Operating System Main Memory Operating System Concepts 8
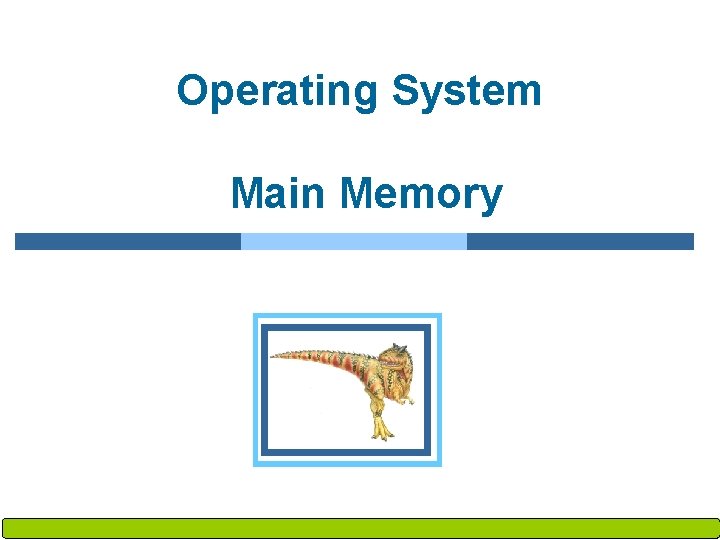
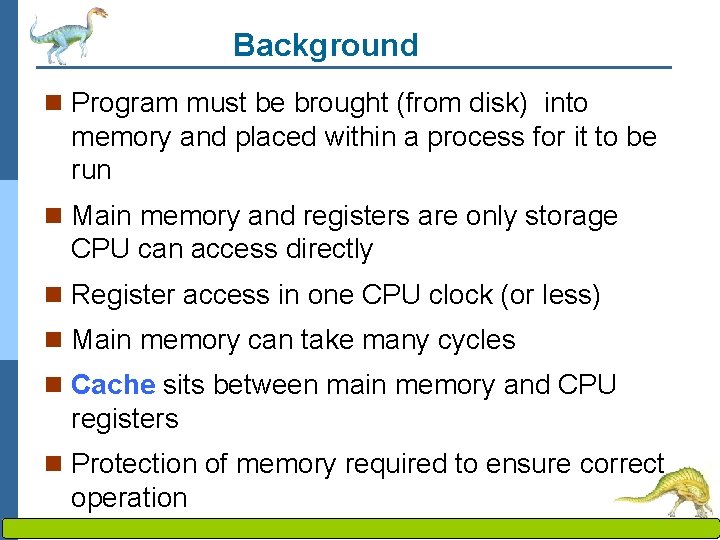
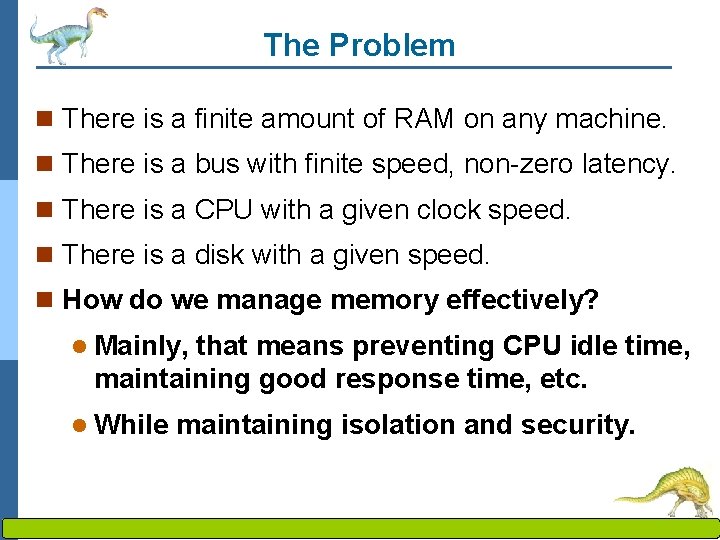
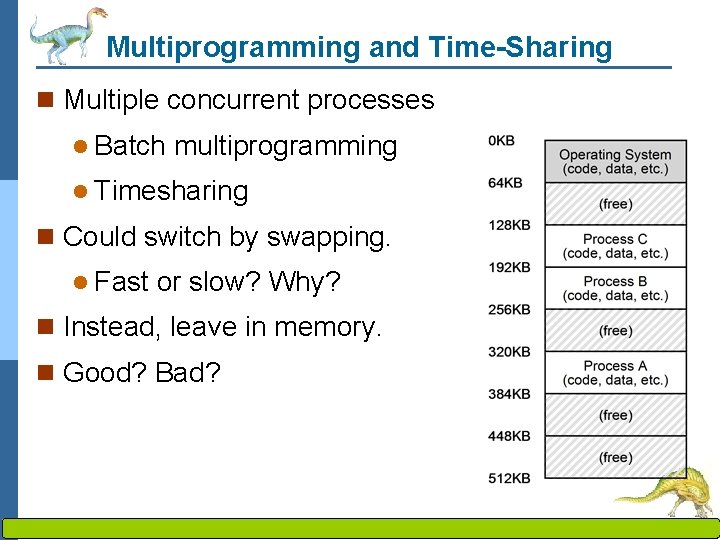
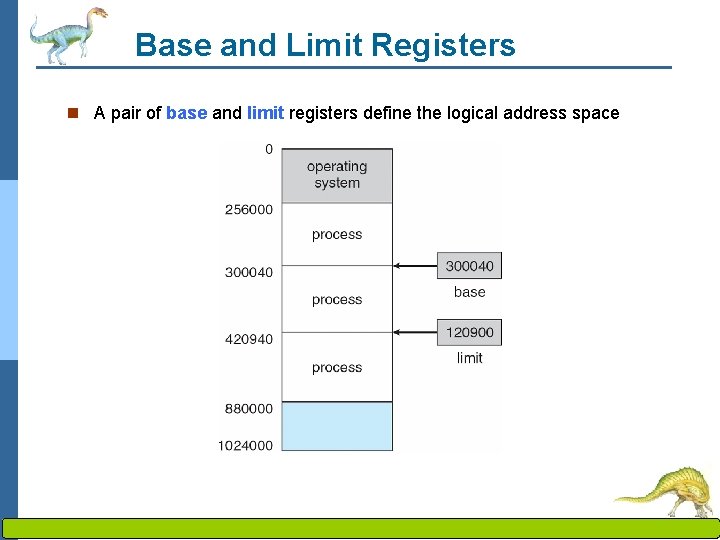
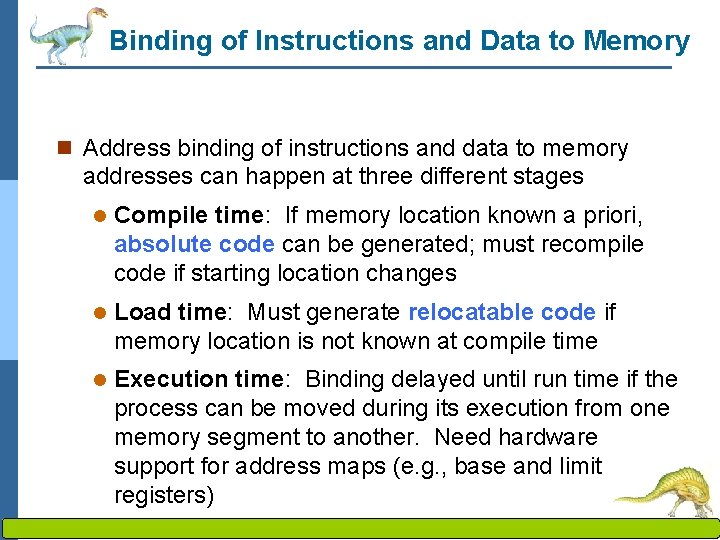
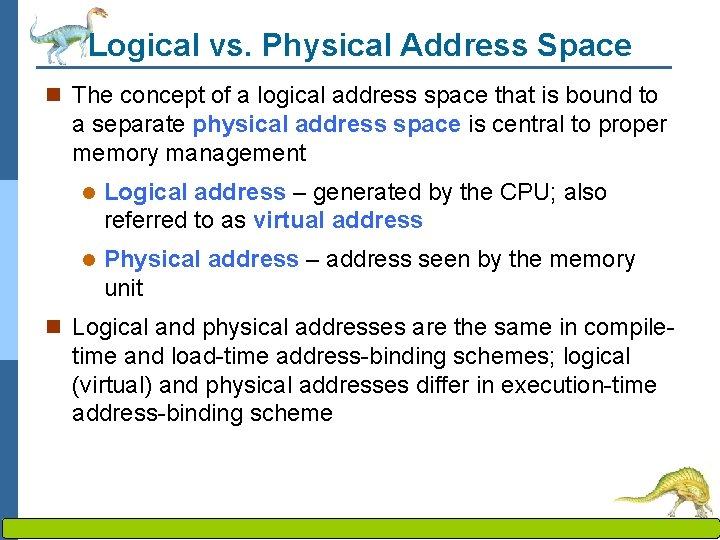
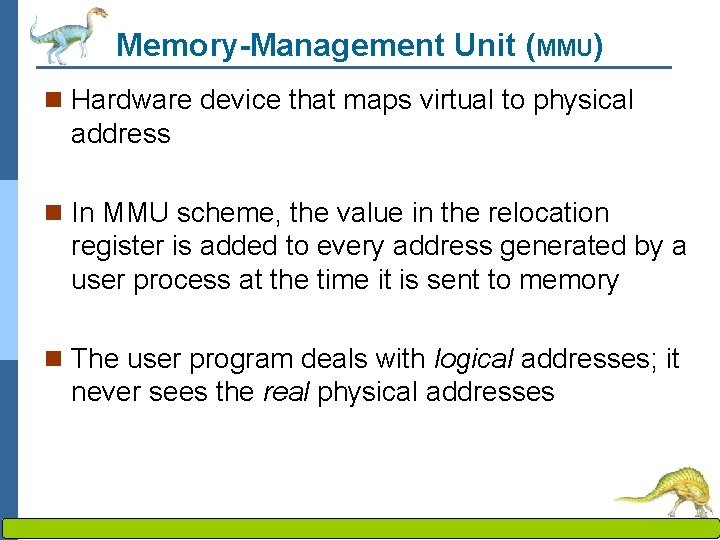
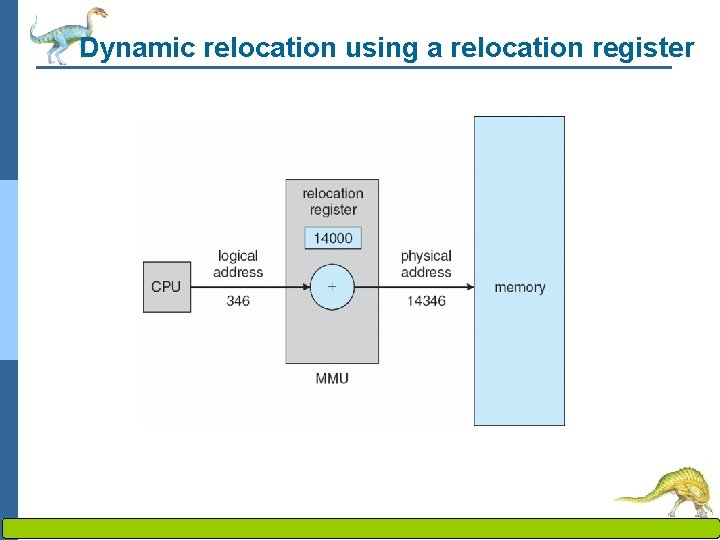
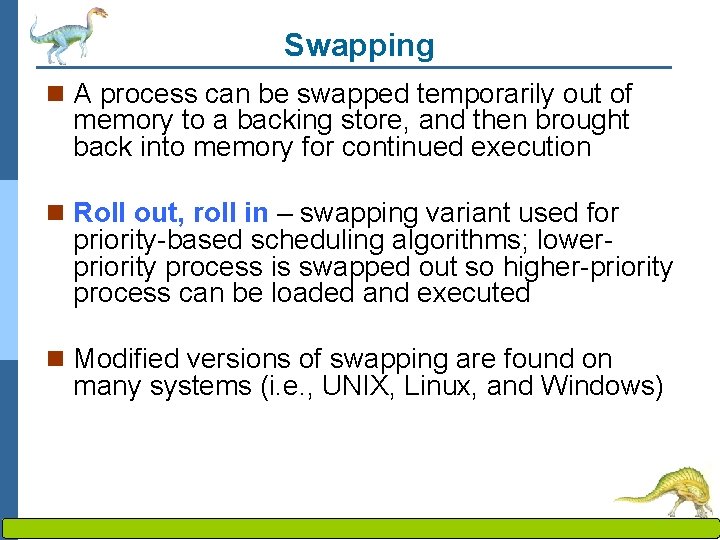
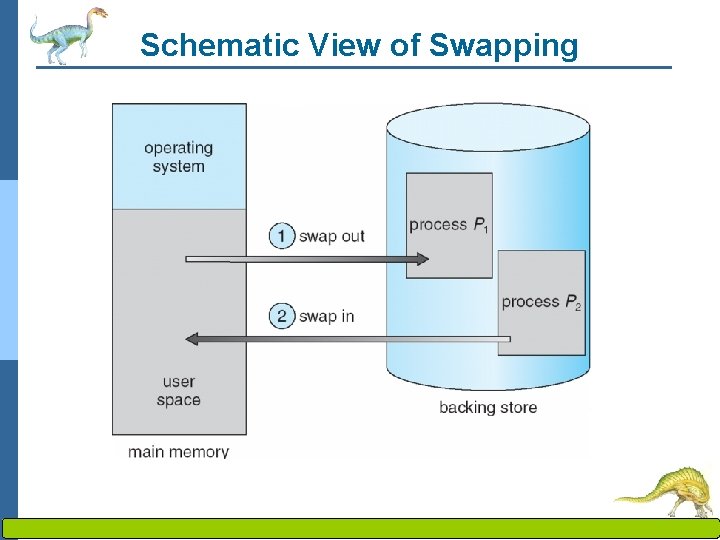
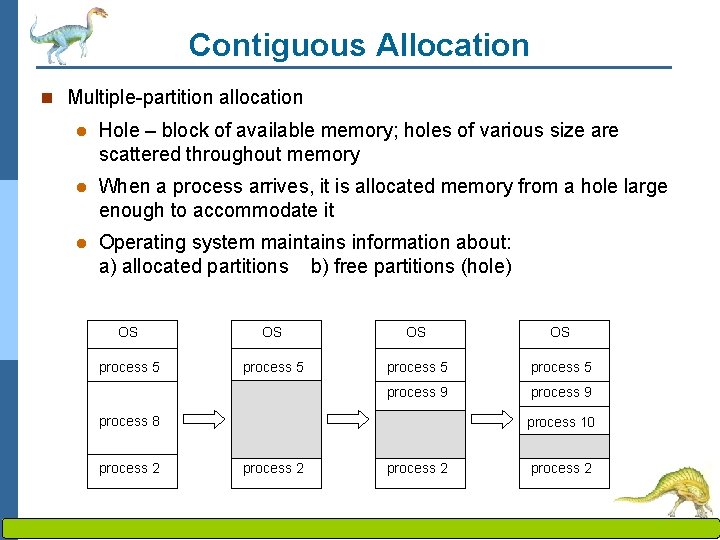
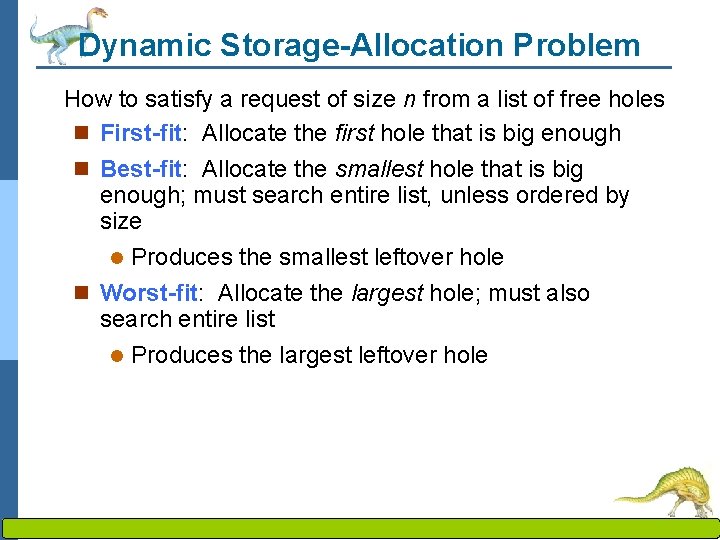
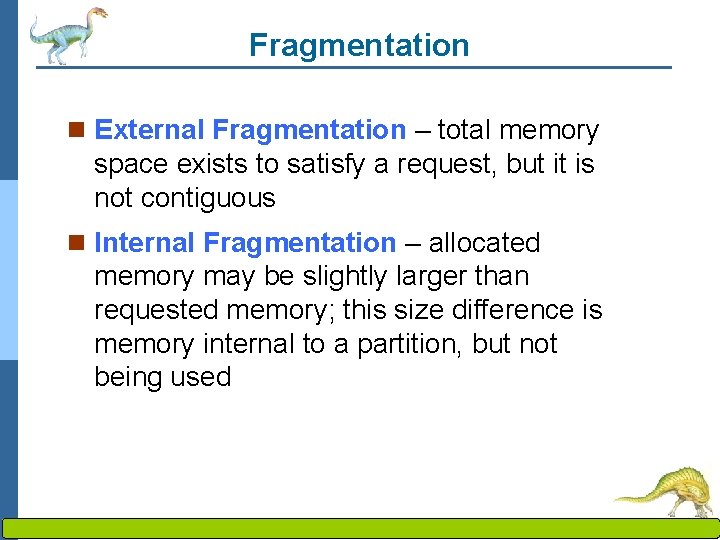
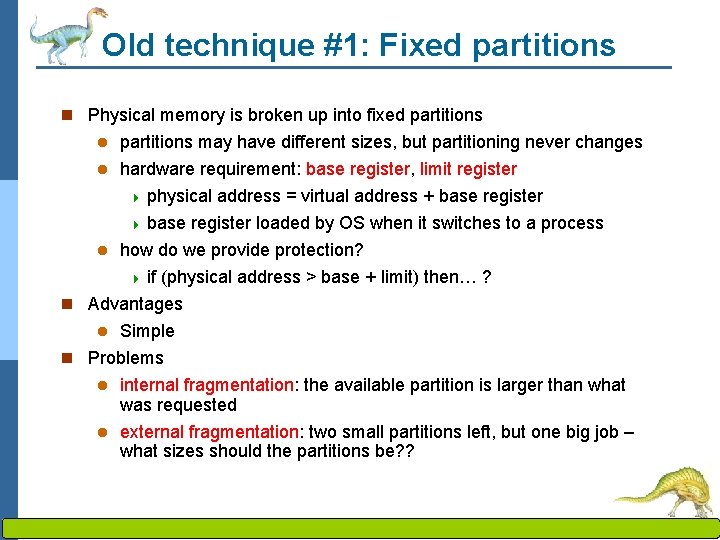
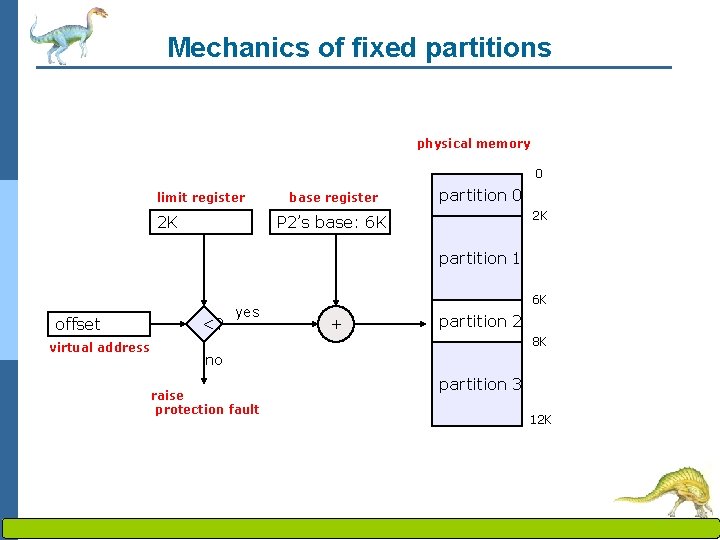
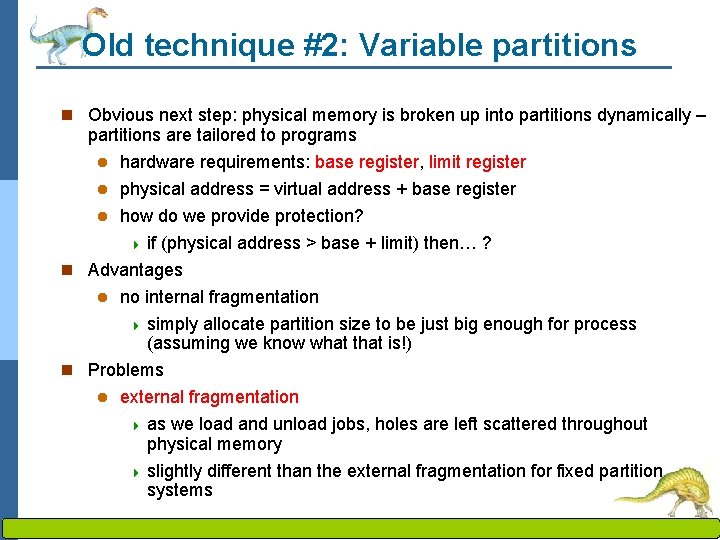
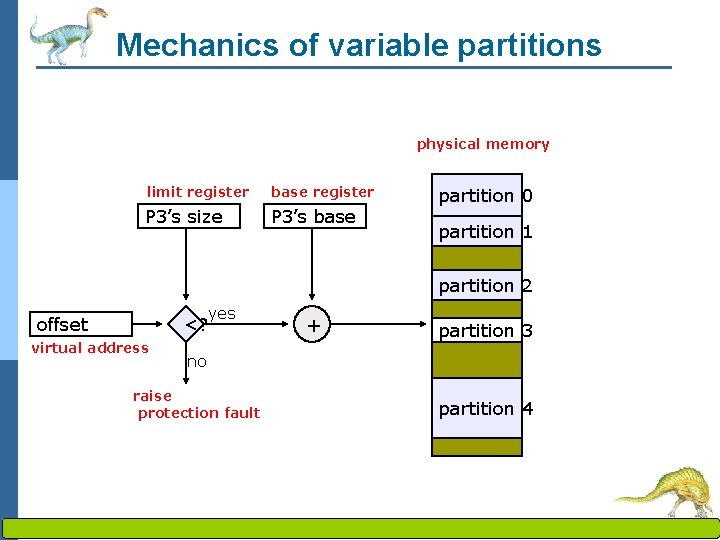
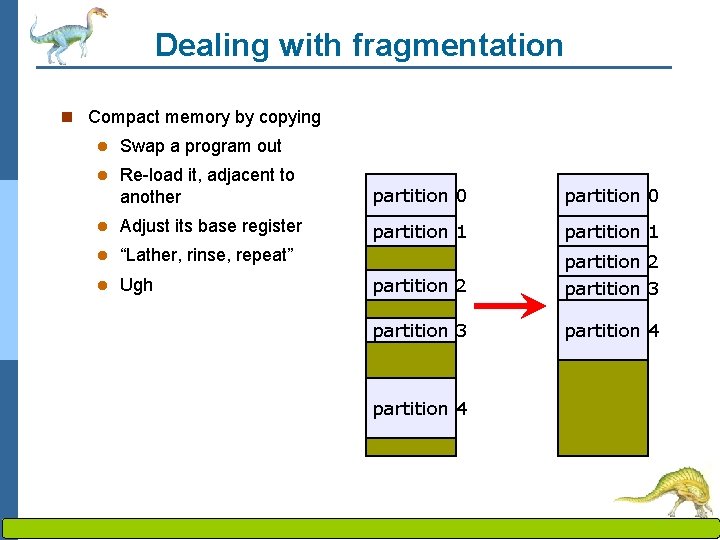
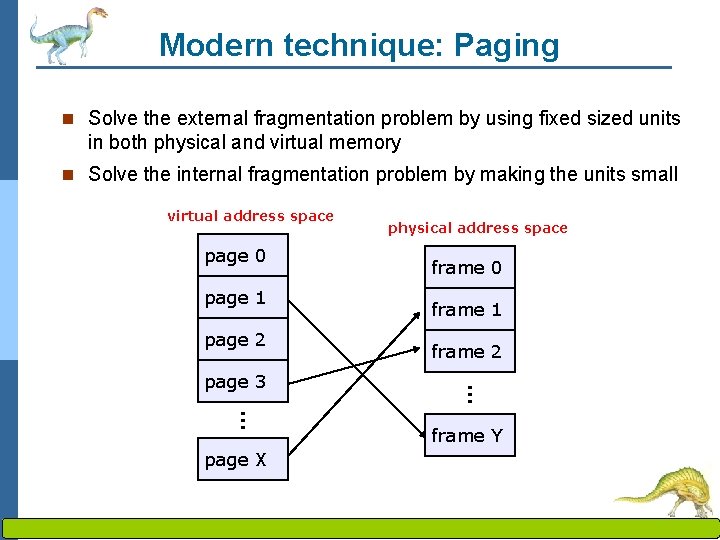
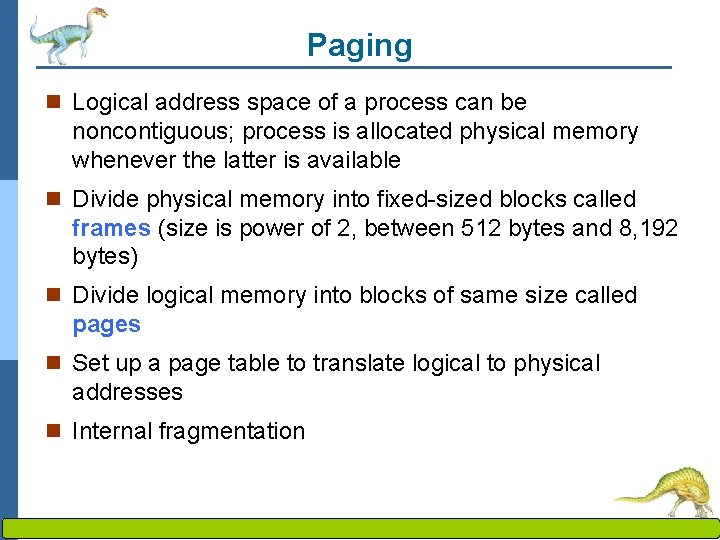
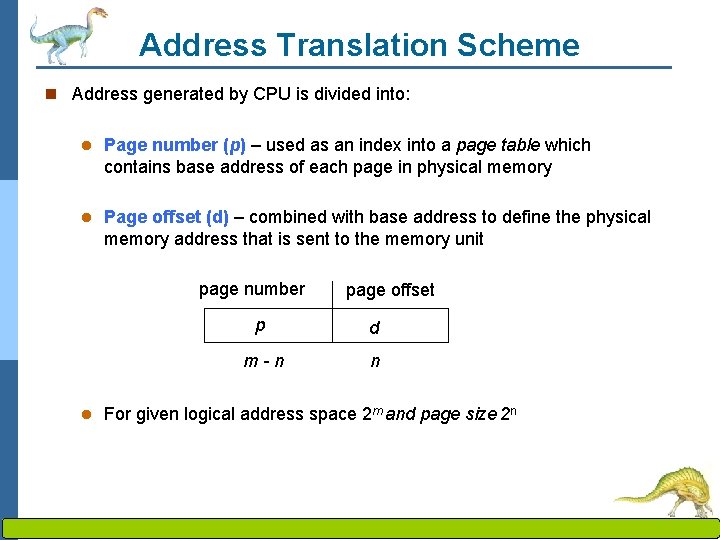
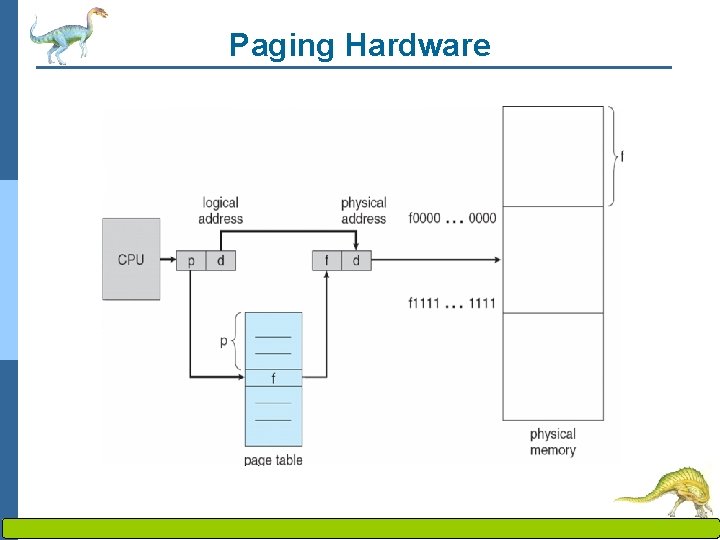
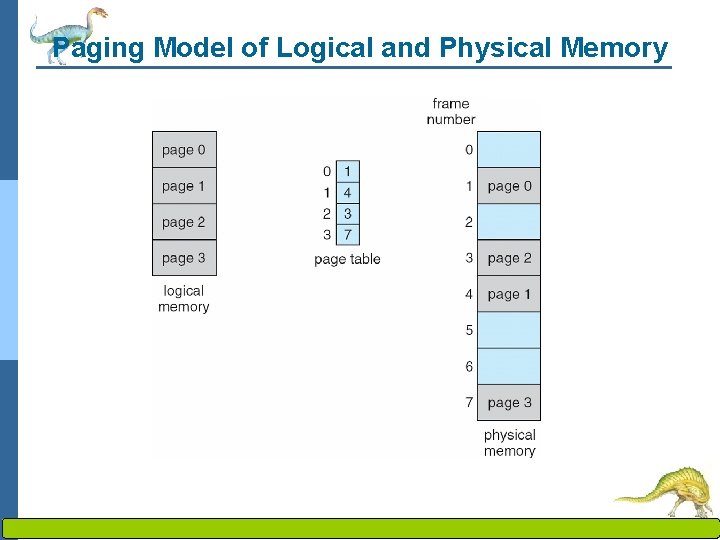
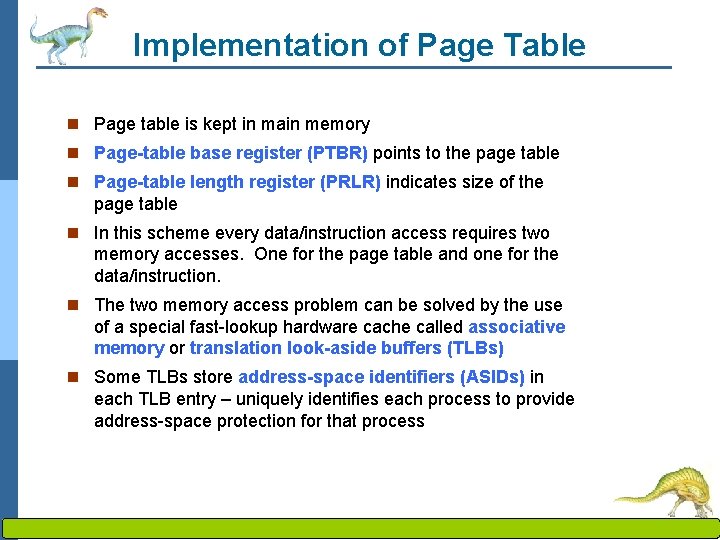
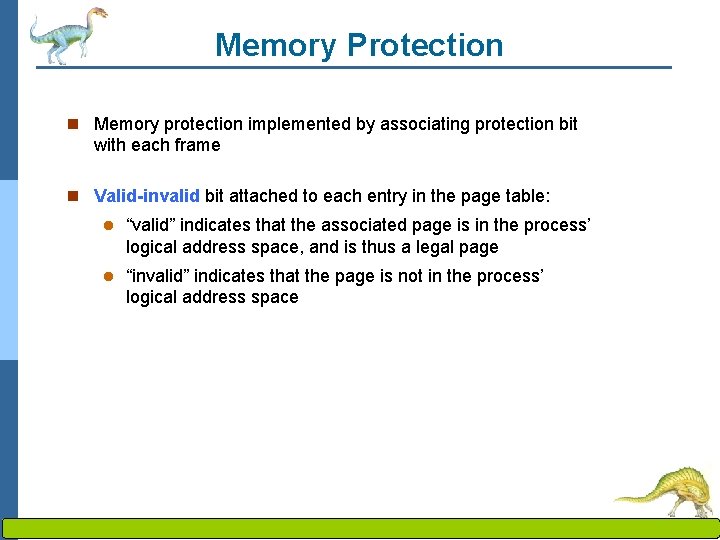
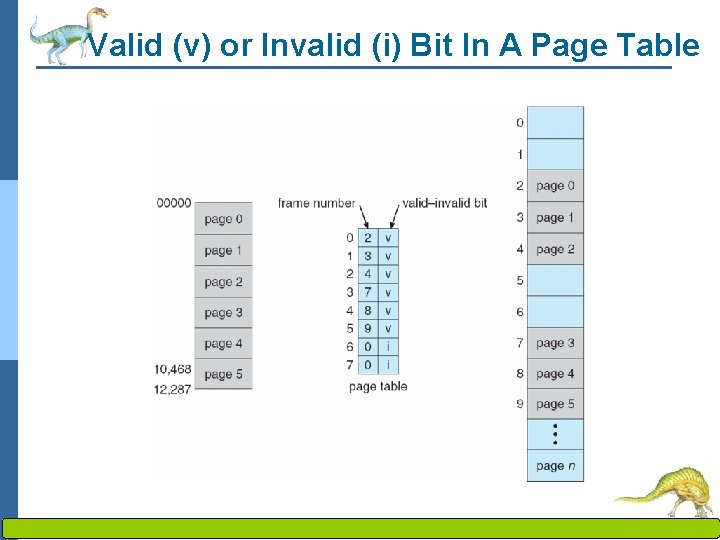
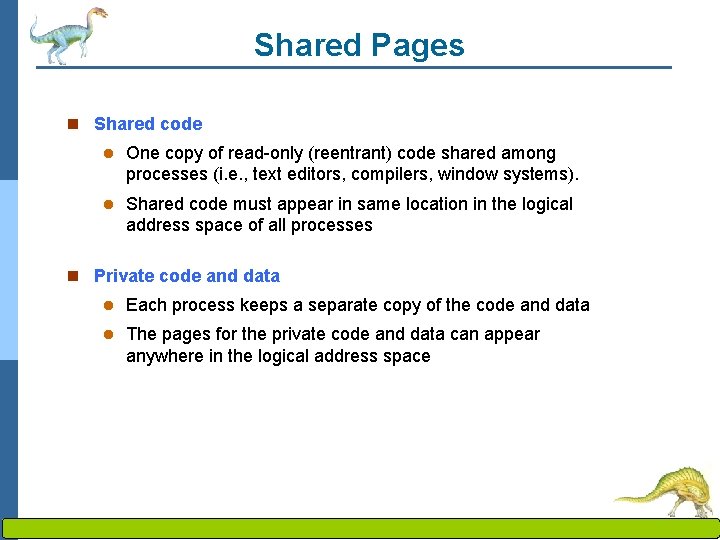
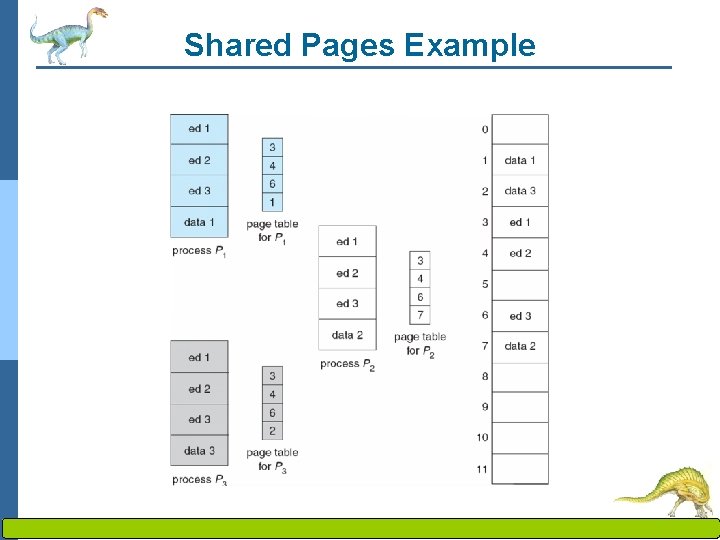
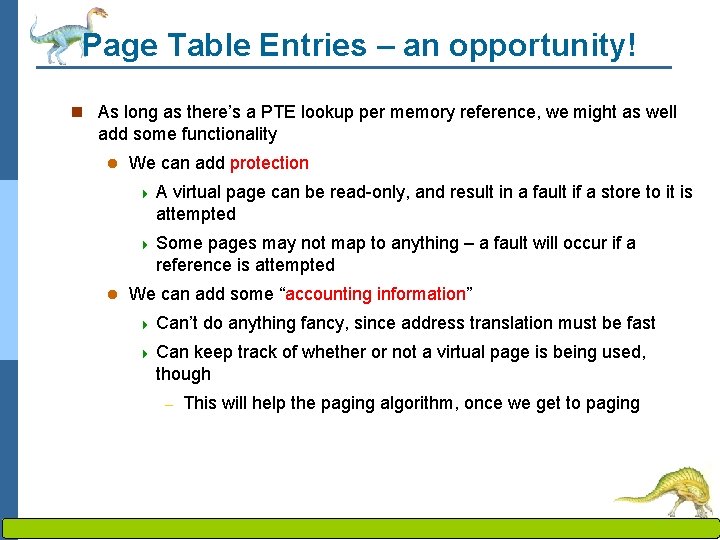
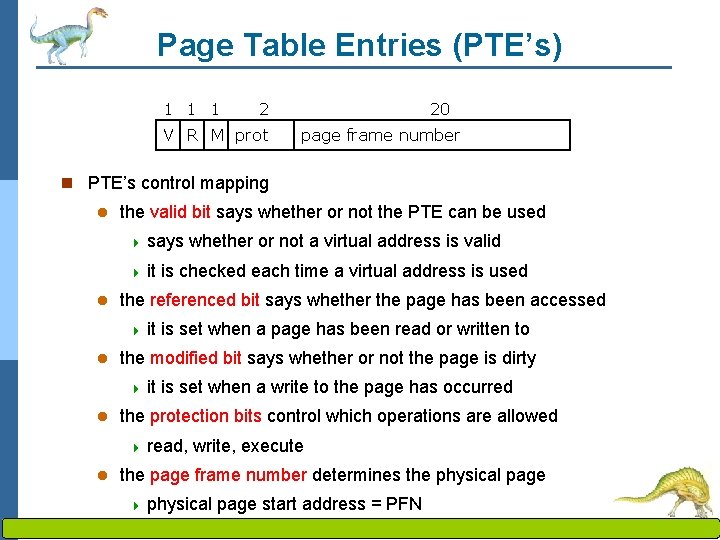
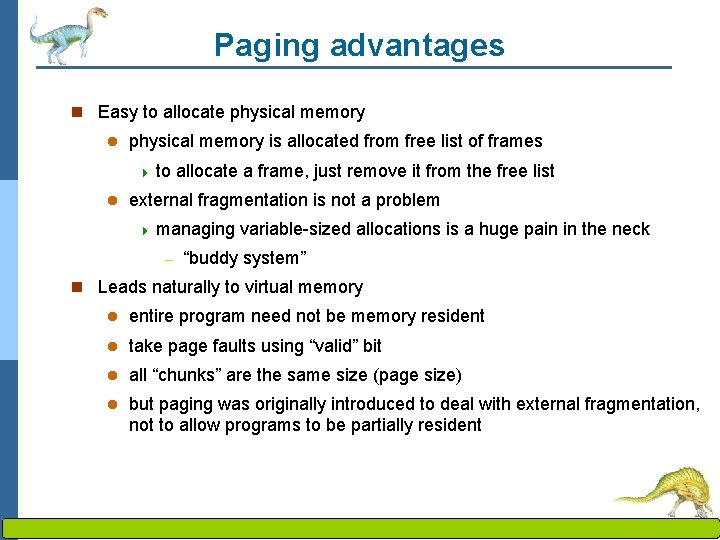
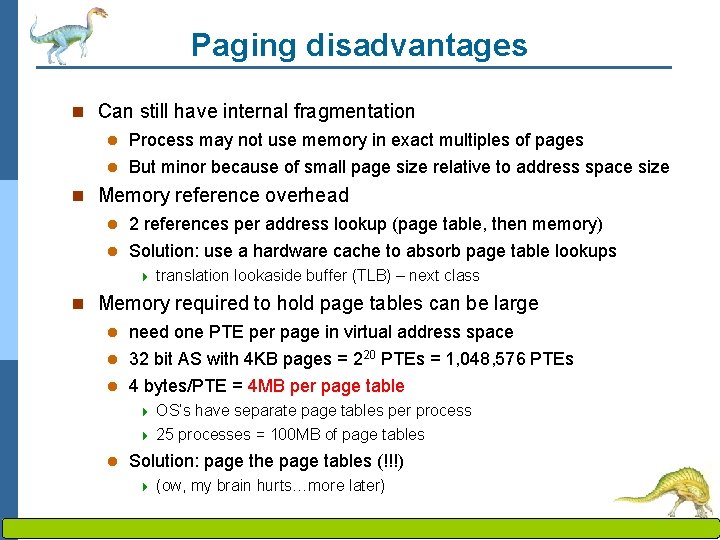
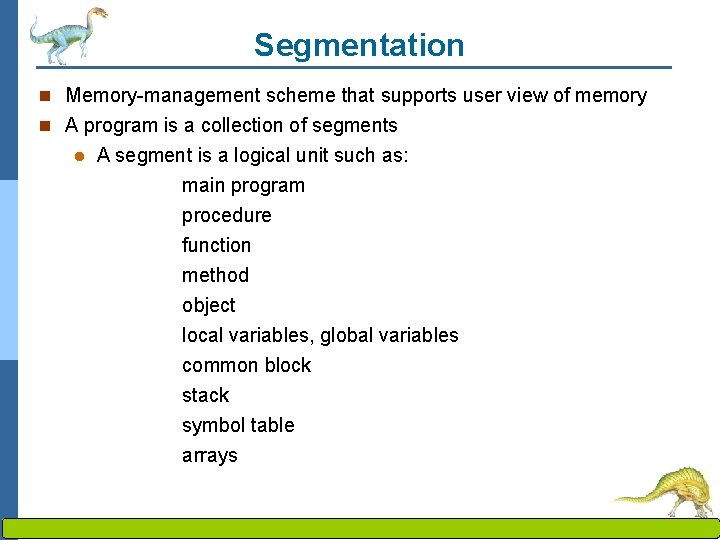
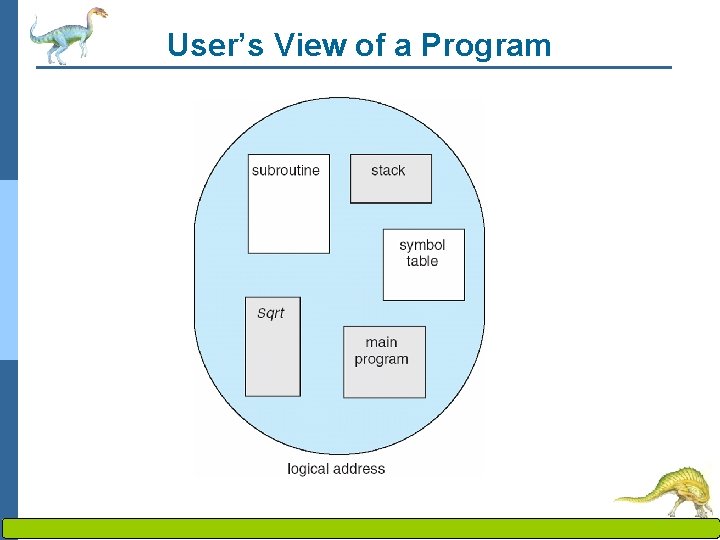
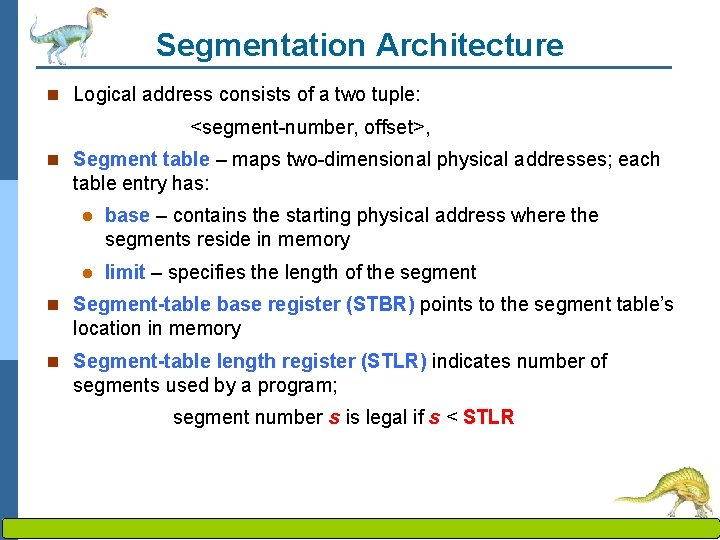
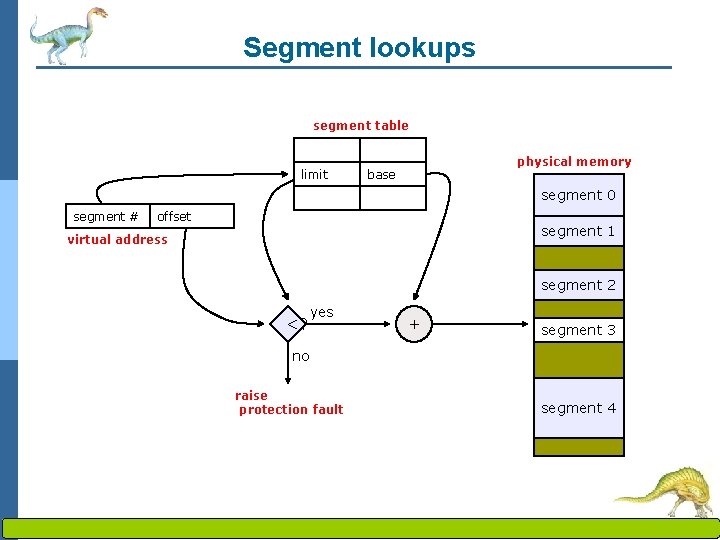
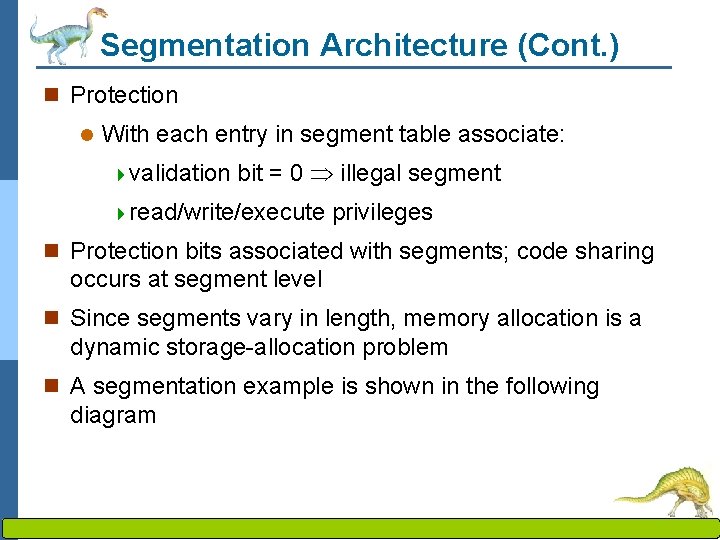
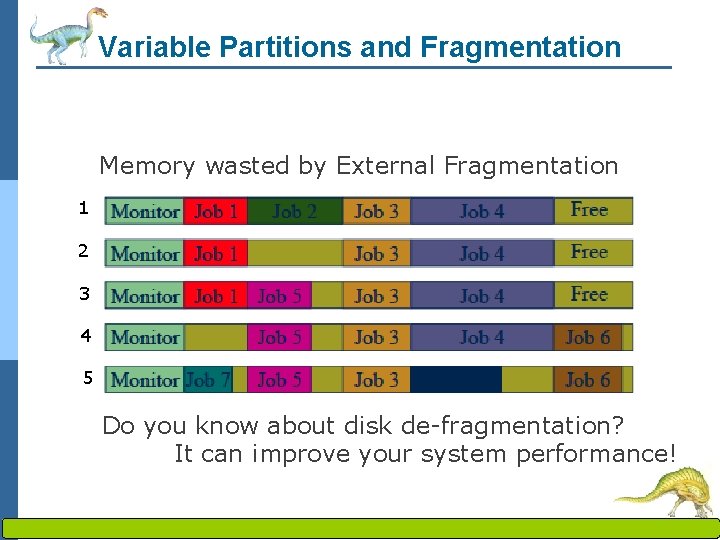
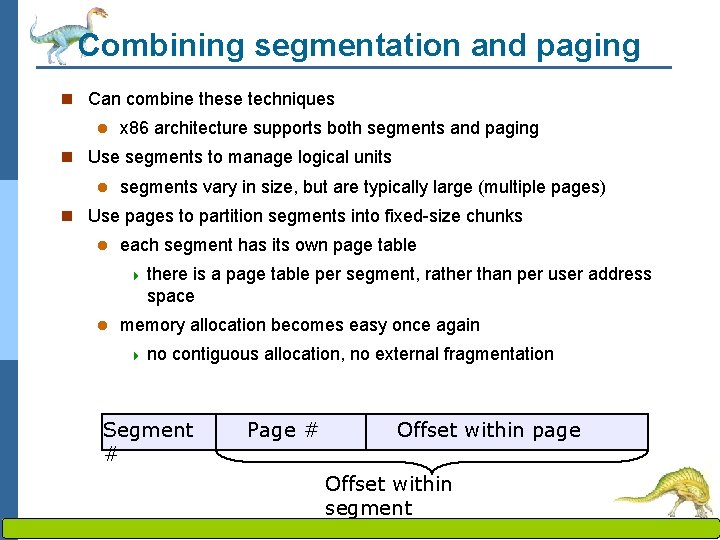
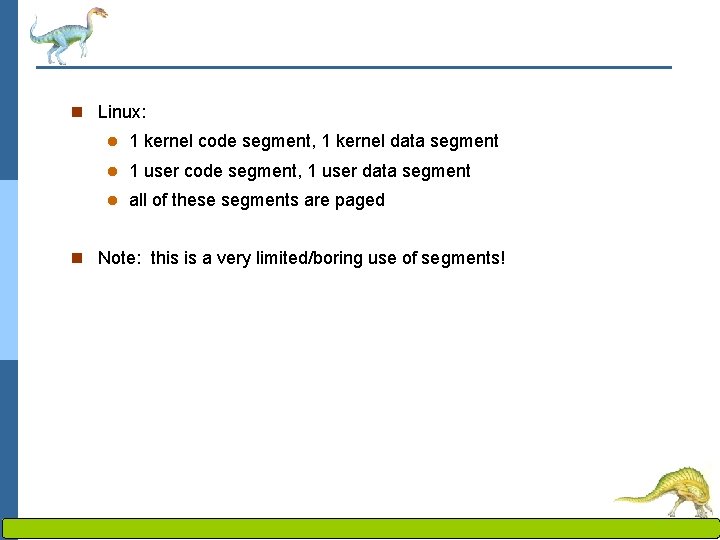
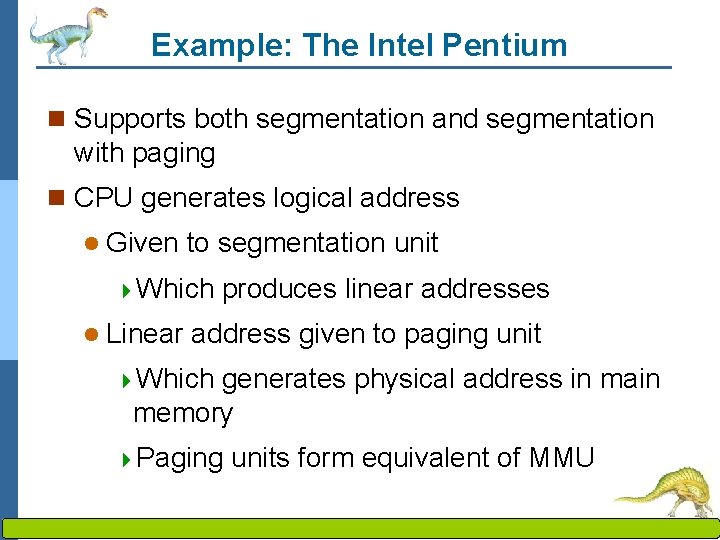
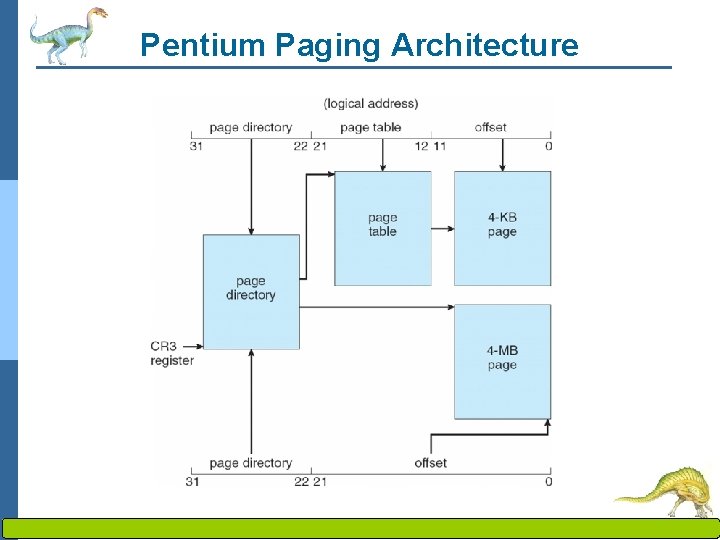
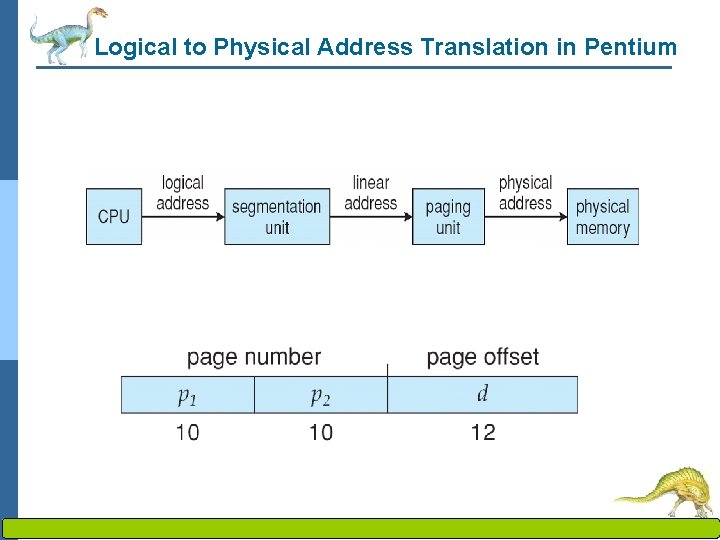
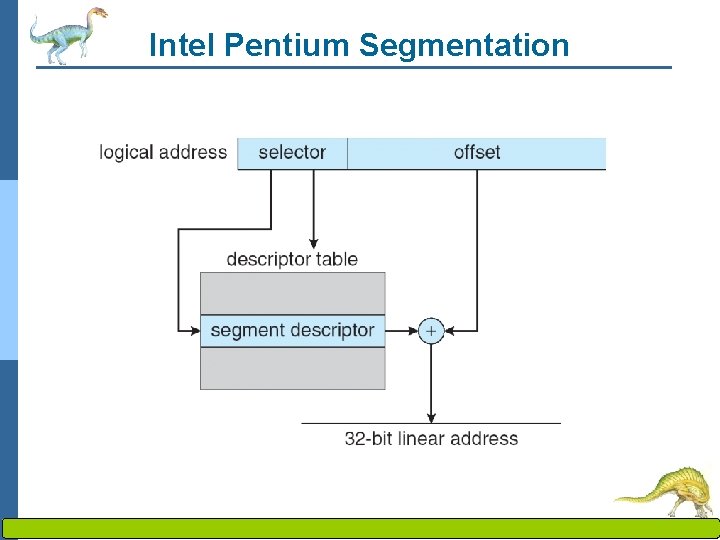
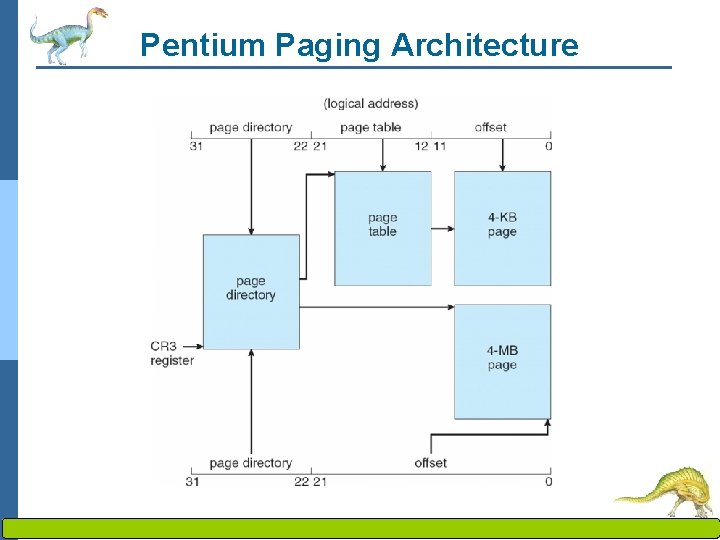
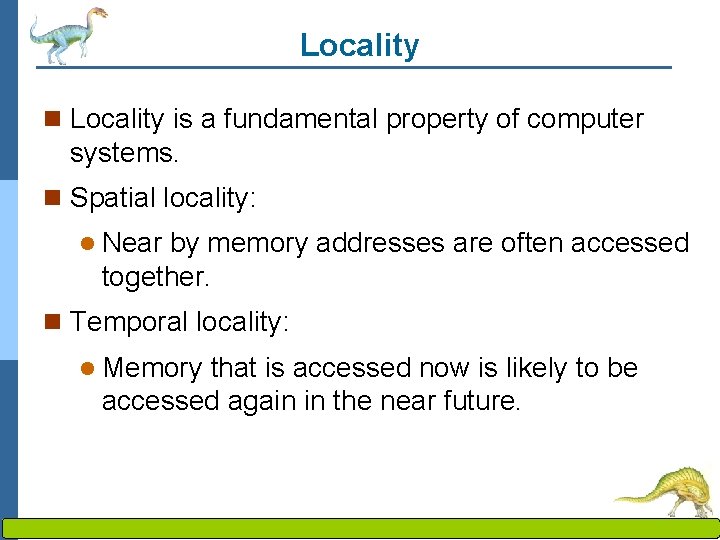
- Slides: 47
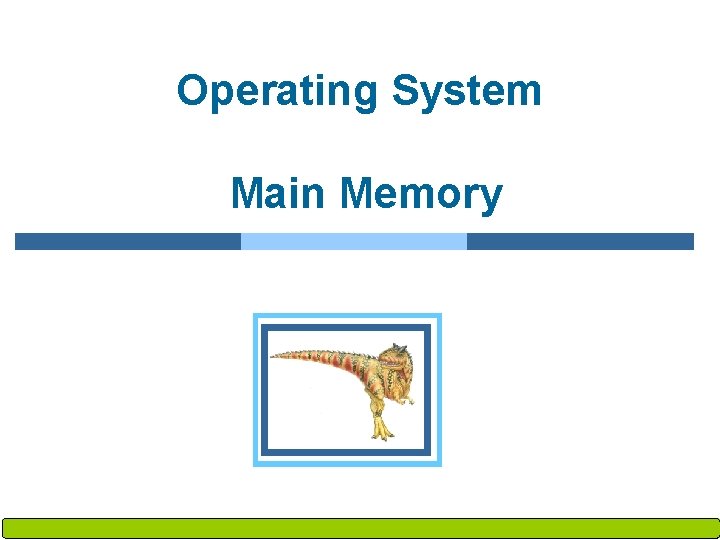
Operating System Main Memory Operating System Concepts – 8 th Edition, Silberschatz, Galvin and Gagne © 2009
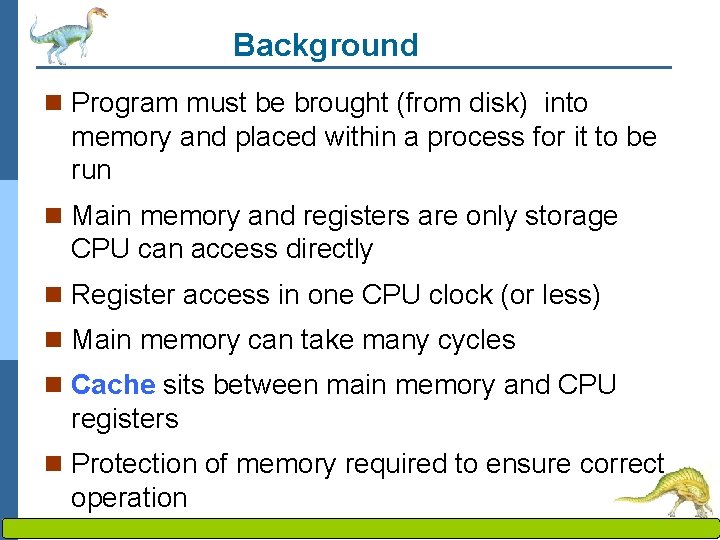
Background n Program must be brought (from disk) into memory and placed within a process for it to be run n Main memory and registers are only storage CPU can access directly n Register access in one CPU clock (or less) n Main memory can take many cycles n Cache sits between main memory and CPU registers n Protection of memory required to ensure correct operation Operating System Concepts – 8 th Edition 8. 2 Silberschatz, Galvin and Gagne © 2009
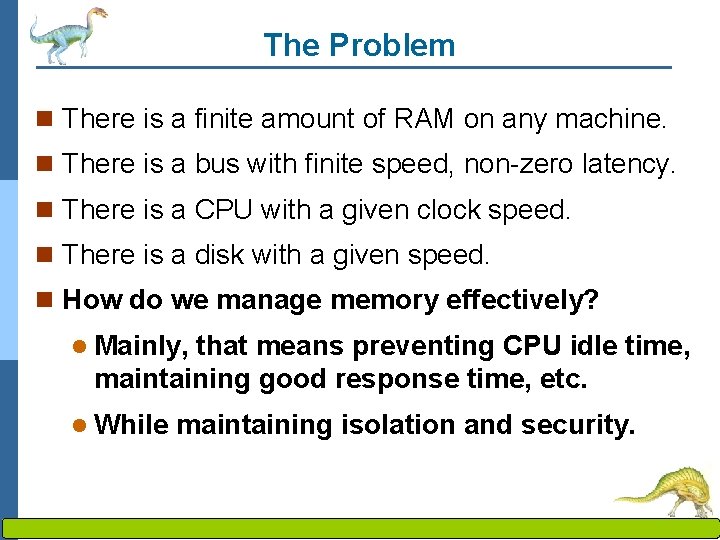
The Problem n There is a finite amount of RAM on any machine. n There is a bus with finite speed, non-zero latency. n There is a CPU with a given clock speed. n There is a disk with a given speed. n How do we manage memory effectively? l Mainly, that means preventing CPU idle time, maintaining good response time, etc. l While maintaining isolation and security. Operating System Concepts – 8 th Edition 8. 3 Silberschatz, Galvin and Gagne © 2009
