The Pesky Neutrino David E Jaffe Physics Department
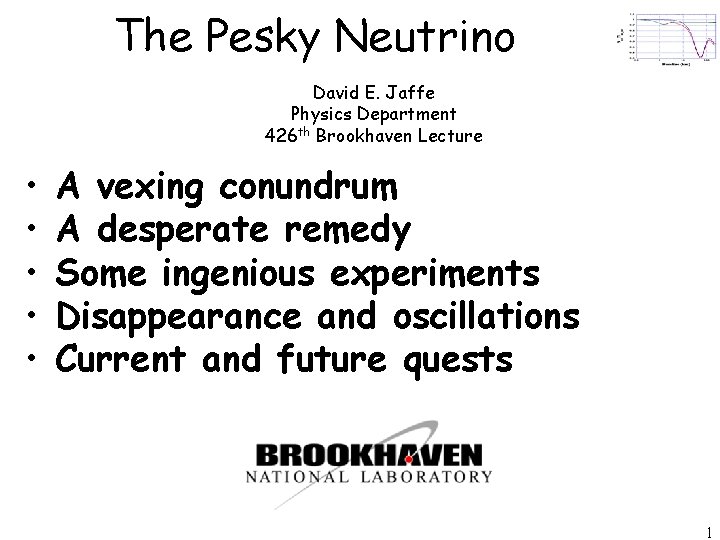
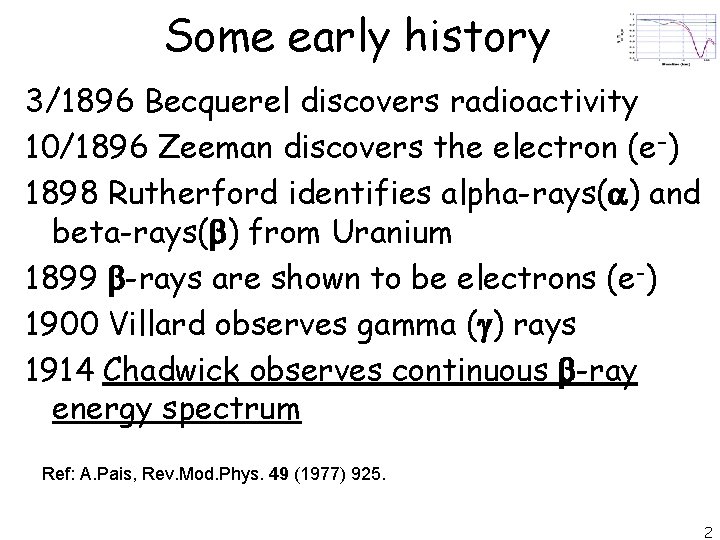
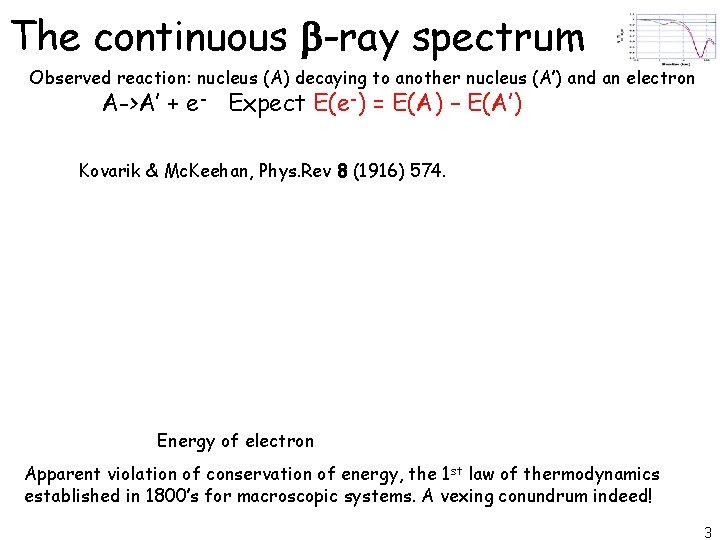
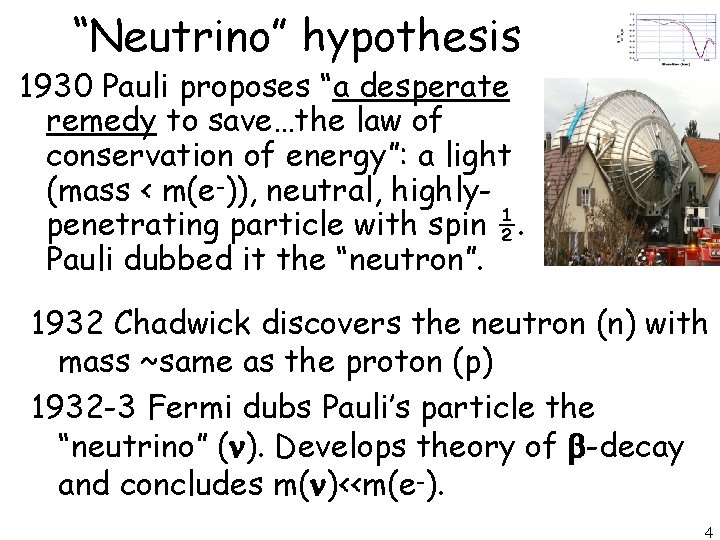
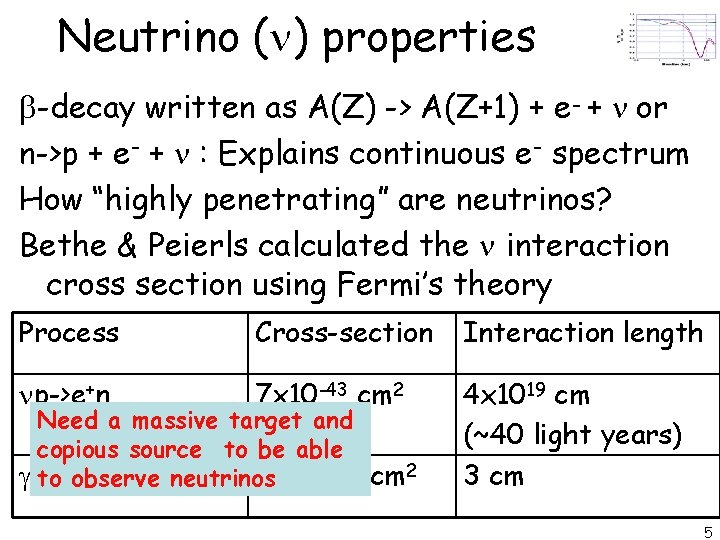
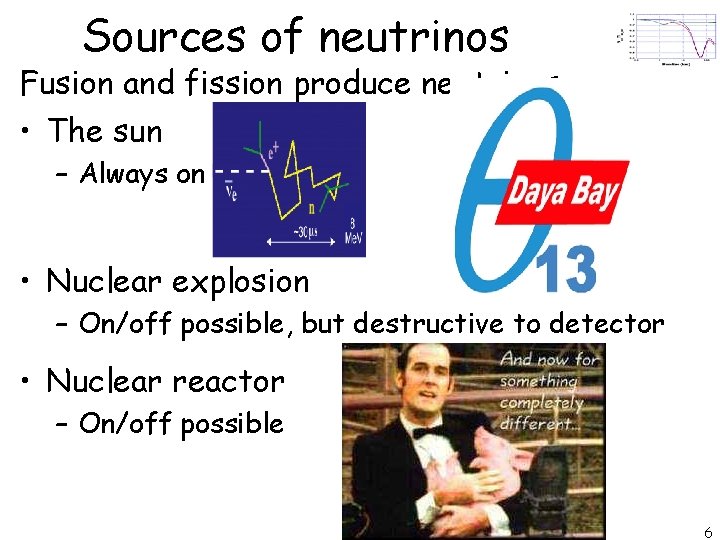
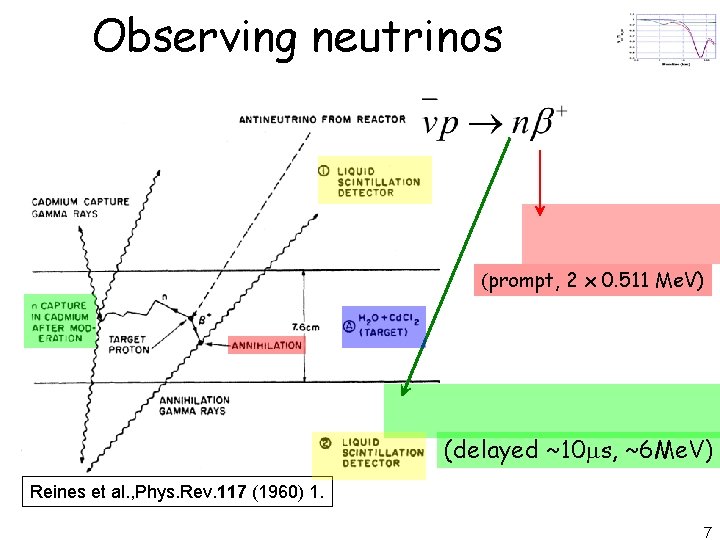
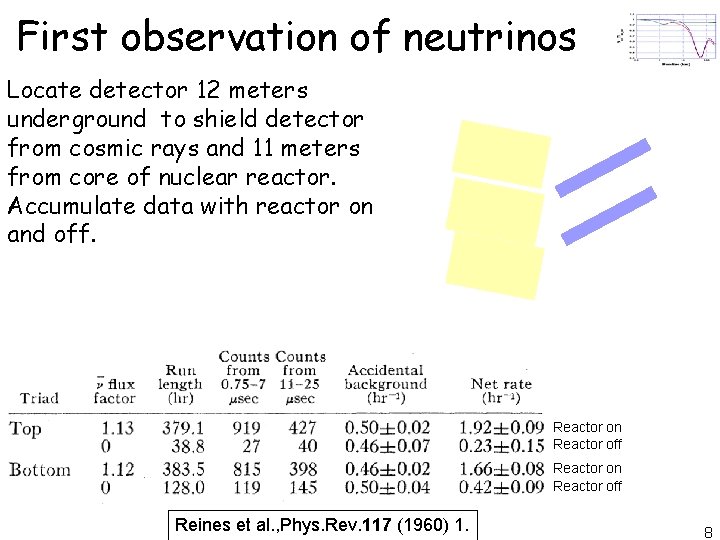
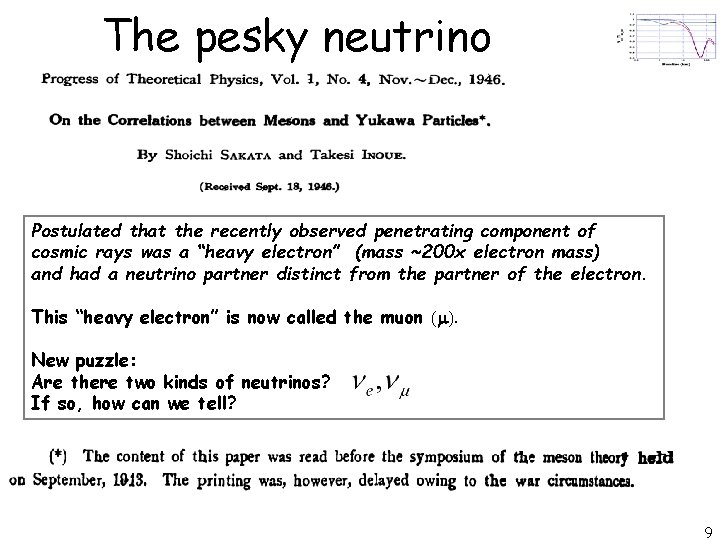
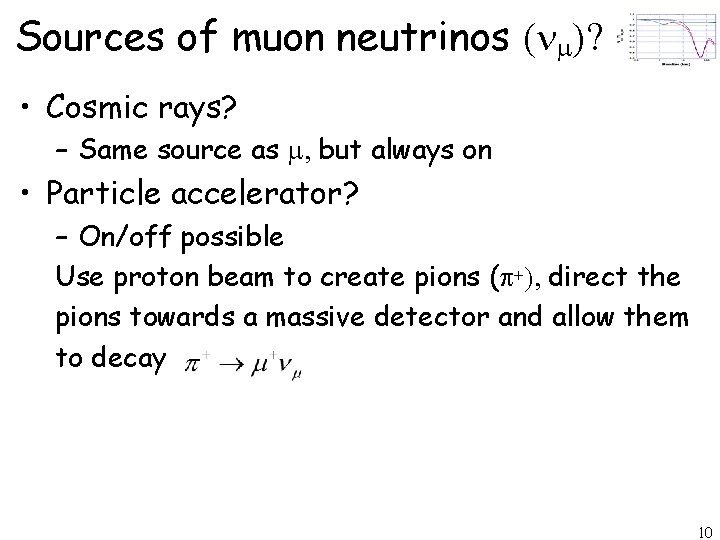
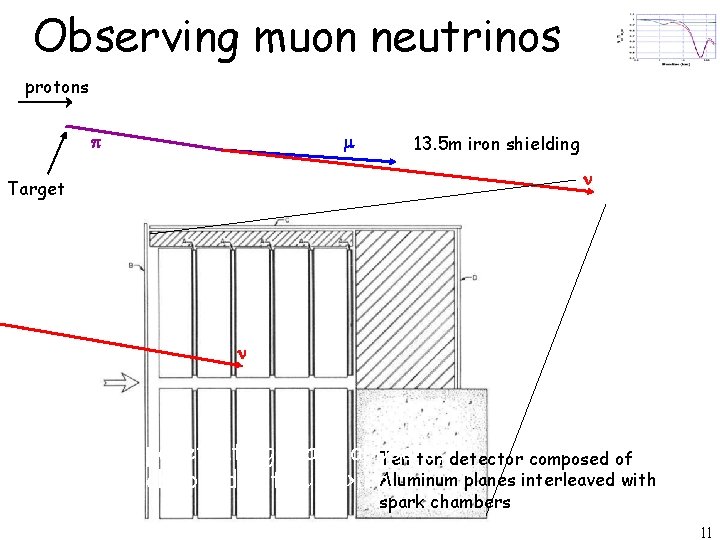
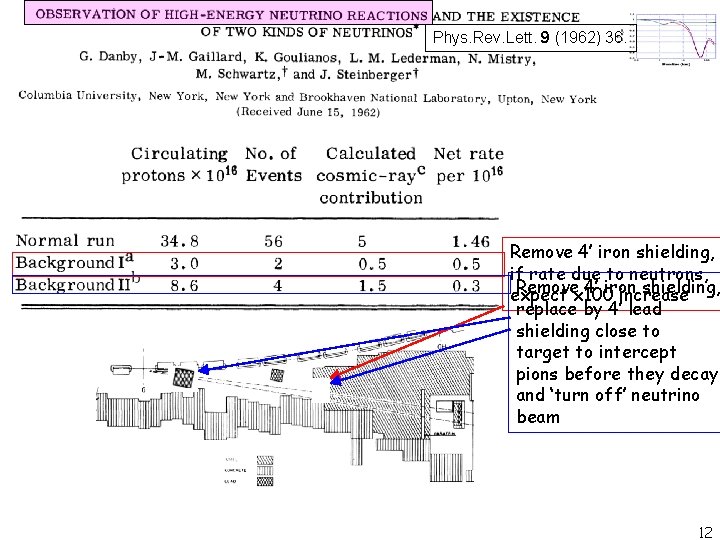
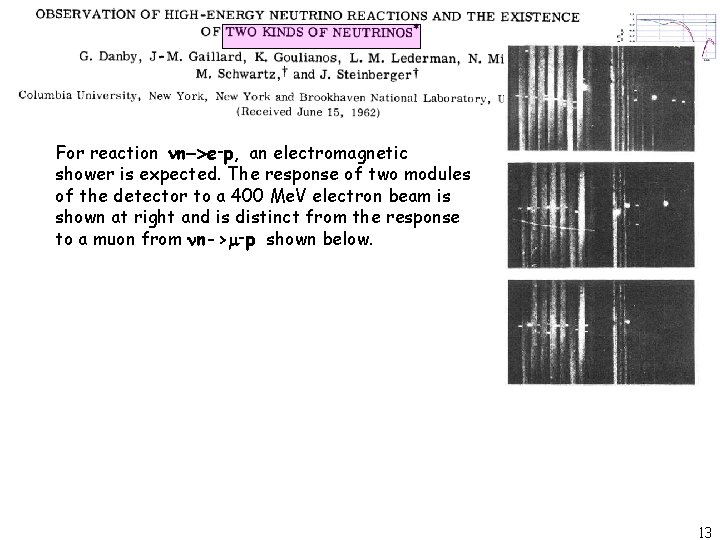
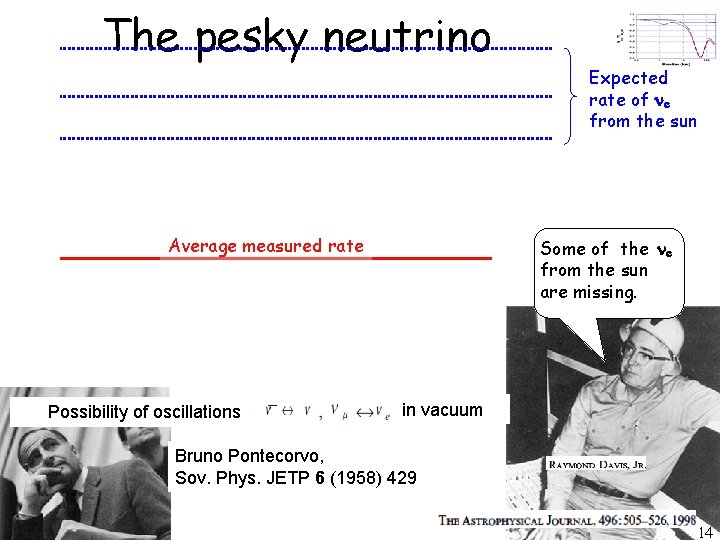
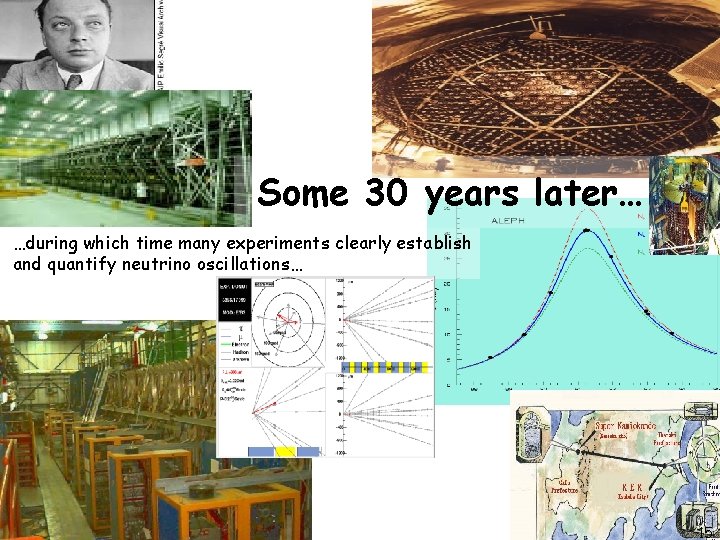
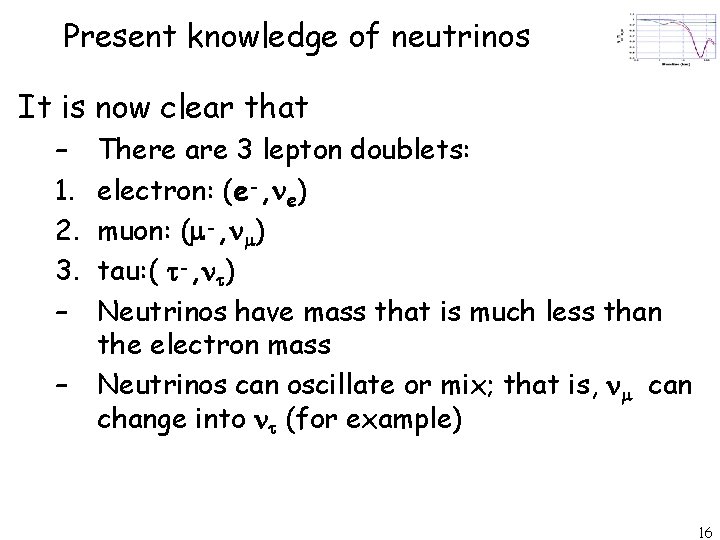
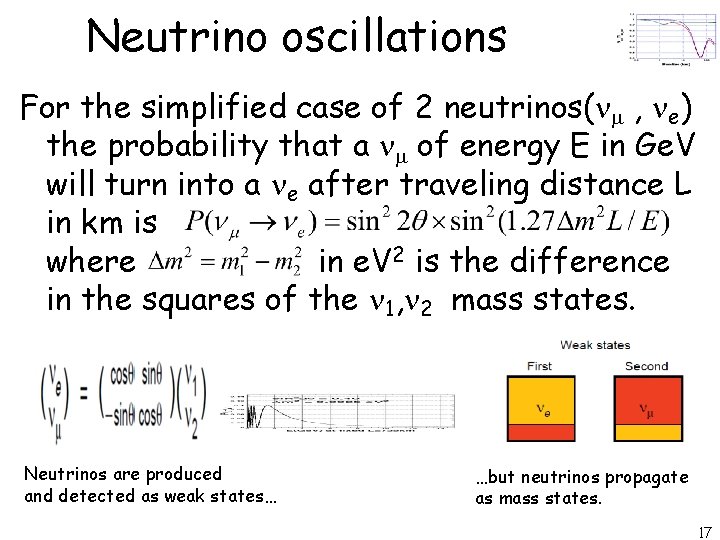
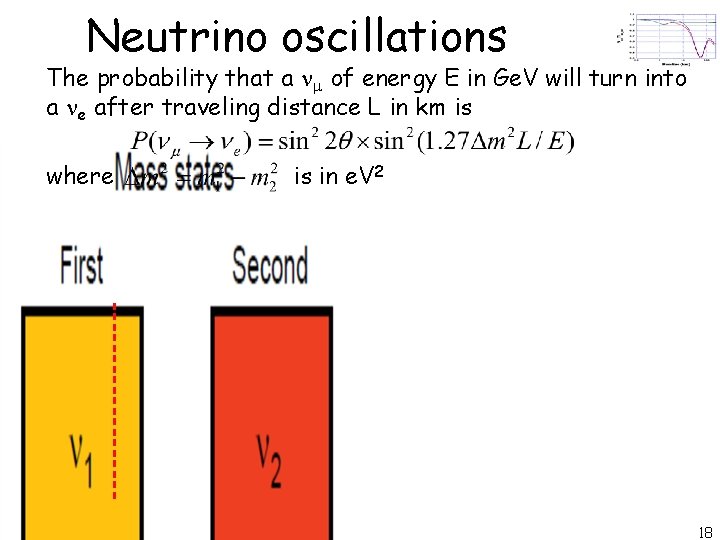
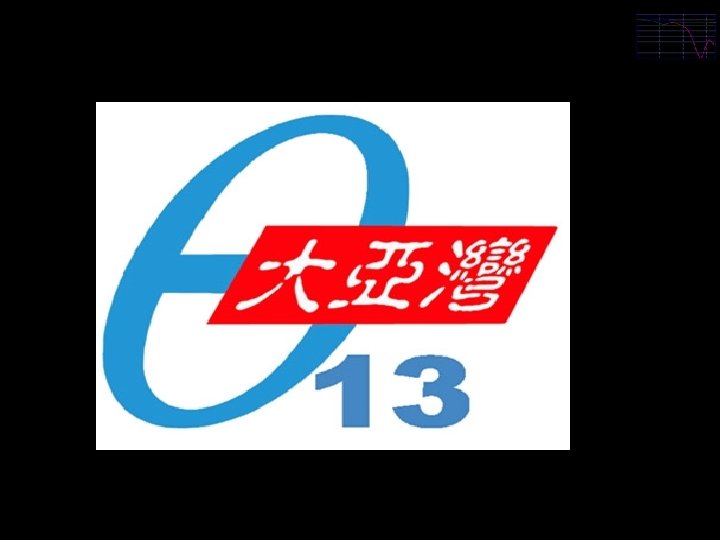
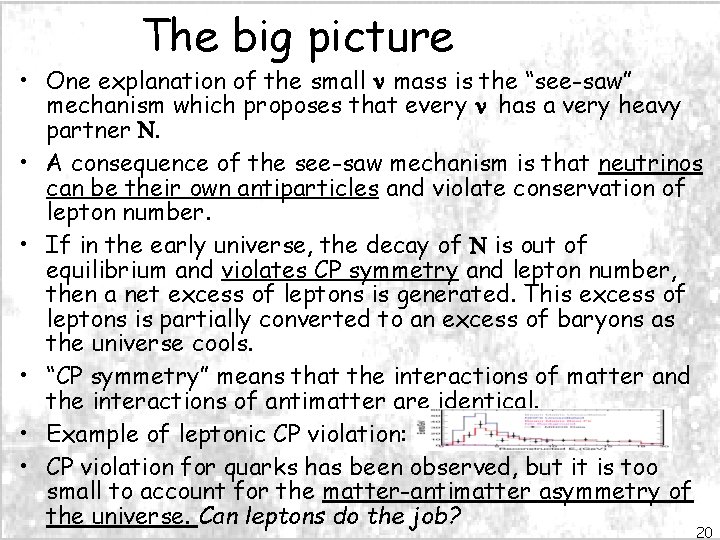
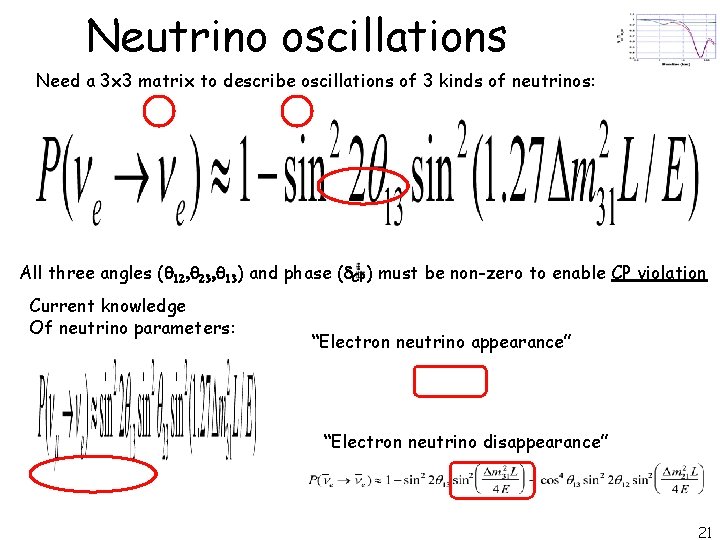
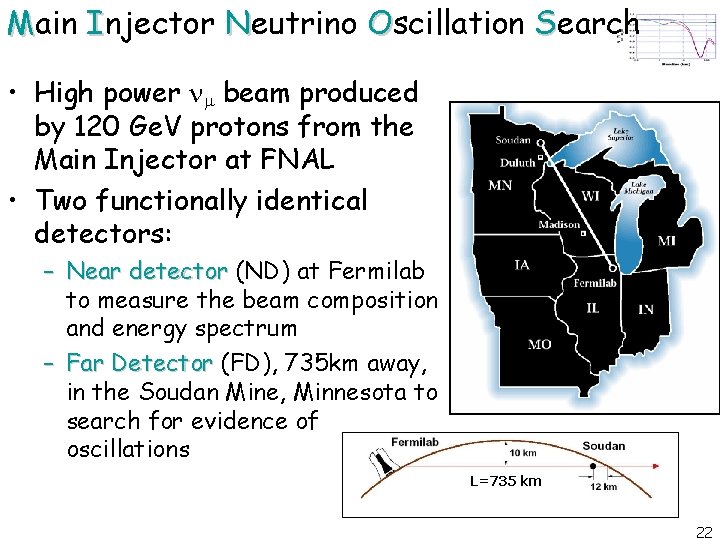
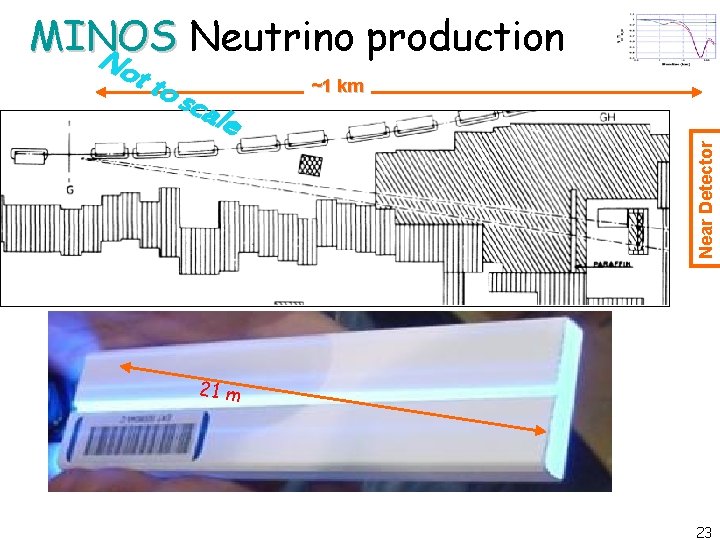
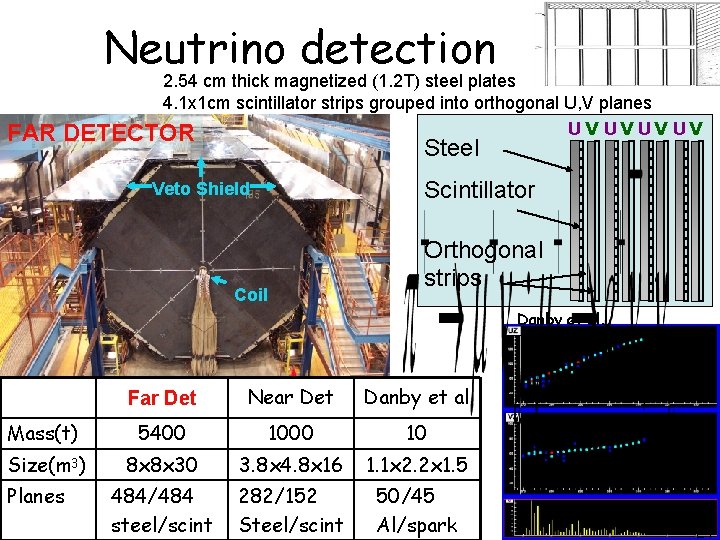
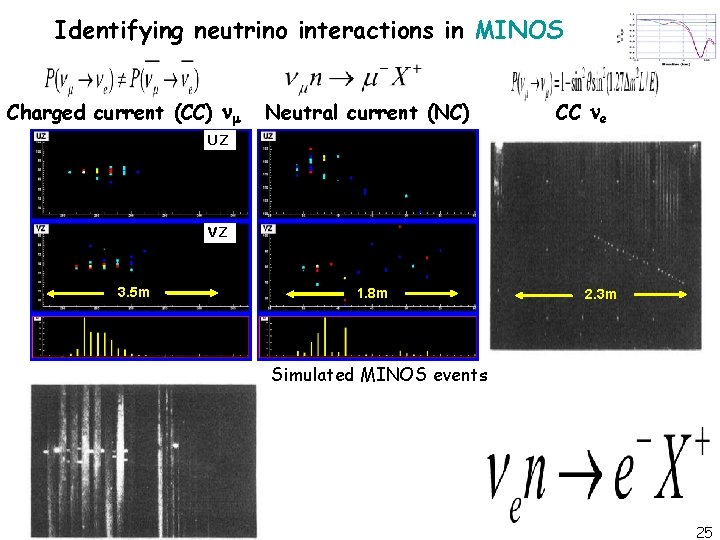
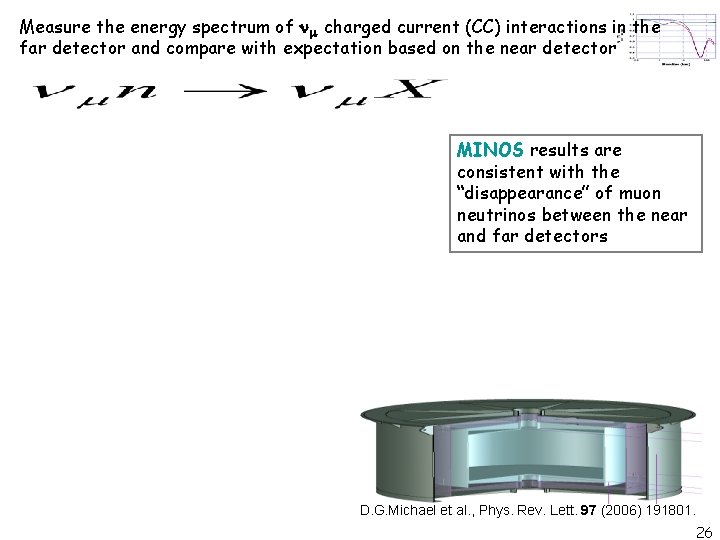
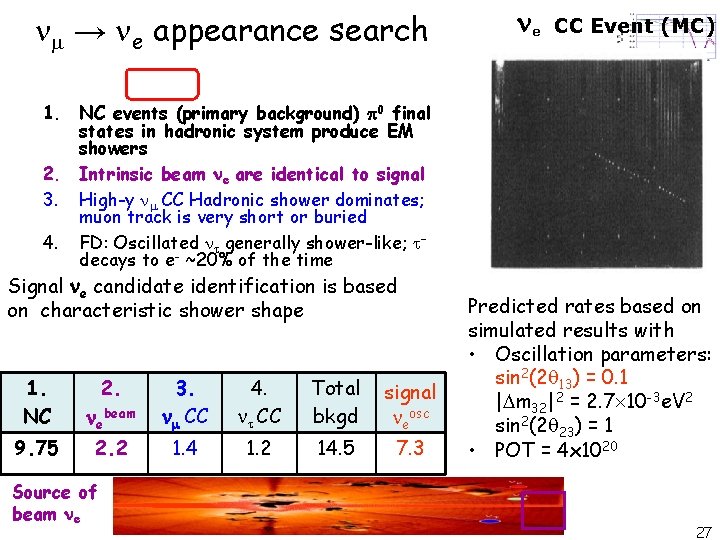
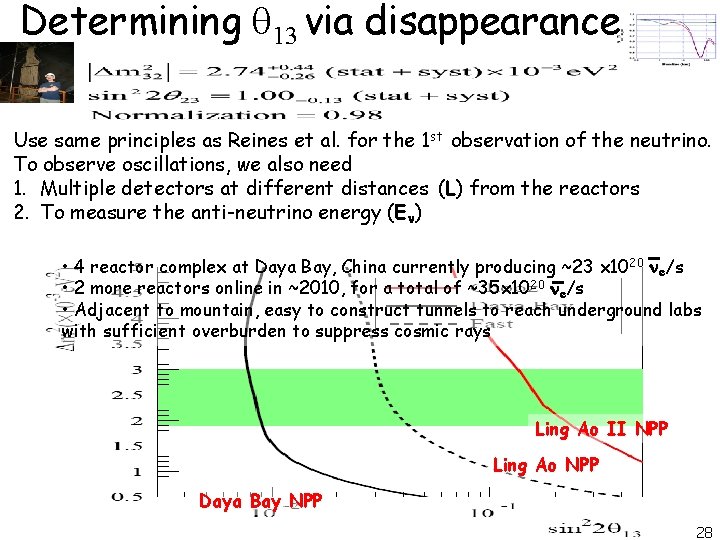
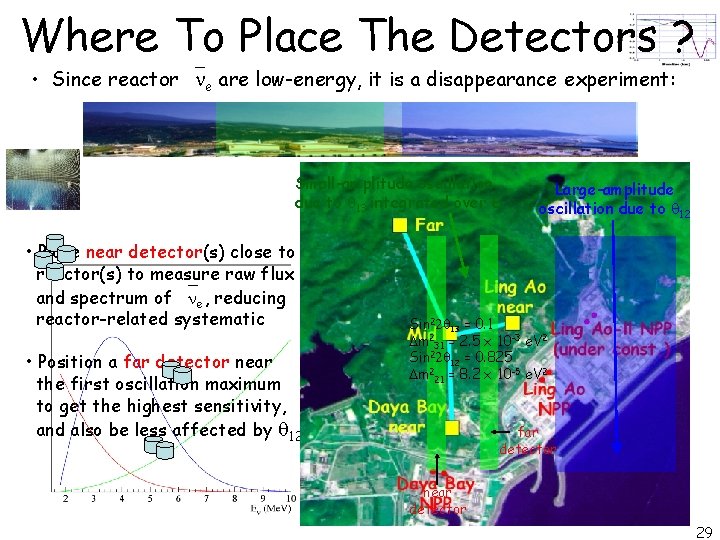
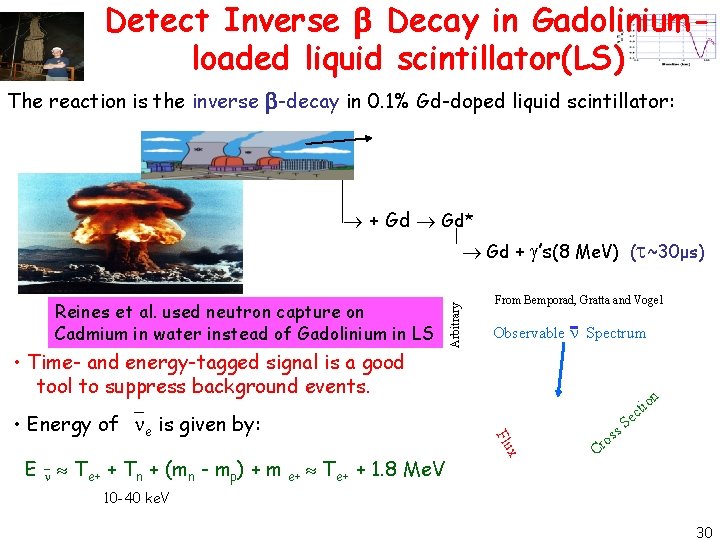
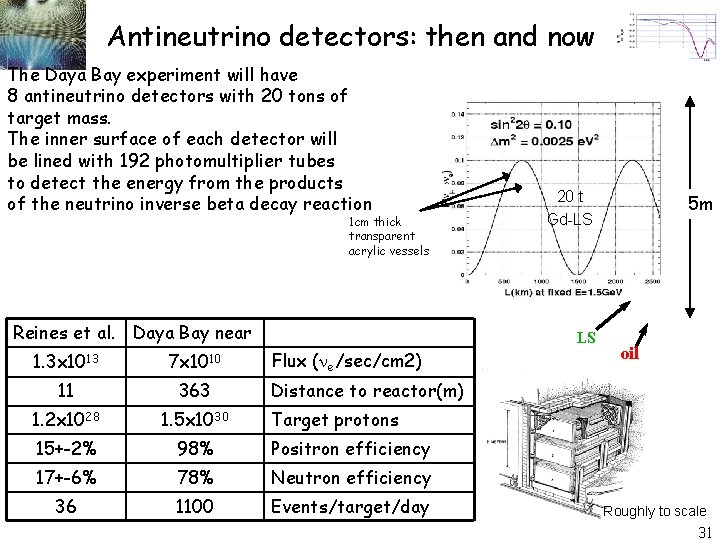
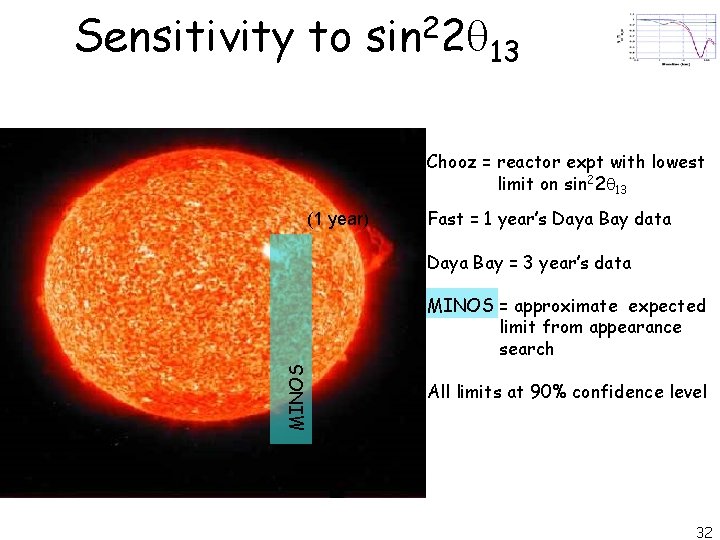
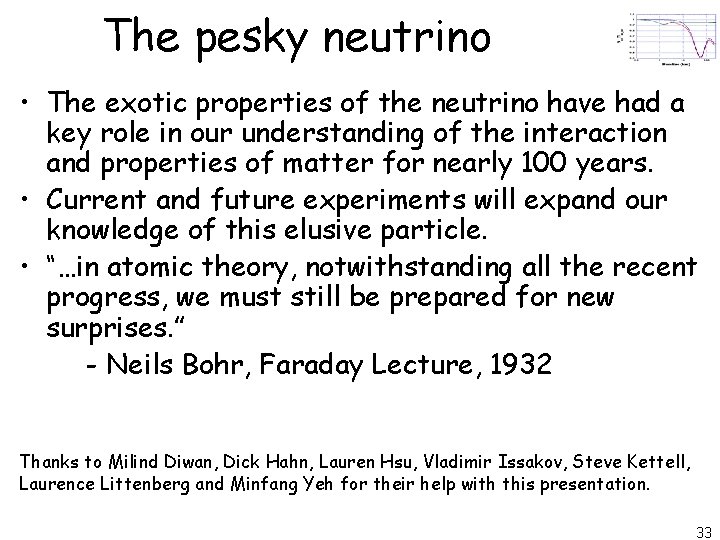
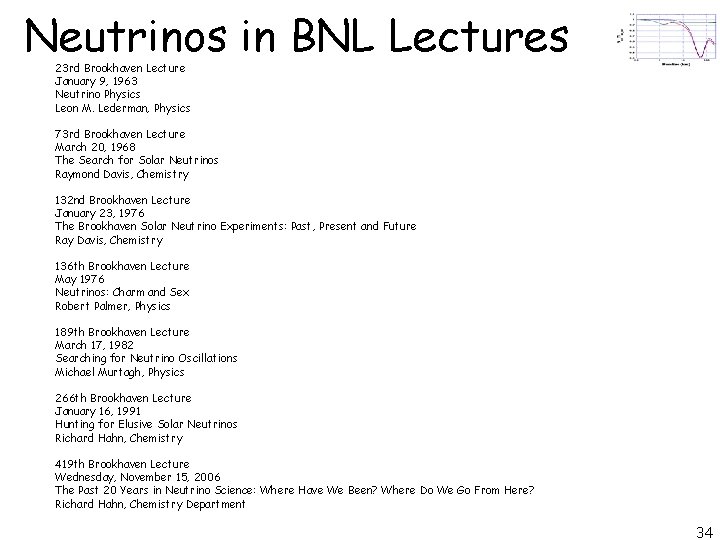
- Slides: 34
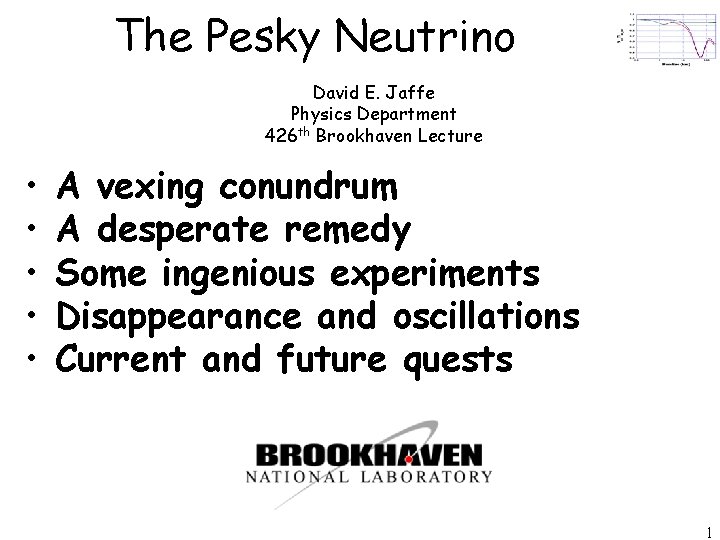
The Pesky Neutrino David E. Jaffe Physics Department 426 th Brookhaven Lecture • • • A vexing conundrum A desperate remedy Some ingenious experiments Disappearance and oscillations Current and future quests 1
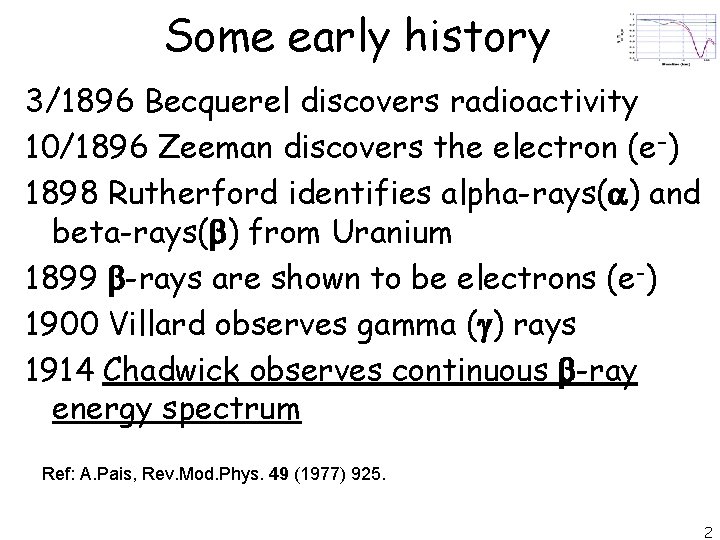
