Why ice matters Mathematical modelling of ice crystals
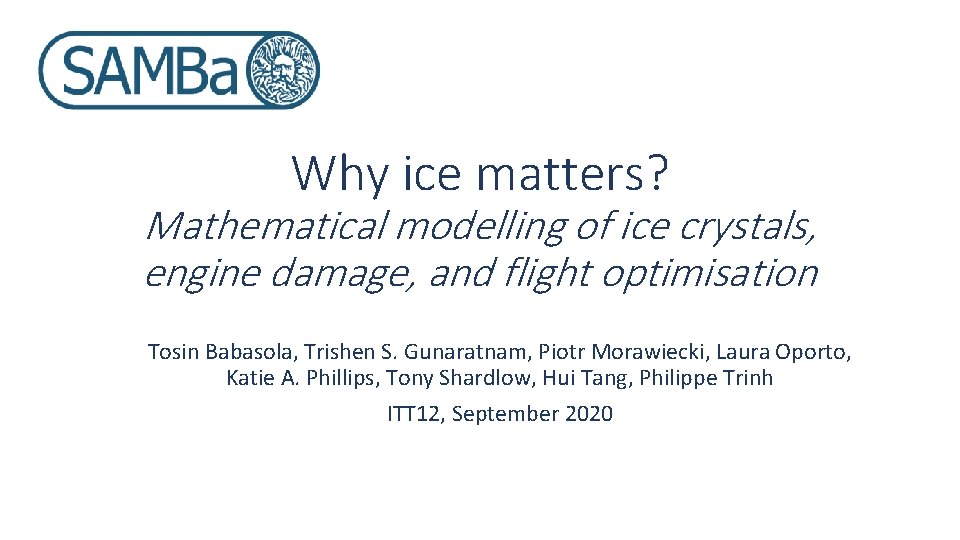
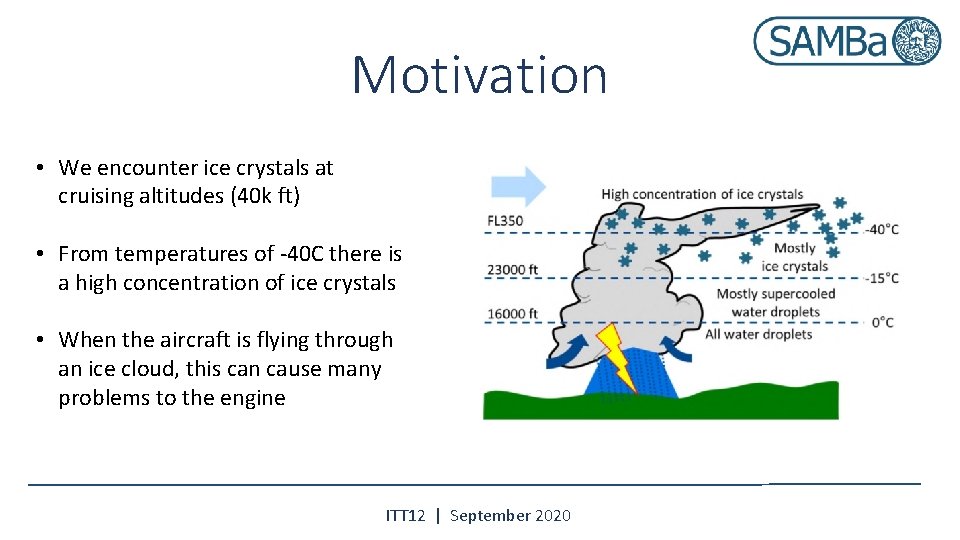
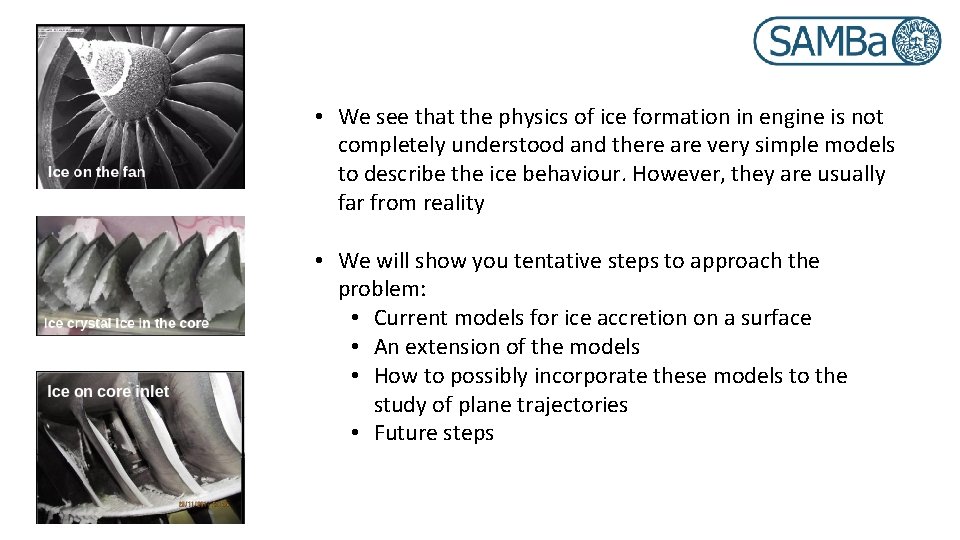
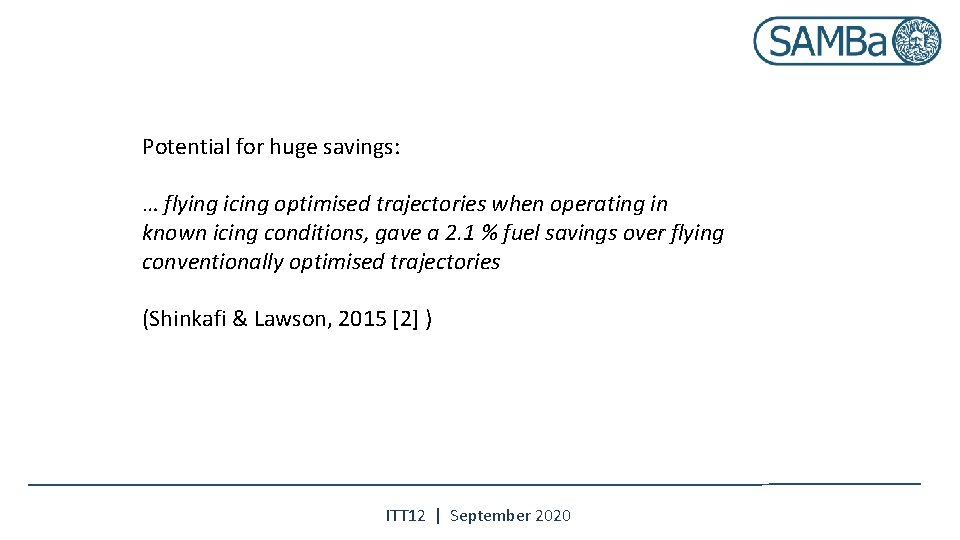
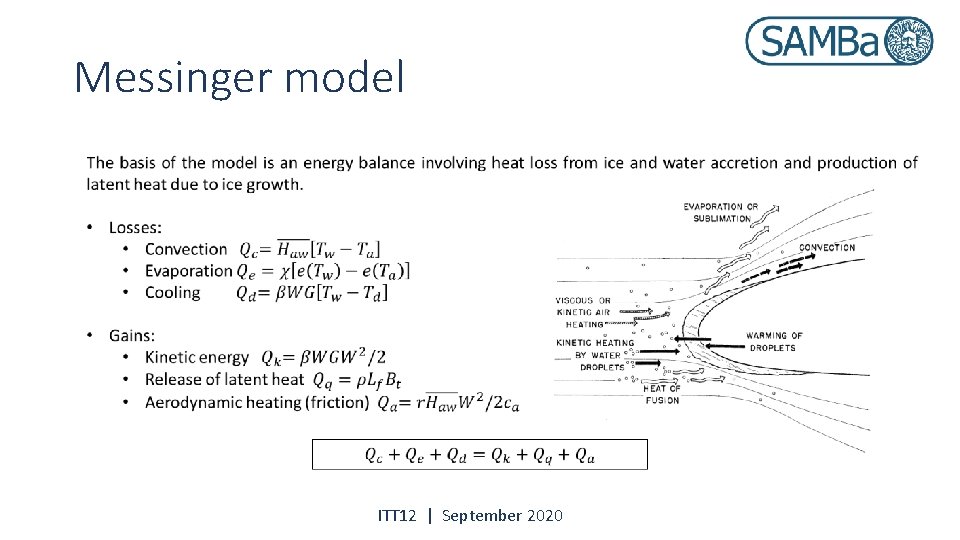
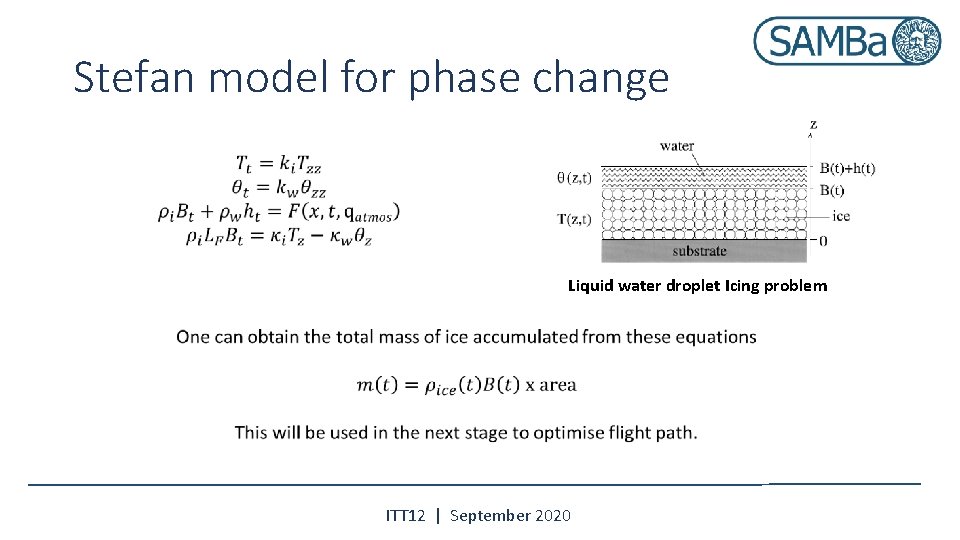
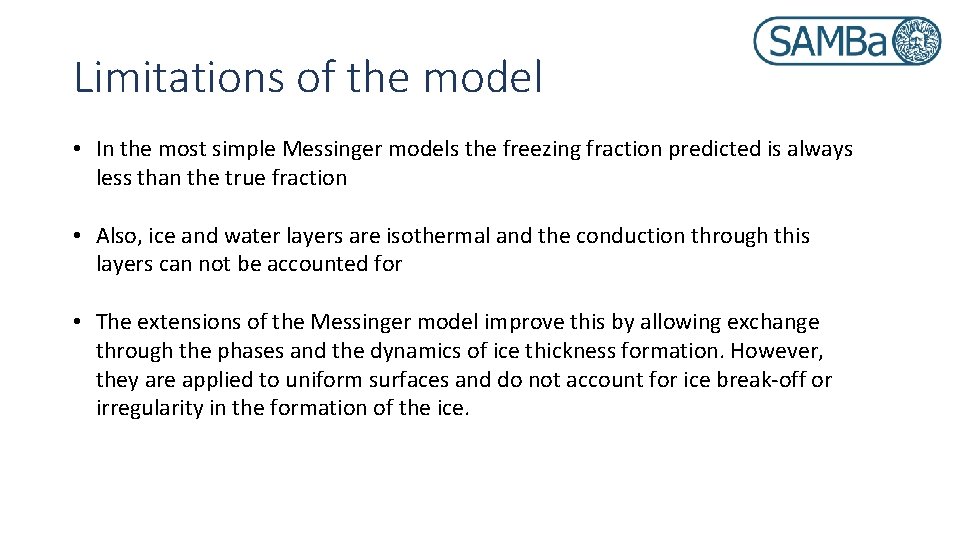
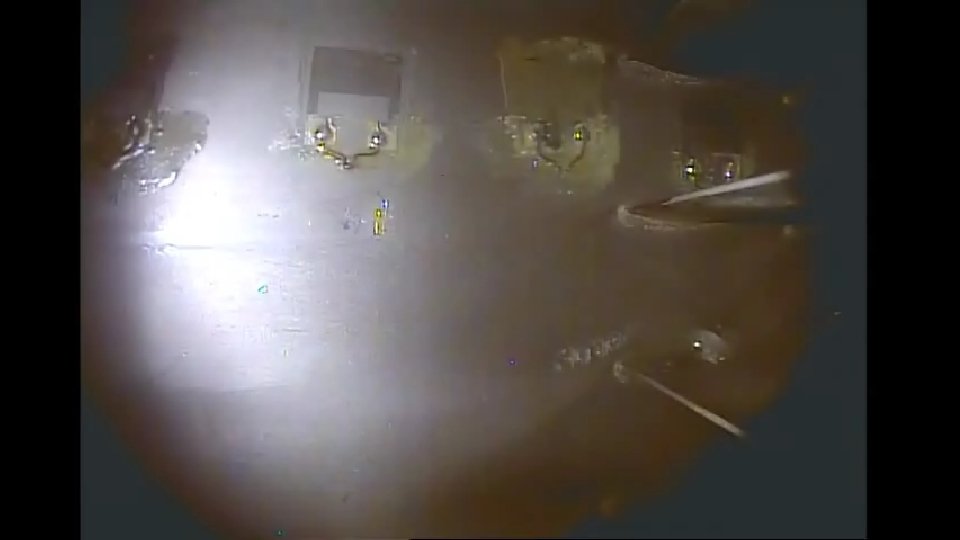
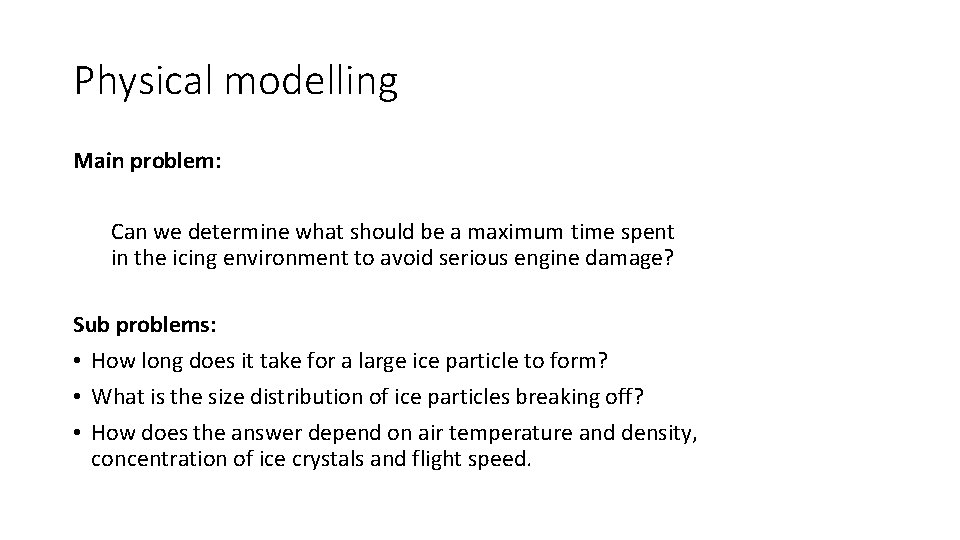
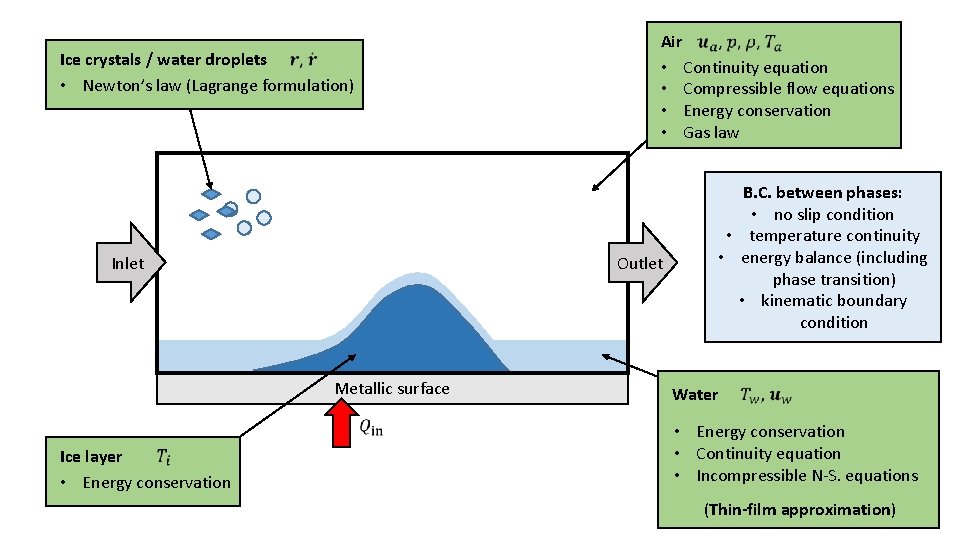
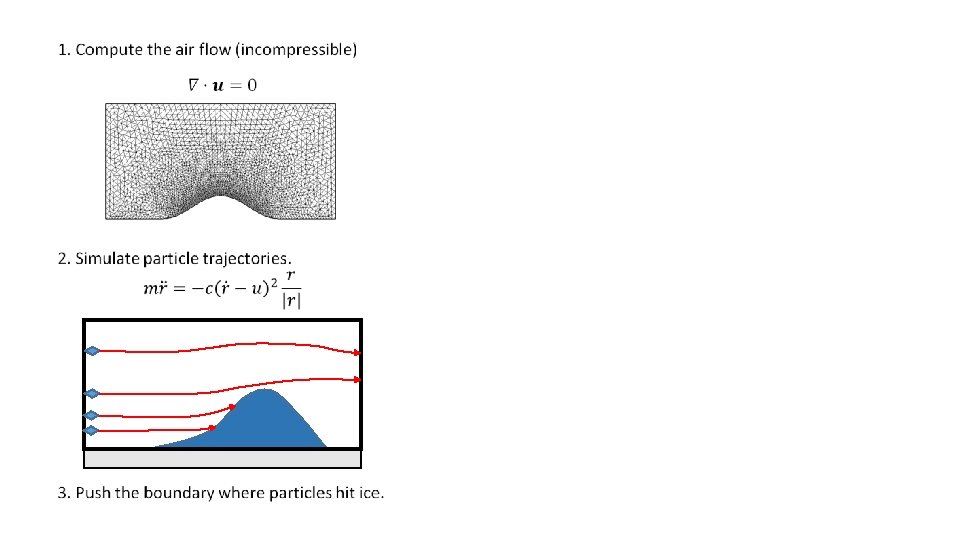
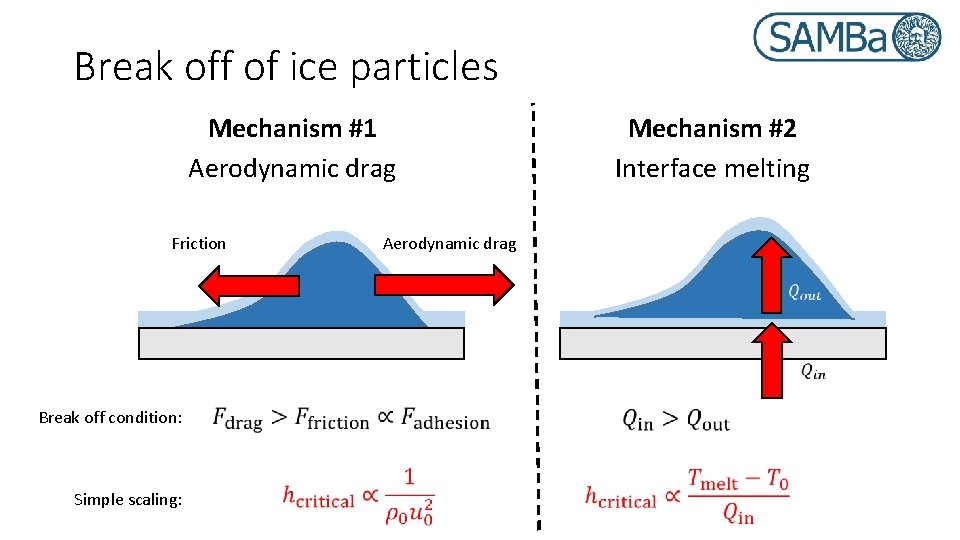
