Chapter 7 Messenger RNA 7 1 Introduction All
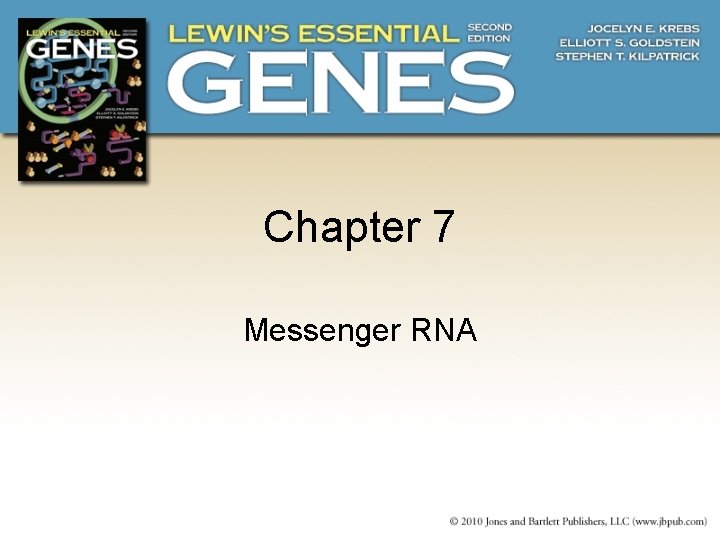
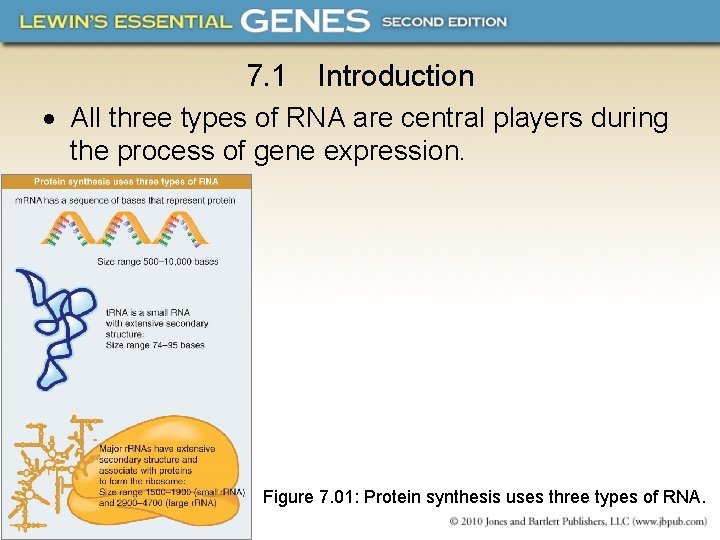
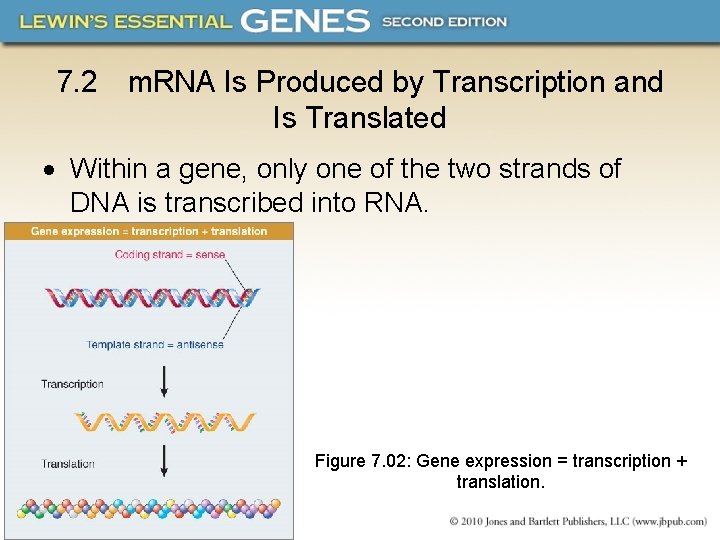
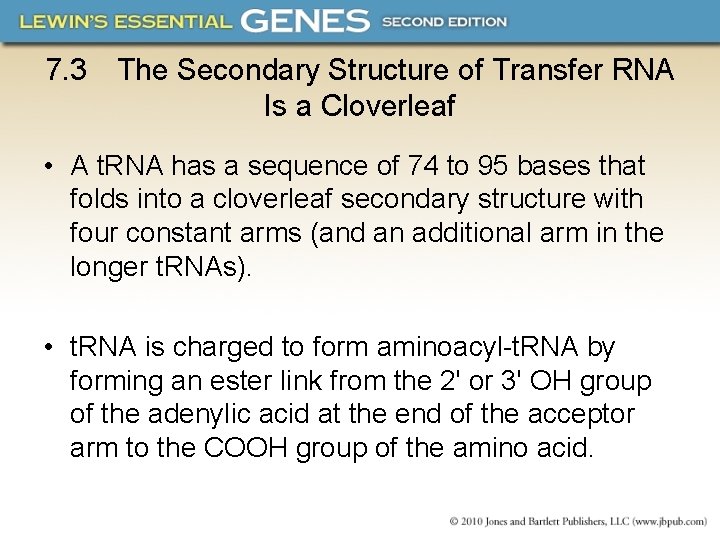
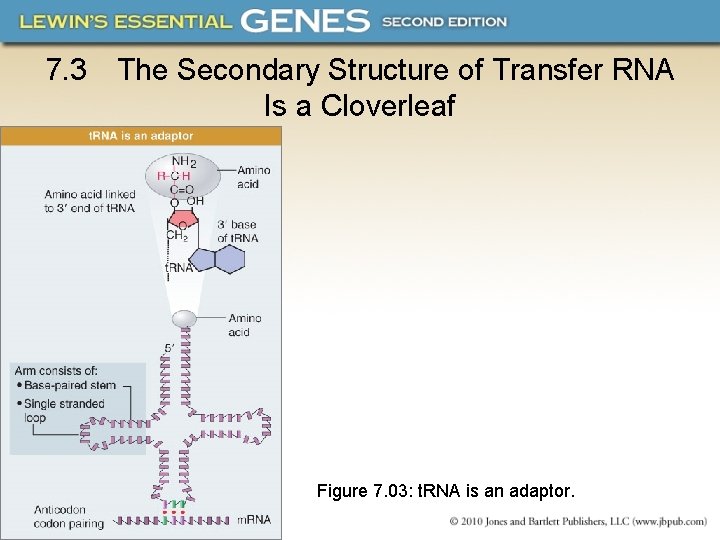
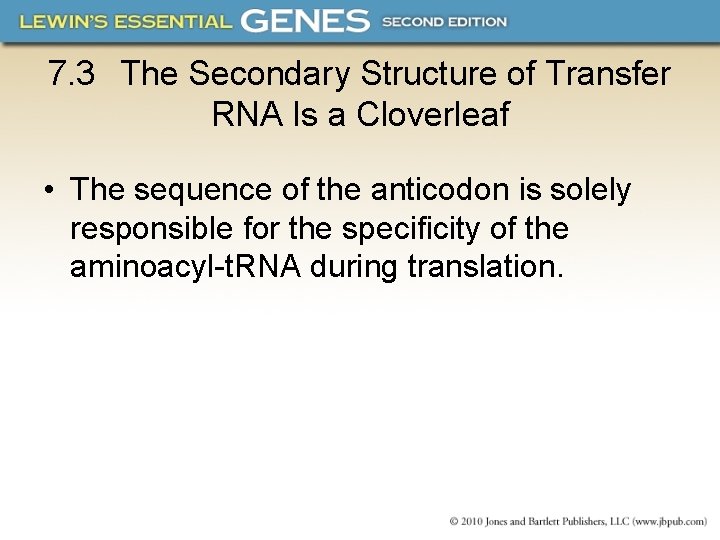
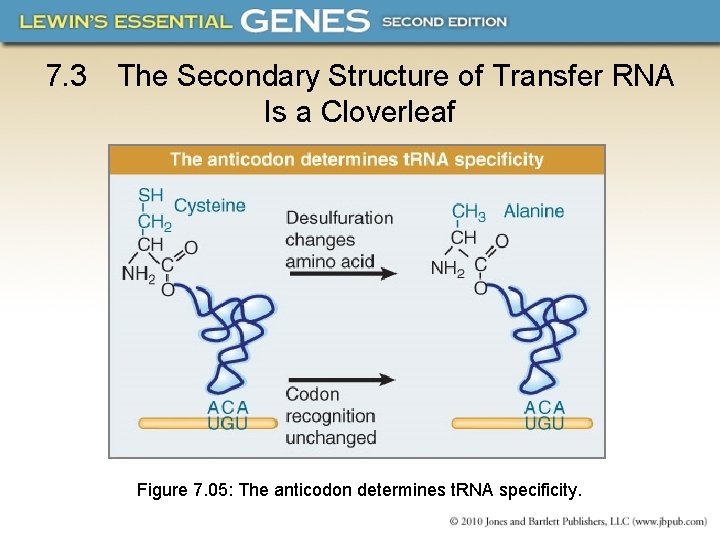
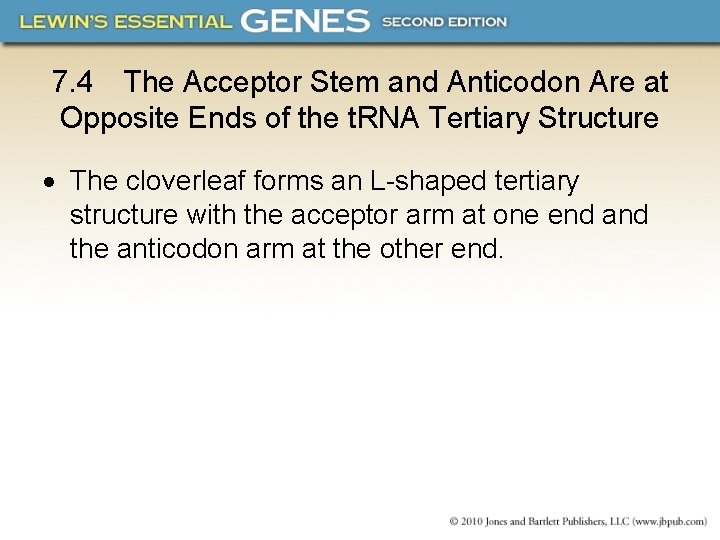
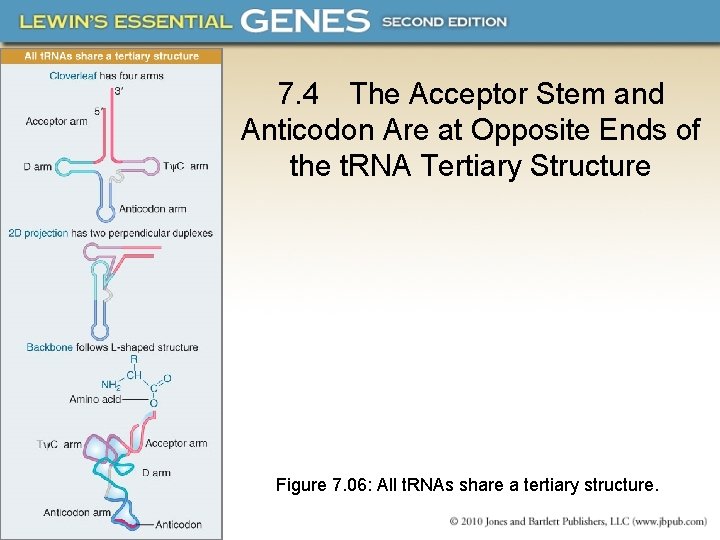
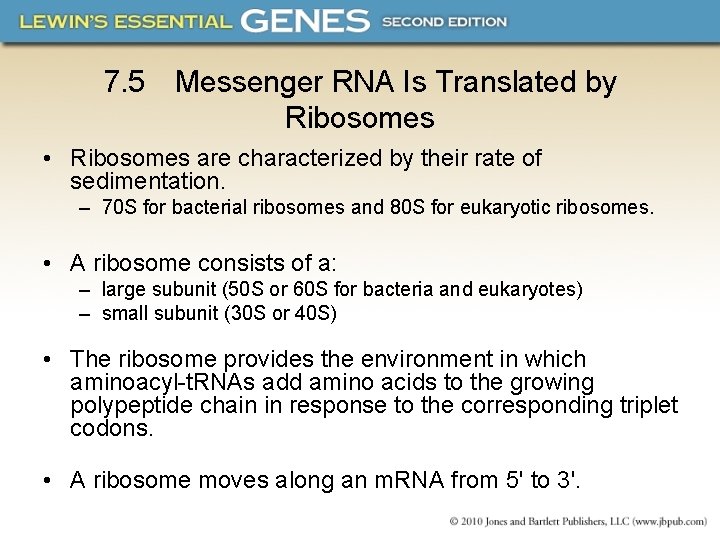
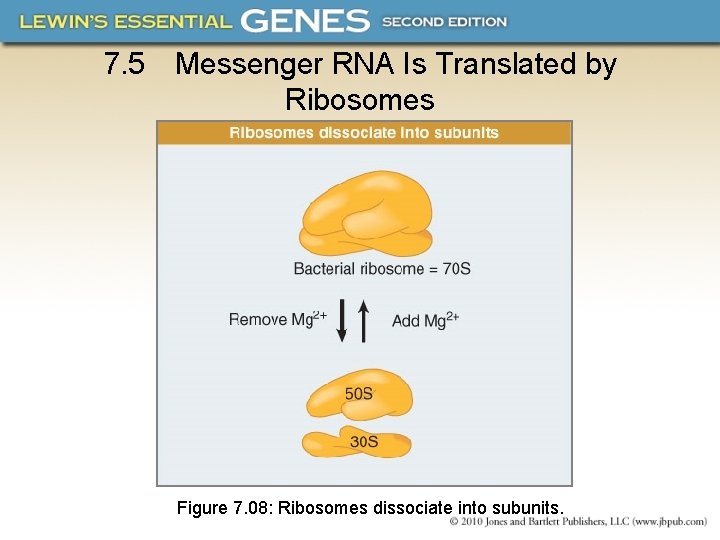
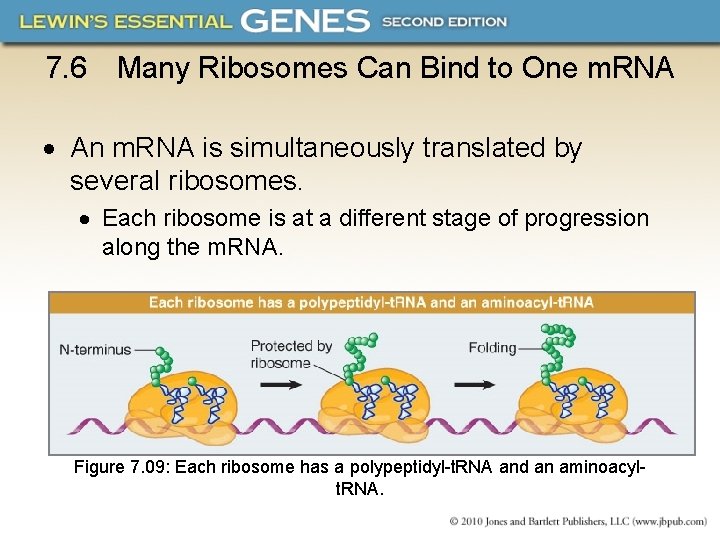
