Results of Experiments in Akeno Kenji SHINOZAKI MaxPlanckInstitut
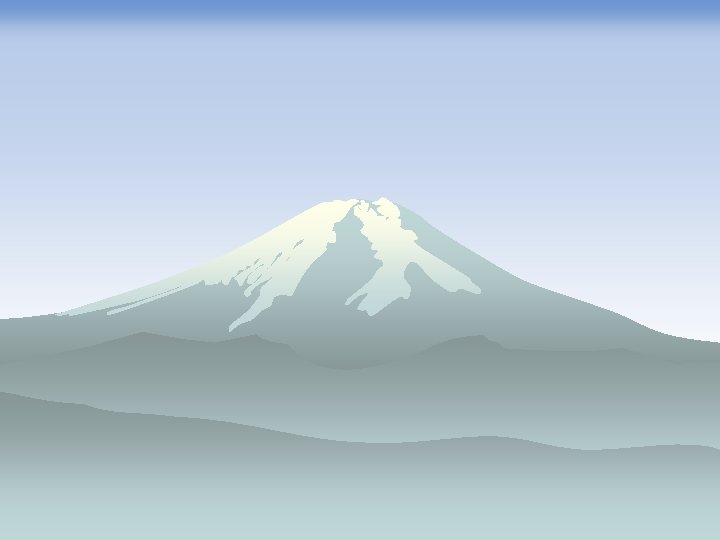
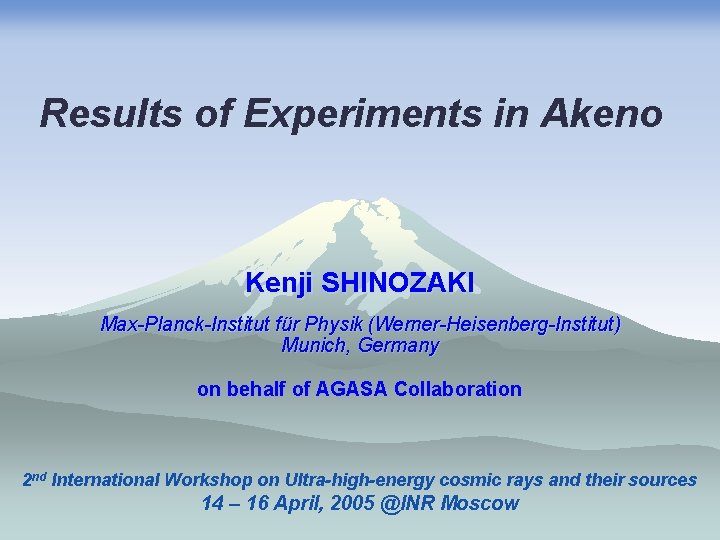
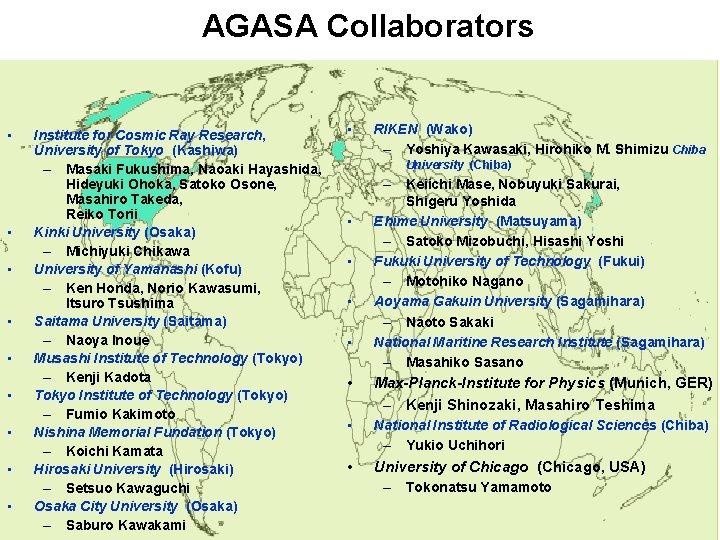
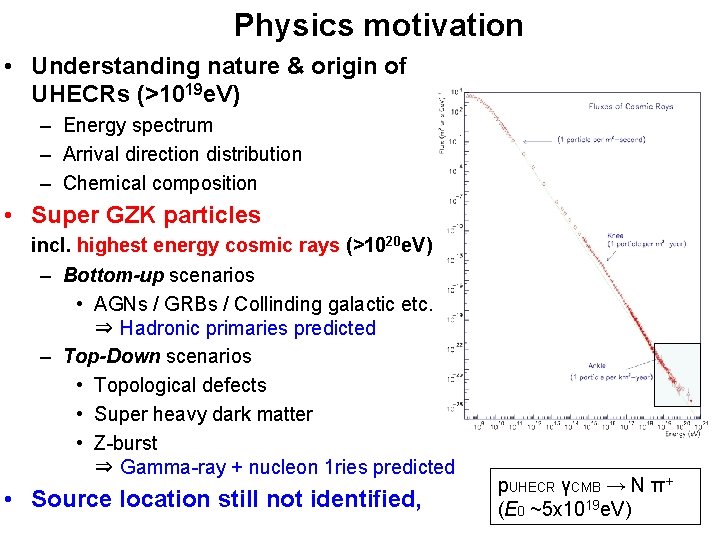
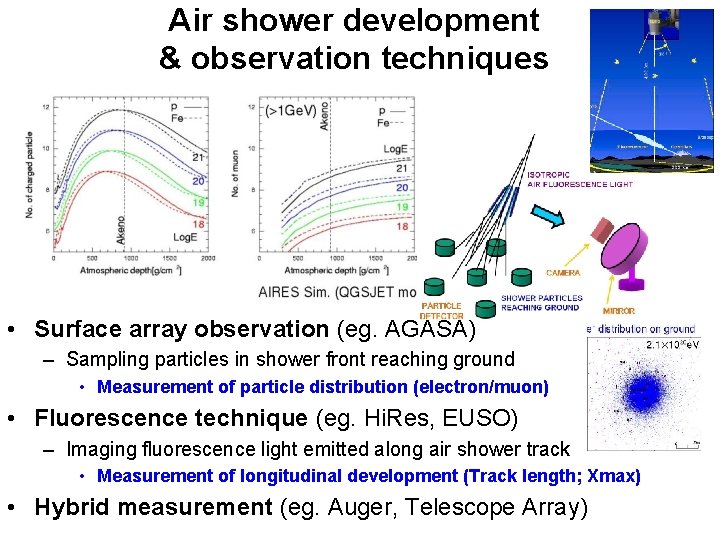
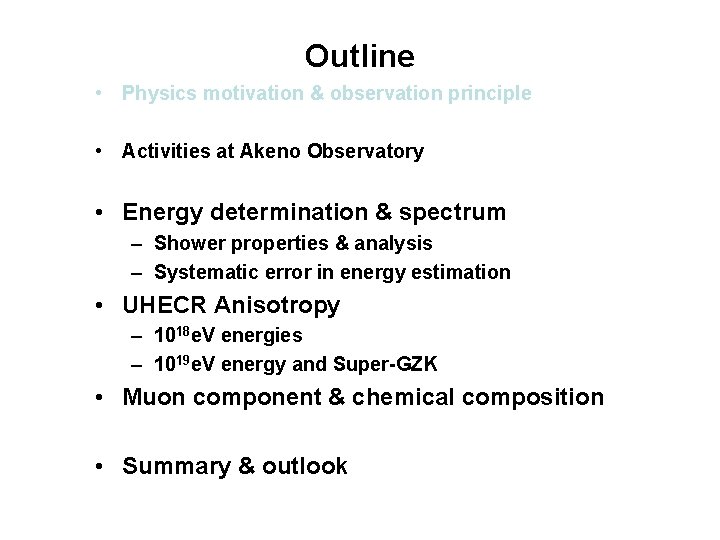
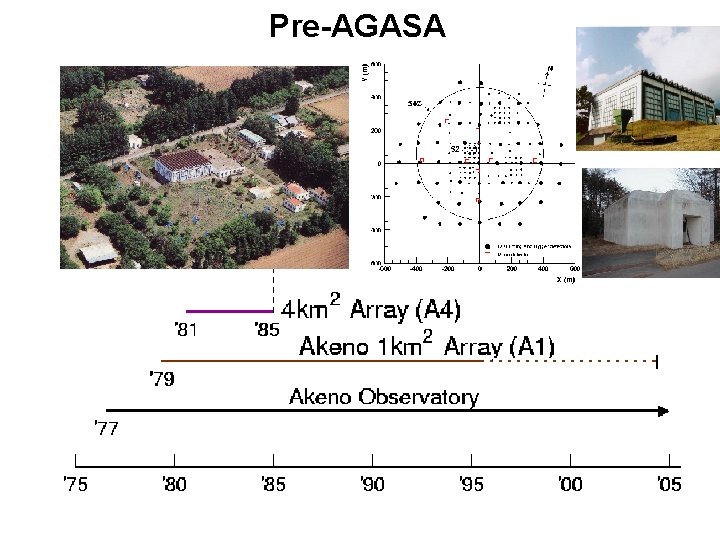
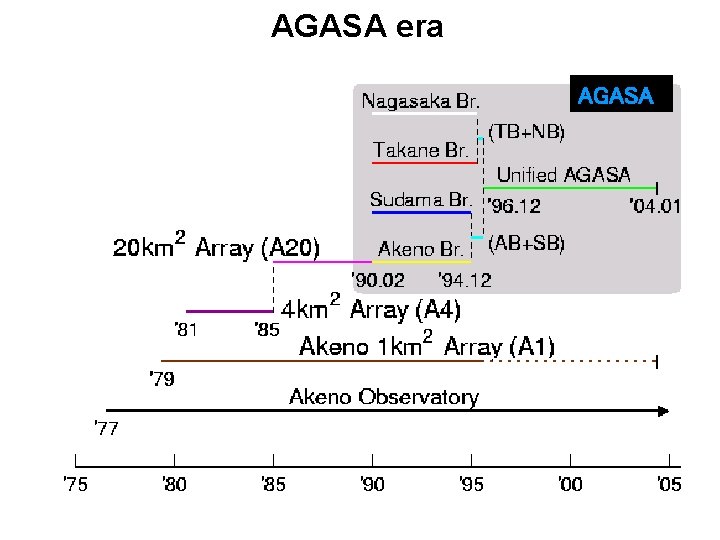
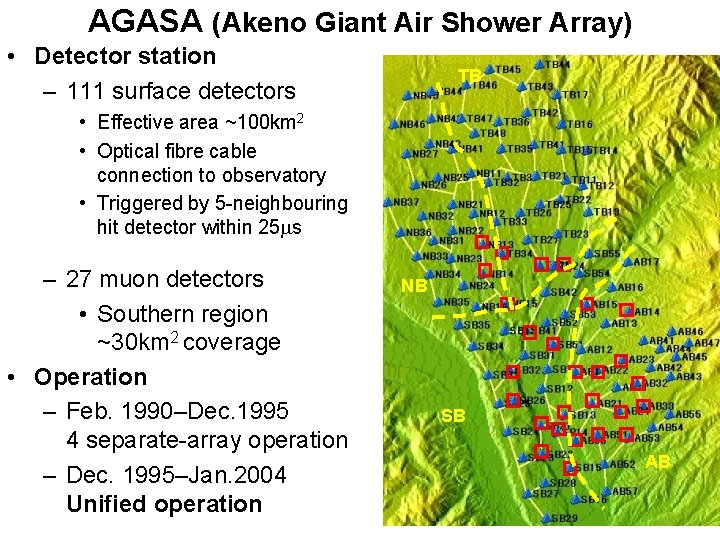
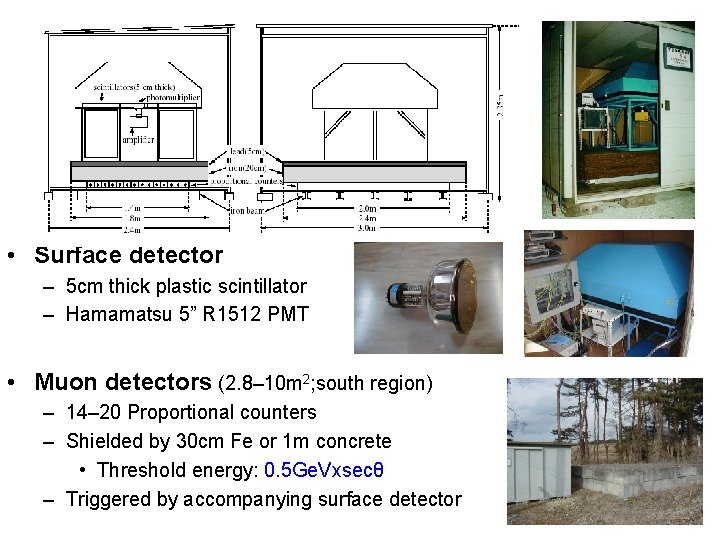
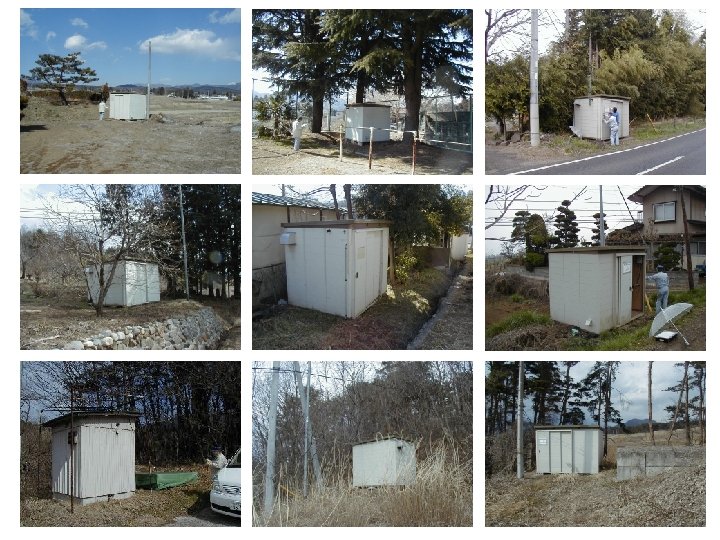
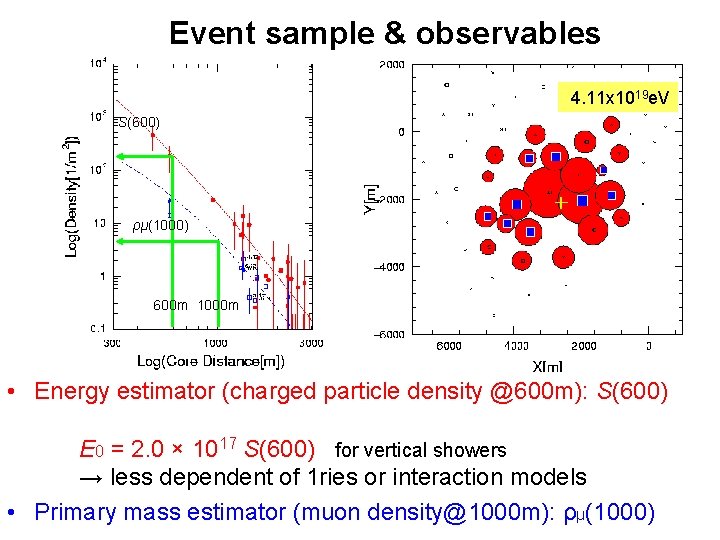
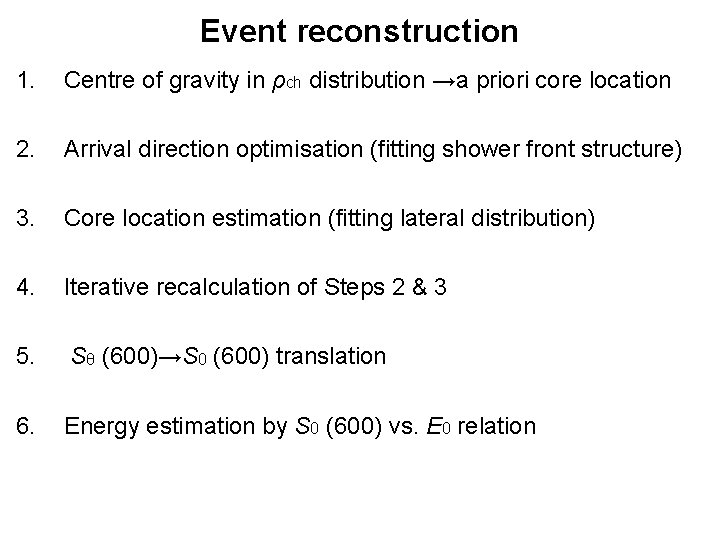
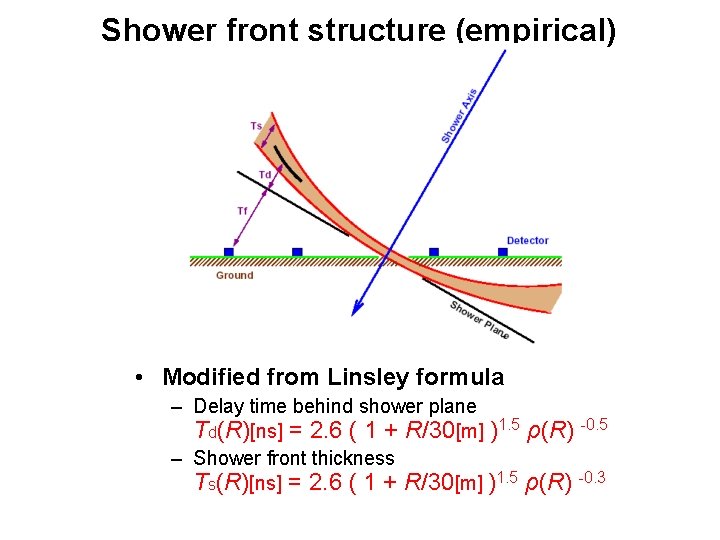
![Lateral distribution (empirical) secθ≤ 1. 1 S(600)=10, 30[m 2] • Modified Linsley formula ρ(R) Lateral distribution (empirical) secθ≤ 1. 1 S(600)=10, 30[m 2] • Modified Linsley formula ρ(R)](https://slidetodoc.com/presentation_image_h2/4ffb27cc0f2edb05f880e0d0f5a294f1/image-15.jpg)
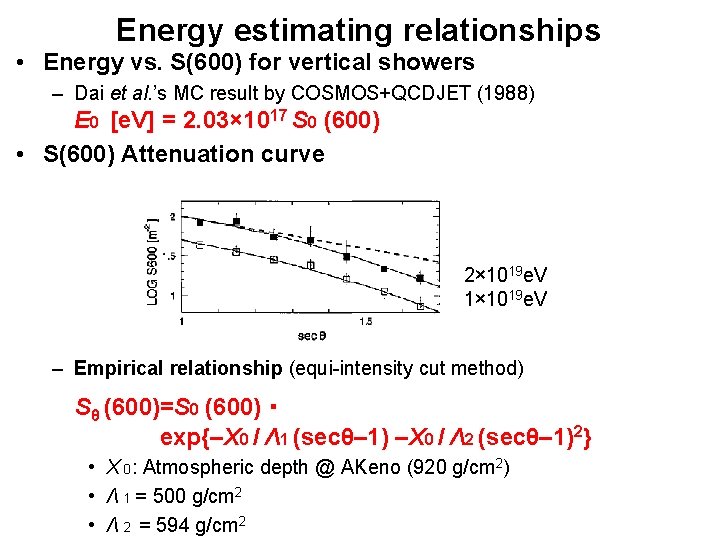
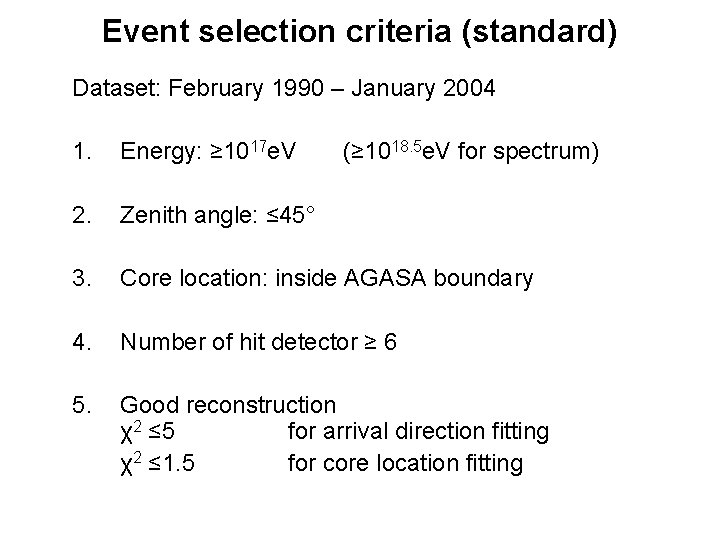
![Reconstruction accuracy (Energy resolution, Angular resolution) 8 Open angle Δθ[º] Counts [%/bin] 20 15 Reconstruction accuracy (Energy resolution, Angular resolution) 8 Open angle Δθ[º] Counts [%/bin] 20 15](https://slidetodoc.com/presentation_image_h2/4ffb27cc0f2edb05f880e0d0f5a294f1/image-18.jpg)
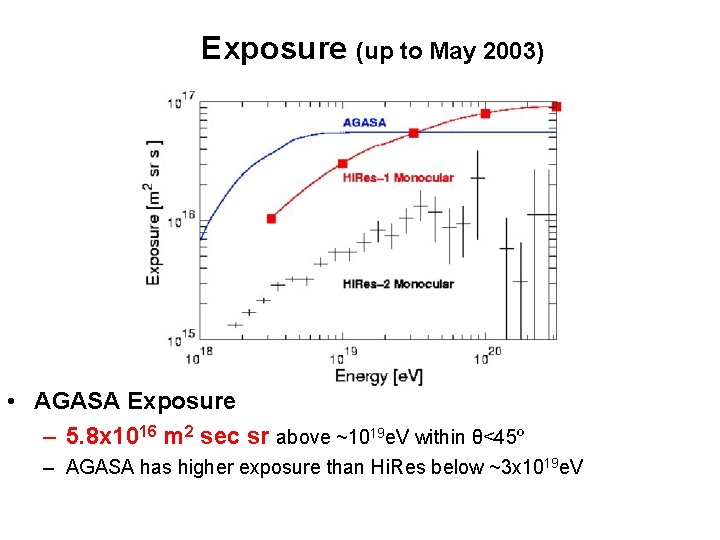
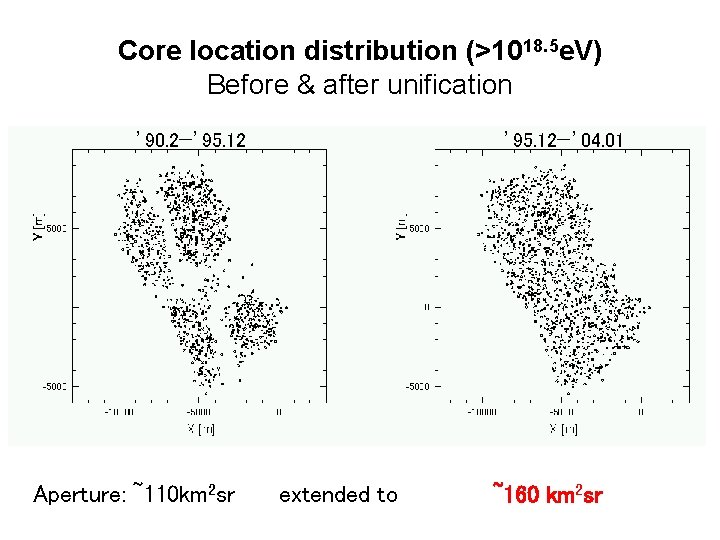
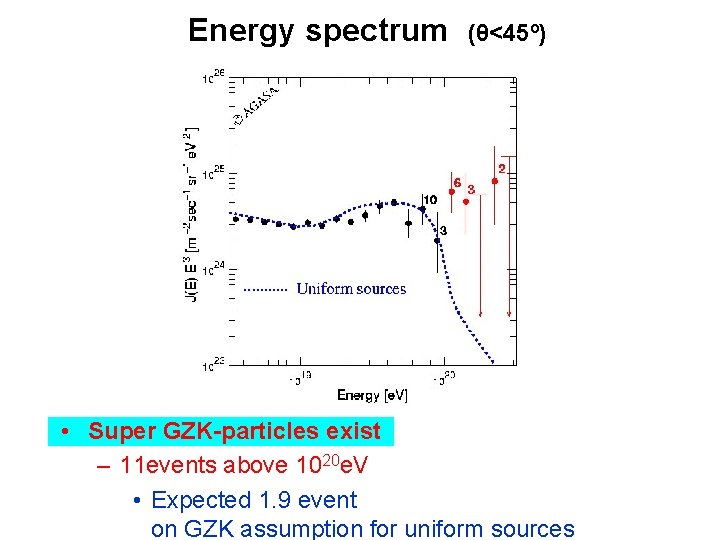
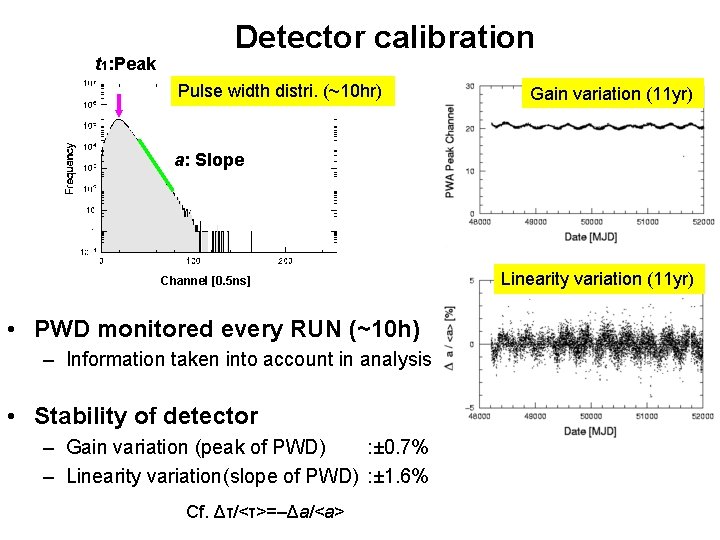
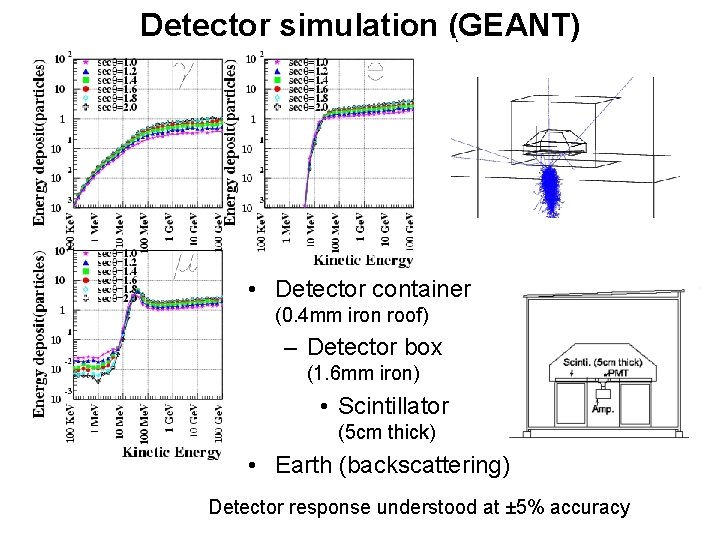
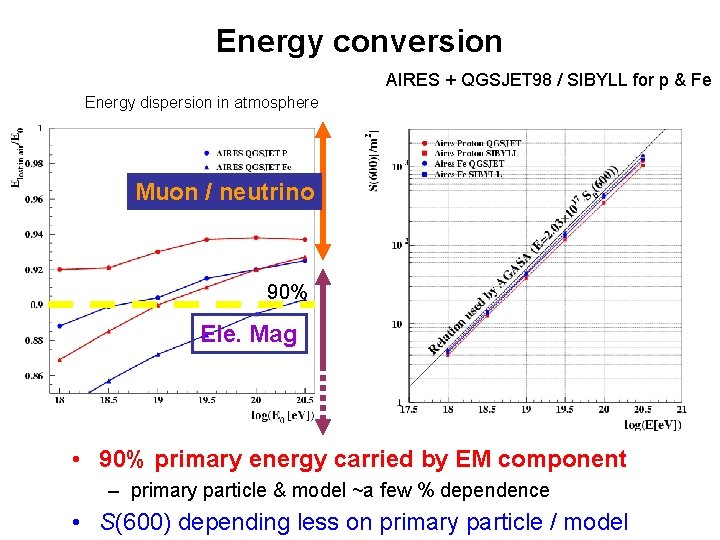
![Energy conversion factor E 0 = a [1017 e. V]x S(600) b Ref. Dai Energy conversion factor E 0 = a [1017 e. V]x S(600) b Ref. Dai](https://slidetodoc.com/presentation_image_h2/4ffb27cc0f2edb05f880e0d0f5a294f1/image-25.jpg)
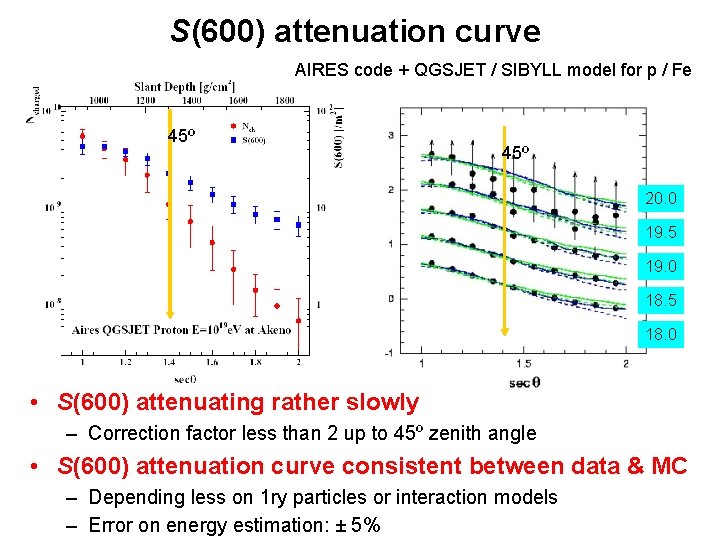
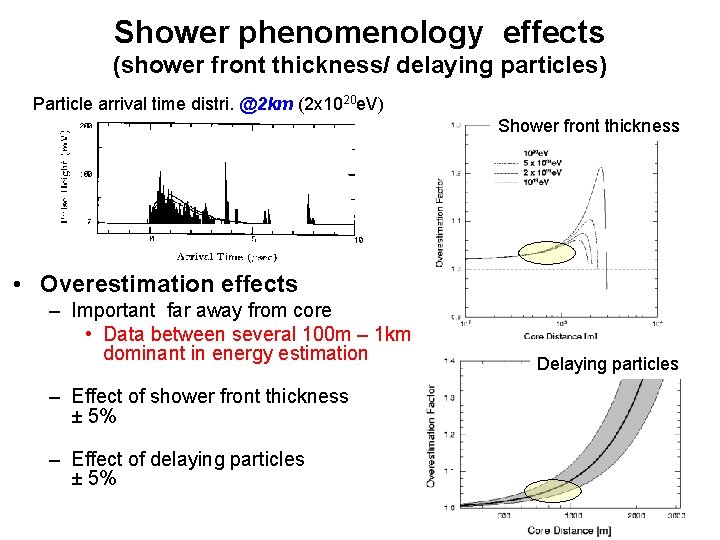
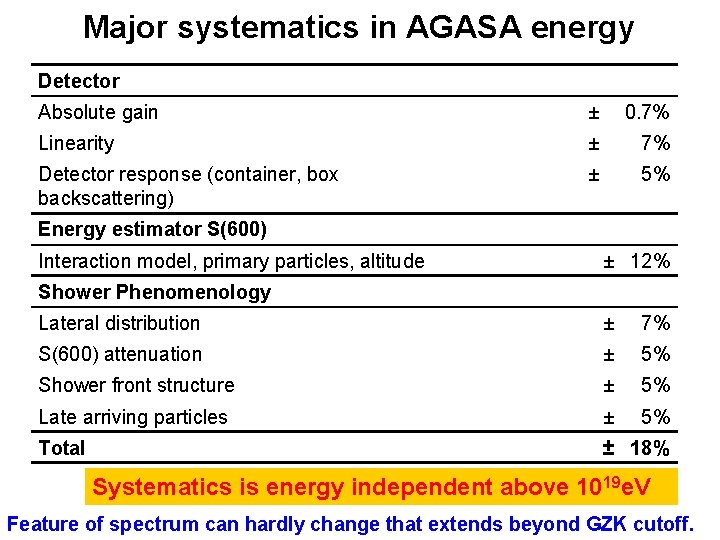
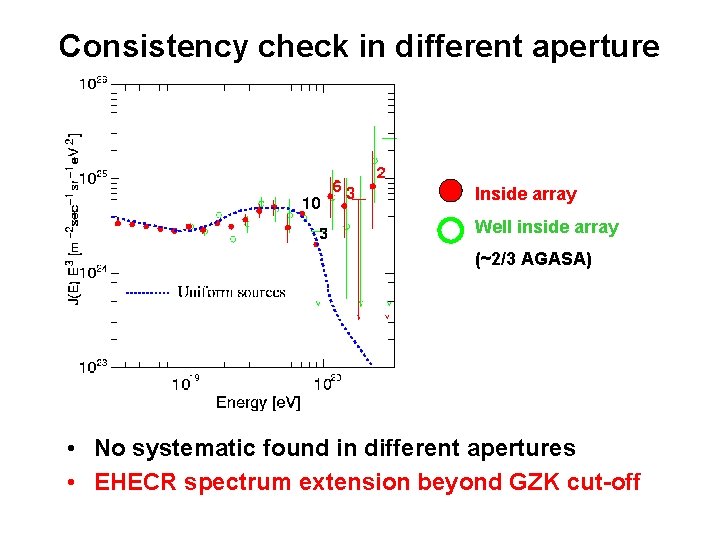
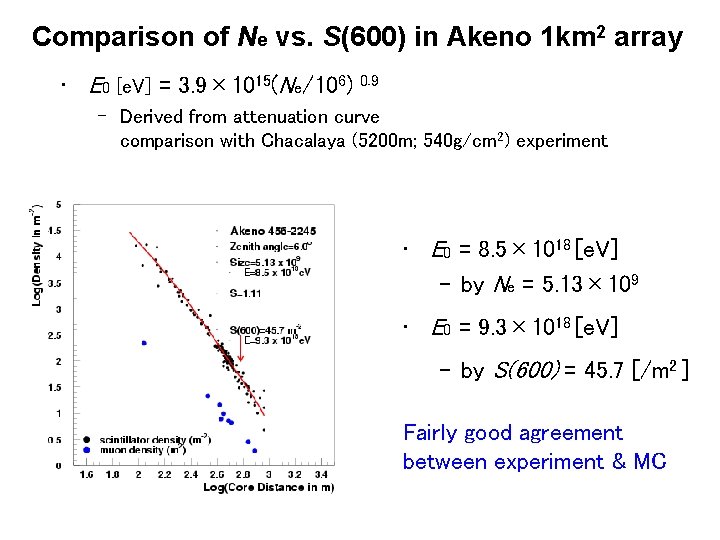
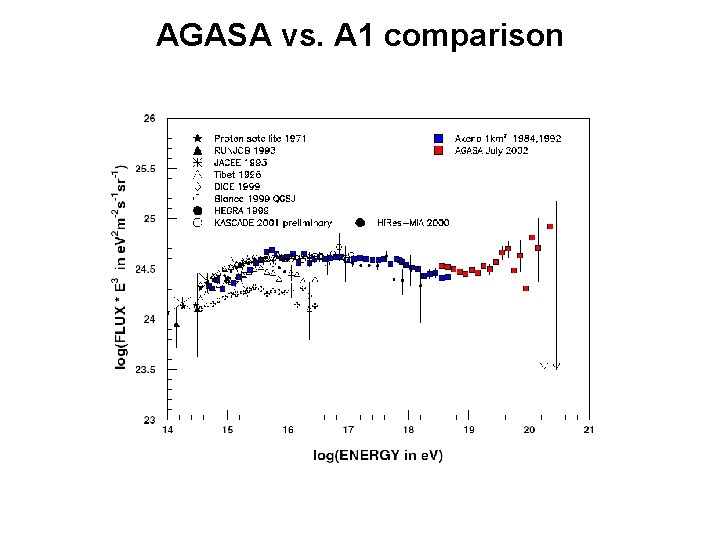
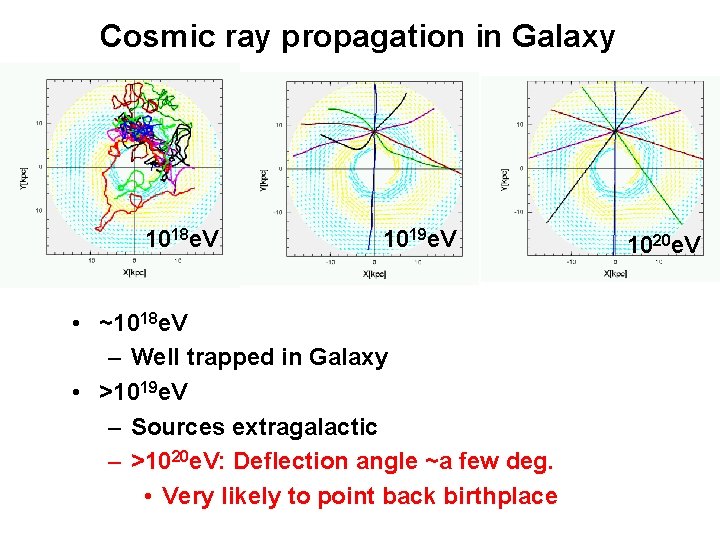
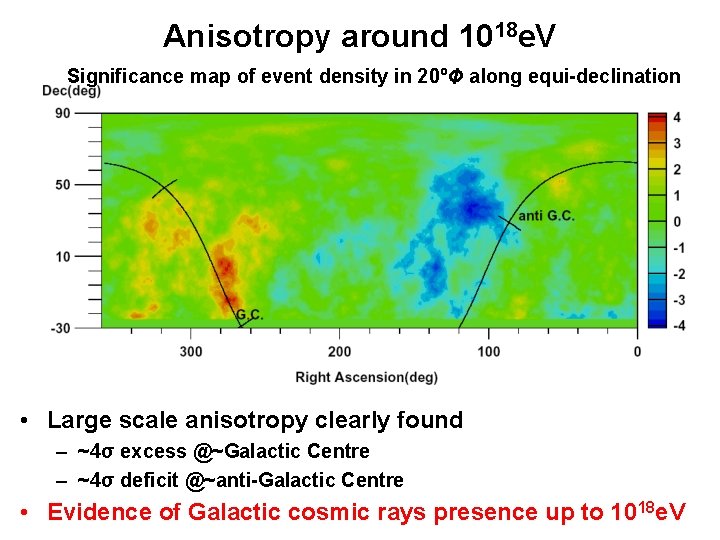
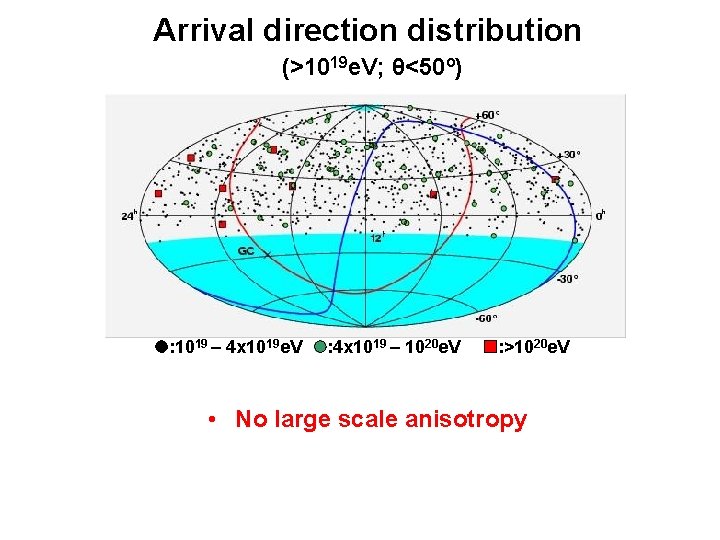
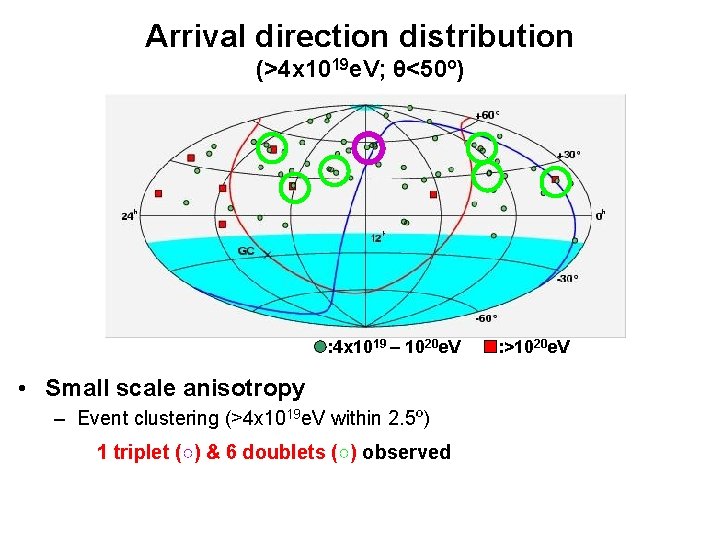
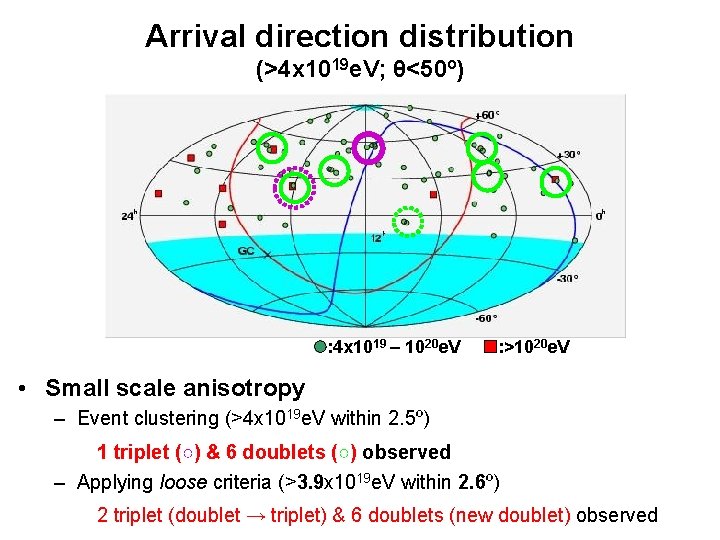
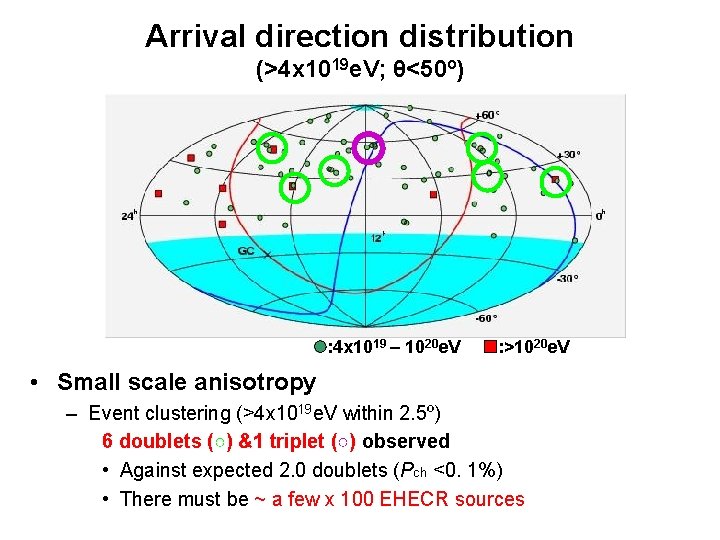
