MaxPlanckInstitut fr Plasmaphysik Advances in stellarator gyrokinetics Per
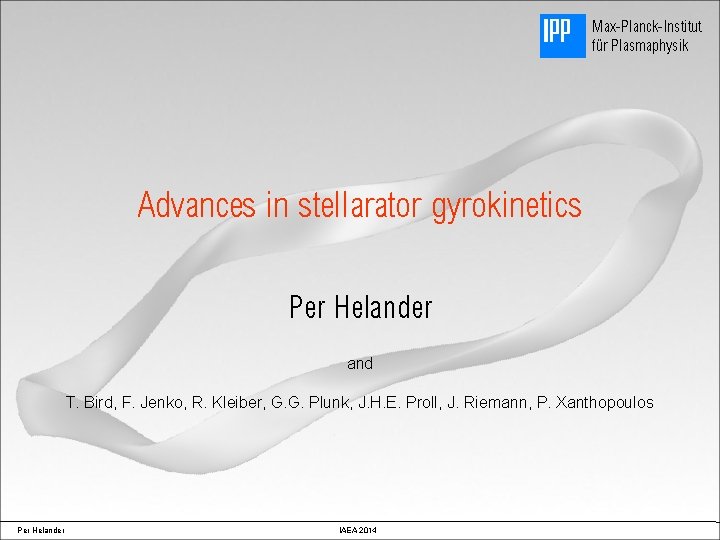
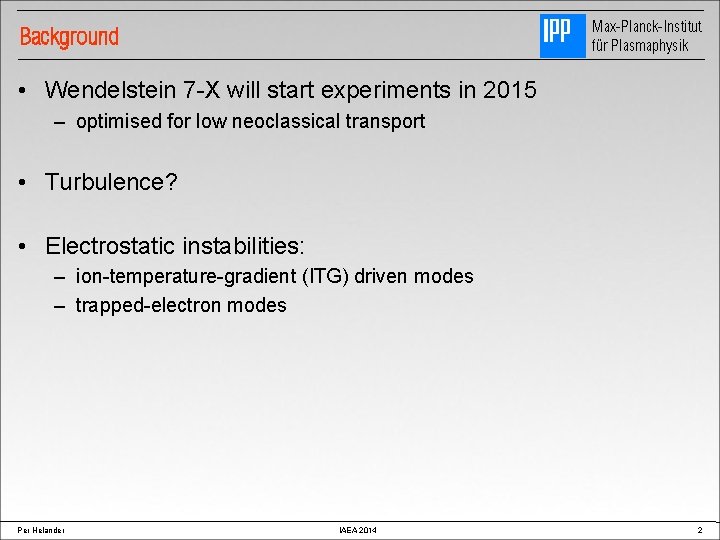
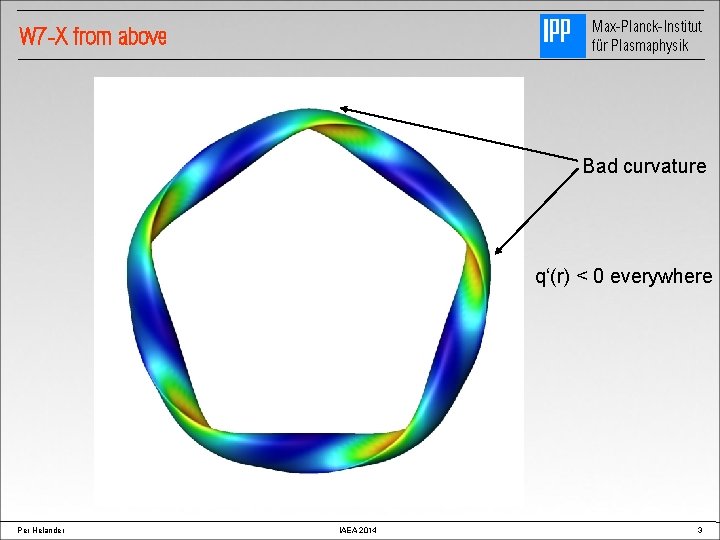
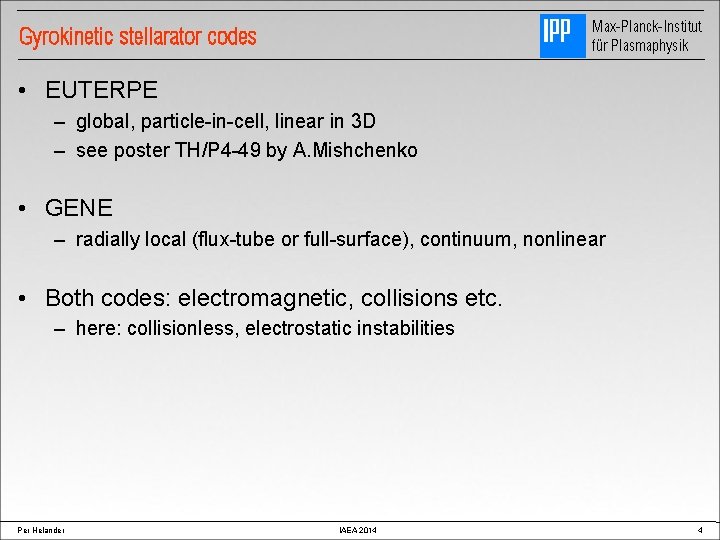
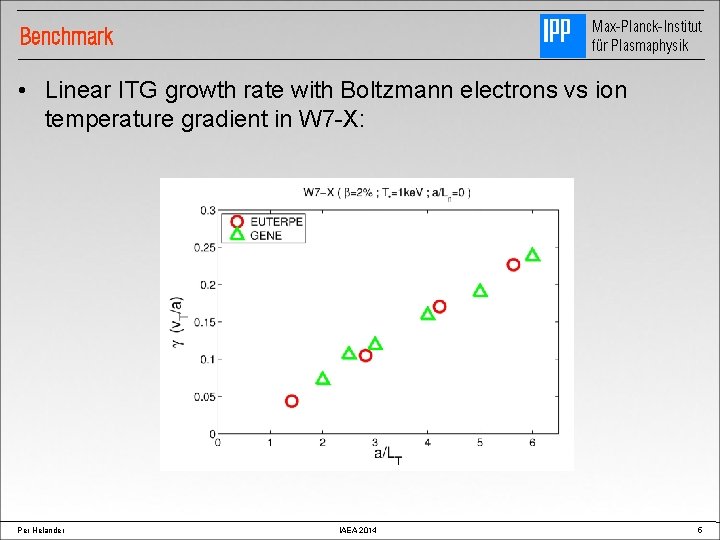
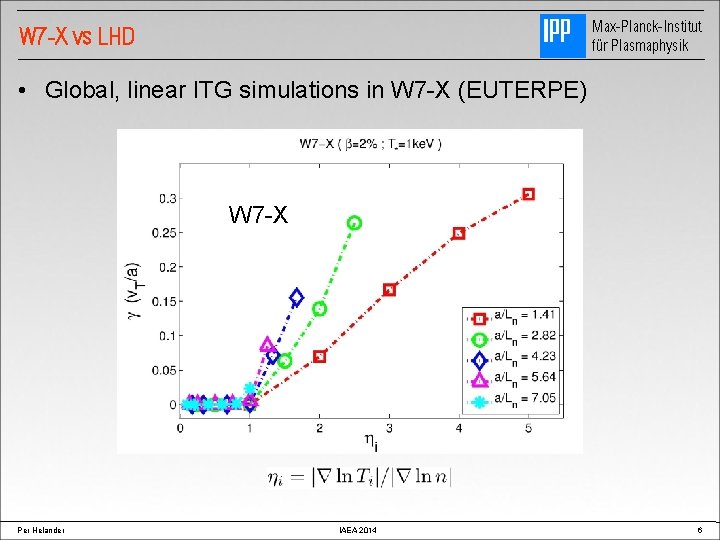
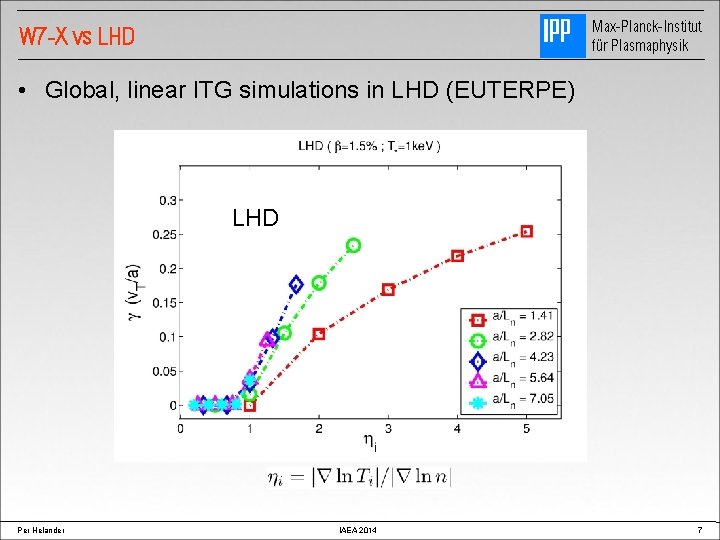
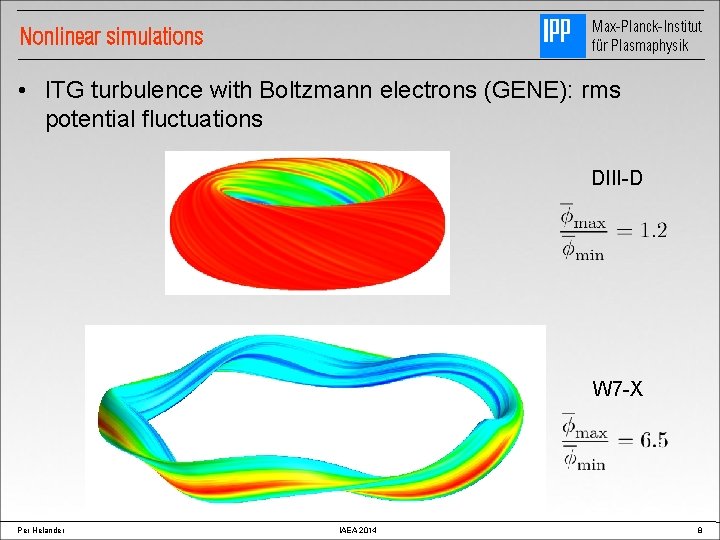
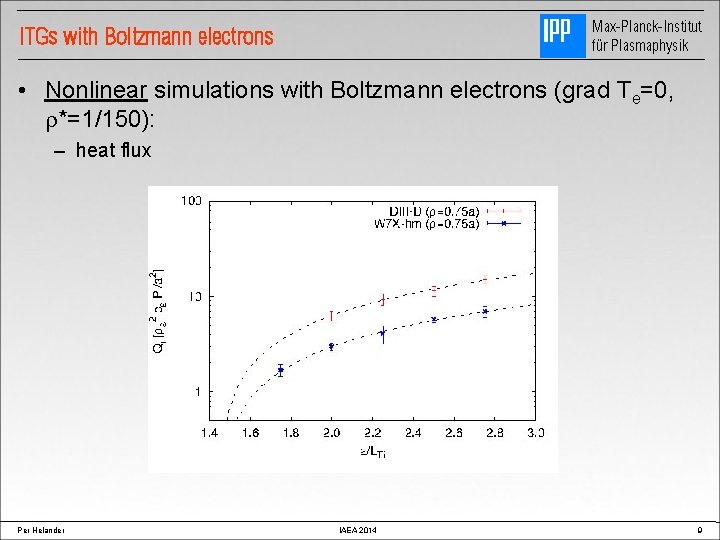
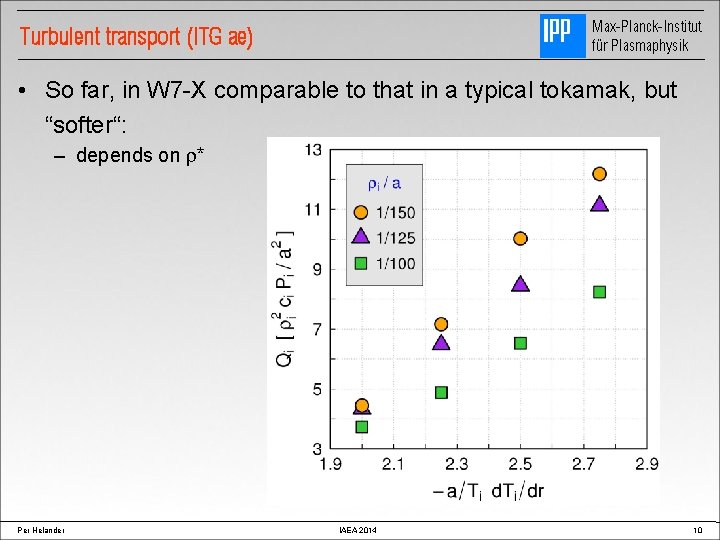
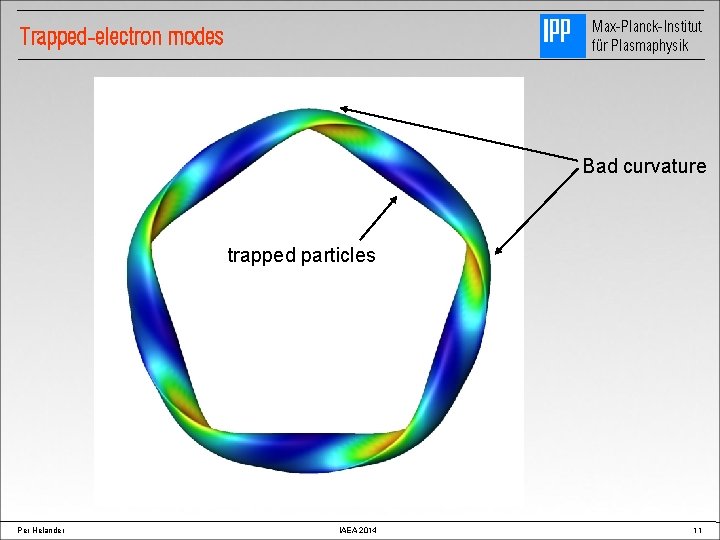
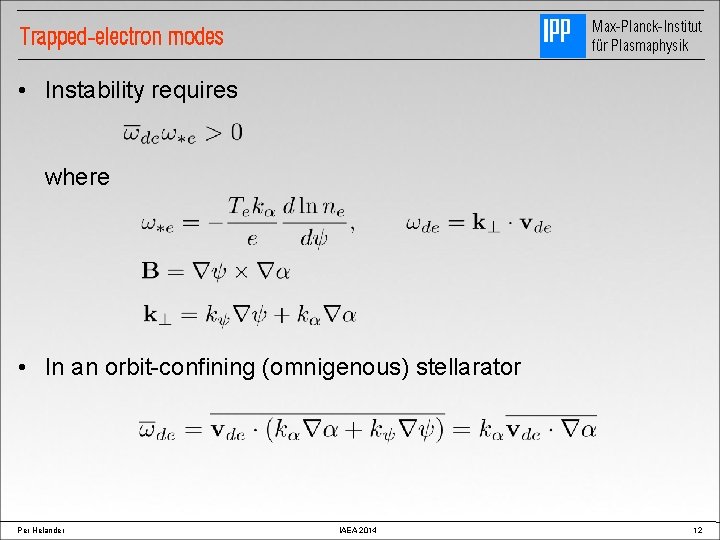
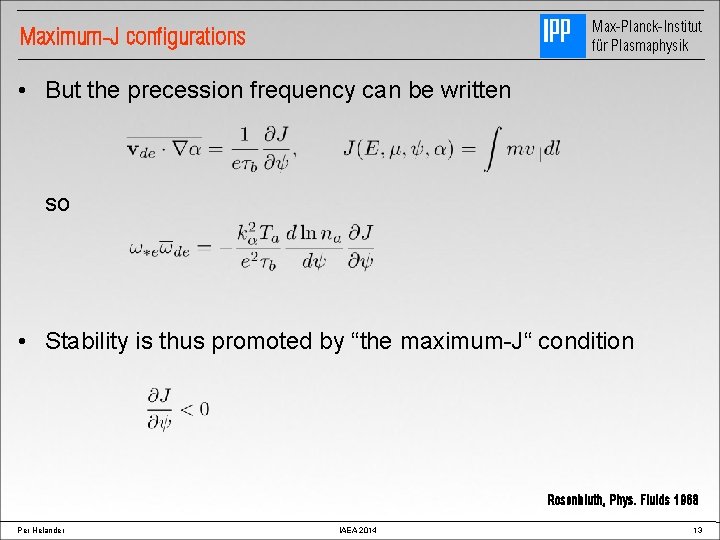
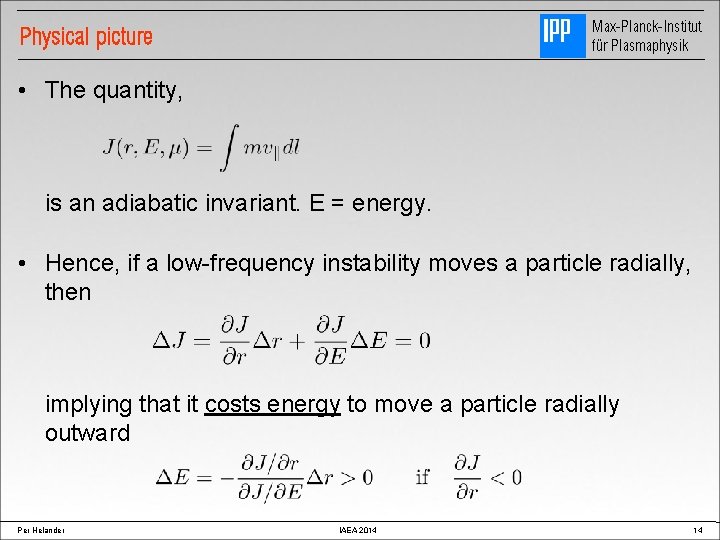
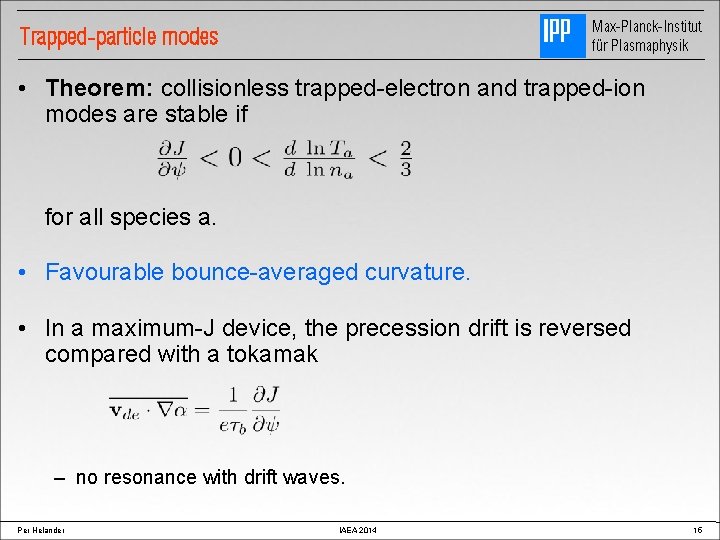
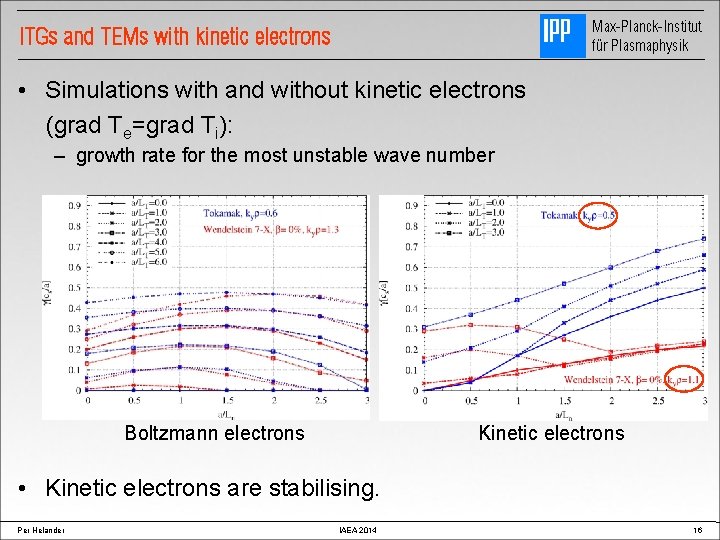
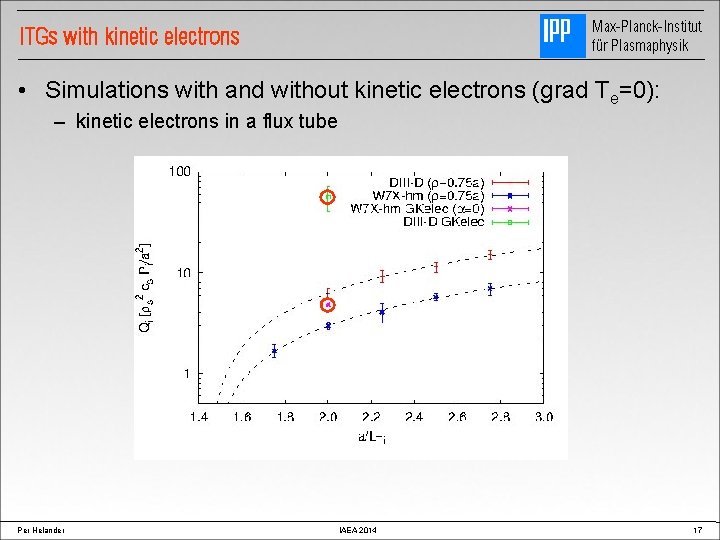
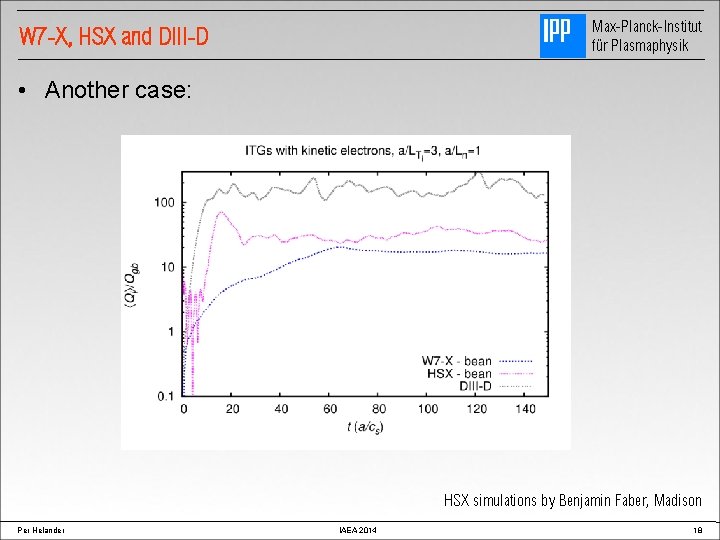
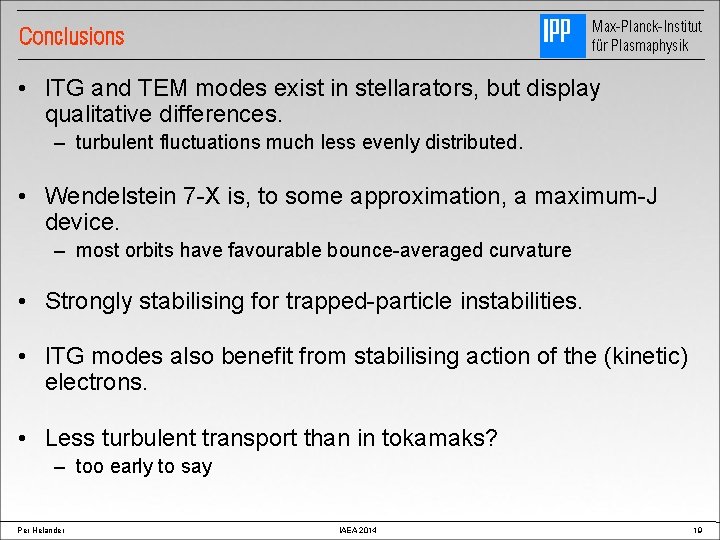
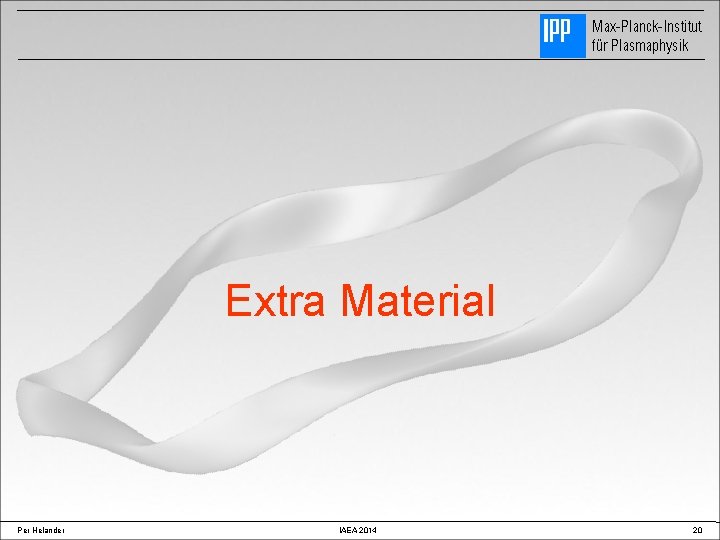
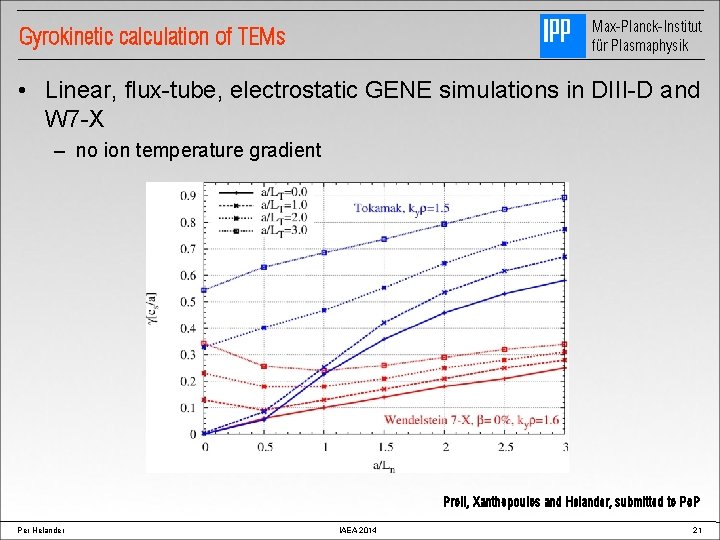
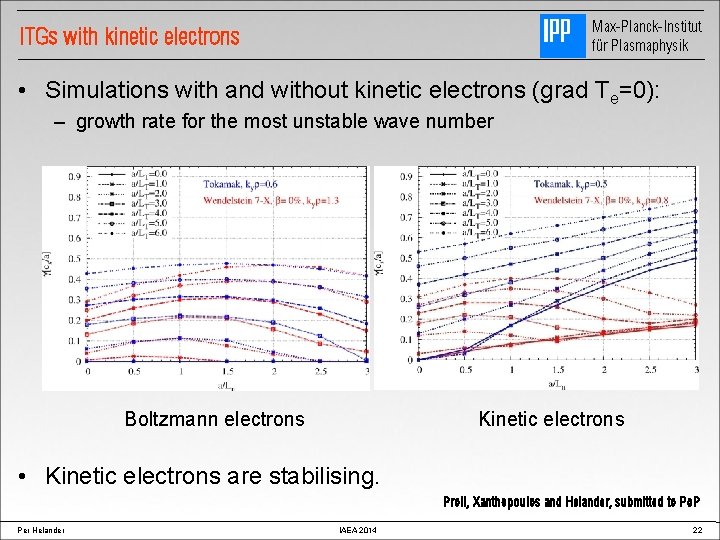
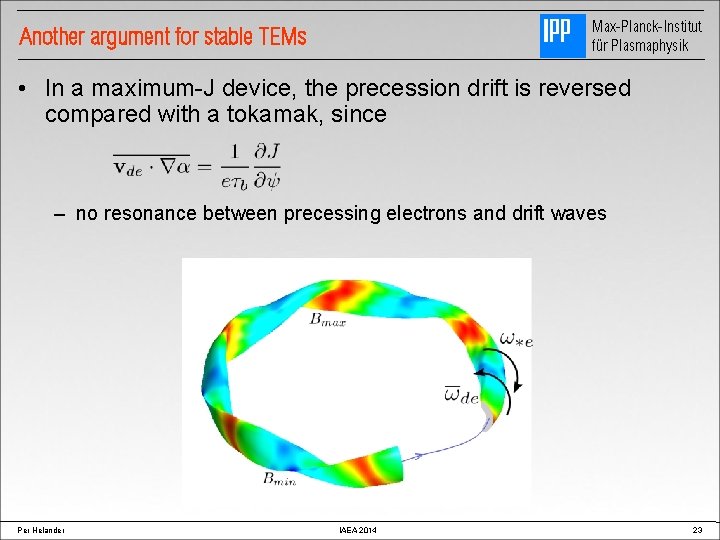
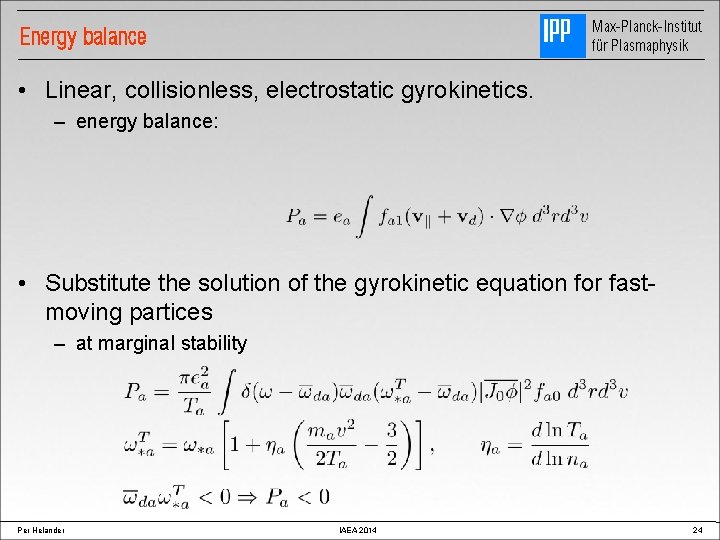
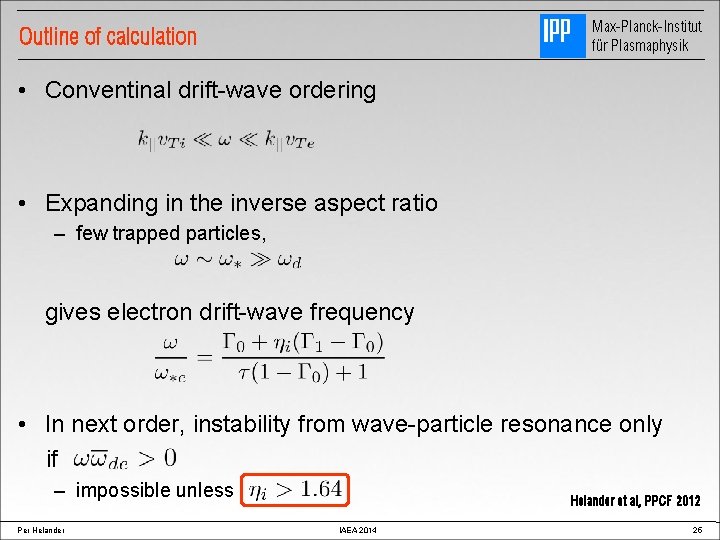
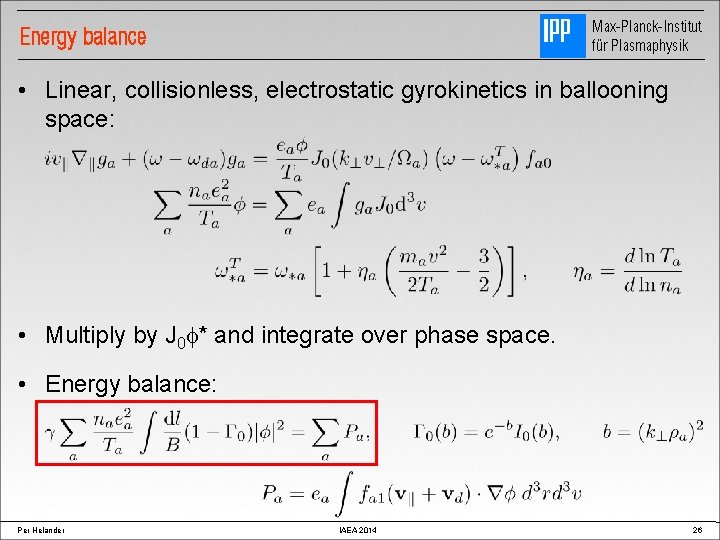
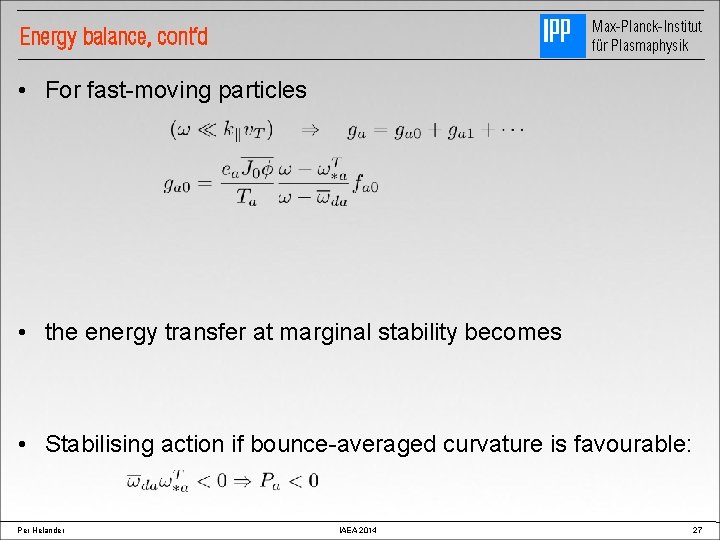
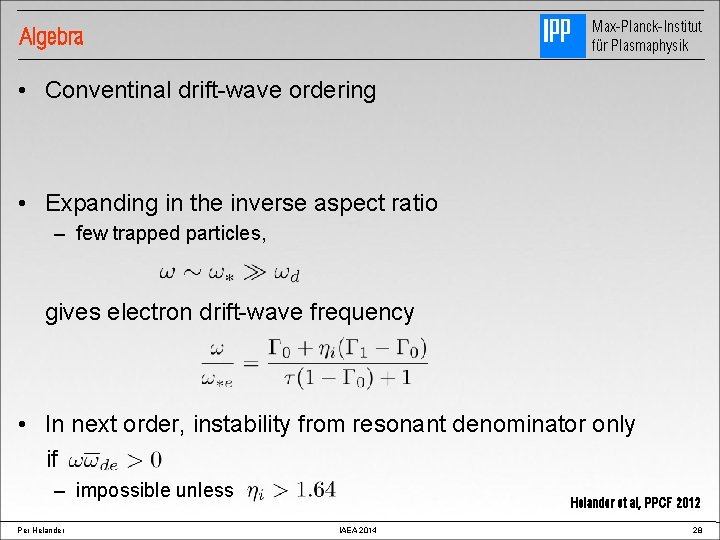
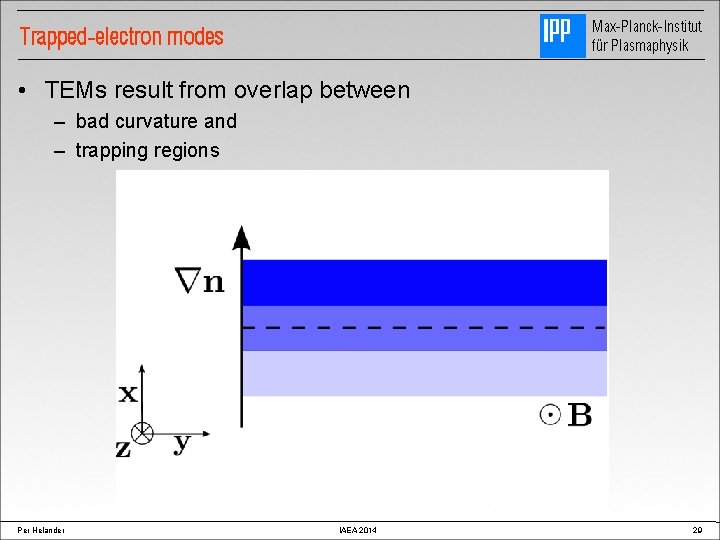
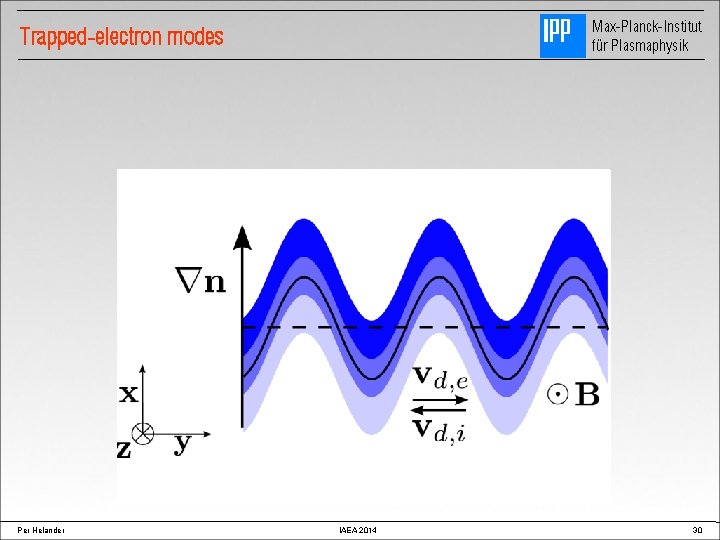
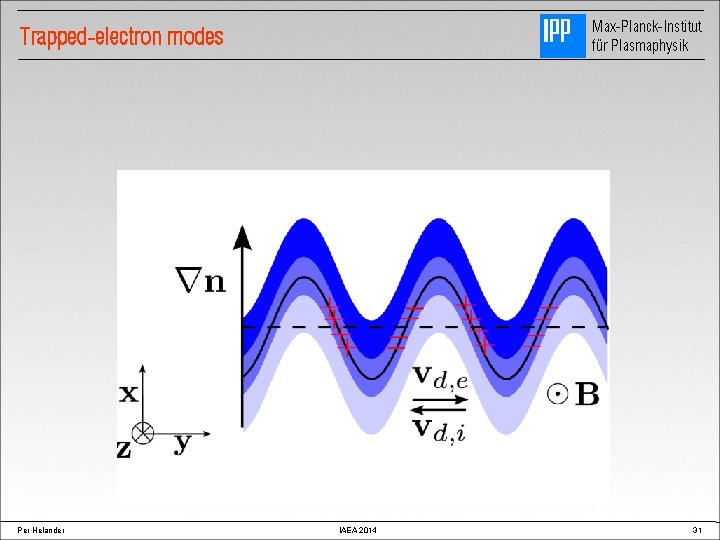
