The Earth I The Mantle Lecture 41 Structure
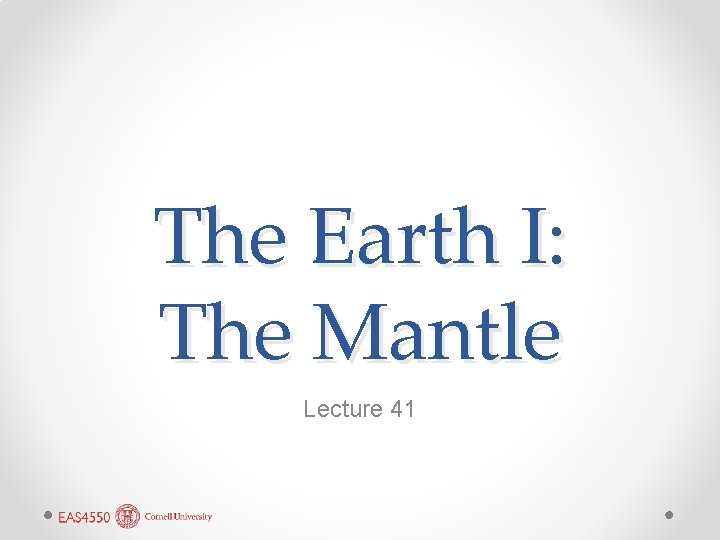
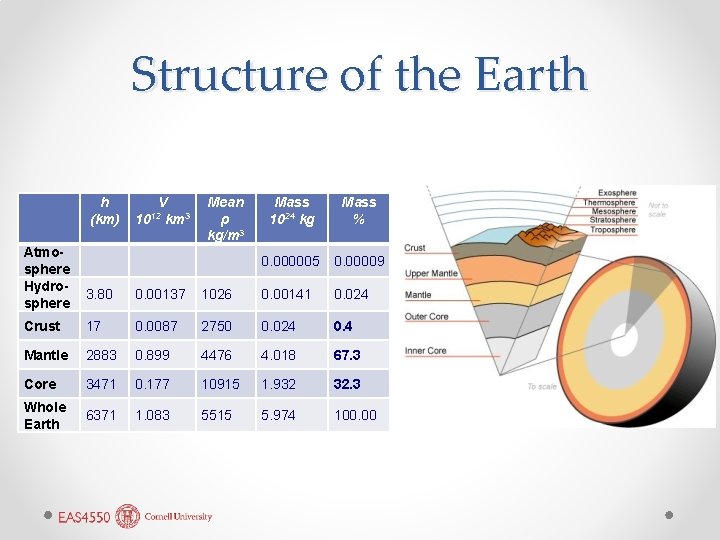
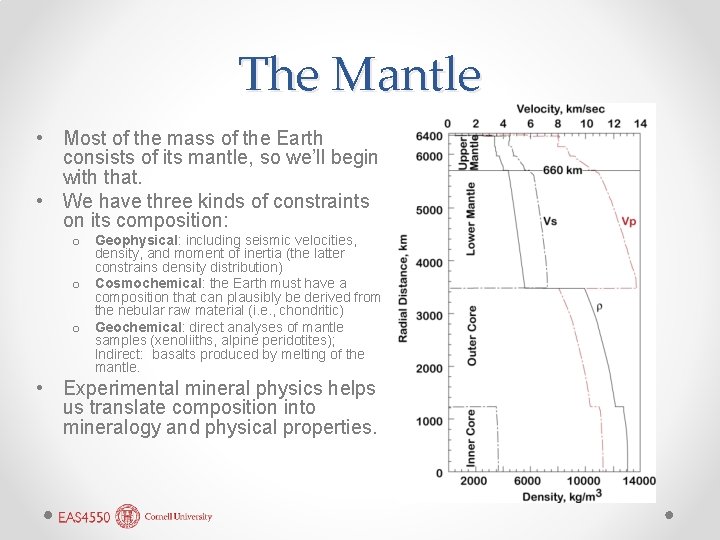
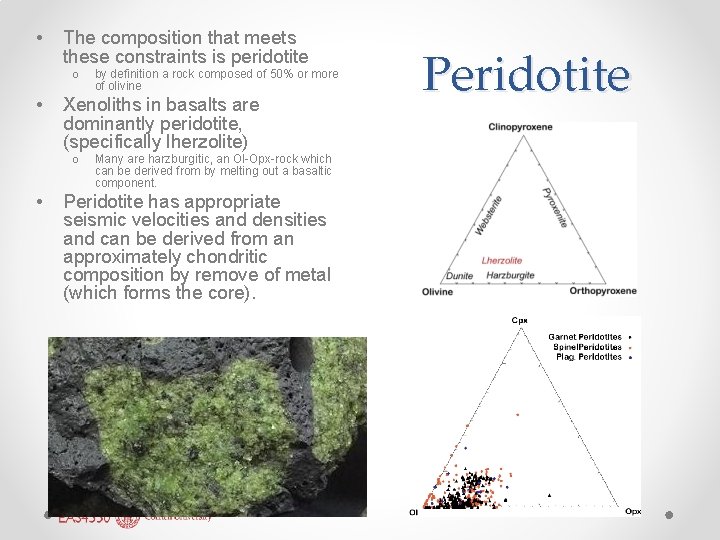
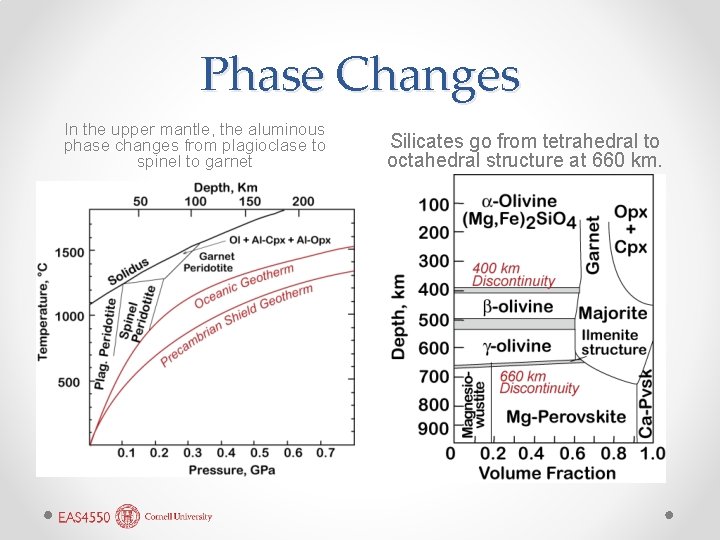
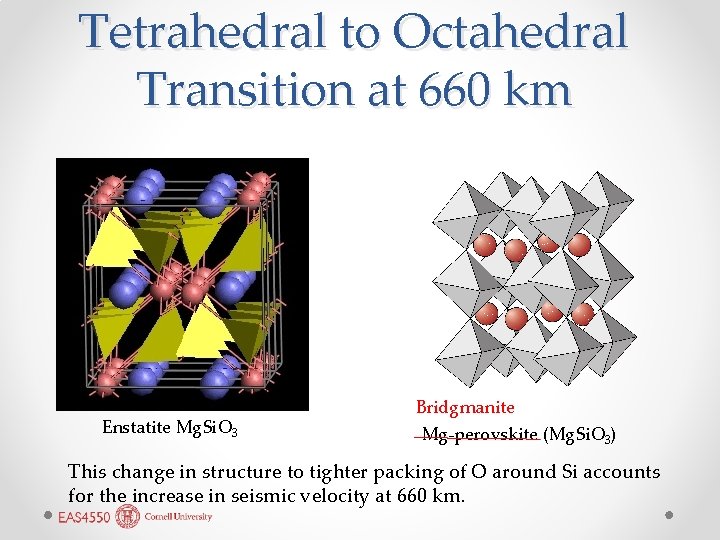
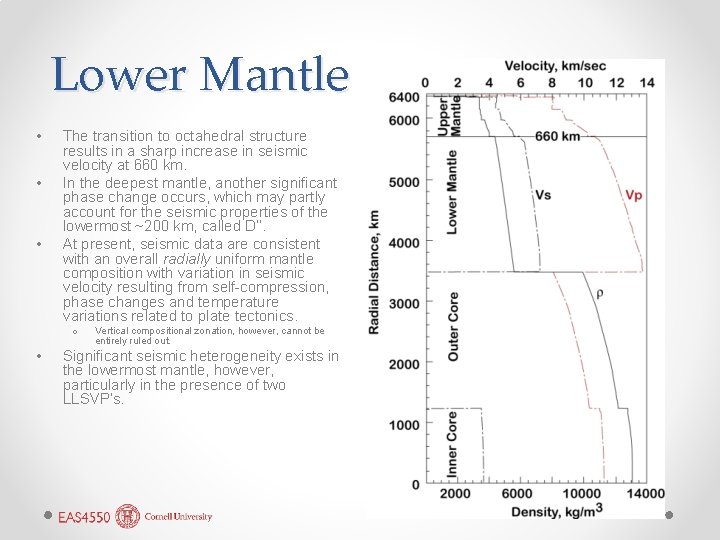
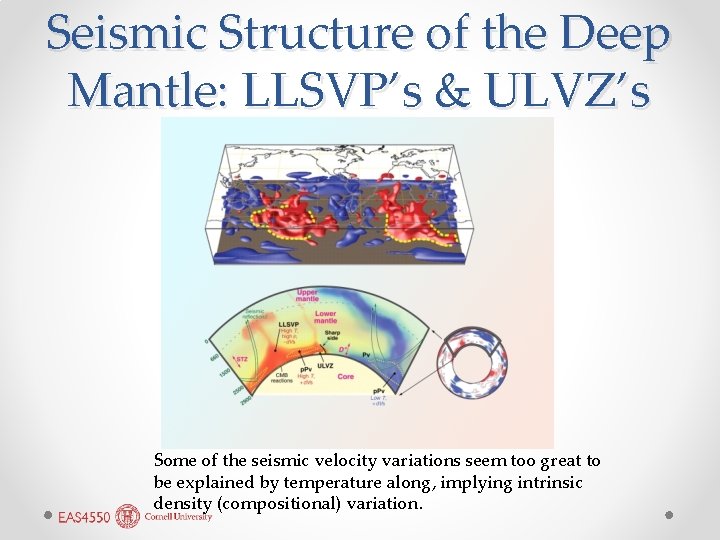
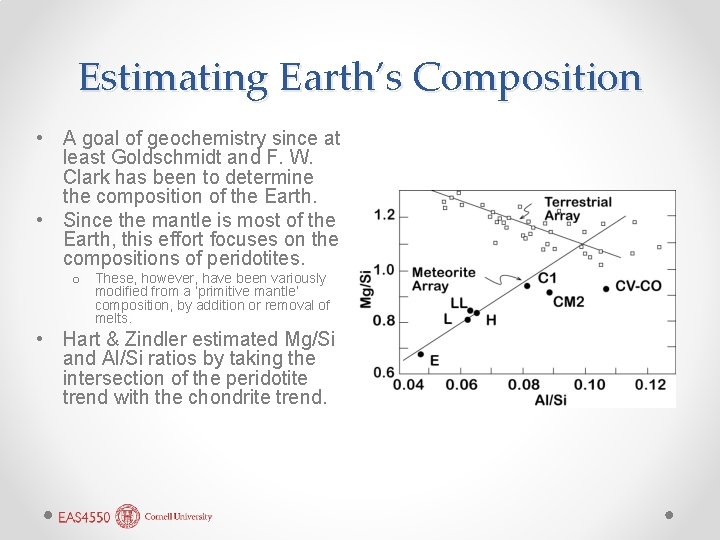
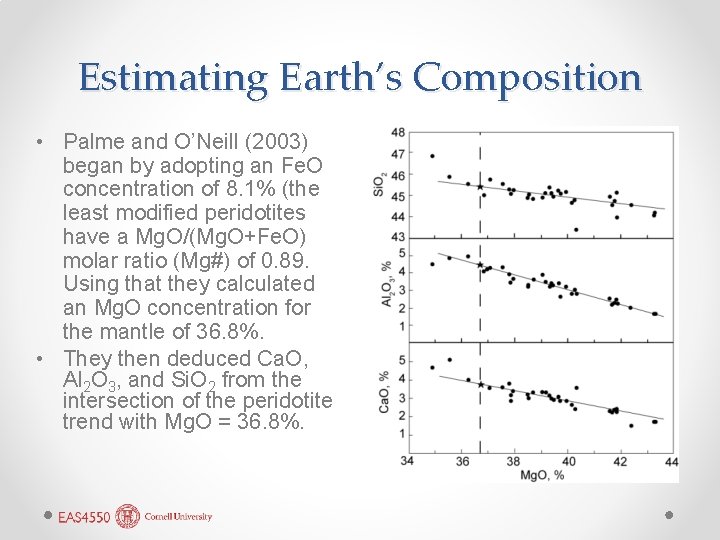
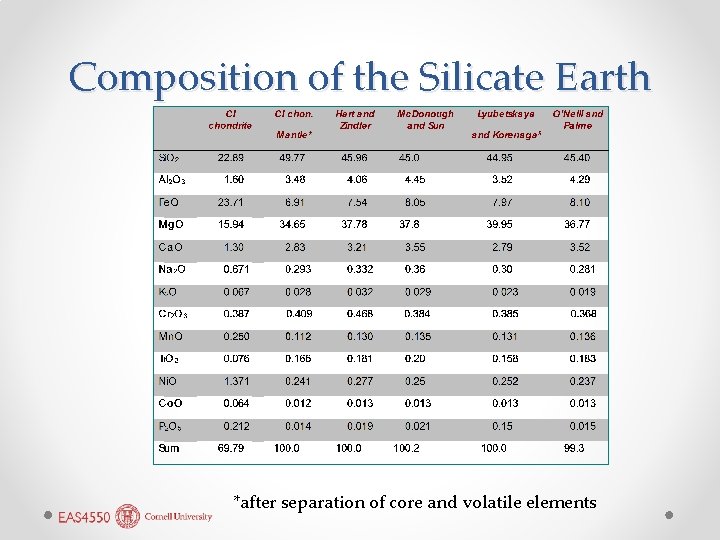
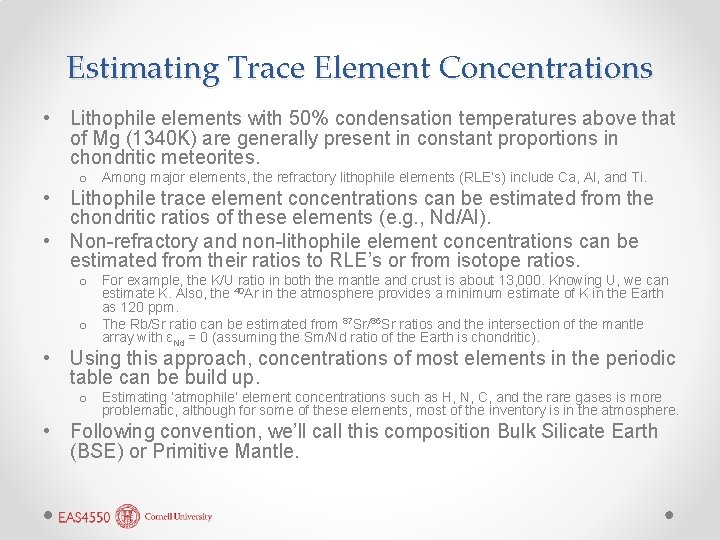
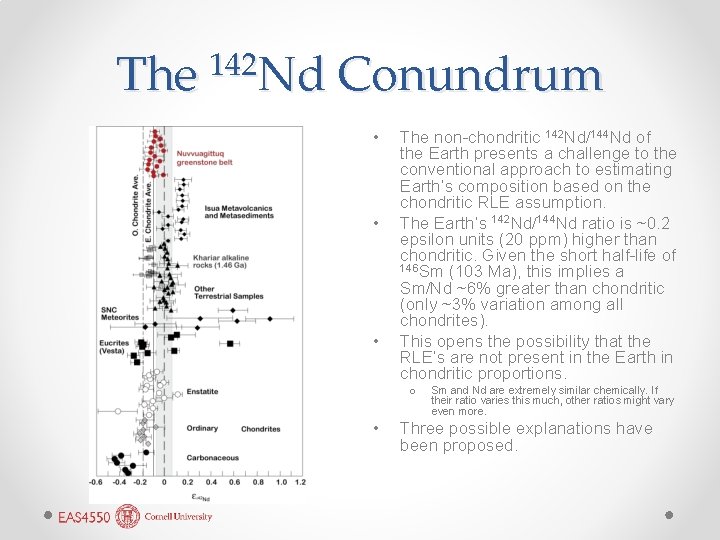
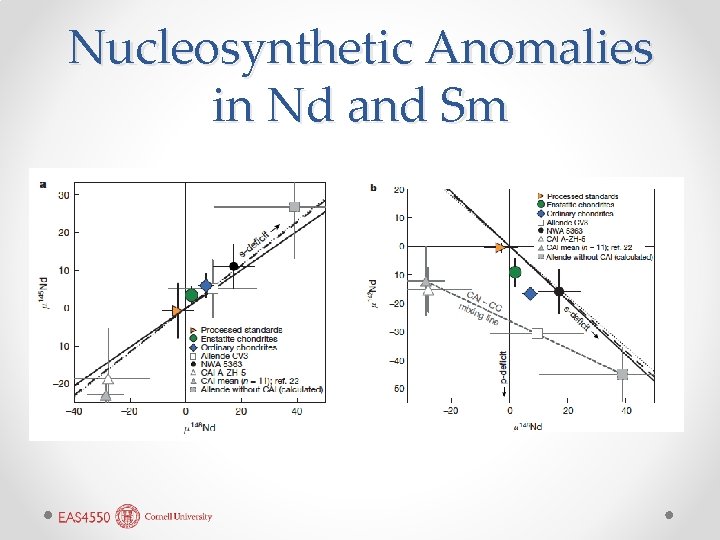
