Today Lecture 16 The Josephson effect magnetic field
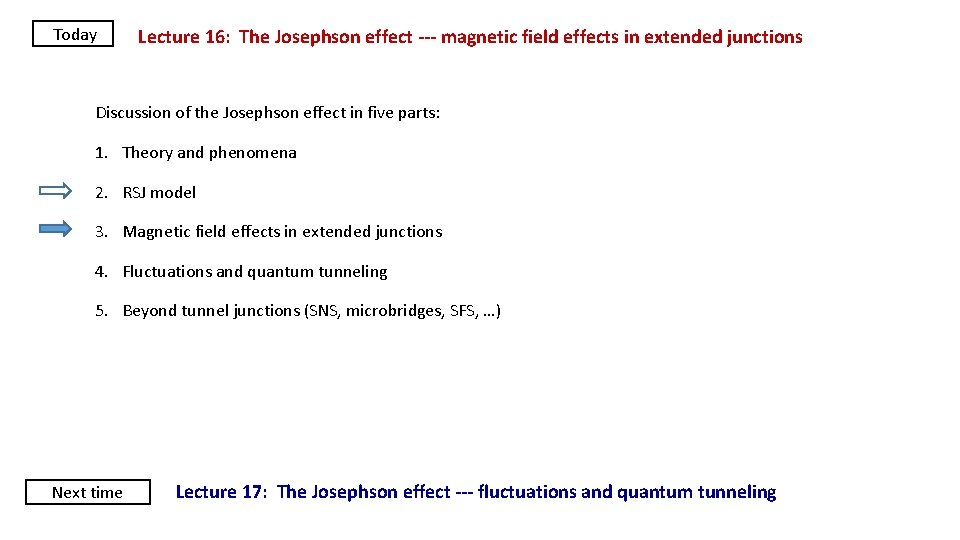
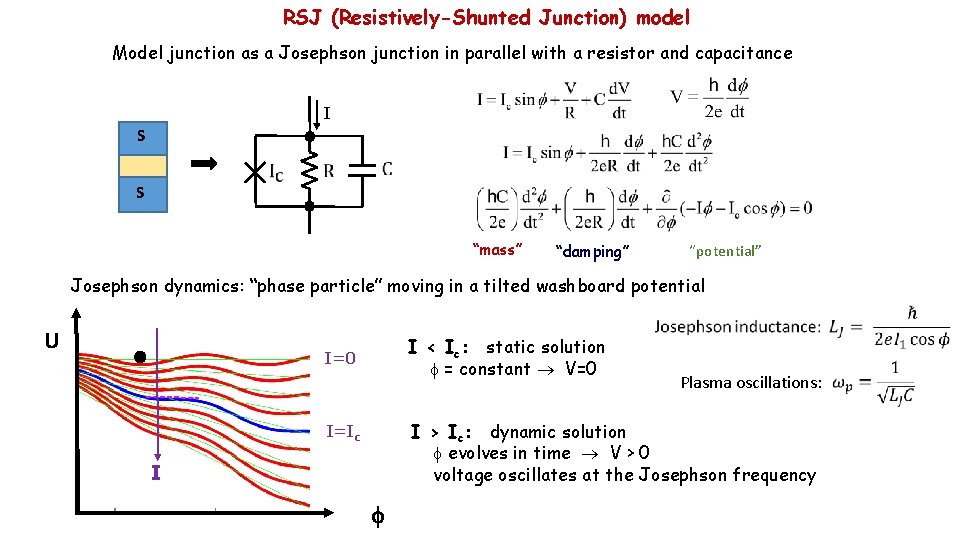
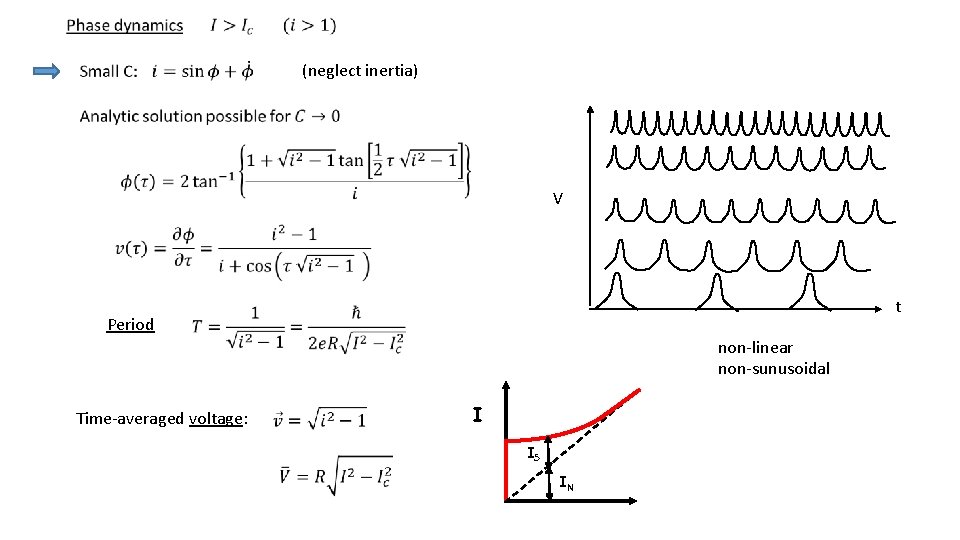
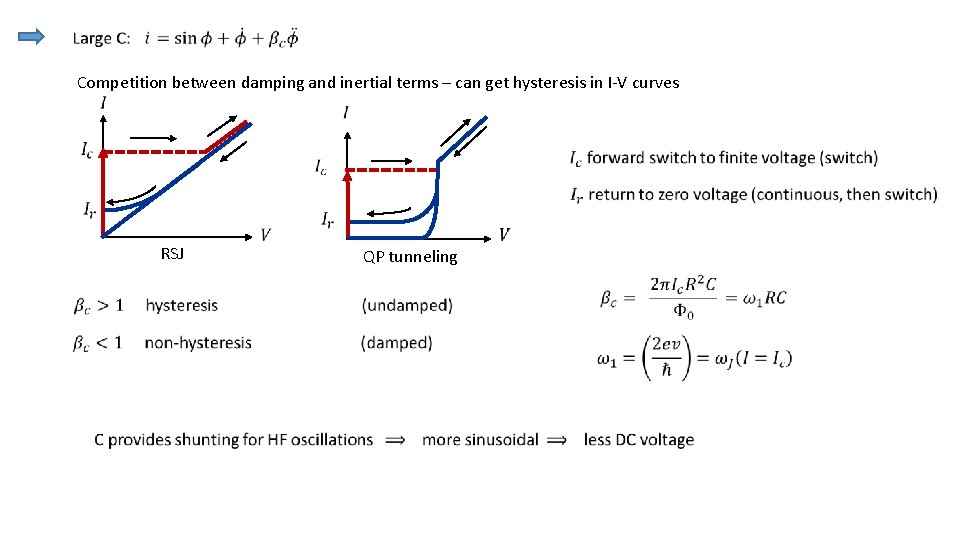
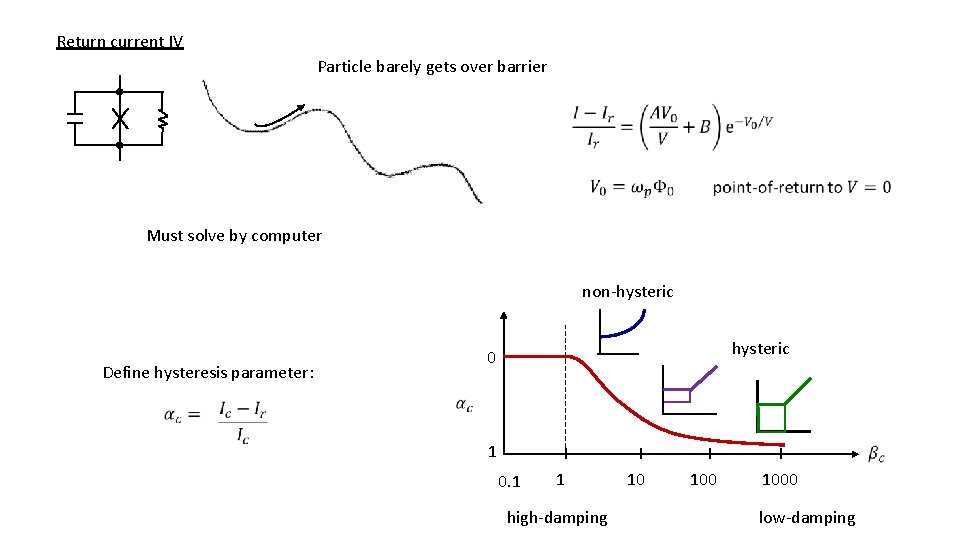
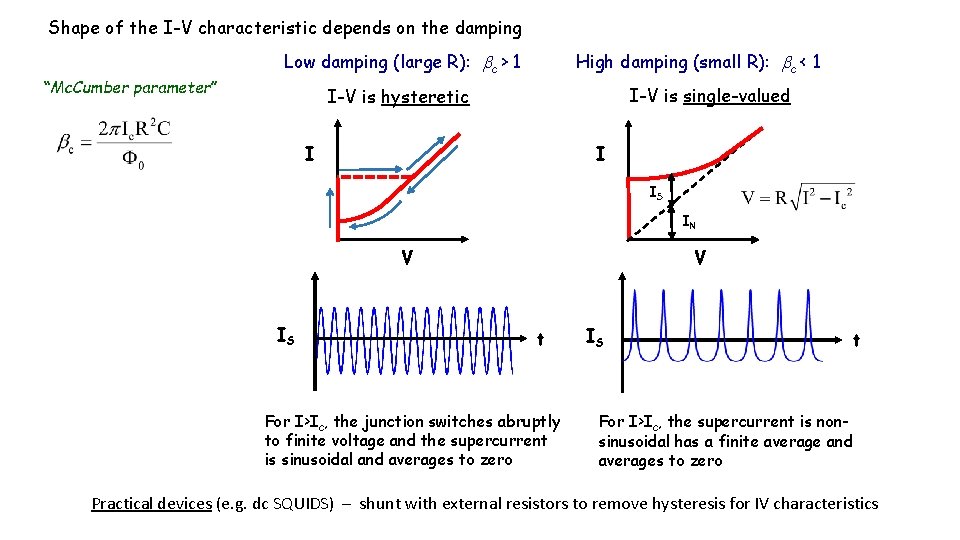
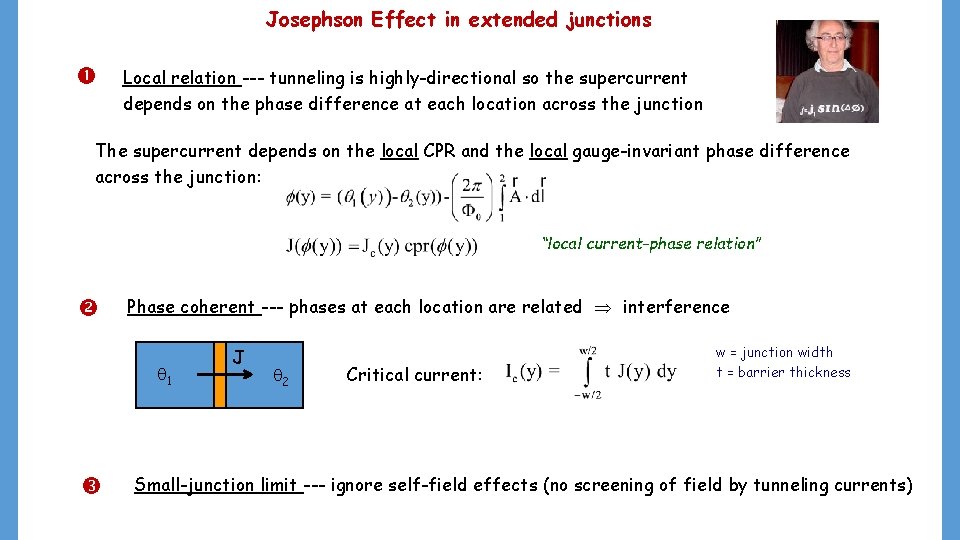
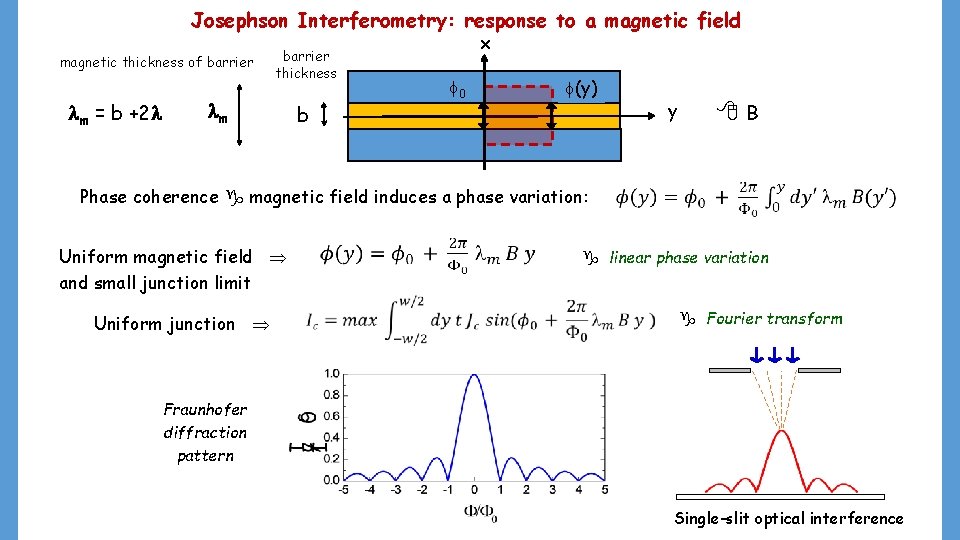
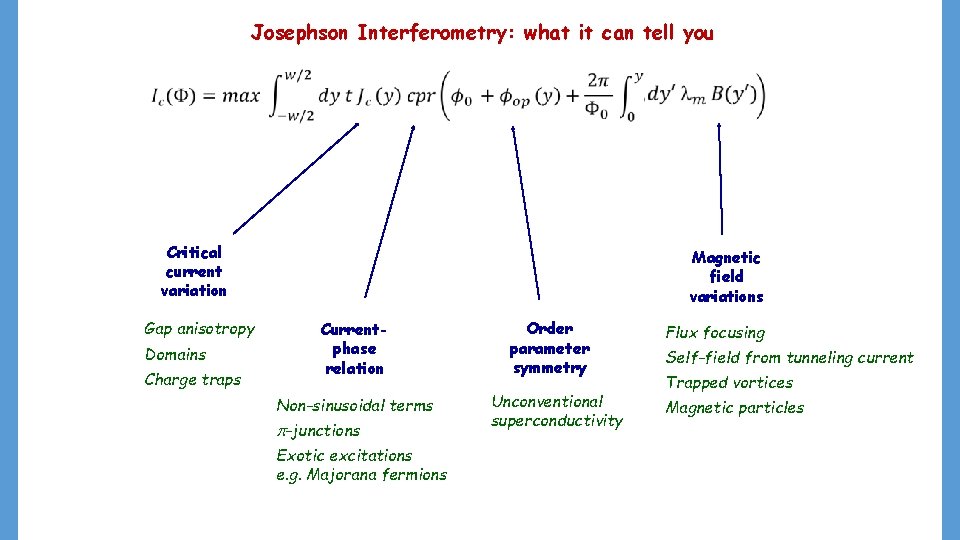
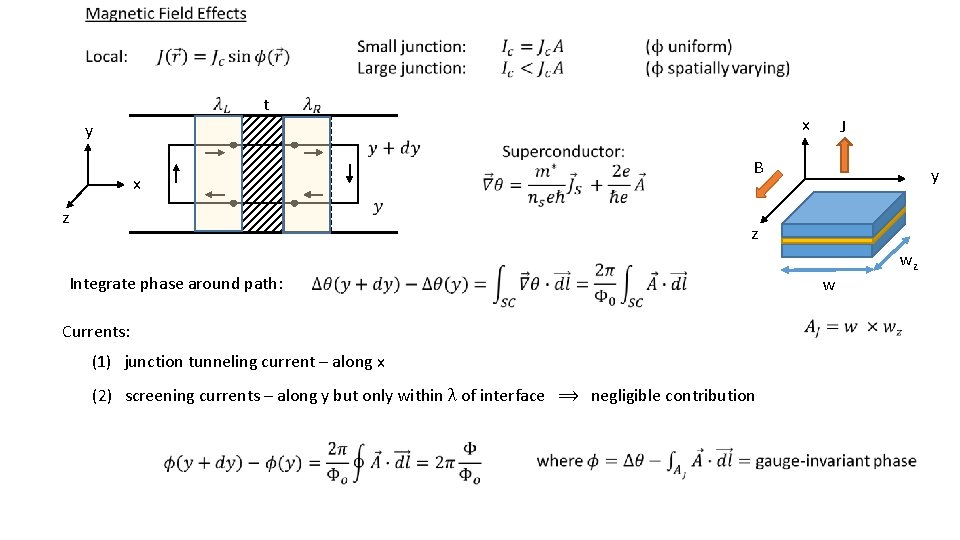
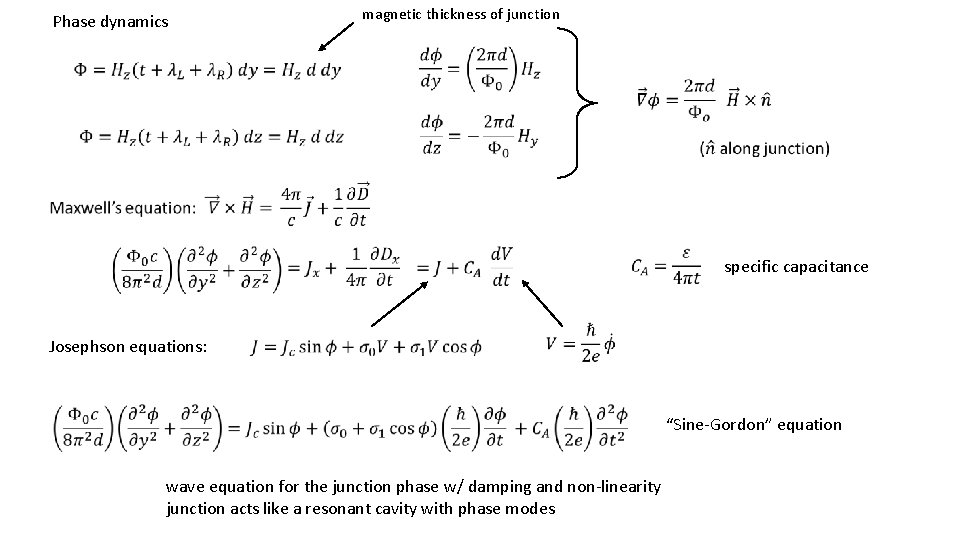
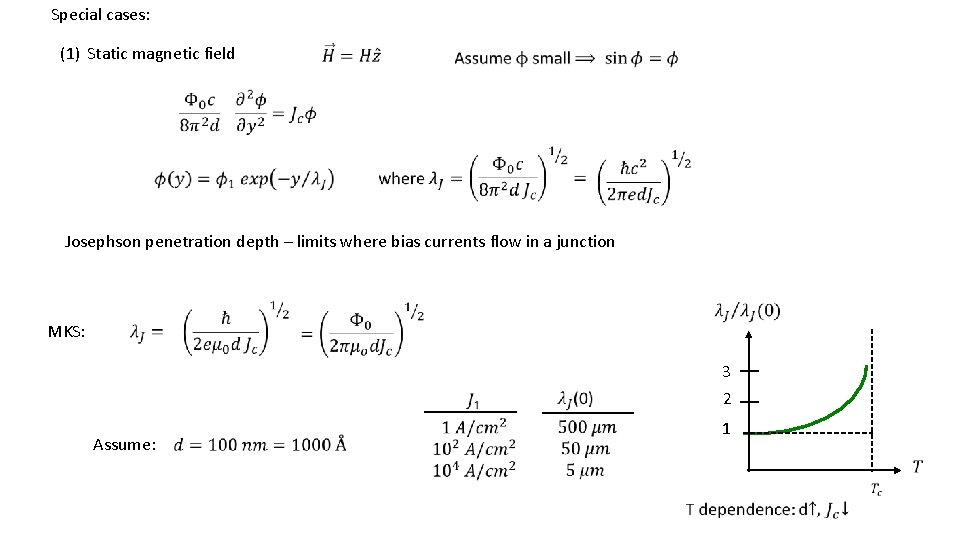
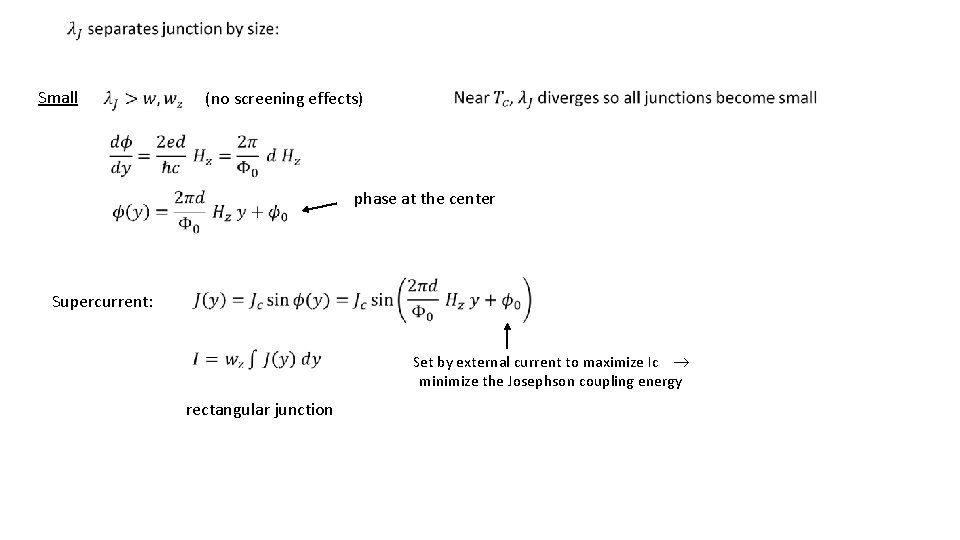
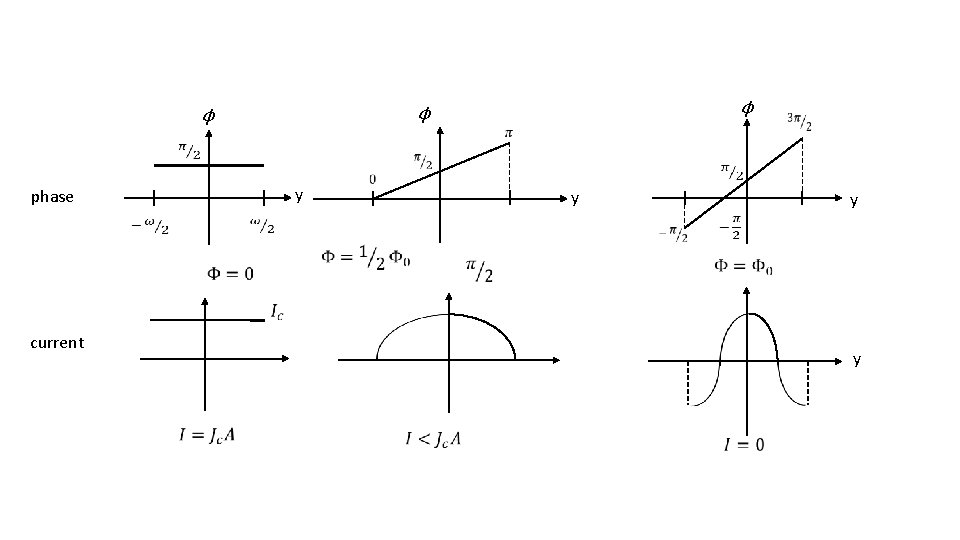
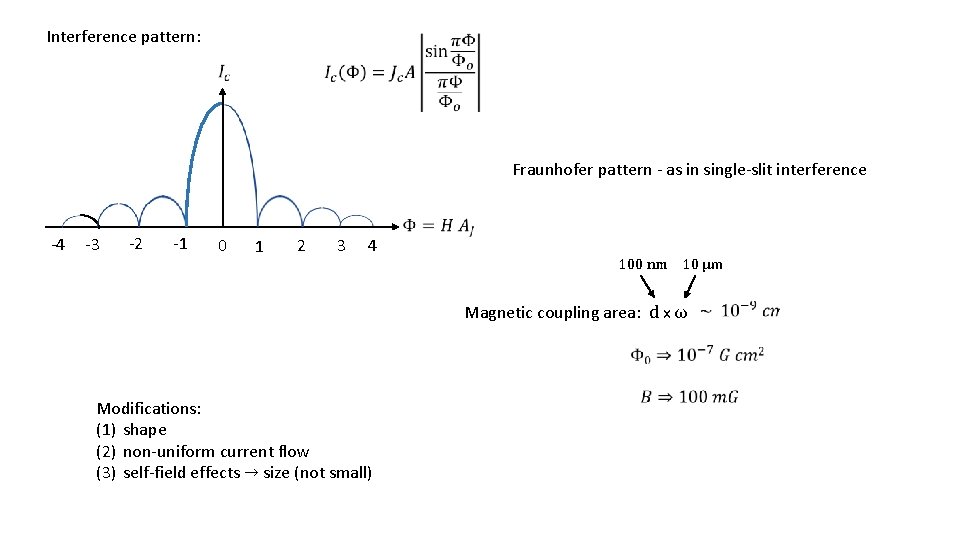
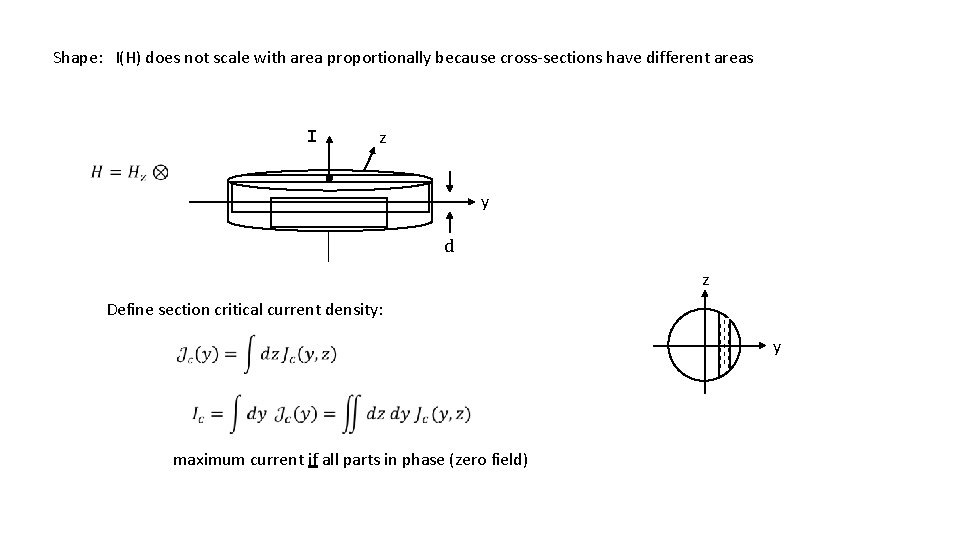
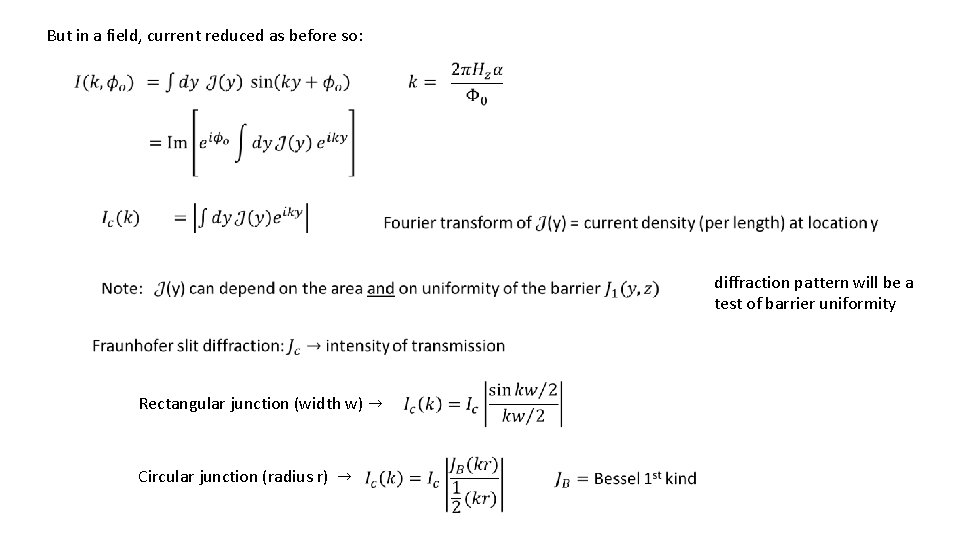
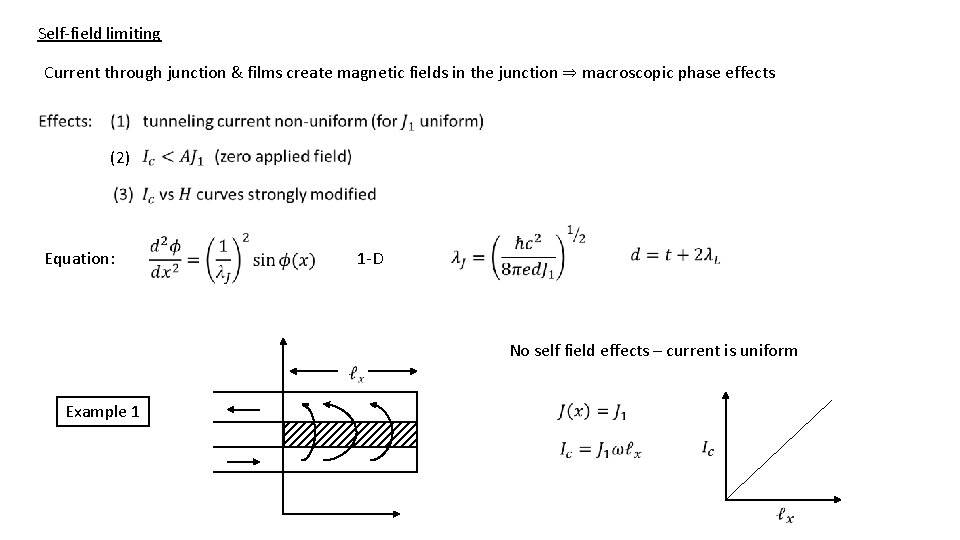
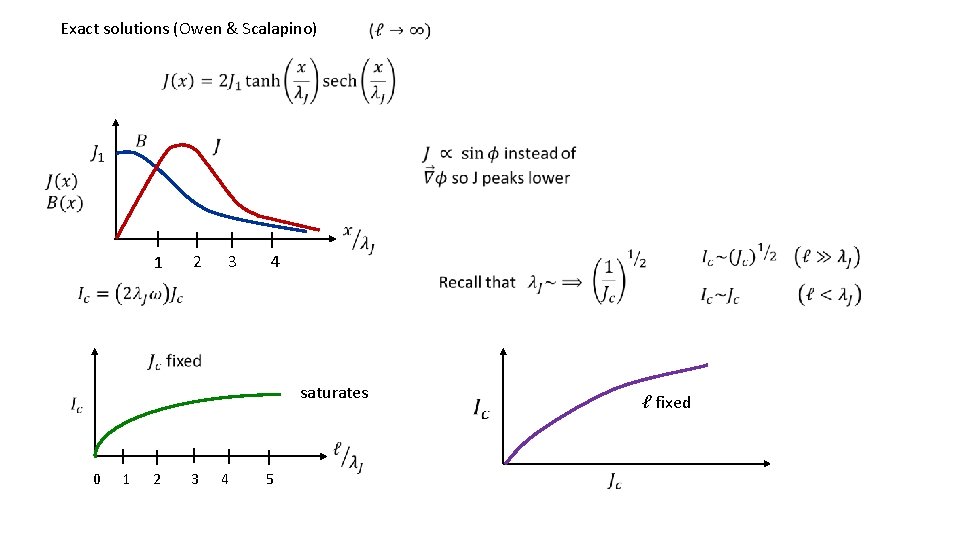
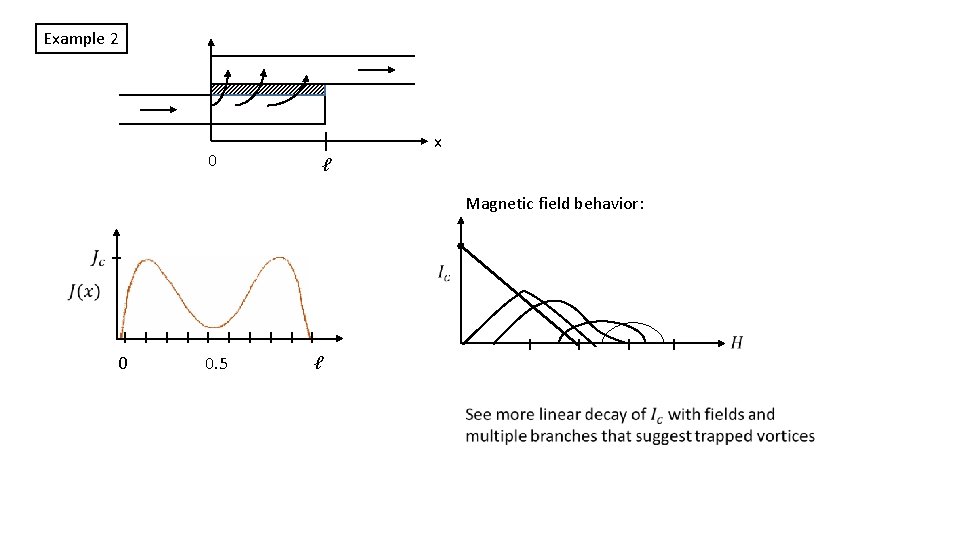
