Fourier Transform Microwave Spectroscopic Studies of Dimethyl Ether
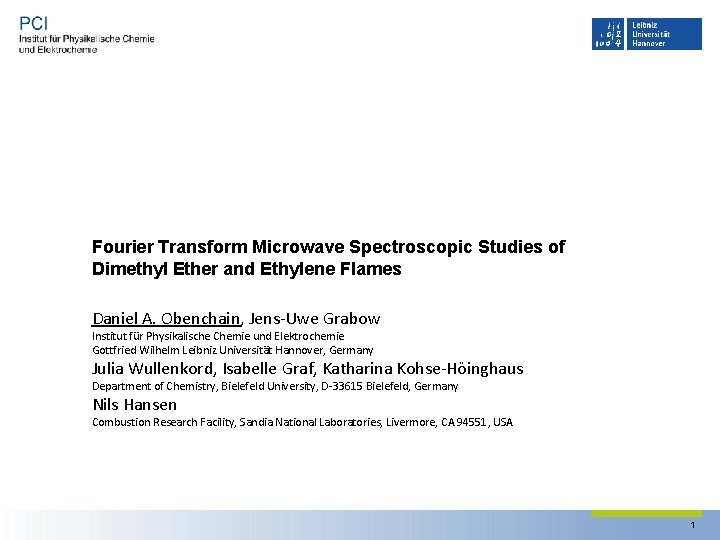
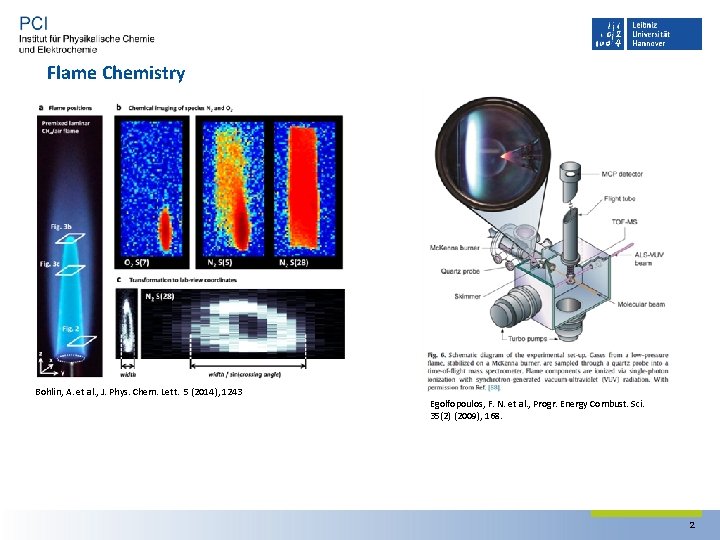
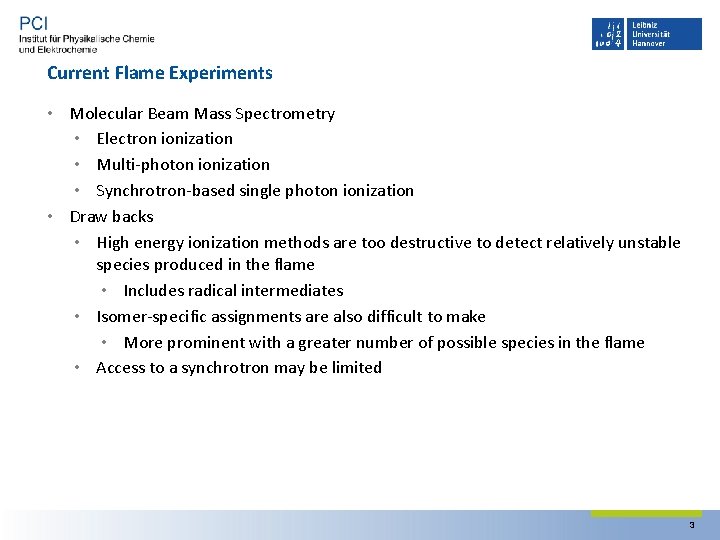
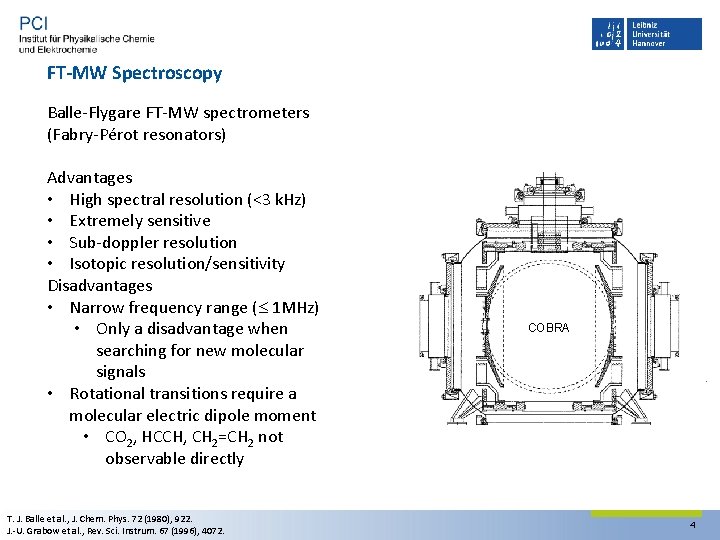
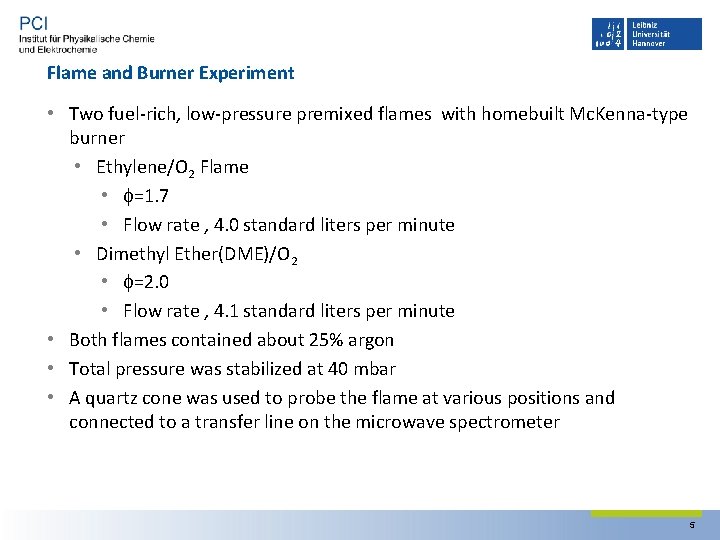
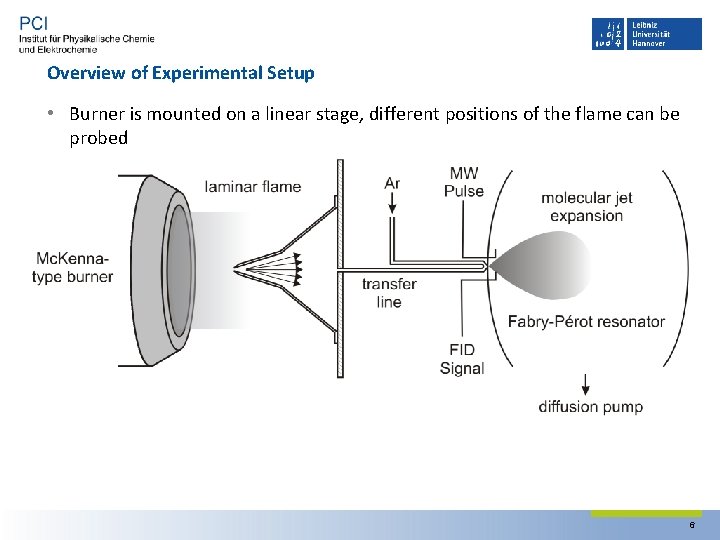
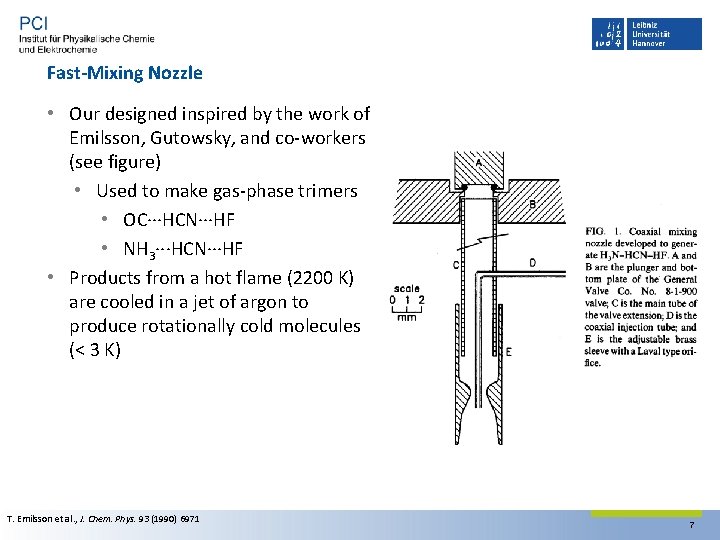
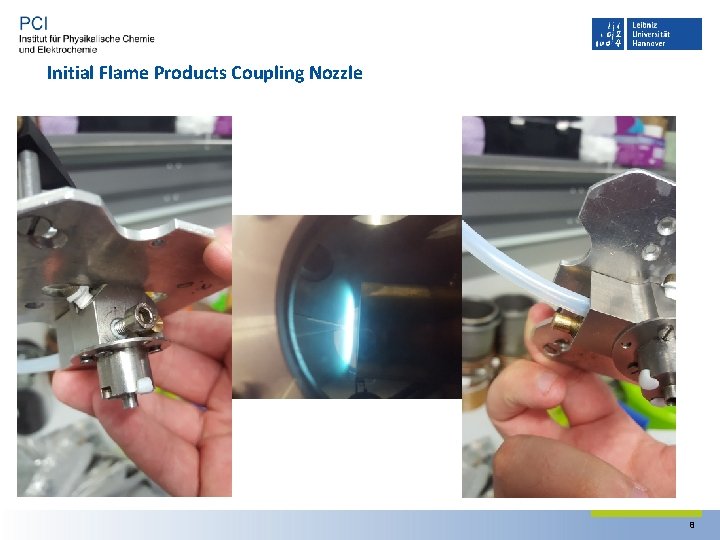
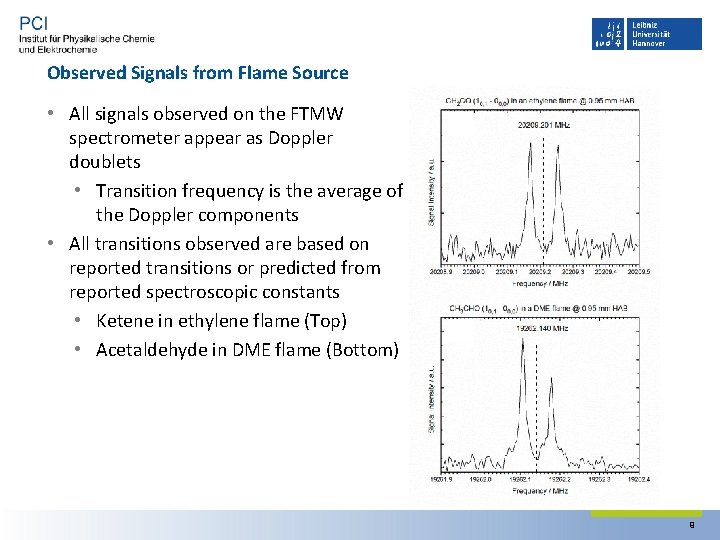
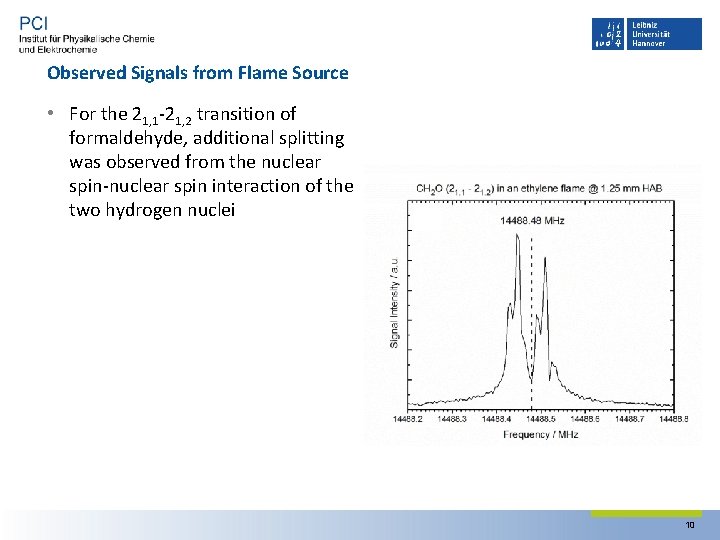
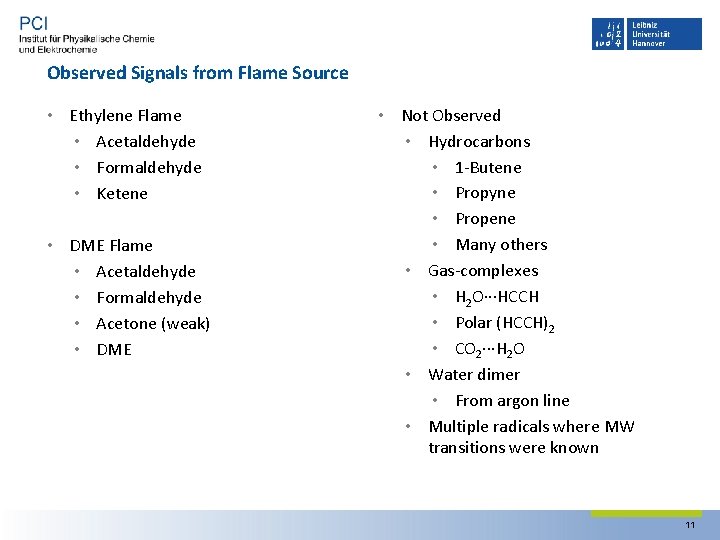
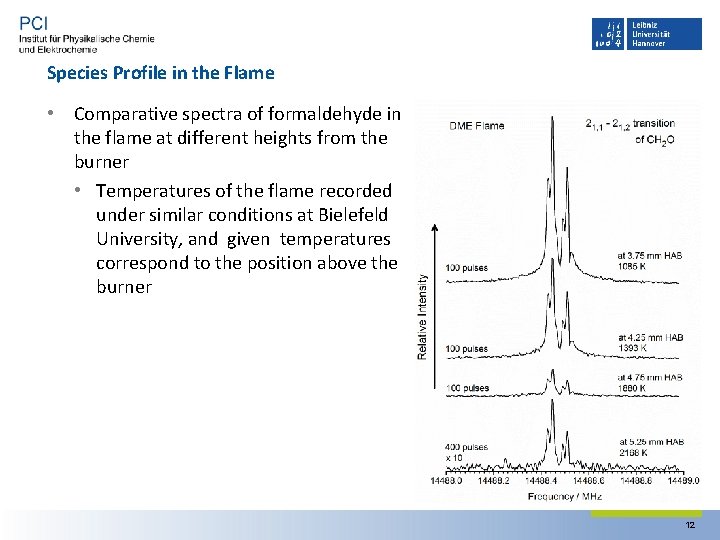
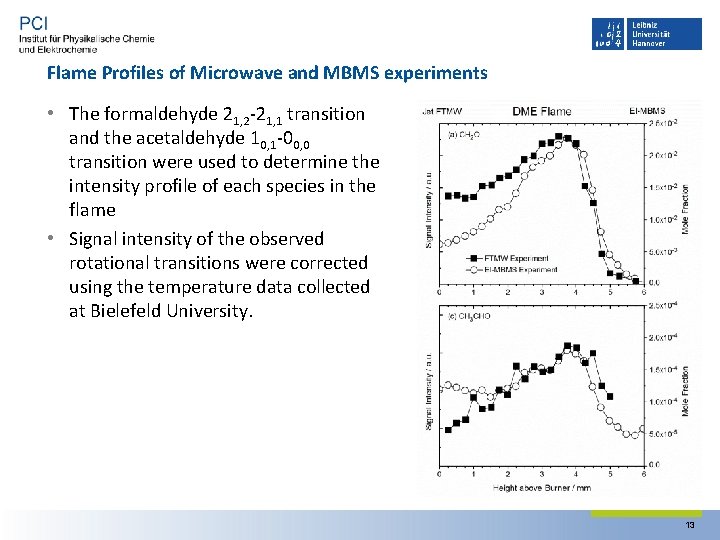
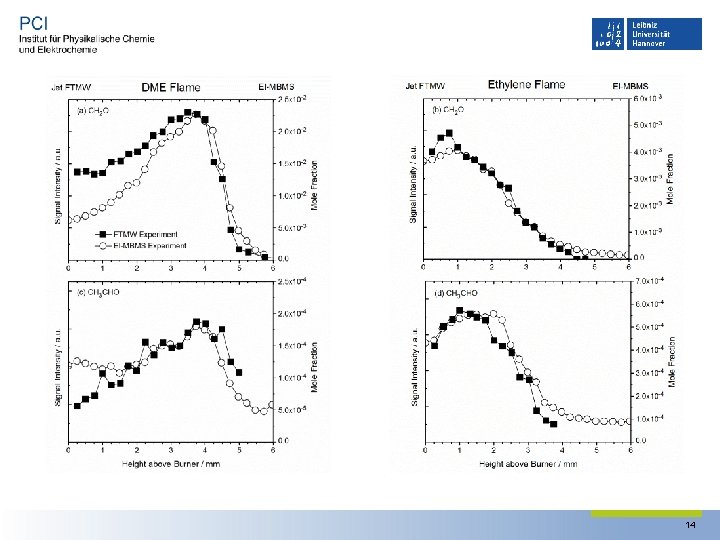
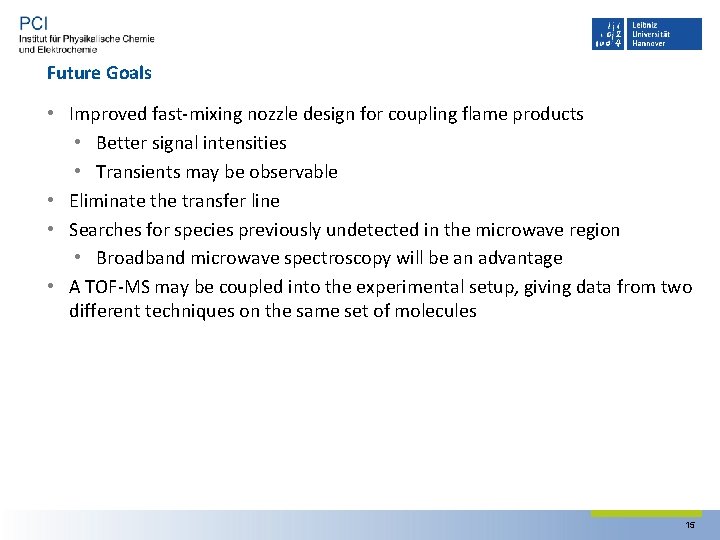
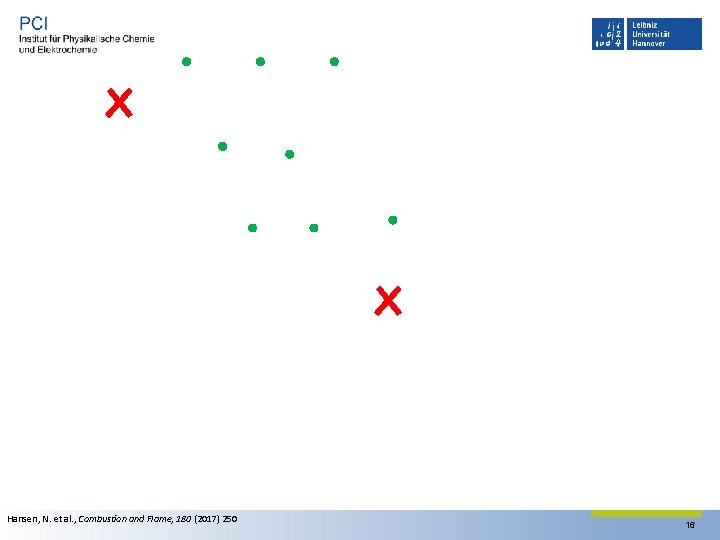
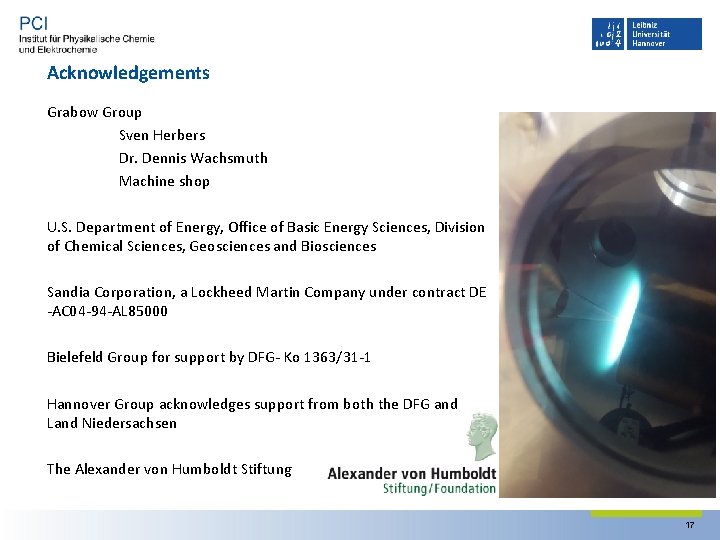
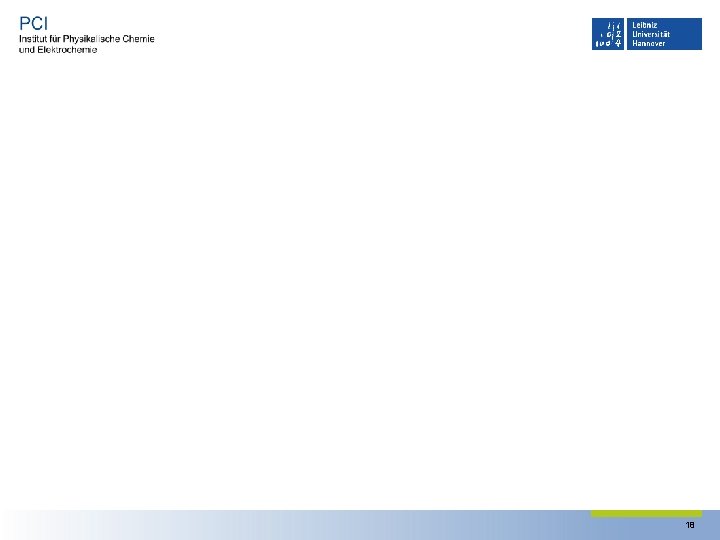
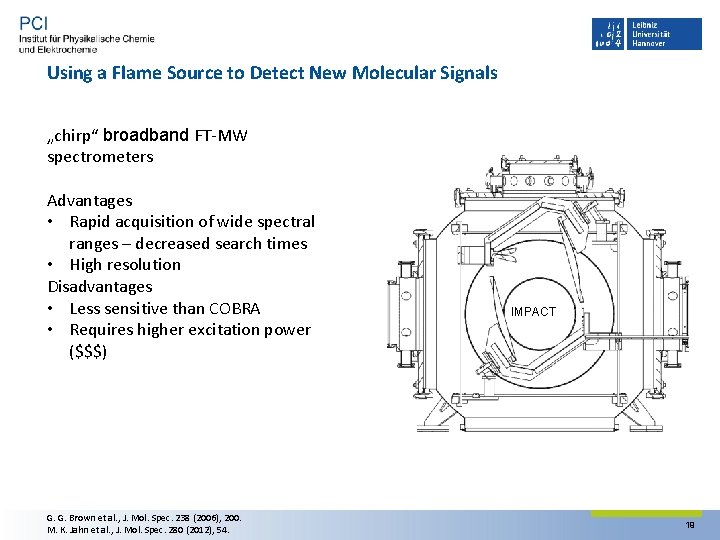
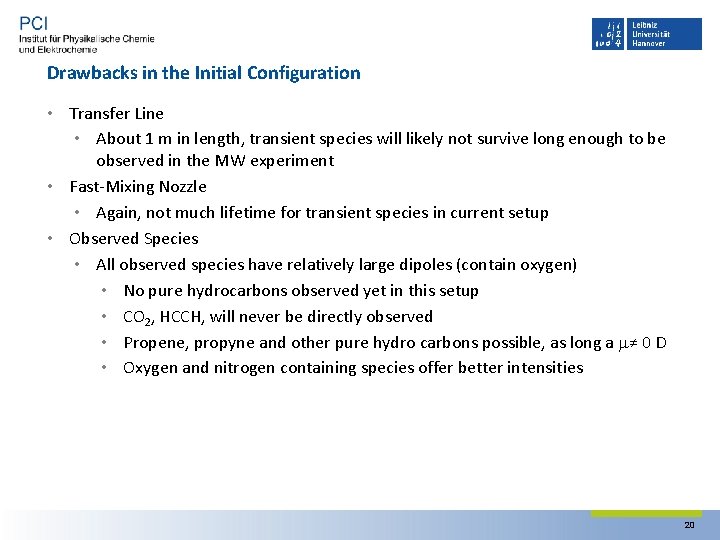
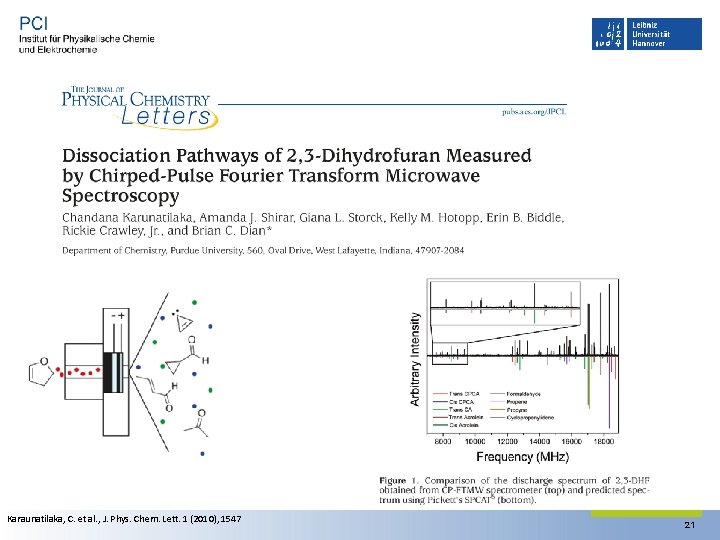
- Slides: 21
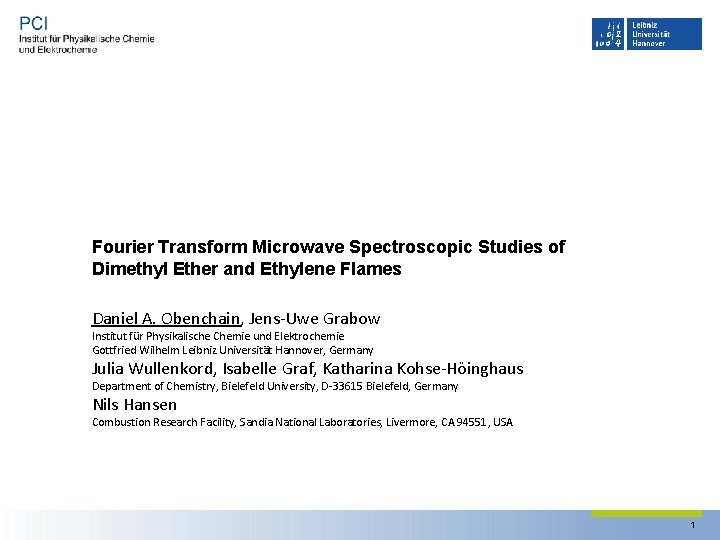