Some early history 3/1896 Becquerel discovers radioactivity 10/1896 Zeeman discovers the electron (e-) 1898 Rutherford identifies alpha-rays(a) and beta-rays( ) from Uranium 1899 -rays are shown to be electrons (e-) 1900 Villard observes gamma (g) rays 1914 Chadwick observes continuous -ray energy spectrum Ref: A. Pais, Rev. Mod. Phys. 49 (1977) 925. 2
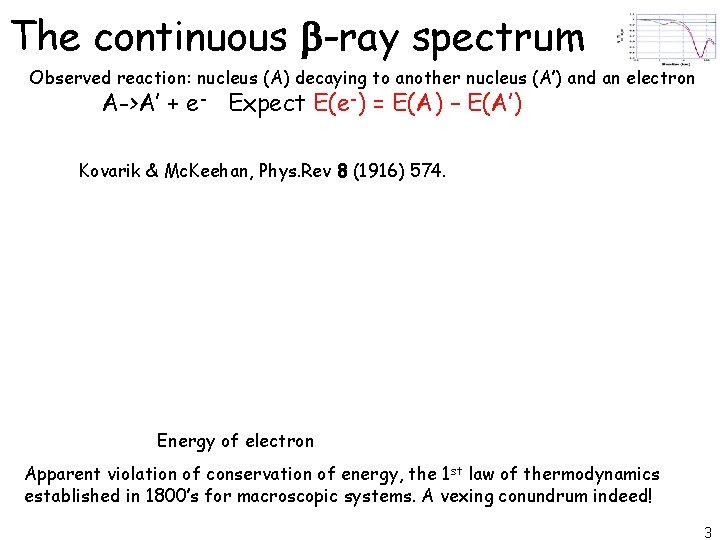
The continuous -ray spectrum Observed reaction: nucleus (A) decaying to another nucleus (A’) and an electron A->A’ + e- Expect E(e-) = E(A) – E(A’) Kovarik & Mc. Keehan, Phys. Rev 8 (1916) 574. Energy of electron Apparent violation of conservation of energy, the 1 st law of thermodynamics established in 1800’s for macroscopic systems. A vexing conundrum indeed! 3
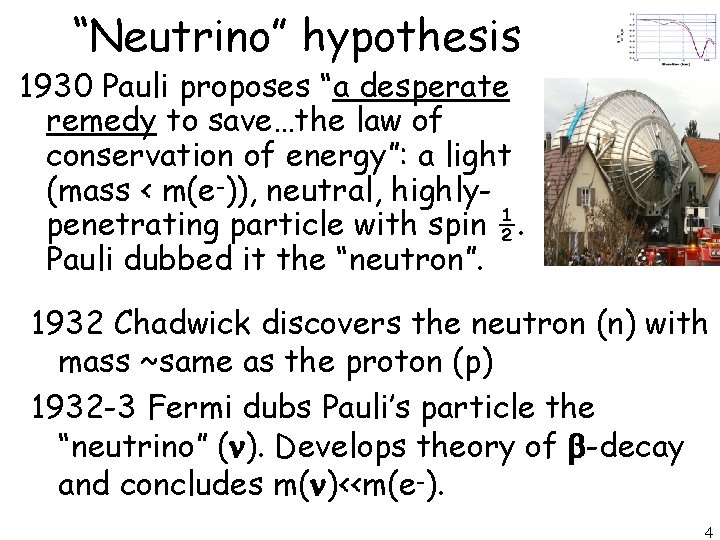
“Neutrino” hypothesis 1930 Pauli proposes “a desperate remedy to save…the law of conservation of energy”: a light (mass < m(e-)), neutral, highlypenetrating particle with spin ½. Pauli dubbed it the “neutron”. 1932 Chadwick discovers the neutron (n) with mass ~same as the proton (p) 1932 -3 Fermi dubs Pauli’s particle the “neutrino” ( ). Develops theory of -decay and concludes m( )<<m(e-). 4
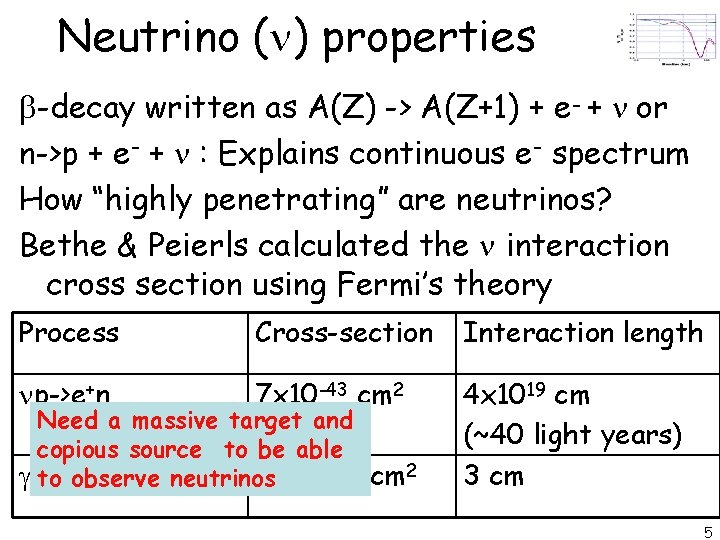
Neutrino ( ) properties b-decay written as A(Z) -> A(Z+1) + e- + or n->p + e- + : Explains continuous e- spectrum How “highly penetrating” are neutrinos? Bethe & Peierls calculated the interaction cross section using Fermi’s theory Process Cross-section Interaction length p->e+n 7 x 10 -43 cm 2 4 x 1019 cm (~40 light years) 3 cm Need a massive target and copious source to be able Pb 10 x 10 -24 cm 2 to observe neutrinos 5
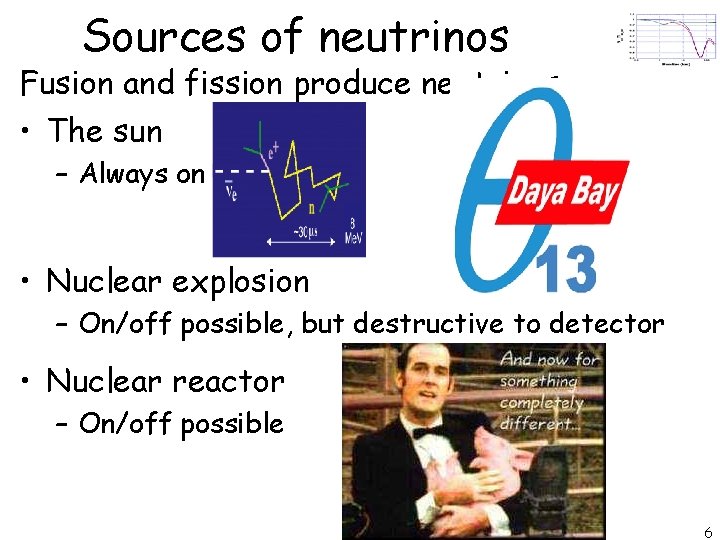
Sources of neutrinos Fusion and fission produce neutrinos • The sun – Always on • Nuclear explosion – On/off possible, but destructive to detector • Nuclear reactor – On/off possible 6
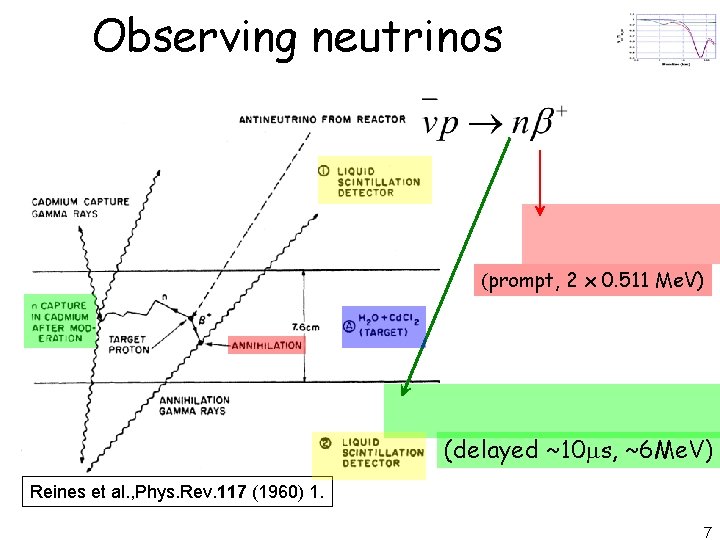
Observing neutrinos (prompt, 2 x 0. 511 Me. V) (delayed ~10 ms, ~6 Me. V) Reines et al. , Phys. Rev. 117 (1960) 1. 7
