Electrostatic Fields Lecture 2 E 8409 High Voltage
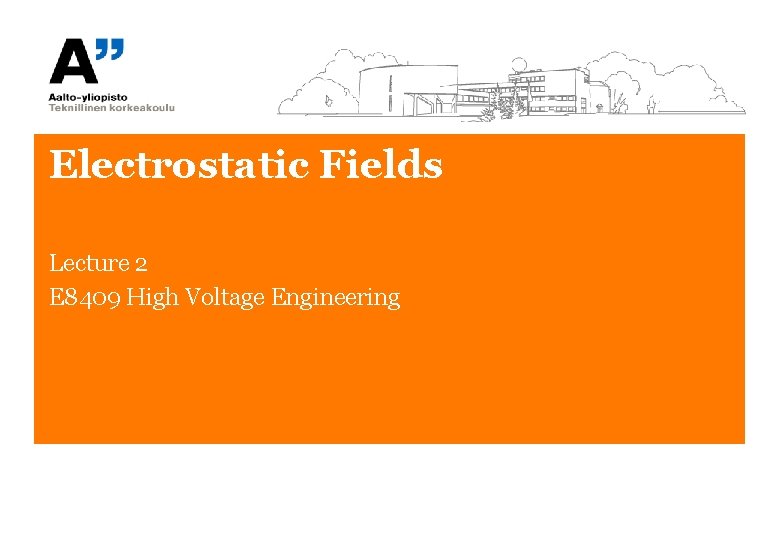
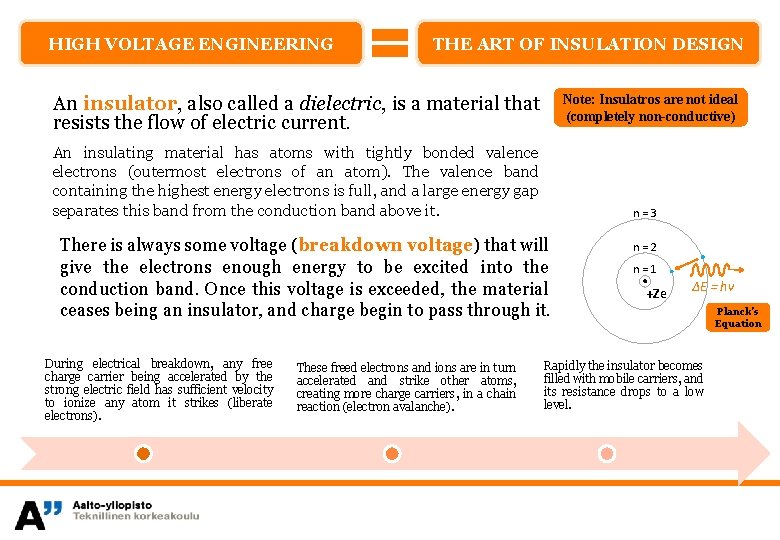
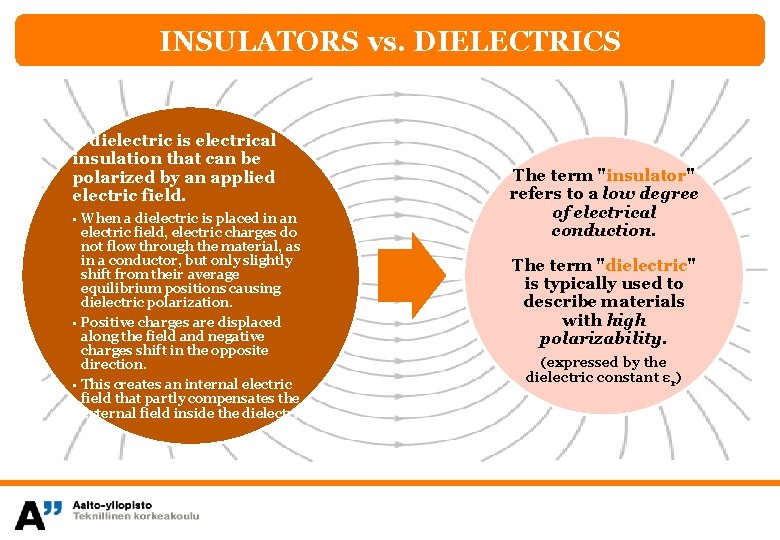
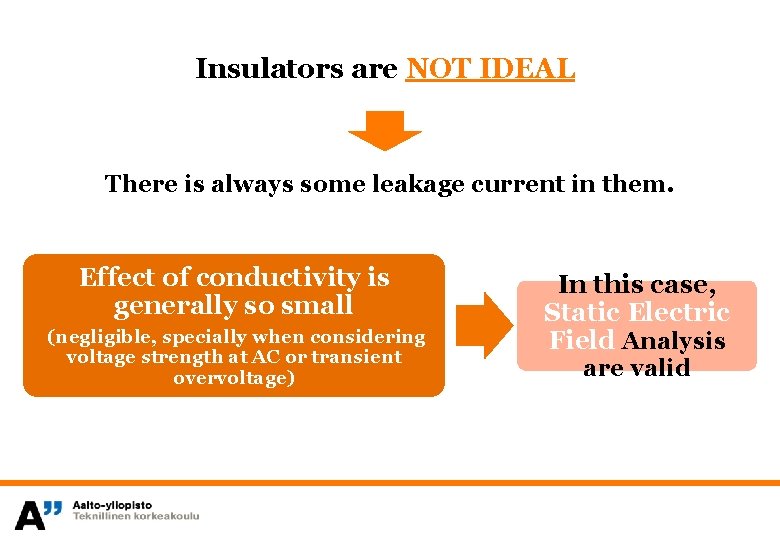
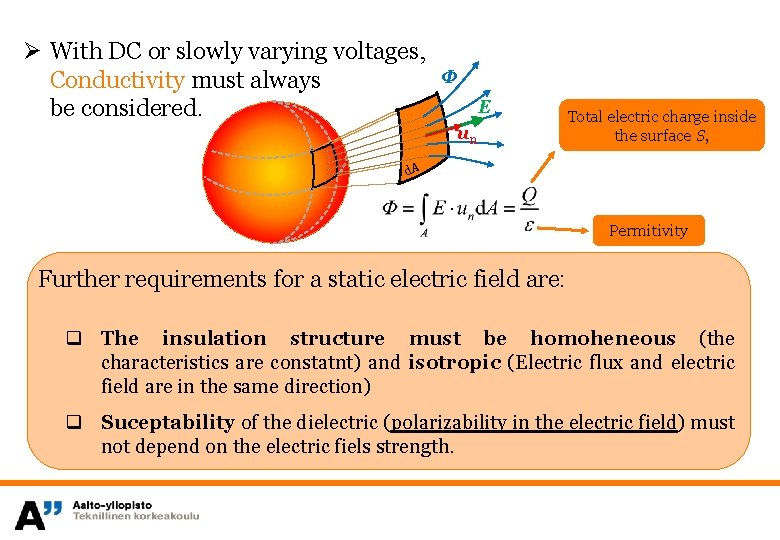
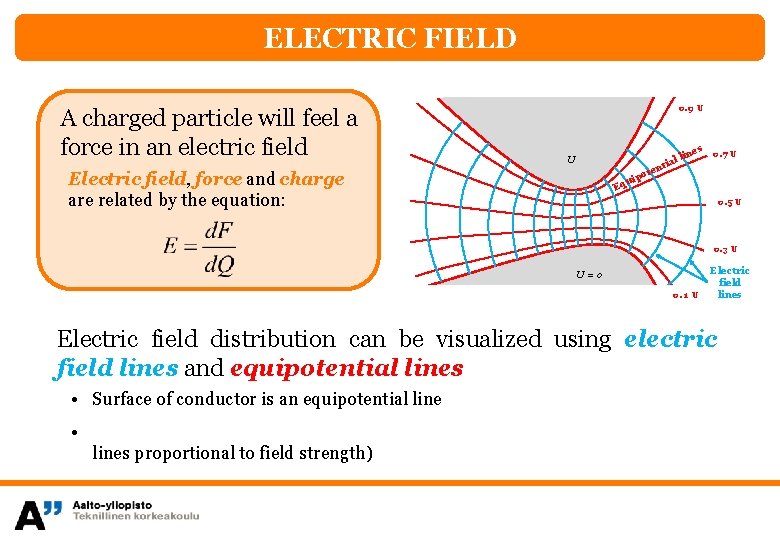
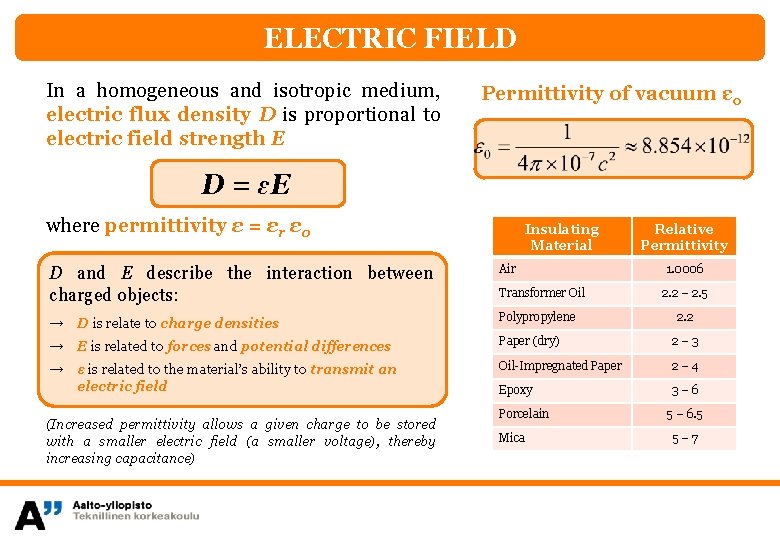
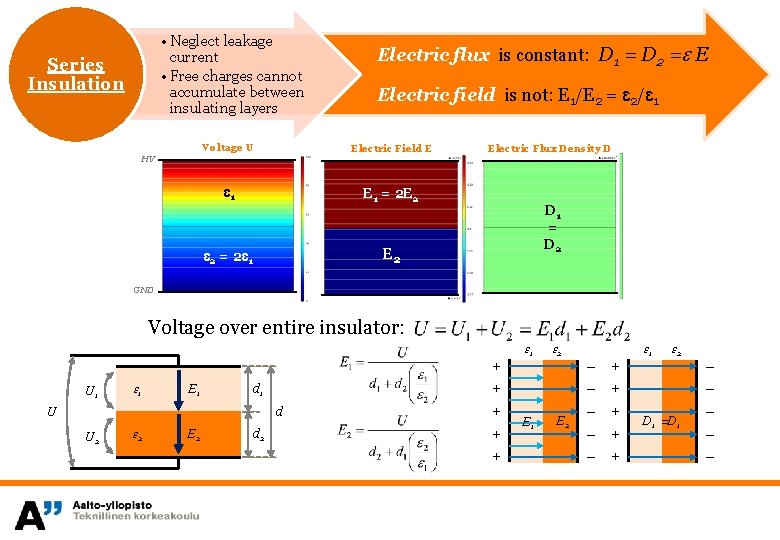
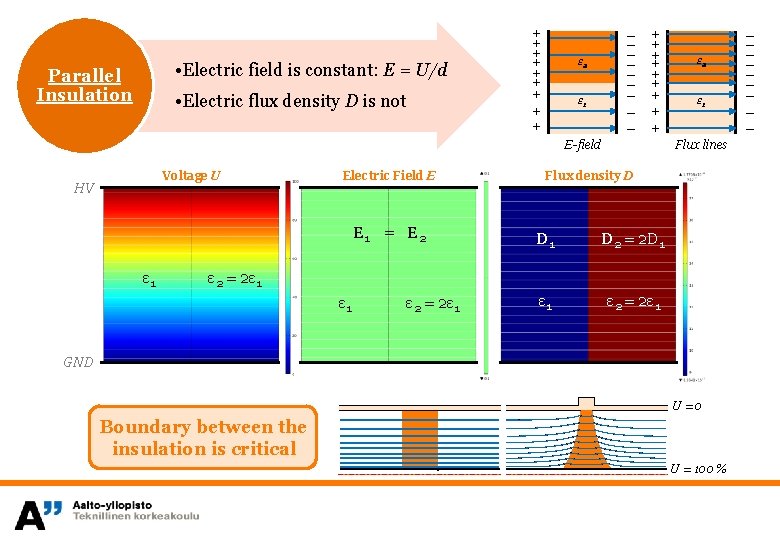
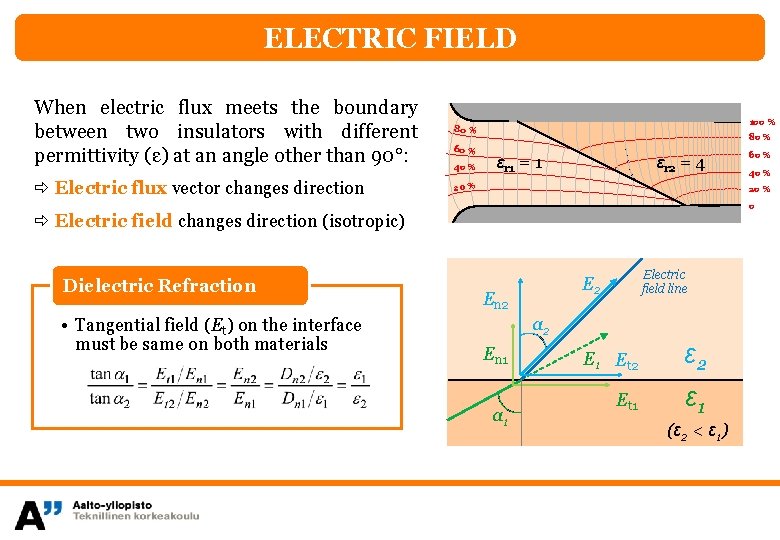
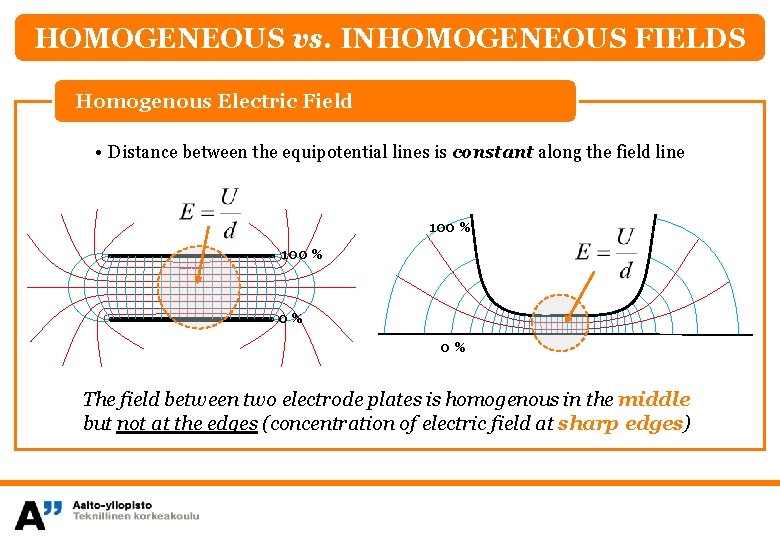
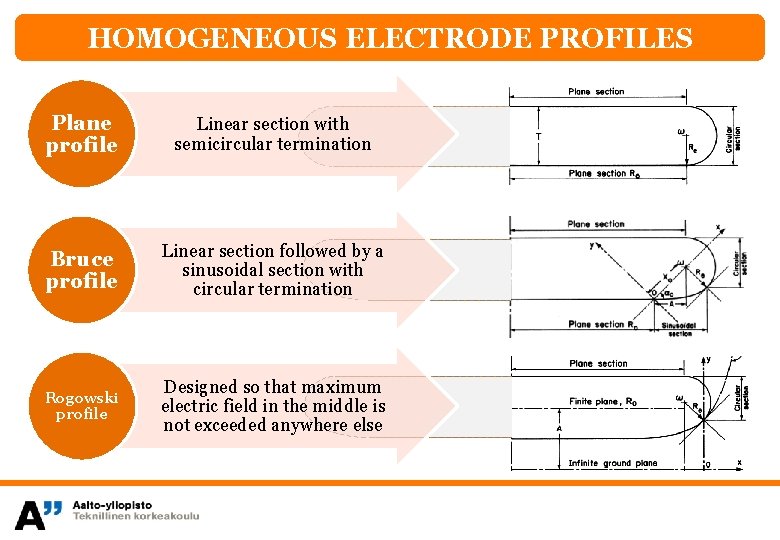
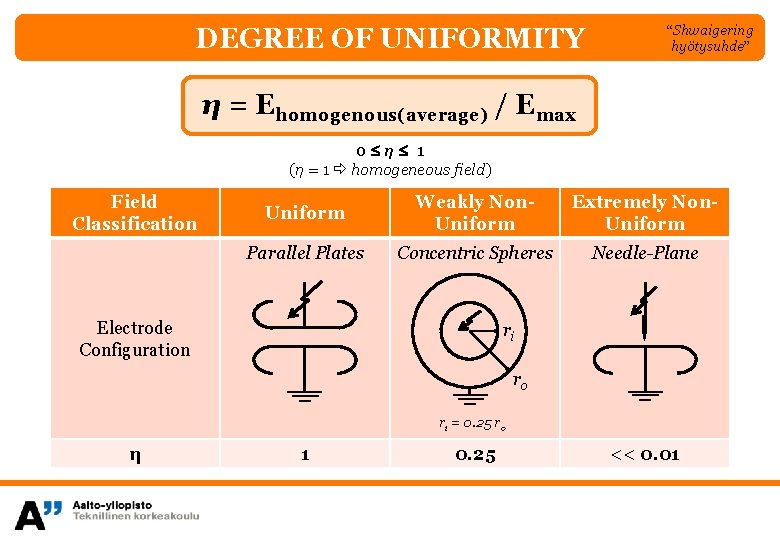
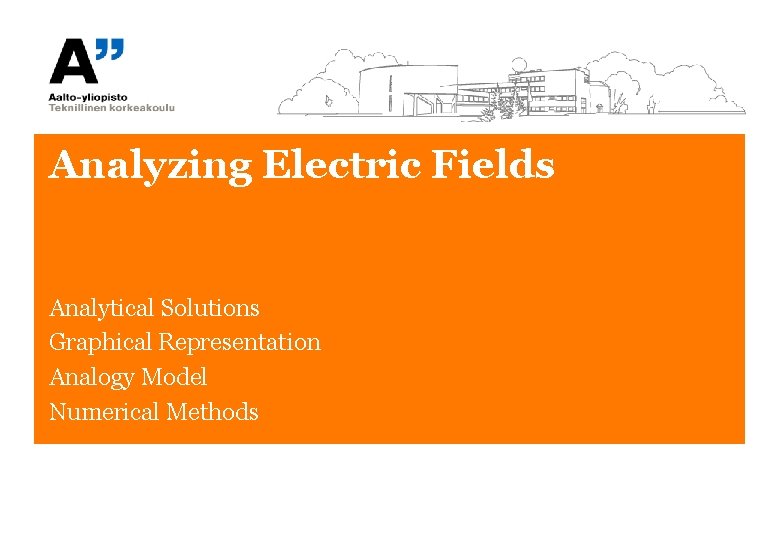
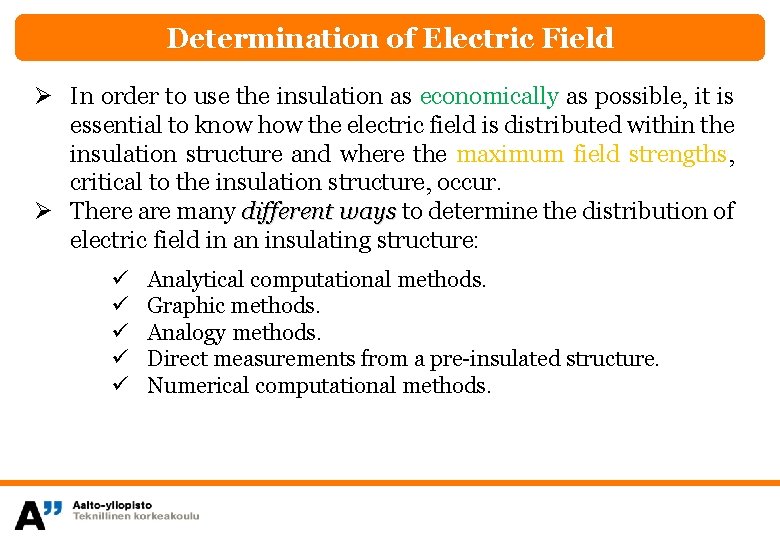
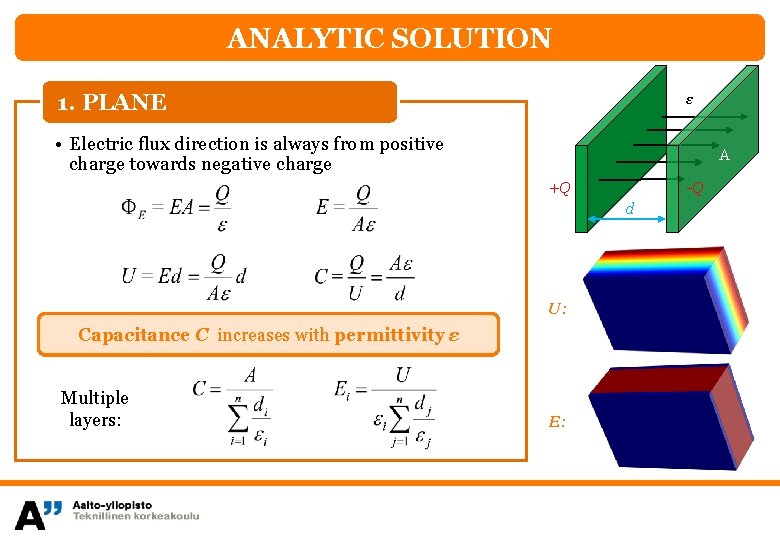
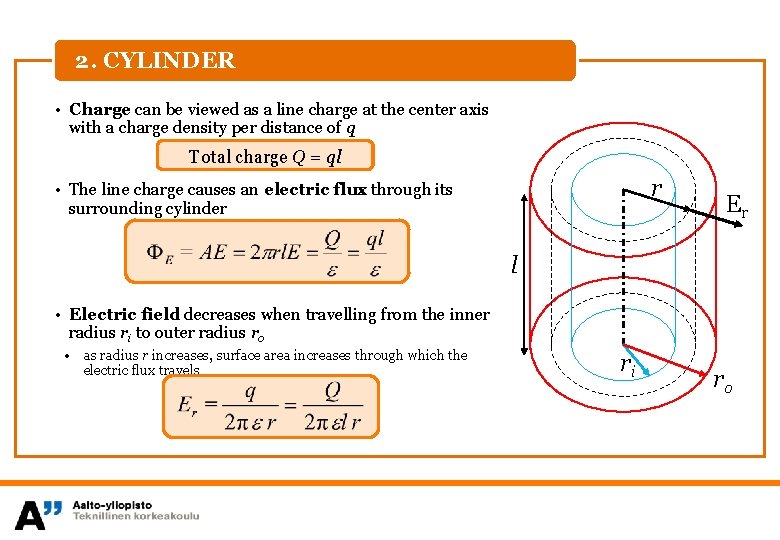
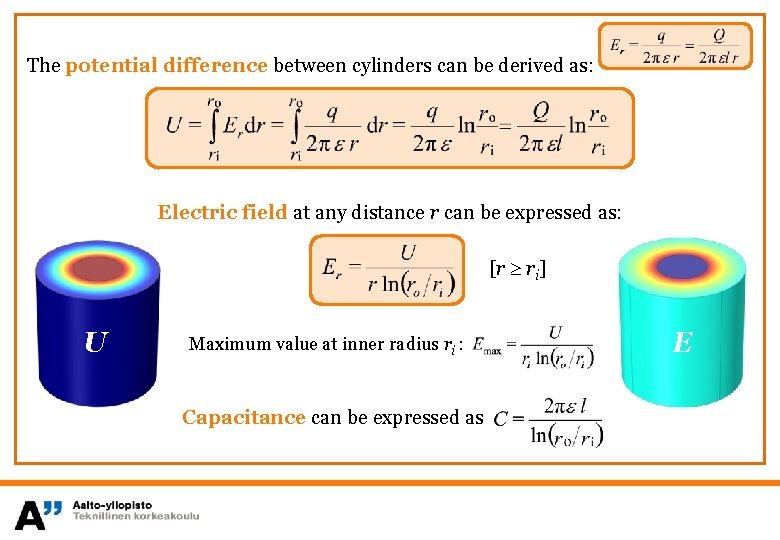
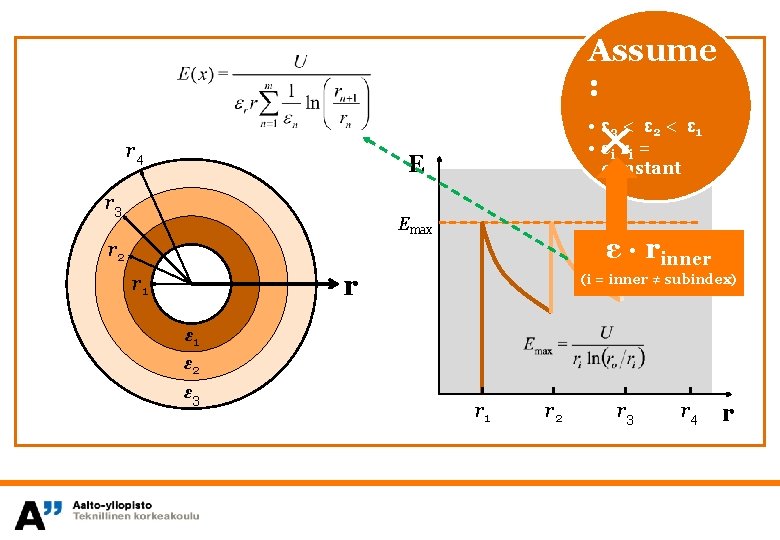
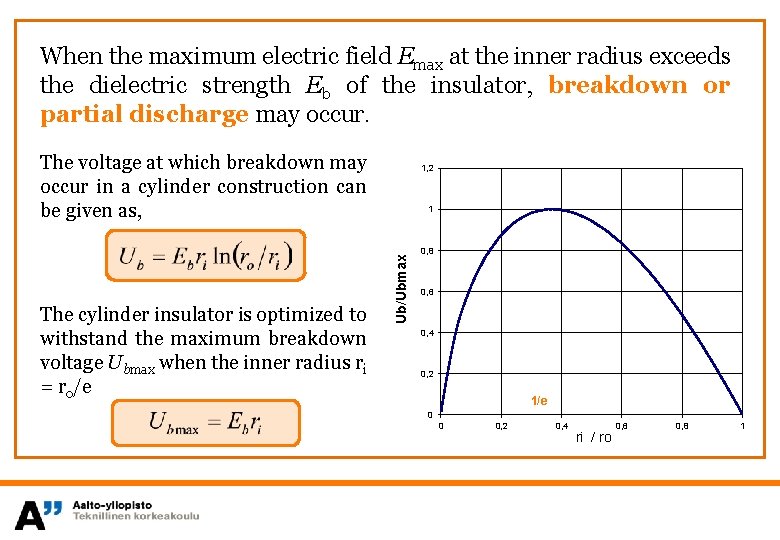
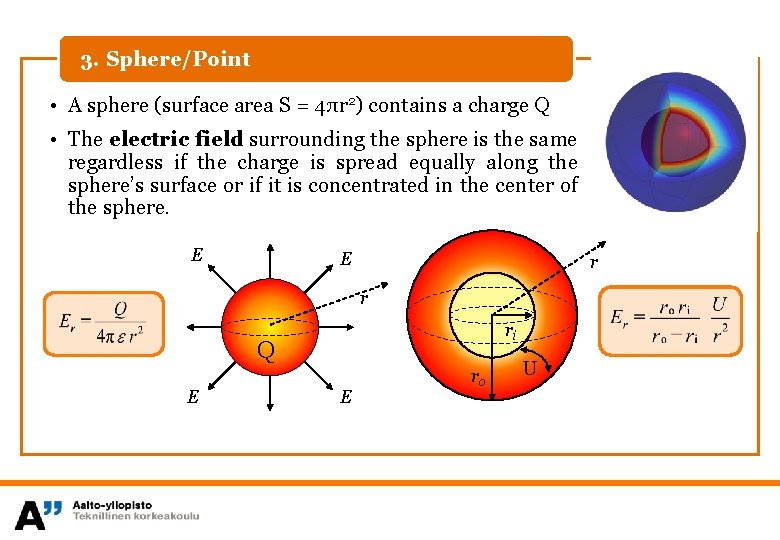
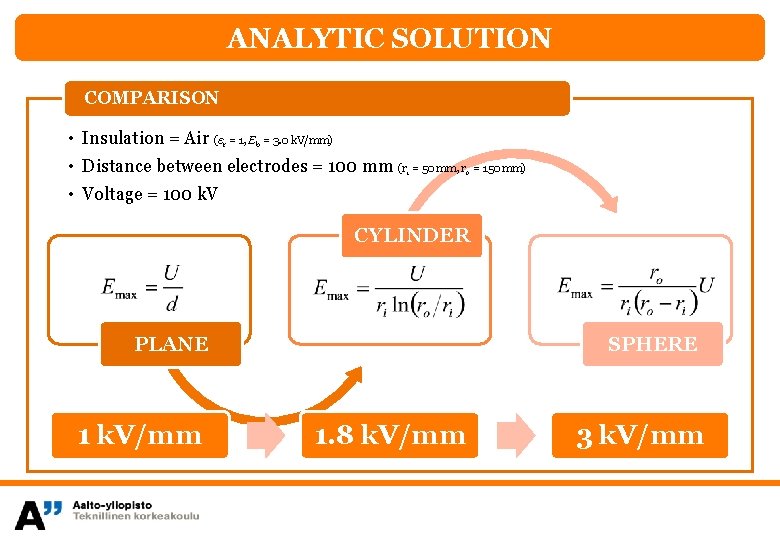
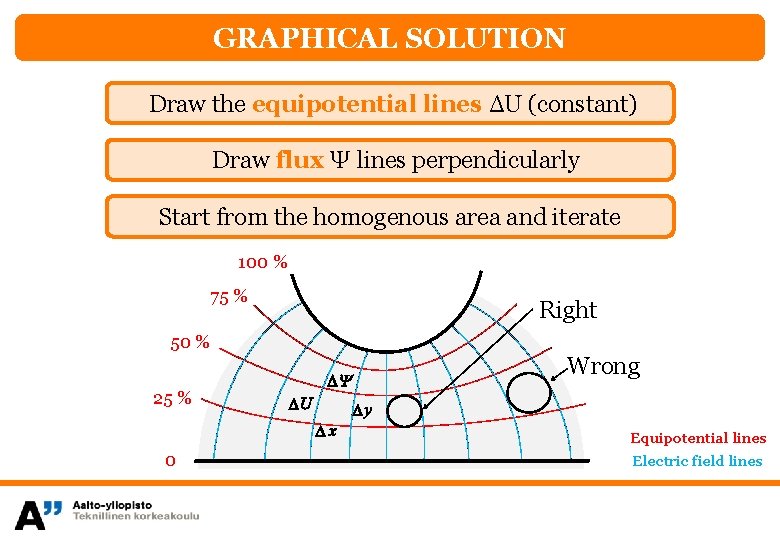
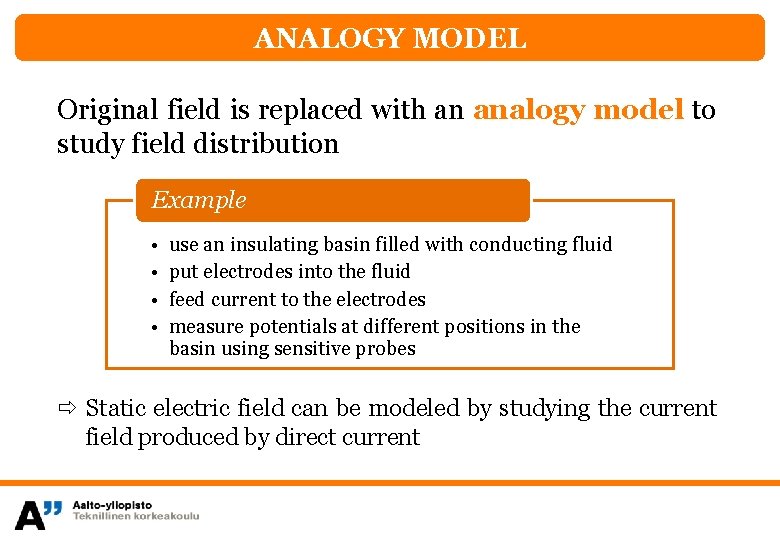
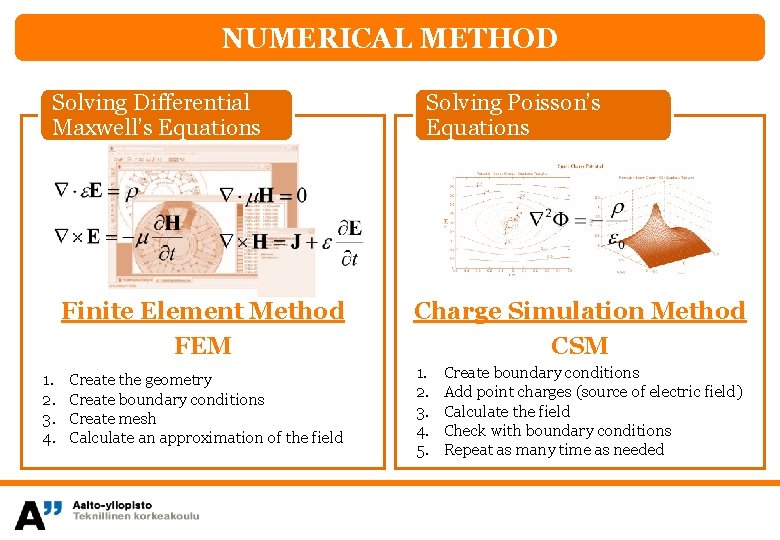
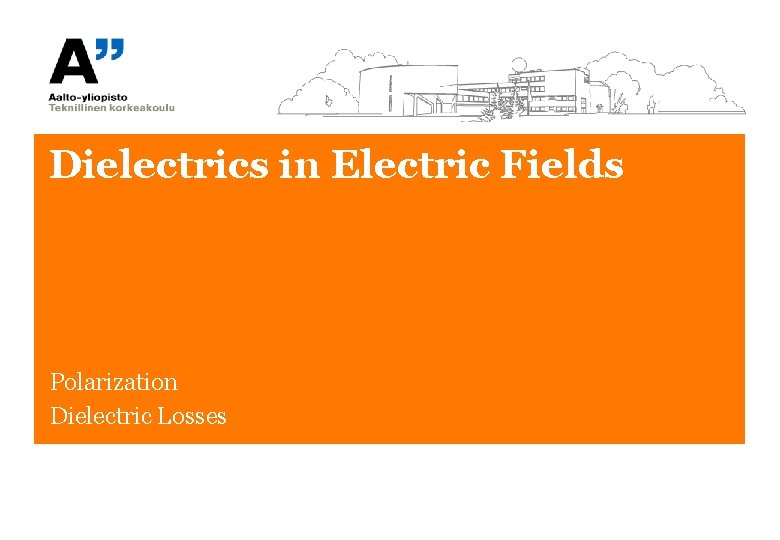
