Xray sources Purpose of Xray diffraction phase composition
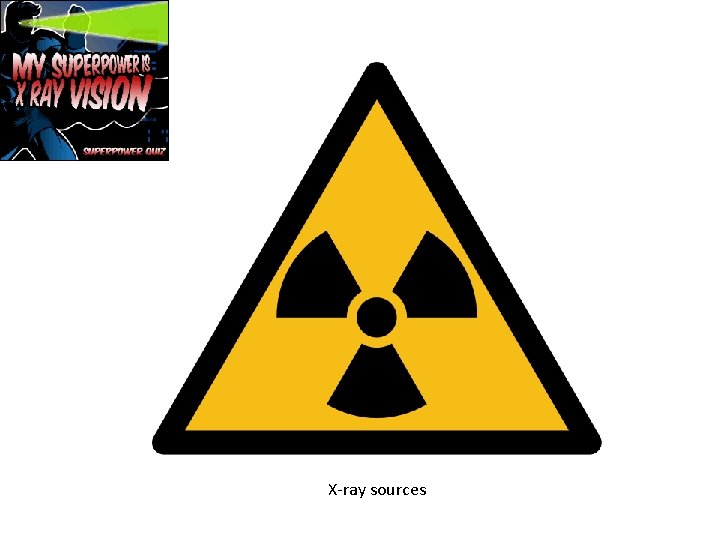
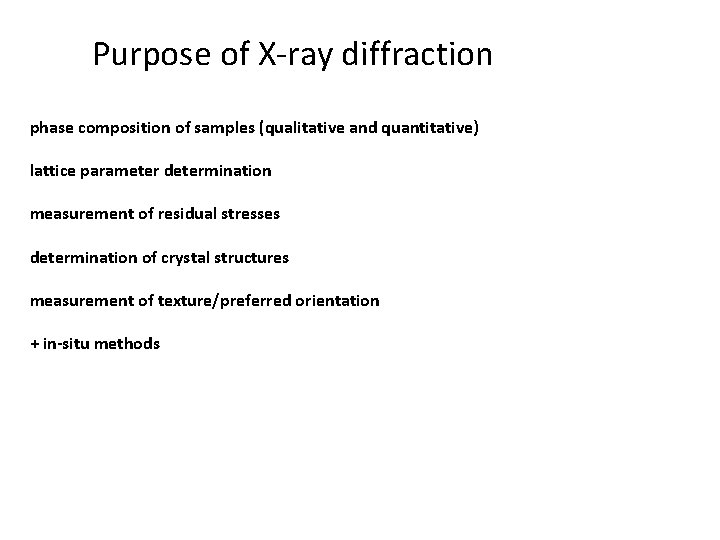
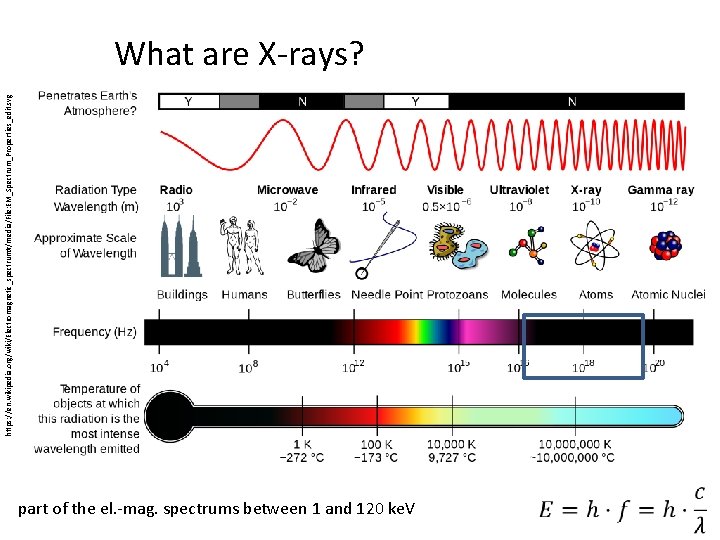
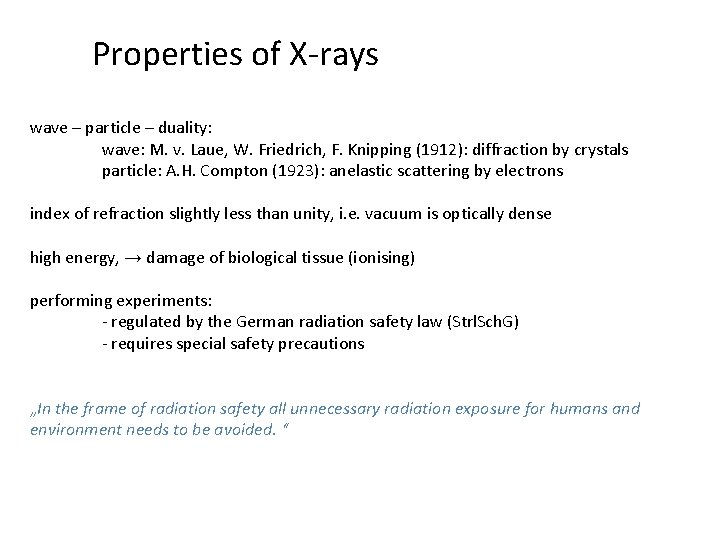
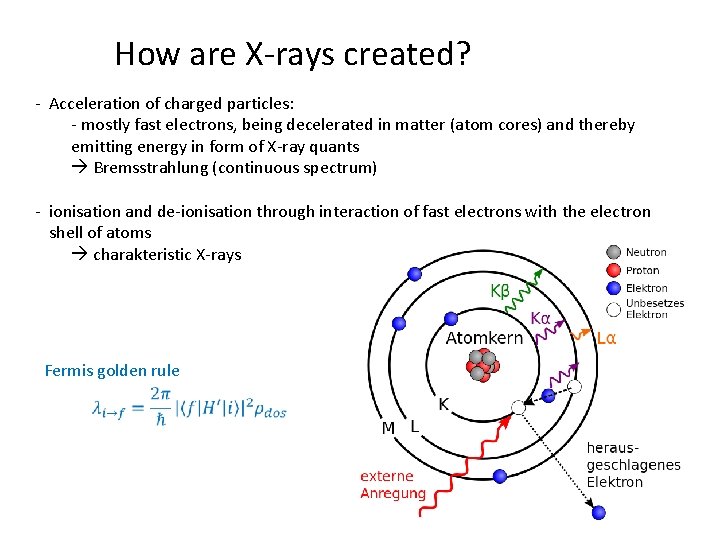
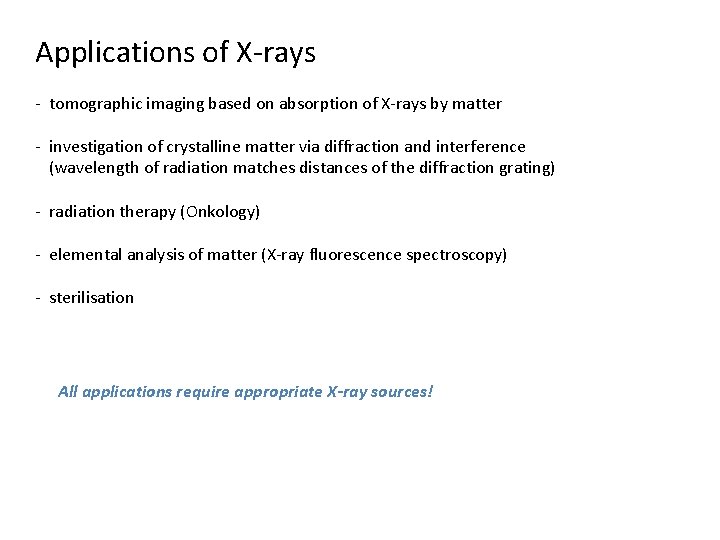
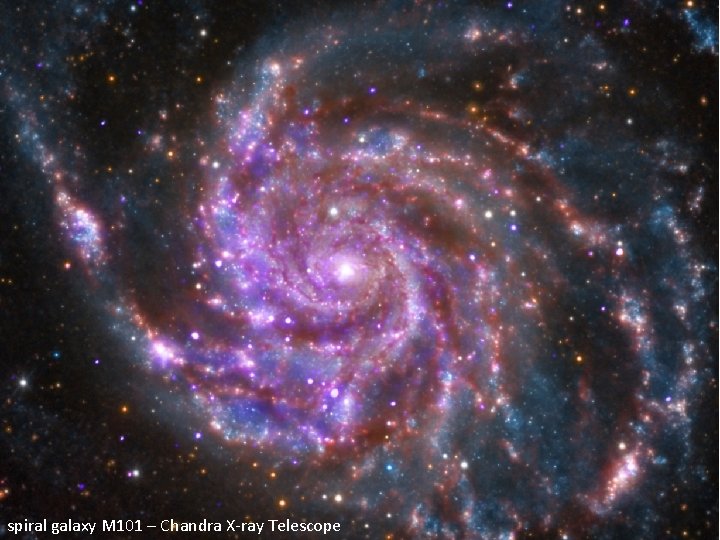
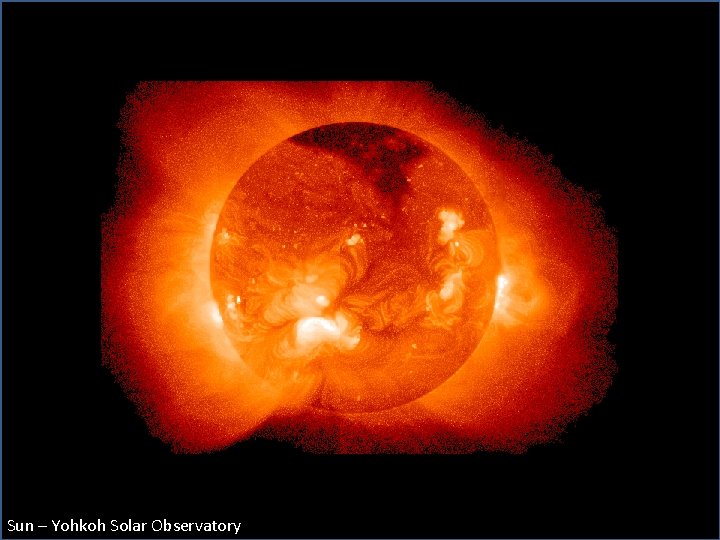
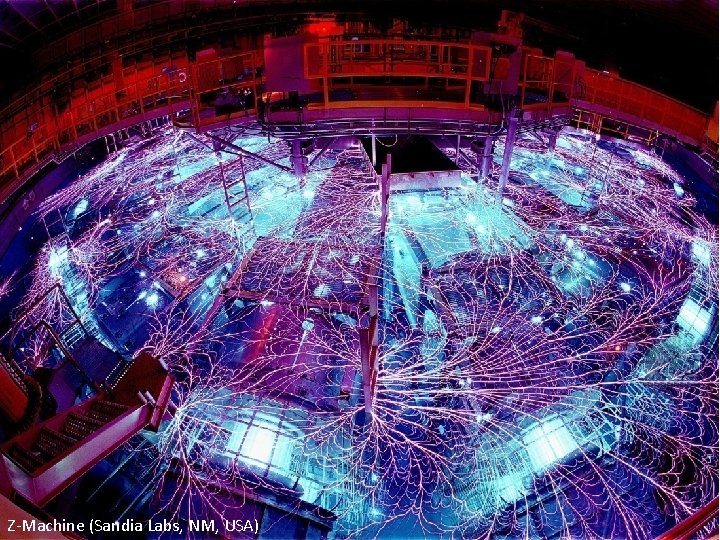
![X-ray sources for laboratories William D. Coolidge [1873 -1975] X-ray sources for laboratories William D. Coolidge [1873 -1975]](https://slidetodoc.com/presentation_image_h/28cd7c381ac8e7abf8c4f27948882bde/image-10.jpg)
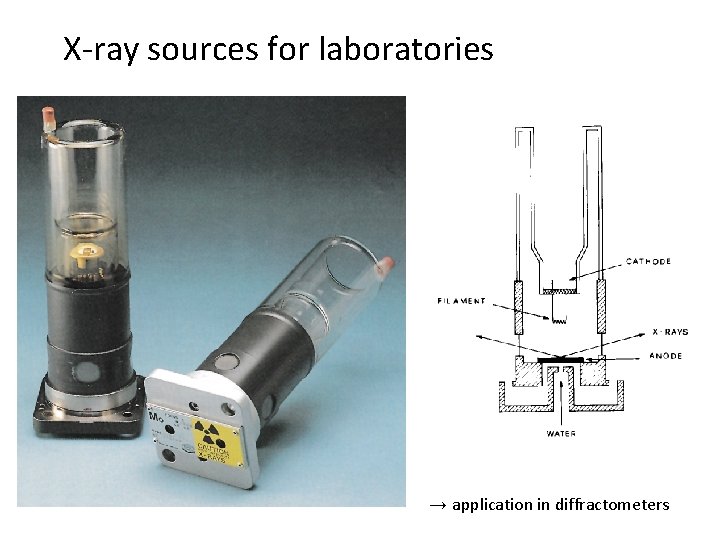
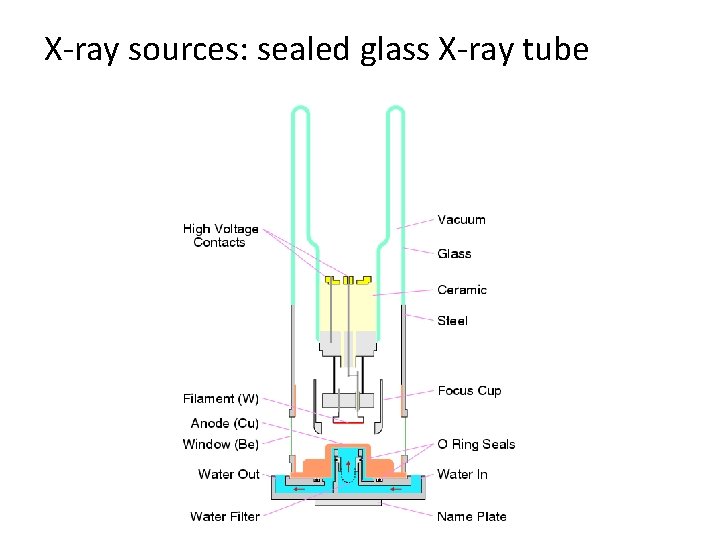
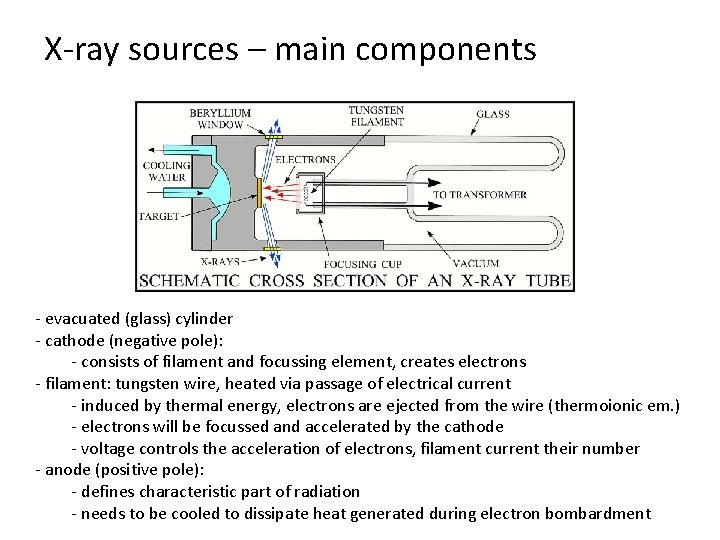
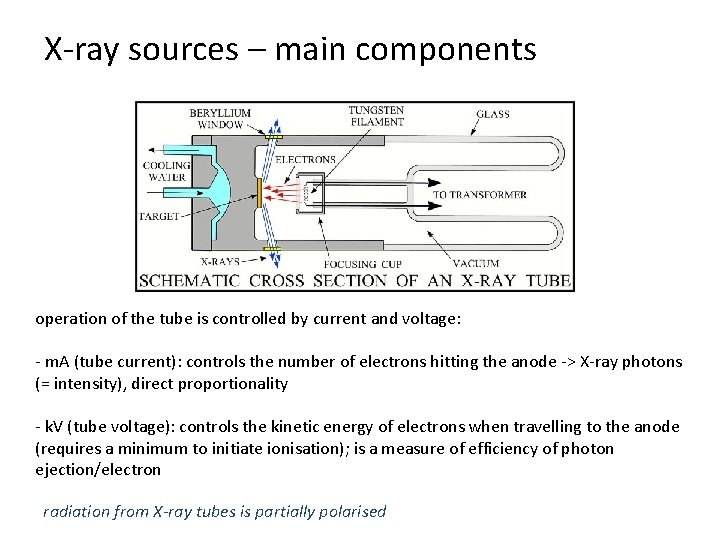
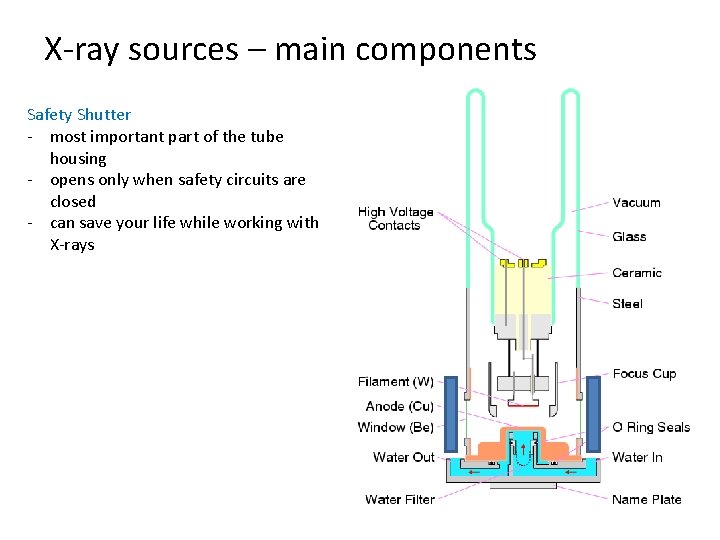
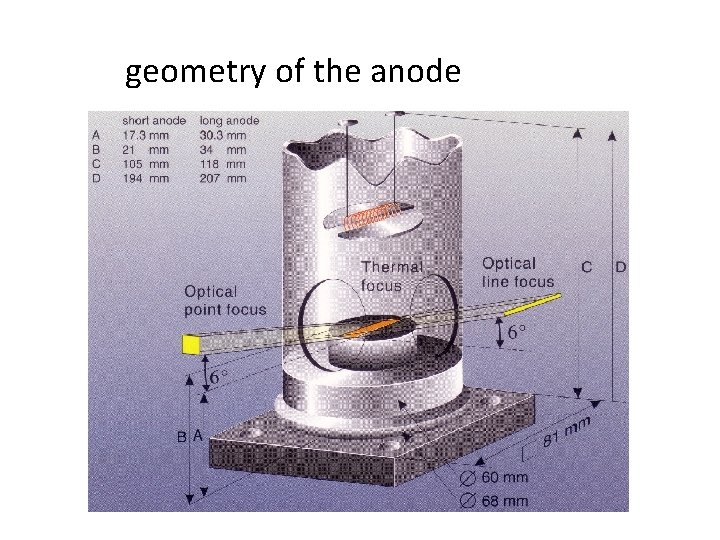
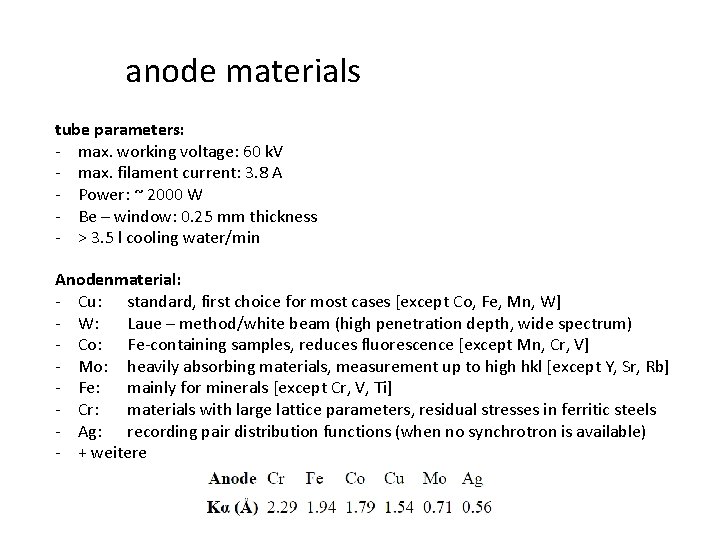
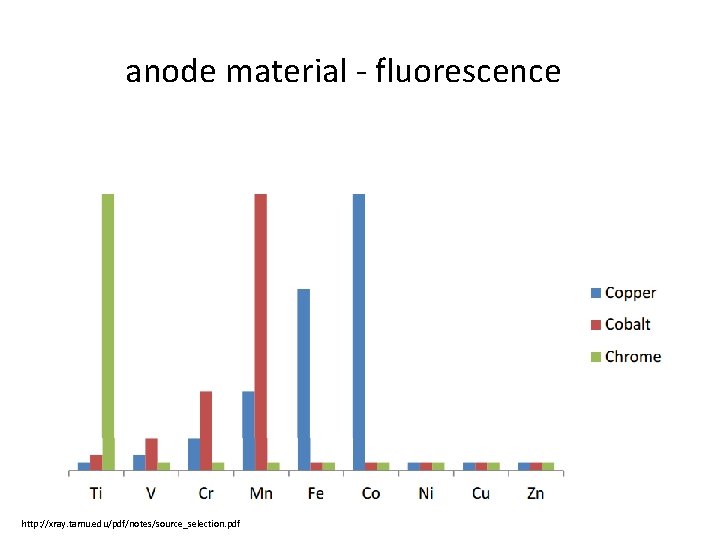
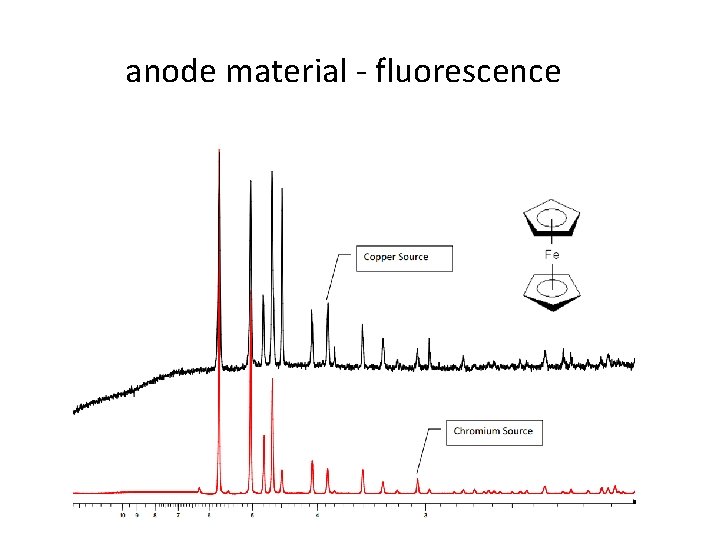
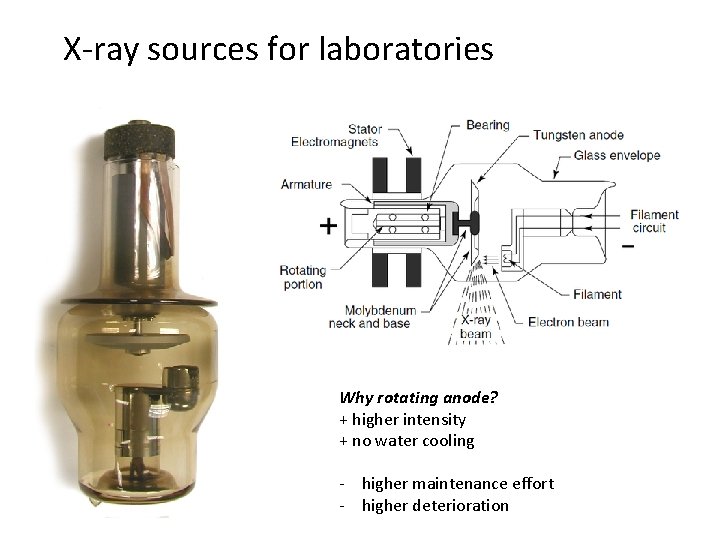
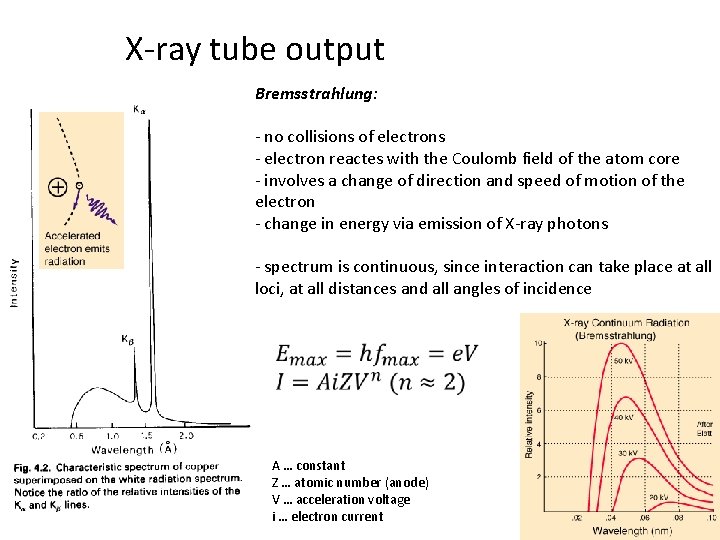
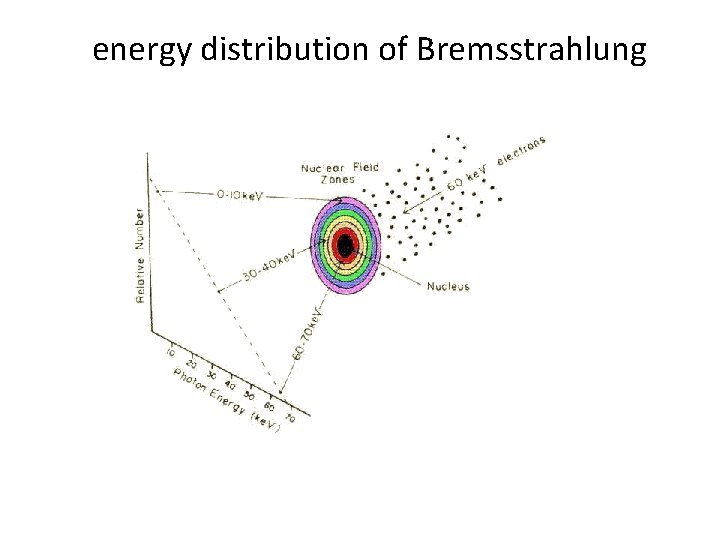
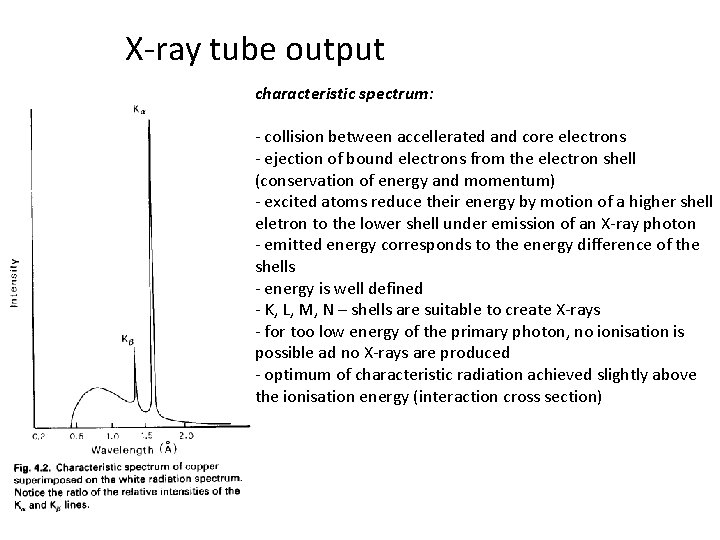
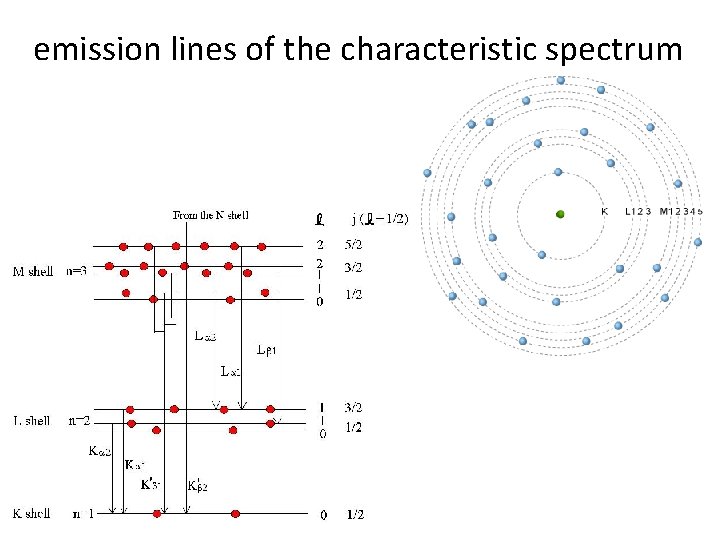
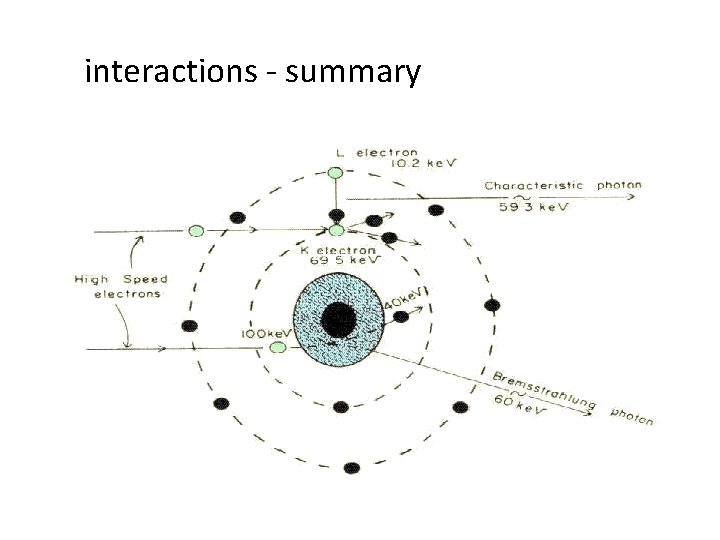
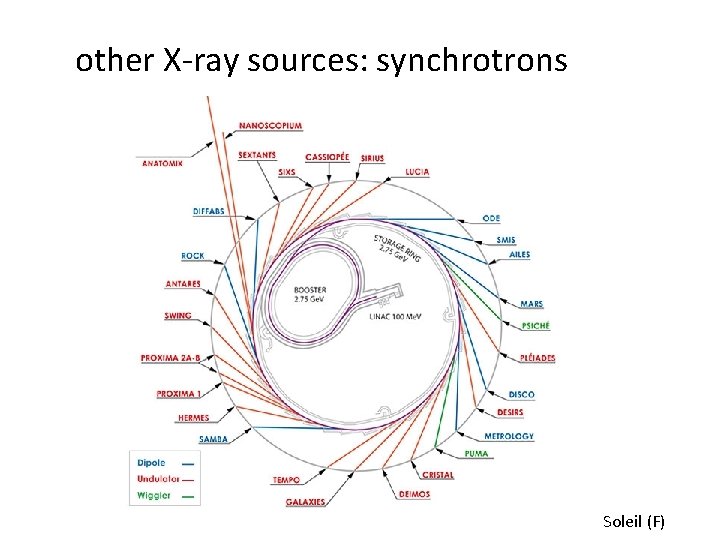
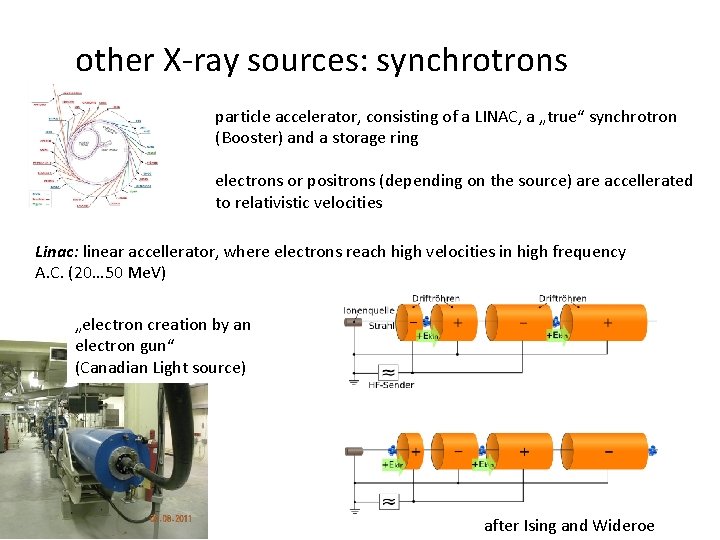
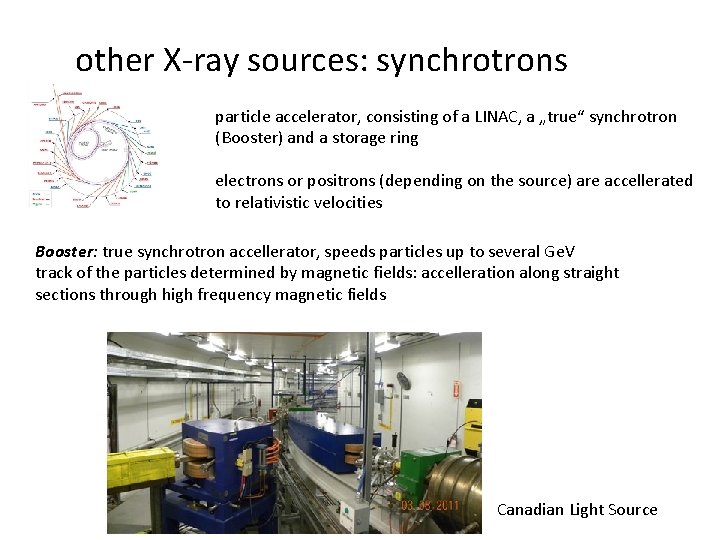
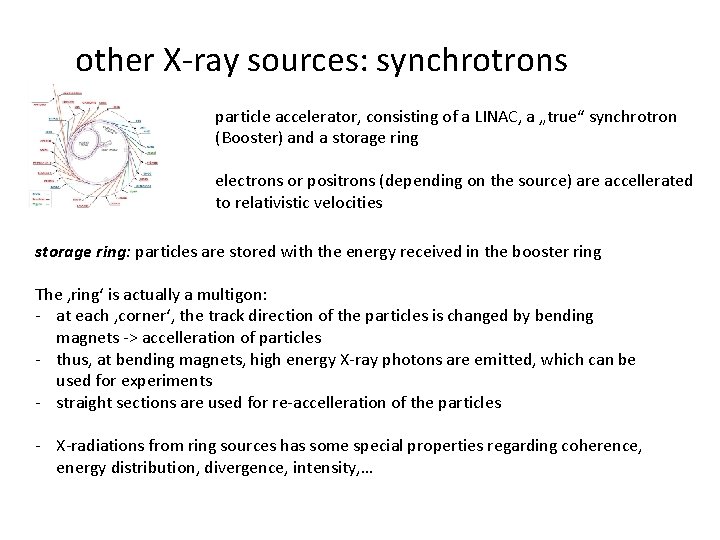
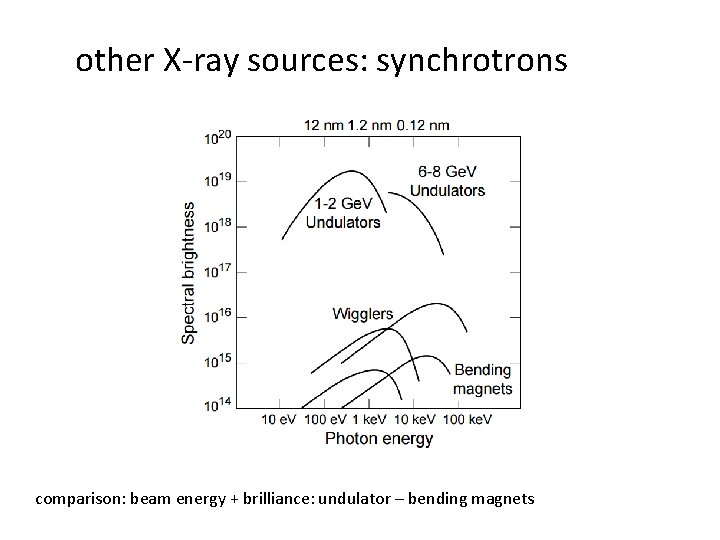
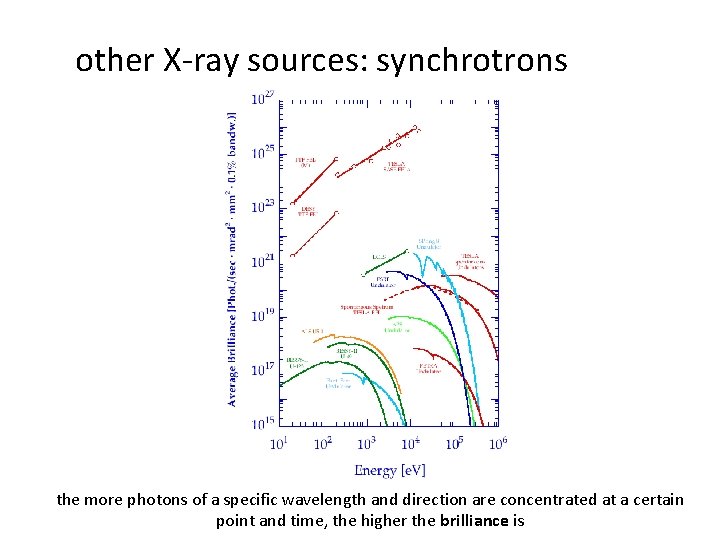
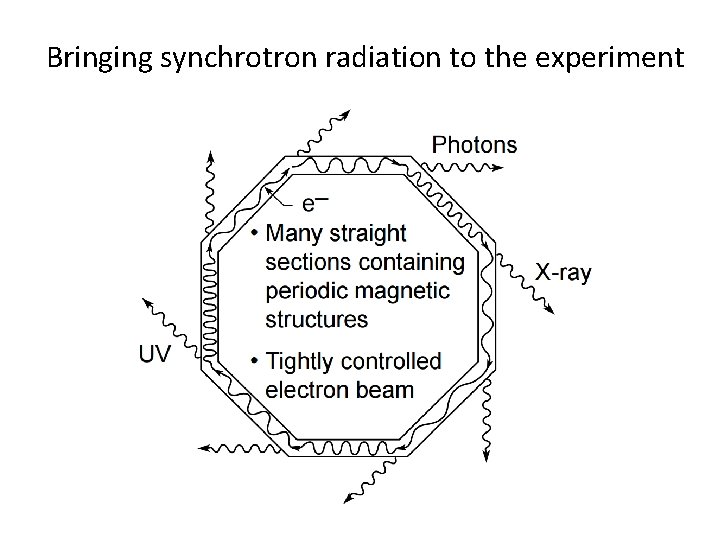
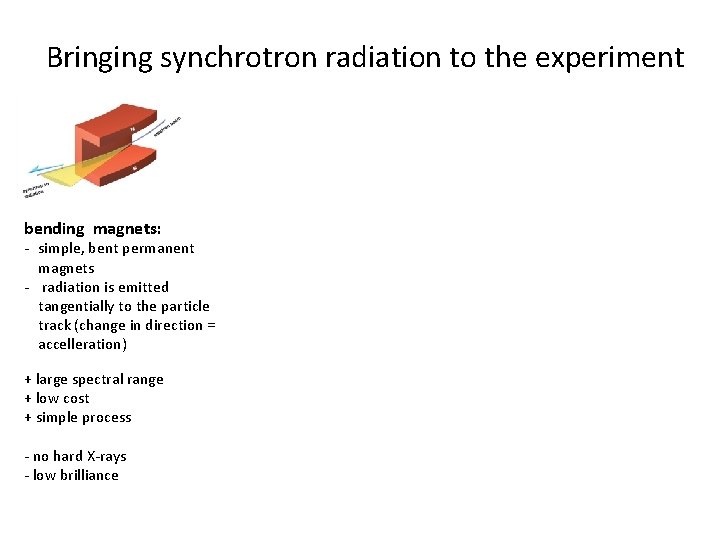
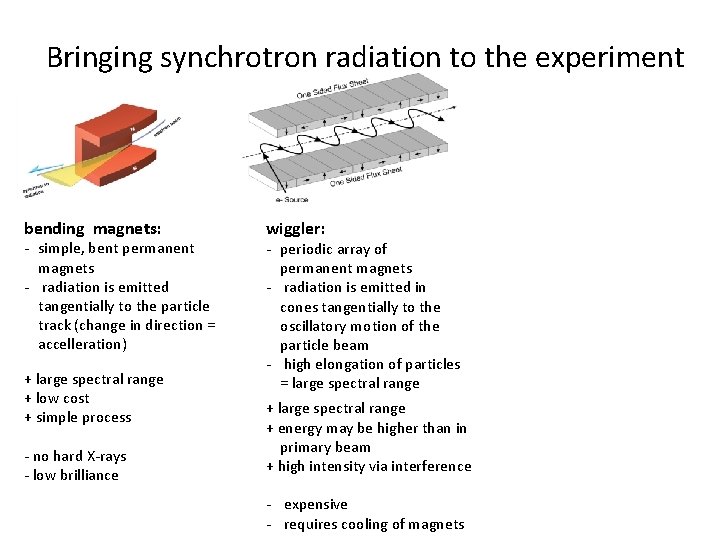
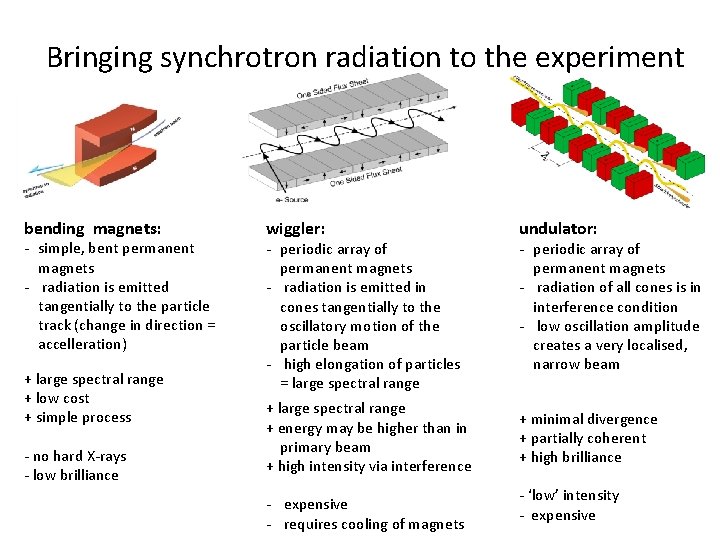
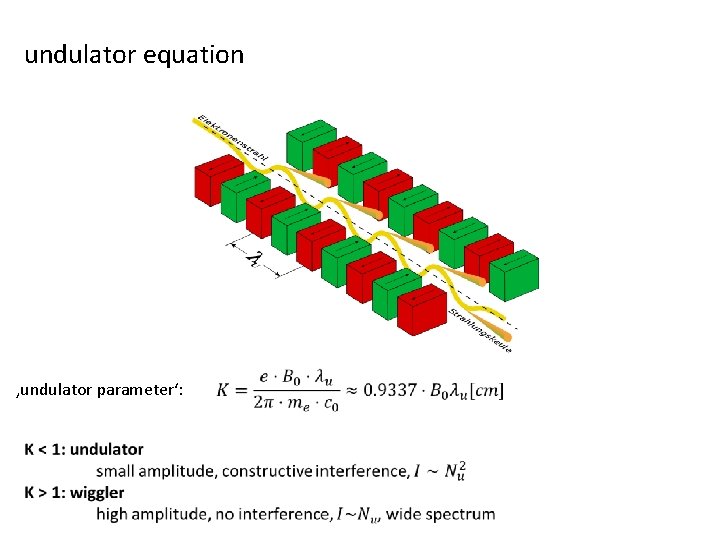
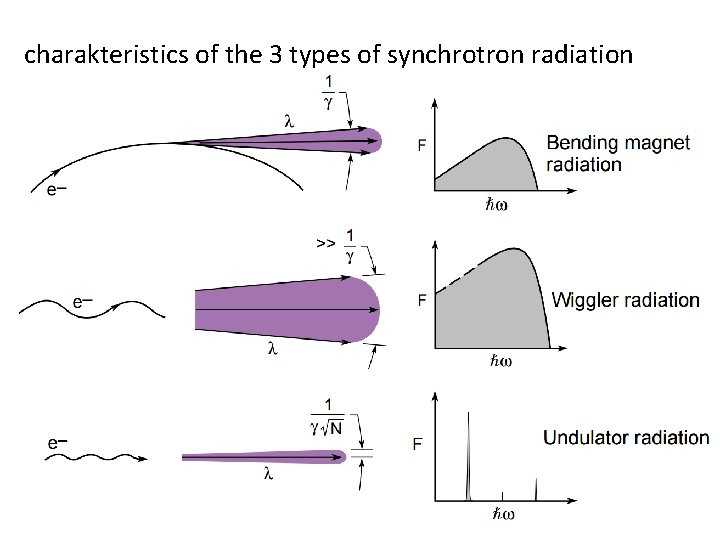
