TECHNICAL UNIVERSITY OF KOICE FACULTY OF ELECTRIC ENGINEERING
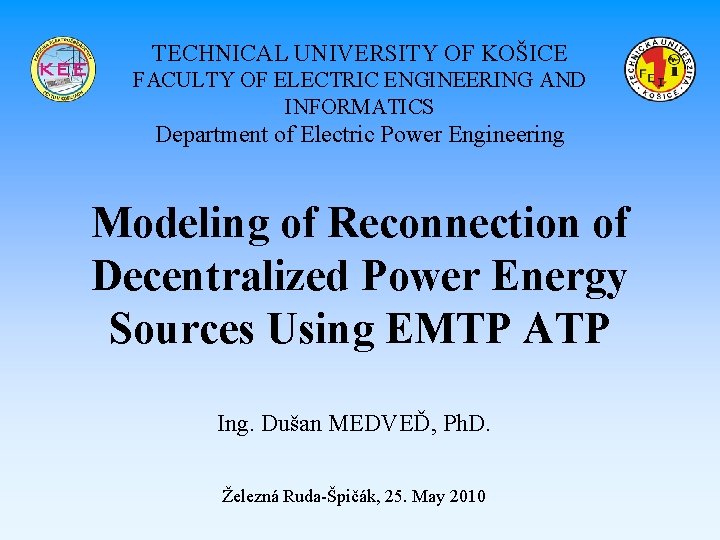
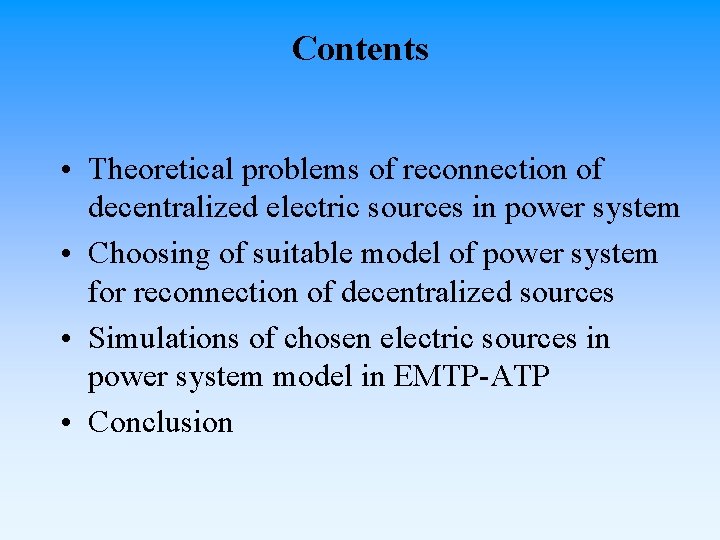
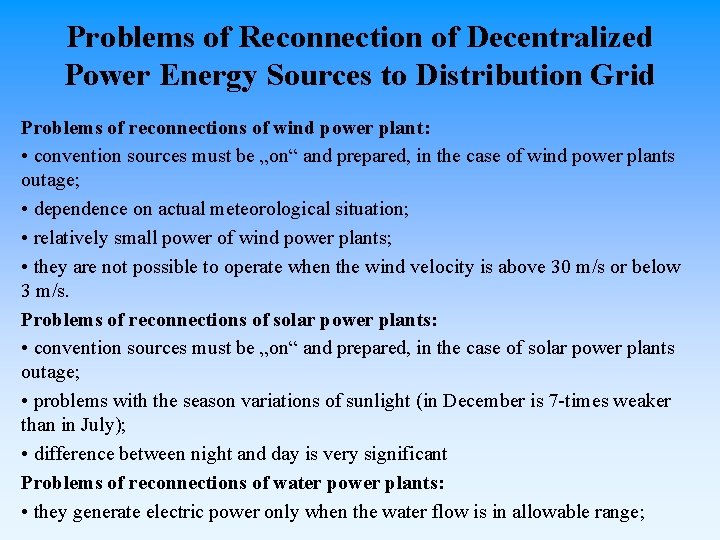
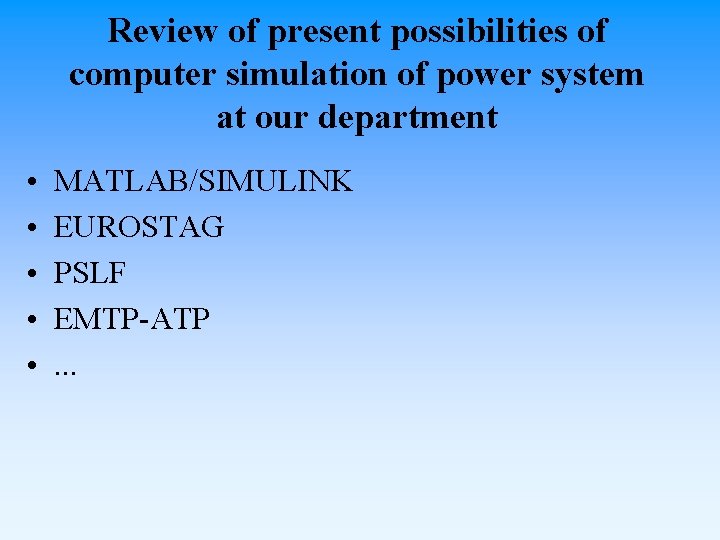
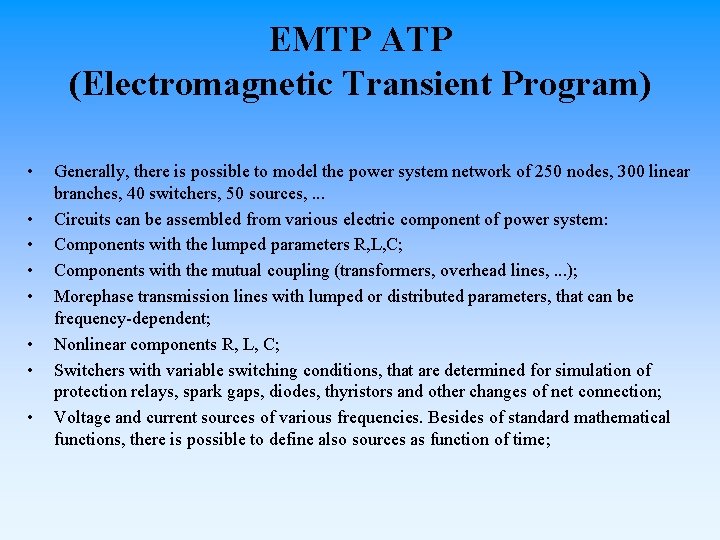
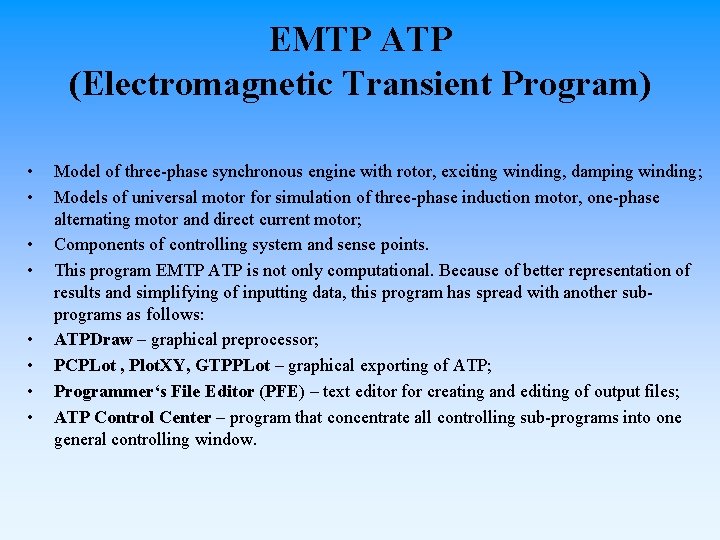
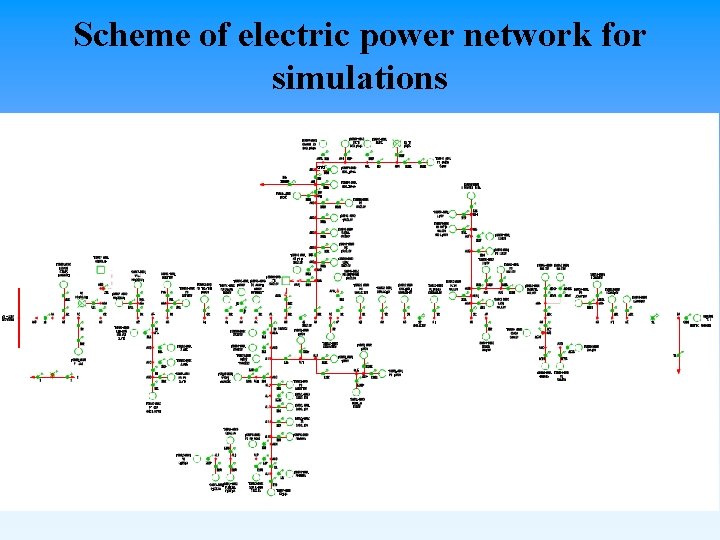
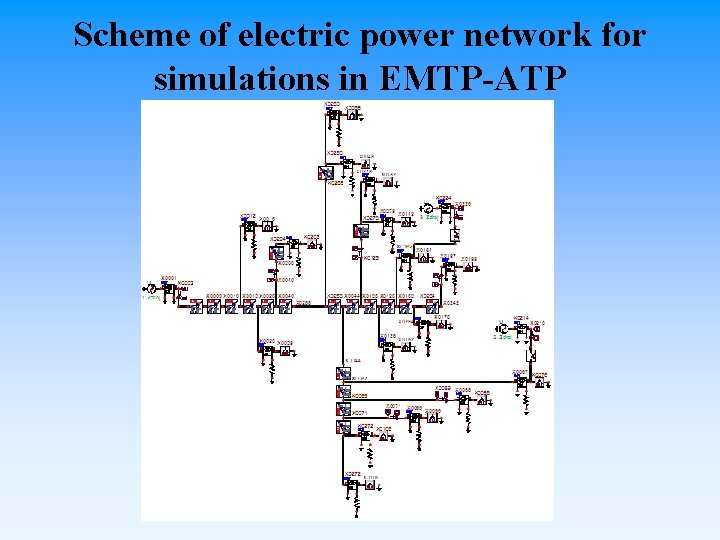
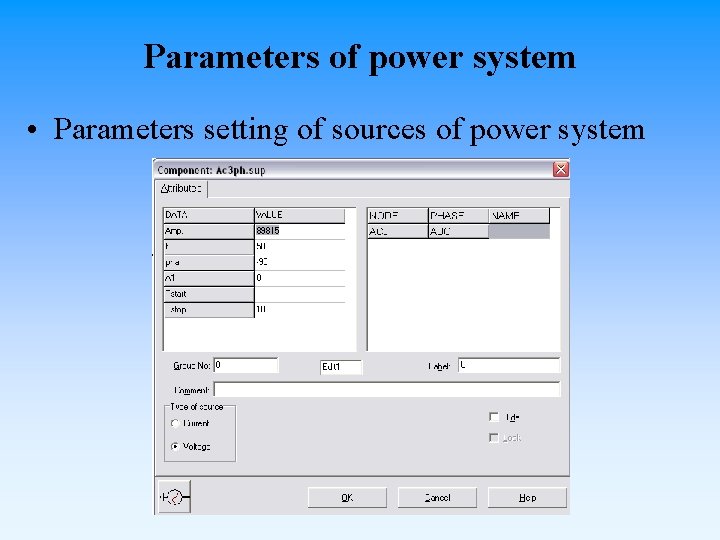
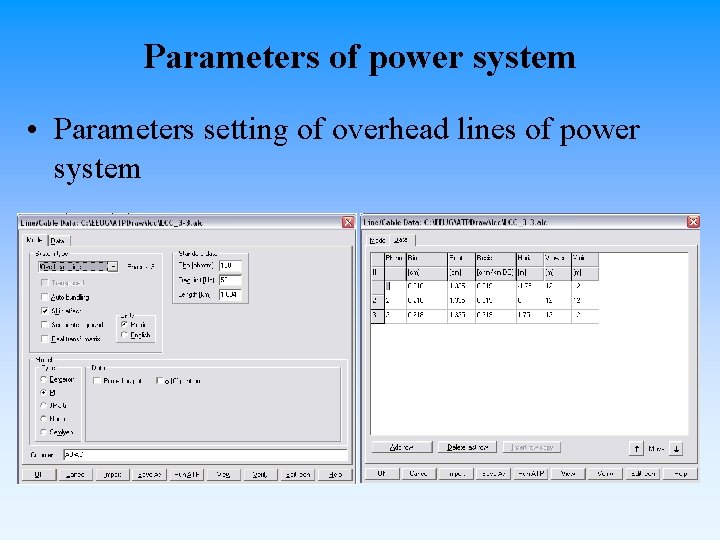
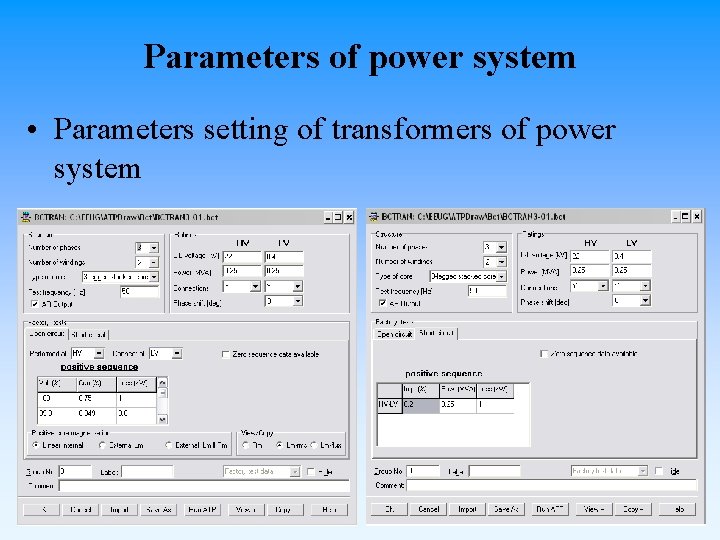
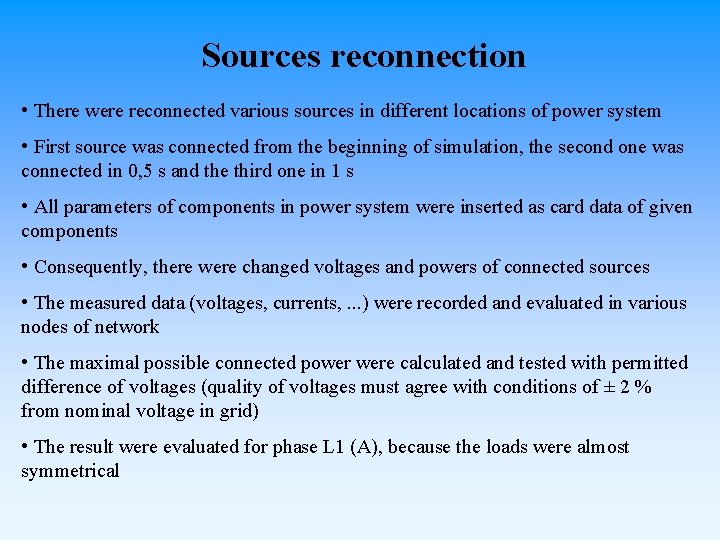
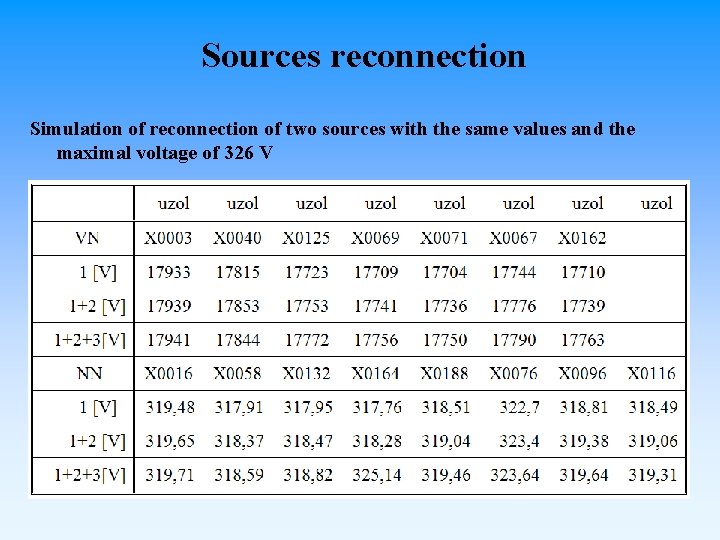
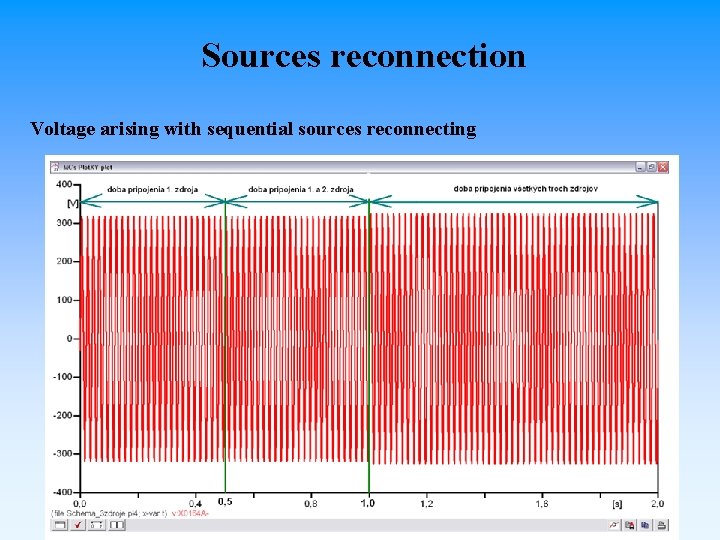
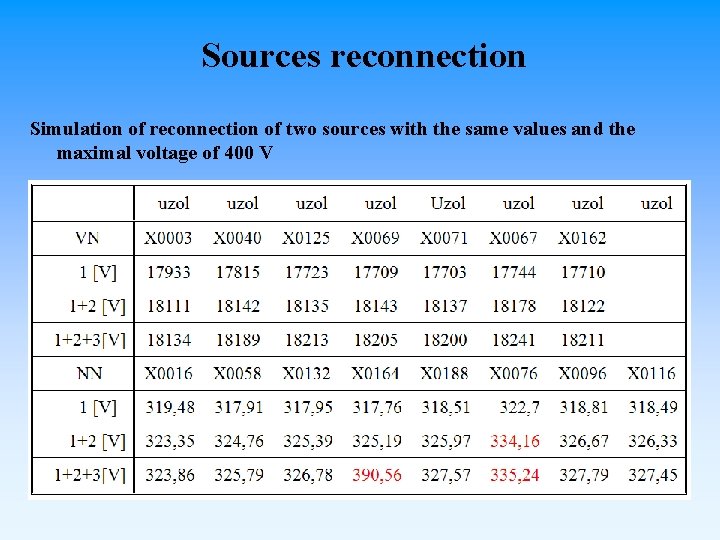
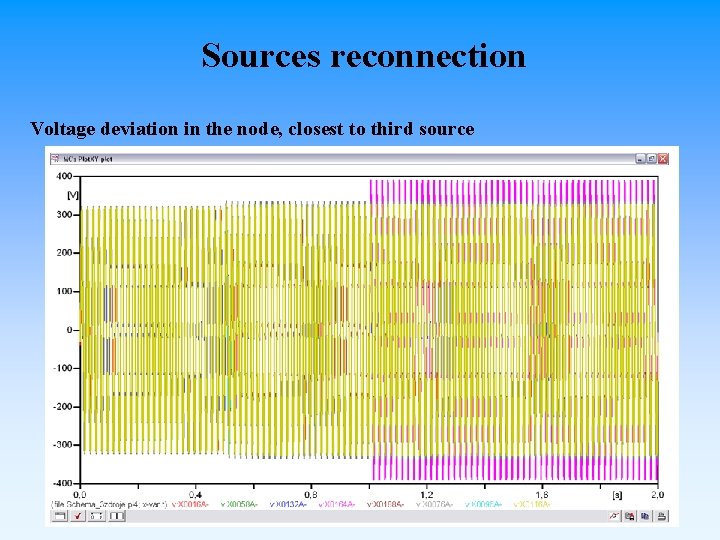
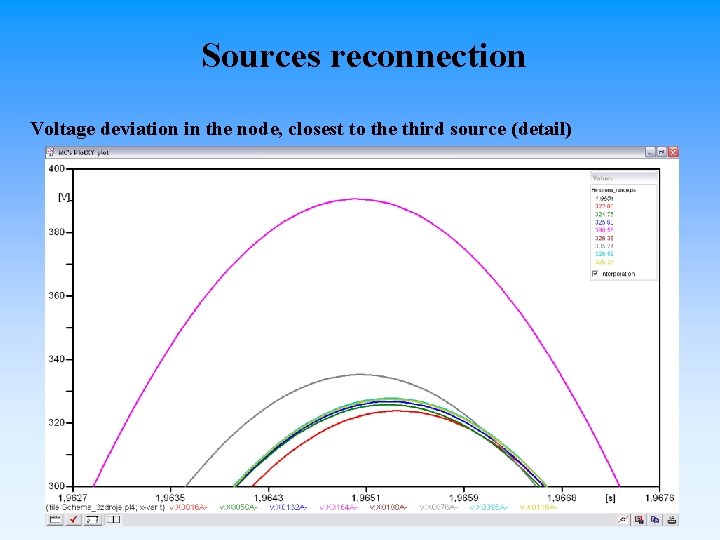
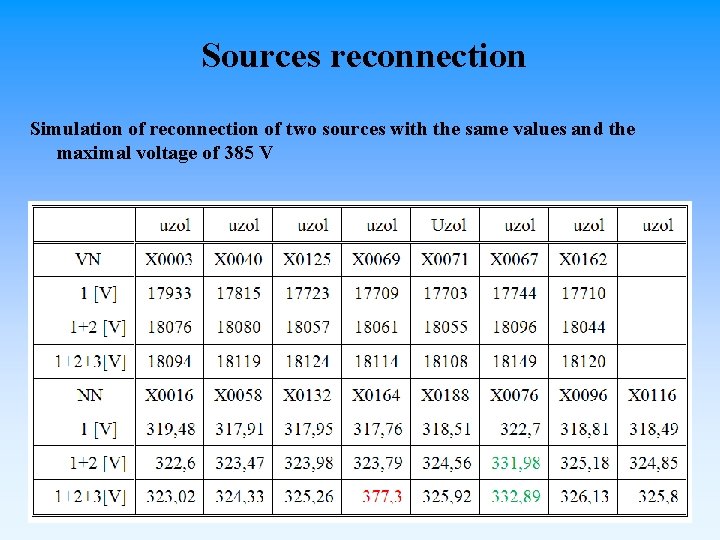
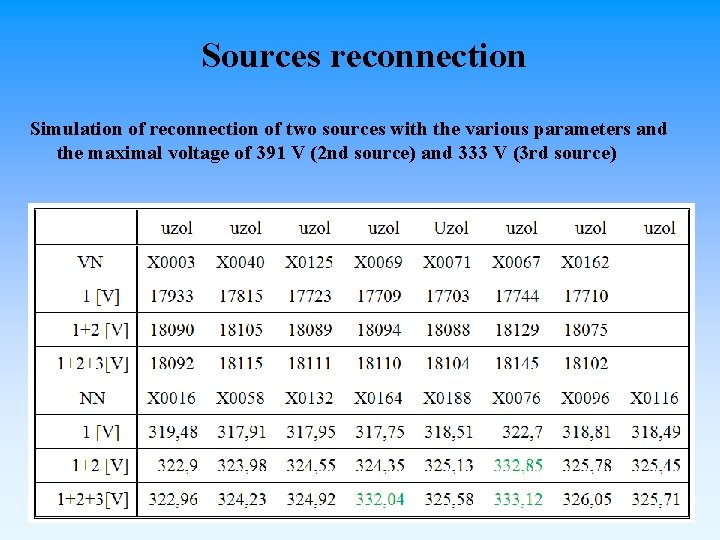
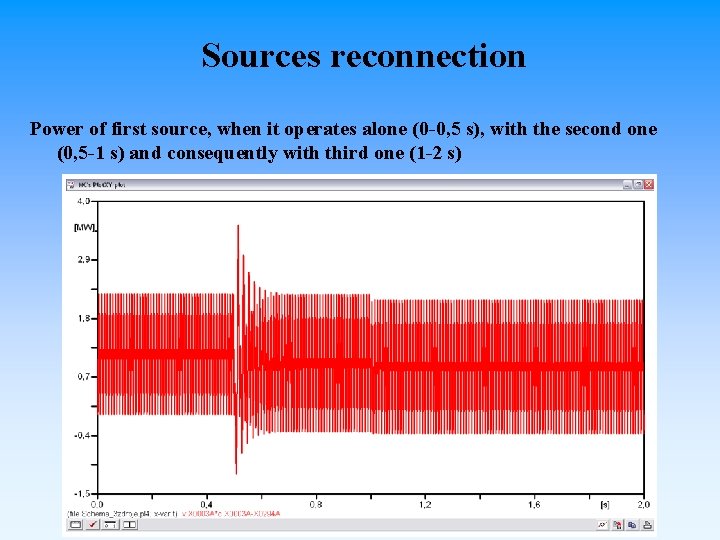
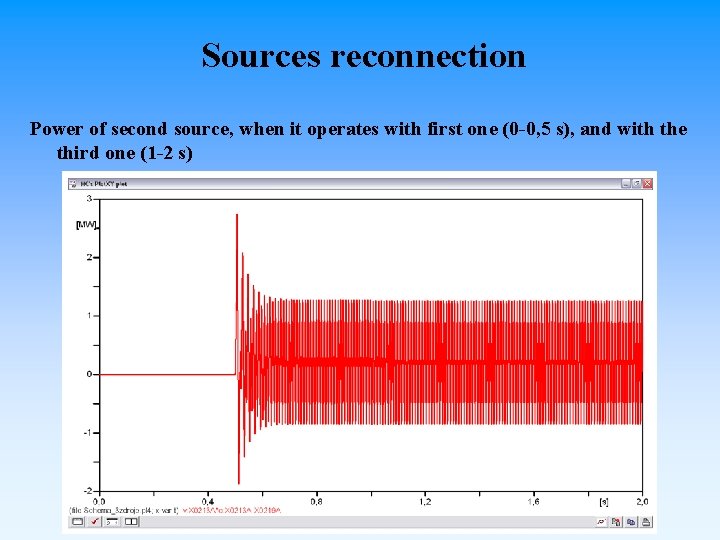
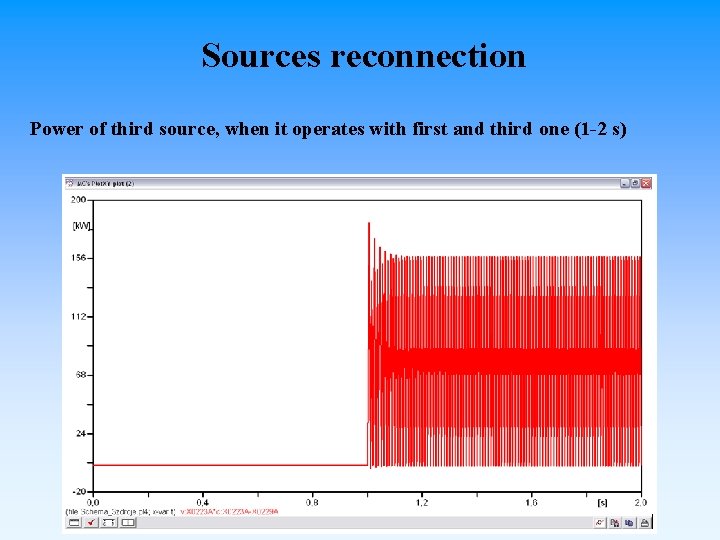
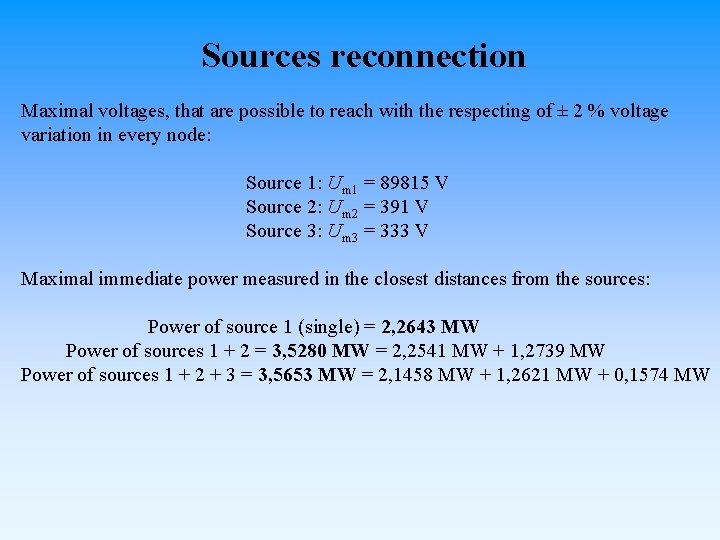
