Ministry of Higher Education scientific Research Mustansiriyah University
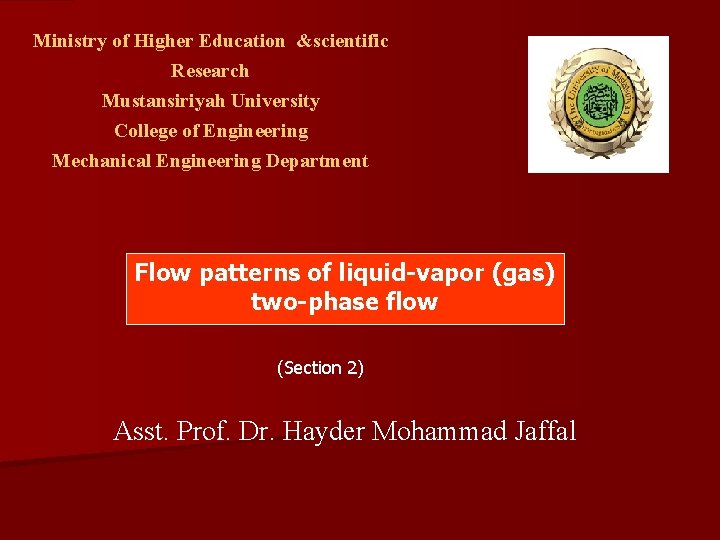
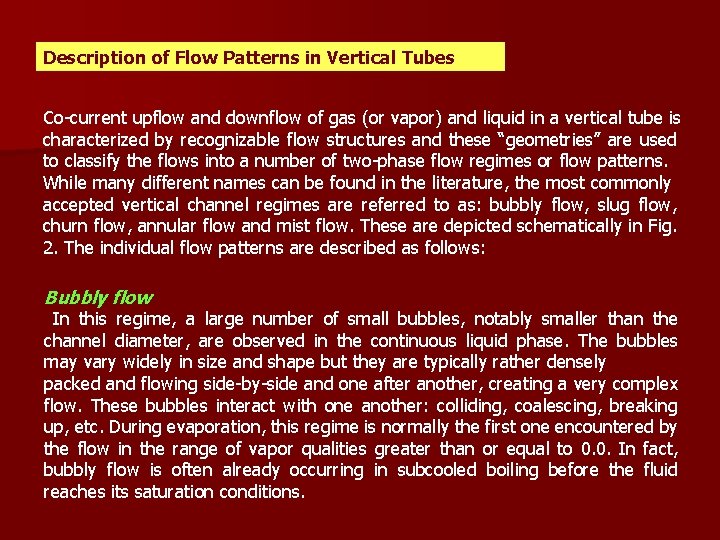
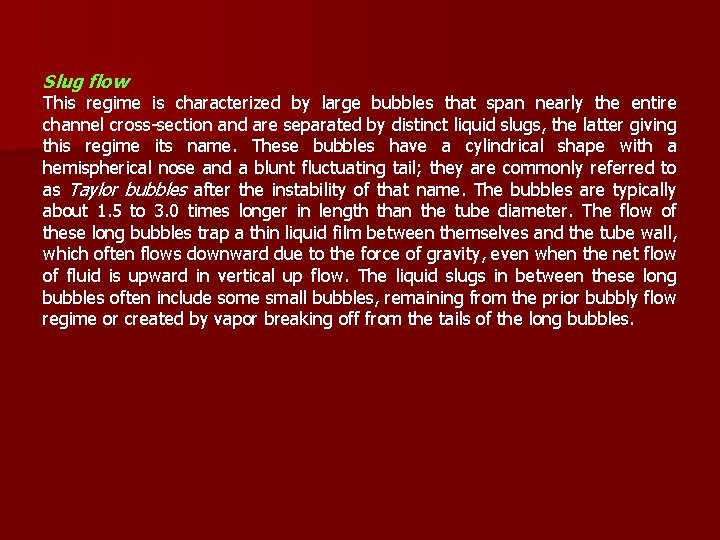
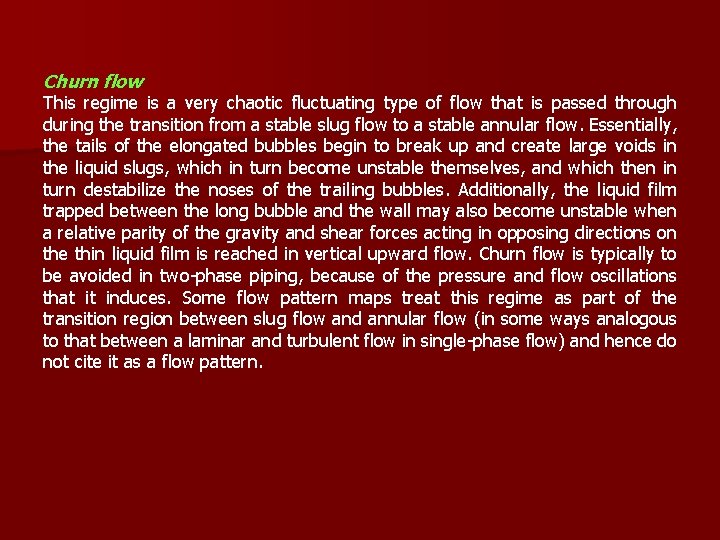
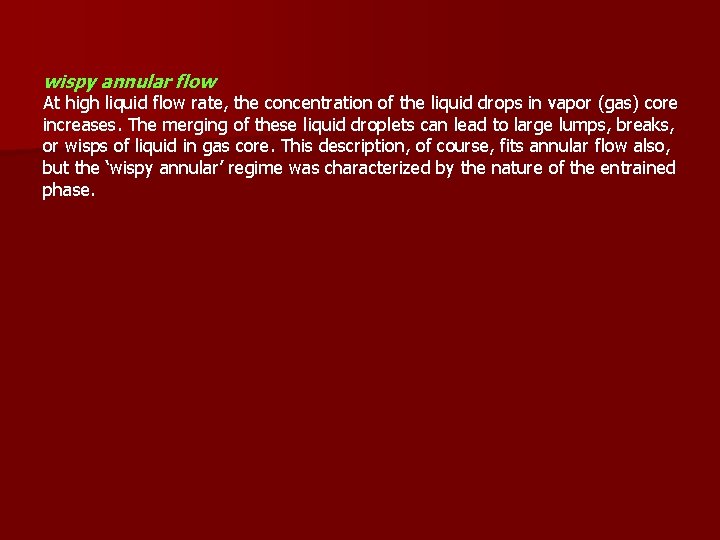
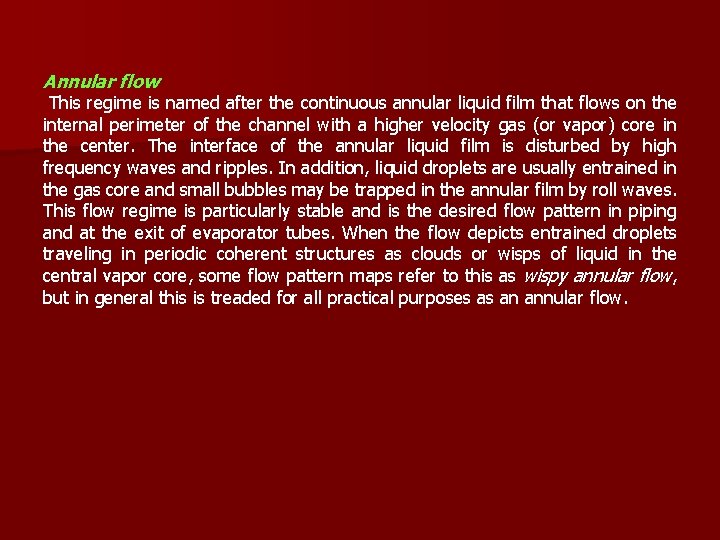
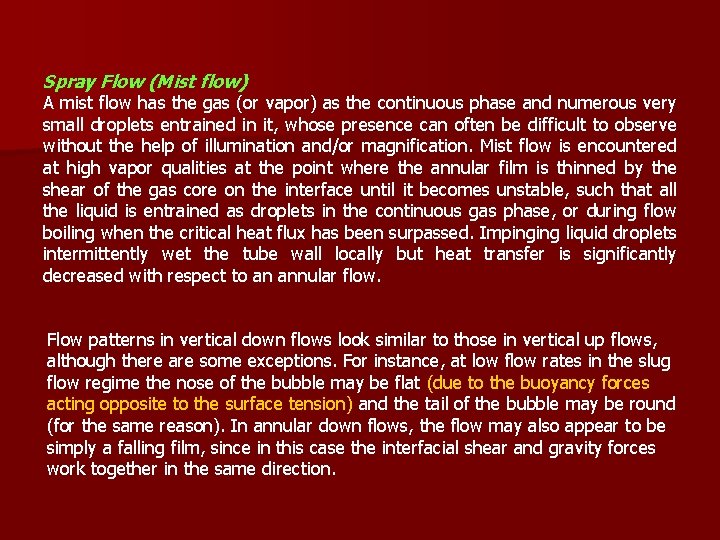
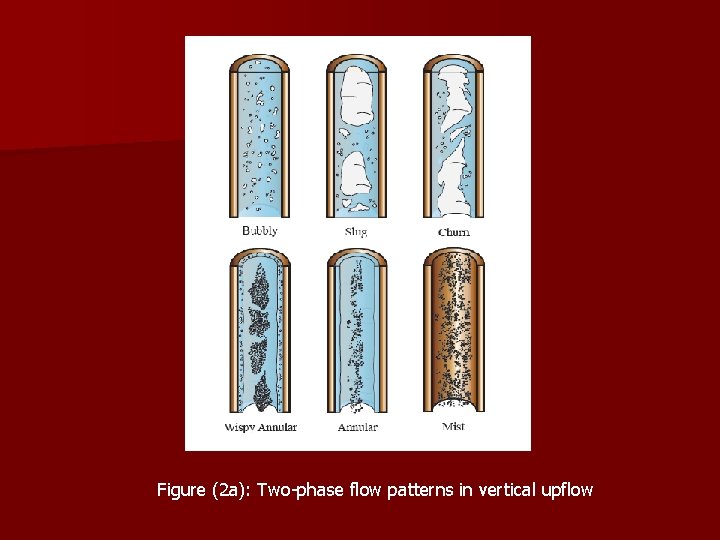
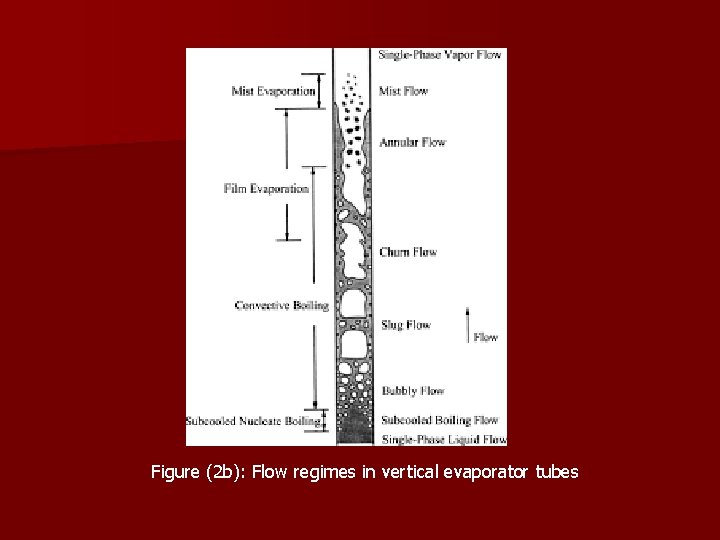
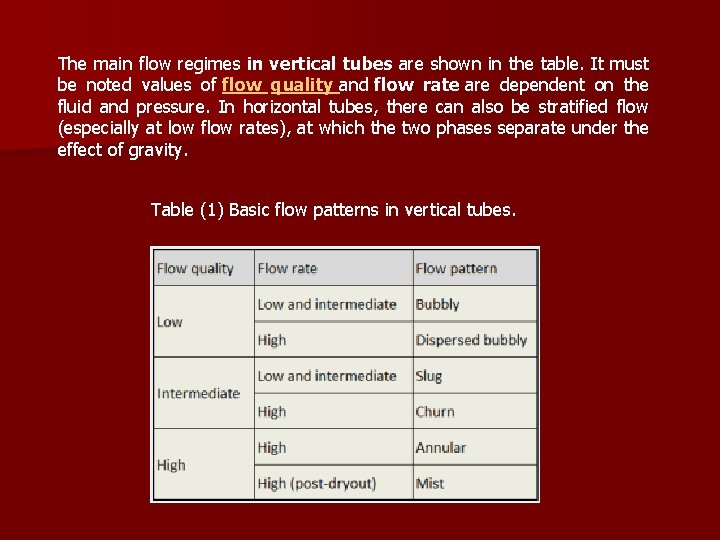
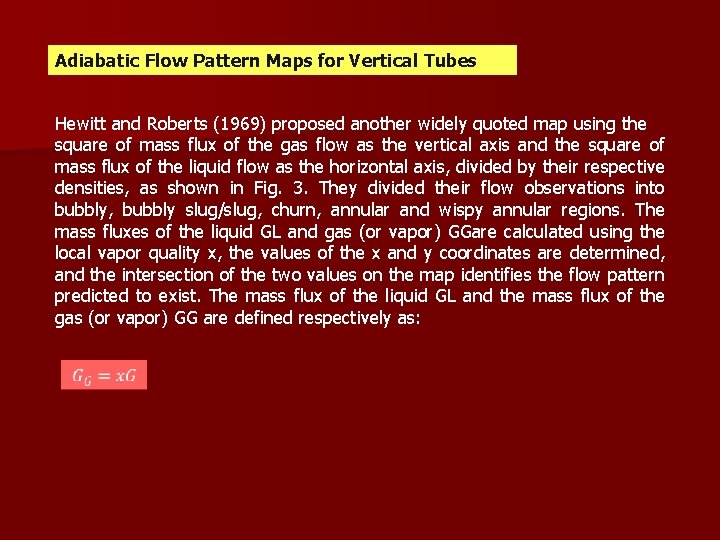
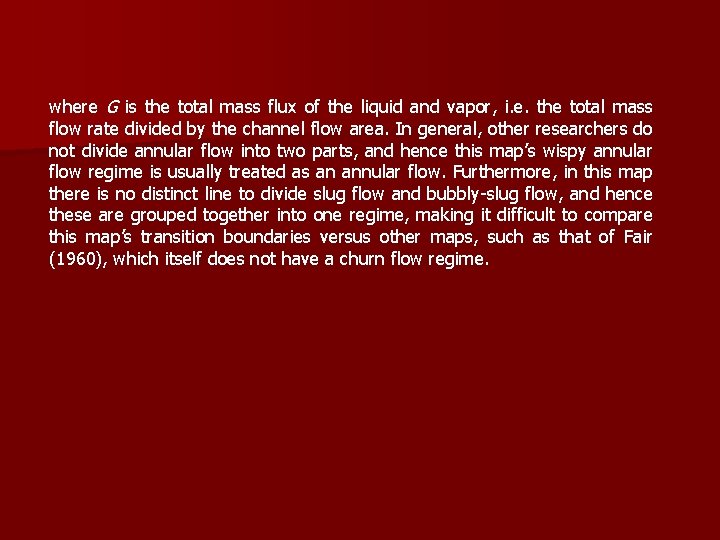
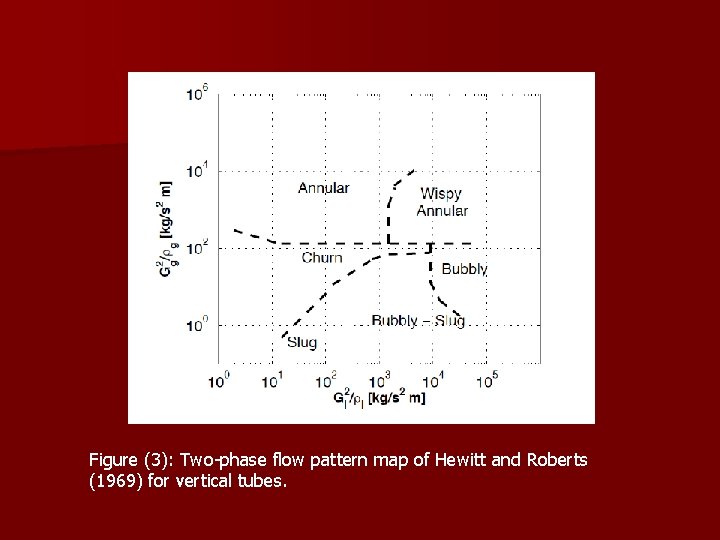
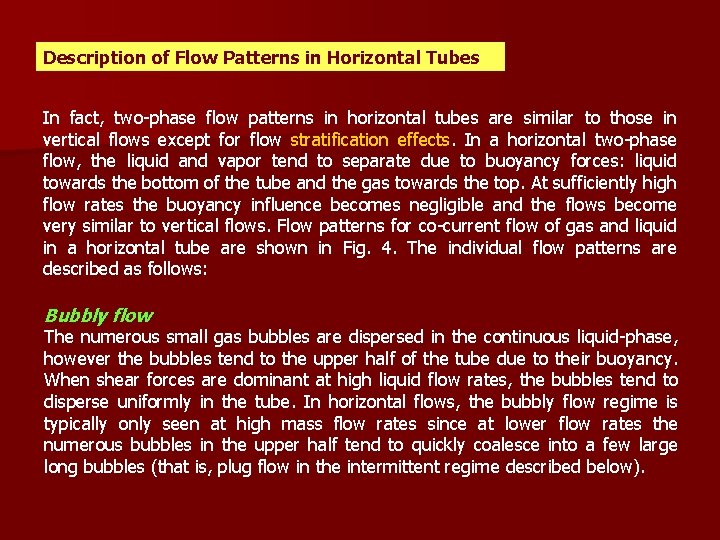
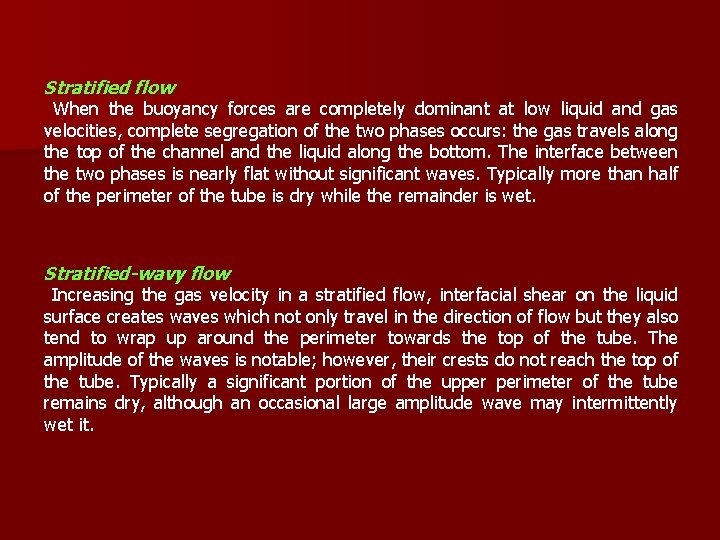
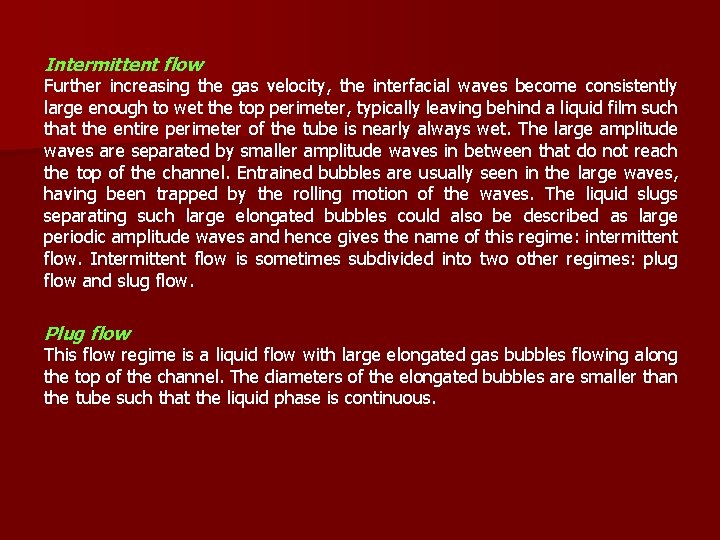
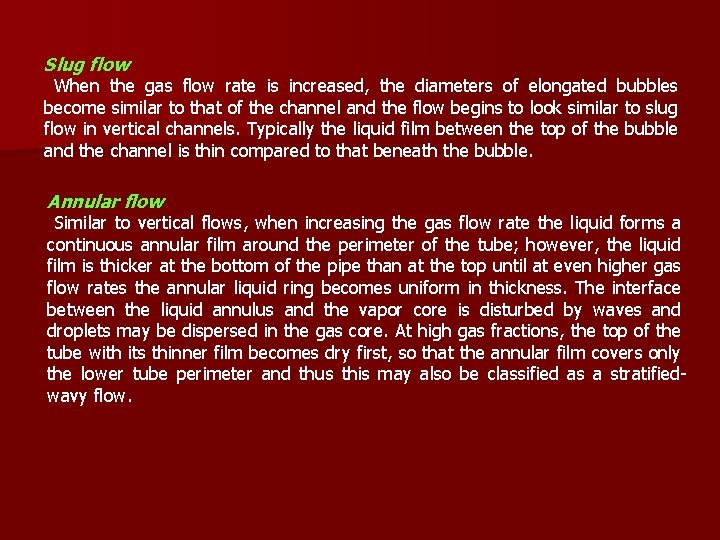
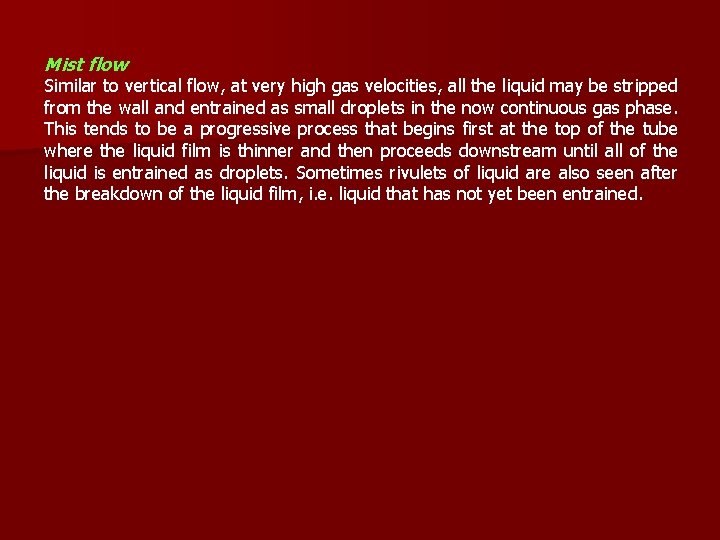
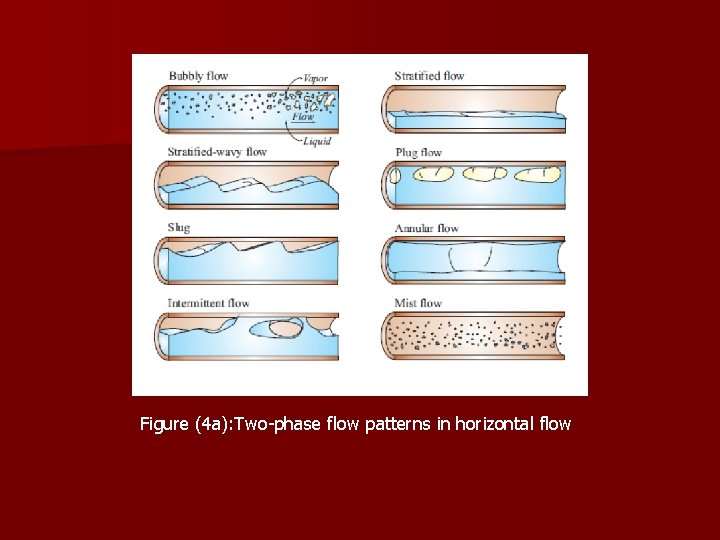
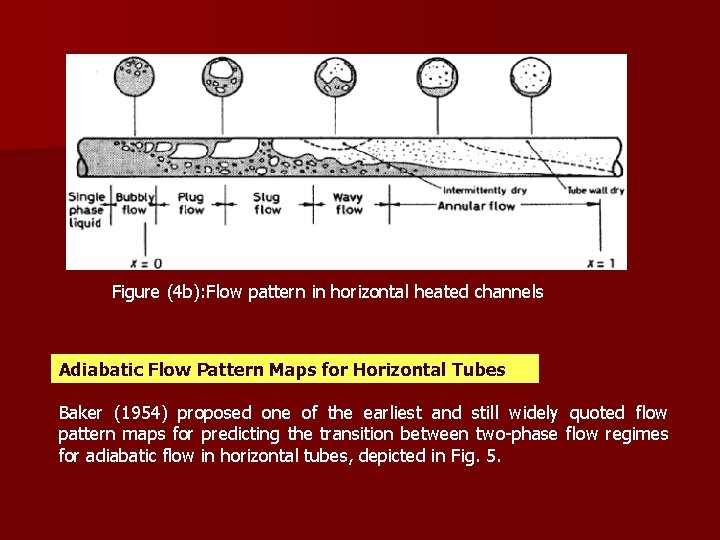
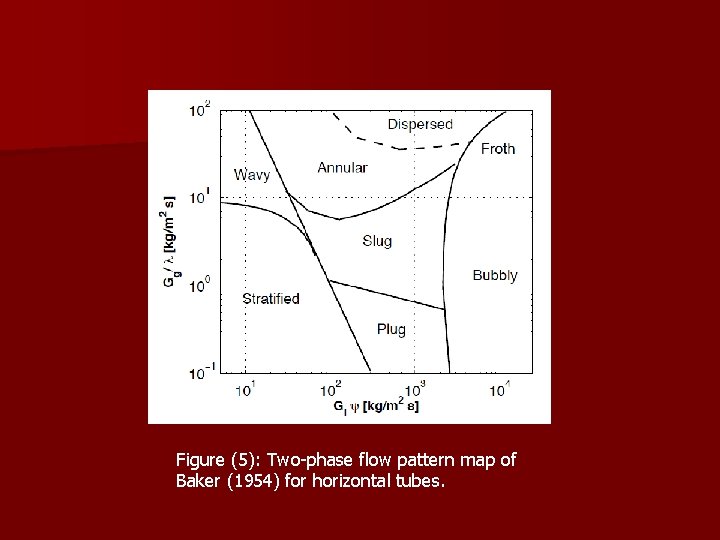
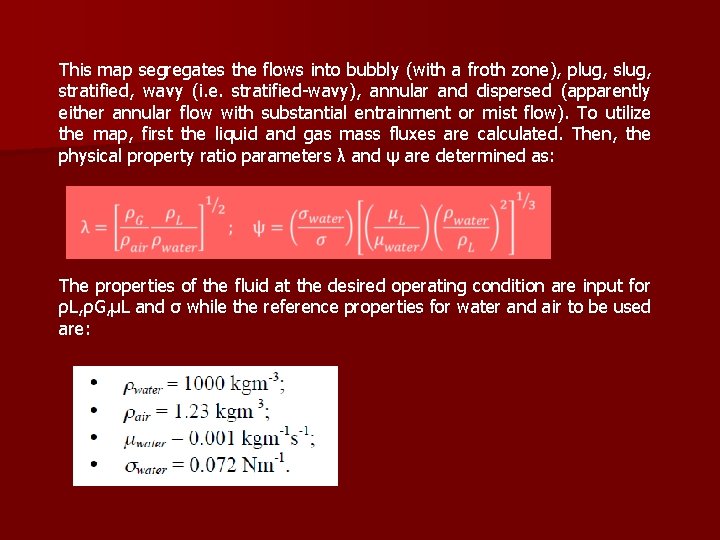
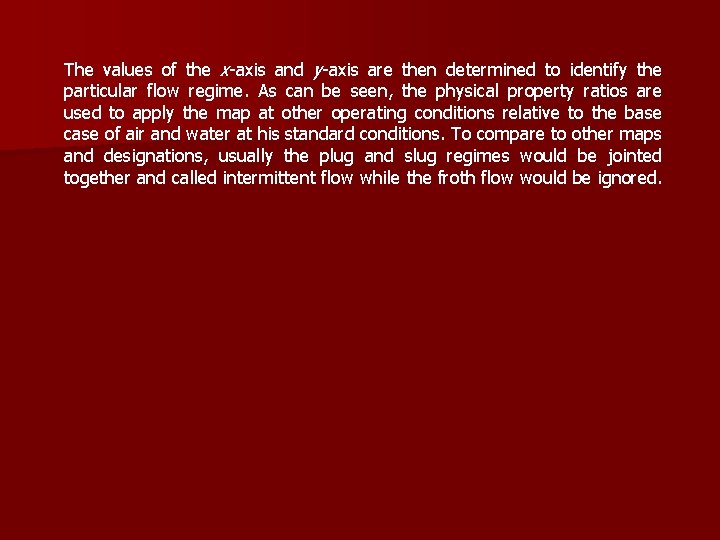
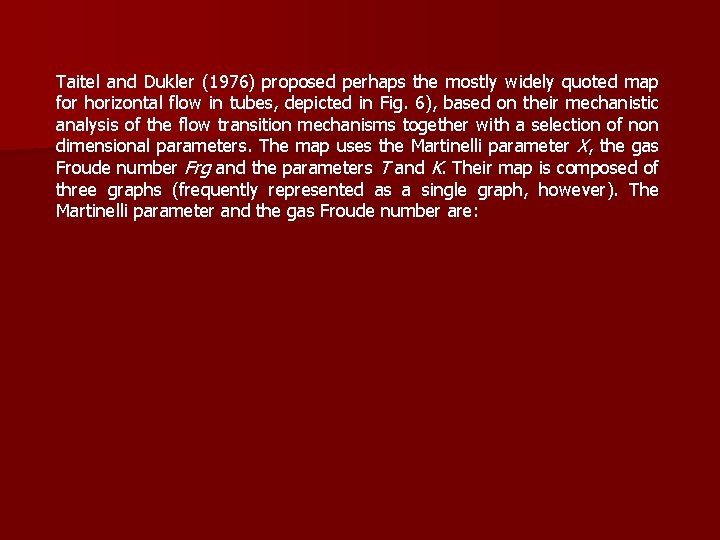
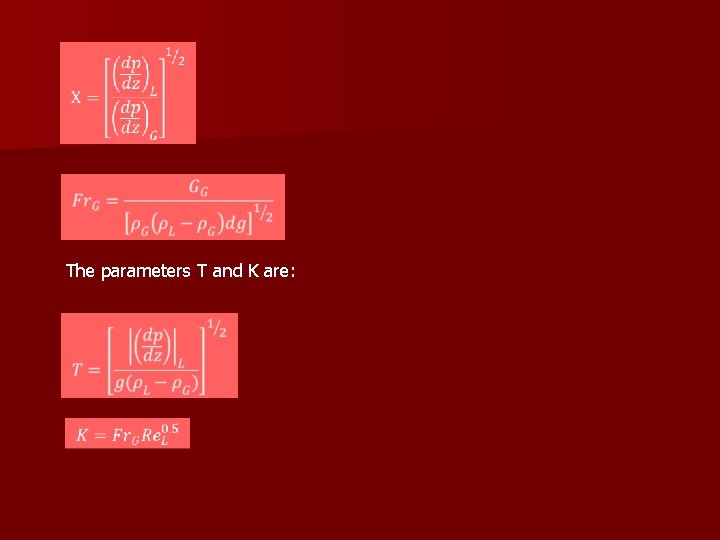
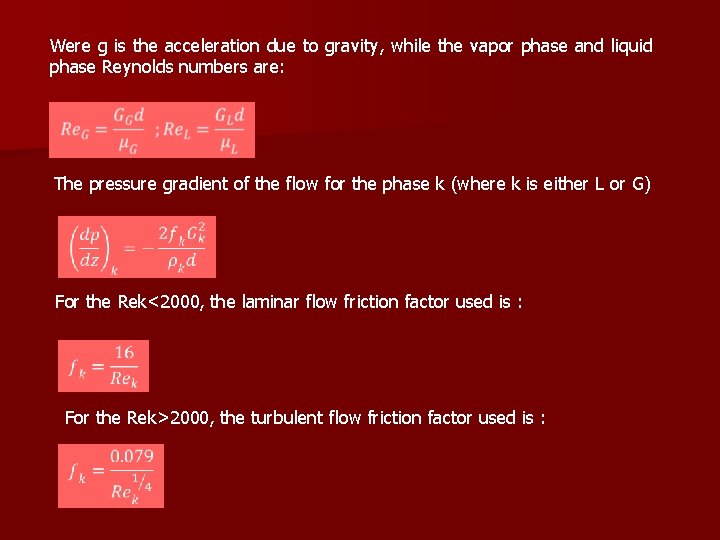
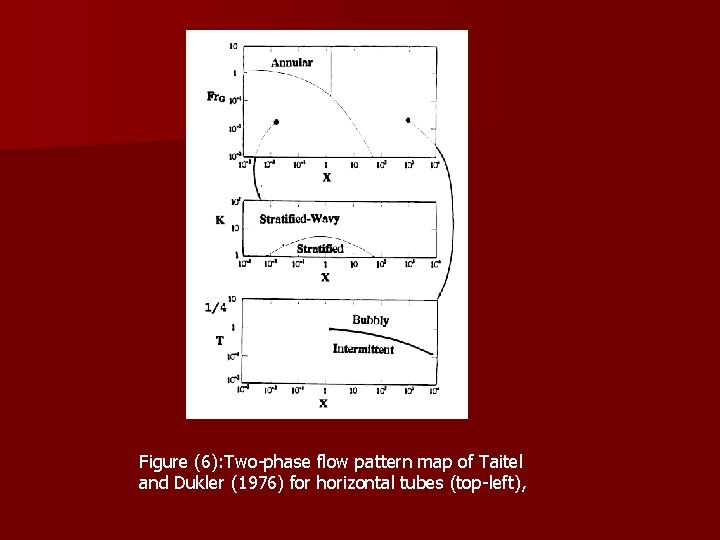
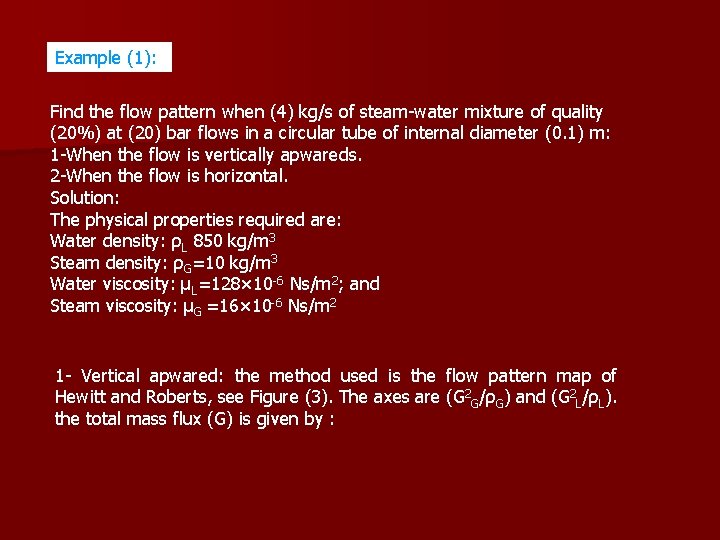
