ZLY 106 NERVOUS SYSTEM DR O A IYIOLA
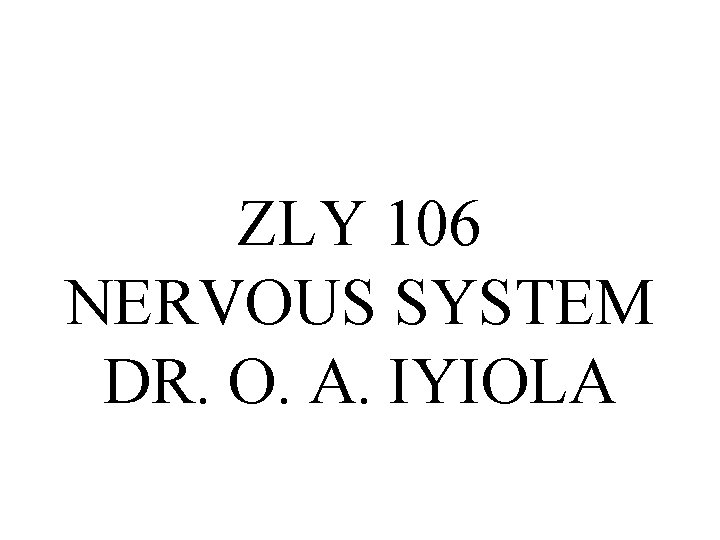
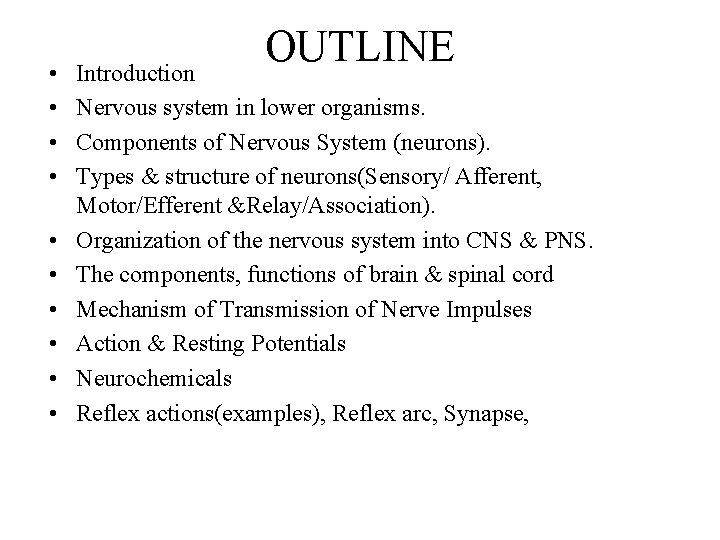
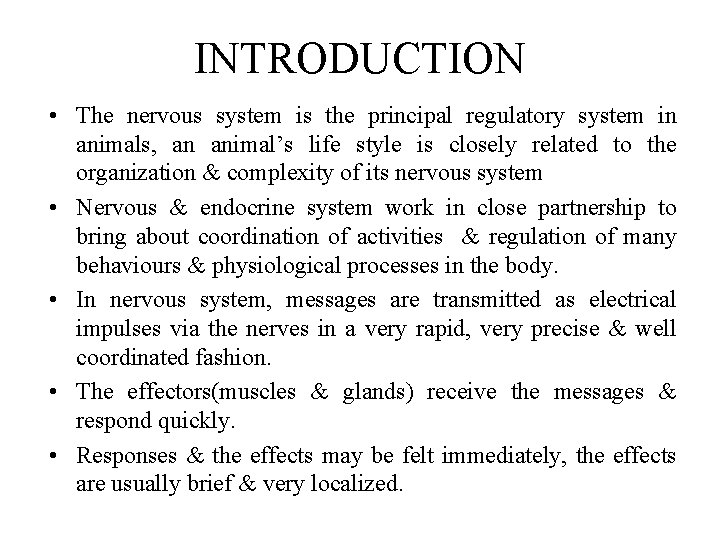
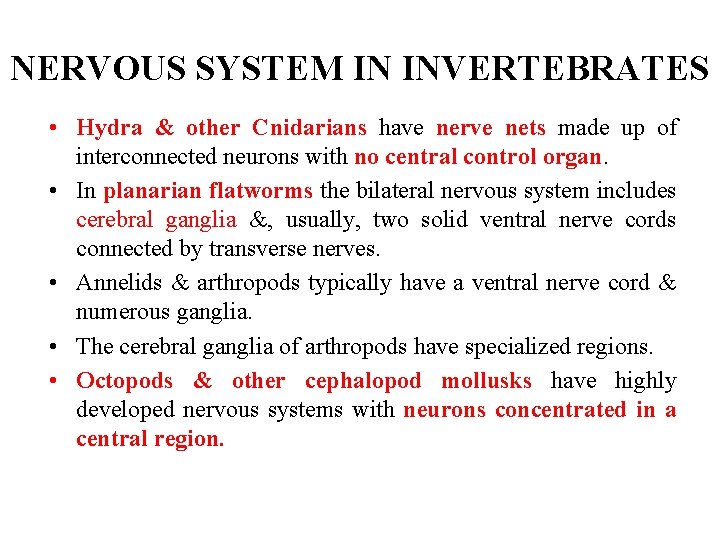
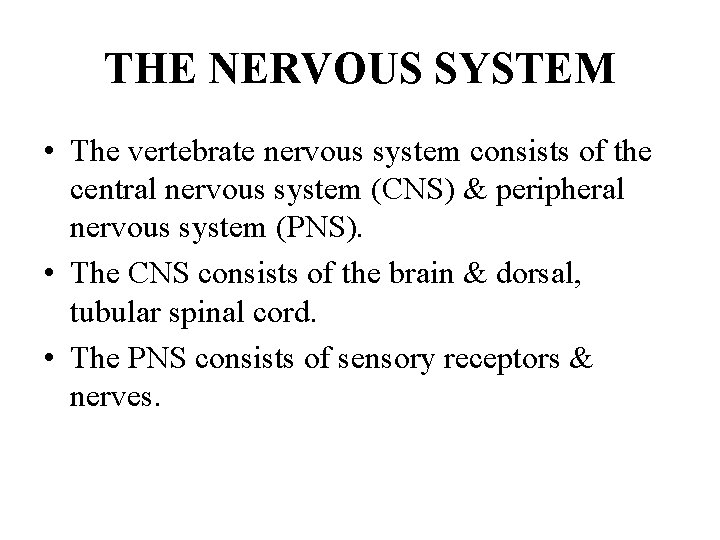
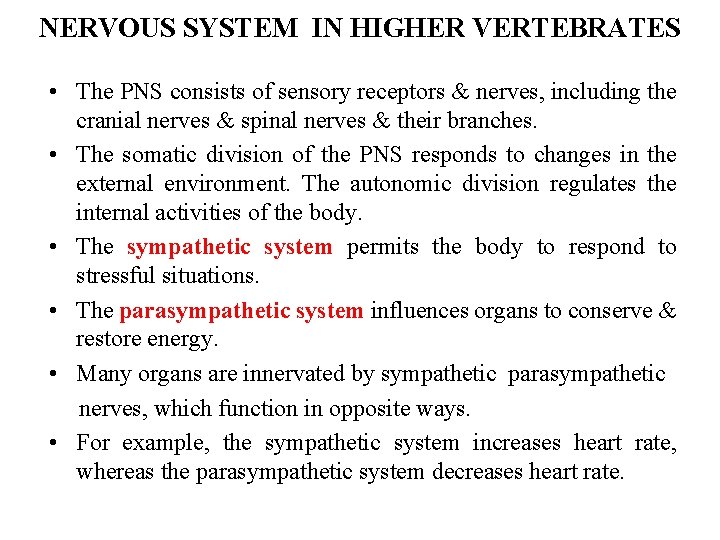
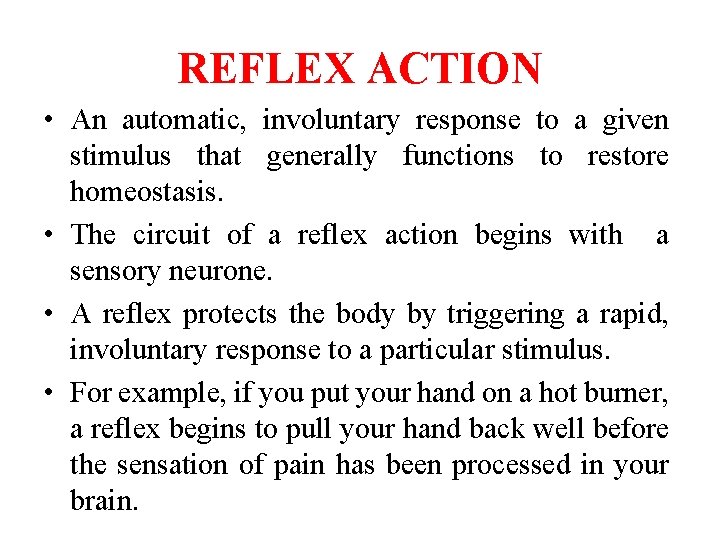
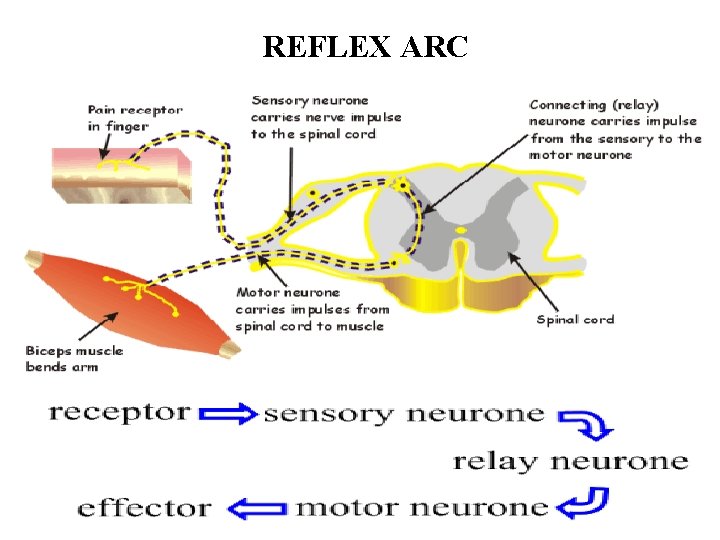
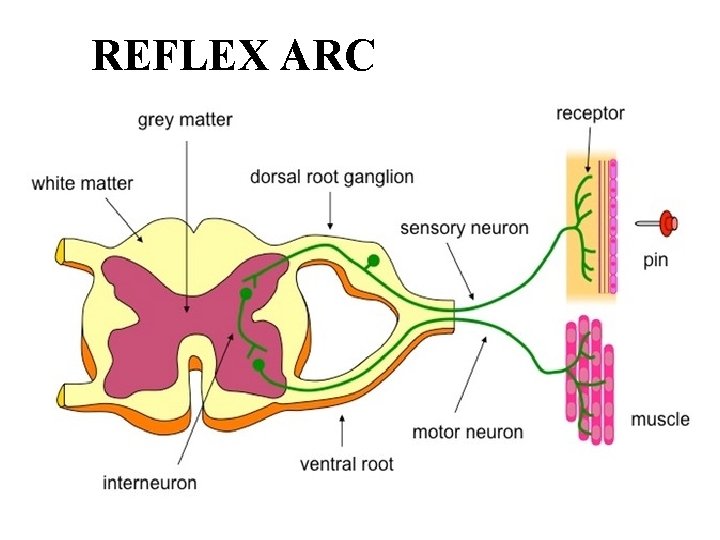
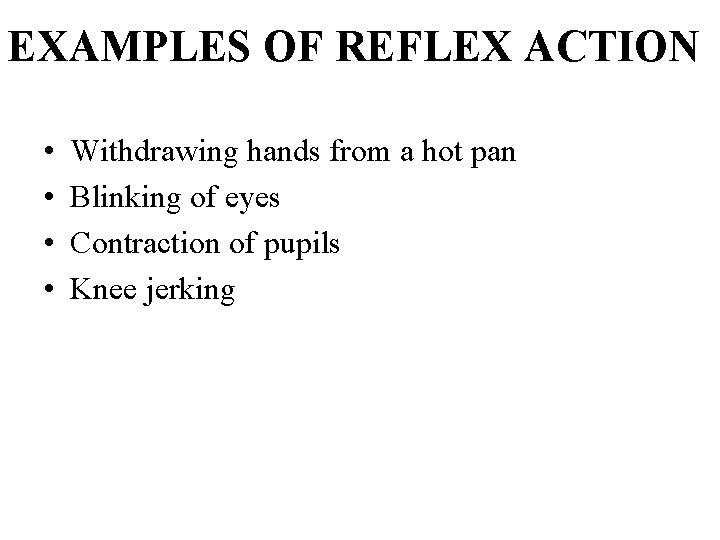
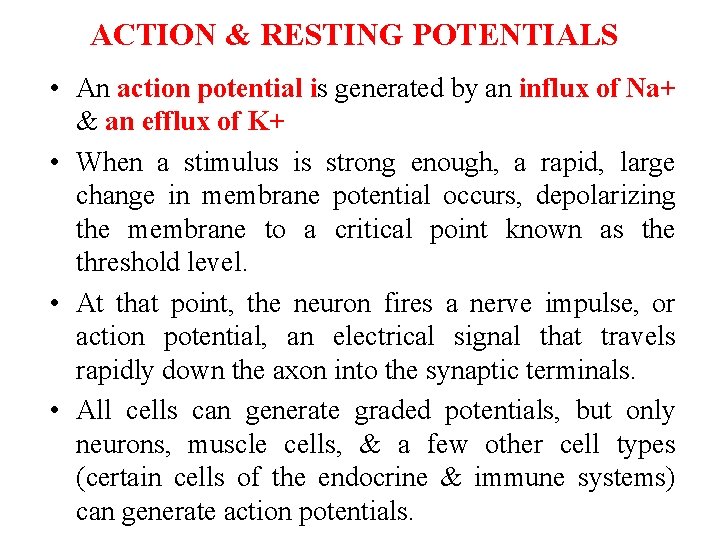
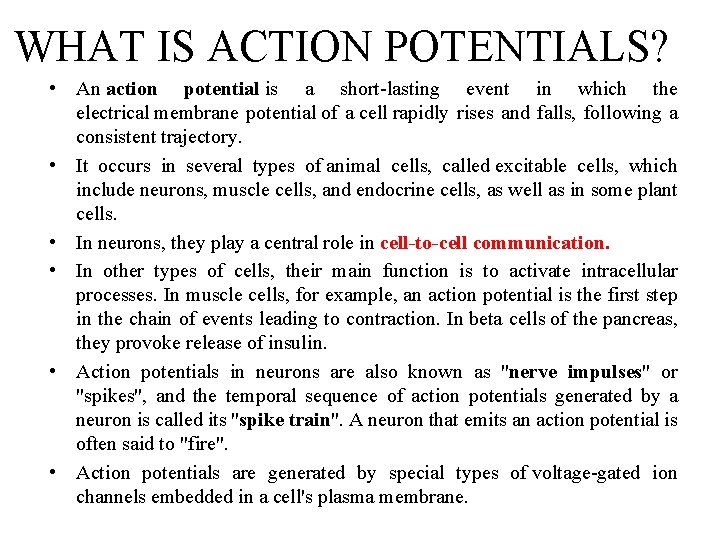
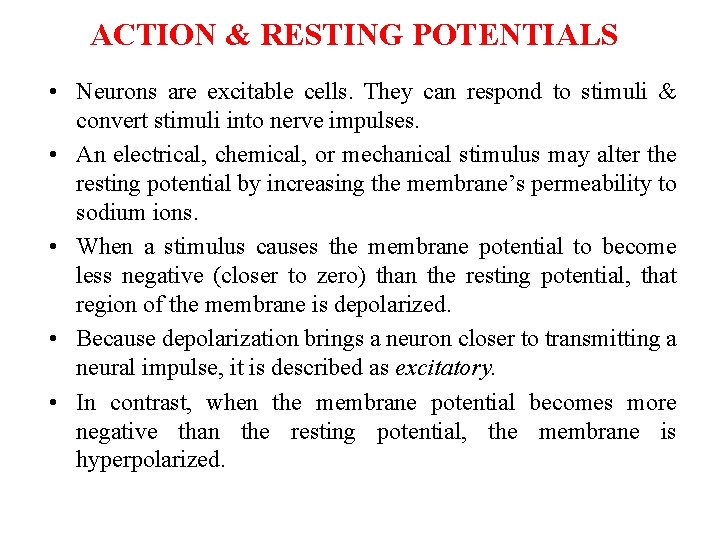
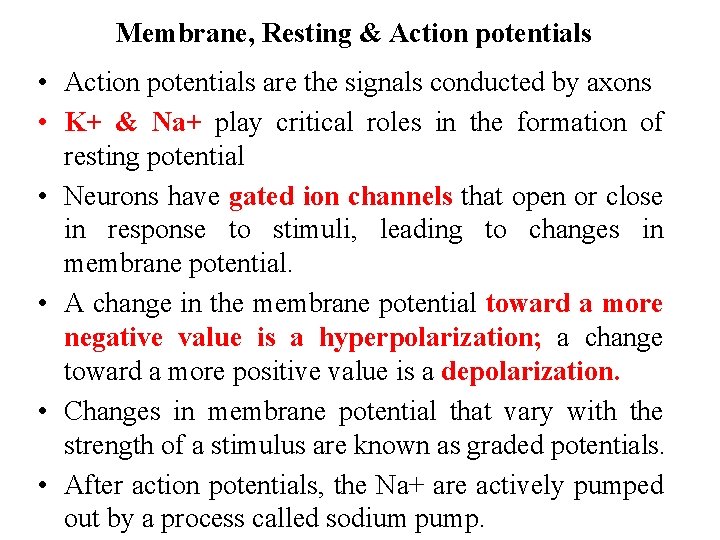
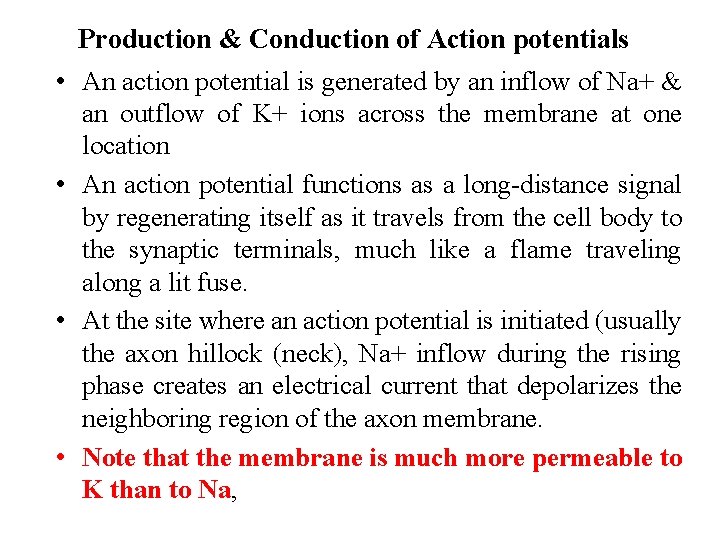
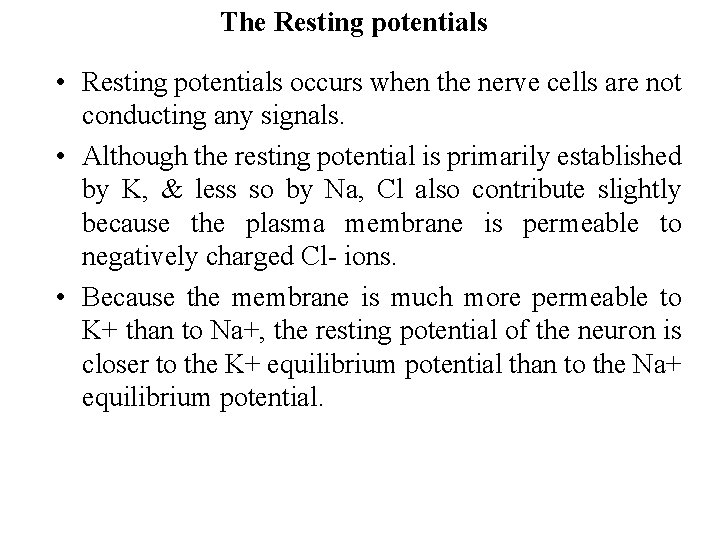
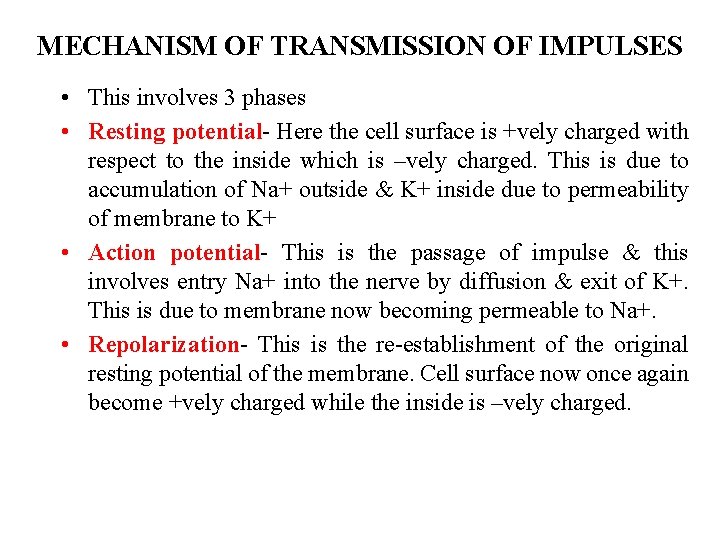
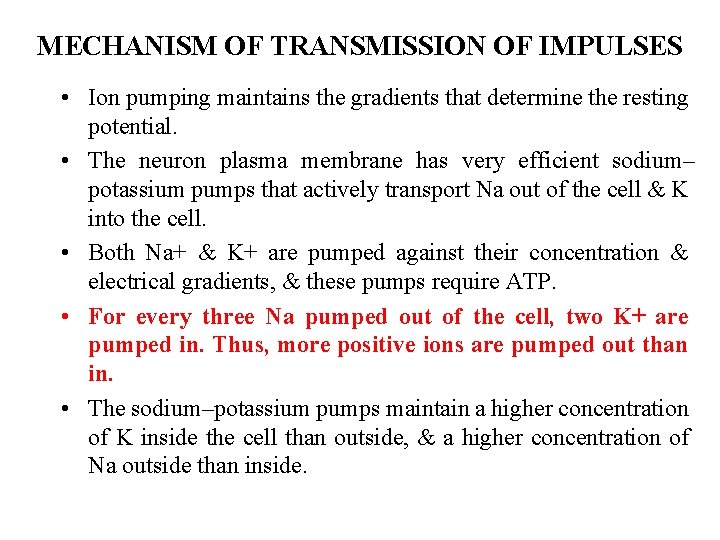
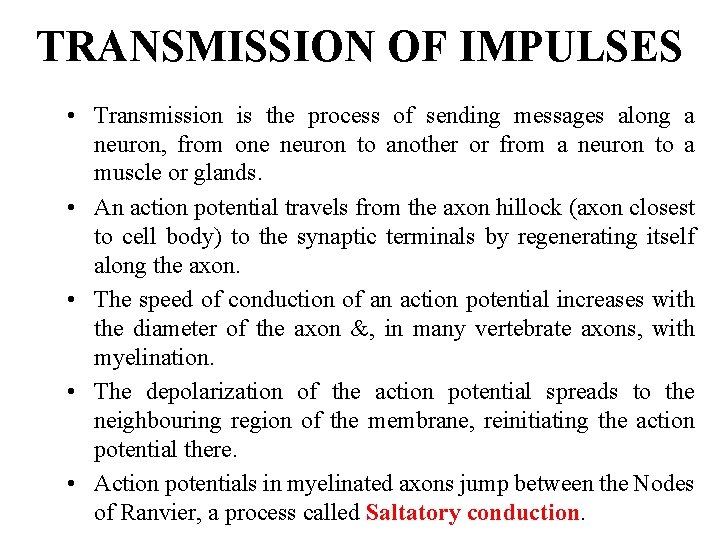
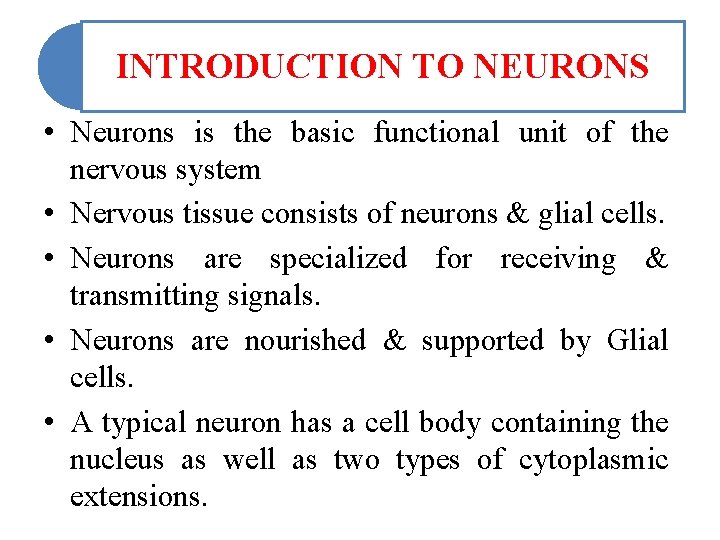
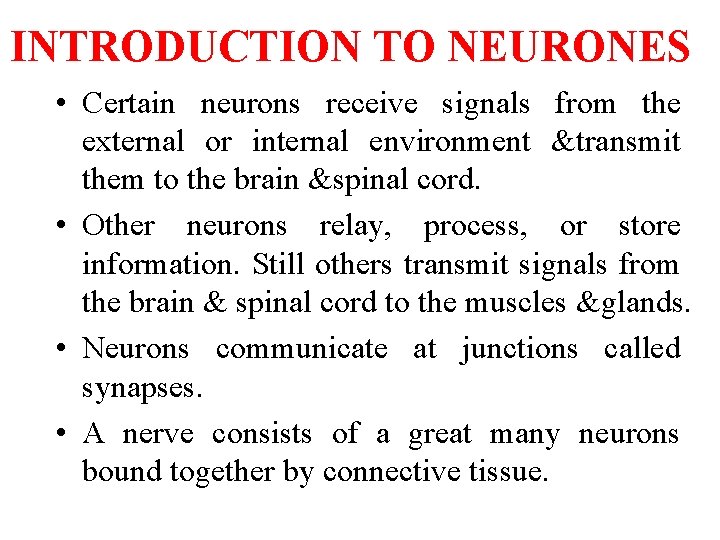
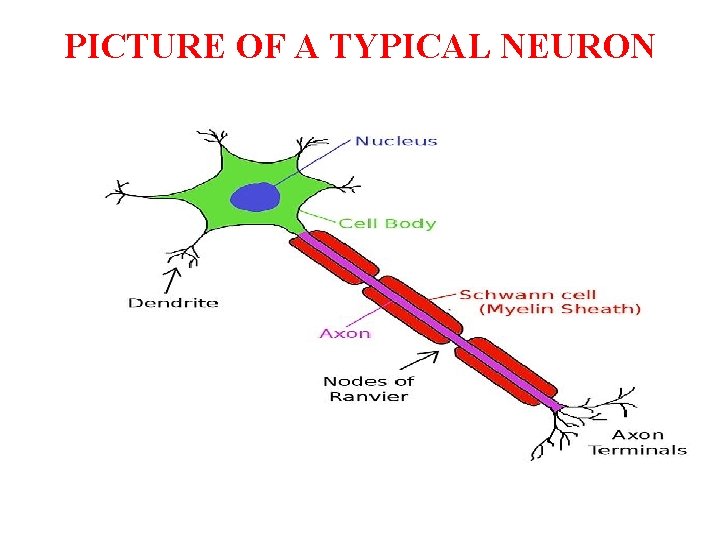
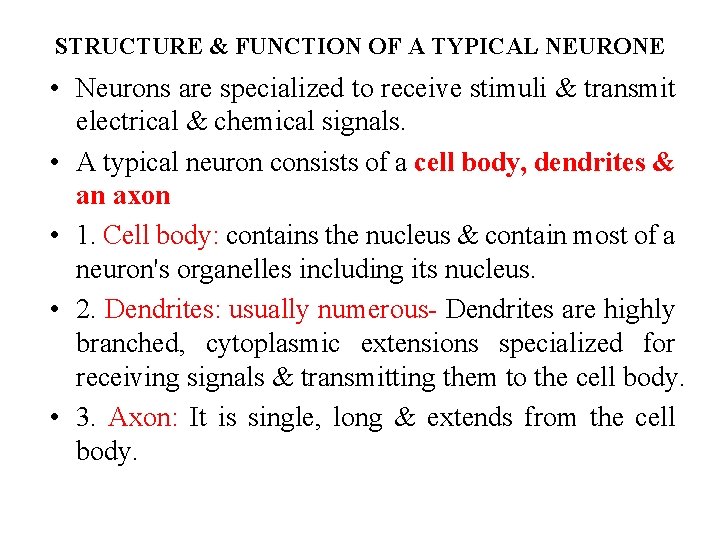
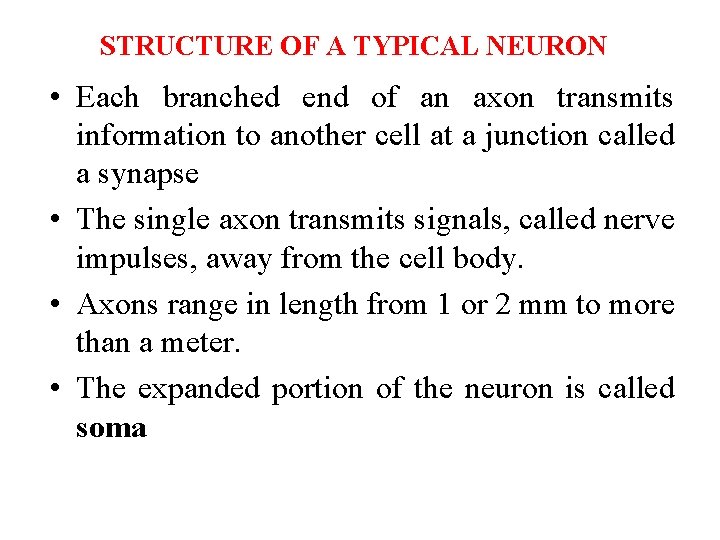
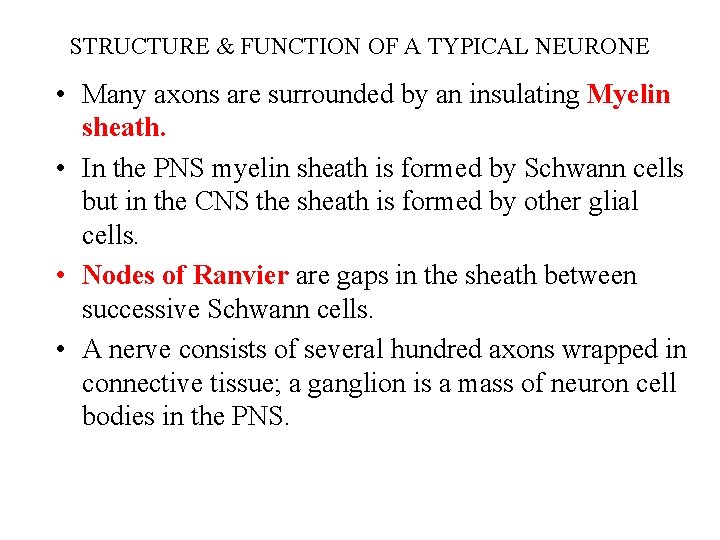
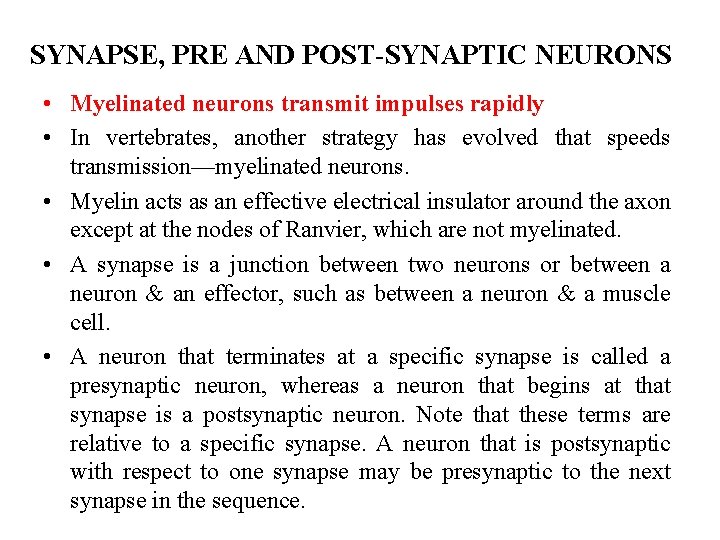
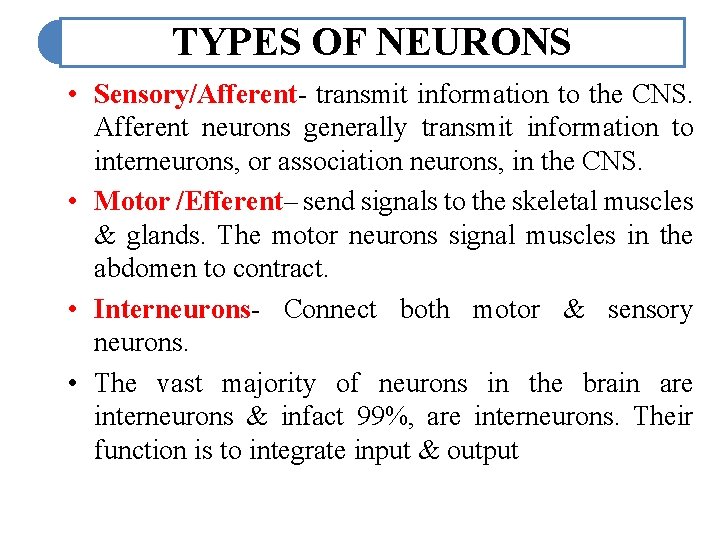