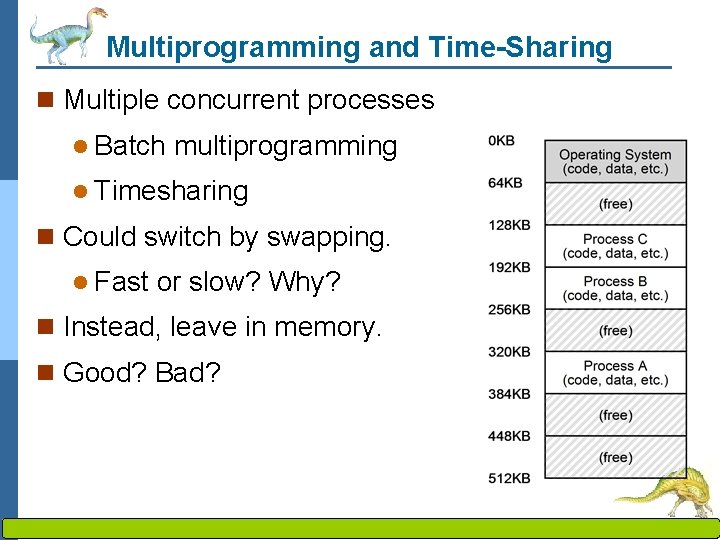
Multiprogramming and Time-Sharing n Multiple concurrent processes l Batch multiprogramming l Timesharing n Could switch by swapping. l Fast or slow? Why? n Instead, leave in memory. n Good? Bad? Operating System Concepts – 8 th Edition 8. 4 Silberschatz, Galvin and Gagne © 2009
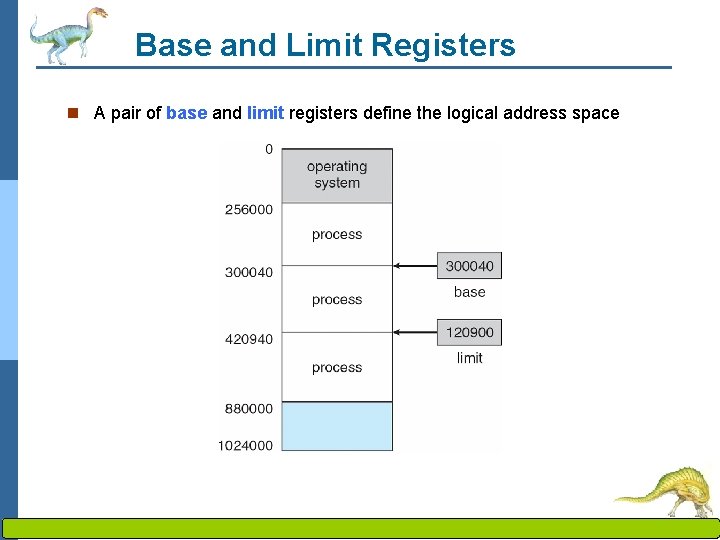
Base and Limit Registers n A pair of base and limit registers define the logical address space Operating System Concepts – 8 th Edition 8. 5 Silberschatz, Galvin and Gagne © 2009
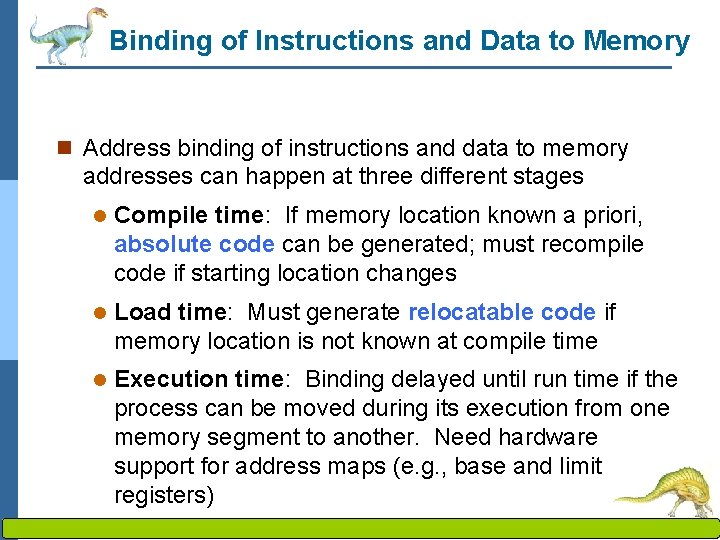
Binding of Instructions and Data to Memory n Address binding of instructions and data to memory addresses can happen at three different stages l Compile time: If memory location known a priori, absolute code can be generated; must recompile code if starting location changes l Load time: Must generate relocatable code if memory location is not known at compile time l Execution time: Binding delayed until run time if the process can be moved during its execution from one memory segment to another. Need hardware support for address maps (e. g. , base and limit registers) Operating System Concepts – 8 th Edition 8. 6 Silberschatz, Galvin and Gagne © 2009
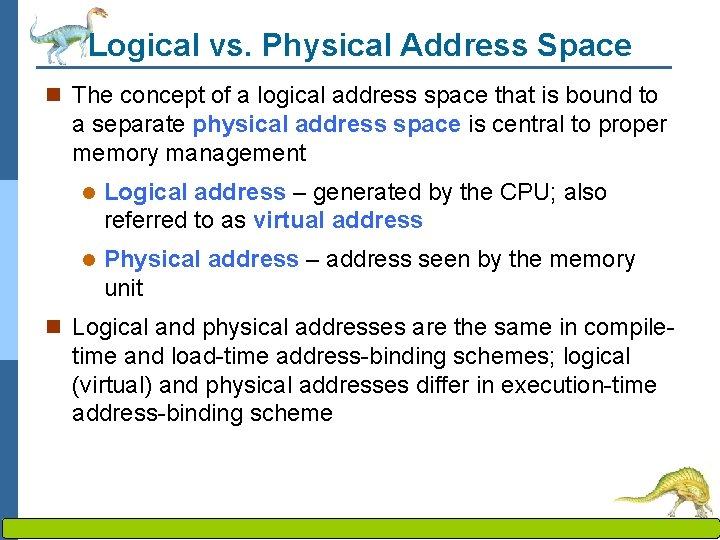
Logical vs. Physical Address Space n The concept of a logical address space that is bound to a separate physical address space is central to proper memory management l Logical address – generated by the CPU; also referred to as virtual address l Physical address – address seen by the memory unit n Logical and physical addresses are the same in compile- time and load-time address-binding schemes; logical (virtual) and physical addresses differ in execution-time address-binding scheme Operating System Concepts – 8 th Edition 8. 7 Silberschatz, Galvin and Gagne © 2009
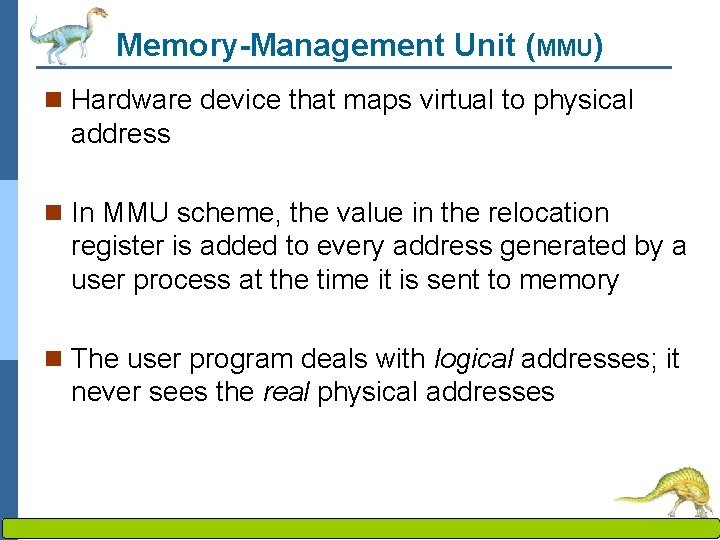
Memory-Management Unit (MMU) n Hardware device that maps virtual to physical address n In MMU scheme, the value in the relocation register is added to every address generated by a user process at the time it is sent to memory n The user program deals with logical addresses; it never sees the real physical addresses Operating System Concepts – 8 th Edition 8. 8 Silberschatz, Galvin and Gagne © 2009
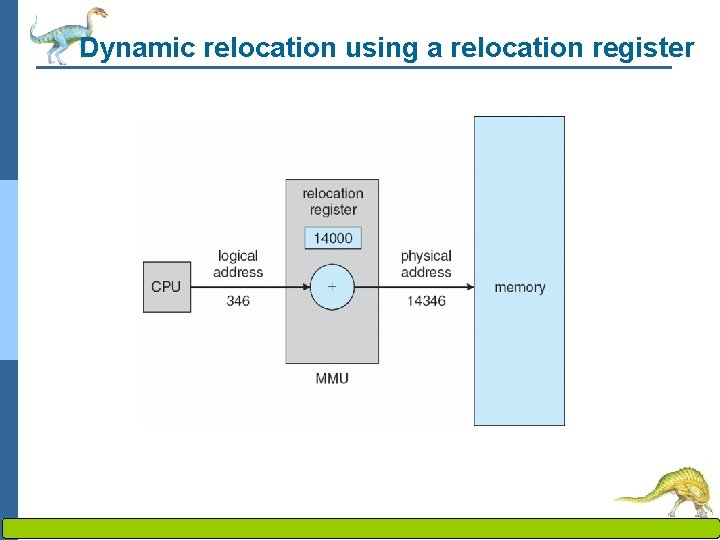
Dynamic relocation using a relocation register Operating System Concepts – 8 th Edition 8. 9 Silberschatz, Galvin and Gagne © 2009
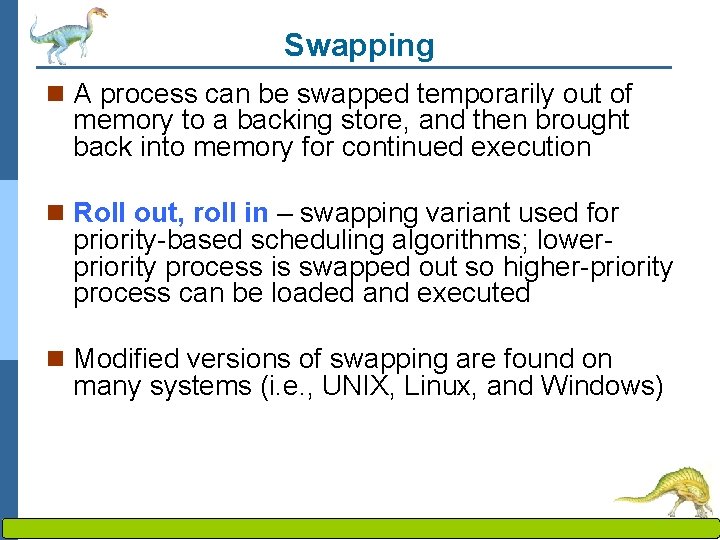
