Precision measurements at the LHC ATLAS and CMS
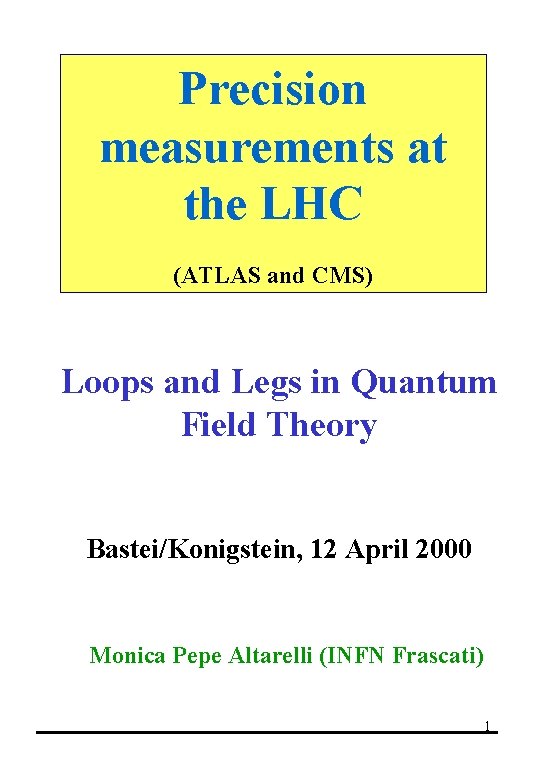
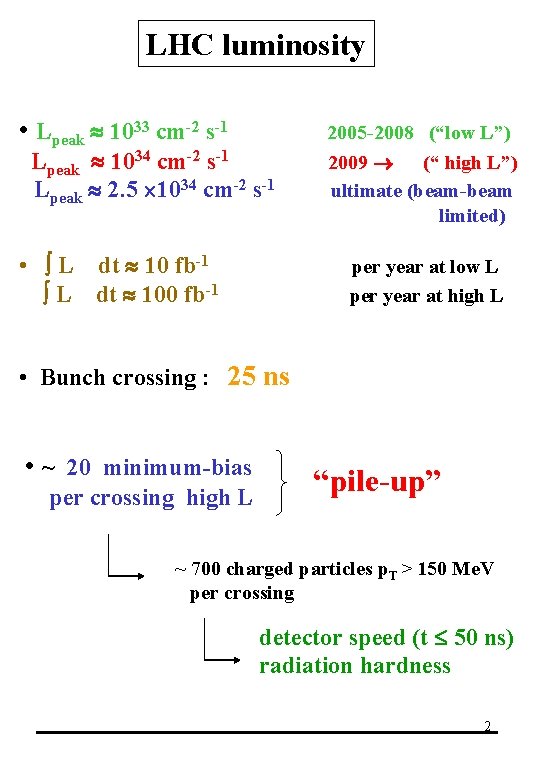
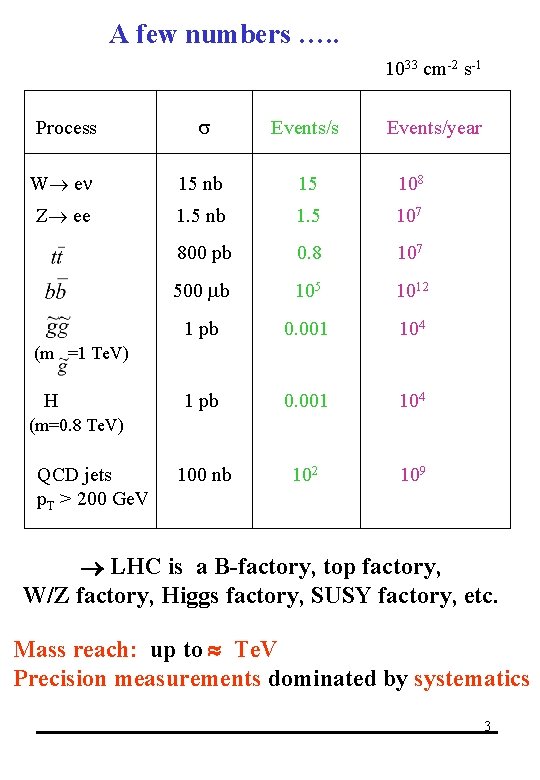
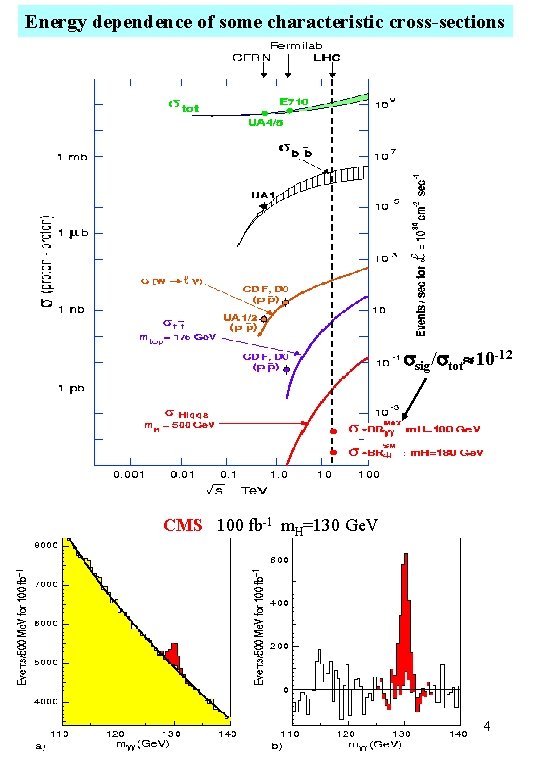
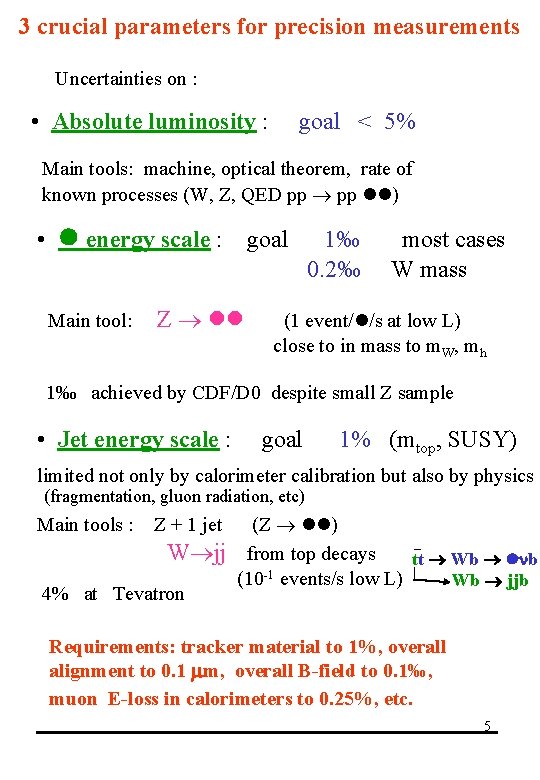
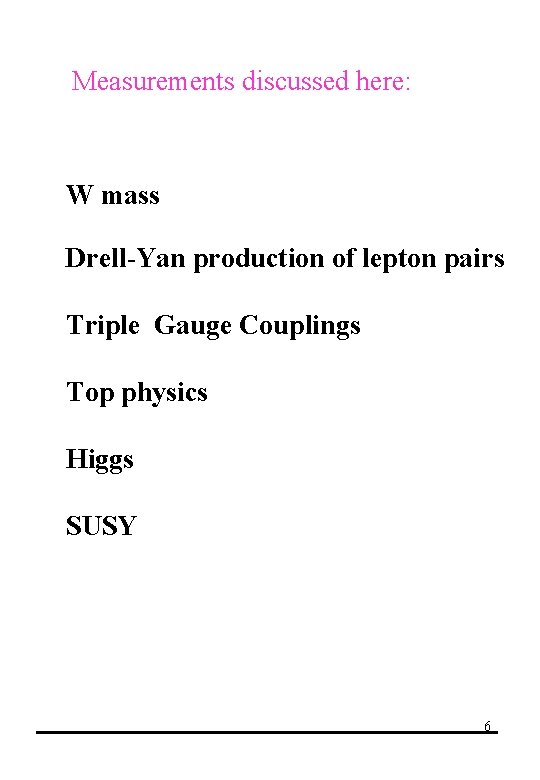
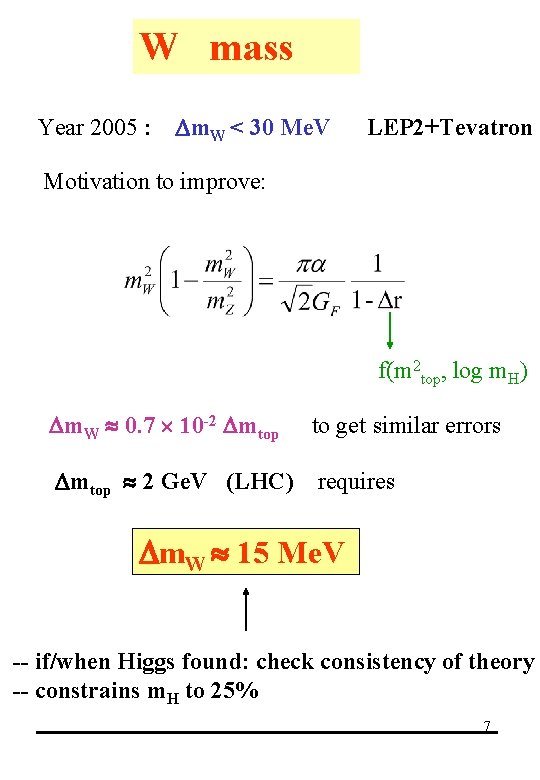
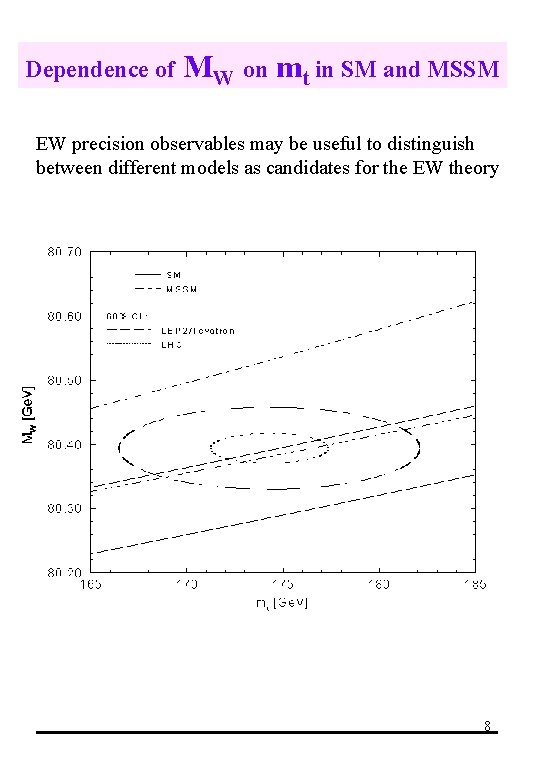
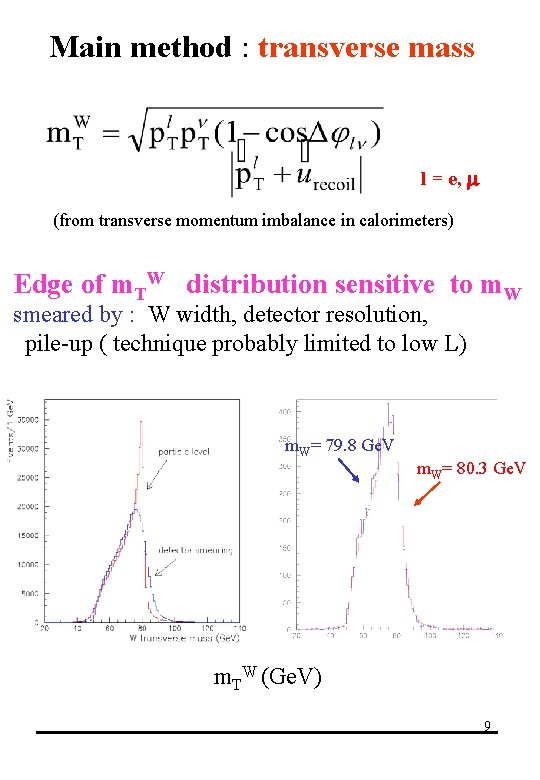
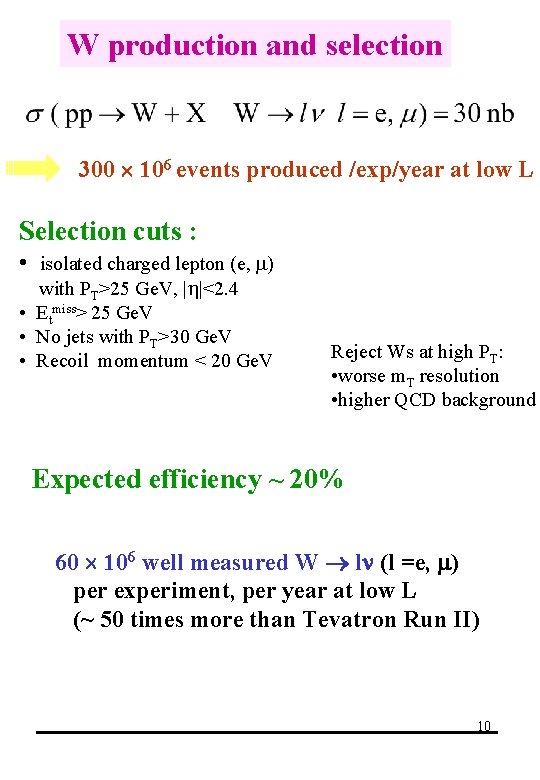
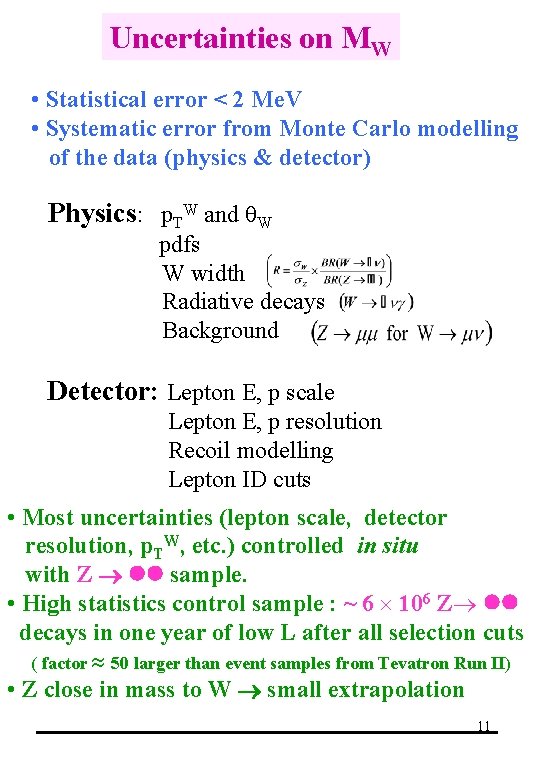
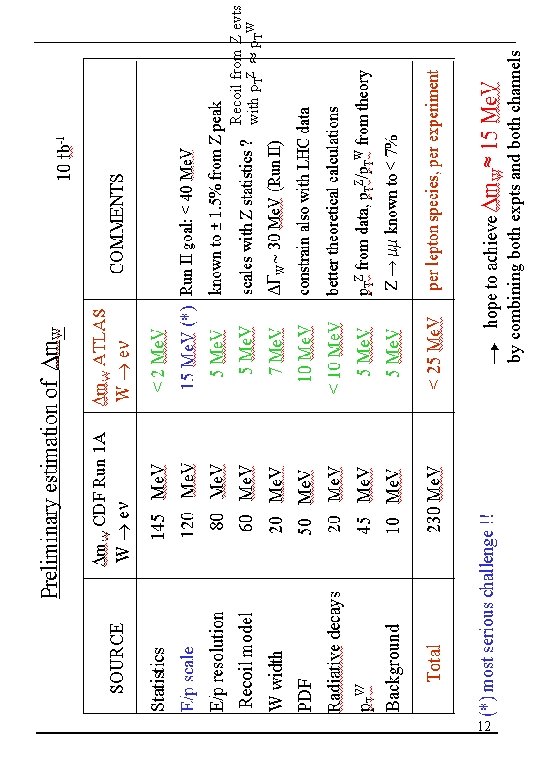
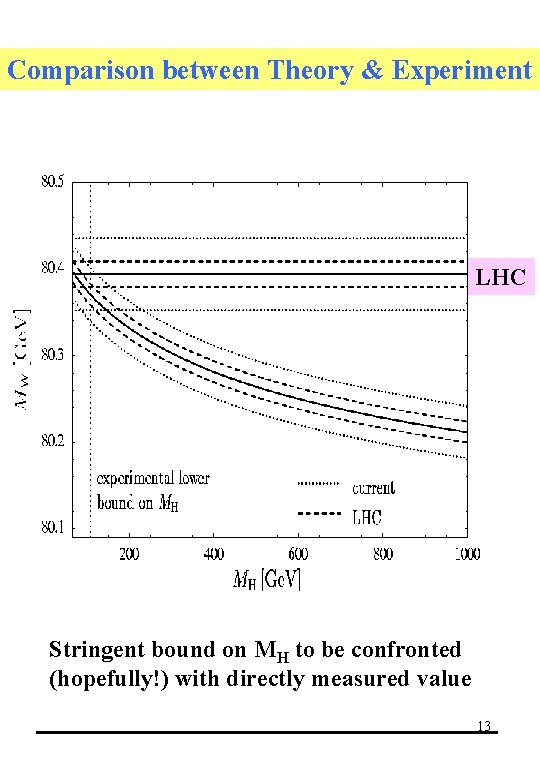
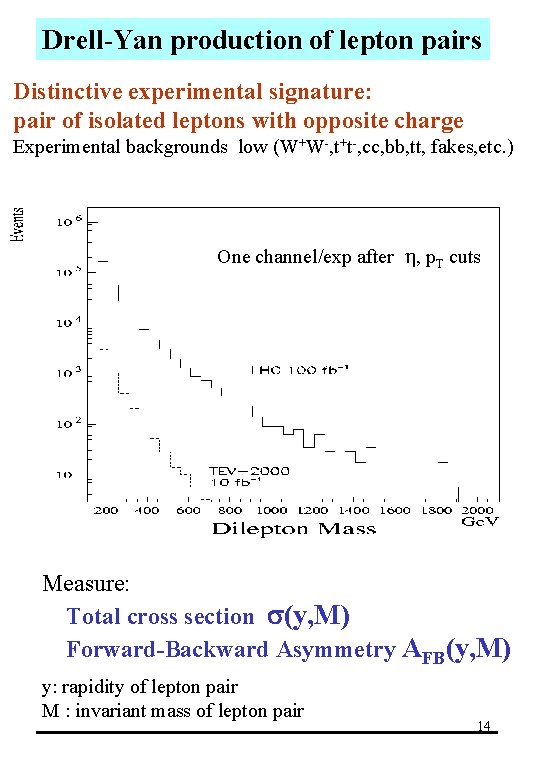
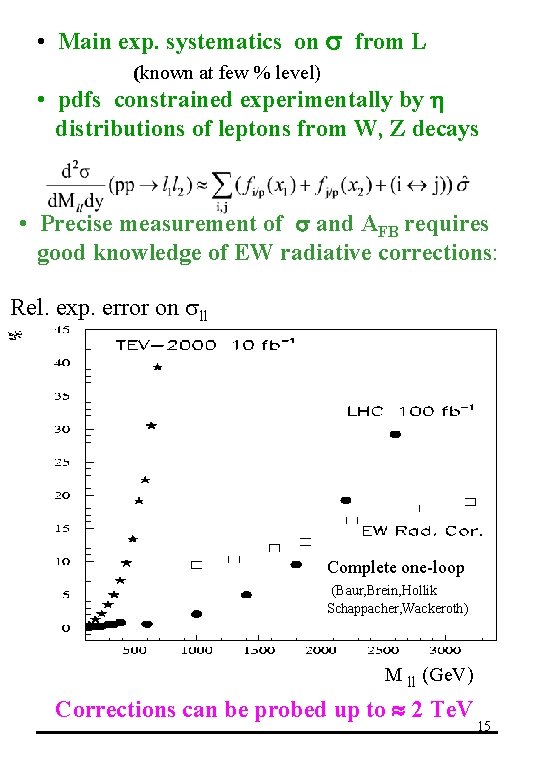
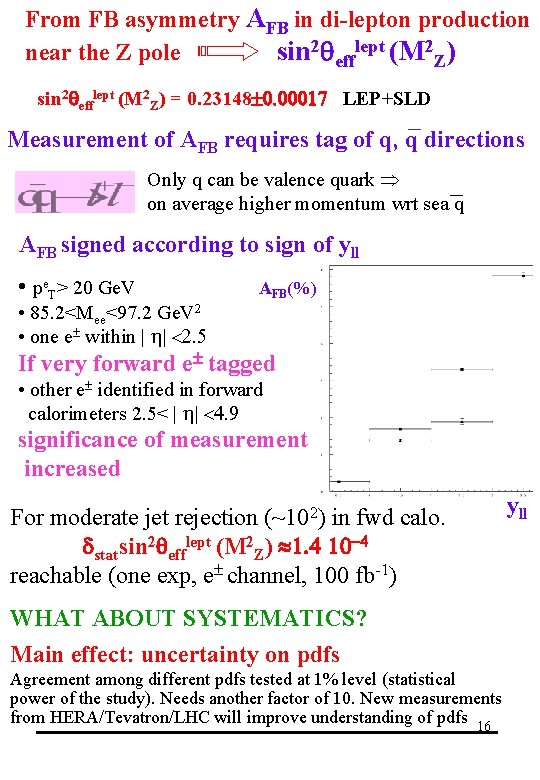
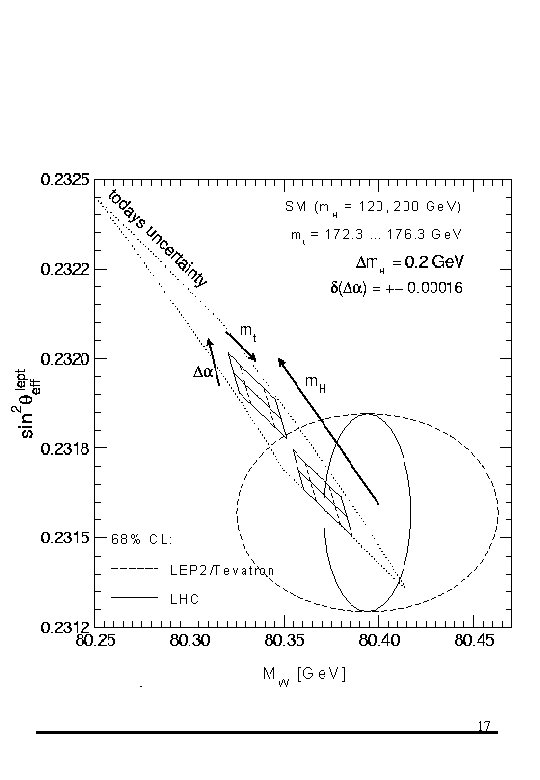
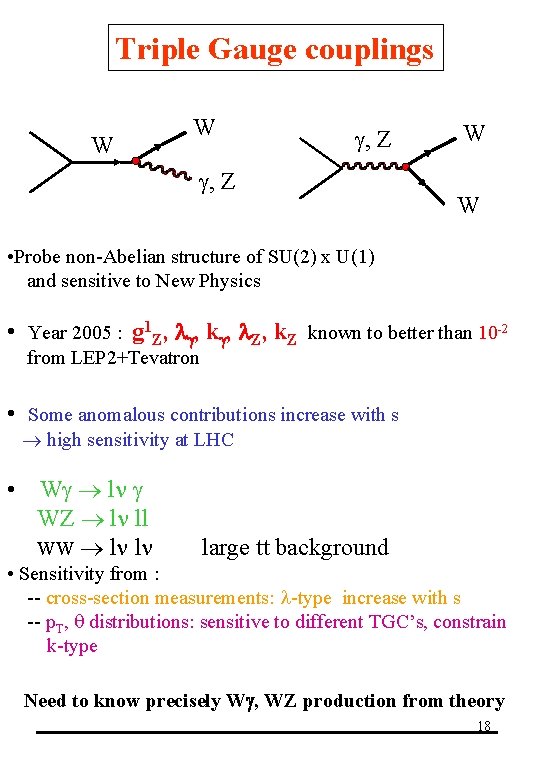
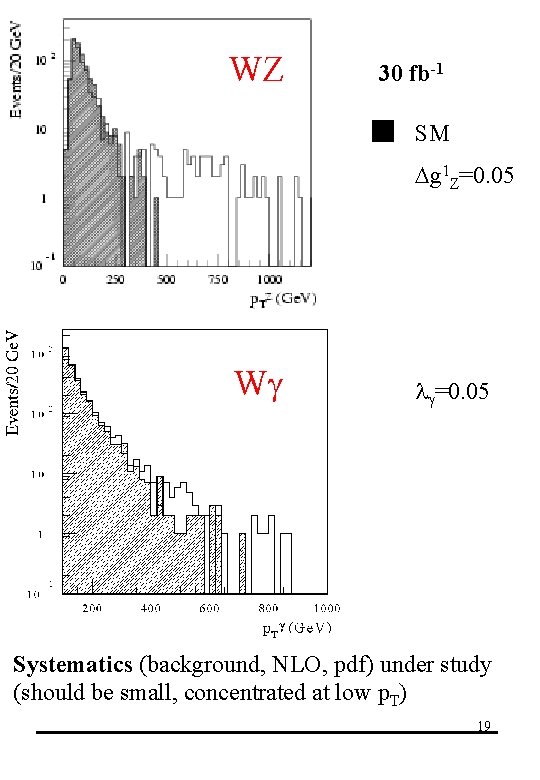
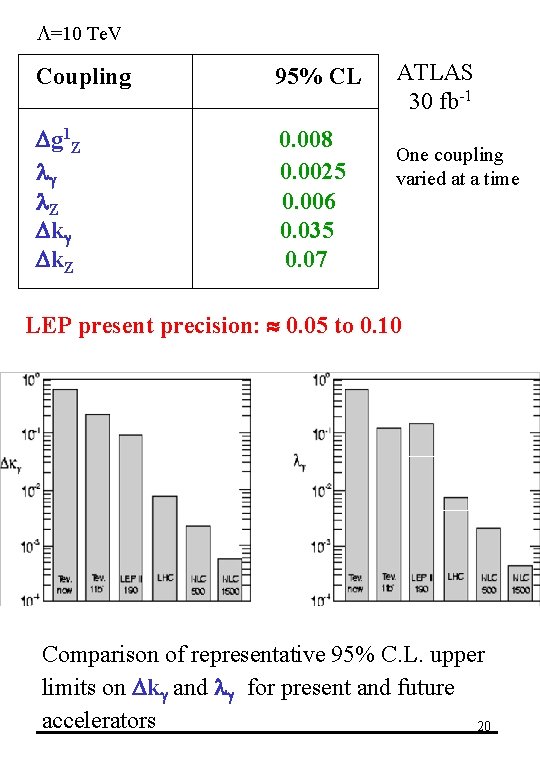
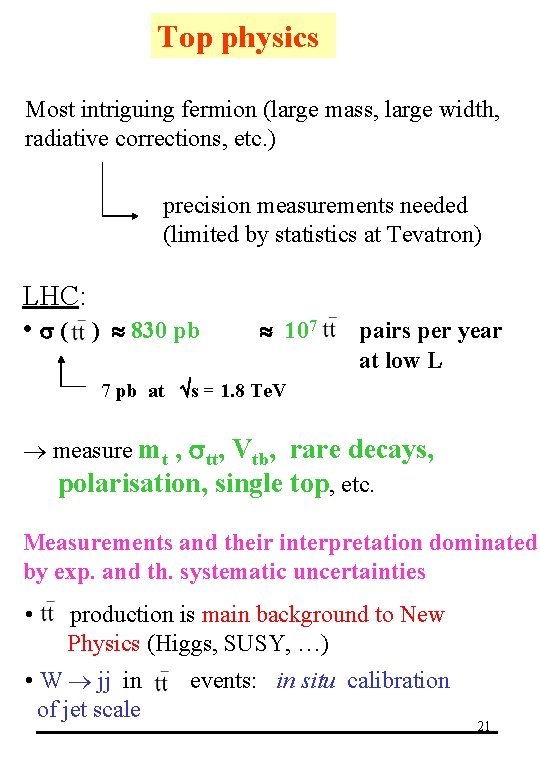
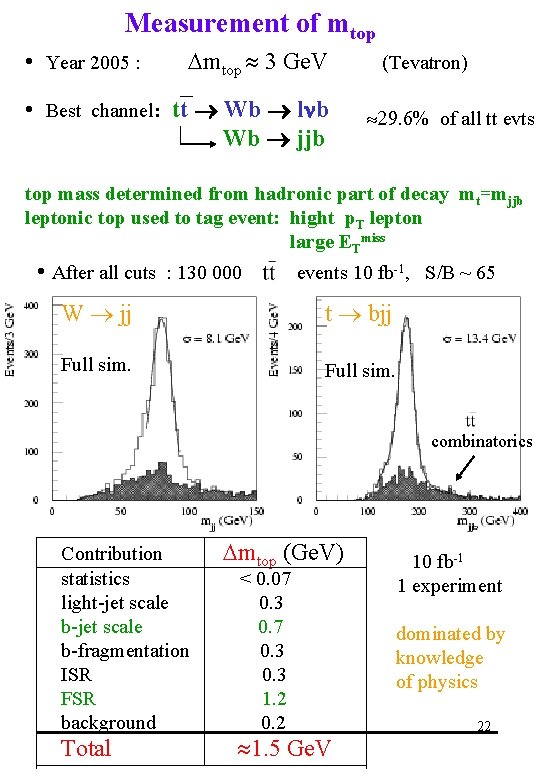
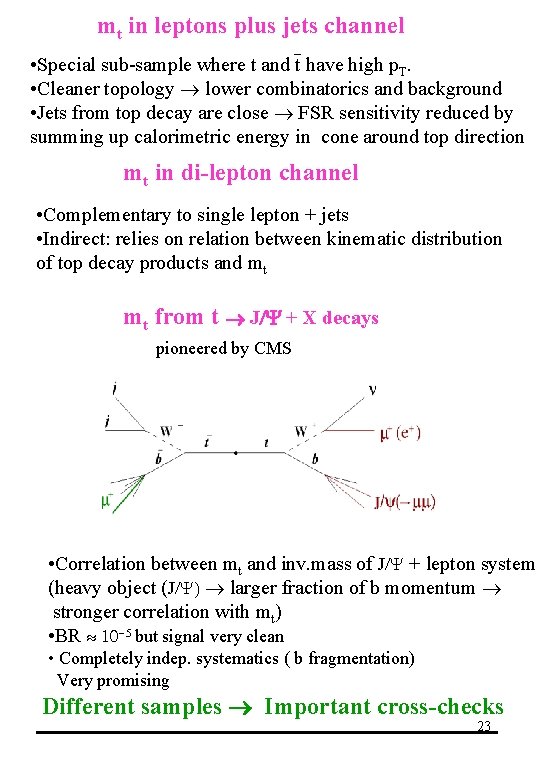
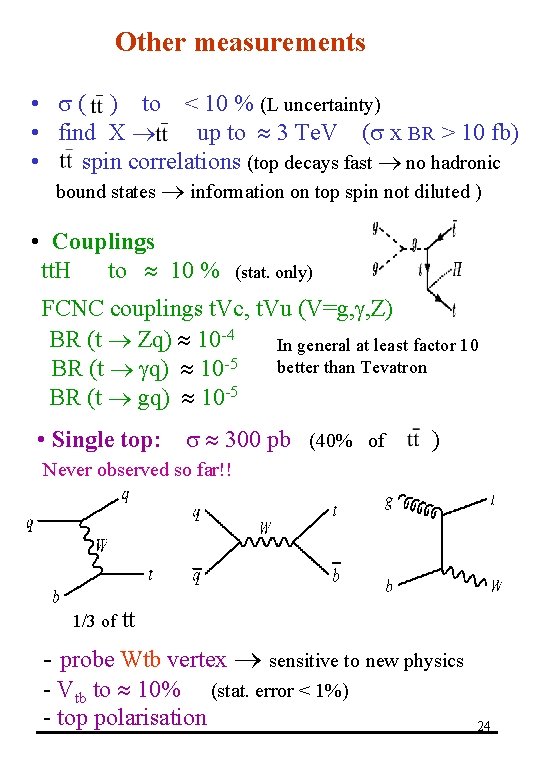
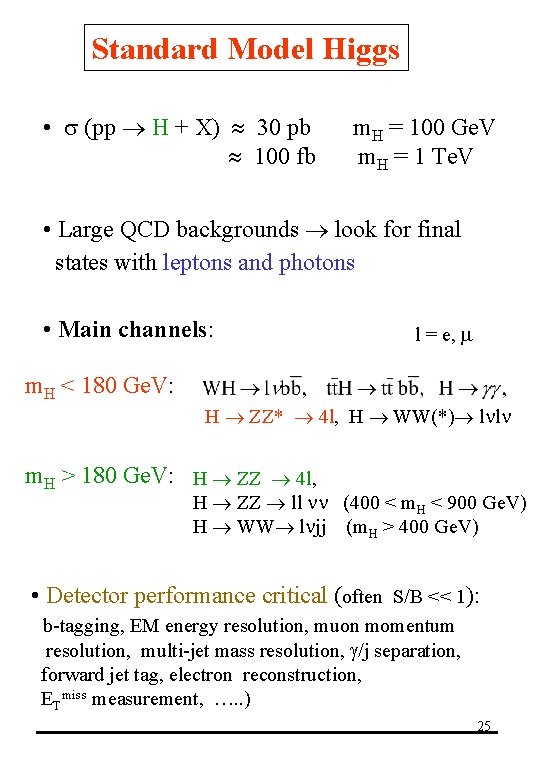
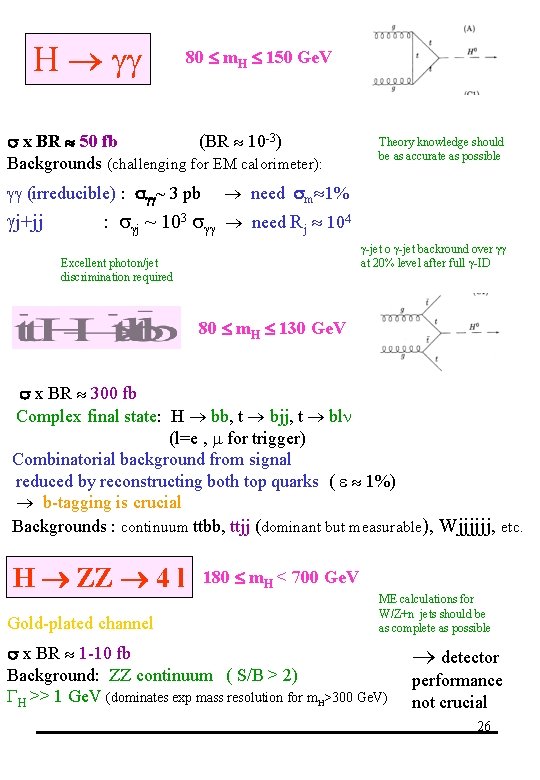
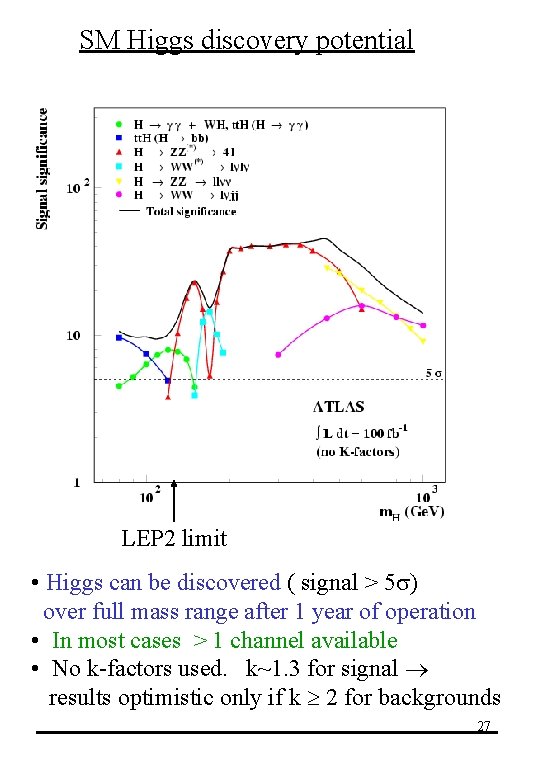
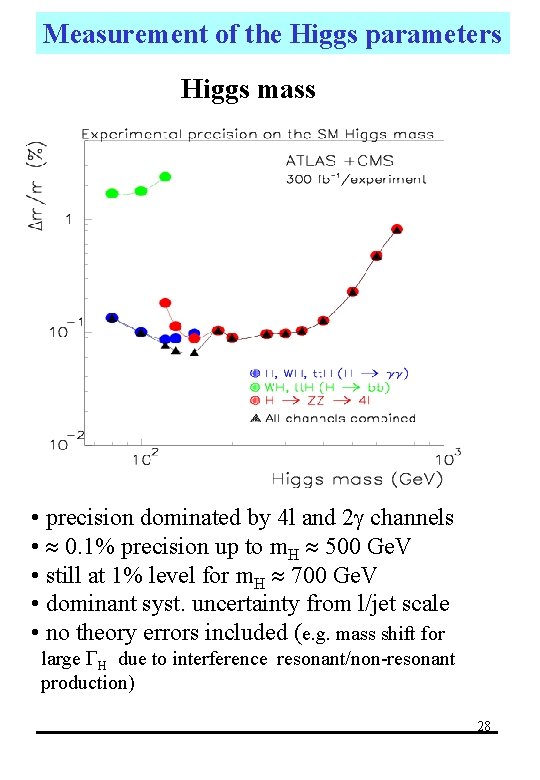
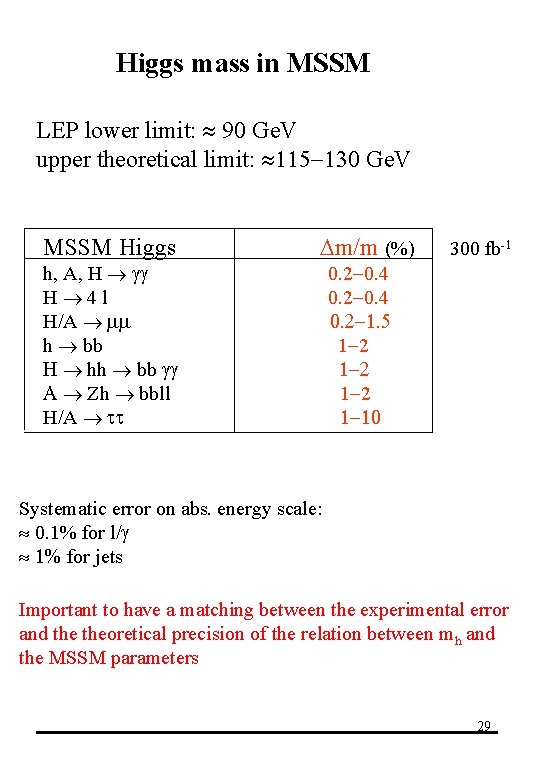
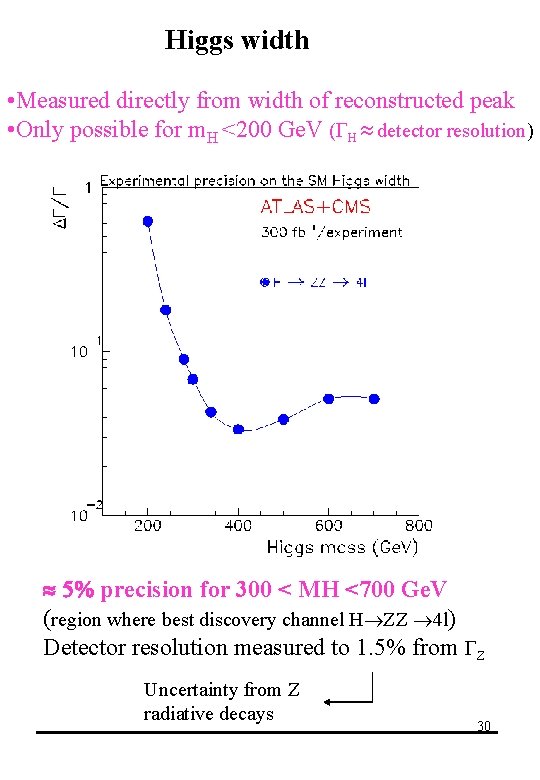
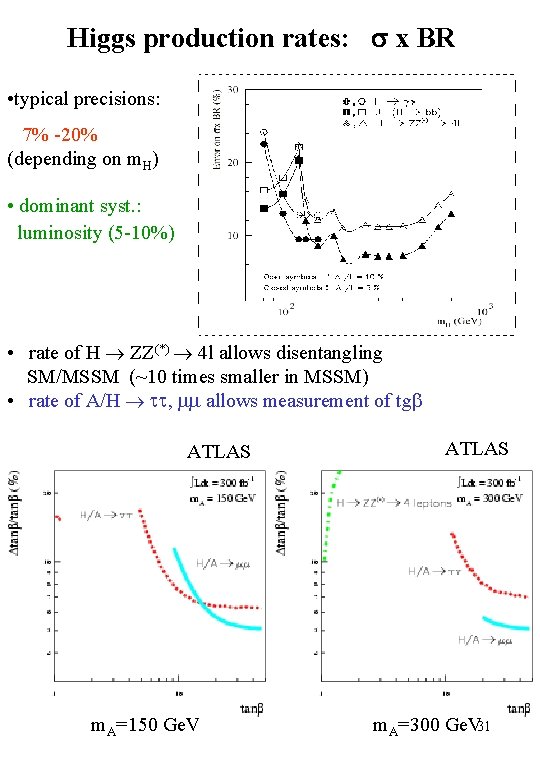
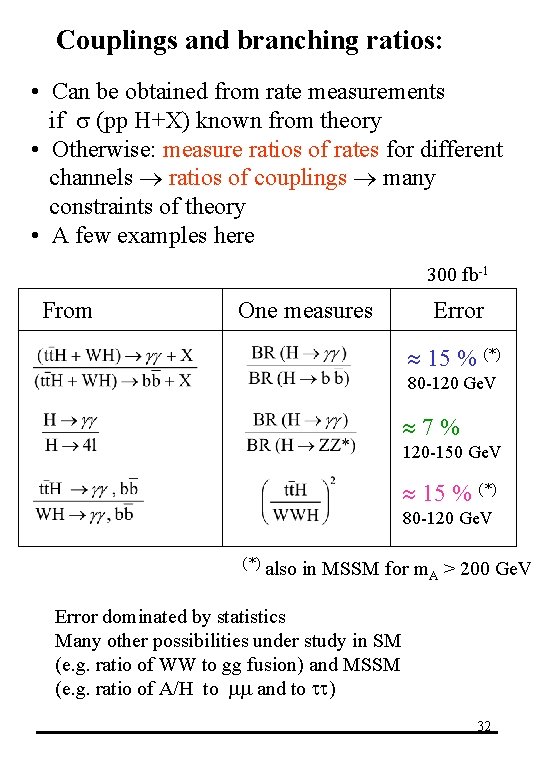
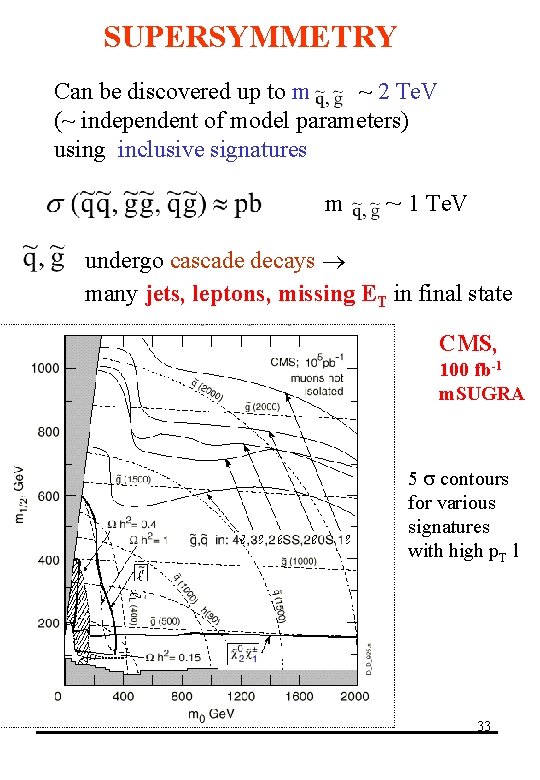
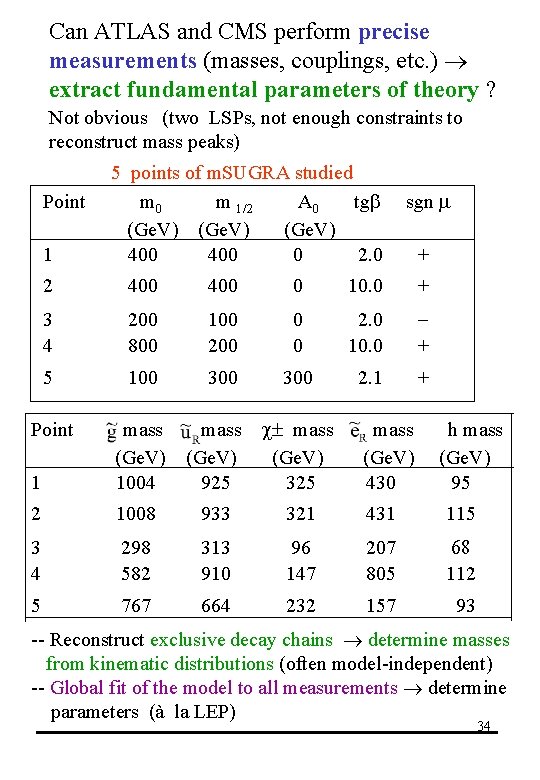
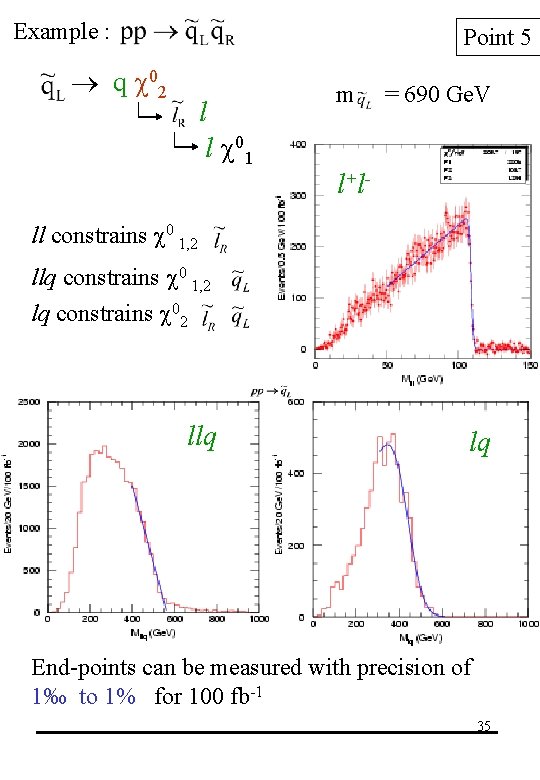
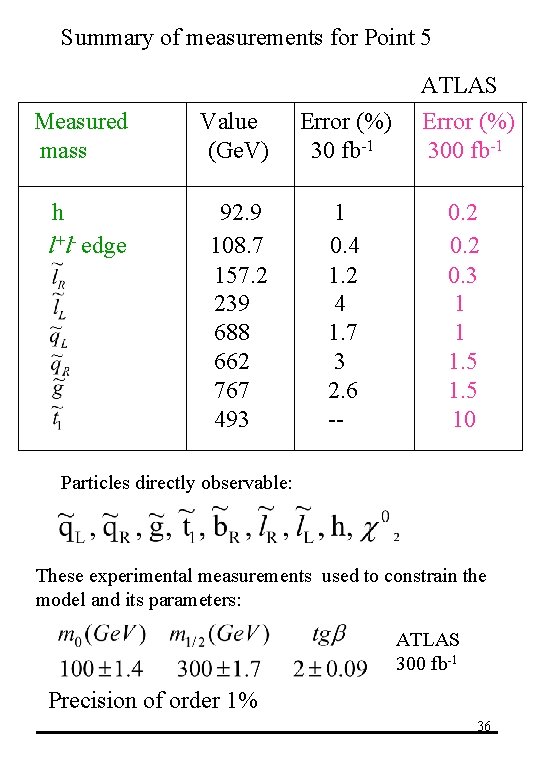
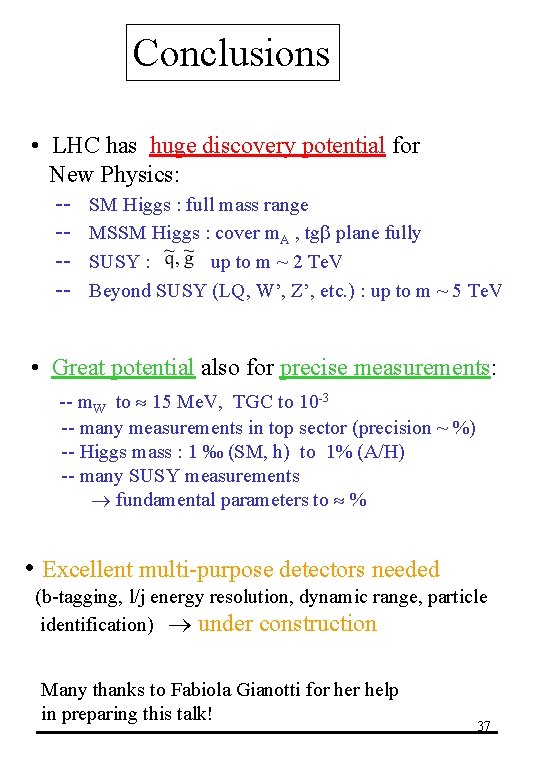
- Slides: 37
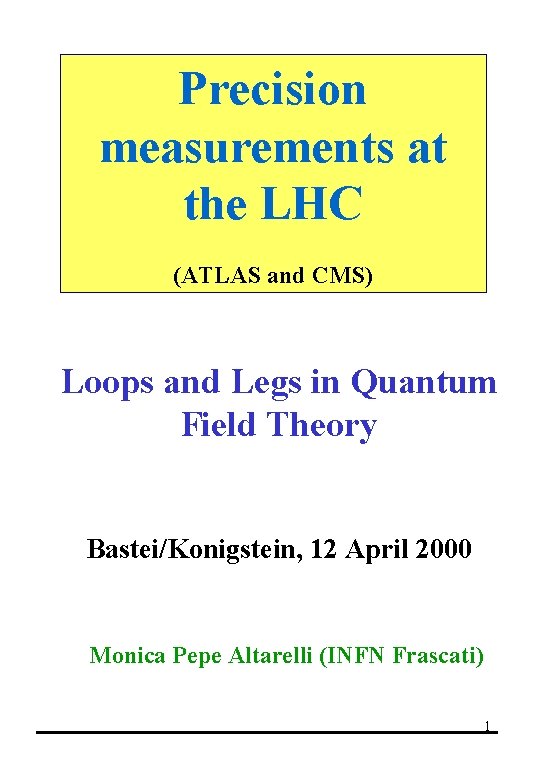
Precision measurements at the LHC (ATLAS and CMS) Loops and Legs in Quantum Field Theory Bastei/Konigstein, 12 April 2000 Monica Pepe Altarelli (INFN Frascati) 1
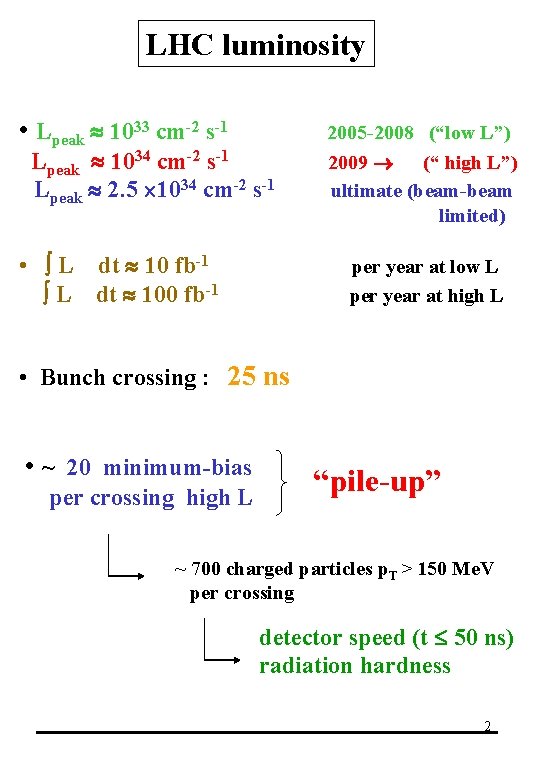
LHC luminosity • Lpeak 1033 cm-2 s-1 2005 -2008 (“low L”) 2009 (“ high L”) ultimate (beam-beam limited) • L dt 10 fb-1 L dt 100 fb-1 per year at low L per year at high L Lpeak 1034 cm-2 s-1 Lpeak 2. 5 1034 cm-2 s-1 • Bunch crossing : 25 ns • ~ 20 minimum-bias per crossing high L “pile-up” ~ 700 charged particles p. T > 150 Me. V per crossing detector speed (t 50 ns) radiation hardness 2
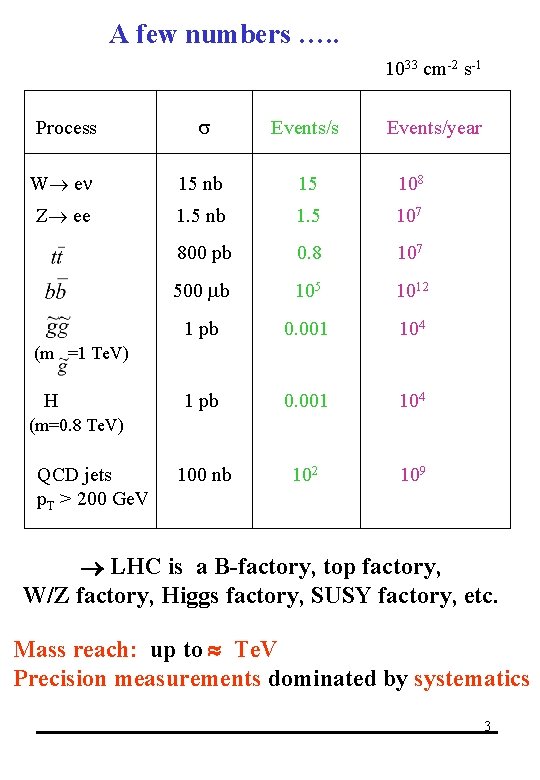