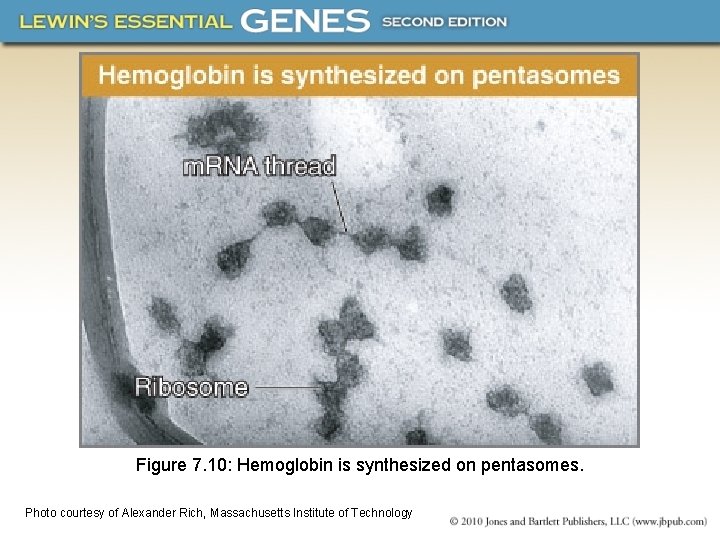
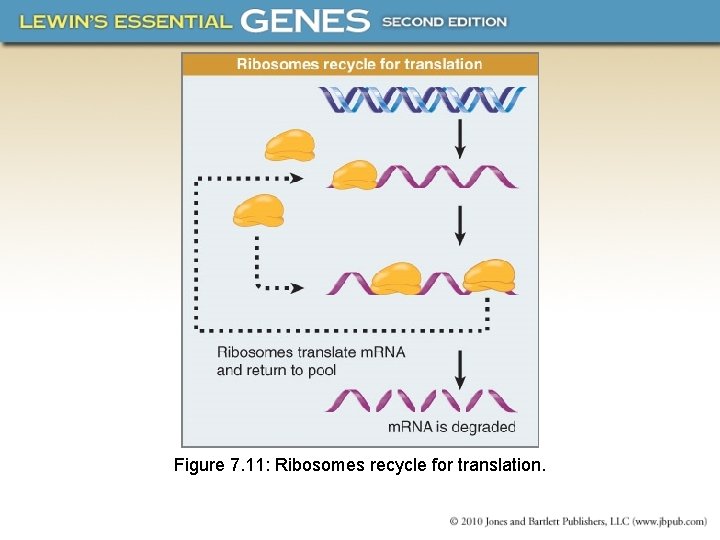
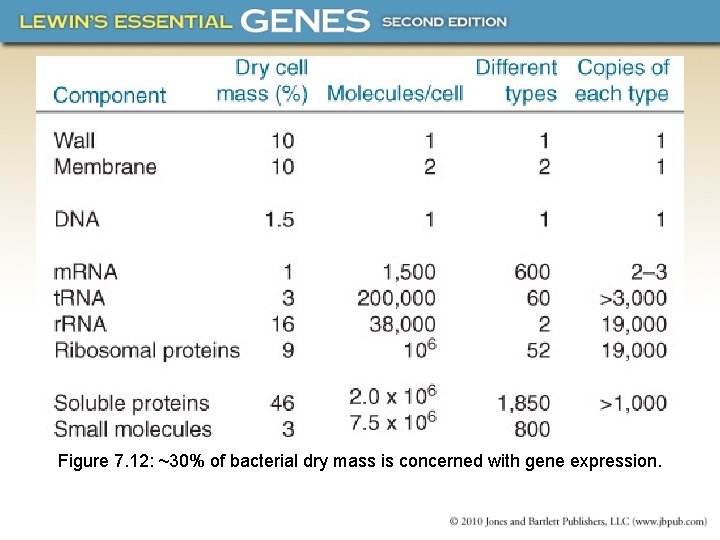
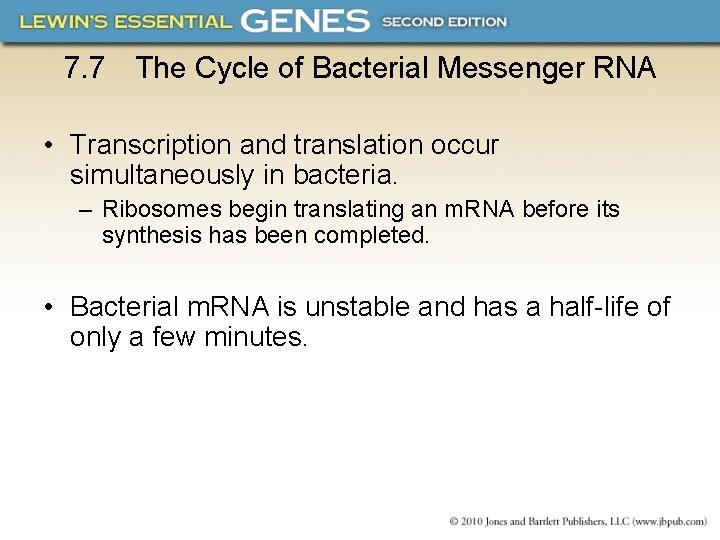
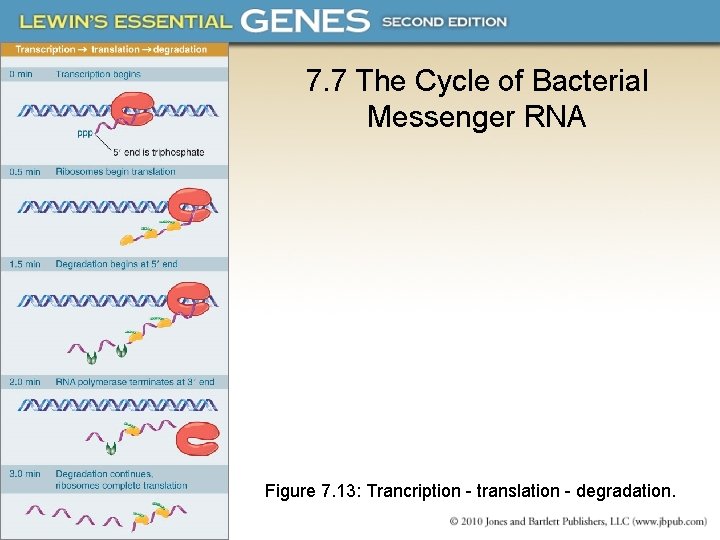
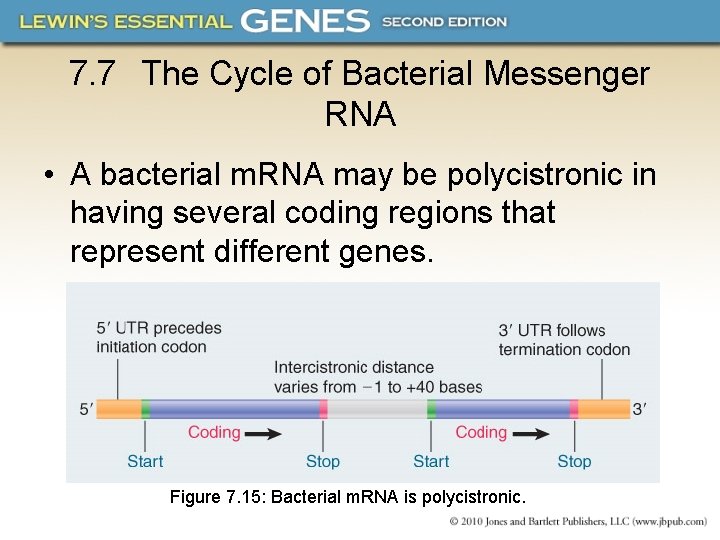
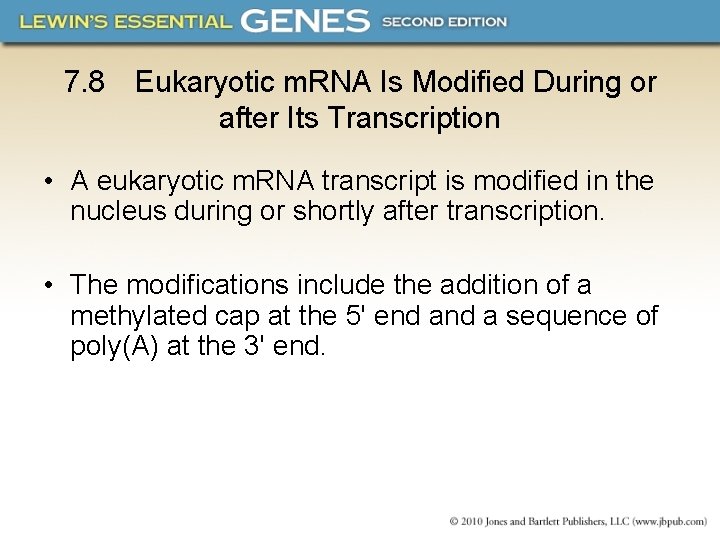
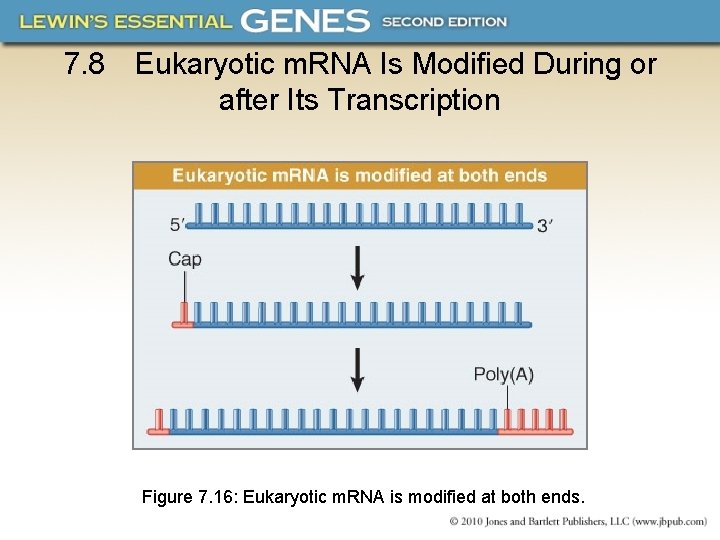
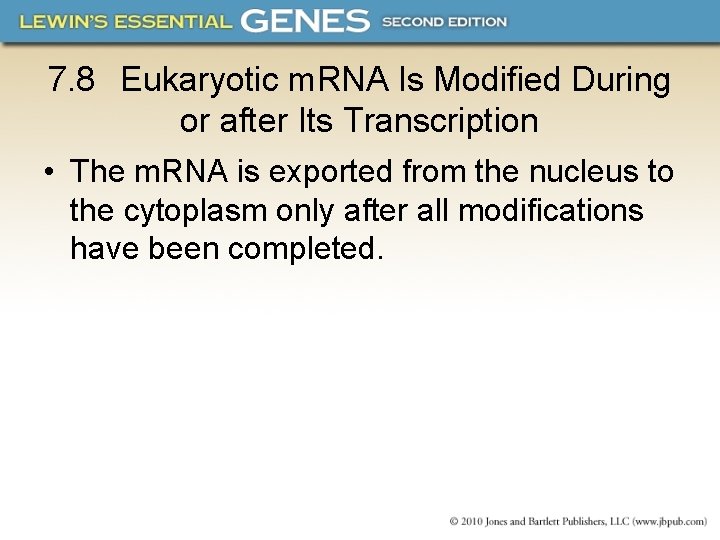
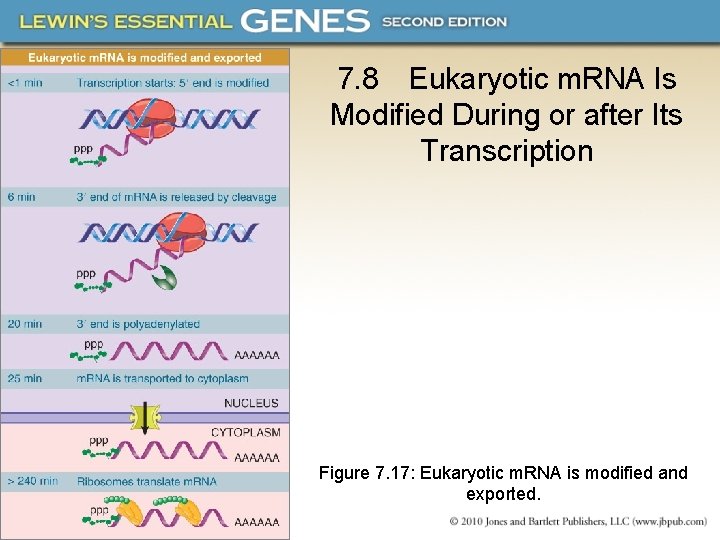
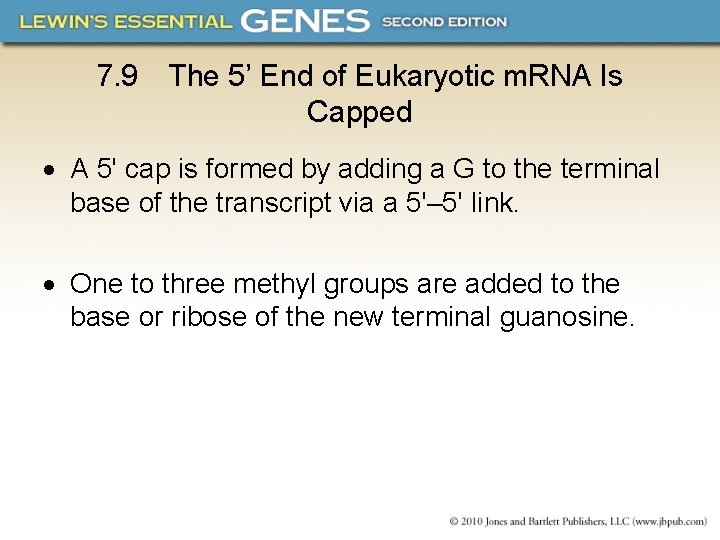
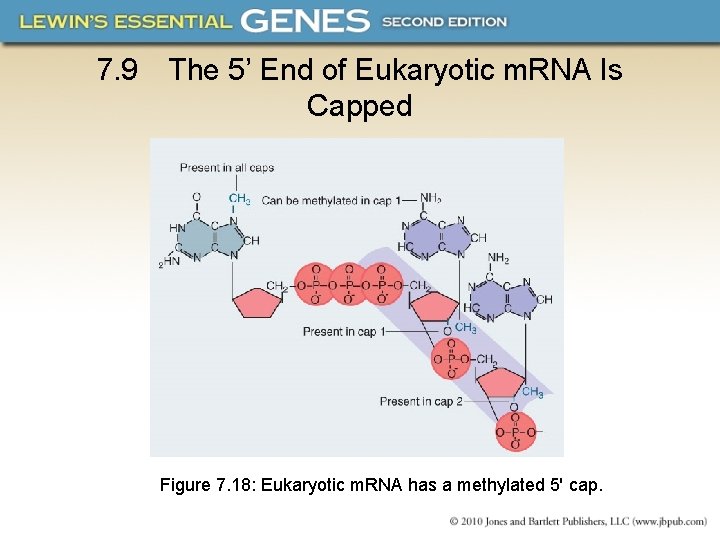
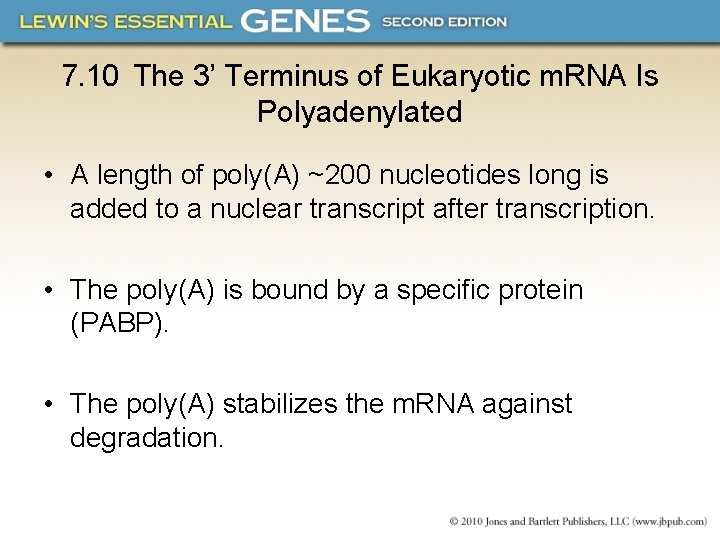
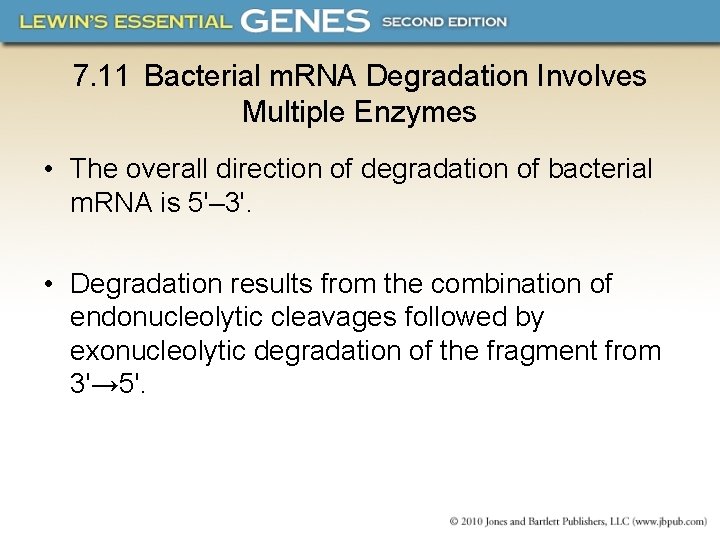