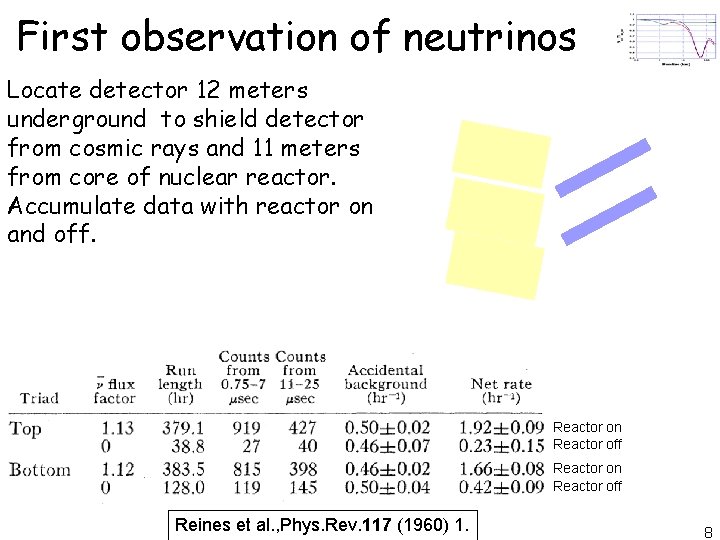
First observation of neutrinos Locate detector 12 meters underground to shield detector from cosmic rays and 11 meters from core of nuclear reactor. Accumulate data with reactor on and off. Reactor on Reactor off Reines et al. , Phys. Rev. 117 (1960) 1. 8
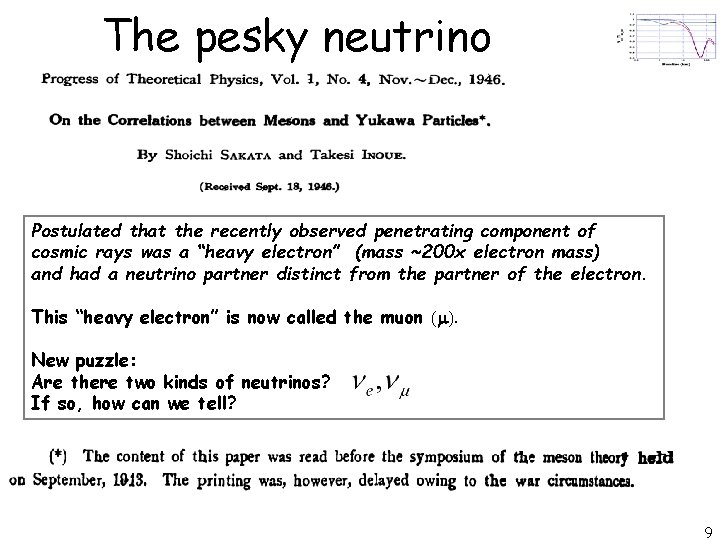
The pesky neutrino Postulated that the recently observed penetrating component of cosmic rays was a “heavy electron” (mass ~200 x electron mass) and had a neutrino partner distinct from the partner of the electron. This “heavy electron” is now called the muon ( ). New puzzle: Are there two kinds of neutrinos? If so, how can we tell? 9
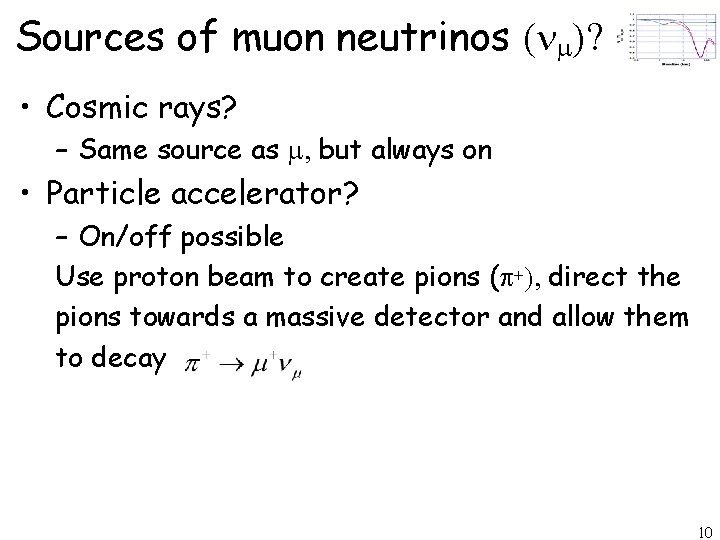
Sources of muon neutrinos ( m)? • Cosmic rays? – Same source as m, but always on • Particle accelerator? – On/off possible Use proton beam to create pions (p+), direct the pions towards a massive detector and allow them to decay 10
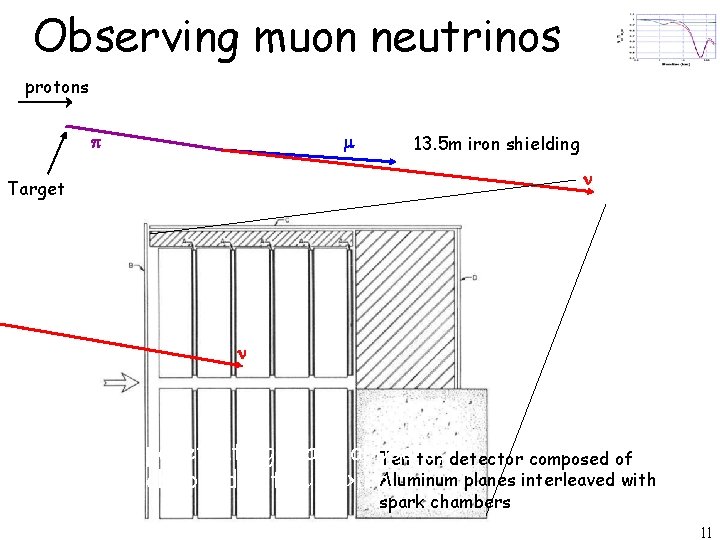
Observing muon neutrinos protons p 13. 5 m iron shielding Target A penetrating track appears, Ten ton detector composed of probably due to mn->m-p. Aluminum planes interleaved with spark chambers 11
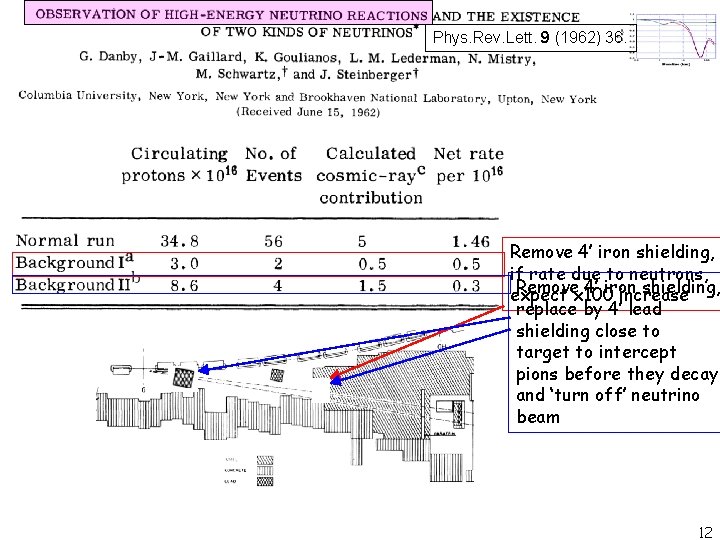
Phys. Rev. Lett. 9 (1962) 36. Remove 4’ iron shielding, if rate due to neutrons, Removex 100 4’ iron shielding, expect increase replace by 4’ lead shielding close to target to intercept pions before they decay and ‘turn off’ neutrino beam 12
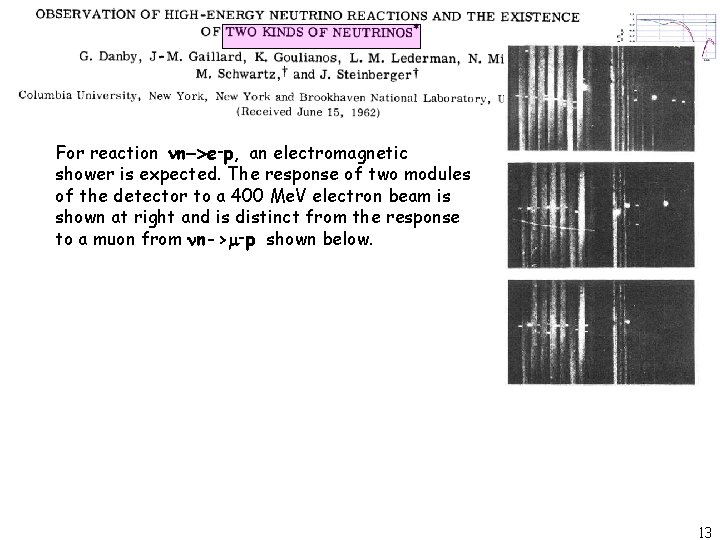
For reaction n->e-p, an electromagnetic shower is expected. The response of two modules of the detector to a 400 Me. V electron beam is shown at right and is distinct from the response to a muon from n-> -p shown below. A penetrating track appears, probably due to mn->m-p 13
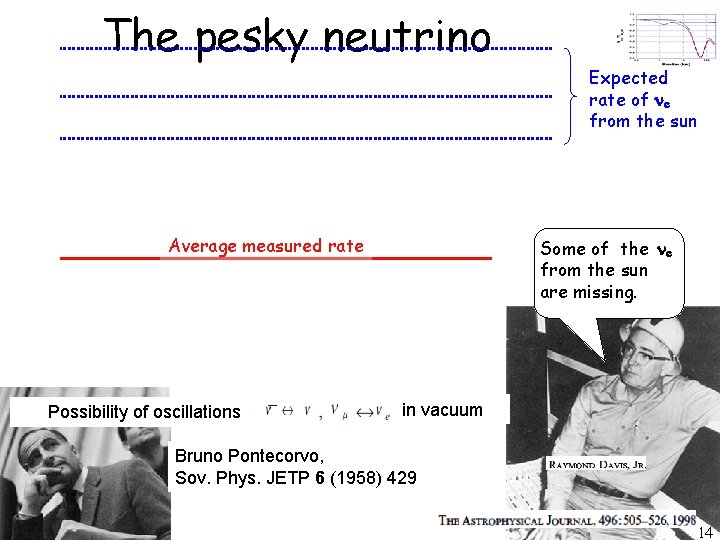