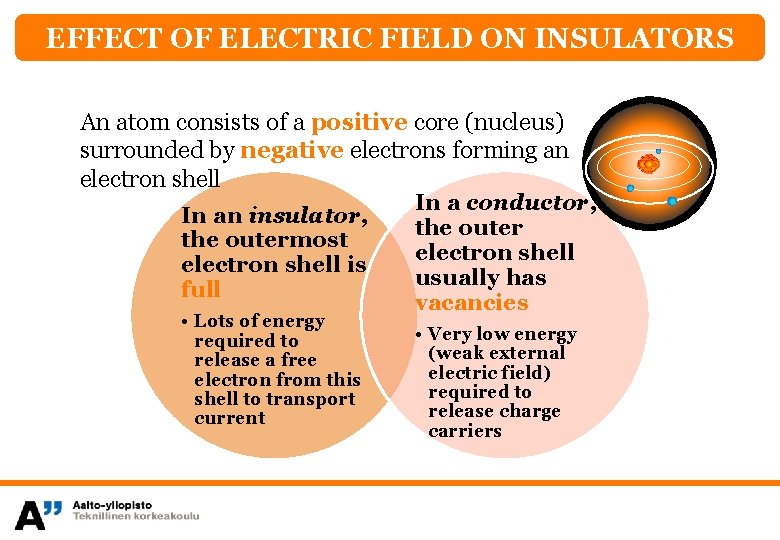
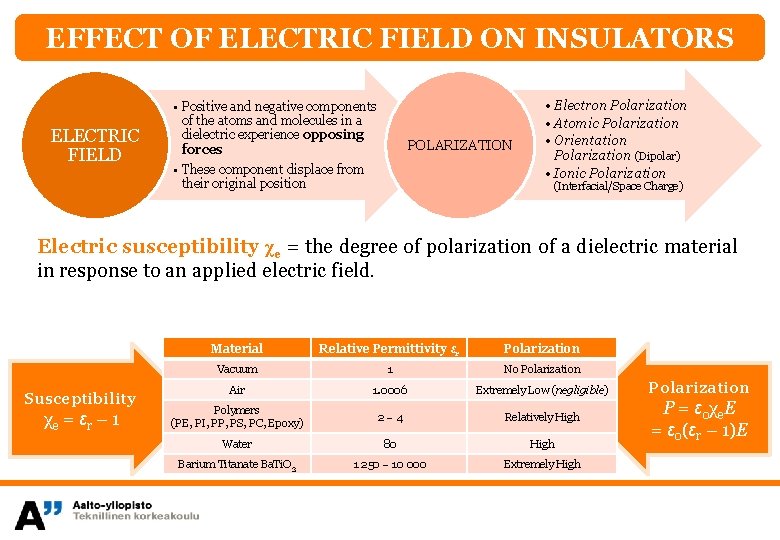
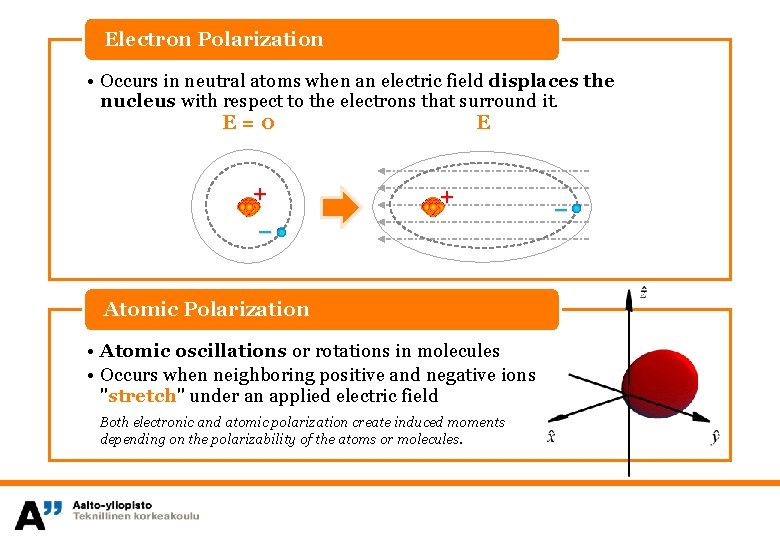
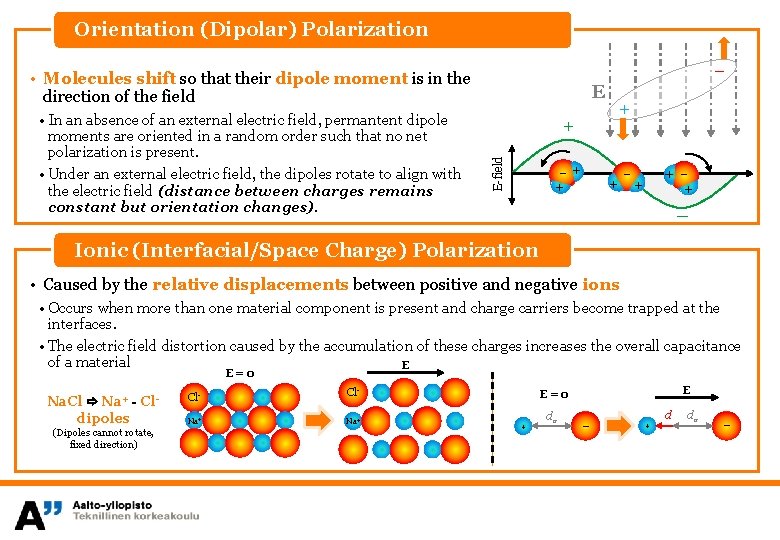
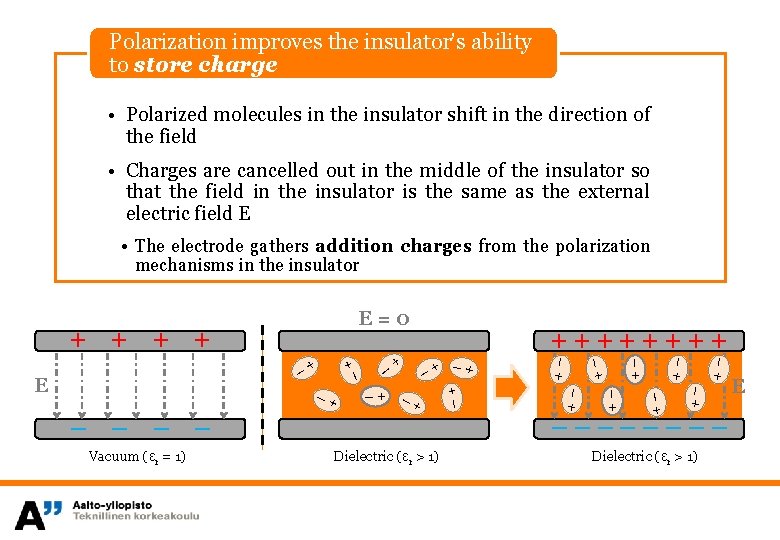
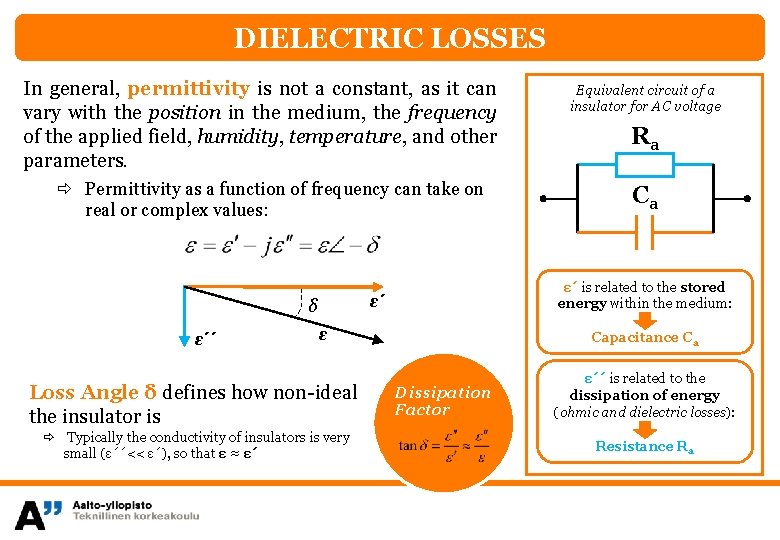
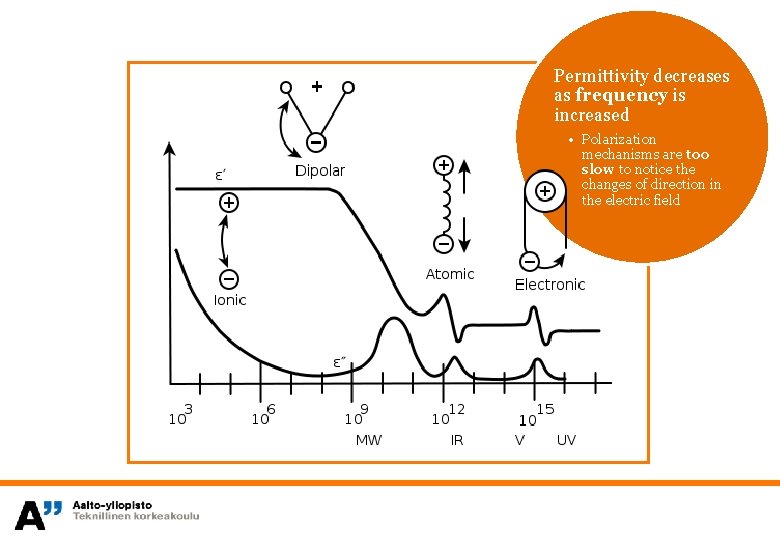
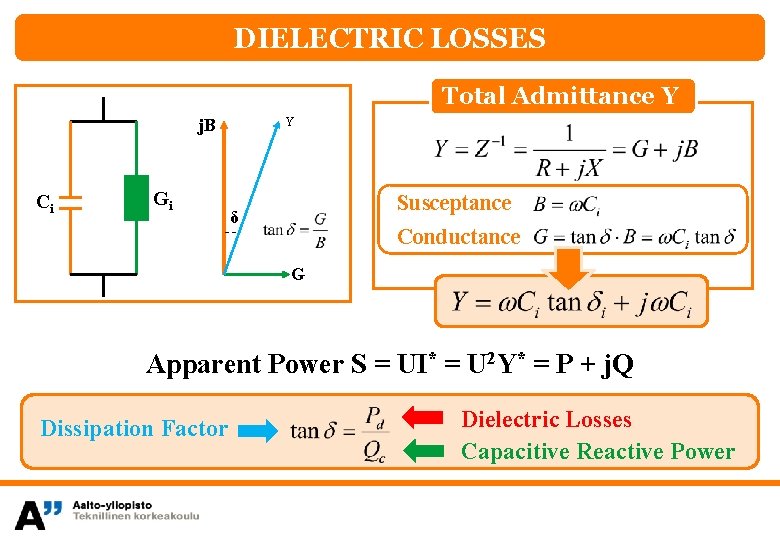
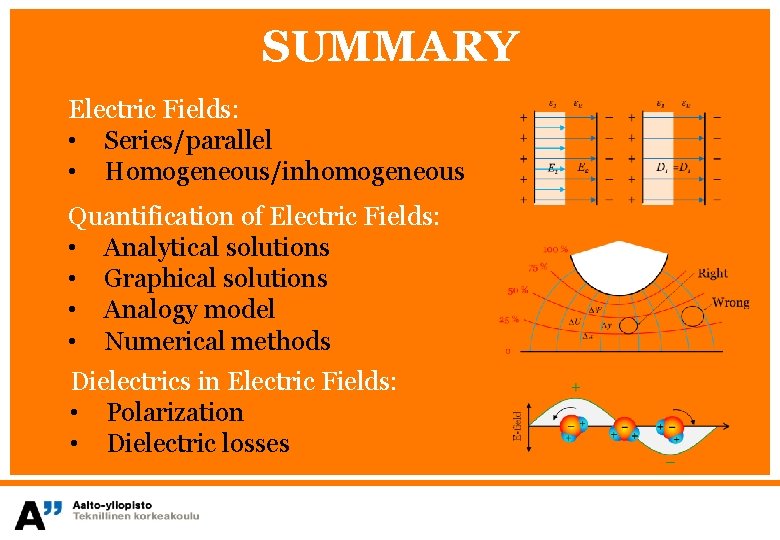
- Slides: 35
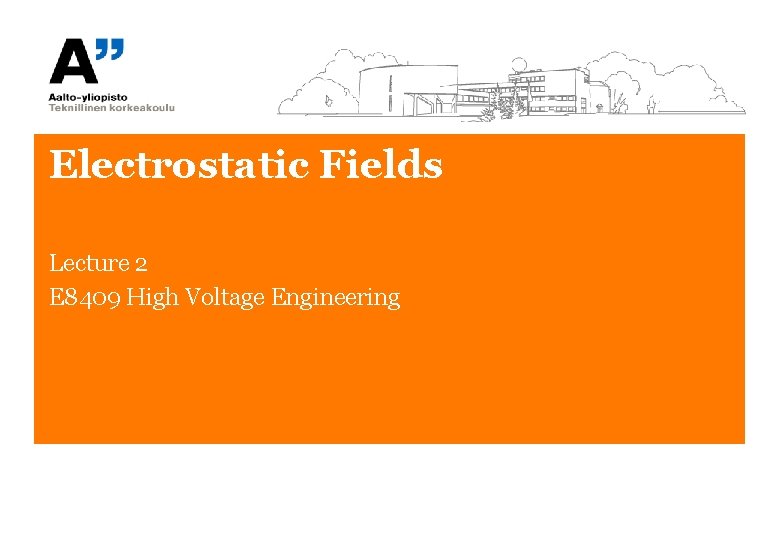
Electrostatic Fields Lecture 2 E 8409 High Voltage Engineering
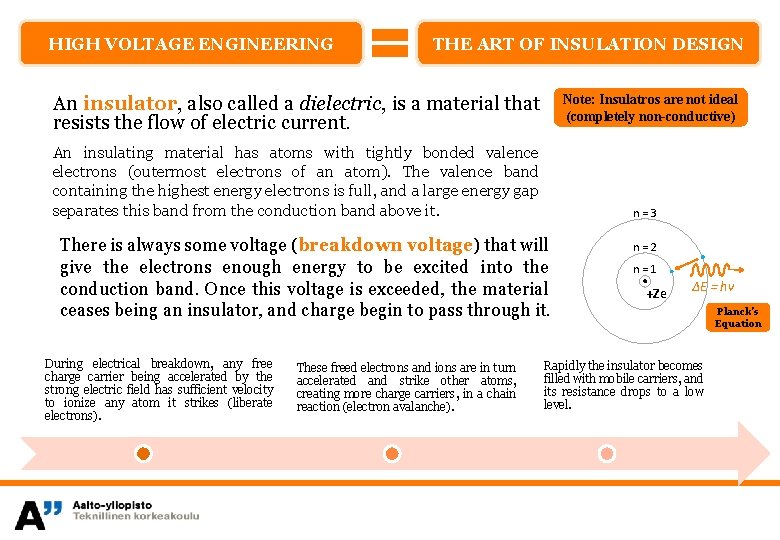
HIGH VOLTAGE ENGINEERING THE ART OF INSULATION DESIGN An insulator, also called a dielectric, is a material that resists the flow of electric current. Note: Insulatros are not ideal (completely non-conductive) An insulating material has atoms with tightly bonded valence electrons (outermost electrons of an atom). The valence band containing the highest energy electrons is full, and a large energy gap separates this band from the conduction band above it. n=3 There is always some voltage (breakdown voltage) that will give the electrons enough energy to be excited