Ultrafast Laser Driven Wakefield Accelerators Oleg Korovyanko 01122009
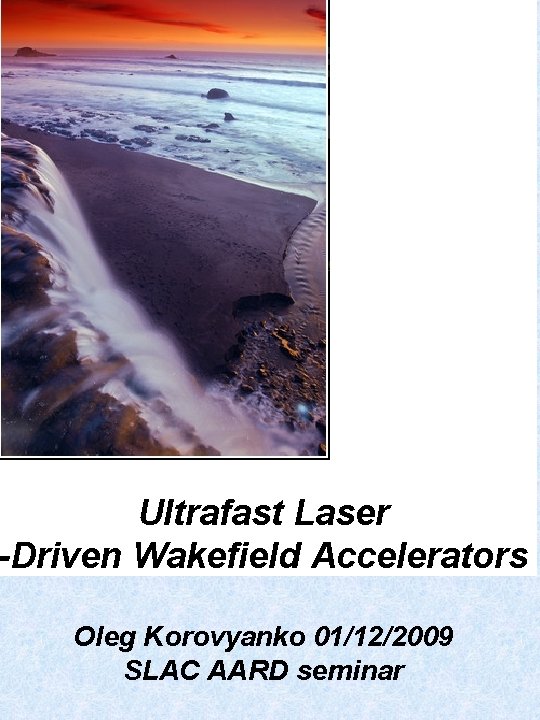
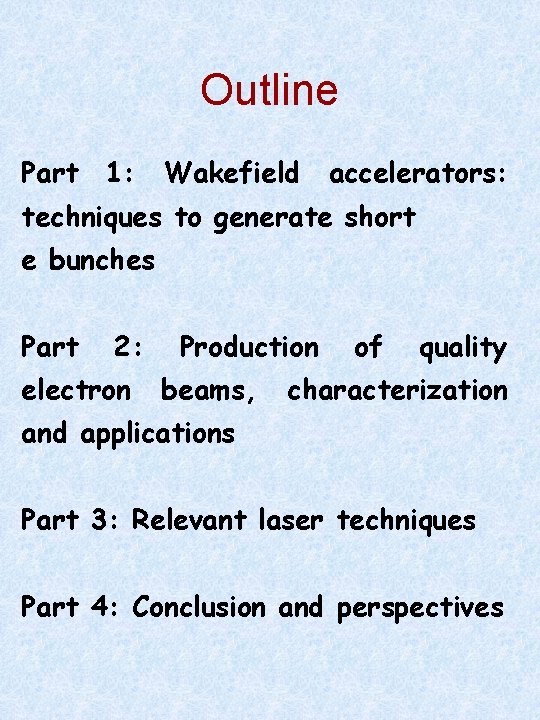
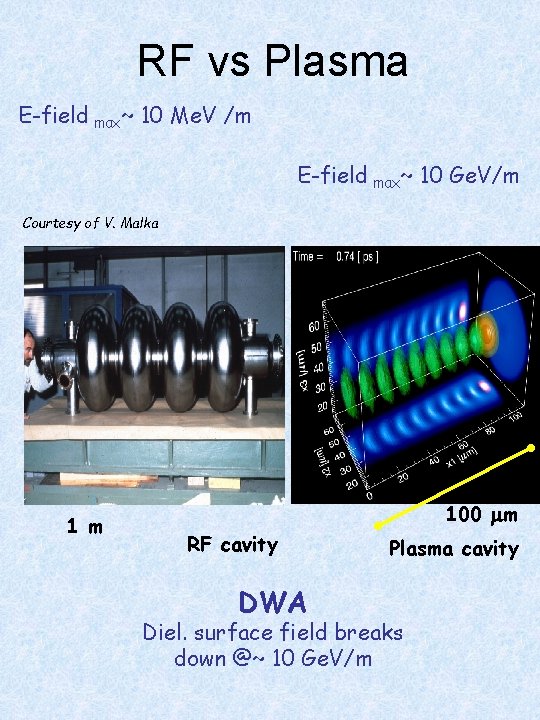
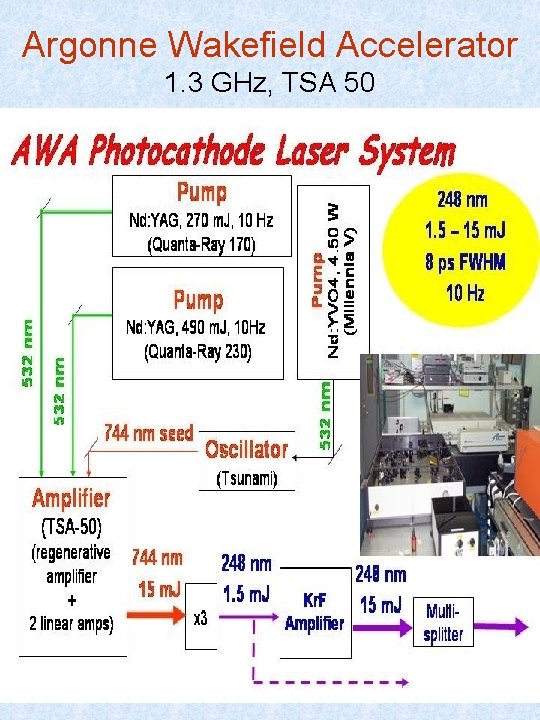
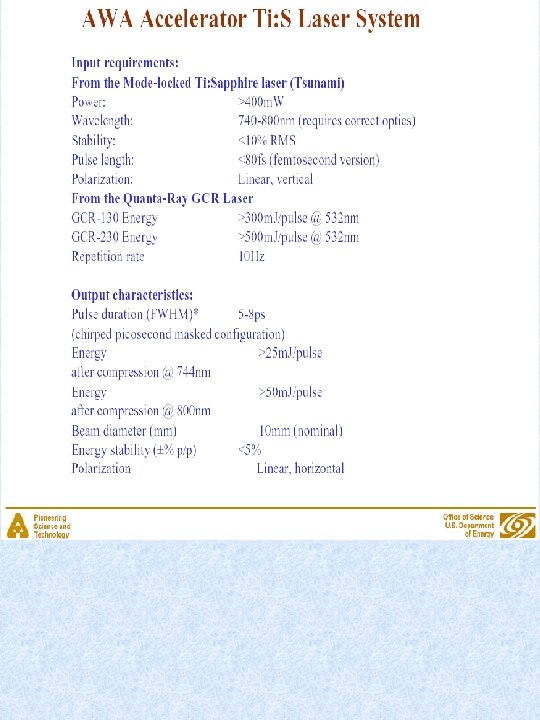
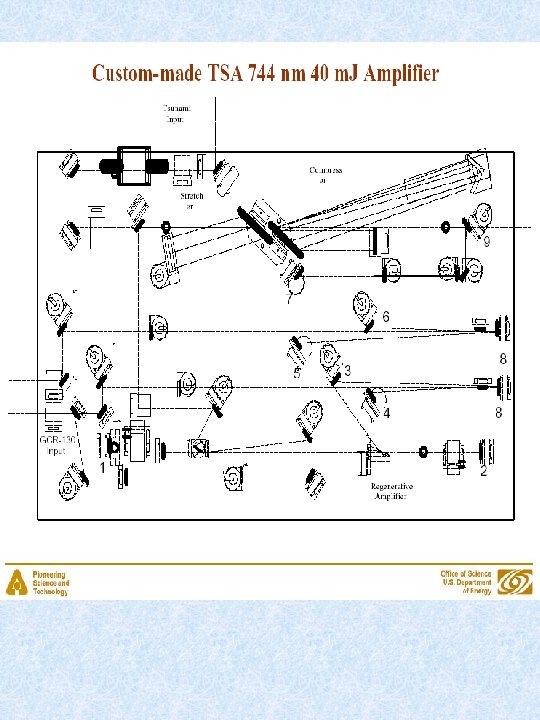
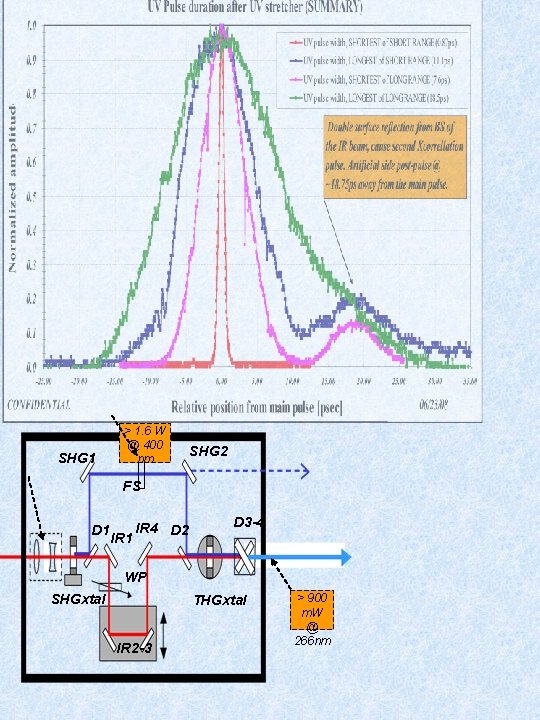
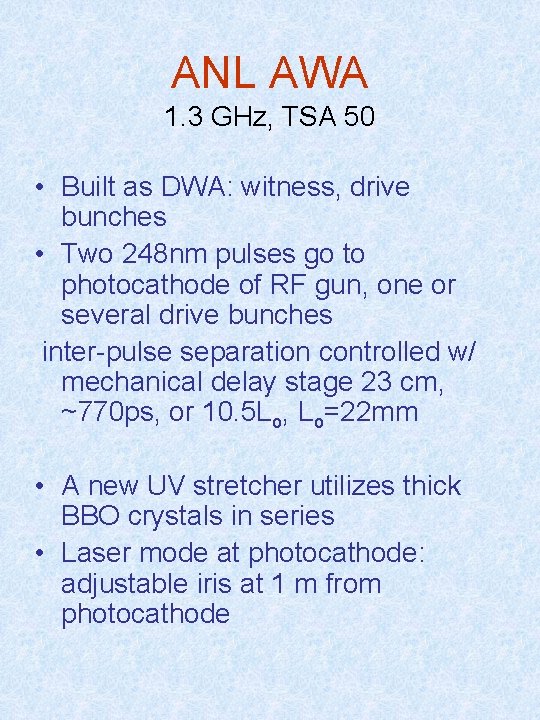
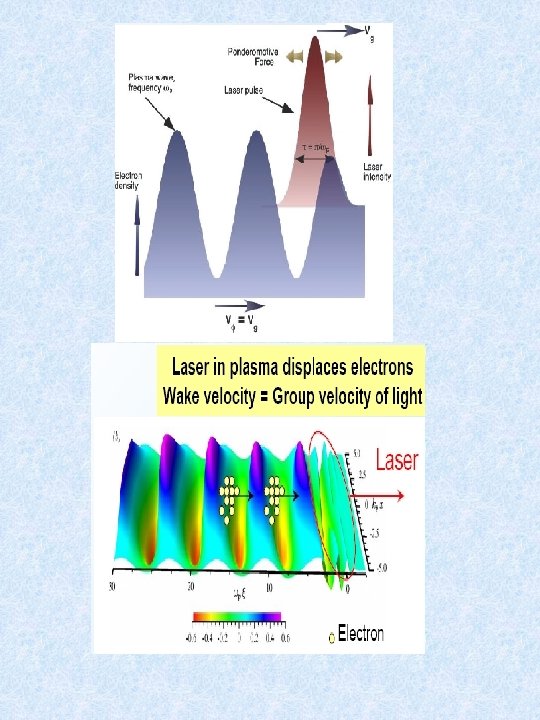
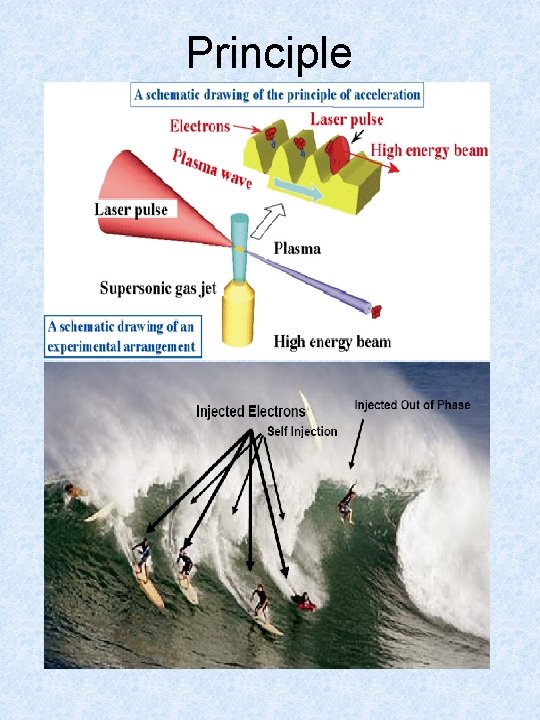
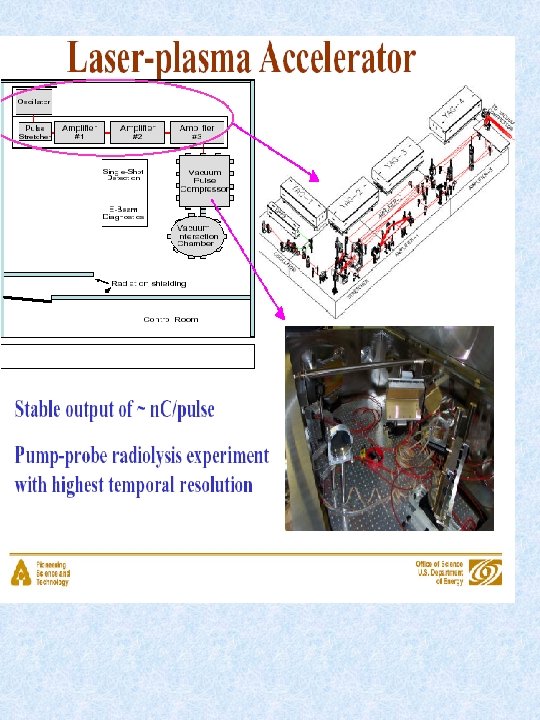
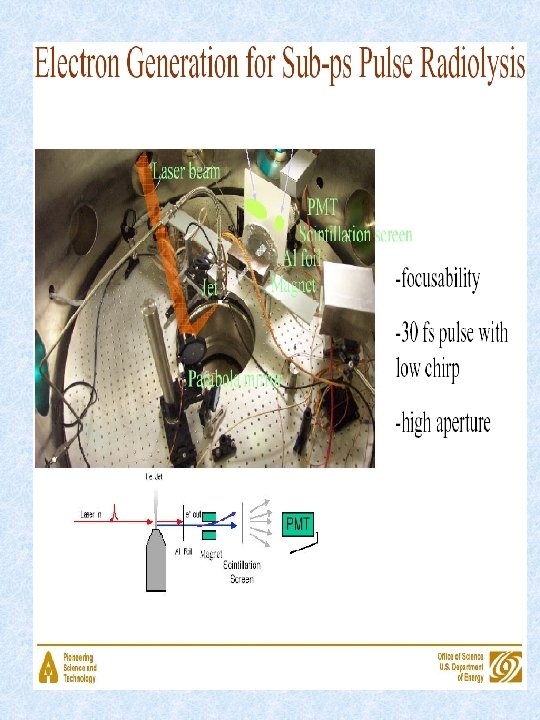
![Monoenergetic Beams from Literature Name Article Lab Energy d. E/E [Me. V] [%] Mangles Monoenergetic Beams from Literature Name Article Lab Energy d. E/E [Me. V] [%] Mangles](https://slidetodoc.com/presentation_image_h2/0e5d78b6dfb1ca51eabf21fa96ddcd92/image-13.jpg)
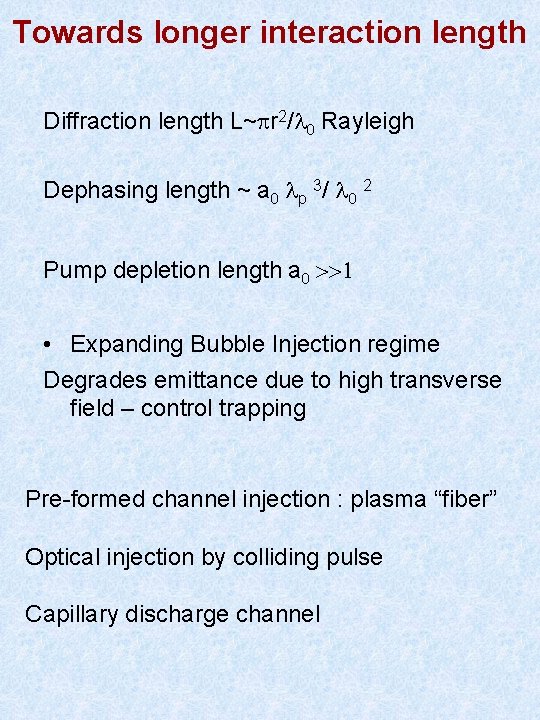
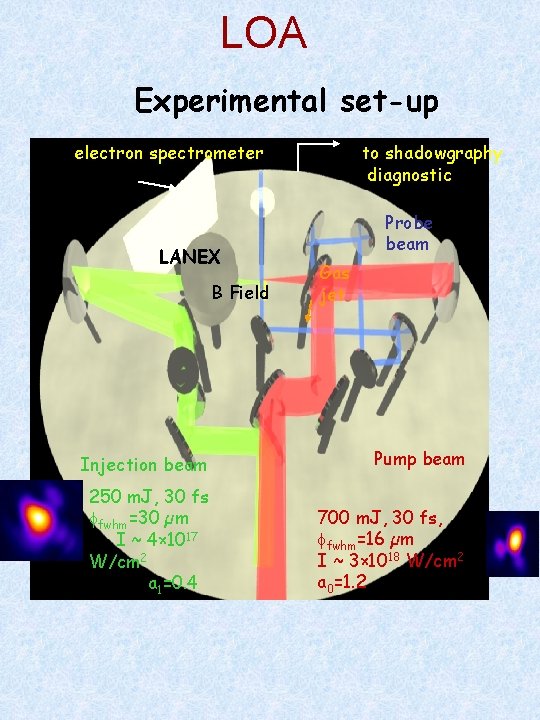
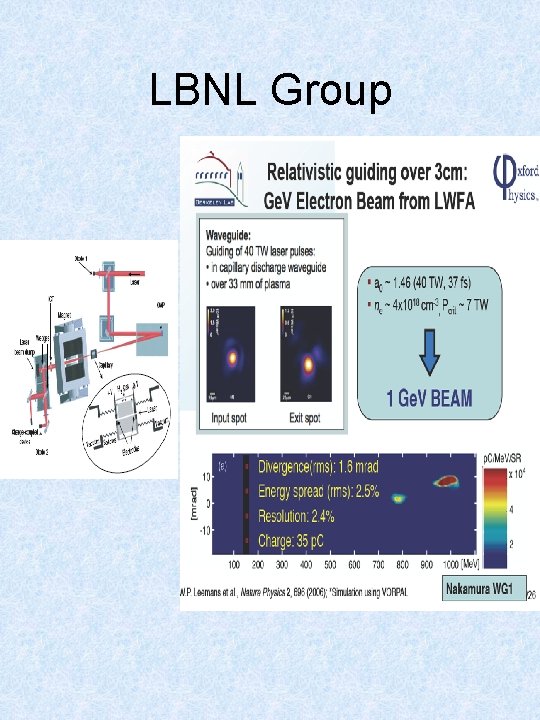
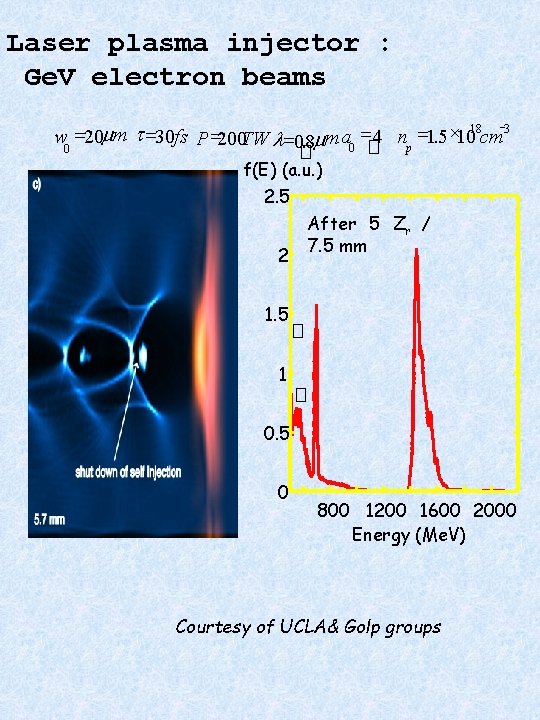
