EUROPEAN PLASMA RESEARCH ACCELERATOR WITH EXCELLENCE IN APPLICATIONS
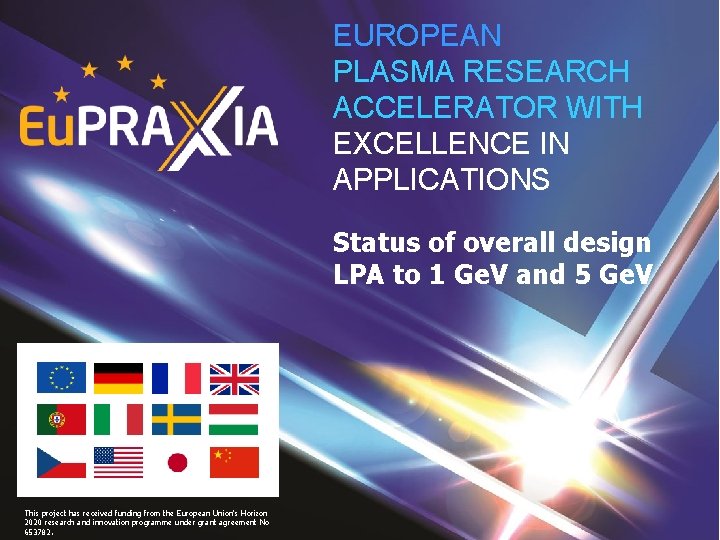
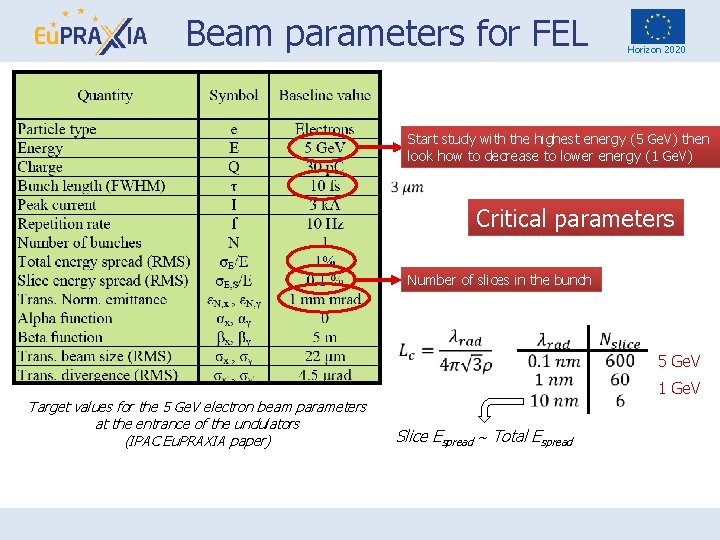
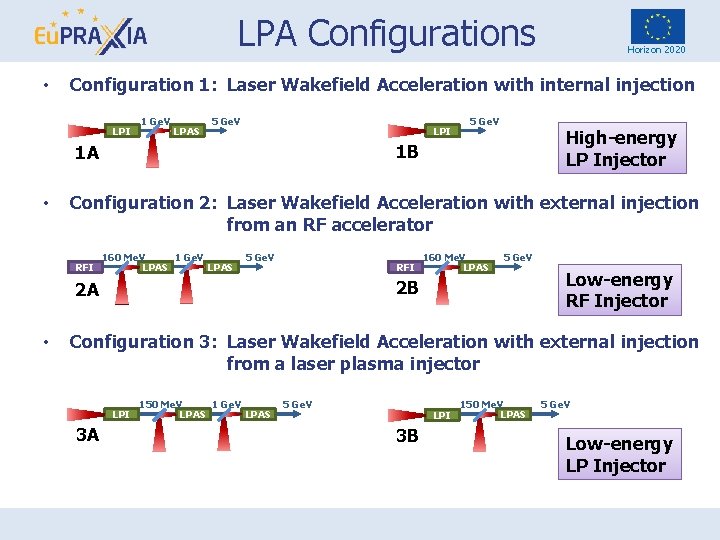
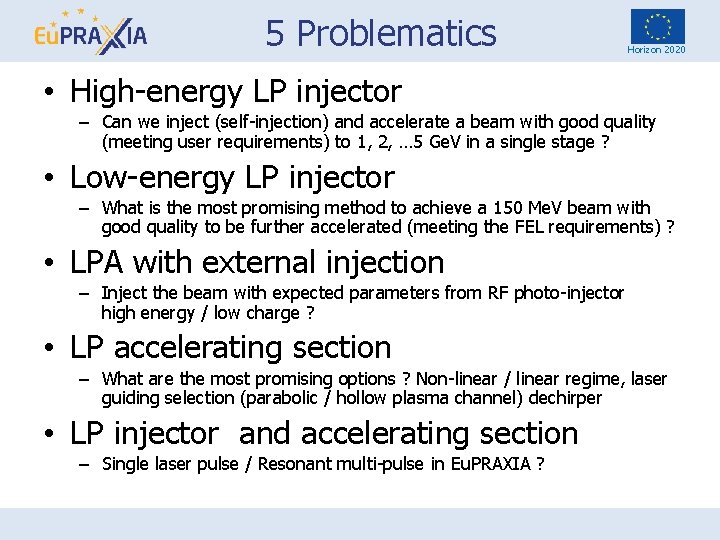
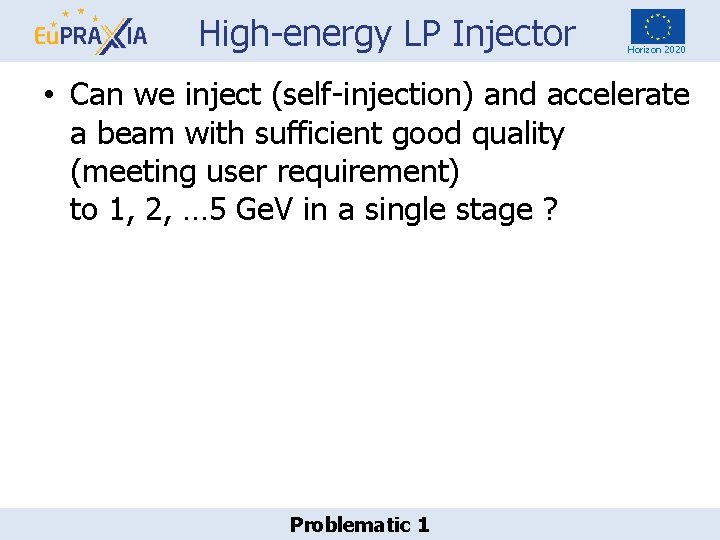
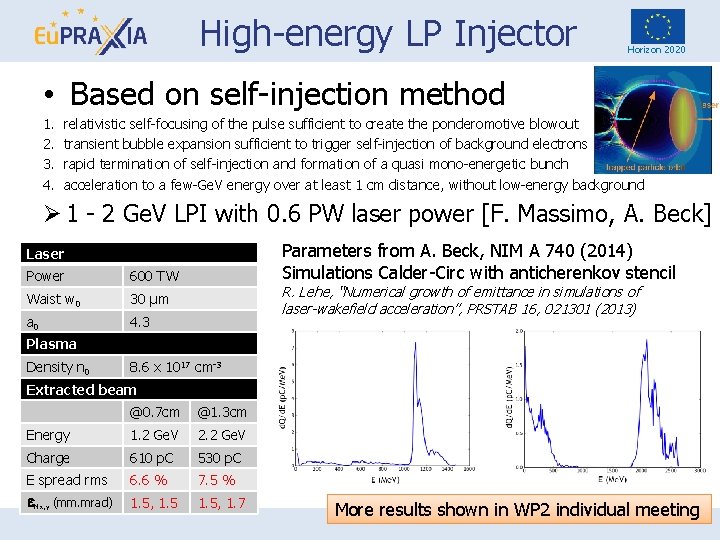
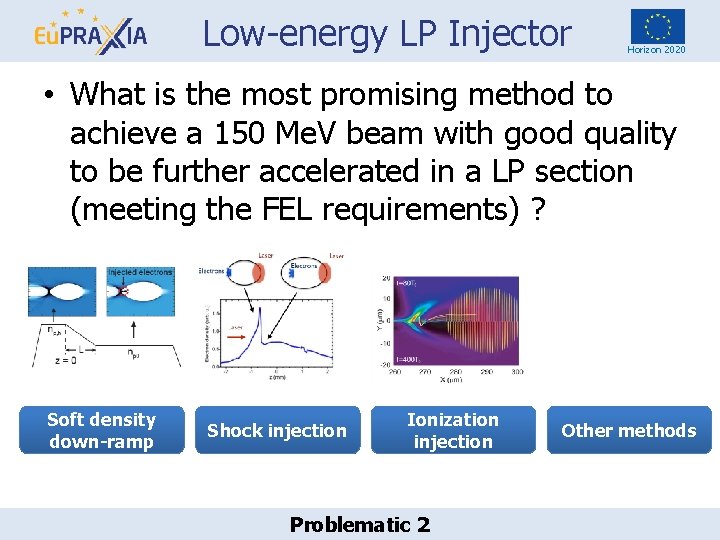
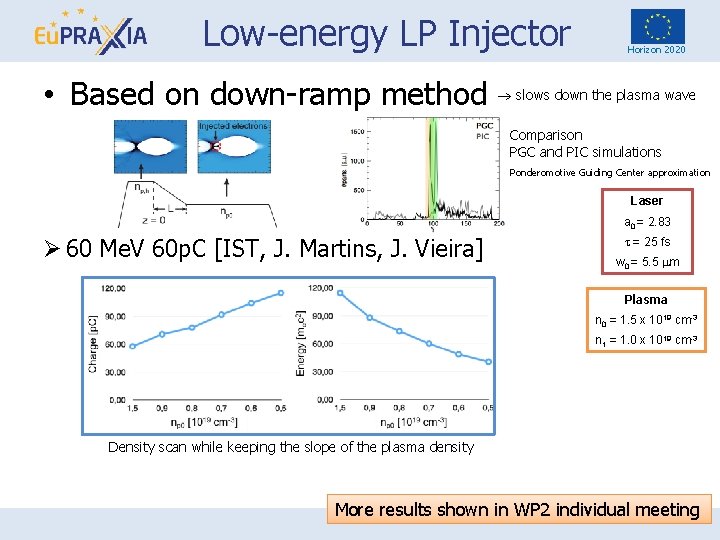
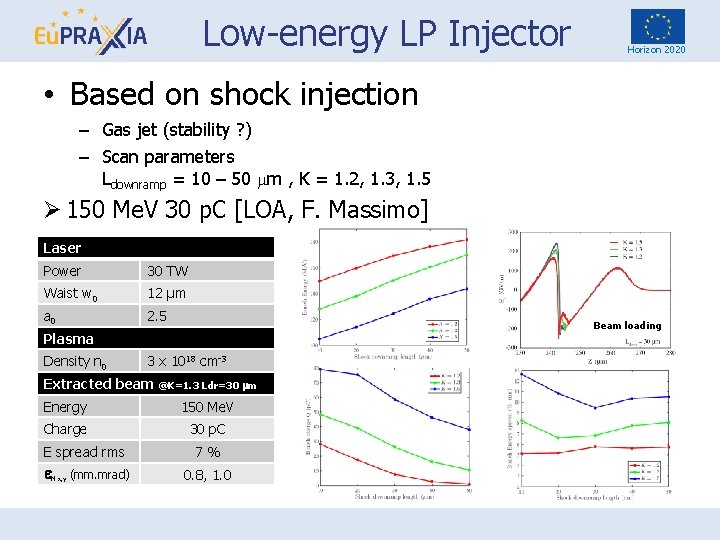
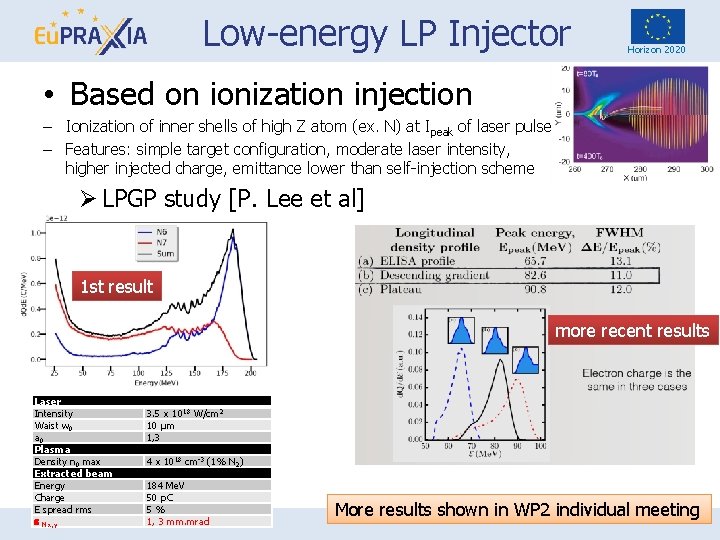
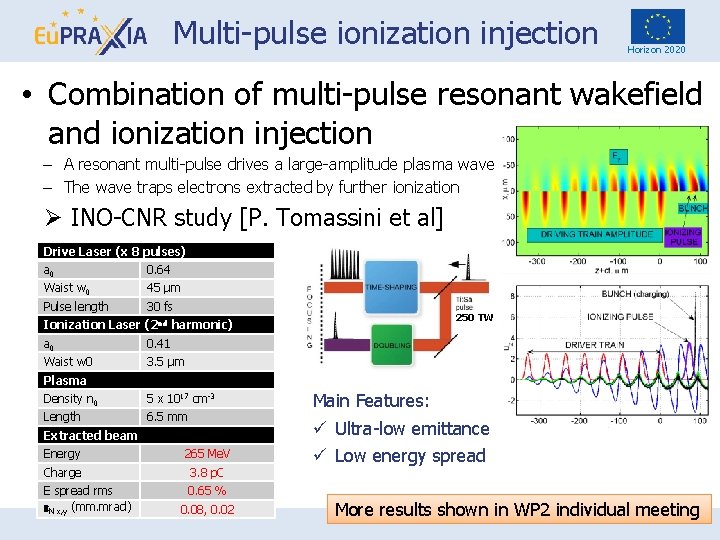
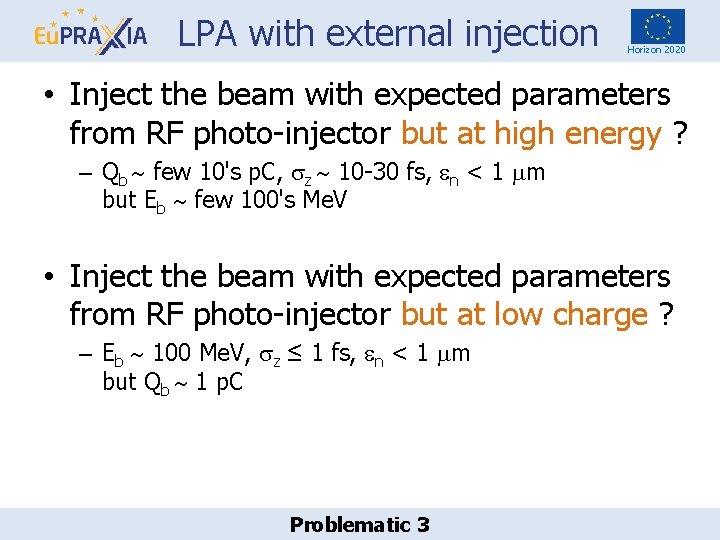
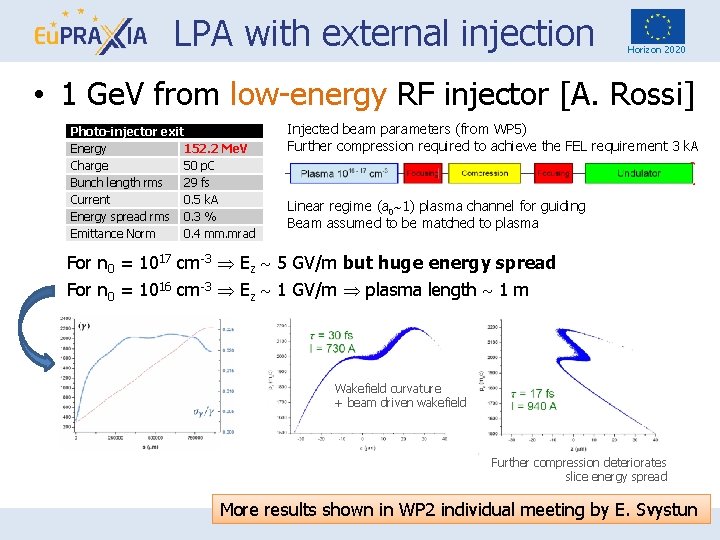
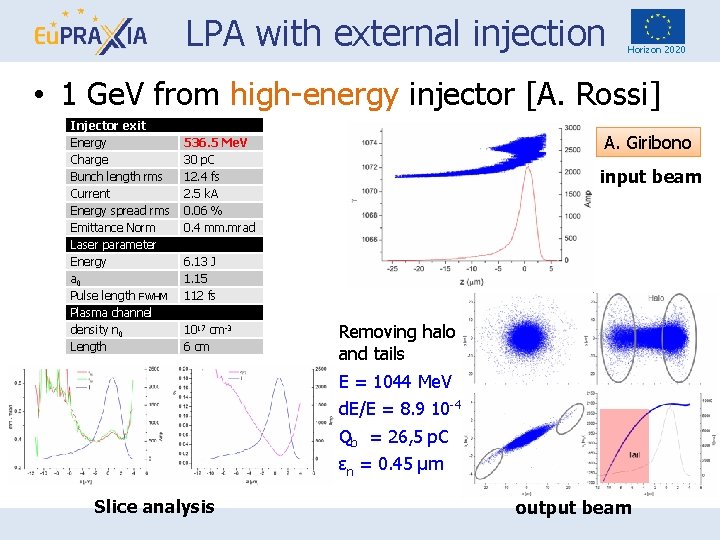
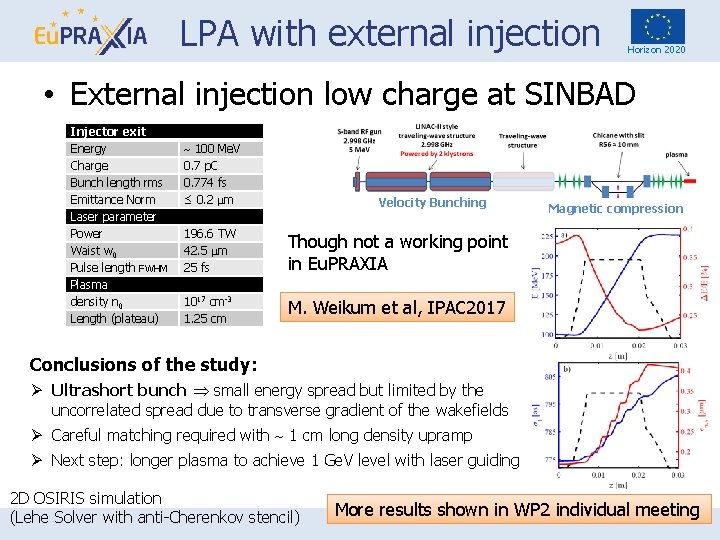
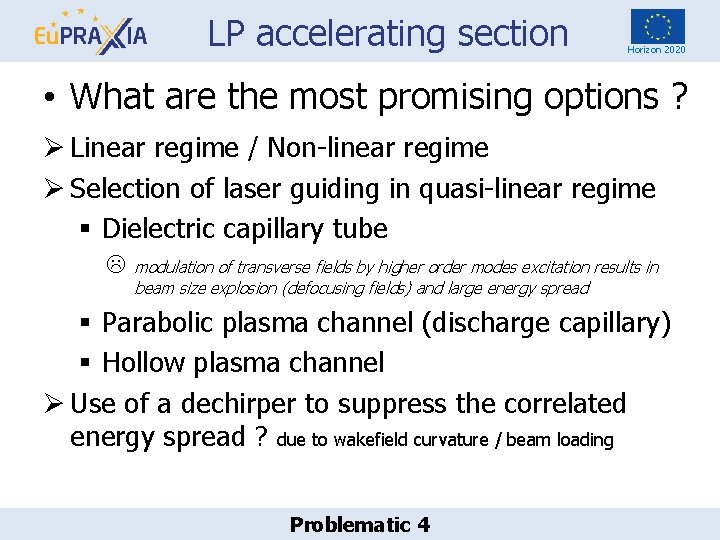
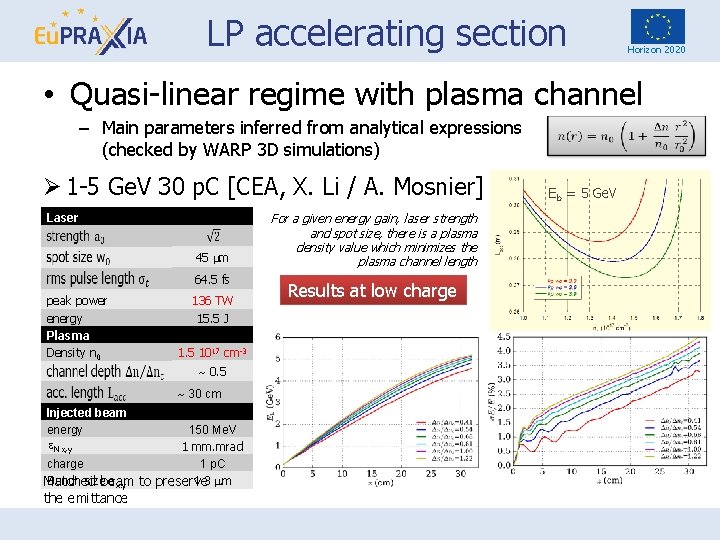