Swapping n A process can be swapped temporarily out of memory to a backing store, and then brought back into memory for continued execution n Roll out, roll in – swapping variant used for priority-based scheduling algorithms; lowerpriority process is swapped out so higher-priority process can be loaded and executed n Modified versions of swapping are found on many systems (i. e. , UNIX, Linux, and Windows) Operating System Concepts – 8 th Edition 8. 10 Silberschatz, Galvin and Gagne © 2009
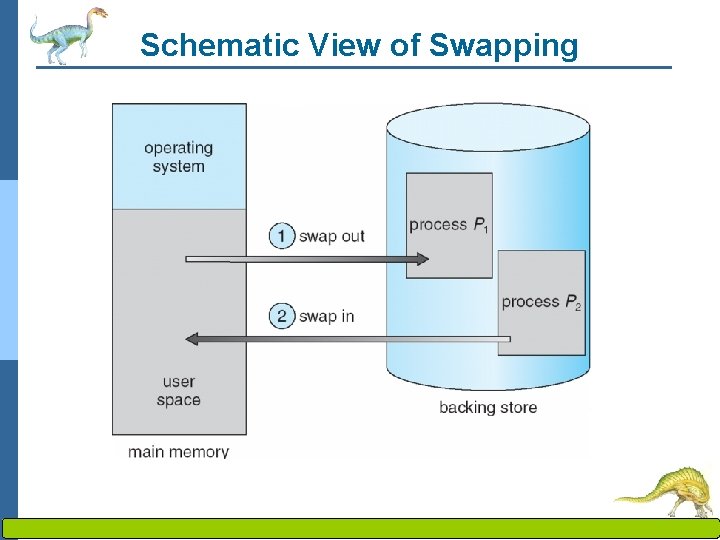
Schematic View of Swapping Operating System Concepts – 8 th Edition 8. 11 Silberschatz, Galvin and Gagne © 2009
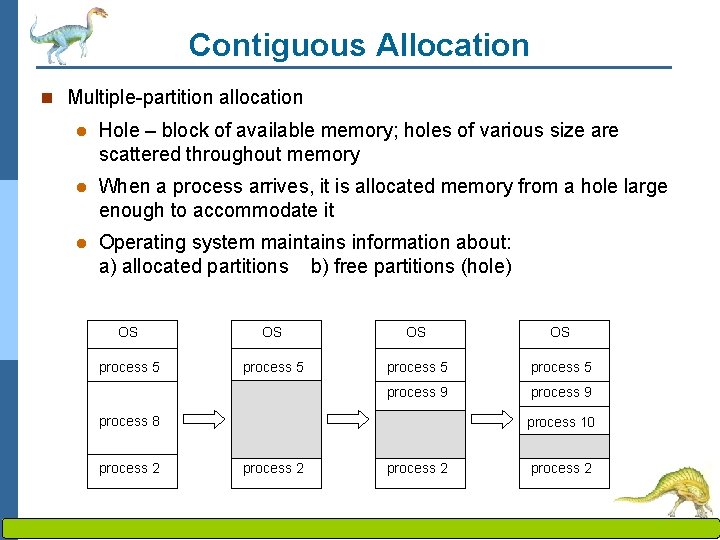
Contiguous Allocation n Multiple-partition allocation l Hole – block of available memory; holes of various size are scattered throughout memory l When a process arrives, it is allocated memory from a hole large enough to accommodate it l Operating system maintains information about: a) allocated partitions b) free partitions (hole) OS OS process 5 process 9 process 8 process 2 Operating System Concepts – 8 th Edition process 10 process 2 8. 12 process 2 Silberschatz, Galvin and Gagne © 2009
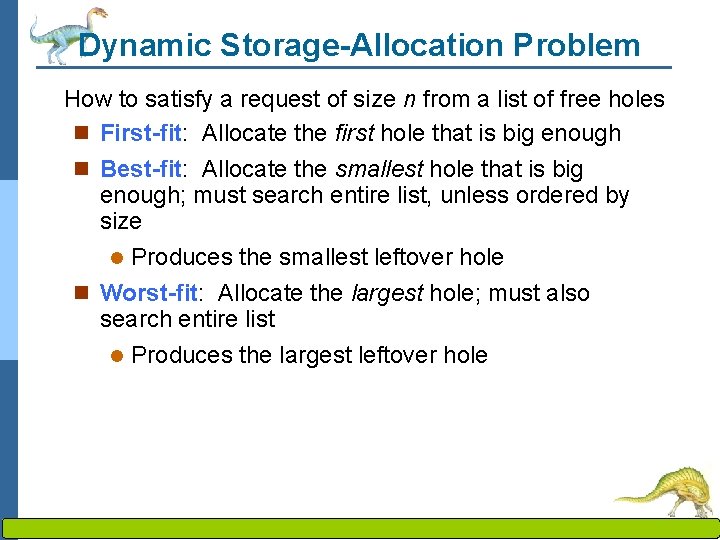
Dynamic Storage-Allocation Problem How to satisfy a request of size n from a list of free holes n First-fit: Allocate the first hole that is big enough n Best-fit: Allocate the smallest hole that is big enough; must search entire list, unless ordered by size l Produces the smallest leftover hole n Worst-fit: Allocate the largest hole; must also search entire list l Produces the largest leftover hole Operating System Concepts – 8 th Edition 8. 13 Silberschatz, Galvin and Gagne © 2009
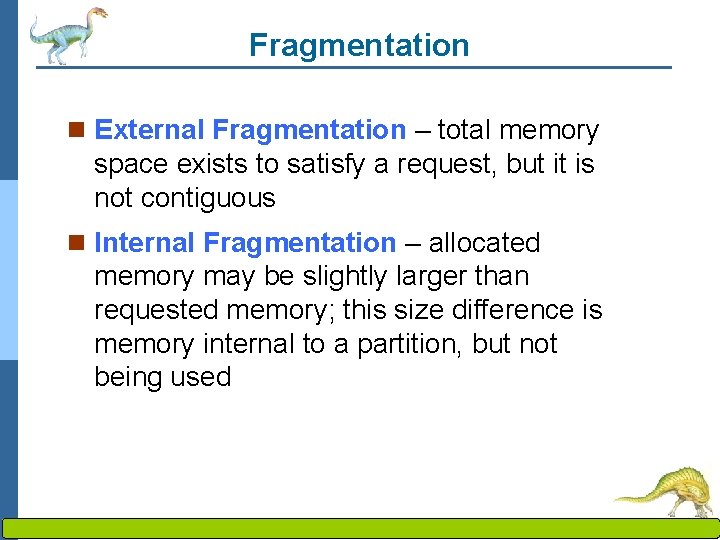
Fragmentation n External Fragmentation – total memory space exists to satisfy a request, but it is not contiguous n Internal Fragmentation – allocated memory may be slightly larger than requested memory; this size difference is memory internal to a partition, but not being used Operating System Concepts – 8 th Edition 8. 14 Silberschatz, Galvin and Gagne © 2009
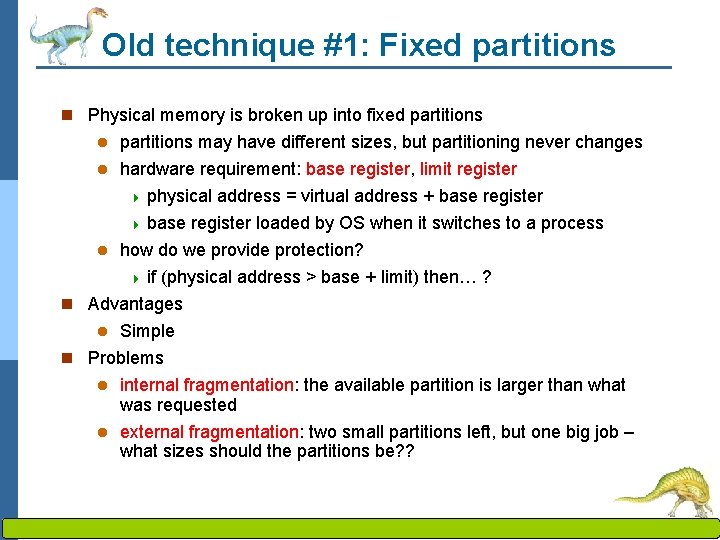
Old technique #1: Fixed partitions n Physical memory is broken up into fixed partitions may have different sizes, but partitioning never changes l hardware requirement: base register, limit register 4 physical address = virtual address + base register 4 base register loaded by OS when it switches to a process l how do we provide protection? 4 if (physical address > base + limit) then… ? n Advantages l Simple l n Problems internal fragmentation: the available partition is larger than what was requested l external fragmentation: two small partitions left, but one big job – what sizes should the partitions be? ? l Operating System Concepts – 8 th Edition 8. 15 Silberschatz, Galvin and Gagne © 2009
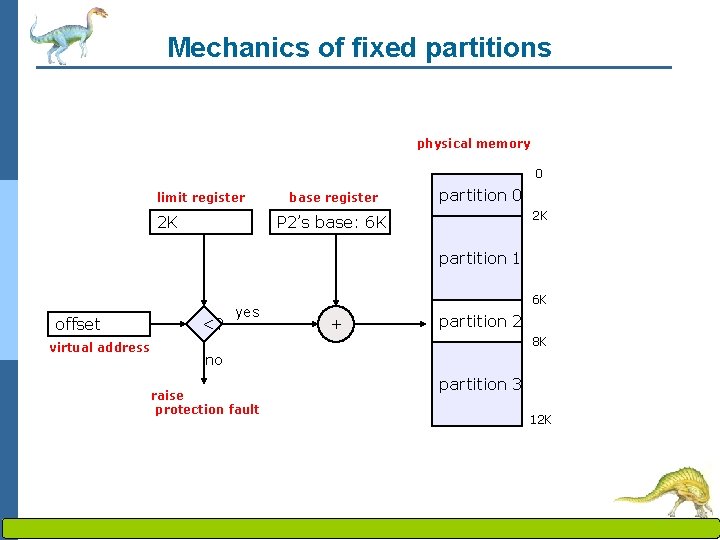
Mechanics of fixed partitions physical memory 0 limit register 2 K base register partition 0 2 K P 2’s base: 6 K partition 1 offset virtual address <? yes 6 K + partition 2 8 K no partition 3 raise protection fault Operating System Concepts – 8 th Edition 12 K 8. 16 Silberschatz, Galvin and Gagne © 2009