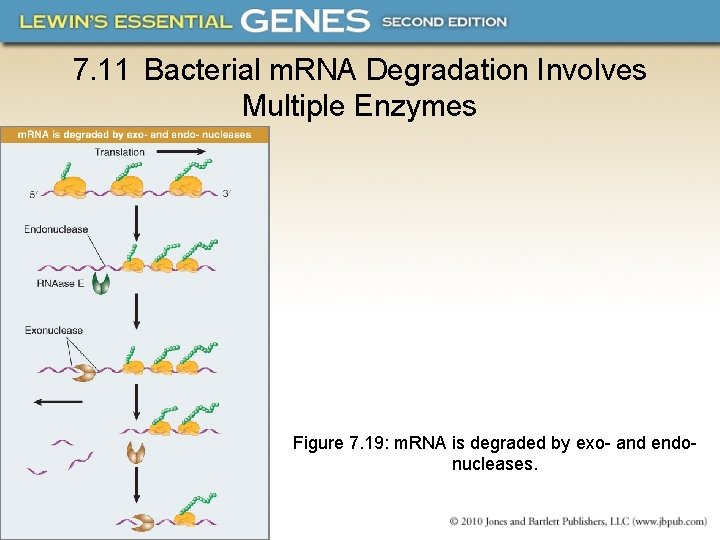
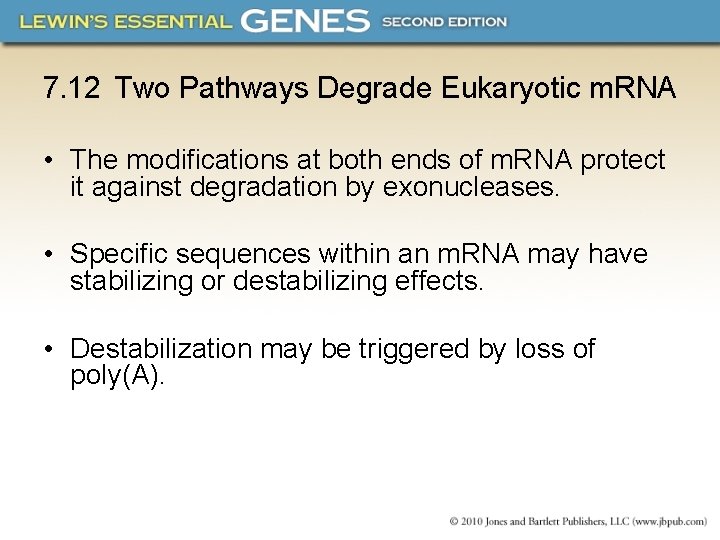
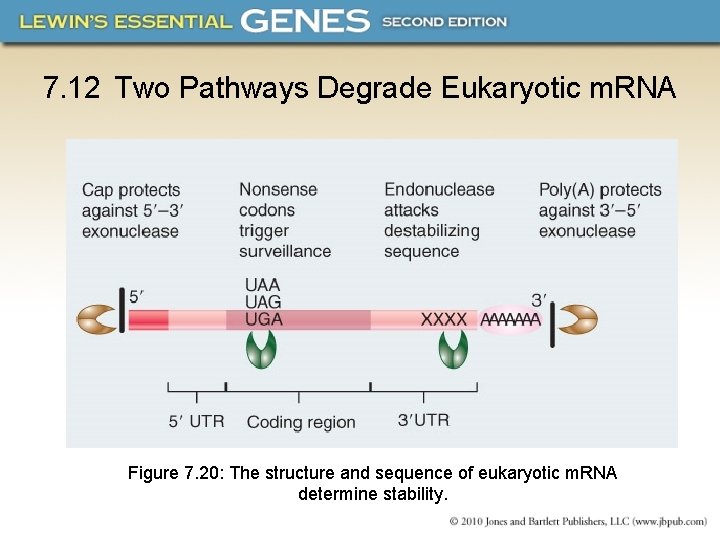
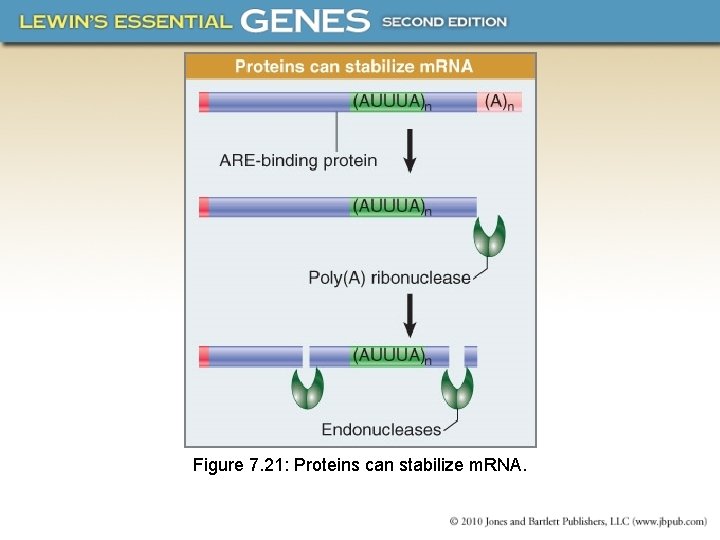
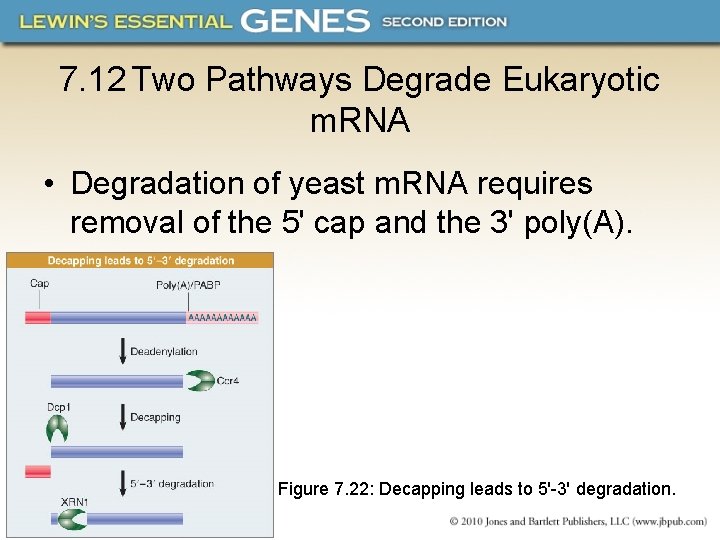
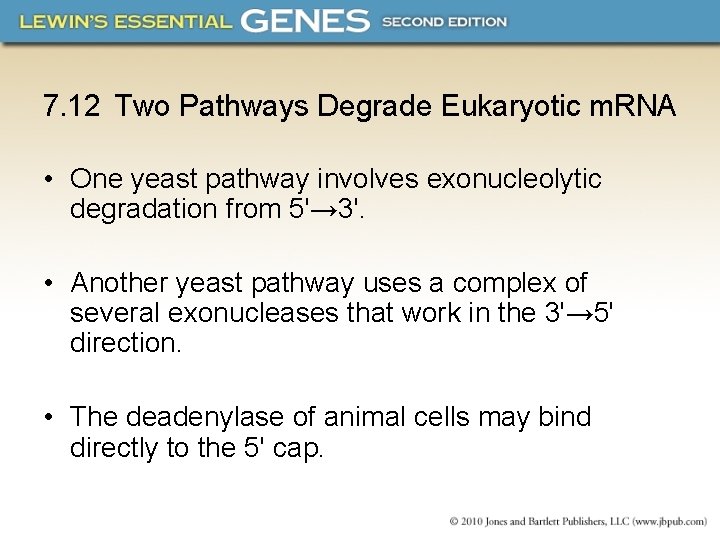
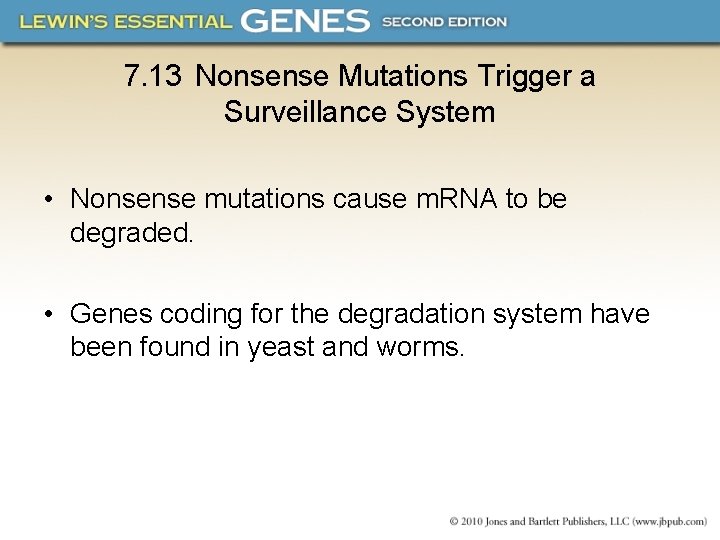
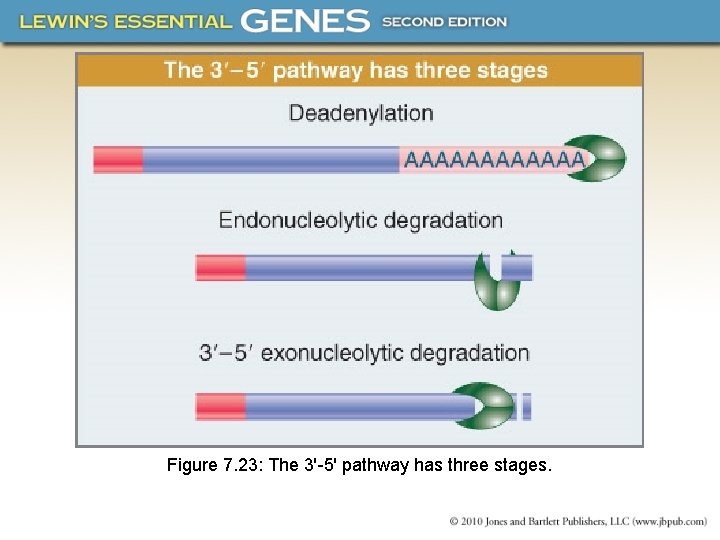
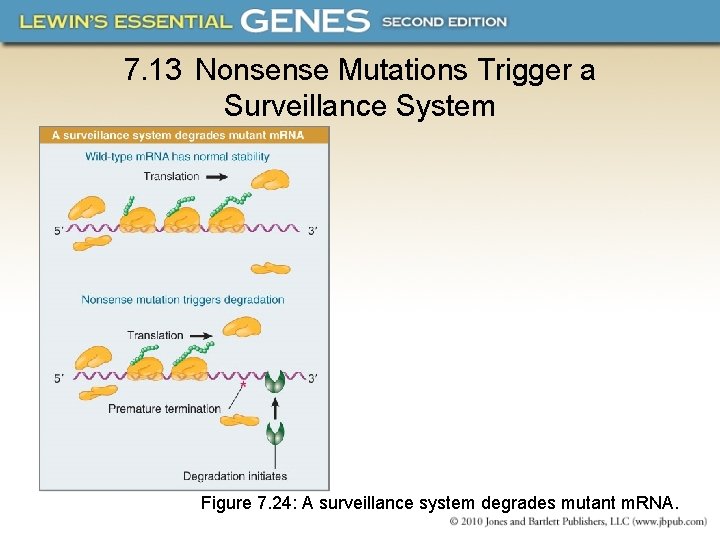
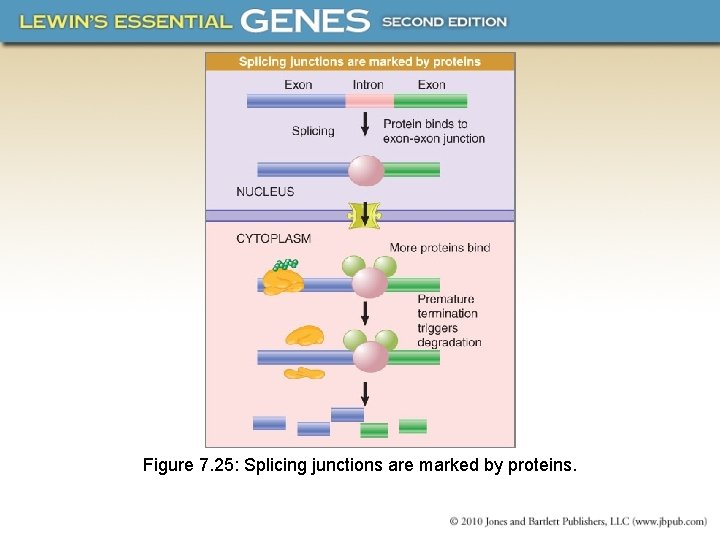
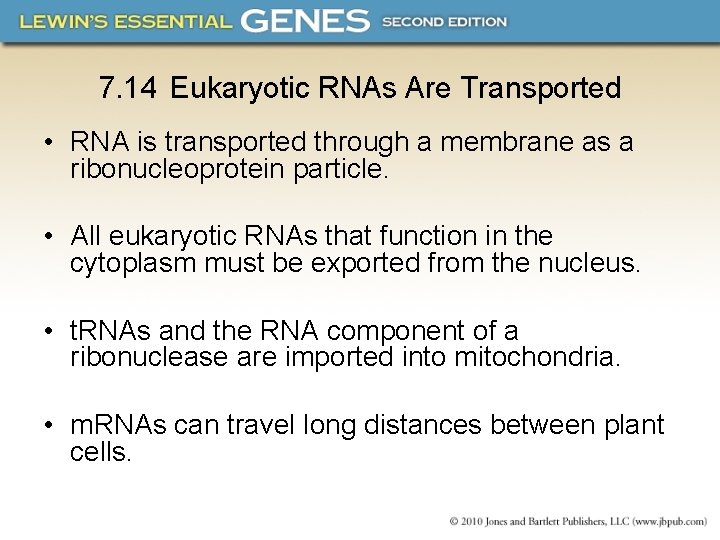
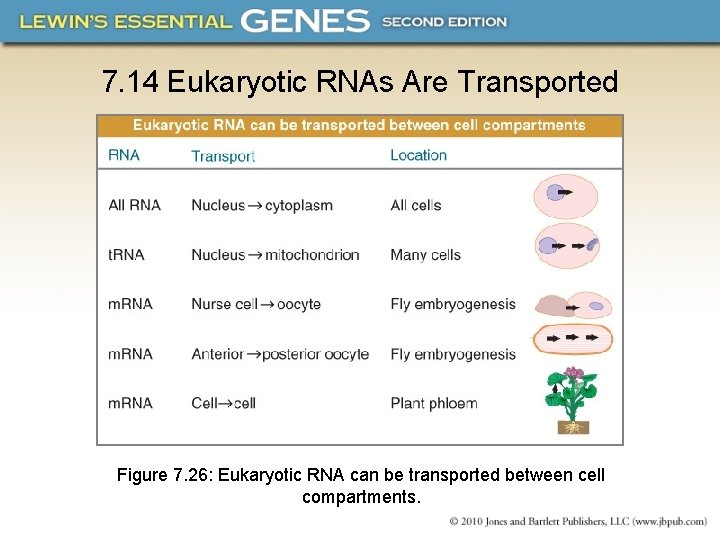
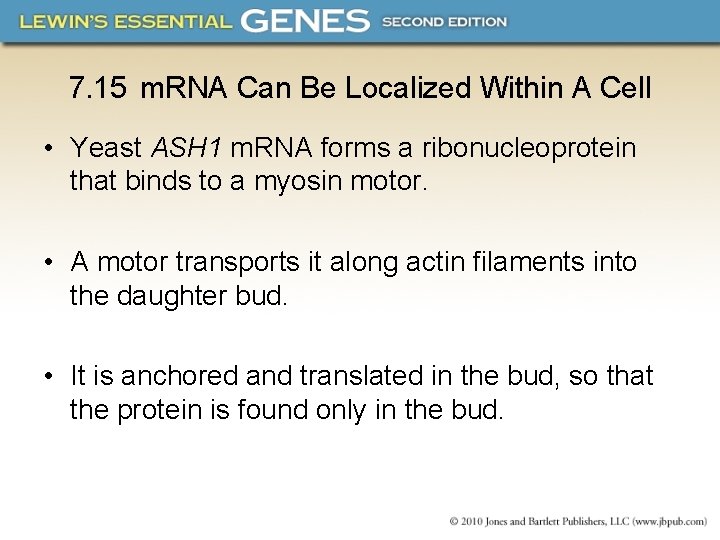
