Complex Plasmas as a Model for the QuarkGluonPlasma
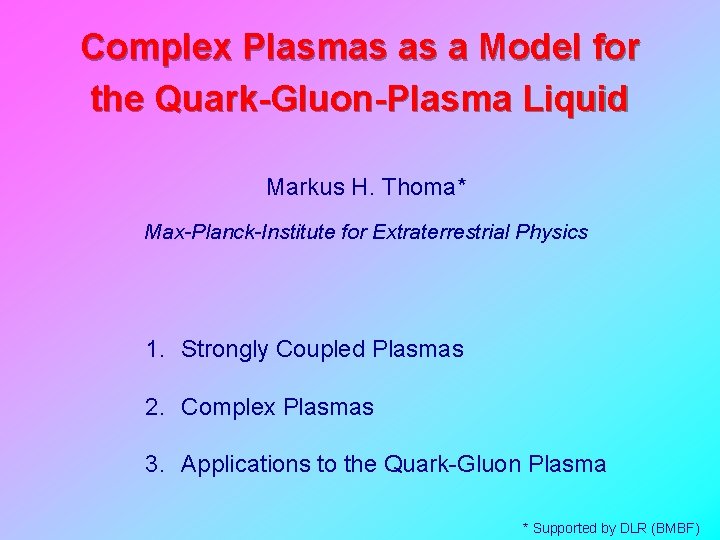
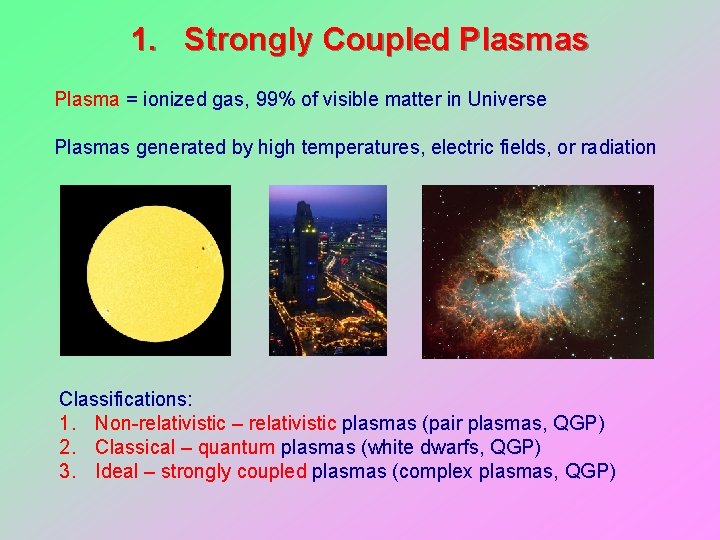
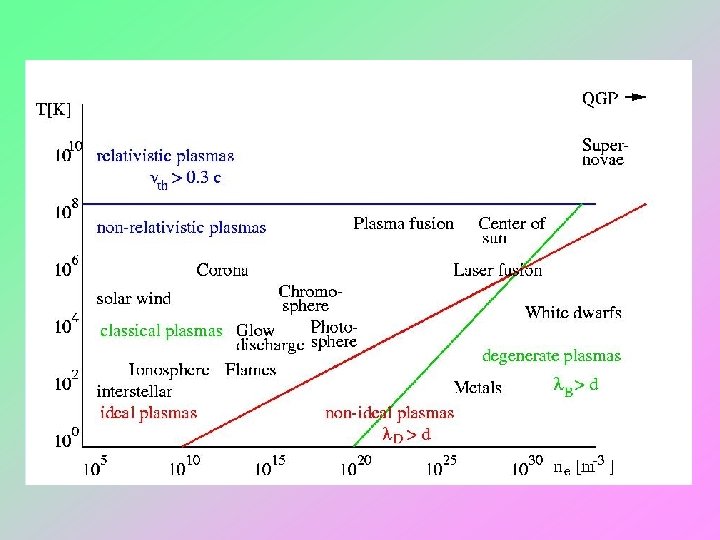
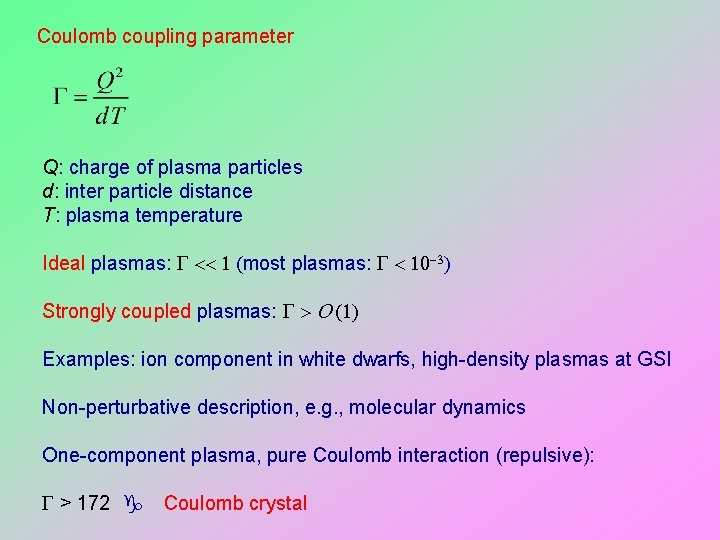
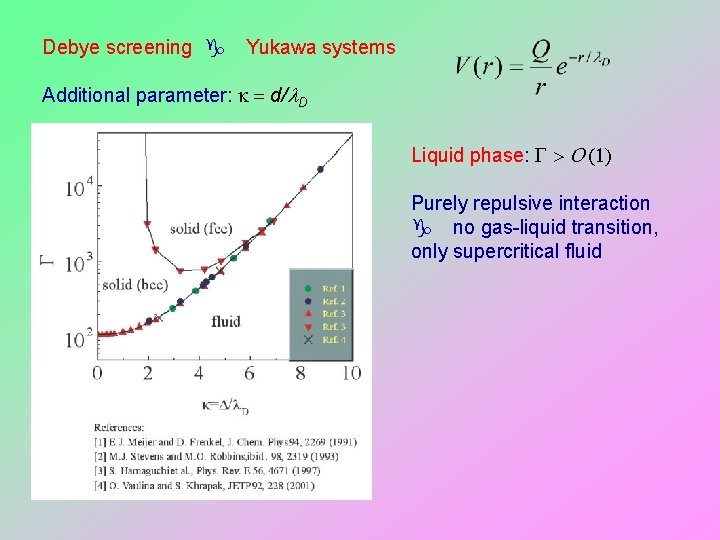
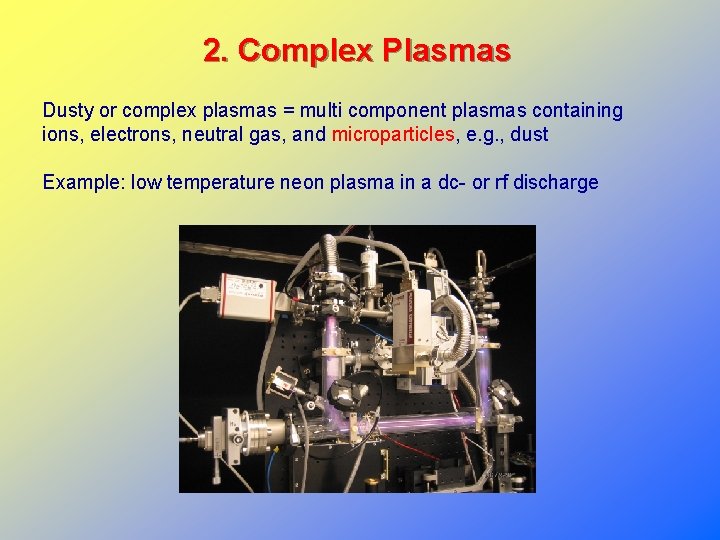
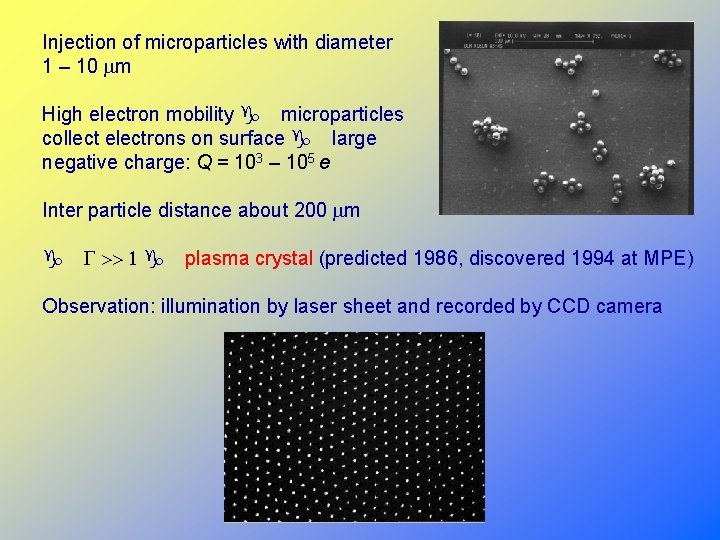
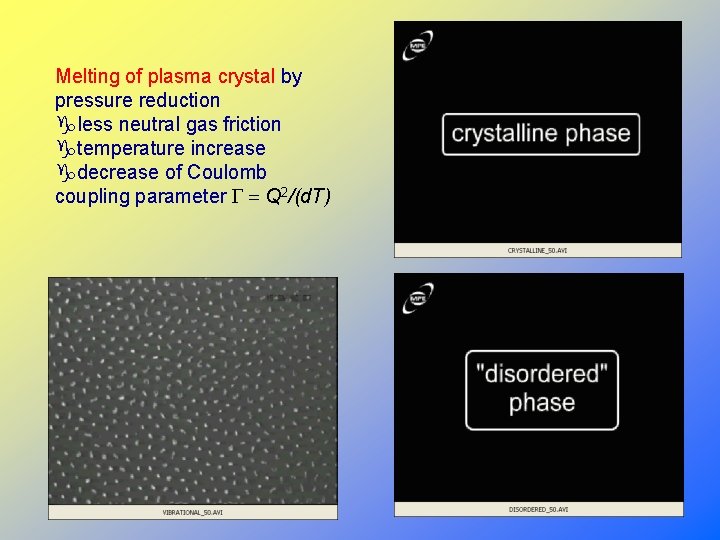
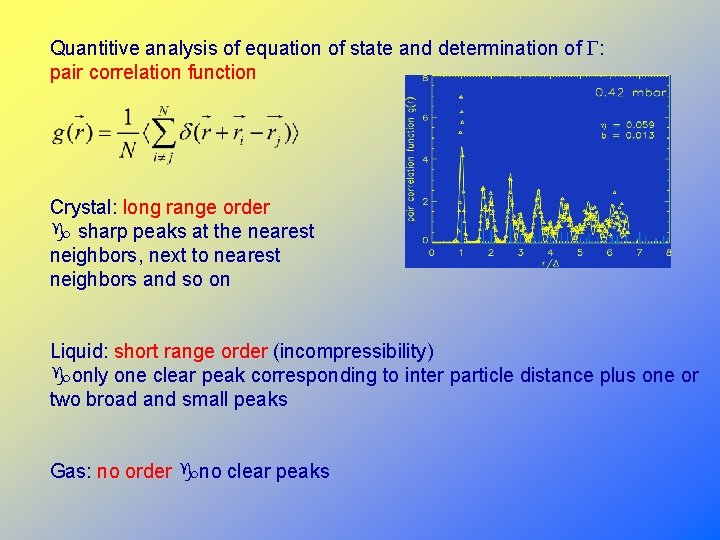
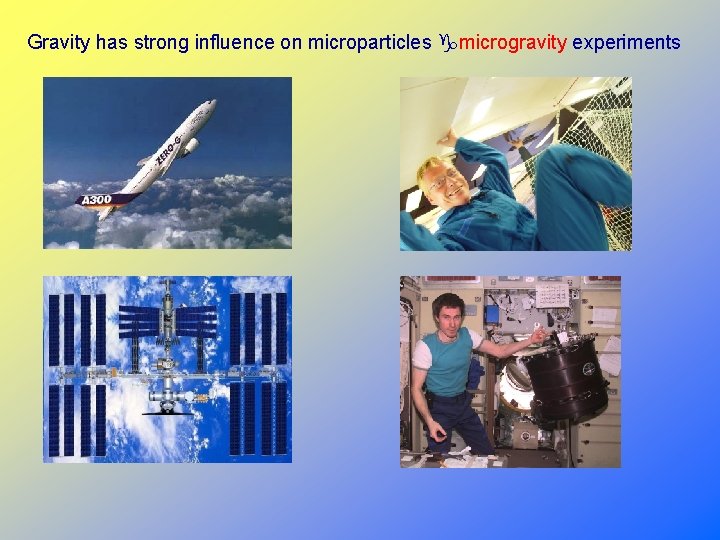
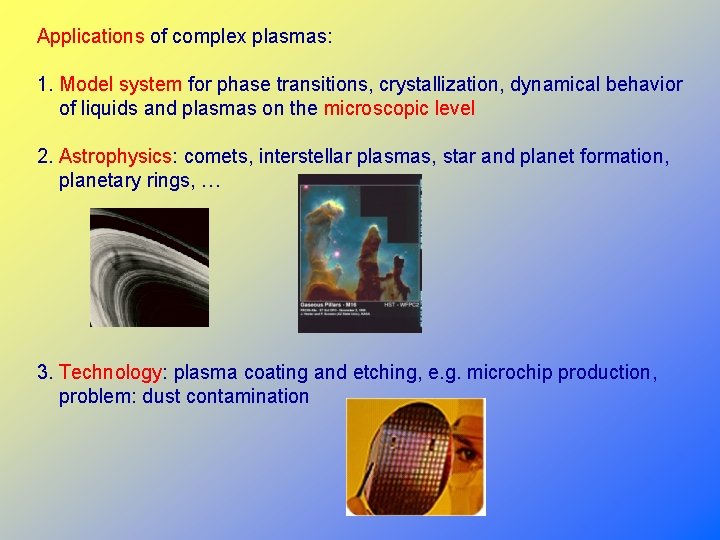
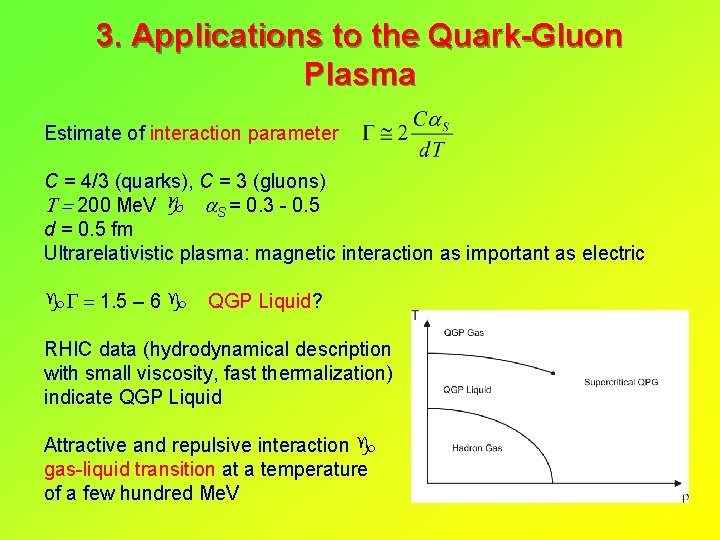
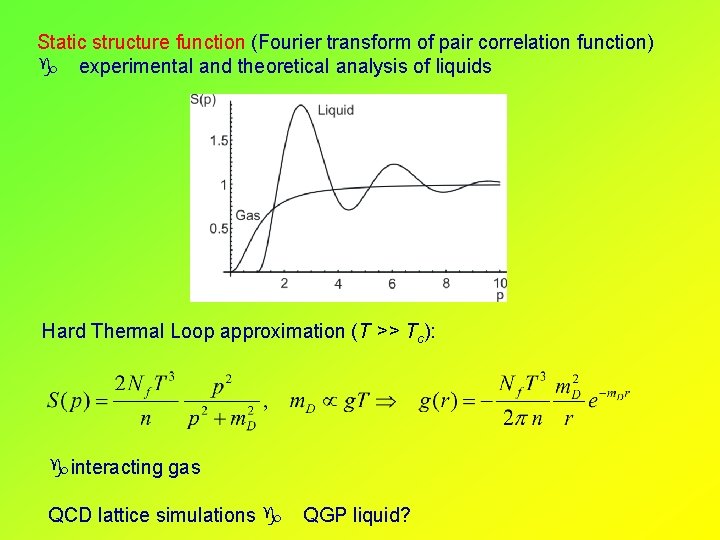
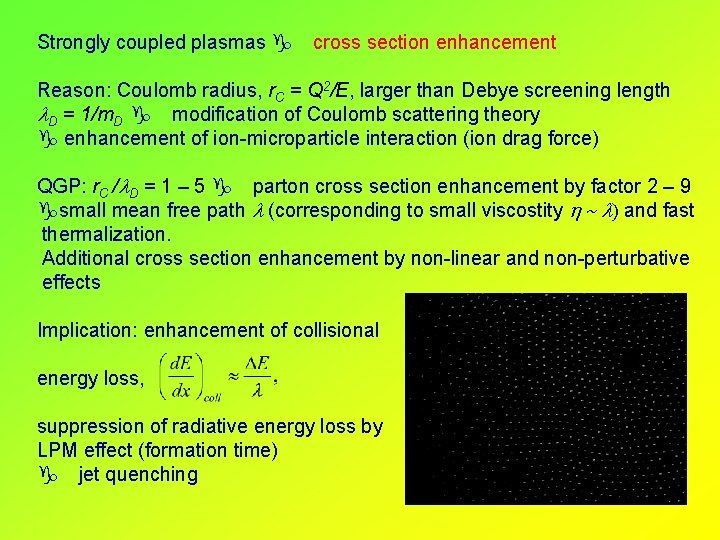
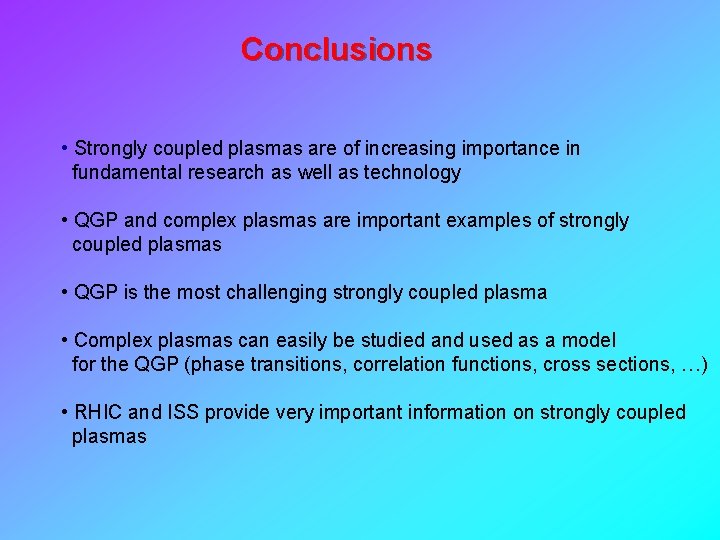
