11 th Mini Lecture CERN Switzerland 26 11
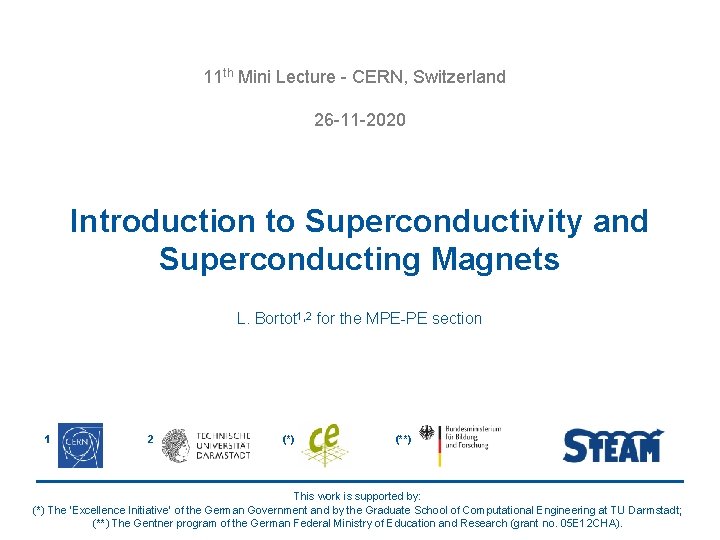
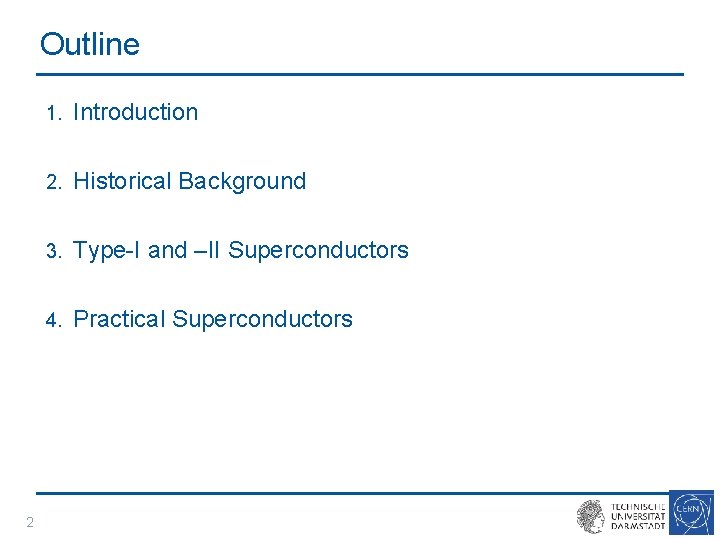
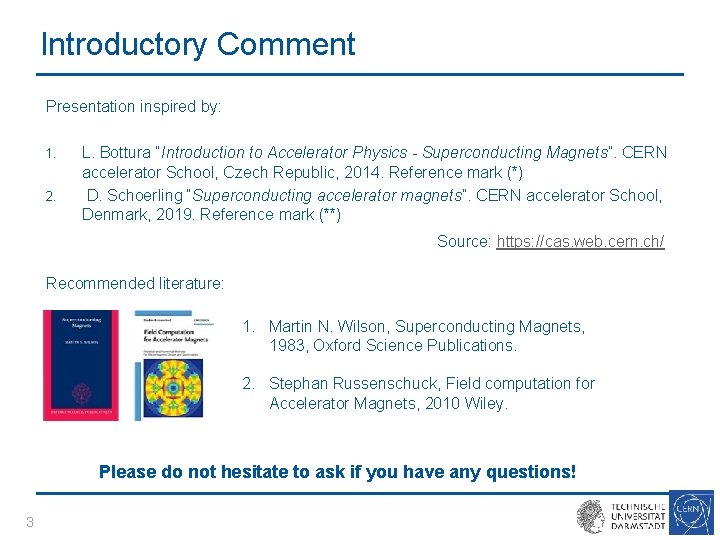
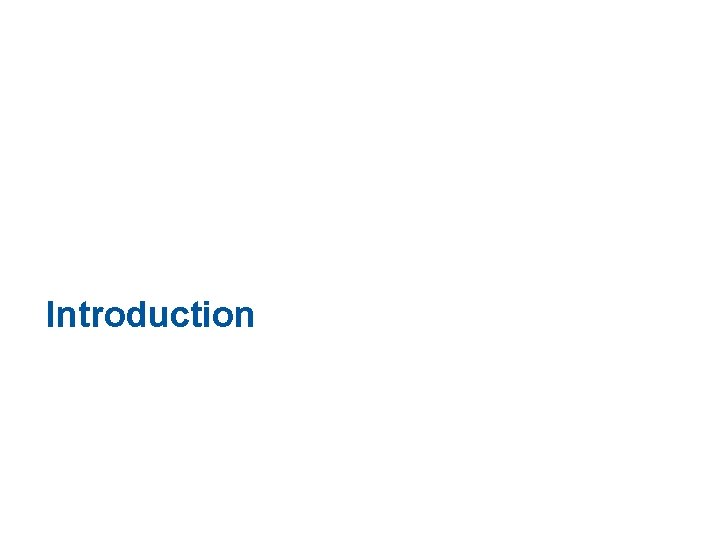
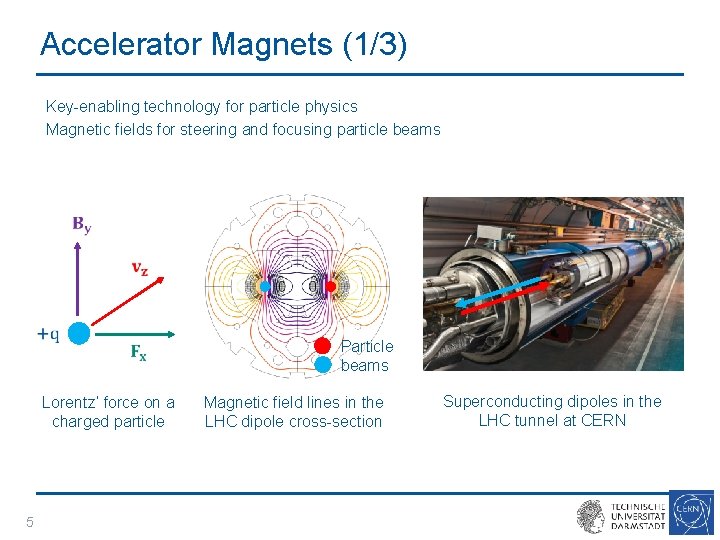
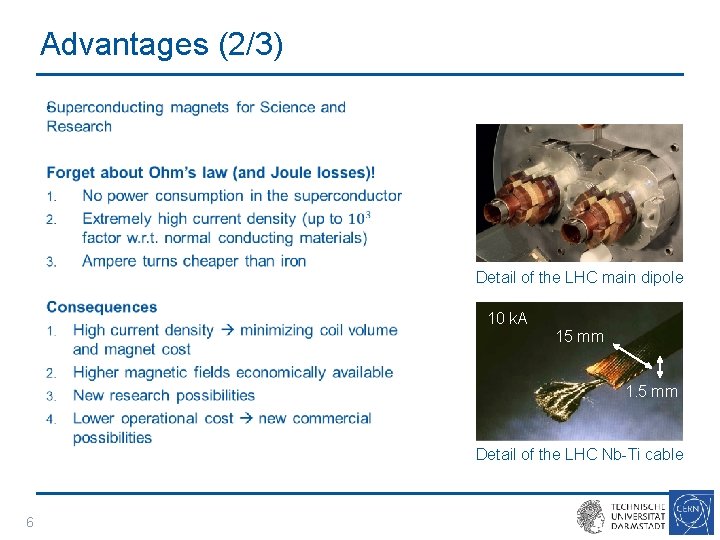
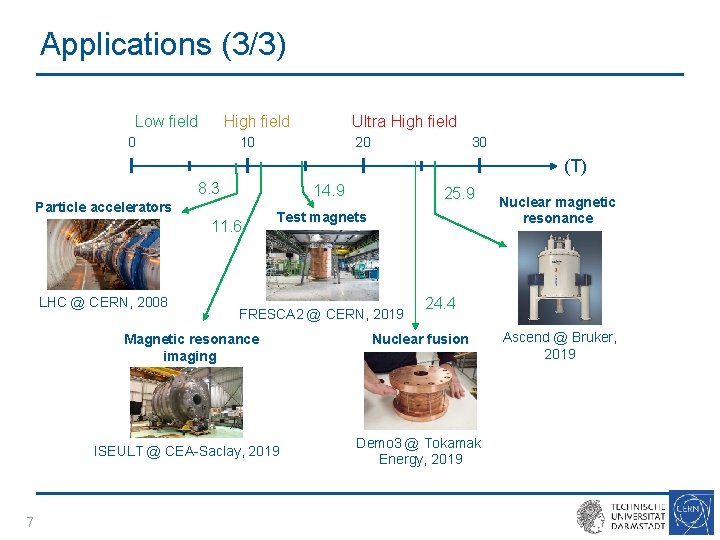
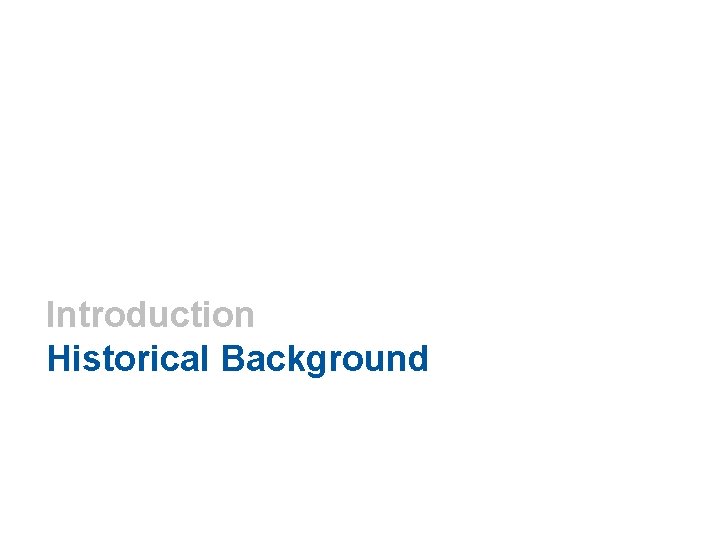
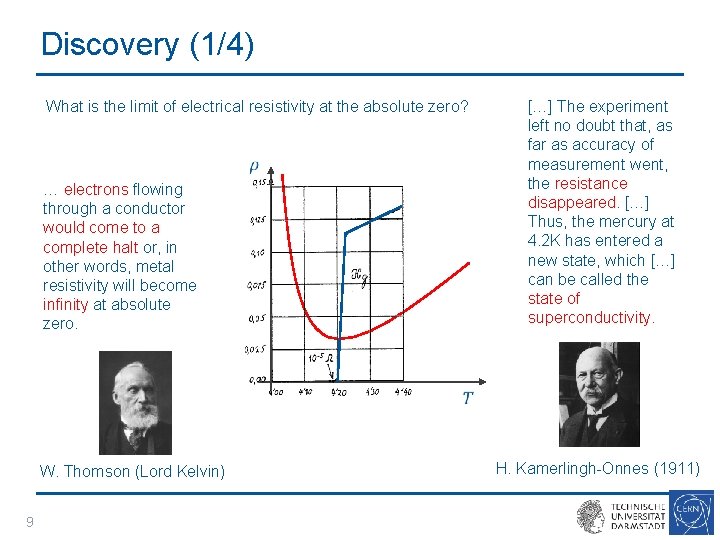
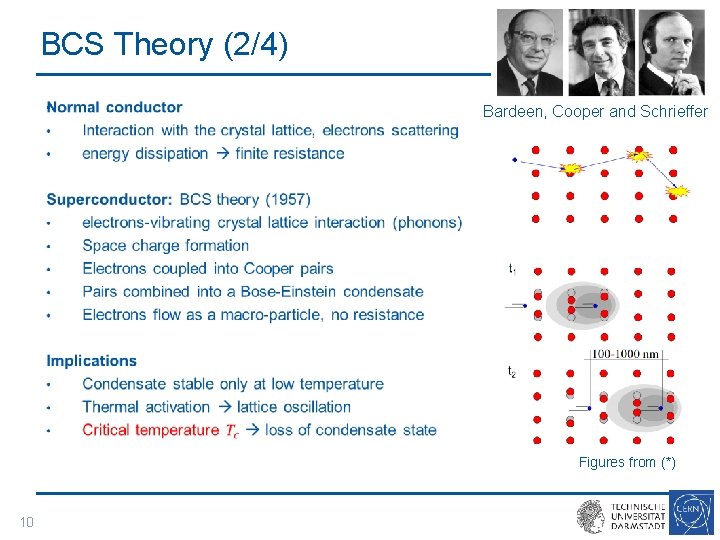