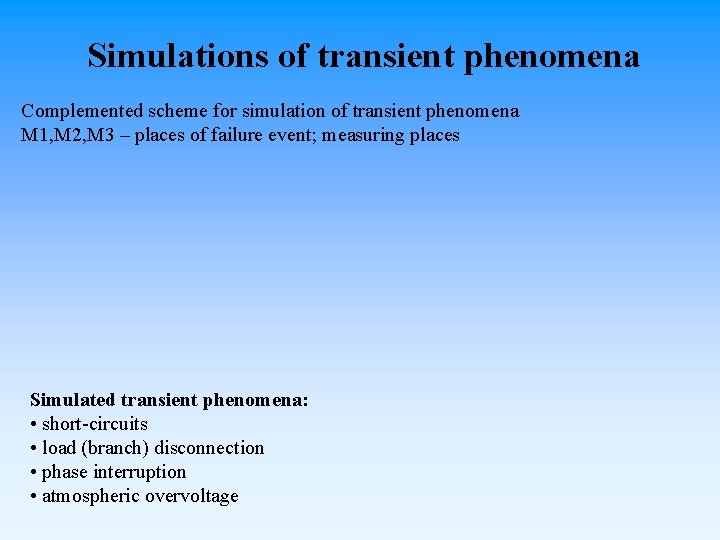
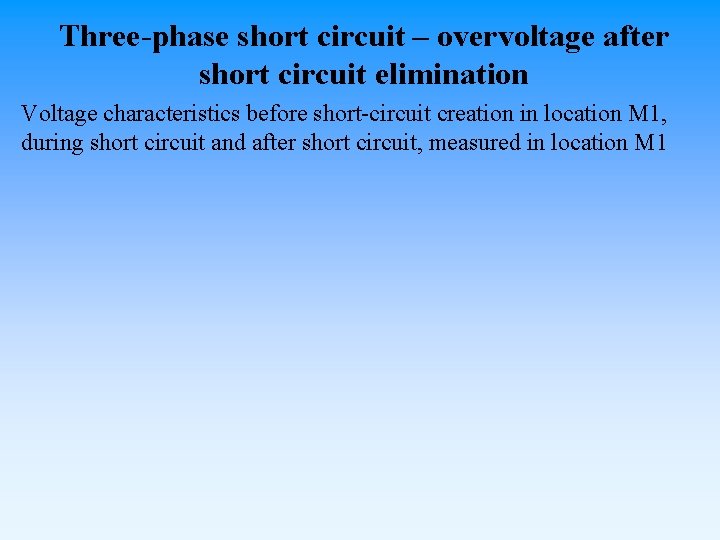
![Three-phase short circuit – measured results Measured place Location M 1 Mutual distances [km] Three-phase short circuit – measured results Measured place Location M 1 Mutual distances [km]](https://slidetodoc.com/presentation_image_h2/e59a9485275f3373820b906bc6eab98c/image-26.jpg)
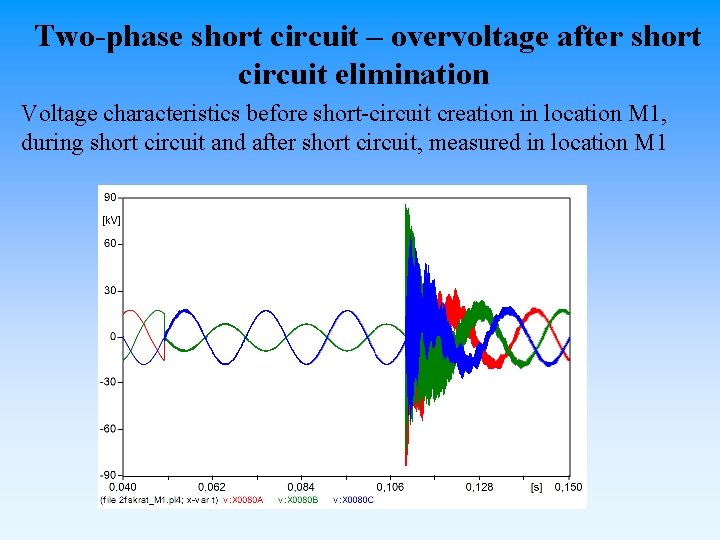
![Two-phase short circuit – measured results Measured place Location M 1 Mutual distances [km] Two-phase short circuit – measured results Measured place Location M 1 Mutual distances [km]](https://slidetodoc.com/presentation_image_h2/e59a9485275f3373820b906bc6eab98c/image-28.jpg)
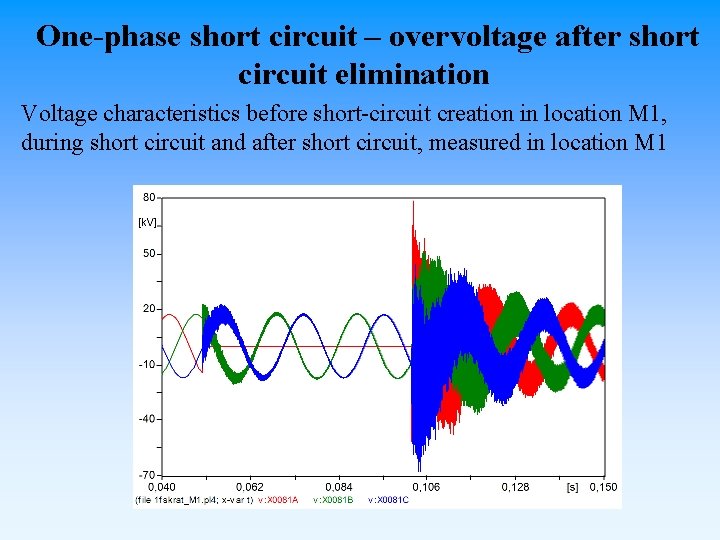
![One-phase short circuit – measured results Measured place Location M 1 Mutual distances [km] One-phase short circuit – measured results Measured place Location M 1 Mutual distances [km]](https://slidetodoc.com/presentation_image_h2/e59a9485275f3373820b906bc6eab98c/image-30.jpg)
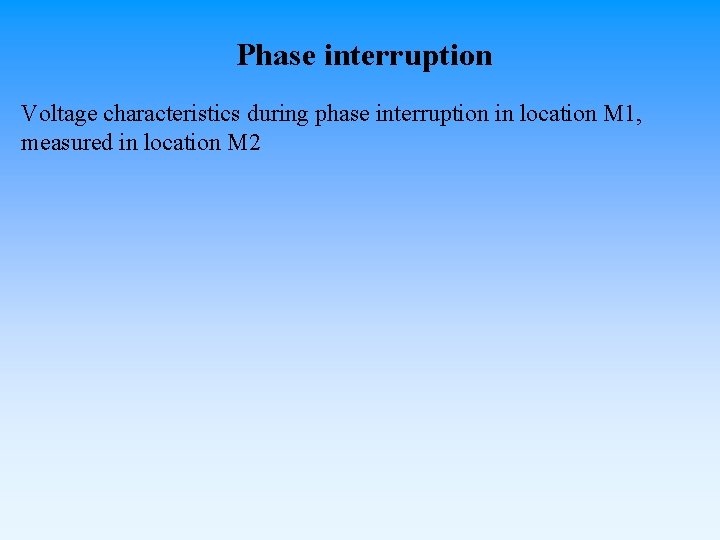