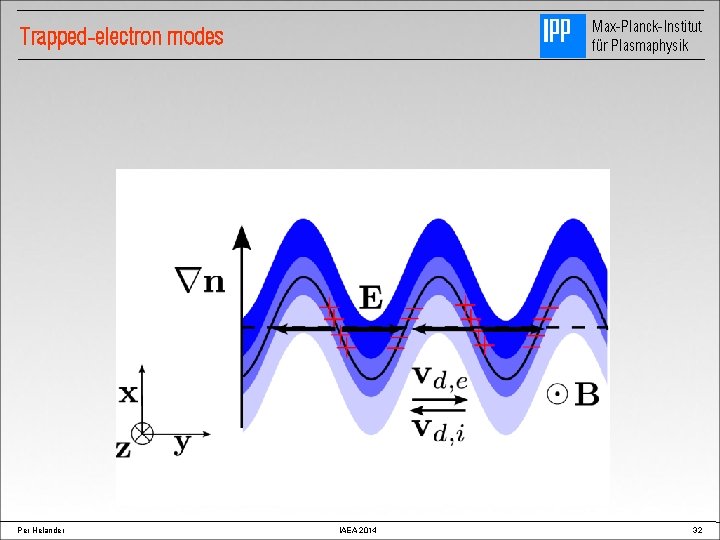
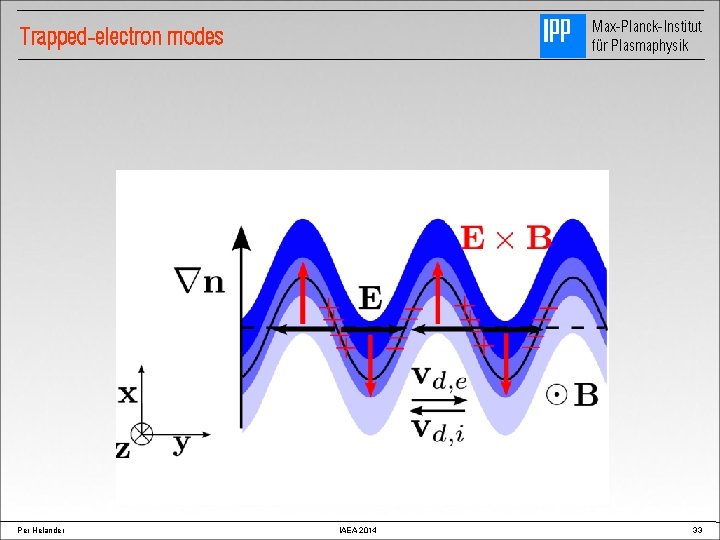
- Slides: 33
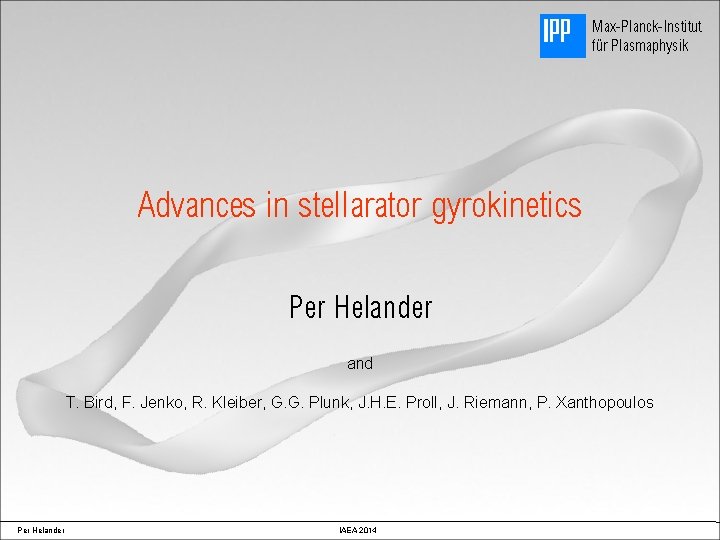
Max-Planck-Institut für Plasmaphysik Advances in stellarator gyrokinetics Per Helander and T. Bird, F. Jenko, R. Kleiber, G. G. Plunk, J. H. E. Proll, J. Riemann, P. Xanthopoulos Per Helander IAEA 2014
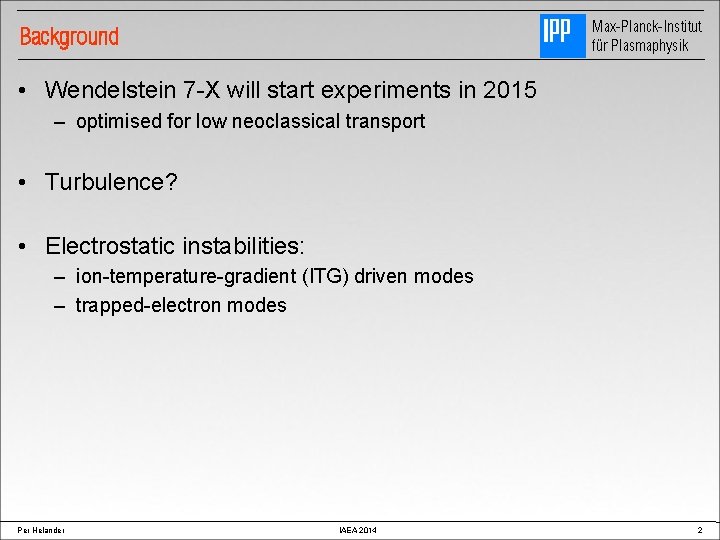
Max-Planck-Institut für Plasmaphysik Background • Wendelstein 7 -X will start experiments in 2015 – optimised for low neoclassical transport • Turbulence? • Electrostatic instabilities: – ion-temperature-gradient (ITG) driven modes – trapped-electron modes Per Helander IAEA 2014 2
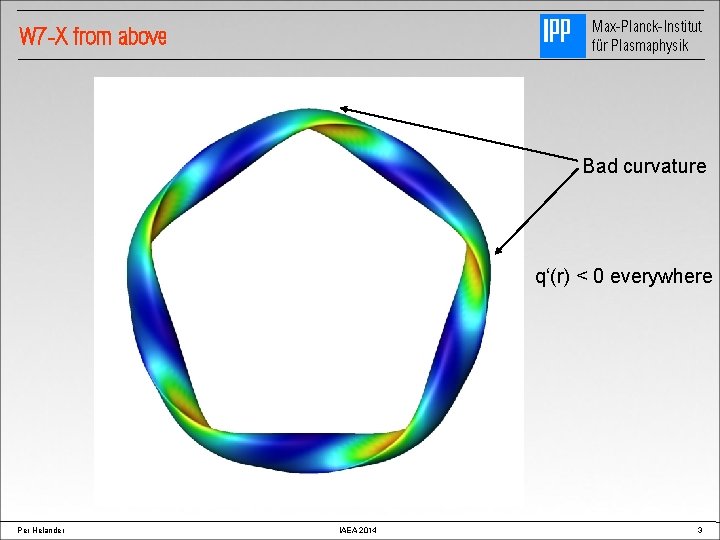
Max-Planck-Institut für Plasmaphysik W 7 -X from above Bad curvature q‘(r) < 0 everywhere Per Helander IAEA 2014 3
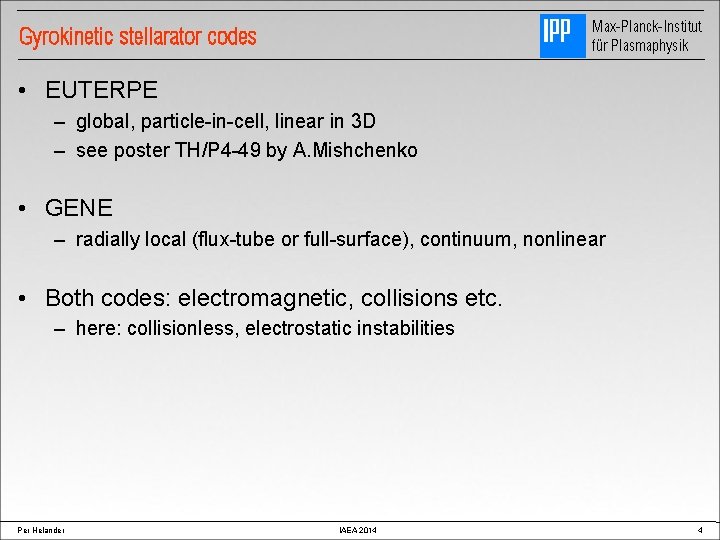
Max-Planck-Institut für Plasmaphysik Gyrokinetic stellarator codes • EUTERPE – global, particle-in-cell, linear in 3 D – see poster TH/P 4 -49 by A. Mishchenko • GENE – radially local (flux-tube or full-surface), continuum, nonlinear • Both codes: electromagnetic, collisions etc. – here: collisionless, electrostatic instabilities Per Helander IAEA 2014 4
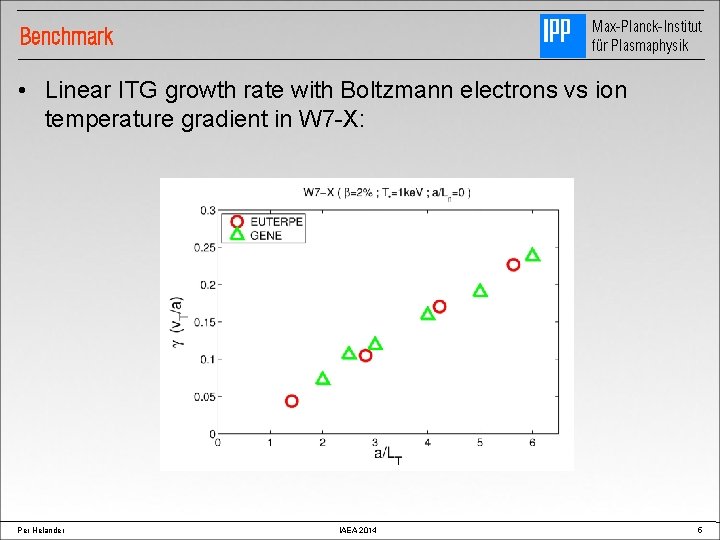
