Atom Optics for Gravitational Wave Detection Holger Mller
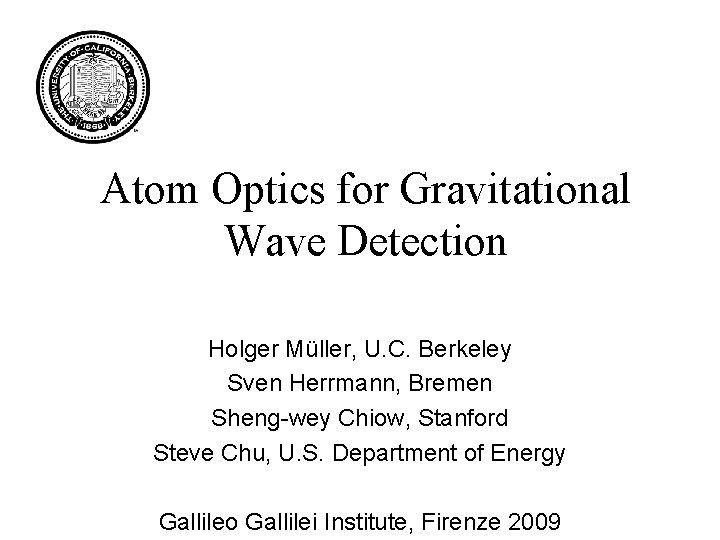
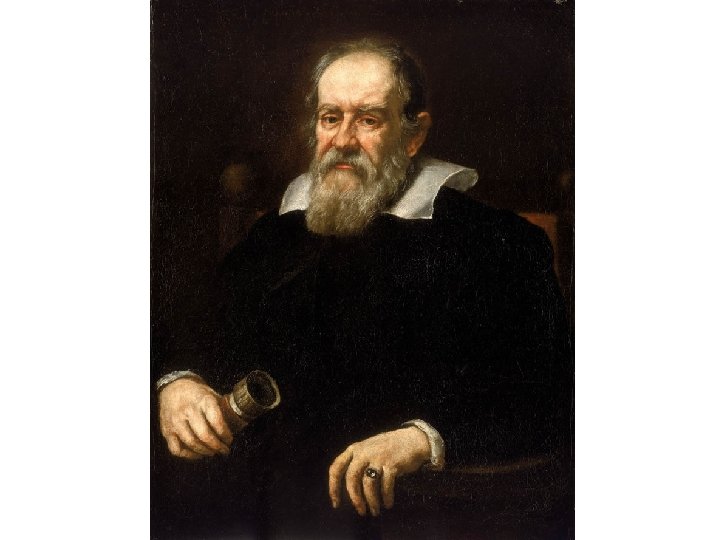
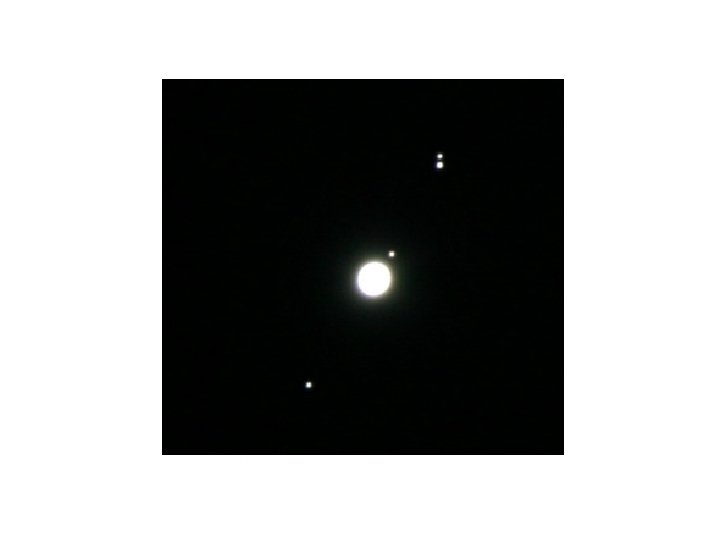
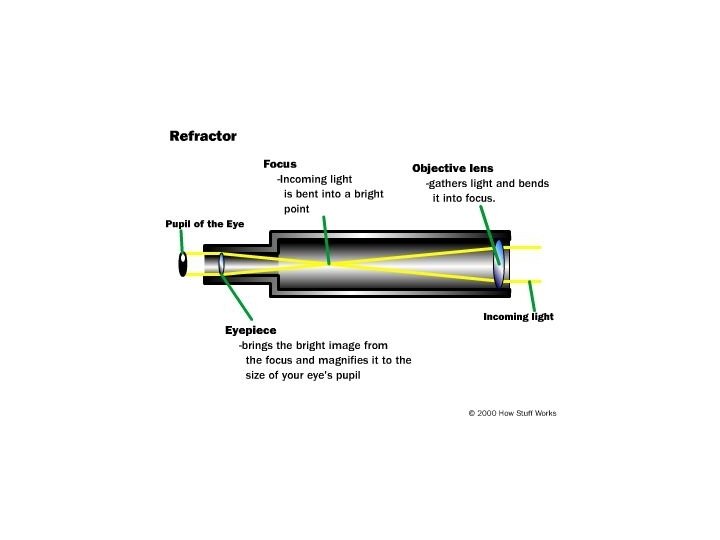
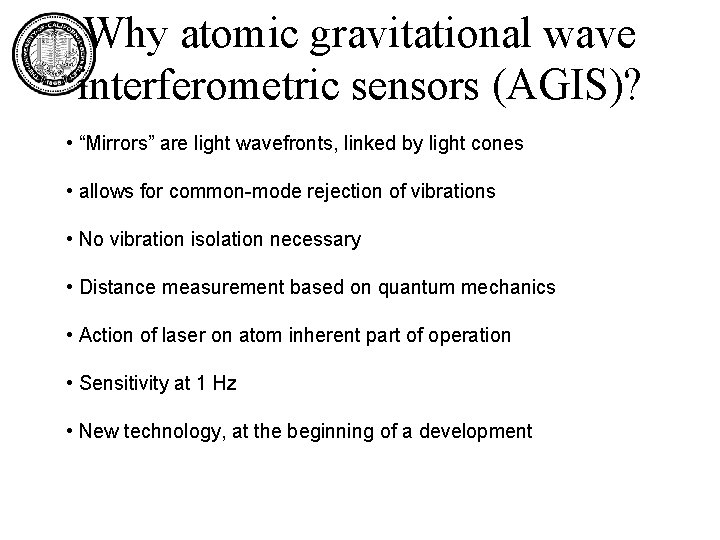
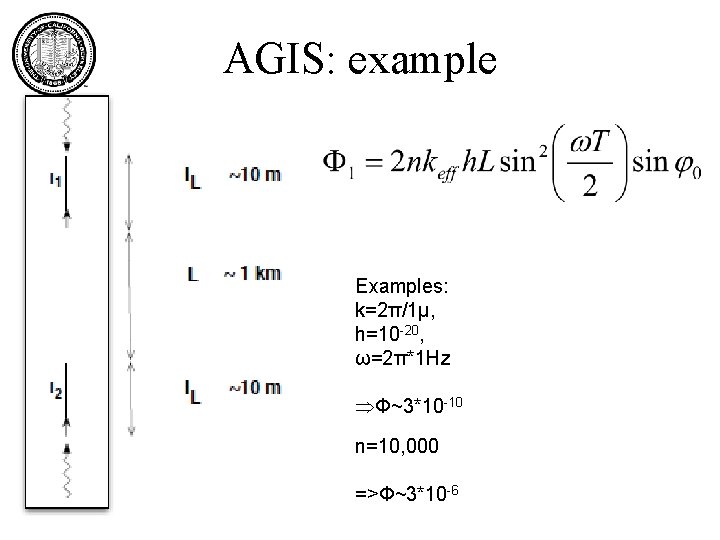
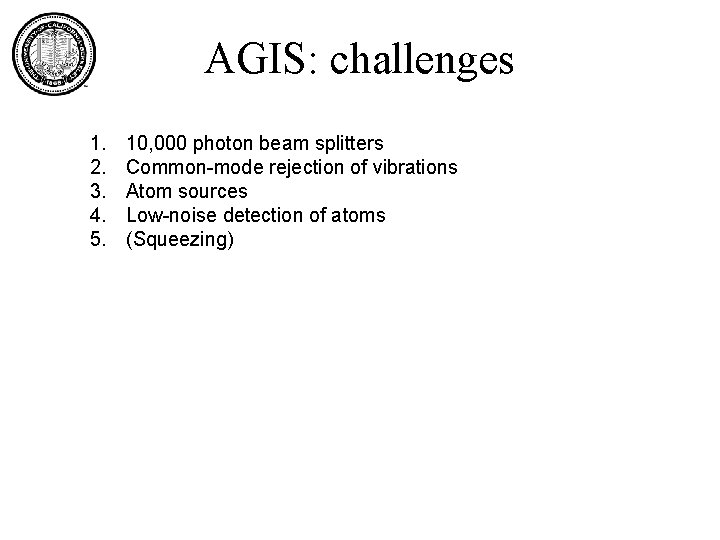
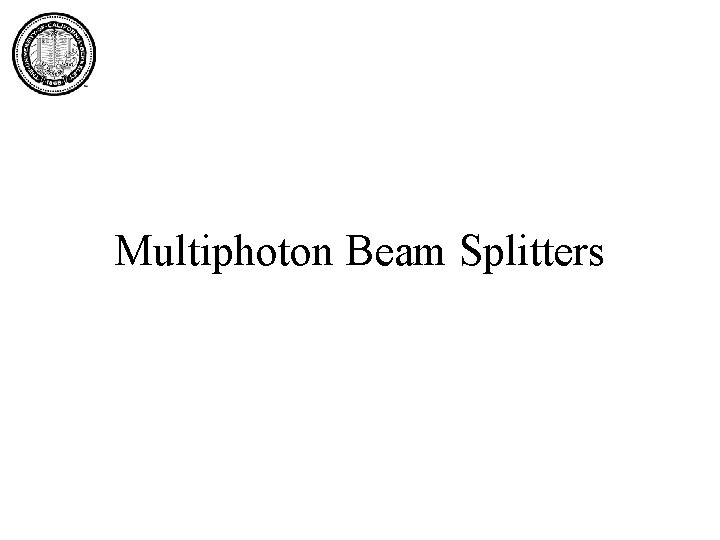
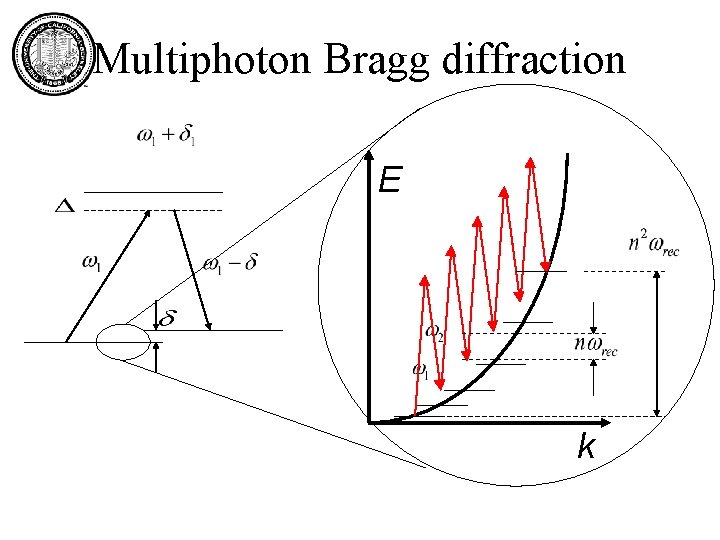
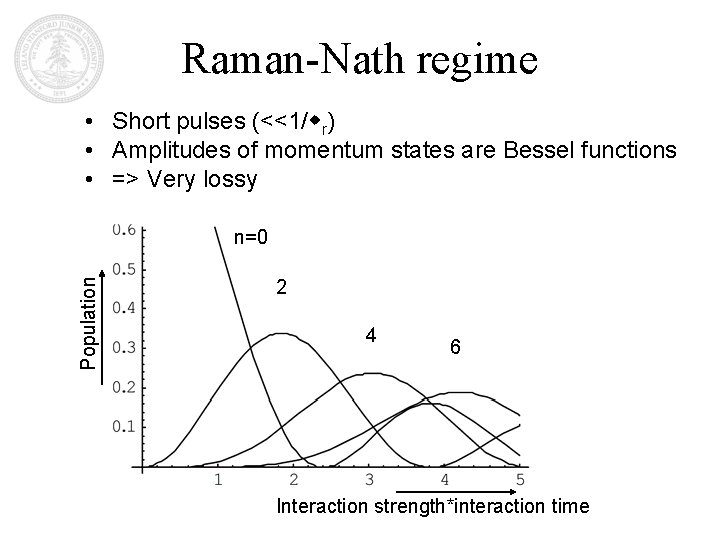
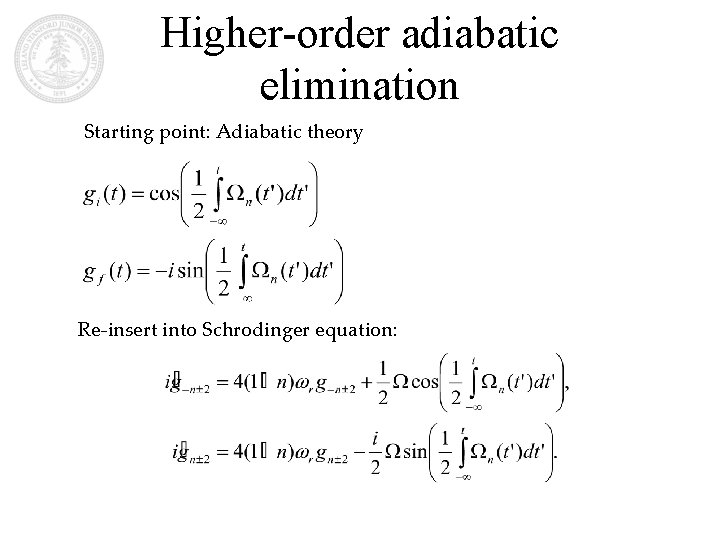
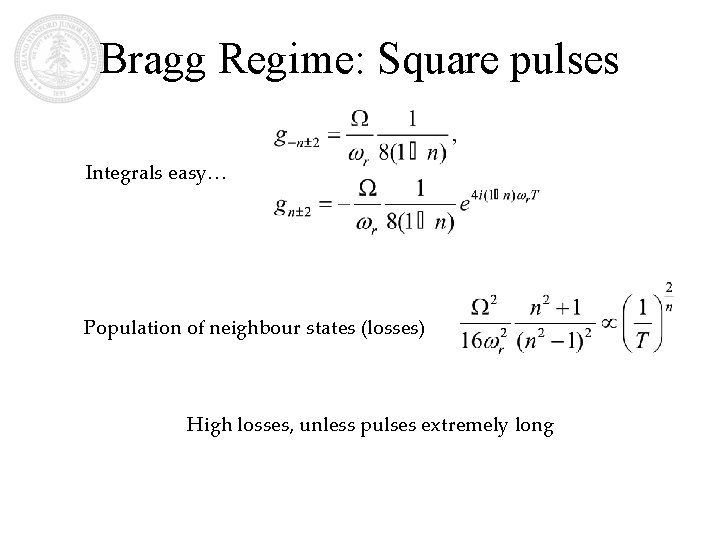
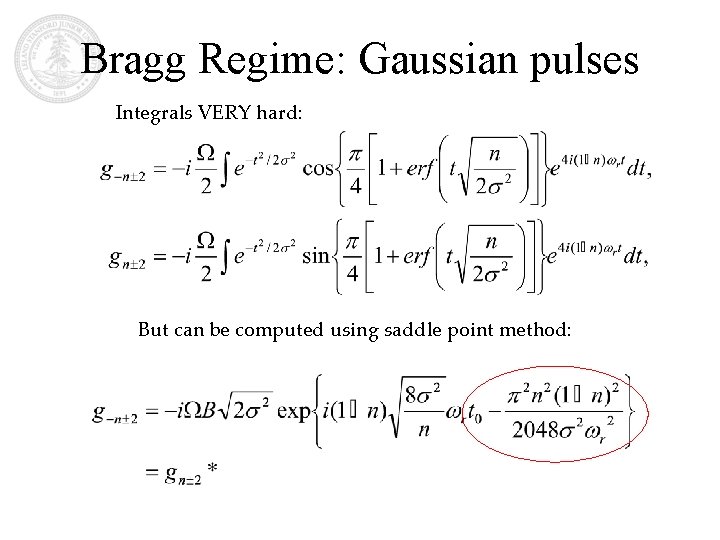
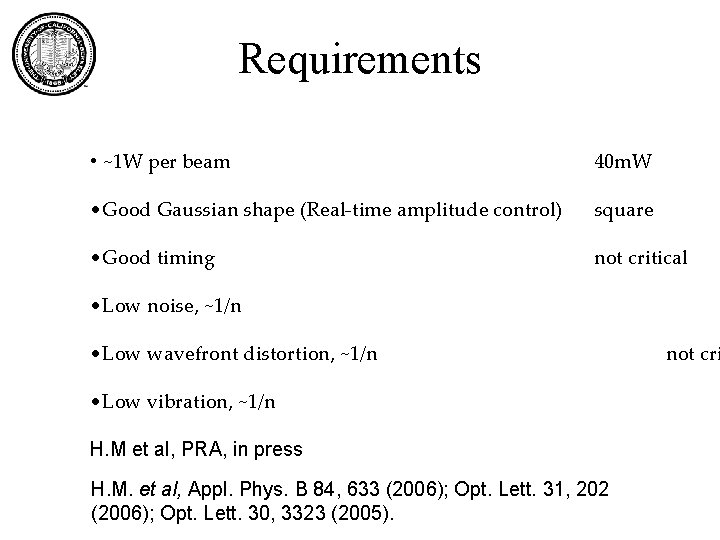
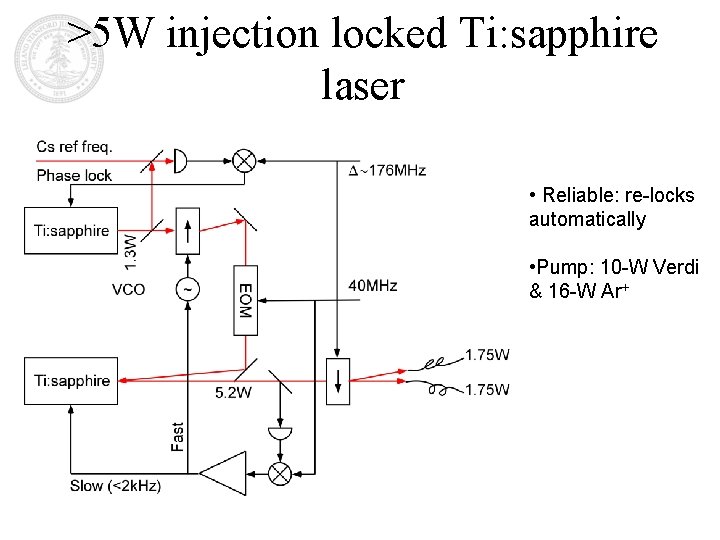
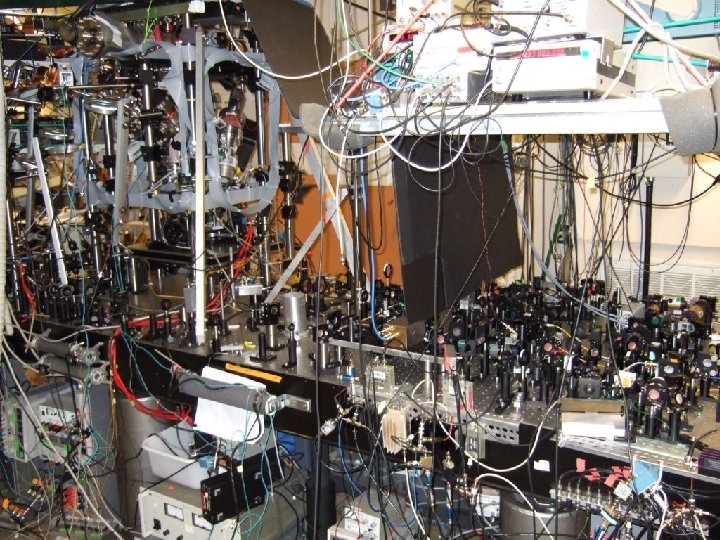
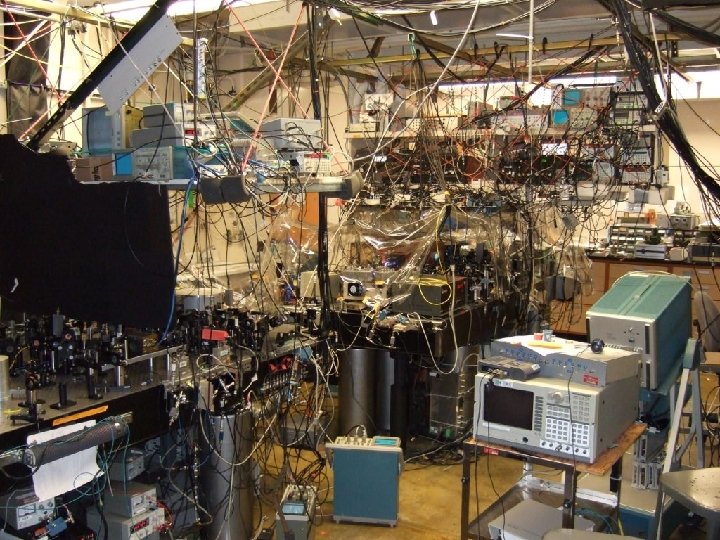
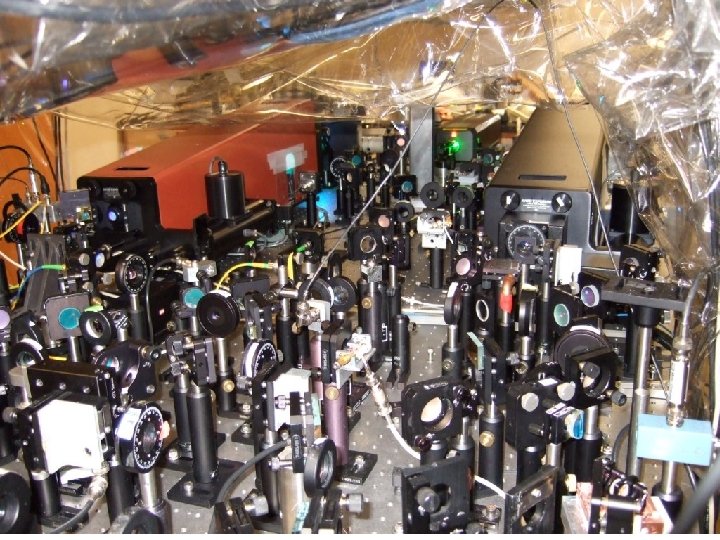
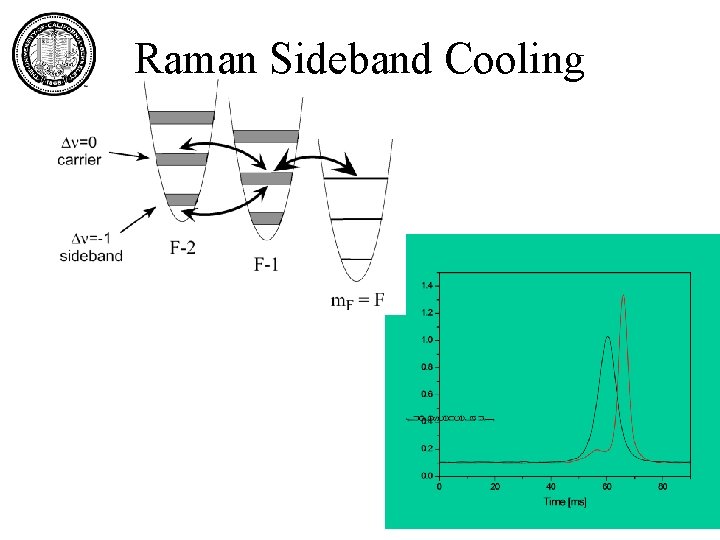
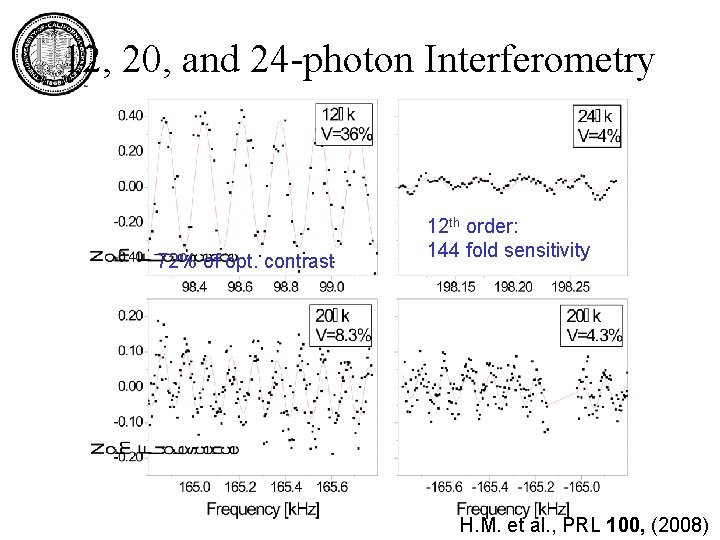
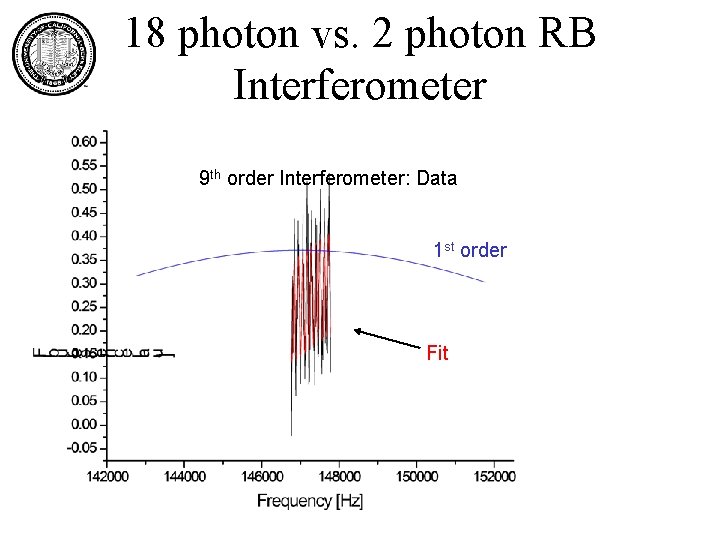
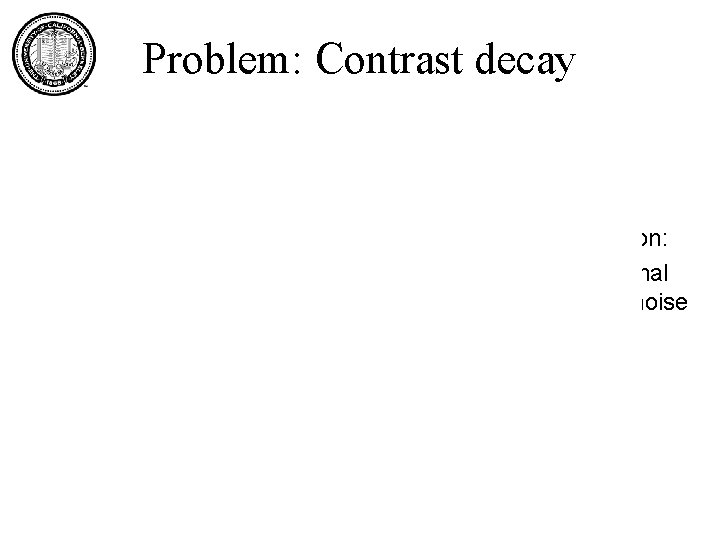
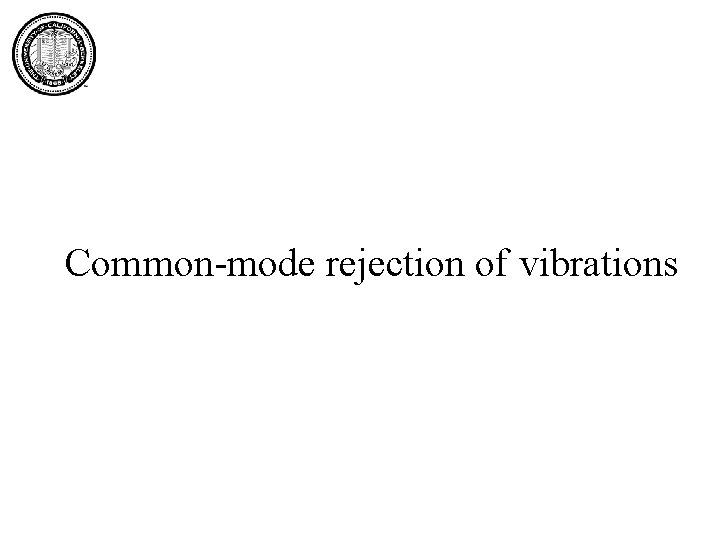
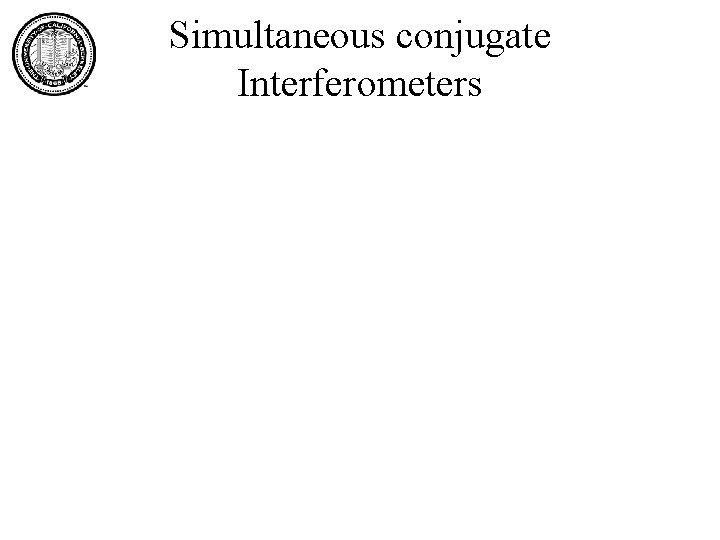
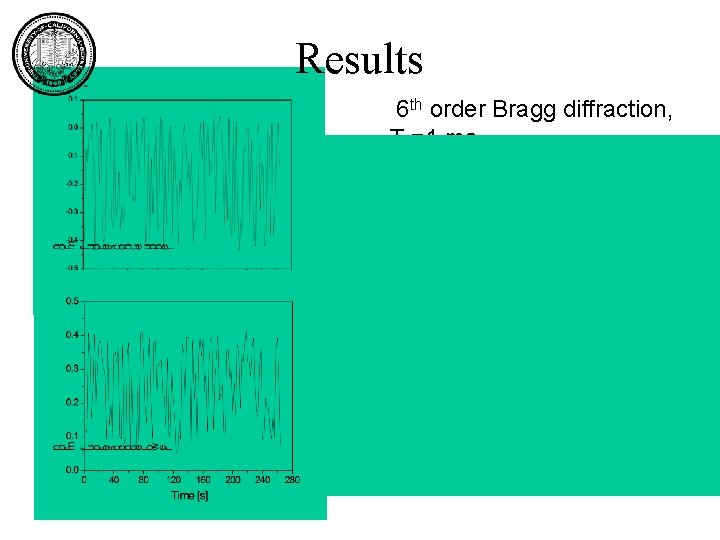
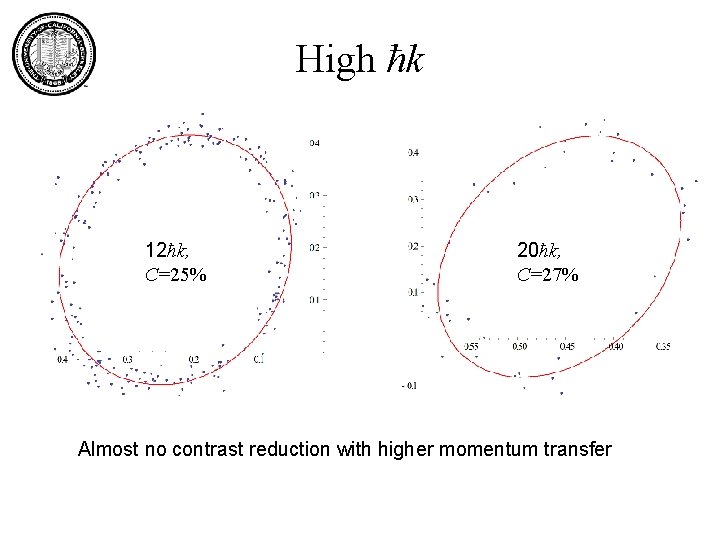