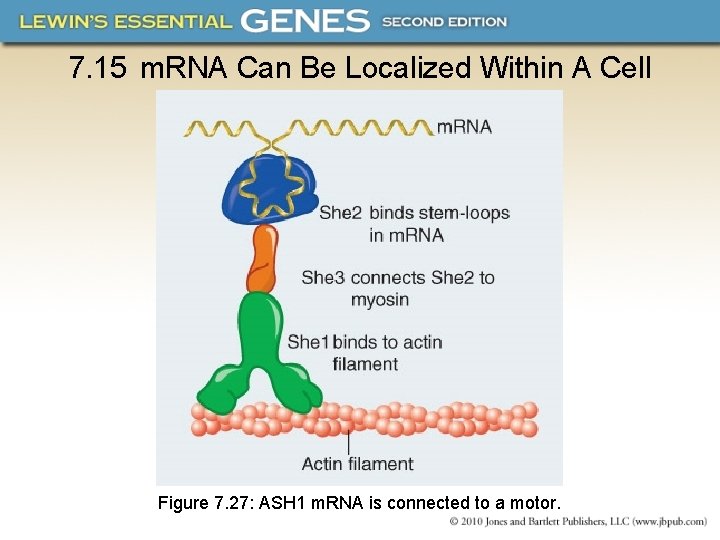
- Slides: 40
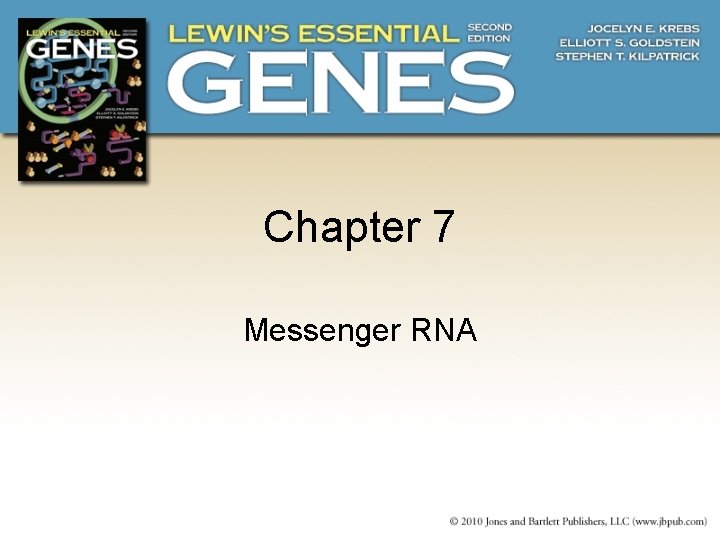
Chapter 7 Messenger RNA
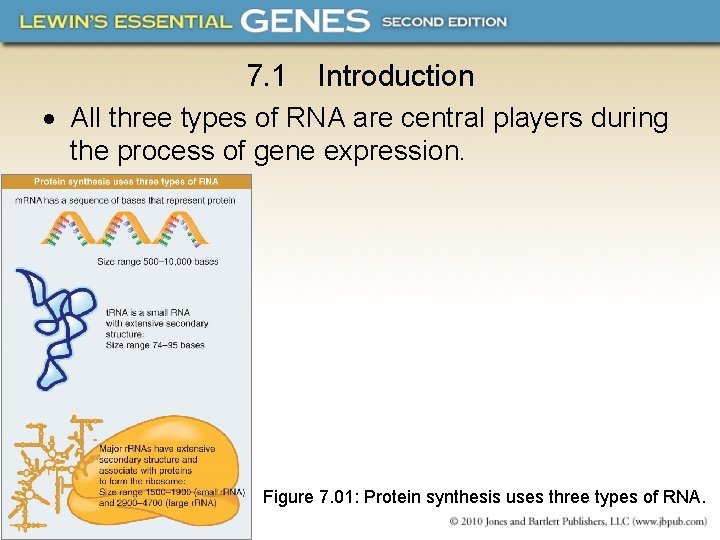
7. 1 Introduction All three types of RNA are central players during the process of gene expression. Figure 7. 01: Protein synthesis uses three types of RNA.
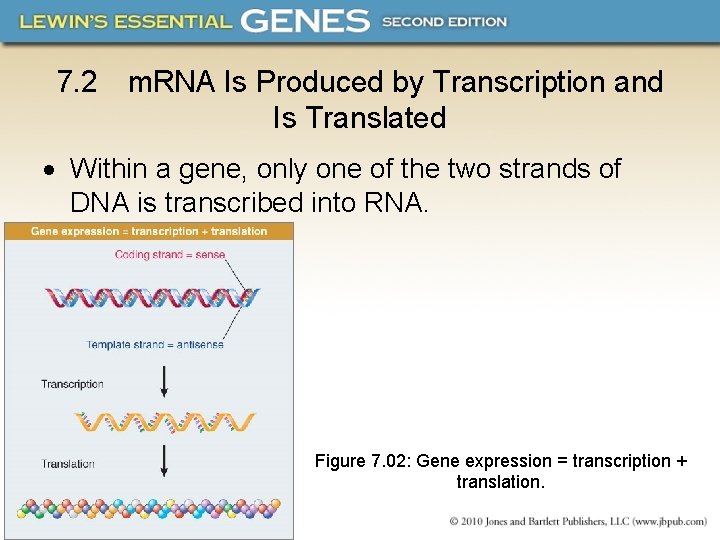
7. 2 m. RNA Is Produced by Transcription and Is Translated Within a gene, only one of the two strands of DNA is transcribed into RNA. Figure 7. 02: Gene expression = transcription + translation.