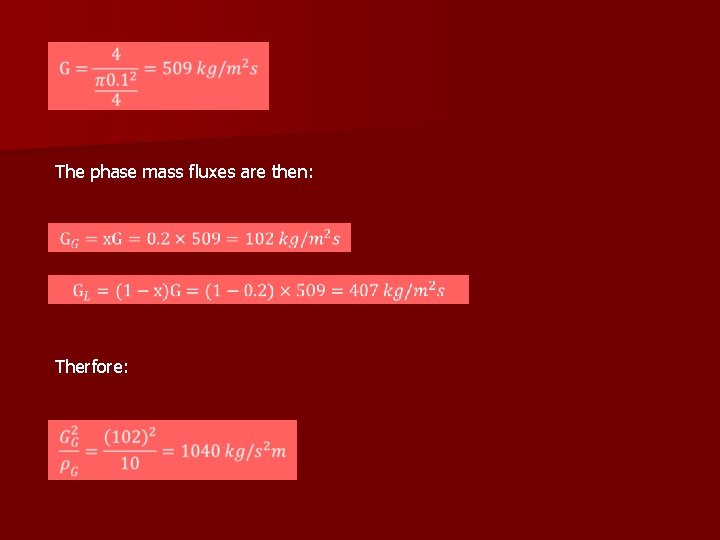
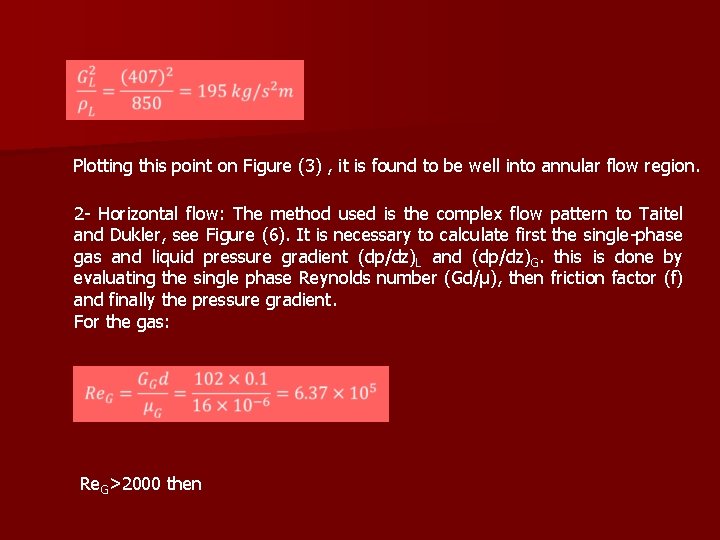
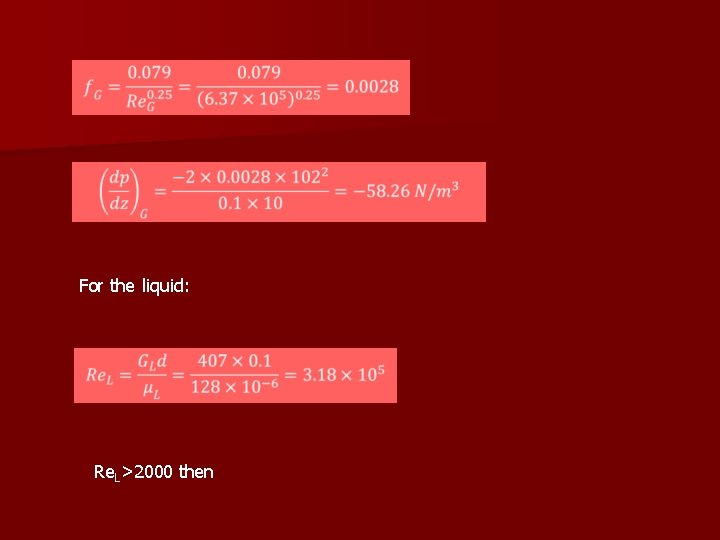
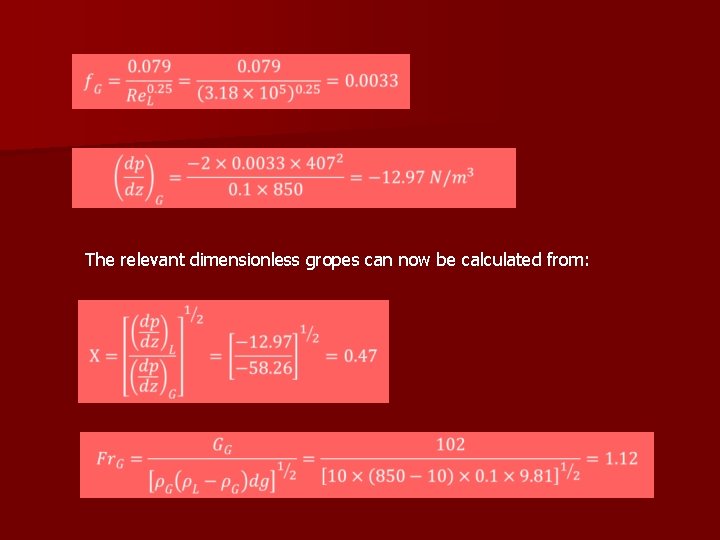
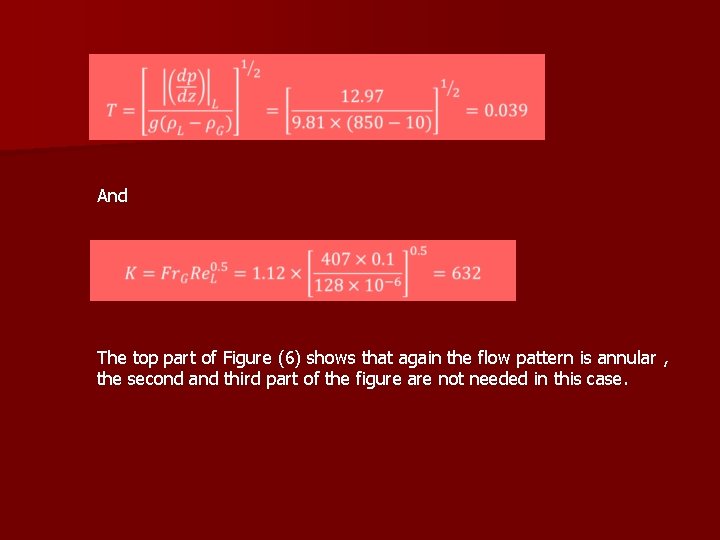
- Slides: 33
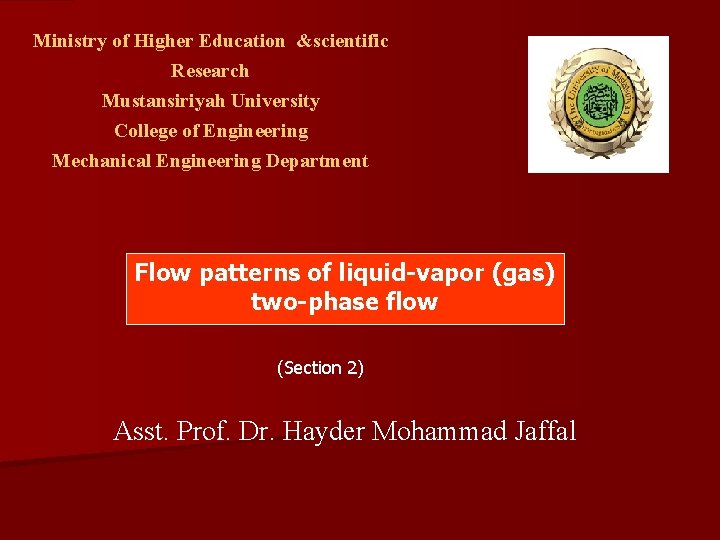
Ministry of Higher Education &scientific Research Mustansiriyah University College of Engineering Mechanical Engineering Department Flow patterns of liquid-vapor (gas) two-phase flow (Section 2) Asst. Prof. Dr. Hayder Mohammad Jaffal
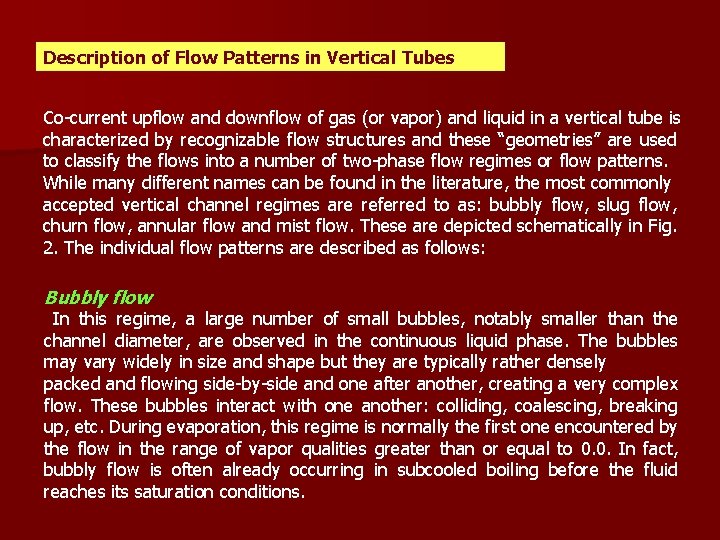
Description of Flow Patterns in Vertical Tubes Co-current upflow and downflow of gas (or vapor) and liquid in a vertical tube is characterized by recognizable flow structures and these “geometries” are used to classify the flows into a number of two-phase flow regimes or flow patterns. While many different names can be found in the literature, the most commonly accepted vertical channel regimes are referred to as: bubbly flow, slug flow, churn flow, annular flow and mist flow. These are depicted schematically in Fig. 2. The individual flow patterns are described as follows: Bubbly flow In this regime, a large number of small bubbles, notably smaller than the channel diameter, are observed in the continuous