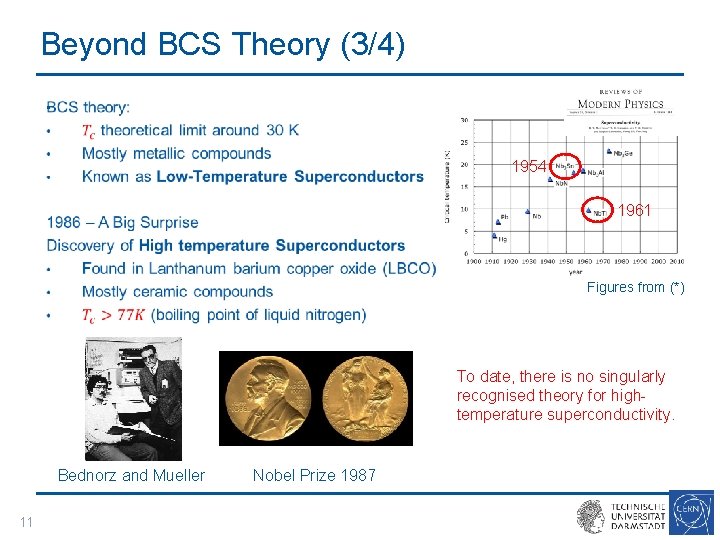
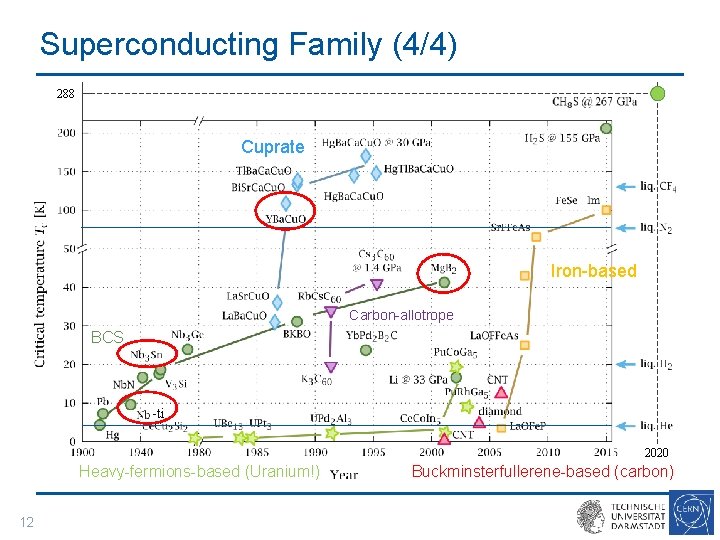
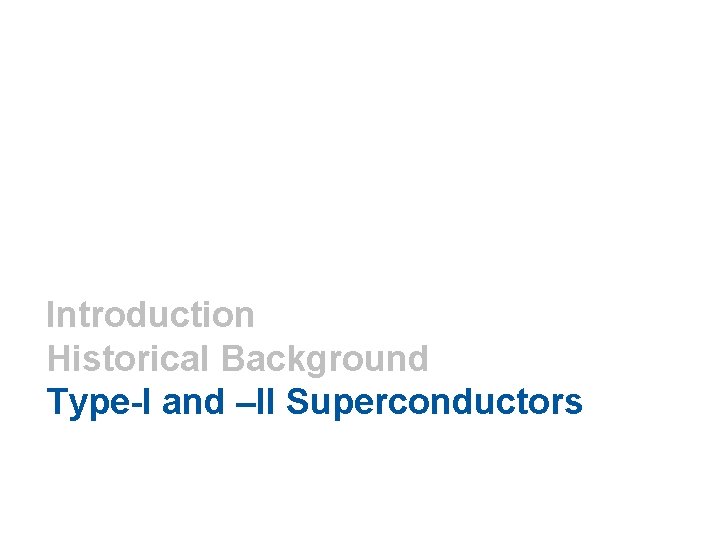
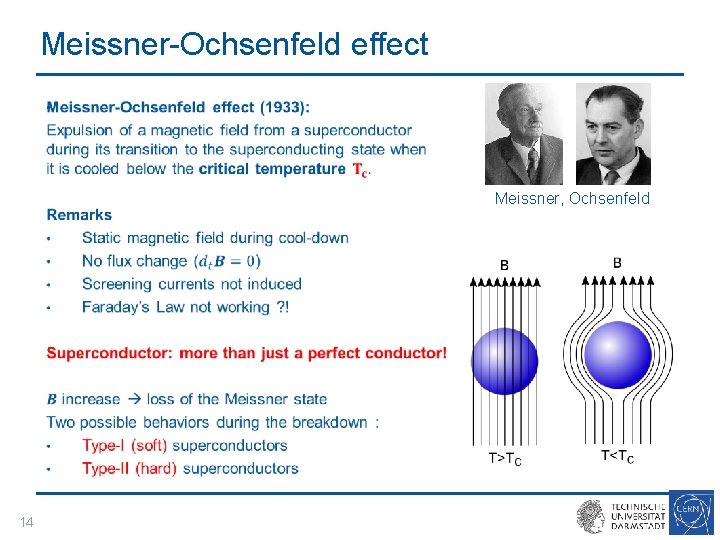
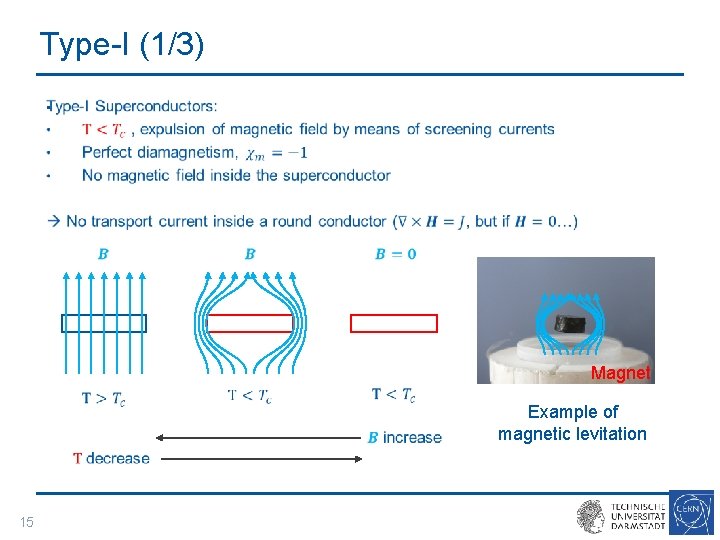
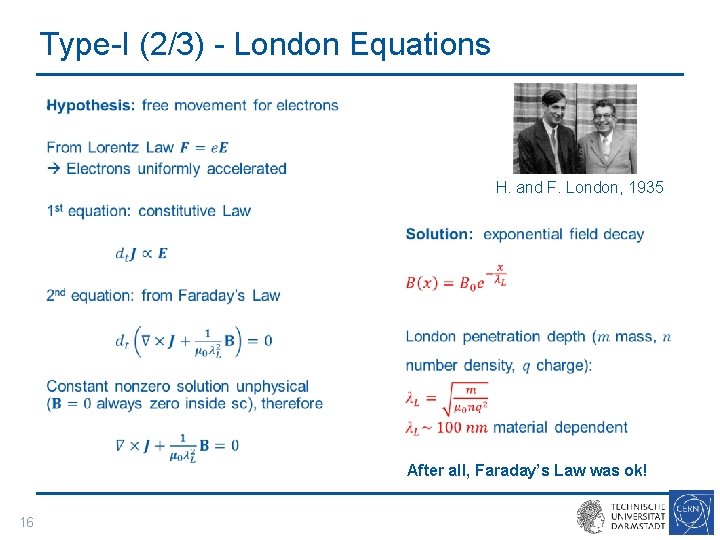
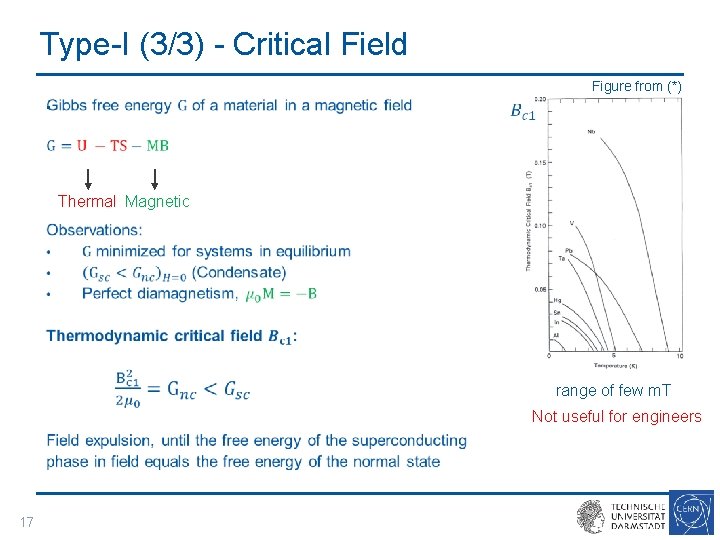
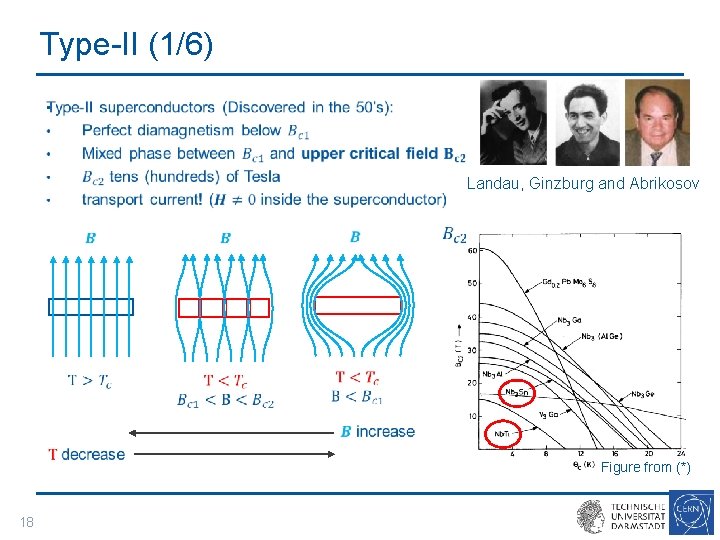
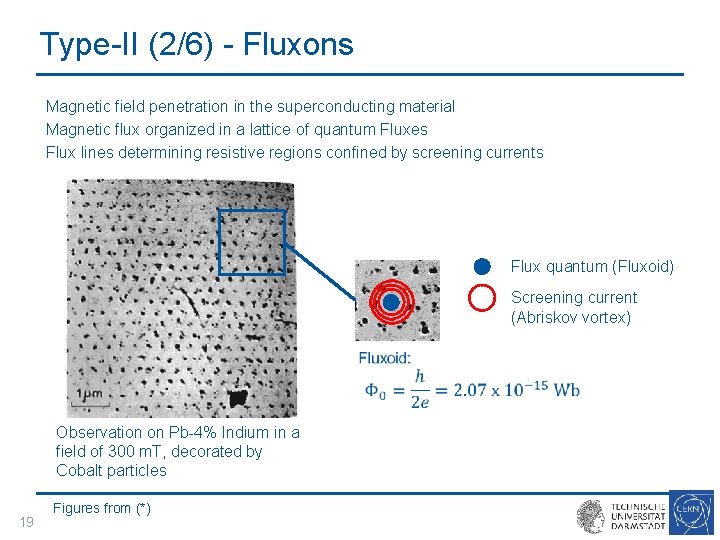
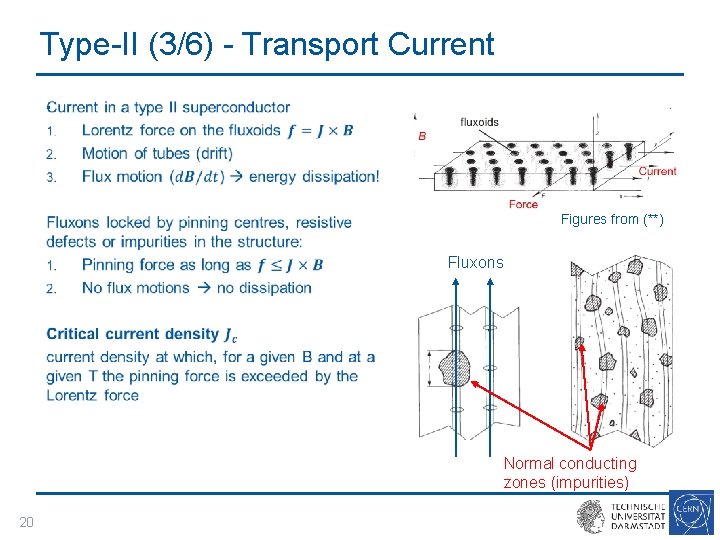
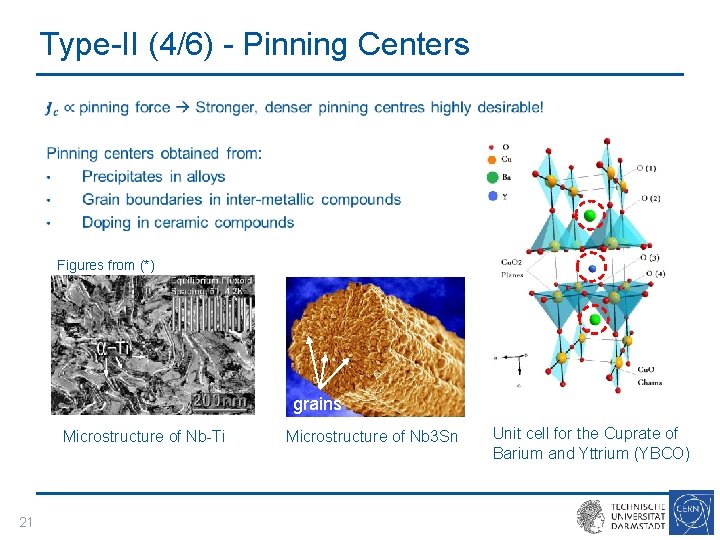