Max-Planck-Institut für Plasmaphysik Benchmark • Linear ITG growth rate with Boltzmann electrons vs ion temperature gradient in W 7 -X: Per Helander IAEA 2014 5
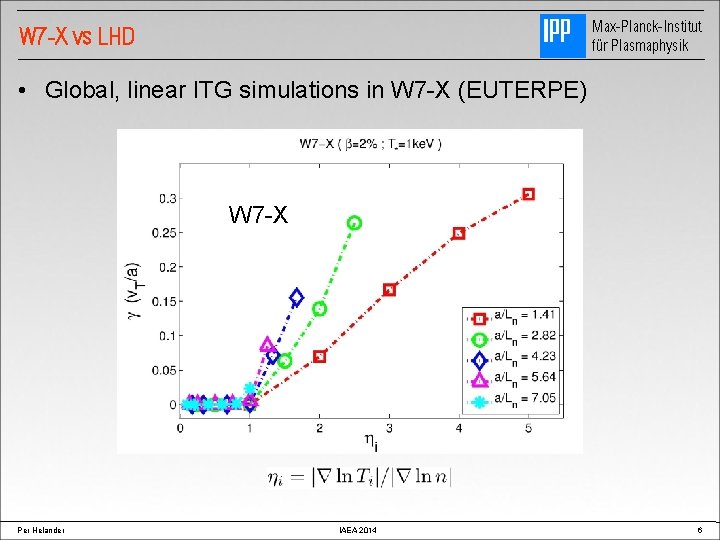
Max-Planck-Institut für Plasmaphysik W 7 -X vs LHD • Global, linear ITG simulations in W 7 -X (EUTERPE) W 7 -X Per Helander IAEA 2014 6
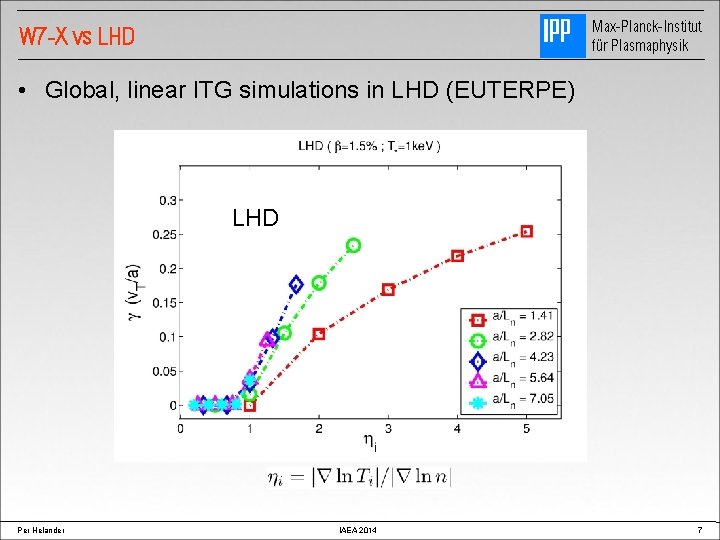
Max-Planck-Institut für Plasmaphysik W 7 -X vs LHD • Global, linear ITG simulations in LHD (EUTERPE) LHD Per Helander IAEA 2014 7
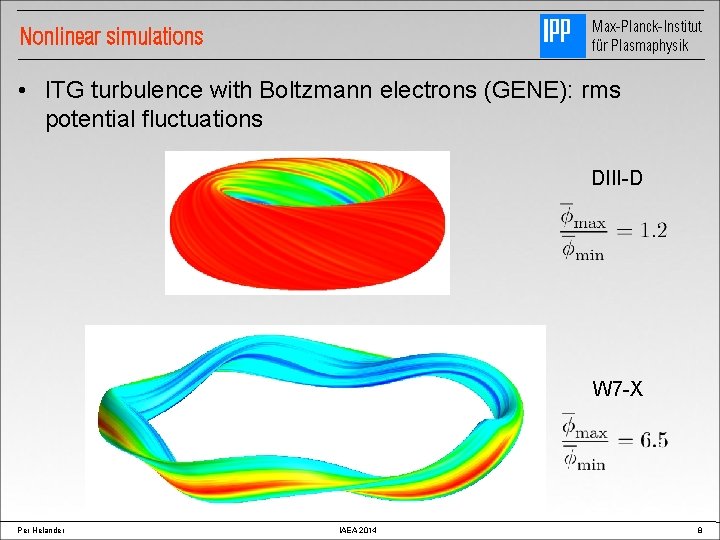
Max-Planck-Institut für Plasmaphysik Nonlinear simulations • ITG turbulence with Boltzmann electrons (GENE): rms potential fluctuations DIII-D W 7 -X Per Helander IAEA 2014 8
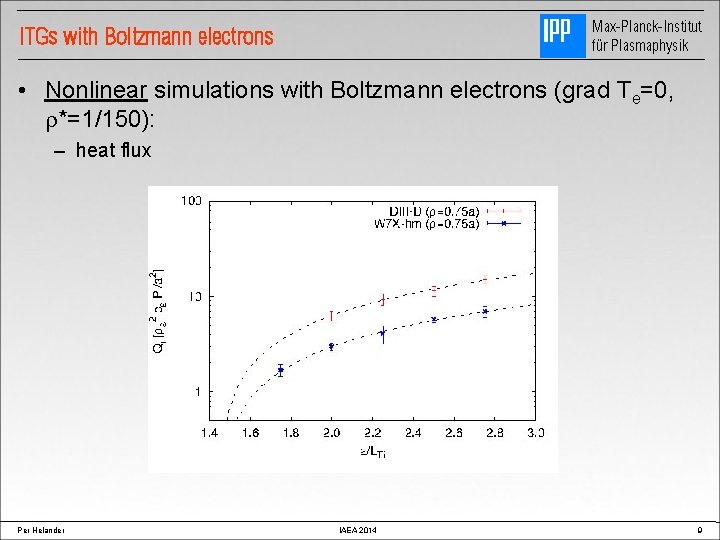
Max-Planck-Institut für Plasmaphysik ITGs with Boltzmann electrons • Nonlinear simulations with Boltzmann electrons (grad Te=0, r*=1/150): – heat flux Per Helander IAEA 2014 9
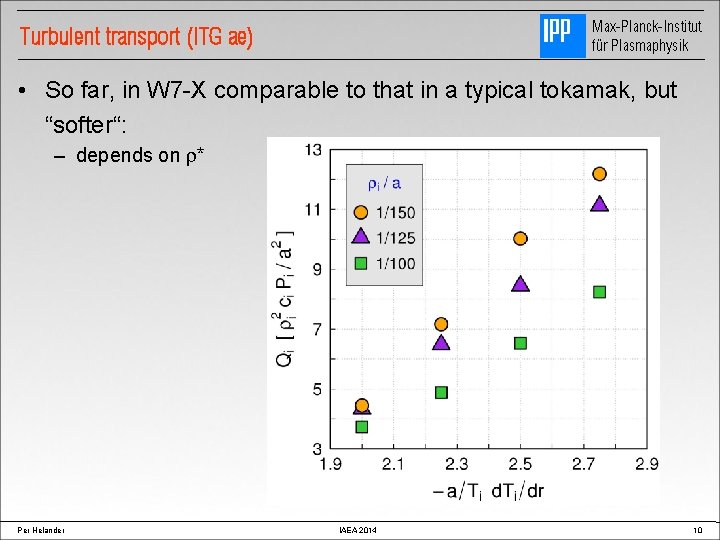
Max-Planck-Institut für Plasmaphysik Turbulent transport (ITG ae) • So far, in W 7 -X comparable to that in a typical tokamak, but “softer“: – depends on r* Per Helander IAEA 2014 10
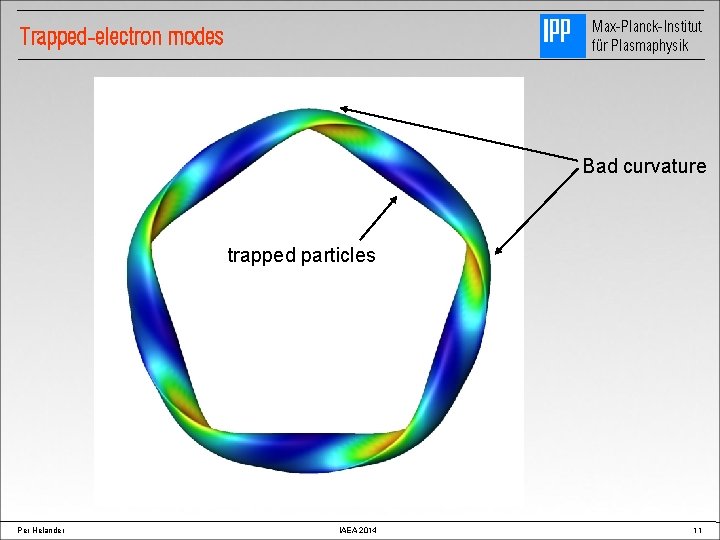
Max-Planck-Institut für Plasmaphysik Trapped-electron modes Bad curvature trapped particles Per Helander IAEA 2014 11
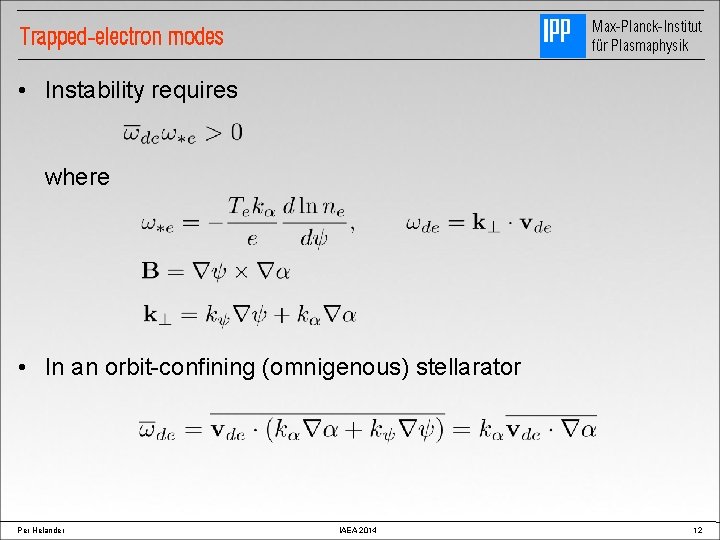
