Electrical Signals of Nerve Cells Resting potential how
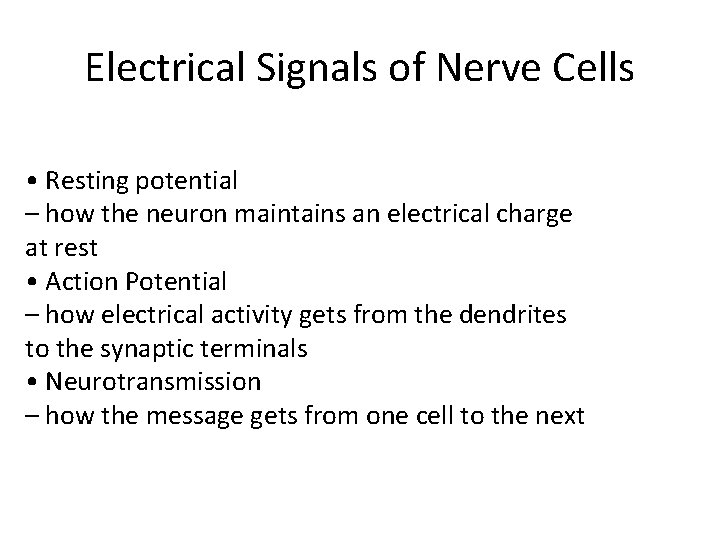
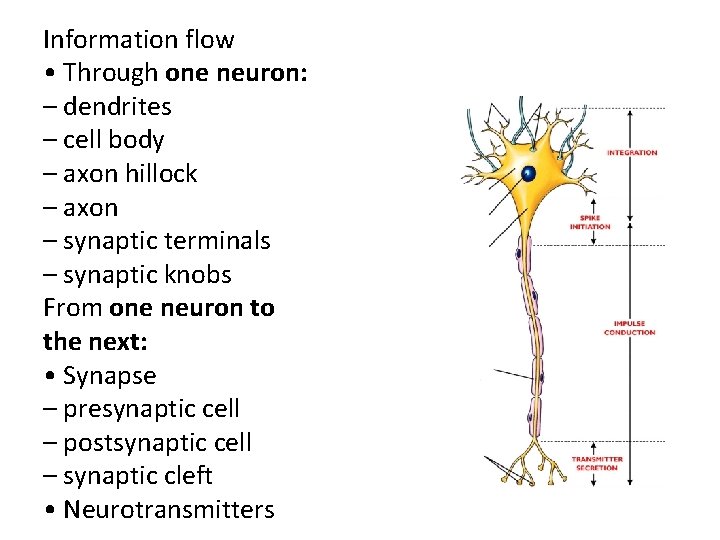
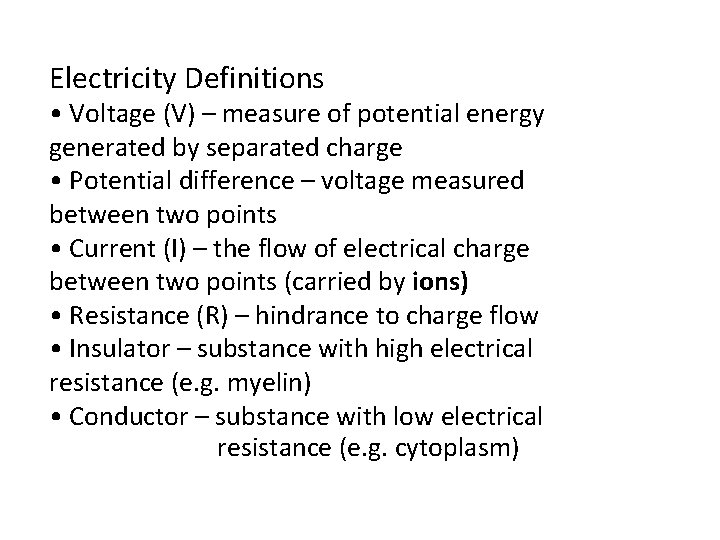
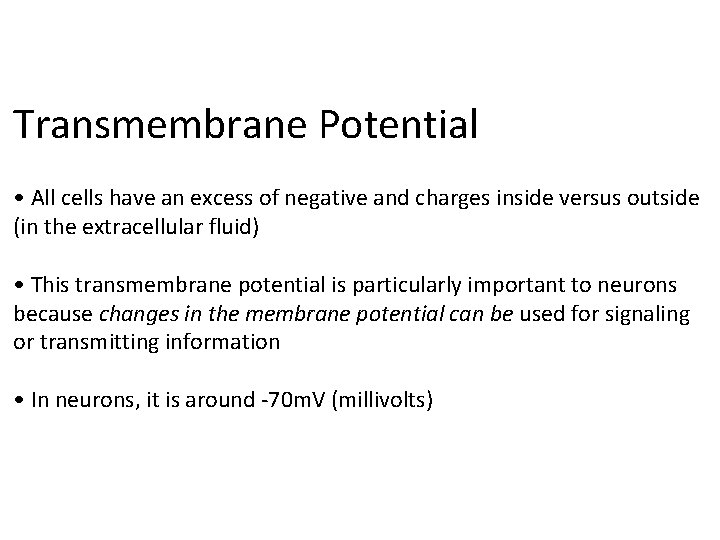
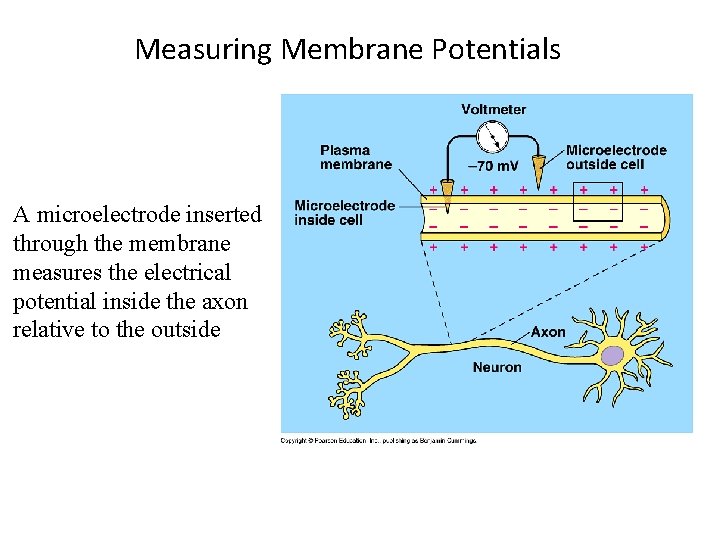
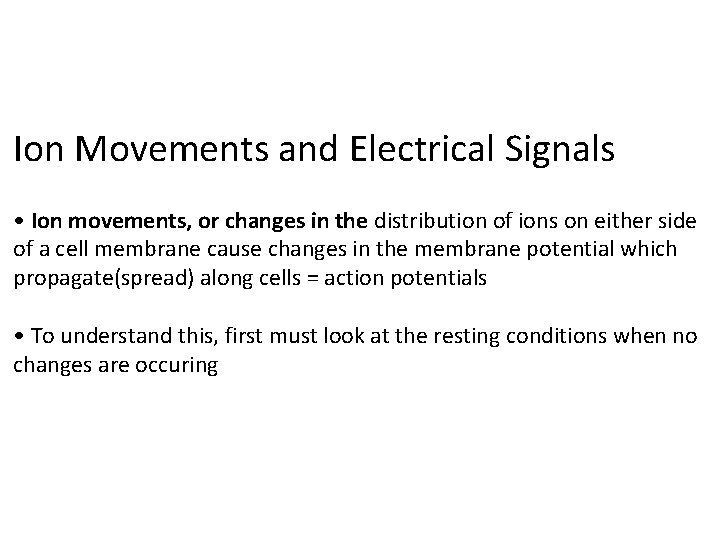
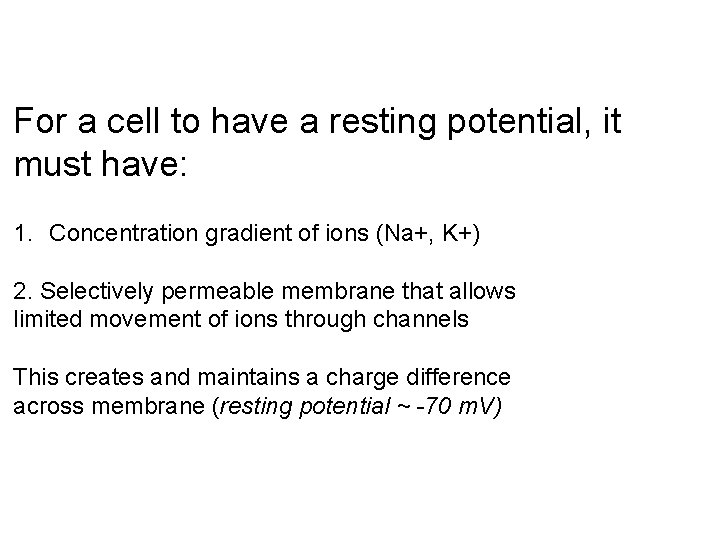
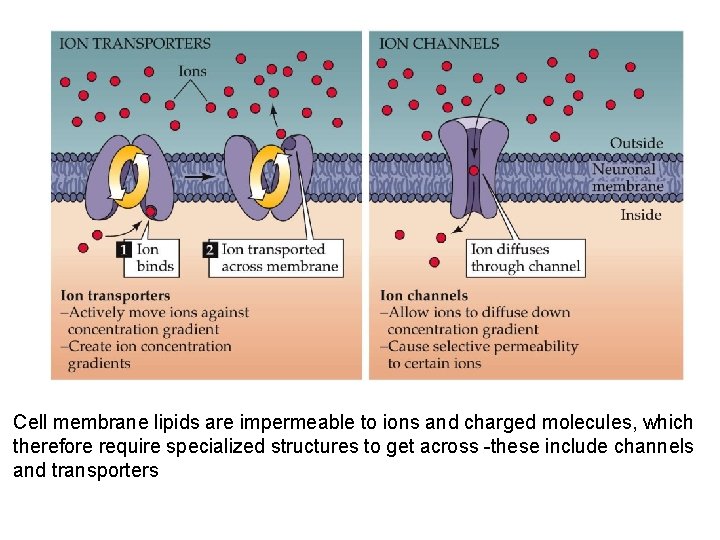
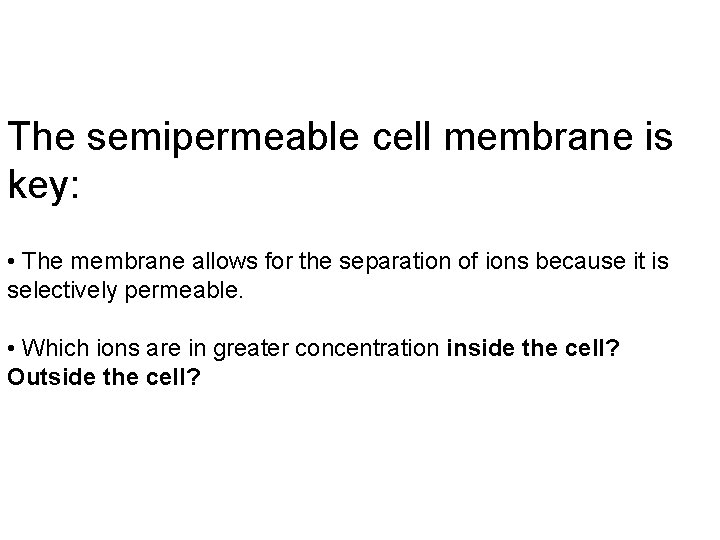
![Membrane concentrations INSIDE cells: • High [K+] • High [proteins] (negatively charged) • Low Membrane concentrations INSIDE cells: • High [K+] • High [proteins] (negatively charged) • Low](https://slidetodoc.com/presentation_image/43a34c6cad430190dbfcf085aaaf60b5/image-10.jpg)
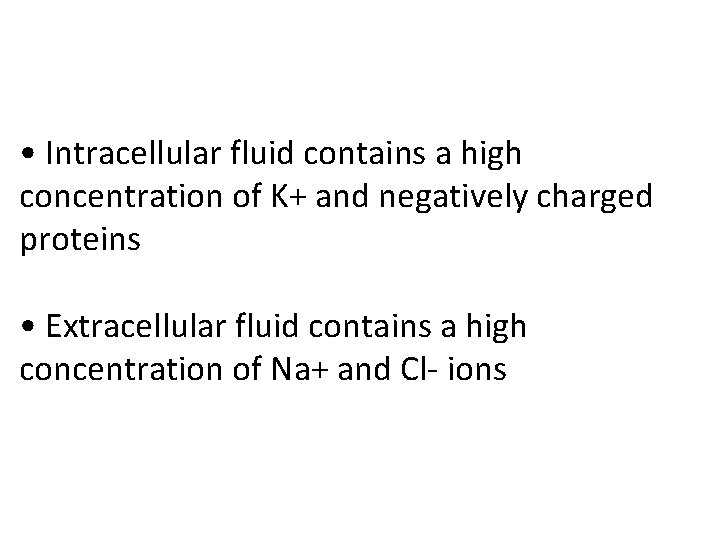
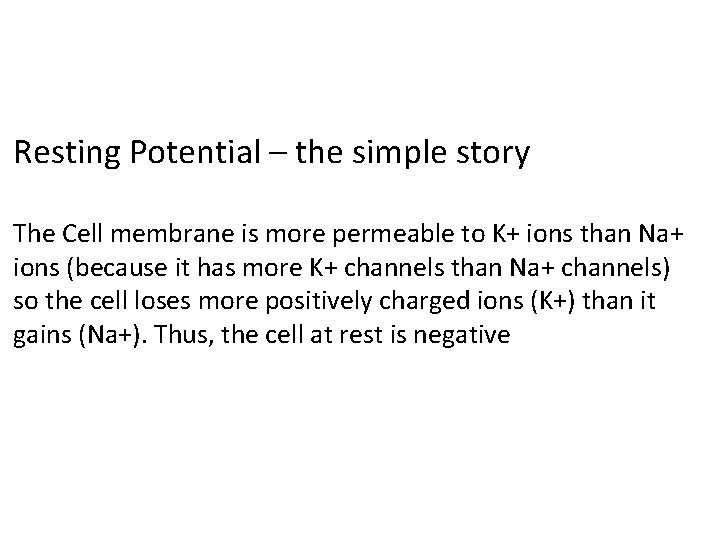
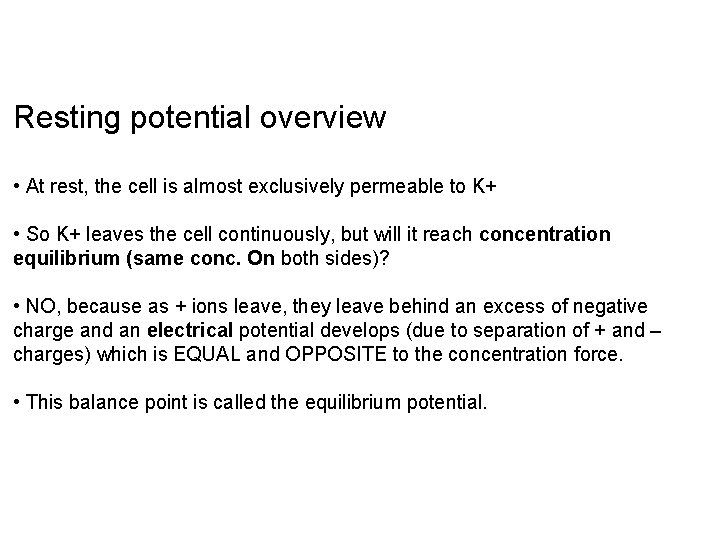
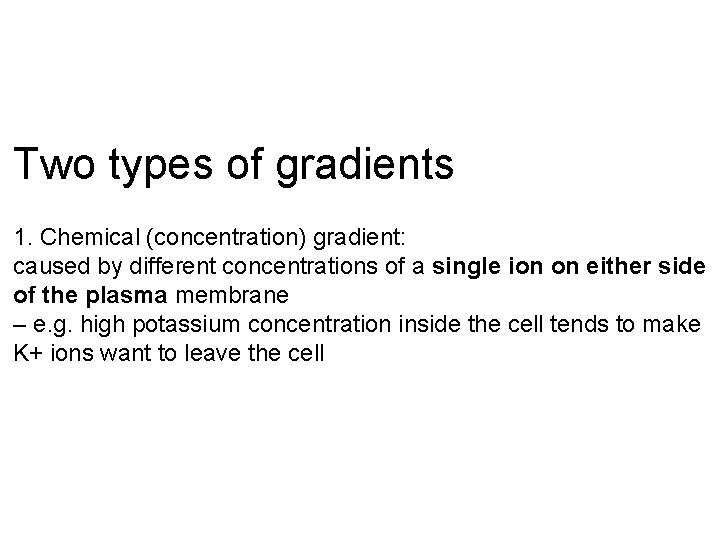
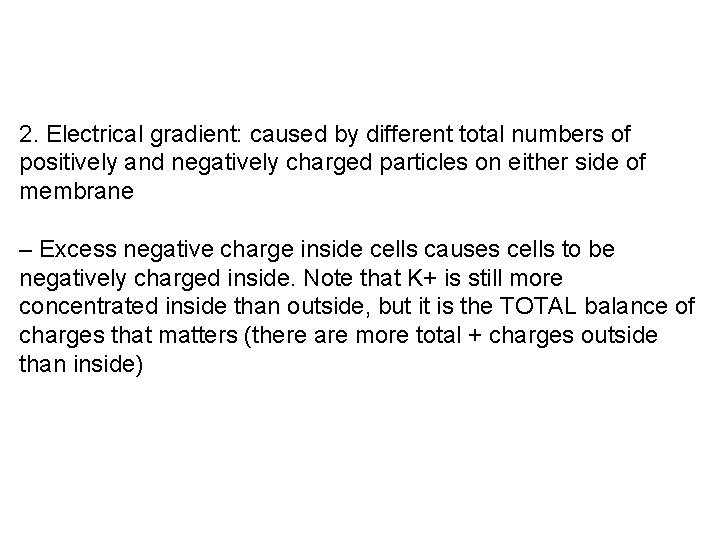
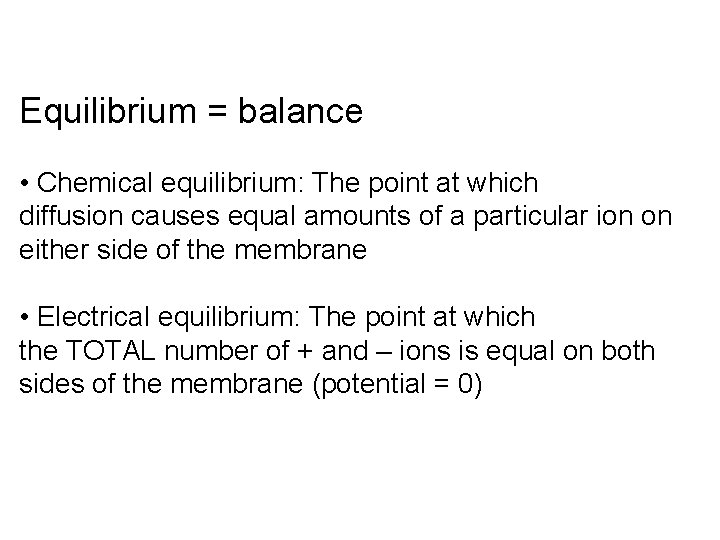
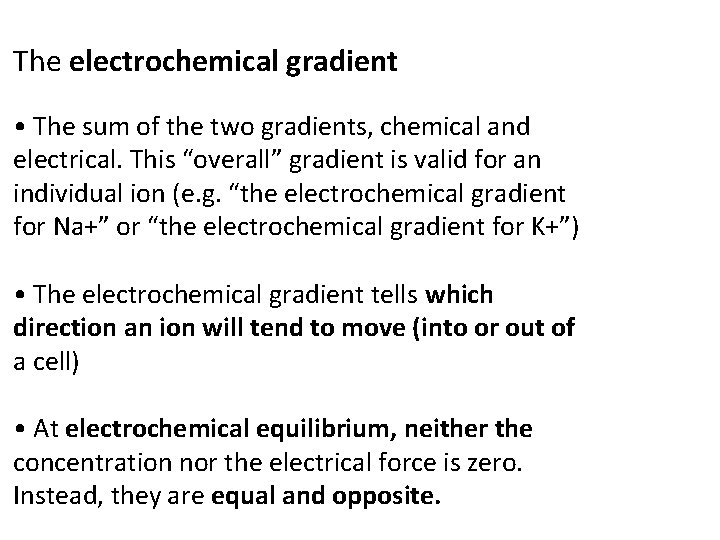
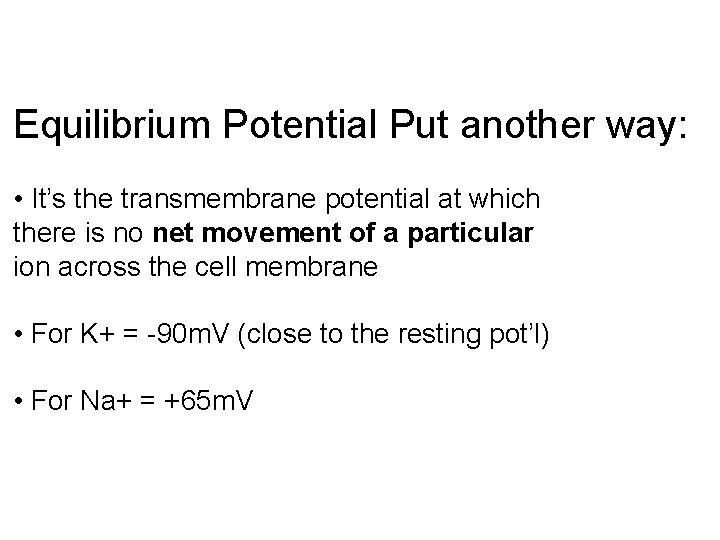
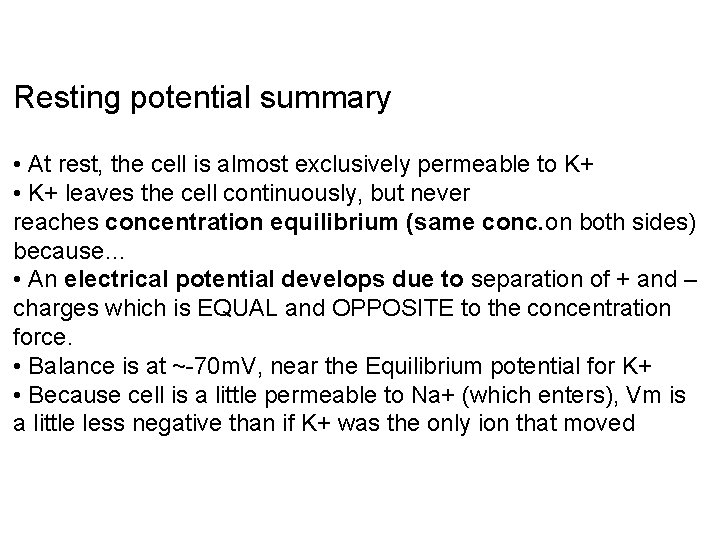
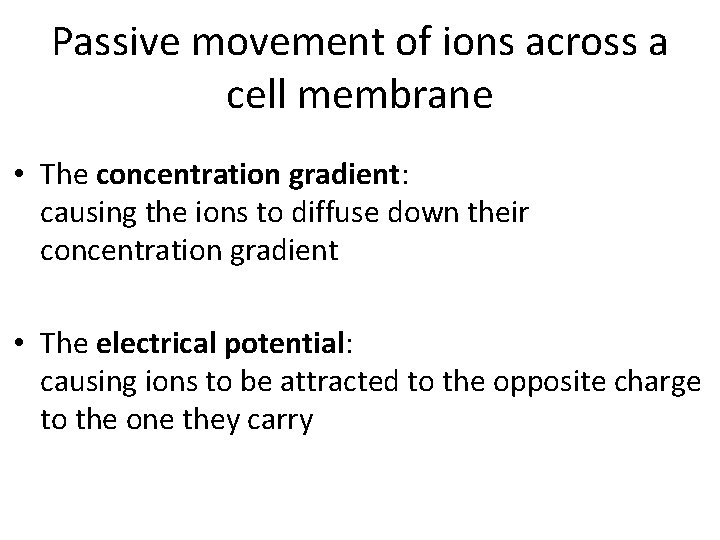
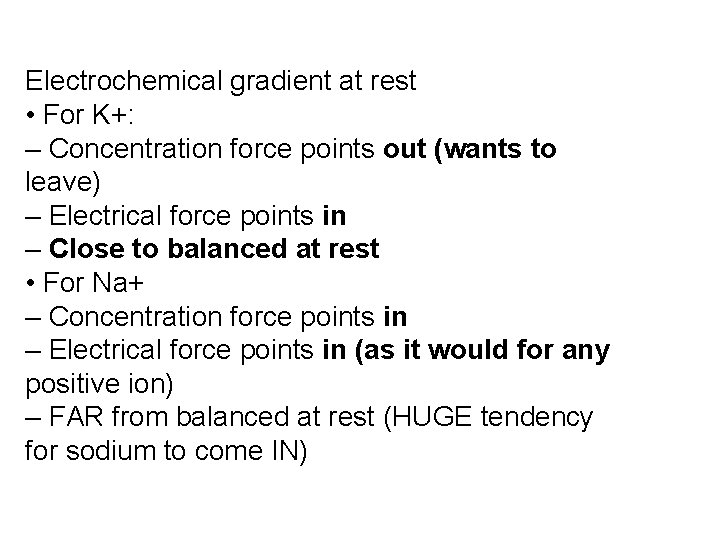
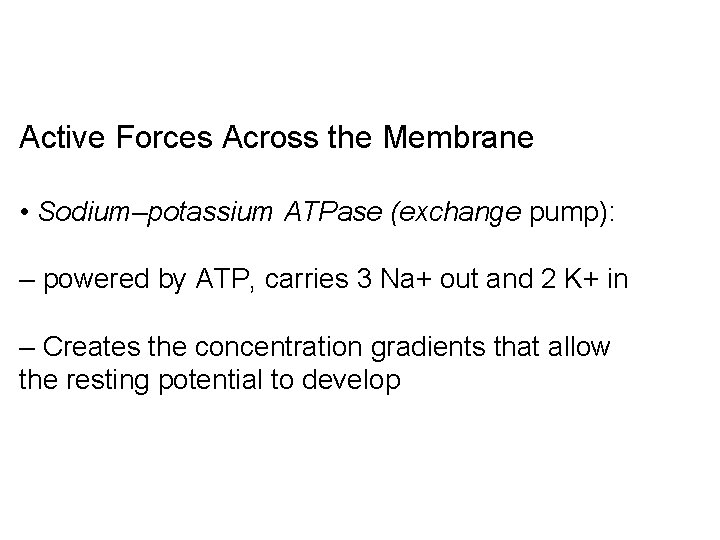
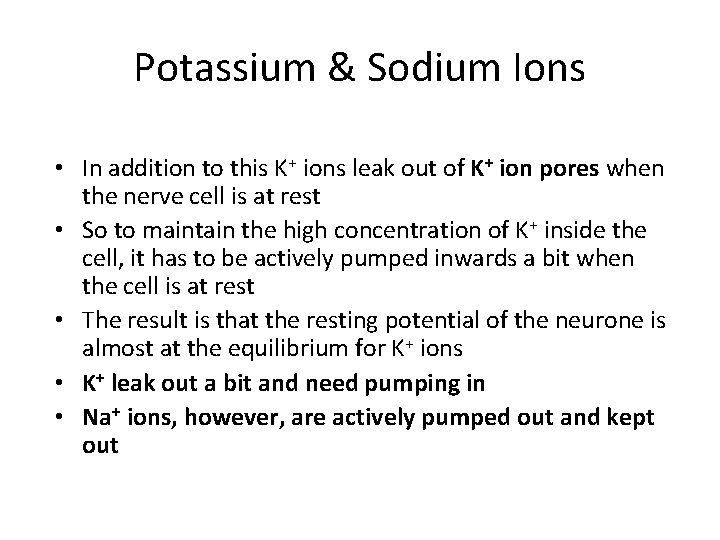
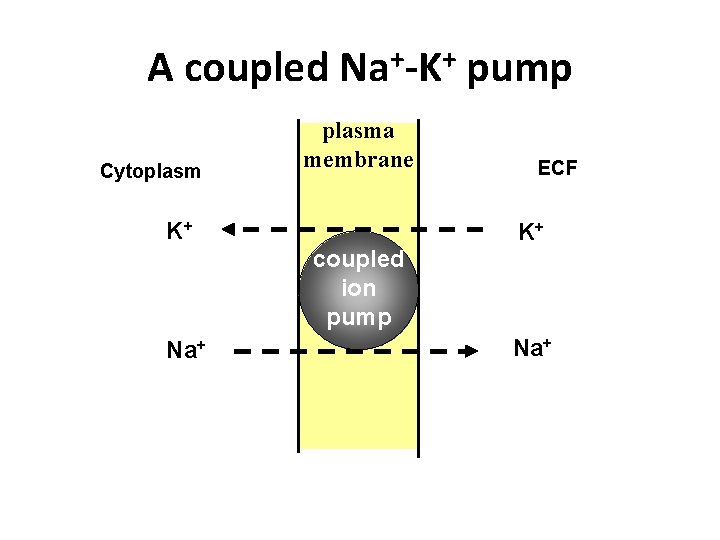
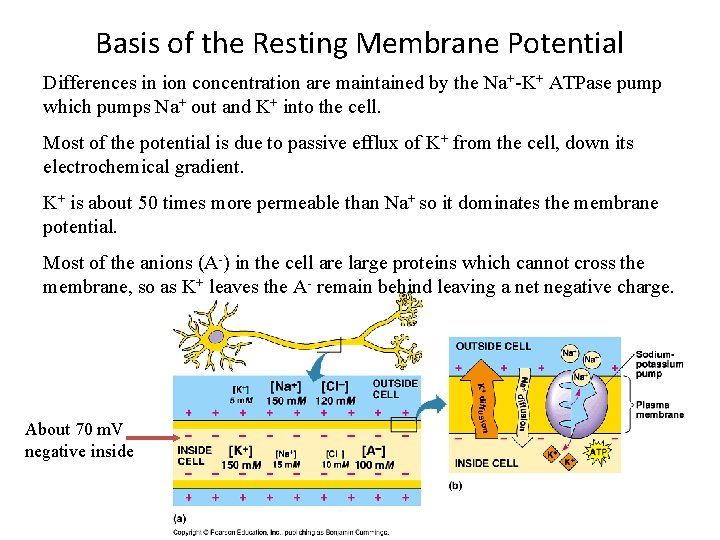