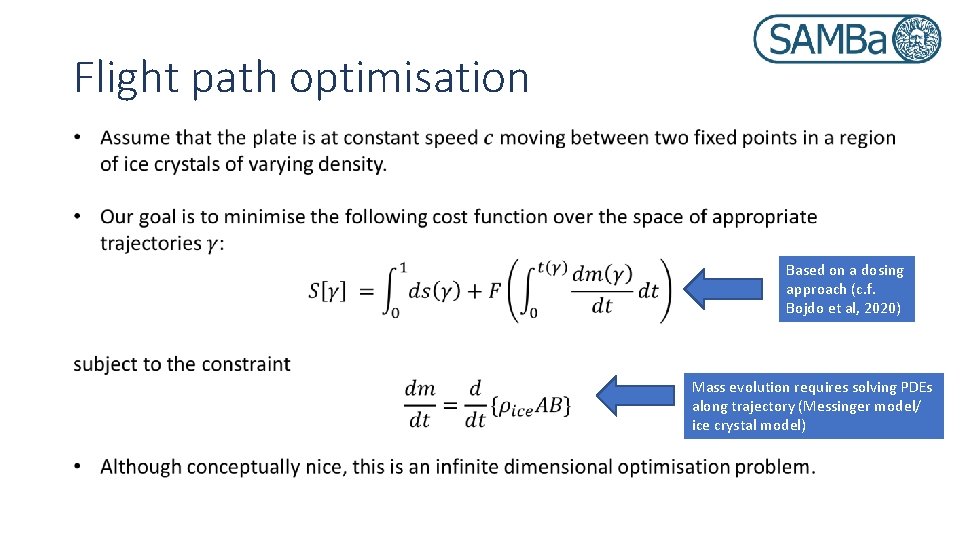
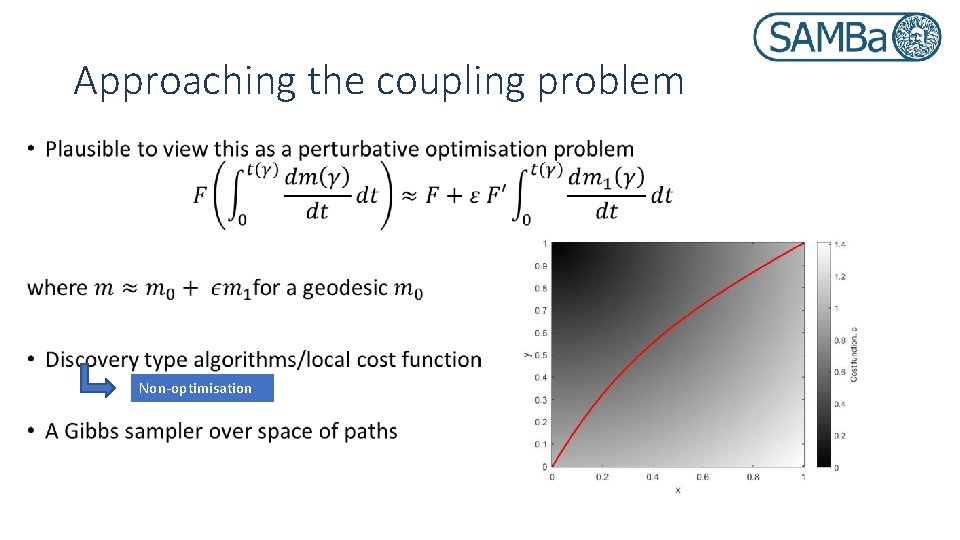
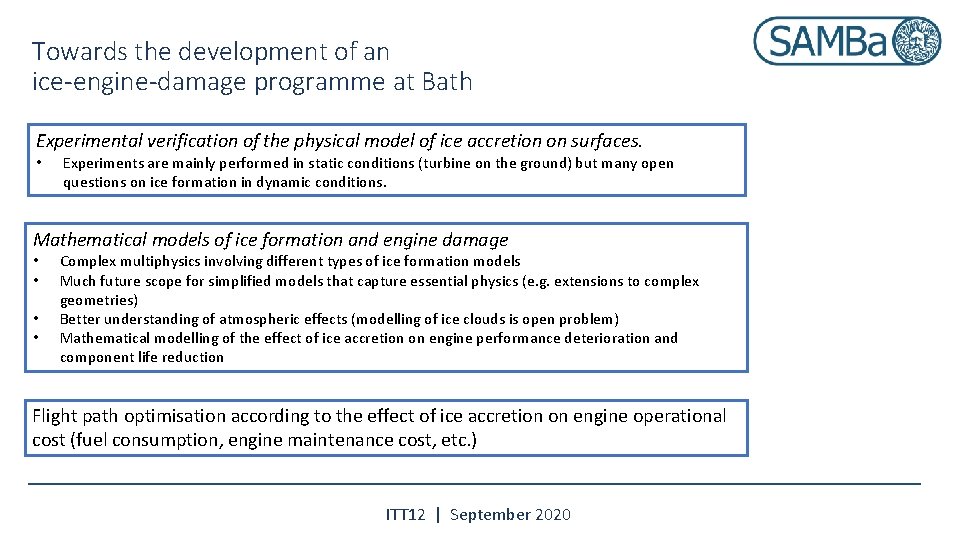
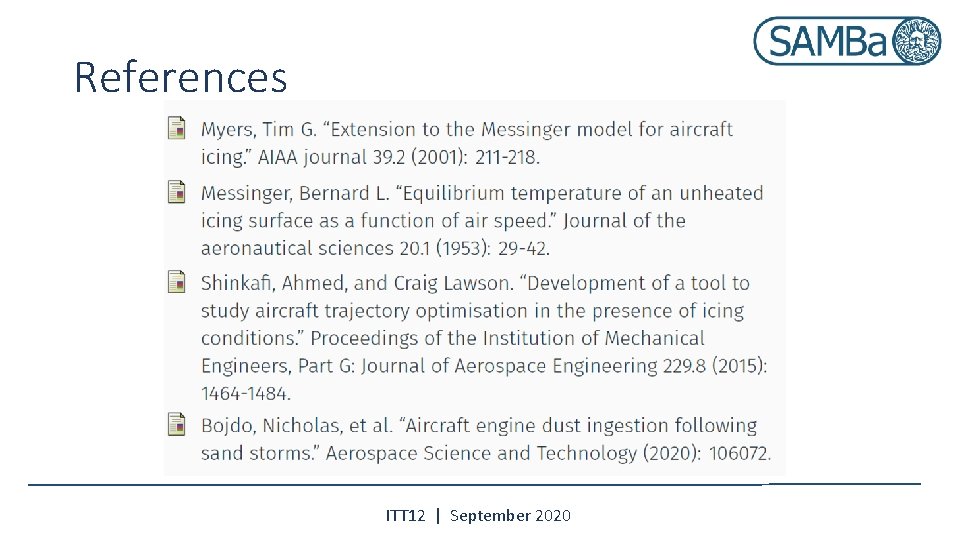
- Slides: 16
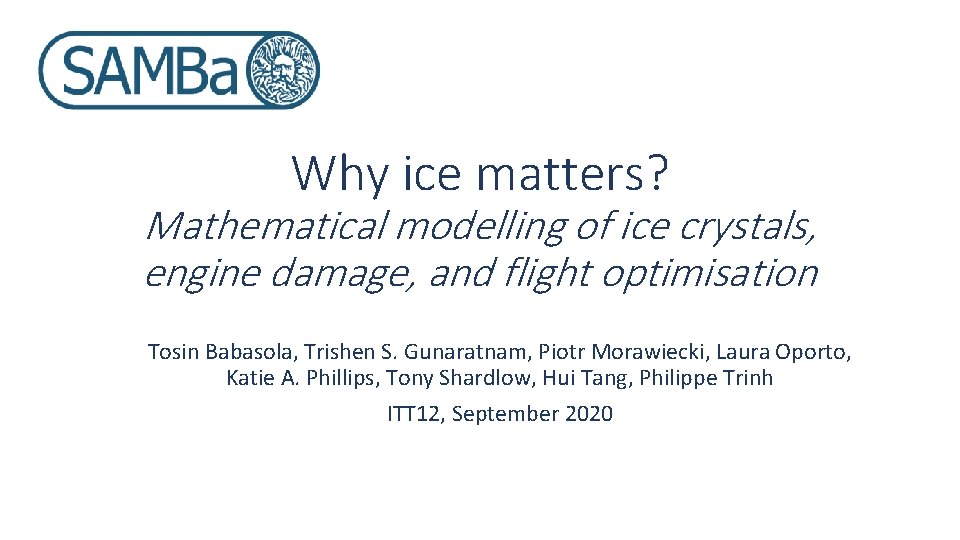
Why ice matters? Mathematical modelling of ice crystals, engine damage, and flight optimisation Tosin Babasola, Trishen S. Gunaratnam, Piotr Morawiecki, Laura Oporto, Katie A. Phillips, Tony Shardlow, Hui Tang, Philippe Trinh ITT 12, September 2020
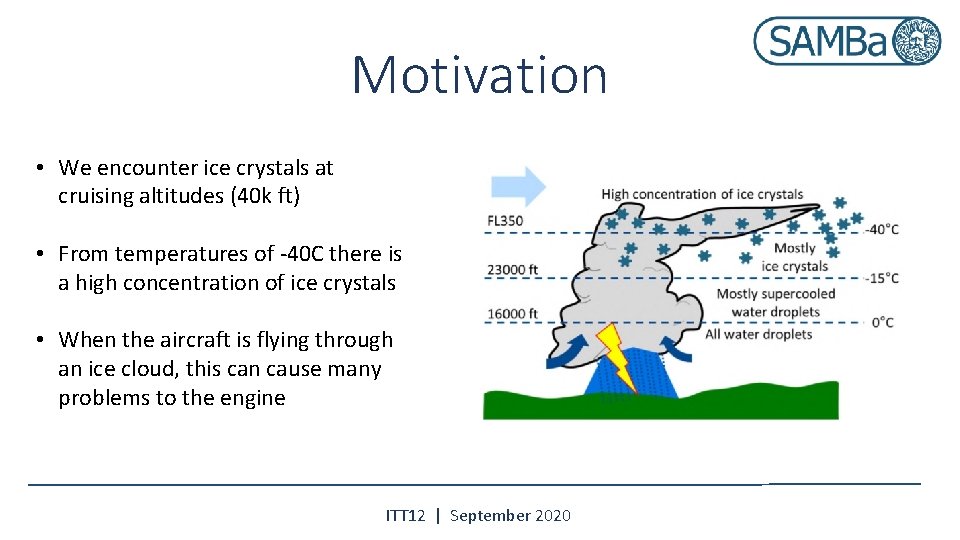
