Reducing Crosstalk in Vertically Integrated CMOS Image Sensors
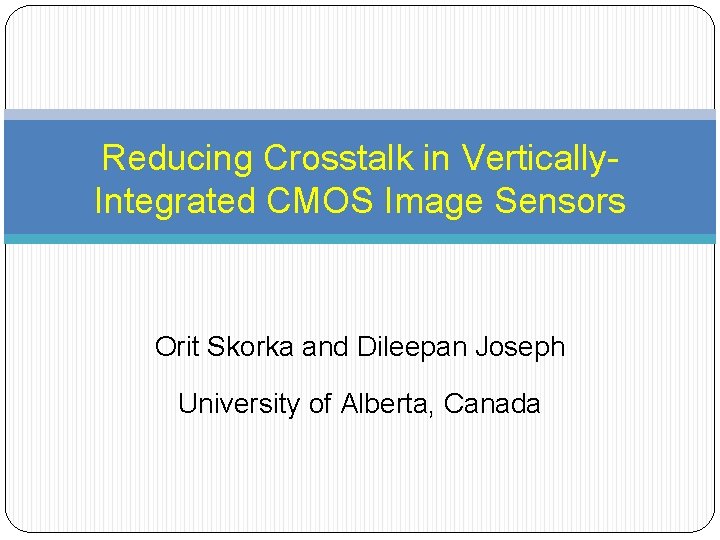
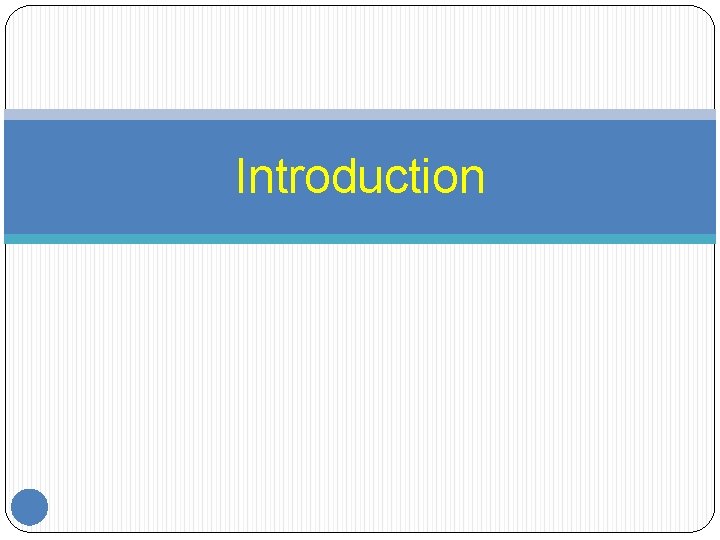
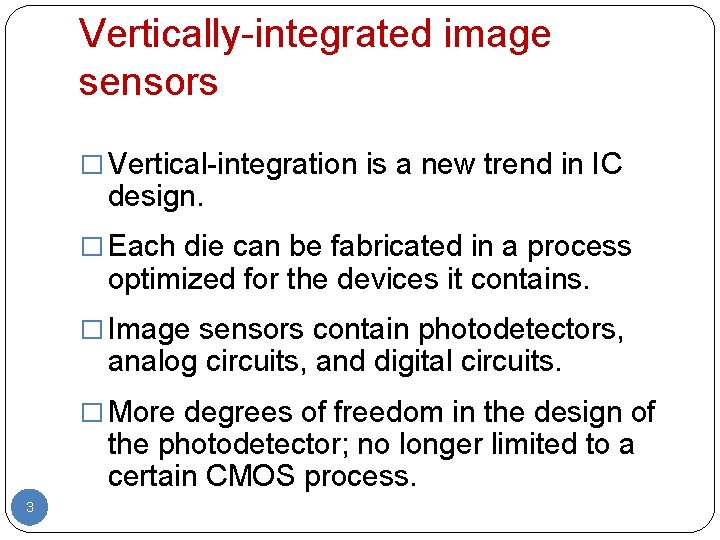
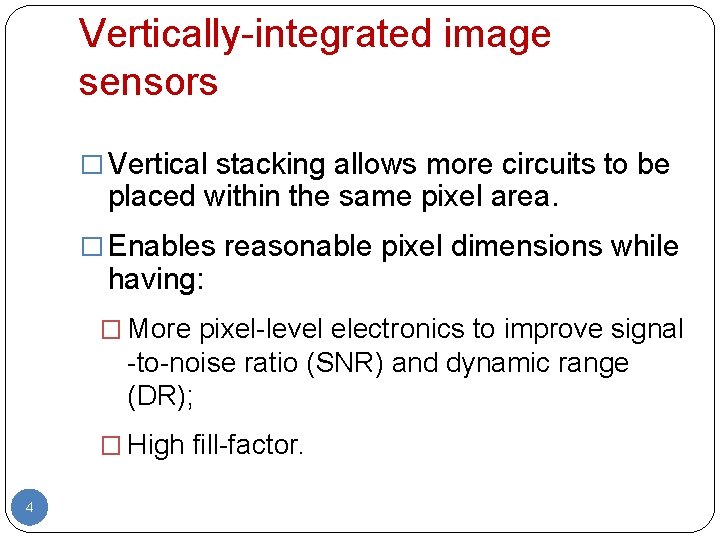
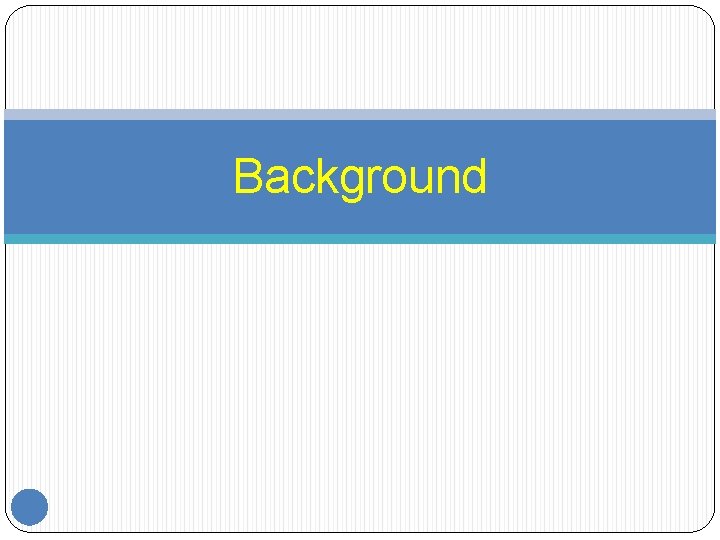
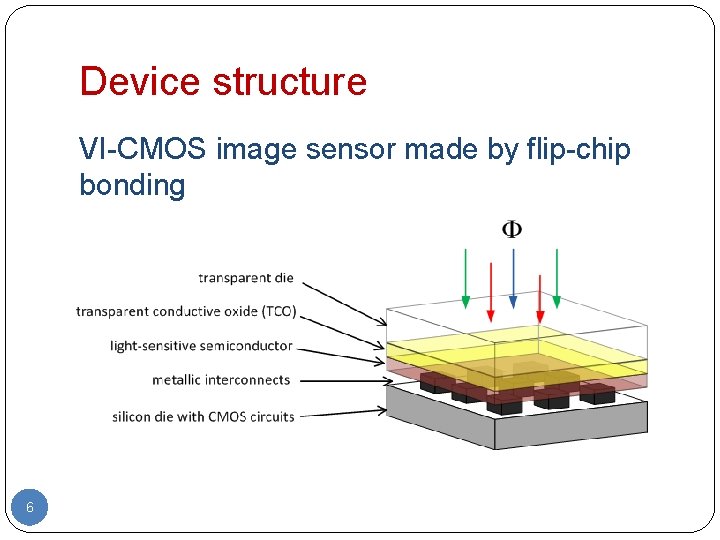
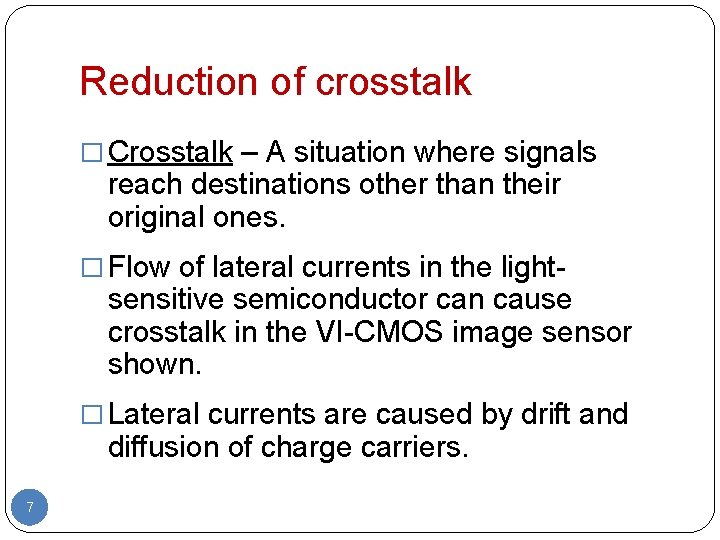
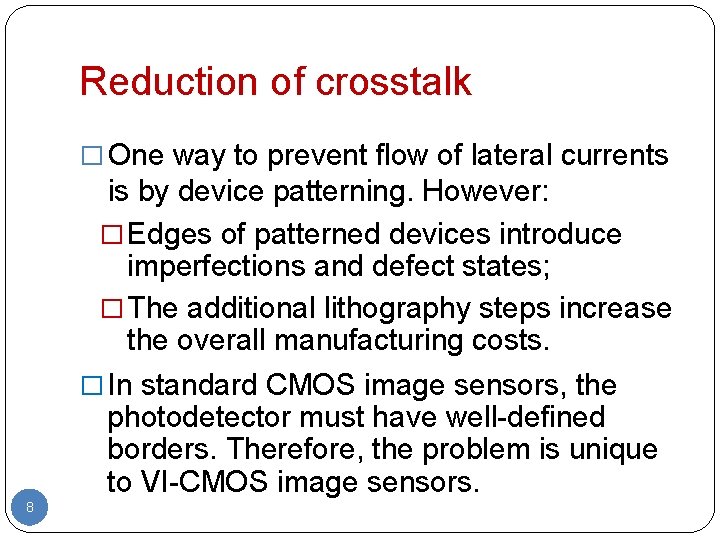
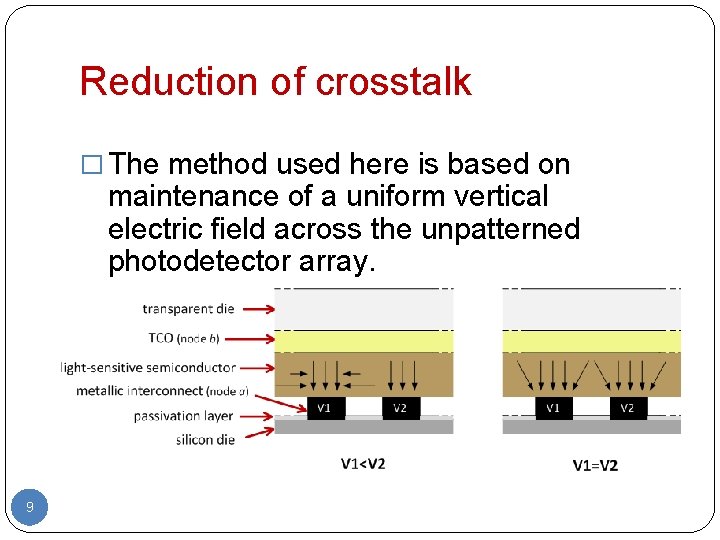
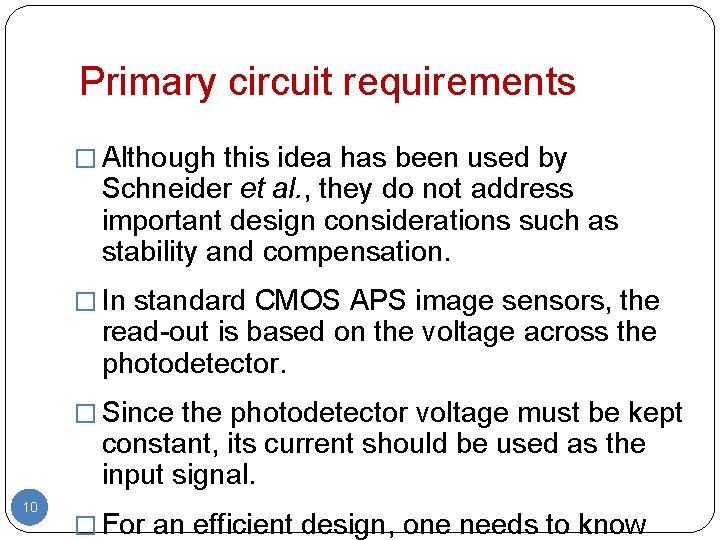
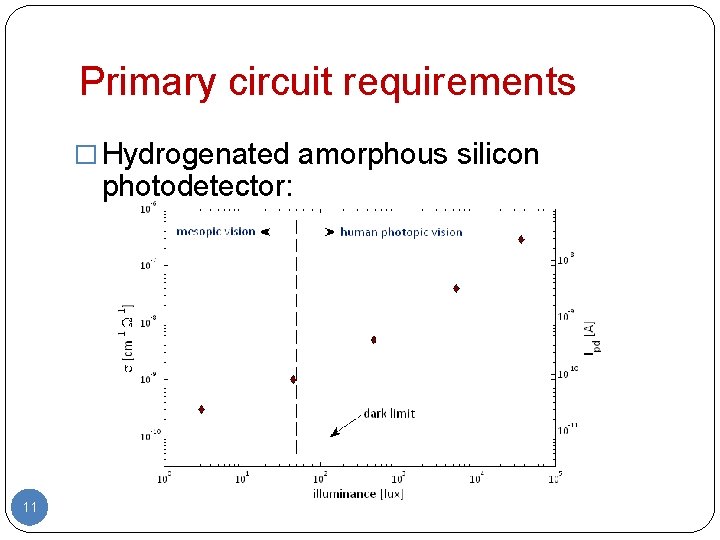
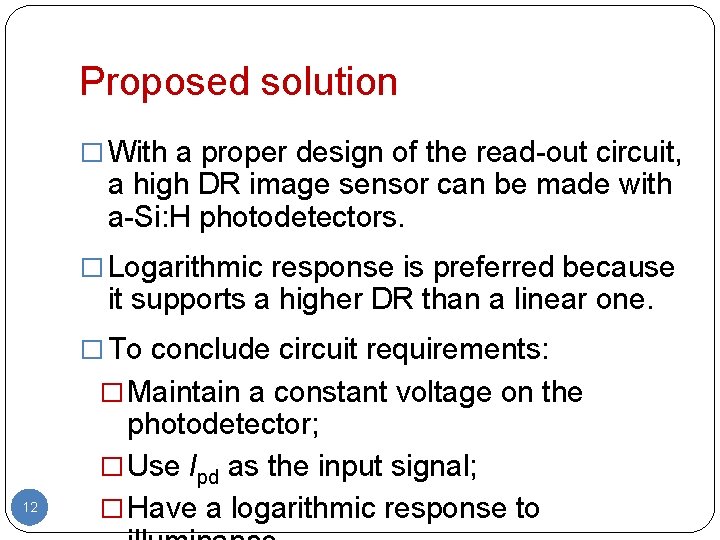
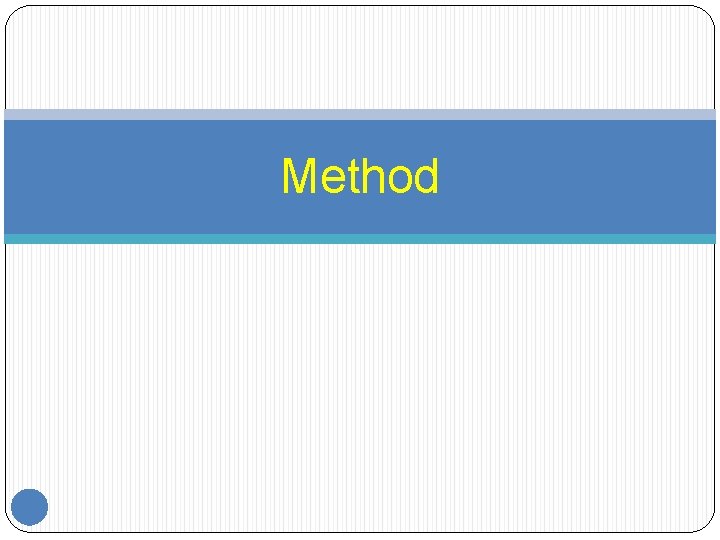
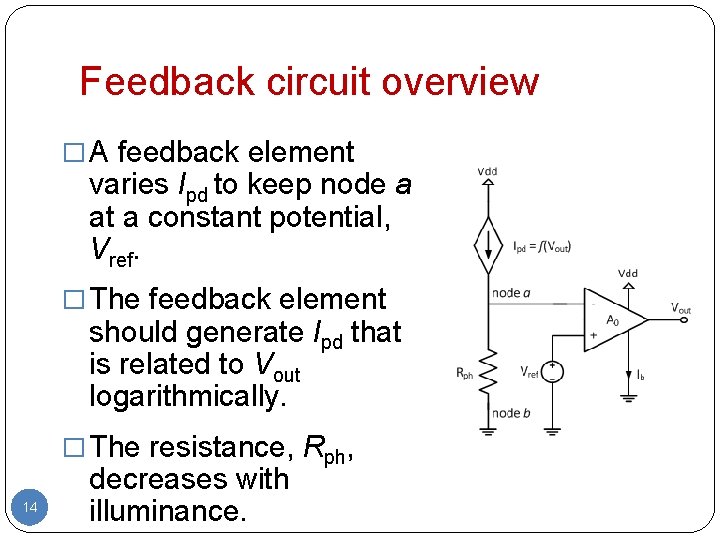
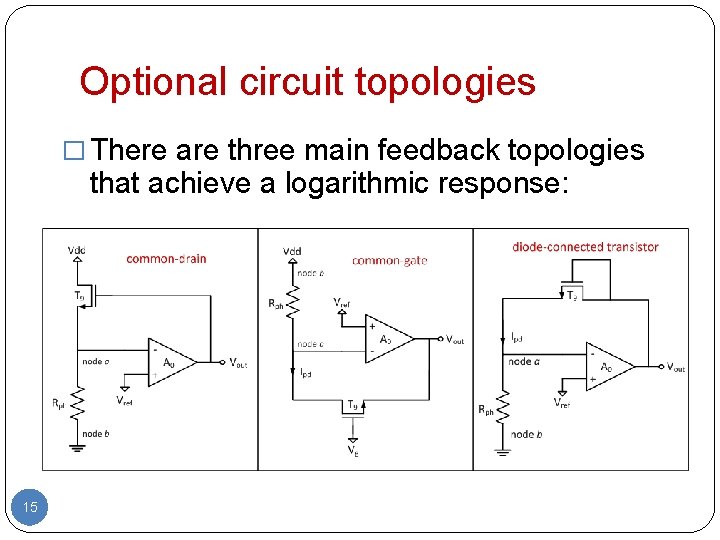
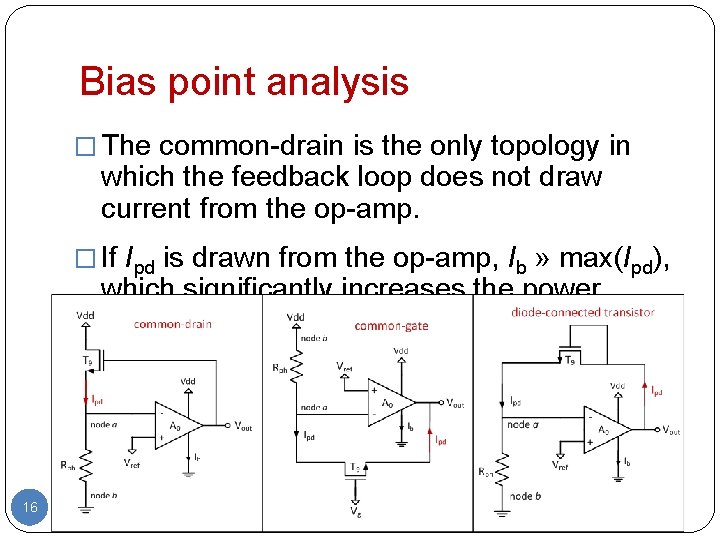
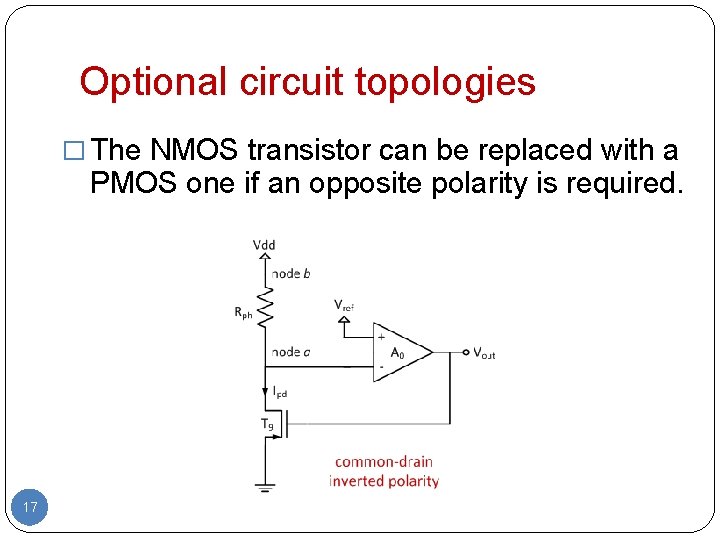
