Elementary Particle Physics Status and Prospects Mogens Dam
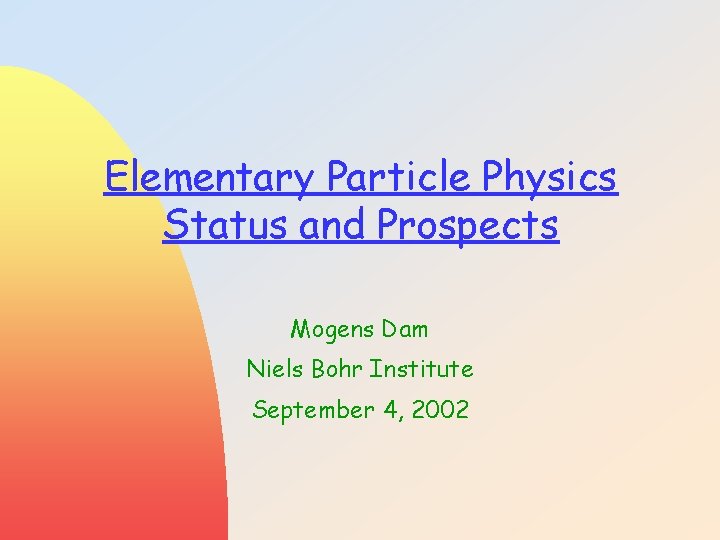
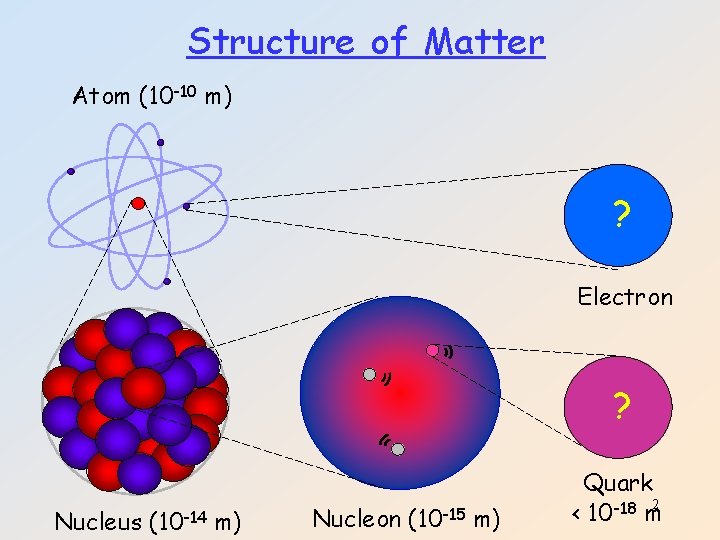
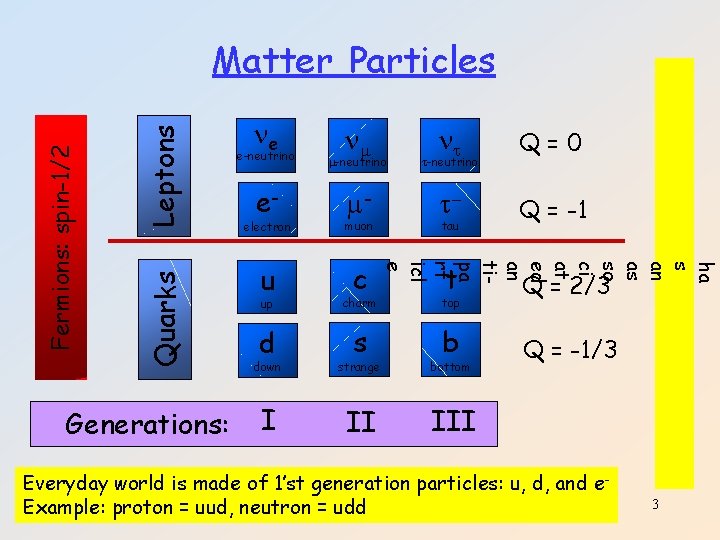
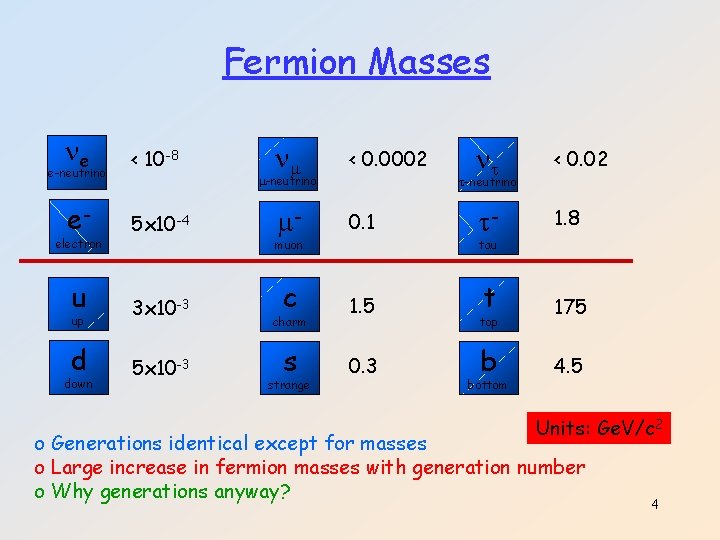
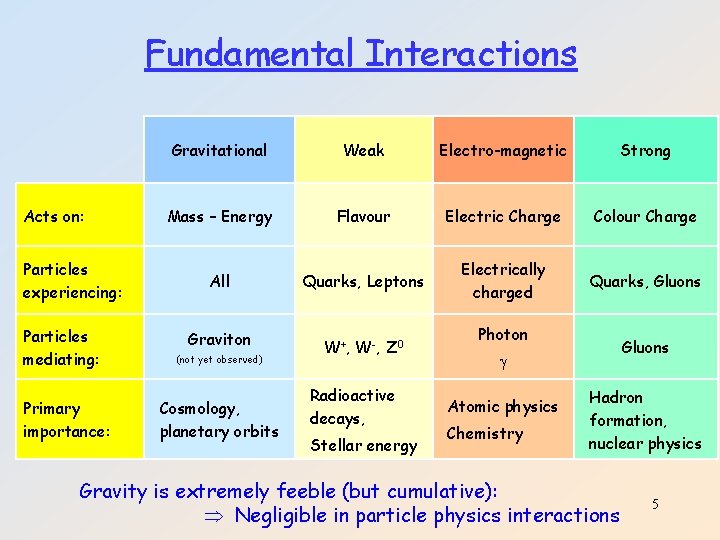
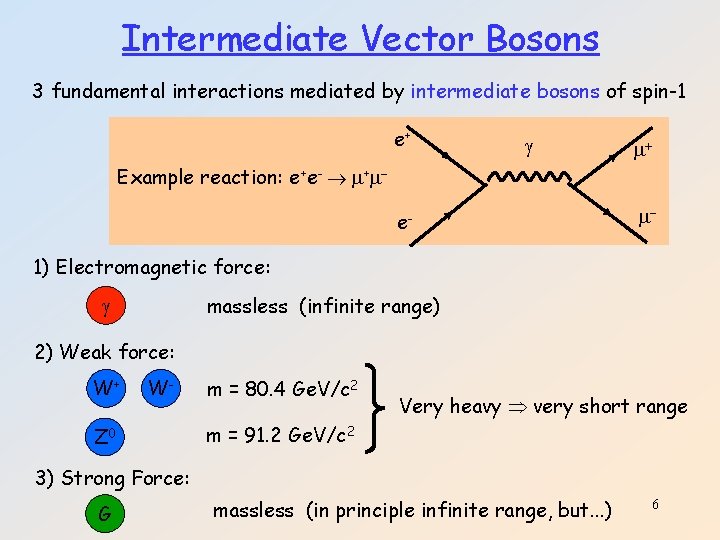
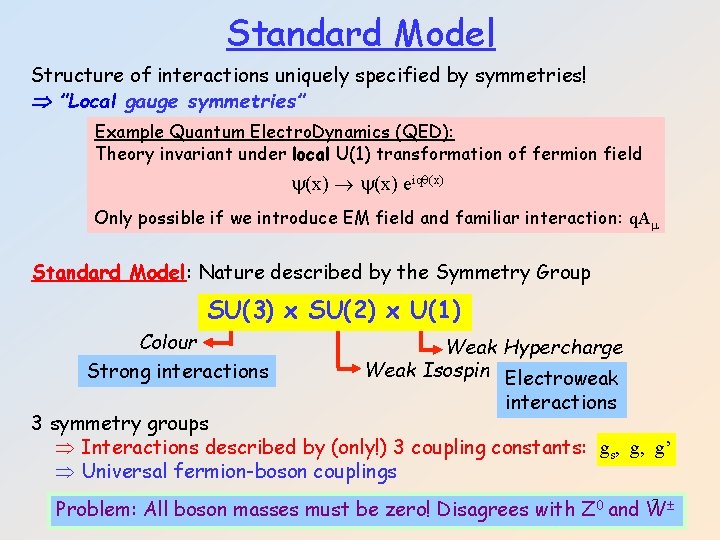
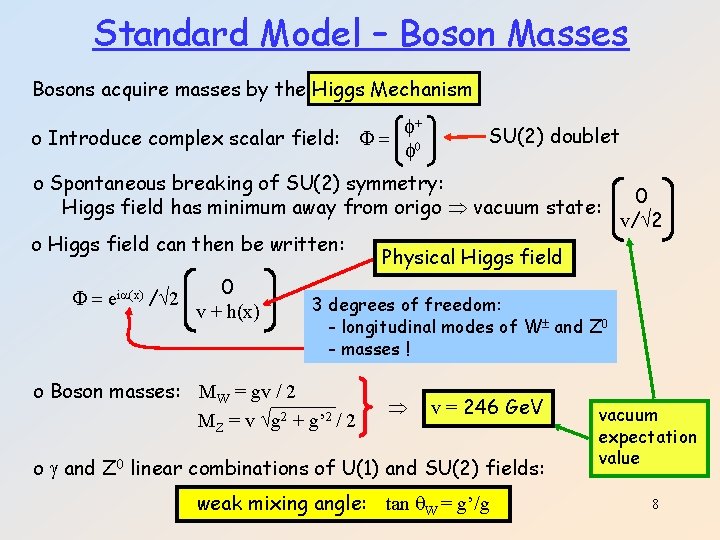
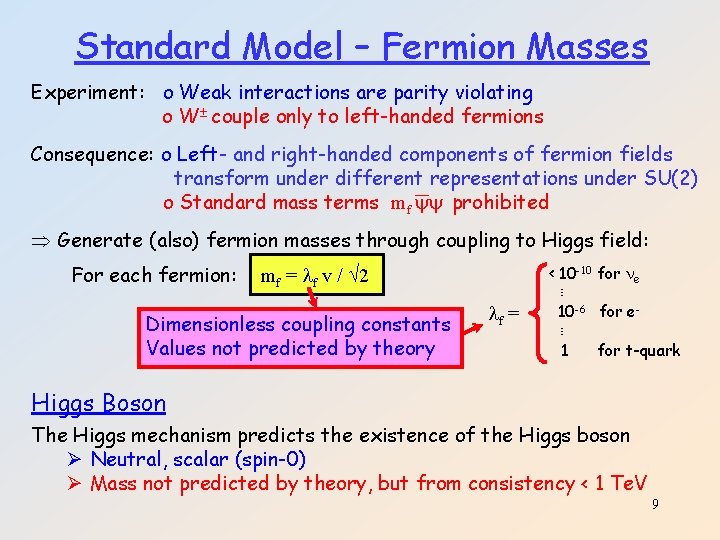
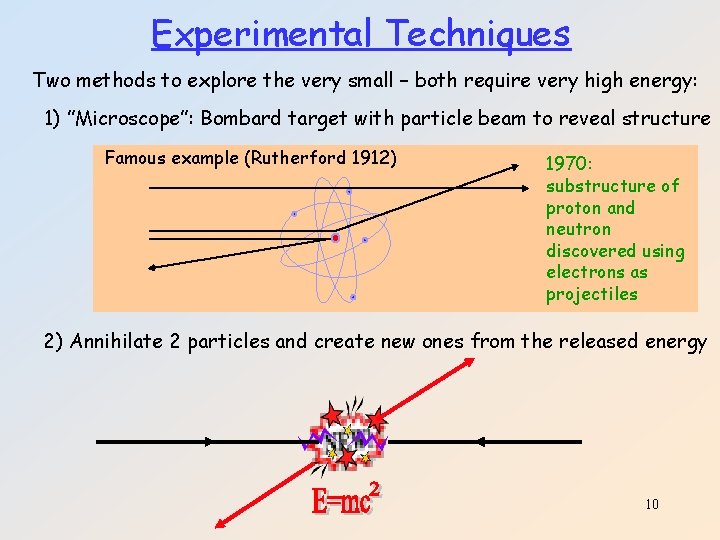
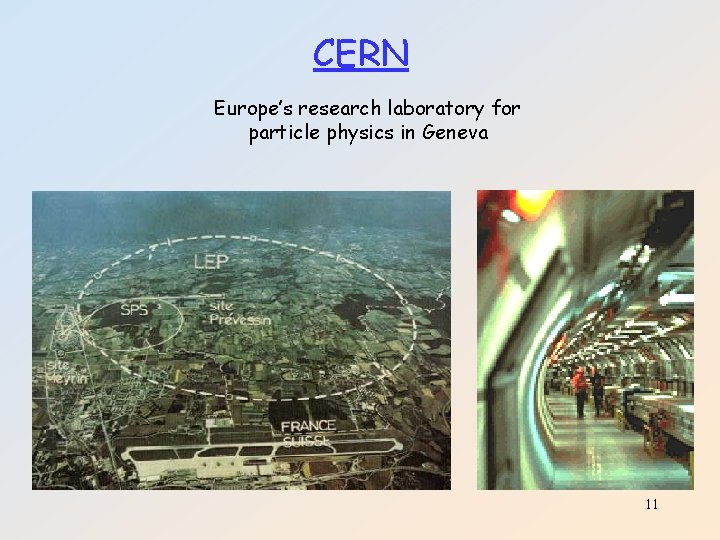
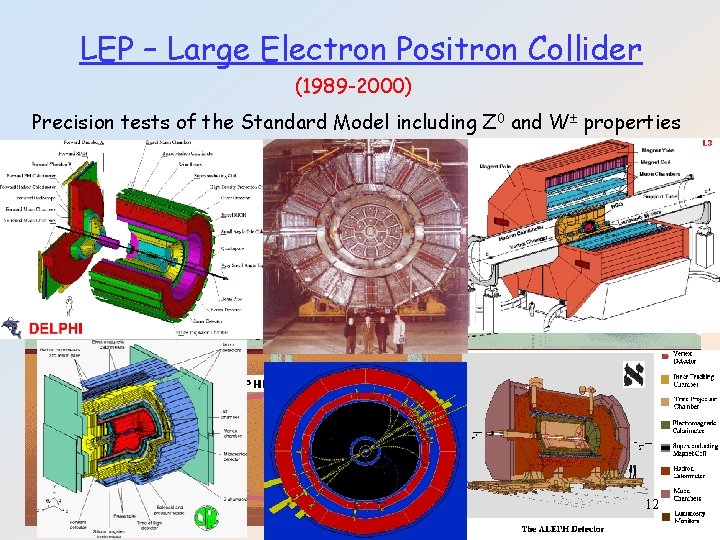
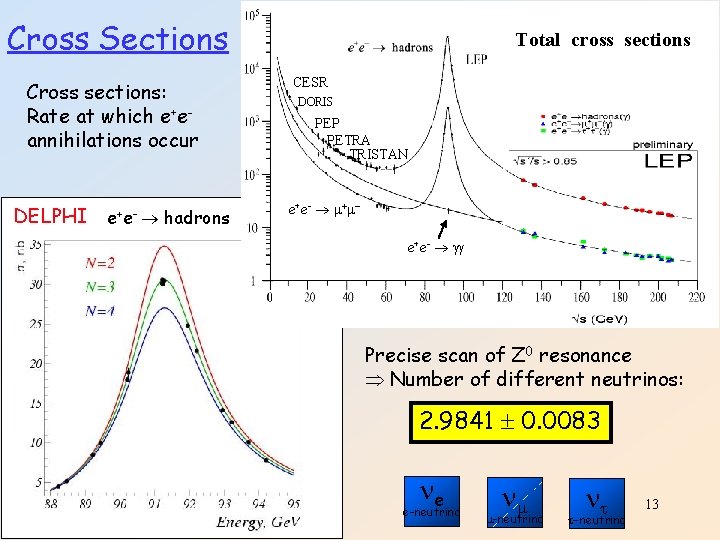
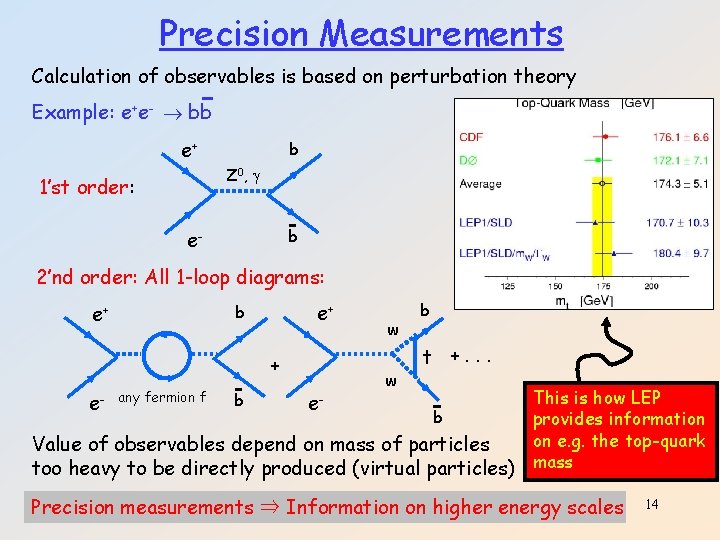
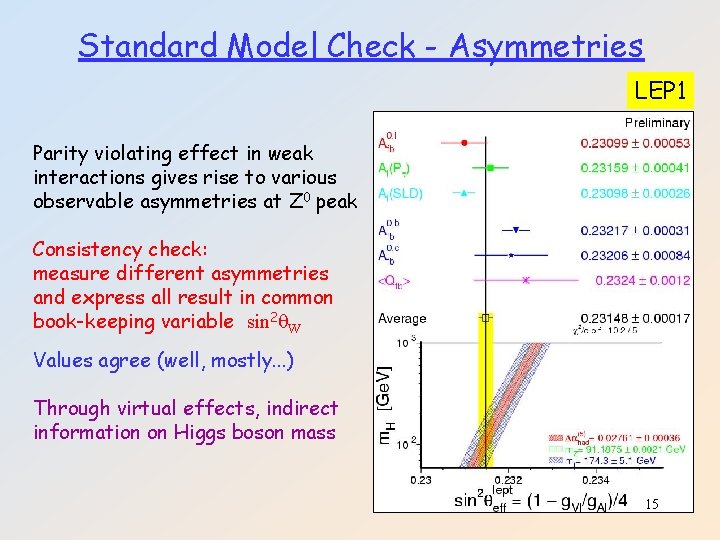
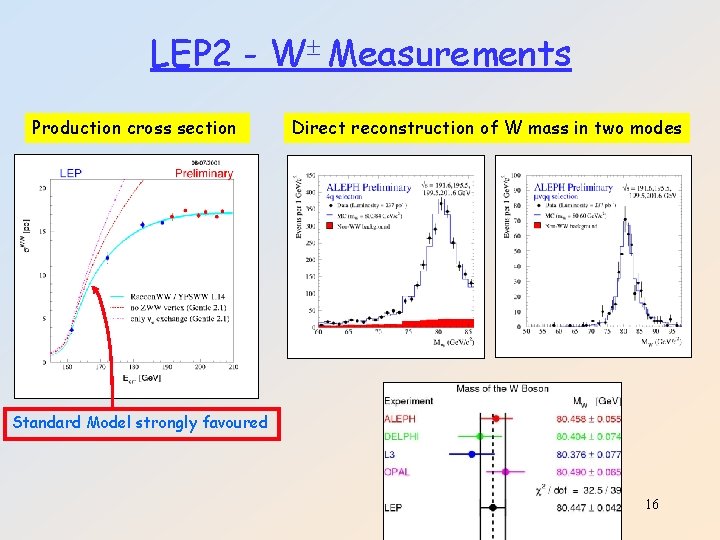
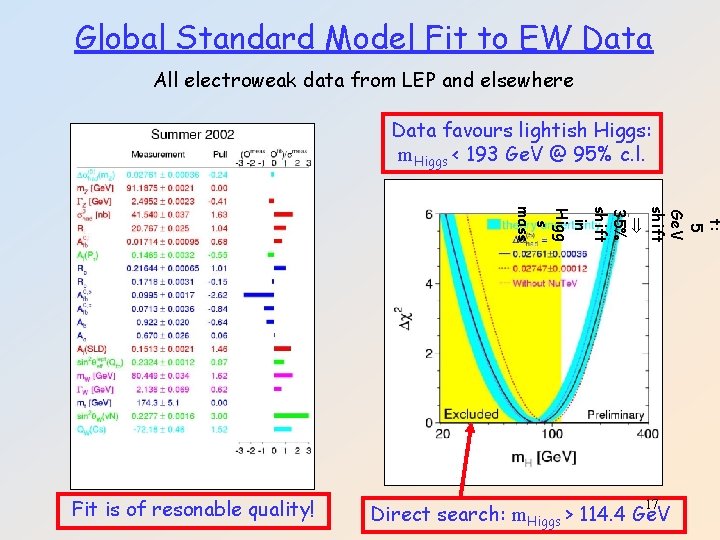
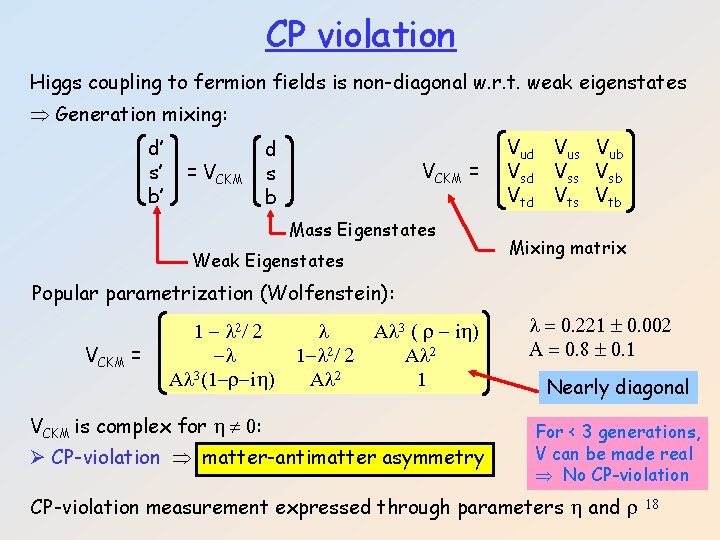
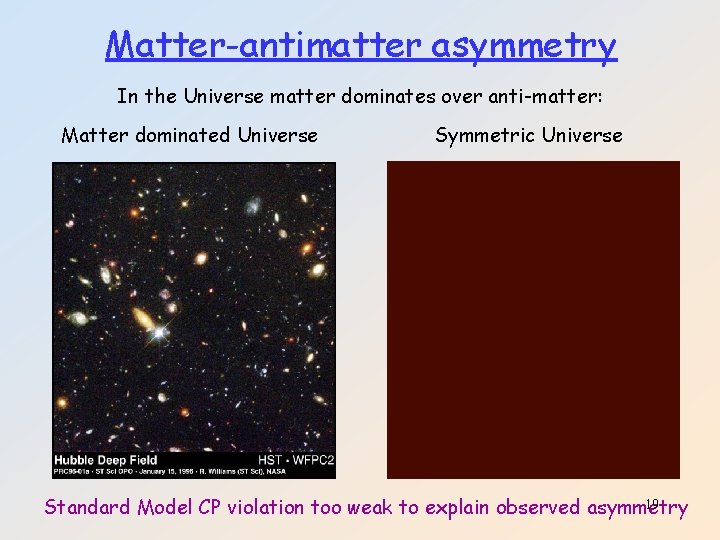
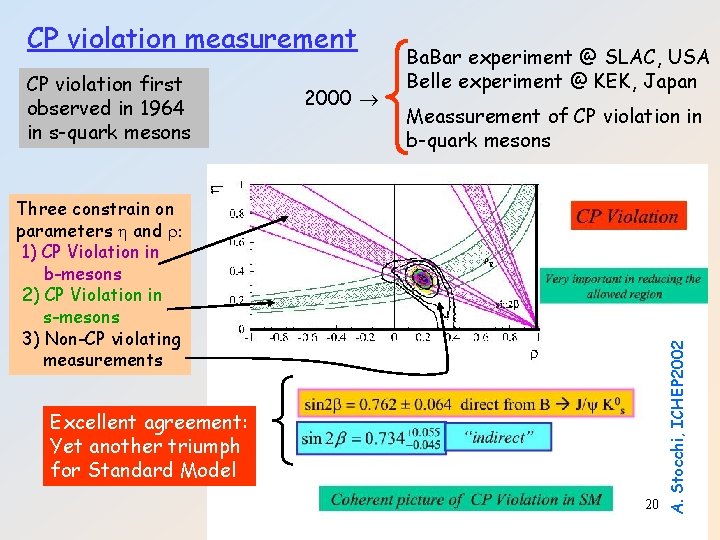
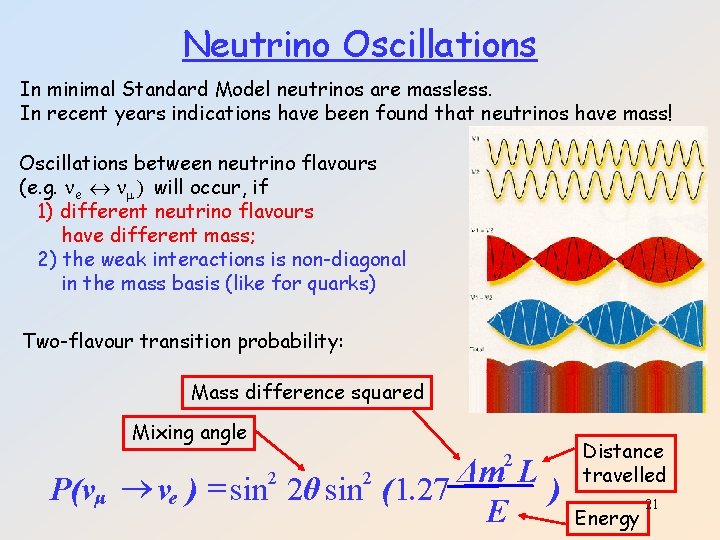
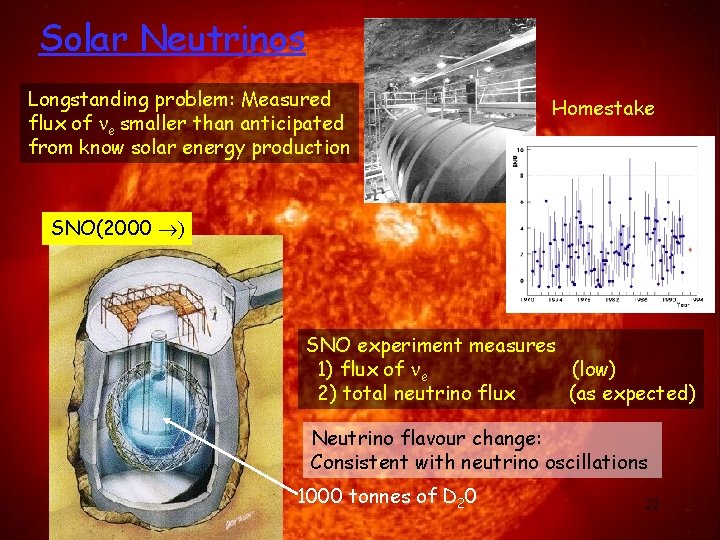
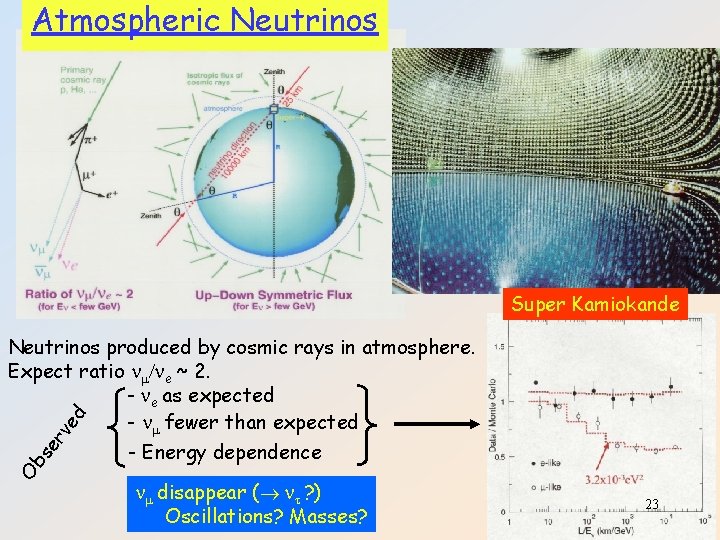
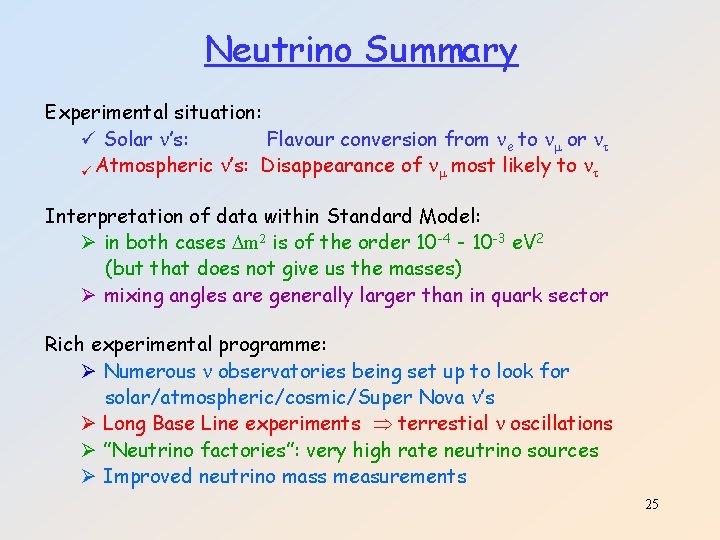
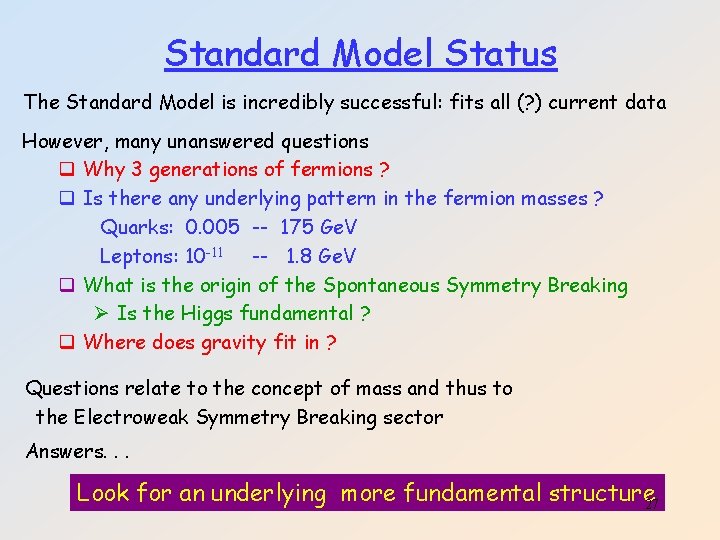
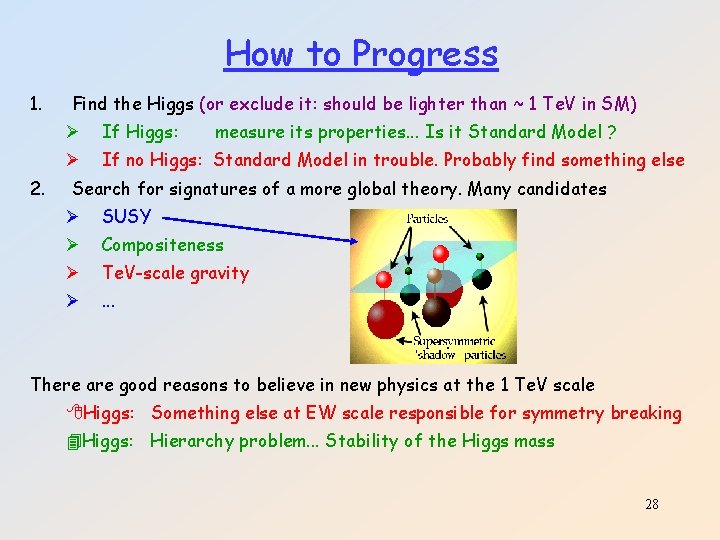
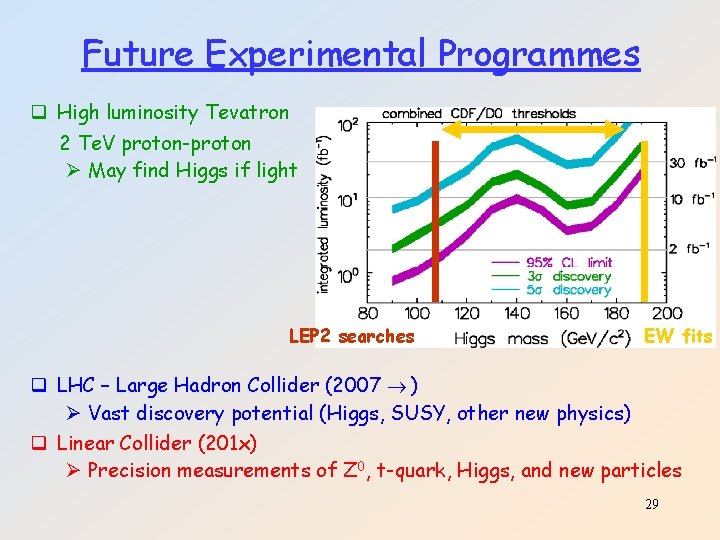
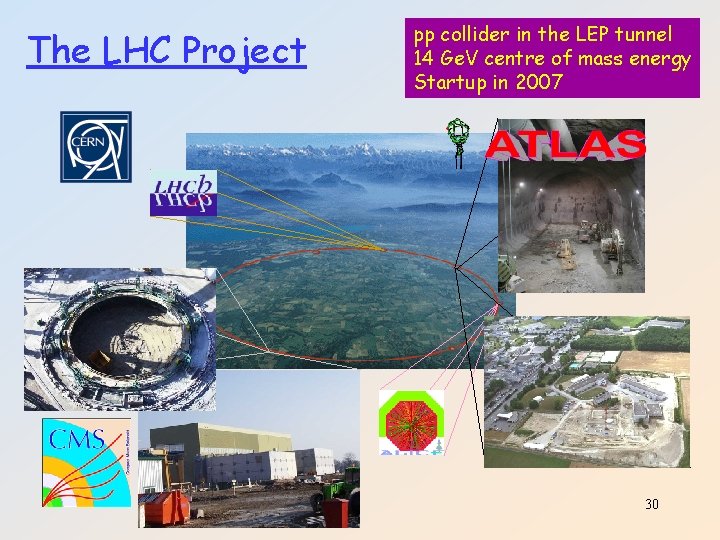
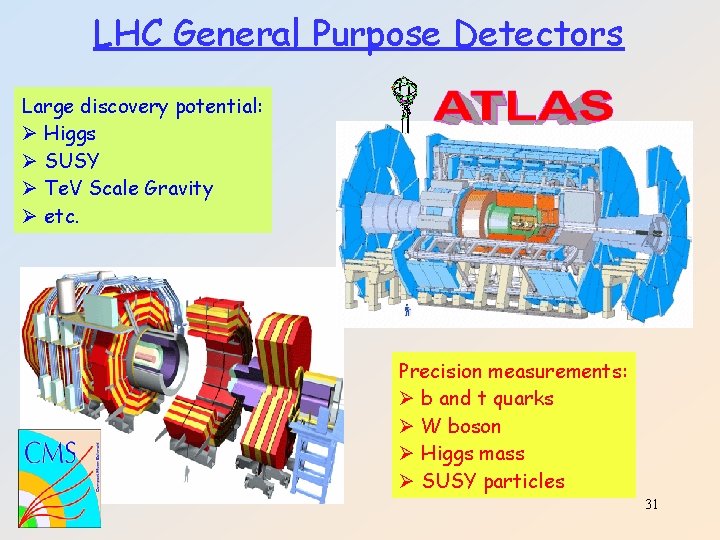
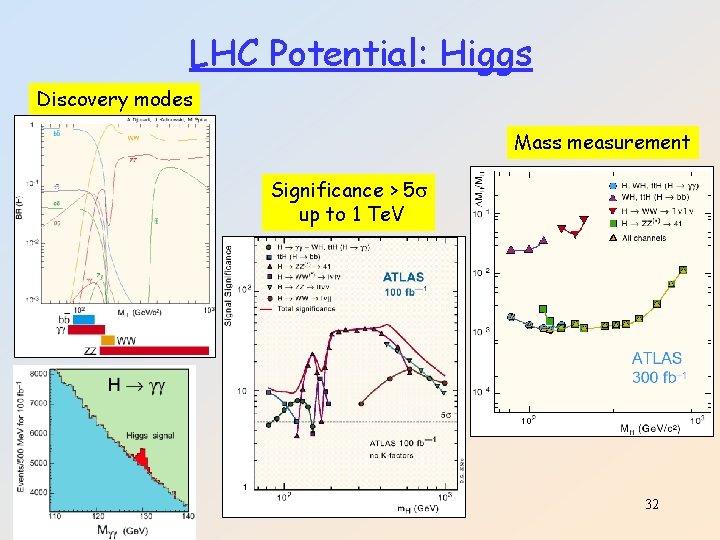
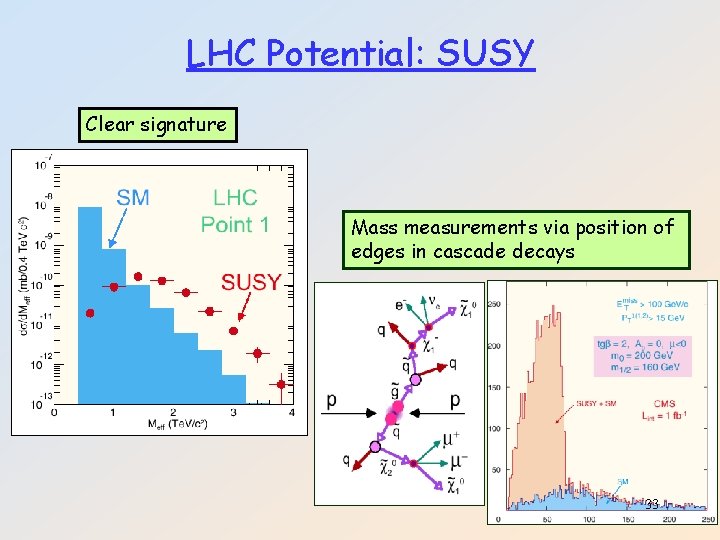
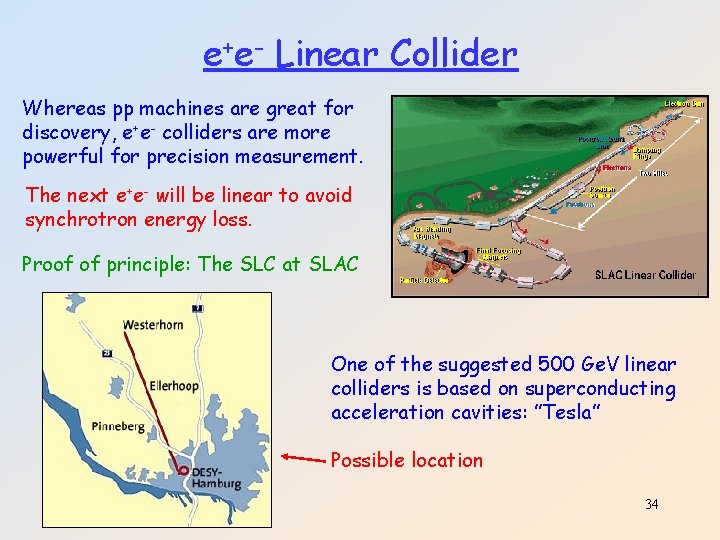
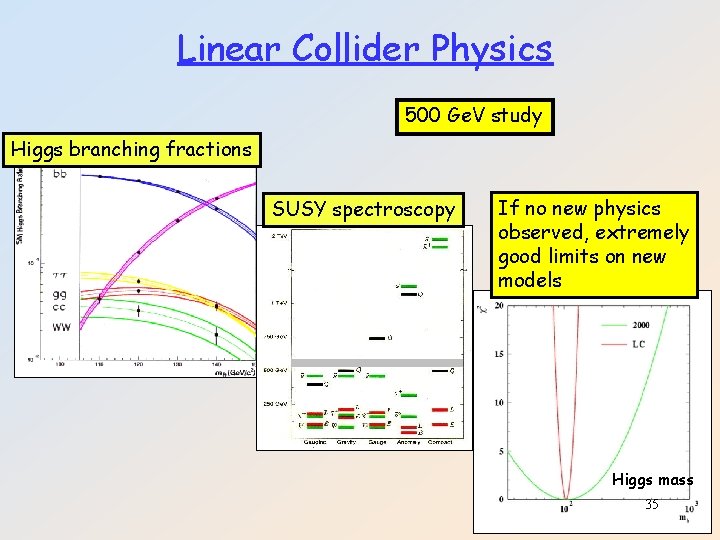
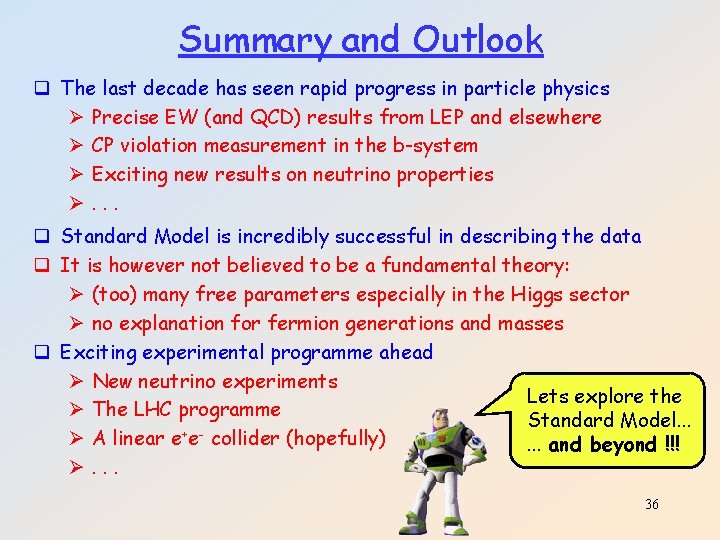
- Slides: 34
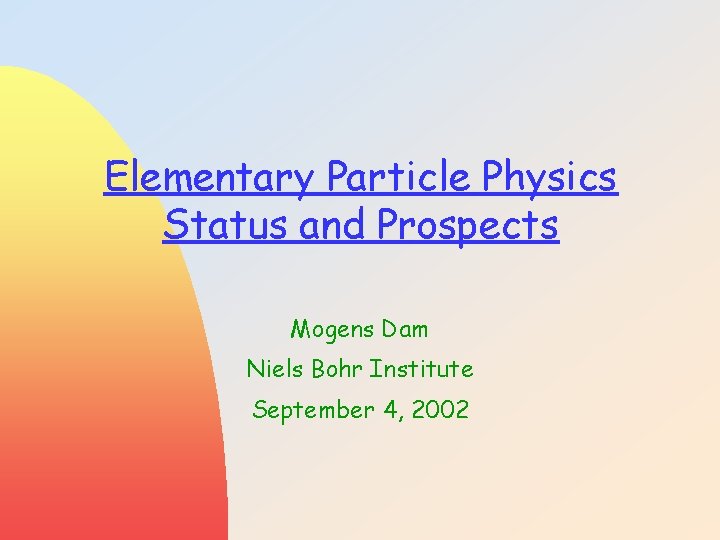
Elementary Particle Physics Status and Prospects Mogens Dam Niels Bohr Institute September 4, 2002
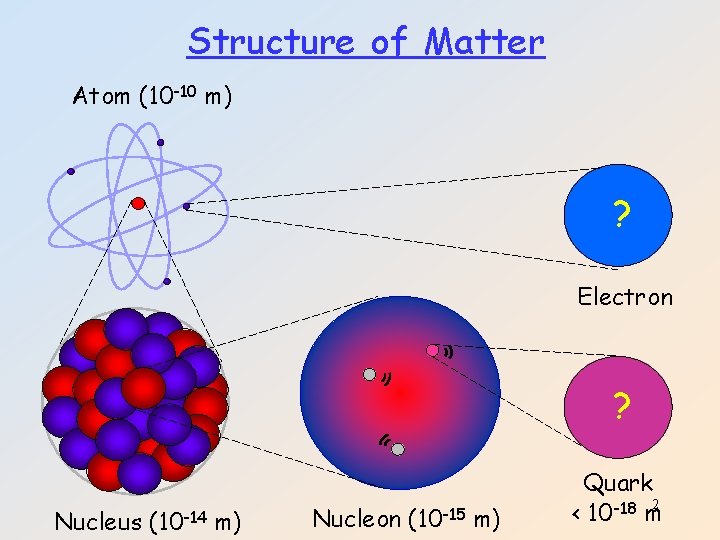
Structure of Matter Atom (10 -10 m) ? Electron ? Nucleus (10 -14 m) Nucleon (10 -15 m) Quark < 10 -18 m 2
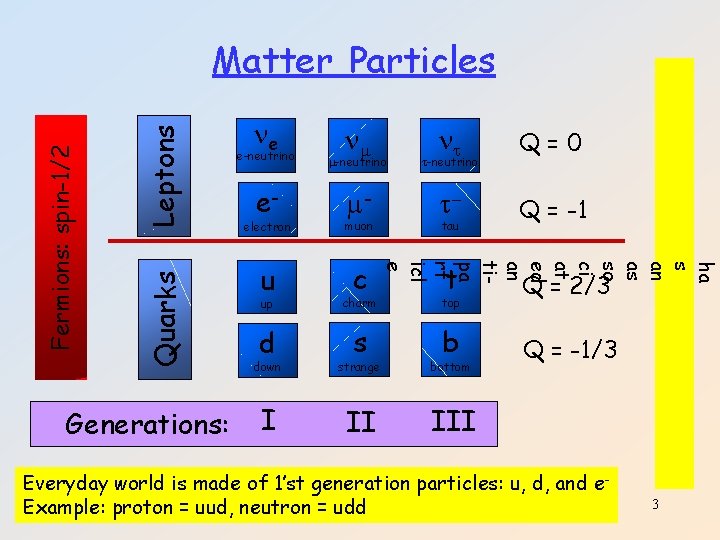
