CMOS Detector Technology Markus Loose Alan Hoffman Vyshnavi
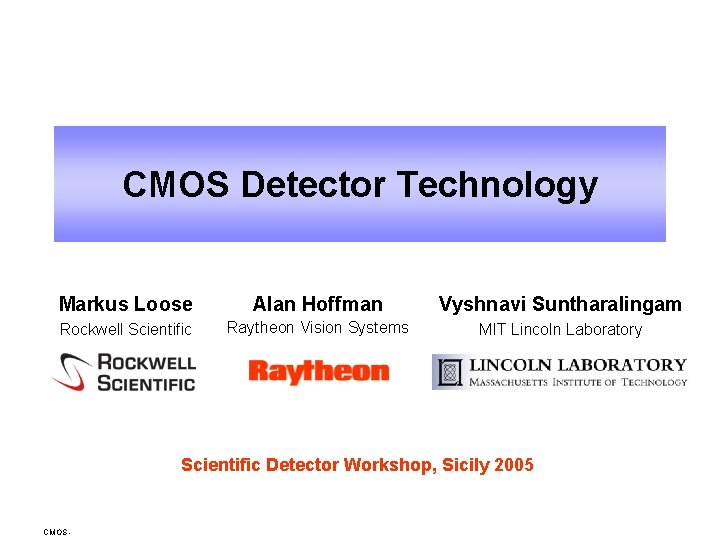
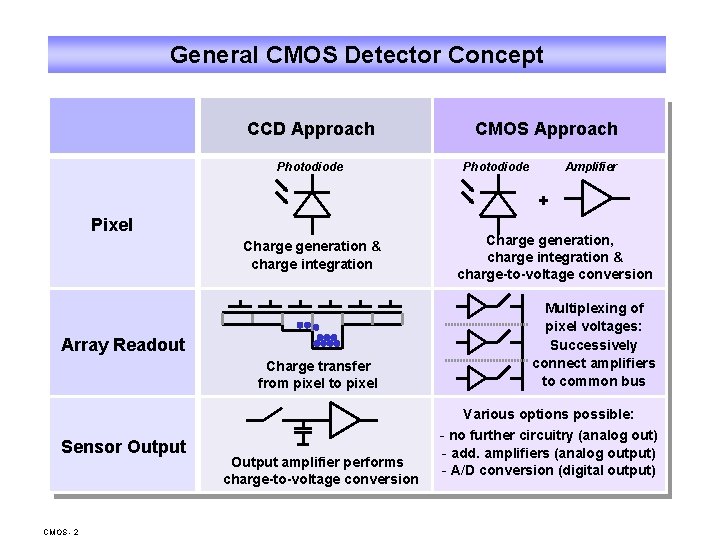
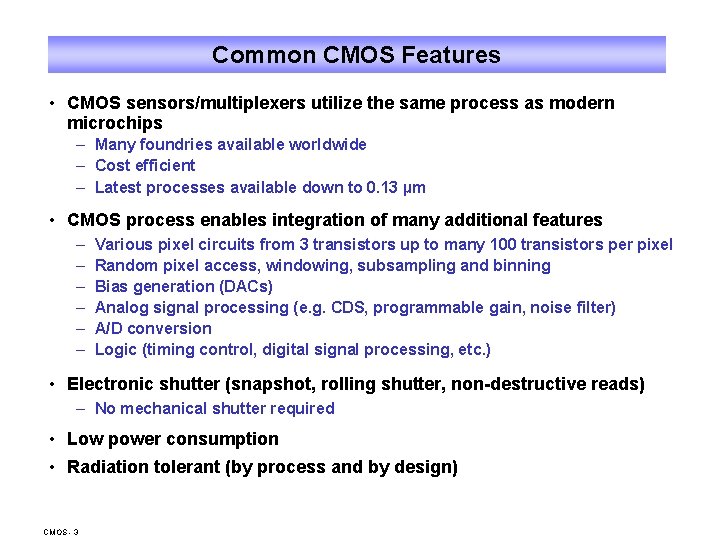
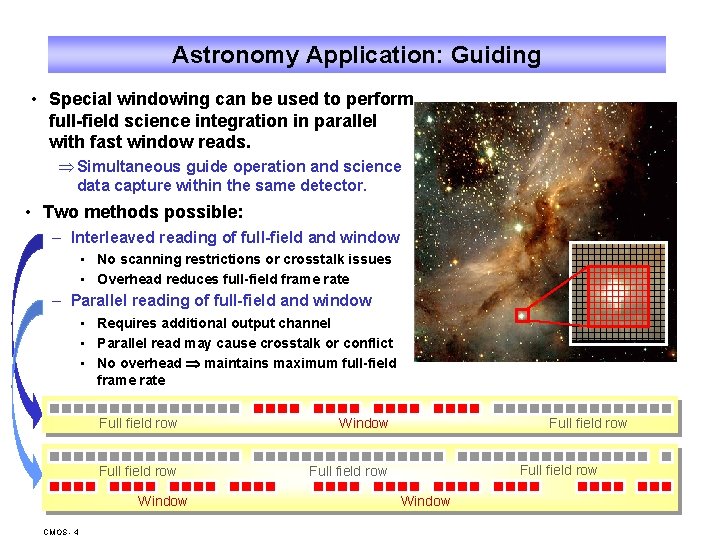
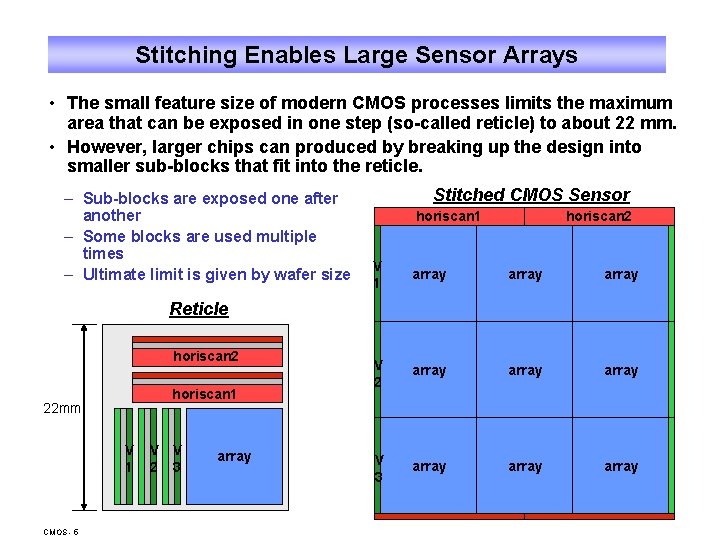
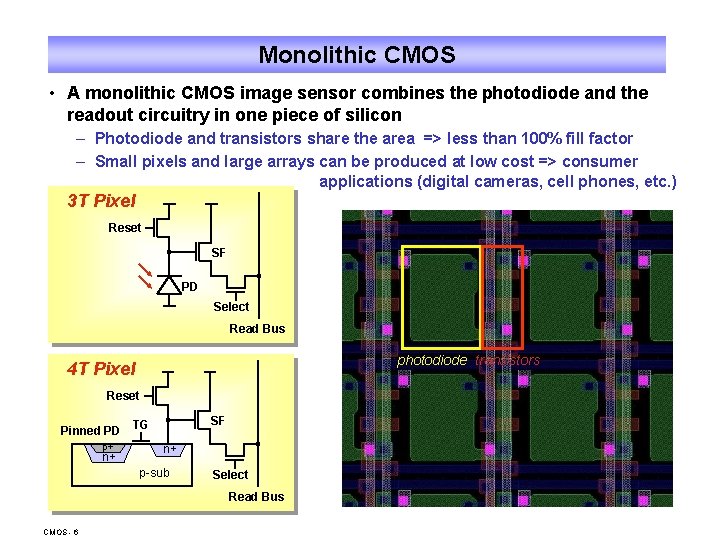
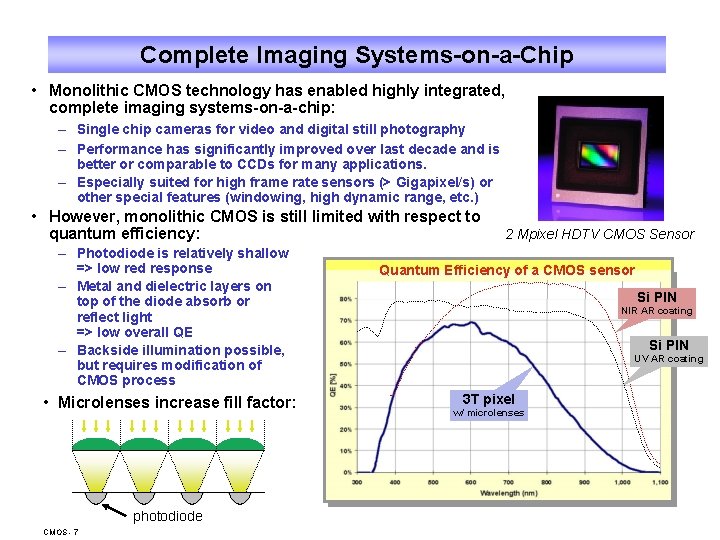
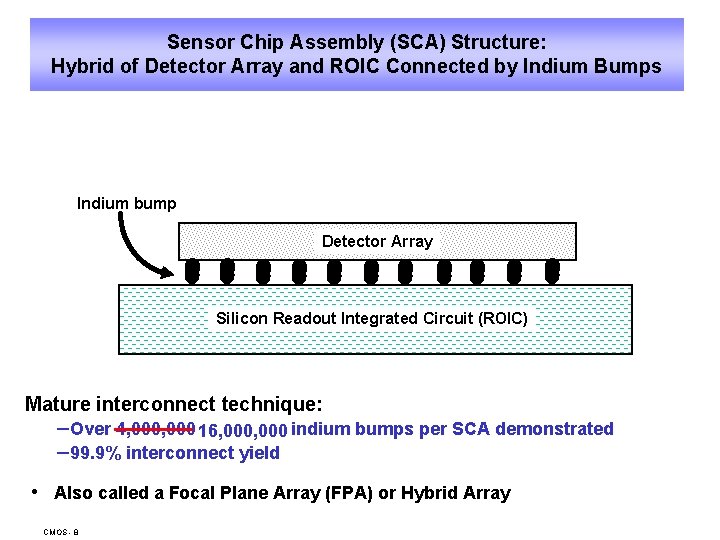
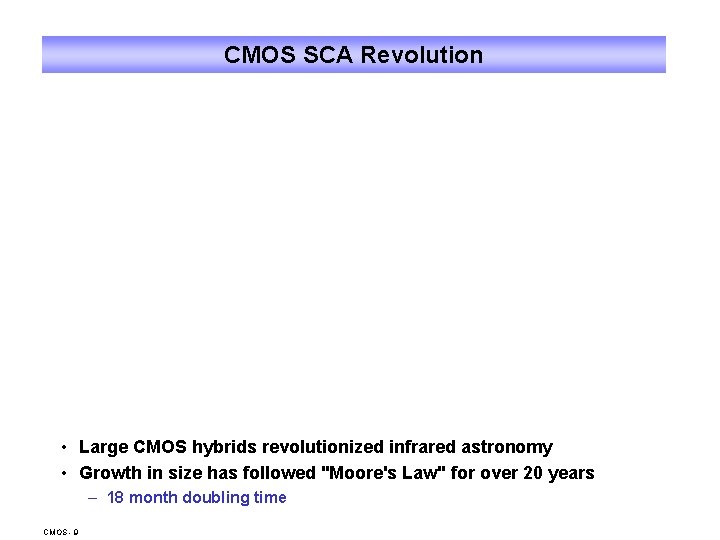
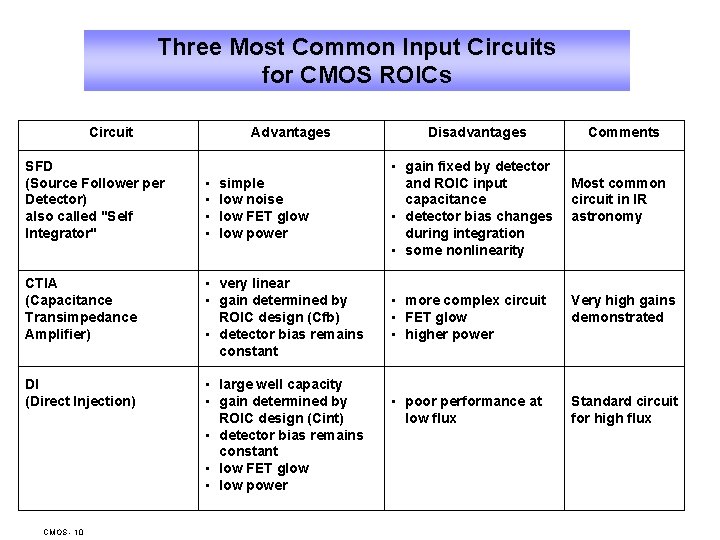
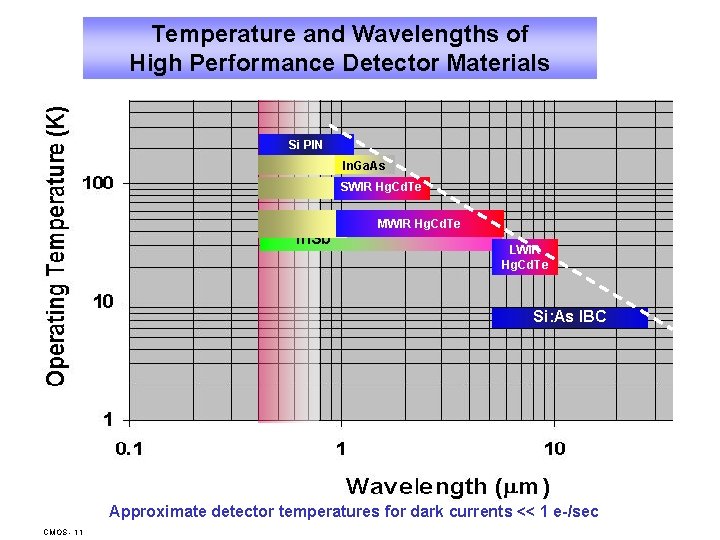
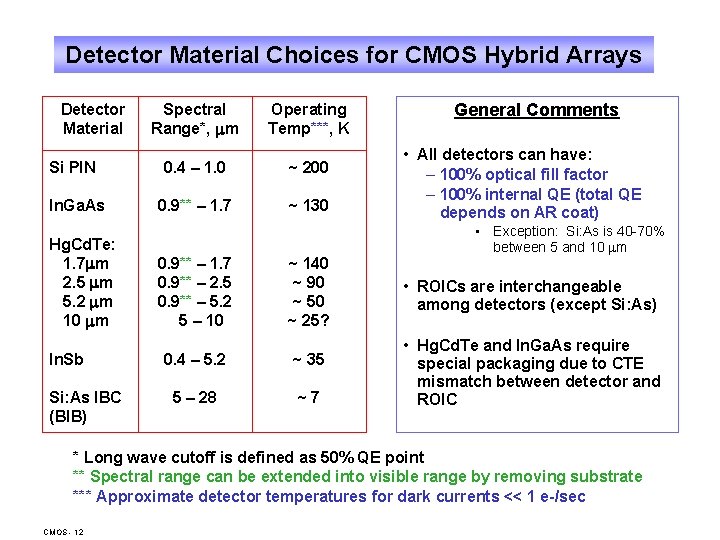
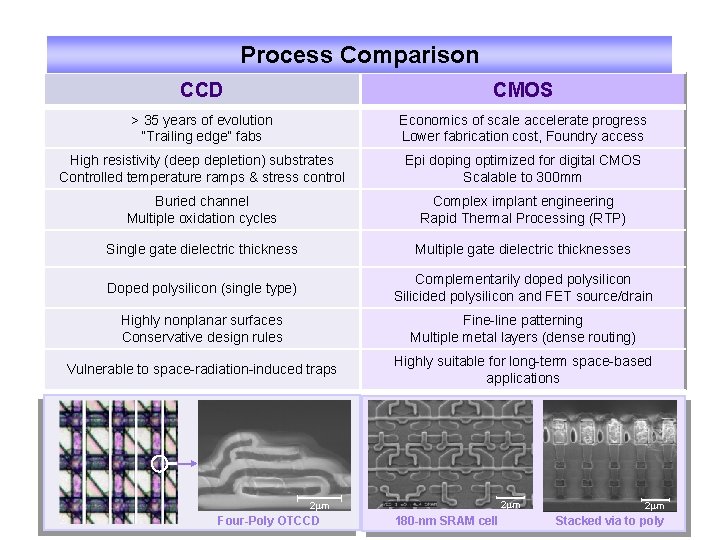
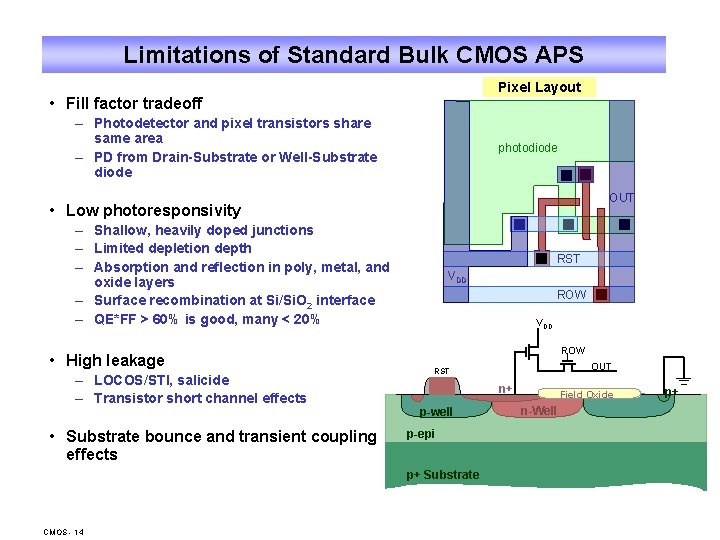
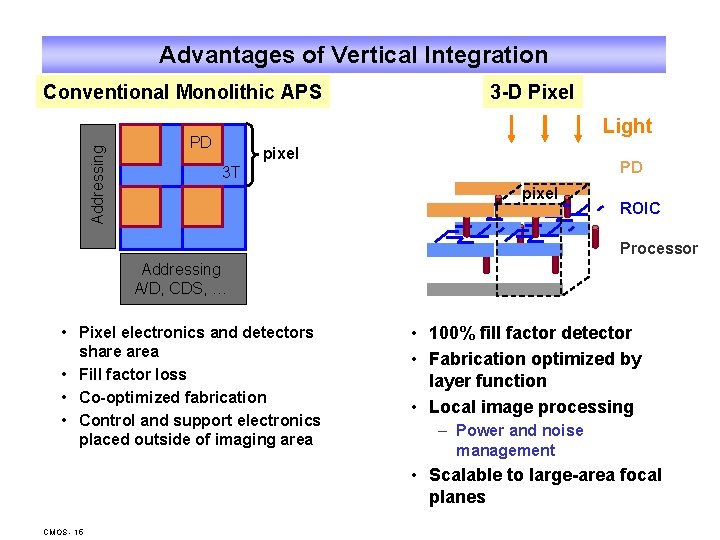
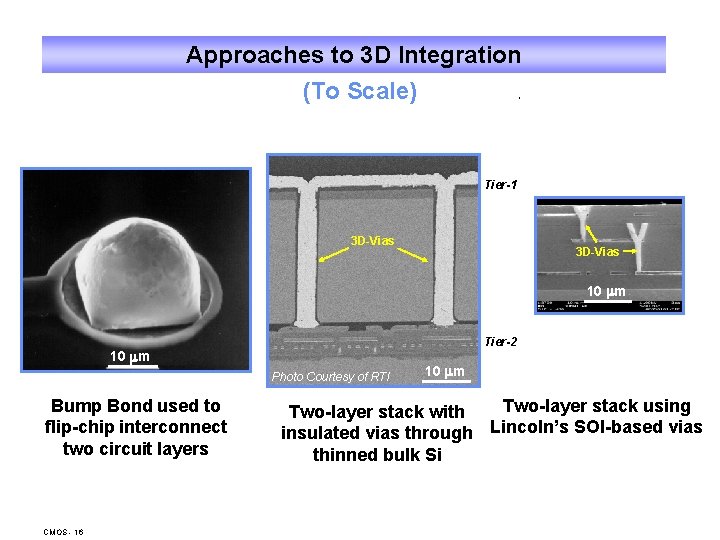
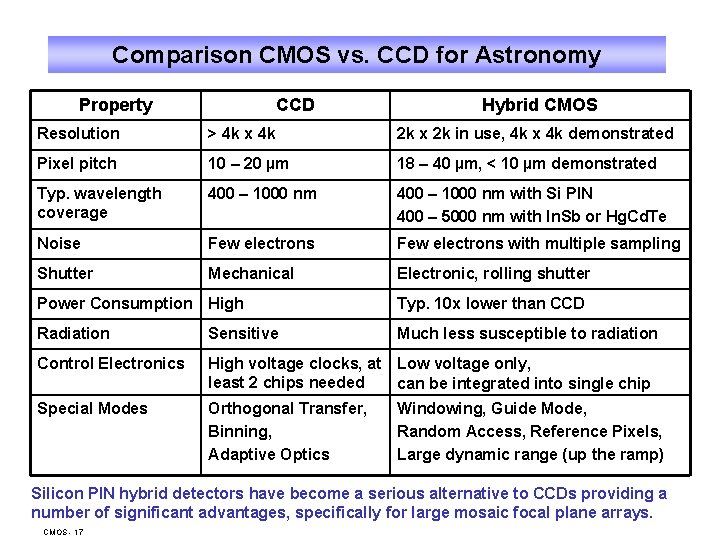
- Slides: 17
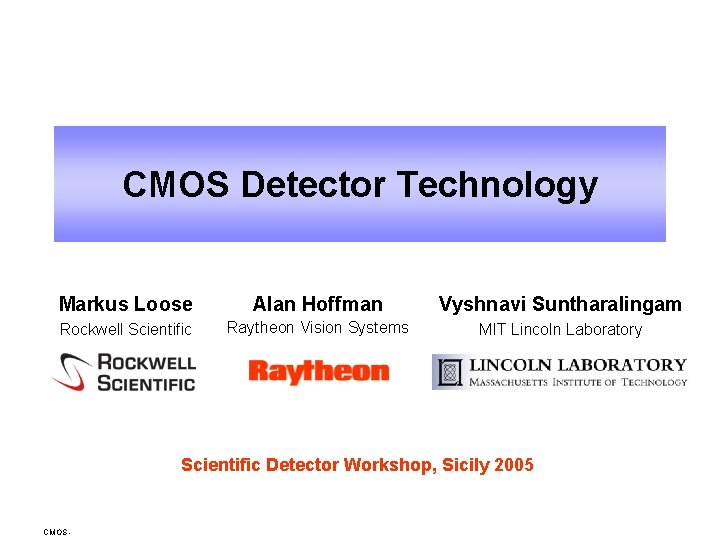
CMOS Detector Technology Markus Loose Alan Hoffman Vyshnavi Suntharalingam Rockwell Scientific Raytheon Vision Systems MIT Lincoln Laboratory Scientific Detector Workshop, Sicily 2005 CMOS -
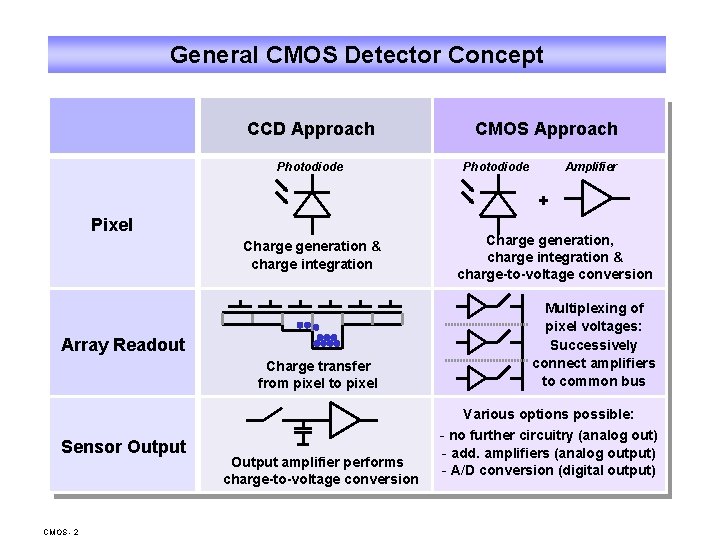
General CMOS Detector Concept CCD Approach Photodiode CMOS Approach Photodiode Amplifier + Pixel Charge generation & charge integration Array Readout Charge transfer from pixel to pixel Sensor Output CMOS - 2 Output amplifier performs charge-to-voltage conversion Charge generation, charge integration & charge-to-voltage conversion Multiplexing of pixel voltages: Successively connect amplifiers to common bus Various options possible: - no further circuitry (analog out) - add. amplifiers (analog output) - A/D conversion (digital output)