A few numbers …. . 1033 cm-2 s-1 Process s Events/s W e 15 nb 15 108 Z ee 1. 5 nb 1. 5 107 800 pb 0. 8 107 500 mb 105 1012 1 pb 0. 001 104 100 nb 102 109 Events/year (m =1 Te. V) H (m=0. 8 Te. V) QCD jets p. T > 200 Ge. V LHC is a B-factory, top factory, W/Z factory, Higgs factory, SUSY factory, etc. Mass reach: up to Te. V Precision measurements dominated by systematics 3
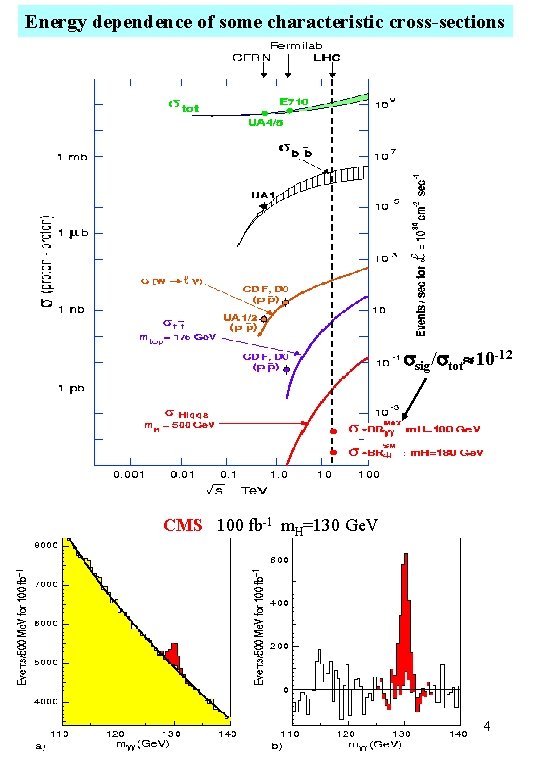
Energy dependence of some characteristic cross-sections ssig/stot 10 -12 CMS 100 fb-1 m. H=130 Ge. V 4
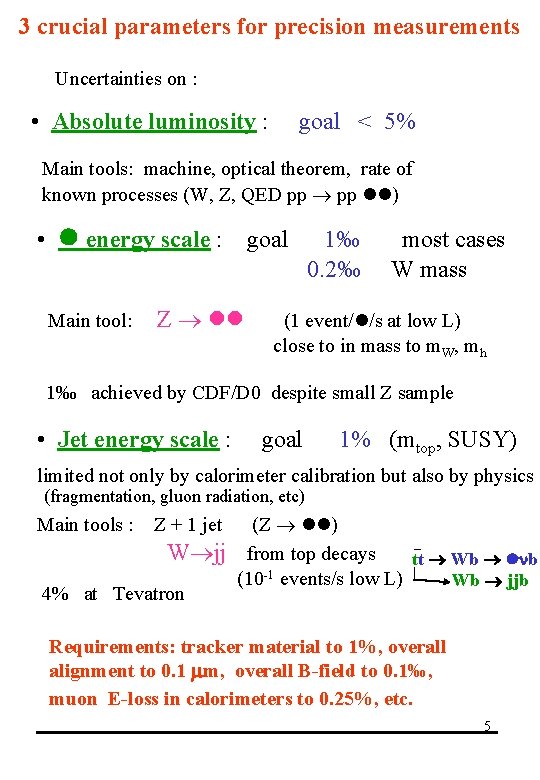
3 crucial parameters for precision measurements Uncertainties on : • Absolute luminosity : goal < 5% Main tools: machine, optical theorem, rate of known processes (W, Z, QED pp ) • energy scale : goal Main tool: Z 1‰ 0. 2‰ most cases W mass (1 event/ /s at low L) close to in mass to m. W, mh 1‰ achieved by CDF/D 0 despite small Z sample • Jet energy scale : goal 1% (mtop, SUSY) limited not only by calorimeter calibration but also by physics (fragmentation, gluon radiation, etc) Main tools : Z + 1 jet W jj 4% at Tevatron (Z ) from top decays tt Wb b -1 (10 events/s low L) Wb jjb Requirements: tracker material to 1%, overall alignment to 0. 1 mm, overall B-field to 0. 1‰, muon E-loss in calorimeters to 0. 25%, etc. 5
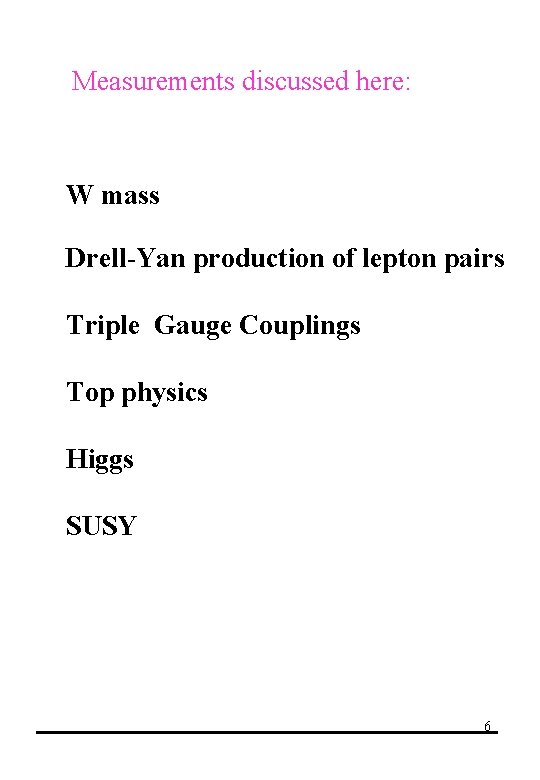
Measurements discussed here: W mass Drell-Yan production of lepton pairs Triple Gauge Couplings Top physics Higgs SUSY 6
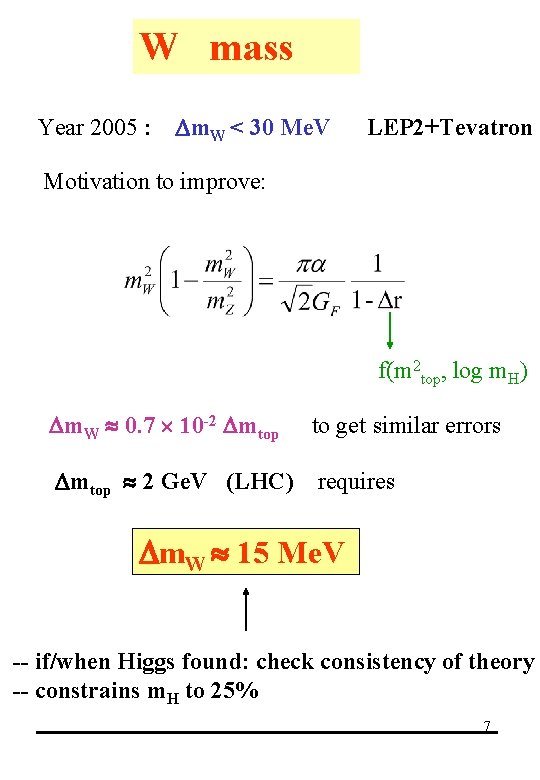
W mass Year 2005 : Dm. W < 30 Me. V LEP 2+Tevatron Motivation to improve: f(m 2 top, log m. H) Dm. W 0. 7 10 -2 Dmtop to get similar errors Dmtop 2 Ge. V (LHC) requires Dm. W 15 Me. V -- if/when Higgs found: check consistency of theory -- constrains m. H to 25% 7
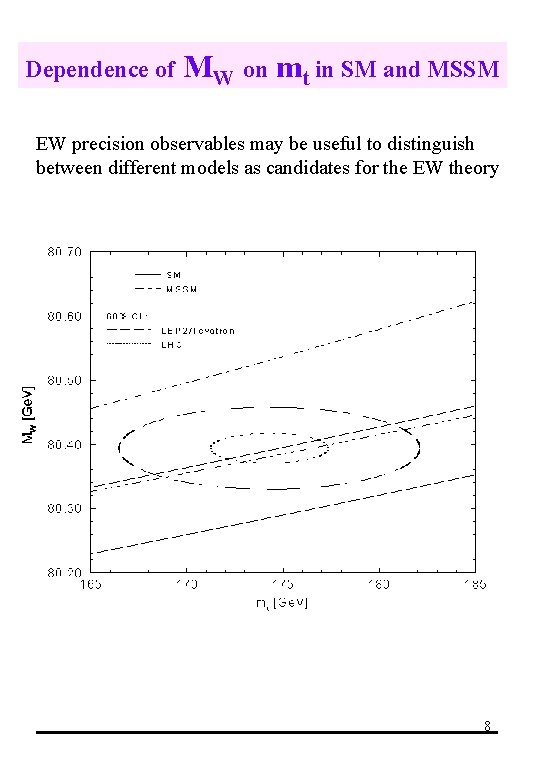
Dependence of MW on mt in SM and MSSM EW precision observables may be useful to distinguish between different models as candidates for the EW theory 8
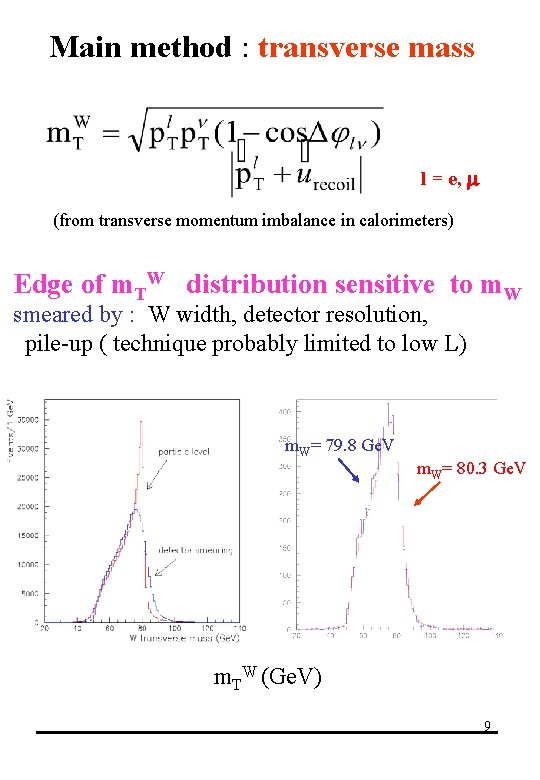
Main method : transverse mass l = e, m (from transverse momentum imbalance in calorimeters) Edge of m. TW distribution sensitive to m. W smeared by : W width, detector resolution, pile-up ( technique probably limited to low L) m. W= 79. 8 Ge. V m. W= 80. 3 Ge. V m. TW (Ge. V) 9