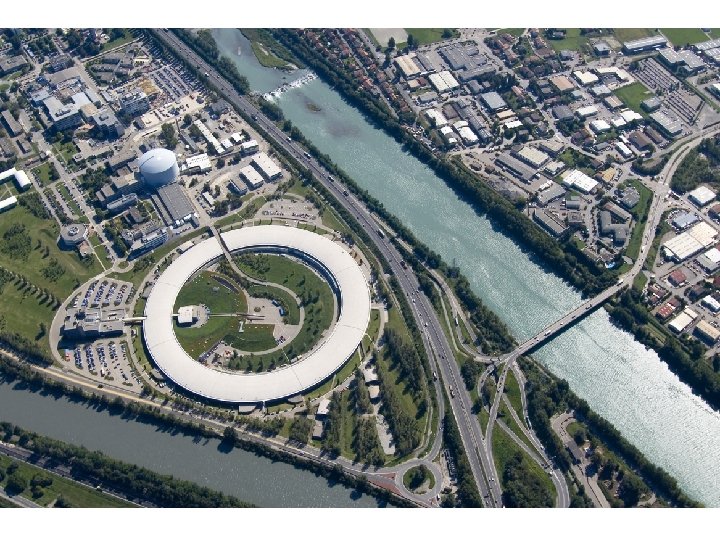
- Slides: 38
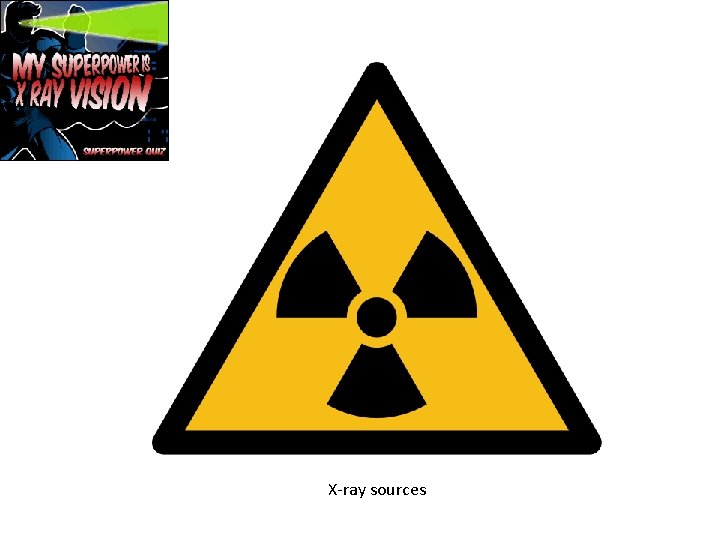
X-ray sources
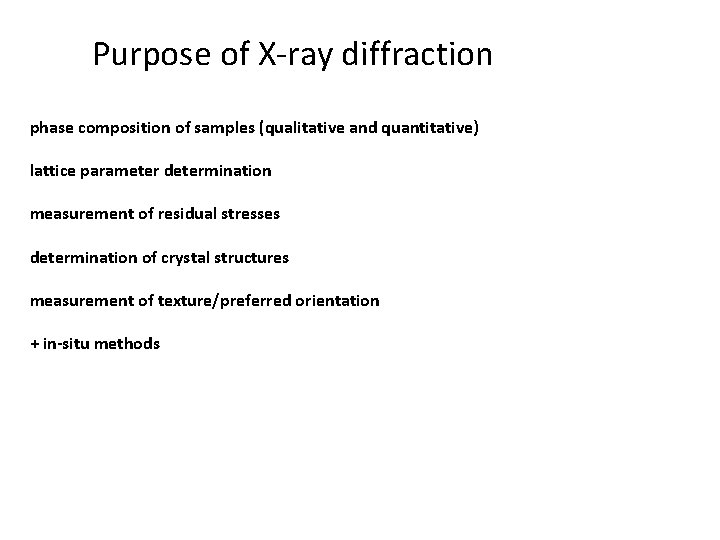
Purpose of X-ray diffraction phase composition of samples (qualitative and quantitative) lattice parameter determination measurement of residual stresses determination of crystal structures measurement of texture/preferred orientation + in-situ methods
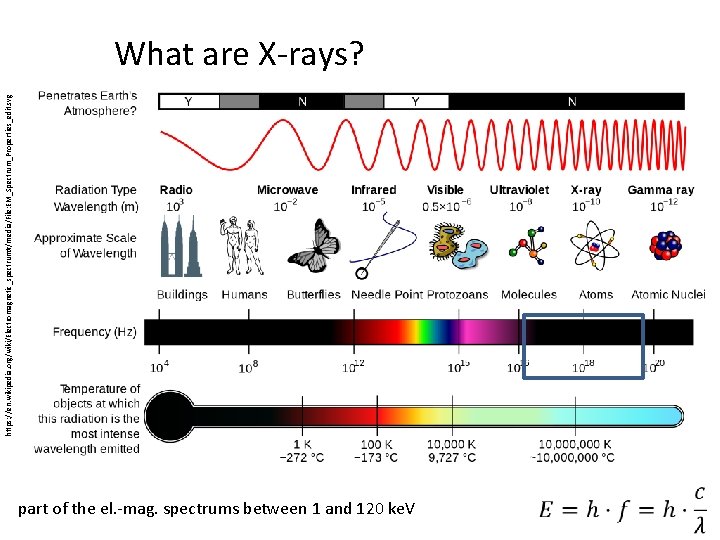
https: //en. wikipedia. org/wiki/Electromagnetic_spectrum#/media/File: EM_Spectrum_Properties_edit. svg What are X-rays? part of the el. -mag. spectrums between 1 and 120 ke. V
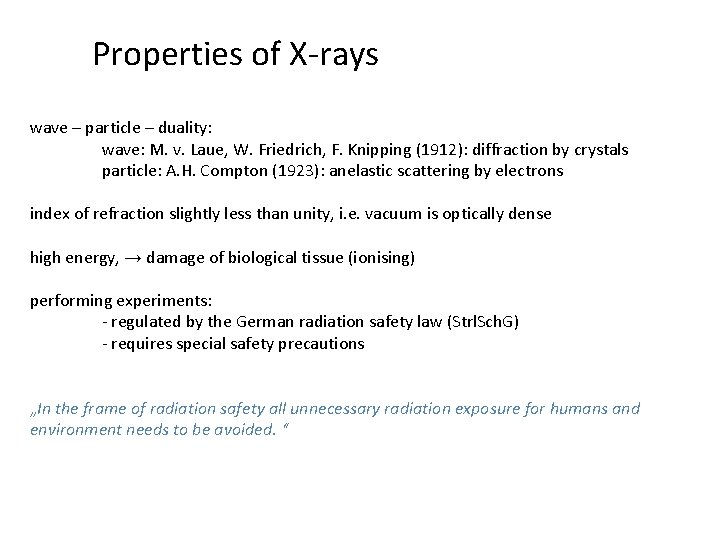
Properties of X-rays wave – particle – duality: wave: M. v. Laue, W. Friedrich, F. Knipping (1912): diffraction by crystals particle: A. H. Compton (1923): anelastic scattering by electrons index of refraction slightly less than unity, i. e. vacuum is optically dense high energy, → damage of biological tissue (ionising) performing experiments: - regulated by the German radiation safety law (Strl. Sch. G) - requires special safety precautions „In the frame of radiation safety all unnecessary radiation exposure for humans and environment needs to be avoided. “
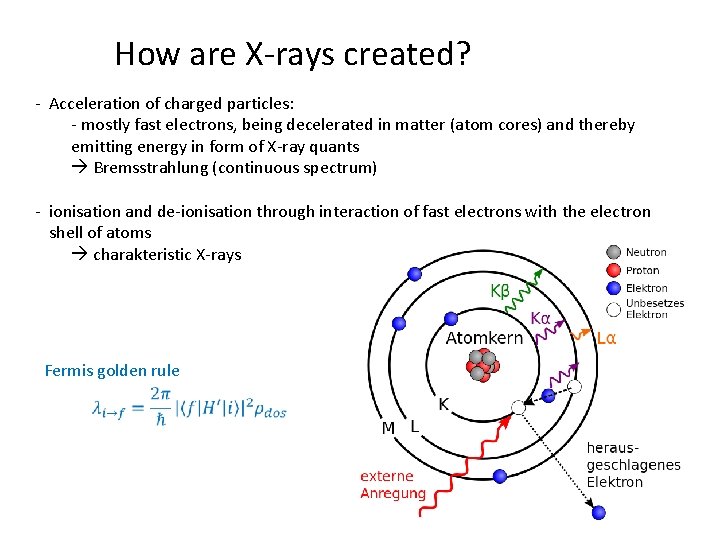
How are X-rays created? - Acceleration of charged particles: - mostly fast electrons, being decelerated in matter (atom cores) and thereby emitting energy in form of X-ray quants Bremsstrahlung (continuous spectrum) - ionisation and de-ionisation through interaction of fast electrons with the electron shell of atoms charakteristic X-rays Fermis golden rule
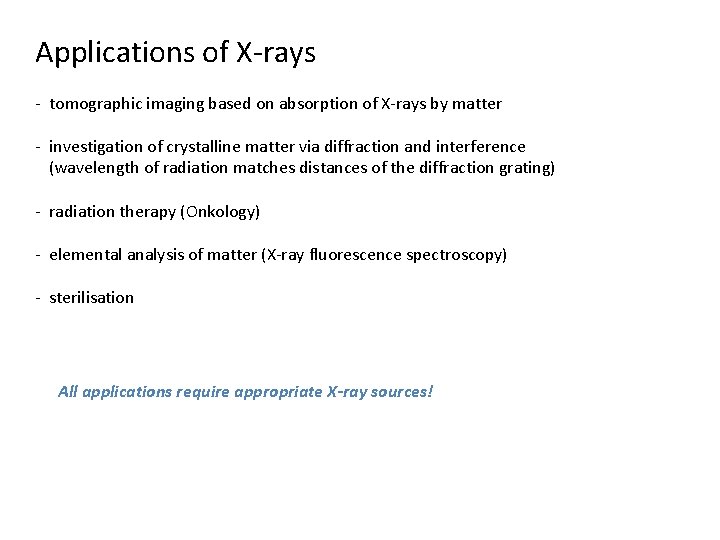
