Electronic Structure of Strongly Correlated Electron Materials A
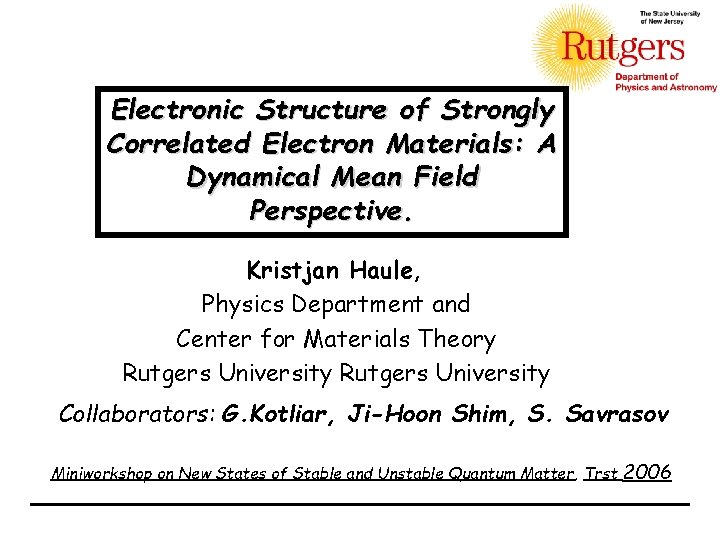
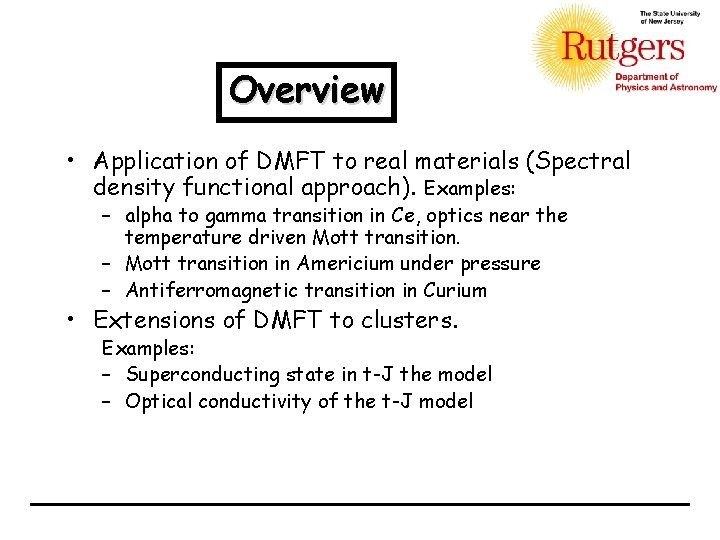
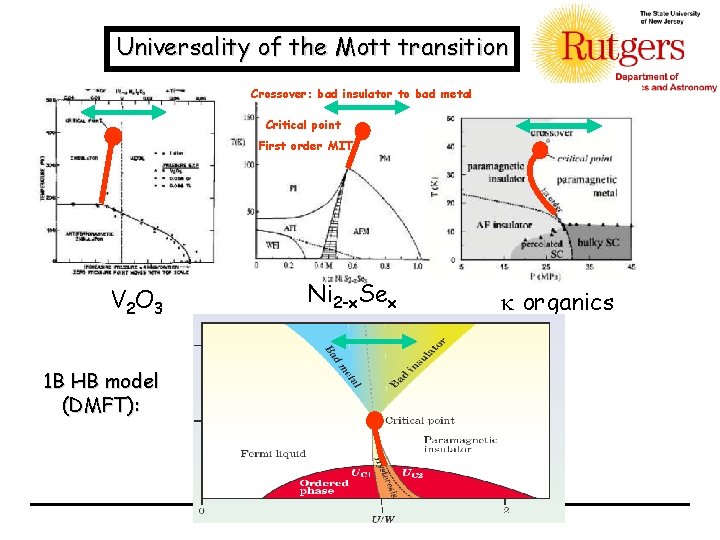
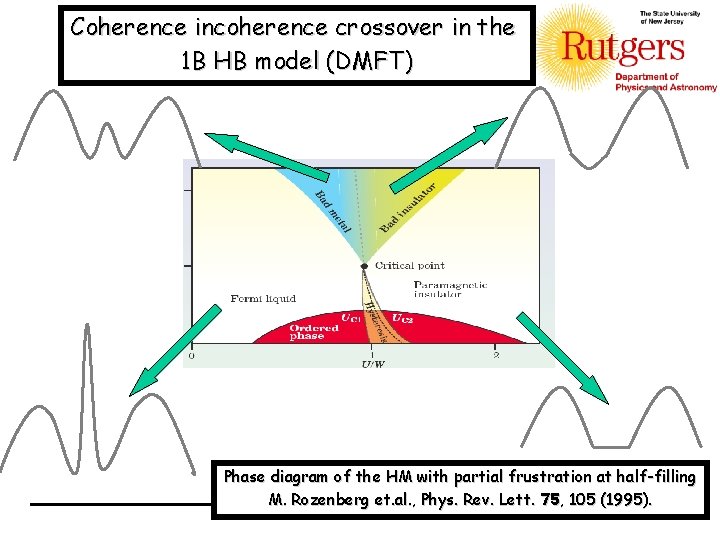
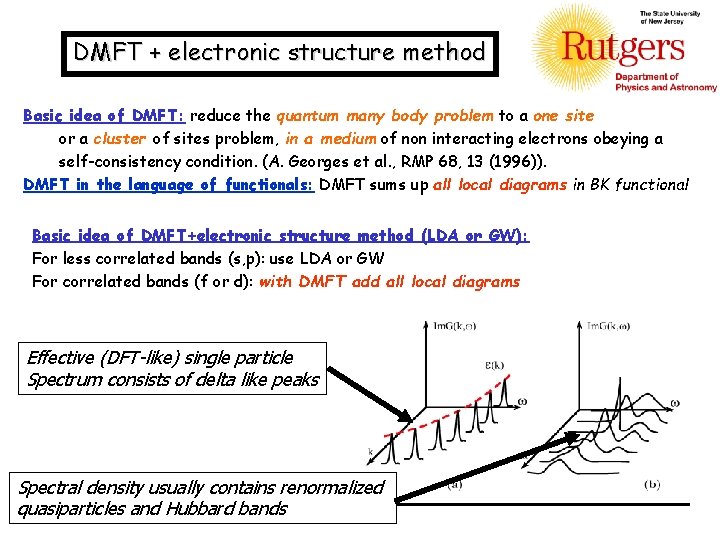
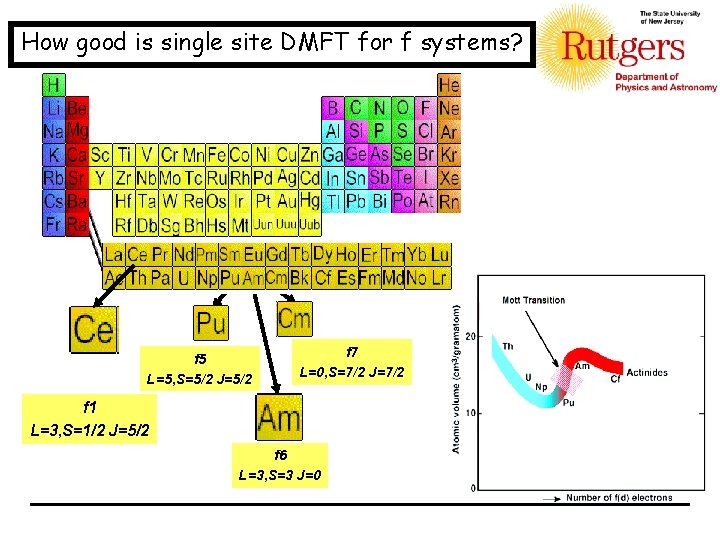
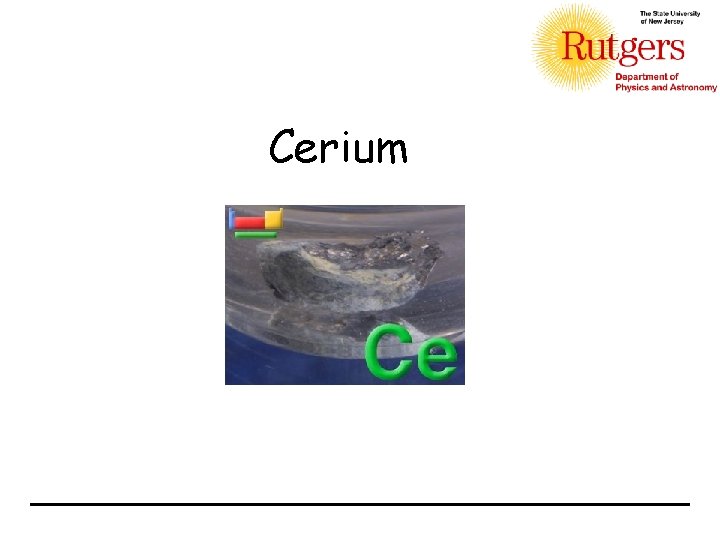
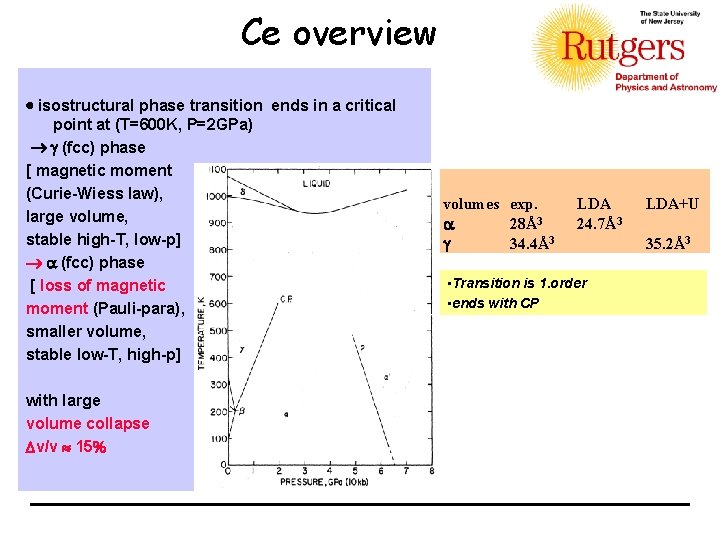
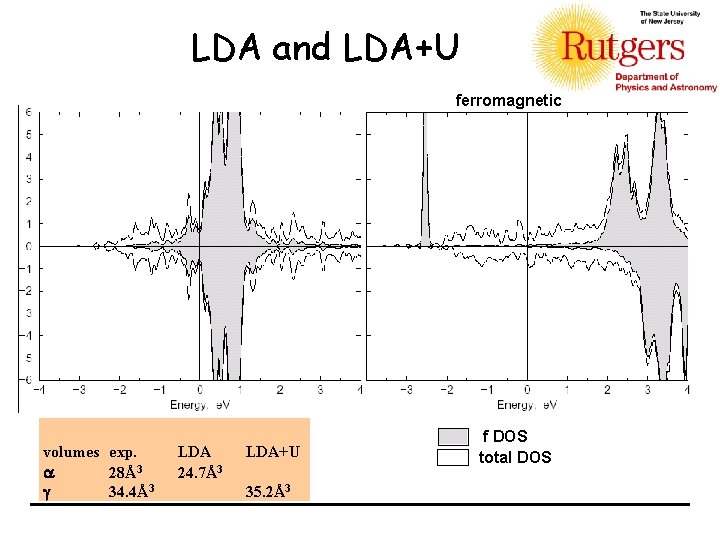
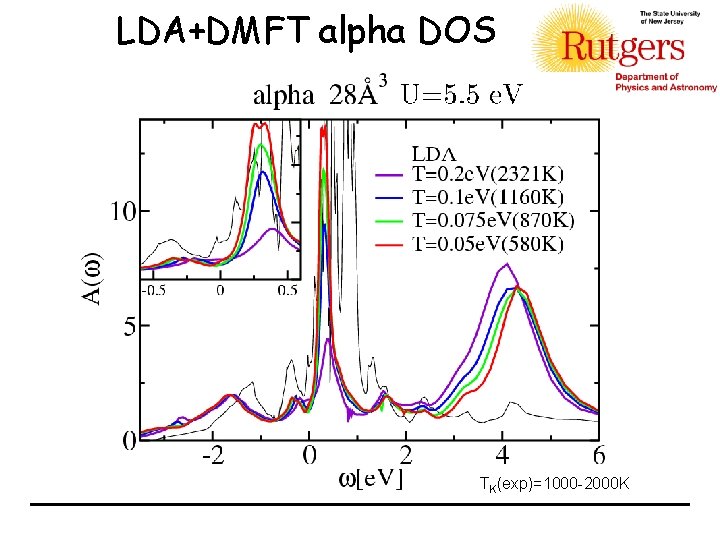
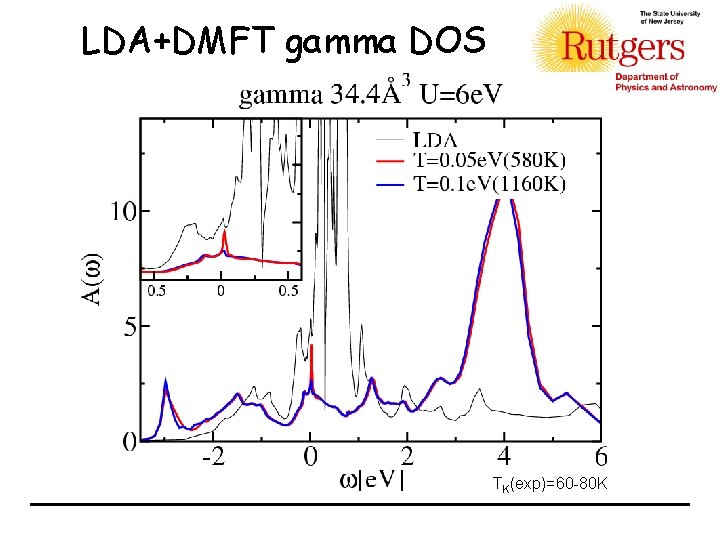
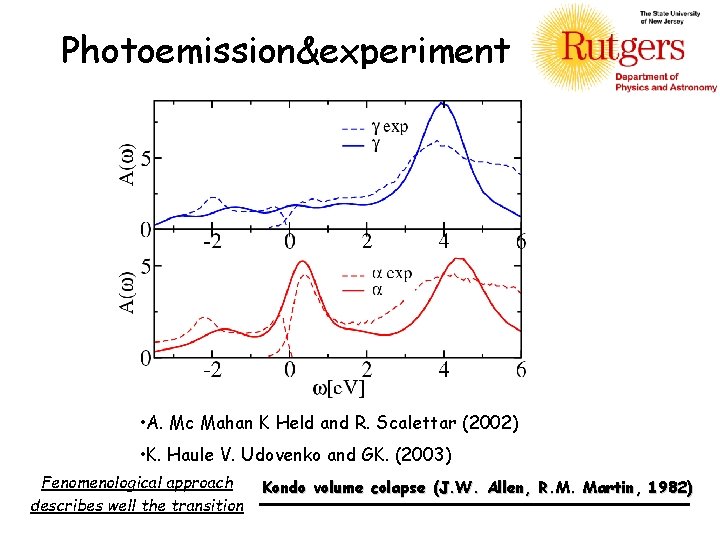
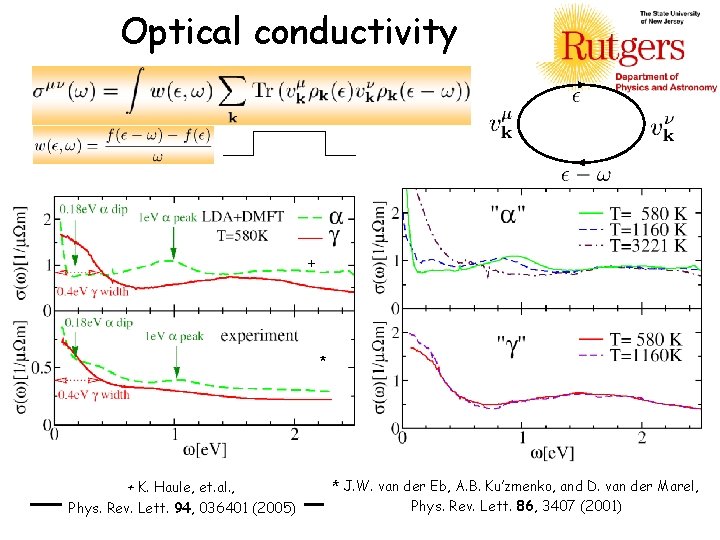
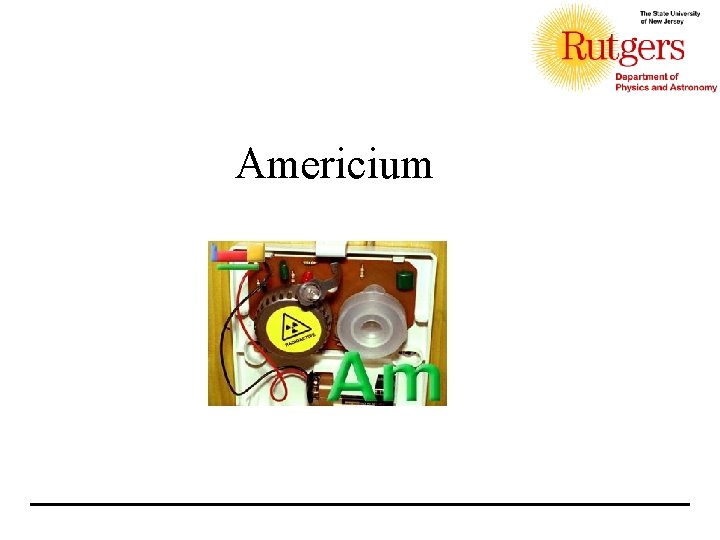
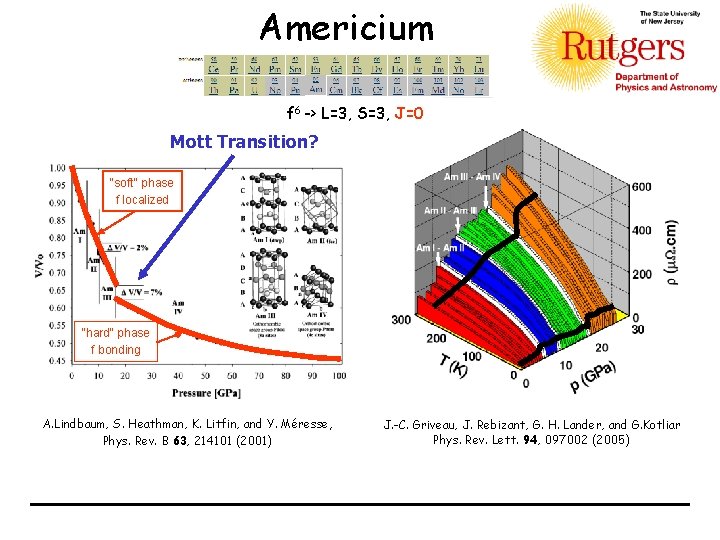