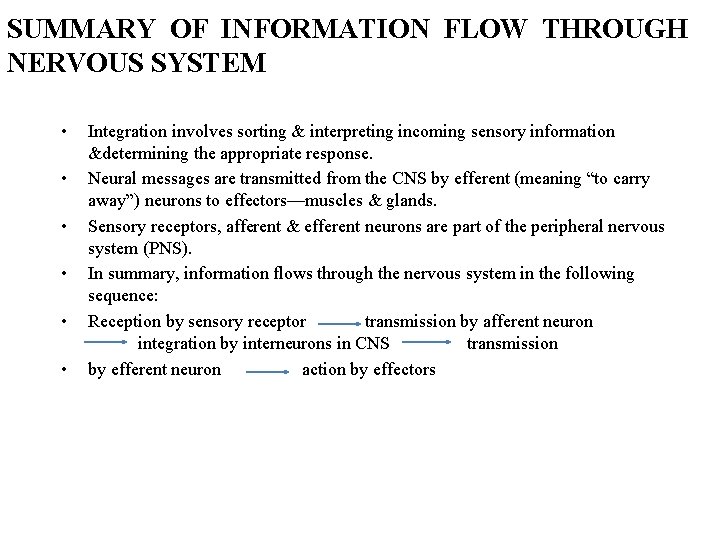
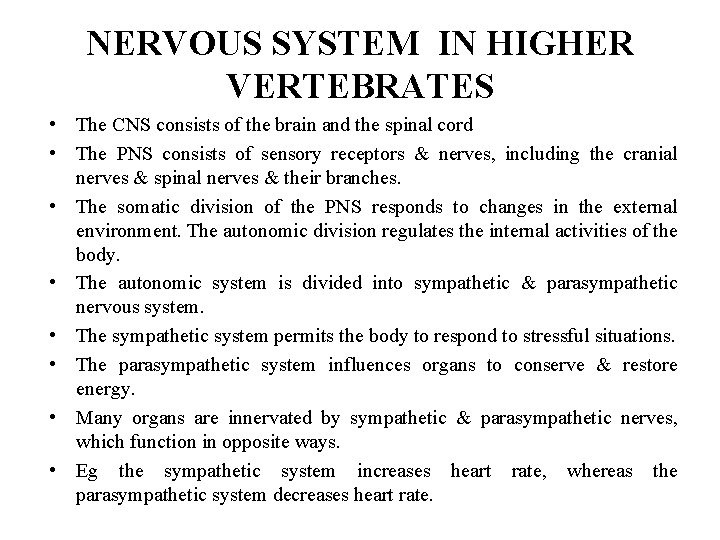
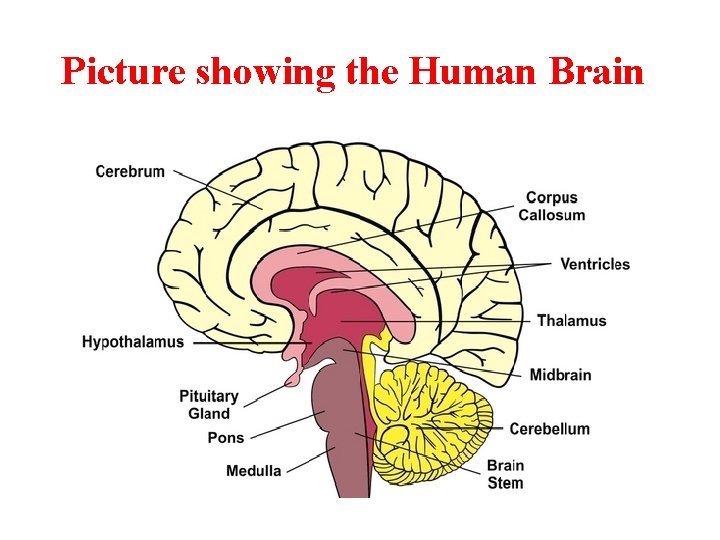
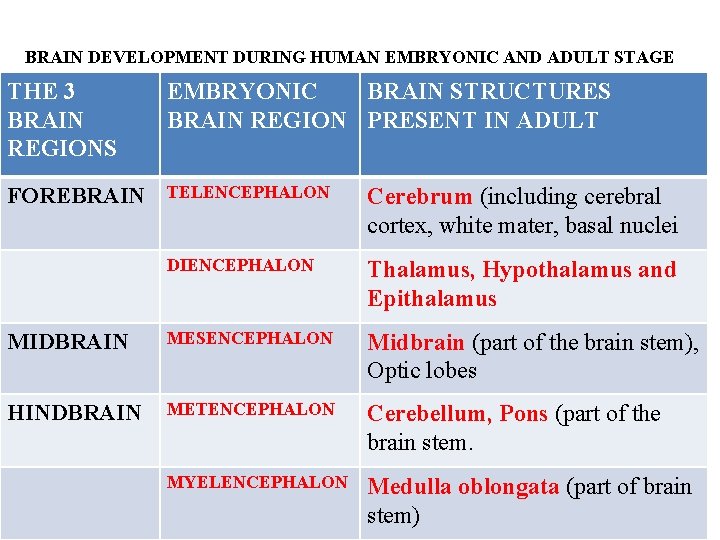
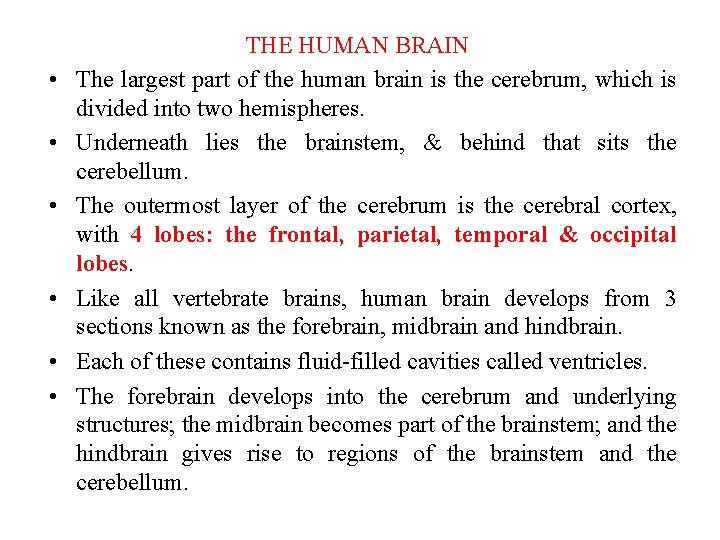
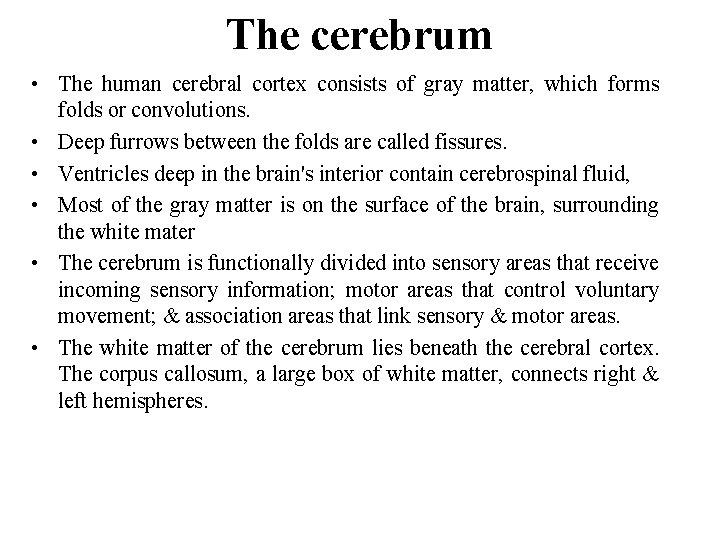
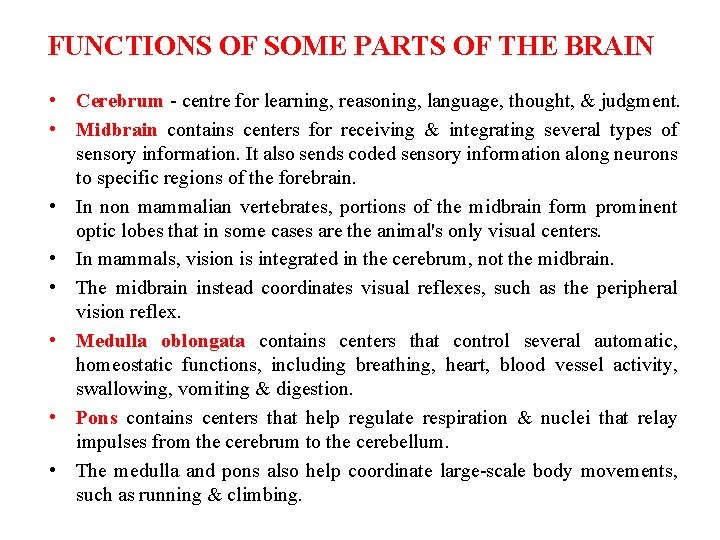
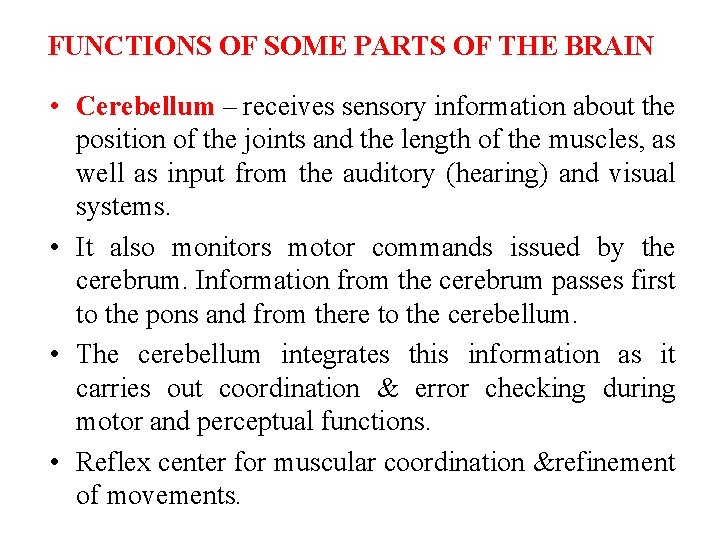
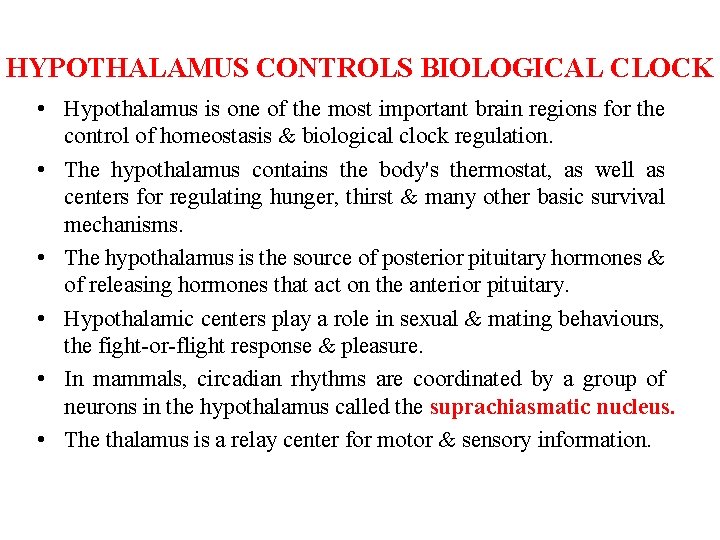
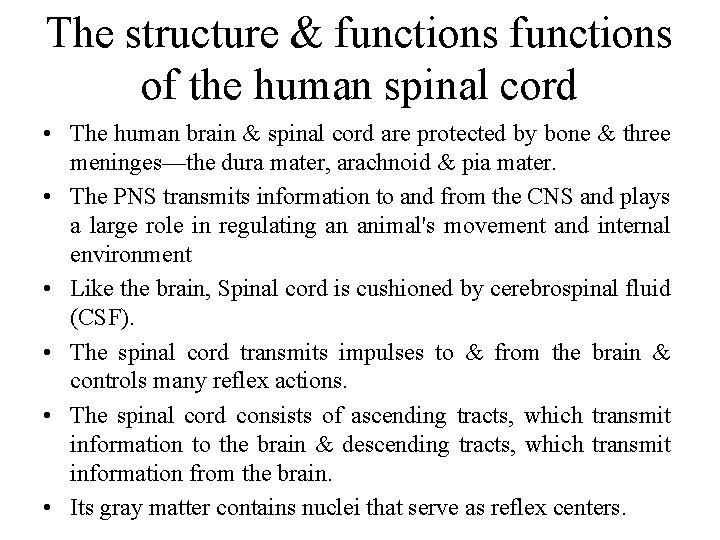
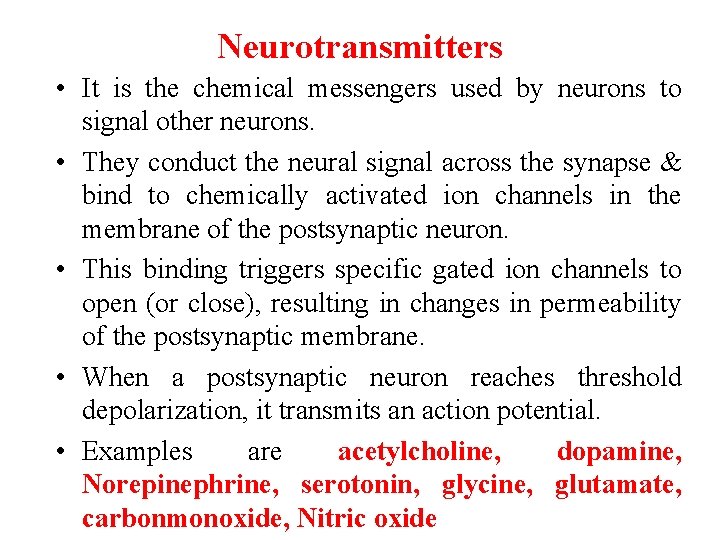
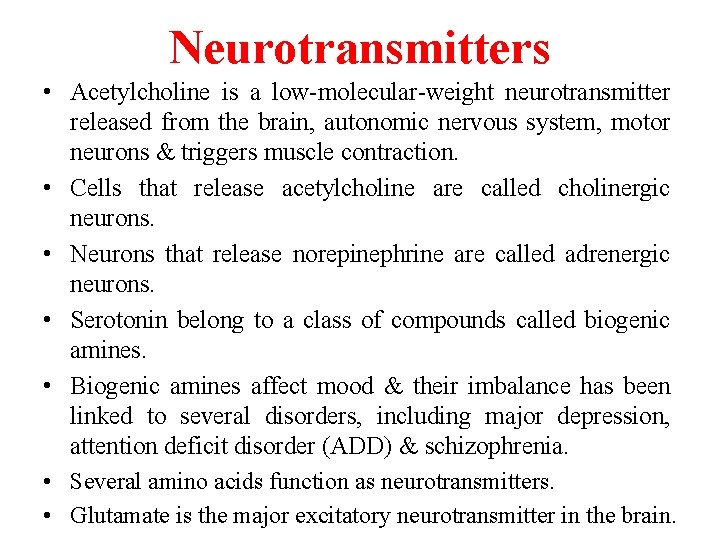
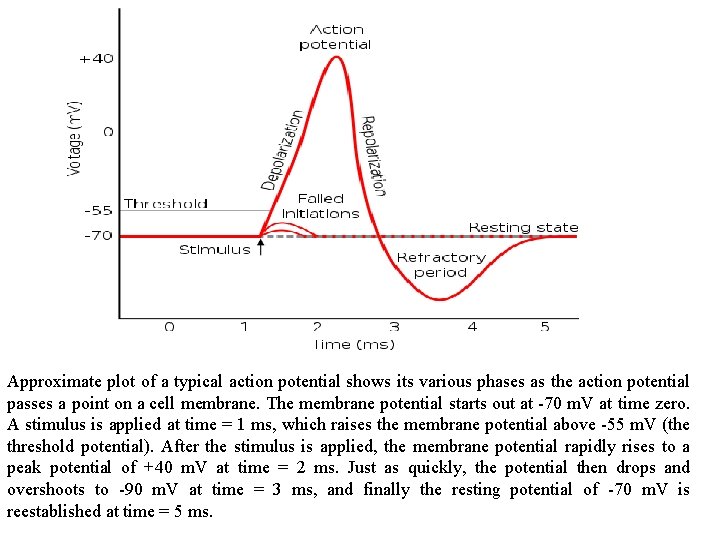
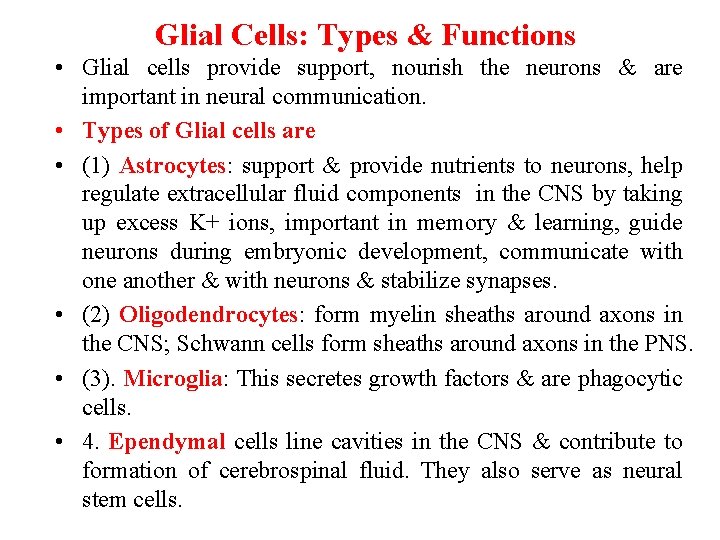
- Slides: 41
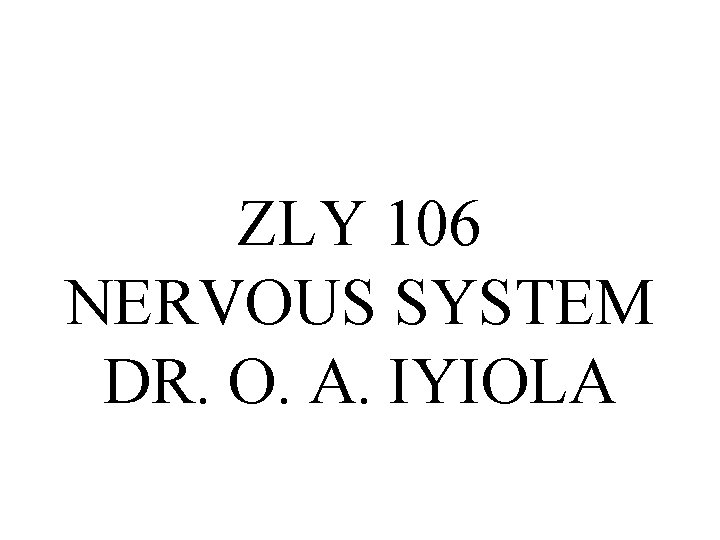
ZLY 106 NERVOUS SYSTEM DR. O. A. IYIOLA
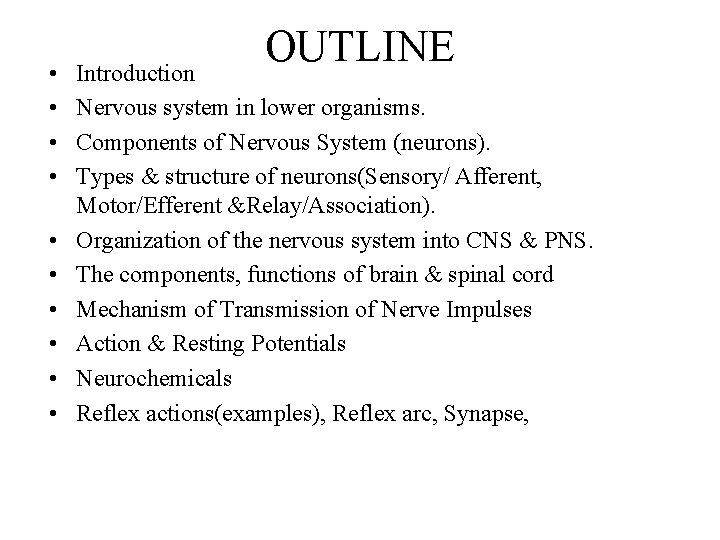
• • • OUTLINE Introduction Nervous system in lower organisms. Components of Nervous System (neurons). Types & structure of neurons(Sensory/ Afferent, Motor/Efferent &Relay/Association). Organization of the nervous system into CNS & PNS. The components, functions of brain & spinal cord Mechanism of Transmission of Nerve Impulses Action & Resting Potentials Neurochemicals Reflex actions(examples), Reflex arc, Synapse,