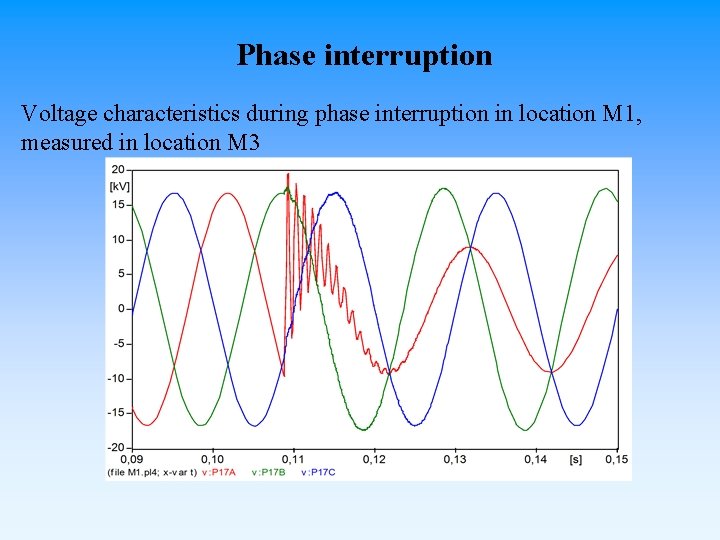
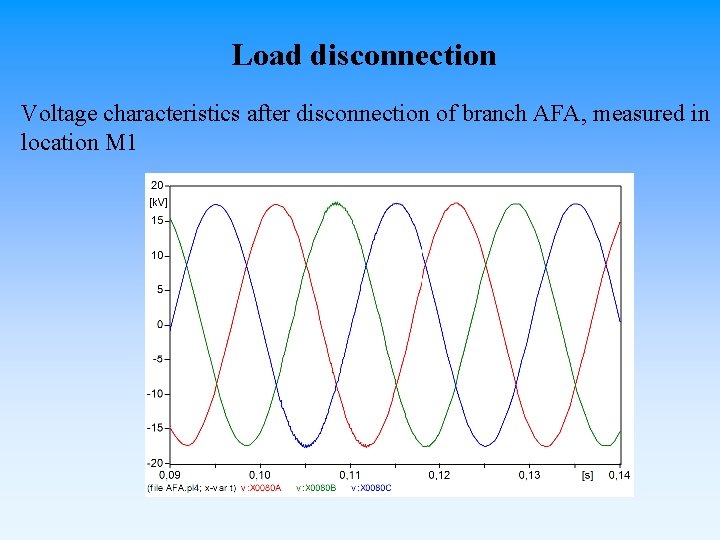
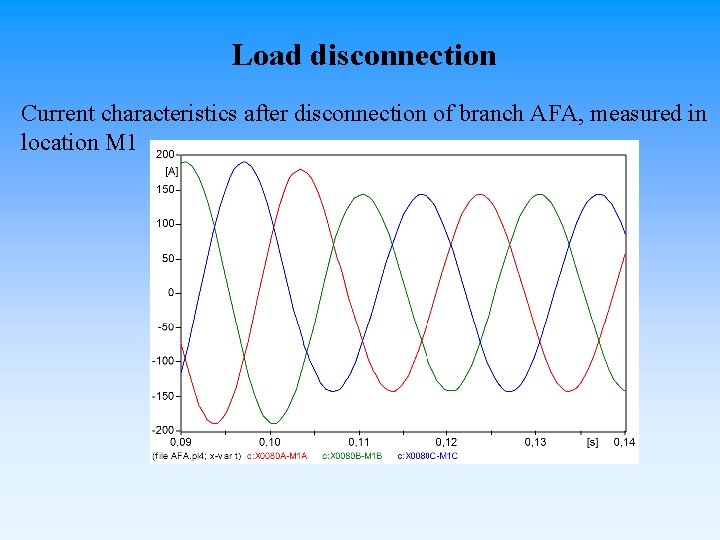
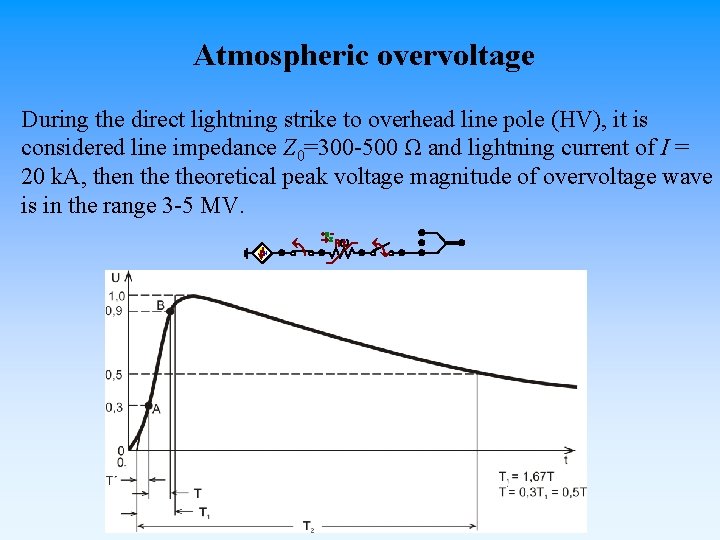
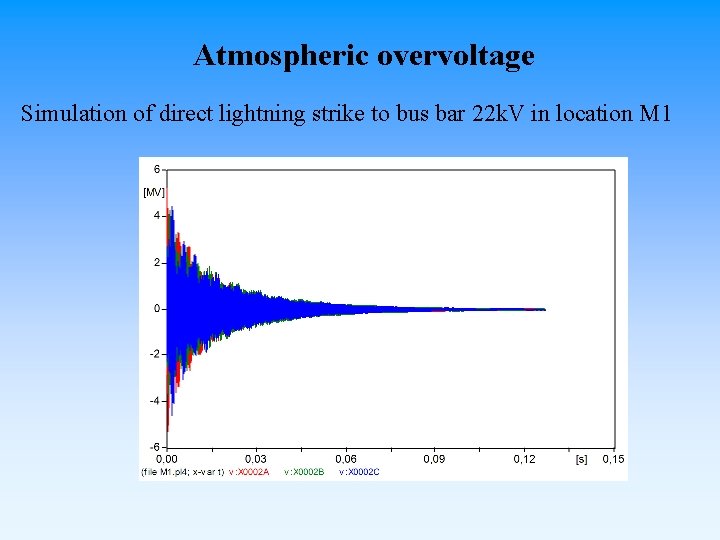
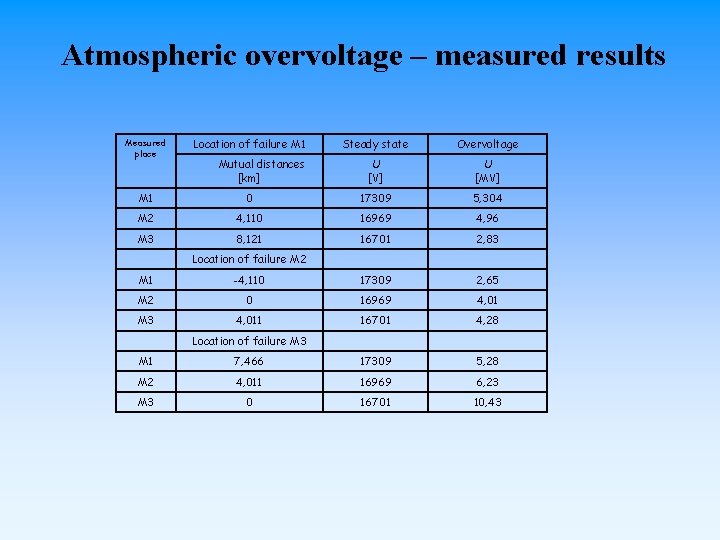
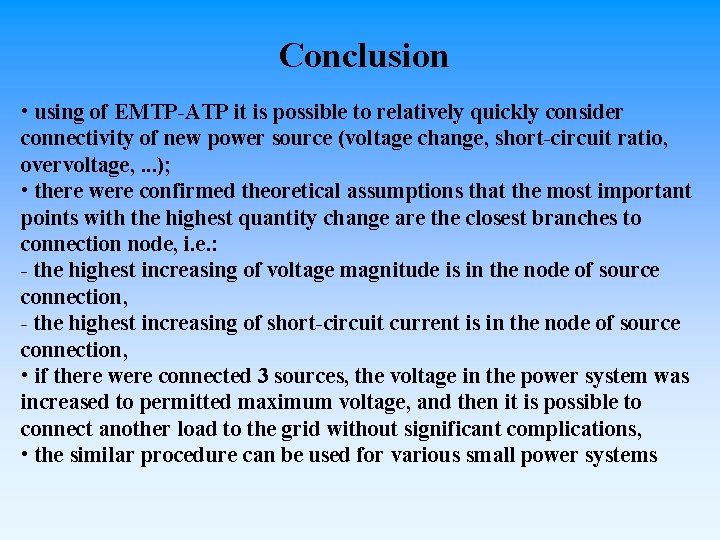
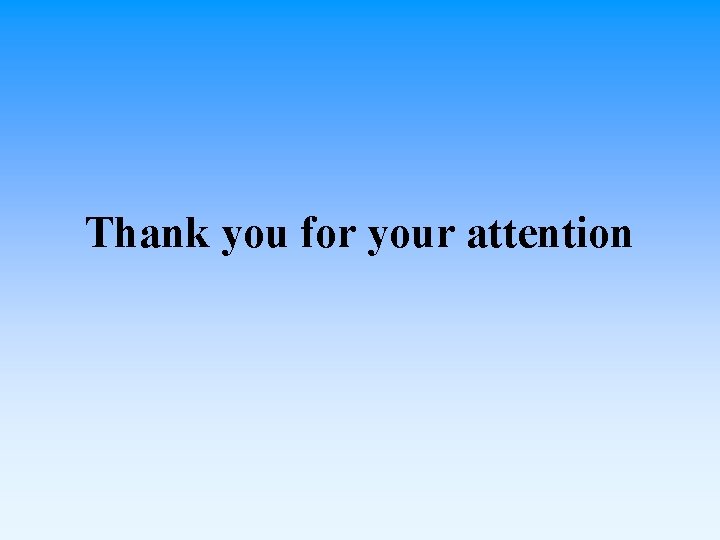
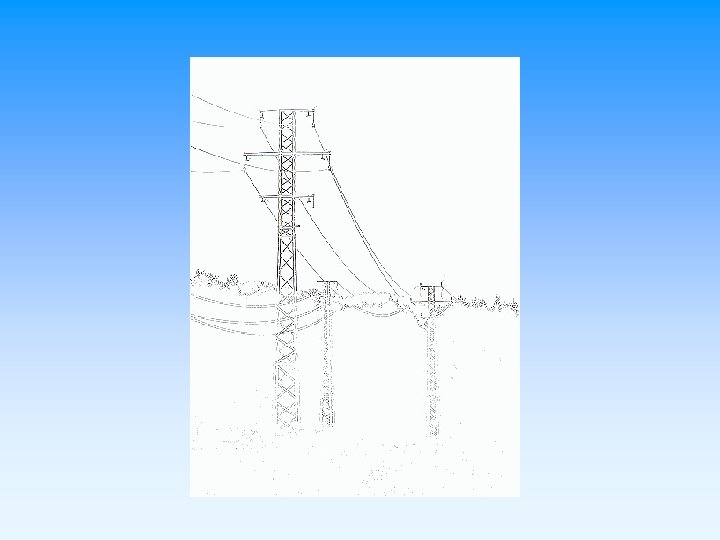
- Slides: 40
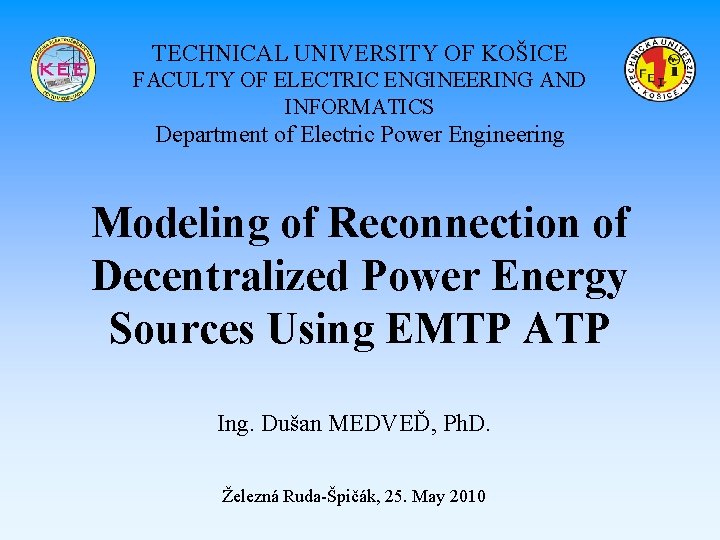
TECHNICAL UNIVERSITY OF KOŠICE FACULTY OF ELECTRIC ENGINEERING AND INFORMATICS Department of Electric Power Engineering Modeling of Reconnection of Decentralized Power Energy Sources Using EMTP ATP Ing. Dušan MEDVEĎ, Ph. D. Železná Ruda-Špičák, 25. May 2010
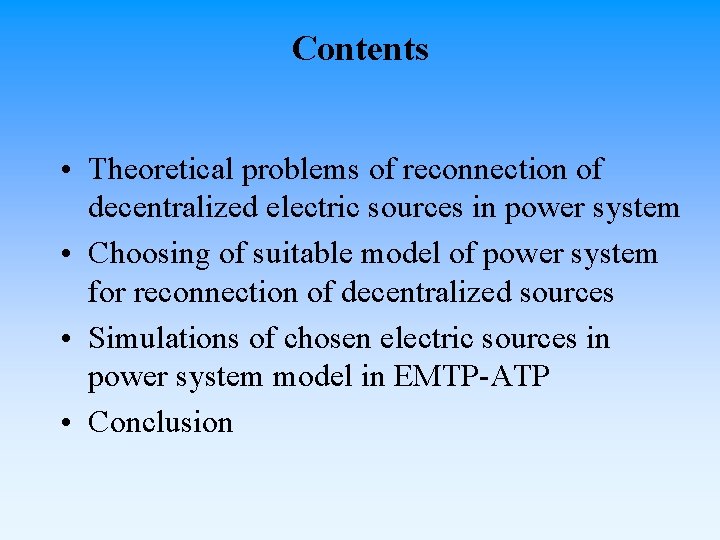
Contents • Theoretical problems of reconnection of decentralized electric sources in power system • Choosing of suitable model of power system for reconnection of decentralized sources • Simulations of chosen electric sources in power system model in EMTP-ATP • Conclusion
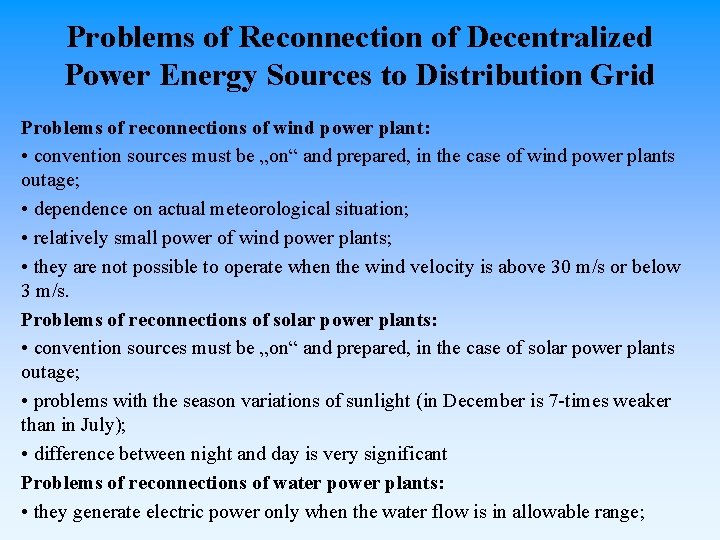