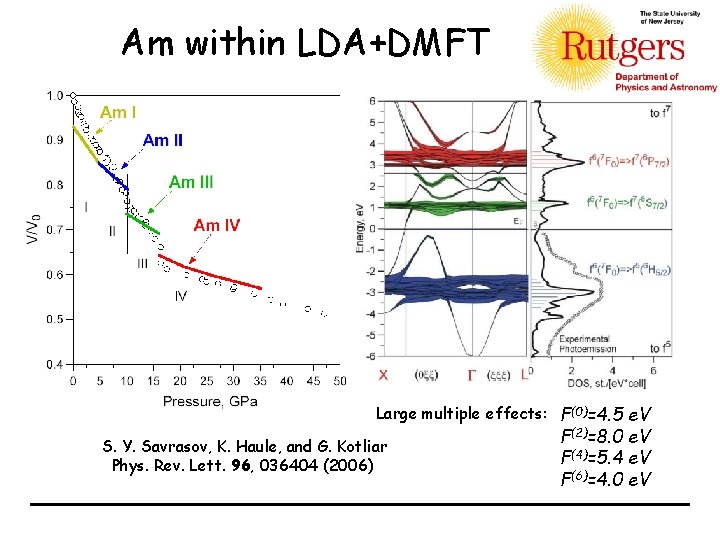
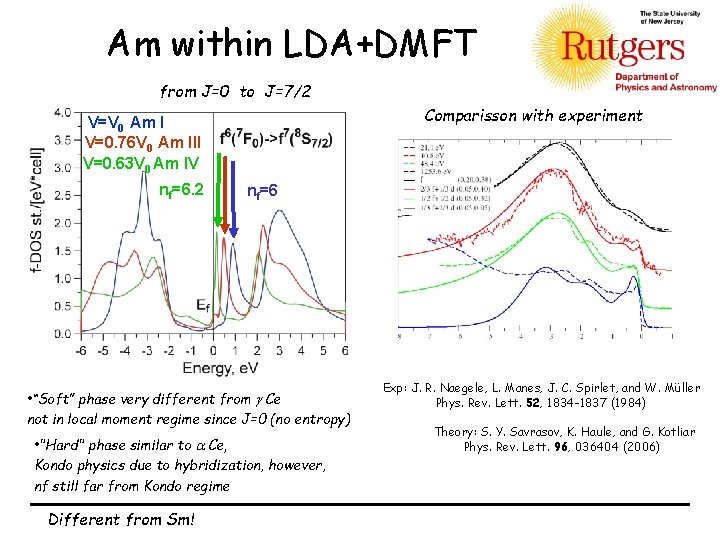
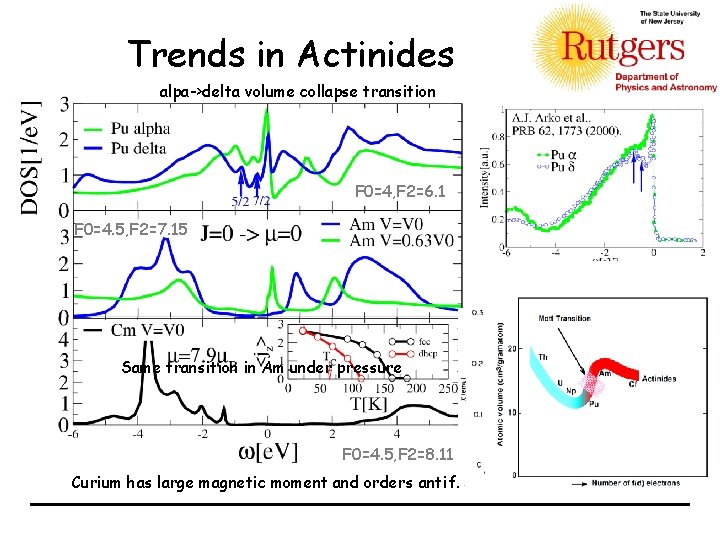
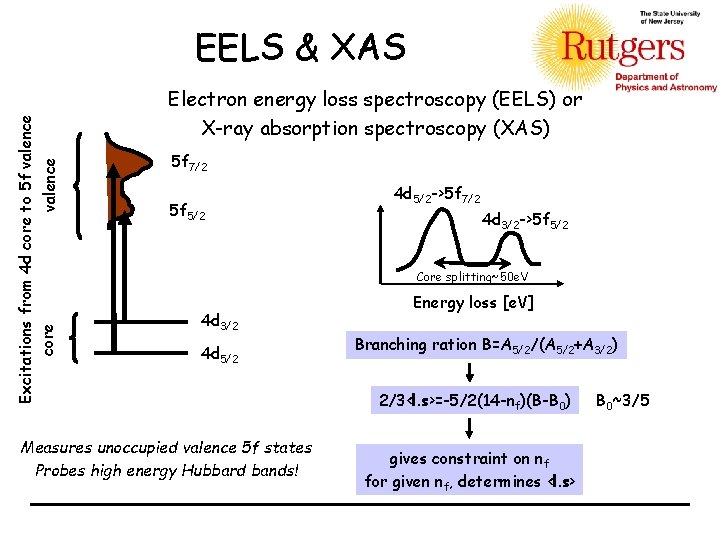
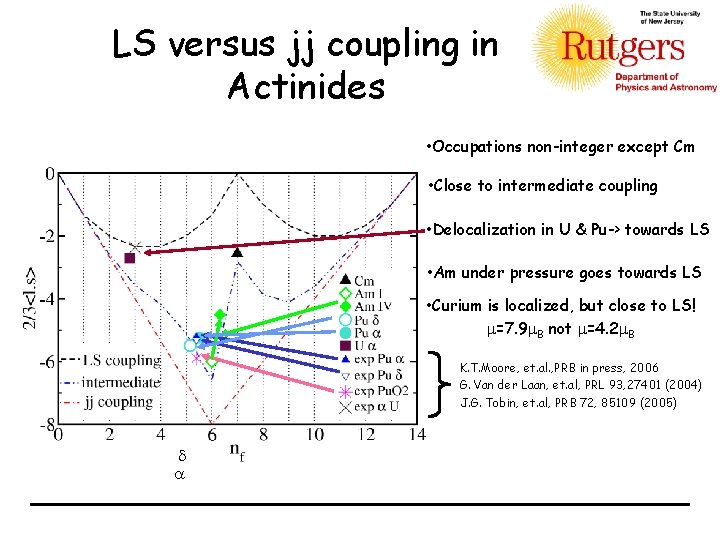
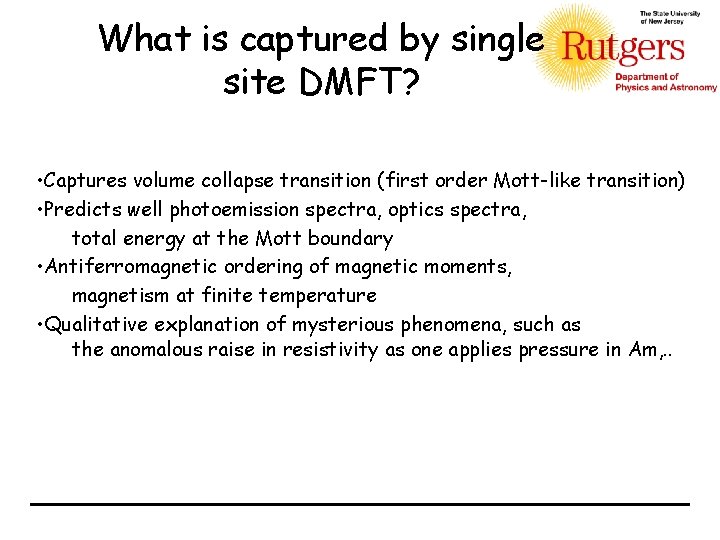
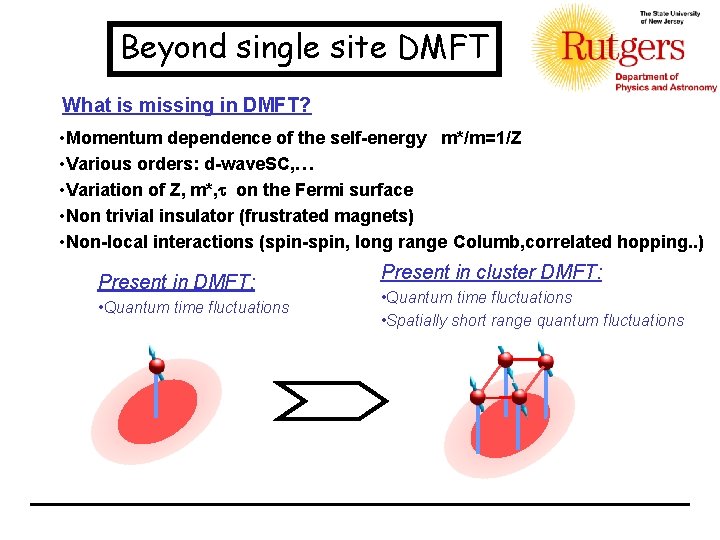
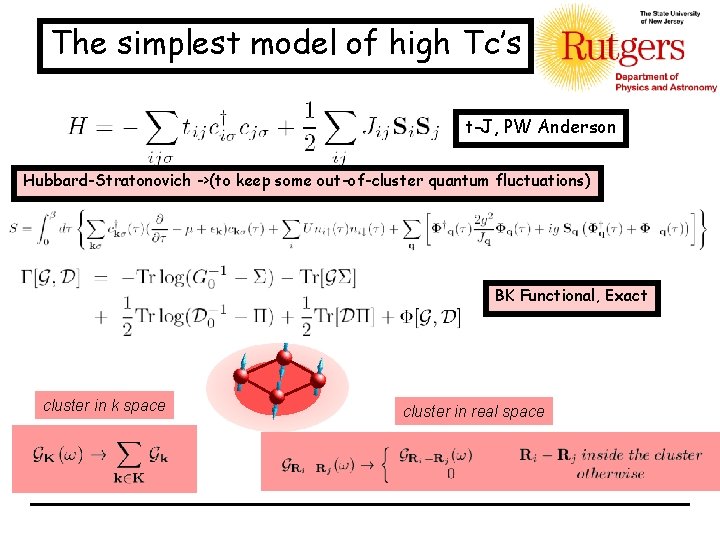
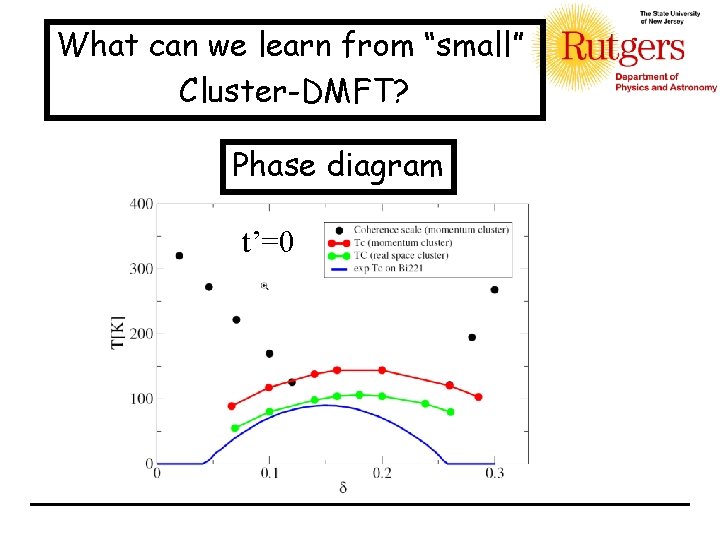
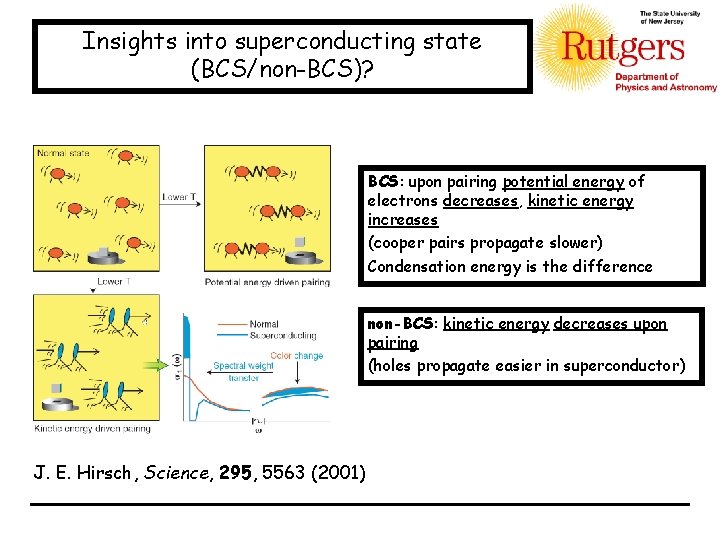
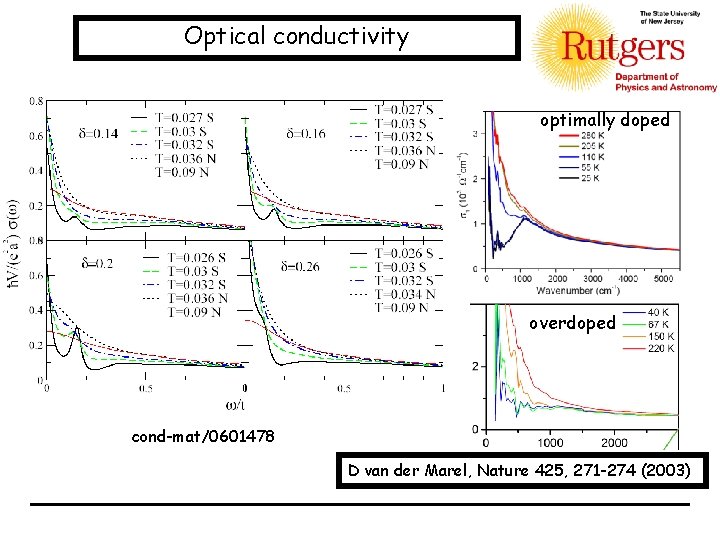
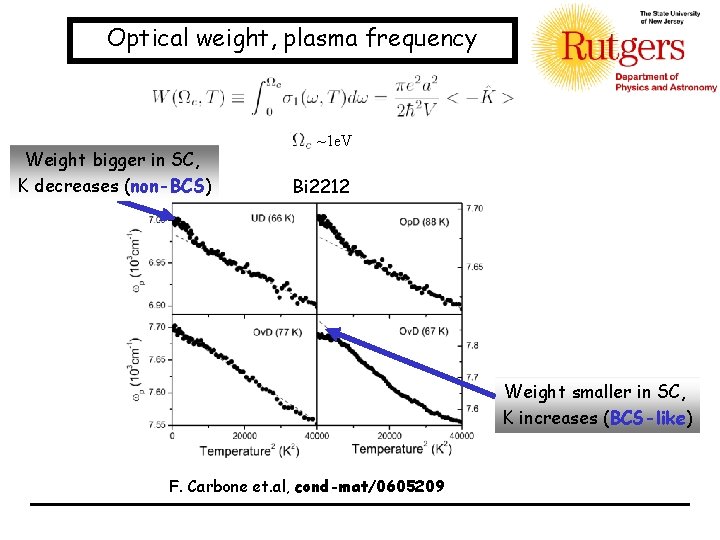
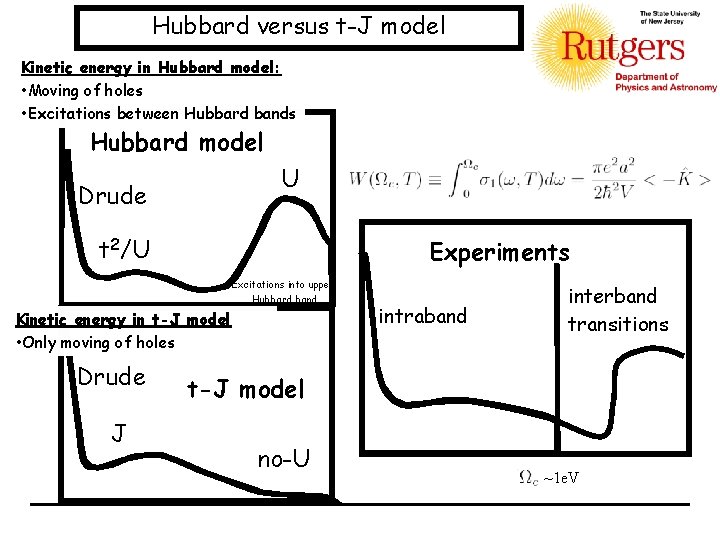
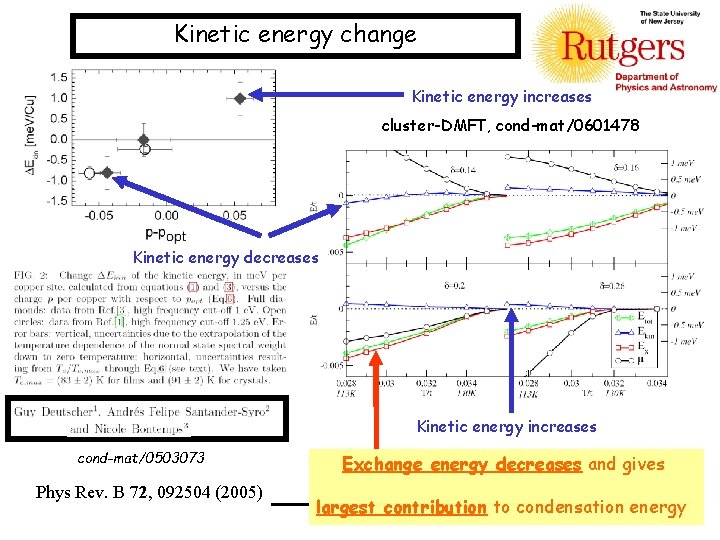
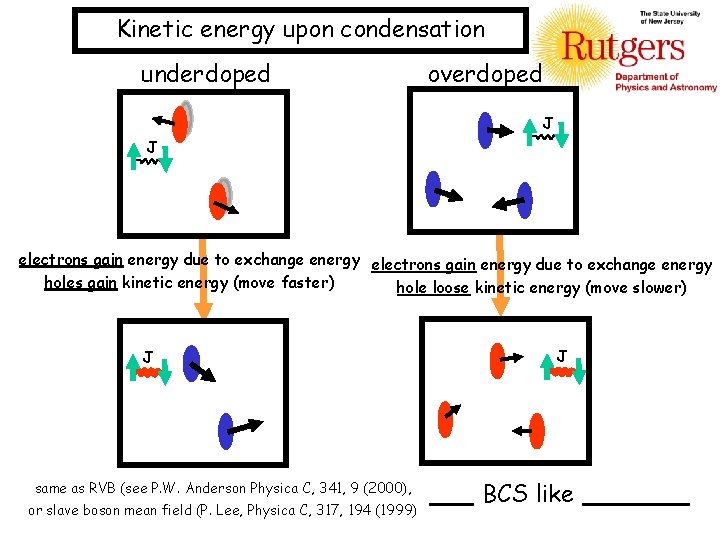
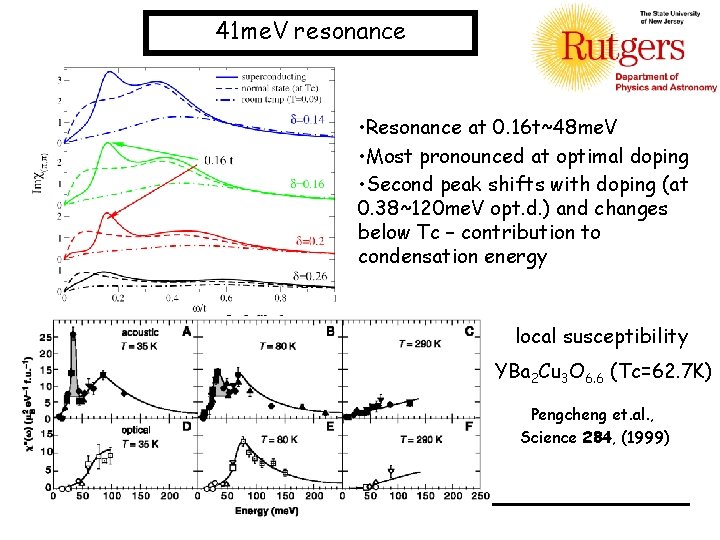
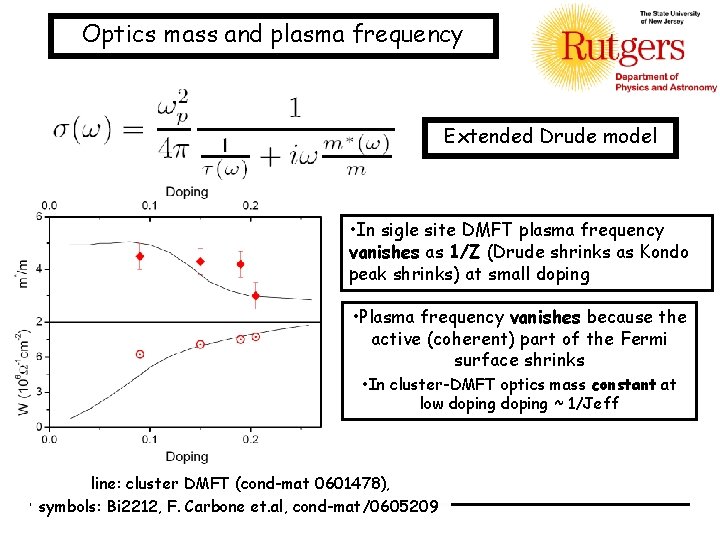