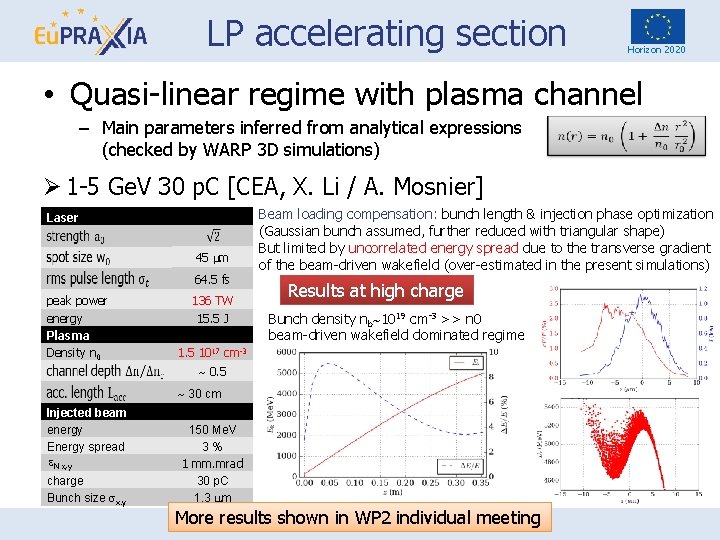
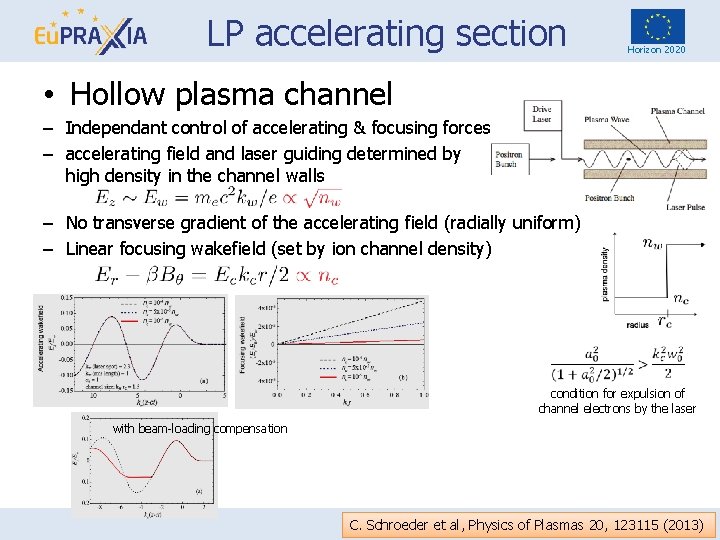
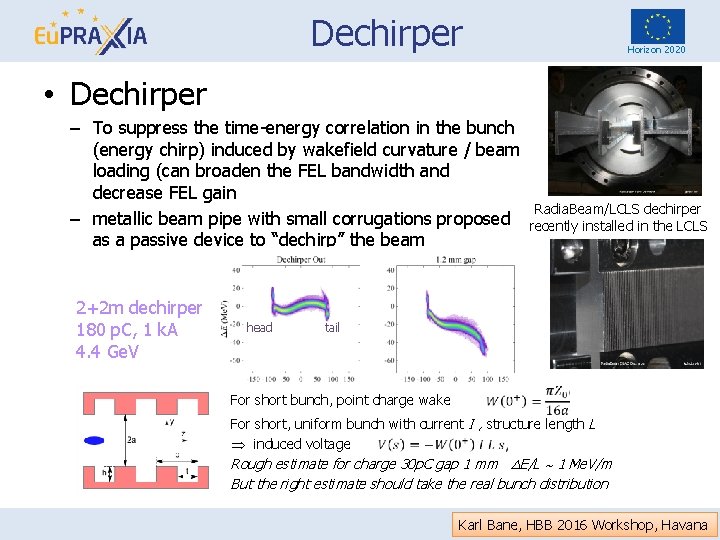
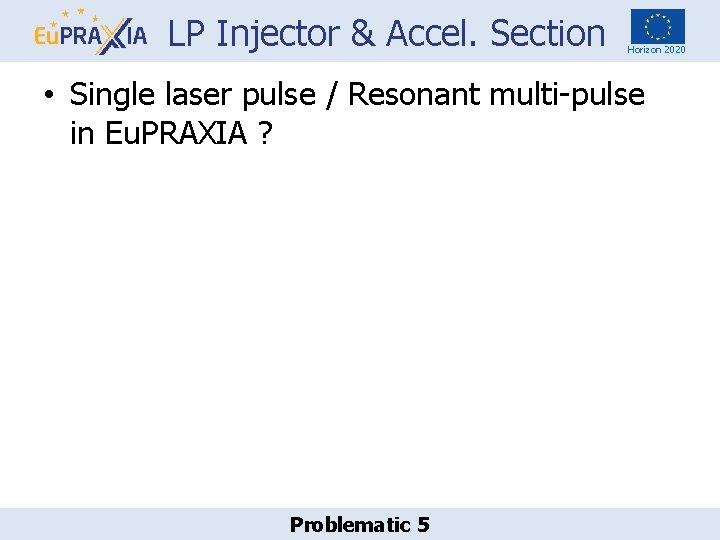
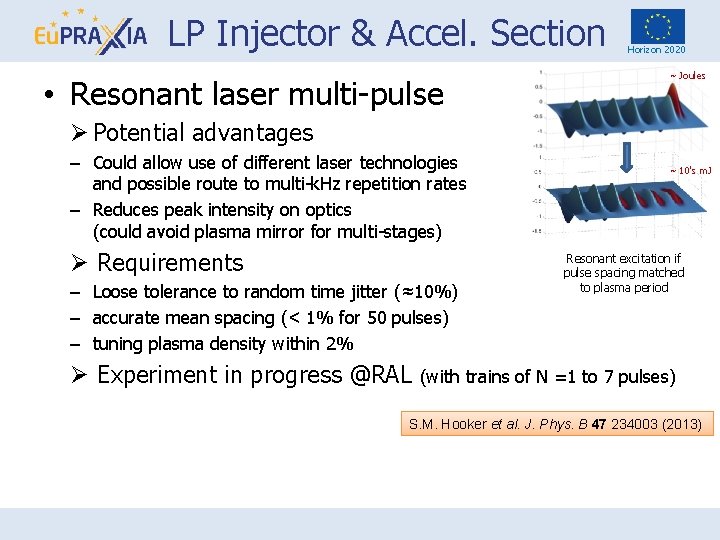
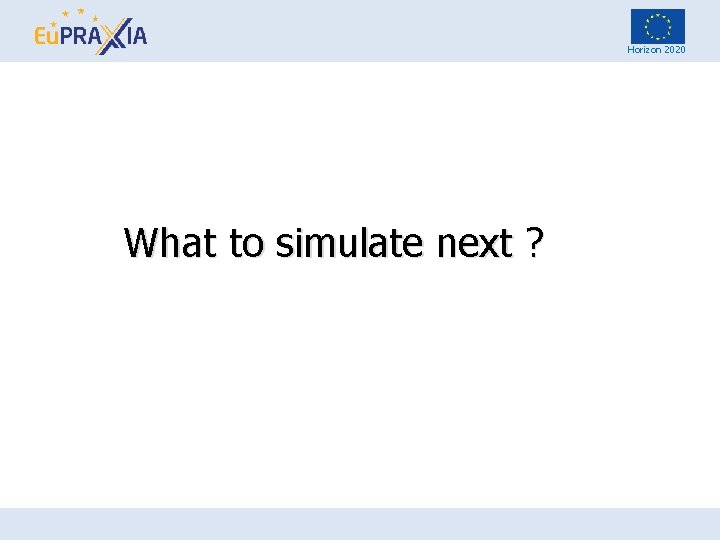
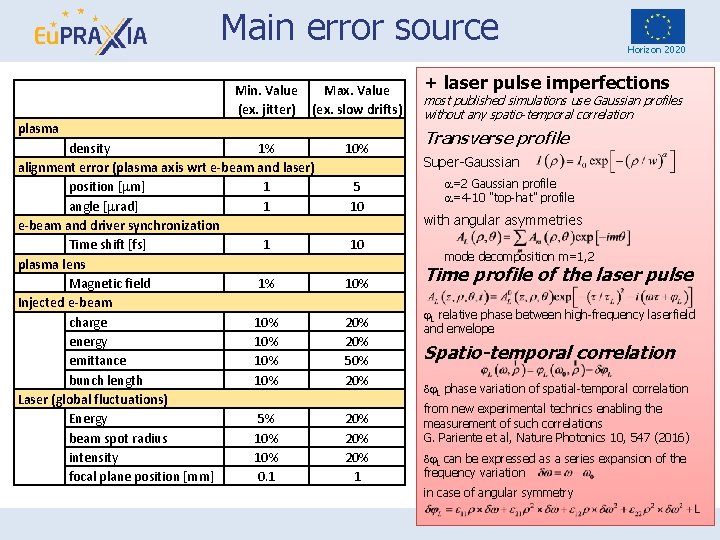
- Slides: 24
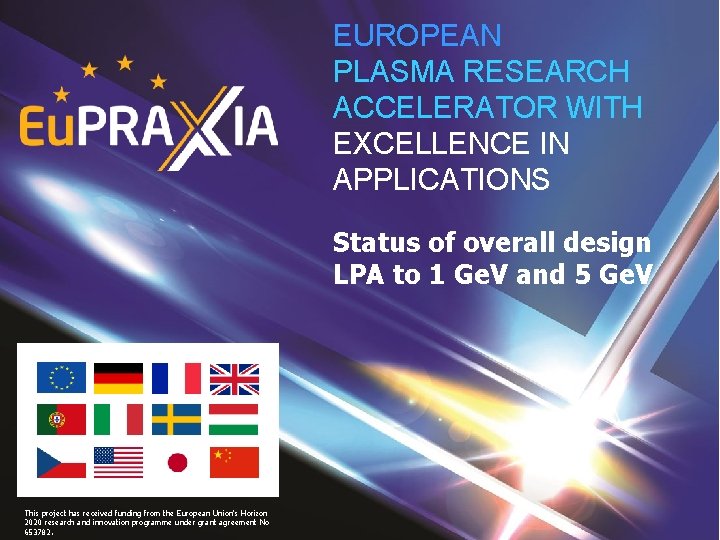
EUROPEAN PLASMA RESEARCH ACCELERATOR WITH EXCELLENCE IN APPLICATIONS Status of overall design LPA to 1 Ge. V and 5 Ge. V This project has received funding from the European Union’s Horizon 2020 research and innovation programme under grant agreement No 653782.
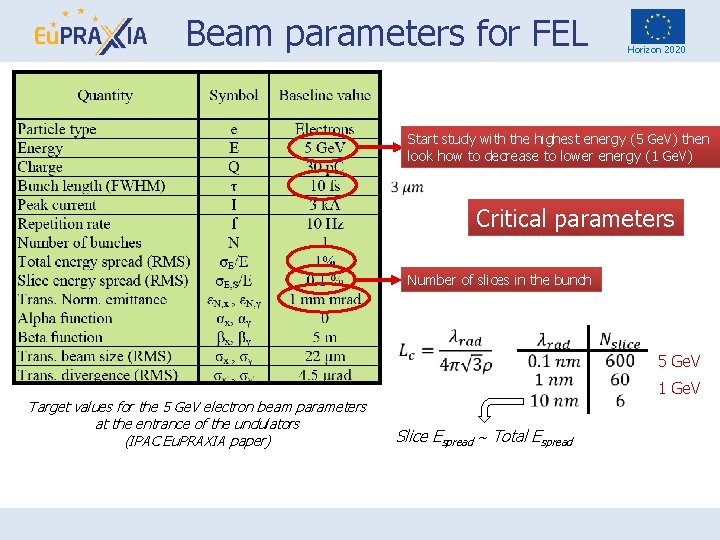
Beam parameters for FEL Horizon 2020 Start study with the highest energy (5 Ge. V) then look how to decrease to lower energy (1 Ge. V) Critical parameters