EXTRA CELLULAR MATRIX PLANT LIGNIN PECTIN Lignin is
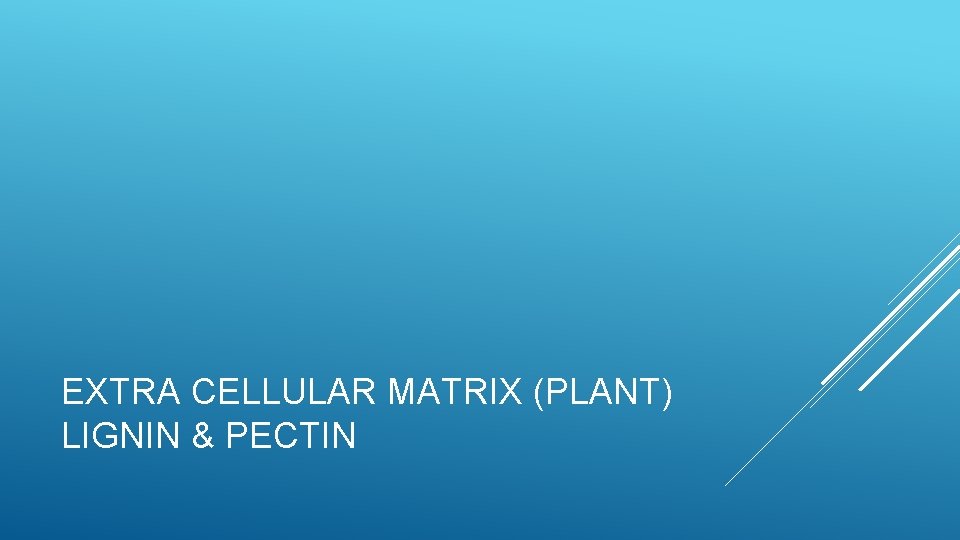
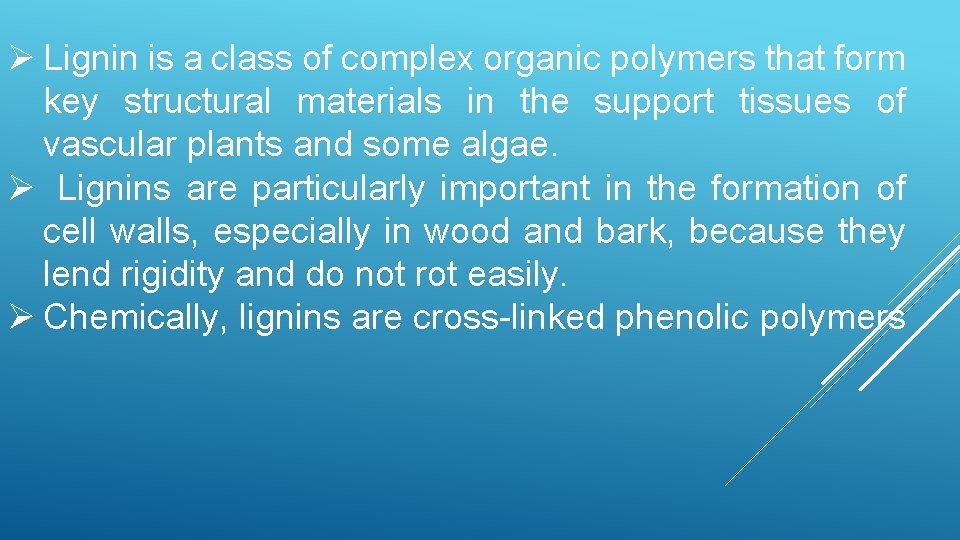
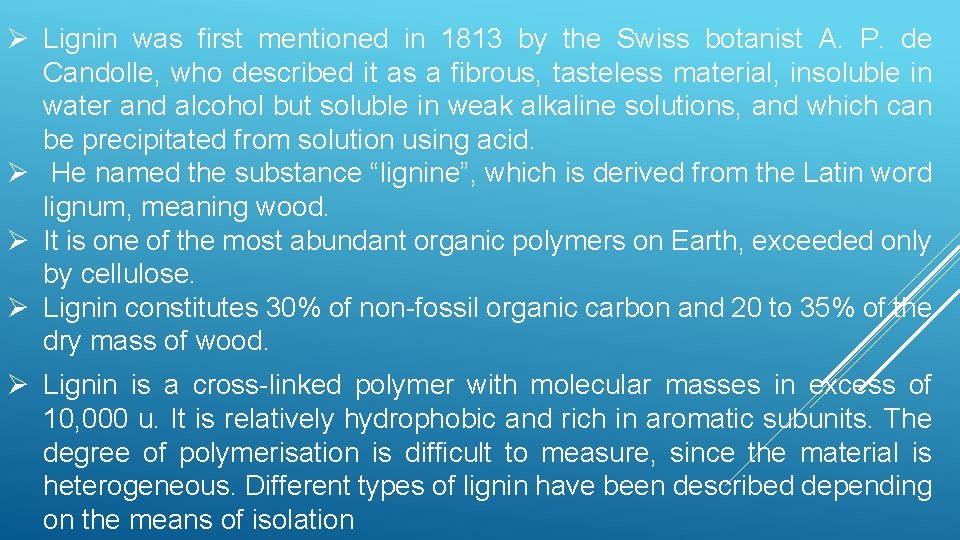
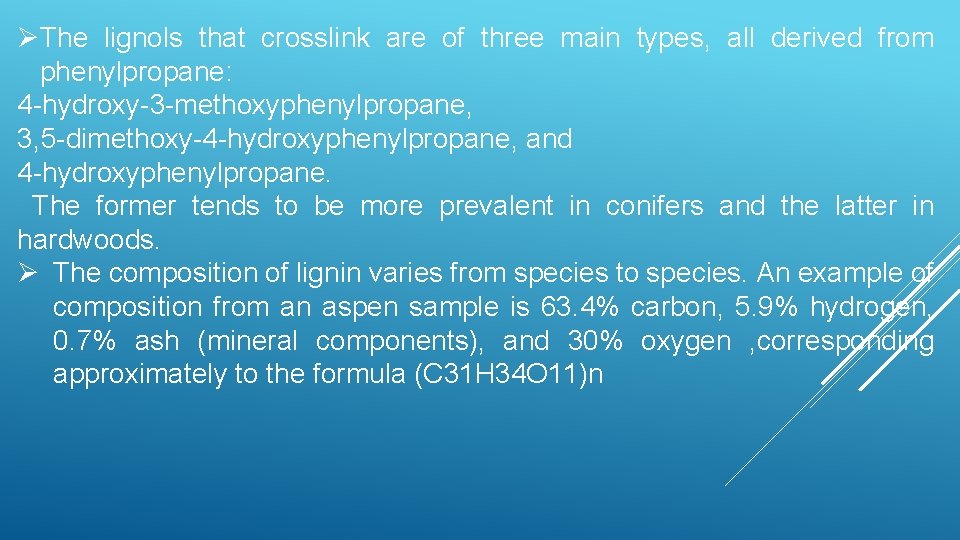
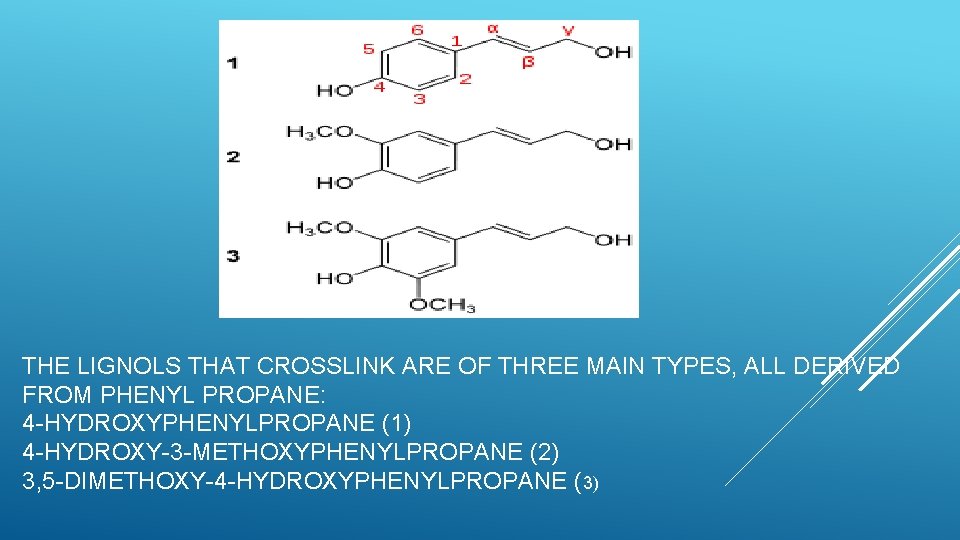
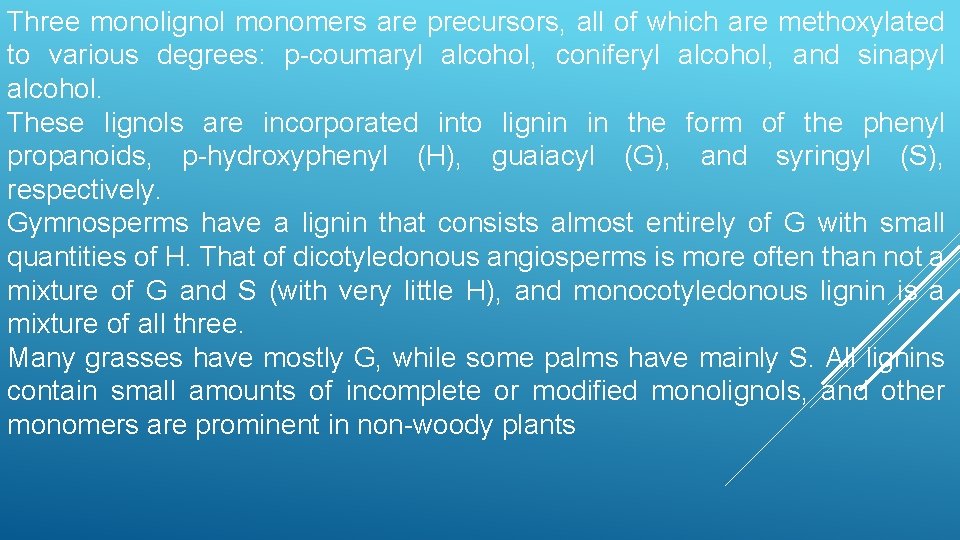
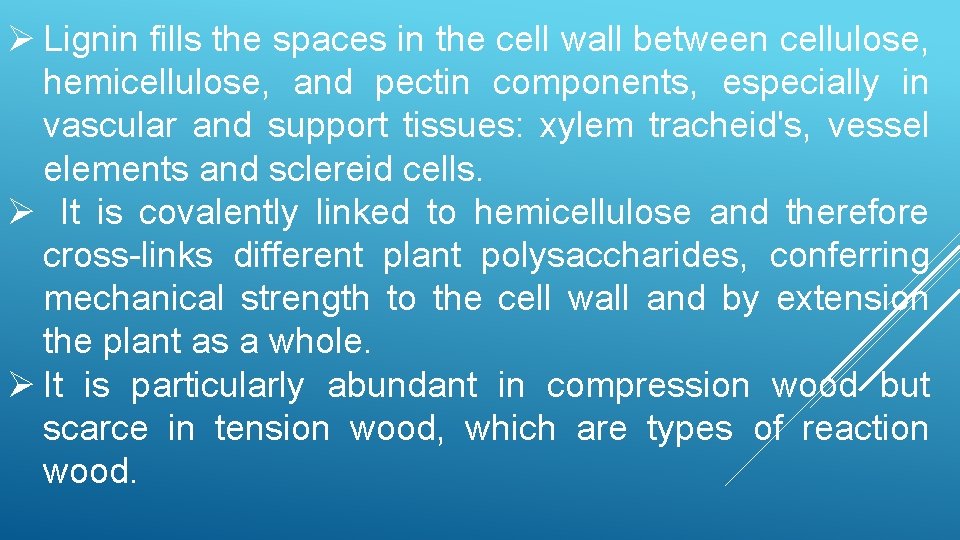
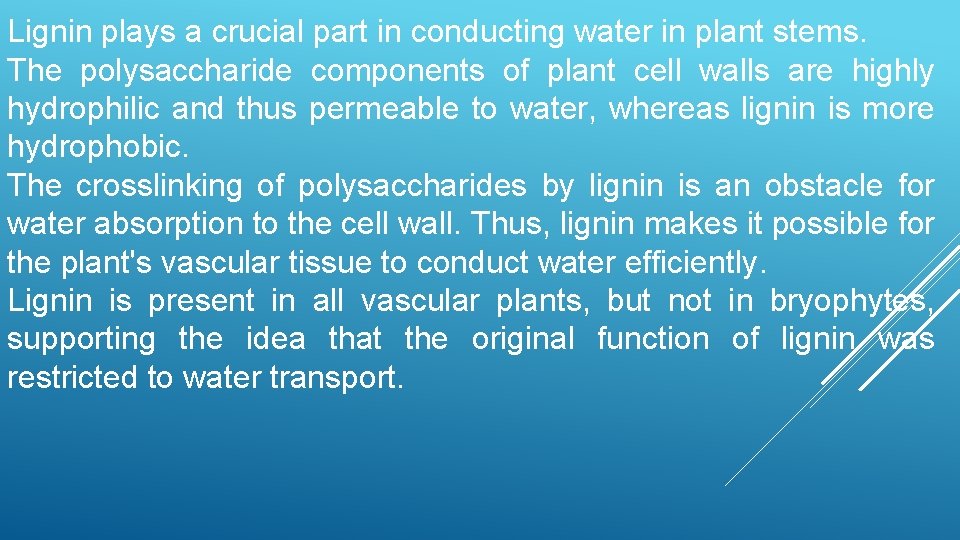
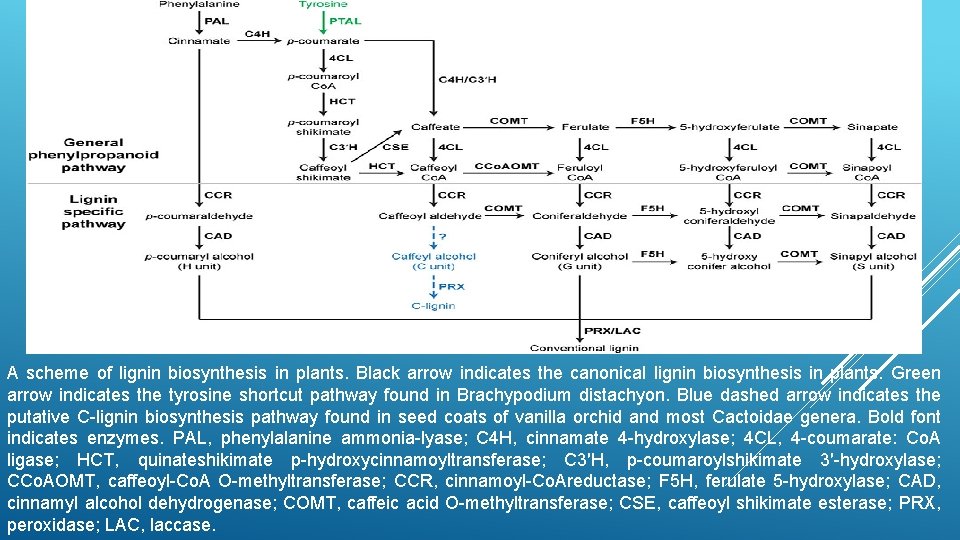
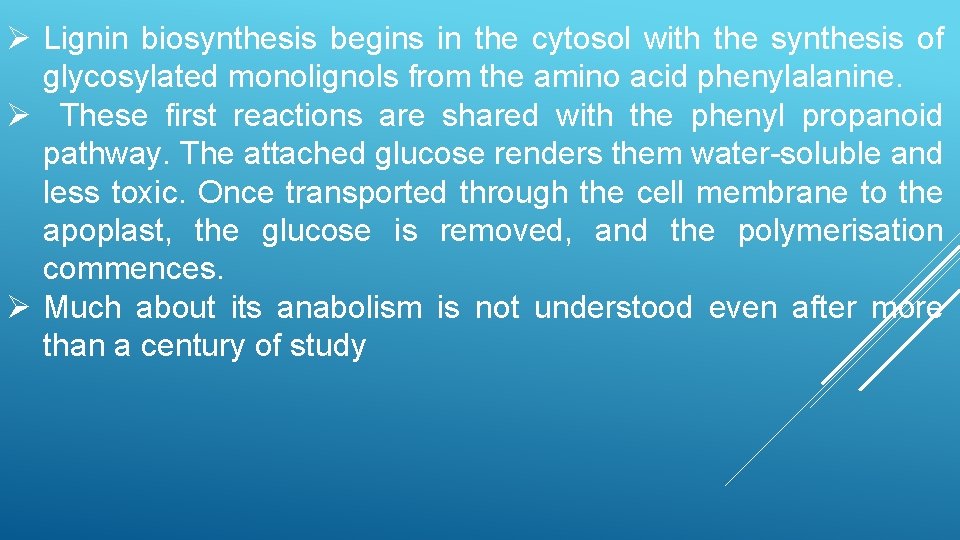
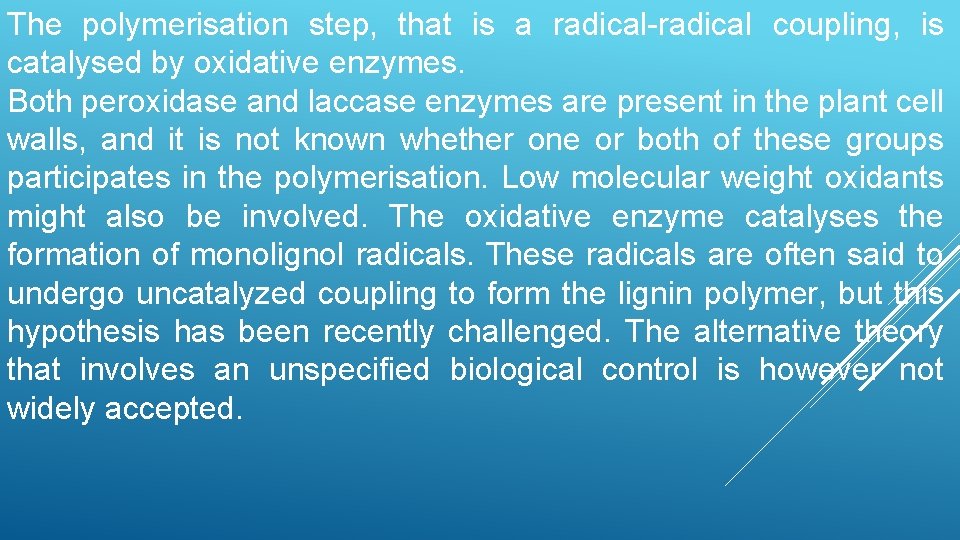
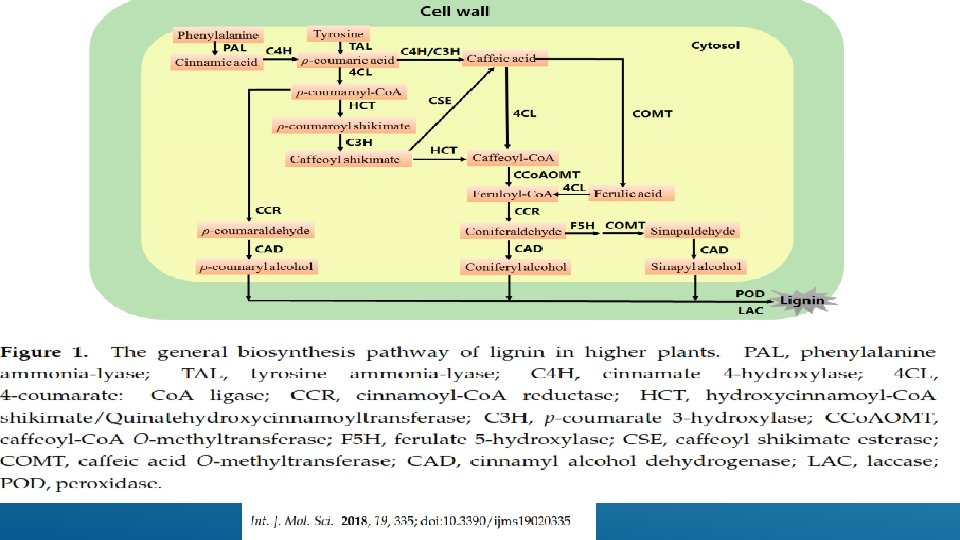
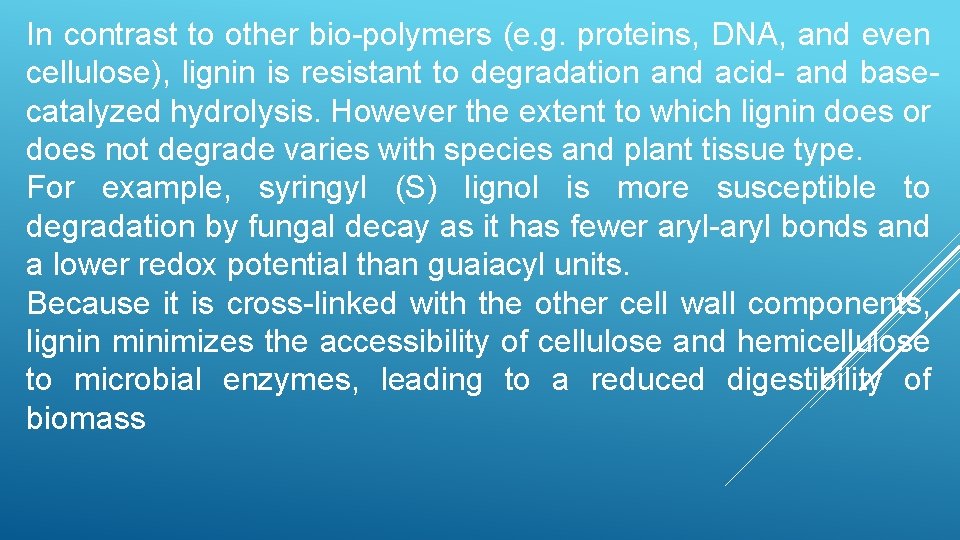
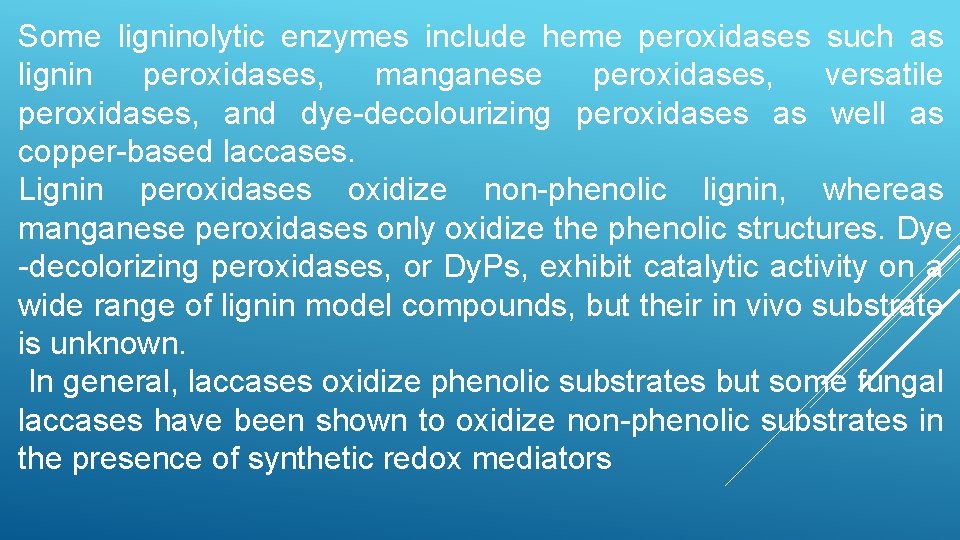
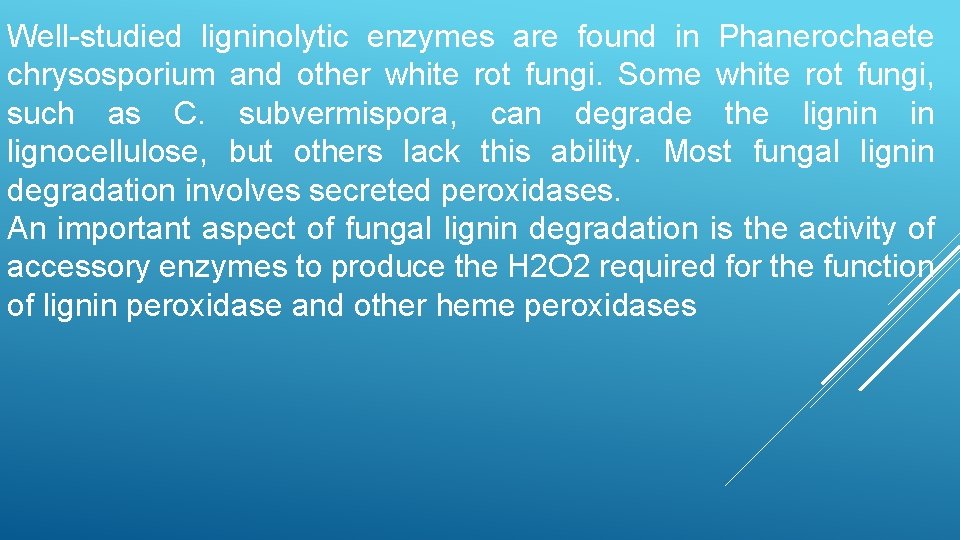
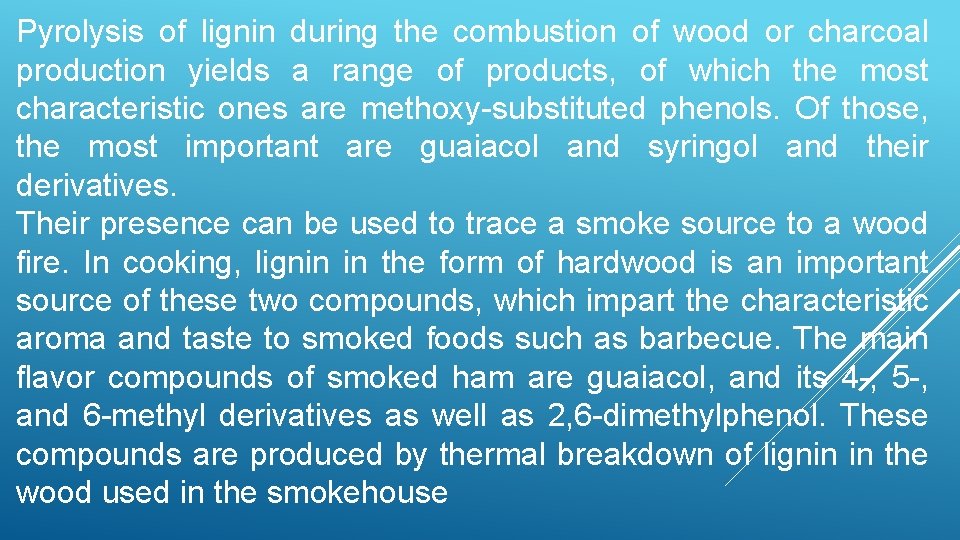
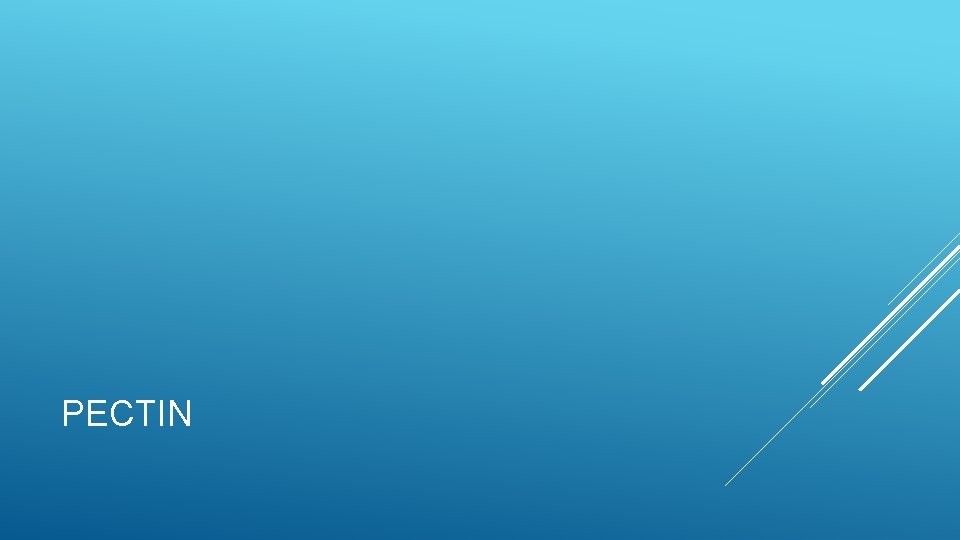
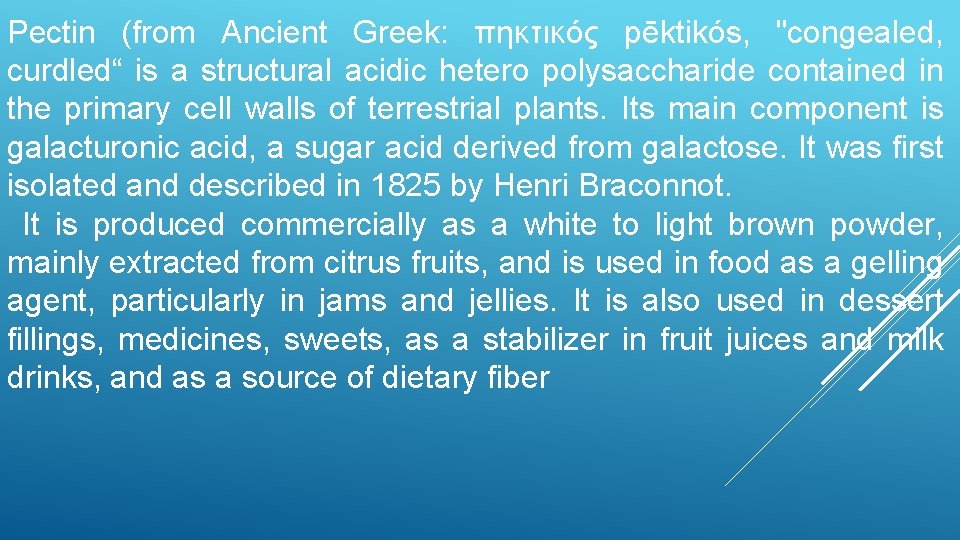
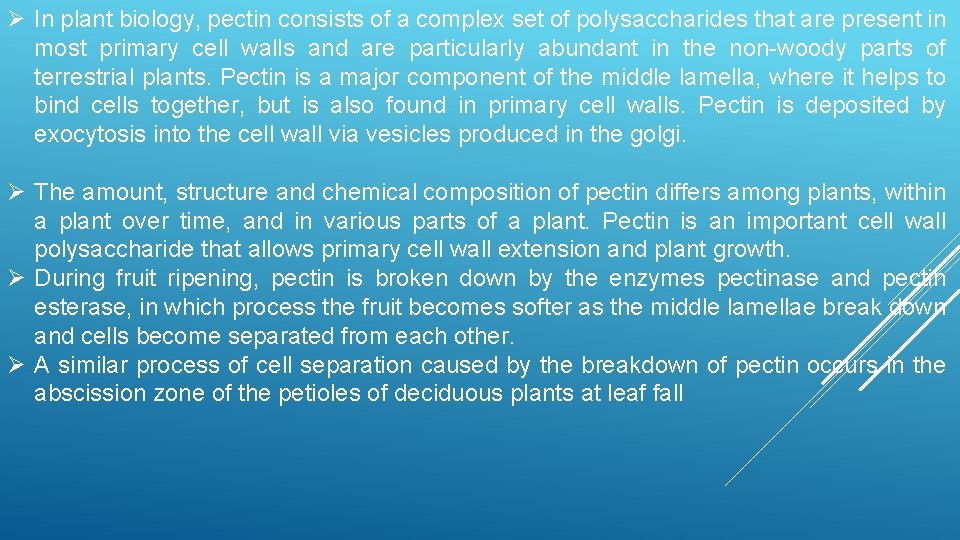
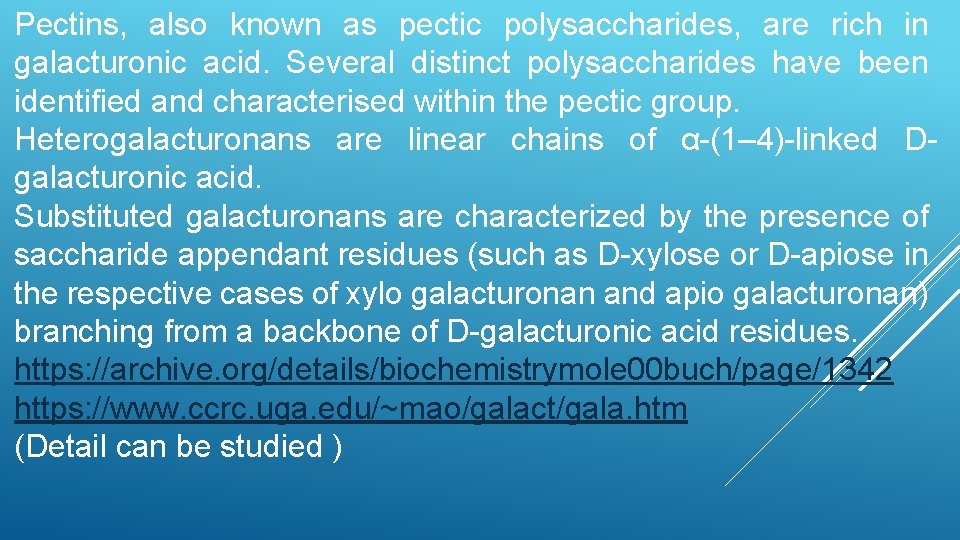
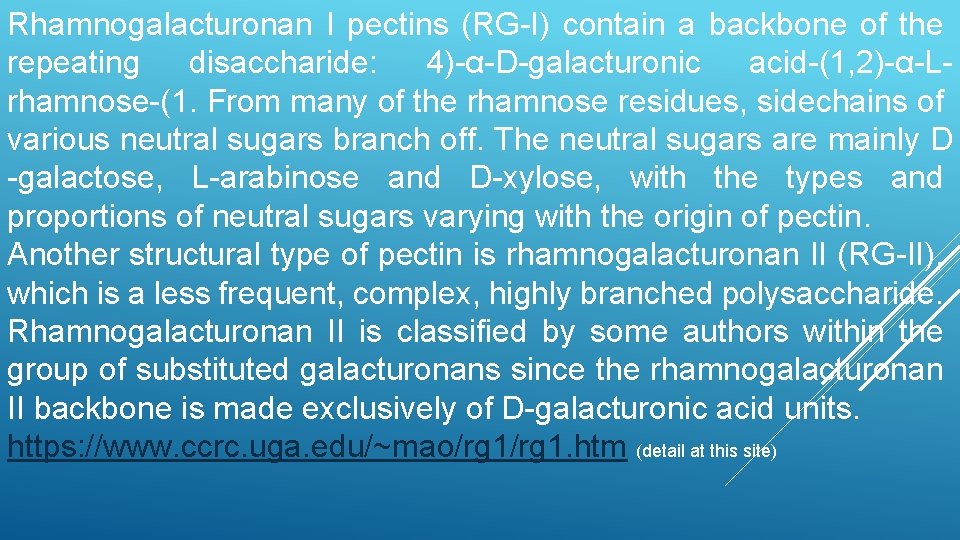
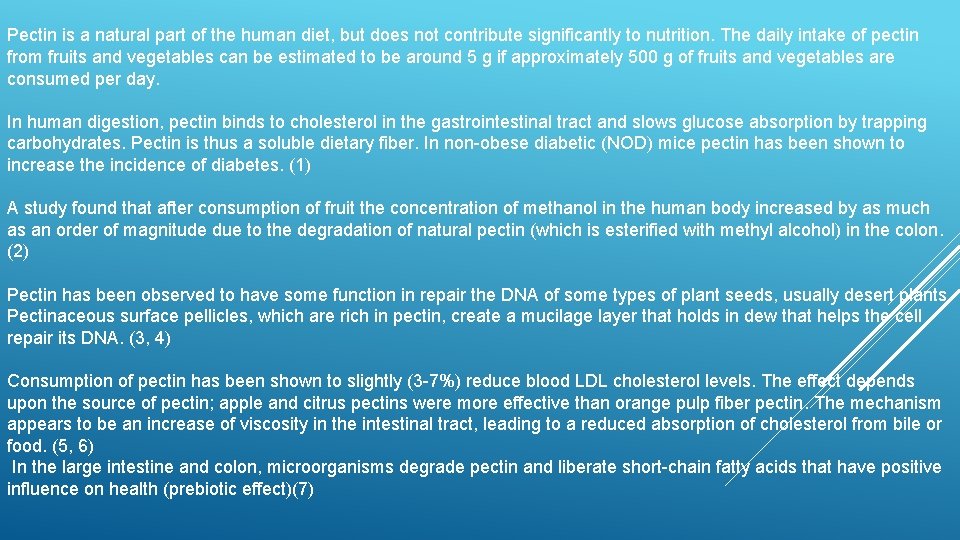
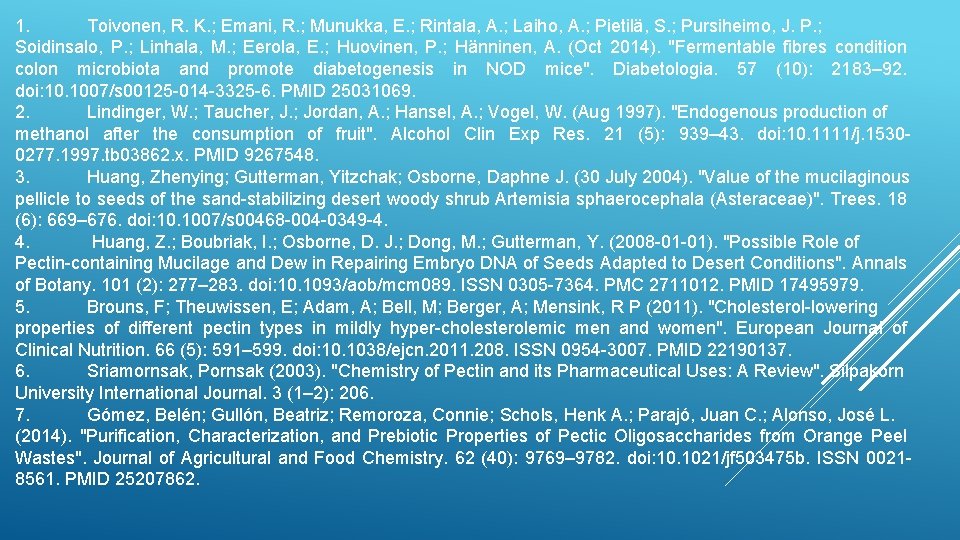
- Slides: 23
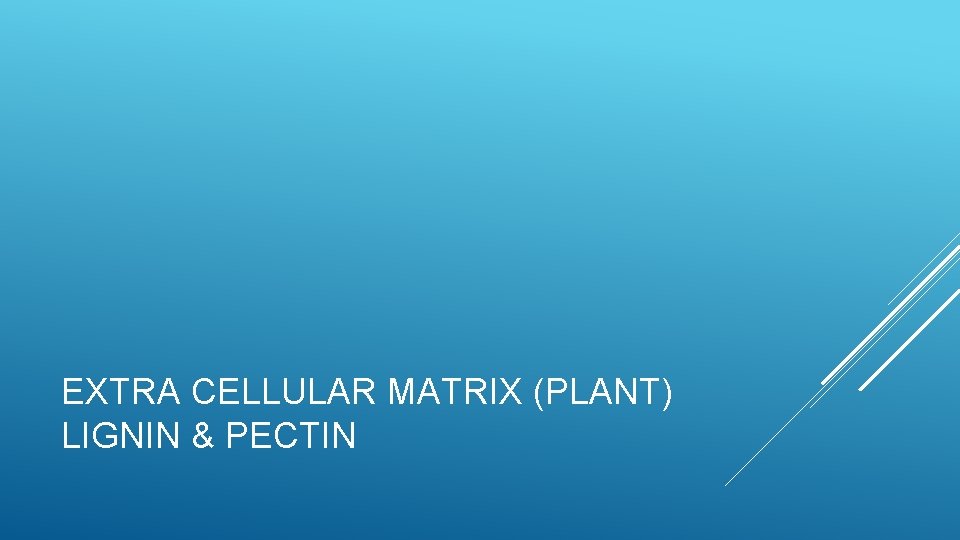
EXTRA CELLULAR MATRIX (PLANT) LIGNIN & PECTIN
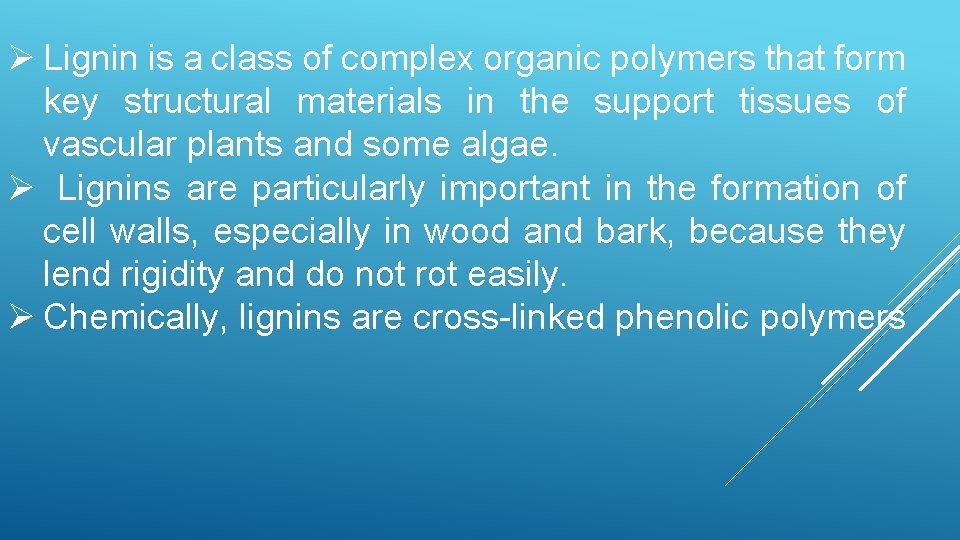
