THREEPHASE INDUCTION MOTORS Study The Variations In Torquespeed
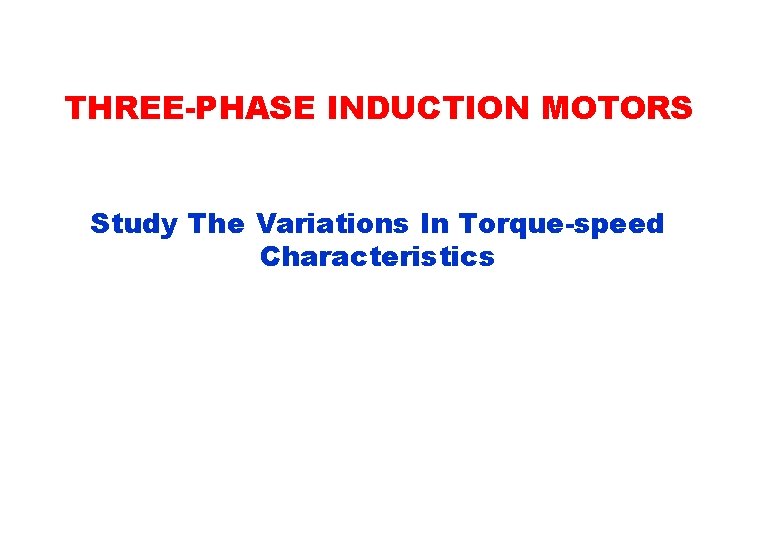
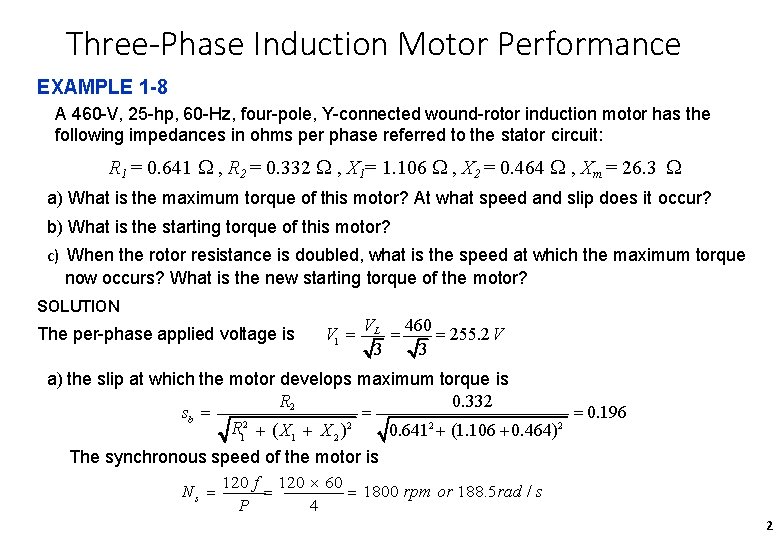
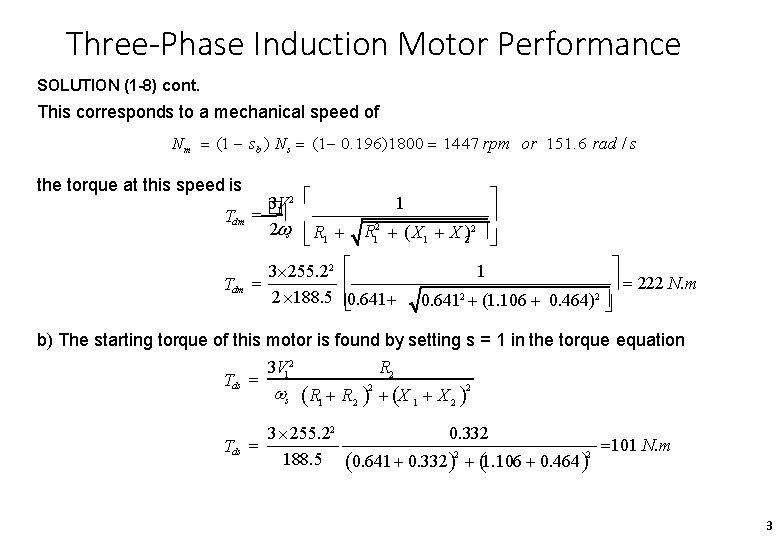
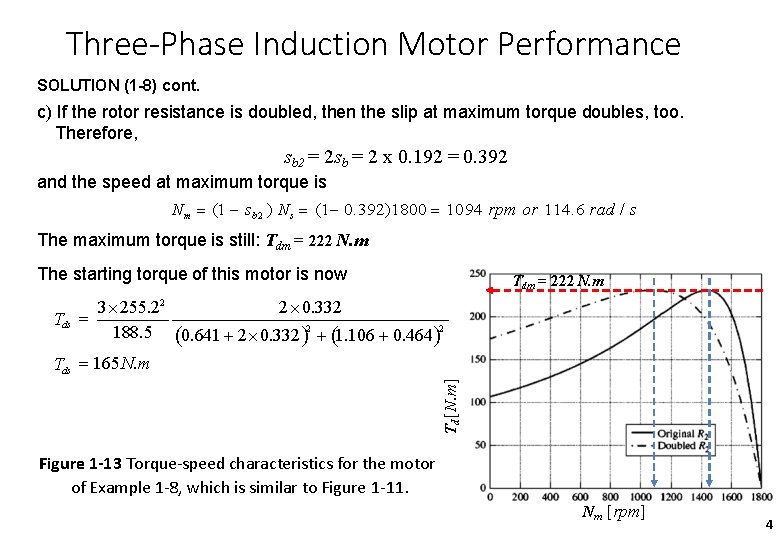
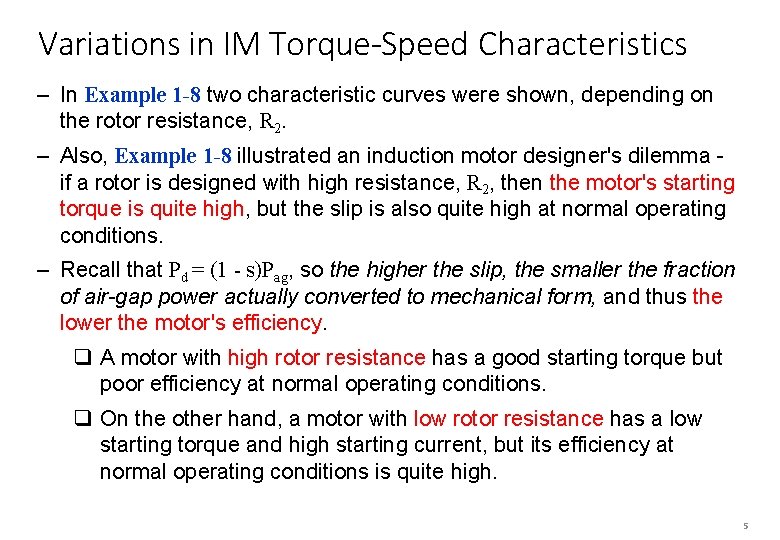
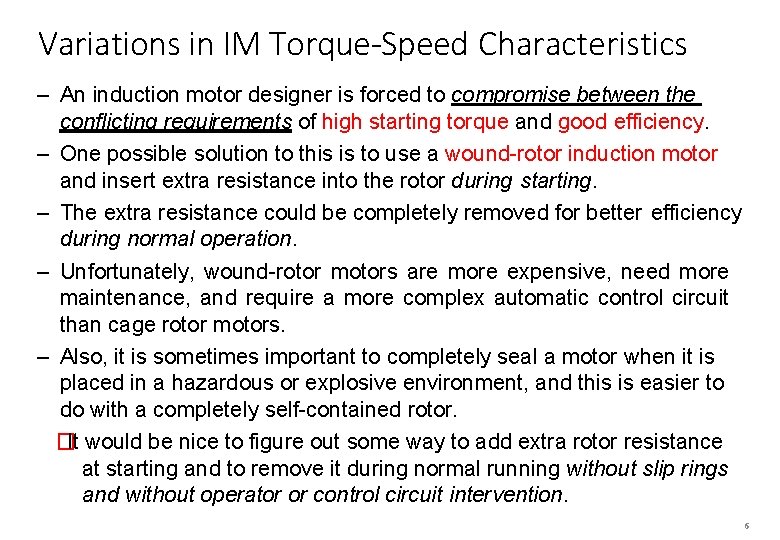
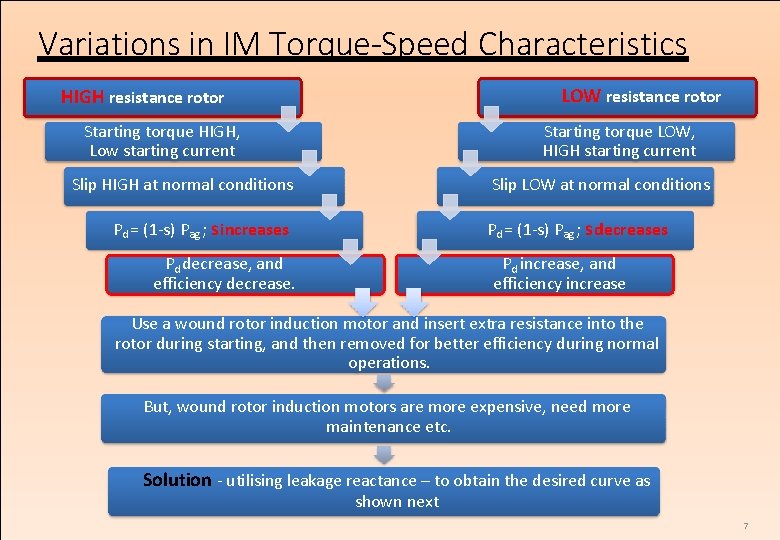
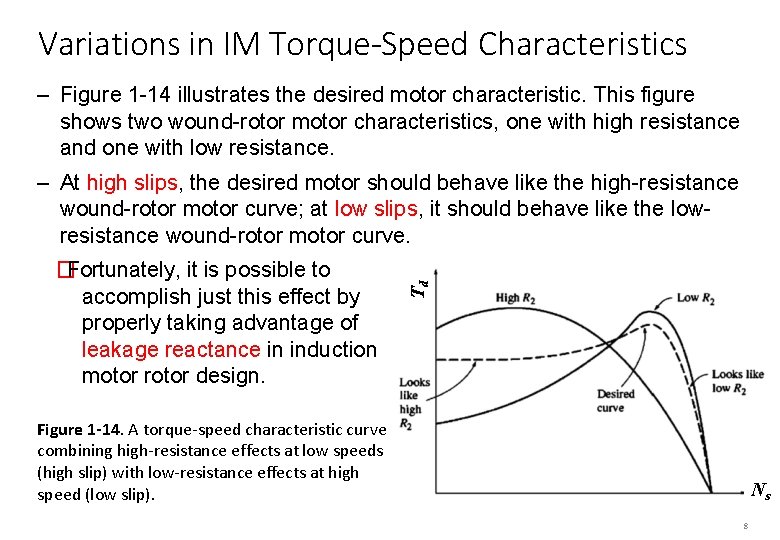
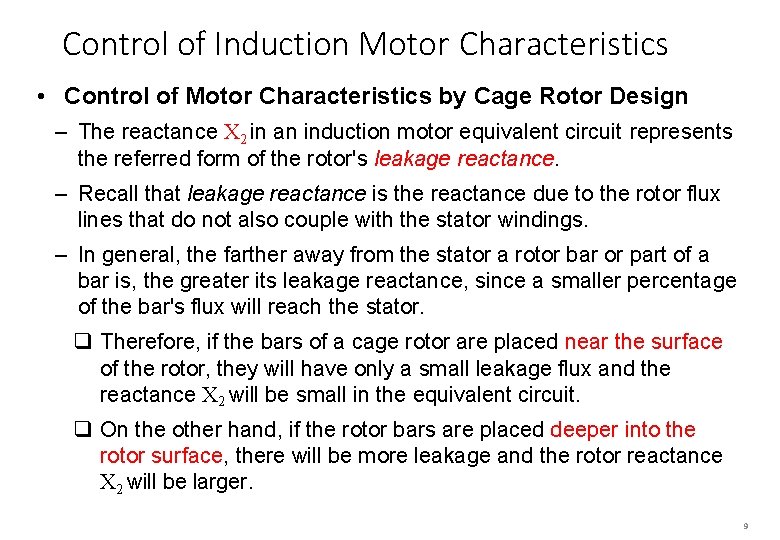
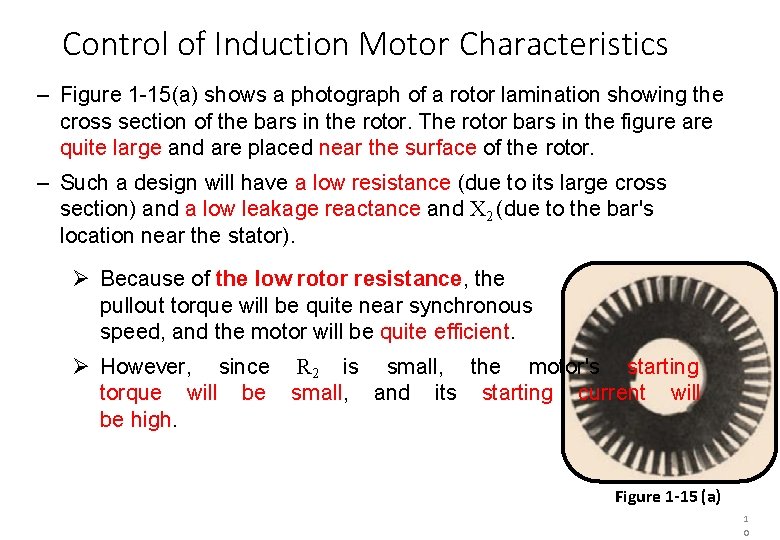
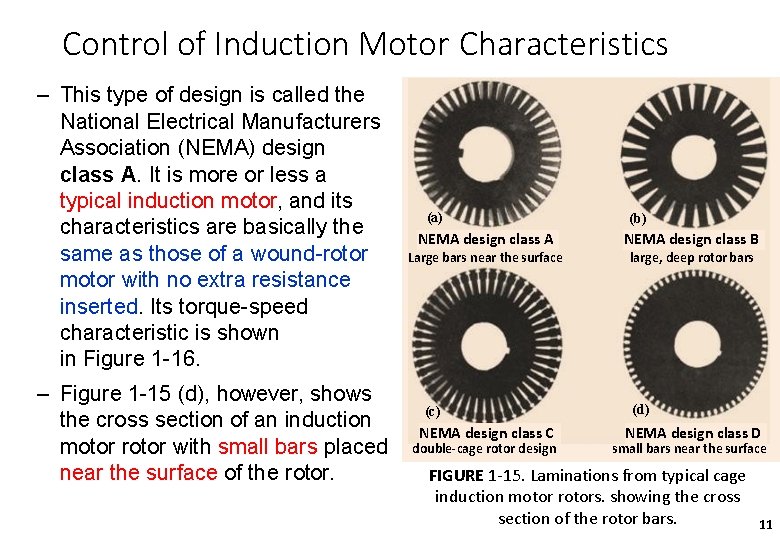
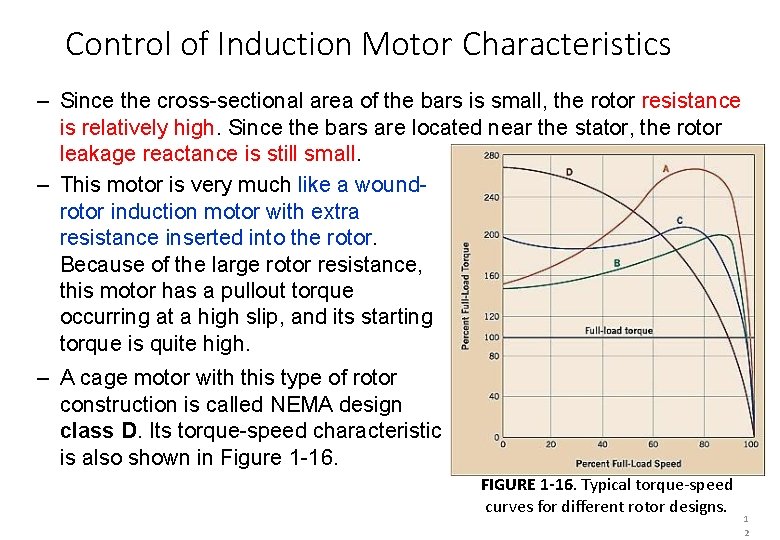
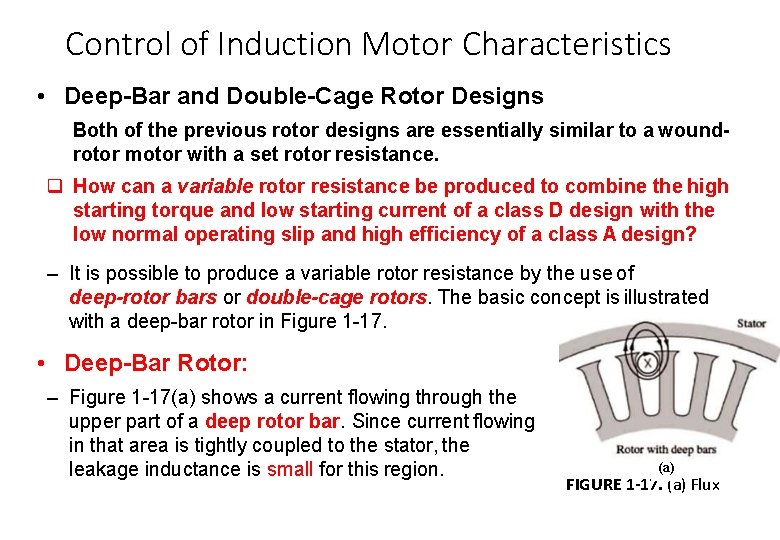
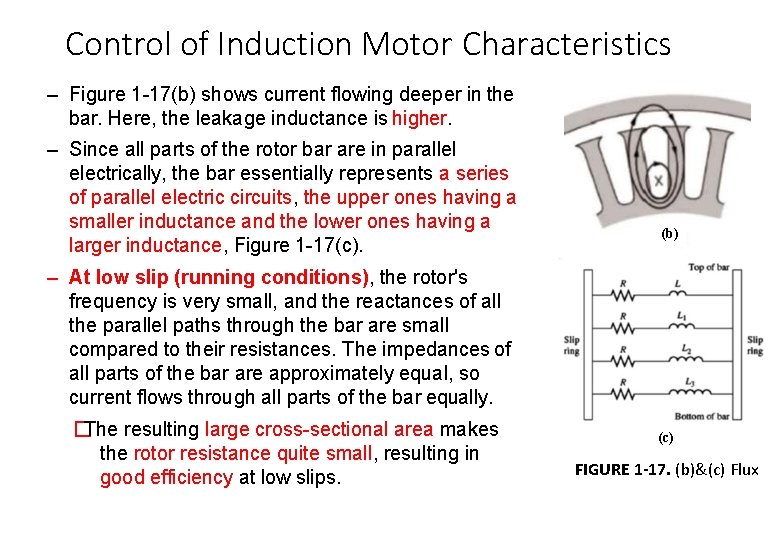
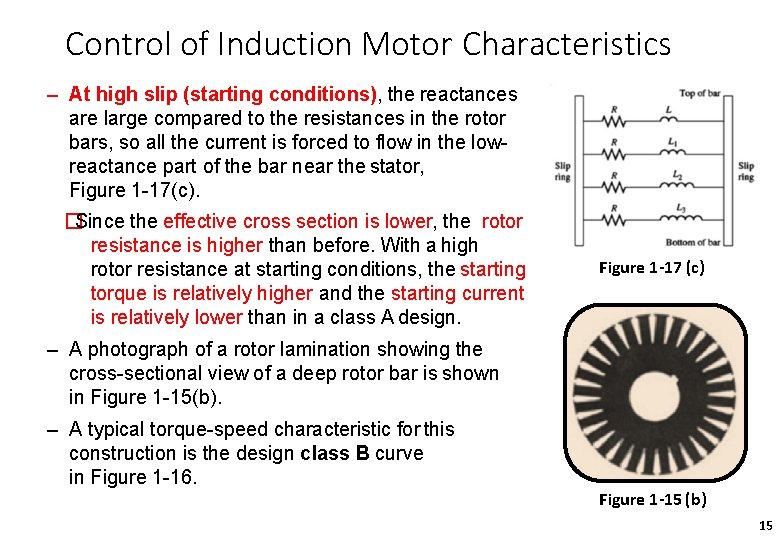
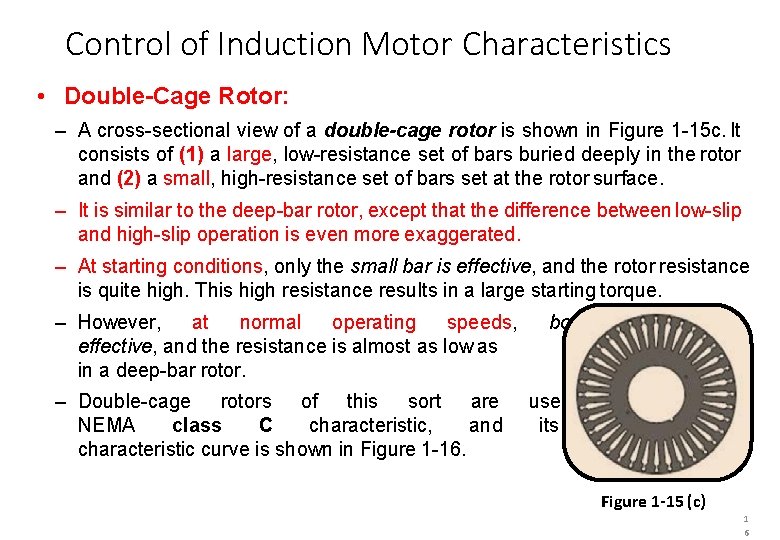
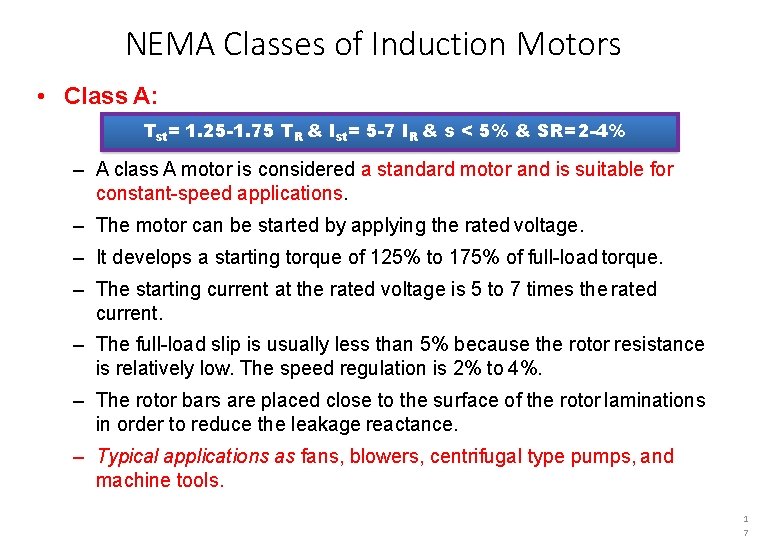
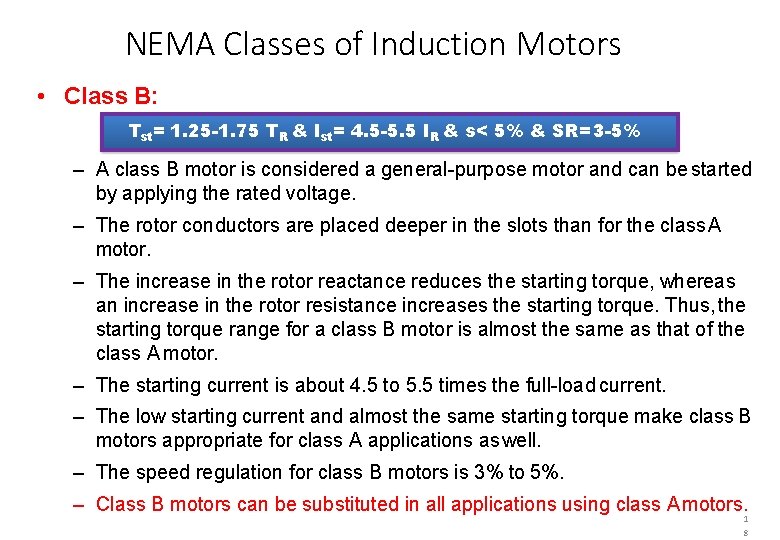
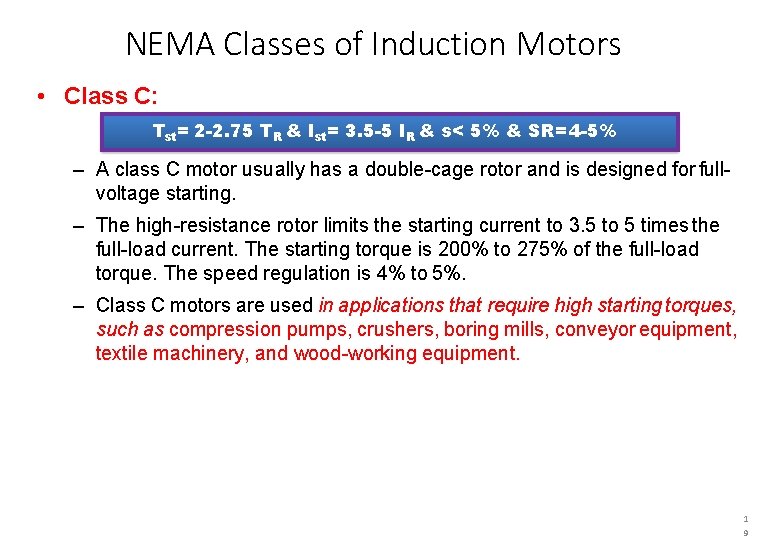
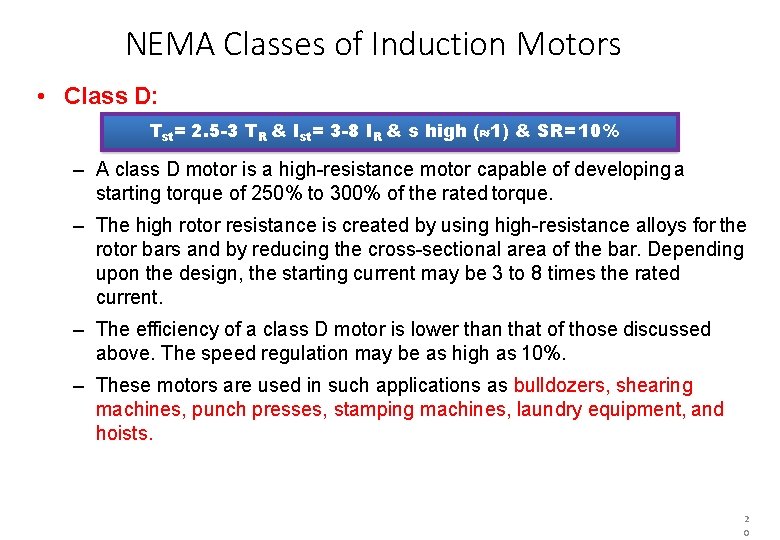
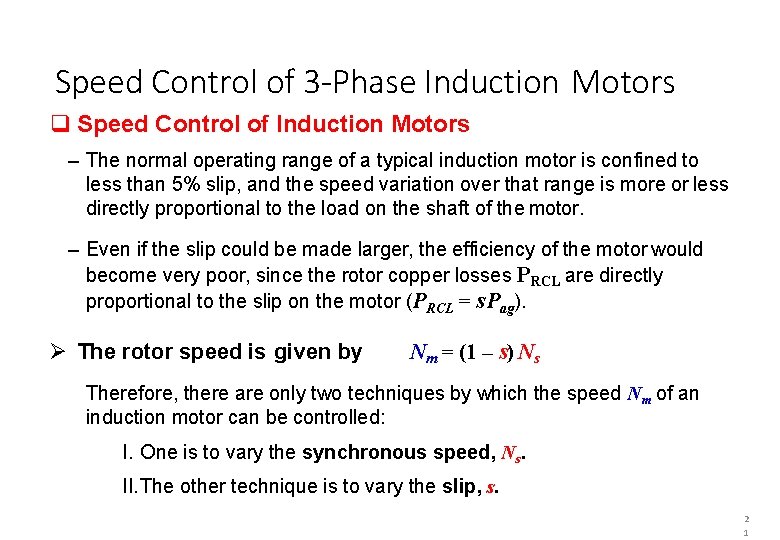
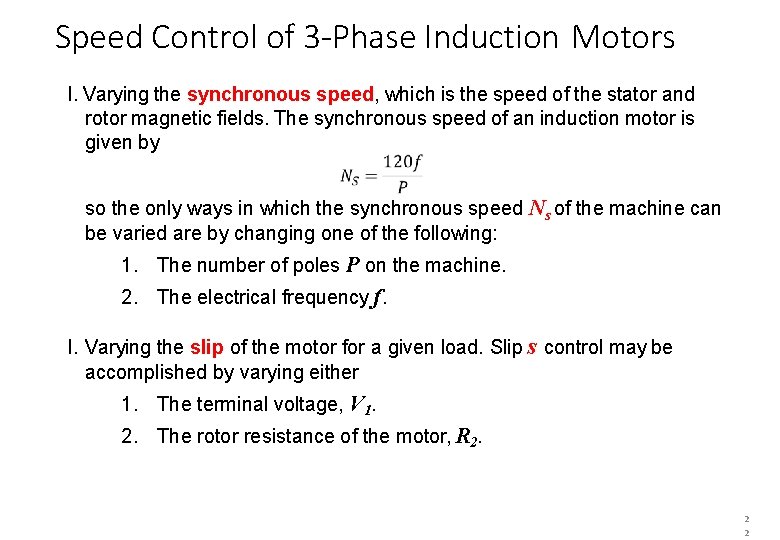
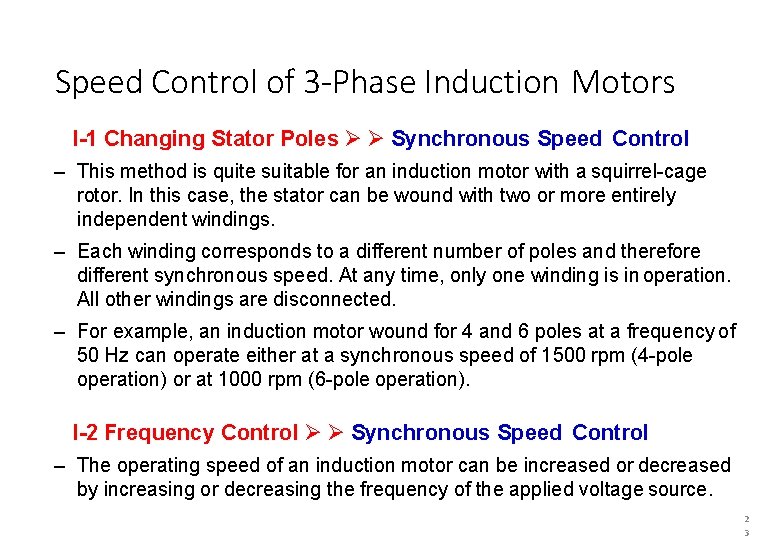
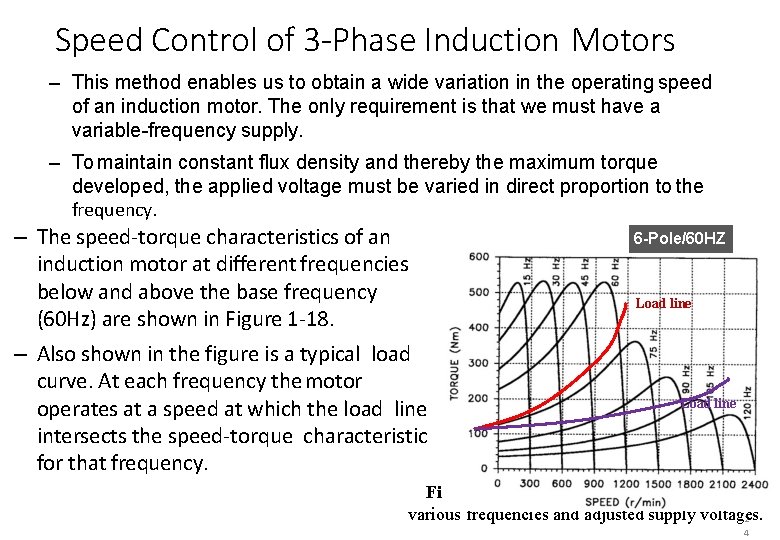
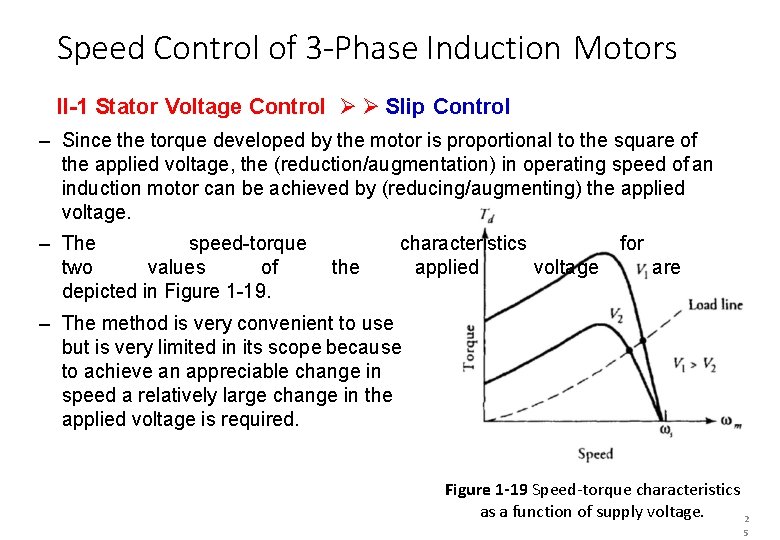
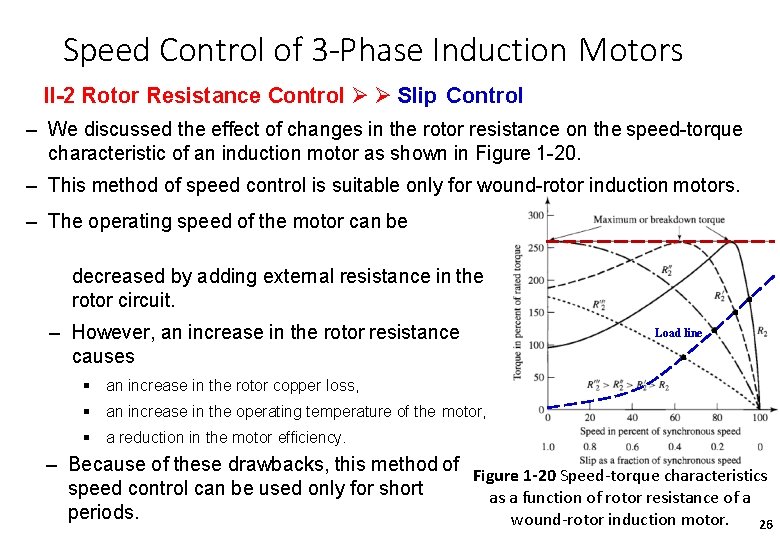
- Slides: 26
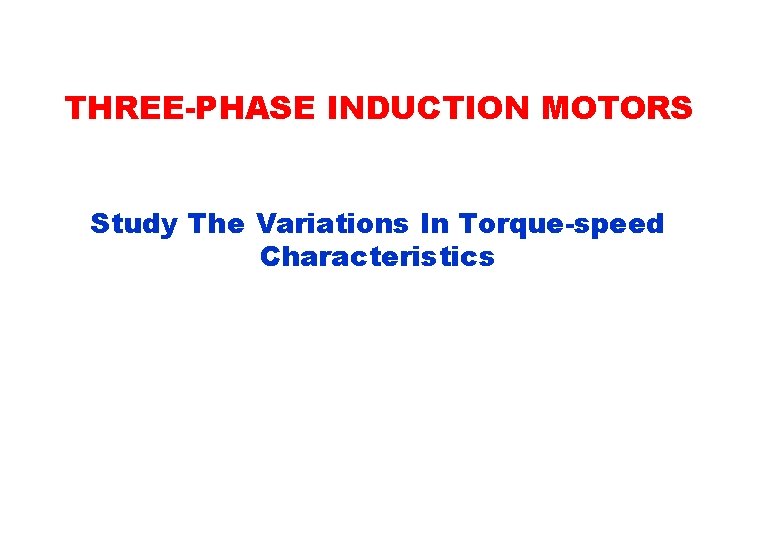
THREE-PHASE INDUCTION MOTORS Study The Variations In Torque-speed Characteristics
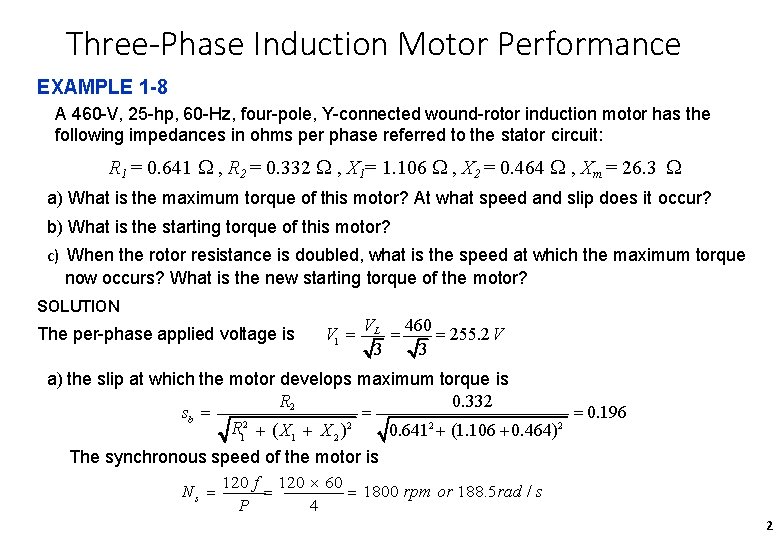
Three-Phase Induction Motor Performance EXAMPLE 1 -8 A 460 -V, 25 -hp, 60 -Hz, four-pole, Y-connected wound-rotor induction motor has the following impedances in ohms per phase referred to the stator circuit: R 1 = 0. 641 , R 2 = 0. 332 , X 1= 1. 106 , X 2 = 0. 464 , Xm = 26. 3 a) What is the maximum torque of this motor? At what speed and slip does it occur? b) What is the starting torque of this motor? c) When the rotor resistance is doubled, what is the speed at which the maximum torque now occurs? What is the new starting torque of the motor? SOLUTION The per-phase applied voltage is V 1 VL 460 255. 2 V 3 3 a) the slip at which the motor develops maximum torque is R 2 0. 332 sb 0. 196 2 2 R 1 ( X 1 X 2 ) 0. 641 (1. 106 0. 464) The synchronous speed of the motor is 120 f 120 60 Ns 1800 rpm or 188. 5 rad / s P 4 2
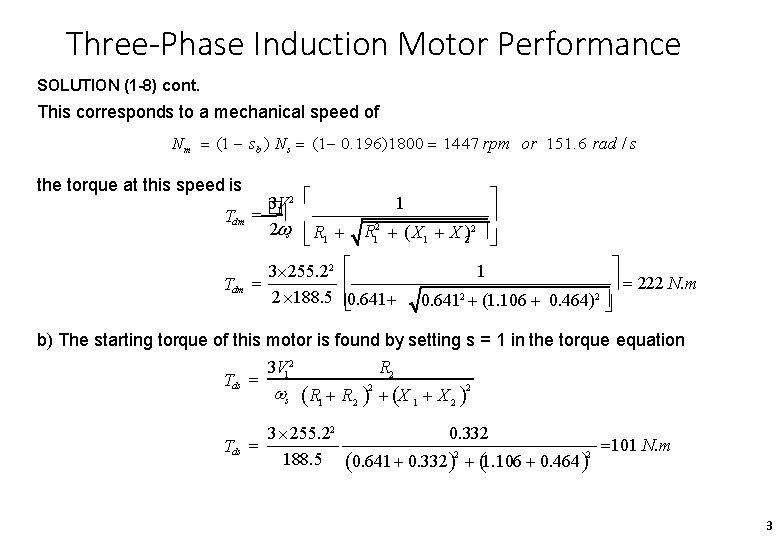
Three-Phase Induction Motor Performance SOLUTION (1 -8) cont. This corresponds to a mechanical speed of N m (1 sb ) N s (1 0. 196)1800 1447 rpm or 151. 6 rad / s the torque at this speed is Tdm 2 3 V � 1 2 s R 1 Tdm 3 255. 22 2 188. 5 2 2 R 1 ( X 1 X )2 1 0. 641 1 0. 6412 (1. 106 0. 464)2 222 N. m b) The starting torque of this motor is found by setting s = 1 in the torque equation 3 V 12 R 2 Tds s R 1 R 2 2 X 1 X 2 2 3 255. 22 0. 332 101 N. m Tds 2 2 188. 5 0. 641 0. 332 1. 106 0. 464 3
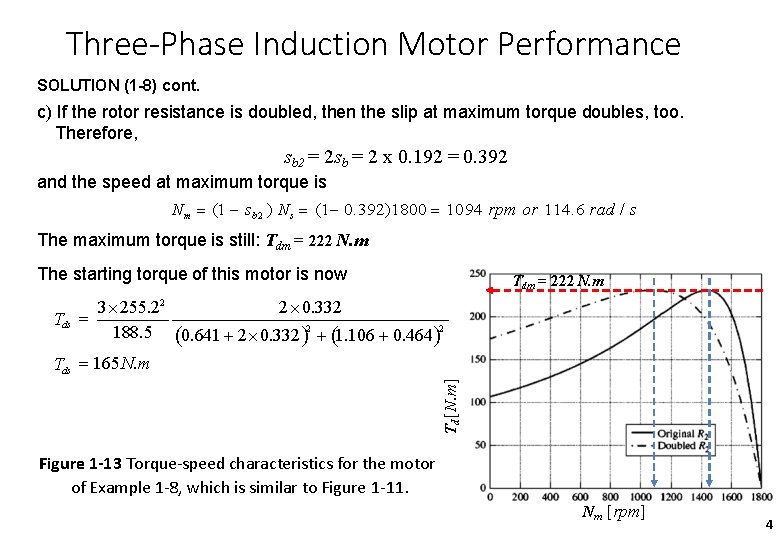