Max-Planck-Institut für Plasmaphysik Trapped-electron modes • Instability requires where • In an orbit-confining (omnigenous) stellarator Per Helander IAEA 2014 12
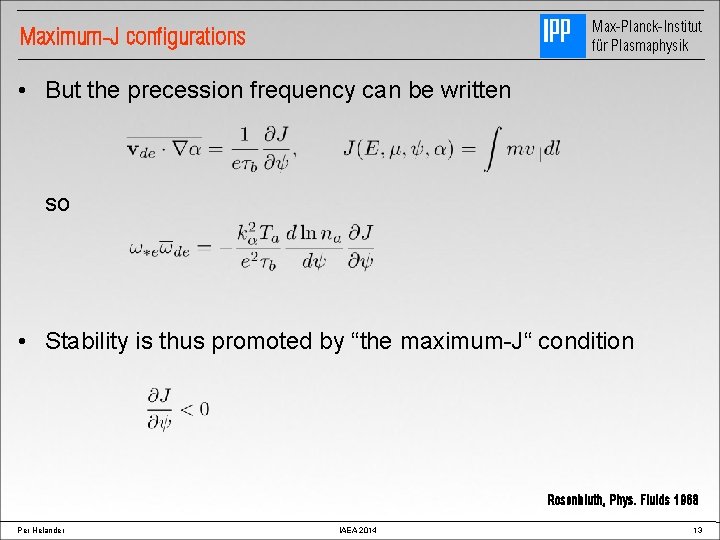
Max-Planck-Institut für Plasmaphysik Maximum-J configurations • But the precession frequency can be written so • Stability is thus promoted by “the maximum-J“ condition Rosenbluth, Phys. Fluids 1968 Per Helander IAEA 2014 13
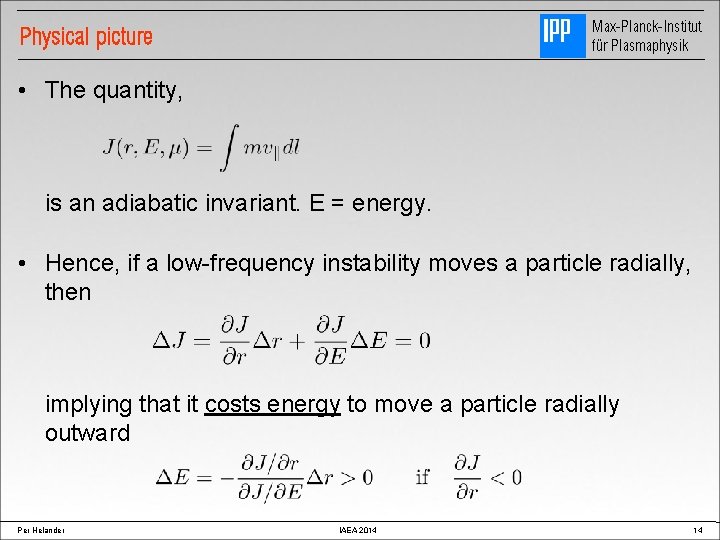
Max-Planck-Institut für Plasmaphysik Physical picture • The quantity, is an adiabatic invariant. E = energy. • Hence, if a low-frequency instability moves a particle radially, then implying that it costs energy to move a particle radially outward Per Helander IAEA 2014 14
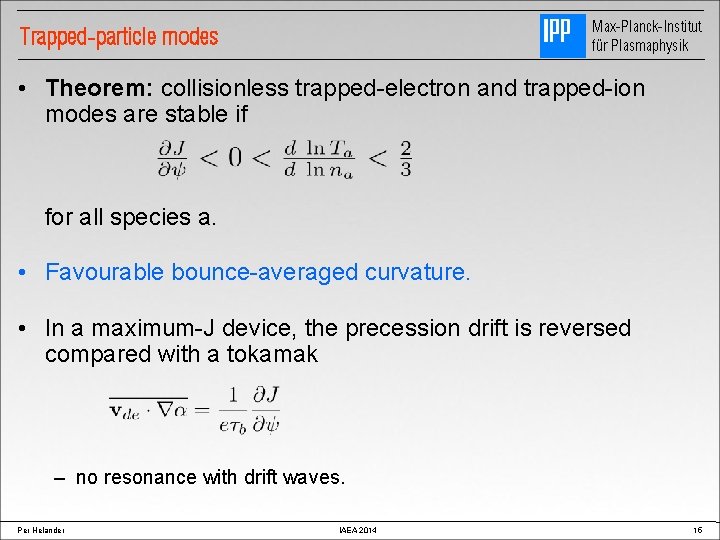
Max-Planck-Institut für Plasmaphysik Trapped-particle modes • Theorem: collisionless trapped-electron and trapped-ion modes are stable if for all species a. • Favourable bounce-averaged curvature. • In a maximum-J device, the precession drift is reversed compared with a tokamak – no resonance with drift waves. Per Helander IAEA 2014 15
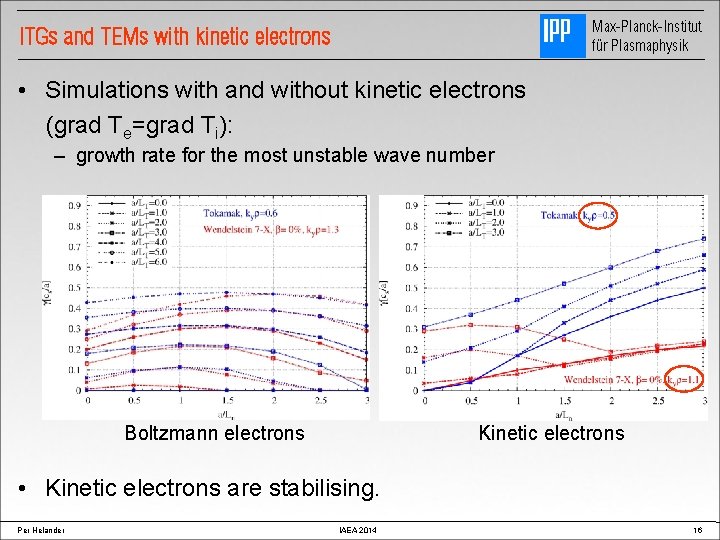
Max-Planck-Institut für Plasmaphysik ITGs and TEMs with kinetic electrons • Simulations with and without kinetic electrons (grad Te=grad Ti): – growth rate for the most unstable wave number Kinetic electrons Boltzmann electrons • Kinetic electrons are stabilising. Per Helander IAEA 2014 16