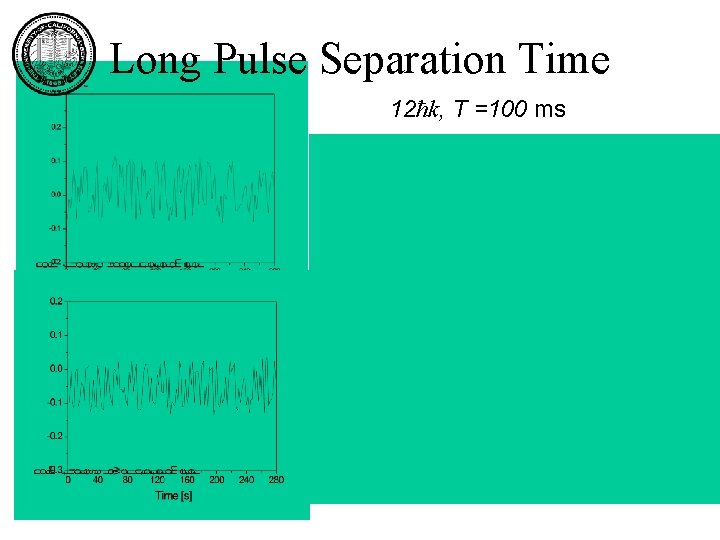
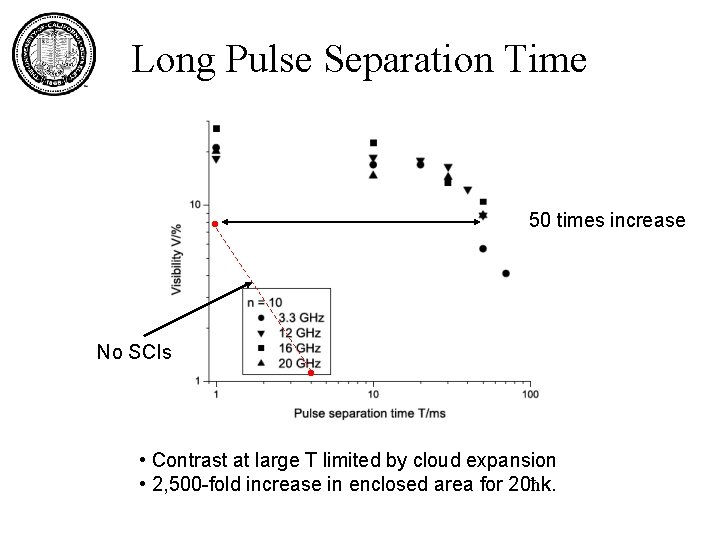
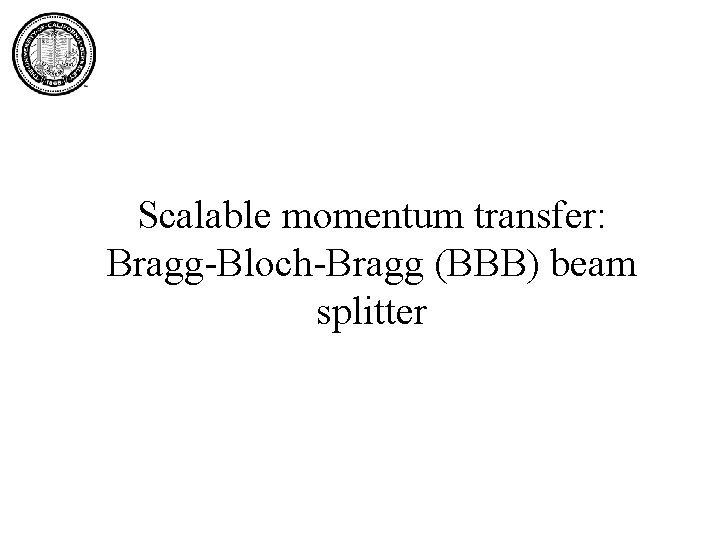
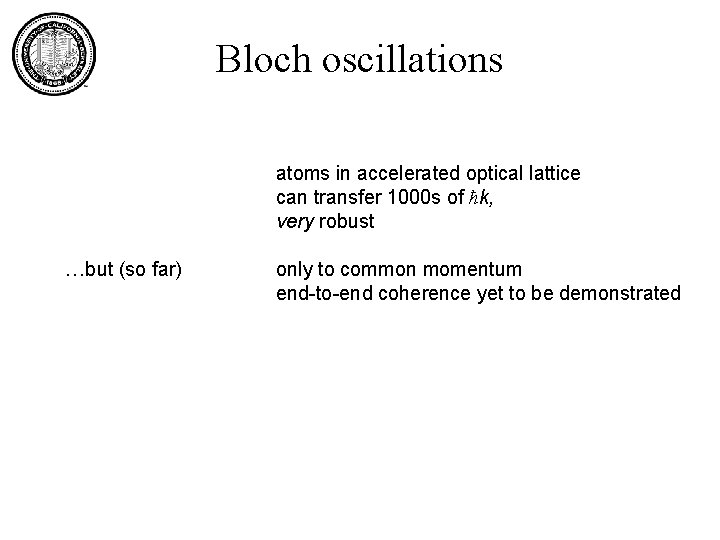
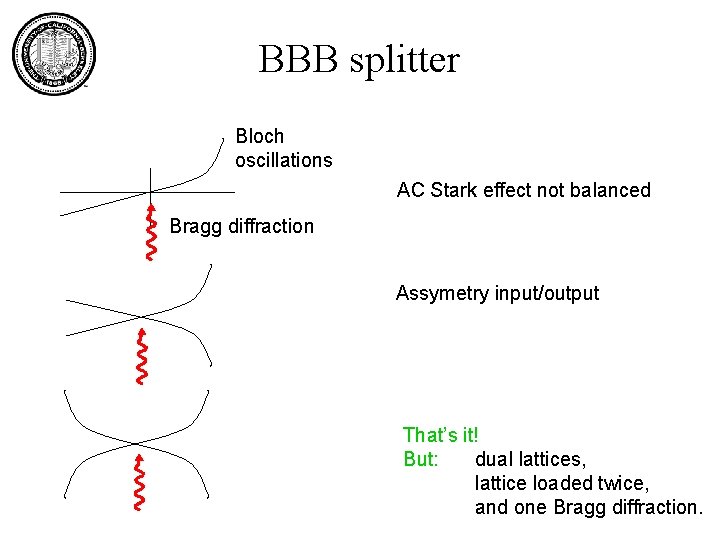
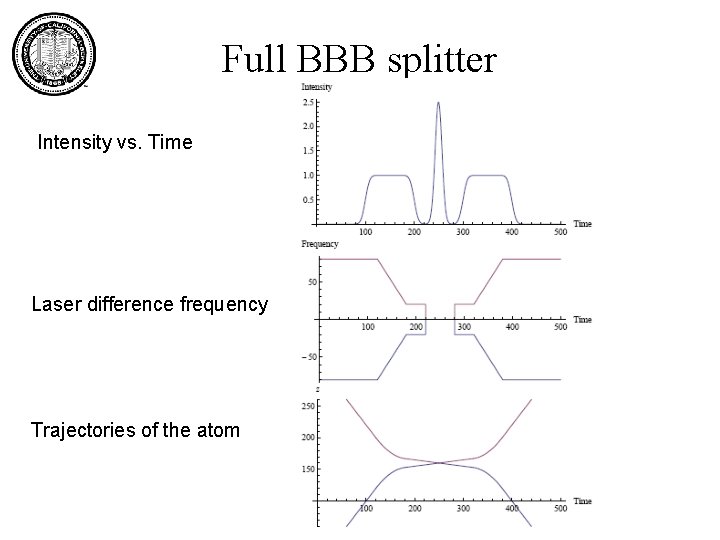
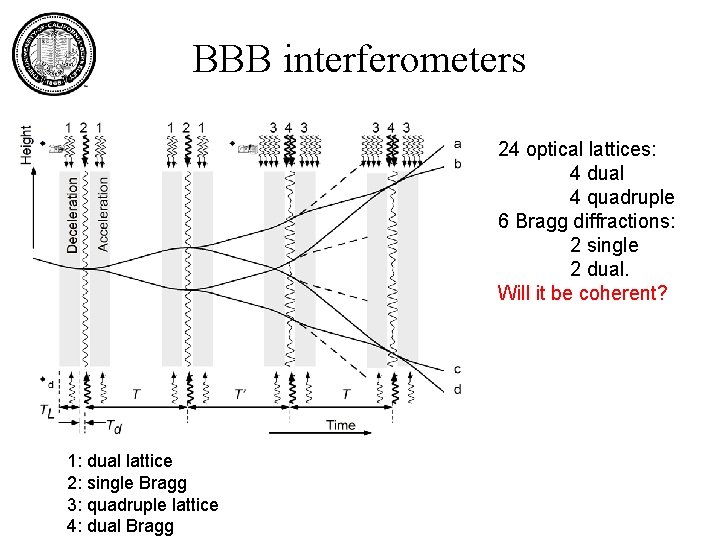
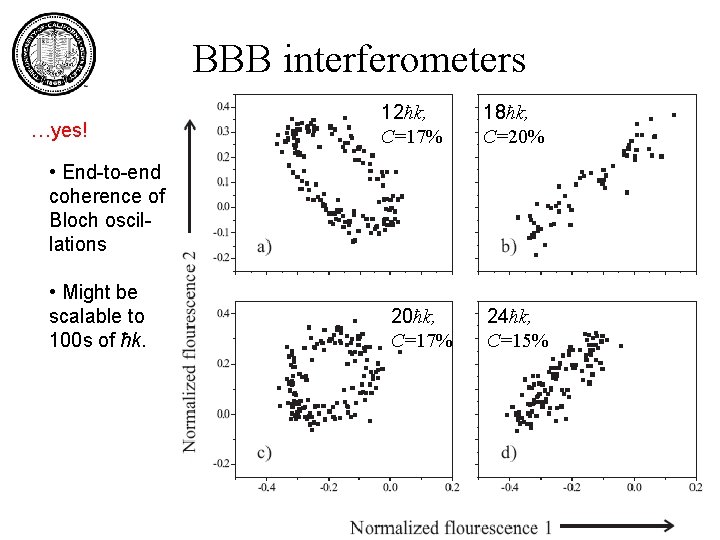
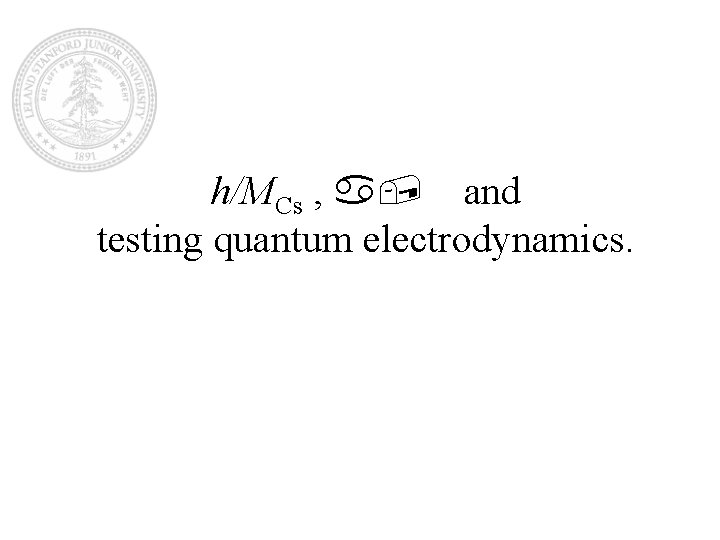
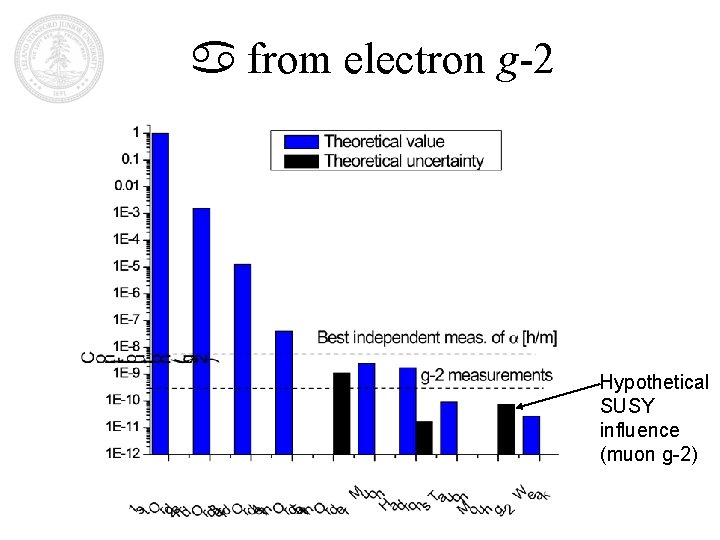
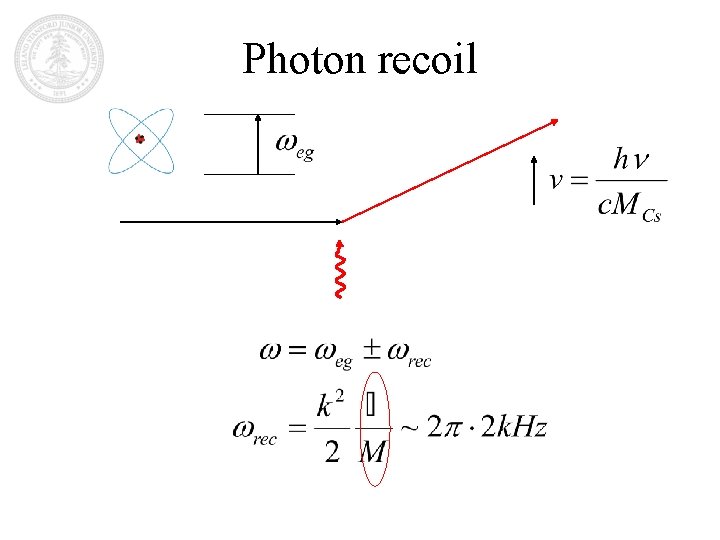
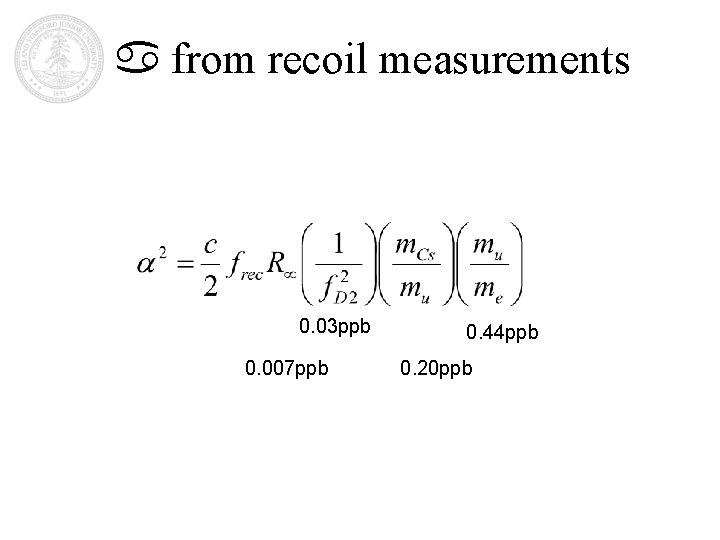
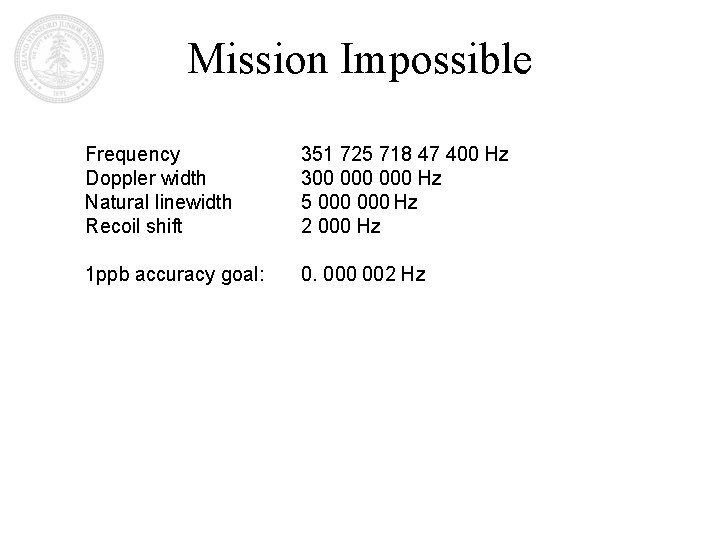
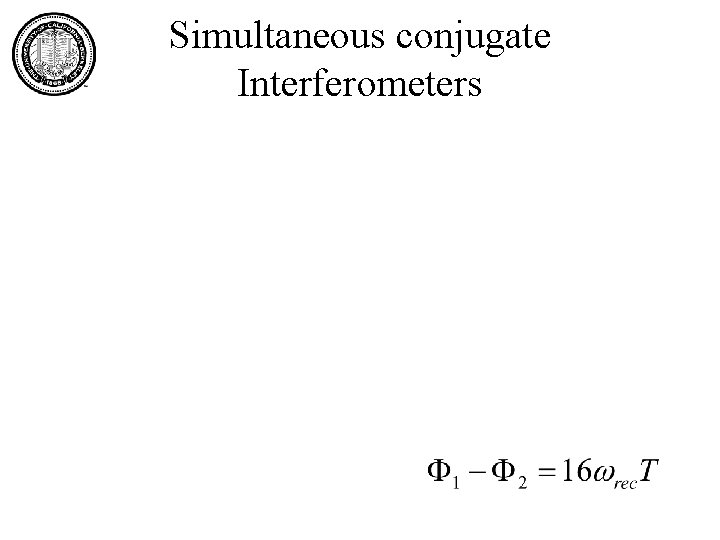
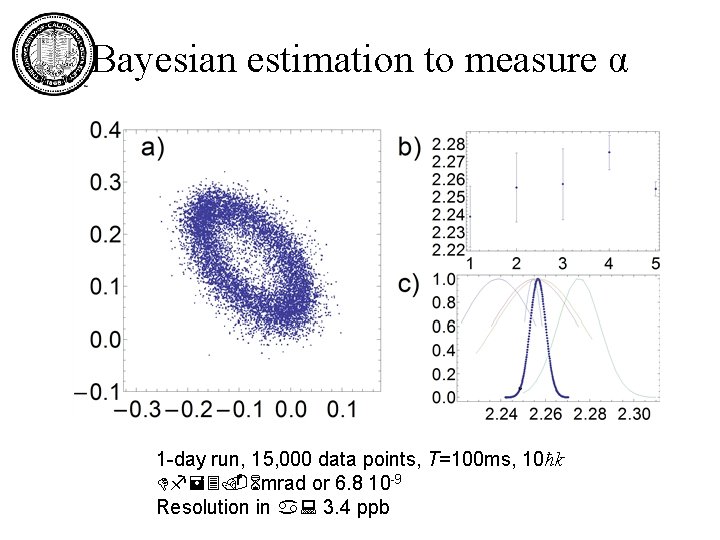
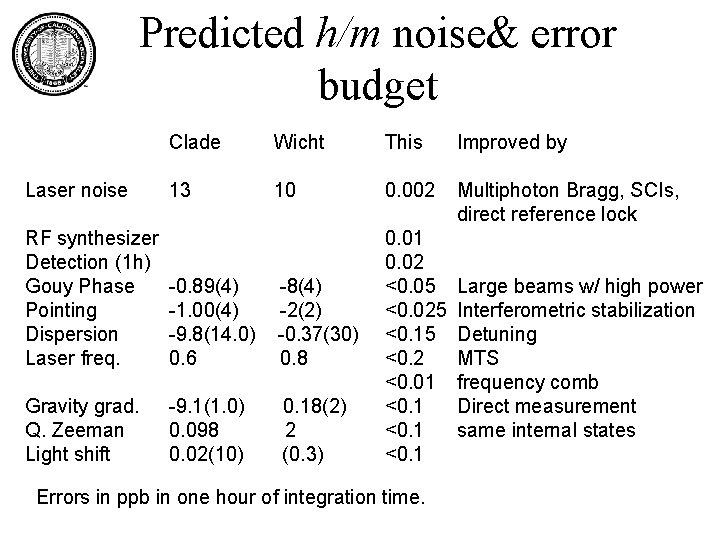
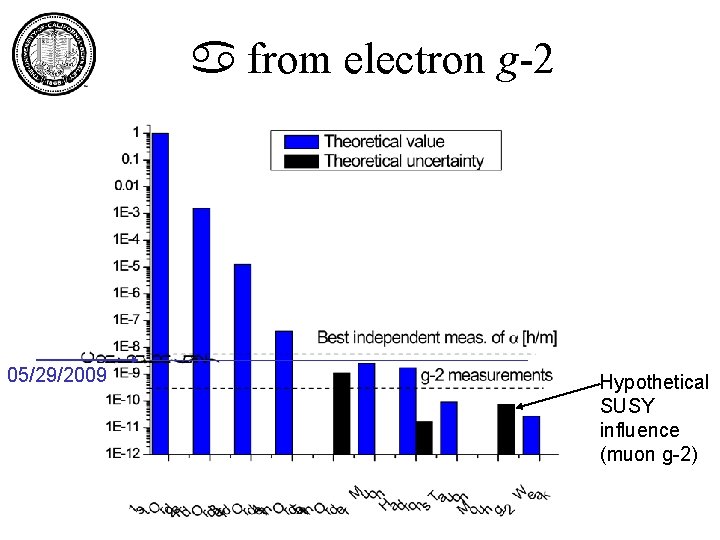
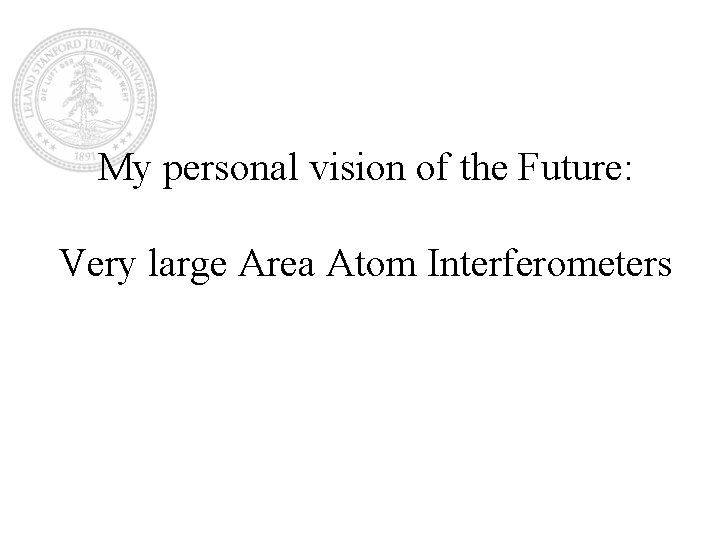
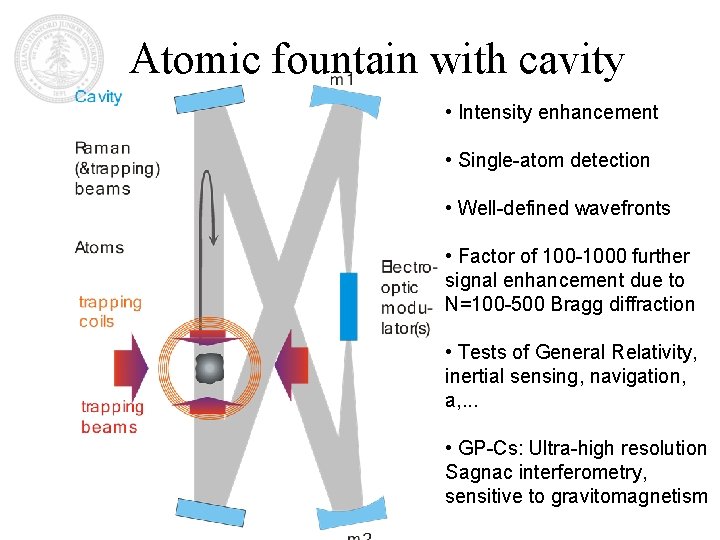
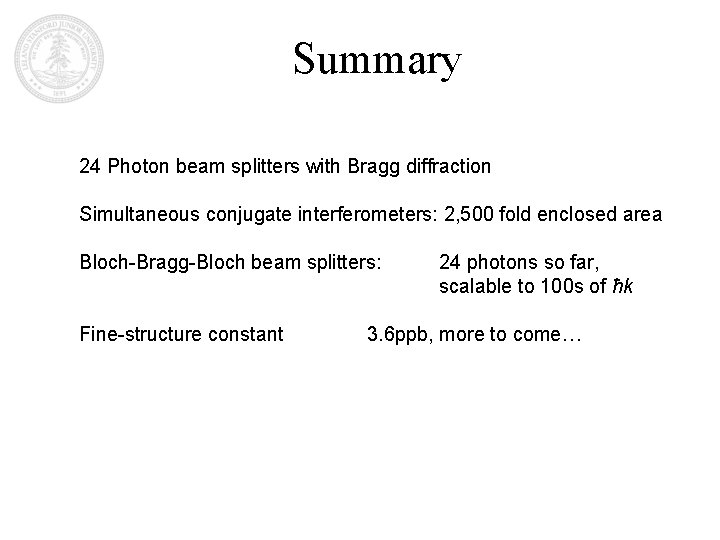
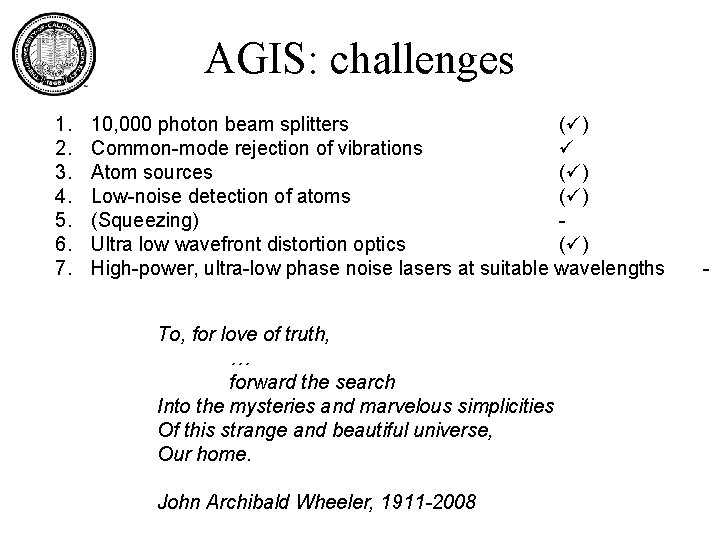
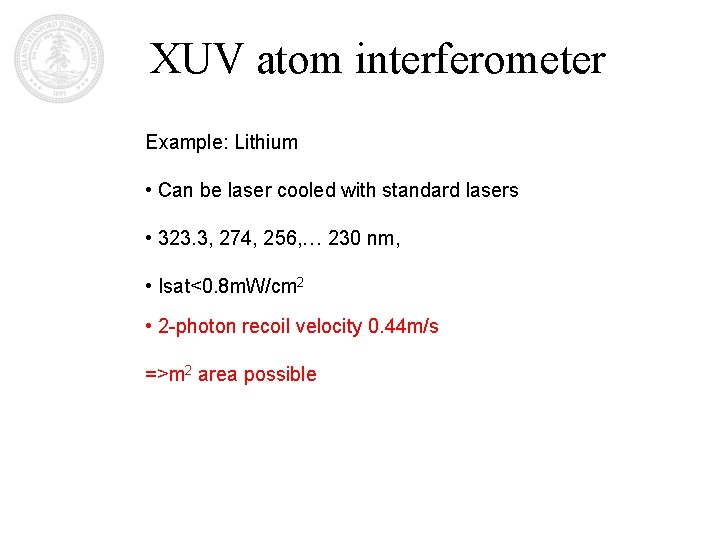
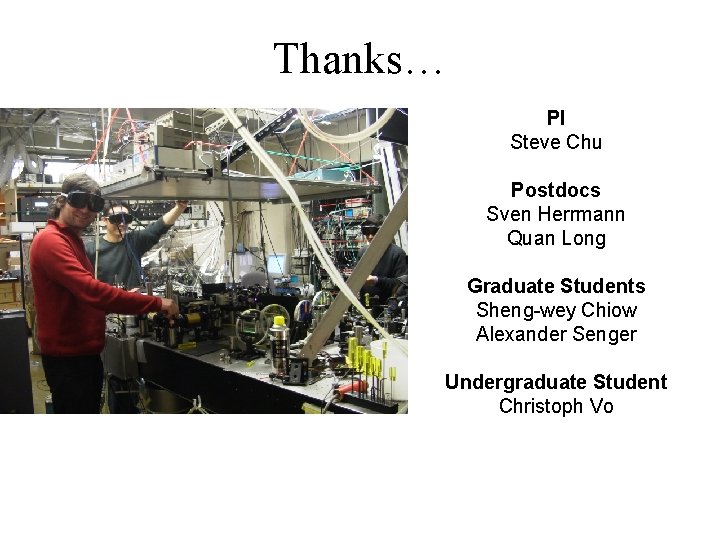
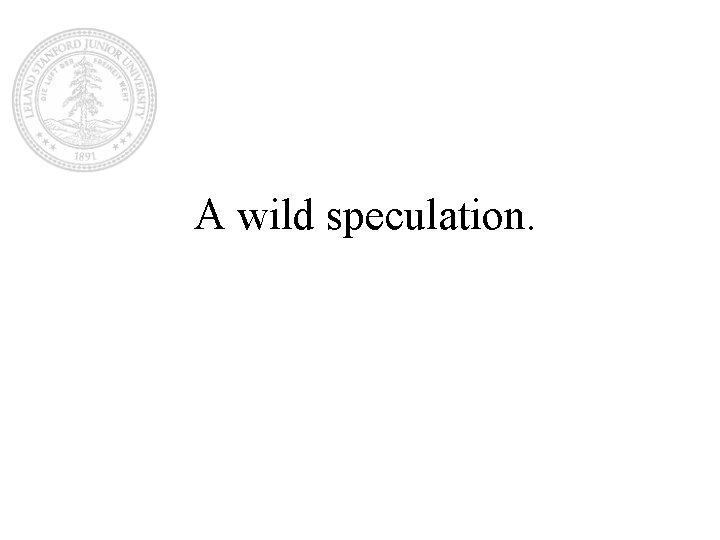
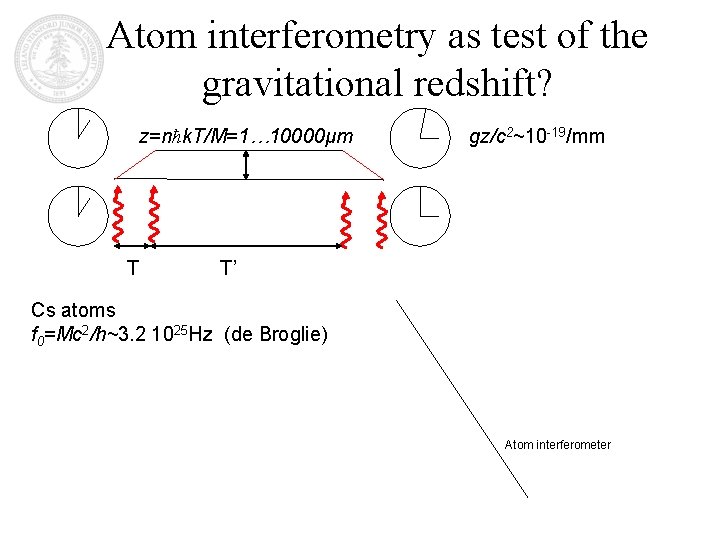
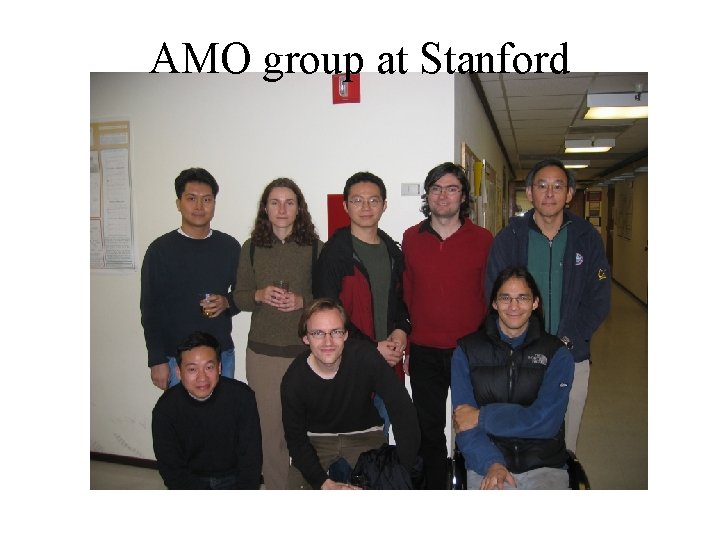
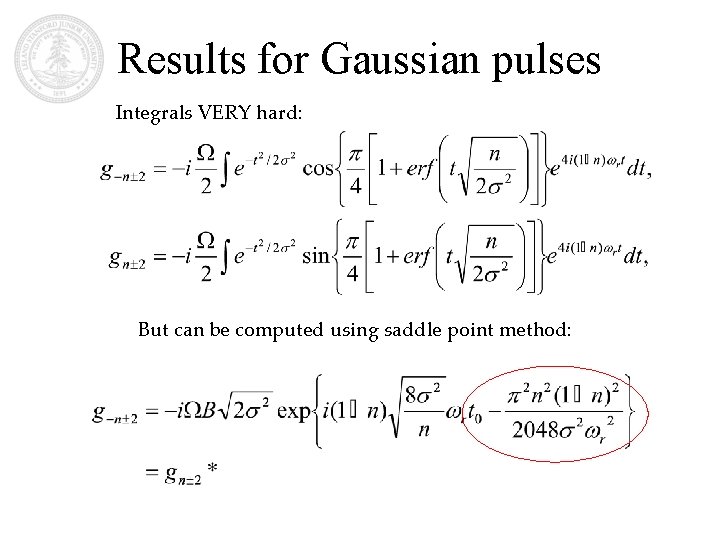
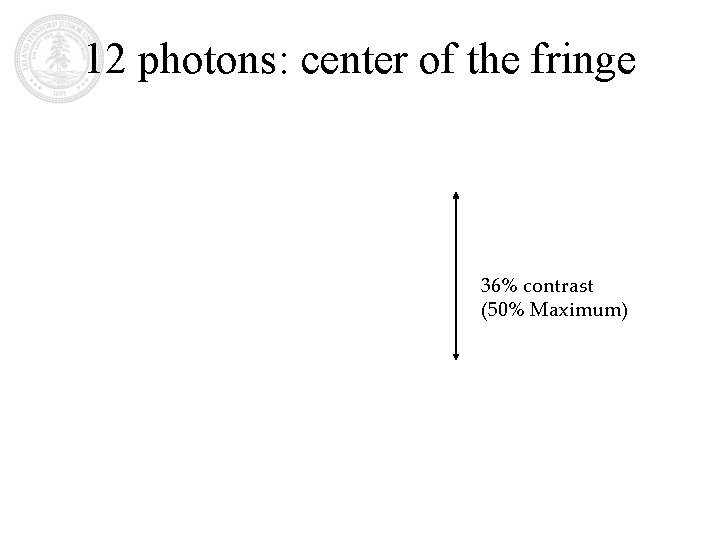