Three-Phase Induction Motor Performance SOLUTION (1 -8) cont. c) If the rotor resistance is doubled, then the slip at maximum torque doubles, too. Therefore, sb 2 = 2 sb = 2 x 0. 192 = 0. 392 and the speed at maximum torque is N m (1 sb 2 ) N s (1 0. 392)1800 1094 rpm or 114. 6 rad / s The maximum torque is still: Tdm = 222 N. m The starting torque of this motor is now Tdm = 222 N. m 3 255. 22 2 0. 332 Tds 188. 5 0. 641 2 0. 332 2 1. 106 0. 464 2 Td [N. m] Tds 165 N. m Figure 1 -13 Torque-speed characteristics for the motor of Example 1 -8, which is similar to Figure 1 -11. Nm [rpm] 4
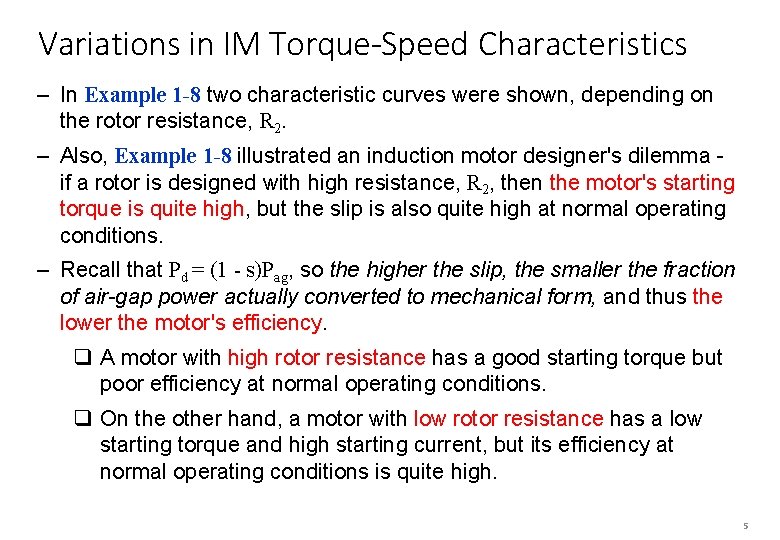
Variations in IM Torque-Speed Characteristics – In Example 1 -8 two characteristic curves were shown, depending on the rotor resistance, R 2. – Also, Example 1 -8 illustrated an induction motor designer's dilemma if a rotor is designed with high resistance, R 2, then the motor's starting torque is quite high, but the slip is also quite high at normal operating conditions. – Recall that Pd = (1 - s)Pag, so the higher the slip, the smaller the fraction of air-gap power actually converted to mechanical form, and thus the lower the motor's efficiency. A motor with high rotor resistance has a good starting torque but poor efficiency at normal operating conditions. On the other hand, a motor with low rotor resistance has a low starting torque and high starting current, but its efficiency at normal operating conditions is quite high. 5
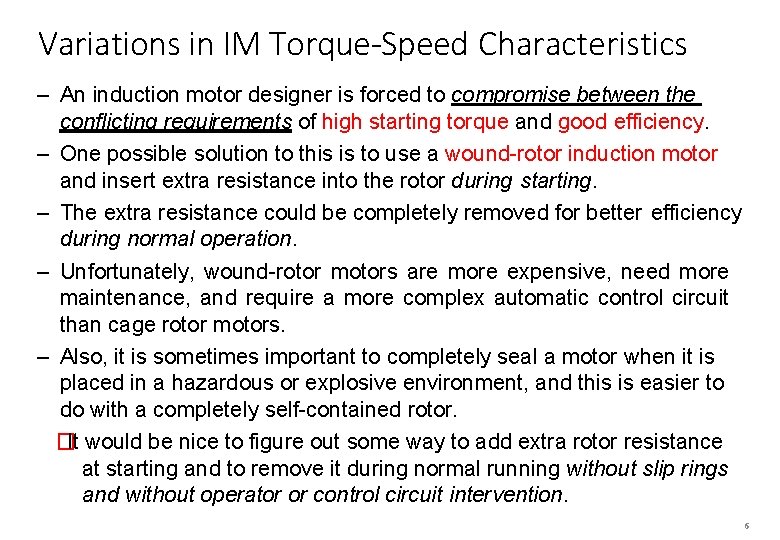
Variations in IM Torque-Speed Characteristics – An induction motor designer is forced to compromise between the conflicting requirements of high starting torque and good efficiency. – One possible solution to this is to use a wound-rotor induction motor and insert extra resistance into the rotor during starting. – The extra resistance could be completely removed for better efficiency during normal operation. – Unfortunately, wound-rotor motors are more expensive, need more maintenance, and require a more complex automatic control circuit than cage rotor motors. – Also, it is sometimes important to completely seal a motor when it is placed in a hazardous or explosive environment, and this is easier to do with a completely self-contained rotor. �It would be nice to figure out some way to add extra rotor resistance at starting and to remove it during normal running without slip rings and without operator or control circuit intervention. 6
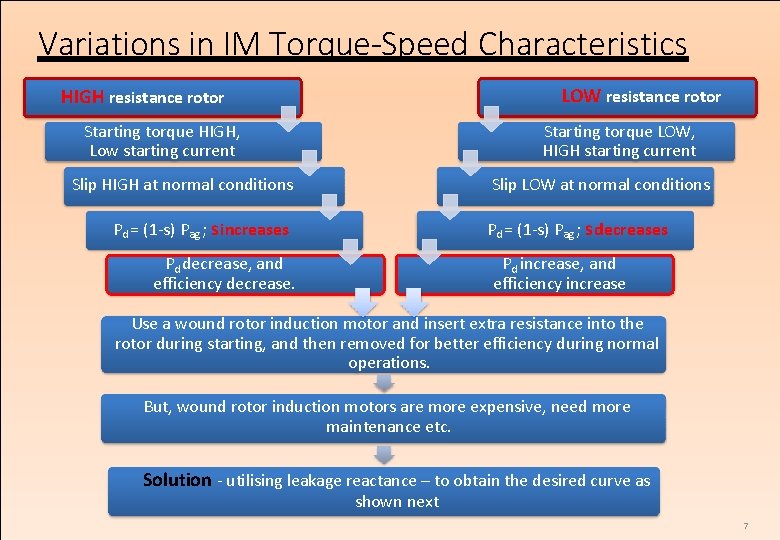