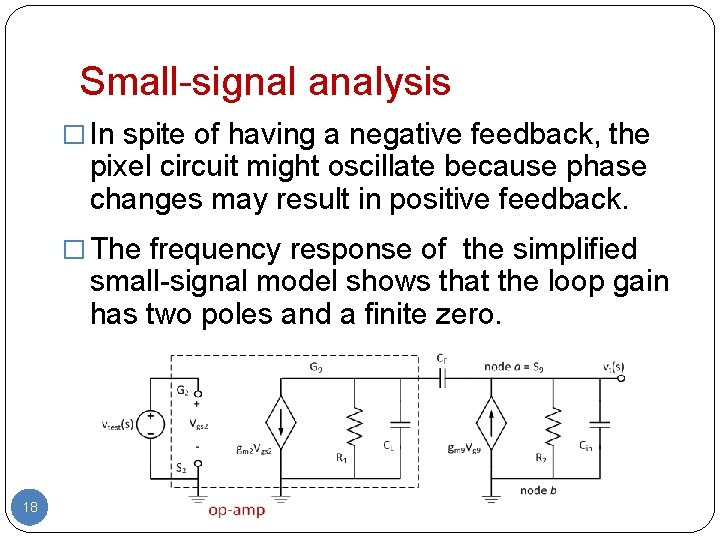
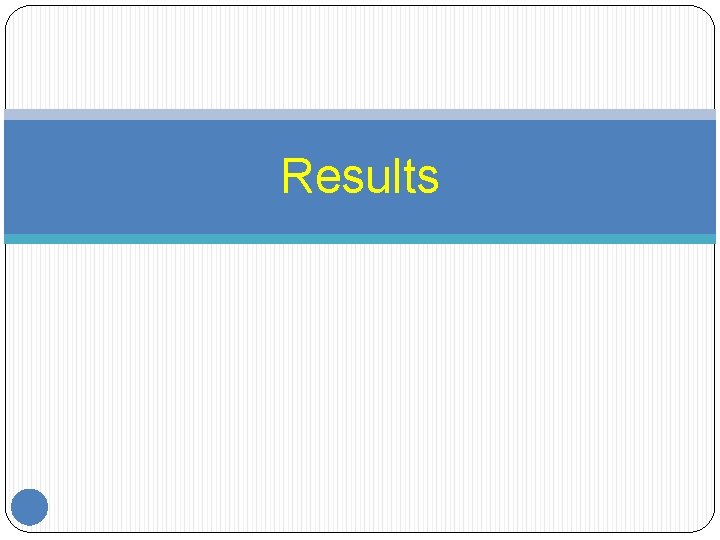
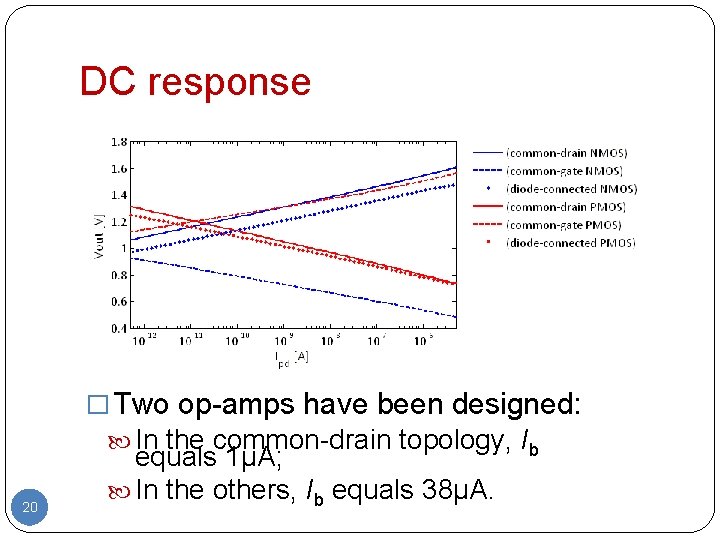
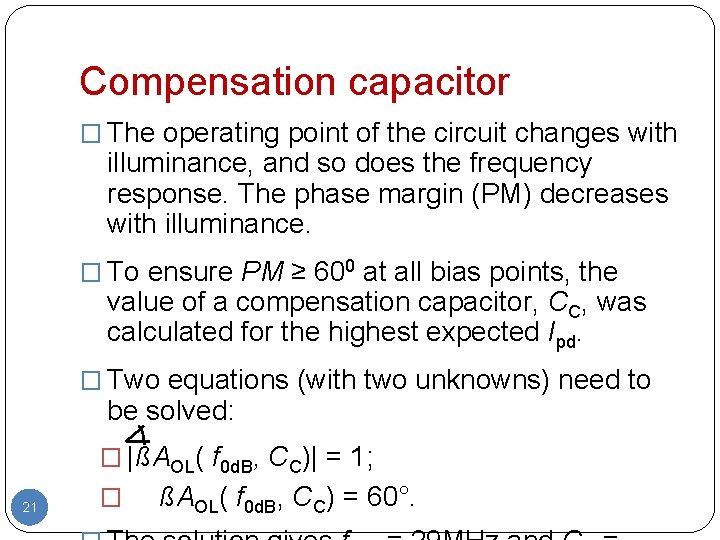
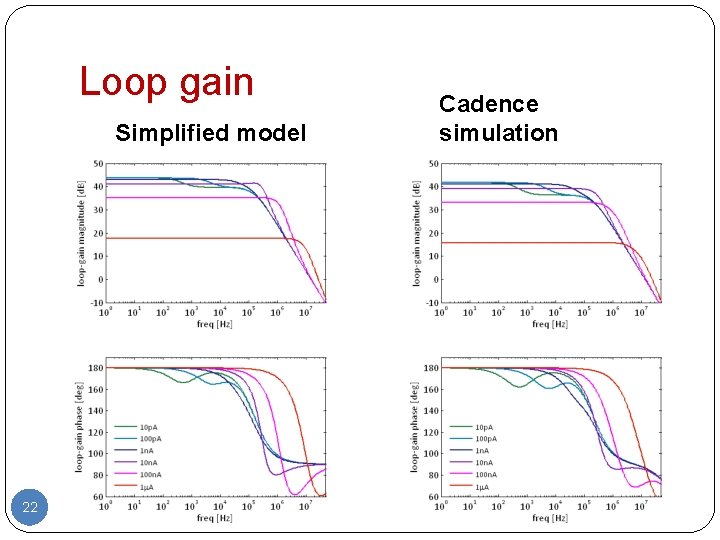
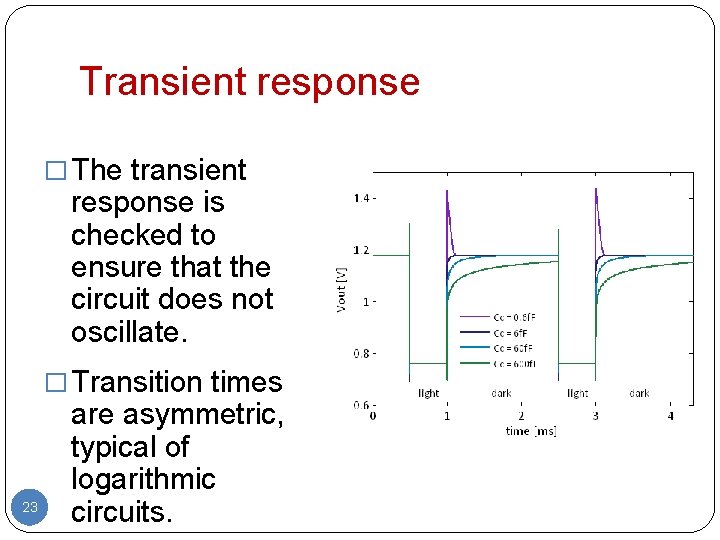
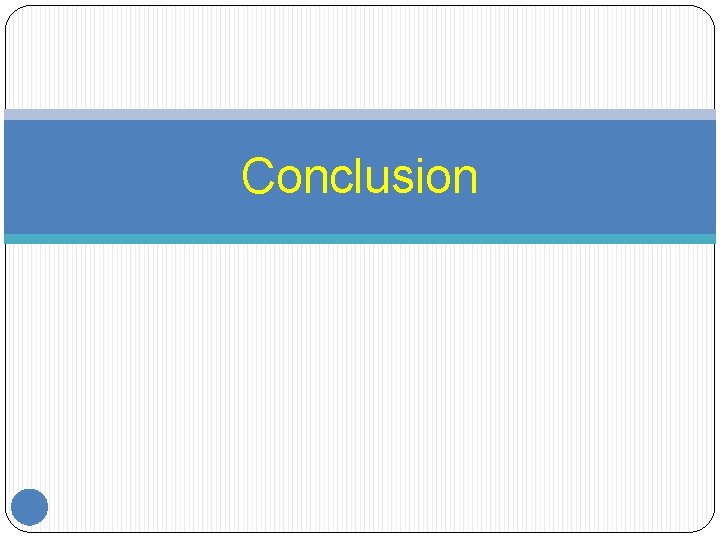
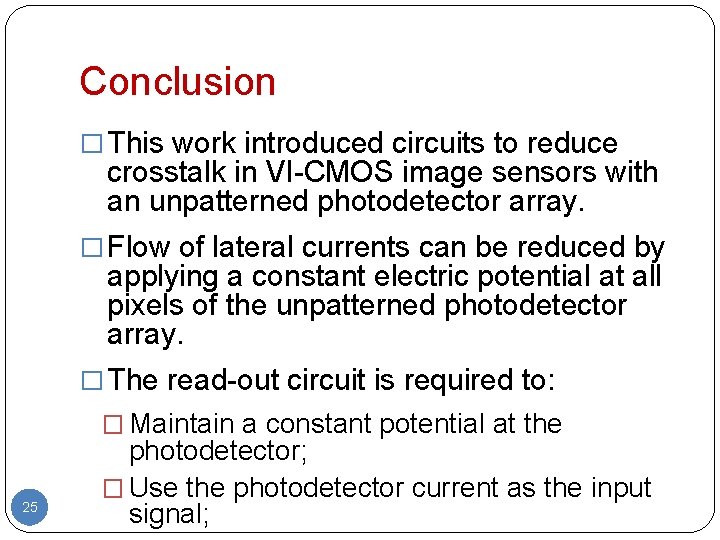
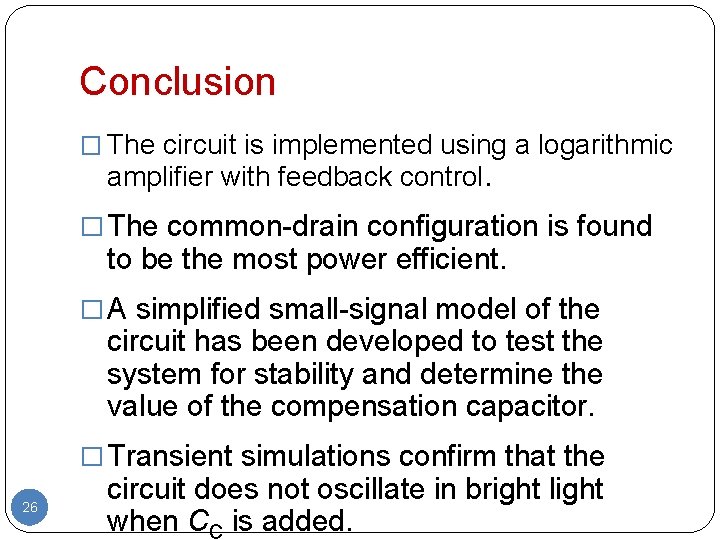
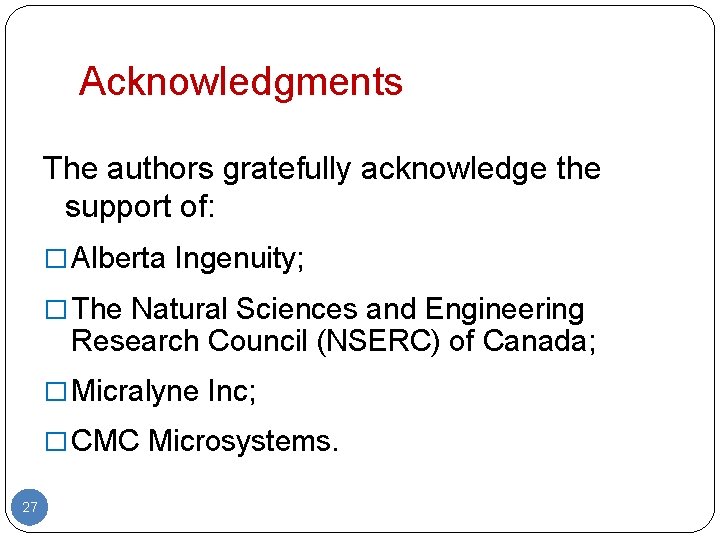
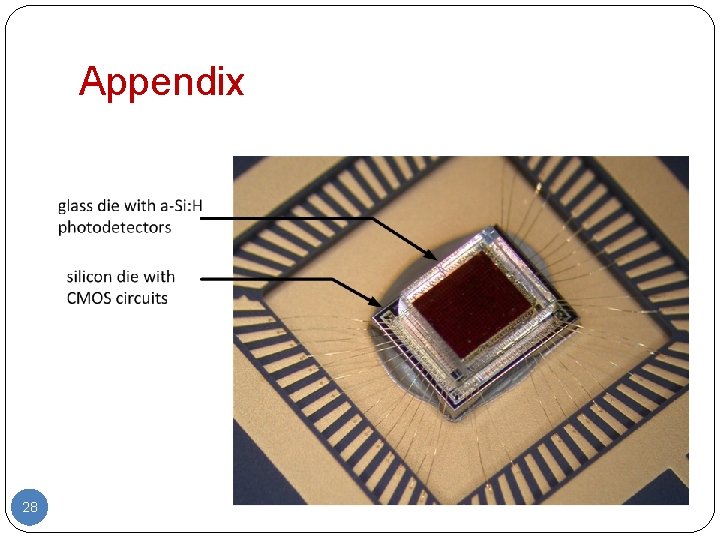
- Slides: 28
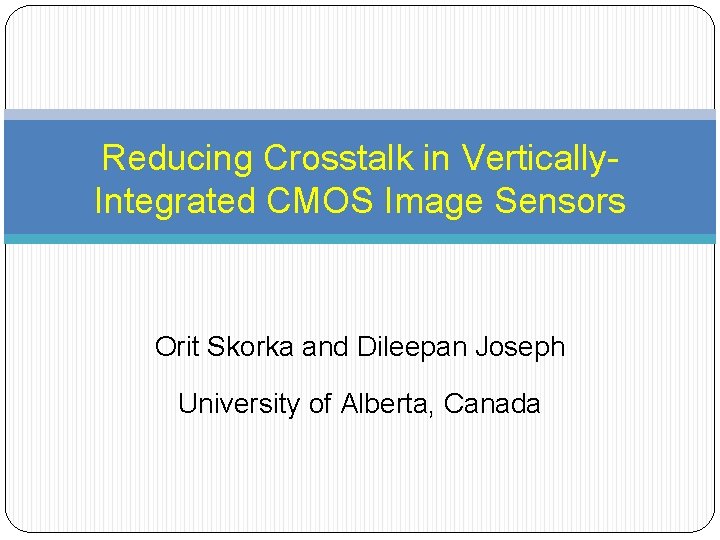
Reducing Crosstalk in Vertically. Integrated CMOS Image Sensors Orit Skorka and Dileepan Joseph University of Alberta, Canada
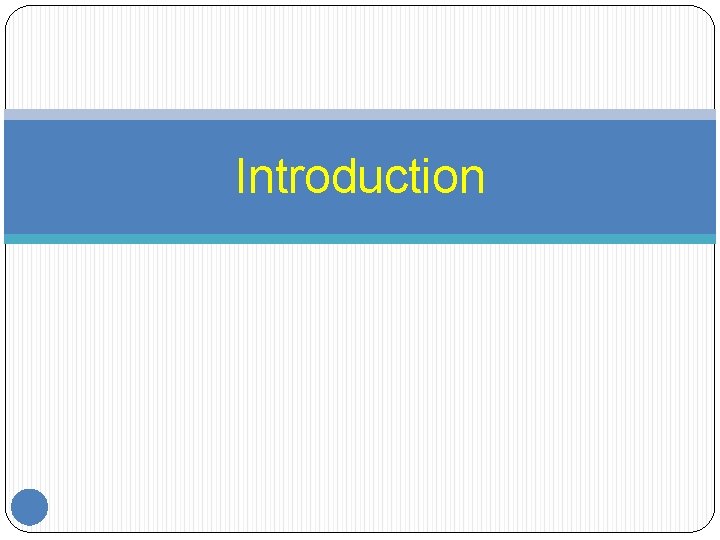
Introduction
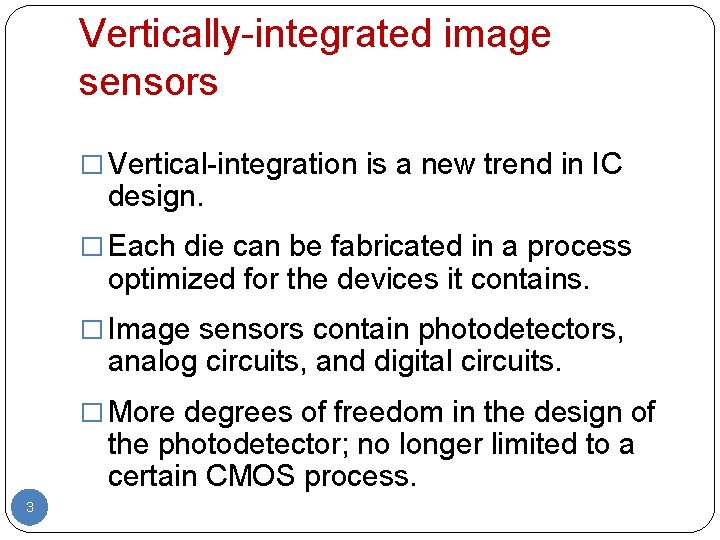