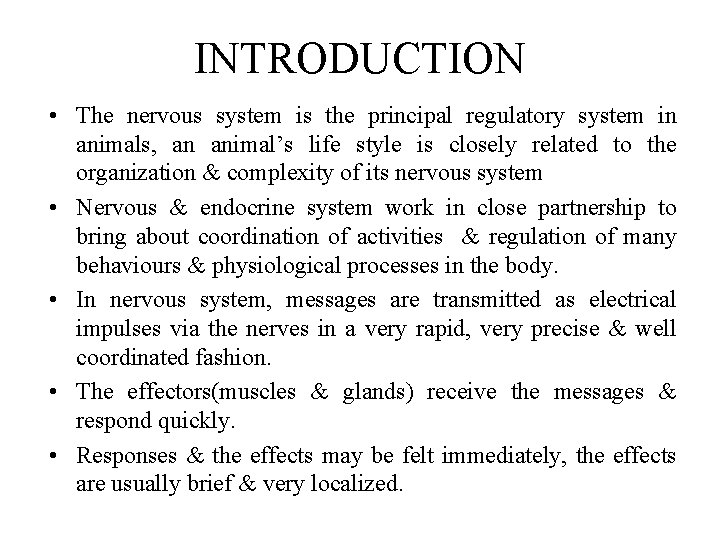
INTRODUCTION • The nervous system is the principal regulatory system in animals, an animal’s life style is closely related to the organization & complexity of its nervous system • Nervous & endocrine system work in close partnership to bring about coordination of activities & regulation of many behaviours & physiological processes in the body. • In nervous system, messages are transmitted as electrical impulses via the nerves in a very rapid, very precise & well coordinated fashion. • The effectors(muscles & glands) receive the messages & respond quickly. • Responses & the effects may be felt immediately, the effects are usually brief & very localized.
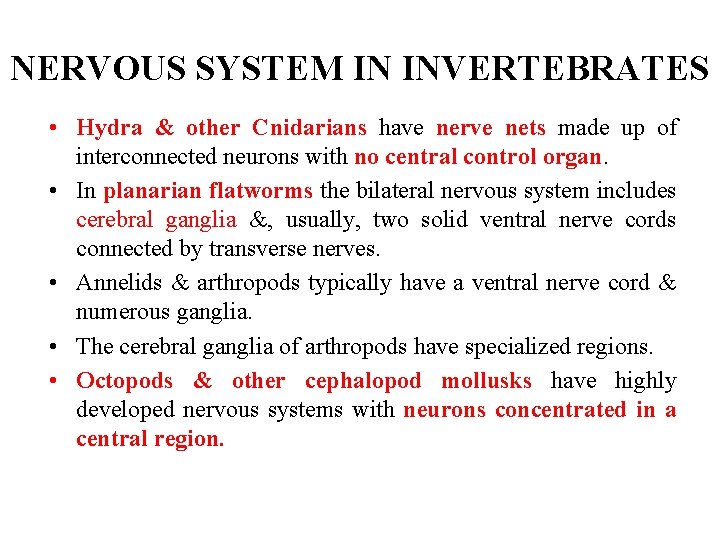
NERVOUS SYSTEM IN INVERTEBRATES • Hydra & other Cnidarians have nerve nets made up of interconnected neurons with no central control organ. • In planarian flatworms the bilateral nervous system includes cerebral ganglia &, usually, two solid ventral nerve cords connected by transverse nerves. • Annelids & arthropods typically have a ventral nerve cord & numerous ganglia. • The cerebral ganglia of arthropods have specialized regions. • Octopods & other cephalopod mollusks have highly developed nervous systems with neurons concentrated in a central region.