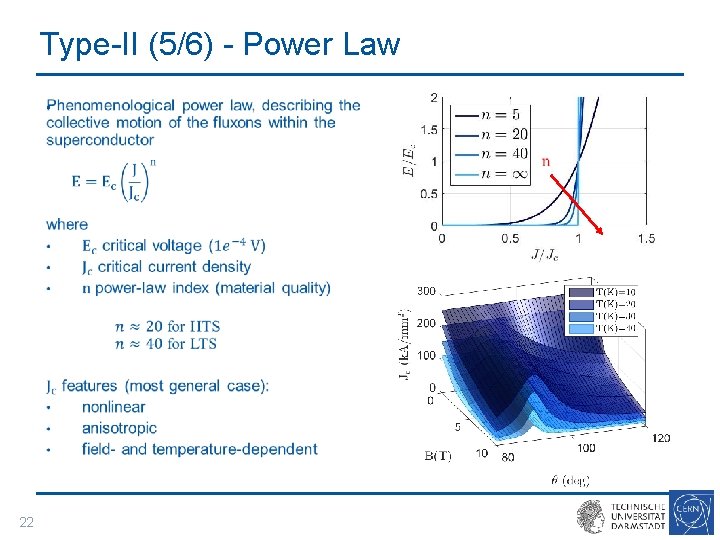
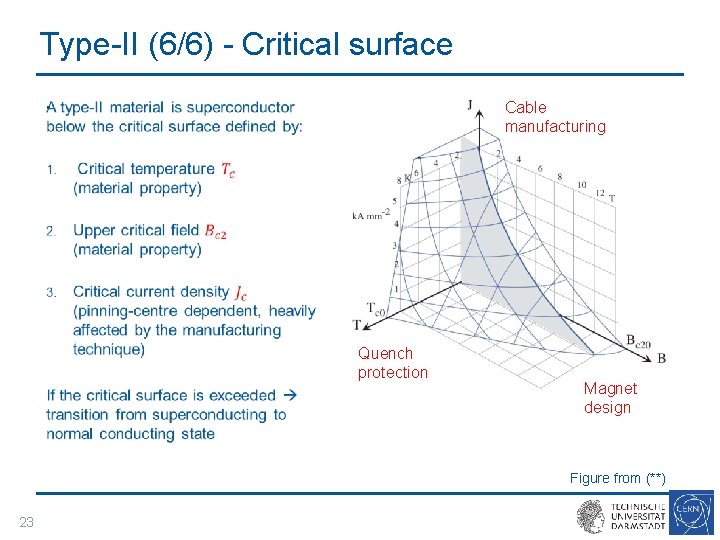
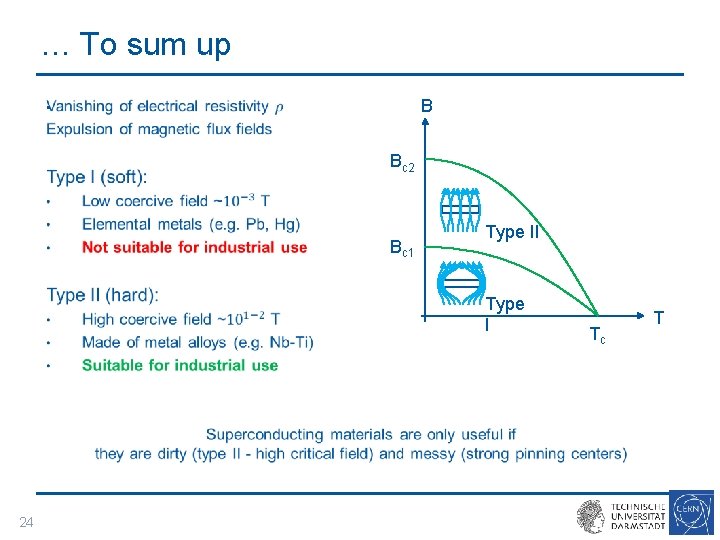
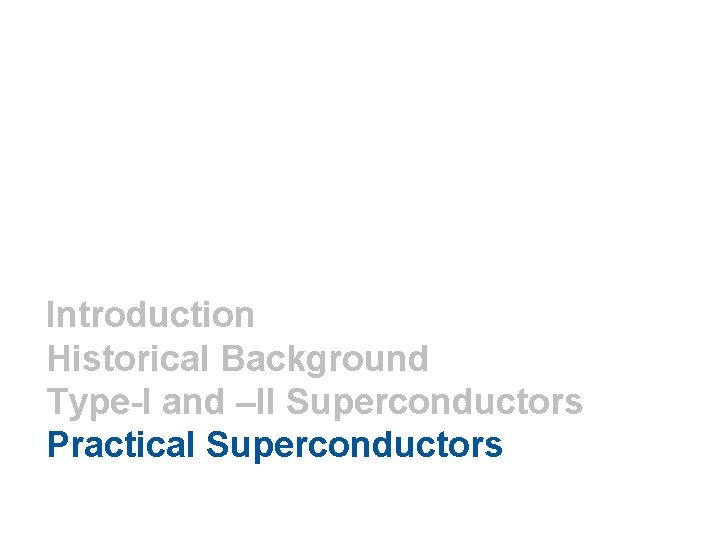
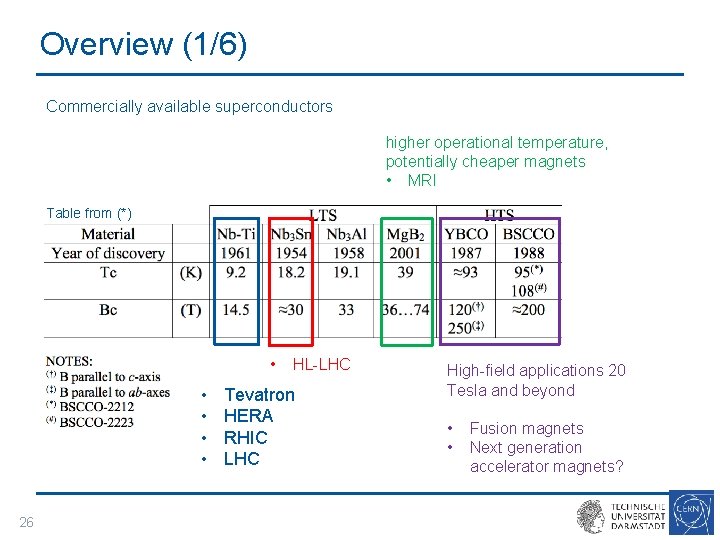
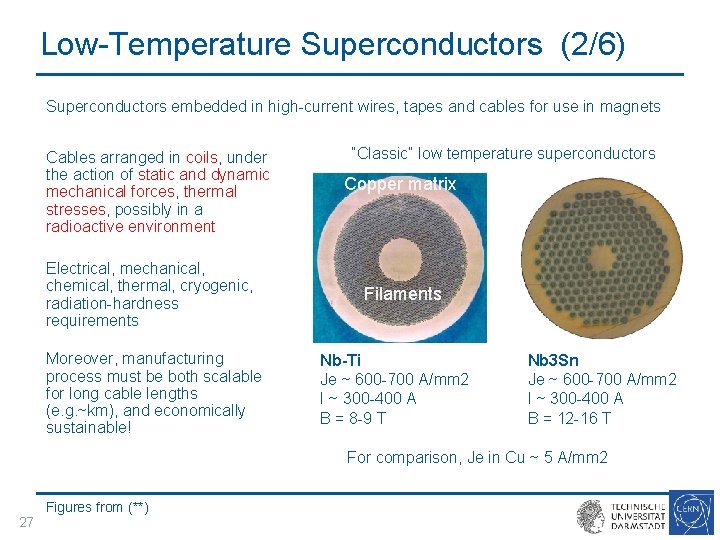
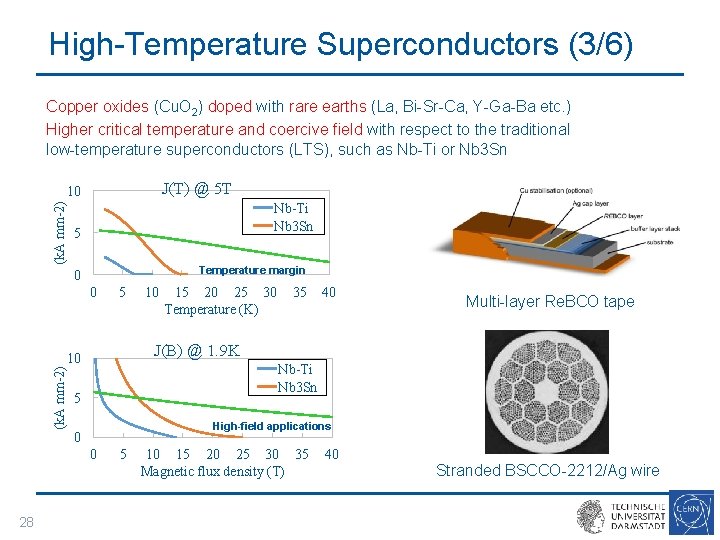
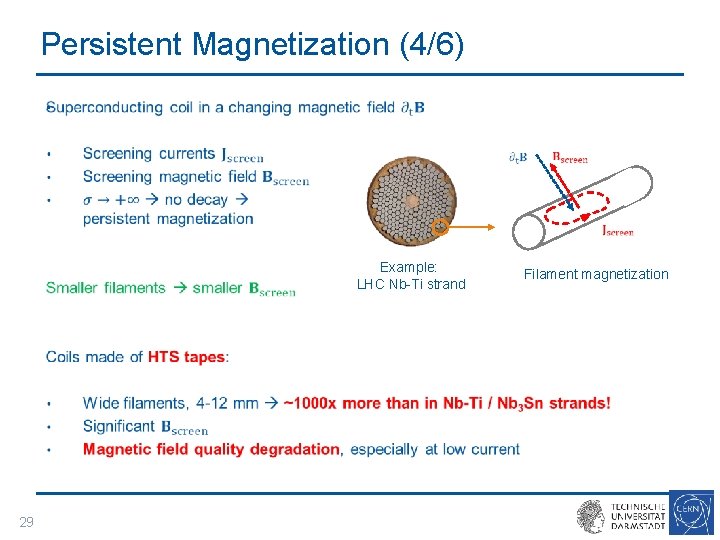
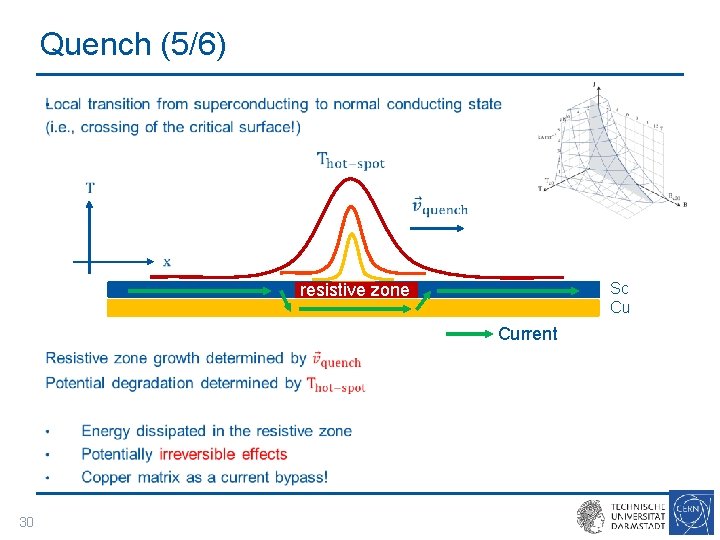
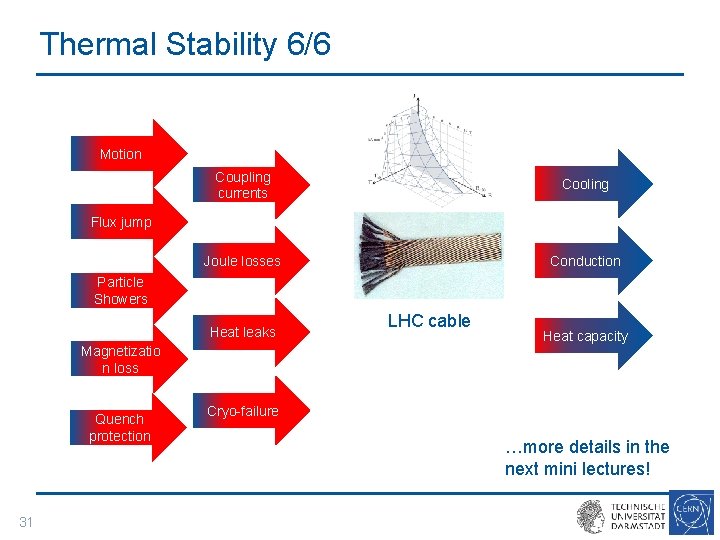
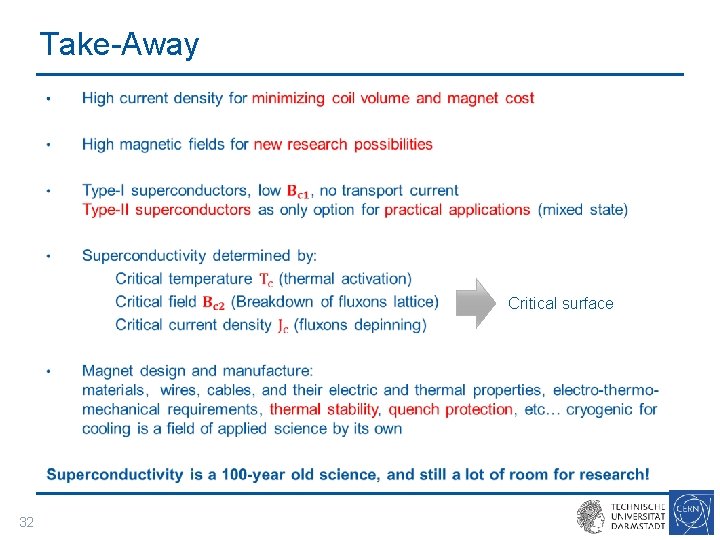
- Slides: 32