Vertically-integrated image sensors � Vertical-integration is a new trend in IC design. � Each die can be fabricated in a process optimized for the devices it contains. � Image sensors contain photodetectors, analog circuits, and digital circuits. � More degrees of freedom in the design of the photodetector; no longer limited to a certain CMOS process. 3
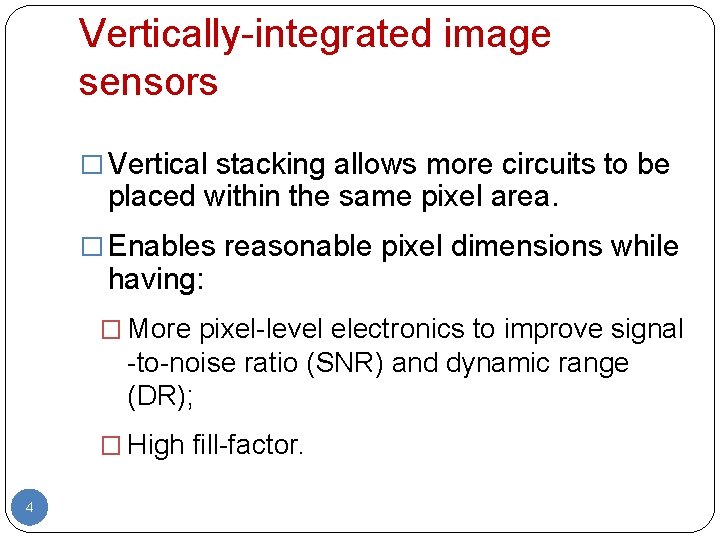
Vertically-integrated image sensors � Vertical stacking allows more circuits to be placed within the same pixel area. � Enables reasonable pixel dimensions while having: � More pixel-level electronics to improve signal -to-noise ratio (SNR) and dynamic range (DR); � High fill-factor. 4
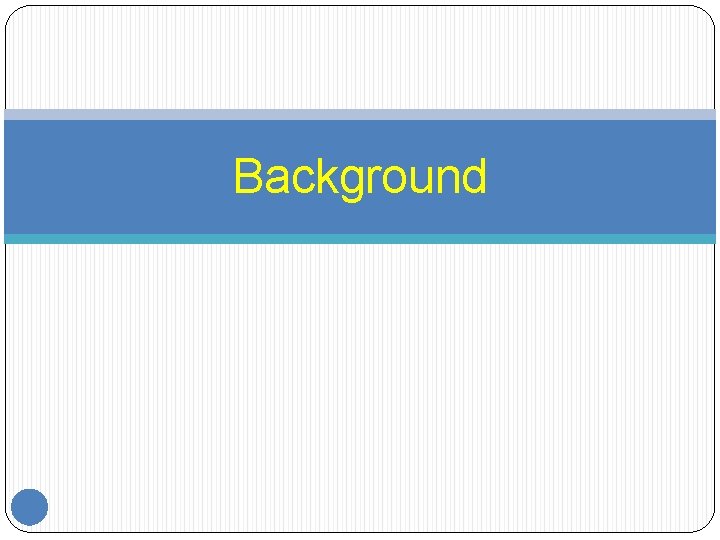
Background
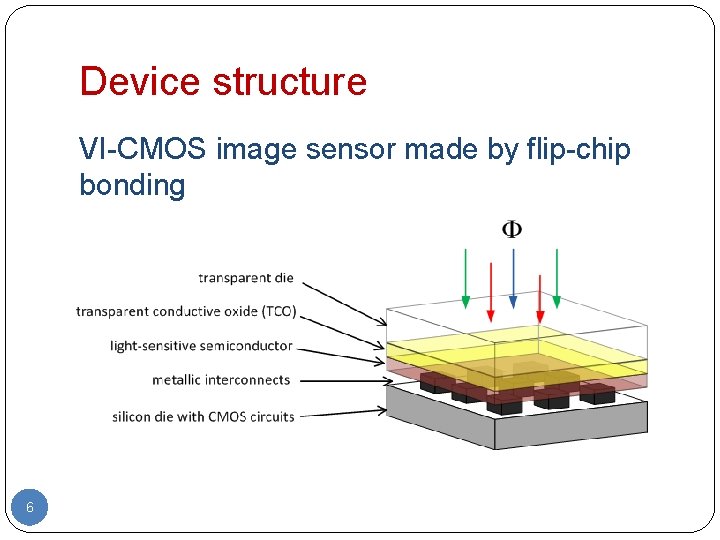
Device structure VI-CMOS image sensor made by flip-chip bonding 6