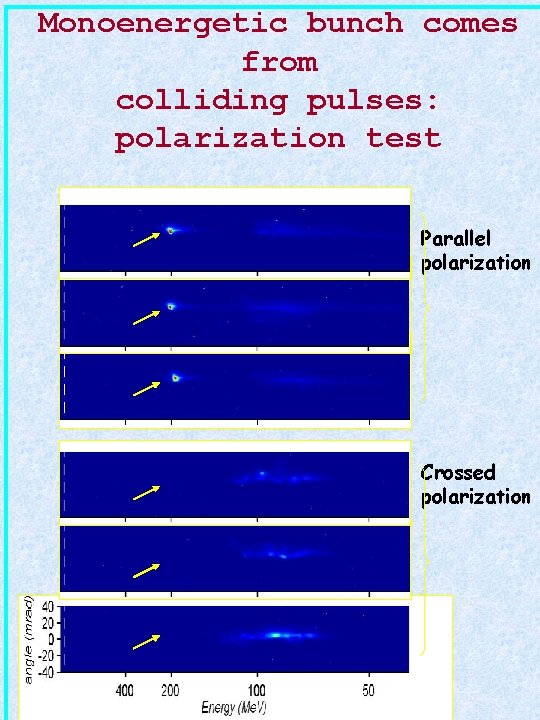
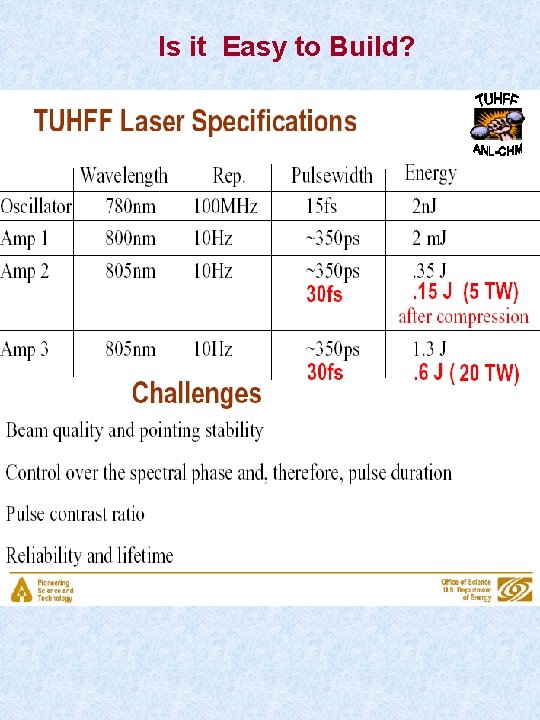
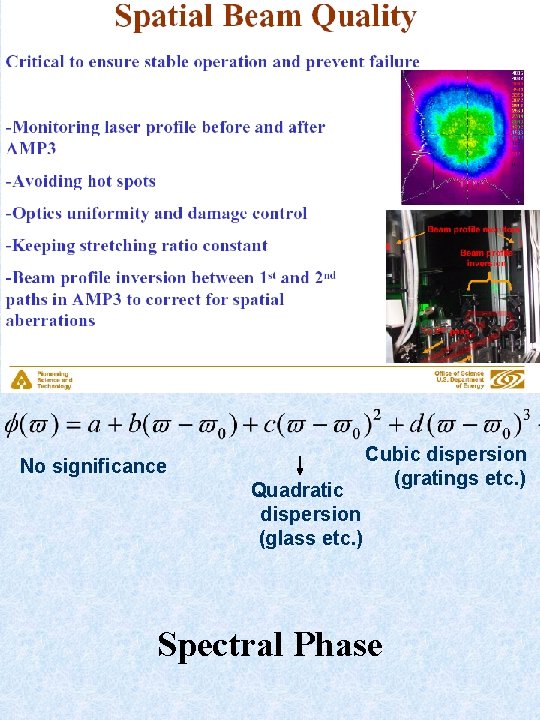
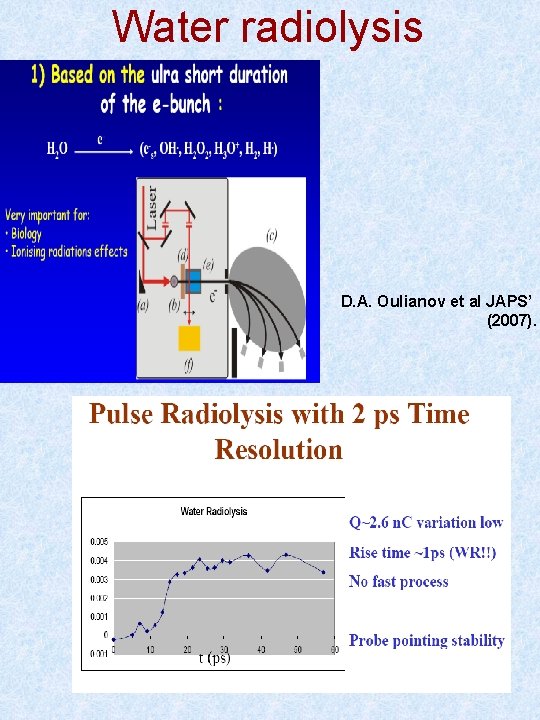
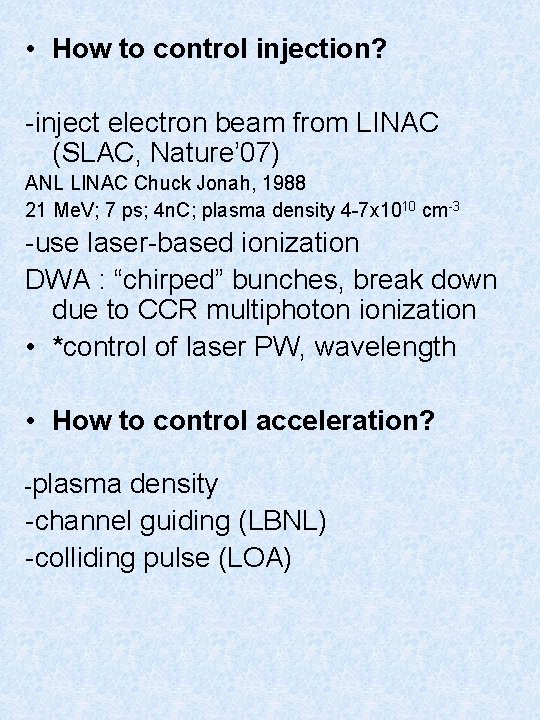
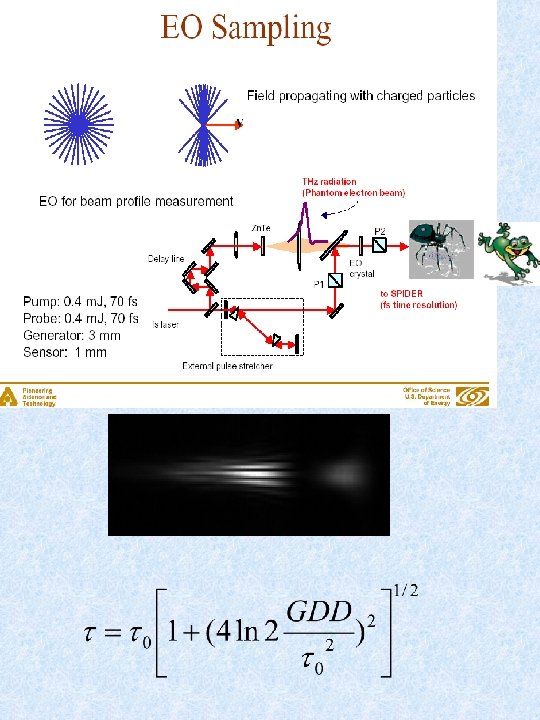
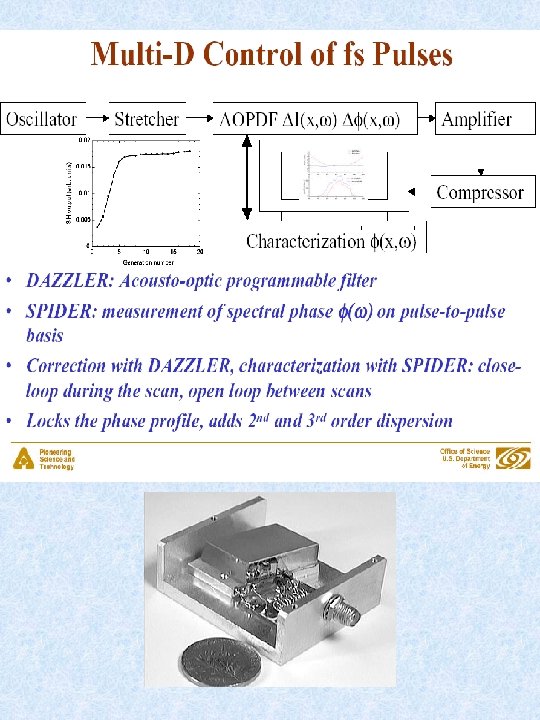
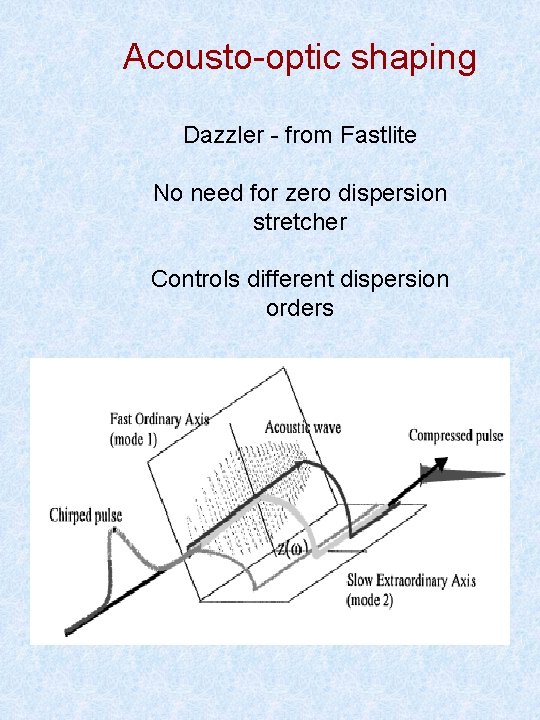
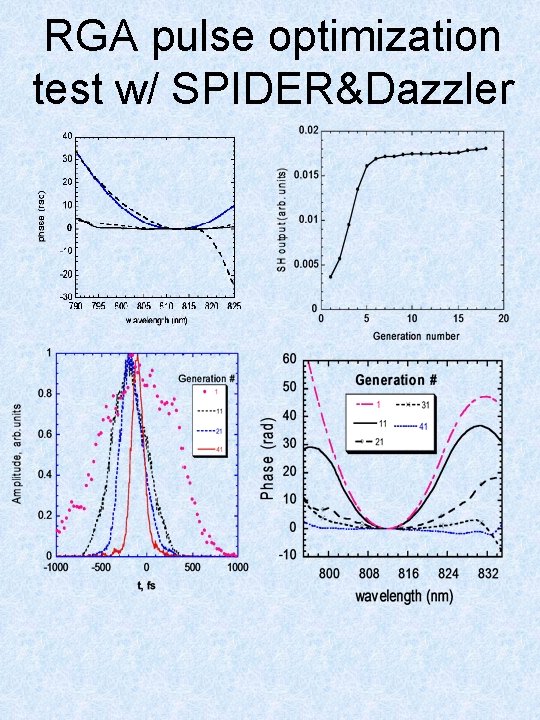
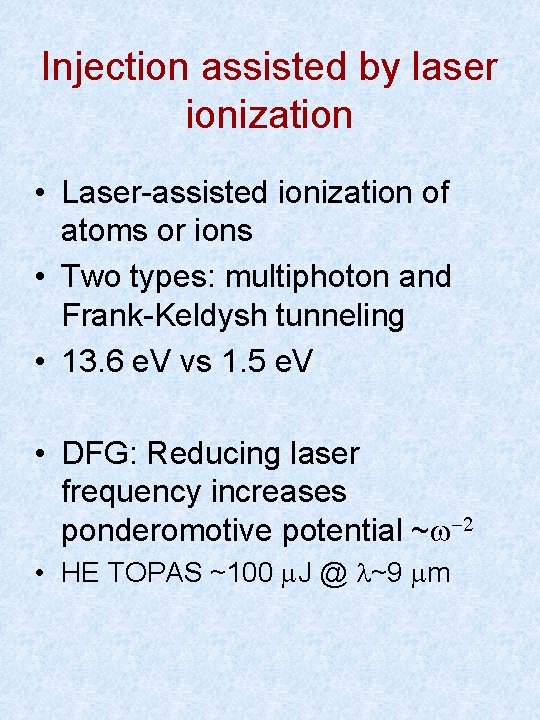
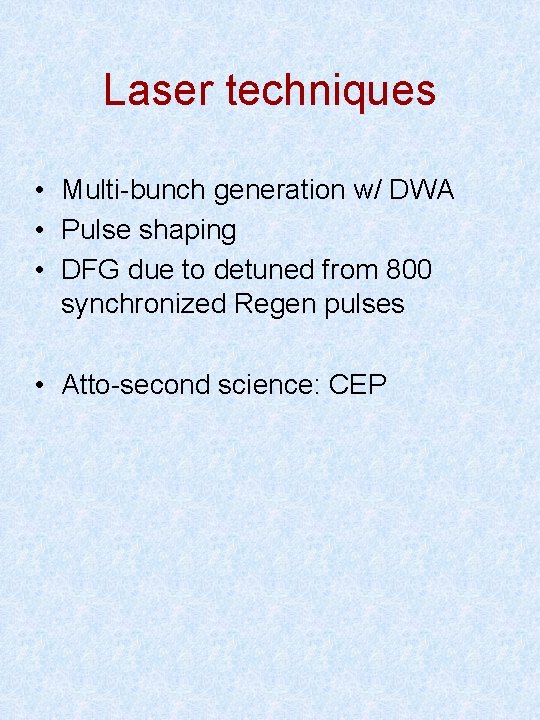
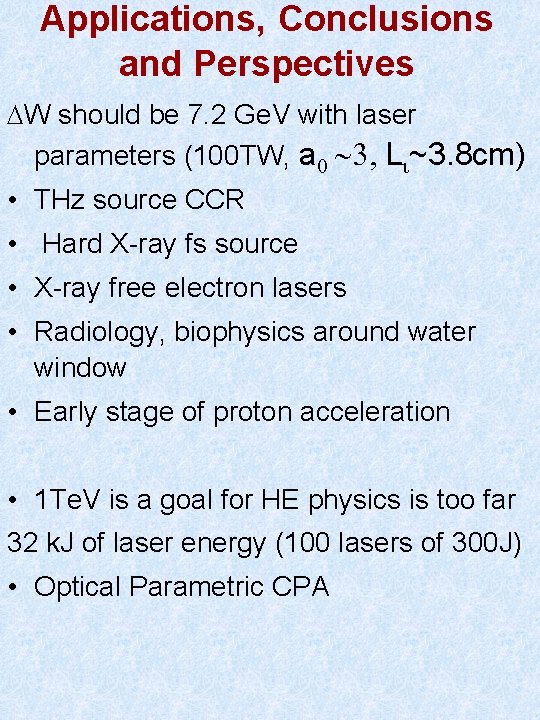
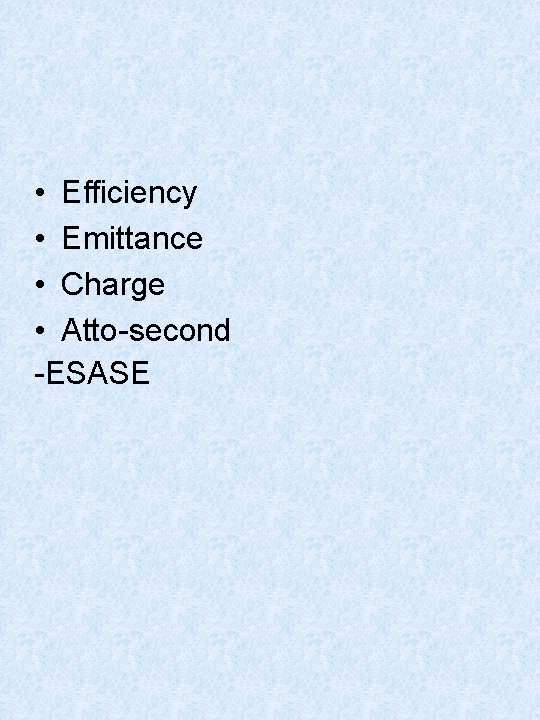
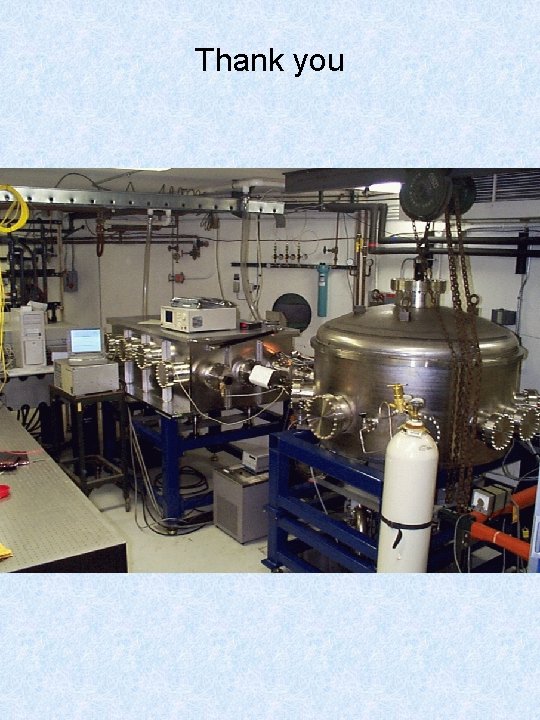
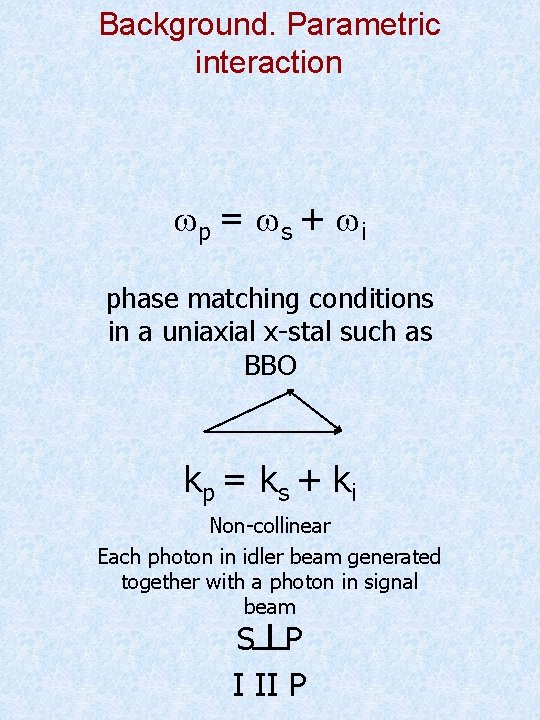
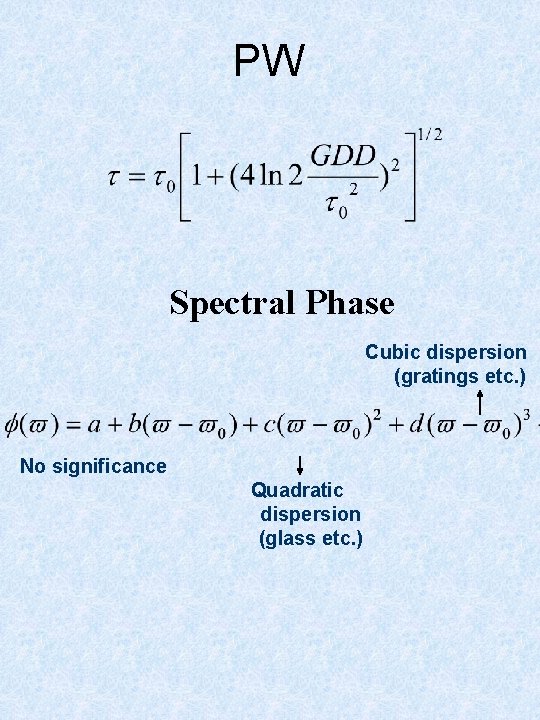
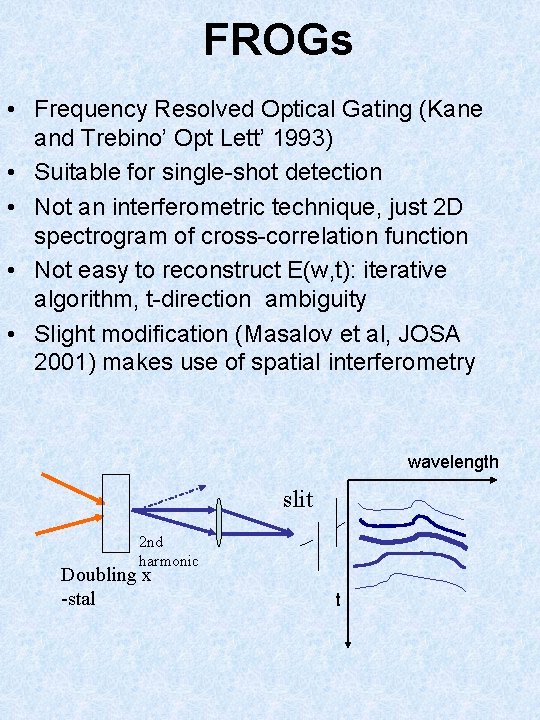
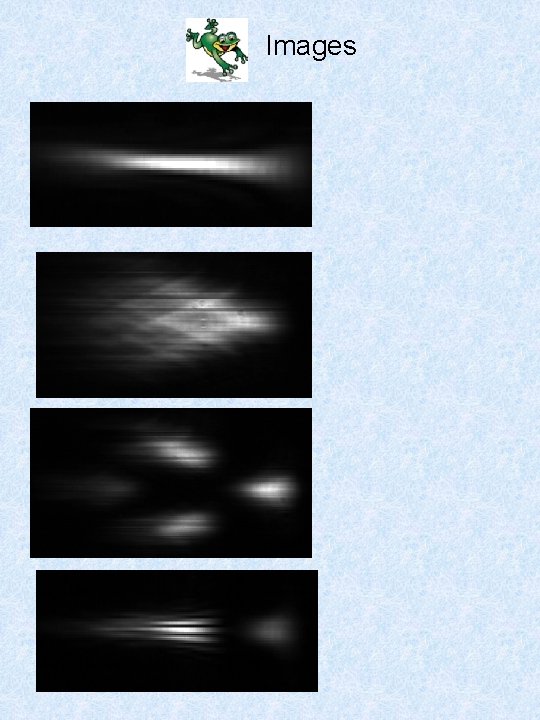
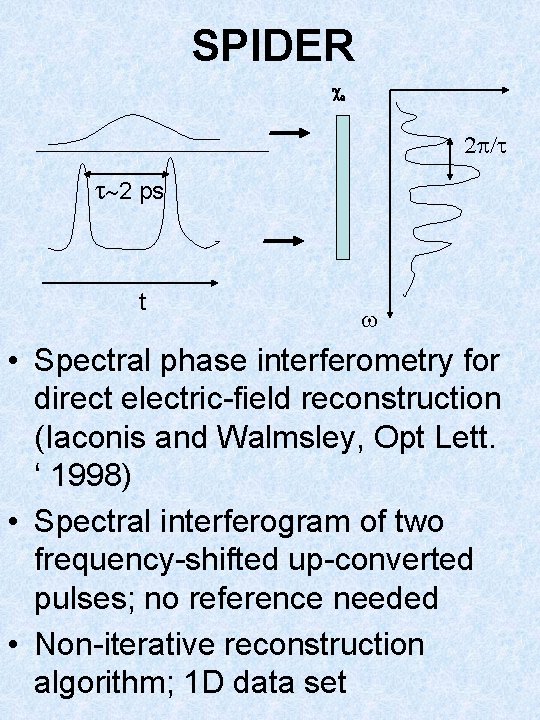
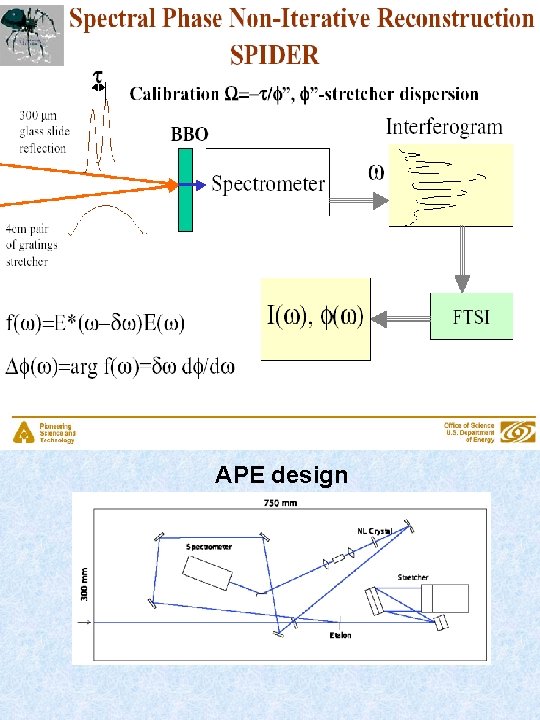
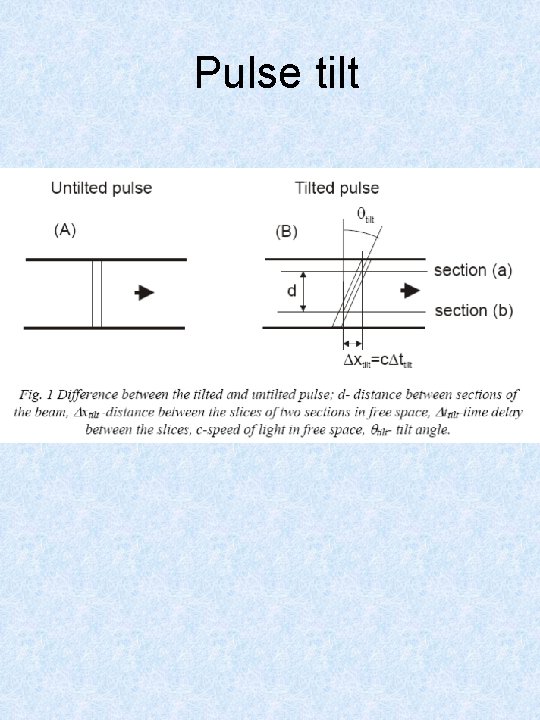
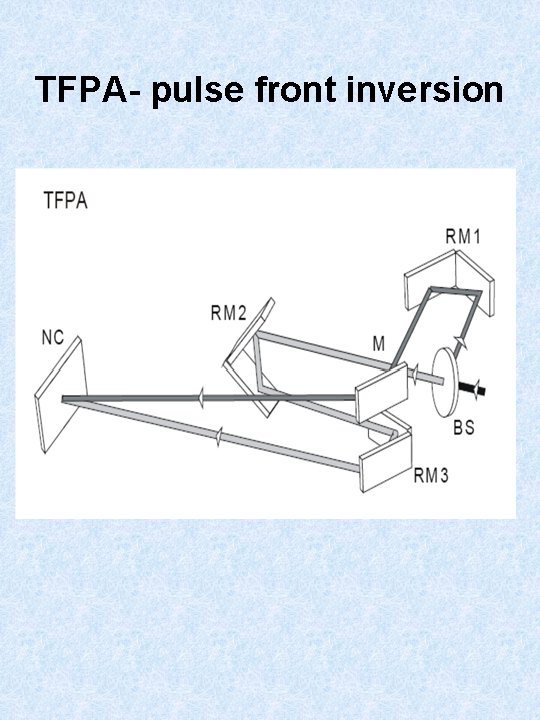
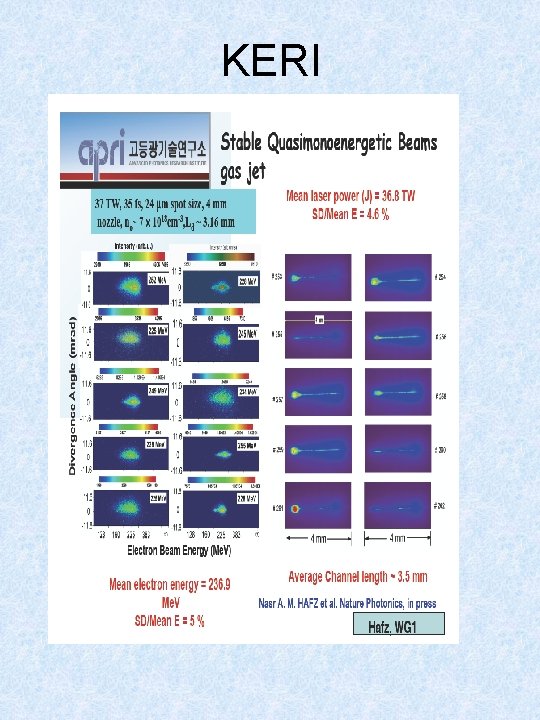
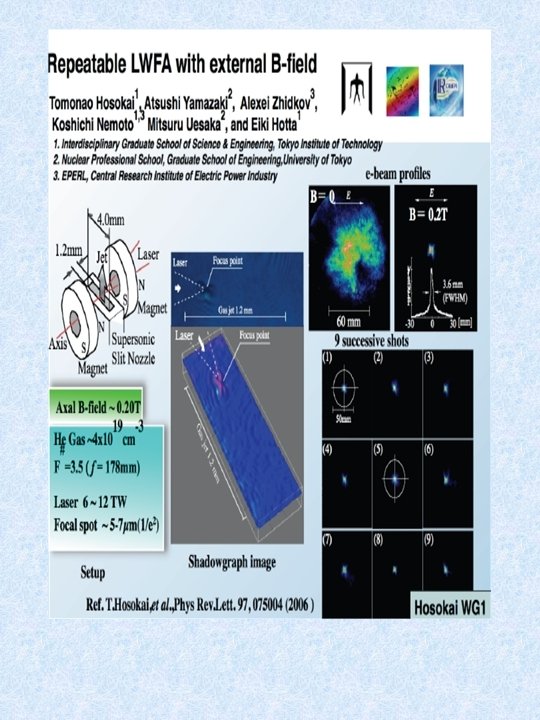
