Towards more efficient klystron designs Chiara Marrelli TIARA
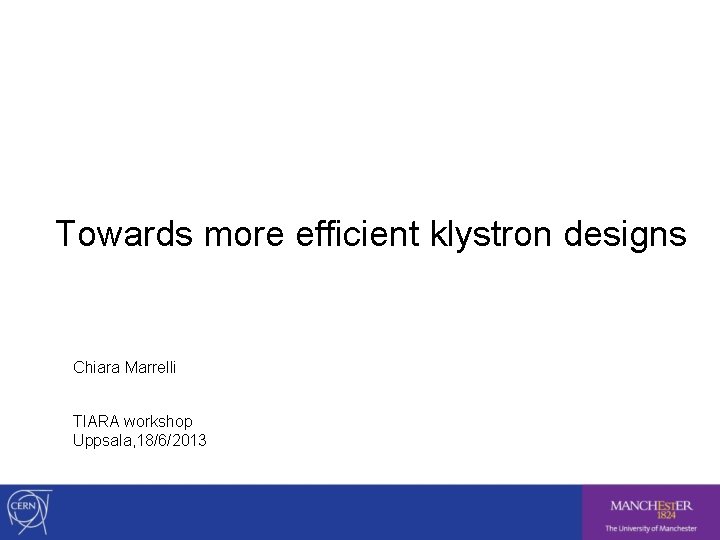
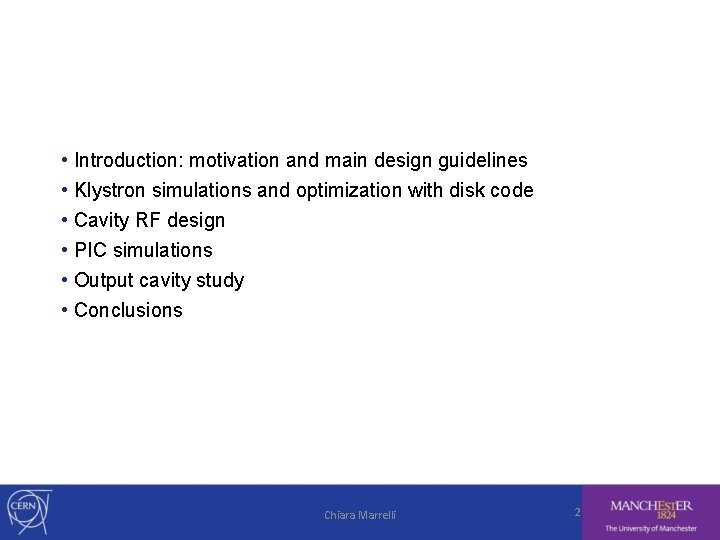
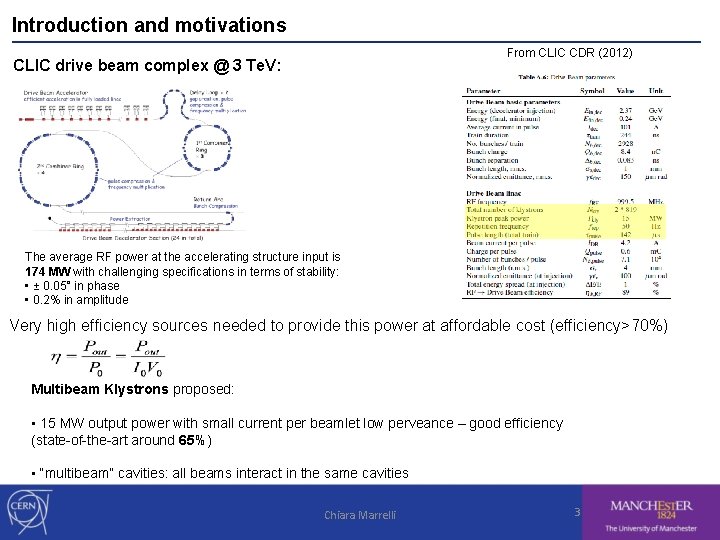
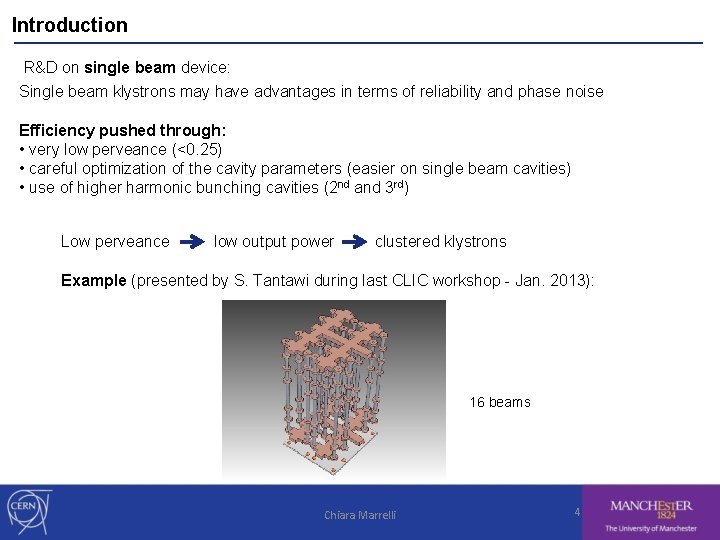
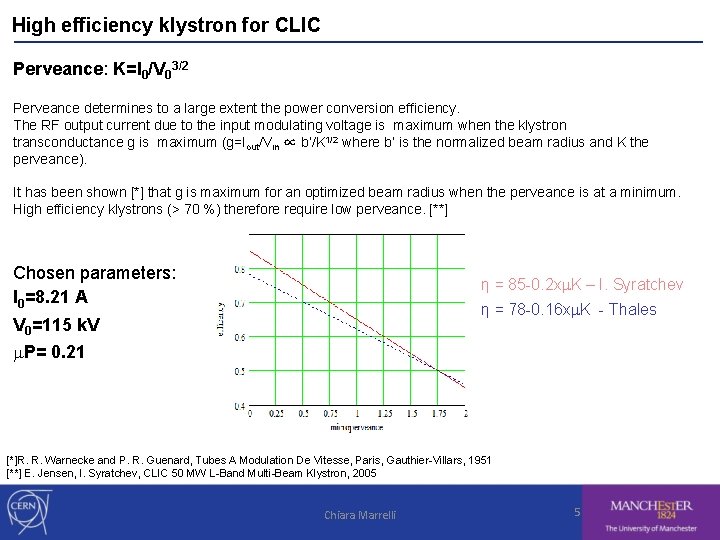
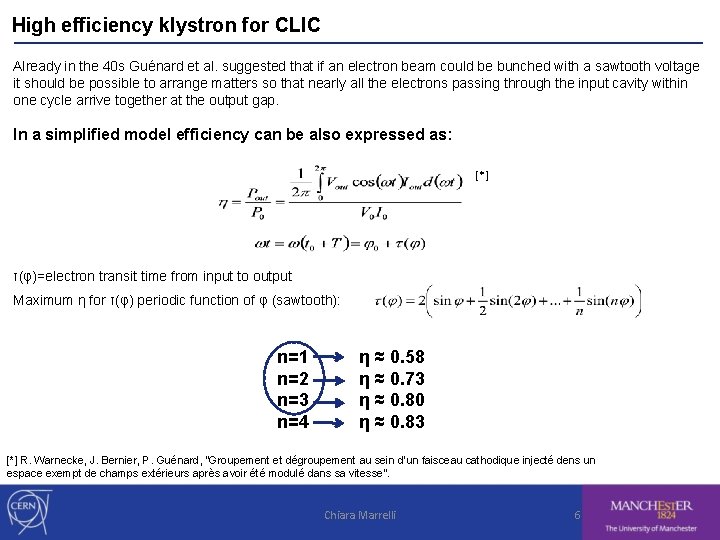
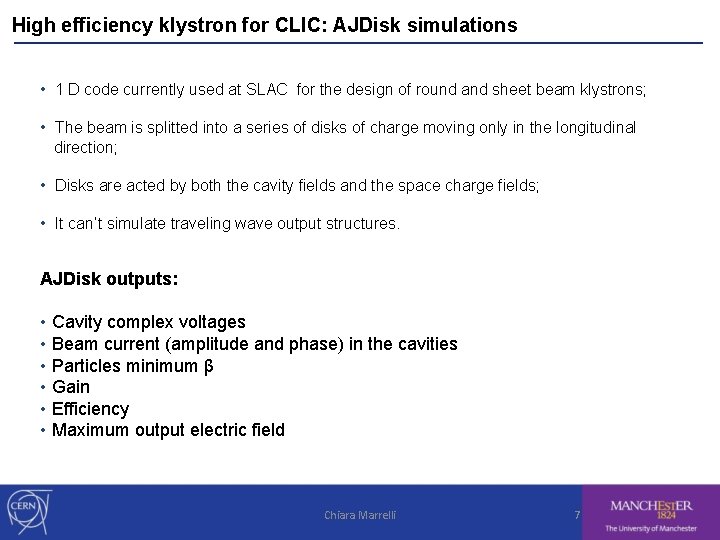
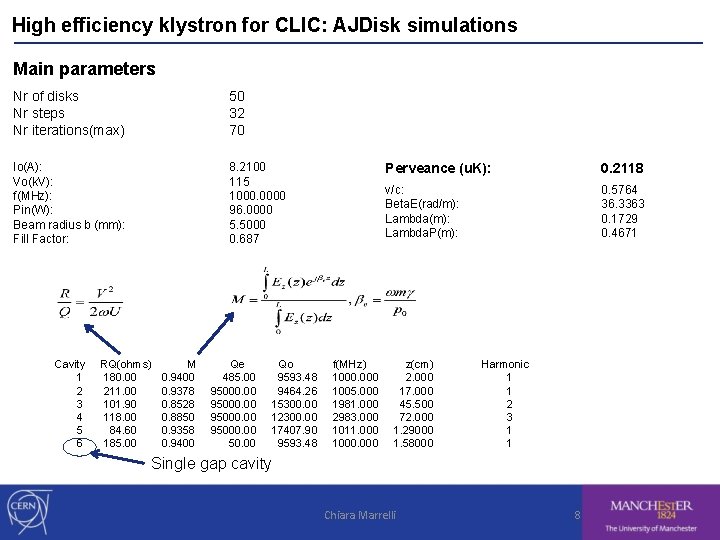
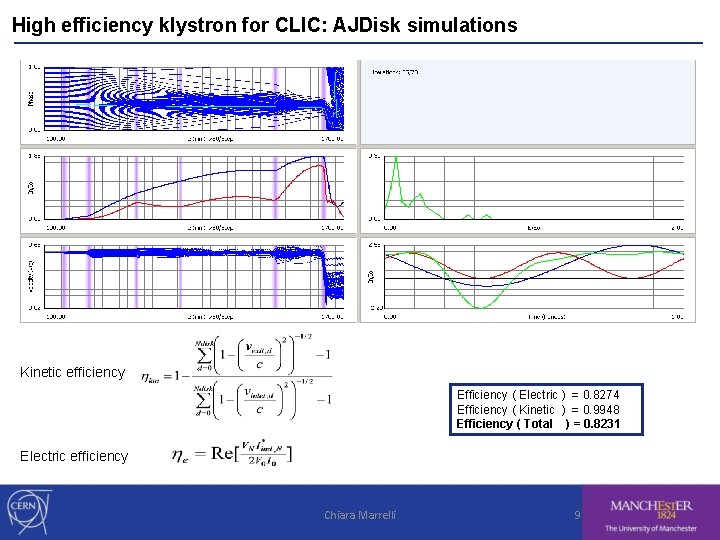
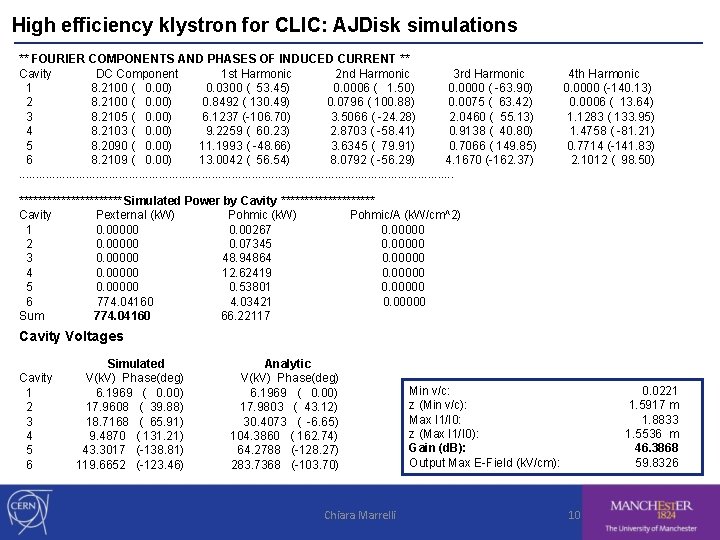
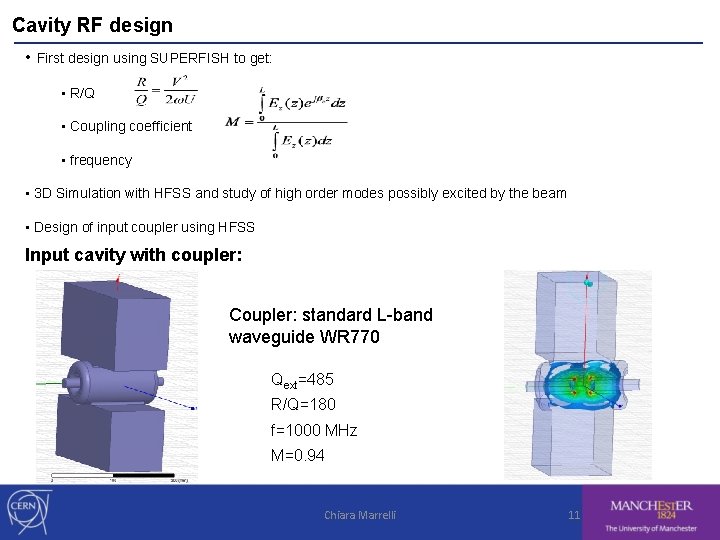
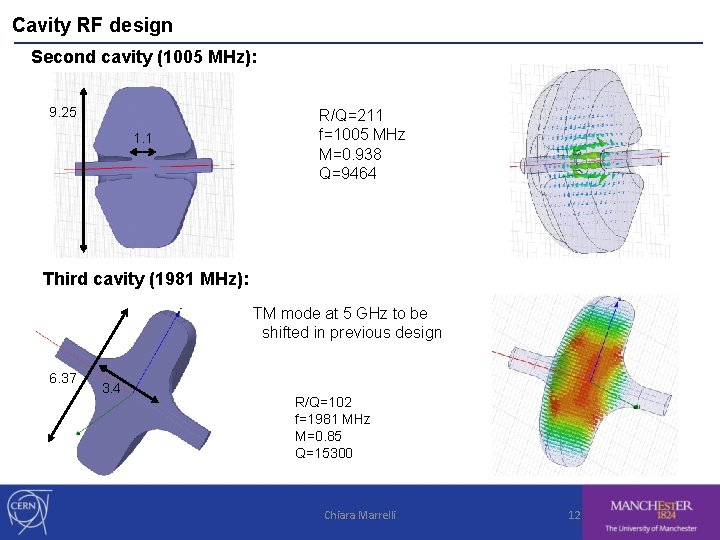
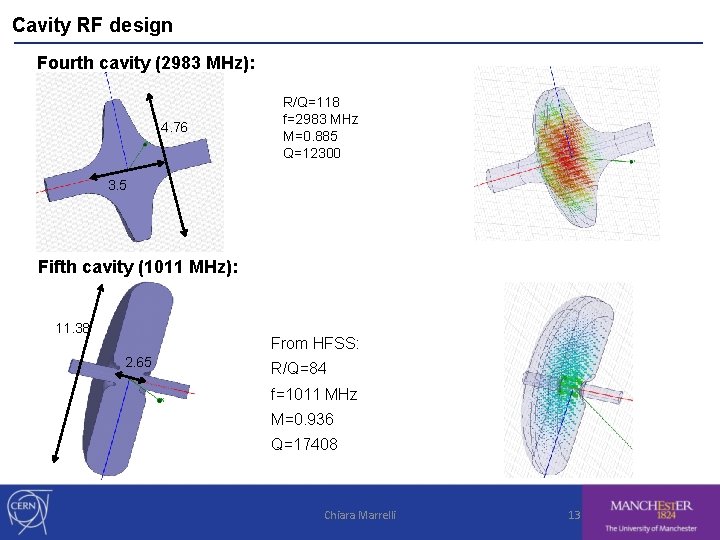
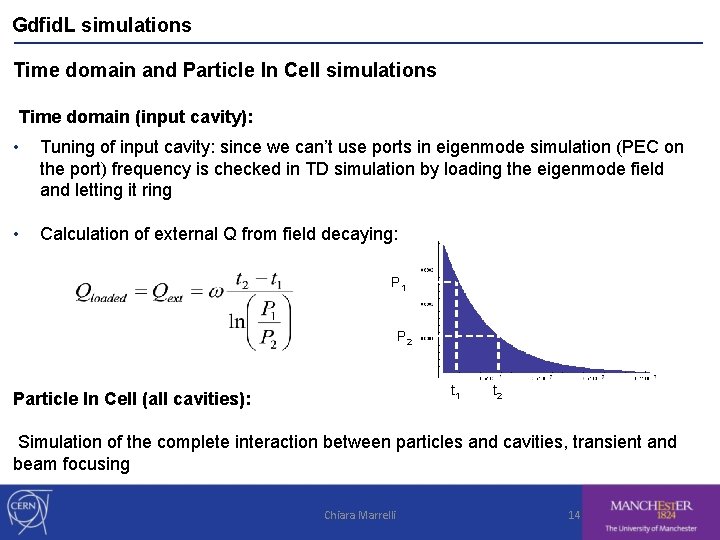
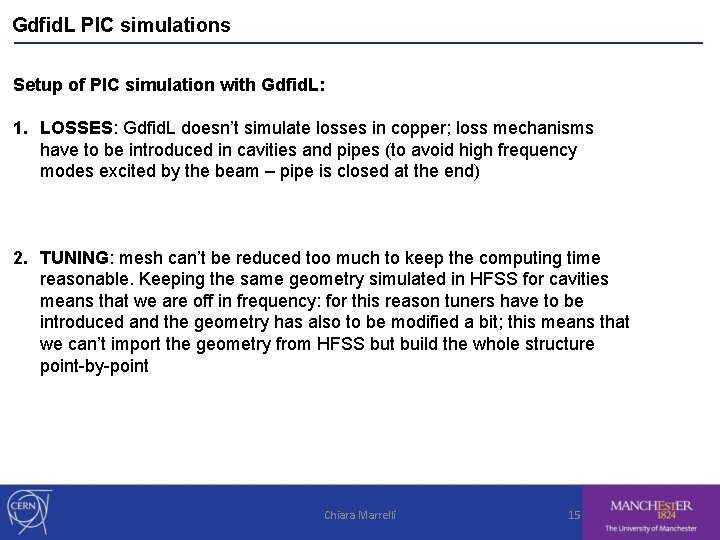
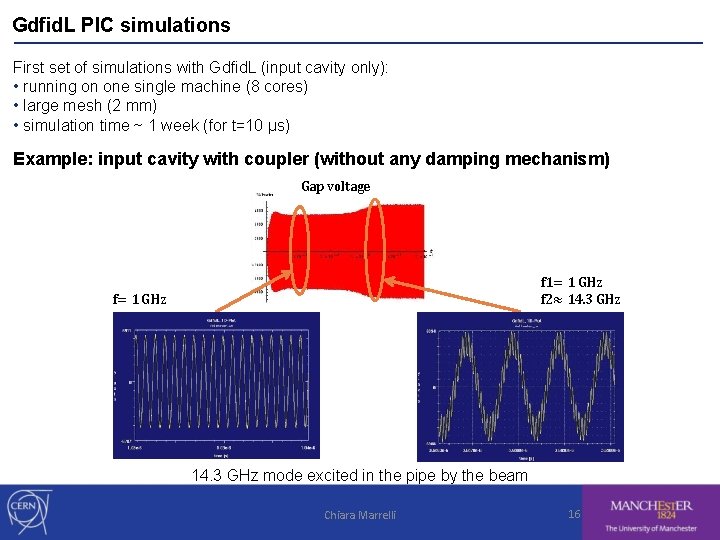
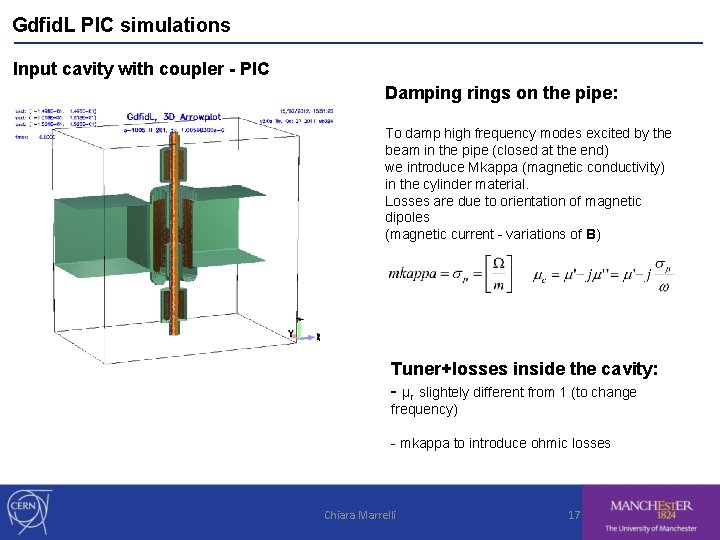
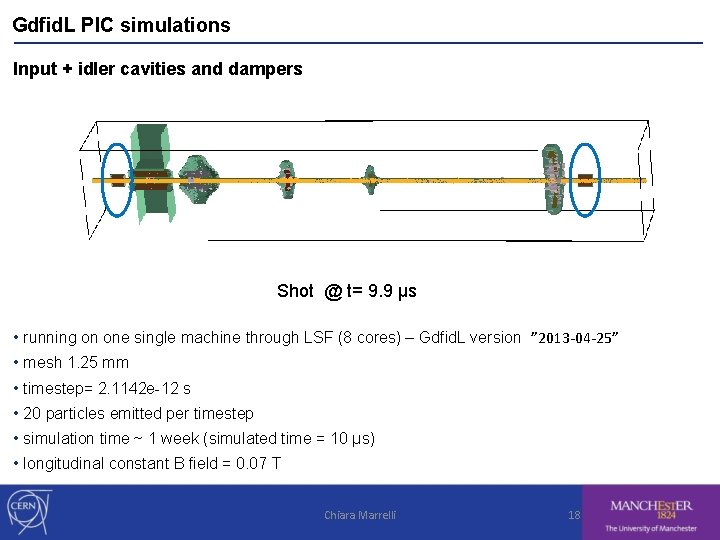
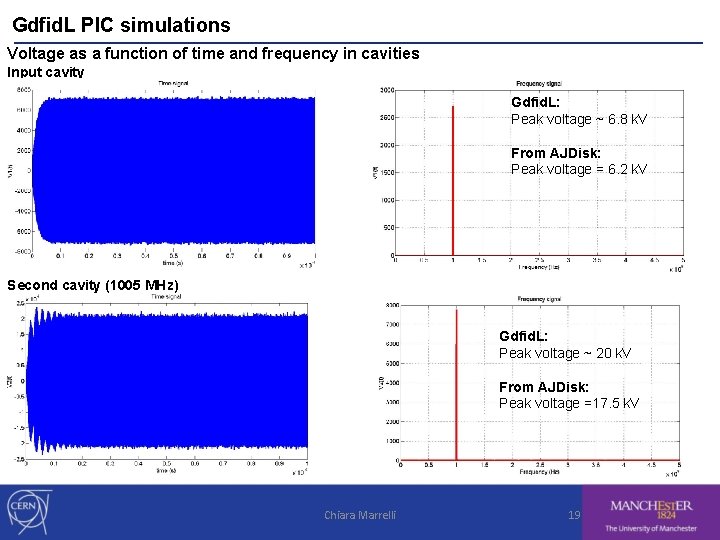
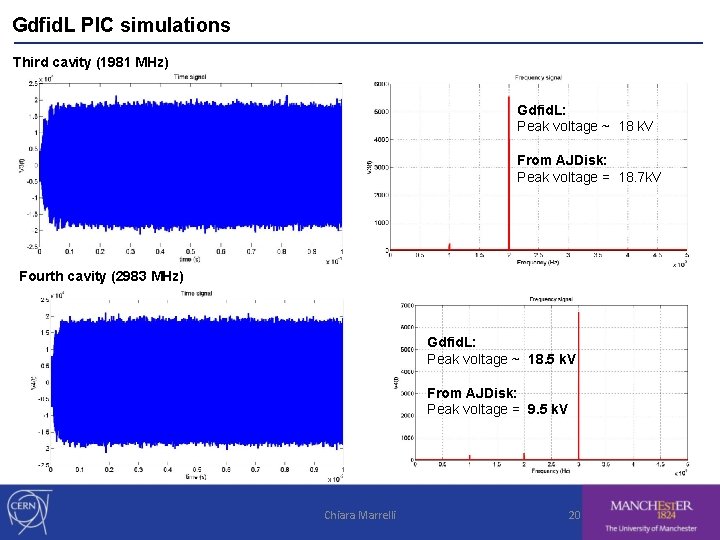
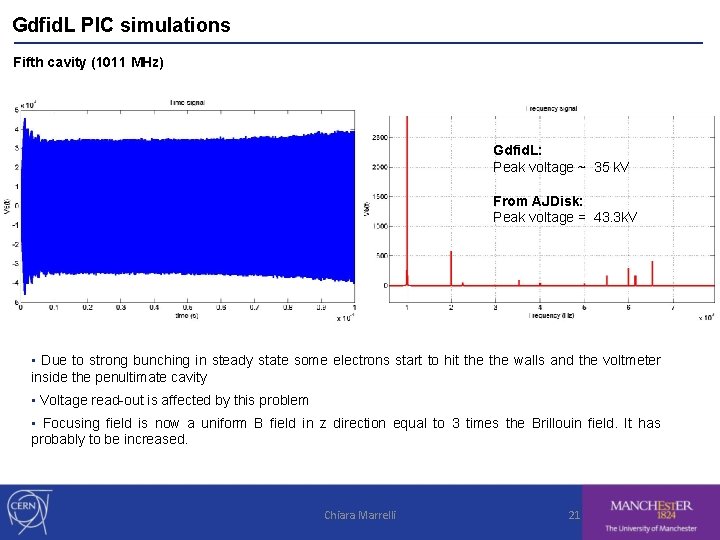
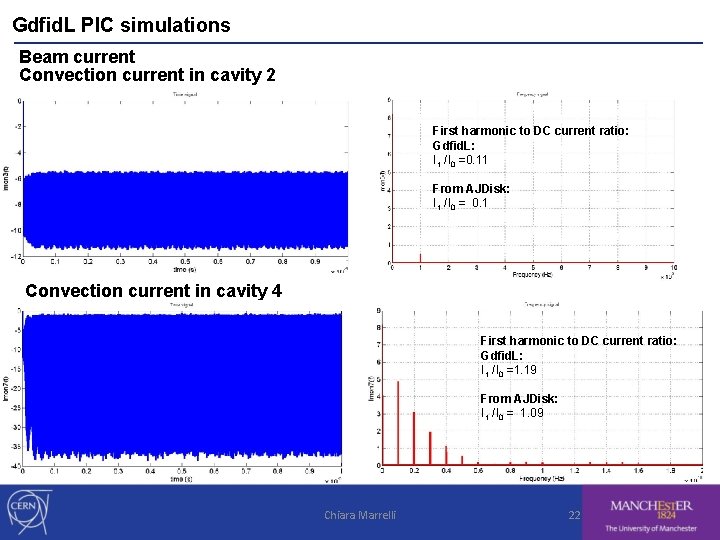
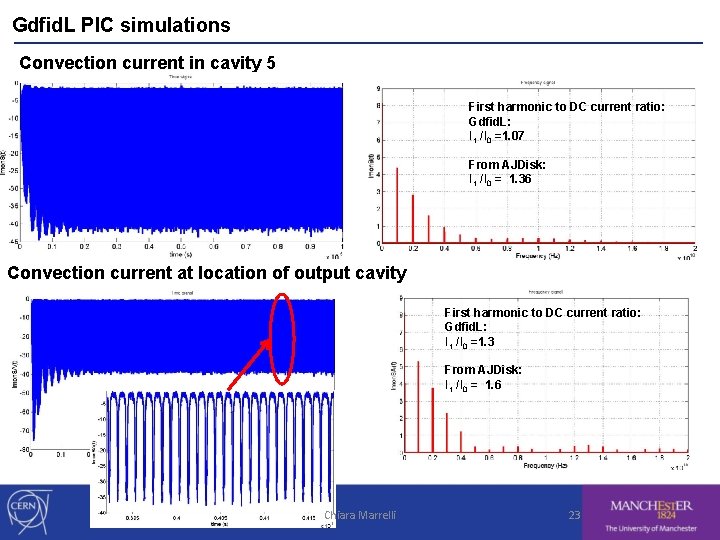
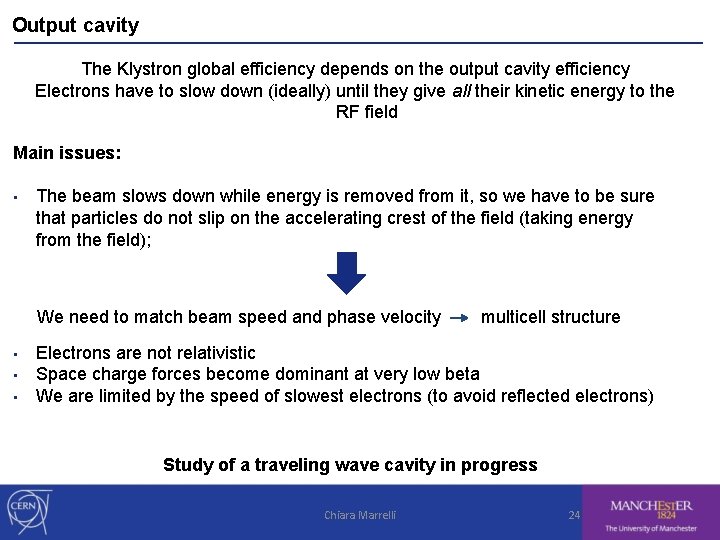
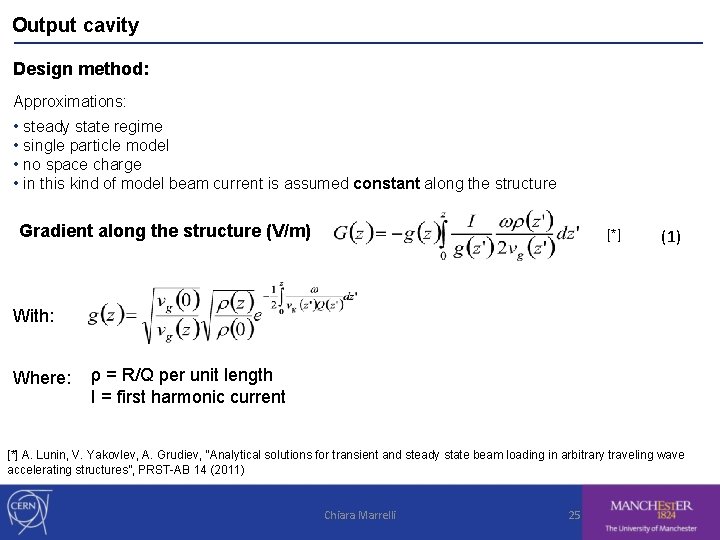
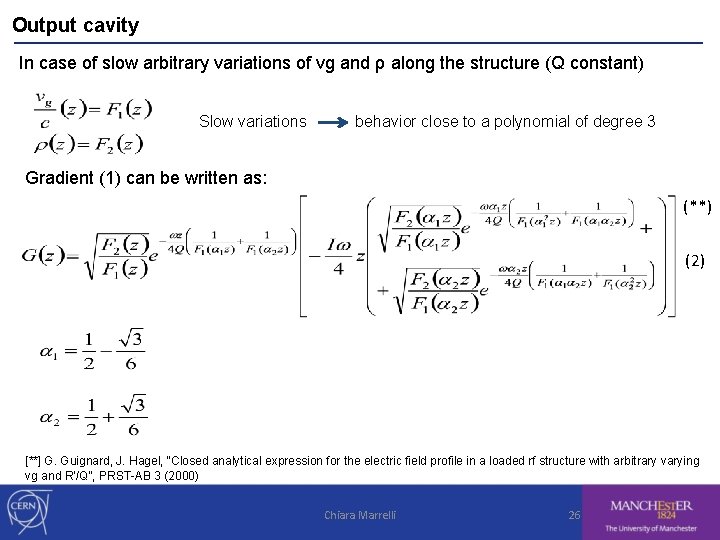
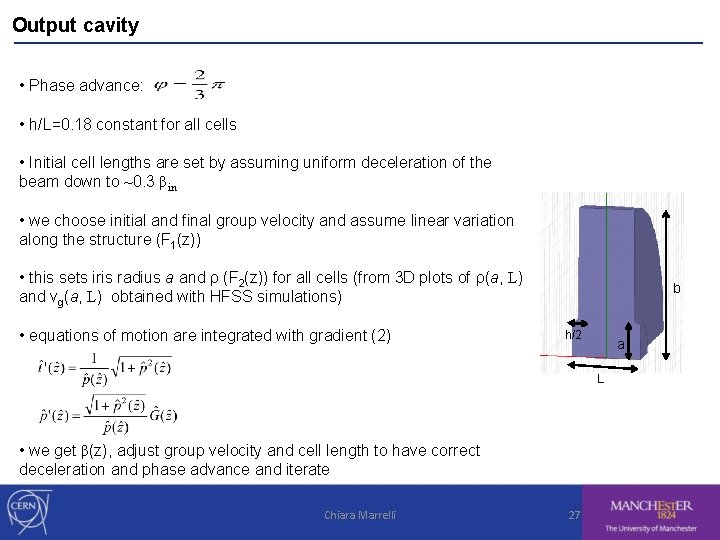
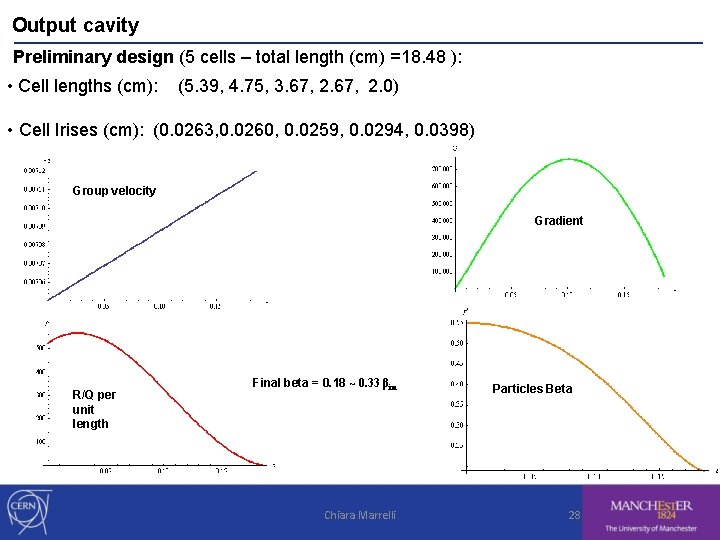
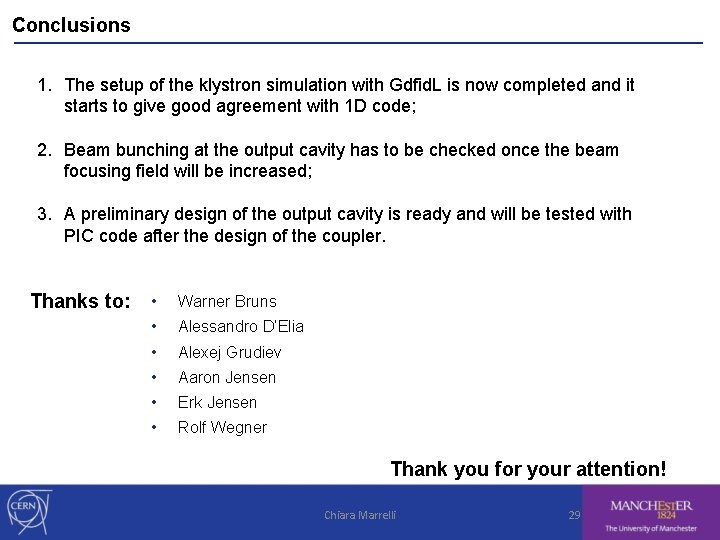
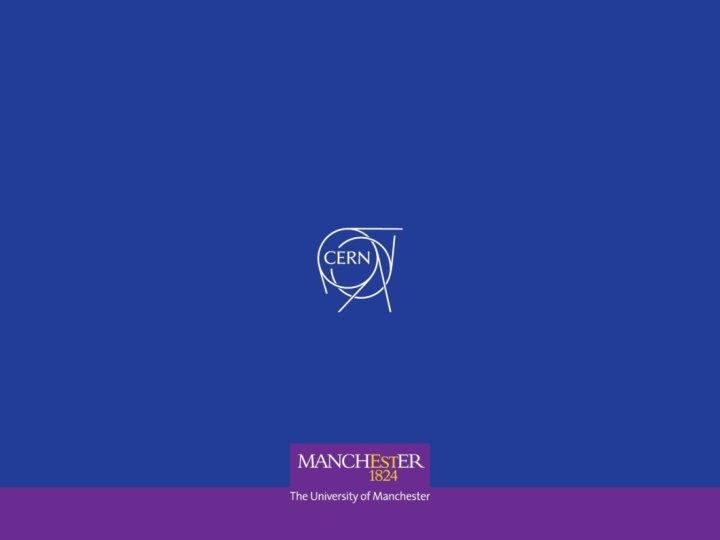
- Slides: 30
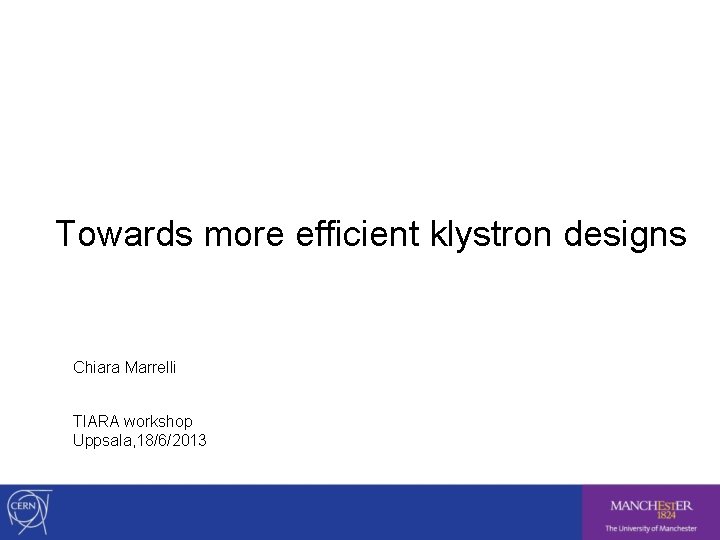
Towards more efficient klystron designs Chiara Marrelli TIARA workshop Uppsala, 18/6/2013
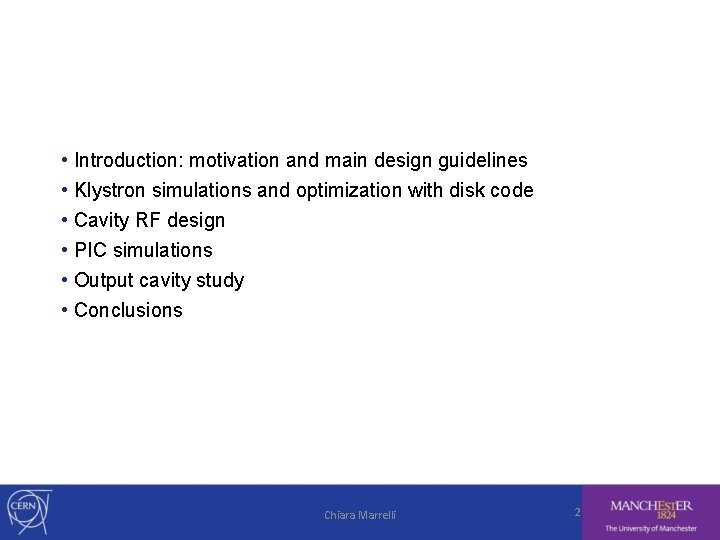
• Introduction: motivation and main design guidelines • Klystron simulations and optimization with disk code • Cavity RF design • PIC simulations • Output cavity study • Conclusions Chiara Marrelli 2
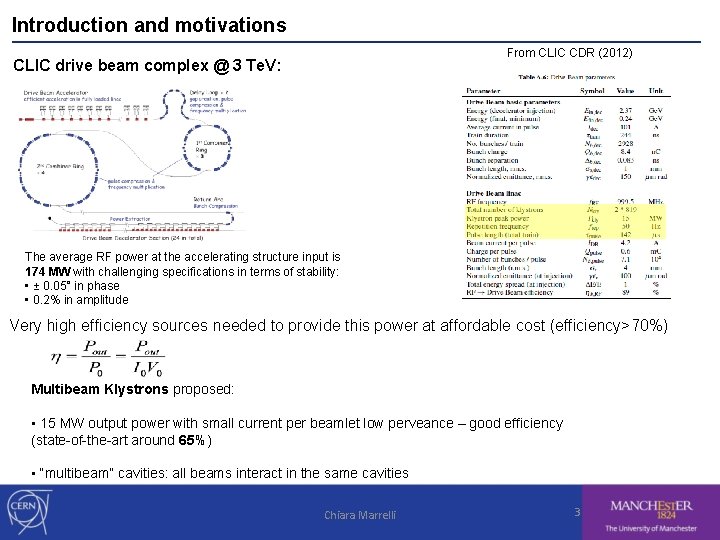
Introduction and motivations From CLIC CDR (2012) CLIC drive beam complex @ 3 Te. V: The average RF power at the accelerating structure input is 174 MW with challenging specifications in terms of stability: • ± 0. 05° in phase • 0. 2% in amplitude Very high efficiency sources needed to provide this power at affordable cost (efficiency>70%) Multibeam Klystrons proposed: • 15 MW output power with small current per beamlet low perveance – good efficiency (state-of-the-art around 65%) • “multibeam” cavities: all beams interact in the same cavities Chiara Marrelli 3
