Elastic Scattering HansJrgen Wollersheim Rutherford scattering discovery of
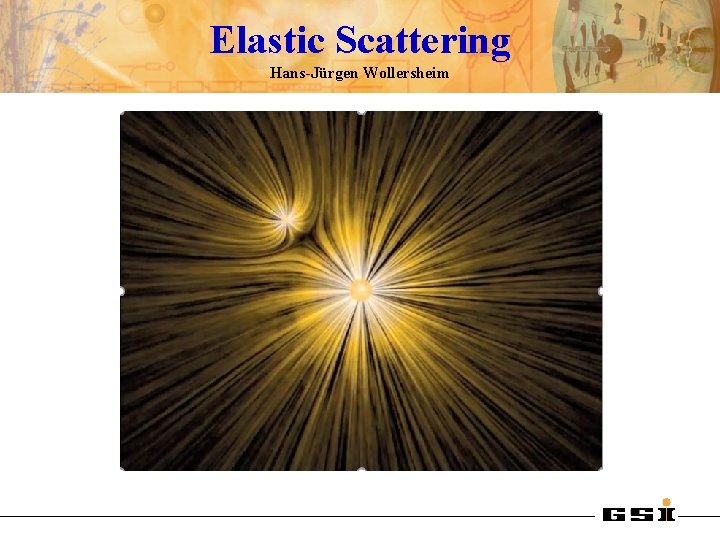
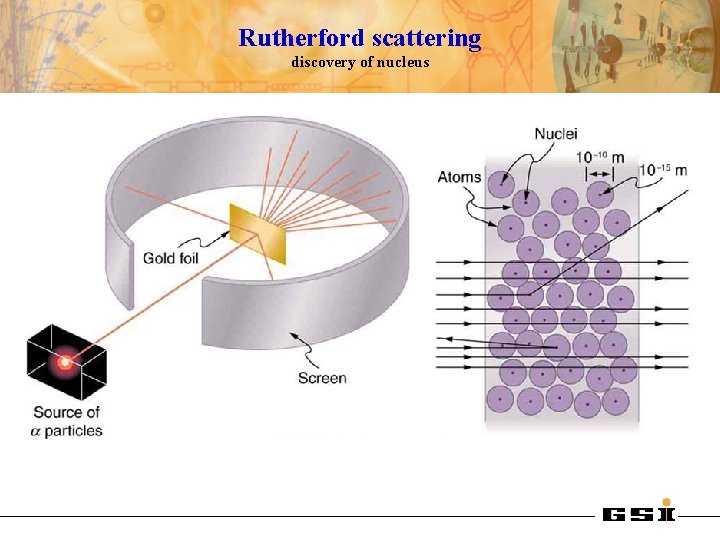
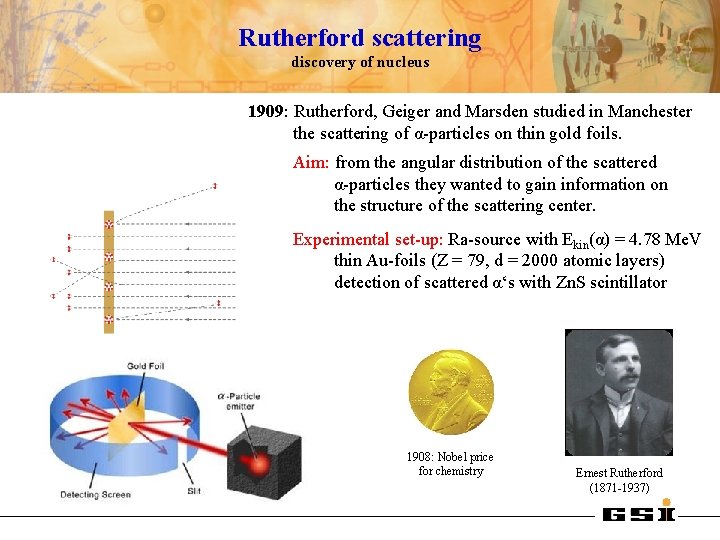
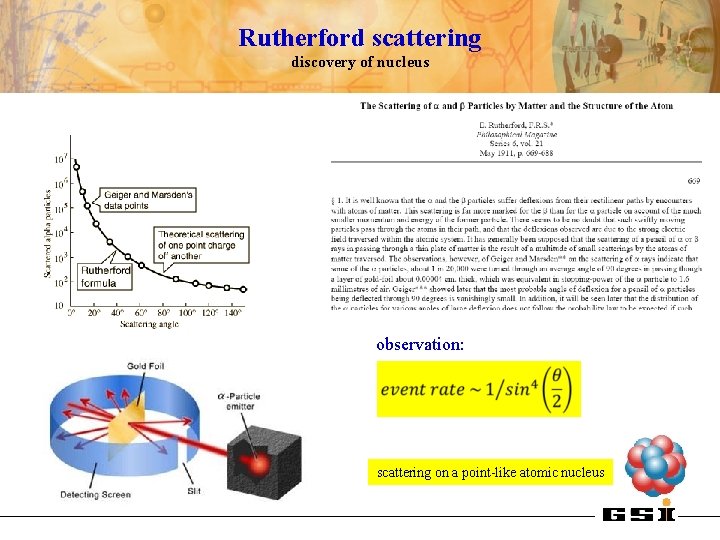
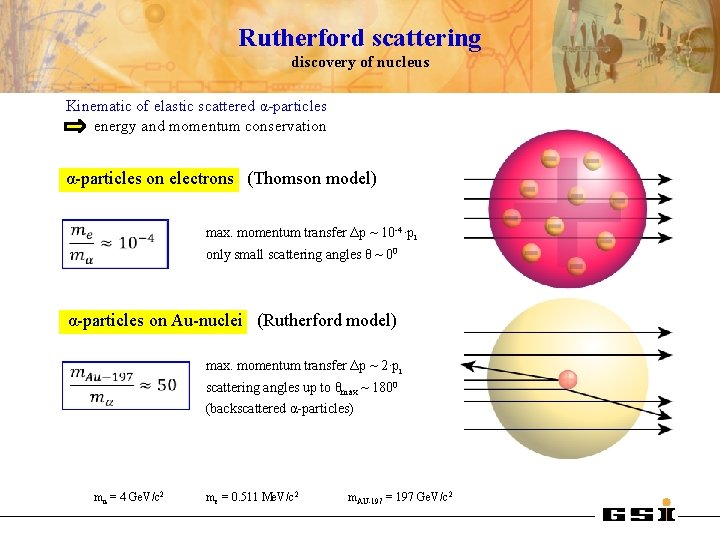
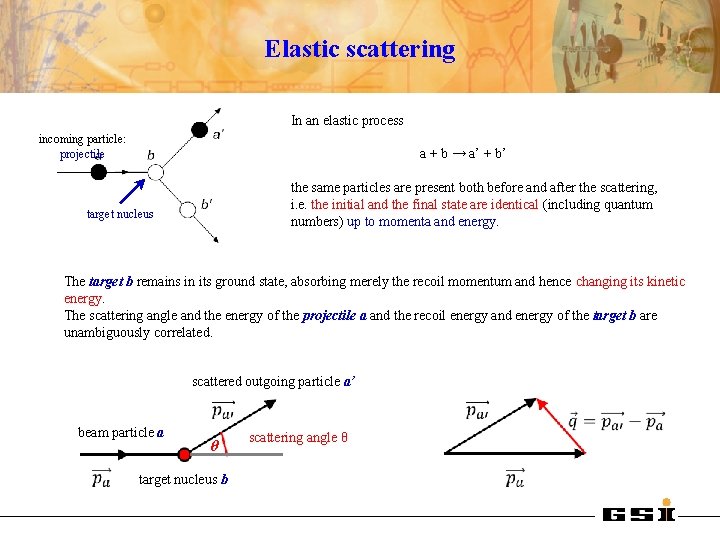
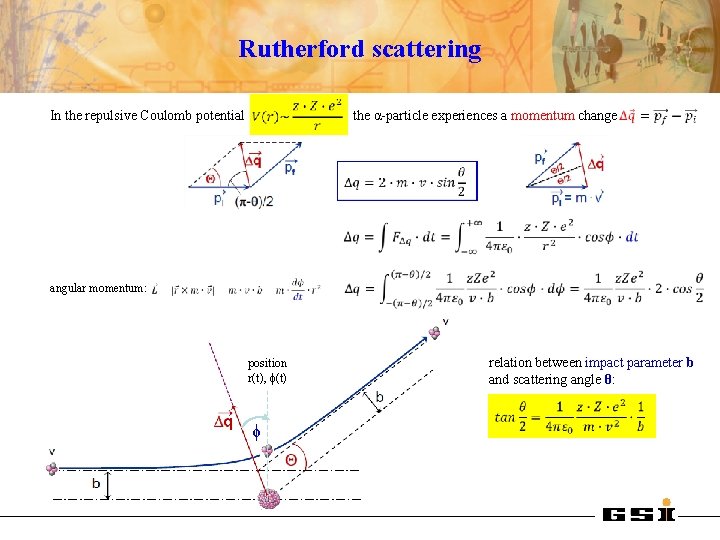
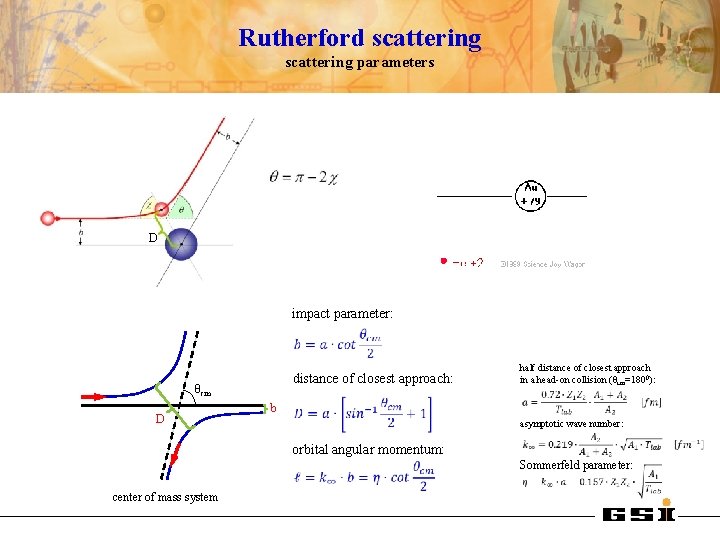
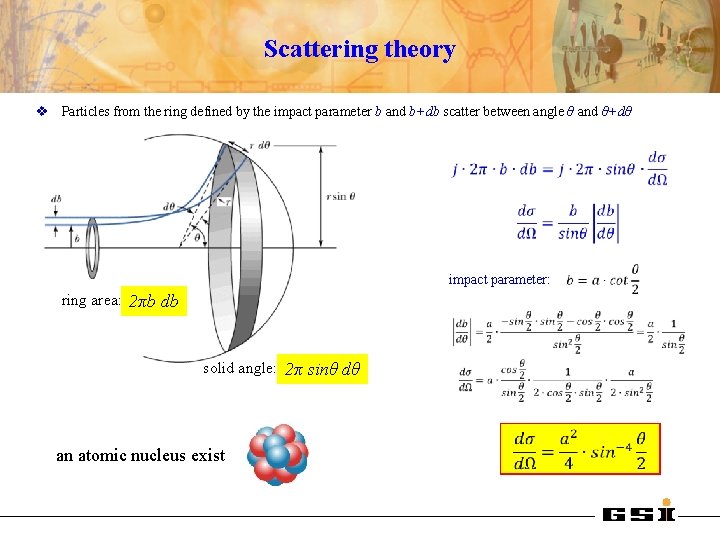
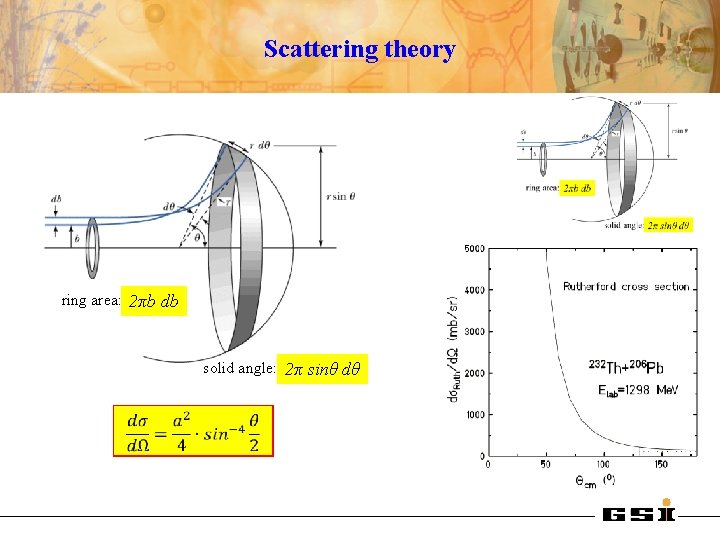
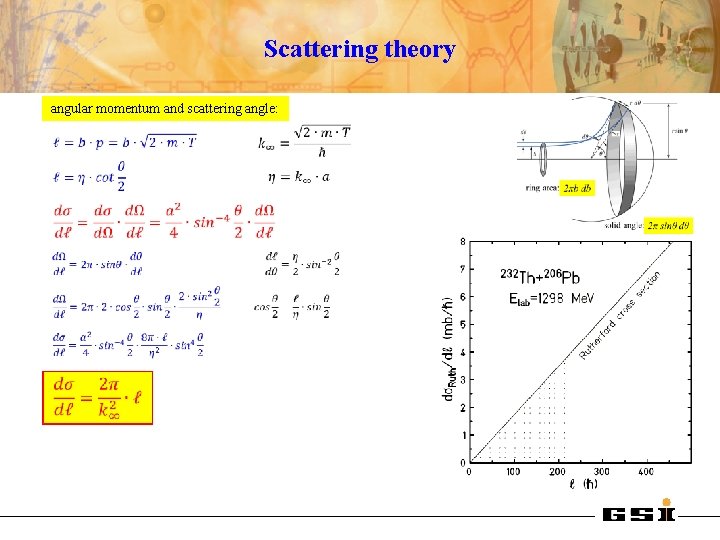
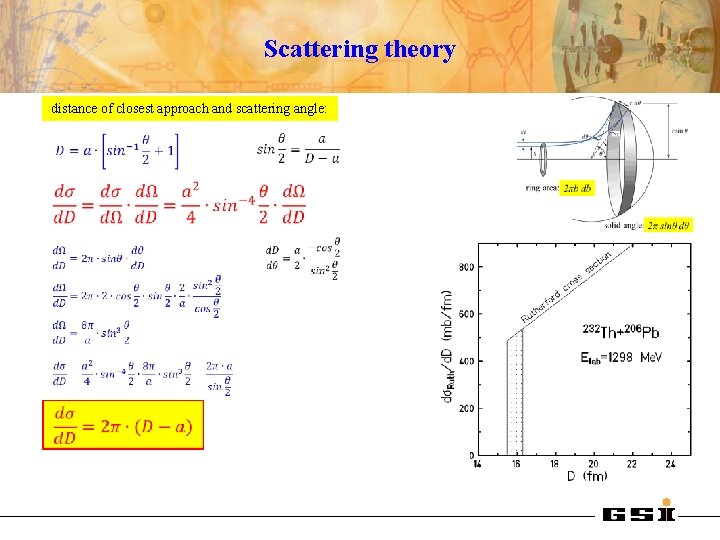
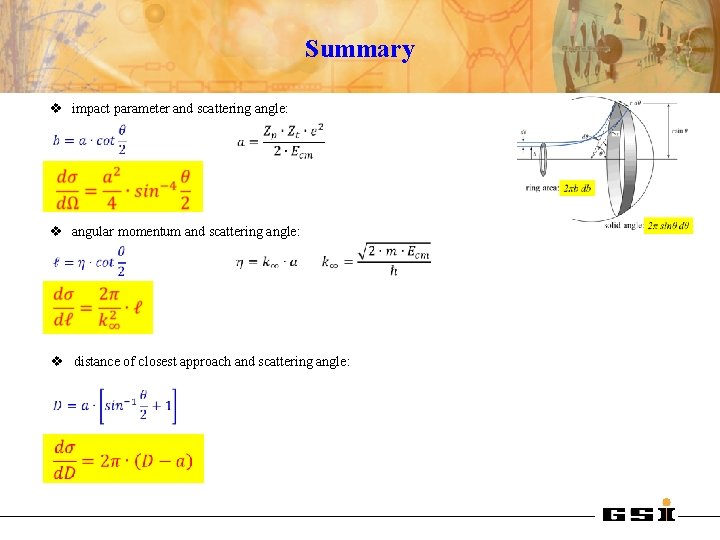
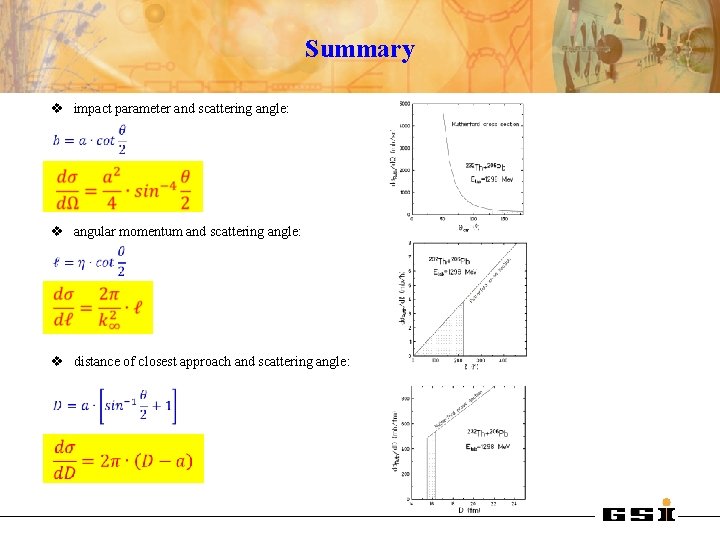
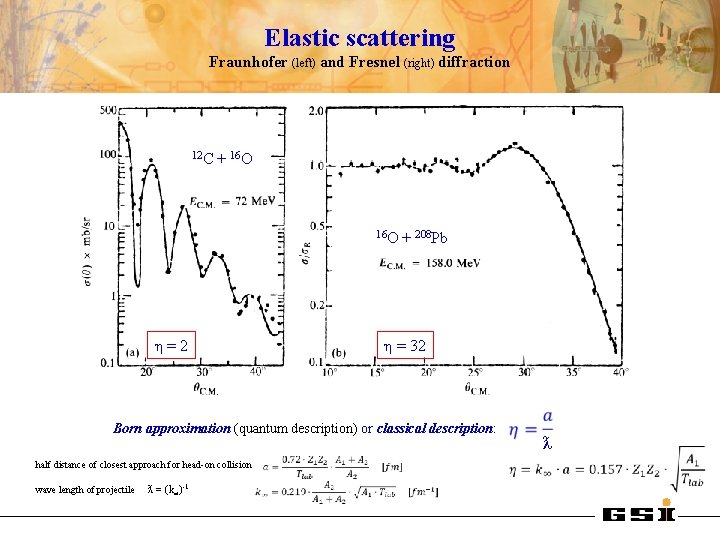
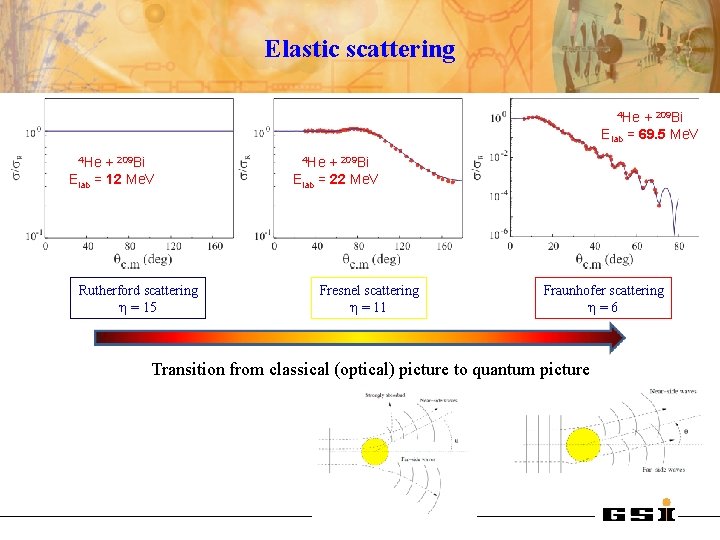
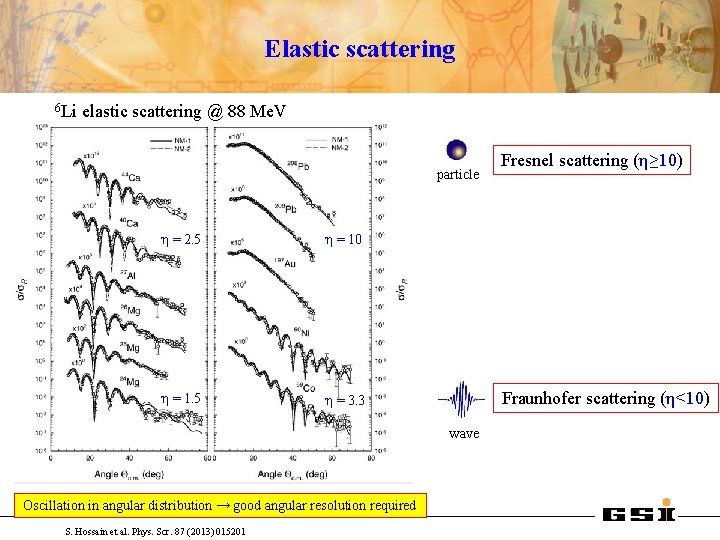
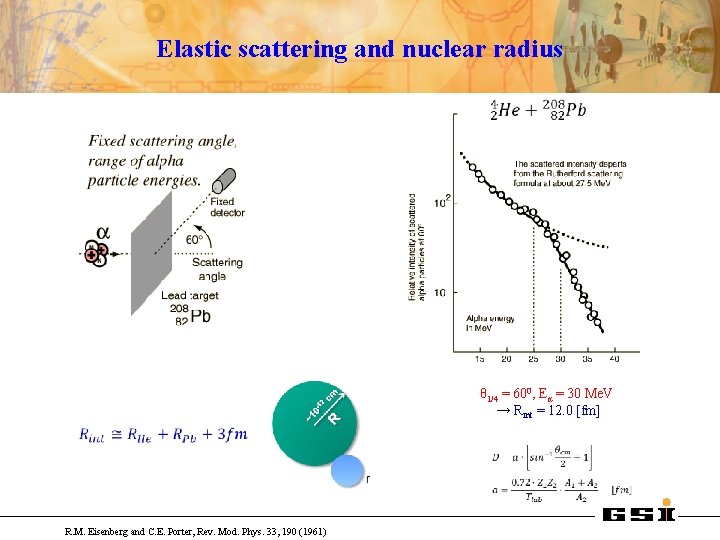
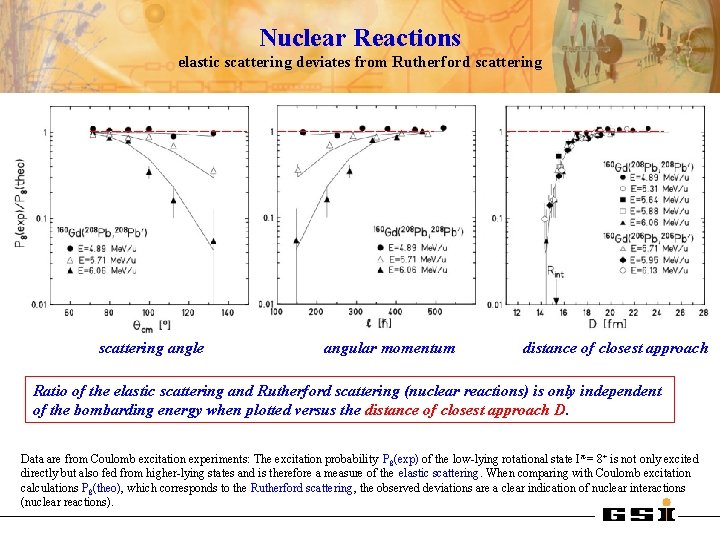
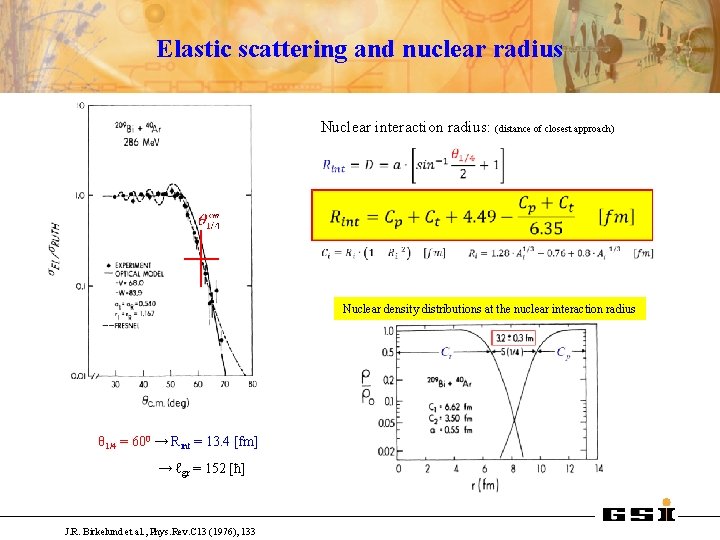
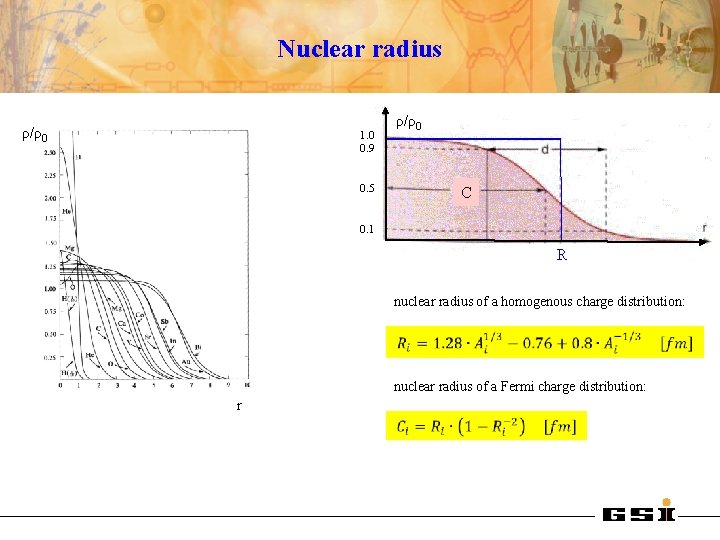
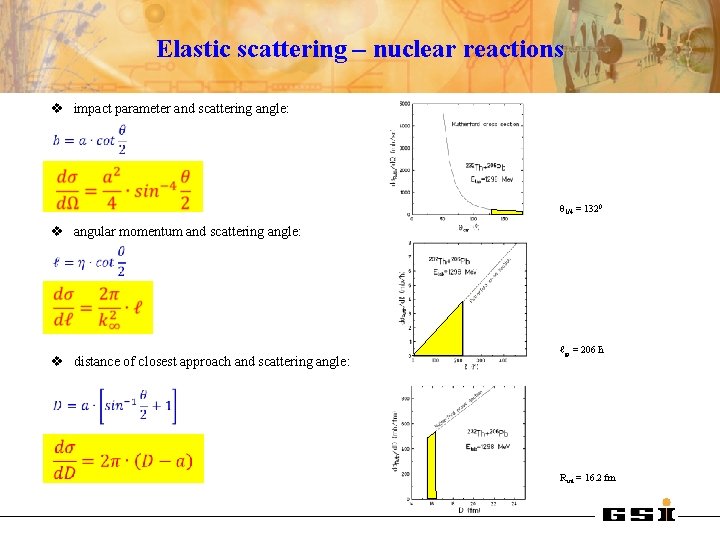
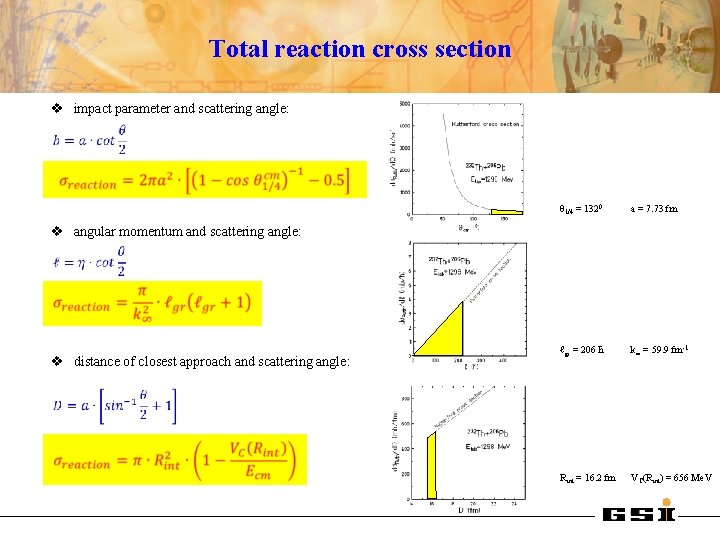
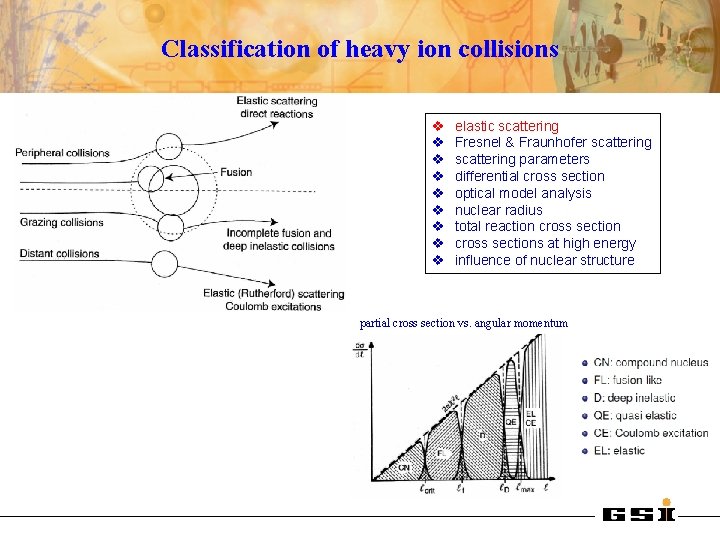
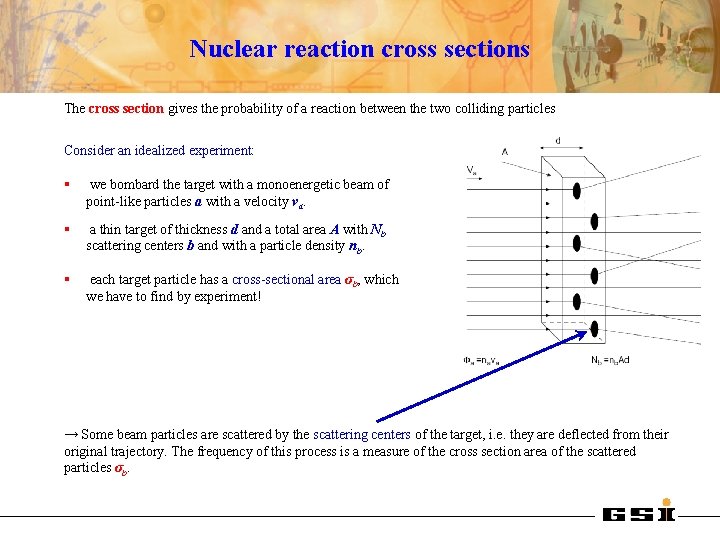
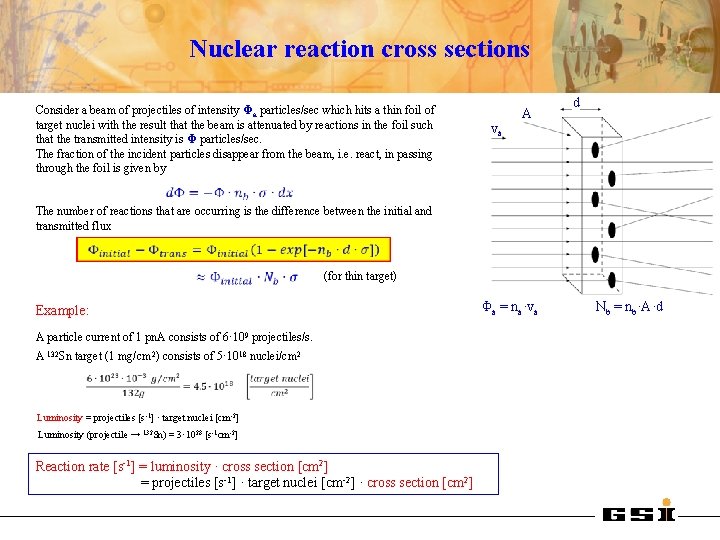
- Slides: 26
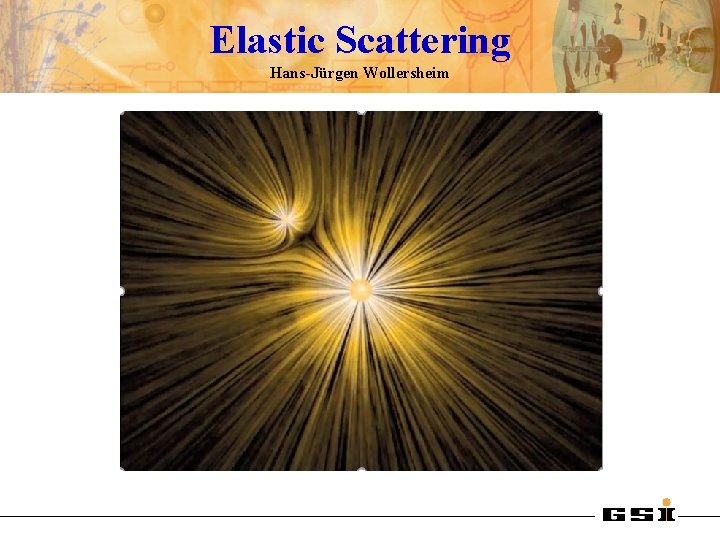
Elastic Scattering Hans-Jürgen Wollersheim
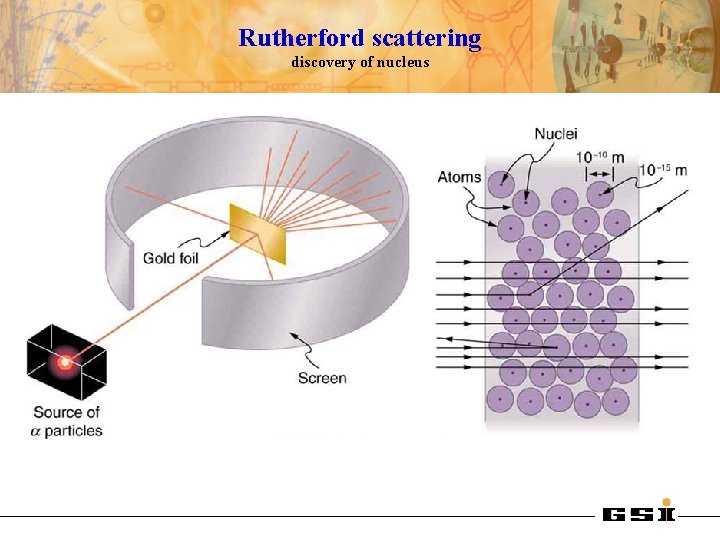