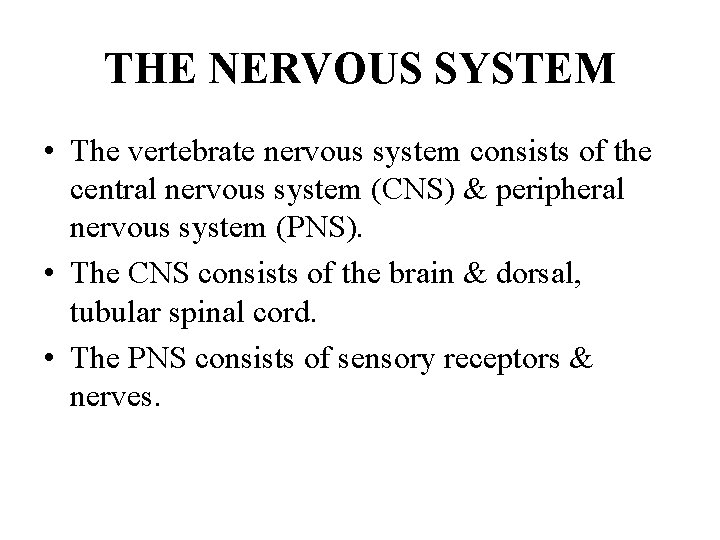
THE NERVOUS SYSTEM • The vertebrate nervous system consists of the central nervous system (CNS) & peripheral nervous system (PNS). • The CNS consists of the brain & dorsal, tubular spinal cord. • The PNS consists of sensory receptors & nerves.
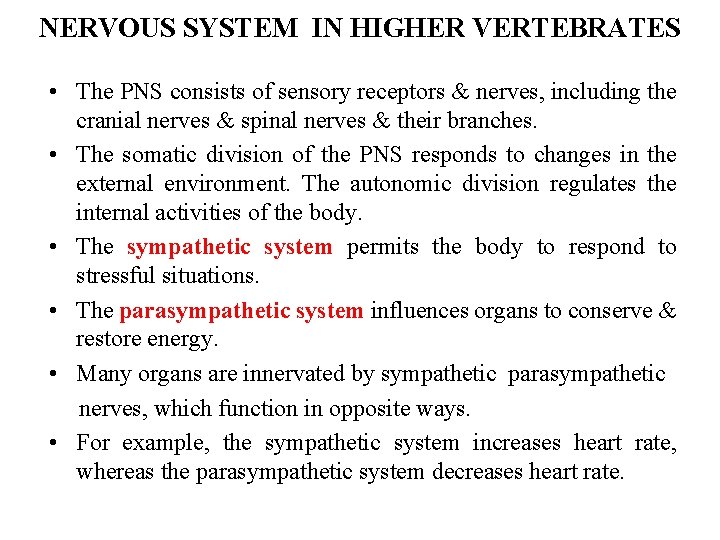
NERVOUS SYSTEM IN HIGHER VERTEBRATES • The PNS consists of sensory receptors & nerves, including the cranial nerves & spinal nerves & their branches. • The somatic division of the PNS responds to changes in the external environment. The autonomic division regulates the internal activities of the body. • The sympathetic system permits the body to respond to stressful situations. • The parasympathetic system influences organs to conserve & restore energy. • Many organs are innervated by sympathetic parasympathetic nerves, which function in opposite ways. • For example, the sympathetic system increases heart rate, whereas the parasympathetic system decreases heart rate.