- Slides: 41
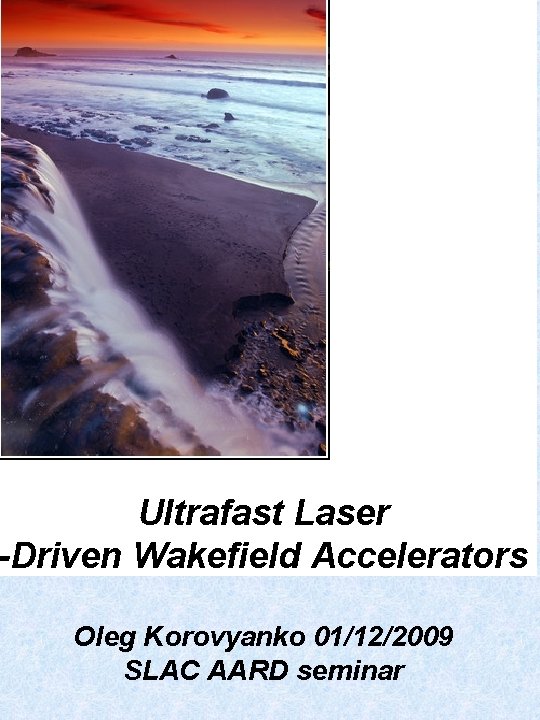
Ultrafast Laser -Driven Wakefield Accelerators Oleg Korovyanko 01/12/2009 SLAC AARD seminar
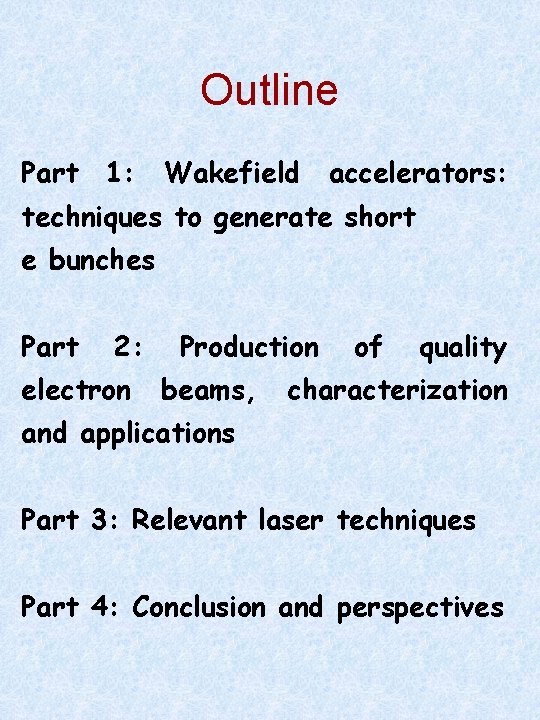
Outline Part 1: Wakefield accelerators: techniques to generate short e bunches Part 2: electron Production beams, of quality characterization and applications Part 3: Relevant laser techniques Part 4: Conclusion and perspectives
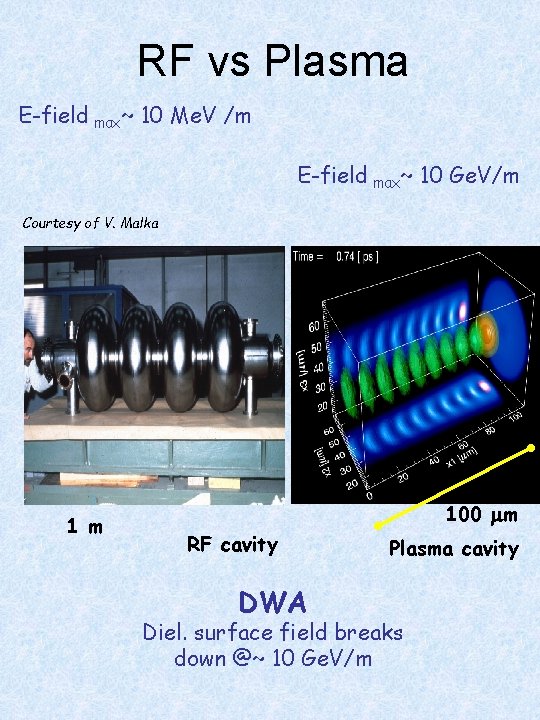
RF vs Plasma E-field max~ 10 Me. V /m E-field max~ 10 Ge. V/m Courtesy of V. Malka 1 m 100 mm RF cavity DWA Plasma cavity Diel. surface field breaks down @~ 10 Ge. V/m
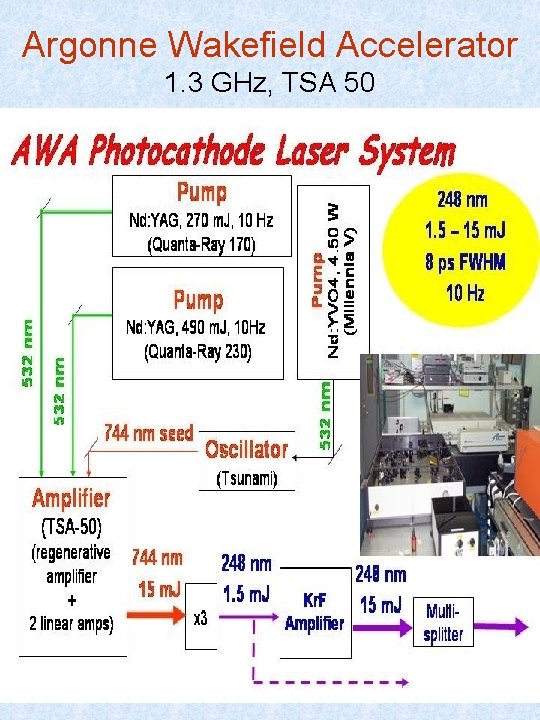
Argonne Wakefield Accelerator 1. 3 GHz, TSA 50
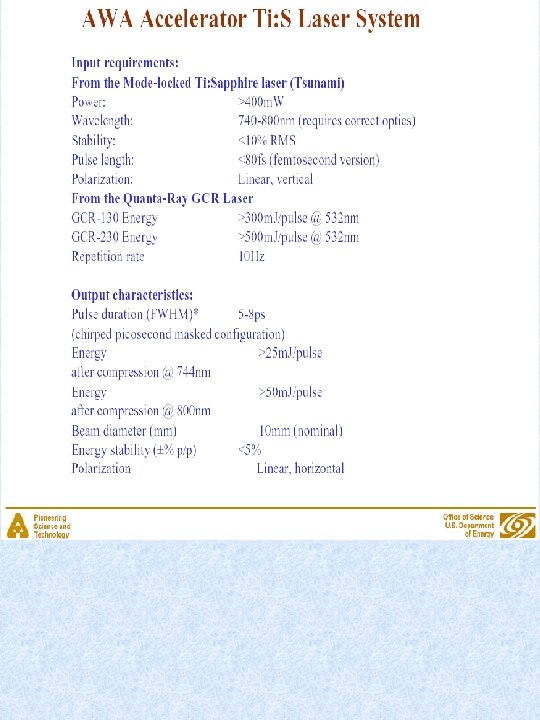
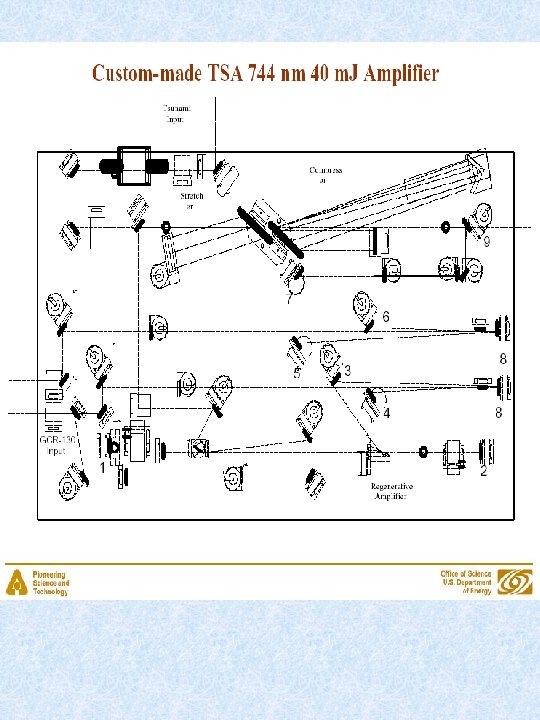
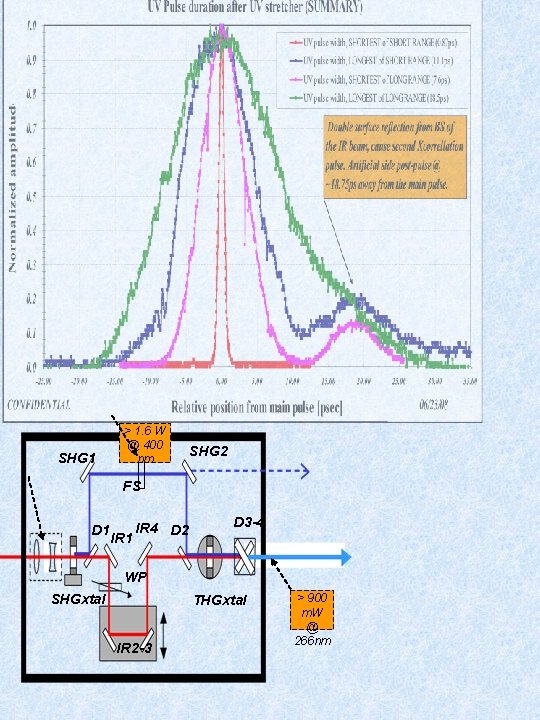
SHG 1 > 1. 6 W @ 400 nm SHG 2 FS D 1 IR 4 D 2 D 3 -4 WP SHGxtal THGxtal IR 2 -3 > 900 m. W @ 266 nm
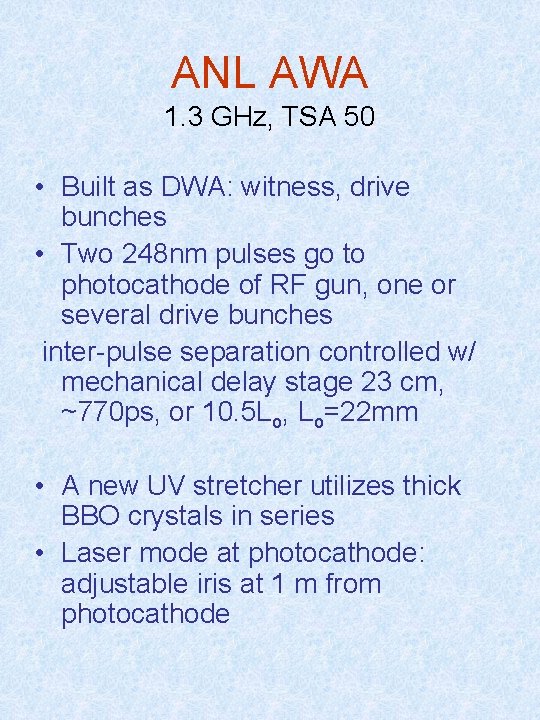