Problems of Reconnection of Decentralized Power Energy Sources to Distribution Grid Problems of reconnections of wind power plant: • convention sources must be „on“ and prepared, in the case of wind power plants outage; • dependence on actual meteorological situation; • relatively small power of wind power plants; • they are not possible to operate when the wind velocity is above 30 m/s or below 3 m/s. Problems of reconnections of solar power plants: • convention sources must be „on“ and prepared, in the case of solar power plants outage; • problems with the season variations of sunlight (in December is 7 -times weaker than in July); • difference between night and day is very significant Problems of reconnections of water power plants: • they generate electric power only when the water flow is in allowable range;
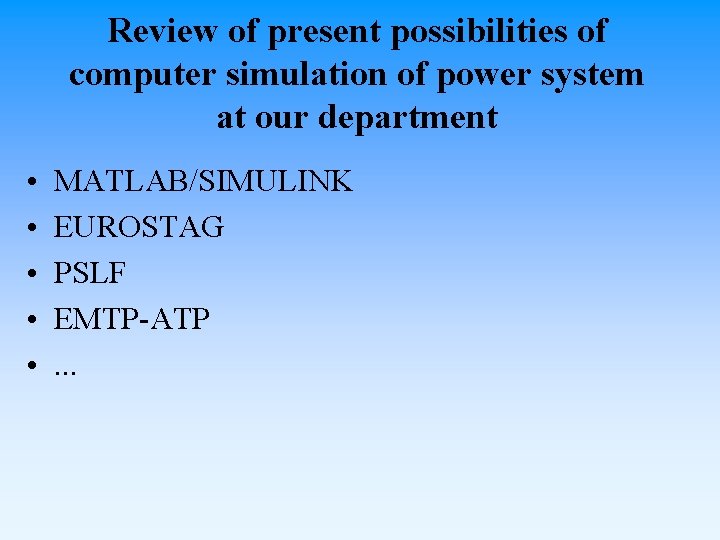
Review of present possibilities of computer simulation of power system at our department • • • MATLAB/SIMULINK EUROSTAG PSLF EMTP-ATP. . .