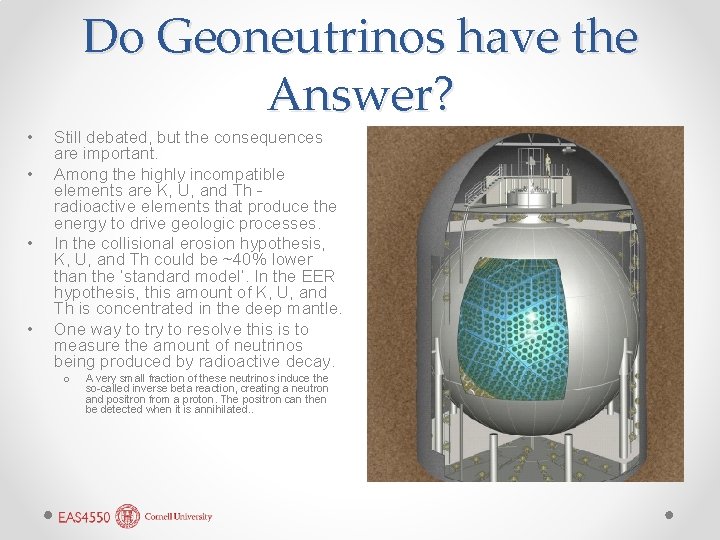
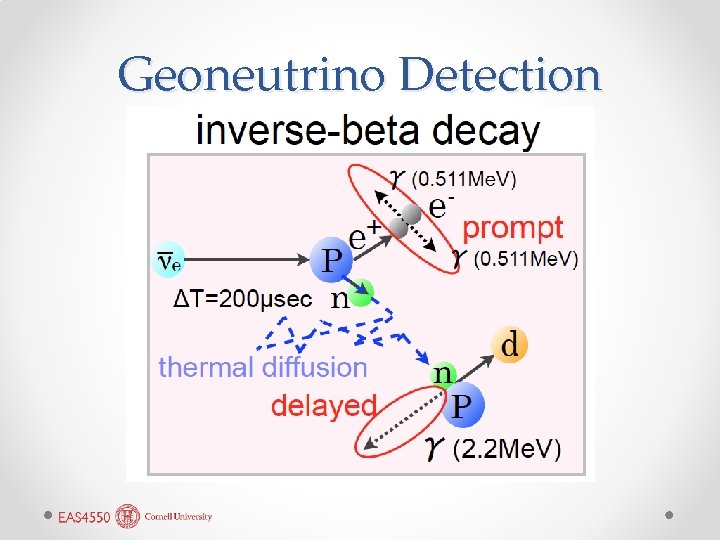
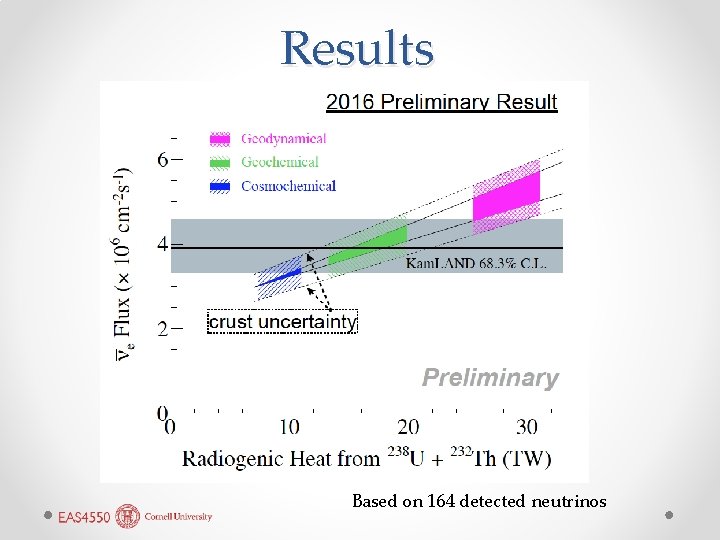
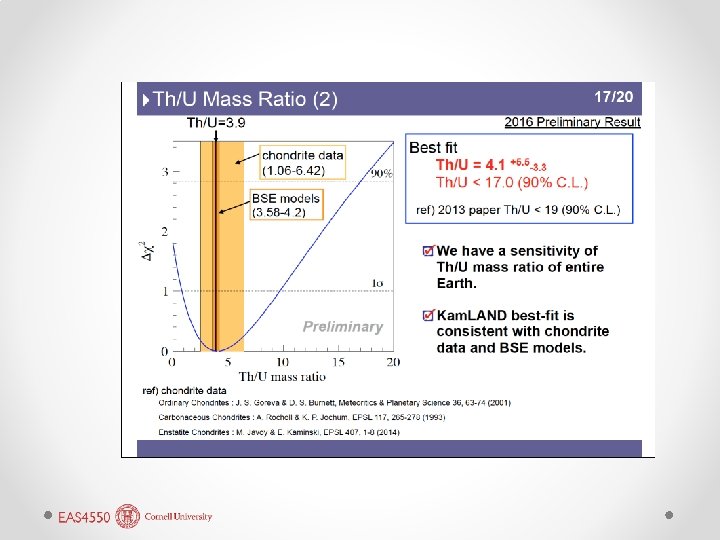
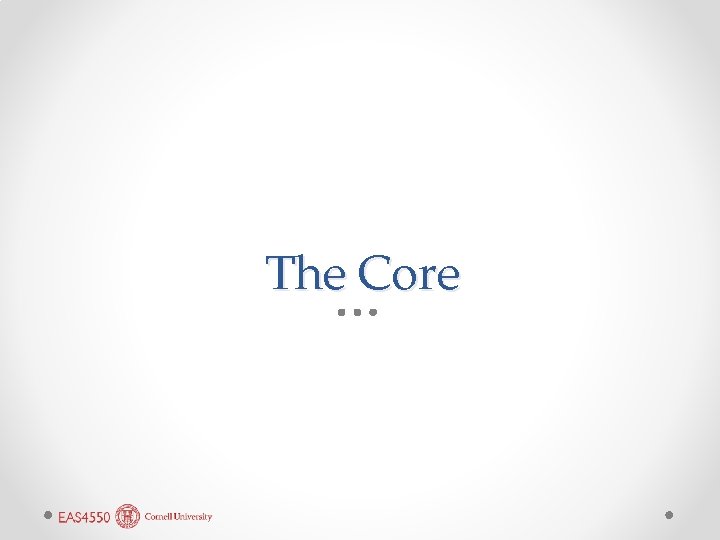
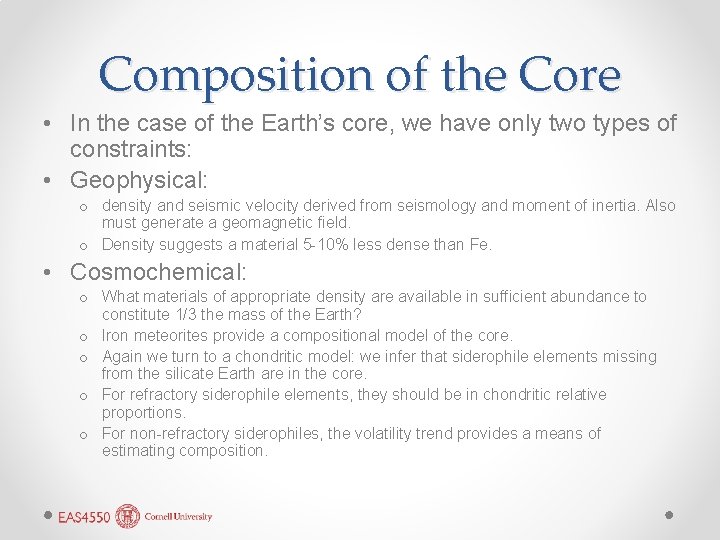
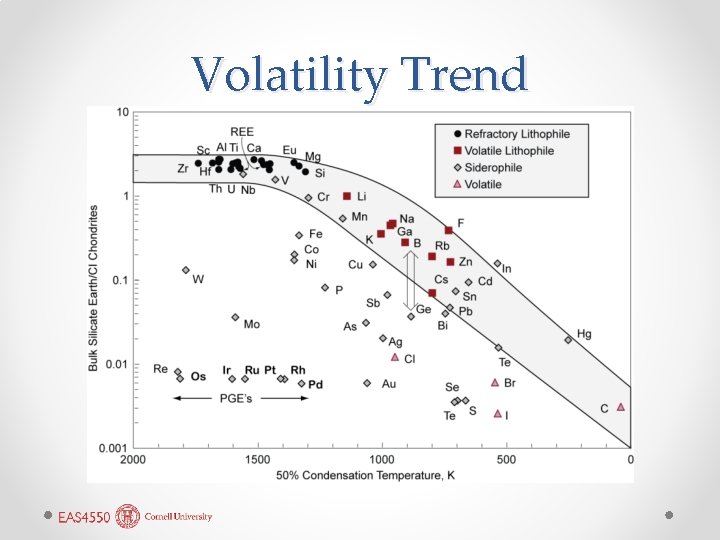
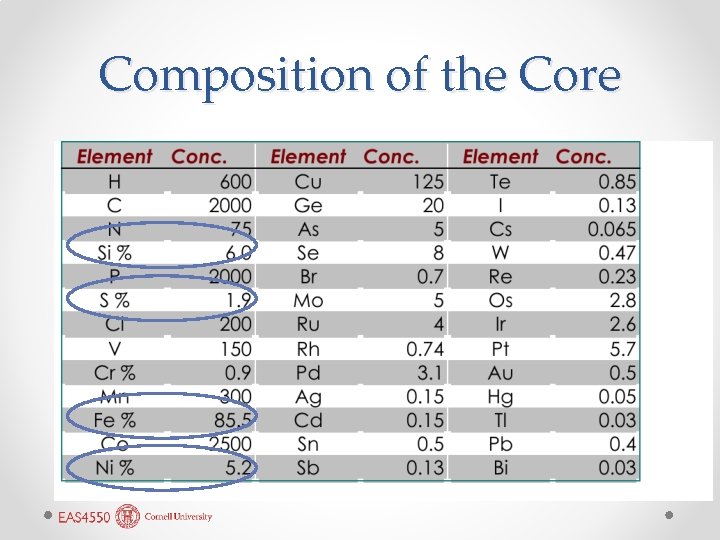
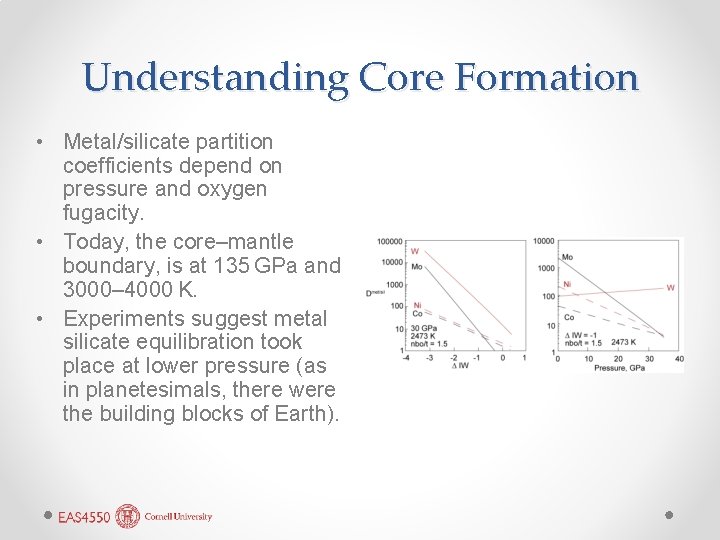
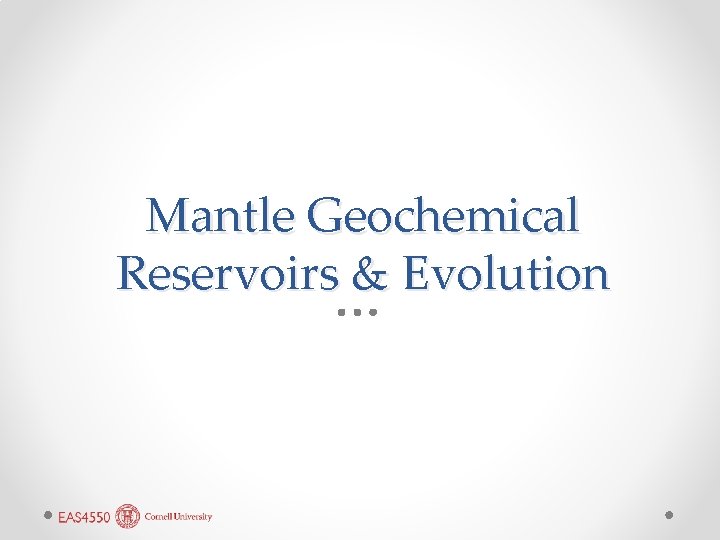
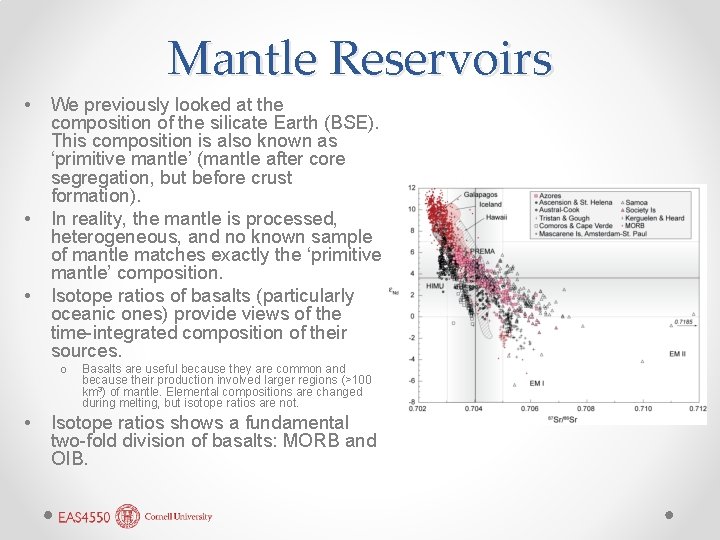
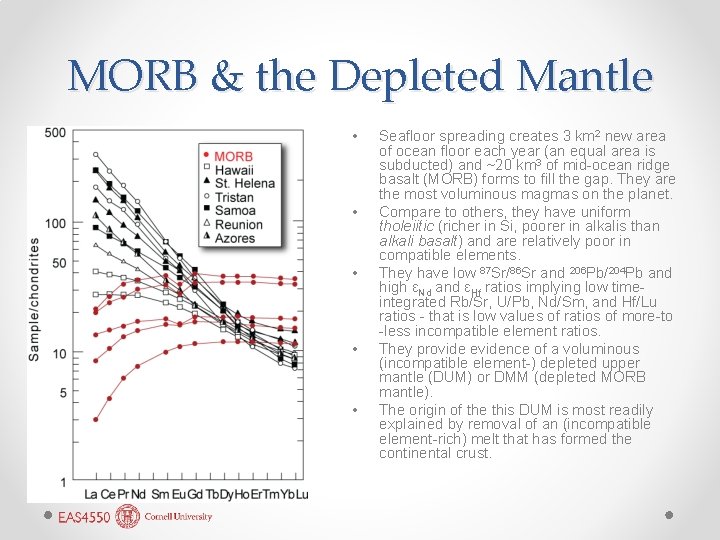
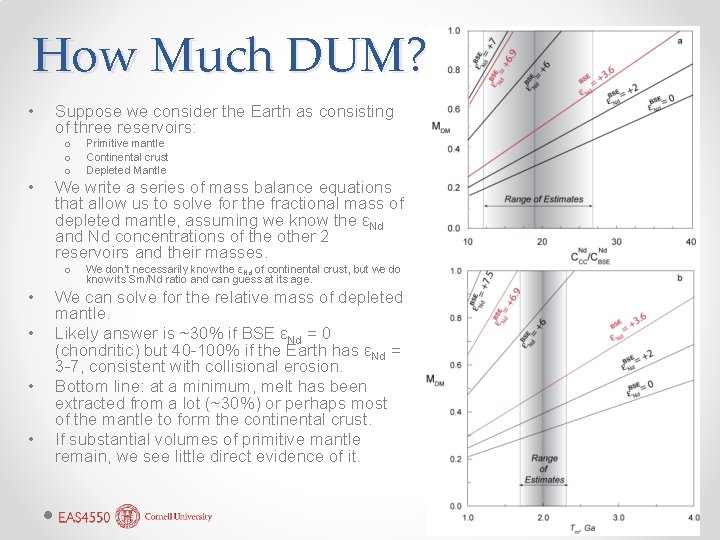
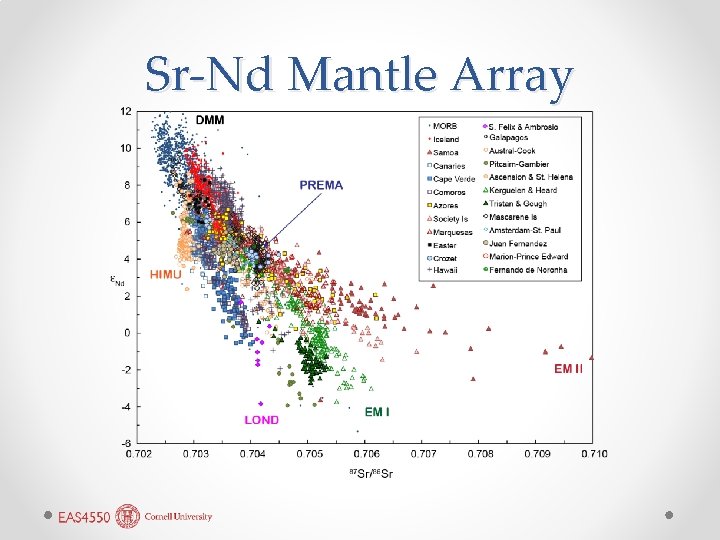
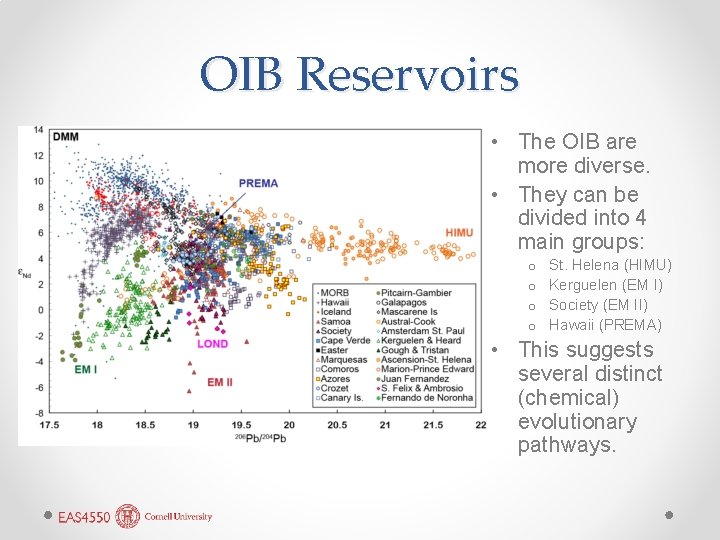
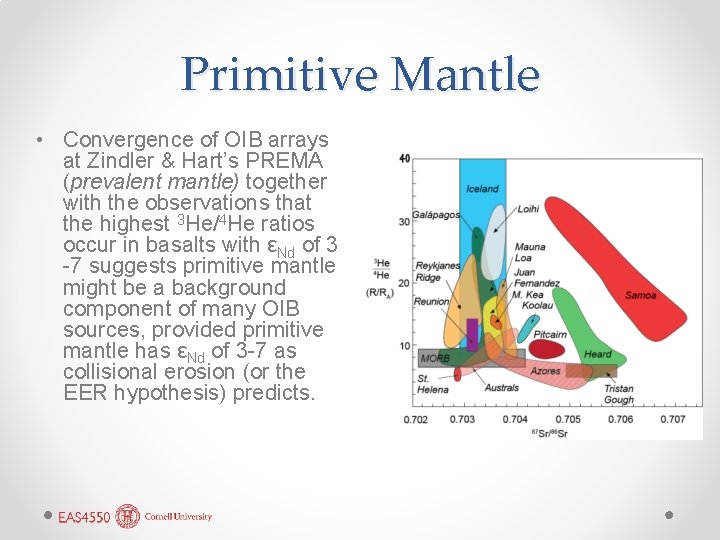