into the conduction band. Once this voltage is exceeded, the material ceases being an insulator, and charge begin to pass through it. During electrical breakdown, any free charge carrier being accelerated by the strong electric field has sufficient velocity to ionize any atom it strikes (liberate electrons). These freed electrons and ions are in turn accelerated and strike other atoms, creating more charge carriers, in a chain reaction (electron avalanche). n=2 n=1 +Ze ∆E = hv Rapidly the insulator becomes filled with mobile carriers, and its resistance drops to a low level. Planck’s Equation
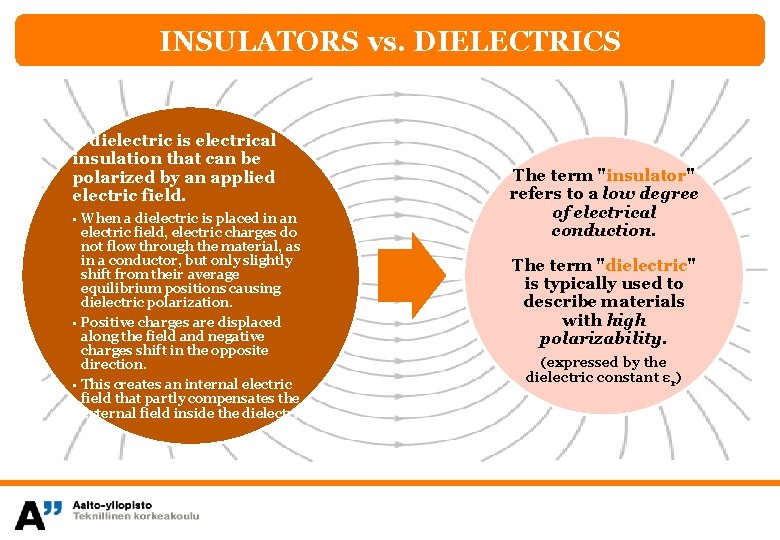
INSULATORS vs. DIELECTRICS A dielectric is electrical insulation that can be polarized by an applied electric field. • When a dielectric is placed in an electric field, electric charges do not flow through the material, as in a conductor, but only slightly shift from their average equilibrium positions causing dielectric polarization. • Positive charges are displaced along the field and negative charges shift in the opposite direction. • This creates an internal