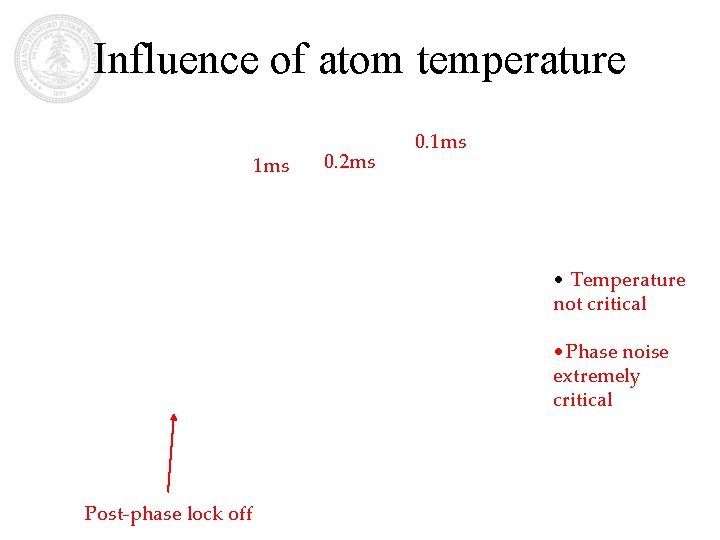
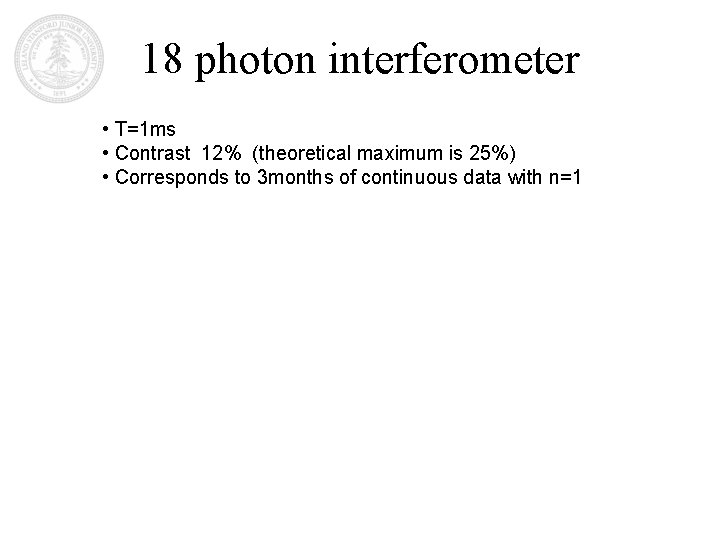
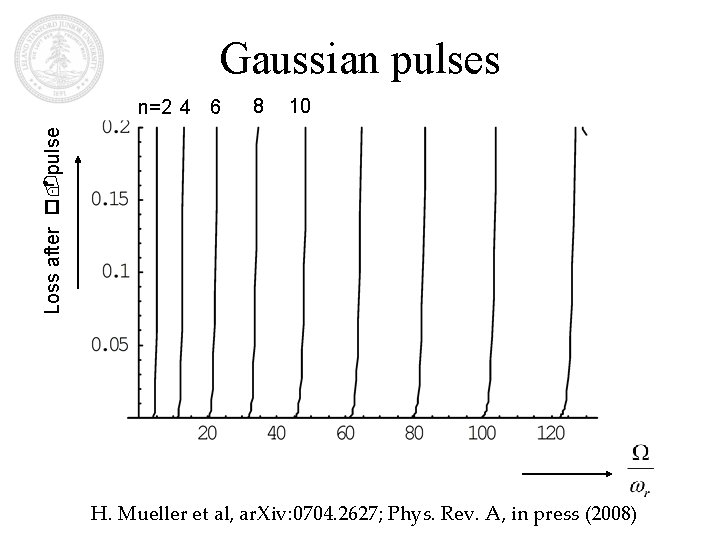
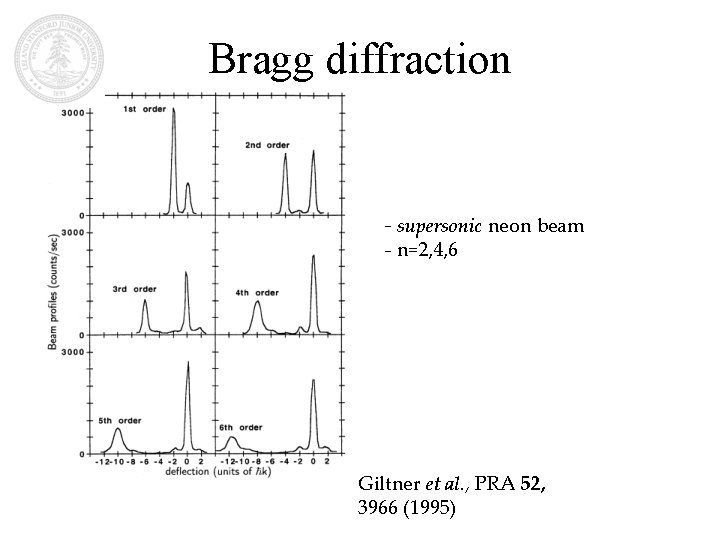
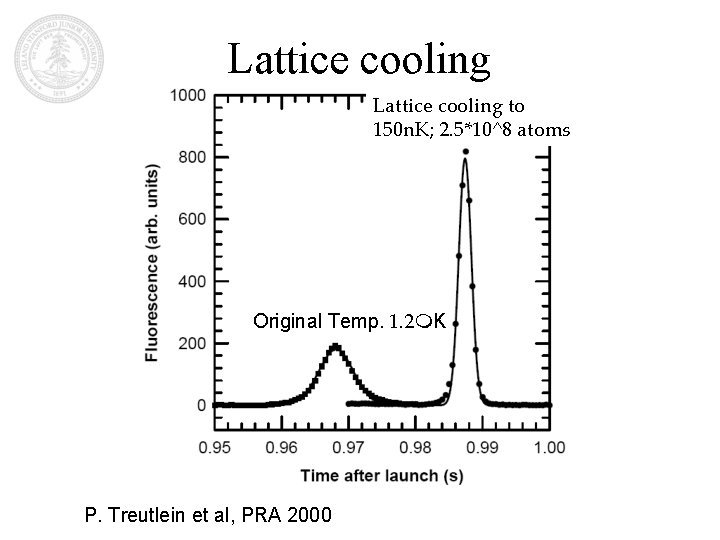
- Slides: 59
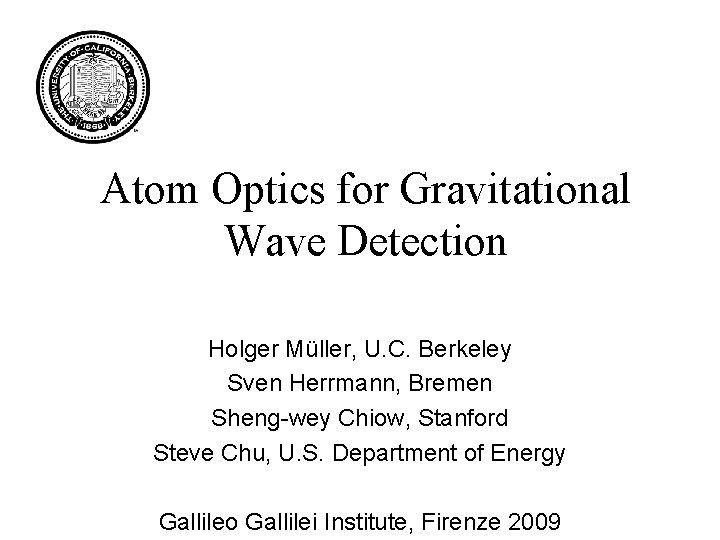
Atom Optics for Gravitational Wave Detection Holger Müller, U. C. Berkeley Sven Herrmann, Bremen Sheng-wey Chiow, Stanford Steve Chu, U. S. Department of Energy Gallileo Gallilei Institute, Firenze 2009
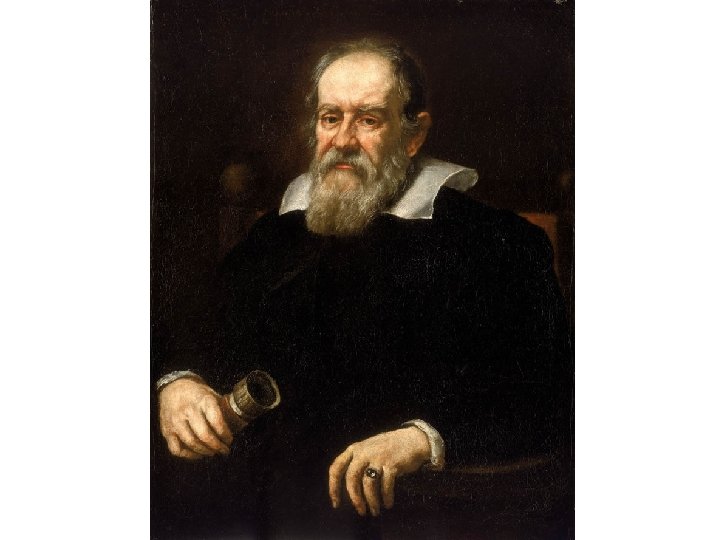
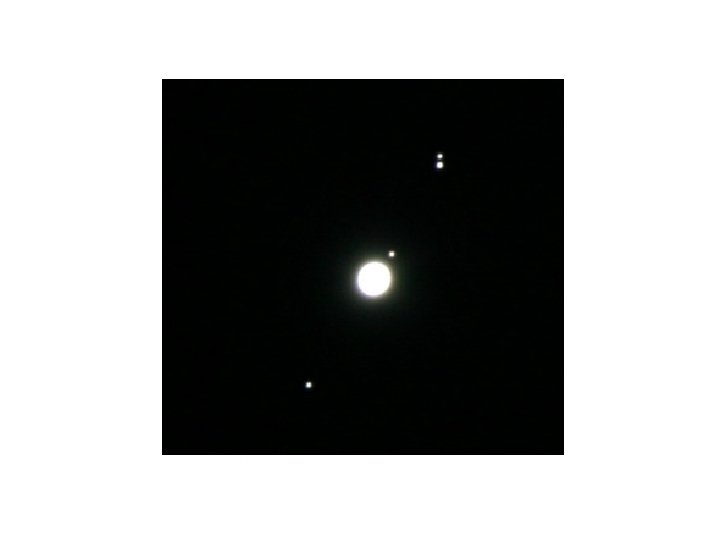
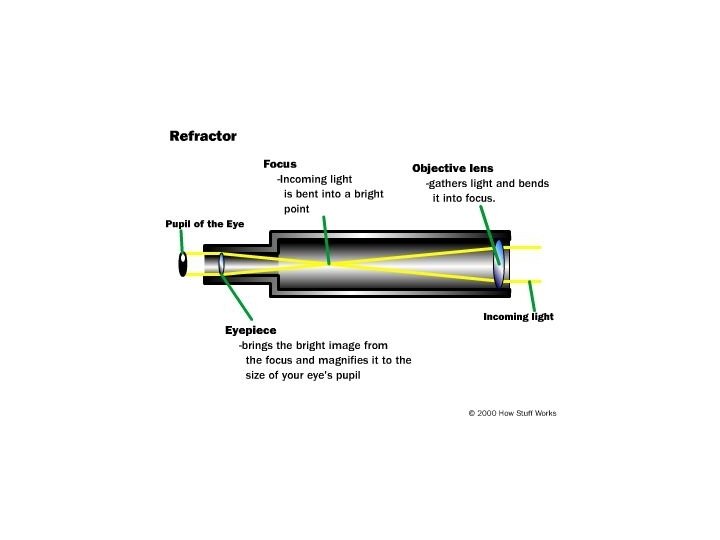
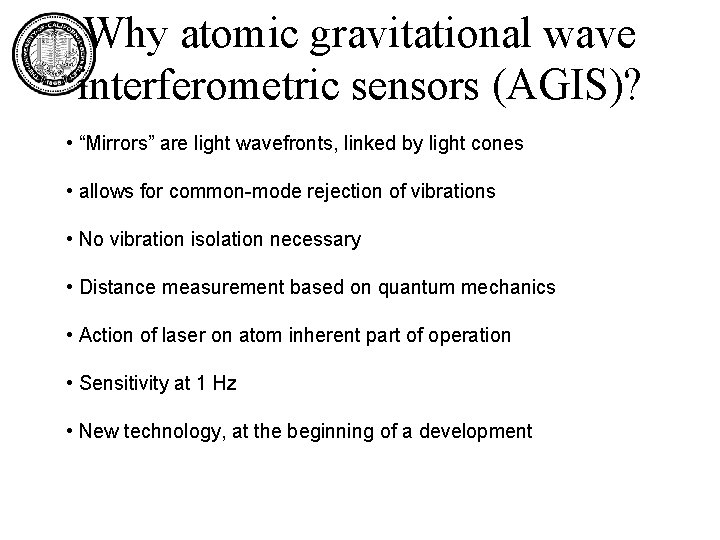
Why atomic gravitational wave interferometric sensors (AGIS)? • “Mirrors” are light wavefronts, linked by light cones • allows for common-mode rejection of vibrations • No vibration isolation necessary • Distance measurement based on quantum mechanics • Action of laser on atom inherent part of operation • Sensitivity at 1 Hz • New technology, at the beginning of a development
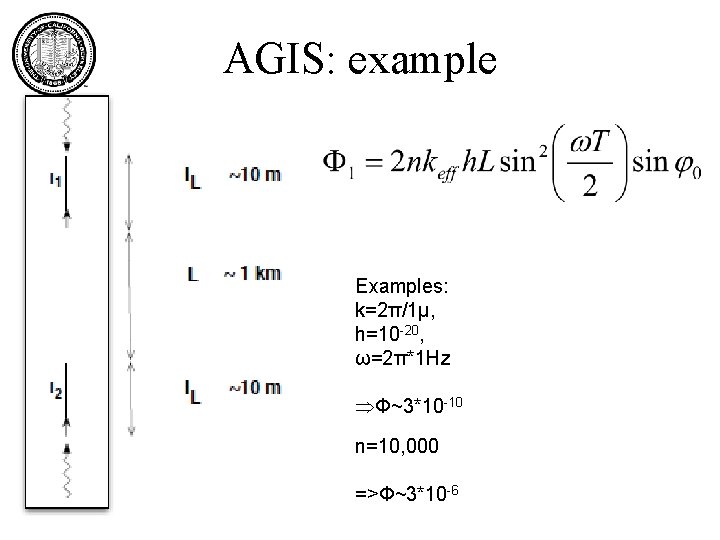
AGIS: example Examples: k=2π/1μ, h=10 -20, ω=2π*1 Hz ÞΦ~3*10 -10 n=10, 000 =>Φ~3*10 -6