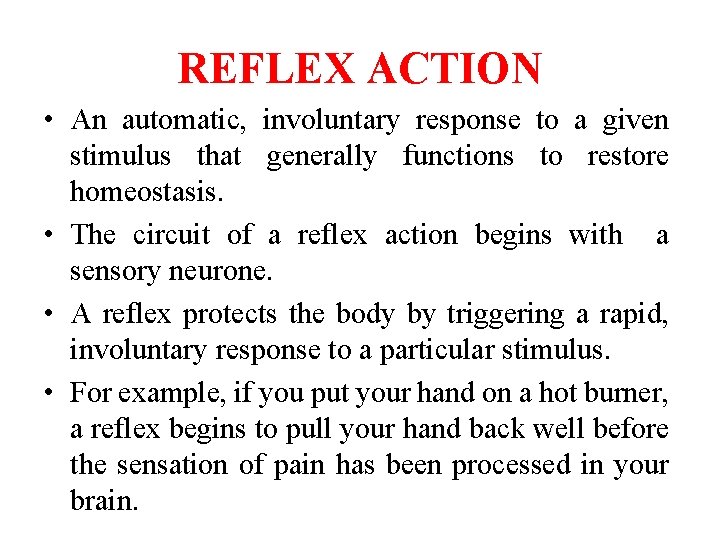
REFLEX ACTION • An automatic, involuntary response to a given stimulus that generally functions to restore homeostasis. • The circuit of a reflex action begins with a sensory neurone. • A reflex protects the body by triggering a rapid, involuntary response to a particular stimulus. • For example, if you put your hand on a hot burner, a reflex begins to pull your hand back well before the sensation of pain has been processed in your brain.
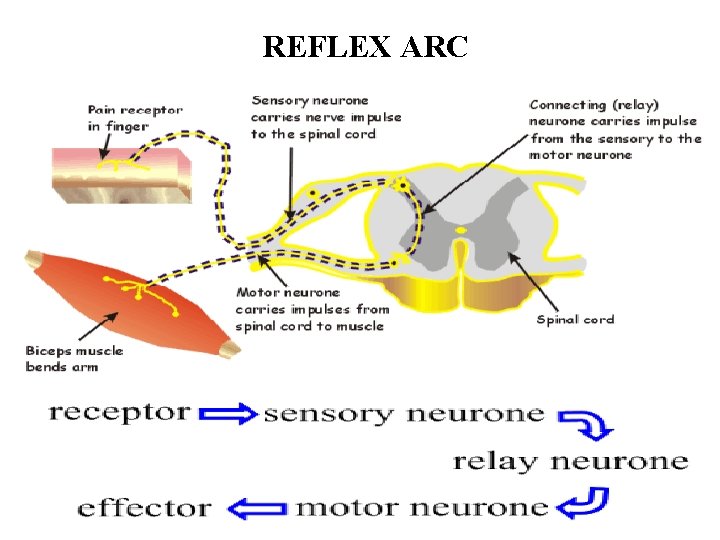
REFLEX ARC
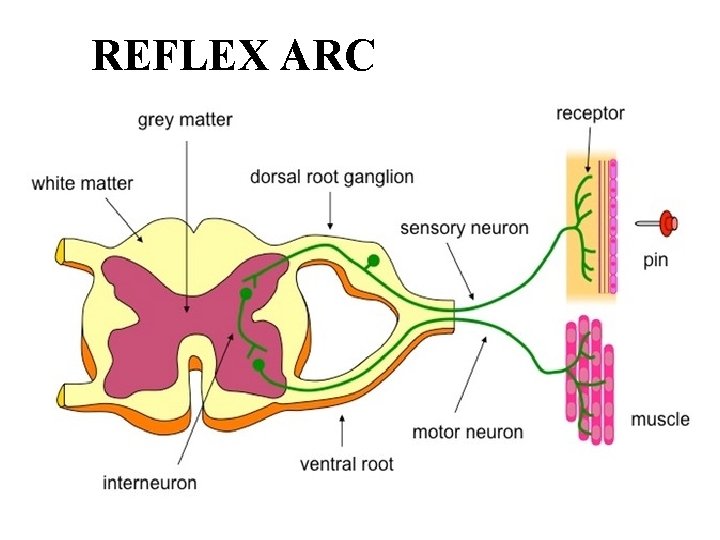
REFLEX ARC
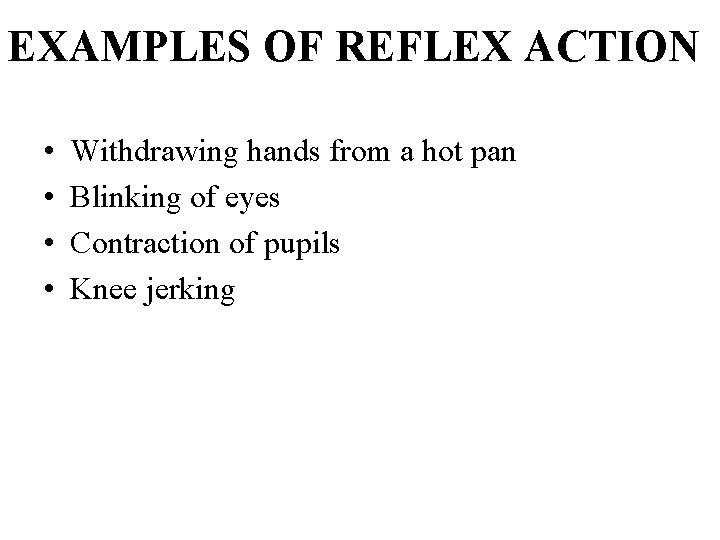
EXAMPLES OF REFLEX ACTION • • Withdrawing hands from a hot pan Blinking of eyes Contraction of pupils Knee jerking
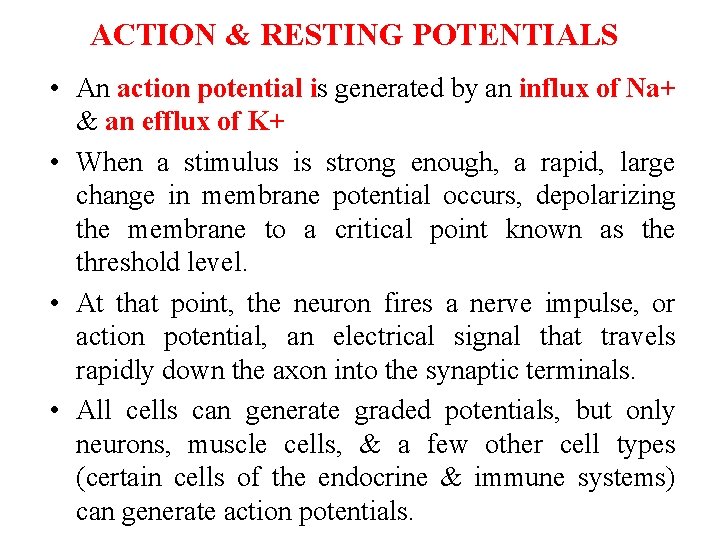
ACTION & RESTING POTENTIALS • An action potential is generated by an influx of Na+ & an efflux of K+ • When a stimulus is strong enough, a rapid, large change in membrane potential occurs, depolarizing the membrane to a critical point known as the threshold level. • At that point, the neuron fires a nerve impulse, or action potential, an electrical signal that travels rapidly down the axon into the synaptic terminals. • All cells can generate graded potentials, but only neurons, muscle cells, & a few other cell types (certain cells of the endocrine & immune systems) can generate action potentials.
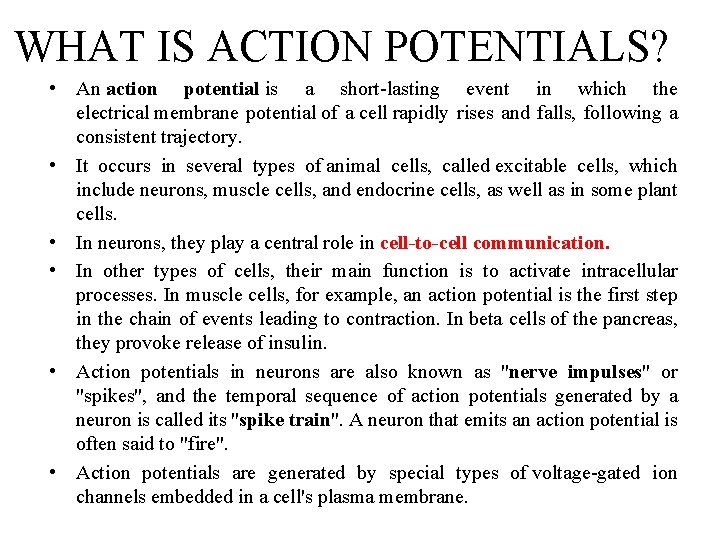
WHAT IS ACTION POTENTIALS? • An action potential is a short-lasting event in which the electrical membrane potential of a cell rapidly rises and falls, following a consistent trajectory. • It occurs in several types of animal cells, called excitable cells, which include neurons, muscle cells, and endocrine cells, as well as in some plant cells. • In neurons, they play a central role in cell-to-cell communication. • In other types of cells, their main function is to activate intracellular processes. In muscle cells, for example, an action potential is the first step in the chain of events leading to contraction. In beta cells of the pancreas, they provoke release of insulin. • Action potentials in neurons are also known as "nerve impulses" or "spikes", and the temporal sequence of action potentials generated by a neuron is called its "spike train". A neuron that emits an action potential is often said to "fire". • Action potentials are generated by special types of voltage-gated ion channels embedded in a cell's plasma membrane.
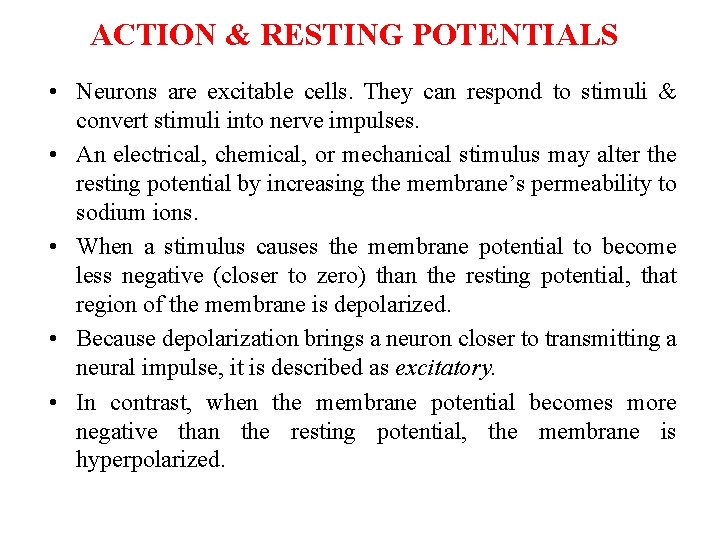
ACTION & RESTING POTENTIALS • Neurons are excitable cells. They can respond to stimuli & convert stimuli into nerve impulses. • An electrical, chemical, or mechanical stimulus may alter the resting potential by increasing the membrane’s permeability to sodium ions. • When a stimulus causes the membrane potential to become less negative (closer to zero) than the resting potential, that region of the membrane is depolarized. • Because depolarization brings a neuron closer to transmitting a neural impulse, it is described as excitatory. • In contrast, when the membrane potential becomes more negative than the resting potential, the membrane is hyperpolarized.
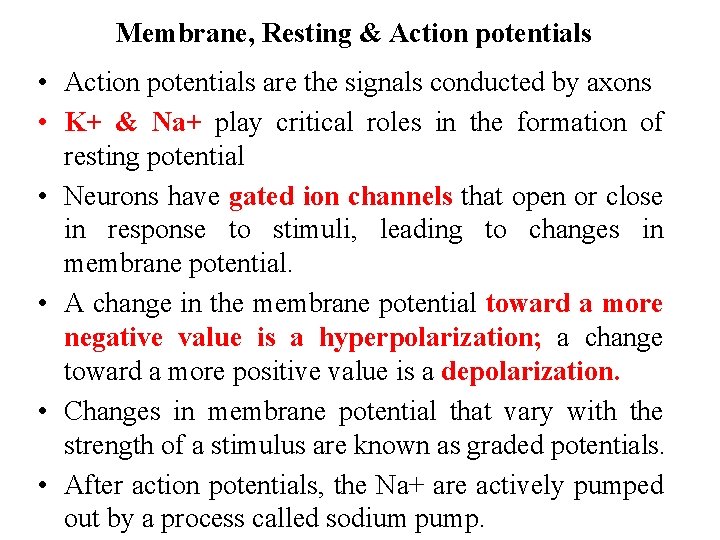
Membrane, Resting & Action potentials • Action potentials are the signals conducted by axons • K+ & Na+ play critical roles in the formation of resting potential • Neurons have gated ion channels that open or close in response to stimuli, leading to changes in membrane potential. • A change in the membrane potential toward a more negative value is a hyperpolarization; a change toward a more positive value is a depolarization. • Changes in membrane potential that vary with the strength of a stimulus are known as graded potentials. • After action potentials, the Na+ are actively pumped out by a process called sodium pump.
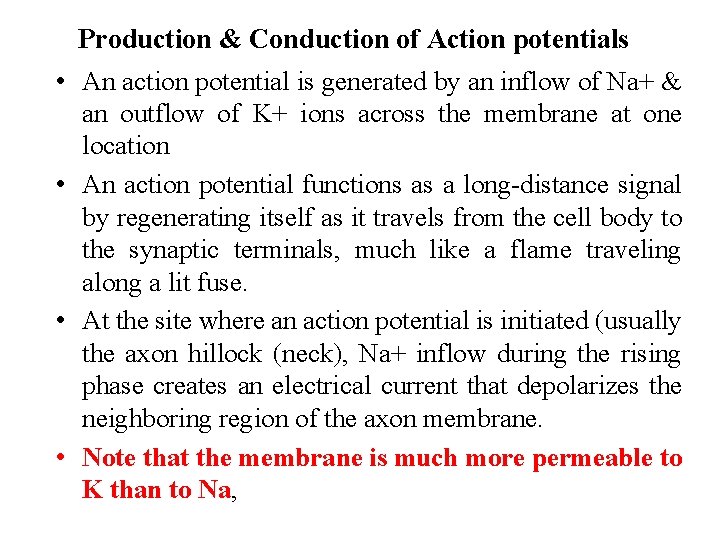
• • Production & Conduction of Action potentials An action potential is generated by an inflow of Na+ & an outflow of K+ ions across the membrane at one location An action potential functions as a long-distance signal by regenerating itself as it travels from the cell body to the synaptic terminals, much like a flame traveling along a lit fuse. At the site where an action potential is initiated (usually the axon hillock (neck), Na+ inflow during the rising phase creates an electrical current that depolarizes the neighboring region of the axon membrane. Note that the membrane is much more permeable to K than to Na,
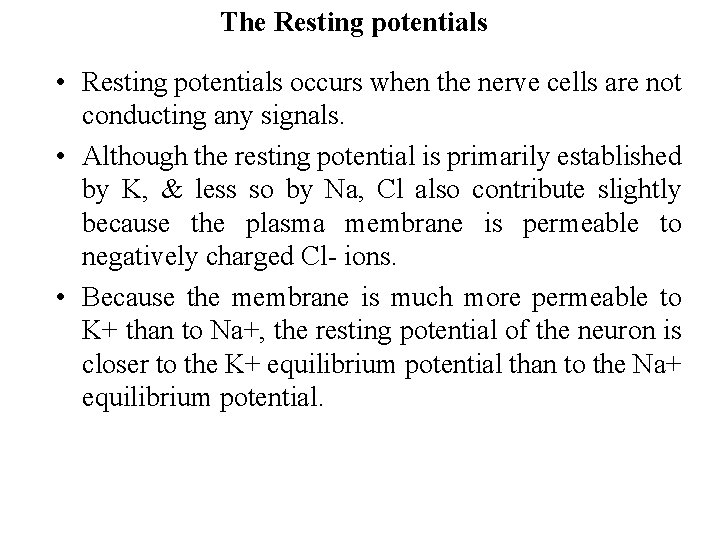
The Resting potentials • Resting potentials occurs when the nerve cells are not conducting any signals. • Although the resting potential is primarily established by K, & less so by Na, Cl also contribute slightly because the plasma membrane is permeable to negatively charged Cl- ions. • Because the membrane is much more permeable to K+ than to Na+, the resting potential of the neuron is closer to the K+ equilibrium potential than to the Na+ equilibrium potential.