Fourier Transform Microwave Spectroscopic Studies of Dimethyl Ether and Ethylene Flames Daniel A. Obenchain, Jens‐Uwe Grabow Institut für Physikalische Chemie und Elektrochemie Gottfried Wilhelm Leibniz Universität Hannover, Germany Julia Wullenkord, Isabelle Graf, Katharina Kohse‐Höinghaus Department of Chemistry, Bielefeld University, D‐ 33615 Bielefeld, Germany Nils Hansen Combustion Research Facility, Sandia National Laboratories, Livermore, CA 94551, USA 1
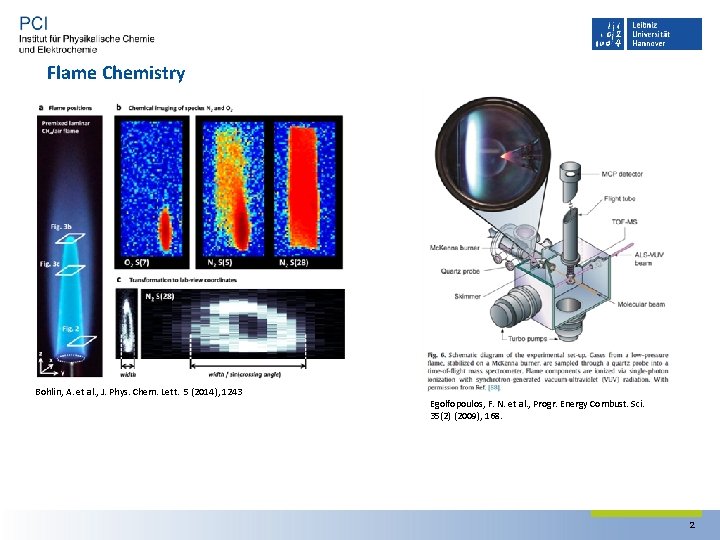
Flame Chemistry Bohlin, A. et al. , J. Phys. Chem. Lett. 5 (2014), 1243 Egolfopoulos, F. N. et al. , Progr. Energy Combust. Sci. 35(2) (2009), 168. 2
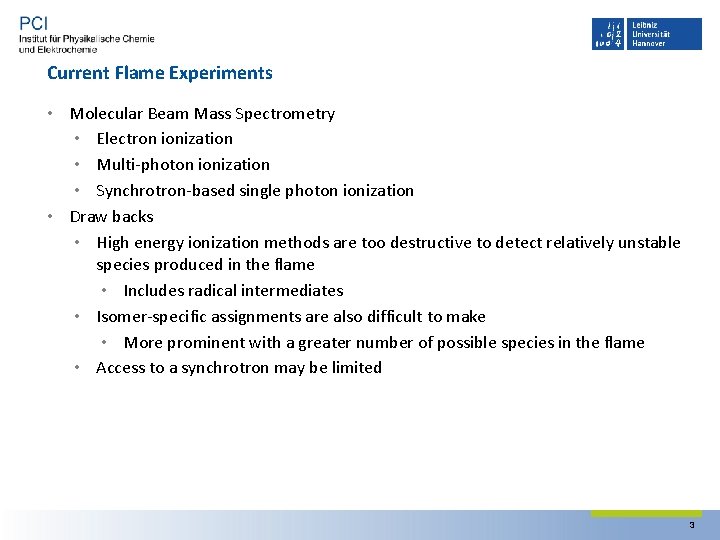
Current Flame Experiments • Molecular Beam Mass Spectrometry • Electron ionization • Multi‐photon ionization • Synchrotron‐based single photon ionization • Draw backs • High energy ionization methods are too destructive to detect relatively unstable species produced in the flame • Includes radical intermediates • Isomer‐specific assignments are also difficult to make • More prominent with a greater number of possible species in the flame • Access to a synchrotron may be limited 3