- Slides: 15
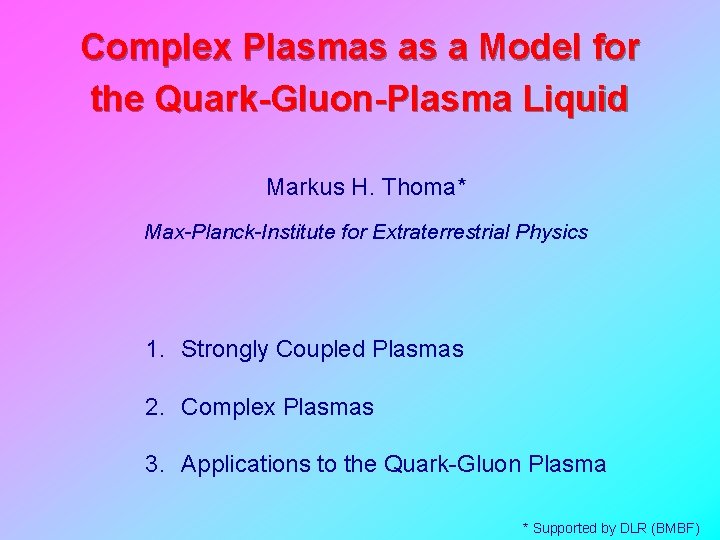
Complex Plasmas as a Model for the Quark-Gluon-Plasma Liquid Markus H. Thoma* Max-Planck-Institute for Extraterrestrial Physics 1. Strongly Coupled Plasmas 2. Complex Plasmas 3. Applications to the Quark-Gluon Plasma * Supported by DLR (BMBF)
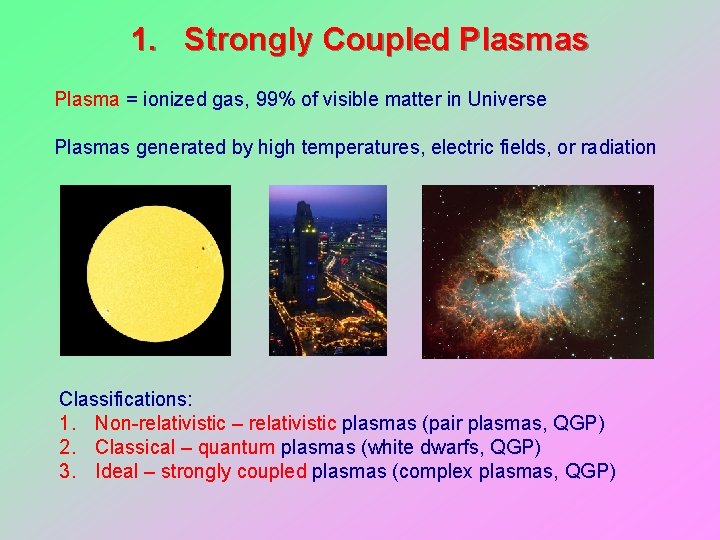
1. Strongly Coupled Plasmas Plasma = ionized gas, 99% of visible matter in Universe Plasmas generated by high temperatures, electric fields, or radiation Classifications: 1. Non-relativistic – relativistic plasmas (pair plasmas, QGP) 2. Classical – quantum plasmas (white dwarfs, QGP) 3. Ideal – strongly coupled plasmas (complex plasmas, QGP)