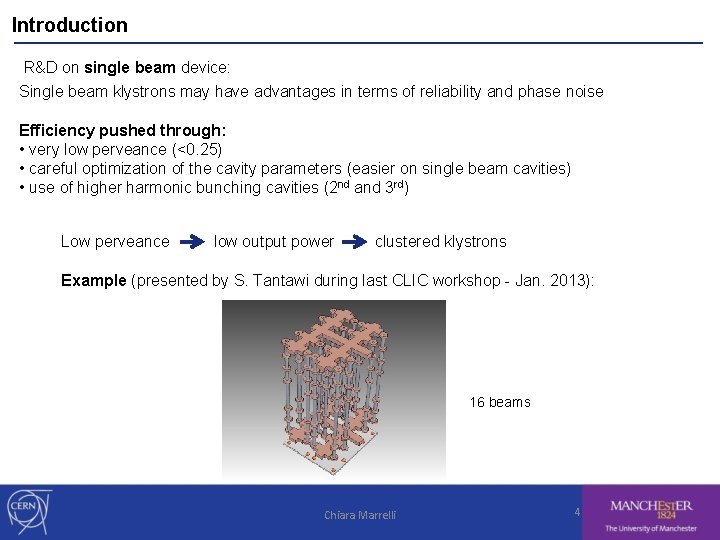
Introduction R&D on single beam device: Single beam klystrons may have advantages in terms of reliability and phase noise Efficiency pushed through: • very low perveance (<0. 25) • careful optimization of the cavity parameters (easier on single beam cavities) • use of higher harmonic bunching cavities (2 nd and 3 rd) Low perveance low output power clustered klystrons Example (presented by S. Tantawi during last CLIC workshop - Jan. 2013): 16 beams Chiara Marrelli 4
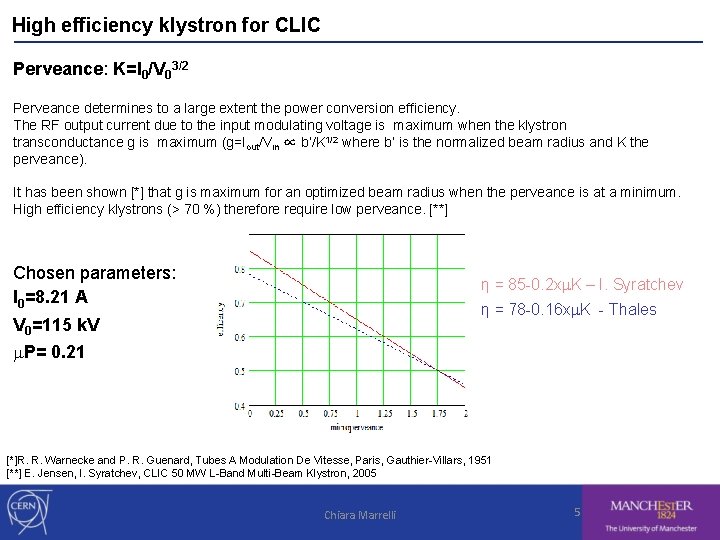
High efficiency klystron for CLIC Perveance: K=I 0/V 03/2 Perveance determines to a large extent the power conversion efficiency. The RF output current due to the input modulating voltage is maximum when the klystron transconductance g is maximum (g=Iout/Vin ∝ b’/K 1/2 where b’ is the normalized beam radius and K the perveance). It has been shown [*] that g is maximum for an optimized beam radius when the perveance is at a minimum. High efficiency klystrons (> 70 %) therefore require low perveance. [**] Chosen parameters: I 0=8. 21 A η = 85 -0. 2 x K – I. Syratchev η = 78 -0. 16 x K - Thales V 0=115 k. V P= 0. 21 [*]R. R. Warnecke and P. R. Guenard, Tubes A Modulation De Vitesse, Paris, Gauthier-Villars, 1951 [**] E. Jensen, I. Syratchev, CLIC 50 MW L-Band Multi-Beam Klystron, 2005 Chiara Marrelli 5
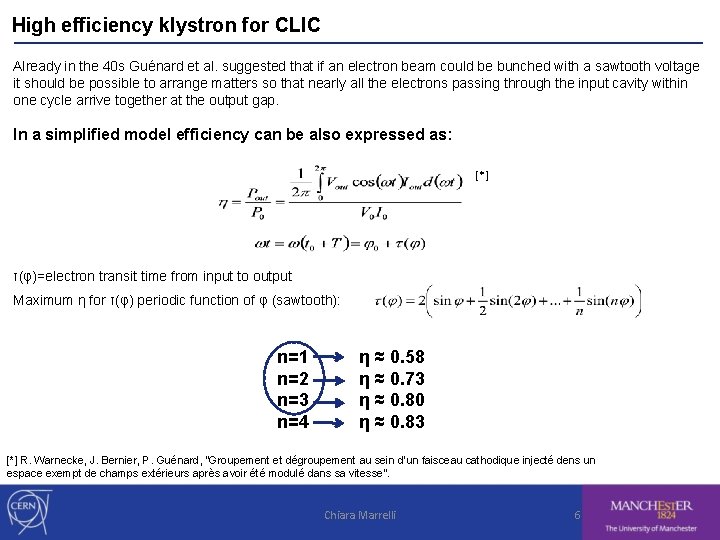
High efficiency klystron for CLIC Already in the 40 s Guénard et al. suggested that if an electron beam could be bunched with a sawtooth voltage it should be possible to arrange matters so that nearly all the electrons passing through the input cavity within one cycle arrive together at the output gap. In a simplified model efficiency can be also expressed as: [*] τ(φ)=electron transit time from input to output Maximum η for τ(φ) periodic function of φ (sawtooth): n=1 n=2 n=3 n=4 η ≈ 0. 58 η ≈ 0. 73 η ≈ 0. 80 η ≈ 0. 83 [*] R. Warnecke, J. Bernier, P. Guénard, “Groupement et dégroupement au sein d’un faisceau cathodique injecté dens un espace exempt de champs extérieurs après avoir été modulé dans sa vitesse”. Chiara Marrelli 6