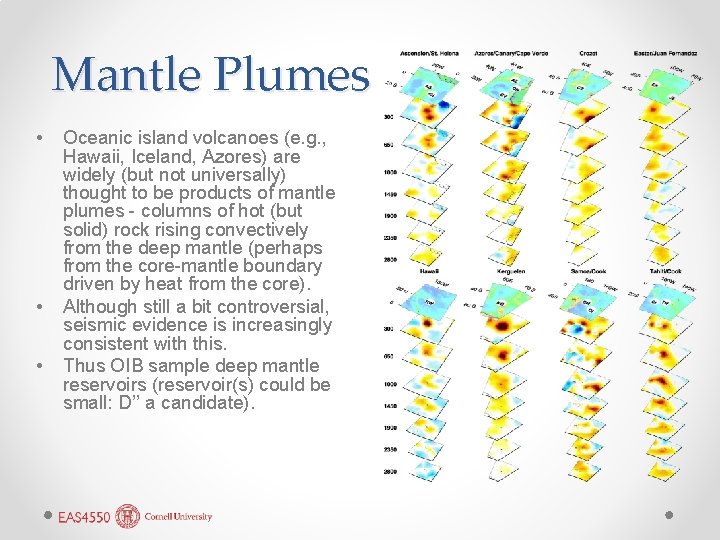
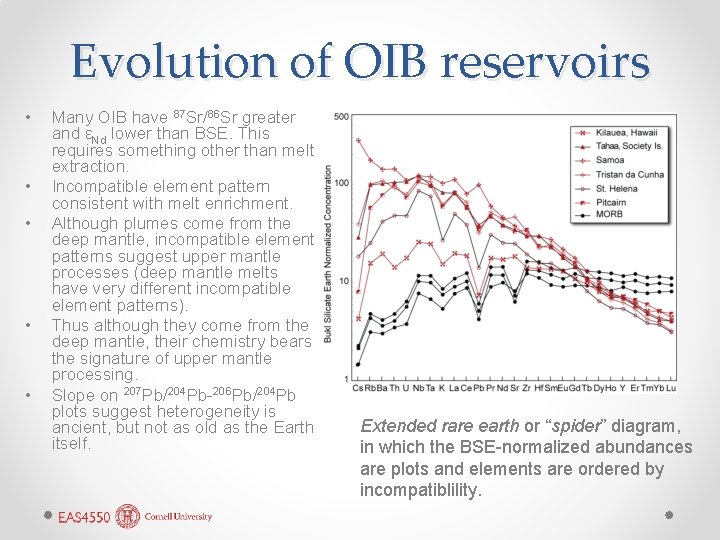
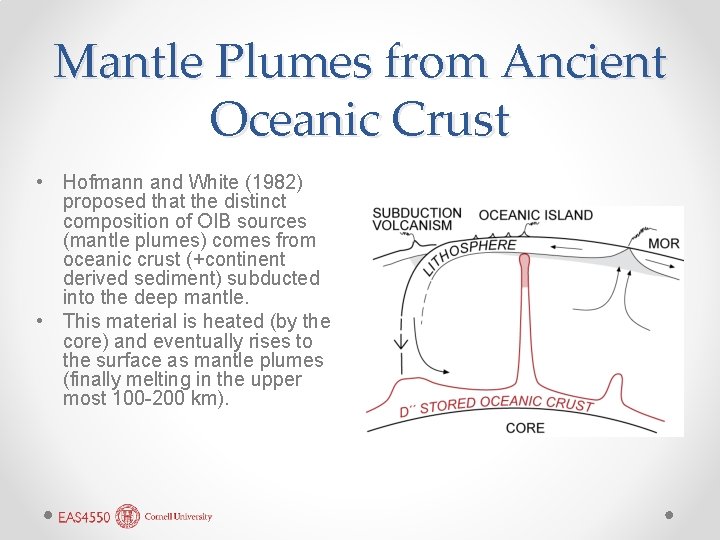
- Slides: 33
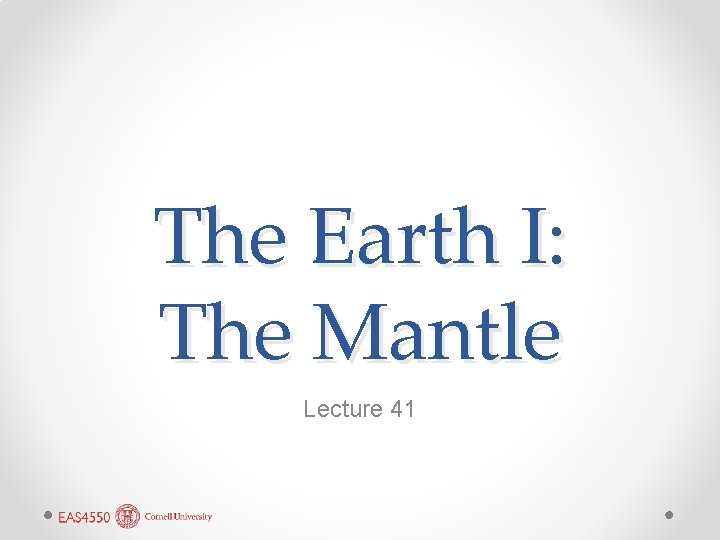
The Earth I: The Mantle Lecture 41
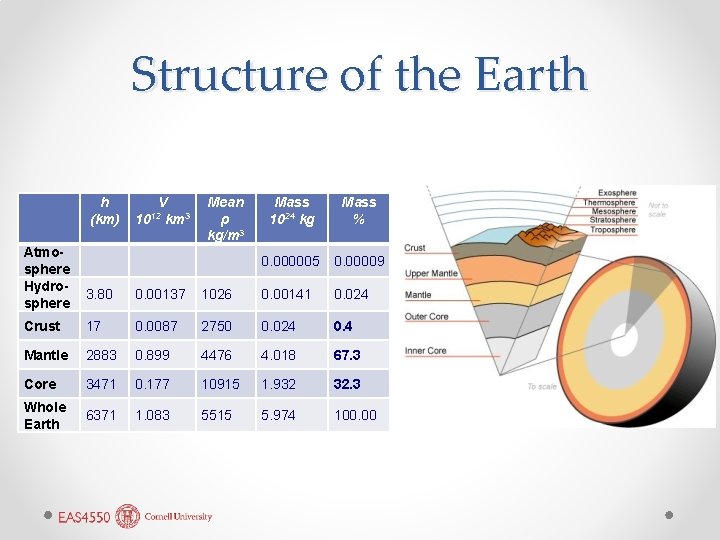
Structure of the Earth h (km) Atmosphere Hydrosphere V km 3 1012 Mean ρ kg/m 3 Mass 1024 kg Mass % 0. 000005 0. 00009 3. 80 0. 00137 1026 0. 00141 0. 024 Crust 17 0. 0087 2750 0. 024 0. 4 Mantle 2883 0. 899 4476 4. 018 67. 3 Core 3471 0. 177 10915 1. 932 32. 3 Whole Earth 6371 1. 083 5515 5. 974 100. 00
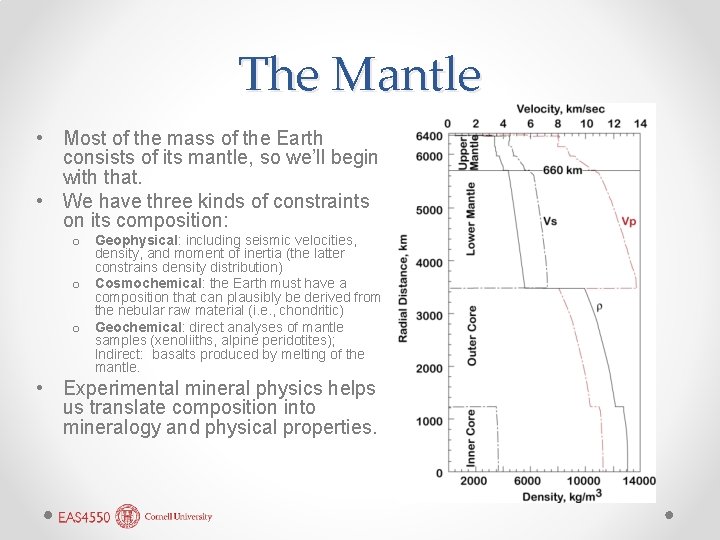
The Mantle • Most of the mass of the Earth consists of its mantle, so we’ll begin with that. • We have three kinds of constraints on its composition: o Geophysical: including seismic velocities, density, and moment of inertia (the latter constrains density distribution) o Cosmochemical: the Earth must have a composition that can plausibly be derived from the nebular raw material (i. e. , chondritic) o Geochemical: direct analyses of mantle samples (xenoliiths, alpine peridotites); Indirect: basalts produced by melting of the mantle. • Experimental mineral physics helps us translate composition into mineralogy and physical properties.