Ø Lignin is a class of complex organic polymers that form key structural materials in the support tissues of vascular plants and some algae. Ø Lignins are particularly important in the formation of cell walls, especially in wood and bark, because they lend rigidity and do not rot easily. Ø Chemically, lignins are cross-linked phenolic polymers
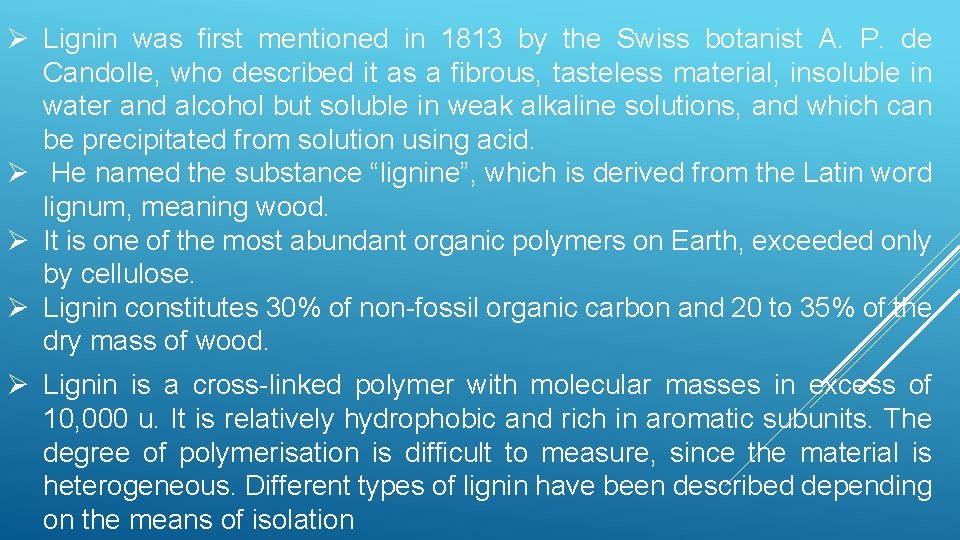
Ø Lignin was first mentioned in 1813 by the Swiss botanist A. P. de Candolle, who described it as a fibrous, tasteless material, insoluble in water and alcohol but soluble in weak alkaline solutions, and which can be precipitated from solution using acid. Ø He named the substance “lignine”, which is derived from the Latin word lignum, meaning wood. Ø It is one of the most abundant organic polymers on Earth, exceeded only by cellulose. Ø Lignin constitutes 30% of non-fossil organic carbon and 20 to 35% of the dry mass of wood. Ø Lignin is a cross-linked polymer with molecular masses in excess of 10, 000 u. It is relatively hydrophobic and rich in aromatic subunits. The degree of polymerisation is difficult to measure, since the material is heterogeneous. Different types of lignin have been described depending on the means of isolation