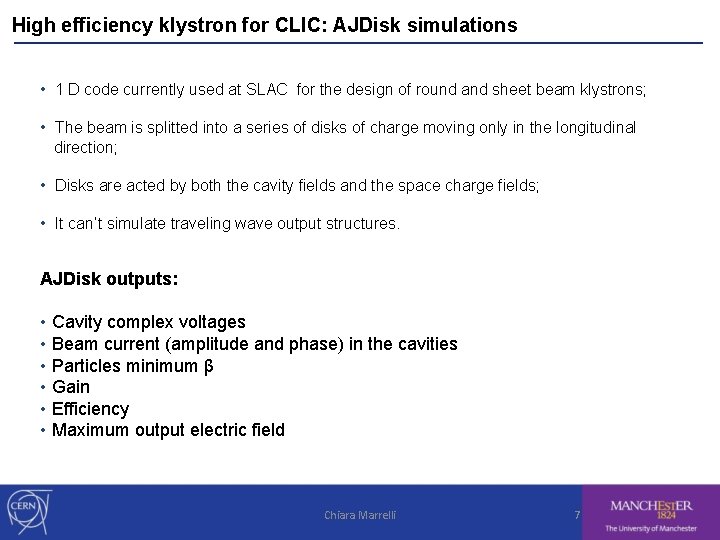
High efficiency klystron for CLIC: AJDisk simulations • 1 D code currently used at SLAC for the design of round and sheet beam klystrons; • The beam is splitted into a series of disks of charge moving only in the longitudinal direction; • Disks are acted by both the cavity fields and the space charge fields; • It can’t simulate traveling wave output structures. AJDisk outputs: • Cavity complex voltages • Beam current (amplitude and phase) in the cavities • Particles minimum β • Gain • Efficiency • Maximum output electric field Chiara Marrelli 7
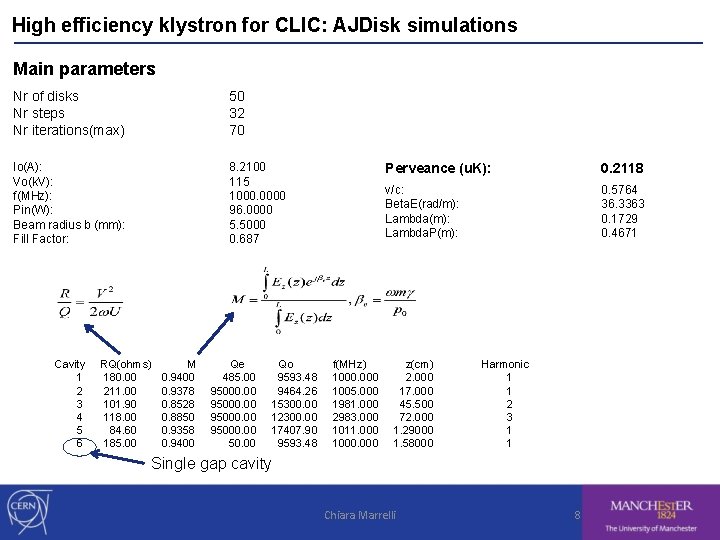
High efficiency klystron for CLIC: AJDisk simulations Main parameters Nr of disks Nr steps Nr iterations(max) 50 32 70 Io(A): Vo(k. V): f(MHz): Pin(W): Beam radius b (mm): Fill Factor: 8. 2100 115 1000. 0000 96. 0000 5. 5000 0. 687 Cavity 1 2 3 4 5 6 RQ(ohms) 180. 00 211. 00 101. 90 118. 00 84. 60 185. 00 M 0. 9400 0. 9378 0. 8528 0. 8850 0. 9358 0. 9400 Qe 485. 00 95000. 00 50. 00 Qo 9593. 48 9464. 26 15300. 00 12300. 00 17407. 90 9593. 48 f(MHz) 1000. 000 1005. 000 1981. 000 2983. 000 1011. 000 1000. 000 Perveance (u. K): 0. 2118 v/c: Beta. E(rad/m): Lambda(m): Lambda. P(m): 0. 5764 36. 3363 0. 1729 0. 4671 z(cm) 2. 000 17. 000 45. 500 72. 000 1. 29000 1. 58000 Harmonic 1 1 2 3 1 1 Single gap cavity Chiara Marrelli 8
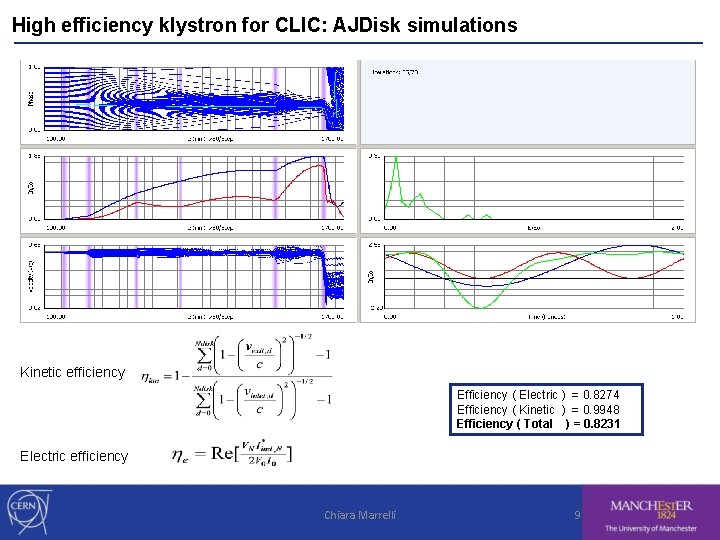
High efficiency klystron for CLIC: AJDisk simulations Kinetic efficiency Efficiency ( Electric ) = 0. 8274 Efficiency ( Kinetic ) = 0. 9948 Efficiency ( Total ) = 0. 8231 Electric efficiency Chiara Marrelli 9
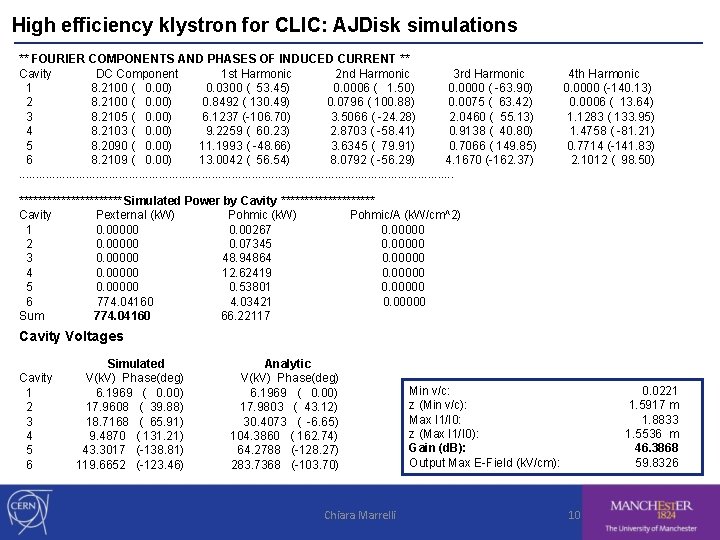
