Monitoraggio Geodetico e Telerilevamento 5 Radar Imaging Interferometric
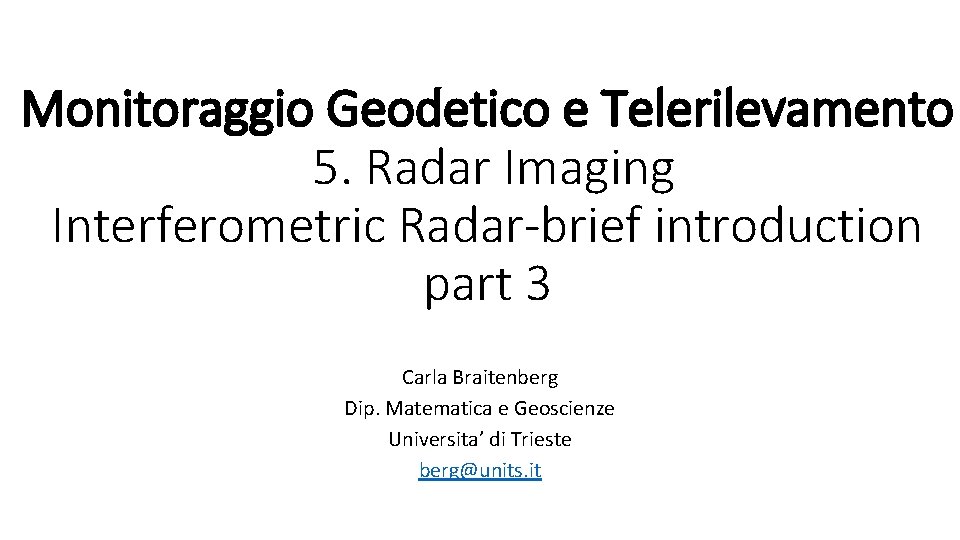
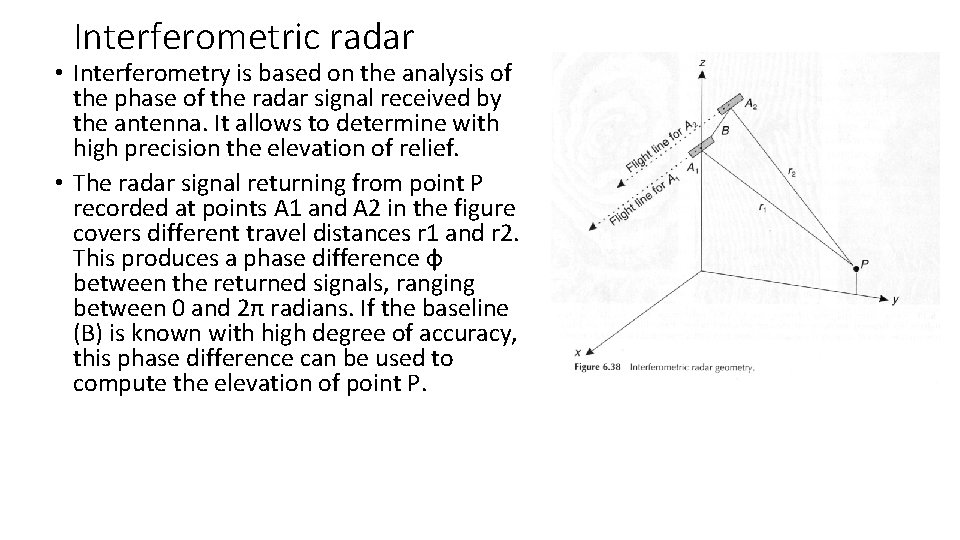
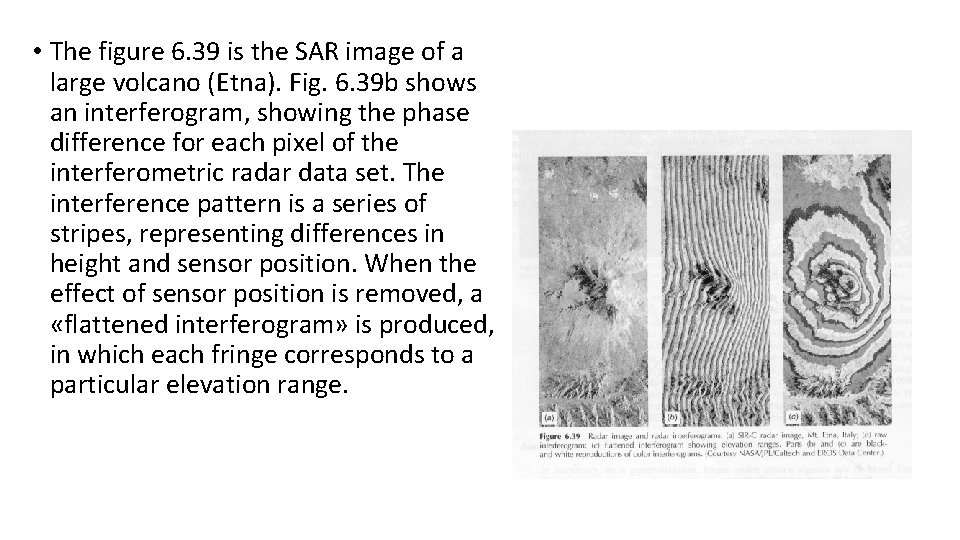
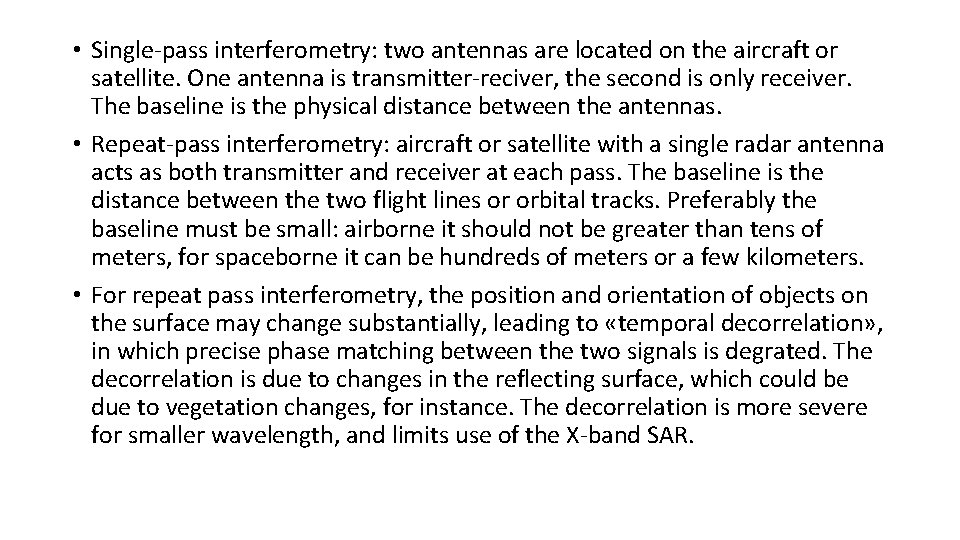
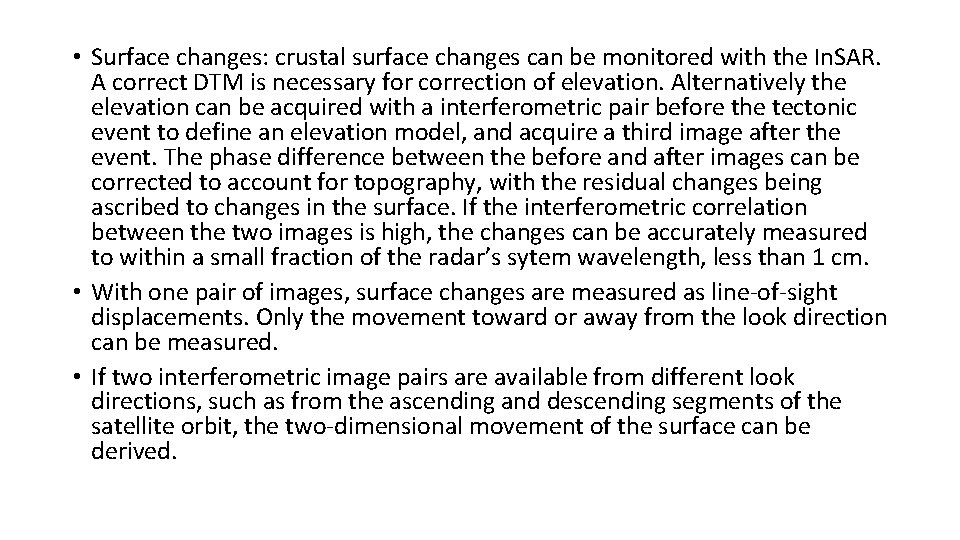
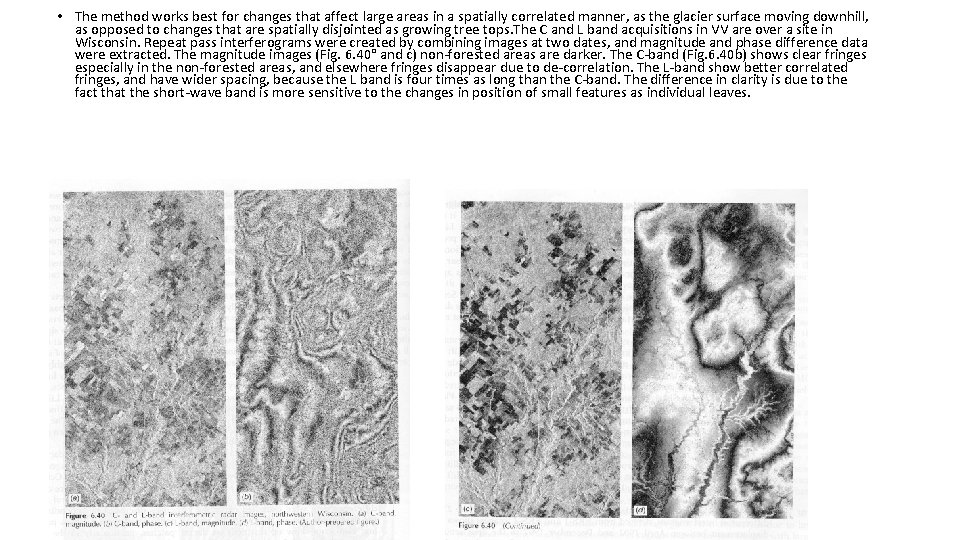
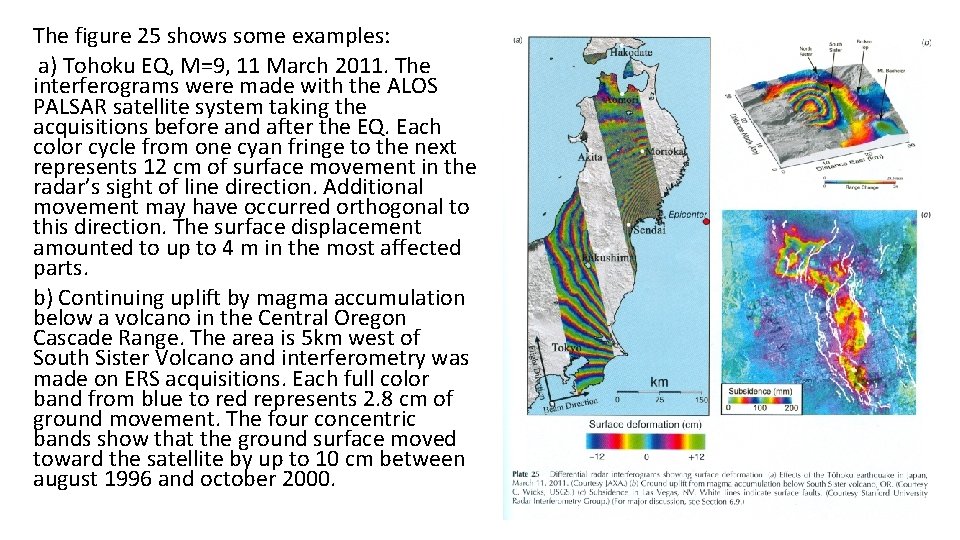