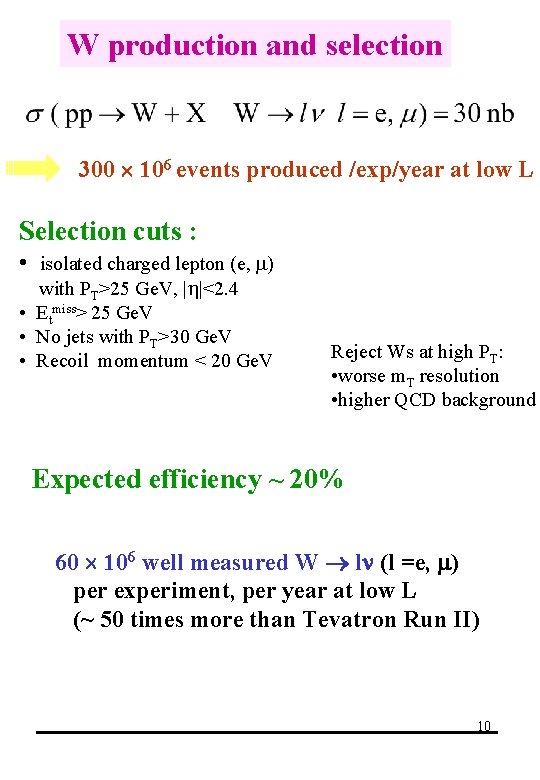
W production and selection 300 106 events produced /exp/year at low L Selection cuts : • isolated charged lepton (e, m) with PT>25 Ge. V, |h|<2. 4 • Etmiss> 25 Ge. V • No jets with PT>30 Ge. V • Recoil momentum < 20 Ge. V Reject Ws at high PT: • worse m. T resolution • higher QCD background Expected efficiency ~ 20% 60 106 well measured W l (l =e, m) per experiment, per year at low L (~ 50 times more than Tevatron Run II) 10
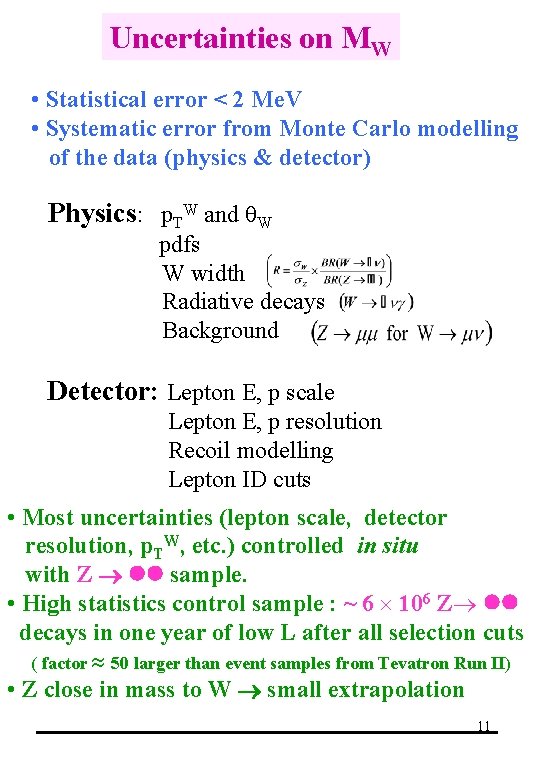
Uncertainties on MW • Statistical error < 2 Me. V • Systematic error from Monte Carlo modelling of the data (physics & detector) Physics: p. TW and W pdfs W width Radiative decays Background Detector: Lepton E, p scale Lepton E, p resolution Recoil modelling Lepton ID cuts • Most uncertainties (lepton scale, detector resolution, p. TW, etc. ) controlled in situ with Z sample. • High statistics control sample : ~ 6 ´ 106 Z decays in one year of low L after all selection cuts ( factor 50 larger than event samples from Tevatron Run II) • Z close in mass to W small extrapolation 11
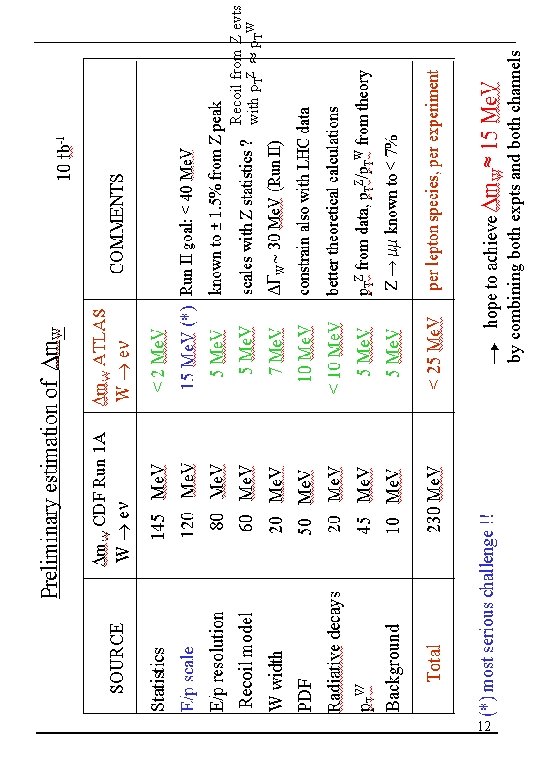
12 by combining both expts and both channels Recoil from Z evts with p. TZ p. TW
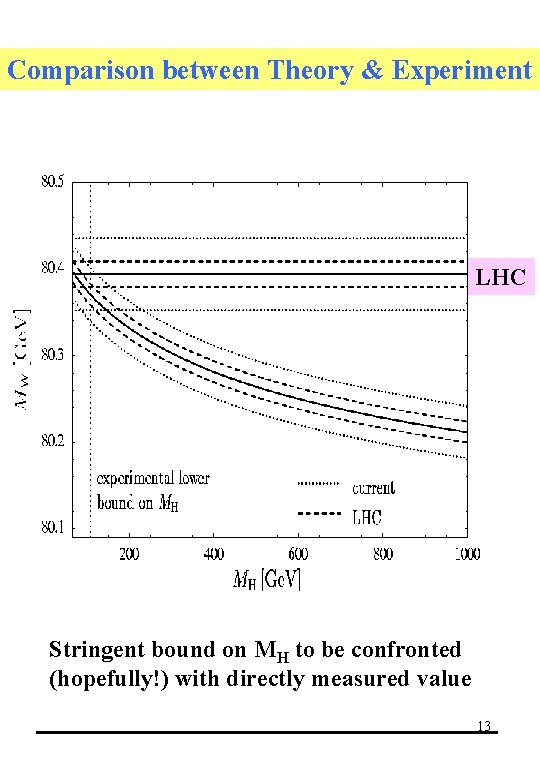
Comparison between Theory & Experiment LHC Stringent bound on MH to be confronted (hopefully!) with directly measured value 13
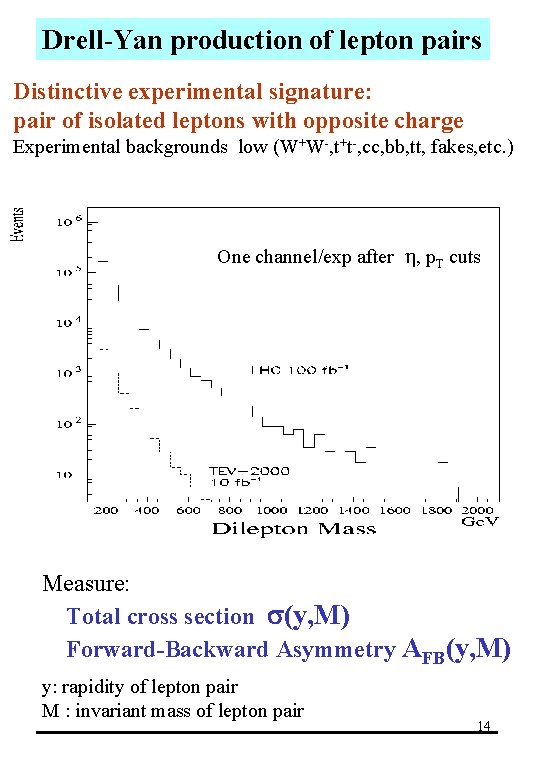
Drell-Yan production of lepton pairs Distinctive experimental signature: pair of isolated leptons with opposite charge Experimental backgrounds low (W+W-, t+t-, cc, bb, tt, fakes, etc. ) One channel/exp after h, p. T cuts Measure: Total cross section s(y, M) Forward-Backward Asymmetry AFB(y, M) y: rapidity of lepton pair M : invariant mass of lepton pair 14
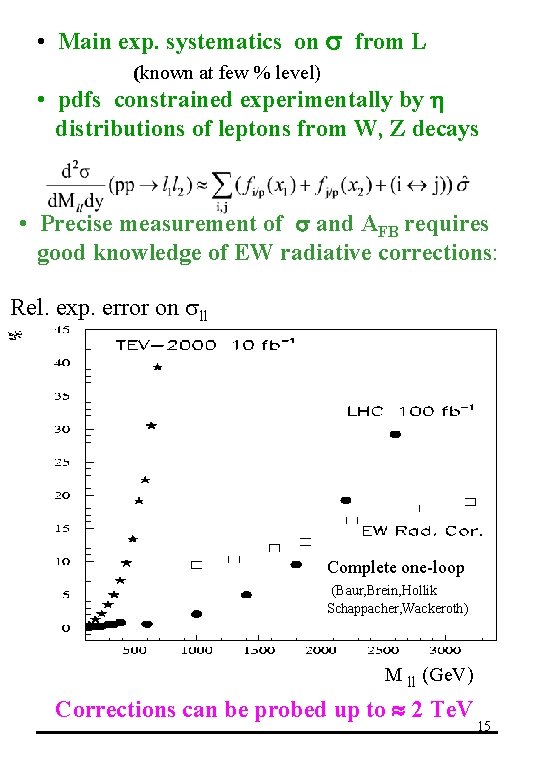
• Main exp. systematics on s from L (known at few % level) • pdfs constrained experimentally by h distributions of leptons from W, Z decays • Precise measurement of s and AFB requires good knowledge of EW radiative corrections: Rel. exp. error on sll Complete one-loop (Baur, Brein, Hollik Schappacher, Wackeroth) M ll (Ge. V) Corrections can be probed up to 2 Te. V 15
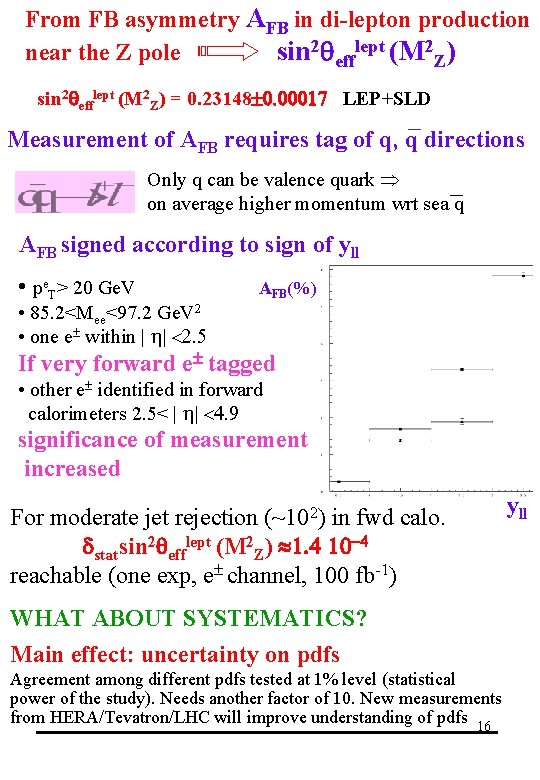