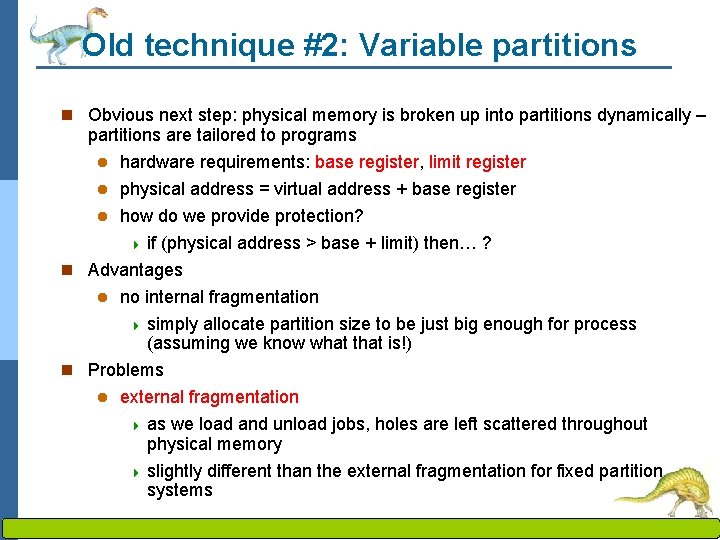
Old technique #2: Variable partitions n Obvious next step: physical memory is broken up into partitions dynamically – partitions are tailored to programs l hardware requirements: base register, limit register l physical address = virtual address + base register l how do we provide protection? 4 if (physical address > base + limit) then… ? n Advantages l no internal fragmentation 4 simply allocate partition size to be just big enough for process (assuming we know what that is!) n Problems l external fragmentation 4 as we load and unload jobs, holes are left scattered throughout physical memory 4 slightly different than the external fragmentation for fixed partition systems Operating System Concepts – 8 th Edition 8. 17 Silberschatz, Galvin and Gagne © 2009
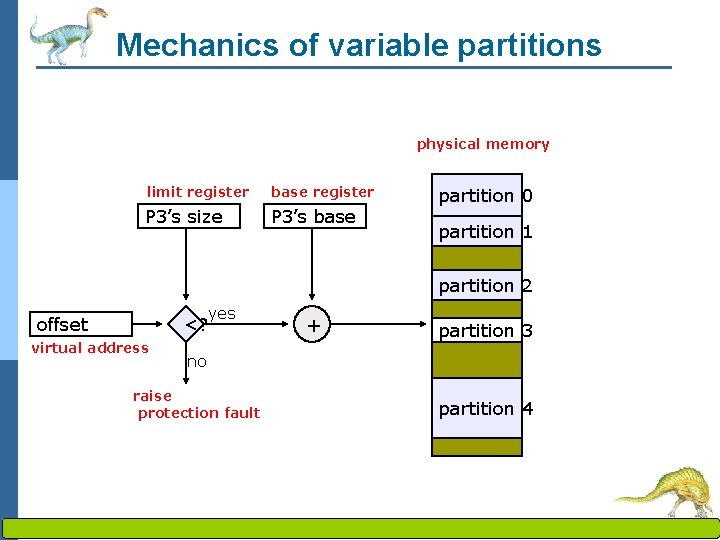
Mechanics of variable partitions physical memory limit register base register P 3’s size P 3’s base partition 0 partition 1 partition 2 yes offset <? virtual address + partition 3 no raise protection fault Operating System Concepts – 8 th Edition partition 4 8. 18 Silberschatz, Galvin and Gagne © 2009
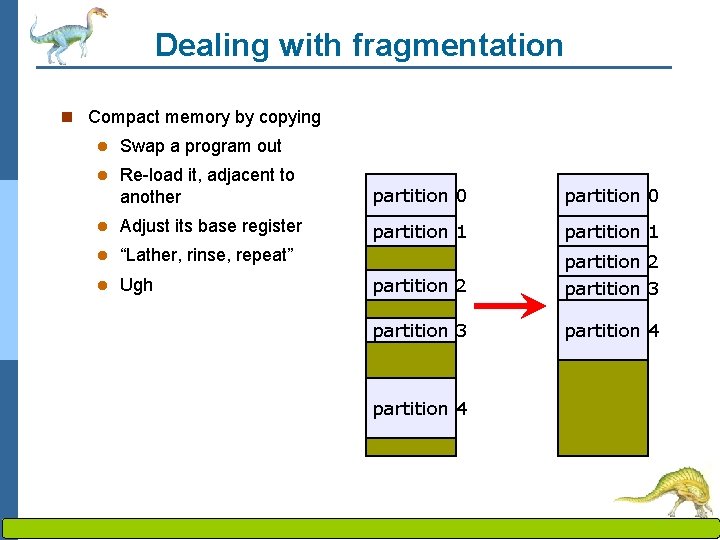
Dealing with fragmentation n Compact memory by copying l Swap a program out l Re-load it, adjacent to another partition 0 l Adjust its base register partition 1 l “Lather, rinse, repeat” l Ugh partition 2 partition 3 partition 4 Operating System Concepts – 8 th Edition 8. 19 Silberschatz, Galvin and Gagne © 2009
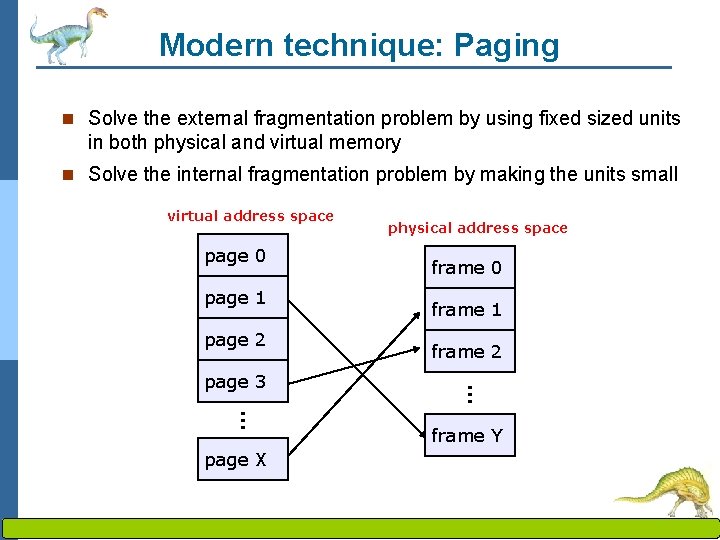
Modern technique: Paging n Solve the external fragmentation problem by using fixed sized units in both physical and virtual memory n Solve the internal fragmentation problem by making the units small virtual address space physical address space page 0 frame 0 page 1 frame 1 page 2 frame 2 … … page 3 frame Y page X Operating System Concepts – 8 th Edition 8. 20 Silberschatz, Galvin and Gagne © 2009
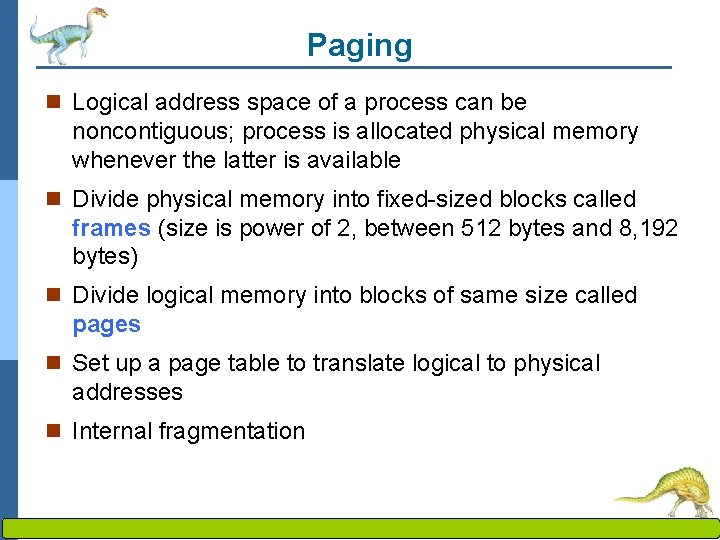
Paging n Logical address space of a process can be noncontiguous; process is allocated physical memory whenever the latter is available n Divide physical memory into fixed-sized blocks called frames (size is power of 2, between 512 bytes and 8, 192 bytes) n Divide logical memory into blocks of same size called pages n Set up a page table to translate logical to physical addresses n Internal fragmentation Operating System Concepts – 8 th Edition 8. 21 Silberschatz, Galvin and Gagne © 2009
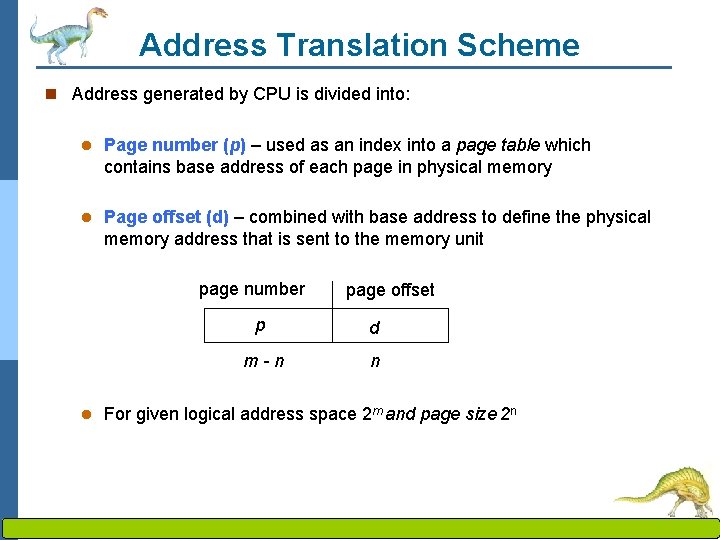
Address Translation Scheme n Address generated by CPU is divided into: l Page number (p) – used as an index into a page table which contains base address of each page in physical memory l Page offset (d) – combined with base address to define the physical memory address that is sent to the memory unit page number l page offset p d m-n n For given logical address space 2 m and page size 2 n Operating System Concepts – 8 th Edition 8. 22 Silberschatz, Galvin and Gagne © 2009