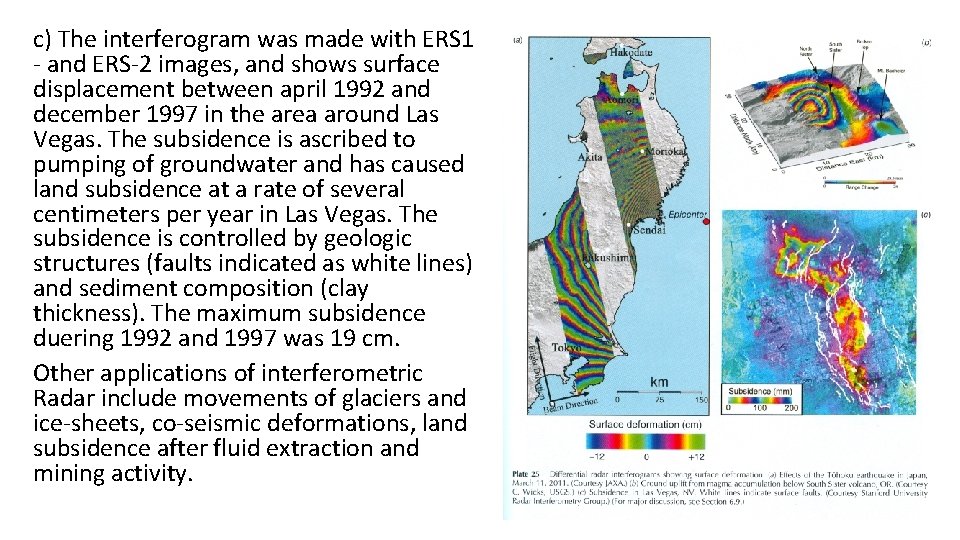
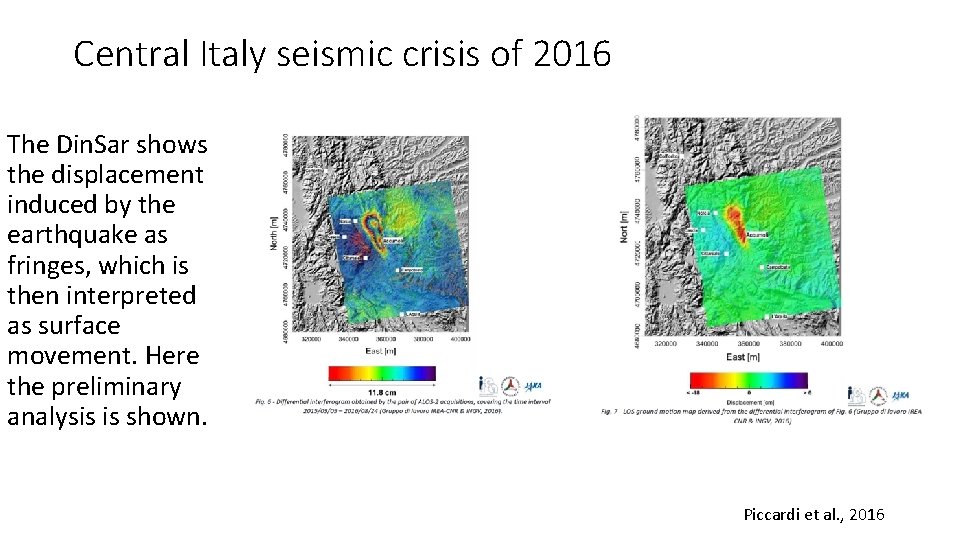
- Slides: 9
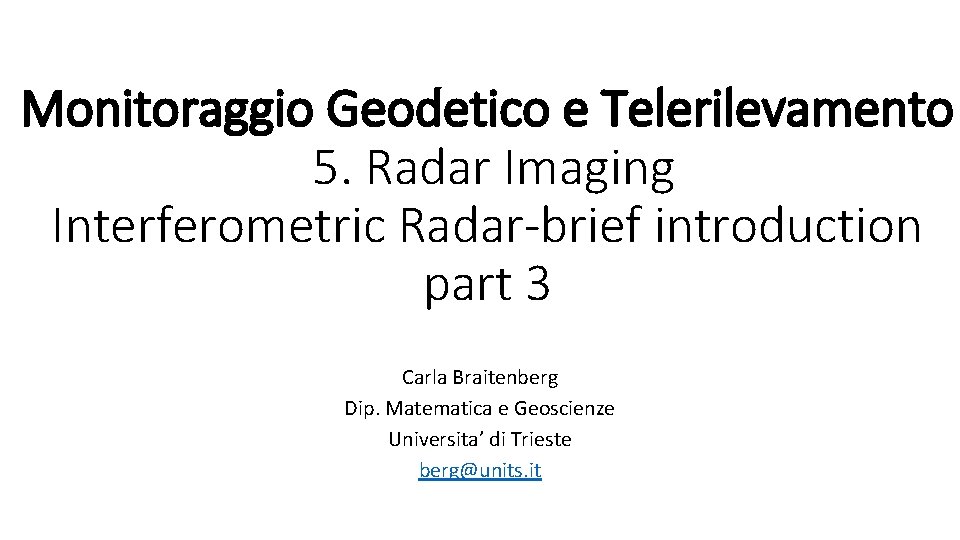
Monitoraggio Geodetico e Telerilevamento 5. Radar Imaging Interferometric Radar-brief introduction part 3 Carla Braitenberg Dip. Matematica e Geoscienze Universita’ di Trieste berg@units. it