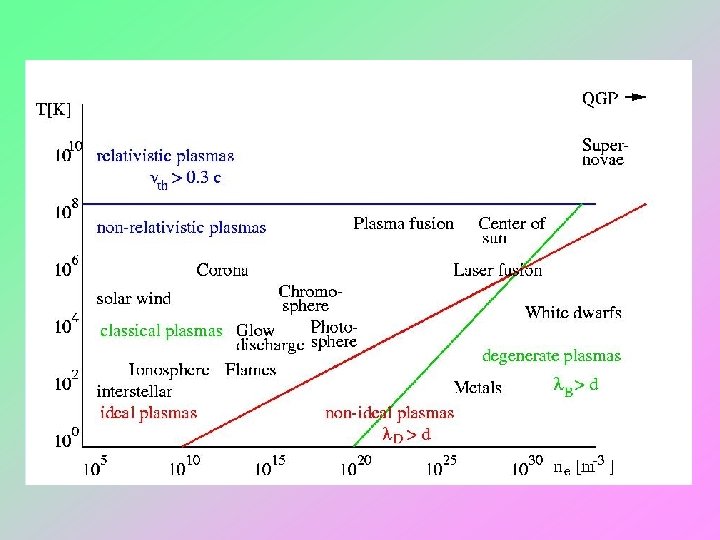
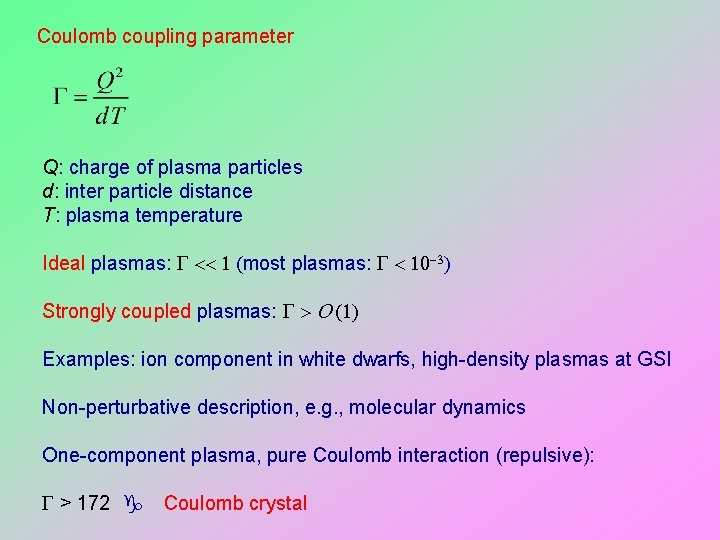
Coulomb coupling parameter Q: charge of plasma particles d: inter particle distance T: plasma temperature Ideal plasmas: G << 1 (most plasmas: G < 10 -3) Strongly coupled plasmas: G > O (1) Examples: ion component in white dwarfs, high-density plasmas at GSI Non-perturbative description, e. g. , molecular dynamics One-component plasma, pure Coulomb interaction (repulsive): G > 172 g Coulomb crystal
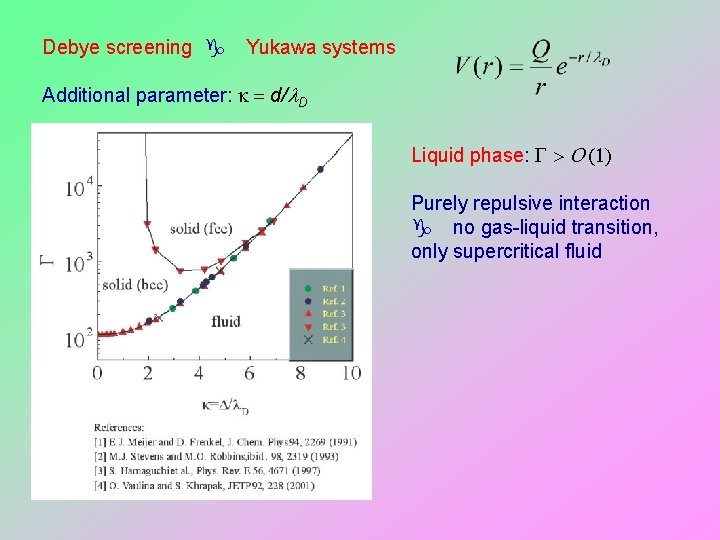