Number of slices in the bunch 5 Ge. V 1 Ge. V Target values for the 5 Ge. V electron beam parameters at the entrance of the undulators (IPAC Eu. PRAXIA paper) Slice Espread Total Espread
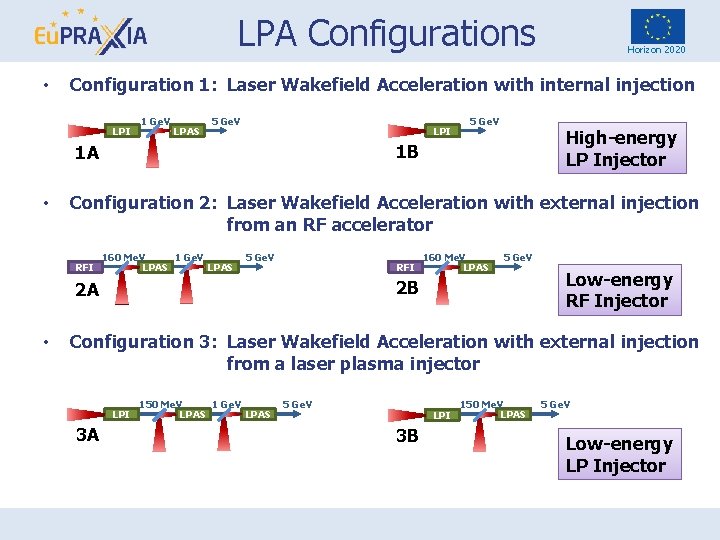
LPA Configurations • Configuration 1: Laser Wakefield Acceleration with internal injection LPI 1 Ge. V LPAS 5 Ge. V LPI High-energy LP Injector Configuration 2: Laser Wakefield Acceleration with external injection from an RF accelerator RFI 160 Me. V 1 Ge. V LPAS 5 Ge. V RFI 160 Me. V LPAS 5 Ge. V 2 B 2 A • 5 Ge. V 1 B 1 A • Horizon 2020 Low-energy RF Injector Configuration 3: Laser Wakefield Acceleration with external injection from a laser plasma injector LPI 3 A 150 Me. V 1 Ge. V LPAS 5 Ge. V LPI 3 B 150 Me. V LPAS 5 Ge. V Low-energy LP Injector