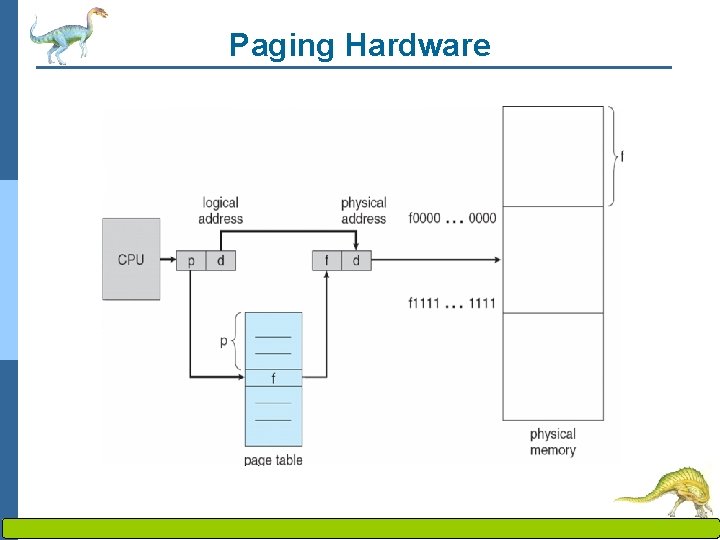
Paging Hardware Operating System Concepts – 8 th Edition 8. 23 Silberschatz, Galvin and Gagne © 2009
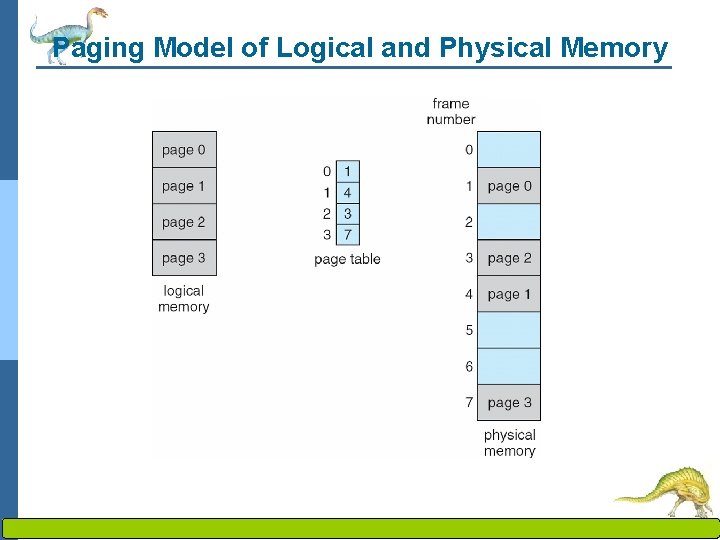
Paging Model of Logical and Physical Memory Operating System Concepts – 8 th Edition 8. 24 Silberschatz, Galvin and Gagne © 2009
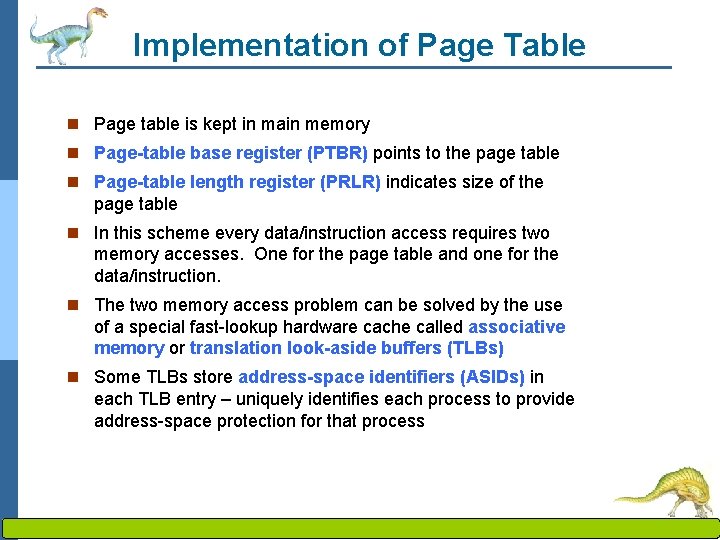
Implementation of Page Table n Page table is kept in main memory n Page-table base register (PTBR) points to the page table n Page-table length register (PRLR) indicates size of the page table n In this scheme every data/instruction access requires two memory accesses. One for the page table and one for the data/instruction. n The two memory access problem can be solved by the use of a special fast-lookup hardware cache called associative memory or translation look-aside buffers (TLBs) n Some TLBs store address-space identifiers (ASIDs) in each TLB entry – uniquely identifies each process to provide address-space protection for that process Operating System Concepts – 8 th Edition 8. 25 Silberschatz, Galvin and Gagne © 2009
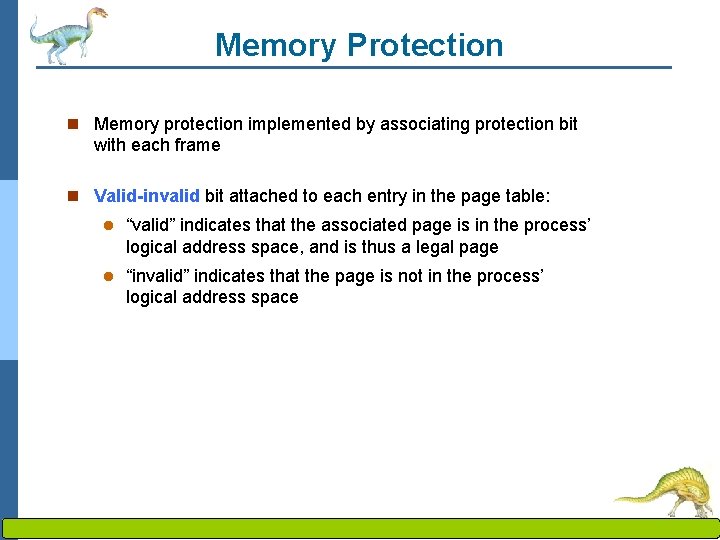
Memory Protection n Memory protection implemented by associating protection bit with each frame n Valid-invalid bit attached to each entry in the page table: l “valid” indicates that the associated page is in the process’ logical address space, and is thus a legal page l “invalid” indicates that the page is not in the process’ logical address space Operating System Concepts – 8 th Edition 8. 26 Silberschatz, Galvin and Gagne © 2009
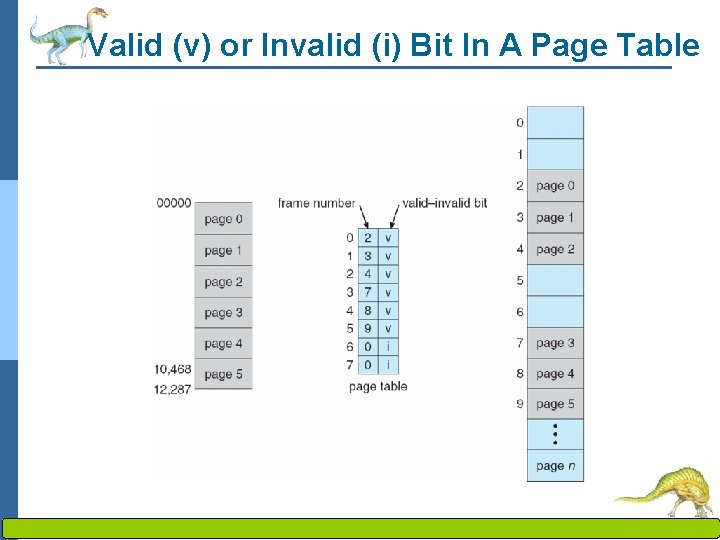
Valid (v) or Invalid (i) Bit In A Page Table Operating System Concepts – 8 th Edition 8. 27 Silberschatz, Galvin and Gagne © 2009
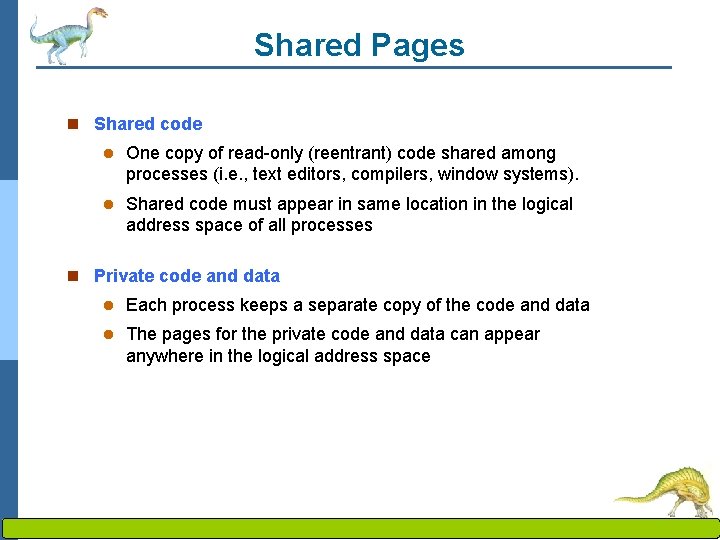
Shared Pages n Shared code l One copy of read-only (reentrant) code shared among processes (i. e. , text editors, compilers, window systems). l Shared code must appear in same location in the logical address space of all processes n Private code and data l Each process keeps a separate copy of the code and data l The pages for the private code and data can appear anywhere in the logical address space Operating System Concepts – 8 th Edition 8. 28 Silberschatz, Galvin and Gagne © 2009
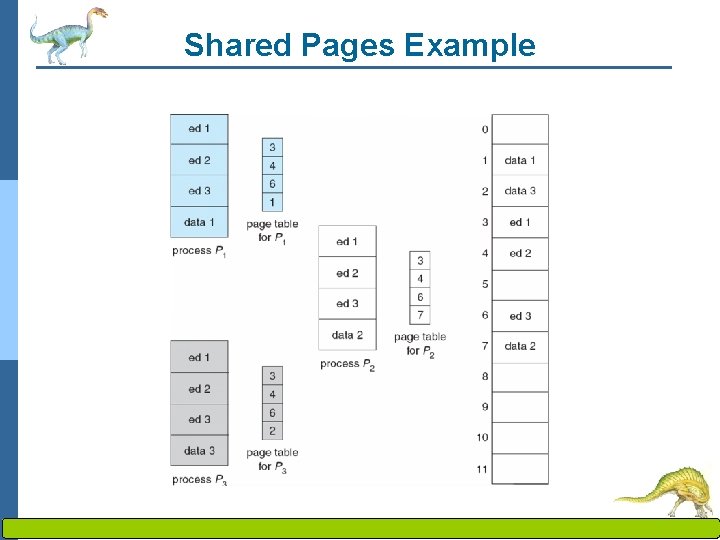
Shared Pages Example Operating System Concepts – 8 th Edition 8. 29 Silberschatz, Galvin and Gagne © 2009
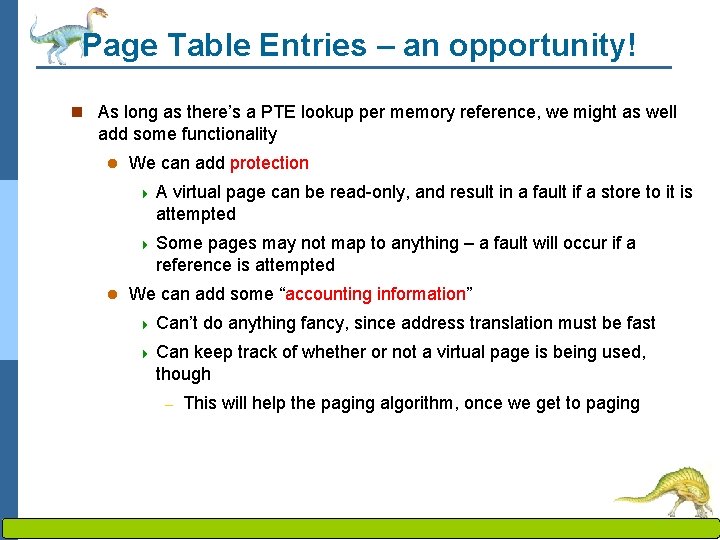
Page Table Entries – an opportunity! n As long as there’s a PTE lookup per memory reference, we might as well add some functionality l We can add protection 4 A virtual page can be read-only, and result in a fault if a store to it is attempted 4 Some pages may not map to anything – a fault will occur if a reference is attempted l We can add some “accounting information” 4 Can’t do anything fancy, since address translation must be fast 4 Can keep track of whether or not a virtual page is being used, though – This will help the paging algorithm, once we get to paging Operating System Concepts – 8 th Edition 8. 30 Silberschatz, Galvin and Gagne © 2009