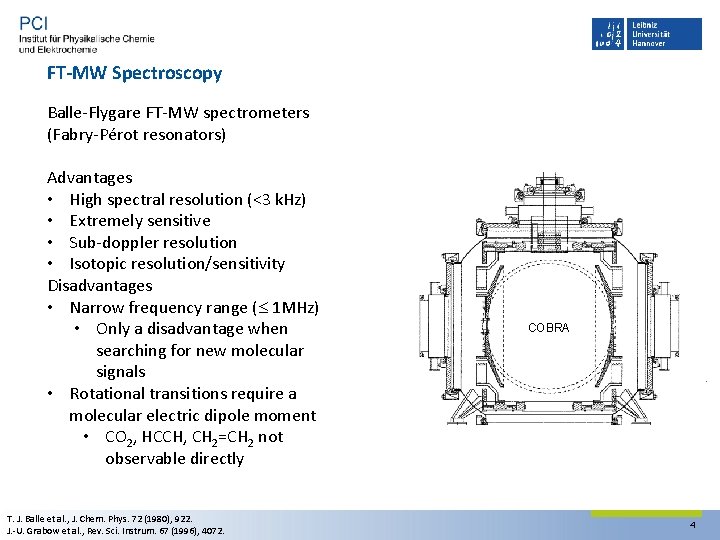
FT-MW Spectroscopy Balle‐Flygare FT‐MW spectrometers (Fabry‐Pérot resonators) Advantages • High spectral resolution (<3 k. Hz) • Extremely sensitive • Sub‐doppler resolution • Isotopic resolution/sensitivity Disadvantages • Narrow frequency range ( 1 MHz) • Only a disadvantage when searching for new molecular signals • Rotational transitions require a molecular electric dipole moment • CO 2, HCCH, CH 2=CH 2 not observable directly T. J. Balle et al. , J. Chem. Phys. 72 (1980), 922. J. ‐U. Grabow et al. , Rev. Sci. Instrum. 67 (1996), 4072. COBRA 4
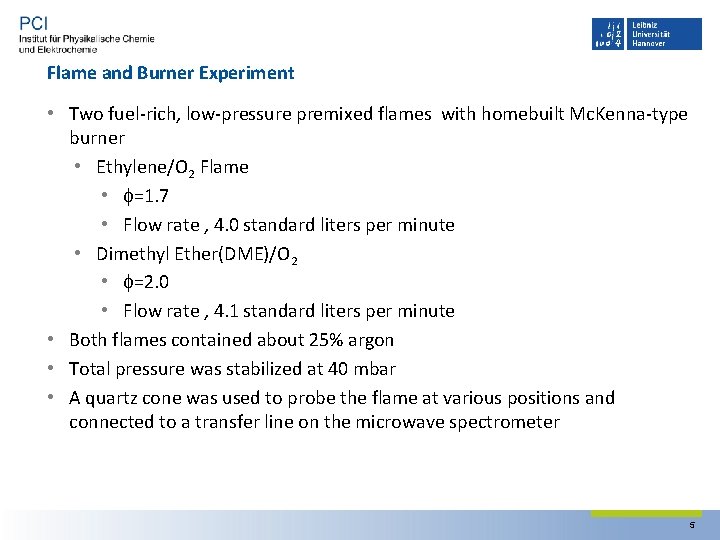
Flame and Burner Experiment • Two fuel‐rich, low‐pressure premixed flames with homebuilt Mc. Kenna‐type burner • Ethylene/O 2 Flame • =1. 7 • Flow rate , 4. 0 standard liters per minute • Dimethyl Ether(DME)/O 2 • =2. 0 • Flow rate , 4. 1 standard liters per minute • Both flames contained about 25% argon • Total pressure was stabilized at 40 mbar • A quartz cone was used to probe the flame at various positions and connected to a transfer line on the microwave spectrometer 5