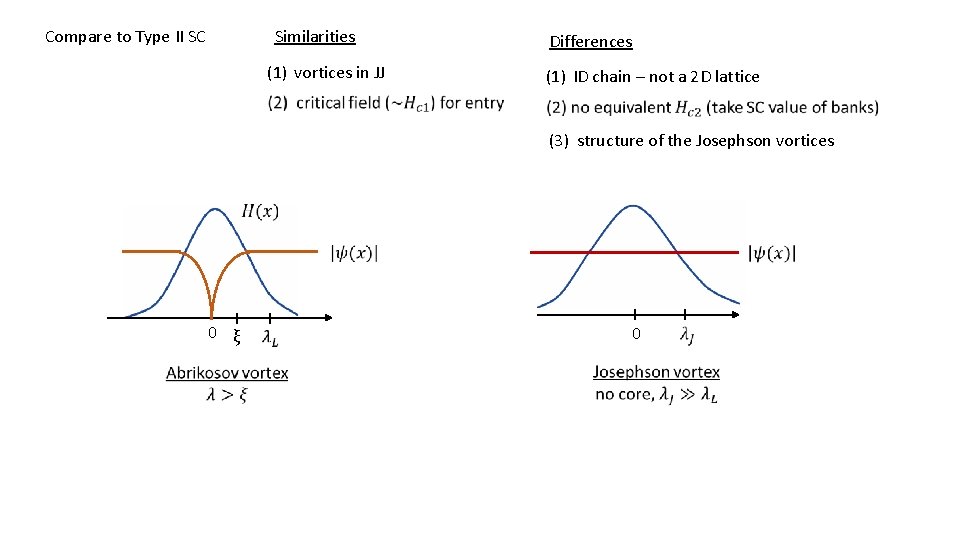
- Slides: 21
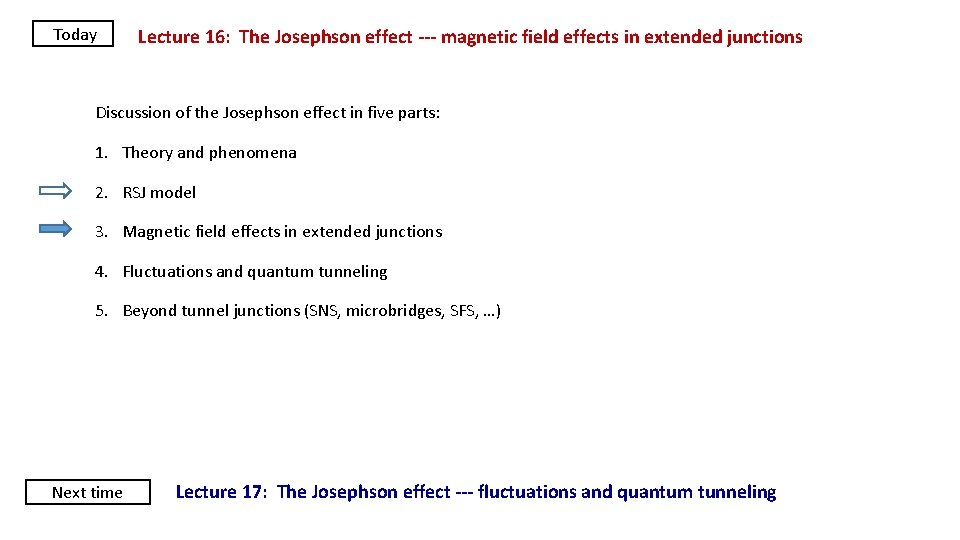
Today Lecture 16: The Josephson effect --- magnetic field effects in extended junctions Discussion of the Josephson effect in five parts: 1. Theory and phenomena 2. RSJ model 3. Magnetic field effects in extended junctions 4. Fluctuations and quantum tunneling 5. Beyond tunnel junctions (SNS, microbridges, SFS, …) Next time Lecture 17: The Josephson effect --- fluctuations and quantum tunneling
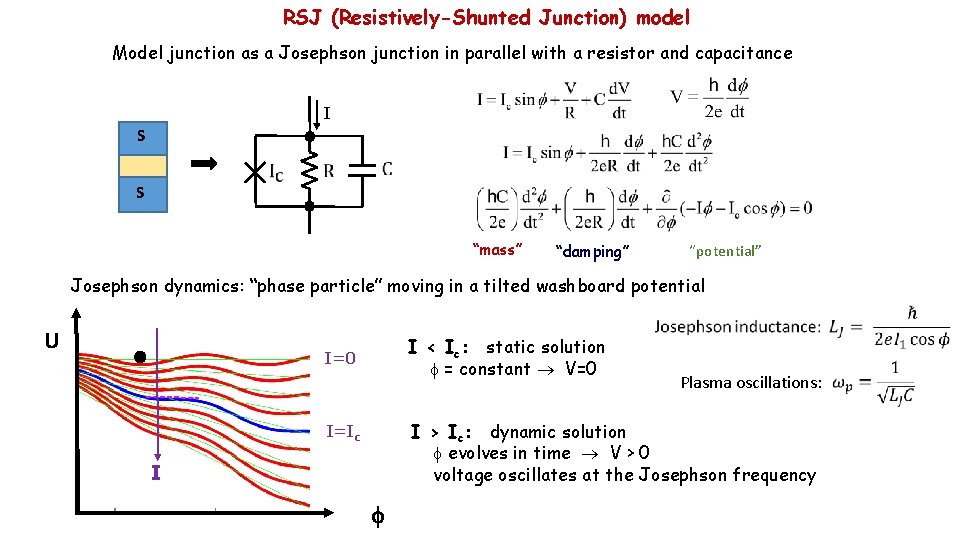
Model junction as a Josephson junction in parallel with a resistor and capacitance I S “mass” “potential” “damping” Josephson dynamics: “phase particle” moving in a tilted washboard potential I < Ic: static solution I=0 = constant V=0 Plasma oscillations: I=I I > Ic: dynamic solution c evolves in time V > 0 I voltage oscillates at the Josephson frequency (Resistively-Shunted Junction) model RSJ U
