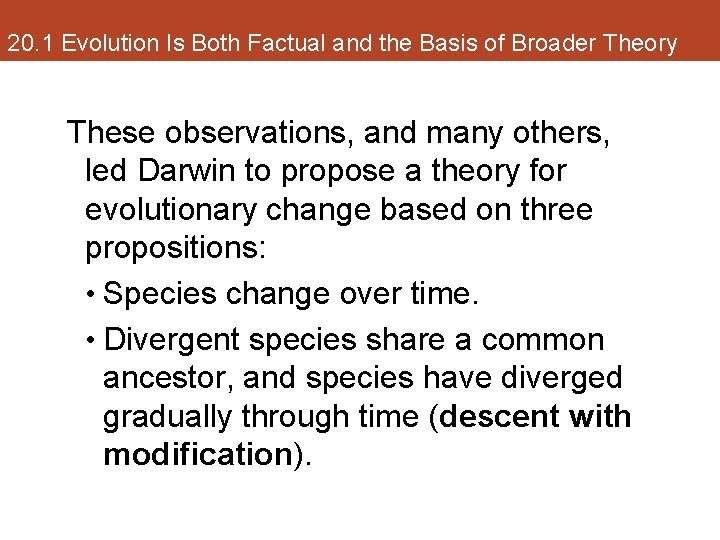
20. 1 Evolution Is Both Factual and the Basis of Broader Theory These observations, and many others, led Darwin to propose a theory for evolutionary change based on three propositions: • Species change over time. • Divergent species share a common ancestor, and species have diverged gradually through time (descent with modification).
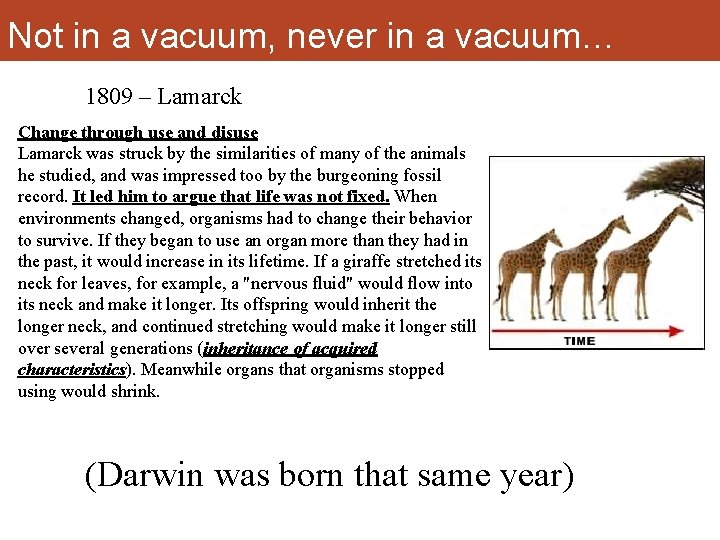
Not in a vacuum, never in a vacuum… 1809 – Lamarck Change through use and disuse Lamarck was struck by the similarities of many of the animals he studied, and was impressed too by the burgeoning fossil record. It led him to argue that life was not fixed. When environments changed, organisms had to change their behavior to survive. If they began to use an organ more than they had in the past, it would increase in its lifetime. If a giraffe stretched its neck for leaves, for example, a "nervous fluid" would flow into its neck and make it longer. Its offspring would inherit the longer neck, and continued stretching would make it longer still over several generations (inheritance of acquired characteristics). Meanwhile organs that organisms stopped using would shrink. (Darwin was born that same year)
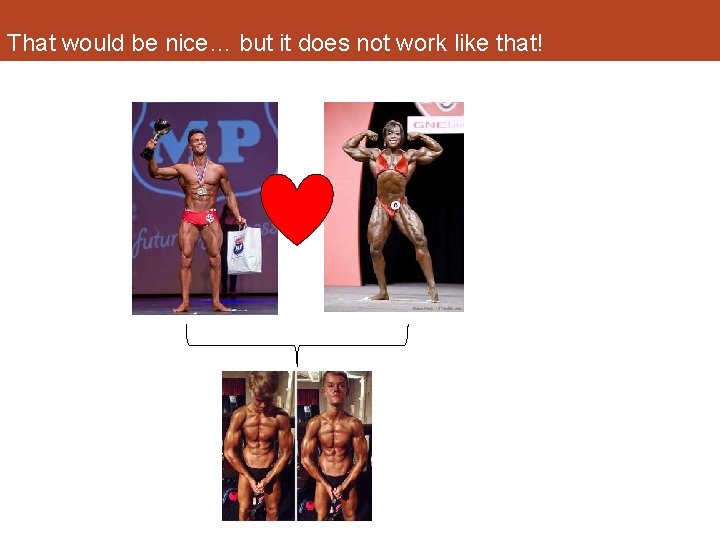
That would be nice… but it does not work like that!
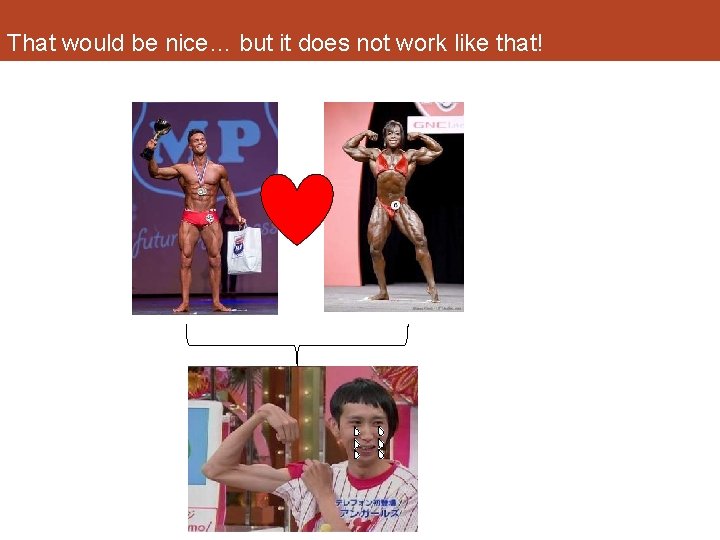
That would be nice… but it does not work like that!
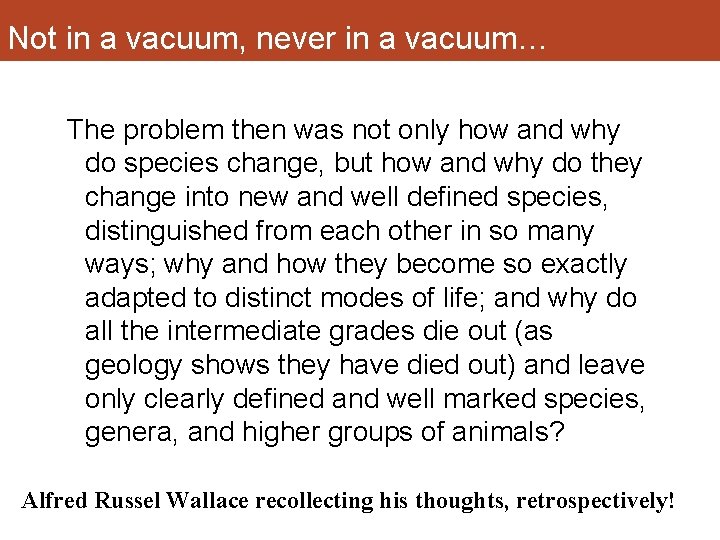
Not in a vacuum, never in a vacuum… The problem then was not only how and why do species change, but how and why do they change into new and well defined species, distinguished from each other in so many ways; why and how they become so exactly adapted to distinct modes of life; and why do all the intermediate grades die out (as geology shows they have died out) and leave only clearly defined and well marked species, genera, and higher groups of animals? Alfred Russel Wallace recollecting his thoughts, retrospectively!
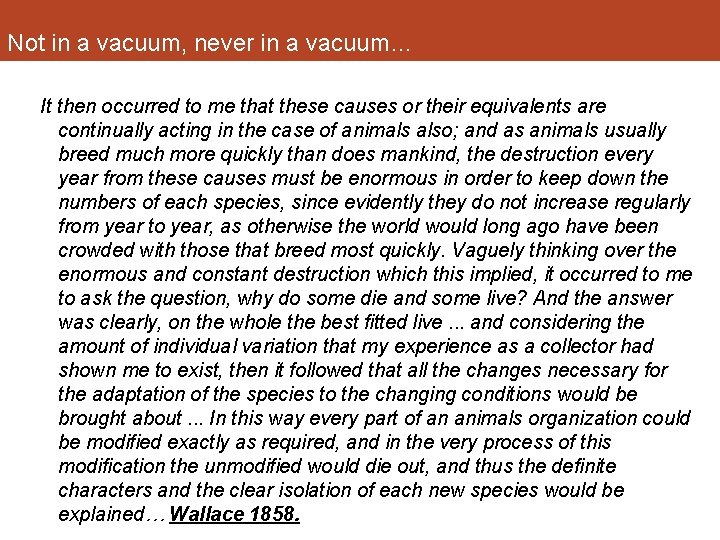
Not in a vacuum, never in a vacuum… It then occurred to me that these causes or their equivalents are continually acting in the case of animals also; and as animals usually breed much more quickly than does mankind, the destruction every year from these causes must be enormous in order to keep down the numbers of each species, since evidently they do not increase regularly from year to year, as otherwise the world would long ago have been crowded with those that breed most quickly. Vaguely thinking over the enormous and constant destruction which this implied, it occurred to me to ask the question, why do some die and some live? And the answer was clearly, on the whole the best fitted live. . . and considering the amount of individual variation that my experience as a collector had shown me to exist, then it followed that all the changes necessary for the adaptation of the species to the changing conditions would be brought about. . . In this way every part of an animals organization could be modified exactly as required, and in the very process of this modification the unmodified would die out, and thus the definite characters and the clear isolation of each new species would be explained… Wallace 1858.
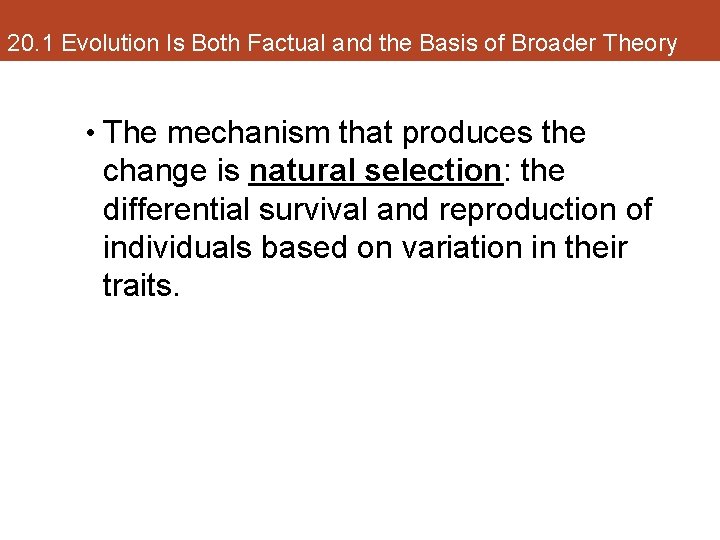
20. 1 Evolution Is Both Factual and the Basis of Broader Theory • The mechanism that produces the change is natural selection: the differential survival and reproduction of individuals based on variation in their traits.
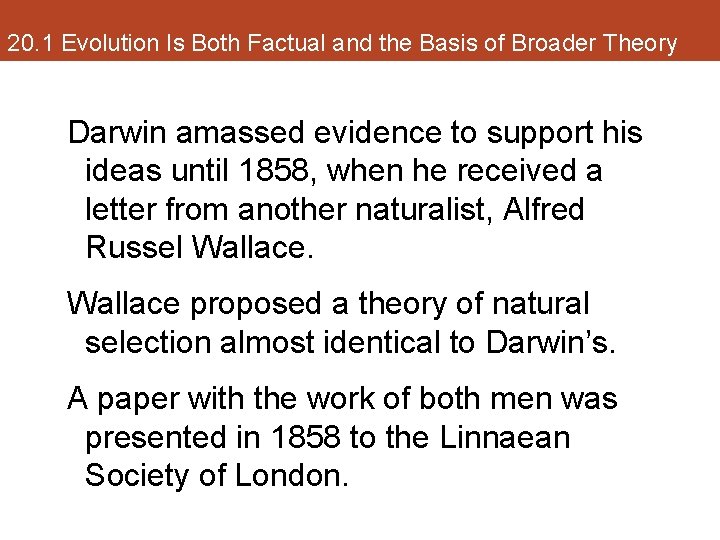
20. 1 Evolution Is Both Factual and the Basis of Broader Theory Darwin amassed evidence to support his ideas until 1858, when he received a letter from another naturalist, Alfred Russel Wallace proposed a theory of natural selection almost identical to Darwin’s. A paper with the work of both men was presented in 1858 to the Linnaean Society of London.
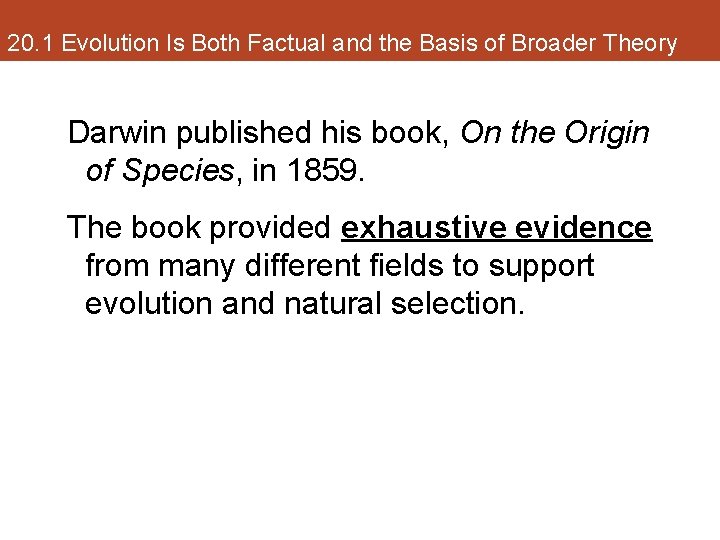
20. 1 Evolution Is Both Factual and the Basis of Broader Theory Darwin published his book, On the Origin of Species, in 1859. The book provided exhaustive evidence from many different fields to support evolution and natural selection.
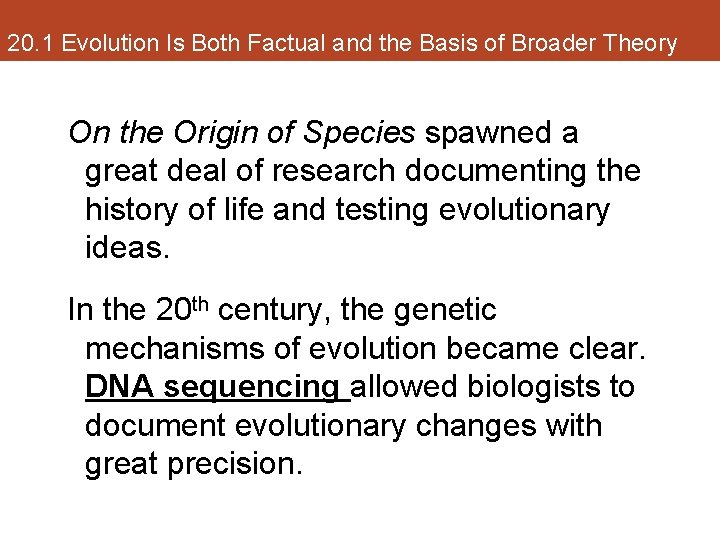
20. 1 Evolution Is Both Factual and the Basis of Broader Theory On the Origin of Species spawned a great deal of research documenting the history of life and testing evolutionary ideas. In the 20 th century, the genetic mechanisms of evolution became clear. DNA sequencing allowed biologists to document evolutionary changes with great precision.
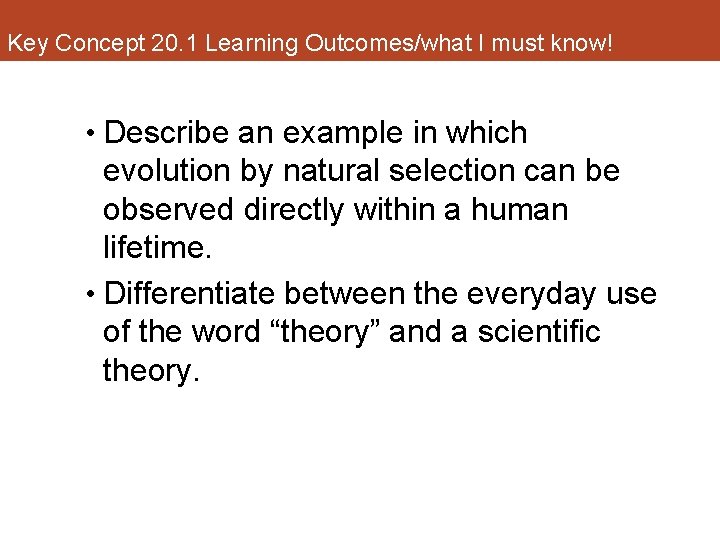
Key Concept 20. 1 Learning Outcomes/what I must know! • Describe an example in which evolution by natural selection can be observed directly within a human lifetime. • Differentiate between the everyday use of the word “theory” and a scientific theory.
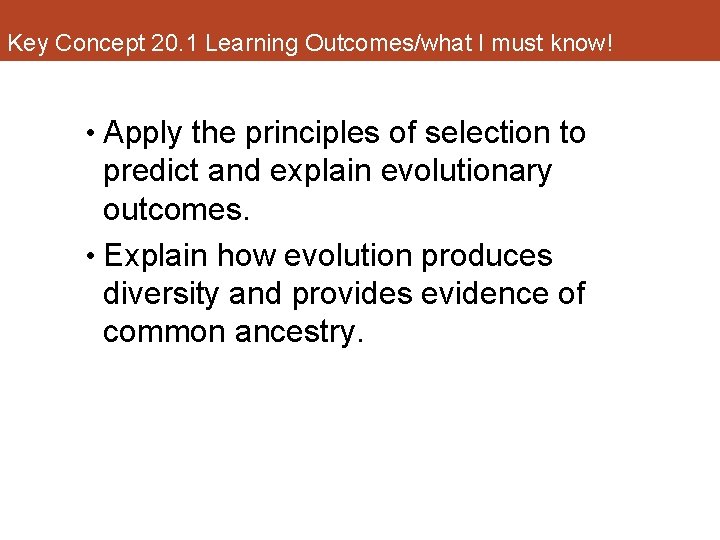
Key Concept 20. 1 Learning Outcomes/what I must know! • Apply the principles of selection to predict and explain evolutionary outcomes. • Explain how evolution produces diversity and provides evidence of common ancestry.
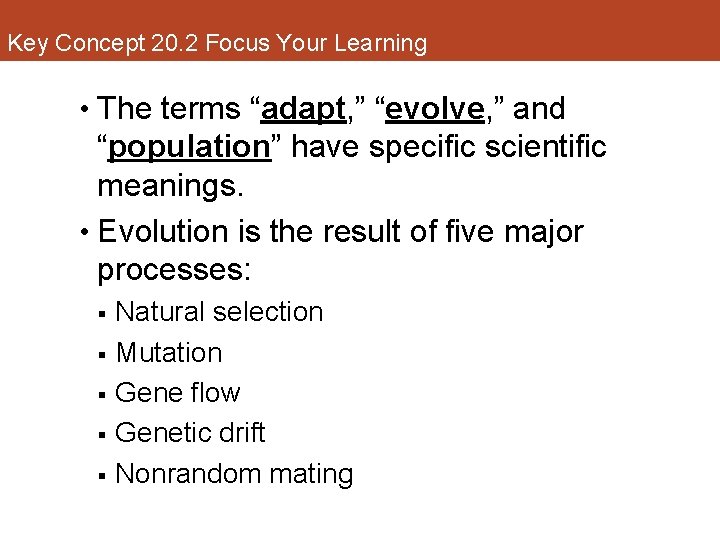
Key Concept 20. 2 Focus Your Learning • The terms “adapt, ” “evolve, ” and “population” have specific scientific meanings. • Evolution is the result of five major processes: § § § Natural selection Mutation Gene flow Genetic drift Nonrandom mating
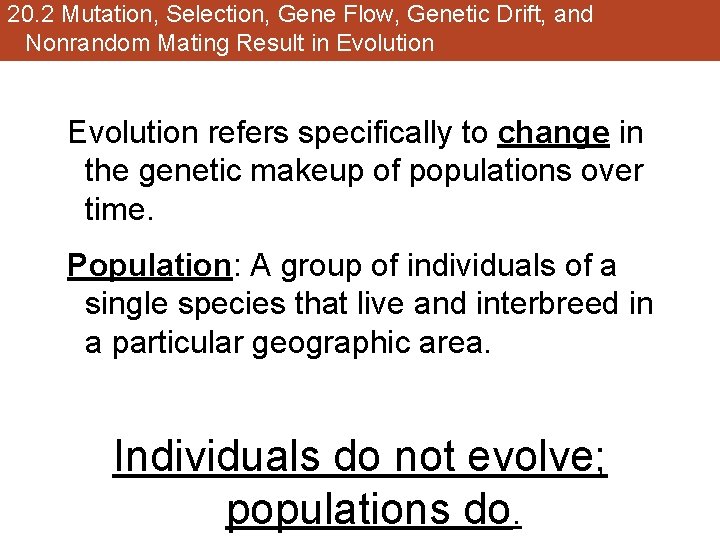
20. 2 Mutation, Selection, Gene Flow, Genetic Drift, and Nonrandom Mating Result in Evolution refers specifically to change in the genetic makeup of populations over time. Population: A group of individuals of a single species that live and interbreed in a particular geographic area. Individuals do not evolve; populations do.