From FB asymmetry AFB in di-lepton production near the Z pole sin 2 qefflept (M 2 Z) = 0. 23148± 0. 00017 LEP+SLD Measurement of AFB requires tag of q, q directions Only q can be valence quark Þ on average higher momentum wrt sea q AFB signed according to sign of yll • pe. T> 20 Ge. V • 85. 2<Mee<97. 2 Ge. V 2 • one e within | h| <2. 5 AFB(%) If very forward e± tagged • other e identified in forward calorimeters 2. 5< | h| <4. 9 significance of measurement increased For moderate jet rejection (~102) in fwd calo. dstatsin 2 qefflept (M 2 Z) 1. 4 10 -4 reachable (one exp, e channel, 100 fb-1) WHAT ABOUT SYSTEMATICS? Main effect: uncertainty on pdfs Agreement among different pdfs tested at 1% level (statistical power of the study). Needs another factor of 10. New measurements from HERA/Tevatron/LHC will improve understanding of pdfs 16 yll
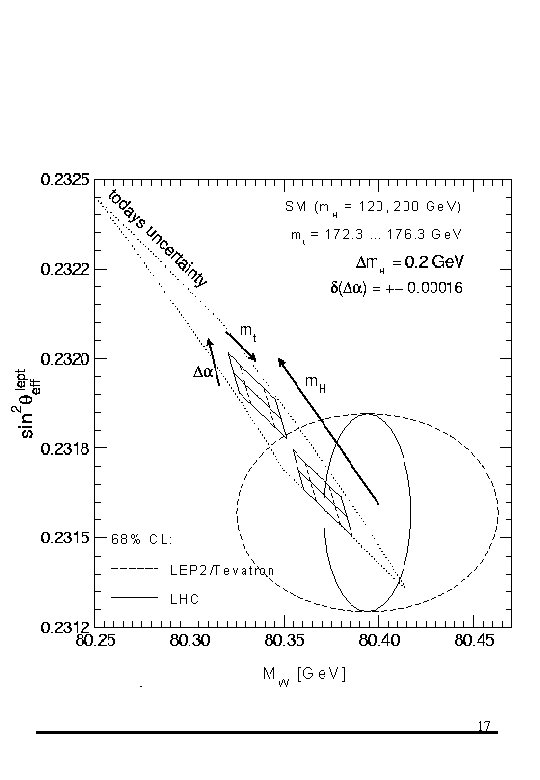
17
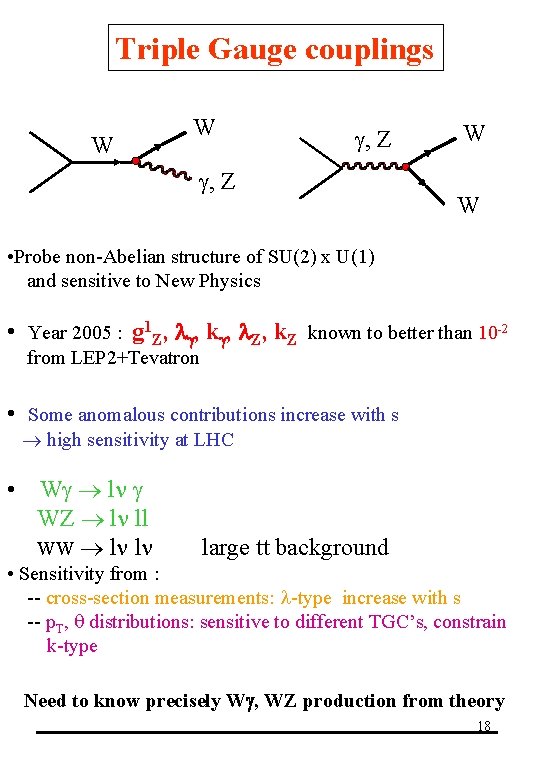
Triple Gauge couplings W W , Z W W • Probe non-Abelian structure of SU(2) x U(1) and sensitive to New Physics • Year 2005 : g 1 Z, lg, kg, l. Z, k. Z known to better than 10 -2 from LEP 2+Tevatron • Some anomalous contributions increase with s high sensitivity at LHC • W l WZ l ll WW l l large tt background • Sensitivity from : -- cross-section measurements: l-type increase with s -- p. T, distributions: sensitive to different TGC’s, constrain k-type Need to know precisely Wg, WZ production from theory 18
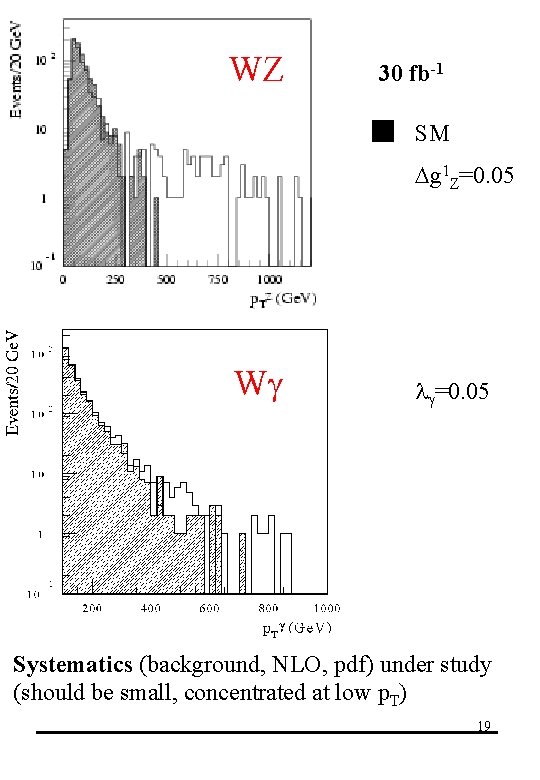
WZ 30 fb-1 SM Dg 1 Z=0. 05 W l =0. 05 Systematics (background, NLO, pdf) under study (should be small, concentrated at low p. T) 19
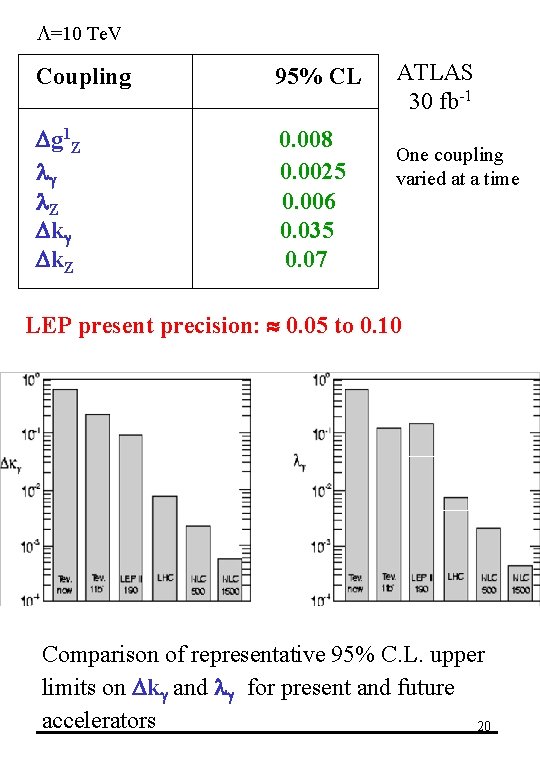
L=10 Te. V Coupling 95% CL Dg 1 Z lg l. Z Dkg Dk. Z 0. 008 0. 0025 0. 006 0. 035 0. 07 ATLAS 30 fb-1 One coupling varied at a time LEP present precision: 0. 05 to 0. 10 Comparison of representative 95% C. L. upper limits on Dkg and lg for present and future accelerators 20
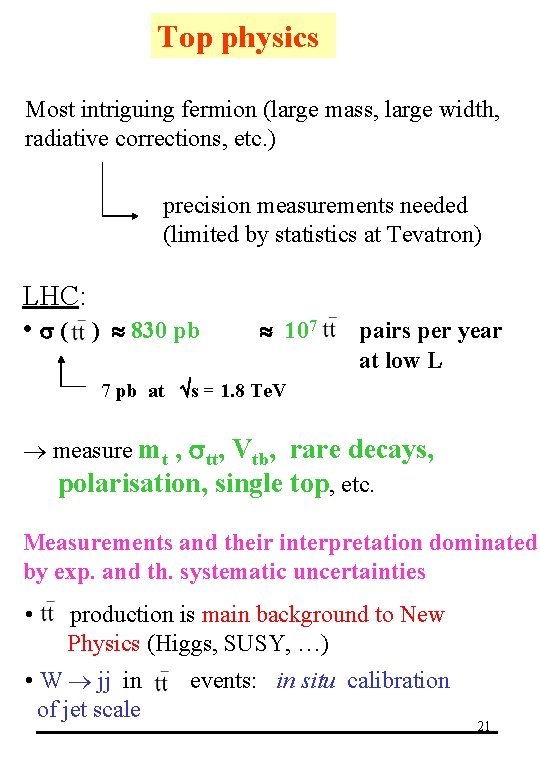
Top physics Most intriguing fermion (large mass, large width, radiative corrections, etc. ) precision measurements needed (limited by statistics at Tevatron) LHC: • s ( ) 830 pb 107 pairs per year at low L 7 pb at s = 1. 8 Te. V measure mt , stt, Vtb, rare decays, polarisation, single top, etc. Measurements and their interpretation dominated by exp. and th. systematic uncertainties • production is main background to New Physics (Higgs, SUSY, …) • W jj in of jet scale events: in situ calibration 21