Rutherford scattering discovery of nucleus
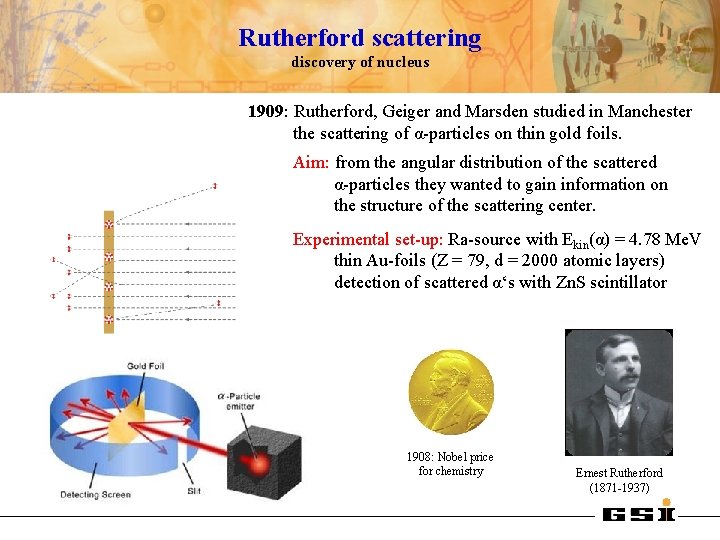
Rutherford scattering discovery of nucleus 1909: Rutherford, Geiger and Marsden studied in Manchester the scattering of α-particles on thin gold foils. Aim: from the angular distribution of the scattered α-particles they wanted to gain information on the structure of the scattering center. Experimental set-up: Ra-source with Ekin(α) = 4. 78 Me. V thin Au-foils (Z = 79, d = 2000 atomic layers) detection of scattered α‘s with Zn. S scintillator 1908: Nobel price for chemistry Ernest Rutherford (1871 -1937)
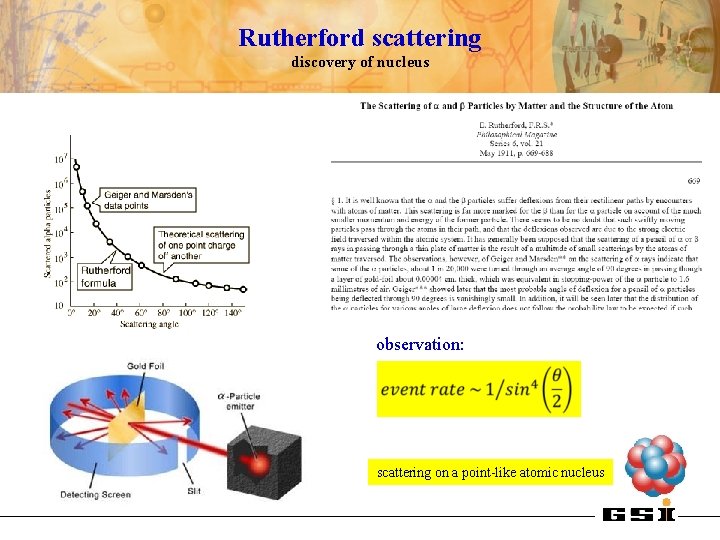
Rutherford scattering discovery of nucleus observation: scattering on a point-like atomic nucleus
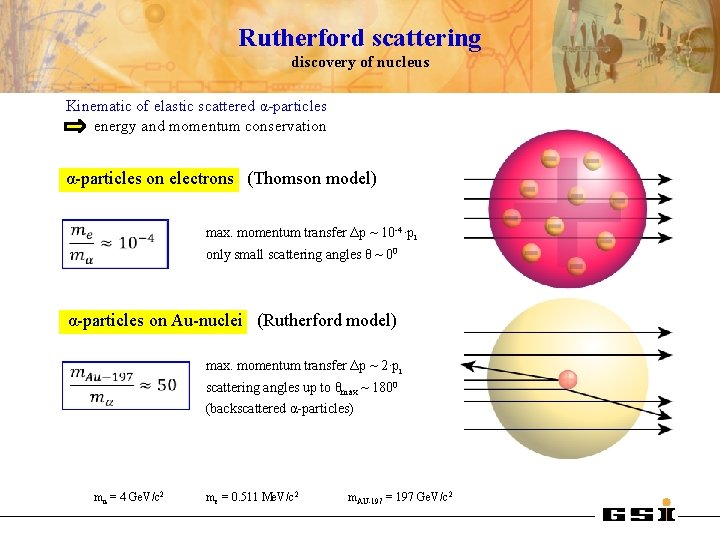
Rutherford scattering discovery of nucleus Kinematic of elastic scattered α-particles energy and momentum conservation α-particles on electrons (Thomson model) max. momentum transfer Δp ~ 10 -4·pi only small scattering angles θ ~ 00 α-particles on Au-nuclei (Rutherford model) max. momentum transfer Δp ~ 2·pi scattering angles up to θmax ~ 1800 (backscattered α-particles) mα = 4 Ge. V/c 2 me = 0. 511 Me. V/c 2 m. AU-197 = 197 Ge. V/c 2
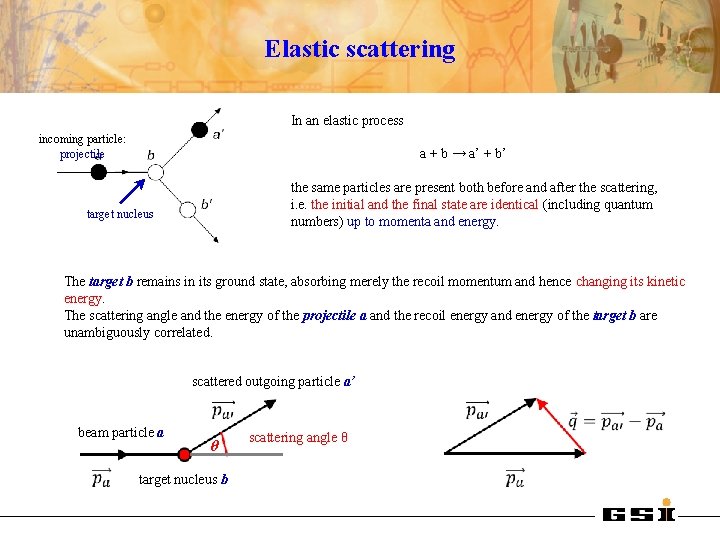