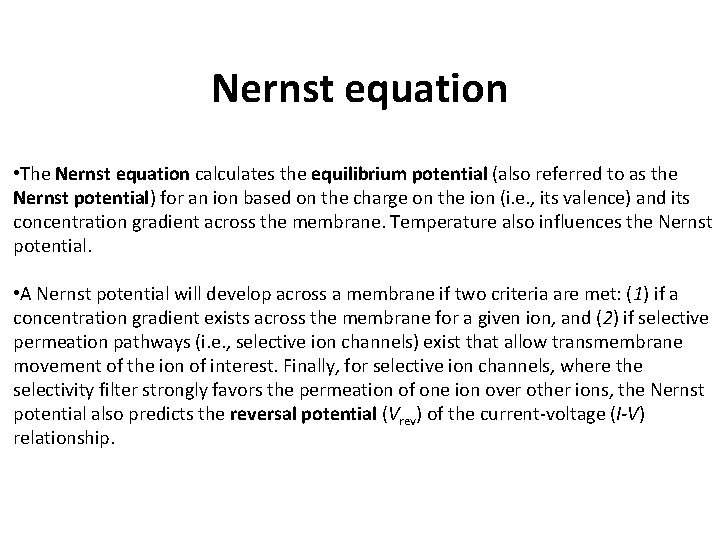
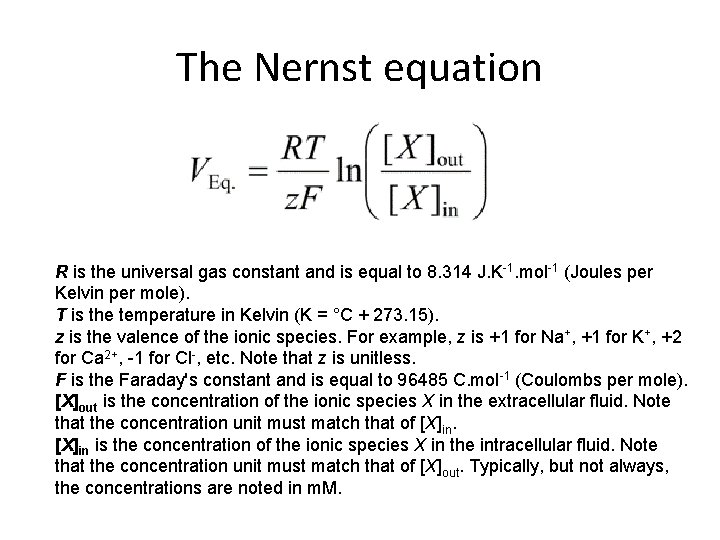
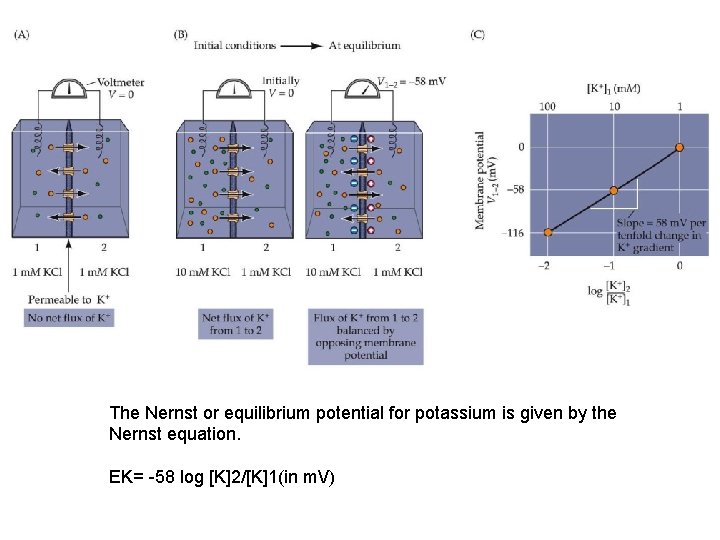
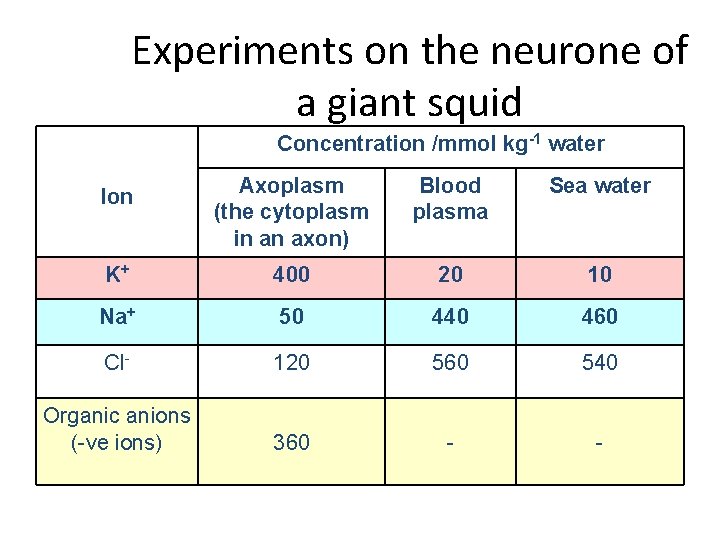
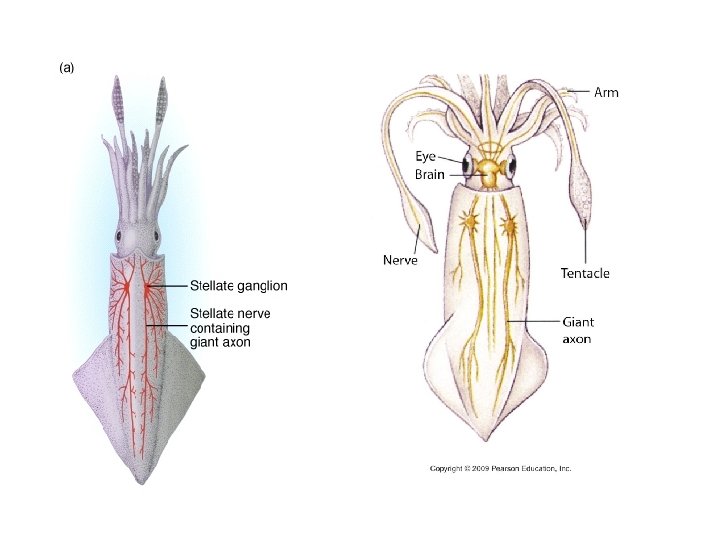
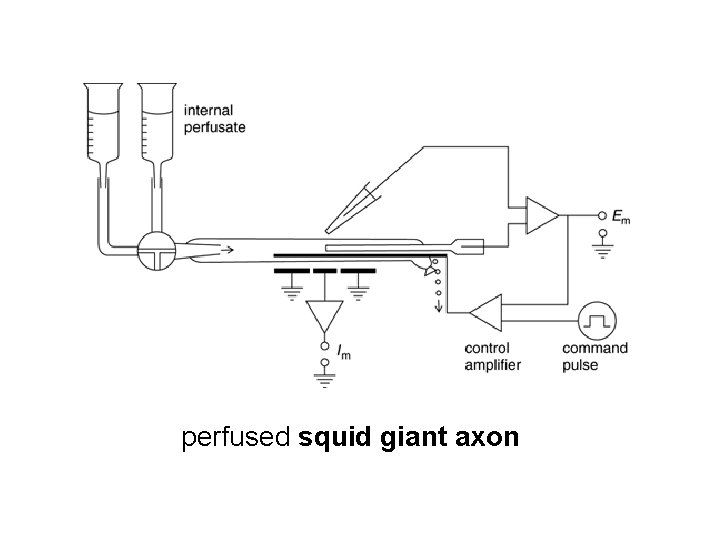
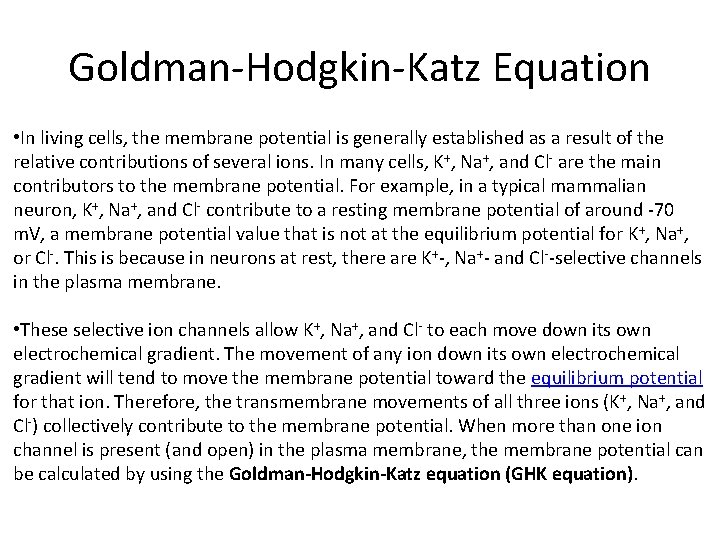
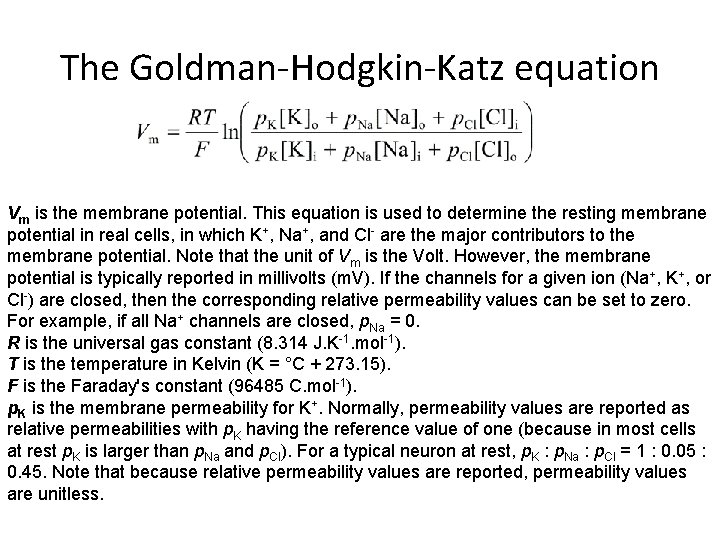
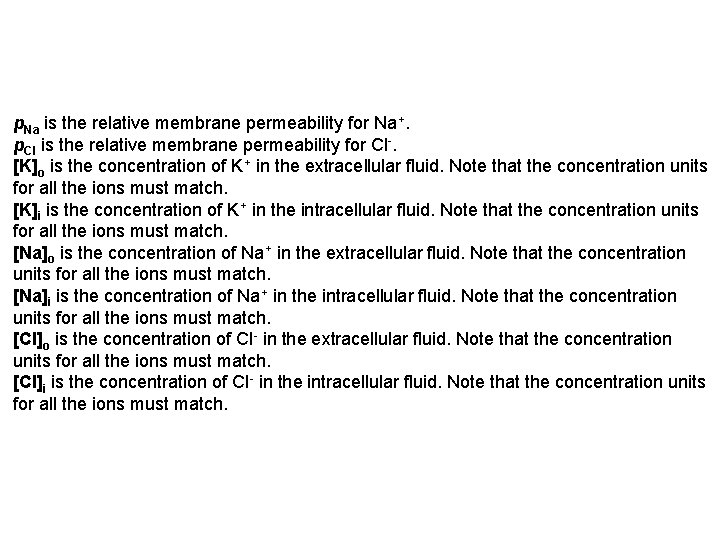
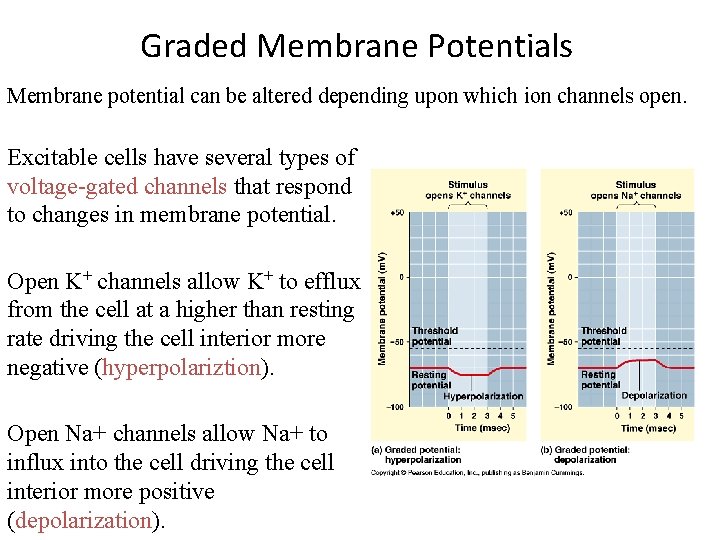
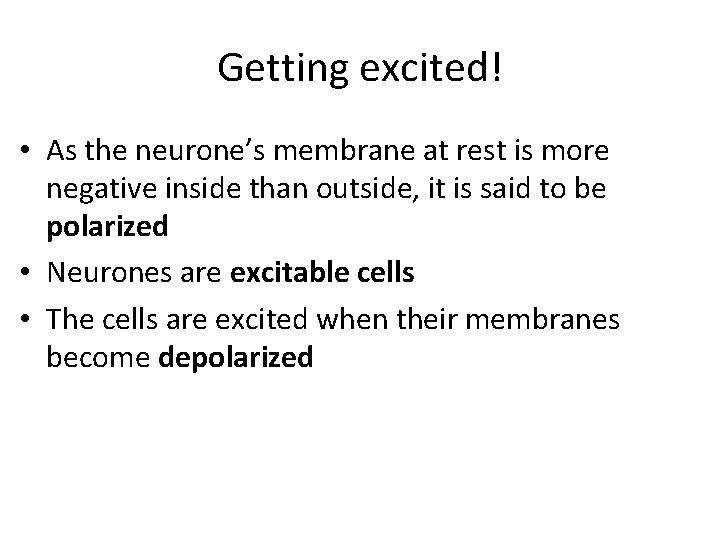
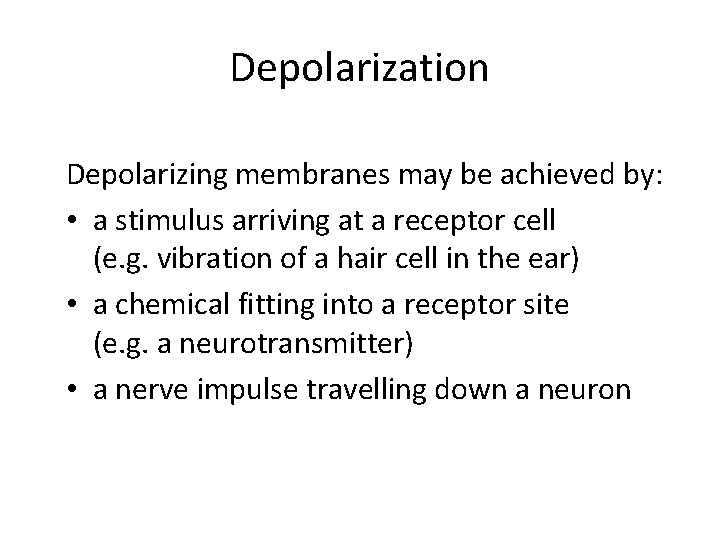
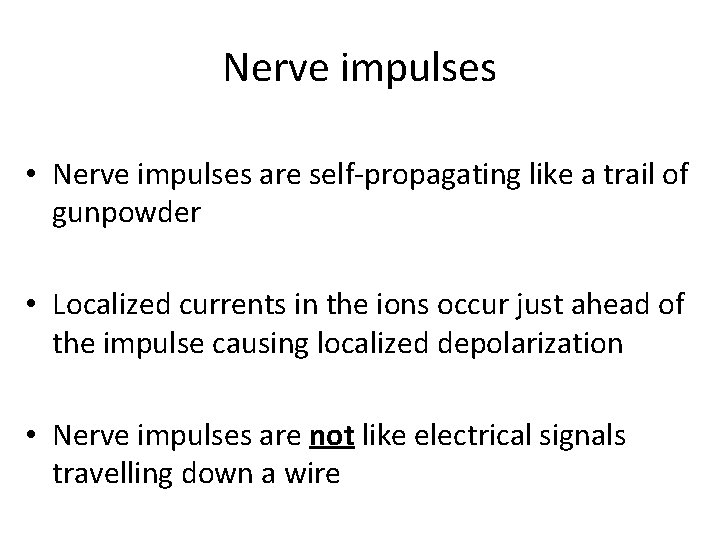
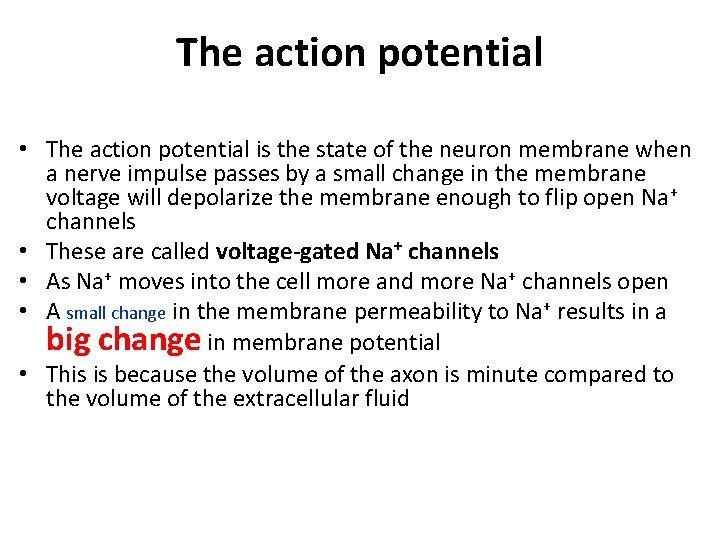
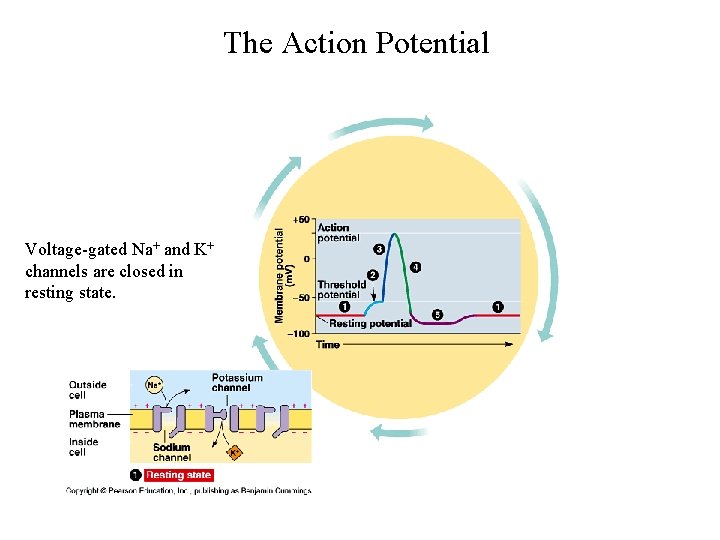
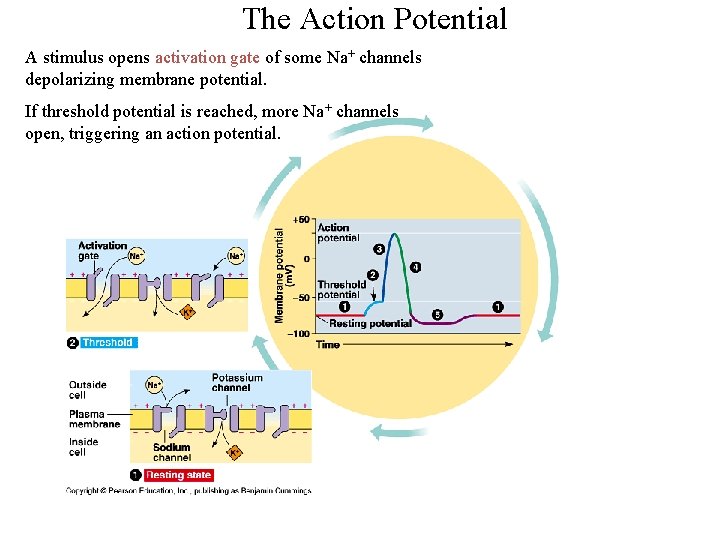
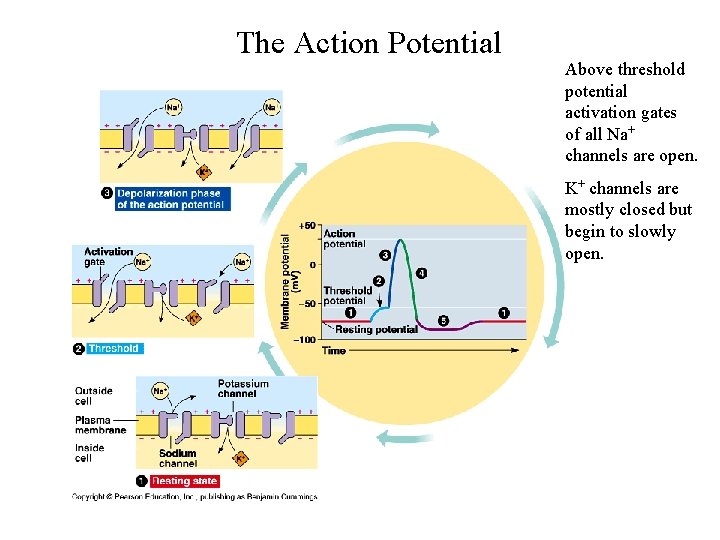
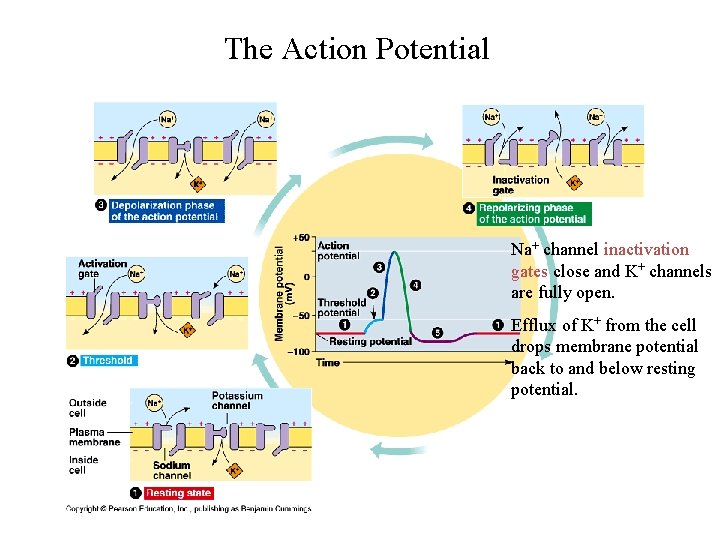
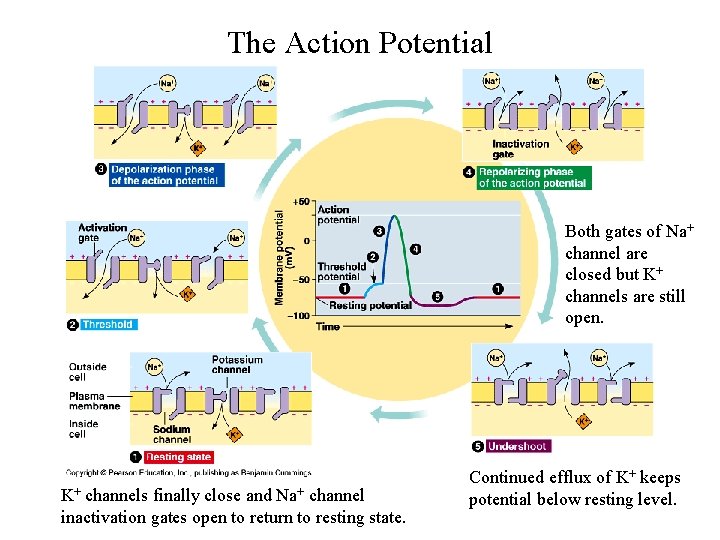
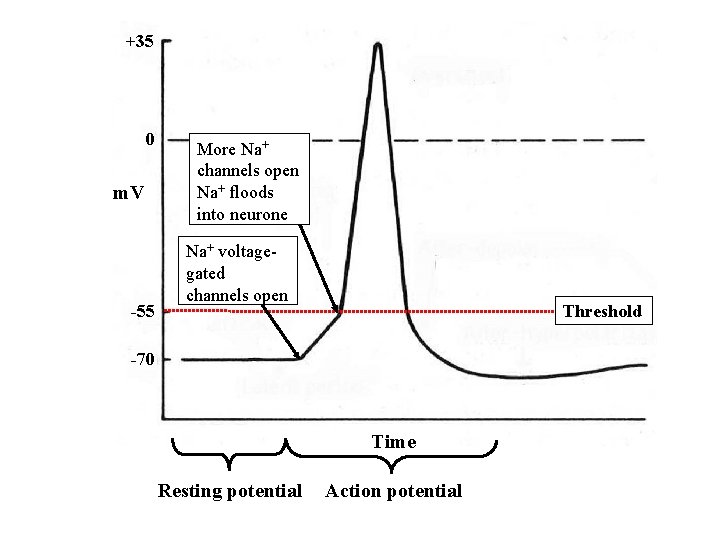
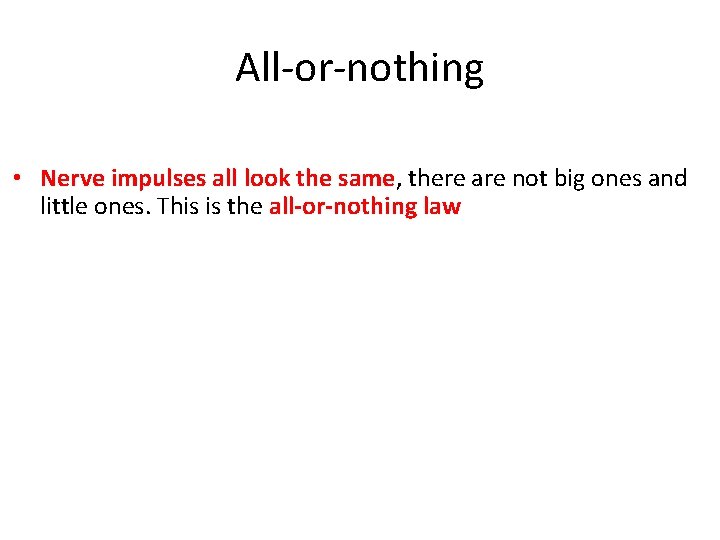
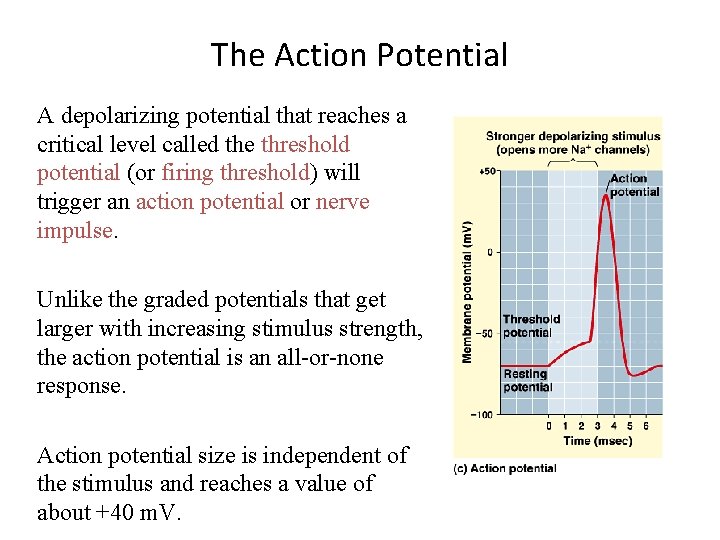
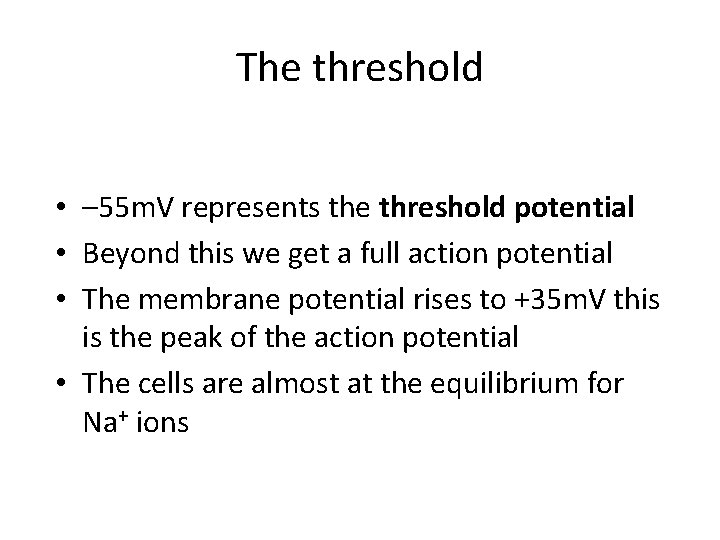
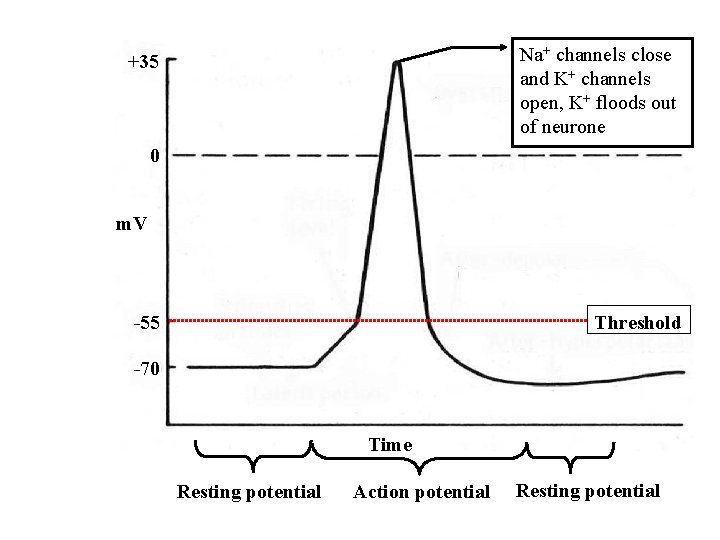
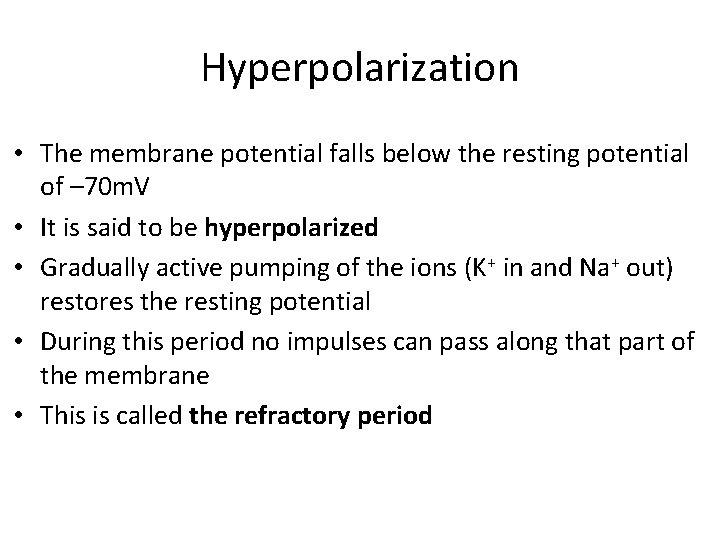
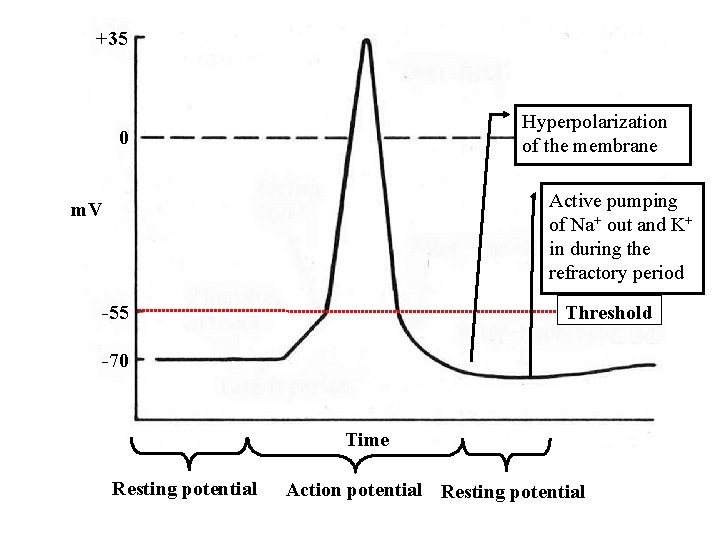
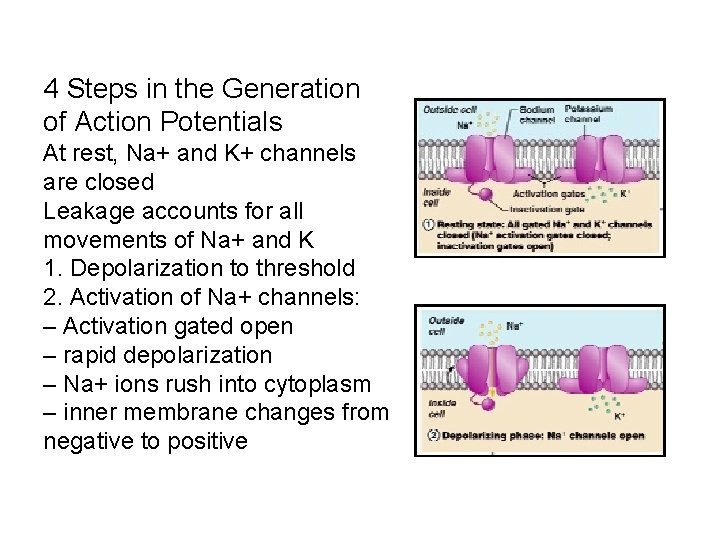
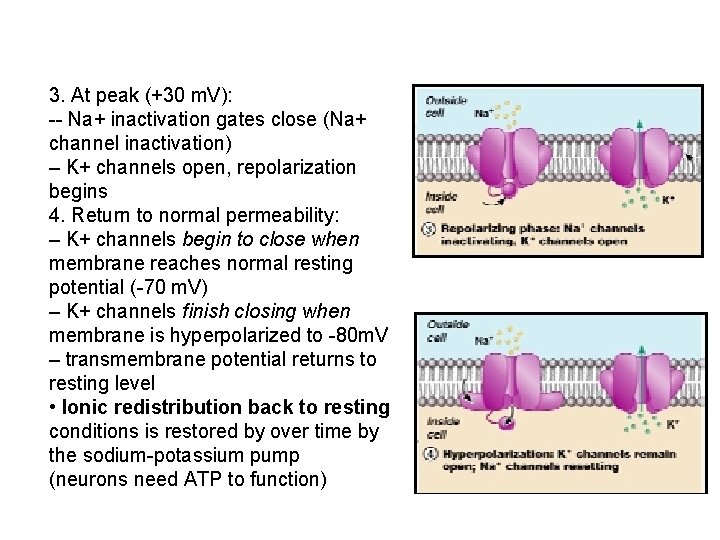
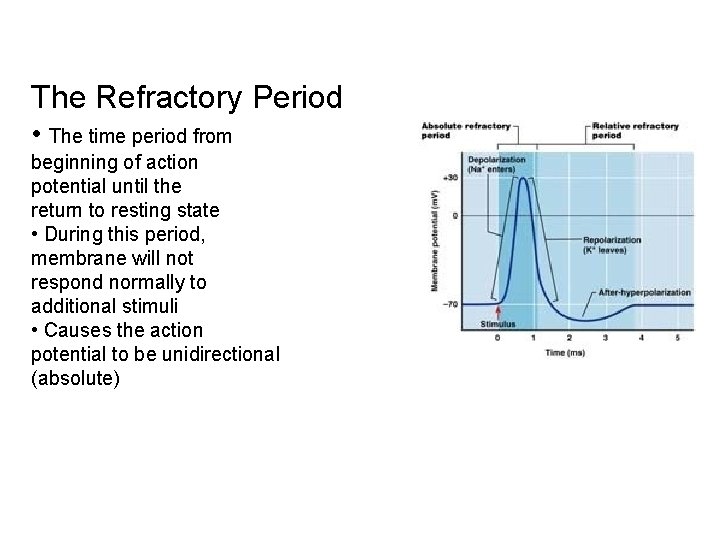
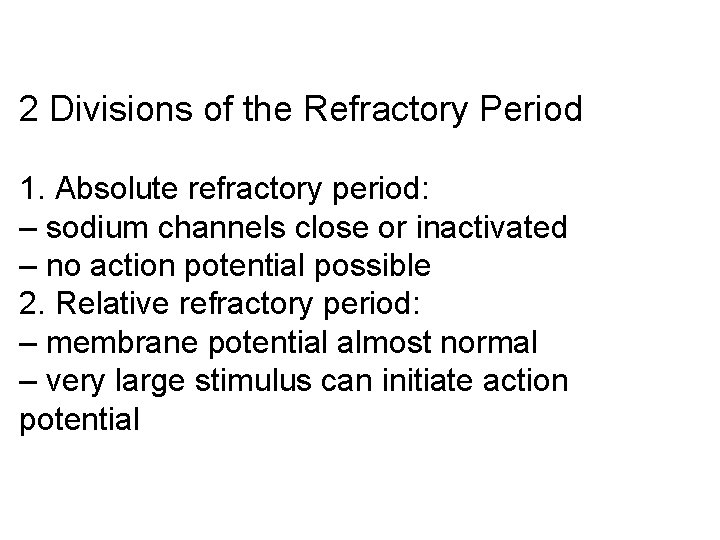
- Slides: 55
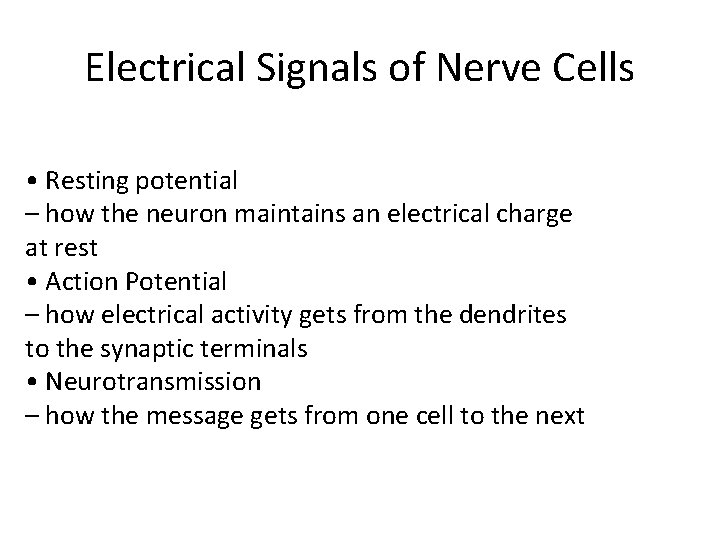