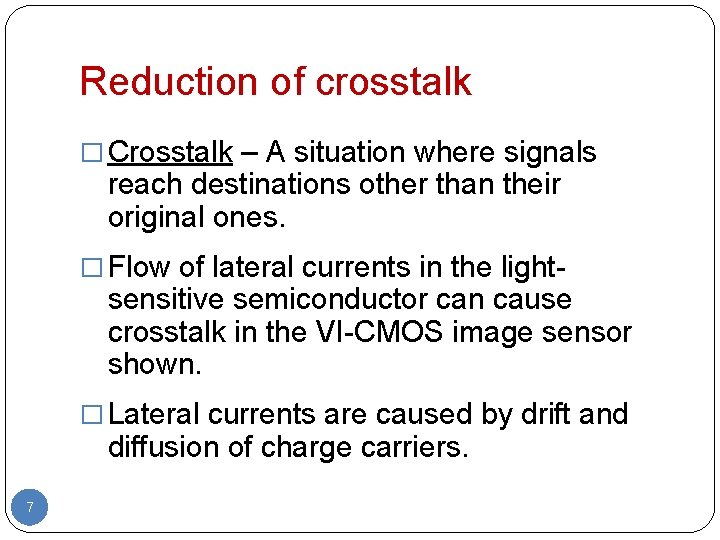
Reduction of crosstalk � Crosstalk – A situation where signals reach destinations other than their original ones. � Flow of lateral currents in the light- sensitive semiconductor can cause crosstalk in the VI-CMOS image sensor shown. � Lateral currents are caused by drift and diffusion of charge carriers. 7
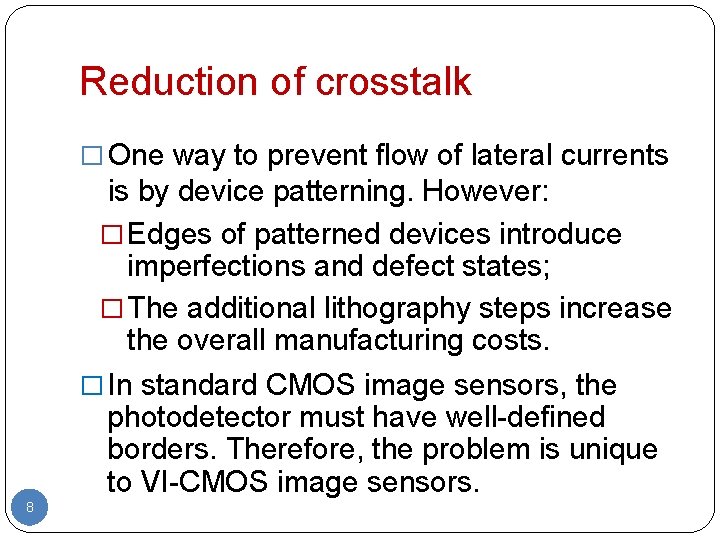
Reduction of crosstalk � One way to prevent flow of lateral currents is by device patterning. However: � Edges of patterned devices introduce imperfections and defect states; � The additional lithography steps increase the overall manufacturing costs. � In standard CMOS image sensors, the photodetector must have well-defined borders. Therefore, the problem is unique to VI-CMOS image sensors. 8