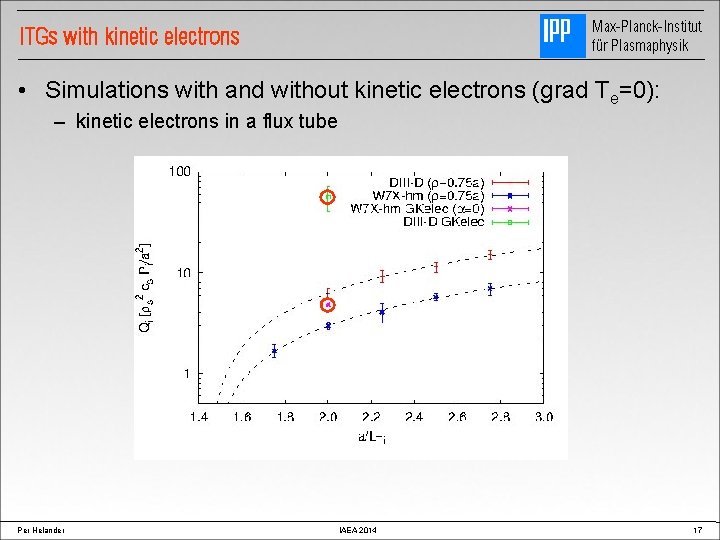
Max-Planck-Institut für Plasmaphysik ITGs with kinetic electrons • Simulations with and without kinetic electrons (grad Te=0): – kinetic electrons in a flux tube Per Helander IAEA 2014 17
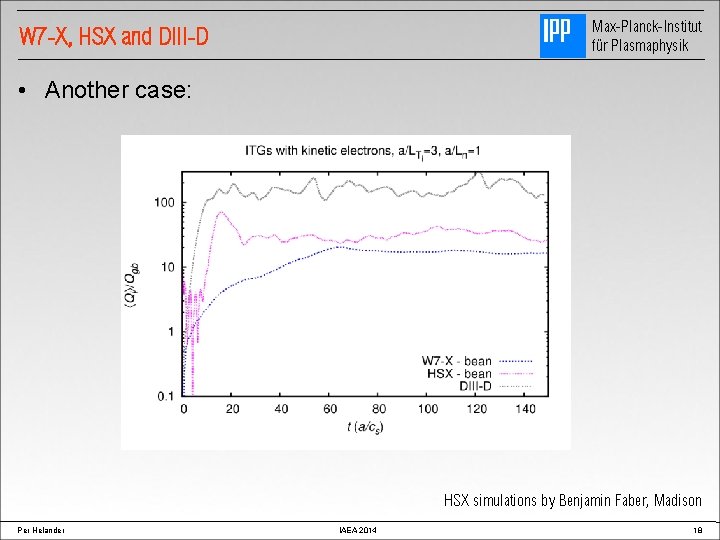
Max-Planck-Institut für Plasmaphysik W 7 -X, HSX and DIII-D • Another case: HSX simulations by Benjamin Faber, Madison Per Helander IAEA 2014 18
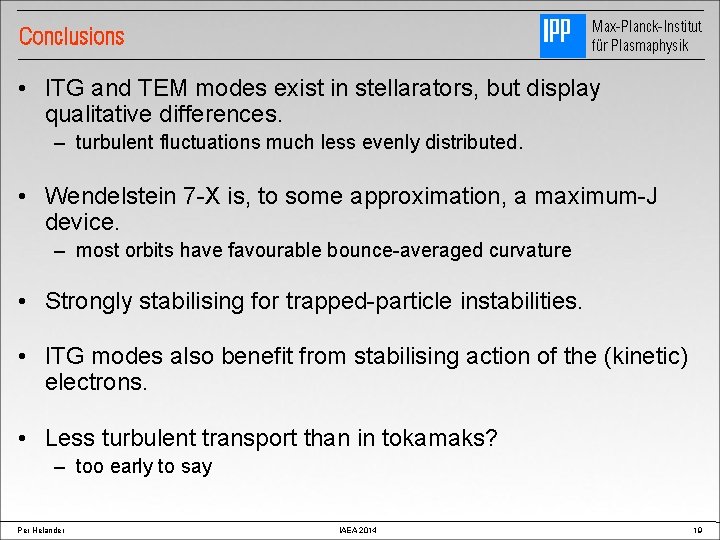
Max-Planck-Institut für Plasmaphysik Conclusions • ITG and TEM modes exist in stellarators, but display qualitative differences. – turbulent fluctuations much less evenly distributed. • Wendelstein 7 -X is, to some approximation, a maximum-J device. – most orbits have favourable bounce-averaged curvature • Strongly stabilising for trapped-particle instabilities. • ITG modes also benefit from stabilising action of the (kinetic) electrons. • Less turbulent transport than in tokamaks? – too early to say Per Helander IAEA 2014 19
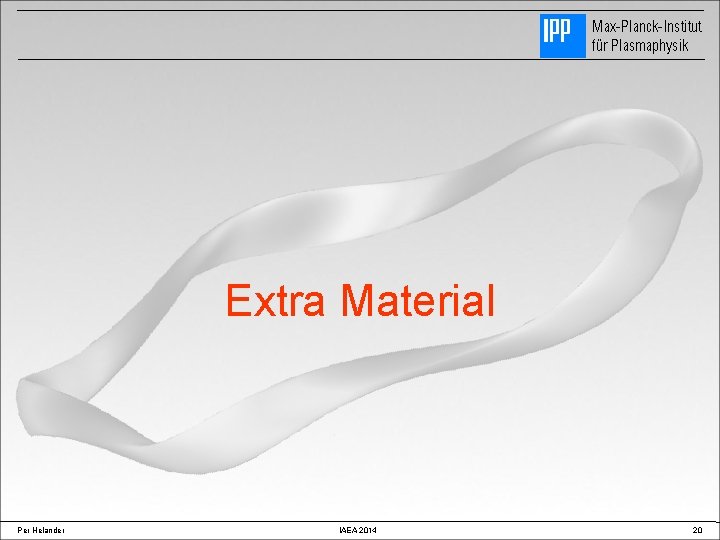
Max-Planck-Institut für Plasmaphysik Extra Material Per Helander IAEA 2014 20
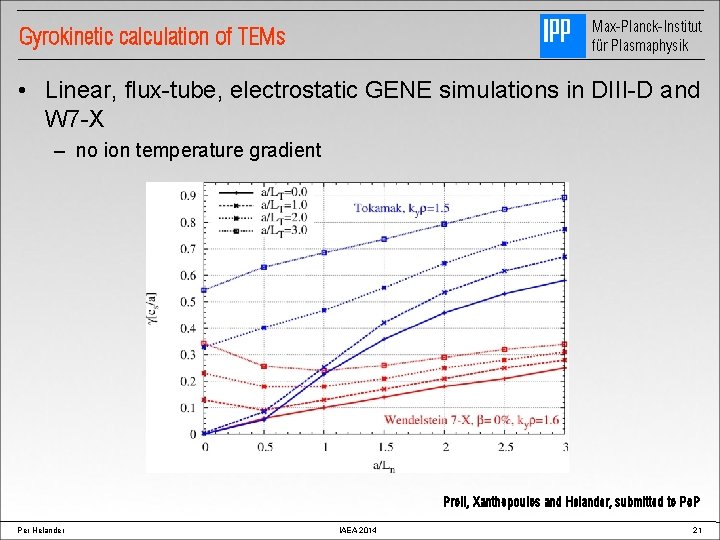