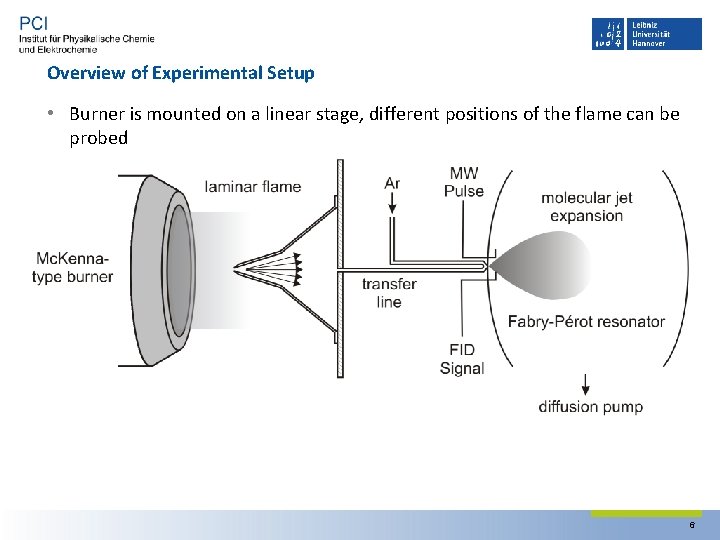
Overview of Experimental Setup • Burner is mounted on a linear stage, different positions of the flame can be probed 6
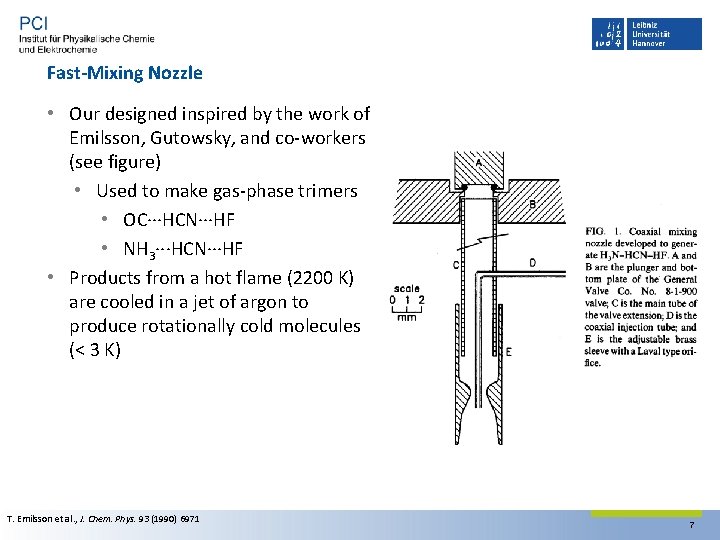
Fast-Mixing Nozzle • Our designed inspired by the work of Emilsson, Gutowsky, and co‐workers (see figure) • Used to make gas‐phase trimers • OC···HCN···HF • NH 3···HCN···HF • Products from a hot flame (2200 K) are cooled in a jet of argon to produce rotationally cold molecules (< 3 K) T. Emilsson et al. , J. Chem. Phys. 93 (1990) 6971 7
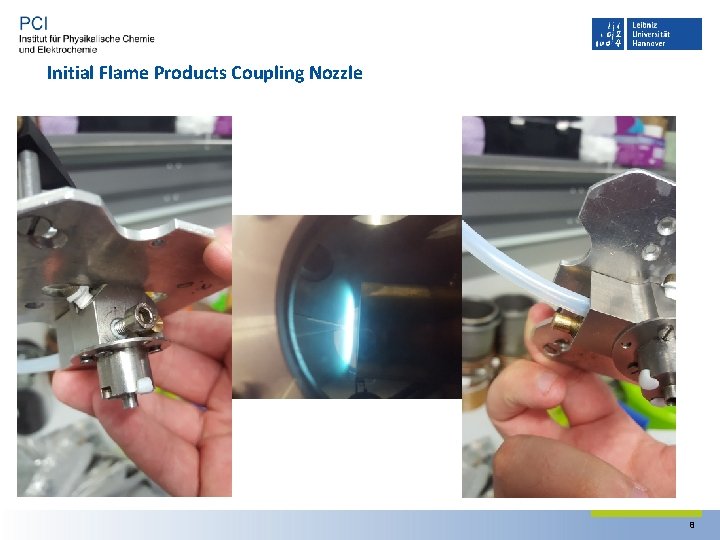
Initial Flame Products Coupling Nozzle 8
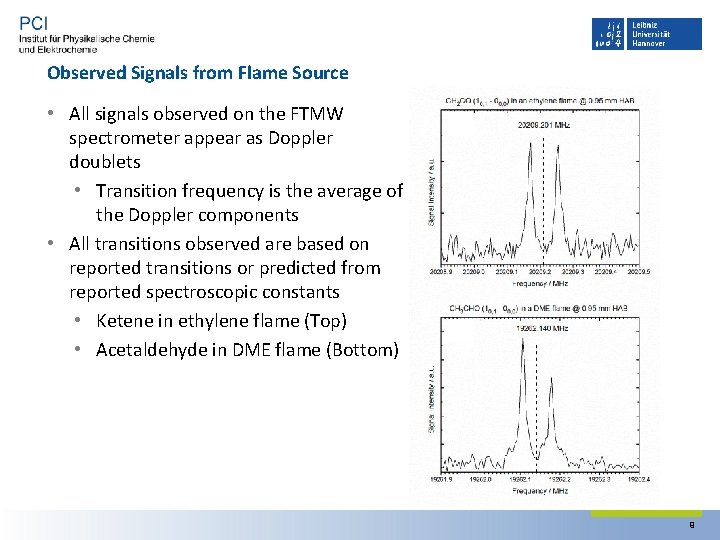
Observed Signals from Flame Source • All signals observed on the FTMW spectrometer appear as Doppler doublets • Transition frequency is the average of the Doppler components • All transitions observed are based on reported transitions or predicted from reported spectroscopic constants • Ketene in ethylene flame (Top) • Acetaldehyde in DME flame (Bottom) 9
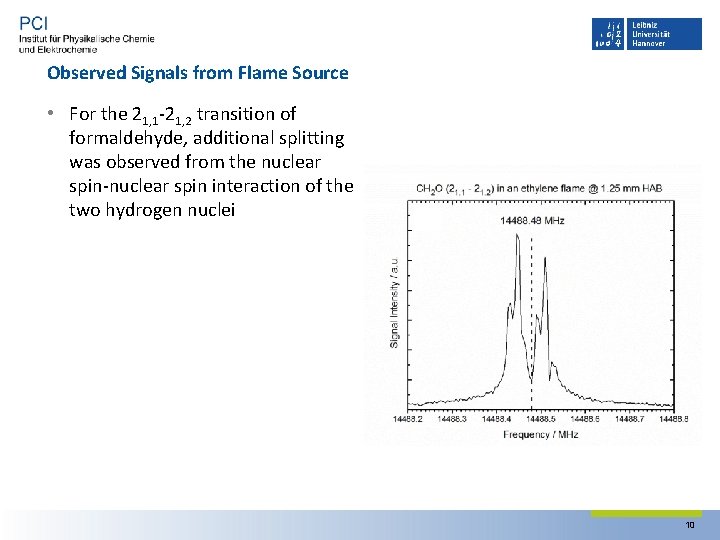