Elastic scattering In an elastic process incoming particle: projectile a + b → a’ + b’ the same particles are present both before and after the scattering, i. e. the initial and the final state are identical (including quantum numbers) up to momenta and energy. target nucleus The target b remains in its ground state, absorbing merely the recoil momentum and hence changing its kinetic energy. The scattering angle and the energy of the projectile a and the recoil energy and energy of the target b are unambiguously correlated. scattered outgoing particle a’ beam particle a θ target nucleus b scattering angle θ
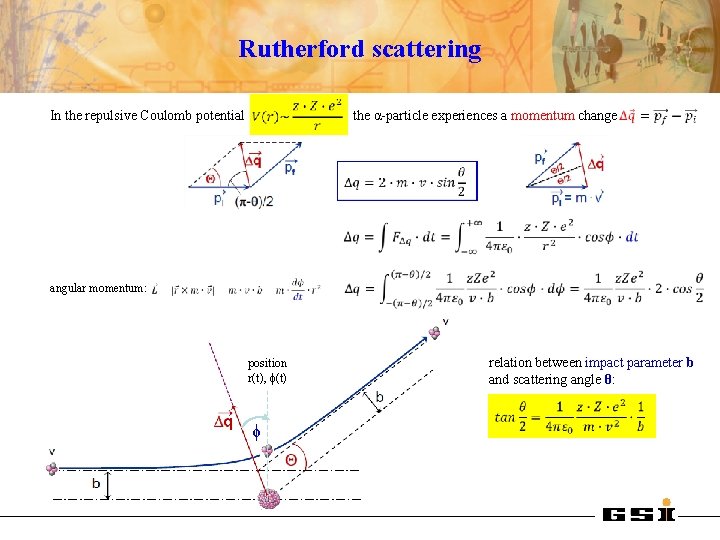
Rutherford scattering In the repulsive Coulomb potential the α-particle experiences a momentum change angular momentum: position r(t), ϕ(t) ϕ relation between impact parameter b and scattering angle θ:
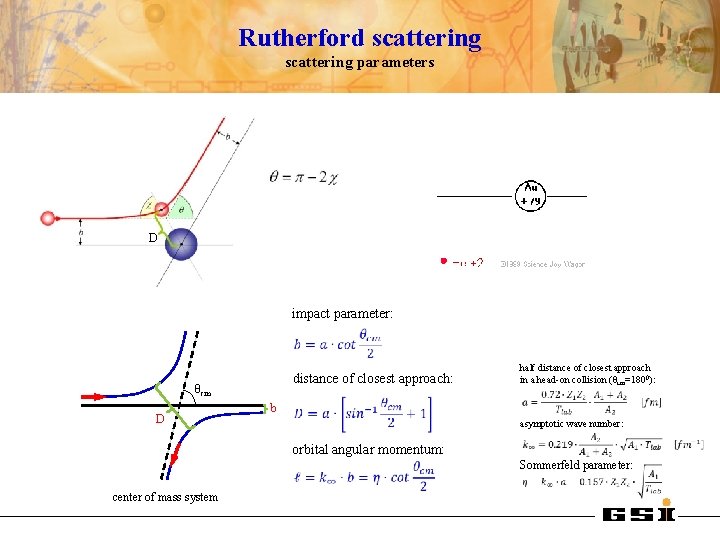
Rutherford scattering parameters D impact parameter: distance of closest approach: θcm D half distance of closest approach in a head-on collision (θcm=1800): b asymptotic wave number: orbital angular momentum: Sommerfeld parameter: center of mass system
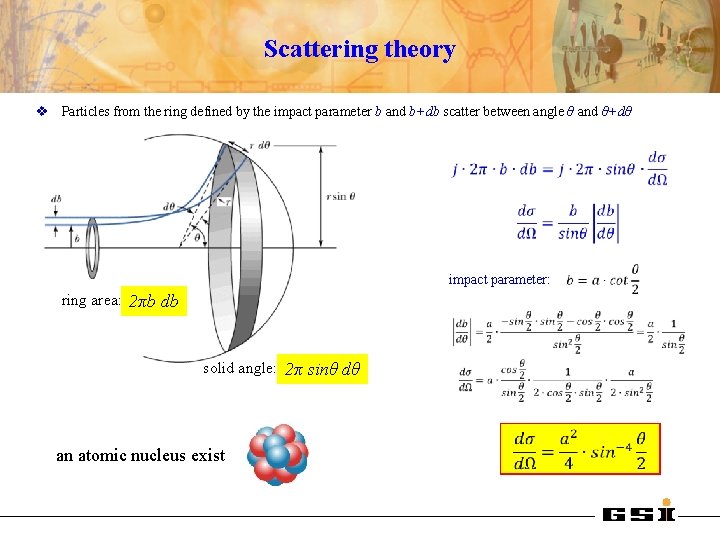