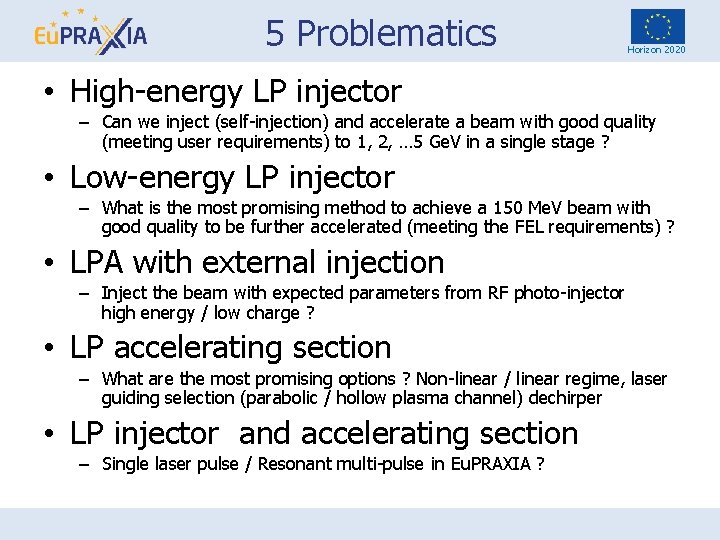
5 Problematics Horizon 2020 • High-energy LP injector – Can we inject (self-injection) and accelerate a beam with good quality (meeting user requirements) to 1, 2, … 5 Ge. V in a single stage ? • Low-energy LP injector – What is the most promising method to achieve a 150 Me. V beam with good quality to be further accelerated (meeting the FEL requirements) ? • LPA with external injection – Inject the beam with expected parameters from RF photo-injector high energy / low charge ? • LP accelerating section – What are the most promising options ? Non-linear / linear regime, laser guiding selection (parabolic / hollow plasma channel) dechirper • LP injector and accelerating section – Single laser pulse / Resonant multi-pulse in Eu. PRAXIA ?