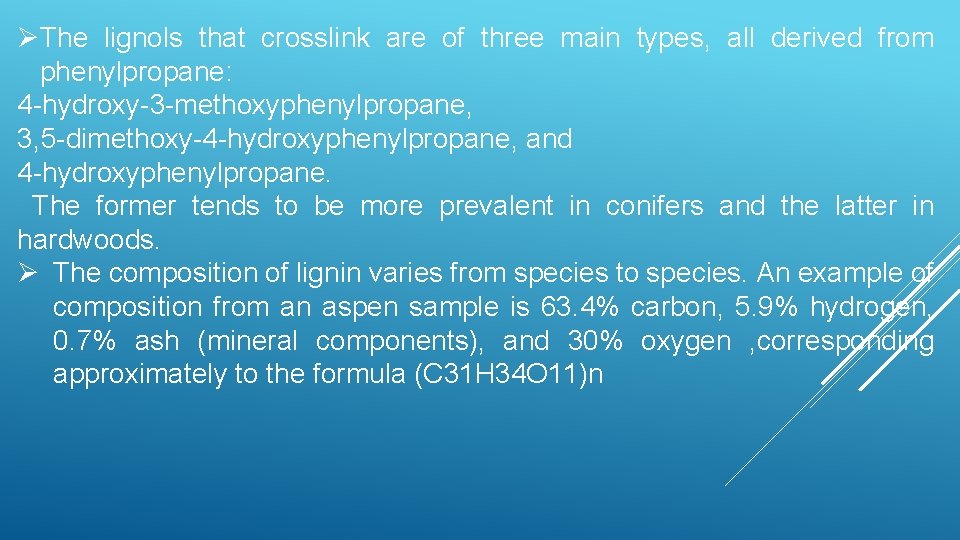
ØThe lignols that crosslink are of three main types, all derived from phenylpropane: 4 -hydroxy-3 -methoxyphenylpropane, 3, 5 -dimethoxy-4 -hydroxyphenylpropane, and 4 -hydroxyphenylpropane. The former tends to be more prevalent in conifers and the latter in hardwoods. Ø The composition of lignin varies from species to species. An example of composition from an aspen sample is 63. 4% carbon, 5. 9% hydrogen, 0. 7% ash (mineral components), and 30% oxygen , corresponding approximately to the formula (C 31 H 34 O 11)n
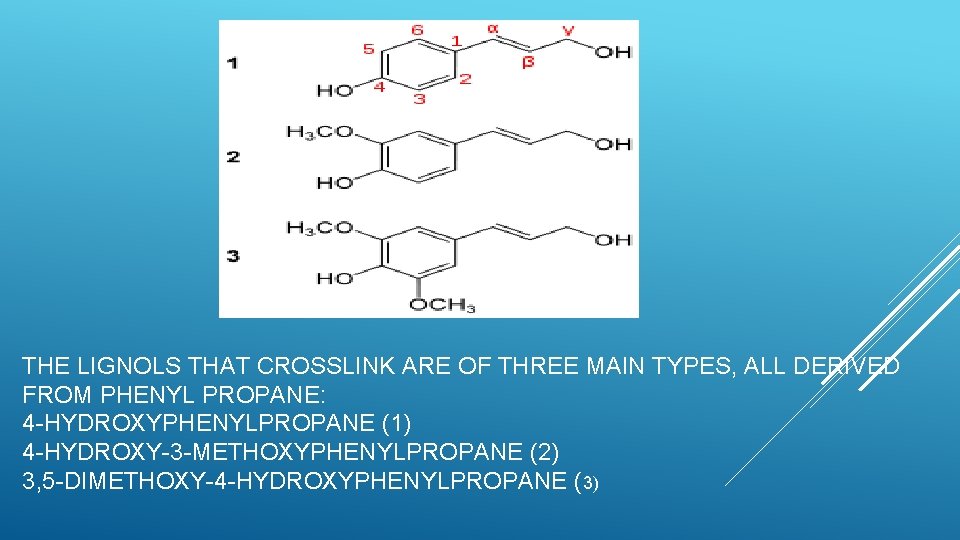
THE LIGNOLS THAT CROSSLINK ARE OF THREE MAIN TYPES, ALL DERIVED FROM PHENYL PROPANE: 4 -HYDROXYPHENYLPROPANE (1) 4 -HYDROXY-3 -METHOXYPHENYLPROPANE (2) 3, 5 -DIMETHOXY-4 -HYDROXYPHENYLPROPANE (3)
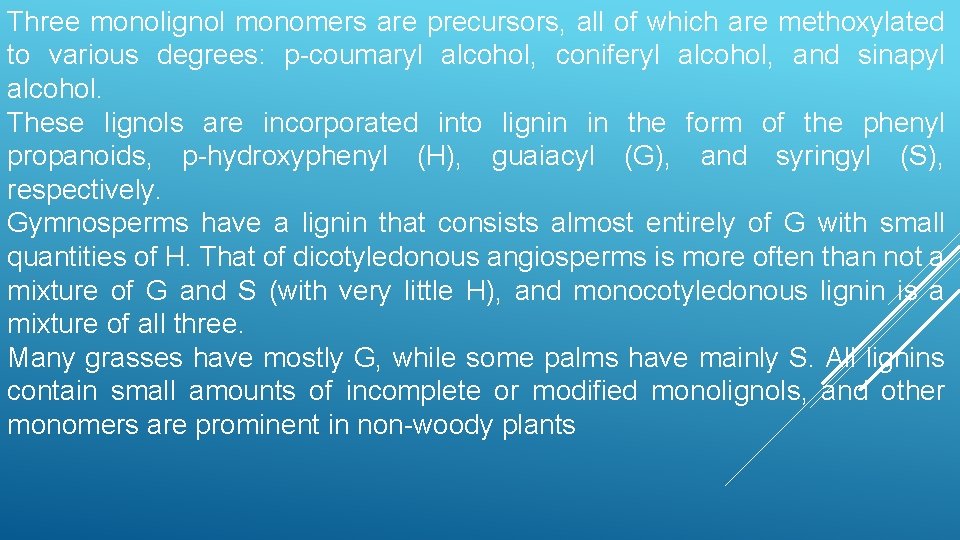
Three monolignol monomers are precursors, all of which are methoxylated to various degrees: p-coumaryl alcohol, coniferyl alcohol, and sinapyl alcohol. These lignols are incorporated into lignin in the form of the phenyl propanoids, p-hydroxyphenyl (H), guaiacyl (G), and syringyl (S), respectively. Gymnosperms have a lignin that consists almost