Leptons Quarks Generations: e -neutrino Q=0 electron - muon - Q = -1 u up c charm t top Q = 2/3 d s b Q = -1/3 e-neutrino e- tau ha s an as so ci at ed an tipa rt icl e Fermions: spin-1/2 Matter Particles down strange bottom I II III Everyday world is made of 1’st generation particles: u, d, and e. Example: proton = uud, neutron = udd 3
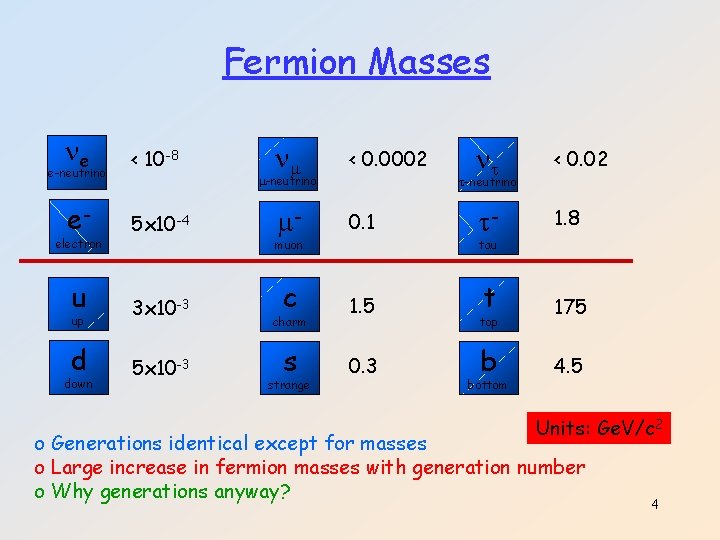
Fermion Masses e e-neutrino < 10 -8 5 x 10 -4 u 3 x 10 -3 d 5 x 10 -3 up down < 0. 0002 - 0. 1 c 1. 5 s 0. 3 -neutrino e- electron strange < 0. 02 - 1. 8 t 175 b 4. 5 -neutrino tau muon charm top bottom Units: Ge. V/c 2 o Generations identical except for masses o Large increase in fermion masses with generation number o Why generations anyway? 4
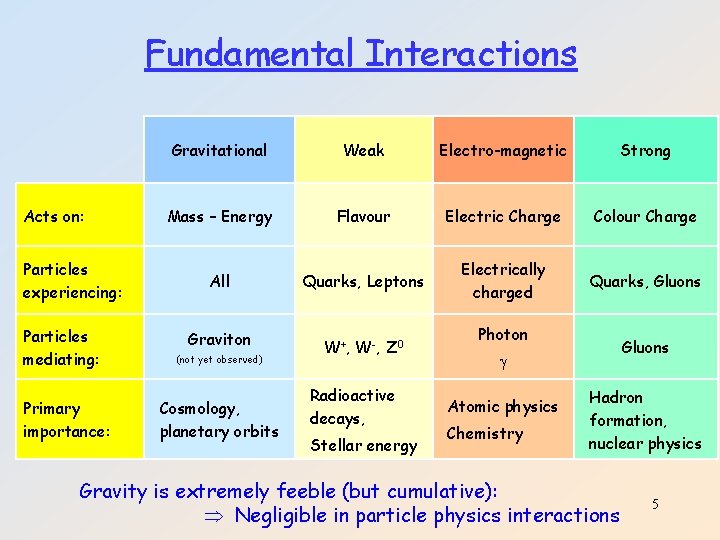
Fundamental Interactions Acts on: Particles experiencing: Particles mediating: Primary importance: Gravitational Weak Electro-magnetic Strong Mass – Energy Flavour Electric Charge Colour Charge All Quarks, Leptons Electrically charged Quarks, Gluons Graviton (not yet observed) Cosmology, planetary orbits W+ , W-, Z 0 Radioactive decays, Stellar energy Photon Gluons g Atomic physics Chemistry Hadron formation, nuclear physics Gravity is extremely feeble (but cumulative): Negligible in particle physics interactions 5
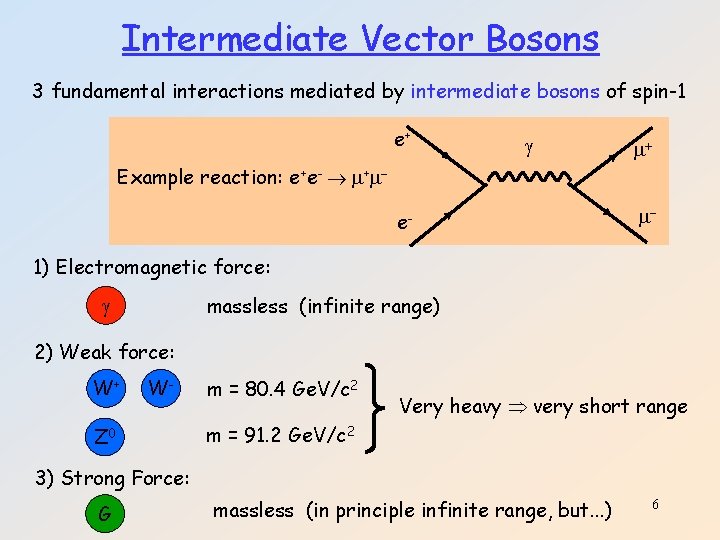
Intermediate Vector Bosons 3 fundamental interactions mediated by intermediate bosons of spin-1 e+ g + Example reaction: e+e- + e- - 1) Electromagnetic force: massless (infinite range) g 2) Weak force: W+ W- Z 0 m = 80. 4 Ge. V/c 2 Very heavy very short range m = 91. 2 Ge. V/c 2 3) Strong Force: G massless (in principle infinite range, but. . . ) 6
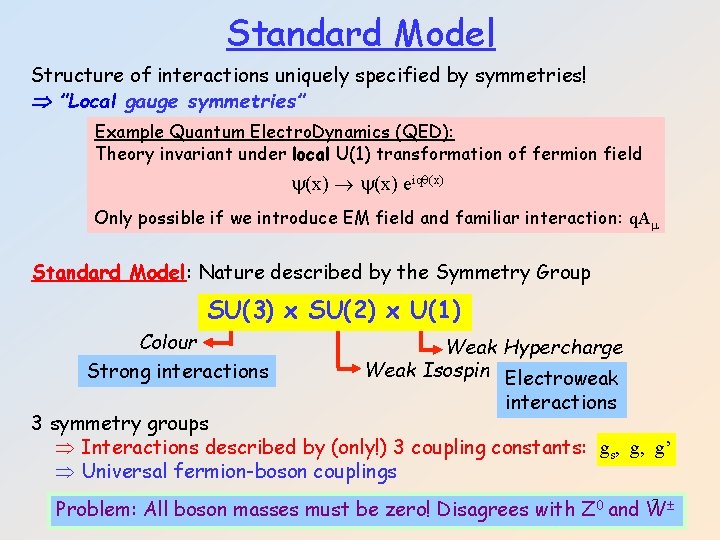
Standard Model Structure of interactions uniquely specified by symmetries! ”Local gauge symmetries” Example Quantum Electro. Dynamics (QED): Theory invariant under local U(1) transformation of fermion field y(x) eiqq(x) Only possible if we introduce EM field and familiar interaction: q. A Standard Model: Nature described by the