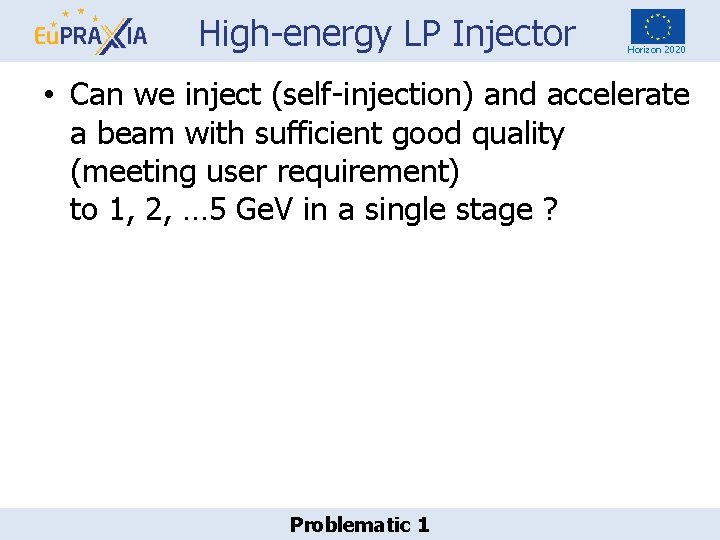
High-energy LP Injector Horizon 2020 • Can we inject (self-injection) and accelerate a beam with sufficient good quality (meeting user requirement) to 1, 2, … 5 Ge. V in a single stage ? Problematic 1
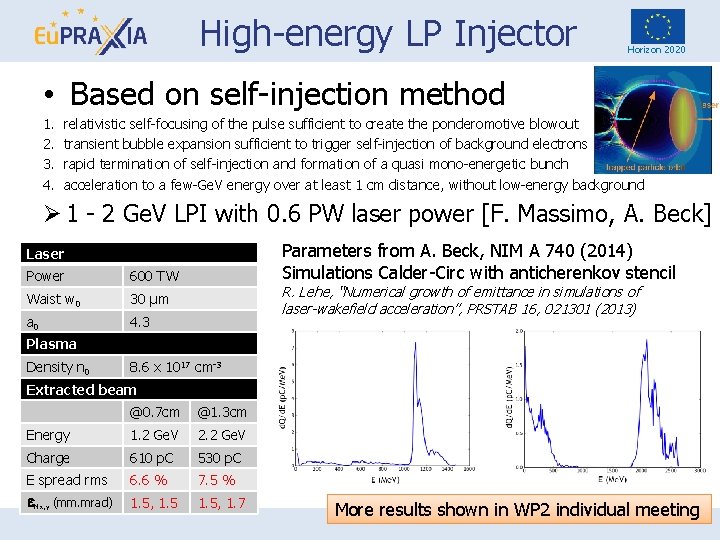
High-energy LP Injector Horizon 2020 • Based on self-injection method 1. 2. 3. 4. relativistic self-focusing of the pulse sufficient to create the ponderomotive blowout transient bubble expansion sufficient to trigger self-injection of background electrons rapid termination of self-injection and formation of a quasi mono-energetic bunch acceleration to a few-Ge. V energy over at least 1 cm distance, without low-energy background Ø 1 - 2 Ge. V LPI with 0. 6 PW laser power [F. Massimo, A. Beck] Parameters from A. Beck, NIM A 740 (2014) Simulations Calder-Circ with anticherenkov stencil Laser Power 600 TW Waist w 0 30 μm a 0 4. 3 R. Lehe, “Numerical growth of emittance in simulations of laser-wakefield acceleration”, PRSTAB 16, 021301 (2013) Plasma Density n 0 8. 6 x 1017 cm-3 Extracted beam @0. 7 cm @1. 3 cm Energy 1. 2 Ge. V 2. 2 Ge. V Charge 610 p. C 530 p. C E spread rms 6. 6 % 7. 5 % N x, y (mm. mrad) 1. 5, 1. 7 More results shown in WP 2 individual meeting
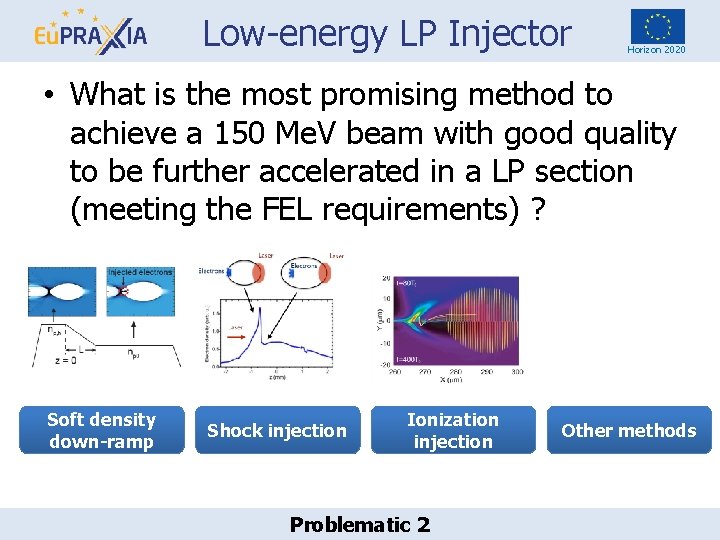
Low-energy LP Injector Horizon 2020 • What is the most promising method to achieve a 150 Me. V beam with good quality to be further accelerated in a LP section (meeting the FEL requirements) ? Soft density down-ramp Shock injection Ionization injection Problematic 2 Other methods
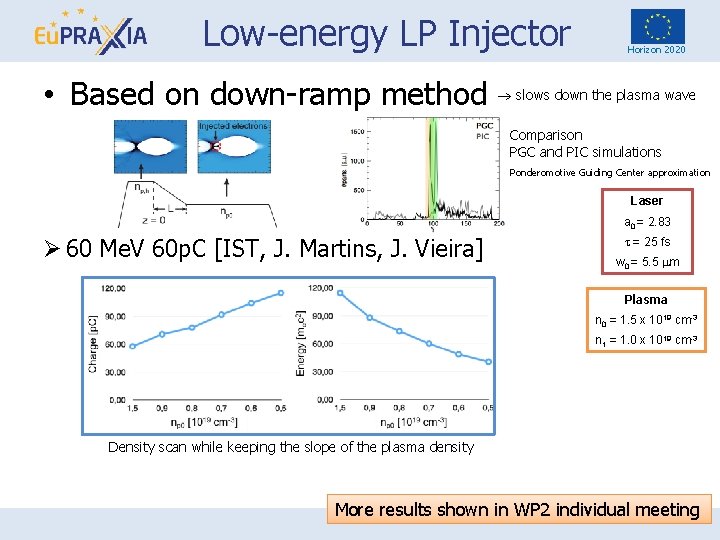
Low-energy LP Injector • Based on down-ramp method Horizon 2020 slows down the plasma wave Comparison PGC and PIC simulations Ponderomotive Guiding Center approximation Laser a 0 = 2. 83 Ø 60 Me. V 60 p. C [IST, J. Martins, J. Vieira] = 25 fs w 0 = 5. 5 m Plasma n 0 = 1. 5 x 1019 cm-3 n 1 = 1. 0 x 1019 cm-3 Density scan while keeping the slope of the plasma density More results shown in WP 2 individual meeting
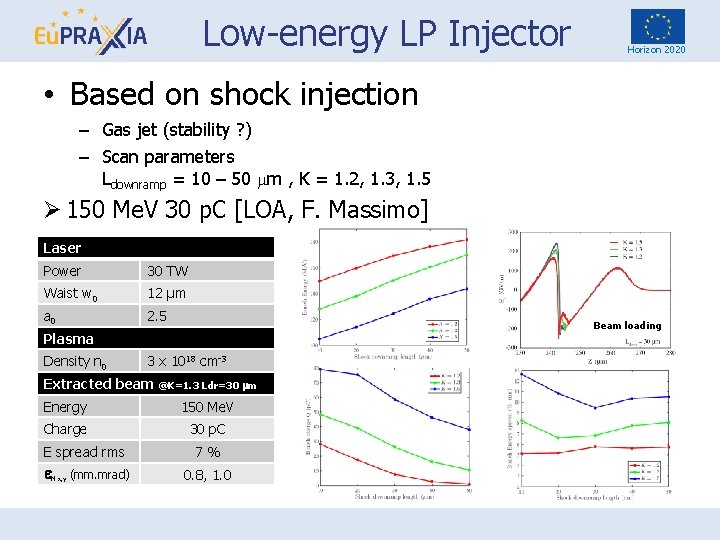
Low-energy LP Injector Horizon 2020 • Based on shock injection – Gas jet (stability ? ) – Scan parameters Ldownramp = 10 – 50 m , K = 1. 2, 1. 3, 1. 5 Ø 150 Me. V 30 p. C [LOA, F. Massimo] Laser Power 30 TW Waist w 0 12 μm a 0 2. 5 Beam loading Plasma Density n 0 3 x 1018 cm-3 Extracted beam @K=1. 3 Ldr=30 m Energy 150 Me. V Charge 30 p. C E spread rms 7% N x, y (mm. mrad) 0. 8, 1. 0
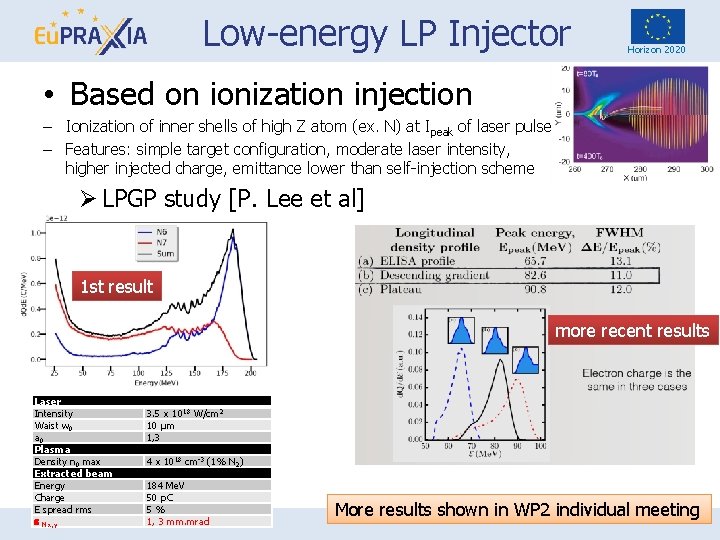
Low-energy LP Injector Horizon 2020 • Based on ionization injection – Ionization of inner shells of high Z atom (ex. N) at Ipeak of laser pulse – Features: simple target configuration, moderate laser intensity, higher injected charge, emittance lower than self-injection scheme Ø LPGP study [P. Lee et al] 1 st result more recent results Laser Intensity Waist w 0 a 0 Plasma Density n 0 max Extracted beam Energy Charge E spread rms N x, y 3. 5 x 1018 W/cm 2 10 μm 1, 3 4 x 1018 cm-3 (1% N 2) 184 Me. V 50 p. C 5% 1, 3 mm. mrad More results shown in WP 2 individual meeting
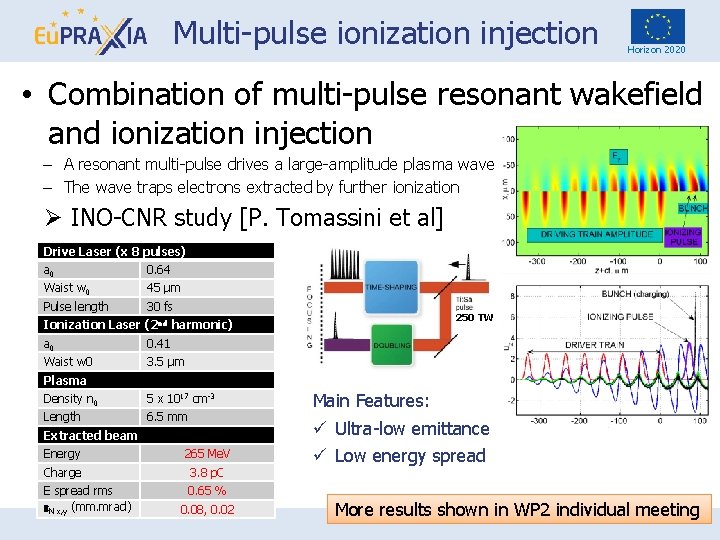