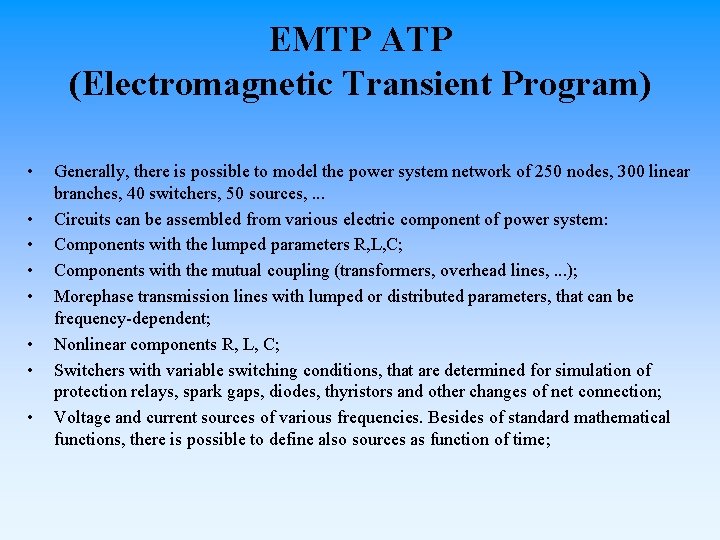
EMTP ATP (Electromagnetic Transient Program) • • Generally, there is possible to model the power system network of 250 nodes, 300 linear branches, 40 switchers, 50 sources, . . . Circuits can be assembled from various electric component of power system: Components with the lumped parameters R, L, C; Components with the mutual coupling (transformers, overhead lines, . . . ); Morephase transmission lines with lumped or distributed parameters, that can be frequency-dependent; Nonlinear components R, L, C; Switchers with variable switching conditions, that are determined for simulation of protection relays, spark gaps, diodes, thyristors and other changes of net connection; Voltage and current sources of various frequencies. Besides of standard mathematical functions, there is possible to define also sources as function of time;
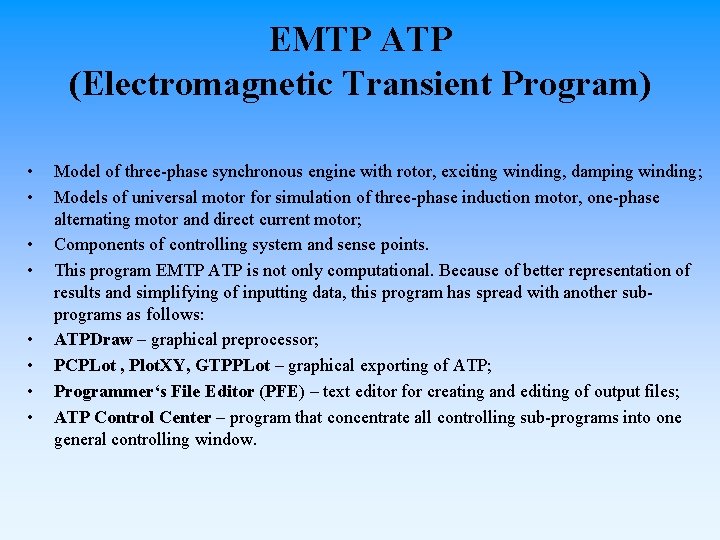
EMTP ATP (Electromagnetic Transient Program) • • Model of three-phase synchronous engine with rotor, exciting winding, damping winding; Models of universal motor for simulation of three-phase induction motor, one-phase alternating motor and direct current motor; Components of controlling system and sense points. This program EMTP ATP is not only computational. Because of better representation of results and simplifying of inputting data, this program has spread with another subprograms as follows: ATPDraw – graphical preprocessor; PCPLot , Plot. XY, GTPPLot – graphical exporting of ATP; Programmer‘s File Editor (PFE) – text editor for creating and editing of output files; ATP Control Center – program that concentrate all controlling sub-programs into one general controlling window.
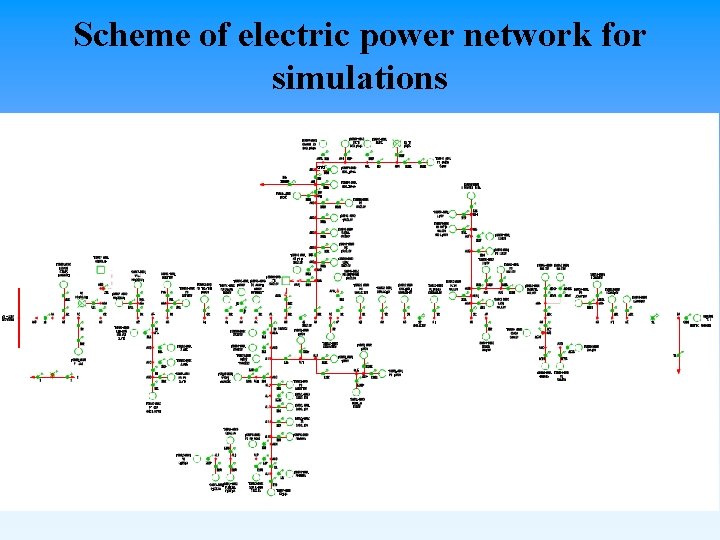
Scheme of electric power network for simulations
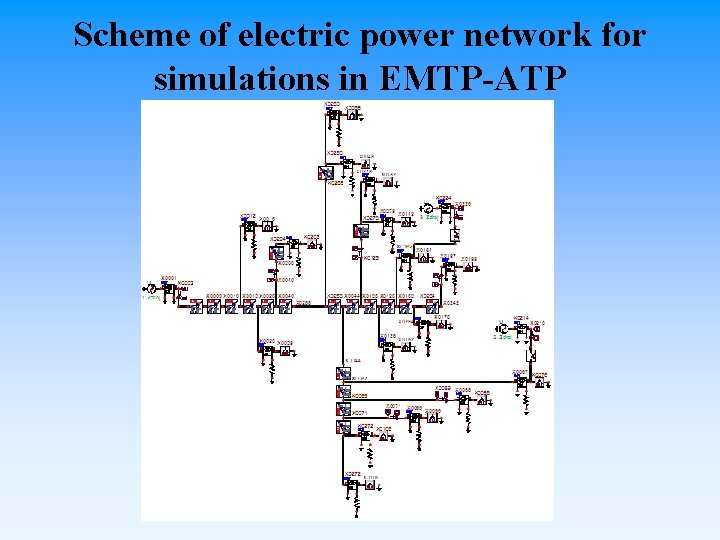
Scheme of electric power network for simulations in EMTP-ATP
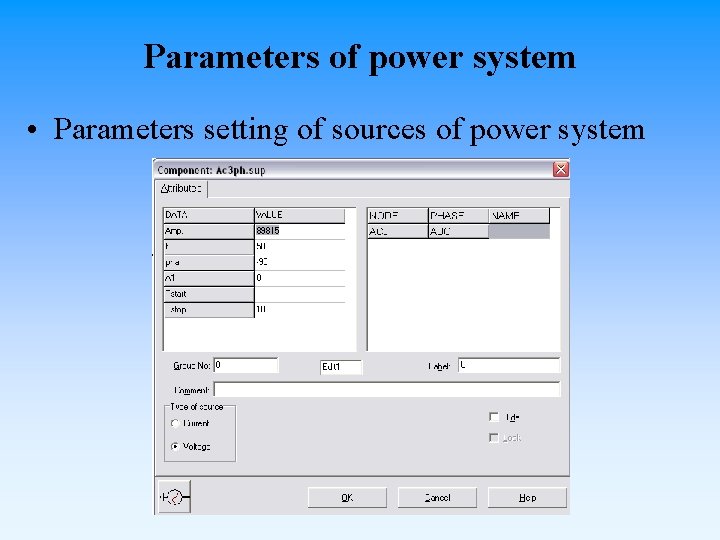
Parameters of power system • Parameters setting of sources of power system
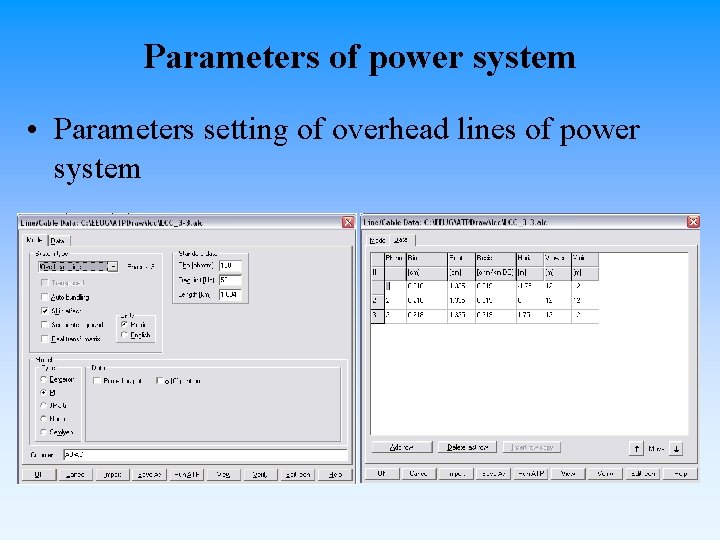
Parameters of power system • Parameters setting of overhead lines of power system
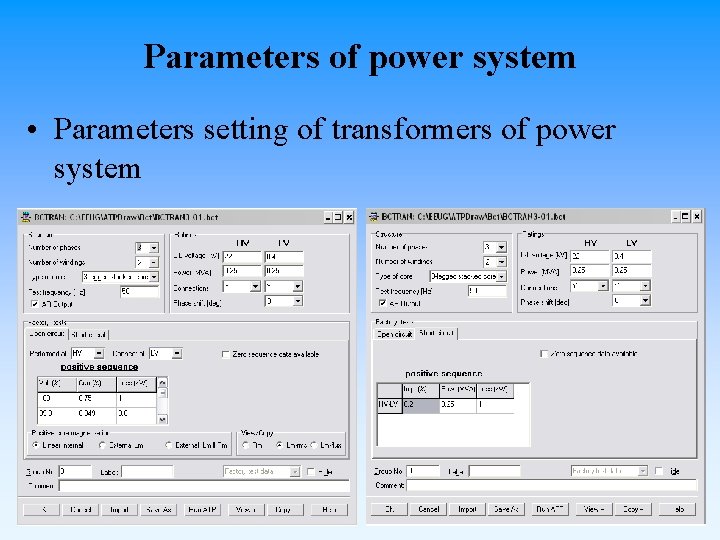
Parameters of power system • Parameters setting of transformers of power system
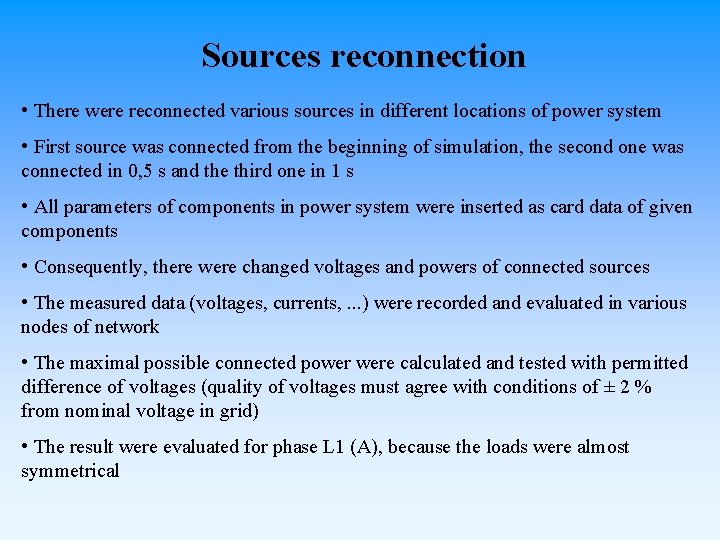
Sources reconnection • There were reconnected various sources in different locations of power system • First source was connected from the beginning of simulation, the second one was connected in 0, 5 s and the third one in 1 s • All parameters of components in power system were inserted as card data of given components • Consequently, there were changed voltages and powers of connected sources • The measured data (voltages, currents, . . . ) were recorded and evaluated in various nodes of network • The maximal possible connected power were calculated and tested with permitted difference of voltages (quality of voltages must agree with conditions of ± 2 % from nominal voltage in grid) • The result were evaluated for phase L 1 (A), because the loads were almost symmetrical
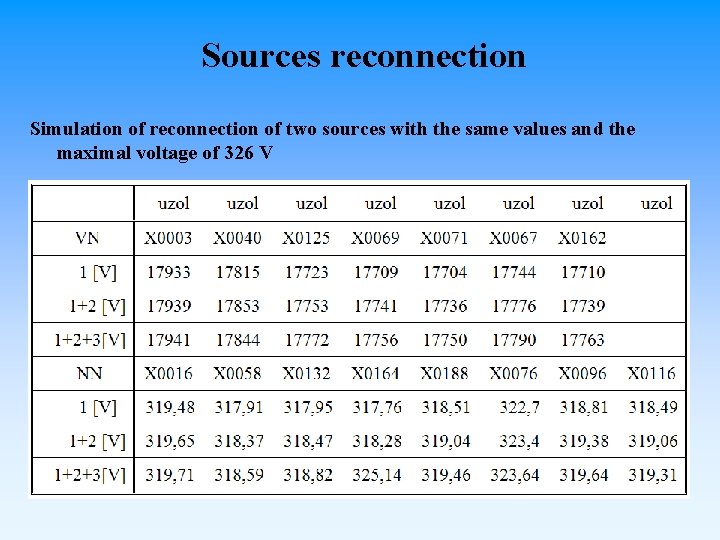
Sources reconnection Simulation of reconnection of two sources with the same values and the maximal voltage of 326 V