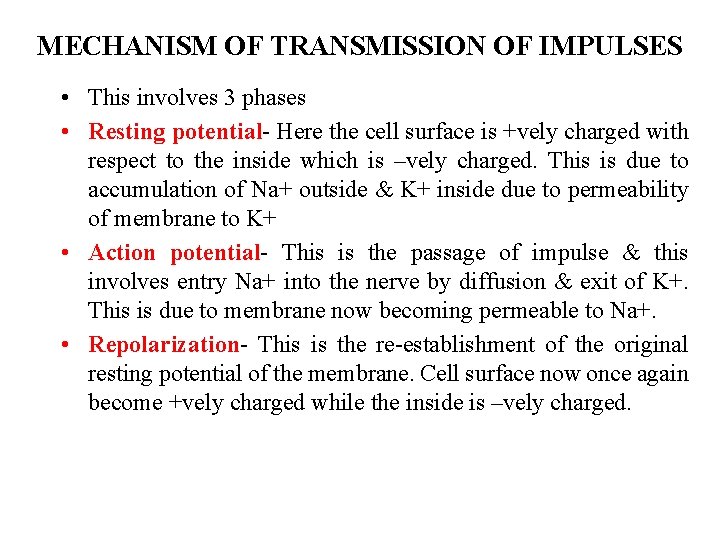
MECHANISM OF TRANSMISSION OF IMPULSES • This involves 3 phases • Resting potential- Here the cell surface is +vely charged with respect to the inside which is –vely charged. This is due to accumulation of Na+ outside & K+ inside due to permeability of membrane to K+ • Action potential- This is the passage of impulse & this involves entry Na+ into the nerve by diffusion & exit of K+. This is due to membrane now becoming permeable to Na+. • Repolarization- This is the re-establishment of the original resting potential of the membrane. Cell surface now once again become +vely charged while the inside is –vely charged.
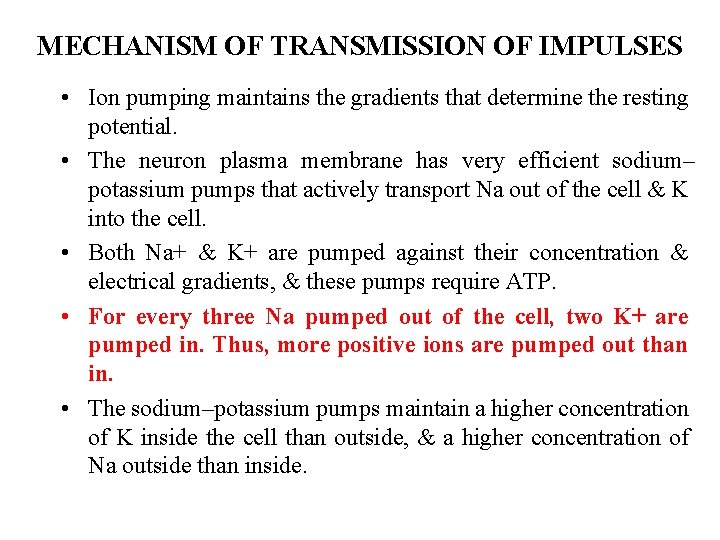
MECHANISM OF TRANSMISSION OF IMPULSES • Ion pumping maintains the gradients that determine the resting potential. • The neuron plasma membrane has very efficient sodium– potassium pumps that actively transport Na out of the cell & K into the cell. • Both Na+ & K+ are pumped against their concentration & electrical gradients, & these pumps require ATP. • For every three Na pumped out of the cell, two K+ are pumped in. Thus, more positive ions are pumped out than in. • The sodium–potassium pumps maintain a higher concentration of K inside the cell than outside, & a higher concentration of Na outside than inside.
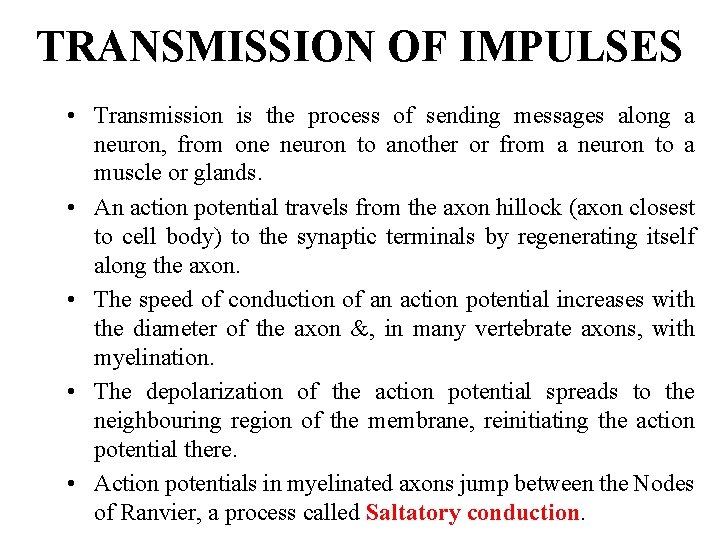
TRANSMISSION OF IMPULSES • Transmission is the process of sending messages along a neuron, from one neuron to another or from a neuron to a muscle or glands. • An action potential travels from the axon hillock (axon closest to cell body) to the synaptic terminals by regenerating itself along the axon. • The speed of conduction of an action potential increases with the diameter of the axon &, in many vertebrate axons, with myelination. • The depolarization of the action potential spreads to the neighbouring region of the membrane, reinitiating the action potential there. • Action potentials in myelinated axons jump between the Nodes of Ranvier, a process called Saltatory conduction.
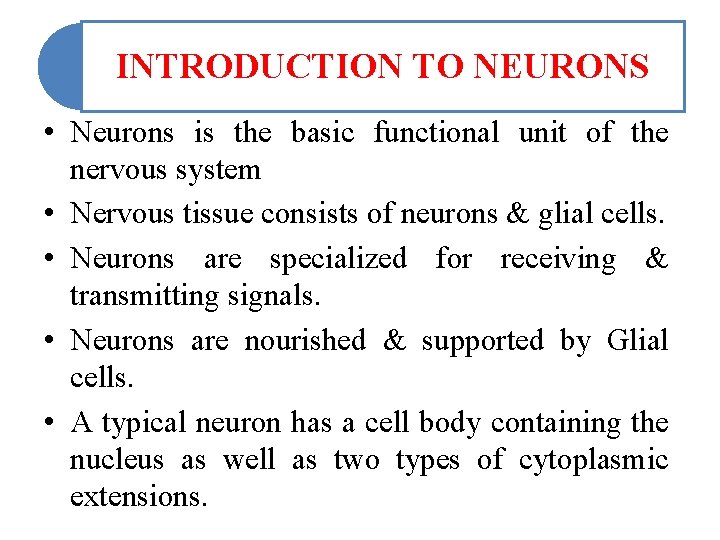
INTRODUCTION TO NEURONS • Neurons is the basic functional unit of the nervous system • Nervous tissue consists of neurons & glial cells. • Neurons are specialized for receiving & transmitting signals. • Neurons are nourished & supported by Glial cells. • A typical neuron has a cell body containing the nucleus as well as two types of cytoplasmic extensions.
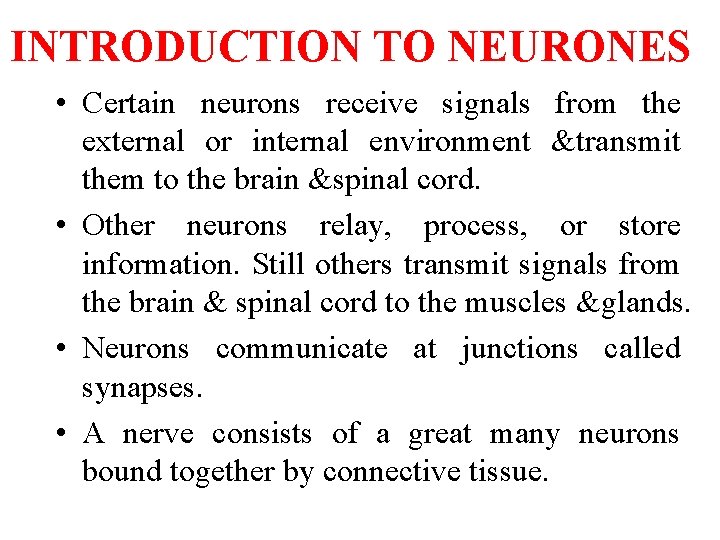
INTRODUCTION TO NEURONES • Certain neurons receive signals from the external or internal environment &transmit them to the brain &spinal cord. • Other neurons relay, process, or store information. Still others transmit signals from the brain & spinal cord to the muscles &glands. • Neurons communicate at junctions called synapses. • A nerve consists of a great many neurons bound together by connective tissue.
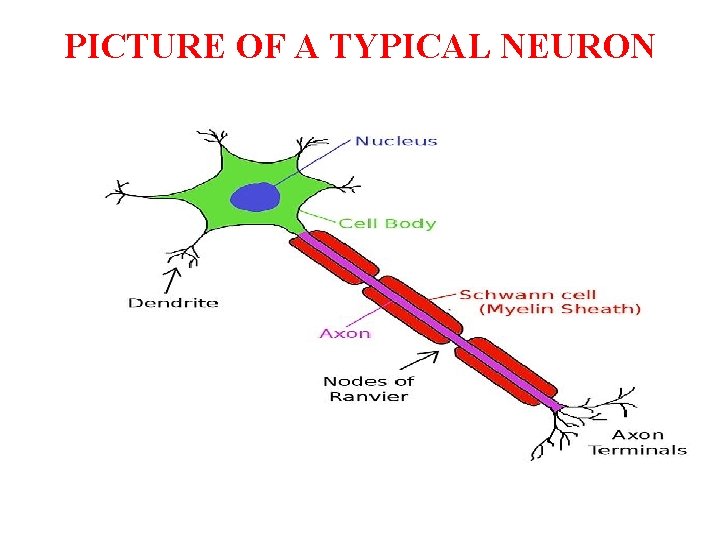
PICTURE OF A TYPICAL NEURON
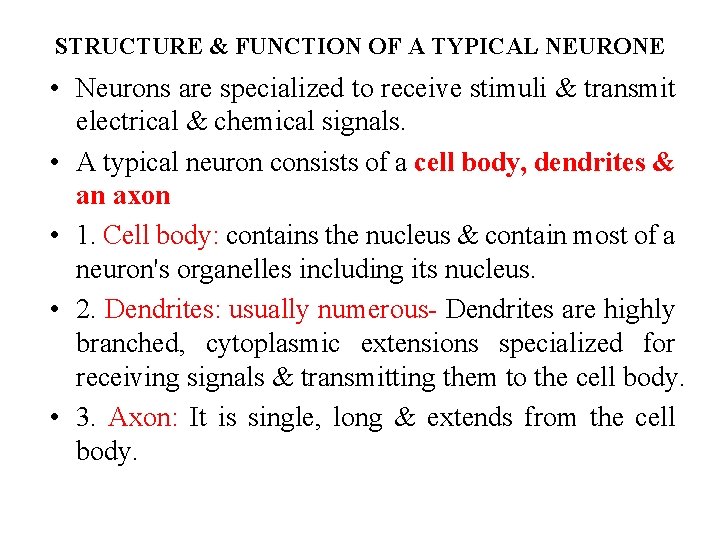
STRUCTURE & FUNCTION OF A TYPICAL NEURONE • Neurons are specialized to receive stimuli & transmit electrical & chemical signals. • A typical neuron consists of a cell body, dendrites & an axon • 1. Cell body: contains the nucleus & contain most of a neuron's organelles including its nucleus. • 2. Dendrites: usually numerous- Dendrites are highly branched, cytoplasmic extensions specialized for receiving signals & transmitting them to the cell body. • 3. Axon: It is single, long & extends from the cell body.
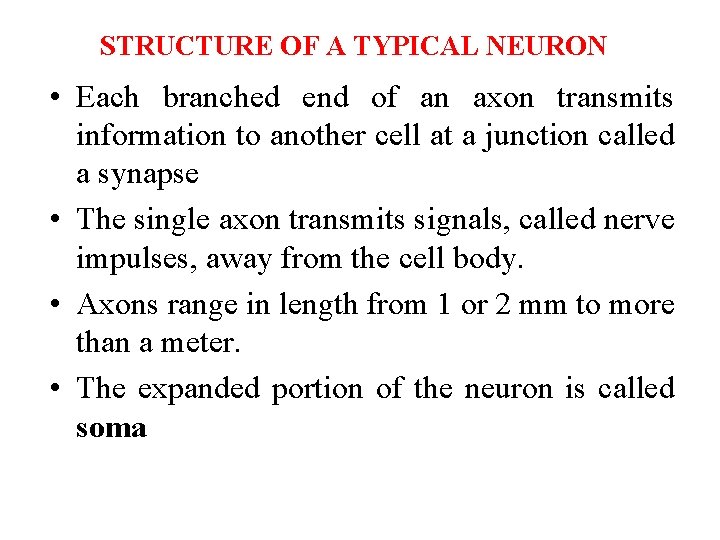
STRUCTURE OF A TYPICAL NEURON • Each branched end of an axon transmits information to another cell at a junction called a synapse • The single axon transmits signals, called nerve impulses, away from the cell body. • Axons range in length from 1 or 2 mm to more than a meter. • The expanded portion of the neuron is called soma
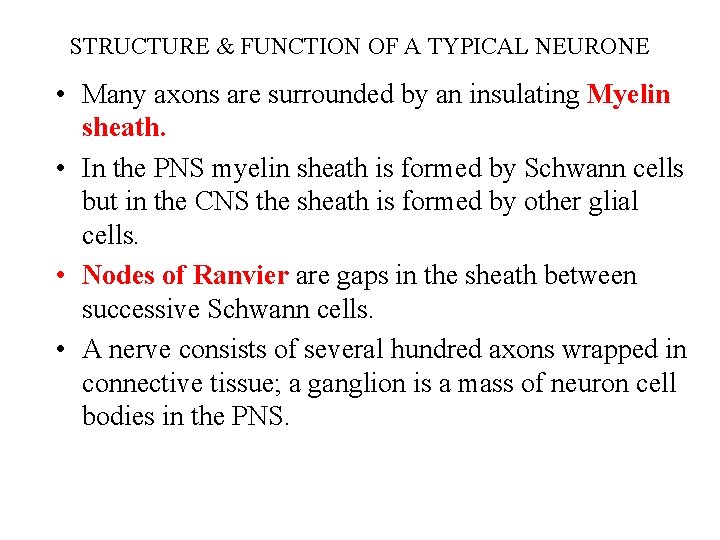
STRUCTURE & FUNCTION OF A TYPICAL NEURONE • Many axons are surrounded by an insulating Myelin sheath. • In the PNS myelin sheath is formed by Schwann cells but in the CNS the sheath is formed by other glial cells. • Nodes of Ranvier are gaps in the sheath between successive Schwann cells. • A nerve consists of several hundred axons wrapped in connective tissue; a ganglion is a mass of neuron cell bodies in the PNS.