Applications of X-rays - tomographic imaging based on absorption of X-rays by matter - investigation of crystalline matter via diffraction and interference (wavelength of radiation matches distances of the diffraction grating) - radiation therapy (Onkology) - elemental analysis of matter (X-ray fluorescence spectroscopy) - sterilisation All applications require appropriate X-ray sources!
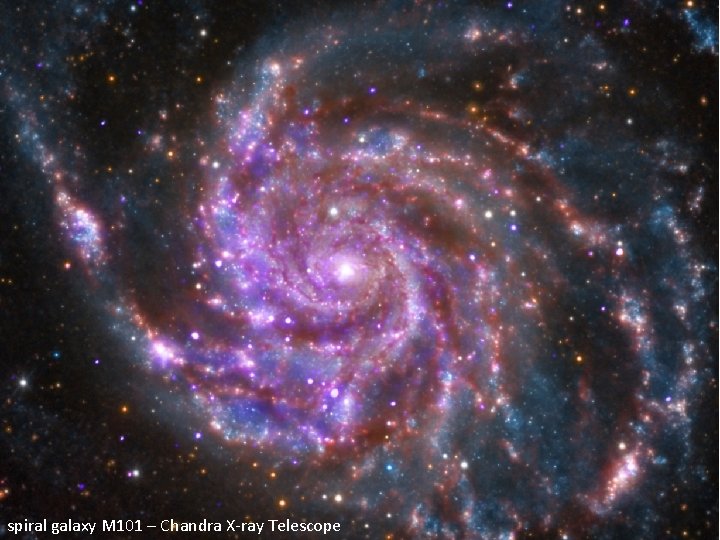
spiral galaxy M 101 – Chandra X-ray Telescope
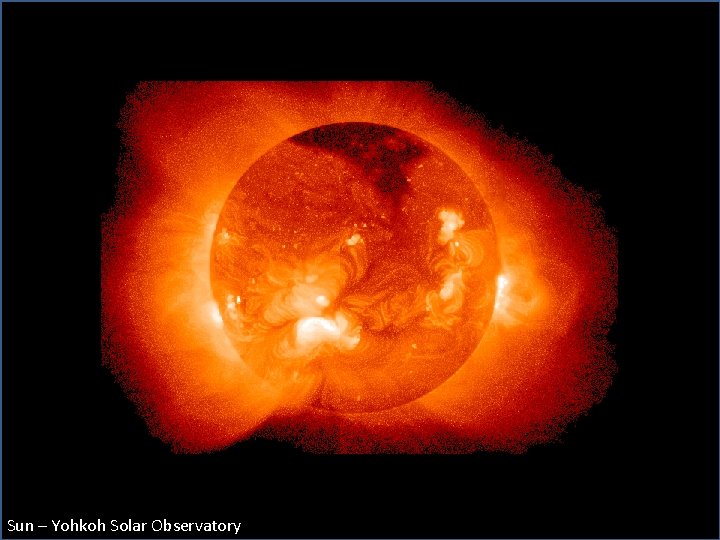
Sun – Yohkoh Solar Observatory
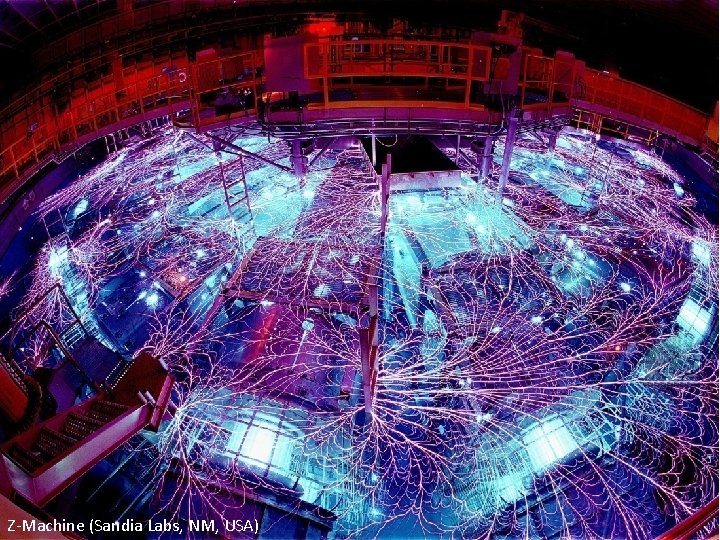
Z-Machine (Sandia Labs, NM, USA)
![Xray sources for laboratories William D Coolidge 1873 1975 X-ray sources for laboratories William D. Coolidge [1873 -1975]](https://slidetodoc.com/presentation_image_h/28cd7c381ac8e7abf8c4f27948882bde/image-10.jpg)
X-ray sources for laboratories William D. Coolidge [1873 -1975]
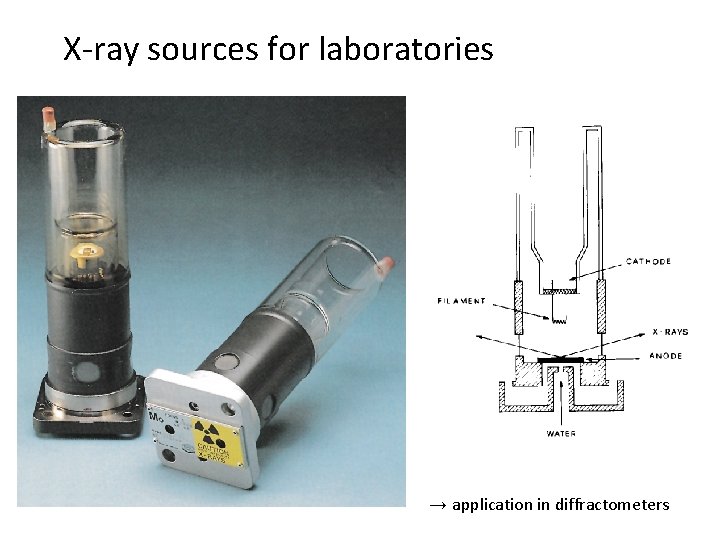
X-ray sources for laboratories → application in diffractometers
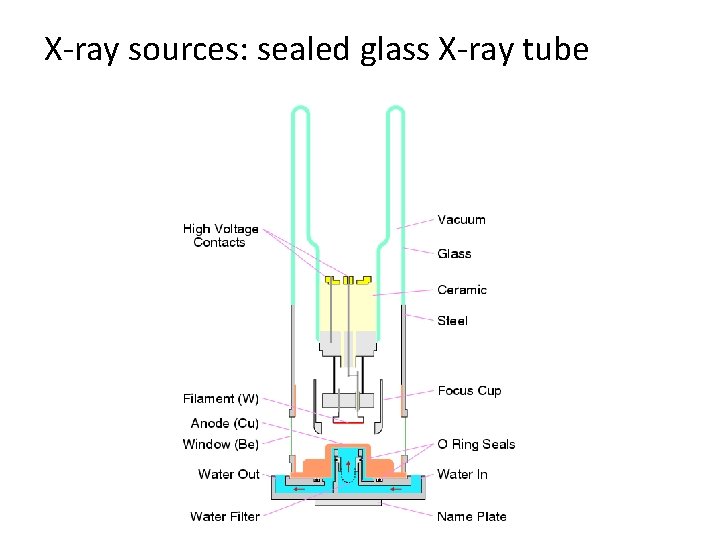
X-ray sources: sealed glass X-ray tube
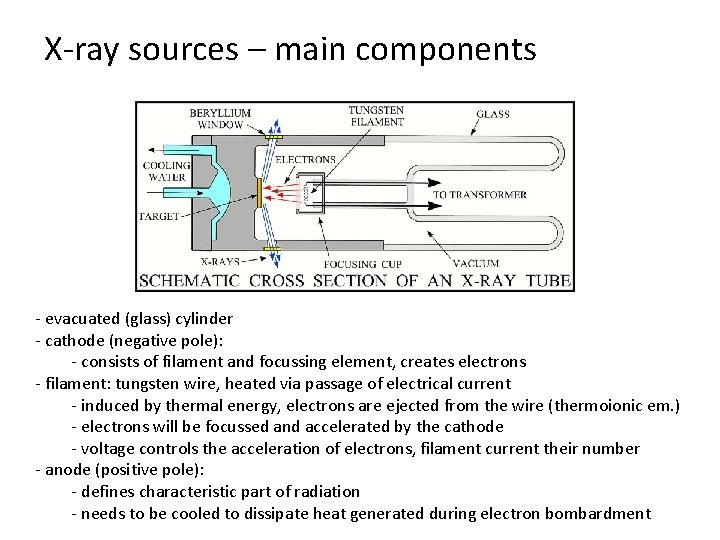
X-ray sources – main components - evacuated (glass) cylinder - cathode (negative pole): - consists of filament and focussing element, creates electrons - filament: tungsten wire, heated via passage of electrical current - induced by thermal energy, electrons are ejected from the wire (thermoionic em. ) - electrons will be focussed and accelerated by the cathode - voltage controls the acceleration of electrons, filament current their number - anode (positive pole): - defines characteristic part of radiation - needs to be cooled to dissipate heat generated during electron bombardment
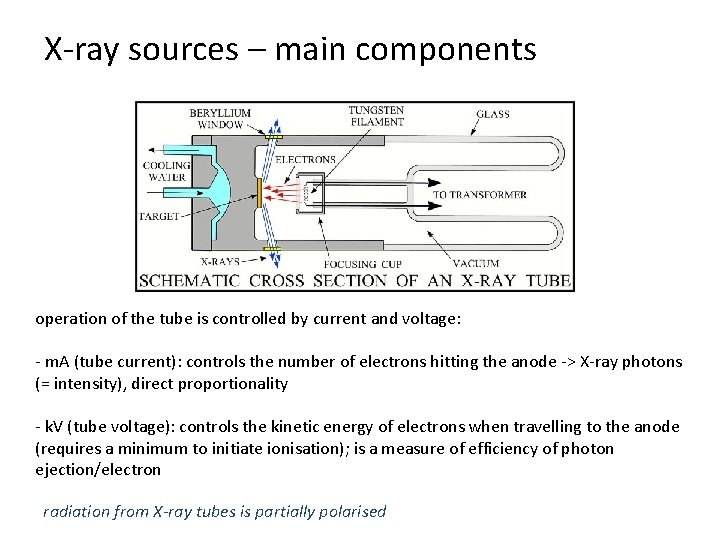