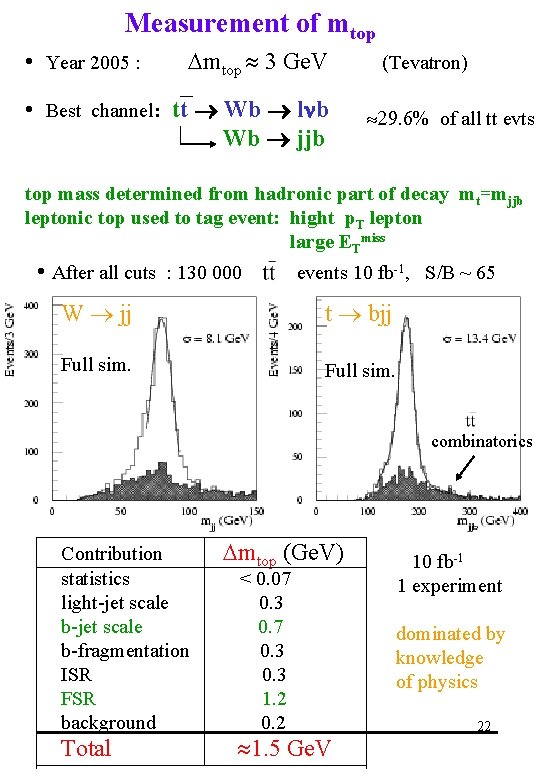
Measurement of mtop • Year 2005 : Dmtop 3 Ge. V • Best channel: tt Wb l b Wb jjb (Tevatron) 29. 6% of all tt evts top mass determined from hadronic part of decay mt=mjjb leptonic top used to tag event: hight p. T lepton large ETmiss • After all cuts : 130 000 events 10 fb-1, S/B ~ 65 W jj t bjj Full sim. combinatorics Contribution statistics light-jet scale b-fragmentation ISR FSR background Total Dmtop (Ge. V) < 0. 07 0. 3 1. 2 0. 2 1. 5 Ge. V 10 fb-1 1 experiment dominated by knowledge of physics 22
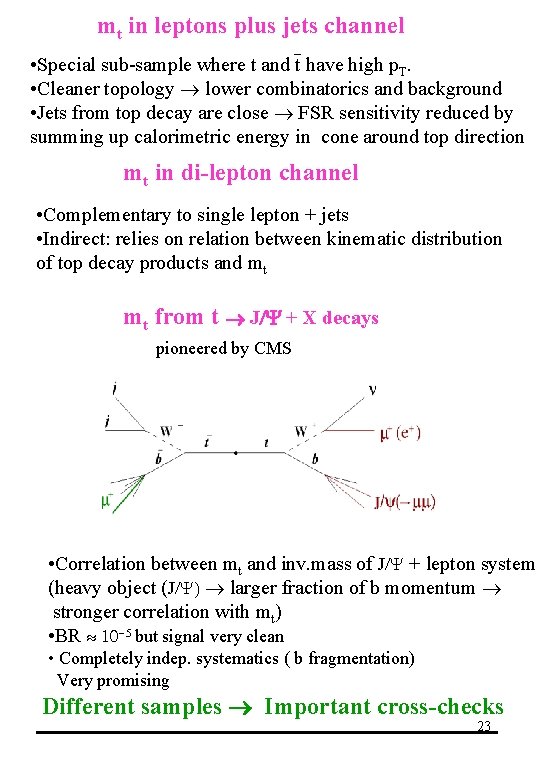
mt in leptons plus jets channel • Special sub-sample where t and t have high p. T. • Cleaner topology lower combinatorics and background • Jets from top decay are close FSR sensitivity reduced by summing up calorimetric energy in cone around top direction mt in di-lepton channel • Complementary to single lepton + jets • Indirect: relies on relation between kinematic distribution of top decay products and mt mt from t J/Y + X decays pioneered by CMS • Correlation between mt and inv. mass of J/Y + lepton system (heavy object (J/Y) larger fraction of b momentum stronger correlation with mt) • BR 10 -5 but signal very clean • Completely indep. systematics ( b fragmentation) Very promising Different samples Important cross-checks 23
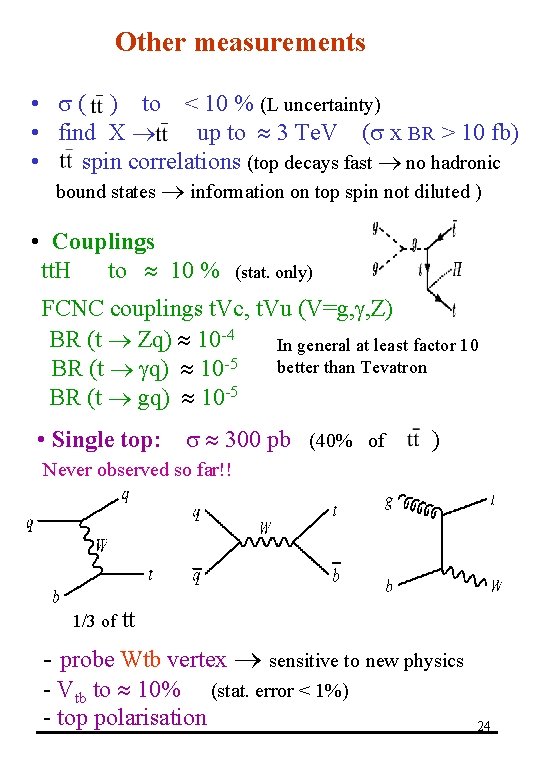
Other measurements • s ( ) to < 10 % (L uncertainty) • find X up to 3 Te. V (s x BR > 10 fb) • spin correlations (top decays fast no hadronic bound states information on top spin not diluted ) • Couplings tt. H to 10 % (stat. only) FCNC couplings t. Vc, t. Vu (V=g, , Z) BR (t Zq) 10 -4 In general at least factor 10 better than Tevatron BR (t q) 10 -5 BR (t gq) 10 -5 • Single top: s 300 pb (40% of ) Never observed so far!! 1/3 of tt - probe Wtb vertex sensitive to new physics - Vtb to 10% (stat. error < 1%) - top polarisation 24
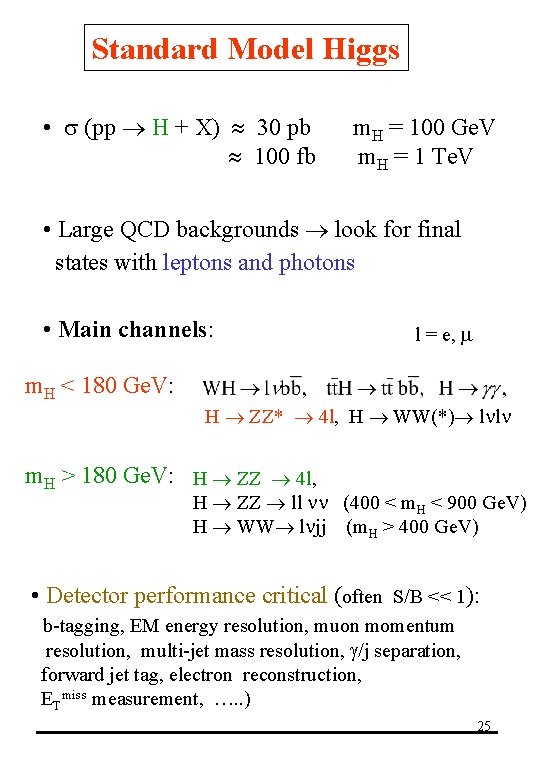