Debye screening g Yukawa systems Additional parameter: k = d/l. D Liquid phase: G > O (1) Purely repulsive interaction g no gas-liquid transition, only supercritical fluid
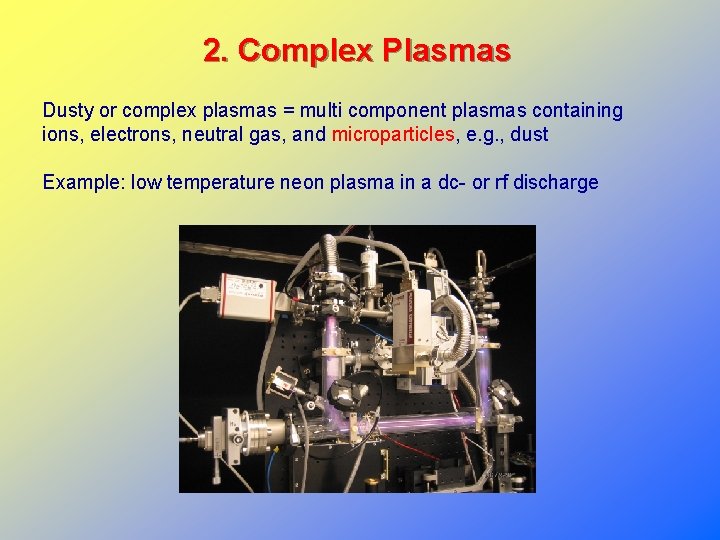
2. Complex Plasmas Dusty or complex plasmas = multi component plasmas containing ions, electrons, neutral gas, and microparticles, e. g. , dust Example: low temperature neon plasma in a dc- or rf discharge
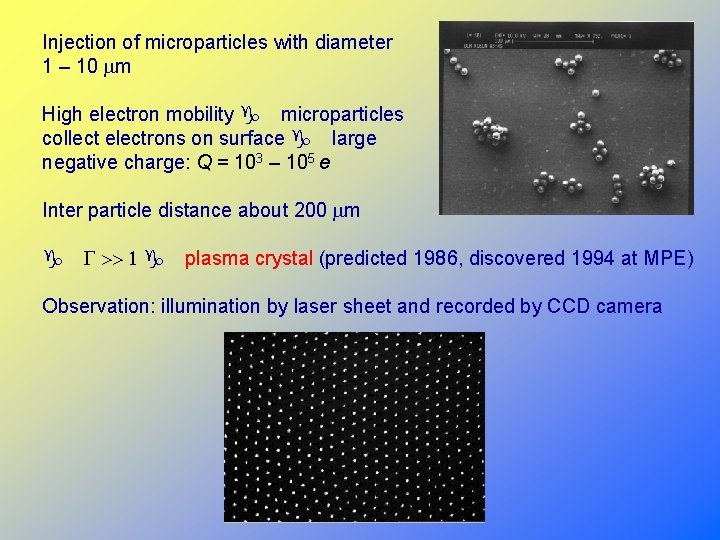
Injection of microparticles with diameter 1 – 10 mm High electron mobility g microparticles collect electrons on surface g large negative charge: Q = 103 – 105 e Inter particle distance about 200 mm g G >> 1 g plasma crystal (predicted 1986, discovered 1994 at MPE) Observation: illumination by laser sheet and recorded by CCD camera