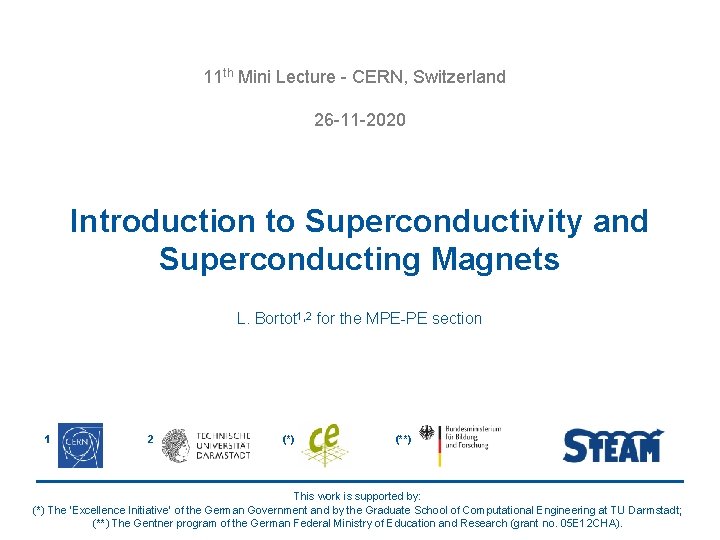
11 th Mini Lecture - CERN, Switzerland 26 -11 -2020 Introduction to Superconductivity and Superconducting Magnets L. Bortot 1, 2 for the MPE-PE section 1 2 (*) (**) This work is supported by: (*) The ‘Excellence Initiative’ of the German Government and by the Graduate School of Computational Engineering at TU Darmstadt; (**) The Gentner program of the German Federal Ministry of Education and Research (grant no. 05 E 12 CHA).