liquid phase. The bubbles may vary widely in size and shape but they are typically rather densely packed and flowing side-by-side and one after another, creating a very complex flow. These bubbles interact with one another: colliding, coalescing, breaking up, etc. During evaporation, this regime is normally the first one encountered by the flow in the range of vapor qualities greater than or equal to 0. 0. In fact, bubbly flow is often already occurring in subcooled boiling before the fluid reaches its saturation conditions.
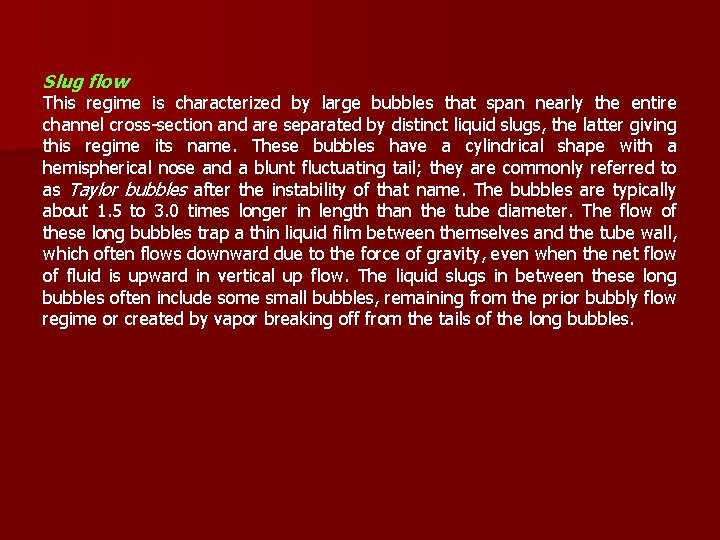
Slug flow This regime is characterized by large bubbles that span nearly the entire channel cross-section and are separated by distinct liquid slugs, the latter giving this regime its name. These bubbles have a cylindrical shape with a hemispherical nose and a blunt fluctuating tail; they are commonly referred to as Taylor bubbles after the instability of that name. The bubbles are typically about 1. 5 to 3. 0 times longer in length than the tube diameter. The flow of these long bubbles trap a thin liquid film between themselves and the tube wall, which often flows downward due to the force of gravity, even when the net flow of fluid is upward in vertical