![Event density [a. u. ] 0 0 Event density [a. u. ] Log E>19. Event density [a. u. ] 0 0 Event density [a. u. ] Log E>19.](https://slidetodoc.com/presentation_image_h2/4ffb27cc0f2edb05f880e0d0f5a294f1/image-38.jpg)
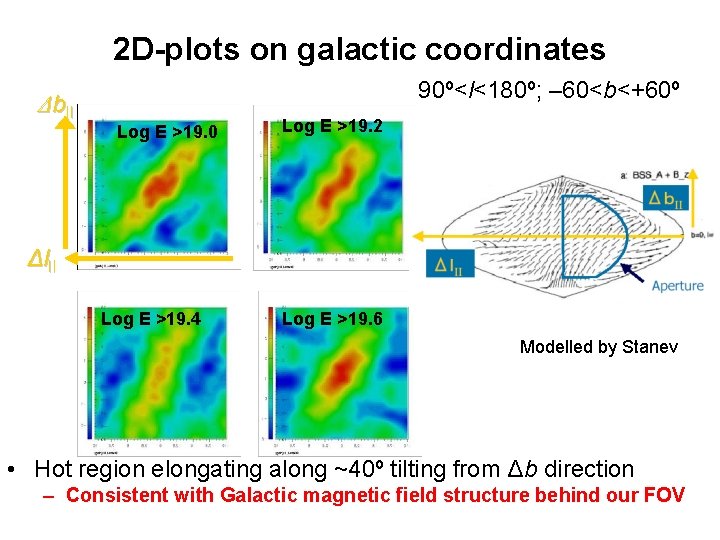
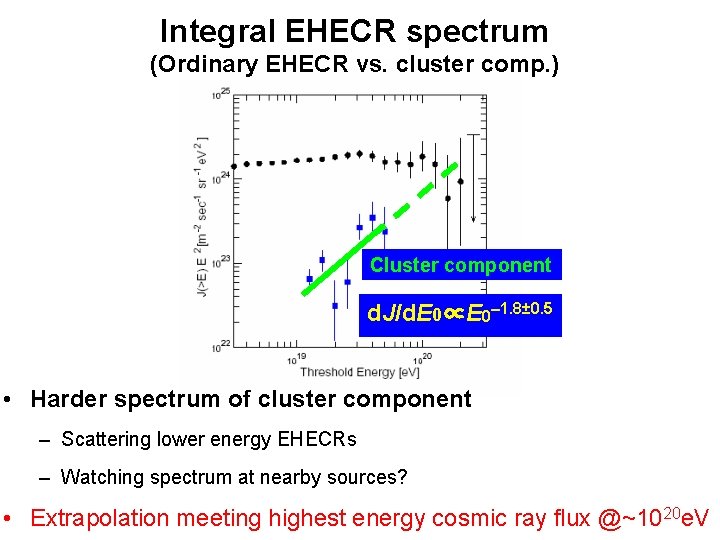
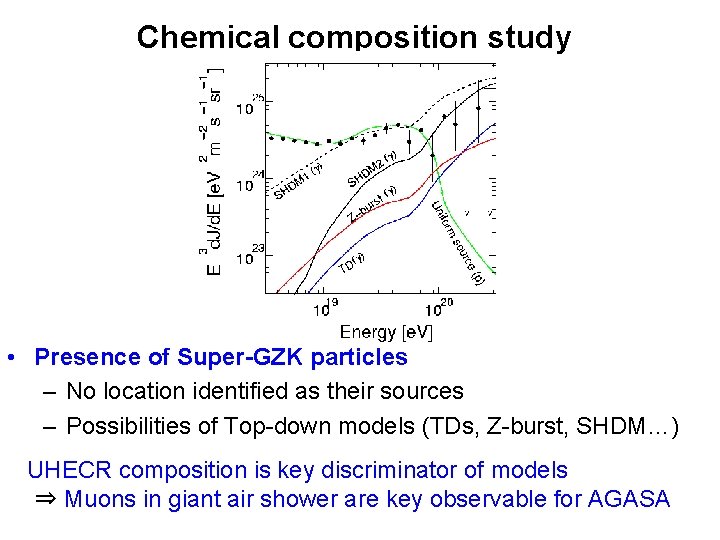
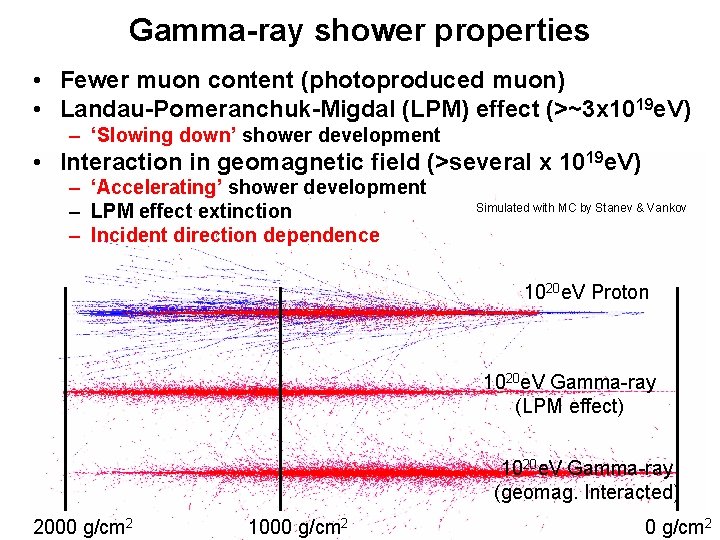
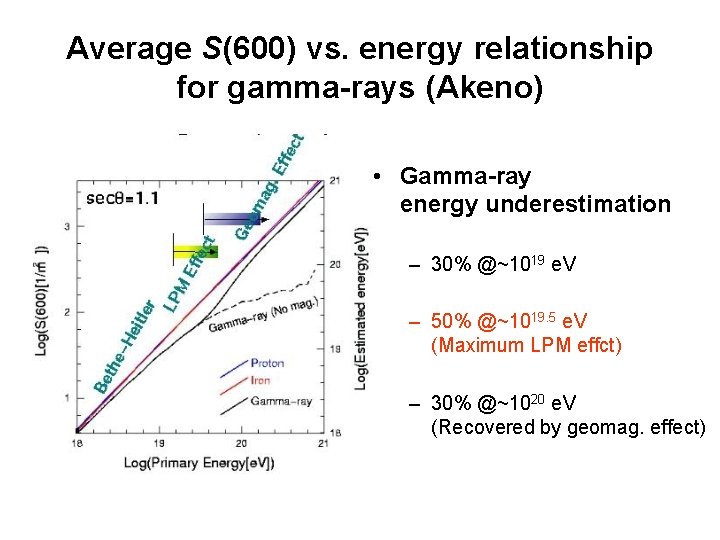
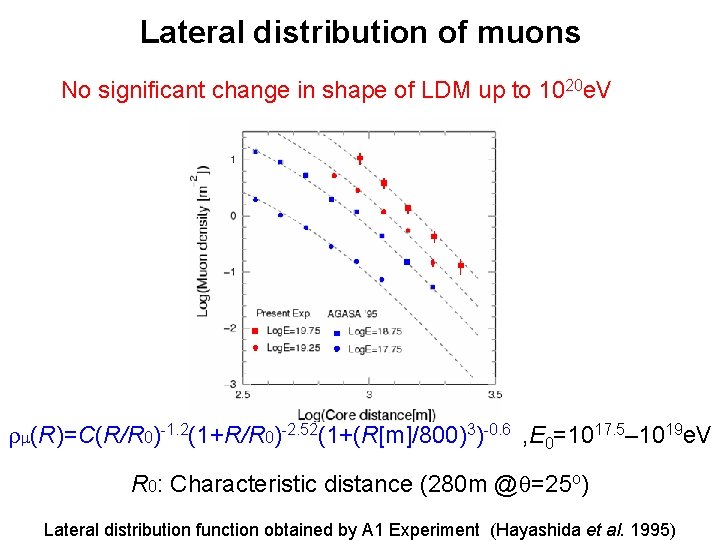
![Primary mass estimator E 0=1. 8 x 1020 e. V rm(1000)=2. 4[/m 2] Lateral Primary mass estimator E 0=1. 8 x 1020 e. V rm(1000)=2. 4[/m 2] Lateral](https://slidetodoc.com/presentation_image_h2/4ffb27cc0f2edb05f880e0d0f5a294f1/image-45.jpg)
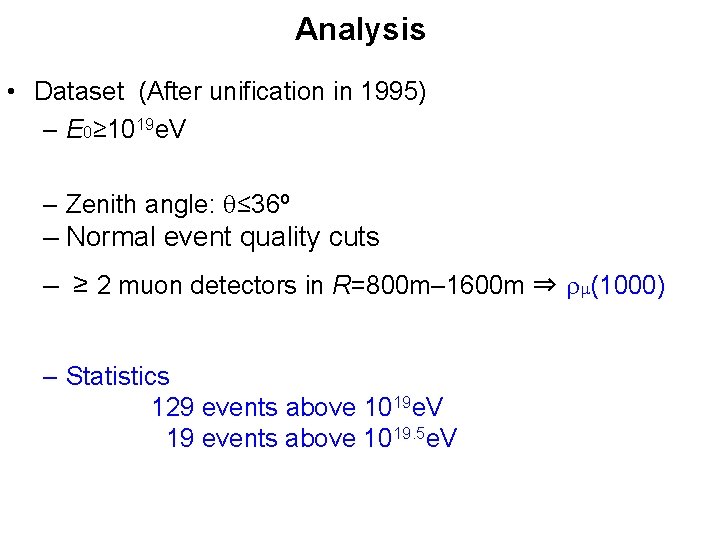
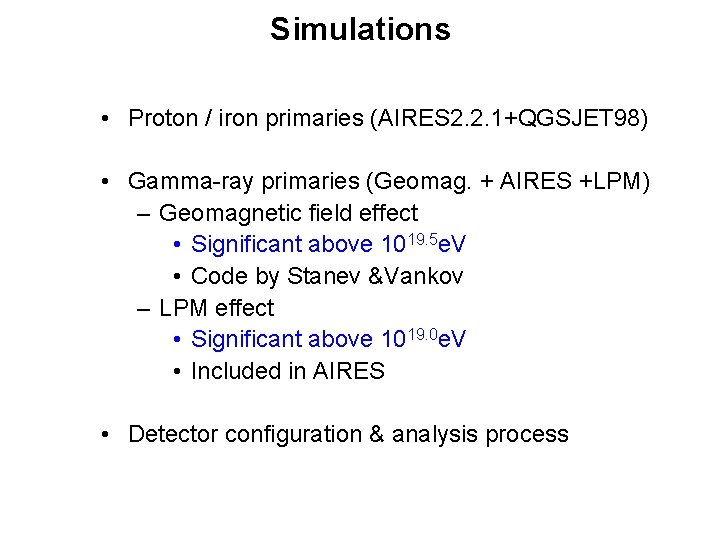
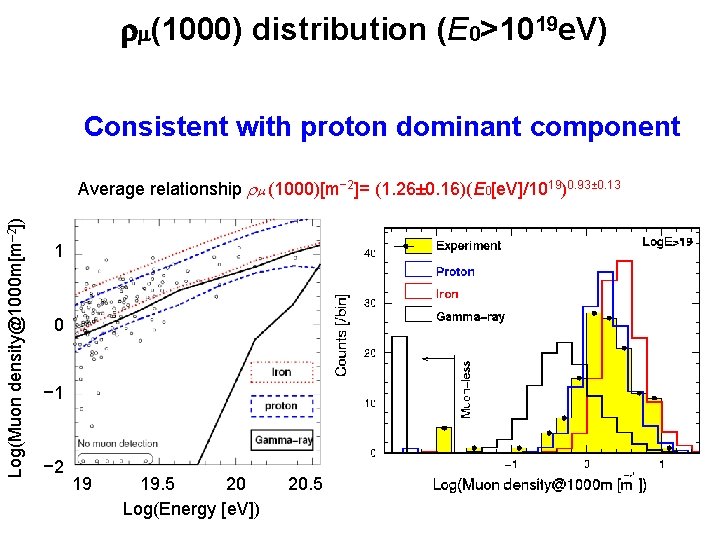
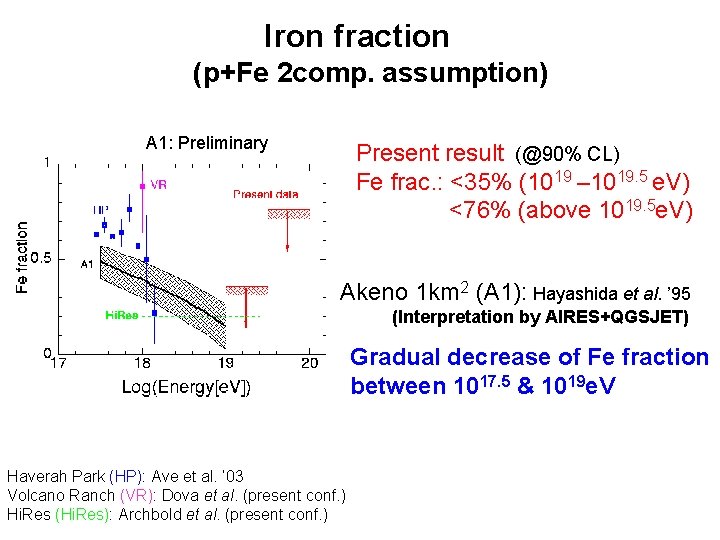
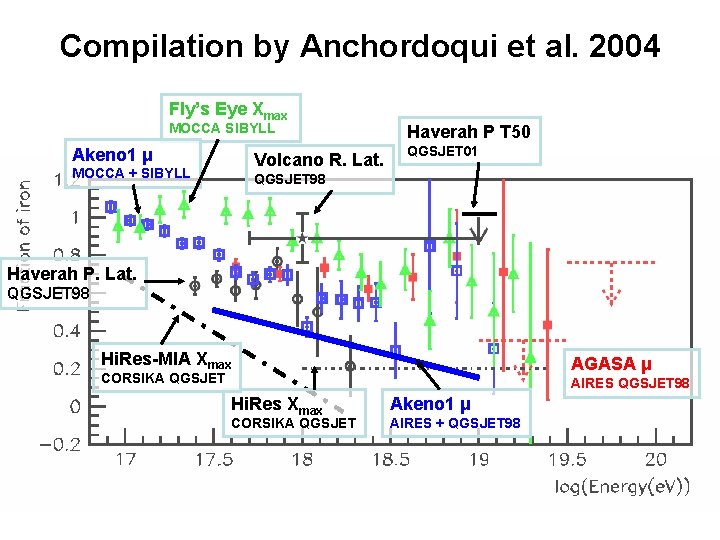
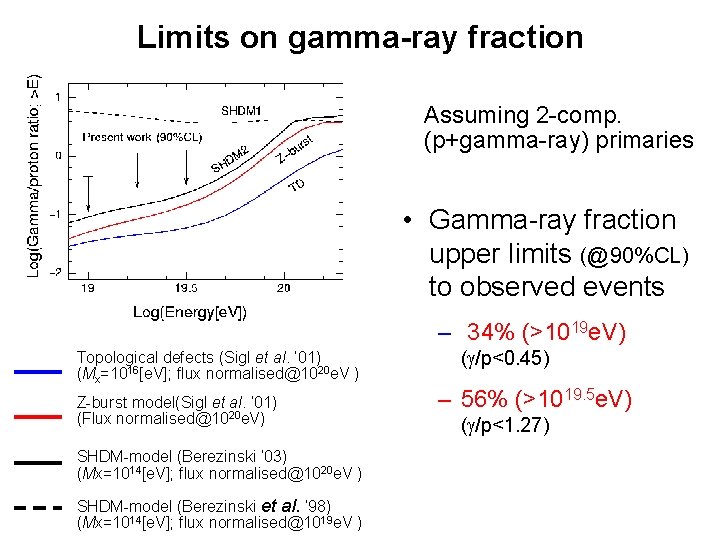
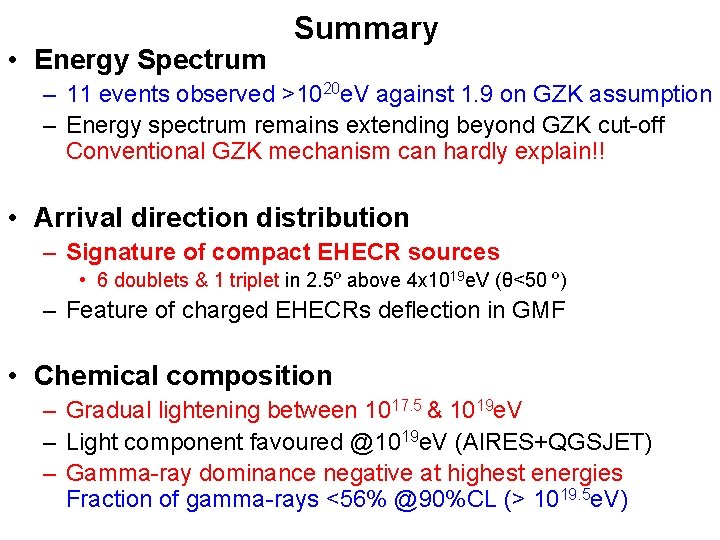
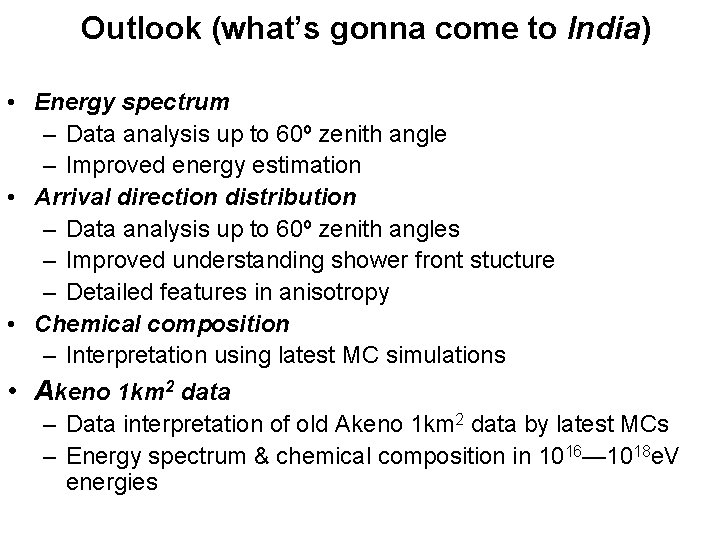
- Slides: 53
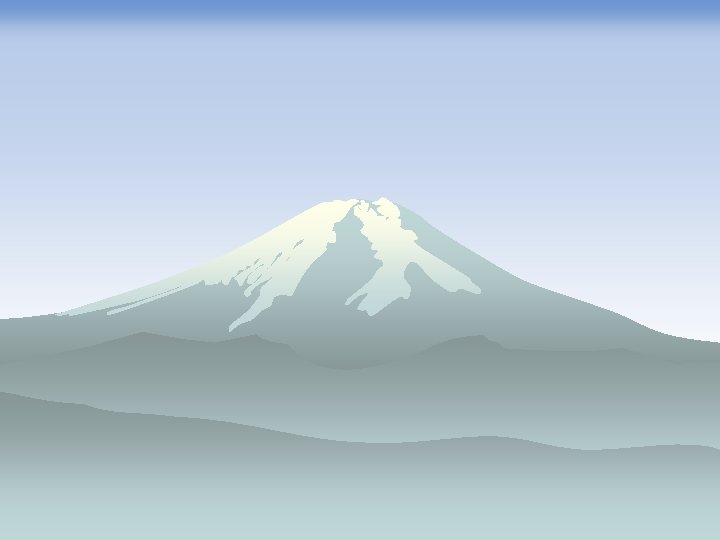
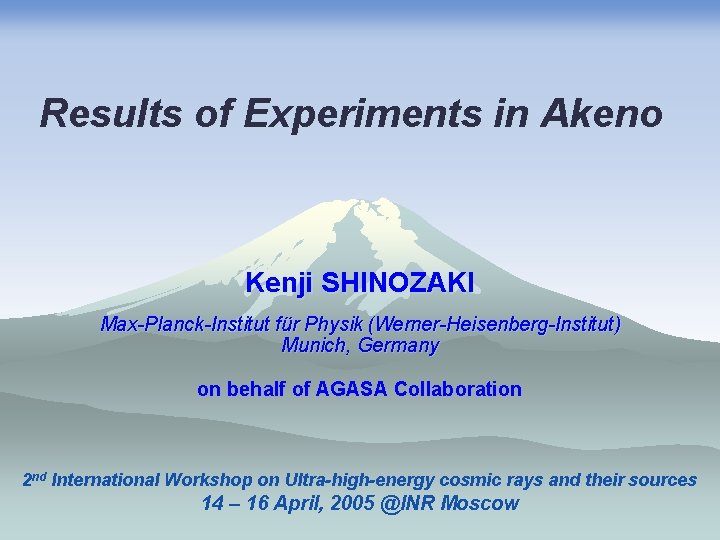
Results of Experiments in Akeno Kenji SHINOZAKI Max-Planck-Institut für Physik (Werner-Heisenberg-Institut) Munich, Germany on behalf of AGASA Collaboration 2 nd International Workshop on Ultra-high-energy cosmic rays and their sources 14 – 16 April, 2005 @INR Moscow
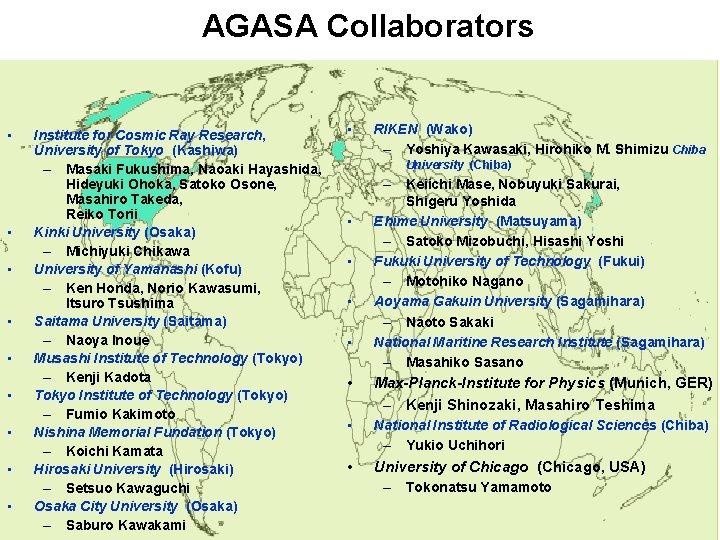
AGASA Collaborators • • • Institute for Cosmic Ray Research, University of Tokyo (Kashiwa) – Masaki Fukushima, Naoaki Hayashida, Hideyuki Ohoka, Satoko Osone, Masahiro Takeda, Reiko Torii Kinki University (Osaka) – Michiyuki Chikawa University of Yamanashi (Kofu) – Ken Honda, Norio Kawasumi, Itsuro Tsushima Saitama University (Saitama) – Naoya Inoue Musashi Institute of Technology (Tokyo) – Kenji Kadota Tokyo Institute of Technology (Tokyo) – Fumio Kakimoto Nishina Memorial Fundation (Tokyo) – Koichi Kamata Hirosaki University (Hirosaki) – Setsuo Kawaguchi Osaka City University (Osaka) – Saburo Kawakami • RIKEN (Wako) – Yoshiya Kawasaki, Hirohiko M. Shimizu Chiba University (Chiba) • • – Keiichi Mase, Nobuyuki Sakurai, Shigeru Yoshida Ehime University (Matsuyama) – Satoko Mizobuchi, Hisashi Yoshi Fukuki University of Technology (Fukui) – Motohiko Nagano Aoyama Gakuin University (Sagamihara) – Naoto Sakaki National Maritine Research Institute (Sagamihara) – Masahiko Sasano Max-Planck-Institute for Physics (Munich, GER) – Kenji Shinozaki, Masahiro Teshima National Institute of Radiological Sciences (Chiba) – Yukio Uchihori University of Chicago (Chicago, USA) – Tokonatsu Yamamoto