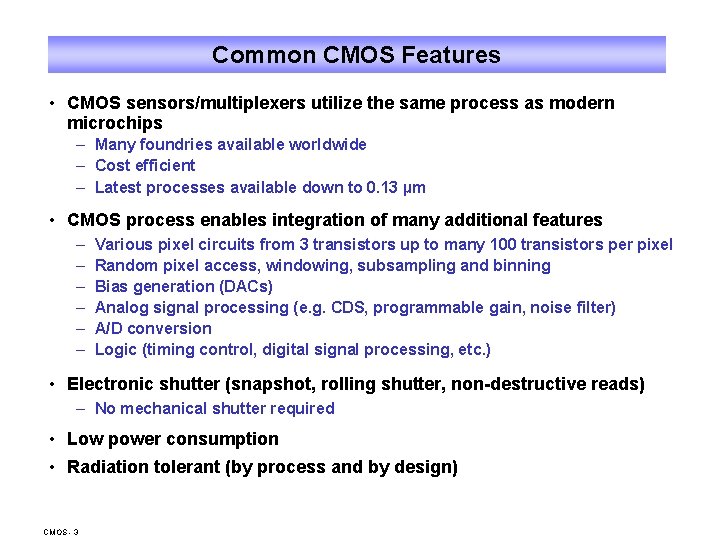
Common CMOS Features • CMOS sensors/multiplexers utilize the same process as modern microchips – Many foundries available worldwide – Cost efficient – Latest processes available down to 0. 13 µm • CMOS process enables integration of many additional features – – – Various pixel circuits from 3 transistors up to many 100 transistors per pixel Random pixel access, windowing, subsampling and binning Bias generation (DACs) Analog signal processing (e. g. CDS, programmable gain, noise filter) A/D conversion Logic (timing control, digital signal processing, etc. ) • Electronic shutter (snapshot, rolling shutter, non-destructive reads) – No mechanical shutter required • Low power consumption • Radiation tolerant (by process and by design) CMOS - 3
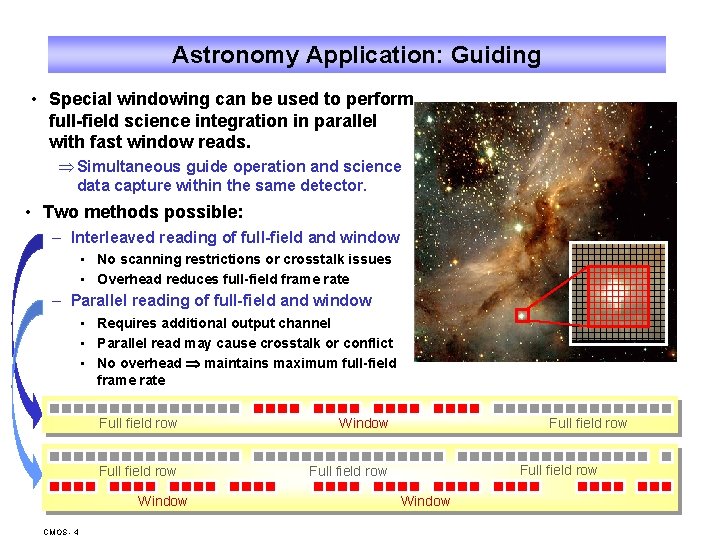
Astronomy Application: Guiding • Special windowing can be used to perform full-field science integration in parallel with fast window reads. Þ Simultaneous guide operation and science data capture within the same detector. • Two methods possible: – Interleaved reading of full-field and window • No scanning restrictions or crosstalk issues • Overhead reduces full-field frame rate – Parallel reading of full-field and window • Requires additional output channel • Parallel read may cause crosstalk or conflict • No overhead maintains maximum full-field frame rate Full field row Window CMOS - 4 Full field row Window