entirely of G with small quantities of H. That of dicotyledonous angiosperms is more often than not a mixture of G and S (with very little H), and monocotyledonous lignin is a mixture of all three. Many grasses have mostly G, while some palms have mainly S. All lignins contain small amounts of incomplete or modified monolignols, and other monomers are prominent in non-woody plants
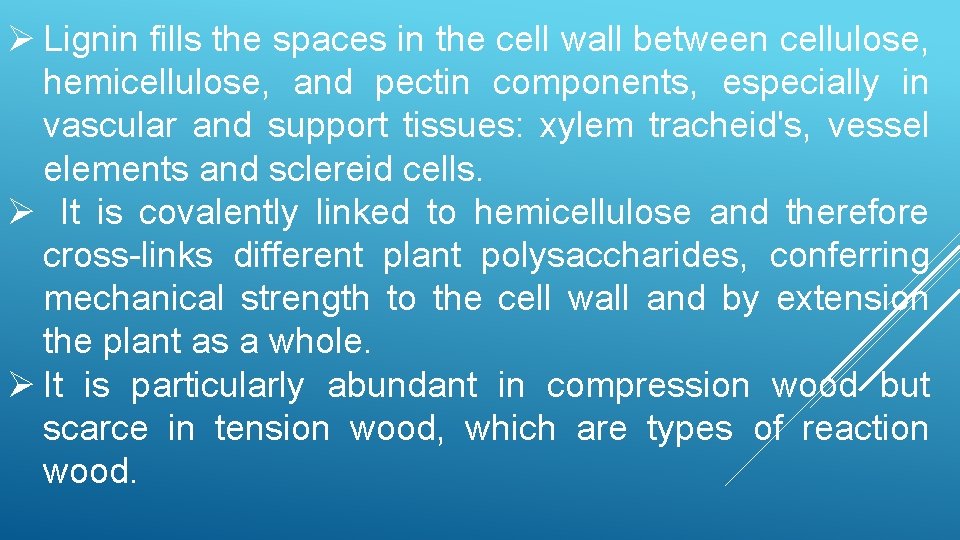
Ø Lignin fills the spaces in the cell wall between cellulose, hemicellulose, and pectin components, especially in vascular and support tissues: xylem tracheid's, vessel elements and sclereid cells. Ø It is covalently linked to hemicellulose and therefore cross-links different plant polysaccharides, conferring mechanical strength to the cell wall and by extension the plant as a whole. Ø It is particularly abundant in compression wood but scarce in tension wood, which are types of reaction wood.
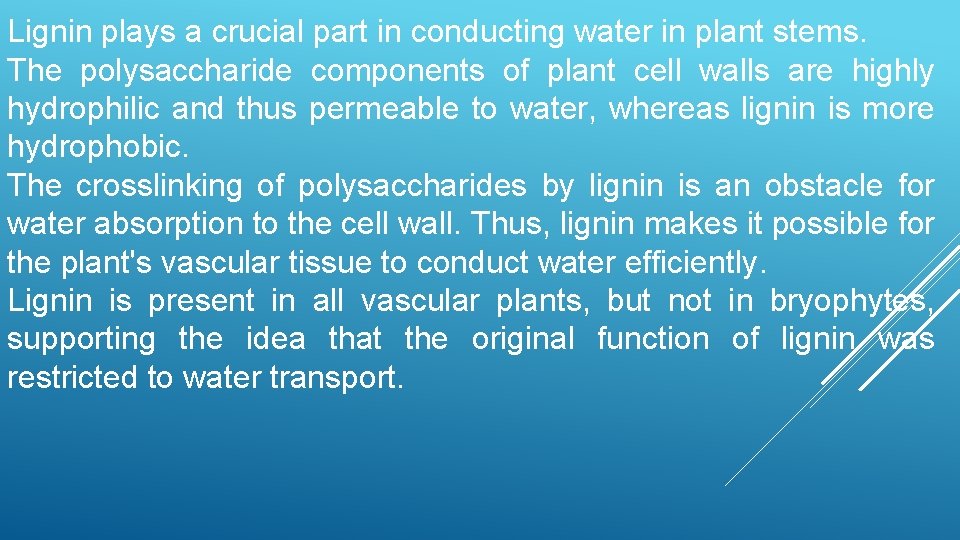
Lignin plays a crucial part in conducting water in plant stems. The polysaccharide components of plant cell walls are highly hydrophilic and thus permeable to water, whereas lignin is more hydrophobic. The crosslinking