High efficiency klystron for CLIC: AJDisk simulations ** FOURIER COMPONENTS AND PHASES OF INDUCED CURRENT ** Cavity DC Component 1 st Harmonic 2 nd Harmonic 3 rd Harmonic 1 8. 2100 ( 0. 00) 0. 0300 ( 53. 45) 0. 0006 ( 1. 50) 0. 0000 ( -63. 90) 2 8. 2100 ( 0. 00) 0. 8492 ( 130. 49) 0. 0796 ( 100. 88) 0. 0075 ( 63. 42) 3 8. 2105 ( 0. 00) 6. 1237 (-106. 70) 3. 5066 ( -24. 28) 2. 0460 ( 55. 13) 4 8. 2103 ( 0. 00) 9. 2259 ( 60. 23) 2. 8703 ( -58. 41) 0. 9138 ( 40. 80) 5 8. 2090 ( 0. 00) 11. 1993 ( -48. 66) 3. 6345 ( 79. 91) 0. 7066 ( 149. 85) 6 8. 2109 ( 0. 00) 13. 0042 ( 56. 54) 8. 0792 ( -56. 29) 4. 1670 (-162. 37). . . . . . . . . 4 th Harmonic 0. 0000 (-140. 13) 0. 0006 ( 13. 64) 1. 1283 ( 133. 95) 1. 4758 ( -81. 21) 0. 7714 (-141. 83) 2. 1012 ( 98. 50) *********** Simulated Power by Cavity ********** Cavity Pexternal (k. W) Pohmic/A (k. W/cm^2) 1 0. 00000 0. 00267 0. 00000 2 0. 00000 0. 07345 0. 00000 3 0. 00000 48. 94864 0. 00000 12. 62419 0. 00000 5 0. 00000 0. 53801 0. 00000 6 774. 04160 4. 03421 0. 00000 Sum 774. 04160 66. 22117 Cavity Voltages Cavity 1 2 3 4 5 6 Simulated V(k. V) Phase(deg) 6. 1969 ( 0. 00) 17. 9608 ( 39. 88) 18. 7168 ( 65. 91) 9. 4870 ( 131. 21) 43. 3017 (-138. 81) 119. 6652 (-123. 46) Analytic V(k. V) Phase(deg) 6. 1969 ( 0. 00) 17. 9803 ( 43. 12) 30. 4073 ( -6. 65) 104. 3860 ( 162. 74) 64. 2788 (-128. 27) 283. 7368 (-103. 70) Chiara Marrelli Min v/c: z (Min v/c): Max I 1/I 0: z (Max I 1/I 0): Gain (d. B): Output Max E-Field (k. V/cm): 0. 0221 1. 5917 m 1. 8833 1. 5536 m 46. 3868 59. 8326 10
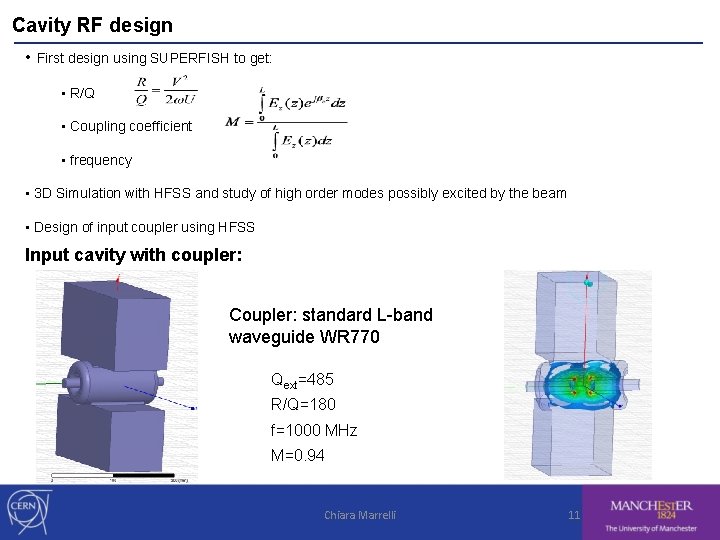
Cavity RF design • First design using SUPERFISH to get: • R/Q • Coupling coefficient • frequency • 3 D Simulation with HFSS and study of high order modes possibly excited by the beam • Design of input coupler using HFSS Input cavity with coupler: Coupler: standard L-band waveguide WR 770 Qext=485 R/Q=180 f=1000 MHz M=0. 94 Chiara Marrelli 11
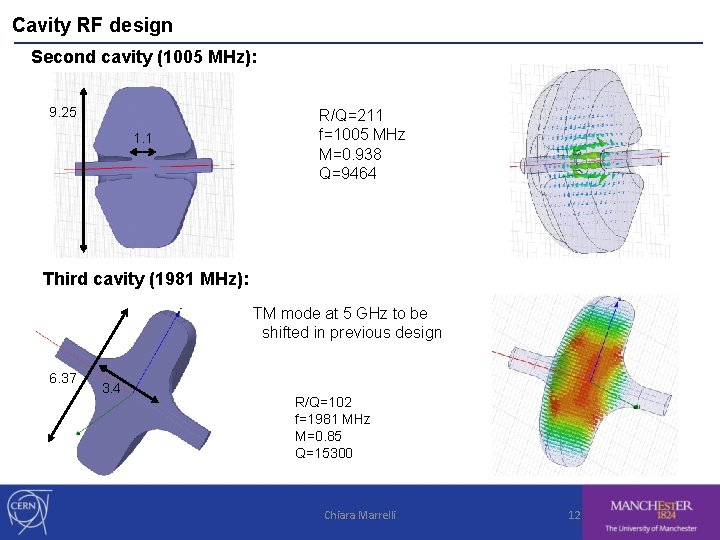
Cavity RF design Second cavity (1005 MHz): 9. 25 Idler cavities: 1. 1 R/Q=211 f=1005 MHz M=0. 938 Q=9464 Third cavity (1981 MHz): TM mode at 5 GHz to be shifted in previous design 6. 37 3. 4 R/Q=102 f=1981 MHz M=0. 85 Q=15300 Chiara Marrelli 12
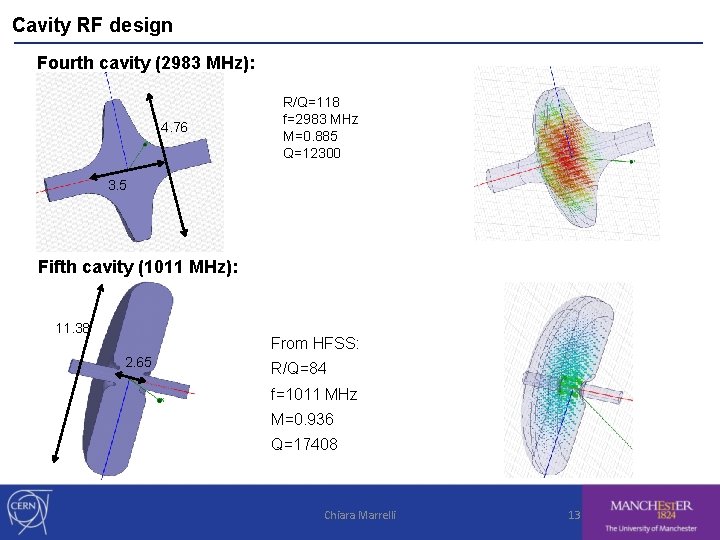