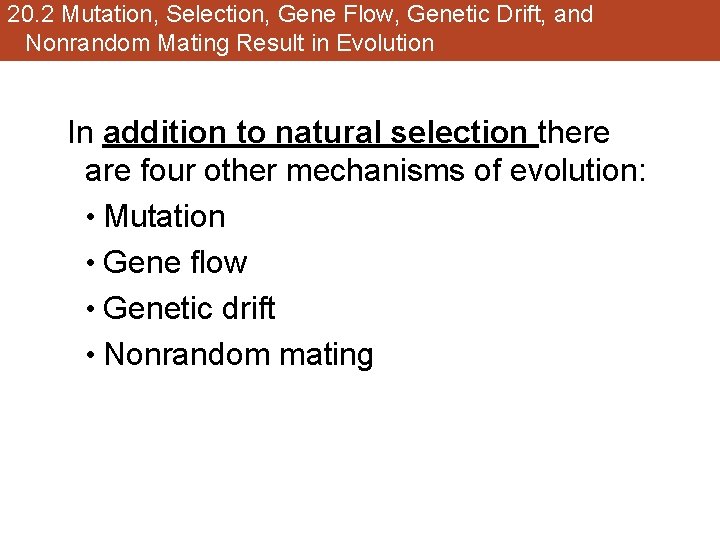
20. 2 Mutation, Selection, Gene Flow, Genetic Drift, and Nonrandom Mating Result in Evolution In addition to natural selection there are four other mechanisms of evolution: • Mutation • Gene flow • Genetic drift • Nonrandom mating
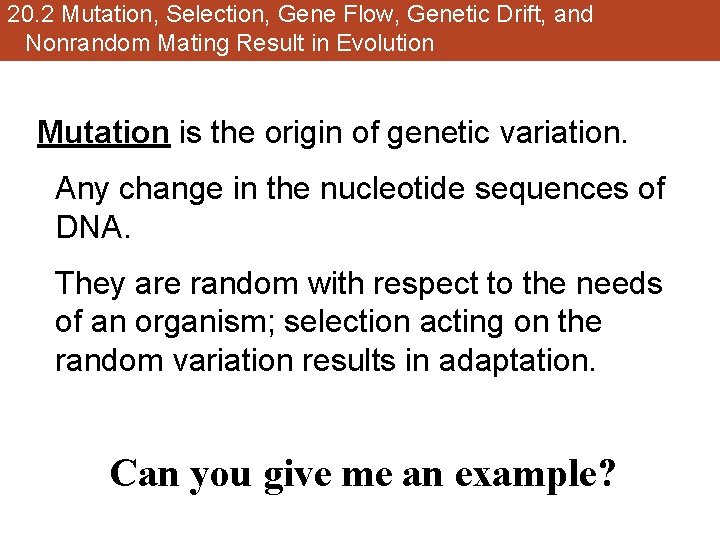
20. 2 Mutation, Selection, Gene Flow, Genetic Drift, and Nonrandom Mating Result in Evolution Mutation is the origin of genetic variation. Any change in the nucleotide sequences of DNA. They are random with respect to the needs of an organism; selection acting on the random variation results in adaptation. Can you give me an example?
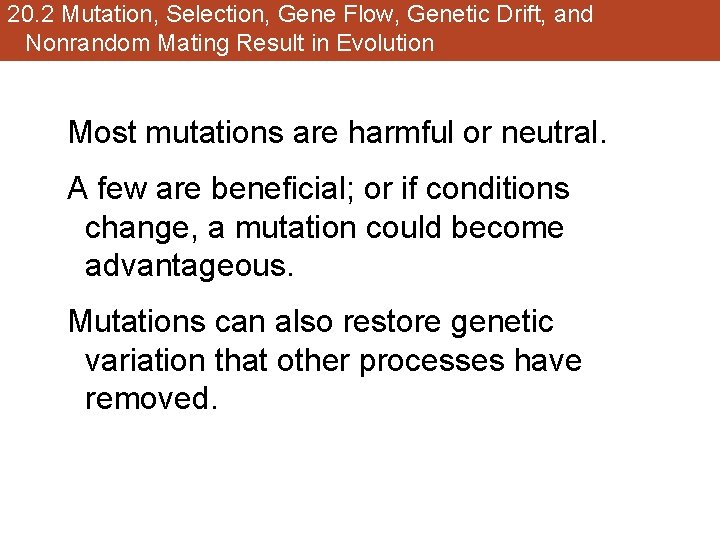
20. 2 Mutation, Selection, Gene Flow, Genetic Drift, and Nonrandom Mating Result in Evolution Most mutations are harmful or neutral. A few are beneficial; or if conditions change, a mutation could become advantageous. Mutations can also restore genetic variation that other processes have removed.
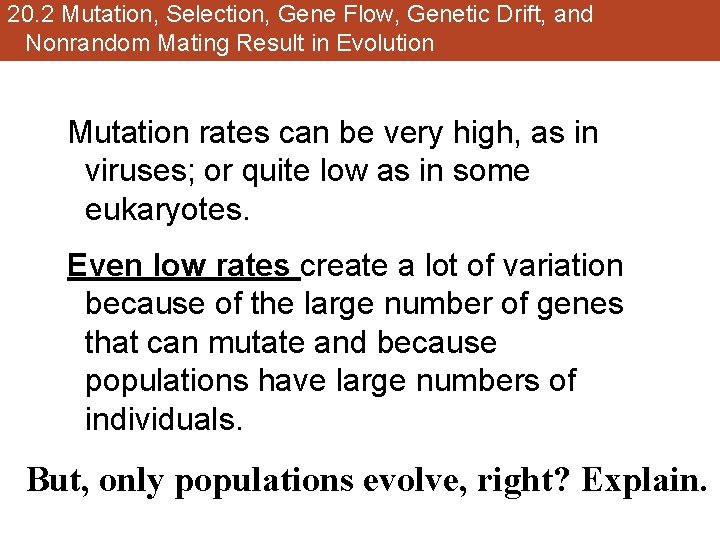
20. 2 Mutation, Selection, Gene Flow, Genetic Drift, and Nonrandom Mating Result in Evolution Mutation rates can be very high, as in viruses; or quite low as in some eukaryotes. Even low rates create a lot of variation because of the large number of genes that can mutate and because populations have large numbers of individuals. But, only populations evolve, right? Explain.
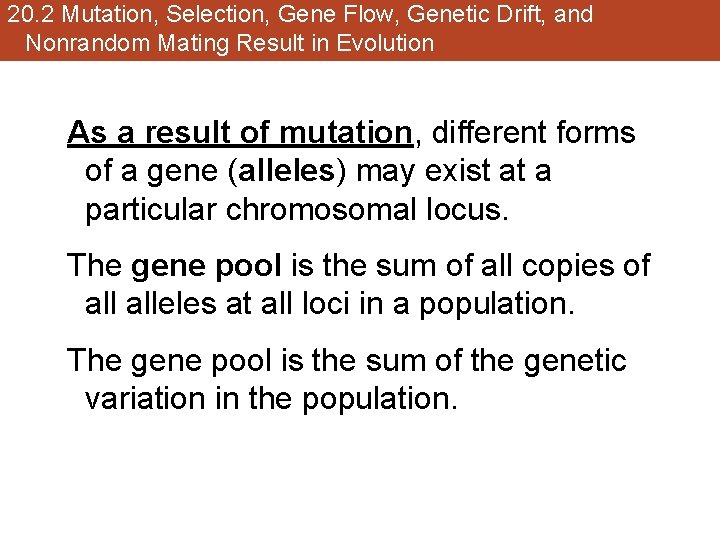
20. 2 Mutation, Selection, Gene Flow, Genetic Drift, and Nonrandom Mating Result in Evolution As a result of mutation, different forms of a gene (alleles) may exist at a particular chromosomal locus. The gene pool is the sum of all copies of alleles at all loci in a population. The gene pool is the sum of the genetic variation in the population.
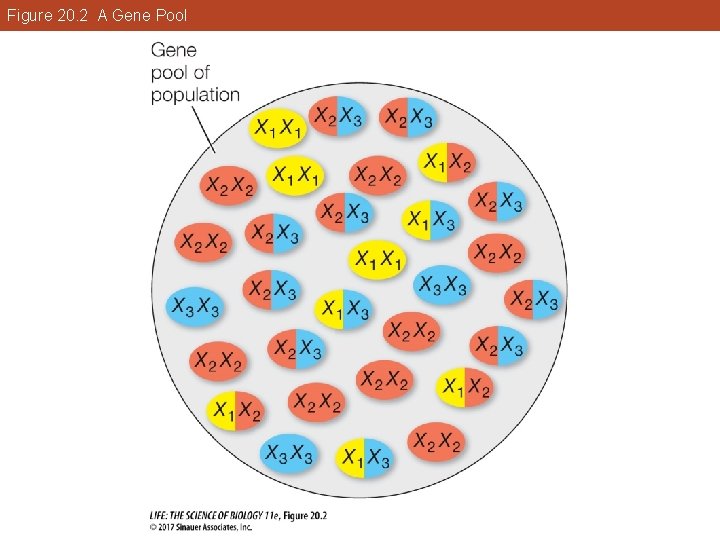
Figure 20. 2 A Gene Pool
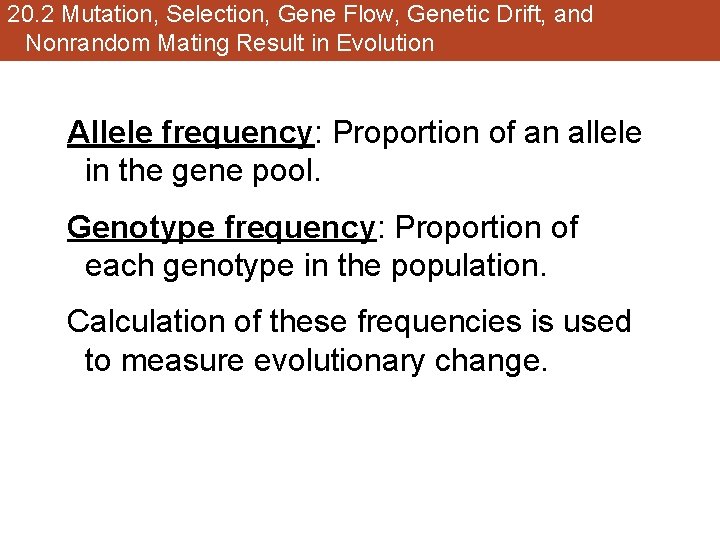
20. 2 Mutation, Selection, Gene Flow, Genetic Drift, and Nonrandom Mating Result in Evolution Allele frequency: Proportion of an allele in the gene pool. Genotype frequency: Proportion of each genotype in the population. Calculation of these frequencies is used to measure evolutionary change.
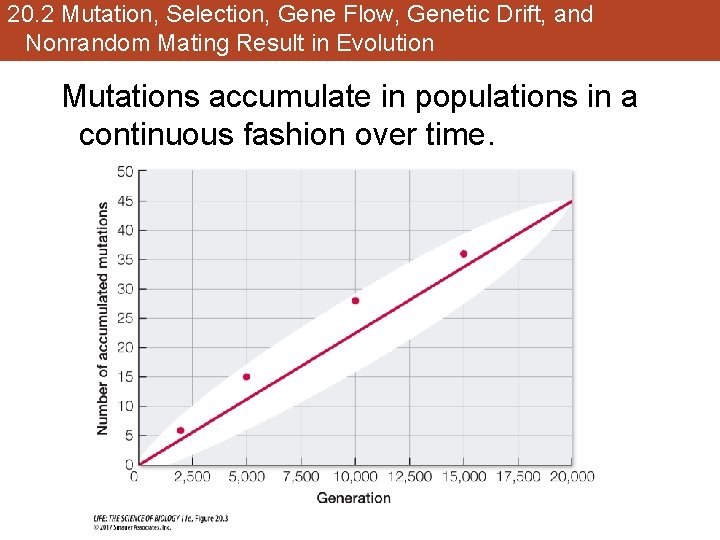
20. 2 Mutation, Selection, Gene Flow, Genetic Drift, and Nonrandom Mating Result in Evolution Mutations accumulate in populations in a continuous fashion over time.
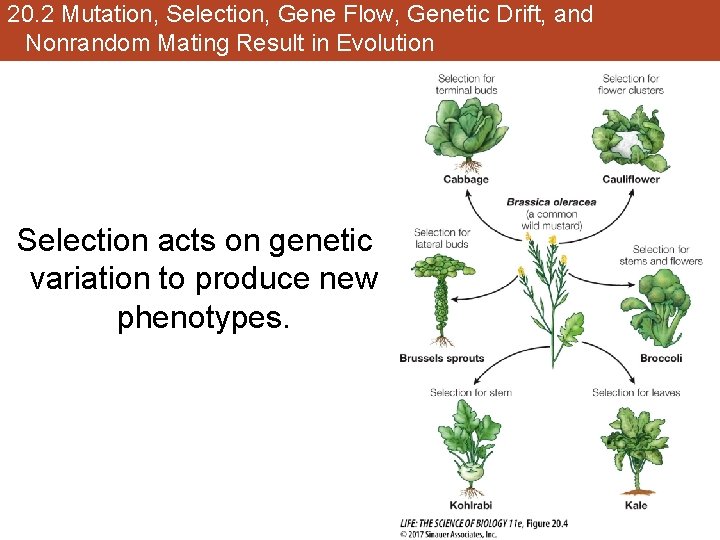
20. 2 Mutation, Selection, Gene Flow, Genetic Drift, and Nonrandom Mating Result in Evolution Selection acts on genetic variation to produce new phenotypes.
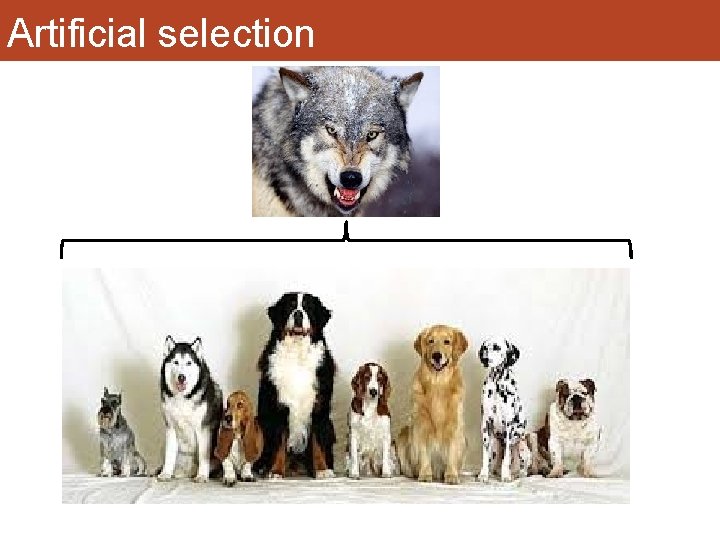
Artificial selection
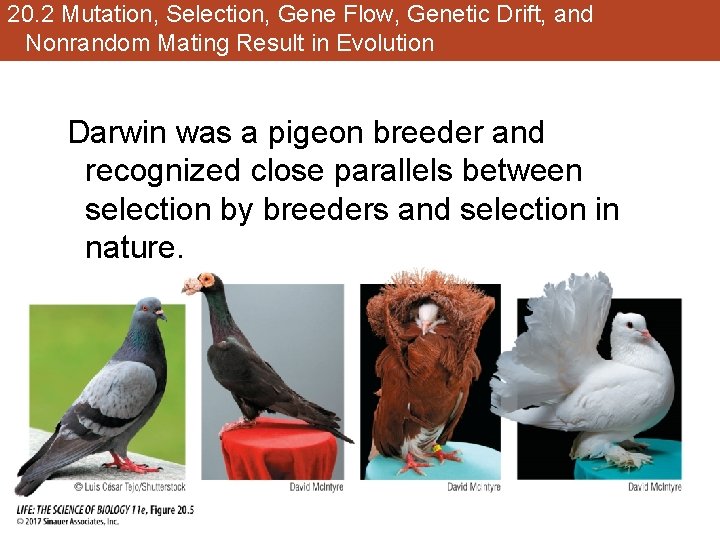
20. 2 Mutation, Selection, Gene Flow, Genetic Drift, and Nonrandom Mating Result in Evolution Darwin was a pigeon breeder and recognized close parallels between selection by breeders and selection in nature.
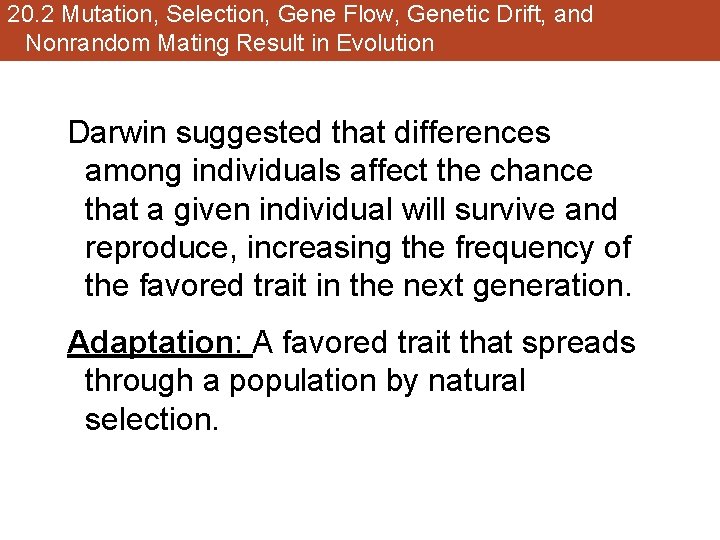
20. 2 Mutation, Selection, Gene Flow, Genetic Drift, and Nonrandom Mating Result in Evolution Darwin suggested that differences among individuals affect the chance that a given individual will survive and reproduce, increasing the frequency of the favored trait in the next generation. Adaptation: A favored trait that spreads through a population by natural selection.
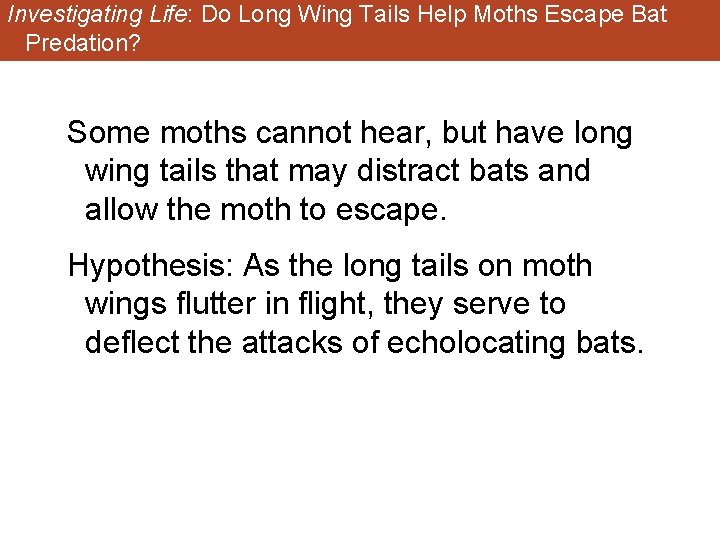
Investigating Life: Do Long Wing Tails Help Moths Escape Bat Predation? Some moths cannot hear, but have long wing tails that may distract bats and allow the moth to escape. Hypothesis: As the long tails on moth wings flutter in flight, they serve to deflect the attacks of echolocating bats.
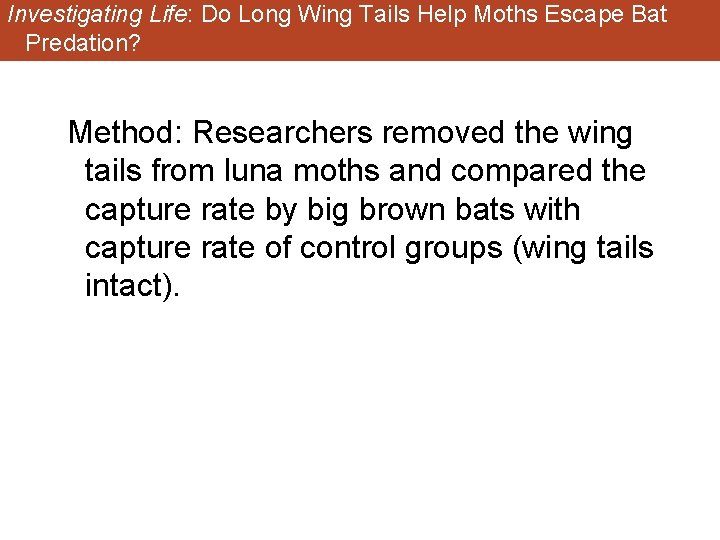
Investigating Life: Do Long Wing Tails Help Moths Escape Bat Predation? Method: Researchers removed the wing tails from luna moths and compared the capture rate by big brown bats with capture rate of control groups (wing tails intact).