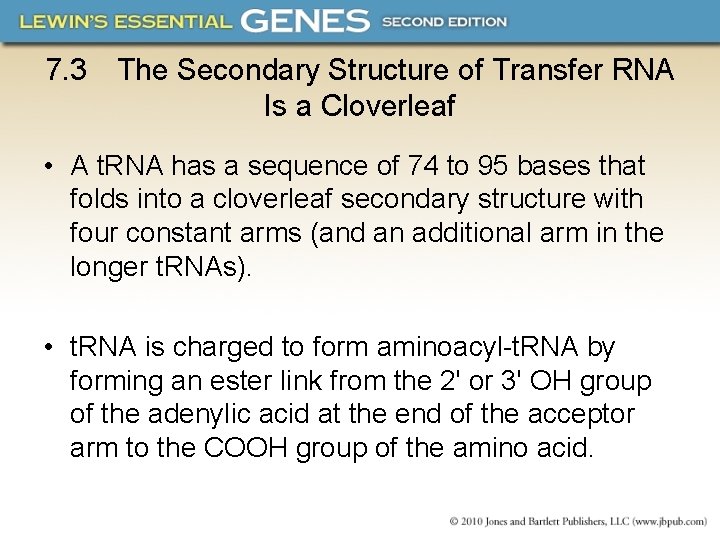
7. 3 The Secondary Structure of Transfer RNA Is a Cloverleaf • A t. RNA has a sequence of 74 to 95 bases that folds into a cloverleaf secondary structure with four constant arms (and an additional arm in the longer t. RNAs). • t. RNA is charged to form aminoacyl-t. RNA by forming an ester link from the 2' or 3' OH group of the adenylic acid at the end of the acceptor arm to the COOH group of the amino acid.
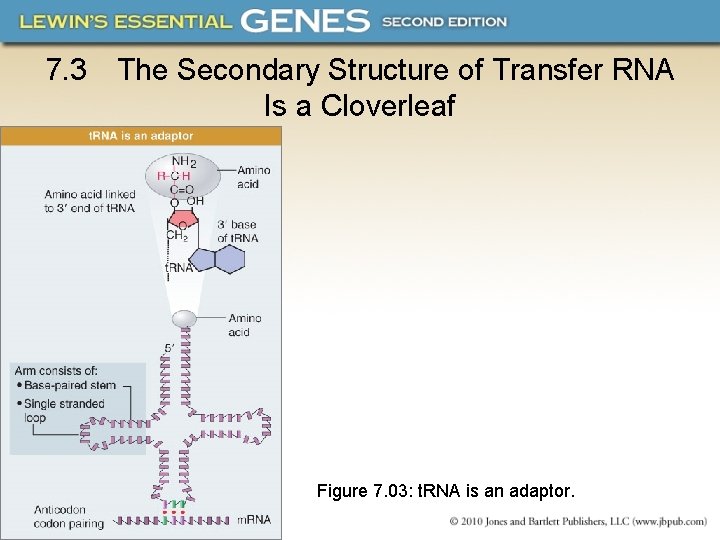
7. 3 The Secondary Structure of Transfer RNA Is a Cloverleaf Figure 7. 03: t. RNA is an adaptor.
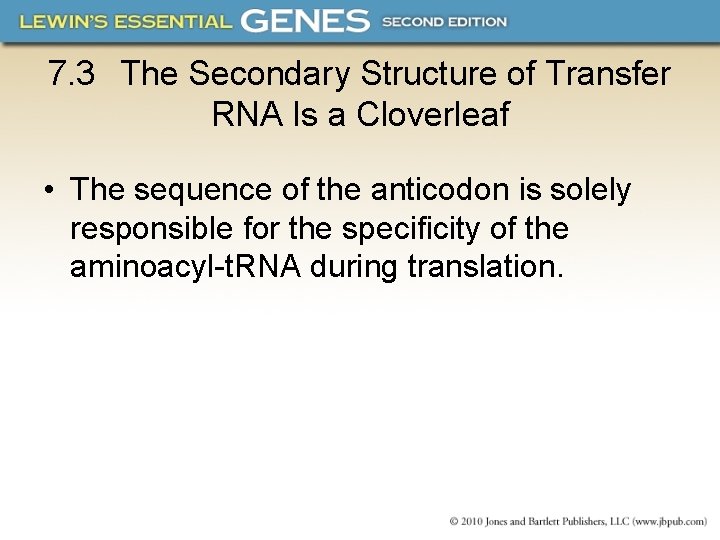
7. 3 The Secondary Structure of Transfer RNA Is a Cloverleaf • The sequence of the anticodon is solely responsible for the specificity of the aminoacyl-t. RNA during translation.
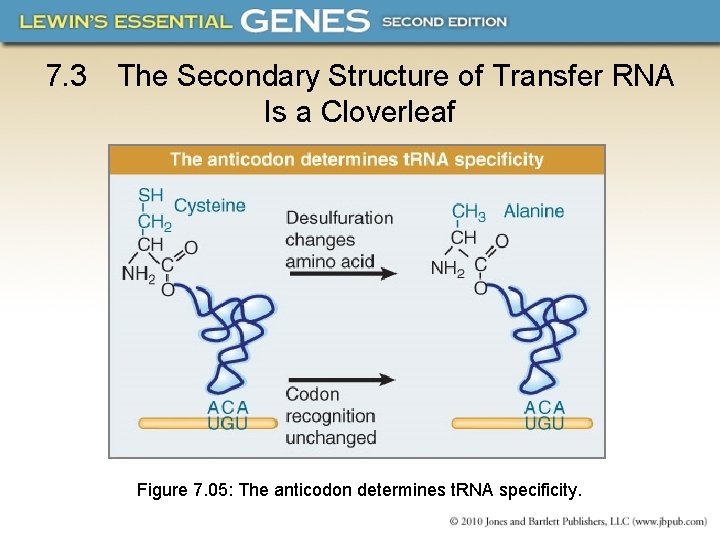
7. 3 The Secondary Structure of Transfer RNA Is a Cloverleaf Figure 7. 05: The anticodon determines t. RNA specificity.
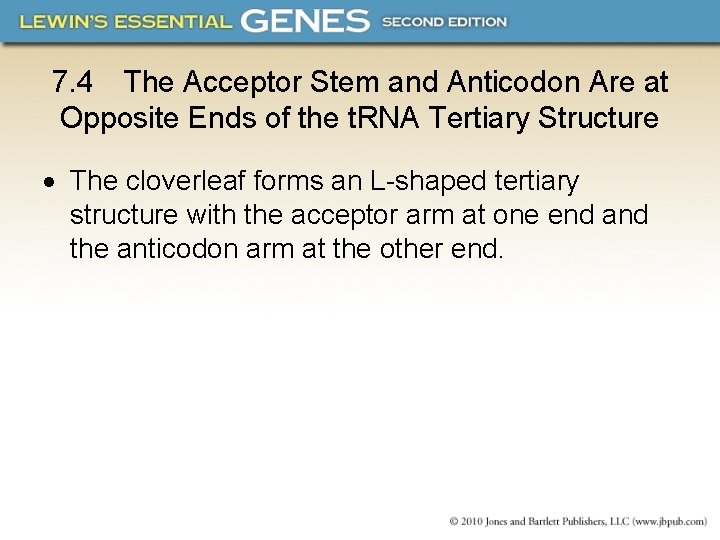
7. 4 The Acceptor Stem and Anticodon Are at Opposite Ends of the t. RNA Tertiary Structure The cloverleaf forms an L-shaped tertiary structure with the acceptor arm at one end and the anticodon arm at the other end.
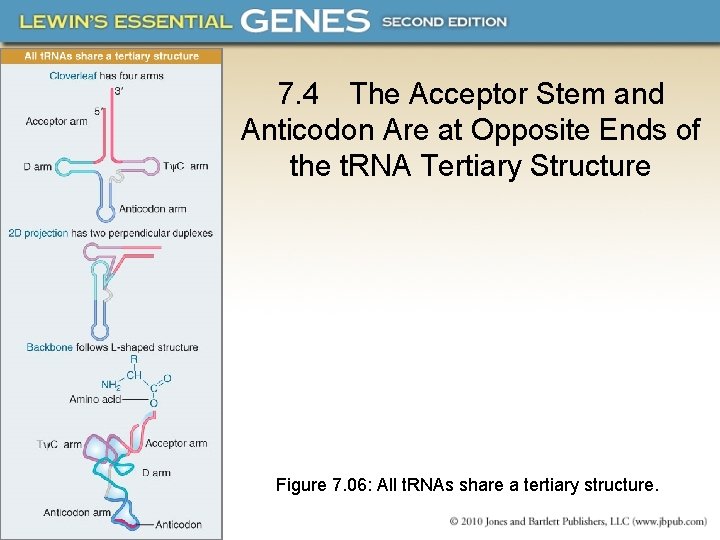
7. 4 The Acceptor Stem and Anticodon Are at Opposite Ends of the t. RNA Tertiary Structure Figure 7. 06: All t. RNAs share a tertiary structure.
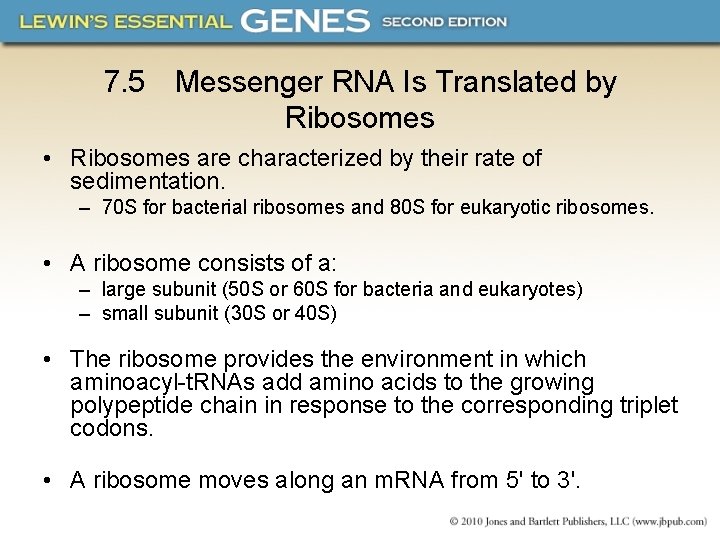
7. 5 Messenger RNA Is Translated by Ribosomes • Ribosomes are characterized by their rate of sedimentation. – 70 S for bacterial ribosomes and 80 S for eukaryotic ribosomes. • A ribosome consists of a: – large subunit (50 S or 60 S for bacteria and eukaryotes) – small subunit (30 S or 40 S) • The ribosome provides the environment in which aminoacyl-t. RNAs add amino acids to the growing polypeptide chain in response to the corresponding triplet codons. • A ribosome moves along an m. RNA from 5' to 3'.
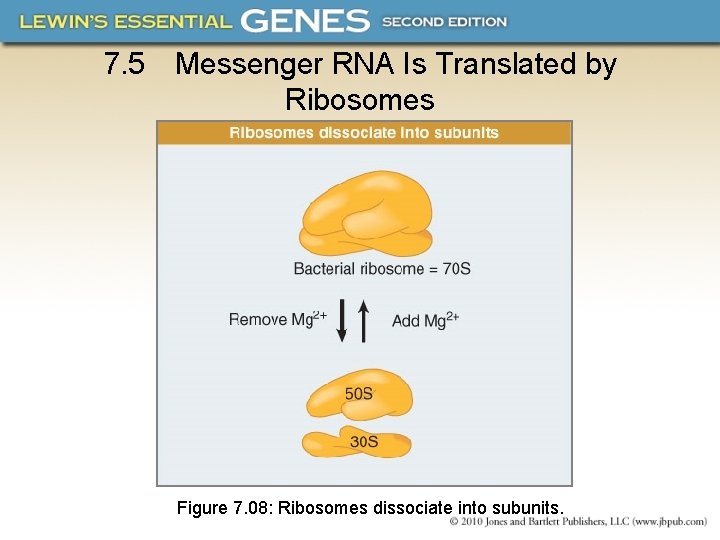
7. 5 Messenger RNA Is Translated by Ribosomes Figure 7. 08: Ribosomes dissociate into subunits.
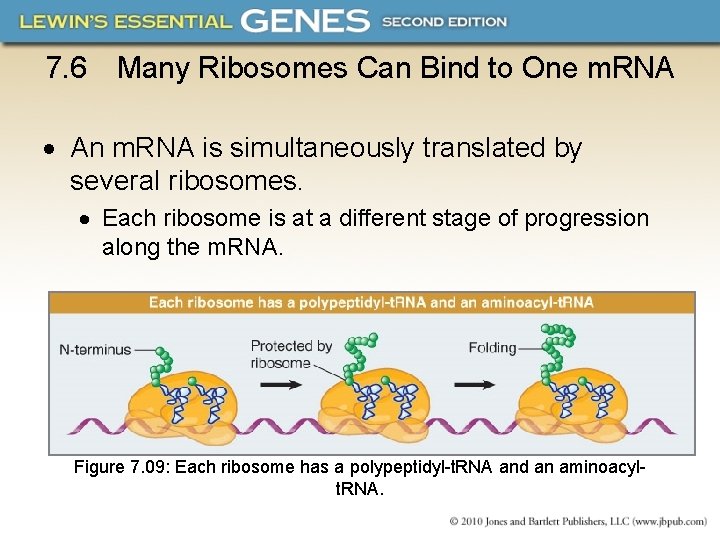
7. 6 Many Ribosomes Can Bind to One m. RNA An m. RNA is simultaneously translated by several ribosomes. Each ribosome is at a different stage of progression along the m. RNA. Figure 7. 09: Each ribosome has a polypeptidyl-t. RNA and an aminoacylt. RNA.