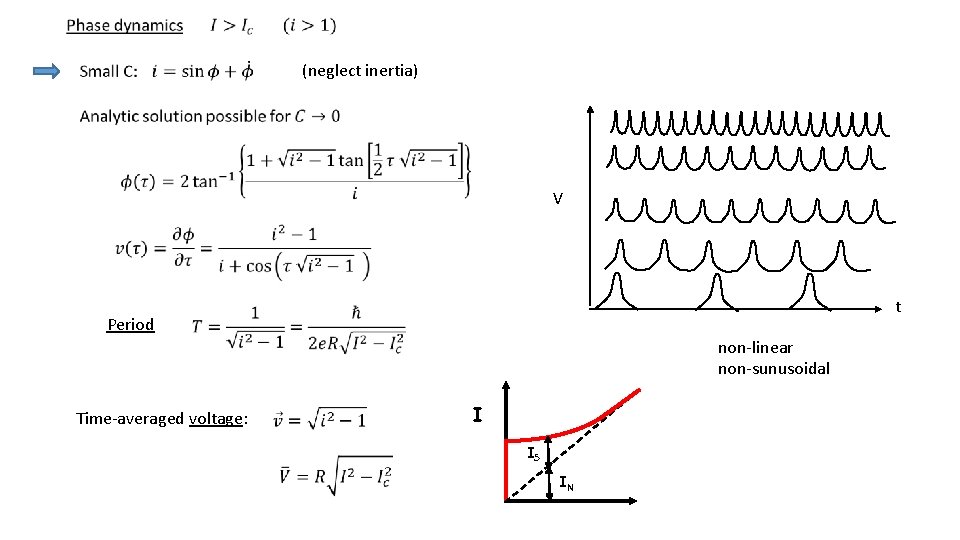
(neglect inertia) V Period t non-linear non-sunusoidal Time-averaged voltage: I IS IN
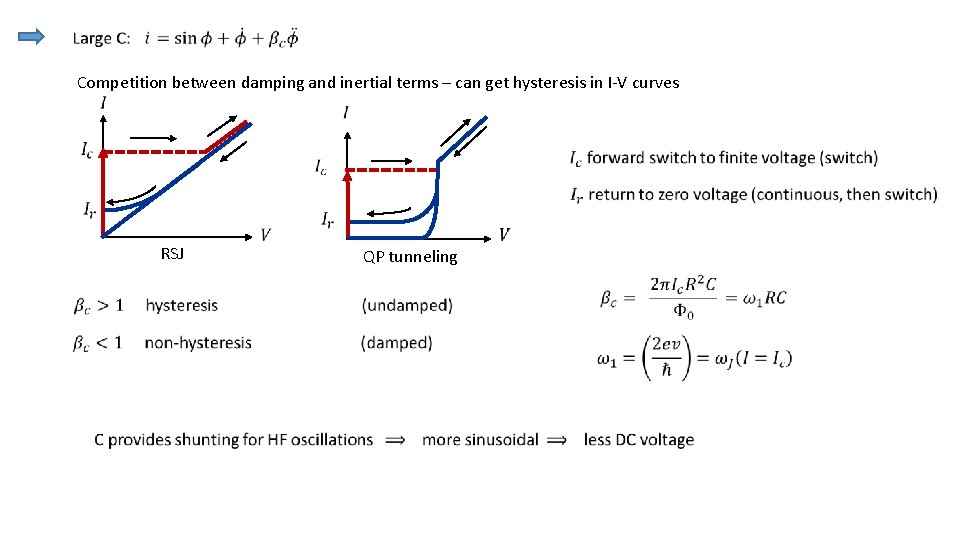
Competition between damping and inertial terms – can get hysteresis in I-V curves RSJ QP tunneling
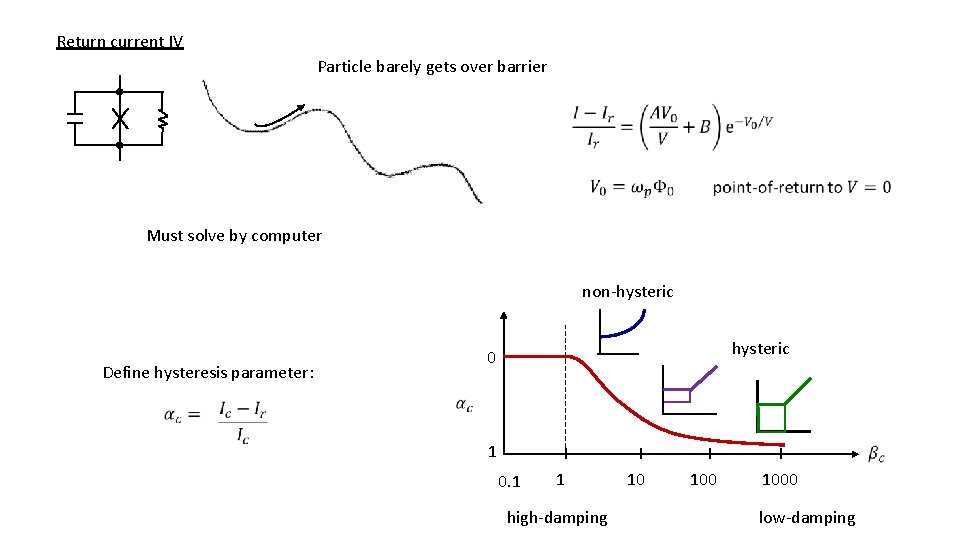
Return current IV Particle barely gets over barrier Must solve by computer non-hysteric Define hysteresis parameter: hysteric 0 1 I I I 1 100 0. 1 high-damping I 1000 low-damping
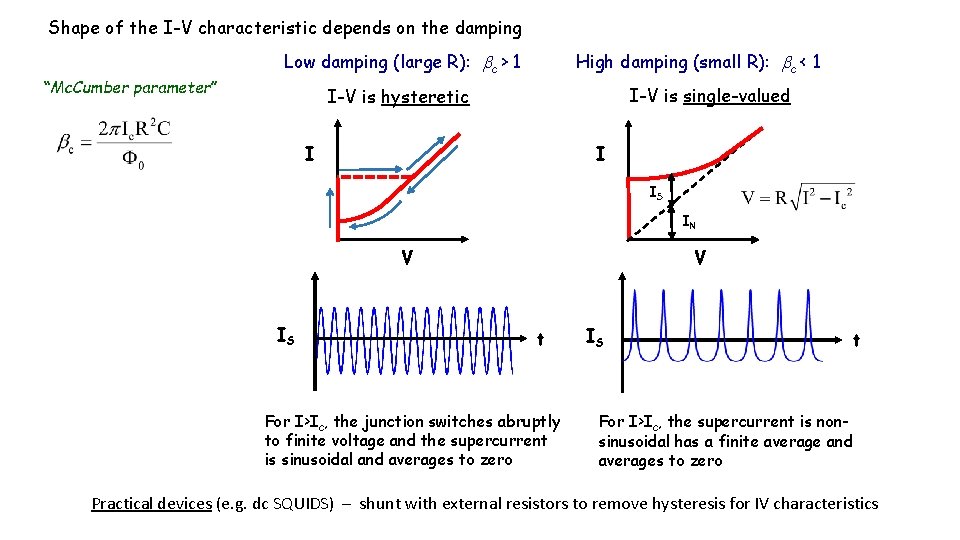
Shape of the I-V characteristic depends on the damping “Mc. Cumber parameter” Low damping (large R): c > 1 High damping (small R): c < 1 I-V is single-valued I-V is hysteretic I I IS IN V IS V t For I>Ic, the junction switches abruptly to finite voltage and the supercurrent is sinusoidal and averages to zero IS t For I>Ic, the