Symmetry Group SU(3) x SU(2) x U(1) Colour Strong interactions Weak Hypercharge Weak Isospin Electroweak interactions 3 symmetry groups Interactions described by (only!) 3 coupling constants: gs, g, g’ Universal fermion-boson couplings 7 Problem: All boson masses must be zero! Disagrees with Z 0 and W
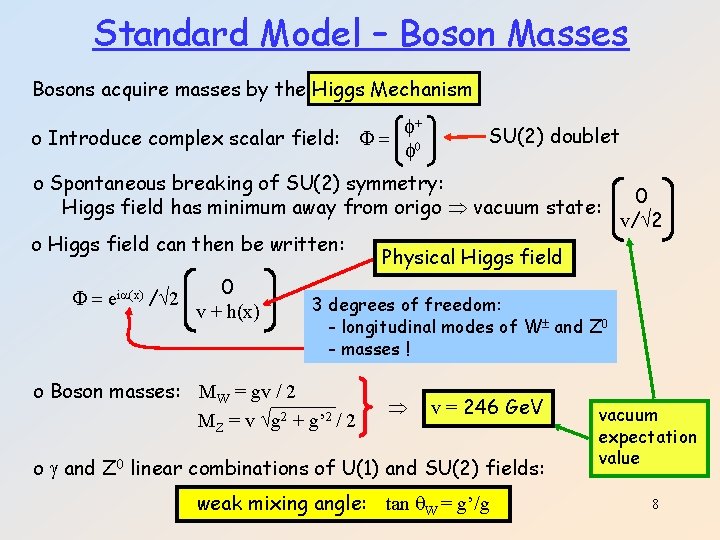
Standard Model – Boson Masses Bosons acquire masses by the Higgs Mechanism f+ o Introduce complex scalar field: F = f SU(2) doublet o Spontaneous breaking of SU(2) symmetry: Higgs field has minimum away from origo vacuum state: o Higgs field can then be written: F = eia(x) / 2 0 v + h(x) 0 v/ 2 Physical Higgs field 3 degrees of freedom: - longitudinal modes of W and Z 0 - masses ! o Boson masses: MW = gv / 2 MZ = v g 2 + g’ 2 / 2 v = 246 Ge. V o g and Z 0 linear combinations of U(1) and SU(2) fields: weak mixing angle: tan q. W = g’/g vacuum expectation value 8
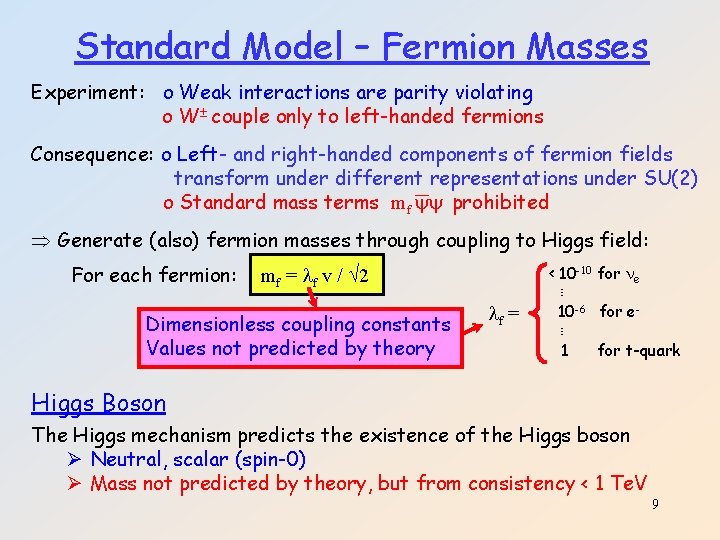
Standard Model – Fermion Masses Experiment: o Weak interactions are parity violating o W couple only to left-handed fermions Consequence: o Left- and right-handed components of fermion fields transform under different representations under SU(2) o Standard mass terms mf yy prohibited Generate (also) fermion masses through coupling to Higgs field: For each fermion: mf = lf v / 2 Dimensionless coupling constants Values not predicted by theory lf = < 10 -10 for e ⋮ 10 -6 for e⋮ 1 for t-quark Higgs Boson The Higgs mechanism predicts the existence of the Higgs boson Ø Neutral, scalar (spin-0) Ø Mass not predicted by theory, but from consistency < 1 Te. V 9
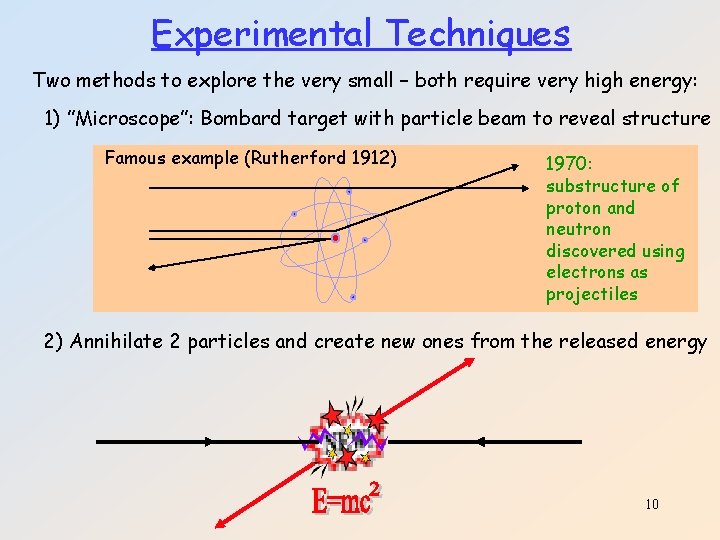
Experimental Techniques Two methods to explore the very small – both require very high energy: 1) ”Microscope”: Bombard target with particle beam to reveal structure Famous example (Rutherford 1912) 1970: substructure of proton and neutron discovered using electrons as projectiles 2) Annihilate 2 particles and create new ones from the released energy 10