Standard Model Higgs • s (pp H + X) 30 pb 100 fb m. H = 100 Ge. V m. H = 1 Te. V • Large QCD backgrounds look for final states with leptons and photons • Main channels: l = e, m m. H < 180 Ge. V: H ZZ* 4 l, H WW(*) l l m. H > 180 Ge. V: H ZZ 4 l, H ZZ ll (400 < m. H < 900 Ge. V) H WW l jj (m. H > 400 Ge. V) • Detector performance critical (often S/B << 1): b-tagging, EM energy resolution, muon momentum resolution, multi-jet mass resolution, /j separation, forward jet tag, electron reconstruction, ETmiss measurement, …. . ) 25
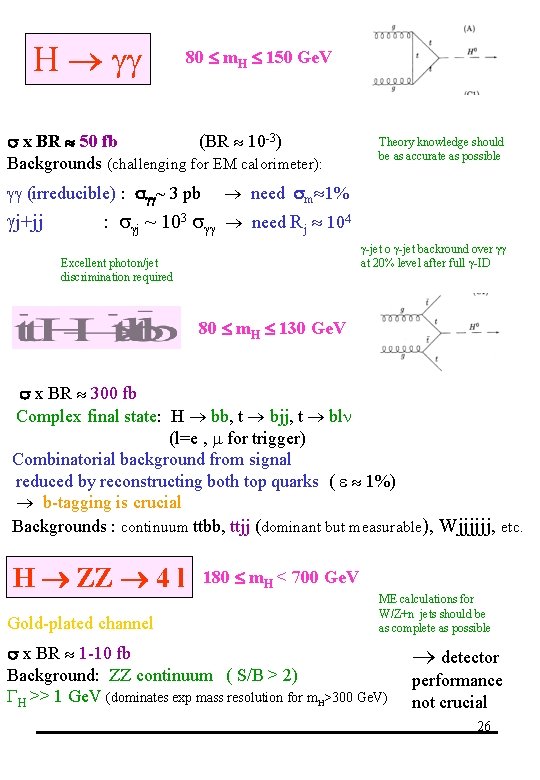
H 80 m. H 150 Ge. V s x BR 50 fb (BR 10 -3) Backgrounds (challenging for EM calorimeter): (irreducible) : sgg~ 3 pb j+jj Theory knowledge should be as accurate as possible need sm 1% : s j ~ 103 s need Rj 104 -jet o -jet backround over at 20% level after full -ID Excellent photon/jet discrimination required 80 m. H 130 Ge. V s x BR 300 fb Complex final state: H bb, t bjj, t bl (l=e , m for trigger) Combinatorial background from signal reduced by reconstructing both top quarks ( e 1%) b-tagging is crucial Backgrounds : continuum ttbb, ttjj (dominant but measurable), Wjjjjjj, etc. H ZZ 4 l Gold-plated channel 180 m. H < 700 Ge. V ME calculations for W/Z+n jets should be as complete as possible s x BR 1 -10 fb Background: ZZ continuum ( S/B > 2) GH >> 1 Ge. V (dominates exp mass resolution for m. H>300 Ge. V) detector performance not crucial 26
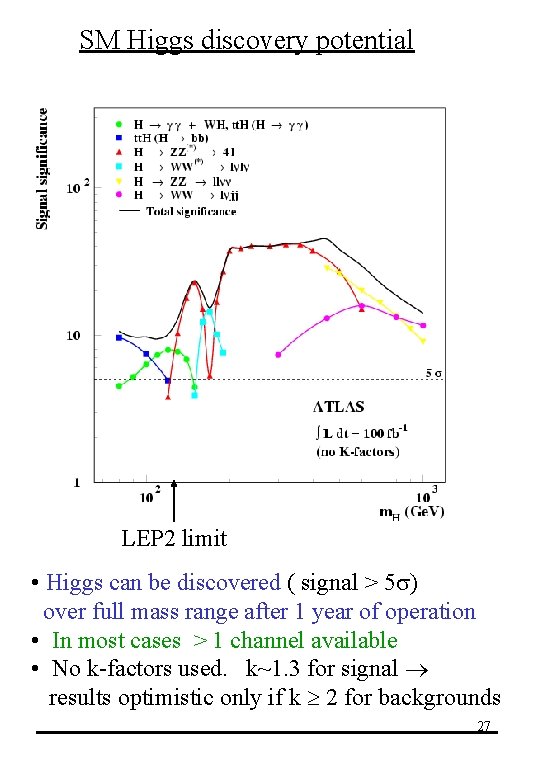
SM Higgs discovery potential LEP 2 limit • Higgs can be discovered ( signal > 5 s) over full mass range after 1 year of operation • In most cases > 1 channel available • No k-factors used. k~1. 3 for signal results optimistic only if k 2 for backgrounds 27
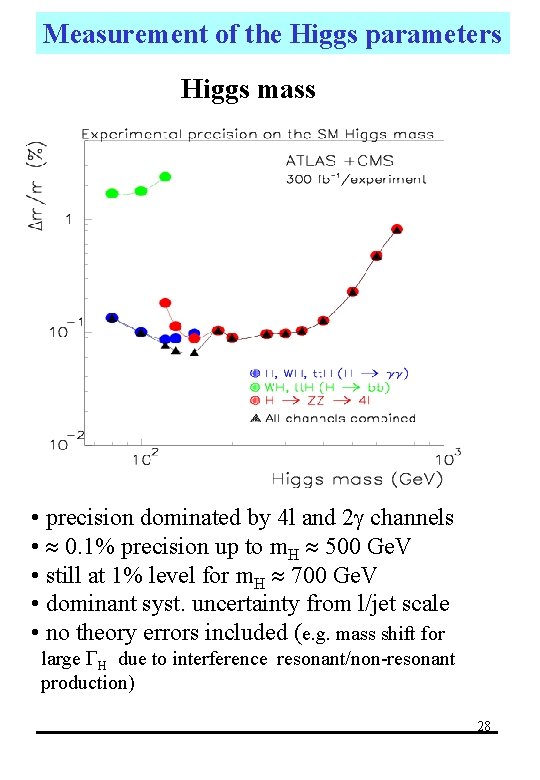
Measurement of the Higgs parameters Higgs mass • precision dominated by 4 l and 2 channels • 0. 1% precision up to m. H 500 Ge. V • still at 1% level for m. H 700 Ge. V • dominant syst. uncertainty from l/jet scale • no theory errors included (e. g. mass shift for large GH due to interference resonant/non-resonant production) 28
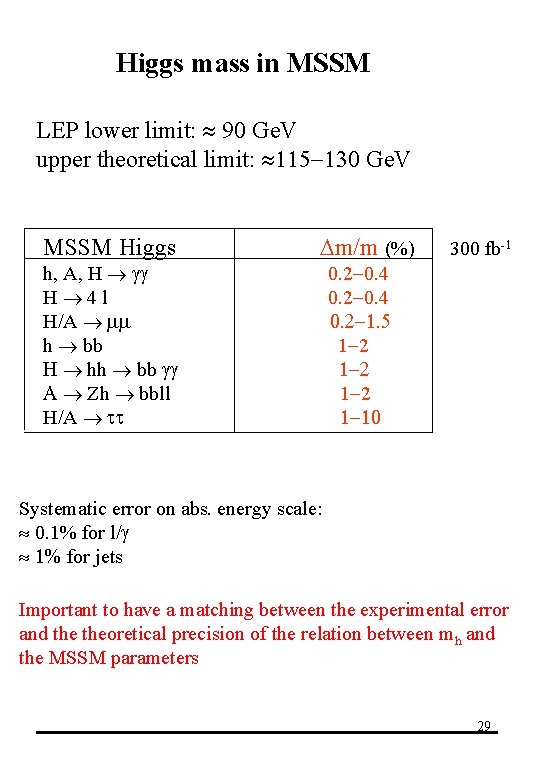