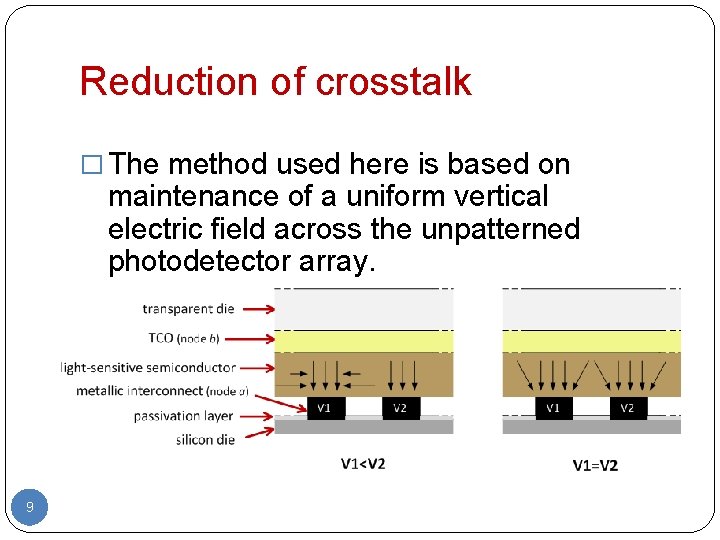
Reduction of crosstalk � The method used here is based on maintenance of a uniform vertical electric field across the unpatterned photodetector array. 9
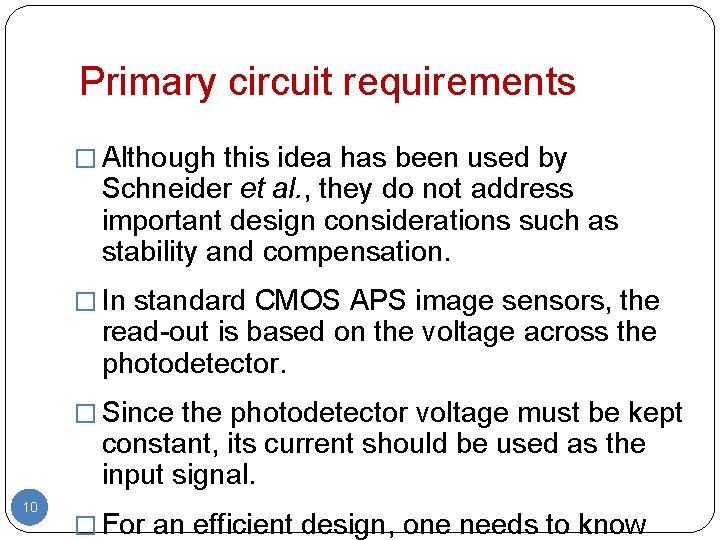
Primary circuit requirements � Although this idea has been used by Schneider et al. , they do not address important design considerations such as stability and compensation. � In standard CMOS APS image sensors, the read-out is based on the voltage across the photodetector. � Since the photodetector voltage must be kept constant, its current should be used as the input signal. 10 � For an efficient design, one needs to know
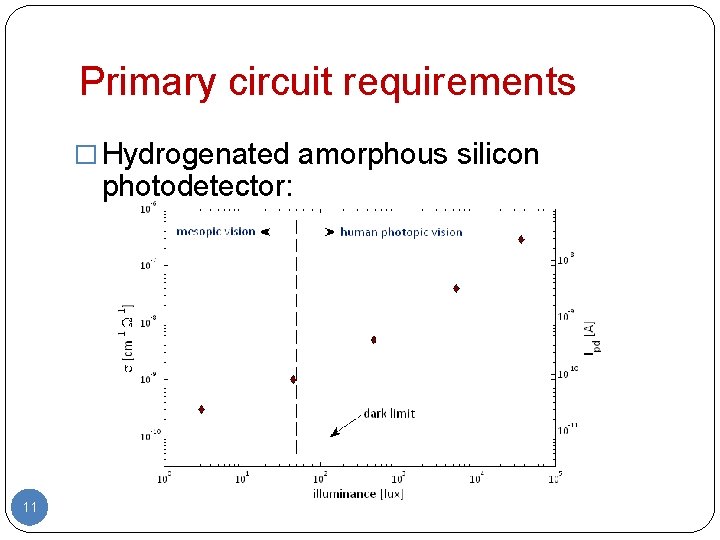
Primary circuit requirements � Hydrogenated amorphous silicon photodetector: 11