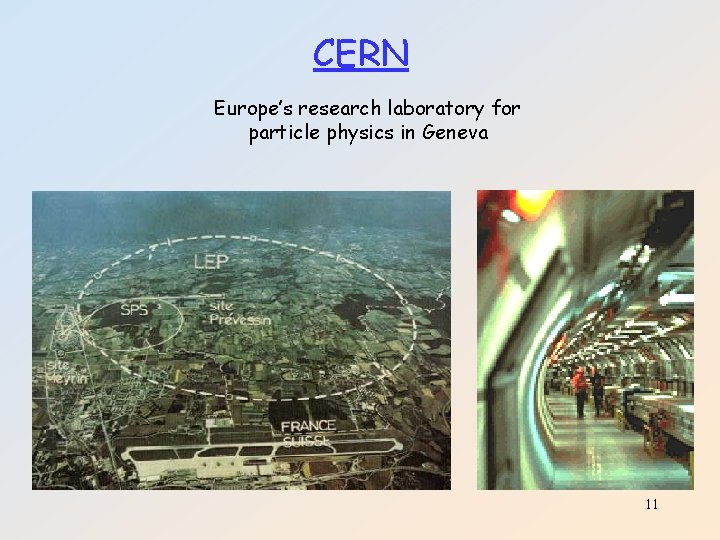
CERN Europe’s research laboratory for particle physics in Geneva 11
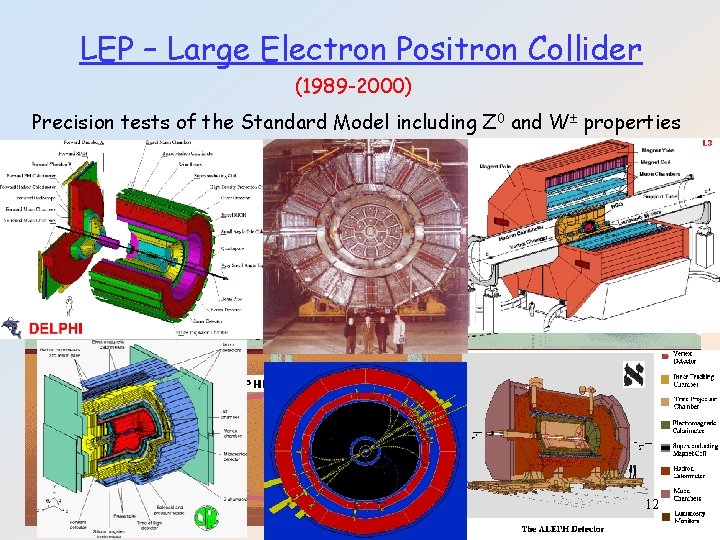
LEP – Large Electron Positron Collider (1989 -2000) Precision tests of the Standard Model including Z 0 and W properties 12
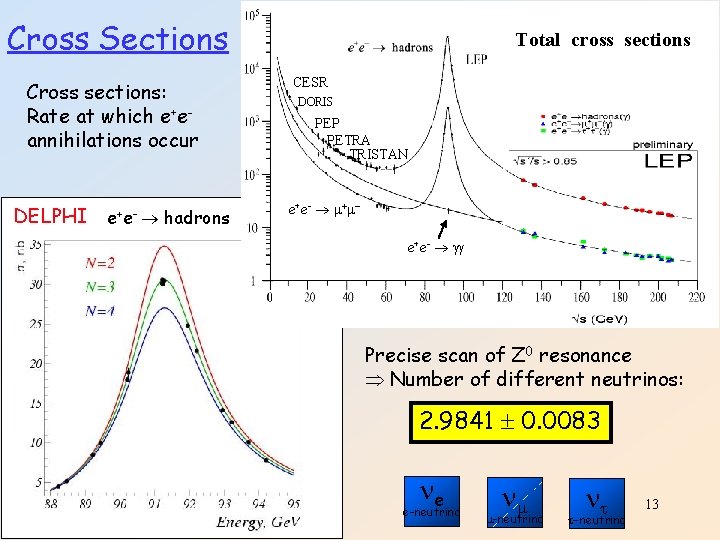
Cross Sections Cross sections: Rate at which e+eannihilations occur DELPHI e+e- hadrons Total cross sections CESR DORIS PEP PETRA TRISTAN e + e - + e+e- gg Precise scan of Z 0 resonance Number of different neutrinos: 2. 9841 0. 0083 e e-neutrino 13
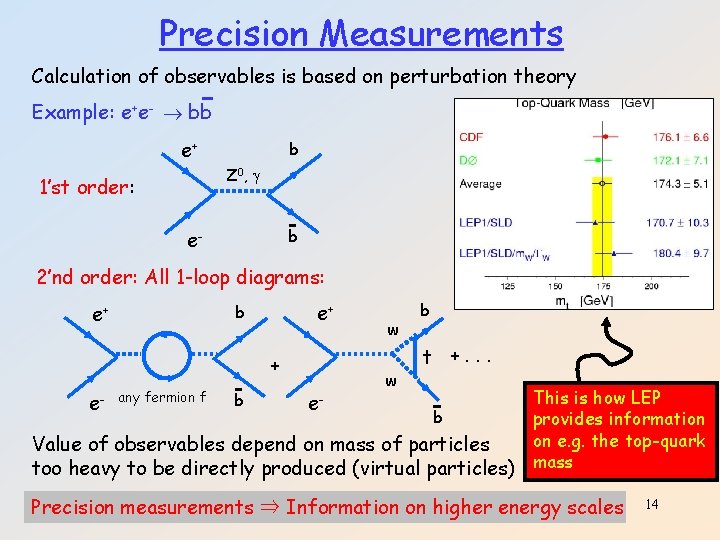
Precision Measurements Calculation of observables is based on perturbation theory Example: e+e- bb e+ b Z 0, g 1’st order: e- b 2’nd order: All 1 -loop diagrams: e+ e+ b + w w b t +. . . This is how LEP b provides information on e. g. the top-quark Value of observables depend on mass of particles too heavy to be directly produced (virtual particles) mass e- any fermion f b e- Precision measurements ⇒ Information on higher energy scales 14
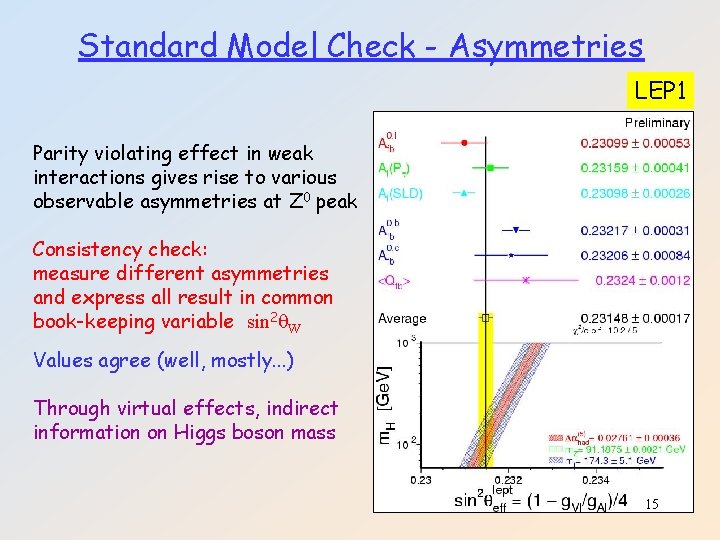
Standard Model Check - Asymmetries LEP 1 Parity violating effect in weak interactions gives rise to various observable asymmetries at Z 0 peak Consistency check: measure different asymmetries and express all result in common book-keeping variable sin 2 q. W Values agree (well, mostly. . . ) Through virtual effects, indirect information on Higgs boson mass 15
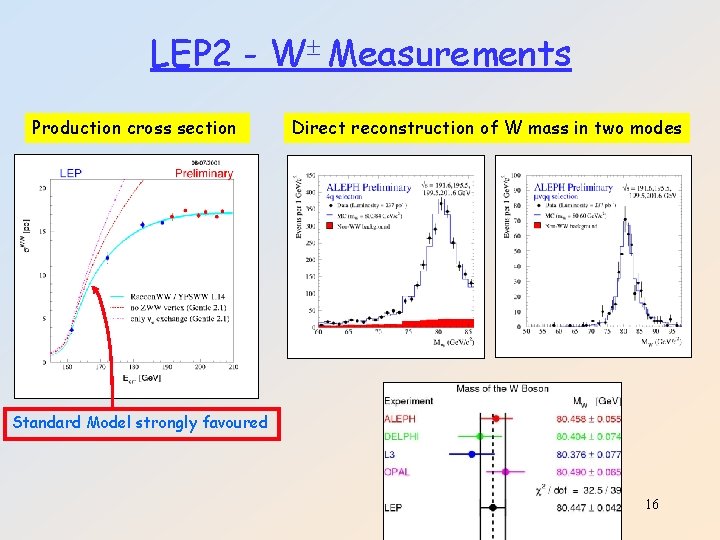
LEP 2 - W Measurements Production cross section Direct reconstruction of W mass in two modes Standard Model strongly favoured 16
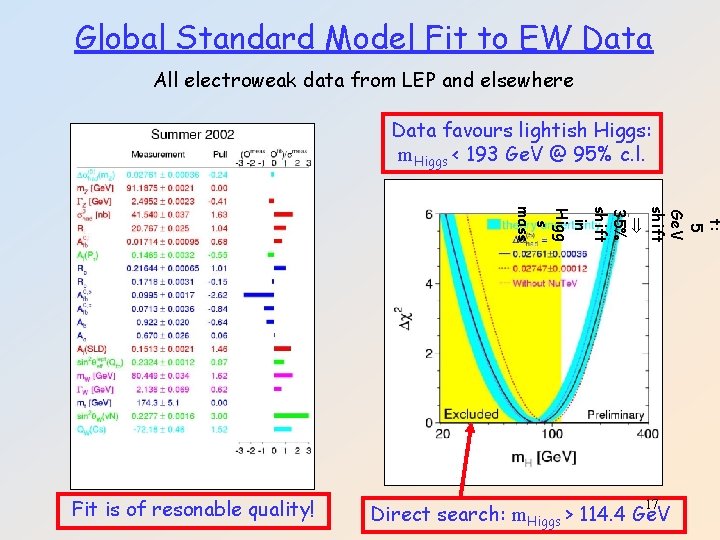
Global Standard Model Fit to EW Data All electroweak data from LEP and elsewhere Data favours lightish Higgs: m. Higgs < 193 Ge. V @ 95% c. l. t: 5 Ge. V shift 35% shift in Higg s mass Fit is of resonable quality! 17 Direct search: m. Higgs > 114. 4 Ge. V