up flow. The liquid slugs in between these long bubbles often include some small bubbles, remaining from the prior bubbly flow regime or created by vapor breaking off from the tails of the long bubbles.
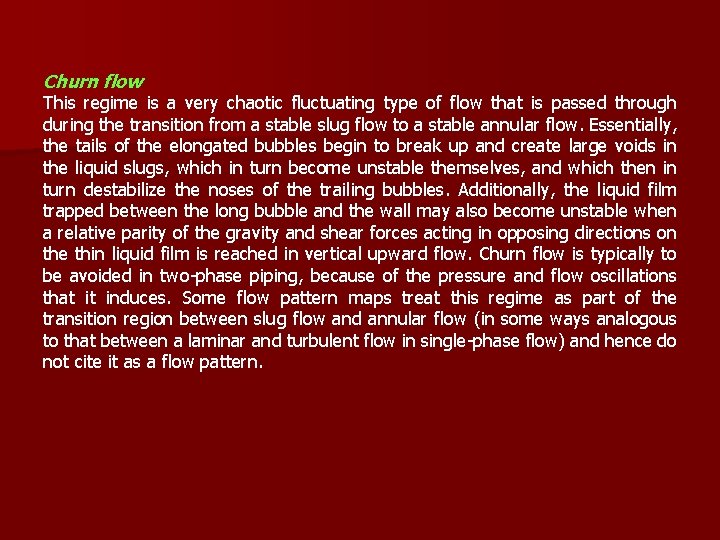
Churn flow This regime is a very chaotic fluctuating type of flow that is passed through during the transition from a stable slug flow to a stable annular flow. Essentially, the tails of the elongated bubbles begin to break up and create large voids in the liquid slugs, which in turn become unstable themselves, and which then in turn destabilize the noses of the trailing bubbles. Additionally, the liquid film trapped between the long bubble and the wall may also become unstable when a relative parity of the gravity and shear forces acting in opposing directions on the thin liquid film is reached in vertical upward flow. Churn flow is typically to be avoided in two-phase piping, because of the pressure and flow oscillations that it induces. Some flow pattern maps treat this regime as part of the transition region between slug flow and annular flow (in some ways analogous to that between a laminar and turbulent flow in single-phase flow) and hence do not cite it as a flow pattern.