Higgs mass in MSSM LEP lower limit: 90 Ge. V upper theoretical limit: 115 -130 Ge. V MSSM Higgs h, A, H H 4 l H/A mm h bb H hh bb A Zh bbll H/A tt Dm/m (%) 300 fb-1 0. 2 -0. 4 0. 2 -1. 5 1 -2 1 -2 1 -10 Systematic error on abs. energy scale: 0. 1% for l/ 1% for jets Important to have a matching between the experimental error and theoretical precision of the relation between mh and the MSSM parameters 29
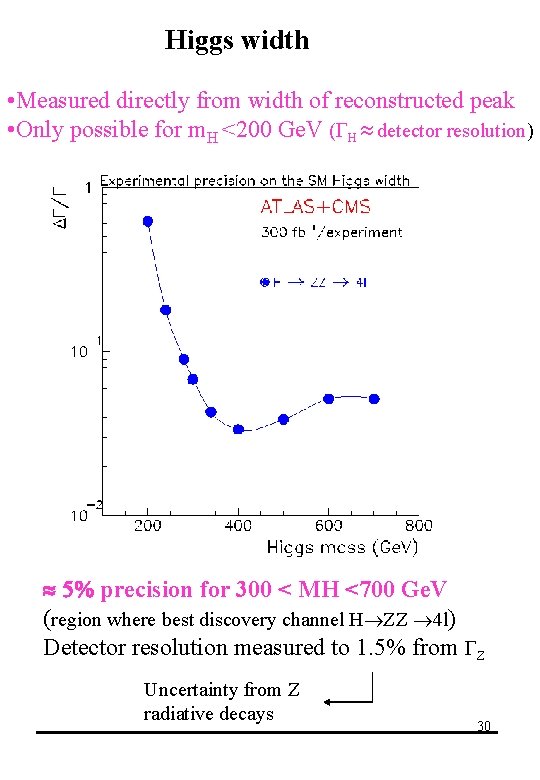
Higgs width • Measured directly from width of reconstructed peak • Only possible for m. H <200 Ge. V (GH detector resolution) 5% precision for 300 < MH <700 Ge. V (region where best discovery channel H ZZ 4 l) Detector resolution measured to 1. 5% from GZ Uncertainty from Z radiative decays 30
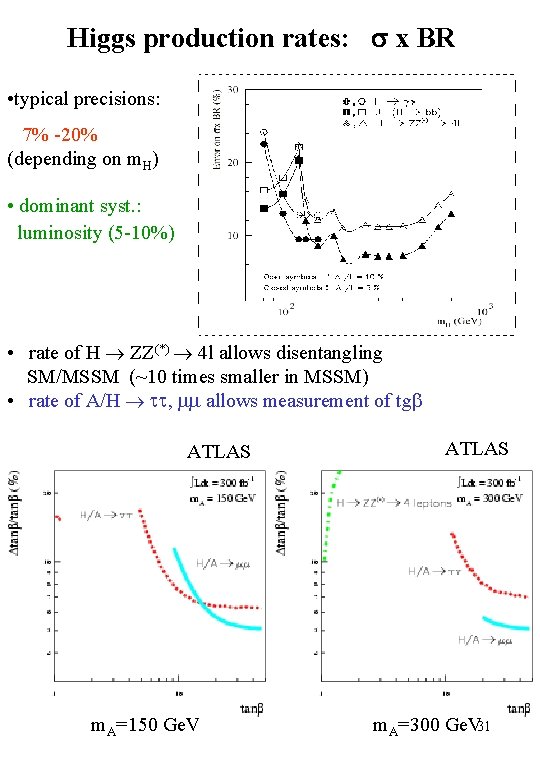
Higgs production rates: s x BR • typical precisions: 7% -20% (depending on m. H) • dominant syst. : luminosity (5 -10%) • rate of H ZZ(*) 4 l allows disentangling SM/MSSM (~10 times smaller in MSSM) • rate of A/H tt, mm allows measurement of tgb ATLAS m. A=150 Ge. V ATLAS m. A=300 Ge. V 31
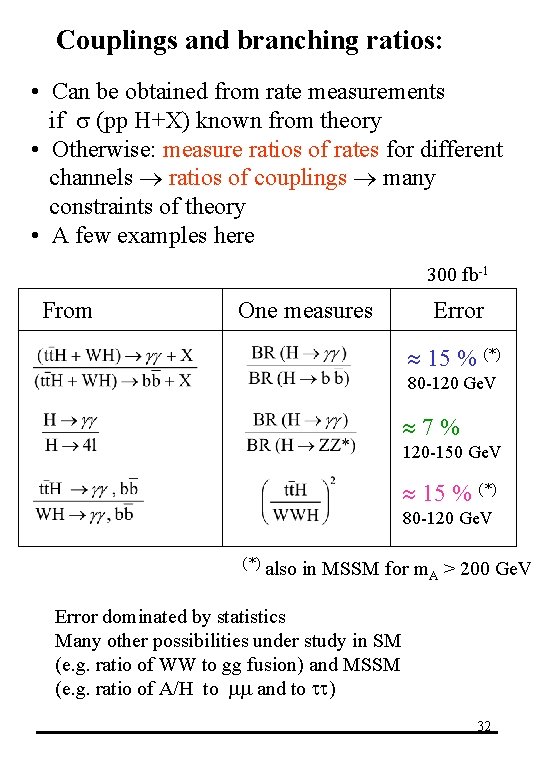
Couplings and branching ratios: • Can be obtained from rate measurements if s (pp H+X) known from theory • Otherwise: measure ratios of rates for different channels ratios of couplings many constraints of theory • A few examples here 300 fb-1 From One measures Error 15 % (*) 80 -120 Ge. V 7% 120 -150 Ge. V 15 % (*) 80 -120 Ge. V (*) also in MSSM for m. A > 200 Ge. V Error dominated by statistics Many other possibilities under study in SM (e. g. ratio of WW to gg fusion) and MSSM (e. g. ratio of A/H to mm and to tt) 32
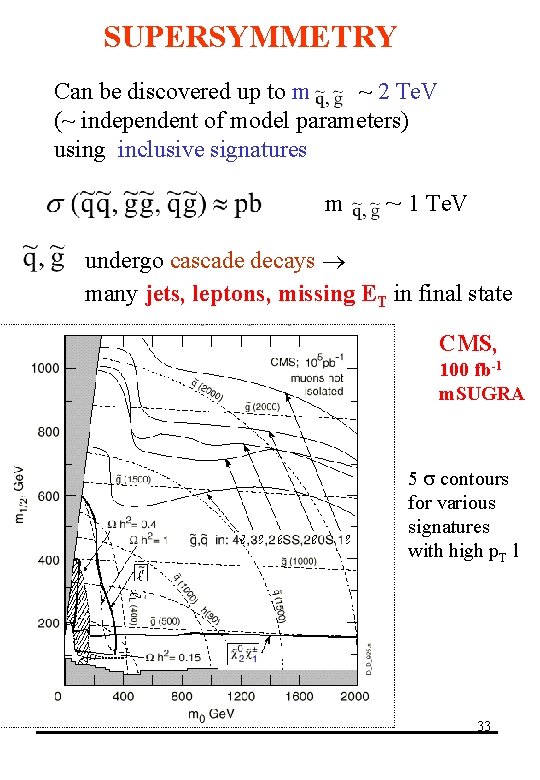
SUPERSYMMETRY Can be discovered up to m ~ 2 Te. V (~ independent of model parameters) using inclusive signatures m ~ 1 Te. V undergo cascade decays many jets, leptons, missing ET in final state CMS, 100 fb-1 m. SUGRA 5 s contours for various signatures with high p. T l 33
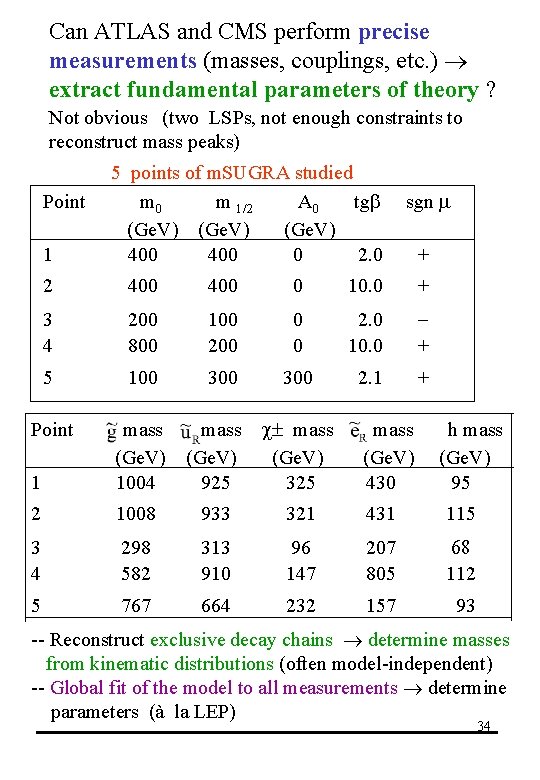
Can ATLAS and CMS perform precise measurements (masses, couplings, etc. ) extract fundamental parameters of theory ? Not obvious (two LSPs, not enough constraints to reconstruct mass peaks) Point 1 5 points of m. SUGRA studied m 0 m 1/2 A 0 tgb (Ge. V) 400 0 2. 0 sgn m + 2 400 0 10. 0 + 3 4 200 800 100 200 0 0 2. 0 10. 0 + 5 100 300 2. 1 + Point 1 mass (Ge. V) 1004 mass (Ge. V) 925 mass (Ge. V) 325 2 1008 933 321 431 115 3 4 298 582 313 910 96 147 207 805 68 112 5 767 664 232 157 93 mass (Ge. V) 430 h mass (Ge. V) 95 -- Reconstruct exclusive decay chains determine masses from kinematic distributions (often model-independent) -- Global fit of the model to all measurements determine parameters (à la LEP) 34