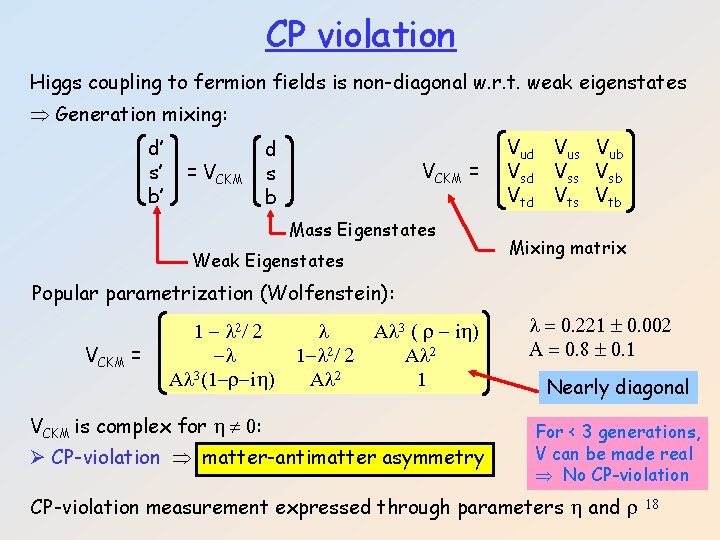
CP violation Higgs coupling to fermion fields is non-diagonal w. r. t. weak eigenstates Generation mixing: d’ s’ b’ = VCKM d s b VCKM = Mass Eigenstates Weak Eigenstates Vud Vus Vub Vsd Vss Vsb Vtd Vts Vtb Mixing matrix Popular parametrization (Wolfenstein): VCKM = 1 - l 2/ 2 l Al 3 ( r - i ) -l 1 -l 2/ 2 Al 2 Al 3(1 -r-i ) Al 2 1 VCKM is complex for : Ø CP-violation matter-antimatter asymmetry l = . 221 . 2 A = . 8 . 1 Nearly diagonal For < 3 generations, V can be made real No CP-violation measurement expressed through parameters and r 18
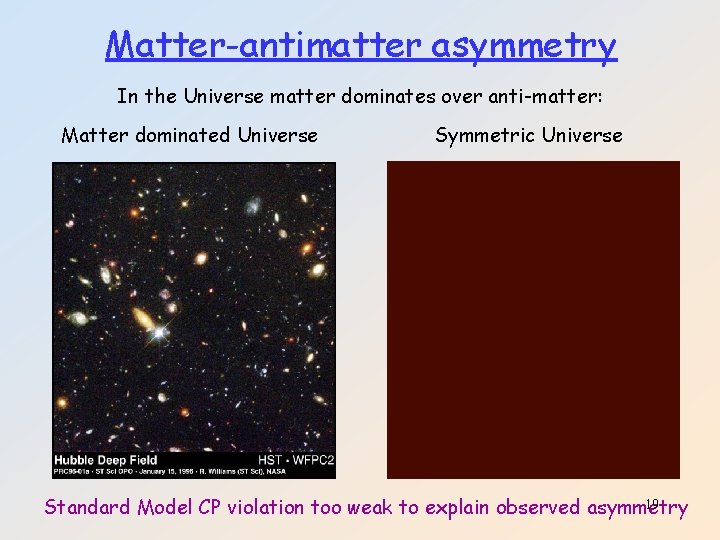
Matter-antimatter asymmetry In the Universe matter dominates over anti-matter: Matter dominated Universe Symmetric Universe 19 Standard Model CP violation too weak to explain observed asymmetry
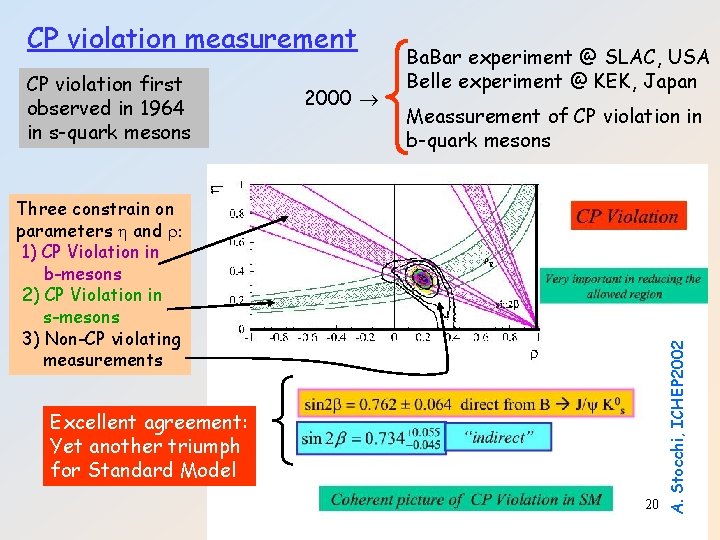
CP violation first observed in 1964 in s-quark mesons 2000 Ba. Bar experiment @ SLAC, USA Belle experiment @ KEK, Japan Meassurement of CP violation in b-quark mesons Three constrain on parameters and r: 1) CP Violation in b-mesons 2) CP Violation in s-mesons 3) Non-CP violating measurements Excellent agreement: Yet another triumph for Standard Model 20 A. Stocchi, ICHEP 2002 CP violation measurement
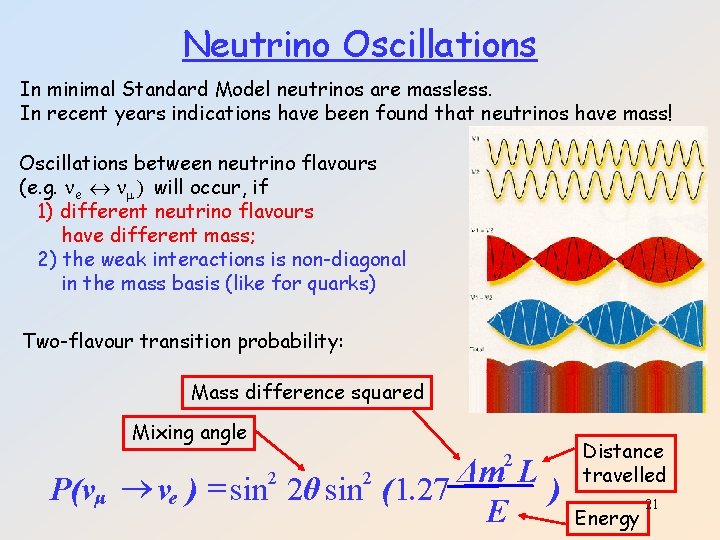
Neutrino Oscillations In minimal Standard Model neutrinos are massless. In recent years indications have been found that neutrinos have mass! Oscillations between neutrino flavours (e. g. e ) will occur, if 1) different neutrino flavours have different mass; 2) the weak interactions is non-diagonal in the mass basis (like for quarks) Two-flavour transition probability: Mass difference squared Mixing angle 2 Δm L ) P(νμ νe ) = sin 2θ sin ( 1. 27 E 2 2 Distance travelled Energy 21
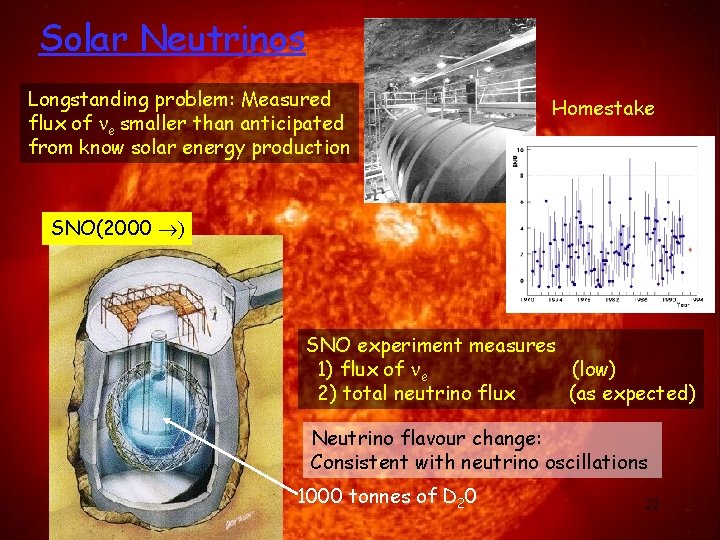