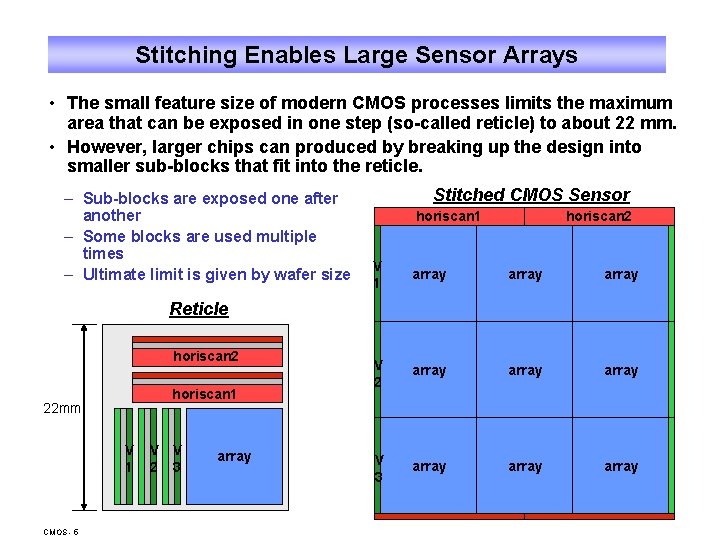
Stitching Enables Large Sensor Arrays • The small feature size of modern CMOS processes limits the maximum area that can be exposed in one step (so-called reticle) to about 22 mm. • However, larger chips can produced by breaking up the design into smaller sub-blocks that fit into the reticle. – Sub-blocks are exposed one after another – Some blocks are used multiple times – Ultimate limit is given by wafer size Stitched CMOS Sensor horiscan 1 horiscan 2 V 1 array V 2 array V 3 array Reticle horiscan 2 horiscan 1 22 mm V 1 CMOS - 5 V V 2 3 array
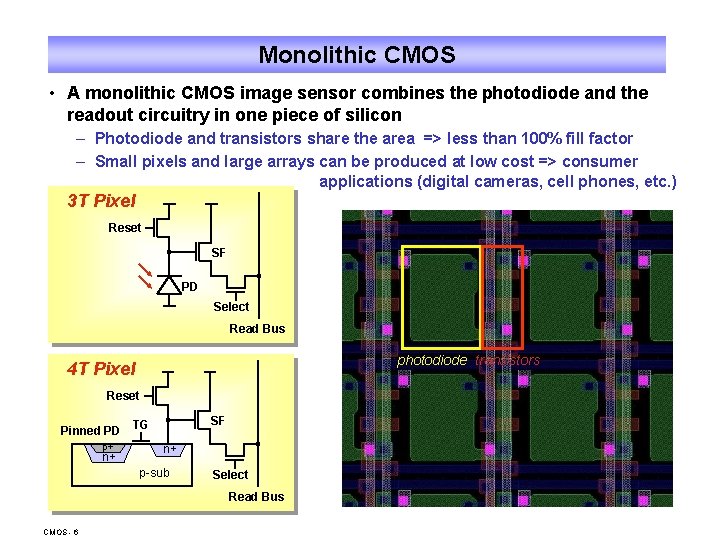
Monolithic CMOS • A monolithic CMOS image sensor combines the photodiode and the readout circuitry in one piece of silicon – Photodiode and transistors share the area => less than 100% fill factor – Small pixels and large arrays can be produced at low cost => consumer applications (digital cameras, cell phones, etc. ) 3 T Pixel Reset SF PD Select Read Bus photodiode transistors 4 T Pixel Reset Pinned PD p+ n+ SF TG n+ p-sub Select Read Bus CMOS - 6
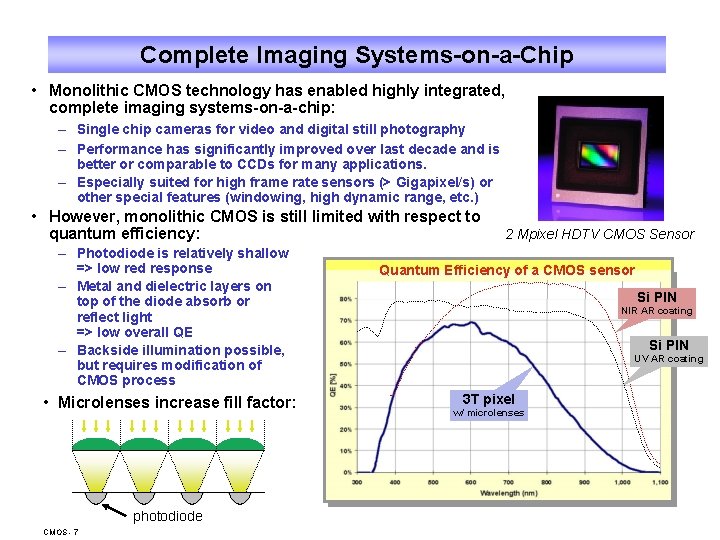