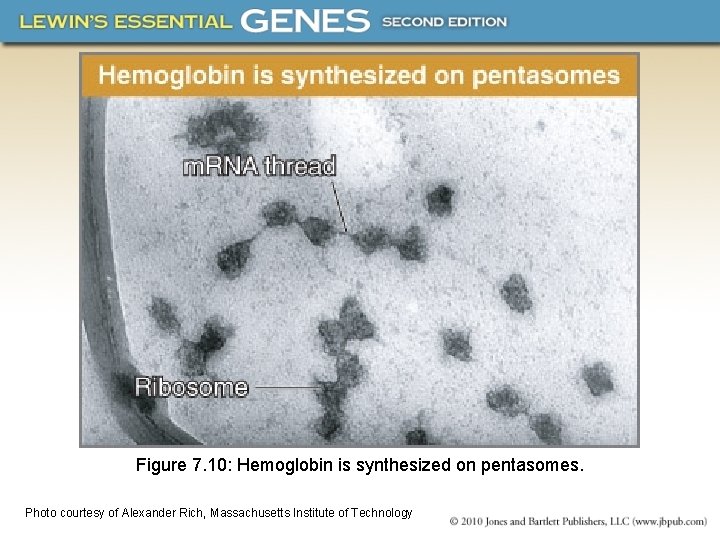
Figure 7. 10: Hemoglobin is synthesized on pentasomes. Photo courtesy of Alexander Rich, Massachusetts Institute of Technology
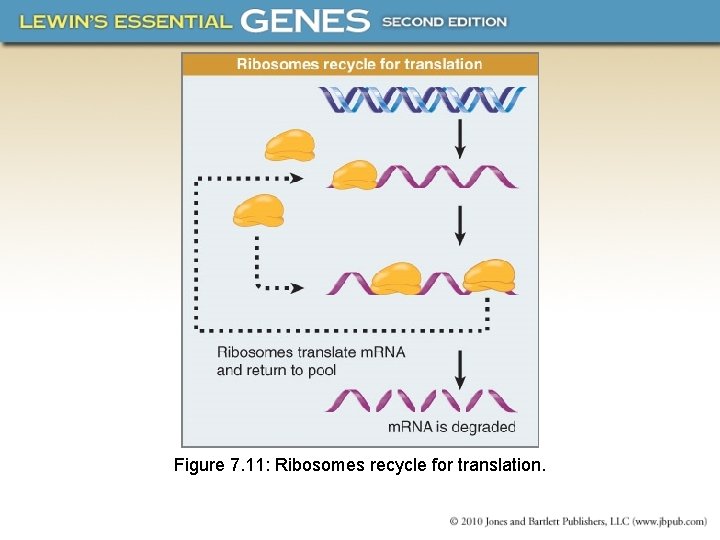
Figure 7. 11: Ribosomes recycle for translation.
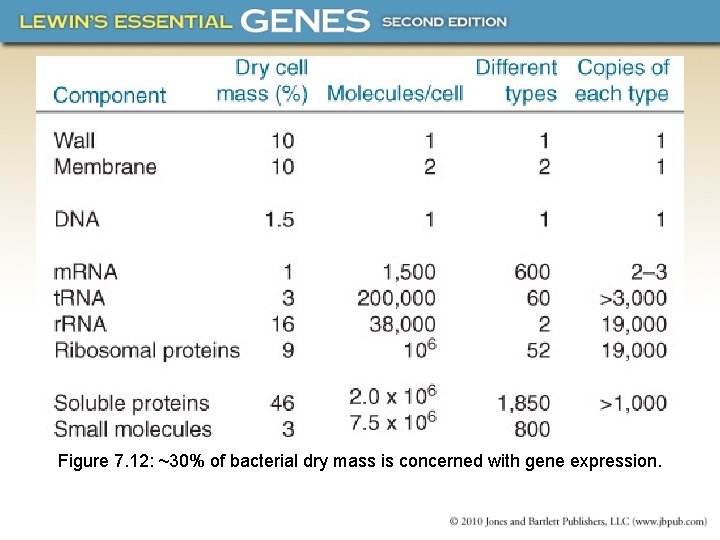
Figure 7. 12: ~30% of bacterial dry mass is concerned with gene expression.
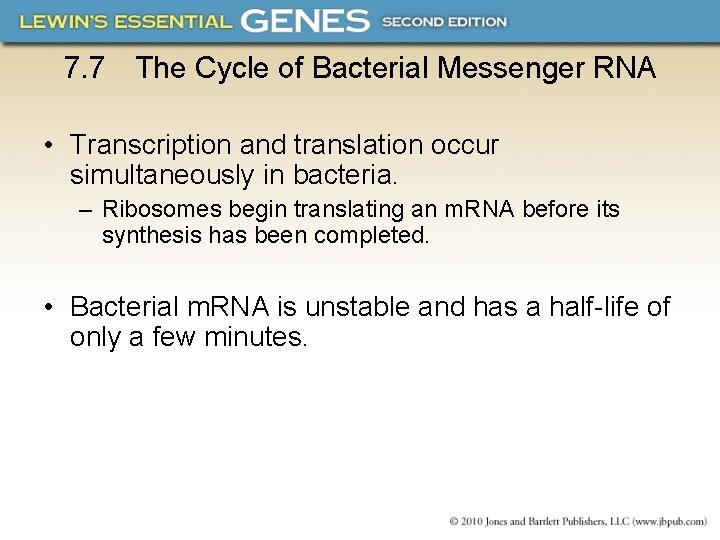
7. 7 The Cycle of Bacterial Messenger RNA • Transcription and translation occur simultaneously in bacteria. – Ribosomes begin translating an m. RNA before its synthesis has been completed. • Bacterial m. RNA is unstable and has a half-life of only a few minutes.
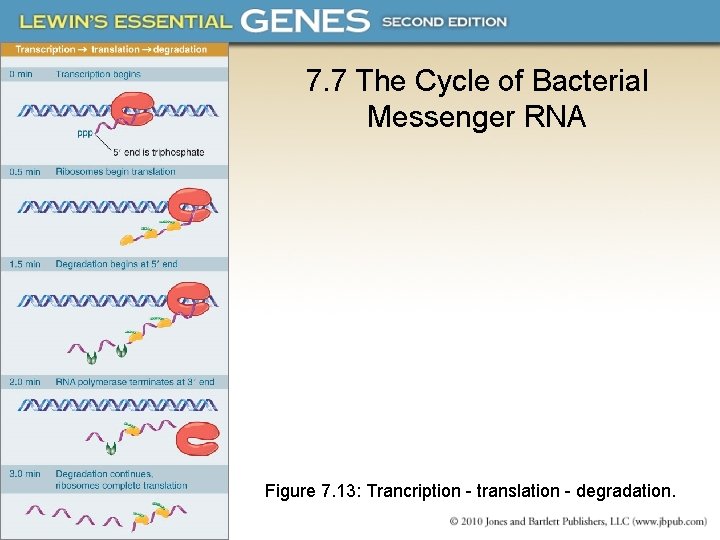
7. 7 The Cycle of Bacterial Messenger RNA Figure 7. 13: Trancription - translation - degradation.
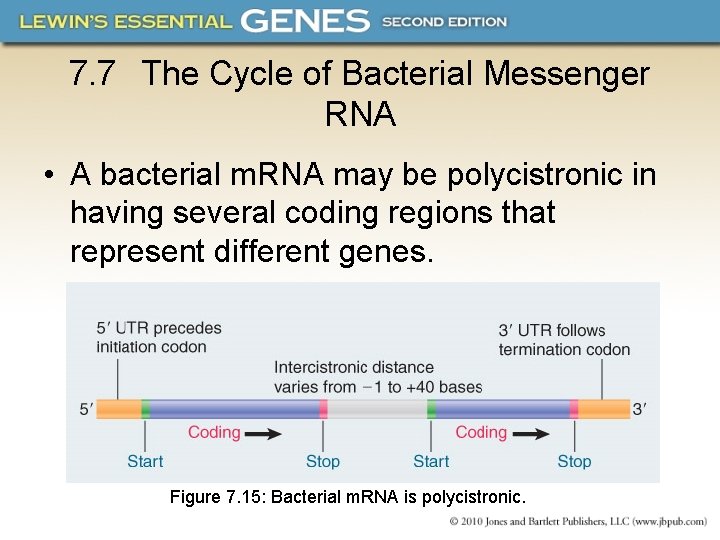
7. 7 The Cycle of Bacterial Messenger RNA • A bacterial m. RNA may be polycistronic in having several coding regions that represent different genes. Figure 7. 15: Bacterial m. RNA is polycistronic.
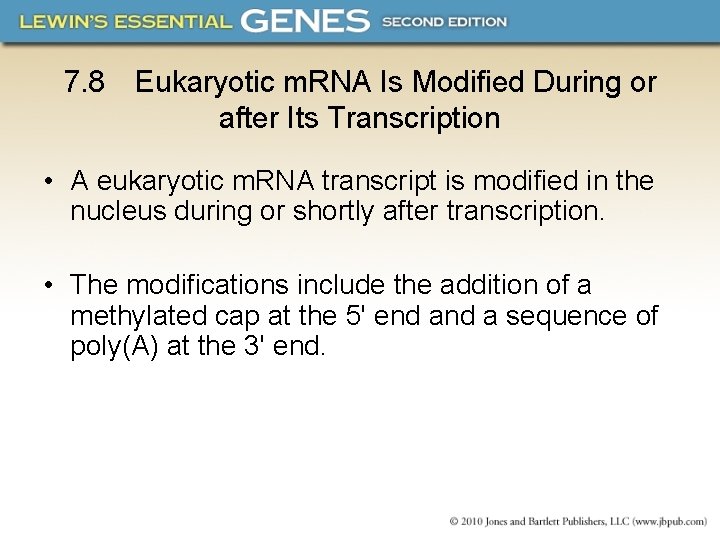
7. 8 Eukaryotic m. RNA Is Modified During or after Its Transcription • A eukaryotic m. RNA transcript is modified in the nucleus during or shortly after transcription. • The modifications include the addition of a methylated cap at the 5' end a sequence of poly(A) at the 3' end.
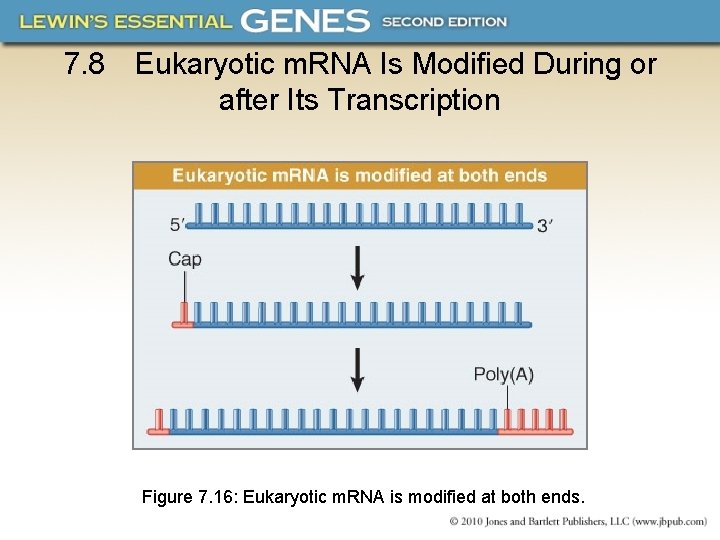
7. 8 Eukaryotic m. RNA Is Modified During or after Its Transcription Figure 7. 16: Eukaryotic m. RNA is modified at both ends.
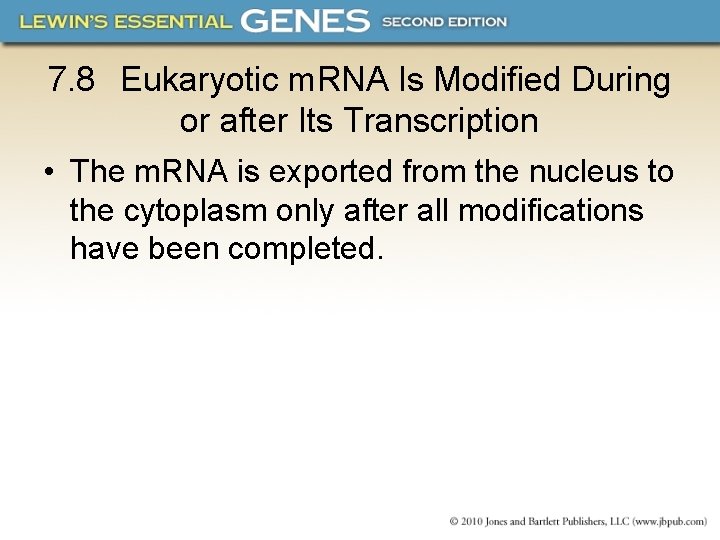
7. 8 Eukaryotic m. RNA Is Modified During or after Its Transcription • The m. RNA is exported from the nucleus to the cytoplasm only after all modifications have been completed.
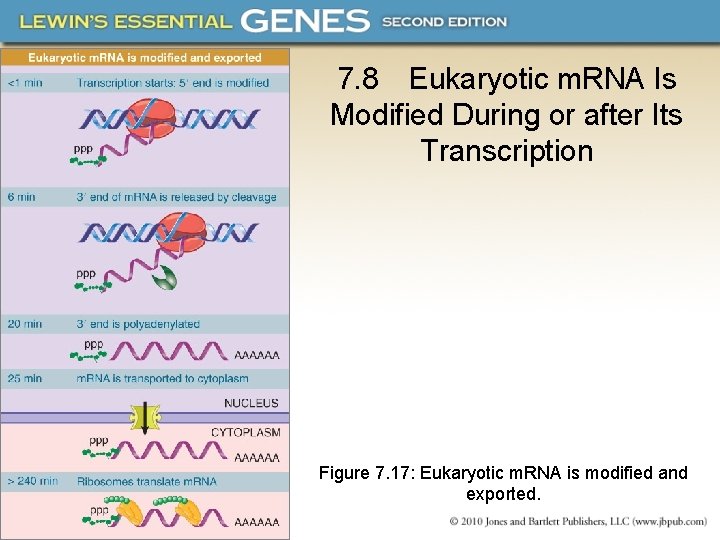
7. 8 Eukaryotic m. RNA Is Modified During or after Its Transcription Figure 7. 17: Eukaryotic m. RNA is modified and exported.