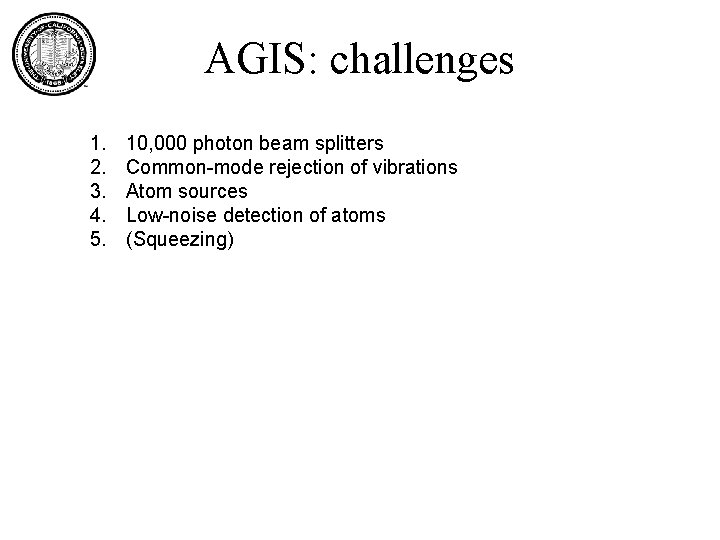
AGIS: challenges 1. 2. 3. 4. 5. 10, 000 photon beam splitters Common-mode rejection of vibrations Atom sources Low-noise detection of atoms (Squeezing)
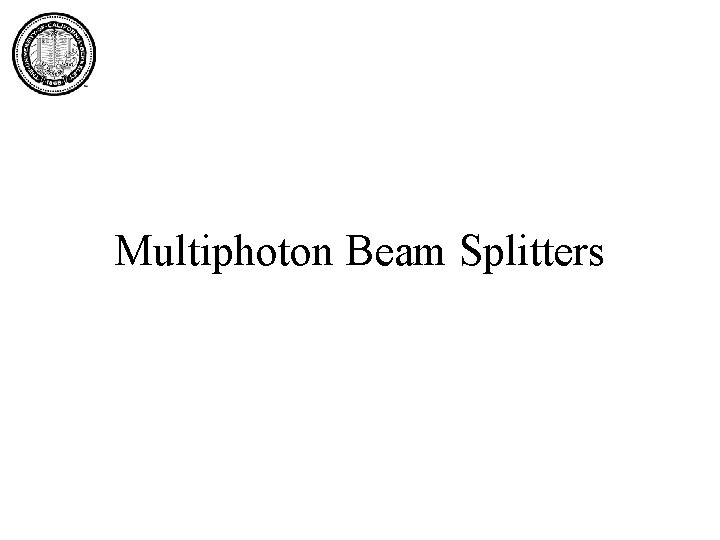
Multiphoton Beam Splitters
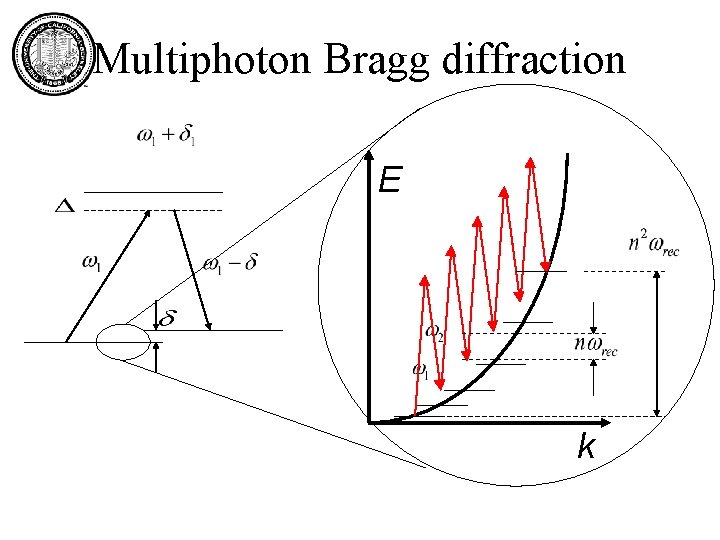
Multiphoton Bragg diffraction E k
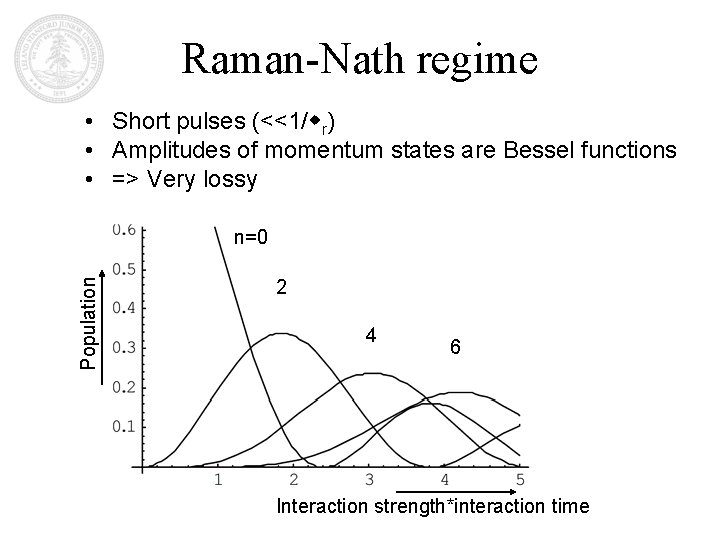
Raman-Nath regime • Short pulses (<<1/wr) • Amplitudes of momentum states are Bessel functions • => Very lossy Population n=0 2 4 6 Interaction strength*interaction time
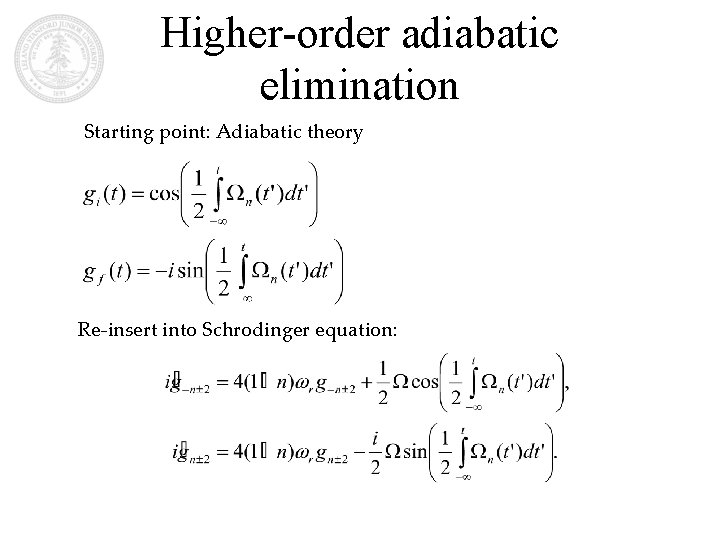
Higher-order adiabatic elimination Starting point: Adiabatic theory Re-insert into Schrodinger equation:
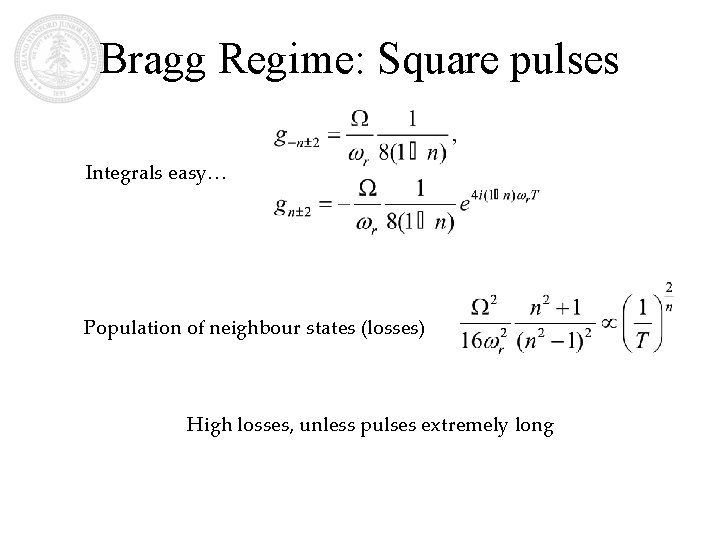
Bragg Regime: Square pulses Integrals easy… Population of neighbour states (losses) High losses, unless pulses extremely long
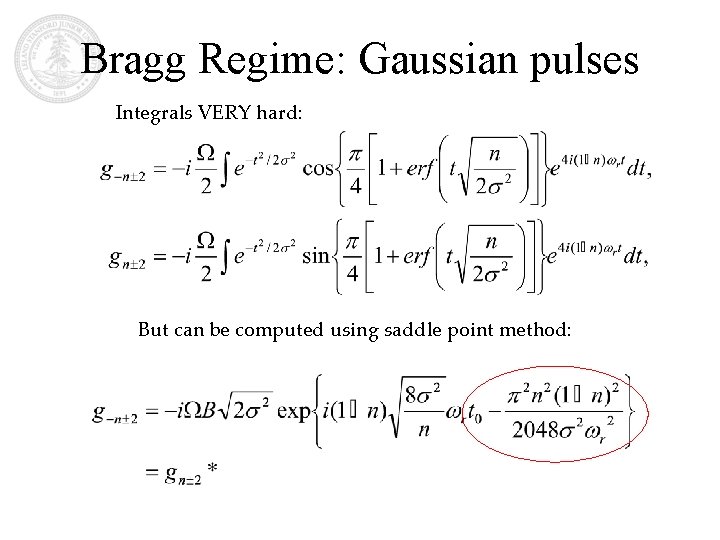
Bragg Regime: Gaussian pulses Integrals VERY hard: But can be computed using saddle point method:
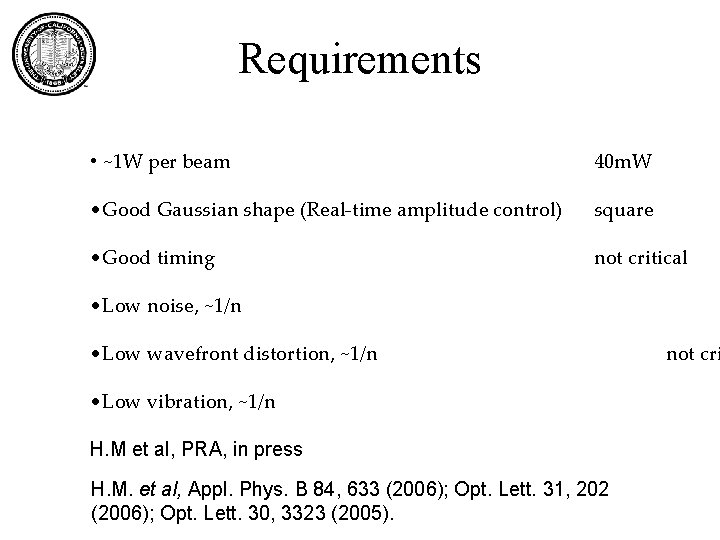