Motivation • We encounter ice crystals at cruising altitudes (40 k ft) • From temperatures of -40 C there is a high concentration of ice crystals • When the aircraft is flying through an ice cloud, this can cause many problems to the engine ITT 12 | September 2020
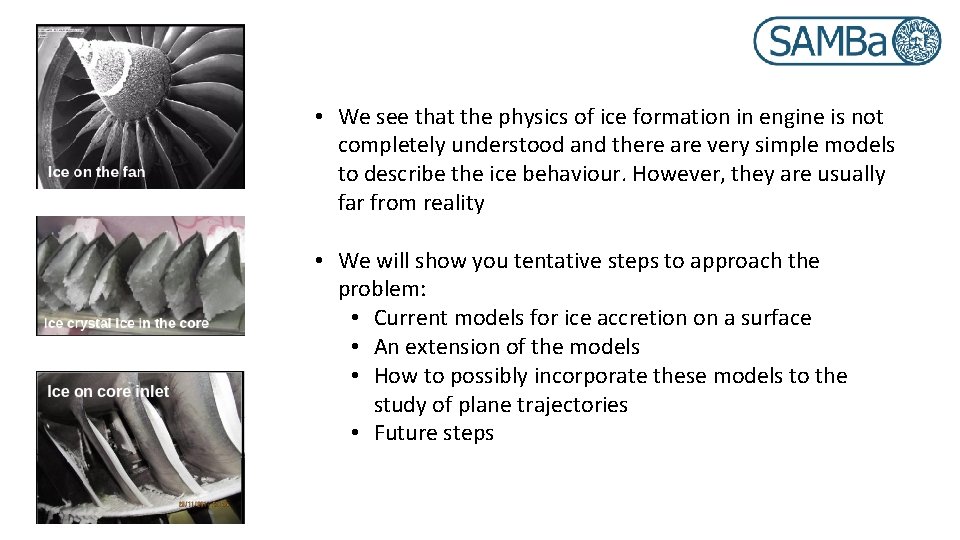
• We see that the physics of ice formation in engine is not completely understood and there are very simple models to describe the ice behaviour. However, they are usually far from reality • We will show you tentative steps to approach the problem: • Current models for ice accretion