of polysaccharides by lignin is an obstacle for water absorption to the cell wall. Thus, lignin makes it possible for the plant's vascular tissue to conduct water efficiently. Lignin is present in all vascular plants, but not in bryophytes, supporting the idea that the original function of lignin was restricted to water transport.
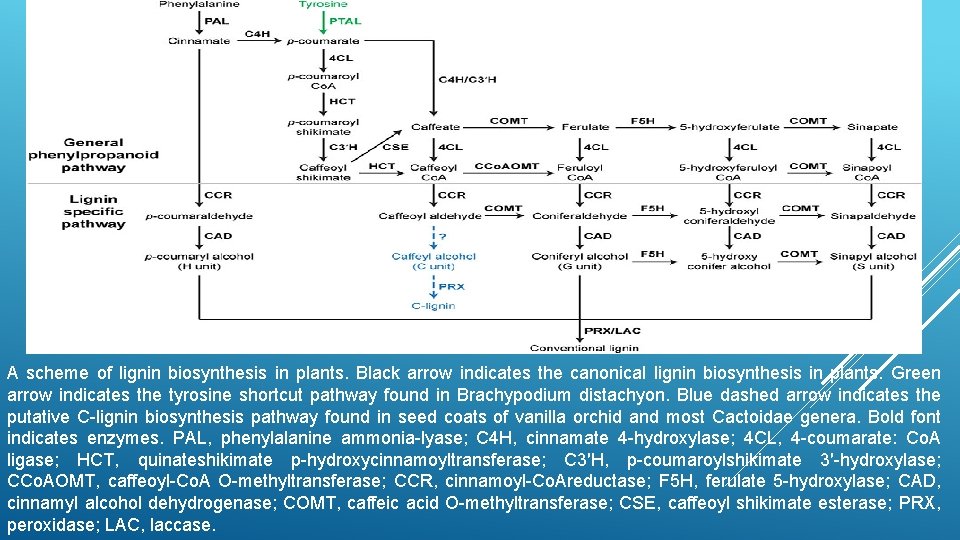
A scheme of lignin biosynthesis in plants. Black arrow indicates the canonical lignin biosynthesis in plants. Green arrow indicates the tyrosine shortcut pathway found in Brachypodium distachyon. Blue dashed arrow indicates the putative C-lignin biosynthesis pathway found in seed coats of vanilla orchid and most Cactoidae genera. Bold font indicates enzymes. PAL, phenylalanine ammonia-lyase; C 4 H, cinnamate 4 -hydroxylase; 4 CL, 4 -coumarate: Co. A ligase; HCT, quinateshikimate p-hydroxycinnamoyltransferase; C 3′H, p-coumaroylshikimate 3′-hydroxylase; CCo. AOMT, caffeoyl-Co. A O-methyltransferase; CCR, cinnamoyl-Co. Areductase; F 5 H, ferulate 5 -hydroxylase; CAD, cinnamyl alcohol dehydrogenase; COMT, caffeic acid O-methyltransferase; CSE, caffeoyl shikimate esterase; PRX, peroxidase; LAC, laccase.