Cavity RF design Fourth cavity (2983 MHz): 4. 76 R/Q=118 f=2983 MHz M=0. 885 Q=12300 3. 5 Fifth cavity (1011 MHz): 11. 38 From HFSS: 2. 65 R/Q=84 f=1011 MHz M=0. 936 Q=17408 Chiara Marrelli 13
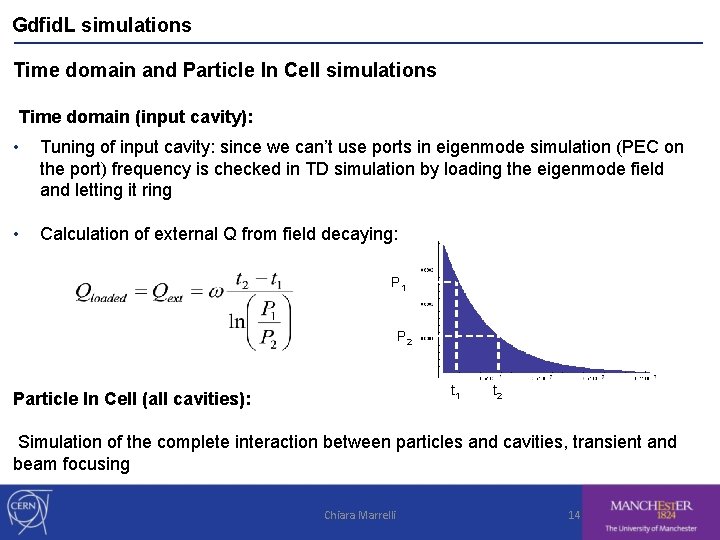
Gdfid. L simulations Time domain and Particle In Cell simulations Time domain (input cavity): • Tuning of input cavity: since we can’t use ports in eigenmode simulation (PEC on the port) frequency is checked in TD simulation by loading the eigenmode field and letting it ring • Calculation of external Q from field decaying: P 1 P 2 t 1 Particle In Cell (all cavities): t 2 Simulation of the complete interaction between particles and cavities, transient and beam focusing Chiara Marrelli 14
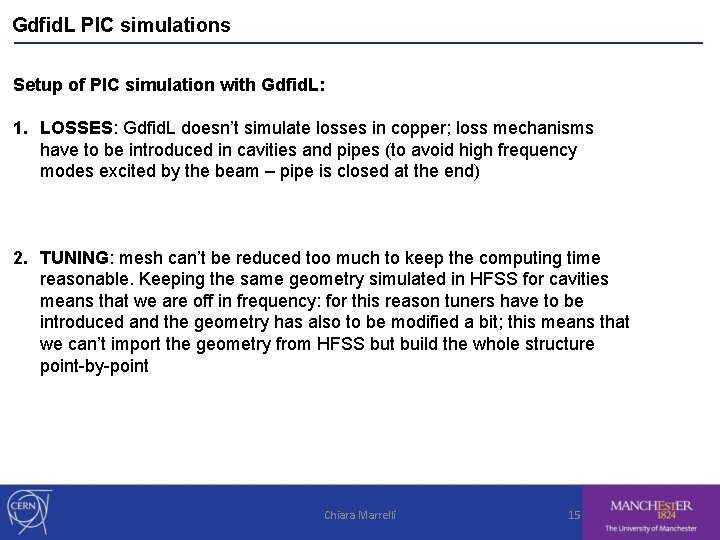
Gdfid. L PIC simulations Setup of PIC simulation with Gdfid. L: 1. LOSSES: Gdfid. L doesn’t simulate losses in copper; loss mechanisms have to be introduced in cavities and pipes (to avoid high frequency modes excited by the beam – pipe is closed at the end) 2. TUNING: mesh can’t be reduced too much to keep the computing time reasonable. Keeping the same geometry simulated in HFSS for cavities means that we are off in frequency: for this reason tuners have to be introduced and the geometry has also to be modified a bit; this means that we can’t import the geometry from HFSS but build the whole structure point-by-point Chiara Marrelli 15
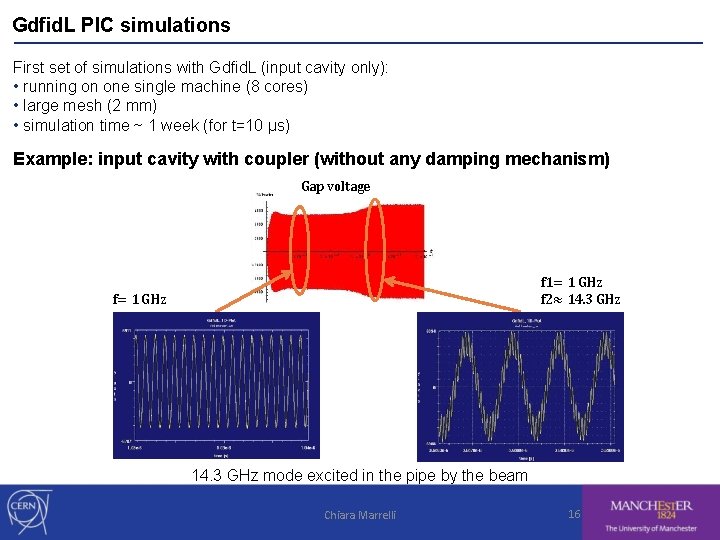
Gdfid. L PIC simulations First set of simulations with Gdfid. L (input cavity only): • running on one single machine (8 cores) • large mesh (2 mm) • simulation time ~ 1 week (for t=10 μs) Example: input cavity with coupler (without any damping mechanism) Gap voltage f 1= 1 GHz f 2≈ 14. 3 GHz f= 1 GHz 14. 3 GHz mode excited in the pipe by the beam Chiara Marrelli 16
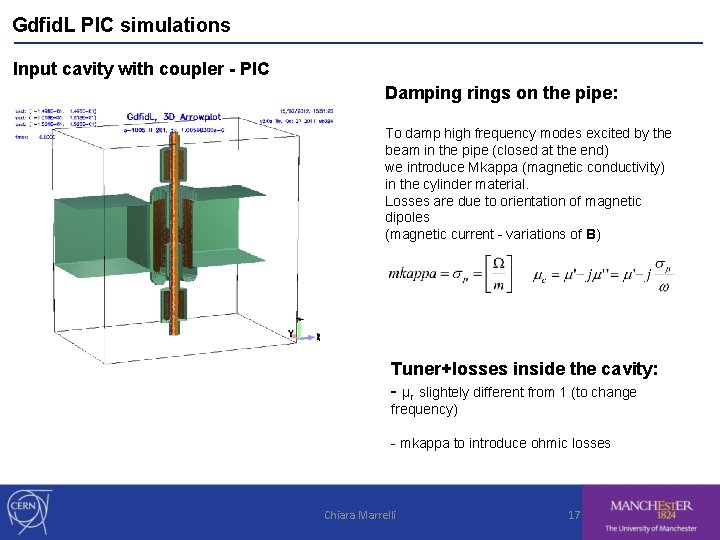
Gdfid. L PIC simulations Input cavity with coupler - PIC Damping rings on the pipe: To damp high frequency modes excited by the beam in the pipe (closed at the end) we introduce Mkappa (magnetic conductivity) in the cylinder material. Losses are due to orientation of magnetic dipoles (magnetic current - variations of B) Tuner+losses inside the cavity: - μr slightely different from 1 (to change frequency) - mkappa to introduce ohmic losses Chiara Marrelli 17