Observed Signals from Flame Source • For the 21, 1‐ 21, 2 transition of formaldehyde, additional splitting was observed from the nuclear spin‐nuclear spin interaction of the two hydrogen nuclei 10
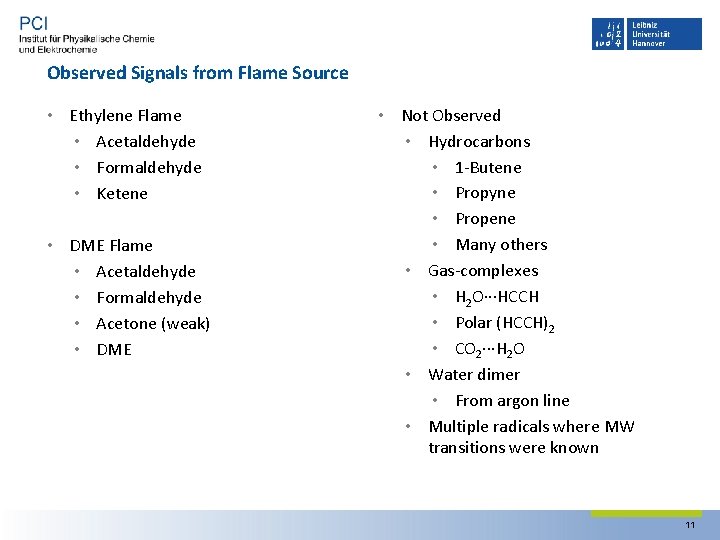
Observed Signals from Flame Source • Ethylene Flame • Acetaldehyde • Formaldehyde • Ketene • DME Flame • Acetaldehyde • Formaldehyde • Acetone (weak) • DME • Not Observed • Hydrocarbons • 1‐Butene • Propyne • Propene • Many others • Gas‐complexes • H 2 O···HCCH • Polar (HCCH)2 • CO 2···H 2 O • Water dimer • From argon line • Multiple radicals where MW transitions were known 11
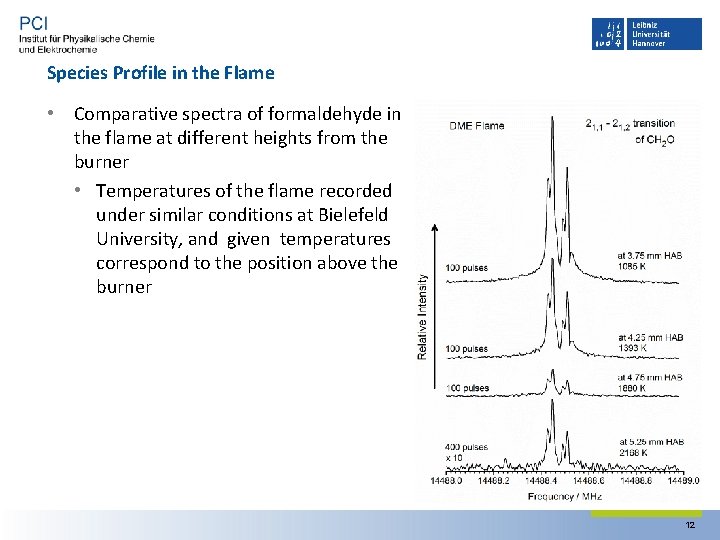
Species Profile in the Flame • Comparative spectra of formaldehyde in the flame at different heights from the burner • Temperatures of the flame recorded under similar conditions at Bielefeld University, and given temperatures correspond to the position above the burner 12
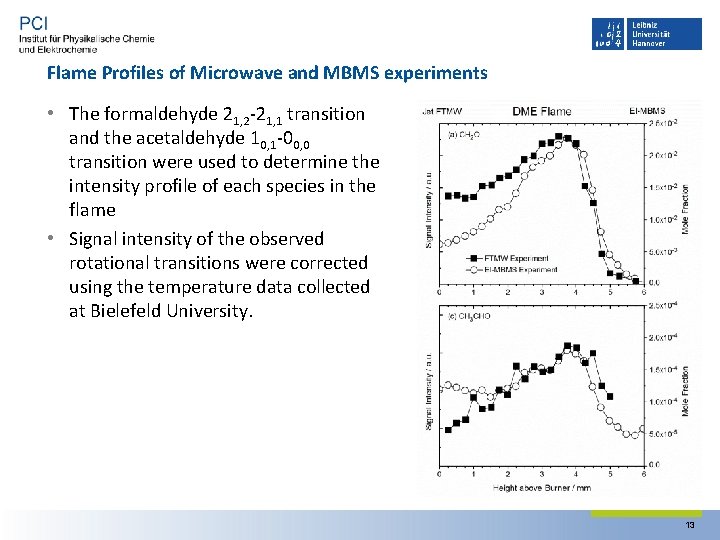