Scattering theory v Particles from the ring defined by the impact parameter b and b+db scatter between angle θ and θ+dθ impact parameter: ring area: 2πb db solid angle: 2π sinθ dθ an atomic nucleus exist
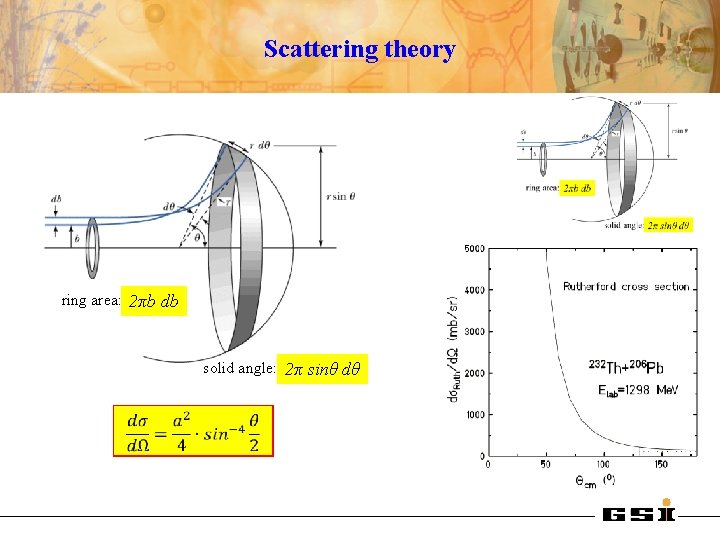
Scattering theory ring area: 2πb db solid angle: 2π sinθ dθ
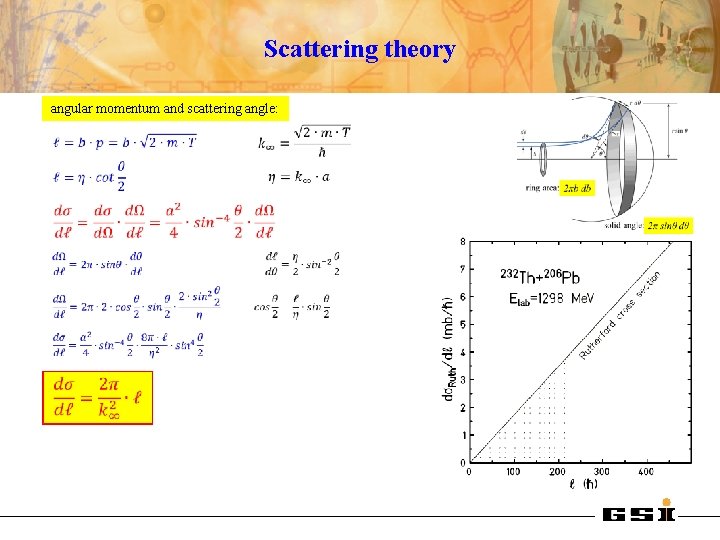
Scattering theory angular momentum and scattering angle:
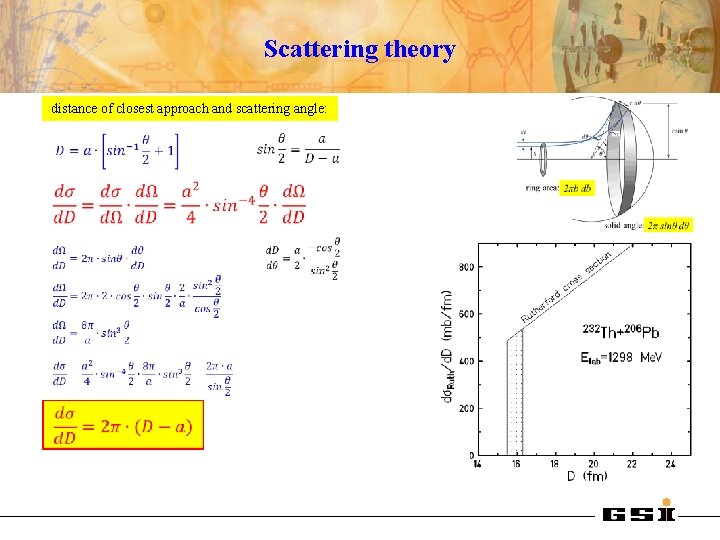
Scattering theory distance of closest approach and scattering angle:
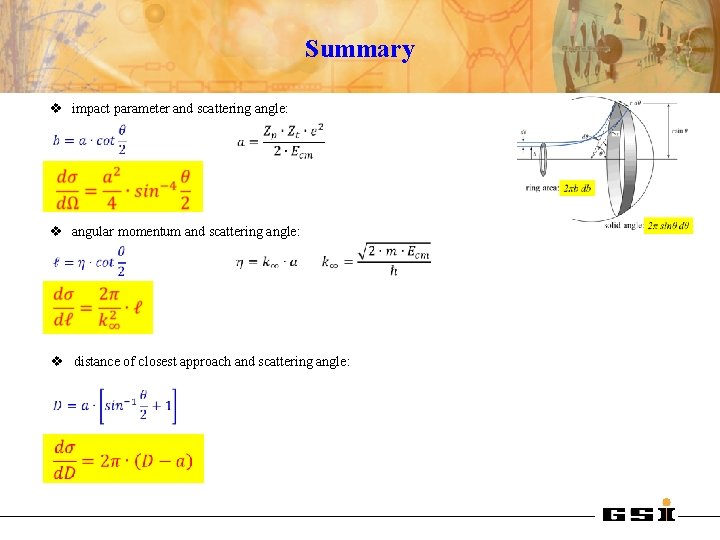
Summary v impact parameter and scattering angle: v angular momentum and scattering angle: v distance of closest approach and scattering angle:
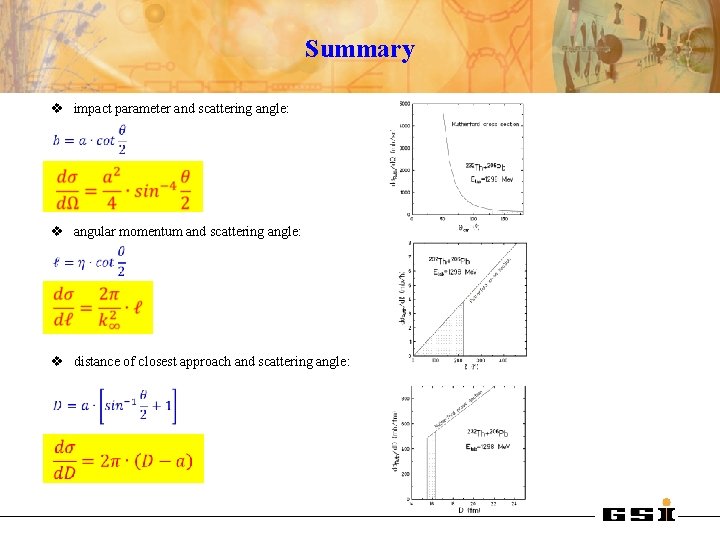
Summary v impact parameter and scattering angle: v angular momentum and scattering angle: v distance of closest approach and scattering angle:
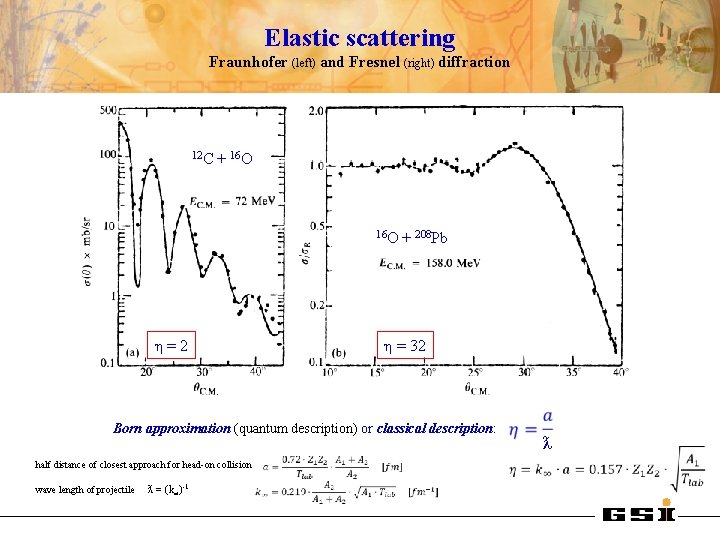
Elastic scattering Fraunhofer (left) and Fresnel (right) diffraction 12 C + 16 O η=2 + 208 Pb η = 32 Born approximation (quantum description) or classical description: half distance of closest approach for head-on collision wave length of projectile ƛ = (k∞)-1 ƛ
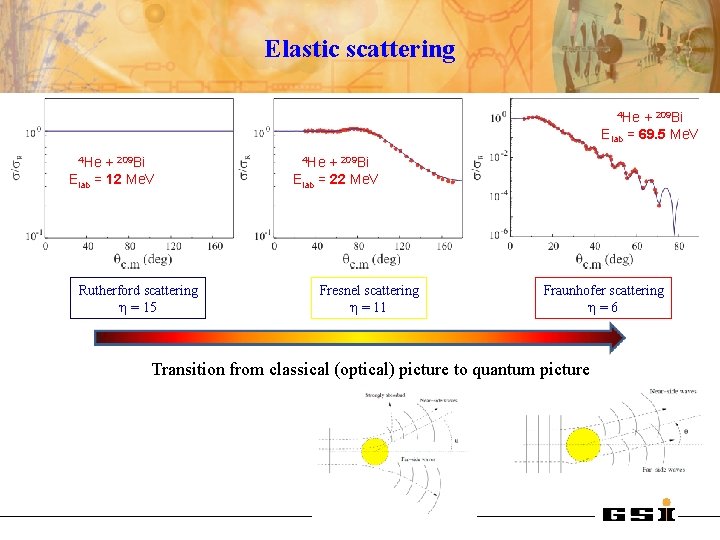
Elastic scattering 4 He + 209 Bi Elab = 69. 5 Me. V 4 He + 209 Bi Elab = 12 Me. V Rutherford scattering η = 15 4 He + 209 Bi Elab = 22 Me. V Fresnel scattering η = 11 Fraunhofer scattering η=6 Transition from classical (optical) picture to quantum picture