supercurrent is nonsinusoidal has a finite average and averages to zero Practical devices (e. g. dc SQUIDS) – shunt with external resistors to remove hysteresis for IV characteristics
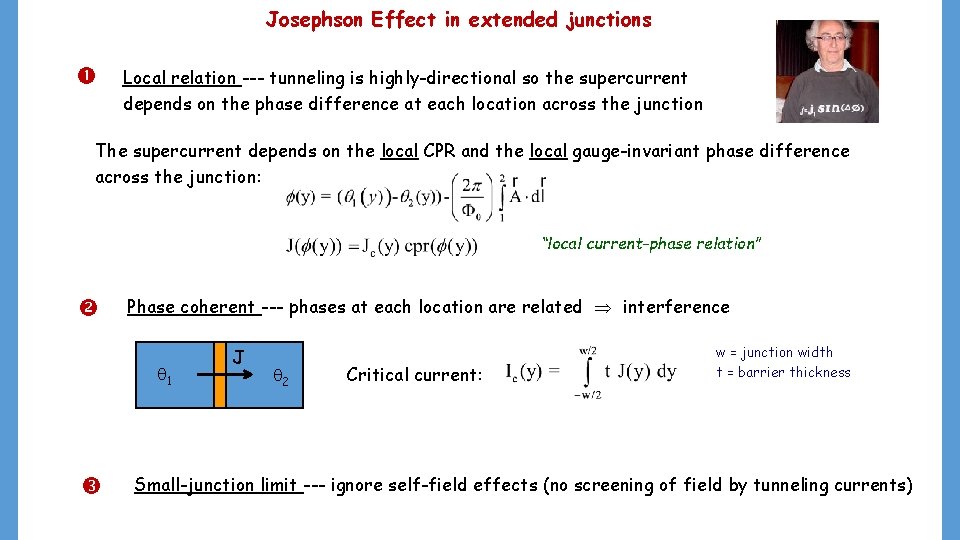
Josephson Effect in extended junctions Local relation --- tunneling is highly-directional so the supercurrent depends on the phase difference at each location across the junction The supercurrent depends on the local CPR and the local gauge-invariant phase difference across the junction: “local current-phase relation” Phase coherent --- phases at each location are related interference 1 J 2 Critical current: w = junction width t = barrier thickness Small-junction limit --- ignore self-field effects (no screening of field by tunneling currents)
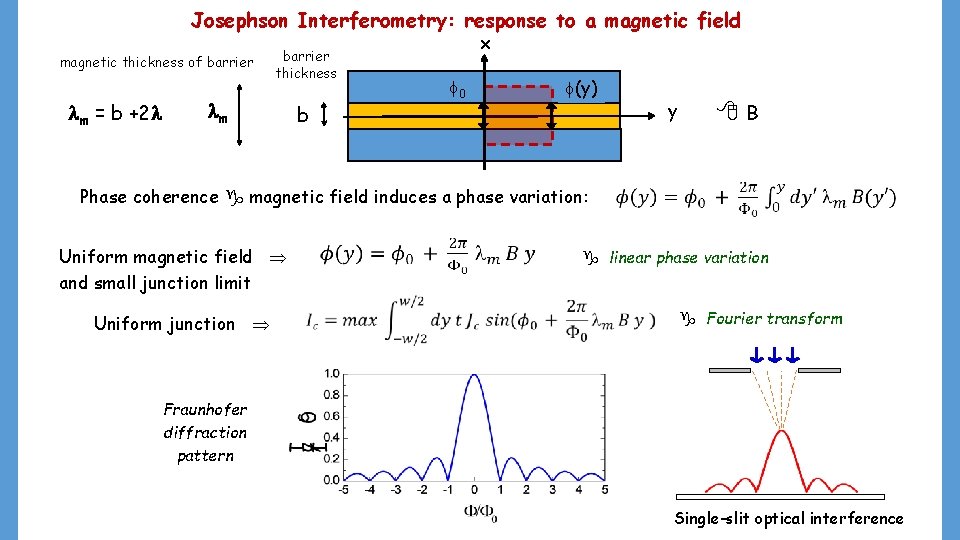