Complete Imaging Systems-on-a-Chip • Monolithic CMOS technology has enabled highly integrated, complete imaging systems-on-a-chip: – Single chip cameras for video and digital still photography – Performance has significantly improved over last decade and is better or comparable to CCDs for many applications. – Especially suited for high frame rate sensors (> Gigapixel/s) or other special features (windowing, high dynamic range, etc. ) • However, monolithic CMOS is still limited with respect to quantum efficiency: – Photodiode is relatively shallow => low red response – Metal and dielectric layers on top of the diode absorb or reflect light => low overall QE – Backside illumination possible, but requires modification of CMOS process • Microlenses increase fill factor: photodiode CMOS - 7 2 Mpixel HDTV CMOS Sensor Quantum Efficiency of a CMOS sensor Si PIN NIR AR coating Si PIN UV AR coating 3 T pixel w/ microlenses
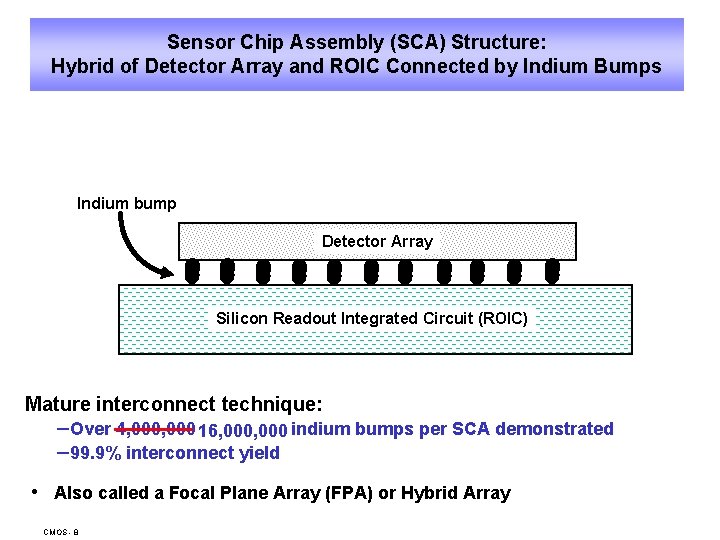
Sensor Chip Assembly (SCA) Structure: Hybrid of Detector Array and ROIC Connected by Indium Bumps Detector Array Indium bump Detector Array Silicon Readout Integrated Circuit (ROIC) Mature interconnect technique: – Over 4, 000 16, 000 indium bumps per SCA demonstrated – 99. 9% interconnect yield • Also called a Focal Plane Array (FPA) or Hybrid Array CMOS - 8
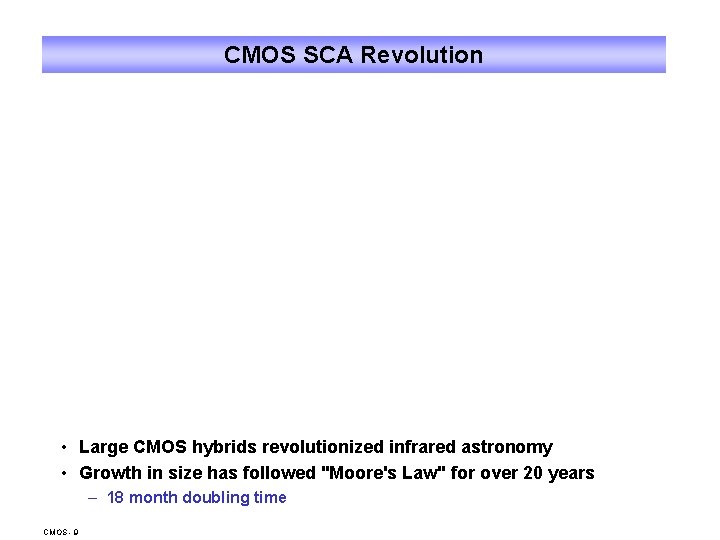
CMOS SCA Revolution • Large CMOS hybrids revolutionized infrared astronomy • Growth in size has followed "Moore's Law" for over 20 years – 18 month doubling time CMOS - 9