X-ray sources – main components operation of the tube is controlled by current and voltage: - m. A (tube current): controls the number of electrons hitting the anode -> X-ray photons (= intensity), direct proportionality - k. V (tube voltage): controls the kinetic energy of electrons when travelling to the anode (requires a minimum to initiate ionisation); is a measure of efficiency of photon ejection/electron radiation from X-ray tubes is partially polarised
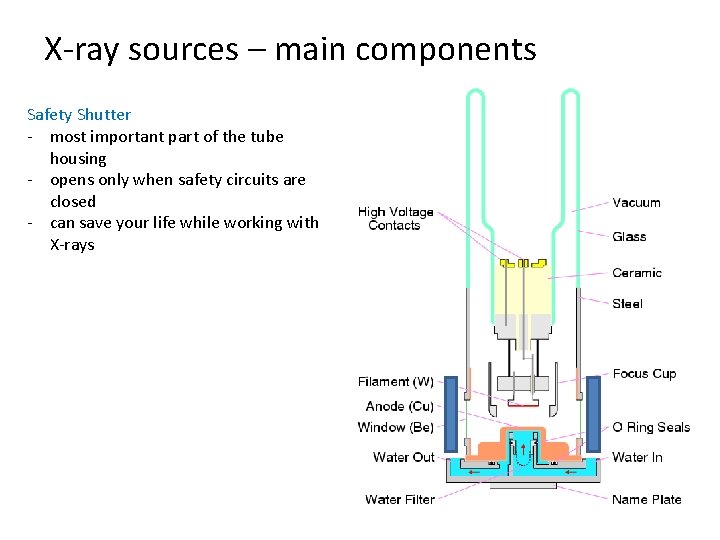
X-ray sources – main components Safety Shutter - most important part of the tube housing - opens only when safety circuits are closed - can save your life while working with X-rays
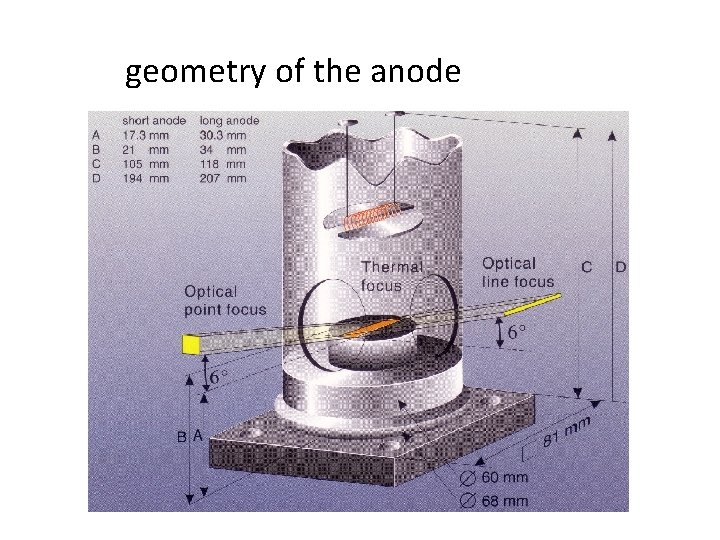
geometry of the anode
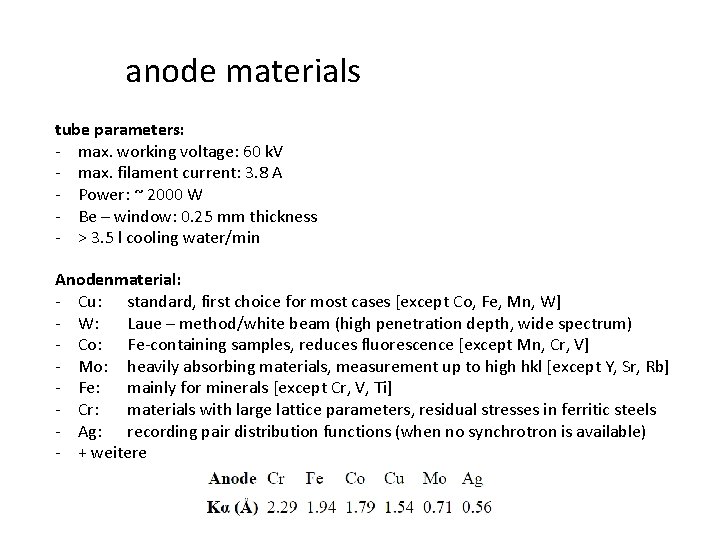
anode materials tube parameters: - max. working voltage: 60 k. V - max. filament current: 3. 8 A - Power: ~ 2000 W - Be – window: 0. 25 mm thickness - > 3. 5 l cooling water/min Anodenmaterial: - Cu: standard, first choice for most cases [except Co, Fe, Mn, W] - W: Laue – method/white beam (high penetration depth, wide spectrum) - Co: Fe-containing samples, reduces fluorescence [except Mn, Cr, V] - Mo: heavily absorbing materials, measurement up to high hkl [except Y, Sr, Rb] - Fe: mainly for minerals [except Cr, V, Ti] - Cr: materials with large lattice parameters, residual stresses in ferritic steels - Ag: recording pair distribution functions (when no synchrotron is available) - + weitere
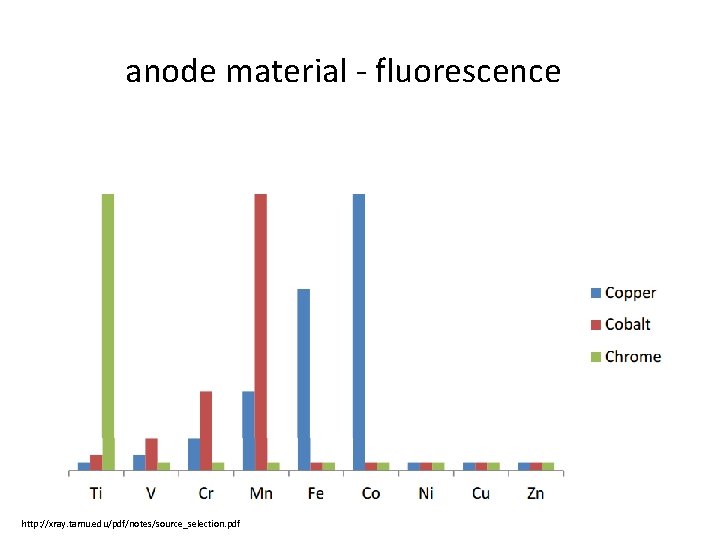
anode material - fluorescence http: //xray. tamu. edu/pdf/notes/source_selection. pdf
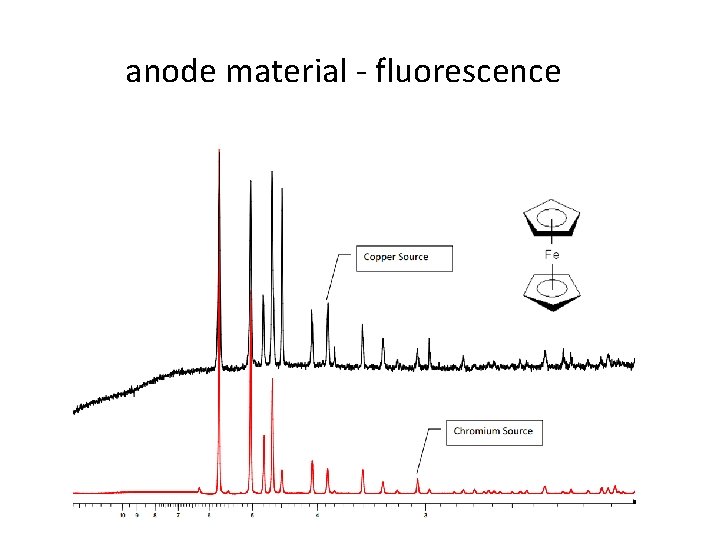
anode material - fluorescence
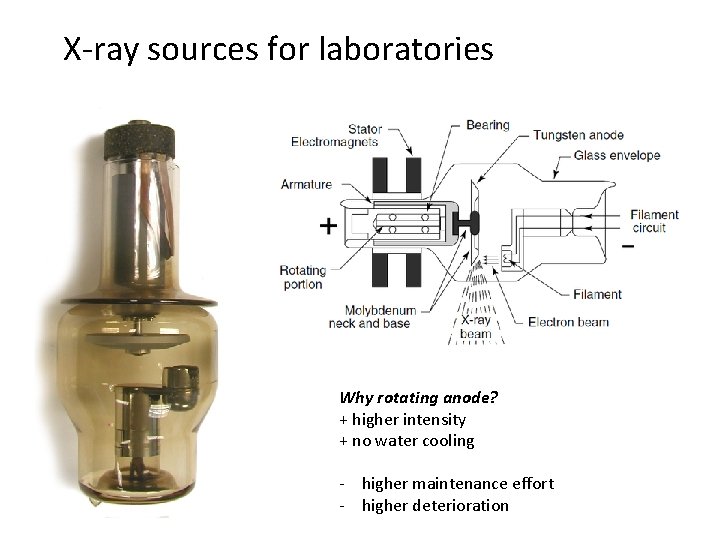
X-ray sources for laboratories Why rotating anode? + higher intensity + no water cooling - higher maintenance effort - higher deterioration