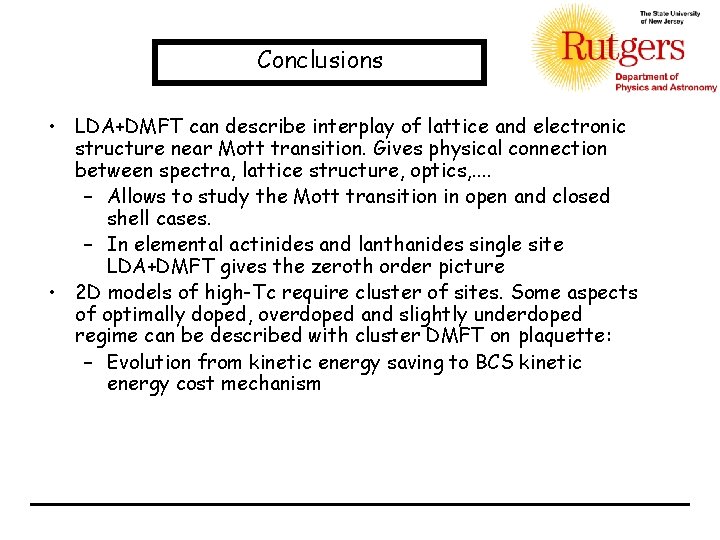
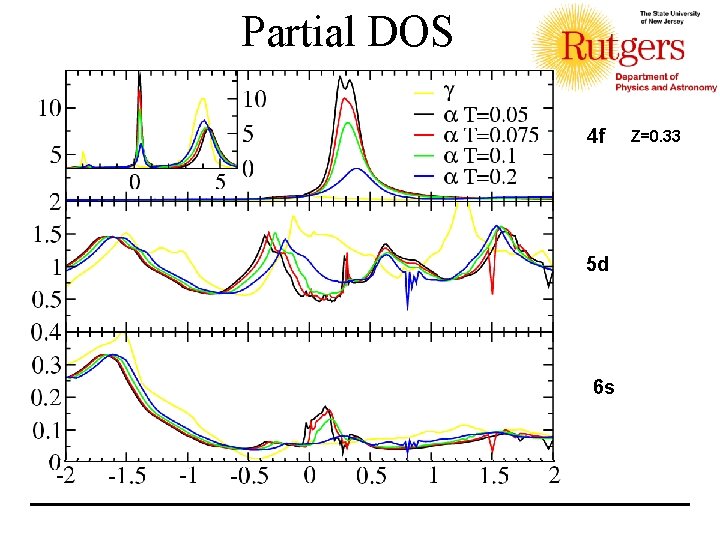
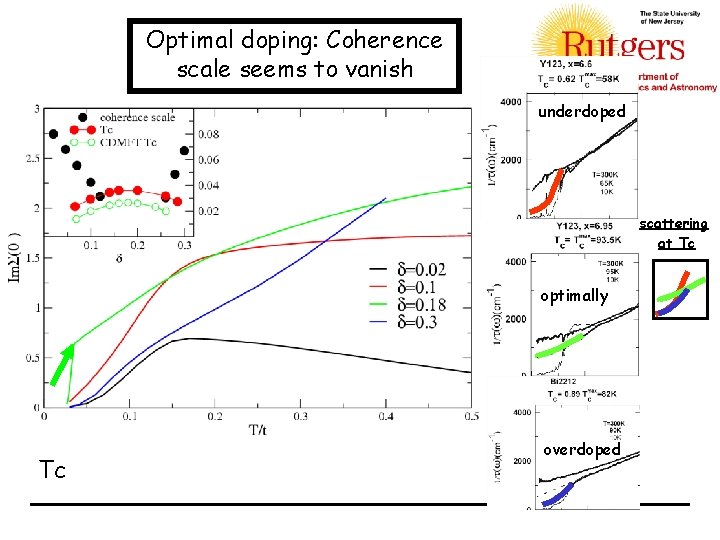
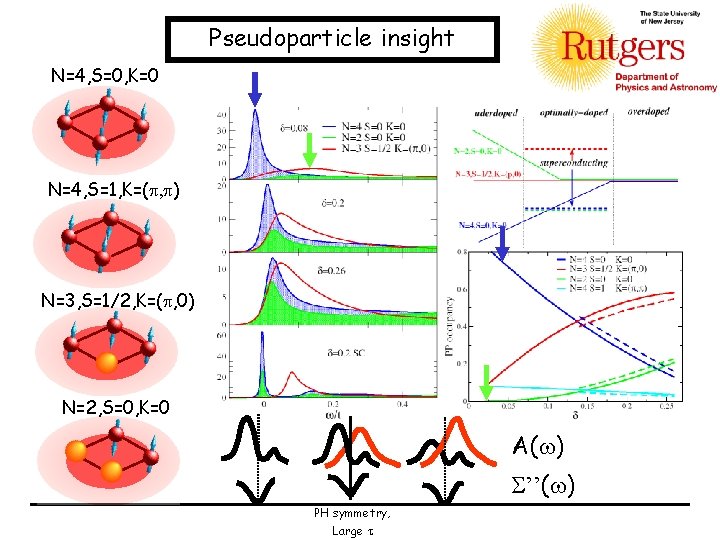
- Slides: 36
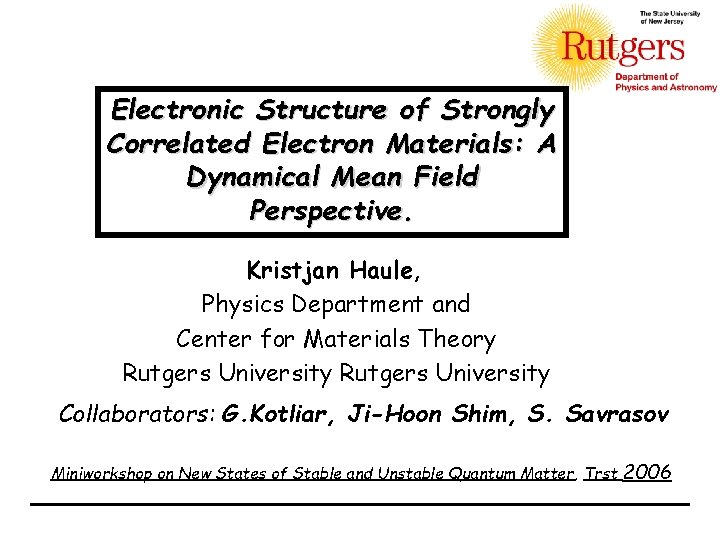
Electronic Structure of Strongly Correlated Electron Materials: A Dynamical Mean Field Perspective. Kristjan Haule, Physics Department and Center for Materials Theory Rutgers University Collaborators: G. Kotliar, Ji-Hoon Shim, S. Savrasov Miniworkshop on New States of Stable and Unstable Quantum Matter, Trst 2006
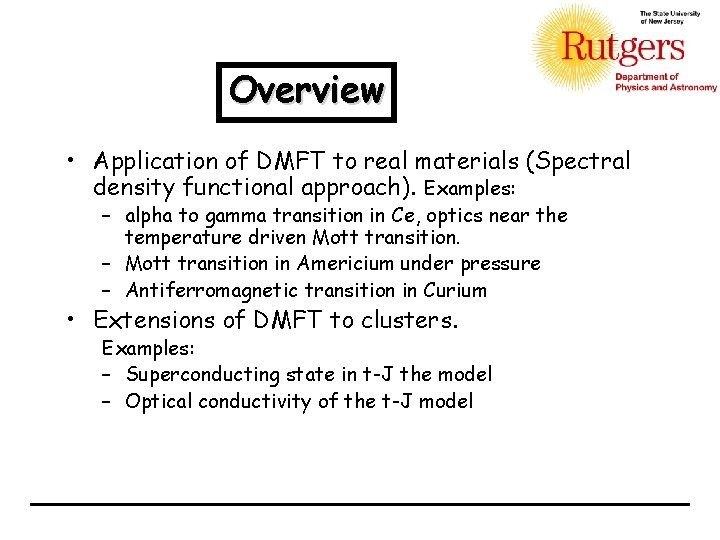
Overview • Application of DMFT to real materials (Spectral density functional approach). Examples: – alpha to gamma transition