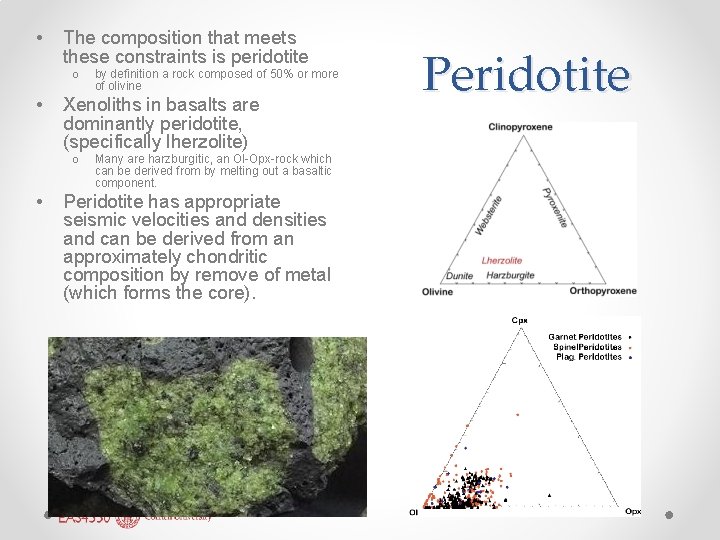
• The composition that meets these constraints is peridotite o • Xenoliths in basalts are dominantly peridotite, (specifically lherzolite) o • by definition a rock composed of 50% or more of olivine Many are harzburgitic, an Ol-Opx-rock which can be derived from by melting out a basaltic component. Peridotite has appropriate seismic velocities and densities and can be derived from an approximately chondritic composition by remove of metal (which forms the core). Peridotite
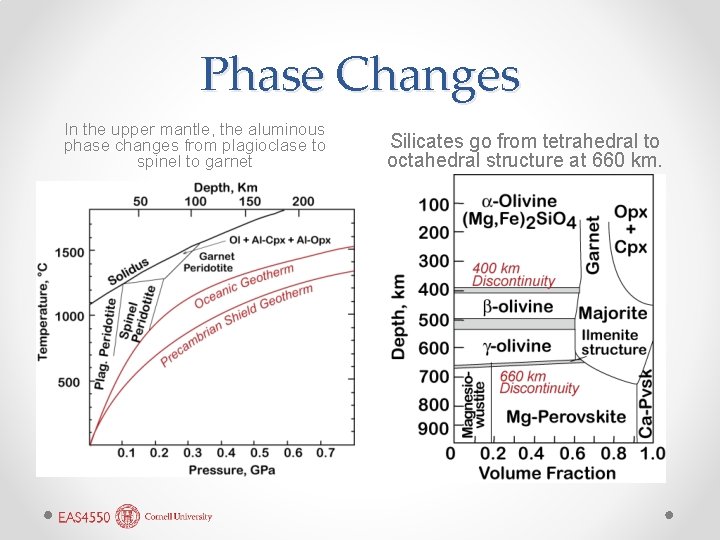
Phase Changes In the upper mantle, the aluminous phase changes from plagioclase to spinel to garnet Silicates go from tetrahedral to octahedral structure at 660 km.
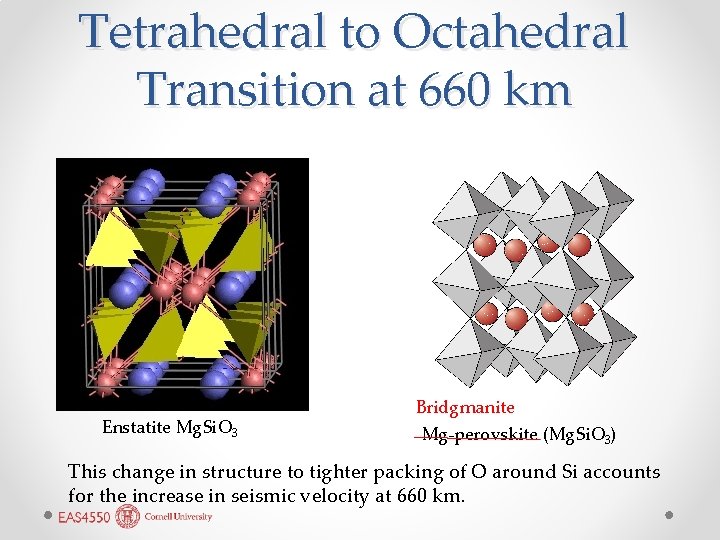
Tetrahedral to Octahedral Transition at 660 km Enstatite Mg. Si. O 3 Bridgmanite Mg-perovskite (Mg. Si. O 3) This change in structure to tighter packing of O around Si accounts for the increase in seismic velocity at 660 km.
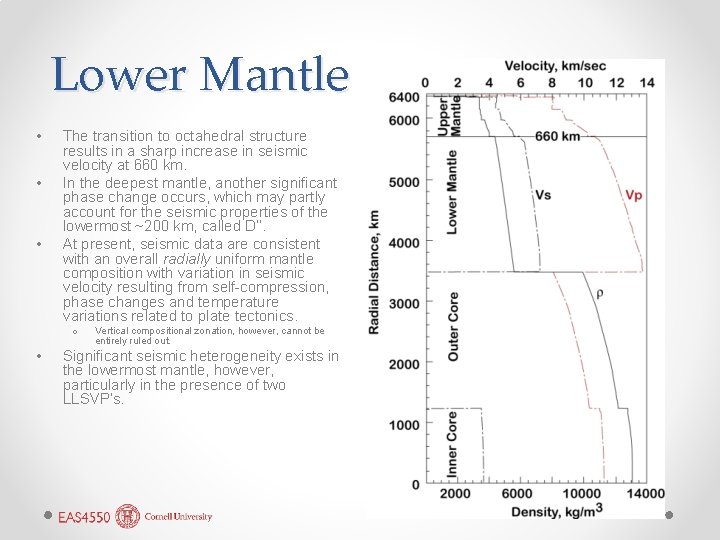
Lower Mantle • • • The transition to octahedral structure results in a sharp increase in seismic velocity at 660 km. In the deepest mantle, another significant phase change occurs, which may partly account for the seismic properties of the lowermost ~200 km, called D’’. At present, seismic data are consistent with an overall radially uniform mantle composition with variation in seismic velocity resulting from self-compression, phase changes and temperature variations related to plate tectonics. o • Vertical compositional zonation, however, cannot be entirely ruled out. Significant seismic heterogeneity exists in the lowermost mantle, however, particularly in the presence of two LLSVP’s. In the lower mantle, the dominant mineral is bridgmanite: Mg. Si. O 3 with the structure of Ca. Ti. O 3. Next most abundant mineral is Mg, Fe. O (magnesiowüstite or ferripericlase)
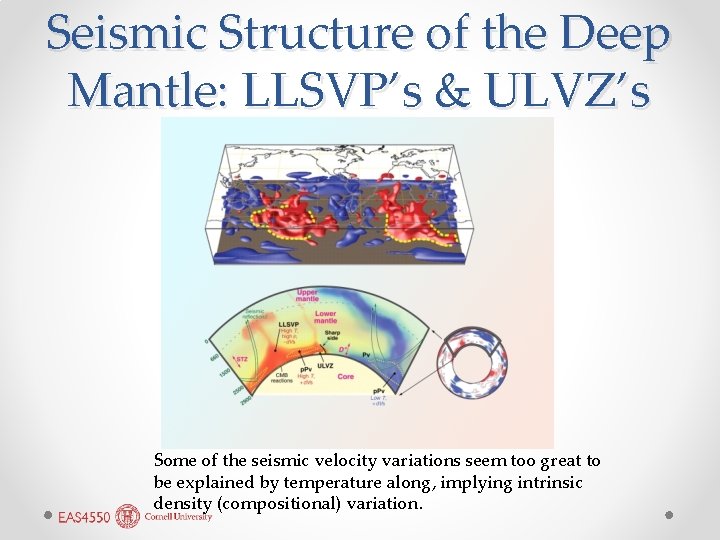
Seismic Structure of the Deep Mantle: LLSVP’s & ULVZ’s Some of the seismic velocity variations seem too great to be explained by temperature along, implying intrinsic density (compositional) variation.
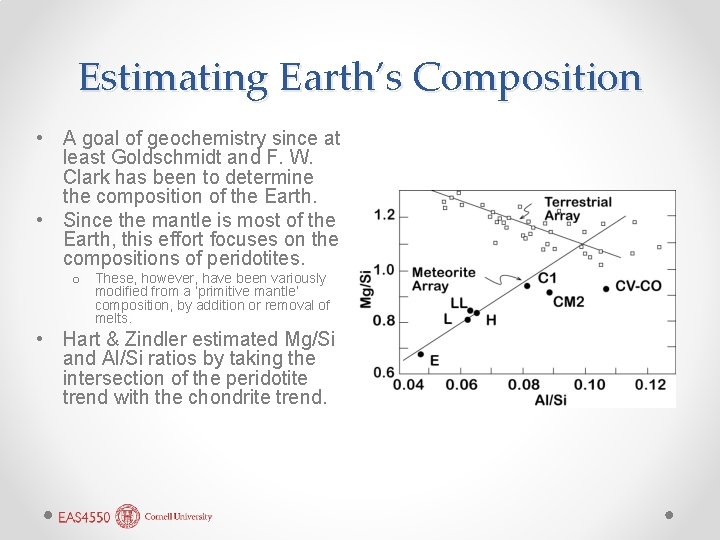
Estimating Earth’s Composition • A goal of geochemistry since at least Goldschmidt and F. W. Clark has been to determine the composition of the Earth. • Since the mantle is most of the Earth, this effort focuses on the compositions of peridotites. o These, however, have been variously modified from a ‘primitive mantle’ composition, by addition or removal of melts. • Hart & Zindler estimated Mg/Si and Al/Si ratios by taking the intersection of the peridotite trend with the chondrite trend.
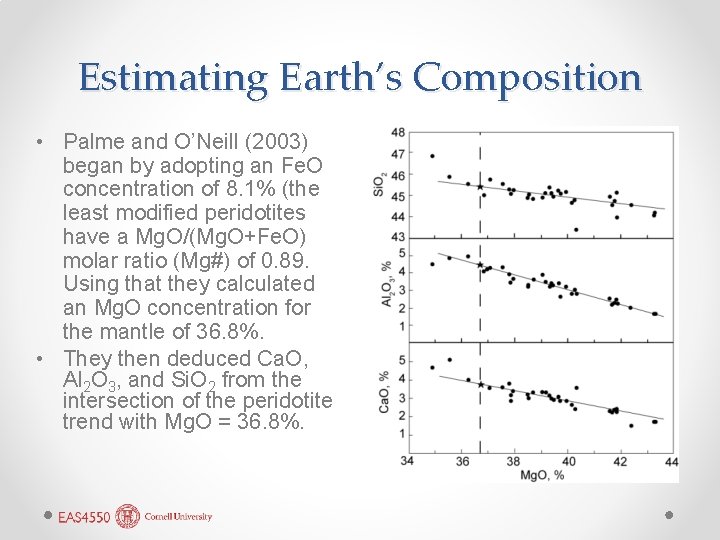
Estimating Earth’s Composition • Palme and O’Neill (2003) began by adopting an Fe. O concentration of 8. 1% (the least modified peridotites have a Mg. O/(Mg. O+Fe. O) molar ratio (Mg#) of 0. 89. Using that they calculated an Mg. O concentration for the mantle of 36. 8%. • They then deduced Ca. O, Al 2 O 3, and Si. O 2 from the intersection of the peridotite trend with Mg. O = 36. 8%.