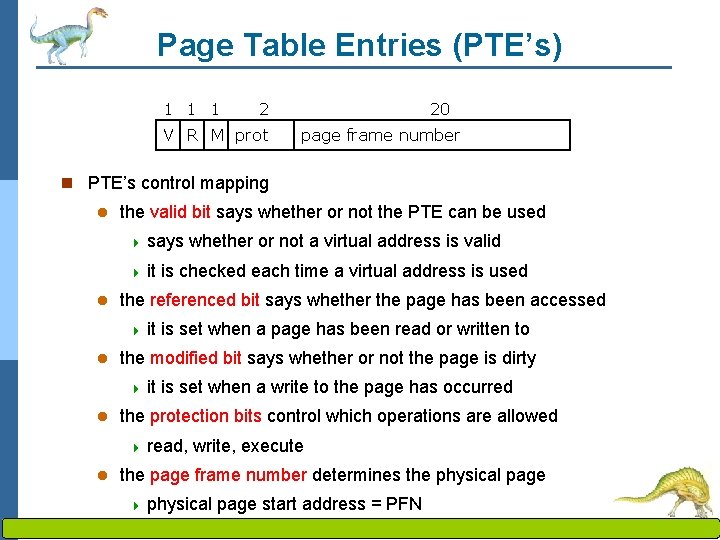
Page Table Entries (PTE’s) 1 1 1 2 V R M prot 20 page frame number n PTE’s control mapping l the valid bit says whether or not the PTE can be used 4 says 4 it l is set when a page has been read or written to the modified bit says whether or not the page is dirty 4 it l is checked each time a virtual address is used the referenced bit says whether the page has been accessed 4 it l is set when a write to the page has occurred the protection bits control which operations are allowed 4 read, l whether or not a virtual address is valid write, execute the page frame number determines the physical page 4 physical Operating System Concepts – 8 th Edition page start address = PFN 8. 31 Silberschatz, Galvin and Gagne © 2009
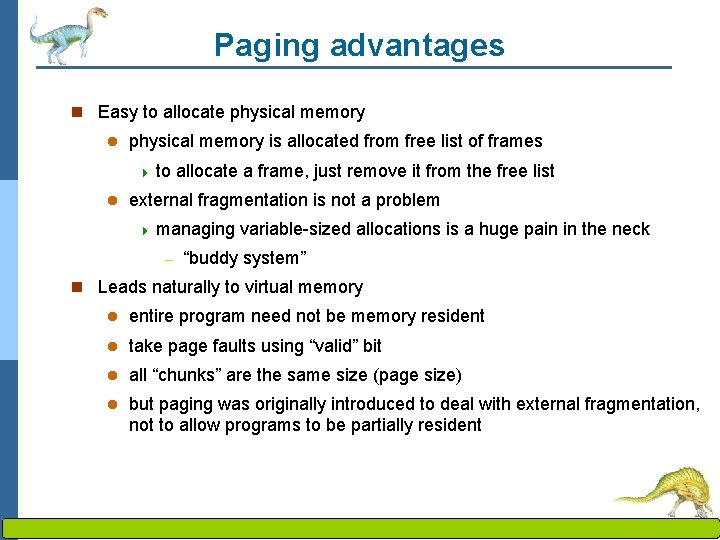
Paging advantages n Easy to allocate physical memory l physical memory is allocated from free list of frames 4 to l allocate a frame, just remove it from the free list external fragmentation is not a problem 4 managing – variable-sized allocations is a huge pain in the neck “buddy system” n Leads naturally to virtual memory l entire program need not be memory resident l take page faults using “valid” bit l all “chunks” are the same size (page size) l but paging was originally introduced to deal with external fragmentation, not to allow programs to be partially resident Operating System Concepts – 8 th Edition 8. 32 Silberschatz, Galvin and Gagne © 2009
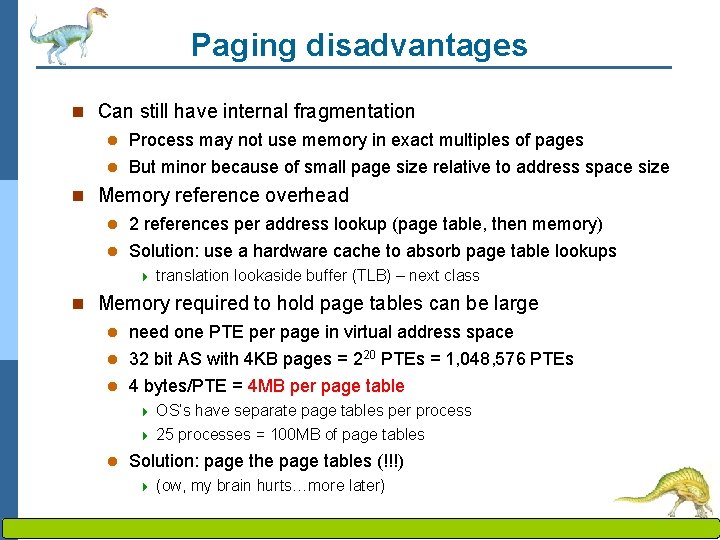
Paging disadvantages n Can still have internal fragmentation l Process may not use memory in exact multiples of pages l But minor because of small page size relative to address space size n Memory reference overhead l 2 references per address lookup (page table, then memory) l Solution: use a hardware cache to absorb page table lookups 4 translation lookaside buffer (TLB) – next class n Memory required to hold page tables can be large l need one PTE per page in virtual address space l 32 bit AS with 4 KB pages = 220 PTEs = 1, 048, 576 PTEs l 4 bytes/PTE = 4 MB per page table 4 4 l OS’s have separate page tables per process 25 processes = 100 MB of page tables Solution: page the page tables (!!!) 4 (ow, my brain hurts…more later) Operating System Concepts – 8 th Edition 8. 33 Silberschatz, Galvin and Gagne © 2009
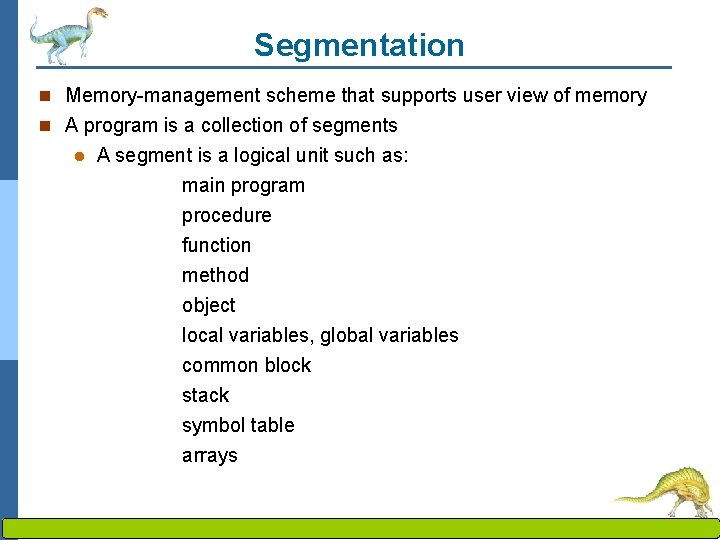
Segmentation n Memory-management scheme that supports user view of memory n A program is a collection of segments l A segment is a logical unit such as: main program procedure function method object local variables, global variables common block stack symbol table arrays Operating System Concepts – 8 th Edition 8. 34 Silberschatz, Galvin and Gagne © 2009