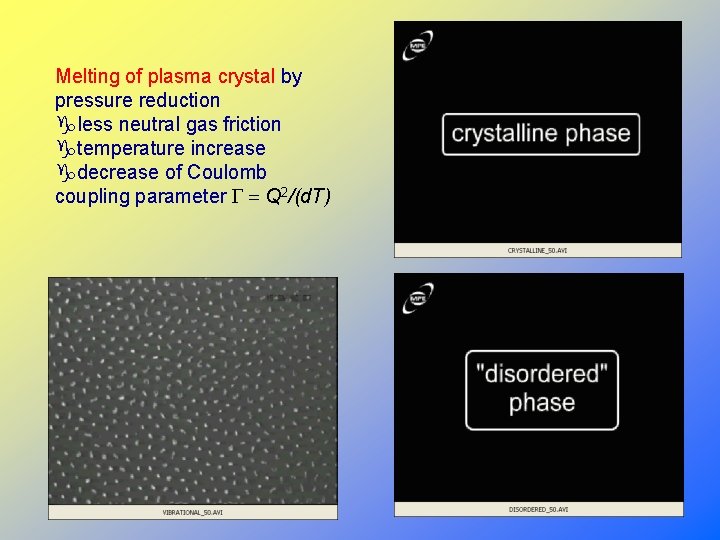
Melting of plasma crystal by pressure reduction gless neutral gas friction gtemperature increase gdecrease of Coulomb coupling parameter G = Q 2/(d. T)
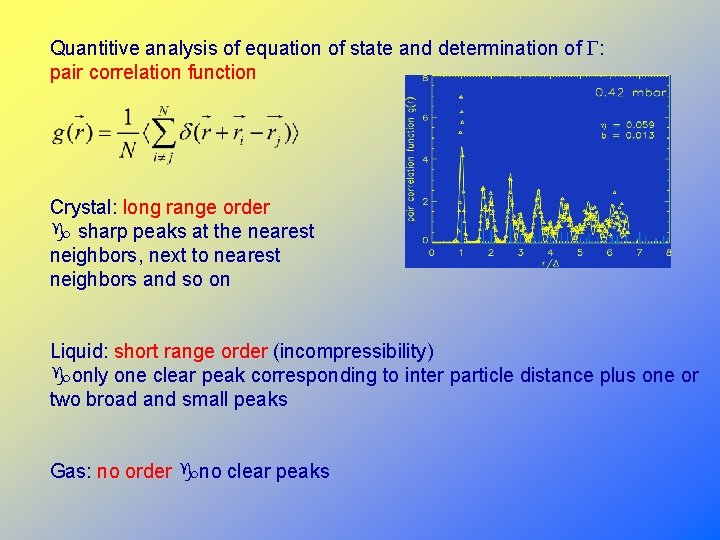
Quantitive analysis of equation of state and determination of G: pair correlation function Crystal: long range order g sharp peaks at the nearest neighbors, next to nearest neighbors and so on Liquid: short range order (incompressibility) gonly one clear peak corresponding to inter particle distance plus one or two broad and small peaks Gas: no order gno clear peaks