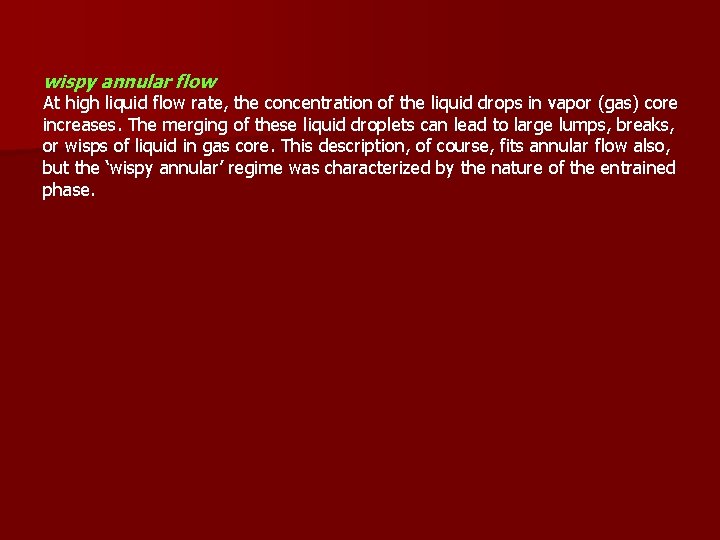
wispy annular flow At high liquid flow rate, the concentration of the liquid drops in vapor (gas) core increases. The merging of these liquid droplets can lead to large lumps, breaks, or wisps of liquid in gas core. This description, of course, fits annular flow also, but the ‘wispy annular’ regime was characterized by the nature of the entrained phase.
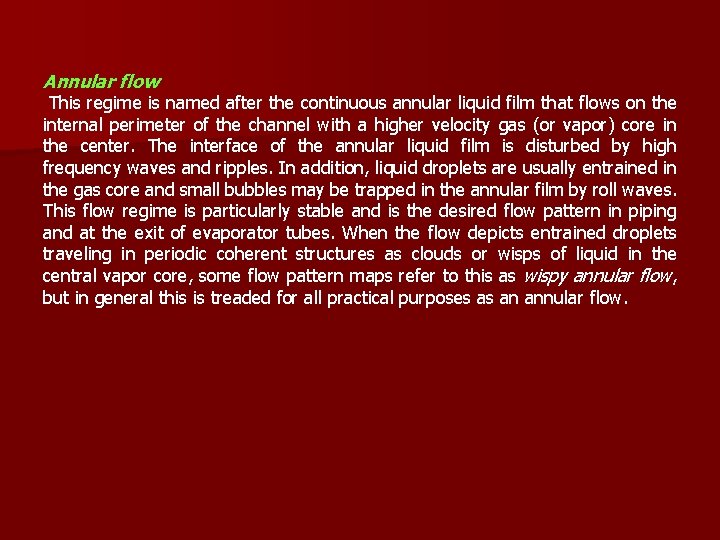
Annular flow This regime is named after the continuous annular liquid film that flows on the internal perimeter of the channel with a higher velocity gas (or vapor) core in the center. The interface of the annular liquid film is disturbed by high frequency waves and ripples. In addition, liquid droplets are usually entrained in the gas core and small bubbles may be trapped in the annular film by roll waves. This flow regime is particularly stable and is the desired flow pattern in piping and at the exit of evaporator tubes. When the flow depicts entrained droplets traveling in periodic coherent structures as clouds or wisps of liquid in the central vapor core, some flow pattern maps refer to this as wispy annular flow, but in general this is treaded for all practical purposes as an annular flow.
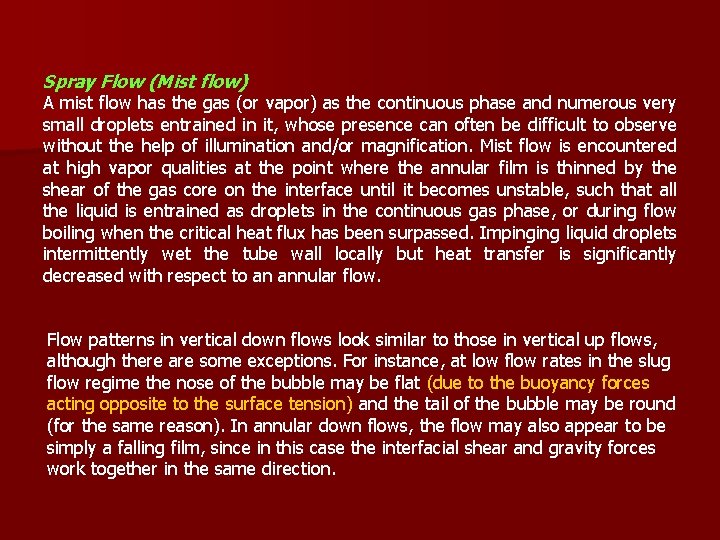
Spray Flow (Mist flow) A mist flow has the gas (or vapor) as the continuous phase and numerous very small droplets entrained in it, whose presence can often be difficult to observe without the help of illumination and/or magnification. Mist flow is encountered at high vapor qualities at the point where the annular film is thinned by the shear of the gas core on the interface until it becomes unstable, such that all the liquid is entrained as droplets in the continuous gas phase, or during flow boiling when the critical heat flux has been surpassed. Impinging liquid droplets intermittently wet the tube wall locally but heat transfer is significantly decreased with respect to an annular flow. Flow patterns in vertical down flows look similar to those in vertical up flows, although there are some exceptions. For instance, at low flow rates in the slug flow regime the nose of the bubble may be flat (due to the buoyancy forces acting opposite to the surface tension) and the tail of the bubble may be round (for the same reason). In annular down flows, the flow may also appear to be simply a falling film, since in this case the interfacial shear and gravity forces work together in the same direction.
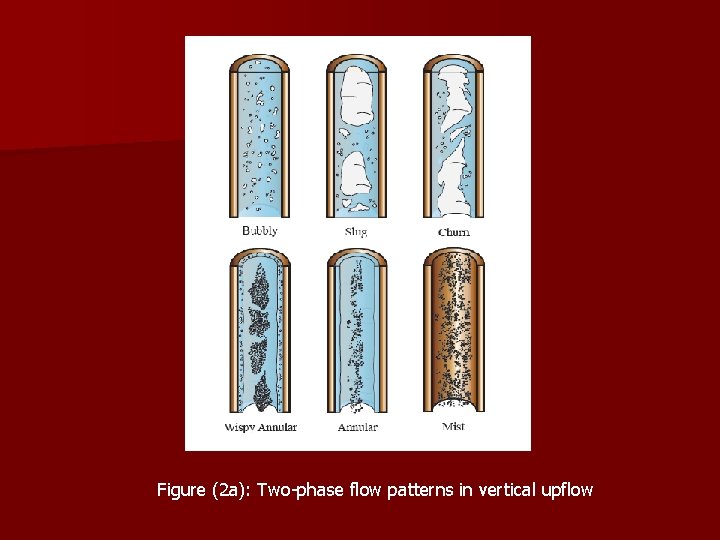
Figure (2 a): Two-phase flow patterns in vertical upflow
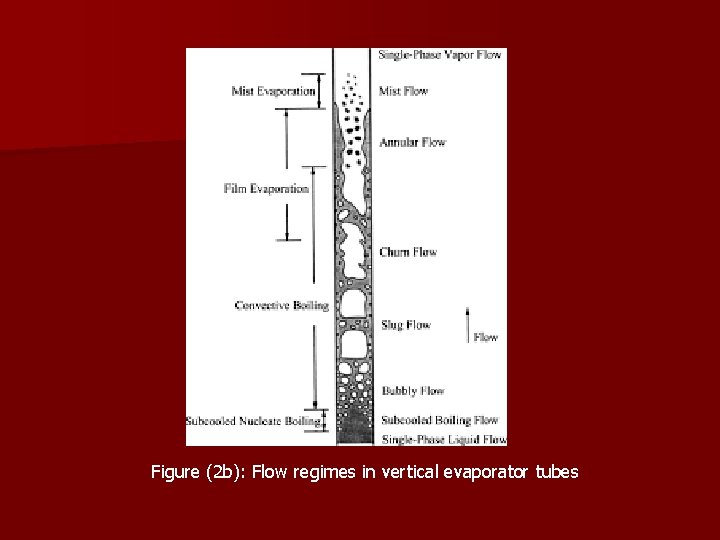
Figure (2 b): Flow regimes in vertical evaporator tubes
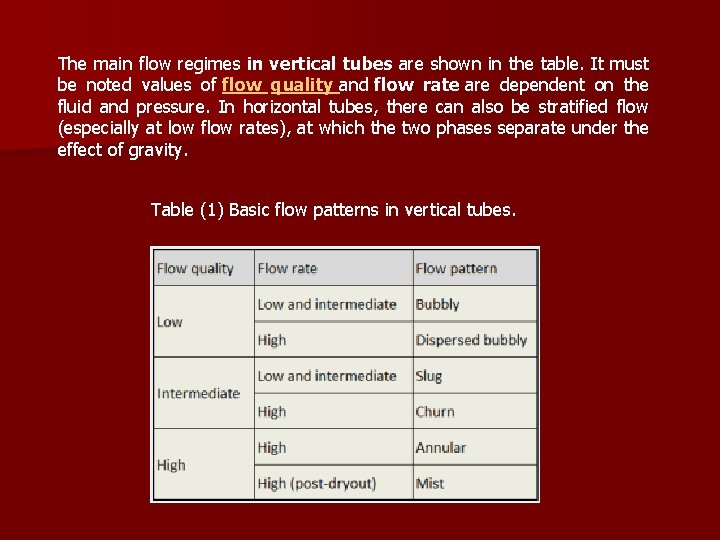
The main flow regimes in vertical tubes are shown in the table. It must be noted values of flow quality and flow rate are dependent on the fluid and pressure. In horizontal tubes, there can also be stratified flow (especially at low flow rates), at which the two phases separate under the effect of gravity. Table (1) Basic flow patterns in vertical tubes.