Electrical Signals of Nerve Cells • Resting potential – how the neuron maintains an electrical charge at rest • Action Potential – how electrical activity gets from the dendrites to the synaptic terminals • Neurotransmission – how the message gets from one cell to the next
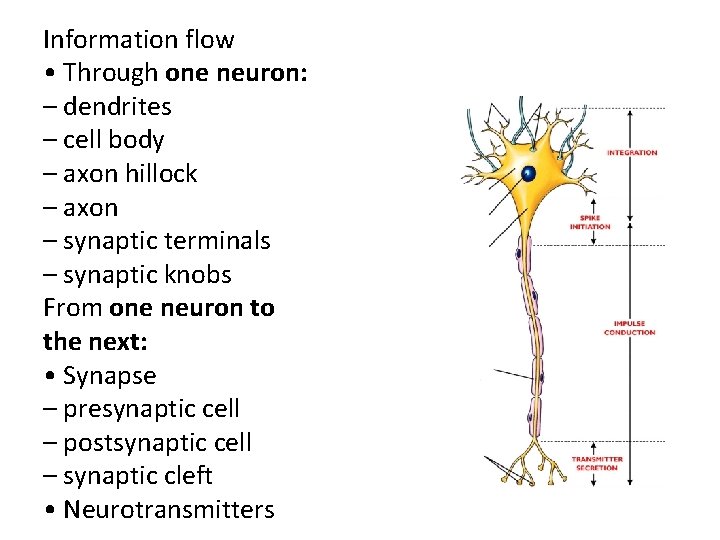
Information flow • Through one neuron: – dendrites – cell body – axon hillock – axon – synaptic terminals – synaptic knobs From one neuron to the next: • Synapse – presynaptic cell – postsynaptic cell – synaptic cleft • Neurotransmitters
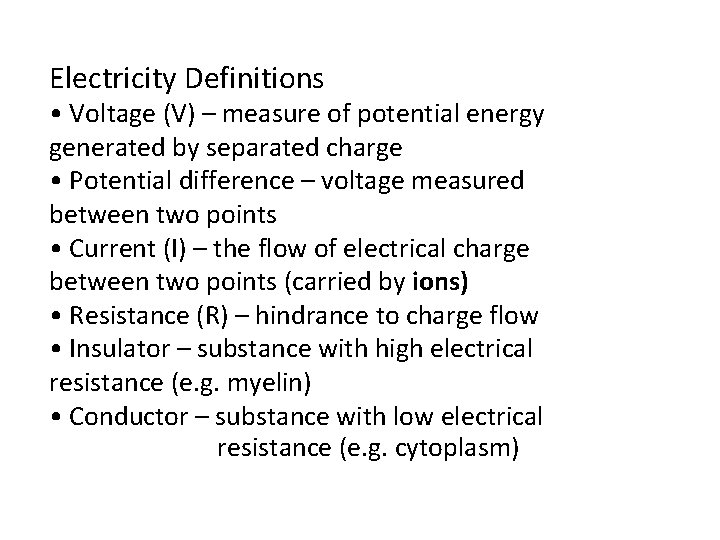
Electricity Definitions • Voltage (V) – measure of potential energy generated by separated charge • Potential difference – voltage measured between two points • Current (I) – the flow of electrical charge between two points (carried by ions) • Resistance (R) – hindrance to charge flow • Insulator – substance with high electrical resistance (e. g. myelin) • Conductor – substance with low electrical resistance (e. g. cytoplasm)
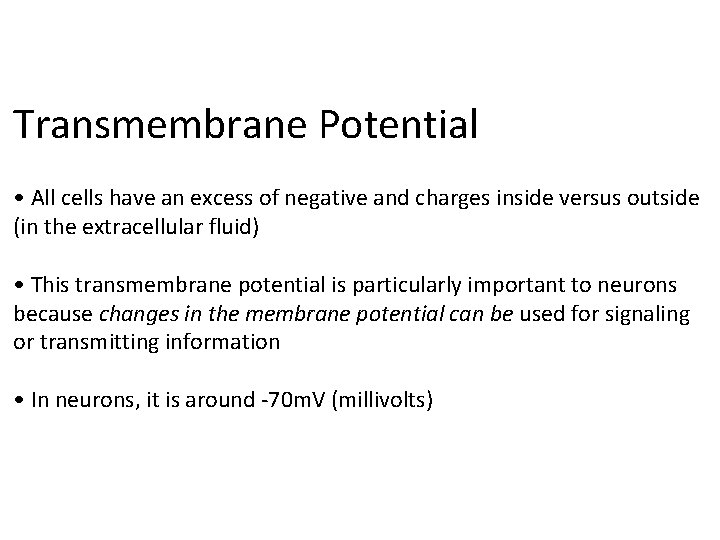
Transmembrane Potential • All cells have an excess of negative and charges inside versus outside (in the extracellular fluid) • This transmembrane potential is particularly important to neurons because changes in the membrane potential can be used for signaling or transmitting information • In neurons, it is around -70 m. V (millivolts)