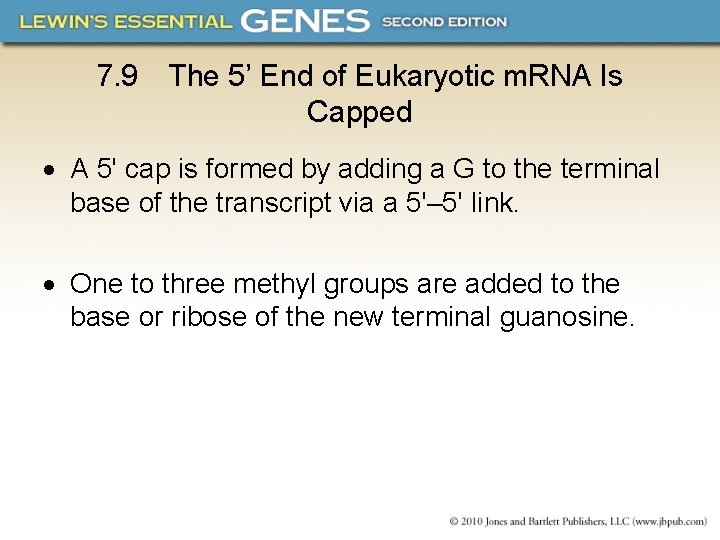
7. 9 The 5’ End of Eukaryotic m. RNA Is Capped A 5' cap is formed by adding a G to the terminal base of the transcript via a 5'– 5' link. One to three methyl groups are added to the base or ribose of the new terminal guanosine.
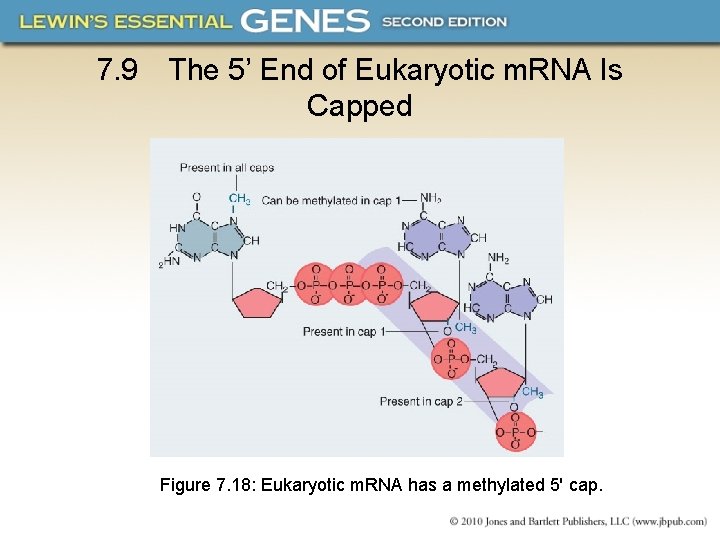
7. 9 The 5’ End of Eukaryotic m. RNA Is Capped Figure 7. 18: Eukaryotic m. RNA has a methylated 5' cap.
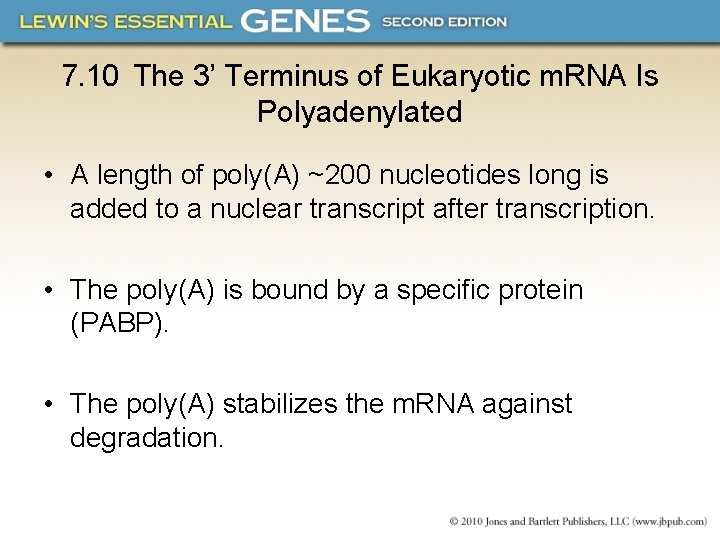
7. 10 The 3’ Terminus of Eukaryotic m. RNA Is Polyadenylated • A length of poly(A) ~200 nucleotides long is added to a nuclear transcript after transcription. • The poly(A) is bound by a specific protein (PABP). • The poly(A) stabilizes the m. RNA against degradation.
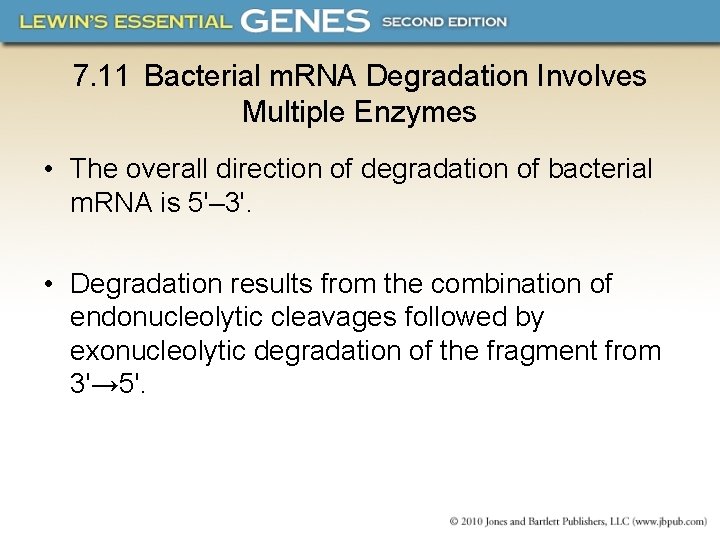
7. 11 Bacterial m. RNA Degradation Involves Multiple Enzymes • The overall direction of degradation of bacterial m. RNA is 5'– 3'. • Degradation results from the combination of endonucleolytic cleavages followed by exonucleolytic degradation of the fragment from 3'→ 5'.
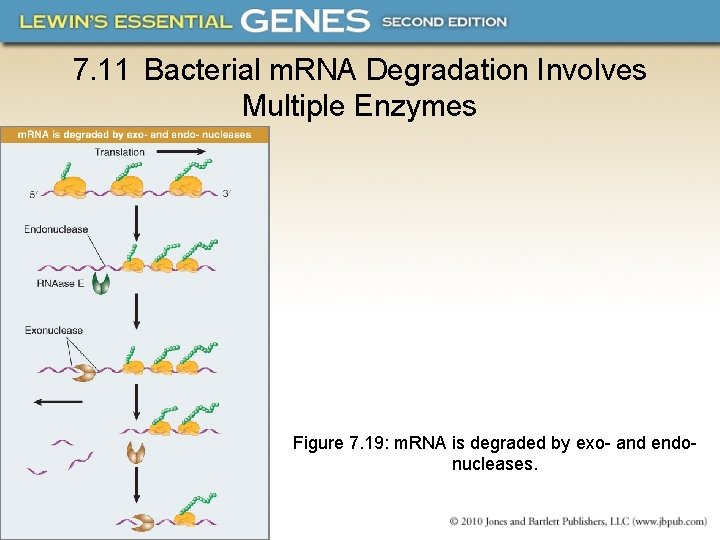
7. 11 Bacterial m. RNA Degradation Involves Multiple Enzymes Figure 7. 19: m. RNA is degraded by exo- and endonucleases.
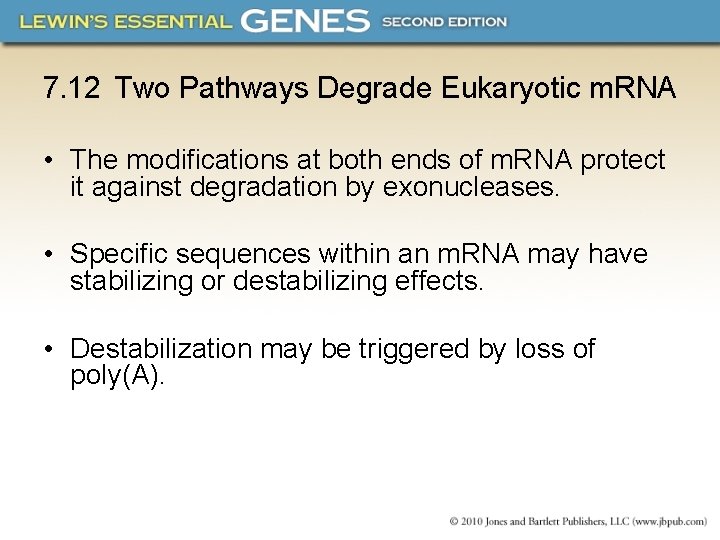
7. 12 Two Pathways Degrade Eukaryotic m. RNA • The modifications at both ends of m. RNA protect it against degradation by exonucleases. • Specific sequences within an m. RNA may have stabilizing or destabilizing effects. • Destabilization may be triggered by loss of poly(A).
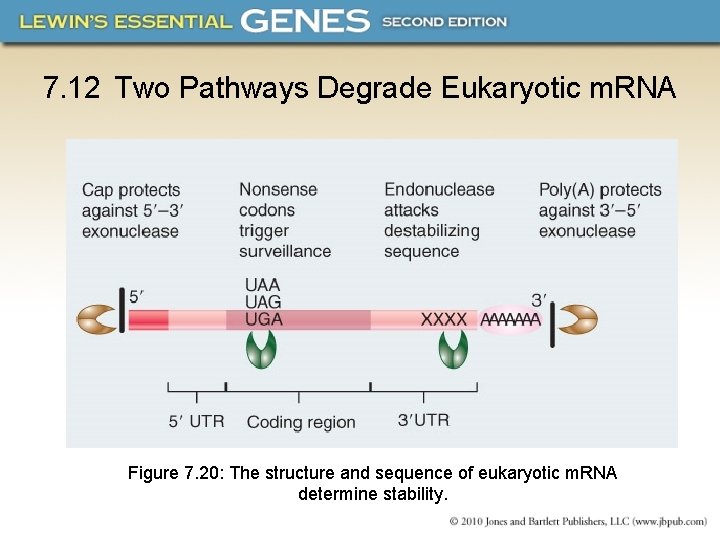
7. 12 Two Pathways Degrade Eukaryotic m. RNA Figure 7. 20: The structure and sequence of eukaryotic m. RNA determine stability.
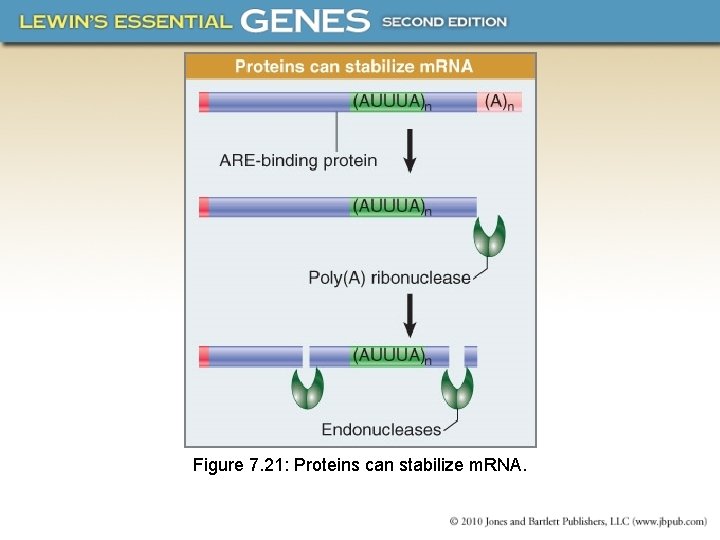
Figure 7. 21: Proteins can stabilize m. RNA.
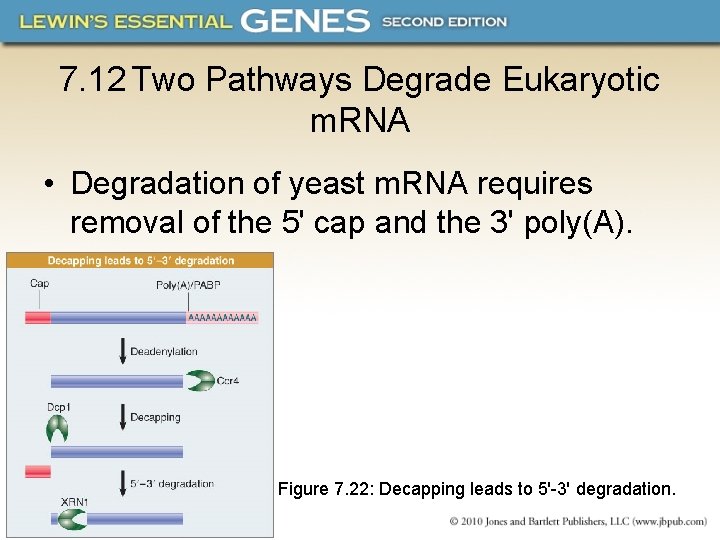
7. 12 Two Pathways Degrade Eukaryotic m. RNA • Degradation of yeast m. RNA requires removal of the 5' cap and the 3' poly(A). Figure 7. 22: Decapping leads to 5'-3' degradation.