ANL AWA 1. 3 GHz, TSA 50 • Built as DWA: witness, drive bunches • Two 248 nm pulses go to photocathode of RF gun, one or several drive bunches inter-pulse separation controlled w/ mechanical delay stage 23 cm, ~770 ps, or 10. 5 Lo, Lo=22 mm • A new UV stretcher utilizes thick BBO crystals in series • Laser mode at photocathode: adjustable iris at 1 m from photocathode
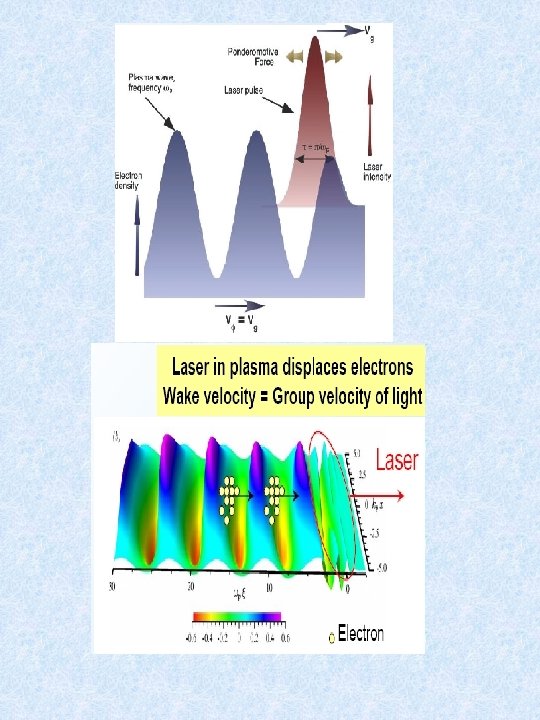
Escalations
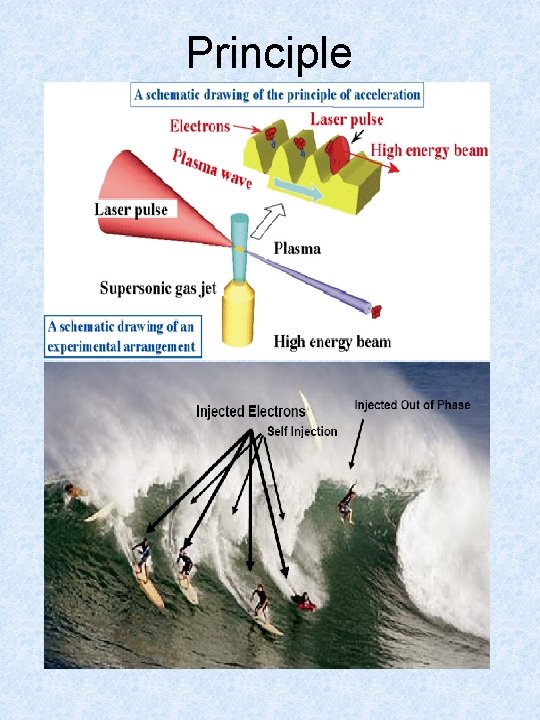
Principle
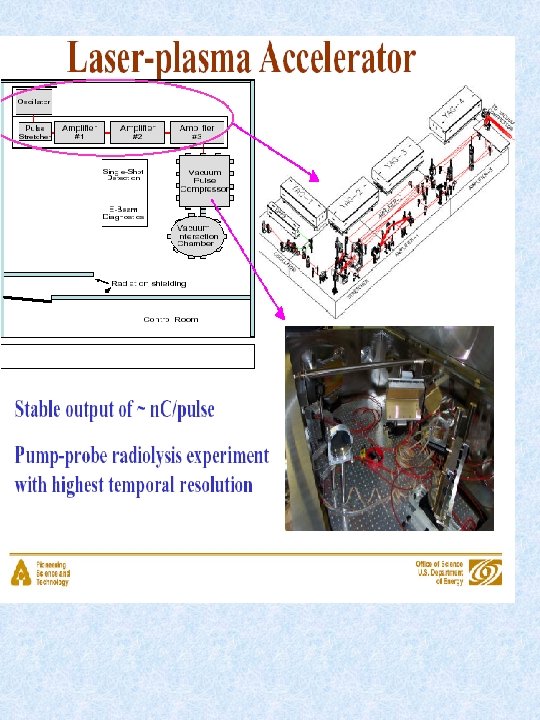
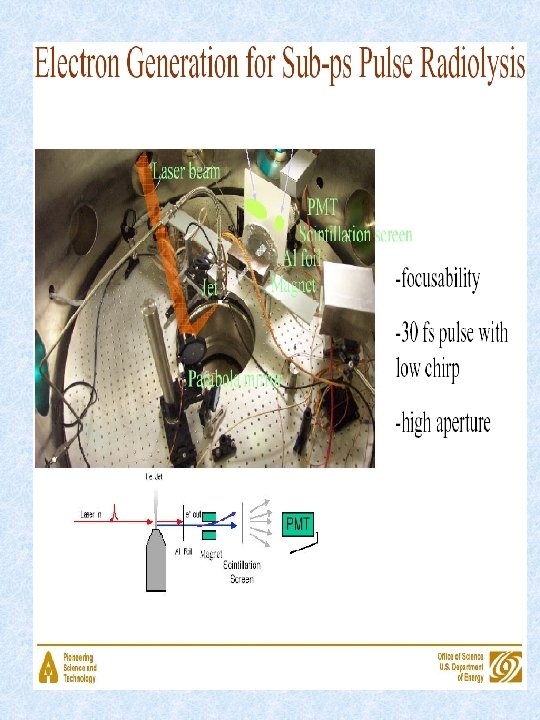
![Monoenergetic Beams from Literature Name Article Lab Energy d EE Me V Mangles Monoenergetic Beams from Literature Name Article Lab Energy d. E/E [Me. V] [%] Mangles](https://slidetodoc.com/presentation_image_h2/0e5d78b6dfb1ca51eabf21fa96ddcd92/image-13.jpg)
Monoenergetic Beams from Literature Name Article Lab Energy d. E/E [Me. V] [%] Mangles Nature (04) RAL t. L/Tp Charge Ne[p. C] Intensity 3 18 2 ] x 1018 /cm x 