Flame Profiles of Microwave and MBMS experiments • The formaldehyde 21, 2‐ 21, 1 transition and the acetaldehyde 10, 1‐ 00, 0 transition were used to determine the intensity profile of each species in the flame • Signal intensity of the observed rotational transitions were corrected using the temperature data collected at Bielefeld University. 13
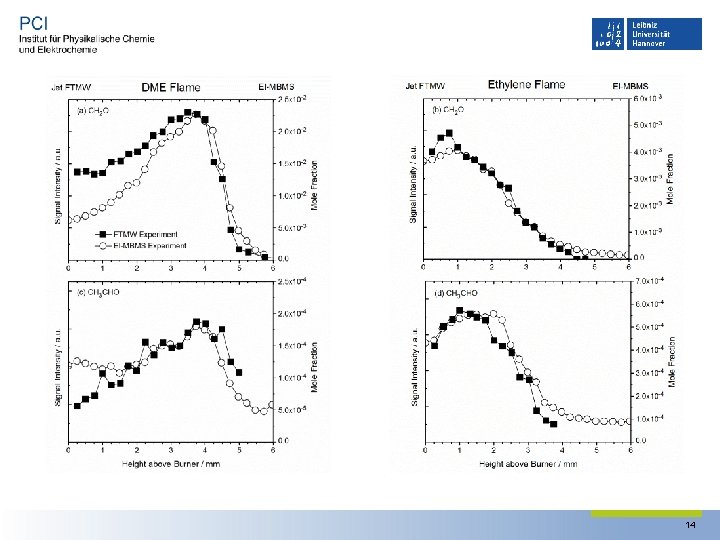
14
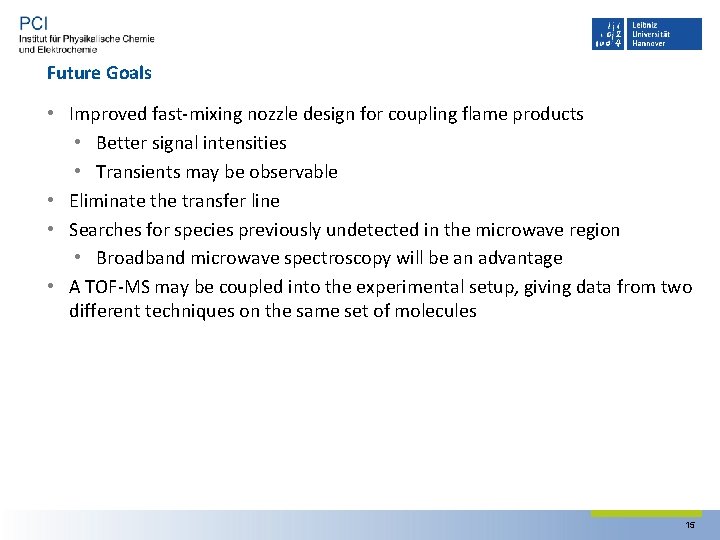
Future Goals • Improved fast‐mixing nozzle design for coupling flame products • Better signal intensities • Transients may be observable • Eliminate the transfer line • Searches for species previously undetected in the microwave region • Broadband microwave spectroscopy will be an advantage • A TOF‐MS may be coupled into the experimental setup, giving data from two different techniques on the same set of molecules 15
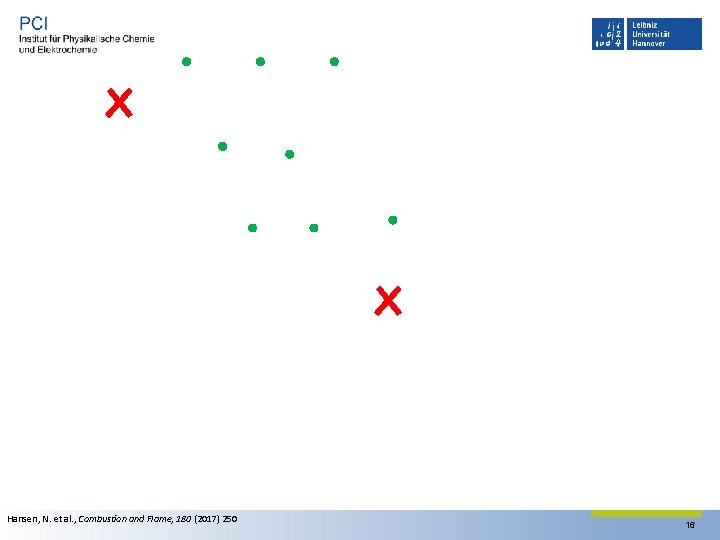
Hansen, N. et al. , Combustion and Flame, 180 (2017) 250 16
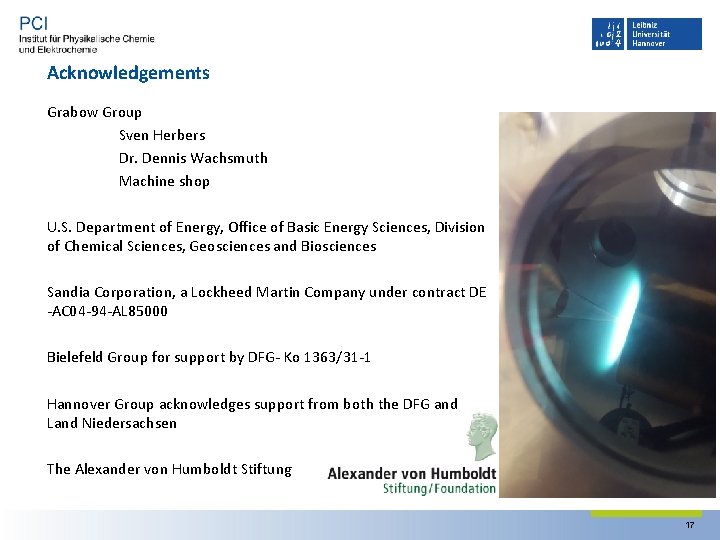
Acknowledgements Grabow Group Sven Herbers Dr. Dennis Wachsmuth Machine shop U. S. Department of Energy, Office of Basic Energy Sciences, Division of Chemical Sciences, Geosciences