Multi-pulse ionization injection Horizon 2020 • Combination of multi-pulse resonant wakefield and ionization injection – A resonant multi-pulse drives a large-amplitude plasma wave – The wave traps electrons extracted by further ionization Ø INO-CNR study [P. Tomassini et al] Drive Laser (x 8 pulses) a 0 0. 64 Waist w 0 45 μm Pulse length 30 fs 250 TW Ionization Laser (2 nd harmonic) a 0 0. 41 Waist w 0 3. 5 μm Plasma Density n 0 5 x 1017 cm-3 Length 6. 5 mm Extracted beam Energy 265 Me. V Charge 3. 8 p. C E spread rms N x, y (mm. mrad) 0. 65 % 0. 08, 0. 02 Main Features: ü Ultra-low emittance ü Low energy spread More results shown in WP 2 individual meeting
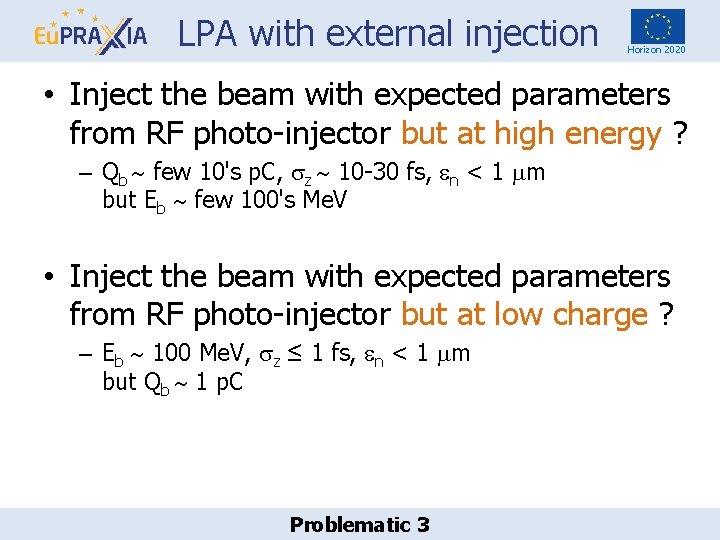
LPA with external injection Horizon 2020 • Inject the beam with expected parameters from RF photo-injector but at high energy ? – Qb few 10's p. C, z 10 -30 fs, n < 1 m but Eb few 100's Me. V • Inject the beam with expected parameters from RF photo-injector but at low charge ? – Eb 100 Me. V, z ≤ 1 fs, n < 1 m but Qb 1 p. C Problematic 3
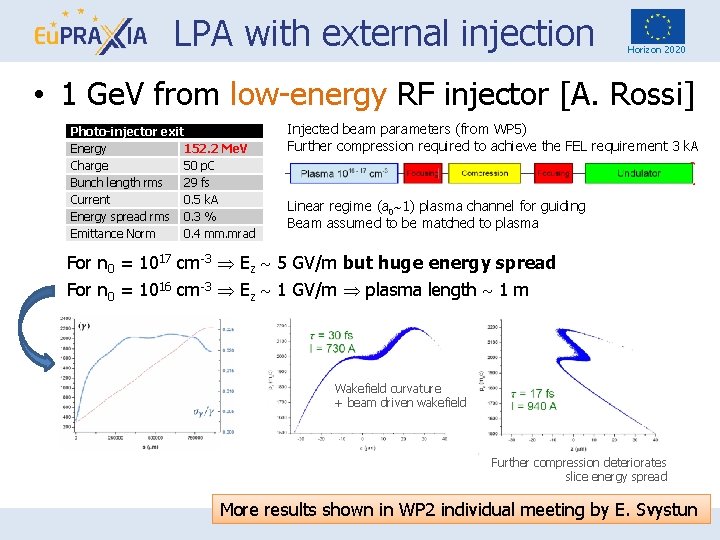
LPA with external injection Horizon 2020 • 1 Ge. V from low-energy RF injector [A. Rossi] Photo-injector exit Energy 152. 2 Me. V Charge 50 p. C Bunch length rms 29 fs Current 0. 5 k. A Energy spread rms 0. 3 % Emittance Norm 0. 4 mm. mrad Injected beam parameters (from WP 5) Further compression required to achieve the FEL requirement 3 k. A Linear regime (a 0 1) plasma channel for guiding Beam assumed to be matched to plasma For n 0 = 1017 cm-3 Ez 5 GV/m but huge energy spread For n 0 = 1016 cm-3 Ez 1 GV/m plasma length 1 m Wakefield curvature + beam driven wakefield Further compression deteriorates slice energy spread More results shown in WP 2 individual meeting by E. Svystun
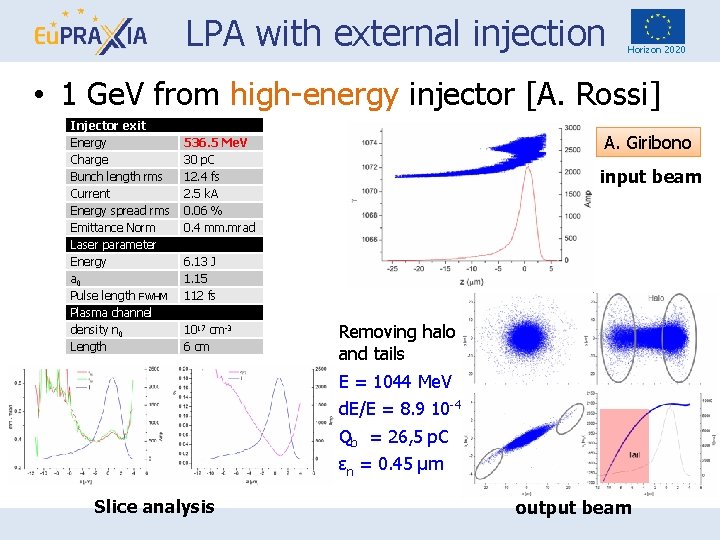
LPA with external injection Horizon 2020 • 1 Ge. V from high-energy injector [A. Rossi] Injector exit Energy Charge Bunch length rms Current Energy spread rms Emittance Norm Laser parameter Energy a 0 Pulse length FWHM Plasma channel density n 0 Length A. Giribono 536. 5 Me. V 30 p. C 12. 4 fs 2. 5 k. A 0. 06 % 0. 4 mm. mrad input beam 6. 13 J 1. 15 112 fs 1017 cm-3 6 cm Removing halo and tails E = 1044 Me. V d. E/E = 8. 9 10 -4 Qb = 26, 5 p. C εn = 0. 45 μm Slice analysis output beam
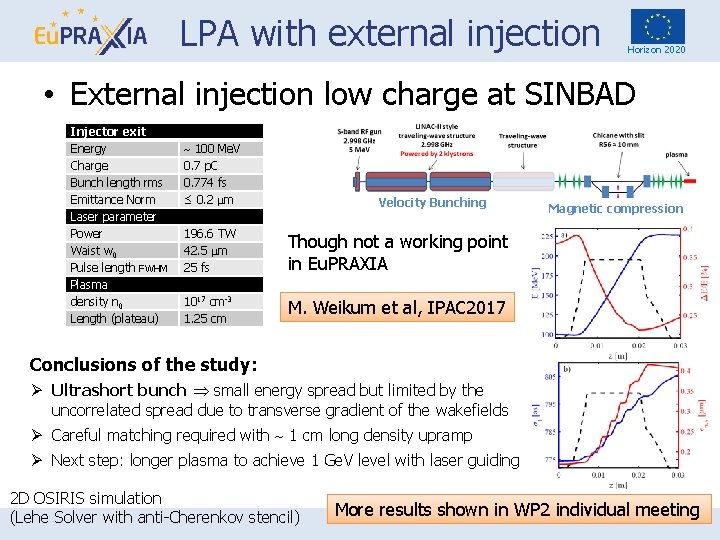
LPA with external injection Horizon 2020 • External injection low charge at SINBAD Injector exit Energy Charge Bunch length rms Emittance Norm Laser parameter Power Waist w 0 Pulse length FWHM Plasma density n 0 Length (plateau) 100 Me. V 0. 7 p. C 0. 774 fs ≤ 0. 2 m Velocity Bunching 196. 6 TW 42. 5 m 25 fs Though not a working point in Eu. PRAXIA 1017 cm-3 1. 25 cm M. Weikum et al, IPAC 2017 Magnetic compression Conclusions of the study: Ø Ultrashort bunch small energy spread but limited by the uncorrelated spread due to transverse gradient of the wakefields Ø Careful matching required with 1 cm long density upramp Ø Next step: longer plasma to achieve 1 Ge. V level with laser guiding 2 D OSIRIS simulation (Lehe Solver with anti-Cherenkov stencil) More results shown in WP 2 individual meeting