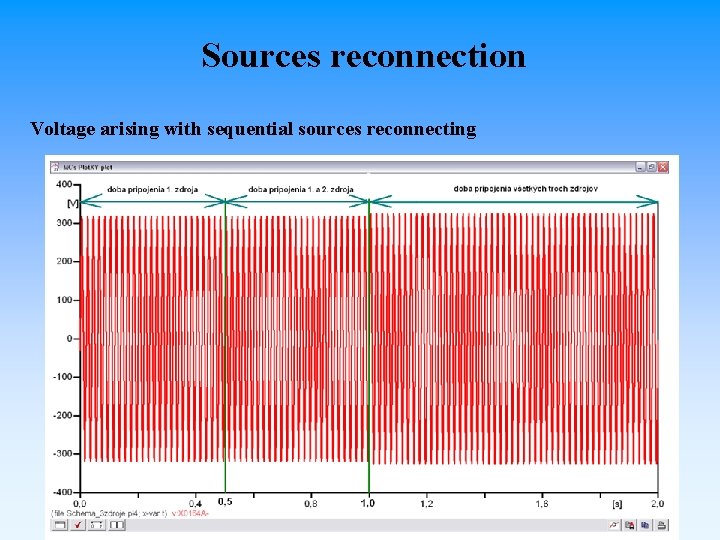
Sources reconnection Voltage arising with sequential sources reconnecting
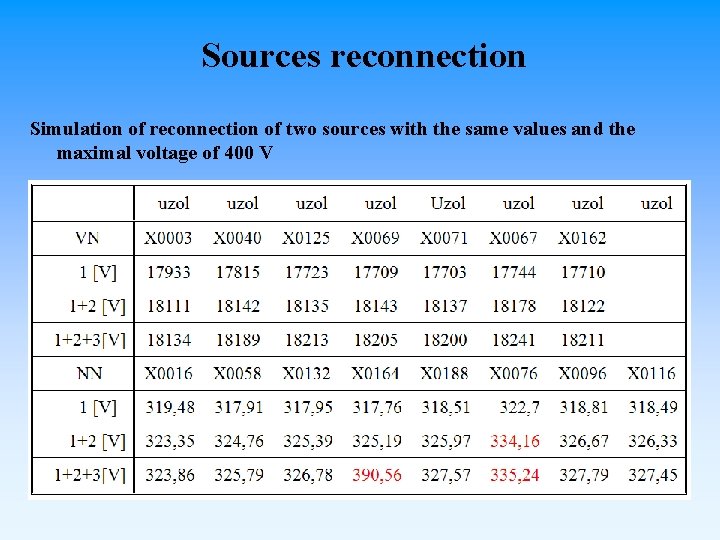
Sources reconnection Simulation of reconnection of two sources with the same values and the maximal voltage of 400 V
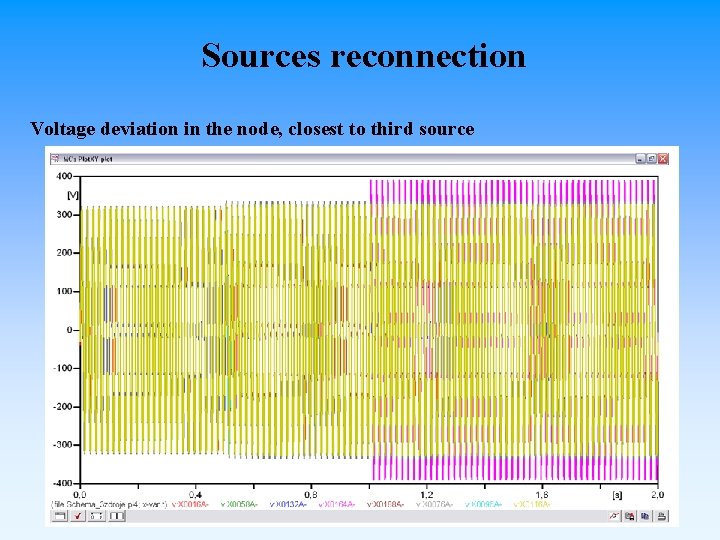
Sources reconnection Voltage deviation in the node, closest to third source
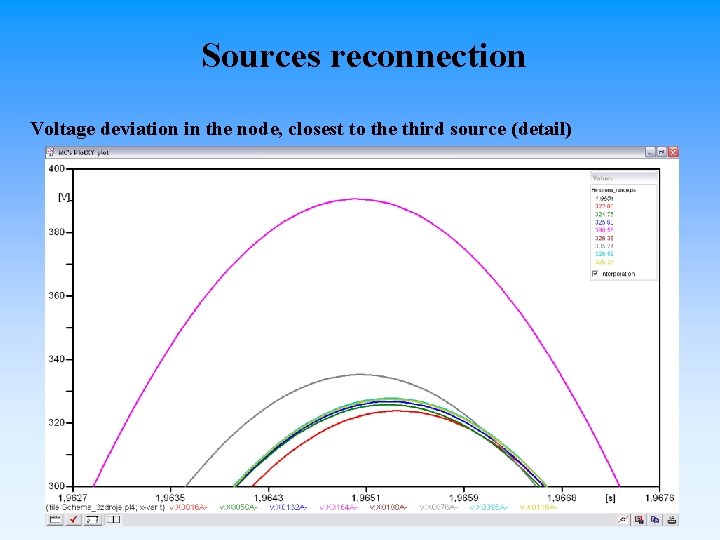
Sources reconnection Voltage deviation in the node, closest to the third source (detail)
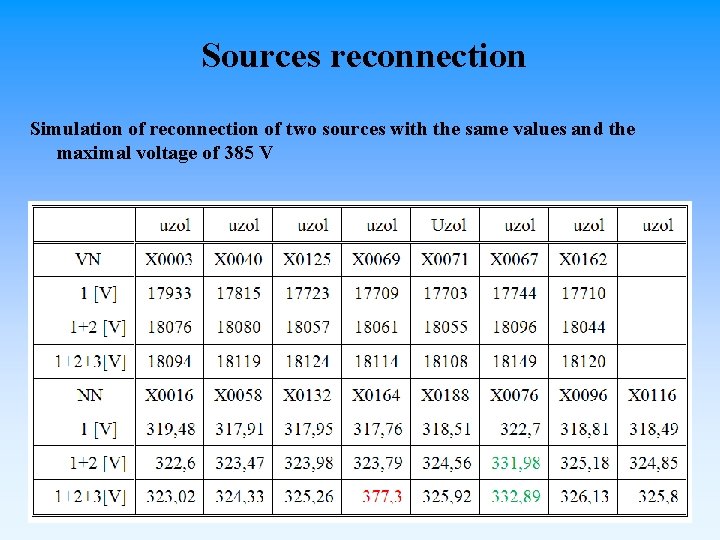
Sources reconnection Simulation of reconnection of two sources with the same values and the maximal voltage of 385 V
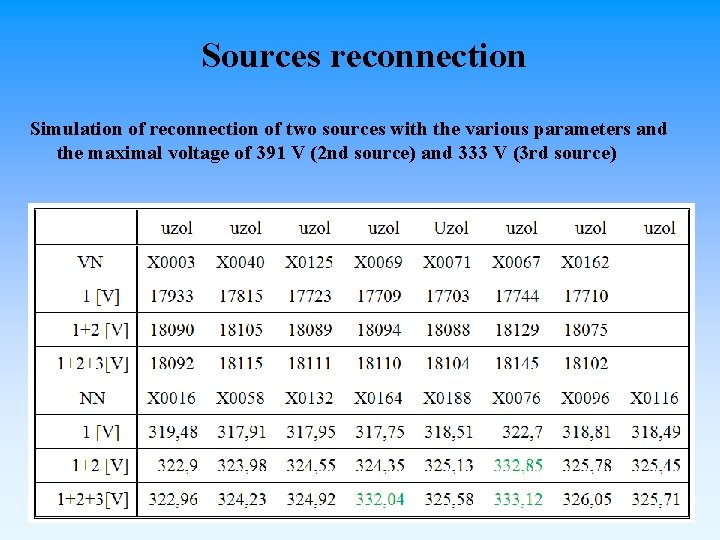
Sources reconnection Simulation of reconnection of two sources with the various parameters and the maximal voltage of 391 V (2 nd source) and 333 V (3 rd source)
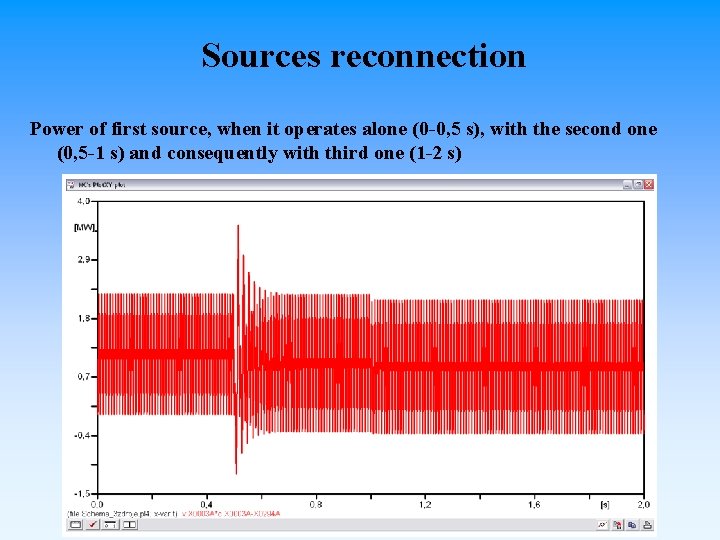
Sources reconnection Power of first source, when it operates alone (0 -0, 5 s), with the second one (0, 5 -1 s) and consequently with third one (1 -2 s)
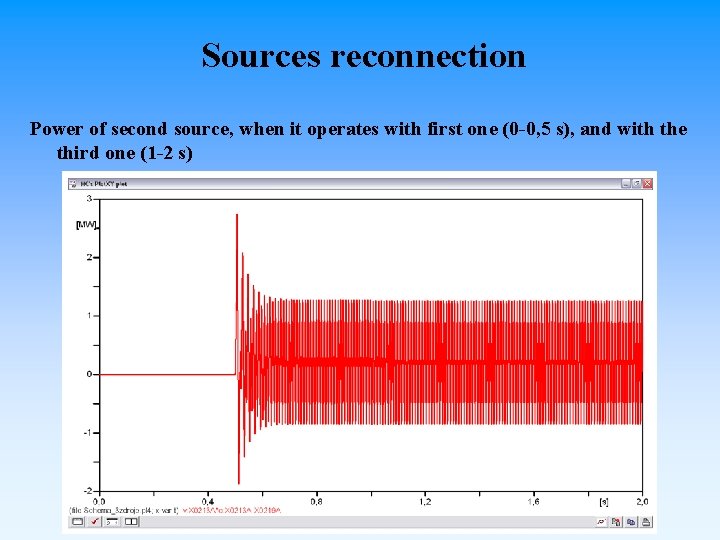
Sources reconnection Power of second source, when it operates with first one (0 -0, 5 s), and with the third one (1 -2 s)
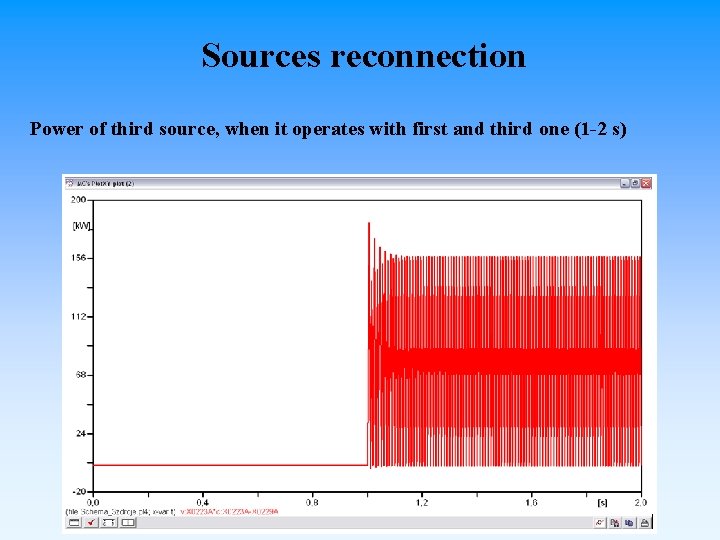
Sources reconnection Power of third source, when it operates with first and third one (1 -2 s)
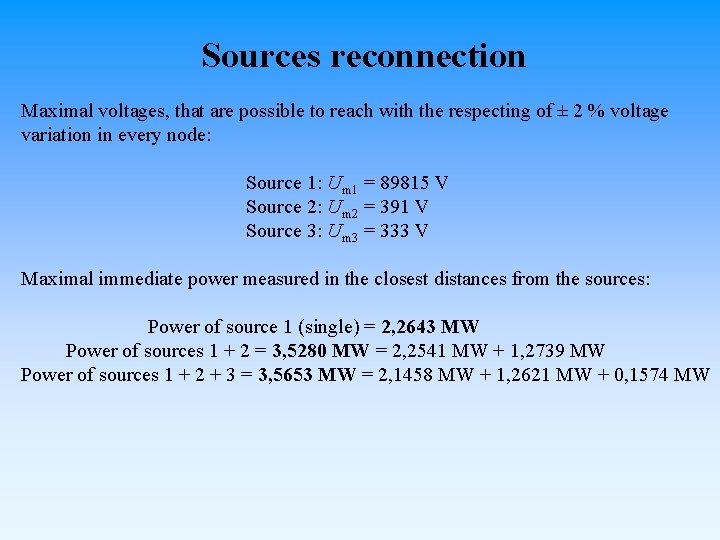
Sources reconnection Maximal voltages, that are possible to reach with the respecting of ± 2 % voltage variation in every node: Source 1: Um 1 = 89815 V Source 2: Um 2 = 391 V Source 3: Um 3 = 333 V Maximal immediate power measured in the closest distances from the sources: Power of source 1 (single) = 2, 2643 MW Power of sources 1 + 2 = 3, 5280 MW = 2, 2541 MW + 1, 2739 MW Power of sources 1 + 2 + 3 = 3, 5653 MW = 2, 1458 MW + 1, 2621 MW + 0, 1574 MW
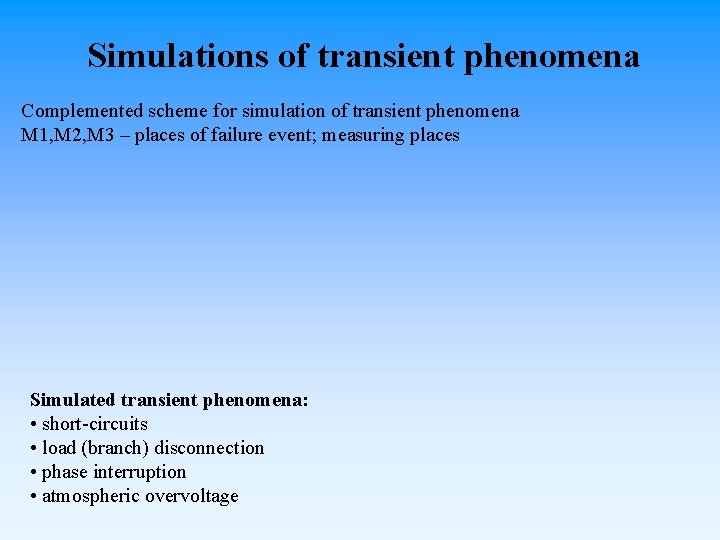
Simulations of transient phenomena Complemented scheme for simulation of transient phenomena M 1, M 2, M 3 – places of failure event; measuring places Simulated transient phenomena: • short-circuits • load (branch) disconnection • phase interruption • atmospheric overvoltage
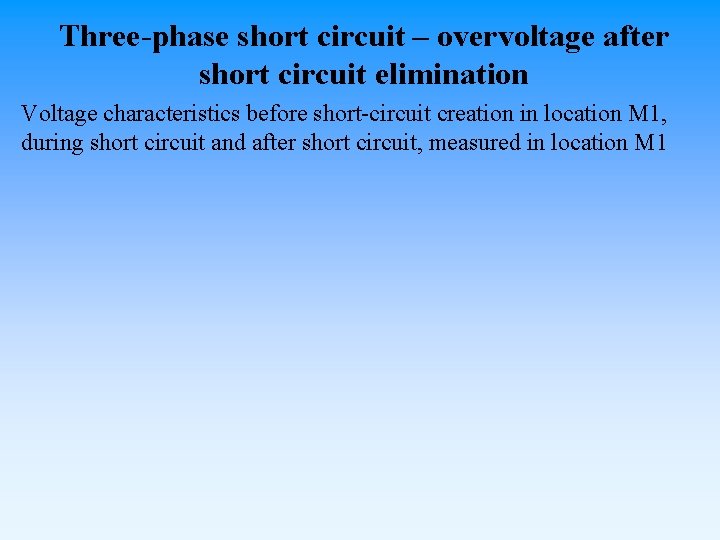
Three-phase short circuit – overvoltage after short circuit elimination Voltage characteristics before short-circuit creation in location M 1, during short circuit and after short circuit, measured in location M 1
![Threephase short circuit measured results Measured place Location M 1 Mutual distances km Three-phase short circuit – measured results Measured place Location M 1 Mutual distances [km]](https://slidetodoc.com/presentation_image_h2/e59a9485275f3373820b906bc6eab98c/image-26.jpg)
Three-phase short circuit – measured results Measured place Location M 1 Mutual distances [km] Steady state Overvoltage after shot-ciruit interuption Peak current during short-circuit U [V] ip [A] M 1 0 17309 88722 6533, 5 M 2 4, 110 16969 69337 203, 5 M 3 8, 121 16701 71948 36, 453 Steady state Overvoltage after shot-ciruit interuption Peak current during short-circuit U [V] ip [A] Measured place Location M 2 Mutual distances [km] M 1 -4, 110 17309 178560 3813, 5 M 2 0 16969 182420 3840, 9 M 3 4, 011 16701 172670 36, 373 Steady state Overvoltage after shot-ciruit interuption Peak current during short-circuit U [V] ip [A] Location M 3 Measured place Mutual distances [km] M 1 7, 466 17309 45529 2453, 3 M 2 4, 011 16969 48449 2432, 2 M 3 0 16701 57918 2402, 8