10 W/cm 73 6 22 20 2, 5 1, 6 Geddes Nature (04) L'OASIS 86 2 320 19 11 2, 2 Faure Nature (04) LOA 170 25 500 6 3 0, 7 9 0, 32 40 50 4, 6 336 40 Hidding PRL (2006) JETI 47 Hsieh IAMS 55 PRL (2006) 2, 6 Hosokai PRE (2006) U. Tokyo 11, 5 10 10 80 22 3, 0 Miura APL (2005) AIST 7 20 432 E-6 130 5 5, 1 Hafz PRE (2006) KERI 4, 3 93 200 28 1 33, 4 Mori Ar. Xiv (06) JAERI 20 24 0, 8 50 0, 9 4, 5 Lund LC 150 20 20 5 1, 4 Mangles PRL (2006) State-of-art gradient 27 Ge. V/m, SLAC, 27 Ge. V drive, Nature’ 2007
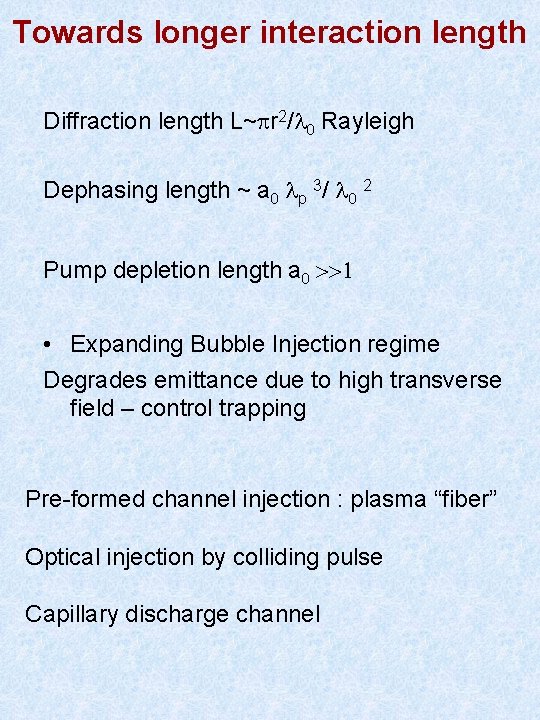
Towards longer interaction length Diffraction length L~pr 2/l 0 Rayleigh Dephasing length ~ a 0 lp 3/ l 0 2 Pump depletion length a 0 >>1 • Expanding Bubble Injection regime Degrades emittance due to high transverse field – control trapping Pre-formed channel injection : plasma “fiber” Optical injection by colliding pulse Capillary discharge channel
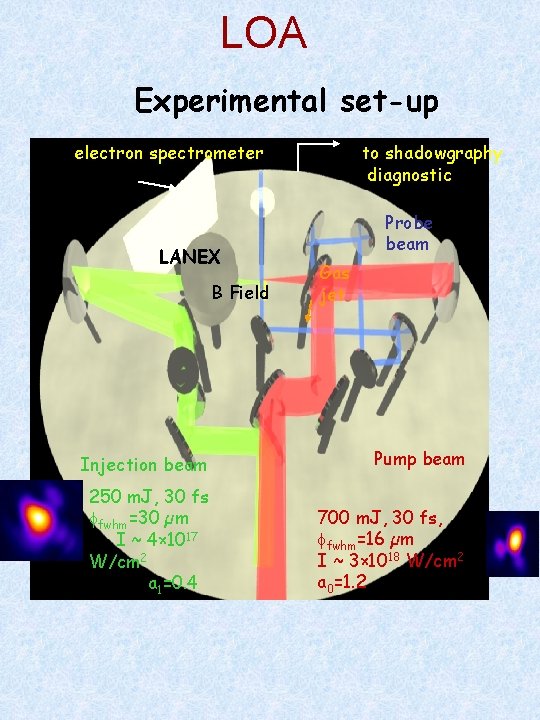