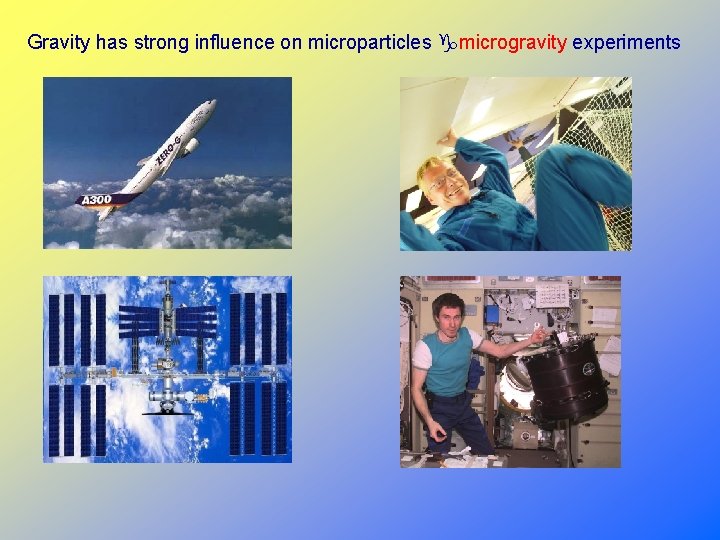
Gravity has strong influence on microparticles gmicrogravity experiments
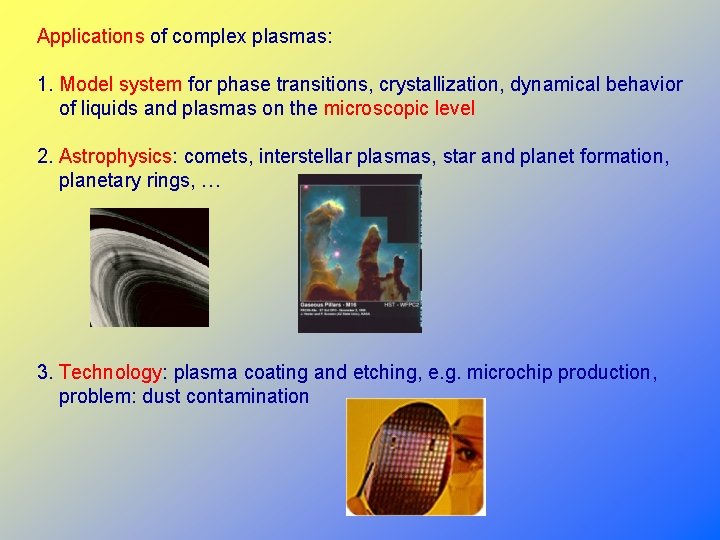
Applications of complex plasmas: 1. Model system for phase transitions, crystallization, dynamical behavior of liquids and plasmas on the microscopic level 2. Astrophysics: comets, interstellar plasmas, star and planet formation, planetary rings, … 3. Technology: plasma coating and etching, e. g. microchip production, problem: dust contamination
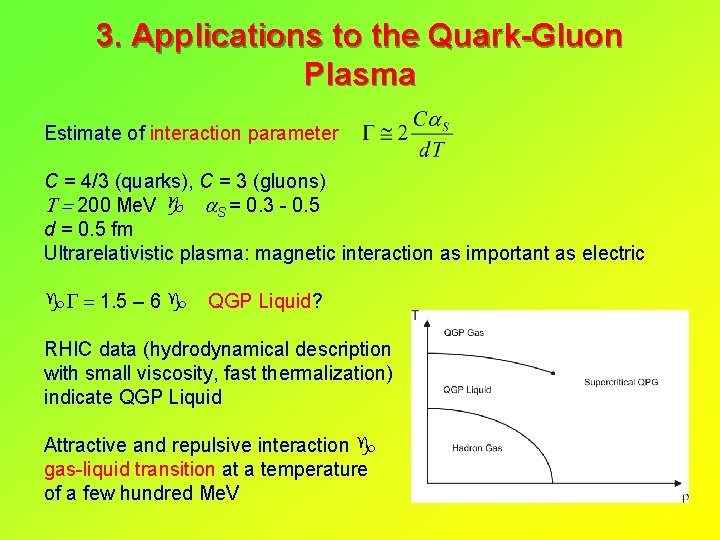