Josephson Interferometry: response to a magnetic field magnetic thickness of barrier m = b +2 barrier thickness m b x 0 (y) y B Phase coherence magnetic field induces a phase variation: Uniform magnetic field and small junction limit Uniform junction linear phase variation Fourier transform Fraunhofer diffraction pattern Single-slit optical interference
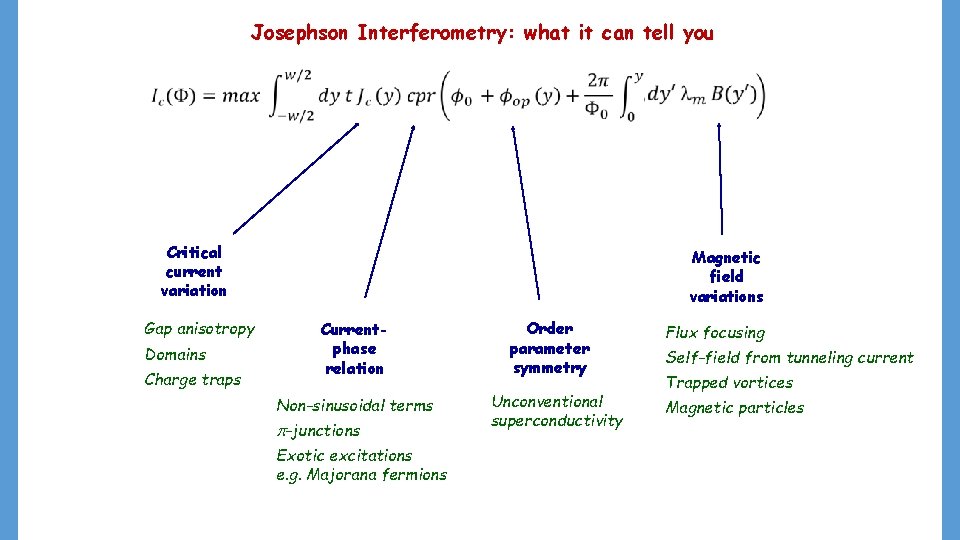
Josephson Interferometry: what it can tell you Critical current variation Gap anisotropy Domains Charge traps Magnetic field variations Currentphase relation Non-sinusoidal terms -junctions Exotic excitations e. g. Majorana fermions Order parameter symmetry Unconventional superconductivity Flux focusing Self-field from tunneling current Trapped vortices Magnetic particles
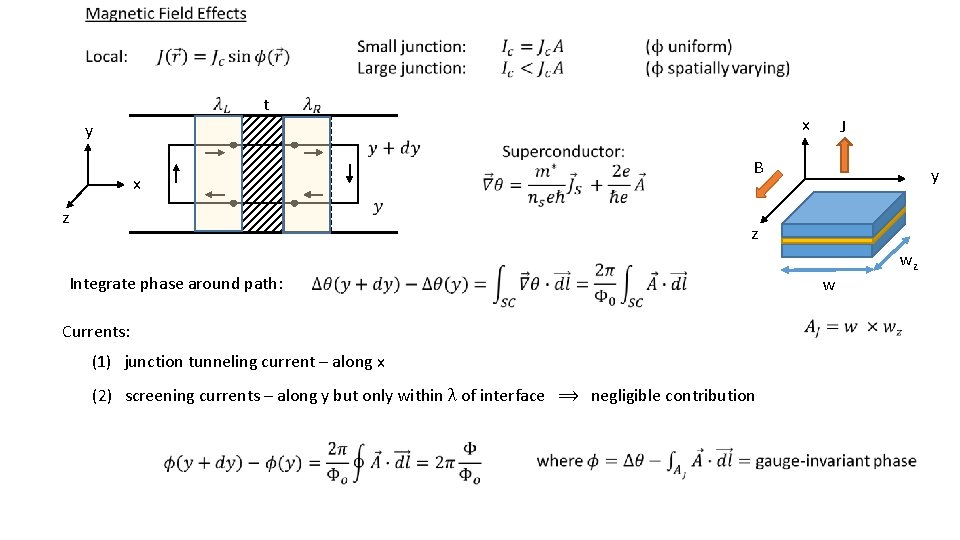
t y x J B x y z z Integrate phase around path: wz w Currents: (1) junction tunneling current – along x (2) screening currents – along y but only within λ of interface ⟹ negligible contribution