in Ce, optics near the temperature driven Mott transition. – Mott transition in Americium under pressure – Antiferromagnetic transition in Curium • Extensions of DMFT to clusters. Examples: – Superconducting state in t-J the model – Optical conductivity of the t-J model
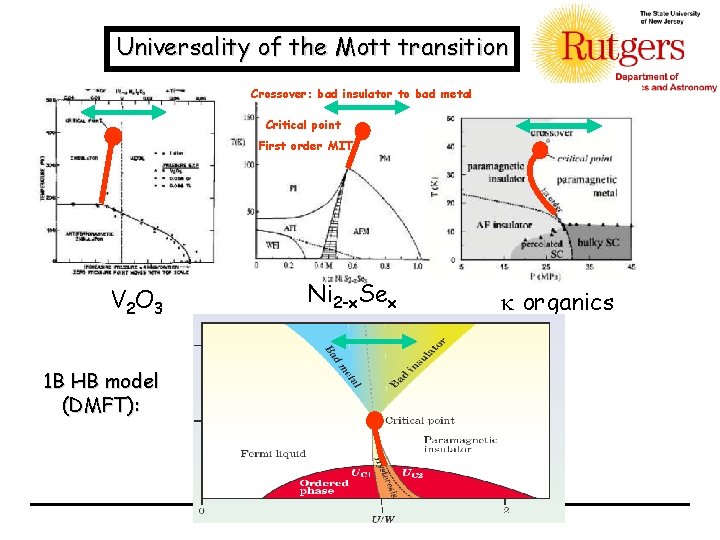
Universality of the Mott transition Crossover: bad insulator to bad metal Critical point First order MIT V 2 O 3 1 B HB model (DMFT): Ni 2 -x. Sex k organics
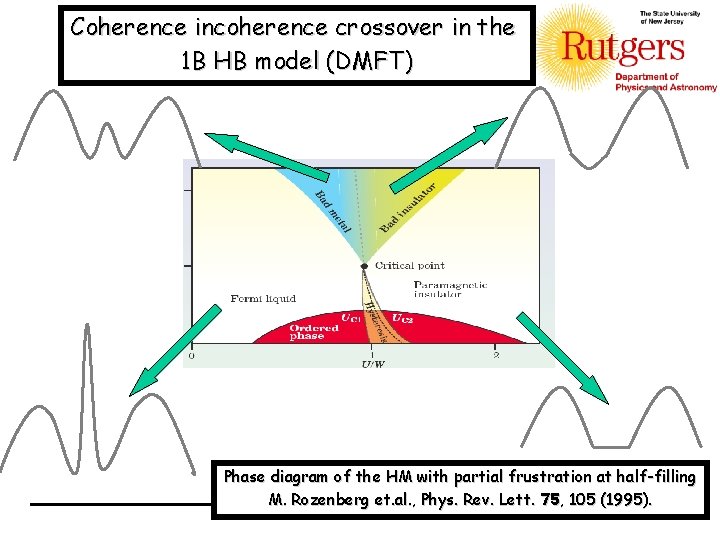
Coherence incoherence crossover in the 1 B HB model (DMFT) Phase diagram of the HM with partial frustration at half-filling M. Rozenberg et. al. , Phys. Rev. Lett. 75, 105 (1995).