3. Applications to the Quark-Gluon Plasma Estimate of interaction parameter C = 4/3 (quarks), C = 3 (gluons) T = 200 Me. V g a. S = 0. 3 - 0. 5 d = 0. 5 fm Ultrarelativistic plasma: magnetic interaction as important as electric g. G = 1. 5 – 6 g QGP Liquid? RHIC data (hydrodynamical description with small viscosity, fast thermalization) indicate QGP Liquid Attractive and repulsive interaction g gas-liquid transition at a temperature of a few hundred Me. V
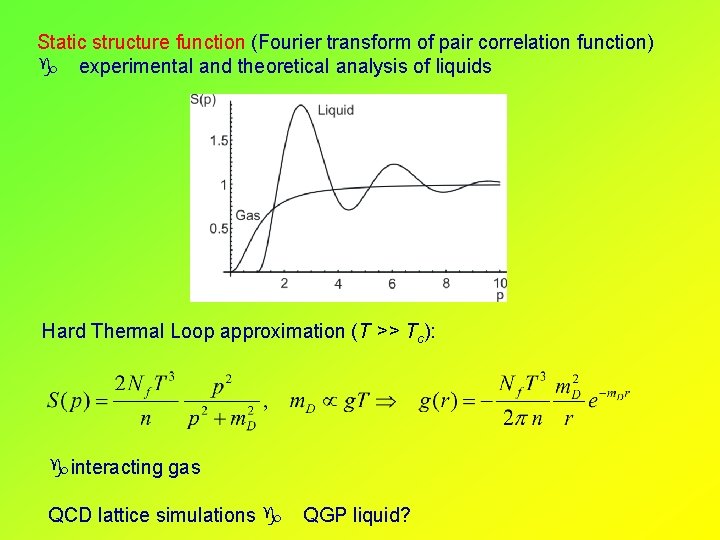
Static structure function (Fourier transform of pair correlation function) g experimental and theoretical analysis of liquids Hard Thermal Loop approximation (T >> Tc): ginteracting gas QCD lattice simulations g QGP liquid?