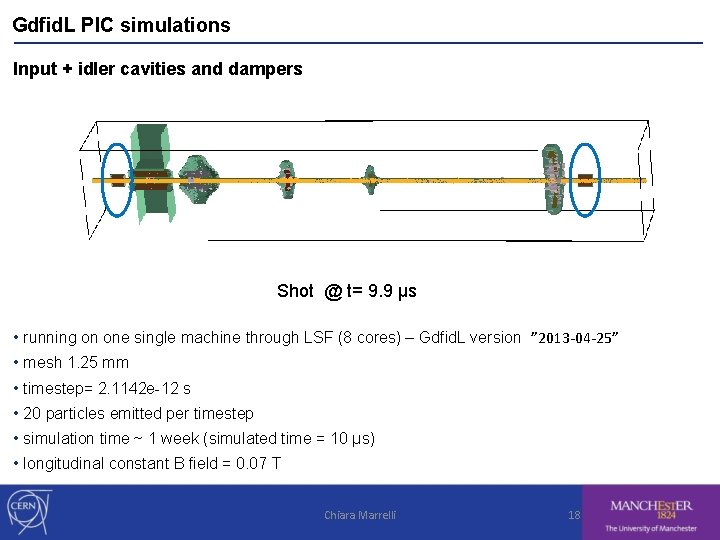
Gdfid. L PIC simulations Input + idler cavities and dampers Shot @ t= 9. 9 μs • running on one single machine through LSF (8 cores) – Gdfid. L version ” 2013 -04 -25” • mesh 1. 25 mm • timestep= 2. 1142 e-12 s • 20 particles emitted per timestep • simulation time ~ 1 week (simulated time = 10 μs) • longitudinal constant B field = 0. 07 T Chiara Marrelli 18
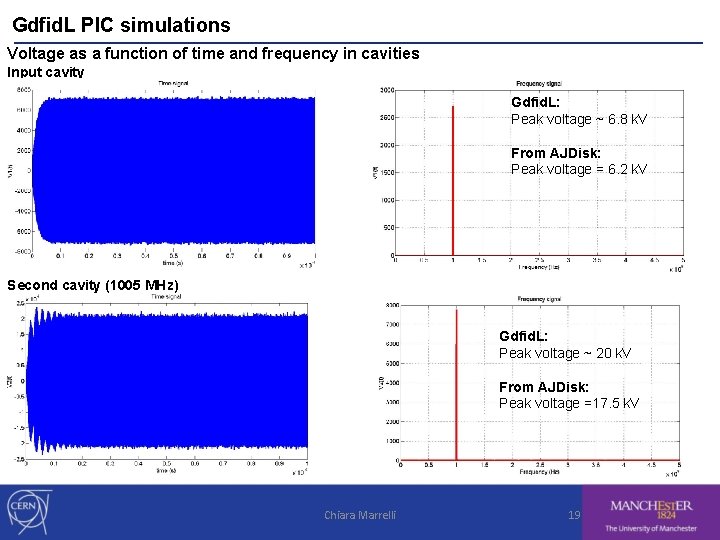
Gdfid. L PIC simulations Voltage as a function of time and frequency in cavities Input cavity Gdfid. L: Peak voltage ~ 6. 8 k. V From AJDisk: Peak voltage = 6. 2 k. V Second cavity (1005 MHz) Gdfid. L: Peak voltage ~ 20 k. V From AJDisk: Peak voltage =17. 5 k. V Chiara Marrelli 19
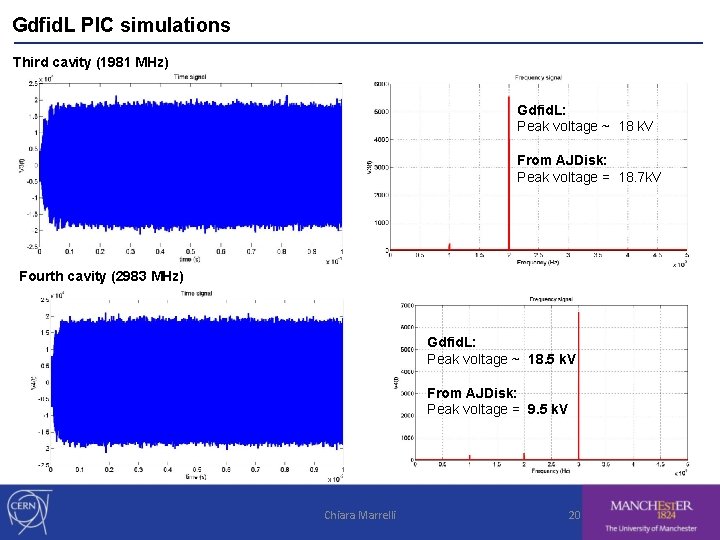
Gdfid. L PIC simulations Third cavity (1981 MHz) Gdfid. L: Peak voltage ~ 18 k. V From AJDisk: Peak voltage = 18. 7 k. V Fourth cavity (2983 MHz) Gdfid. L: Peak voltage ~ 18. 5 k. V From AJDisk: Peak voltage = 9. 5 k. V Chiara Marrelli 20
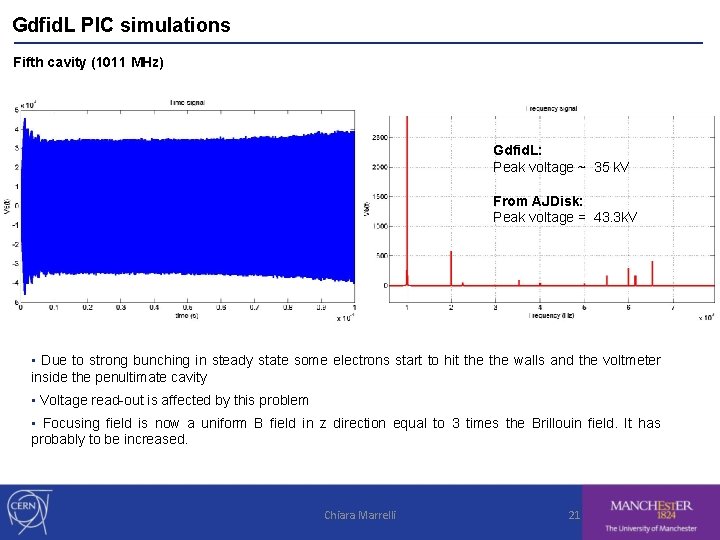
Gdfid. L PIC simulations Fifth cavity (1011 MHz) Gdfid. L: Peak voltage ~ 35 k. V From AJDisk: Peak voltage = 43. 3 k. V • Due to strong bunching in steady state some electrons start to hit the walls and the voltmeter inside the penultimate cavity • Voltage read-out is affected by this problem • Focusing field is now a uniform B field in z direction equal to 3 times the Brillouin field. It has probably to be increased. Chiara Marrelli 21
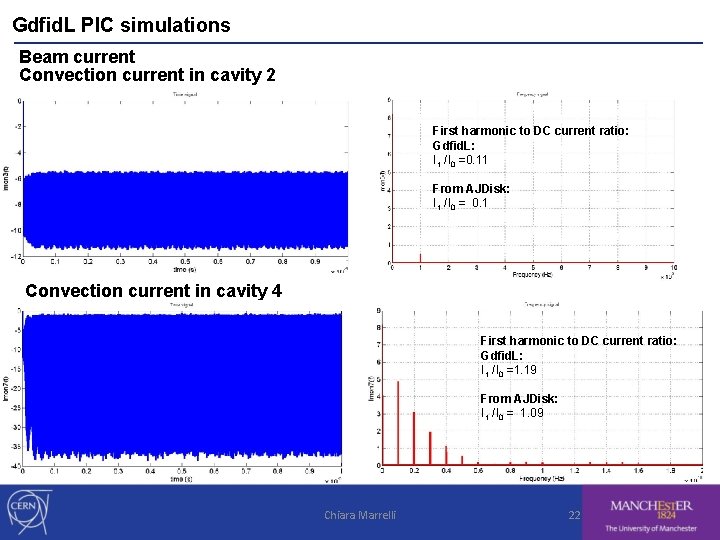
Gdfid. L PIC simulations Beam current Convection current in cavity 2 First harmonic to DC current ratio: Gdfid. L: I 1 /I 0 =0. 11 From AJDisk: I 1 /I 0 = 0. 1 Convection current in cavity 4 First harmonic to DC current ratio: Gdfid. L: I 1 /I 0 =1. 19 From AJDisk: I 1 /I 0 = 1. 09 Chiara Marrelli 22
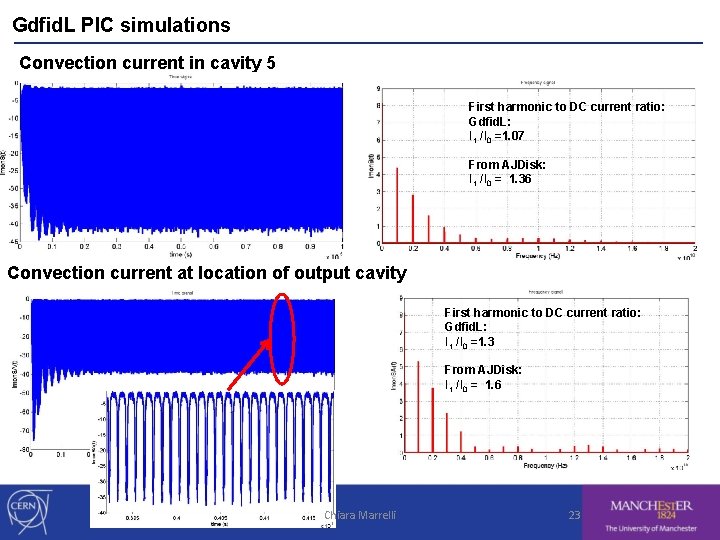