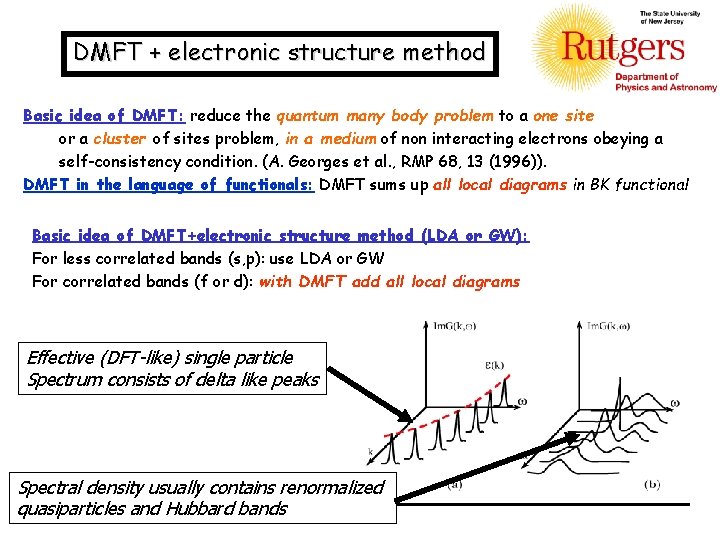
DMFT + electronic structure method Basic idea of DMFT: reduce the quantum many body problem to a one site or a cluster of sites problem, in a medium of non interacting electrons obeying a self-consistency condition. (A. Georges et al. , RMP 68, 13 (1996)). DMFT in the language of functionals: DMFT sums up all local diagrams in BK functional Basic idea of DMFT+electronic structure method (LDA or GW): For less correlated bands (s, p): use LDA or GW For correlated bands (f or d): with DMFT add all local diagrams Effective (DFT-like) single particle Spectrum consists of delta like peaks Spectral density usually contains renormalized quasiparticles and Hubbard bands
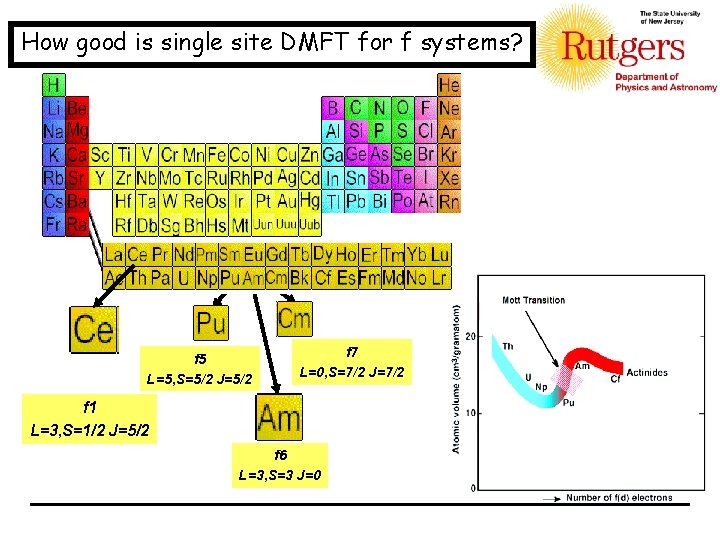
How good is single site DMFT for f systems? f 5 L=5, S=5/2 J=5/2 f 7 L=0, S=7/2 J=7/2 f 1 L=3, S=1/2 J=5/2 f 6 L=3, S=3 J=0
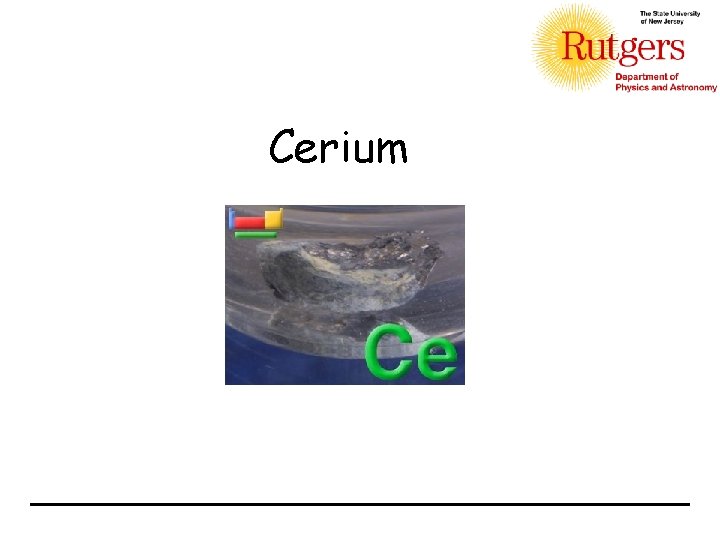
Cerium
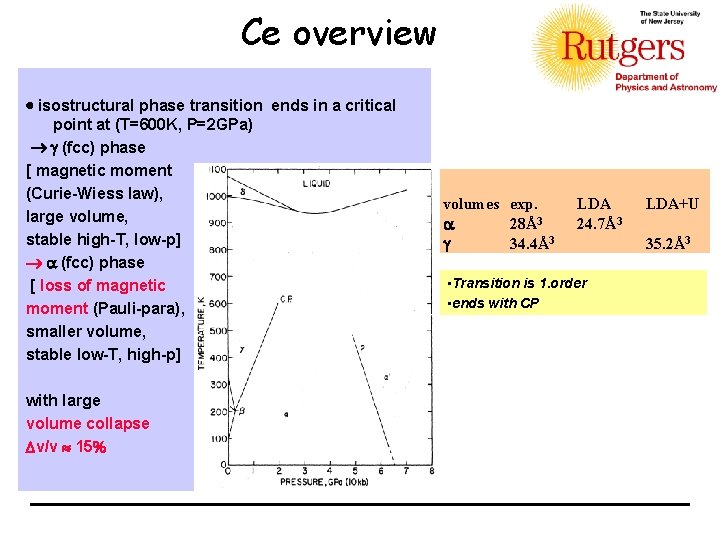
Ce overview isostructural phase transition ends in a critical point at (T=600 K, P=2 GPa) (fcc) phase [ magnetic moment (Curie-Wiess law), large volume, stable high-T, low-p] (fcc) phase [ loss of magnetic moment (Pauli-para), smaller volume, stable low-T, high-p] with large volume collapse v/v 15 volumes exp. 28Å3 34. 4Å3 LDA 24. 7Å3 • Transition is 1. order • ends with CP LDA+U 35. 2Å3
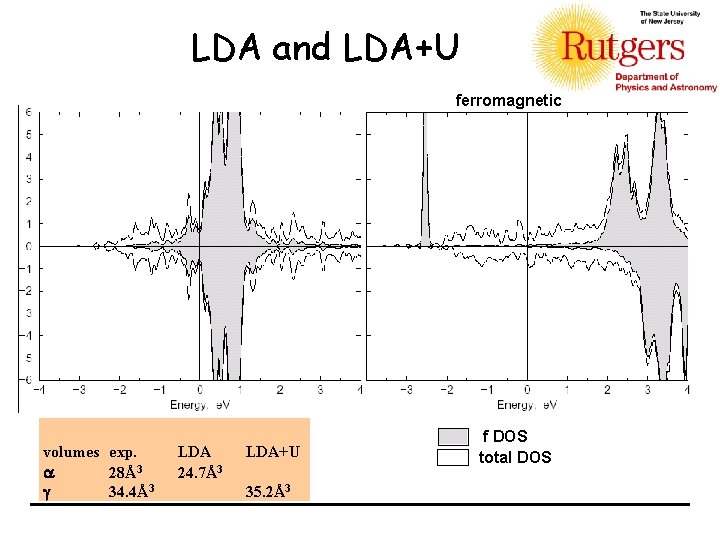
LDA and LDA+U ferromagnetic volumes exp. 28Å3 34. 4Å3 LDA 24. 7Å3 LDA+U 35. 2Å3 f DOS total DOS
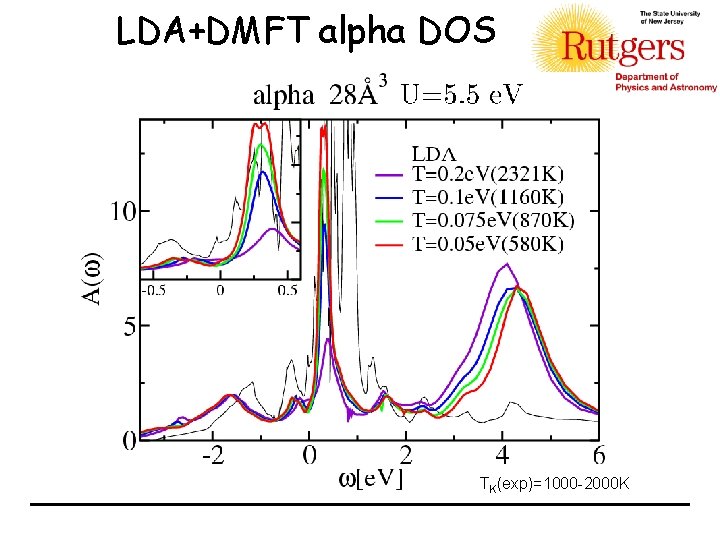
LDA+DMFT alpha DOS TK(exp)=1000 -2000 K
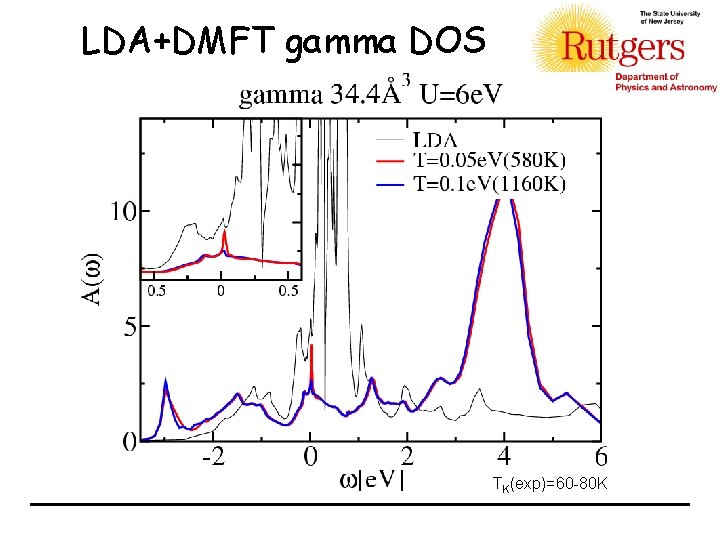
LDA+DMFT gamma DOS TK(exp)=60 -80 K
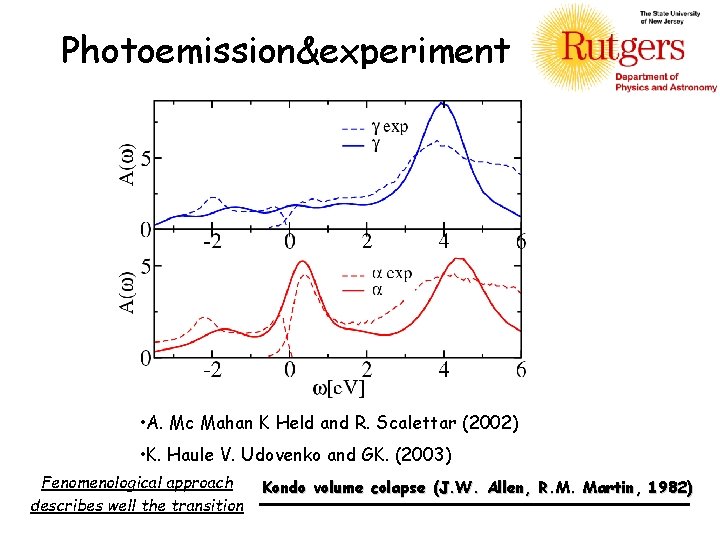
Photoemission&experiment • A. Mc Mahan K Held and R. Scalettar (2002) • K. Haule V. Udovenko and GK. (2003) Fenomenological approach describes well the transition Kondo volume colapse (J. W. Allen, R. M. Martin, 1982)
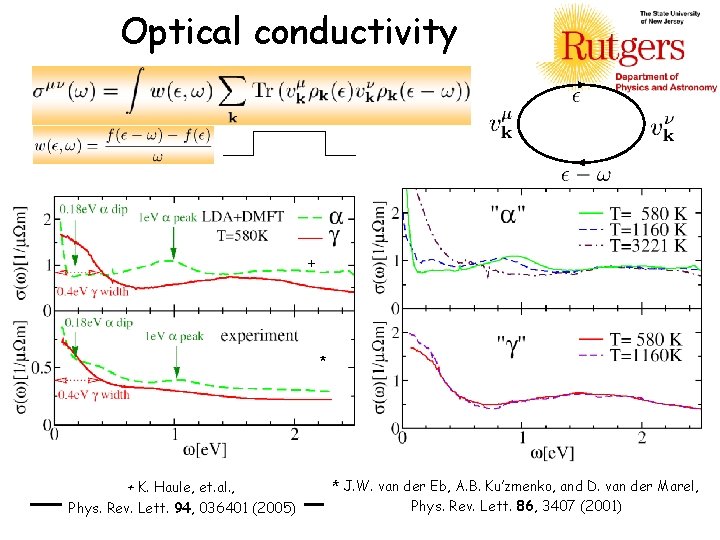
Optical conductivity + * + K. Haule, et. al. , Phys. Rev. Lett. 94, 036401 (2005) * J. W. van der Eb, A. B. Ku’zmenko, and D. van der Marel, Phys. Rev. Lett. 86, 3407 (2001)
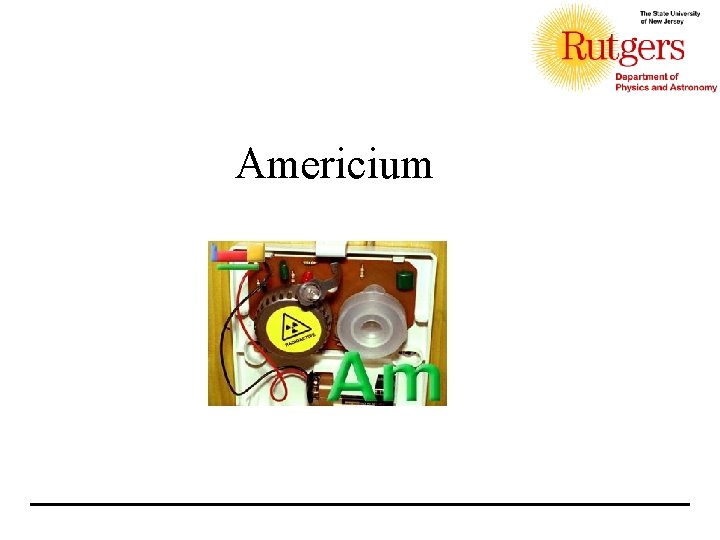
Americium
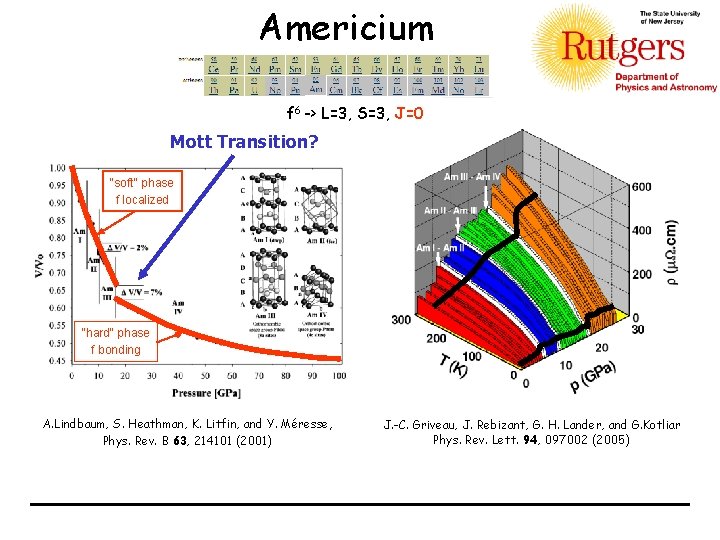
Americium f 6 -> L=3, S=3, J=0 Mott Transition? "soft" phase f localized "hard" phase f bonding A. Lindbaum, S. Heathman, K. Litfin, and Y. Méresse, Phys. Rev. B 63, 214101 (2001) J. -C. Griveau, J. Rebizant, G. H. Lander, and G. Kotliar Phys. Rev. Lett. 94, 097002 (2005)