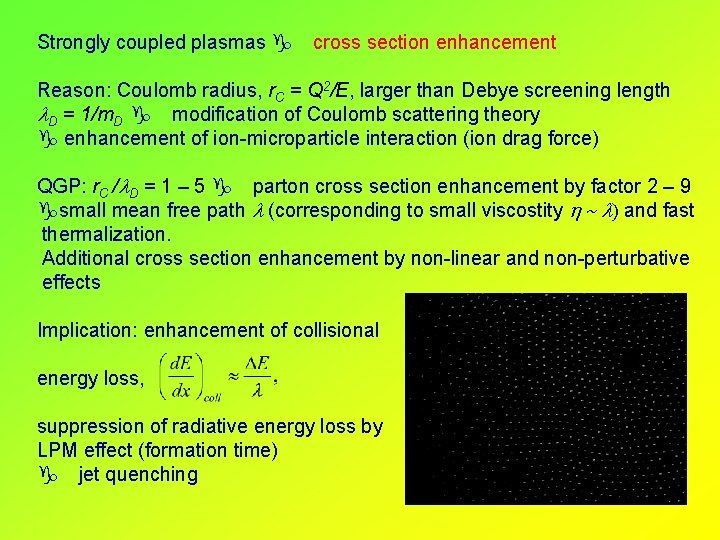
Strongly coupled plasmas g cross section enhancement Reason: Coulomb radius, r. C = Q 2/E, larger than Debye screening length l. D = 1/m. D g modification of Coulomb scattering theory g enhancement of ion-microparticle interaction (ion drag force) QGP: r. C /l. D = 1 – 5 g parton cross section enhancement by factor 2 – 9 gsmall mean free path l (corresponding to small viscostity h ~ l) and fast thermalization. Additional cross section enhancement by non-linear and non-perturbative effects Implication: enhancement of collisional energy loss, suppression of radiative energy loss by LPM effect (formation time) g jet quenching
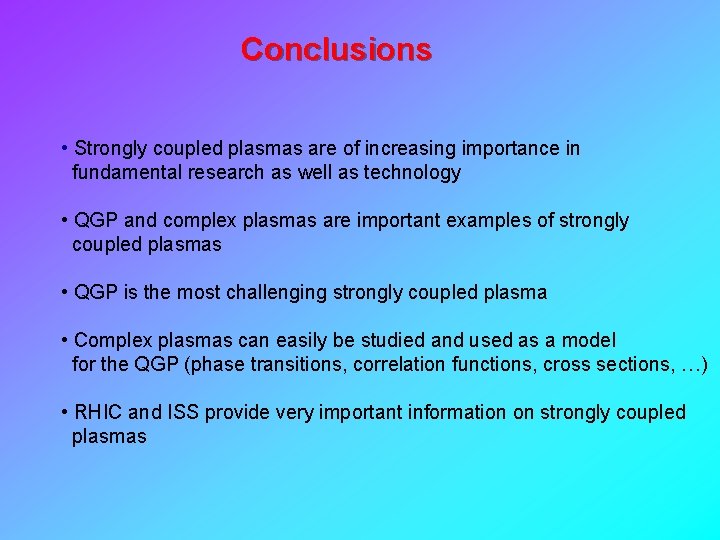
Conclusions • Strongly coupled plasmas are of increasing importance in fundamental research as well as technology • QGP and complex plasmas are important examples of strongly coupled plasmas • QGP is the most challenging strongly coupled plasma • Complex plasmas can easily be studied and used as a model for the QGP (phase transitions, correlation functions, cross sections, …) • RHIC and ISS provide very important information on strongly coupled plasmas
Frequency formula
Matter is
Pauline and bruno have a big argument
Miliary tb
Simple compound complex sentences quiz
Electra complex vs oedipus complex
Psychodynamic theory examples
Thematic apperception
Iso 22301 utbildning
Typiska drag för en novell
Tack för att ni lyssnade bild
Vad står k.r.å.k.a.n för
Varför kallas perioden 1918-1939 för mellankrigstiden
En lathund för arbete med kontinuitetshantering
Adressändring ideell förening
Tidbok yrkesförare