on a surface • An extension of the models • How to possibly incorporate these models to the study of plane trajectories • Future steps
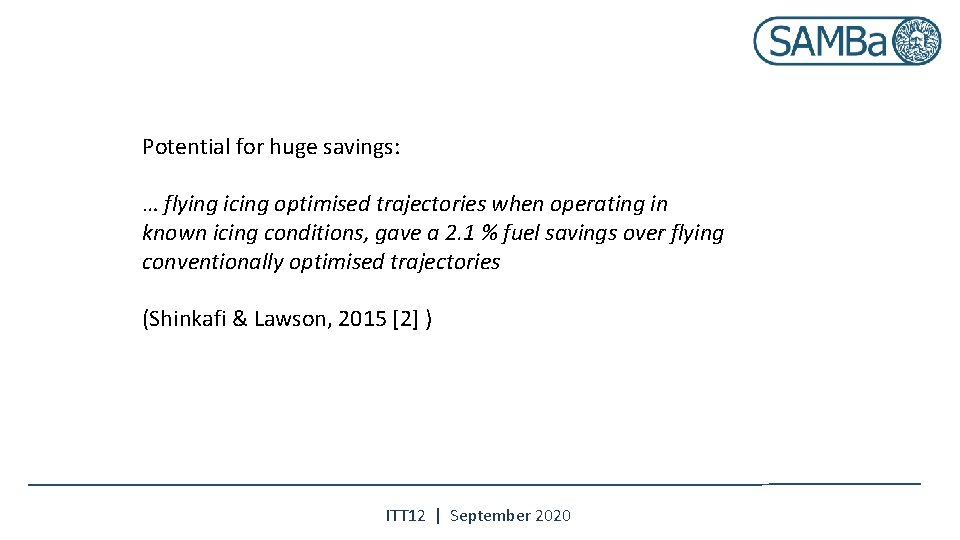
Potential for huge savings: … flying icing optimised trajectories when operating in known icing conditions, gave a 2. 1 % fuel savings over flying conventionally optimised trajectories (Shinkafi & Lawson, 2015 [2] ) ITT 12 | September 2020
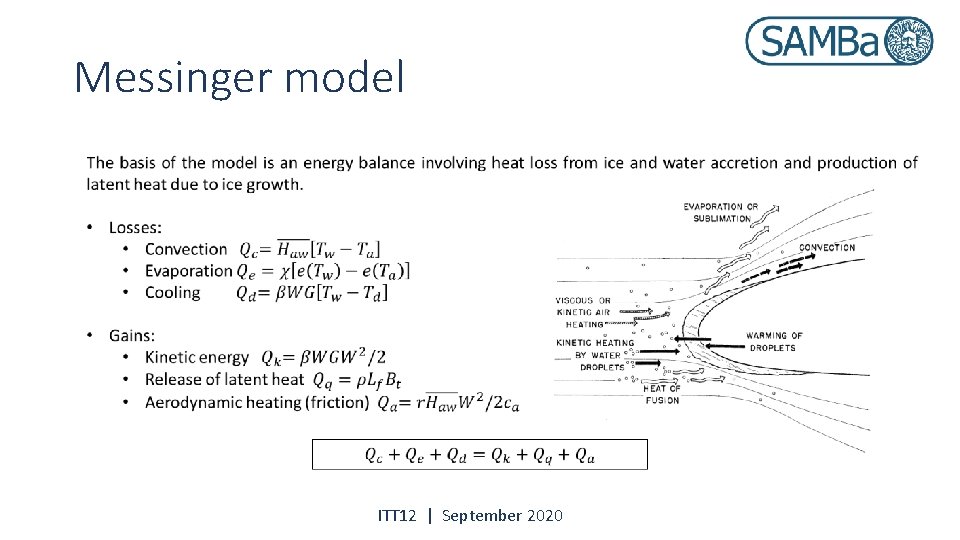
Messinger model ITT 12 | September 2020
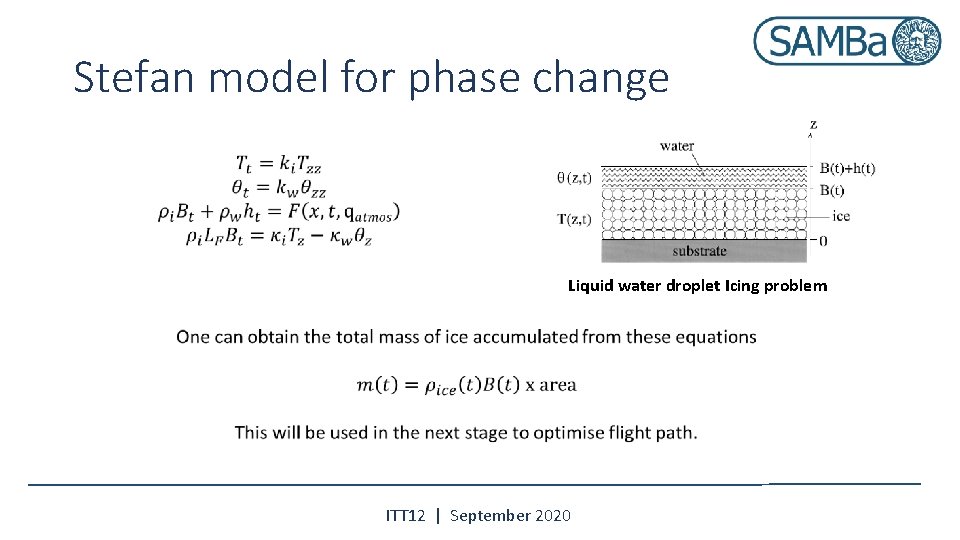