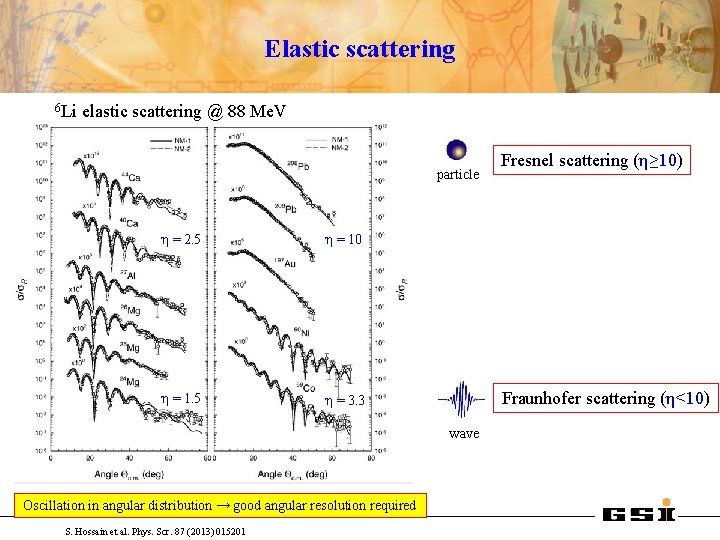
Elastic scattering 6 Li elastic scattering @ 88 Me. V particle η = 2. 5 η = 10 η = 1. 5 η = 3. 3 Fraunhofer scattering (η<10) wave Oscillation in angular distribution → good angular resolution required S. Hossain et al. Phys. Scr. 87 (2013) 015201 Fresnel scattering (η≥ 10)
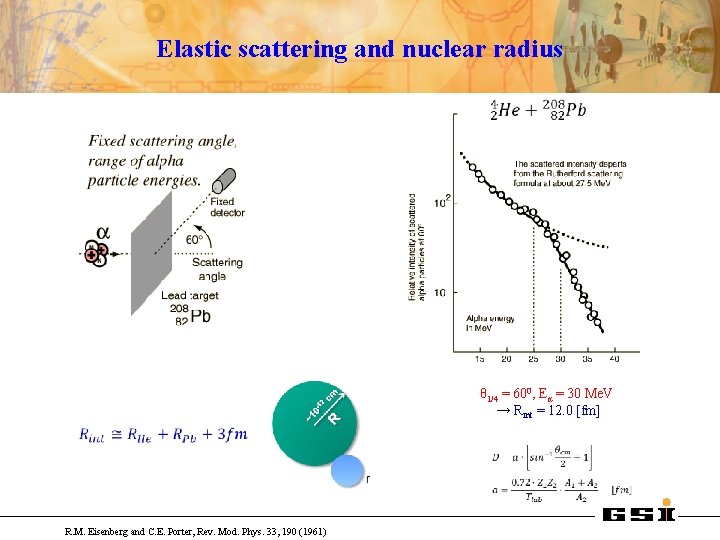
Elastic scattering and nuclear radius θ 1/4 = 600, Eα = 30 Me. V → Rint = 12. 0 [fm] R. M. Eisenberg and C. E. Porter, Rev. Mod. Phys. 33, 190 (1961)
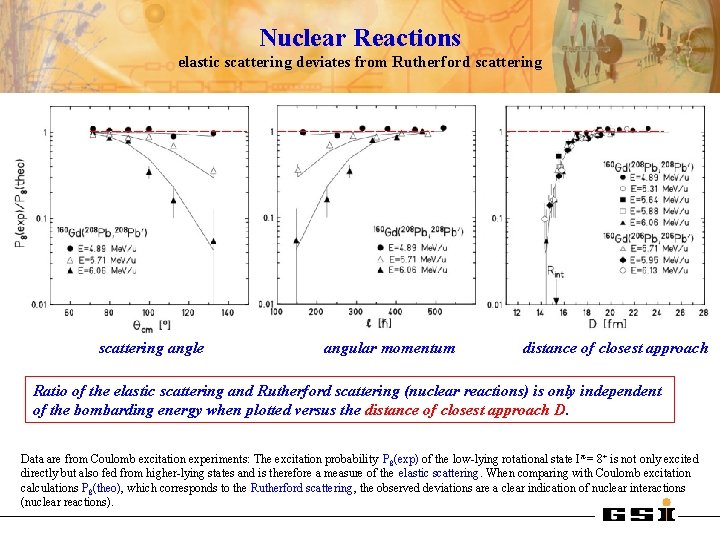
Nuclear Reactions elastic scattering deviates from Rutherford scattering angle angular momentum distance of closest approach Ratio of the elastic scattering and Rutherford scattering (nuclear reactions) is only independent of the bombarding energy when plotted versus the distance of closest approach D. Data are from Coulomb excitation experiments: The excitation probability P 8(exp) of the low-lying rotational state I π = 8+ is not only excited directly but also fed from higher-lying states and is therefore a measure of the elastic scattering. When comparing with Coulomb excitation calculations P 8(theo), which corresponds to the Rutherford scattering, the observed deviations are a clear indication of nuclear interactions (nuclear reactions).