Max-Planck-Institut für Plasmaphysik Gyrokinetic calculation of TEMs • Linear, flux-tube, electrostatic GENE simulations in DIII-D and W 7 -X – no ion temperature gradient Proll, Xanthopoulos and Helander, submitted to Po. P Per Helander IAEA 2014 21
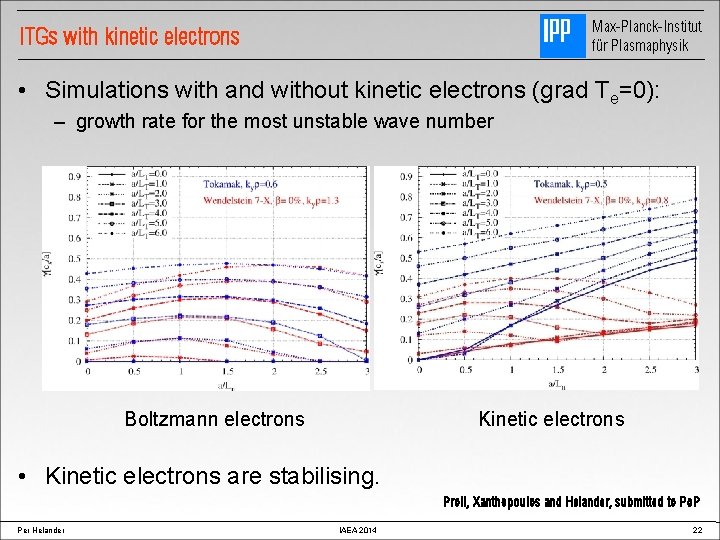
Max-Planck-Institut für Plasmaphysik ITGs with kinetic electrons • Simulations with and without kinetic electrons (grad Te=0): – growth rate for the most unstable wave number Kinetic electrons Boltzmann electrons • Kinetic electrons are stabilising. Proll, Xanthopoulos and Helander, submitted to Po. P Per Helander IAEA 2014 22
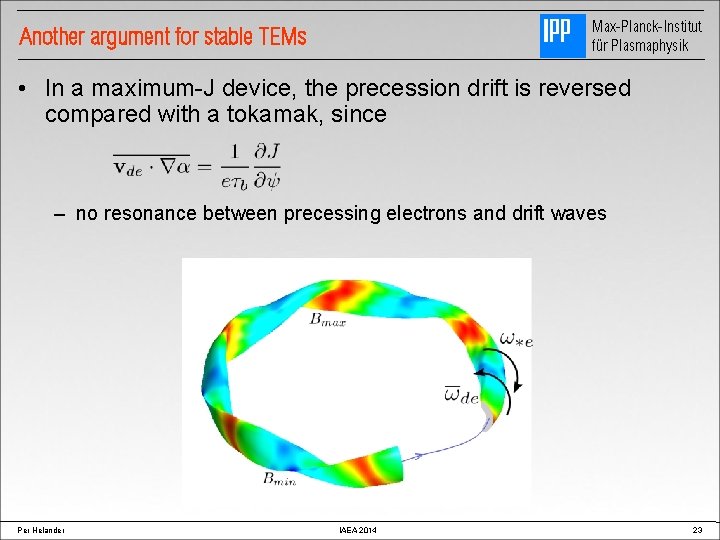
Max-Planck-Institut für Plasmaphysik Another argument for stable TEMs • In a maximum-J device, the precession drift is reversed compared with a tokamak, since – no resonance between precessing electrons and drift waves Per Helander IAEA 2014 23
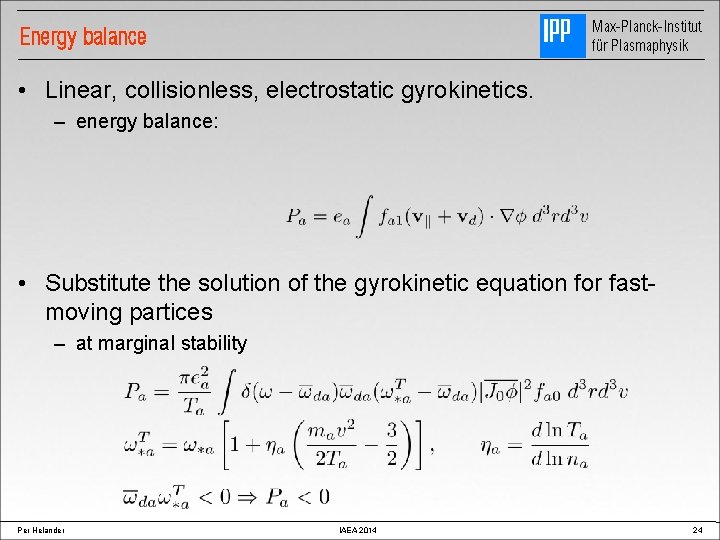
Max-Planck-Institut für Plasmaphysik Energy balance • Linear, collisionless, electrostatic gyrokinetics. – energy balance: • Substitute the solution of the gyrokinetic equation for fastmoving partices – at marginal stability Per Helander IAEA 2014 24
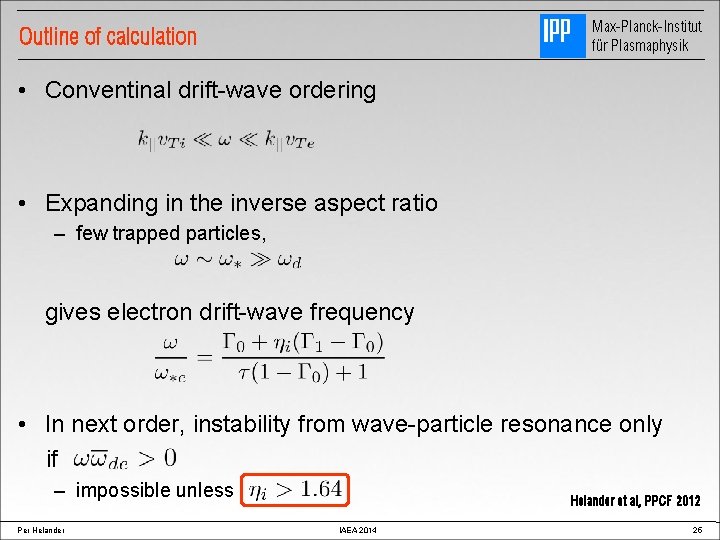
Max-Planck-Institut für Plasmaphysik Outline of calculation • Conventinal drift-wave ordering • Expanding in the inverse aspect ratio – few trapped particles, gives electron drift-wave frequency • In next order, instability from wave-particle resonance only if – impossible unless Per Helander et al, PPCF 2012 IAEA 2014 25