Requirements • ~1 W per beam 40 m. W • Good Gaussian shape (Real-time amplitude control) square • Good timing not critical • Low noise, ~1/n • Low wavefront distortion, ~1/n • Low vibration, ~1/n H. M et al, PRA, in press H. M. et al, Appl. Phys. B 84, 633 (2006); Opt. Lett. 31, 202 (2006); Opt. Lett. 30, 3323 (2005). not cri
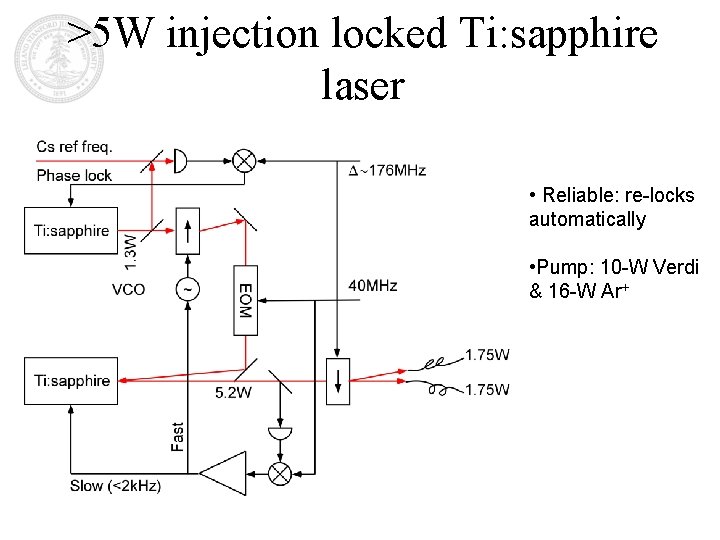
>5 W injection locked Ti: sapphire laser • Reliable: re-locks automatically • Pump: 10 -W Verdi & 16 -W Ar+
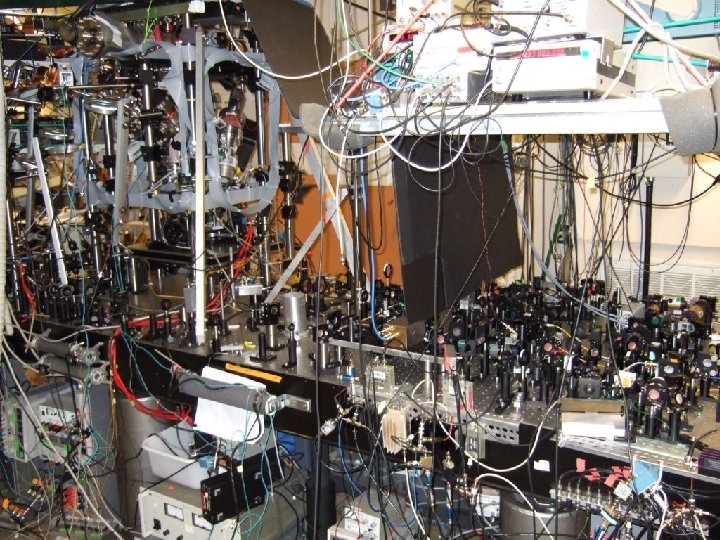
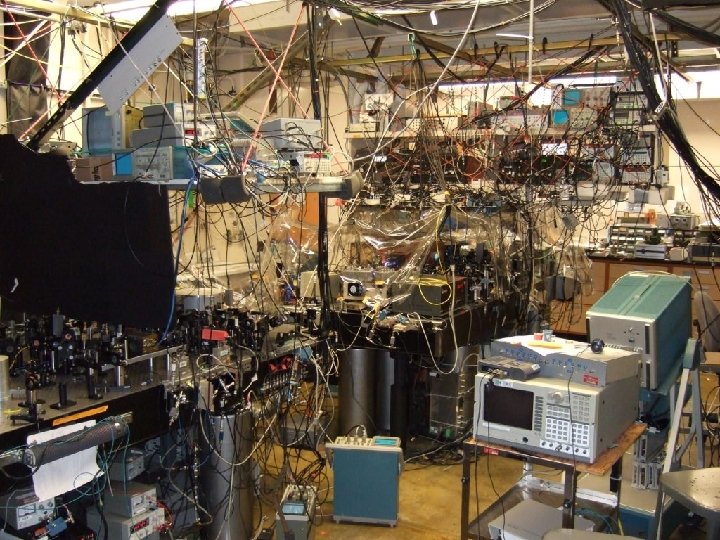
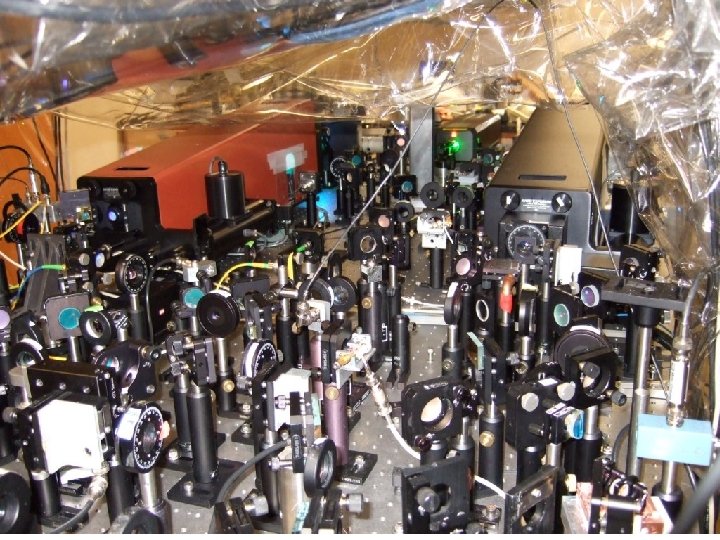
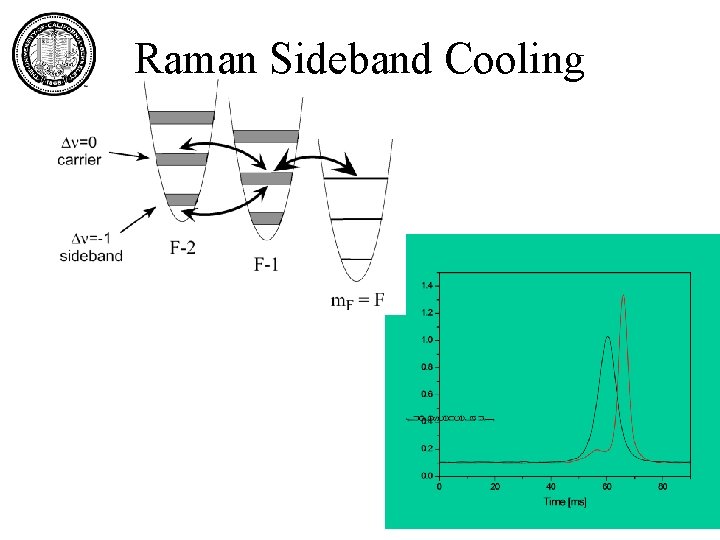
Raman Sideband Cooling Next steps: Lattice cooling
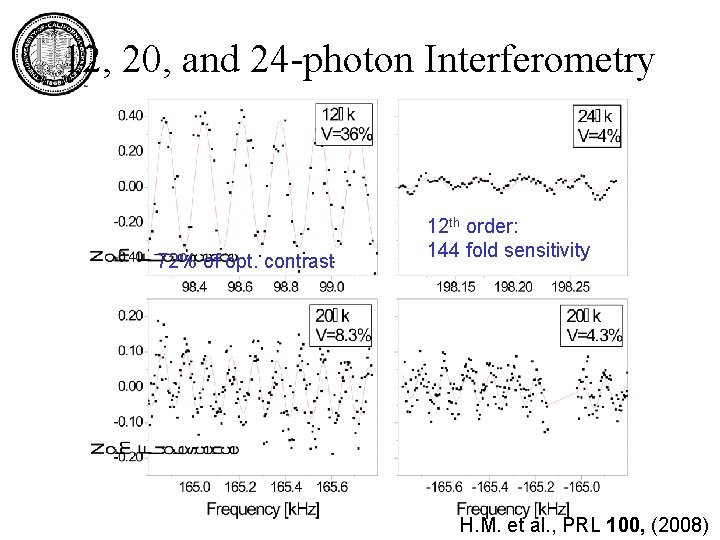
12, 20, and 24 -photon Interferometry 72% of opt. contrast 12 th order: 144 fold sensitivity H. M. et al. , PRL 100, (2008)
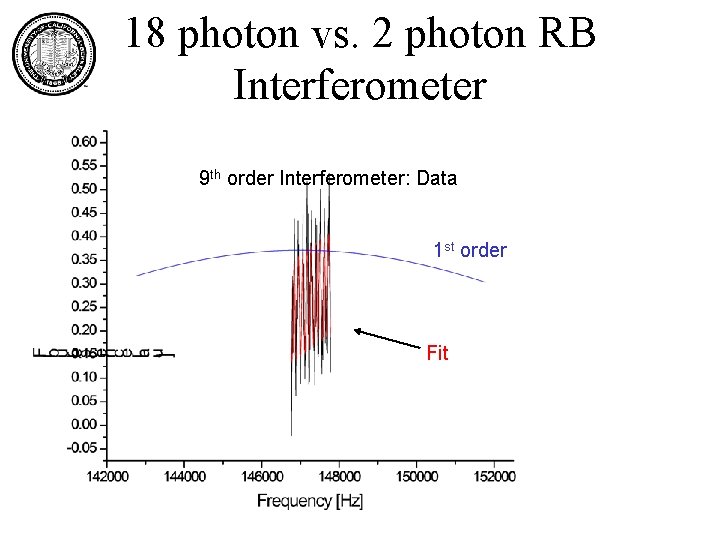
18 photon vs. 2 photon RB Interferometer 9 th order Interferometer: Data 1 st order Fit
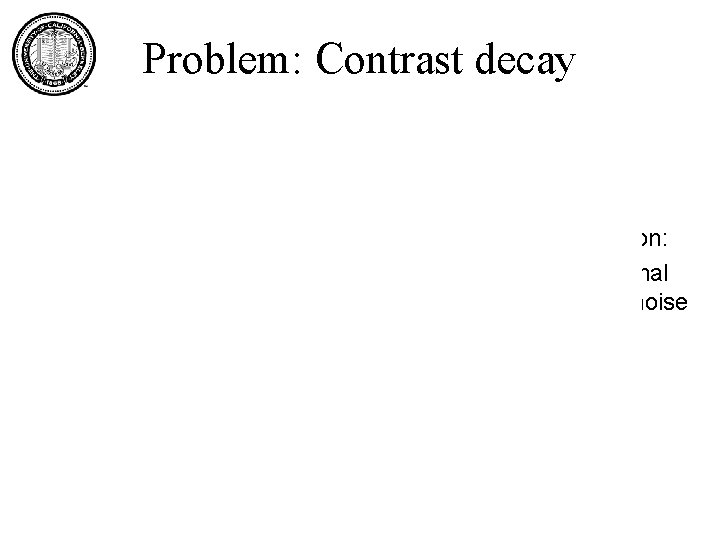
Problem: Contrast decay Visibility vs. pulse separation Vibration Isolation cutoff Limitation: vibrational phase noise
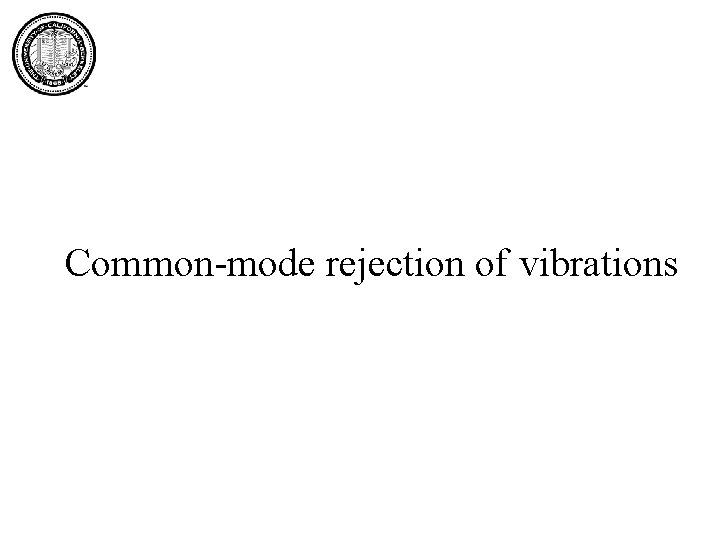
Common-mode rejection of vibrations