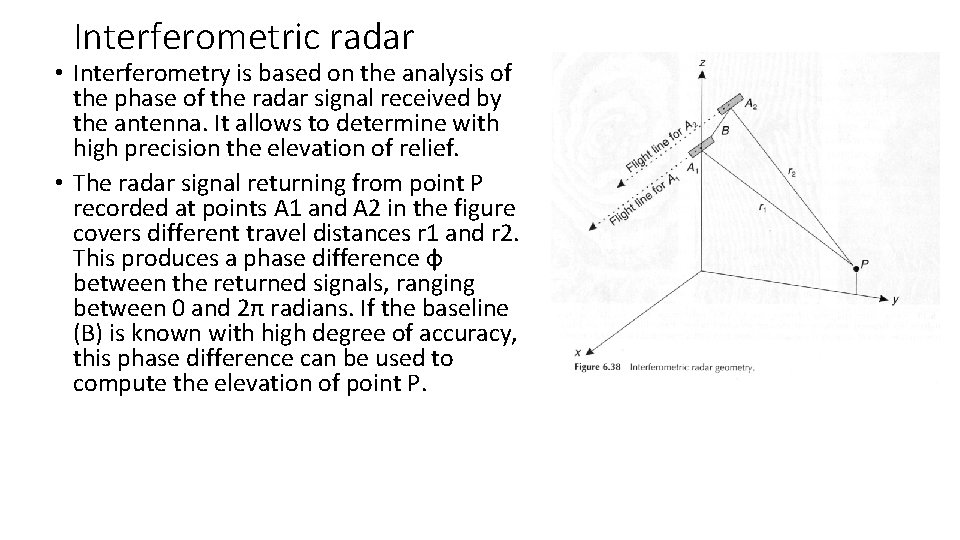
Interferometric radar • Interferometry is based on the analysis of the phase of the radar signal received by the antenna. It allows to determine with high precision the elevation of relief. • The radar signal returning from point P recorded at points A 1 and A 2 in the figure covers different travel distances r 1 and r 2. This