Variations in IM Torque-Speed Characteristics LOW resistance rotor HIGH resistance rotor Starting torque HIGH, Low starting current Starting torque LOW, HIGH starting current Slip HIGH at normal conditions Slip LOW at normal conditions Pd = (1 -s) Pag ; s increases Pd = (1 -s) Pag ; s decreases Pd decrease, and efficiency decrease. Pd increase, and efficiency increase Use a wound rotor induction motor and insert extra resistance into the rotor during starting, and then removed for better efficiency during normal operations. But, wound rotor induction motors are more expensive, need more maintenance etc. Solution - utilising leakage reactance – to obtain the desired curve as shown next 7
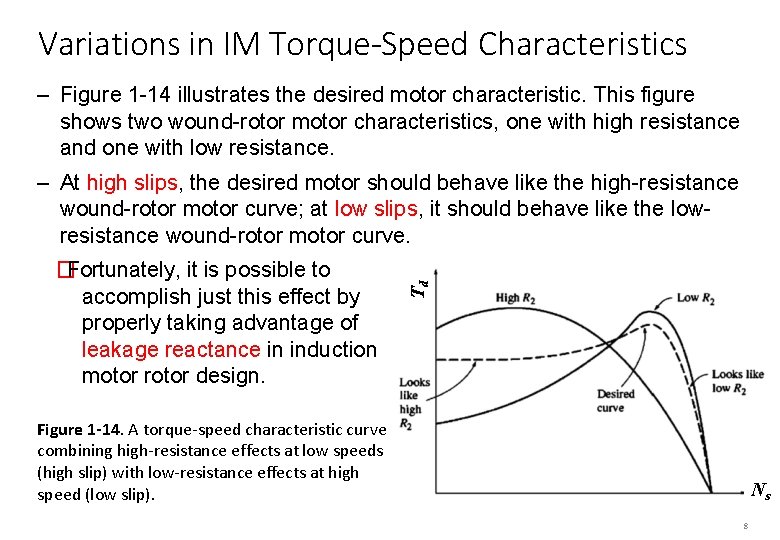
Variations in IM Torque-Speed Characteristics – Figure 1 -14 illustrates the desired motor characteristic. This figure shows two wound-rotor motor characteristics, one with high resistance and one with low resistance. �Fortunately, it is possible to accomplish just this effect by properly taking advantage of leakage reactance in induction motor rotor design. Td – At high slips, the desired motor should behave like the high-resistance wound-rotor motor curve; at low slips, it should behave like the lowresistance wound-rotor motor curve. Figure 1 -14. A torque-speed characteristic curve combining high-resistance effects at low speeds (high slip) with low-resistance effects at high speed (low slip). Ns 8
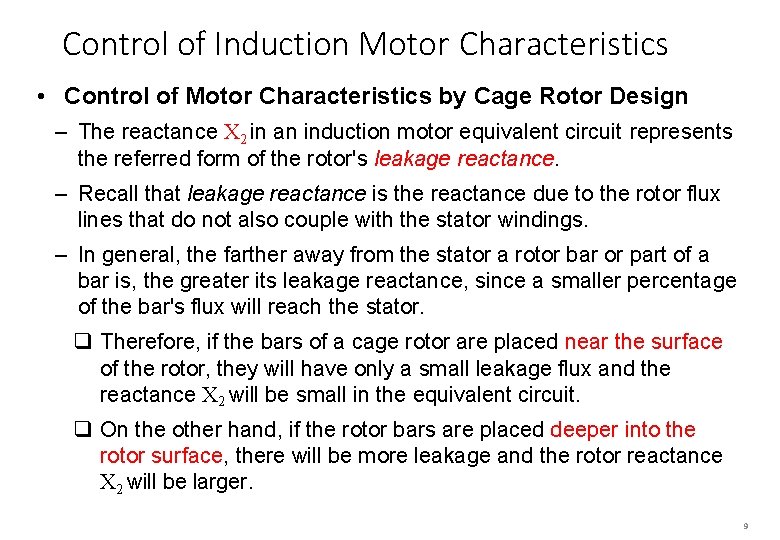
Control of Induction Motor Characteristics • Control of Motor Characteristics by Cage Rotor Design – The reactance X 2 in an induction motor equivalent circuit represents the referred form of the rotor's leakage reactance. – Recall that leakage reactance is the reactance due to the rotor flux lines that do not also couple with the stator windings. – In general, the farther away from the stator a rotor bar or part of a bar is, the greater its leakage reactance, since a smaller percentage of the bar's flux will reach the stator. Therefore, if the bars of a cage rotor are placed near the surface of the rotor, they will have only a small leakage flux and the reactance X 2 will be small in the equivalent circuit. On the other hand, if the rotor bars are placed deeper into the rotor surface, there will be more leakage and the rotor reactance X 2 will be larger. 9
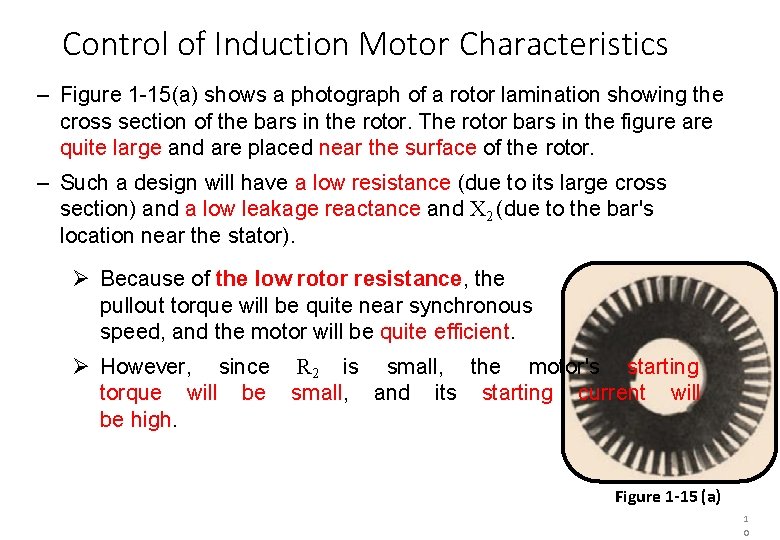
Control of Induction Motor Characteristics – Figure 1 -15(a) shows a photograph of a rotor lamination showing the cross section of the bars in the rotor. The rotor bars in the figure are quite large and are placed near the surface of the rotor. – Such a design will have a low resistance (due to its large cross section) and a low leakage reactance and X 2 (due to the bar's location near the stator). Because of the low rotor resistance, the pullout torque will be quite near synchronous speed, and the motor will be quite efficient. However, since R 2 is small, the motor's starting torque will be small, and its starting current will be high. Figure 1 -15 (a) 1 0