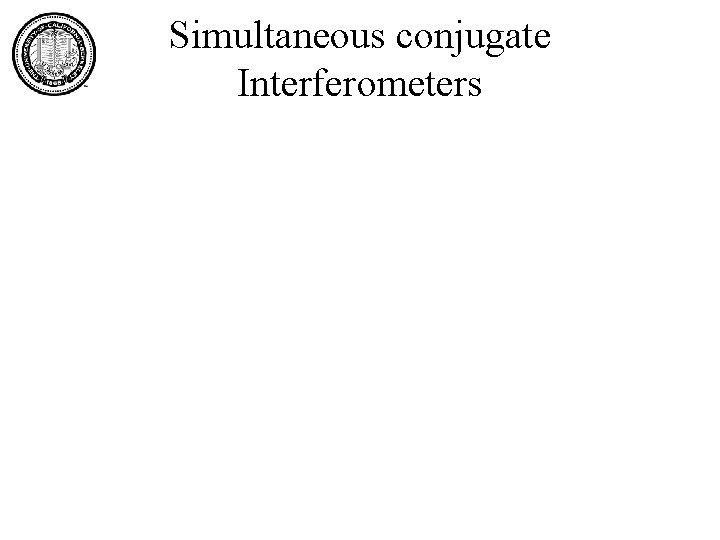
Simultaneous conjugate Interferometers
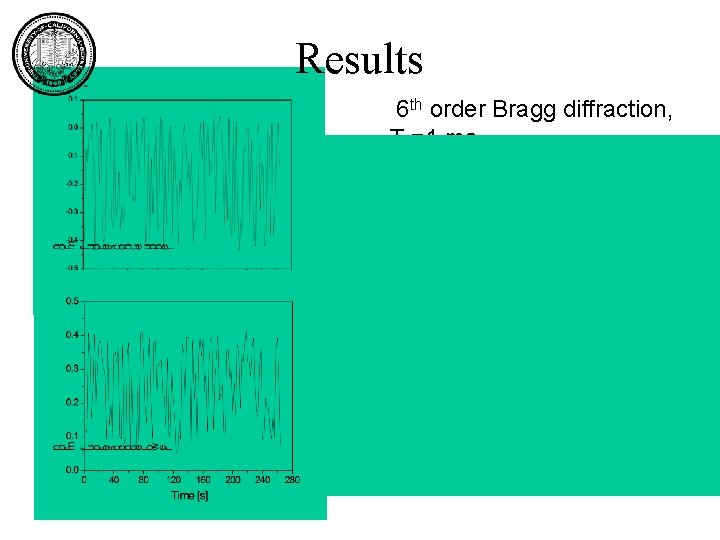
Results 6 order Bragg diffraction, th T =1 ms
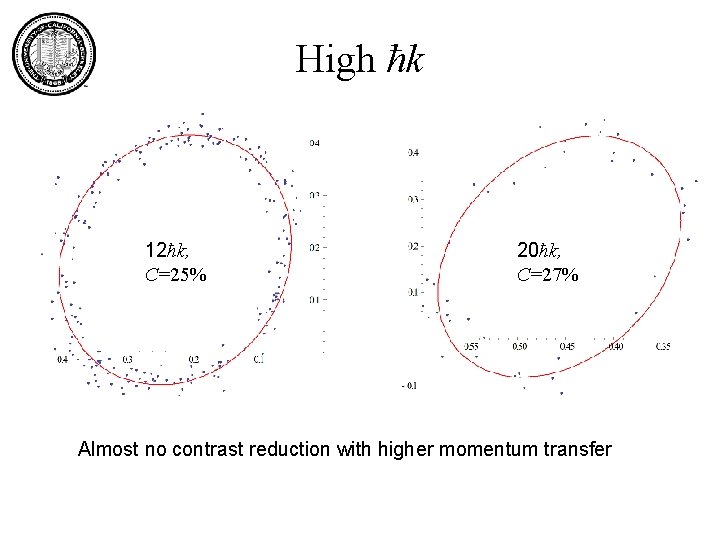
High ħk 12ħk, C=25% 20ħk, C=27% Almost no contrast reduction with higher momentum transfer
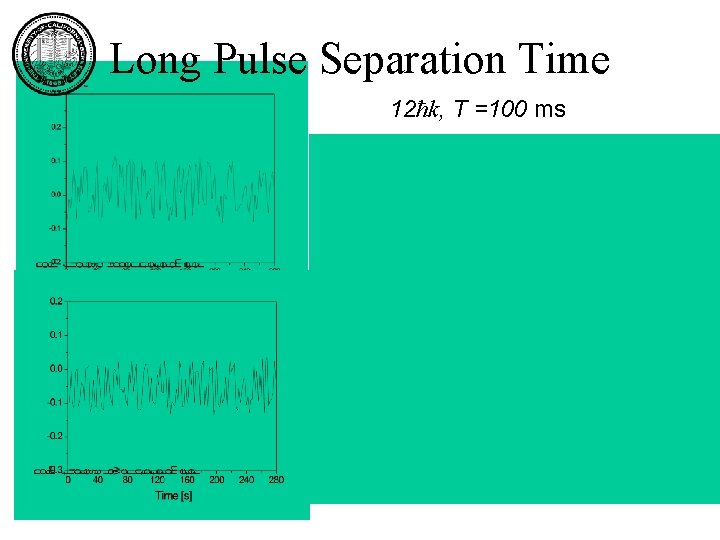
Long Pulse Separation Time Results 12ħk, T =100 ms
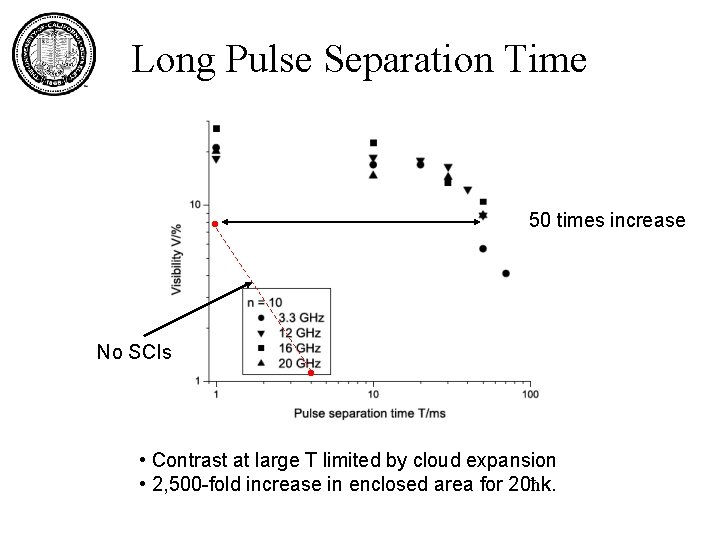
Long Pulse Separation Time 50 times increase • No SCIs • • Contrast at large T limited by cloud expansion • 2, 500 -fold increase in enclosed area for 20ħk.
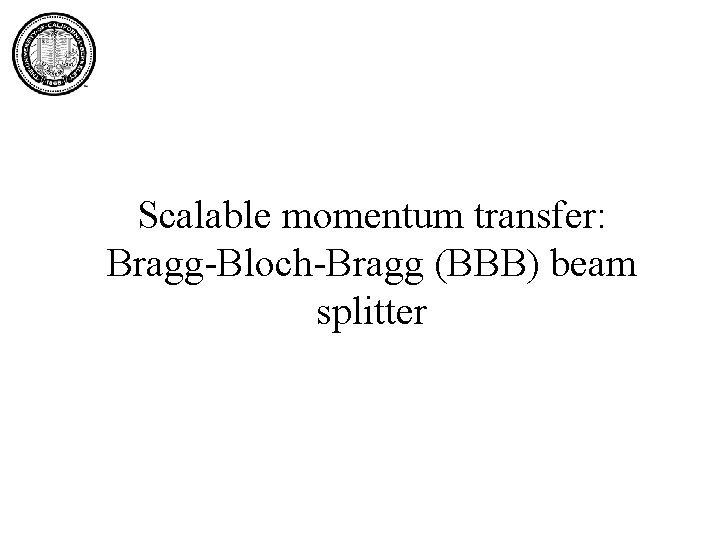
Scalable momentum transfer: Bragg-Bloch-Bragg (BBB) beam splitter
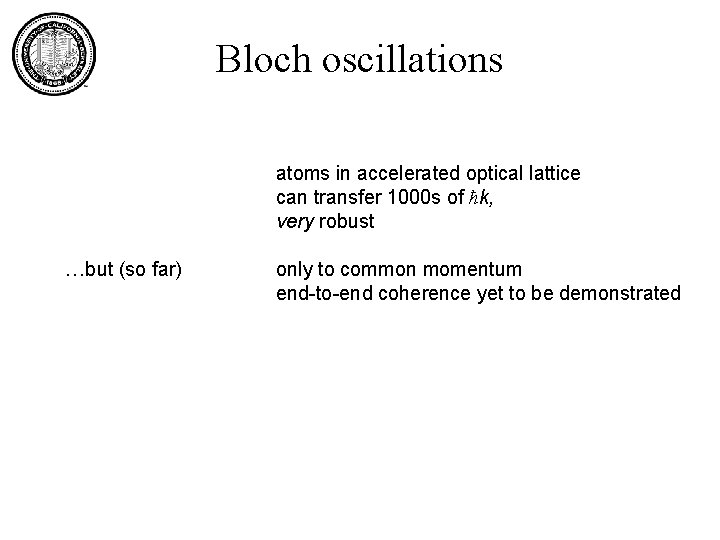
Bloch oscillations atoms in accelerated optical lattice can transfer 1000 s of ħk, very robust …but (so far) only to common momentum end-to-end coherence yet to be demonstrated
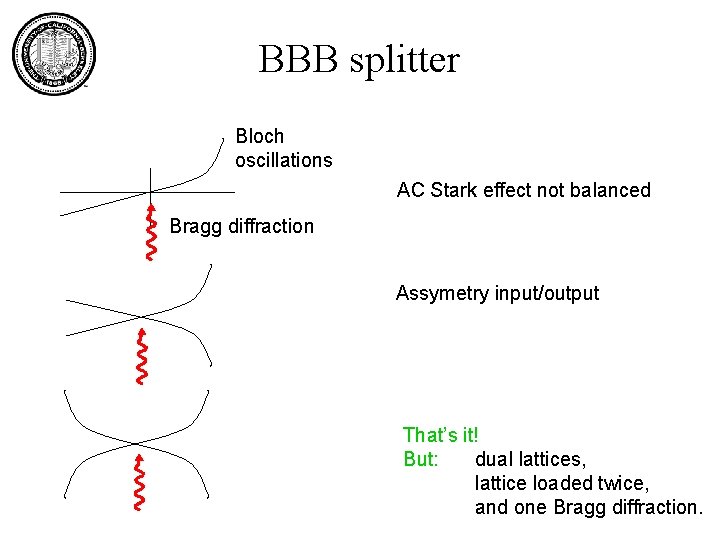
BBB splitter Bloch oscillations AC Stark effect not balanced Bragg diffraction Assymetry input/output That’s it! But: dual lattices, lattice loaded twice, and one Bragg diffraction.