The pesky neutrino Average measured rate Possibility of oscillations Expected rate of e from the sun Some of the e from the sun are missing. in vacuum Bruno Pontecorvo, Sov. Phys. JETP 6 (1958) 429 14
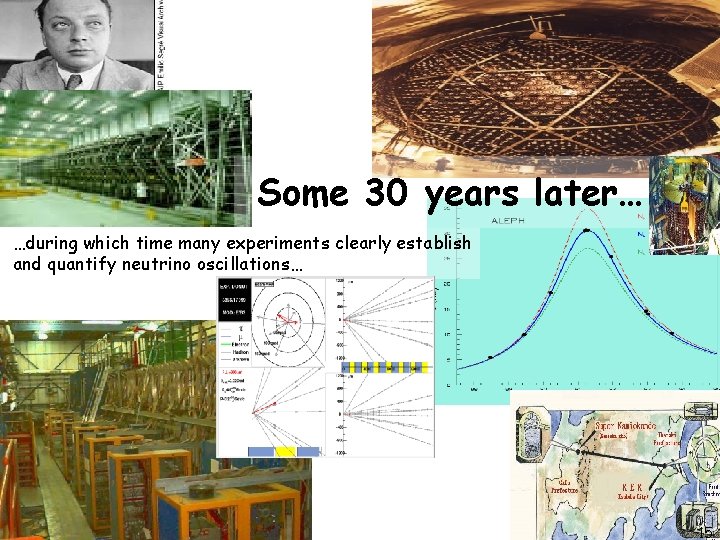
Some 30 years later… …during which time many experiments clearly establish and quantify neutrino oscillations… 15
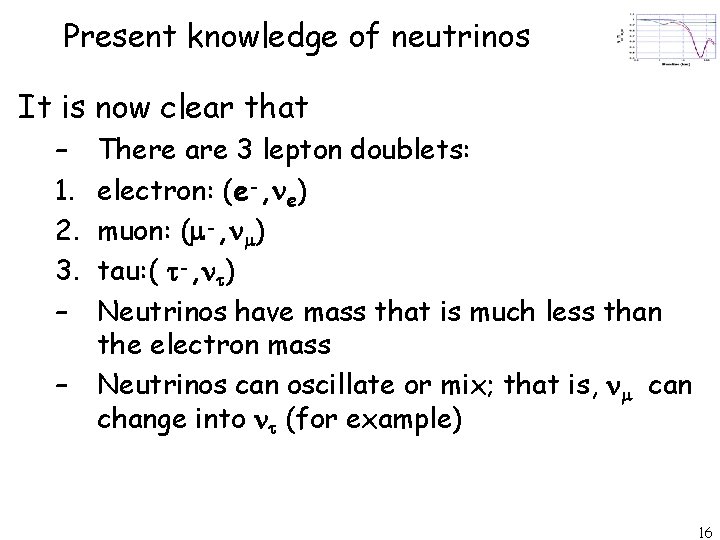
Present knowledge of neutrinos It is now clear that – 1. 2. 3. – – There are 3 lepton doublets: electron: (e-, e) muon: ( -, ) tau: ( t-, t) Neutrinos have mass that is much less than the electron mass Neutrinos can oscillate or mix; that is, can change into t (for example) 16
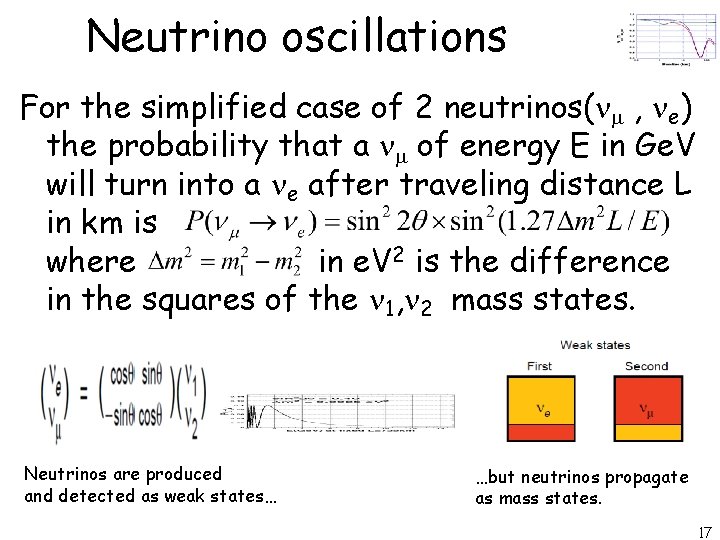
Neutrino oscillations For the simplified case of 2 neutrinos( m , e) the probability that a m of energy E in Ge. V will turn into a e after traveling distance L in km is where in e. V 2 is the difference in the squares of the 1, 2 mass states. Neutrinos are produced and detected as weak states… …but neutrinos propagate as mass states. 17
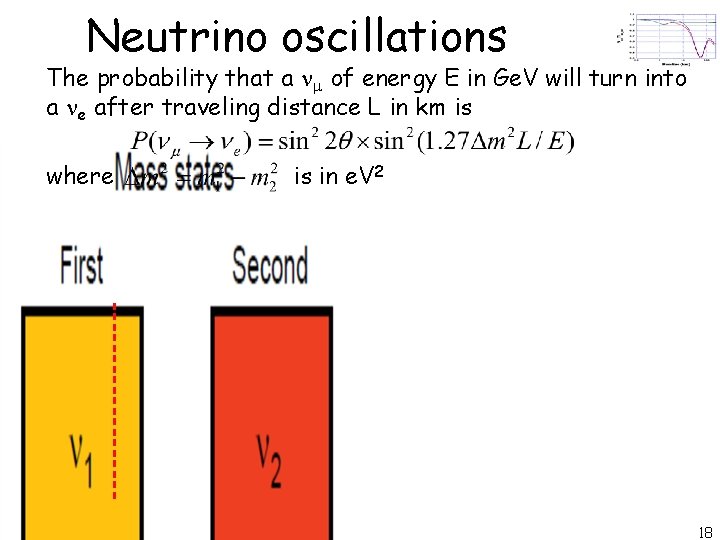
Neutrino oscillations The probability that a m of energy E in Ge. V will turn into a e after traveling distance L in km is where is in e. V 2 18
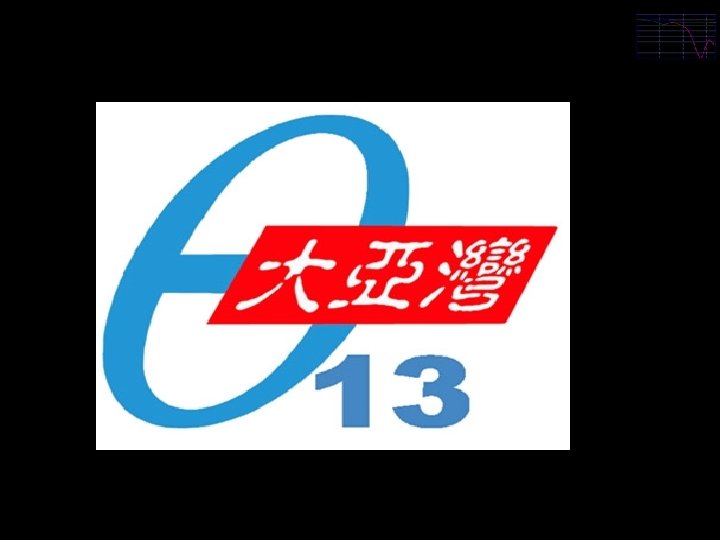
19
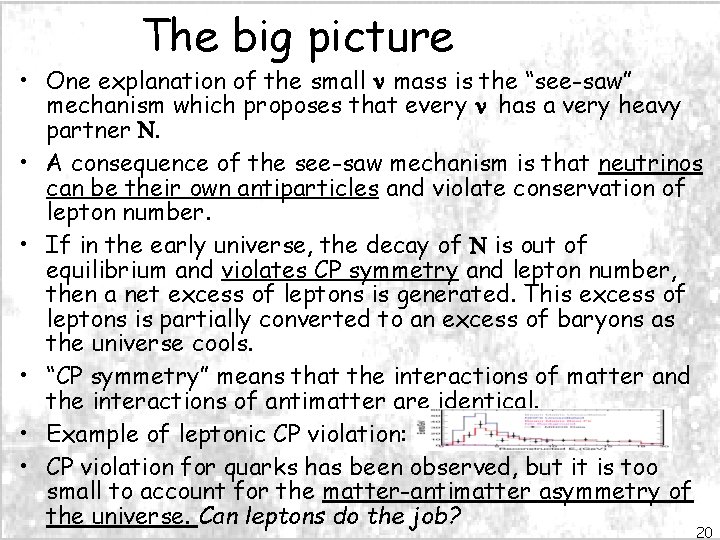
The big picture • One explanation of the small mass is the “see-saw” mechanism which proposes that every has a very heavy partner N. • A consequence of the see-saw mechanism is that neutrinos can be their own antiparticles and violate conservation of lepton number. • If in the early universe, the decay of N is out of equilibrium and violates CP symmetry and lepton number, then a net excess of leptons is generated. This excess of leptons is partially converted to an excess of baryons as the universe cools. • “CP symmetry” means that the interactions of matter and the interactions of antimatter are identical. • Example of leptonic CP violation: • CP violation for quarks has been observed, but it is too small to account for the matter-antimatter asymmetry of the universe. Can leptons do the job? 20