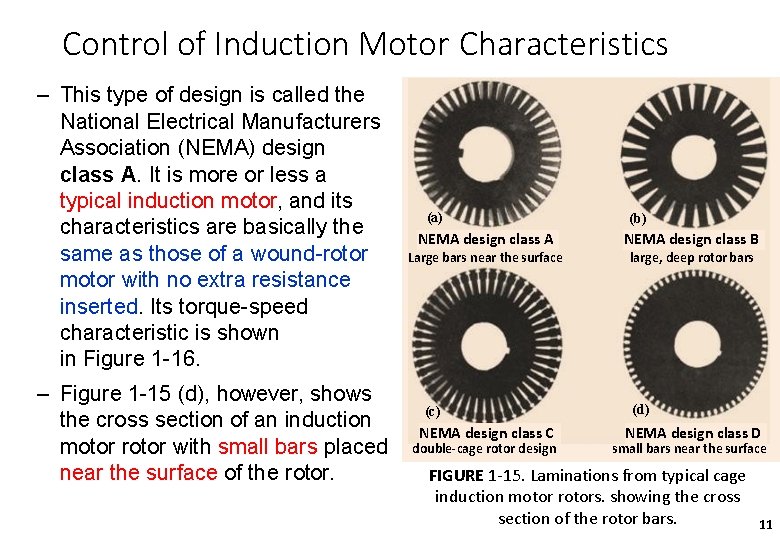
Control of Induction Motor Characteristics – This type of design is called the National Electrical Manufacturers Association (NEMA) design class A. It is more or less a typical induction motor, and its characteristics are basically the same as those of a wound-rotor motor with no extra resistance inserted. Its torque-speed characteristic is shown in Figure 1 -16. – Figure 1 -15 (d), however, shows the cross section of an induction motor rotor with small bars placed near the surface of the rotor. (a) NEMA design class A Large bars near the surface (c) NEMA design class C double-cage rotor design (b) NEMA design class B large, deep rotor bars (d) NEMA design class D small bars near the surface FIGURE 1 -15. Laminations from typical cage induction motor rotors. showing the cross section of the rotor bars. 11
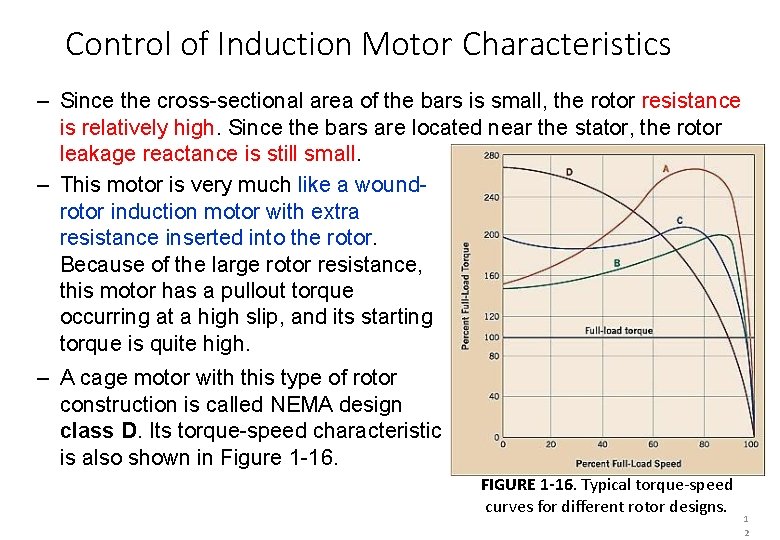
Control of Induction Motor Characteristics – Since the cross-sectional area of the bars is small, the rotor resistance is relatively high. Since the bars are located near the stator, the rotor leakage reactance is still small. – This motor is very much like a woundrotor induction motor with extra resistance inserted into the rotor. Because of the large rotor resistance, this motor has a pullout torque occurring at a high slip, and its starting torque is quite high. – A cage motor with this type of rotor construction is called NEMA design class D. Its torque-speed characteristic is also shown in Figure 1 -16. FIGURE 1 -16. Typical torque-speed curves for different rotor designs. 1 2
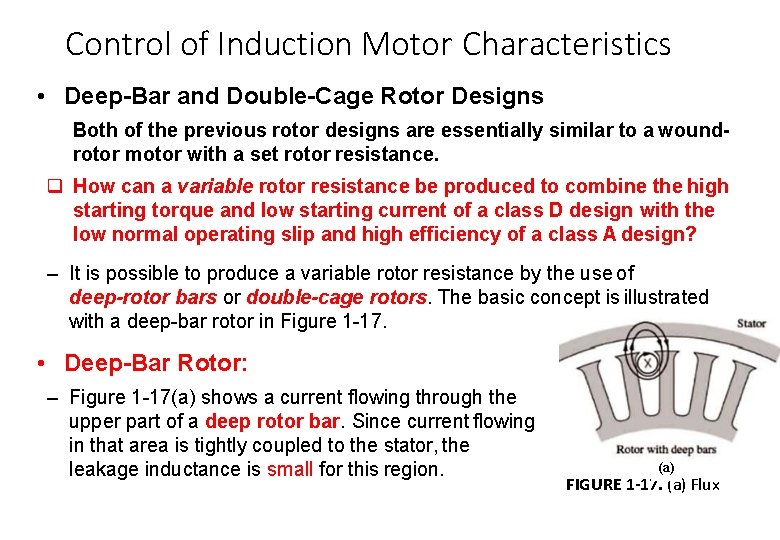
Control of Induction Motor Characteristics • Deep-Bar and Double-Cage Rotor Designs Both of the previous rotor designs are essentially similar to a woundrotor motor with a set rotor resistance. How can a variable rotor resistance be produced to combine the high starting torque and low starting current of a class D design with the low normal operating slip and high efficiency of a class A design? – It is possible to produce a variable rotor resistance by the use of deep-rotor bars or double-cage rotors. The basic concept is illustrated with a deep-bar rotor in Figure 1 -17. • Deep-Bar Rotor: – Figure 1 -17(a) shows a current flowing through the upper part of a deep rotor bar. Since current flowing in that area is tightly coupled to the stator, the leakage inductance is small for this region. (a) FIGURE 1 -17. (a) Flux
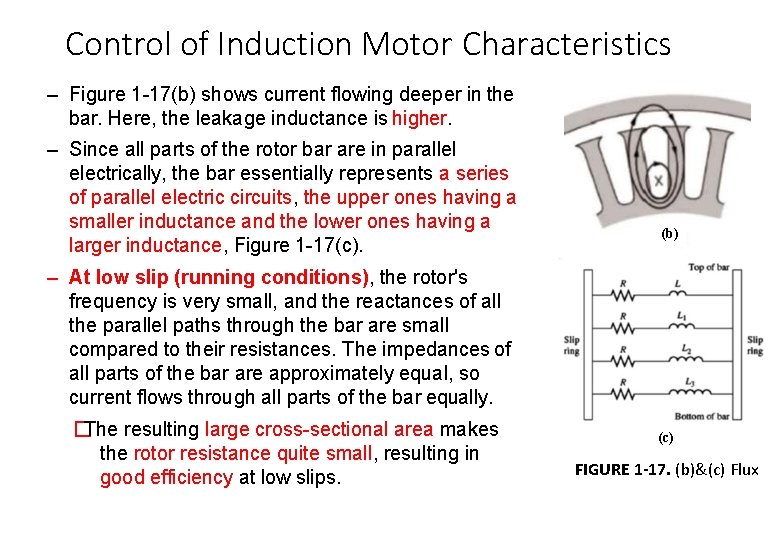