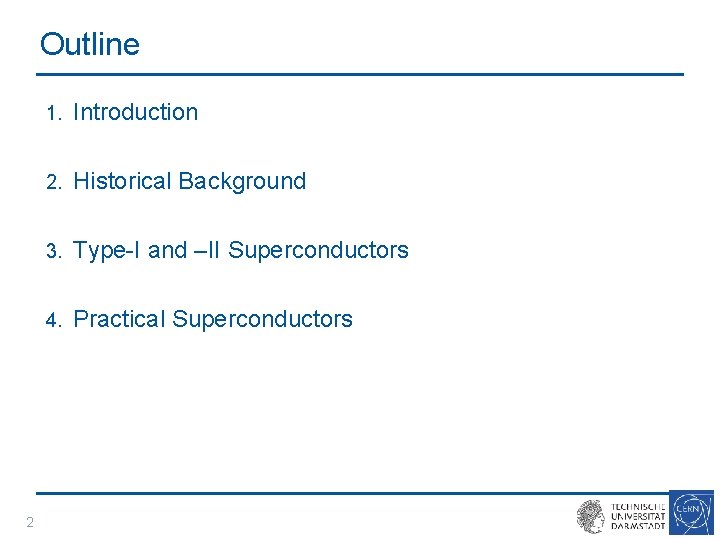
Outline 2 1. Introduction 2. Historical Background 3. Type-I and –II Superconductors 4. Practical Superconductors
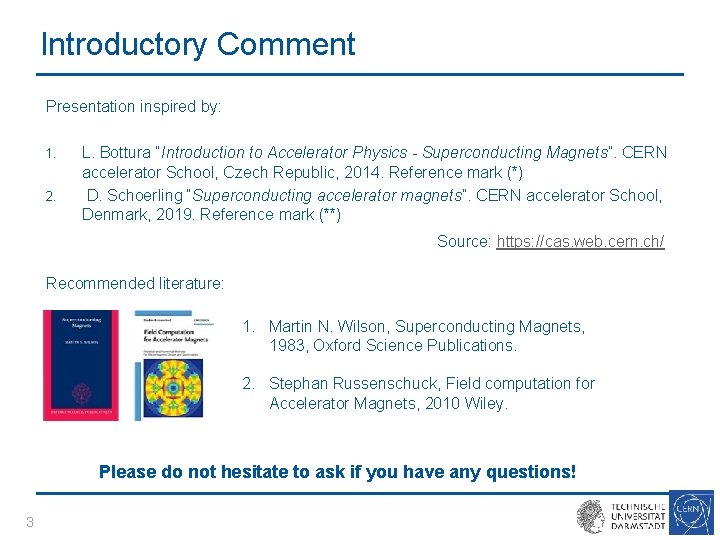
Introductory Comment Presentation inspired by: 1. 2. L. Bottura “Introduction to Accelerator Physics - Superconducting Magnets”. CERN accelerator School, Czech Republic, 2014. Reference mark (*) D. Schoerling “Superconducting accelerator magnets”. CERN accelerator School, Denmark, 2019. Reference mark (**) Source: https: //cas. web. cern. ch/ Recommended literature: 1. Martin N. Wilson, Superconducting Magnets, 1983, Oxford Science Publications. 2. Stephan Russenschuck, Field computation for Accelerator Magnets, 2010 Wiley. Please do not hesitate to ask if you have any questions! 3
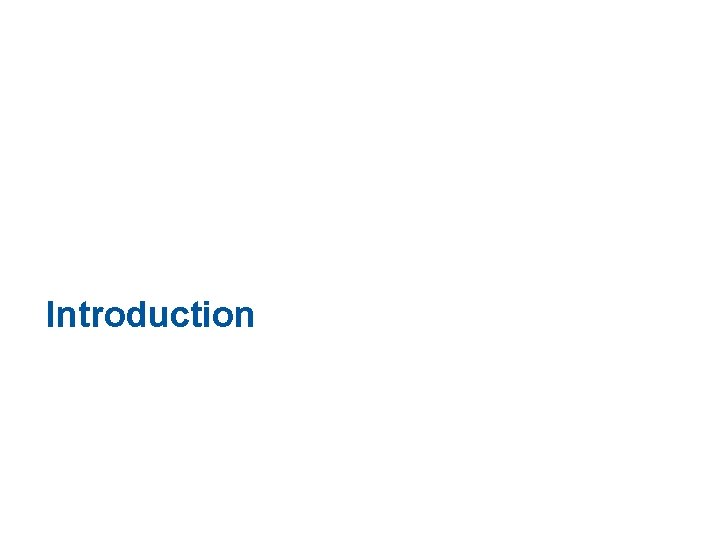
Introduction
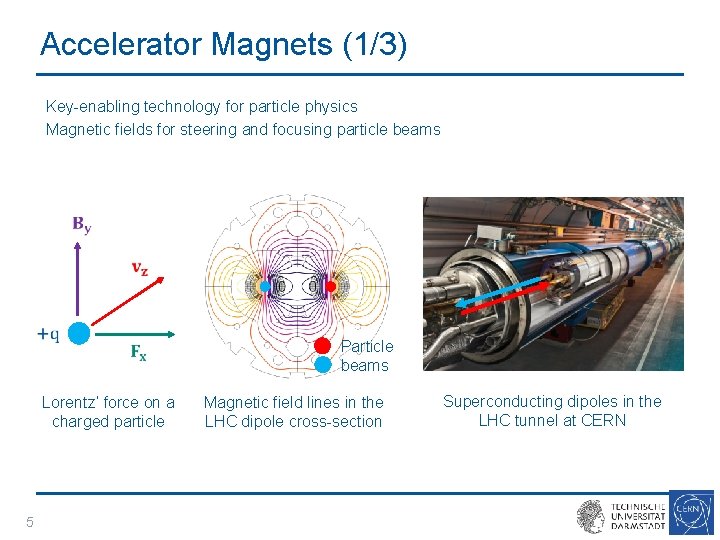
Accelerator Magnets (1/3) Key-enabling technology for particle physics Magnetic fields for steering and focusing particle beams Lorentz’ force on a charged particle 5 Particle beams Magnetic field lines in the LHC dipole cross-section Superconducting dipoles in the LHC tunnel at CERN
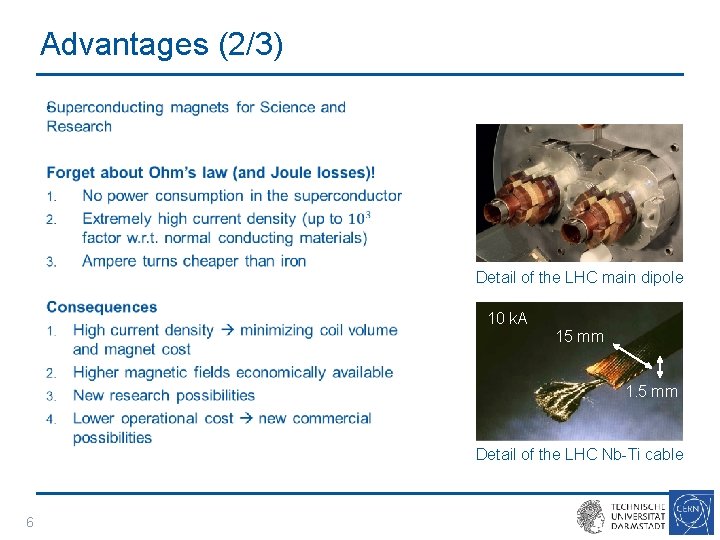
Advantages (2/3) • Detail of the LHC main dipole 10 k. A 15 mm 1. 5 mm Detail of the LHC Nb-Ti cable 6
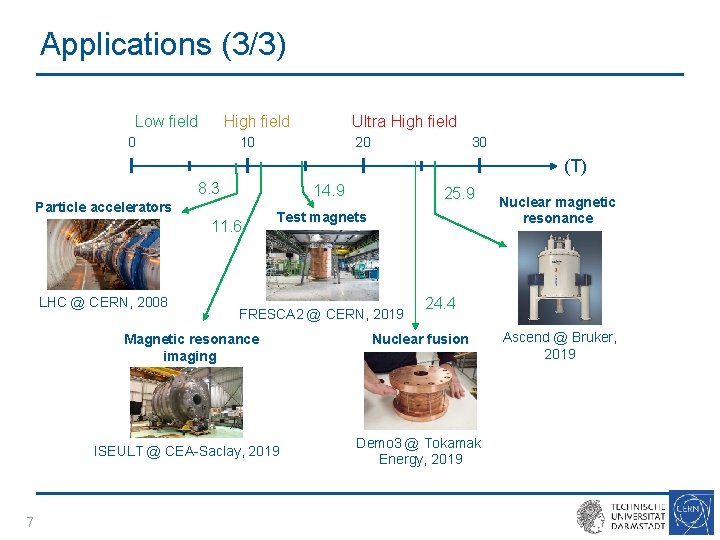
Applications (3/3) Low field High field 0 Ultra High field 10 20 30 (T) 8. 3 14. 9 Particle accelerators 11. 6 LHC @ CERN, 2008 Test magnets FRESCA 2 @ CERN, 2019 Magnetic resonance imaging ISEULT @ CEA-Saclay, 2019 7 25. 9 Nuclear magnetic resonance 24. 4 Nuclear fusion Demo 3 @ Tokamak Energy, 2019 Ascend @ Bruker, 2019