produces a phase difference φ between the returned signals, ranging between 0 and 2π radians. If the baseline (B) is known with high degree of accuracy, this phase difference can be used to compute the elevation of point P.
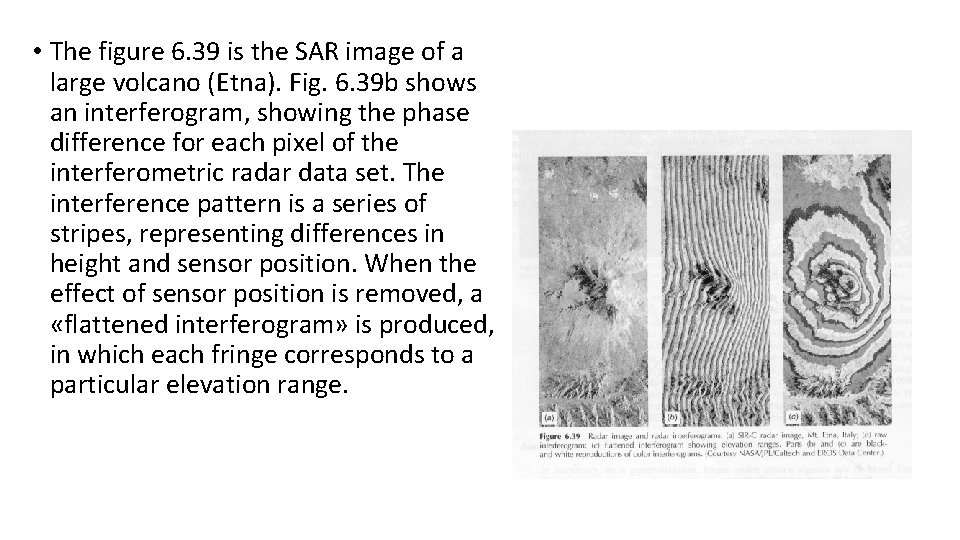
• The figure 6. 39 is the SAR image of a large volcano (Etna). Fig. 6. 39 b shows an interferogram, showing the phase difference for each pixel of the interferometric radar data set. The interference pattern is a series of stripes, representing differences in height and sensor position. When the effect of sensor position is removed, a «flattened interferogram» is produced, in which each fringe corresponds to a particular elevation range.