Solar Neutrinos Longstanding problem: Measured flux of e smaller than anticipated from know solar energy production Homestake SNO(2000 ) SNO experiment measures 1) flux of e (low) 2) total neutrino flux (as expected) Neutrino flavour change: Consistent with neutrino oscillations 1000 tonnes of D 20 22
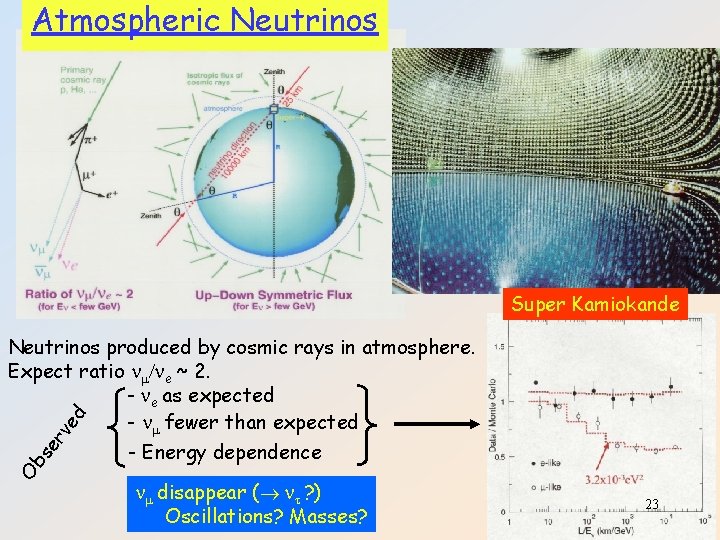
Atmospheric Neutrinos Super Kamiokande O bs er ve d Neutrinos produced by cosmic rays in atmosphere. Expect ratio / e ~ 2. - e as expected - fewer than expected - Energy dependence disappear ( ? ) Oscillations? Masses? 23
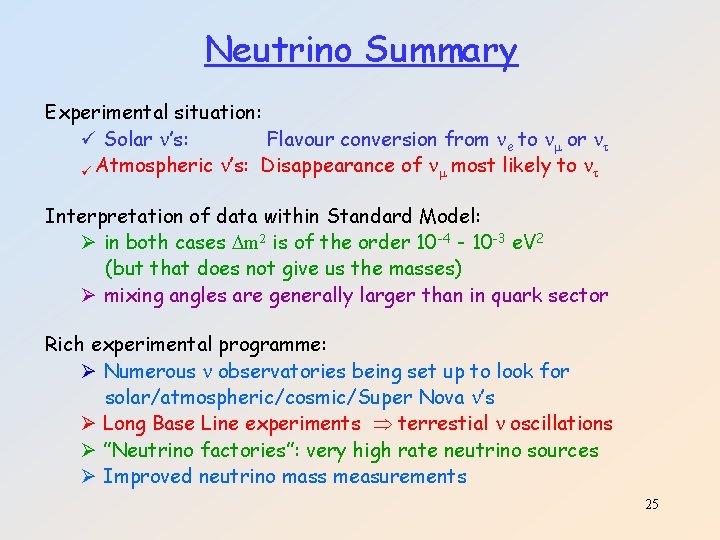
Neutrino Summary Experimental situation: ü Solar ’s: Flavour conversion from e to or ü Atmospheric ’s: Disappearance of most likely to Interpretation of data within Standard Model: Ø in both cases Dm 2 is of the order 10 -4 - 10 -3 e. V 2 (but that does not give us the masses) Ø mixing angles are generally larger than in quark sector Rich experimental programme: Ø Numerous observatories being set up to look for solar/atmospheric/cosmic/Super Nova ’s Ø Long Base Line experiments terrestial oscillations Ø ”Neutrino factories”: very high rate neutrino sources Ø Improved neutrino mass measurements 25
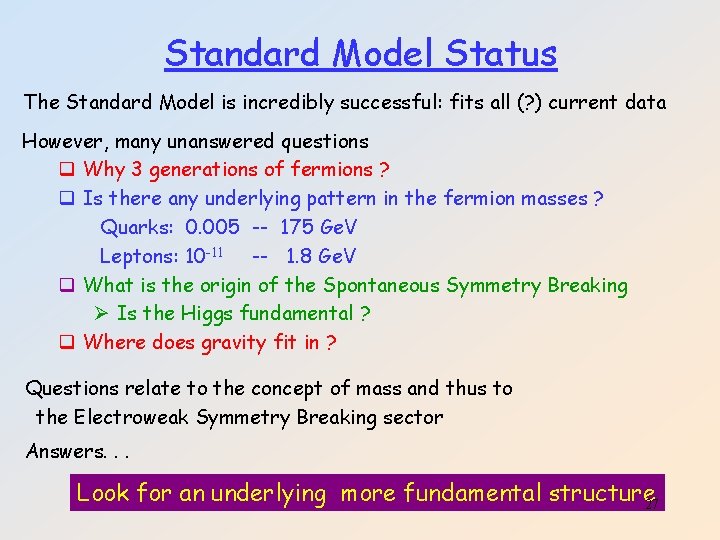
Standard Model Status The Standard Model is incredibly successful: fits all (? ) current data However, many unanswered questions q Why 3 generations of fermions ? q Is there any underlying pattern in the fermion masses ? Quarks: 0. 005 -- 175 Ge. V Leptons: 10 -11 -- 1. 8 Ge. V q What is the origin of the Spontaneous Symmetry Breaking Ø Is the Higgs fundamental ? q Where does gravity fit in ? Questions relate to the concept of mass and thus to the Electroweak Symmetry Breaking sector Answers. . . Look for an underlying more fundamental structure 27
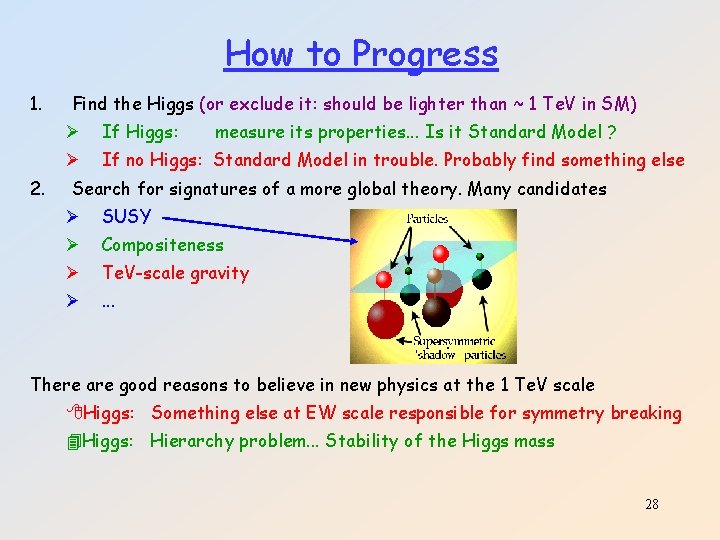