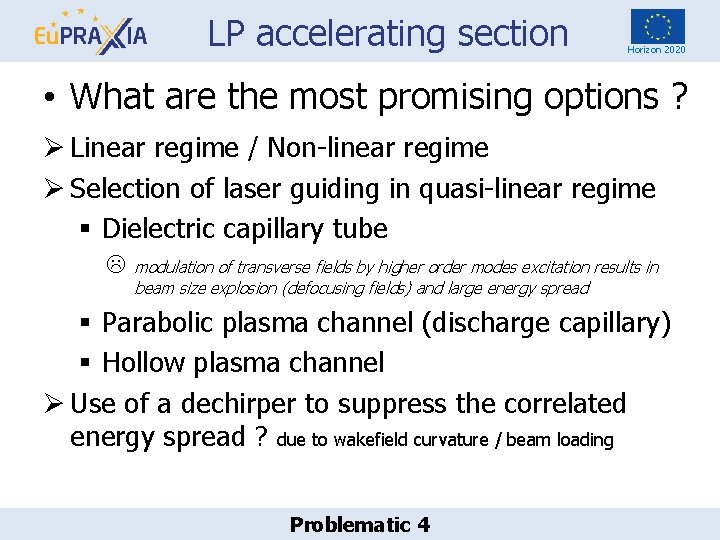
LP accelerating section Horizon 2020 • What are the most promising options ? Ø Linear regime / Non-linear regime Ø Selection of laser guiding in quasi-linear regime § Dielectric capillary tube modulation of transverse fields by higher order modes excitation results in beam size explosion (defocusing fields) and large energy spread § Parabolic plasma channel (discharge capillary) § Hollow plasma channel Ø Use of a dechirper to suppress the correlated energy spread ? due to wakefield curvature / beam loading Problematic 4
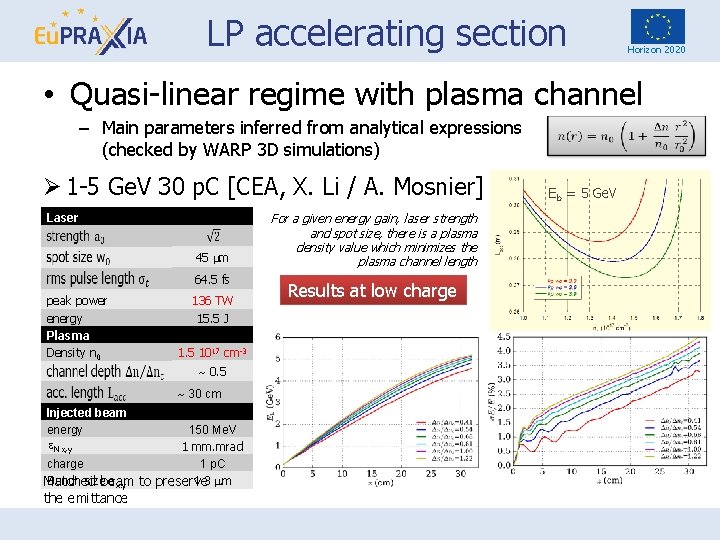
LP accelerating section Horizon 2020 • Quasi-linear regime with plasma channel – Main parameters inferred from analytical expressions (checked by WARP 3 D simulations) Ø 1 -5 Ge. V 30 p. C [CEA, X. Li / A. Mosnier] Laser 45 m 64. 5 fs peak power energy Plasma Density n 0 136 TW 15. 5 J 1. 5 1017 cm-3 0. 5 30 cm Injected beam energy N x, y 150 Me. V 1 mm. mrad charge 1 p. C Bunch sizebeam x, y to preserve 1. 3 m Matched the emittance For a given energy gain, laser strength and spot size, there is a plasma density value which minimizes the plasma channel length Results at low charge Eb = 5 Ge. V
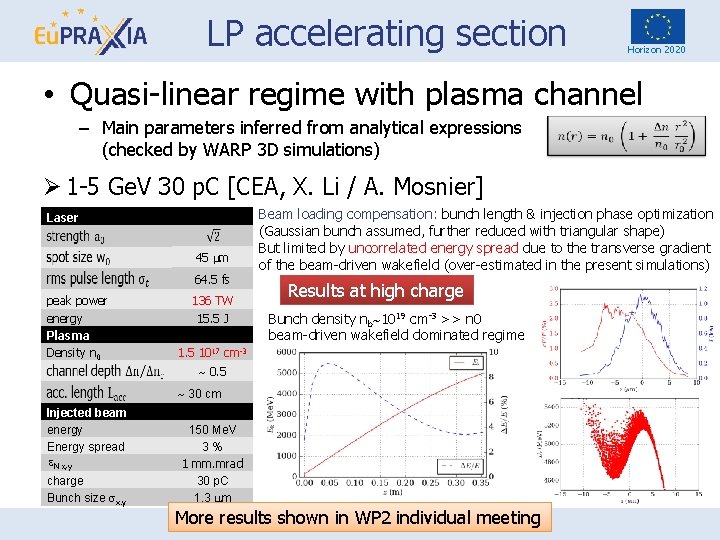
LP accelerating section Horizon 2020 • Quasi-linear regime with plasma channel – Main parameters inferred from analytical expressions (checked by WARP 3 D simulations) Ø 1 -5 Ge. V 30 p. C [CEA, X. Li / A. Mosnier] Laser 45 m 64. 5 fs peak power energy Plasma Density n 0 136 TW 15. 5 J Beam loading compensation: bunch length & injection phase optimization (Gaussian bunch assumed, further reduced with triangular shape) But limited by uncorrelated energy spread due to the transverse gradient of the beam-driven wakefield (over-estimated in the present simulations) Results at high charge Bunch density nb 1019 cm-3 >> n 0 beam-driven wakefield dominated regime 1. 5 1017 cm-3 0. 5 30 cm Injected beam energy Energy spread N x, y charge Bunch size x, y 150 Me. V 3% 1 mm. mrad 30 p. C 1. 3 m More results shown in WP 2 individual meeting
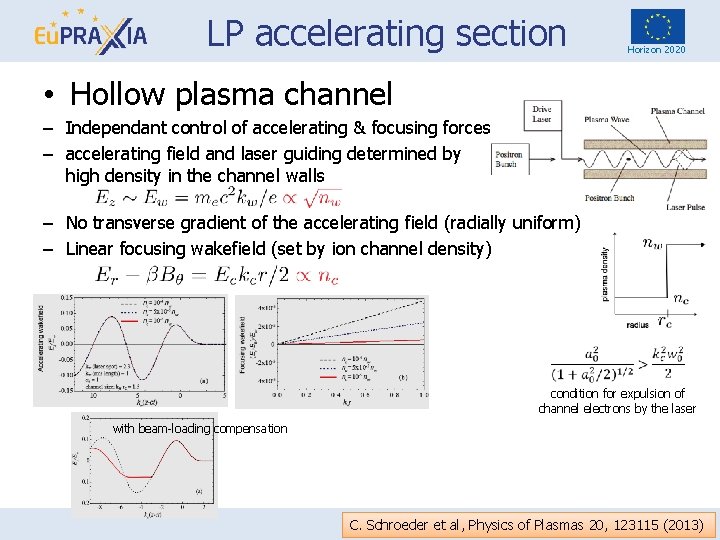
LP accelerating section Horizon 2020 • Hollow plasma channel – Independant control of accelerating & focusing forces – accelerating field and laser guiding determined by high density in the channel walls – No transverse gradient of the accelerating field (radially uniform) – Linear focusing wakefield (set by ion channel density) condition for expulsion of channel electrons by the laser with beam-loading compensation C. Schroeder et al, Physics of Plasmas 20, 123115 (2013)
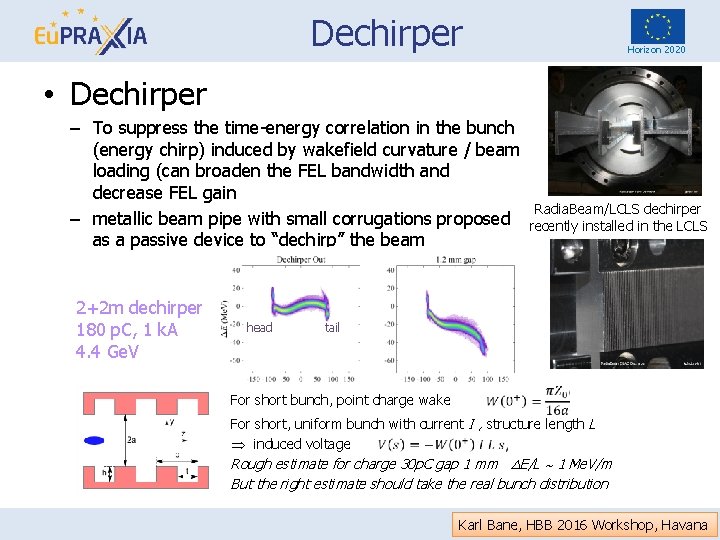
Dechirper Horizon 2020 • Dechirper – To suppress the time-energy correlation in the bunch (energy chirp) induced by wakefield curvature / beam loading (can broaden the FEL bandwidth and decrease FEL gain – metallic beam pipe with small corrugations proposed as a passive device to “dechirp” the beam 2+2 m dechirper 180 p. C, 1 k. A 4. 4 Ge. V head Radia. Beam/LCLS dechirper recently installed in the LCLS tail For short bunch, point charge wake For short, uniform bunch with current I , structure length L induced voltage Rough estimate for charge 30 p. C gap 1 mm E/L 1 Me. V/m But the right estimate should take the real bunch distribution Karl Bane, HBB 2016 Workshop, Havana