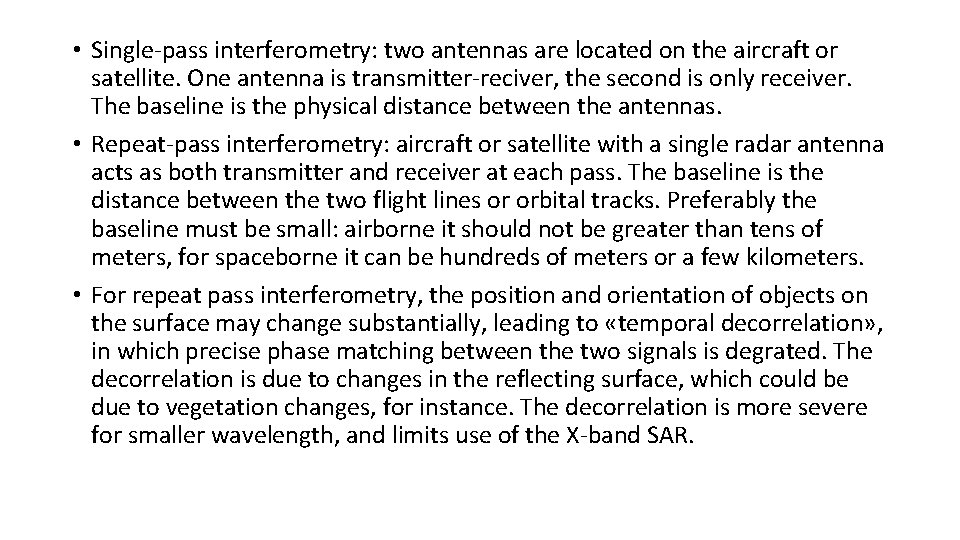
• Single-pass interferometry: two antennas are located on the aircraft or satellite. One antenna is transmitter-reciver, the second is only receiver. The baseline is the physical distance between the antennas. • Repeat-pass interferometry: aircraft or satellite with a single radar antenna acts as both transmitter and receiver at each pass. The baseline is the distance between the two flight lines or orbital tracks. Preferably the baseline must be small: airborne it should not be greater than tens of meters, for spaceborne it can be hundreds of meters or a few kilometers. • For repeat pass interferometry, the position and orientation of objects on the surface may change substantially, leading to «temporal decorrelation» , in which precise phase matching between the two signals is degrated. The decorrelation is due to changes in the reflecting surface, which could be due to vegetation changes, for instance. The decorrelation is more severe for smaller wavelength, and limits use of the X-band SAR.
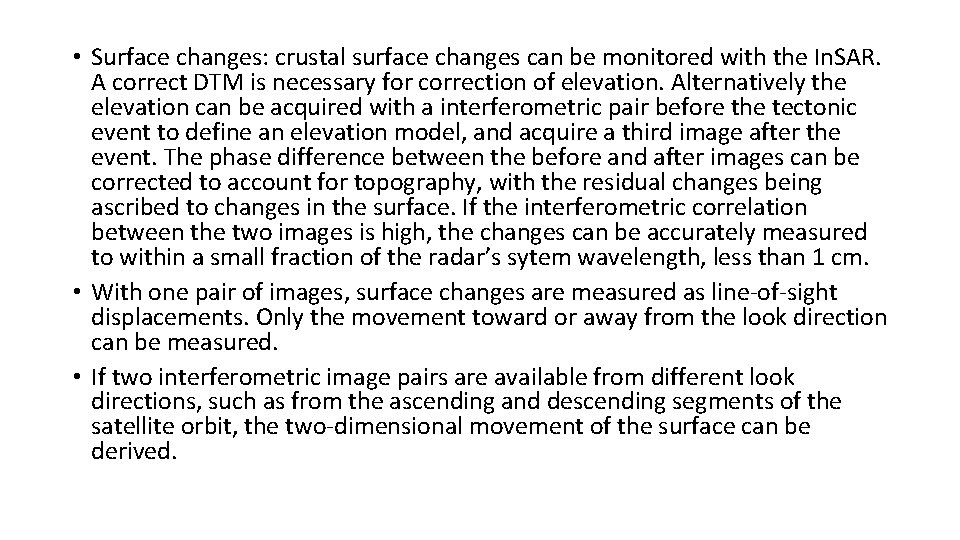
• Surface changes: crustal surface changes can be monitored with the In. SAR. A correct DTM is necessary for correction of elevation. Alternatively the elevation can be acquired with a interferometric pair before the tectonic event to define an elevation model, and acquire a third image after the event. The phase difference between the before and after images can be corrected to account for topography, with the residual changes being ascribed to changes in the surface. If the interferometric correlation between the two images is high, the changes can be accurately measured to within a small fraction of the radar’s sytem wavelength, less than 1 cm. • With one pair of images, surface changes are measured as line-of-sight displacements. Only the movement toward or away from the look direction can be measured. • If two interferometric image pairs are available from different look directions, such as from the ascending and descending segments of the satellite orbit, the two-dimensional movement of the surface can be derived.