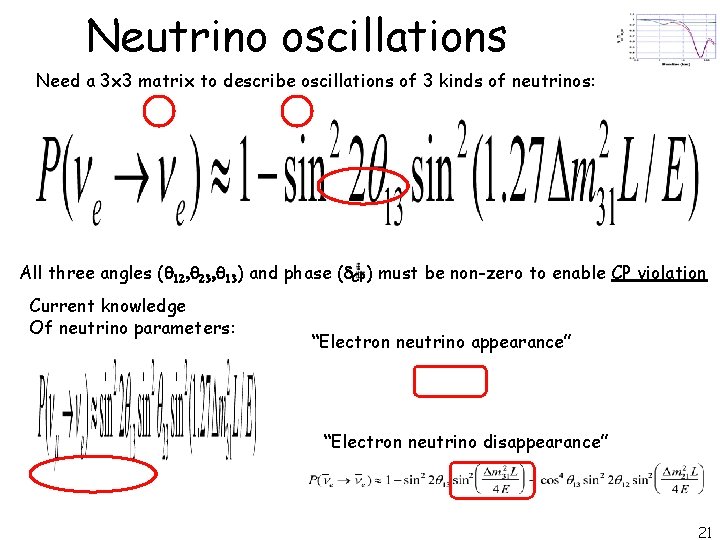
Neutrino oscillations Need a 3 x 3 matrix to describe oscillations of 3 kinds of neutrinos: All three angles (q 12, q 23, q 13) and phase (d. CP) must be non-zero to enable CP violation Current knowledge Of neutrino parameters: “Electron neutrino appearance” “Electron neutrino disappearance” 21
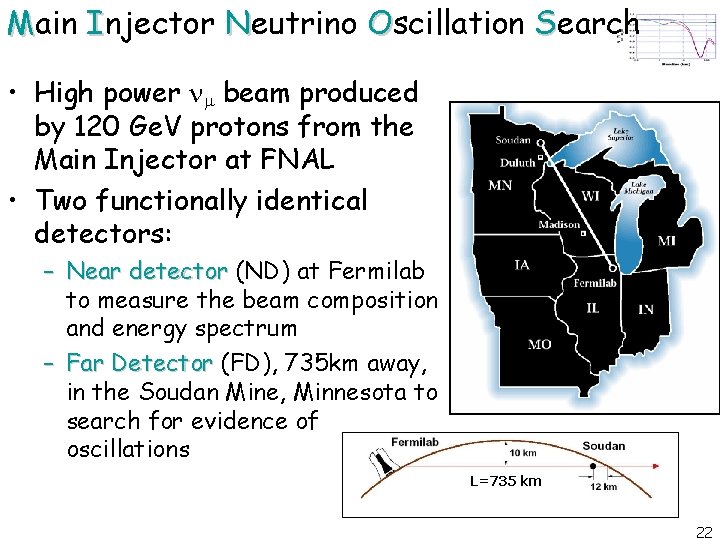
Main Injector Neutrino Oscillation Search • High power m beam produced by 120 Ge. V protons from the Main Injector at FNAL • Two functionally identical detectors: – Near detector (ND) at Fermilab to measure the beam composition and energy spectrum – Far Detector (FD), 735 km away, in the Soudan Mine, Minnesota to search for evidence of oscillations L=735 km 22
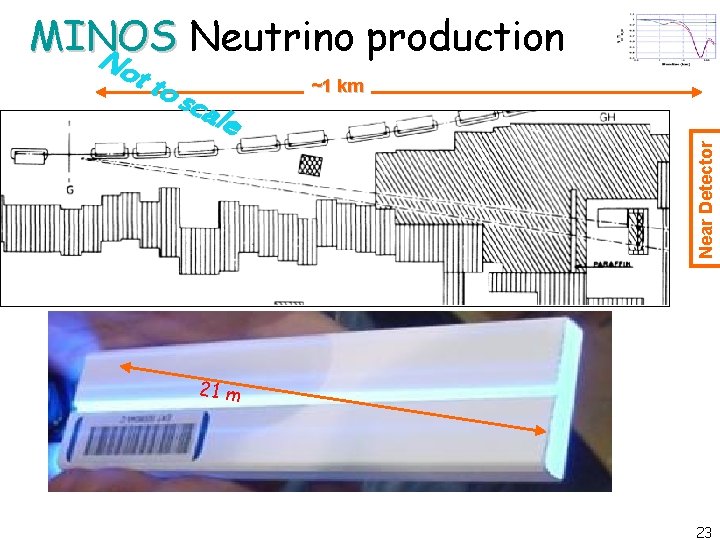
MINOS Neutrino production N o sc ale ~1 km Near Detector ot t 21 m 23
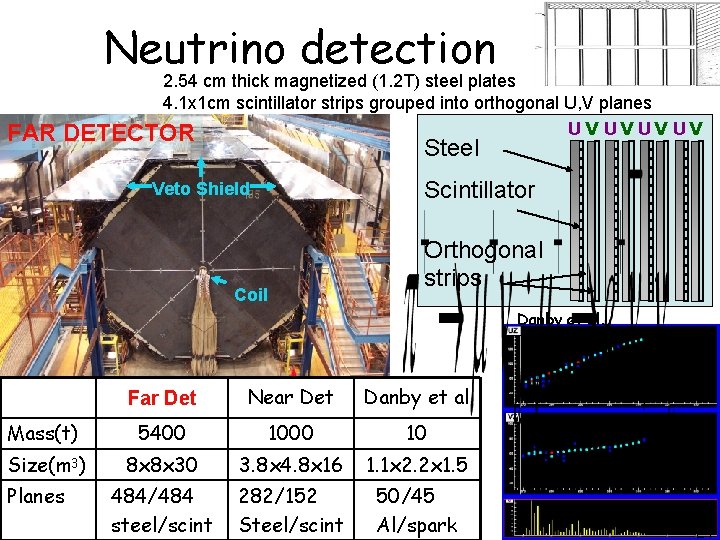
Neutrino detection FAR 2. 54 cm thick magnetized (1. 2 T) steel plates 4. 1 x 1 cm scintillator strips grouped into orthogonal U, V planes UV UV DETECTOR Steel Scintillator Veto Shield Orthogonal strips Coil Danby et al. Far Det Near Det Danby et al. Mass(t) 5400 10 Size(m 3) 8 x 8 x 30 3. 8 x 4. 8 x 16 1. 1 x 2. 2 x 1. 5 484/484 steel/scint 282/152 Steel/scint 50/45 Al/spark Planes 24
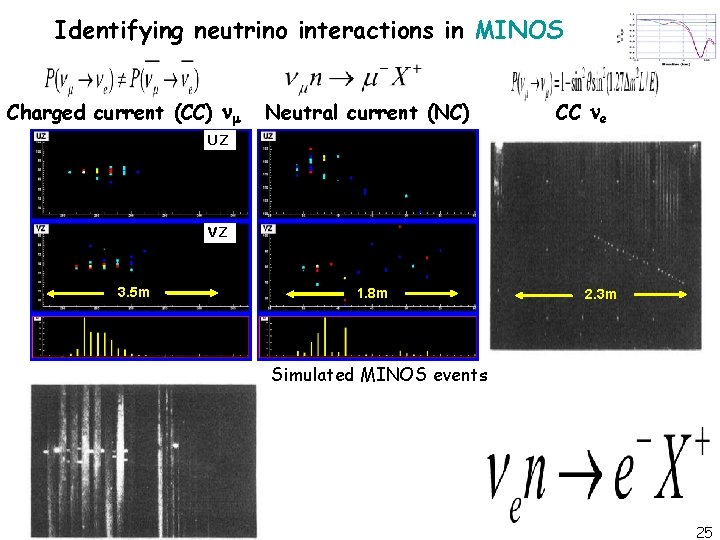
Identifying neutrino interactions in MINOS Charged current (CC) Neutral current (NC) CC e UZ VZ 3. 5 m 1. 8 m 2. 3 m Simulated MINOS events 25
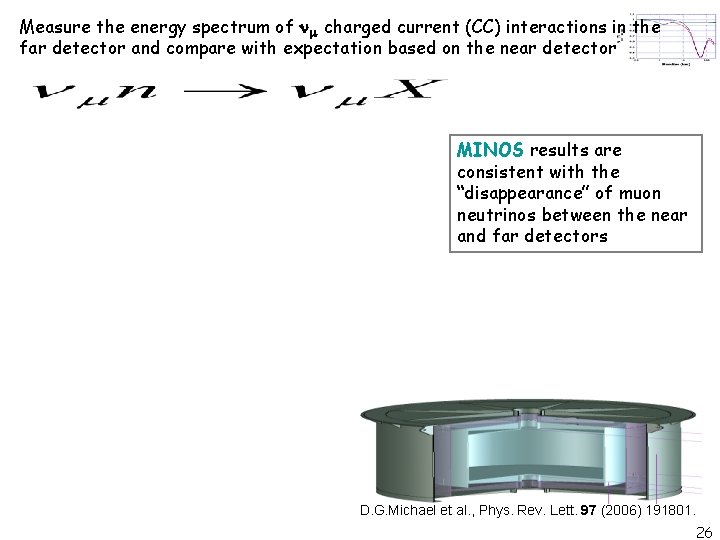