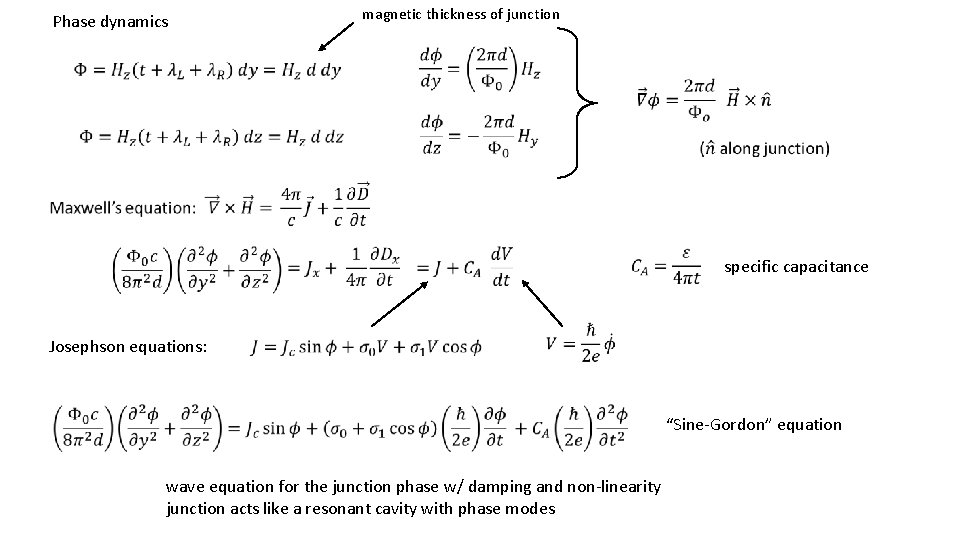
magnetic thickness of junction Phase dynamics Josephson equations: specific capacitance “Sine-Gordon” equation wave equation for the junction phase w/ damping and non-linearity junction acts like a resonant cavity with phase modes
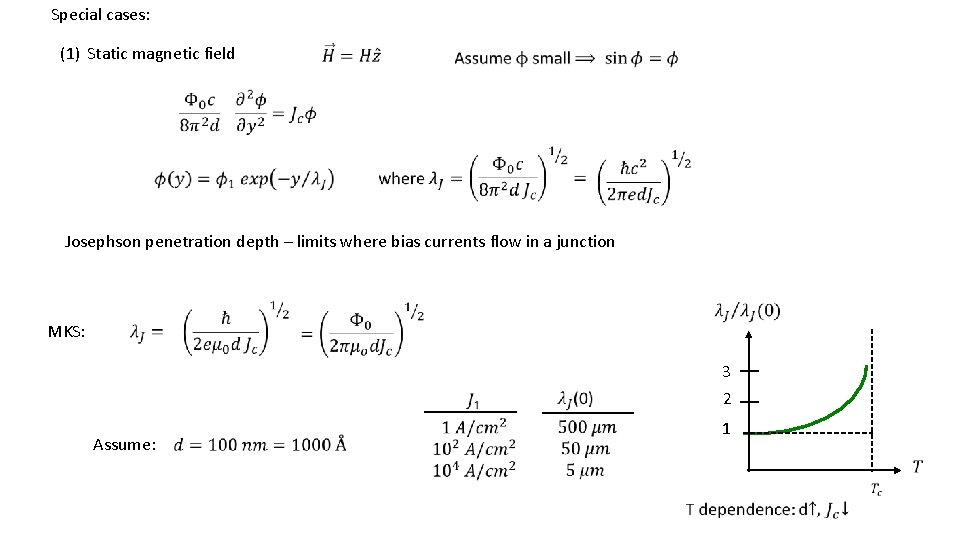
Special cases: (1) Static magnetic field Josephson penetration depth – limits where bias currents flow in a junction MKS: 3 2 1 Assume:
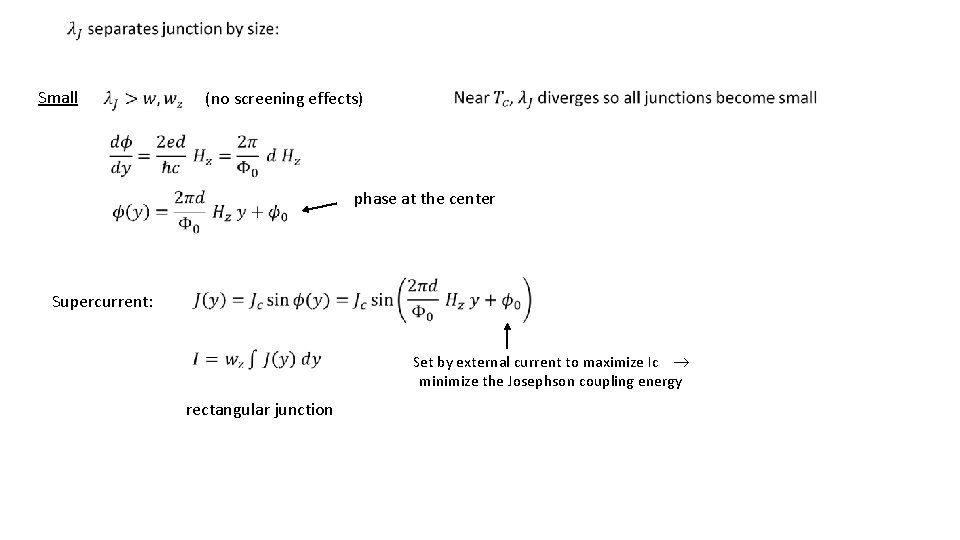
Small (no screening effects) phase at the center Supercurrent: rectangular junction Set by external current to maximize Ic minimize the Josephson coupling energy
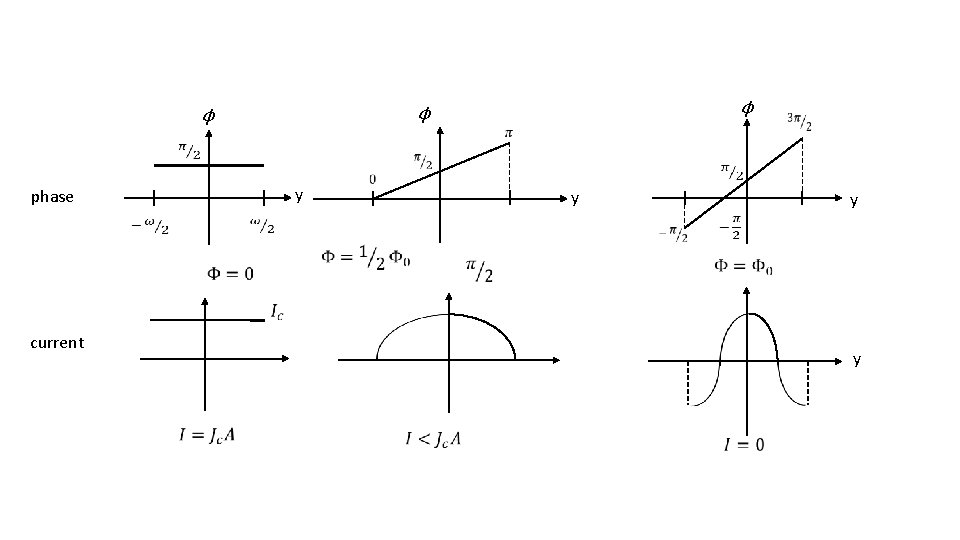
ϕ ϕ ϕ y phase y current y
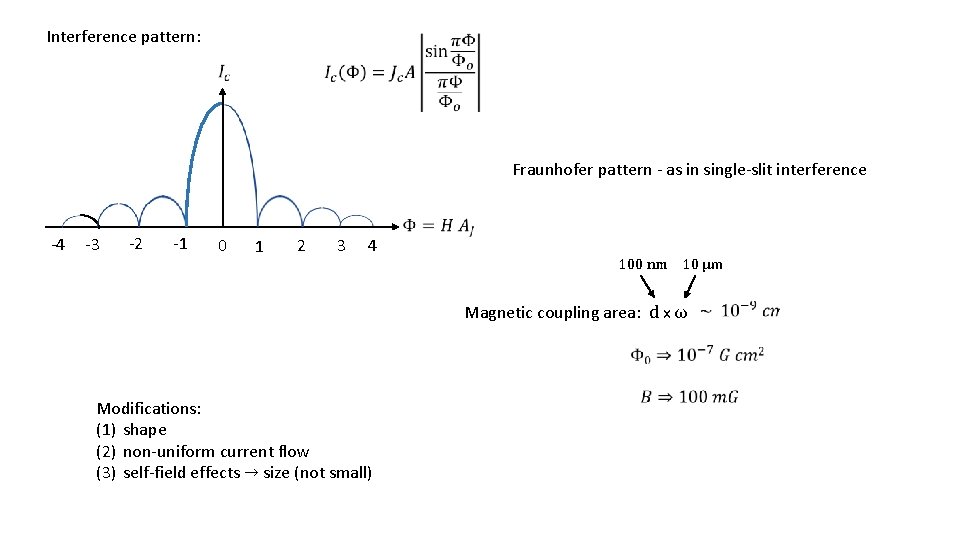