LOA Experimental set-up electron spectrometer LANEX B Field to shadowgraphy diagnostic Probe beam Gas jet Injection beam Pump beam 250 m. J, 30 fs ffwhm=30 µm I ~ 4× 1017 W/cm 2 a 1=0. 4 700 m. J, 30 fs, ffwhm=16 µm I ~ 3× 1018 W/cm 2 a 0=1. 2
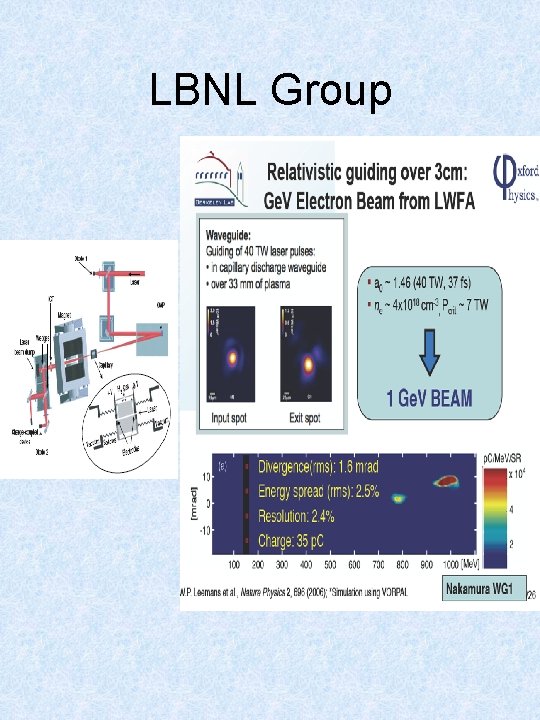
LBNL Group
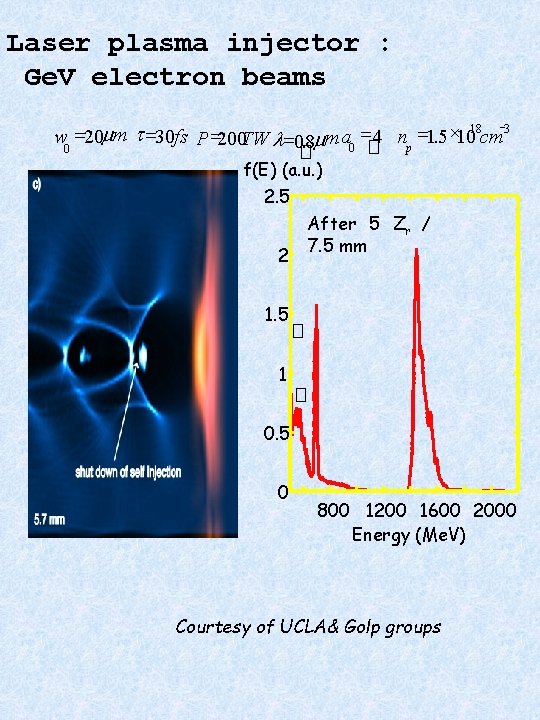
Laser plasma injector : Ge. V electron beams 18 -3 w 0 =20 mm t =30 fs P =200 TW l =0. 8 mm a 0 = 4 np =1. 5 × 10 cm � � f(E) (a. u. ) 2. 5 After 5 Zr / 7. 5 mm 2 1. 5 1 � � 0. 5 0 800 1200 1600 2000 Energy (Me. V) Courtesy of UCLA& Golp groups
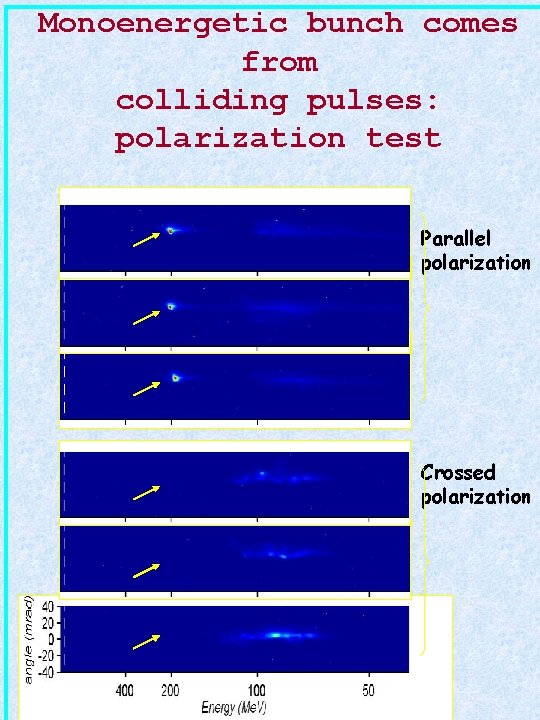
Monoenergetic bunch comes from colliding pulses: polarization test Parallel polarization Crossed polarization
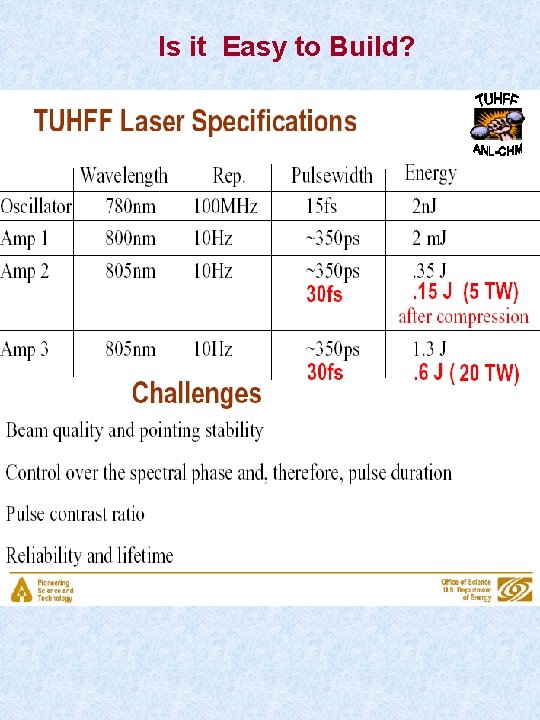
Is it Easy to Build?
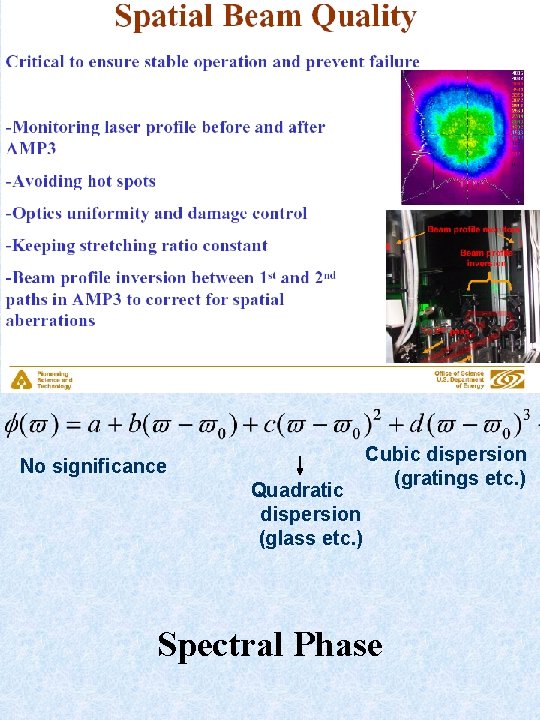
No significance Quadratic dispersion (glass etc. ) Cubic dispersion (gratings etc. ) Spectral Phase
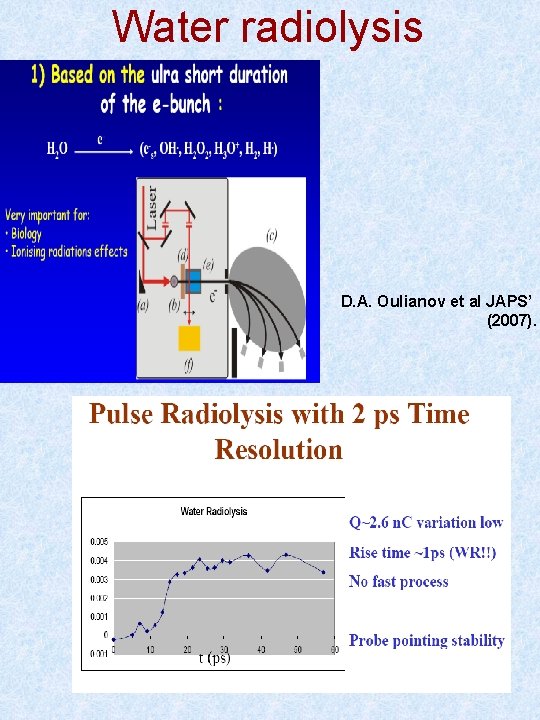
Water radiolysis D. A. Oulianov et al JAPS’ (2007).