Stefan model for phase change Liquid water droplet Icing problem ITT 12 | September 2020
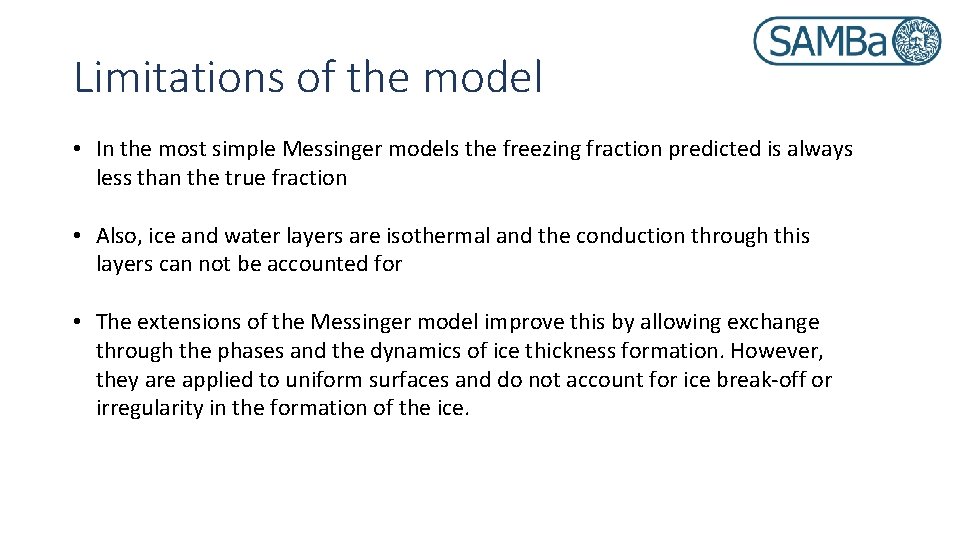
Limitations of the model • In the most simple Messinger models the freezing fraction predicted is always less than the true fraction • Also, ice and water layers are isothermal and the conduction through this layers can not be accounted for • The extensions of the Messinger model improve this by allowing exchange through the phases and the dynamics of ice thickness formation. However, they are applied to uniform surfaces and do not account for ice break-off or irregularity in the formation of the ice.