Control of Induction Motor Characteristics – Figure 1 -17(b) shows current flowing deeper in the bar. Here, the leakage inductance is higher. – Since all parts of the rotor bar are in parallel electrically, the bar essentially represents a series of parallel electric circuits, the upper ones having a smaller inductance and the lower ones having a larger inductance, Figure 1 -17(c). (b) – At low slip (running conditions), the rotor's frequency is very small, and the reactances of all the parallel paths through the bar are small compared to their resistances. The impedances of all parts of the bar are approximately equal, so current flows through all parts of the bar equally. �The resulting large cross-sectional area makes the rotor resistance quite small, resulting in good efficiency at low slips. (c) FIGURE 1 -17. (b)&(c) Flux
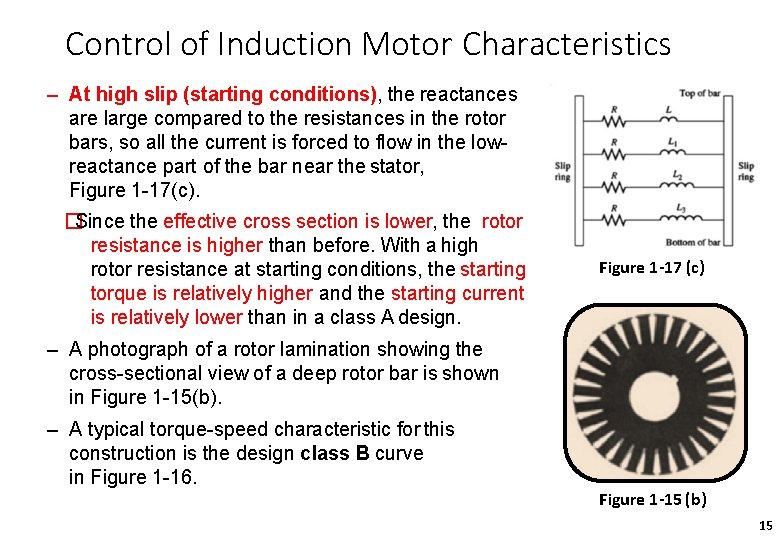
Control of Induction Motor Characteristics – At high slip (starting conditions), the reactances are large compared to the resistances in the rotor bars, so all the current is forced to flow in the lowreactance part of the bar near the stator, Figure 1 -17(c). �Since the effective cross section is lower, the rotor resistance is higher than before. With a high rotor resistance at starting conditions, the starting torque is relatively higher and the starting current is relatively lower than in a class A design. Figure 1 -17 (c) – A photograph of a rotor lamination showing the cross-sectional view of a deep rotor bar is shown in Figure 1 -15(b). – A typical torque-speed characteristic for this construction is the design class B curve in Figure 1 -16. Figure 1 -15 (b) 15
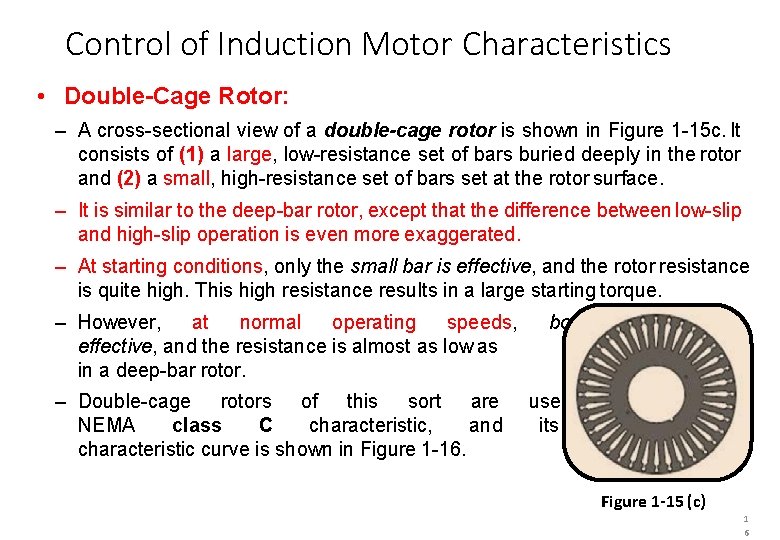
Control of Induction Motor Characteristics • Double-Cage Rotor: – A cross-sectional view of a double-cage rotor is shown in Figure 1 -15 c. It consists of (1) a large, low-resistance set of bars buried deeply in the rotor and (2) a small, high-resistance set of bars set at the rotor surface. – It is similar to the deep-bar rotor, except that the difference between low-slip and high-slip operation is even more exaggerated. – At starting conditions, only the small bar is effective, and the rotor resistance is quite high. This high resistance results in a large starting torque. – However, at normal operating speeds, effective, and the resistance is almost as low as in a deep-bar rotor. – Double-cage rotors of this sort are NEMA class C characteristic, and characteristic curve is shown in Figure 1 -16. both used its bars are to produce torque-speed Figure 1 -15 (c) 1 6
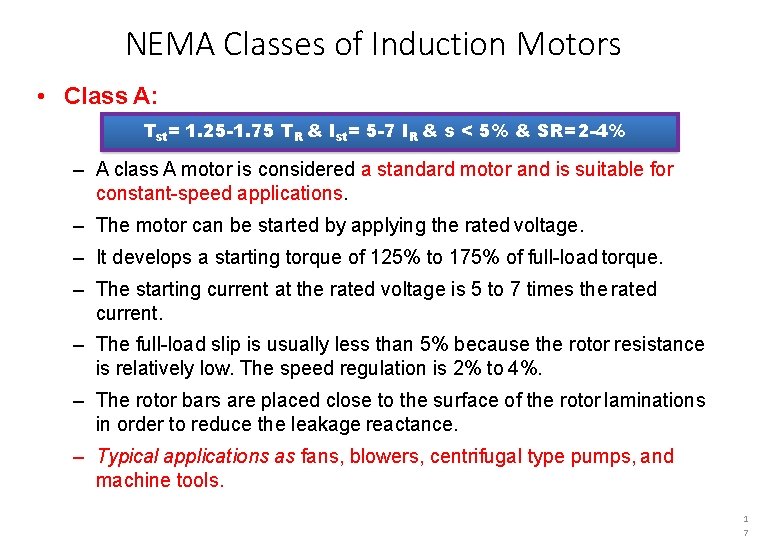