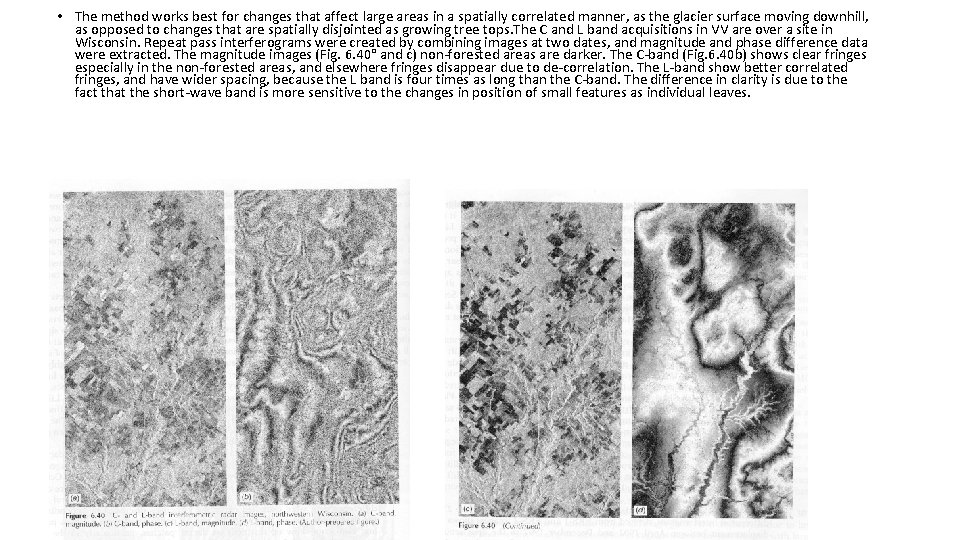
• The method works best for changes that affect large areas in a spatially correlated manner, as the glacier surface moving downhill, as opposed to changes that are spatially disjointed as growing tree tops. The C and L band acquisitions in VV are over a site in Wisconsin. Repeat pass interferograms were created by combining images at two dates, and magnitude and phase difference data were extracted. The magnitude images (Fig. 6. 40° and c) non-forested areas are darker. The C-band (Fig. 6. 40 b) shows clear fringes especially in the non-forested areas, and elsewhere fringes disappear due to de-correlation. The L-band show better correlated fringes, and have wider spacing, because the L band is four times as long than the C-band. The difference in clarity is due to the fact that the short-wave band is more sensitive to the changes in position of small features as individual leaves.
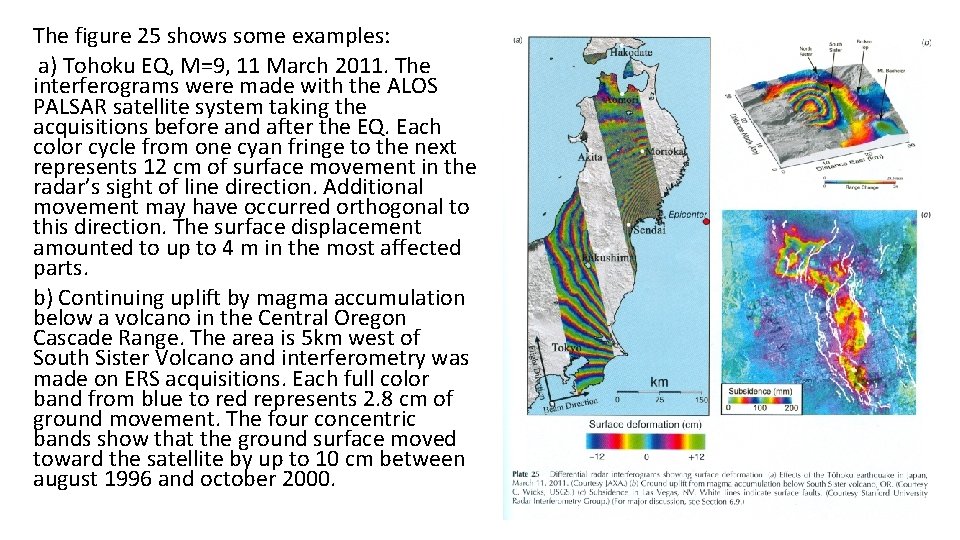
The figure 25 shows some examples: a) Tohoku EQ, M=9, 11 March 2011. The interferograms were made with the ALOS PALSAR satellite system taking the acquisitions before and after the EQ. Each color cycle from one cyan fringe to the next represents 12 cm of surface movement in the radar’s sight of line direction. Additional movement may have occurred orthogonal to this direction. The surface displacement amounted to up to 4 m in the most affected parts. b) Continuing uplift by magma accumulation below a volcano in the Central Oregon Cascade Range. The area is 5 km west of South Sister Volcano and interferometry was made on ERS acquisitions. Each full color band from blue to red represents 2. 8 cm of ground movement. The four concentric bands show that the ground surface moved toward the satellite by up to 10 cm between august 1996 and october 2000.