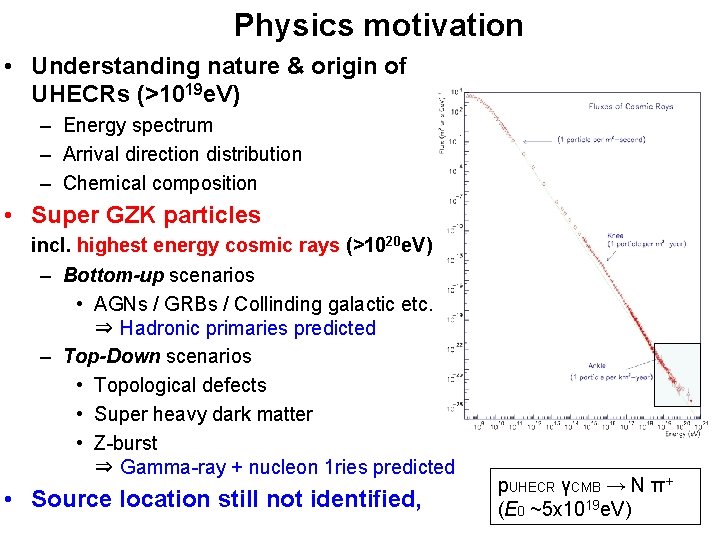
Physics motivation • Understanding nature & origin of UHECRs (>1019 e. V) – Energy spectrum – Arrival direction distribution – Chemical composition • Super GZK particles incl. highest energy cosmic rays (>1020 e. V) – Bottom-up scenarios • AGNs / GRBs / Collinding galactic etc. ⇒ Hadronic primaries predicted – Top-Down scenarios • Topological defects • Super heavy dark matter • Z-burst ⇒ Gamma-ray + nucleon 1 ries predicted • Source location still not identified, p. UHECR γCMB → N π+ (E 0 ~5 x 1019 e. V)
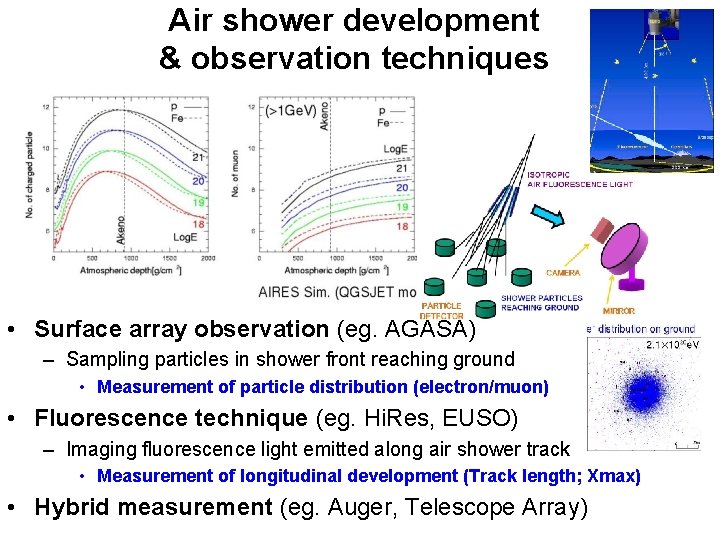
Air shower development & observation techniques • Surface array observation (eg. AGASA) – Sampling particles in shower front reaching ground • Measurement of particle distribution (electron/muon) • Fluorescence technique (eg. Hi. Res, EUSO) – Imaging fluorescence light emitted along air shower track • Measurement of longitudinal development (Track length; Xmax) • Hybrid measurement (eg. Auger, Telescope Array)
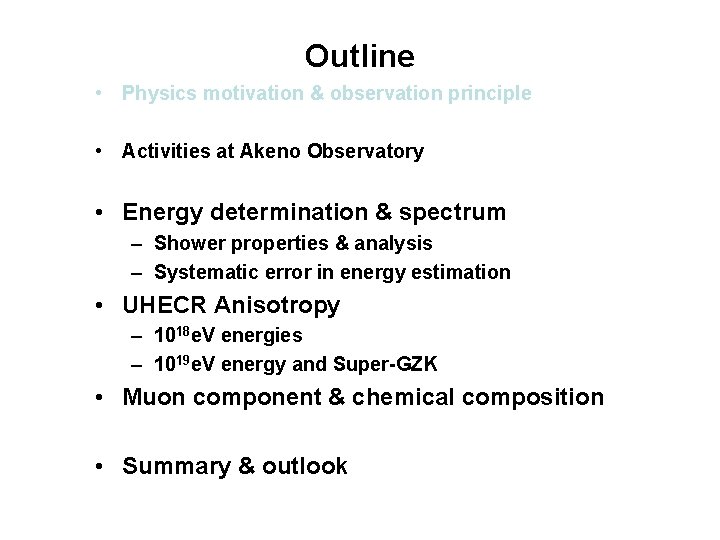
Outline • Physics motivation & observation principle • Activities at Akeno Observatory • Energy determination & spectrum – Shower properties & analysis – Systematic error in energy estimation • UHECR Anisotropy – 1018 e. V energies – 1019 e. V energy and Super-GZK • Muon component & chemical composition • Summary & outlook
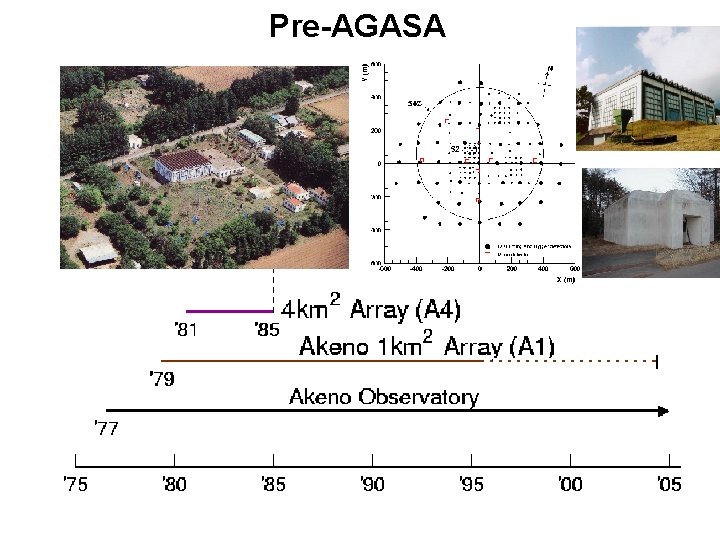
Pre-AGASA
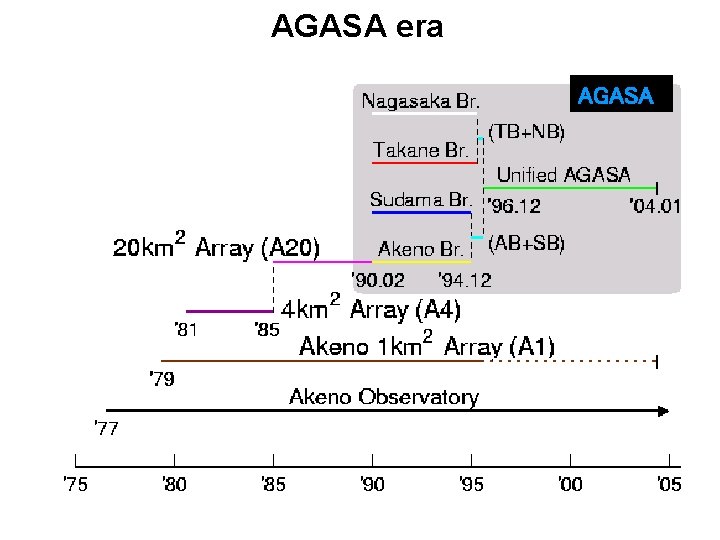
AGASA era AGASA
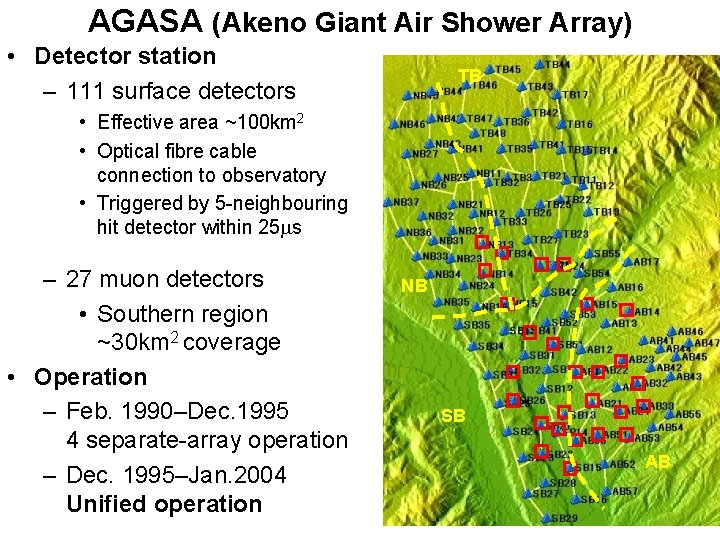
AGASA (Akeno Giant Air Shower Array) • Detector station – 111 surface detectors TB • Effective area ~100 km 2 • Optical fibre cable connection to observatory • Triggered by 5 -neighbouring hit detector within 25 ms – 27 muon detectors • Southern region ~30 km 2 coverage • Operation – Feb. 1990–Dec. 1995 4 separate-array operation – Dec. 1995–Jan. 2004 Unified operation NB SB AB
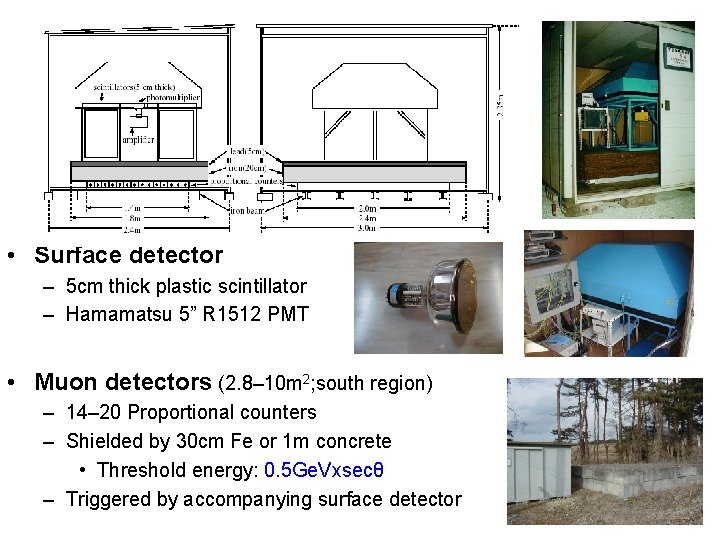