electric field that partly compensates the external field inside the dielectric. The term "insulator" refers to a low degree of electrical conduction. The term "dielectric" is typically used to describe materials with high polarizability. (expressed by the dielectric constant εr)
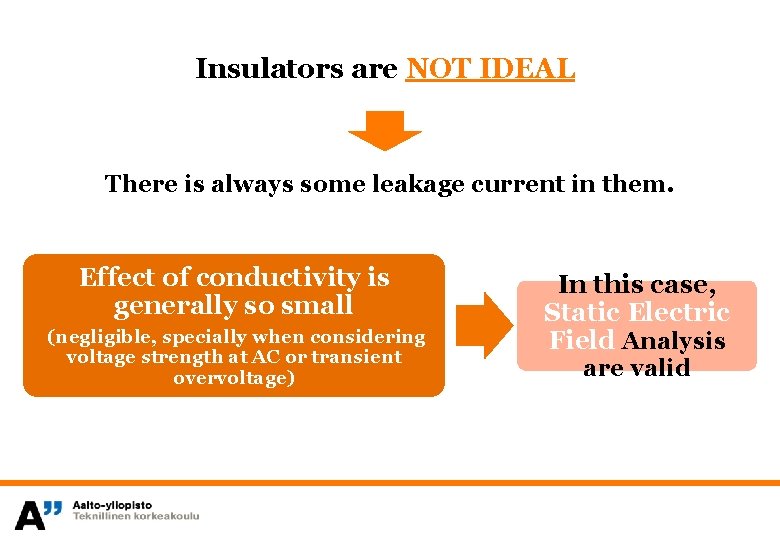
Insulators are NOT IDEAL There is always some leakage current in them. Effect of conductivity is generally so small (negligible, specially when considering voltage strength at AC or transient overvoltage) In this case, Static Electric Field Analysis are valid
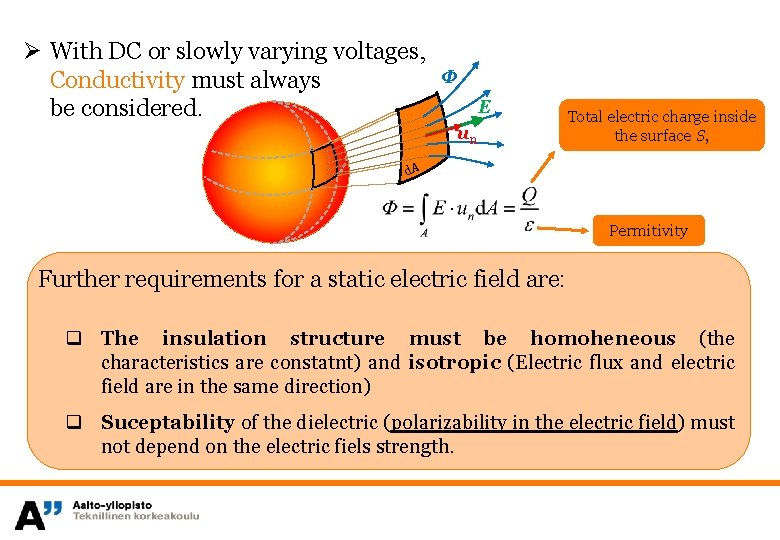
Ø With DC or slowly varying voltages, Conductivity must always be considered. Φ E un Total electric charge inside the surface S, d. A Permitivity Further requirements for a static electric field are: q The insulation structure must be homoheneous (the characteristics are constatnt) and isotropic (Electric flux and electric field are in the same direction) q Suceptability of the dielectric (polarizability in the electric field) must not depend on the electric fiels strength.