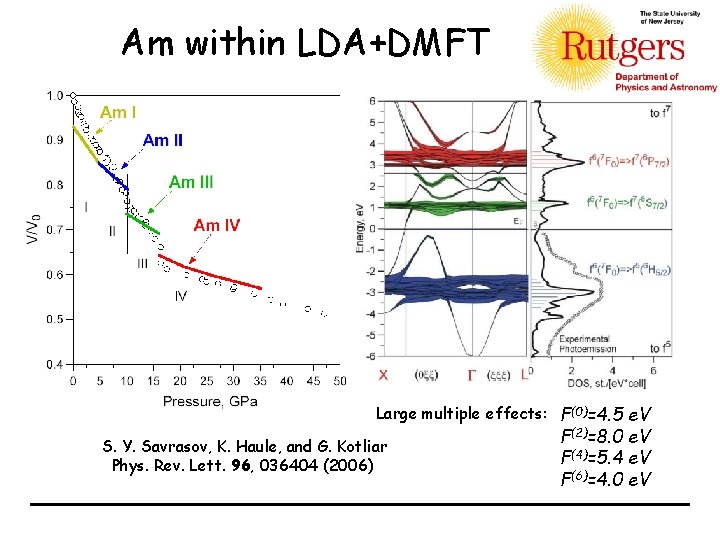
Am within LDA+DMFT Large multiple effects: F(0)=4. 5 e. V S. Y. Savrasov, K. Haule, and G. Kotliar Phys. Rev. Lett. 96, 036404 (2006) F(2)=8. 0 e. V F(4)=5. 4 e. V F(6)=4. 0 e. V
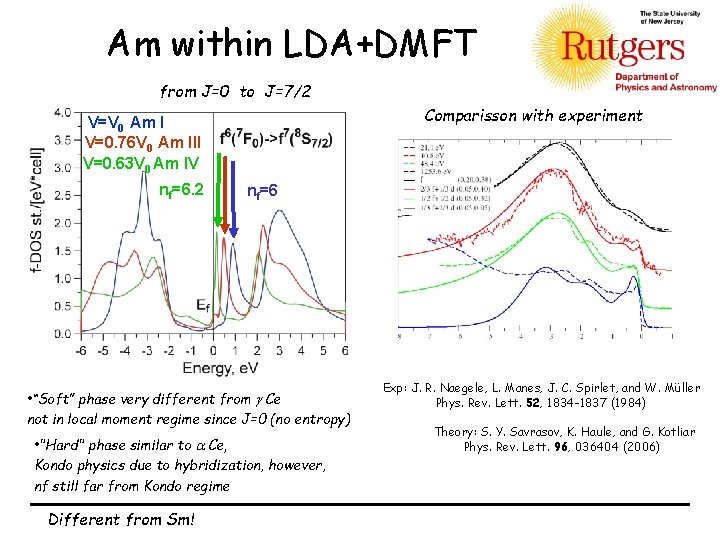
Am within LDA+DMFT from J=0 to J=7/2 Comparisson with experiment V=V 0 Am I V=0. 76 V 0 Am III V=0. 63 V 0 Am IV nf=6. 2 nf=6 • “Soft” phase very different from g Ce not in local moment regime since J=0 (no entropy) • "Hard" phase similar to a Ce, Kondo physics due to hybridization, however, nf still far from Kondo regime Different from Sm! Exp: J. R. Naegele, L. Manes, J. C. Spirlet, and W. Müller Phys. Rev. Lett. 52, 1834 -1837 (1984) Theory: S. Y. Savrasov, K. Haule, and G. Kotliar Phys. Rev. Lett. 96, 036404 (2006)
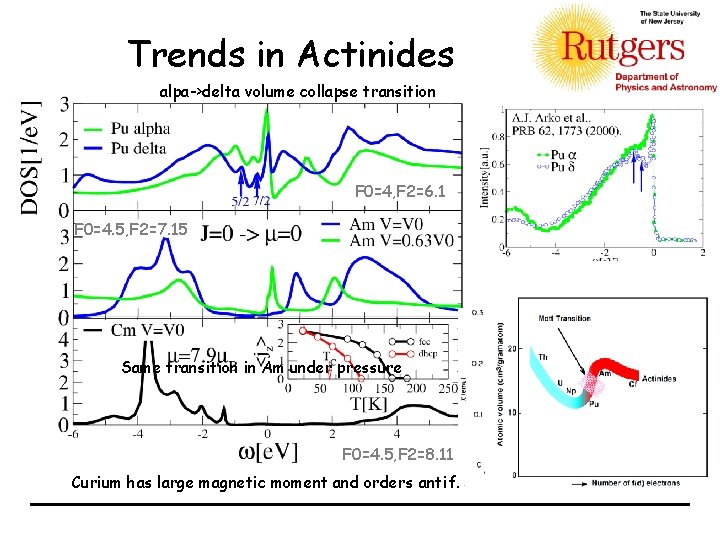
Trends in Actinides alpa->delta volume collapse transition F 0=4, F 2=6. 1 F 0=4. 5, F 2=7. 15 Curie-Weiss Same transition in Am under pressure F 0=4. 5, F 2=8. 11 Curium has large magnetic moment and orders antif. Tc
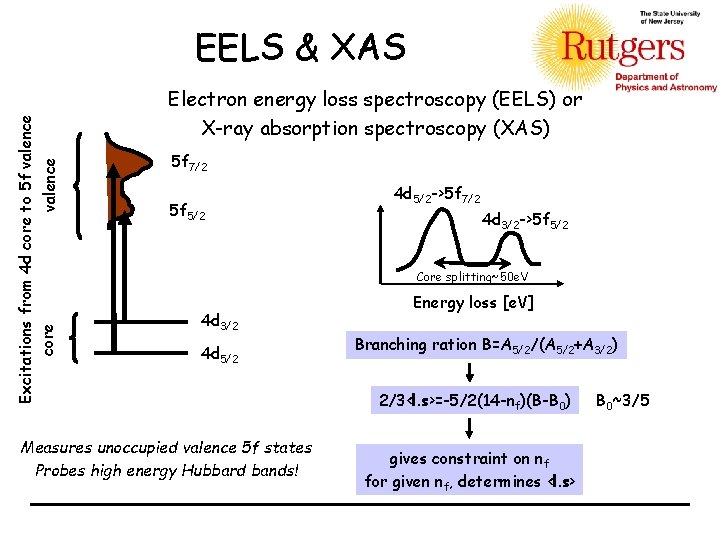
Excitations from 4 d core to 5 f valence core valence EELS & XAS Electron energy loss spectroscopy (EELS) or X-ray absorption spectroscopy (XAS) 5 f 7/2 5 f 5/2 4 d 5/2 ->5 f 7/2 4 d 3/2 ->5 f 5/2 Core splitting~50 e. V 4 d 3/2 4 d 5/2 Measures unoccupied valence 5 f states Probes high energy Hubbard bands! Energy loss [e. V] Branching ration B=A 5/2/(A 5/2+A 3/2) 2/3<l. s>=-5/2(14 -nf)(B-B 0) gives constraint on nf for given nf, determines <l. s> B 0~3/5
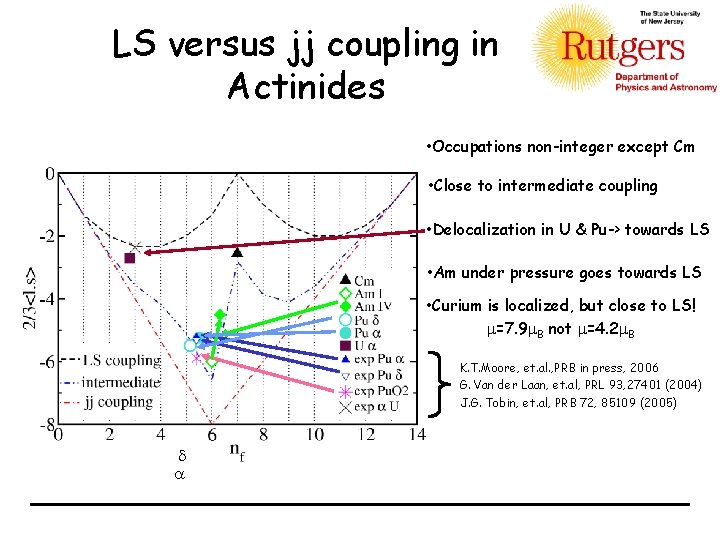
LS versus jj coupling in Actinides • Occupations non-integer except Cm • Close to intermediate coupling • Delocalization in U & Pu-> towards LS • Am under pressure goes towards LS • Curium is localized, but close to LS! m=7. 9 m. B not m=4. 2 m. B K. T. Moore, et. al. , PRB in press, 2006 G. Van der Laan, et. al, PRL 93, 27401 (2004) J. G. Tobin, et. al, PRB 72, 85109 (2005) d a
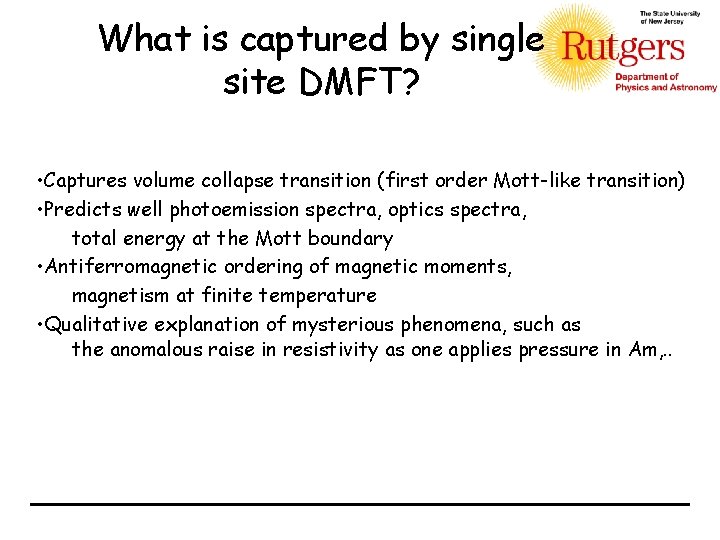
What is captured by single site DMFT? • Captures volume collapse transition (first order Mott-like transition) • Predicts well photoemission spectra, optics spectra, total energy at the Mott boundary • Antiferromagnetic ordering of magnetic moments, magnetism at finite temperature • Qualitative explanation of mysterious phenomena, such as the anomalous raise in resistivity as one applies pressure in Am, . .