• Surface detector – 5 cm thick plastic scintillator – Hamamatsu 5” R 1512 PMT • Muon detectors (2. 8– 10 m 2; south region) – 14– 20 Proportional counters – Shielded by 30 cm Fe or 1 m concrete • Threshold energy: 0. 5 Ge. Vxsecθ – Triggered by accompanying surface detector
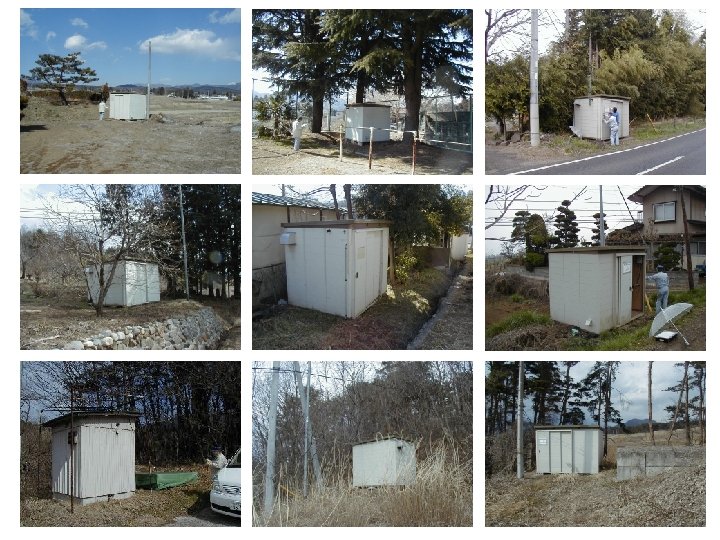
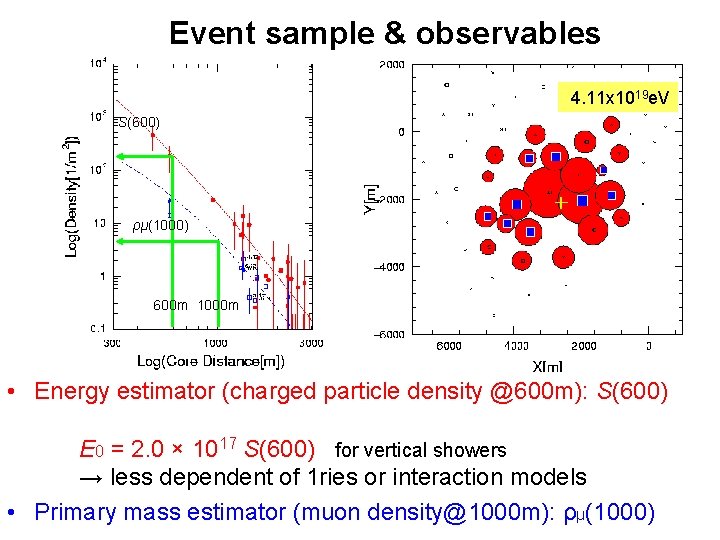
Event sample & observables 4. 11 x 1019 e. V S(600) ρμ(1000) 600 m 1000 m • Energy estimator (charged particle density @600 m): S(600) E 0 = 2. 0 × 1017 S(600) for vertical showers → less dependent of 1 ries or interaction models • Primary mass estimator (muon density@1000 m): ρμ(1000)
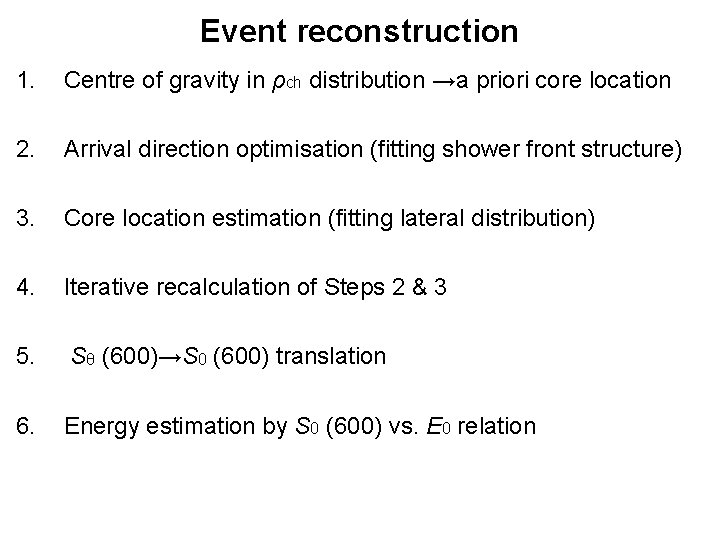
Event reconstruction 1. Centre of gravity in ρch distribution →a priori core location 2. Arrival direction optimisation (fitting shower front structure) 3. Core location estimation (fitting lateral distribution) 4. Iterative recalculation of Steps 2 & 3 5. Sθ (600)→S 0 (600) translation 6. Energy estimation by S 0 (600) vs. E 0 relation
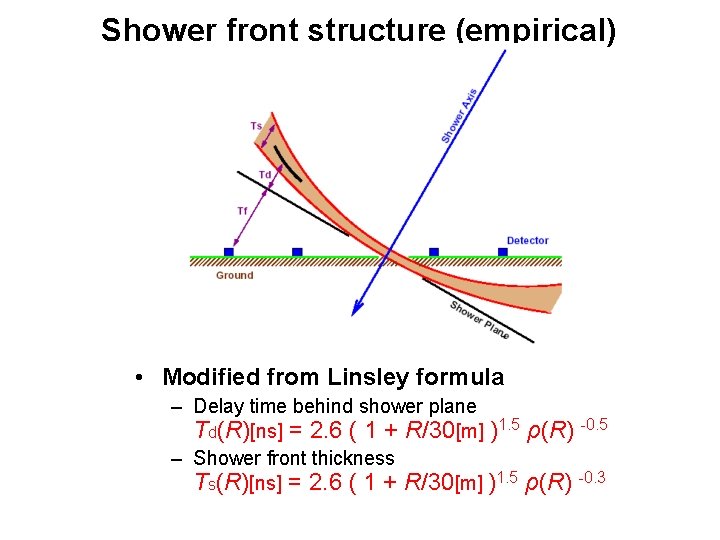
Shower front structure (empirical) • Modified from Linsley formula – Delay time behind shower plane Td(R)[ns] = 2. 6 ( 1 + R/30[m] )1. 5 ρ(R) -0. 5 – Shower front thickness Ts(R)[ns] = 2. 6 ( 1 + R/30[m] )1. 5 ρ(R) -0. 3
![Lateral distribution empirical secθ 1 1 S60010 30m 2 Modified Linsley formula ρR Lateral distribution (empirical) secθ≤ 1. 1 S(600)=10, 30[m 2] • Modified Linsley formula ρ(R)](https://slidetodoc.com/presentation_image_h2/4ffb27cc0f2edb05f880e0d0f5a294f1/image-15.jpg)
Lateral distribution (empirical) secθ≤ 1. 1 S(600)=10, 30[m 2] • Modified Linsley formula ρ(R) = C (R/RM) –α (1+R/RM) –(η–α) {1+(R/1000)2} –δ • C: Normalisation constant, α=1. 2, δ=0. 6 • RM: Moliere unit @ Akeno (=91. 6 m) • η = (3. 97± 0. 13) – (1. 79± 0. 62) (secθ – 1) • Fluctuation of observed particle number σρ2 = ρ + 0. 25 ρ2 + ρ (= σscin 2 + σrest 2 + σstat 2)
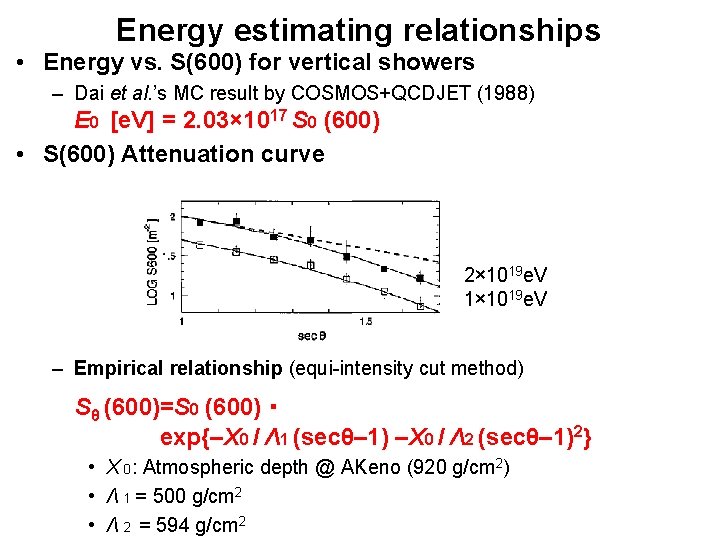
Energy estimating relationships • Energy vs. S(600) for vertical showers – Dai et al. ’s MC result by COSMOS+QCDJET (1988) E 0 [e. V] = 2. 03× 1017 S 0 (600) • S(600) Attenuation curve 2× 1019 e. V 1× 1019 e. V – Empirical relationship (equi-intensity cut method) Sθ (600)=S 0 (600)・ exp{–X 0 / Λ 1 (secθ– 1) –X 0 / Λ 2 (secθ– 1)2} • X 0: Atmospheric depth @ AKeno (920 g/cm 2) • Λ 1 = 500 g/cm 2 • Λ 2 = 594 g/cm 2