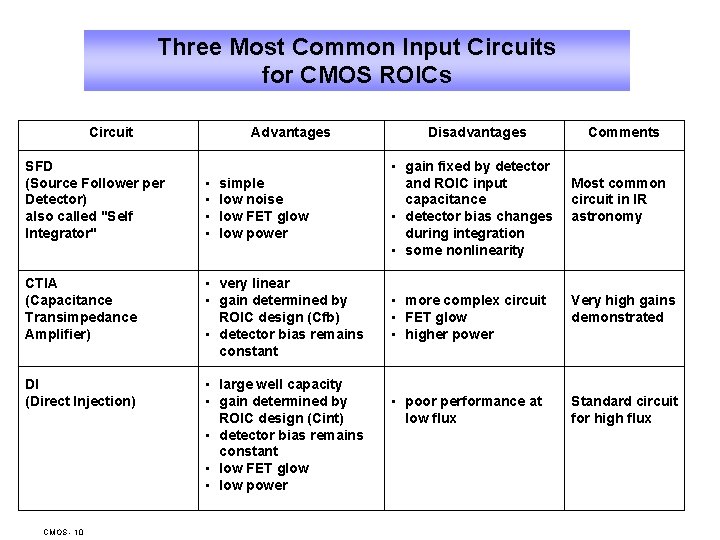
Three Most Common Input Circuits for CMOS ROICs Circuit SFD (Source Follower per Detector) also called "Self Integrator" Advantages • • simple low noise low FET glow power CTIA (Capacitance Transimpedance Amplifier) • very linear • gain determined by ROIC design (Cfb) • detector bias remains constant DI (Direct Injection) • large well capacity • gain determined by ROIC design (Cint) • detector bias remains constant • low FET glow • low power CMOS - 10 Disadvantages • gain fixed by detector and ROIC input capacitance • detector bias changes during integration • some nonlinearity Comments Most common circuit in IR astronomy • more complex circuit • FET glow • higher power Very high gains demonstrated • poor performance at low flux Standard circuit for high flux
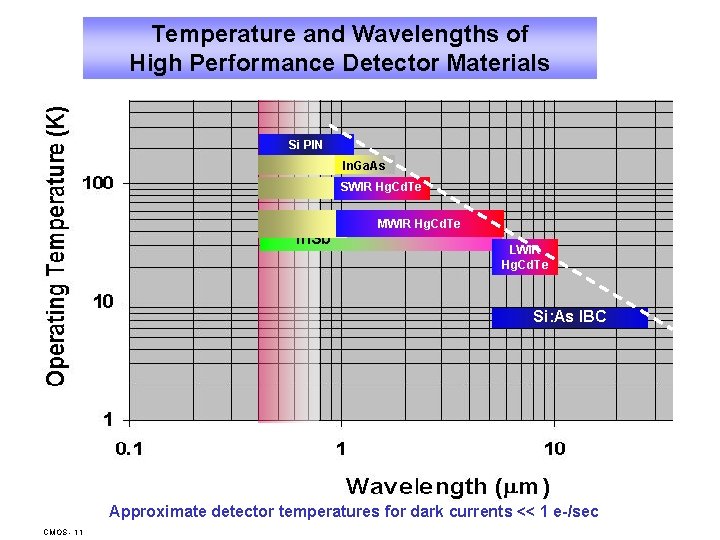
Temperature and Wavelengths of High Performance Detector Materials Si PIN In. Ga. As SWIR Hg. Cd. Te In. Sb MWIR Hg. Cd. Te LWIR Hg. Cd. Te Si: As IBC Approximate detector temperatures for dark currents << 1 e-/sec CMOS - 11
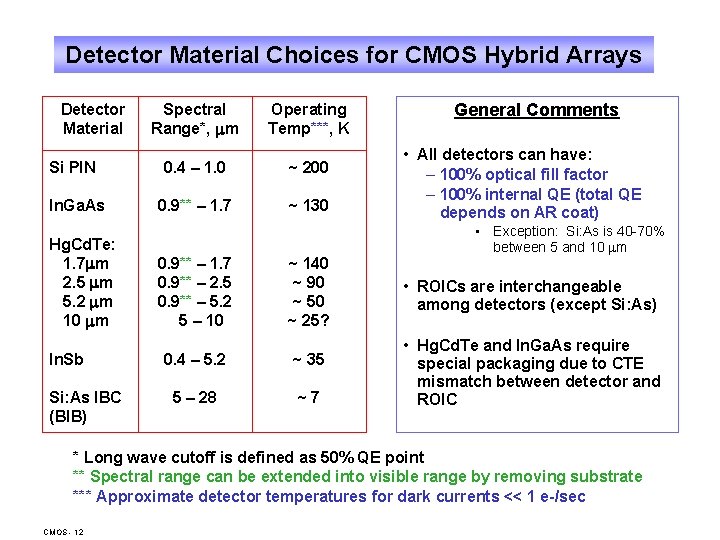
Detector Material Choices for CMOS Hybrid Arrays Detector Material Si PIN In. Ga. As Hg. Cd. Te: 1. 7 m 2. 5 m 5. 2 m 10 m In. Sb Si: As IBC (BIB) Spectral Range*, m Operating Temp***, K 0. 4 – 1. 0 ~ 200 0. 9** – 1. 7 ~ 130 0. 9** – 1. 7 0. 9** – 2. 5 0. 9** – 5. 2 5 – 10 ~ 140 ~ 90 ~ 50 ~ 25? 