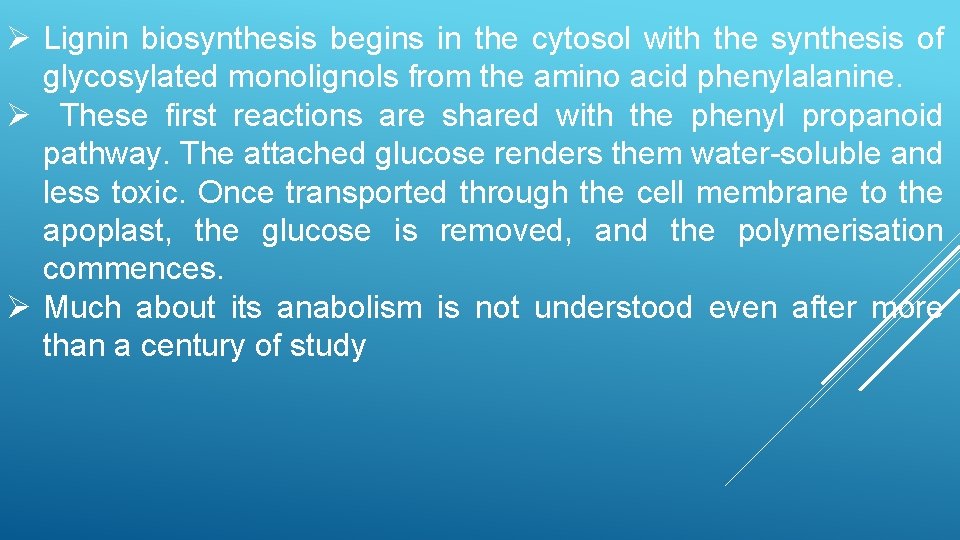
Ø Lignin biosynthesis begins in the cytosol with the synthesis of glycosylated monolignols from the amino acid phenylalanine. Ø These first reactions are shared with the phenyl propanoid pathway. The attached glucose renders them water-soluble and less toxic. Once transported through the cell membrane to the apoplast, the glucose is removed, and the polymerisation commences. Ø Much about its anabolism is not understood even after more than a century of study
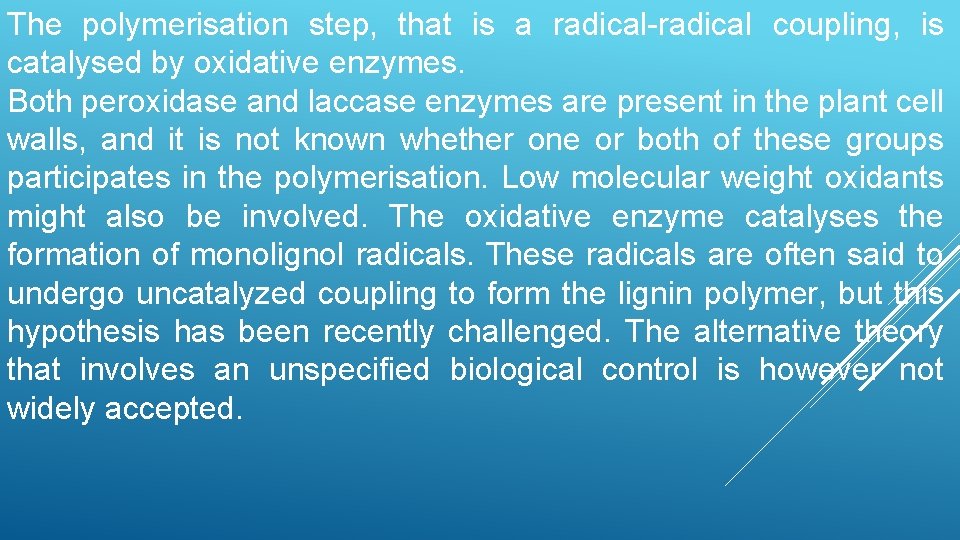
The polymerisation step, that is a radical-radical coupling, is catalysed by oxidative enzymes. Both peroxidase and laccase enzymes are present in the plant cell walls, and it is not known whether one or both of these groups participates in the polymerisation. Low molecular weight oxidants might also be involved. The oxidative enzyme catalyses the formation of monolignol radicals. These radicals are often said to undergo uncatalyzed coupling to form the lignin polymer, but this hypothesis has been recently challenged. The alternative theory that involves an unspecified biological control is however not widely accepted.
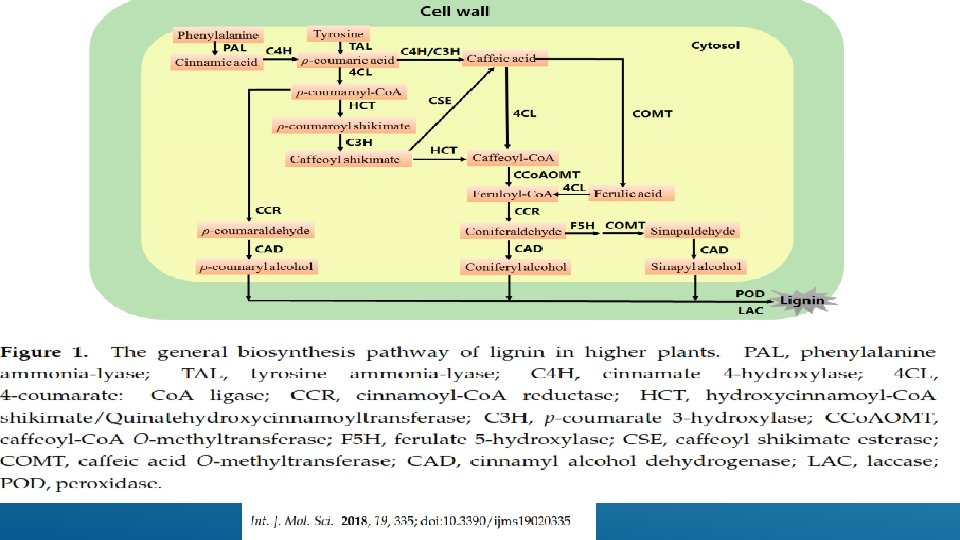
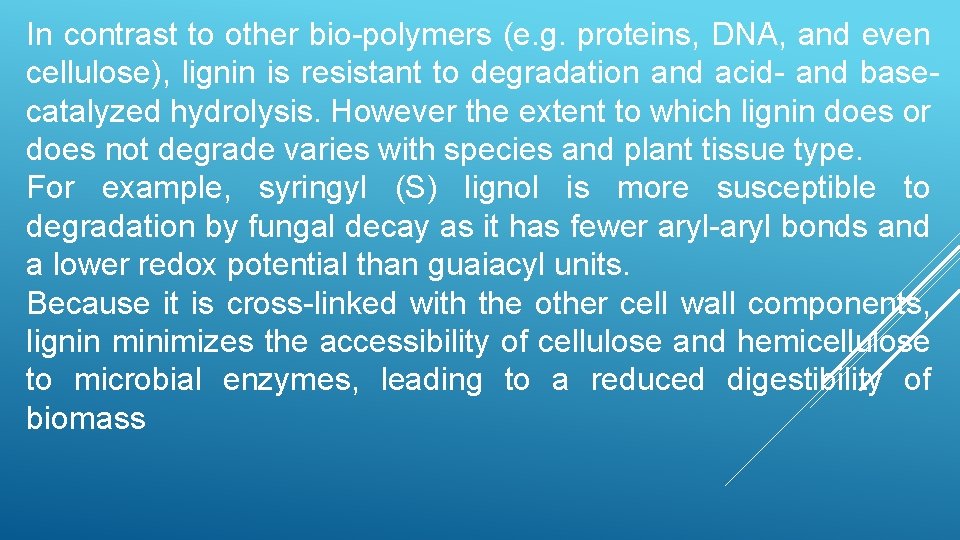
In contrast to other bio-polymers (e. g. proteins, DNA, and even cellulose), lignin is resistant to degradation and acid- and basecatalyzed hydrolysis. However the extent to which lignin does or does not degrade varies with species and plant tissue type. For example, syringyl (S) lignol is more susceptible to degradation by fungal decay as it has fewer aryl-aryl bonds and a lower redox potential than guaiacyl units. Because it is cross-linked with the other cell wall components, lignin minimizes the accessibility of cellulose and hemicellulose to microbial enzymes, leading to a reduced digestibility of biomass
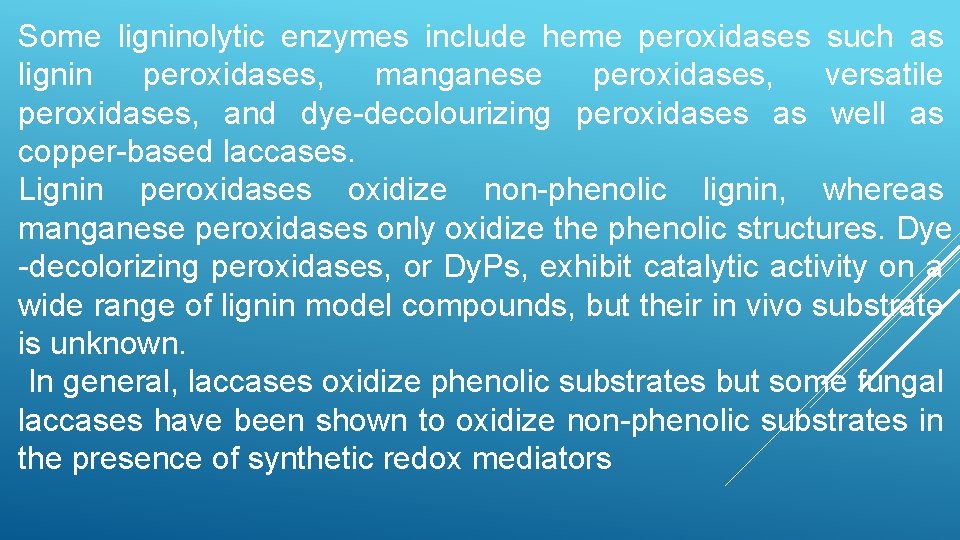
Some ligninolytic enzymes include heme peroxidases such as lignin peroxidases, manganese peroxidases, versatile peroxidases, and dye-decolourizing peroxidases as well as copper-based laccases. Lignin peroxidases oxidize non-phenolic lignin, whereas manganese peroxidases only oxidize the phenolic structures. Dye -decolorizing peroxidases, or Dy. Ps, exhibit catalytic activity on a wide range of lignin model compounds, but their in vivo substrate is unknown. In general, laccases oxidize phenolic substrates but some fungal laccases have been shown to oxidize non-phenolic substrates in the presence of synthetic redox mediators
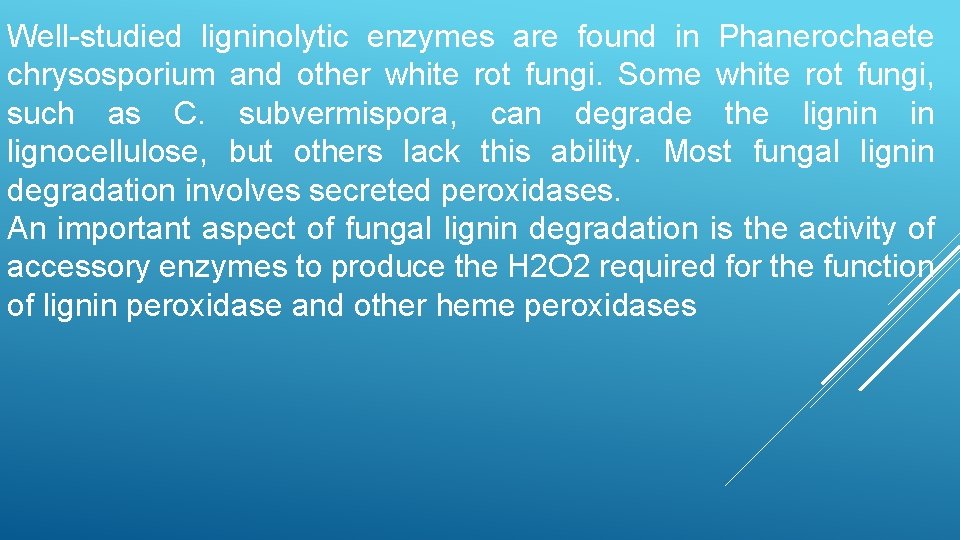
Well-studied ligninolytic enzymes are found in Phanerochaete chrysosporium and other white rot fungi. Some white rot fungi, such as C. subvermispora, can degrade the lignin in lignocellulose, but others lack this ability. Most fungal lignin degradation involves secreted peroxidases. An important aspect of fungal lignin degradation is the activity of accessory enzymes to produce the H 2 O 2 required for the function of lignin peroxidase and other heme peroxidases
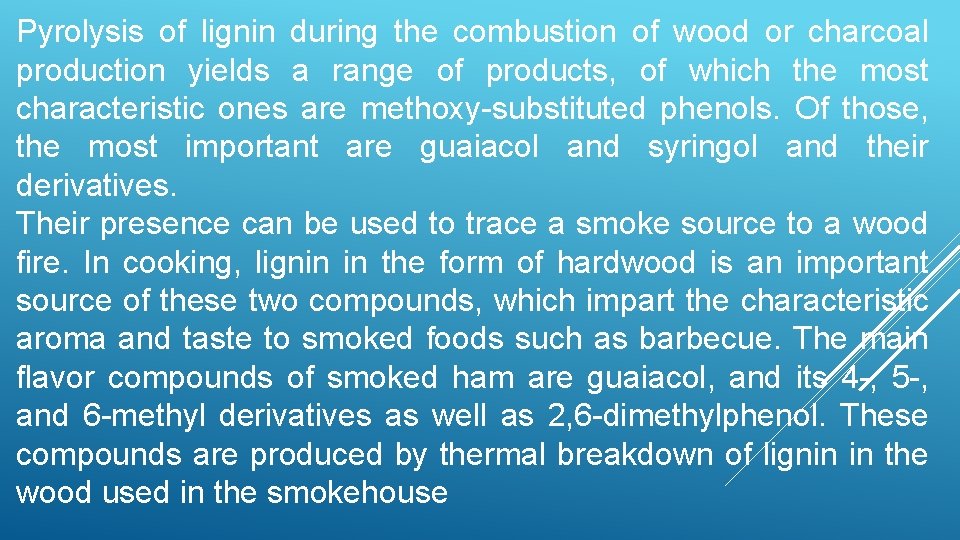
Pyrolysis of lignin during the combustion of wood or charcoal production yields a range of products, of which the most characteristic ones are methoxy-substituted phenols. Of those, the most important are guaiacol and syringol and their derivatives. Their presence can be used to trace a smoke source to a wood fire. In cooking, lignin in the form of hardwood is an important source of these two compounds, which impart the characteristic aroma and taste to smoked foods such as barbecue. The main flavor compounds of smoked ham are guaiacol, and its 4 -, 5 -, and 6 -methyl derivatives as well as 2, 6 -dimethylphenol. These compounds are produced by thermal breakdown of lignin in the wood used in the smokehouse