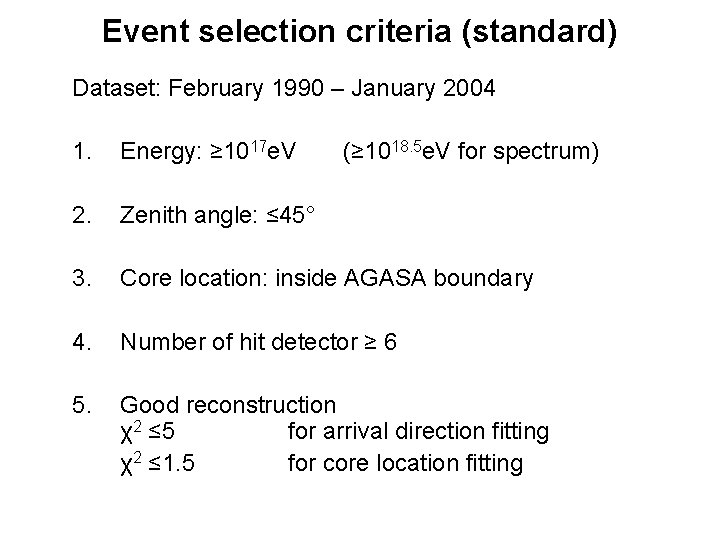
Event selection criteria (standard) Dataset: February 1990 – January 2004 1. Energy: ≥ 1017 e. V 2. Zenith angle: ≤ 45° 3. Core location: inside AGASA boundary 4. Number of hit detector ≥ 6 5. Good reconstruction χ2 ≤ 5 for arrival direction fitting χ2 ≤ 1. 5 for core location fitting (≥ 1018. 5 e. V for spectrum)
![Reconstruction accuracy Energy resolution Angular resolution 8 Open angle Δθº Counts bin 20 15 Reconstruction accuracy (Energy resolution, Angular resolution) 8 Open angle Δθ[º] Counts [%/bin] 20 15](https://slidetodoc.com/presentation_image_h2/4ffb27cc0f2edb05f880e0d0f5a294f1/image-18.jpg)
Reconstruction accuracy (Energy resolution, Angular resolution) 8 Open angle Δθ[º] Counts [%/bin] 20 15 10 5 0 – 1. 0 0. 0 1. 0 ΔLog(Energy[e. V]) • Energy resolution – ΔE 0/E 0=± 30% @1019. 5 e. V – ΔE 0/E 0=± 25% @1020 e. V 6 4 90% 2 0 68% 18 19 20 Log(Energy[e. V]) • Angular resolution – Δθ=2. 0º @1019. 5 e. V – Δθ=1. 3º @1020 e. V
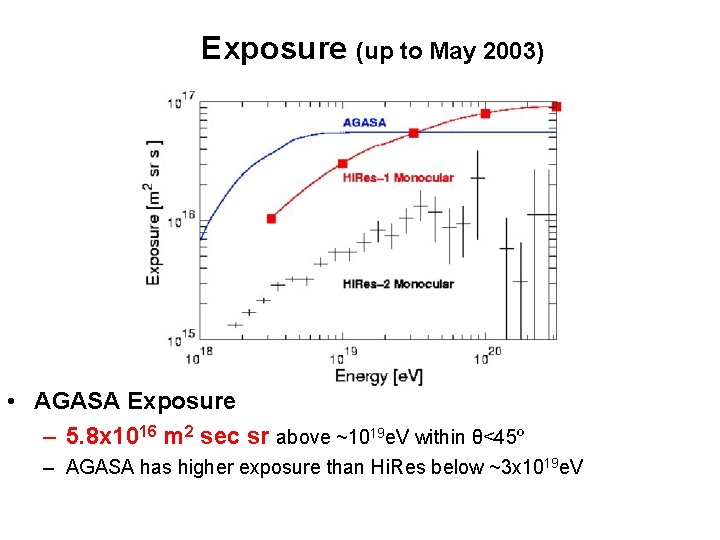
Exposure (up to May 2003) • AGASA Exposure – 5. 8 x 1016 m 2 sec sr above ~1019 e. V within θ<45º – AGASA has higher exposure than Hi. Res below ~3 x 1019 e. V
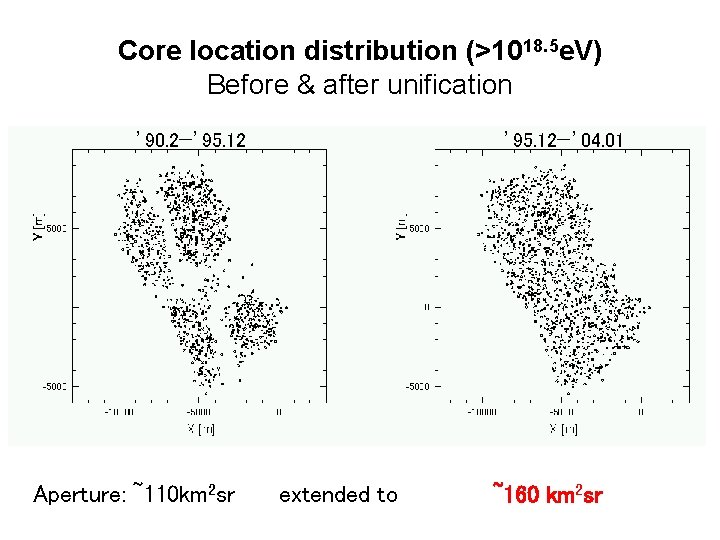
Core location distribution (>1018. 5 e. V) Before & after unification ’ 90. 2—’ 95. 12 Aperture: ~110 km 2 sr ’ 95. 12—’ 04. 01 extended to ~160 km 2 sr
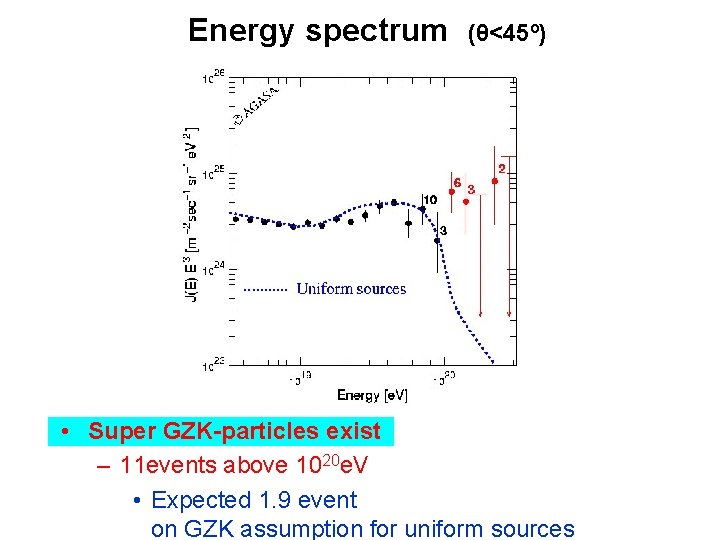
Energy spectrum (θ<45º) • Super GZK-particles exist – 11 events above 1020 e. V • Expected 1. 9 event on GZK assumption for uniform sources
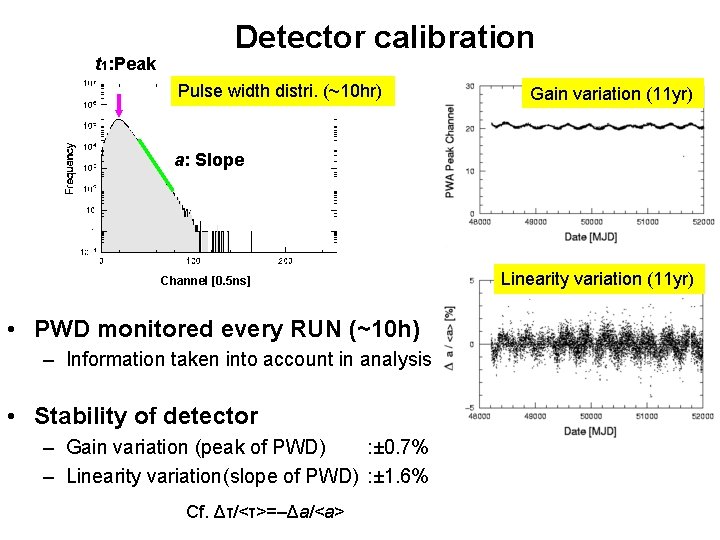
t 1: Peak Detector calibration Pulse width distri. (~10 hr) Gain variation (11 yr) a: Slope Channel [0. 5 ns] • PWD monitored every RUN (~10 h) – Information taken into account in analysis • Stability of detector – Gain variation (peak of PWD) : ± 0. 7% – Linearity variation(slope of PWD) : ± 1. 6% Cf. Δτ/<τ>=–Δa/<a> Linearity variation (11 yr)
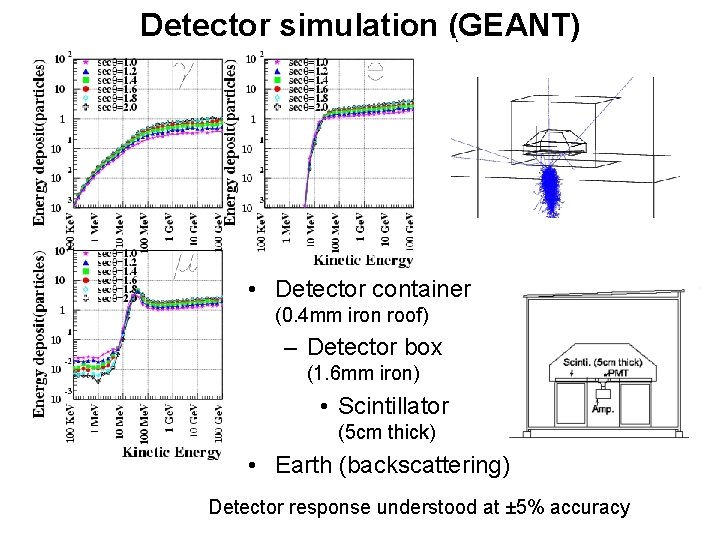
Detector simulation (GEANT) • Detector container (0. 4 mm iron roof) – Detector box (1. 6 mm iron) • Scintillator (5 cm thick) • Earth (backscattering) Detector response understood at ± 5% accuracy
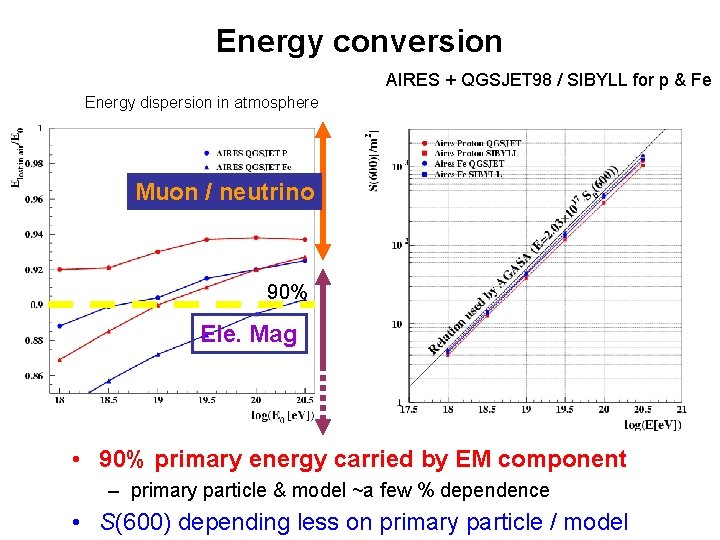