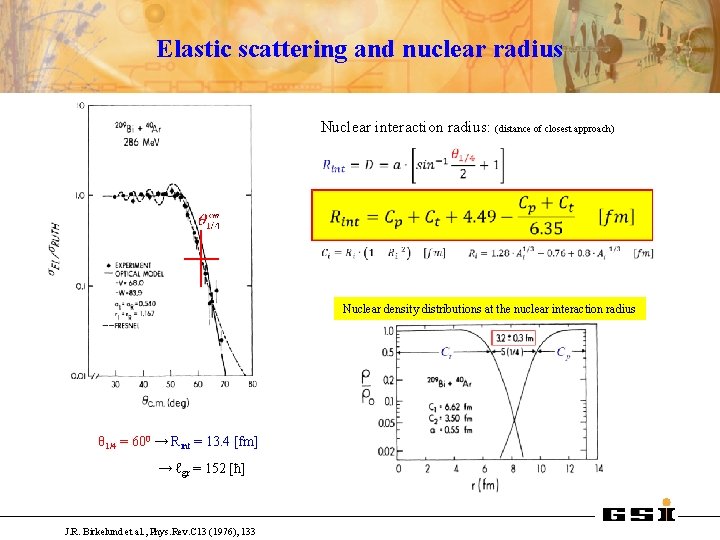
Elastic scattering and nuclear radius Nuclear interaction radius: (distance of closest approach) Nuclear density distributions at the nuclear interaction radius θ 1/4 = 600 → Rint = 13. 4 [fm] → ℓgr = 152 [ħ] J. R. Birkelund et al. , Phys. Rev. C 13 (1976), 133
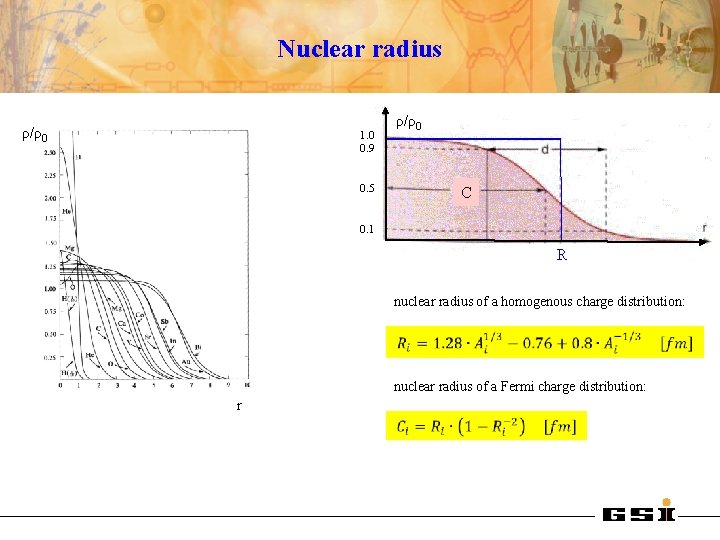
Nuclear radius ρ/ρ0 1. 0 0. 9 0. 5 ρ/ρ0 C 0. 1 R nuclear radius of a homogenous charge distribution: nuclear radius of a Fermi charge distribution: r
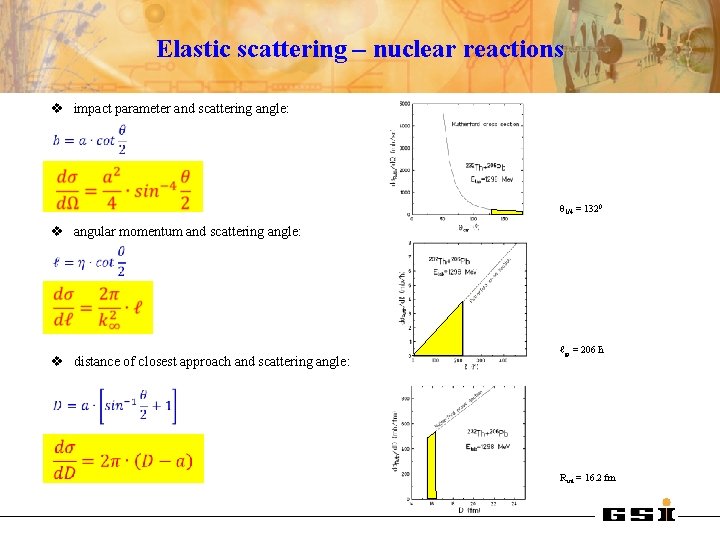
Elastic scattering – nuclear reactions v impact parameter and scattering angle: θ 1/4 = 1320 v angular momentum and scattering angle: v distance of closest approach and scattering angle: ℓgr = 206 ħ Rint = 16. 2 fm
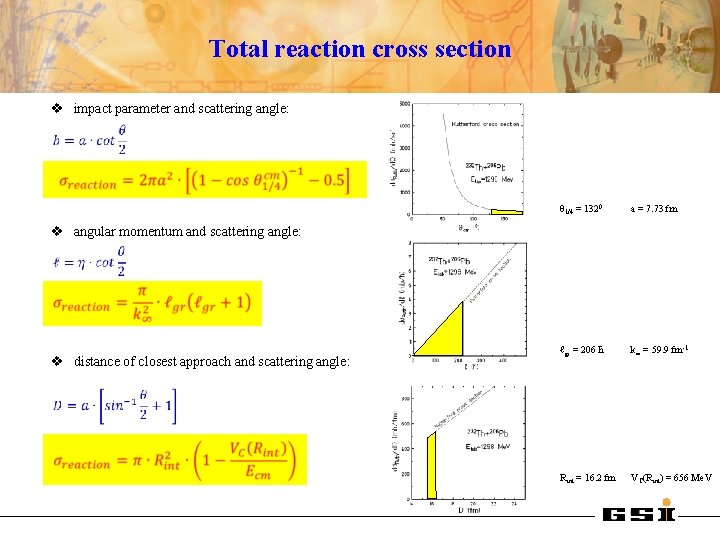
Total reaction cross section v impact parameter and scattering angle: θ 1/4 = 1320 a = 7. 73 fm ℓgr = 206 ħ k∞ = 59. 9 fm-1 Rint = 16. 2 fm VC(Rint) = 656 Me. V v angular momentum and scattering angle: v distance of closest approach and scattering angle:
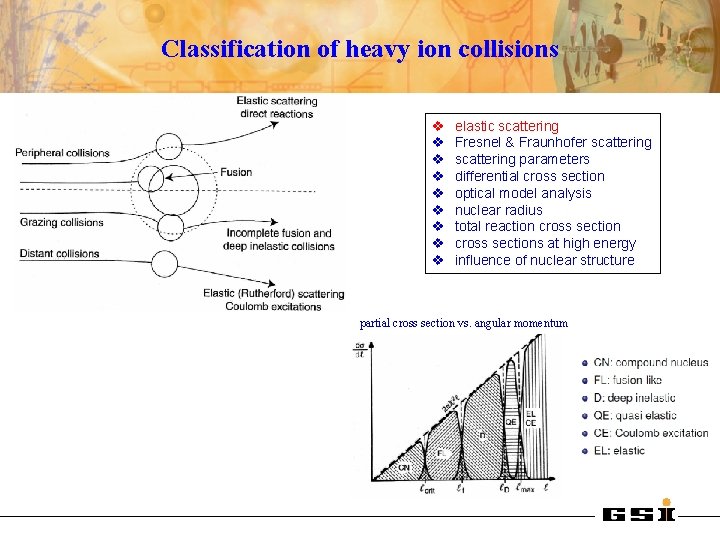