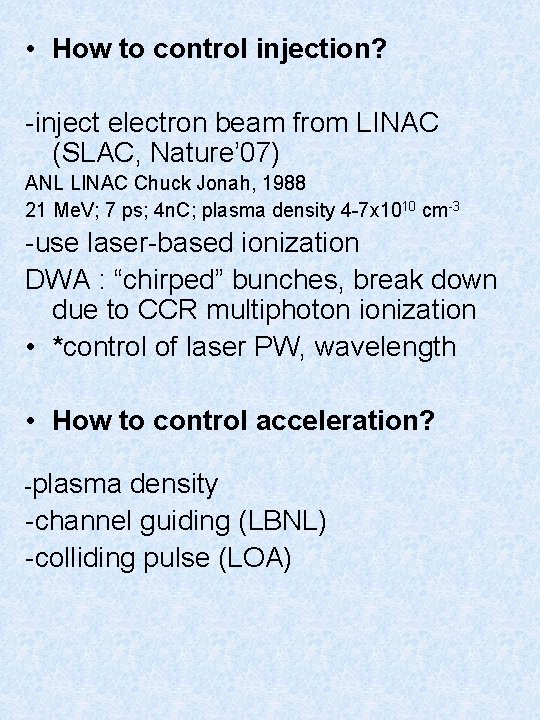
• How to control injection? -inject electron beam from LINAC (SLAC, Nature’ 07) ANL LINAC Chuck Jonah, 1988 21 Me. V; 7 ps; 4 n. C; plasma density 4 -7 x 1010 cm-3 -use laser-based ionization DWA : “chirped” bunches, break down due to CCR multiphoton ionization • *control of laser PW, wavelength • How to control acceleration? -plasma density -channel guiding (LBNL) -colliding pulse (LOA)
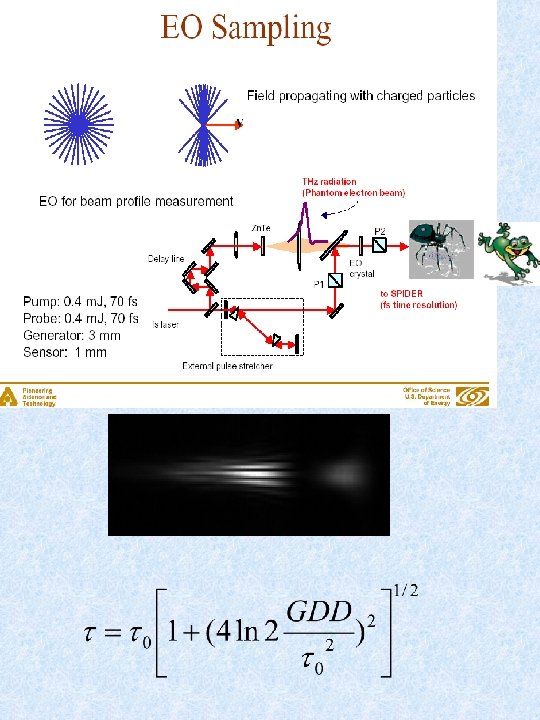
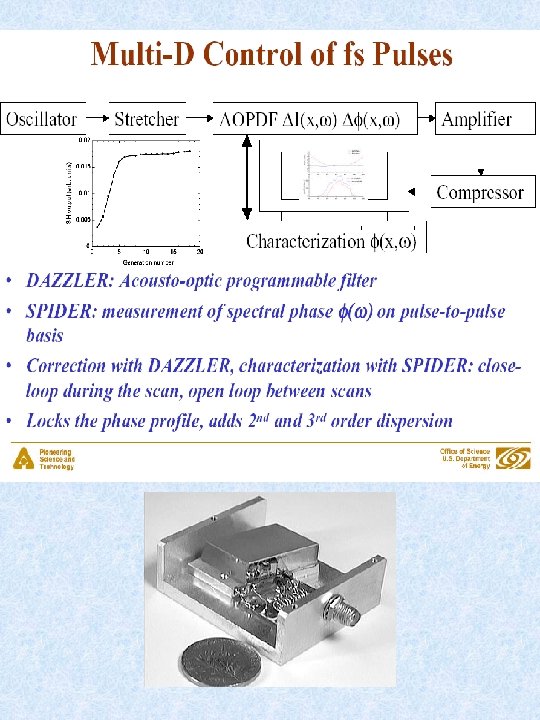
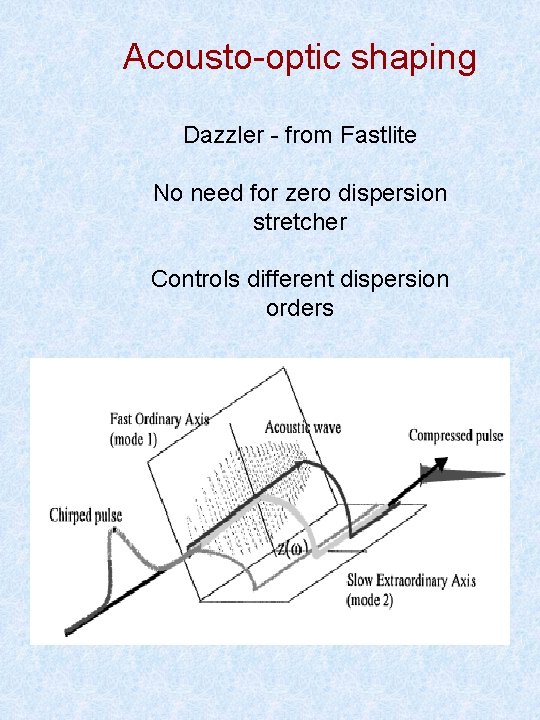
Acousto-optic shaping Dazzler - from Fastlite No need for zero dispersion stretcher Controls different dispersion orders
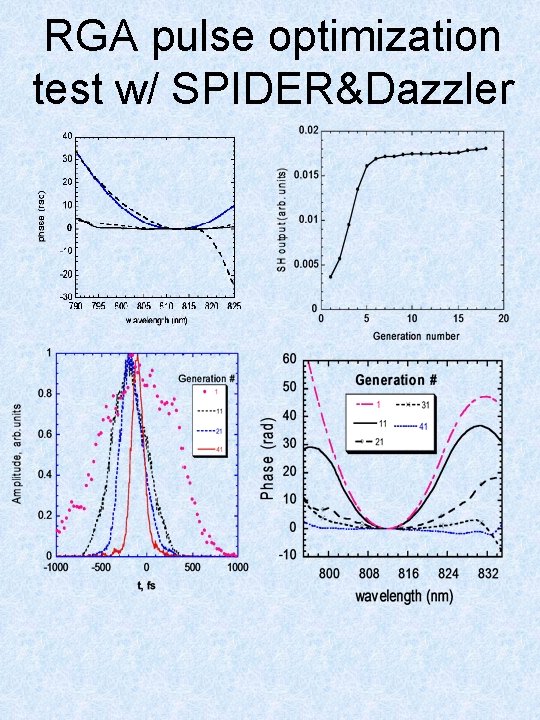
RGA pulse optimization test w/ SPIDER&Dazzler
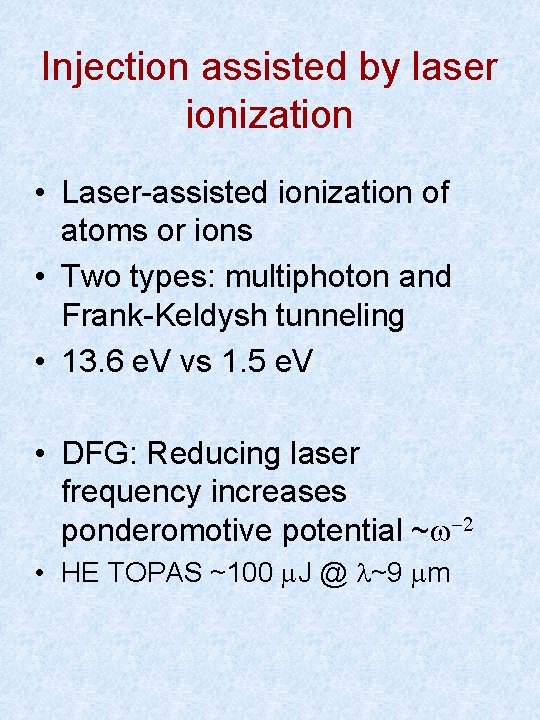
Injection assisted by laser ionization • Laser-assisted ionization of atoms or ions • Two types: multiphoton and Frank-Keldysh tunneling • 13. 6 e. V vs 1. 5 e. V • DFG: Reducing laser frequency increases ponderomotive potential ~w-2 • HE TOPAS ~100 m. J @ l~9 mm
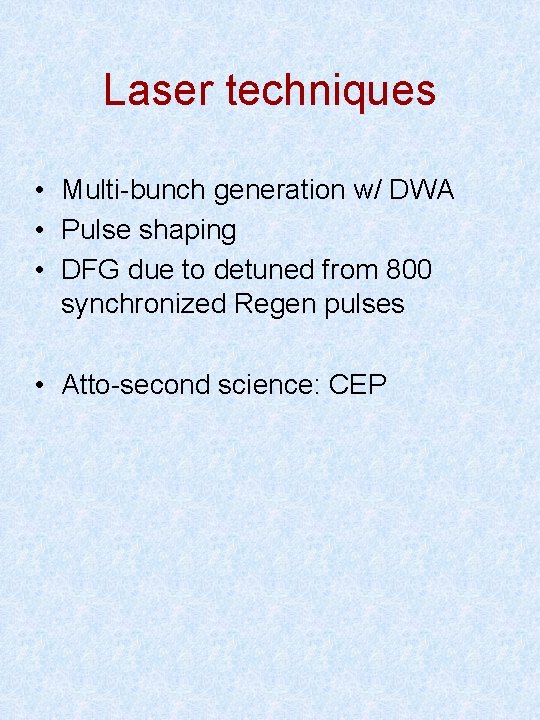
Laser techniques • Multi-bunch generation w/ DWA • Pulse shaping • DFG due to detuned from 800 synchronized Regen pulses • Atto-second science: CEP
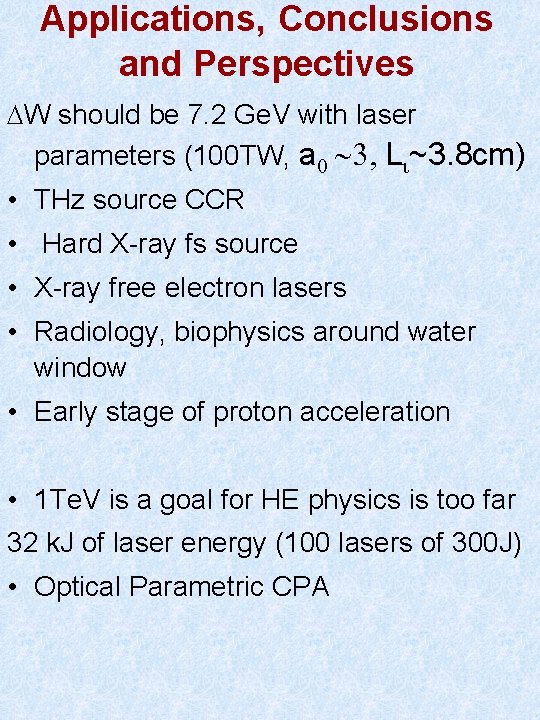
Applications, Conclusions and Perspectives DW should be 7. 2 Ge. V with laser parameters (100 TW, a 0 ~3, Li~3. 8 cm) • THz source CCR • Hard X-ray fs source • X-ray free electron lasers • Radiology, biophysics around water window • Early stage of proton acceleration • 1 Te. V is a goal for HE physics is too far 32 k. J of laser energy (100 lasers of 300 J) • Optical Parametric CPA
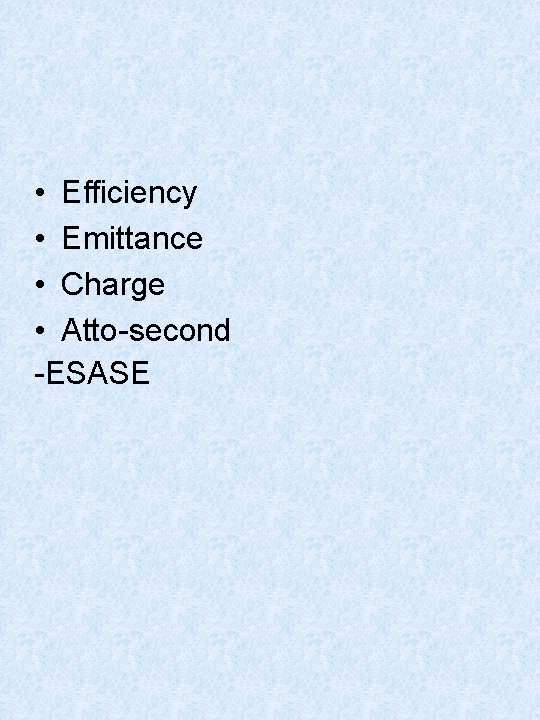
• Efficiency • Emittance • Charge • Atto-second -ESASE
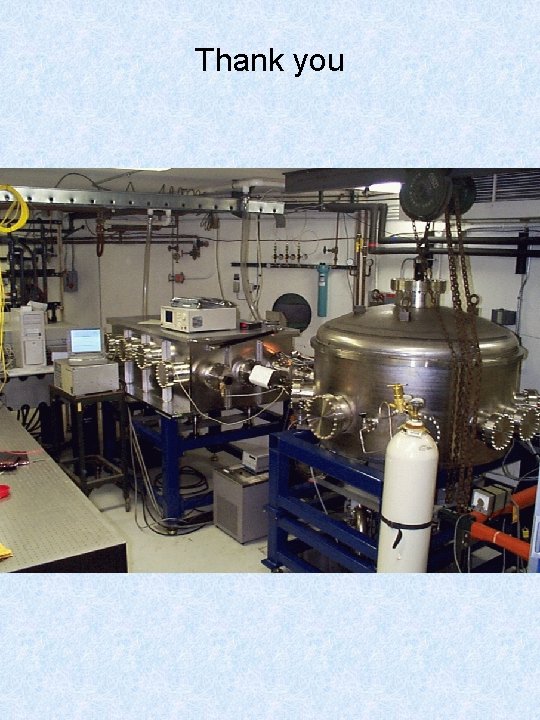
Thank you
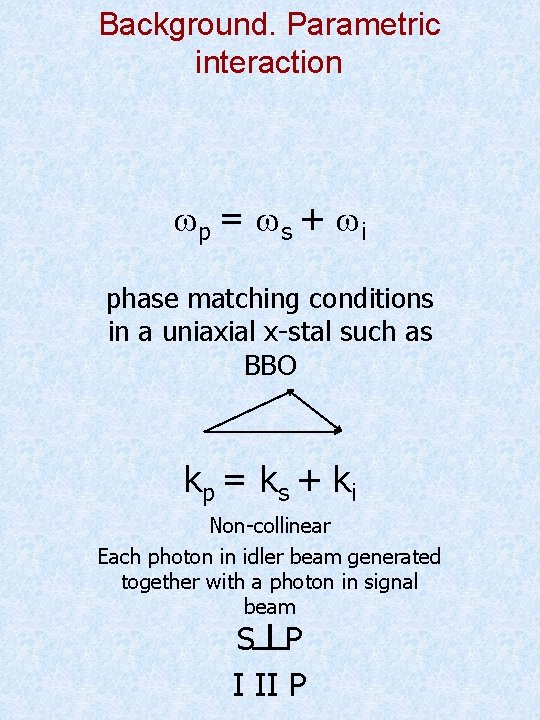
Background. Parametric interaction wp = ws + wi phase matching conditions in a uniaxial x-stal such as BBO kp = ks + ki Non-collinear Each photon in idler beam generated together with a photon in signal beam S P I II P