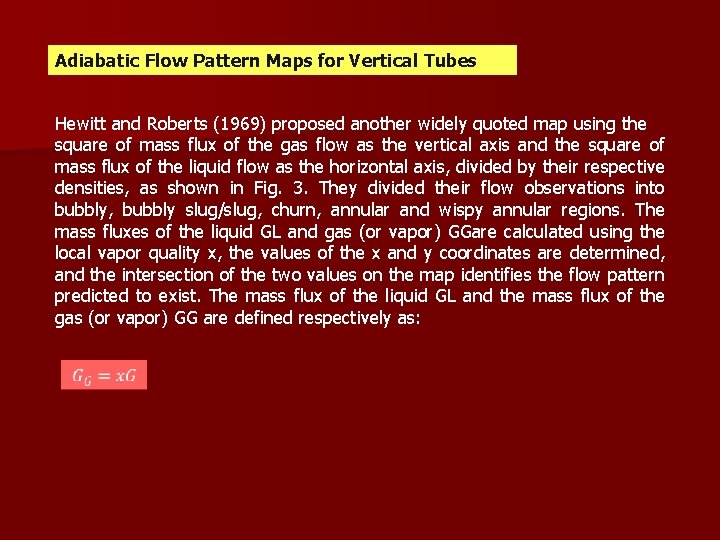
Adiabatic Flow Pattern Maps for Vertical Tubes Hewitt and Roberts (1969) proposed another widely quoted map using the square of mass flux of the gas flow as the vertical axis and the square of mass flux of the liquid flow as the horizontal axis, divided by their respective densities, as shown in Fig. 3. They divided their flow observations into bubbly, bubbly slug/slug, churn, annular and wispy annular regions. The mass fluxes of the liquid GL and gas (or vapor) GGare calculated using the local vapor quality x, the values of the x and y coordinates are determined, and the intersection of the two values on the map identifies the flow pattern predicted to exist. The mass flux of the liquid GL and the mass flux of the gas (or vapor) GG are defined respectively as:
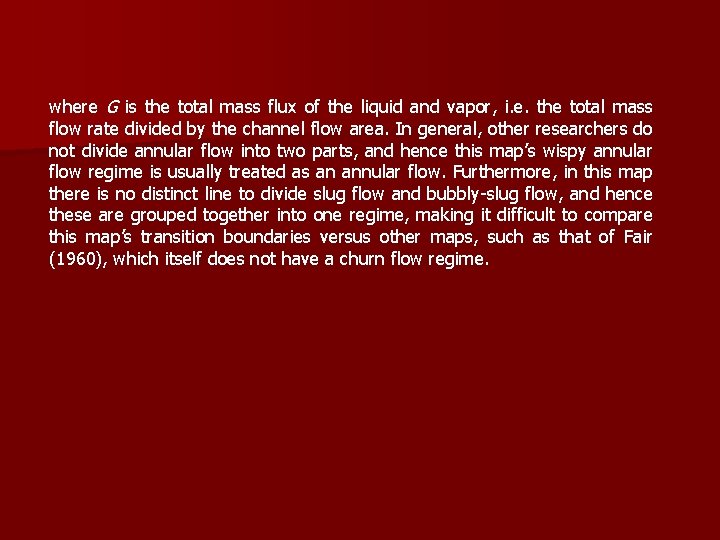
where G is the total mass flux of the liquid and vapor, i. e. the total mass flow rate divided by the channel flow area. In general, other researchers do not divide annular flow into two parts, and hence this map’s wispy annular flow regime is usually treated as an annular flow. Furthermore, in this map there is no distinct line to divide slug flow and bubbly-slug flow, and hence these are grouped together into one regime, making it difficult to compare this map’s transition boundaries versus other maps, such as that of Fair (1960), which itself does not have a churn flow regime.
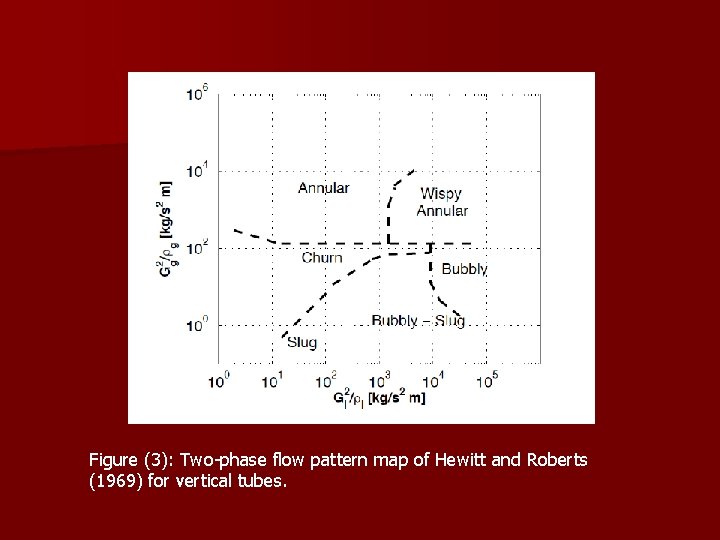
Figure (3): Two-phase flow pattern map of Hewitt and Roberts (1969) for vertical tubes.
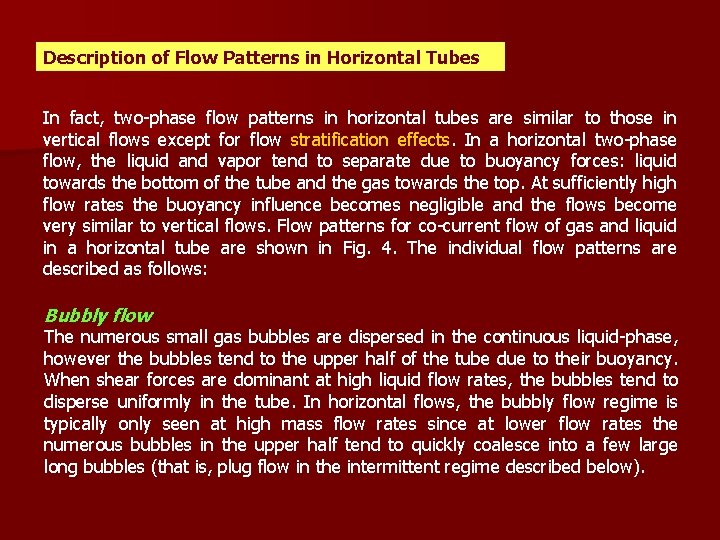
Description of Flow Patterns in Horizontal Tubes In fact, two-phase flow patterns in horizontal tubes are similar to those in vertical flows except for flow stratification effects. In a horizontal two-phase flow, the liquid and vapor tend to separate due to buoyancy forces: liquid towards the bottom of the tube and the gas towards the top. At sufficiently high flow rates the buoyancy influence becomes negligible and the flows become very similar to vertical flows. Flow patterns for co-current flow of gas and liquid in a horizontal tube are shown in Fig. 4. The individual flow patterns are described as follows: Bubbly flow The numerous small gas bubbles are dispersed in the continuous liquid-phase, however the bubbles tend to the upper half of the tube due to their buoyancy. When shear forces are dominant at high liquid flow rates, the bubbles tend to disperse uniformly in the tube. In horizontal flows, the bubbly flow regime is typically only seen at high mass flow rates since at lower flow rates the numerous bubbles in the upper half tend to quickly coalesce into a few large long bubbles (that is, plug flow in the intermittent regime described below).
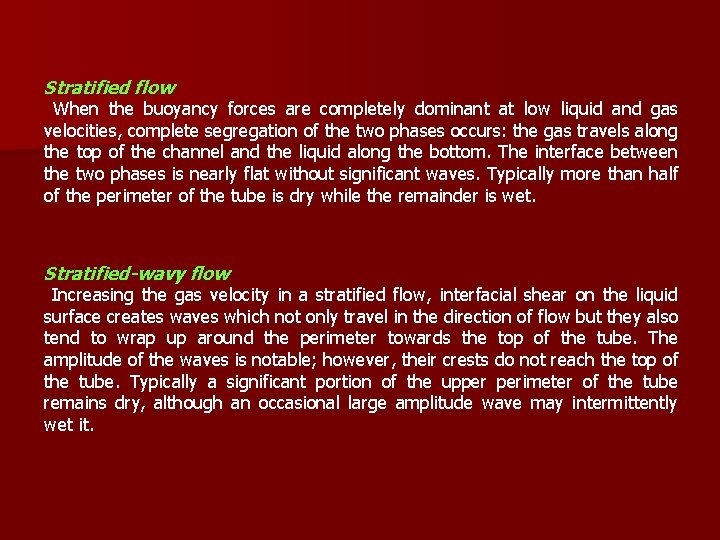
Stratified flow When the buoyancy forces are completely dominant at low liquid and gas velocities, complete segregation of the two phases occurs: the gas travels along the top of the channel and the liquid along the bottom. The interface between the two phases is nearly flat without significant waves. Typically more than half of the perimeter of the tube is dry while the remainder is wet. Stratified-wavy flow Increasing the gas velocity in a stratified flow, interfacial shear on the liquid surface creates waves which not only travel in the direction of flow but they also tend to wrap up around the perimeter towards the top of the tube. The amplitude of the waves is notable; however, their crests do not reach the top of the tube. Typically a significant portion of the upper perimeter of the tube remains dry, although an occasional large amplitude wave may intermittently wet it.
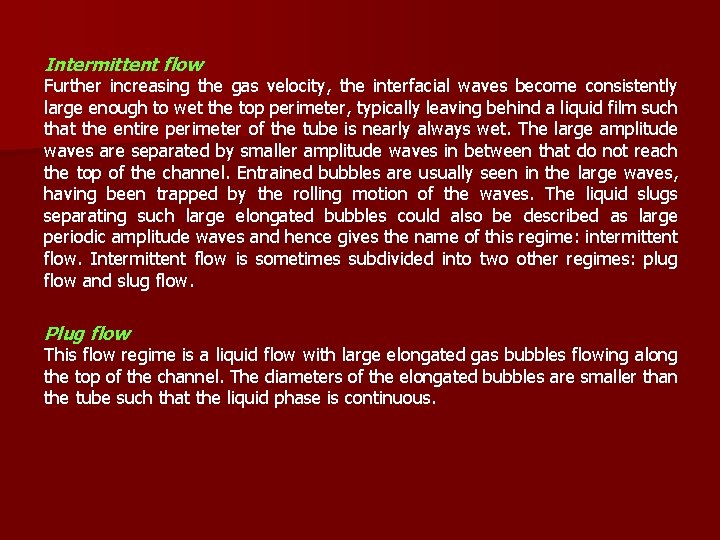
Intermittent flow Further increasing the gas velocity, the interfacial waves become consistently large enough to wet the top perimeter, typically leaving behind a liquid film such that the entire perimeter of the tube is nearly always wet. The large amplitude waves are separated by smaller amplitude waves in between that do not reach the top of the channel. Entrained bubbles are usually seen in the large waves, having been trapped by the rolling motion of the waves. The liquid slugs separating such large elongated bubbles could also be described as large periodic amplitude waves and hence gives the name of this regime: intermittent flow. Intermittent flow is sometimes subdivided into two other regimes: plug flow and slug flow. Plug flow This flow regime is a liquid flow with large elongated gas bubbles flowing along the top of the channel. The diameters of the elongated bubbles are smaller than the tube such that the liquid phase is continuous.
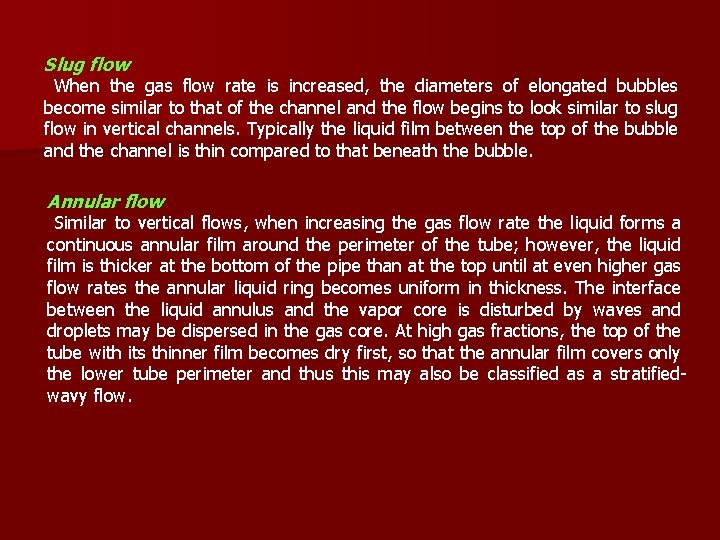
Slug flow When the gas flow rate is increased, the diameters of elongated bubbles become similar to that of the channel and the flow begins to look similar to slug flow in vertical channels. Typically the liquid film between the top of the bubble and the channel is thin compared to that beneath the bubble. Annular flow Similar to vertical flows, when increasing the gas flow rate the liquid forms a continuous annular film around the perimeter of the tube; however, the liquid film is thicker at the bottom of the pipe than at the top until at even higher gas flow rates the annular liquid ring becomes uniform in thickness. The interface between the liquid annulus and the vapor core is disturbed by waves and droplets may be dispersed in the gas core. At high gas fractions, the top of the tube with its thinner film becomes dry first, so that the annular film covers only the lower tube perimeter and thus this may also be classified as a stratifiedwavy flow.