Classification of heavy ion collisions v v v v v elastic scattering Fresnel & Fraunhofer scattering parameters differential cross section optical model analysis nuclear radius total reaction cross sections at high energy influence of nuclear structure partial cross section vs. angular momentum
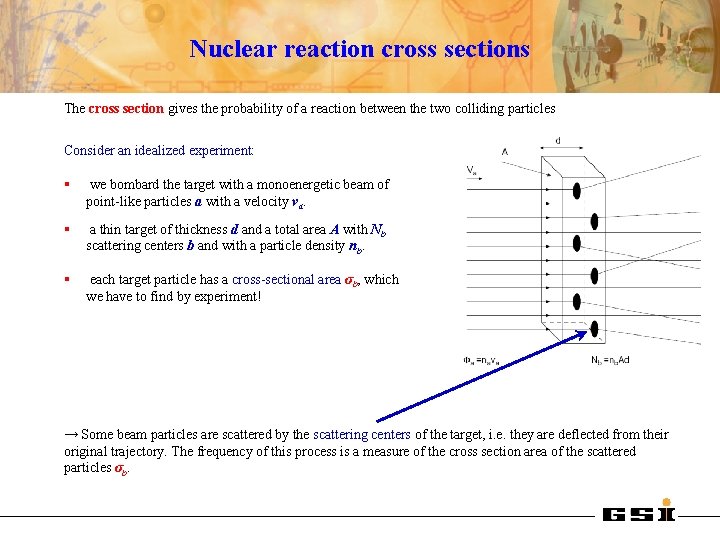
Nuclear reaction cross sections The cross section gives the probability of a reaction between the two colliding particles Consider an idealized experiment: § we bombard the target with a monoenergetic beam of point-like particles a with a velocity va. § a thin target of thickness d and a total area A with Nb scattering centers b and with a particle density nb. § each target particle has a cross-sectional area σb, which we have to find by experiment! → Some beam particles are scattered by the scattering centers of the target, i. e. they are deflected from their original trajectory. The frequency of this process is a measure of the cross section area of the scattered particles σb.
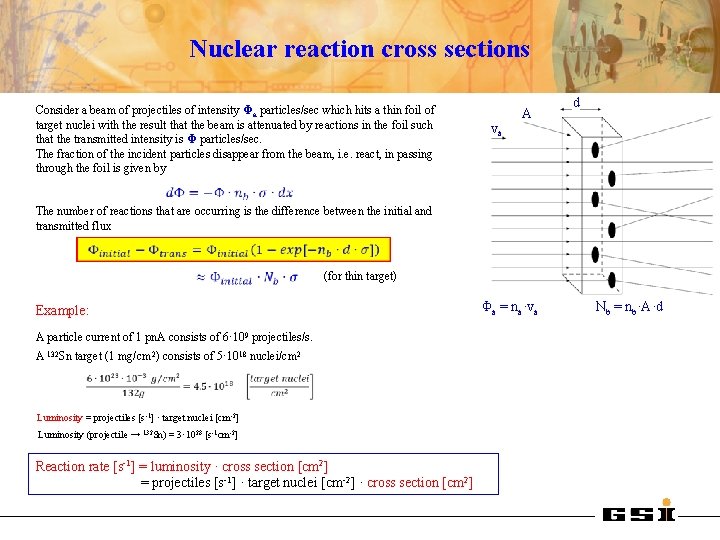
Nuclear reaction cross sections Consider a beam of projectiles of intensity Φa particles/sec which hits a thin foil of target nuclei with the result that the beam is attenuated by reactions in the foil such that the transmitted intensity is