NEMA Classes of Induction Motors • Class A: Tst= 1. 25 -1. 75 TR & Ist= 5 -7 IR & s < 5% & SR= 2 -4% – A class A motor is considered a standard motor and is suitable for constant-speed applications. – The motor can be started by applying the rated voltage. – It develops a starting torque of 125% to 175% of full-load torque. – The starting current at the rated voltage is 5 to 7 times the rated current. – The full-load slip is usually less than 5% because the rotor resistance is relatively low. The speed regulation is 2% to 4%. – The rotor bars are placed close to the surface of the rotor laminations in order to reduce the leakage reactance. – Typical applications as fans, blowers, centrifugal type pumps, and machine tools. 1 7
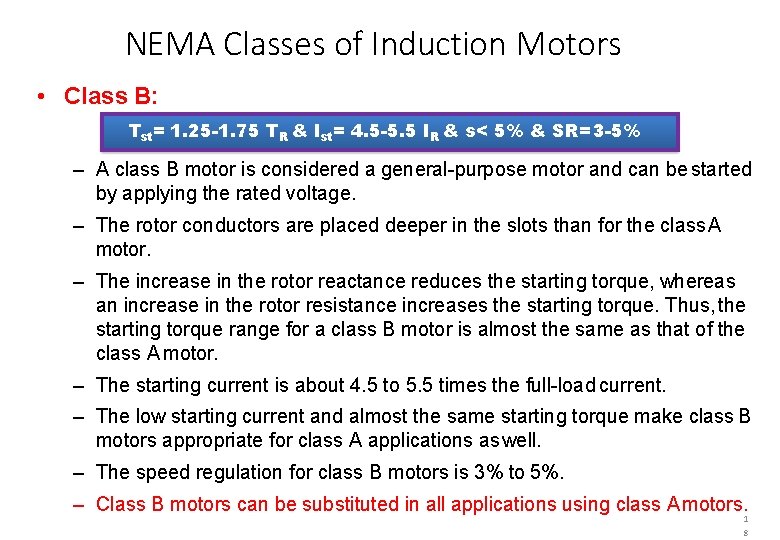
NEMA Classes of Induction Motors • Class B: Tst= 1. 25 -1. 75 TR & Ist= 4. 5 -5. 5 IR & s< 5% & SR= 3 -5% – A class B motor is considered a general-purpose motor and can be started by applying the rated voltage. – The rotor conductors are placed deeper in the slots than for the class A motor. – The increase in the rotor reactance reduces the starting torque, whereas an increase in the rotor resistance increases the starting torque. Thus, the starting torque range for a class B motor is almost the same as that of the class A motor. – The starting current is about 4. 5 to 5. 5 times the full-load current. – The low starting current and almost the same starting torque make class B motors appropriate for class A applications as well. – The speed regulation for class B motors is 3% to 5%. – Class B motors can be substituted in all applications using class A motors. 1 8
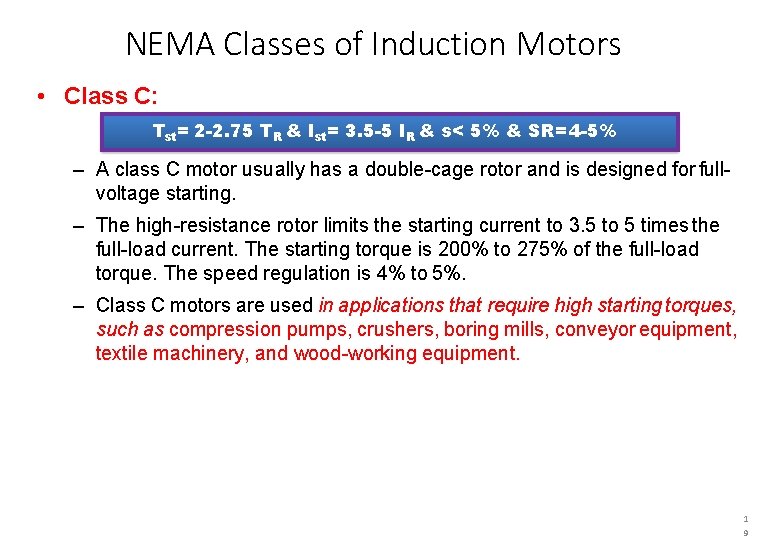
NEMA Classes of Induction Motors • Class C: Tst= 2 -2. 75 TR & Ist= 3. 5 -5 IR & s< 5% & SR= 4 -5% – A class C motor usually has a double-cage rotor and is designed for fullvoltage starting. – The high-resistance rotor limits the starting current to 3. 5 to 5 times the full-load current. The starting torque is 200% to 275% of the full-load torque. The speed regulation is 4% to 5%. – Class C motors are used in applications that require high starting torques, such as compression pumps, crushers, boring mills, conveyor equipment, textile machinery, and wood-working equipment. 1 9
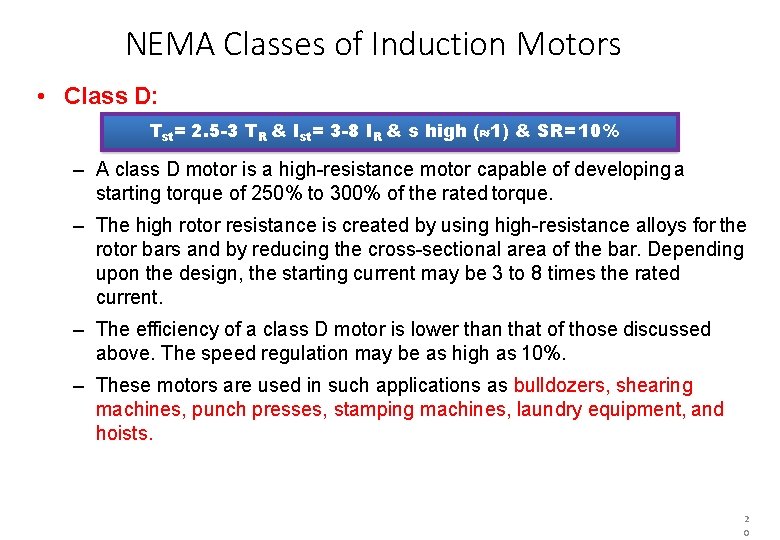
NEMA Classes of Induction Motors • Class D: Tst= 2. 5 -3 TR & Ist= 3 -8 IR & s high ( 1) & SR= 10% – A class D motor is a high-resistance motor capable of developing a starting torque of 250% to 300% of the rated torque. – The high rotor resistance is created by using high-resistance alloys for the rotor bars and by reducing the cross-sectional area of the bar. Depending upon the design, the starting current may be 3 to 8 times the rated current. – The efficiency of a class D motor is lower than that of those discussed above. The speed regulation may be as high as 10%. – These motors are used in such applications as bulldozers, shearing machines, punch presses, stamping machines, laundry equipment, and hoists. 2 0