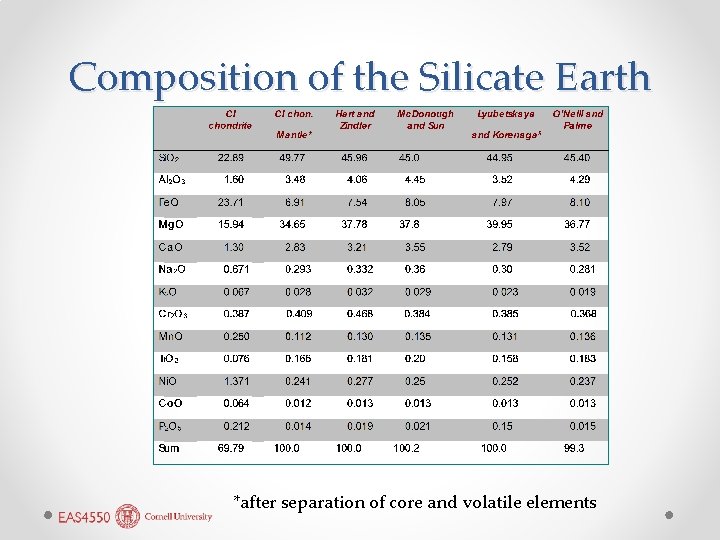
Composition of the Silicate Earth *after separation of core and volatile elements
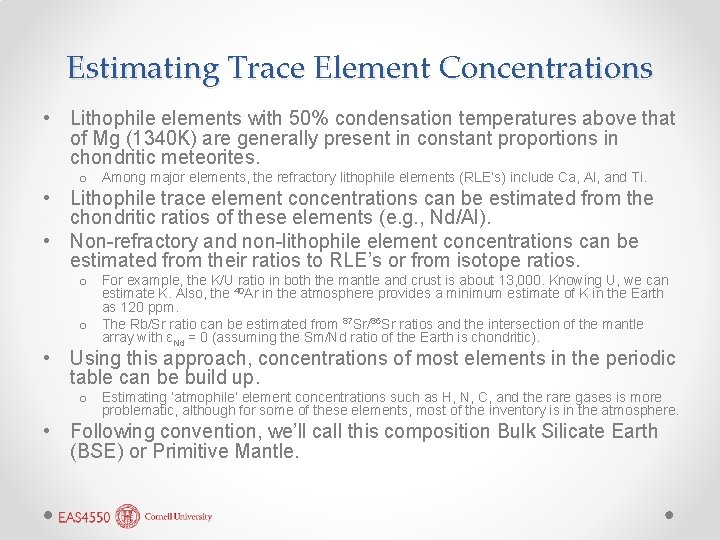
Estimating Trace Element Concentrations • Lithophile elements with 50% condensation temperatures above that of Mg (1340 K) are generally present in constant proportions in chondritic meteorites. o Among major elements, the refractory lithophile elements (RLE’s) include Ca, Al, and Ti. • Lithophile trace element concentrations can be estimated from the chondritic ratios of these elements (e. g. , Nd/Al). • Non-refractory and non-lithophile element concentrations can be estimated from their ratios to RLE’s or from isotope ratios. o For example, the K/U ratio in both the mantle and crust is about 13, 000. Knowing U, we can estimate K. Also, the 40 Ar in the atmosphere provides a minimum estimate of K in the Earth as 120 ppm. o The Rb/Sr ratio can be estimated from 87 Sr/86 Sr ratios and the intersection of the mantle array with εNd = 0 (assuming the Sm/Nd ratio of the Earth is chondritic). • Using this approach, concentrations of most elements in the periodic table can be build up. o Estimating ‘atmophile’ element concentrations such as H, N, C, and the rare gases is more problematic, although for some of these elements, most of the inventory is in the atmosphere. • Following convention, we’ll call this composition Bulk Silicate Earth (BSE) or Primitive Mantle.
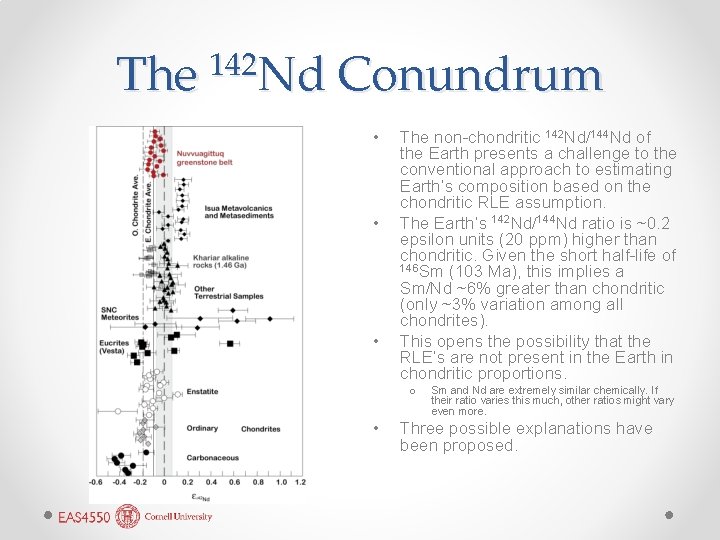
The 142 Nd Conundrum • • • The non-chondritic 142 Nd/144 Nd of the Earth presents a challenge to the conventional approach to estimating Earth’s composition based on the chondritic RLE assumption. The Earth’s 142 Nd/144 Nd ratio is ~0. 2 epsilon units (20 ppm) higher than chondritic. Given the short half-life of 146 Sm (103 Ma), this implies a Sm/Nd ~6% greater than chondritic (only ~3% variation among all chondrites). This opens the possibility that the RLE’s are not present in the Earth in chondritic proportions. o • Sm and Nd are extremely similar chemically. If their ratio varies this much, other ratios might vary even more. Three possible explanations have been proposed.
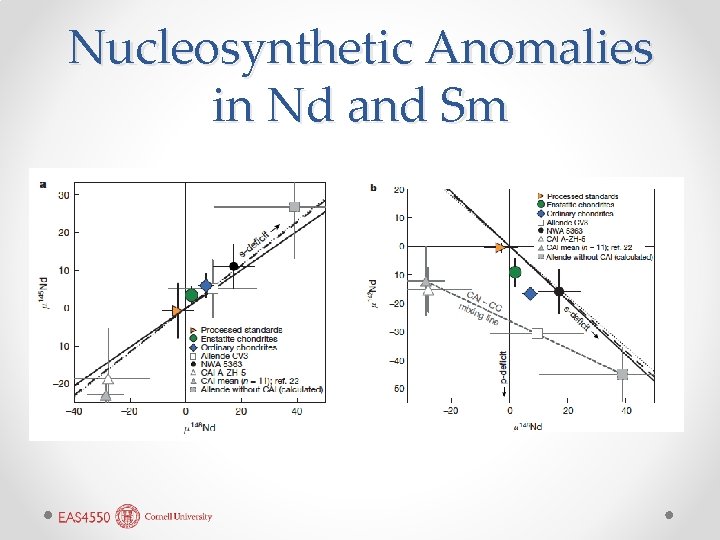
Nucleosynthetic Anomalies in Nd and Sm
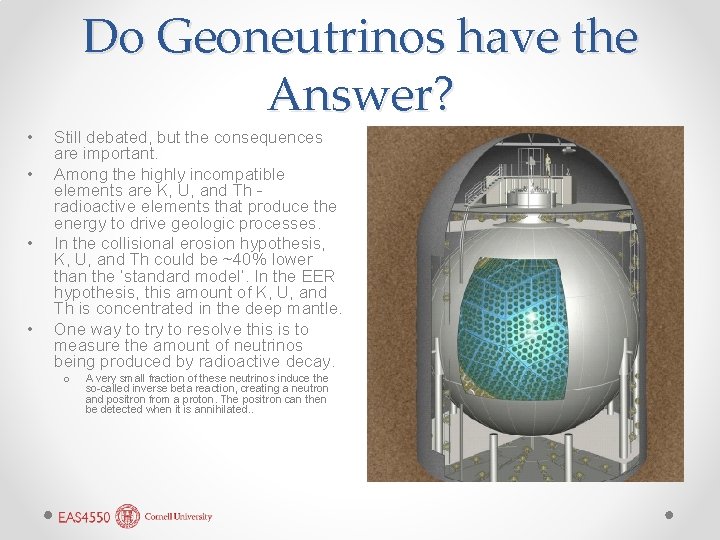
Do Geoneutrinos have the Answer? • • Still debated, but the consequences are important. Among the highly incompatible elements are K, U, and Th radioactive elements that produce the energy to drive geologic processes. In the collisional erosion hypothesis, K, U, and Th could be ~40% lower than the ‘standard model’. In the EER hypothesis, this amount of K, U, and Th is concentrated in the deep mantle. One way to try to resolve this is to measure the amount of neutrinos being produced by radioactive decay. o A very small fraction of these neutrinos induce the so-called inverse beta reaction, creating a neutron and positron from a proton. The positron can then be detected when it is annihilated. .
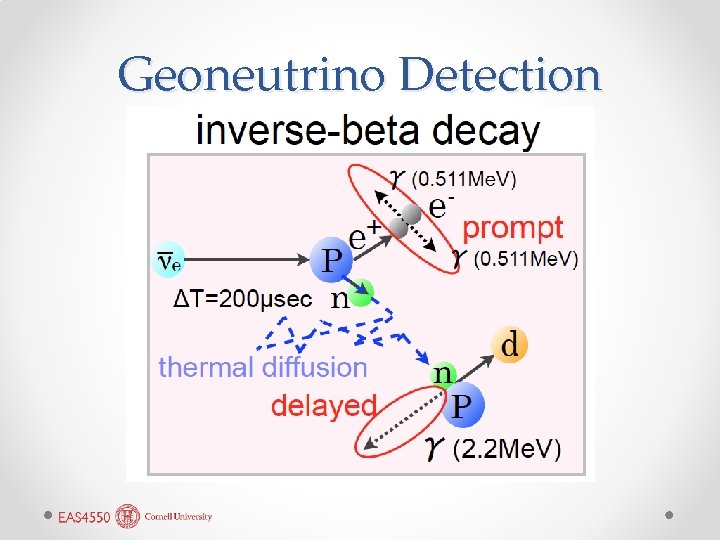
Geoneutrino Detection
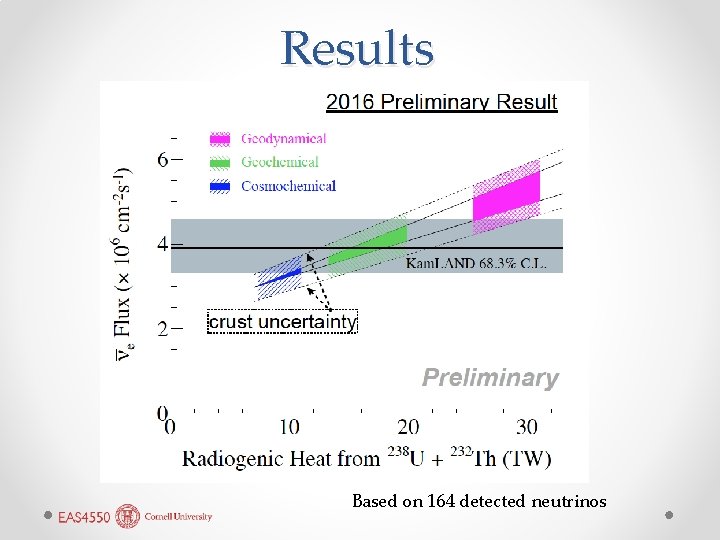
Results Based on 164 detected neutrinos
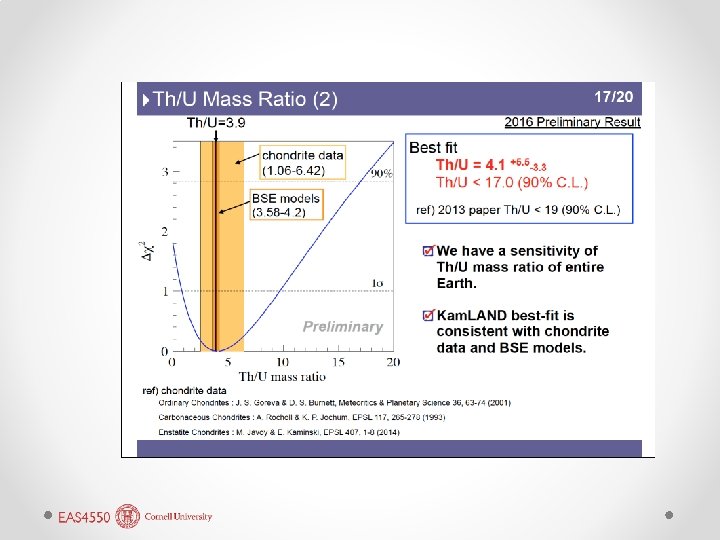
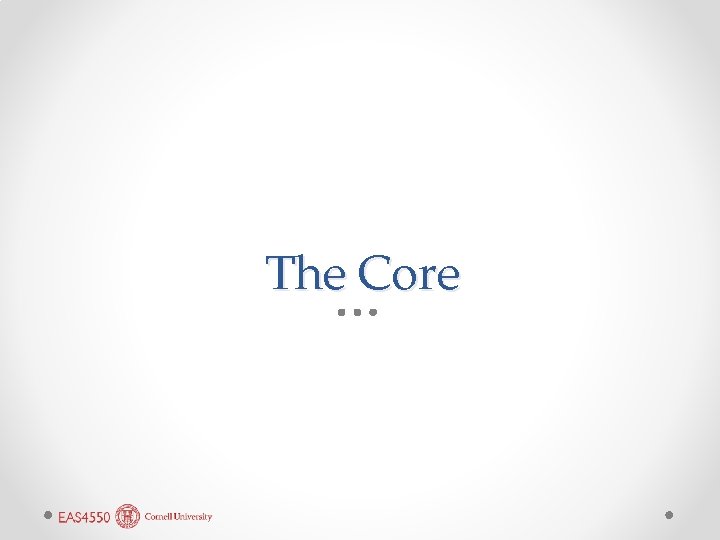
The Core
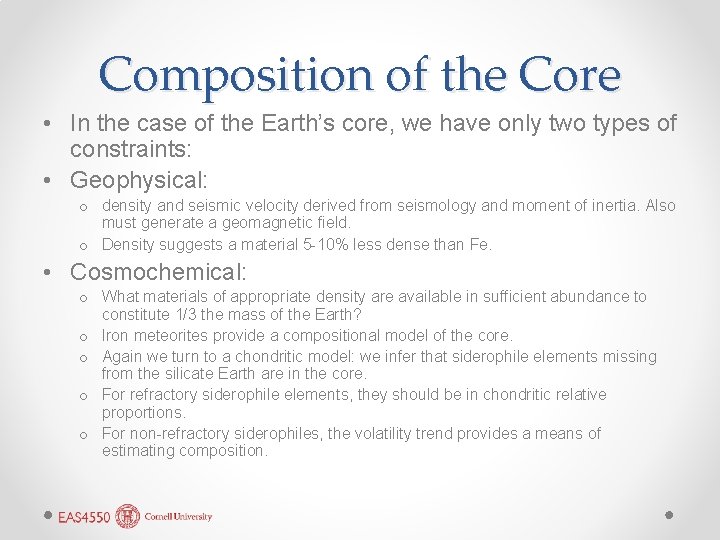
Composition of the Core • In the case of the Earth’s core, we have only two types of constraints: • Geophysical: o density and seismic velocity derived from seismology and moment of inertia. Also must generate a geomagnetic field. o Density suggests a material 5 -10% less dense than Fe. • Cosmochemical: o What materials of appropriate density are available in sufficient abundance to constitute 1/3 the mass of the Earth? o Iron meteorites provide a compositional model of the core. o Again we turn to a chondritic model: we infer that siderophile elements missing from the silicate Earth are in the core. o For refractory siderophile elements, they should be in chondritic relative proportions. o For non-refractory siderophiles, the volatility trend provides a means of estimating composition.