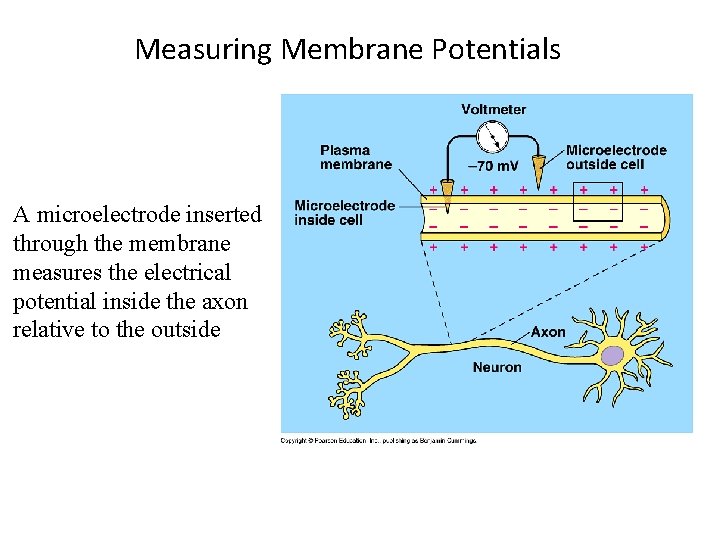
Measuring Membrane Potentials A microelectrode inserted through the membrane measures the electrical potential inside the axon relative to the outside
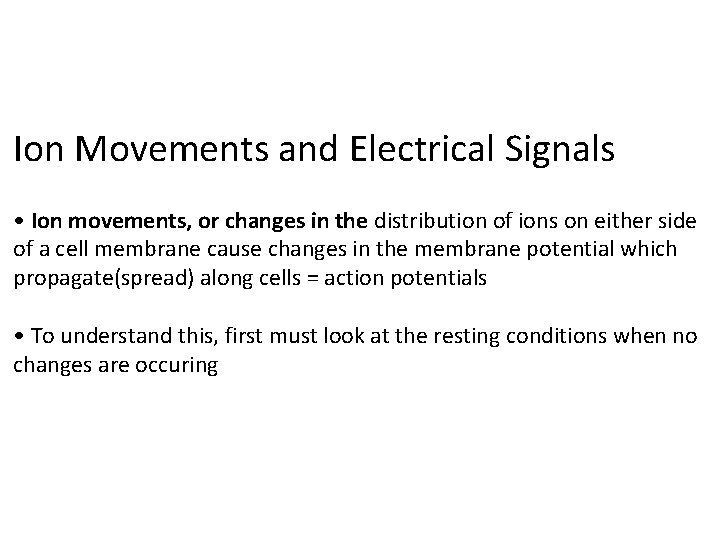
Ion Movements and Electrical Signals • Ion movements, or changes in the distribution of ions on either side of a cell membrane cause changes in the membrane potential which propagate(spread) along cells = action potentials • To understand this, first must look at the resting conditions when no changes are occuring
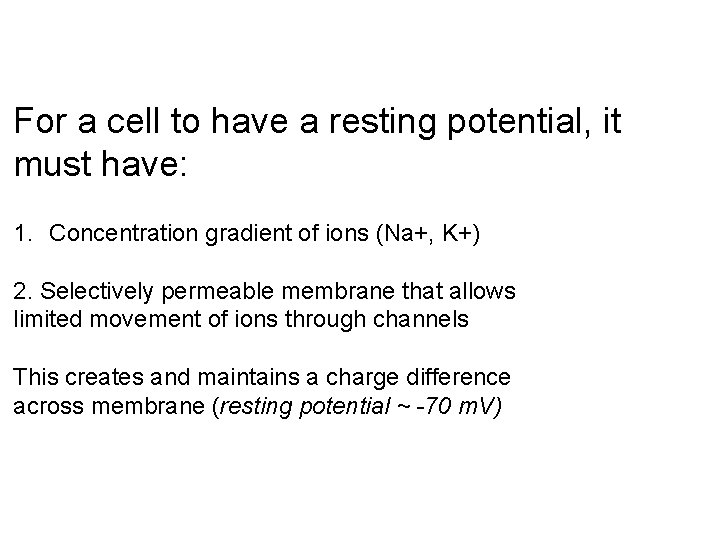
For a cell to have a resting potential, it must have: 1. Concentration gradient of ions (Na+, K+) 2. Selectively permeable membrane that allows limited movement of ions through channels This creates and maintains a charge difference across membrane (resting potential ~ -70 m. V)
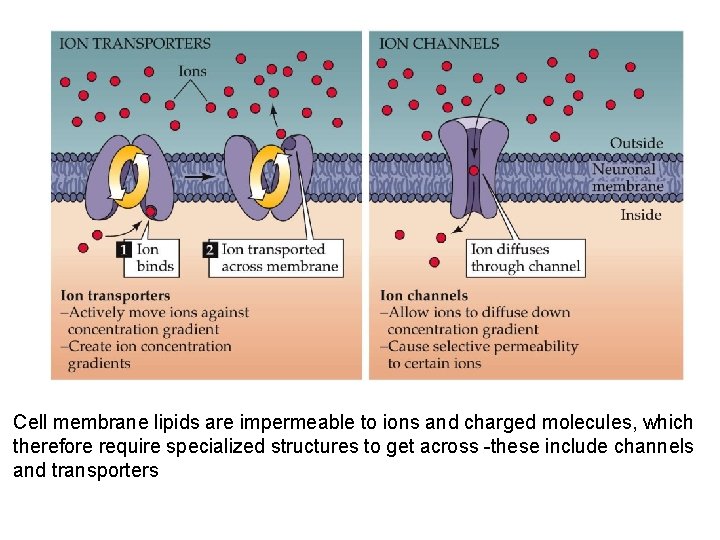
Cell membrane lipids are impermeable to ions and charged molecules, which therefore require specialized structures to get across -these include channels and transporters
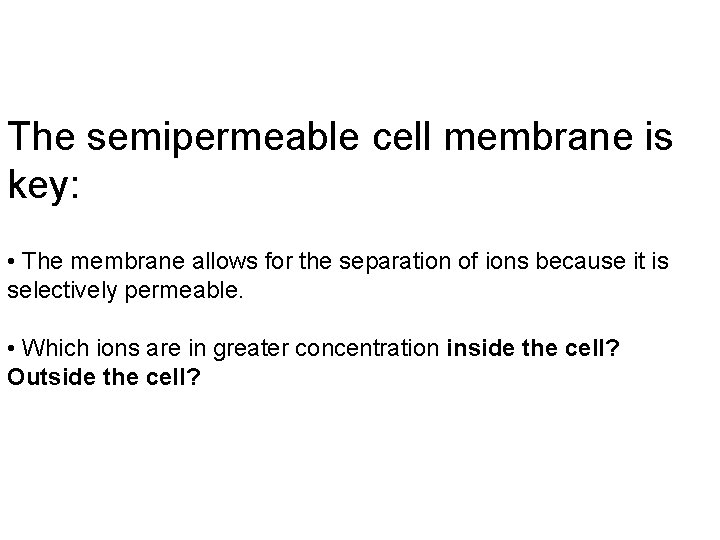
The semipermeable cell membrane is key: • The membrane allows for the separation of ions because it is selectively permeable. • Which ions are in greater concentration inside the cell? Outside the cell?