Measure the energy spectrum of charged current (CC) interactions in the far detector and compare with expectation based on the near detector MINOS results are consistent with the “disappearance” of muon neutrinos between the near and far detectors D. G. Michael et al. , Phys. Rev. Lett. 97 (2006) 191801. 26
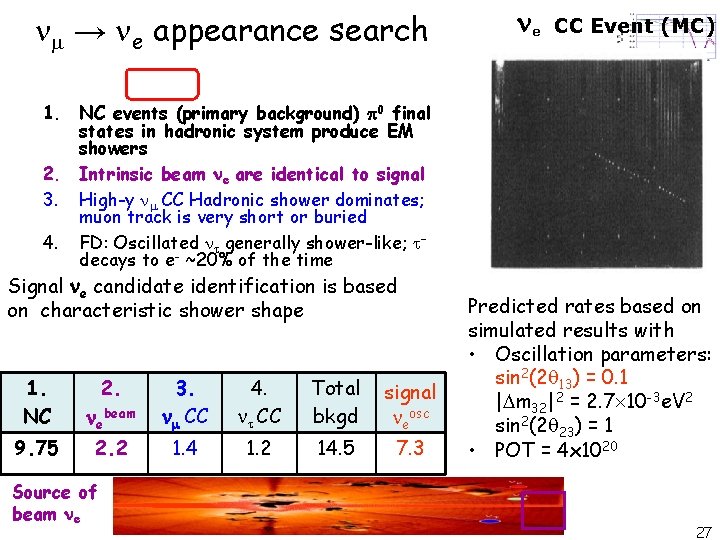
m → e appearance search e CC Event (MC) 1. NC events (primary background) p 0 final states in hadronic system produce EM showers 2. Intrinsic beam e are identical to signal 3. High-y m CC Hadronic shower dominates; muon track is very short or buried 4. FD: Oscillated generally shower-like; decays to e- ~20% of the time Signal e candidate identification is based on characteristic shower shape 1. NC 9. 75 2. ebeam 2. 2 Source of beam e 3. CC 4. CC Total bkgd 1. 4 1. 2 14. 5 signal eosc 7. 3 Predicted rates based on simulated results with • Oscillation parameters: sin 2(2 13) = 0. 1 | m 32|2 = 2. 7 10 -3 e. V 2 sin 2(2 23) = 1 • POT = 4 x 1020 27
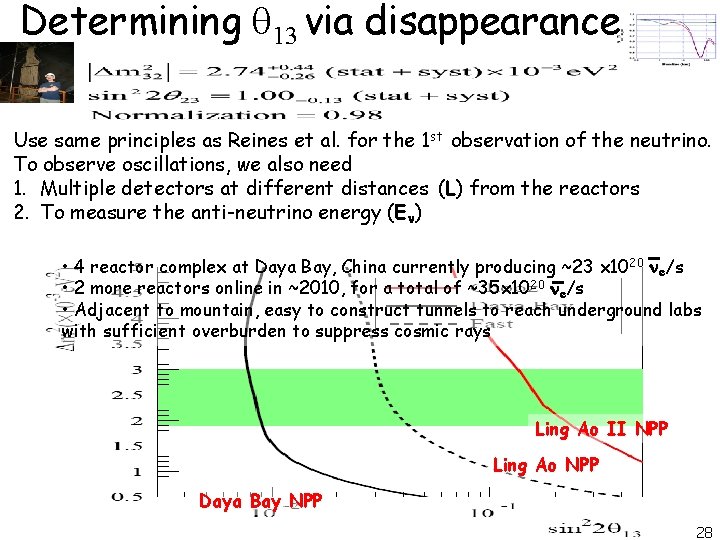
Determining 13 via disappearance Use same principles as Reines et al. for the 1 st observation of the neutrino. To observe oscillations, we also need 1. Multiple detectors at different distances (L) from the reactors 2. To measure the anti-neutrino energy (E ) • 4 reactor complex at Daya Bay, China currently producing ~23 x 1020 e/s • 2 more reactors online in ~2010, for a total of ~35 x 1020 e/s • Adjacent to mountain, easy to construct tunnels to reach underground labs with sufficient overburden to suppress cosmic rays Ling Ao II NPP Ling Ao NPP Daya Bay NPP 28
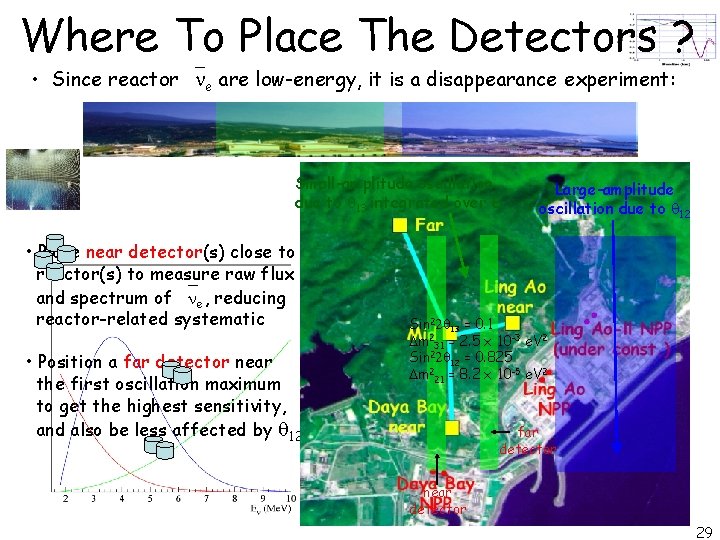
Where To Place The Detectors ? • Since reactor e are low-energy, it is a disappearance experiment: Small-amplitude oscillation due to 13 integrated over E • Place near detector(s) close to reactor(s) to measure raw flux and spectrum of e, reducing reactor-related systematic • Position a far detector near the first oscillation maximum to get the highest sensitivity, and also be less affected by 12 Large-amplitude oscillation due to 12 Sin 22 13 = 0. 1 m 231 = 2. 5 x 10 -3 e. V 2 Sin 22 12 = 0. 825 m 221 = 8. 2 x 10 -5 e. V 2 far detector near detector 29