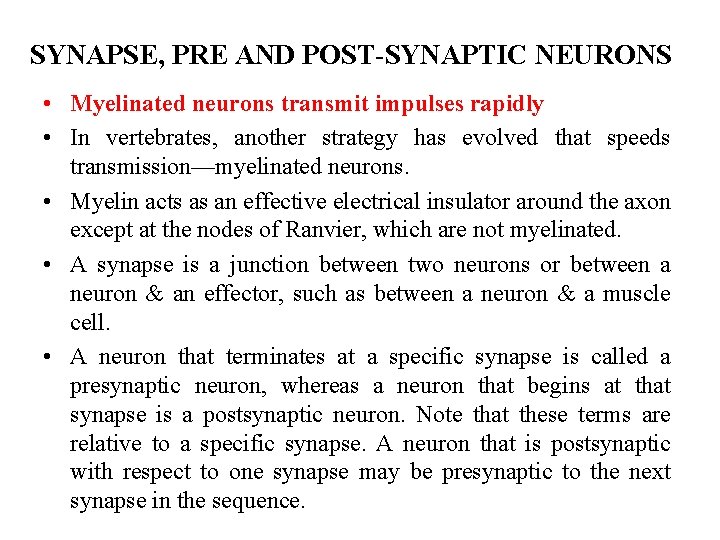
SYNAPSE, PRE AND POST-SYNAPTIC NEURONS • Myelinated neurons transmit impulses rapidly • In vertebrates, another strategy has evolved that speeds transmission—myelinated neurons. • Myelin acts as an effective electrical insulator around the axon except at the nodes of Ranvier, which are not myelinated. • A synapse is a junction between two neurons or between a neuron & an effector, such as between a neuron & a muscle cell. • A neuron that terminates at a specific synapse is called a presynaptic neuron, whereas a neuron that begins at that synapse is a postsynaptic neuron. Note that these terms are relative to a specific synapse. A neuron that is postsynaptic with respect to one synapse may be presynaptic to the next synapse in the sequence.
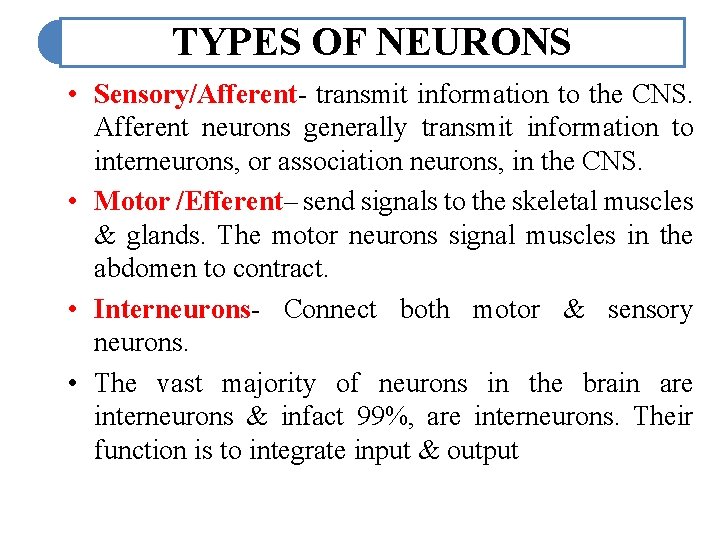
TYPES OF NEURONS • Sensory/Afferent- transmit information to the CNS. Afferent neurons generally transmit information to interneurons, or association neurons, in the CNS. • Motor /Efferent– send signals to the skeletal muscles & glands. The motor neurons signal muscles in the abdomen to contract. • Interneurons- Connect both motor & sensory neurons. • The vast majority of neurons in the brain are interneurons & infact 99%, are interneurons. Their function is to integrate input & output
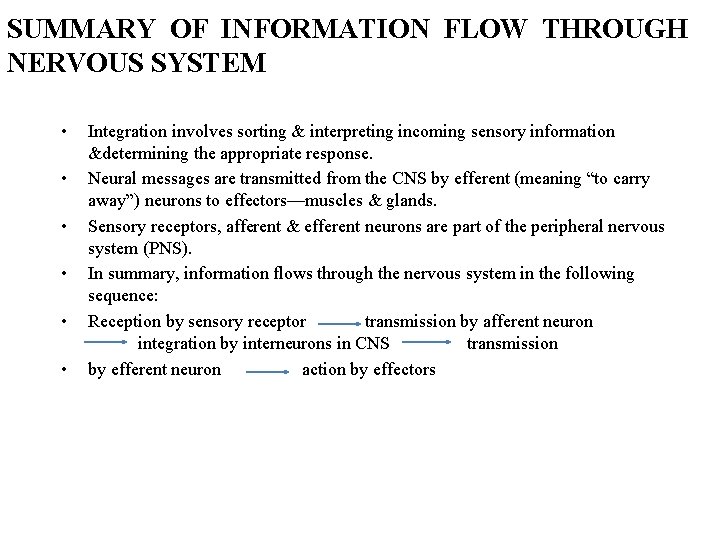
SUMMARY OF INFORMATION FLOW THROUGH NERVOUS SYSTEM • • • Integration involves sorting & interpreting incoming sensory information &determining the appropriate response. Neural messages are transmitted from the CNS by efferent (meaning “to carry away”) neurons to effectors—muscles & glands. Sensory receptors, afferent & efferent neurons are part of the peripheral nervous system (PNS). In summary, information flows through the nervous system in the following sequence: Reception by sensory receptor transmission by afferent neuron integration by interneurons in CNS transmission by efferent neuron action by effectors
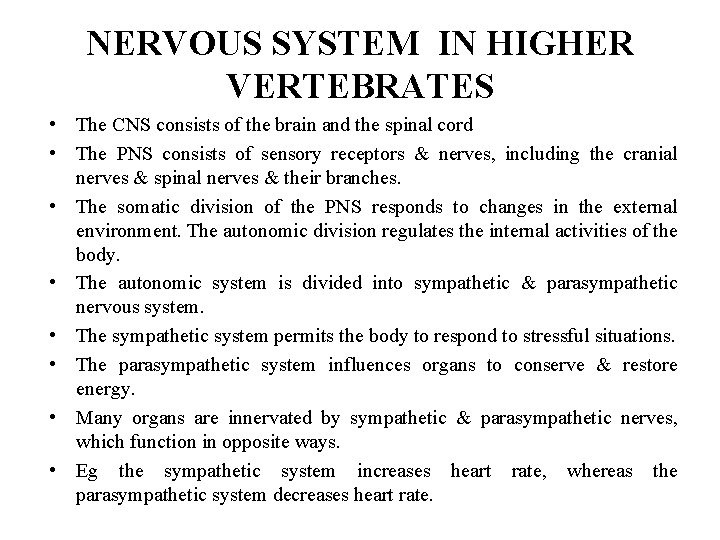
NERVOUS SYSTEM IN HIGHER VERTEBRATES • The CNS consists of the brain and the spinal cord • The PNS consists of sensory receptors & nerves, including the cranial nerves & spinal nerves & their branches. • The somatic division of the PNS responds to changes in the external environment. The autonomic division regulates the internal activities of the body. • The autonomic system is divided into sympathetic & parasympathetic nervous system. • The sympathetic system permits the body to respond to stressful situations. • The parasympathetic system influences organs to conserve & restore energy. • Many organs are innervated by sympathetic & parasympathetic nerves, which function in opposite ways. • Eg the sympathetic system increases heart rate, whereas the parasympathetic system decreases heart rate.
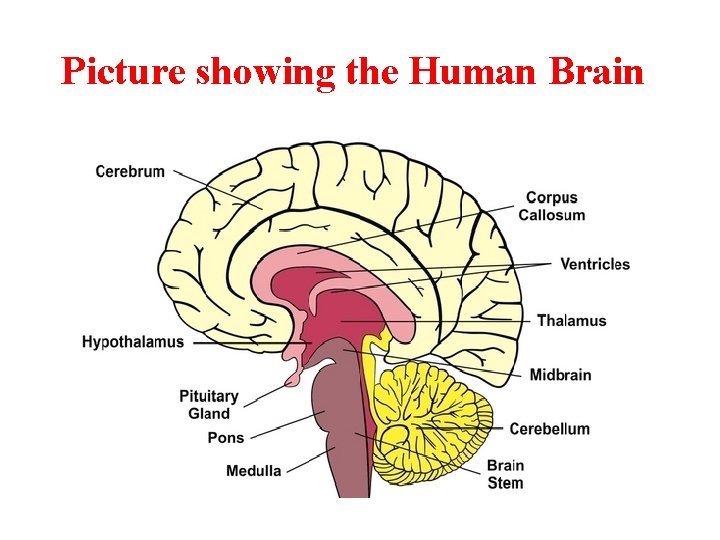
Picture showing the Human Brain
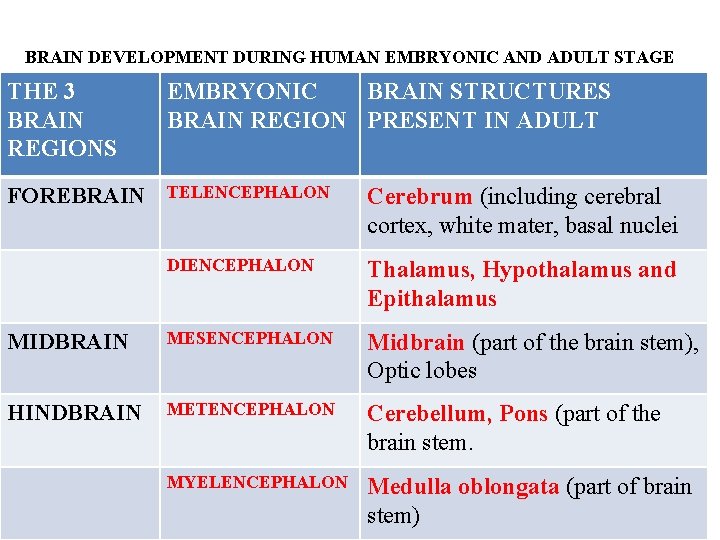
BRAIN DEVELOPMENT DURING HUMAN EMBRYONIC AND ADULT STAGE THE 3 BRAIN REGIONS EMBRYONIC BRAIN STRUCTURES BRAIN REGION PRESENT IN ADULT FOREBRAIN TELENCEPHALON Cerebrum (including cerebral cortex, white mater, basal nuclei DIENCEPHALON Thalamus, Hypothalamus and Epithalamus MIDBRAIN MESENCEPHALON Midbrain (part of the brain stem), Optic lobes HINDBRAIN METENCEPHALON Cerebellum, Pons (part of the brain stem. MYELENCEPHALON Medulla oblongata (part of brain stem)
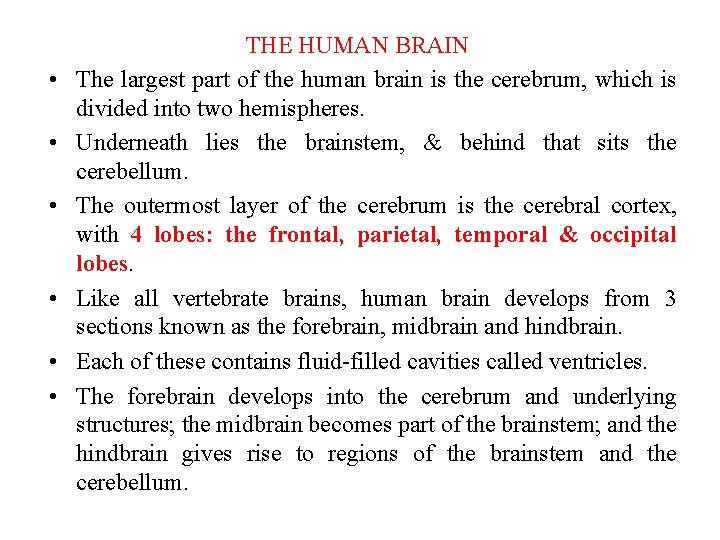
• • • THE HUMAN BRAIN The largest part of the human brain is the cerebrum, which is divided into two hemispheres. Underneath lies the brainstem, & behind that sits the cerebellum. The outermost layer of the cerebrum is the cerebral cortex, with 4 lobes: the frontal, parietal, temporal & occipital lobes. Like all vertebrate brains, human brain develops from 3 sections known as the forebrain, midbrain and hindbrain. Each of these contains fluid-filled cavities called ventricles. The forebrain develops into the cerebrum and underlying structures; the midbrain becomes part of the brainstem; and the hindbrain gives rise to regions of the brainstem and the cerebellum.
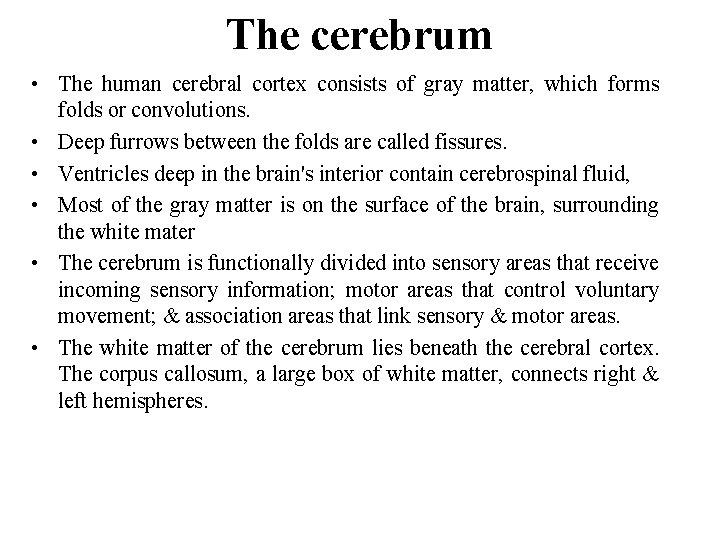
The cerebrum • The human cerebral cortex consists of gray matter, which forms folds or convolutions. • Deep furrows between the folds are called fissures. • Ventricles deep in the brain's interior contain cerebrospinal fluid, • Most of the gray matter is on the surface of the brain, surrounding the white mater • The cerebrum is functionally divided into sensory areas that receive incoming sensory information; motor areas that control voluntary movement; & association areas that link sensory & motor areas. • The white matter of the cerebrum lies beneath the cerebral cortex. The corpus callosum, a large box of white matter, connects right & left hemispheres.