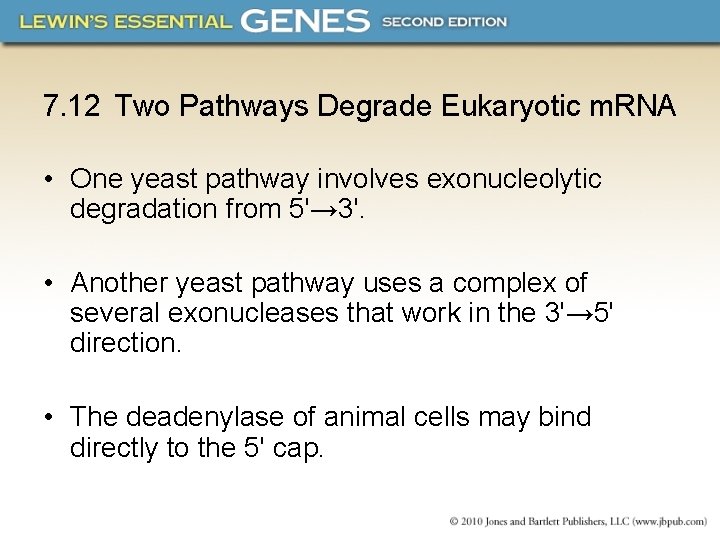
7. 12 Two Pathways Degrade Eukaryotic m. RNA • One yeast pathway involves exonucleolytic degradation from 5'→ 3'. • Another yeast pathway uses a complex of several exonucleases that work in the 3'→ 5' direction. • The deadenylase of animal cells may bind directly to the 5' cap.
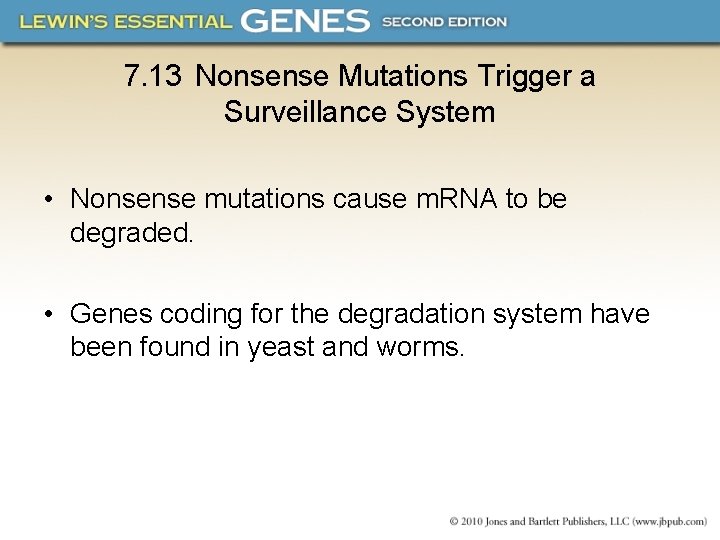
7. 13 Nonsense Mutations Trigger a Surveillance System • Nonsense mutations cause m. RNA to be degraded. • Genes coding for the degradation system have been found in yeast and worms.
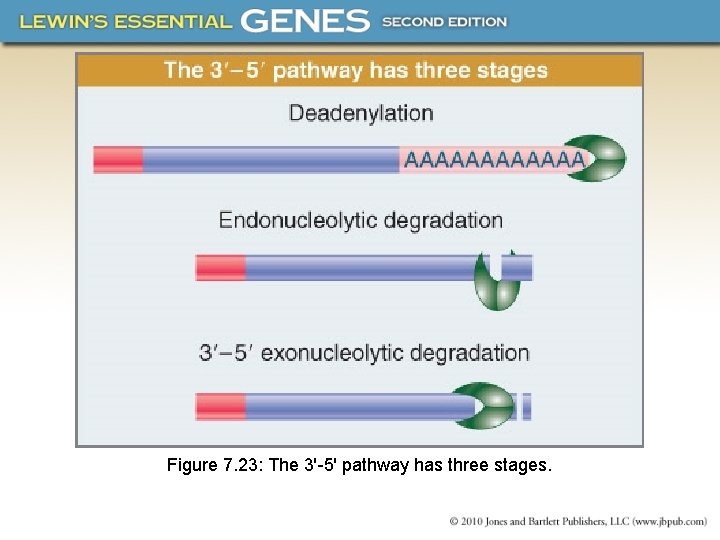
Figure 7. 23: The 3'-5' pathway has three stages.
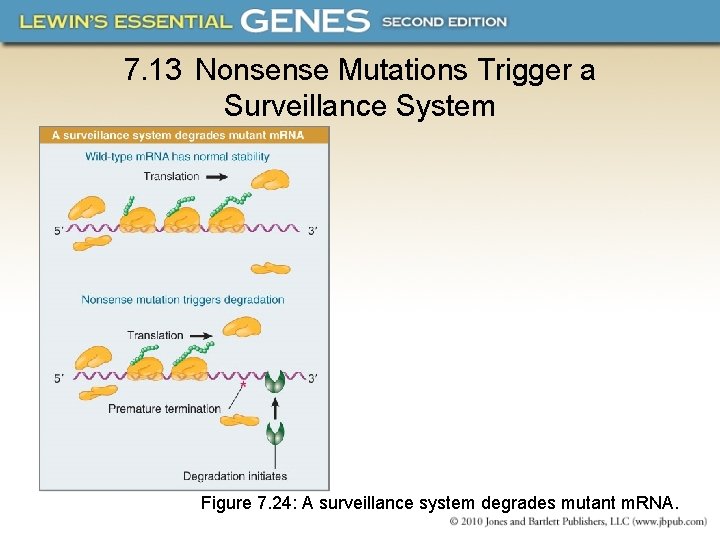
7. 13 Nonsense Mutations Trigger a Surveillance System Figure 7. 24: A surveillance system degrades mutant m. RNA.
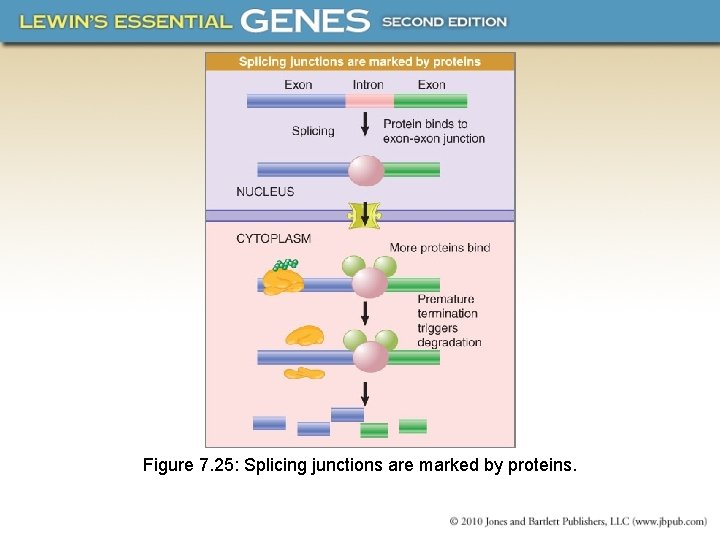
Figure 7. 25: Splicing junctions are marked by proteins.
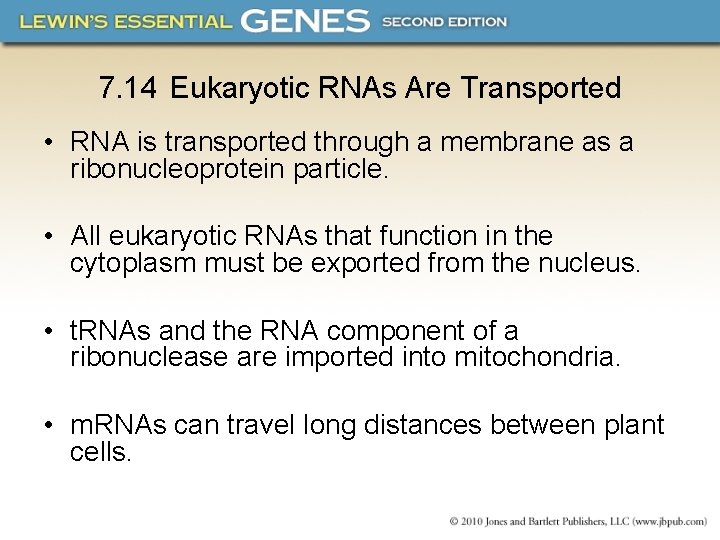
7. 14 Eukaryotic RNAs Are Transported • RNA is transported through a membrane as a ribonucleoprotein particle. • All eukaryotic RNAs that function in the cytoplasm must be exported from the nucleus. • t. RNAs and the RNA component of a ribonuclease are imported into mitochondria. • m. RNAs can travel long distances between plant cells.
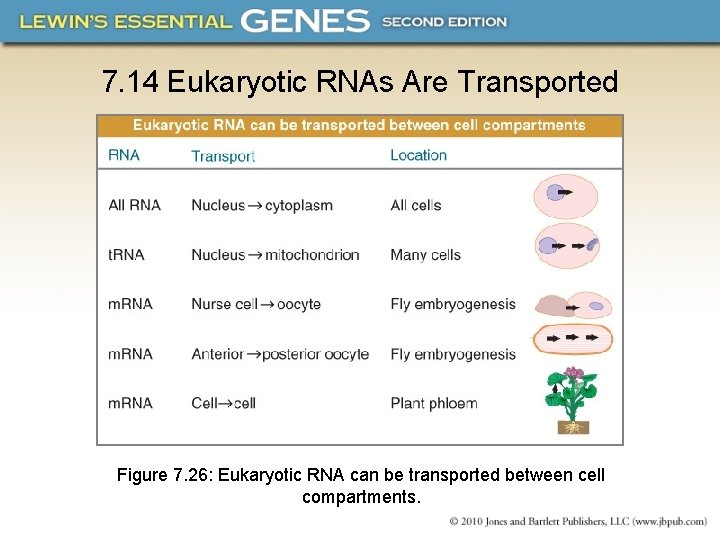
7. 14 Eukaryotic RNAs Are Transported Figure 7. 26: Eukaryotic RNA can be transported between cell compartments.
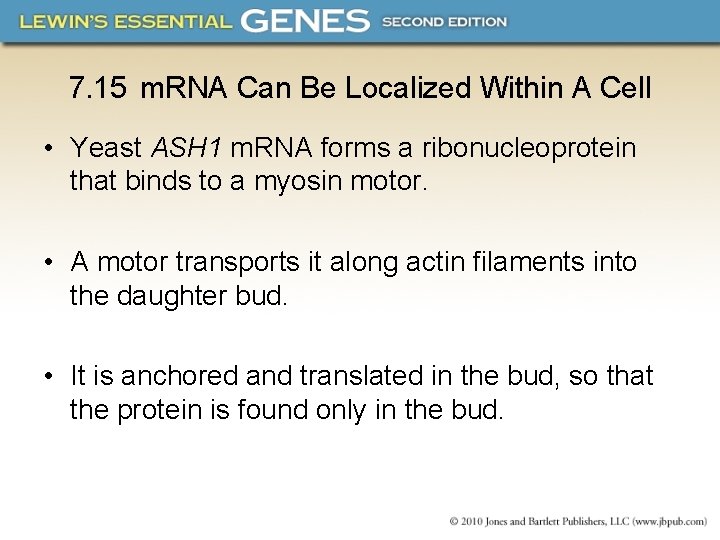
7. 15 m. RNA Can Be Localized Within A Cell • Yeast ASH 1 m. RNA forms a ribonucleoprotein that binds to a myosin motor. • A motor transports it along actin filaments into the daughter bud. • It is anchored and translated in the bud, so that the protein is found only in the bud.
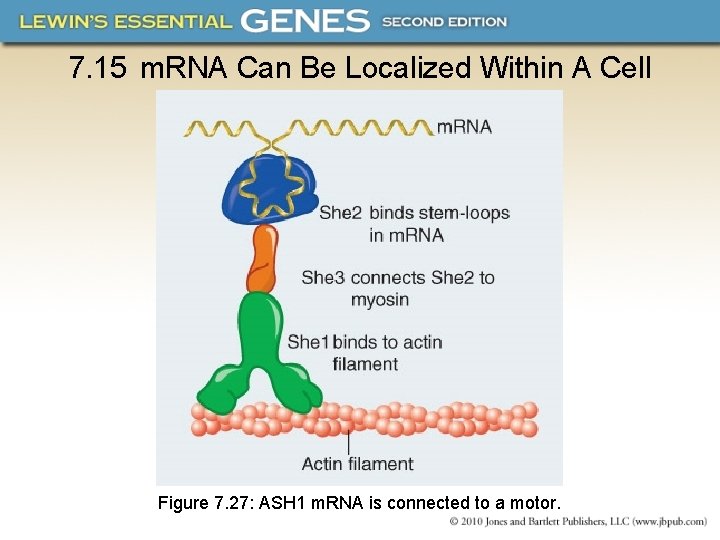
7. 15 m. RNA Can Be Localized Within A Cell Figure 7. 27: ASH 1 m. RNA is connected to a motor.
Messenger rna sequence
Gene interaction example
Transcription and translation
Messenger rna sequence
Protein synthesis
Name
Chapter 12 dna and rna
Chapter 12 section 3 dna rna and protein
Chapter grabber
On and off
Characterization ed the messenger
Greek messenger
Instant messenger msn
Squiggle chat
Whose great days are like ghosts gone
Messenger
Kairos messenger
Indeed in the messenger of allah
Angieville
Real life calculator
Msn messenger chat rooms
Msn messenger 7
Dynamic messenger
What does oedipus announce to the theban people
Jonah 1:2-12
Messenger of god planet
Acromegaly
Secondary messenger
Messenger
Rsview 32
Second messenger hypothesis
Distributed database projects source code
Messenger
Calculator messenger
Yahoo messenger
Histamine
The messenger trainer
Bebo messenger
Messenger
2 corinthians 12 7-10
Messenger brak ankiety