![Membrane concentrations INSIDE cells High K High proteins negatively charged Low Membrane concentrations INSIDE cells: • High [K+] • High [proteins] (negatively charged) • Low](https://slidetodoc.com/presentation_image/43a34c6cad430190dbfcf085aaaf60b5/image-10.jpg)
Membrane concentrations INSIDE cells: • High [K+] • High [proteins] (negatively charged) • Low [Cl-] • Low [Na+] OUTSIDE cells: • High [Na+] • High [Cl-] • Low [K+]
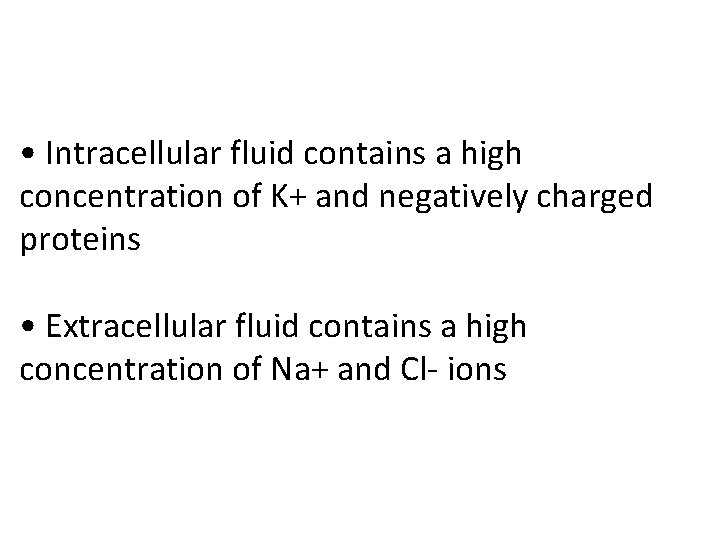
• Intracellular fluid contains a high concentration of K+ and negatively charged proteins • Extracellular fluid contains a high concentration of Na+ and Cl- ions
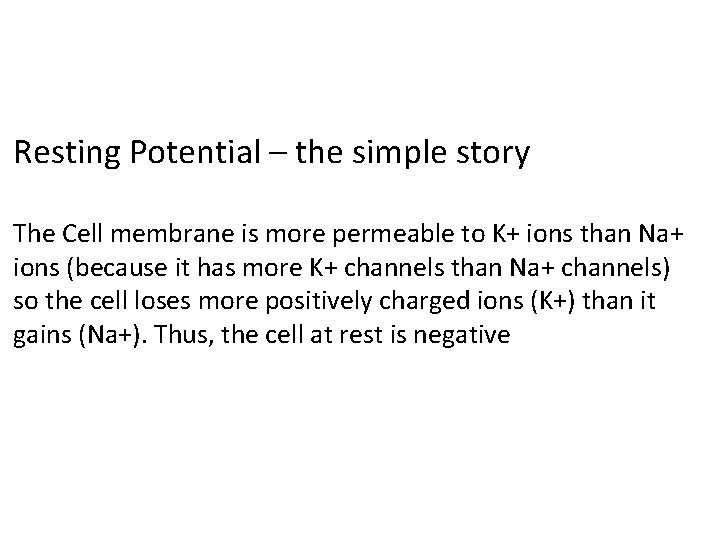
Resting Potential – the simple story The Cell membrane is more permeable to K+ ions than Na+ ions (because it has more K+ channels than Na+ channels) so the cell loses more positively charged ions (K+) than it gains (Na+). Thus, the cell at rest is negative
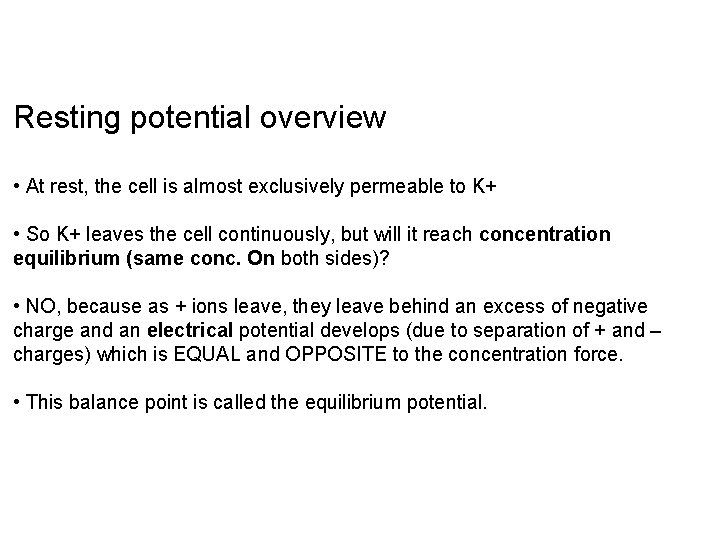
Resting potential overview • At rest, the cell is almost exclusively permeable to K+ • So K+ leaves the cell continuously, but will it reach concentration equilibrium (same conc. On both sides)? • NO, because as + ions leave, they leave behind an excess of negative charge and an electrical potential develops (due to separation of + and – charges) which is EQUAL and OPPOSITE to the concentration force. • This balance point is called the equilibrium potential.
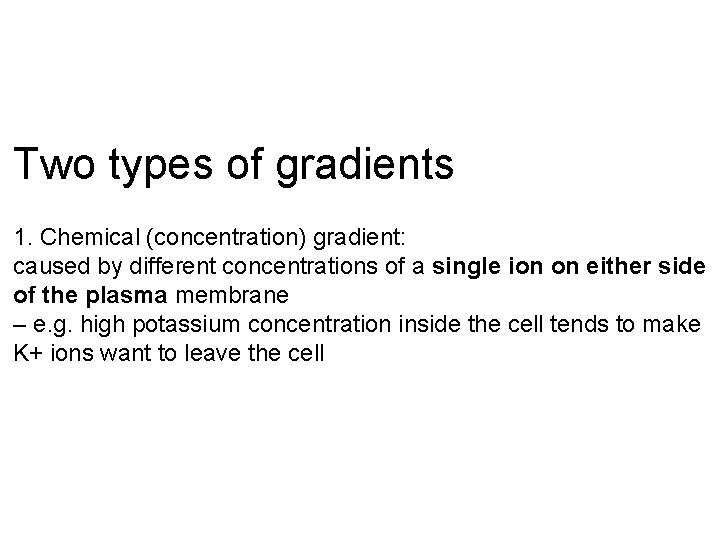
Two types of gradients 1. Chemical (concentration) gradient: caused by different concentrations of a single ion on either side of the plasma membrane – e. g. high potassium concentration inside the cell tends to make K+ ions want to leave the cell
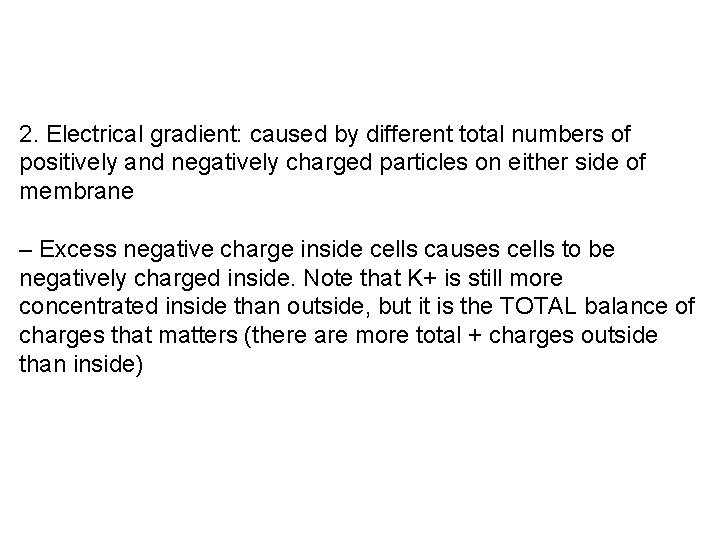
2. Electrical gradient: caused by different total numbers of positively and negatively charged particles on either side of membrane – Excess negative charge inside cells causes cells to be negatively charged inside. Note that K+ is still more concentrated inside than outside, but it is the TOTAL balance of charges that matters (there are more total + charges outside than inside)
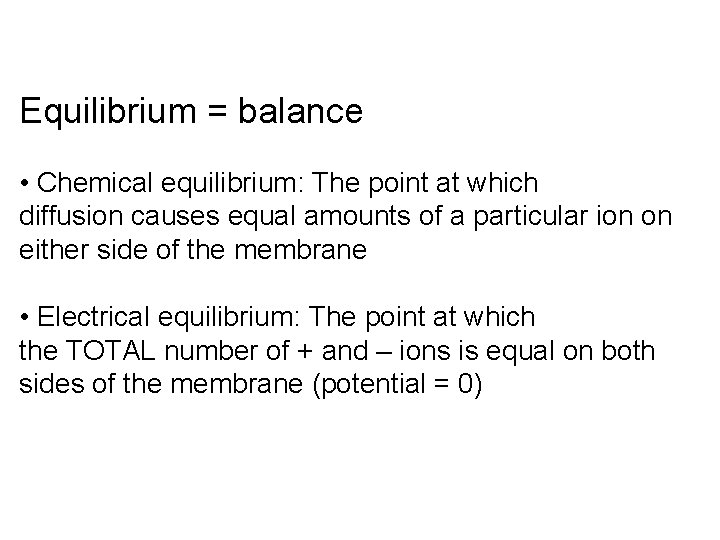
Equilibrium = balance • Chemical equilibrium: The point at which diffusion causes equal amounts of a particular ion on either side of the membrane • Electrical equilibrium: The point at which the TOTAL number of + and – ions is equal on both sides of the membrane (potential = 0)
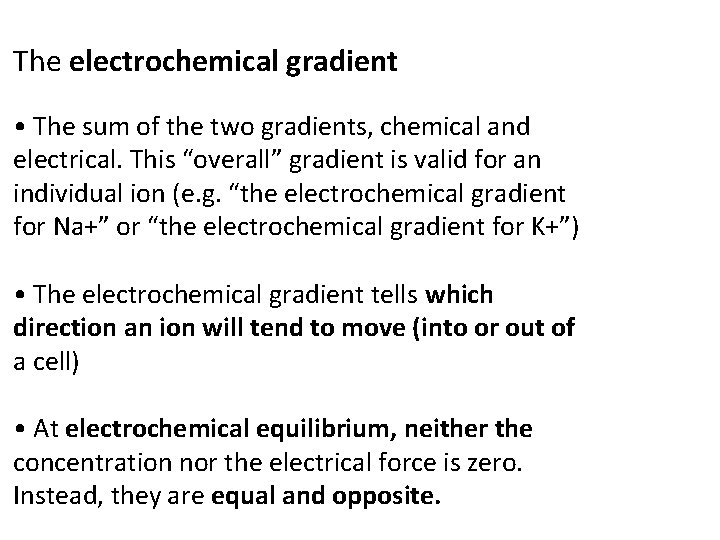
The electrochemical gradient • The sum of the two gradients, chemical and electrical. This “overall” gradient is valid for an individual ion (e. g. “the electrochemical gradient for Na+” or “the electrochemical gradient for K+”) • The electrochemical gradient tells which direction an ion will tend to move (into or out of a cell) • At electrochemical equilibrium, neither the concentration nor the electrical force is zero. Instead, they are equal and opposite.