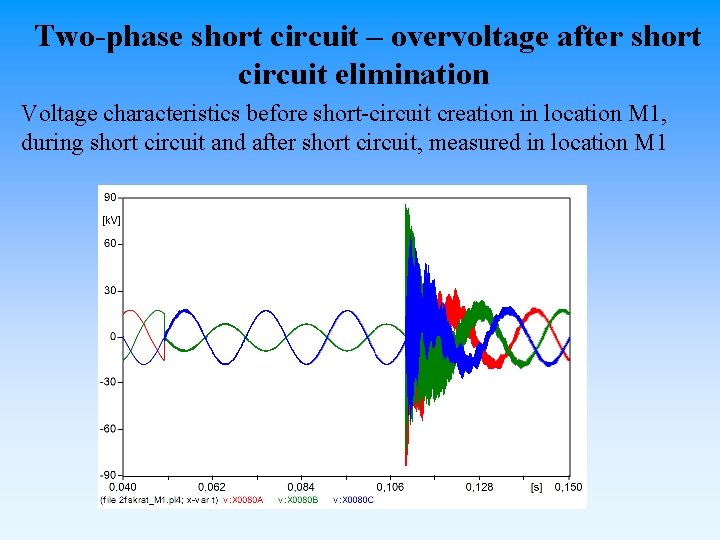
Two-phase short circuit – overvoltage after short circuit elimination Voltage characteristics before short-circuit creation in location M 1, during short circuit and after short circuit, measured in location M 1
![Twophase short circuit measured results Measured place Location M 1 Mutual distances km Two-phase short circuit – measured results Measured place Location M 1 Mutual distances [km]](https://slidetodoc.com/presentation_image_h2/e59a9485275f3373820b906bc6eab98c/image-28.jpg)
Two-phase short circuit – measured results Measured place Location M 1 Mutual distances [km] Steady state Overvoltage after shot-ciruit interuption Peak current during short-circuit U [V] ip [A] M 1 0 17309 86268 3122, 3 M 2 4, 110 16969 71133 197, 08 M 3 8, 121 16701 69032 36, 822 Steady state Overvoltage after shot-ciruit interuption Peak current during short-circuit U [V] ip [A] Measured place Location M 2 Mutual distances [km] M 1 -4, 110 17309 167630 2536, 6 M 2 0 16969 178690 2697, 5 M 3 4, 011 16701 163660 36, 975 Steady state Overvoltage after shot-ciruit interuption Peak current during short-circuit U [V] ip [A] Measured place Location M 3 Mutual distances [km] M 1 7, 466 17309 69409 1881, 2 M 2 4, 011 16969 67746 1854 M 3 0 16701 98249 1938
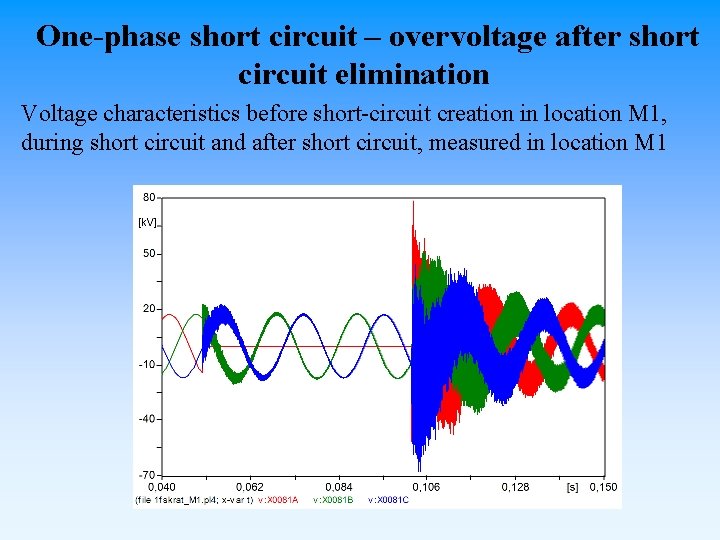
One-phase short circuit – overvoltage after short circuit elimination Voltage characteristics before short-circuit creation in location M 1, during short circuit and after short circuit, measured in location M 1
![Onephase short circuit measured results Measured place Location M 1 Mutual distances km One-phase short circuit – measured results Measured place Location M 1 Mutual distances [km]](https://slidetodoc.com/presentation_image_h2/e59a9485275f3373820b906bc6eab98c/image-30.jpg)
One-phase short circuit – measured results Measured place Location M 1 Mutual distances [km] Steady state Overvoltage after shot-ciruit interuption Peak current during short-circuit U [V] ip [A] M 1 0 17309 78221 5100, 5 M 2 4, 110 16969 47897 157, 81 M 3 8, 121 16701 55168 36, 45 Steady state Overvoltage after shot-ciruit interuption Peak current during short-circuit U [V] ip [A] Measured place Location M 2 Mutual distances [km] M 1 -4, 110 17309 129130 2607, 7 M 2 0 16969 147370 2711, 3 M 3 4, 011 16701 144980 36, 43 Steady state Overvoltage after shot-ciruit interuption Peak current during short-circuit U [V] ip [A] Measured place Location M 3 Mutual distances [km] M 1 7, 466 17309 55513 1672, 5 M 2 4, 011 16969 59507 1641, 2 M 3 0 16701 91992 1676, 2
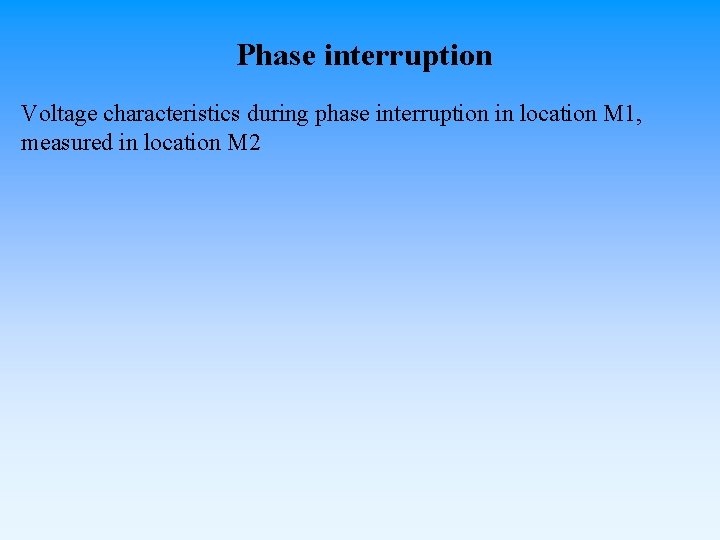
Phase interruption Voltage characteristics during phase interruption in location M 1, measured in location M 2
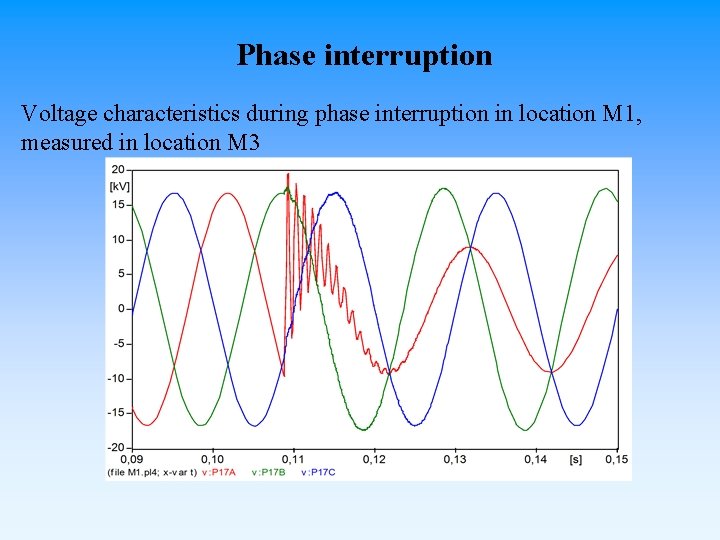
Phase interruption Voltage characteristics during phase interruption in location M 1, measured in location M 3
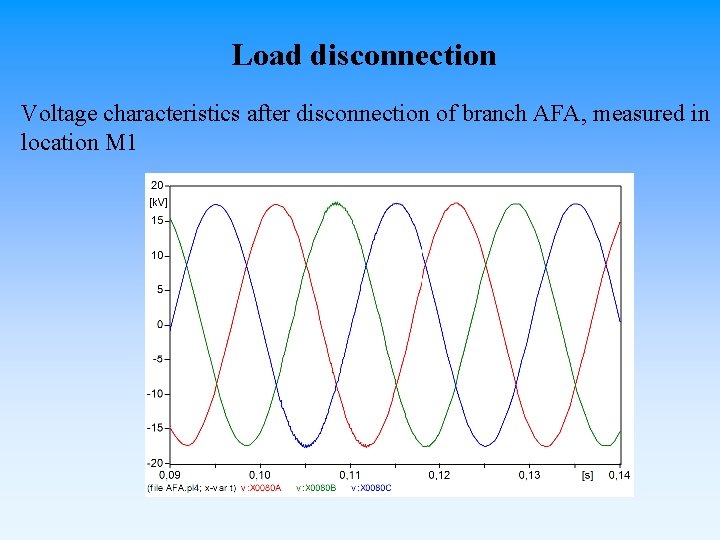