Energy conversion AIRES + QGSJET 98 / SIBYLL for p & Fe Energy dispersion in atmosphere Muon / neutrino 90% Ele. Mag • 90% primary energy carried by EM component – primary particle & model ~a few % dependence • S(600) depending less on primary particle / model
![Energy conversion factor E 0 a 1017 e Vx S600 b Ref Dai Energy conversion factor E 0 = a [1017 e. V]x S(600) b Ref. Dai](https://slidetodoc.com/presentation_image_h2/4ffb27cc0f2edb05f880e0d0f5a294f1/image-25.jpg)
Energy conversion factor E 0 = a [1017 e. V]x S(600) b Ref. Dai et al. ’ 88 COSMOS Model 1 ry a b QCDJET p 2. 03 1. 02 QGSJET 98 p 2. 07 1. 03 Fe 2. 34 1. 00 p 2. 30 1. 03 Fe 2. 19 1. 03 p 2. 17 1. 01 Fe 2. 15 1. 03 p 2. 34 1. 04 Fe 2. 24 1. 02 Single=electron (900 m) Nagano et al. ’ 99 (CORSIKA 5. 621) Single= PH peak (900 m) SIBYLL 1. 6 Sakaki et al. ’ 01 (AIRES 2. 2. 1) QGSJET 98 Single= PW peak (667 m) SIBYLL 1. 6 • Presently assigned primary energy: – 10% ± 1 2% – Most conservative (We need to push up current energy)
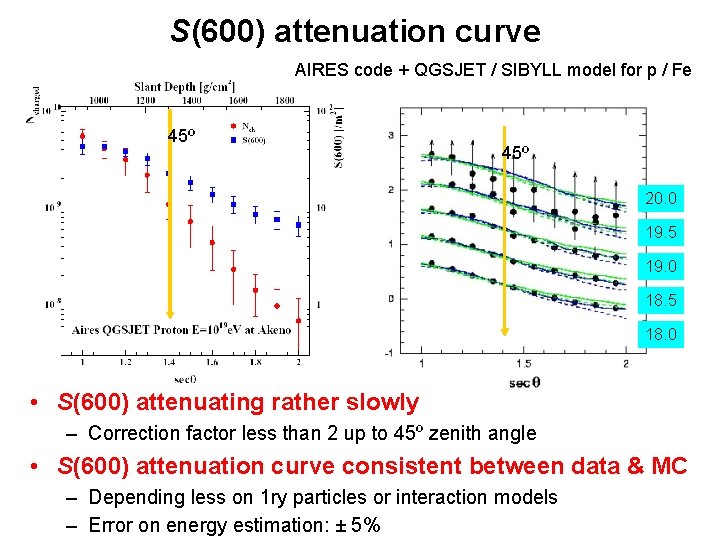
S(600) attenuation curve AIRES code + QGSJET / SIBYLL model for p / Fe 45º 20. 0 19. 5 19. 0 18. 5 18. 0 • S(600) attenuating rather slowly – Correction factor less than 2 up to 45º zenith angle • S(600) attenuation curve consistent between data & MC – Depending less on 1 ry particles or interaction models – Error on energy estimation: ± 5%
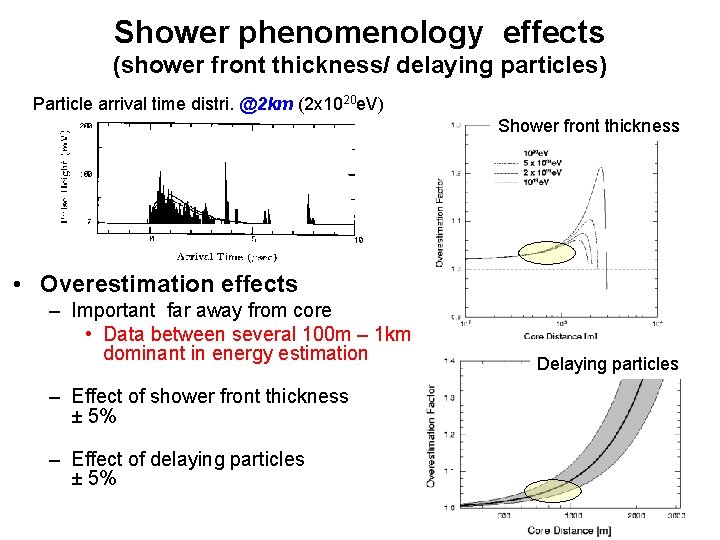
Shower phenomenology effects (shower front thickness/ delaying particles) Particle arrival time distri. @2 km (2 x 1020 e. V) Shower front thickness • Overestimation effects – Important far away from core • Data between several 100 m – 1 km dominant in energy estimation – Effect of shower front thickness ± 5% – Effect of delaying particles ± 5% Delaying particles
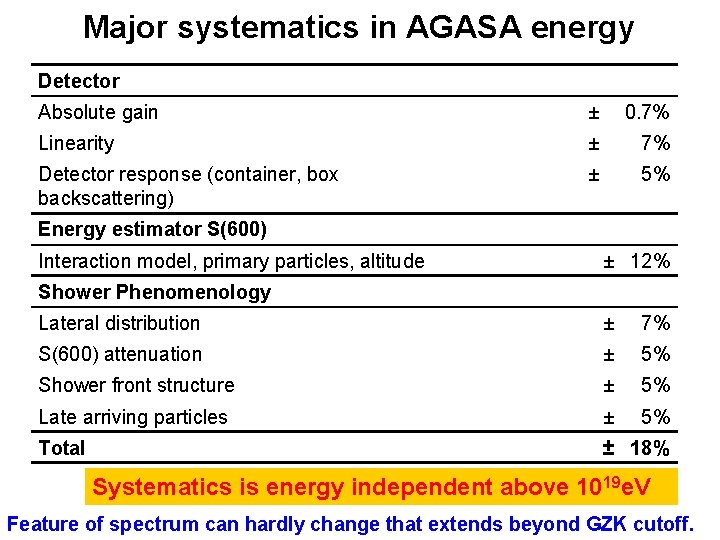
Major systematics in AGASA energy Detector Absolute gain ± 0. 7% Linearity ± 7% Detector response (container, box backscattering) ± 5% Energy estimator S(600) Interaction model, primary particles, altitude ± 12% Shower Phenomenology Lateral distribution ± 7% S(600) attenuation ± 5% Shower front structure ± 5% Late arriving particles ± 5% Total ± 18% Systematics is energy independent above 1019 e. V Feature of spectrum can hardly change that extends beyond GZK cutoff.