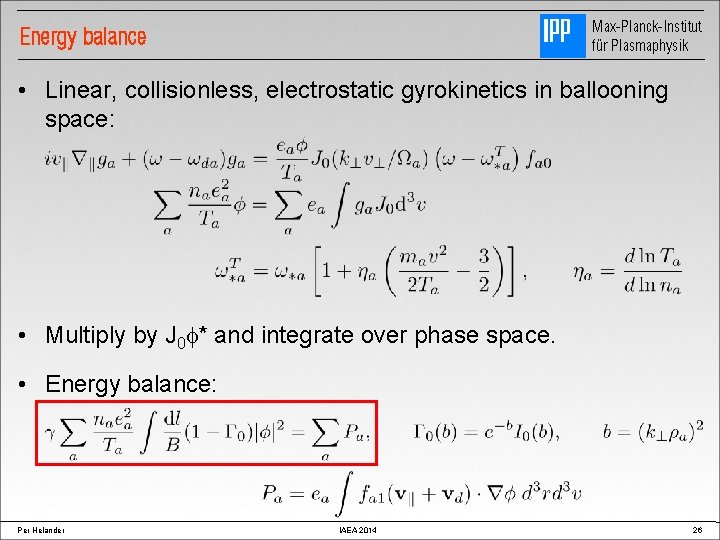
Max-Planck-Institut für Plasmaphysik Energy balance • Linear, collisionless, electrostatic gyrokinetics in ballooning space: • Multiply by J 0 f* and integrate over phase space. • Energy balance: Per Helander IAEA 2014 26
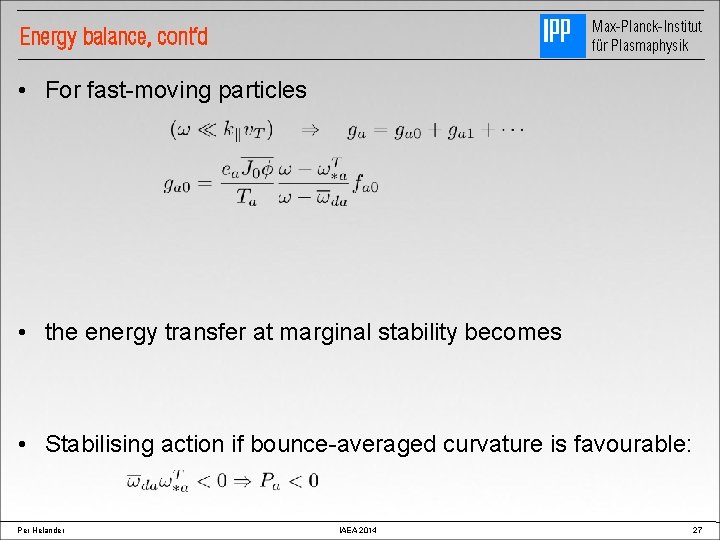
Max-Planck-Institut für Plasmaphysik Energy balance, cont‘d • For fast-moving particles • the energy transfer at marginal stability becomes • Stabilising action if bounce-averaged curvature is favourable: Per Helander IAEA 2014 27
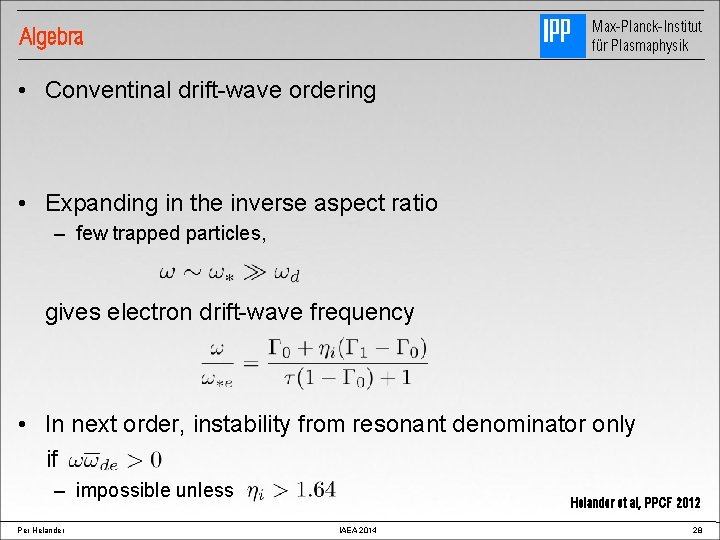
Max-Planck-Institut für Plasmaphysik Algebra • Conventinal drift-wave ordering • Expanding in the inverse aspect ratio – few trapped particles, gives electron drift-wave frequency • In next order, instability from resonant denominator only if – impossible unless Per Helander et al, PPCF 2012 IAEA 2014 28
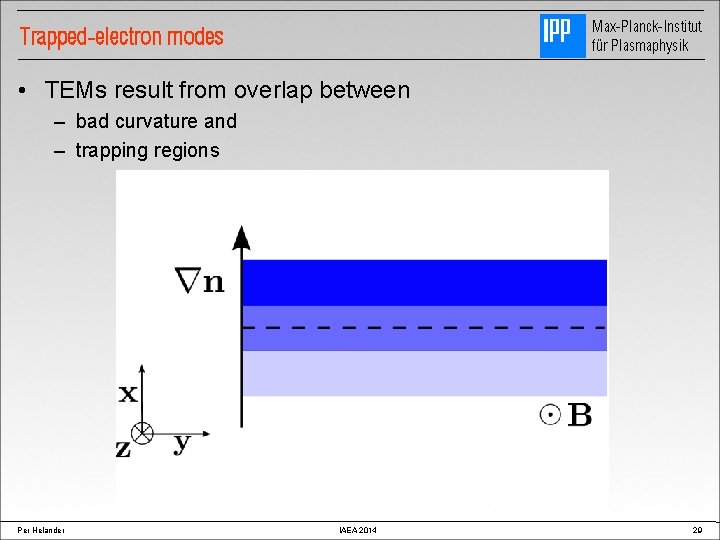
Max-Planck-Institut für Plasmaphysik Trapped-electron modes • TEMs result from overlap between – bad curvature and – trapping regions Per Helander IAEA 2014 29
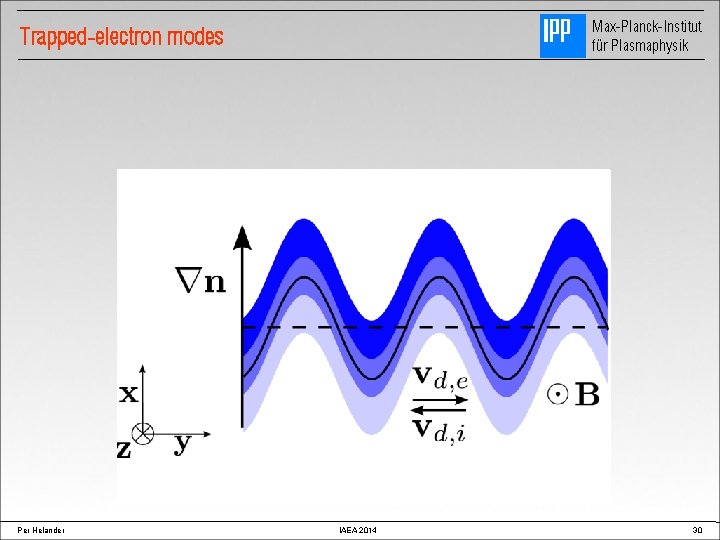
Max-Planck-Institut für Plasmaphysik Trapped-electron modes Per Helander IAEA 2014 30