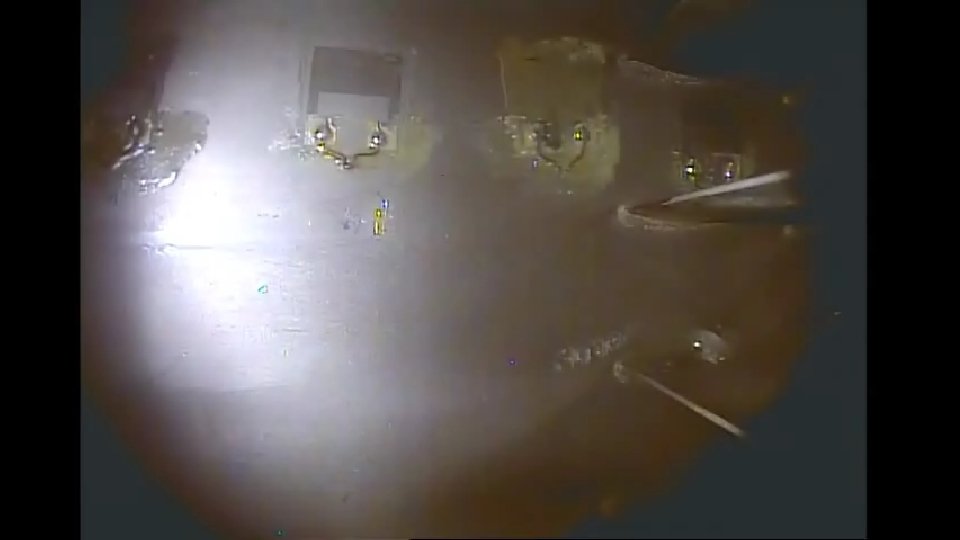
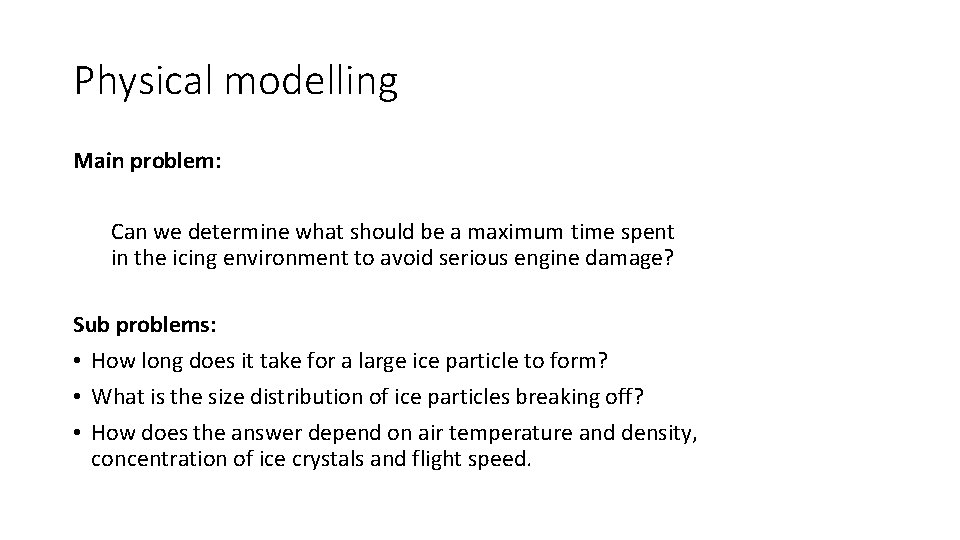
Physical modelling Main problem: Can we determine what should be a maximum time spent in the icing environment to avoid serious engine damage? Sub problems: • How long does it take for a large ice particle to form? • What is the size distribution of ice particles breaking off? • How does the answer depend on air temperature and density, concentration of ice crystals and flight speed.