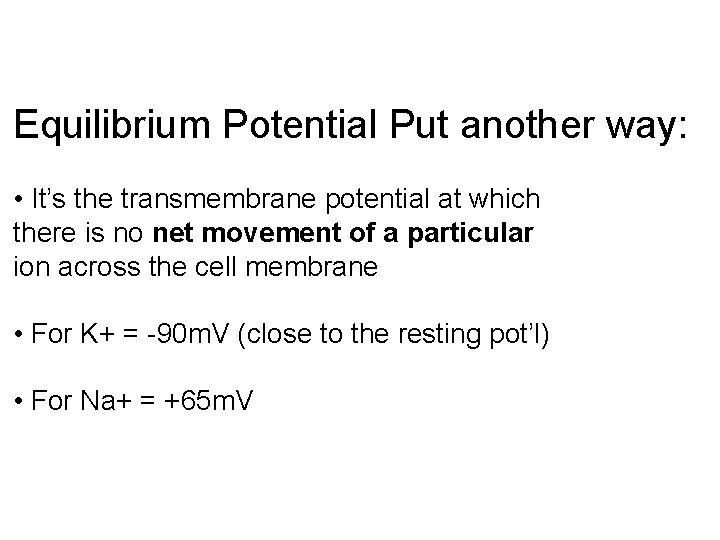
Equilibrium Potential Put another way: • It’s the transmembrane potential at which there is no net movement of a particular ion across the cell membrane • For K+ = -90 m. V (close to the resting pot’l) • For Na+ = +65 m. V
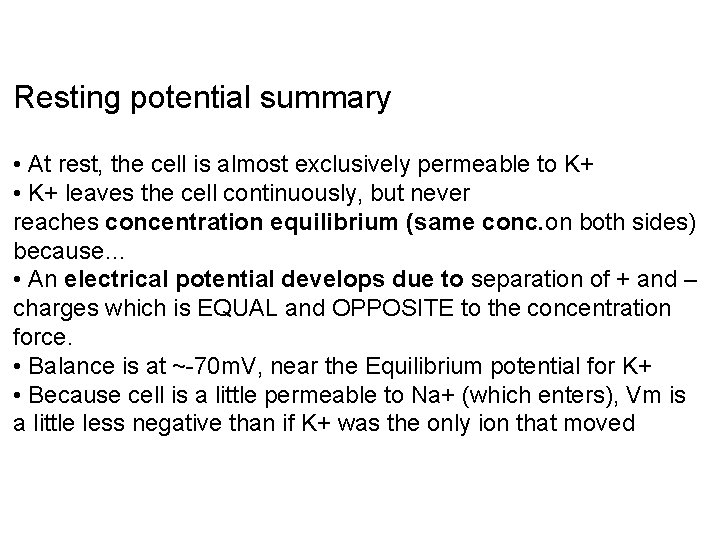
Resting potential summary • At rest, the cell is almost exclusively permeable to K+ • K+ leaves the cell continuously, but never reaches concentration equilibrium (same conc. on both sides) because… • An electrical potential develops due to separation of + and – charges which is EQUAL and OPPOSITE to the concentration force. • Balance is at ~-70 m. V, near the Equilibrium potential for K+ • Because cell is a little permeable to Na+ (which enters), Vm is a little less negative than if K+ was the only ion that moved
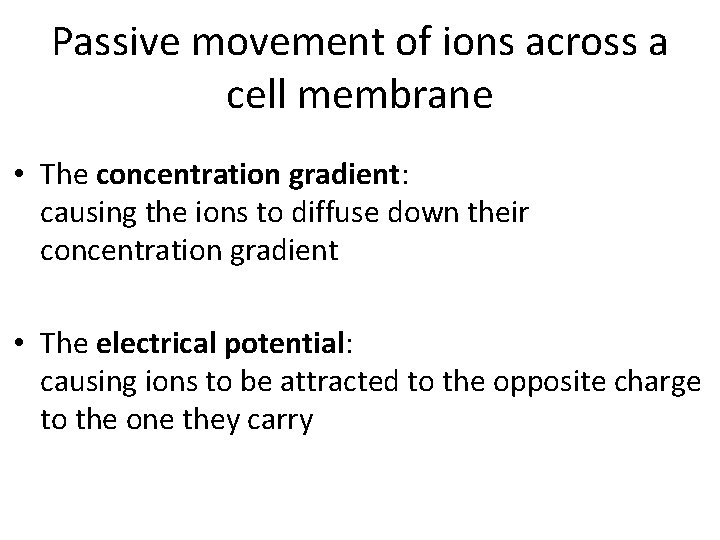
Passive movement of ions across a cell membrane • The concentration gradient: causing the ions to diffuse down their concentration gradient • The electrical potential: causing ions to be attracted to the opposite charge to the one they carry
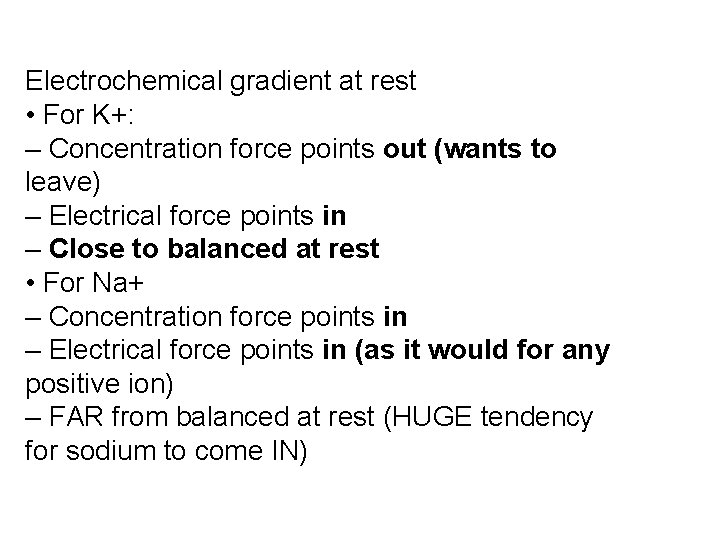
Electrochemical gradient at rest • For K+: – Concentration force points out (wants to leave) – Electrical force points in – Close to balanced at rest • For Na+ – Concentration force points in – Electrical force points in (as it would for any positive ion) – FAR from balanced at rest (HUGE tendency for sodium to come IN)
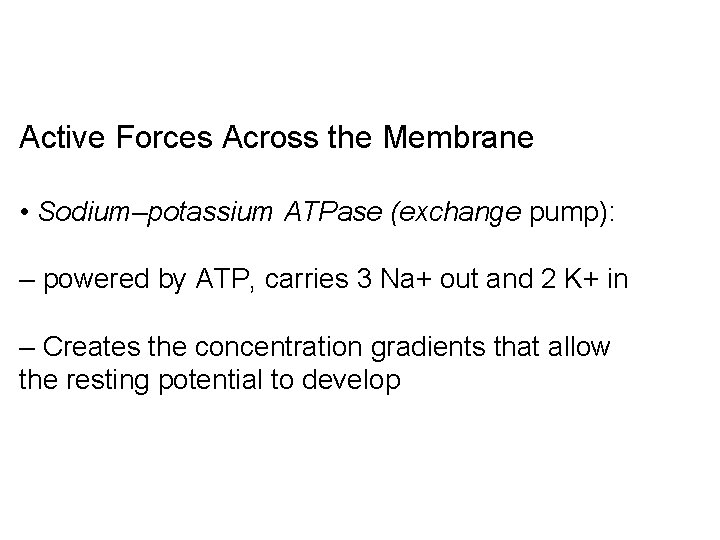
Active Forces Across the Membrane • Sodium–potassium ATPase (exchange pump): – powered by ATP, carries 3 Na+ out and 2 K+ in – Creates the concentration gradients that allow the resting potential to develop
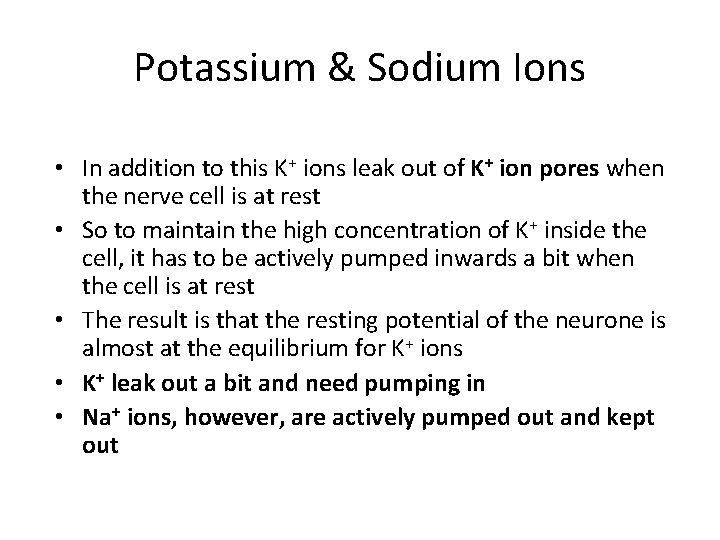
Potassium & Sodium Ions • In addition to this K+ ions leak out of K+ ion pores when the nerve cell is at rest • So to maintain the high concentration of K+ inside the cell, it has to be actively pumped inwards a bit when the cell is at rest • The result is that the resting potential of the neurone is almost at the equilibrium for K+ ions • K+ leak out a bit and need pumping in • Na+ ions, however, are actively pumped out and kept out
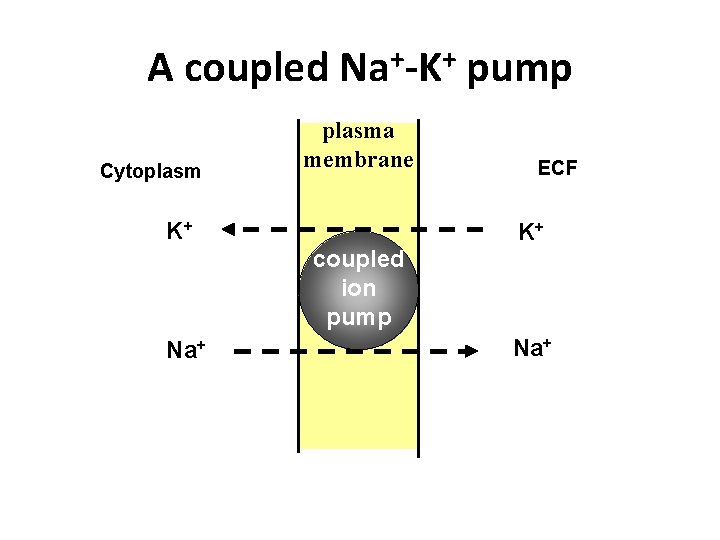
A coupled Na+-K+ pump Cytoplasma membrane K+ coupled ion pump Na+ ECF K+ Na+
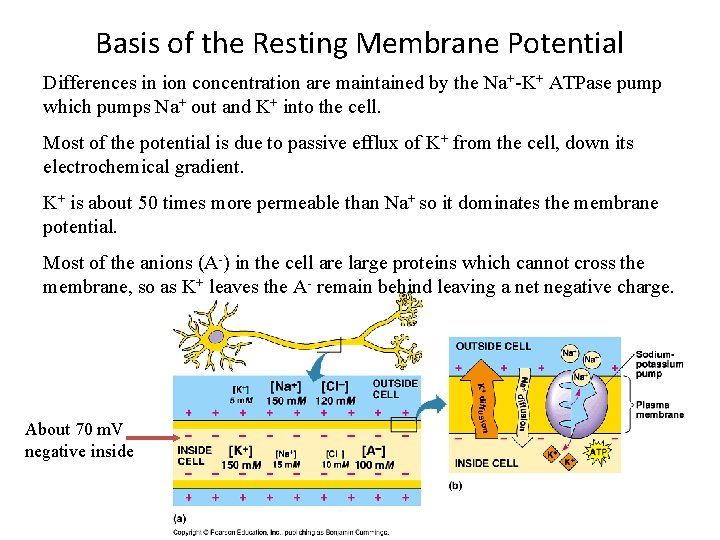
Basis of the Resting Membrane Potential Differences in ion concentration are maintained by the Na+-K+ ATPase pump which pumps Na+ out and K+ into the cell. Most of the potential is due to passive efflux of K+ from the cell, down its electrochemical gradient. K+ is about 50 times more permeable than Na+ so it dominates the membrane potential. Most of the anions (A-) in the cell are large proteins which cannot cross the membrane, so as K+ leaves the A- remain behind leaving a net negative charge. About 70 m. V negative inside
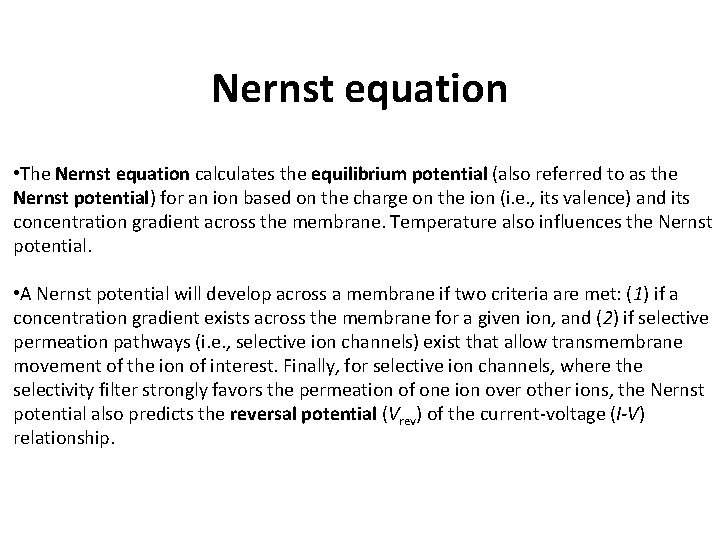
Nernst equation • The Nernst equation calculates the equilibrium potential (also referred to as the Nernst potential) for an ion based on the charge on the ion (i. e. , its valence) and its concentration gradient across the membrane. Temperature also influences the Nernst potential. • A Nernst potential will develop across a membrane if two criteria are met: (1) if a concentration gradient exists across the membrane for a given ion, and (2) if selective permeation pathways (i. e. , selective ion channels) exist that allow transmembrane movement of the ion of interest. Finally, for selective ion channels, where the selectivity filter strongly favors the permeation of one ion over other ions, the Nernst potential also predicts the reversal potential (Vrev) of the current-voltage (I-V) relationship.
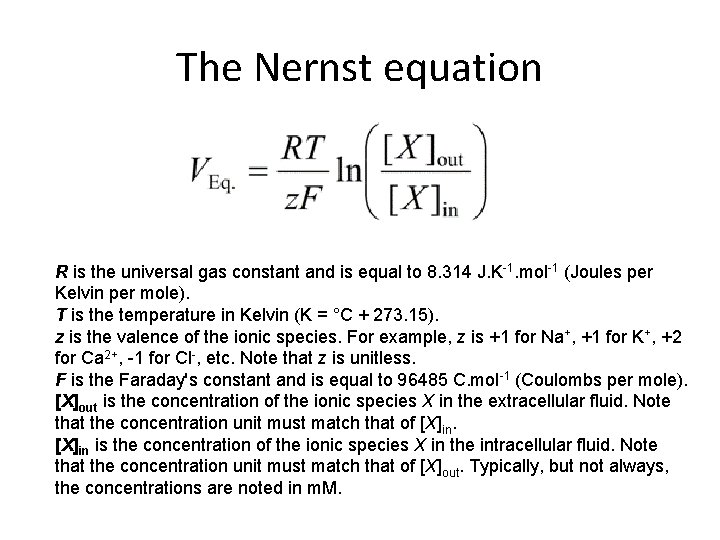
The Nernst equation R is the universal gas constant and is equal to 8. 314 J. K-1. mol-1 (Joules per Kelvin per mole). T is the temperature in Kelvin (K = °C + 273. 15). z is the valence of the ionic species. For example, z is +1 for Na+, +1 for K+, +2 for Ca 2+, -1 for Cl-, etc. Note that z is unitless. F is the Faraday's constant and is equal to 96485 C. mol-1 (Coulombs per mole). [X]out is the concentration of the ionic species X in the extracellular fluid. Note that the concentration unit must match that of [X]in is the concentration of the ionic species X in the intracellular fluid. Note that the concentration unit must match that of [X]out. Typically, but not always, the concentrations are noted in m. M.
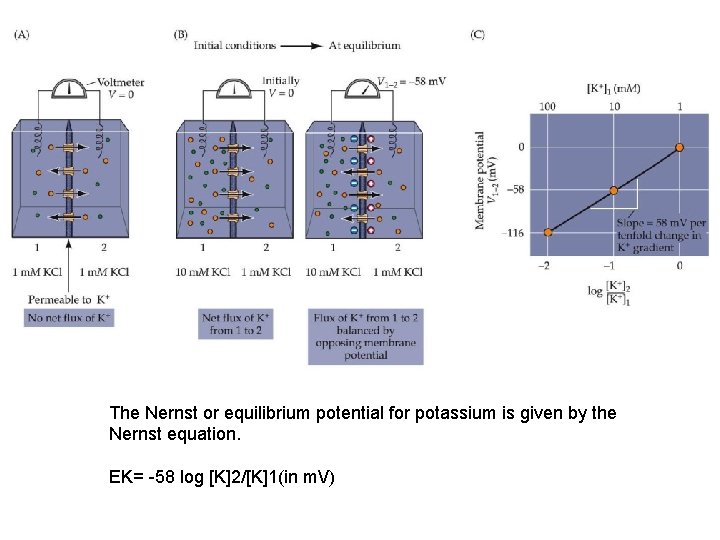
The Nernst or equilibrium potential for potassium is given by the Nernst equation. EK= -58 log [K]2/[K]1(in m. V)
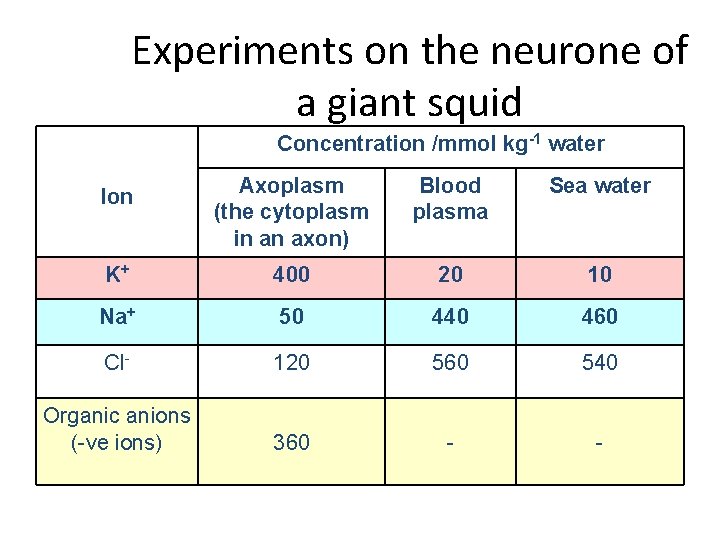
Experiments on the neurone of a giant squid Concentration /mmol kg-1 water Ion Axoplasm (the cytoplasm in an axon) Blood plasma Sea water K+ 400 20 10 Na+ 50 440 460 Cl- 120 560 540 Organic anions (-ve ions) 360 - -
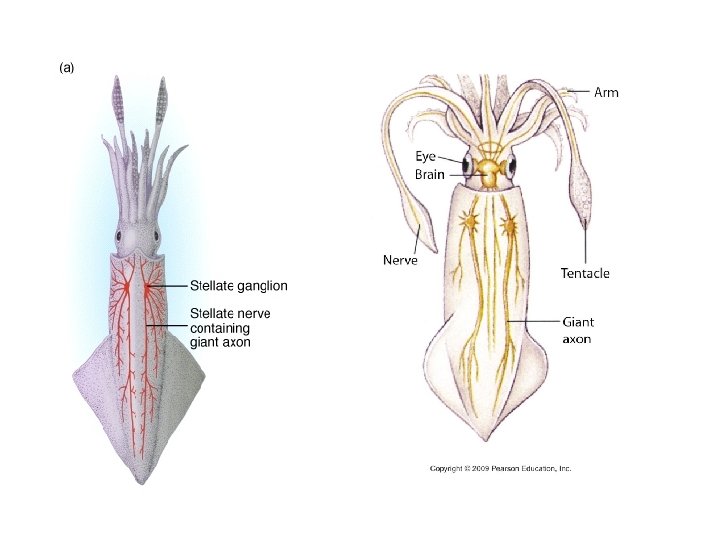
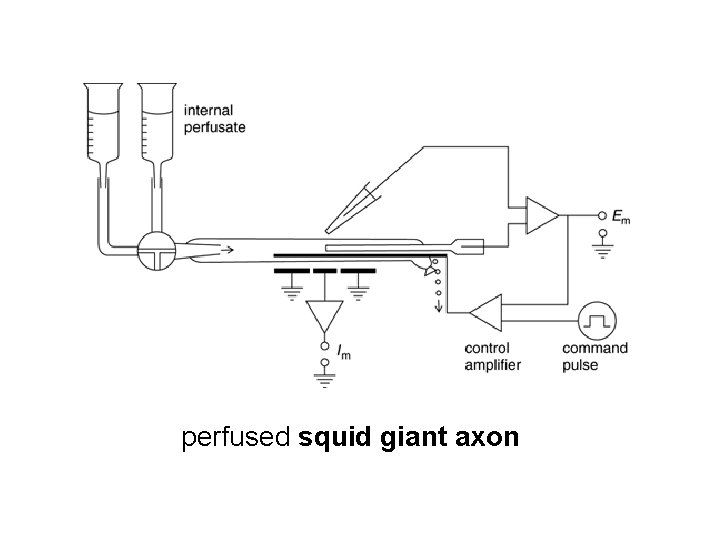
perfused squid giant axon
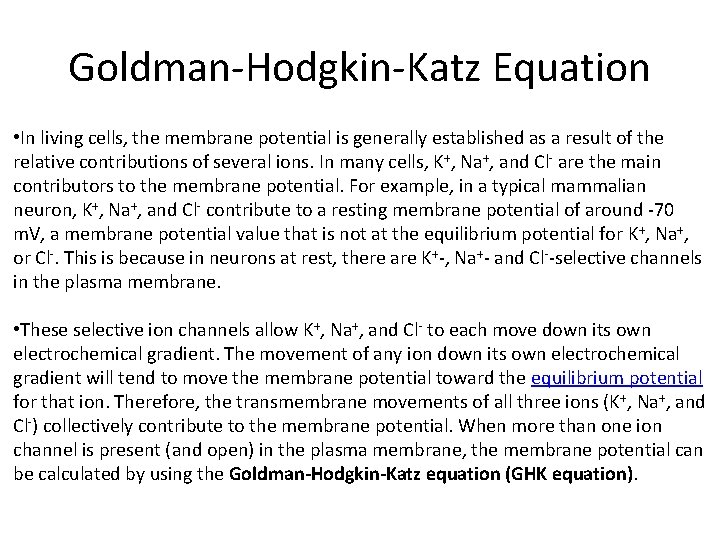
Goldman-Hodgkin-Katz Equation • In living cells, the membrane potential is generally established as a result of the relative contributions of several ions. In many cells, K+, Na+, and Cl- are the main contributors to the membrane potential. For example, in a typical mammalian neuron, K+, Na+, and Cl- contribute to a resting membrane potential of around -70 m. V, a membrane potential value that is not at the equilibrium potential for K +, Na+, or Cl-. This is because in neurons at rest, there are K+-, Na+- and Cl--selective channels in the plasma membrane. • These selective ion channels allow K+, Na+, and Cl- to each move down its own electrochemical gradient. The movement of any ion down its own electrochemical gradient will tend to move the membrane potential toward the equilibrium potential for that ion. Therefore, the transmembrane movements of all three ions (K +, Na+, and Cl-) collectively contribute to the membrane potential. When more than one ion channel is present (and open) in the plasma membrane, the membrane potential can be calculated by using the Goldman-Hodgkin-Katz equation (GHK equation).