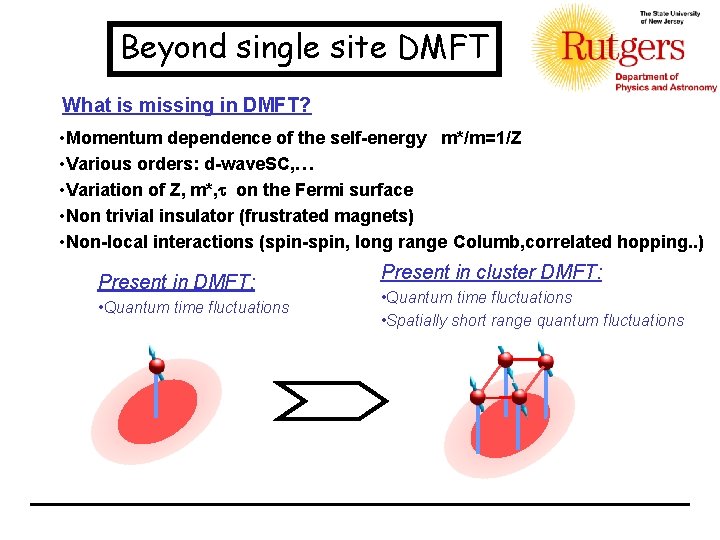
Beyond single site DMFT What is missing in DMFT? • Momentum dependence of the self-energy m*/m=1/Z • Various orders: d-wave. SC, … • Variation of Z, m*, t on the Fermi surface • Non trivial insulator (frustrated magnets) • Non-local interactions (spin-spin, long range Columb, correlated hopping. . ) Present in DMFT: • Quantum time fluctuations Present in cluster DMFT: • Quantum time fluctuations • Spatially short range quantum fluctuations
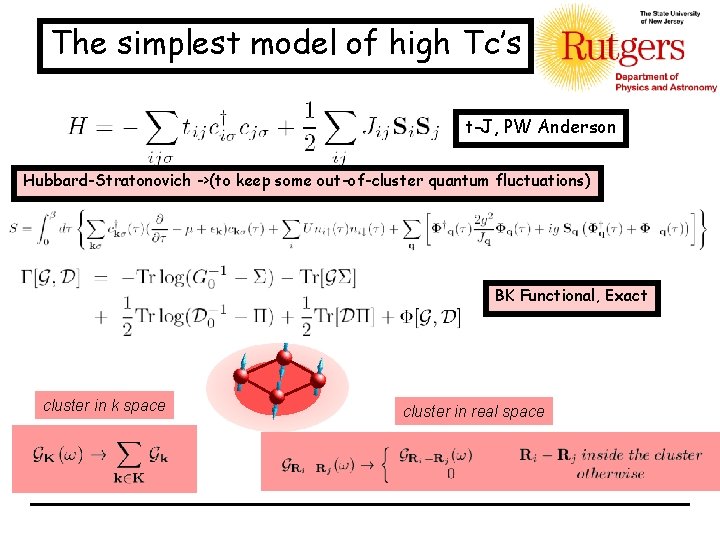
The simplest model of high Tc’s t-J, PW Anderson Hubbard-Stratonovich ->(to keep some out-of-cluster quantum fluctuations) BK Functional, Exact cluster in k space cluster in real space
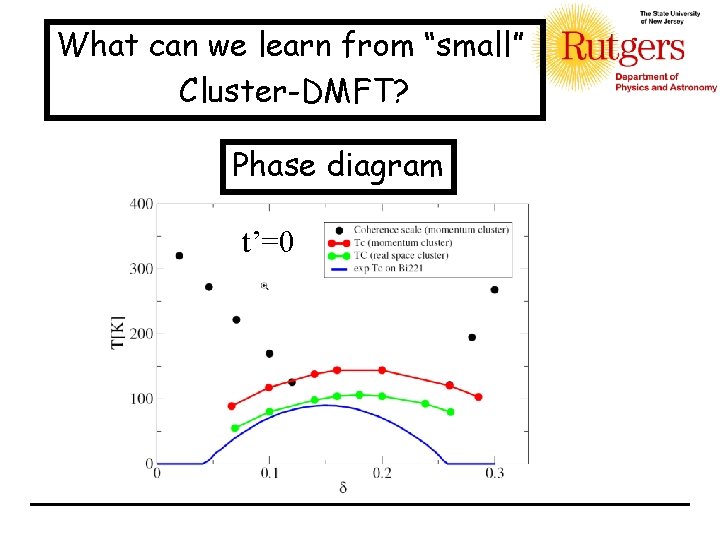
What can we learn from “small” Cluster-DMFT? Phase diagram t’=0
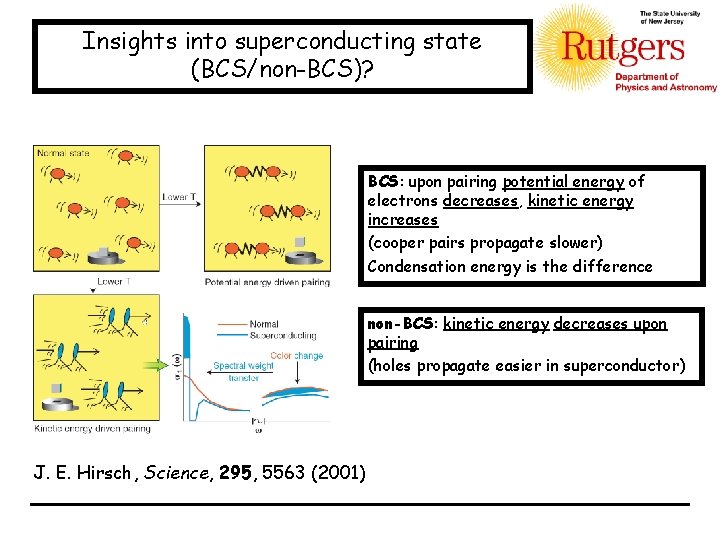
Insights into superconducting state (BCS/non-BCS)? BCS: upon pairing potential energy of electrons decreases, kinetic energy increases (cooper pairs propagate slower) Condensation energy is the difference non-BCS: kinetic energy decreases upon pairing (holes propagate easier in superconductor) J. E. Hirsch, Science, 295, 5563 (2001)
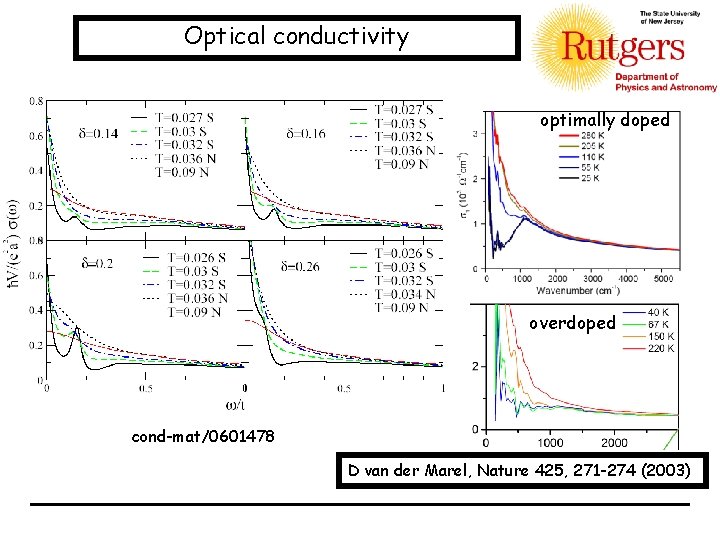
Optical conductivity optimally doped overdoped cond-mat/0601478 D van der Marel, Nature 425, 271 -274 (2003)
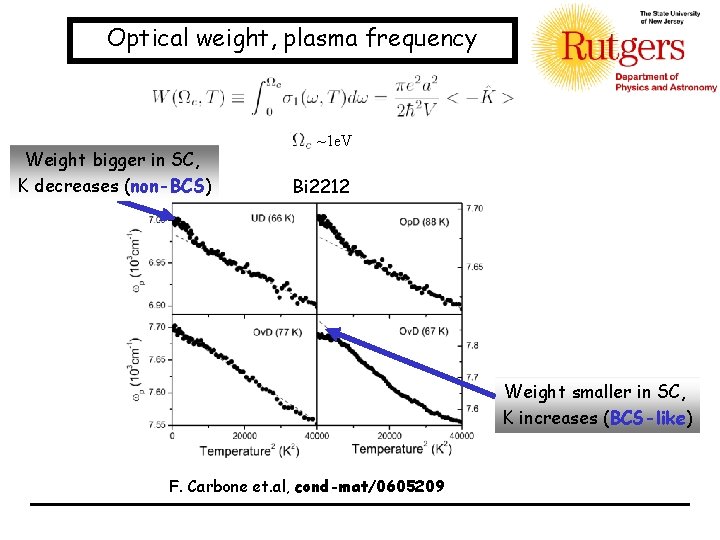
Optical weight, plasma frequency Weight bigger in SC, K decreases (non-BCS) ~1 e. V Bi 2212 Weight smaller in SC, K increases (BCS-like) F. Carbone et. al, cond-mat/0605209
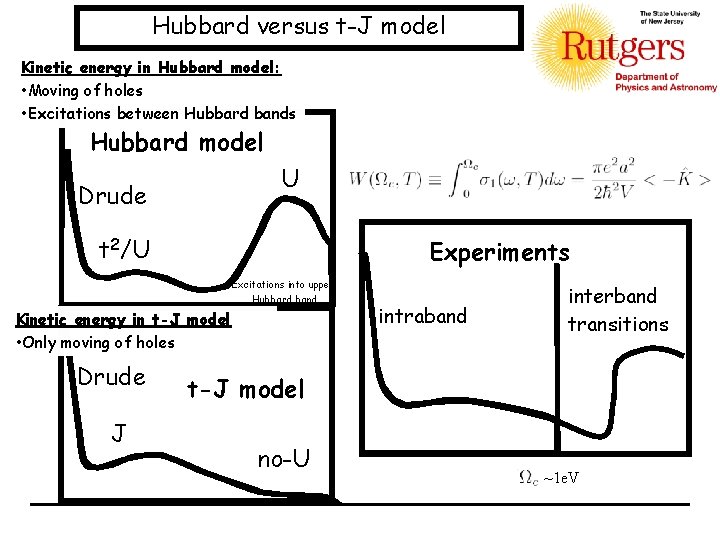
Hubbard versus t-J model Kinetic energy in Hubbard model: • Moving of holes • Excitations between Hubbard bands Hubbard model U Drude t 2/U Experiments Excitations into upper Hubbard band Kinetic energy in t-J model • Only moving of holes Drude J intraband interband transitions t-J model no-U ~1 e. V
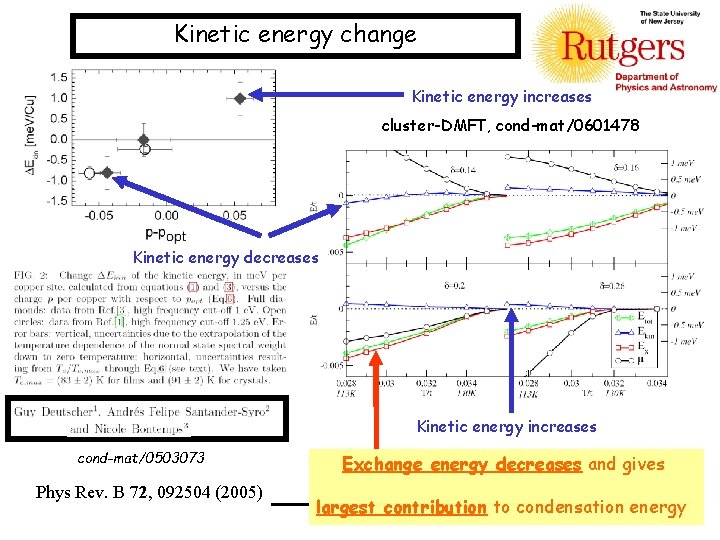
Kinetic energy change Kinetic energy increases cluster-DMFT, cond-mat/0601478 Kinetic energy decreases Kinetic energy increases cond-mat/0503073 Phys Rev. B 72, 092504 (2005) Exchange energy decreases and gives largest contribution to condensation energy
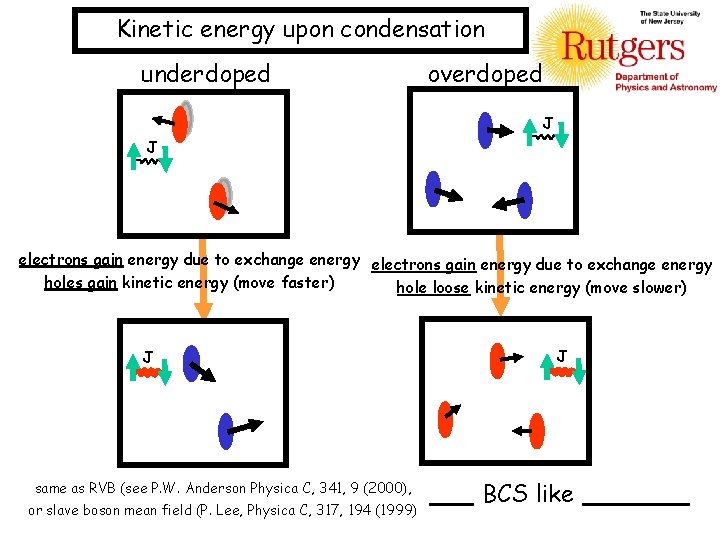
Kinetic energy upon condensation underdoped overdoped J J electrons gain energy due to exchange energy holes gain kinetic energy (move faster) hole loose kinetic energy (move slower) J same as RVB (see P. W. Anderson Physica C, 341, 9 (2000), or slave boson mean field (P. Lee, Physica C, 317, 194 (1999) J BCS like
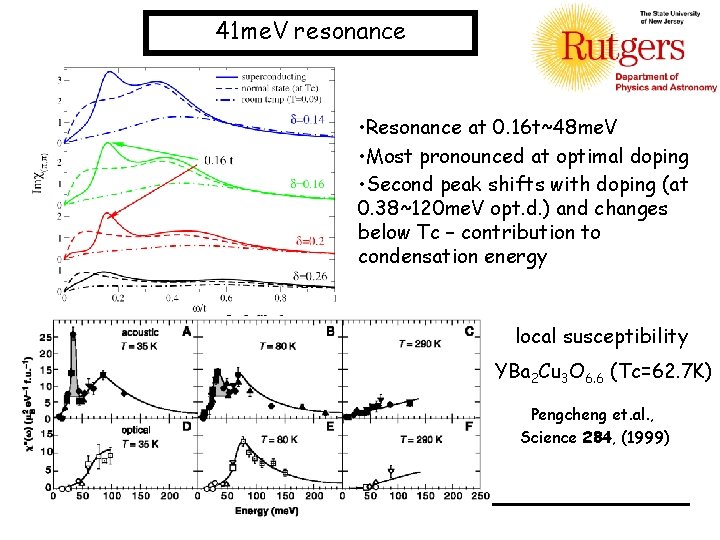