0. 4 – 5. 2 ~ 35 5 – 28 ~7 General Comments • All detectors can have: – 100% optical fill factor – 100% internal QE (total QE depends on AR coat) • Exception: Si: As is 40 -70% between 5 and 10 m • ROICs are interchangeable among detectors (except Si: As) • Hg. Cd. Te and In. Ga. As require special packaging due to CTE mismatch between detector and ROIC * Long wave cutoff is defined as 50% QE point ** Spectral range can be extended into visible range by removing substrate *** Approximate detector temperatures for dark currents << 1 e-/sec CMOS - 12
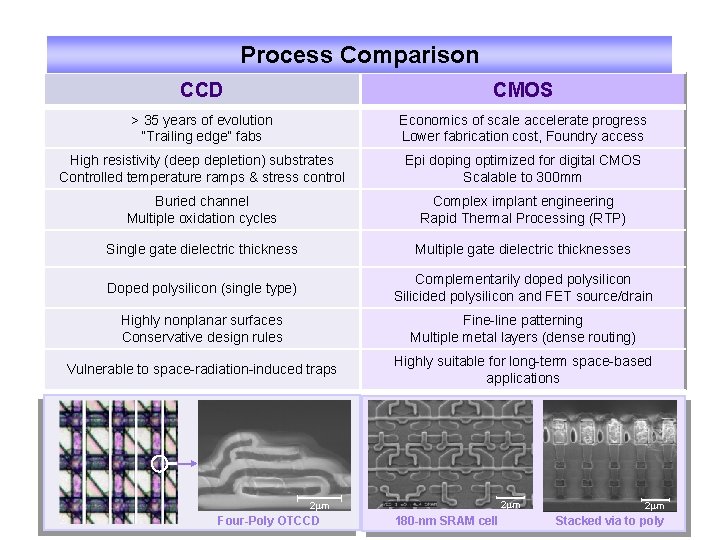
Process Comparison CCD CMOS > 35 years of evolution “Trailing edge” fabs Economics of scale accelerate progress Lower fabrication cost, Foundry access High resistivity (deep depletion) substrates Controlled temperature ramps & stress control Epi doping optimized for digital CMOS Scalable to 300 mm Buried channel Multiple oxidation cycles Complex implant engineering Rapid Thermal Processing (RTP) Single gate dielectric thickness Multiple gate dielectric thicknesses Doped polysilicon (single type) Complementarily doped polysilicon Silicided polysilicon and FET source/drain Highly nonplanar surfaces Conservative design rules Fine-line patterning Multiple metal layers (dense routing) Vulnerable to space-radiation-induced traps Highly suitable for long-term space-based applications 2 m Four-Poly OTCCD CMOS - 13 180 -nm SRAM cell 2 m Stacked via to poly