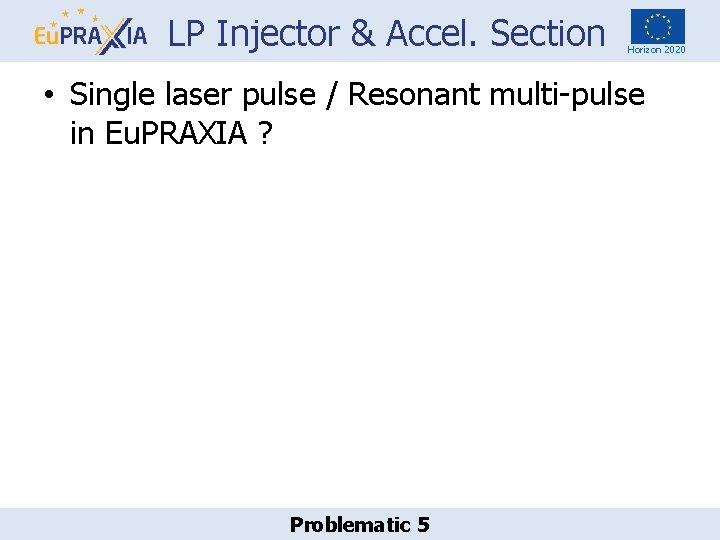
LP Injector & Accel. Section Horizon 2020 • Single laser pulse / Resonant multi-pulse in Eu. PRAXIA ? Problematic 5
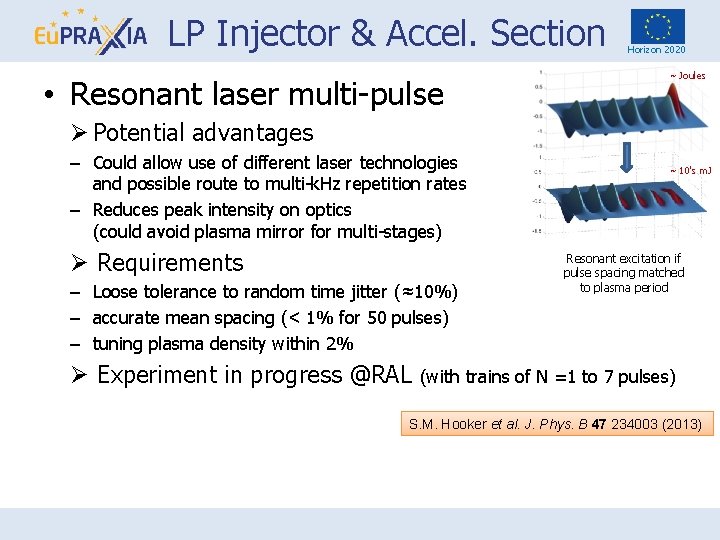
LP Injector & Accel. Section • Resonant laser multi-pulse Horizon 2020 Joules Ø Potential advantages – Could allow use of different laser technologies and possible route to multi-k. Hz repetition rates – Reduces peak intensity on optics (could avoid plasma mirror for multi-stages) Ø Requirements – Loose tolerance to random time jitter (≈10%) – accurate mean spacing (< 1% for 50 pulses) – tuning plasma density within 2% Ø Experiment in progress @RAL 10's m. J Resonant excitation if pulse spacing matched to plasma period (with trains of N =1 to 7 pulses) S. M. Hooker et al. J. Phys. B 47 234003 (2013)
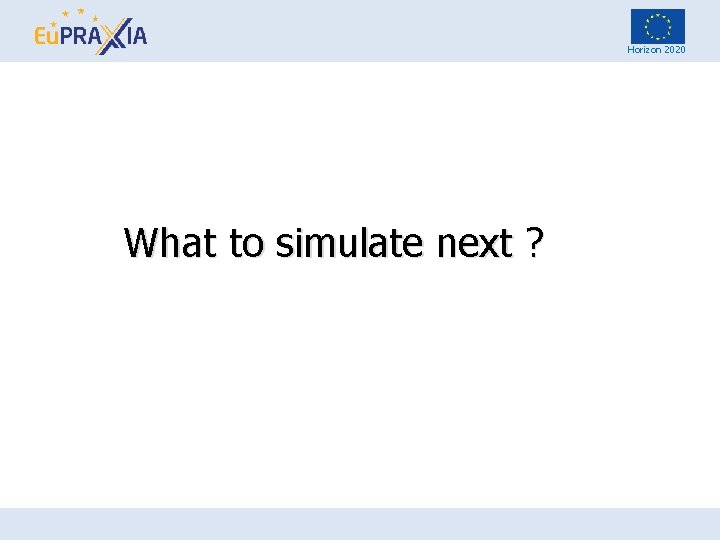
Horizon 2020 What to simulate next ?
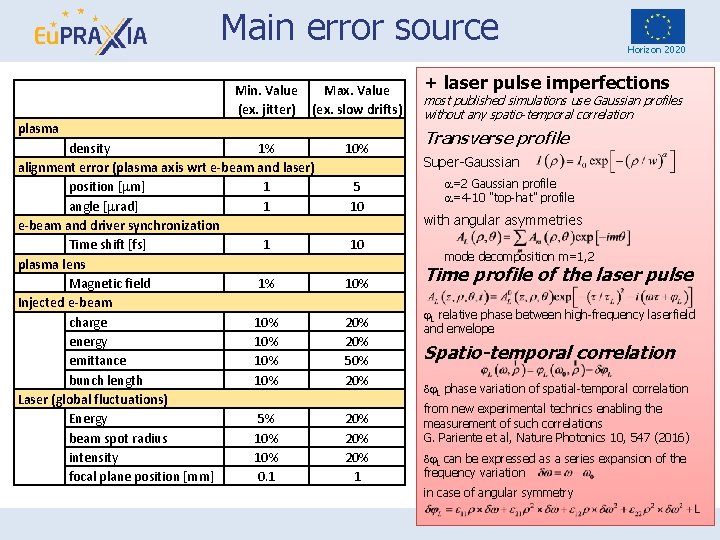
Main error source Min. Value (ex. jitter) Max. Value (ex. slow drifts) plasma density 1% alignment error (plasma axis wrt e-beam and laser) position [ m] 1 angle [ rad] 1 e-beam and driver synchronization Time shift [fs] 1 plasma lens Magnetic field 1% Injected e-beam charge 10% energy 10% emittance 10% bunch length 10% Laser (global fluctuations) Energy 5% beam spot radius 10% intensity 10% focal plane position [mm] 0. 1 10% 5 10 10 10% 20% 50% 20% 20% 1 Horizon 2020 + laser pulse imperfections most published simulations use Gaussian profiles without any spatio-temporal correlation Transverse profile Super-Gaussian =2 Gaussian profile =4 -10 "top-hat" profile with angular asymmetries mode decomposition m=1, 2 Time profile of the laser pulse L relative phase between high-frequency laserfield and envelope Spatio-temporal correlation L phase variation of spatial-temporal correlation from new experimental technics enabling the measurement of such correlations G. Pariente et al, Nature Photonics 10, 547 (2016) L can be expressed as a series expansion of the frequency variation in case of angular symmetry
Eden european destinations of excellence
Abu dhabi award for research excellence (aare)
European strategy forum on research infrastructures
European commission community research
Transformational leadership questionnaire (tlq)
Limitations of accelerator theory of investment
Biztalk accelerator for swift
Netbackup accelerator
Fiscal policy ib
Accelerator coherency port
Financial accelerator
Offshore wind accelerator
Particle accelerator formula
Vm backup best practices
Accelerator mass spectrometry
Open capi
Extensor: an accelerator for sparse tensor algebra
Avamar overview
Charter network accelerator
Accelerator
Accelerator
Microsoft virtual machine converter download
United way social innovation accelerator
Uplogix 5000
Cern accelerator complex