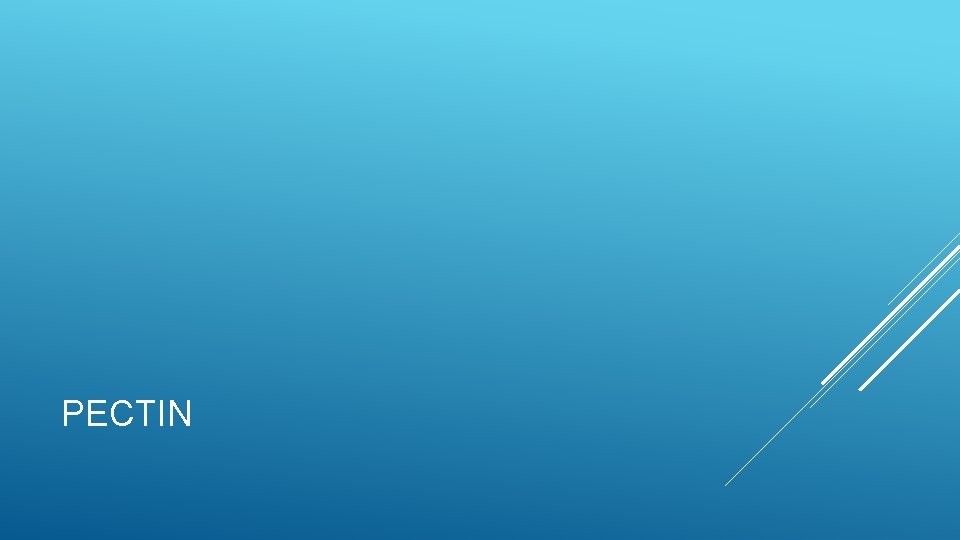
PECTIN
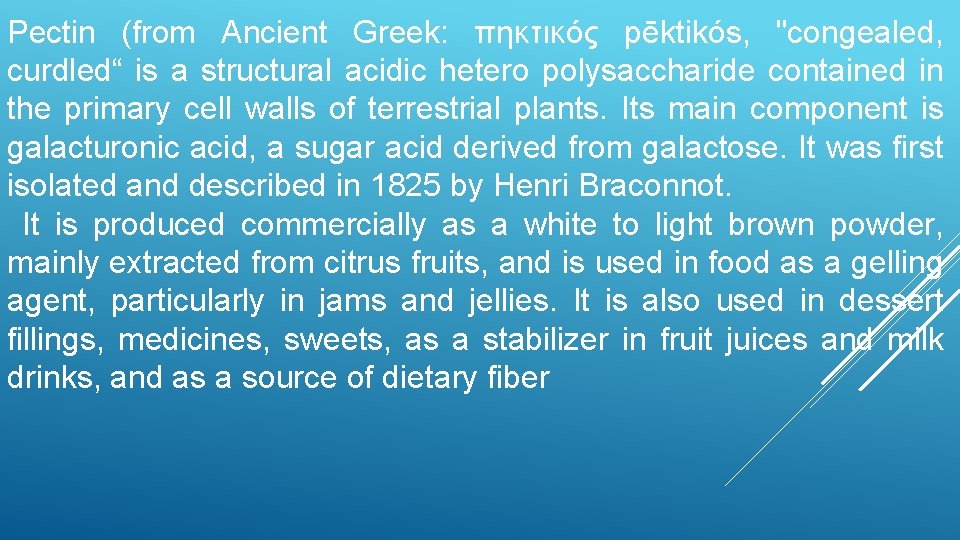
Pectin (from Ancient Greek: πηκτικός pēktikós, "congealed, curdled“ is a structural acidic hetero polysaccharide contained in the primary cell walls of terrestrial plants. Its main component is galacturonic acid, a sugar acid derived from galactose. It was first isolated and described in 1825 by Henri Braconnot. It is produced commercially as a white to light brown powder, mainly extracted from citrus fruits, and is used in food as a gelling agent, particularly in jams and jellies. It is also used in dessert fillings, medicines, sweets, as a stabilizer in fruit juices and milk drinks, and as a source of dietary fiber
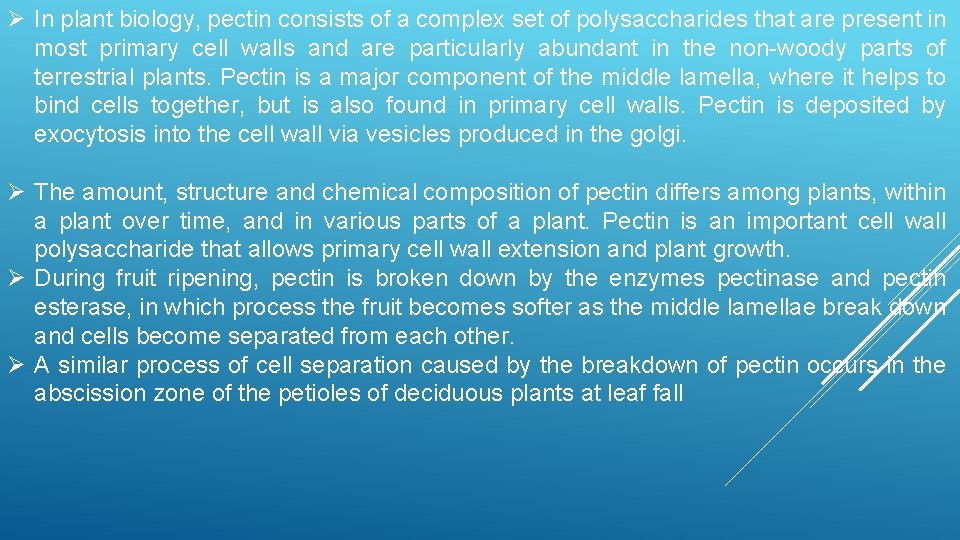
Ø In plant biology, pectin consists of a complex set of polysaccharides that are present in most primary cell walls and are particularly abundant in the non-woody parts of terrestrial plants. Pectin is a major component of the middle lamella, where it helps to bind cells together, but is also found in primary cell walls. Pectin is deposited by exocytosis into the cell wall via vesicles produced in the golgi. Ø The amount, structure and chemical composition of pectin differs among plants, within a plant over time, and in various parts of a plant. Pectin is an important cell wall polysaccharide that allows primary cell wall extension and plant growth. Ø During fruit ripening, pectin is broken down by the enzymes pectinase and pectin esterase, in which process the fruit becomes softer as the middle lamellae break down and cells become separated from each other. Ø A similar process of cell separation caused by the breakdown of pectin occurs in the abscission zone of the petioles of deciduous plants at leaf fall
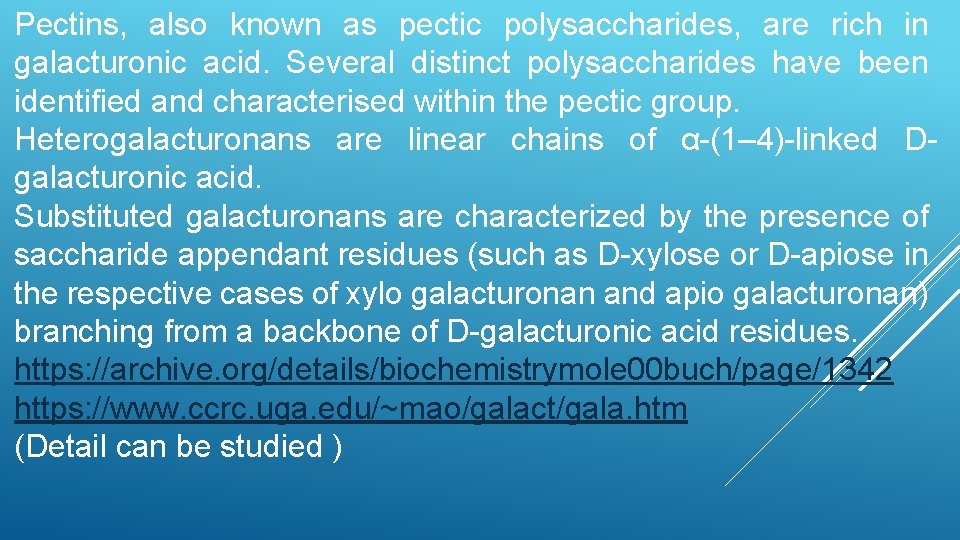
Pectins, also known as pectic polysaccharides, are rich in galacturonic acid. Several distinct polysaccharides have been identified and characterised within the pectic group. Heterogalacturonans are linear chains of α-(1– 4)-linked Dgalacturonic acid. Substituted galacturonans are characterized by the presence of saccharide appendant residues (such as D-xylose or D-apiose in the respective cases of xylo galacturonan and apio galacturonan) branching from a backbone of D-galacturonic acid residues. https: //archive. org/details/biochemistrymole 00 buch/page/1342 https: //www. ccrc. uga. edu/~mao/galact/gala. htm (Detail can be studied )
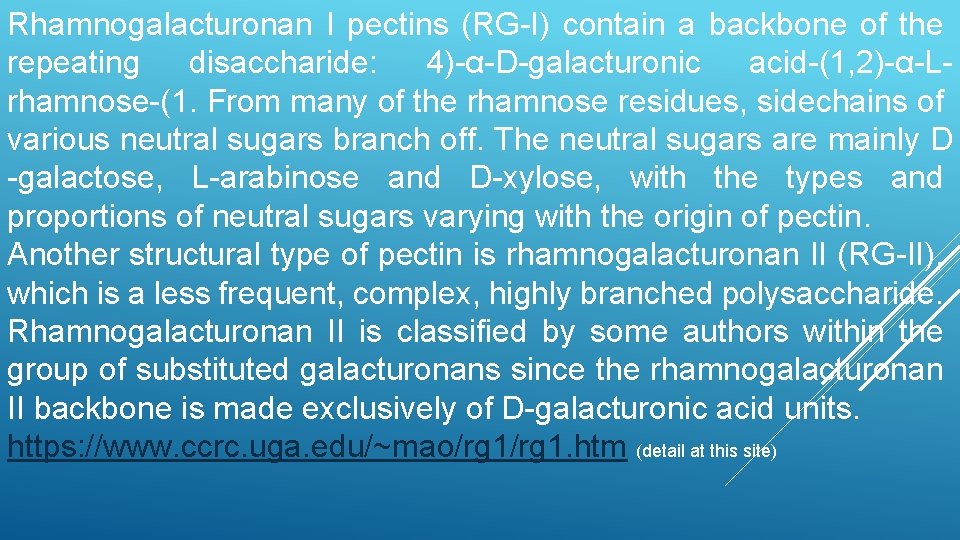
Rhamnogalacturonan I pectins (RG-I) contain a backbone of the repeating disaccharide: 4)-α-D-galacturonic acid-(1, 2)-α-Lrhamnose-(1. From many of the rhamnose residues, sidechains of various neutral sugars branch off. The neutral sugars are mainly D -galactose, L-arabinose and D-xylose, with the types and proportions of neutral sugars varying with the origin of pectin. Another structural type of pectin is rhamnogalacturonan II (RG-II), which is a less frequent, complex, highly branched polysaccharide. Rhamnogalacturonan II is classified by some authors within the group of substituted galacturonans since the rhamnogalacturonan II backbone is made exclusively of D-galacturonic acid units. https: //www. ccrc. uga. edu/~mao/rg 1. htm (detail at this site)
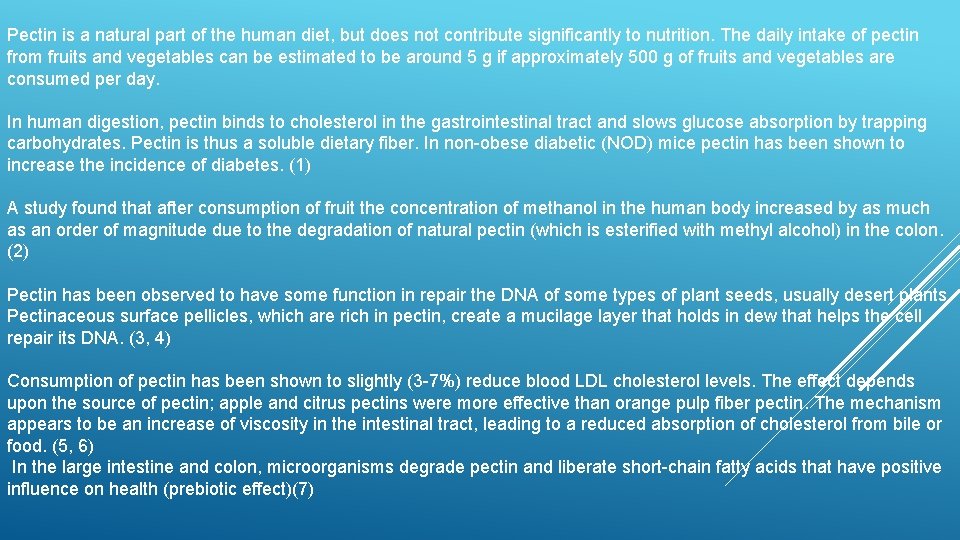