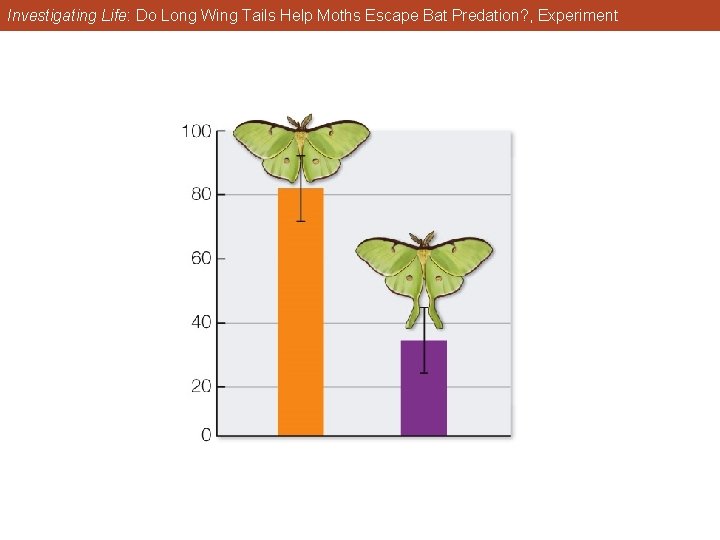
Investigating Life: Do Long Wing Tails Help Moths Escape Bat Predation? , Experiment
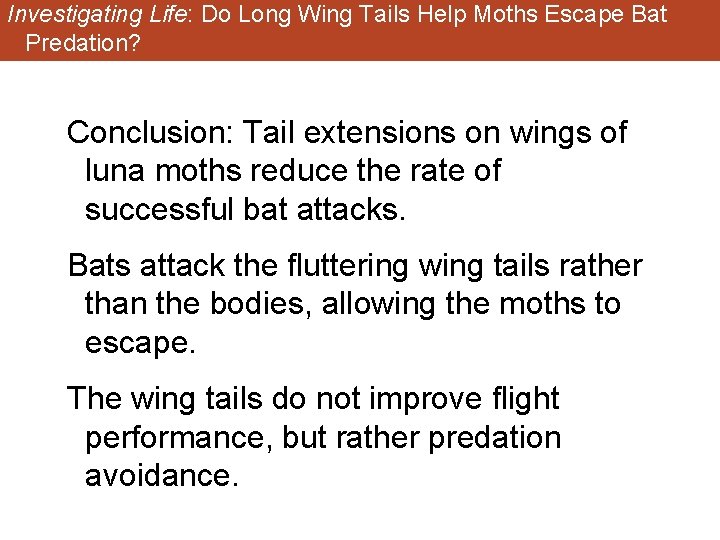
Investigating Life: Do Long Wing Tails Help Moths Escape Bat Predation? Conclusion: Tail extensions on wings of luna moths reduce the rate of successful bat attacks. Bats attack the fluttering wing tails rather than the bodies, allowing the moths to escape. The wing tails do not improve flight performance, but rather predation avoidance.
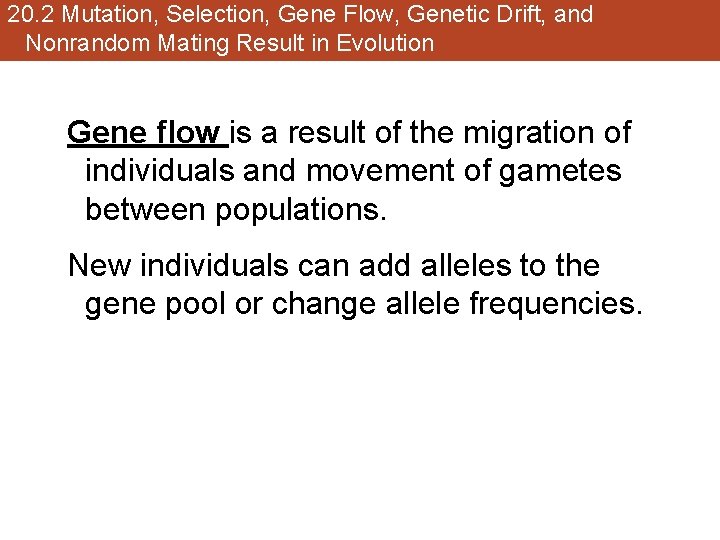
20. 2 Mutation, Selection, Gene Flow, Genetic Drift, and Nonrandom Mating Result in Evolution Gene flow is a result of the migration of individuals and movement of gametes between populations. New individuals can add alleles to the gene pool or change allele frequencies.
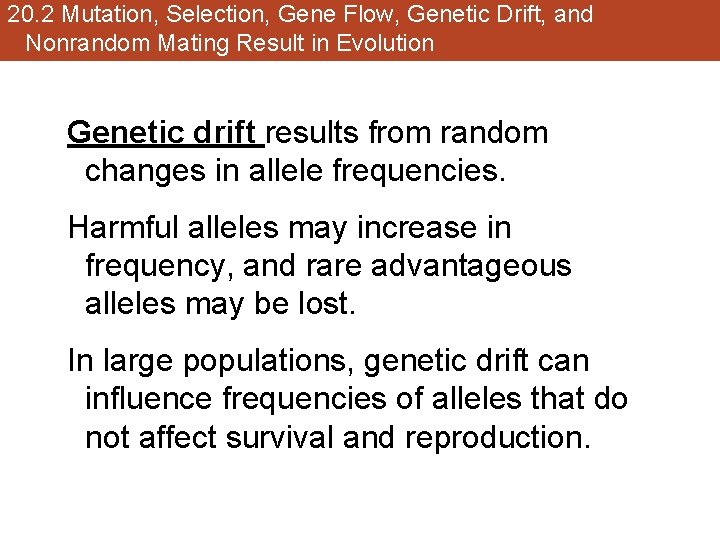
20. 2 Mutation, Selection, Gene Flow, Genetic Drift, and Nonrandom Mating Result in Evolution Genetic drift results from random changes in allele frequencies. Harmful alleles may increase in frequency, and rare advantageous alleles may be lost. In large populations, genetic drift can influence frequencies of alleles that do not affect survival and reproduction.
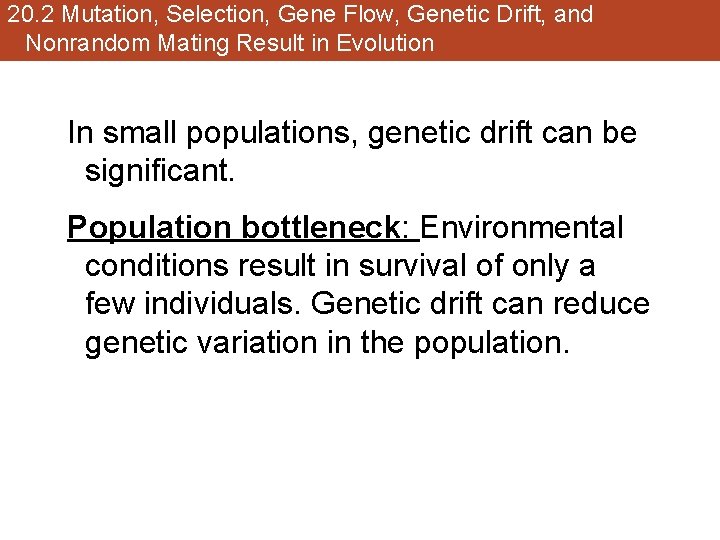
20. 2 Mutation, Selection, Gene Flow, Genetic Drift, and Nonrandom Mating Result in Evolution In small populations, genetic drift can be significant. Population bottleneck: Environmental conditions result in survival of only a few individuals. Genetic drift can reduce genetic variation in the population.
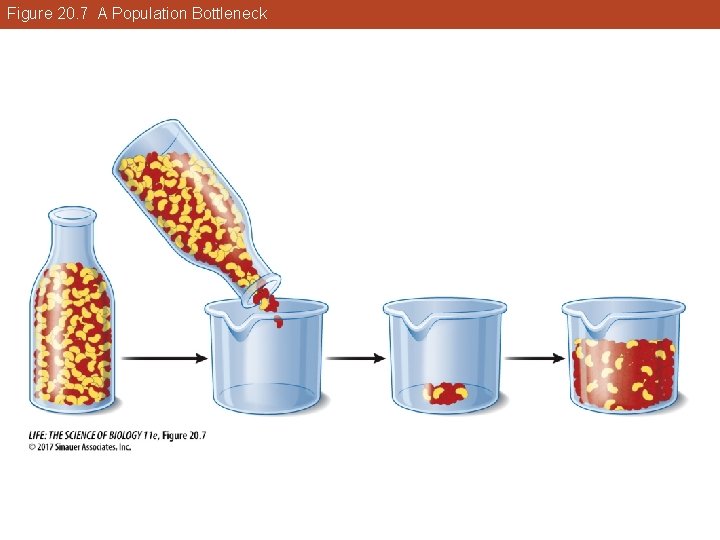
Figure 20. 7 A Population Bottleneck
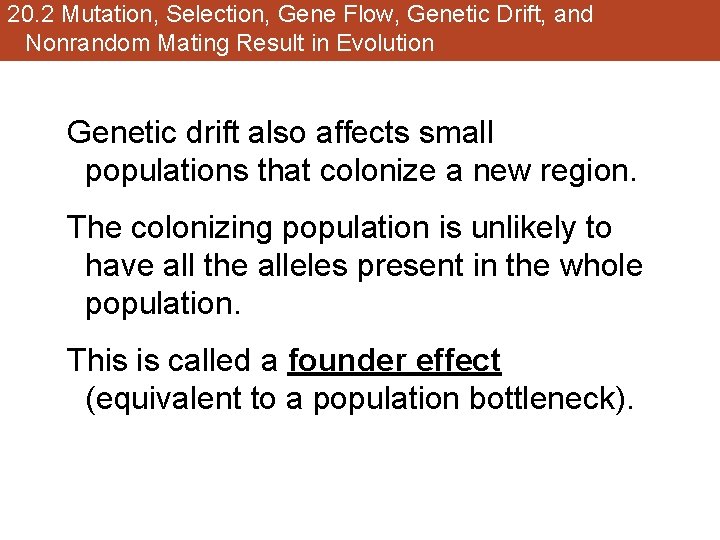
20. 2 Mutation, Selection, Gene Flow, Genetic Drift, and Nonrandom Mating Result in Evolution Genetic drift also affects small populations that colonize a new region. The colonizing population is unlikely to have all the alleles present in the whole population. This is called a founder effect (equivalent to a population bottleneck).
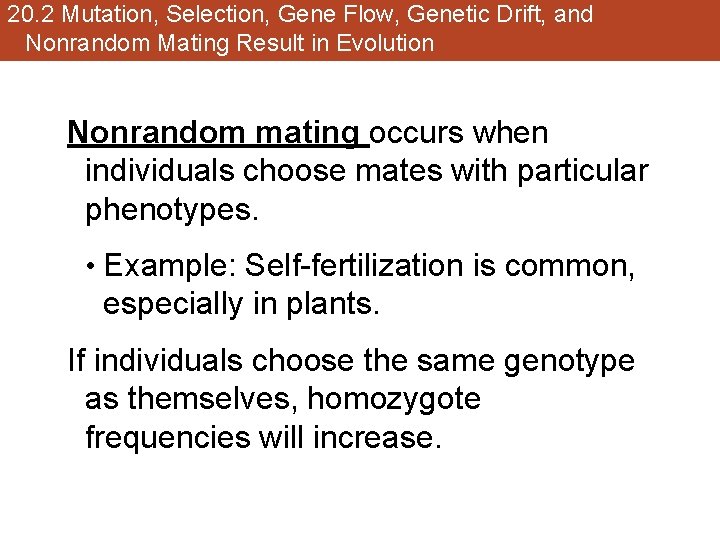
20. 2 Mutation, Selection, Gene Flow, Genetic Drift, and Nonrandom Mating Result in Evolution Nonrandom mating occurs when individuals choose mates with particular phenotypes. • Example: Self-fertilization is common, especially in plants. If individuals choose the same genotype as themselves, homozygote frequencies will increase.
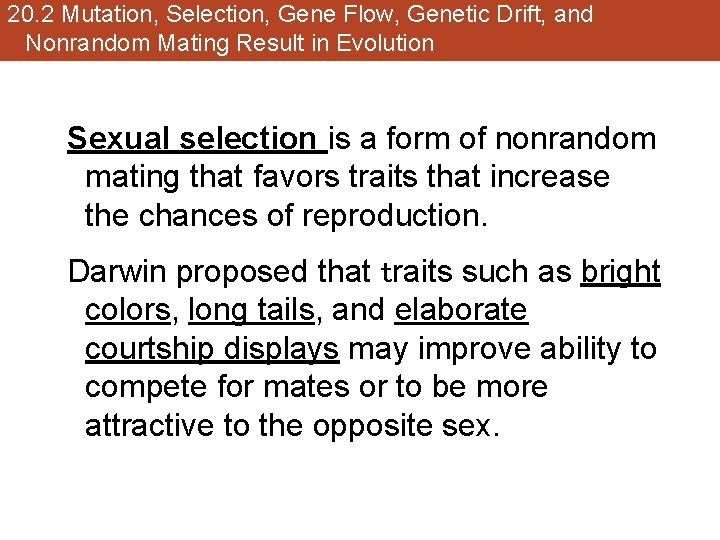
20. 2 Mutation, Selection, Gene Flow, Genetic Drift, and Nonrandom Mating Result in Evolution Sexual selection is a form of nonrandom mating that favors traits that increase the chances of reproduction. Darwin proposed that traits such as bright colors, long tails, and elaborate courtship displays may improve ability to compete for mates or to be more attractive to the opposite sex.
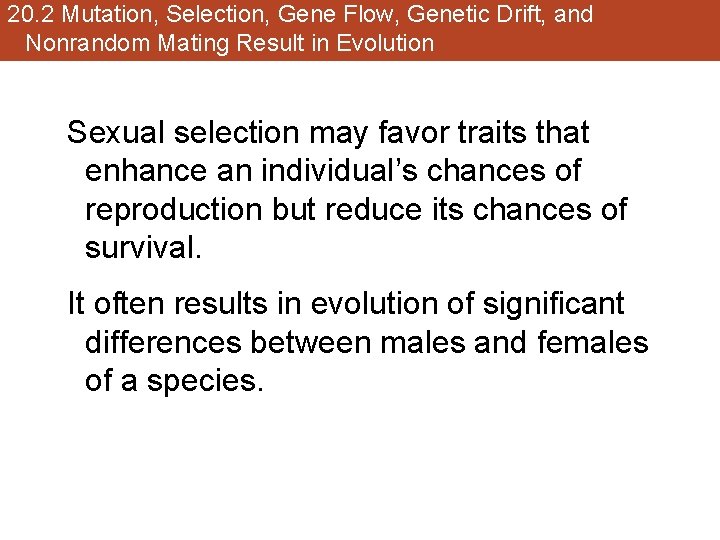
20. 2 Mutation, Selection, Gene Flow, Genetic Drift, and Nonrandom Mating Result in Evolution Sexual selection may favor traits that enhance an individual’s chances of reproduction but reduce its chances of survival. It often results in evolution of significant differences between males and females of a species.
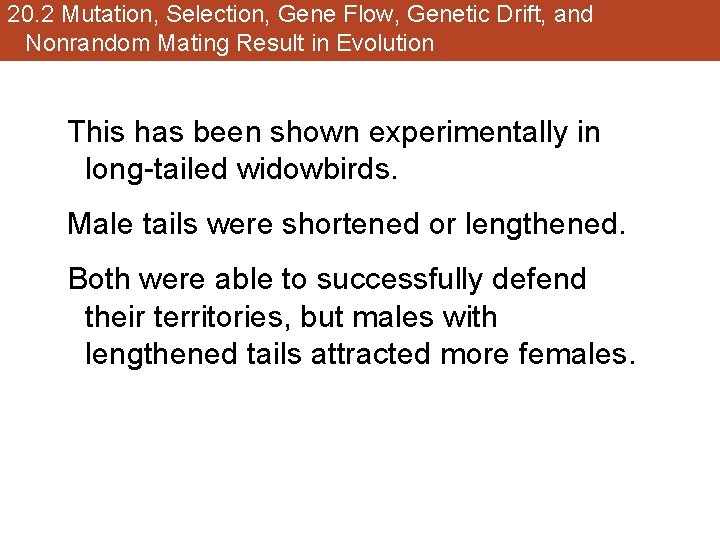
20. 2 Mutation, Selection, Gene Flow, Genetic Drift, and Nonrandom Mating Result in Evolution This has been shown experimentally in long-tailed widowbirds. Male tails were shortened or lengthened. Both were able to successfully defend their territories, but males with lengthened tails attracted more females.
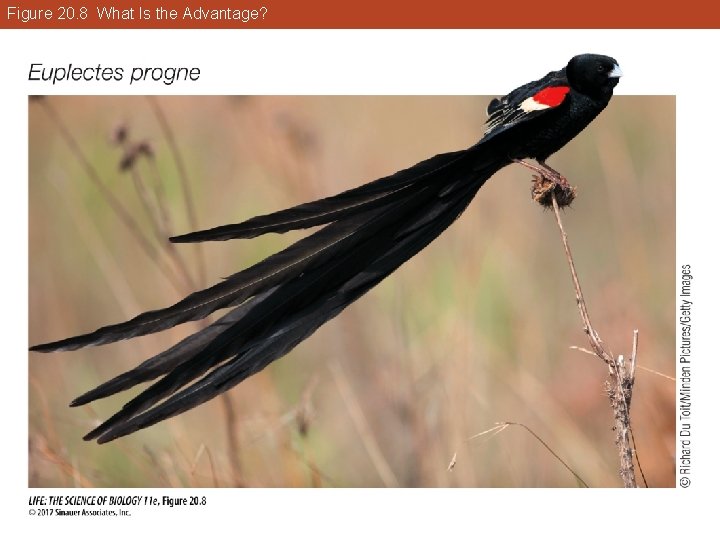
Figure 20. 8 What Is the Advantage?
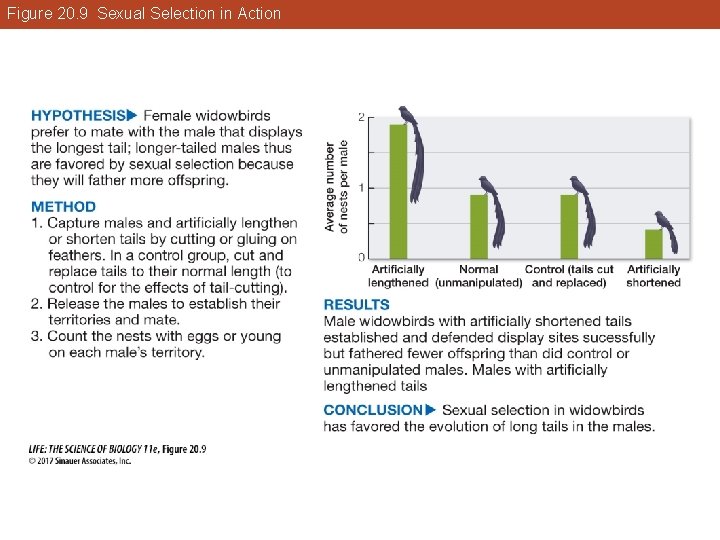
Figure 20. 9 Sexual Selection in Action
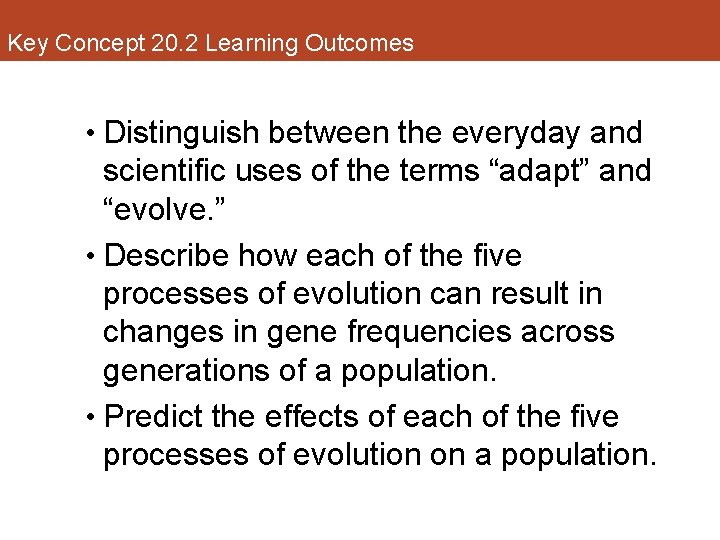
Key Concept 20. 2 Learning Outcomes • Distinguish between the everyday and scientific uses of the terms “adapt” and “evolve. ” • Describe how each of the five processes of evolution can result in changes in gene frequencies across generations of a population. • Predict the effects of each of the five processes of evolution on a population.