Interference pattern: Fraunhofer pattern - as in single-slit interference -4 -3 -2 -1 0 1 2 3 4 100 nm 10 μm Magnetic coupling area: d x ω Modifications: (1) shape (2) non-uniform current flow (3) self-field effects → size (not small)
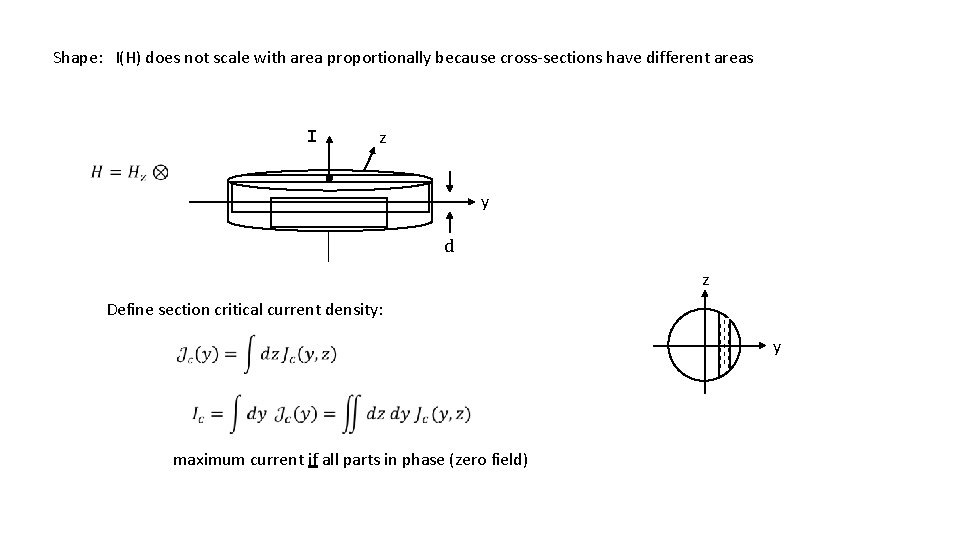
Shape: I(H) does not scale with area proportionally because cross-sections have different areas I z y d z Define section critical current density: maximum current if all parts in phase (zero field) y
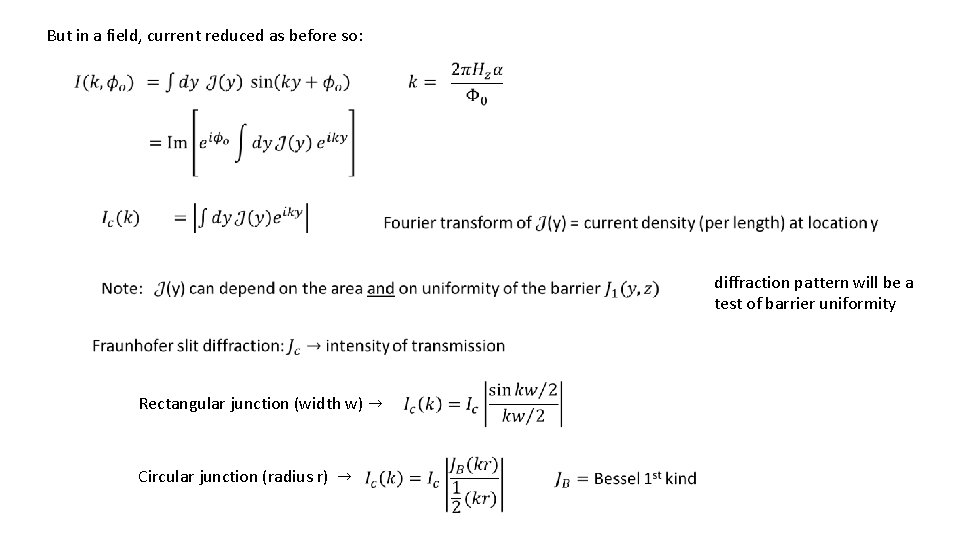
But in a field, current reduced as before so: diffraction pattern will be a test of barrier uniformity Rectangular junction (width w) → Circular junction (radius r) →
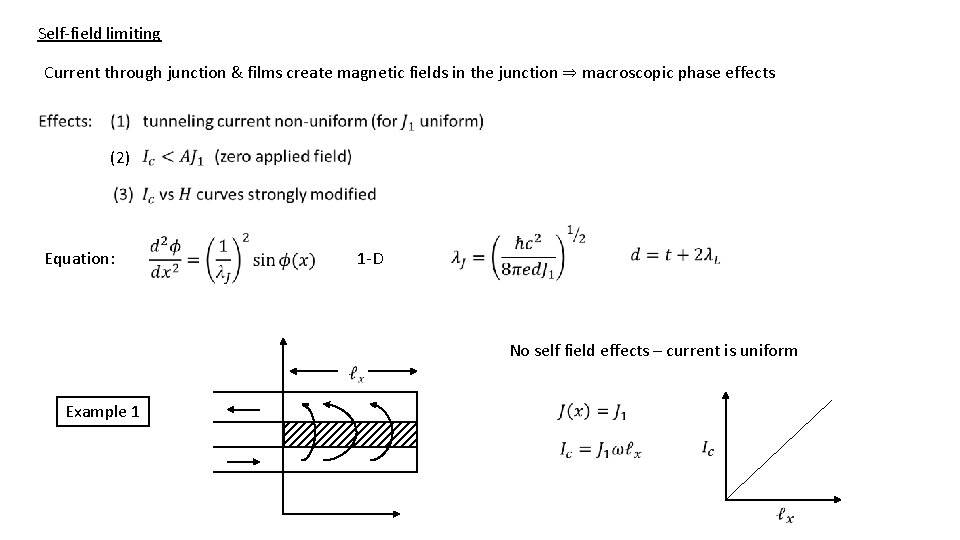
Self-field limiting Current through junction & films create magnetic fields in the junction ⇒ macroscopic phase effects (2) Equation: Example 1 1 -D No self field effects – current is uniform
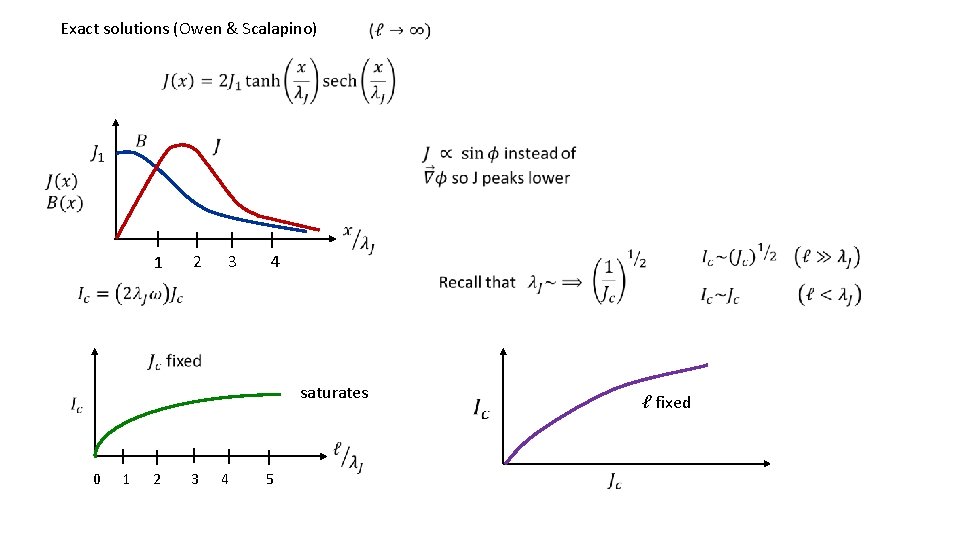