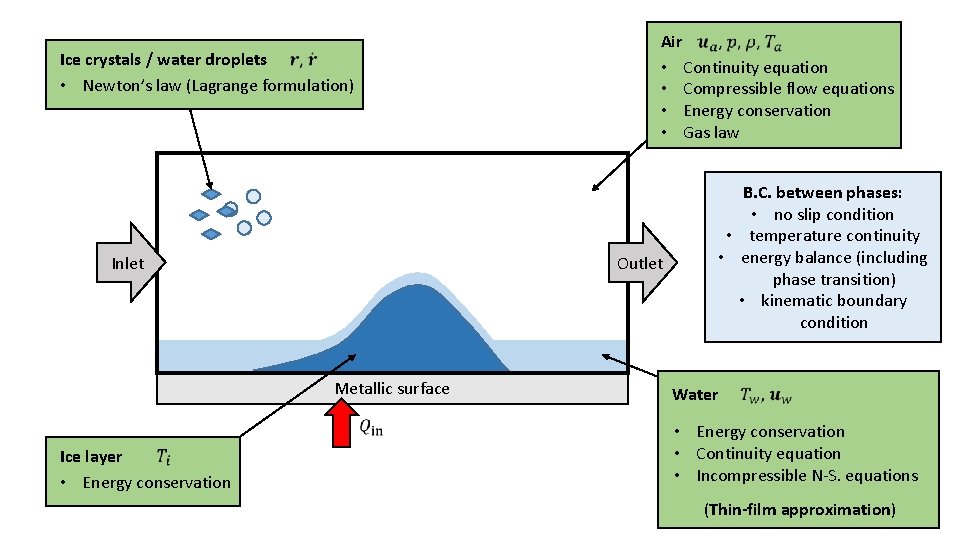
Air • Continuity equation • Compressible flow equations • Energy conservation • Gas law Ice crystals / water droplets • Newton’s law (Lagrange formulation) Outlet Inlet Metallic surface Ice layer • Energy conservation B. C. between phases: • no slip condition • temperature continuity • energy balance (including phase transition) • kinematic boundary condition Water • Energy conservation • Continuity equation • Incompressible N-S. equations (Thin-film approximation)
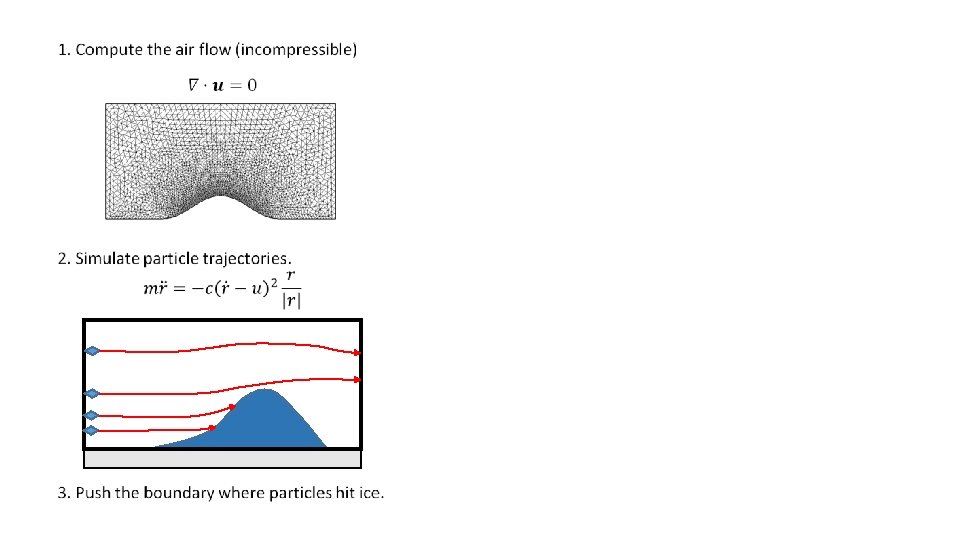
Simulation done using Finite Element Method in Free. FEM++
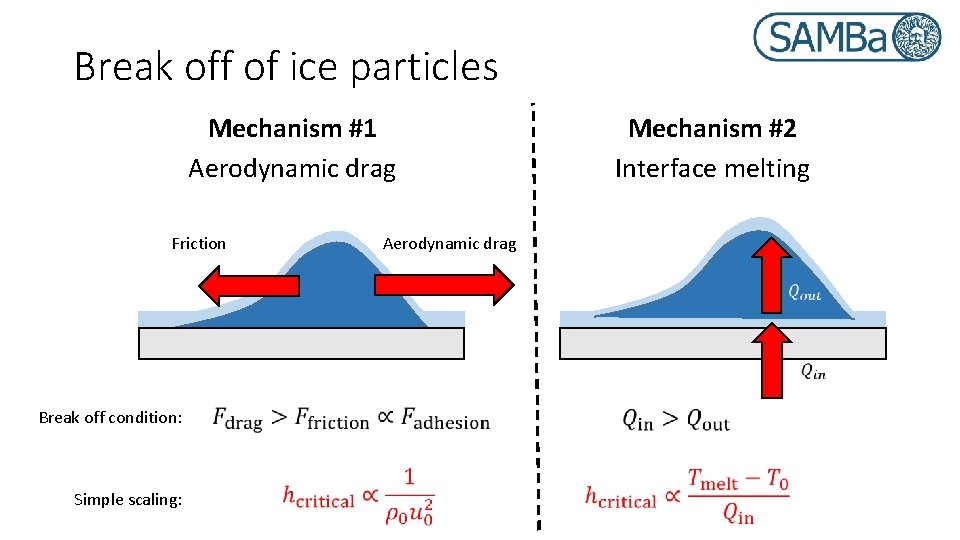
Break off of ice particles Mechanism #1 Aerodynamic drag Friction Mechanism #2 Interface melting Aerodynamic drag Break off condition: Simple scaling:
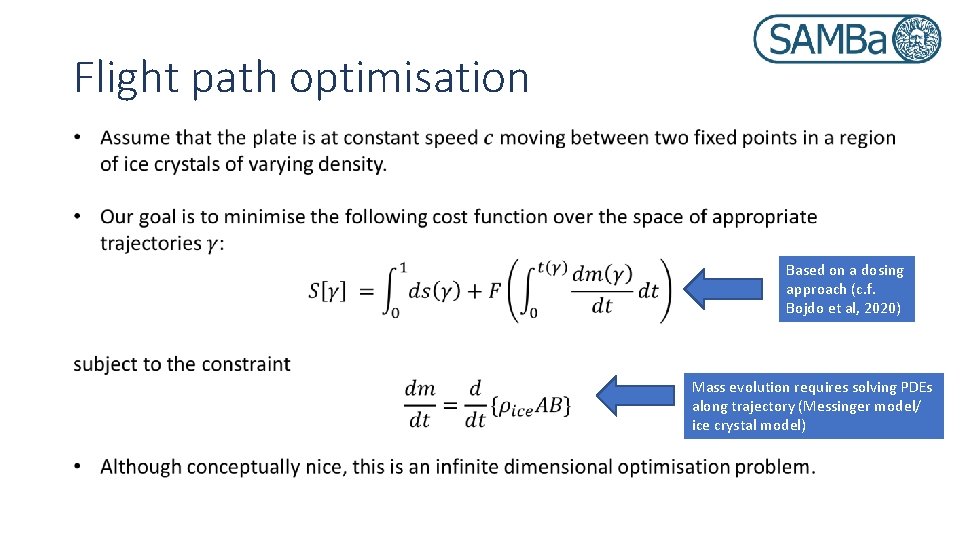
Flight path optimisation Based on a dosing approach (c. f. Bojdo et al, 2020) Mass evolution requires solving PDEs along trajectory (Messinger model/ ice crystal model)
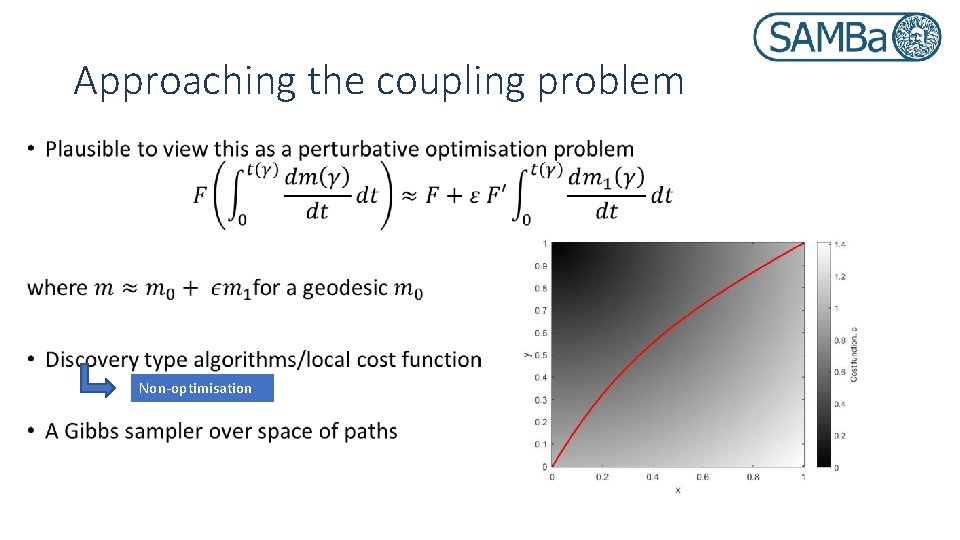
Approaching the coupling problem • Non-optimisation
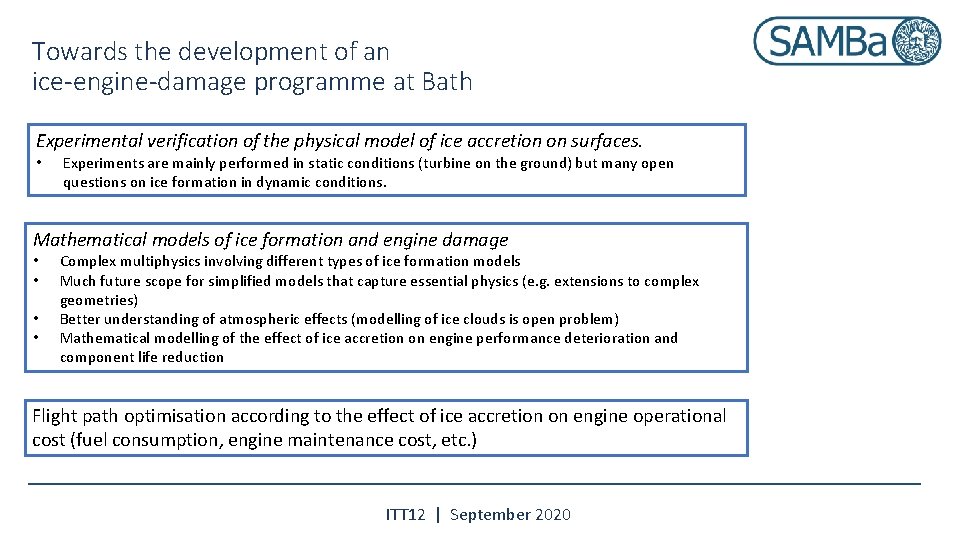