Gdfid. L PIC simulations Convection current in cavity 5 First harmonic to DC current ratio: Gdfid. L: I 1 /I 0 =1. 07 From AJDisk: I 1 /I 0 = 1. 36 Convection current at location of output cavity First harmonic to DC current ratio: Gdfid. L: I 1 /I 0 =1. 3 From AJDisk: I 1 /I 0 = 1. 6 Chiara Marrelli 23
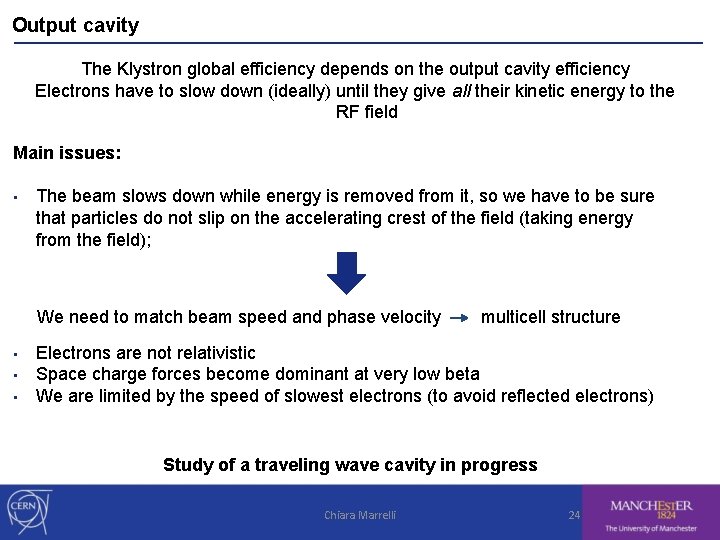
Output cavity The Klystron global efficiency depends on the output cavity efficiency Electrons have to slow down (ideally) until they give all their kinetic energy to the RF field Main issues: • The beam slows down while energy is removed from it, so we have to be sure that particles do not slip on the accelerating crest of the field (taking energy from the field); We need to match beam speed and phase velocity • • • multicell structure Electrons are not relativistic Space charge forces become dominant at very low beta We are limited by the speed of slowest electrons (to avoid reflected electrons) Study of a traveling wave cavity in progress Chiara Marrelli 24
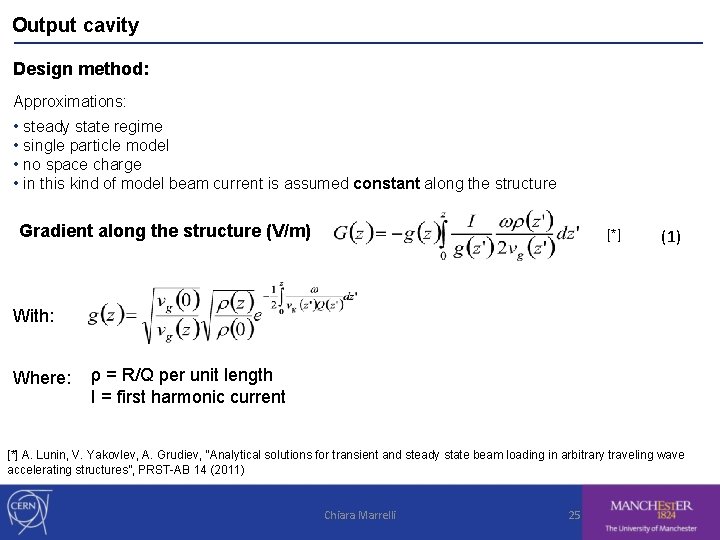
Output cavity Design method: Approximations: • steady state regime • single particle model • no space charge • in this kind of model beam current is assumed constant along the structure Gradient along the structure (V/m) [*] (1) With: Where: ρ = R/Q per unit length I = first harmonic current [*] A. Lunin, V. Yakovlev, A. Grudiev, “Analytical solutions for transient and steady state beam loading in arbitrary traveling wave accelerating structures”, PRST-AB 14 (2011) Chiara Marrelli 25
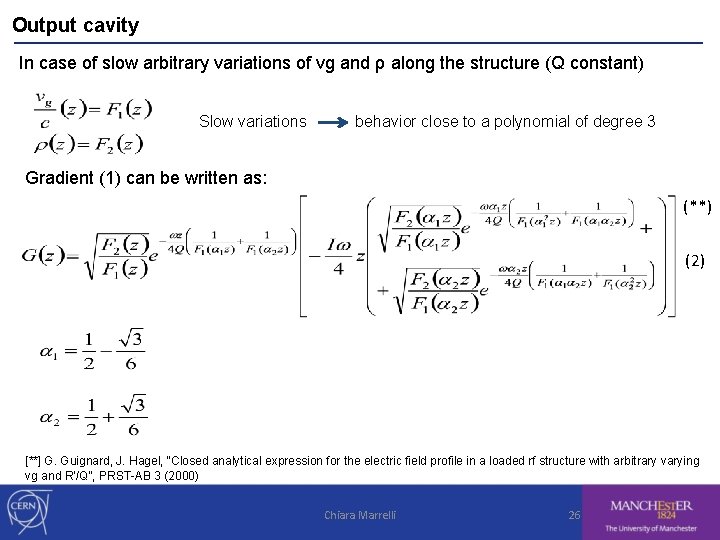
Output cavity In case of slow arbitrary variations of vg and ρ along the structure (Q constant) Slow variations behavior close to a polynomial of degree 3 Gradient (1) can be written as: (**) (2) [**] G. Guignard, J. Hagel, “Closed analytical expression for the electric field profile in a loaded rf structure with arbitrary varying vg and R’/Q”, PRST-AB 3 (2000) Chiara Marrelli 26
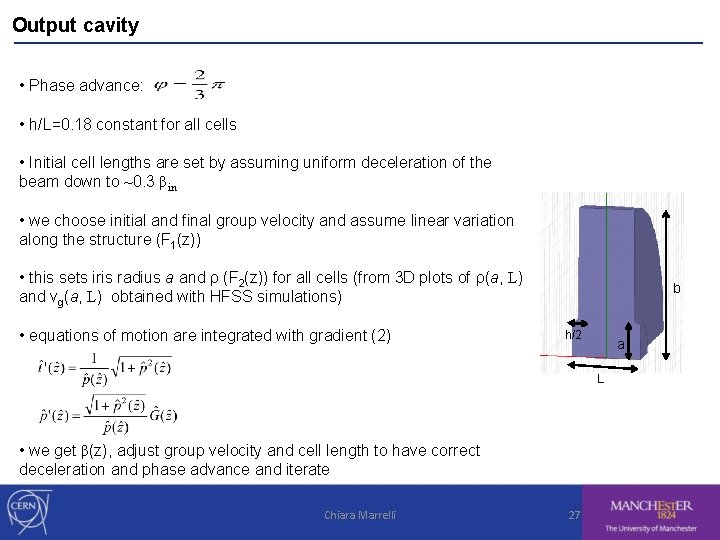