and Biosciences Sandia Corporation, a Lockheed Martin Company under contract DE ‐AC 04‐ 94‐AL 85000 Bielefeld Group for support by DFG‐ Ko 1363/31‐ 1 Hannover Group acknowledges support from both the DFG and Land Niedersachsen The Alexander von Humboldt Stiftung 17
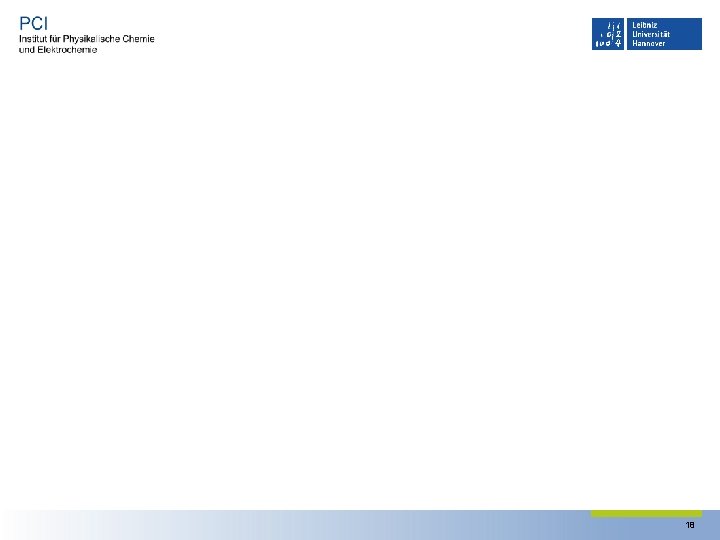
18
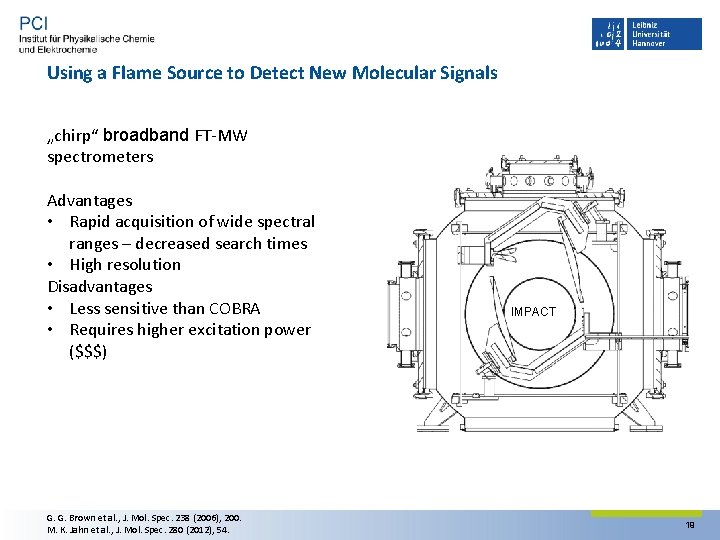
Using a Flame Source to Detect New Molecular Signals „chirp“ broadband FT‐MW spectrometers Advantages • Rapid acquisition of wide spectral ranges – decreased search times • High resolution Disadvantages • Less sensitive than COBRA • Requires higher excitation power ($$$) G. G. Brown et al. , J. Mol. Spec. 238 (2006), 200. M. K. Jahn et al. , J. Mol. Spec. 280 (2012), 54. IMPACT 19
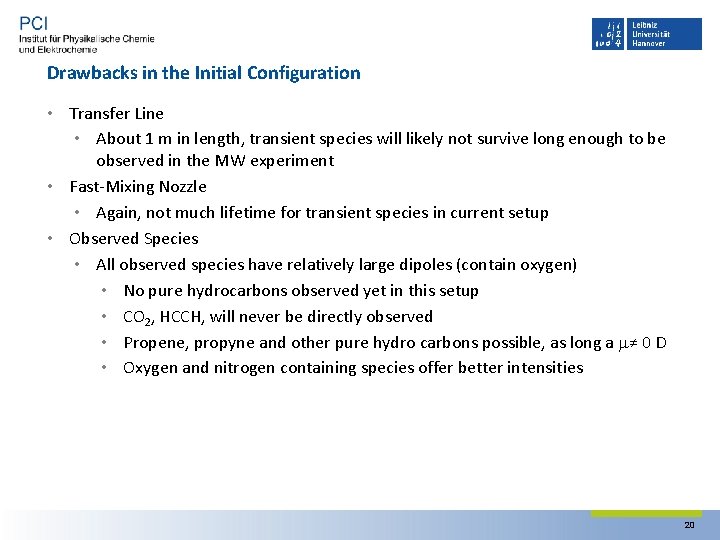
Drawbacks in the Initial Configuration • Transfer Line • About 1 m in length, transient species will likely not survive long enough to be observed in the MW experiment • Fast‐Mixing Nozzle • Again, not much lifetime for transient species in current setup • Observed Species • All observed species