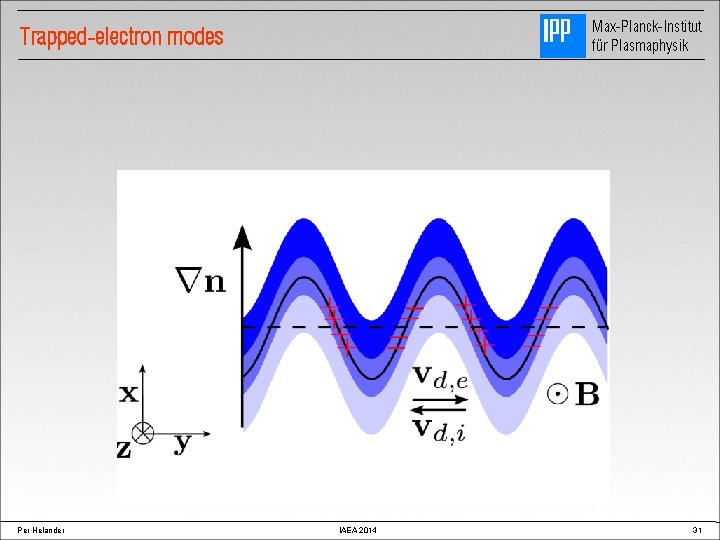
Max-Planck-Institut für Plasmaphysik Trapped-electron modes Per Helander IAEA 2014 31
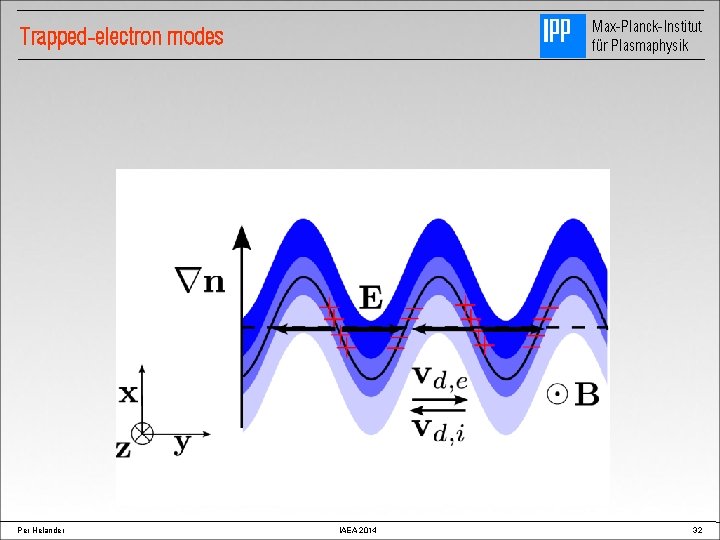
Max-Planck-Institut für Plasmaphysik Trapped-electron modes Per Helander IAEA 2014 32
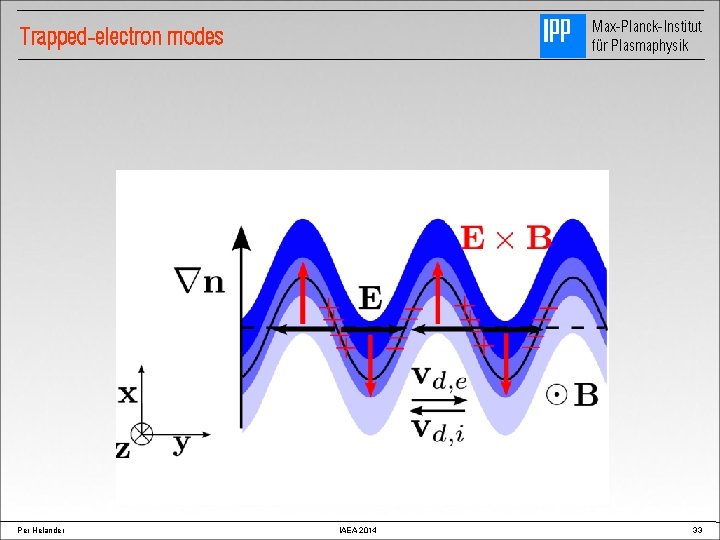
Max-Planck-Institut für Plasmaphysik Trapped-electron modes Per Helander IAEA 2014 33
Stellarator
Stellarator
Stellarator
Classification of advances as per rbi
Recent advances in ceramics
Taly payroll
Coherent scattering
Lurbinectedin posologie
Advances in technology during wwii
Short term loans and advances
Advances in memory technology
Lesson 9.1 intellectual advances in the first year
Opto-electronic advances
Axis powers
Advances in real time rendering
Longum iter est
Una voce il mio diletto eccolo viene saltando per i monti
1800dam/jam berapa hm/menit
Per capita vs per stirpes
Auksu mesta sidabru austa
Per stirpes v per capita
Approssimazione per difetto e per eccesso
Catullus 84 translation
27 miles per gallon into kilometers per liter
Multas per gentes et multa per aequora vectus
Coop per te
Is 60 seconds 1 minute
Per knudsen
Somma per colore excel
Subarachnoid space
Costante dielettrica nel vuoto formula
Coulomb force potential energy
Father of physical education in germany
Foglio a quadretti per lim