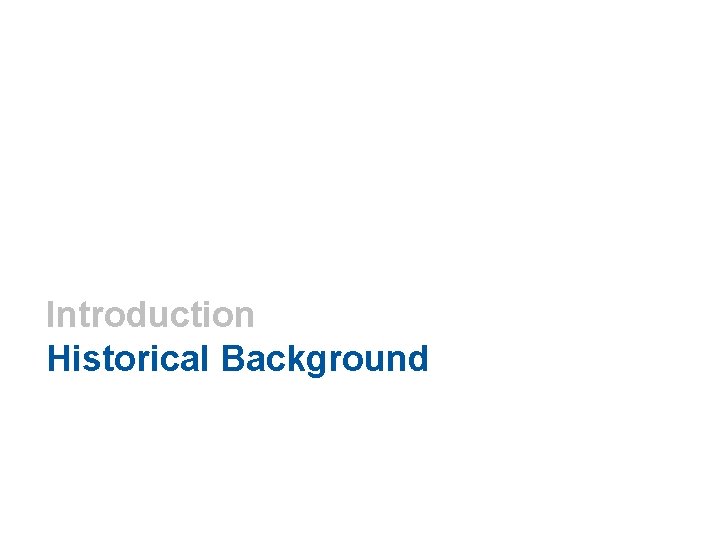
Introduction Historical Background
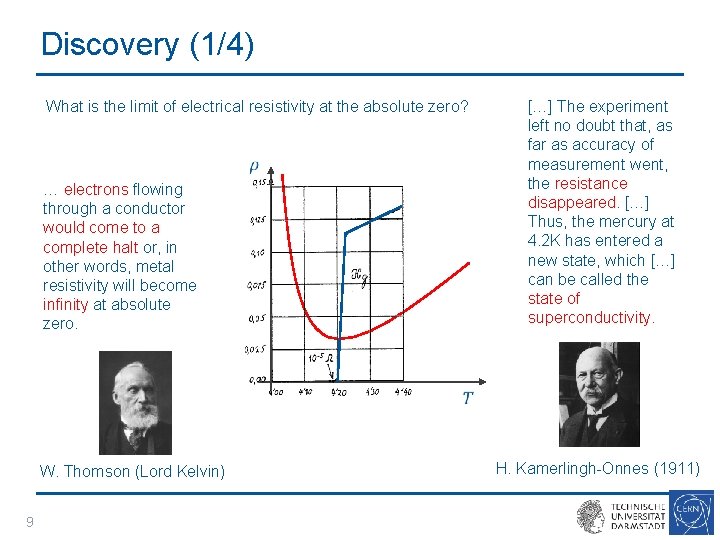
Discovery (1/4) What is the limit of electrical resistivity at the absolute zero? … electrons flowing through a conductor would come to a complete halt or, in other words, metal resistivity will become infinity at absolute zero. […] The experiment left no doubt that, as far as accuracy of measurement went, the resistance disappeared. […] Thus, the mercury at 4. 2 K has entered a new state, which […] can be called the state of superconductivity. W. Thomson (Lord Kelvin) 9 H. Kamerlingh-Onnes (1911)
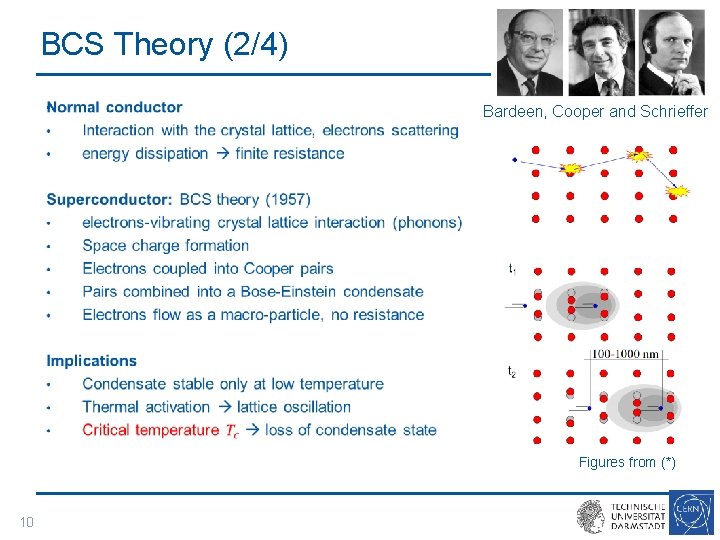
BCS Theory (2/4) • Bardeen, Cooper and Schrieffer Figures from (*) 10
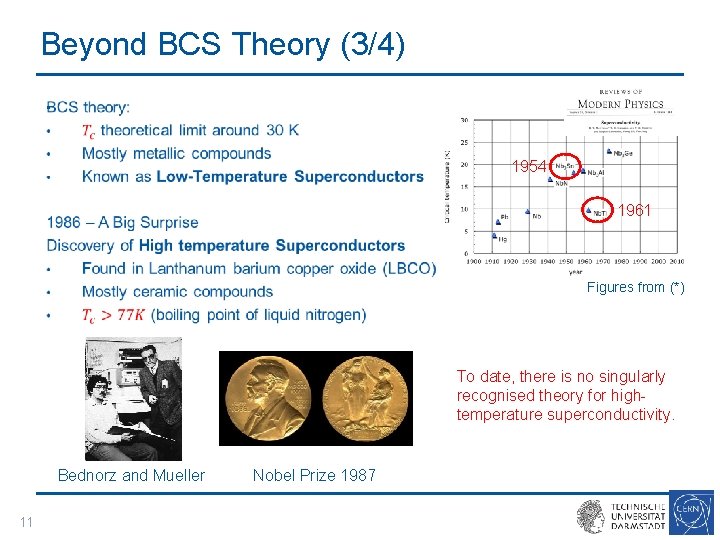
Beyond BCS Theory (3/4) • 1954 1961 Figures from (*) To date, there is no singularly recognised theory for hightemperature superconductivity. Bednorz and Mueller 11 Nobel Prize 1987
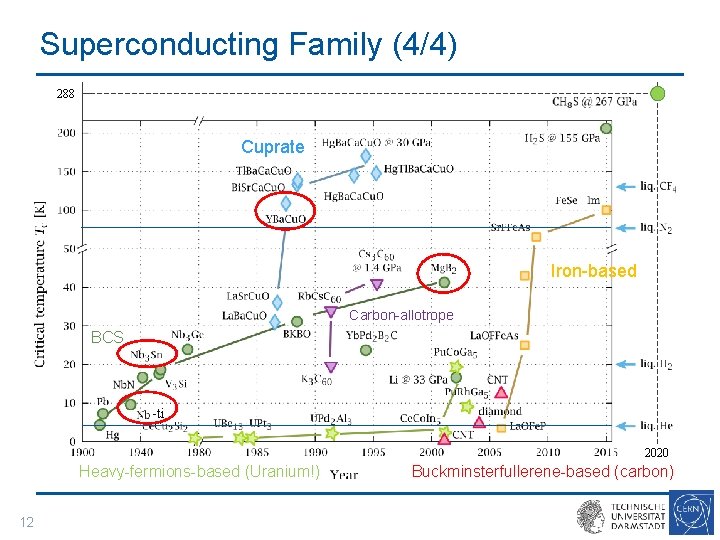
Superconducting Family (4/4) 288 Cuprate Iron-based Carbon-allotrope BCS -ti 2020 Heavy-fermions-based (Uranium!) 12 Buckminsterfullerene-based (carbon)
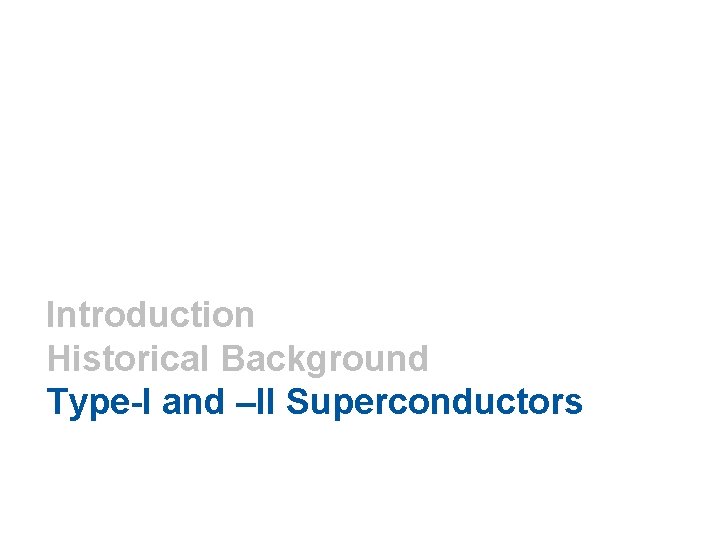
Introduction Historical Background Type-I and –II Superconductors
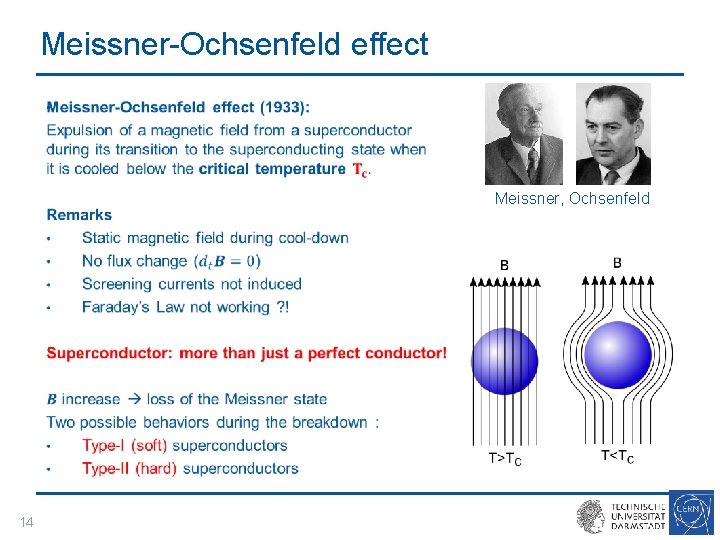
Meissner-Ochsenfeld effect • Meissner, Ochsenfeld 14
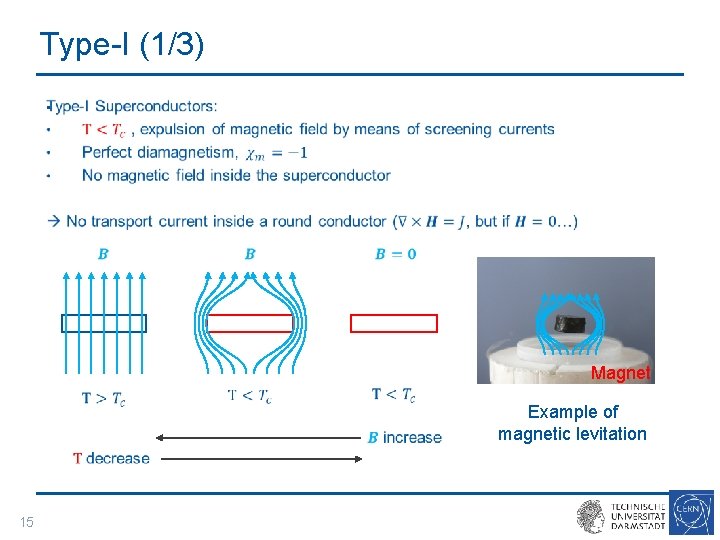
Type-I (1/3) • Magnet 15 Example of magnetic levitation
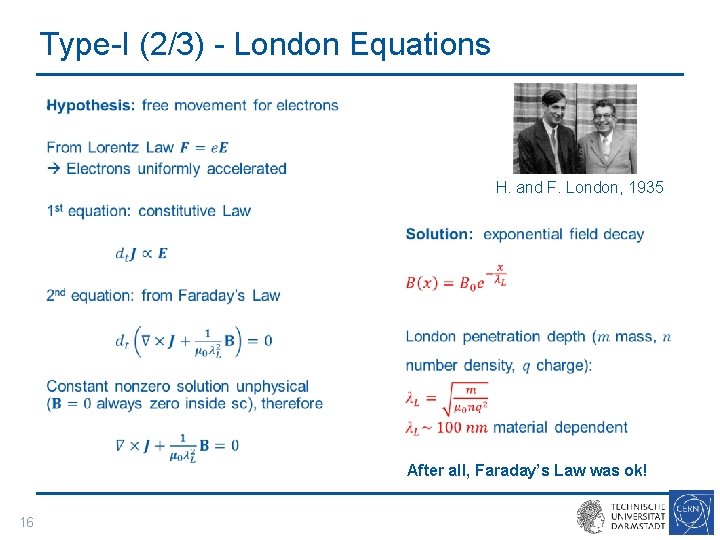
Type-I (2/3) - London Equations • H. and F. London, 1935 After all, Faraday’s Law was ok! 16
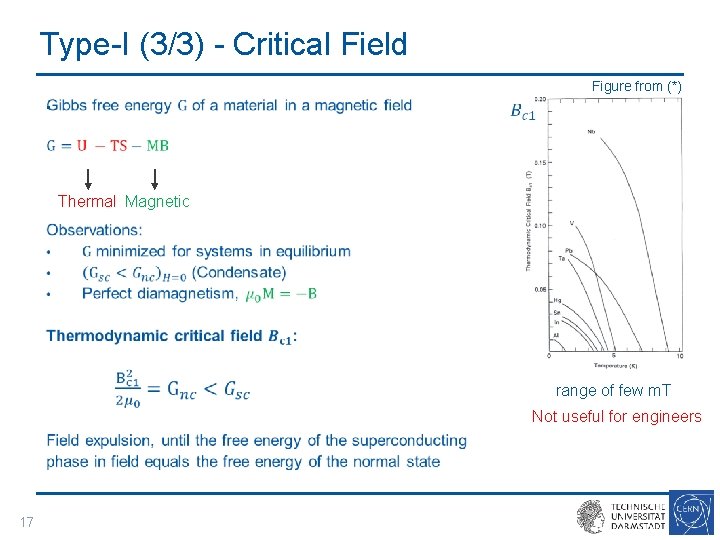
Type-I (3/3) - Critical Field Figure from (*) • Thermal Magnetic range of few m. T Not useful for engineers 17