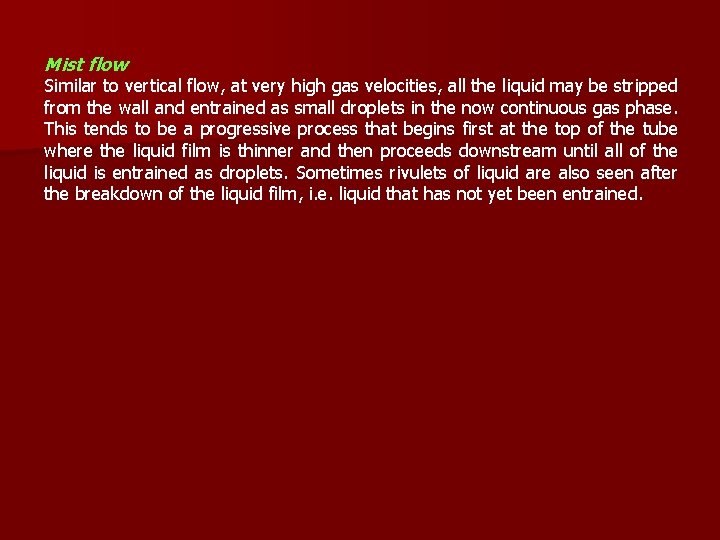
Mist flow Similar to vertical flow, at very high gas velocities, all the liquid may be stripped from the wall and entrained as small droplets in the now continuous gas phase. This tends to be a progressive process that begins first at the top of the tube where the liquid film is thinner and then proceeds downstream until all of the liquid is entrained as droplets. Sometimes rivulets of liquid are also seen after the breakdown of the liquid film, i. e. liquid that has not yet been entrained.
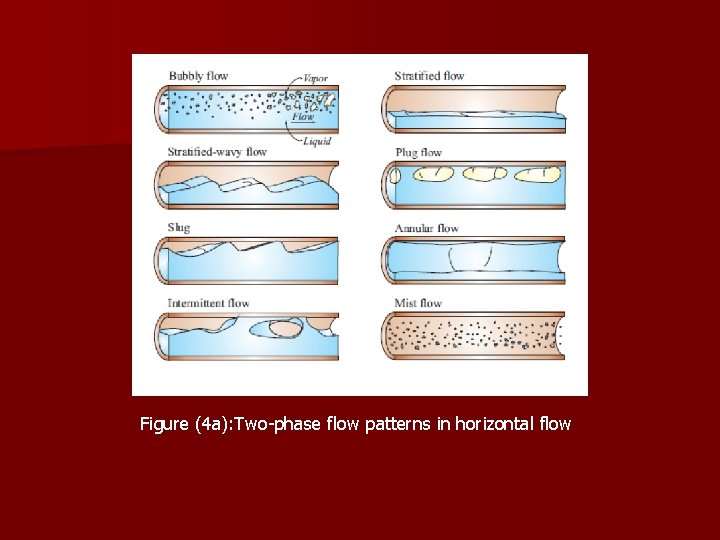
Figure (4 a): Two-phase flow patterns in horizontal flow
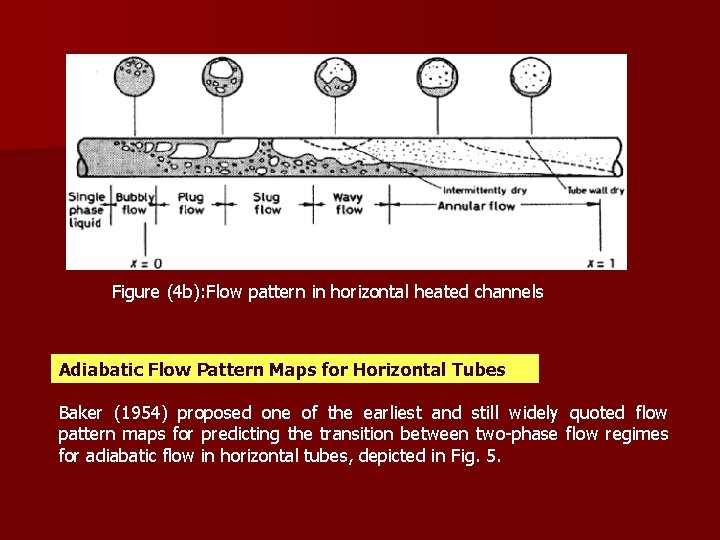
Figure (4 b): Flow pattern in horizontal heated channels Adiabatic Flow Pattern Maps for Horizontal Tubes Baker (1954) proposed one of the earliest and still widely quoted flow pattern maps for predicting the transition between two-phase flow regimes for adiabatic flow in horizontal tubes, depicted in Fig. 5.
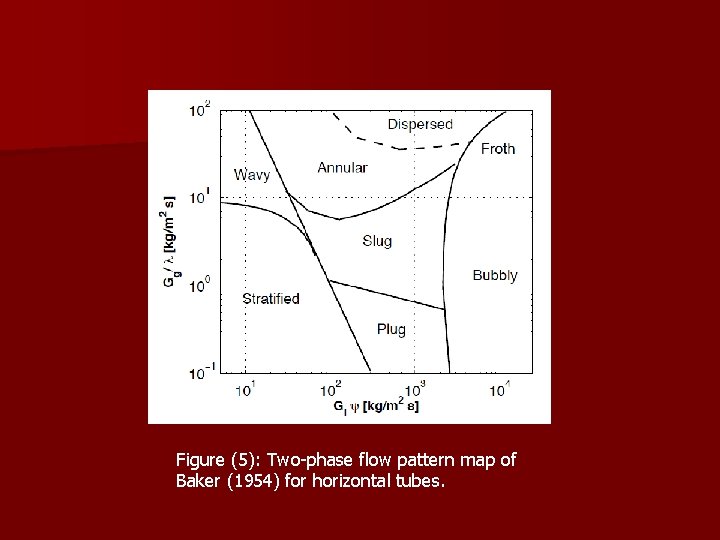
Figure (5): Two-phase flow pattern map of Baker (1954) for horizontal tubes.
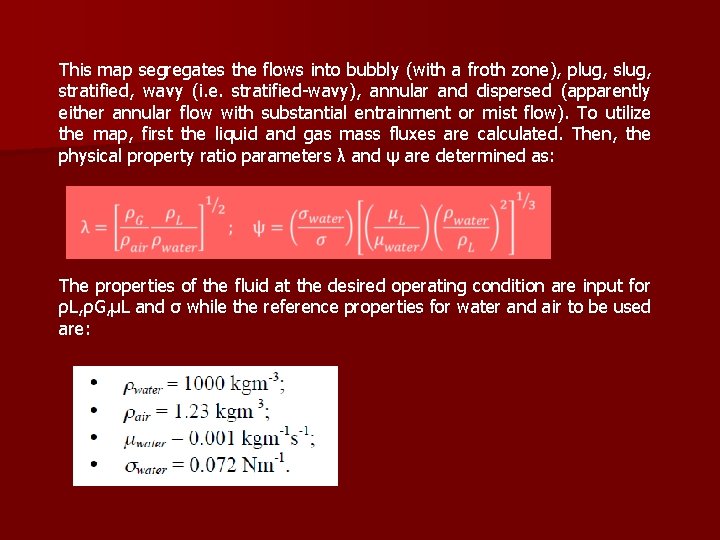
This map segregates the flows into bubbly (with a froth zone), plug, stratified, wavy (i. e. stratified-wavy), annular and dispersed (apparently either annular flow with substantial entrainment or mist flow). To utilize the map, first the liquid and gas mass fluxes are calculated. Then, the physical property ratio parameters λ and ψ are determined as: The properties of the fluid at the desired operating condition are input for ρL, ρG, μL and σ while the reference properties for water and air to be used are:
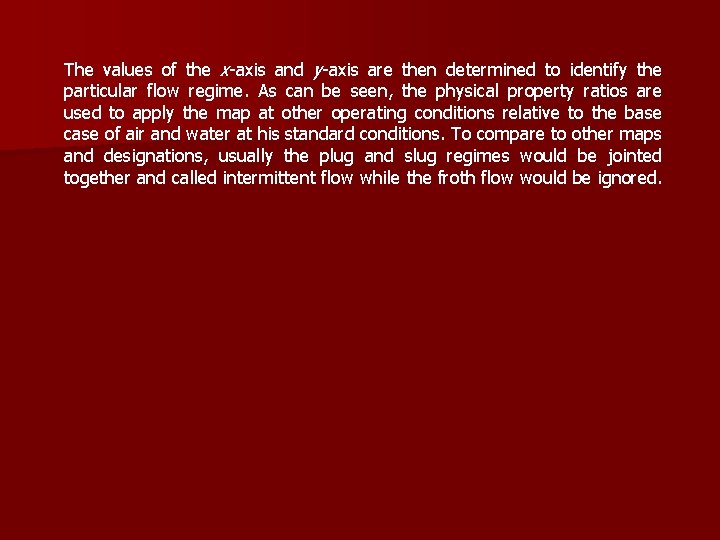
The values of the x-axis and y-axis are then determined to identify the particular flow regime. As can be seen, the physical property ratios are used to apply the map at other operating conditions relative to the base case of air and water at his standard conditions. To compare to other maps and designations, usually the plug and slug regimes would be jointed together and called intermittent flow while the froth flow would be ignored.