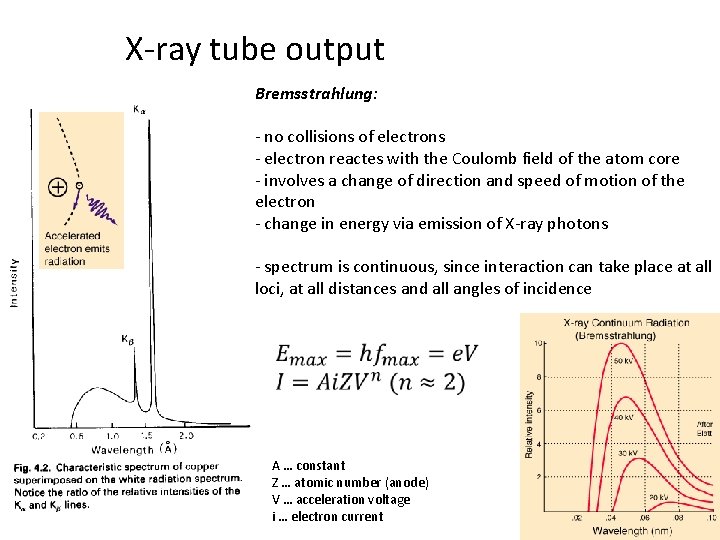
X-ray tube output Bremsstrahlung: - no collisions of electrons - electron reactes with the Coulomb field of the atom core - involves a change of direction and speed of motion of the electron - change in energy via emission of X-ray photons - spectrum is continuous, since interaction can take place at all loci, at all distances and all angles of incidence A … constant Z … atomic number (anode) V … acceleration voltage i … electron current
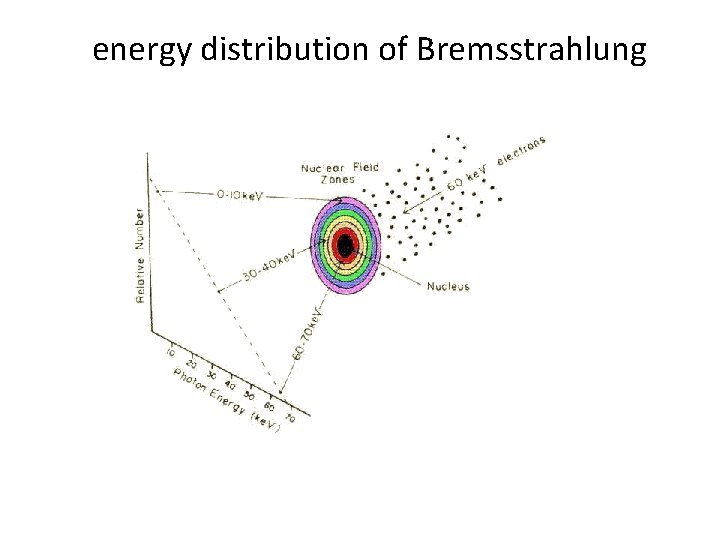
energy distribution of Bremsstrahlung
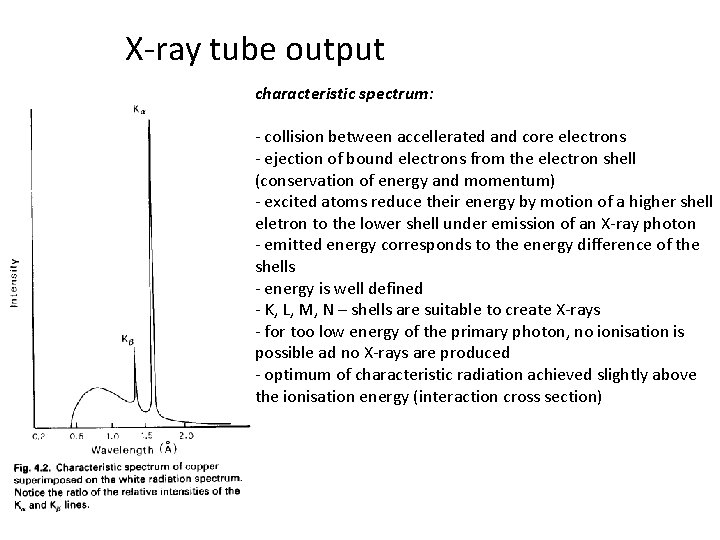
X-ray tube output characteristic spectrum: - collision between accellerated and core electrons - ejection of bound electrons from the electron shell (conservation of energy and momentum) - excited atoms reduce their energy by motion of a higher shell eletron to the lower shell under emission of an X-ray photon - emitted energy corresponds to the energy difference of the shells - energy is well defined - K, L, M, N – shells are suitable to create X-rays - for too low energy of the primary photon, no ionisation is possible ad no X-rays are produced - optimum of characteristic radiation achieved slightly above the ionisation energy (interaction cross section)
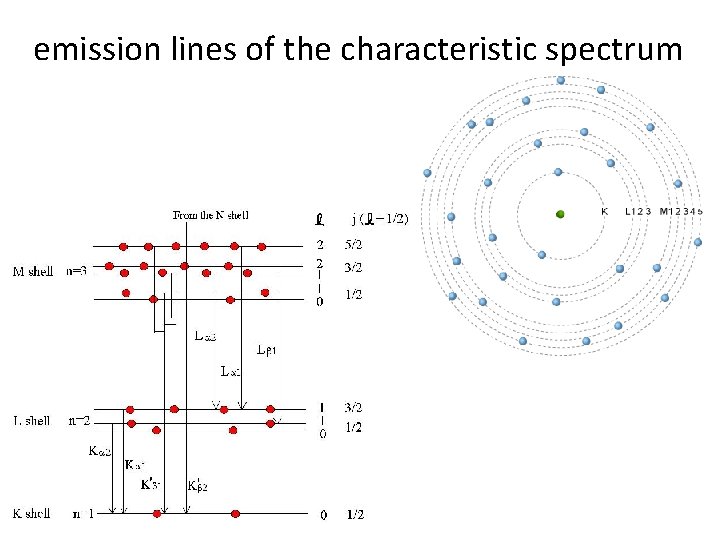
emission lines of the characteristic spectrum
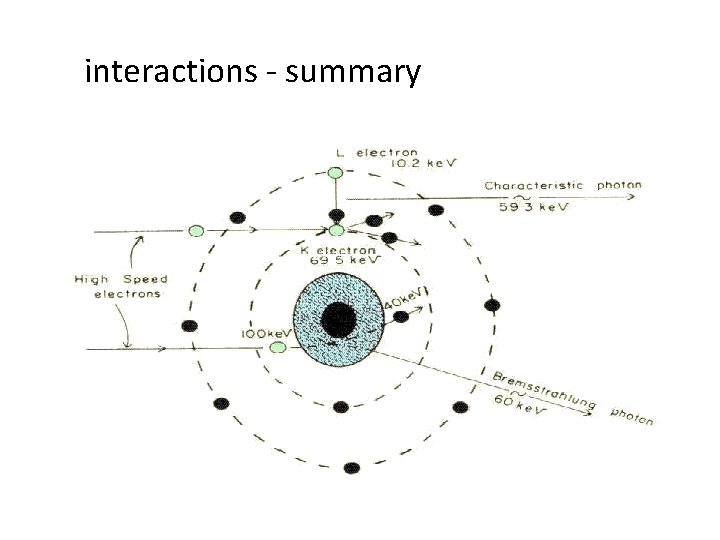
interactions - summary
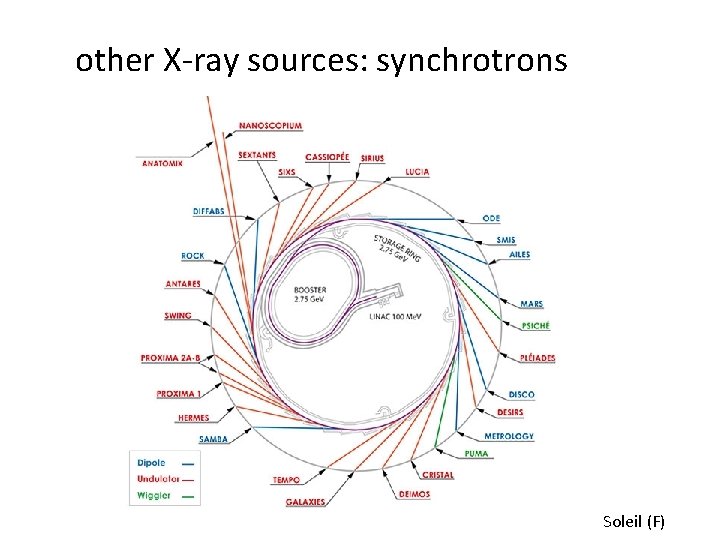
other X-ray sources: synchrotrons Soleil (F)
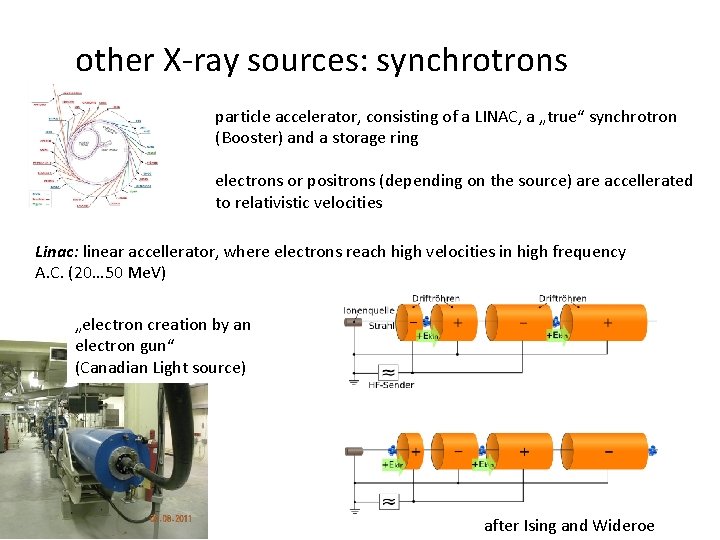