41 me. V resonance • Resonance at 0. 16 t~48 me. V • Most pronounced at optimal doping • Second peak shifts with doping (at 0. 38~120 me. V opt. d. ) and changes below Tc – contribution to condensation energy local susceptibility YBa 2 Cu 3 O 6. 6 (Tc=62. 7 K) Pengcheng et. al. , Science 284, (1999)
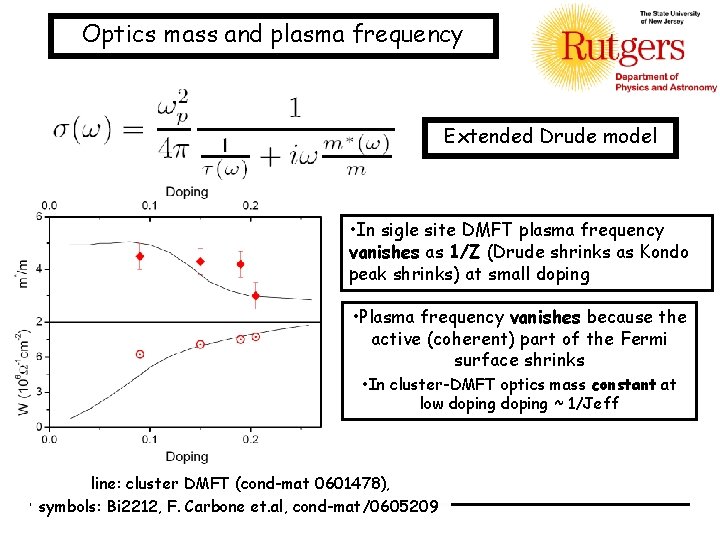
Optics mass and plasma frequency Extended Drude model • In sigle site DMFT plasma frequency vanishes as 1/Z (Drude shrinks as Kondo peak shrinks) at small doping • Plasma frequency vanishes because the active (coherent) part of the Fermi surface shrinks • In cluster-DMFT optics mass constant at low doping ~ 1/Jeff line: cluster DMFT (cond-mat 0601478), symbols: Bi 2212, F. Carbone et. al, cond-mat/0605209
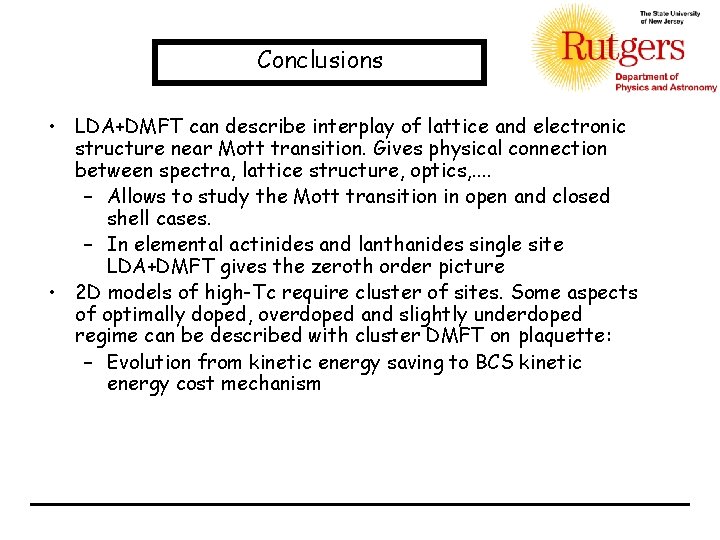
Conclusions • LDA+DMFT can describe interplay of lattice and electronic structure near Mott transition. Gives physical connection between spectra, lattice structure, optics, . . – Allows to study the Mott transition in open and closed shell cases. – In elemental actinides and lanthanides single site LDA+DMFT gives the zeroth order picture • 2 D models of high-Tc require cluster of sites. Some aspects of optimally doped, overdoped and slightly underdoped regime can be described with cluster DMFT on plaquette: – Evolution from kinetic energy saving to BCS kinetic energy cost mechanism
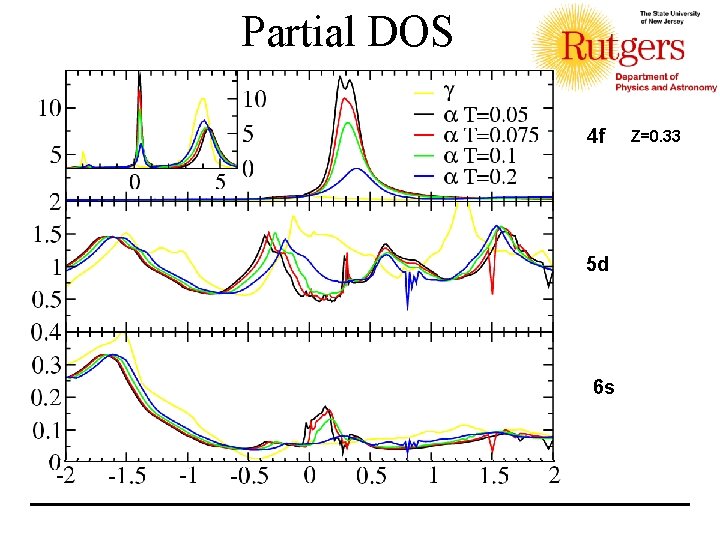
Partial DOS 4 f 5 d 6 s Z=0. 33
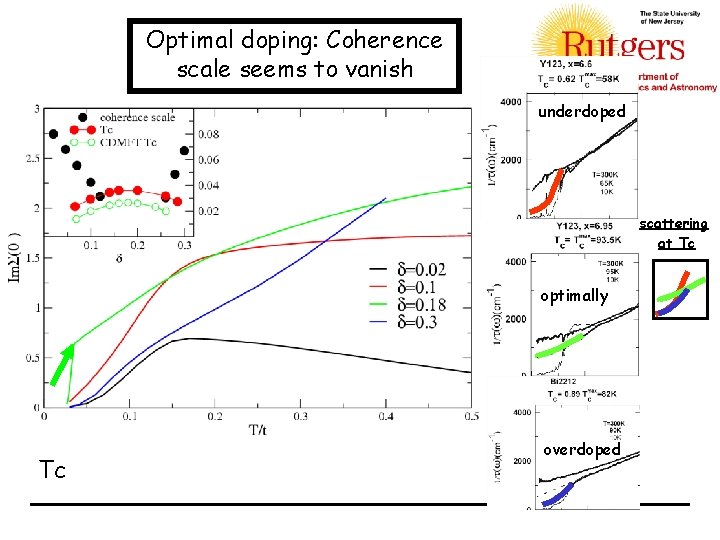
Optimal doping: Coherence scale seems to vanish underdoped scattering at Tc optimally Tc overdoped
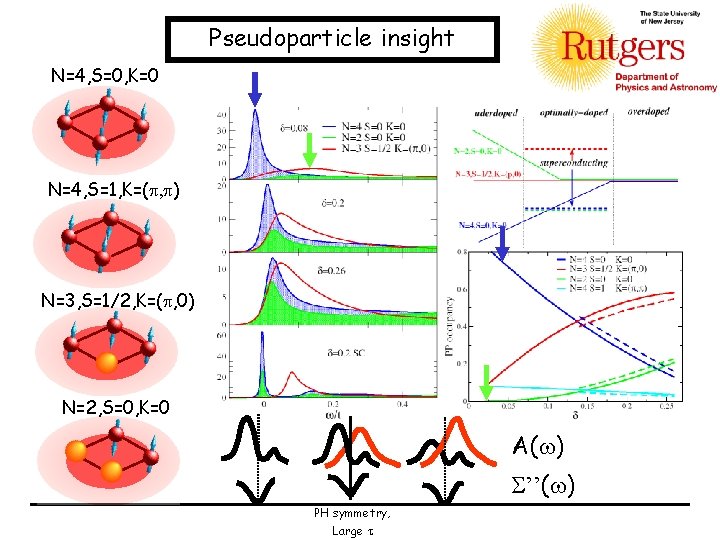
Pseudoparticle insight N=4, S=0, K=0 N=4, S=1, K=(p, p) N=3, S=1/2, K=(p, 0) N=2, S=0, K=0 A(w) S’’(w) PH symmetry, Large t
The future of the correlated electron problem
Correlated equilibrium
Line of best fit
Correlated equilibrium
Correlated equilibrium
Correlated equilibrium
Conitzer
Correlated equilibrium
Correlated group design
Dominant strategy
Correlated equilibrium
Correlated equilibrium
Rights and duties are correlated
Correlated group design
Mass m, volume v and density d are correlated as
Correlated curriculum
Scrip exchange
Electronic news gathering and electronic field production
Taisil electronic materials corporation
Cant stop the feeling go noodle
Title useful and harmful materials at home
Natural man made
Adopting and adapting teaching materials
Direct materials budget with multiple materials
Vegetables u
Types of programming languages
Strongly connected graph
Hawthorne motivation theory
Strongly connected components
Strongly connected components
Latitude strongly influences climate because
Difference between strongly and weakly typed languages
Acid and carbonate reaction
8 vegetable classifications
India is strongly influenced by monsoon winds
Ethos rhetoric
5-2 limits to growth