Pectin is a natural part of the human diet, but does not contribute significantly to nutrition. The daily intake of pectin from fruits and vegetables can be estimated to be around 5 g if approximately 500 g of fruits and vegetables are consumed per day. In human digestion, pectin binds to cholesterol in the gastrointestinal tract and slows glucose absorption by trapping carbohydrates. Pectin is thus a soluble dietary fiber. In non-obese diabetic (NOD) mice pectin has been shown to increase the incidence of diabetes. (1) A study found that after consumption of fruit the concentration of methanol in the human body increased by as much as an order of magnitude due to the degradation of natural pectin (which is esterified with methyl alcohol) in the colon. (2) Pectin has been observed to have some function in repair the DNA of some types of plant seeds, usually desert plants. Pectinaceous surface pellicles, which are rich in pectin, create a mucilage layer that holds in dew that helps the cell repair its DNA. (3, 4) Consumption of pectin has been shown to slightly (3 -7%) reduce blood LDL cholesterol levels. The effect depends upon the source of pectin; apple and citrus pectins were more effective than orange pulp fiber pectin. The mechanism appears to be an increase of viscosity in the intestinal tract, leading to a reduced absorption of cholesterol from bile or food. (5, 6) In the large intestine and colon, microorganisms degrade pectin and liberate short-chain fatty acids that have positive influence on health (prebiotic effect)(7)
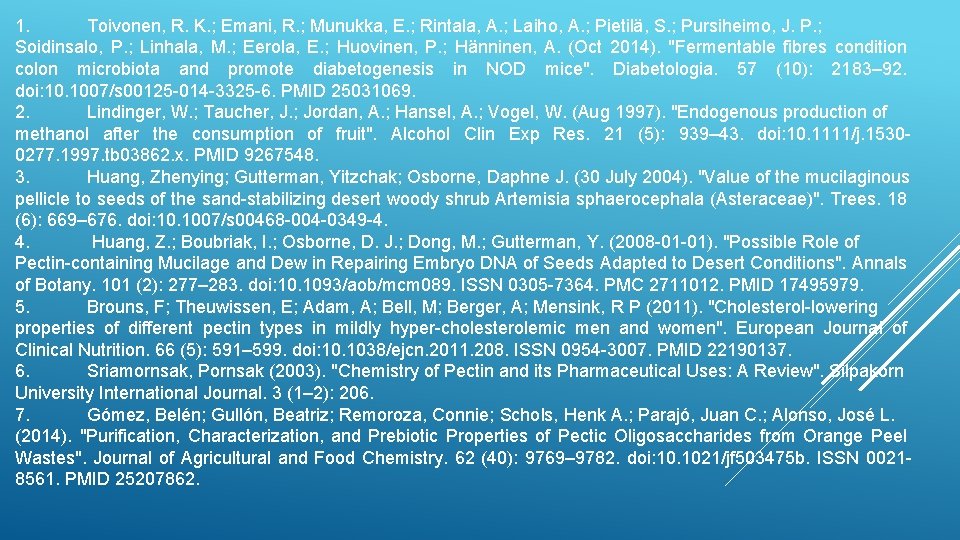
1. Toivonen, R. K. ; Emani, R. ; Munukka, E. ; Rintala, A. ; Laiho, A. ; Pietilä, S. ; Pursiheimo, J. P. ; Soidinsalo, P. ; Linhala, M. ; Eerola, E. ; Huovinen, P. ; Hänninen, A. (Oct 2014). "Fermentable fibres condition colon microbiota and promote diabetogenesis in NOD mice". Diabetologia. 57 (10): 2183– 92. doi: 10. 1007/s 00125 -014 -3325 -6. PMID 25031069. 2. Lindinger, W. ; Taucher, J. ; Jordan, A. ; Hansel, A. ; Vogel, W. (Aug 1997). "Endogenous production of methanol after the consumption of fruit". Alcohol Clin Exp Res. 21 (5): 939– 43. doi: 10. 1111/j. 15300277. 1997. tb 03862. x. PMID 9267548. 3. Huang, Zhenying; Gutterman, Yitzchak; Osborne, Daphne J. (30 July 2004). "Value of the mucilaginous pellicle to seeds of the sand-stabilizing desert woody shrub Artemisia sphaerocephala (Asteraceae)". Trees. 18 (6): 669– 676. doi: 10. 1007/s 00468 -004 -0349 -4. 4. Huang, Z. ; Boubriak, I. ; Osborne, D. J. ; Dong, M. ; Gutterman, Y. (2008 -01 -01). "Possible Role of Pectin-containing Mucilage and Dew in Repairing Embryo DNA of Seeds Adapted to Desert Conditions". Annals of Botany. 101 (2): 277– 283. doi: 10. 1093/aob/mcm 089. ISSN 0305 -7364. PMC 2711012. PMID 17495979. 5. Brouns, F; Theuwissen, E; Adam, A; Bell, M; Berger, A; Mensink, R P (2011). "Cholesterol-lowering properties of different pectin types in mildly hyper-cholesterolemic men and women". European Journal of Clinical Nutrition. 66 (5): 591– 599. doi: 10. 1038/ejcn. 2011. 208. ISSN 0954 -3007. PMID 22190137. 6. Sriamornsak, Pornsak (2003). "Chemistry of Pectin and its Pharmaceutical Uses: A Review". Silpakorn University International Journal. 3 (1– 2): 206. 7. Gómez, Belén; Gullón, Beatriz; Remoroza, Connie; Schols, Henk A. ; Parajó, Juan C. ; Alonso, José L. (2014). "Purification, Characterization, and Prebiotic Properties of Pectic Oligosaccharides from Orange Peel Wastes". Journal of Agricultural and Food Chemistry. 62 (40): 9769– 9782. doi: 10. 1021/jf 503475 b. ISSN 00218561. PMID 25207862.
Polysakarida
Example of pectin
Structure of lignin
Drevo
Purehemp technology
Pektin lignin
Chlorenchyma
Extra cell matrix
Objective of plant breeding
Plant introduction in plant breeding
Plant introduction in plant breeding
Tronsmo plant pathology and plant diseases download
Tronsmo plant pathology and plant diseases download
Albugo eye
Rectangular matrix
Transpose of matrix product
Symmetric matrix
Agnes csaki semmelweis
Product of orthogonal matrices
Simple matching coefficient
Orthogonal matrix example
Critics of ansoff's matrix mention that the matrix does not
Fluid matrix of blood
Radazin herbicid