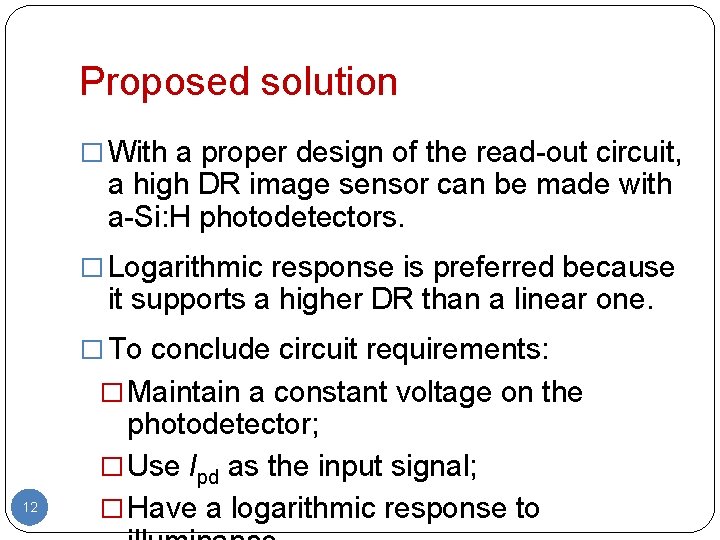
Proposed solution � With a proper design of the read-out circuit, a high DR image sensor can be made with a-Si: H photodetectors. � Logarithmic response is preferred because it supports a higher DR than a linear one. � To conclude circuit requirements: � Maintain a constant voltage on the 12 photodetector; � Use Ipd as the input signal; � Have a logarithmic response to
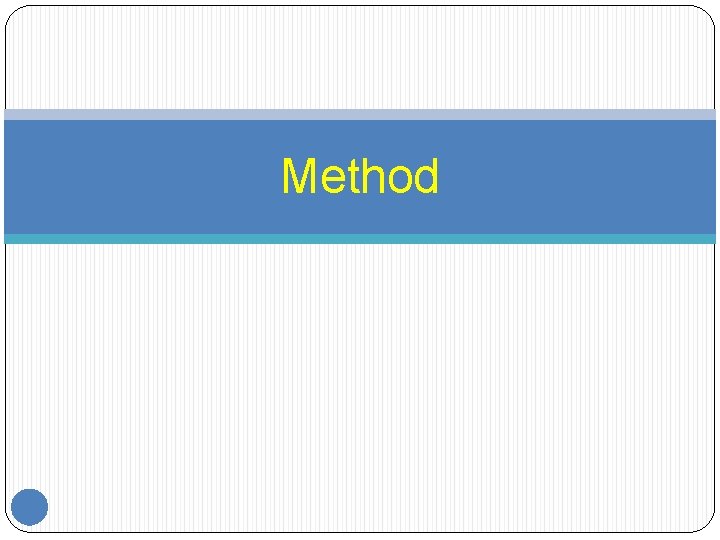
Method
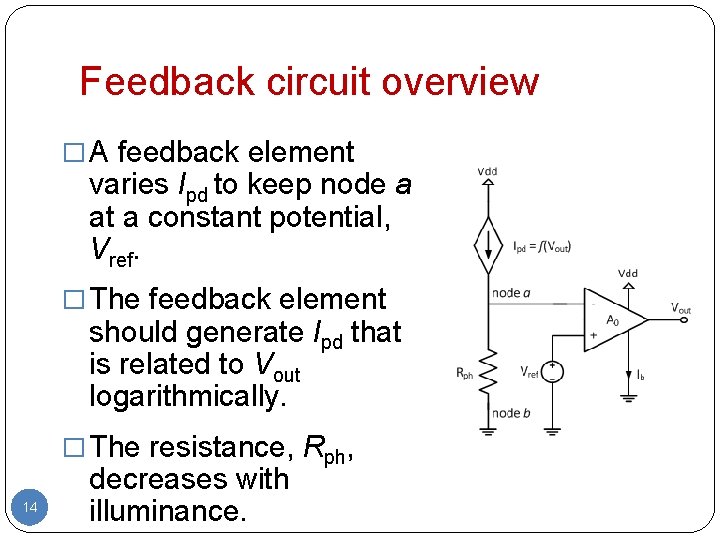
Feedback circuit overview � A feedback element varies Ipd to keep node a at a constant potential, Vref. � The feedback element should generate Ipd that is related to Vout logarithmically. � The resistance, Rph, 14 decreases with illuminance.