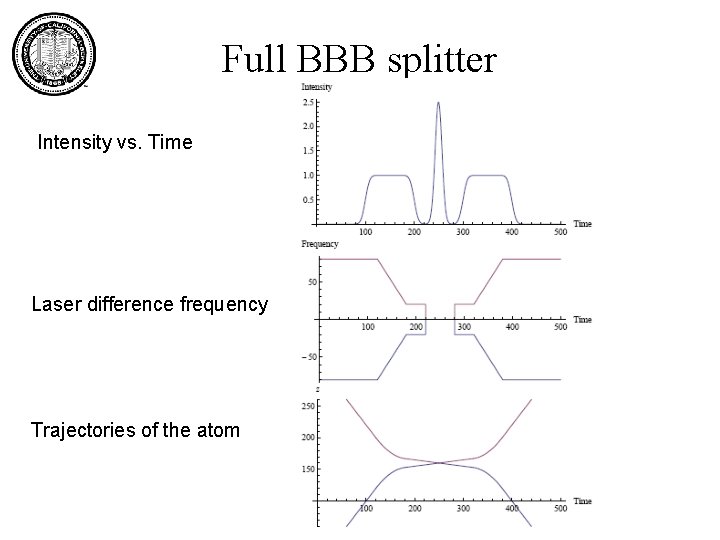
Full BBB splitter Intensity vs. Time Laser difference frequency Trajectories of the atom
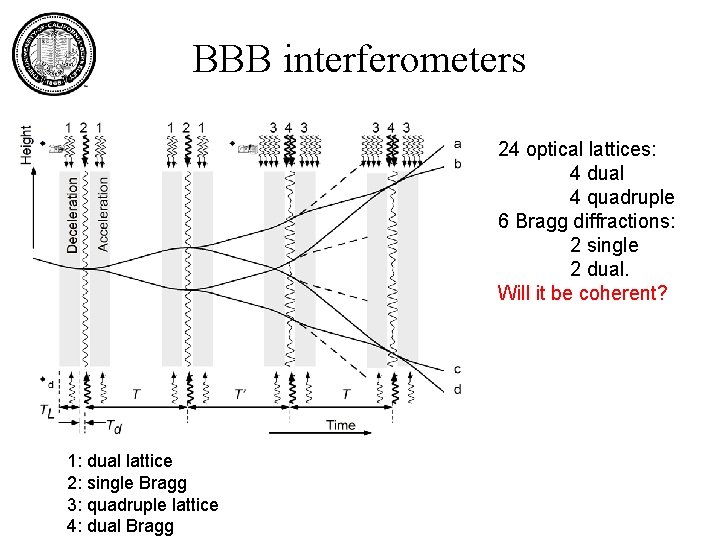
BBB interferometers 24 optical lattices: 4 dual 4 quadruple 6 Bragg diffractions: 2 single 2 dual. Will it be coherent? 1: dual lattice 2: single Bragg 3: quadruple lattice 4: dual Bragg
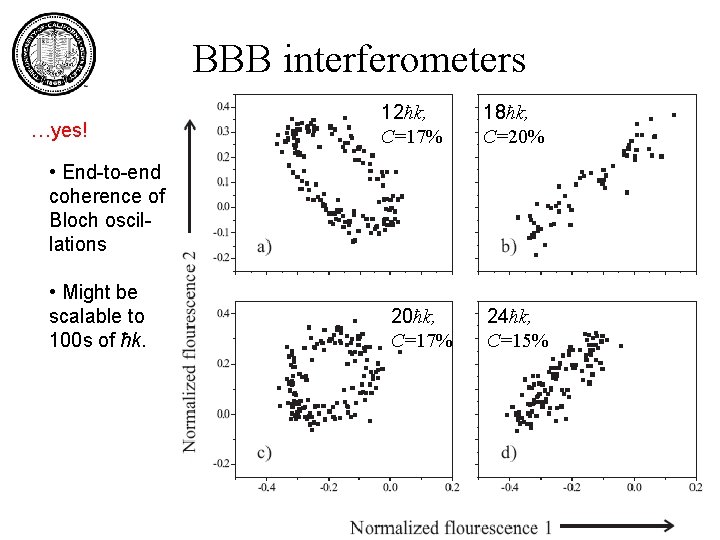
BBB interferometers …yes! 12ħk, C=17% 18ħk, C=20% • End-to-end coherence of Bloch oscillations • Might be scalable to 100 s of ħk. 20ħk, C=17% 24ħk, C=15%
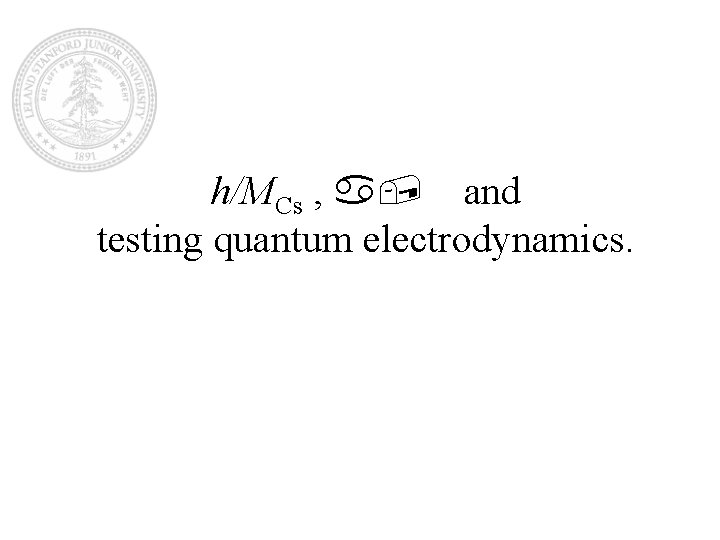
h/MCs , a, and testing quantum electrodynamics.
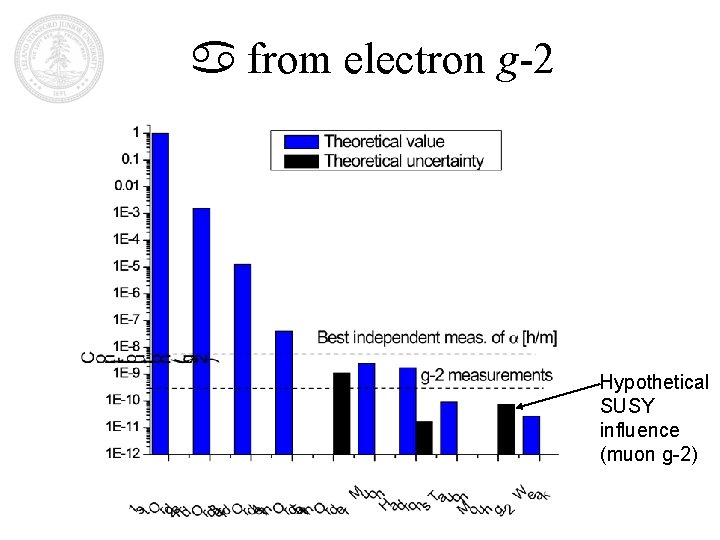
a from electron g-2 Hypothetical SUSY influence (muon g-2)
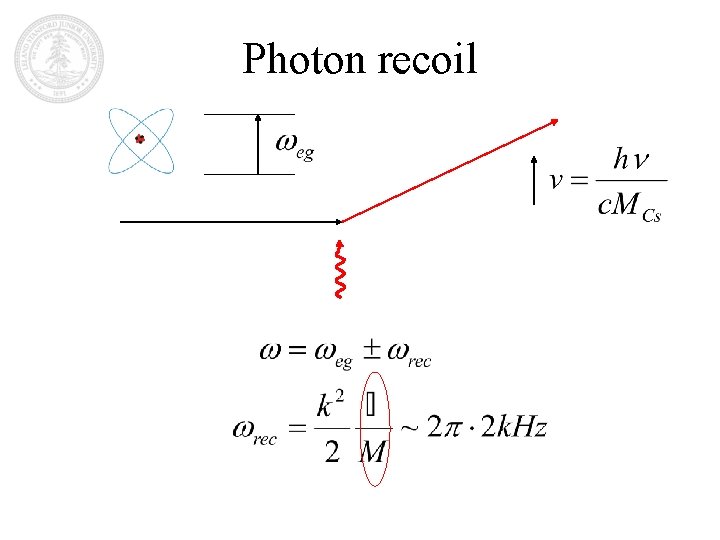
Photon recoil
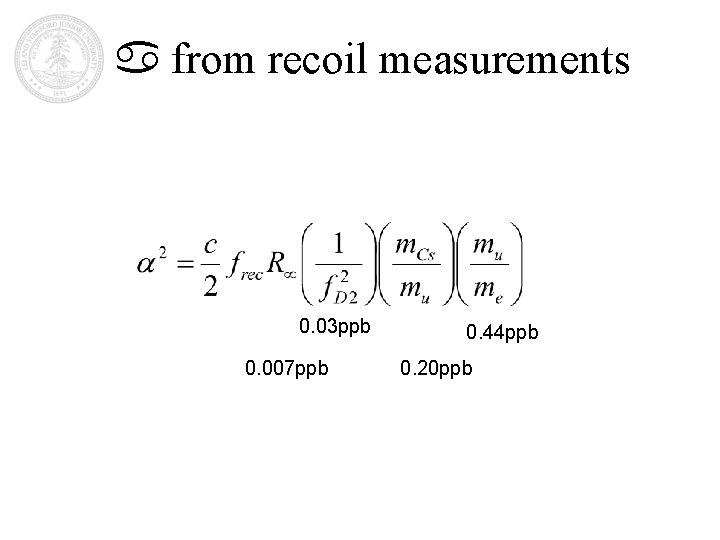
a from recoil measurements 0. 03 ppb 0. 007 ppb 0. 44 ppb 0. 20 ppb
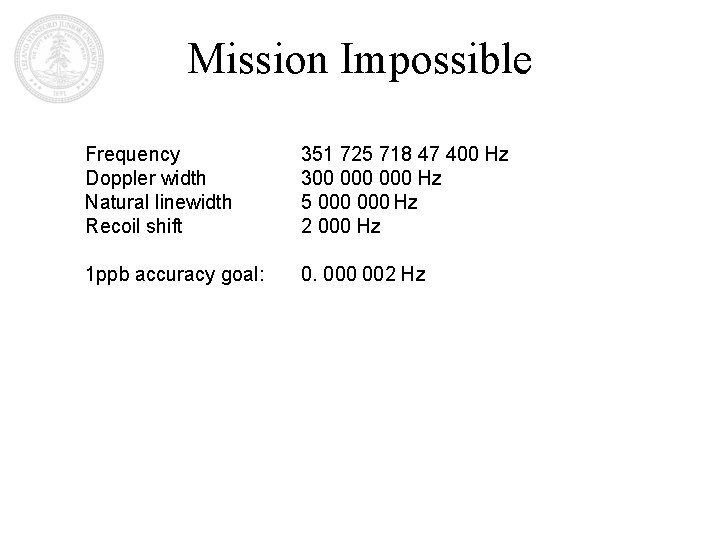
Mission Impossible Frequency Doppler width Natural linewidth Recoil shift 351 725 718 47 400 Hz 300 000 Hz 5 000 Hz 2 000 Hz 1 ppb accuracy goal: 0. 000 002 Hz
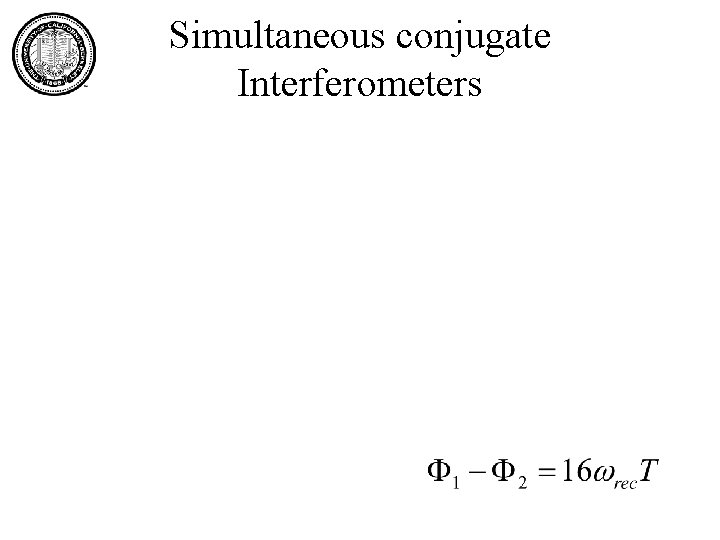
Simultaneous conjugate Interferometers
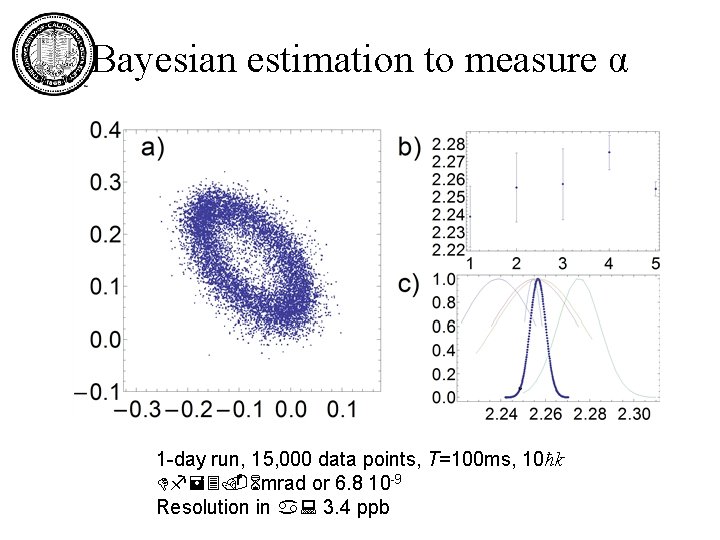
Bayesian estimation to measure α 1 -day run, 15, 000 data points, T=100 ms, 10ħk Df=3. 6 mrad or 6. 8 10 -9 Resolution in a: 3. 4 ppb
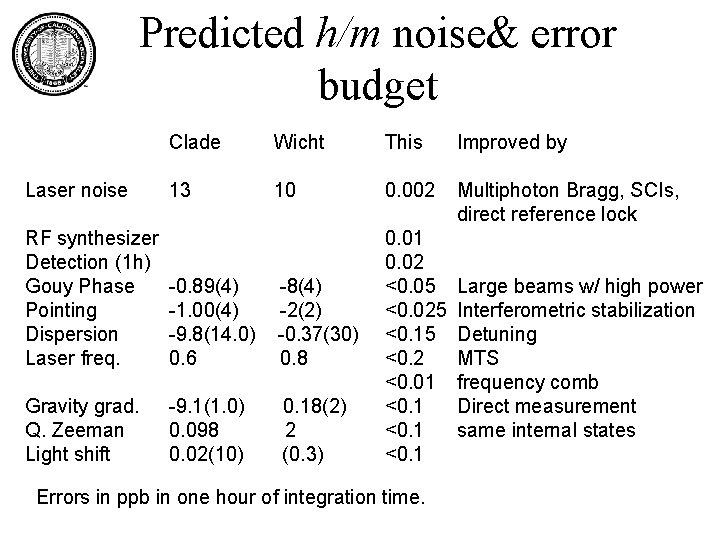
Predicted h/m noise& error budget Laser noise Clade Wicht This Improved by 13 10 0. 002 Multiphoton Bragg, SCIs, direct reference lock RF synthesizer Detection (1 h) Gouy Phase Pointing Dispersion Laser freq. -0. 89(4) -1. 00(4) -9. 8(14. 0) 0. 6 -8(4) -2(2) -0. 37(30) 0. 8 Gravity grad. Q. Zeeman Light shift -9. 1(1. 0) 0. 098 0. 02(10) 0. 18(2) 2 (0. 3) 0. 01 0. 02 <0. 05 <0. 025 <0. 15 <0. 2 <0. 01 <0. 1 Errors in ppb in one hour of integration time. Large beams w/ high power Interferometric stabilization Detuning MTS frequency comb Direct measurement same internal states
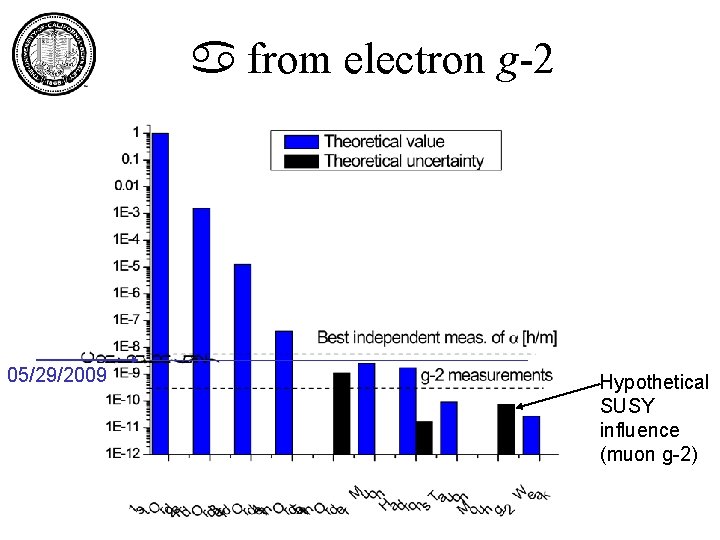
a from electron g-2 05/29/2009 Hypothetical SUSY influence (muon g-2)
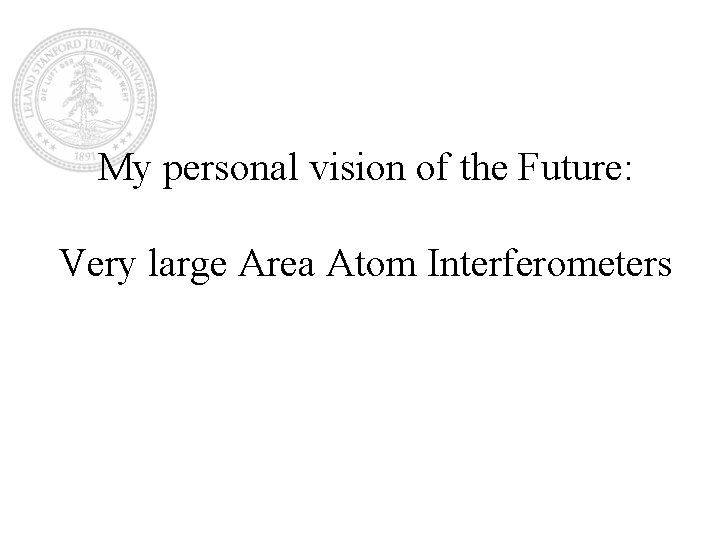
My personal vision of the Future: Very large Area Atom Interferometers
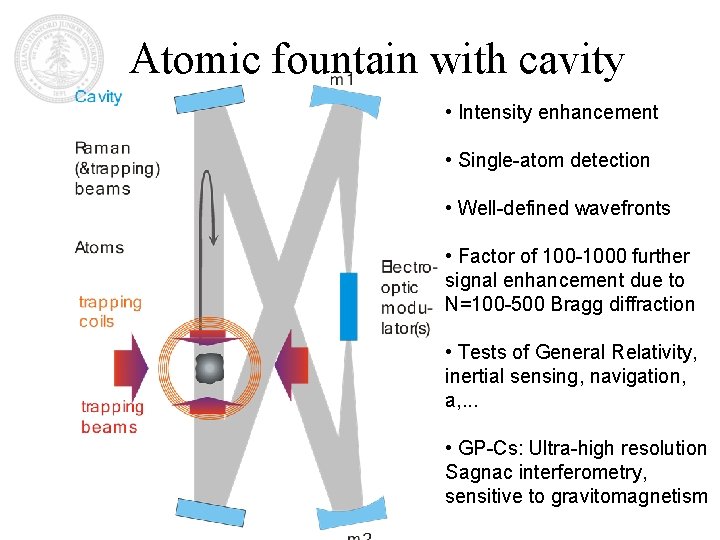
Atomic fountain with cavity • Intensity enhancement • Single-atom detection • Well-defined wavefronts • Factor of 100 -1000 further signal enhancement due to N=100 -500 Bragg diffraction • Tests of General Relativity, inertial sensing, navigation, a, . . . • GP-Cs: Ultra-high resolution Sagnac interferometry, sensitive to gravitomagnetism
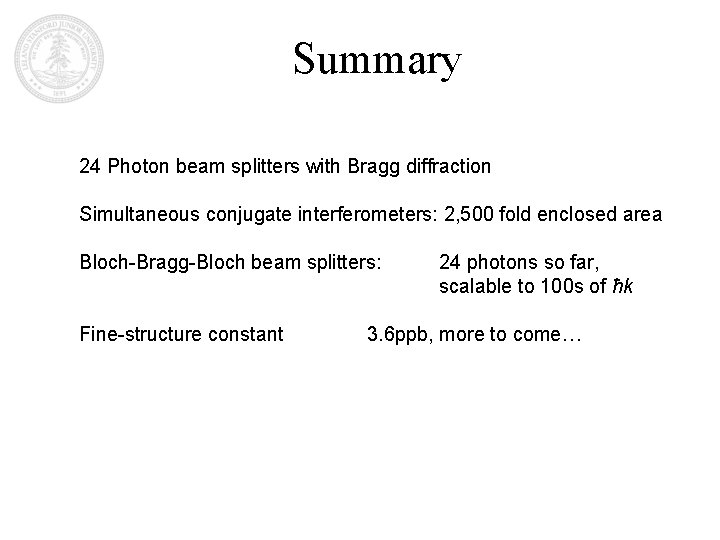
Summary 24 Photon beam splitters with Bragg diffraction Simultaneous conjugate interferometers: 2, 500 fold enclosed area Bloch-Bragg-Bloch beam splitters: Fine-structure constant 24 photons so far, scalable to 100 s of ħk 3. 6 ppb, more to come…
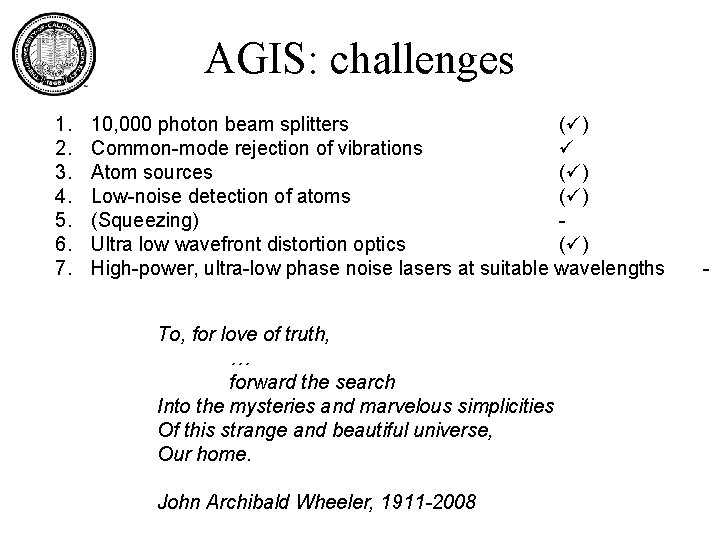
AGIS: challenges 1. 2. 3. 4. 5. 6. 7. 10, 000 photon beam splitters ( ) Common-mode rejection of vibrations Atom sources ( ) Low-noise detection of atoms ( ) (Squeezing) Ultra low wavefront distortion optics ( ) High-power, ultra-low phase noise lasers at suitable wavelengths To, for love of truth, … forward the search Into the mysteries and marvelous simplicities Of this strange and beautiful universe, Our home. John Archibald Wheeler, 1911 -2008 -
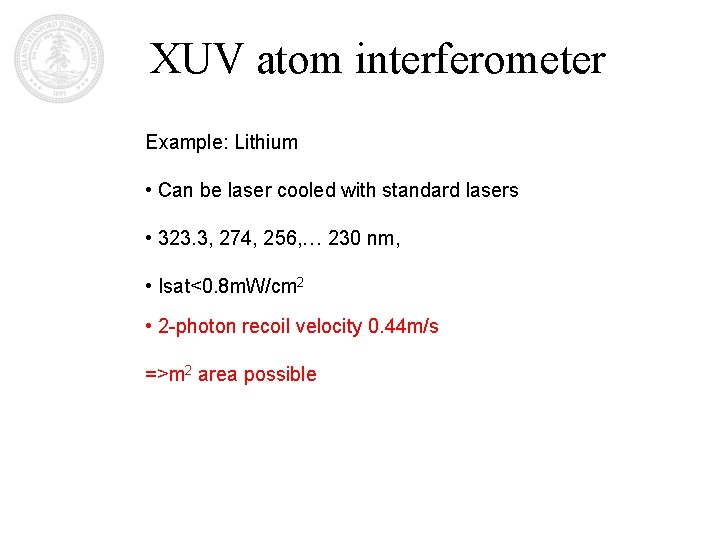
XUV atom interferometer Example: Lithium • Can be laser cooled with standard lasers • 323. 3, 274, 256, … 230 nm, • Isat<0. 8 m. W/cm 2 • 2 -photon recoil velocity 0. 44 m/s =>m 2 area possible
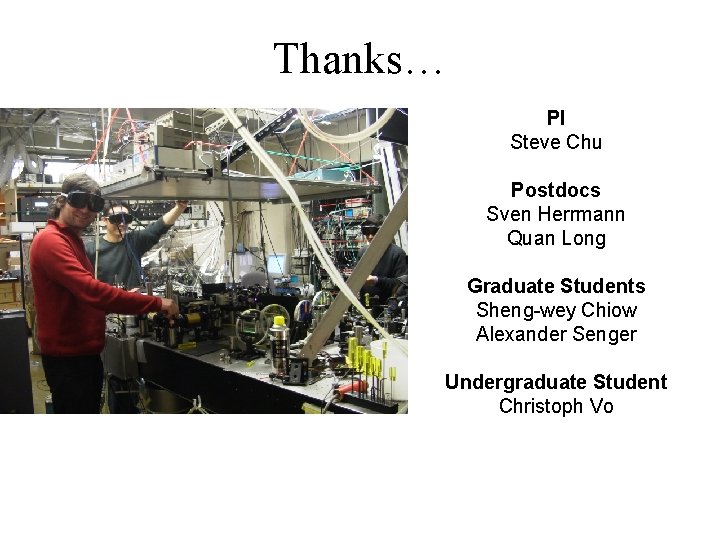
Thanks… PI Steve Chu Postdocs Sven Herrmann Quan Long Graduate Students Sheng-wey Chiow Alexander Senger Undergraduate Student Christoph Vo
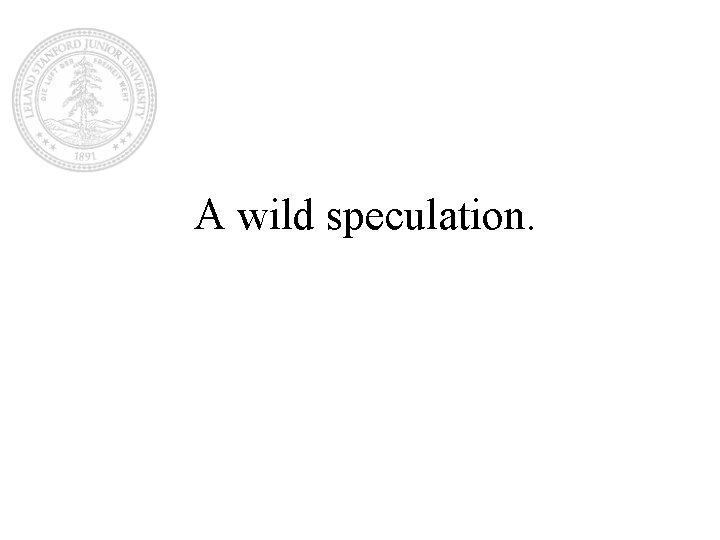
A wild speculation.