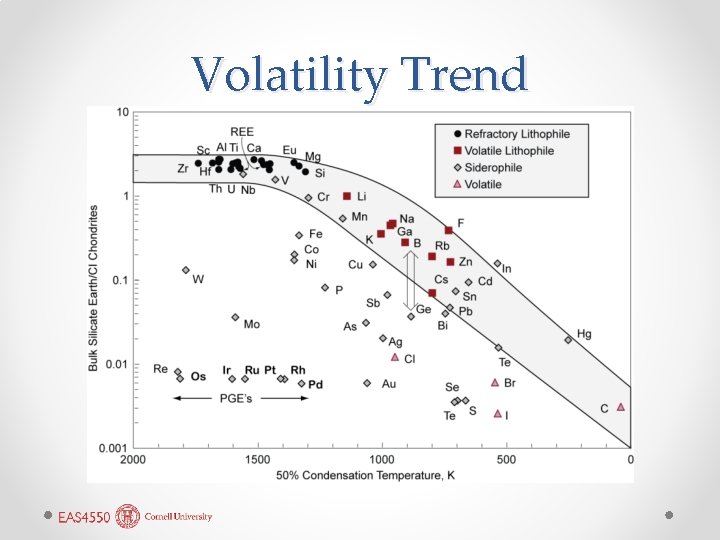
Volatility Trend
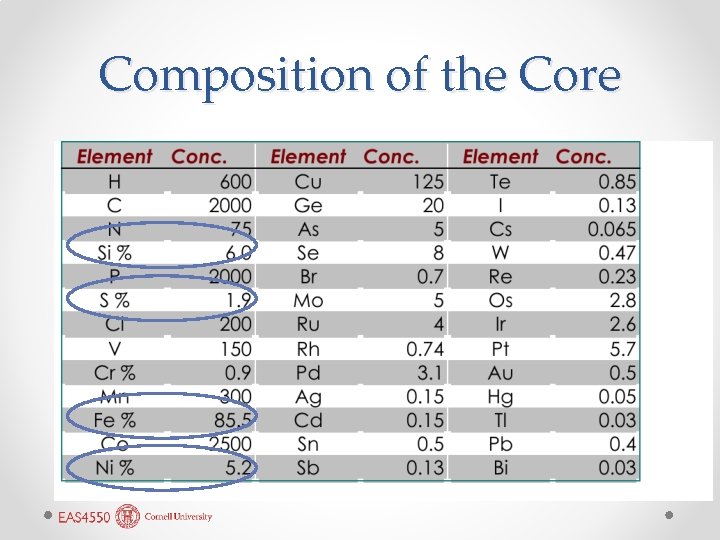
Composition of the Core
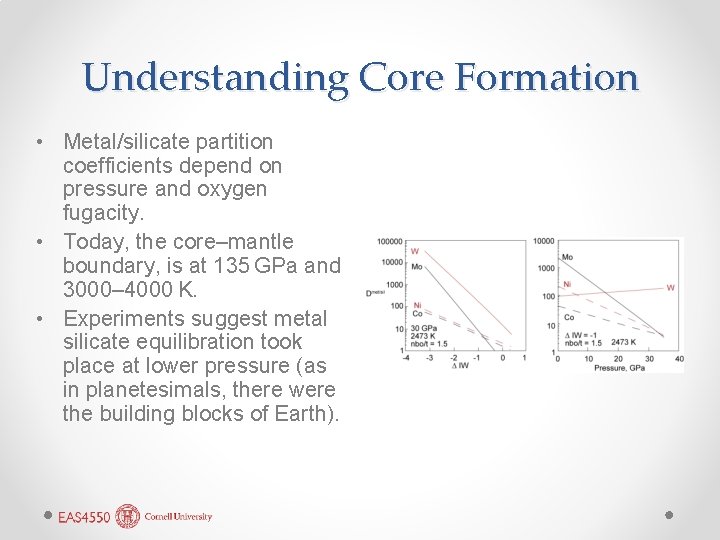
Understanding Core Formation • Metal/silicate partition coefficients depend on pressure and oxygen fugacity. • Today, the core–mantle boundary, is at 135 GPa and 3000– 4000 K. • Experiments suggest metal silicate equilibration took place at lower pressure (as in planetesimals, there were the building blocks of Earth).
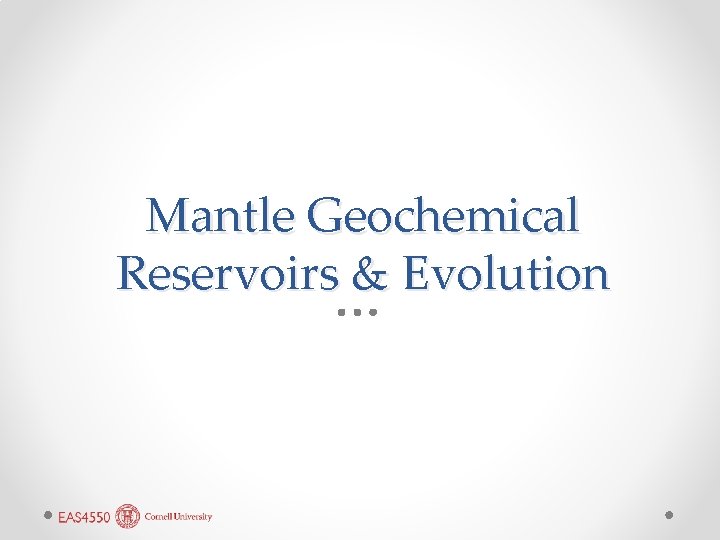
Mantle Geochemical Reservoirs & Evolution
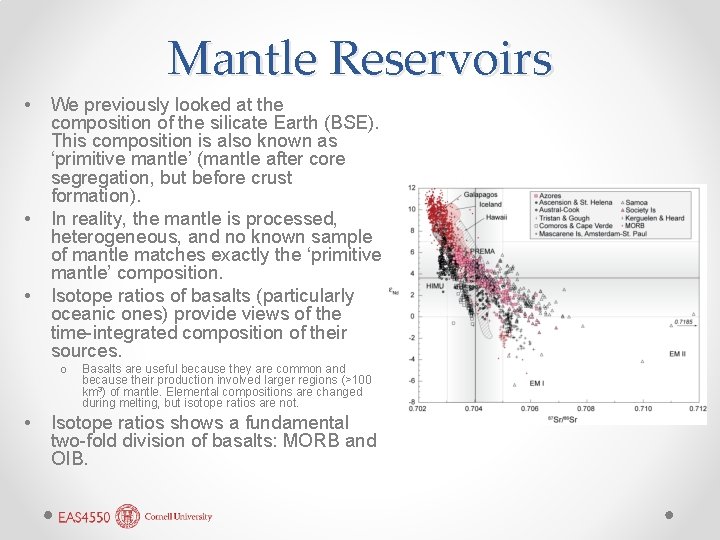
Mantle Reservoirs • • • We previously looked at the composition of the silicate Earth (BSE). This composition is also known as ‘primitive mantle’ (mantle after core segregation, but before crust formation). In reality, the mantle is processed, heterogeneous, and no known sample of mantle matches exactly the ‘primitive mantle’ composition. Isotope ratios of basalts (particularly oceanic ones) provide views of the time-integrated composition of their sources. o • Basalts are useful because they are common and because their production involved larger regions (>100 km 3) of mantle. Elemental compositions are changed during melting, but isotope ratios are not. Isotope ratios shows a fundamental two-fold division of basalts: MORB and OIB.
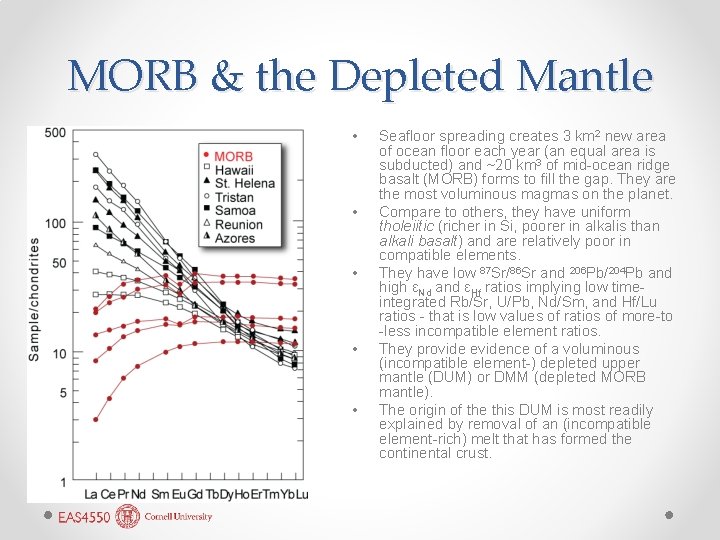
MORB & the Depleted Mantle • • • Seafloor spreading creates 3 km 2 new area of ocean floor each year (an equal area is subducted) and ~20 km 3 of mid-ocean ridge basalt (MORB) forms to fill the gap. They are the most voluminous magmas on the planet. Compare to others, they have uniform tholeiitic (richer in Si, poorer in alkalis than alkali basalt) and are relatively poor in compatible elements. They have low 87 Sr/86 Sr and 206 Pb/204 Pb and high εNd and εHf ratios implying low timeintegrated Rb/Sr, U/Pb, Nd/Sm, and Hf/Lu ratios - that is low values of ratios of more-to -less incompatible element ratios. They provide evidence of a voluminous (incompatible element-) depleted upper mantle (DUM) or DMM (depleted MORB mantle). The origin of the this DUM is most readily explained by removal of an (incompatible element-rich) melt that has formed the continental crust.