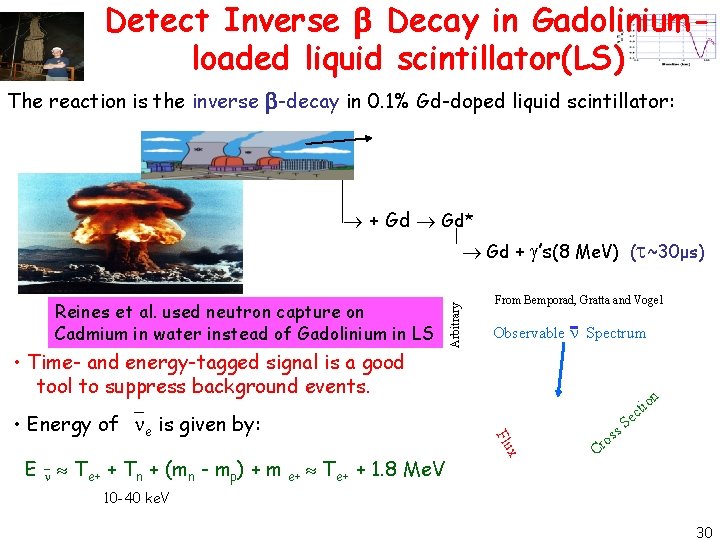
Detect Inverse Decay in Gadoliniumloaded liquid scintillator(LS) The reaction is the inverse -decay in 0. 1% Gd-doped liquid scintillator: + Gd Gd* Reines et al. used neutron capture on Cadmium in water instead of Gadolinium in LS • Time- and energy-tagged signal is a good tool to suppress background events. Observable Spectrum n e+ Te+ + 1. 8 Me. V x E Te+ + Tn + (mn - mp) + m From Bemporad, Gratta and Vogel Flu • Energy of e is given by: Arbitrary Gd + ’s(8 Me. V) ( ~30μs) s C s ro tio c Se 10 -40 ke. V 30
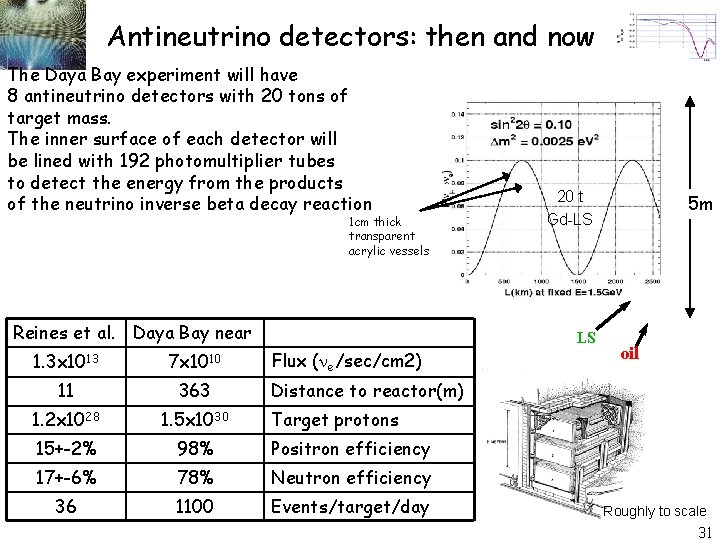
Antineutrino detectors: then and now The Daya Bay experiment will have 8 antineutrino detectors with 20 tons of target mass. The inner surface of each detector will be lined with 192 photomultiplier tubes to detect the energy from the products of the neutrino inverse beta decay reaction 1 cm thick transparent acrylic vessels Reines et al. Daya Bay near 1. 3 x 1013 7 x 1010 11 363 1. 2 x 1028 1. 5 x 1030 15+-2% 98% Positron efficiency 17+-6% 78% Neutron efficiency 36 1100 Events/target/day 20 t Gd-LS LS Flux ( e/sec/cm 2) 5 m oil Distance to reactor(m) Target protons Roughly to scale 31
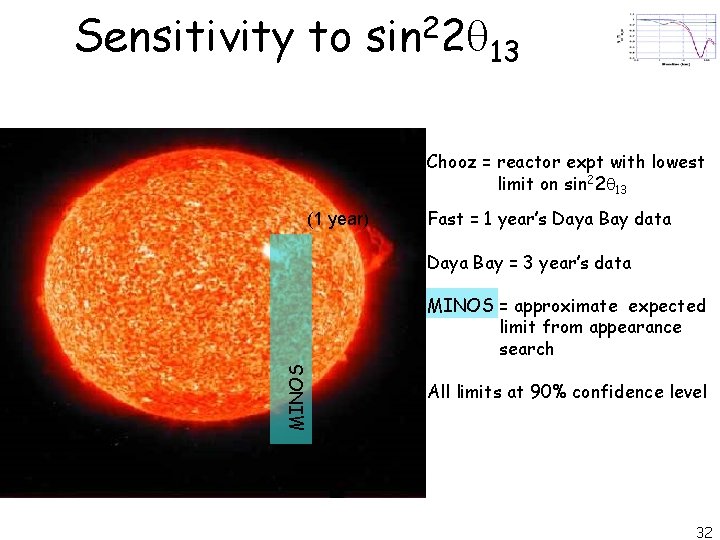
Sensitivity to sin 22 13 Chooz = reactor expt with lowest limit on sin 22 13 (1 year) Fast = 1 year’s Daya Bay data Daya Bay = 3 year’s data MINOS = approximate expected limit from appearance search All limits at 90% confidence level 32
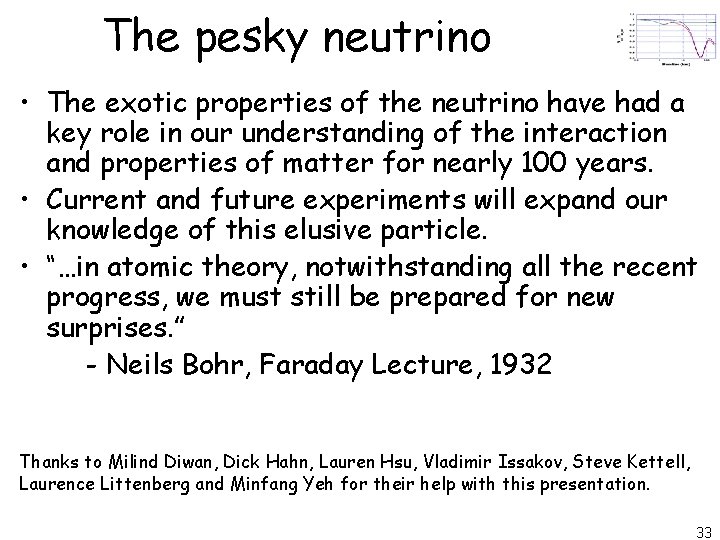