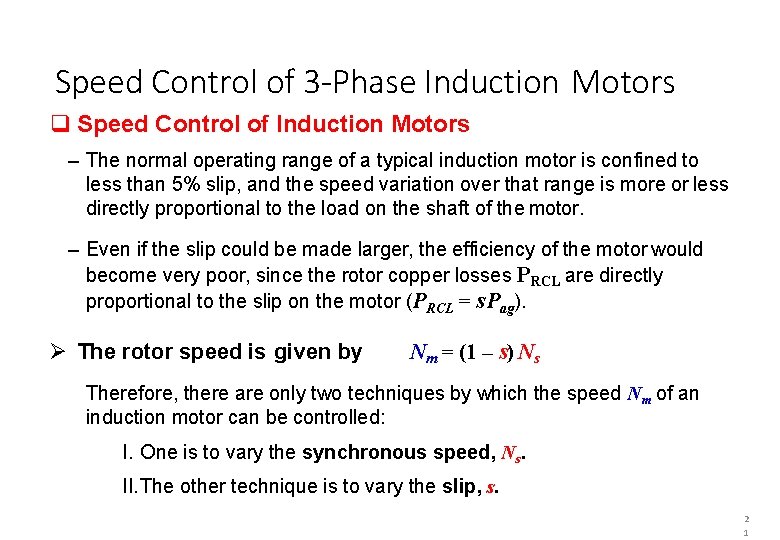
Speed Control of 3 -Phase Induction Motors Speed Control of Induction Motors – The normal operating range of a typical induction motor is confined to less than 5% slip, and the speed variation over that range is more or less directly proportional to the load on the shaft of the motor. – Even if the slip could be made larger, the efficiency of the motor would become very poor, since the rotor copper losses PRCL are directly proportional to the slip on the motor (PRCL = s Pag). The rotor speed is given by Nm = (1 – s) Ns Therefore, there are only two techniques by which the speed Nm of an induction motor can be controlled: I. One is to vary the synchronous speed, Ns. II. The other technique is to vary the slip, s. 2 1
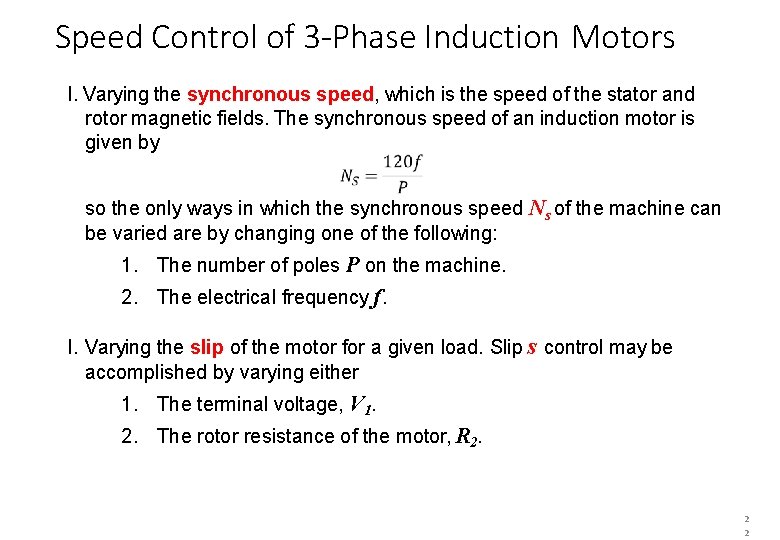
Speed Control of 3 -Phase Induction Motors I. Varying the synchronous speed, which is the speed of the stator and rotor magnetic fields. The synchronous speed of an induction motor is given by so the only ways in which the synchronous speed Ns of the machine can be varied are by changing one of the following: 1. The number of poles P on the machine. 2. The electrical frequency f. I. Varying the slip of the motor for a given load. Slip s control may be accomplished by varying either 1. The terminal voltage, V 1. 2. The rotor resistance of the motor, R 2. 2 2
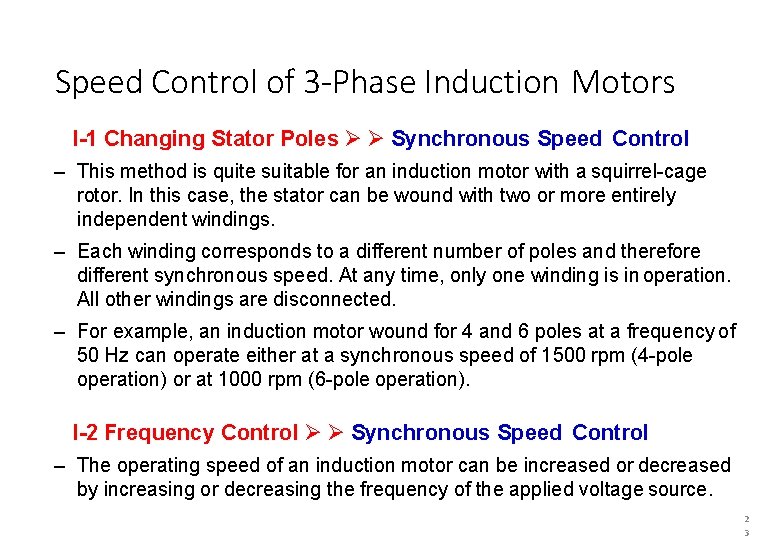
Speed Control of 3 -Phase Induction Motors I-1 Changing Stator Poles Synchronous Speed Control – This method is quite suitable for an induction motor with a squirrel-cage rotor. In this case, the stator can be wound with two or more entirely independent windings. – Each winding corresponds to a different number of poles and therefore different synchronous speed. At any time, only one winding is in operation. All other windings are disconnected. – For example, an induction motor wound for 4 and 6 poles at a frequency of 50 Hz can operate either at a synchronous speed of 1500 rpm (4 -pole operation) or at 1000 rpm (6 -pole operation). I-2 Frequency Control Synchronous Speed Control – The operating speed of an induction motor can be increased or decreased by increasing or decreasing the frequency of the applied voltage source. 2 3
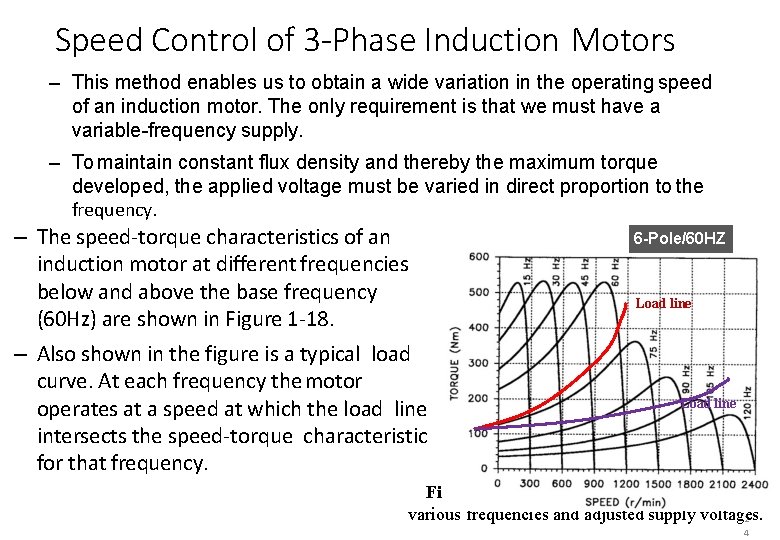