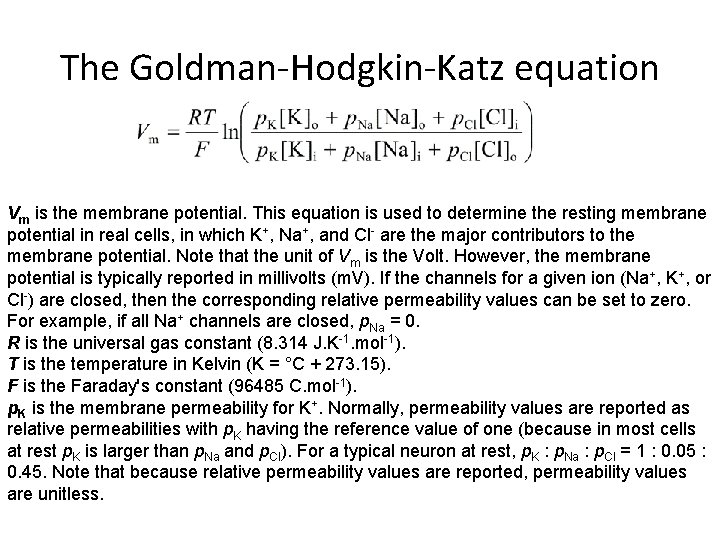
The Goldman-Hodgkin-Katz equation Vm is the membrane potential. This equation is used to determine the resting membrane potential in real cells, in which K+, Na+, and Cl- are the major contributors to the membrane potential. Note that the unit of Vm is the Volt. However, the membrane potential is typically reported in millivolts (m. V). If the channels for a given ion (Na+, K+, or Cl-) are closed, then the corresponding relative permeability values can be set to zero. For example, if all Na+ channels are closed, p. Na = 0. R is the universal gas constant (8. 314 J. K-1. mol-1). T is the temperature in Kelvin (K = °C + 273. 15). F is the Faraday's constant (96485 C. mol-1). p. K is the membrane permeability for K+. Normally, permeability values are reported as relative permeabilities with p. K having the reference value of one (because in most cells at rest p. K is larger than p. Na and p. Cl). For a typical neuron at rest, p. K : p. Na : p. Cl = 1 : 0. 05 : 0. 45. Note that because relative permeability values are reported, permeability values are unitless.
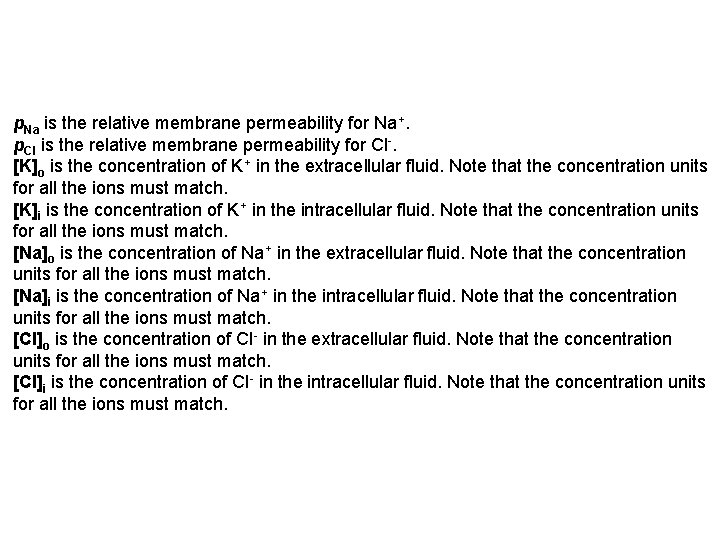
p. Na is the relative membrane permeability for Na+. p. Cl is the relative membrane permeability for Cl-. [K]o is the concentration of K+ in the extracellular fluid. Note that the concentration units for all the ions must match. [K]i is the concentration of K+ in the intracellular fluid. Note that the concentration units for all the ions must match. [Na]o is the concentration of Na+ in the extracellular fluid. Note that the concentration units for all the ions must match. [Na]i is the concentration of Na+ in the intracellular fluid. Note that the concentration units for all the ions must match. [Cl]o is the concentration of Cl- in the extracellular fluid. Note that the concentration units for all the ions must match. [Cl]i is the concentration of Cl- in the intracellular fluid. Note that the concentration units for all the ions must match.
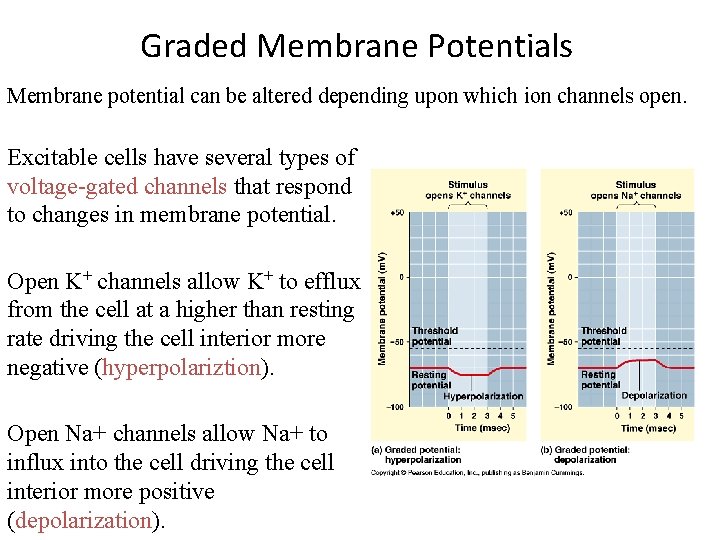
Graded Membrane Potentials Membrane potential can be altered depending upon which ion channels open. Excitable cells have several types of voltage-gated channels that respond to changes in membrane potential. Open K+ channels allow K+ to efflux from the cell at a higher than resting rate driving the cell interior more negative (hyperpolariztion). Open Na+ channels allow Na+ to influx into the cell driving the cell interior more positive (depolarization).
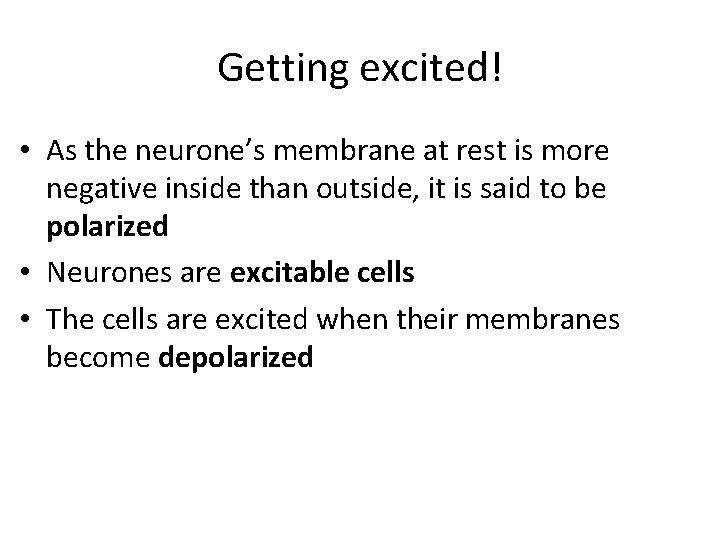
Getting excited! • As the neurone’s membrane at rest is more negative inside than outside, it is said to be polarized • Neurones are excitable cells • The cells are excited when their membranes become depolarized
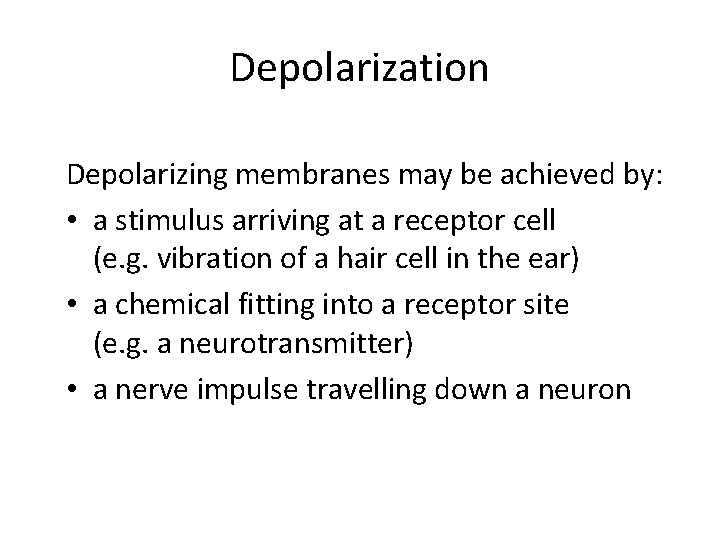
Depolarization Depolarizing membranes may be achieved by: • a stimulus arriving at a receptor cell (e. g. vibration of a hair cell in the ear) • a chemical fitting into a receptor site (e. g. a neurotransmitter) • a nerve impulse travelling down a neuron
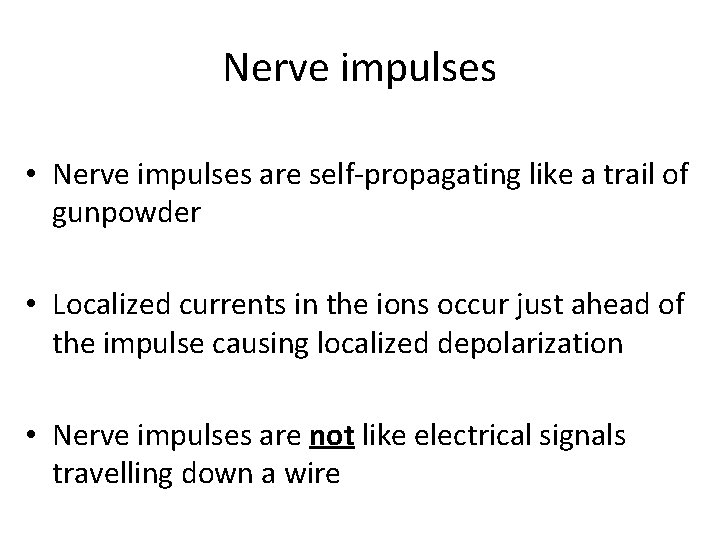
Nerve impulses • Nerve impulses are self-propagating like a trail of gunpowder • Localized currents in the ions occur just ahead of the impulse causing localized depolarization • Nerve impulses are not like electrical signals travelling down a wire
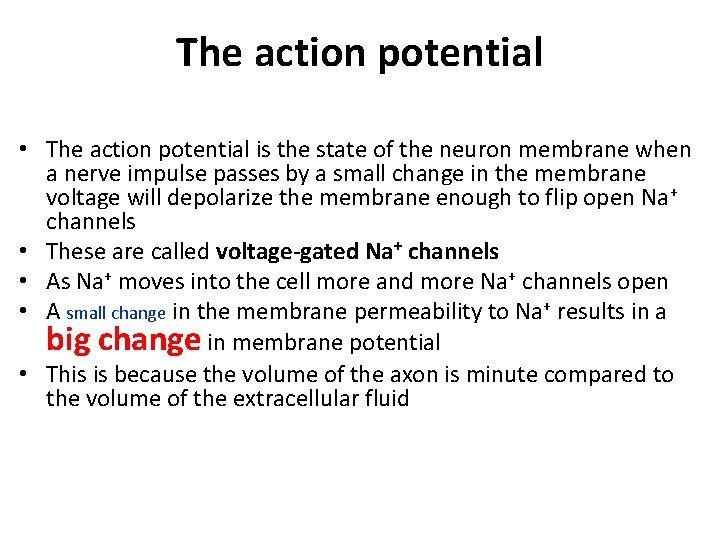
The action potential • The action potential is the state of the neuron membrane when a nerve impulse passes by a small change in the membrane voltage will depolarize the membrane enough to flip open Na+ channels • These are called voltage-gated Na+ channels • As Na+ moves into the cell more and more Na+ channels open • A small change in the membrane permeability to Na+ results in a big change in membrane potential • This is because the volume of the axon is minute compared to the volume of the extracellular fluid
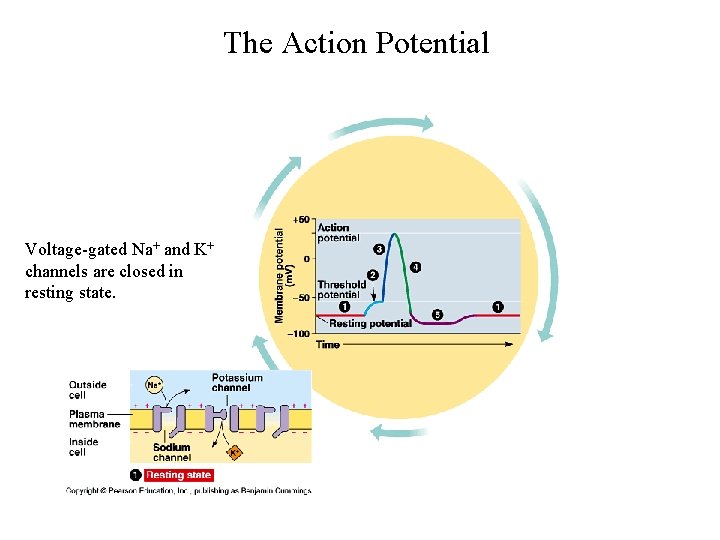
The Action Potential Voltage-gated Na+ and K+ channels are closed in resting state.
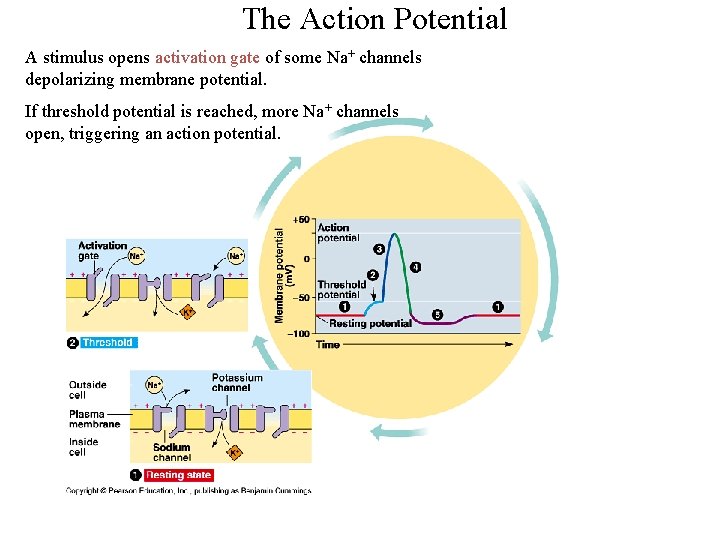
The Action Potential A stimulus opens activation gate of some Na+ channels depolarizing membrane potential. If threshold potential is reached, more Na+ channels open, triggering an action potential.
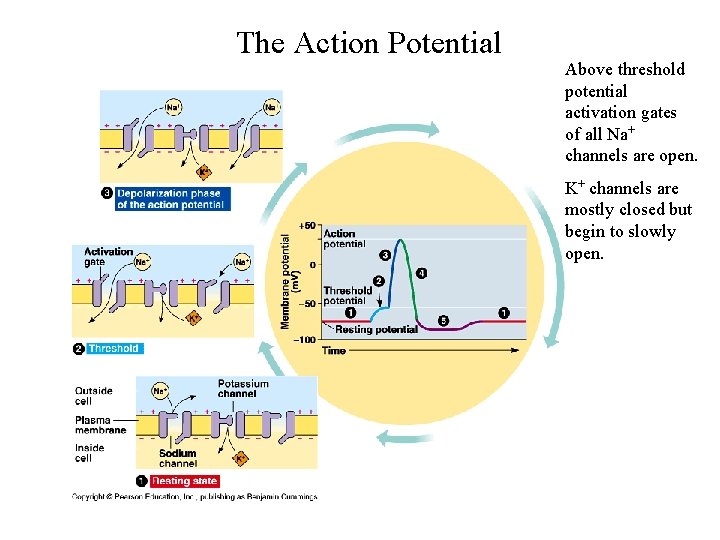
The Action Potential Above threshold potential activation gates of all Na+ channels are open. K+ channels are mostly closed but begin to slowly open.
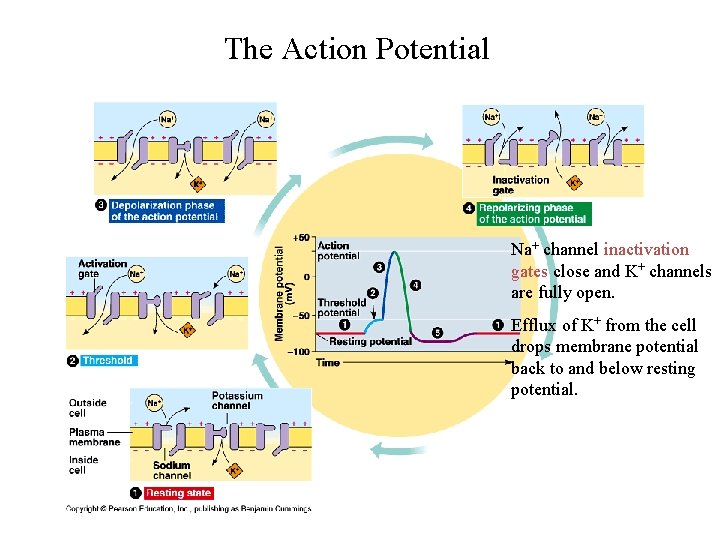
The Action Potential Na+ channel inactivation gates close and K+ channels are fully open. Efflux of K+ from the cell drops membrane potential back to and below resting potential.