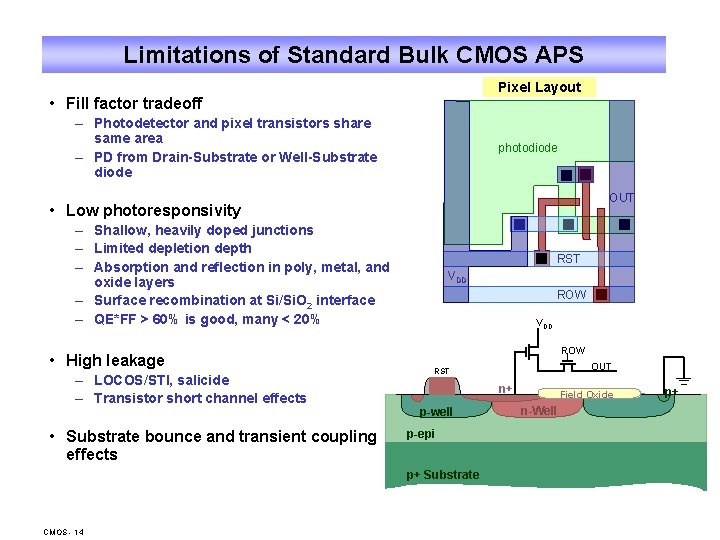
Limitations of Standard Bulk CMOS APS Pixel Layout • Fill factor tradeoff – Photodetector and pixel transistors share same area – PD from Drain-Substrate or Well-Substrate diode photodiode OUT • Low photoresponsivity – Shallow, heavily doped junctions – Limited depletion depth – Absorption and reflection in poly, metal, and oxide layers – Surface recombination at Si/Si. O 2 interface – QE*FF > 60% is good, many < 20% • High leakage – LOCOS/STI, salicide – Transistor short channel effects • Substrate bounce and transient coupling effects RST VDD ROW n+ p-well p-epi p+ Substrate CMOS - 14 OUT RST Field Oxide n-Well p+
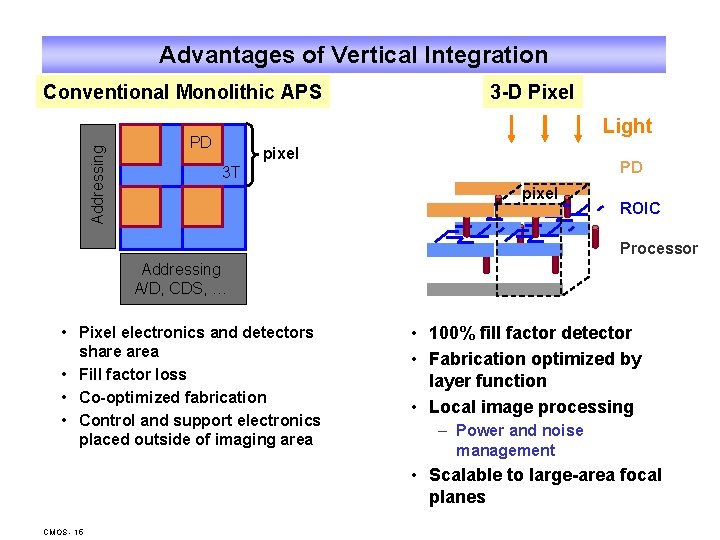
Advantages of Vertical Integration Addressing Conventional Monolithic APS 3 -D Pixel Light PD pixel PD 3 T pixel ROIC Processor Addressing A/D, CDS, … • Pixel electronics and detectors share area • Fill factor loss • Co-optimized fabrication • Control and support electronics placed outside of imaging area • 100% fill factor detector • Fabrication optimized by layer function • Local image processing – Power and noise management • Scalable to large-area focal planes CMOS - 15
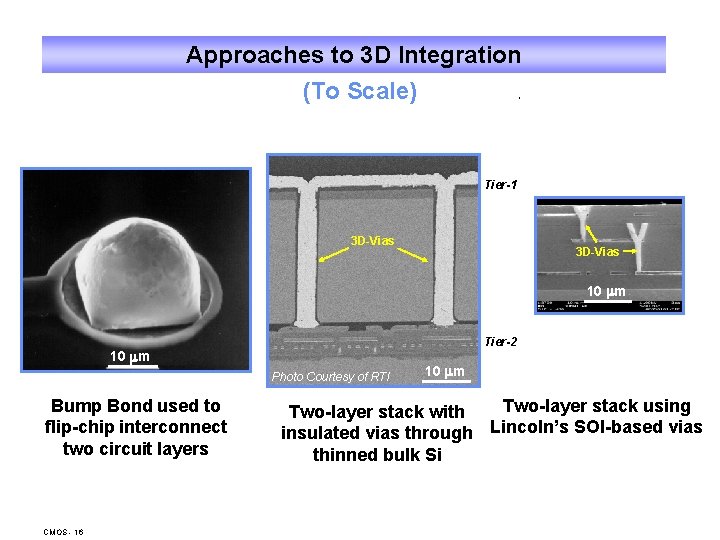
Approaches to 3 D Integration (To Scale) Tier-1 3 D-Vias 10 m Tier-2 10 m Photo Courtesy of RTI Bump Bond used to flip-chip interconnect two circuit layers CMOS - 16 10 m Two-layer stack using Two-layer stack with insulated vias through Lincoln’s SOI-based vias thinned bulk Si