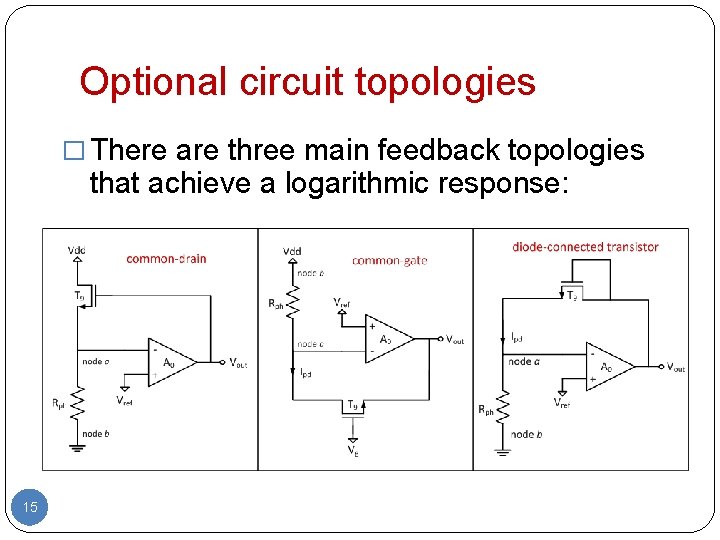
Optional circuit topologies � There are three main feedback topologies that achieve a logarithmic response: 15
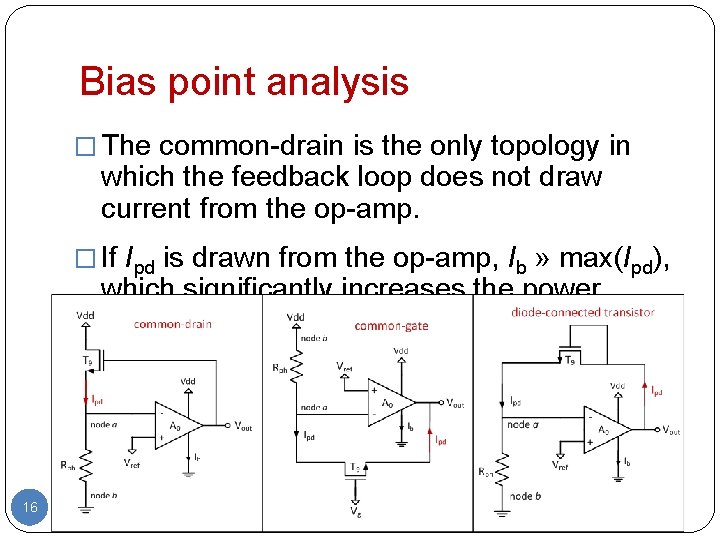
Bias point analysis � The common-drain is the only topology in which the feedback loop does not draw current from the op-amp. � If Ipd is drawn from the op-amp, Ib » max(Ipd), which significantly increases the power consumption. 16
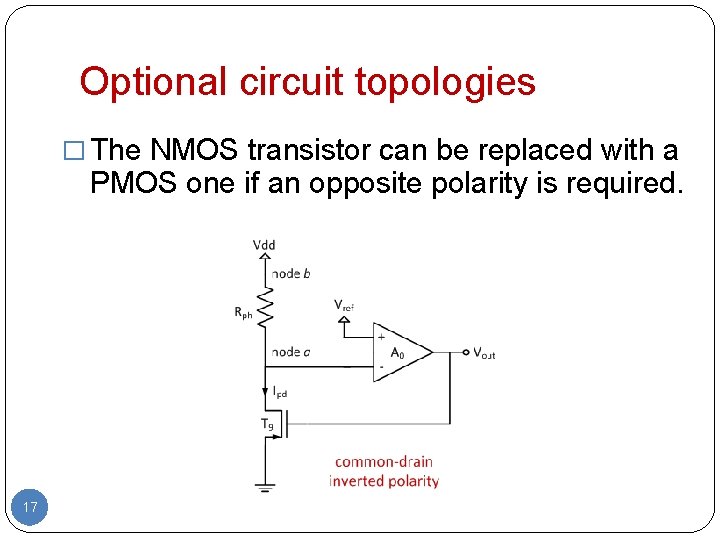
Optional circuit topologies � The NMOS transistor can be replaced with a PMOS one if an opposite polarity is required. 17
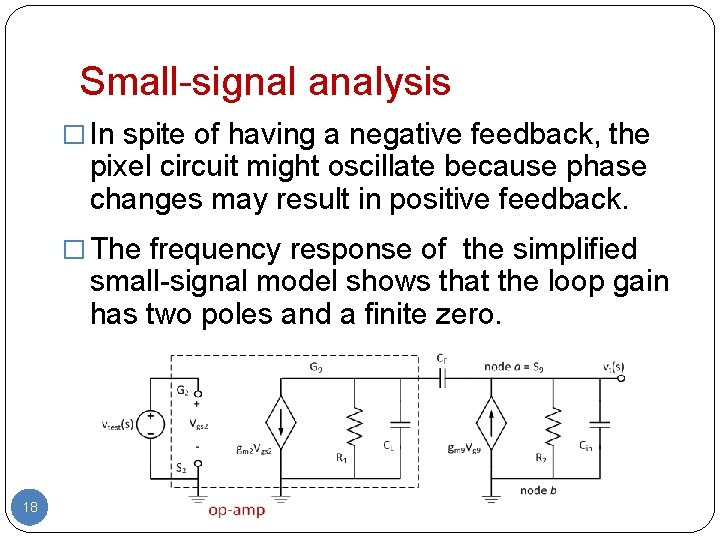
Small-signal analysis � In spite of having a negative feedback, the pixel circuit might oscillate because phase changes may result in positive feedback. � The frequency response of the simplified small-signal model shows that the loop gain has two poles and a finite zero. 18
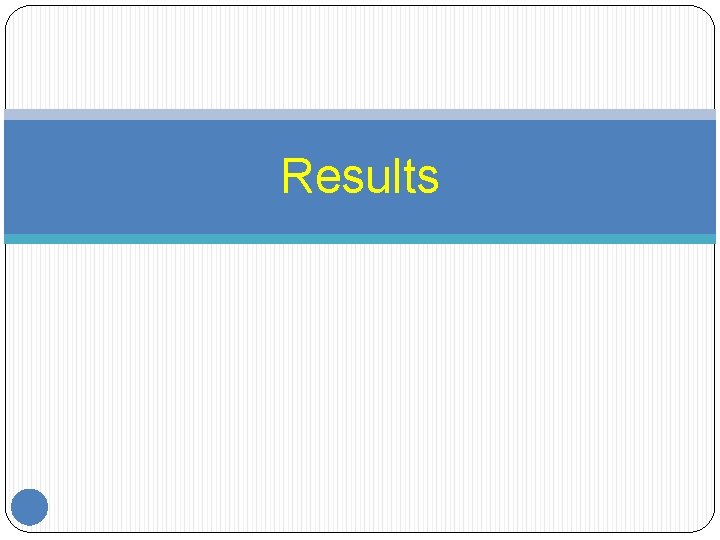
Results
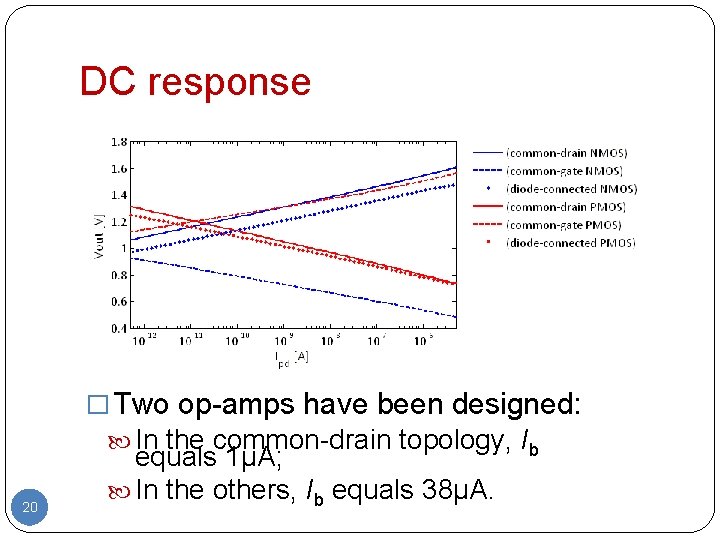
DC response � Two op-amps have been designed: In the common-drain topology, Ib 20 equals 1µA; In the others, Ib equals 38µA.
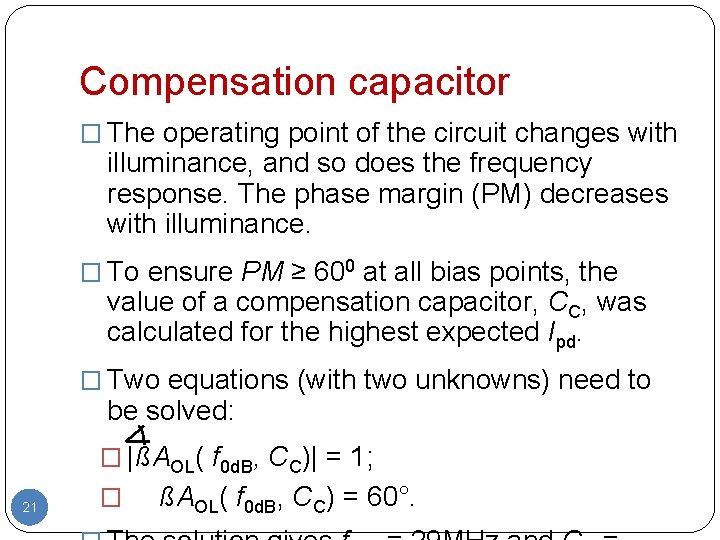
Compensation capacitor � The operating point of the circuit changes with illuminance, and so does the frequency response. The phase margin (PM) decreases with illuminance. � To ensure PM ≥ 600 at all bias points, the value of a compensation capacitor, CC, was calculated for the highest expected Ipd. � Two equations (with two unknowns) need to be solved: � |ßAOL( f 0 d. B, CC)| = 1; 21 � ßAOL( f 0 d. B, CC) = 60°.