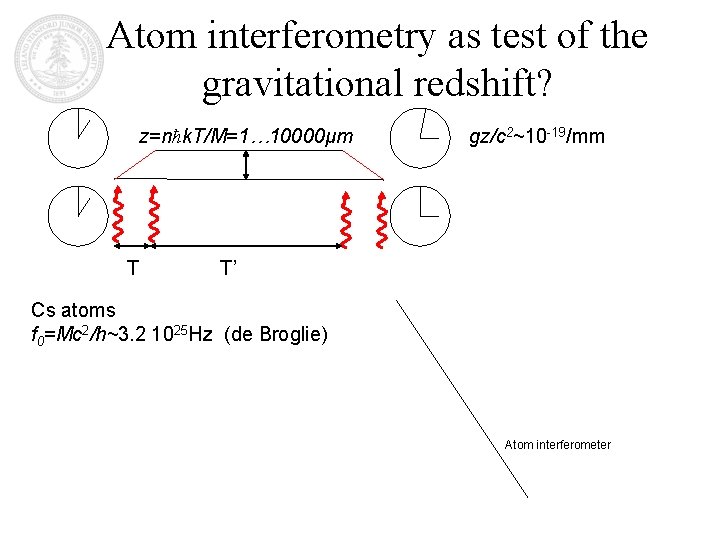
Atom interferometry as test of the gravitational redshift? z=nħk. T/M=1… 10000μm T gz/c 2~10 -19/mm T’ Cs atoms f 0=Mc 2/h~3. 2 1025 Hz (de Broglie) Atom interferometer
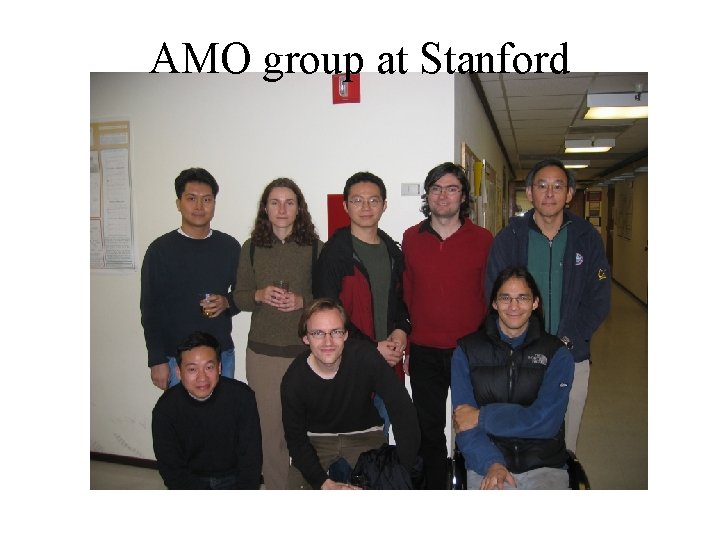
AMO group at Stanford
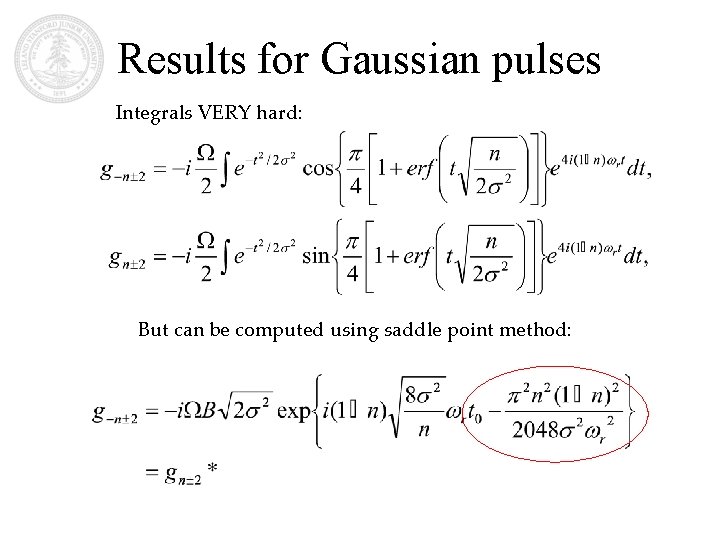
Results for Gaussian pulses Integrals VERY hard: But can be computed using saddle point method:
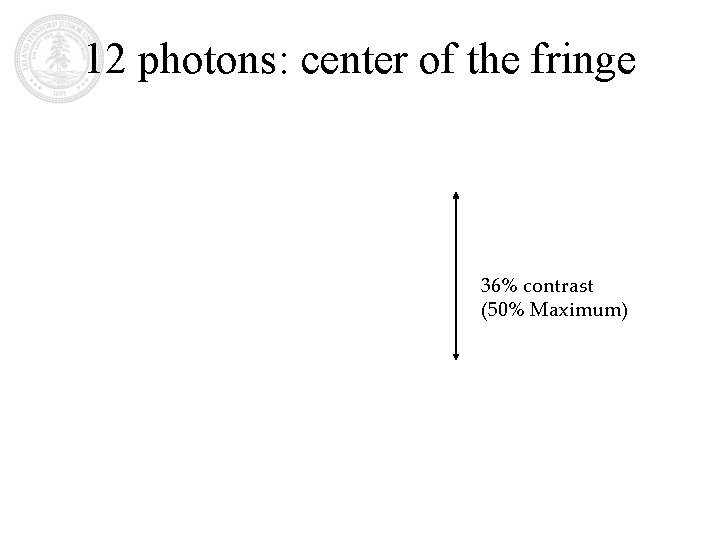
12 photons: center of the fringe 36% contrast (50% Maximum)
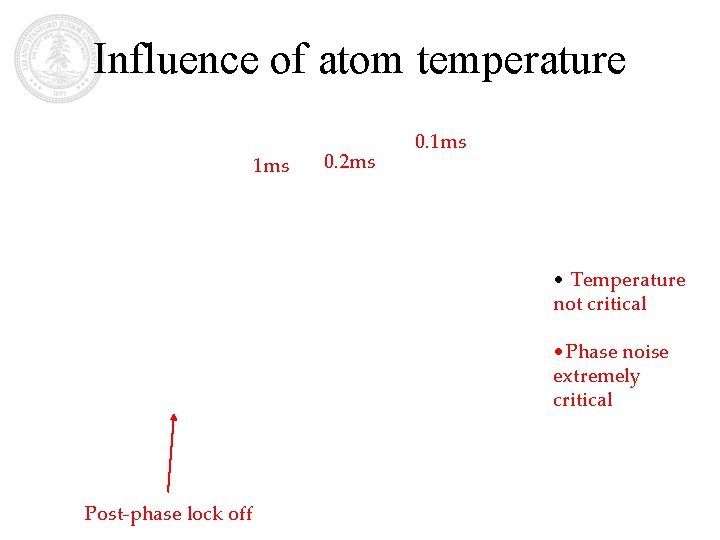
Influence of atom temperature 1 ms 0. 2 ms 0. 1 ms • Temperature not critical • Phase noise extremely critical Post-phase lock off
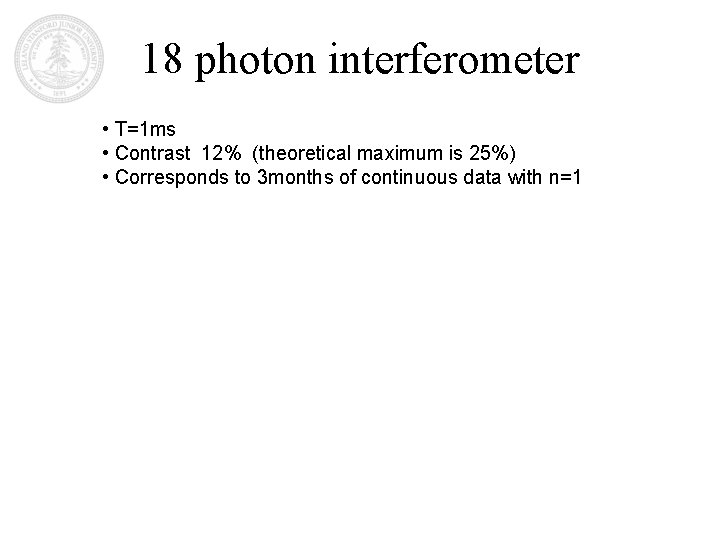
18 photon interferometer • T=1 ms • Contrast 12% (theoretical maximum is 25%) • Corresponds to 3 months of continuous data with n=1
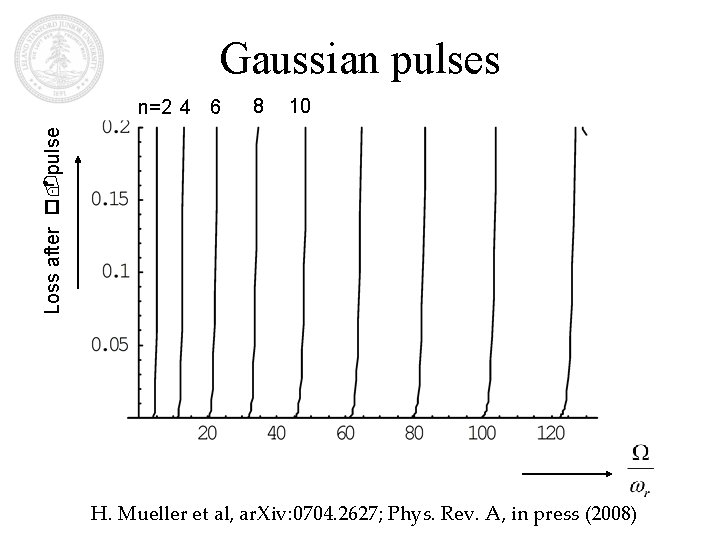
Gaussian pulses 8 10 Loss after p-pulse n=2 4 6 H. Mueller et al, ar. Xiv: 0704. 2627; Phys. Rev. A, in press (2008)
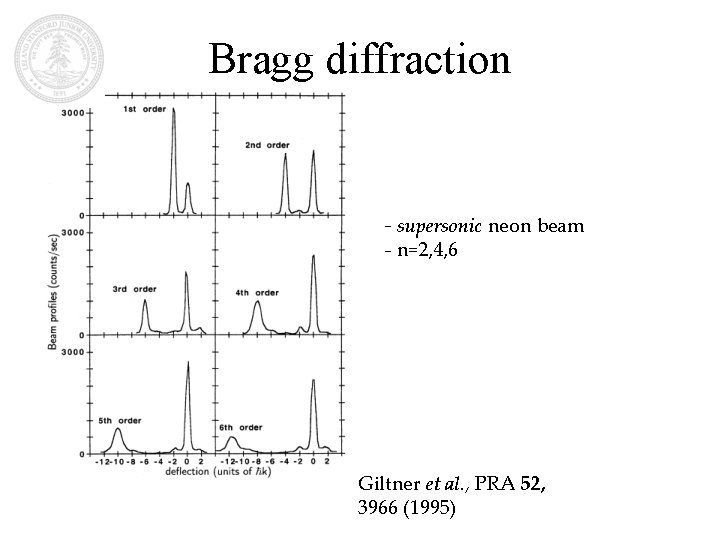
Bragg diffraction - supersonic neon beam - n=2, 4, 6 Giltner et al. , PRA 52, 3966 (1995)
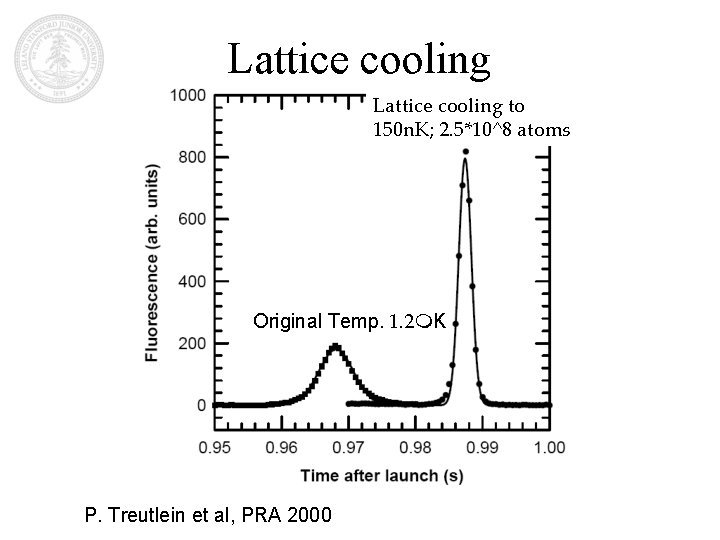
Lattice cooling to 150 n. K; 2. 5*10^8 atoms Original Temp. 1. 2 m. K P. Treutlein et al, PRA 2000
Difference between ray optics and wave optics
Reflection and refraction venn diagram
Http //vsg.quasihome.com
Wave optics b.sc physics
Gravitational wave open science center
Gravitational wave hear murmurs universe
Gravitational wave
Gravitational wave
Giant gravitational wave murmurs universe
The structure of the atom section 2 defining the atom
Sejarah perkembangan model atom
Holger kallehauge
Holger witte
Holger hunger
Paradigmen
Holger feindel
Holger feindel
Holger brock
Dr beneder
Hf finanzconsulting
Holger witte
Holger kreckel
Kısmi havayolu tıkanmasındaki bulgular
Holger nielsen metodu ne için kullanılır
Holger kallehauge
Holger krawinkel
Rds rightsizing
Holger schlarb
Holger hoos
Structural health monitoring aircraft
Hc-cargo catalogue pdf
Holger kallehauge
Holger schmidt fh dortmund
Holger diessel
P and s wave chart
Velocity frequency wavelength triangle
Quarter-wave symmetry
Transverse and longitudnal waves
Full wave rectified sine wave fourier series
Longitudinal wave vs transverse wave
Examples of half wave rectifier
Wave wave repeating
Mechanical and electromagnetic waves
Short wave vs long wave radiation
Bridge type rectifier circuit
Wave wave repeating
Mechanical waves and electromagnetic waves similarities
Difference between full wave and half wave rectifier
Geometric optics
Fiber optics richard sanders
Geometric optics ppt
Iso 10110-7
The computational complexity of linear optics
Optics
Grade 10 optics review
Nonlinear optics
Optics poynting's theorem
Free space optics
Cauchy formula optics
Free space optics