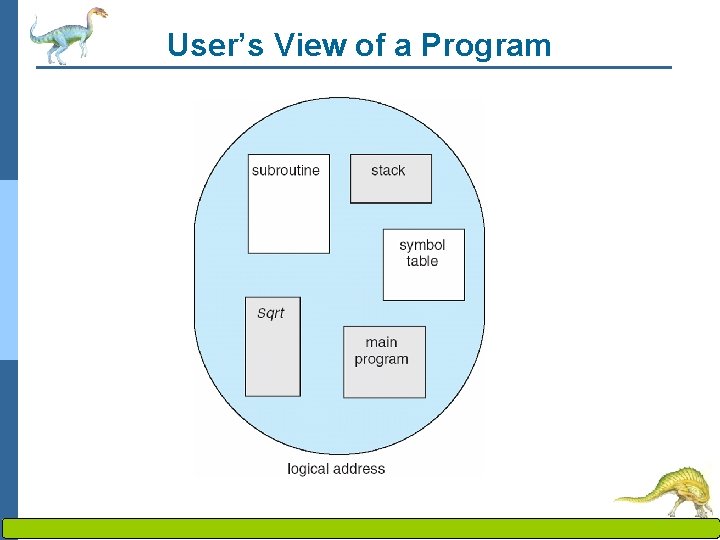
User’s View of a Program Operating System Concepts – 8 th Edition 8. 35 Silberschatz, Galvin and Gagne © 2009
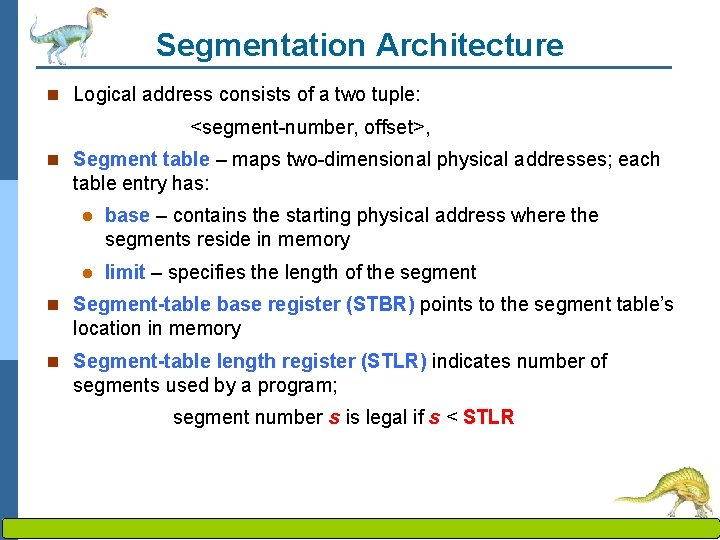
Segmentation Architecture n Logical address consists of a two tuple: <segment-number, offset>, n Segment table – maps two-dimensional physical addresses; each table entry has: l base – contains the starting physical address where the segments reside in memory l limit – specifies the length of the segment n Segment-table base register (STBR) points to the segment table’s location in memory n Segment-table length register (STLR) indicates number of segments used by a program; segment number s is legal if s < STLR Operating System Concepts – 8 th Edition 8. 36 Silberschatz, Galvin and Gagne © 2009
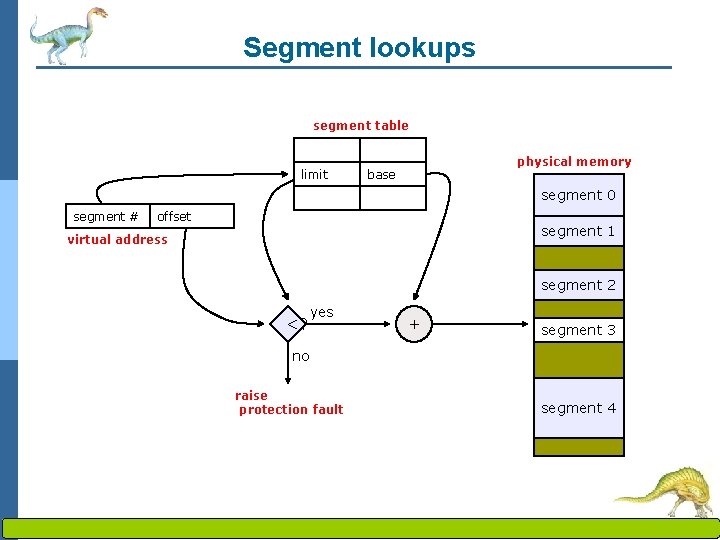
Segment lookups segment table limit physical memory base segment 0 segment # offset segment 1 virtual address segment 2 <? yes + segment 3 no raise protection fault Operating System Concepts – 8 th Edition 8. 37 segment 4 Silberschatz, Galvin and Gagne © 2009
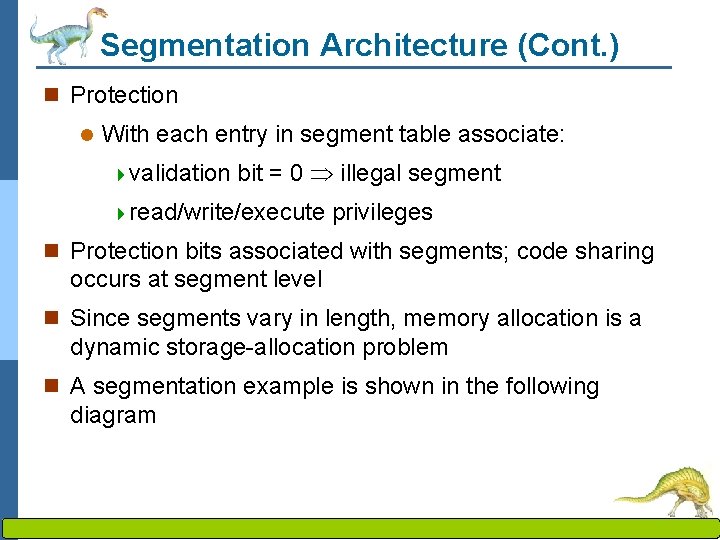
Segmentation Architecture (Cont. ) n Protection l With each entry in segment table associate: 4 validation bit = 0 illegal segment 4 read/write/execute privileges n Protection bits associated with segments; code sharing occurs at segment level n Since segments vary in length, memory allocation is a dynamic storage-allocation problem n A segmentation example is shown in the following diagram Operating System Concepts – 8 th Edition 8. 38 Silberschatz, Galvin and Gagne © 2009
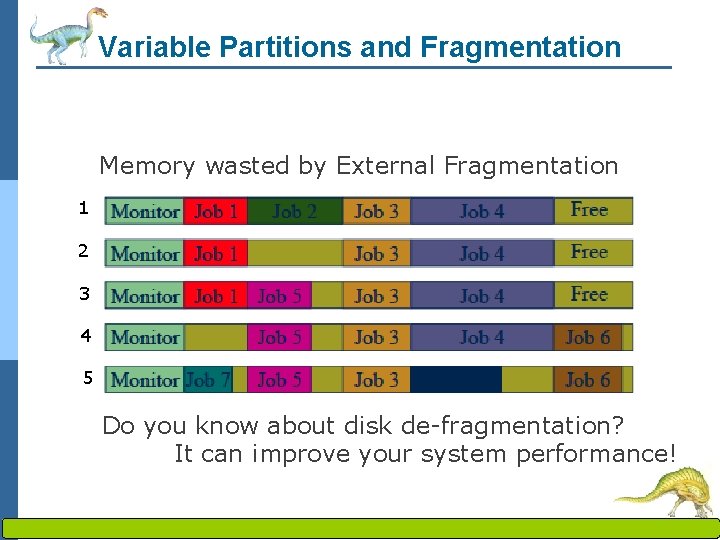
Variable Partitions and Fragmentation Memory wasted by External Fragmentation 1 2 3 4 5 Do you know about disk de-fragmentation? It can improve your system performance! Operating System Concepts – 8 th Edition 8. 39 Silberschatz, Galvin and Gagne © 2009
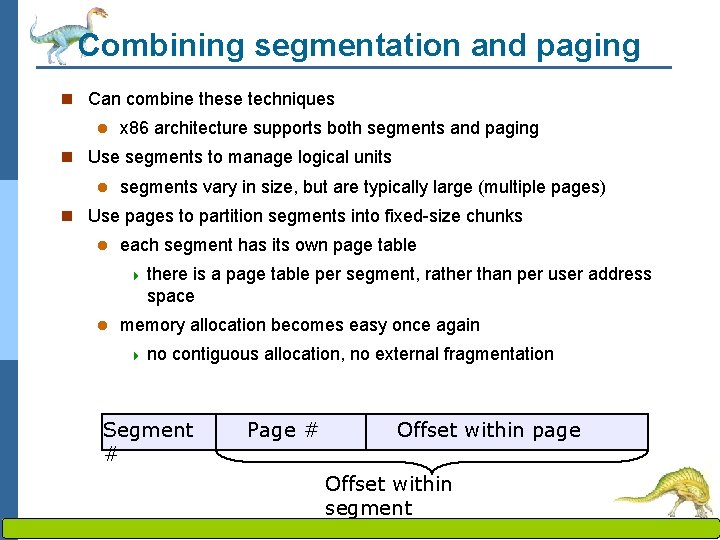
Combining segmentation and paging n Can combine these techniques l x 86 architecture supports both segments and paging n Use segments to manage logical units l segments vary in size, but are typically large (multiple pages) n Use pages to partition segments into fixed-size chunks l each segment has its own page table 4 there is a page table per segment, rather than per user address space l memory allocation becomes easy once again 4 no contiguous allocation, no external fragmentation Segment # Page # Offset within page Offset within segment Operating System Concepts – 8 th Edition 8. 40 Silberschatz, Galvin and Gagne © 2009
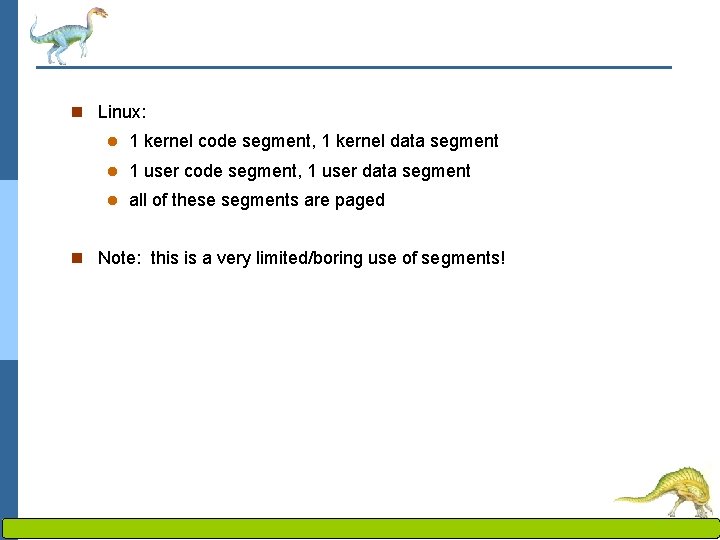
n Linux: l 1 kernel code segment, 1 kernel data segment l 1 user code segment, 1 user data segment l all of these segments are paged n Note: this is a very limited/boring use of segments! Operating System Concepts – 8 th Edition 8. 41 Silberschatz, Galvin and Gagne © 2009