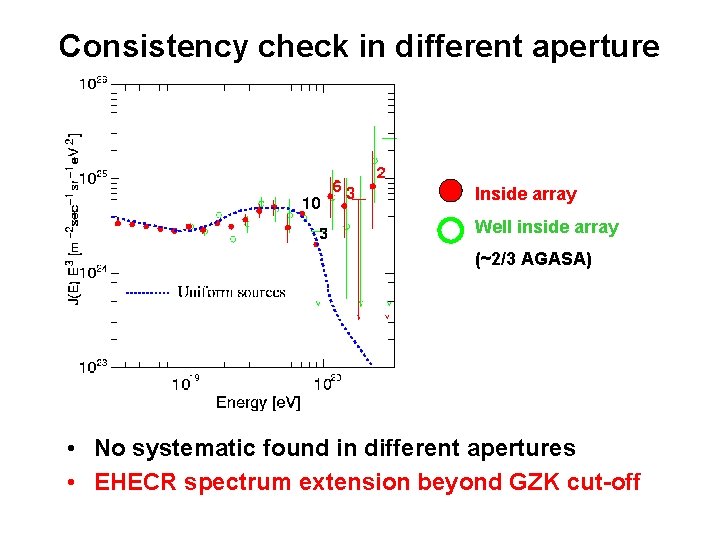
Consistency check in different aperture Inside array Well inside array (~2/3 AGASA) • No systematic found in different apertures • EHECR spectrum extension beyond GZK cut-off
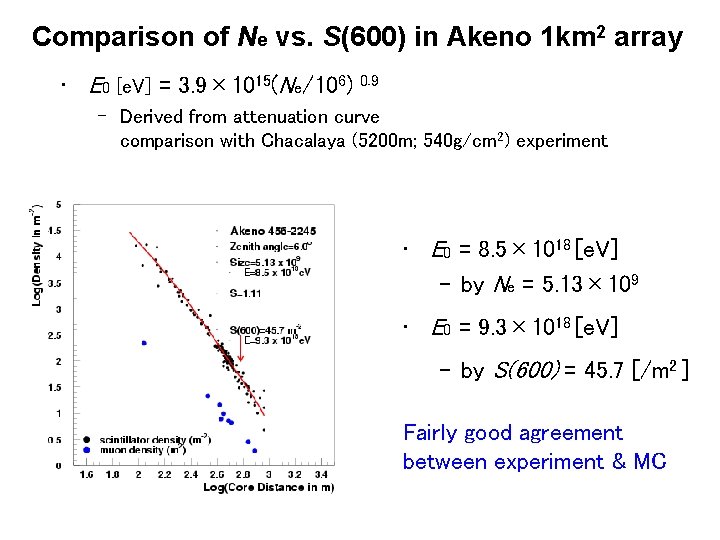
Comparison of Ne vs. S(600) in Akeno 1 km 2 array • E 0 [e. V] = 3. 9× 1015(Ne/106) 0. 9 – Derived from attenuation curve comparison with Chacalaya (5200 m; 540 g/cm 2) experiment • E 0 = 8. 5× 1018 [e. V] – by Ne = 5. 13× 109 • E 0 = 9. 3× 1018 [e. V] – by S(600) = 45. 7 [/m 2 ] Fairly good agreement between experiment & MC
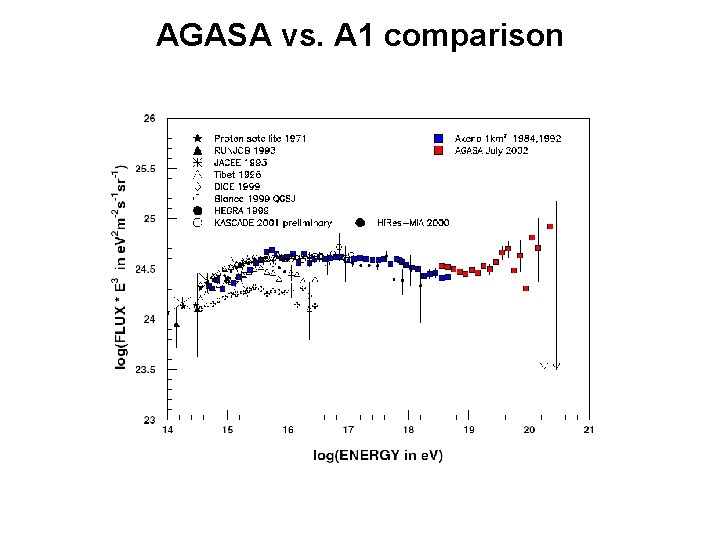
AGASA vs. A 1 comparison
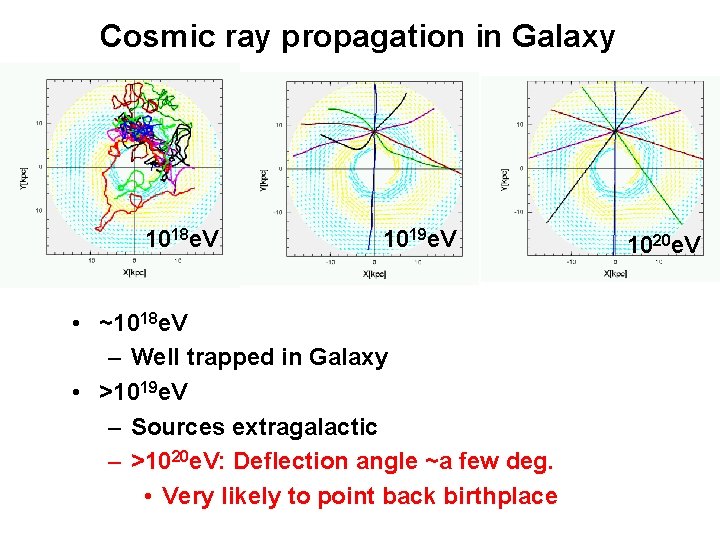
Cosmic ray propagation in Galaxy 1018 e. V 1019 e. V • ~1018 e. V – Well trapped in Galaxy • >1019 e. V – Sources extragalactic – >1020 e. V: Deflection angle ~a few deg. • Very likely to point back birthplace 1020 e. V
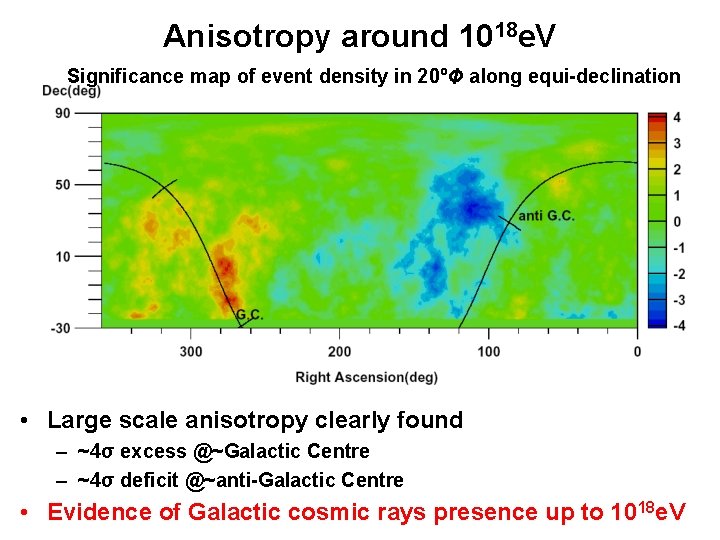
Anisotropy around 1018 e. V Significance map of event density in 20ºΦ along equi-declination • Large scale anisotropy clearly found – ~4σ excess @~Galactic Centre – ~4σ deficit @~anti-Galactic Centre • Evidence of Galactic cosmic rays presence up to 1018 e. V
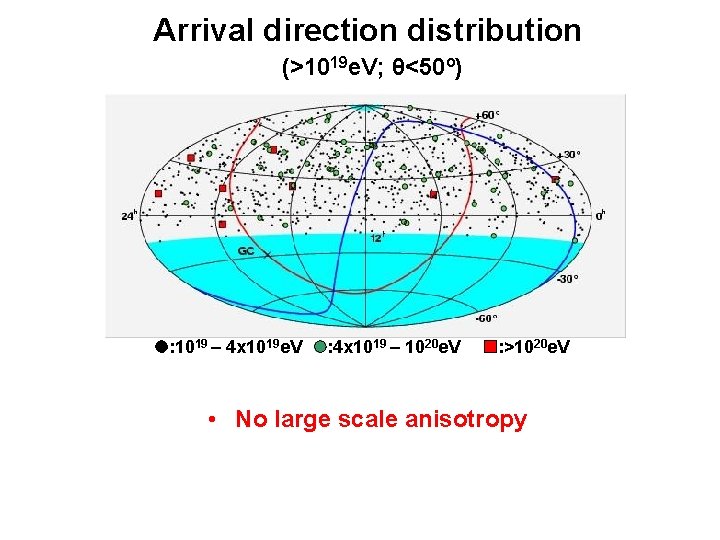
Arrival direction distribution (>1019 e. V; θ<50º) : 1019 – 4 x 1019 e. V : 4 x 1019 – 1020 e. V : >1020 e. V • No large scale anisotropy
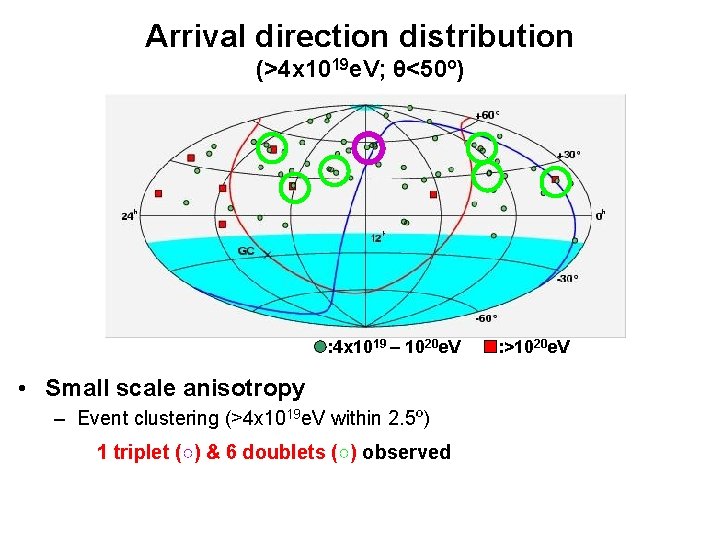
Arrival direction distribution (>4 x 1019 e. V; θ<50º) : 4 x 1019 – 1020 e. V • Small scale anisotropy – Event clustering (>4 x 1019 e. V within 2. 5º) 1 triplet (○) & 6 doublets (○) observed : >1020 e. V
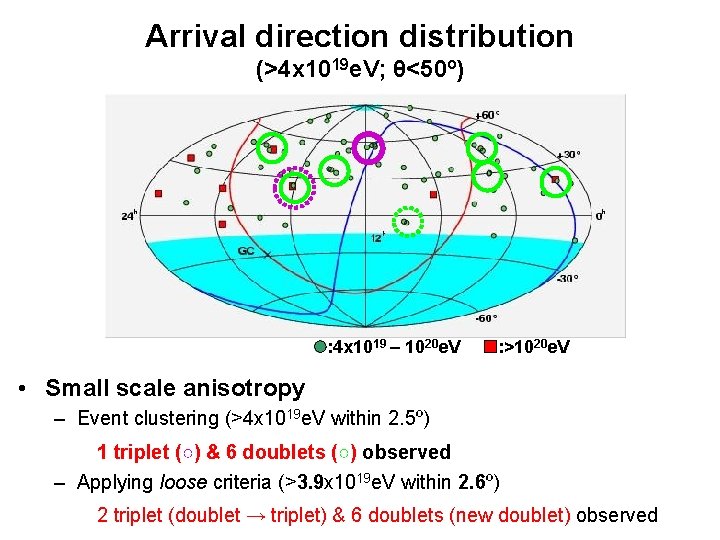
Arrival direction distribution (>4 x 1019 e. V; θ<50º) : 4 x 1019 – 1020 e. V : >1020 e. V • Small scale anisotropy – Event clustering (>4 x 1019 e. V within 2. 5º) 1 triplet (○) & 6 doublets (○) observed – Applying loose criteria (>3. 9 x 1019 e. V within 2. 6º) 2 triplet (doublet → triplet) & 6 doublets (new doublet) observed
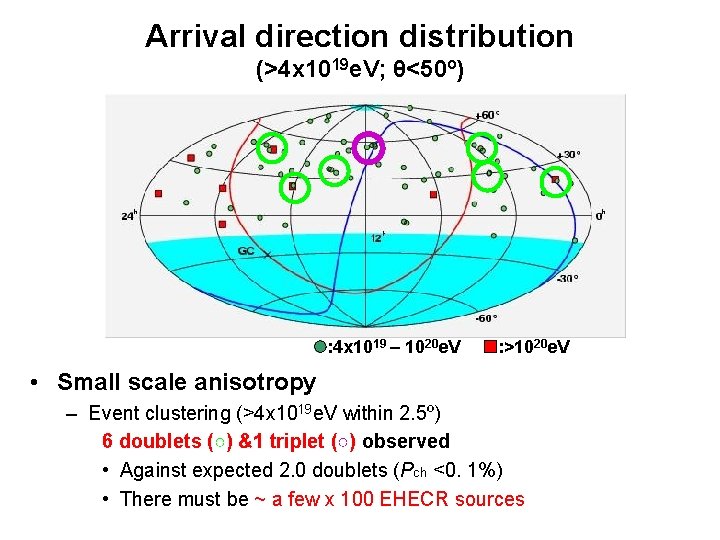
Arrival direction distribution (>4 x 1019 e. V; θ<50º) : 4 x 1019 – 1020 e. V : >1020 e. V • Small scale anisotropy – Event clustering (>4 x 1019 e. V within 2. 5º) 6 doublets (○) &1 triplet (○) observed • Against expected 2. 0 doublets (Pch <0. 1%) • There must be ~ a few x 100 EHECR sources
![Event density a u 0 0 Event density a u Log E19 Event density [a. u. ] 0 0 Event density [a. u. ] Log E>19.](https://slidetodoc.com/presentation_image_h2/4ffb27cc0f2edb05f880e0d0f5a294f1/image-38.jpg)
Event density [a. u. ] 0 0 Event density [a. u. ] Log E>19. 0 3. 4σ 20 40 Space angle [º] 60 Log E>19. 4 2. 0σ 20 40 Space angle [º] 60 0 Log E>19. 2 3. 0σ 20 40 Space angle [º] Event density [a. u. ] Space angle distribution of events 0 60 Log E>19. 6 4. 4σ 20 40 Space angle [º] 60 • Significant peak @ 0 degree – implying presence of compact EHECR sources
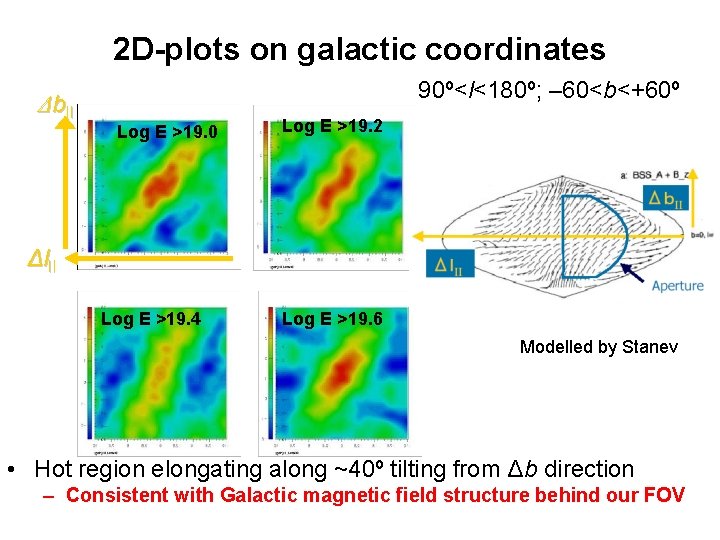
2 D-plots on galactic coordinates Δb. II 90º<l<180º; – 60<b<+60º Log E >19. 0 Log E >19. 2 Δl. II Log E >19. 4 Log E >19. 6 Modelled by Stanev • Hot region elongating along ~40º tilting from Δb direction – Consistent with Galactic magnetic field structure behind our FOV
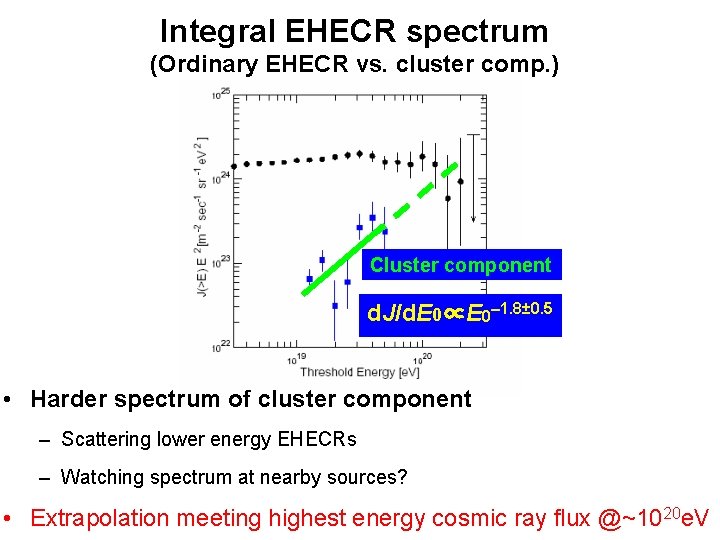
Integral EHECR spectrum (Ordinary EHECR vs. cluster comp. ) Cluster component d. J/d. E 0∝E 0– 1. 8± 0. 5 • Harder spectrum of cluster component – Scattering lower energy EHECRs – Watching spectrum at nearby sources? • Extrapolation meeting highest energy cosmic ray flux @~1020 e. V
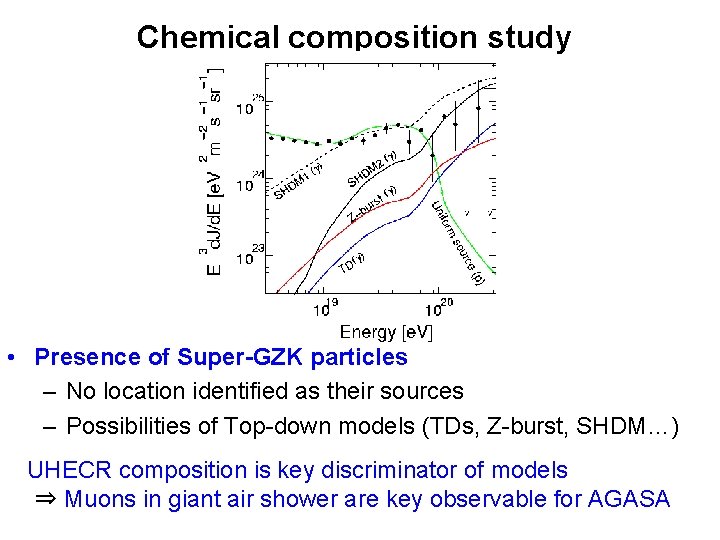
Chemical composition study • Presence of Super-GZK particles – No location identified as their sources – Possibilities of Top-down models (TDs, Z-burst, SHDM…) UHECR composition is key discriminator of models ⇒ Muons in giant air shower are key observable for AGASA
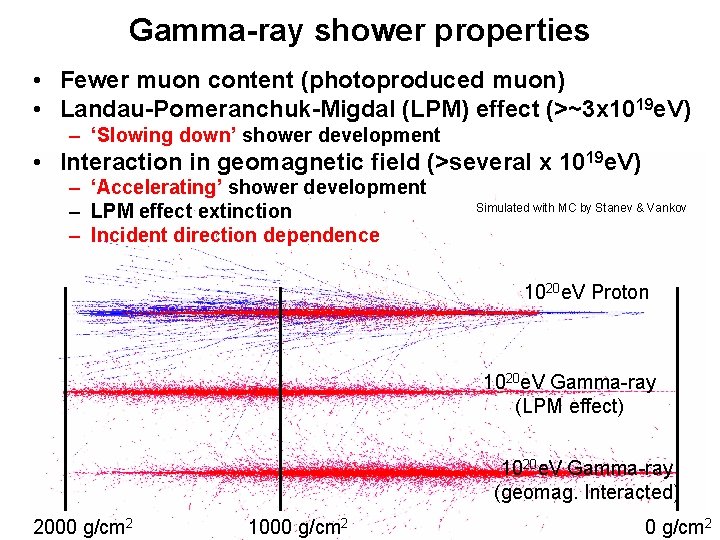
Gamma-ray shower properties • Fewer muon content (photoproduced muon) • Landau-Pomeranchuk-Migdal (LPM) effect (>~3 x 1019 e. V) – ‘Slowing down’ shower development • Interaction in geomagnetic field (>several x 1019 e. V) – ‘Accelerating’ shower development – LPM effect extinction – Incident direction dependence Simulated with MC by Stanev & Vankov 1020 e. V Proton 1020 e. V Gamma-ray (LPM effect) 1020 e. V Gamma-ray (geomag. Interacted) 2000 g/cm 2 1000 g/cm 2
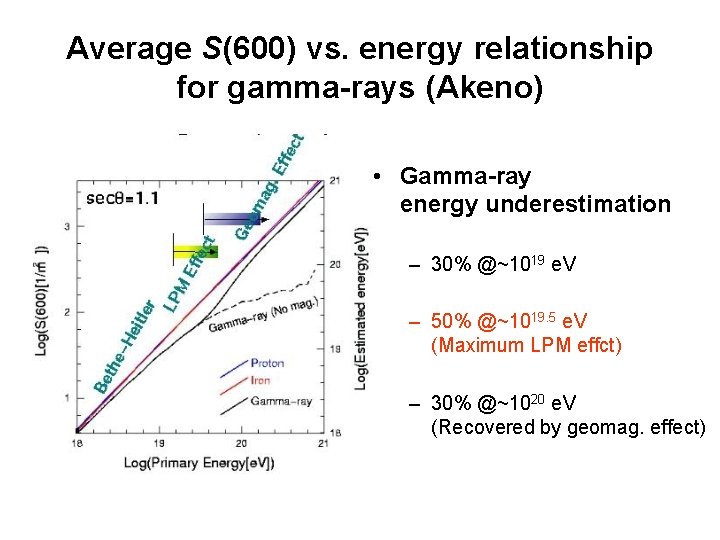
Average S(600) vs. energy relationship for gamma-rays (Akeno) • Gamma-ray energy underestimation – 30% @~1019 e. V – 50% @~1019. 5 e. V (Maximum LPM effct) – 30% @~1020 e. V (Recovered by geomag. effect)
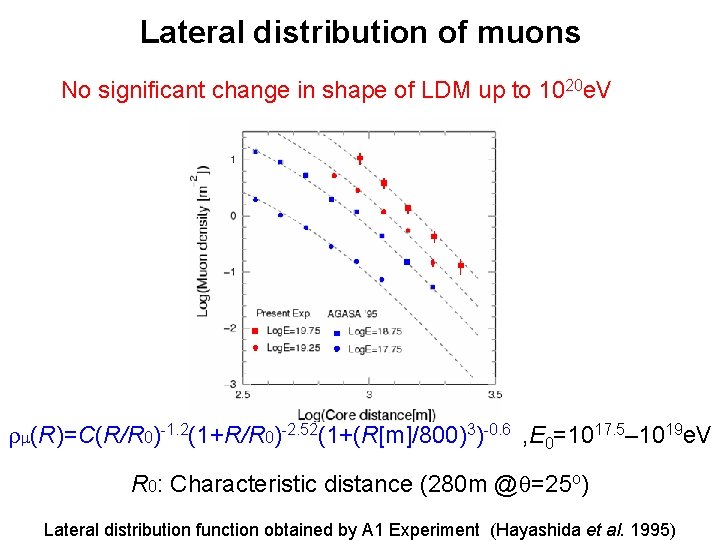
Lateral distribution of muons No significant change in shape of LDM up to 1020 e. V rm(R)=C(R/R 0)-1. 2(1+R/R 0)-2. 52(1+(R[m]/800)3)-0. 6 , E 0=1017. 5– 1019 e. V R 0: Characteristic distance (280 m @q=25 o) Lateral distribution function obtained by A 1 Experiment (Hayashida et al. 1995)
![Primary mass estimator E 01 8 x 1020 e V rm10002 4m 2 Lateral Primary mass estimator E 0=1. 8 x 1020 e. V rm(1000)=2. 4[/m 2] Lateral](https://slidetodoc.com/presentation_image_h2/4ffb27cc0f2edb05f880e0d0f5a294f1/image-45.jpg)