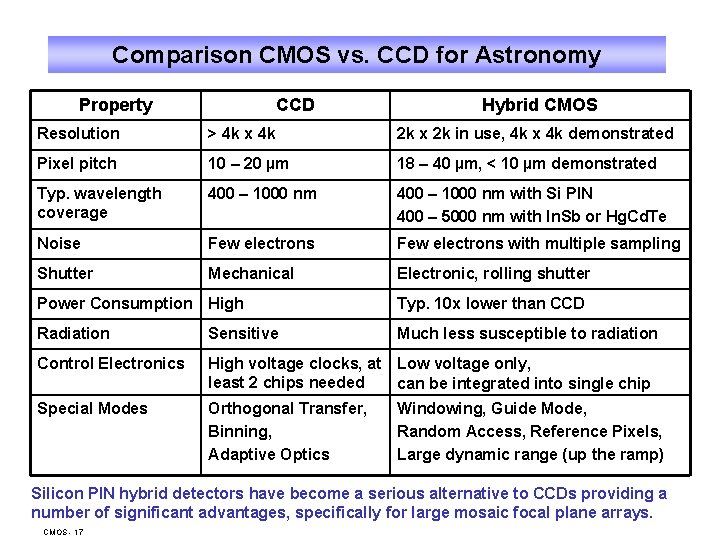
Comparison CMOS vs. CCD for Astronomy Property CCD Hybrid CMOS Resolution > 4 k x 4 k 2 k x 2 k in use, 4 k x 4 k demonstrated Pixel pitch 10 – 20 µm 18 – 40 µm, < 10 µm demonstrated Typ. wavelength coverage 400 – 1000 nm with Si PIN 400 – 5000 nm with In. Sb or Hg. Cd. Te Noise Few electrons with multiple sampling Shutter Mechanical Electronic, rolling shutter Power Consumption High Typ. 10 x lower than CCD Radiation Sensitive Much less susceptible to radiation Control Electronics High voltage clocks, at Low voltage only, least 2 chips needed can be integrated into single chip Special Modes Orthogonal Transfer, Binning, Adaptive Optics Windowing, Guide Mode, Random Access, Reference Pixels, Large dynamic range (up the ramp) Silicon PIN hybrid detectors have become a serious alternative to CCDs providing a number of significant advantages, specifically for large mosaic focal plane arrays. CMOS - 17
Cmos technology
Cmos technology
Wa4nc
Hans christian andersen życiorys
Mark hoffman auburn
Unit 5 meeting individual needs
Chloe hoffman
Bifid nipple breastfeeding
Jay r hoffman
Mark hoffman auburn
Mark oreglia
Michael b hoffman
Marta hoffman
Hoffman calculus
The infernal desire machines of doctor hoffman
Tiina hoffman
Katrina hoffman
Salvino darmate