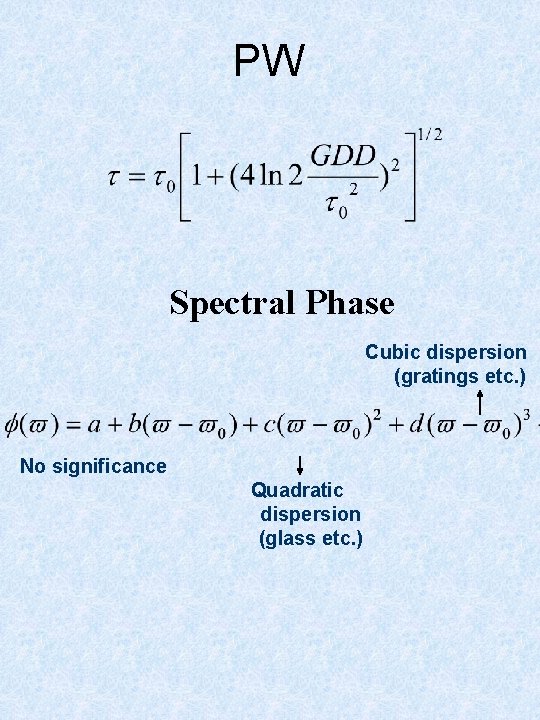
PW Spectral Phase Cubic dispersion (gratings etc. ) No significance Quadratic dispersion (glass etc. )
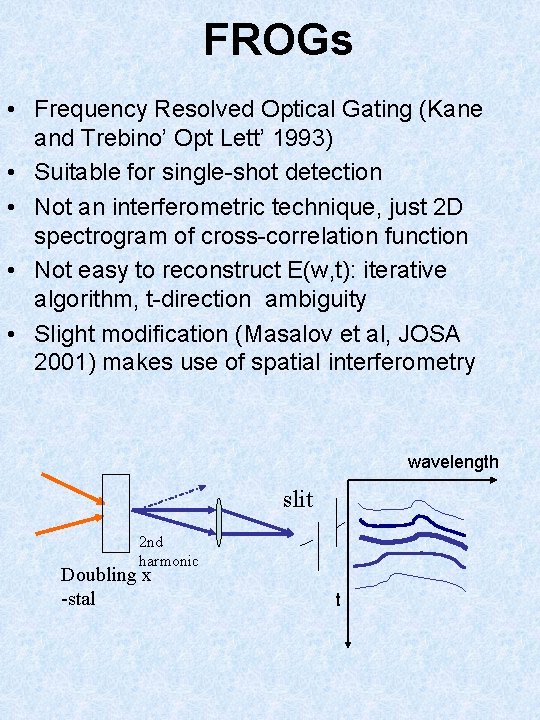
FROGs • Frequency Resolved Optical Gating (Kane and Trebino’ Opt Lett’ 1993) • Suitable for single-shot detection • Not an interferometric technique, just 2 D spectrogram of cross-correlation function • Not easy to reconstruct E(w, t): iterative algorithm, t-direction ambiguity • Slight modification (Masalov et al, JOSA 2001) makes use of spatial interferometry wavelength slit 2 nd harmonic Doubling x -stal t
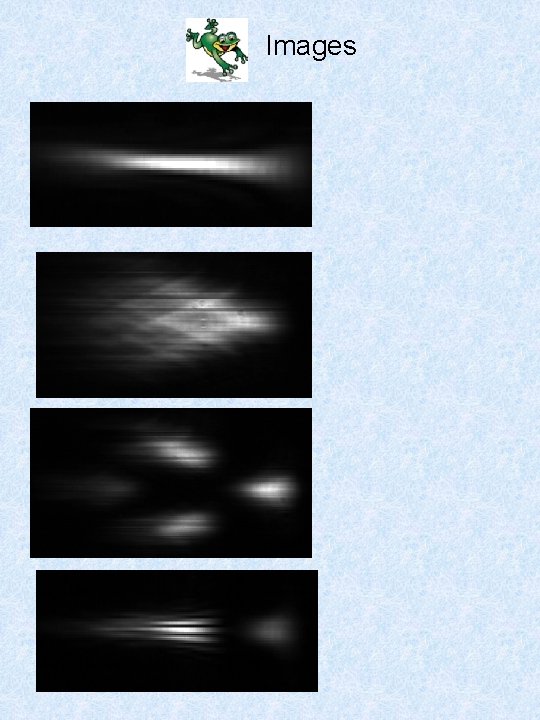
Images
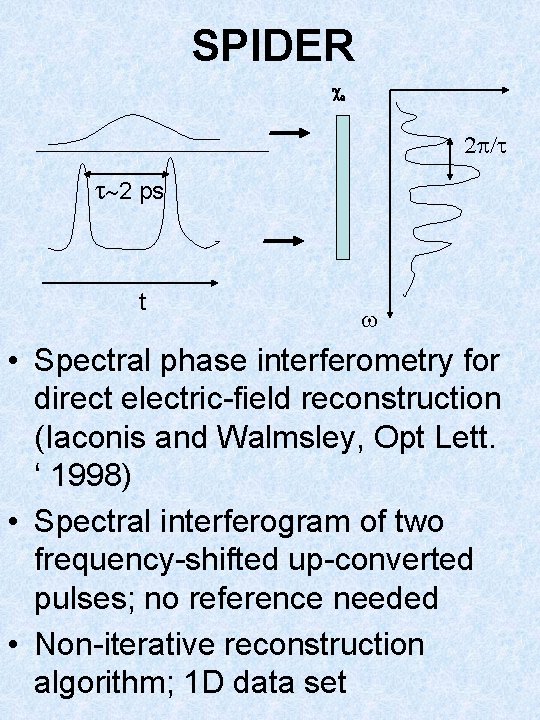
SPIDER c 2 2 p/t t~2 ps t w • Spectral phase interferometry for direct electric-field reconstruction (Iaconis and Walmsley, Opt Lett. ‘ 1998) • Spectral interferogram of two frequency-shifted up-converted pulses; no reference needed • Non-iterative reconstruction algorithm; 1 D data set
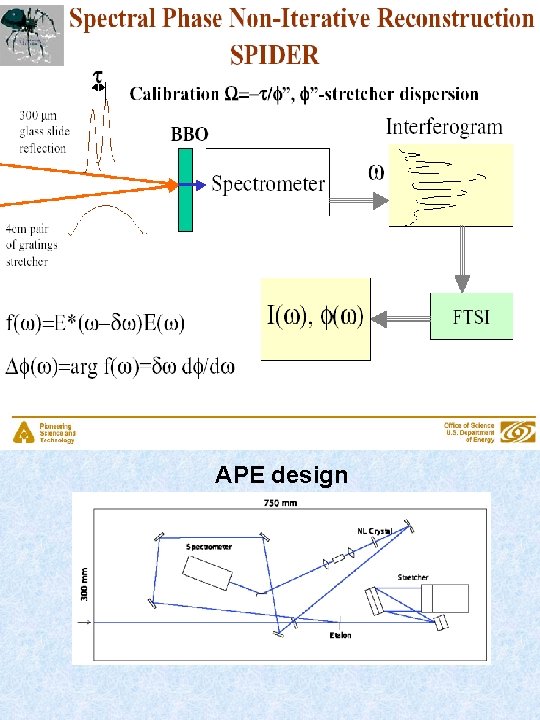
APE design
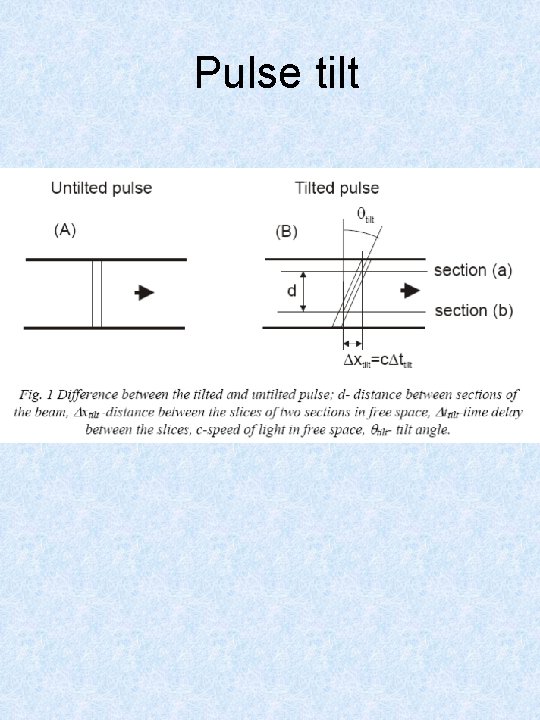
Pulse tilt
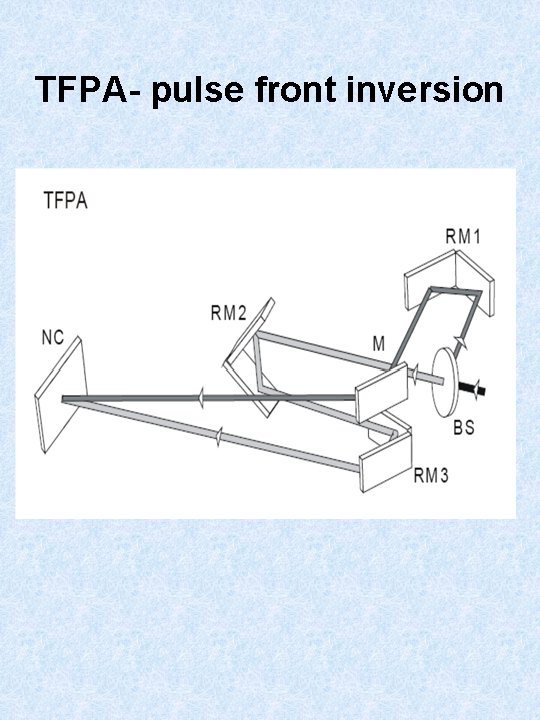
TFPA- pulse front inversion
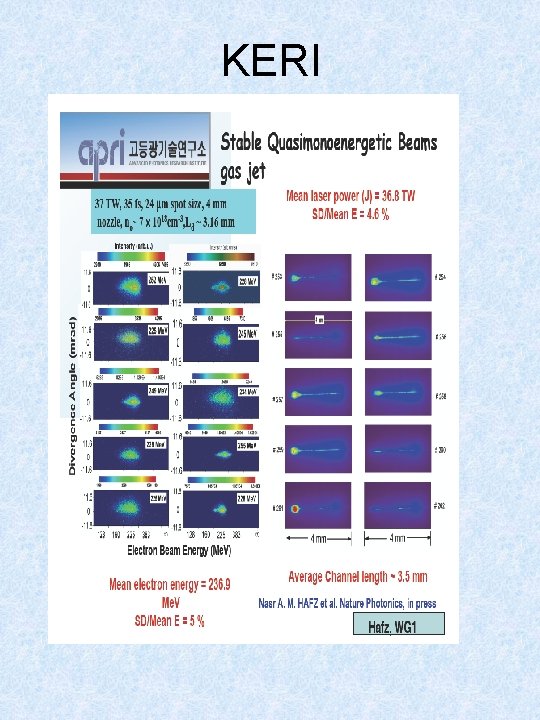
KERI
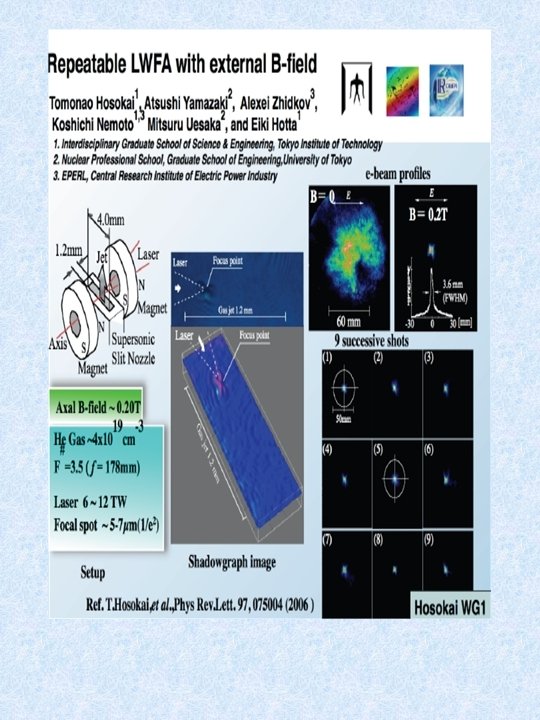
Plasma wakefield acceleration
Ultrafast
Ultrafast demagnetization
Ultrafast magnetism
The long-term future of particle accelerators
Good to great chapter 7 summary
Accelerators computer architecture
Cosmic super accelerators
Slidetodoc
Set current query acceleration
Good to great technology accelerators
Oleg kolesnikov
Oleg lodygensky
Pintor ucraniano
Moldvia
Oleg deev
Oleg grachov
Oleg kouznetsov
Oleg deev
Oleg shuplyak freud voyeur
Kníže oleg
Moldvia
Oleg rokhlenko
Maro tashkan
Butirka
Led light working principle
Oleg kolesnikov
Oleg kouznetsov
Oleg figovsky
For teen
Dr tamekia wakefield
Wakefield middle school bell schedule
Brady's place weymouth ma
Wakefield youth offending team
Hmp wakefield
Rank and wakefield classification of wound
Brendan white wakefield
Public health mdc
Solearth
Laser de electrones libres
Laser interferometer space antenna
Population inversion laser