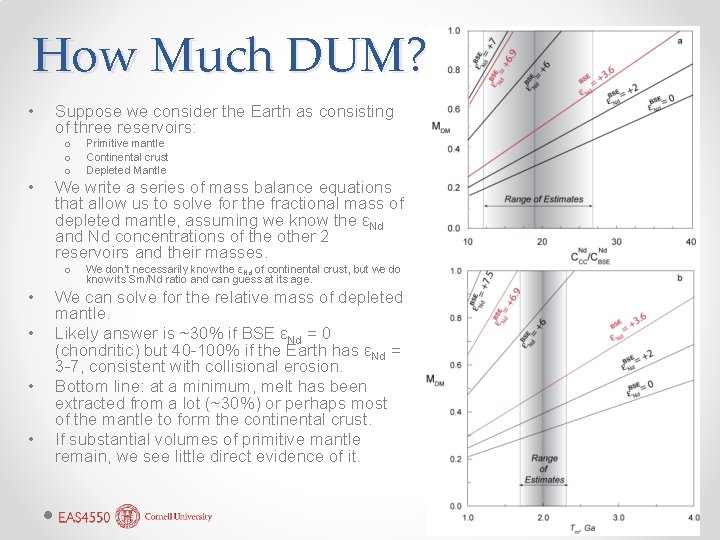
How Much DUM? • Suppose we consider the Earth as consisting of three reservoirs: o o o • We write a series of mass balance equations that allow us to solve for the fractional mass of depleted mantle, assuming we know the εNd and Nd concentrations of the other 2 reservoirs and their masses. o • • Primitive mantle Continental crust Depleted Mantle We don’t necessarily know the εNd of continental crust, but we do know its Sm/Nd ratio and can guess at its age. We can solve for the relative mass of depleted mantle. Likely answer is ~30% if BSE εNd = 0 (chondritic) but 40 -100% if the Earth has εNd = 3 -7, consistent with collisional erosion. Bottom line: at a minimum, melt has been extracted from a lot (~30%) or perhaps most of the mantle to form the continental crust. If substantial volumes of primitive mantle remain, we see little direct evidence of it.
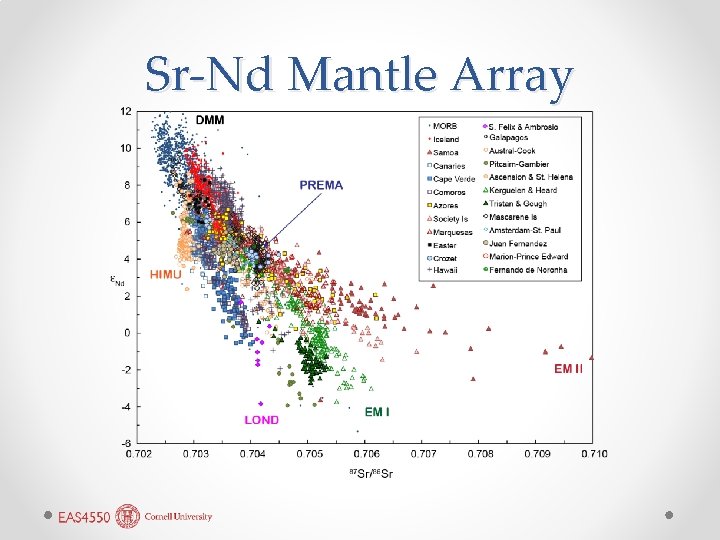
Sr-Nd Mantle Array
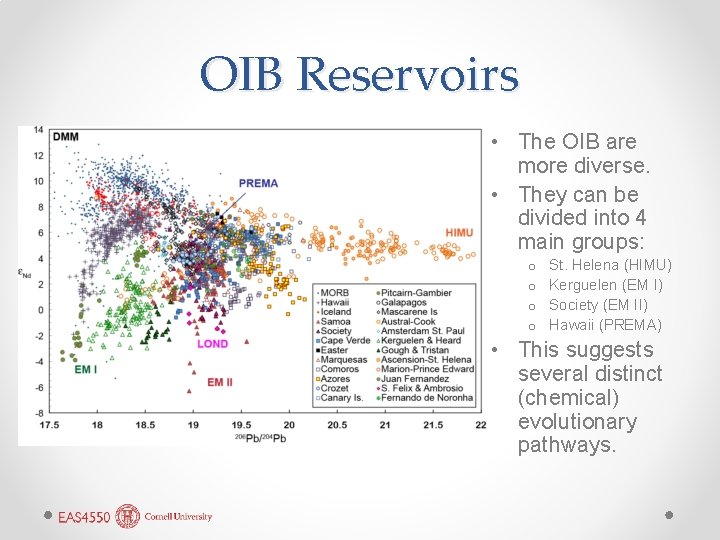
OIB Reservoirs • The OIB are more diverse. • They can be divided into 4 main groups: o o St. Helena (HIMU) Kerguelen (EM I) Society (EM II) Hawaii (PREMA) • This suggests several distinct (chemical) evolutionary pathways.
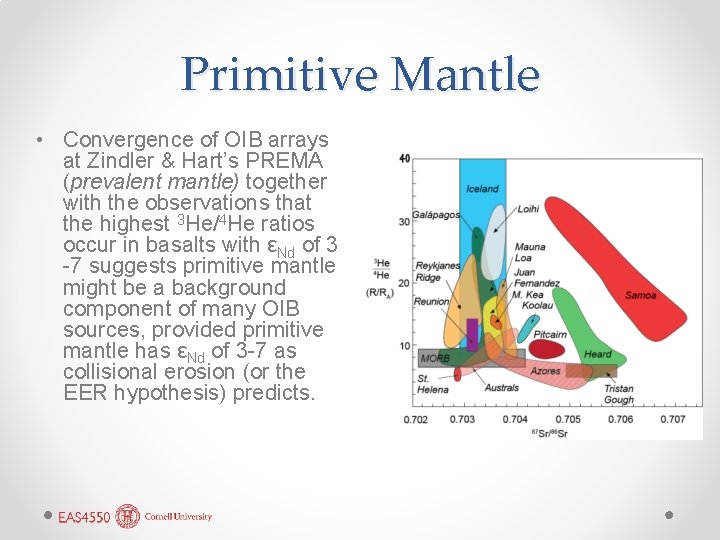
Primitive Mantle • Convergence of OIB arrays at Zindler & Hart’s PREMA (prevalent mantle) together with the observations that the highest 3 He/4 He ratios occur in basalts with εNd of 3 -7 suggests primitive mantle might be a background component of many OIB sources, provided primitive mantle has εNd of 3 -7 as collisional erosion (or the EER hypothesis) predicts.
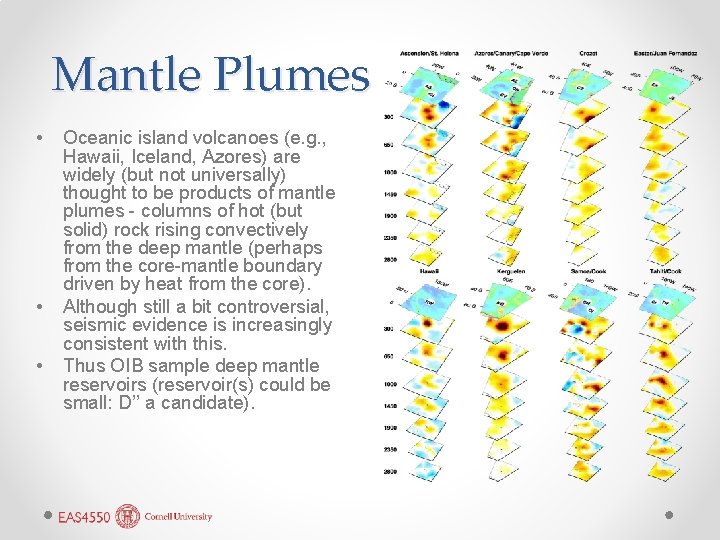
Mantle Plumes • • • Oceanic island volcanoes (e. g. , Hawaii, Iceland, Azores) are widely (but not universally) thought to be products of mantle plumes - columns of hot (but solid) rock rising convectively from the deep mantle (perhaps from the core-mantle boundary driven by heat from the core). Although still a bit controversial, seismic evidence is increasingly consistent with this. Thus OIB sample deep mantle reservoirs (reservoir(s) could be small: D’’ a candidate).
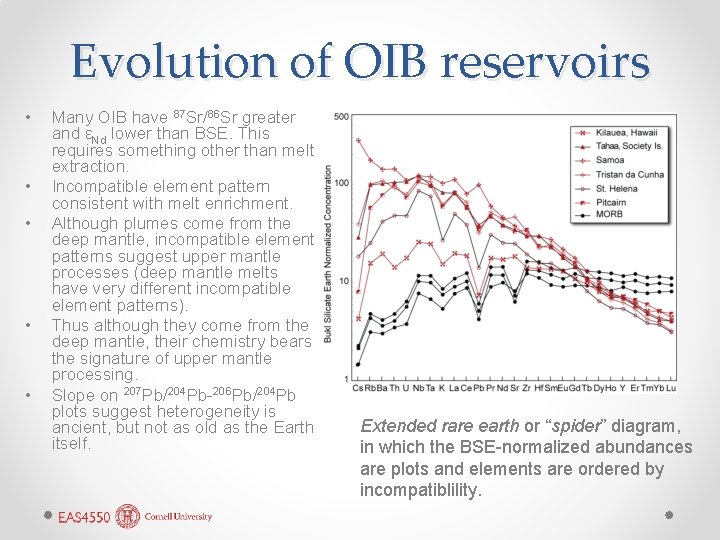
Evolution of OIB reservoirs • • • Many OIB have 87 Sr/86 Sr greater and εNd lower than BSE. This requires something other than melt extraction. Incompatible element pattern consistent with melt enrichment. Although plumes come from the deep mantle, incompatible element patterns suggest upper mantle processes (deep mantle melts have very different incompatible element patterns). Thus although they come from the deep mantle, their chemistry bears the signature of upper mantle processing. Slope on 207 Pb/204 Pb-206 Pb/204 Pb plots suggest heterogeneity is ancient, but not as old as the Earth itself. Extended rare earth or “spider” diagram, in which the BSE-normalized abundances are plots and elements are ordered by incompatiblility.
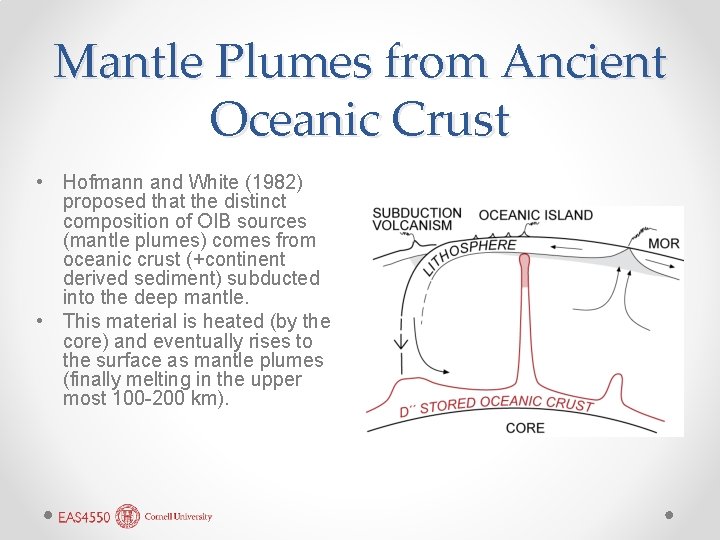
Mantle Plumes from Ancient Oceanic Crust • Hofmann and White (1982) proposed that the distinct composition of OIB sources (mantle plumes) comes from oceanic crust (+continent derived sediment) subducted into the deep mantle. • This material is heated (by the core) and eventually rises to the surface as mantle plumes (finally melting in the upper most 100 -200 km).
Characteristics of continental crust
What best describes the mantle of earth
Earth mantle
Cause of convection currents
Earth's mantle
01:640:244 lecture notes - lecture 15: plat, idah, farad
Mantle made of
Elisha mantle
Mantle convection and plate tectonics
Crust mantle core
Mantle made of
Extreme earth
Basic layers of the earth
Heating mantle risk assessment
Mechanical layers of the earth
Tasty tectonics answer key
Rbac mantle cell lymphoma
Oreo cookies plate tectonics
Mantle wedge
2 types of earth's crust
Mantle magma
Mantle biblical definition
Mantle magma
Plate tectonics test review answer key
Core mantle crust
Mantle layer
Core crust mantle
Normal fault
Pangea explanation
Maintenance rituximab mantle cell lymphoma
Mantle convection diagram
Structures of the earth
Rocks are classified according to their
Pass the mantle