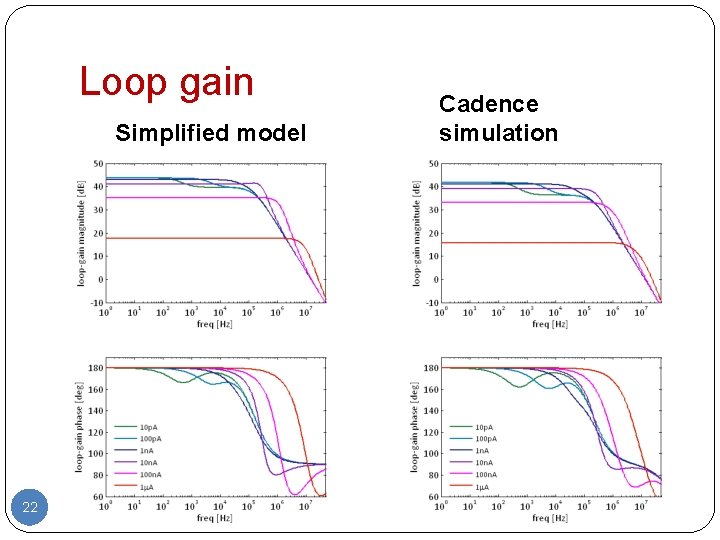
Loop gain Simplified model 22 Cadence simulation
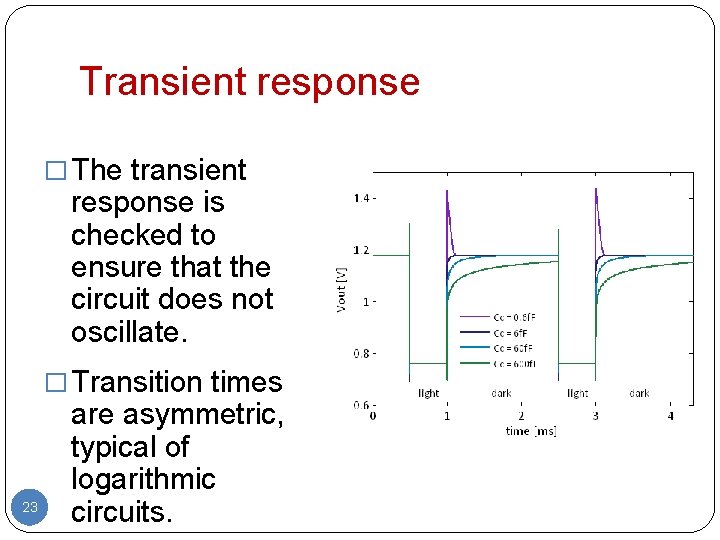
Transient response � The transient response is checked to ensure that the circuit does not oscillate. � Transition times 23 are asymmetric, typical of logarithmic circuits.
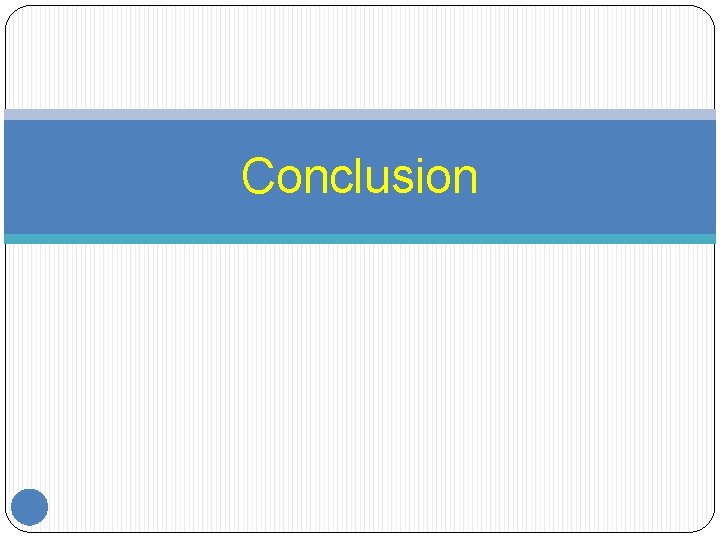
Conclusion
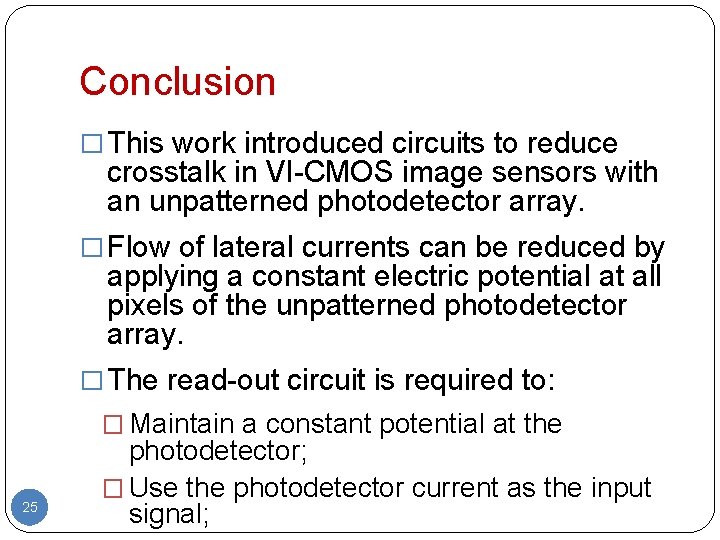
Conclusion � This work introduced circuits to reduce crosstalk in VI-CMOS image sensors with an unpatterned photodetector array. � Flow of lateral currents can be reduced by applying a constant electric potential at all pixels of the unpatterned photodetector array. � The read-out circuit is required to: � Maintain a constant potential at the 25 photodetector; � Use the photodetector current as the input signal;
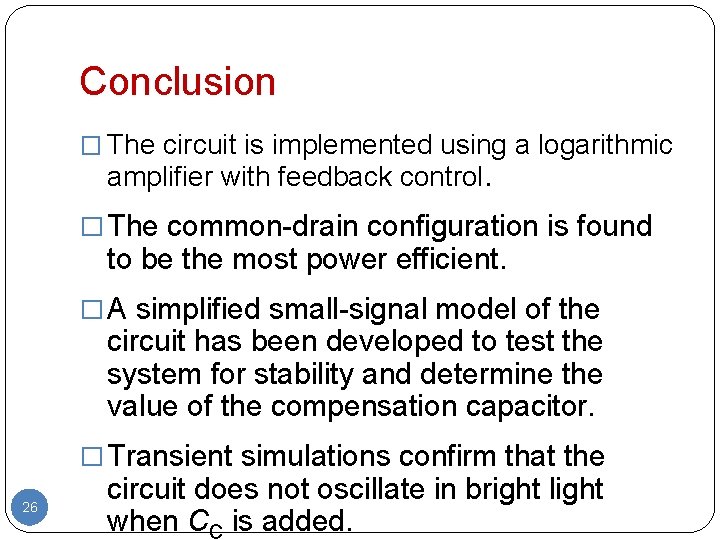
Conclusion � The circuit is implemented using a logarithmic amplifier with feedback control. � The common-drain configuration is found to be the most power efficient. � A simplified small-signal model of the circuit has been developed to test the system for stability and determine the value of the compensation capacitor. � Transient simulations confirm that the 26 circuit does not oscillate in bright light when CC is added.
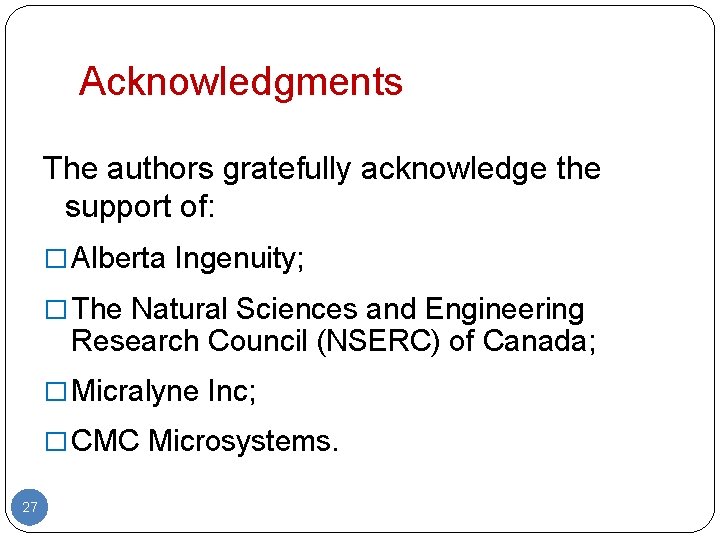
Acknowledgments The authors gratefully acknowledge the support of: � Alberta Ingenuity; � The Natural Sciences and Engineering Research Council (NSERC) of Canada; � Micralyne Inc; � CMC Microsystems. 27
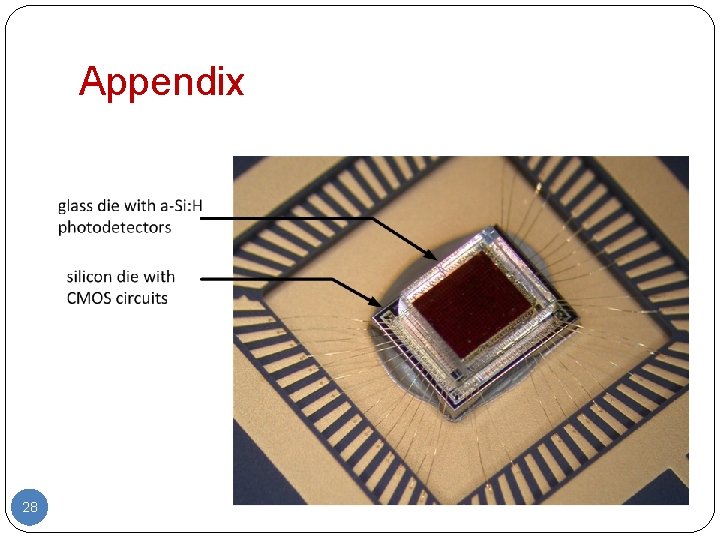
Appendix 28
Wireless integrated network sensors
Wireless integrated network sensors
Horizontal monopoly
Vertically integrated
Vertically integrated
Oled crosstalk
Crosstalk equation
Crosstalk programa de televisión
Crosstalk utp
Near end crosstalk
Crosstalk
Near end crosstalk
Glucose seliwanoff test
Phosphorylase vs kinase
Iodine test positive result
Reducing vs. non-reducing sugars
Zener diode equations
Wearable inertial sensors
Ergonomic+sensors
Washroom
Conclusion of smart sensors
Oil debris monitoring system
Types of negotiations
Peas description for house cleaning robot
Density of sea water
Ieee sensors journal submission
Gds sensors
Actuator with hall effect sensors
Chemical sensors ppt