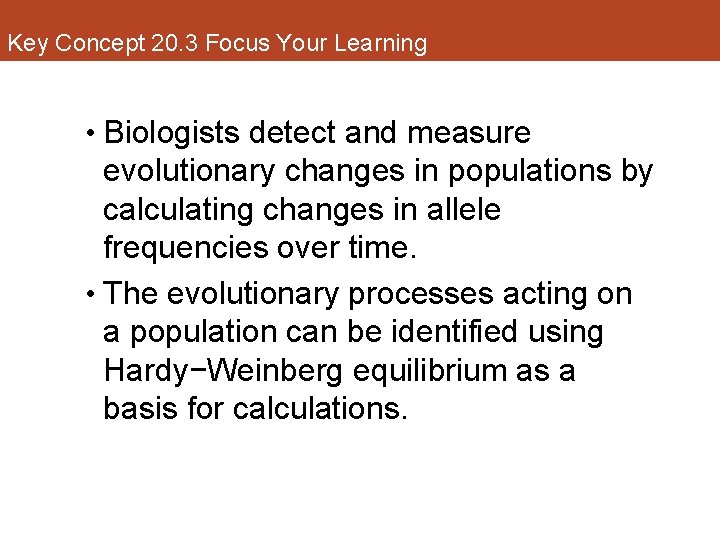
Key Concept 20. 3 Focus Your Learning • Biologists detect and measure evolutionary changes in populations by calculating changes in allele frequencies over time. • The evolutionary processes acting on a population can be identified using Hardy−Weinberg equilibrium as a basis for calculations.
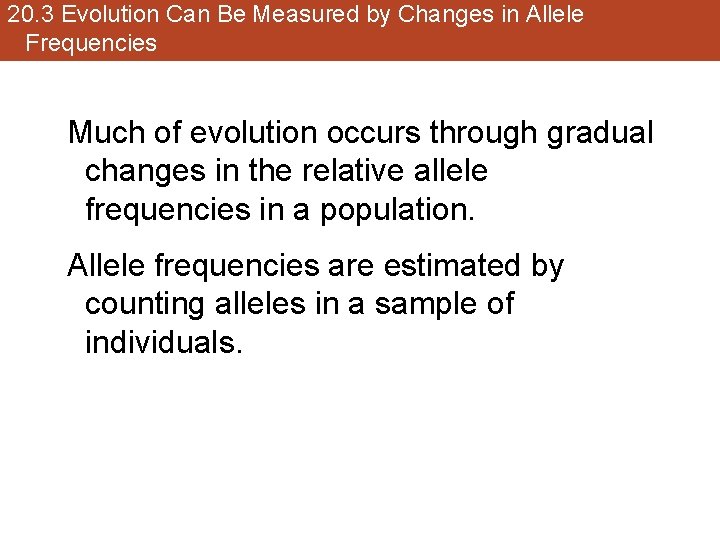
20. 3 Evolution Can Be Measured by Changes in Allele Frequencies Much of evolution occurs through gradual changes in the relative allele frequencies in a population. Allele frequencies are estimated by counting alleles in a sample of individuals.
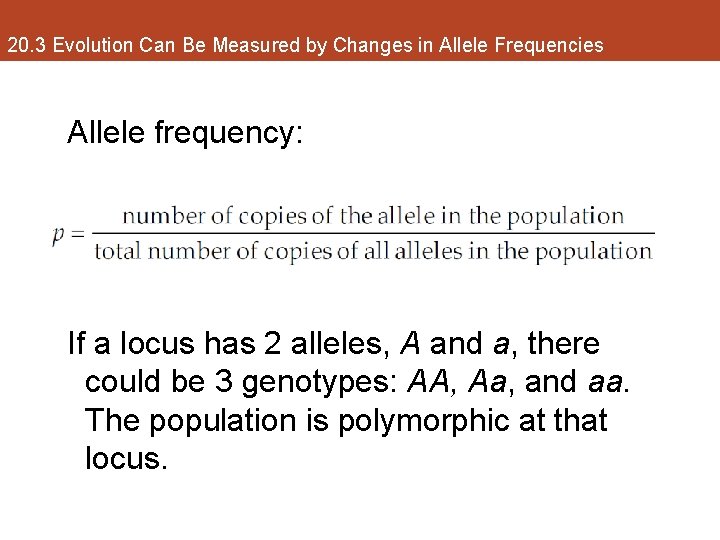
20. 3 Evolution Can Be Measured by Changes in Allele Frequencies Allele frequency: If a locus has 2 alleles, A and a, there could be 3 genotypes: AA, Aa, and aa. The population is polymorphic at that locus.
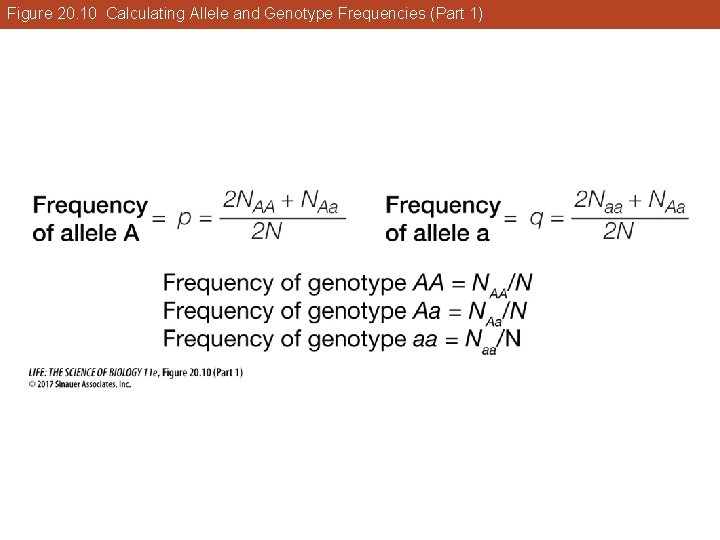
Figure 20. 10 Calculating Allele and Genotype Frequencies (Part 1)
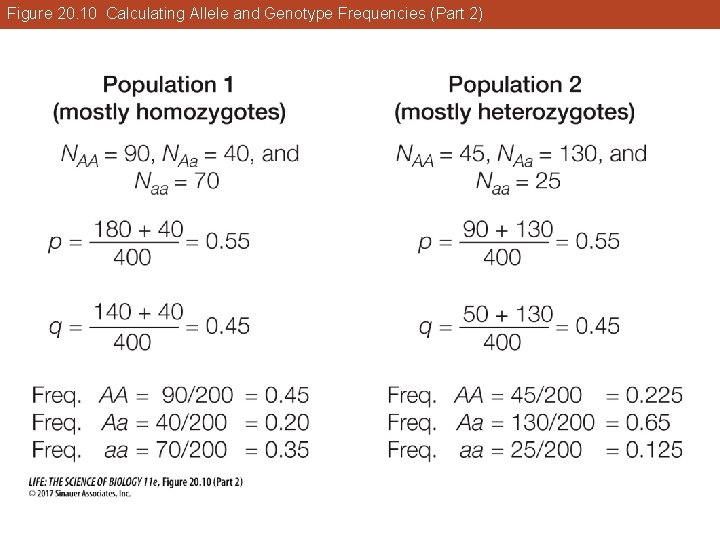
Figure 20. 10 Calculating Allele and Genotype Frequencies (Part 2)
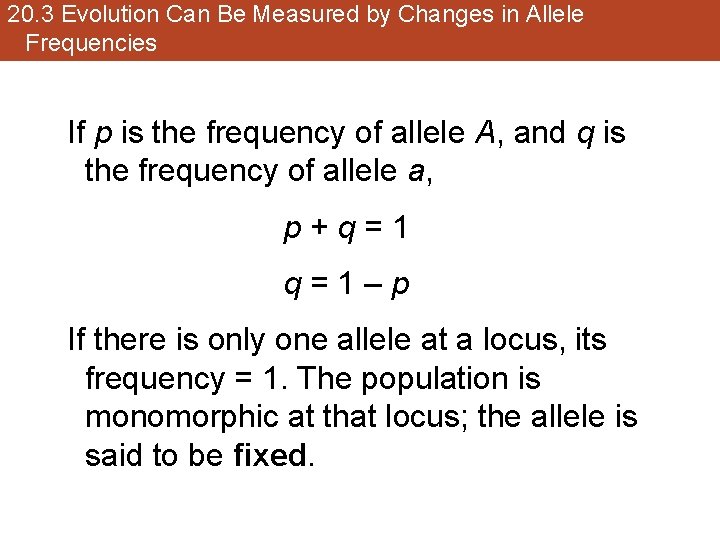
20. 3 Evolution Can Be Measured by Changes in Allele Frequencies If p is the frequency of allele A, and q is the frequency of allele a, p+q=1 q=1–p If there is only one allele at a locus, its frequency = 1. The population is monomorphic at that locus; the allele is said to be fixed.
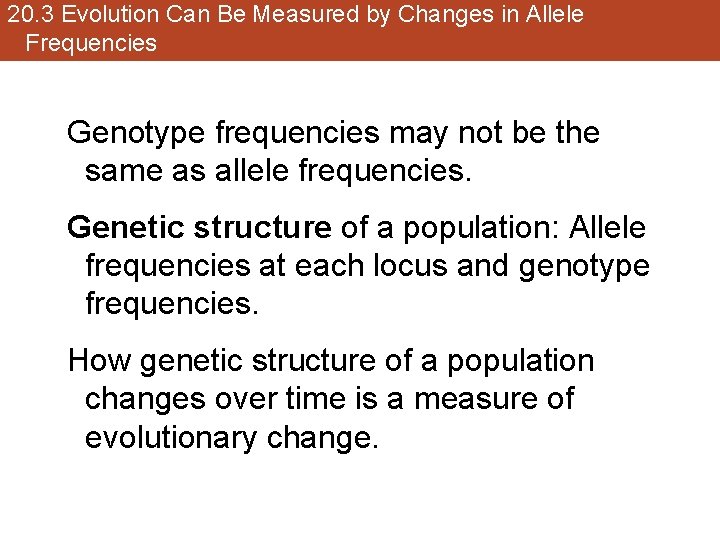
20. 3 Evolution Can Be Measured by Changes in Allele Frequencies Genotype frequencies may not be the same as allele frequencies. Genetic structure of a population: Allele frequencies at each locus and genotype frequencies. How genetic structure of a population changes over time is a measure of evolutionary change.
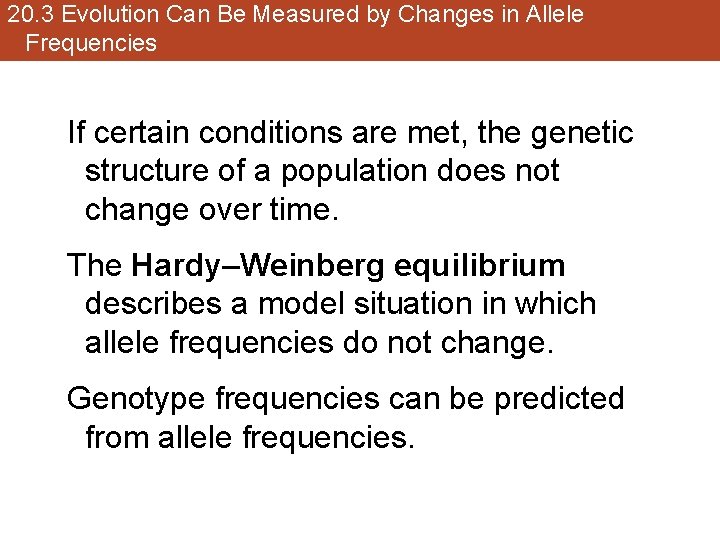
20. 3 Evolution Can Be Measured by Changes in Allele Frequencies If certain conditions are met, the genetic structure of a population does not change over time. The Hardy–Weinberg equilibrium describes a model situation in which allele frequencies do not change. Genotype frequencies can be predicted from allele frequencies.
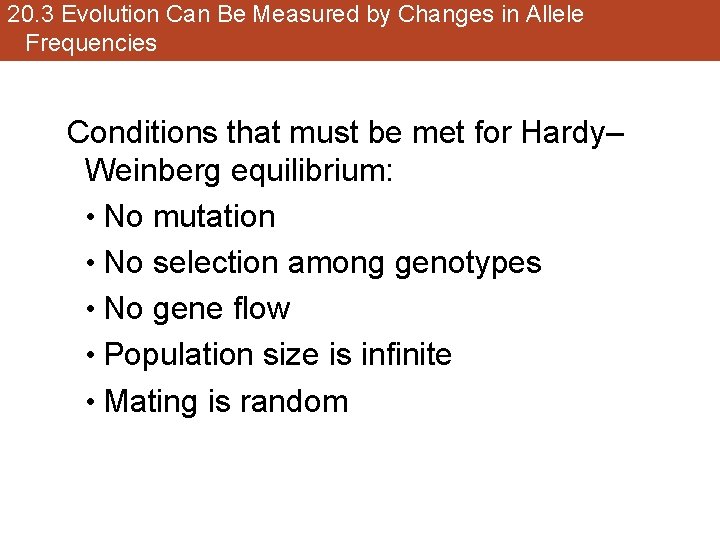
20. 3 Evolution Can Be Measured by Changes in Allele Frequencies Conditions that must be met for Hardy– Weinberg equilibrium: • No mutation • No selection among genotypes • No gene flow • Population size is infinite • Mating is random
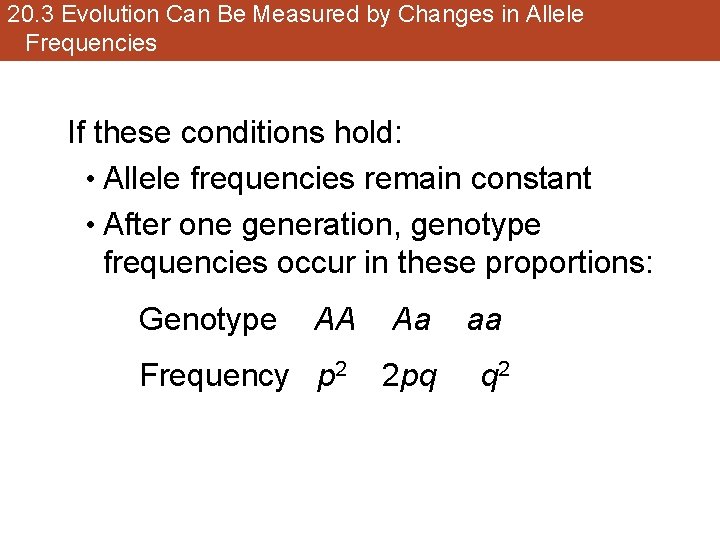
20. 3 Evolution Can Be Measured by Changes in Allele Frequencies If these conditions hold: • Allele frequencies remain constant • After one generation, genotype frequencies occur in these proportions: Genotype AA Aa aa Frequency p 2 2 pq q 2
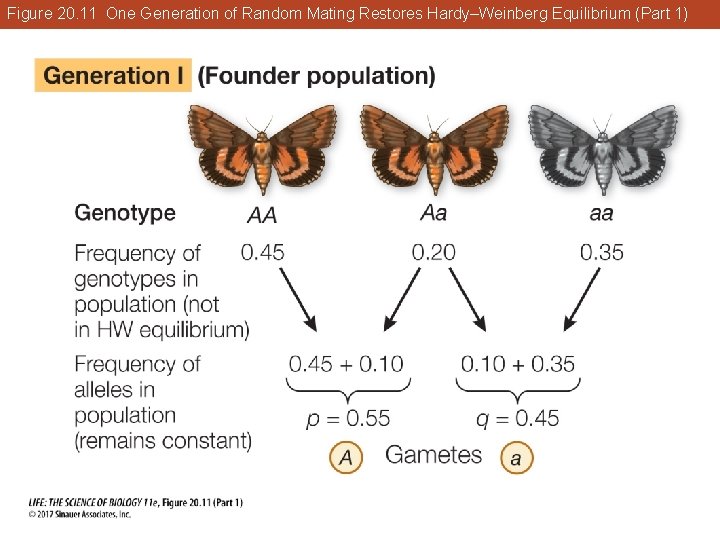
Figure 20. 11 One Generation of Random Mating Restores Hardy–Weinberg Equilibrium (Part 1)
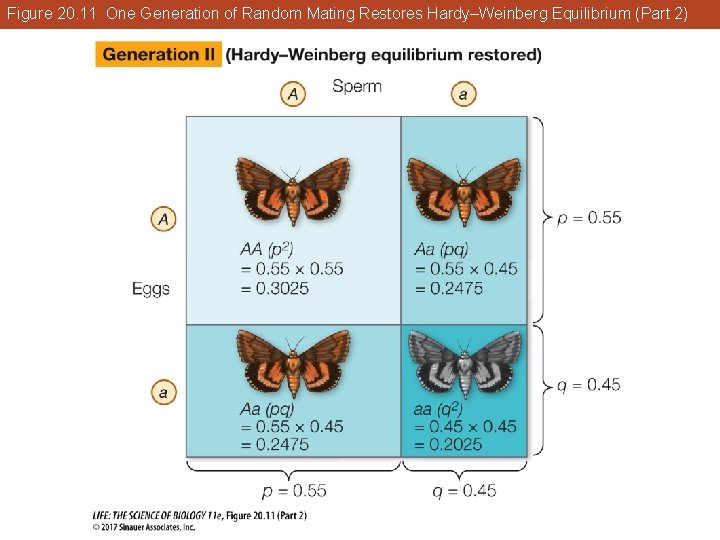
Figure 20. 11 One Generation of Random Mating Restores Hardy–Weinberg Equilibrium (Part 2)
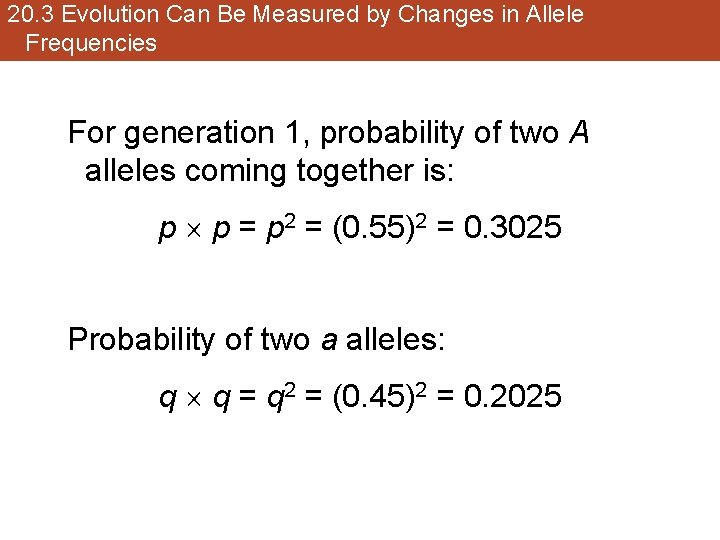
20. 3 Evolution Can Be Measured by Changes in Allele Frequencies For generation 1, probability of two A alleles coming together is: p p = p 2 = (0. 55)2 = 0. 3025 Probability of two a alleles: q q = q 2 = (0. 45)2 = 0. 2025
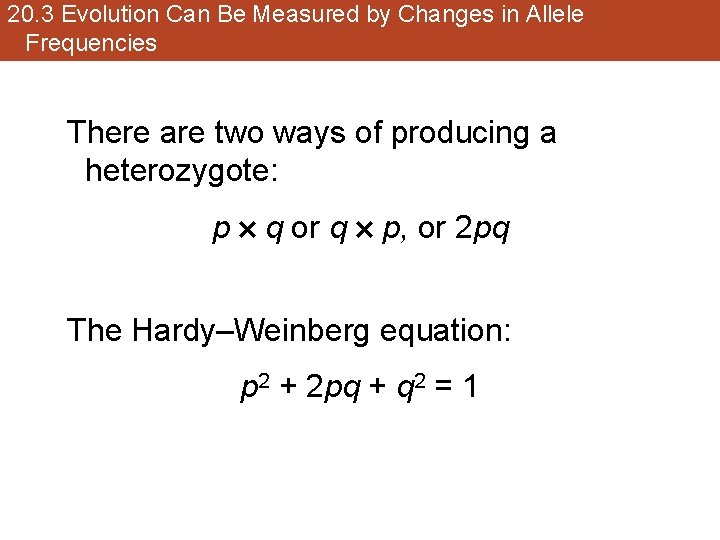
20. 3 Evolution Can Be Measured by Changes in Allele Frequencies There are two ways of producing a heterozygote: p q or q p, or 2 pq The Hardy–Weinberg equation: p 2 + 2 pq + q 2 = 1
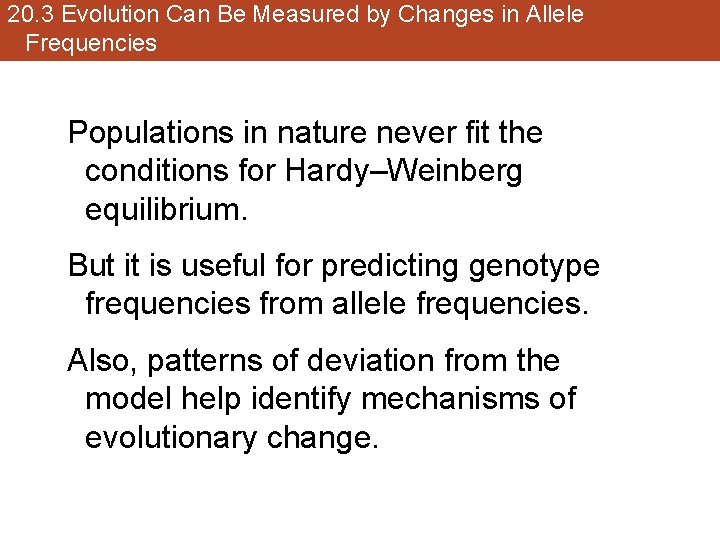
20. 3 Evolution Can Be Measured by Changes in Allele Frequencies Populations in nature never fit the conditions for Hardy–Weinberg equilibrium. But it is useful for predicting genotype frequencies from allele frequencies. Also, patterns of deviation from the model help identify mechanisms of evolutionary change.