Exact solutions (Owen & Scalapino) 1 2 3 4 saturates ℓ fixed 0 1 2 3 4 5
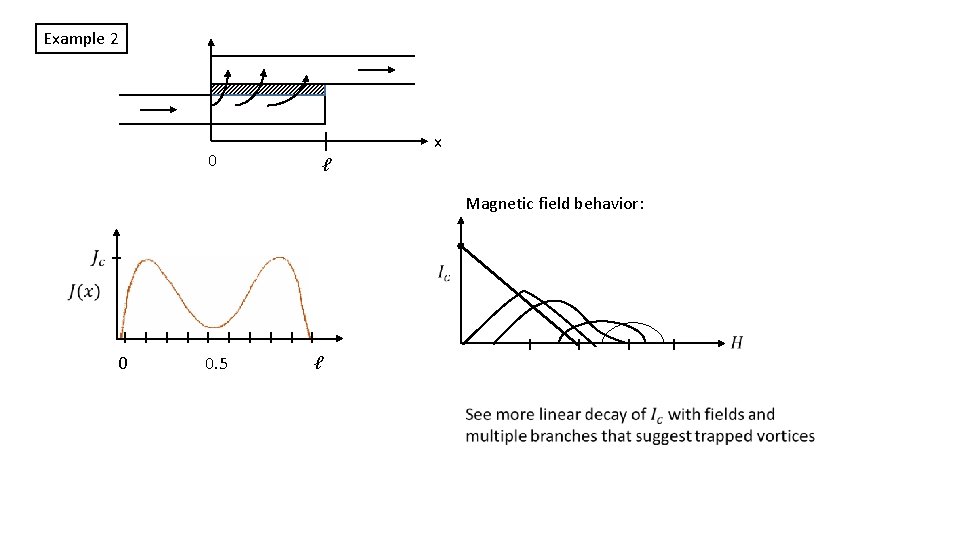
Example 2 0 x ℓ Magnetic field behavior: – I I I 0 ℓ 0. 5 I I I
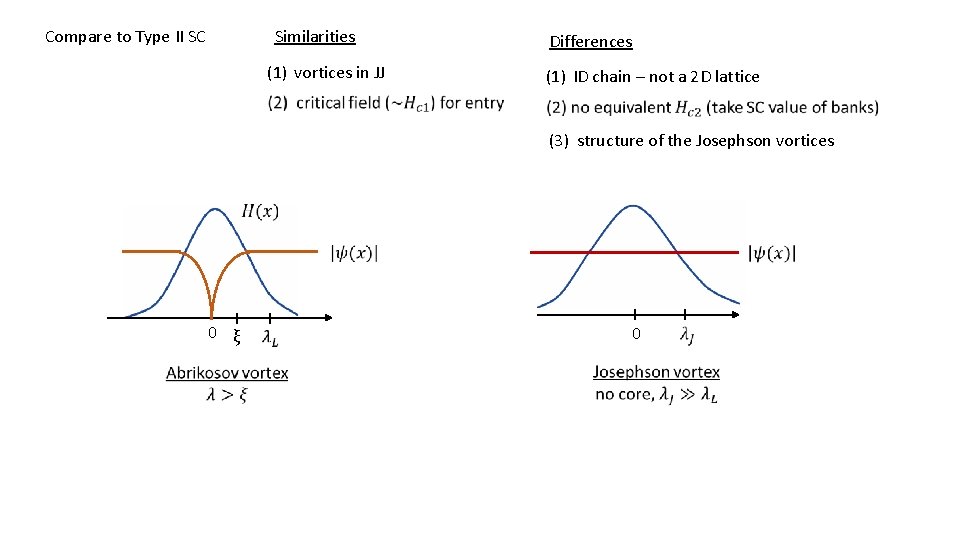
Compare to Type II SC Similarities Differences (1) vortices in JJ (1) ID chain – not a 2 D lattice (3) structure of the Josephson vortices I I 0 ξ
Josephson effect
Josephson effect
Magnetic moment and magnetic field relation
Magnetic field density
Magnetic field and magnetic force
Magnitude of magnetic force
Distinguish between magnetic and nonmagnetic materials
Magnetic field
Vi/vt vereckei
Josephson devices
Josephson sign
Michael josephson ethics
Josephson junction
Josephson junction
Josephson junction
Chris josephson
Josephson junctions
Josephson institute of ethics
Josephson c700s
Hysteresis loop magnetism
01:640:244 lecture notes - lecture 15: plat, idah, farad
For today's meeting