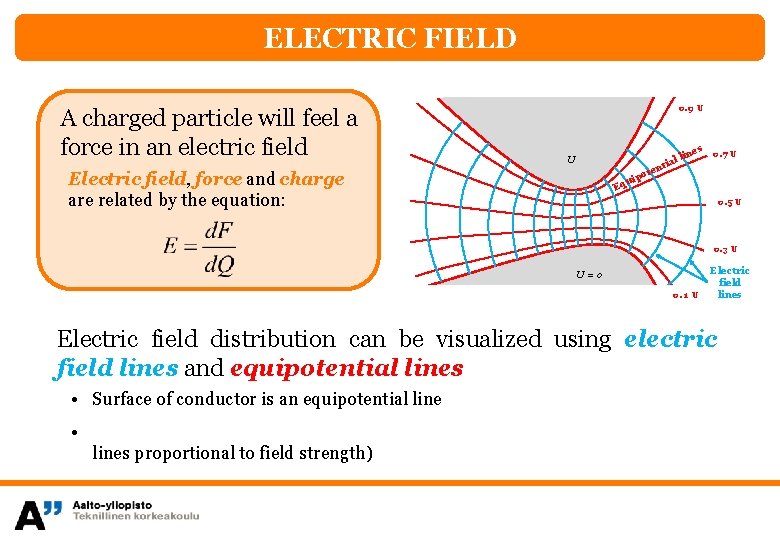
ELECTRIC FIELD A charged particle will feel a force in an electric field 0. 9 U s ne l li tia n ote U Electric field, force and charge are related by the equation: 0. 7 U uip Eq 0. 5 U 0. 3 U U=0 0. 1 U Electric field lines Electric field distribution can be visualized using electric field lines and equipotential lines • Surface of conductor is an equipotential line • lines proportional to field strength)
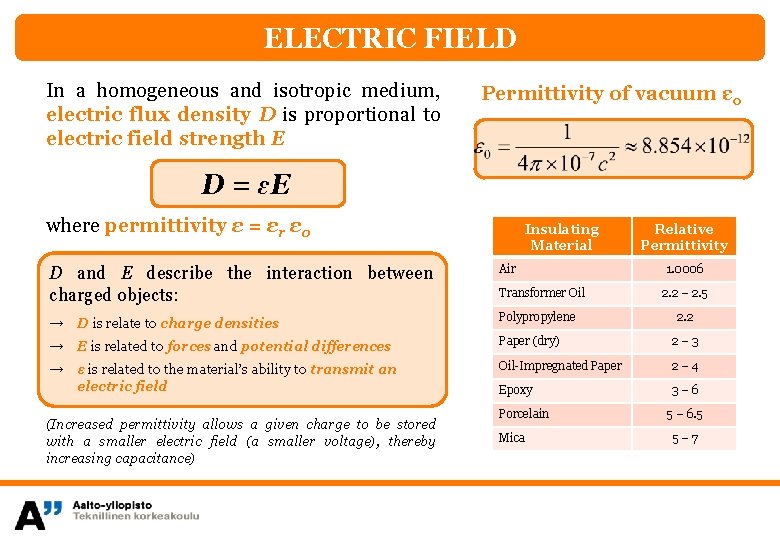
ELECTRIC FIELD In a homogeneous and isotropic medium, electric flux density D is proportional to electric field strength E Permittivity of vacuum ε 0 D = ɛE where permittivity ε = εr ε 0 Insulating Material Relative Permittivity D and E describe the interaction between charged objects: Air → D is relate to charge densities Polypropylene → E is related to forces and potential differences Paper (dry) 2– 3 → ε is related to the material’s ability to transmit an electric field Oil-Impregnated Paper 2– 4 Epoxy 3– 6 (Increased permittivity allows a given charge to be stored with a smaller electric field (a smaller voltage), thereby increasing capacitance) Transformer Oil Porcelain Mica 1. 0006 2. 2 – 2. 5 2. 2 5 – 6. 5 5– 7
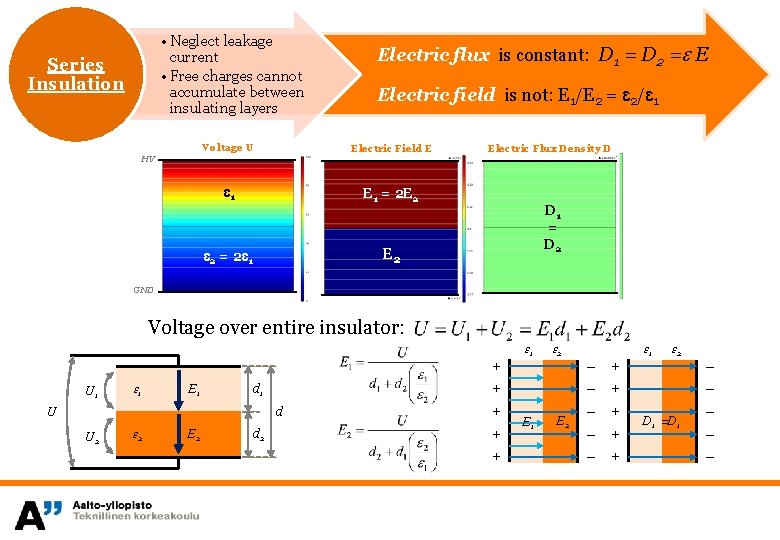
• Neglect leakage current • Free charges cannot accumulate between insulating layers Series Insulation HV Electric flux is constant: D 1 = D 2 = E Electric field is not: E 1/E 2 = ɛ 2/ɛ 1 Voltage U Electric Field E ɛ 1 E 1 = 2 E 2 Electric Flux Density D D 1 = D 2 E 2 ɛ 2 = 2ɛ 1 GND Voltage over entire insulator: + U 1 ɛ 1 E 1 d U 2 ɛ 2 E 2 d 2 ɛ 2 + d 1 U ɛ 1 + + + E 1 E 2 – + – + – + ɛ 1 ɛ 2 – – D 1 =D 1 – – –
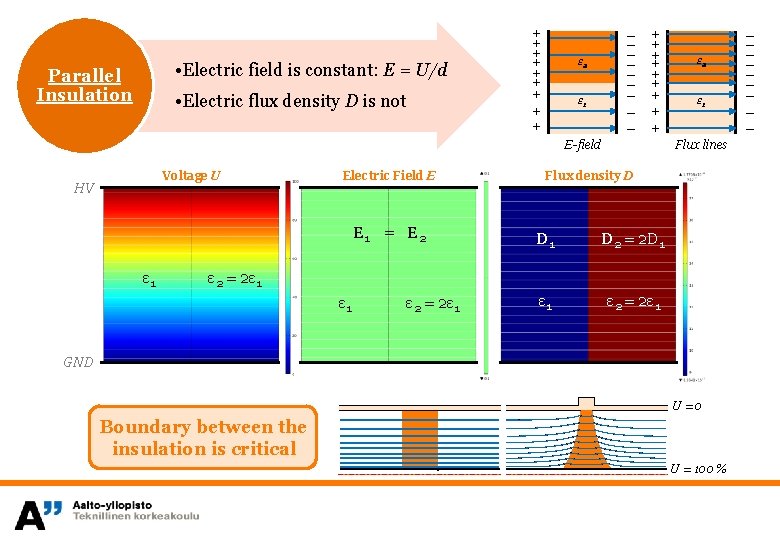
• Electric field is constant: E = U/d Parallel Insulation • Electric flux density D is not + + + + + ɛ 2 ɛ 1 – – – – – + + + + + E-field Voltage U HV Electric Field E E 1 = E 2 ε 1 ɛ 2 ɛ 1 Flux lines Flux density D D 1 D 2 = 2 D 1 ε 2 = 2ε 1 ε 1 ε 2 = 2ε 1 GND U=0 Boundary between the insulation is critical U = 100 % – – – – –
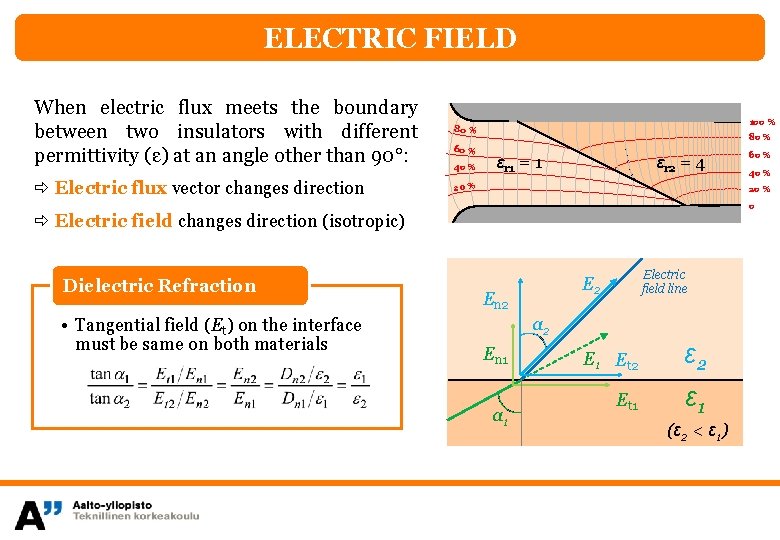
ELECTRIC FIELD When electric flux meets the boundary between two insulators with different permittivity (ε) at an angle other than 90°: Electric flux vector changes direction 100 % 80 % 60 % 40 % 80 % ɛr 1 = 1 ɛr 2 = 4 20 % • Tangential field (Et) on the interface must be same on both materials 40 % 20 % 0 Electric field changes direction (isotropic) Dielectric Refraction 60 % Electric field line E 2 En 2 α 2 En 1 α 1 Et 2 ɛ 2 Et 1 ɛ 1 (ɛ 2 < ɛ 1)
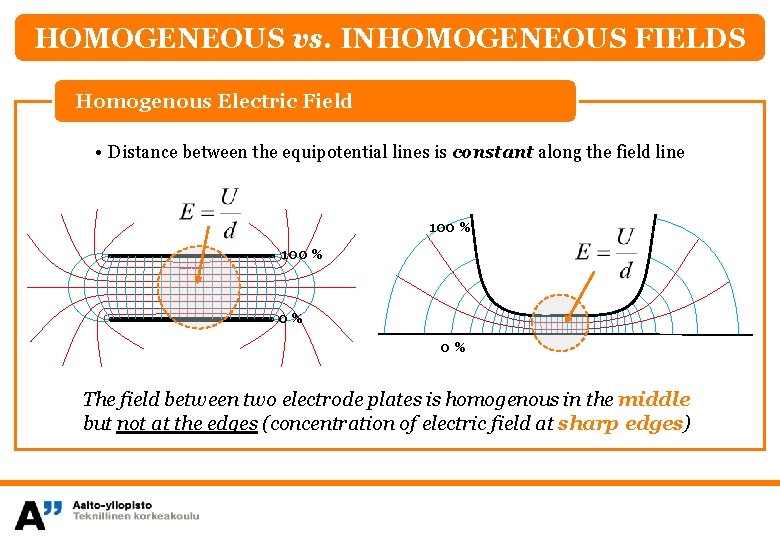
HOMOGENEOUS vs. INHOMOGENEOUS FIELDS Homogenous Electric Field • Distance between the equipotential lines is constant along the field line 100 % 0% 0% The field between two electrode plates is homogenous in the middle but not at the edges (concentration of electric field at sharp edges)
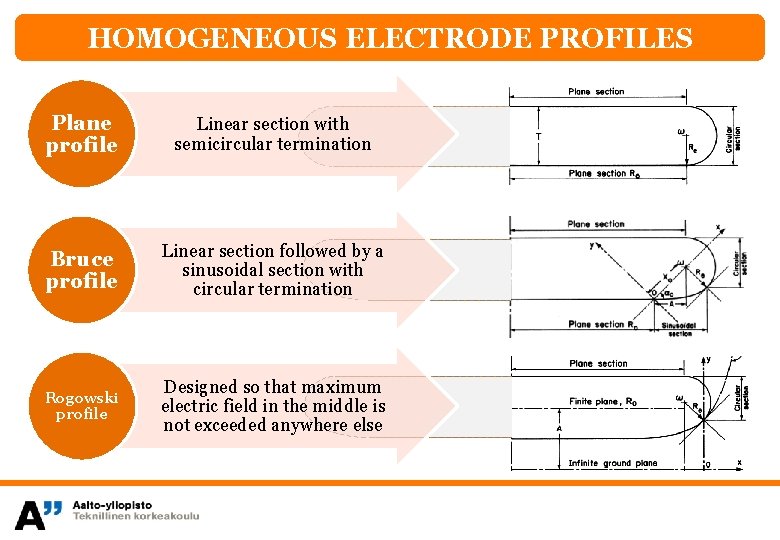
HOMOGENEOUS ELECTRODE PROFILES Plane profile Linear section with semicircular termination Bruce profile Linear section followed by a sinusoidal section with circular termination Rogowski profile Designed so that maximum electric field in the middle is not exceeded anywhere else
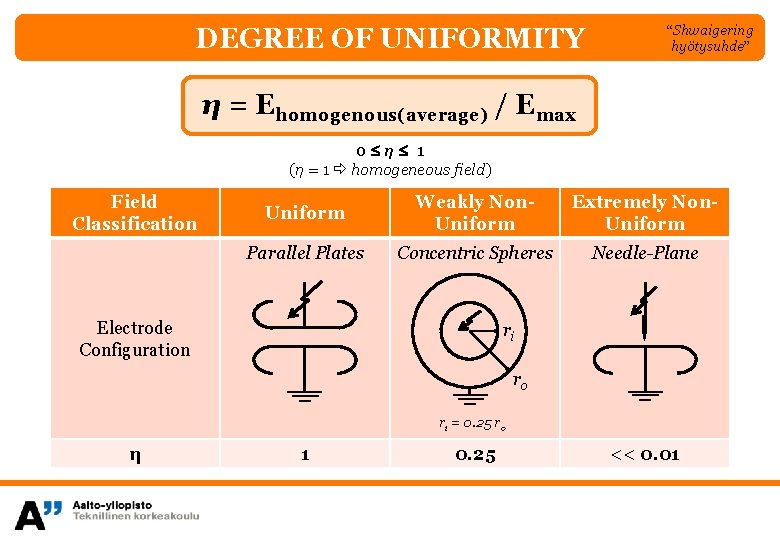