have relatively large dipoles (contain oxygen) • No pure hydrocarbons observed yet in this setup • CO 2, HCCH, will never be directly observed • Propene, propyne and other pure hydro carbons possible, as long a ≠ 0 D • Oxygen and nitrogen containing species offer better intensities 20
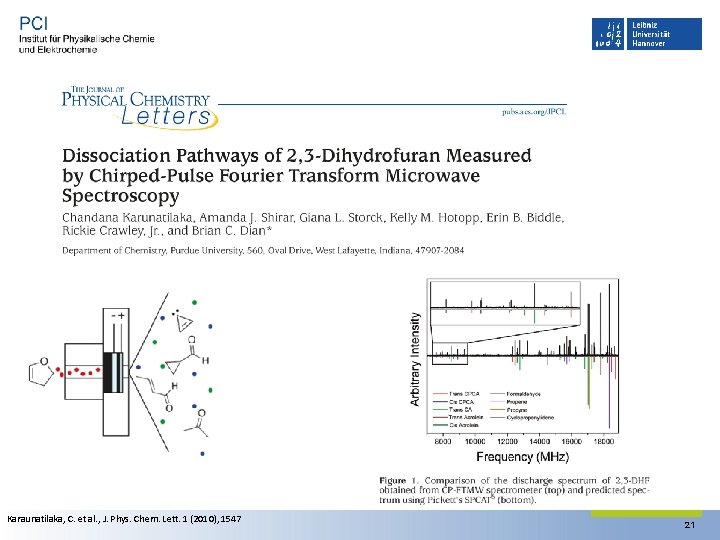
Karaunatilaka, C. et al. , J. Phys. Chem. Lett. 1 (2010), 1547 21
Intermolecular forces in dimethyl ether
Chirped pulse fourier transform microwave spectroscopy
Free ion terms
What is the mulliken symbols for 'f' spectroscopic term in
Spectroscopic notation
Spectroscopic data in chemistry
Dimethyl tetraphenylphthalate boiling point
3 6-diethyl-2 4-dimethyl-4-propylokta-1 7-dien
Isobutylnonane
3 6-diethyl-2 4-dimethyl-4-propylokta-1 7-dien
Quantum fourier transform applications
Unit step function fourier transform
Fourier transform solver
Nate conger
Rect(t-1/2)
Duality of fourier transform
State a laplace transform function and explain the meaning
Fourier transform
Fourier
Impulse train fourier transform
Fourier transform seismic
Math review