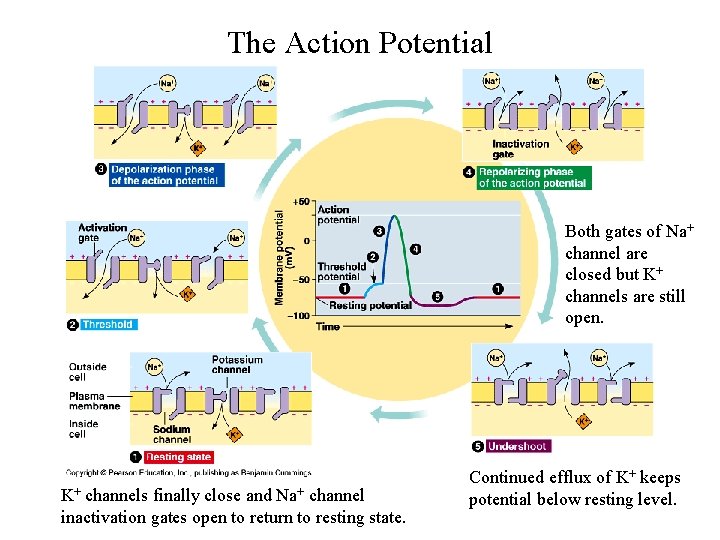
The Action Potential Both gates of Na+ channel are closed but K+ channels are still open. K+ channels finally close and Na+ channel inactivation gates open to return to resting state. Continued efflux of K+ keeps potential below resting level.
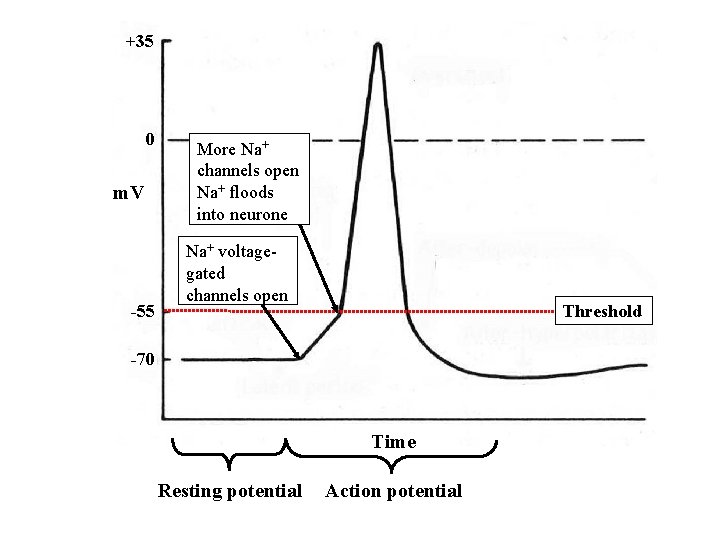
+35 0 m. V -55 More Na+ channels open Na+ floods into neurone Na+ voltagegated channels open Threshold -70 Time Resting potential Action potential
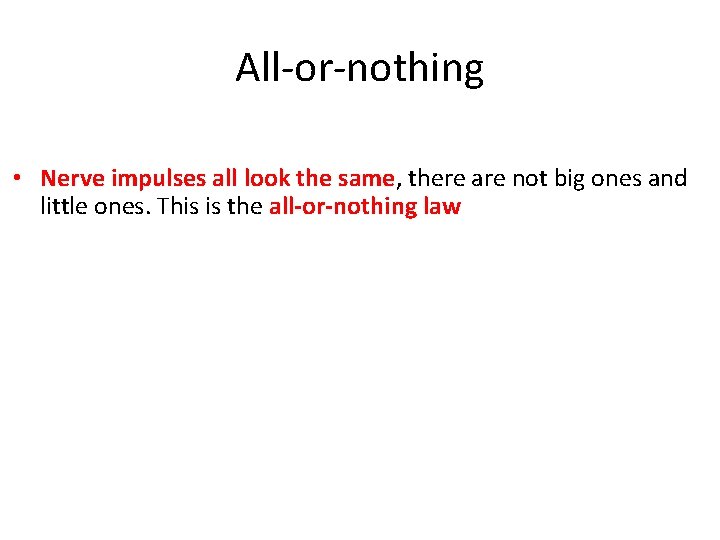
All-or-nothing • Nerve impulses all look the same, there are not big ones and little ones. This is the all-or-nothing law
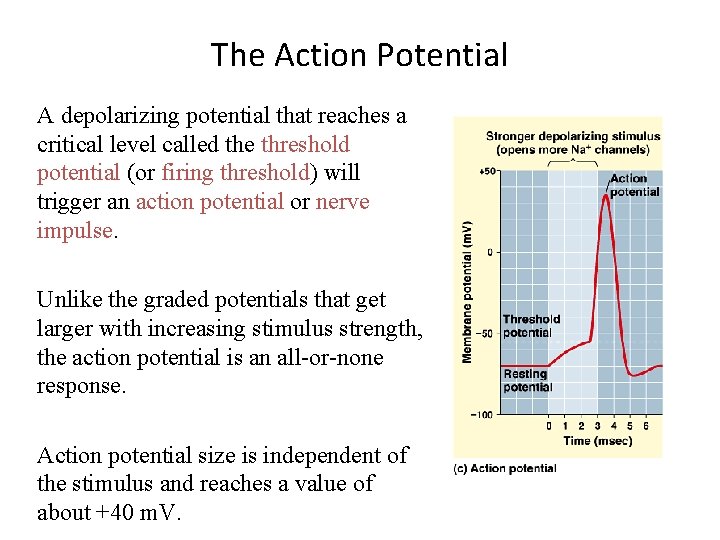
The Action Potential A depolarizing potential that reaches a critical level called the threshold potential (or firing threshold) will trigger an action potential or nerve impulse. Unlike the graded potentials that get larger with increasing stimulus strength, the action potential is an all-or-none response. Action potential size is independent of the stimulus and reaches a value of about +40 m. V.
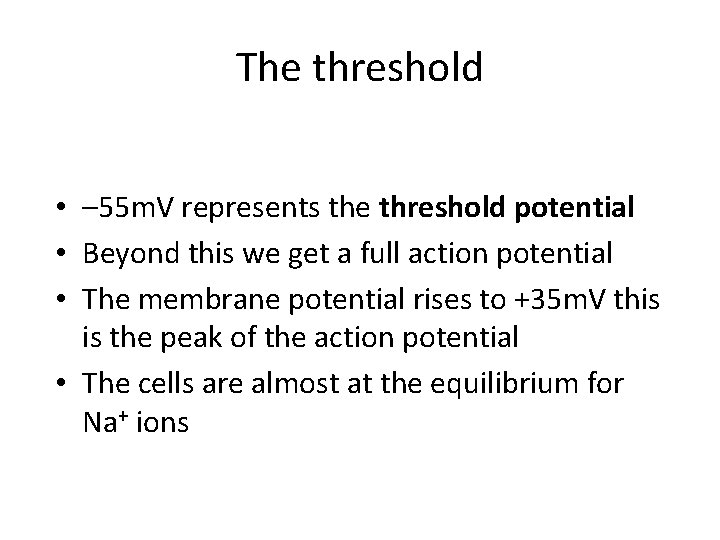
The threshold • – 55 m. V represents the threshold potential • Beyond this we get a full action potential • The membrane potential rises to +35 m. V this is the peak of the action potential • The cells are almost at the equilibrium for Na+ ions
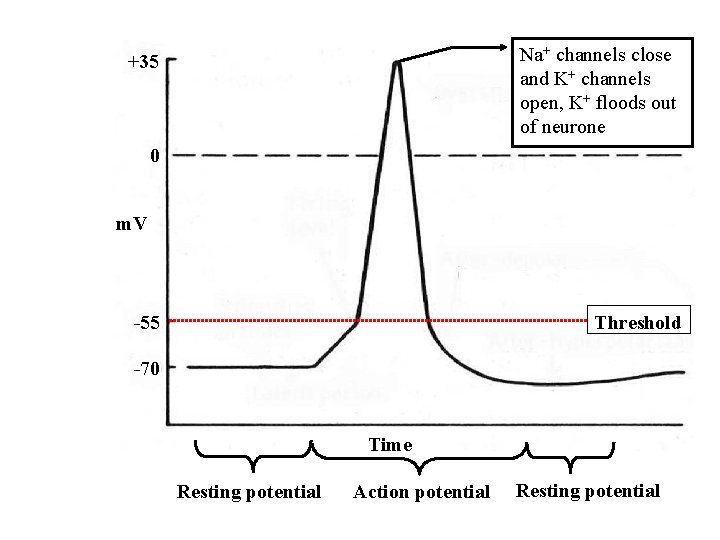
Na+ channels close and K+ channels open, K+ floods out of neurone +35 0 m. V -55 Threshold -70 Time Resting potential Action potential Resting potential
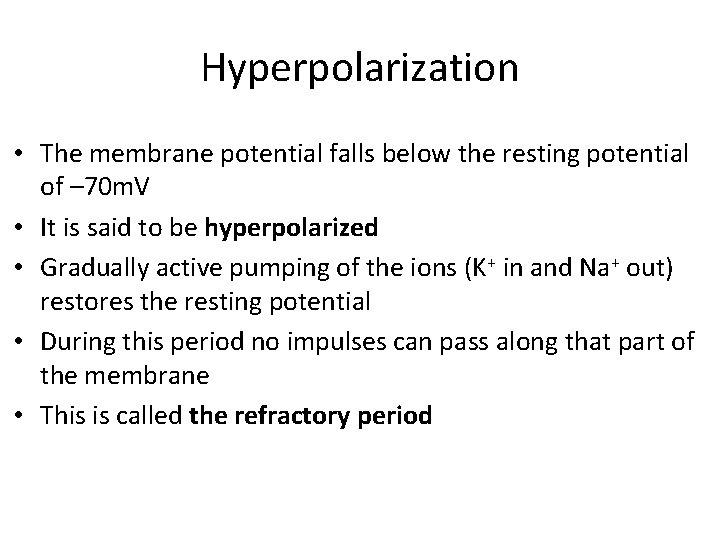
Hyperpolarization • The membrane potential falls below the resting potential of – 70 m. V • It is said to be hyperpolarized • Gradually active pumping of the ions (K+ in and Na+ out) restores the resting potential • During this period no impulses can pass along that part of the membrane • This is called the refractory period
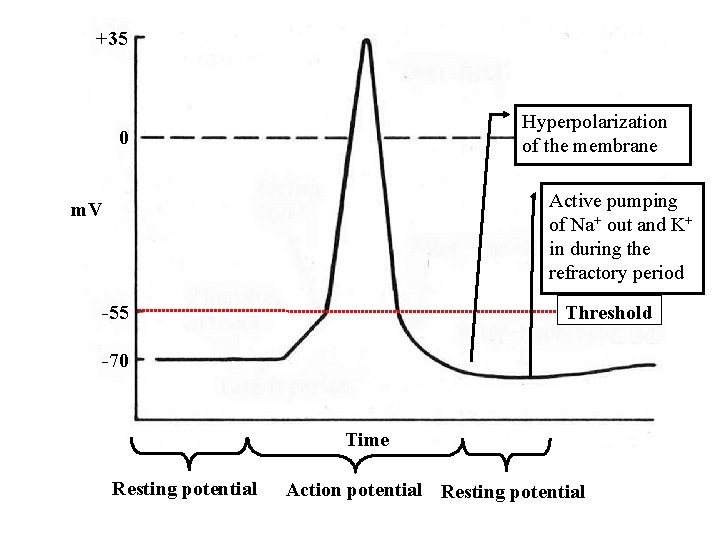
+35 Hyperpolarization of the membrane 0 Active pumping of Na+ out and K+ in during the refractory period m. V -55 Threshold -70 Time Resting potential Action potential Resting potential
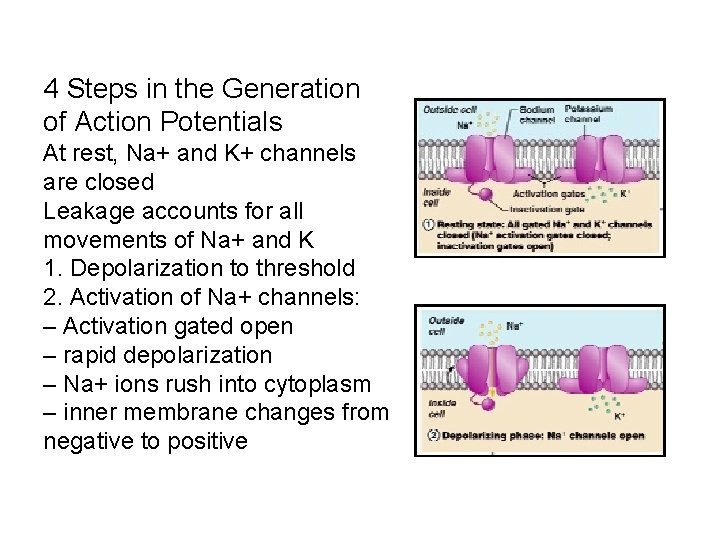
4 Steps in the Generation of Action Potentials At rest, Na+ and K+ channels are closed Leakage accounts for all movements of Na+ and K 1. Depolarization to threshold 2. Activation of Na+ channels: – Activation gated open – rapid depolarization – Na+ ions rush into cytoplasm – inner membrane changes from negative to positive
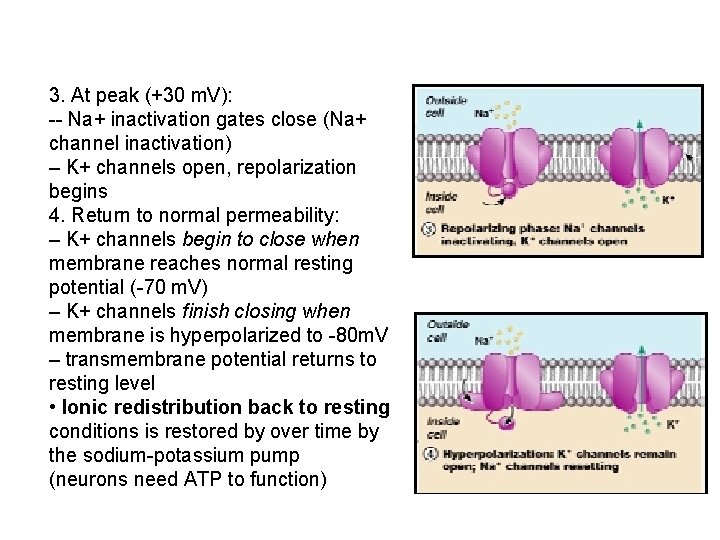
3. At peak (+30 m. V): -- Na+ inactivation gates close (Na+ channel inactivation) – K+ channels open, repolarization begins 4. Return to normal permeability: – K+ channels begin to close when membrane reaches normal resting potential (-70 m. V) – K+ channels finish closing when membrane is hyperpolarized to -80 m. V – transmembrane potential returns to resting level • Ionic redistribution back to resting conditions is restored by over time by the sodium-potassium pump (neurons need ATP to function)
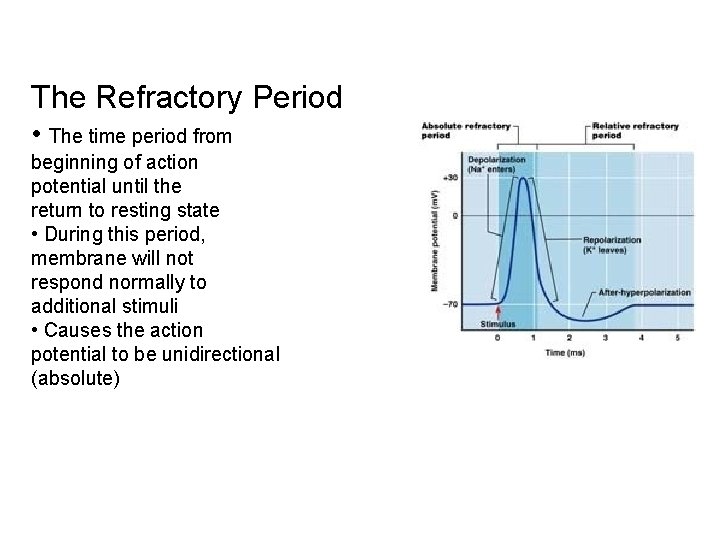
The Refractory Period • The time period from beginning of action potential until the return to resting state • During this period, membrane will not respond normally to additional stimuli • Causes the action potential to be unidirectional (absolute)
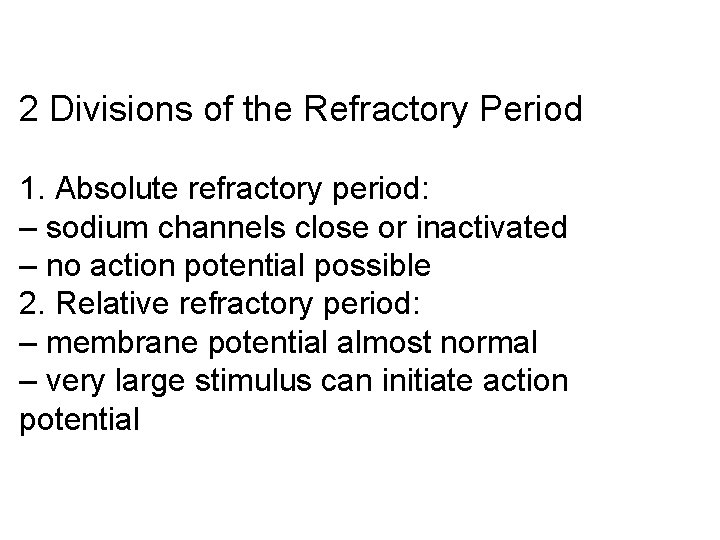
2 Divisions of the Refractory Period 1. Absolute refractory period: – sodium channels close or inactivated – no action potential possible 2. Relative refractory period: – membrane potential almost normal – very large stimulus can initiate action potential
Action potential resting potential
Action potential resting potential
Axon hillock
Resting membrane potential of neurons
Relaxation and contraction of muscles
What is resting membrane potential
Steps of an action potential
Refractory period
Resting membrane potential
Resting membrane potential
Communicative signals and informative signals
Duality in linguistics
Communicative and informative signals
Binary uses electrical signals
Trigeminal nerve which cranial nerve
Properties of nerve
Cranial nerve
Graded potentials
Masses of cells form and steal nutrients from healthy cells
Is a staphylococcus cell prokaryotic or eukaryotic
Haploid and diploid venn diagram
What is an organelle
Pns water view position
Chapter 8 cellular reproduction cells from cells
Label
Somatic cells vs germ cells
Prokaryotes vs eukaryotes venn diagram
Alpha intercalated cell
Cells cells they're made of organelles meme
Chlorocruorin
Cell organelle jeopardy
4 types of eukaryotic cells
Parafollicular cells vs follicular cells
Prokaryotic cells vs eukaryotic cells
Electric potential si unit
Electric potential difference
Electrostatic potential
Potential energy to electrical energy
Energy electric field equation
Potential energy
Potential energy of capacitor
Electron volt to volt
Electrostatic potential
What is the potential at point a
Potential due to a dipole
If a battery provides a high voltage it can
Action potential in contractile cells
Water potential definition biology
Electric potential
Action potential definition
Neuromuscular junction
Water potential equation
Refractory period in action potential
Sales potential vs market potential
V = pe/q
Graded potential vs action potential