DEGREE OF UNIFORMITY “Shwaigering hyötysuhde” η = Ehomogenous(average) / Emax 0 η 1 (η = 1 homogeneous field) Field Classification Uniform Weakly Non. Uniform Extremely Non. Uniform Parallel Plates Concentric Spheres Needle-Plane Electrode Configuration ri ro ri = 0. 25 ro η 1 0. 25 << 0. 01
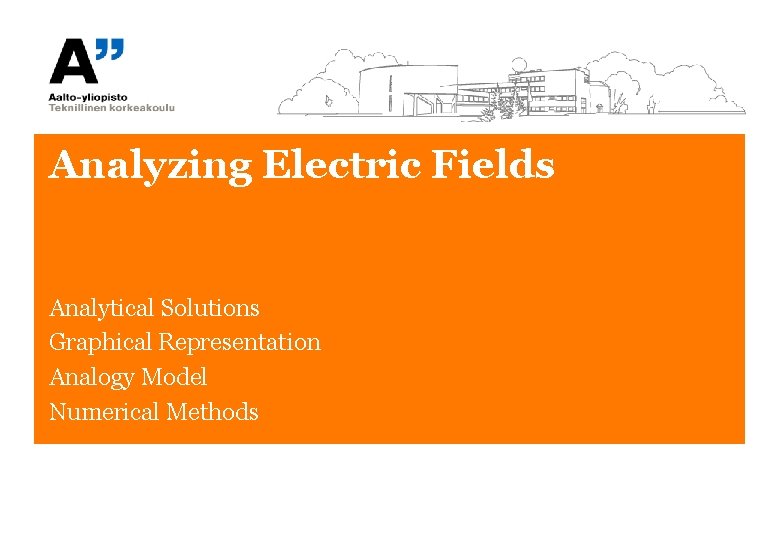
Analyzing Electric Fields Analytical Solutions Graphical Representation Analogy Model Numerical Methods
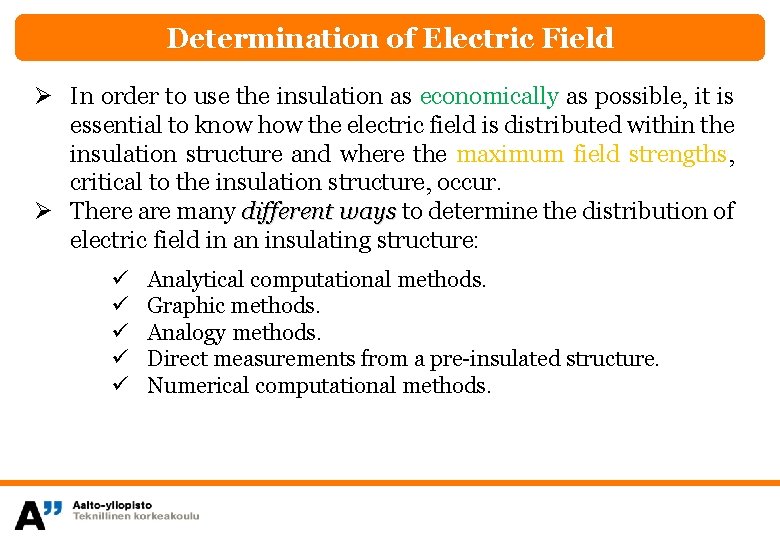
Determination of Electric Field Ø In order to use the insulation as economically as possible, it is essential to know how the electric field is distributed within the insulation structure and where the maximum field strengths, critical to the insulation structure, occur. Ø There are many different ways to determine the distribution of electric field in an insulating structure: ü ü ü Analytical computational methods. Graphic methods. Analogy methods. Direct measurements from a pre-insulated structure. Numerical computational methods.
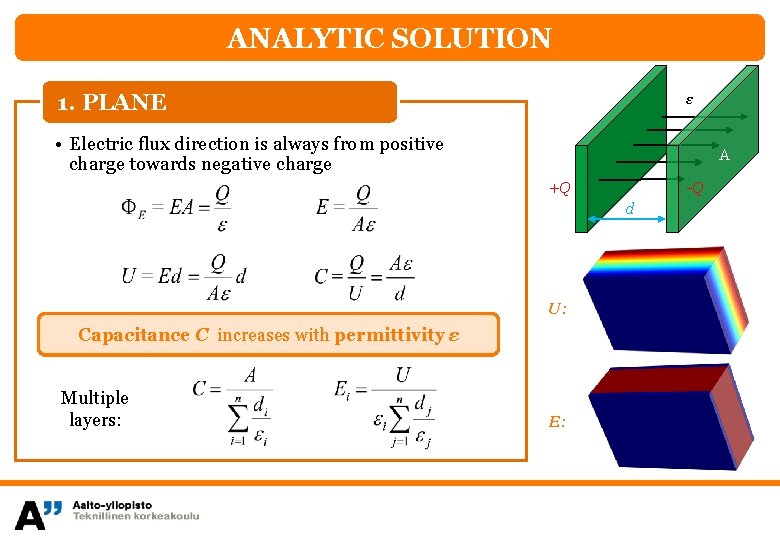
ANALYTIC SOLUTION 1. PLANE ɛ • Electric flux direction is always from positive charge towards negative charge A +Q -Q d U: Capacitance C increases with permittivity ε Multiple layers: E:
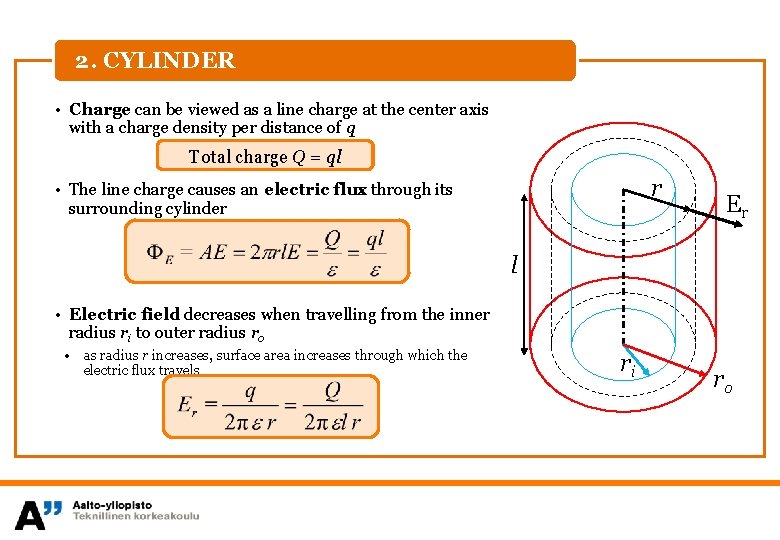
2. CYLINDER • Charge can be viewed as a line charge at the center axis with a charge density per distance of q Total charge Q = ql r • The line charge causes an electric flux through its surrounding cylinder Er l • Electric field decreases when travelling from the inner radius ri to outer radius ro • as radius r increases, surface area increases through which the electric flux travels ri ro
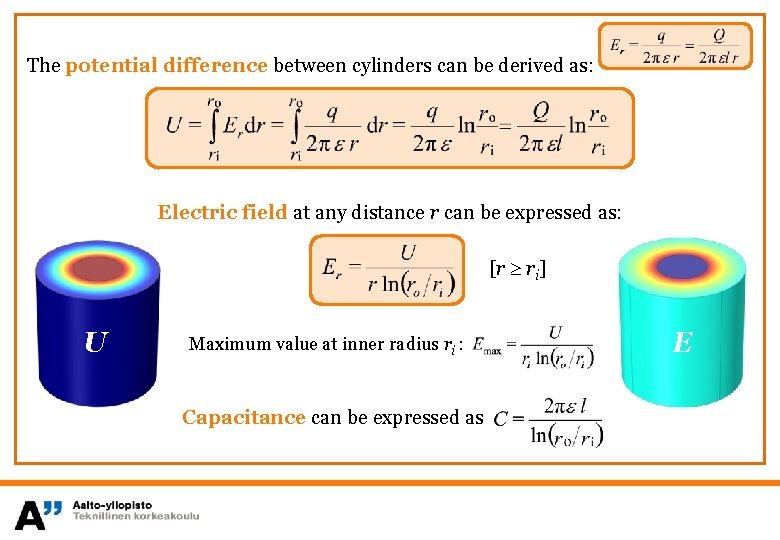
The potential difference between cylinders can be derived as: Electric field at any distance r can be expressed as: [r ri] U Maximum value at inner radius ri : Capacitance can be expressed as E
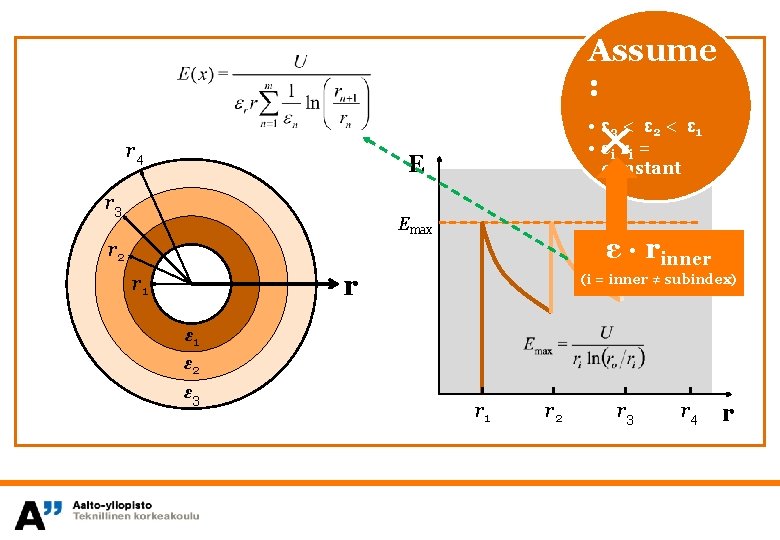
Assume : r 4 • ɛ 3 < ɛ 2 < ɛ 1 • εi r i = constant E r 3 Emax ε ∙ rinner r 2 r r 1 ɛ 2 ɛ 3 (i = inner ≠ subindex) r 1 r 2 r 3 r 4 r
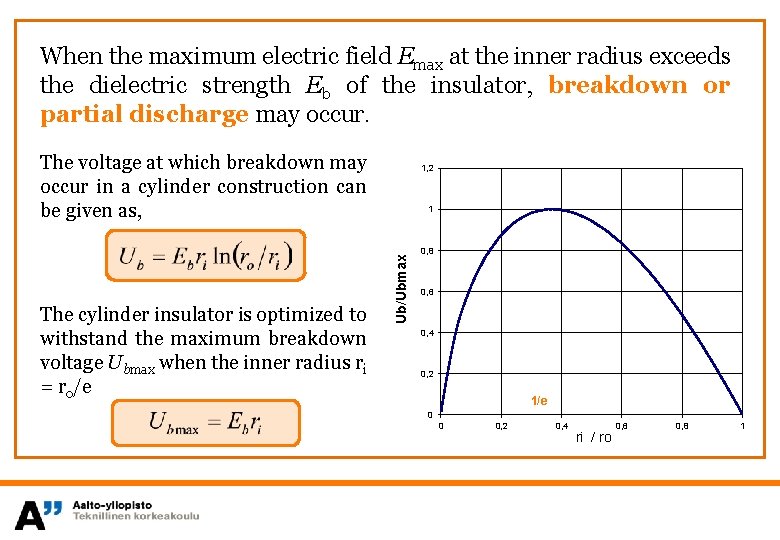
When the maximum electric field Emax at the inner radius exceeds the dielectric strength Eb of the insulator, breakdown or partial discharge may occur. The voltage at which breakdown may occur in a cylinder construction can be given as, 1 Ub/Ubmax The cylinder insulator is optimized to withstand the maximum breakdown voltage Ubmax when the inner radius ri = ro/e 1, 2 0, 8 0, 6 0, 4 0, 2 1/e 0 0 0, 2 0, 4 ri / ro 0, 6 0, 8 1
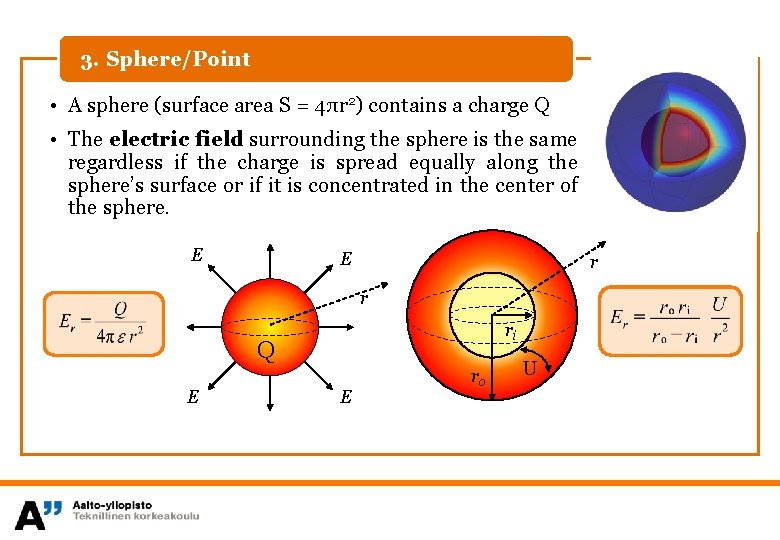
3. Sphere/Point • A sphere (surface area S = 4πr 2) contains a charge Q • The electric field surrounding the sphere is the same regardless if the charge is spread equally along the sphere’s surface or if it is concentrated in the center of the sphere. E E r r ri Q E E ro U
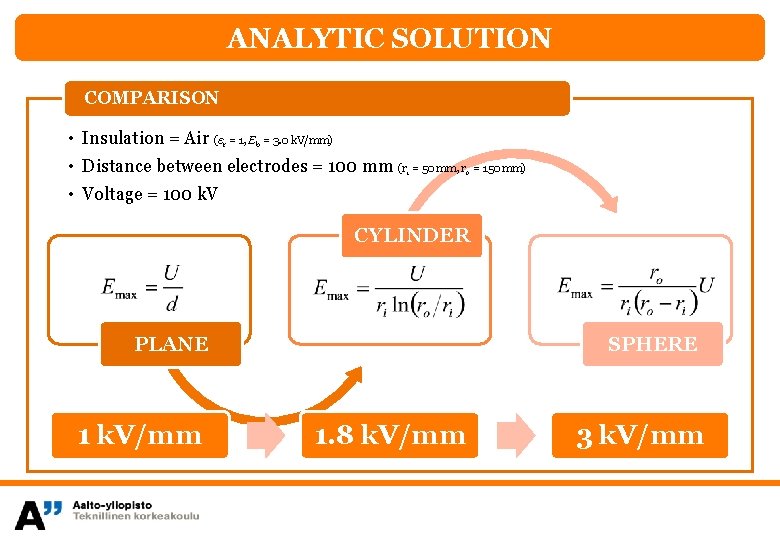
ANALYTIC SOLUTION COMPARISON • Insulation = Air (ε = 1, E = 3. 0 k. V/mm) • Distance between electrodes = 100 mm (r = 50 mm, r • Voltage = 100 k. V r b i o = 150 mm) CYLINDER PLANE 1 k. V/mm SPHERE 1. 8 k. V/mm 3 k. V/mm
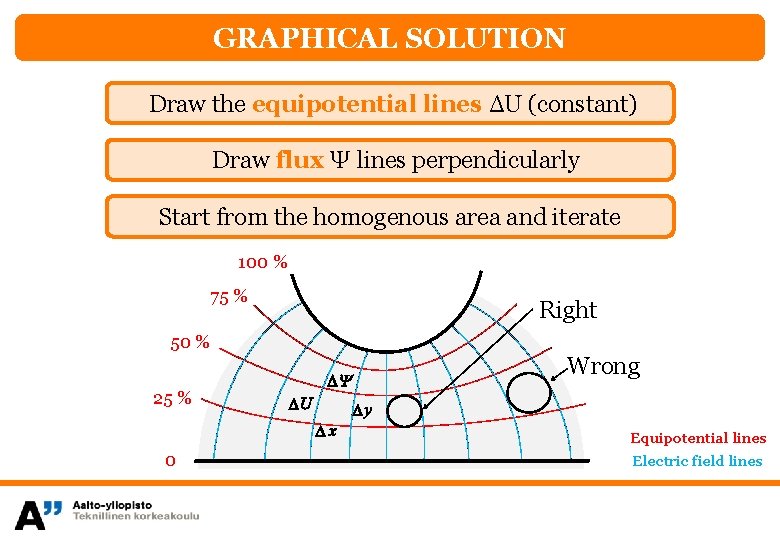
GRAPHICAL SOLUTION Draw the equipotential lines ΔU (constant) Draw flux Ψ lines perpendicularly Start from the homogenous area and iterate 100 % 75 % Right 50 % Wrong 25 % Equipotential lines 0 Electric field lines
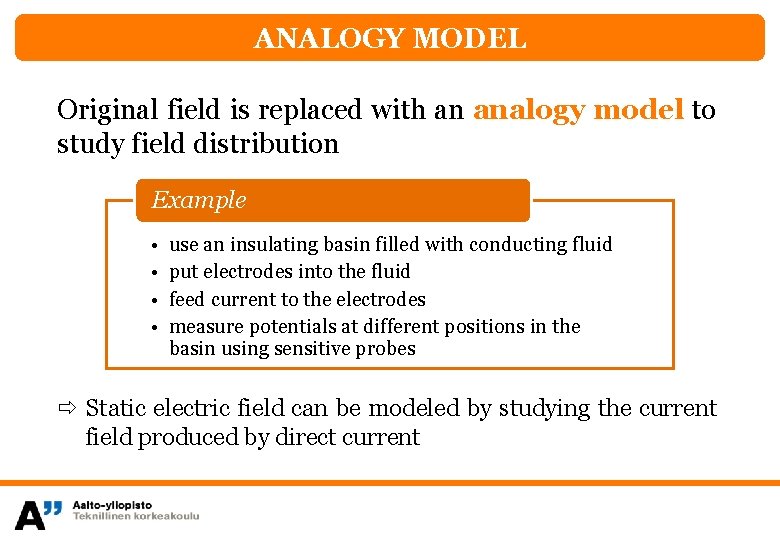