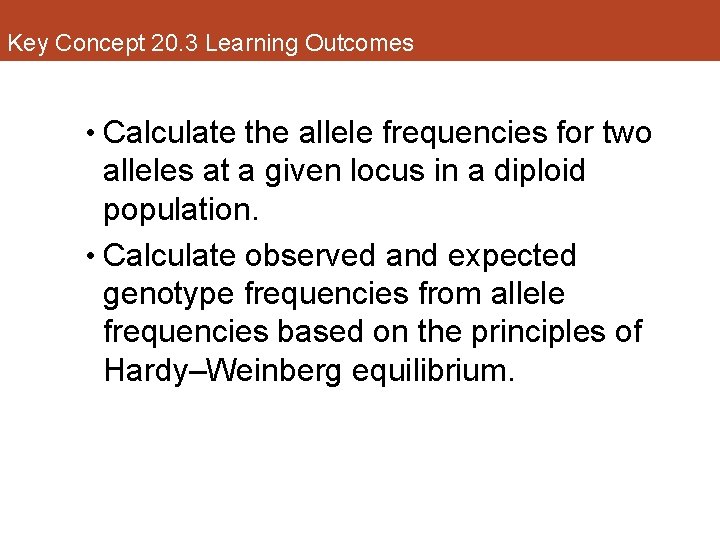
Key Concept 20. 3 Learning Outcomes • Calculate the allele frequencies for two alleles at a given locus in a diploid population. • Calculate observed and expected genotype frequencies from allele frequencies based on the principles of Hardy–Weinberg equilibrium.
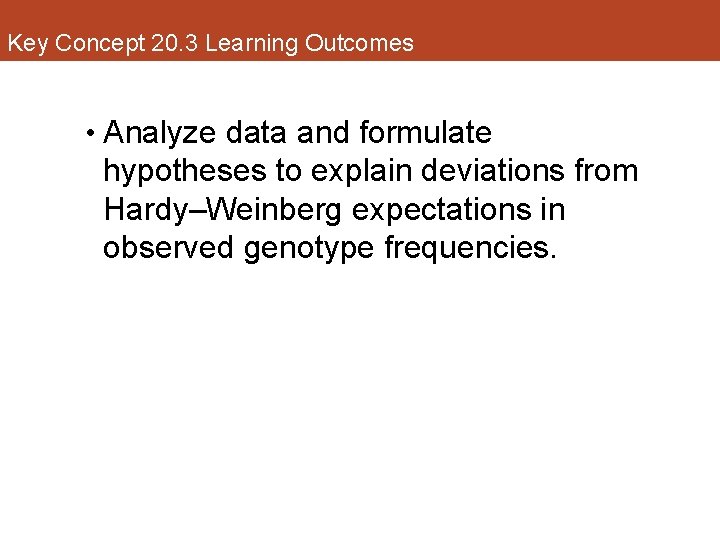
Key Concept 20. 3 Learning Outcomes • Analyze data and formulate hypotheses to explain deviations from Hardy–Weinberg expectations in observed genotype frequencies.
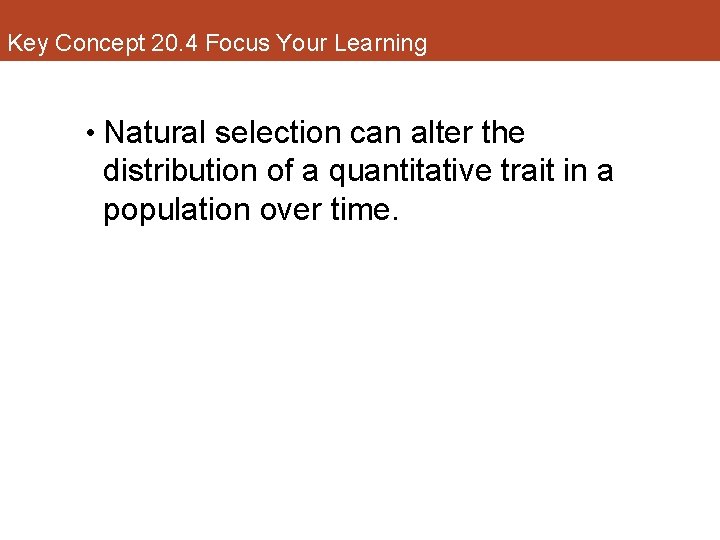
Key Concept 20. 4 Focus Your Learning • Natural selection can alter the distribution of a quantitative trait in a population over time.
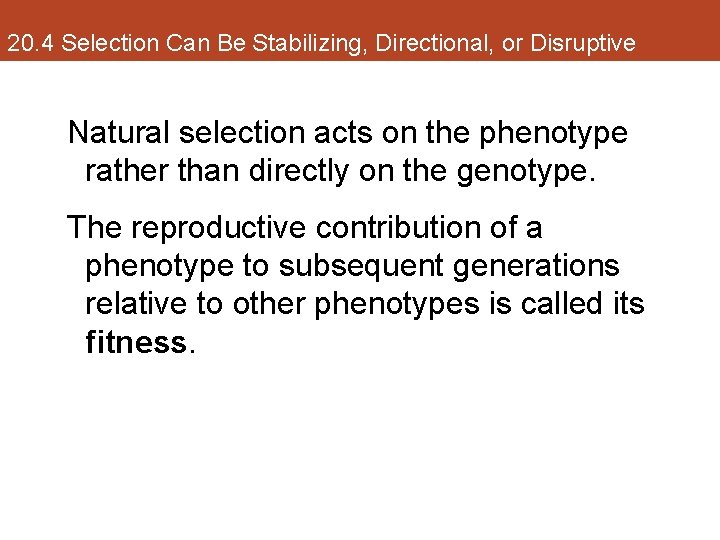
20. 4 Selection Can Be Stabilizing, Directional, or Disruptive Natural selection acts on the phenotype rather than directly on the genotype. The reproductive contribution of a phenotype to subsequent generations relative to other phenotypes is called its fitness.
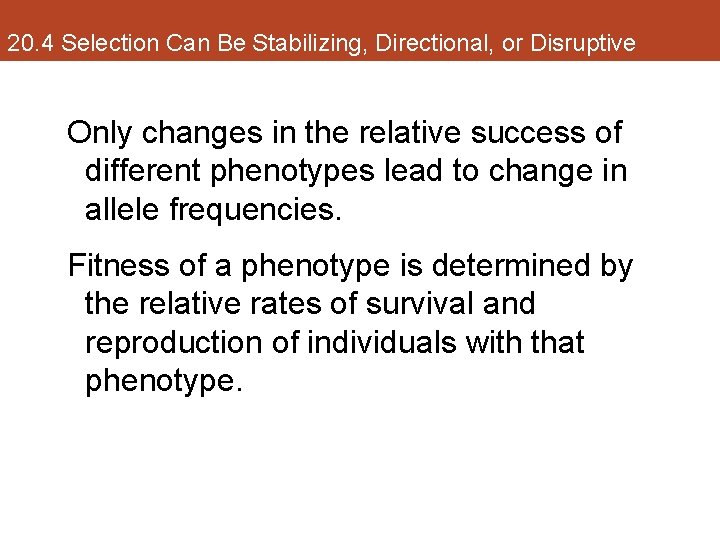
20. 4 Selection Can Be Stabilizing, Directional, or Disruptive Only changes in the relative success of different phenotypes lead to change in allele frequencies. Fitness of a phenotype is determined by the relative rates of survival and reproduction of individuals with that phenotype.
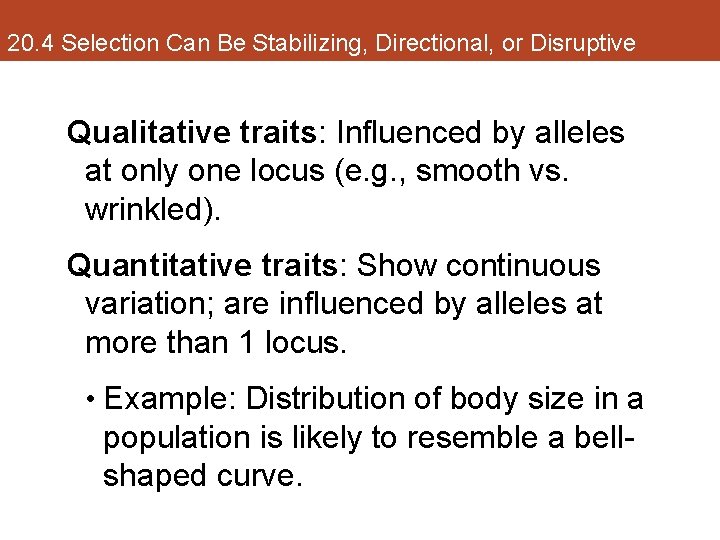
20. 4 Selection Can Be Stabilizing, Directional, or Disruptive Qualitative traits: Influenced by alleles at only one locus (e. g. , smooth vs. wrinkled). Quantitative traits: Show continuous variation; are influenced by alleles at more than 1 locus. • Example: Distribution of body size in a population is likely to resemble a bellshaped curve.
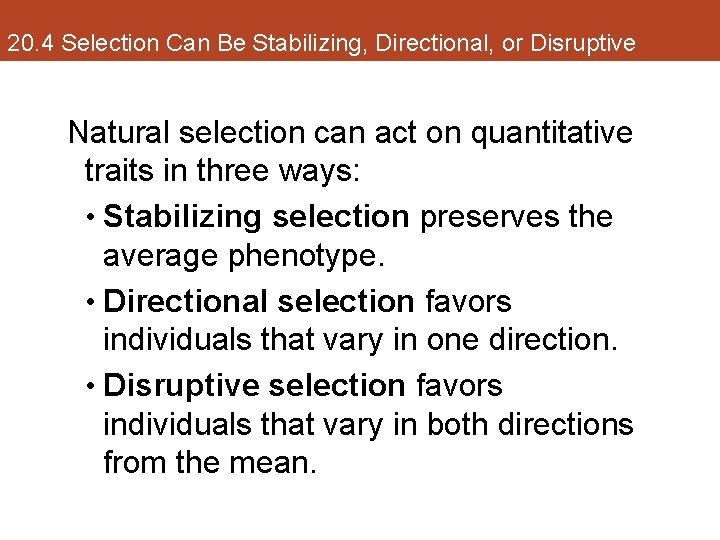
20. 4 Selection Can Be Stabilizing, Directional, or Disruptive Natural selection can act on quantitative traits in three ways: • Stabilizing selection preserves the average phenotype. • Directional selection favors individuals that vary in one direction. • Disruptive selection favors individuals that vary in both directions from the mean.
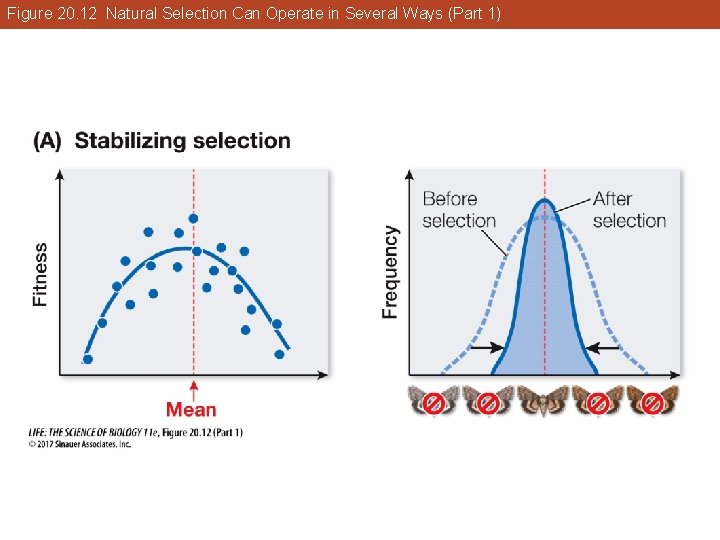
Figure 20. 12 Natural Selection Can Operate in Several Ways (Part 1)
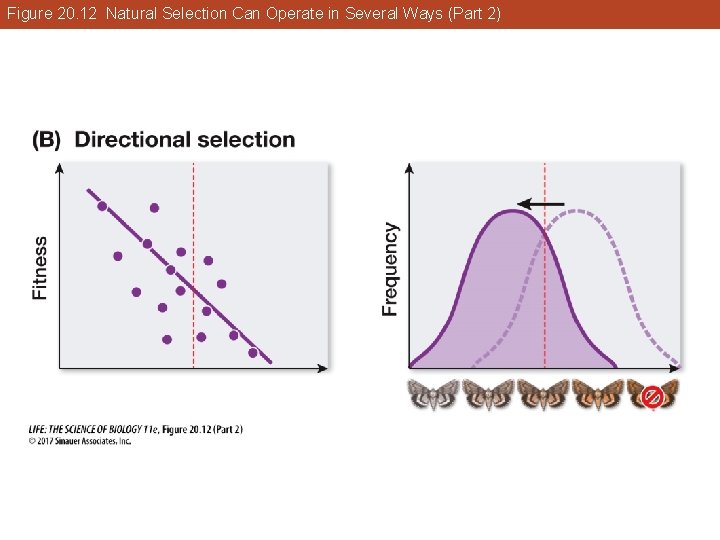
Figure 20. 12 Natural Selection Can Operate in Several Ways (Part 2)
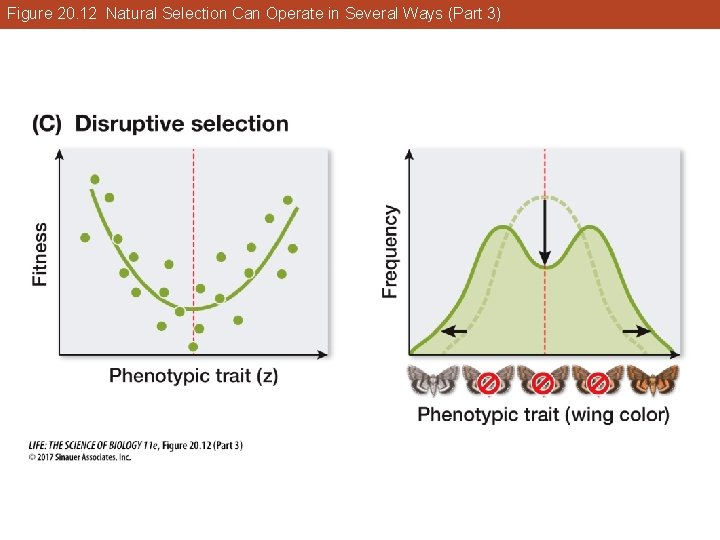
Figure 20. 12 Natural Selection Can Operate in Several Ways (Part 3)
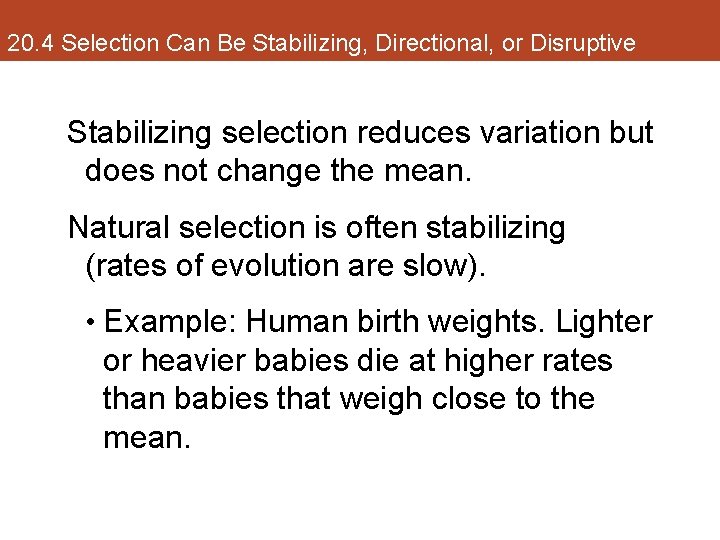
20. 4 Selection Can Be Stabilizing, Directional, or Disruptive Stabilizing selection reduces variation but does not change the mean. Natural selection is often stabilizing (rates of evolution are slow). • Example: Human birth weights. Lighter or heavier babies die at higher rates than babies that weigh close to the mean.
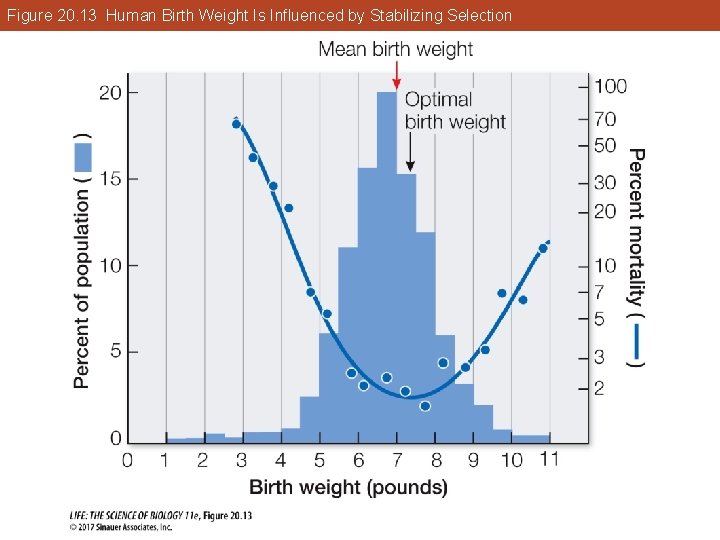
Figure 20. 13 Human Birth Weight Is Influenced by Stabilizing Selection
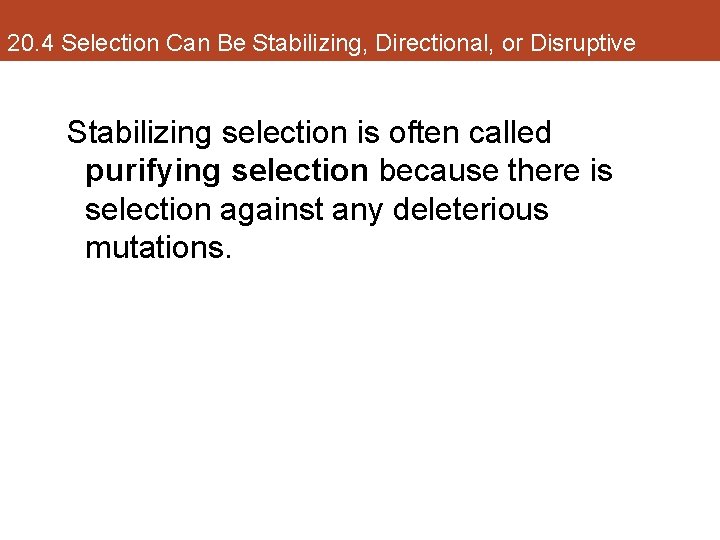
20. 4 Selection Can Be Stabilizing, Directional, or Disruptive Stabilizing selection is often called purifying selection because there is selection against any deleterious mutations.
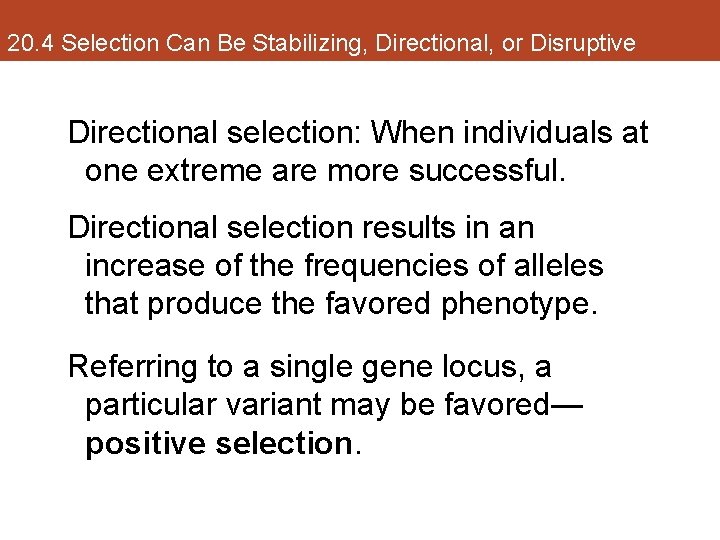
20. 4 Selection Can Be Stabilizing, Directional, or Disruptive Directional selection: When individuals at one extreme are more successful. Directional selection results in an increase of the frequencies of alleles that produce the favored phenotype. Referring to a single gene locus, a particular variant may be favored— positive selection.