Load disconnection Voltage characteristics after disconnection of branch AFA, measured in location M 1
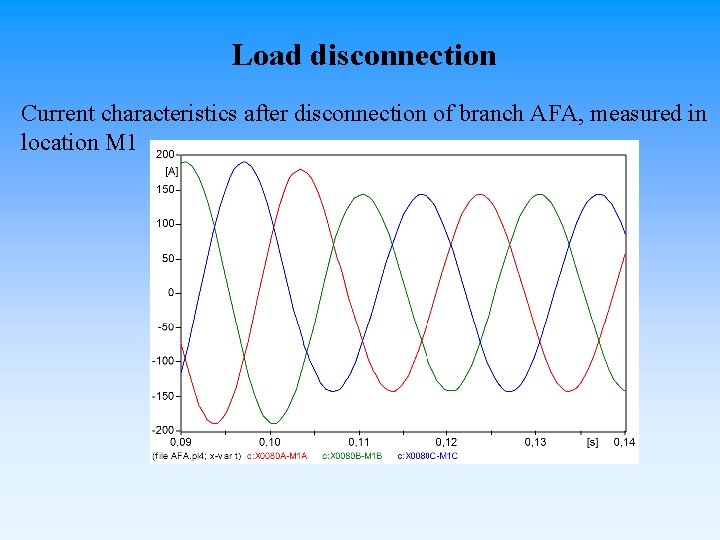
Load disconnection Current characteristics after disconnection of branch AFA, measured in location M 1
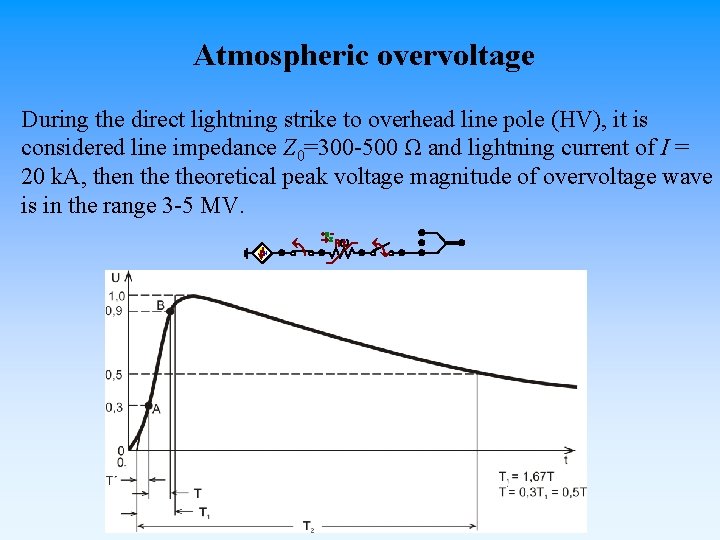
Atmospheric overvoltage During the direct lightning strike to overhead line pole (HV), it is considered line impedance Z 0=300 -500 Ω and lightning current of I = 20 k. A, then theoretical peak voltage magnitude of overvoltage wave is in the range 3 -5 MV. H
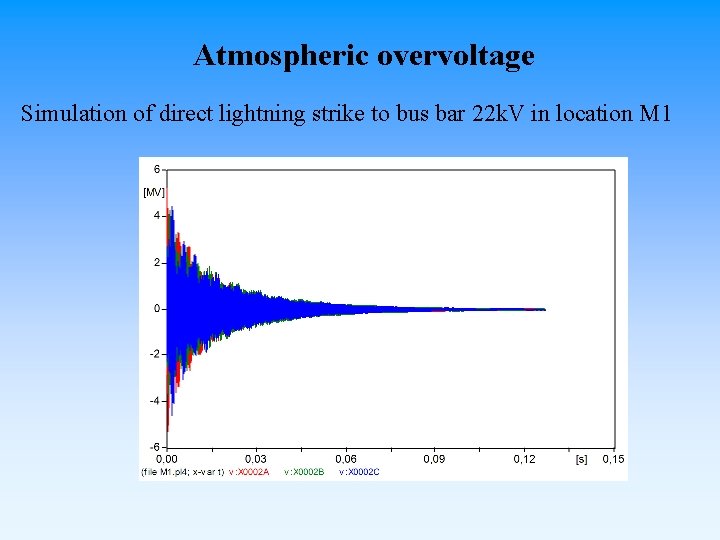
Atmospheric overvoltage Simulation of direct lightning strike to bus bar 22 k. V in location M 1
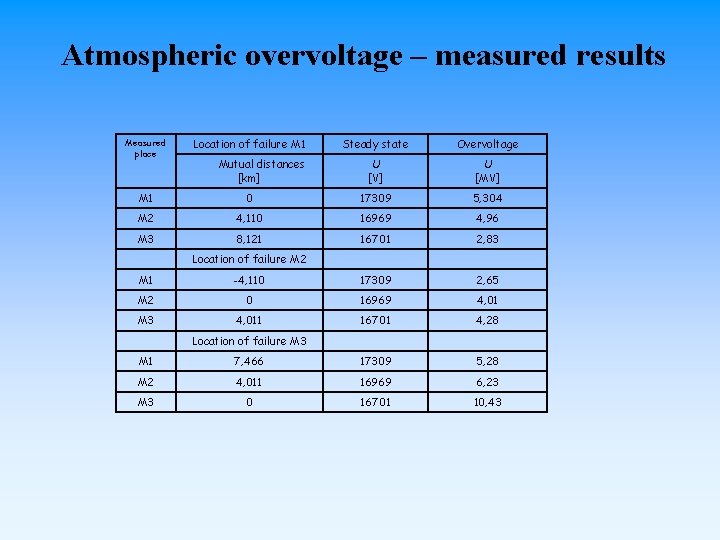
Atmospheric overvoltage – measured results Measured place Location of failure M 1 Steady state Overvoltage U [V] U [MV] M 1 0 17309 5, 304 M 2 4, 110 16969 4, 96 M 3 8, 121 16701 2, 83 Mutual distances [km] Location of failure M 2 M 1 -4, 110 17309 2, 65 M 2 0 16969 4, 01 M 3 4, 011 16701 4, 28 Location of failure M 3 M 1 7, 466 17309 5, 28 M 2 4, 011 16969 6, 23 M 3 0 16701 10, 43
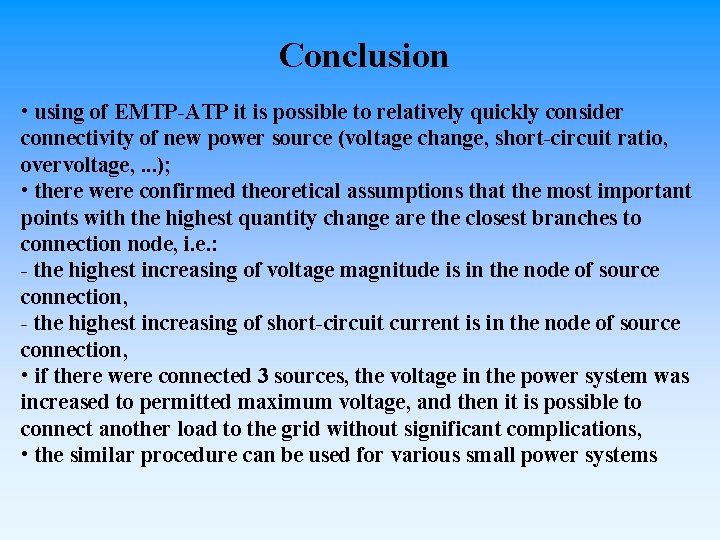
Conclusion • using of EMTP-ATP it is possible to relatively quickly consider connectivity of new power source (voltage change, short-circuit ratio, overvoltage, . . . ); • there were confirmed theoretical assumptions that the most important points with the highest quantity change are the closest branches to connection node, i. e. : - the highest increasing of voltage magnitude is in the node of source connection, - the highest increasing of short-circuit current is in the node of source connection, • if there were connected 3 sources, the voltage in the power system was increased to permitted maximum voltage, and then it is possible to connect another load to the grid without significant complications, • the similar procedure can be used for various small power systems
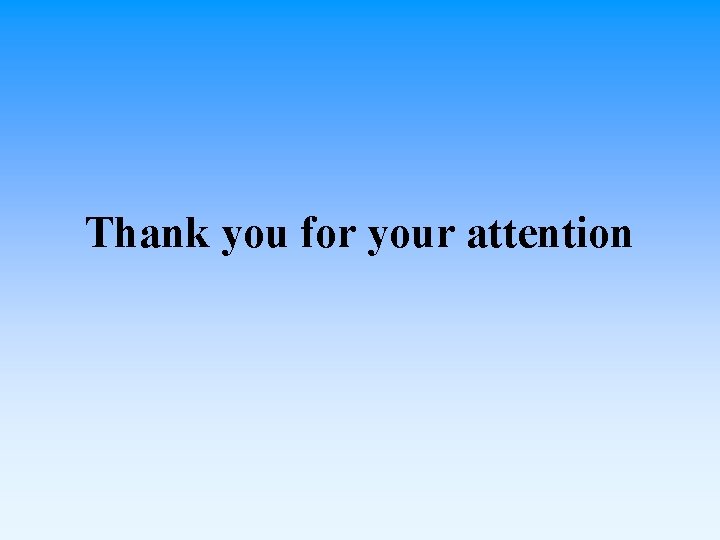
Thank you for your attention
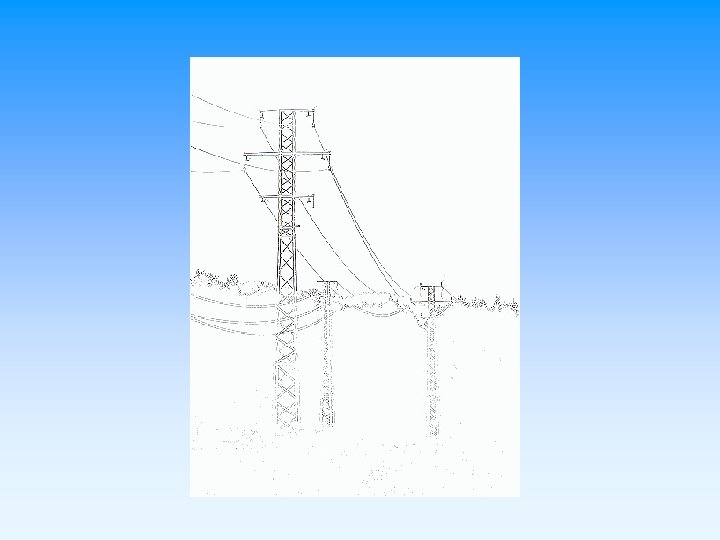
University of kragujevac faculty of technical sciences
Faculty of engineering university of porto
Roumieh university
Ece clemson
Faculty of mechanical engineering thammasat university
Koice
Skauti
Koice
Koice
Koice
Koice
Koice
Erasmus ytu
Czech technical university mechanical engineering
Faculty of civil engineering ctu prague
Czech technical university in prague civil engineering
Lee kong chian faculty of engineering and science
Faculty of engineering shoubra
Usf canvas
Civil engineering faculty
St anns college chirala
Igor dujlovic
Hijjawi faculty for engineering technology
Lee kong chian faculty of engineering and science
Mun engineering complementary studies
Studis fe
University of split faculty of maritime studies
University of bridgeport computer science faculty
University of bridgeport computer science faculty
Hubert kairuki memorial university faculty of medicine
Semmelweis
Applied medical sciences
Mch fsu
Mendel university - faculty of business and economics
Singularity university faculty
Agnes csaki semmelweis
Masaryk university medical faculty
Ldap cuni
Faculty of veterinary medicine cairo university logo
Faculty of law of the university of zagreb
University of montenegro faculty of law