other X-ray sources: synchrotrons particle accelerator, consisting of a LINAC, a „true“ synchrotron (Booster) and a storage ring electrons or positrons (depending on the source) are accellerated to relativistic velocities Linac: linear accellerator, where electrons reach high velocities in high frequency A. C. (20… 50 Me. V) „electron creation by an electron gun“ (Canadian Light source) after Ising and Wideroe
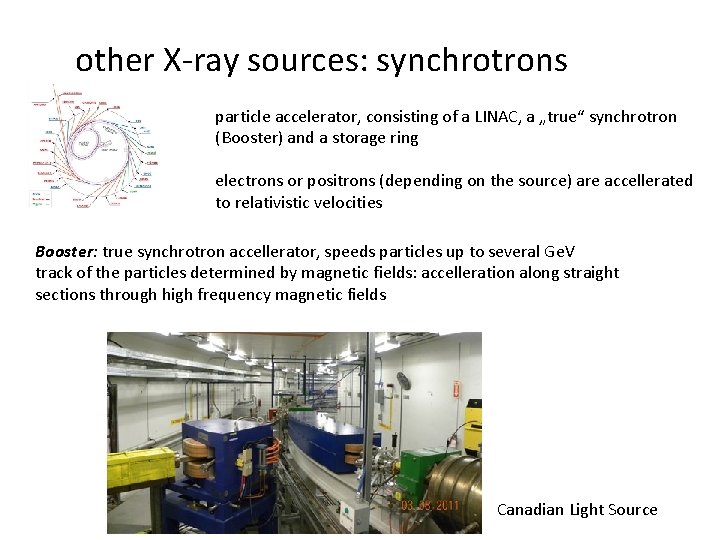
other X-ray sources: synchrotrons particle accelerator, consisting of a LINAC, a „true“ synchrotron (Booster) and a storage ring electrons or positrons (depending on the source) are accellerated to relativistic velocities Booster: true synchrotron accellerator, speeds particles up to several Ge. V track of the particles determined by magnetic fields: accelleration along straight sections through high frequency magnetic fields Canadian Light Source
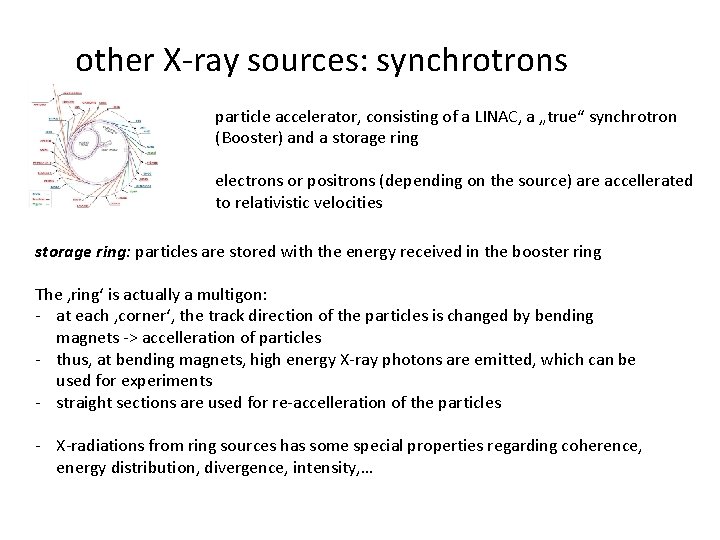
other X-ray sources: synchrotrons particle accelerator, consisting of a LINAC, a „true“ synchrotron (Booster) and a storage ring electrons or positrons (depending on the source) are accellerated to relativistic velocities storage ring: particles are stored with the energy received in the booster ring The ‚ring‘ is actually a multigon: - at each ‚corner‘, the track direction of the particles is changed by bending magnets -> accelleration of particles - thus, at bending magnets, high energy X-ray photons are emitted, which can be used for experiments - straight sections are used for re-accelleration of the particles - X-radiations from ring sources has some special properties regarding coherence, energy distribution, divergence, intensity, …
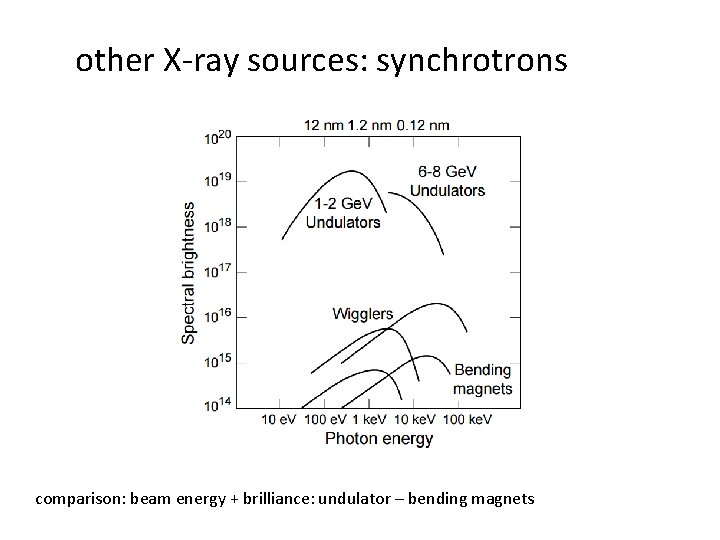
other X-ray sources: synchrotrons comparison: beam energy + brilliance: undulator – bending magnets
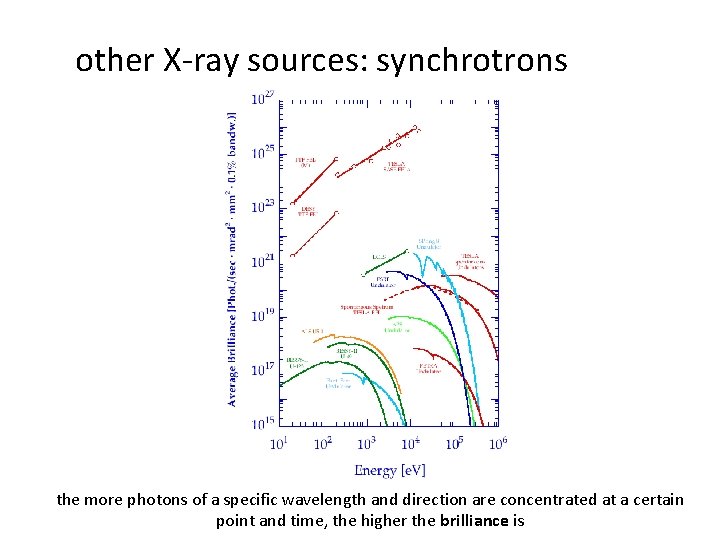