The pesky neutrino • The exotic properties of the neutrino have had a key role in our understanding of the interaction and properties of matter for nearly 100 years. • Current and future experiments will expand our knowledge of this elusive particle. • “…in atomic theory, notwithstanding all the recent progress, we must still be prepared for new surprises. ” - Neils Bohr, Faraday Lecture, 1932 Thanks to Milind Diwan, Dick Hahn, Lauren Hsu, Vladimir Issakov, Steve Kettell, Laurence Littenberg and Minfang Yeh for their help with this presentation. 33
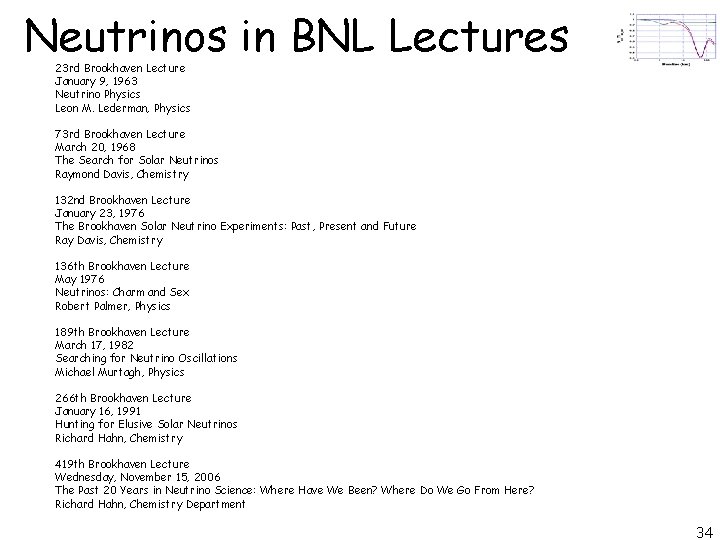
Neutrinos in BNL Lectures 23 rd Brookhaven Lecture January 9, 1963 Neutrino Physics Leon M. Lederman, Physics 73 rd Brookhaven Lecture March 20, 1968 The Search for Solar Neutrinos Raymond Davis, Chemistry 132 nd Brookhaven Lecture January 23, 1976 The Brookhaven Solar Neutrino Experiments: Past, Present and Future Ray Davis, Chemistry 136 th Brookhaven Lecture May 1976 Neutrinos: Charm and Sex Robert Palmer, Physics 189 th Brookhaven Lecture March 17, 1982 Searching for Neutrino Oscillations Michael Murtagh, Physics 266 th Brookhaven Lecture January 16, 1991 Hunting for Elusive Solar Neutrinos Richard Hahn, Chemistry 419 th Brookhaven Lecture Wednesday, November 15, 2006 The Past 20 Years in Neutrino Science: Where Have We Been? Where Do We Go From Here? Richard Hahn, Chemistry Department 34
Lee pesky learning center
Pesky sentence
Hawley appliance with expansion screw
Elliot jaffe net worth
Harold jaffe cdc
Charles jaffe md
Neutrino beteckning
The leptons
Neutrino lepton number
Neutrino
Neutrino
Lekka cząstka elementarna mion elektron lub neutrino
Who discovered neutrino
Neutrino
Neutrino
Neutrino density
Neutrino
Paris traceroute
Neutrino
Neutrino symbol
Ice cube status
Neutrino
Cerenkov
Neutrino
Solar neutrino
Nakamura
Neutrino mass
Neutrino
Neutrino interaction with matter
Neutrino mass
Princeton physics department
Physics 121 njit
Sputonik
Warwick astro
Bhu physics department