Φ particles/sec. The fraction of the incident particles disappear from the beam, i. e. react, in passing through the foil is given by va A d The number of reactions that are occurring is the difference between the initial and transmitted flux (for thin target) Example: A particle current of 1 pn. A consists of 6· 109 projectiles/s. A 132 Sn target (1 mg/cm 2) consists of 5· 1018 nuclei/cm 2 Luminosity = projectiles [s-1] · target nuclei [cm -2] Luminosity (projectile → 132 Sn) = 3· 1028 [s-1 cm-2] Reaction rate [s-1] = luminosity · cross section [cm 2] = projectiles [s-1] · target nuclei [cm-2] · cross section [cm 2] Φa = na·va Nb = nb·A·d
Non elastic scattering
Rutherford scattering
Scattering di rutherford
Lacunae in bone
Small angle scattering
Photoelectric effect สรุป
Scattering matrix
Rayleigh vs mie
Double parton scattering
Double scattering
Rayleigh scattering formula
Diffraction and scattering
Scattering matrix
Dynamic light scattering 원리
Scattering of light in suspension
Compton effect
Elastic energy
Pauli blocking of light scattering in degenerate fermions
Mie scattering vs rayleigh
Scattering efficiency
Contoh refraksi gelombang
Horizontal
Liquid crystal display
Light scattering
Scattering in a central force field
Mie plot
Reciprocal network