ANALOGY MODEL Original field is replaced with an analogy model to study field distribution Example • • use an insulating basin filled with conducting fluid put electrodes into the fluid feed current to the electrodes measure potentials at different positions in the basin using sensitive probes Static electric field can be modeled by studying the current field produced by direct current
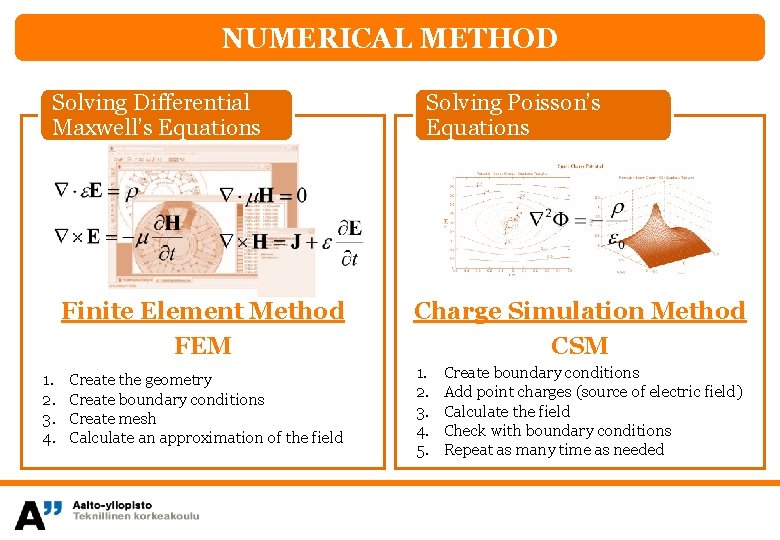
NUMERICAL METHOD Solving Differential Maxwell’s Equations Finite Element Method FEM 1. 2. 3. 4. Create the geometry Create boundary conditions Create mesh Calculate an approximation of the field Solving Poisson’s Equations Charge Simulation Method CSM 1. 2. 3. 4. 5. Create boundary conditions Add point charges (source of electric field) Calculate the field Check with boundary conditions Repeat as many time as needed
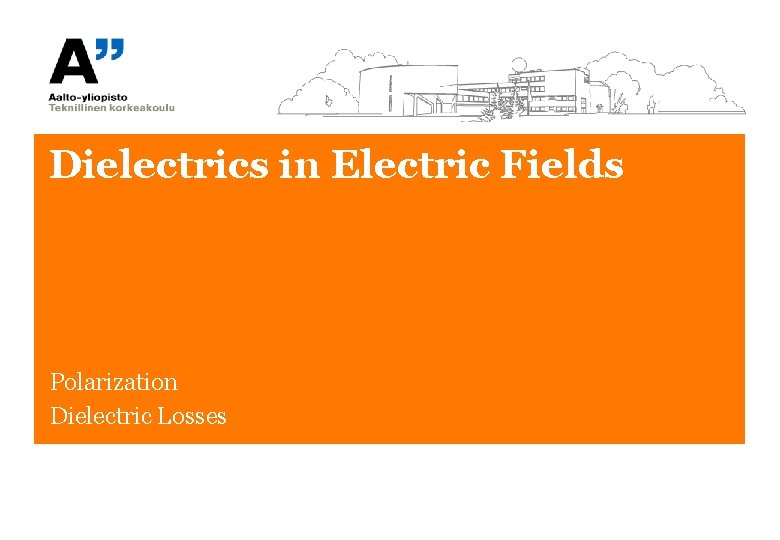
Dielectrics in Electric Fields Polarization Dielectric Losses
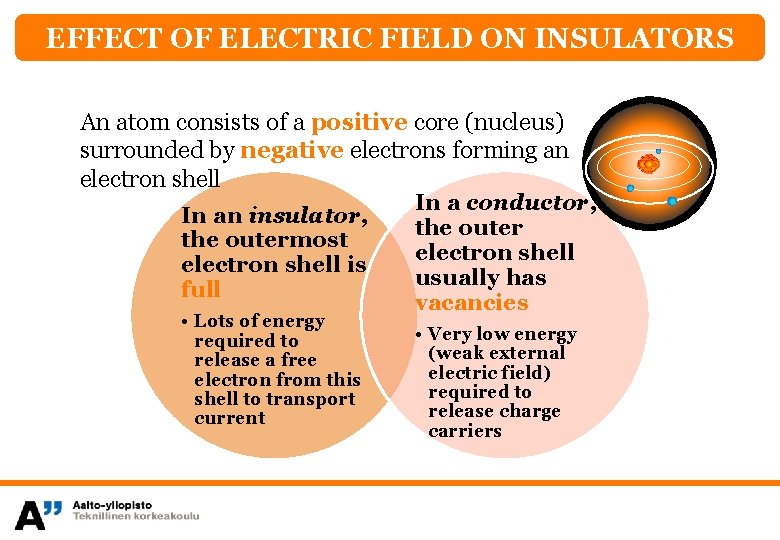
EFFECT OF ELECTRIC FIELD ON INSULATORS An atom consists of a positive core (nucleus) surrounded by negative electrons forming an electron shell In a conductor, In an insulator, the outermost electron shell is usually has full vacancies • Lots of energy required to release a free electron from this shell to transport current • Very low energy (weak external electric field) required to release charge carriers
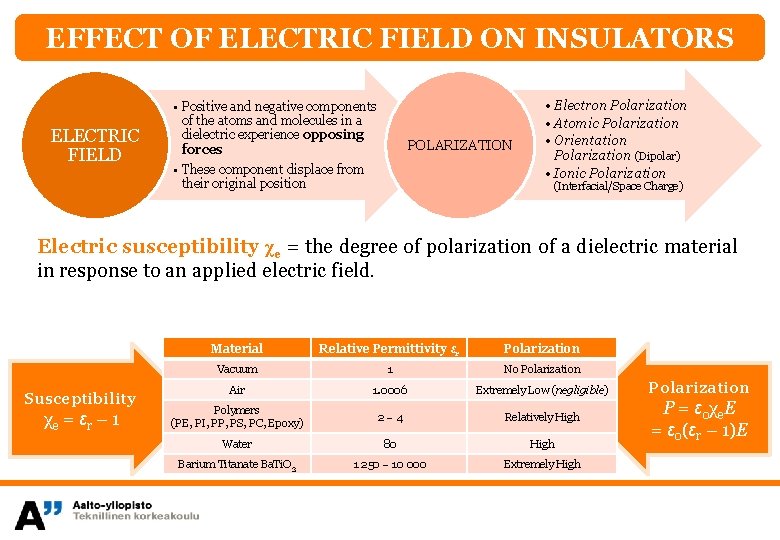
EFFECT OF ELECTRIC FIELD ON INSULATORS ELECTRIC FIELD • Positive and negative components of the atoms and molecules in a dielectric experience opposing forces • These component displace from their original position POLARIZATION • Electron Polarization • Atomic Polarization • Orientation Polarization (Dipolar) • Ionic Polarization (Interfacial/Space Charge) Electric susceptibility χe = the degree of polarization of a dielectric material in response to an applied electric field. Susceptibility χe = ɛ r – 1 Material Relative Permittivity ɛr Polarization Vacuum 1 No Polarization Air 1. 0006 Extremely Low (negligible) Polarization Polymers (PE, PI, PP, PS, PC, Epoxy) 2– 4 Relatively High Water 80 High P = ɛ 0χe. E = ɛ 0(ɛr – 1)E Barium Titanate Ba. Ti. O 3 1 250 – 10 000 Extremely High
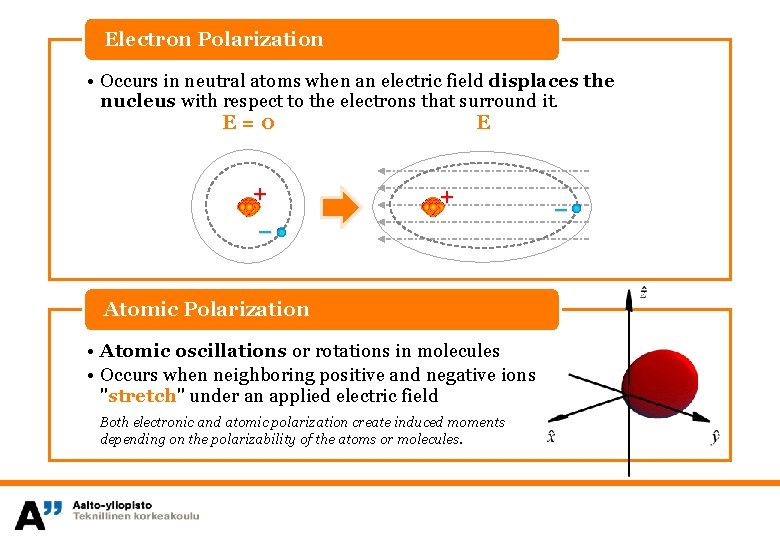
Electron Polarization • Occurs in neutral atoms when an electric field displaces the nucleus with respect to the electrons that surround it. E E=0 + + – Atomic Polarization • Atomic oscillations or rotations in molecules • Occurs when neighboring positive and negative ions "stretch" under an applied electric field Both electronic and atomic polarization create induced moments depending on the polarizability of the atoms or molecules. –
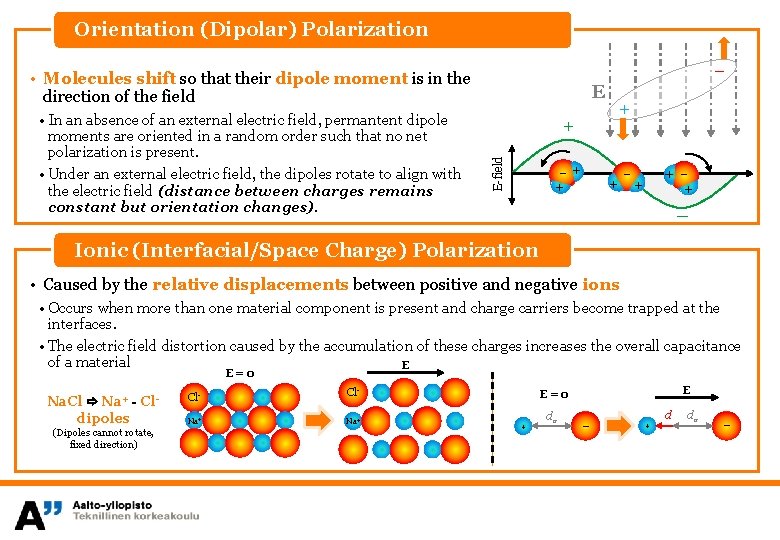
Orientation (Dipolar) Polarization – • Molecules shift so that their dipole moment is in the direction of the field + + E-field • In an absence of an external electric field, permantent dipole moments are oriented in a random order such that no net polarization is present. • Under an external electric field, the dipoles rotate to align with the electric field (distance between charges remains constant but orientation changes). E – + + + – Ionic (Interfacial/Space Charge) Polarization • Caused by the relative displacements between positive and negative ions • Occurs when more than one material component is present and charge carriers become trapped at the interfaces. • The electric field distortion caused by the accumulation of these charges increases the overall capacitance of a material E E=0 Na. Cl Na+ - Cldipoles (Dipoles cannot rotate, fixed direction) Cl. Na+ E E=0 + d 0 – + d d 0 –