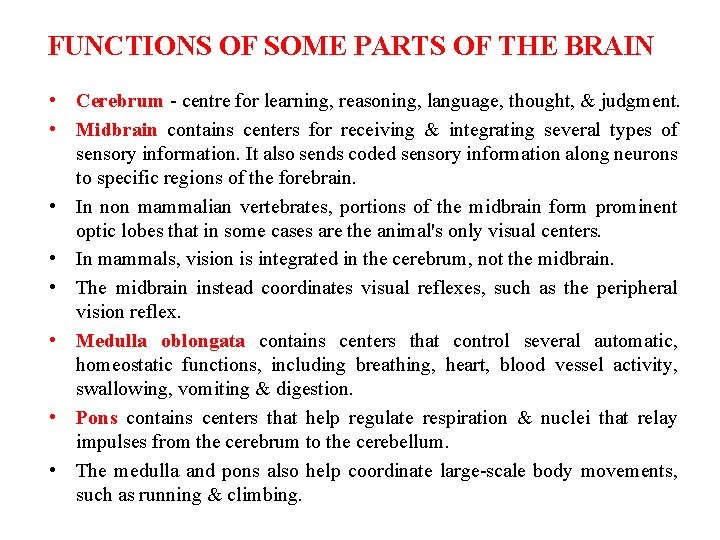
FUNCTIONS OF SOME PARTS OF THE BRAIN • Cerebrum - centre for learning, reasoning, language, thought, & judgment. • Midbrain contains centers for receiving & integrating several types of sensory information. It also sends coded sensory information along neurons to specific regions of the forebrain. • In non mammalian vertebrates, portions of the midbrain form prominent optic lobes that in some cases are the animal's only visual centers. • In mammals, vision is integrated in the cerebrum, not the midbrain. • The midbrain instead coordinates visual reflexes, such as the peripheral vision reflex. • Medulla oblongata contains centers that control several automatic, homeostatic functions, including breathing, heart, blood vessel activity, swallowing, vomiting & digestion. • Pons contains centers that help regulate respiration & nuclei that relay impulses from the cerebrum to the cerebellum. • The medulla and pons also help coordinate large-scale body movements, such as running & climbing.
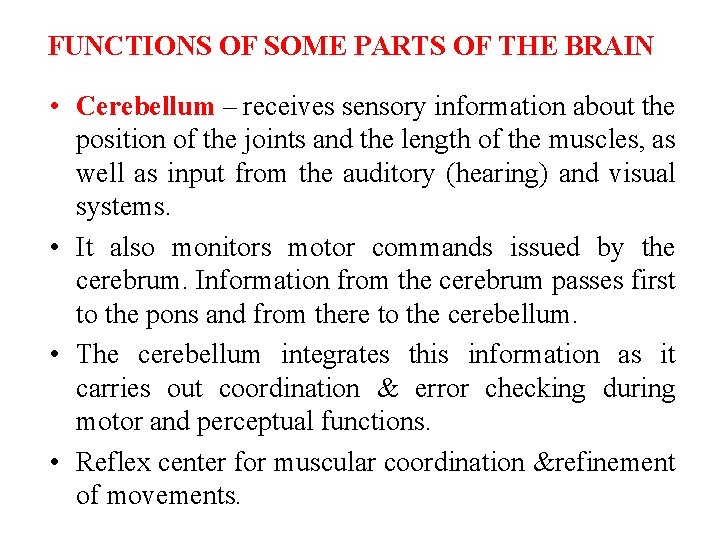
FUNCTIONS OF SOME PARTS OF THE BRAIN • Cerebellum – receives sensory information about the position of the joints and the length of the muscles, as well as input from the auditory (hearing) and visual systems. • It also monitors motor commands issued by the cerebrum. Information from the cerebrum passes first to the pons and from there to the cerebellum. • The cerebellum integrates this information as it carries out coordination & error checking during motor and perceptual functions. • Reflex center for muscular coordination &refinement of movements.
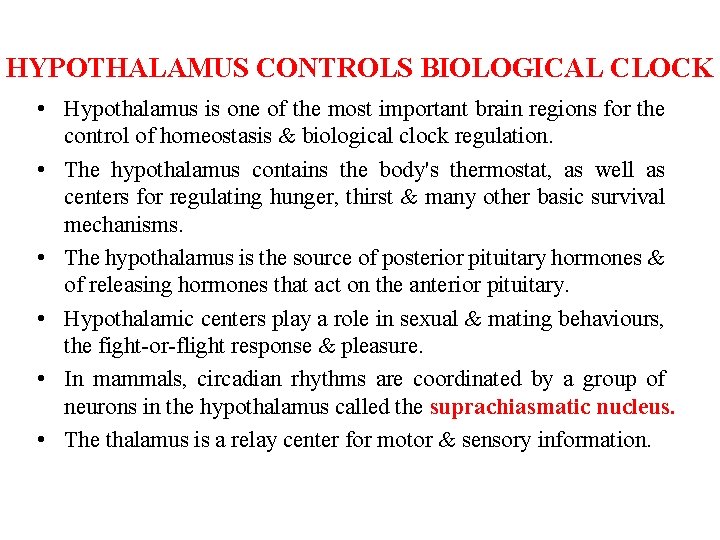
HYPOTHALAMUS CONTROLS BIOLOGICAL CLOCK • Hypothalamus is one of the most important brain regions for the control of homeostasis & biological clock regulation. • The hypothalamus contains the body's thermostat, as well as centers for regulating hunger, thirst & many other basic survival mechanisms. • The hypothalamus is the source of posterior pituitary hormones & of releasing hormones that act on the anterior pituitary. • Hypothalamic centers play a role in sexual & mating behaviours, the fight-or-flight response & pleasure. • In mammals, circadian rhythms are coordinated by a group of neurons in the hypothalamus called the suprachiasmatic nucleus. • The thalamus is a relay center for motor & sensory information.
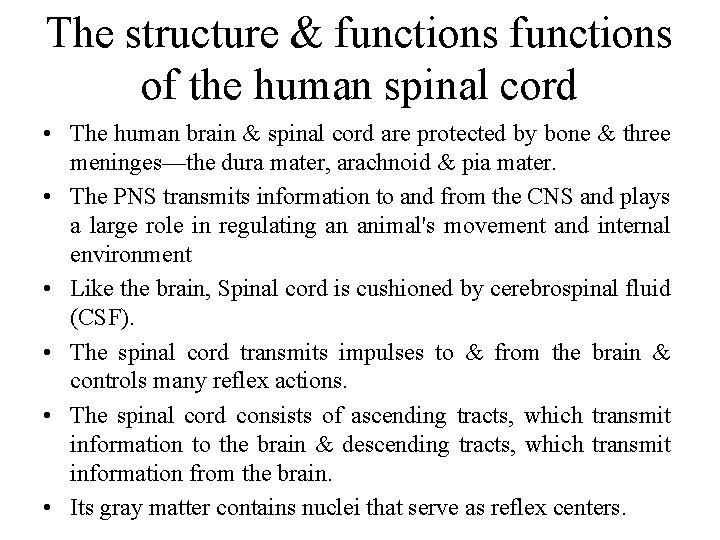
The structure & functions of the human spinal cord • The human brain & spinal cord are protected by bone & three meninges—the dura mater, arachnoid & pia mater. • The PNS transmits information to and from the CNS and plays a large role in regulating an animal's movement and internal environment • Like the brain, Spinal cord is cushioned by cerebrospinal fluid (CSF). • The spinal cord transmits impulses to & from the brain & controls many reflex actions. • The spinal cord consists of ascending tracts, which transmit information to the brain & descending tracts, which transmit information from the brain. • Its gray matter contains nuclei that serve as reflex centers.
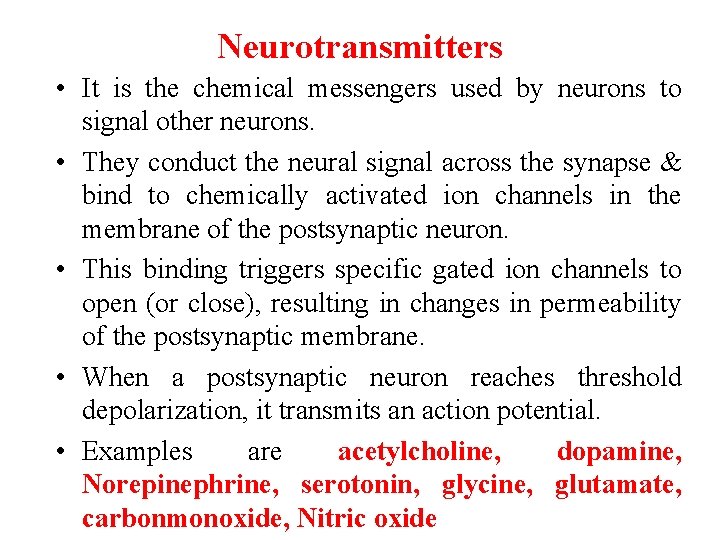
Neurotransmitters • It is the chemical messengers used by neurons to signal other neurons. • They conduct the neural signal across the synapse & bind to chemically activated ion channels in the membrane of the postsynaptic neuron. • This binding triggers specific gated ion channels to open (or close), resulting in changes in permeability of the postsynaptic membrane. • When a postsynaptic neuron reaches threshold depolarization, it transmits an action potential. • Examples are acetylcholine, dopamine, Norepinephrine, serotonin, glycine, glutamate, carbonmonoxide, Nitric oxide
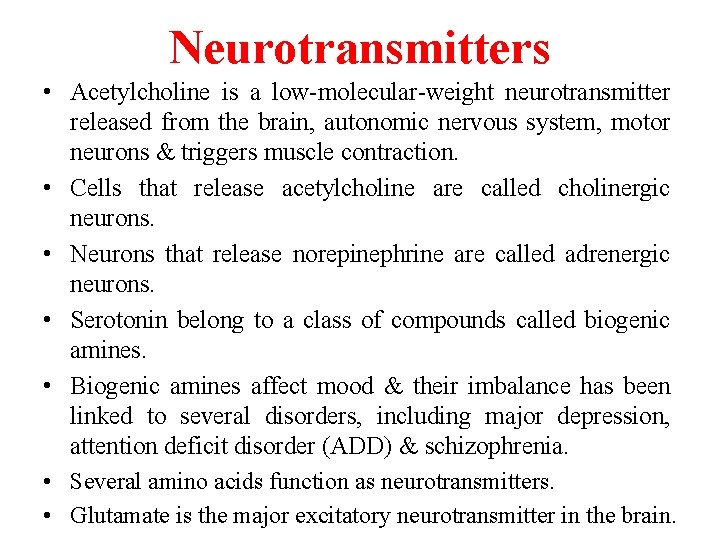
Neurotransmitters • Acetylcholine is a low-molecular-weight neurotransmitter released from the brain, autonomic nervous system, motor neurons & triggers muscle contraction. • Cells that release acetylcholine are called cholinergic neurons. • Neurons that release norepinephrine are called adrenergic neurons. • Serotonin belong to a class of compounds called biogenic amines. • Biogenic amines affect mood & their imbalance has been linked to several disorders, including major depression, attention deficit disorder (ADD) & schizophrenia. • Several amino acids function as neurotransmitters. • Glutamate is the major excitatory neurotransmitter in the brain.
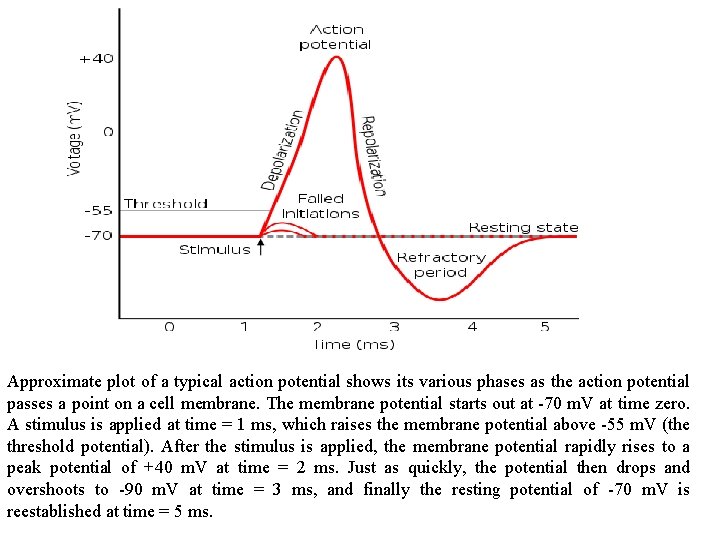
Approximate plot of a typical action potential shows its various phases as the action potential passes a point on a cell membrane. The membrane potential starts out at -70 m. V at time zero. A stimulus is applied at time = 1 ms, which raises the membrane potential above -55 m. V (the threshold potential). After the stimulus is applied, the membrane potential rapidly rises to a peak potential of +40 m. V at time = 2 ms. Just as quickly, the potential then drops and overshoots to -90 m. V at time = 3 ms, and finally the resting potential of -70 m. V is reestablished at time = 5 ms.
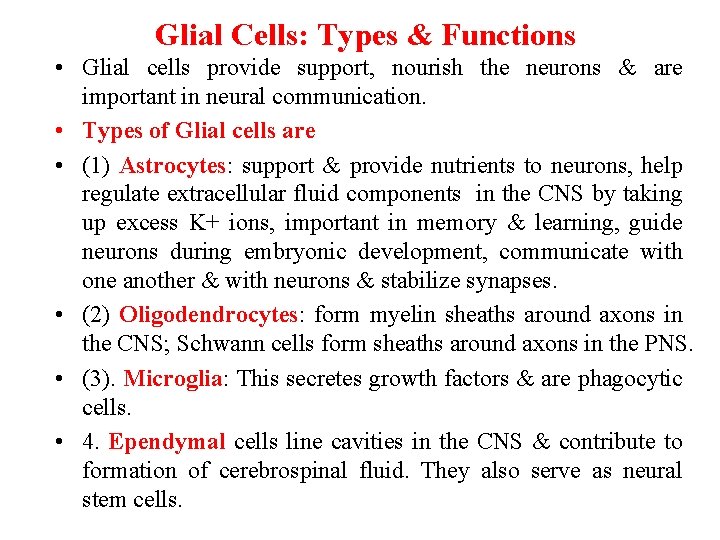
Glial Cells: Types & Functions • Glial cells provide support, nourish the neurons & are important in neural communication. • Types of Glial cells are • (1) Astrocytes: support & provide nutrients to neurons, help regulate extracellular fluid components in the CNS by taking up excess K+ ions, important in memory & learning, guide neurons during embryonic development, communicate with one another & with neurons & stabilize synapses. • (2) Oligodendrocytes: form myelin sheaths around axons in the CNS; Schwann cells form sheaths around axons in the PNS. • (3). Microglia: This secretes growth factors & are phagocytic cells. • 4. Ependymal cells line cavities in the CNS & contribute to formation of cerebrospinal fluid. They also serve as neural stem cells.
What are the characteristics of nervous tissue
Nervous
Fundamentals of the nervous system and nervous tissue
Oheň je dobrý sluha ale zlý pán projekt
Dobrý sluha ale zlý pán chemie
Spider phylum
Ohen dobry sluha zly pan
Nervous system and digestive system
Endocrine system vs nervous system
Amino acid-based hormones
Endocrine system
106 spelled out
Fahrenheit 451 coloring by number answer key
Cs 106 a
Irig chapter 10
Mae 106 uci
Plt 106
106 pal
106 signal brigade
Error n 106
Section 106 compliance
Salmo 106
Fas 87 pension
Kj 106
Iec 62334
Iat 106
Round the factors to estimate the products 597×52
Roberts building 106
Round the factors to estimate the products
生命聖詩106
101 102 103
101 prime number
Sqte-106
Multiway search tree
Glucosio 106 a digiuno
Col 106
105 106 107
Lusíadas canto v estância 92 a 100 recursos expressivos
Nur 106
106 - 43
Tempo 106
Iat 106