How to Progress 1. 2. Find the Higgs (or exclude it: should be lighter than ~ 1 Te. V in SM) Ø If Higgs: measure its properties. . . Is it Standard Model ? Ø If no Higgs: Standard Model in trouble. Probably find something else Search for signatures of a more global theory. Many candidates Ø SUSY Ø Compositeness Ø Te. V-scale gravity Ø . . . There are good reasons to believe in new physics at the 1 Te. V scale Higgs: Something else at EW scale responsible for symmetry breaking Higgs: Hierarchy problem. . . Stability of the Higgs mass 28
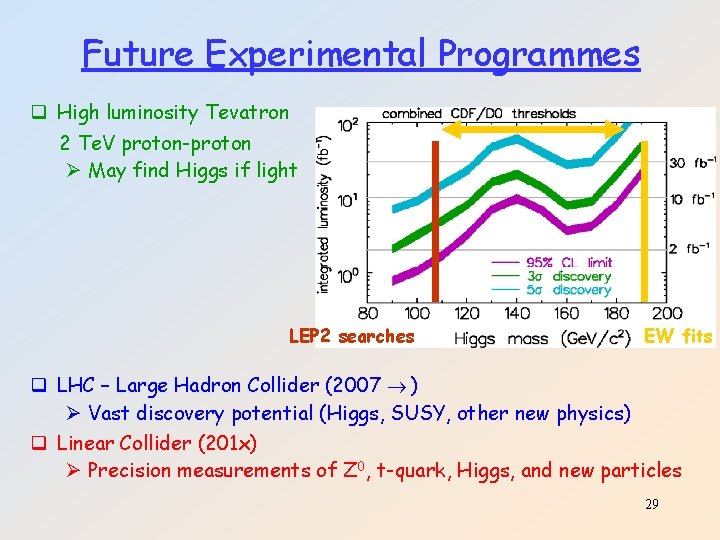
Future Experimental Programmes q High luminosity Tevatron 2 Te. V proton-proton Ø May find Higgs if light LEP 2 searches EW fits q LHC – Large Hadron Collider (2007 ) Ø Vast discovery potential (Higgs, SUSY, other new physics) q Linear Collider (201 x) Ø Precision measurements of Z 0, t-quark, Higgs, and new particles 29
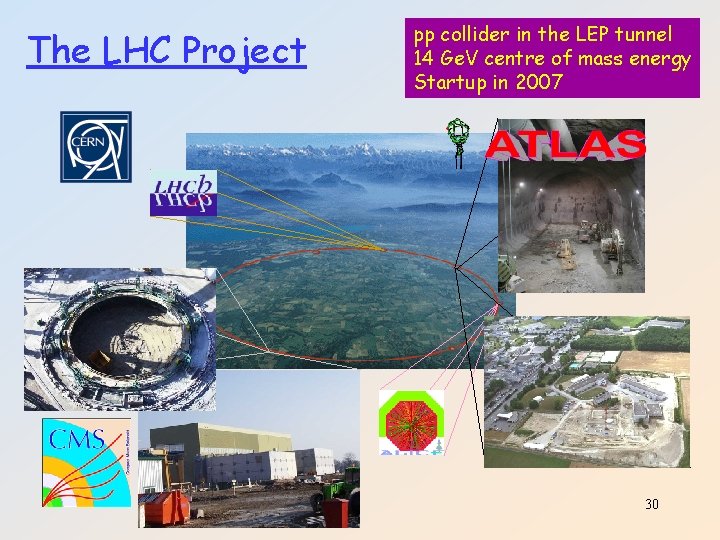
The LHC Project pp collider in the LEP tunnel 14 Ge. V centre of mass energy Startup in 2007 30
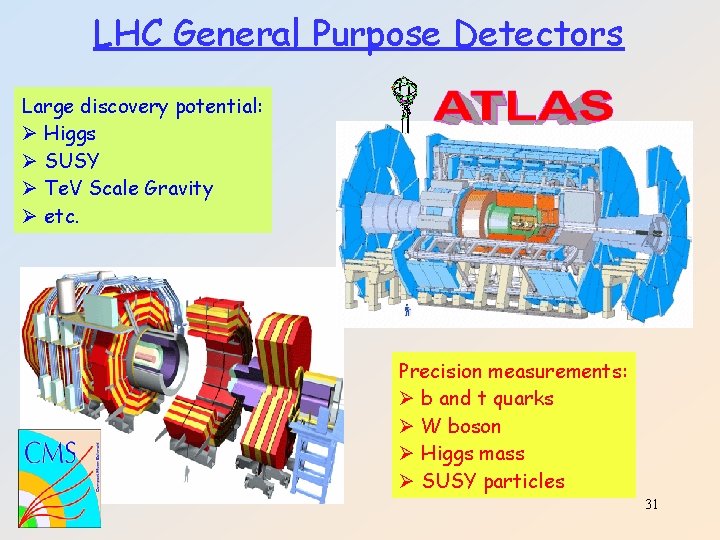
LHC General Purpose Detectors Large discovery potential: Ø Higgs Ø SUSY Ø Te. V Scale Gravity Ø etc. Precision measurements: Ø b and t quarks Ø W boson Ø Higgs mass Ø SUSY particles 31
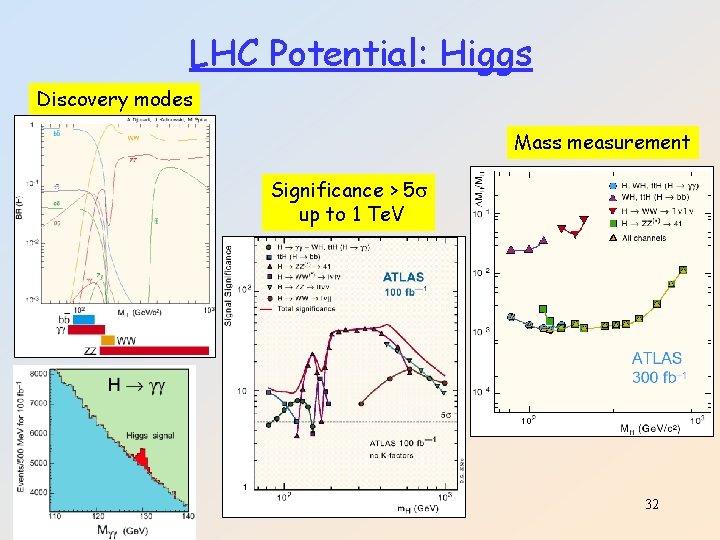
LHC Potential: Higgs Discovery modes Mass measurement Significance > 5 s up to 1 Te. V 32
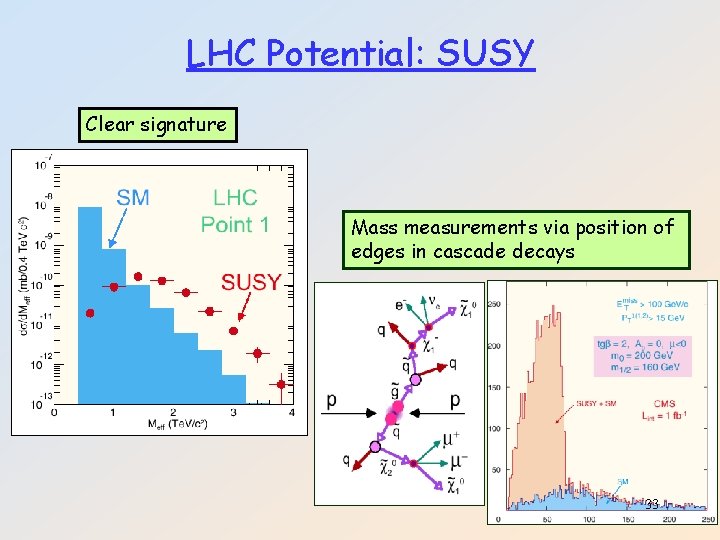
LHC Potential: SUSY Clear signature Mass measurements via position of edges in cascade decays 33
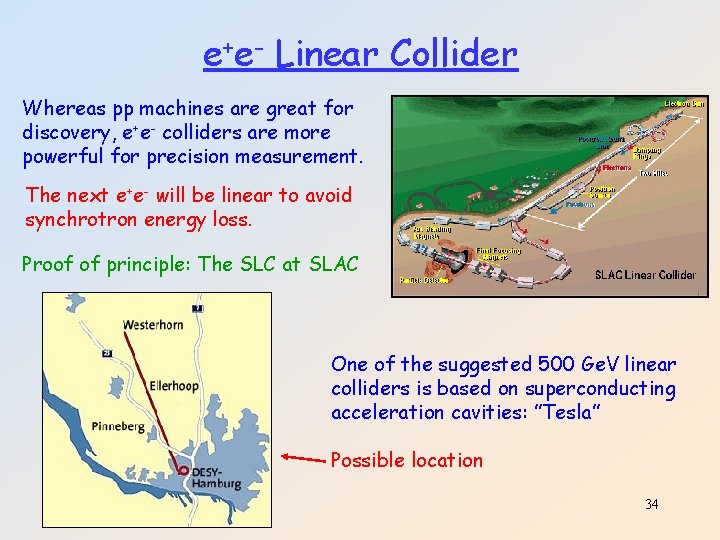
e+e- Linear Collider Whereas pp machines are great for discovery, e+e- colliders are more powerful for precision measurement. The next e+e- will be linear to avoid synchrotron energy loss. Proof of principle: The SLC at SLAC One of the suggested 500 Ge. V linear colliders is based on superconducting acceleration cavities: ”Tesla” Possible location 34
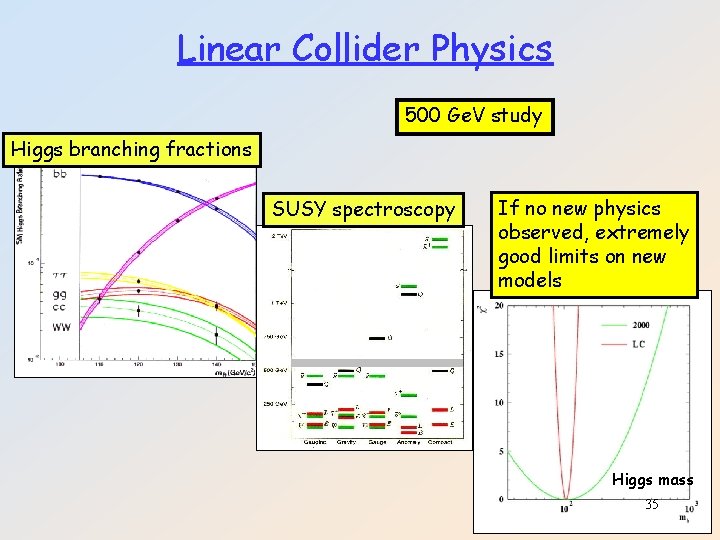