Output cavity • Phase advance: • h/L=0. 18 constant for all cells • Initial cell lengths are set by assuming uniform deceleration of the beam down to ~0. 3 βin • we choose initial and final group velocity and assume linear variation along the structure (F 1(z)) • this sets iris radius a and ρ (F 2(z)) for all cells (from 3 D plots of ρ(a, L) and vg(a, L) obtained with HFSS simulations) • equations of motion are integrated with gradient (2) b h/2 a L • we get β(z), adjust group velocity and cell length to have correct deceleration and phase advance and iterate Chiara Marrelli 27
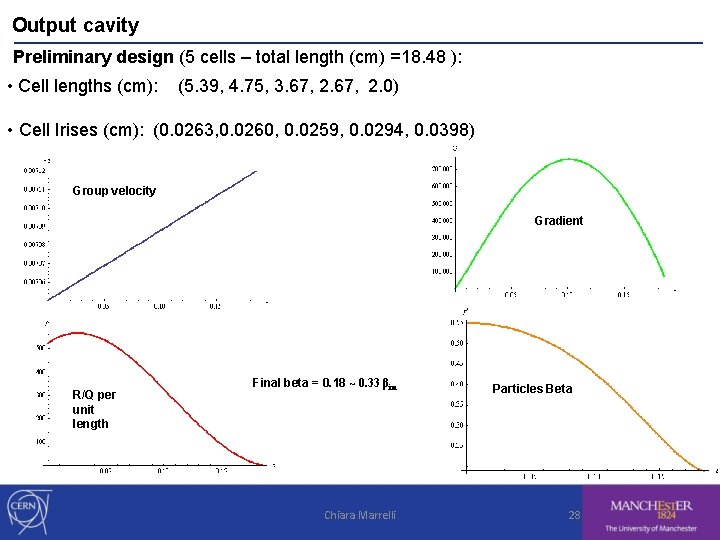
Output cavity Preliminary design (5 cells – total length (cm) =18. 48 ): • Cell lengths (cm): (5. 39, 4. 75, 3. 67, 2. 0) • Cell Irises (cm): (0. 0263, 0. 0260, 0. 0259, 0. 0294, 0. 0398) Group velocity Gradient R/Q per unit length Final beta = 0. 18 ~ 0. 33 βin Chiara Marrelli Particles Beta 28
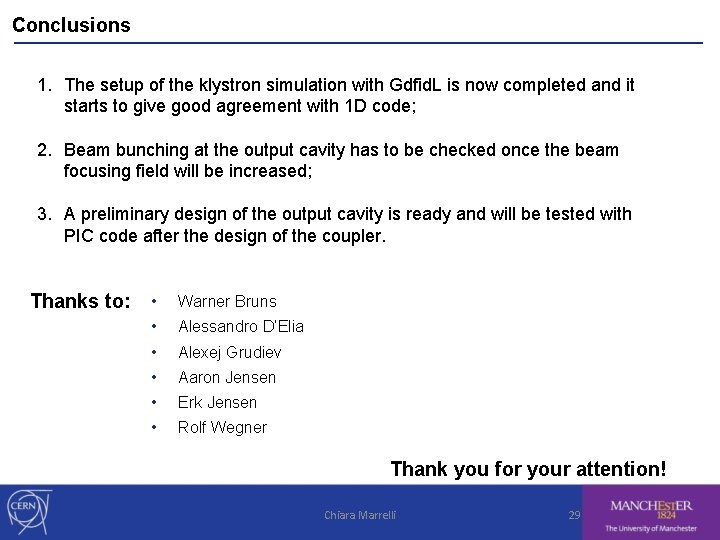
Conclusions 1. The setup of the klystron simulation with Gdfid. L is now completed and it starts to give good agreement with 1 D code; 2. Beam bunching at the output cavity has to be checked once the beam focusing field will be increased; 3. A preliminary design of the output cavity is ready and will be tested with PIC code after the design of the coupler. Thanks to: • Warner Bruns • Alessandro D’Elia • Alexej Grudiev • Aaron Jensen • Erk Jensen • Rolf Wegner Thank you for your attention! Chiara Marrelli 29
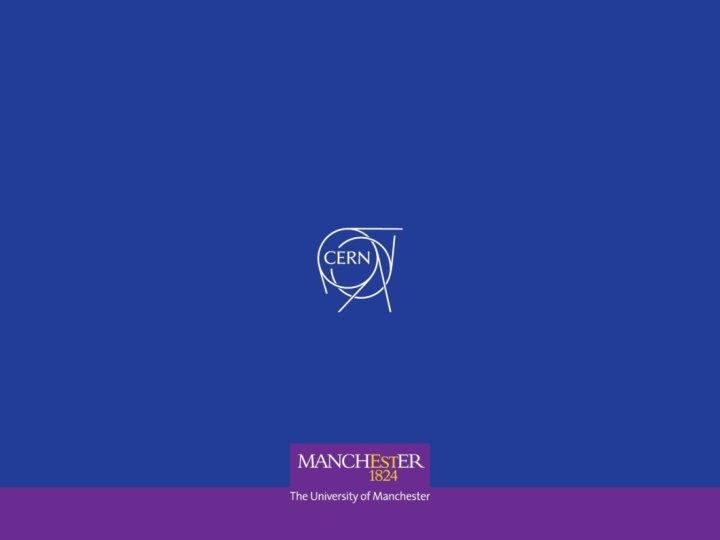
Lirik lagu more more more we praise you
More more more i want more more more more we praise you
Magnet marrelli
Allocative efficiency
C b a d
Allocative efficiency vs productive efficiency
Productively efficient vs allocatively efficient
Allocative efficiency vs productive efficiency
Shapes.io levels
Juliette plouin
Gchf.pk
Klystron design
Flatining
Klystron thales
Thales klystron
Thales klystron
Klystron power amplifier
Klystron price
Tiara virus
Halal tv channel
Lamp list example
Aspire not to
More choices more chances
When dishes remain on a table when you yank
Human history becomes more and more a race
The more you take the more you leave behind
Knowing more remembering more
The more you study the more you learn
More love to thee o lord
Chiara speranza
Chiara capretti