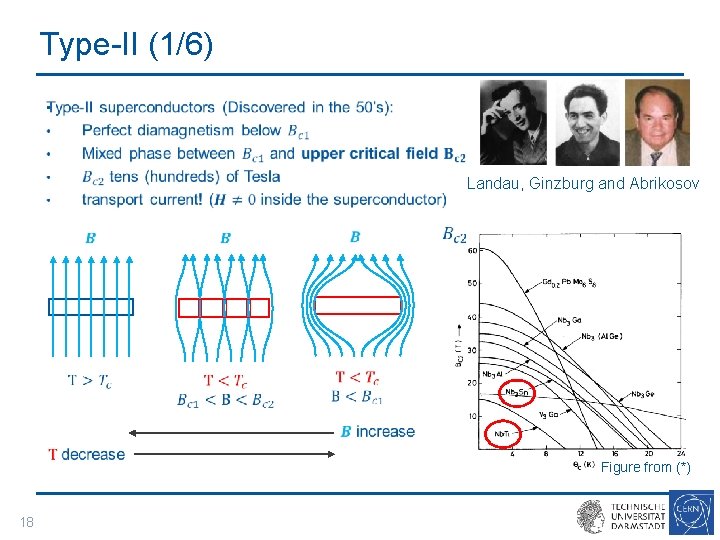
Type-II (1/6) • Landau, Ginzburg and Abrikosov 18 Figure from (*)
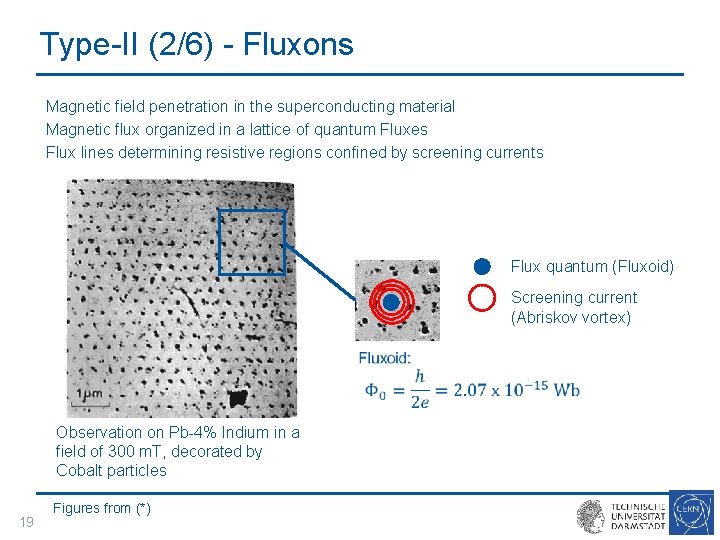
Type-II (2/6) - Fluxons Magnetic field penetration in the superconducting material Magnetic flux organized in a lattice of quantum Fluxes Flux lines determining resistive regions confined by screening currents Flux quantum (Fluxoid) Screening current (Abriskov vortex) Observation on Pb-4% Indium in a field of 300 m. T, decorated by Cobalt particles 19 Figures from (*)
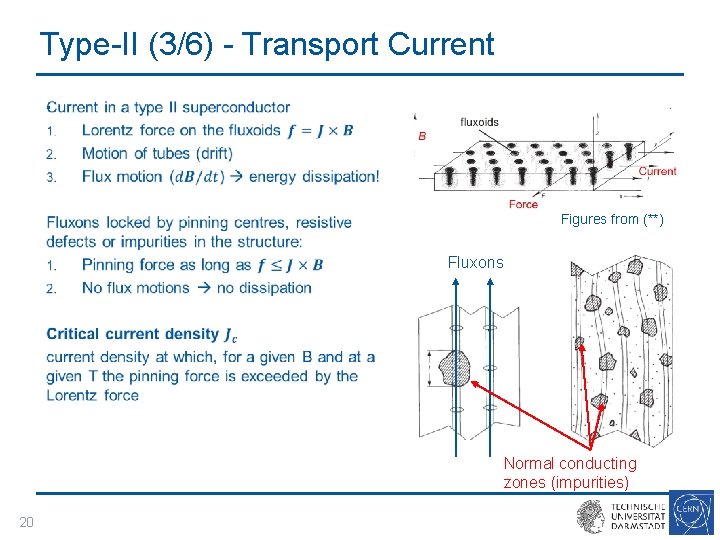
Type-II (3/6) - Transport Current • Figures from (**) Fluxons Normal conducting zones (impurities) 20
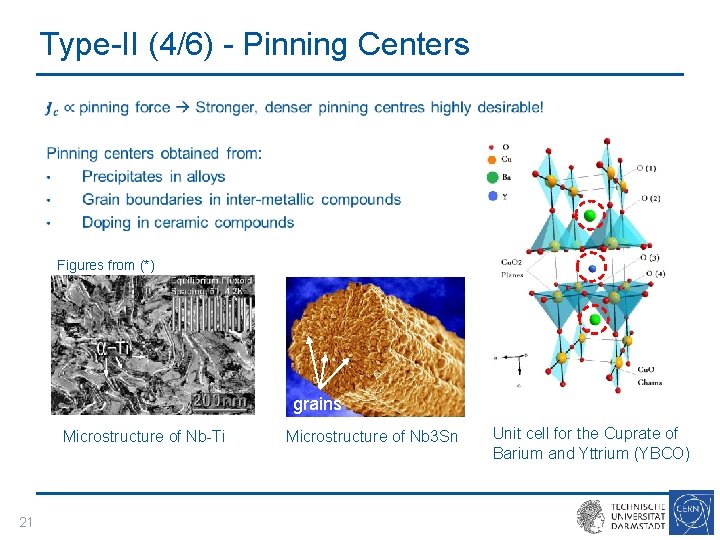
Type-II (4/6) - Pinning Centers • Figures from (*) grains Microstructure of Nb-Ti 21 Microstructure of Nb 3 Sn Unit cell for the Cuprate of Barium and Yttrium (YBCO)
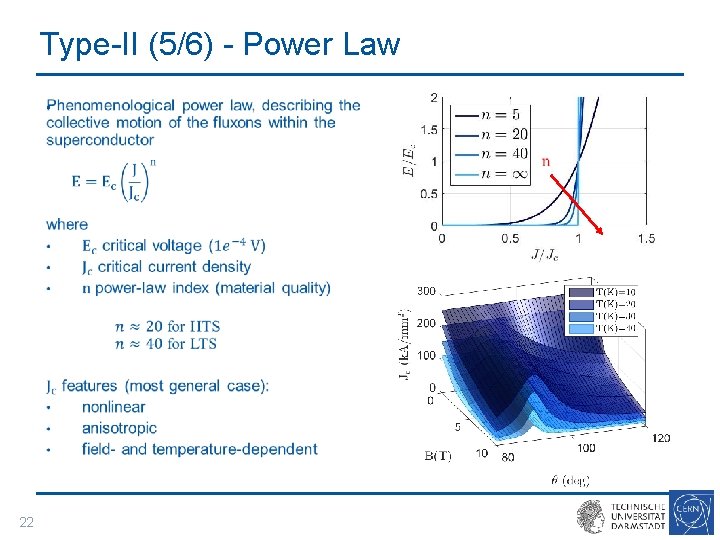
Type-II (5/6) - Power Law • 22
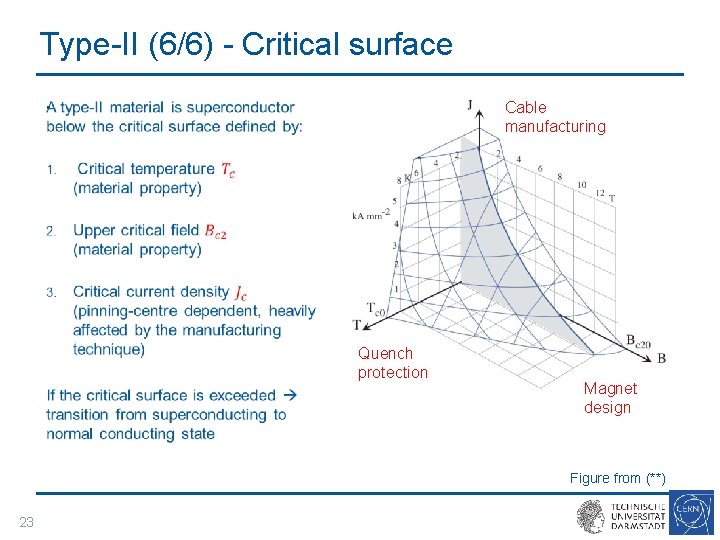
Type-II (6/6) - Critical surface • Cable manufacturing Quench protection Magnet design Figure from (**) 23
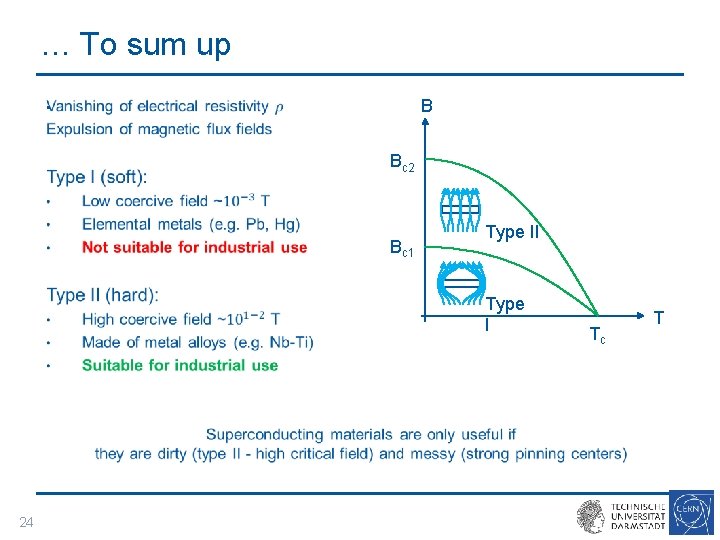
… To sum up • B Bc 2 Bc 1 Type II Type I 24 Tc T
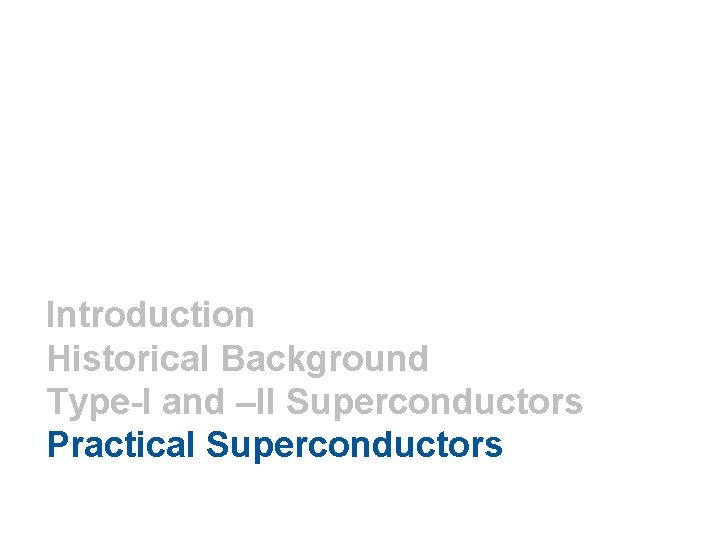
Introduction Historical Background Type-I and –II Superconductors Practical Superconductors
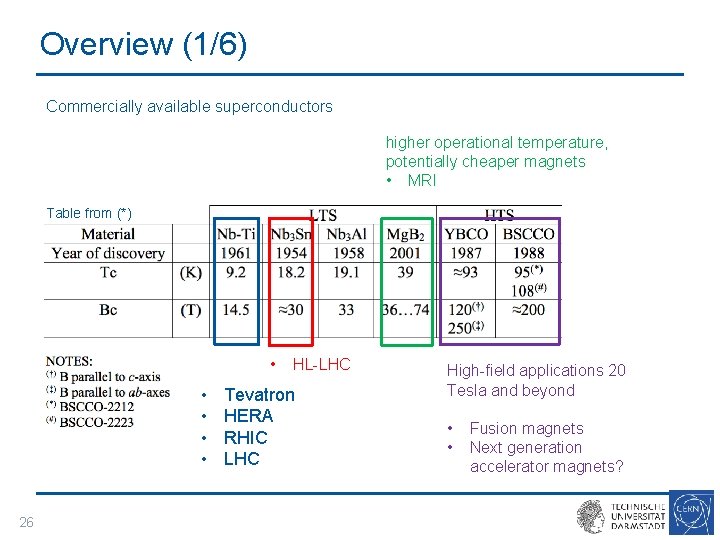
Overview (1/6) Commercially available superconductors higher operational temperature, potentially cheaper magnets • MRI Table from (*) • • • 26 HL-LHC Tevatron HERA RHIC LHC High-field applications 20 Tesla and beyond • • Fusion magnets Next generation accelerator magnets?