Linear Collider Physics 500 Ge. V study Higgs branching fractions SUSY spectroscopy If no new physics observed, extremely good limits on new models Higgs mass 35
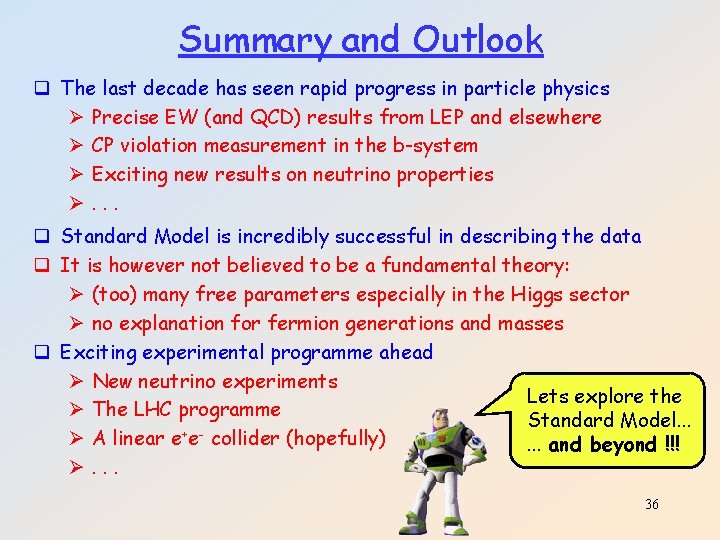
Summary and Outlook q The last decade has seen rapid progress in particle physics Ø Precise EW (and QCD) results from LEP and elsewhere Ø CP violation measurement in the b-system Ø Exciting new results on neutrino properties Ø. . . q Standard Model is incredibly successful in describing the data q It is however not believed to be a fundamental theory: Ø (too) many free parameters especially in the Higgs sector Ø no explanation for fermion generations and masses q Exciting experimental programme ahead Ø New neutrino experiments Lets explore the Ø The LHC programme Standard Model. . . + Ø A linear e e collider (hopefully). . . and beyond !!! Ø. . . 36
Mogens dam
Rigid and non rigid dams
Mogens bak hansen
Mogens bak hansen
Nikolaj jacobsen sille jacobsen
Perbedaan sabo dam dan check dam
Quantum computing: progress and prospects
In line 2 impatient of is best interpreted as meaning
Prospects of agriculture in bangladesh
World population prospects
Prospects preposition
Three gorges dam
Basic particles
Pmt particle physics
Modern particle physics mark thomson
Particle physics
Particle physics practice quiz
Particle physics timeline
Concezio bozzi
Cern particle physics
European laboratory for particle physics
Cern particle physics
Particle physics
Form factor particle physics
International masterclasses hands on particle physics
Modern physics vs classical physics
University physics with modern physics fifteenth edition
Ib physics hl ia examples
Dental dam forceps are positioned with the beaks facing
Dam benefits and problems
Advantages and disadvantages of dam
Difference between particle and rigid body
Particle equilibrium in 2d and 3d engineering mechanics
Kinetics of a particle: force and acceleration
Kinetics of a particle: impulse and momentum