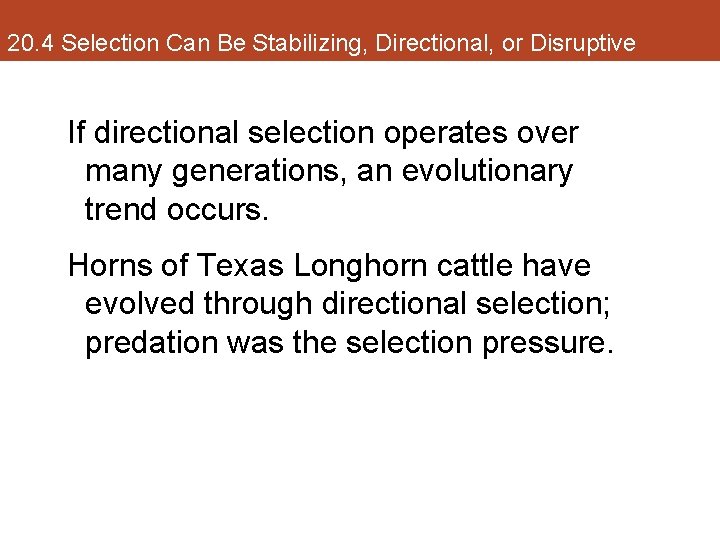
20. 4 Selection Can Be Stabilizing, Directional, or Disruptive If directional selection operates over many generations, an evolutionary trend occurs. Horns of Texas Longhorn cattle have evolved through directional selection; predation was the selection pressure.
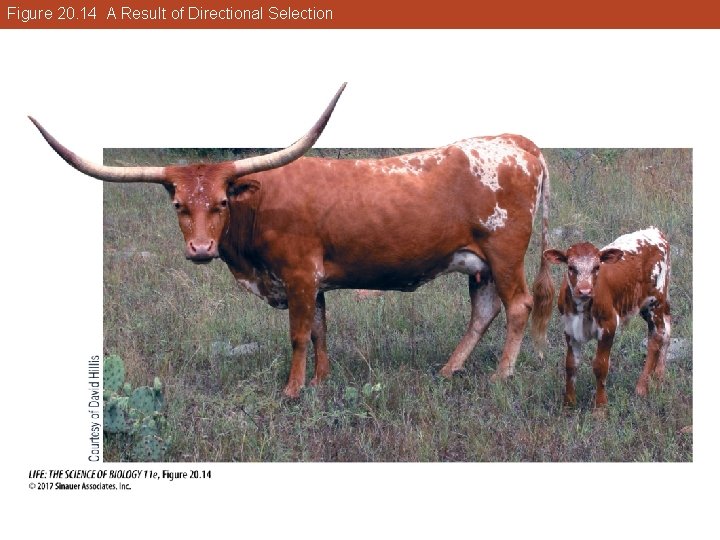
Figure 20. 14 A Result of Directional Selection
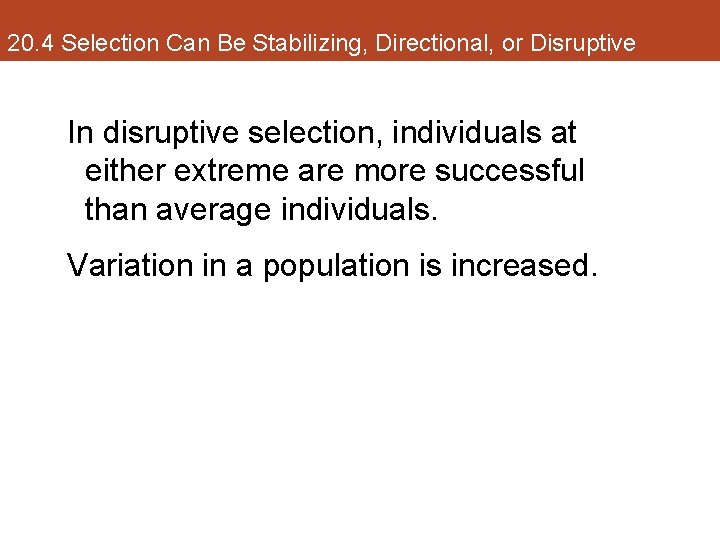
20. 4 Selection Can Be Stabilizing, Directional, or Disruptive In disruptive selection, individuals at either extreme are more successful than average individuals. Variation in a population is increased.
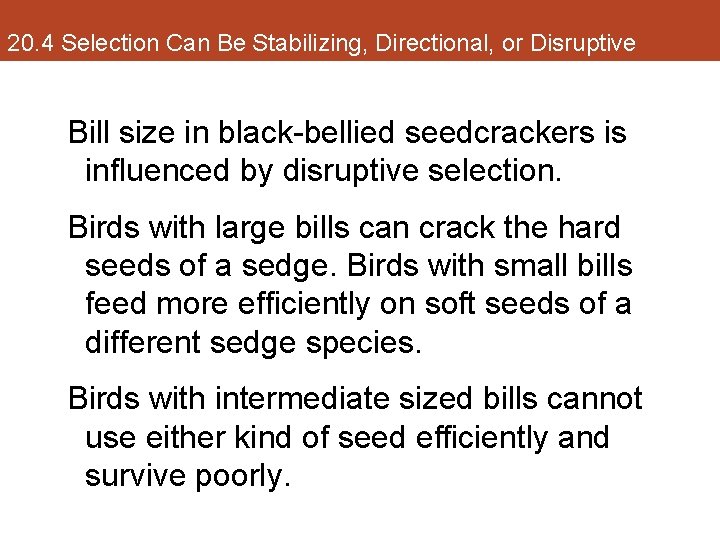
20. 4 Selection Can Be Stabilizing, Directional, or Disruptive Bill size in black-bellied seedcrackers is influenced by disruptive selection. Birds with large bills can crack the hard seeds of a sedge. Birds with small bills feed more efficiently on soft seeds of a different sedge species. Birds with intermediate sized bills cannot use either kind of seed efficiently and survive poorly.
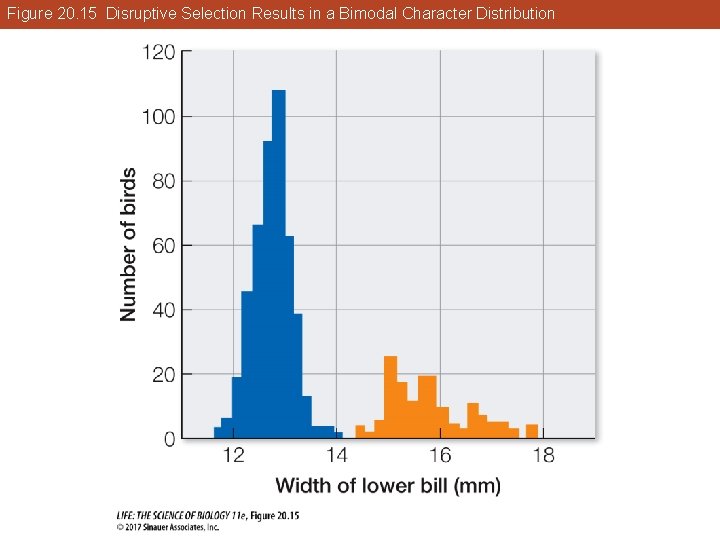
Figure 20. 15 Disruptive Selection Results in a Bimodal Character Distribution
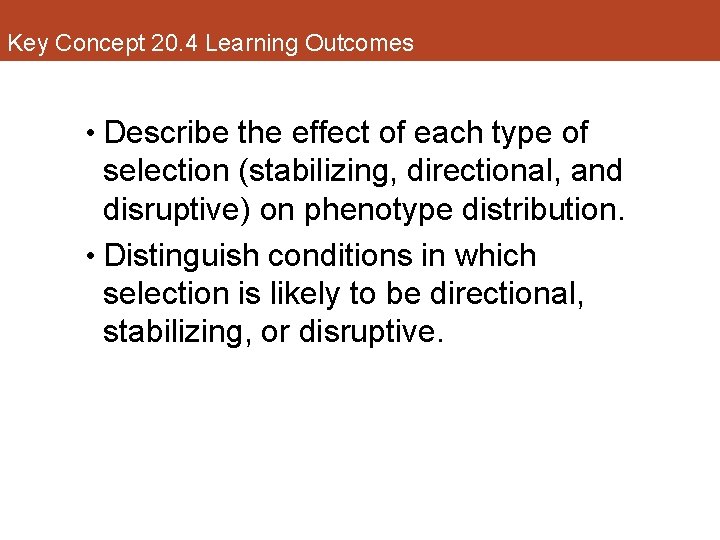
Key Concept 20. 4 Learning Outcomes • Describe the effect of each type of selection (stabilizing, directional, and disruptive) on phenotype distribution. • Distinguish conditions in which selection is likely to be directional, stabilizing, or disruptive.
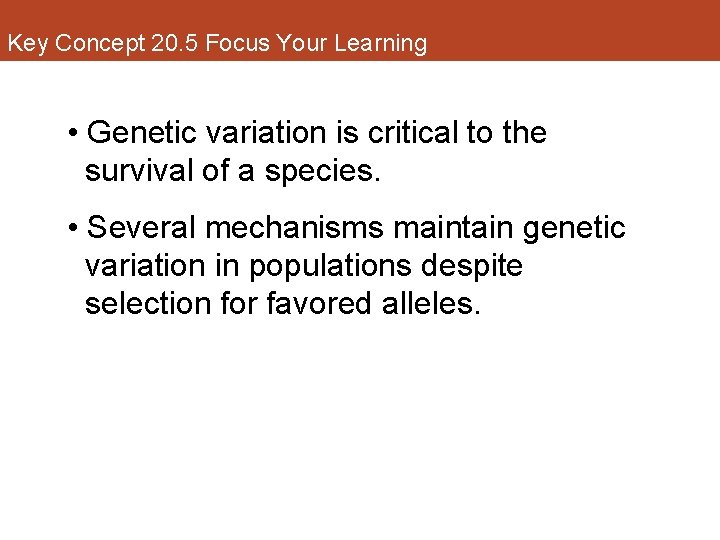
Key Concept 20. 5 Focus Your Learning • Genetic variation is critical to the survival of a species. • Several mechanisms maintain genetic variation in populations despite selection for favored alleles.
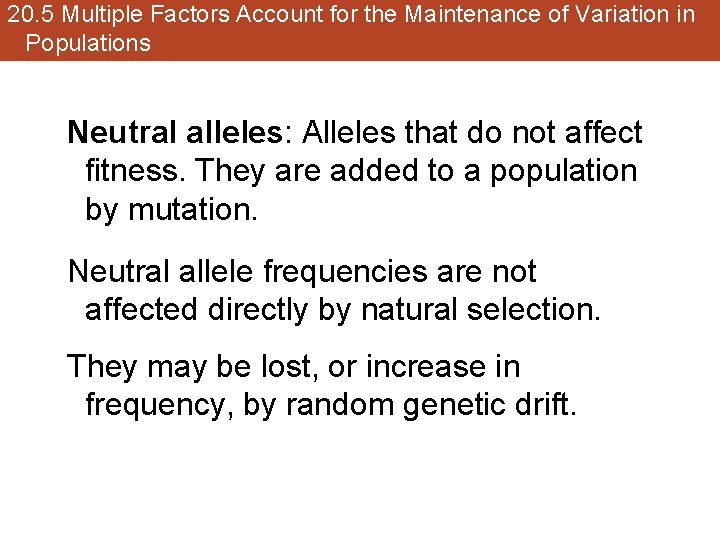
20. 5 Multiple Factors Account for the Maintenance of Variation in Populations Neutral alleles: Alleles that do not affect fitness. They are added to a population by mutation. Neutral allele frequencies are not affected directly by natural selection. They may be lost, or increase in frequency, by random genetic drift.
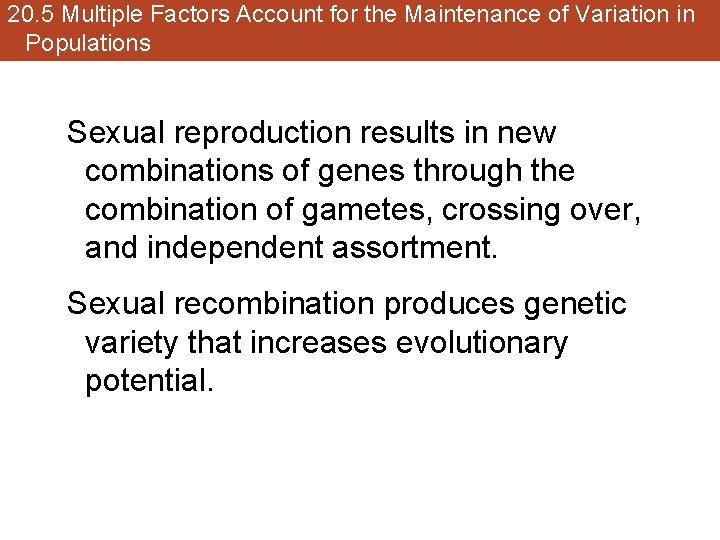
20. 5 Multiple Factors Account for the Maintenance of Variation in Populations Sexual reproduction results in new combinations of genes through the combination of gametes, crossing over, and independent assortment. Sexual recombination produces genetic variety that increases evolutionary potential.
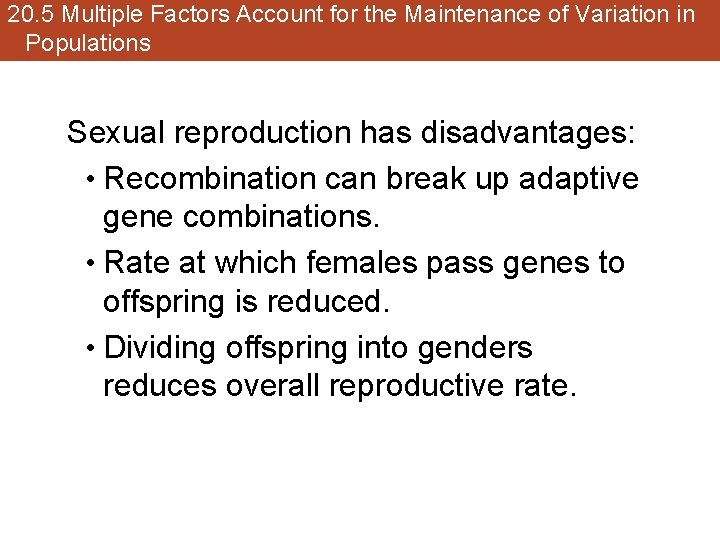
20. 5 Multiple Factors Account for the Maintenance of Variation in Populations Sexual reproduction has disadvantages: • Recombination can break up adaptive gene combinations. • Rate at which females pass genes to offspring is reduced. • Dividing offspring into genders reduces overall reproductive rate.
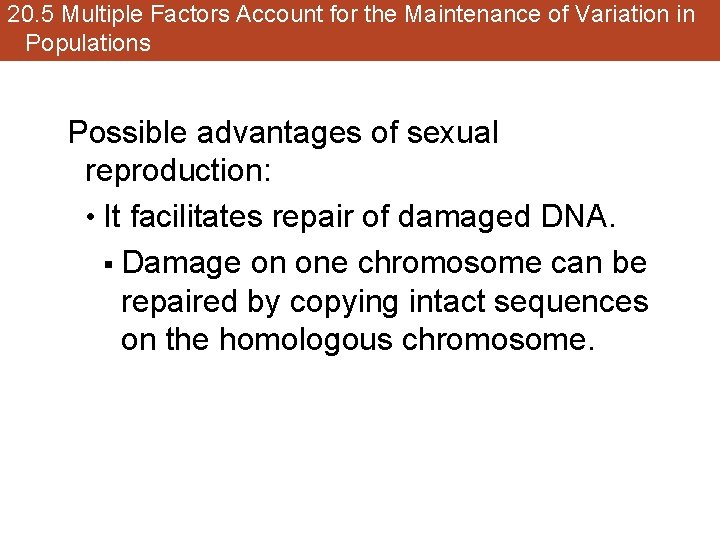
20. 5 Multiple Factors Account for the Maintenance of Variation in Populations Possible advantages of sexual reproduction: • It facilitates repair of damaged DNA. § Damage on one chromosome can be repaired by copying intact sequences on the homologous chromosome.
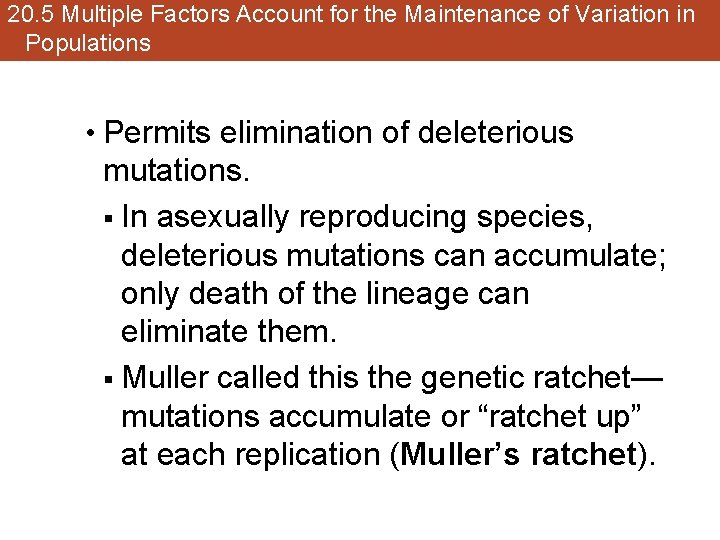
20. 5 Multiple Factors Account for the Maintenance of Variation in Populations • Permits elimination of deleterious mutations. § In asexually reproducing species, deleterious mutations can accumulate; only death of the lineage can eliminate them. § Muller called this the genetic ratchet— mutations accumulate or “ratchet up” at each replication (Muller’s ratchet).
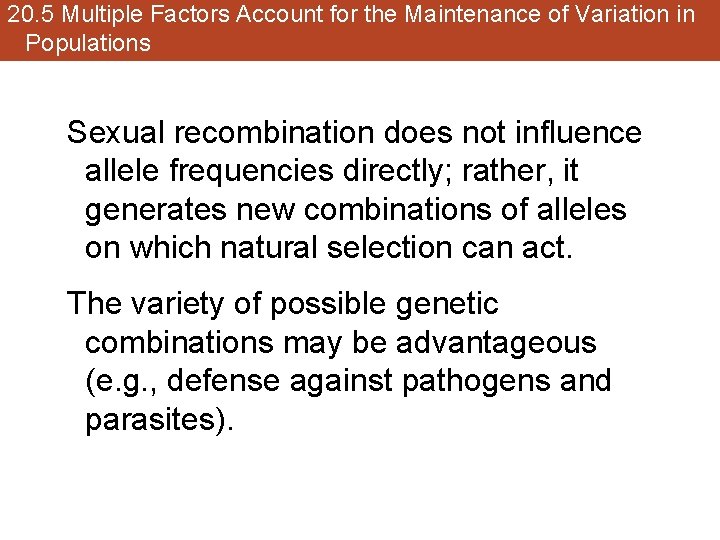
20. 5 Multiple Factors Account for the Maintenance of Variation in Populations Sexual recombination does not influence allele frequencies directly; rather, it generates new combinations of alleles on which natural selection can act. The variety of possible genetic combinations may be advantageous (e. g. , defense against pathogens and parasites).
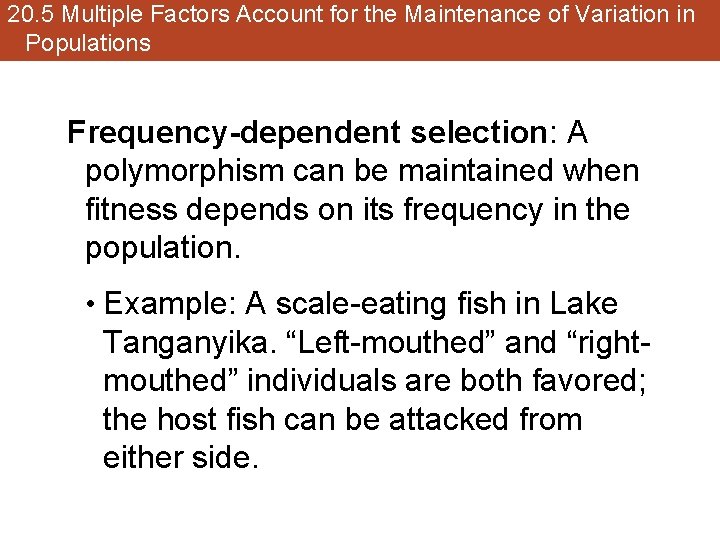
20. 5 Multiple Factors Account for the Maintenance of Variation in Populations Frequency-dependent selection: A polymorphism can be maintained when fitness depends on its frequency in the population. • Example: A scale-eating fish in Lake Tanganyika. “Left-mouthed” and “rightmouthed” individuals are both favored; the host fish can be attacked from either side.