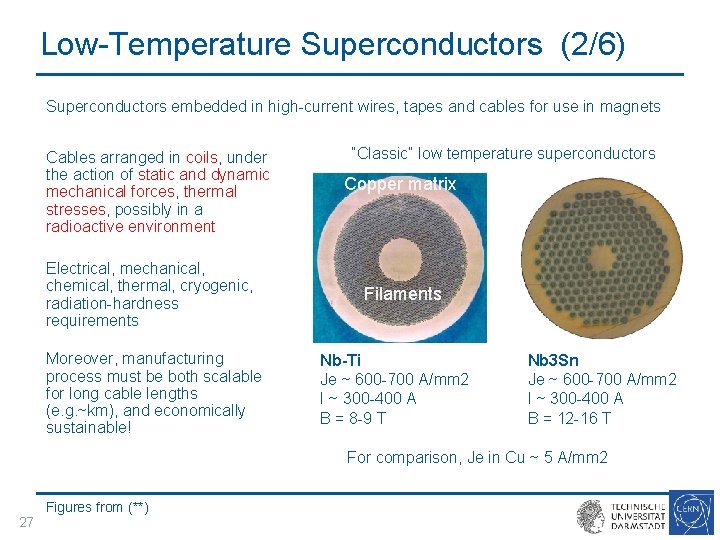
Low-Temperature Superconductors (2/6) Superconductors embedded in high-current wires, tapes and cables for use in magnets Cables arranged in coils, under the action of static and dynamic mechanical forces, thermal stresses, possibly in a radioactive environment Electrical, mechanical, chemical, thermal, cryogenic, radiation-hardness requirements Moreover, manufacturing process must be both scalable for long cable lengths (e. g. ~km), and economically sustainable! “Classic” low temperature superconductors Copper matrix Filaments Nb-Ti Je ~ 600 -700 A/mm 2 I ~ 300 -400 A B = 8 -9 T Nb 3 Sn Je ~ 600 -700 A/mm 2 I ~ 300 -400 A B = 12 -16 T For comparison, Je in Cu ~ 5 A/mm 2 Figures from (**) 27
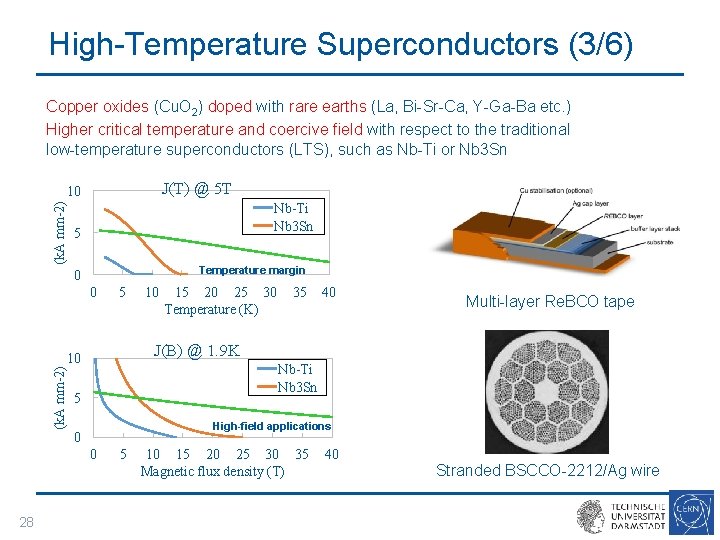
High-Temperature Superconductors (3/6) Copper oxides (Cu. O 2) doped with rare earths (La, Bi-Sr-Ca, Y-Ga-Ba etc. ) Higher critical temperature and coercive field with respect to the traditional low-temperature superconductors (LTS), such as Nb-Ti or Nb 3 Sn J(T) @ 5 T (k. A mm-2) 10 Nb-Ti Nb 3 Sn 5 Temperature margin 0 (k. A mm-2) 0 5 15 20 25 30 Temperature (K) 35 40 Multi-layer Re. BCO tape J(B) @ 1. 9 K 10 Nb-Ti Nb 3 Sn 5 High-field applications 0 0 28 10 5 10 15 20 25 30 35 Magnetic flux density (T) 40 Stranded BSCCO-2212/Ag wire
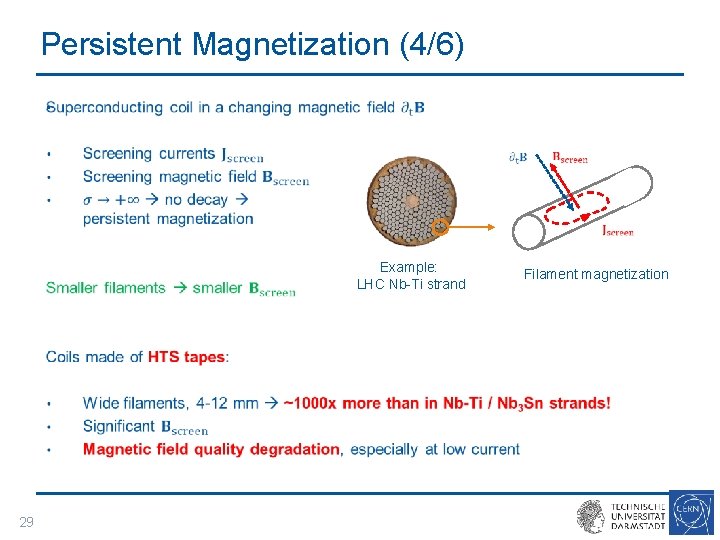
Persistent Magnetization (4/6) • Example: LHC Nb-Ti strand 29 Filament magnetization
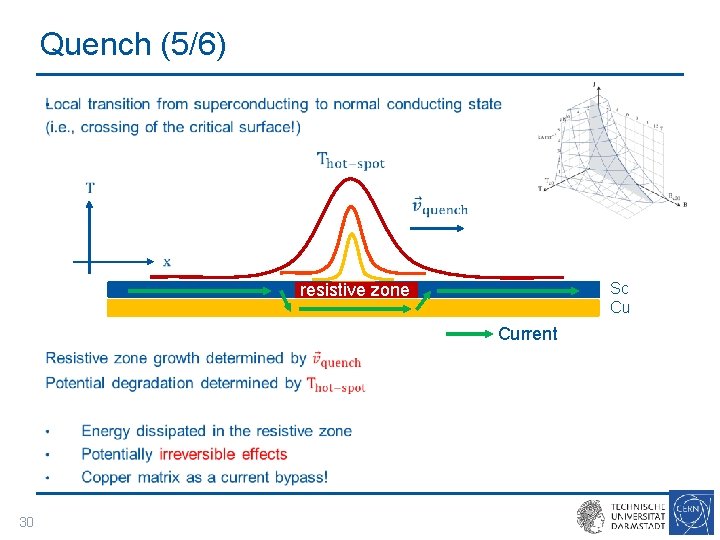
Quench (5/6) • Sc Cu resistive zone Current 30
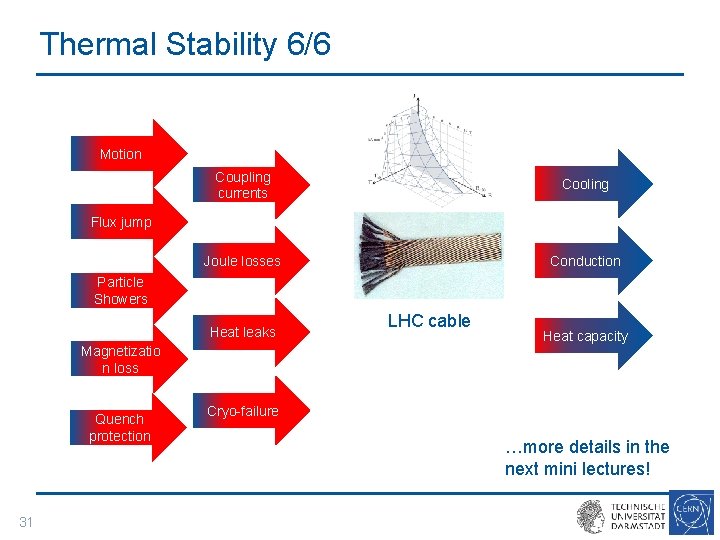
Thermal Stability 6/6 Motion Coupling currents Cooling Joule losses Conduction Flux jump Particle Showers Heat leaks Magnetizatio n loss Quench protection 31 LHC cable Heat capacity Cryo-failure …more details in the next mini lectures!
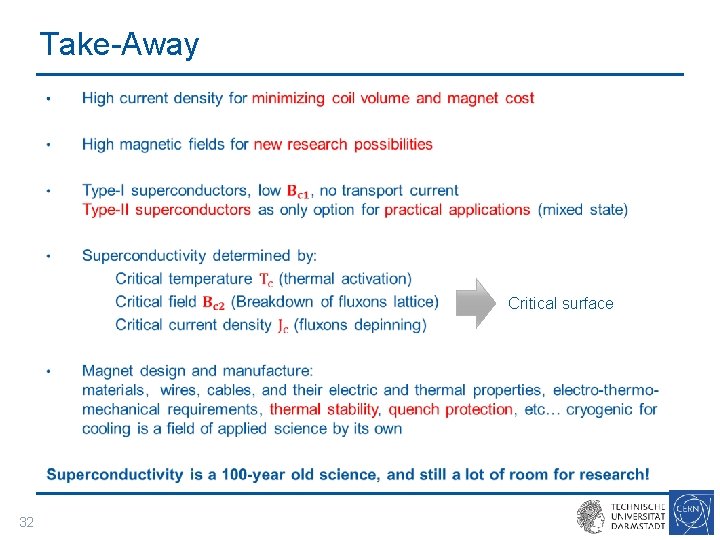
Take-Away • Critical surface 32
Analisis foda maxi mini ejemplos
Foda de una persona con mentalidad positiva
Ejemplo de análisis foda de una institución pública
01:640:244 lecture notes - lecture 15: plat, idah, farad
Atom tunnel switzerland
Abms degree
5232 villigen
National working group on swiss franc reference rates
Cern users office
Is switzerland a landlocked country
Ang unang paglalakbay ni rizal
G. bopp usa, inc.
Verdingkinder definition
Switzerland
Egypt caller id
Caller id certificate
Swiss point middle
Switzerland continent
Door to door luggage service switzerland
Schweiz suisse svizzera svizra switzerland
Bern wikivoyage
Thermal baths vals switzerland
Eures jobs switzerland
Ecotourism in switzerland
Ikea switzerland
Switzerland ada dimana
Elog switzerland
National working group on swiss franc reference rates
Switzerland middle school
Squin sa switzerland
Mini daq
Why is the book of isaiah called a mini bible
Exemple de sommaire d'un projet