Towards the development of an ice-engine-damage programme at Bath Experimental verification of the physical model of ice accretion on surfaces. • Experiments are mainly performed in static conditions (turbine on the ground) but many open questions on ice formation in dynamic conditions. Mathematical models of ice formation and engine damage • • Complex multiphysics involving different types of ice formation models Much future scope for simplified models that capture essential physics (e. g. extensions to complex geometries) Better understanding of atmospheric effects (modelling of ice clouds is open problem) Mathematical modelling of the effect of ice accretion on engine performance deterioration and component life reduction Flight path optimisation according to the effect of ice accretion on engine operational cost (fuel consumption, engine maintenance cost, etc. ) ITT 12 | September 2020
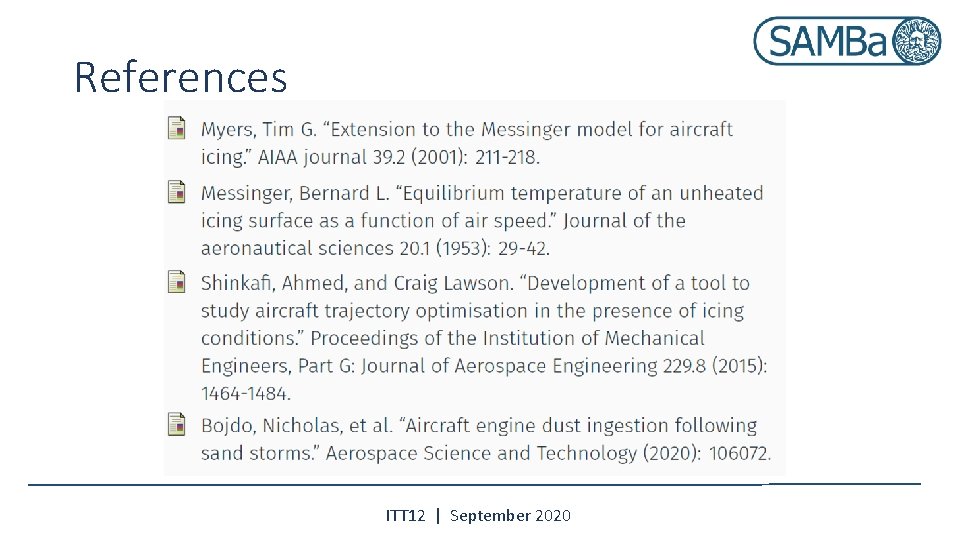
References ITT 12 | September 2020
Mathematical modeling of electrical systems
Vibration engineering
Image of water cycle
Hey hey bye bye
Why do ionic bonds form crystals
Econ213
Punctuation is important
Why productivity matters
Standard data quality dimensions kpmg
Why was every child matters scrapped
Why patient experience matters
Closing the word gap
Biosocial framework
Why data governance matters
Why productivity matters
Why motivation matters
Masonic civility