other X-ray sources: synchrotrons the more photons of a specific wavelength and direction are concentrated at a certain point and time, the higher the brilliance is
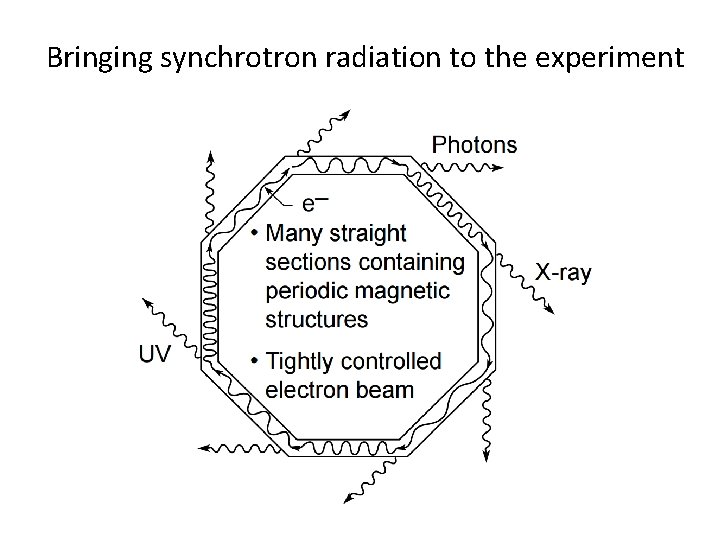
Bringing synchrotron radiation to the experiment
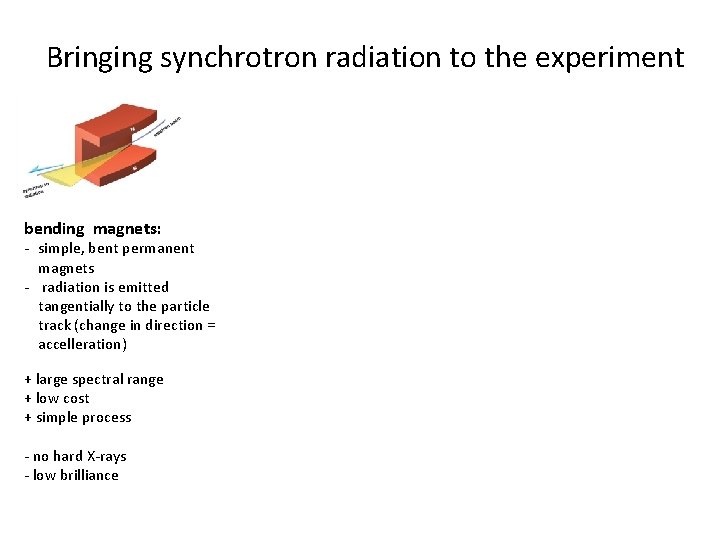
Bringing synchrotron radiation to the experiment bending magnets: - simple, bent permanent magnets - radiation is emitted tangentially to the particle track (change in direction = accelleration) + large spectral range + low cost + simple process - no hard X-rays - low brilliance
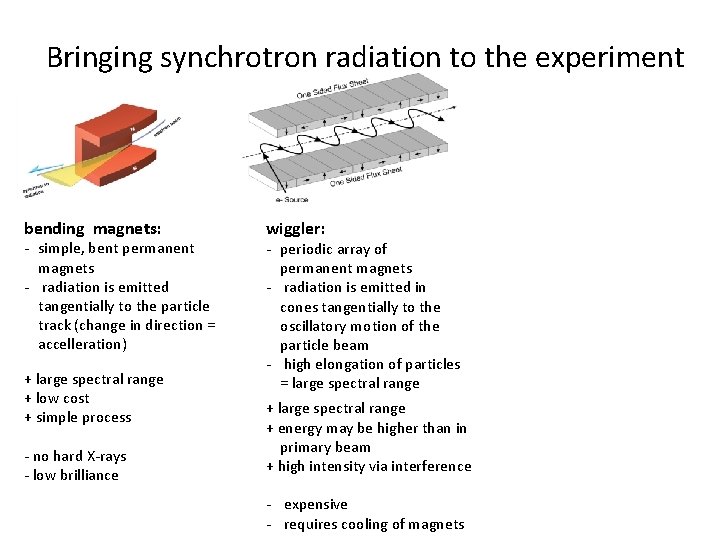
Bringing synchrotron radiation to the experiment bending magnets: - simple, bent permanent magnets - radiation is emitted tangentially to the particle track (change in direction = accelleration) + large spectral range + low cost + simple process - no hard X-rays - low brilliance wiggler: - periodic array of permanent magnets - radiation is emitted in cones tangentially to the oscillatory motion of the particle beam - high elongation of particles = large spectral range + energy may be higher than in primary beam + high intensity via interference - expensive - requires cooling of magnets
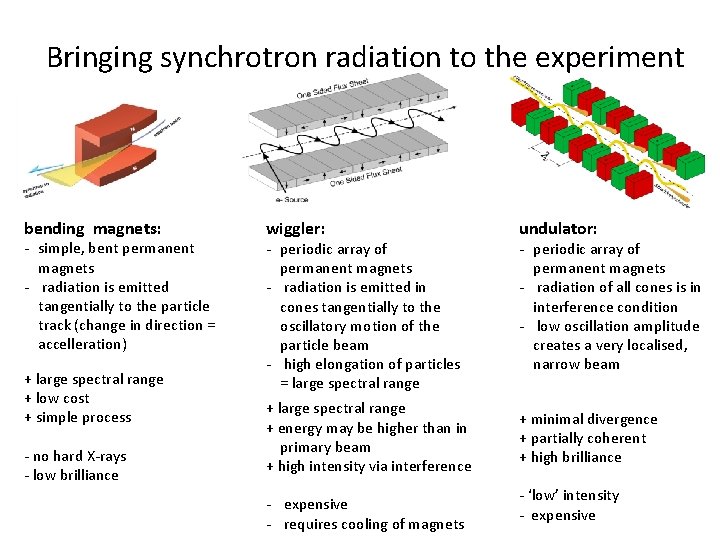
Bringing synchrotron radiation to the experiment bending magnets: - simple, bent permanent magnets - radiation is emitted tangentially to the particle track (change in direction = accelleration) + large spectral range + low cost + simple process - no hard X-rays - low brilliance wiggler: undulator: + large spectral range + energy may be higher than in primary beam + high intensity via interference + minimal divergence + partially coherent + high brilliance - periodic array of permanent magnets - radiation is emitted in cones tangentially