Speed Control of 3 -Phase Induction Motors – This method enables us to obtain a wide variation in the operating speed of an induction motor. The only requirement is that we must have a variable-frequency supply. – To maintain constant flux density and thereby the maximum torque developed, the applied voltage must be varied in direct proportion to the frequency. – The speed-torque characteristics of an induction motor at different frequencies below and above the base frequency (60 Hz) are shown in Figure 1 -18. – Also shown in the figure is a typical load curve. At each frequency the motor operates at a speed at which the load line intersects the speed-torque characteristic for that frequency. 6 -Pole/60 HZ Load line Figure 1 -18 Speed-torque characteristics for various frequencies and adjusted supply voltages. 2 4
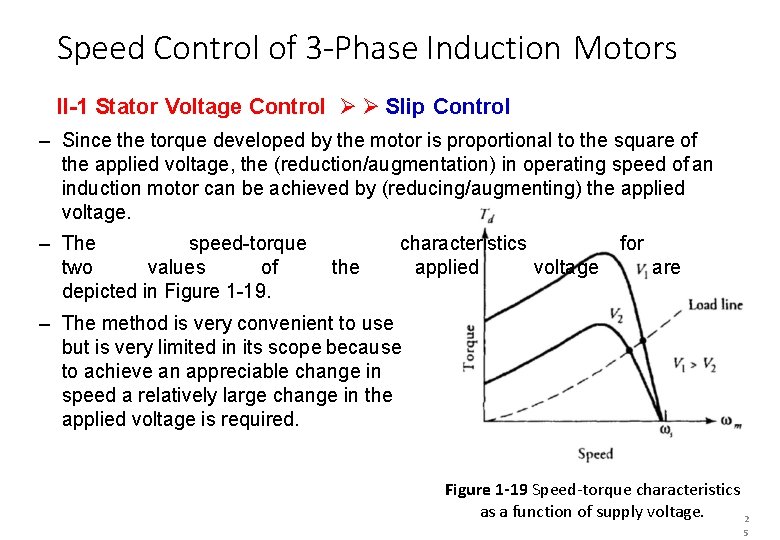
Speed Control of 3 -Phase Induction Motors II-1 Stator Voltage Control Slip Control – Since the torque developed by the motor is proportional to the square of the applied voltage, the (reduction/augmentation) in operating speed of an induction motor can be achieved by (reducing/augmenting) the applied voltage. – The speed-torque two values of depicted in Figure 1 -19. the characteristics applied voltage for are – The method is very convenient to use but is very limited in its scope because to achieve an appreciable change in speed a relatively large change in the applied voltage is required. Figure 1 -19 Speed-torque characteristics as a function of supply voltage. 2 5
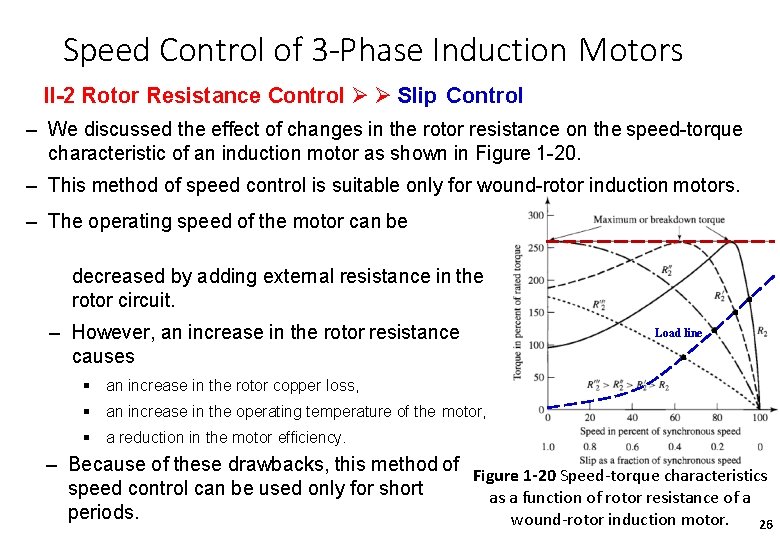
Speed Control of 3 -Phase Induction Motors II-2 Rotor Resistance Control Slip Control – We discussed the effect of changes in the rotor resistance on the speed-torque characteristic of an induction motor as shown in Figure 1 -20. – This method of speed control is suitable only for wound-rotor induction motors. – The operating speed of the motor can be decreased by adding external resistance in the rotor circuit. – However, an increase in the rotor resistance causes Load line an increase in the rotor copper loss, an increase in the operating temperature of the motor, a reduction in the motor efficiency. – Because of these drawbacks, this method of Figure 1 -20 Speed-torque characteristics speed control can be used only for short as a function of rotor resistance of a periods. wound-rotor induction motor. 26
Induction motors procurement
Variations pathologiques de la température
Example of inverse variation graph
Variations
Social variation differences
Language
Thermal stability in bjt
Independent assortment of chromosomes
Variations in psychological attributes
Kwl chart benefits
Variations
Possible variations
Super bowl squares variations
Valuation of variations
Snow white 1812
Possible variations
What is sine and cosine rule
Cultural variations in attachment
Natural variations operations management
Linked list variations
Joint variation equation examples
Kinds of variation
Forms of productive capacity
Variations de stock
Possible variations
Spec rating formula in computer organization
Is a direct variation a linear function