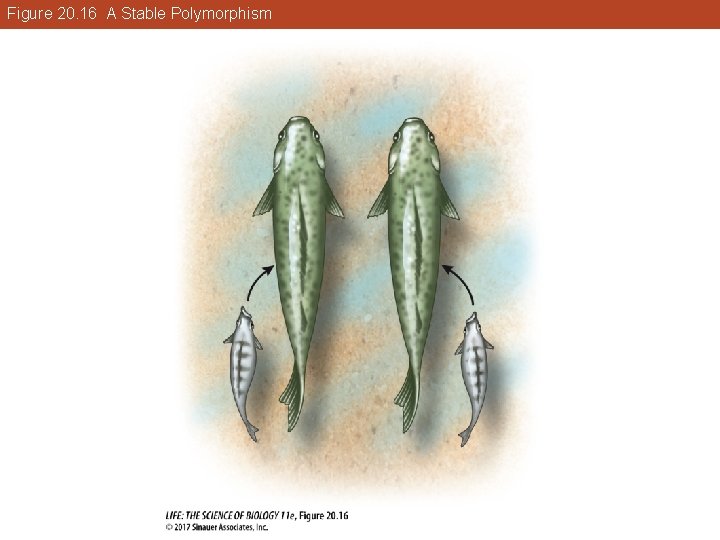
Figure 20. 16 A Stable Polymorphism
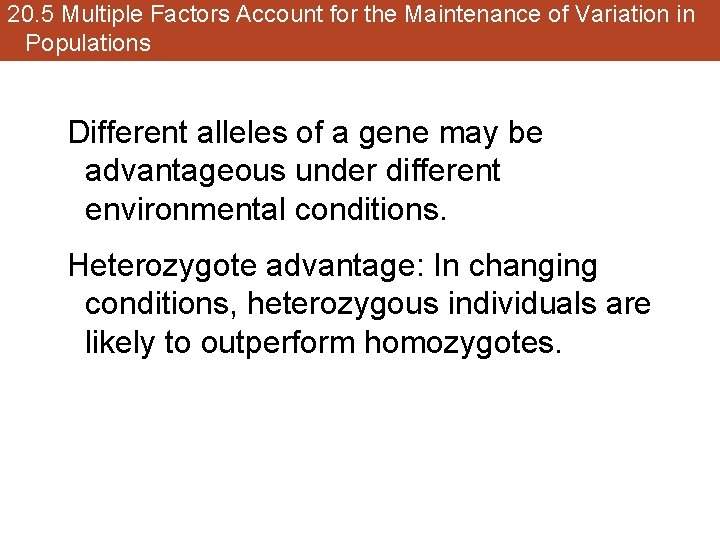
20. 5 Multiple Factors Account for the Maintenance of Variation in Populations Different alleles of a gene may be advantageous under different environmental conditions. Heterozygote advantage: In changing conditions, heterozygous individuals are likely to outperform homozygotes.
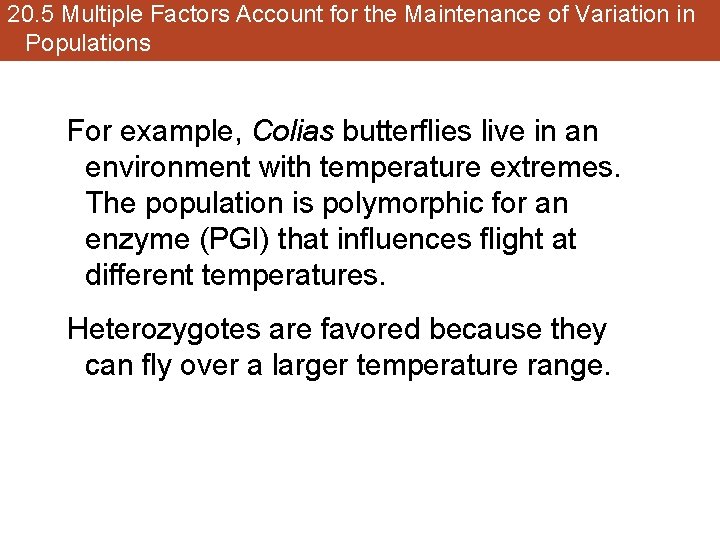
20. 5 Multiple Factors Account for the Maintenance of Variation in Populations For example, Colias butterflies live in an environment with temperature extremes. The population is polymorphic for an enzyme (PGI) that influences flight at different temperatures. Heterozygotes are favored because they can fly over a larger temperature range.
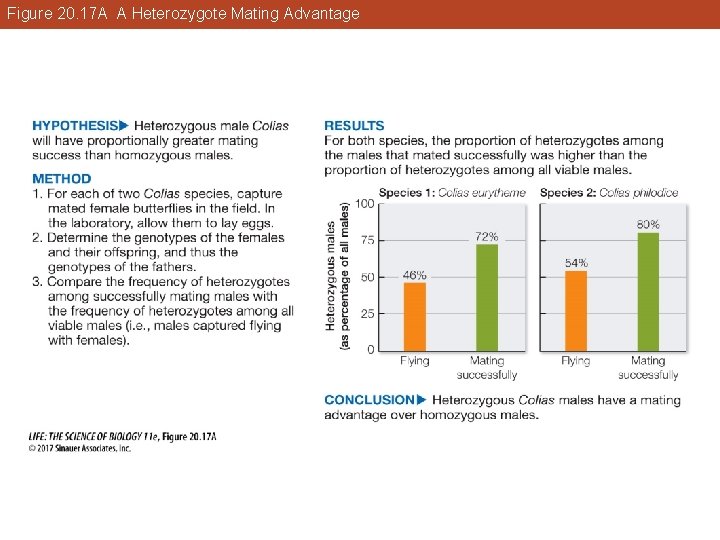
Figure 20. 17 A A Heterozygote Mating Advantage
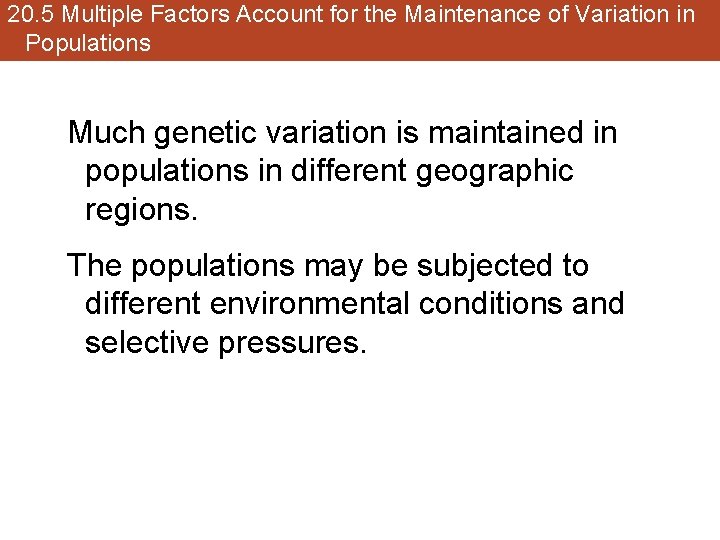
20. 5 Multiple Factors Account for the Maintenance of Variation in Populations Much genetic variation is maintained in populations in different geographic regions. The populations may be subjected to different environmental conditions and selective pressures.
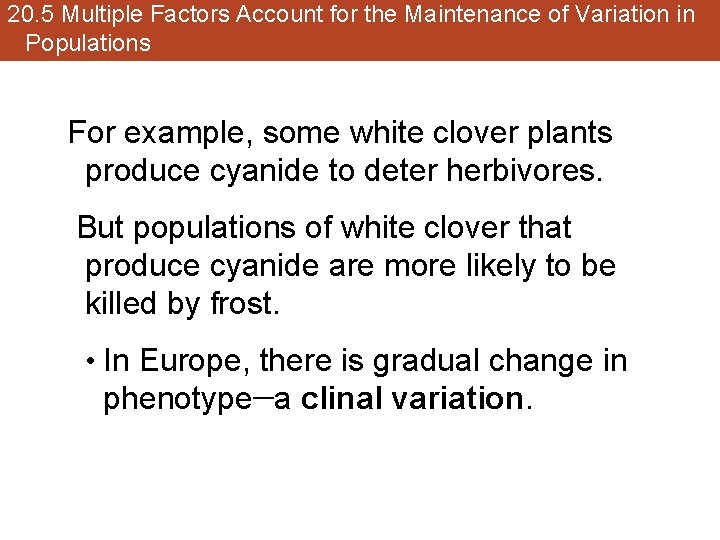
20. 5 Multiple Factors Account for the Maintenance of Variation in Populations For example, some white clover plants produce cyanide to deter herbivores. But populations of white clover that produce cyanide are more likely to be killed by frost. • In Europe, there is gradual change in phenotype—a clinal variation.
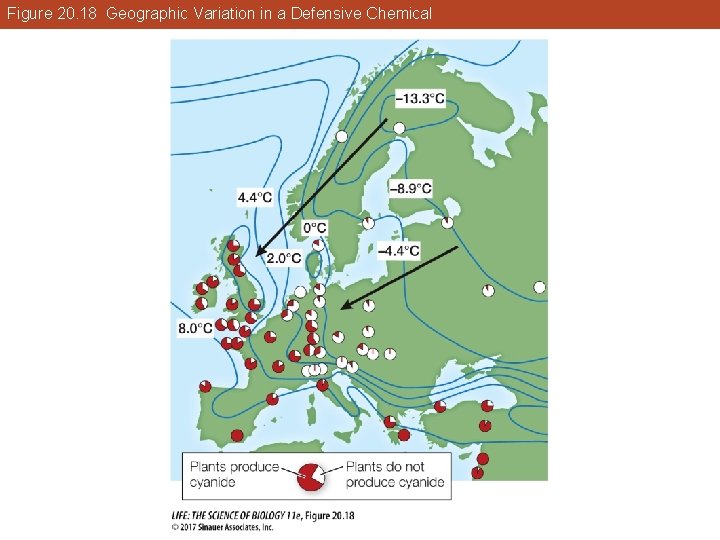
Figure 20. 18 Geographic Variation in a Defensive Chemical
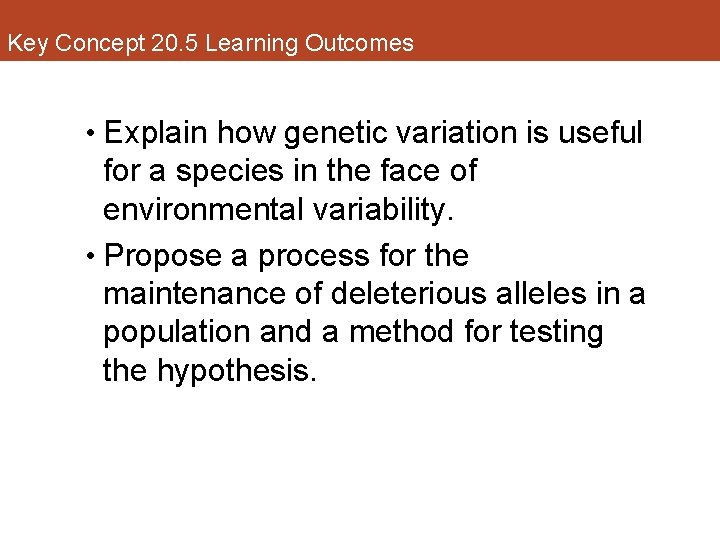
Key Concept 20. 5 Learning Outcomes • Explain how genetic variation is useful for a species in the face of environmental variability. • Propose a process for the maintenance of deleterious alleles in a population and a method for testing the hypothesis.
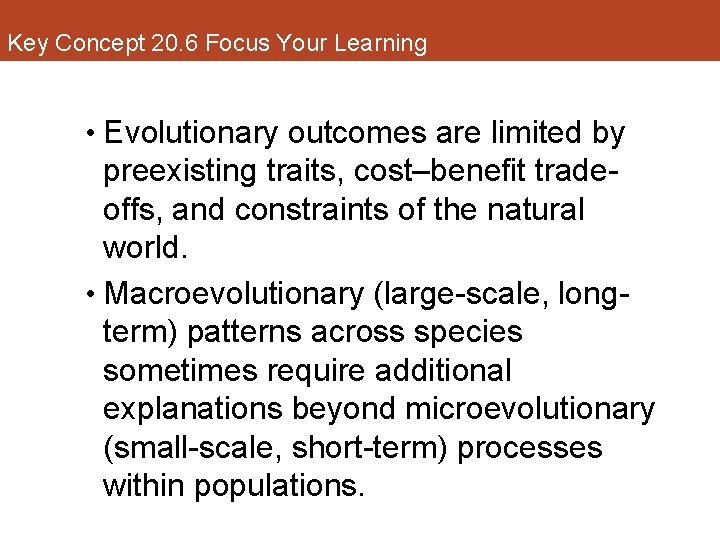
Key Concept 20. 6 Focus Your Learning • Evolutionary outcomes are limited by preexisting traits, cost–benefit tradeoffs, and constraints of the natural world. • Macroevolutionary (large-scale, longterm) patterns across species sometimes require additional explanations beyond microevolutionary (small-scale, short-term) processes within populations.
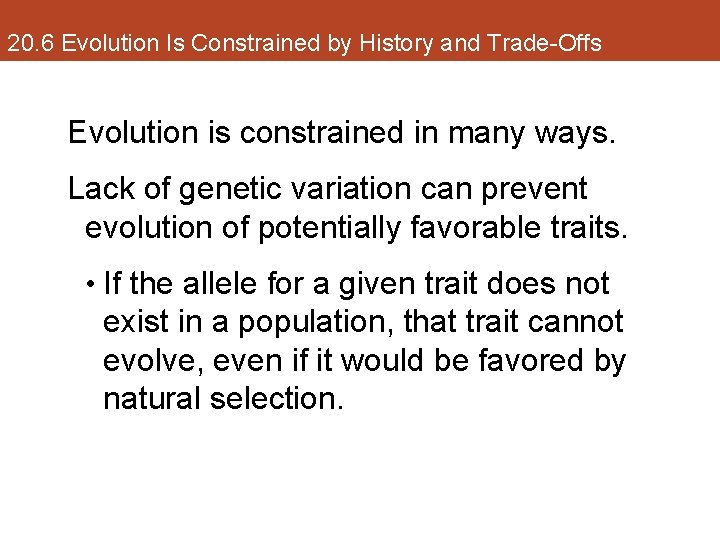
20. 6 Evolution Is Constrained by History and Trade-Offs Evolution is constrained in many ways. Lack of genetic variation can prevent evolution of potentially favorable traits. • If the allele for a given trait does not exist in a population, that trait cannot evolve, even if it would be favored by natural selection.
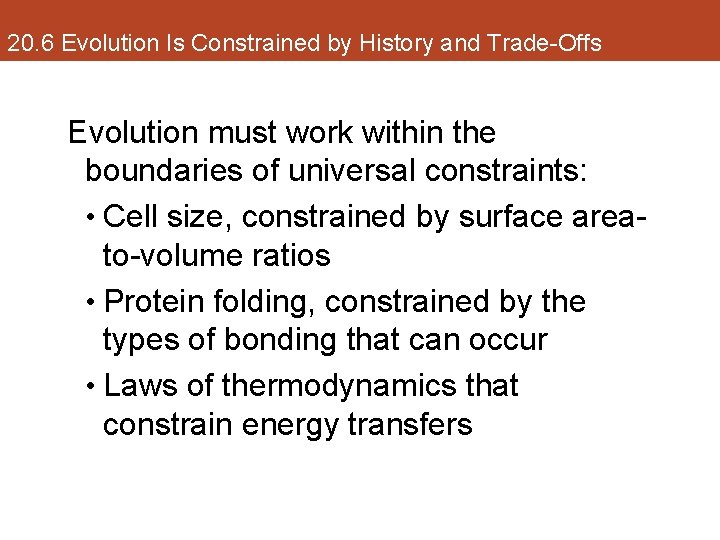
20. 6 Evolution Is Constrained by History and Trade-Offs Evolution must work within the boundaries of universal constraints: • Cell size, constrained by surface areato-volume ratios • Protein folding, constrained by the types of bonding that can occur • Laws of thermodynamics that constrain energy transfers
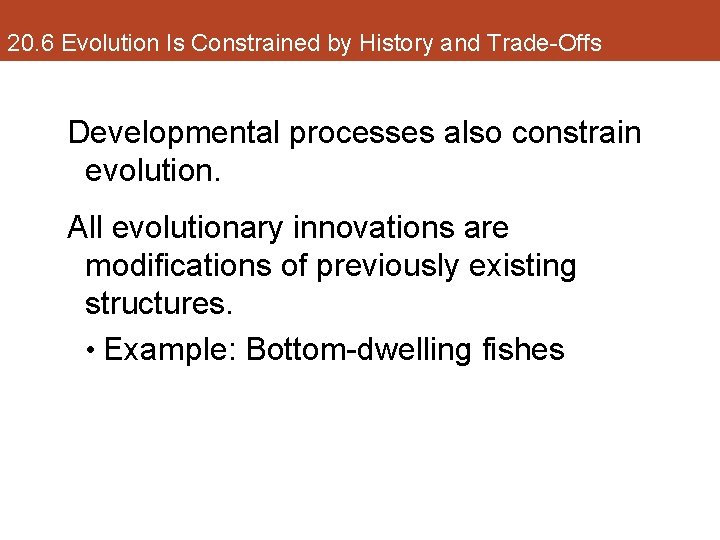
20. 6 Evolution Is Constrained by History and Trade-Offs Developmental processes also constrain evolution. All evolutionary innovations are modifications of previously existing structures. • Example: Bottom-dwelling fishes
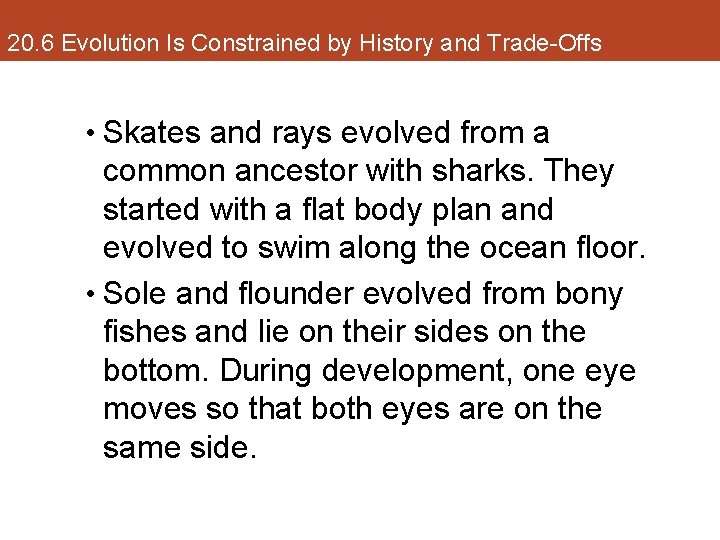
20. 6 Evolution Is Constrained by History and Trade-Offs • Skates and rays evolved from a common ancestor with sharks. They started with a flat body plan and evolved to swim along the ocean floor. • Sole and flounder evolved from bony fishes and lie on their sides on the bottom. During development, one eye moves so that both eyes are on the same side.