Primary mass estimator E 0=1. 8 x 1020 e. V rm(1000)=2. 4[/m 2] Lateral distribution SAMPLE • Muon density at 1000 m rm(1000) – Fitting muon data in R=800 -1600 m to LDM – Error~± 40% Muon: Charged particle: Empirical formulae
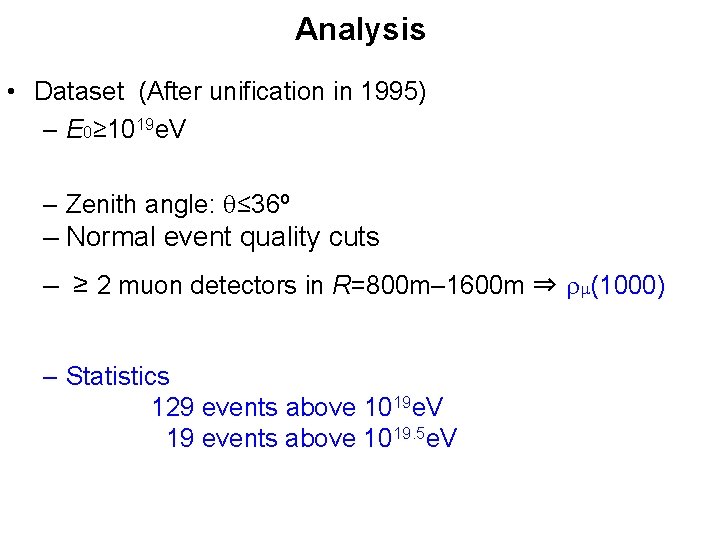
Analysis • Dataset (After unification in 1995) – E 0≥ 1019 e. V – Zenith angle: q≤ 36º – Normal event quality cuts – ≥ 2 muon detectors in R=800 m– 1600 m ⇒ rm(1000) – Statistics 129 events above 1019 e. V 19 events above 1019. 5 e. V
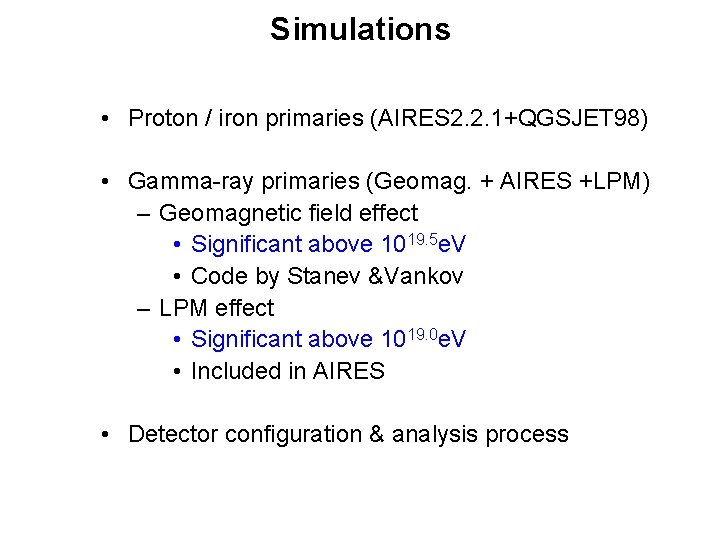
Simulations • Proton / iron primaries (AIRES 2. 2. 1+QGSJET 98) • Gamma-ray primaries (Geomag. + AIRES +LPM) – Geomagnetic field effect • Significant above 1019. 5 e. V • Code by Stanev &Vankov – LPM effect • Significant above 1019. 0 e. V • Included in AIRES • Detector configuration & analysis process
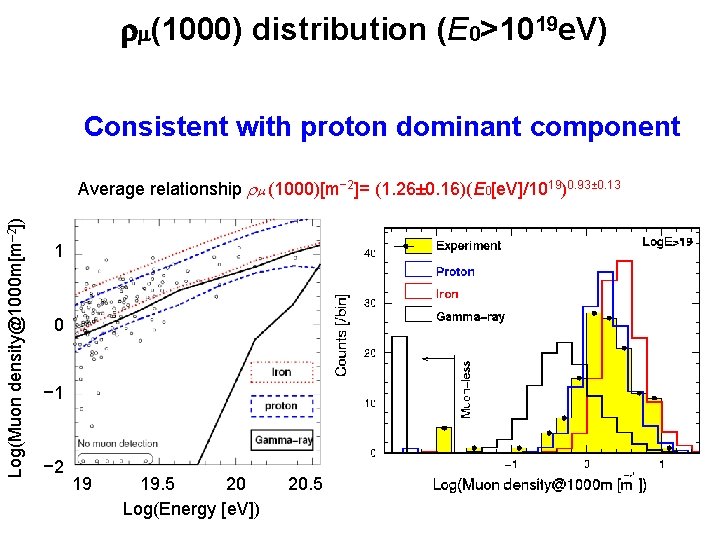
rm(1000) distribution (E 0>1019 e. V) Consistent with proton dominant component Log(Muon density@1000 m[m– 2]) Average relationship rm (1000)[m− 2]= (1. 26± 0. 16)(E 0[e. V]/1019)0. 93± 0. 13 1 0 − 1 − 2 19 19. 5 20 Log(Energy [e. V]) 20. 5
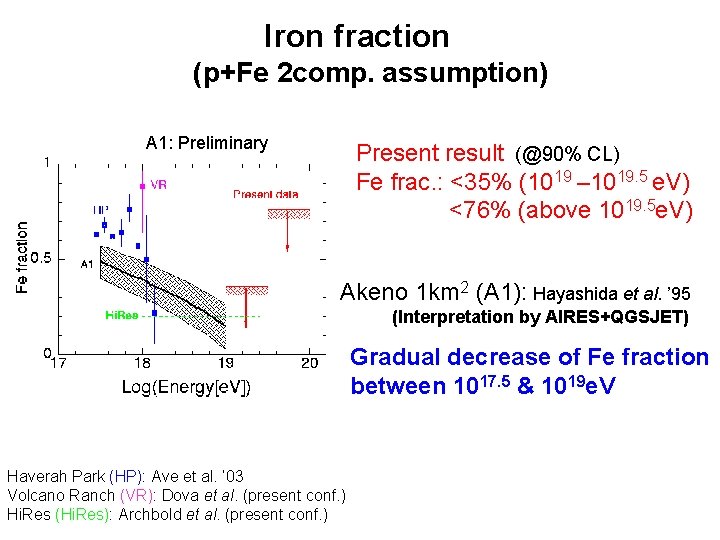
Iron fraction (p+Fe 2 comp. assumption) A 1: Preliminary A 1: PRELIMINARY Present result (@90% CL) Fe frac. : <35% (1019 – 1019. 5 e. V) <76% (above 1019. 5 e. V) Akeno 1 km 2 (A 1): Hayashida et al. ’ 95 (Interpretation by AIRES+QGSJET) Gradual decrease of Fe fraction between 1017. 5 & 1019 e. V Haverah Park (HP): Ave et al. ’ 03 Volcano Ranch (VR): Dova et al. (present conf. ) Hi. Res (Hi. Res): Archbold et al. (present conf. )
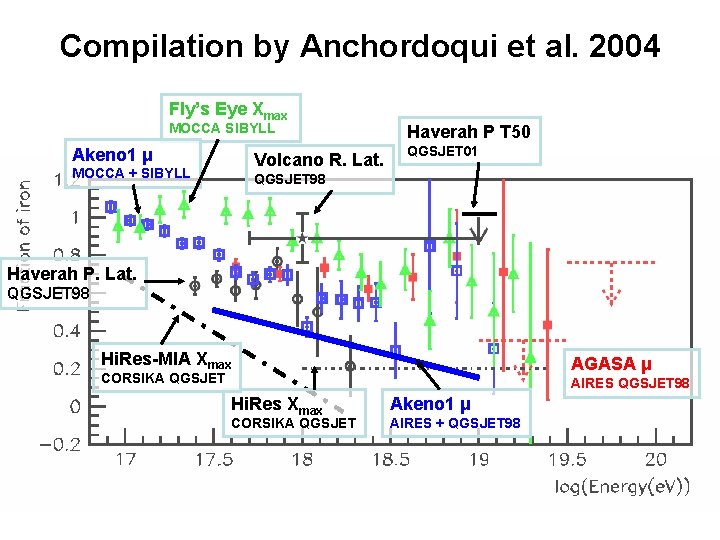
Compilation by Anchordoqui et al. 2004 Fly’s Eye Xmax MOCCA SIBYLL Akeno 1 μ Volcano R. Lat. MOCCA + SIBYLL Haverah P T 50 QGSJET 01 QGSJET 98 Haverah P. Lat. QGSJET 98 Hi. Res-MIA Xmax AGASA μ CORSIKA QGSJET AIRES QGSJET 98 Hi. Res Xmax CORSIKA QGSJET Akeno 1 μ AIRES + QGSJET 98
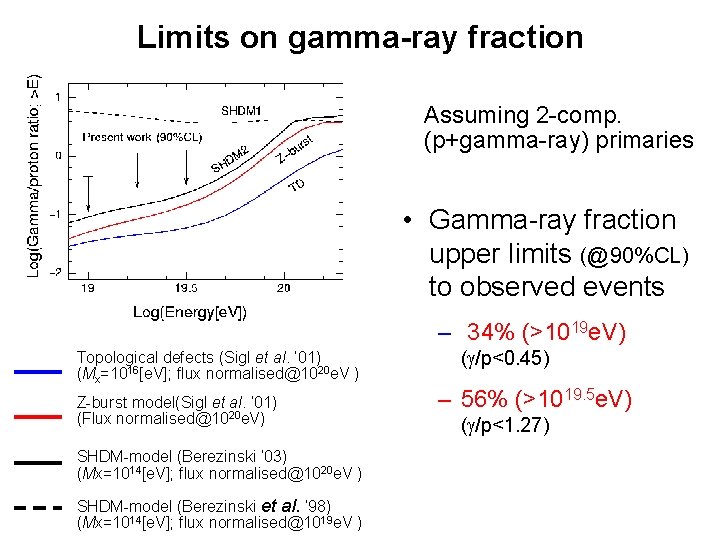
Limits on gamma-ray fraction Assuming 2 -comp. (p+gamma-ray) primaries • Gamma-ray fraction upper limits (@90%CL) to observed events – 34% (>1019 e. V) Topological defects (Sigl et al. ‘ 01) (Mx=1016[e. V]; flux normalised@1020 e. V ) Z-burst model(Sigl et al. ‘ 01) (Flux normalised@1020 e. V) SHDM-model (Berezinski ‘ 03) (Mx=1014[e. V]; flux normalised@1020 e. V ) SHDM-model (Berezinski et al. ‘ 98) (Mx=1014[e. V]; flux normalised@1019 e. V ) (g/p<0. 45) – 56% (>1019. 5 e. V) (g/p<1. 27)
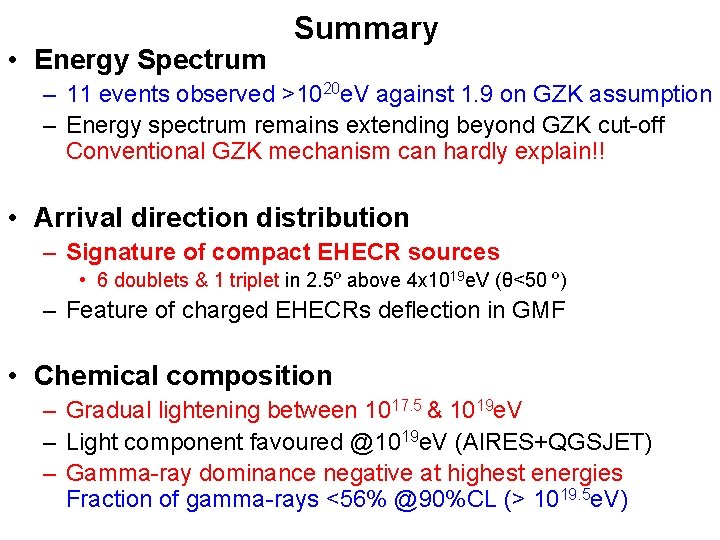
• Energy Spectrum Summary – 11 events observed >1020 e. V against 1. 9 on GZK assumption – Energy spectrum remains extending beyond GZK cut-off Conventional GZK mechanism can hardly explain!! • Arrival direction distribution – Signature of compact EHECR sources • 6 doublets & 1 triplet in 2. 5º above 4 x 1019 e. V (θ<50 º) – Feature of charged EHECRs deflection in GMF • Chemical composition – Gradual lightening between 1017. 5 & 1019 e. V – Light component favoured @1019 e. V (AIRES+QGSJET) – Gamma-ray dominance negative at highest energies Fraction of gamma-rays <56% @90%CL (> 1019. 5 e. V)
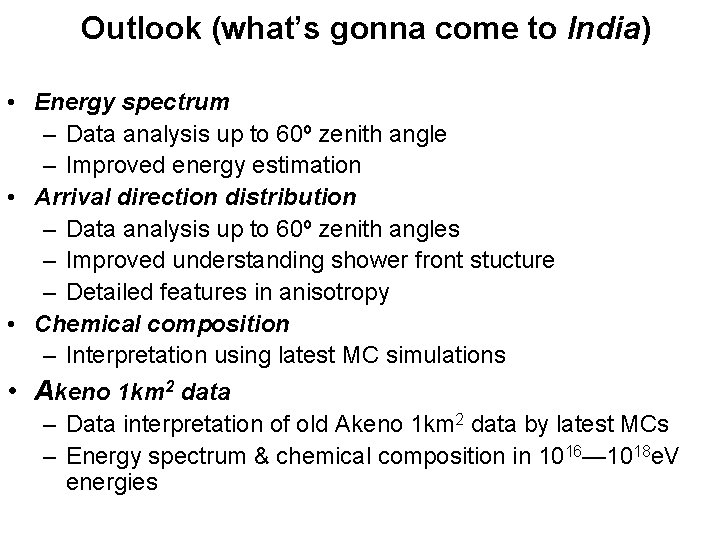
Outlook (what’s gonna come to India) • Energy spectrum – Data analysis up to 60º zenith angle – Improved energy estimation • Arrival direction distribution – Data analysis up to 60º zenith angles – Improved understanding shower front stucture – Detailed features in anisotropy • Chemical composition – Interpretation using latest MC simulations • Akeno 1 km 2 data – Data interpretation of old Akeno 1 km 2 data by latest MCs – Energy spectrum & chemical composition in 1016— 1018 e. V energies
Kenji morita
Kenji shinoda
Kenji osafune
Kenji kadota
Kenji kaneda
Kenji kitao
Kenji doya
Kenji sagae
Joakim nivre
Frederick griffith 1928
Six sigma betekenis
Experiments on animals pros and cons
Liz sneddon experiments
Miller and urey's experiments attempted to demonstrate
Observational study examples statistics
Design of experiments quality management pmp
Viking experiments
Jmp doe analysis
Viking experiments
Eyewitness testimony video experiments
Chapter 2 american experiments
If acid is splashed on your skin wash at once with
Abnormal psychology experiments
George a miller ap psychology
Core flooding experiment
Rerandomization to improve covariate balance in experiments
Design of experiments doe
Tom campbell virtual reality
Split brain experiments
Paper presentation design
Piaget 1896
Experiments in goodness
Nn torrent
Survey experiment observation
Checklist for good practices in laboratory experiments
Computer science experiments
How do scientist test hypothesis? *
2k factorial experiments and fractions
Twins separated at birth nature vs nurture
[http://earthobservatory.nasa.gov/experiments/biome/]
Mass interviewing programme
What is radial conduction
In his transformation experiments what did griffith observe
Design of experiments
Integrated science labs
Orthogonal array
Observational studies vs experiments
Counting rule for permutations
Colorimetry involve
Examples of binomial experiments
Dream experiments
Czech experiments
Science experiment risk assessment
Design of experiments pmp