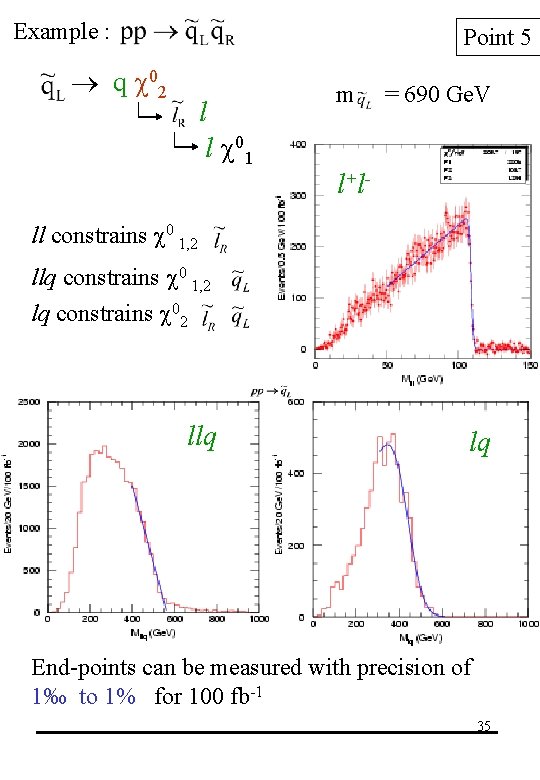
Example : Point 5 q 02 l l 01 m = 690 Ge. V l +l ll constrains 0 1, 2 llq constrains 0 1, 2 lq constrains 02 llq lq End-points can be measured with precision of 1‰ to 1% for 100 fb-1 35
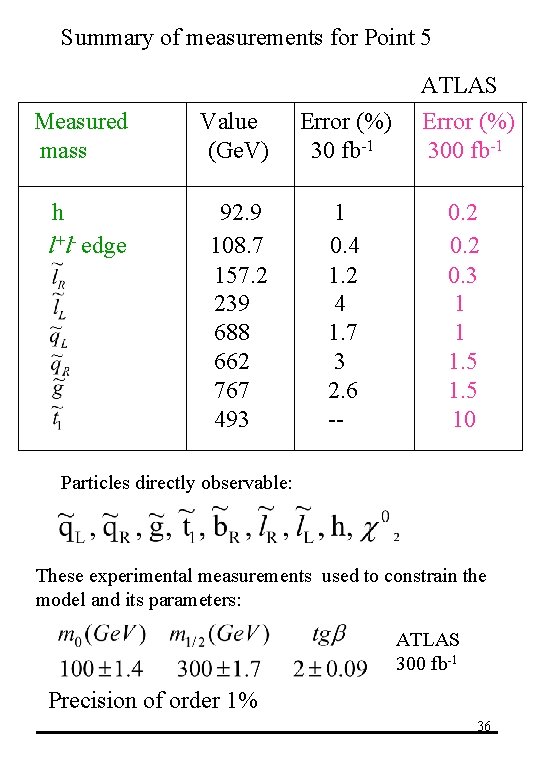
Summary of measurements for Point 5 ATLAS Measured mass Value (Ge. V) Error (%) 30 fb-1 Error (%) 300 fb-1 h l+l- edge 92. 9 108. 7 157. 2 239 688 662 767 493 1 0. 4 1. 2 4 1. 7 3 2. 6 -- 0. 2 0. 3 1 1 1. 5 10 Particles directly observable: These experimental measurements used to constrain the model and its parameters: ATLAS 300 fb-1 Precision of order 1% 36
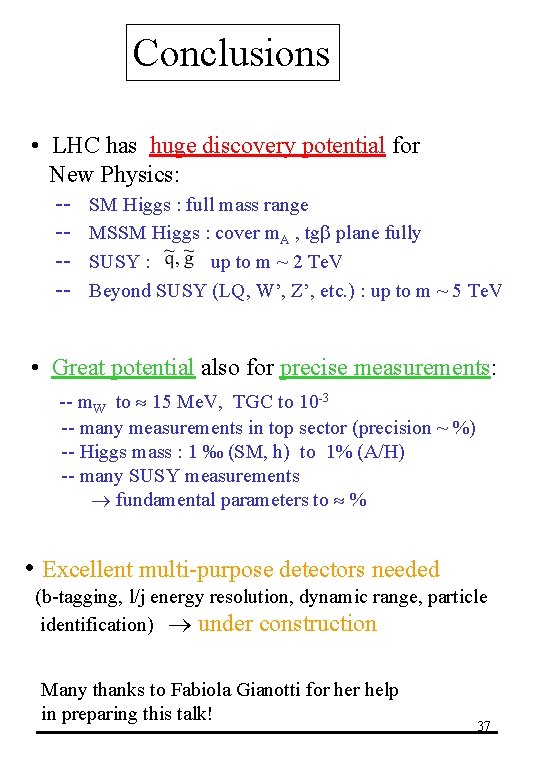
Conclusions • LHC has huge discovery potential for New Physics: -- SM Higgs : full mass range -- MSSM Higgs : cover m. A , tgb plane fully -- SUSY : up to m ~ 2 Te. V -- Beyond SUSY (LQ, W’, Z’, etc. ) : up to m ~ 5 Te. V • Great potential also for precise measurements: -- m. W to 15 Me. V, TGC to 10 -3 -- many measurements in top sector (precision ~ %) -- Higgs mass : 1 ‰ (SM, h) to 1% (A/H) -- many SUSY measurements fundamental parameters to % • Excellent multi-purpose detectors needed (b-tagging, l/j energy resolution, dynamic range, particle identification) under construction Many thanks to Fabiola Gianotti for help in preparing this talk! 37
Precision and non precision instruments
Precision vs semi precision attachment
Bcd gösterimi
Tevatron vs lhc
Lhc housing
Hl-lhc schedule
Lhc forum
Lhc logbook
Lhc morning meeting
Worldwide lhc computing grid
Lhc budget
Lhc schedule 2022
Lhc performance workshop
Lhc performance workshop
Lhc intranet
Hl-lhc schedule
Lhc performance workshop
Lhc filling scheme
Gant chart
"lhc"
Lhc
Lhc:8001692
Lhc beam dump
Lhc quench
Lhc beam dump
Forum lhc
"lhc"
"lhc"
Damien lafarge
Hadrons
Ti8 schedule
Lhc technical coordination
Hl-lhc schedule
Finsler geometry
Kme copper
Lhc plan
Measurements equivalents and adjustments
Chapter 2 measurements and calculations