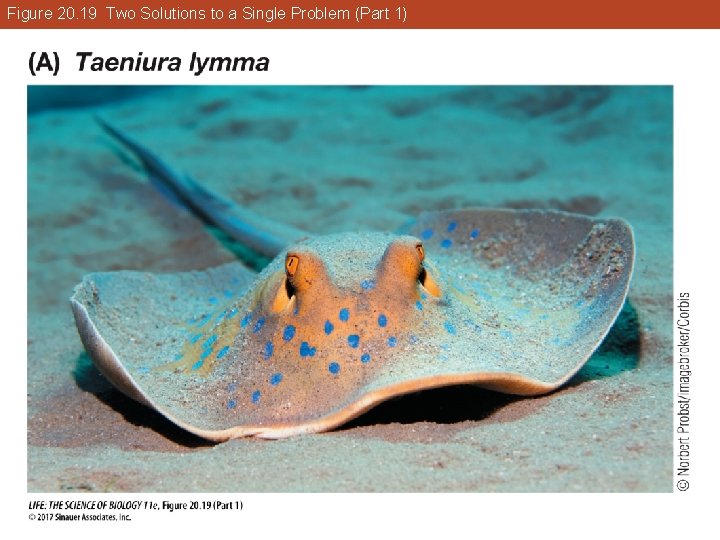
Figure 20. 19 Two Solutions to a Single Problem (Part 1)
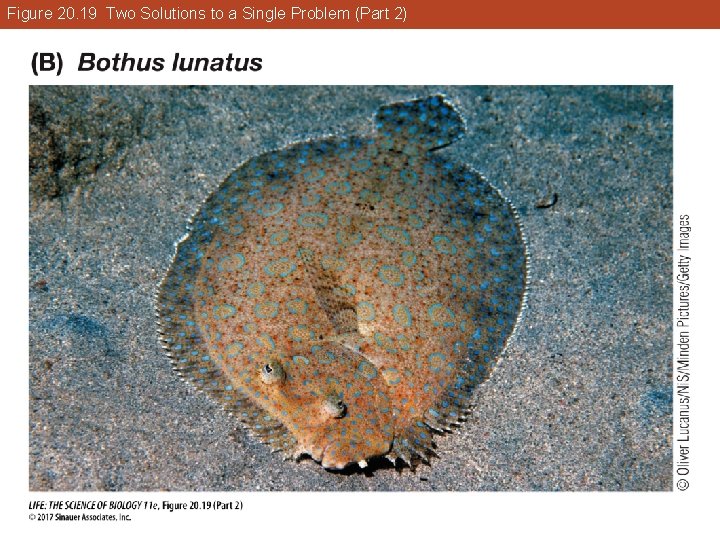
Figure 20. 19 Two Solutions to a Single Problem (Part 2)
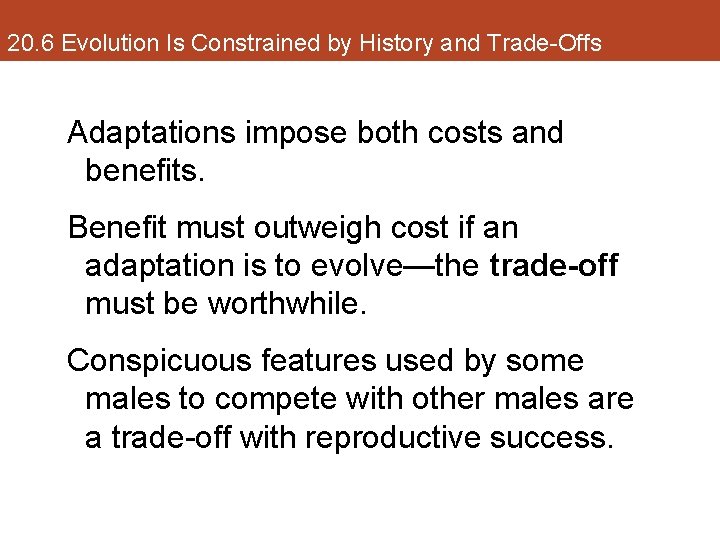
20. 6 Evolution Is Constrained by History and Trade-Offs Adaptations impose both costs and benefits. Benefit must outweigh cost if an adaptation is to evolve—the trade-off must be worthwhile. Conspicuous features used by some males to compete with other males are a trade-off with reproductive success.
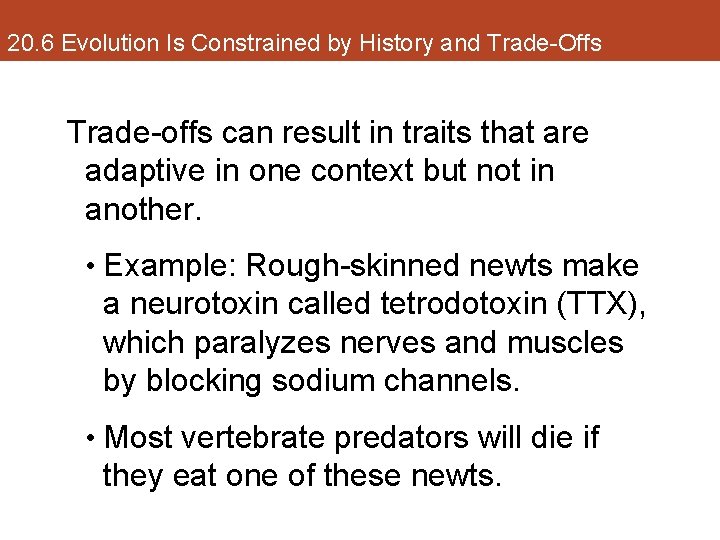
20. 6 Evolution Is Constrained by History and Trade-Offs Trade-offs can result in traits that are adaptive in one context but not in another. • Example: Rough-skinned newts make a neurotoxin called tetrodotoxin (TTX), which paralyzes nerves and muscles by blocking sodium channels. • Most vertebrate predators will die if they eat one of these newts.
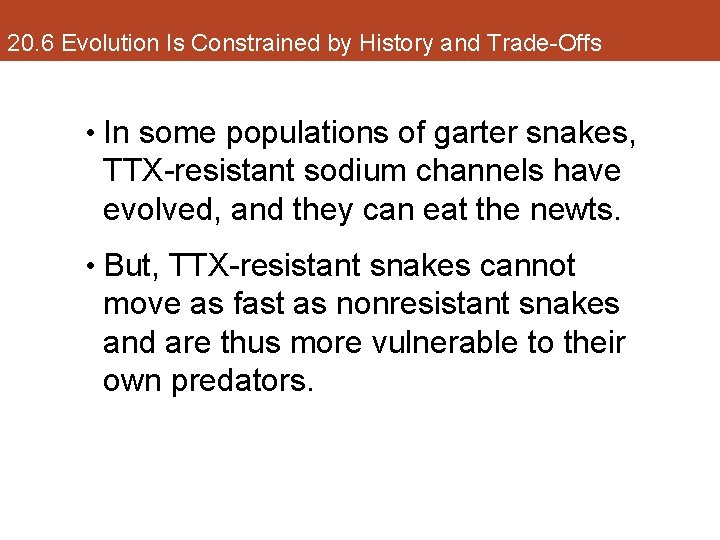
20. 6 Evolution Is Constrained by History and Trade-Offs • In some populations of garter snakes, TTX-resistant sodium channels have evolved, and they can eat the newts. • But, TTX-resistant snakes cannot move as fast as nonresistant snakes and are thus more vulnerable to their own predators.
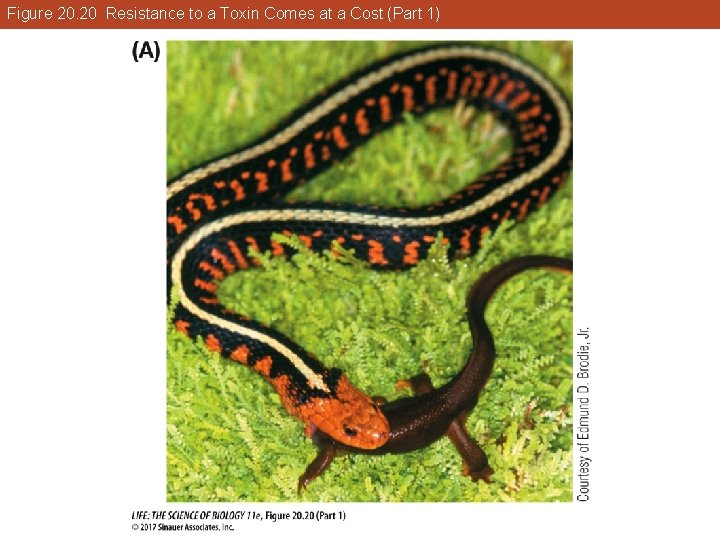
Figure 20. 20 Resistance to a Toxin Comes at a Cost (Part 1)
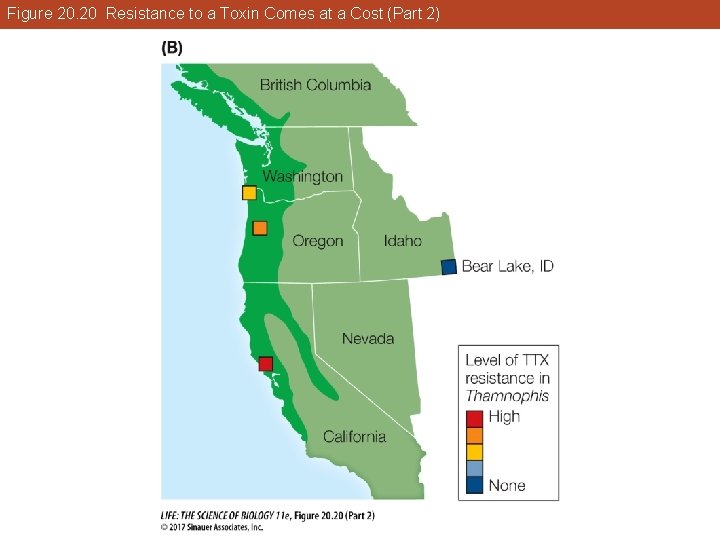
Figure 20. 20 Resistance to a Toxin Comes at a Cost (Part 2)
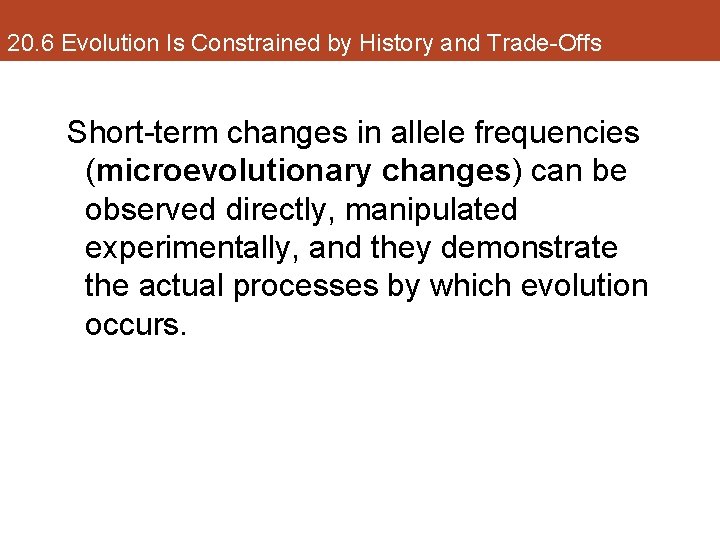
20. 6 Evolution Is Constrained by History and Trade-Offs Short-term changes in allele frequencies (microevolutionary changes) can be observed directly, manipulated experimentally, and they demonstrate the actual processes by which evolution occurs.
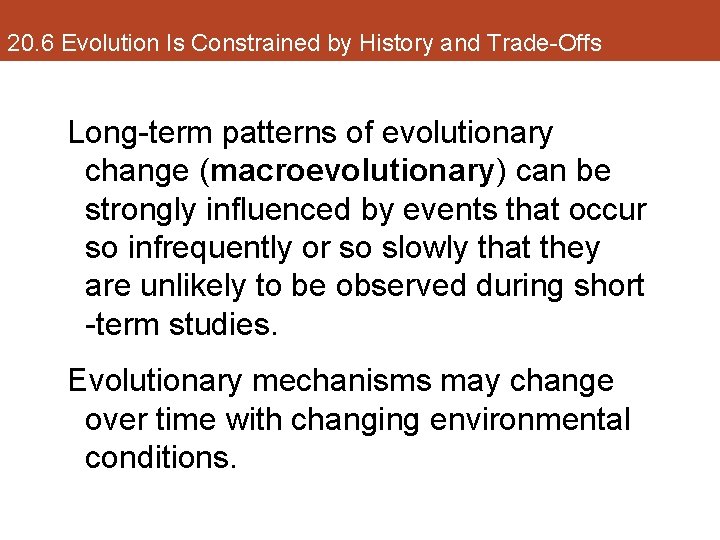
20. 6 Evolution Is Constrained by History and Trade-Offs Long-term patterns of evolutionary change (macroevolutionary) can be strongly influenced by events that occur so infrequently or so slowly that they are unlikely to be observed during short -term studies. Evolutionary mechanisms may change over time with changing environmental conditions.
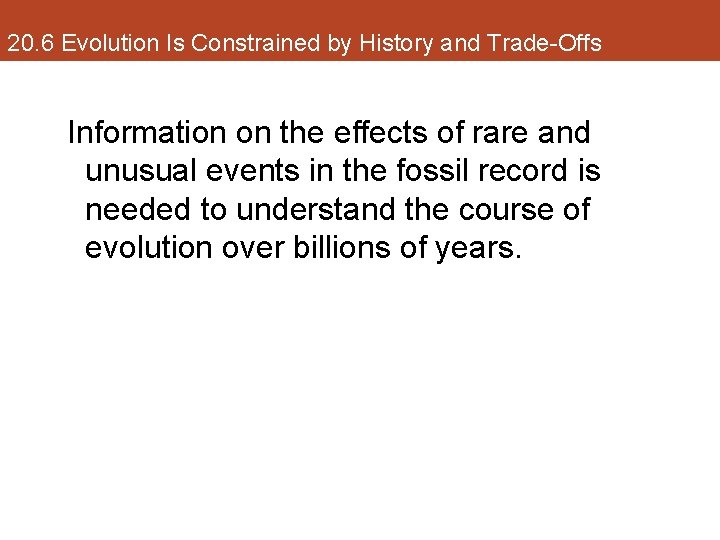
20. 6 Evolution Is Constrained by History and Trade-Offs Information on the effects of rare and unusual events in the fossil record is needed to understand the course of evolution over billions of years.
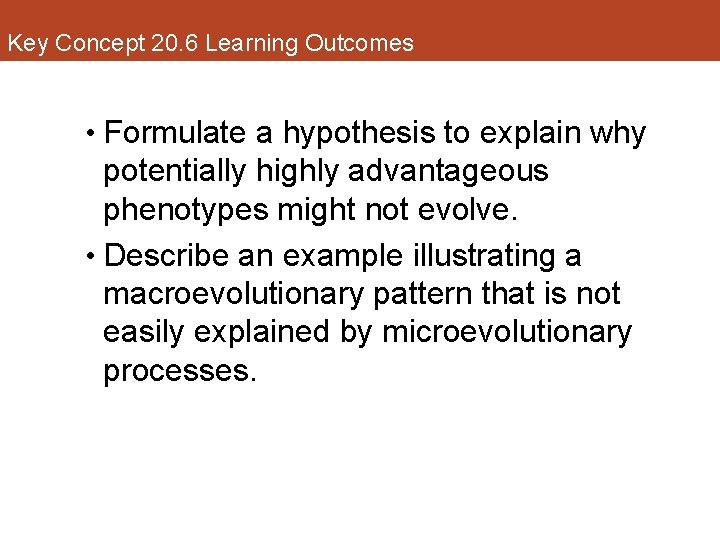
Key Concept 20. 6 Learning Outcomes • Formulate a hypothesis to explain why potentially highly advantageous phenotypes might not evolve. • Describe an example illustrating a macroevolutionary pattern that is not easily explained by microevolutionary processes.
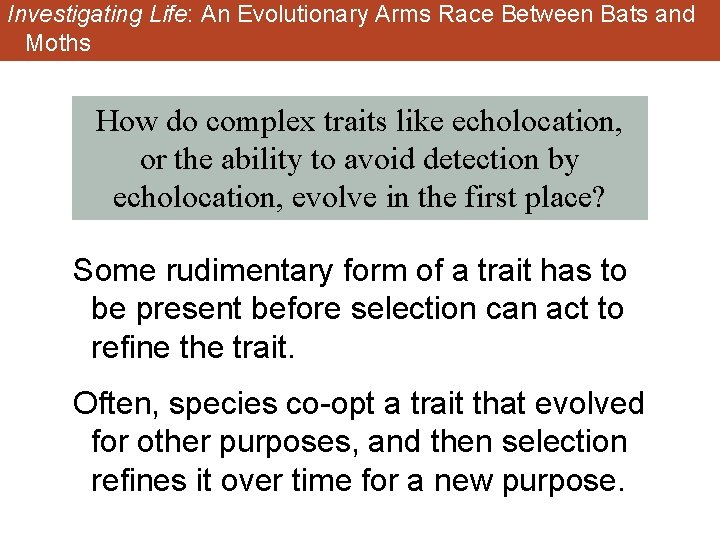
Investigating Life: An Evolutionary Arms Race Between Bats and Moths How do complex traits like echolocation, or the ability to avoid detection by echolocation, evolve in the first place? Some rudimentary form of a trait has to be present before selection can act to refine the trait. Often, species co-opt a trait that evolved for other purposes, and then selection refines it over time for a new purpose.
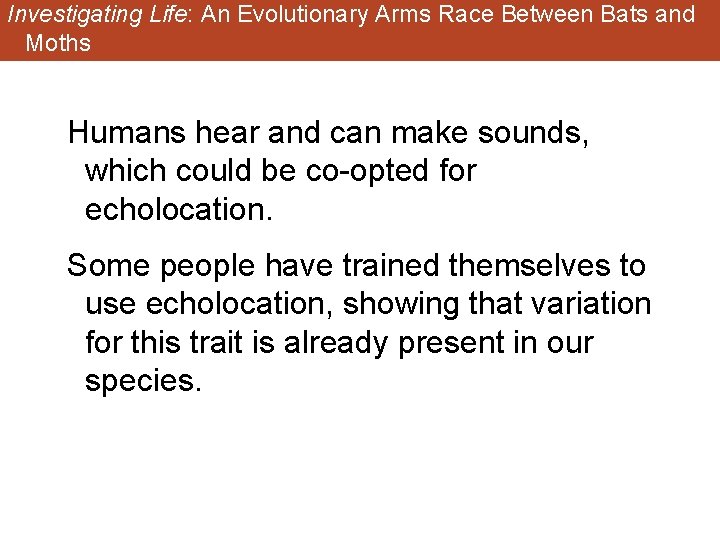
Investigating Life: An Evolutionary Arms Race Between Bats and Moths Humans hear and can make sounds, which could be co-opted for echolocation. Some people have trained themselves to use echolocation, showing that variation for this trait is already present in our species.
Course number and title
Chalmers course evaluation
Idea course evaluations
Strategy evaluation course
Half brick wall
Course interne moyenne externe
Chapter 9 admission transfer discharge and physical exams
Admission transfer discharge and physical exams
Poem on exams
Finland exams
Adit exams
Comprehensive exams
Prayer to st joseph of cupertino for students
Prayer before a big exam
Equipment used for physical examination
New york state teacher certification exams
Example of exam
Berkeley law exams
English official exams
Judy believes that her fate
Conditionals finish the sentences
Byod exams
What is strategic staffing
Phys 172
Peoplecert online proctoring
Cambridge exam invigilator
Exam questions on human resource management
Ku leuven ombuds
York uni vle
Exams actesting
Are the keystone exams mandatory
Vce physics past exams
Rcpch exams
Compassionate consideration
Indiana teacher license test
Derived grade exams
Yale placement tests
Decas exams
Paragraph about exams
Myp cards
Ib history of the americas study guide
How many rakats in each prayer
Washback definition
Www.awinfosys.com/eassessment/exams sample.htm
Aspec exams
Progress and performance measurement and evaluation
What is visitor pre registration in picme
Progress and performance measurement and evaluation
Simons and chabris evaluation
M&e cycle
Comparison between monitoring and evaluation
Project evaluation and control
Project evaluation and control
What are the principles of monitoring and evaluation
Monitoring and evaluation dashboard
Monitoring and evaluation of family planning programs
Concept map of measurement assessment and evaluation
Knowledge management monitoring and evaluation
Eti isef
Importance of planning, monitoring and evaluation
Hrd program implementation
Gulf of evaluation
Kcapanse
M & e plan template
Macro evaluation
Community health planning and implementation
Application synthesis evaluation
What is transformational advertising
User interface design and evaluation
Principles of monitoring and evaluation
Free will and determinism evaluation
Exploration and evaluation assets shall be classified as
Measurement and evaluation in human performance 5e download
What is hseep
Basics of monitoring and evaluation
Internal and external evaluation
Tindak lanjut bimbingan konseling
Fedex balanced scorecard
Chapter 6 implementation and evaluation
Country evaluation and selection
Project analysis and evaluation
Chapter 11 assessment and evaluation of sports injuries
Monitoring and evaluation challenges
Centre for drug evaluation and research
Centre for evaluation and monitoring
Counseling outcome research and evaluation
Cognitive walkthrough and heuristic evaluation
Placement, formative, diagnostic and summative assessment
How to measure standing vertical jump
Continuity assessment record and evaluation
Sensory evaluation of dairy products
Types of project monitoring
Monitoring and evaluation in advocacy
Model evaluation and selection
Loftus and palmer (1974)
Test evaluation master plan
What is continuous and comprehensive evaluation
Richard rumelt strategy evaluation
Evaluation paradigms
Project formulation and evaluation
Kim marshall rubric
Strategic measurement and evaluation
Budget monitoring and evaluation
Monitoring and evaluation image
Concept of measurement assessment and evaluation
Introduction to monitoring and evaluation
Evaluation test for face powder and compact
Strategy evaluation techniques
Difference between assessment and evaluation
Monitoring and evaluation tool sample deped
Bandura sample size
Research methods in monitoring and evaluation
Conclusion of continuous and comprehensive evaluation
Example of monitoring and evaluation in project proposal
Shaala siddhi mission statement example
Difference between monitoring and evaluation
Example of monitoring and evaluation in project proposal
Me framework
Siklus interaksi
What is formative assessment
School monitoring, evaluation and plan adjustment sample
Penilaian formatif
Forest and range evaluation program
Fatality assessment and control evaluation
Sip/aip based monitoring and evaluation instrument tool
Reading for understanding analysis and evaluation