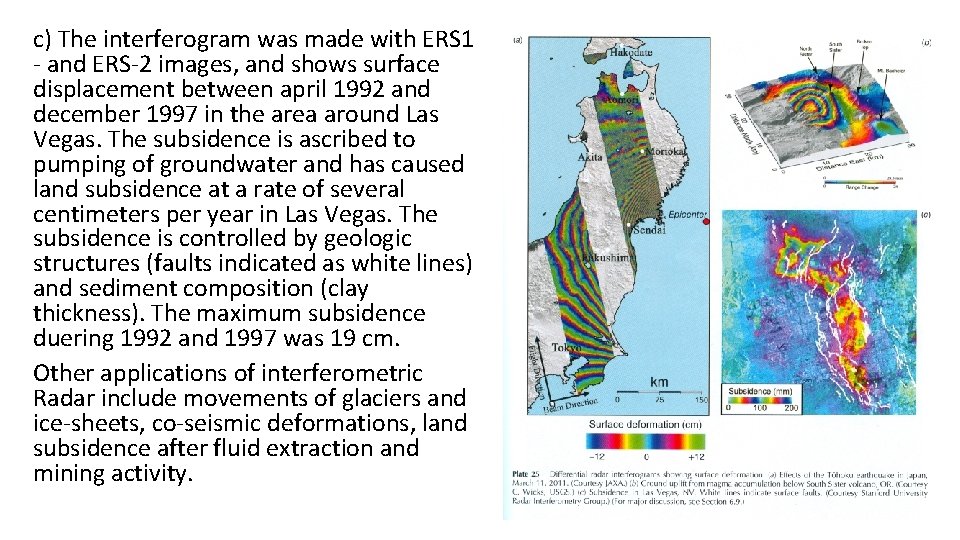
c) The interferogram was made with ERS 1 - and ERS-2 images, and shows surface displacement between april 1992 and december 1997 in the area around Las Vegas. The subsidence is ascribed to pumping of groundwater and has caused land subsidence at a rate of several centimeters per year in Las Vegas. The subsidence is controlled by geologic structures (faults indicated as white lines) and sediment composition (clay thickness). The maximum subsidence duering 1992 and 1997 was 19 cm. Other applications of interferometric Radar include movements of glaciers and ice-sheets, co-seismic deformations, land subsidence after fluid extraction and mining activity.
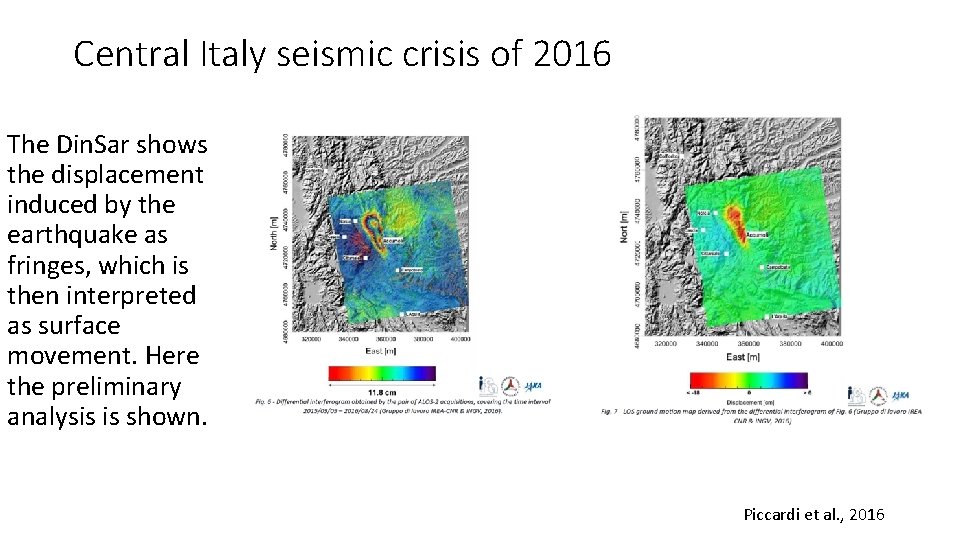
Central Italy seismic crisis of 2016 The Din. Sar shows the displacement induced by the earthquake as fringes, which is then interpreted as surface movement. Here the preliminary analysis is shown. Piccardi et al. , 2016