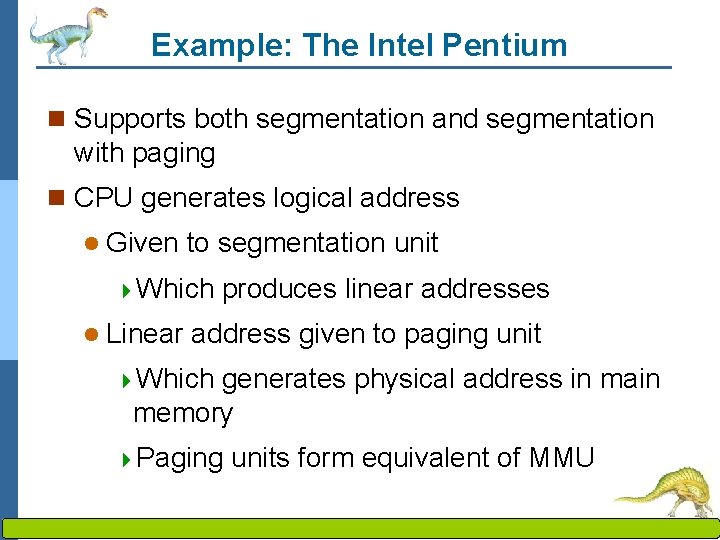
Example: The Intel Pentium n Supports both segmentation and segmentation with paging n CPU generates logical address l Given to segmentation unit 4 Which l Linear produces linear addresses address given to paging unit 4 Which generates physical address in main memory 4 Paging Operating System Concepts – 8 th Edition units form equivalent of MMU 8. 42 Silberschatz, Galvin and Gagne © 2009
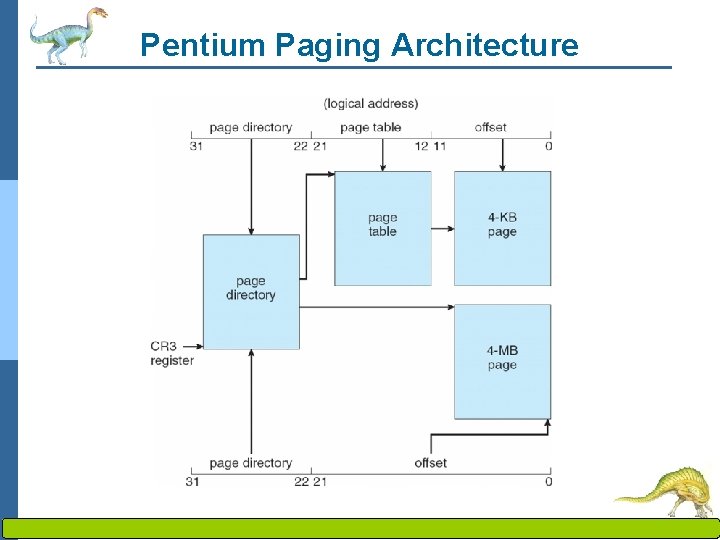
Pentium Paging Architecture Operating System Concepts – 8 th Edition 8. 43 Silberschatz, Galvin and Gagne © 2009
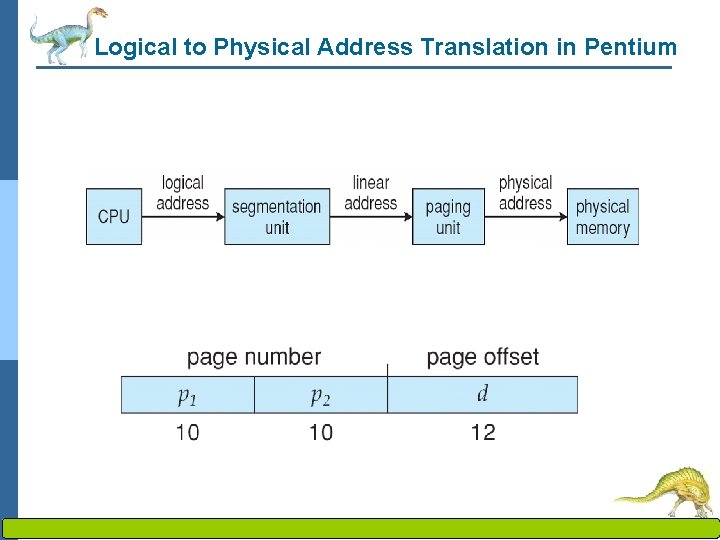
Logical to Physical Address Translation in Pentium Operating System Concepts – 8 th Edition 8. 44 Silberschatz, Galvin and Gagne © 2009
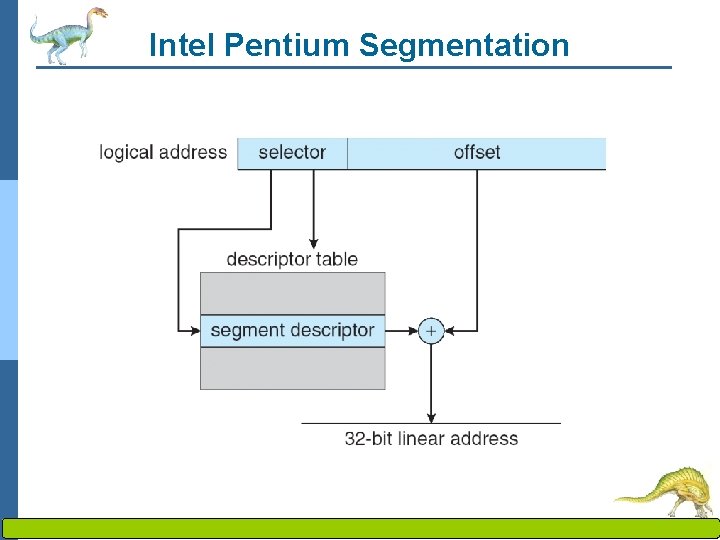
Intel Pentium Segmentation Operating System Concepts – 8 th Edition 8. 45 Silberschatz, Galvin and Gagne © 2009
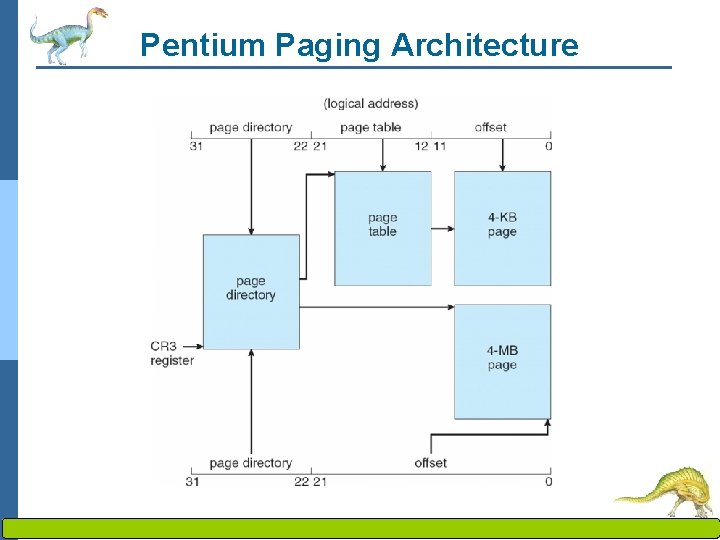
Pentium Paging Architecture Operating System Concepts – 8 th Edition 8. 46 Silberschatz, Galvin and Gagne © 2009
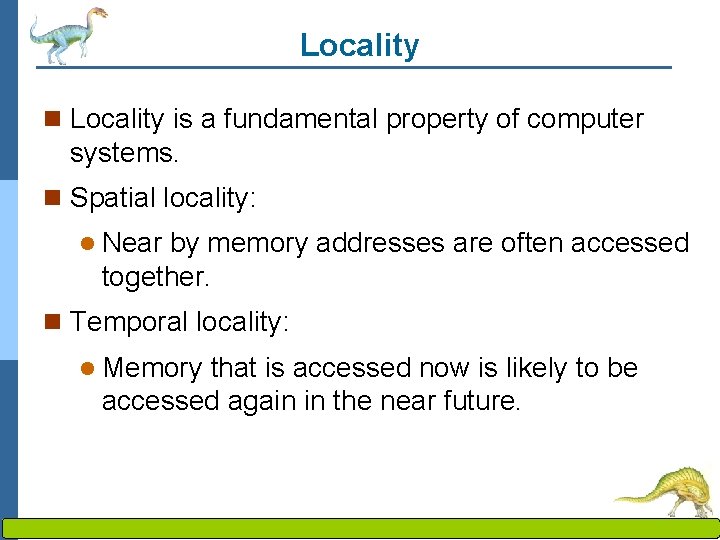
Locality n Locality is a fundamental property of computer systems. n Spatial locality: l Near by memory addresses are often accessed together. n Temporal locality: l Memory that is accessed now is likely to be accessed again in the near future. Operating System Concepts – 8 th Edition 8. 47 Silberschatz, Galvin and Gagne © 2009
Basic concept of memory
Operating system concepts, 10th edition
Linux operating system concepts
Operating system concepts with java
Operating system concepts 6th edition
Operating system concepts 9
Realtime operating system
Operating system concepts 9판 한국어판
Operating system concepts chapter 8 solutions
Operating system concepts chapter 5 solutions
Operating system concepts chapter 5 solutions
Basic operating system concepts
Operating system concepts 11th
Operating system concepts essentials
Operating system concepts essentials
Galvin os
Virtual memory in operating system
Single partition allocation
Alpha axp page table entry
Main function of operating system
Main function of operating system
Basic concepts of memory
Semantic knowledge
Explicit memory
Long term memory vs short term memory
Internal memory and external memory
Primary memory and secondary memory
Logical memory is broken into
Which memory is the actual working memory?
Virtual memory
Virtual memory in memory hierarchy consists of
Eidetic memory vs iconic memory
Shared memory vs distributed memory
Operating systems
Advantages and disadvantages of direct mapping cache
Two kinds of main memory are
Internal memory rom
Major components of computer
Main memory
Main memory
What is the main idea of hope despair and memory
Future tense of watch
Implied main idea
Void main int main
Buddy system in os
File system in operating system
Ufs4.0
File system in operating system