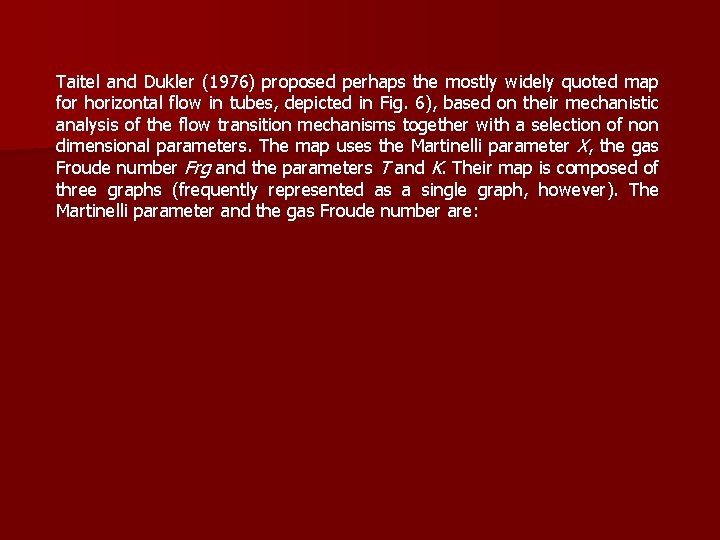
Taitel and Dukler (1976) proposed perhaps the mostly widely quoted map for horizontal flow in tubes, depicted in Fig. 6), based on their mechanistic analysis of the flow transition mechanisms together with a selection of non dimensional parameters. The map uses the Martinelli parameter X, the gas Froude number Frg and the parameters T and K. Their map is composed of three graphs (frequently represented as a single graph, however). The Martinelli parameter and the gas Froude number are:
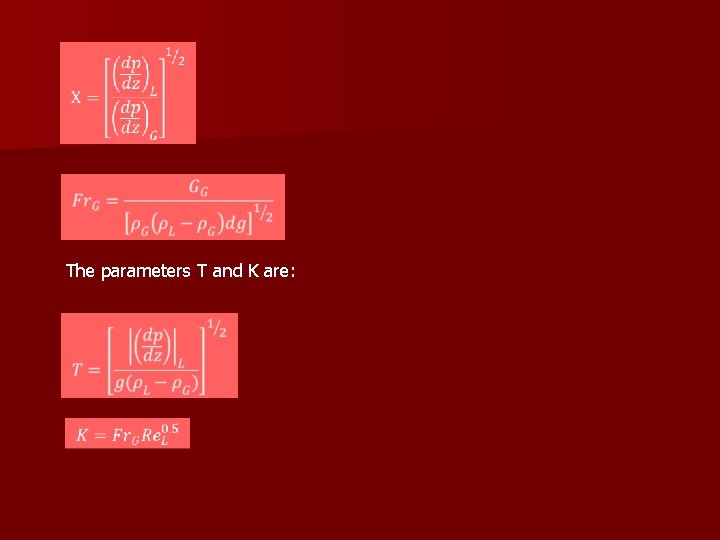
The parameters T and K are:
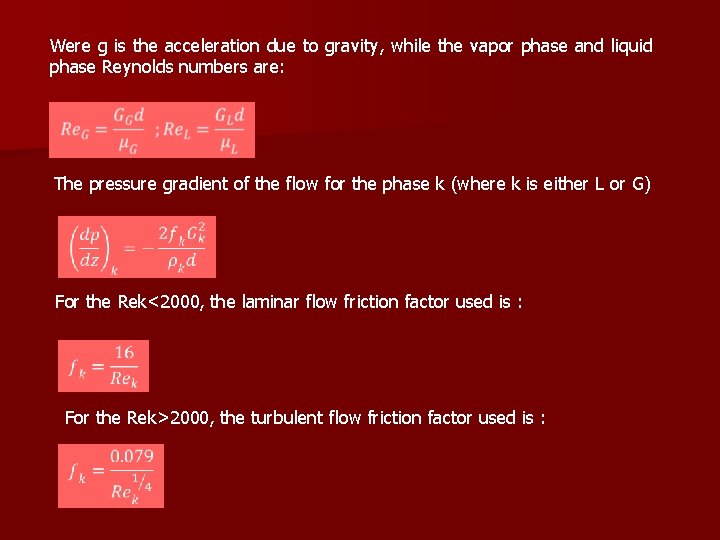
Were g is the acceleration due to gravity, while the vapor phase and liquid phase Reynolds numbers are: The pressure gradient of the flow for the phase k (where k is either L or G) For the Rek<2000, the laminar flow friction factor used is : For the Rek>2000, the turbulent flow friction factor used is :
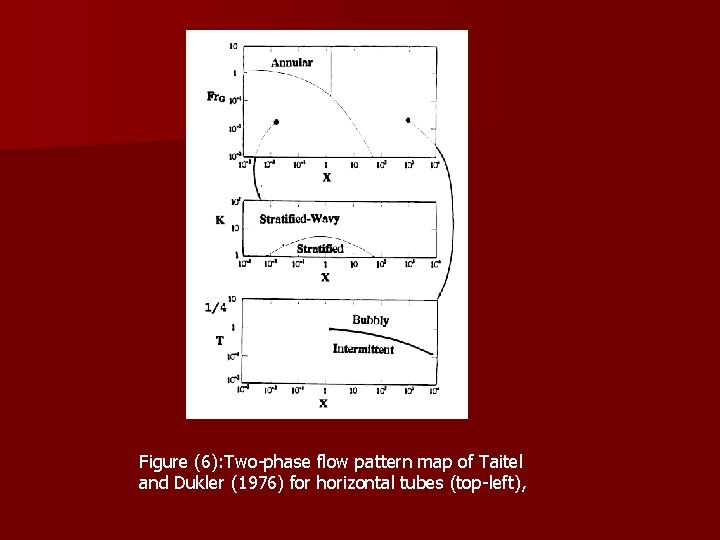
Figure (6): Two-phase flow pattern map of Taitel and Dukler (1976) for horizontal tubes (top-left),
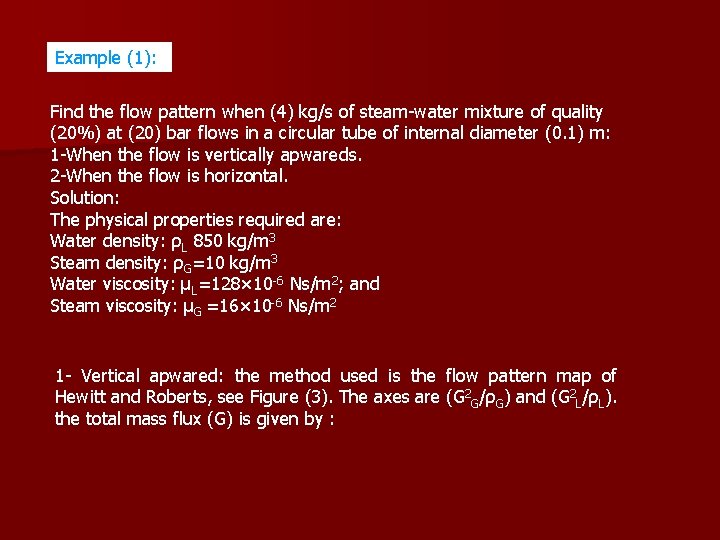
Example (1): Find the flow pattern when (4) kg/s of steam-water mixture of quality (20%) at (20) bar flows in a circular tube of internal diameter (0. 1) m: 1 -When the flow is vertically apwareds. 2 -When the flow is horizontal. Solution: The physical properties required are: Water density: ρL 850 kg/m 3 Steam density: ρG=10 kg/m 3 Water viscosity: μL=128× 10 -6 Ns/m 2; and Steam viscosity: μG =16× 10 -6 Ns/m 2 1 - Vertical apwared: the method used is the flow pattern map of Hewitt and Roberts, see Figure (3). The axes are (G 2 G/ρG) and (G 2 L/ρL). the total mass flux (G) is given by :
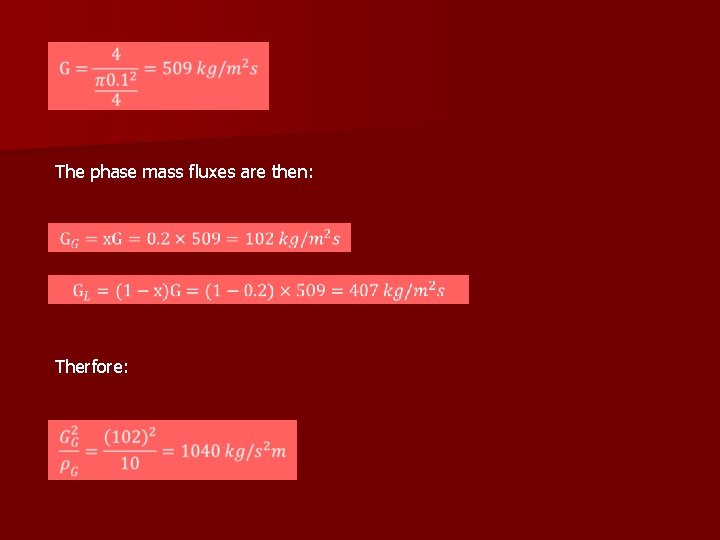
The phase mass fluxes are then: Therfore:
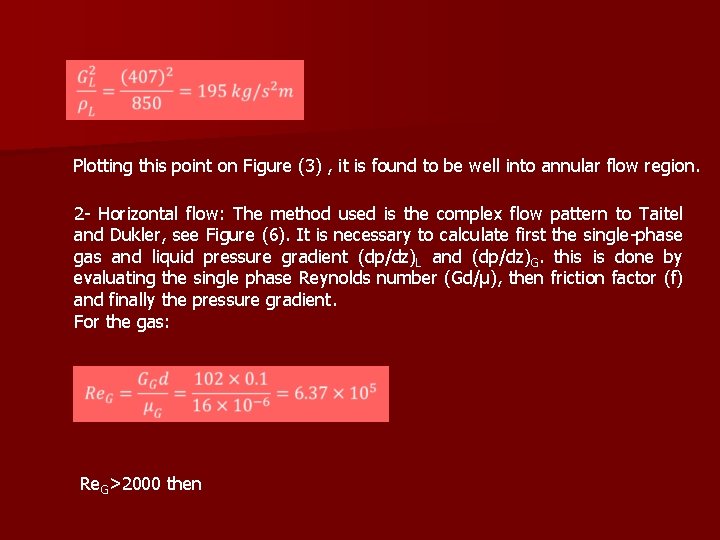
Plotting this point on Figure (3) , it is found to be well into annular flow region. 2 - Horizontal flow: The method used is the complex flow pattern to Taitel and Dukler, see Figure (6). It is necessary to calculate first the single-phase gas and liquid pressure gradient (dp/dz)L and (dp/dz)G. this is done by evaluating the single phase Reynolds number (Gd/μ), then friction factor (f) and finally the pressure gradient. For the gas: Re. G>2000 then
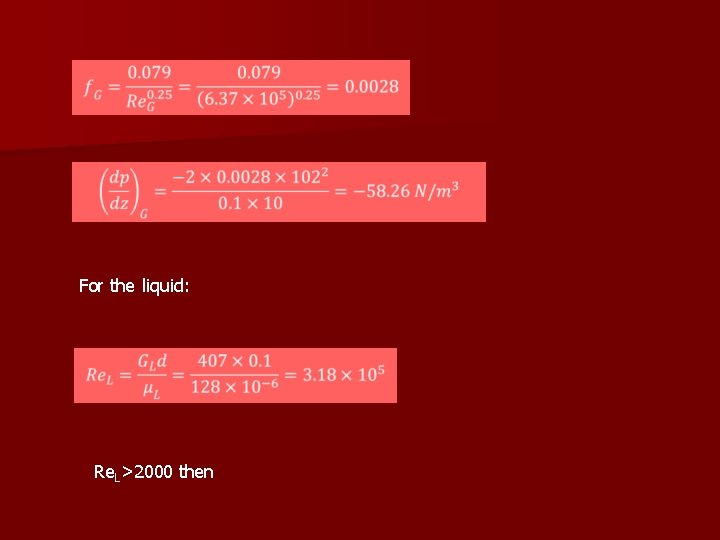
For the liquid: Re. L>2000 then
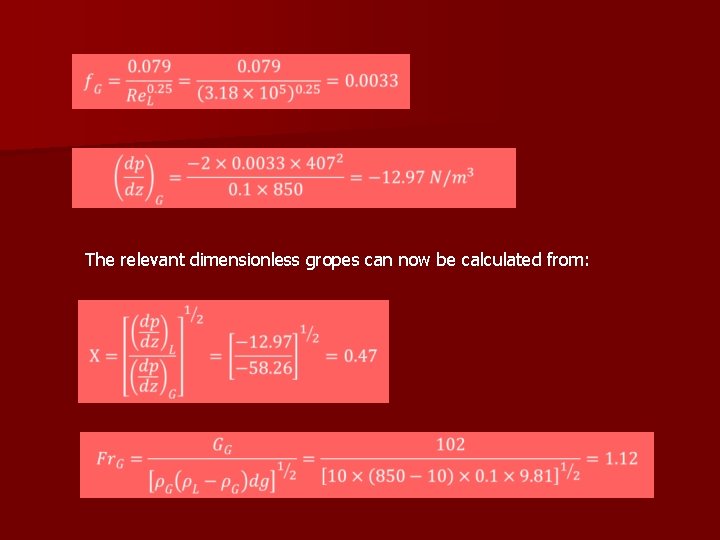
The relevant dimensionless gropes can now be calculated from:
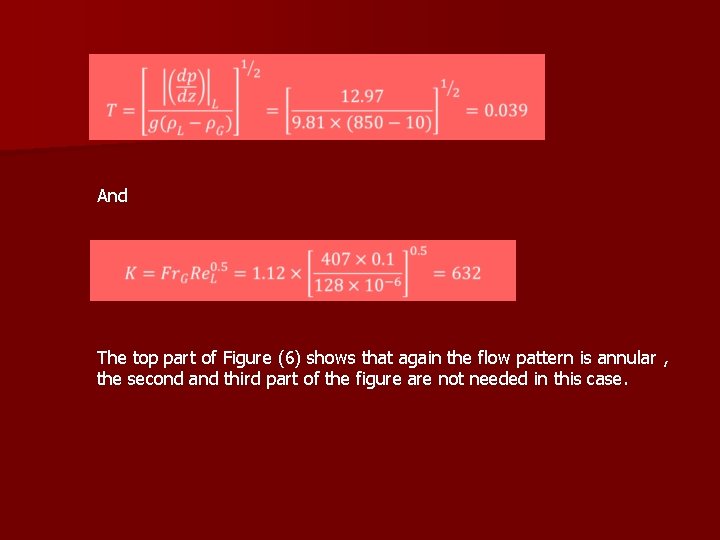
And The top part of Figure (6) shows that again the flow pattern is annular , the second and third part of the figure are not needed in this case.
Ministry of higher education (afghanistan)
Kurdistan
Krg ministry of higher education
Kurdistan
Ministry of higher education saudi arabia
Ministry of higher education erbil
Ministry of higher education tunisia
Ministry of higher education saudi
Ministry of education romania
Ministry of education and research romania
Seattle university school of theology and ministry
Ministry of education serbia
Kuwait ministry of education
Matriculation certificate
Ministry of education armenia
التعليم الخاص مبارك الكبير
Ministry of education iceland
Ministry of education and culture cyprus
Ministry of national education morocco
Life skills
Ministry of education
Ministry of education peru
Secondary engagement programme
Hellenic ministry of education and religious affairs
Ministry of education
Ministry of education
Ministry of education and science of the russian federation
Ministry of national education romania
Ministry of education moldova
Ministry of education zone 1
Information gathered during an experiment
How is a scientific law different from a scientific theory?
Web analytics in higher education
Capacity building in the field of higher education