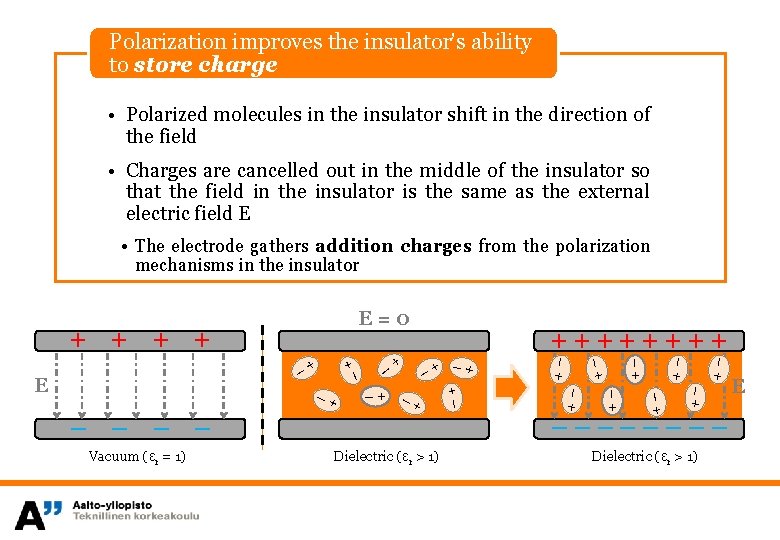
Polarization improves the insulator’s ability to store charge • Polarized molecules in the insulator shift in the direction of the field • Charges are cancelled out in the middle of the insulator so that the field in the insulator is the same as the external electric field E • The electrode gathers addition charges from the polarization mechanisms in the insulator + + –+ –+ –+ Vacuum (ɛr = 1) –+ – + –+ –+ – –+ E + –+ – ++++ –+ + E=0 –––– – Dielectric (ɛr > 1) E
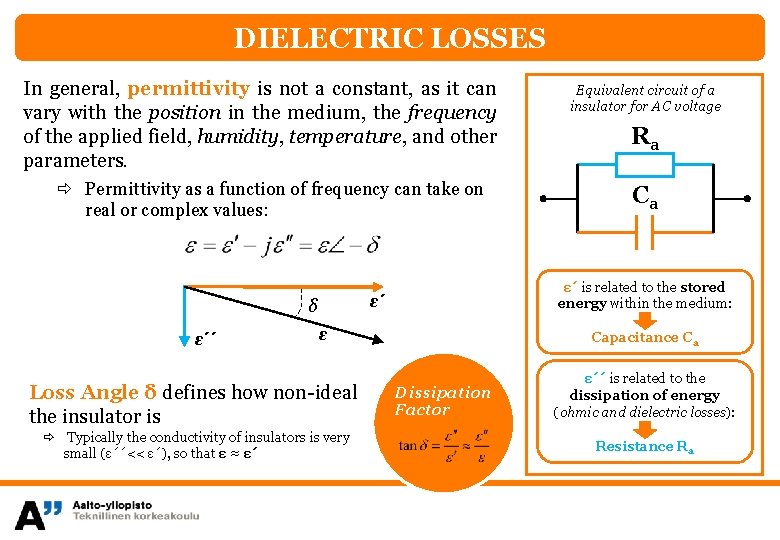
DIELECTRIC LOSSES In general, permittivity is not a constant, as it can vary with the position in the medium, the frequency of the applied field, humidity, temperature, and other parameters. Permittivity as a function of frequency can take on real or complex values: ɛ´´ ɛ Loss Angle δ defines how non-ideal the insulator is Typically the conductivity of insulators is very small (ε´´<< ε´), so that ε ≈ ε´ Ra Ca ε´ is related to the stored energy within the medium: ɛ´ δ Equivalent circuit of a insulator for AC voltage Capacitance Ca Dissipation Factor ε´´ is related to the dissipation of energy (ohmic and dielectric losses): Resistance Ra
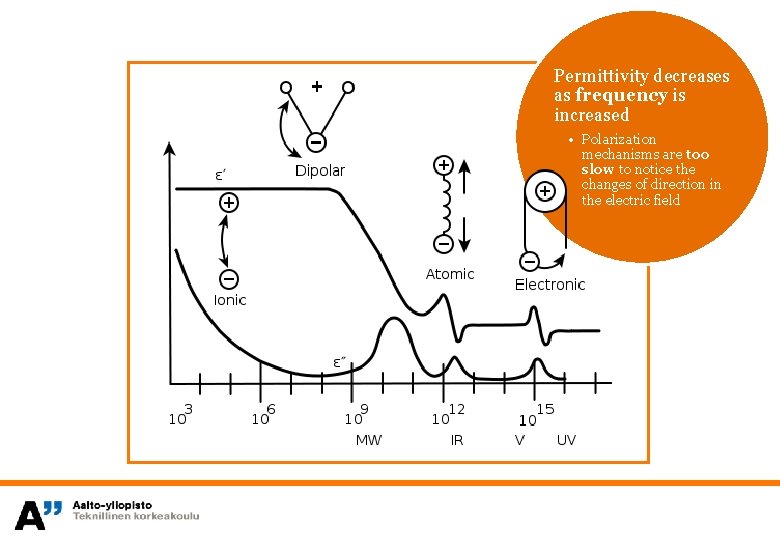
Permittivity decreases as frequency is increased • Polarization mechanisms are too slow to notice the changes of direction in the electric field
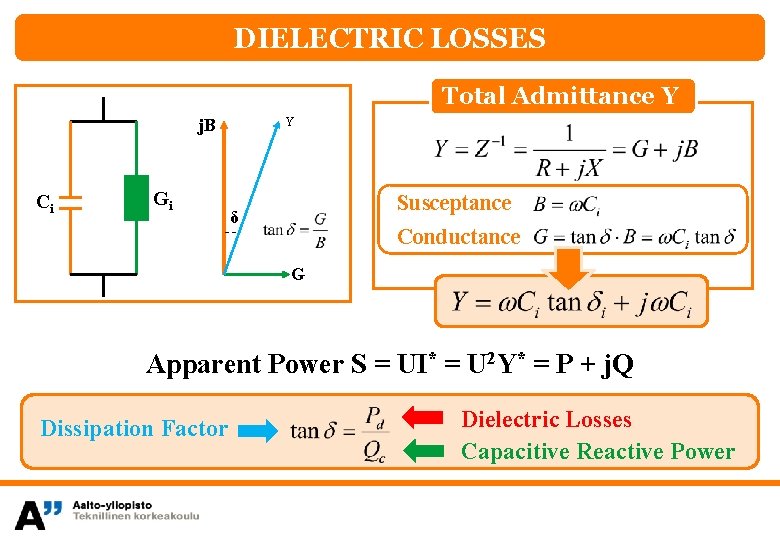
DIELECTRIC LOSSES Total Admittance Y Y j. B Ci Gi Susceptance δ Conductance G Apparent Power S = UI* = U 2 Y* = P + j. Q Dissipation Factor Dielectric Losses Capacitive Reactive Power
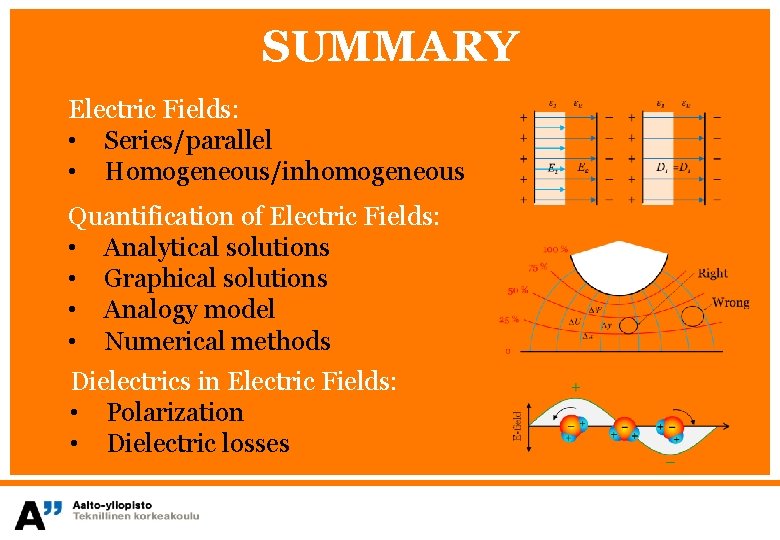
SUMMARY Electric Fields: • Series/parallel • Homogeneous/inhomogeneous Quantification of Electric Fields: • Analytical solutions • Graphical solutions • Analogy model • Numerical methods Dielectrics in Electric Fields: • Polarization • Dielectric losses
Maxwell's two equations for electrostatic fields
Red fields
What is considered high voltage
Ac voltage controller applications
Line current and phase current
Rms to peak conversion
The objective of earthing or grounding is
01:640:244 lecture notes - lecture 15: plat, idah, farad
High voltage testing of electrical apparatus
High voltage mux
If a battery provides a high voltage it can
If a battery provides a high voltage, it can ____.
Electric field stress in high voltage engineering
Generation of high ac voltage
High voltage leak detection
Insulation coordination in high voltage engineering
High voltage testing of electrical apparatus
Spellman hv
High ohmic series resistance with microammeter
Ee2353 high voltage engineering
High voltage disconnect fsae
Electrostatic disinfection chelsea
Triboelectric series
Electrostatic energy
Electrostatic equilibrium
What is electrostatic series
Electrostatic forces
Electric force meaning
Electric field and voltage
Amber effect
Coulomb's law in electric field
Electric flux through a closed surface
Surface integral of electric field
Boundary conditions for electric field
Electrostatic lens pdf
Smog hog electrostatic precipitators nyc