to the oscillatory motion of the particle beam - high elongation of particles = large spectral range - expensive - requires cooling of magnets - periodic array of permanent magnets - radiation of all cones is in interference condition - low oscillation amplitude creates a very localised, narrow beam - ‘low’ intensity - expensive
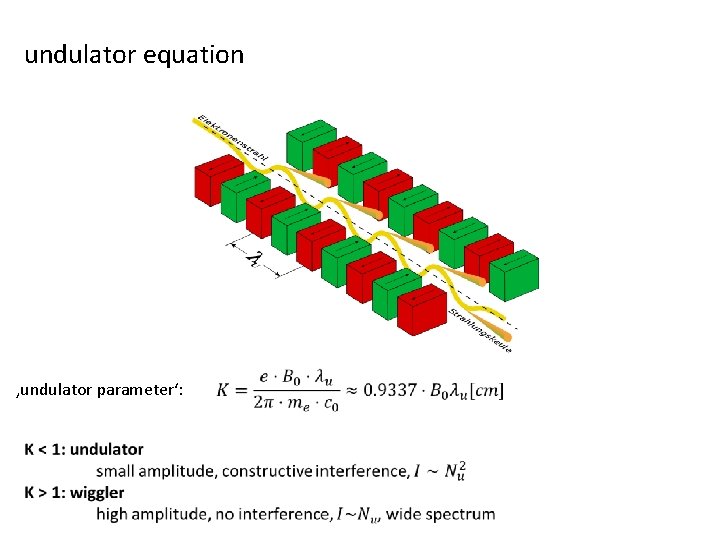
undulator equation ‚undulator parameter‘:
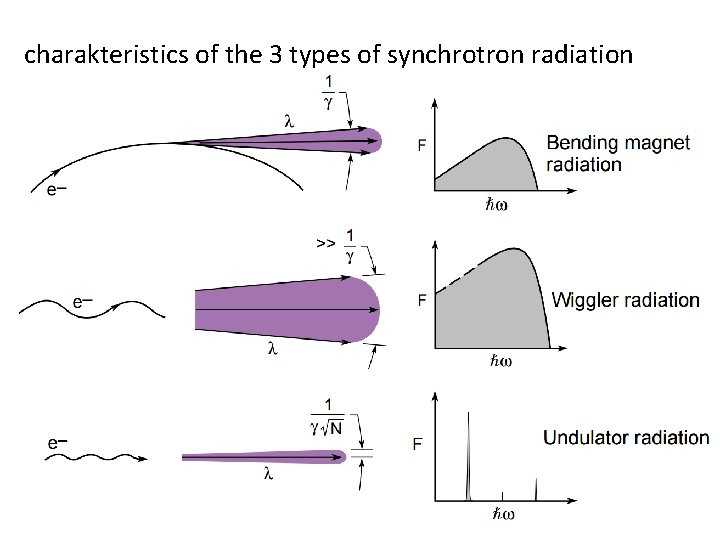
charakteristics of the 3 types of synchrotron radiation
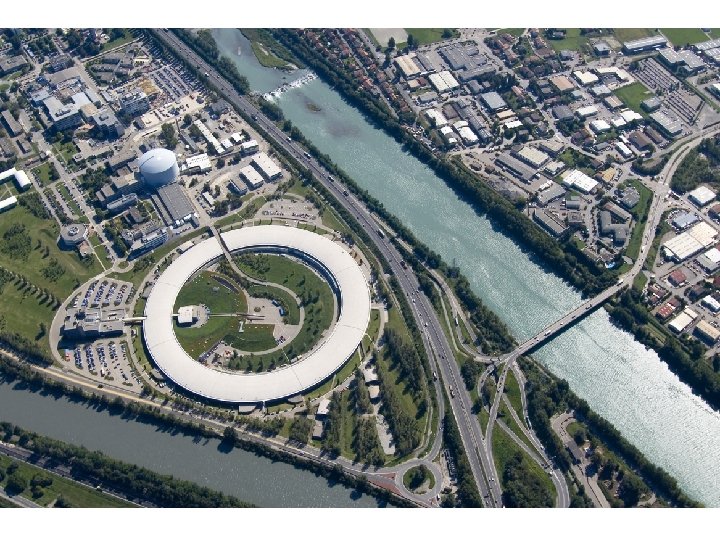
Print sources and web sources
Important of water management
Pressure composition phase diagram
Normal phase vs reverse phase chromatography
Tswett pronunciation
Mobile phase and stationary phase
Stationary phase and mobile phase in hplc
Normal phase vs reverse phase chromatography
Difference between phase voltage and line voltage
Chromatography mobile phase and stationary phase
In a ∆-connected source feeding a y-connected load
Broad phase vs narrow phase
First minima in diffraction
Reflection refraction diffraction interference
Refraction vs diffraction
Multiple slits diffraction
Magnets for neutron diffraction
Absent spectra in diffraction grating
Define dispersive power of grating
Missing order in diffraction
Sound diffraction
Fresnel and fraunhofer diffraction difference
Diffraction
Fresnel diffraction
Missing order in diffraction
Diffraction grating
Diffraction in a sentence
Von laue
Diffraction and polarization
Slit diffraction
Bragg law in reciprocal lattice
Diffraction examples
Reflection wave behavior
Powder diffraction database
Fresnel and fraunhofer diffraction difference
Laser diffraction spectroscopy
Huygens principle
Diffraction of light
Electromagnetic waves in water